- 1The Manta Trust, Dorchester, United Kingdom
- 2School of Marine Science and Engineering, University of Plymouth, Plymouth, United Kingdom
- 3Centre for Ecology and Conservation, University of Exeter, Penryn, United Kingdom
- 4Department of Environment and Geography, University of York, York, United Kingdom
Manta ray populations worldwide are vulnerable to sublethal injuries resulting from human activities, e.g., entanglement in fishing line and boat strikes, which have the potential to impact an individual’s health, fitness, and behaviour. Sublethal injuries and physical abnormalities also occur naturally from predation events, deformity, parasites, and disease. To determine the type and frequency of anthropogenic and natural originated injury events affecting Mobula alfredi and M. birostris in the Maldives, we examined data from the Manta Trust’s Maldivian Manta Ray Project (MMRP) database, which contains 73,638 photo-identification (photo-ID) sightings of the two manta ray species from 1987 to 2019. The likely origin of each injury or physical abnormality was determined based on visual assessment of the photo-ID images. Multiple injuries to an individual originating from the same event were grouped for analysis. Generalised linear mixed models (GLMM) were used to investigate the relationship between the occurrence of injury events and the explanatory variables sex and maturity status for both species, with the additional variable site function (cleaning, feeding, cruising) investigated for M. alfredi. Spatial and temporal variations in M. alfredi injury events, and their origin and type, were investigated by calculating the percentage of injury events per sighted individual at each Maldivian atoll, and per re-sighted individual in each year from 2005 to 2019. For both species, injury events were predominantly of natural origin, with predatory bites being the most frequent type. The most common anthropogenic injury type was entanglement in fishing line. Injuries to M. alfredi were significantly more likely to be observed on juveniles than adults, males than females, and at cleaning stations as opposed to feeding or cruising sites. Neither sex nor maturity status were significant explanatory variables for the occurrence of injuries to M. birostris. Highest percentages of anthropogenic injuries per sighted M. alfredi were recorded in North Malé, South Malé, Baa, Addu, and Laamu Atolls, where boat traffic, fishing, and tourism activities are concentrated. Overall, this work greatly improves understanding of the sublethal threats faced by manta rays in the Maldives; identifying focus areas where conservation management actions are required to ensure more effective protection of this threatened species group.
Introduction
The zooplanktivorous reef and oceanic manta rays (Mobula alfredi and M. birostris, respectively) are two of the ocean’s largest species (Marshall et al., 2009; White et al., 2018). Fragmented populations of M. alfredi are widely distributed throughout the tropical and sub-tropical waters of the Indo-West Pacific Oceans, where they frequent coastal reef habitats, but also use offshore environments and the mesopelagic zone (Kashiwagi et al., 2011; Couturier et al., 2012; Braun et al., 2014; Jaine et al., 2014; Stevens et al., 2018a; Hosegood, 2020). Mobula birostris are distributed throughout all tropical oceans and also range into temperate waters. They are more oceanic in habitat use than M. alfredi, visiting shallow coastal areas infrequently (Kashiwagi et al., 2011; Couturier et al., 2012; Stewart et al., 2016a; Stevens et al., 2018a). Both species demonstrate long-term site fidelity, and form seasonal aggregations at key habitats (Dewar et al., 2008; Jaine et al., 2012; Braun et al., 2015; Stewart et al., 2016b, 2018a; Couturier et al., 2018; Setyawan et al., 2018; Germanov et al., 2019; Perryman et al., 2019; Harris et al., 2020; Pate and Marshall, 2020).
As large-bodied, slow growing, late maturing animals, manta rays are among the least fecund of all vertebrates (Dulvy et al., 2014; Stevens, 2016; Stewart et al., 2018b). These life history traits make manta rays particularly vulnerable to increased mortality rates, as populations cannot easily recover from depletion (Dulvy et al., 2014; Lawson et al., 2017). The predominant threat to manta rays worldwide is overexploitation by fisheries, which have, in part, been driven by the high demand for mobulid gill plates in Asian markets (Ward-Paige et al., 2013; Croll et al., 2016; Lawson et al., 2017; O’Malley et al., 2017). To address the growing threat of the gill plate trade, both manta species were listed on Appendix II of the Convention on International Trade in Endangered Species in 2013, and they are also listed on Appendices I and II of the Convention on the Conservation of Migratory Species (Lawson et al., 2017). Despite these protective measures, targeted and incidental bycatch of manta rays in small- and large-scale fisheries remains a persistent threat (Dulvy et al., 2014; Croll et al., 2016; Lawson et al., 2017; Fernando and Stewart, 2021). Less directly, impacts of the climate crisis and reef degradation threaten manta ray food supply and habitat (Richardson, 2008; Stevens and Froman, 2019), while unregulated tourism operations can impact feeding, alter behaviour, and inflict lethal and sublethal boat strikes (Anderson et al., 2011a; Venables, 2013; Venables et al., 2016; Murray et al., 2020). These anthropogenic threats, coupled with the vulnerable life history traits of the species, have led to population declines in recent decades (Lewis et al., 2015; White et al., 2015; Rohner et al., 2017). As a result, M. alfredi is listed as Vulnerable to extinction on the IUCN’s Red List of Threatened Species (Marshall et al., 2019), with M. birostris recently uplisted to Endangered (Marshall et al., 2020).
Manta ray populations are also threatened by sublethal injuries (Stewart et al., 2018b), which can originate directly from anthropogenic activities, such as fishing and tourism, or occur naturally through predation, disease, or deformity (Stevens et al., 2018a; Stewart et al., 2018b; Stevens and Froman, 2019). Anthropogenic originated injuries are apparent in every monitored mobulid population across the world, predominantly resulting from interactions with fishing gear and vessel strikes (Deakos et al., 2011; Stewart et al., 2018b). Entanglement in fishing line, nets, and mooring ropes can cause serious injury and death (Couturier et al., 2012; Carpentier et al., 2019). Spending considerable time at the surface (Braun et al., 2014, 2015), e.g., while feeding, manta rays are especially susceptible to severe injuries from boat strikes and from contact with propellers (McGregor et al., 2019; Stevens and Froman, 2019).
Due to their large size, only large predatory sharks (e.g., tiger Galeocerdo cuvier and bull Carcharhinus leucas) and some cetaceans (e.g., false killer whales Pseudorca crassidens and orca Orcinus orca) are known to predate on adult manta rays (Gannier, 2002; Visser and Bonoccorso, 2003; Alava and Merlen, 2009; Marshall and Bennett, 2010; Stevens et al., 2018a). Unsuccessful predation attempts can leave permanent injuries (Marshall and Bennett, 2010; Stevens et al., 2018a) ranging from small quick-healing flesh wounds, with little or no tissue loss, to severe bites which truncate or disfigure pectoral fins (Marshall and Bennett, 2010; Stevens et al., 2018a).
External wounds in elasmobranchs are known to heal well (Towner et al., 2012; Chin et al., 2015; McGregor et al., 2019; Pate and Marshall, 2020; Womersley et al., 2021), likely due in part to their unique adaptive immune systems (Marra et al., 2017). Manta rays have shown resilience to a range of sublethal injuries (Marshall and Bennett, 2010; Pate and Marshall, 2020), including wounds from boat propellers (McGregor et al., 2019). However, such recovery will likely incur significant energy cost and conduct certain metabolic processes, which may shift energy allocation from reproductive effort, growth, and ability to feed, thereby reducing individual fitness (Archie, 2013; Chin et al., 2015; Harvey-Carroll et al., 2021; Womersley et al., 2021). Stress-responses to injury, entanglement, noise pollution, or tourist interactions in elasmobranchs and other marine megafauna taxon are often high energy behaviours, thus also detrimental to fitness (Pankhurst and Van der Kraak, 1997; Renshaw et al., 2012; Wilson et al., 2014; Rolland et al., 2017; Harvey-Carroll et al., 2021), and may even compromise wound healing (Archie, 2013). Moreover, as manta rays often inhabit areas of high, and increasing, human activity, the resulting sublethal injuries and/or physiological stress inflicted (Stewart et al., 2018b; Stevens and Froman, 2019; Pate and Marshall, 2020) are of increasing concern for the conservation management of these threatened species (Stewart et al., 2018b).
Assessment of the origins of sublethal injury to individuals provides a method for investigating the relative impact of different types of threats to a species (Archibald and James, 2018). Researchers have investigated sublethal injuries to M. alfredi in Mozambique, Hawaii, French Polynesia, Australia, and Indonesia (Marshall and Bennett, 2010; Deakos et al., 2011; Carpentier et al., 2019; Germanov et al., 2019; McGregor et al., 2019), and to M. birostris in southeast Florida, United States (Pate and Marshall, 2020). Previous studies have analysed only a single injury origin type in detail, e.g., predatory bites in Mozambique (Marshall and Bennett, 2010) and boat strikes in Australia (McGregor et al., 2019), or provided only a limited quantification of the injuries observed (e.g., Deakos et al., 2011 in Hawaii). Moreover, variations in the temporal, spatial, and demographic trends in the frequency and type of sublethal injuries have not been examined in detail. Furthermore, the fitness cost of sublethal injuries to manta ray individuals and populations is currently unclear and has been identified as an important knowledge gap in mobulid research and conservation efforts (Couturier et al., 2012; Stewart et al., 2018b).
Studies of other marine megafauna species identify various consequences for the health, fitness, and behaviour of an individual, which may impact post-injury survival (Johnson et al., 2005; Andersen et al., 2008; Bansemer and Bennett, 2010; Cassoff et al., 2011; Moore and Van der Hoop, 2012; Moore et al., 2013; Wilson et al., 2014). For example, foraging ability can be significantly impaired when fishing gear damages, or is attached to, a cetacean’s mouth, and has led to starvation in some cases (Andersen et al., 2008; Cassoff et al., 2011). Increased drag from carrying fishing gear can incur considerable energetic costs (Moore and Van der Hoop, 2012; Van der Hoop et al., 2016), while severe tissue damage can result in haemorrhage or debilitation (Cassoff et al., 2011; Moore and Van der Hoop, 2012), and open, unresolved wounds can lead to serious infection (Borucinska et al., 2002; Cassoff et al., 2011). Injured whale sharks (Rhincodon typus) exhibit less evasive behaviours toward tourists and boats than non-injured individuals (Quiros, 2007; Haskell et al., 2015), which suggests that injuries may reduce their agility, or that individuals are choosing warmer surface waters, where tourism activities are concentrated, to aid wound healing (Womersley et al., 2021). Injuries have also been found to disrupt social behaviours of bottlenose dolphins (Tursiops truncatus), which may compromise their long-term survival, or make them more vulnerable to predation (Greenfield et al., 2021). The presence of sublethal injuries is not thought to substantially impair reproductive capacity, unless the reproductive organs are damaged (Jessop et al., 2004; Andersen et al., 2008; Wells et al., 2008; Germanov et al., 2019). However, it is unclear whether injury has an impact on the rate of reproduction or level of reproductive success, which could have implications for population health (Heithaus, 2001b; Andersen et al., 2008; Wells et al., 2008). For example, major injuries to an organism can delay the age at sexual maturity (Harris, 1989), and physiological stress (e.g., from capture or entanglement) can lead to abortion in elasmobranchs (Adams et al., 2018).
The Republic of Maldives in the Indian Ocean (Figure 1) supports the world’s largest known population of M. alfredi, which occur throughout all 26 geographical atolls of the archipelago (Kitchen-Wheeler et al., 2012; Stevens, 2016; Harris et al., 2020). Mobula alfredi predictably migrate across the archipelago following areas of enhanced zooplankton availability, driven by the biannual reversal of the South Asian Monsoon winds (Anderson et al., 2011b; Harris et al., 2020). Aggregations of M. alfredi occur at locations where food becomes seasonally abundant, often in shallow bays and channels (Armstrong et al., 2016, 2021a; Harris et al., 2020). They also visit nearby cleaning stations where cleaner fishes remove parasites and clean wounds (Foster, 1985; Marshall, 2008), and where courtship and mating interactions occur (Stevens, 2016; Stevens et al., 2018b). Predator avoidance and thermoregulation may also be functions of shallow coral reef site use (Stevens, 2016). Mobula birostris are less frequently sighted, except during a few months each year (March–April) at Addu and Fuvahmulah, the two southernmost atolls of the archipelago (Stevens, 2016; Maldvian Manta Ray Project [MMRP], 2019a; Nicholson-Jack et al., 2021). These areas are close to deep-water oceanic habitat (Stevens, 2016), where M. birostris are most commonly encountered throughout their range (Kashiwagi et al., 2011; Stewart et al., 2016a). In the Maldives, individual M. birostris are rarely re-sighted, which suggests the population is transient, and predominantly uses habitat away from the reef systems there (Maldvian Manta Ray Project [MMRP], 2019a).
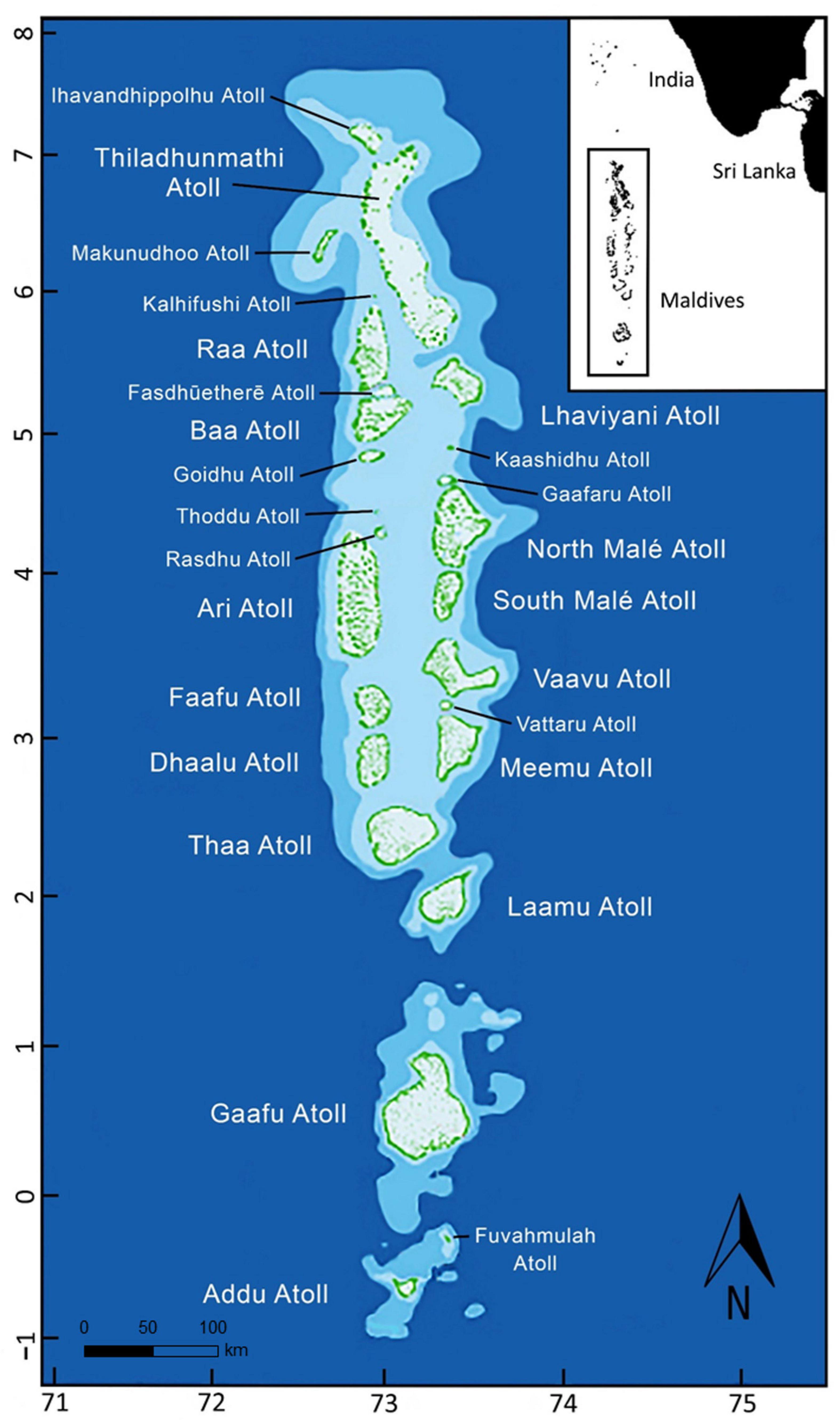
Figure 1. Map of the Maldives archipelago located to the southwest of India. Diagram shows the 26 geographical atolls illustrated in green.
