Foraging Paths of Breeding Leach’s Storm-Petrels in Relation to Offshore Oil Platforms, Breeding Stage, and Year
- 1Cognitive and Behavioral Ecology, Departments of Biology and Psychology, Memorial University of Newfoundland and Labrador, St. John’s, NL, Canada
- 2Wildlife Research Division, Environment and Climate Change Canada, Mount Pearl, NL, Canada
The global population of Leach’s Storm-Petrels (Hydrobates leucorhous), the smallest and most abundant breeding seabird species in eastern Canada, has declined substantially in recent decades. The species is listed as “Threatened” by the Committee On the Status of Endangered Wildlife in Canada (COSEWIC) and as “Vulnerable” by the International Union for Conservation of Nature (IUCN). Fatal attraction to anthropogenic light is a major risk for Leach’s Storm-Petrels and many other nocturnal seabirds. From May to September each year, Leach’s Storm-Petrels in eastern Canada breed in island colonies and travel many hundreds of kilometers to obtain prey for themselves and their chick. At the species’ largest colonies in eastern Newfoundland, brightly illuminated oil production platforms intersect breeding storm-petrels’ foraging paths. The level of risk posed by these platforms is poorly understood. GPS tracking from 2016 to 2021 at one of the world’s largest colonies revealed considerable similarity in foraging trip distance, location, and behavior (inferred from Hidden Markov Models) among years, and a decrease in trip distance and duration between incubation and chick-rearing. Leach’s Storm-Petrels flew within the light catch-basin of an oil platform in 17.5% of trips, and the birds tended to transit rapidly past platforms during the day when light attraction is minimal. Exposure to oil platforms at night occurred in only 1.1% of trips. Despite our findings, Leach’s Storm-Petrels are known to strand on oil platforms in large numbers, especially during the fledging period. In addition, storm-petrels migrate over great distances and are likely exposed to brightly illuminated oceanic oil platforms outside the breeding season. Our results emphasize the need to focus conservation research on risks during migration and winter, and on juveniles and immature birds.
Introduction
Globally, seabirds are declining, with nearly 50% of species classified as Near Threatened or worse, and 50% of the at-risk species having earned this classification due to recent rapid declines (Croxall et al., 2012; Dias et al., 2019). Brightly lit offshore oil production platforms are a known risk to many seabird species (Wiese et al., 2001; Burke et al., 2012; Montevecchi et al., 2012; Ronconi et al., 2015), which may be at risk from attraction (positive phototaxis, see Reed et al., 1985; Miles et al., 2010; Rodríguez et al., 2014) to these structures and the flares they produce. In Atlantic Canada, where large populations of seabirds breed, episodic mortality events are ongoing at oil platforms (Davis et al., 2017; Gjerdrum et al., 2021) and can result from collisions, incineration in flares, oiling, injury by machinery, increased predation due to artificial reef attraction, or disorientation and excessive energy expenditure while circling the platform (Wiese et al., 2001; Montevecchi, 2006; Burke et al., 2012; Ronconi et al., 2015; Dierschke et al., 2016).
Procellariiformes, one of the most threatened groups of seabirds (Croxall et al., 2012), are particularly at risk because they aggregate around sources of anthropogenic light (Le Corre et al., 2002; Miles et al., 2010; Davis et al., 2017; Rodríguez et al., 2017b; COSEWIC, 2020; Gjerdrum et al., 2021). Leach’s Storm-Petrels (Hydrobates leucorhous) are the most commonly observed species in stranding events on oil platforms in Atlantic Canada (Davis et al., 2017; COSEWIC, 2020; Gjerdrum et al., 2021). This species is listed as “Vulnerable” on the International Union for Conservation of Nature (IUCN) Red List and “Threatened” by the Committee On the Status of Endangered Wildlife In Canada (COSEWIC) as a result of a 54% decline in northwestern Atlantic colonies over three generations (approximately 44 years; BirdLife International, 2018; Bird et al., 2020; COSEWIC, 2020). The species’ largest colonies, located in Atlantic Canada, are experiencing the most drastic declines (Montevecchi and McFarlane, 2019; Duda et al., 2020; d’Entremont et al., 2020; Wilhelm et al., 2020), with lower estimated annual survival than conspecifics in the Pacific Ocean (Fife et al., 2015; Rennie et al., 2020). These birds are highly pelagic and spend the non-breeding period on the open ocean (Pollet et al., 2021). During the breeding season, Leach’s Storm-Petrels are central place foragers (Halpin et al., 2018) and often travel more than 2,000 km during a single foraging trip to feed over deep off-shelf waters (Pollet et al., 2014b; Hedd et al., 2018). The core foraging areas of birds from several Atlantic Canadian colonies intersect offshore oil production platforms (Hedd et al., 2018), so it is vital to better understand the risks platforms pose.
While previous studies using geolocation devices have detailed the colony-level intersection of storm-petrel foraging areas with platforms, the coarse nature of these data precluded detailed investigation of exposure risk. Leach’s Storm-Petrels depart and return to the colony almost exclusively at night (Collins, 2021; Pollet et al., 2021), and their primary prey, myctophid fishes, occur in mesopelagic waters beyond the continental shelf and are only available to surface feeding storm-petrels at night owing to their diel vertical migration (Hedd and Montevecchi, 2006; Hedd et al., 2009; Watanuki and Thiebot, 2018; Pollet et al., 2021). Hence, the behavior of these birds, particularly the location and timing of their foraging and nest attendance, appears to be temporally constrained. Bird timing and behavior near platforms is also a determinant of the level of exposure and risk. These factors, as well as other aspects of foraging trip structure (duration, total distance, and location), may also vary within and among years. Annual differences in foraging location or behavior may reflect attraction to or avoidance of lit structures (e.g., Dierschke et al., 2016; Syposz et al., 2021), or could reflect shifts in prey distribution or availability (e.g., Burke and Montevecchi, 2009; Garthe et al., 2011). Within-year differences could result from changing energetic demands through offspring development.
Dietary changes, adult energetic requirements, and the frequency at which they return to the burrow likely influence the duration, distance, and location of foraging trips. Adults usually incubate in 3–4 day shifts but can incubate for up to 6 days (Gross, 1935; Pollet et al., 2021). Once chicks have hatched, adults rarely stay in the burrow during the day, but individual parents return to the burrow nocturnally to feed their chick every one to four nights (Ricklefs et al., 1985), and the chick-feeding interval increases as chicks age (Pollet et al., 2021). During chick-rearing, adult mass is less, and metabolic rate is higher than during incubation (Montevecchi et al., 1992; Niizuma and Watanuki, 1997; Niizuma et al., 2001). Adults also consume more pelagic fishes and crustaceans during chick-rearing (Watanuki, 1985; Vermeer et al., 1988). These dietary and energetic changes may influence trip structure and area use within a breeding season. Previous studies have found consistent foraging area use by incubating Leach’s Storm-Petrels among years (Pollet et al., 2014b; Hedd et al., 2018). Foraging information for Leach’s Storm-Petrels during chick-rearing is limited, but differences in the foraging strategies of other Procellariiformes are informative. European Storm-Petrels (H. pelagicus), Mediterranean Storm-Petrels (H. pelagicus melitensus), and Wandering Albatrosses (Diomedea exulans) reduce the total distance traveled, area covered, and duration of foraging trips during chick-rearing (Weimerskirch et al., 1993; Bolton, 2021; De Pascalis et al., 2021), while Antarctic Prions (Pachyptila desolata) and Blue Petrels (Halobaena caerulea) alternate between long and short trips while raising young (Chaurand and Weimerskirch, 1994; Weimerskirch et al., 1999). Because other Procellariiformes, and storm-petrels in particular, reduce trip metrics during chick-rearing, Leach’s Storm-Petrels might be expected to do the same.
Here, we use a global positioning system (GPS) tracking dataset spanning 2016–2021 from the population of Leach’s Storm-Petrels nesting on Gull Island, Witless Bay, Newfoundland and Labrador, Canada to investigate light exposure from oil production platforms occurring within their foraging range on the Grand Bank. The number and location of oil platforms remained constant throughout the study period. As foraging behavior can vary both within and among years, we investigate temporal differences in foraging trip structure to better understand risk exposure. We predict that (1) foraging adult Leach’s Storm-Petrels will pass near oil platforms on the Grand Bank (as in Hedd et al., 2018), (2) foraging trip structure will remain consistent among years (as in Pollet et al., 2014b; Hedd et al., 2018), and (3a) foraging trip structure will change during the breeding season with storm-petrels making shorter trips during chick-rearing than during incubation (based on observations of other Procellariiformes; Weimerskirch et al., 1993; Bolton, 2021; De Pascalis et al., 2021), (3b) resulting in differences in risk exposure. We also predict that, owing to obligate nocturnality at the colony and on the foraging ground (Collins, 2021; Pollet et al., 2021), (4) storm-petrels will pass by oil platforms mostly during the day.
Materials and Methods
Field Methods
Study Site
We studied the Leach’s Storm-Petrel population on Gull Island (47.26265, -52.77187) in the Witless Bay Ecological Reserve, Newfoundland and Labrador, Canada. The island is approximately 0.74 km2 in area and Leach’s Storm-Petrel burrows cover more than 60% of the island. The most recent population estimate for this colony (2017) is 179 743 pairs, down from a 2001 estimate of 351 866 pairs (Robertson et al., 2006; Wilhelm, 2017). This colony has been monitored for more than 10 years for dietary trends, foraging and migratory behavior, survival, and reproductive success. The island is mostly forested, with open fern areas and grassy slopes around the perimeter.
GPS Deployment and Retrieval
GPS devices (Pathtrack nanoFix GEO-Mini devices, 1.31–2.74% body mass, Figure 1) were deployed on breeding adult Leach’s Storm-Petrels to track and map foraging area use. GPS device deployments (2016–2021, n = 127, Table 1) occurred within an area of approximately 200 m radius within the colony. These archival devices can log a maximum of 160–300 data points (depending on the year of production). The sampling interval for all GPS devices was 2 h (except 3 h in 2019, n = 7). When attempting to determine a location, the GPS devices searched for satellite signals for up to 12 s (10 s in 2016 and 2018).
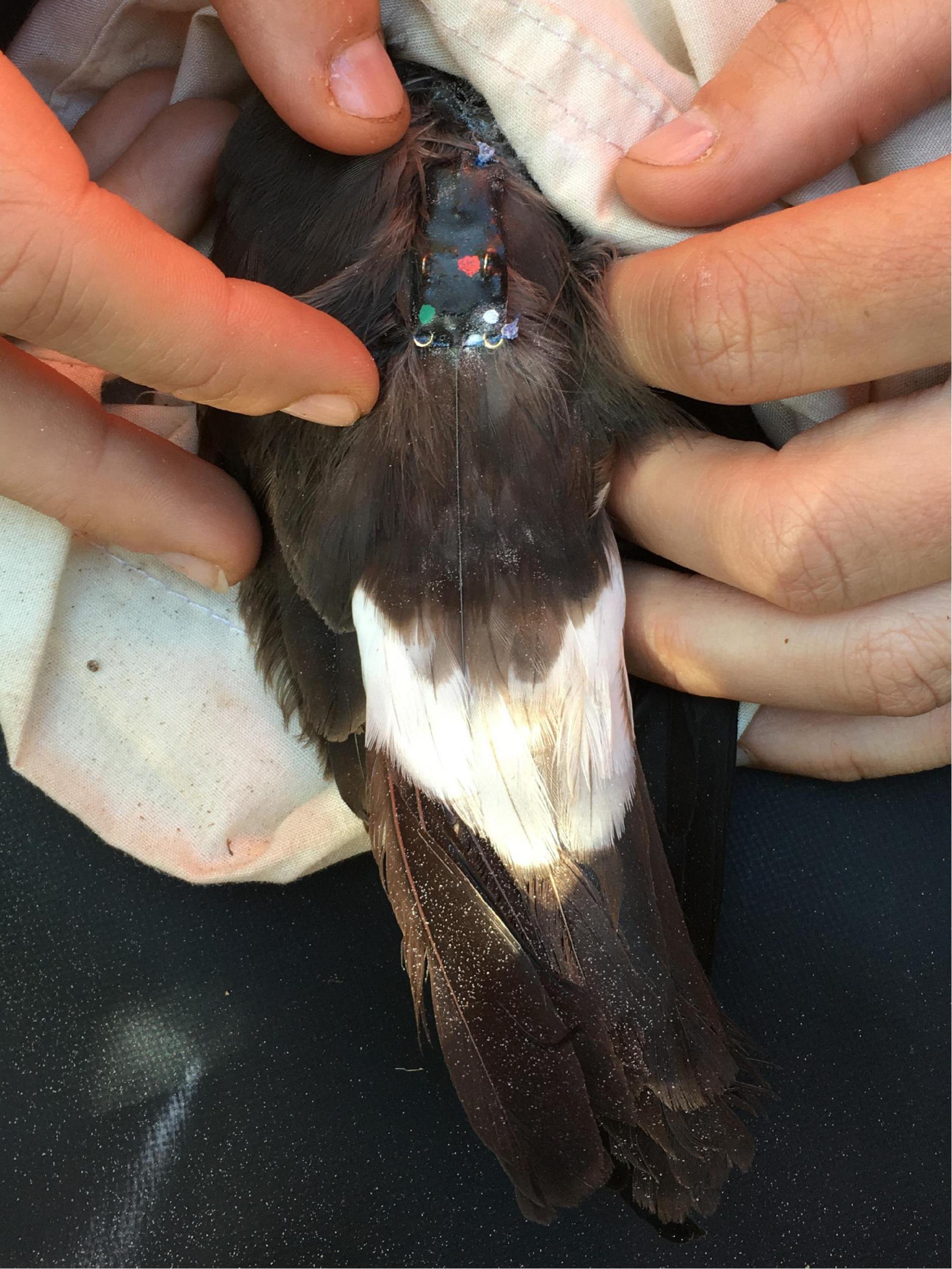
Figure 1. Adult Leach’s Storm-Petrel with a GPS sutured to its back (photo taken by Sydney M. Collins).
