- 1Department of Marine Biotechnology, Stazione Zoologica Anton Dohrn, Naples, Italy
- 2Fundación MEDINA, Centro de Excelencia en Investigación de Medicamentos Innovadores en Andalucía, Granada, Spain
Dunaliella tertiolecta is a green flagellated microalga with a high tolerance to salinity and high production of pigments such as zeaxanthin, a xanthophyll carotenoid present in higher plants known for its antioxidant potential. In the current study, the antiproliferative activity of raw extracts and fractions of D. tertiolecta (clone CCMP 1320) was evaluated against four different human cancer cell lines: melanoma, hepatocellular liver carcinoma, and two lung adenocarcinoma cell lines. In addition, a normal cell line (lung fibroblast) was used as toxicity control. The activity was evaluated by treatment with the extracts/fractions following the MTT colorimetric assay procedures. HPLC-UV-HRMS based dereplication helped to identify the bioactive metabolites. A glycoglycerolipid was identified in the active fraction, being involved in the bioactivity of this microalga. This compound, glycerol 1-(9Z,12Z,15Z-octadecatrienoate)-2-(4Z,7Z,10Z,13Z-hexadecatetraenoate)-3-O-β-D-galactopyranoside (1), was purified and its antiproliferative activity was confirmed. This work gives new insights on the antiproliferative activity of the green alga D. tertiolecta and its potential industrial applications.
Introduction
Cancer represents a huge threat for human health linked to the unrestrained cellular growth of different types of tissues in the human body. There are more than 100 types of cancer, and this is why it is considered a conglomerate of diseases and not a single disease, with the disadvantage that it can spread all over the human body via metastasis. The number of cases in Europe (EU-27 countries) rose to approximately 3 million new cases registered in 2020 according to the European Cancer Observatory.1 The demand for new drugs for the treatment of cancer diseases has triggered a growing interest in marine sources for the biodiscovery of new molecules with the potential to become anticancer drugs (Jaspars et al., 2016; Romano et al., 2017). Among all marine sources with potential in drug discovery, marine microalgae represent a poorly explored resource for drug discovery but with huge potential for their exploitation (Mimouni et al., 2012; Samarakoon et al., 2013; Lauritano et al., 2016, 2018, 2019, 2020; Lauritano and Ianora, 2016; Park et al., 2017; Brillatz et al., 2018; Giordano et al., 2018; Martínez Andrade et al., 2018; Guzmán et al., 2019; Riccio et al., 2020a,b; Riccio and Lauritano, 2020; Saide et al., 2020). They have several advantages throughout the early stage drug discovery pipeline, as they can be easily cultivated in photo-bioreactors to obtain large volumes of biomass, and allow for an environmentally friendly approach for drug discovery by overcoming problems associated with the over-utilization of marine resources and the use of destructive collection practices (Lauritano et al., 2016). Additionally, marine microalgae have already demonstrated their potential as valuable sources of secondary metabolites with anticancer properties (Martínez Andrade et al., 2018; Abd El-Hack et al., 2019), and their metabolic plasticity is a crucial property that increases the probabilities to find compounds with applications in pharmaceutical industries (Lauritano et al., 2016; Romano et al., 2017). In particular, the marine microalga Dunaliella tertiolecta is a flagellated green alga that belongs to the class Chlorophyceae, and Dunaliella species are known for their high salinity tolerance (Pick, 2002) as well as their ability to produce commercially valuable products such as pigments (Wasanasathian and Peng, 2007; Moura et al., 2020), products that may have an application in cancer treatment or prevention. For instance, violaxanthin is one of the pigments produced by D. tertiolecta clone CCMP364 (NCMA Bigelow), highlighted as the source of anticancer activity observed in raw extracts and fractions by Pasquet et al. (2011). Authors showed that a fraction from D. tertiolecta CCMP364 containing 95% of violaxanthin displayed 50% growth inhibition on the MCF7 breast cancer cell line at a concentration of 11.7 μg/mL. Within this work, and considering the discussed background, another D. tertiolecta clone (CCMP 1320) was selected and tested against several cancer cell lines, and a bioactivity-guided fractionation approach allowed to identify the source of the biological activity observed within the extracts of this green alga.
Materials and Methods
General Experimental Procedures
Fractions from D. tertiolecta extracts were analyzed by HPLC-UV-HRESIMS on an Agilent 1200 RR coupled to a Bruker maXis QToF spectrometer with electrospray ionization, as reported by Martín et al. (2014). Data were analyzed following the guidelines developed by Fundación MEDINA (Perez-Victoria et al., 2016), and compared with the data available on the Fundación MEDINA internal database and the Dictionary of Natural Products database. 1H NMR and HSQC spectra were recorded on a Bruker Avance III spectrometer (500 MHz for 1H NMR) equipped with a 1.7 mm TCI MicroCryoProbe, using the signal of the residual solvent as internal reference (δH 7.24 and δC 77.23 ppm for CDCl3).
Dunaliella tertiolecta Culturing
Dunaliella tertiolecta was bought from NCMA Bigelow (code CCMP 1320) in September 2010 and has been maintained in the culture collection of the Stazione Zoologica Anton Dohrn since then. The medium used to maintain and grow this green alga was Guillard’s f/2 medium (Guillard, 1975) with silica depletion. Briefly, D. tertiolecta was inoculated in a volume of 30 mL and further scaled up to 2 L at an initial concentration of 5,000 cells/mL to produce enough biomass for chemical and biological analyses. Culture growth was monitored daily from samples fixed with one drop of Lugol (final concentration of about 2%) and counted in a Bürker counting chamber under an Axioskop 2 microscope (20×) (Carl Zeiss GmbH, Jena, Germany) (Orefice et al., 2015). Once the stationary phase was reached (day 15), aliquots of 50 mL were harvested by centrifugation at 3,000 rpm and 4°C, for 10 min. The wet biomass harvested was immediately used for chemical extraction. A biological triplicate was performed in order to generate solid results.
Chemical Extraction
The wet biological material (80% water content) was extracted by soaking in methanol (proportion 1:5, w/v) and 30 min of maceration. The organic mixture was then vortexed for 1 min, sonicated with three bursts of 30 s in an icy water bath to lysate the cells, and centrifuged at 3,000 rpm and 4°C to precipitate the solid material in suspension that was discarded. The organic phase was then transferred to a rounded Pyrex flask and evaporated under reduced pressure. The extraction method was performed according to the protocol described by Cutignano et al. (2015), a specific method developed to avoid or at least ameliorate many of the difficulties associated with screening of marine natural extracts while extracting the most common classes of natural products present in such samples.
