- 1Institute of Marine Science, The University of Auckland, Auckland, New Zealand
- 2Conservation International Aotearoa, The University of Auckland, Auckland, New Zealand
- 3Bird’s Head Seascape Program, Konservasi Indonesia, Sorong, Indonesia
- 4School of Veterinary and Life Sciences, Murdoch University, Perth, WA, Australia
- 5School of Biological Sciences, The University of Auckland, Auckland, New Zealand
- 6Department of Statistics, The University of Auckland, Auckland, New Zealand
- 7Integrated Marine Observing System (IMOS) Animal Tracking Facility, Sydney Institute of Marine Science, Mosman, NSW, Australia
- 8School of Natural Sciences, Macquarie University, Sydney, NSW, Australia
The behaviour and spatial use patterns of juvenile manta rays within their critical nursery habitats remain largely undocumented. Here, we report on the horizontal movements and residency of juvenile reef manta rays (Mobula alfredi) at a recently discovered nursery site in the Wayag lagoon, Raja Ampat, Indonesia. Using a multi-disciplinary approach, we provide further corroborative evidence that the lagoon serves as an important M. alfredi nursery. A total of 34 juvenile rays were photo-identified from 47 sightings in the sheltered nursery between 2013–2021. Five (14.7%) of these individuals were resighted for at least 486 days (~1.3 years), including two juveniles resighted after 641 and 649 days (~1.7 years), still using the nursery. Visually estimated (n=34) disc widths (DW) of juveniles using the nursery site ranged from 150–240 cm (mean ± SD: 199 ± 19), and the DW of two juveniles measured using drones were 218 and 219 cm. Five juveniles were tracked using GPS-enabled satellite transmitters for 12–69 days (mean ± SD: 37 ± 22) in 2015 and 2017, and nine juveniles were tracked using passive acoustic transmitters for 69–439 days (mean ± SD: 182 ± 109) from May 2019–September 2021. Satellite-tracked individuals exhibited restricted movements within Wayag lagoon. The minimum core activity space (50% Utilisation Distribution-UD) estimated for these five individuals ranged from 1.1–181.8 km2 and the extent of activity space (95% UD) between 5.3–1,195.4 km2 in area. All acoustically tagged individuals displayed high residency within the nursery area, with no acoustic detections recorded outside the lagoon in the broader Raja Ampat region. These juveniles were detected by receivers in the lagoon throughout the 24 h diel cycle, with more detections recorded at night and different patterns of spatial use of the lagoon between day and night. The observed long-term residency of juvenile M. alfredi provides further compelling evidence that the Wayag lagoon is an important nursery area for this globally vulnerable species. These important findings have been used to underpin the formulation of management strategies to specifically protect the Wayag lagoon, which will be instrumental for the survival and recovery of M. alfredi populations in Raja Ampat region.
1 Introduction
Although nursery areas have been identified for a variety of elasmobranch species (Heupel et al., 2019), few studies have specifically examined the benefits of nursery areas for newborn and juvenile elasmobranchs, such as improved fitness and increased survival. Many elasmobranch species, including manta rays and other mobulid rays (Mobula spp.), use shallow and sheltered habitats like lagoons as nursery areas for newborns (Heupel et al., 2007; Stewart et al., 2018b). Reef lagoons provide several benefits for juvenile elasmobranchs, such as calm sea conditions, protection from large predators, reliable food availability, and opportunities for social interaction with conspecifics (Guttridge et al., 2011; Jacoby et al., 2012; McCauley et al., 2014; Rojas et al., 2014; Heupel et al., 2019). Occupying sheltered nursery areas may also contribute to higher chances of newborn survival by enabling individuals to grow in a safe environment and become better equipped to later escape predators and find diffuse prey (Branstetter, 1990).
In the last five decades, global populations of oceanic sharks and rays, including the reef manta ray Mobula alfredi, have declined significantly (Pacoureau et al., 2021). To promote the recovery and persistence of manta ray populations, Stewart et al. (2018a) highlighted the importance of identifying critical habitats, including pupping and nursery areas, as an urgent priority to support conservation management efforts. While the majority of literature on the spatial ecology of M. alfredi has focused primarily on large or sexually mature individuals, with juveniles included opportunistically (Jaine et al., 2014; Braun et al., 2015; Kessel et al., 2017; Couturier et al., 2018; Peel et al., 2019; Lassauce et al., 2020; Peel et al., 2020; Venables et al., 2020), the ecology and ontogeny of juvenile M. alfredi remain understudied. Information on juvenile movements, residency, and habitat use in nursery areas is urgently required to inform the planning and management of existing marine protected areas (MPAs), specifically to develop the most appropriate strategies and regulations to safeguard this vulnerable species (Stewart et al., 2018a).
Several locations around the globe have been proposed as manta ray nurseries. The Flower Garden Banks National Marine Sanctuary in the northwestern Gulf of Mexico has been suggested as a nursery habitat for Caribbean manta rays M. cf. birostris (Childs, 2001; Stewart et al., 2018b). Similarly, Pate and Marshall (2020) suggested a coastal region of southeastern Florida may also function as a nursery for that species. Additionally, several potential nursery areas for M. alfredi have been suggested in the Maldives (Kitchen-Wheeler et al., 2011; Stevens, 2016), Palmyra Atoll (McCauley et al., 2014) and Nusa Penida in southern Indonesia (Germanov et al., 2019).
In the Raja Ampat archipelago of West Papua, Indonesia, four areas have also been identified as potential M. alfredi nurseries (Setyawan et al., 2020), based upon the three criteria proposed by Heupel et al. (2007) to define elasmobranch nurseries. These criteria, as applied to M. alfredi, include (1) Young-of-the-Year (YoY) and juvenile animals are more commonly encountered in the nursery area than in other areas; (2) YoY and juveniles remain in the nursery area for extended periods; and (3) the nursery area is used repeatedly by YoY and juveniles across years.
Of the four proposed M. alfredi nursery areas in Raja Ampat, the Wayag Lagoon has been the focus of the most intense research efforts. Based upon surveys in the Wayag lagoon between 2013-2019, Setyawan et al. (2020) provided evidence that the area fulfils Criteria (1) and (3) of Heupel et al. (2007) in functioning as a M. alfredi nursery. Specifically, those authors showed that YoY and juvenile animals [defined as individuals ≤2.0 m DW and ≤2.4 m DW (Peel et al., 2019), respectively] are more commonly observed within the Wayag lagoon than in the general population. YoY and juvenile M. alfredi comprise 47.6% and 95.2% of individuals recorded from Wayag lagoon, compared to only 4.7% and 11.1% of the 4,052 sightings recorded for the entire Raja Ampat population. Moreover, they reported that YoY and juvenile M. alfredi were observed on all 26 surveys conducted over the seven-year period, confirming Criterion (3) that the nursery is used repeatedly across years.
As noted by Heupel et al. (2019), assessing Criterion (2) of their elasmobranch nursery definition (residency within the nursery for extended periods) is best conducted using acoustic or satellite telemetry. Preliminary results of a pilot study using a Wildlife Computers SPOT5 satellite tag showed a YoY M. alfredi remained in and near the Wayag lagoon continuously for 6.5 months (Setyawan, Unpubl. Data). Here, we expand upon that study to assess Criterion (2) of the elasmobranch nursery definition using a combination of photo-identification (photo-ID), satellite telemetry, and passive acoustic telemetry to further describe the movement patterns and residency of juvenile M. alfredi in and around the Wayag lagoon. For the purposes of this study, we use the definition proposed by Chapman et al. (2015) that residency represents a nearly continuous occupancy by an individual in a restricted area for an extended period of time. Finally, we describe the home-range and habitat use patterns of the tracked juvenile M. alfredi in relation to the Wayag lagoon nursery area.
2 Materials and Methods
2.1 Study Area
The Raja Ampat archipelago in West Papua, Indonesia, is home to large populations of both M. alfredi and oceanic manta ray (M. birostris) that appear to be in a healthy state with high survival rates and reproductive periodicity (Beale et al., 2019; Setyawan et al., 2020). Both species have been fully protected in this region since the Raja Ampat government designated the entire archipelago as Southeast Asia’s first shark and ray sanctuary in 2012 (Dharmadi and Satria, 2015; Setyawan et al., 2022a). Wayag (S 0.172995°, E 130.035316°), located in the northwest of the Raja Ampat archipelago (Figure 1), is an island comprised of mountainous limestone karst. It is part of the SAP (Suaka Alam Perairan – marine reserve) Waigeo Barat MPA, established in 2011 and covering an area of 1,550 km2 (Mangubhai et al., 2012). The Wayag lagoon covers an area of ca. 14 km2 and has been identified as a potential M. alfredi nursery area (Erdmann, 2014; Setyawan et al., 2020).
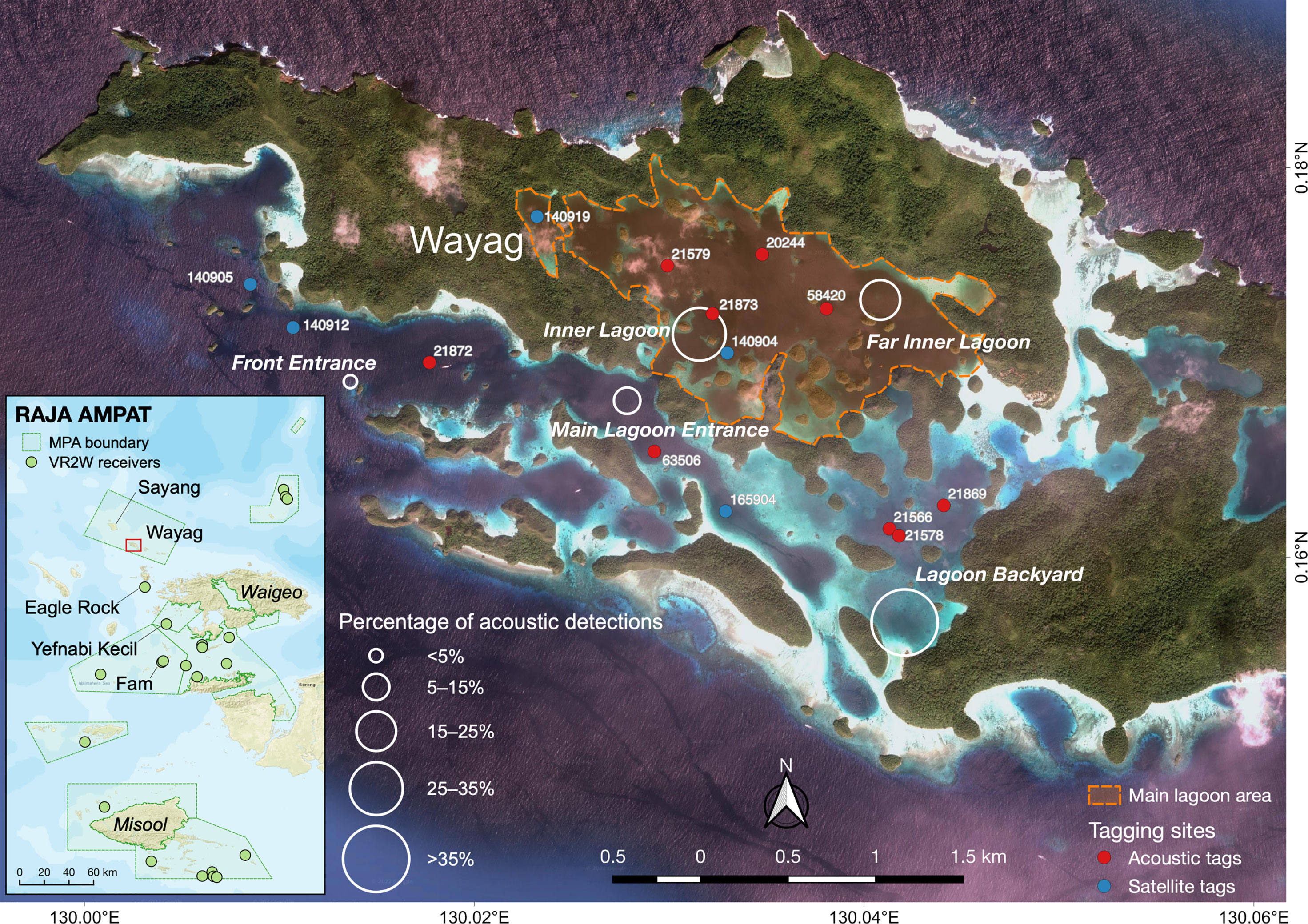
Figure 1 The Raja Ampat, West Papua, Indonesia region (inset) and Wayag lagoon denoted in the red box. Green points on the inset map depict the location of acoustic receivers deployed throughout the Raja Ampat archipelago. White circles on the main map indicate the location of the passive acoustic telemetry array deployed in the study area to monitor juvenile M. alfredi residency and habitat use. The size of circles indicates the proportion of tagged M. alfredi acoustic detections recorded by each receiver throughout the study period. Red and blue points on the main map indicated the deployment locations of all transmitters.
2.2 Data Collection
2.2.1 Photo-Identification
Between January 2013 and May 2021, surveys were undertaken every three to six months in Wayag. In all these surveys, we collected photo-ID images of M. alfredi in the Wayag lagoon via 1) underwater surveys by free diving and deploying GoPro camera traps at cleaning stations, and 2) starting in 2019, opportunistic aerial surveys of somersault-feeding manta rays using drones (Setyawan et al., 2020; Setyawan et al., 2022b). Individuals were identified using the unique spot patterns on their ventral side (Marshall and Pierce, 2012; Stevens et al., 2018) and visually matched to catalogued individuals in the “Bird’s Head Seascape M. alfredi Photo-ID Database” (Setyawan et al., 2020) to determine whether each juvenile was a newly-sighted individual or a resighting. For each photo-ID, we recorded date, time, location, sex, colour morph, and estimated disc width (DW) to the nearest 10 cm (Setyawan et al., 2020). The DW of juvenile M. alfredi was also measured opportunistically to the nearest 1 cm using a novel photogrammetry method using drones (Setyawan et al., 2022b). The sex of each individual was determined through observation of claspers on males and lack thereof for females (Marshall and Bennett, 2010; Stevens, 2016).
