- 1Department of Environmental, Earth and Physical Sciences, University of Siena, Siena, Italy
- 2Institute of Organic Chemistry (IQOG), CSIC, Madrid, Spain
- 3Centro Interuniversitario di Ricerca sui Cetacei (CIRCE), c/o Department of Environmental, Earth and Physical Sciences, University of Siena, Siena, Italy
- 4Tethys Research Institute, Milan, Italy
- 5Italian Institute for Environmental Protection and Research (ISPRA), Rome, Italy
Among killer whale forms, type C is a fish-eating form and is the most common in the Ross Sea. In the austral summer 2015, a study was conducted to evaluate the toxicological hazard these marine mammals face in the Antarctic ecosystem. Seven biopsy samples were collected from adult individuals (five males and two females) in the surroundings of the Italian Research Station Mario Zucchelli, Terra Nova Bay, by remote dart sampling from the pack ice. The accumulation levels of persistent organic pollutants (POPs) such as legacy (DDTs, PCBs, and HCB) and emerging (PBDEs and DP) were measured. Moreover, the protein expression of cytochrome P450 (CYP1A1 and 2B) and the mRNA level variations of the peroxisome proliferator-activated receptors α and γ (PPARα-γ) and the estrogen receptor α (ERα), aryl hydrocarbon receptor (AhR), and Cyp1a were evaluated. Twenty PCB congeners, six DDTs, HCB, three HCHs, and fourteen brominated BDEs and DP-syn and anti-isomers were analyzed on freeze-dried blubber biopsy samples by GC-MS. The protein expression was evaluated by Western Blot and the mRNA levels were quantified by quantitative real-time PCR. The average abundance pattern for the contaminants was DDTs > PCBs > HCB > HCHs ≈ PBDEs >> DP. Contaminant levels resulted to be lower when compared to the existing data from the Antarctic type C killer whales from the McMurdo Sound (Ross Sea) and those reported for fish-eating killer whales worldwide. The mRNA levels of the five target genes were successfully quantified, but no statistical correlation was found with POP levels, suggesting that either the low levels of quantified POPs in blubber may not significantly affect the biological responses investigated, or that other stressors could contribute to the alterations of the molecular biomarkers. Although the results showed a lower risk related to contamination compared to more impacted areas, this study provides baseline data for the conservation of this species in an area with high ecological value, recently declared as the largest Marine Protected Area in Antarctica, where pollutants should remain at minimum levels despite increasing multiple stresses existing in the region.
Introduction
Killer whales (Orcinus orca) can be found in all marine regions, ranging from the polar areas to the equator (Pitman and Ensor, 2003; Lauriano et al., 2011). Despite O. orca being considered a single species, there are suggestions of taxonomic diversity (Morin et al., 2015), supported by the presence of specialized ecotypes in several locations in both hemispheres (Foote et al., 2016).
Differences in habitat, as well as prey preferences, diet specialization, behavior, and group size are elements that make it possible to distinguish five killer whale ecotypes in the Southern Ocean (Pitman et al., 2018). Among these five types A, B (1 and 2), C, and D, the most common ecotype in the Ross Sea is the C form, known as the Ross sea killer whale (RSKW). The RSKW is the smallest killer whale form, easily recognizable through its small and dorsal-oriented post ocular eye patch and the dorsal patch.
The RSKW is distributed in the coastal area, where it is known to search for prey, mainly consisting of fish (Krahn et al., 2008; Lauriano et al., 2020). Occurrence and movements of the RSKW are described for the Mc Murdo Sound area (Ainley et al., 2017; Pitman et al., 2018), where the ecotype is known to occur in mid-November to benefit from prey availability in the icebreaker channel to catch the Antarctic toothfish (Dissostichus mawsoni). In Terranova Bay (TNB), the presence of RSKW is delayed compared to the nearby Mc Murdo area (250 Km south of TNB), being reported in mid-January (Lauriano et al., 2011, 2020). A recent study from Lauriano et al. (2020) revealed RSKW movements and inferred the presence of feeding grounds in the eastern Ross Sea coastal area and a traveling behavior outside the polar front.
The Antarctic toothfish has been considered a major diet component of this fish-eating killer whale; in TNB a stable isotope study revealed that the Notothenoid species accounted for 35% of the ingested biomass in the sampled individuals (Lauriano et al., 2020).