There has never been a targeted commercial fishery for manta rays in the Maldives, and in 2014 the Maldives government declared all species of ray protected nationally (MEPA, 2014; Stevens, 2016). However, like manta ray populations worldwide, they are still vulnerable to sublethal injuries and associated stressors resulting from human activities (Stevens et al., 2018a; Stevens and Froman, 2019). As such, this study offers an opportunity to assess the sublethal threats to an unfished population, but one which is still affected by issues such as bycatch, tourism, and natural predation (Stevens and Froman, 2019). The level of impact from these issues, and the measures required to mitigate their effects, may vary depending on the locations frequented by the manta rays, temporal visitation patterns, and the sex and maturity status of the individuals. Therefore, we use photographic identification data to investigate the origin and type of sublethal injuries (and physical abnormalities) observed in M. alfredi and M. birostris in the Maldives, and if these injuries vary demographically, spatially, or temporally. This information will enhance understanding of the likely impact of sublethal injury to manta rays, and highlight what conservation action is required to address the problem.
Materials and Methods
Injury Identification
Surveys to record sightings of M. alfredi and M. birostris were performed via SCUBA or freediving by trained Manta Trust Maldivian Manta Ray Project (MMRP) staff1 and citizen science contributors between 1987 and 2019. Surveys were carried out across the whole archipelago throughout the year, in all study years, although known M. alfredi and M. birostris aggregation sites were surveyed most frequently, creating some sampling bias.
Photographs collected during surveys were compiled into a photographic identification (photo-ID) database which records all manta ray sightings. A “sighting” is defined as a confirmed photo-ID (an image/video which captures the ray’s unique ventral spot pattern) of an individual manta ray on a given day at a defined location (Marshall, 2008; Marshall and Pierce, 2012; Stevens, 2016; Harris et al., 2020). During each sighting, the primary behavioural activity of the manta ray (cleaning, feeding, courtship, cruising, or breaching) (Stevens, 2016, Stevens et al., 2018b) was recorded, as well as the individual’s species (Marshall et al., 2009), sex, size (approx. disc width), and maturity status (Stevens, 2016; Harris et al., 2020). Sex was determined by the presence of claspers in males, which are absent in females (Deakos et al., 2011; Stevens, 2016). Males were considered sexually mature only when their claspers extended well past the posterior edge of the pelvic fins and were fully calcified (Stevens, 2016). Females were considered mature if they were visibly pregnant, if dorsal mating scars or ventral mating wounds or scars were observed, or if the animal was estimated to be > 320 cm in disc width (Deakos et al., 2011; Stevens, 2016). Manta rays of unknown sex and maturity status were excluded from all analyses.
All photographs were visually analysed for the presence of permanent sublethal injuries or physical abnormalities (collectively referred to as injuries hereafter) which, once healed, leave substantial permanent scars, disfigurements, or missing tissue that remains visible for the rest of an animal’s life (Figures 2, 3; Marshall and Bennett, 2010). Superficial injuries or abnormalities, such as small cuts or scars, fibropapillomatosis growths, or the presence of a lightly embedded fishing hook, were excluded from all analyses. Injuries were categorised according to their likely origin (natural or anthropogenic) and type (Table 1 and Figures 2, 3). Categorisation criteria (Table 1) was determined by the characteristics and placement of the injury on a manta ray’s body, based on two decades of direct observations by the study authors on thousands of the individuals included in this study. Injury types of natural origin include semi-circular bite wounds or scarring from predation attempts; lesions or scarring resulting from infections, diseases, or parasites; and birth deformities which could not be attributed to any other injury type, e.g., a misshapen head, cephalic lobes, or tail. Anthropogenic injury types include distinctive lacerations or scars attributed to boat strikes; straight knife-life cuts, slices, or scars from entanglement in fishing line; small, equally spaced cuts or scars from net entanglement; and thicker, more localised cuts or scars caused by entanglement in rope. If the origin and type could not be determined, for example, if poor image quality precluded identification, it was recorded as “unknown (Supplementary Figure S1).” To accurately record sublethal events throughout a manta ray’s life, multiple injuries, if determined to originate from a single incident, were grouped together and classed as one injury “event” (e.g., damage to the right cephalic fin and right pectoral fin resulting from a single fishing line entanglement). Thus, an injury event may consist of multiple injuries of the same type, or of just a single injury. Each injury was only recorded once, during the first sighting it was observed on the individual. Multiple injury events may be recorded during a single sighting if the injury types are determined to be different (e.g., one predation bite and one boat strike scar are present), or if the injury events occurred at different times (e.g., two predation bites were present; one a healed scar, the other a fresh wound).
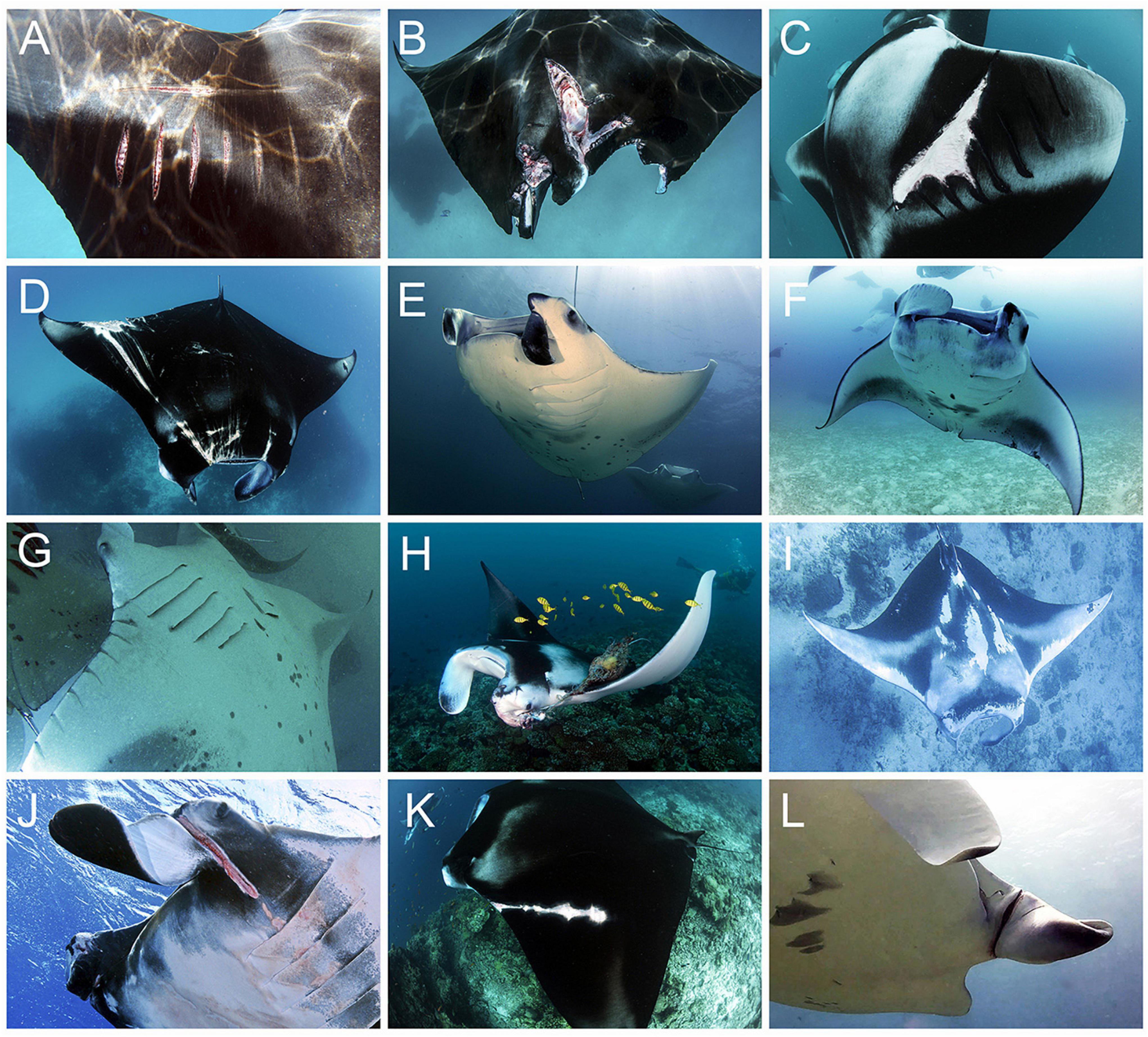
Figure 2. Anthropogenic Mobula alfredi and M. birostris sublethal injury types: (A–C) Boat strike. (A,B) Fresh wounds within days of strike, and (C) several weeks after strike. (D–F) Fishing line/hook. (D) Fresh wound days after line cut free, (E) left cephalic fin three-quarters severed and functionless, and (F) left cephalic fin amputated and scarring around mouth, down gill slits and on trailing edge of left pectoral fin. (G–I) Net entanglement. (G) Regularly spaced gill net scarring down ventral surface of right pectoral fin, (H) ghost net entangled in left cephalic fin, and (I) scarring to entire dorsal body surface, increasing around body edges and extremities. (J–L) Rope entanglement. (J,L) Left cephalic fins with deep laceration (injuries recorded directly after rope was cut free), and (K) large scarring from rope entanglement over left shoulder. All images collected in the Maldives during this study. Images © Manta Trust.
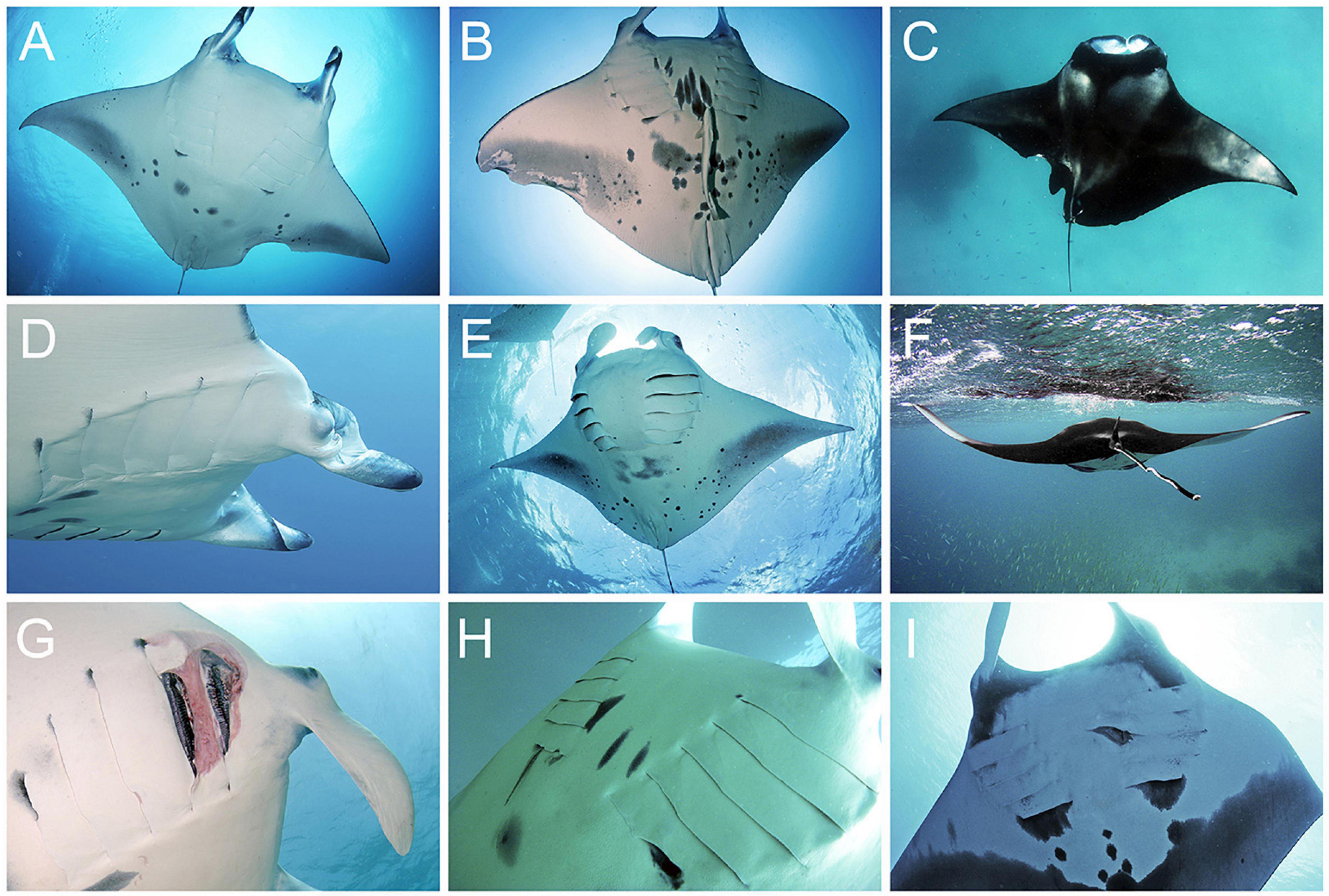
Figure 3. Natural Mobula alfredi and M. birostris sublethal injury types: (A–C) Predatory bites. (A) Large semi-circular shark bite scar to left pectoral fin, (B) predation bite amputation of right pectoral fin, and (C) very large shark bite scar to left pectoral fin, resulting in a large section of missing fin. (D–F) Deformity. (D) Right eye and head severely deformed, (E) left cephalic fin smaller, bent and with reduced functionality, and (F) tail abnormally bent along length. (G–I) Disease, infection, and parasitism. (G) First and second gill slits severely damaged and gill chamber infected, (H) fifth right and left gill slits scarred and damaged by juvenile sharksucker remora (Echeneis naucrates) seeking shelter inside the ray’s gill cavity, and (I) second left gill slit with a large wound resulting from repeated intrusion into the gill cavity by a giant remora (Remora remora). All images collected in the Maldives during this study. Images © Manta Trust.