2.2.2 Transmitter Deployments
We equipped five juvenile M. alfredi with SPLASH10-F-321A satellite transmitters (Wildlife Computers, Redmond, USA) in the Wayag lagoon in 2015 (n = 3) and 2017 (n = 2) (Table 1; Figure 1). Additionally, nine juvenile M. alfredi were tagged using V16-5H acoustic transmitters (Innovasea, Halifax, CA) operating at 69 kHz frequency and transmitting pings randomly every 60–130 s. The transmitters were deployed in Wayag lagoon (Figure 1) over four different periods: May 2019 (n = 2), October 2019 (n = 2), January 2020 (n = 2), and May 2021 (n = 3) (Table 2), following Setyawan et al. (2018). Briefly, each transmitter was attached to a titanium dart via a 25 cm (satellite tags) or 12 cm (acoustic tags) stainless steel tether coated with heat shrink tubing. Both satellite and acoustic transmitters were coated with non-toxic silicone based Propspeed™ ablative coating to prevent fouling of the transmitters and antennae. Transmitters were deployed while free diving using a pole spear to insert the titanium dart tip into the dorsum of the ray in the muscle band between the right pectoral fin and the body cavity. Prior to tagging, we also collected identification photographs of each juvenile and sexed them, whenever possible.
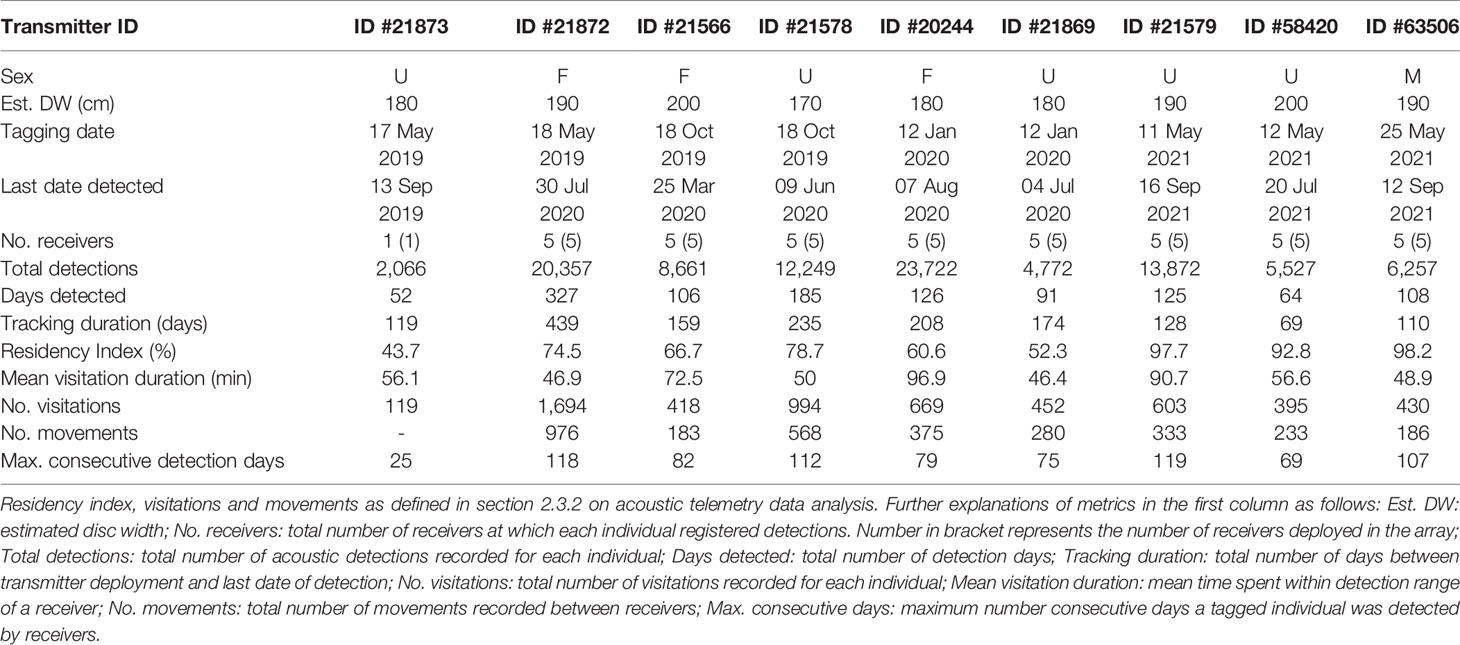
Table 2 Summary details for the nine juvenile M. alfredi tracked within the Wayag lagoon using passive acoustic telemetry.
The SPLASH10-F-321A satellite transmitters were programmed to remain attached for 180 days to collect Fastloc GPS locations every hour with a maximum of 24 locations per day. Upon surfacing, the SPLASH10-F-321A satellite tags transmitted location data, including both ARGOS and Fastloc GPS locations. For subsequent analyses, we only report on GPS positions based on their higher accuracy for estimating home range and fine-scale habitat use patterns (Dujon et al., 2014; Thomson et al., 2017). Additionally, the satellite transmitters were programmed to record and archive dive-depth, light levels, and ambient sea temperature. In this study, however, we only focused on horizontal movements and therefore don’t report on these other data.
2.2.3 Acoustic Receiver Deployments
To record detections transmitted by the V16-5H acoustic transmitters, we deployed Innovasea VR2W-69 kHz acoustic receivers at five sites, approximately 550-1900 m apart, inside the Wayag lagoon (Figure 1). The receivers were deployed at depths ranging from 8–26 m, and they were securely cable-tied to buoyed moorings that were directly attached to the substrate using galvanised chain anchors and ropes, with each receiver approximately 1.5 m above the surrounding substrate (Setyawan et al., 2018). Four of the receivers were deployed in areas where juvenile M. alfredi had been previously observed feeding or cleaning (Setyawan, Unpubl. Data), while the “Front Entrance” (Figure 1) receiver was strategically placed to record any manta rays leaving the lagoon through the main channel connecting the lagoon to the deeper waters outside of Wayag. One receiver (“Main Lagoon Entrance”) was deployed in May 2019, while the other four were deployed in January 2020, for a period of 325–460 days (Table 3). At the same time, a larger array of 23 VR2W-69 kHz acoustic receivers were deployed throughout the Raja Ampat archipelago. These receivers were part of a broader study on manta rays and were located 35 (Eagle Rock) to 280 km (Misool) away from the Wayag lagoon receivers (Figure 1).
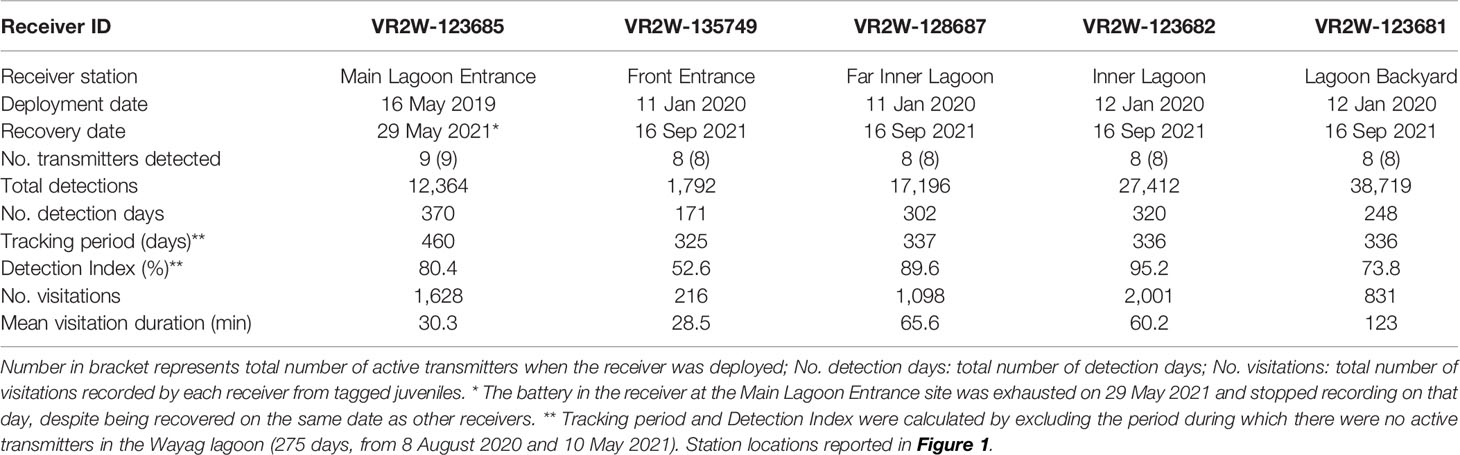
Table 3 Summary of acoustic receiver deployments within the Wayag lagoon, Raja Ampat. Further explanations of metrics in the first column as follows: No. transmitters detected: Total number of transmitters that were detected by the receiver.
Importantly, the detection range of each acoustic receiver can vary dramatically depending upon ambient noise levels, receiver biofouling, and environmental conditions, and has been estimated to vary between ~0–800 m (Heupel et al., 2008; Kessel et al., 2014; Huveneers et al., 2016). Receivers were serviced and downloaded every 3-6 months when our team was on site, and thus biofouling did not impact receiver performance. In order to quantify the detection range of the receivers in our array, a basic range test was conducted at the “Lagoon Backyard” receiver (Figure 1). Limited time and resources prevented us from undertaking a rigorous range test at all receiver sites. The range test was undertaken by deploying a fixed delay transmitter for one hour during the day at each of the following distances from the receiver: 0, 100, 150, 175, 200, 300, and 400 m. The tag was secured at 2 m depth by a rope attached to an anchor and buoy. The shallow (<8 m), sandy bottom location in the vicinity of that receiver was most likely to restrict detection range and hence this receiver was considered to be the “worst case scenario” and a conservative estimate for range detection for the five receivers in the array (Babin et al., 2019). No detections were recorded by this receiver from the fixed delay transmitter placed further than 150 m from the receiver. This test indicated reliable detection when a transmitter was within ~150 m of the receiver, which is similar to the detection range estimated in a study using the same types of receivers and transmitters in other areas of Raja Ampat (Setyawan et al., 2018).
One undeployed acoustic transmitter (#21839), that accidently fell off and was not able to be recovered, was continuously detected by the receiver at MLE from 16–29 May 2021 (the receiver battery was exhausted and therefore the receiver stopped recording on 29 May 2021). We used the detection data from this lost ‘sentinel tag’ to assess the temporal variation of detections recorded by a receiver throughout the 24-hour cycle, as well as to examine receiver ability to detect a transmitter when the tagged juveniles were absent from areas where receivers had been deployed (Couturier et al., 2018).
2.3 Data Analyses
2.3.1 Satellite Telemetry Data Analysis
Each SPLASH10-F-321A satellite transmitter was equipped with a Fastloc GPS receiver that takes a “snapshot” of the radio signals produced by all GPS satellites orbiting above the transmitter at any given time the manta ray is on the surface and the transmitter’s GPS antenna exposed to the air. Each snapshot was compressed onboard the transmitter and the data were transmitted to the Wildlife Computers Data Portal via the ARGOS satellite network. GPS location datasets were initially processed using the Wildlife Computers’ Fastloc GPS Processor as described in the “Location Processing (GPE3 & Fastloc GPS)” in the Wildlife Computers Data Portal User Guide (v.202007). GPS locations were further processed in Movebank (https://www.movebank.org) in order to allow us to manually remove outliers based on a maximum plausible swimming speed of 2 m/s. Furthermore, any GPS locations situated on land and further than 70 m inland from shore, based upon the accuracy of Fastloc GPS locations (Dujon et al., 2014), were also removed. These processed and filtered data (Setyawan et al., 2022c) were then used to track the patterns and scale of movements of juvenile M. alfredi.
Given the small scale and very fractioned landscape in Wayag, we did not restrict movement tracks to preclude movement over land masses. We calculated the minimum distance (including crossing land) travelled by the tagged juveniles using the ‘move’ package (Kranstauber et al., 2021) in R version 4.1.2 (R Core Team, 2021). To estimate core activity space (50% UD) and the extent of activity space (95% UD), we fitted an optimally weighted Autocorrelated Kernel Density Estimator (AKDE) (Fleming et al., 2018) on the filtered GPS locations using the ‘ctmm’ R package (Calabrese et al., 2016). The optimally weighted AKDE takes into account the autocorrelation of GPS locations obtained from satellite-tagged individuals and the highly irregular nature of location data collection in the marine environment (which, if ignored, typically leads to underestimation of home range size), and has been demonstrated to be applicable for M. alfredi satellite tag data (Fleming et al., 2018).
Despite the satellite transmitters being programmed to collect GPS locations every hour, the resulting GPS data were obtained irregularly, with time difference between subsequent GPS locations across tagged individuals ranging from 2.2–11.0 hours (mean ± SD: 6.2 ± 4.4 hours) due to the unpredictable nature of manta ray surfacing behaviour. Given the irregularity of the available GPS location data, we fitted a state-space model on the GPS location data to estimate the most likely movement tracks for each individual using the ‘foieGras’ R package (Jonsen and Patterson, 2020). We applied correlated random walks with a six-hour time step to produce estimated locations every six hours. We used the move persistence index between estimated locations to characterise the likely behaviours of the tagged individuals during the tracking period (Jonsen et al., 2019). The move persistence index, which captures autocorrelation in both movement speed and direction, ranges between 0 and 1, with a low index suggestive of correlated random walks or Area Restricted Search (ARS) behaviour, and higher index values indicative of uncorrelated movement steps or likely transiting behaviour (Jonsen et al., 2019).