As top predators with long life spans, generally killer whales are prone to accumulate high concentrations of environmental contaminants of toxicological concern, such as persistent organic pollutants (POPs); hence, the population worldwide has been predicted to decline due to POP accumulation (Desforges et al., 2016, 2018; Pedro et al., 2019). Among the most relevant and studied POPs, there are organochlorine pesticides such as dichlorodiphenyltrichloroethane (DDT) and its metabolites, hexachlorobenzene (HCB), or hexachlorocyclohexanes (HCHs), as well as the well-known polychlorinated biphenyls (PCBs) or halogenated flame retardants, such as polybrominated diphenyl ethers (PBDEs) or the highly chlorinated Dechlorane plus (DP). Generally, POPs experience biomagnification within food webs since dietary intake acts as the main route for contaminant accumulation. Thus, high levels of POPs have been documented in killer whales from different geographical areas (mostly from the northern hemisphere; Desforges et al., 2018; Andvik et al., 2020; Lawson et al., 2020; Remili et al., 2021). Substantial differences due to their ecotype (based on prey specialization) influence the accumulation of contaminants in killer whales, which may exert different effects on different populations worldwide (Desforges et al., 2018). Robust data from killer whale populations inhabiting pristine areas such as Antarctica are much less abundant. However, there is an increasing concern about the potential effects of POPs on polar environments and polar food webs. The polar regions can represent the final sink for POPs, which are detected in polar marine, terrestrial ecosystems, and biota (Corsolini, 2009; Corsolini et al., 2017), despite their low level of anthropization and distance from the main pollution sources. In addition, polar regions are sensitive to cumulative stress, mostly related to climate change, with modifying sea-ice dynamics and temperature, which can affect organisms’ health in these environments (Hückstädt et al., 2017). The Ross Sea has been recently recognized for its ecological importance as a large marine protected area (MPA) in Antarctica since it is an important breeding site of several penguins’ species and fish species (e.g., Antarctic silverfish). Moreover, this ecosystem sustains many predators (including seabirds and marine mammals) due to high primary production (Ballard et al., 2012).
The Antarctic type C killer whale population has been classified at low risk of exposure to pollutants due to relatively low concentration of PCBs when compared to other populations worldwide (Desforges et al., 2018). However, very few data are available on the accumulation of both legacy and emerging contaminants in this ecotype and, specifically, no data are available on the ecotoxicological effects due to contaminants exposure and cumulative effects due to other stressors, such as prey depletion and climate change. Thus, the conservation status of this population is still nearly unknown.
Alterations in the expression profiles of mRNA and proteins related to the exposure to POPs and the complex mixture of contaminants in the environment represent a valid tool to assess the health effects on wild populations including marine mammals (Godard-Codding and Fossi, 2018). Several molecular warning signals are investigated to evaluate the cumulative effects due to the exposure to different classes of contaminants or other environmental stressors.
Since a key component of PPAR-dependent transcriptional activity is the binding of an endogenous ligand, environmental contaminants can act as peroxisome proliferator-activated receptor (PPARs) agonists (e.g., per- and polyfluoroalkyl substances, PFAS). There are three isoforms of PPARs (PPARα, PPARδ, and PPARγ) that share a similar structure, but they are expressed in different tissues and vary in their physiological roles. The physiological functioning of these receptors is crucial for mammals because they regulate adipogenesis and the storage of lipids (Rosen et al., 1999; Lühmann et al., 2020), inflammation, adipogenesis, and reproduction (Schupp and Lazar, 2010). The endocrine system is fundamental to the health of marine mammals, which are exposed to many classes of pollutants defined as endocrine-disrupting chemicals in humans and wildlife. Tartu et al. (2017) reported increasing transcript levels of PPARγ and its target genes with increasing POP concentrations in polar bears (Ursus maritimus). Agonistic or antagonistic effects of chlorinated and brominated POPs and PFAS have been shown in mammalian PPARγ, and several studies have reported significant correlations between transcript levels of nuclear receptors and concentrations of POPs in marine mammals (Routti et al., 2016; Fossi et al., 2018; Kurtz et al., 2019).
Moreover, a compound with endocrine disruptor capability can act by altering the mRNA levels of the estrogen receptors (ERs), which are nuclear ligand-inducible transcription factors. POPs may exert estrogenic and antiestrogenic effects interfering with endogenous ligand-binding (Kester et al., 2000) and altering the hormone-dependent transcription of ER-inducible genes, including Cyp1a1.
Several environmental pollutants modulate the transcriptional activity of these nuclear receptors at environmentally relevant concentrations for whales (Lühmann et al., 2020) as well as the aryl hydrocarbon receptor (AhR), which is a ligand-activated transcription factor that regulates several genes involved in the metabolism of several classes of contaminants (also known as AhR-inducers) and mediates the activation of CYP1a transcription (Wilson et al., 2007). AhR, along with the cytochrome P450 (CYP450) isoforms, has been considered a biomarker of chemical exposure in marine mammals (Buckman et al., 2011; Noël et al., 2014; Godard-Codding and Fossi, 2018). CYP1A1 and CYP2B proteins have been detected in cetacean skin. The different expression of these CYP isoforms has been related to the exposure to lipophilic contaminants (AhR inducers) such as organochlorine compounds [OCs, polycyclic aromatic hydrocarbons (PAHs), and PBDEs both ex vivo and in in-field studies (Godard, 2004; Baini et al., 2020)].
Although POP concentrations have been measured before in RSKW blubber (Krahn et al., 2008) and that the population size and trend and conservation status of RSKW is still unknown, there are no published studies to date investigating the possible health effects of POPs in RSKW. The few studies available that measure health effects at the molecular level have attempted to relate biomarkers of toxicological effects to pollutant concentrations in other KW populations worldwide (e.g., Buckman et al., 2011; Fossi et al., 2014), but none on this population.
For these reasons, the main aim of this study was to investigate the potential ecotoxicological effects in RSKW population related to POP exposure and other environmental stressors occurring in this ecologically important area. This is the first study to profile the expression of molecular biomarkers in this Antarctic ecotype, and it paves the way for future biomarker research in these marine sentinels of the Ross Sea ecosystem, especially after the recent establishment of the Ross Sea Region MPA and the recent concern on the global killer whale population collapse (Jepson et al., 2016; Desforges et al., 2018).