Data Analysis
Generalised Linear Mixed Models
To investigate the relationship between the occurrence of M. alfredi injury events and explanatory variables, generalised linear mixed models (GLMM) for sex, maturity status, and site function (cleaning, feeding, or cruising) were used via the “lme4” R package (Bates et al., 2020). Site function was determined by the predominant primary behavioural activity of manta rays sighted there. A binary response was established for each sighting; with injury event (=1), and no injury event (=0). Each model was fitted with a logit link function and contained the manta-ID as a random intercept to account for any correlation due to individual manta rays being repeatedly observed. Three separate models were built, with the response variables (1) all injury events recorded (natural, anthropogenic, and unknown), (2) natural injury events only, and (3) anthropogenic injury events only. All combinations of sex, maturity status, and site function were tested to identify the most informative explanatory variables (Supplementary Table S1). Model performance was assessed using Akaike information criterion (AIC) test statistic (Burnham and Anderson, 2002) using the “MuMin” R package (Kamil Bartoń, 2018). The model with the lowest AIC value for each response variable was interpreted in terms of odds ratios (ORs), and the significance of each explanatory variable was determined by the 95% confidence interval (CI). There is a significantly lower likelihood of an injury event being recorded if the CI range is below one, and a significantly higher likelihood of an injury event being recorded if above one. A CI that crossed one is considered non-significant. Any ORs with p > 0.05 are not reported. These values were then converted to percentage likelihood using (OR−1)x 100. The same analysis was conducted for M. birostris sightings, but without the inclusion of site function (as it could not be established) in the GLMM.
Spatial and Temporal Trends
Spatial variations in M. alfredi injury events, their origin, and type were assessed by calculating the percentage of injury events per sighted individual at each atoll (total number of injury events in each atoll/total number of individual manta rays sighted in the atoll × 100). Atolls where < 50 individual manta rays were sighted during the study period were excluded from spatial analysis to reduce bias (Supplementary Table S2).
Temporal trends were analysed by calculating the percentage of injury events per re-sighted individual each year between 2005 and 2019. Pre-existing injuries recorded on a manta ray’s first sighting are not reported for temporal variations, as it was not possible to estimate the years in which these injury events first occurred. Only injury events which were recorded on re-sightings of an individual (i.e., new injury events) are reported. Years in which < 100 individual manta rays were sighted were excluded from temporal analysis to reduce bias.
Spatial and temporal trends in M. birostris injury events were not investigated as most sightings were recorded in one atoll and very few re-sightings have occurred.
Results
Injury Profile for Mobula alfredi
A total of 4,901 M. alfredi were individually identified (male = 2,442, 49.8%, female = 2,459, 50.2%). Of these, 3,746 were sighted more than once and 44 were sighted as both a juvenile and an adult. Overall, 1,432 individual M. alfredi were observed with injuries, which equates to 29% of the population, of which 683 (48%) were males (adult = 569, juvenile = 114) and 749 (52%) were female. Of the 749 females, one was observed with injuries as both a juvenile and an adult. Therefore, when summarised by life stage, adult females = 412, and juvenile females = 338. The number of injury events per injured M. alfredi individual ranged from one to five, with 13% (n = 180) of injured individuals having suffered two or more. The mean number of events for injured individuals was 1.14 (SD 0.40), while this figure for the population as a whole was 0.33 (SD 0.56).
A total of 1,635 injury events were documented from 1,597 of the 72,912 (2%) sightings recorded between 1987 and 2019. Of the sightings where injury events were recorded, 489 were of adult females (31%), of which five individuals were observed to have injuries from both a natural and anthropogenic origin. Juvenile females were sighted with injuries on 364 (23%) occasions, of which three individuals were observed to have injuries of both natural and anthropogenic origins. Injury events were recorded for adult males during 625 (39%) sightings, of which nine individuals were observed to have injuries from both a natural and anthropogenic origin. Juvenile males were sighted with injuries on 119 (7%) occasions, of which one individual was observed to have injuries from both a natural and anthropogenic origin.
Of the 1,635 injury events recorded for M. alfredi (Figure 4), 207 (13%) were of unknown type and origin, 518 (32%) were of anthropogenic origin, and 910 (55%) originated naturally. Of the identified injury types (n = 1,428), the most frequently observed injuries were predatory bites, with 789 (55%) injury events recorded, while injuries caused by fishing line or hooks accounted for 32% (n = 456) of injury events and were the most frequently observed anthropogenic injury type. A total of 53 (4%) boat strike injury events were also recorded.
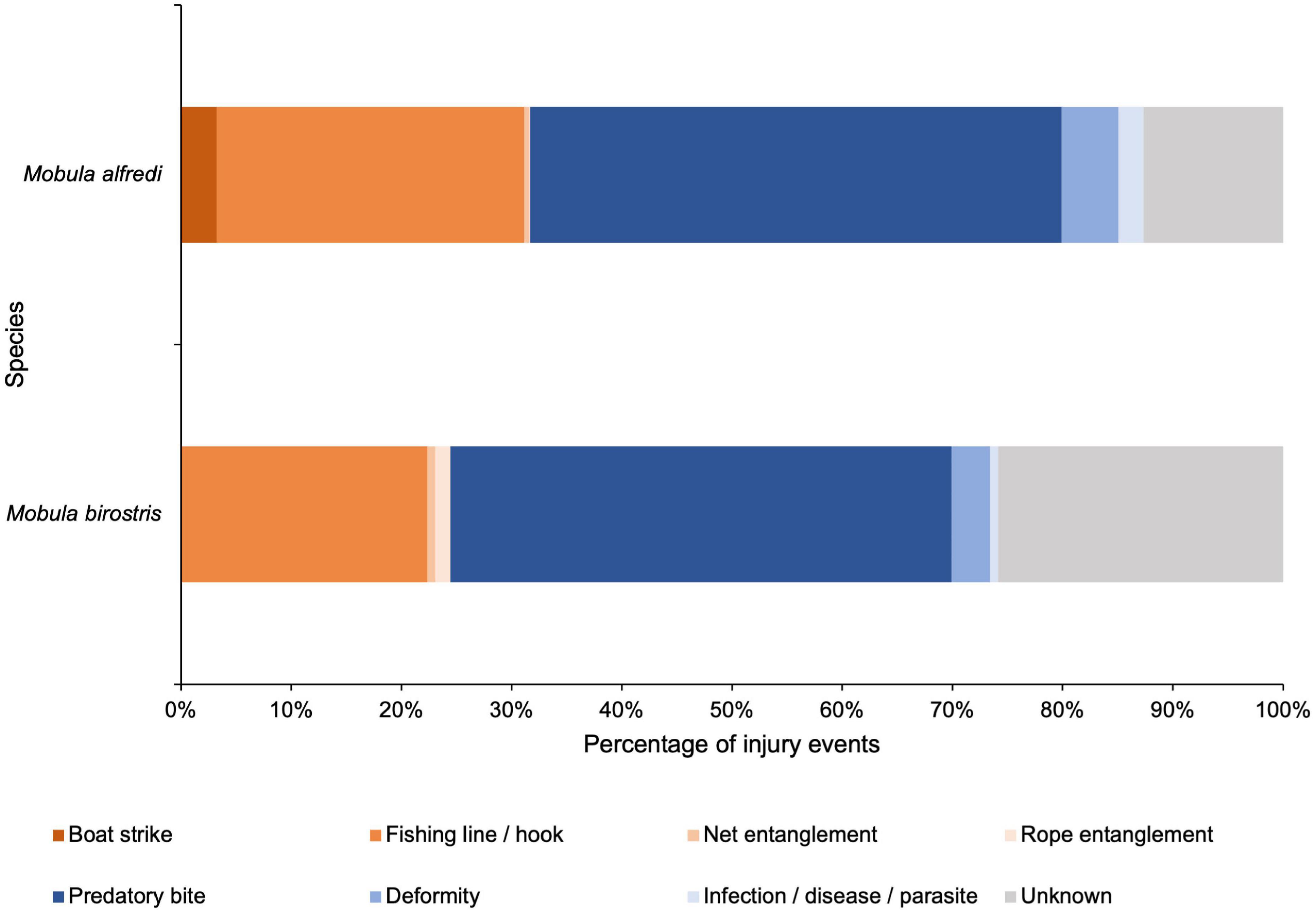
Figure 4. Breakdown of sublethal injury events to Mobula alfredi and M. birostris by origin and type. A comparison of the percentage of each injury event type, of either anthropogenic (oranges), natural (blues), or unknown (grey) origin, recorded for M. alfredi (n = 1,635) and M. birostris (n = 143) in the Maldives.
Generalised Linear Mixed Models: Mobula alfredi
All Injury Events
The best fit GLMM model included all three explanatory variables (sex, maturity status, and site function). The results indicate that injuries were most likely to be observed at cleaning stations; 35% more likely than at feeding areas (OR = 0.65) (Figure 5). Injuries were also more likely to be observed on juveniles (OR = 1.2), which were 20% more likely to have an injury than adults, and males were 25% (OR = 1.25) more likely to have an injury when sighted than females.
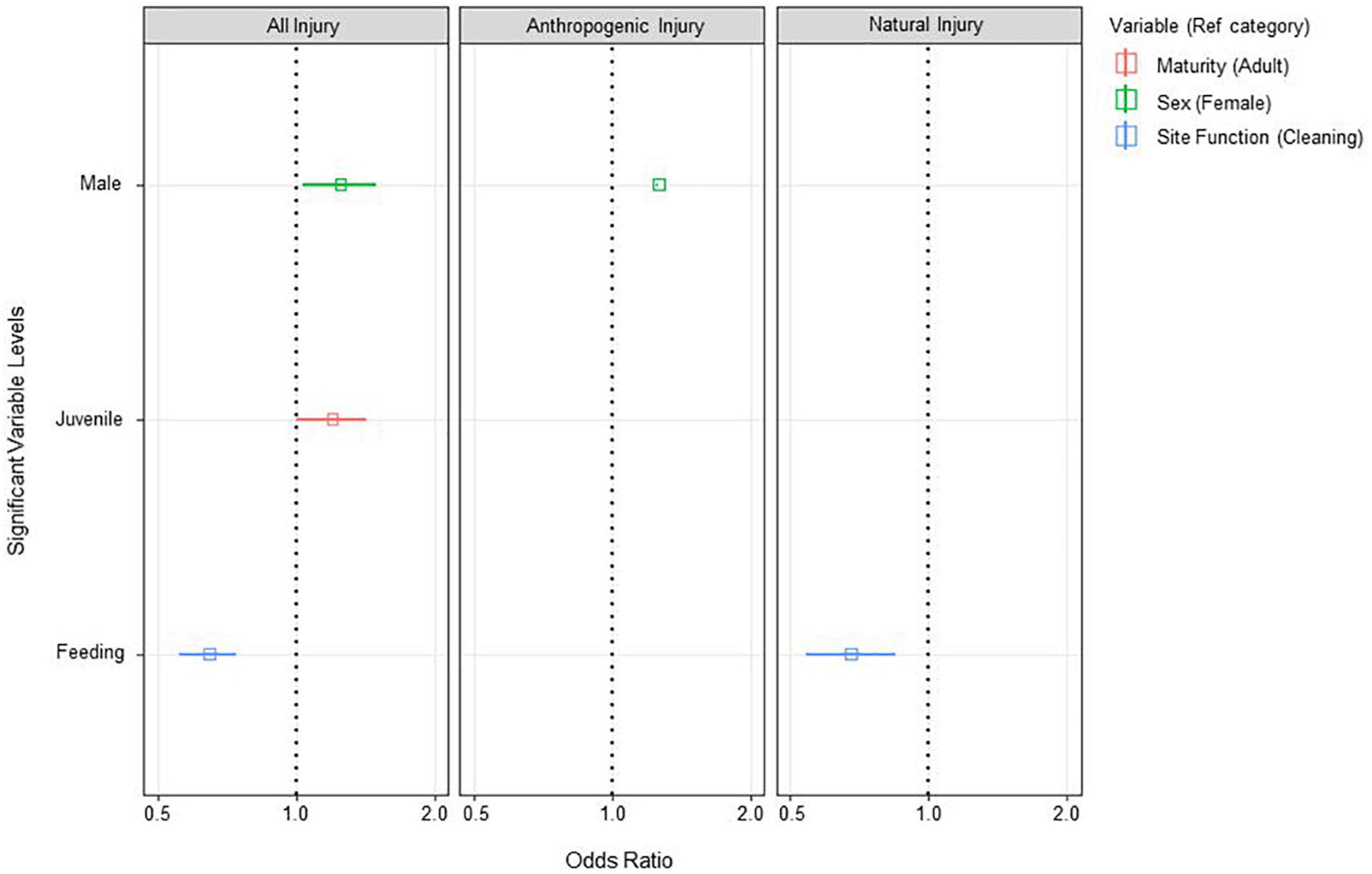
Figure 5. GLMM odds ratio plot for Mobula alfredi injuries. Relationship between injury events and significant explanatory variables (p < 0.05). Plots show relationship for all injury events (left), anthropogenic injury events only (middle), and natural injury events only (right). Results are plotted in terms of odds ratio (OR) indicating the likelihood of presence in comparison with the reference category shown in the legend. Odds ratio values are plotted with 95% confidence intervals (CI) where applicable (CI; solid horizontal lines). Where the CI does not span 1, the explanatory variable is significantly more likely when OR > 1, and significantly less likely when OR < 1.
Anthropogenic Injury Events
The best fit GLMM model included only sex. The results (Figure 5) suggest anthropogenic injuries were more likely to be observed on males (OR = 1.26), which were 26% more likely to have an injury than females.
Natural Injury Events
The best fit GLMM model included only site function. The results (Figure 5) indicate that natural injuries were most likely to be observed at cleaning stations, which was 32% more likely than at feeding areas (OR = 0.68).
Injury Profile for Mobula birostris
A total of 663 M. birostris were individually identified (male = 363, 54.8%, female = 300, 45.2%), of these, 52 were sighted more than once. Overall, 134 individuals were observed with injuries, which equates to 20% of the population, of which 76 (57%) were males (adult = 68, juvenile = 8) and 58 (43%) were female (adult = 42, juvenile = 16). The number of injury events per injured M. birostris was either one or two (recorded for 7%, n = 9, of injured individuals) with a mean of 1.07 (SD 0.25) for injured individuals and 0.22 (SD 0.44) for the entire population.
A total of 143 injury events were recorded during 134 of 726 (18%) sightings between 1996 and 2019. Of the sightings where injury events were recorded, 42 (31%) were of adult females, of which one individual was observed to have injuries of both natural and anthropogenic origins. Juvenile females were sighted with injuries on 16 (12%) occasions. Injury events were recorded for adult males during 68 sightings (51%), of which two individuals were observed to have injuries from both a natural and anthropogenic origin. Juvenile males were sighted with injuries on 8 (6%) occasions, of which one individual was observed to have both natural and anthropogenic injuries.
Of the 143 injury events recorded for M. birostris (Figure 4), 37 (26%) were from an unknown origin and type, 35 (24%) were of anthropogenic origin, and 71 (50%) originated naturally. Of the identified injury types (n = 106), the most frequently observed injuries were predatory bites, with 65 (61%) injury events recorded, while injuries caused by fishing lines or hooks accounted for 30% (n = 32) of injury events and were the most frequently recorded anthropogenic injury type. No boat strike injuries were observed for M. birostris.
Generalised Linear Mixed Models: Mobula birostris
The best fit GLMM for all three models (all injury events, anthropogenic injury events only, and natural injury events only) included sex. However, all three indicated that there was no significant difference (p > 0.05) between the occurrence of injuries on male and female M. birostris.
Spatial and Temporal Trends in Mobula alfredi Injury Events
Between 1987 and 2019, North Malé Atoll had the highest percentage of injury events (anthropogenic, natural, and unknown) per sighted M. alfredi (37%), followed by Laamu (35%), Lhaviyani (28%), Baa (28%), and Thiladhunmathi Atolls (28%) (Figure 6). Injuries caused by fishing lines or hooks were highest per sighted manta in Laamu Atoll (11%), followed by North Malé (10%), Addu (10%), and Baa (9%). Boat strike injury events were recorded in eight atolls, with South Malé having the highest percentage per sighted individual (4%), followed by Addu (1.3%), North Malé (1.2%), and Baa (1.2%). Predatory bites were most frequent at North Malé (19%), Ihavandhippolhu (18%), Lhaviyani (17%), and Thiladhunmathi Atolls (17%). At very low frequencies, injuries from entanglement in fishing nets (n = 8) were recorded in five atolls and rope entanglement (n = 1) in just one. Natural deformities (n = 84) were recorded in 14 of the 15 atolls reported, while scars from infection, disease, or parasites (n = 37) were observed in seven atolls.