2.3.2 Acoustic Telemetry Data Analysis
Acoustic detection data (Setyawan et al., 2020c) were recorded as a timestamped log of transmitter IDs detected by each of the five receivers. We used a two-sample t-test to compare the hourly mean number of acoustic detections between daytime (06:00–18:00) and night-time (18:00–06:00). To examine residency of the tagged juveniles, we used the ‘VTrack’ R package and its Animal Tracking Toolbox (Campbell et al., 2012; Udyawer et al., 2018). A residency index (RI) (Couturier et al., 2018; Andrzejaczek et al., 2020; Venables et al., 2020) was calculated for each tagged juvenile using the following formula:
We used a linear model to examine the correlation between tracking period and RI. In addition to RI for each tagged individual, we calculated a Detection Index (DI) for each acoustic receiver in the array using the following formula:
We also examined the number and duration of visitations at receiver stations. A visitation represents a period when a tagged juvenile was detected continuously by a receiver. It begins when a transmitter is detected by a given receiver and terminates if either the transmitter is not detected again by that receiver within 60 mins or if the transmitter is detected by another receiver (Setyawan et al., 2018). In addition to visitations, we also calculated the number of movements of these juveniles between receivers. Overall, data were visualised using ‘gpplot2’ R package (Wickham, 2016), while the number of movements were visualised using the circular layout in the ‘circlize’ R package (Gu et al., 2014).
3 Results
3.1 Juvenile Reef Manta Ray Sightings
Juvenile M. alfredi were observed at multiple sites within the Wayag lagoon. Most sightings occurred when individuals were somersault feeding near the surface, and some when they visited cleaning stations. A total of 34 individuals were photo-identified from 47 sightings between May 2013–May 2021. Twelve of these (35.2%) were female, 11 (32.4%) were male, and 11 (32.4%) were of unknown sex. Nine of the 34 juveniles (26.5%) were resighted at least once within the Wayag lagoon (Figure 2); five were resighted once and the other four were resighted twice over periods ranging from 1–648 days (~1.7 years), with six of the nine individuals recording sighting spans in excess of 320 days (Supplementary Table 1). None of the remaining 24 photographically identified juveniles from the lagoon have been resighted to date as part of regular visits to the site every three to six months. The size of the 34 juveniles ranged between DW of 150–240 cm at first sighting (mean ± SD: 199 ± 19 cm), with 18 individuals with a DW ≤200 cm at first sighting and thereby considered as YoY (Setyawan et al., 2020) (Supplementary Table 2). The DW of two unidentified juveniles measured using drones were 218 cm (95% CI: 216–220) and 219 cm (95% CI: 218–221). Several of the YoY M. alfredi recorded in the Wayag lagoon appeared to be true newborns, as evidenced by their small size (estimated 150–180 cm DW), unmistakably “clean” and unmarked appearance with no scratches evident (Marshall and Bennett, 2010), and obvious “creases” between pectoral fins and body cavity, presumably from the folding of the fins over the body while in utero (Marshall et al., 2008).
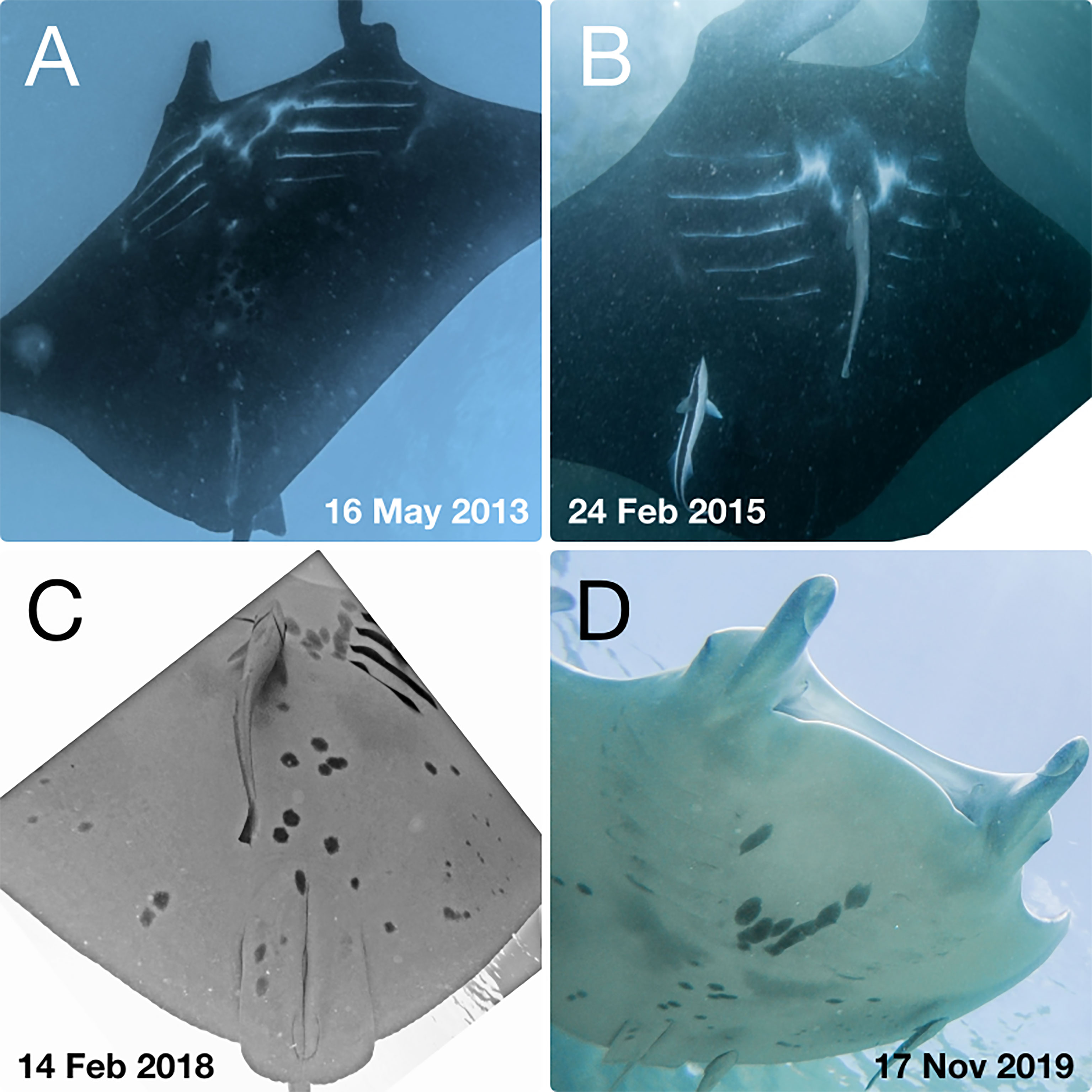
Figure 2 Example of resighted juvenile M. alfredi RA-MA-1322 (A, B) and RA-MA-0525 (C, D) within the Wayag lagoon, Raja Ampat.
3.2 Movements and Regional Habitat Use as Revealed by Satellite Telemetry
3.2.1 Core and the Extent of Activity Space
The tracking duration across all five juvenile M. alfredi, three females and two of unknown sex (Table 1), ranged from 12–69 days (mean ± SD: 37 ± 22 days) between January and April 2017. The filtering procedure resulted in the removal of 15 (1.25%) out of 1,199 Fastloc GPS locations. The minimum straight-line distance travelled, including over land (a result of the complex geography of the lagoon), ranged from 115.0–630.9 km (mean ± SE: 246 ± 96.9 km), with mean daily distances travelled ranging from 3.6–15.5 km (mean ± SE: 7.4 ± 2.25 km).
Despite occasional excursions to areas outside the Wayag lagoon and the MPA boundary (Figure 3, Supplementary Figure 3), the majority of satellite-tracked juveniles demonstrated narrow and restricted core activity space (50% UD) located within the Wayag lagoon or near Wayag Island (Figure 4). The 50% UD core activity space ranged from 1.1–181.8 km2, while the extent of activity space (95% UD) was 5.3–1,195.4 km2. The size of the 95% UD varied between individuals, from just outside Wayag lagoon to areas up to ~45 km away from the lagoon. The smallest 50% and 95% UDs were registered by ID #165904 (1.1 km2 and 5.3 km2), while ID #140905 exhibited the largest (181.8 km2 and 1,195.4 km2) (Table 1) with 50% UD (mean ± SD: 49.3 ± 76.0 km2) and 95% UD (mean ± SD: 329.2 ± 499.8 km2) across all tracked individuals.
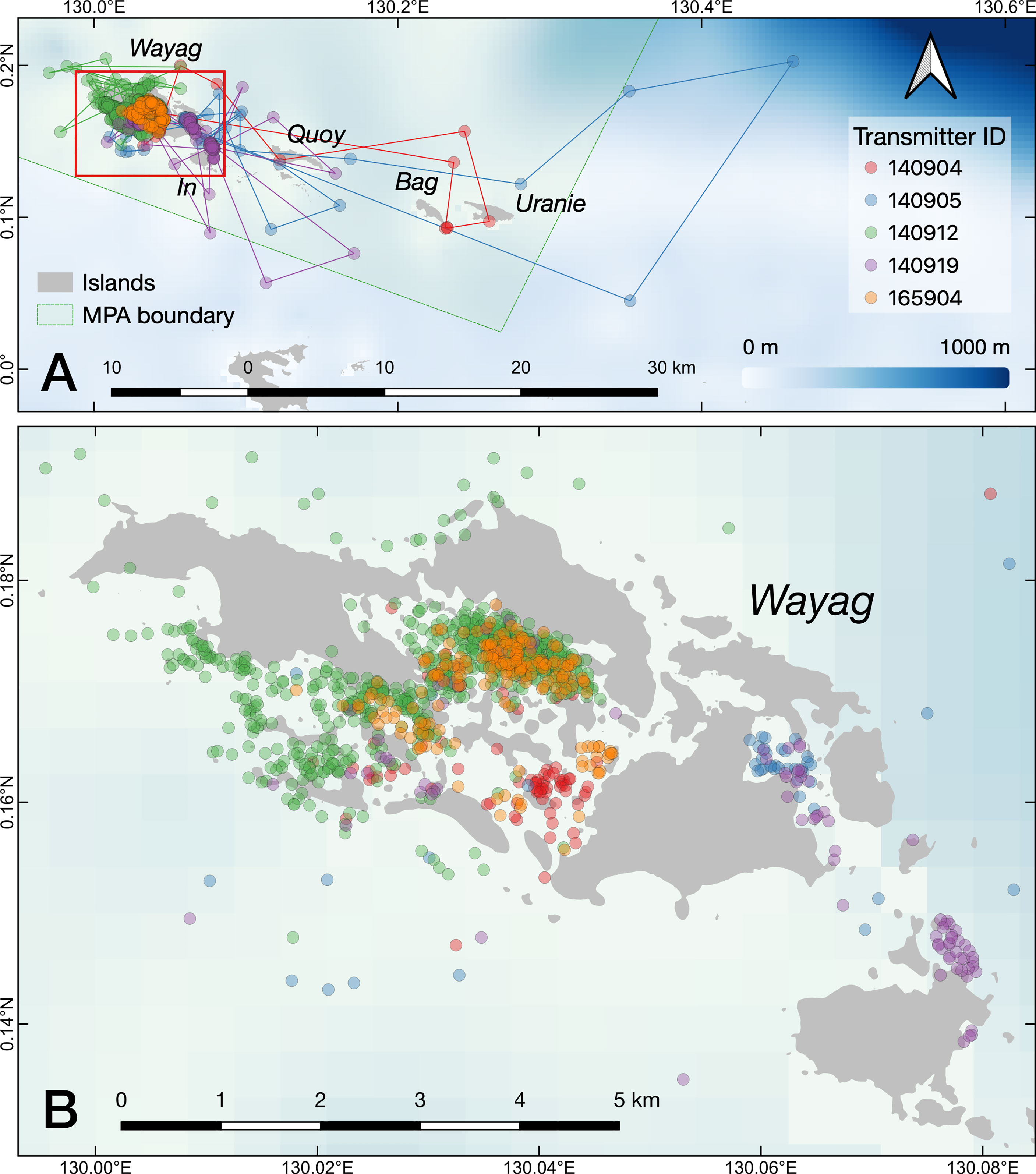
Figure 3 The raw movement tracks (lines) derived from GPS locations (dots) recorded by the satellite transmitters on the five tagged juvenile M. alfredi. (A) the SAP Waigeo Barat MPA with names of the islands; (B) a close up of the Wayag lagoon.