Materials and Methods
Sampling Area and Biopsy Sampling
This study was conducted in the Terranova Bay, between Cape Washington and the Italian research station Mario Zucchelli (MZS; Figure 1), between mid-January and mid-February 2015. Killer whale pods were located by helicopter survey along the ice shelf; once a killer whale pod was sighted, the survey platform moved in their travel direction to anticipate individuals’ movements and to let researchers prepare for the biopsy sampling. A 150-lb recurve crossbow, equipped with a 55 cm long carbon fiber dart with a 8 mm × 60 mm biopsy tip (manufactured by Ceta Dart V/F. Larsen) was used to collect skin biopsies, and the shooting distance ranged between 3 and 15 m. Samples were stored at −20°C for contaminant analysis and in RNAlater™ for protein and mRNA analysis.
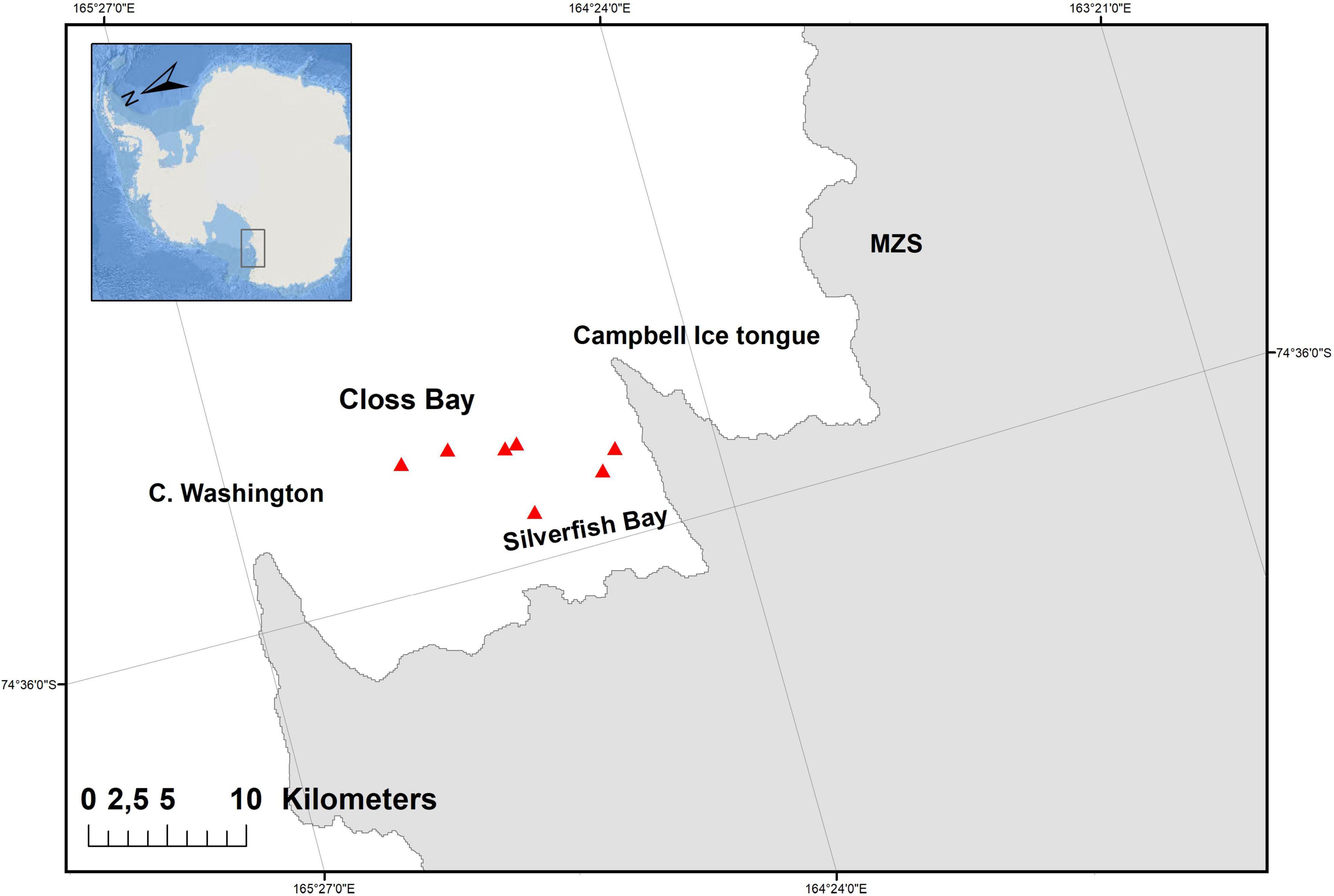
Figure 1. The study area in the Ross Sea and the sampling locations of the killer whales (red triangles).
Seven RSKW skin biopsies (five males and two females) were sampled and a multidisciplinary approach was applied to each individual to successfully study movements, foraging areas, and diving behavior, thanks to the simultaneous application of satellite telemetry devices and biopsy sampling to describe the diet by stable isotope analysis (Lauriano et al., 2020).
A permit to collect biopsy samples was issued by the Italian Antarctic Research Programme on behalf of the Italian Ministry of Foreign Affairs.