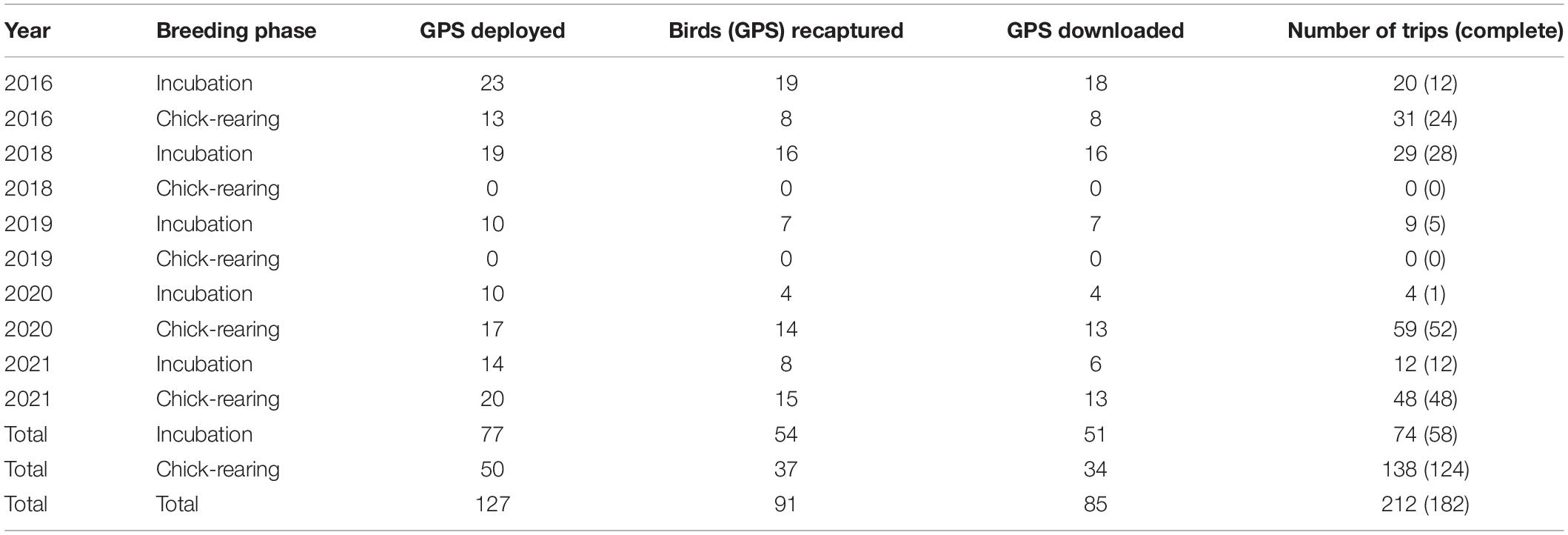
Table 1. Summary of GPS tags deployed, birds (GPS) recaptured, and useable tracks downloaded for each year of the study on Gull Island, Witless Bay, Newfoundland and Labrador, Canada.
Incubating Leach’s Storm-Petrels were captured in burrows by hand during the day. During chick-rearing, when adults only make brief night-time provisioning visits to the burrow, one-way trap doors were inserted into burrow entrances to retain birds until GPS device deployment or retrieval, which occurred either the same night or the following morning. GPS devices were sutured to the back of the birds (as described in Pollet et al., 2014a,b; Figure 1). GPS devices were deployed in late June/early July to record foraging trips during incubation in all study years and different adults were equipped in mid-August to record foraging during chick-rearing in 2016, 2020, and 2021 (Table 1). Two birds were tracked in multiple years. GPS devices were recovered 6–29 days (average 9.4 days during incubation and 15.1 days during chick-rearing) after deployment and recorded one or more foraging trips. For incubation trips, two chicks hatched before GPS device recovery (both during 2021) but were likely < 5 days old as adults were still brooding them. Each tracked adult bird was weighed in a cloth bag (100 g Pesola scale in 2016 and 2018 and Starfrit High Precision Pocket Scale, resolution = 0.1 g in 2019–2021) then returned to the burrow following deployment and recapture of the GPS device. To determine device effects, we conducted a paired t-test for bird mass before deployment and after recapture for all tagged birds. All analyses were performed in R version 4.0.1 (R Core Team, 2020).
Analysis of GPS Data
Trip Delineation
GPS tracks were partitioned into foraging trips (Table 1) using the “track2KBA” package, and the “tripSummary” function in particular (Beal et al., 2020). Trips were delineated based on the proximity of points to the colony, with a “trip” being defined as having a minimum travel distance of 68 km and a minimum duration of 8 h. These values were based on manual examination of a sample of GPS tracks and on return and departure data from Passive Integrated Transponder (PIT) tags (borne by birds that did not have a GPS device) on Gull Island (DF, unpublished data). Trips were classified as complete if the captured GPS points started and ended within approximately 100 km of the colony (based on the observed step lengths in our data, it is possible that a bird could have traveled this far within the 2-h interval). Incomplete trips resulted from our program setup (delayed start times), battery running out, full memory, or damage to the device. We forced each complete trip to start and end at the colony by assigning the start/end times to be 1 h (i.e., half the GPS sampling interval) before/after the first/last recorded trip points. We did not calculate trip distance, duration, or platform proximity for incomplete trips and these trips were not used in the statistical models (see below).
Failed GPS fixes and the variable delay in achieving successful fixes (10–12 s max) result in inconsistent inter-point timing that is unusable for behavioral classification (see below; Patterson et al., 2008). Hence, trip points were interpolated to achieve consistent inter-point intervals of 2 h (3 h in 2019) by mathematically approximating the longitude and latitude of each point at these standardized times, given the assumption of a straight path between each GPS point (as in Raine et al., 2021). These interpolated data were used for all statistics, except for the calculations of total trip distance and trip duration, as the raw data gave more accurate estimations of these values.
Behavioral Classifications
As delineating behavior may inform the level of risk, we assessed bird behavior for each point in a trip and explored differences within and among years. For example, birds spend considerably more time in areas where they are resting or feeding than in areas through which they transit, so their exposure times to any associated risks may vary. The time spent in an area may not be the only factor affecting risk, however. There are numerous ways that oil platforms may pose risk to storm-petrels, and the risk from these factors may differ depending on behavioral state. For example, a transiting bird may be more likely to collide with a platform than a foraging bird, and a foraging or resting bird may be more at risk from increased predation than a transiting bird. Because we do not have a high temporal resolution for our data and it is unknown exactly how and in what proportion different factors may negatively affect birds around oil platforms, we do not attempt to interpret risk from behavioral state beyond exposure time.
Hidden Markov Models (HMMs) are commonly used to categorize points in a GPS track into different states, allowing researchers to infer behavior at sea based on properties of the state (Boyd et al., 2014; Bennison et al., 2018; McClintock and Michelot, 2018). This method first calculates the step length (distance in km) and turning angle between each point. The mean step length and turning angle is then calculated for groups within the data, the number of which is predetermined. Each group represents a distinct behavior known as a “behavioral state.” The chosen number of groups is the number of behaviors that the researchers expect to observe, and can be chosen based on previous studies, and/or by running the model several times with different numbers of groups and comparing the models using AIC.
We constructed all HMMs using the “momentuHMM” package in R (McClintock and Michelot, 2018). This package allows the addition of covariates to account for variations in turning angle within a behavioral state, and for variations in the probability of transitioning between states. We chose to classify the data into three states to align with previous studies (e.g., Boyd et al., 2014; Torres et al., 2017; Bennison et al., 2018), and because a three-state model fit better than a two-state model. We fit models with different combinations of Hour (of day) and Bathymetry (depth of ocean floor) as covariates. Bathymetric data were obtained from the package “marmap” (Pante and Simon-Bouhet, 2013). The HMM with both turning angle and state transition probabilities varying with hour was the best model for the main dataset (Supplementary Figures 1, 2, respectively). For the 2019 dataset, the HMM with hour covarying with transition probabilities was the best model, though it was only marginally better than the same model used for the larger dataset. So, the same model was used for all datasets to ensure consistency. The models showed little deviation from the assumptions involved (no obvious patterns in the pseudoresiduals, no skewness in the normal q-q plot, and no evidence of autocorrelation; Supplementary Figure 3). Three behavioral states were categorized. State 1 is characterized by short step lengths and tighter turning angles (Figure 2). State 2 has intermediate step lengths and turning angles that are wider than state 1 (Figure 2). State 3 is characterized by much longer step lengths and turning angles closer to zero (Figure 2), indicating that the bird was traveling over long distances in a relatively straight path. We therefore consider state 3 as “Transiting.” Because state 1 shows birds spending the most time in a concentrated area, they may be resting or actively feeding, but it is impossible to differentiate between these two behaviors with the 2-h sampling interval. We refer to state 1 as “Intensive Search.” State 2 will be referred to as “Extensive Search,” because birds slow their movement compared to transiting, likely in response to location of a food source or recognition of a feeding area, but cover a wider area compared to intensive search. Because the fit of the model can be improved with covariates and because other studies have deemed the HMM to be one of the best methods for modeling behavioral states in foraging seabirds (Bennison et al., 2018), we use the states produced by the HMM in our analyses.