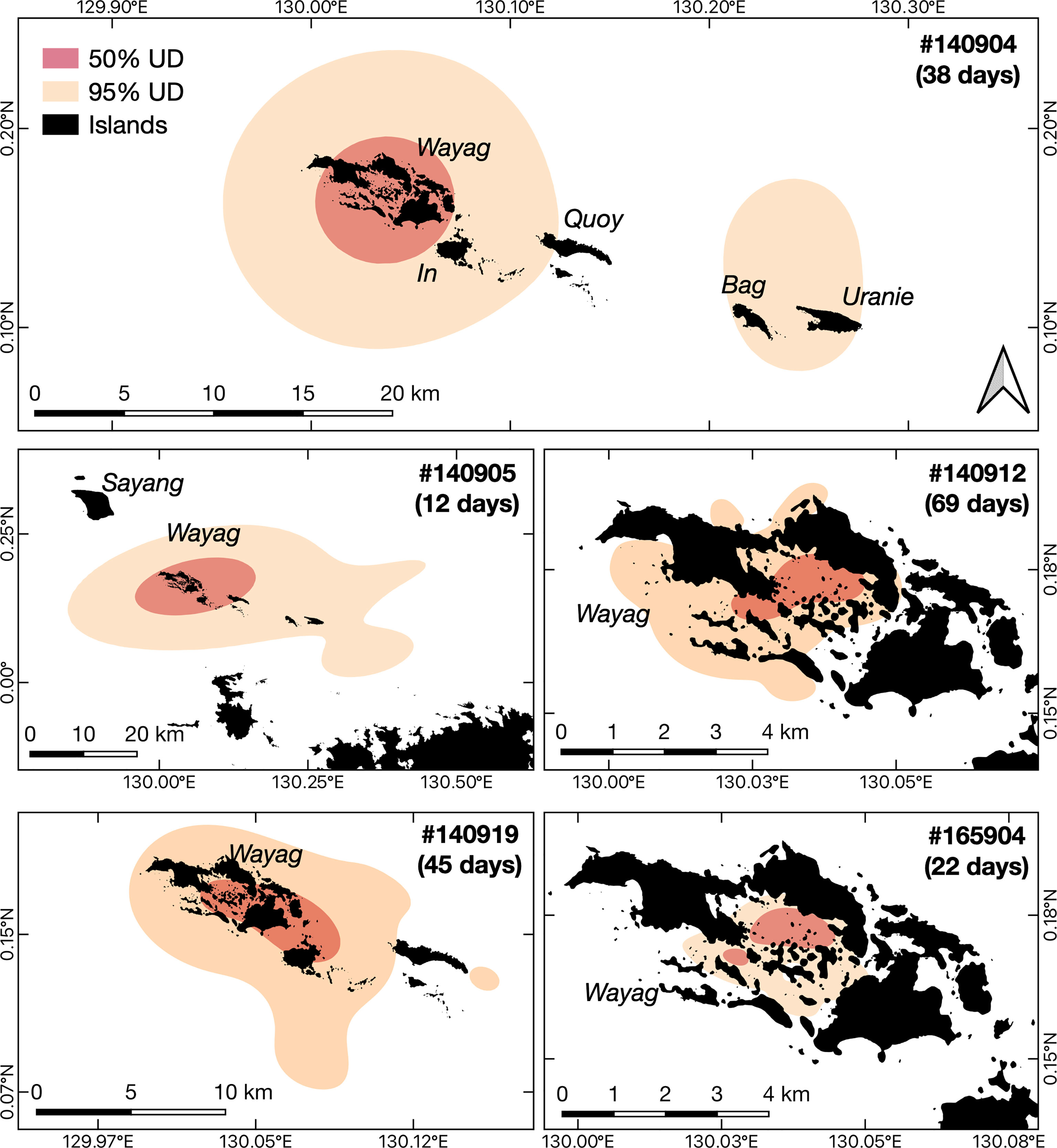
Figure 4 Core activity space and the extent of activity space for each of the five juvenile M. alfredi satellite tracked around Wayag, Raja Ampat. The red polygons (50% UD) and the orange polygons (95% UD) denote the smallest estimated core activity space and the extent of activity space, respectively, where the tagged juveniles were expected to spend their time during the tracking period.
3.2.2 Regional Movements and Residency Within the Wayag Lagoon
The estimated movement tracks derived from the state-space model suggested that all of the tagged M. alfredi spent the majority of their time within the Wayag lagoon, where they were tagged, or in waters adjacent to Wayag (Figure 5A). When in or around the Wayag lagoon, all tagged individuals displayed less persistent and directed movements in their localised tracks, suggesting affinity to this area (Figure 5B). Individuals occasionally made excursions to other areas around the small islands to the east and just outside of the Wayag lagoon. Three juveniles (ID #140904, #140905, and 140919) exhibited movements likely indicative of transiting behaviour, with more directed and faster movements as shown by higher move persistence index values for estimated locations outside of the Wayag lagoon (Figure 5B). Two individuals (ID #140905 and #140919) also travelled to areas outside the MPA boundary. While traveling outside of Wayag, ID #140905 also displayed low move persistence values in Wayag. ID #140912 spent all of its time within 5 km of the Wayag lagoon and showed low move persistence values during the 69-day tracking period.
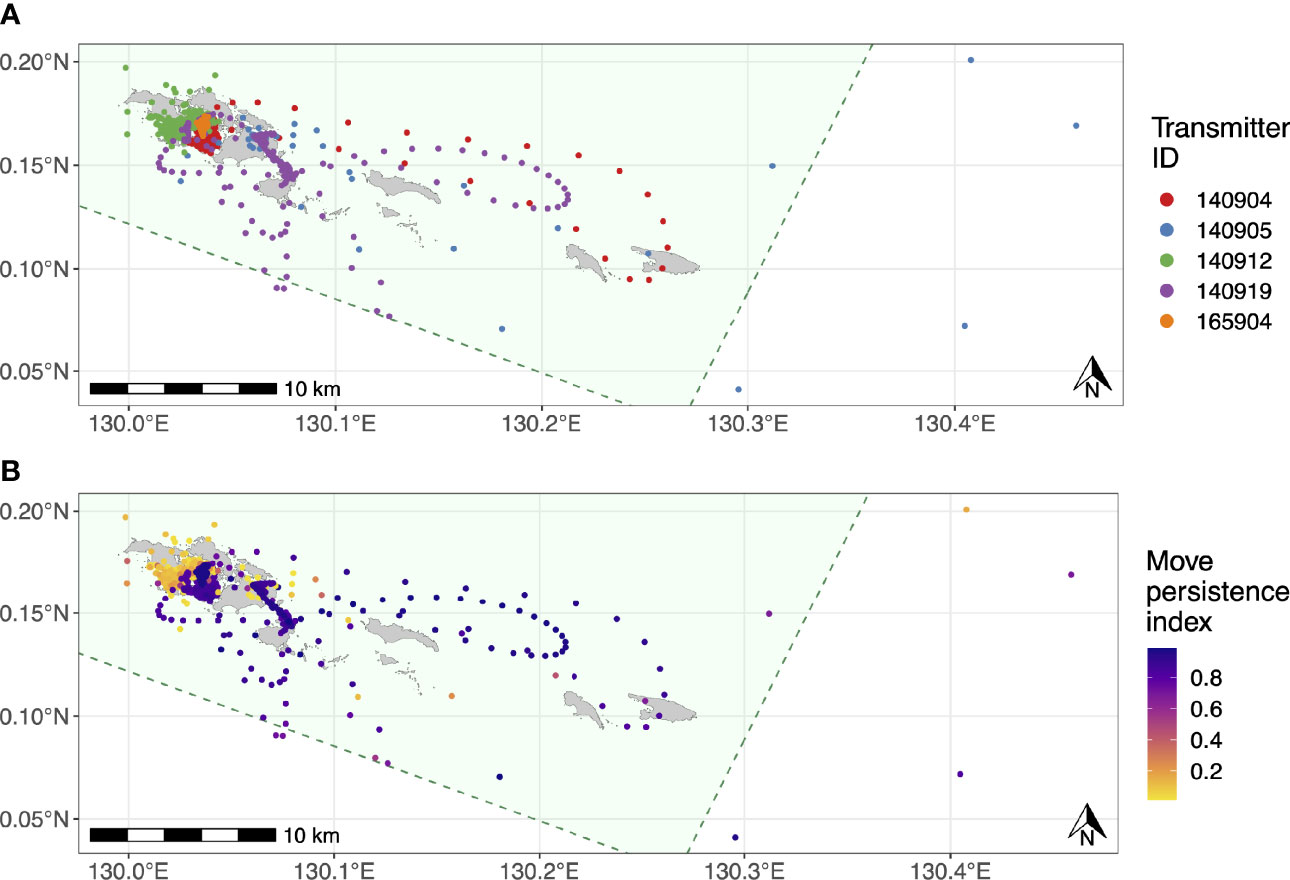
Figure 5 Movement tracks for the five satellite-tracked juvenile M. alfredi estimated using state-space models with six-hour time steps. (A) Most likely track for each tagged individual; (B) Move persistence behavioural indices for all estimated M. alfredi positions. The green polygons in both panels denote SAP Waigeo Barat MPA boundary and the grey polygons represent islands.
3.3 Residency and Fine-Scale Habitat Use Within the Wayag Lagoon as Revealed by Acoustic Telemetry
Between May 2019–September 2021, nine juvenile M. alfredi (size-range = 170–200 DW) were tracked via passive acoustic telemetry (Table 2). Individuals were tracked for periods of 69–439 days (mean ± SD: 182 ± 109 days), with a total of 97,483 detections recorded across the five monitored receiver sites within the Wayag lagoon. Nearly a quarter (23,722 detections; 24.3%) of these detections were registered from ID #20244. The maximum number of consecutive detection days ranged between 25 (ID #21873) and 119 days (ID #21579) (mean ± SD: 87 ± 30 days). Excluding ID #21873 that was only detected by one receiver, the maximum number of consecutive days ranged from 69–119 days (mean ± SD: 95 ± 21 days). In addition, a total of 11,728 detections were recorded from the ‘sentinel tag’ by the ‘Main Lagoon Entrance’ receiver from 16–29 May 2021. Across 24-hour periods, the hourly mean number of detections of the sentinel tag was relatively constant (Supplementary Figure 1). During that period, the mean detection rate at night-time (35.6 detections/hour) was slightly higher than that at daytime (35.3 detections/hour), and the difference (0.38 detections/hour) was not significant (two-sample t-test, p = 0.285).
3.3.1 Detection Patterns and Residency
Detection patterns for the nine tagged M. alfredi varied between sites. The receiver deployed at Lagoon Backyard (Figure 1) recorded the most detections (38,719; 39.7% of all detections) with Front Entrance recording the fewest detections (1,792; 1.8%) (Table 3). The receiver at Main Lagoon Entrance, deployed in May 2019, detected all nine tagged individuals while the other receivers, deployed in January 2020, detected all eight available transmitters (as the first transmitter deployed, ID #21873, was no longer active in the lagoon at the time these four receivers were deployed). Importantly, none of the acoustically tagged M. alfredi were recorded by an extensive array of 23 receivers deployed within the broader Raja Ampat region (Figure 1).
The Detection Index (DI) calculated for acoustic receivers deployed in Wayag lagoon similarly varied between receiver sites. The Front Entrance receiver accounted for the lowest DI of all receivers (56.2%) (Table 3). DIs at receivers inside the main lagoon of Wayag (i.e., the Inner Lagoon and Far Inner Lagoon receivers) were higher than 89% for both receivers, and overall larger than those outside the main lagoon. This suggests that the tagged juveniles were more detectable within the main lagoon, especially around the two receivers, than outside of the main lagoon on a daily basis.
Residency behaviour varied slightly among the nine tagged individuals yet indicated high residency of the tagged rays to the Wayag lagoon. The RI of each tagged juvenile, particularly the eight individuals detected by all five receivers, ranged between 52.3–98.2% (mean ± SD: 77.7 ± 17.4%). The single individual detected by only one receiver accounted for a smaller RI of 43.7%. A linear model showed that even though the tracking period was negatively correlated (R2 = 0.13) with the RI of eight individuals, this correlation was not significant (p = 0.38). On average, juvenile M. alfredi spent between 46.4–96.9 mins around a given acoustic receiver for each recorded visitation (Table 2). Detectability of individuals varied between sites, with the Inner Lagoon receiver recording the highest number of visitations (2,001), and the Front Entrance receiver recording the lowest (216; Table 3). Despite recording the second highest number of visitations, the mean duration of these visitations at the Main Lagoon Entrance receiver was relatively low (30 mins) compared to other sites further into the Wayag lagoon, where the mean duration was over one hour for the Inner Lagoon and Far Inner Lagoon receivers, and up to two hours at the Lagoon Backyard receiver.
Overall, the longest continuous visitation was recorded at the Lagoon Backyard receiver, where ID #20244 remained continuously for 16.6 h (Figure 6), followed by ID #21579 remaining within the detection range of the Inner Lagoon receiver for 10.6 h. At the other receiver sites, the maximum visitation durations were less than half of that in Lagoon Backyard, with Front Entrance receiver recording the same individual for 3.6 h, Main Lagoon Entrance receiver for 7.8 h, and Far Inner Lagoon receiver for 8.3 h.
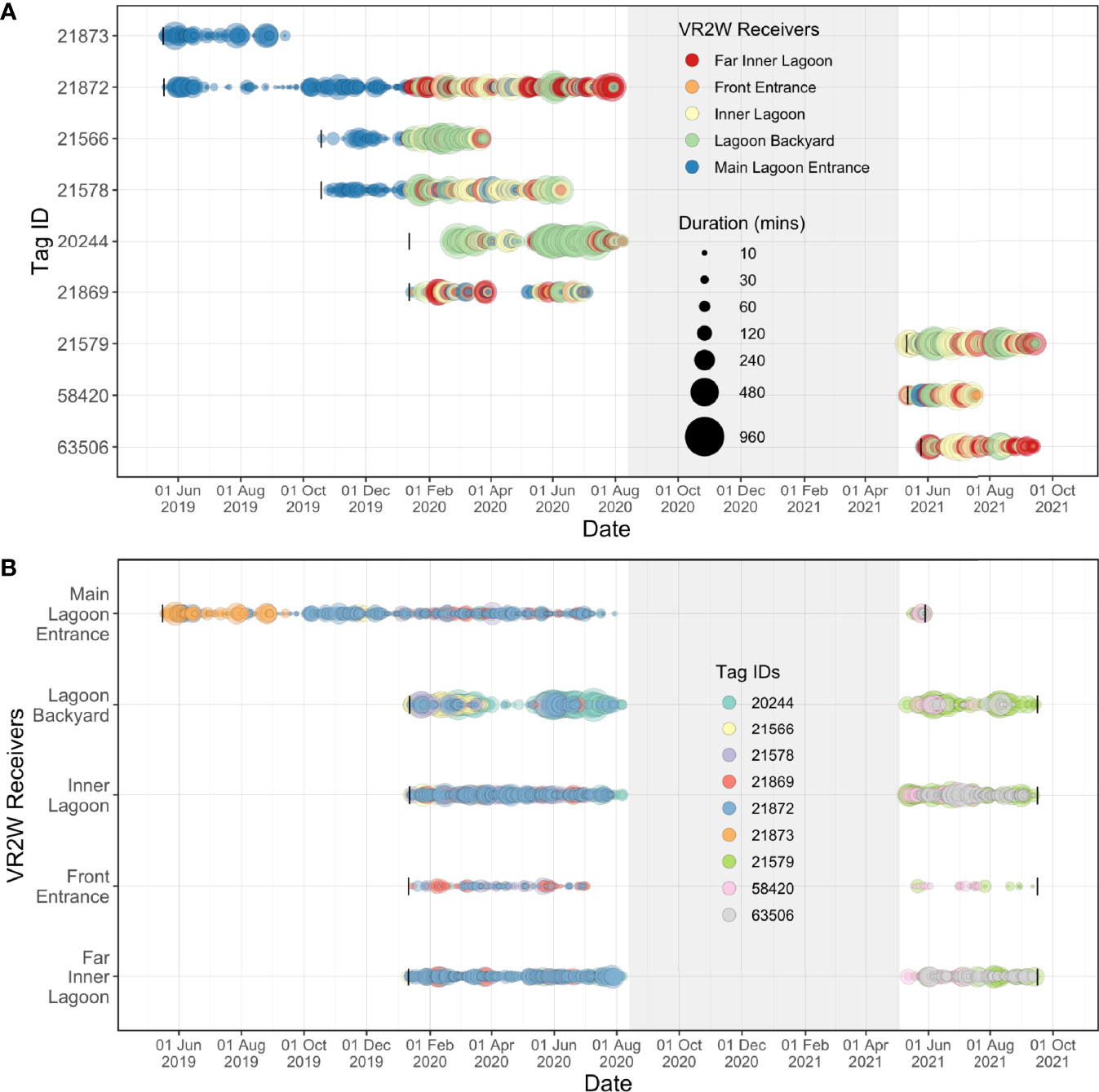
Figure 6 Acoustic detections recorded over time for each tagged juvenile M. alfredi at each receiver deployed in the Wayag lagoon between May 2019–September 2021. The size of the bubbles indicates (A) the duration of visitations recorded by each receiver for each individual; and (B) the duration of visitations recorded for each individual at each receiver site. The grey shaded areas denote the period during which there were no active acoustic transmitters on manta rays in the lagoon, yet the receivers were still deployed in the lagoon. Black vertical lines in Panel A represent deployment dates of each transmitter, while black vertical lines in Panel B represent deployment and recovery dates of each receiver.