Contaminant Analysis
Sample Treatment
The blubber samples of RSKW (∼300 mg on average) were first freeze-dried and subsequently extracted and purified as indicated in Muñoz-Arnanz et al. (2019) with minor variations. In short, freeze-dried samples were mixed with 5 g of anhydrous Na2SO4 and spiked with different quantities of isotopically labeled standards as surrogates. Extraction was performed for 24 h in Soxhlet using precleaned cellulose thimbles with n-hexane:dichloromethane (9:1 v/v). Lipid content was determined gravimetrically for each sample. Extracts were subsequently purified by low-pressure chromatography on multilayer silica gel, reduced down to ∼1 mL by means of a TurboVap ® system, transferred to vials and further reduced to incipient dryness under nitrogen. Samples were reconstituted in a 20 μL solution of internal standards (IS) before instrumental analysis. Full information on materials and standards used can be found in Supplementary Tables 1–3.
Instrumental Determination
Twenty PCB congeners (# 28, 52, 95, 101, 105, 114, 118, 123, 132, 138, 149, 153, 156, 157, 167, 170, 180, 183, 189, and 194), six DDTs (p,p′- and o,p′-DDT, -DDD and -DDE), HCB, and three HCHs (α-, β-, and γ-) were analyzed by gas chromatography low resolution mass spectrometry (GC-LRMS) employing a 7890N gas chromatograph coupled with a 5975C quadrupole mass spectrometer (Agilent, Palo Alto, CA, United States) with electronic impact (EI) as ionization and operating in selected ion monitoring mode (SIM). The isotopic dilution technique was used for the quantitation of these target analytes. Fourteen brominated BDE congeners, from tri- to deca-substituted (#28, 47, 66, 85, 99, 100, 153, 154, 183, 184, 191, 196, 197, and 209) and DP (syn- and anti- isomers), were analyzed by GC-LRMS using a 6890N gas chromatograph coupled with a 5975 quadrupole mass spectrometer (Agilent, Palo Alto, CA, United States) operated in SIM with negative chemical ionization (NCI). Quantitation of PBDEs and DP relied on the use of IS (PBDEs) or the isotopic dilution technique (DP). Comprehensive details on each chromatographic and MS method are indicated in Muñoz-Arnanz et al. (2016, 2019) and in the Supplementary Material.
Sex Determination
The sex was first estimated in the field based on the size and shape of the dorsal fin size. Sex was then confirmed by molecular sex determination based on ZFY/ZFX gene. A total of 20 mg of dermal tissue was homogenized with a Tissue Lyser (Qiagen, Hilden, Germany) to isolate DNA with the Wizard ® SV Genomic DNA Purification Kit (Promega, Madison, WI, United States) following the manufacturer’s instructions. DNA was quantified by Nano-Drop ND-100 UV–Vis spectrophotometer (NanoDrop Technologies Inc., Wilmington, DE, United States), and the purity was assessed by 280/260 and 260/230 nm ratios. Gender was determined using 50–100 ng of DNA in standard PCR reactions following the protocol described by Berube and Palsbøll (1996).
RNA Isolation and Quantitative Real-Time PCR Analysis
Total RNA was isolated using 20–30 mg of the dermal part of the biopsy to analyze transcript levels of five target genes. Skin samples were homogenized using a Tissue Lyser II (Qiagen, Hilden, Germany), and the total RNA was extracted using the Aurum Total Fatty and Fibrous Tissue kit (Bio-Rad Laboratories, Hercules, CA, United States) following the manufacturer’s instructions and stored at −80°C. Genomic DNA was digested by DNase-on-column treatment for each sample. RNA was quantified by Nano-Drop ND-100 UV–Vis spectrophotometer (NanoDrop Technologies Inc., Wilmington, DE, United States). An additional assessment of the integrity of the samples was determined by denaturing agarose gel (1.2%) electrophoresis and ethidium bromide staining. A total of 1 μg of the total RNA for each sample was retrotranscribed using the iScript cDNA Synthesis Kit (Bio-Rad Laboratories, Hercules, CA, United States).
Primers for quantitative real time-PCR (qRT-PCR) for PPARα and γ were both designed on the specific killer whale sequences. Genes were sequenced using killer whale samples available in the University of Siena tissue bank and deposited in GenBank under the accession numbers OL331015 (PPARα) and OL331016 (PPARγ). Primers were then specifically designed using the Beacon Designer v. 8.14 software (Premier Biosoft, Palo Alto, CA, United States). ERα, Cyp1a, and AhR primer sequence were obtained from Panti et al. (2011), and the primer sequences for the reference gene tyrosine 3-monooxygenase/tryptophan 5-monooxygenase activation protein, zeta polypeptide (YWHAZ), and glyceraldehyde-3-phosphate dehydrogenase (GAPDH) were obtained from Spinsanti et al. (2006; Supplementary Table 5). Primer pairs were validated with cetacean samples using traditional PCR and sequencing of the PCR product.
All primers were purchased from (Merck Sigma-Aldrich, Dermstadt, Germany). The efficiency of each primer pair (Supplementary Table 5) for each gene was determined by a calibration curve (1:5 cDNA serial dilutions). Each primer pair presented a melting curve with a sharp peak, indicating no unspecific products or primer dimer formation. The amplicon length was verified on 2% agarose gel with ethidium-bromide staining.