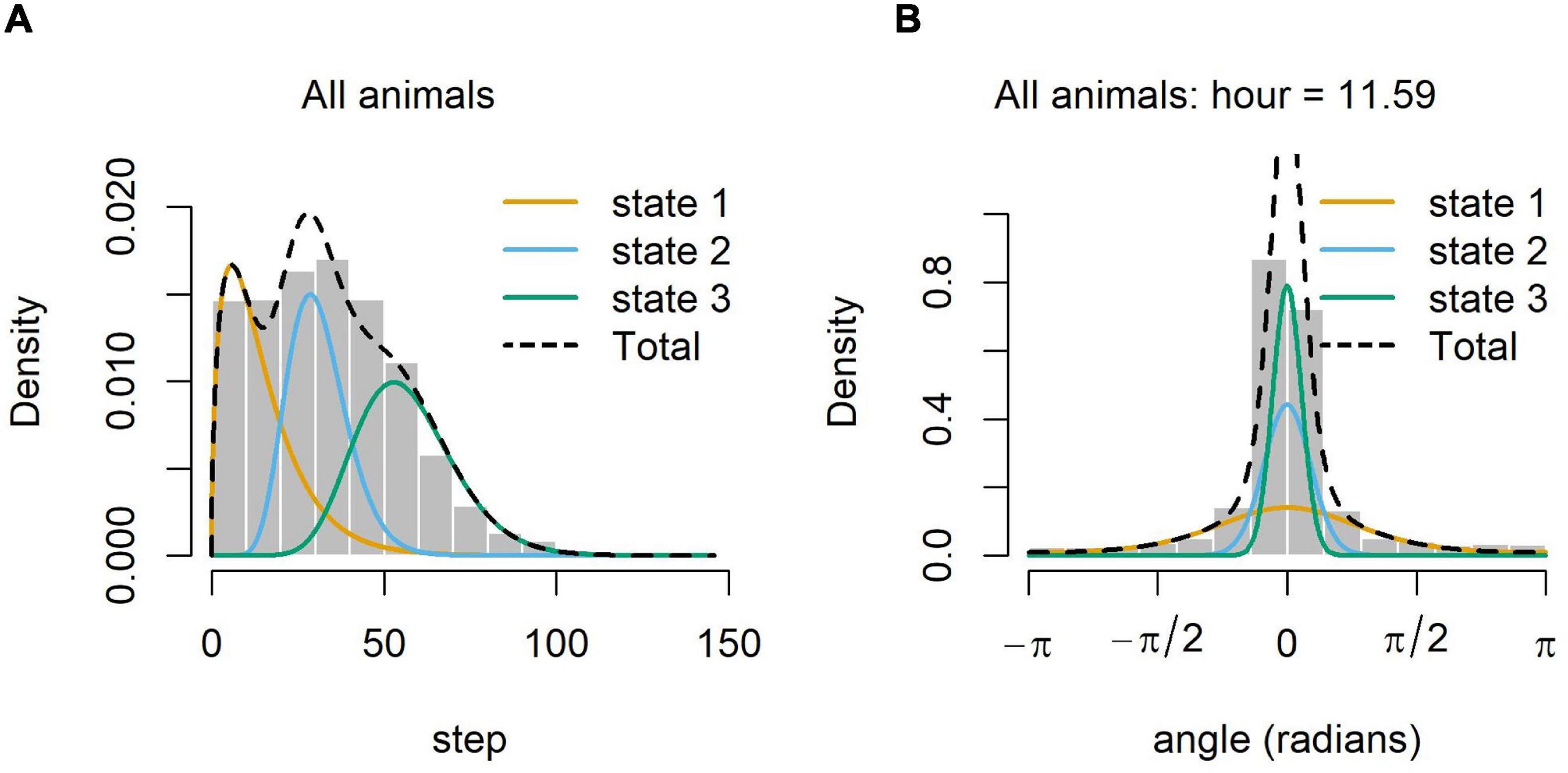
Figure 2. Distributions of step lengths (km) and turning angles (degrees) for each behavioral state. Within each behavioral state, there is a range of step lengths and turning angles that can be associated with each point where the mean is the highest point of the smooth curve in both plots. Gray bars are histograms of the data. Plot (A) shows that the step lengths associated with state 1 are, on average, the shortest and the step lengths associated with state 3 are, on average, the longest. Step lengths associated with state 2 are usually between those of states 1 and 3. In (B), the distributions of turning angles are shown for each state. Although the mean turning angle for all states was around 0, the distribution curve for state 3 is much steeper and tighter around 0 than those of states 1 and 2, which means that the turning angles associated with each state 3 point are most likely to be close to 0. The much flatter curve of state 1 means that sharper turning angles are more likely in this state. Again, the distribution of state 2 is between that of states 1 and 3.
Calculating Distance to Oil Platforms
We calculated the closest distance of each trip (assuming a straight line between each point; hereafter “platform proximity”) to an oil platform, noting both the time and behavioral state at the closest point (determined using the “spDistsN1” function of package “sp”; Pebesma and Bivand, 2005; Bivand et al., 2013). For each trip, sunrise and sunset times were obtained with the package “suncalc” (Thieurmel and Elmarhraoui, 2019), allowing us to determine if birds were closest to the platform at night or during the day. A light catch basin is the area around a lit structure within which light can presumably be seen by an animal and therefore could result in attraction to the structure. We calculated the number of birds that flew within the annual average (average radius = 5.33 km) and maximum (average maximum radius = 10.70 km) light catch basin of the oil platforms on the Grand Bank. These radius values were calculated using the average catch basin areas for platforms on the Grand Bank determined by Gjerdrum et al. (2021), and based on a light radiance cut-off value of 0.75 nW⋅cm−2⋅sr−1, determined from the maximum light radiance in areas distant from any light source and thus considered dark. We chose to use the same radius value for all platforms because the catch basin radius varies between platforms but also for each individual platform based on time, date, and weather factors (Gjerdrum et al., 2021).
To characterize the overlap with and potential risk of oil platform light attraction for breeding Leach’s Storm-Petrels, we calculated the average platform proximity and the proportion of trips in which an individual flew within the light catch-basin. The proportion of trips in which individuals were transiting when closest to an oil platform and the proportion of trips in which individuals were closest to the platform during the night (when the risk of light attraction is assumed to be highest) were also calculated. We estimated the time per trip that individuals spent within the average and maximum light catch basins by calculating the distance traveled within the catch basin and dividing by the average flight speed. Because Leach’s Storm-Petrels travel at different speeds depending on their behavior at sea, we calculated exposure duration with multiple possible flight speeds.
Kernel Home Range Analysis
To determine consistency in area use for Leach’s Storm-Petrels, we used Kernel Home Range Analysis to quantify spatial overlap among behavioral states, individuals, breeding phases, and years. Kernel Home Range analyses (Worton, 1989) were conducted using “adehabitatHR” (Calenge, 2006). We utilized the reference bandwidth smoothing parameter (Worton, 1989), as opposed to the bandwidth that minimizes the least square cross validation score (Worton, 1995; Seaman et al., 1999) as the latter can result in highly fragmented and under-smoothed utilization distributions (UDs) for large datasets with frequent (multiple per day) points (Kie, 2013). The UDs provide a probability estimate of finding a storm-petrel within an area, so we also calculated the minimum utilization distribution that contains all four oil platforms. The 50, 64, and 95% Utilization Distributions (UDs) were constructed for the entire dataset; the 64% UD being the minimum % UD that overlapped will all four oil platforms on the Grand Bank. We used the 50% UDs to estimate consistency in area use, and these were constructed for each year, for the breeding phases (using all incubation and chick-rearing trips), for individuals, and for the behavioral states (Worton, 1989; Fieberg and Kochanny, 2005; Oppel et al., 2018).
Utilization Distributions Overlap
Overlap of 50% UDs were conducted using the “kerneloverlaphr” function of the “adehabitatHR” package (Calenge, 2006). As recommended by Fieberg and Kochanny (2005), we used the Bhattacharyya Affinity Index (BAI) to measure similarity between UDs. The BAI ranges from zero (no similarity) to one (completely similar). We also report the average percent overlap as these values complement the presented maps of the UDs. We first calculated the overlap among behavioral states. Because the behavioral states occurred in distinct regions, we calculated the overlap for individuals, years, and breeding phase separately for transiting and foraging areas. Some individuals were only tracked for a single trip whereas others had up to nine total trips, so for calculations of overlap among individuals, we compared only the UDs of individuals from their first trip to minimize behavioral bias from acclimation to the GPS device. We made annual comparisons of area use separately for each breeding phase, and we compared the breeding phases only in 2016, 2020, and 2021 (no tracking data were collected in 2017, and no chick-rearing data were collected in 2018 or 2019).
Statistical Models
The difference between chick-rearing and incubation in the proportion of time spent in each behavioral state was calculated with a test of equality of proportions. We fit three models to examine the sources of variation in total (1) trip distance, (2) trip duration, and (3) risk exposure (binary) using the package “lme4” (Bates et al., 2015). All regression models were created and assessed following the steps of Zuur and Ieno (2016), and all assumptions were checked according to Zuur et al. (2010). For all models, the trip, not the individual, was the unit of replication.
We looked at the variation of trip distance and trip duration with Year and Breeding Phase and included the individual bird ID as a random intercept (Equations 1 and 2). Although there were low outliers (n = 8) in the data for both models that caused violation of the normality assumption, removal of these points did not change the inference, so the final models included all points. The models of trip distance and trip duration did not violate the other assumptions of Gaussian distributed residuals.
Equation 1. The general linear mixed model relating Total Trip Distance to Year (categorical) and Breeding Phase (categorical), where Total Trip Distanceij is the distance in km for the ith foraging trip of bird j, μij is the mean and σ2 is the variance of Total Trip Distanceij, α is the intercept, βx are the computed regression coefficients, IDj is the random intercept for each bird, εij is the error term, i = 1,…,182, and j = 1,…,70.
Equation 2. The general linear mixed model relating Total Trip Duration to Year (categorical) and Breeding Phase (categorical), where Total Trip Durationij is the time in hours for the ith foraging trip of bird j, μij is the mean and σ2 is the variance of Total Trip Durationij, α is the intercept, βx are the computed regression coefficients, IDj is the random intercept for each bird, εij is the error term, i = 1,…,182, and j = 1,…,70.
We used logistic regression to model variation in risk exposure (binary response: 1—flew within the light catch basin, 0—did not fly within the light catch basin) with Year, Breeding Phase, and Time of Approach (day or night), and included the individual bird ID as a random intercept (Equation 3). The model did not violate the assumptions of logistic regression.
Equation 3. The logistic mixed model relating risk exposure to light catch-basin around an oil platform (binary) to Year (categorical), Breeding Phase (categorical), and Time of Approach (categorical, day or night), where Riskij is the binary qualification of whether bird j flew within the average light catch-basin of an oil platform during the ith foraging trip, πij is the probability of success (bird flies within catch basin), α is the intercept, βx are the computed regression coefficients, IDj is the random intercept for each bird, i = 1,…,182, and j = 1,…,70.
Results
Recapture Rates and Device Effects
Our overall recapture rate was 72%, and data were retrieved from 92% of recaptured devices (Table 1). All recaptured birds had the GPS attached. Devices that did not collect data were likely damaged while being worn by the bird, resulting in water ingress or antenna damage that prevented the device from picking up a satellite signal. Over 5 years, we captured the foraging tracks of 85 individual Leach’s Storm-Petrels from Gull Island, resulting in 212 total trips (182 complete; Table 1). Most complete trips (69%) were during the chick-rearing phase (31% during incubation; Table 1). Bird mass did not change significantly during the deployment period (t = −1.59, df = 86, p = 0.12), indicating that carrying a GPS did not negatively affect the birds’ condition.
Area Use and Utilization Distributions Overlap
Leach’s Storm-Petrels breeding at Gull Island were found across most of the Grand Bank as well as adjacent deeper waters during the study period (Figure 3). Breeding storm-petrels tended to forage over deeper waters off the continental shelf edge (Figure 4). Some individuals also foraged closer to Gull Island, either after returning from a longer trip on which they foraged off-shelf, or on a shorter trip where they foraged exclusively in the area (Figures 4, 5 and Supplementary Figure 4). Individual storm-petrels performed both short and long trips (Figure 5), but there was no obvious alternating pattern (Supplementary Table 1).