Within the lagoon, the tagged juveniles moved numerous times between receivers, ranging from 183–976 movements per individual (mean ± SD: 392 ± 267 movements), with a total of 3,134 movements recorded. No movement was recorded from ID #21873, as only a single receiver was deployed in Wayag during its period of detection (Table 2). Movements recorded between receivers were variable and were generally made between nearby receivers (Figure 7). For example, of the 357 movements starting from the Lagoon Backyard receiver, 41% ended at the Main Lagoon Entrance, 33% at the Inner Lagoon, and 21% at the Far Inner Lagoon receiver.
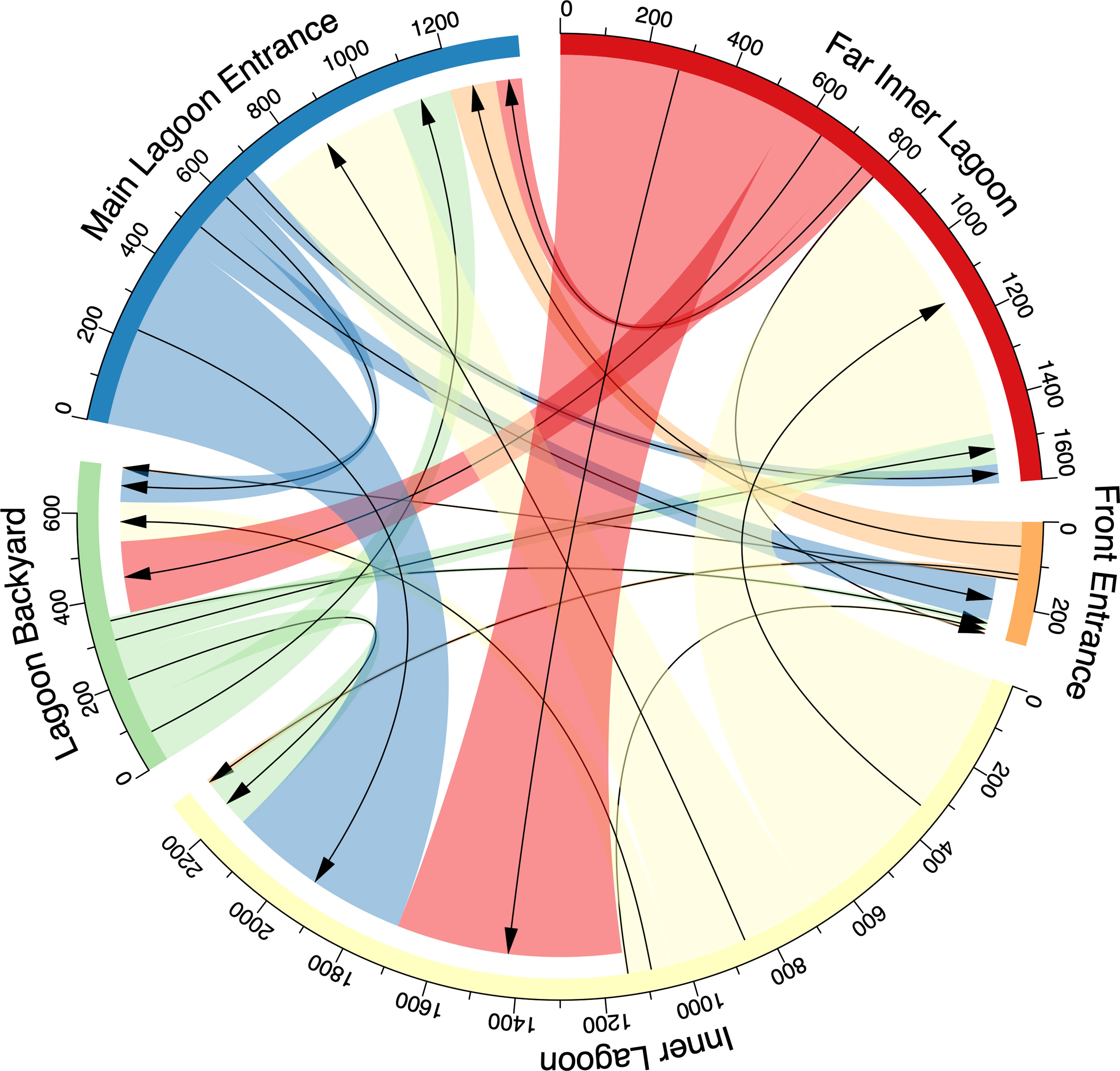
Figure 7 Connectivity plot presenting the number of movements of acoustically tagged juvenile M. alfredi between receivers in the Wayag lagoon. The arrows show the direction of movement from one receiver to another, and the colour-coded receiver location names are outside the circle.
3.3.2 Seasonality and Periodicity in Visitations
The five acoustic receivers deployed within the Wayag lagoon recorded transmitter detections more or less continuously after their initial deployment (Figure 6B). However, some of the juveniles were not detected by any receiver in the array in several instances, suggesting that they may have left the lagoon for brief periods, or at least remained in areas of the lagoon where they were not detectable (Supplementary Figure 2). For example, ID #21869 disappeared from the array in late March 2020 for 40 days before being detected again in early May 2020 (Figure 6A). During the same period, considerable gaps in detection of all tagged individuals were also observed at Lagoon Backyard (though detections were continuous through this time at the other four receivers). Importantly, the six transmitters deployed on juveniles in May and October 2019 and January 2020 all disappeared from the array by early August 2020 (Figure 6). Of these, two (ID #21873 & #21566) were detected for periods of four and six months, respectively, while the other four transmitters all disappeared from the array between early June 2020 and early August 2020 (Figure 6B). No detections occurred on the array between August 2020 and May 2021, until the final deployment of three transmitters in early May 2021. All receivers then continued to record detections until 16 September 2021, when they were downloaded for the final time for this study. Unfortunately, the Main Lagoon Entrance receiver’s battery inexplicably ceased to function on 29 May 2021 (despite having been replaced with a new battery in early May at the time of transmitter deployment).
Overall, the mean hourly number of acoustic detections recorded by all receivers combined was significantly different (two-sample t-test, p = 0.01) between daytime (mean ± SD: 3,817 ± 343 detections) and night-time (mean ± SD: 4,307 ± 494 detections). The juveniles displayed a striking contrast in spatial use of the Wayag lagoon between day and night (Figure 8A). While the Inner Lagoon and Far Inner Lagoon receivers recorded the majority of their detections during daylight hours, the Lagoon Backyard and Main Lagoon Entrance receivers recorded most of their detections during night-time (detections at the Front Entrance receiver were too few to discern a pattern). Every tagged individual was detected by receivers throughout the 24 h diel cycle, with variations in daytime and night-time occupancy among individuals at each hour of the day (Figure 8B).
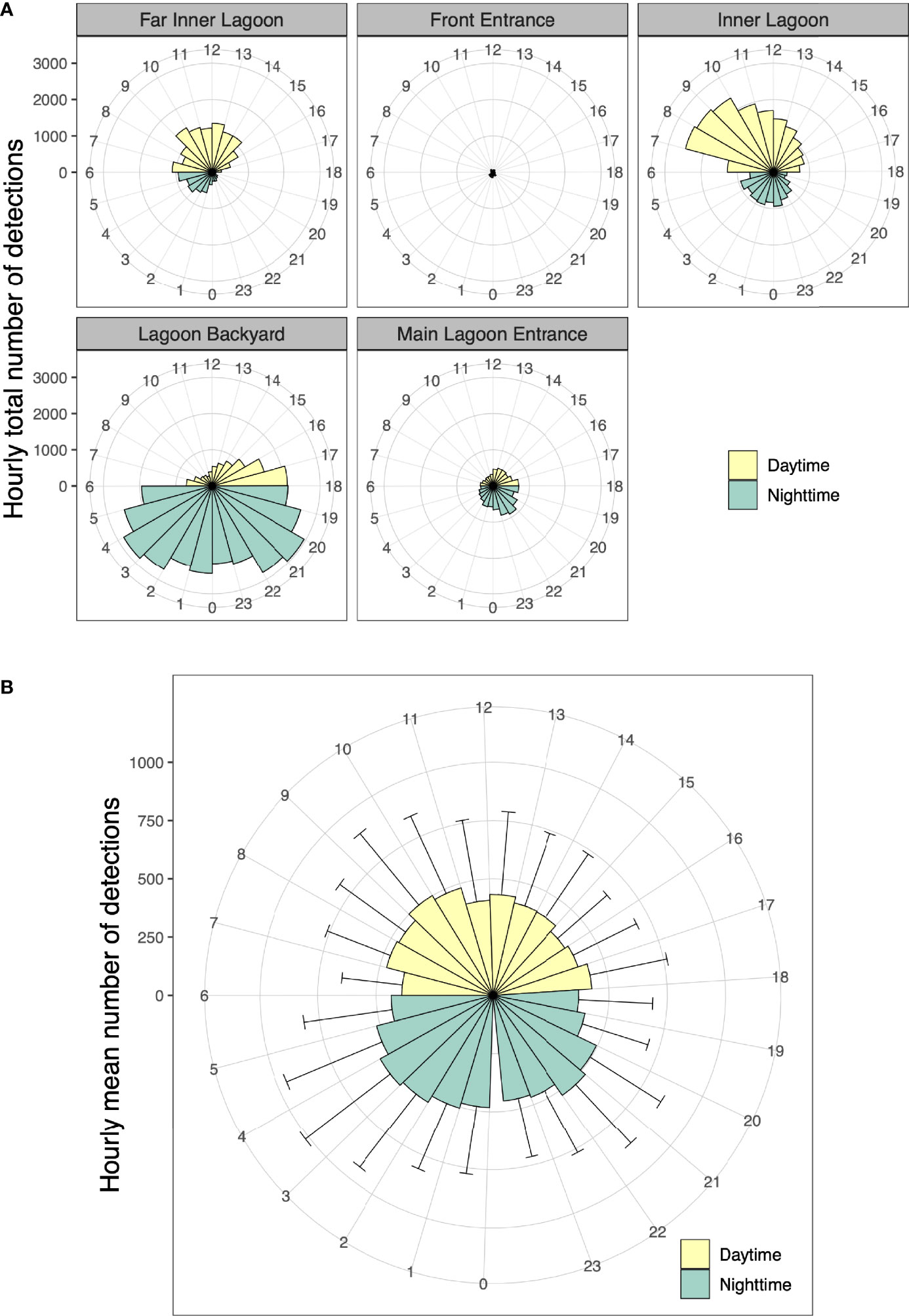
Figure 8 (A) Hourly total number of acoustic detections for 24 h (0–23) in the Wayag lagoon between 17 May 2019 and 16 September 2021 recorded by each receiver, and (B) Hourly average number of detections for all tagged juveniles with error bars showing the variability across individuals. As Wayag is less than 20 km north of the equator, daylight hours are more or less constant throughout the year and denoted here as 06:00 to 18:00.
4 Discussion
Using multiple investigative techniques, this study provides compelling evidence that fulfils Criterion (2) for elasmobranch nursery areas as proposed by Heupel et al. (2007); i.e., demonstrated residency by juveniles within the proposed nursery area for extended periods. We reveal juvenile M. alfredi tend to remain within or briefly leave and return to the Wayag lagoon for extended periods, with only short excursions outside of the study area. Some of the photo-identified juveniles were resighted within the Wayag lagoon up to 1.7 years after their first observation, while satellite and passive acoustic telemetry data revealed restricted movements and near-continuous use of the lagoon for extended periods of up to ~14.5 months. Previously, Setyawan et al. (2020) showed conclusively that the Wayag lagoon fulfils Heupel et al. (2007)’s elasmobranch nursery Criteria (1) and (3); i.e., YoY and juvenile M. alfredi are more commonly encountered in the Wayag lagoon than in other areas and that the lagoon is used repeatedly by YoY and juveniles across years. Taken together, these studies present the most robust assessment to date of a M. alfredi nursery and show conclusively that juveniles use Wayag lagoon in northwestern Raja Ampat as a nursery.