Fractionation of the Raw Extract
Fractionation of each extract (an aliquot with a maximum weight of 20 mg) was performed by solid phase extraction (SPE) using CHROMABOND® HR-X cartridges (6 mL/500 mg) as reported in Cutignano et al. (2015). In this study, the elution process was achieved by gravity. Briefly, the cartridge was washed with 3 mL of methanol and equilibrated with 6 mL of distilled water. The extract was suspended in 1 mL of distilled water and sonicated for a few seconds in a bath of icy water before loading it into the cartridge. After the extract suspension was absorbed by the resin, the following elution steps were performed to produce five different fractions: Washing step 100% H2O (2 mL, discarded); 100% H2O (6 mL, fraction A); CH3OH/H2O (50:50, 9 mL, fraction B); CH3CN/H2O (70:30, 9 mL, fraction C); 100% CH3CN (9 mL, fraction D); CH2Cl2/CH3OH (90:10, 9 mL, fraction E). Each fraction was then evaporated under reduced pressure, weighted, and preserved at −20°C.
In vitro Antiproliferative Assay
Human cells were bought at ATCC.2 Human melanoma cells (A2058; ATCC® CRL-11147™) were cultured in DMEM and human lung adenocarcinoma cells (HCC827; ATCC® CRL-2868™) were cultured in RPMI. Human lung adenocarcinoma cells (Calu-3; ATCC® HTB-55™), human hepatocellular liver carcinoma cells (HepG2; ATCC® HB-8065™) and normal lung fibroblast cells (MRC-5; ATCC® CRL-171™) were cultured in EMEM medium. The media were supplemented with 10% fetal bovine serum, 50 U/mL penicillin, and 50 μg/mL streptomycin (Sigma Aldrich). To estimate the in vitro antiproliferative effects of D. tertiolecta, A2058, HepG2, HCC827, Calu-3, and MRC-5 cells line were seeded in 96-well microtiter plates at a density of 1 × 104 cells/well and incubated at 37°C to allow for cell adhesion in the plates. After 24 h, the medium was replaced with fresh medium containing increasing concentrations of the fractions (10, 50, and 100 μg/mL) dissolved in dimethyl sulfoxide (DMSO) and further incubated for 72 h. The maximum concentration of DMSO used was 1% (v/v). Each concentration was tested at least in triplicate. Doxorubicin, used as positive control, was tested at 5 mM, and a dose response curve with 8-point serial dilution (1:2 dilutions) was performed. Cell viability was 0% at each concentration tested. After 72 h, cell viability was assessed using the MTT test 3-(4,5-dimethyl-2-thizolyl)-2,5-diphenyl-2H-tetrazolium bromide; A2231,0001, Applichem Panreac Tischkalender, Darmstadt, GmbH) (Baudelet et al., 2013; Russo et al., 2016). Briefly, the medium was replaced with medium containing MTT at 0.5 mg/mL and the plates were incubated for 3 h at 37°C. After incubation, cells were treated with isopropyl alcohol (used as MTT solvent) for 30 min at room temperature. Absorbance was measured at OD = 570 nm using a microplate reader (Multiskan™ FC Microplate Photometer, Thermo Fisher Scientific, Waltham, MA, United States) (Sansone et al., 2014). The same mentioned protocol was used to treat the A2058 cell lines with glycerol 1-(9Z,12Z,15Z-octadecatrienoate) 2-(4Z,7Z,10Z,13Z-hexadecatetraenoate)-3-O-β-D-galactopyranoside (1) purified from the raw extracts of D. tertiolecta. Cell survival was expressed as a percentage of viable cells in the presence of the tested samples, with respect to untreated control cultures with only DMSO. Extracts/fractions with cell viabilities above 60% were not considered active.
Purification of Glycerol 1-(9Z,12Z,15Z-Octadecatrienoate)-2-(4Z,7Z,10Z,13Z-Hexadecatetraenoate)-3-O-β-D-Galactopyranoside
Ten grams of lyophilized D. tertiolecta biomass were extracted by sonication and further maceration in methanol as reported in the chemical extraction method. The organic phase was filtered and evaporated at 35°C under reduced pressure, yielding 2.1 g of raw extract. The SPE-method for fractionation of marine extracts reported by Cutignano et al. (2015) was scaled-up for 2.1 g of raw extract and performed to yield five different fractions of decreasing polarity (A–E).
Fractions B to D, containing the compound of interest, were pooled and processed by reversed phase preparative HPLC (Agilent Zorbax SB-C8, 21.2 × 250 mm, 7 μm; 20 mL/min, UV detection at 210 nm) with a linear gradient of CH3CN in water from 5 to 100% over 30 min and a wash step at 100% over 10 min where the fraction containing 1 (7.6 mg) eluted at 30.5 min. This fraction was further purified by reversed phase semipreparative HPLC (XBridge C18, 10 × 150 mm, 5 μm, 3.8 mL/min, UV detection at 210 nm) with a linear gradient of CH3CN in water from 80 to 92% over 37 min to yield 2.4 mg of compound 1 (rT 31 min, >90% purity calculated in terms of area of the peak in the UV 210nm chromatogram of the aliquot analyzed).
Results and Discussion
Antiproliferative Activity of the Raw Extract
The total extract of D. tertiolecta obtained during the stationary growth phase was tested against human cell lines using the MTT assay. In particular, A2058, HepG2, HCC-827, Calu-3, and MRC-5 cells were incubated in the presence or absence of three different concentrations (10, 50, and 100 μg/mL) of D. tertiolecta total extract. After 72 h of incubation at 37°C, cell survival was measured with the MTT assay. The screening was performed using three biological replicates. As shown in Figure 1, total extracts of D. tertiolecta showed antiproliferative activity only on A2058 cells with 44 and 35% of cell viability at 50 and 100 μg/mL concentrations, respectively. On the other hand, no cytotoxic effects were observed for the HepG2, HCC827, Calu-3 cancer cell lines, and for MRC-5 normal cell lines.