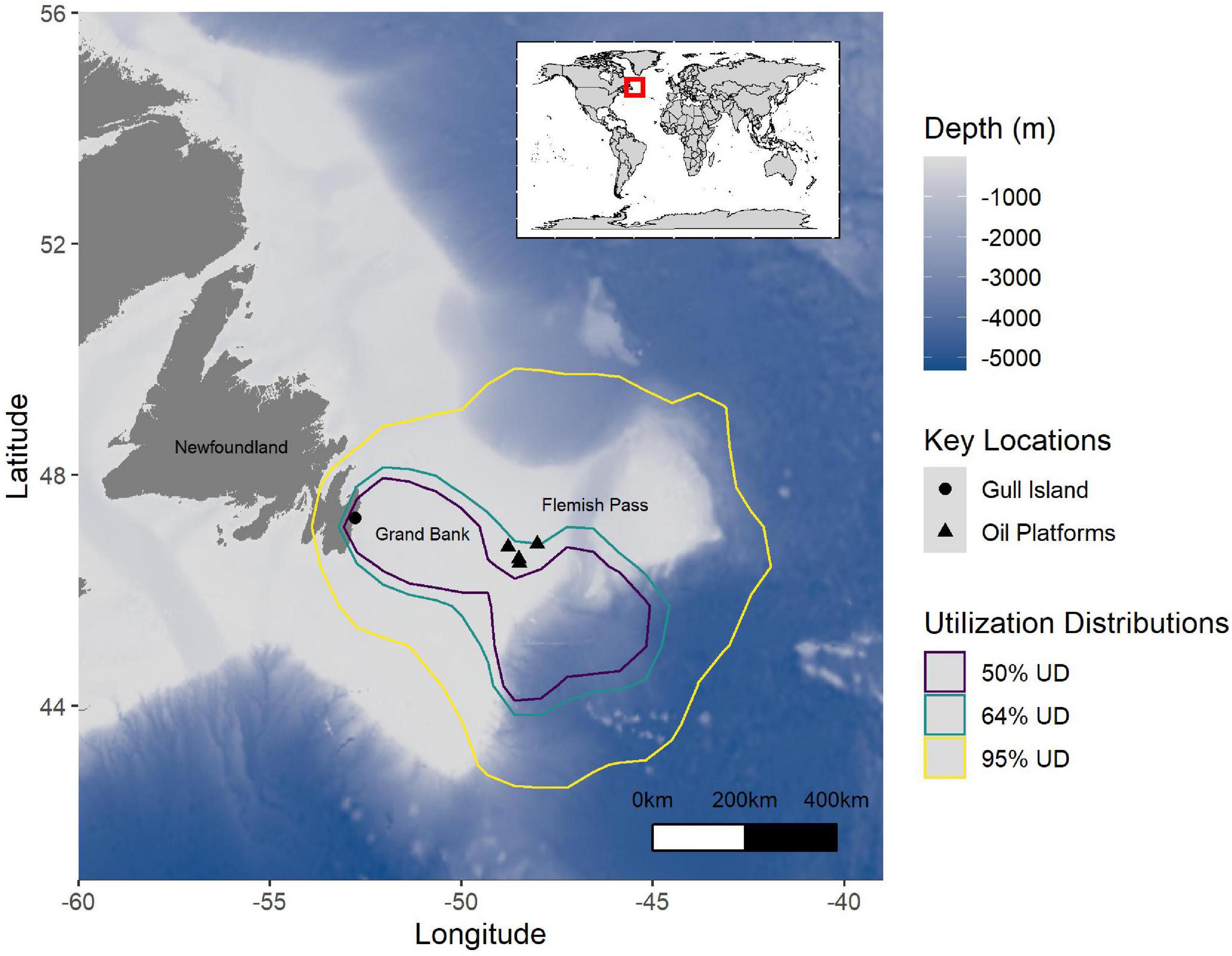
Figure 3. The full Utilization Distributions (50%: purple, 64%: teal, 95%: yellow) for Leach’s Storm-Petrels breeding on Gull Island, Witless Bay, Newfoundland and Labrador, Canada from 2016 to 2021. Note that the 64% Utilization Distribution includes all oil platforms. The large black triangles represent the oil platforms, and the large black circle represents Gull Island. The bathymetry is represented where darker blue indicates deeper water.
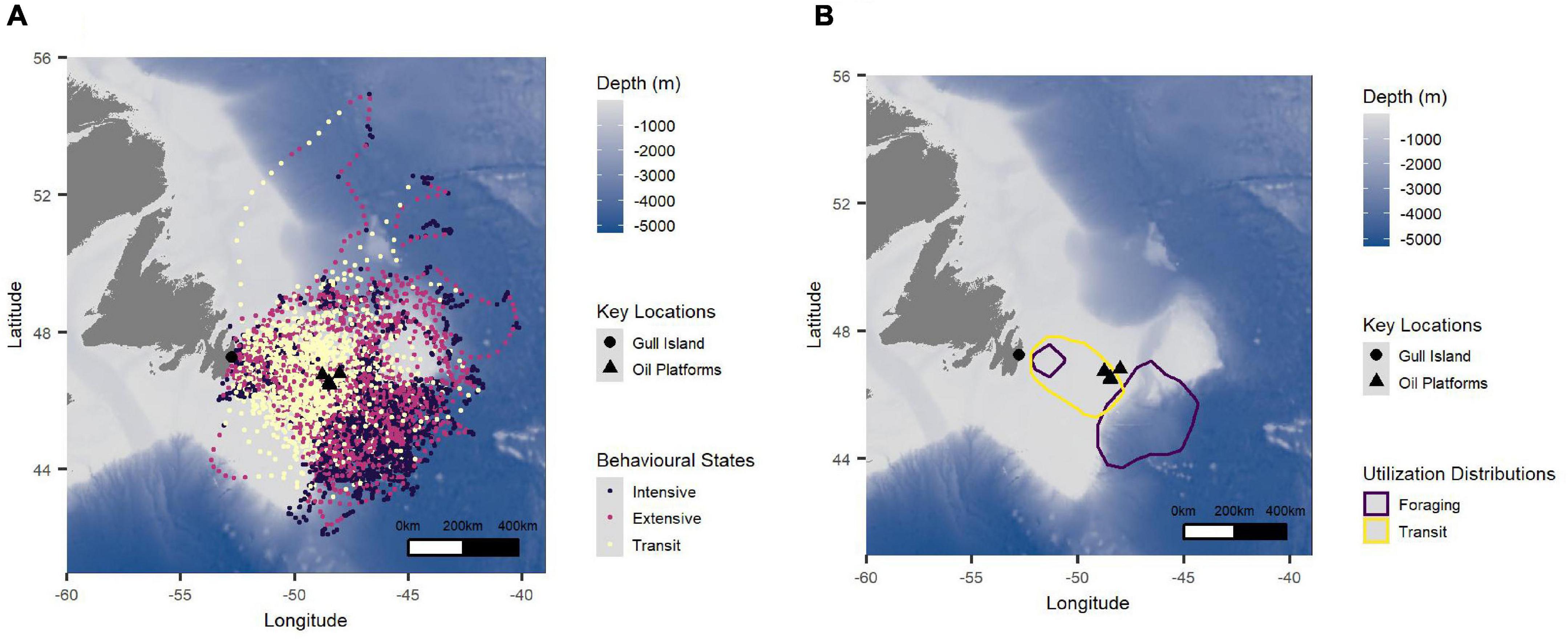
Figure 4. (A) All tracking points colored by behavior (intensive search: black, extensive search: pink, transit: yellow) and (B) 50% utilization distributions of transiting (yellow) and foraging (intensive and extensive states combined; purple) locations for Leach’s Storm-Petrels in the study sample from Gull Island, Witless Bay, Newfoudland and Labrador, Canada from 2016 to 2021. The large black triangles represent the oil platforms, and the large black circle represents Gull Island. The bathymetry is represented where darker blue indicates deeper water. Data from all years and breeding phases are included in these figures.
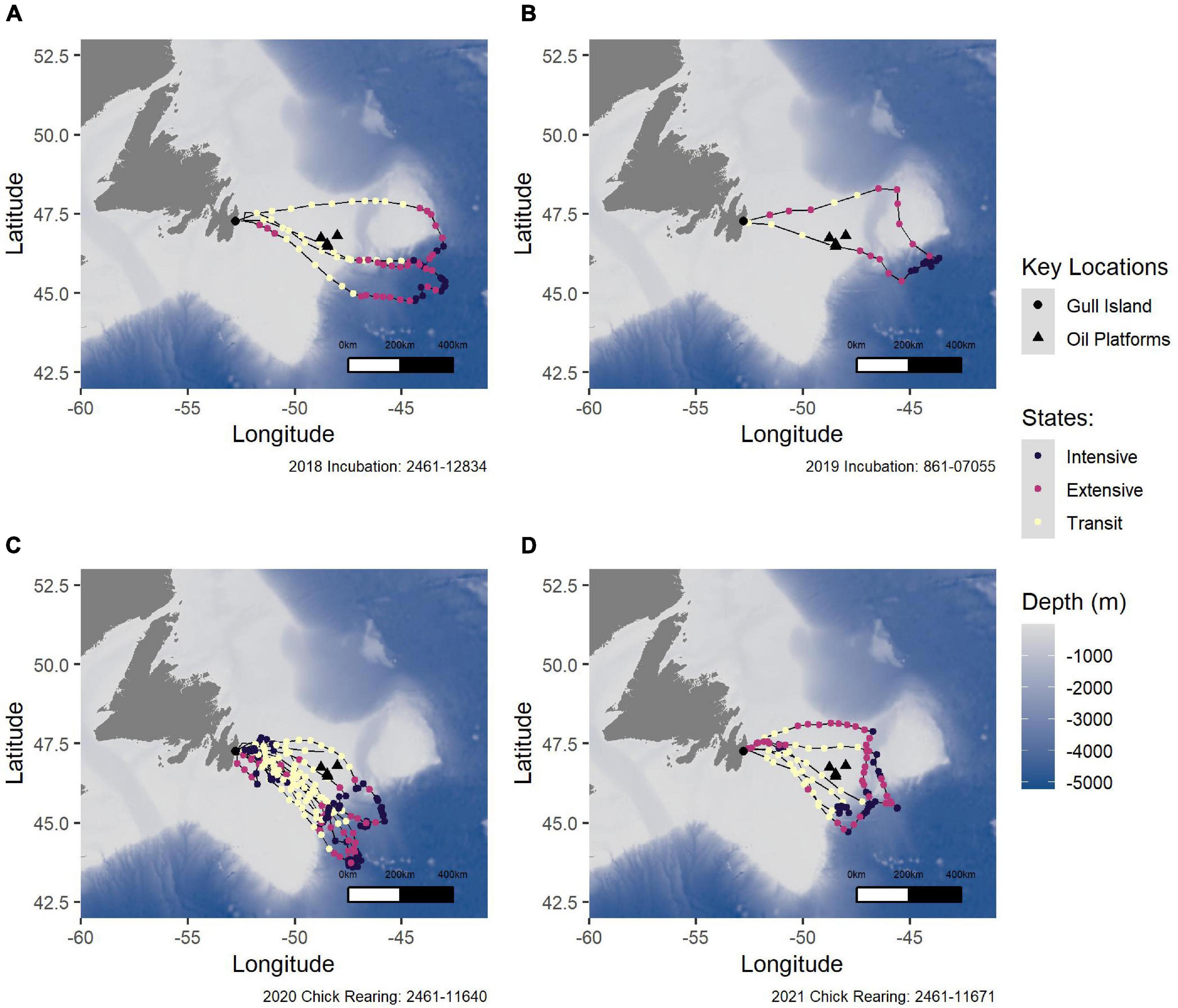
Figure 5. A selection of tracks from individual Leach’s Storm-Petrels in the study sample from incubation in (A) 2018, (B) 2019, and from chick-rearing in (C) 2020, and (D) 2021. Black points represent intensive search, pink points represent extensive search, and yellow points represent transiting. The large black triangles represent the oil platforms, and the large black circle represents Gull Island. The bathymetry is represented where darker blue indicates deeper water. The 8- or 9-digit numbers indicate the ID of each bird.
There was high similarity between the UDs for intensive and extensive search behavioral states (together termed “foraging”), and the UDs for transit and foraging behaviors were more dissimilar (Supplementary Table 2). Because birds did not tend to transit and forage in the same locations, we investigated the overlap of foraging areas and transiting areas separately to better understand consistency in area use. The average similarity between the UD of all individuals (as measured by the average of each calculated BAI or % overlap for every possible pair of individuals) was higher for transiting locations than for foraging locations (Supplementary Table 2). The average similarity in UDs between incubation and chick-rearing was generally high and was higher for transiting areas than for foraging areas (Supplementary Table 2 and Figure 6). Within incubation, foraging areas were more dissimilar than transiting areas among years (Supplementary Table 2 and Figures 6A,C). This difference was less pronounced during chick-rearing (Supplementary Table 2 and Figures 6B,D). Overall, the area used to transit was more consistent (higher overlap among individuals, breeding phases, and years) than the area used to forage (Supplementary Table 2).