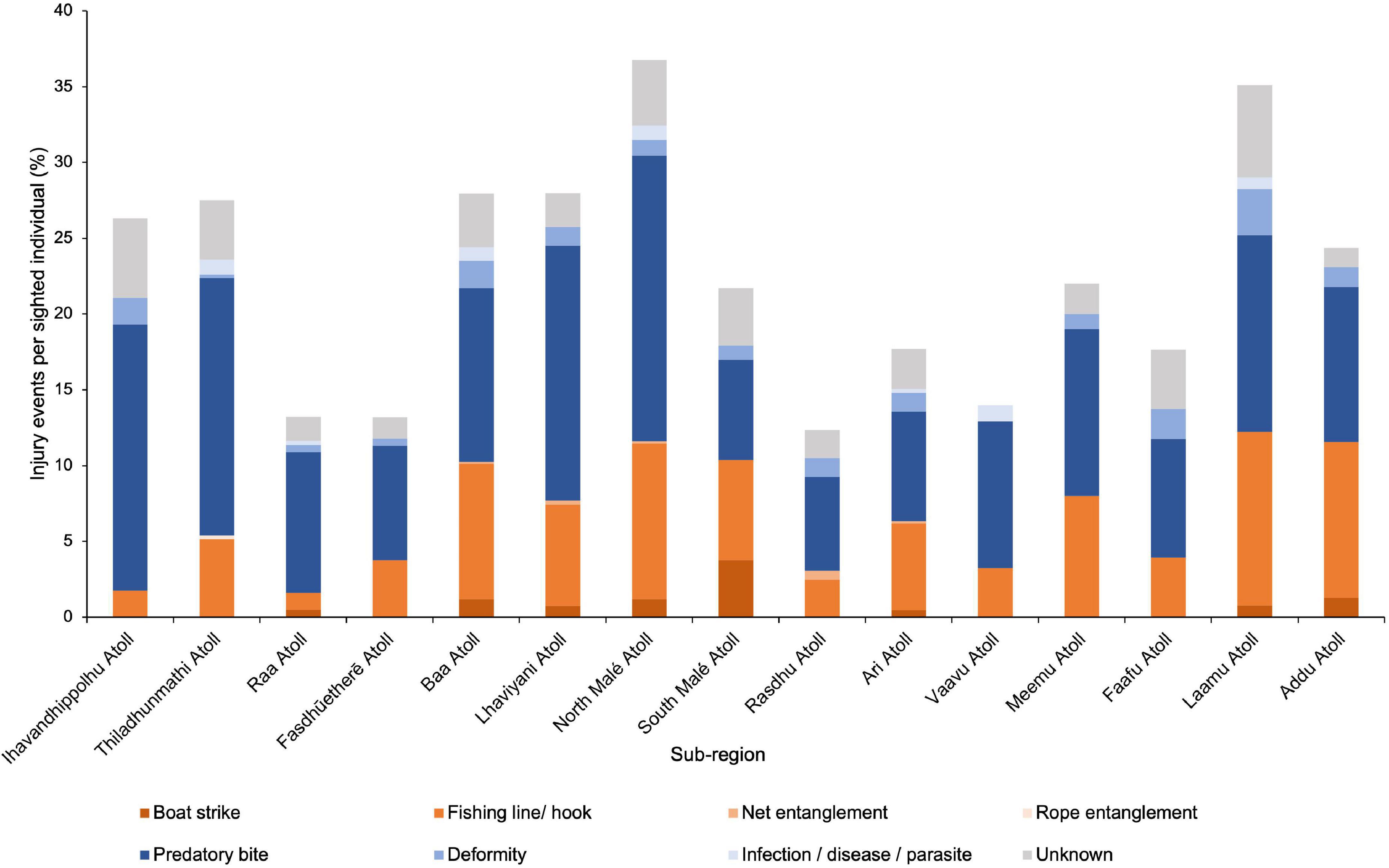
Figure 6. Percentage of injury events per sighted Mobula alfredi throughout the Maldives atolls (1987–2019). Distribution of anthropogenic (oranges), natural (blues), and unknown (grey) injury events (n = 1,635), grouped by injury type, per individual sighted. Records from 15 of the Maldives’ 26 geographical atolls, listed north (left) to south (right).
Overall, there were no clear annual trends in the percentage of injury events per re-sighted M. alfredi between 2005 and 2019 (Figure 7). Injuries caused by fishing line or hooks per re-sighted individual remained consistent between 2005 and 2017, before gradually declining from 2017 (1.3%) to their lowest frequency in 2019 (0.3%). However, a fluctuation in fishing line injury events occurred between 2011 and 2013, declining by a factor of 2.8, from 1.1% in 2011 to 0.4% in 2012, before the figure rose again to 1.3% in 2013. Boat strikes were of comparatively low frequency and fluctuated throughout the study period but were most frequent in 2018 (0.6%). An increase in predatory bites by a factor of 2.9 occurred between 2007 and 2018, rising from 0.8 to 2.3%, before falling to 1.4% in 2019. The remaining natural and anthropogenic types accounted for few injury events on re-sightings in each year (n < 4).
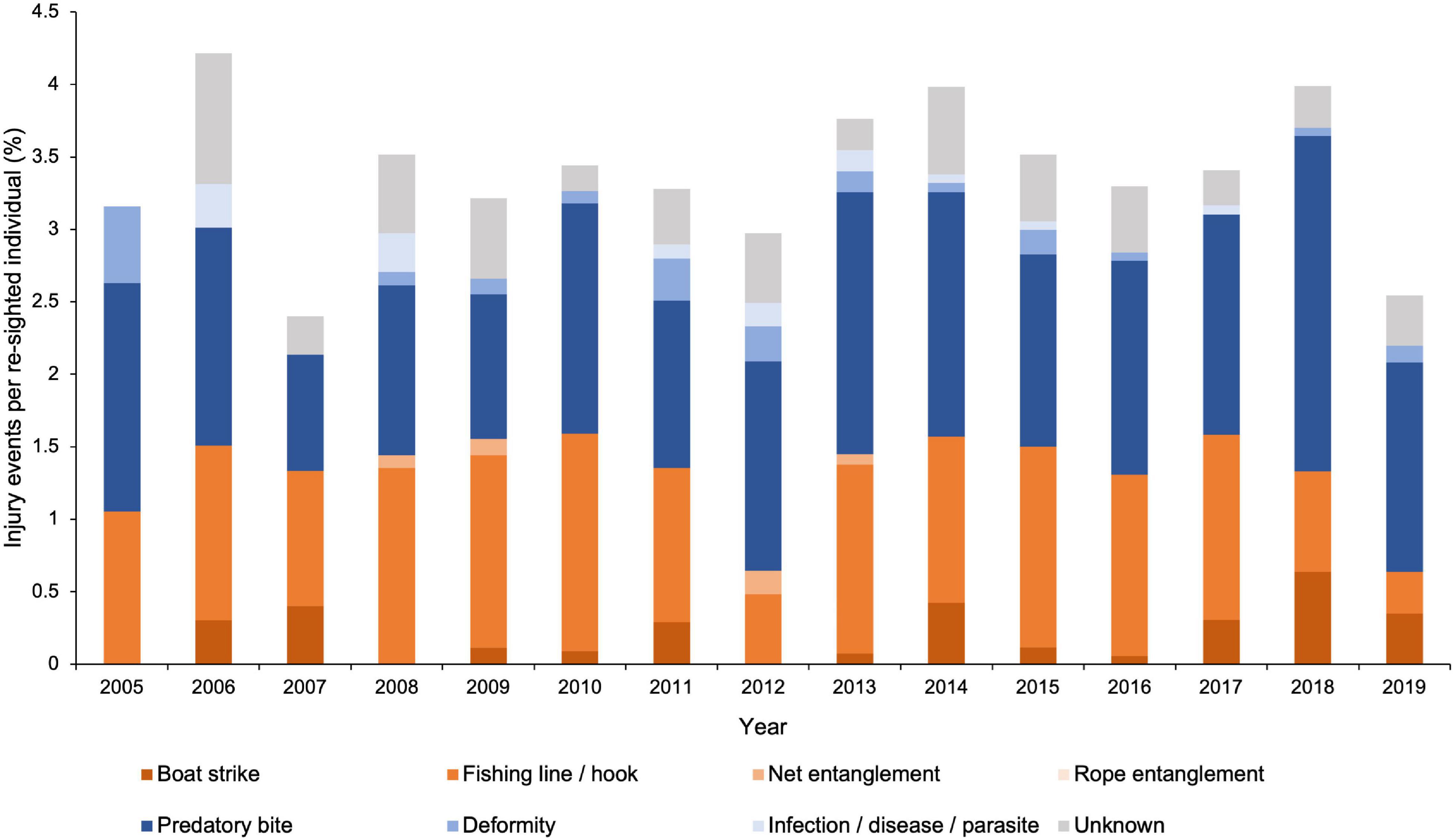
Figure 7. Percentage of annual injury events per re-sighted Mobula alfredi (2005–2019). Distribution of anthropogenic (oranges), natural (blues), and unknown (grey) injury events (n = 623), grouped by injury type, per re-sighted individual.
Discussion
During this study, all permanent sublethal injuries within the Maldives M. alfredi and M. birostris populations were recorded and identified to type and origin (where possible). This is the first time all the sublethal injuries and physical abnormalities of a manta ray population have been studied in this way. Overall, we were able to identify seven types of injuries, accounting for 87% (n = 1,428) of all injury events recorded for M. alfredi and 74% (n = 106) for M. birostris. Unknown injuries which could not be categorised accounted for 13% (n = 207) and 26% (n = 37) of injury events for M. alfredi and M. birostris, respectively. Sublethal injuries were observed on 29% (n = 1,432) of the M. alfredi population and 20% (n = 134) of M. birostris.
For both species, the injury type resulting in the greatest proportion of the total injury events were natural predatory bites (M. alfredi = 48%, M. birostris = 45%). However, despite being the most prevalent sublethal injury in the Maldives, the overall percentage of the populations that exhibited predatory bites (M. alfredi = 15%, M. birostris = 10%) were considerably lower than was recorded in a M. alfredi population in southern Mozambique in 2010 (76%) (Marshall and Bennett, 2010) and 2020 (68%) (Venables, 2020). Predatory pressure on the Mozambique population appears high, especially when compared with other documented M. alfredi populations in Maui, Hawaii, and eastern Australia, where 33% (Deakos et al., 2011) and 23% (Couturier et al., 2014) of individuals were observed to have shark-inflicted injuries. In Ningaloo, Western Australia, just 2.7% of the M. alfredi population had injuries which unambiguously originated from predation events (McGregor et al., 2019), while in French Polynesia, this was the case for just two individuals (Carpentier et al., 2019). Many of these study sites, including in the Maldives, are shallow, protected coastal reefs which favour resident manta ray behaviour (Stevens, 2016; Venables, 2020). It is possible that shallow feeding, cleaning, or nursery sites (e.g., in lagoons or bays) offer manta rays some reduction in predation risk, giving large sharks fewer opportunities to successfully attack because they are less able to approach the ray from below (Heupel et al., 2007; Stevens, 2016; Stevens et al., 2018a; Stewart et al., 2018a). In contrast, the southern Mozambique coastline is predominantly exposed with strong currents and deeper rocky reefs (Venables, 2020), and M. alfredi exhibit wide-ranging movements within the region (Marshall et al., 2011; Venables et al., 2020). It is thought that their major food sources are further offshore in deeper water, so they may be less resident to inshore reefs (Venables, 2020; Venables et al., 2020). Spending more time in open water is likely to increase a manta ray’s exposure to predatory attack (Stevens, 2016). Indeed, predatory attacks on R. typus are thought to mainly occur in the open ocean (Speed et al., 2008; Lester et al., 2020).
Large predatory sharks which are known to attack and consume manta rays, such as C. leucas and G. cuvier (Dicken et al., 2017), are common in southern Mozambique, where predatory attacks on manta rays are relatively common (Marshall and Bennett, 2010; Venables, 2020), and a G. cuvier “hotspot” has been identified (Daly et al., 2018). Throughout most of the Maldives, large predatory shark species are rarely sighted in shallow reef habitats (Clarke et al., 2012; Sattar et al., 2013), which may also contribute to why the proportions of M. alfredi with predatory bites were considerably lower than in Mozambique (Marshall and Bennett, 2010; Venables, 2020). Shark fisheries in the Maldives intensified from the 1970s onward (Anderson and Ahmed, 1993), and pressure from three types of shark fishery led to concerns of overexploitation of shark stocks (Martin and Hakeem, 2006; MRC, 2009) and diminishing shark sightings by divers (Sinan et al., 2011; Ali and Sinan, 2014, 2015). Indeed, Ward-Paige (2017) reported that the Maldives had the highest shark catch per kilometre squared (between 1950 and 2010) when compared with 10 other (now) shark sanctuaries. Various shark management measures were introduced in the Maldives between the late twentieth and early twenty-first century, culminating in a complete ban on killing, capture, and extraction of any shark species in 2010 (Ushan and Wood, 2010; Sinan et al., 2011). Although some illegal shark fishing continues (Ali and Sinan, 2014), shark populations are slowly recovering in most, but not all, atolls (Sattar et al., 2013; Zimmerhackel et al., 2018). An increase in shark numbers, particularly G. cuvier, following the implementation of the shark fishing ban has been reported by local fishers and divers, which suggests conservation efforts are having a positive effect (Maldivian Manta Ray Project [MMRP], 2014, 2015; Zimmerhackel et al., 2018). Therefore, it is possible that the increase in predatory bite injuries per re-sighted M. alfredi found in this study is a result of increasing shark abundance in the region. Moreover, it is important to note that while assessing sublethal predation injuries can provide an indication of predatory pressure for a species, these scars are only a marker of failed predation attempts, so the true frequency of shark attacks on M. alfredi and M. birostris is likely to be much higher than recorded (Heithaus, 2001a,b). Manta rays’ quick healing capacity may also prevent identification of predation attempts in some cases.
In this study, predatory bites per sighted M. alfredi individual were highest in North Malé, Lhaviyani, Ihavandhippolhu, and Thiladhunmathi Atolls. Before the shark fishing ban was announced in 2010, a 10-year moratorium on shark fishing was introduced in seven major tourism atolls, including North Malé and Lhaviyani (Ushan and Wood, 2010; Sinan et al., 2011; Ali and Sinan, 2014). Although these measures were not properly enforced, and some shark fishing did continue in these areas (Martin and Hakeem, 2006; Ushan and Wood, 2010), tourism levels were high, and many resorts were monitoring illegal shark fishing. Therefore, sharks in these areas were subject to some protective measures even before the ban, which could explain why more predatory bites per sighted M. alfredi were recorded there during our study period. Moreover, fewer predatory bites per sighted M. alfredi were recorded in atolls which have historically experienced high shark fishing pressure, such as Raa and Baa Atolls (Anderson and Ahmed, 1993; Sattar et al., 2013). Throughout the 1990s, shark fishing effort was higher in the north-central atolls (Anderson and Waheed, 1999), and studies reported a considerable decline in reef shark numbers there prior to protection (Martin and Hakeem, 2006; Sinan et al., 2011). By 2010, sightings of large predatory shark species in Raa and Baa Atolls were extremely rare, and populations still have not recovered in these areas (G. Stevens, pers. obs.). Following the ban, Sattar et al. (2013) reported that the average number of sharks per survey was among the lowest in Baa Atoll, suggesting that fishing pressure there may have caused a population decline. Moreover, while it is impossible to know for certain where an injury event occurred, M. alfredi exhibit high site fidelity in the region, with 70% of the 4901 individuals in this study sighted in just one atoll, and 23% sighted in two atolls (the second atoll is usually the closest geographically to the first sighted atoll). Therefore, the high levels of residency recorded suggest the sighting location is the area where the recorded injury is most likely to have occurred.