The qRT-PCR assays were carried out in 96-well reaction plates with an iCycler iQ5 (Bio-Rad Laboratories, Hercules, CA, United States) using the SsoAdvanced Universal SYBR Green Supermix (Bio-Rad Laboratories, Hercules, CA, United States) as described in Routti et al. (2019).
The five genes of interest and two reference genes selected for the normalization procedure were amplified for each of the seven samples. Each reaction was run in triplicate and a control with no template was included in each reaction series. The normalized fold expression values are expressed as the mean value of the triplicate for each sample for each gene.
Protein Expression: Cytochrome P450 Analysis
Analysis of cytochrome P450 CYP1A1 and CYP2B, used in this study as a potential marker of POP exposure, were analyzed in the dermal part of the skin biopsies of RSKW using Western Blot (WB) and a semiquantitative analysis of the data according to Fossi et al. (2008). Subsamples of biopsies (about 30 mg) were homogenized using a Tissue Lyser II (Qiagen, Hilden, Germany) in the aryl-hydrocarbon-receptor buffer (1:10), and the lysate processed according to Baini et al. (2020). Semiquantitative analysis was performed using the Quantity One software (1-D Analysis Software, Bio-Rad Laboratories, Hercules, CA, United States) and molecular weights calculated with multiple regression models using the Precision Plus Protein™ Standard (Bio-Rad Laboratories, Hercules, CA, United States).
Data Analysis
Contaminants concentrations below quantification limits were assigned a value of zero and regarded as not detected (ND). The relative gene expression obtained by qRT-PCR was calculated using the ΔΔCt method according to Livak and Schmittgen (2001). Statistical analyses were conducted using the software RStudio v2021.09.0 + 351. Spearman’s rank correlation was adopted to measure the correlation between variables. All statistical analyses were considered significant at p < 0.05. Missing data were handled using pairwise deletion methods, and PCA analysis was performed with Factoshiny-FactoMineR package (R Core Team, 2021).
Results and Discussion
The ecotoxicological study consisted of the quantification of POPs (PCBs, HCH, PBDEs, DDTs, and DP) and the investigation of the molecular effect by quantifying mRNA and protein levels in seven RSKW skin biopsies are listed in Table 1 and sampled during the austral summer of 2015 off the Italian Research Station Mario Zucchelli in TNB, Antarctica.
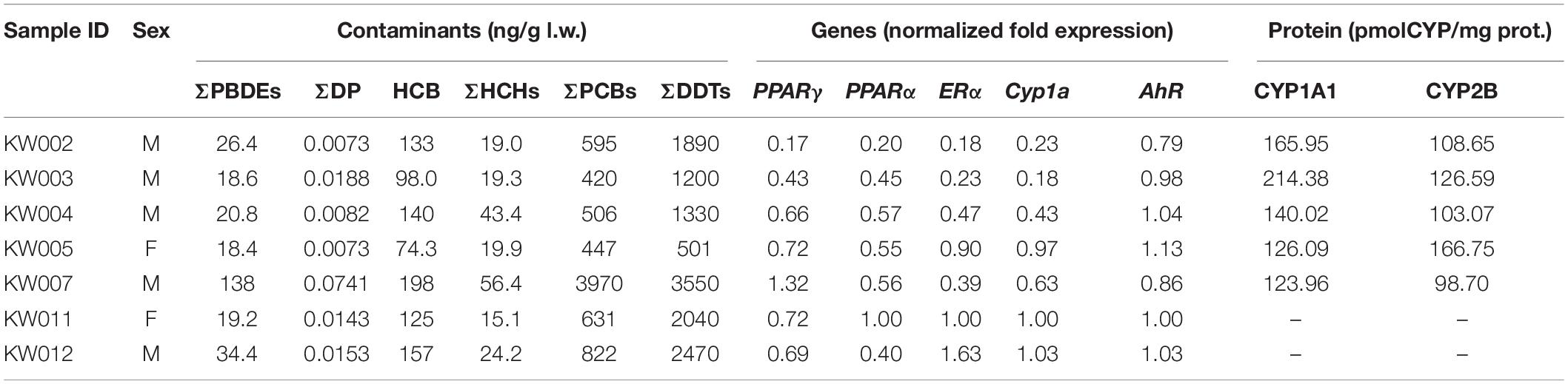
Table 1. Summary of the results on pollutant levels, mRNA levels, and protein levels in the seven Ross Sea Killer Whales (RSKWs).
Pollutant Concentrations
All target contaminants were detected in the killer whale’s samples analyzed in this study. Concentrations on lipid weight (l.w.) basis (ng/g) are summarized in Table 2.