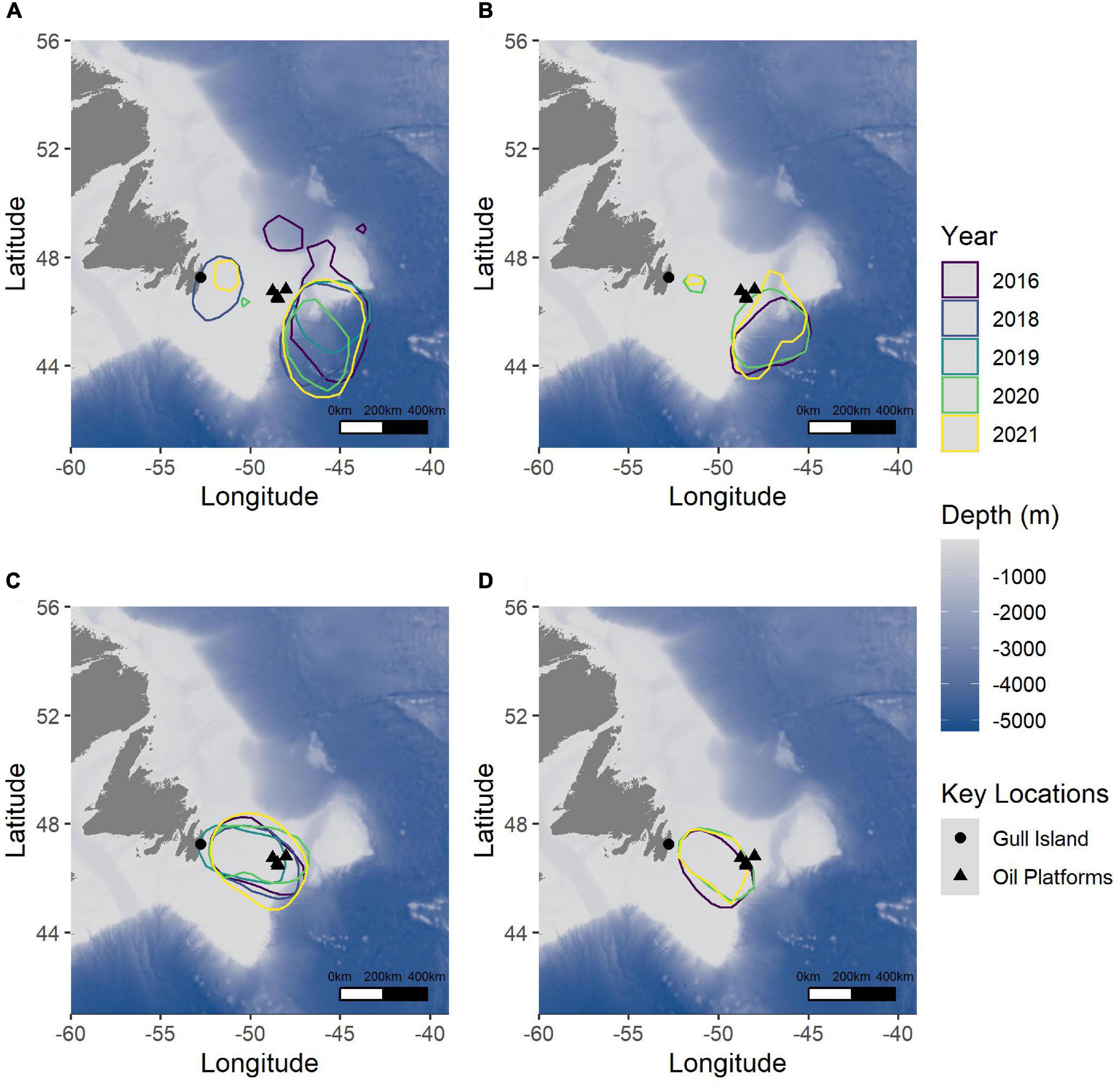
Figure 6. 50% Utilization Distributions of Leach’s Storm-Petrels breeding on Gull Island, Witless Bay, Newfoundland and Labrador, Canada, in 2016–2021 for foraging areas (intensive and extensive) during (A) incubation and (B) chick-rearing, showing reduced foraging areas which are closer to the edge of the Grand Bank during chick-rearing. Transiting areas were not modified between (C) incubation and (D) chick-rearing. The large black triangles represent the oil platforms, and the large black circle represents Gull Island. The bathymetry is represented where darker blue indicates deeper water.
Variation in Trip Structure and Platform Proximity
The proportion of time spent in each behavioral state was similar during incubation and chick-rearing (Intensive: propinc = 0.38, propchick = 0.33, χ2 = 0.047, df = 1, p = 0.83; Extensive: propinc = 0.30, propchick = 0.30, χ2 < 0.001, df = 1, p = 1; Transit: propinc = 0.33, propchick = 0.37, χ2 = 0.045, df = 1, p = 0.83). Total trip distance and trip duration did not vary between years (distance: χ2 = 6.32, df = 4, p = 0.18; duration: χ2 = 3.99, df = 4, p = 0.41), but trips during chick-rearing were significantly shorter in both total distance and duration (distance: mean difference = 86.15 km, χ2 = 4.39, df = 1, p = 0.036; duration: mean difference = 7.78 h, χ2 = 5.20, df = 1, p = 0.023; Table 2 and Supplementary Tables 3, 4).
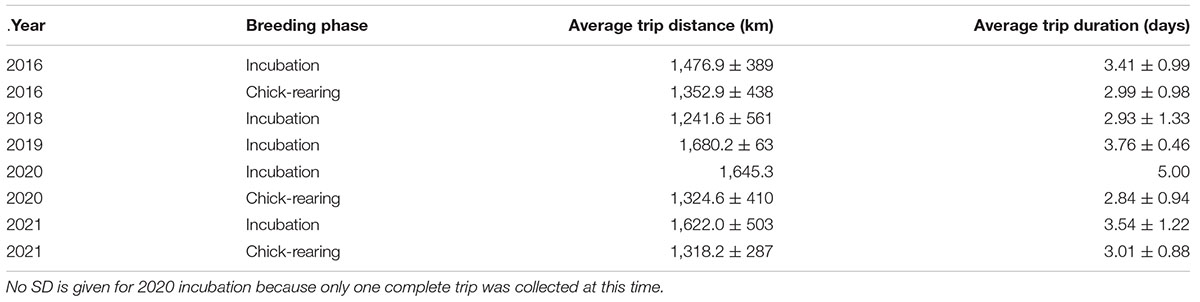
Table 2. Average trip distance ± SD and duration ± SD by year and breeding phase for Leach’s Storm-Petrels breeding at Gull Island, Witless Bay, Newfoundland and Labrador, Canada from 2016–2021.
The average platform proximity was 65 km (range: 0.3–287.5 km; Figure 7), and on 89.0% of all trips, Leach’s Storm-Petrels passed closest by the oil platforms during the day. In 71.4% of all trips, birds were transiting when they flew closest to the oil platforms. Birds flew within the average light catch basin of an oil platform in 17.5% of trips and flew within the maximum average catch basin in 25.8% of trips. In just 1.1% of all trips, birds flew within the average light catch basin at night, while during the remainder of trips (16.4%), they traversed the average light catch basin during the day. Of birds that flew within the average catch basin of the platforms, 71.9% were transiting.
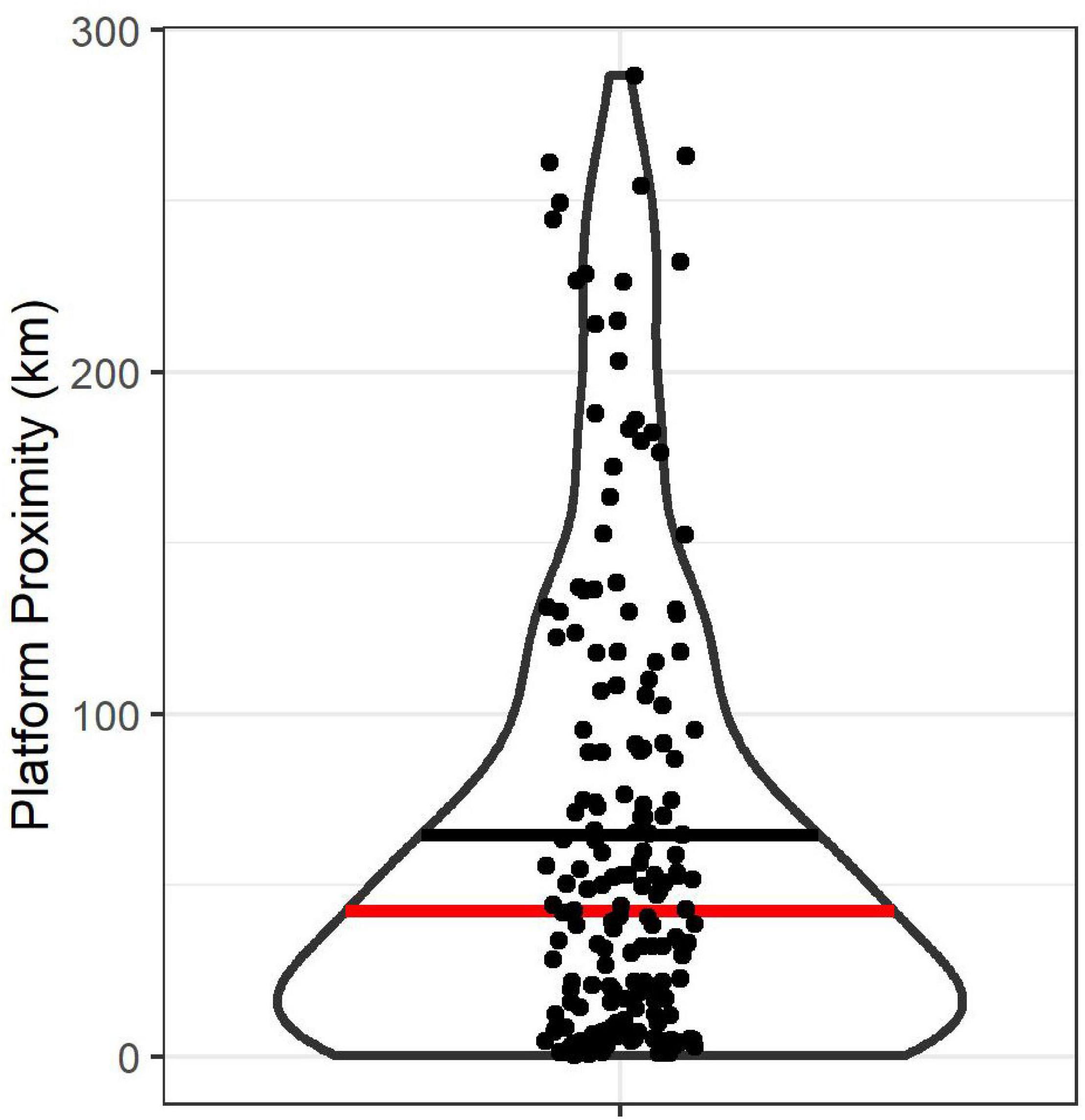
Figure 7. Violin plot of the closest proximity to an oil platform for foraging Leach’s Storm-Petrels breeding at Gull Island, Witless Bay, Newfoundland and Labrador, Canada from 2016 to 2021. The black horizontal line represents the mean proximity (64.7 km) and the red horizontal line is the median (42.7 km).
When considering the average flight speed of transiting storm-petrels (∼30 km/h), birds spent an average of 21 min (60 min in maximum light catch basin) within the light catch basin of an oil platform (Supplementary Table 5). The exposure duration within the average light catch basin ranged from 6 min (minimum for transit flight speed) to 3.4 h (maximum for intensive search flight speed) depending on the flight speed (Supplementary Table 5). The exposure duration within the maximum light catch basin ranged from 2.7 h (minimum for transit flight speed) to 10.6 h (maximum for intensive search flight speed) depending on the flight speed (Supplementary Table 5). 25.0% of the birds that flew within the average light catch basin flew within the light catch basin of more than one oil platform (two maximum). 53.2% of birds exposed to the maximum light catch basin flew within multiple maximum light catch basins (one platform: 46.8%, two platforms: 42.6%, three platforms: 10.6%). The odds of a storm-petrel flying within an average light catch basin did not vary with year (χ2 = 1.46, df = 4, p = 0.83), breeding phase (χ2 = 0.039, df = 1, p = 0.84), or time of day (whether the bird flew within the catch basin during the day or at night; χ2 = 1.35, df = 1, p = 0.25; Supplementary Table 6).