The body size distribution of M. alfredi observed in the Wayag lagoon obtained from visual estimation and drone measurements suggests that the Wayag lagoon not only serves as a primary nursery area used by newborn or YoY individuals, but also serves as a secondary nursery area (Bass, 1978) based on the presence of juveniles sized ≤2.4 m DW (Setyawan et al., 2020). Primary and secondary nurseries occur in the same areas for a number of elasmobranch species (Simpfendorfer and Milward, 1993), and have been identified in several tropical marine regions (Yokota and Lessa, 2006; Palacios et al., 2021). We suggest that the Wayag lagoon may also act as a pupping ground. Despite the general dearth of adult manta ray sightings in the lagoon, since the start of our monitoring program in 2013, three near-term pregnant female M. alfredi have been observed in Wayag, in particular in the channel between the Front Entrance and Main Lagoon Entrance receivers (Setyawan et al., 2020). A pregnant female acoustically tagged in the Dampier Strait region of Raja Ampat (Figure 1) was detected several months later (close to her estimated time of parturition) by a receiver in the Wayag lagoon (Setyawan et al., 2018). Additionally, the estimated sizes of several YoY M. alfredi that appeared to be newborns, are equal to the smallest reported birth size of M. alfredi by Murakumo et al. (2020). Furthermore, the obvious “creases” observed on these individuals, where their pectoral fins would have been dorsally folded over their body cavity within the mother’s uterus, were much like those shown by Marshall et al. (2008) for a late-term M. alfredi foetus in Mozambique.
Whilst all of the satellite-tracked juveniles exhibited sustained and restricted movements inside the Wayag lagoon area, occasional excursions to adjacent areas were also recorded. Individuals equipped with acoustic transmitters occasionally went undetected for a period of weeks by the acoustic receiver array inside Wayag lagoon, particularly from the end of March to early May. We hypothesise that these excursions outside of the nursery area were likely associated with maximising foraging activities in nearby highly productive waters to the east of Wayag at the end of the northwest monsoon. A mature male M. alfredi satellite tagged in Eagle Rock (Figure 1) in Feb 2015 transmitted a substantial number of GPS locations from areas between Quoy and Uranie islands (Figure 3) in March-April 2015 (Setyawan, Unpubl. Data), which might indicate that these locations were associated with extensive surface foraging activities. Additionally, it is possible that the acoustically tagged juveniles that disappeared between the end of March and early May from the array in Wayag visited nearby Sayang and Piai islands, approximately 15 km to the northwest (Figure 1). Juvenile M. alfredi are frequently sighted foraging at the surface along the south coasts of Sayang and Piai islands around March–April (Ferdiel Ballamu pers. comm.) during the transitional period between the northwest and southeast monsoon. These excursions may be evidence of the juvenile manta rays’ exploratory behaviours and developing their foraging behaviours including searching for prey in a more open ocean environment – a necessity for young elasmobranchs like basking sharks (Cetorhinus maximus), which have lower prey encounter success rates than adults (Sims et al., 2006). Such behaviour is reported in a number of ocean-going taxa; for instance, Grecian et al. (2018) found differences in foraging proficiency between mature and immature gannets (Morus bassanus), while younger individuals of narwhals (Monodon monoceros) demonstrated different movement patterns from older individuals, likely associated with increased exploratory behaviours and less developed foraging proficiency (Laidre et al., 2004).
Though M. alfredi are capable of travelling up to several hundred kilometres to visit seasonally-productive sites (Anderson et al., 2011; Couturier et al., 2014; Jaine et al., 2014), the 14 satellite or acoustically-tagged individuals in this study showed high residency to the Wayag lagoon, with a maximum movement of 47 km to the east of the lagoon. None of the acoustically-tagged individuals were detected within the an array of 23 acoustic receivers placed at all known M. alfredi aggregation sites in the Raja Ampat archipelago (Figure 1), including at the heavily-visited cleaning and feeding aggregation site Eagle Rock, just 36 km to the south of the Wayag lagoon (Setyawan et al., 2018).
Despite several movements to areas outside the Wayag lagoon, the tagged juveniles repeatedly returned to and showed a strong residency to the study site. Three of five satellite-tagged juveniles exhibited the extent of activity space (95% UD) that extended less than 10 km from the Wayag lagoon (Figure 4). Notably, the core activity space (50% UD) of all satellite-tagged juveniles mainly encompassed the Wayag lagoon and nearby areas within a 5 km radius. The largest 50% and 95% UDs identified in our study encompassed 182 and 1,195 km2, respectively. These are much smaller than those of a juvenile male in the Red Sea, with 50% and 95% UDs of 414 and 2,457 km2 (Kessel et al., 2017). It is important to note that the activity space estimated in our study was restricted to short periods of satellite tracking (12–69 days), therefore it might realistically be larger than what is currently estimated. We also note that satellite tagged juveniles in our study were mostly females, therefore we were unable to explore sex-linked nuances in the spatial movements of juveniles, though maturity is a more relevant factor than sex when identifying nurseries.
The restricted activity space and low move persistence recorded for satellite-tracked individuals in the vicinity of the Wayag lagoon suggest strong residency within this site. This residency may be driven by the safe habitat for juveniles or could also reflect the reliable availability of prey in this area. For manta rays, which rely on finding large quantities of diffuse zooplankton prey in a dynamic pelagic ocean, sheltered coral reef lagoons may supply reliable and sustained quantities of prey to support the energetic needs of juveniles. Numerous studies have documented large M. alfredi foraging aggregations at isolated coral reefs where local tidal dynamics act to concentrate zooplankton prey (Jaine et al., 2012; Weeks et al., 2015; Armstrong et al., 2016), including inside the lagoons of coral reef atolls (Papastamatiou et al., 2012; Armstrong et al., 2021). Within the Wayag lagoon, juvenile M. alfredi are frequently observed using surface and somersault feeding strategies (Setyawan et al., 2020) similar to other lagoon habitats (McCauley et al., 2014; Stevens, 2016). We did not investigate the taxonomic composition or biomass of the planktonic prey targeted by feeding juvenile manta rays in the Wayag lagoon, though this certainly represents an important future field of study.
Acoustic telemetry detections of the tagged juvenile M. alfredi, in particular eight individuals that were detected by all receivers, indicated high residency indices (RI: 52–98%) at the monitored sites within the Wayag lagoon. Such residency levels are substantially higher than that of individuals (mostly adults) tagged during a previous acoustic tagging study in northern Raja Ampat (RI: 21%) (Setyawan et al., 2018). Similarly, acoustically-tagged juvenile M. alfredi in the Amirantes, Seychelles, also showed a higher residency than adults around key habitats (Peel et al., 2019). In the Red Sea, the residency of small (<2.5 m DW, likely juvenile) M. alfredi was also relatively high at 65% (Braun et al., 2015). Lower residency levels (15–40%) of acoustically-tagged animals have been reported from other populations (Clark, 2010; Couturier et al., 2018; Andrzejaczek et al., 2020; Venables et al., 2020), presumably because the studies tagged adult individuals. It is also possible that our RI may have been affected by the design of our acoustic tracking array. In addition to age-class (Chapman et al., 2015; Peel et al., 2019), the number of receivers, their position and the design of the acoustic array are important considerations (Espinoza et al., 2016; Peel et al., 2019). This artefact of array design is demonstrated by the fact that the lowest RI in our study (43.7%) was registered by ID #21873 when there was only one receiver deployed in the lagoon at the beginning of the study period.
Passive acoustic monitoring of tagged juvenile M. alfredi revealed individual and temporal variability in fine-scale (<4 km) space use and site occupancy within the Wayag lagoon. The nine acoustically tracked individuals exhibited strong site affinity, with high residency times around acoustic receivers and frequent, repeated visits to the sites. The maximum consecutive days of acoustic detections by tagged juveniles of up to 119 days (~4 months), with an average of 95 days (~3 months) of maximum consecutive detection days for tagged juveniles detected by five receivers, clearly indicate that these sites provide important habitat in Wayag lagoon. The long-term residency of juvenile M. alfredi in Wayag lagoon has been documented through individual photo-ID, with some juveniles resighted on several occasions over a 21-month period, as well as by passive acoustic telemetry revealing quasi-continuous occupancy in the nursery for over 14 months. Globally, it is still unclear how long juvenile reef manta rays use a nursery area, and at what age or size they decide to leave the nursery. In another proposed M. alfredi nursery area located in Fam, Raja Ampat (approximately 100 km to the south of Wayag), three juveniles were visually resighted after 26 months, and one resighted after 28 months, still present in the nursery (Setyawan et al., 2020); however, it is unknown whether these individuals had left the area during this time. We provide here the first documented continuous occupancy of juvenile manta rays in a nursery area. Coupled with multi-year presence of the juveniles, their continuous occupancy highlights the importance of this nursery area for their early-stage development. Further studies are required to better understand the ecological function of nursery areas in contributing to recruitment into adult populations of M. alfredi. Setyawan et al. (2020) documented one such recruitment; a juvenile M. alfredi, first sighted in Wayag lagoon as a 180 cm DW YoY male in November 2013, was resighted six years later as a 260 cm DW adult in the South East Misool MPA in southern Raja Ampat, 296 km to the south. Other valuable lines of future investigation include examining the social interactions and bonds created between newborns and juveniles within nurseries and their persistence over time, as well as investigating the “carrying capacity” of the area to serve as a nursery for newborn and juvenile M. alfredi, given the small size of the Wayag lagoon.
Passive acoustic tracking in Wayag lagoon yielded similar proportions of juvenile detections between day (47%) and night (53%), though the number of night-time detections was significantly higher than during the day. This is in sharp contrast with similar studies conducted in several other sites in Indonesia (Dewar et al., 2008; Setyawan et al., 2018) and other countries (Clark, 2010; Couturier et al., 2018; Peel et al., 2019; Andrzejaczek et al., 2020; Venables et al., 2020), in which the numbers of acoustic detections of adult or subadult M. alfredi were significantly lower during the night than during the daytime. Most of these studies reported that manta rays would visit and occupy receiver sites mainly during the day for foraging and cleaning, but at night, tagged individuals would disappear from the tracking arrays, presumably moving to offshore or deeper waters to feed on vertically migrating deep scattering layers or emerging benthic zooplankton (Clark, 2010; Couturier et al., 2013; Braun et al., 2014). This doesn’t appear to be the case in Wayag, where the juveniles were detected in the lagoon throughout both day and night. Furthermore, the distinct variations in the daytime and night-time detections between those receivers inside main lagoon area, that are surrounded by deep water, and Lagoon Backyard, located in shallow water, highlight a potentially interesting distinction in habitat use by juveniles in nursery areas. McCauley et al. (2014) observed that M. alfredi in a sheltered lagoon in Palmyra Atoll continuously used large areas of the lagoon and spent more time at greater depth during the day than at night. Further research into the vertical movements of M. alfredi in and outside of the Wayag lagoon using satellite telemetry will help understand the diel diving behaviour of juveniles.
In contrast to the main lagoon area (Figure 1) that was used extensively by juvenile M. alfredi during the day, the shallow Lagoon Backyard site was primarily visited around dusk and at night, often for extensive periods up to 16 hours. In most other acoustic telemetry studies conducted in reef environments and published in the literature, it is indeed possible that biological noise emanating from the reef at night may have prevented some detections to be recorded by the receivers (Kessel et al., 2014). However, data from the sentinel tag detected at the Main Lagoon Entrance receiver showed no obvious reduction in tag detectability based on time of day, suggesting continuous ability of the receiver to detect transmitters in the absence of tagged juveniles. Therefore, the distinctive diel pattern in visitation at the receivers in Wayag lagoon was likely due to actual juvenile visitations rather than being an artefact of detection range. Sheltered, shallower sites can act as ideal night-time habitats by providing safety from potential predators (Wetherbee et al., 2007; Guttridge et al., 2012) and a potential suitable supply of demersal zooplankton emerging from the shallow seabed (Alldredge and King, 1977; Ohlhorst, 1982). In southern Mozambique, acoustically tagged M. alfredi visited a feeding site mostly at night (Venables et al., 2020), though it is unclear whether they were foraging around the receiver at this site. At Palmyra Atoll in the Line Islands, Papastamatiou et al. (2012) recorded high nocturnal area-restricted search behaviour associated with high zooplankton prey patches at specific sites inside a coral reef lagoon. It is possible that juvenile M. alfredi in Wayag use the Lagoon Backyard site for the same reason. Further research into the night-time behaviour of M. alfredi at this site, potentially using active acoustic tracking, may help ascertain the drivers of the observed high nocturnal residency times in this shallow area of the Wayag lagoon.
Importantly, the findings of this study have been shared with the Raja Ampat MPA Management Authority and have already been used to redesign and improve conservation and management measures for manta ray protection in the SAP Waigeo Barat MPA. Our findings have contributed to the designation of manta rays as one of the primary conservation targets for this MPA due to the importance of the Wayag lagoon as a manta ray nursery. Given the status of manta rays as a conservation target, stricter protection must now be implemented in Wayag; therefore, some areas within the main lagoon of Wayag have recently been designated as a “core conservation zone” with strictly restricted access. The areas outside of this core zone remain designated as tourism zones, where visitors, but no fishing, is allowed. The MPA Management Authority is currently working on finalising the legislation for both the revised zonation system and the management plan for SAP Waigeo Barat MPA, which will include important regulations (e.g., boat speed limits of less than 5 knots inside the main lagoon and the areas around Lagoon Backyard, as well as stipulated mooring areas for liveaboards far from known manta sites) to ensure the nursery function of the Wayag lagoon is not compromised.
5 Conclusions
This study shows conclusively that the Wayag lagoon in Raja Ampat archipelago serves as a nursery for newborn and juvenile M. alfredi and provides the most robust assessments to date of a M. alfredi nursery. It also provides key information on the residency and fine-scale habitat use of M. alfredi in this nursery area. These important findings have been used to underpin the formulation of management strategies to specifically protect the Wayag lagoon and its function as a manta ray nursery. Safeguarding this nursery could ultimately be instrumental for the survival and recovery of M. alfredi populations in the region.