Variations in predation rates between populations and study sites have been reported for other species of marine megafauna. For example, R. typus at Ningaloo Reef had more predatory bites (44% of individuals) than those in Mahé, Seychelles (21%) and southern Mozambique (15%), which was attributed to the abundance of G. cuvier and other species of Carcharhinidae sharks regularly sighted during peak R. typus seasonal sightings (Speed et al., 2008). However, R. typus individuals are highly migratory (Hearn et al., 2016), so healed predation injuries could have occurred in other locations within their range (Speed et al., 2008). Studies investigating shark bite scarring frequencies in dolphins (e.g., Tursiops spp.) have suggested predation pressure may be influenced by the availability of other shark prey (Heithaus, 2001a; Smith et al., 2018), spatial and temporal overlap of dolphins and sharks (Melillo-Sweeting et al., 2021), similar habitat selection (e.g., the use of sheltered semi-enclosed waters) (Sprogis et al., 2018), reduced fishing pressure for large sharks (Castelblanco-Martínez et al., 2021), or differences in dolphin and shark species or sizes, and the resulting probability of a lethal shark encounter (Heithaus, 2001b; Heithaus et al., 2017; Wilkinson et al., 2017).
Injuries originating naturally from infections, diseases, or parasites were recorded for < 1% (n = 37) of the M. alfredi population in the Maldives, and one M. birostris individual. While these types of injuries were not common in either species here, batoid rays infected with parasites can suffer a variety of health consequences, which may prove lethal in some species (Caira and Healy, 2004; Garner, 2013; Murie et al., 2020). These include skin lesions, inflammation, necrosis, bacterial and viral infections, and respiratory disease (Caira and Healy, 2004; Garner, 2013; Murie et al., 2020). However, manta rays are known to visit cleaning stations for the removal of dead or infected tissue (to control infections) and parasites by cleaner fish (Foster, 1985; Grutter, 1999; Marshall, 2008; Armstrong et al., 2021b).
Mobula alfredi and M. birostris are commonly sighted in association with smaller hitchhiker species (e.g., sharksucker remora Echeneis naucrates and giant remora Remora remora) (Nicholson-Jack et al., 2021), which utilise their hosts for benefits such as increased food availability and shelter from predation, in exchange for removing parasites from their hosts’ bodies (Cressey and Lachner, 1970; Flammang et al., 2020; Solleliet-Ferreira et al., 2020). While remoras are often described as a beneficial symbiont for manta rays (Stevens et al., 2018a), this study is the first to describe injuries, sometimes severe, which can result from close association with these hitchhikers. For example, remoras (often juveniles) seeking shelter inside a manta ray’s gill cavity can cause significant damage to the gill slits, particularly if repeated intrusion occurs (Figure 3).
Natural deformities (e.g., misshapen head, cephalic lobe, or severely bent tail) were recorded for 1.7% (n = 83) of M. alfredi and < 1% (n = 5) of M. birostris. It is unclear whether these physical abnormalities have implications for the health or fitness of individuals; however, a misshapen cephalic fin has the potential to reduce feeding efficiency (Deakos et al., 2011).
Of the four anthropogenic sublethal injury types identified in this study, fishing line injuries and boat strikes accounted for 98% of the total for M. alfredi and 91% for M. birostris. Although manta rays are not targeted by Maldivian fisheries (MEPA, 2014; Stevens, 2016), entanglement in fishing line was the most common anthropogenic injury event recorded for both species, with 9% (n = 432) of all recorded M. alfredi, and 5% (n = 32) of all M. birostris having sustained these sublethal injuries. While these figures are comparatively lower than M. alfredi sighted within the Nusa Penida marine protected area (MPA) (∼14%) (Germanov et al., 2019), and M. birostris studied in southeast Florida, United States (27%) (Pate and Marshall, 2020), it indicates that incidental bycatch remains a significant threat to manta rays in the Maldives. Deakos et al. (2011) reported 10% of a M. alfredi population in Hawaii had an amputated or non-functioning cephalic fin, likely caused by entanglement in monofilament fishing line. Here, similar damage to the cephalic fins was recorded in 23% (n = 105) of injury events involving fishing line. Severe injuries to the cephalic fins may impair feeding efficiency and reduce the fitness of those afflicted individuals (Deakos et al., 2011). As in Deakos et al. (2011), individuals in the current study with only one functioning cephalic fin appeared to be healthy, although further research should investigate how the loss of a cephalic fin may affect an individual’s growth rate, size, or reproductive success. Incidental capture by fishers is also likely to cause considerable stress to a manta ray, even if the resulting injuries are minimal (Wilson et al., 2014; Pate and Marshall, 2020).
Injuries from interactions with fishing line were highest per sighted M. alfredi in Laamu, North Malé, Addu, and Baa Atolls. A likely reason for this is that fishing pressure on reef fish species is highest in areas where tourism is concentrated (Sattar et al., 2014). Although the offshore tuna fishery historically maintained low levels of reef fishing in the Maldives, the expansion of the tourism industry and rising demand for reef fish in Asia led to the emergence of a reef fishery in recent decades (Adam, 2004; Jaleel, 2013; Yadav et al., 2020). Kaafu Atoll (the administrative division which includes North and South Malé) was the first atoll where the tourism industry was introduced and developed, and is the most heavily populated, so reef fishing has been carried out within the atoll for longer (Sattar et al., 2014). Big game fishing is also popular among tourists in these atolls, targeting larger pelagic species (e.g., sailfish Istiophorus platypterus, dogtooth tuna Gymosarda unicolor, and wahoo Acanthocybium solandi) (Sattar et al., 2012; Ahusan et al., 2017), and the lines used (e.g., troll lines) are the types manta rays often get caught in (G. Stevens, pers. obs.). Compared with the central atolls, the tourism industry is less developed in the northern atolls and levels of exploitation of reef fish are lower (Sattar et al., 2014), which is likely why fewer fishing line injuries per sighted M. alfredi were recorded in these atolls during our study period. Quantifying the variation in anthropogenic impacts, tourism, and human population across the Maldives may be a useful future study area to further this work.
Overall, the frequency of fishing line injuries per re-sighted M. alfredi individual declined during our study period. However, the temporal variations found in this study should be interpreted cautiously, as calculations could only include injuries which were recorded on re-sightings of individuals, which only accounted for 38% of the total injury events observed for M. alfredi.
Entanglement in fishing nets is considered a key threat to manta rays worldwide (Stewart et al., 2018b). However, in this study, injuries of this type were rarely observed for both manta ray species. Indeed, a recent study on injuries to R. typus observed in South Ari Atoll in the Maldives reported that only 1.1% of injuries recorded for 82 resident individuals originated from entanglement with fishing nets, ropes, and hooks, although the atoll is not a major fishing region (Allen et al., 2021). The Maldives has a ban on all net fishing (Nizar and Ibrahim, 2019), except for the use of small baitfish nets. Although illegal gill net fishing does occur (G. Stevens, pers. obs.), and ghost drift nets are also a problem (e.g., Stelfox et al., 2019, 2020), overall, the impacts of destructive net fishing practices are greatly reduced in the region because of these protective measures. However, the potential for net entanglement to result in the death of an individual may mean that its prevalence is underreported here.
While rope entanglement injuries were rarely observed in this study for both M. alfredi (n = 1) and M. birostris (n = 2), entanglement mortality from boat mooring and buoy lines has been identified as a serious threat (Manta Trust, 2019a). Entanglement in a mooring line, which can easily occur, will most likely lead to asphyxiation and death for a manta ray. Mooring ropes are less frequently used in the Maldives than other locations worldwide (e.g., at dive sites). However, increasing development of resorts, dive, and water-sports centres have led to the installation of hundreds of new mooring lines in the last few years, resulting in an increase in manta ray entanglement, mortality, and mitigation measures (Manta Trust, 2019a,b; Stevens and Froman, 2019). In 2019, the Manta Trust published Manta Ray Entanglement Protocol and simple mitigation measures which help ensure mooring lines are “manta safe” (Manta Trust, 2019a,b). If these measures are widely adopted, the threat of entanglement in a mooring line would be greatly reduced.
Vessel strikes have been identified as a major concern for M. alfredi (Stewart et al., 2018b), and although these kinds of sublethal injuries were not as common in this study (3%, n = 53) as fishing line injuries, they still pose a significant risk to this population, especially as boat traffic continues to rapidly increase in the Maldives (Stevens and Froman, 2019). Indeed, Allen et al. (2021) reported that abrasions and lacerations accounted for 77% of injuries to R. typus resident in South Ari Atoll, with lacerations being the most common type of major injury observed. These injuries were often caused by boat strikes with characteristic propeller marks, and a large proportion can be attributed to high numbers of tourist vessels searching for megafauna in the area (Allen et al., 2021). In this study, the number of boat strike and propeller injuries per re-sighted M. alfredi has increased since 2016. This increase is also likely linked to a rise in boat traffic resulting from increased tourism activities (Anderson et al., 2011a; Venables, 2013; Stevens and Froman, 2019; Murray et al., 2020; Allen et al., 2021). Indeed, the highest percentage of boat strike injuries recorded occurred in some of the busiest tourism atolls (e.g., North Malé and South Malé) (Maldivian Manta Ray Project [MMRP], 2019b). These observations are consistent with a study in French Polynesia, which found M. alfredi were more likely to be injured around inhabited islands with more marine traffic than at remote uninhabited areas (Carpentier et al., 2019). Here, no boat strike injuries were observed for M. birostris, probably because M. birostris in the Maldives, unlike M. alfredi, rarely visit nearshore lagoonal or reef habitats, where heavy boat traffic occurs (Maldvian Manta Ray Project [MMRP], 2019a; Stevens and Froman, 2019).
Boat strike injuries were most frequent per sighted M. alfredi in one lagoon (Guraidhoo Falhu) and channel area in South Malé Atoll. This site is a busy highway for boat traffic and a key aggregation site for juvenile M. alfredi, which exhibit extremely high site fidelity (Couturier et al., 2018; Germanov et al., 2019; Setyawan et al., 2020). Therefore, any injuries to these juvenile M. alfredi are likely to have occurred in the close vicinity of this site. At Guraidhoo Falhu lagoon, the juvenile M. alfredi feed at the surface and unfortunately often get hit by speedboats with outboard engines often traveling at speeds > 30 mph (G. Stevens, pers. obs.). Direct impact with a manta ray at this speed is likely to prove lethal to the ray (Deakos et al., 2011; McGregor et al., 2019). However, quantifying lethal injuries is challenging because dead manta rays sink, and are rarely observed in the field (Deakos et al., 2011). It is also possible that manta rays are experiencing blunt force trauma from boat strikes, without showing obvious external injuries (Pate and Marshall, 2020).
In this study, juvenile M. alfredi were more likely to have injuries than adults, which may be a result of life-stage segregation in habitat-use. Juvenile manta rays in the Maldives (Stevens, 2016), Indonesia (Germanov et al., 2019; Setyawan et al., 2020), Palmyra Atoll (McCauley et al., 2014), southeast Florida, United States (Pate and Marshall, 2020), and the Gulf of Mexico (Stewart et al., 2018a) have been shown to reside in shallow reef habitats for longer periods than adults, and in higher numbers, and exhibit long-term habitat use of these areas. It has been suggested that lagoons serve as important nursery grounds for juvenile manta rays, providing benefits such as reliable food availability, refuge from predators (e.g., large pelagic sharks), or the opportunity for thermoregulation via basking behaviour after deep foraging dives (Heupel et al., 2007; McCauley et al., 2014; Stevens, 2016; Stewart et al., 2018a; Germanov et al., 2019; Pate and Marshall, 2020). However, these sheltered and easily accessed lagoons are often areas of increased human activity, such as coastal development, pollution, fishing, and boat traffic (Blumenthal et al., 2010; Pate and Marshall, 2020). The juveniles which rely on these habitats are, therefore, directly exposed to these threats (McCauley et al., 2014), which would increase the likelihood of injury from anthropogenic sources (Pate and Marshall, 2020). Indeed, in southeast Florida, United States, boat propellers (30%) and fishing line (27%) were the most common sources of injuries to the population of juvenile M. birostris which frequent the shallow coastal waters in the region, where human activity is heavily concentrated (Pate and Marshall, 2020). Unfortunately, throughout the Maldives, M. alfredi, especially juveniles, aggregate in shallow lagoons where increasing boat traffic is likely to lead to greater sublethal injuries and mortality events if protective management measures are not introduced (Stevens, 2016; Maldivian Manta Ray Project [MMRP], 2017; Harris et al., 2020). To reduce the impact of tourism and fishing activities on juvenile M. alfredi, these important nursery aggregation sites should be protected through speed limits, outboard engine restrictions, and no-take fishing zones (Carpentier et al., 2019; Germanov et al., 2019; Stevens and Froman, 2019).
Injuries were also more likely to be observed on male M. alfredi than females. Males reach a smaller maximum disc width than females which may make them more vulnerable to predatory attack (Marshall et al., 2011; Stevens, 2016). Sexual segregation of the use of shallow lagoons may also explain why anthropogenic injuries were more likely to be observed on males than females. Studies have suggested males are more likely to aggregate in these sheltered habitats than females, for reasons like predator avoidance and reliable foraging opportunities (McCauley et al., 2014; Stevens, 2016; Germanov et al., 2019). Thus, adult males may be more vulnerable to human activities than adult females (McCauley et al., 2014), with a higher risk of anthropogenic injury in these shallow areas.
In the current study, injuries were more likely to be observed on M. alfredi at cleaning stations. This is likely because manta rays are known to visit cleaning stations to promote wound healing and remove parasites (Foster, 1985; Marshall, 2008), with individuals regularly returning to specific stations over long periods of time, and sometimes spending hours there during the day (Dewar et al., 2008; O’Shea et al., 2010; McGregor et al., 2019; Armstrong et al., 2021b). Cleaner fish are thought to assist with wound healing by removing injured tissue from their hosts which may prevent further infection (Foster, 1985). It has been suggested that injured manta rays may exhibit greater site fidelity, and may remain in an area to visit cleaning stations more regularly (Marshall, 2008; Marshall et al., 2011).
In contrast to M. alfredi, neither sex nor maturity status were significant explanatory variables for the occurrence of injuries to M. birostris. Potentially, these results reflect the contrasting life history of the species, for example, the more wide-ranging nature of M. birostris compared to M. alfredi (Kashiwagi et al., 2011; Stewart et al., 2016b; Marshall et al., 2020). However, similar to M. alfredi, sex and life-stage segregation of habitat-use by M. birostris has been reported, for example, in southeast Florida (Pate and Marshall, 2020), Indonesia (Beale et al., 2019) and the Gulf of Mexico (Stewart et al., 2018a). Juvenile M. birostris were found to aggregate in shallow reef habitats, which serve as nursery grounds (Stewart et al., 2018a; Pate and Marshall, 2020), and a female bias was found at cleaning stations (Beale et al., 2019). In the Maldives, sightings and re-sightings of this species, particularly of juveniles, are much less frequent than M. alfredi and predominantly occur around Fuvahmulah Atoll (Maldvian Manta Ray Project [MMRP], 2019a). Therefore, future studies would benefit from the inclusion of a more extended and spatially comprehensive dataset, similar to that of M. alfredi, to assess whether the occurrence of injuries to M. birostris is influenced by sex and maturity status.