Discussion
The objective of this study was to examine the exposure of Leach’s Storm-Petrels to brightly lit offshore oil platforms while foraging during the breeding season. To better understand exposure and risk, we examined variation in foraging trip structure, behavior near platforms, and the timing and duration of platform proximity within and between years. Likely owing to their dual temporal constraints involved with nocturnal colony visitation and nocturnal foraging off the shelf-edge, Leach’s Storm-Petrels flew past oil platforms during the day in nearly 90% of trips. Leach’s Storm-Petrels were no more likely to fly within a light catch basin if they passed closest by the platform during the day or at night (Supplementary Table 6). While attraction to artificial light during the day has been demonstrated for some animals (Baik et al., 2020), there is no evidence that storm-petrels are attracted to light during daylight. Still, studies of light attraction in different environmental lighting conditions should be conducted to better understand the risk to seabirds. This is particularly important on the Grand Bank where foggy conditions are common (Isaac et al., 2020). Light may disperse differently in fog, influencing the size of the light catch basin. Additionally, Leach’s Storm-Petrels may be attracted to oil platforms in other ways. For example, the artificial reef effect could be at play, and storm-petrels may navigate toward a potentially increased food source via olfaction or social cues (Nevitt and Haberman, 2003; Dierschke et al., 2016; COSEWIC, 2020). These mechanisms require further study to understand the extent and radius of attraction and risk.
At the population level, Leach’s Storm-Petrels exhibited similar at-sea area use among years and between incubation and chick-rearing, although incubating birds tended to forage farther off the continental shelf than chick-rearing birds (Figure 6). The areas used for transiting were more consistent than those used for foraging (Supplementary Table 2 and Figure 6). This consistency may result from the fact that all tracked birds were from the same colony, as Leach’s Storm-Petrels in Atlantic Canada tend to show colony-level consistency in area use but differences in area use among colonies (Hedd et al., 2018). Birds tended to transit over the Grand Bank, and intensively and extensively searched beyond the edge of the shelf, mainly within the Flemish Pass and east of the Grand Bank, though at times near the colony (Figures 4, 6). There was considerable overlap in the areas in which birds intensively and extensively searched, but lower overlap between foraging areas and transit areas (Supplementary Table 2 and Figure 4B). This suggests that, at the population level, storm-petrels breeding on Gull Island likely do not forage to any great degree over the Grand Bank. The utilization distributions observed in this study are consistent geographically with previous tracking of Leach’s Storm-Petrels from this colony (Hedd et al., 2018). At-sea surveys in the region conducted from 1966–1990 and 1998–1999 also indicate high use of shallow water habitats (near colonies) and deeper waters off the shelf (Hedd et al., 2009). Myctophids, mesopelagic fish that migrate to the surface at night, dominate the diets of Leach’s Storm-Petrels in the region, suggesting why foraging effort is concentrated over deep waters off the continental shelf edge (Hedd and Montevecchi, 2006; Hedd et al., 2009).
At the individual level, there was generally low overlap in area use, and foraging areas tended to be less consistent than transiting areas (Supplementary Table 2). These findings concur with Oppel et al. (2018) who found low individual overlap for several wide-ranging seabird species, including storm-petrels. This result emphasizes that, at the population level, area use tends to be consistent among years and between breeding stages, but individual birds tend to use different foraging areas. This could mean that individuals may experience differing levels of risk, so further research into individual consistency in foraging area use, at-sea behavior, and risk exposure is needed.
Neither trip distance nor trip duration varied significantly among years (Supplementary Tables 3, 4). Within years, however, trips during chick-rearing tended to have shorter duration and total distance (Supplementary Tables 3, 4). This is consistent with our prediction and aligns with findings for other Procellariiformes (Weimerskirch et al., 1993; Hedd et al., 2014; Bolton, 2021; De Pascalis et al., 2021). The most likely explanation for this pattern is that breeding birds are reducing their foraging trips in response to the high energetic demands and need for frequent provisioning of the chick (Ricklefs et al., 1980). Storm-petrels took both short and long trips, but the occurrence of short trips cannot explain the shorter overall trip distance and duration we observed. Short and long trips occurred in no obvious pattern in our sample, and short trips were equally likely in incubation and chick-rearing (Supplementary Table 1). Short trips during chick-rearing likely function primarily to provision chicks (Chaurand and Weimerskirch, 1994), while short trips during incubation likely reflect mates not switching duties when the foraging partner returned to the nest (as in Shy Albatrosses Thalassarche cauta, Hedd and Gales, 2005). This result also cannot be explained by changes in behavioral patterns, as incubating and chick-rearing adults spent proportionally similar time foraging vs. transiting on their trips. Overall, the birds spent about a third of their time in each behavioral state, regardless of breeding stage. Because Leach’s Storm-Petrels are central place foragers, they must optimize energy expenditure when foraging (Burke and Montevecchi, 2009; Elliott et al., 2009). They must spend enough time foraging to make a long trip worthwhile. Digestion, which likely occurs when storm-petrels are in behavioral state 1 (intensive search) and is mutually exclusive from active foraging (Rosen et al., 2007), is also an important and time-consuming component of the foraging trip. Leach’s Storm-Petrels reduce food volume by concentrating ingested prey as high-energy oils which are used by the adult or fed to chicks (Place et al., 1989). Rather than changing the proportion of time spent on specific behaviors, Leach’s Storm-Petrels appear to compensate for increased energetic demands during chick-rearing in different ways. For example, body mass loss during early chick-rearing increases flight efficiency by 14% (Niizuma et al., 2001). While previous research has identified population-level consistency in the foraging locations of Leach’s Storm-Petrels across years and throughout incubation (Hedd et al., 2009, 2018; Pollet et al., 2014b), this is, to our knowledge, the first study to compare foraging locations and behavior between incubation and chick-rearing.
The home range of Leach’s Storm-Petrels overlapped with oil and gas production platforms on the Grand Bank (Figure 3; Hedd et al., 2018). They flew within 65 km of an oil platform on average throughout the breeding period. Overall, storm-petrels flew within the average light catch basin of oil platforms on almost 20% of foraging trips and within the maximum light catch basin on more than 25% of trips. The risk of flying within the light catch basin of an oil platform did not vary among years or between incubation and chick-rearing (Supplementary Table 6). Of birds that flew within the average light catch basin, most (72%) were transiting. When near a platform, storm-petrels are at risk of collision, oiling, incineration in the flare, predation, and exhaustion from flying around the illuminated structure (Wiese et al., 2001; Montevecchi, 2006; Burke et al., 2012; Ronconi et al., 2015; Dierschke et al., 2016). Prolonged exposure likely increases the likelihood of risk. Transiting Leach’s Storm-Petrels spent an average of about 20 min within light catch basins of oil platforms on the Grand Bank, but exposure durations ranged from just a few minutes to more than 3 h, depending on a bird’s behavior when near the platform. A quarter of the birds that flew within a light catch basin were exposed to multiple oil platforms on their foraging trips. Our analyses indicate that the duration of exposure to oil platforms may be minimal given that the birds are usually rapidly transiting to or from the foraging area when they pass.
Our calculations were based on the assumption that storm-petrels fly in a straight line between locations, though they may have flown closer or farther away from the platforms than we calculated. We used 5.33 km for the average light catch basin and 10.70 km for the maximum light catch basin, but the catch basin of gas flares can be much greater than the values found by Gjerdrum et al. (2021). We also used the average annual value, though the size of the catch basin can vary within the year, with flare heights that are higher when oil cannot be offloaded in poor weather, and with other weather conditions (e.g., fog; Gjerdrum et al., 2021). Importantly, we can only report results for the birds that we recaptured. The fate of birds that we did not recapture (about 30%, Table 1) is unknown. We could simply have missed their return, they may have abandoned their nest, or they may have died. Our recapture rates were similar to or better than those of other tracking studies of this species (Pollet et al., 2014b; Hedd et al., 2018; Mohammad Fahmy, unpublished data). Given that strandings of adults on oil platforms during the breeding season are not uncommon (Gjerdrum et al., 2021), it is possible that some of these unrecovered birds died from interactions with oil platforms. Hence, it is possible that the percentage of trips in which storm-petrels from Gull Island fly within the light catch basin of oil platforms at night is higher than 1.1% and that the assumed risk is higher than reported here.
Considerable effort is being invested into risk assessment for Leach’s Storm-Petrels across Atlantic Canada with a focus on breeding adults (e.g., Hedd et al., 2018; d’Entremont et al., 2020; Wilhelm et al., 2020; Collins, 2021; COSEWIC, 2020; Hoeg et al., 2021). Studies indicate that Leach’s Storm-Petrels in the region strand on oil platforms, vessels, and at brightly illuminated coastal sites during the breeding season, but the greatest number of stranded birds are found during the fall when fledglings are departing colonies for the first time (Davis et al., 2017; Gjerdrum et al., 2021; Wilhelm et al., 2021; WM, unpublished data). These circumstances raise concern about risk posed by light attraction for adults and other age-classes outside the breeding period. Light pollution poses a major risk to seabirds globally, with tube-nosed seabirds being especially vulnerable (Reed et al., 1985; Wiese et al., 2001; Montevecchi, 2006; Poot et al., 2008; Burke et al., 2012; Day et al., 2015; Rodríguez et al., 2017a; Rebke et al., 2019). Based on the expansive area covered by Leach’s Storm-Petrels during the non-breeding period (Pollet et al., 2014a,2019), individuals from this and other populations will be exposed to oceanic sources of anthropogenic light such as boats, oil platforms, and drill rigs. Very little is known about juvenile survival, but the majority of seabirds that have been recovered from light-polluted areas are juveniles (Rodríguez et al., 2017b; Atchoi et al., 2020; Wilhelm et al., 2021). The visual system of Leach’s Storm-Petrel chicks develops very slowly, and their eyes may not be fully developed until after fledging (Mitkus et al., 2018). Atchoi et al. (2020) proposed a connection between the slow development of the visual system and the increased level of juvenile attraction to light. Adults and juveniles could, therefore, differ in their level of vulnerability to light attraction, owing to differences in eye structure (Mitkus et al., 2018).
Mitigation efforts have been investigated regarding light sources. Different wavelengths of light appear to differ in their level of attractiveness (Montevecchi, 2006). There is, however, disagreement over the least attractive wavelengths to birds. Some studies have found that white and red light are the most attractive (Poot et al., 2008), whereas others have found that white, green, and blue light attract more migrating birds (Rebke et al., 2019; Syposz et al., 2021). The rapidly increasing use of bright LEDs, which are a major source of short blue wavelength light, is a problematic development (Rodríguez et al., 2017a; Hung et al., 2021). Mitigation strategies such as shielding light from unnecessary skyward projection (Reed et al., 1985), or changing the type of light source (i.e., LED vs. high-pressure sodium; Rodríguez et al., 2017a), have a range of efficacy that depends upon the species and, likely, the weather (Wilhelm et al., 2013).
Continued research on the risks of light attraction to Leach’s Storm-Petrels is needed and should be broadened to include vessel and coastal lighting, to assess the risks at different times of the year (e.g., Wilhelm et al., 2021), and to assess the risks for individuals of different age groups. Further research on the visual system of Leach’s Storm-Petrels will inform best practices moving forward. Researchers and governments should work with corporations that produce oceanic light pollution to develop and implement mitigation strategies to reduce the attraction of marine birds.
Data Availability Statement
The raw data supporting the conclusions of this article will be made available by the authors, without undue reservation.
Ethics Statement
The animal study was reviewed and approved by the Memorial University of Newfoundland Animal Care Committee and by Environment and Climate Change Canada’s Eastern Wildlife Animal Care Committee.
Author Contributions
This research was produced as a part of the Master of Science thesis of SC. SC: conceptualization, methodology, investigation, formal analysis, and writing (both original draft and review and editing). AH: conceptualization, methodology, investigation, formal analysis, and writing (reviews and editing). DF: methodology, investigation, formal analysis, and writing (review and editing). DW: writing (review and editing). WM: conceptualization, methodology, investigation, writing (both original draft and review and editing), and supervision. All authors contributed to the article and approved the submitted version.
Funding
The funding for this work was provided by the Natural Sciences and Engineering Research Council (NSERC) in a Discovery Grant to WM (Grant No: RGPIN/005433-2017) and an Alexander Graham Bell Canada Graduate Scholarship-Master’s (CGS-M) to SC (Grant No: PGSD3-547858-2020). Further funding was provided by Memorial University of Newfoundland and Labrador and Environment and Climate Change Canada.
Conflict of Interest
The authors declare that the research was conducted in the absence of any commercial or financial relationships that could be construed as a potential conflict of interest.
Publisher’s Note
All claims expressed in this article are solely those of the authors and do not necessarily represent those of their affiliated organizations, or those of the publisher, the editors and the reviewers. Any product that may be evaluated in this article, or claim that may be made by its manufacturer, is not guaranteed or endorsed by the publisher.