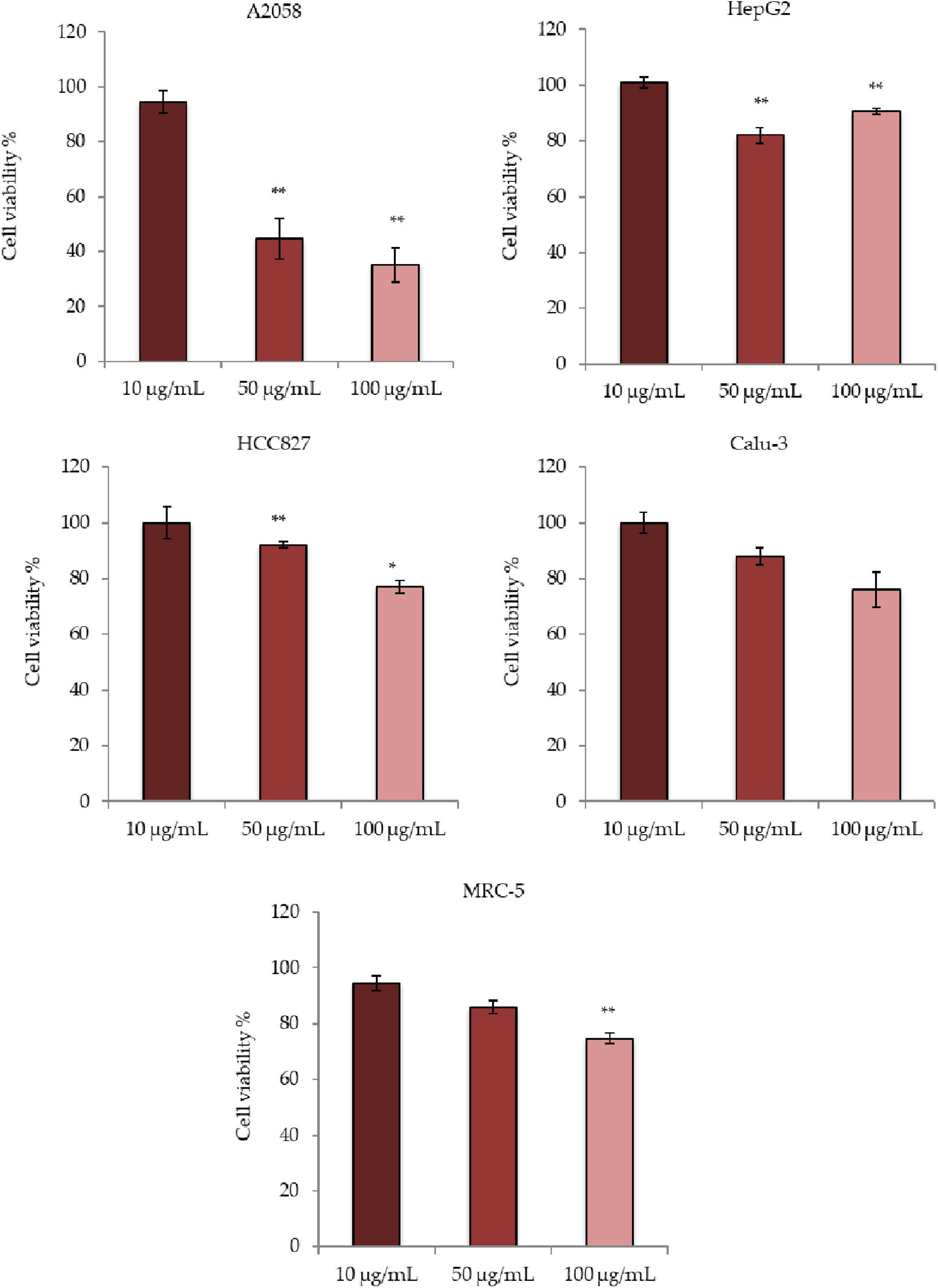
Figure 1. Antiproliferative effects of Dunaliella tertiolecta raw extract on A2058, HepG2, HCC827, Calu-3, and MRC-5 cell lines. Results are expressed as percentage of cell survival after 72 h exposure (n = 3). Cell growth was expressed as the percentage cell viability with respect to the vehicle (DMSO) for each concentration. **p < 0.01, *p < 0.05 vs. untreated cells.
Antiproliferative Activity of the Fractions
D. tertiolecta raw extract was then fractionated to obtain five fractions (Fractions A to E). As reported in the solid phase extraction (SPE) method to fractionate marine organic extracts (Cutignano et al., 2015), the method yielded an amino acid- and saccharide- rich fraction (Fraction A), a nucleoside-rich fraction (Fraction B), a glycol- and phospholipid-rich fraction (Fraction C), a free fatty acid- and sterol-rich fraction (Fraction D), and a triglyceride-rich fraction (Fraction E). Fraction A was not considered for testing since it was mainly composed by amino acids, saccharides (primary metabolites) and remaining salts. For the antiproliferative assay and bioactivity evaluation, the same cell lines (i.e., cancer cell lines A2058, HepG2, HCC827, Calu-3, and MRC-5) were used. Bioactivity testing of the other four fractions identified fractions D and E as the most active against A2058, HepG2, HCC827, and Calu-3 (Figure 2). In particular, cell viability of A2058 cells was reduced to 16% when incubated with Fraction D at 100 μg/mL; 38% in HepG2 cells at 100 μg/mL; 46% in HCC827 cells at 50 μg/mL and 9% at 100 μg/mL; and 21% in Calu-3 cells at 100 μg/mL. Fraction E lowered cell viability to 36% in A2058 cells and 45% in HCC827 cells at 100 μg/mL. Fractions B and C did not show any significant antiproliferative activity on any of the cell lines tested. Regarding toxicity, the fractions did not display any cytotoxic effect against normal cells MRC-5. Activities above 60% cell viability were not considered.