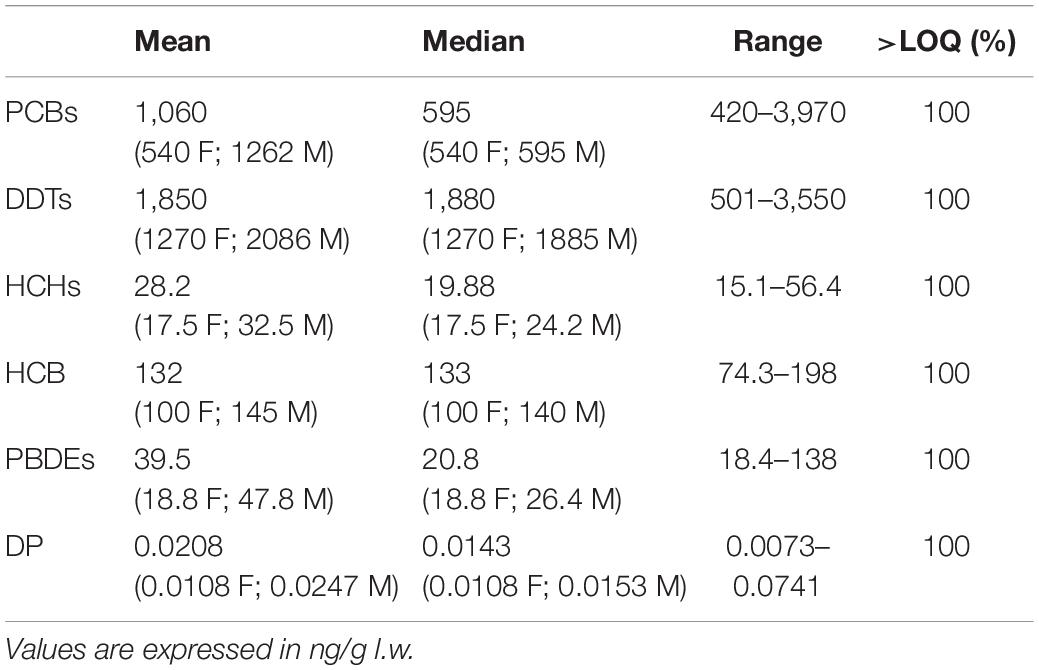
Table 2. Mean (in brackets the mean and median values for females-F and males-M), median, range, and detection frequencies [% >limit of quantification (LOQ)] of target contaminant concentrations.
The relative abundance of the contaminants analyzed followed the order DDTs > PCBs > HCB > HCHs ≈ PBDEs >> DP. This pattern of abundance is similar to what has been described for type C killer whale specimens sampled at the Ross Sea in 2005/2006 and reported by Krahn et al. (2008). However, given the small number of samples in this study, the comparison with values reported by Krahn et al. (2008) should be exerted with caution. Interestingly, this pattern was also similar to that reported for toothfish (Dissostichus mawsoni) from the same area by Corsolini et al. (2017), which is relevant since toothfish is assumed to be among the main prey of type C KW foraging in the Ross Sea (Krahn et al., 2008; Lauriano et al., 2020).
The results underline the importance of top predators as sentinels of ecosystems’ health due to their trophic position and their role as integrators of multiple environmental stressors, allowing us to understand the relationships between exposure and the range of effects.
Factors such as sex, age, or reproductive status highly influence the bioaccumulation of pollutant burdens in marine mammals in general, which can explain the range of values found among the animals sampled in this study, although they were all adults. Nonetheless, in terms of total concentrations and direct comparison with values reported by Krahn et al. (2008) within a time interval of roughly 10 years, our results suggest an important reduction in the area of legacy POPs such as PCBs, DDTs, and HCBs, confirming the results of Hao et al. (2019) in air monitoring, in which PCBs, HCHs, DDTs, and HCB were found in decreasing temporal trends, and there was an equally important increase of “emerged” POPs such as PBDEs.
It is worth noting the detection of DP, which, to the best of our knowledge, has been only been published on killer whales from the Artic (Vorkamp et al., 2019) at very low detection frequencies (∼18%) and concentrations (only anti isomer at <0.037–2.01 ng/g l.w. in blubber). The occurrence of DP in the Antarctic marine biota has been, however, documented before. For instance, Na et al. (2017) studied the trophic magnification of DP in marine food webs of the Fildes Peninsula finding DP concentrations in nine different species ranging from average values (ng/g l.w.) of 0.25 in krill to 6.81 in seals, with intermediate levels for fish species, such as starfish (1.22) or cod (2.36). Thus, the extremely low DP concentrations found in our study, in agreement with the low values in Arctic specimens of killer whale, might indicate a lack of bioaccumulation and biomagnification of this POP, perhaps in relation to this species’ metabolism. This is, however, a hypothesis that needs to be tested and for which further investigation and more data are needed.
Concentrations of the rest of target POPs, while orders of magnitude higher than those of DP, ranked among the lowest values ever found in killer whales from the Northern (summary of values from various locations at Atkinson et al., 2019; Andvik et al., 2020; McCormley et al., 2021) or even the Southern Hemisphere (Noël et al., 2009) and in agreement with that previously reported by Krahn et al. (2008). This probably reflects on the diet of Type C killer whales and a general lower degree of pollution in the Ross Sea area.
Pollutant Profiles
Pollutant profiles were noticeably similar across all sampled specimens. Graphical analysis of each pollutant group can be found in the Supplementary Figures 1–4). The RSKW’s average PCB content (Supplementary Figure 1) was dominated by the contribution of hexachlorinated congeners such as CB-153, -138, and -149, followed by heptachlorinated (CB-180) and pentachlorinated (CB-101 and -95). This pattern of abundance is consistent with what has been generally described in killer whales within an ample range of sampled time and areas, such as the North East Pacific (Ross et al., 2000), the Russian North Pacific (Atkinson et al., 2019), the North of Norway (Andvik et al., 2020, 2021), and Ireland (Schlingermann et al., 2020) or the Southern Indian Ocean (Noël et al., 2009), likely reflecting on the length of killer whales’ food chains as apex predators and the resistance of these congeners to biodegradation (Borja et al., 2005).