Acknowledgments
We thank S. Abbott, M. Norman, and M. Grace, SMC’s undergraduate assistants, whose hard work and dedication facilitated this research. We also thank the following for assistance in the field and for their indispensable contribution to this research, D. Wadley, L. Lake, R. Blackmore, K. Fisher, K. d’Entremont, C. Gjerdrum, M. Fitzsimmons, C. Richards, B. Harkness, C. Burke, M. Montevecchi, and M. Valliant. We thank I. Pratte for help with statistics and R code. We extend a special thanks to R. Ronconi for his contribution in conceptualization of the project. We thank A. Ausems and I. Pollet, whose comments during review greatly improved this manuscript.
Supplementary Material
The Supplementary Material for this article can be found online at: https://www.frontiersin.org/articles/10.3389/fmars.2022.816659/full#supplementary-material
References
Atchoi, E., Mitkus, M., and Rodríguez, A. (2020). Is seabird light-induced mortality explained by the visual system development? Conserv. Sci. Pract. 2:e195. doi: 10.1111/csp2.195
Baik, L. S., Nave, C., Au, D. D., Guda, T., Chevez, J. A., Ray, A., et al. (2020). Circadian regulation of light-evoked attraction and avoidance behaviors in daytime- versus nighttime-biting mosquitoes. Curr. Biol. 30, 3252–3259. doi: 10.1016/j.cub.2020.06.010
Bates, D., Mächler, M., Bolker, B., and Walker, S. (2015). Fitting linear mixed-effects models using lme4. J. Stat. Softw. 67, 1–48. doi: 10.18637/jss.v067.i01
Beal, M., Oppel, S., Handley, J., Pearmain, L., Morera-Pujol, V., Miller, M., et al. (2020). BirdLifeInternational/track2kba: First Release (Version 0.5.0). doi: 10.5281/ZENODO.3823902
Bennison, A., Bearhop, S., Bodey, T. W., Votier, S. C., Grecian, W. J., Wakefield, E. D., et al. (2018). Search and foraging behaviors from movement data: a comparison of methods. Ecol. Evol. 8, 13–24. doi: 10.1002/ece3.3593
Bird, J. P., Martin, R., Akçakaya, H. R., Gilroy, J., Burfield, I. J., Garnett, S. T., et al. (2020). Generation lengths of the world’s birds and their implications for extinction risk. Conserv. Biol. 34, 1252–1261. doi: 10.1111/cobi.13486
BirdLife International (2018). Hydrobates Leucorhous, Leach’s Storm-petrel. IUCN Red List Threat. Species 2018, e.T132438298A132438484. Cambridge: BirdLife International.
Bivand, R. S., Pebesma, E. J., and Gomez-Rubio, V. (2013). Applied Spatial Data Analysis With R, 2nd Edn. New York, NY: Springer.
Bolton, M. (2021). GPS tracking reveals highly consistent use of restricted foraging areas by European Storm-petrels Hydrobates pelagicus breeding at the largest UK colony: implications for conservation management. Bird Conserv. Int. 31, 35–52. doi: 10.1017/S0959270920000374
Boyd, C., Punt, A. E., Weimerskirch, H., and Bertrand, S. (2014). Movement models provide insights into variation in the foraging effort of central place foragers. Ecol. Modell. 286, 13–25. doi: 10.1016/j.ecolmodel.2014.03.015
Burke, C. M., and Montevecchi, W. A. (2009). The foraging decisions of a central place foraging seabird in response to fluctuations in local prey conditions. J. Zool. 278, 354–361. doi: 10.1111/j.1469-7998.2009.00584.x
Burke, C. M., Montevecchi, W. A., and Wiese, F. K. (2012). Inadequate environmental monitoring around offshore oil and gas platforms on the Grand Bank of Eastern Canada: are risks to marine birds known? J. Environ. Manage. 104, 121–126. doi: 10.1016/j.jenvman.2012.02.012
Calenge, C. (2006). The package adehabitat for the R software: tool for the analysis of space and habitat use by animals. Ecol. Modell. 197, 516–519. doi: 10.1016/J.ECOLMODEL.2006.03.017
Chaurand, T., and Weimerskirch, H. (1994). The regular alternation of short and long foraging trips in the Blue Petrel Halobaena caerulea: a previously undescribed strategy of food provisioning in a Pelagic seabird. J. Anim. Ecol. 63:275. doi: 10.2307/5546
Collins, S. M. (2021). Terrestrial and Marine Risks for Leach’s Storm-Petrels During the Breeding Season master’s thesis. St. John’s, NL: Memorial University of Newfoundland.
COSEWIC (2020). COSEWIC Assessment and Status Report 2020 on the Leach’s Storm-Petrel (Atlantic population) Oceanodroma Leucorhoa in Canada. Ottawa, ON: COSEWIC.
Croxall, J. P., Butchart, S. H. M., Lascelles, B., Stattersfield, A. J., Sullivan, B., Symes, A., et al. (2012). Seabird conservation status, threats and priority actions: a global assessment. Bird Conserv. Int. 22, 1–34. doi: 10.1017/S0959270912000020
Davis, R. A., Lang, A. L., and Mactavish, B. (2017). Study of Seabird Attraction to the Hebron Production Platform: A proposed study approach. Rep. No. SA1190. St. John’s, NL: Prepared by LGL Limited.
Day, R. H., Rose, J. R., Prichard, A. K., and Streever, B. (2015). Effects of gas flaring on the behavior of night-migrating birds at an artificial oil-production Island. Arctic Alaska. Arctic 68, 367–379. doi: 10.14430/arctic4507
De Pascalis, F., Pala, D., Pisu, D., Morinay, J., Benvenuti, A., Spano, C., et al. (2021). Searching on the edge: dynamic oceanographic features increase foraging opportunities in a small pelagic seabird. Mar. Ecol. Prog. Ser. 668, 121–132. doi: 10.3354/meps13726
d’Entremont, K. J. N., Minich Zitske, L., Gladwell, A. J., Elliott, N. K., Mauck, R. A., and Ronconi, R. A. (2020). Breeding population decline and associations with nest site use of Leach’s Storm-Petrels on Kent Island, New Brunswick from 2001 to 2018. Avian Conserv. Ecol. 15:11. doi: 10.5751/ace-01526-150111
Dias, M. P., Martin, R., Pearmain, E. J., Burfield, I. J., Small, C., Phillips, R. A., et al. (2019). Threats to seabirds: a global assessment. Biol. Conserv. 237, 525–537. doi: 10.1016/j.biocon.2019.06.033
Dierschke, V., Furness, R. W., and Garthe, S. (2016). Seabirds and offshore wind farms in European waters: avoidance and attraction. Biol. Conserv. 202, 59–68. doi: 10.1016/j.biocon.2016.08.016
Duda, M. P., Robertson, G. J., Lim, J. E., Kissinger, J. A., Eickmeyer, D. C., Grooms, C., et al. (2020). Striking centennial-scale changes in the population size of a threatened seabird. Proc. R. Soc. B 287:20192234. doi: 10.1098/rspb.2019.2234
Elliott, K. H., Woo, K. J., Gaston, A. J., Benvenuti, S., Dall’Antonia, L., and Davoren, G. K. (2009). Central-place foraging in and Arctic seabird provides evidence for Storer-Ashmole’s Halo. Auk 126, 613–625. doi: 10.1525/auk.2009.08245
Fieberg, J., and Kochanny, C. O. (2005). Quantifying home-range overlap: the importance of the utilization distribution. J. Wildl. Manage. 69, 1346–1359. doi: 10.2193/0022-541x(2005)69[1346:qhotio]2.0.co;2
Fife, D. T., Pollet, I. L., Robertson, G. J., Mallory, M. L., and Shutler, D. (2015). Apparent survival of adult Leach’s Storm-petrels (Oceanodroma leucorhoa) breeding on Bon Portage Island, Nova Scotia. Avian Conserv. Ecol. 10:1. doi: 10.5751/ace-00771-100201
Garthe, S., Montevecchi, W. A., and Davoren, G. K. (2011). Inter-annual changes in prey fields trigger different foraging tactics in a large marine predator. Limnol. Oceanogr. 56, 802–812. doi: 10.4319/lo.2011.56.3.0802
Gjerdrum, C., Ronconi, R. A., Turner, K. L., and Hamer, T. E. (2021). Bird strandings and bright lights at coastal and offshore industrial sites in Atlantic Canada. Avian Conserv. Ecol. 16:22. doi: 10.5751/ace-01860-160122
Gross, W. A. O. (1935). The life history cycle of Leach’s Petrel (Oceanodroma leucorhoa leucorhoa) on the Outer Sea Islands of the Bay of Fundy. Auk 52, 382–399. doi: 10.2307/4077511
Halpin, L. R., Pollet, I. L., Lee, C., Morgan, K. H., and Carter, H. R. (2018). Year-round movements of sympatric Fork-tailed (Oceanodroma furcata) and Leach’s (O. leucorhoa) storm-petrels. J. F. Ornithol. 89, 207–220. doi: 10.1111/jofo.12255
Hedd, A., and Gales, R. (2005). Breeding and overwintering ecology of shy albatrosses in Southern Australia: year-round patterns of colony attendance and foraging-trip durations. Condor 107, 375–387. doi: 10.1650/7563
Hedd, A., and Montevecchi, W. A. (2006). Diet and trophic position of Leach’s Storm-Petrel Oceanodroma leucorhoa during breeding and moult, inferred from stable isotope analysis of feathers. Mar. Ecol. Prog. Ser. 322, 291–301. doi: 10.3354/meps322291
Hedd, A., Montevecchi, W. A., Davoren, G. K., and Fifield, D. A. (2009). Diets and distributions of Leach’s Storm-Petrel (Oceanodroma leucorhoa) before and after an ecosystem shift in the Northwest Atlantic. Can. J. Zool. 87, 787–801. doi: 10.1139/Z09-060
Hedd, A., Montevecchi, W. A., Phillips, R. A., and Fifield, D. A. (2014). Seasonal sexual segregation by monomorphic sooty shearwaters Puffinus griseus reflects different reproductive roles during the pre-laying period. PLoS One 9:85572. doi: 10.1371/journal.pone.0085572
Hedd, A., Pollet, I. L., Mauck, R. A., Burke, C. M., Mallory, M. L., McFarlane Tranquilla, L. A., et al. (2018). Foraging areas, offshore habitat use, and colony overlap by incubating Leach’s Storm-Petrels Oceanodroma leucorhoa in the Northwest Atlantic. PLoS One 13:e0194389. doi: 10.1371/journal.pone.0194389
Hoeg, R., Shutler, D., and Pollet, I. L. (2021). Levels of predation at two Leach’s Storm Petrel Hydrobates leucorhous breeding colonies. Mar. Ornithol. 49, 119–125.
Hung, L. W., Anderson, S. J., Pipkin, A., and Fristrup, K. (2021). Changes in night sky brightness after a countywide LED retrofit. J. Environ. Manage. 292:112776. doi: 10.1016/j.jenvman.2021.112776
Isaac, G. A., Bullock, T., Beale, J., and Beale, S. (2020). Characterizing and predicting marine fog offshore Newfoundland and Labrador. Weather Forecast. 35, 347–366. doi: 10.1175/WAF-D-19-0085.1
Kie, J. G. (2013). A rule-based ad hoc method for selecting a bandwidth in kernel home-range analyses. Anim. Biotelem. 1, 1–12. doi: 10.1186/2050-3385-1-13
Le Corre, M., Ollivier, A., Ribes, S., and Jouventin, P. (2002). Light-induced mortality of petrels: a 4-year study from Réunion Island (Indian Ocean). Biol. Conserv. 105, 93–102. doi: 10.1016/S0006-3207(01)00207-5
McClintock, B. T., and Michelot, T. (2018). momentuHMM: R package for generalized hidden Markov models of animal movement. Methods Ecol. Evol. 9, 1518–1530. doi: 10.1111/2041-210X.12995
Miles, W., Money, S., Luxmoore, R., and Furness, R. W. (2010). Effects of artificial lights and moonlight on petrels at St Kilda. Bird Study 57, 244–251. doi: 10.1080/00063651003605064
Mitkus, M., Nevitt, G. A., and Kelber, A. (2018). Development of the visual system in a burrow-nesting seabird: Leach’s Storm-Petrel. Brain Behav. Evol. 91, 4–16. doi: 10.1159/000484080
Montevecchi, W. A. (2006). “Influences of artificial light on marine birds,” in Ecological Consequences of Artificial Night Lighting, eds C. Rich and T. Longcore (Washington, DC: Island Press), 95–113.