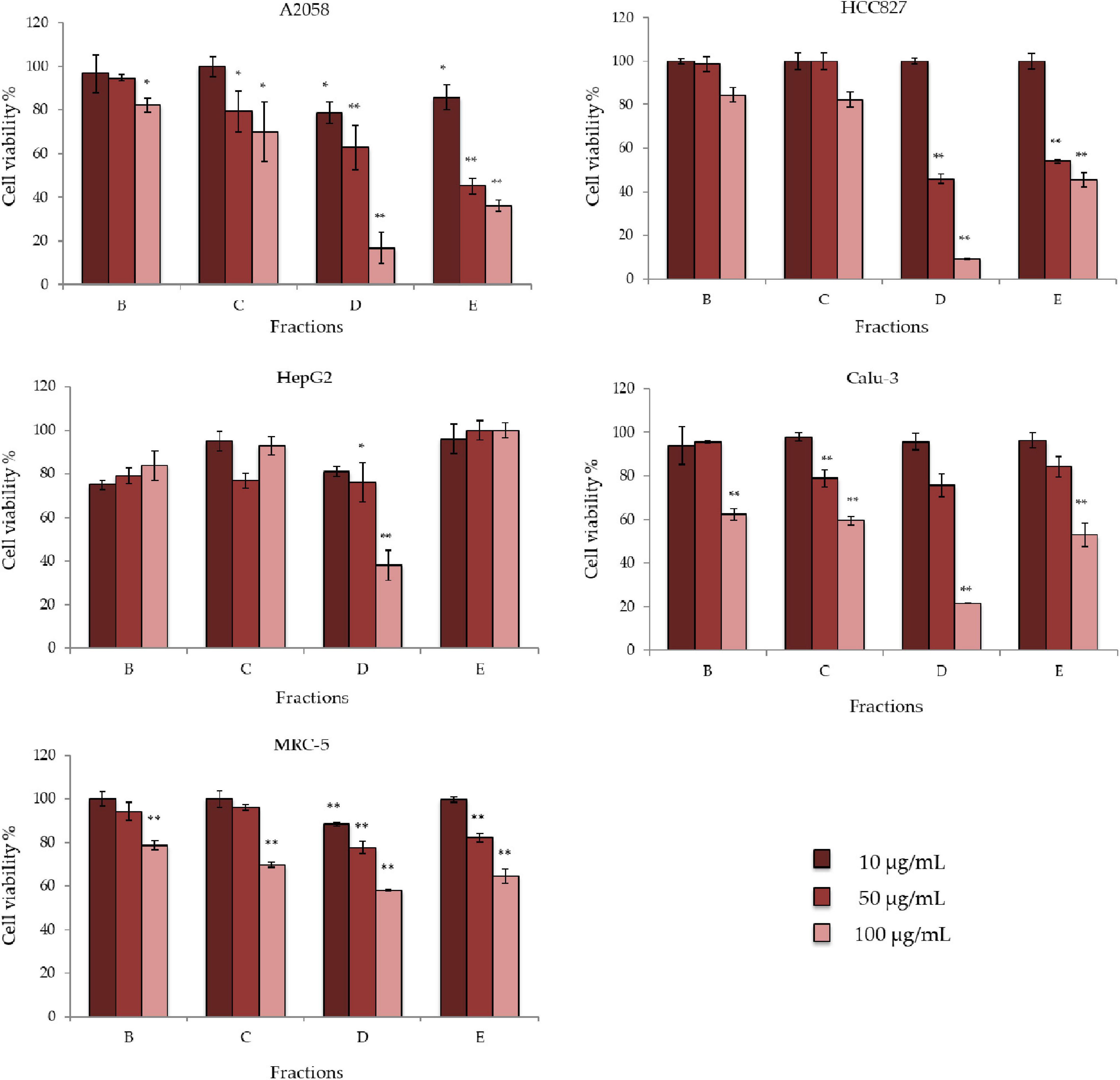
Figure 2. Antiproliferative effects of Dunaliella tertiolecta fractions on A2058, HepG2, HCC827, Calu-3, and MRC-5 cell lines. Results are expressed as percentage of cell survival after 72 h exposure (n = 3). Cell growth was expressed as the percentage of cell viability with respect to the vehicle (DMSO) for each concentration. **p < 0.01, *p < 0.05 vs. untreated cells.
HPLC-UV-HRMS Dereplication of the Fractions
HPLC-UV-HRESIMS based dereplication was used to identify the components in bioactive fractions. LC-UV traces of the most active fractions (D and E) and HRESIMS spectra of the most abundant components are reported in the figures below (Figures 3, 4). The compounds most likely present in fractions D and E were identified according to their mass spectra, UV maxima, natural source (taxonomy) and biological activity data.
Peak 1 (P1), observed in both fraction D and E, was suggested to have a molecular formula of C43H68O10 based on the existence of an [M + NH4]+ ion at m/z 762.5208 (calcd. for C43H72NO10+ 762.5151). The best match in the Dictionary of Natural Products database (DNP) according to the HRMS data, biological source, retention times and UV (λ) maxima, was glycerol-1-(9Z,12Z,15Z-octadecatrienoate)-2-(4Z,7Z,10Z,13Z-hexadecatetraenoate)-3-O-β-D-galactopyranoside (1) (Figure 5). This compound has already been found and isolated from extracts of another two green algae: Dunaliella acidophila (Della Greca et al., 1989) and Tetraselmis chui (Banskota et al., 2013), and it was reported to possess nitric oxide inhibitory activity. However, this is the first time it is tested for its antiproliferative activity on cancer cells. This compound is one of the monogalactosyldiacylglycerols (MGDG, one type of glycoglycerolipid) present in high amounts in the thylakoid membranes of photosynthetic organisms (Boudière et al., 2014) including plants, algae and cyanobacteria. The genes involved in the biosynthesis of MGDGs are present in several microalgal species, as reported in Riccio et al. (2020a). Maeda et al. (2010) studied the effect of a pool of MGDGs from spinach on six different cancer cell lines: lung cancer A549, acute lymphoblastoid leukemia BALL1, colon carcinoma HCT116, cervix cancer HeLa, promyelocytic leukemia HL-60 and stomach cancer NUGC3. The authors extracted this glycoglycerolipid from spinach and demonstrated that this molecule affected the activity of DNA polymerases α, γ, δ and ε (DNA metabolic enzymes). They showed that spinach MGDGs may be able to penetrate cancer cells and reach the nucleus, where the inhibition of the DNA polymerase activities may lead to selective cancer cell growth suppression. MTT assay was used to demonstrate that spinach MGDGs reduced the proliferation of all six cancer cell lines, and promyelocytic leukemia (HL60) cells were the most affected with an IC50 value of 39.2 μg/mL. Such results fall into the same order of magnitude as the results obtained for Fraction D in the current work (for instance, 46% cell viability at 50 μg/mL in HCC827 cell line) even though they didn’t test the individual compounds. An MGDG could hence explain the biological activity observed within Fraction D.