The major contributor to the average PBDE profile was BDE-47, as it is usually reported in marine food webs and found dominant in killer whales (Alava et al., 2016). Major contributors in order of abundance were BDE-85, -99, -209 ≈ -28, -153 ≈ -154 ≈ -100. All of these congeners have been previously found in similar relative abundance in these animals (Rayne et al., 2004; Haraguchi et al., 2009; Schlingermann et al., 2020). It is noteworthy that there was a high contribution of BDE-85, which is an understudied BDE congener for which there are no available data on killer whales or their prey. Furthermore, the relatively high contribution of BDE-209 should be highlighted. This is usually not detected in marine mammals under the general assumption of both reduced bioavailability (owing to preferential binding to particle phase) and bioaccumulation (owing to ready debromination to lower brominated congeners; Alava et al., 2016). At the same time though, most studies on PBDEs in killer whales, and generally in marine biota, have not included this congener in their analytical procedures, it poses the question of whether the earlier assumption should be revisited once data on this specific congener’s occurrence are generated. As an example, BDE-209 very recently was found predominant in two penguin species (Pygoscelis antarticus and Pygoscelis papua) from the Antarctic area (Morales et al., 2022), but also in other organisms in other areas as in sperm whales (Physeter macrocephalus) from the Mediterranean Sea (Zaccaroni et al., 2018) or in whale sharks (Rhincodon typus) from the Gulf of California (Fossi et al., 2017).
Organochlorine pesticides (DDTs and HCHs) were also found in abundance patterns in consonance with that described for killer whales from other areas such British and Irish waters (McHugh et al., 2007; Schlingermann et al., 2020) or the Pacific Coast of Japan (Haraguchi et al., 2009). p,p-DDE showed a heightened contribution of about 80% to the DDT content quantified. The rest of analytes were found in decreasing order with p,p-DDT (∼9%) > o,p-DDT (∼7%) > p,p-DDD (∼3%) > o,p-DDE (1.4%) > o,p-DDD (0.9%). Among HCHs, the α-isomer contributed with almost half to the total content (∼49%), followed by the γ- and β- isomers with ∼34 and ∼17%, respectively.
The DP antiisomer was found in all specimens (median 9.02 and range 3.67–37.0 pg/g l.w.), while the syn-isomer was not detected in two samples (median 3.02 and range 0–37.0 pg/g l.w.). As stated earlier (paragr. 3.1), the presence and trophic magnification of this pollutant has been described in the Antarctica (Na et al., 2017), with the highest levels found in seals (6.81 ng/g l.w.). Moreover, these authors estimated an fanti (calculated as the concentration of anti-DP by the sum of anti-DP and syn-DP) for the different trophic levels studied in the range of 0.23–0.53, lower than the average fanti derived from commercial products (0.68). In our study, not only the concentration of both DP enantiomers was found at about three orders of magnitude lower, but also the median fanti was higher than the commercial signature, reaching a value of 0.75 (in the range 0.50–1.00). These two facts seem to back up the concept of a ready degradation of DP by killer whales, most likely enhanced for the syn-isomer. In contrast with what has been described for other Antarctic marine mammals, this hypothesis should warrant further investigation.
mRNA and Protein Quantification
The analysis of mRNA transcripts was performed in the dermal part of the skin biopsies and analyzed using quantitative real-time PCR. Al the target genes were successfully amplified.
To the best of our knowledge, the expression of five target genes (PPARγ, PPARα, AhR, ERα, and Cyp1a) was evaluated for the first time in RSKWs. The expression of the five target genes varied across the samples, with males generally having lower mRNA levels for all five genes than females (Figure 2). KW002 (male) showed the lowest expressions for all target genes. Generally, the expression of the AhR gene was more stable in the seven individuals compared to the other genes, including Cyp1a. Although the upregulation of AhR may result in metabolic and physiological changes through enhancement of AhR-CYP pathways (Kim et al., 2005), any statistically significant correlation was found among the expression of these two genes, as instead shown in previous studies on hepatic Cyp1a and AhR in pacific killer whales and arctic beluga whales (Buckman et al., 2011; Noël et al., 2014). The mRNA levels of Cyp1a and ERα, instead, were positively correlated (rho = 0.929, p = 0.007; Figure 3). There are some evidence that the upregulation of ERs may affect steroid hormone concentration, but also it can alter the AhR-dependent Cyp1a expression when a ligand (or a toxic compound) activates the ER binding to estrogen response elements can alter the hormone-dependent transcription of ER-inducible genes including cytochromes P450 1A1, 1A2, or 1B (Matthews et al., 2007). The KW012 male individual showed the highest ER mRNA levels, which was 1.7-fold higher than the female mean expression and 5.1-fold higher than the average value for males. Regarding the PPARγ gene, KW007 showed the highest mRNA levels. It is worth noting that the same individual presented the highest accumulation of POPs analyzed (Table 1). It has been demonstrated that exposure to PCBs, DDTs, and PBDEs can increase the PPARγ transcript levels in polar bears (Routti et al., 2016; Tartu et al., 2017). Additionally, the transcriptional activity of PPARγ after the exposure to POPs has also been demonstrated to be activated by agonistic effects in fin whales and blue whales. Since the ligand-binding domain of whale PPARγ is the same as in other cetacean species, similar responses are expected also in killer whales (Lühmann et al., 2020). The PPARα mRNA levels can be modulated by the exposure to POPs and perfluorinated alkyl acids (PFAAs) compounds, and the activation of the receptor, in turn, promotes the transcription of genes responsible for energy metabolism and regulation of lipids (Kurtz et al., 2019; Griffin et al., 2020). The highest PPARα mRNA levels were found in the female KW011 and the lowest in KW002. The mRNA levels varied across the RSKW samples, but any statistical correlation was found with the POP levels. The downregulation of PPARα gene has been shown in killer whale organotypic cell cultures exposed to BPA and PFOA (Fossi et al., 2018), but few studies investigated the expression of this gene in marine mammals. In ringed seals from Baltic Sea, for instance, the expression of PPARα was not related to POP exposure or sampling area, but to fasting and molting period (Castelli et al., 2014), suggesting that the effects of contaminants on the activation of this receptor and the related pathways are negligible if compared to physiological and metabolic status of the organisms.