Data Availability Statement
The datasets generated during and/or analysed during the current study are available in the Movebank Data Repository, which can be found here: https://doi.org/10.5441/001/1.n95c7182 ([Setyawan et al., 2022c]).
Ethics Statement
The animal study was reviewed and approved by The University of Auckland Animal Ethics Committee and was conducted following protocol 002228. Permission to conduct the research was granted by the Raja Ampat Marine Protected Area (MPA) Management Authorities (Balai Kawasan Konservasi Perairan Nasional (BKKPN) Kupang and BLUD UPTD Pengelolaan KKP Kepulauan Raja Ampat).
Author Contributions
ES, ME, RM, AH, and AS collected the data. ES performed the statistical analysis and created figures, tables, and maps with guidance from FJ and BS. ES drafted the manuscript. FJ, ME, RC, and BS provided guidance and supervision, reviewed, and edited drafts of the manuscript. All authors discussed the results, contributed to and approved the final manuscript.
Funding
The research was generously funded by David and Lucile Packard Foundation, MAC3 Impact Philanthropies, Sunbridge Foundation, Wolcott Henry Foundation, SEA Aquarium Singapore, Save the Blue Foundation, Stellar Blue Fund, Sea Sanctuaries Trust, Seth Neiman, Katrine Bosley, Dawn Arnall, Alex and Sybilla Balkanski, Marie-Elizabeth Mali and Daniel Roozen, and the Indonesia Climate Change Trust Fund (ICCTF).
Conflict of Interest
The authors declare that the research was conducted in the absence of any commercial or financial relationships that could be construed as a potential conflict of interest.
The reviewer LF declared a past collaboration with one of the co-authors FJ to the handling editor.
Publisher’s Note
All claims expressed in this article are solely those of the authors and do not necessarily represent those of their affiliated organizations, or those of the publisher, the editors and the reviewers. Any product that may be evaluated in this article, or claim that may be made by its manufacturer, is not guaranteed or endorsed by the publisher.
Acknowledgments
The authors thank the Government of Indonesia (including the Ministry of Marine Affairs and Fisheries and the Ministry of Environment and Forestry), the West Papua Conservation Agency (BBKSDA Papua II), the Raja Ampat MPA Management Authorities (BLUD UPTD Pengelolaan KKP Kepulauan Raja Ampat and Balai Kawasan Konservasi Perairan Nasional Kupang), and in particular the adat people of Raja Ampat for hosting this work. We thank Conservation International donors for their generous support for this project, Sarah Lewis, Shawn Heinrichs, Meity Mongdong, Kristian Thebu, Alberth Nebore, and Iqbal Herwata for their support of this manta ray research and conservation program in Raja Ampat. We thank Indonesia Climate Change Trust Fund (ICCTF) and Reef Check Indonesia for their support for this study. We extend a warm thanks to Urias Tuhumena, Demas Fiay, Muhamad Izuan, Orgenes Ambafen, and Imanuel Mofu for their tireless effort in assisting data collection in the field. Also, thanks to the field support team who ensured boats and logistical supplies were always available for the many long-distance trips to Wayag: Timore Kristiani, Hanrika Leimena, Julius Thonak, Poerwanto, Marselinus Uskono, and Yakonias Thonak. We thank Vinay Udyawer for assistance with home range analysis. E.S. also would like to acknowledge the New Zealand Scholarship (NZAS) award granted to him to undertake a doctoral program at the University of Auckland. Lastly, we also note that this research was made possible thanks to funding to E.S. from WWF’s Russell E. Train Education for Nature Program (EFN).
Supplementary Material
The Supplementary Material for this article can be found online at: https://www.frontiersin.org/articles/10.3389/fmars.2022.815094/full#supplementary-material
References
Alldredge A. L., King J. M. (1977). Distribution, Abundance, and Substrate Preferences of Demersal Reef Zooplankton at Lizard Island Lagoon, Great Barrier Reef. Mar. Biol. 41 (4), 317–333. doi: 10.1007/BF00389098
Anderson R. C., Adam M. S., Goes J. I. (2011). From Monsoons to Mantas: Seasonal Distribution of Manta alfredi in the Maldives. Fish. Oceanogr. 20 (2), 104–113. doi: 10.1111/j.1365-2419.2011.00571.x
Andrzejaczek S., Chapple T. K., Curnick D. J., Carlisle A. B., Castleton M., Jacoby D. M., et al. (2020). Individual Variation in Residency and Regional Movements of Reef Manta Rays Mobula alfredi in a Large Marine Protected Area. Mar. Ecol. Prog. Ser. 639. doi: 10.3389/fmars.2020.00558
Armstrong A. O., Armstrong A. J., Jaine F. R., Couturier L. I., Fiora K., Uribe-Palomino J., et al. (2016). Prey Density Threshold and Tidal Influence on Reef Manta Ray Foraging at an Aggregation Site on the Great Barrier Reef. PloS One 11 (5), e0153393. doi: 10.1371/journal.pone.0153393
Armstrong A. O., Stevens G. M., Townsend K. A., Murray A., Bennett M. B., Armstrong A. J., et al. (2021). Reef Manta Rays Forage on Tidally Driven, High Density Zooplankton Patches in Hanifaru Bay, Maldives. PeerJ 9, e11992. doi: 10.7717/peerj.11992
Babin A., Fitzpatrick L., Linnansaari T., Curry R. A. (2019). Detection Range of Acoustic Receivers in a Large Hydropower Reservoir. Fishes 4 (4), 60. doi: 10.3390/fishes4040060
Bass A. (1978). “Problems in Studies of Sharks in the Southwest Indian Ocean,” in Sensory Biology of Sharks, Skates and Rays. Eds. Hodgson E., Mathewson R. (Arlington, Virginia: Office of Naval Research, Department of the Navy), 545–594.
Beale C. S., Stewart J. D., Setyawan E., Sianipar A. B., Erdmann M. V. (2019). Population Dynamics of Oceanic Manta Rays (Mobula birostris) in the Raja Ampat Archipelago, West Papua, Indonesia, and the Impacts of the El Niño–Southern Oscillation on Their Movement Ecology. Divers. Distrib. 25, 1472–1487. doi: 10.1111/ddi.12962
Branstetter S. (1990). “Early Life-History Implications of Selected Carcharhinoid and Lamnoid Sharks of the Northwest Atlantic,” in Elasmobranchs as Living Resources: Advances in the Biology, Ecology, Systematics, and the Status of the Fisheries. Eds. Pratt H. L. Jr., Gruber S. H., Taninchi T. (Virginia, USA: VIMS Books and Book Chapters), 17–28.
Braun C. D., Skomal G. B., Thorrold S. R., Berumen M. L. (2014). Diving Behavior of the Reef Manta Ray Links Coral Reefs With Adjacent Deep Pelagic Habitats. PloS One 9 (2), e88170. doi: 10.1371/journal.pone.0088170
Braun C. D., Skomal G. B., Thorrold S. R., Berumen M. L. (2015). Movements of the Reef Manta Ray (Manta alfredi) in the Red Sea Using Satellite and Acoustic Telemetry. Mar. Biol. 162 (12), 2351–2362. doi: 10.1007/s00227-015-2760-3
Calabrese J. M., Fleming C. H., Gurarie E. (2016). Ctmm: An R Package for Analyzing Animal Relocation Data as a Continuous-Time Stochastic Process. Methods Ecol. Evol. 7 (9), 1124–1132. doi: 10.1111/2041-210X.12559
Campbell H. A., Watts M. E., Dwyer R. G., Franklin C. E. (2012). V-Track: Software for Analysing and Visualising Animal Movement From Acoustic Telemetry Detections. Mar. Freshw. Res. 63 (9), 815–820. doi: 10.1071/MF12194
Chapman D. D., Feldheim K. A., Papastamatiou Y. P., Hueter R. E. (2015). There and Back Again: A Review of Residency and Return Migrations in Sharks, With Implications for Population Structure and Management. Ann. Rev. Mar. Sci. 7 (1), 547–570. doi: 10.1146/annurev-marine-010814-015730
Childs J. N. (2001). The Occurence, Habitat Use, and Behaviour of Sharks and Rays Associating With Topographic Highs in the Northwestern Gulf of Mexico. Master’s Thesis (Texas: Texas A&M University).
Clark T. B. (2010). Abundance, Home Range, and Movement Patterns of Manta Rays (Manta alfredi, M. birostris) in Hawai’i (Honolulu: PhD Dissertation, University of Hawai’i at Manoa).
Couturier L. I. E., Dudgeon C. L., Pollock K. H., Jaine F. R. A., Bennett M. B., Townsend K. A., et al. (2014). Population Dynamics of the Reef Manta Ray Manta alfredi in Eastern Australia. Coral. Reefs. 33 (2), 329–342. doi: 10.1007/s00338-014-1126-5
Couturier L. I. E., Newman P., Jaine F. R. A., Bennett M. B., Venables W. N., Cagua E. F., et al. (2018). Variation in Occupancy and Habitat Use of Mobula alfredi at a Major Aggregation Site. Mar. Ecol. Prog. Ser. 599, 125–145. doi: 10.3354/meps12610
Couturier L. I., Rohner C. A., Richardson A. J., Marshall A. D., Jaine F. R., Bennett M. B., et al. (2013). Stable Isotope and Signature Fatty Acid Analyses Suggest Reef Manta Rays Feed on Demersal Zooplankton. PloS One 8 (10), e77152. doi: 10.1371/journal.pone.0077152
Dewar H., Mous P., Domeier M., Muljadi A., Pet J., Whitty J. (2008). Movements and Site Fidelity of the Giant Manta Ray, Manta birostris, in the Komodo Marine Park, Indonesia. Mar. Biol. 155 (2), 121–133. doi: 10.1007/s00227-008-0988-x
Dharmadi F., Satria F. (2015). Fisheries Management and Conservation of Sharks in Indonesia. Afr. J. Mar. Sci. 37 (2), 249–258. doi: 10.2989/1814232X.2015.1045431
Dujon A. M., Lindstrom R. T., Hays G. C. (2014). The Accuracy of Fastloc-GPS Locations and Implications for Animal Tracking. Methods Ecol. Evol. 5 (11), 1162–1169. doi: 10.1111/2041-210X.12286
Erdmann M. V. (2014) New MMAF-CI-SEAA Manta Tagging Program Launched. Available at: https://birdsheadseascape.com/conservation-science/new-mmaf-ci-seaa-manta-tagging-program-launched/ (Accessed 14 October 2021).
Espinoza M., Heupel M. R., Tobin A. J., Simpfendorfer C. A. (2016). Evidence of Partial Migration in a Large Coastal Predator: Opportunistic Foraging and Reproduction as Key Drivers? PloS One 11 (2), e0147608. doi: 10.1371/journal.pone.0147608
Fleming C., Sheldon D., Fagan W., Leimgruber P., Mueller T., Nandintsetseg D., et al. (2018). Correcting for Missing and Irregular Data in Home-Range Estimation. Ecol. Appl. 28 (4), 1003–1010. doi: 10.1002/eap.1704
Germanov E. S., Bejder L., Chabanne D. B., Dharmadi D., Hendrawan I. G., Marshall A. D., et al. (2019). Contrasting Habitat Use and Population Dynamics of Reef Manta Rays Within the Nusa Penida Marine Protected Area, Indonesia. Front. Mar. Sci. 6. doi: 10.3389/fmars.2019.00215
Grecian W. J., Lane J. V., Michelot T., Wade H. M., Hamer K. C. (2018). Understanding the Ontogeny of Foraging Behaviour: Insights From Combining Marine Predator Bio-Logging With Satellite-Derived Oceanography in Hidden Markov Models. J. R. Soc. Interface 15 (143), 20180084. doi: 10.1098/rsif.2018.0084
Gu Z., Gu L., Eils R., Schlesner M., Brors B. (2014). Circlize Implements and Enhances Circular Visualization in R. Bioinformatics 30 (19), 2811–2812. doi: 10.1093/bioinformatics/btu393
Guttridge T. L., Gruber S. H., DiBattista J. D., Feldheim K. A., Croft D. P., Krause S., et al. (2011). Assortative Interactions and Leadership in a Free-Ranging Population of Juvenile Lemon Shark Negaprion brevirostris. Mar. Ecol. Prog. Ser. 423, 235–245. doi: 10.3354/meps08929
Guttridge T.L., Gruber S.H., Franks B.R., Kessel S.T., Gledhill K.S., Uphill J., et al. (2012). Deep Danger: Intra-Specific Predation Risk Influences Habitat Use and Aggregation Formation of Juvenile Lemon Sharks Negaprion brevirostris. Mar. Ecol. Prog. Ser. 445, 279–291. doi: 10.3354/meps09423
Heupel M. R., Carlson J. K., Simpfendorfer C. A. (2007). Shark Nursery Areas: Concepts, Definition, Characterization and Assumptions. Mar. Ecol. Prog. Ser. 337, 287–297. doi: 10.3354/meps337287
Heupel M. R., Kanno S., Martins A. P., Simpfendorfer C. A. (2019). Advances in Understanding the Roles and Benefits of Nursery Areas for Elasmobranch Populations. Mar. Freshw. Res. 70 (7), 897–907. doi: 10.1071/MF18081
Heupel M. R., Reiss K. L., Yeiser B. G., Simpfendorfer C. A. (2008). Effects of Biofouling on Performance of Moored Data Logging Acoustic Receivers. Limnol. Oceanogr. Methods 6 (7), 327–335. doi: 10.4319/lom.2008.6.327
Huveneers C., Simpfendorfer C. A., Kim S., Semmens J. M., Hobday A. J., Pederson H., et al. (2016). The Influence of Environmental Parameters on the Performance and Detection Range of Acoustic Receivers. Methods Ecol. Evol. 7 (7), 825–835. doi: 10.1111/2041-210X.12520
Jacoby D. M. P., Croft D. P., Sims D. W. (2012). Social Behaviour in Sharks and Rays: Analysis, Patterns and Implications for Conservation. Fish. Fish. 13 (4), 399–417. doi: 10.1111/j.1467-2979.2011.00436.x
Jaine F. R., Couturier L. I., Weeks S. J., Townsend K. A., Bennett M. B., Fiora K., et al. (2012). When Giants Turn Up: Sighting Trends, Environmental Influences and Habitat Use of the Manta Ray Manta alfredi at a Coral Reef. PloS One 7 (10), e46170. doi: 10.1371/journal.pone.0046170
Jaine F., Rohner C., Weeks S., Couturier L., Bennett M., Townsend K., et al. (2014). Movements and Habitat Use of Reef Manta Rays Off Eastern Australia: Offshore Excursions, Deep Diving and Eddy Affinity Revealed by Satellite Telemetry. Mar. Ecol. Prog. Ser. 510, 73–86. doi: 10.3354/meps10910
Jonsen I. D., McMahon C. R., Patterson T. A., Auger-Méthé M., Harcourt R., Hindell M. A., et al. (2019). Movement Responses to Environment: Fast Inference of Variation Among Southern Elephant Seals With a Mixed Effects Model. Ecology 100 (1), e02566. doi: 10.1002/ecy.2566
Jonsen I., Patterson T. (2020). foieGras: Fit latent Variable Movement Models to Animal Tracking Data for Location Quality Control and Behavioural Inference. doi: 10.5281/zenodo.3899972
Kessel S. T., Cooke S. J., Heupel M. R., Hussey N. E., Simpfendorfer C. A., Vagle S., et al. (2014). A Review of Detection Range Testing in Aquatic Passive Acoustic Telemetry Studies. Rev. Fish. Biol. Fish. 24 (1), 199–218. doi: 10.1007/s11160-013-9328-4
Kessel S. T., Elamin N. A., Yurkowski D. J., Chekchak T., Walter R. P., Klaus R., et al. (2017). Conservation of Reef Manta Rays (Manta alfredi) in a UNESCO World Heritage Site: Large-Scale Island Development or Sustainable Tourism? PloS One 12 (10), e0185419. doi: 10.1371/journal.pone.0185419
Kitchen-Wheeler A., Ari C., Edwards A. J. (2011). Population Estimates of Alfred Mantas (Manta alfredi) in Central Maldives Atolls: North Male, Ari and Baa. Environ. Biol. Fish. 93 (4), 557–575. doi: 10.1007/s10641-011-9950-8
Kranstauber B., Smolla M., Scharf A. K. (2021). Move: Visualizing and Analyzing Animal Track Data. Available at: https://CRAN.R-project.org/package=move.