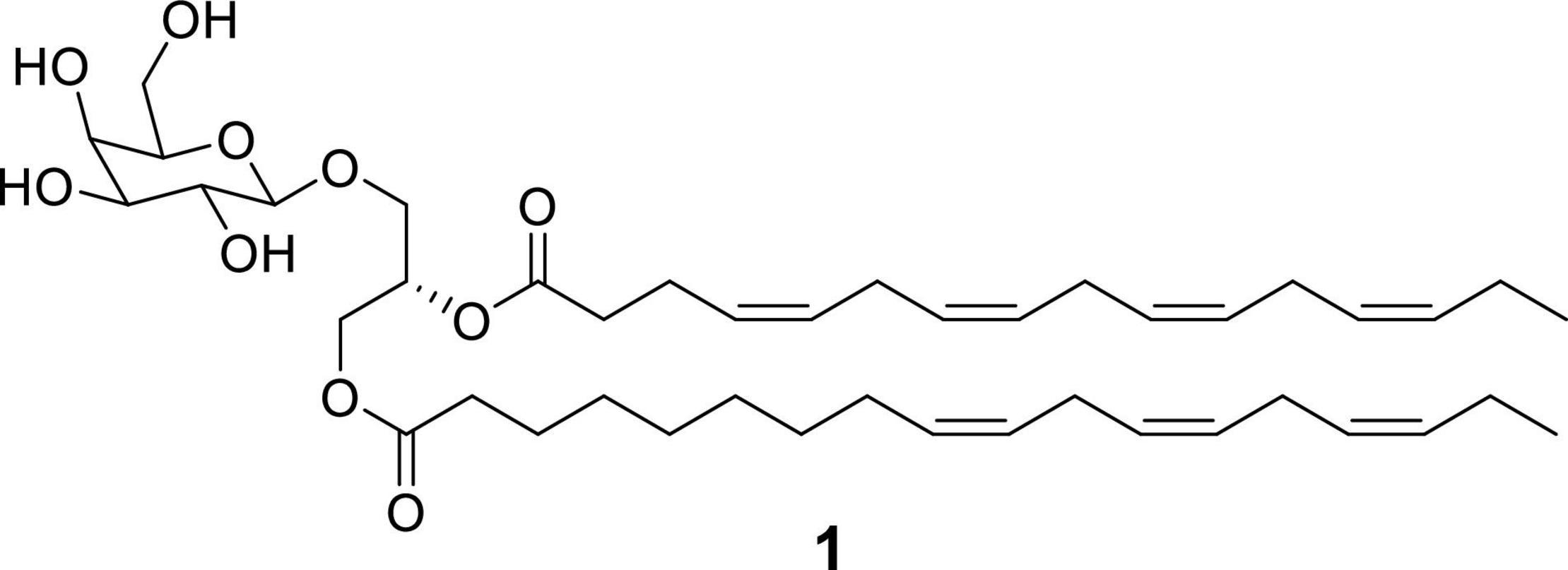
Figure 5. Chemical structure of glycerol 1-(9Z,12Z,15Z-octadecatrienoate) 2-(4Z,7Z,10Z,13Z-hexadecatetraenoate)-3-O-β-D-galactopyranoside (1).
Peak 2 (P2), observed only in fraction E, was suggested to have a molecular formula of C40H54O according to the existence of an [M + H]+ peak at m/z 551.4248 in its HRMS spectrum (calcd. for C40H55O+ 551.4247). The best coincidence in the DNP corresponded to crocoxanthin, according to the HRMS data, biological source, retention times and UV (λ) maxima. Crocoxanthin is a bioactive carotenoid of microalgal origin that was first isolated from the mixotroph marine microalgae Hemiselmis virescens (Chapman, 1966). In particular, de Oliveira-Júnior et al. (2020) demonstrated that crocoxanthin purified from Rhodomonas salina showed antiproliferative activity against A2058 cells, inhibiting cell growth by 50% at 50 μM (IC50). In that study, Crocoxanthin promoted growth inhibition, decreased cell migration, and induced apoptosis and sub-G1 cells accumulation after 72 h of treatment. Since the second component of peak 2 is most likely to be a known pigment with antiproliferative activity on cancer cells, it was not further confirmed nor studied in this work.
Purification and Confirmation of the Identity of Compound 1
Compound 1 was purified from a methanolic extract of the green microalga using solid phase extraction to yield three different fractions containing the compound, which were then processed by low pressure chromatography followed by preparative and semipreparative reversed phase HPLC, as reported in materials and methods. Mass spectrometry and NMR analysis confirmed the structure of the molecule as glycerol 1-(9Z,12Z,15Z-octadecatrienoate)-2-(4Z,7Z,10Z,13Z-hexadecatetraenoate)-3-O-β-D-galactopyranoside (Della Greca et al., 1989; Banskota et al., 2013). 1H NMR, HSQC 2D-NMR, UV-HRMS spectra, MS/MS spectra and fragmentation pathway are reported in Supplementary Figures 1–5. Two tables containing the NMR and MS/MS signals were also included in Supplementary Tables 1, 2. NMR spectra were consistent with bibliography, being identical to the original data by Della Greca et al. (1989), and almost identical to the data published by Banskota et al. (2013) regarding (2S)-1-O-(6Z,9Z,12Z,15Z-octadecatrinoyl)-2-O-(4Z,7Z,10Z,13Z-hexadecatetranoyl)-3-O-D-galactopyranosylglycerol, except for the presence of the extra double bond (6Z). In addition, MS/MS data allowed to build a fragmentation pathway that is consistent with the proposed structure. Metfrag3 was used to assign mass peaks to the corresponding ions.
Antiproliferative Activity of Compound 1
The antiproliferative activity of compound 1 was tested against A2058 melanoma cell line, to confirm that growth inhibition observed for the fraction was displayed by this compound. Compound 1 induced a 50% reduction of cell viability when tested at 50 μg/mL, while at 100 μg/mL cell viability was 10%, respect to the vehicle (Figure 6).