Montevecchi, W. A., Birt-Friesen, V. L., and Cairns, D. K. (1992). Reproductive energetics and prey harvest of Leach’s Storm-Petrels in the Northwest Atlantic. Ecology 73, 823–832. doi: 10.2307/1940160
Montevecchi, W. A., Hedd, A., McFarlane Tranquilla, L., Fifield, D. A., Burke, C. M., Regular, P. M., et al. (2012). Tracking seabirds to identify ecologically important and high-risk marine areas in the western North Atlantic. Biol. Conserv. 156, 62–71. doi: 10.1016/j.biocon.2011.12.001
Montevecchi, W. A., and McFarlane, L. (2019). More than 3 million missing birds: investigating the plight of the Leach’s Storm-Petrel. Birdwatch Can. Spring 87, 10–15.
Nevitt, G. A., and Haberman, K. (2003). Behavioral attraction of Leach’s Storm-Petrels (Oceanodroma leucorhoa) to dimethyl sulfide. J. Exp. Biol. 206, 1497–1501. doi: 10.1242/jeb.00287
Niizuma, Y., Takahashi, A., Sasaki, N., Hayama, S.-I., Tokita, N., and Watanuki, Y. (2001). Benefits of mass reduction for commuting flight with heavy food load in Leach’s Storm-Petrel, Oceanodroma leucorhoa. Ecol. Res. 16, 197–203. doi: 10.1046/j.1440-1703.2001.00386.x
Niizuma, Y., and Watanuki, Y. (1997). Effects of circadian rhythm and breeding stage on resting metabolic rates in fasting Leach’s Storm-Petrels. J. Yamashina Inst. Ornithol. 29, 83–90. doi: 10.3312/jyio1952.29.83
Oppel, S., Bolton, M., Carneiro, A. P. B., Dias, M. P., Green, J. A., Masello, J. F., et al. (2018). Spatial scales of marine conservation management for breeding seabirds. Mar. Pol. 98, 37–46. doi: 10.1016/j.marpol.2018.08.024
Pante, E., and Simon-Bouhet, B. (2013). marmap: a package for importing, plotting and analyzing bathymetric and topographic data in R. PLoS One 8:e73051. doi: 10.1371/journal.pone.0073051
Patterson, T. A., Thomas, L., Wilcox, C., Ovaskainen, O., and Matthiopoulos, J. (2008). State-space models of individual animal movement. Trends Ecol. Evol. 23, 87–94. doi: 10.1016/j.tree.2007.10.009
Place, A. R., Stoyan, N. C., Ricklefs, R. E., and Butler, R. G. (1989). Physiological basis of stomach oil formation in Leach’s Storm-Petrel (Oceanodroma leucorhoa). Auk 106, 687–699.
Pollet, I. L., Bond, A. L., Hedd, A., Huntington, C. E., Butler, R. G., and Mauck, R. (2021). Leach’s Storm-Petrel (Hydrobates leucorhous) in Birds of the World. Ithaca, NY: Cornell Lab of Ornithology, doi: 10.2173/bow.lcspet.01.1
Pollet, I. L., Ronconi, R. A., Jonsen, I. D., Leonard, M. L., Taylor, P. D., and Shutler, D. (2014b). Foraging movements of Leach’s Storm-Petrels Oceanodroma leucorhoa during incubation. J. Avian Biol. 45, 305–314. doi: 10.1111/jav.00361
Pollet, I. L., Hedd, A., Taylor, P. D., Montevecchi, W. A., and Shutler, D. (2014a). Migratory movements and wintering areas of Leach’s Storm-Petrels tracked using geolocators. J. Field Ornithol. 85, 321–328. doi: 10.1111/jofo.12071
Pollet, I. L., Ronconi, R. A., Leonard, M. L., and Shutler, D. (2019). Migration routes and stopover areas of Leach’s Storm-Petrels Oceanodroma leucorhoa. Mar. Ornithol. 47, 55–65.
Poot, H., Ens, B. J., de Vries, H., Donners, M. A. H., Wernand, M. R., and Marquenie, J. M. (2008). Green light for nocturnally migrating birds. Ecol. Soc. 13:47. doi: 10.5751/ES-02720-130247
Raine, A. F., Gjerdrum, C., Pratte, I., Madeiros, J., Felis, J. J., and Adams, J. (2021). Marine distribution and foraging habitat highlight potential threats at sea for the Endangered Bermuda petrel Pterodroma cahow. Endanger. Spp. Res. 45, 337–356. doi: 10.3354/ESR01139
Rebke, M., Dierschke, V., Weiner, C. N., Aumüller, R., Hill, K., and Hill, R. (2019). Attraction of nocturnally migrating birds to artificial light: the influence of colour, intensity and blinking mode under different cloud cover conditions. Biol. Cons. 233, 220–227. doi: 10.1016/j.biocon.2019.02.029
Reed, J. R., Sincock, J. L., and Hailman, J. P. (1985). Light attraction in endangered Procellariiform birds: reduction by shielding upward radiation. Auk 102, 377–383. doi: 10.2307/4086782
Rennie, I. R. F., Green, D. J., Krebs, E. A., and Harfenist, A. (2020). High apparent survival of adult Leach’s Storm-Petrels Oceanodroma leucorhoa in British Columbia. Mar. Ornithol. 48, 133–140.
Ricklefs, R. E., Day, C. H., Huntington, C. E., and Williams, J. B. (1985). Variability in feeding rate and meal size of Leach’s Storm-Petrel at Kent Island, New Brunswick. J. Anim. Ecol. 54:883. doi: 10.2307/4385
Ricklefs, R. E., White, S. C., and Cullen, J. (1980). Energetics of postnatal growth in Leach’s Storm-Petrel. Auk 97, 566–575. doi: 10.1007/978-1-4613-0425-8_13
Robertson, G. J., Russell, J., Bryant, R., Fifield, D. A., and Stenhouse, I. J. (2006). Size and trends of Leach’s Storm-Petrel Oceanodroma leucorhoa breeding populations in Newfoundland. Atl. Seabirds 8, 41–50.
Rodríguez, A., Burgan, G., Dann, P., Jessop, R., Negro, J. J., and Chiaradia, A. (2014). Fatal attraction of Short-tailed Shearwaters to artificial lights. PLoS One 9:e0110114. doi: 10.1371/journal.pone.0110114
Rodríguez, A., Moffett, J., Revoltós, A., Wasiak, P., McIntosh, R. R., Sutherland, D. R., et al. (2017b). Light pollution and seabird fledglings: targeting efforts in rescue programs. J. Wildl. Manage. 81, 734–741. doi: 10.1002/jwmg.21237
Rodríguez, A., Dann, P., and Chiaradia, A. (2017a). Reducing light-induced mortality of seabirds: high pressure sodium lights decrease the fatal attraction of shearwaters. J. Nat. Cons. 39, 68–72. doi: 10.1016/j.jnc.2017.07.001
Ronconi, R. A., Allard, K. A., and Taylor, P. D. (2015). Bird interactions with offshore oil and gas platforms: review of impacts and monitoring techniques. J. Environ. Manage. 147, 34–45. doi: 10.1016/j.jenvman.2014.07.031
Rosen, D. A. S., Winship, A. J., and Hoopes, L. A. (2007). Thermal and digestive constraints to foraging behaviour in marine mammals. Philos. Trans. R. Soc. B Biol. Sci. 362, 2151–2168. doi: 10.1098/rstb.2007.2108
Seaman, D. E., Millspaugh, J. J., Kernohan, B. J., Brundige, G. C., Raedeke, K. J., and Gitzen, R. A. (1999). Effects of sample size on kernel home range estimates. J. Wildl. Manage. 63:739. doi: 10.2307/3802664
Syposz, M., Padget, O., Willis, J., Van Doren, B. M., Gillies, N., Fayet, A. L., et al. (2021). Avoidance of different durations, colours and intensities of artificial light by adult seabirds. Sci. Rep. 11:18941. doi: 10.1038/s41598-021-97986-x
Thieurmel, B., and Elmarhraoui, A. (2019). suncalc: Compute sun Position, Sunlight Phases, Moon Position and Lunar Phase. Source?.
Torres, L. G., Orben, R. A., Tolkova, I., and Thompson, D. R. (2017). Classification of animal movement behavior through residence in space and time. PLoS One 12:e0168513. doi: 10.1371/journal.pone.0168513
Vermeer, K., Devito, K., and Rankin, L. (1988). Comparison of nesting biology of Fork-tailed and Leach’s Storm-Petrels. Colon. Waterbirds 11, 46–57. doi: 10.2307/1521169
Watanuki, Y. (1985). Food of breeding Leach’s Storm-Petrels (Oceanodroma leucorhoa). Auk 102, 884–886. doi: 10.2307/4086731
Watanuki, Y., and Thiebot, J. B. (2018). Factors affecting the importance of myctophids in the diet of the world’s seabirds. Mar. Biol. 165:79. doi: 10.1007/s00227-018-3334-y
Weimerskirch, H., Fradet, G., and Cherel, Y. (1999). Natural and experimental changes in chick provisioning in a long-lived seabird, the Antarctic Prion. J. Avian Biol. 30:165. doi: 10.2307/3677126
Weimerskirch, H., Salamolard, M., Sarrazin, F., and Jouventin, P. (1993). Foraging strategy of Wandering Albatrosses through the breeding season: a study using satellite telemetry. Auk 110, 325–342. doi: 10.2307/4088559
Wiese, F. K., Montevecchi, W. A., Davoren, G. K., Huettmann, F., Diamond, A. W., and Linke, J. (2001). Seabirds at risk around offshore oil platforms in the North-west Atlantic. Mar. Pollut. Bull. 42, 1285–1290. doi: 10.1016/S0025-326X(01)00096-0
Wilhelm, S. I. (2017). CWS: Waterbird Colony Database (Atlantic region). Dartmouth, NS: Bedford Institute of Oceanography.
Wilhelm, S. I., Dooley, S. M., Corbett, E. P., Fitzsimmons, M. G., Ryan, P. C., and Robertson, G. J. (2021). Effects of land-based light pollution on two species of burrow-nesting seabirds in Newfoundland and Labrador. Canada. Avian Conserv. Ecol. 16:12.
Wilhelm, S. I., Hedd, A., Robertson, G. J., Mailhiot, J., Regular, P. M., Ryan, P. C., et al. (2020). The world’s largest breeding colony of Leach’s Storm-Petrel Hydrobates leucorhous has declined. Bird Cons. Int. 30, 40–57. doi: 10.1017/S0959270919000248
Wilhelm, S. I., Schau, J. J., Schau, E., Dooley, S. M., Wiseman, D. L., and Hogan, H. A. (2013). Atlantic Puffins are attracted to coastal communities in Eastern Newfoundland. Northeast. Nat. 20, 624–630. doi: 10.1656/045.020.0409
Worton, B. J. (1989). Kernel methods for estimating the utilization distribution in home- range studies. Ecology 70, 164–168. doi: 10.2307/1938423
Worton, B. J. (1995). Using Monte Carlo simulation to evaluate kernel-based home range estimators. J. Wildl. Manage. 59:794. doi: 10.2307/3801959
Zuur, A. F., and Ieno, E. N. (2016). A protocol for conducting and presenting results of regression-type analyses. Meth. Ecol. Evol. 7, 636–645. doi: 10.1111/2041-210X.12577
Keywords: foraging, risk, tracking, oil platform, light attraction, Hydrobates leucorhous
Citation: Collins SM, Hedd A, Fifield DA, Wilson DR and Montevecchi WA (2022) Foraging Paths of Breeding Leach’s Storm-Petrels in Relation to Offshore Oil Platforms, Breeding Stage, and Year. Front. Mar. Sci. 9:816659. doi: 10.3389/fmars.2022.816659
Received: 18 November 2021; Accepted: 14 January 2022;
Published: 25 February 2022.
Edited by:
Ryan Rudolf Reisinger, University of Southampton, United KingdomCopyright © 2022 Collins, Hedd, Fifield, Wilson and Montevecchi. This is an open-access article distributed under the terms of the Creative Commons Attribution License (CC BY). The use, distribution or reproduction in other forums is permitted, provided the original author(s) and the copyright owner(s) are credited and that the original publication in this journal is cited, in accordance with accepted academic practice. No use, distribution or reproduction is permitted which does not comply with these terms.
*Correspondence: Sydney M. Collins, smcollins@mun.ca