Laidre K. L., Heide-Jørgensen M. P., Logsdon M. L., Hobbs R. C., Dietz R., VanBlaricom G. R. (2004). Fractal Analysis of Narwhal Space Use Patterns. Zoology 107 (1), 3–11. doi: 10.1016/j.zool.2003.09.001
Lassauce H., Chateau O., Erdmann M. V., Wantiez L. (2020). Diving Behavior of the Reef Manta Ray (Mobula alfredi) in New Caledonia: More Frequent and Deeper Night-Time Diving to 672 Meters. PloS One 15 (3), e0228815. doi: 10.1371/journal.pone.0228815
Mangubhai S., Erdmann M. V., Wilson J. R., Huffard C. L., Ballamu F., Hidayat N. I., et al. (2012). Papuan Bird’s Head Seascape: Emerging Threats and Challenges in the Global Center of Marine Biodiversity. Mar. Pollut. Bull. 64 (11), 2279–2295. doi: 10.1016/j.marpolbul.2012.07.024
Marshall A. D., Bennett M. B. (2010). Reproductive Ecology of the Reef Manta Ray Manta alfredi in Southern Mozambique. J. Fish. Biol. 77 (1), 169–190. doi: 10.1111/j.1095-8649.2010.02669.x
Marshall A. D., Pierce S. J. (2012). The Use and Abuse of Photographic Identification in Sharks and Rays. J. Fish. Biol. 80 (5), 1361–1379. doi: 10.1111/j.1095-8649.2012.03244.x
Marshall A. D., Pierce S. J., Bennett M. B. (2008). Morphological Measurements of Manta Rays (Manta birostris) With a Description of a Foetus From the East Coast of Southern Africa. Zootaxa 1717 (1717), 24–30. doi: 10.11646/zootaxa.1717.1.2
McCauley D. J., DeSalles P. A., Young H. S., Papastamatiou Y. P., Caselle J. E., Deakos M. H., et al. (2014). Reliance of Mobile Species on Sensitive Habitats: A Case Study of Manta Rays (Manta alfredi) and Lagoons. Mar. Biol. 161 (9), 1987–1998. doi: 10.1007/s00227-014-2478-7
Murakumo K., Matsumoto R., Tomita T., Matsumoto Y., Ueda K. (2020). The Power of Ultrasound: Observation of Nearly the Entire Gestation and Embryonic Developmental Process of Captive Reef Manta Rays (Mobula alfredi). Fish. Bull. 118 (1), 1–8. doi: 10.7755/fb.118.1.1
Ohlhorst S. L. (1982). Diel Migration Patterns of Demersal Reef Zooplankton. J. Exp. Mar. Biol. Ecol. 60 (1), 1–15. doi: 10.1016/0022-0981(81)90176-3
Pacoureau N., Rigby C. L., Kyne P. M., Sherley R. B., Winker H., Carlson J. K., et al. (2021). Half a Century of Global Decline in Oceanic Sharks and Rays. Nature 589 (7843), 567–571. doi: 10.1038/s41586-020-03173-9
Palacios M. D., Hoyos-Padilla E. M., Trejo-Ramírez A., Croll D. A., Galván-Magaña F., Zilliacus K. M., et al. (2021). Description of First Nursery Area for a Pygmy Devil Ray Species (Mobula munkiana) in the Gulf of California, Mexico. Sci. Rep. 11 (1), 132. doi: 10.1038/s41598-020-80506-8
Papastamatiou Y. P., DeSalles P. A., McCauley D. J. (2012). Area-Restricted Searching by Manta Rays and Their Response to Spatial Scale in Lagoon Habitats. Mar. Ecol. Prog. Ser. 456, 233–244. doi: 10.3354/meps09721
Pate J. H., Marshall A. D. (2020). Urban Manta Rays: Potential Manta Ray Nursery Habitat Along a Highly Developed Florida Coastline. Endanger. Species. Res. 43, 51–64. doi: 10.3354/esr01054
Peel L. R., Stevens G. M. W., Daly R., Daly C. A. K., Lea J. S. E., Clarke C. R., et al. (2019). Movement and Residency Patterns of Reef Manta Rays Mobula alfredi in the Amirante Islands, Seychelles. Mar. Ecol. Prog. Ser. 621, 169–184. doi: 10.3354/meps12995
Peel L. R., Stevens G. M., Daly R., Keating Daly C. A., Collin S. P., Nogués J., et al. (2020). Regional Movements of Reef Manta Rays (Mobula alfredi) in Seychelles Waters. Front. Mar. Sci. 7. doi: 10.3389/fmars.2020.00558
R Core Team (2021). “R: A Language and Environment for Statistical Computing,” (Vienna, Austria: R Foundation for Statistical Computing)Available at: http://www.Rproject.org/.
Rojas Y. E. T., Osuna F. P., Herrera A. H., Magaña F. G., García S. A., Villalobos Ortíz H., et al. (2014). Feeding Grounds of Juvenile Scalloped Hammerhead Sharks (Sphyrna lewini) in the South-Eastern Gulf of California. Hydrobiologia 726 (1), 81–94. doi: 10.1007/s10750-013-1753-9
Setyawan E., Erdmann M., Gunadharma N., Gunawan T., Hasan A., Izuan M., et al. (2022a). A Holistic Approach to Manta Ray Conservation in the Papuan Bird’s Head Seascape: Resounding Success, Ongoing Challenges. Mar. Policy 137, 104953. doi: 10.1016/j.marpol.2021.104953
Setyawan E., Erdmann M. V., Lewis S. A., Mambrasar R., Hasan A. W., Templeton S., et al. (2020). Natural History of Manta Rays in the Bird's Head Seascape, Indonesia, With an Analysis of the Demography and Spatial Ecology of Mobula alfredi (Elasmobranchii: Mobulidae). J. Ocean. Sci. Found. 36, 49–83. doi: 10.5281/zenodo.4396260
Setyawan E., Sianipar A. B., Erdmann M. V., Fischer A. M., Haddy J. A., Beale C. S., et al. (2018). Site Fidelity and Movement Patterns of Reef Manta Rays (Mobula alfredi): Mobulidae Using Passive Acoustic Telemetry in Northern Raja Ampat, Indonesia. Nat. Conserv. Res. 3 (4), 17–31. doi: 10.24189/ncr.2018.043
Setyawan E., Stevenson B. C., Izuan M., Constantine R., Erdmann M. V. (2022b). How Big Is That Manta Ray? A Novel and Non-Invasive Method for Measuring Reef Manta Rays Using Small Drones. Drones 6 (3), 63. doi: 10.3390/drones6030063
Setyawan E., Mambrasar R., Sianipar A.B., Lewis S., Mofu I., Ambafen O, et al (2022c). Data from: Residency and use of an important nursery habitat, Raja Ampat’s Wayag lagoon, by juvenile reef manta rays (Mobula alfredi). Movebank Data Repository. doi: 10.5441/001/1.n95c7182
Simpfendorfer C. A., Milward N. E. (1993). Utilisation of a Tropical Bay as a Nursery Area by Sharks of the Families Carcharhinidae and Sphyrnidae. Environ. Biol. Fish. 37 (4), 337–345. doi: 10.1007/BF00005200
Sims D. W., Witt M. J., Richardson A. J., Southall E. J., Metcalfe J. D. (2006). Encounter Success of Free-Ranging Marine Predator Movements Across a Dynamic Prey Landscape. Proc. R. Soc. B.: Biol. Sci. 273 (1591), 1195–1201. doi: 10.1098/rspb.2005.3444
Stevens G. M. W. (2016). Conservation and Population Ecology of Manta Rays in the Maldives (York: PhD Dissertation, University of York).
Stevens G. M. W., Hawkins J. P., Roberts C. M. (2018). Courtship and Mating Behaviour of Manta Rays Mobula alfredi and M. birostris in the Maldives. J. Fish. Biol. 93 (2), 344–359. doi: 10.1111/jfb.13768
Stewart J. D., Jaine F. R. A., Armstrong A. J., Armstrong A. O., Bennett M. B., Burgess K. B., et al. (2018a). Research Priorities to Support Effective Manta and Devil Ray Conservation. Front. Mar. Sci. 5. doi: 10.3389/fmars.2018.00314
Stewart J. D., Nuttall M., Hickerson E. L., Johnston M. A. (2018b). Important Juvenile Manta Ray Habitat at Flower Garden Banks National Marine Sanctuary in the Northwestern Gulf of Mexico. Mar. Biol. 165 (7), 111. doi: 10.1007/s00227-018-3364-5
Thomson J. A., Börger L., Christianen M. J. A., Esteban N., Laloë J. O., Hays G. C. (2017). Implications of Location Accuracy and Data Volume for Home Range Estimation and Fine-Scale Movement Analysis: Comparing Argos and Fastloc-GPS Tracking Data. Mar. Biol. 164 (10), 204. doi: 10.1007/s00227-017-3225-7
Udyawer V., Dwyer R. G., Hoenner X., Babcock R. C., Brodie S., Campbell H. A., et al. (2018). A Standardised Framework for Analysing Animal Detections From Automated Tracking Arrays. Anim. Biotelemet. 6 (1), 17. doi: 10.1186/s40317-018-0162-2
Venables S. K., Duinkerken D. I., Rohner C., Marshall A. D. (2020). Habitat Use and Movement Patterns of Reef Manta Rays Mobula alfredi in Southern Mozambique. Mar. Ecol. Prog. Ser. 634, 99–114. doi: 10.3354/meps13178
Weeks S. J., Magno-Canto M. M., Jaine F. R. A., Brodie J., Richardson A. J. (2015). Unique Sequence of Events Triggers Manta Ray Feeding Frenzy in the Southern Great Barrier Reef, Australia. Remote Sens. 7 (3), 3138–3152. doi: 10.3390/rs70303138
Wetherbee B. M., Gruber S. H., Rosa R. S (2007). Movement Patterns of Juvenile Lemon Sharks Negaprion brevirostris Within Atol das Rocas, Brazil: A Nursery Characterized by Tidal Extremes Mar. Ecol. Prog. Ser. 343, 283–293. doi: 10.3354/meps06920
Wickham H. (2016). Ggplot2: Elegant Graphics for Data Analysis. Springer-Verlag New York. Available at: https://ggplot2.tidyverse.org.
Keywords: movements, coral reefs, marine megafauna, home range, satellite telemetry, passive acoustic telemetry, photo-identification, spatial ecology
Citation: Setyawan E, Erdmann MV, Mambrasar R, Hasan AW, Sianipar AB, Constantine R, Stevenson BC and Jaine FRA (2022) Residency and Use of an Important Nursery Habitat, Raja Ampat’s Wayag Lagoon, by Juvenile Reef Manta Rays (Mobula alfredi). Front. Mar. Sci. 9:815094. doi: 10.3389/fmars.2022.815094
Received: 15 November 2021; Accepted: 19 April 2022;
Published: 26 May 2022.
Edited by:
Yan Ropert-Coudert, UMR7372 Centre d’études biologiques de Chizé (CEBC), FranceReviewed by:
Luciana C. Ferreira, Australian Institute of Marine Science (AIMS), AustraliaElitza Germanov, Marine Megafauna Foundation, United States
Copyright © 2022 Setyawan, Erdmann, Mambrasar, Hasan, Sianipar, Constantine, Stevenson and Jaine. This is an open-access article distributed under the terms of the Creative Commons Attribution License (CC BY). The use, distribution or reproduction in other forums is permitted, provided the original author(s) and the copyright owner(s) are credited and that the original publication in this journal is cited, in accordance with accepted academic practice. No use, distribution or reproduction is permitted which does not comply with these terms.
*Correspondence: Edy Setyawan, edysetyawan@gmail.com