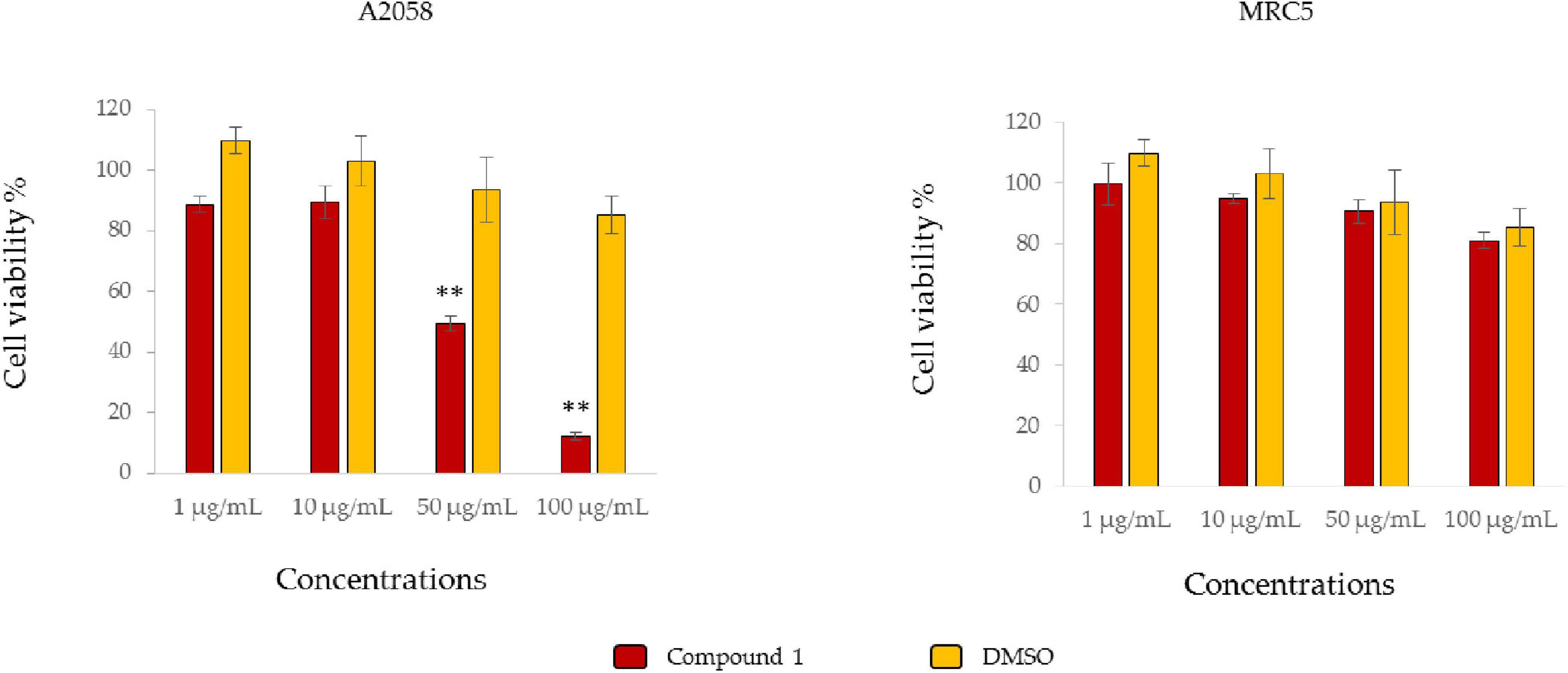
Figure 6. Antiproliferative assay. The histograms show antiproliferative effects of Compound 1 on A2058 cell line (melanoma) and MRC5 (normal cell line) and the respective controls only with the vehicle (DMSO). Results are expressed as percentage of cell survival after 72 h exposure (n = 3). Cell growth was expressed as the percentage of cell viability with respect to the vehicle for each concentration **p < 0.01.
Conclusion
Here we present for the first time the antiproliferative activity of extracts of the microalga D. tertiolecta (clone CCMP 1320) on different cancer cell lines. Raw extracts were active only against the A2058 human melanoma cell line, inducing a 44% reduction in cell viability at 50 μg/mL and 35% at 100 μg/mL. However, once the raw extracts were fractionated to obtain enriched fractions, two of these fractions (D and E) induced more potent antiproliferative effects. In particular, fraction D was active against A2058, HepG2, HCC827, and Calu-3 cell lines (cell viability ranged between 9 and 38% at 100 μg/mL), while fraction E was active against A2058 and HCC827 cell lines (36 and 45% cell viability at 100 μg/mL, respectively). An HPLC-UV_HRMS dereplication step of the bioactive fractions was performed and, as a result, glycerol 1-(9Z,12Z,15Z-octadecatrienoate) 2-(4Z,7Z,10Z,13Z-hexadecatetraenoate)-3-O-β-D-galactopyranoside (1) and a compound most likely to be the pigment crocoxanthin were identified in fraction E, while compound 1 was the only component of fraction D. The identity of compound 1 was confirmed by its biological source, UV absorbance, retention time, NMR spectra, HRMS and MS/MS data. This is the first time 1 is tested against cancer cells, even if a pool of related MGDGs have already been tested for its antiproliferative activity (Maeda et al., 2010; Hussein and Abdullah, 2020). The antiproliferative of the compound 1 was determined against the A2058 melanoma cell line. This is also the first case that this compound has been detected in fractions of the green alga D. tertiolecta. The present study provides new insights on the bioactivity of the green alga D. tertiolecta (clone CCMP 1320), and its possible new application for melanoma cancer prevention and treatment.
Data Availability Statement
The original contributions presented in the study are included in the article/Supplementary Material, further inquiries can be directed to the corresponding author.
Author Contributions
KAM, AS, and CL: conceptualization and writing—original draft. KAM and AS: data curation. KAM, AS, and JM: methodology. KAM, AS, GC, JM, GR, FR, and CL: resources. FR, CL, and AI: supervision. KAM, AS, FR, CL, and AI: writing—review and editing. All authors contributed to the article and approved the submitted version.
Funding
This project received funding from the Marie Skłodowska-Curie Actions “MarPipe” project of the European Union (H2020-MSCA-ITN-2016). AS was supported by a research grant of the project “Antitumor Drugs and Vaccines from the Sea (ADViSE)” (CUP B43D18000240007–SURF 17061BP000000011, PG/2018/0494374) funded by POR Campania FESR 2014–2020 “Technology Platform for Therapeutic Strategies against Cancer”–Action 1.1.2 and 1.2.2.