Although injured manta rays have high wound healing capacities (McGregor et al., 2019; Pate and Marshall, 2020), sublethal injuries have the potential to affect their long-term health and fitness. For example, truncated pectoral fins or a trailing fishing line could impair a manta ray’s swimming efficiency, or their ability to evade predation (Germanov et al., 2019); a slower, weaker manta ray is also more of a target. An amputated tail may reduce an individual’s ability to detect predators approaching from behind (Stevens et al., 2018a). Damage to a manta ray’s sexual organs can impact, or even prevent, reproductive success (Marshall and Bennett, 2010). Loss or reduced functioning of the cephalic fins from entanglement in monofilament line may impair feeding success (Deakos et al., 2011). Capture, entanglement, and predation may also induce premature birth or abortion in elasmobranchs, which could have implications at the population level (Adams et al., 2018).
Conclusion
Globally, sublethal injuries to manta rays add pressure to populations which are already under threat from targeted fisheries and incidental bycatch (Stewart et al., 2018b), the climate crisis, tourism pressures, and reef degradation, which combined greatly impact their food supply, reproductive opportunities, and suitable habitat (Richardson, 2008; Stevens and Froman, 2019). This is the first time a study has attempted to categorise all sublethal injuries and physical abnormalities for these species. Although manta rays in the Maldives are protected nationally and have never been targeted by a commercial fishery in the region (MEPA, 2014; Stevens, 2016), here we show that incidental bycatch and boat traffic present a significant threat to these animals. Overall, higher incidences of anthropogenic injuries to M. alfredi were recorded at Baa, North Malé, South Malé, Laamu, and Addu Atolls, which corresponds to where tourism activities, fishing (commercial and leisure), and boat traffic are more concentrated. As the tourism industry in the Maldives continues to expand, so too will the demand for recreational fishing and fish as a source of food (Sattar et al., 2014). Although individual manta rays have shown resilience to a range of sublethal injuries (Marshall and Bennett, 2010; McGregor et al., 2019; Pate and Marshall, 2020), the continued increase in marine traffic and fishing activities in the region will likely lead to more frequent injuries, which could have implications for the health and fitness of these populations.
While the Maldives contains 42 MPAs, these only cover 0.5% of the country’s total area (Stevens and Froman, 2019), and only 3 of 48 key manta ray aggregation sites fall within an MPA with active enforcement (Harris et al., 2020). To help safeguard these vulnerable species, all mooring and buoy lines in manta ray aggregation areas should be modified to reduce the risk of entanglement, which often proves lethal (Manta Trust, 2019a). The establishment of no-take fishing zones and speedboat exclusion (or restriction) zones in areas of critical manta ray habitat (e.g., feeding and cleaning aggregation sites, and juvenile nursery habitat) would greatly help reduce the frequency that these animals become entangled in fishing line, or hit by vessels (Carpentier et al., 2019; Germanov et al., 2019; Stevens and Froman, 2019).
Data Availability Statement
The datasets presented in this study can be found in online repositories. The names of the repository/repositories and accession number(s) can be found below: http://idthemanta-intg.eu-west-2.elasticbeanstalk.com/home#!/home.
Ethics Statement
Ethical review and approval was not required for the animal study because no invasive work was undertaken in the data collection.
Author Contributions
ES and GS contributed to the conception of the study, and the project administration. ES and JLH performed the formal analysis and wrote the first draft of the manuscript. GS and JPH acquired funding and provided supervision of the project. ES, JLH, and GS conducted the investigation. ES, JLH, KB, and GS contributed to the methodology. GS contributed to data curation and provided the resources. All authors contributed to manuscript revision, read, and approved the submitted version.
Funding
The authors declare that grant support for fieldwork for this study was provided by Save Our Seas Foundation https://saveourseas.com/. Author who received the award: GS. Funding for open access publication fee provided by University of Exeter. The funders had no role in study design, data collection and analysis, decision to publish, or preparation of the manuscript.
Conflict of Interest
The authors declare that the research was conducted in the absence of any commercial or financial relationships that could be construed as a potential conflict of interest.
The reviewer AA declared a past co-authorship with the author GS to the handling editor.
Publisher’s Note
All claims expressed in this article are solely those of the authors and do not necessarily represent those of their affiliated organizations, or those of the publisher, the editors and the reviewers. Any product that may be evaluated in this article, or claim that may be made by its manufacturer, is not guaranteed or endorsed by the publisher.
Acknowledgments
We thank the Maldives Government for granting us permission to undertake the research (annually renewable permit: PA/2020/PSR-M07). We thank the Manta Trust’s resort and dive operator partners in the Maldives for their overall support with the study. We thank all Manta Trust staff, students, and volunteers in the Maldives (past and present, especially Tam Sawers, and Niv Froman), as well as the marine biologists, water sports and dive teams throughout the country who contributed huge amounts of photo-ID data to this study. We also thank Peter McGregor. Finally, we would like to thank all the members of the public who submitted images to the Manta Trust for this study. We could not have undertaken this work without all your help. Content of this work has previously appeared online in a master’s thesis (Strike, 2020), available from: https://www.mantatrust.org/resources.
Supplementary Material
The Supplementary Material for this article can be found online at: https://www.frontiersin.org/articles/10.3389/fmars.2022.773897/full#supplementary-material
Footnotes
References
Adam, M. S. (2004). Country Review: Maldives. Review of the State of World Marine Capture Fisheries Management: FAO Fisheries Technical Paper, Vol. 488. Rome: Food and Agriculture Organization of the United Nations, 383–391.
Adams, K. R., Fetterplace, L. C., Davis, A. R., Taylor, M. D., and Knott, N. A. (2018). Sharks, rays and abortion: the prevalence of capture-induced parturition in elasmobranchs. Biol. Conserv. 217, 11–27. doi: 10.1016/j.biocon.2017.10.010
Ahusan, M., Adam, M. S., Ziyad, A., Ali, K., and Shifaz, A. (2017). Maldives National Report Submitted to the Indian Ocean Tuna Commission Scientific Committee – 2017. Male’: Marine Research Centre, Ministry of Fisheries and Agriculture, 2–17.
Alava, J. J., and Merlen, G. (2009). Video-documentation of a killer whale (Orcinus orca) predatory attack on a Giant Manta (Manta birostris) in the Galapagos Islands. Lat. Am. J. Aquat. Mamm. 7, 81–84. doi: 10.5597/lajam00139
Ali, K., and Sinan, H. (2014). Shark ban in its infancy: successes, challenges and lessons learned. J. Mar. Biol. Ass. India 56, 34–40. doi: 10.6024/jmbai.2014.56.1.01750s-05
Ali, K., and Sinan, H. (2015). National Plan of Action: For the Conservation and Management of Sharks in the Maldives. Male’: Ministry of Fisheries and Agriculture, 1–47.
Allen, H. L., Stewart, B. D., Mcclean, C. J., Hancock, J., and Rees, R. (2021). Anthropogenic injury and site fidelity in Maldivian whale sharks (Rhincodon typus). Aquat. Conserv: Mar Freshw Ecosyst. 31, 1429–1442. doi: 10.1002/aqc.3524
Andersen, M. S., Forney, K. A., Cole, T. V., Eagle, T., Angliss, R., Long, K., et al. (2008). Differentiating Serious and non-Serious Injury of Marine Mammals: Report of the Serious Injury Technical Workshop. NOAA Tech. Memo. NMFS-OPR, Vol. 39. Seattle, WA: NOAA Fisheries, 94.
Anderson, R. C., Adam, M. S., Kitchen-Wheeler, A.-M., and Stevens, G. (2011a). Extent and economic value of Manta ray watching in Maldives. Tour. Mar. Environ. 7, 15–27. doi: 10.3727/154427310X12826772784793
Anderson, R. C., Adam, M. S., and Goes, J. I. (2011b). From monsoons to Mantas: seasonal distribution of Manta alfredi in the Maldives. Fish. Oceanogr. 20, 104–113. doi: 10.1111/j.1365-2419.2011.00571.x
Anderson, R. C., and Waheed, Z. (1999). “Management of shark fisheries in the Maldives,” in Case Studies in the Management of Elasmobranch Fisheries. FAO Fisheries Technical Paper 378 (Parts 1 & 2), ed. R. Shotton (Rome: FAO), 367–401.
Archibald, D. W., and James, M. C. (2018). Prevalence of visible injuries to leatherback sea turtles Dermochelys coriacea in the Northwest Atlantic. Endanger. Species Res. 37, 149–163. doi: 10.3354/esr00920
Archie, E. A. (2013). Wound healing in the wild: stress, sociality and energetic costs affect wound healing in natural populations. Parasite Immunol. 35, 374–385. doi: 10.1111/pim.12048
Armstrong, A. O., Stevens, G. M. W., Townsend, K. A., Murray, A., Bennett, M. B., Armstrong, A. J., et al. (2021a). Reef Manta rays forage on tidally driven, high density zooplankton patches in Hanifaru Bay, Maldives. PeerJ 9:e11992. doi: 10.7717/peerj.11992
Armstrong, A. O., Armstrong, A. J., Bennett, M. B., Richardson, A. J., Townsend, K. A., Everett, J. D., et al. (2021b). Mutualism promotes site selection in a large marine planktivore. Ecol. Evol. 11, 5606–5623. doi: 10.1002/ece3.7464
Armstrong, A. O., Armstrong, A. J., Jaine, F. R. A., Couturier, L. I. E., Fiora, K., Uribe-Palomino, J., et al. (2016). Prey density threshold and tidal influence on reef Manta ray foraging at an aggregation site on the Great Barrier Reef. PLoS One 11:e0153393. doi: 10.1371/journal.pone.0153393
Bansemer, C. S., and Bennett, M. B. (2010). Retained fishing gear and associated injuries in the east Australian grey nurse sharks (Carcharias taurus): implications for population recovery. Mar. Freshw. Res. 61, 97–103. doi: 10.1071/MF08362
Bates, A. D., Maechler, M., Bolker, B., Walker, S., Haubo, R., Christensen, B., et al. (2020). Linear Mixed-Effects Models using “Eigen” and S4 Package ‘lme4’.
Beale, C. S., Stewart, J. D., Setyawan, E., Sianipar, A. B., and Erdmann, M. V. (2019). Population dynamics of oceanic Manta rays (Mobula birostris) in the Raja Ampat Archipelago, West Papua, Indonesia, and the impacts of the El Niño–Southern Oscillation on their movement ecology. Divers. Distrib. 25, 1472–1487. doi: 10.1111/ddi.12962
Blumenthal, J. M., Austin, T. J., Bothwell, J. B., Broderick, A. C., Ebanks-Petrie, G., Olynik, J. R., et al. (2010). Life in (and out of) the lagoon: fine-scale movements of green turtles tracked using time-depth recorders. Aquat. Biol. 9, 113–121. doi: 10.3354/ab00222
Borucinska, J., Kohler, N., Natanson, L., and Skomal, G. (2002). Pathology associated with retained fishing hooks in blue sharks, Prionace glauca (L.), with implications for their conservation. J. Fish Dis. 25, 515–521. doi: 10.1046/j.1365-2761.2002.00396.x
Braun, C. D., Skomal, G. B., Thorrold, S. R., and Berumen, M. L. (2014). Diving behavior of the reef Manta ray links coral reefs with adjacent deep pelagic habitats. PLoS One 9:e88170. doi: 10.1371/journal.pone.0088170
Braun, C. D., Skomal, G. B., Thorrold, S. R., and Berumen, M. L. (2015). Movements of the reef Manta ray (Manta alfredi) in the Red Sea using satellite and acoustic telemetry. Mar. Biol. 162, 2351–2362. doi: 10.1007/s00227-015-2760-
Burnham, K., and Anderson, D. (2002). Model Selection and Multimodel Inference: A Practical Information-Theoretic Approach, 2nd Edn. New York, NY: Springer. doi: 10.1016/j.ecolmodel.2003.11.004
Caira, J. N., and Healy, C. J. (2004). “Elasmobranchs as hosts of metazoan parasites,” in Biology of Sharks and their Relatives, eds J. C. Carrier, J. A. Musick, and M. R. Heithaus (Boca Raton, FL: CRC Press), 523–551. doi: 10.1201/9780203491317.ch18
Carpentier, A. S., Berthe, C., Ender, I., Jaine, F. R. A., Mourier, J., Stevens, G., et al. (2019). Preliminary insights into the population characteristics and distribution of reef (Mobula alfredi) and oceanic (M. birostris) Manta rays in French Polynesia. Coral Reefs 38, 1197–1210. doi: 10.1007/s00338-019-01854-0
Cassoff, R. M., Moore, K. M., Mclellan, W. A., Barco, S. G., Rotstein, D. S., and Moore, M. J. (2011). Lethal entanglement in baleen whales. Dis. Aquat. Org. 96, 175–185. doi: 10.3354/dao02385
Castelblanco-Martínez, D. N., Ramos, E. A., Kiszka, J. J., Blanco-Parra, M. P., Padilla-Saldívar, J. A., García, J., et al. (2021). Spatial patterns of shark-inflicted injuries on coastal bottlenose dolphins in the Mesoamerican Reef System. Stud. Neotrop. Fauna Environ. 1–7. doi: 10.1080/01650521.2021.187739
Chin, A., Mourier, J., and Rummer, J. L. (2015). Blacktip reef sharks (Carcharhinus melanopterus) show high capacity for wound healing and recovery following injury. Conserv. Physiol. 3:cov062. doi: 10.1093/conphys/cov062
Clarke, C., Lea, J., and Ormond, R. (2012). “Comparative abundance of reef sharks in the Western Indian Ocean,” in Proceedings of the 12th international coral reef symposium, Cairns, QLD, 9–13. doi: 10.1002/ece3.8492
Couturier, L. I. E., Dudgeon, C. L., Pollock, K. H., Jaine, F. R. A., Bennett, M. B., Townsend, K., et al. (2014). Population dynamics of the reef Manta ray Manta alfredi in eastern Australia. Coral Reefs. 33, 329–342. doi: 10.1007/s00338-014-1126-5
Couturier, L. I. E., Marshall, A. D., Jaine, F. R. A., Kashiwagi, T., Pierce, S. J., Townsend, K. A., et al. (2012). Biology, ecology and conservation of the Mobulidae. J. Fish Biol. 80, 1075–1119. doi: 10.1111/j.1095-8649.2012.03264.x
Couturier, L. I. E., Newman, P., Jaine, F. R. A., Bennett, M. B., Venables, W. N., Cagua, E. F., et al. (2018). Variation in occupancy and habitat use of Mobula alfredi at a major aggregation site. Mar. Ecol. Prog. Ser. 599, 125–145. doi: 10.3354/meps12610
Cressey, R. F., and Lachner, E. A. (1970). The parasitic copepod diet and life history of diskfishes (Echeneidae). Copeia 1970, 310–318. doi: 10.2307/1441652
Croll, D. A., Dewar, H., Dulvy, N. K., Fernando, D., Francis, M. P., Galván-Magaña, F., et al. (2016). Vulnerabilities and fisheries impacts: the uncertain future of Manta and devil rays. Aquat. Conserv. Mar. Freshw. Ecosyst. 26, 562–575. doi: 10.1002/aqc.2591
Daly, R., Smale, M. J., Singh, S., Anders, D., Shivji, M., Daly, C. A. K., et al. (2018). Refuges and risks: evaluating the benefits of an expanded MPA network for mobile apex predators. Divers. Distrib. 24, 1217–1230. doi: 10.1111/ddi.12758
Deakos, M. H., Baker, J. D., and Bejder, L. (2011). Characteristics of a Manta ray Manta alfredi –population off Maui, Hawaii, and implications for management. Mar. Ecol. Prog. Ser. 429, 245–260. doi: 10.3354/meps09085
Dewar, H., Mous, P., Domeier, M., Muljadi, A., Pet, J., and Whitty, J. (2008). Movements and site fidelity of the giant Manta ray, Manta birostris, in the Komodo Marine Park, Indonesia. Mar. Biol. 155, 121–133. doi: 10.1007/s00227-008-0988-
Dicken, M. L., Hussey, N. E., Christiansen, H. M., Smale, M. J., Nkabi, N., Cliff, G., et al. (2017). Diet and trophic ecology of the tiger shark (Galeocerdo cuvier) from South African waters. PLoS One 12:e0177897. doi: 10.1371/journal.pone.0177897
Dulvy, N. K., Pardo, S. A., Simpfendorfer, C. A., and Carlson, J. K. (2014). Diagnosing the dangerous demography of Manta rays using life history theory. PeerJ 2:e400. doi: 10.7717/peerj.400
Fernando, D., and Stewart, J. D. (2021). High bycatch rates of manta and devil rays in the “small-scale” artisanal fisheries of Sri Lanka. PeerJ 9:e11994. doi: 10.7717/peerj.11994
Flammang, B. E., Marras, S., Anderson, E. J., Lehmkuhl, O., Mukherjee, A., Cade, D. E., et al. (2020). Remoras pick where they stick on blue whales. J. Exp. Biol. 223:jeb226654. doi: 10.1242/jeb.226654
Foster, S. A. (1985). Wound healing: a possible role of cleaning stations. Copeia 1985, 875–880. doi: 10.2307/1445236
Gannier, A. (2002). Cetaceans of the Marquesas Islands (French Polynesia): distribution and relative abundance as obtained from a small boat dedicated survey. Aquat. Mamm. 28, 198–210.
Garner, M. M. (2013). A retrospective study of disease in elasmobranchs. Vet. Pathol. 50, 377–389. doi: 10.1177/0300985813482147
Germanov, E. S., Bejder, L., Chabanne, D. B. H., Dharmadi, D., Hendrawan, I. G., Marshall, A., et al. (2019). Contrasting habitat use and population dynamics of reef Manta rays within the Nusa Penida Marine Protected Area, Indonesia. Front. Mar. Sci. 6:215. doi: 10.3389/fmars.2019.00215
Greenfield, M. R., Mchugh, K. A., Wells, R. S., and Rubenstein, D. I. (2021). Anthropogenic injuries disrupt social associations of common bottlenose dolphins (Tursiops truncates) in Sarasota Bay, Florida. Mar. Mamm. Sci. 37, 29–44. doi: 10.1111/mms.12729
Harris, J. L., Mcgregor, P. K., Oates, Y., and Stevens, G. M. W. (2020). Gone with the wind: seasonal distribution and habitat use by the reef Manta ray (Mobula alfredi) in the Maldives, implications for conservation. Aquat. Conserv: Mar. Freshw. Ecosyst. 30, 1649–1664. doi: 10.1002/aqc.3350
Harris, R. N. (1989). Nonlethal injury to organisms as a mechanism of population regulation. Am. Nat. 134, 835–847. doi: 10.1086/285016
Harvey-Carroll, J., Stewart, J. D., Carroll, D., Mohamed, B., Shameel, I., Zareer, I. H., et al. (2021). The impact of injury on apparent survival of whale sharks (Rhincodon typus) in South Ari Atoll Marine Protected Area, Maldives. Sci. Rep. 11:937. doi: 10.1038/s41598-020-79101-8
Haskell, P., McGowan, A., Westling, A., Méndez-Jiménez, A., Rohner, C., Collins, K., et al. (2015). Monitoring the effects of tourism on whale shark Rhincodon typus behaviour in Mozambique. Oryx 49, 492–499. doi: 10.1017/S0030605313001257
Hearn, A. R., Green, J., Román, M. H., Acuña-Marrero, D., Espinoza, E., and Klimley, A. P. (2016). Adult female whale sharks make long-distance movements past Darwin Island (Galapagos, Ecuador) in the Eastern Tropical Pacific. Mar. Biol. 163:214. doi: 10.1007/s00227-016-2991-y
Heithaus, M. R. (2001a). Predator–prey and competitive interactions between sharks (order Selachii) and dolphins (suborder Odontoceti): a review. J. Zool. 253, 53–68. doi: 10.1017/S0952836901000061
Heithaus, M. R. (2001b). Shark attacks on bottlenose dolphins (Tursiops aduncus) in Shark Bay, Western Australia: attack rate, bite scar frequencies, and attack seasonality. Mar. Mamm. Sci. 17, 526–539. doi: 10.1111/j.1748-7692.2001.tb01002.x
Heithaus, M. R., Kiszka, J. J., Cadinouche, A., Dulau-Drouot, V., Boucaud, V., Perez-Jorge, S., et al. (2017). Spatial variation in shark-inflicted injuries to Indo-Pacific bottlenose dolphins (Tursiops aduncus) of the southwestern Indian Ocean. Mar. Mamm. Sci. 33, 335–341. doi: 10.1111/mms.12346
Heupel, M. R., Carlson, J. K., and Simpfendorfer, C. A. (2007). Shark nursery areas: concepts, definition, characterization and assumptions. Mar. Ecol. Prog. Ser. 337, 287–297. doi: 10.3354/meps337287
Hosegood, J. (2020). Genomic Tools for Conservation and Management of Manta and Devil Rays (Mobula spp.). Ph.D. thesis. Bangor: Bangor University.
Jaine, F. R. A., Couturier, L. I. E., Weeks, S. J., Townsend, K. A., Bennett, M. B., Fiora, K., et al. (2012). When giants turn up: sighting trends, environmental influences and habitat use of the Manta ray Manta alfredi at a coral reef. PLoS One 7:e46170. doi: 10.1371/journal.pone.0046170
Jaine, F. R. A., Rohner, C. A., Weeks, S. J., Couturier, L. I. E., Bennett, M. B., Townsend, K. A., et al. (2014). Movements and habitat use of reef Manta rays off Eastern Australia: offshore excursions, deep diving and eddy affinity revealed by satellite telemetry. Mar. Ecol. Prog. Ser. 510, 73–86. doi: 10.3354/meps10910
Jaleel, A. (2013). The status of the coral reefs and the management approaches: the case of the Maldives. Ocean Coast. Manag. 82, 104–118. doi: 10.1016/j.ocecoaman.2013.05.009
Jessop, T., Sumner, J., Lance, V., and Limpus, C. (2004). Reproduction in shark–attacked sea turtles is supported by stress–reduction mechanisms. Proc. R. Soc. B. 271, S91–S94. doi: 10.1098/rsbl.2003.0102
Johnson, A., Salvador, G., Kenney, J., Robbins, J., Kraus, S., Landry, S., et al. (2005). Fishing gear involved in entanglements of right and humpback whales. Mar. Mamm. Sci. 21, 635–645. doi: 10.1111/j.1748-7692.2005.tb01256.x
Kashiwagi, T., Marshall, A. D., Bennett, M. B., and Ovenden, J. R. (2011). Habitat segregation and mosaic sympatry of the two species of manta ray in the Indian and Pacific Oceans: Manta alfredi and M. birostris. Mar. Biodivers. Rec. 4:e53. doi: 10.1017/S1755267211000479
Kitchen-Wheeler, A.-M., Ari, C., and Edwards, A. J. (2012). Population estimates of Alfred mantas (Manta alfredi) in central Maldives atolls: North Male, Ari and Baa. Environ. Biol. Fish. 93, 557–575. doi: 10.1007/s10641-011-9950-8
Lawson, J. M., Fordham, S. V., O’Malley, M. P., Davidson, L. N. K., Walls, R. H. L., Heupel, M. R., et al. (2017). Sympathy for the devil: a conservation strategy for devil and manta rays. PeerJ. 5:e3027. doi: 10.7717/peerj.3027
Lester, E., Meekan, M. G., Barnes, P., Raudino, H., Rob, D., Waples, K., et al. (2020). Multi-year patterns in scarring, survival and residency of whale sharks in Ningaloo Marine Park, Western Australia. Mar. Ecol. Prog. Ser. 634, 115–125. doi: 10.3354/meps13173
Lewis, S. A., Setiasih, N., Fahmi, Dharmadi, D., O’Malley, M. P., Campbell, S. J., et al. (2015). Assessing Indonesian manta and devil ray populations through historical landings and fishing community interviews. PeerJ Prepr. 6:e1334v1. doi: 10.7287/peerj.preprints.1334v1
Maldivian Manta Ray Project [MMRP] (2014). The Value of Traditional Knowledge in Manta ray Conservation in the Maldives. Manta Trust. Available online at: https://static1.squarespace.com/static/5a196500914e6b09132e911f/t/5d82097c57e3753573d71651/1568803214358/MT_MMRP_Thesis+Report+2014_The+Value+of+Traditional+Knowledge_Tam+Sawers.pdf (accessed March 15, 2021).
Maldivian Manta Ray Project [MMRP] (2015). The Value of Diver Knowledge for Manta Ray Conservation in the Maldives. Manta Trust. Available online at: https://static1.squarespace.com/static/5a196500914e6b09132e911f/t/5d8211ecf9ae04297be8f4b4/1568805370800/MT_MMRP_Thesis+Report+2015_The+Value+of+Diver+Knowledge_Nicola+Bassett.pdf (accessed March 15, 2021).
Maldivian Manta Ray Project [MMRP] (2017). North and South Malé atoll Report 1987-2017. Manta Trust. Available online at: https://static1.squarespace.com/static/5a196500914e6b09132e911f/t/5dc54d1c6f569d05a9724bdd/1573211591533/MT_MMRP_North+and+South+Male+Atoll+Report_1987-2017_FINAL.pdf (accessed September 14, 2020).
Maldivian Manta Ray Project [MMRP] (2019b). North and South Malé atoll report 2019. Manta Trust. Available online at: https://static1.squarespace.com/static/5a196500914e6b09132e911f/t/5fc51f0d4e98326c02b4a7ec/1606754094379/MT_MMRP_Annual+Report_N%26S+Male+Atolls_2019_FINAL.pdf (accessed January 20, 2021).
Maldvian Manta Ray Project [MMRP] (2019a). Oceanic Manta Ray Summary report 2019. The Manta Trust. Available online at: https://www.mantatrust.org/resources (accessed April 1, 2021).
Manta Trust (2019a). Mooring Line Entanglement Mitigation. Manta Trust. Available online at: https://static1.squarespace.com/static/5a196500914e6b09132e911f/t/5dfe1e20b02a616945eb5772/1576934948296/MT_MMRP_Entanglement+Mitigation_2019_FINAL.pdf (accessed January 20, 2021).
Manta Trust (2019b). Manta Ray Entanglement Protocol. Manta Trust. Available online at: https://static1.squarespace.com/static/5a196500914e6b09132e911f/t/5dfe2e70229b17607ddeb2c9/1576939123128/MT_MMRP_Entanglement+Protocol_2019_FINAL.pdf (accessed March 2, 2021).
Marra, N. J., Richards, V. P., Early, A., Bogdanowicz, S. M., Pavinski Bitar, P. D., Stanhope, M. J., et al. (2017). Comparative transcriptomics of elasmobranchs and teleosts highlight important processes in adaptive immunity and regional endothermy. BMC Genom. 18:87. doi: 10.1186/s12864-016-3411-x
Marshall, A. (2008). Biology and Population Ecology of Manta birostris in Southern Mozambique. Ph.D. thesis. Brisbane, QLD: University of Queensland.
Marshall, A. D., and Bennett, M. B. (2010). The frequency and effect of shark-inflicted bite injuries to the reef manta ray Manta alfredi. Afr. J. Mar. Sci. 32, 573–580. doi: 10.2989/1814232X.2010.538152
Marshall, A. D., and Pierce, S. J. (2012). The use and abuse of photographic identification in sharks and rays. J. Fish Biol. 80, 1361–1379. doi: 10.1111/j.1095-8649.2012.03244.x
Marshall, A. D., Compagno, L. J., and Bennett, M. B. (2009). Redescription of the genus Manta with resurrection of Manta alfredi (Krefft, 1868) (Chondrichthyes; Myliobatoidei; Mobulidae). Zootaxa. 2301, 24–30. doi: 10.11646/zootaxa.2301.1.1
Marshall, A. D., Dudgeon, C. L., and Bennett, M. B. (2011). Size and structure of a photographically identified population of manta rays Manta alfredi in southern Mozambique. Mar. Biol. 158, 1111–1124. doi: 10.1007/s00227-011-1634-6
Marshall, A., Barreto, R., Carlson, J., Fernando, D., Fordham, S., Francis, M. P., et al. (2019). Mobula alfredi. The IUCN Red List of Threatened Species 2019. doi: 10.2305/IUCN.UK.2019-3.RLTS.T195459A68632178.en
Marshall, A., Barreto, R., Carlson, J., Fernando, D., Fordham, S., Francis, M. P., et al. (2020). Mobula birostris. The IUCN Red List of Threatened Species 2020. doi: 10.2305/IUCN.UK.2020-3.RLTS.T198921A68632946.en
Martin, R. A., and Hakeem, A. A. A. (2006). Development of a sustainable shark diving ecotourism industry in the maldives: challenges and opportunities. Maldives Mar. Res. Bull. 9:20.
McCauley, D. J., Desalles, P. A., Young, H. S., Papastamatiou, Y. P., Caselle, J. E., Deakos, M. H., et al. (2014). Reliance of mobile species on sensitive habitats: a case study of manta rays (Manta alfredi) and lagoons. Mar. Biol. 161, 1987–1998. doi: 10.1007/s00227-014-2478-7
McGregor, F., Richardson, A. J., Armstrong, A. J., Armstrong, A. O., and Dudgeon, C. L. (2019). Rapid wound healing in a reef manta ray masks the extent of vessel strike. PLoS One 14:e0225681. doi: 10.1371/journal.pone.0225681
Melillo-Sweeting, K., Maust-Mohl, M., and Smukall, M. J. (2021). Examining shark bite scars on dolphins off Bimini, The Bahamas: comparisons between bottlenose and Atlantic spotted dolphins. Mar. Mamm. Sci. 38, 18–28. doi: 10.1111/mms.12840
MEPA (2014). Batoidea Maldives Protection Gazette No. (IUL) 438-ECAS/438/2014/81. 1. Available online at: https://www.gazette.gov.mv/iulaan/view/15844 (accessed Febuary 04, 2021).
Moore, M. J., and Van der Hoop, J. M. (2012). The painful side of trap and fixed net fisheries: chronic entanglement of large whales. J. Mar. Biol. 2012:230653. doi: 10.1155/2012/230653
Moore, M. J., Van der Hoop, J., Barco, S. G., Costidis, A. M., Gulland, F. M., Jepson, P. D., et al. (2013). Criteria and case definitions for serious injury and death of pinnipeds and cetaceans caused by anthropogenic trauma. Dis. Aquat. Org. 103, 229–264. doi: 10.3354/dao02566
MRC (2009). Status of the Shark Fishery of the Maldives. Male’: Marine Research Centre, Ministry of Fisheries, Agriculture and Marine Resources.