Conflict of Interest
The authors declare that the research was conducted in the absence of any commercial or financial relationships that could be construed as a potential conflict of interest.
Publisher’s Note
All claims expressed in this article are solely those of the authors and do not necessarily represent those of their affiliated organizations, or those of the publisher, the editors and the reviewers. Any product that may be evaluated in this article, or claim that may be made by its manufacturer, is not guaranteed or endorsed by the publisher.
Acknowledgments
We thank Massimo Perna and Mariano Amoroso for algal medium preparation. We thank the Stazione Zoologica Anton Dohrn-Open University Ph.D. program (XIX Cycle) for the academic support to KAM.
Supplementary Material
The Supplementary Material for this article can be found online at: https://www.frontiersin.org/articles/10.3389/fmars.2022.778108/full#supplementary-material
Footnotes
- ^ https://ecis.jrc.ec.europa.eu/
- ^ https://www.lgcstandards-atcc.org
- ^ https://ipb-halle.github.io/MetFrag/
References
Abd El-Hack, M. E., Abdelnour, S., Alagawany, M., Abdo, M., Sakr, M. A., Khafaga, A. F., et al. (2019). Microalgae in modern cancer therapy: current knowledge. Biomed. Pharmacother. 111, 42–50. doi: 10.1016/j.biopha.2018.12.069
Banskota, A. H., Gallant, P., Stefanova, R., Melanson, R., and Oleary, S. J. B. (2013). Monogalactosyldiacylglycerols, potent nitric oxide inhibitors from the marine microalga Tetraselmis chui. Nat. Prod. Res. 27, 1084–1090. doi: 10.1080/14786419.2012.717285
Baudelet, P. H., Gagez, A. L., Bérard, J. B., Juin, C., Bridiau, N., Kaas, R., et al. (2013). Antiproliferative activity of Cyanophora paradoxa pigments in melanoma, breast and lung cancer cells. Mar. Drugs 11, 4390–4406. doi: 10.3390/md11114390
Boudière, L., Michaud, M., Petroutsos, D., Rébeillé, F., Falconet, D., Bastien, O., et al. (2014). Glycerolipids in photosynthesis: composition, synthesis and trafficking. Biochim. Biophys. Acta Bioenerg. 1837, 470–480. doi: 10.1016/j.bbabio.2013.09.007
Brillatz, T., Lauritano, C., Jacmin, M., Khamma, S., Marcourt, L., Righi, D., et al. (2018). Zebrafish-based identification of the antiseizure nucleoside inosine from the marine diatom Skeletonema marinoi. PLoS One 13:e0196195. doi: 10.1371/journal.pone.0196195
Chapman, D. J. (1966). Three new carotenoids isolated from algae. Phytochemistry 5, 1331–1333. doi: 10.1016/S0031-9422(00)86131-2
Cutignano, A., Nuzzo, G., Ianora, A., Luongo, E., Romano, G., Gallo, C., et al. (2015). Development and application of a novel SPE-method for bioassay-guided fractionation of marine extracts. Mar. Drugs 13, 5736–5749. doi: 10.3390/md13095736
de Oliveira-Júnior, R. G., Nicolau, E., Bonnet, A., Prunier, G., Beaugeard, L., Joguet, N., et al. (2020). Carotenoids from Rhodomonas salina Induce Apoptosis and Sensitize A2058 Melanoma Cells to Chemotherapy. Rev. Bras. Farmacogn. 30, 155–168. doi: 10.1007/s43450-020-00036-2
Della Greca, M., Monaco, P., Previtera, L., and Parrilli, M. (1989). Structure determination of the main monogalactodiglyceride of Dunaliella acidophila. Gazz. Chim. Ital. 119, 549–551.
Giordano, D., Costantini, M., Coppola, D., Lauritano, C., Núñez Pons, L., Ruocco, N., et al. (2018). Biotechnological applications of bioactive peptides from marine sources. Adv. Microb. Physiol. 73, 171–220. doi: 10.1016/bs.ampbs.2018.05.002
Guillard, R. R. L. (1975). “Culture of phytoplankton for feeding marine invertebrates,” in Culture of Marine Invertebrate Animals, eds W. L. Smith and M. H. Chanley (Boston: Springer), 29–60. doi: 10.1007/978-1-4615-8714-9_3
Guzmán, F., Wong, G., Román, T., Cárdenas, C., Alvárez, C., Schmitt, P., et al. (2019). Identification of antimicrobial peptides from the microalgae Tetraselmis suecica (Kylin) butcher and bactericidal activity improvement. Mar. Drugs 17:453. doi: 10.3390/md17080453
Hussein, H. A., and Abdullah, M. A. (2020). Anticancer compounds derived from marine diatoms. Mar. Drugs 18:356. doi: 10.3390/md18070356
Jaspars, M., De Pascale, D., Andersen, J. H., Reyes, F., Crawford, A. D., and Ianora, A. (2016). The marine biodiscovery pipeline and ocean medicines of tomorrow. J. Mar. Biol. Assoc. U. K. 96, 151–158. doi: 10.1017/S0025315415002106
Lauritano, C., Andersen, J. H., Hansen, E., Albrigtsen, M., Escalera, L., Esposito, F., et al. (2016). Bioactivity screening of microalgae for antioxidant, anti-inflammatory, anticancer, anti-diabetes and antibacterial activities. Front. Mar. Sci. 3:68. doi: 10.3389/fmars.2016.00068
Lauritano, C., Ferrante, M. I., and Rogato, A. (2019). Marine natural products from microalgae: an -omics overview. Mar. Drugs 17:269. doi: 10.3390/md17050269
Lauritano, C., Helland, K., Riccio, G., Andersen, J. H., Ianora, A., and Hansen, E. H. (2020). Lysophosphatidylcholines and Chlorophyll-Derived molecules from the diatom Cylindrotheca closterium with anti-inflammatory activity. Mar. Drugs 18:166. doi: 10.3390/md18030166
Lauritano, C., and Ianora, A. (2016). Marine organisms with anti-diabetes properties. Mar. Drugs 14:220. doi: 10.3390/md14120220
Lauritano, C., Martín, J., De La Cruz, M., Reyes, F., Romano, G., and Ianora, A. (2018). First identification of marine diatoms with anti-tuberculosis activity. Sci. Rep. 8:2284. doi: 10.1038/s41598-018-20611-x