Murie, C., Spencer, M., and Oliver, S. P. (2020). Current strength, temperature, and bodyscape modulate cleaning services for giant manta rays. Mar. Biol. 167:54. doi: 10.1007/s00227-020-3674-2
Murray, A., Garrud, E., Ender, I., Lee-Brooks, K., Atkins, R., Lynam, R., et al. (2020). Protecting the million-dollar mantas; creating an evidence-based code of conduct for manta ray tourism interactions. J. Ecotour. 19, 132–147. doi: 10.1080/14724049.2019.1659802
Nicholson-Jack, A. E., Harris, J. L., Ballard, K., Turner, K. M. E., and Stevens, G. M. W. (2021). A hitchhiker guide to manta rays: patterns of association between Mobula alfredi, M. birostris, their symbionts, and other fishes in the Maldives. PLoS One 16:e0253704. doi: 10.1371/journal.pone.0253704
Nizar, H. R., and Ibrahim, M. (2019). Fishermen’s Forum 2019: On the occasion of the 39th Fishermen’s Day of the Maldives. Malé: Ministry of Fisheries, Marine Resources and Agriculture.
O’Malley, M. P., Townsend, K. A., Hilton, P., Heinrichs, S., and Stewart, J. D. (2017). Characterization of the trade in manta and devil ray gill plates in China and South-east Asia through trader surveys. Aquat. Conserv. Mar Freshw Ecosyst. 27, 394–413. doi: 10.1002/aqc.2670
O’Shea, O. R., Kingsford, M. J., and Seymour, J. (2010). Tide-related periodicity of manta rays and sharks to cleaning stations on a coral reef. Mar. Freshw. Res. 61, 65–73. doi: 10.1071/MF08301
Pankhurst, N. W., and Van der Kraak, G. (1997). Effects of Stress On Reproduction and Growth of Fish. Cambridge: Cambridge University Press.
Pate, J. H., and Marshall, A. D. (2020). Urban manta rays: potential manta ray nursery habitat along a highly developed Florida coastline. Endanger. Species Res. 43, 51–64. doi: 10.3354/esr01054
Perryman, R. J. Y., Venables, S. K., Tapilatu, R. F., Marshall, A. D., Brown, C., and Franks, D. W. (2019). Social preferences and network structure in a population of reef manta rays. Behav. Ecol. Sociobiol. 73:114. doi: 10.1007/s00265-019-2720-x
Quiros, A. L. (2007). Tourist compliance to a Code of Conduct and the resulting effects on whale shark (Rhincodon typus) behavior in Donsol, Philippines. Fish. Res. 84, 102–108. doi: 10.1016/j.fishres.2006.11.017
Renshaw, G. M. C., Kutek, A. K., Grant, G. D., and Anoopkumar-Dukie, S. (2012). Forecasting elasmobranch survival following exposure to severe stressors. Comp. Biochem. Physiol. Part A Mol. Integr. Physiol. 162, 101–112. doi: 10.1016/j.cbpa.2011.08.001
Richardson, A. J. (2008). In hot water: zooplankton and climate change. ICES J. Mar. Sci. 65, 279–295. doi: 10.1093/icesjms/fsn028
Rohner, C. A., Flam, A. L., Pierce, S. J., and Marshall, A. D. (2017). Steep declines in sightings of manta rays and devilrays (Mobulidae) in southern Mozambique. PeerJ Prepr. 5:e3051v1. doi: 10.7287/peerj.preprints.3051v1
Rolland, R. M., Mclellan, W. A., Moore, M. J., Harms, C. A., Burgess, E. A., and Hunt, K. E. (2017). Fecal glucocorticoids and anthropogenic injury and mortality in North Atlantic right whales Eubalaena glacialis. Endanger. Species Res. 34, 417–429. doi: 10.3354/esr00866
Sattar, S. A., Wood, E., Islam, F., and Najeeb, A. (2014). Current Status of the Reef Fisheries of Maldives and Recommendations of Management: Darwin Reef Fish Project. Malé: Marine Research Centre.
Sattar, S. A., Wood, E., Ushan, M., and Ali, K. (2013). Overview of the Sharkwatch Programme 2009 – 2013. Malé: Marine Research Centre.
Sattar, S., Andrefouet, S., Ahsan, M., Adam, S., Anderson, C. R., and Scott, L. (2012). Status of the coral reef fishery in an atoll country under tourism development: the case of Central Maldives. Atoll Res. Bull. 590, 163–186.
Setyawan, E., Erdmann, M. V., Lewis, S. A., Mambrasar, R., Hasan, A. W., Templeton, S., et al. (2020). Natural history of manta rays in the Bird’s Head Seascape, Indonesia, with an analysis of the demography and spatial ecology of Mobula alfredi (Elasmobranchii: Mobulidae). J. Ocean Sci. Found. 36, 49–83. doi: 10.5281/zenodo.4396260
Setyawan, E., Sianipar, A. B., Erdmann, M. V., Fischer, A. M., Haddy, J. A., Beale, C. S., et al. (2018). Site fidelity and movement patterns of reef manta rays (Mobula alfredi: Mobulidae) using passive acoustic telemetry in northern Raja Ampat, Indonesia. Nat. Conserv. Res. 3, 17–31. doi: 10.24189/ncr.2018.043
Sinan, H., Adam, M., and Anderson, R. C. (2011). Status of Shark Fisheries in the Maldives. Paper presented at Seventh Working Party on Ecosystems and Bycatch, Maldives 24-27 October 2011. IOTC–2011–WPEB07–56.9. Available online at: https://www.iotc.org/sites/default/files/documents/proceedings/2011/wpeb/IOTC-2011-WPEB07-56.pdf (accessed 1 Mar 2021).
Smith, F., Allen, S. J., Bejder, L., and Brown, A. M. (2018). Shark bite injuries on three inshore dolphin species in tropical northwestern Australia. Mar. Mamm. Sci. 34, 87–99. doi: 10.1111/mms.12435
Solleliet-Ferreira, S., Macena, B. C. L., Laglbauer, B. J. L., Sobral, A. F., Afonso, P., and Fontes, J. (2020). Sicklefin devilray and common remora prey jointly on baitfish. Environ. Biol. Fishes. 103, 993–1000. doi: 10.1007/s10641-020-00990-9
Speed, C. W., Meekan, M. G., Rowat, D., Pierce, S. J., Marshall, A. D., and Bradshaw, C. J. A. (2008). Scarring patterns and relative mortality rates of Indian Ocean whale sharks. J. Fish Biol. 72, 1488–1503. doi: 10.1111/j.1095-8649.2008.01810.x
Sprogis, K. R., King, C., Bejder, L., and Loneragan, N. R. (2018). Frequency and temporal trends of shark predation attempts on bottlenose dolphins (Tursiops aduncus) in temperate Australian waters. J. Exp. Mar. Bio. Ecol. 508, 35–43. doi: 10.1016/j.jembe.2018.08.008
Stelfox, M., Bulling, M., and Sweet, M. (2019). Untangling the origin of ghost gear within the Maldivian archipelago and its impact on olive ridley (Lepidochelys olivacea) populations. Endanger. Species Res. 40, 309–320. doi: 10.3354/esr00990
Stelfox, M., Burian, A., Shanker, K., Rees, A. F., Jean, C., Willson, M. S., et al. (2020). Tracing the origin of olive ridley turtles entangled in ghost nets in the Maldives: a phylogeographic assessment of populations at risk. Biol. Conserv. 245:108499. doi: 10.1016/j.biocon.2020.108499
Stevens, G. M. W. (2016). Conservation and Population Ecology of Manta Rays in the Maldives. Ph.D. thesis. York: University of York.
Stevens, G. M. W., and Froman, N. (2019). “The Maldives Archipelago,” in World Seas: An Environmental Evaluation: The Indian Ocean to the Pacific, 2nd Edn, Vol. II, ed. C. Sheppard (London: Academic Press), 211–236.
Stevens, G. M. W., Fernando, D., Dando, M., and Di Sciara, G. N. (2018a). Guide to the Manta and Devil Rays of the World. Plymouth: Wild Nature Press.
Stevens, G. M. W., Hawkins, J. P., and Roberts, C. M. (2018b). Courtship and mating behaviour of manta rays Mobula alfredi and M. birostris in the Maldives. J. Fish Biol. 93, 344–359. doi: 10.1111/jfb.13768
Stewart, J. D., Hoyos-Padilla, E. M., Kumli, K. R., and Rubin, R. D. (2016a). Deep-water feeding and behavioral plasticity in Manta birostris revealed by archival tags and submersible observations. Zoology 119, 406–413. doi: 10.1016/j.zool.2016.05.010
Stewart, J. D., Beale, C. S., Fernando, D., Sianipar, A. B., Burton, R. S., Semmens, B. X., et al. (2016b). Spatial ecology and conservation of Manta birostris in the Indo-Pacific. Biol. Conserv. 200, 178–183. doi: 10.1016/j.biocon.2016.05.016
Stewart, J. D., Nuttall, M., Hickerson, E. L., and Johnston, M. A. (2018a). Important juvenile manta ray habitat at Flower Garden Banks National Marine Sanctuary in the northwestern Gulf of Mexico. Mar. Biol. 165:111. doi: 10.1007/s00227-018-3364-5
Stewart, J. D., Jaine, F. R. A., Armstrong, A. J., Armstrong, A. O., Bennett, M. B., Burgess, K. B., et al. (2018b). Research priorities to support effective Manta and Devil Ray conservation. Front. Mar. Sci. 5:314. doi: 10.3389/fmars.2018.00314
Strike, E. M. (2020). Variations in Sub-Lethal Injuries to Manta Rays in the Maldives. Master’s thesis. York: University of York.
Towner, A., Smale, M. J., and Jewell, O. (2012). “Boat strike wound healing in Carcharodon carcharias,” in Global Perspectives on the Biology and Life History of the White Shark, ed. M. L. Domeier (Boca Rotan, FL: CRC Press), 77–84.
Ushan, M., and Wood, E. M. (2010). Maldives Sharkwatch Report 2009 to 2010. Malé: Marine Research Centre, Marine Conservation Society.
Van der Hoop, J. M., Corkeron, P., Kenney, J., Landry, S., Morin, D., Smith, J., et al. (2016). Drag from fishing gear entangling north Atlantic right whales. Mar. Mamm. Sci. 32, 619–642. doi: 10.1111/mms.12292
Venables, S. (2013). Short term Behavioural Responses of Manta rays, Manta alfredi, to Tourism Interactions in Coral Bay, Western Australia. Honours thesis. Perth, WA: Murdoch University.
Venables, S. K. (2020). Ecology and Conservation of a Threatened Reef Manta Ray (Mobula alfredi) Population in Southern Mozambique. Ph.D. thesis. Perth, WA: University of Western Australia, doi: 10.26182/c5ng-sf57
Venables, S. K., Van Duinkerken, D. I., Rohner, C. A., and Marshall, A. D. (2020). Habitat use and movement patterns of reef manta rays Mobula alfredi in southern Mozambique. Mar. Ecol. Prog. Ser. 634, 99–114. doi: 10.3354/meps13178
Venables, S., Mcgregor, F., Brain, L., and Van Keulen, M. (2016). Manta ray tourism management, precautionary strategies for a growing industry: a case study from the Ningaloo Marine Park, Western Australia. Pac. Conserv. Biol. 22, 295–300. doi: 10.1071/PC16003
Visser, I., and Bonoccorso, F. (2003). New observations and a review of killer whale (Orcinus orca) sightings in Papua New Guinea waters. Aquat. Mamm. 29, 150–172. doi: 10.1578/016754203101024004
Ward-Paige, C. A. (2017). A global overview of shark sanctuary regulations and their impact on shark fisheries. Mar. Policy. 82, 87–97. doi: 10.1016/j.marpol.2017.05.004
Ward-Paige, C. A., Davis, B., and Worm, B. (2013). Global population trends and human use patterns of manta and mobula rays. PLoS One 8:e74835. doi: 10.1371/journal.pone.0074835
Wells, R. S., Allen, J. B., Hofmann, S., Bassos-Hull, K., Fauquier, D. A., Barros, N. B., et al. (2008). Consequences of injuries on survival and reproduction of common bottlenose dolphins (Tursiops truncatus) along the west coast of Florida. Mar. Mamm. Sci. 24, 774–794. doi: 10.1111/j.1748-7692.2008.00212.x
White, E. R., Myers, M. C., Flemming, J. M., and Baum, J. K. (2015). Shifting elasmobranch community assemblage at Cocos Island—an isolated marine protected area. Conserv. Biol. 29, 1186–1197. doi: 10.1111/cobi.12478
White, W. T., Corrigan, S., Yang, L., Henderson, A. C., Bazinet, A. L., Swofford, D. L., et al. (2018). Phylogeny of the manta and devil rays (Chondrichthyes: mobulidae), with an updated taxonomic arrangement for the family. Zool. J. Linnean Soc. 182, 50–75. doi: 10.1093/zoolinnean/zlx018
Wilkinson, K. A., Wells, R. S., Pine Iii, W. E., and Borkhataria, R. R. (2017). Shark bite scar frequency in resident common bottlenose dolphins (Tursiops truncatus) in Sarasota Bay, Florida. Mar. Mamm. Sci. 33, 678–686. doi: 10.1111/mms.12385
Wilson, S. M., Raby, G. D., Burnett, N. J., Hinch, S. G., and Cooke, S. J. (2014). Looking beyond the mortality of bycatch: sublethal effects of incidental capture on marine animals. Biol. Conserv. 171, 61–72. doi: 10.1016/j.biocon.2014.01.020
Womersley, F., Hancock, J., Perry, C. T., and Rowat, D. (2021). Wound-healing capabilities of whale sharks (Rhincodon typus) and implications for conservation management. Conserv. Physiol. 9:coaa120. doi: 10.1093/conphys/coaa120
Yadav, S., Abdulla, A., Bertz, N., and Mawyer, A. (2020). King tuna: Indian Ocean trade, offshore fishing, and coral reef resilience in the Maldives archipelago. ICES J. Mar. Sci. 77, 398–407. doi: 10.1093/icesjms/fsz170
Keywords: entanglement, boat strike, anthropogenic threats, natural predation, mobulid, GLMM, bycatch
Citation: Strike EM, Harris JL, Ballard KL, Hawkins JP, Crockett J and Stevens GMW (2022) Sublethal Injuries and Physical Abnormalities in Maldives Manta Rays, Mobula alfredi and Mobula birostris. Front. Mar. Sci. 9:773897. doi: 10.3389/fmars.2022.773897
Received: 10 September 2021; Accepted: 09 February 2022;
Published: 08 March 2022.
Edited by:
Vitor H. Paiva, University of Coimbra, PortugalReviewed by:
Jessica Pate, Marine Megafauna Foundation, United StatesAmelia Armstrong, University of Queensland, Australia
Bernabé Moreno, Institute of Oceanology (PAN), Poland
Copyright © 2022 Strike, Harris, Ballard, Hawkins, Crockett and Stevens. This is an open-access article distributed under the terms of the Creative Commons Attribution License (CC BY). The use, distribution or reproduction in other forums is permitted, provided the original author(s) and the copyright owner(s) are credited and that the original publication in this journal is cited, in accordance with accepted academic practice. No use, distribution or reproduction is permitted which does not comply with these terms.
*Correspondence: Elspeth M. Strike, ZWxzcGV0aC5zdHJpa2VAbWFudGF0cnVzdC5vcmc=
†These authors have contributed equally to this work