Maeda, N., Matsubara, K., Yoshida, H., and Mizushina, Y. (2010). Anti-cancer effect of spinach glycoglycerolipids as angiogenesis inhibitors based on the selective inhibition of DNA polymerase activity. Mini Rev. Med. Chem. 11, 32–38. doi: 10.2174/138955711793564042
Martín, J., Crespo, G., González-Menéndez, V., Pérez-Moreno, G., Sánchez-Carrasco, P., Pérez-Victoria, I., et al. (2014). MDN-0104, an antiplasmodial betaine lipid from Heterospora chenopodii. J. Nat. Prod. 77, 2118–2123. doi: 10.1021/np500577v
Martínez Andrade, K. A., Lauritano, C., Romano, G., and Ianora, A. (2018). Marine microalgae with anti-cancer properties. Mar. Drugs 16:165. doi: 10.3390/md16050165
Mimouni, V., Ulmann, L., Pasquet, V., Mathieu, M., Picot, L., Bougaran, G., et al. (2012). The potential of microalgae for the production of bioactive molecules of pharmaceutical interest. Curr. Pharm. Biotechnol. 13, 2733–2750. doi: 10.2174/138920112804724828
Moura, Y. A. S., Viana-Marques, D., de, A., Porto, A. L. F., Bezerra, R. P., and Converti, A. (2020). Pigments production, growth kinetics, and bioenergetic patterns in Dunaliella tertiolecta (Chlorophyta) in response to different culture media. Energies 13:5347. doi: 10.3390/en13205347
Orefice, I., Lauritano, C., Procaccini, G., Ianora, A., and Romano, G. (2015). Insights into possible cell-death markers in the diatom Skeletonema marinoi in response to senescence and silica starvation. Mar. Genomics 24, 81–88. doi: 10.1016/j.margen.2015.06.008
Park, G. T., Go, R. E., Lee, H. M., Lee, G. A., Kim, C. W., Seo, J. W., et al. (2017). Potential anti-proliferative and immunomodulatory effects of marine microalgal exopolysaccharide on various human cancer cells and lymphocytes in vitro. Mar. Biotechnol. 19, 136–146. doi: 10.1007/s10126-017-9735-y
Pasquet, V., Morisset, P., Ihammouine, S., Chepied, A., Aumailley, L., Berard, J. B., et al. (2011). Antiproliferative activity of violaxanthin isolated from bioguided fractionation of Dunaliella tertiolecta extracts. Mar. Drugs 9, 819–831. doi: 10.3390/md9050819
Perez-Victoria, I., Martin, J., and Reyes, F. (2016). Combined LC/UV/MS and NMR strategies for the dereplication of marine natural products. Planta Med. 82, 857–871. doi: 10.1055/s-0042-101763
Pick, U. (2002). “Adaptation of the halotolerant alga Dunaliella to high salinity,” in Salinity: Environment - Plants - Molecules, eds A. Läuchli and U. Lüttge (Dordrecht: Springer), 97–112. doi: 10.1007/0-306-48155-3_5
Riccio, G., De Luca, D., and Lauritano, C. (2020a). Monogalactosyldiacylglycerol and sulfolipid synthesis in microalgae. Mar. Drugs 18:237. doi: 10.3390/md18050237
Riccio, G., Ruocco, N., Mutalipassi, M., Costantini, M., Zupo, V., Coppola, D., et al. (2020b). Ten-year research update review: antiviral activities from marine organisms. Biomolecules 10:1007. doi: 10.3390/biom10071007
Riccio, G., and Lauritano, C. (2020). Microalgae with immunomodulatory activities. Mar. Drugs 18:2. doi: 10.3390/md18010002
Romano, G., Costantini, M., Sansone, C., Lauritano, C., Ruocco, N., and Ianora, A. (2017). Marine microorganisms as a promising and sustainable source of bioactive molecules. Mar. Environ. Res. 128, 58–69. doi: 10.1016/j.marenvres.2016.05.002
Russo, A., Saide, A., Cagliani, R., Cantile, M., Botti, G., and Russo, G. (2016). rpL3 promotes the apoptosis of p53 mutated lung cancer cells by down- regulating CBS and NF κ B upon 5-FU treatment. Sci. Rep. 6:38369. doi: 10.1038/srep38369
Saide, A., Lauritano, C., and Ianora, A. (2020). Pheophorbide A: state of the art. Mar. Drugs 18:257. doi: 10.3390/md18050257
Samarakoon, K. W., Ko, J. Y., Shah, M. M. R., Lee, J. H., Kang, M. C., O-Nam, K., et al. (2013). In vitro studies of anti-inflammatory and anticancer activities of organic solvent extracts from cultured marine microalgae. Algae 28, 111–119. doi: 10.4490/algae.2013.28.1.111
Sansone, C., Braca, A., Ercolesi, E., Romano, G., Palumbo, A., Casotti, R., et al. (2014). Diatom-derived polyunsaturated aldehydes activate cell death in human cancer cell lines but not normal cells. PLoS One 9:e101220. doi: 10.1371/journal.pone.0101220
Keywords: marine microalgae, Dunaliella tertiolecta, green algae, drug discovery, antiproliferative activity, dereplication
Citation: Martínez KA, Saide A, Crespo G, Martín J, Romano G, Reyes F, Lauritano C and Ianora A (2022) Promising Antiproliferative Compound From the Green Microalga Dunaliella tertiolecta Against Human Cancer Cells. Front. Mar. Sci. 9:778108. doi: 10.3389/fmars.2022.778108
Received: 16 September 2021; Accepted: 27 January 2022;
Published: 17 February 2022.
Edited by:
Carlos Fernando Sanchez-Arcos, University of Cologne, GermanyReviewed by:
Marta Correia-da-Silva, University of Porto, PortugalMatteo Francavilla, University of Foggia, Italy
Copyright © 2022 Martínez, Saide, Crespo, Martín, Romano, Reyes, Lauritano and Ianora. This is an open-access article distributed under the terms of the Creative Commons Attribution License (CC BY). The use, distribution or reproduction in other forums is permitted, provided the original author(s) and the copyright owner(s) are credited and that the original publication in this journal is cited, in accordance with accepted academic practice. No use, distribution or reproduction is permitted which does not comply with these terms.
*Correspondence: Chiara Lauritano, Y2hpYXJhLmxhdXJpdGFub0Bzem4uaXQ=