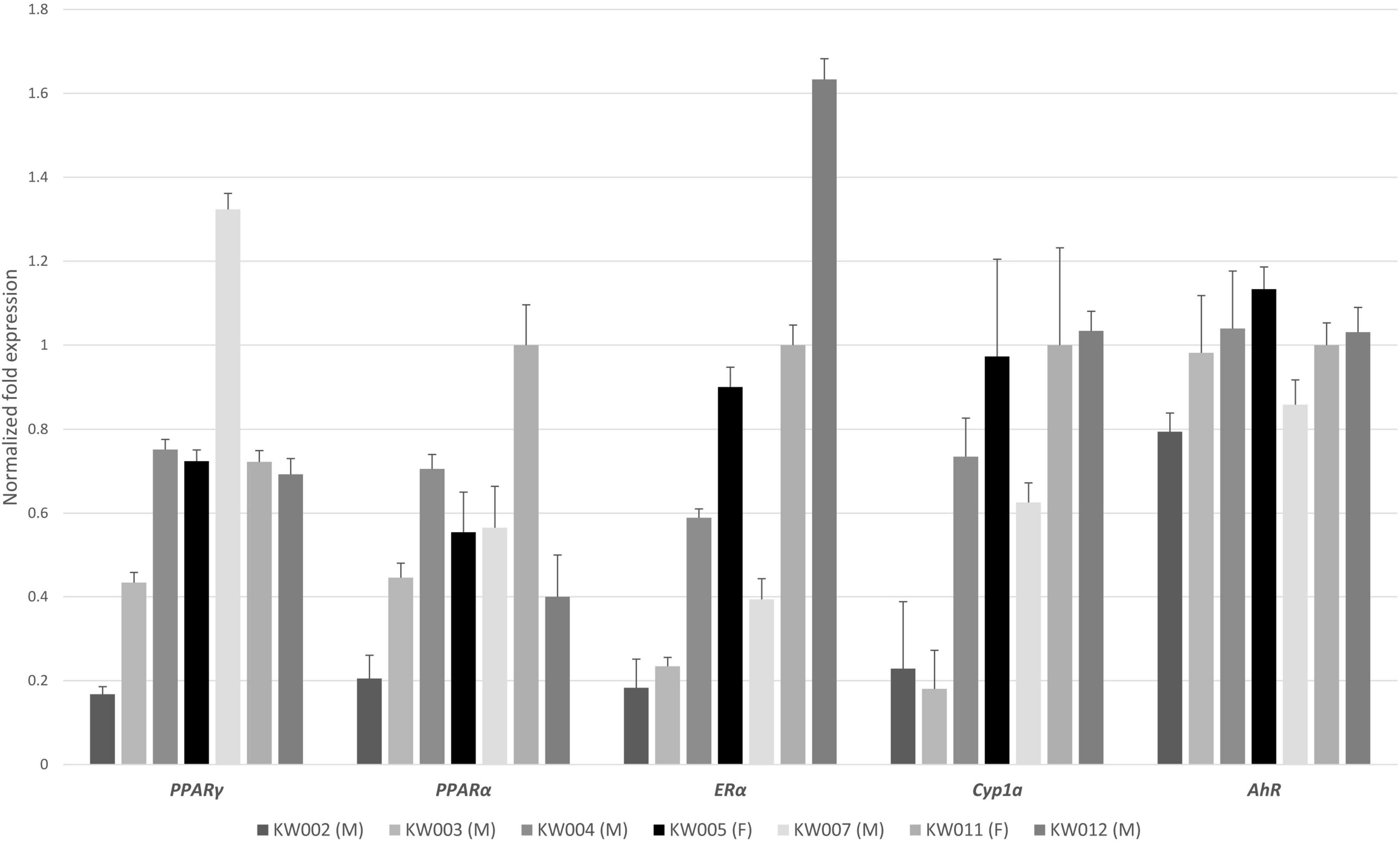
Figure 2. mRNA expression of five genes (PPARγ, PPARα, ERα, Cyp1a, and AhR) in the dermal part of RSKW skin biopsies for each individual (M = male, F = female). Relative quantities normalized to GAPDH and YWHAZ genes. The values are expressed as normalized fold expression ± standard deviation.
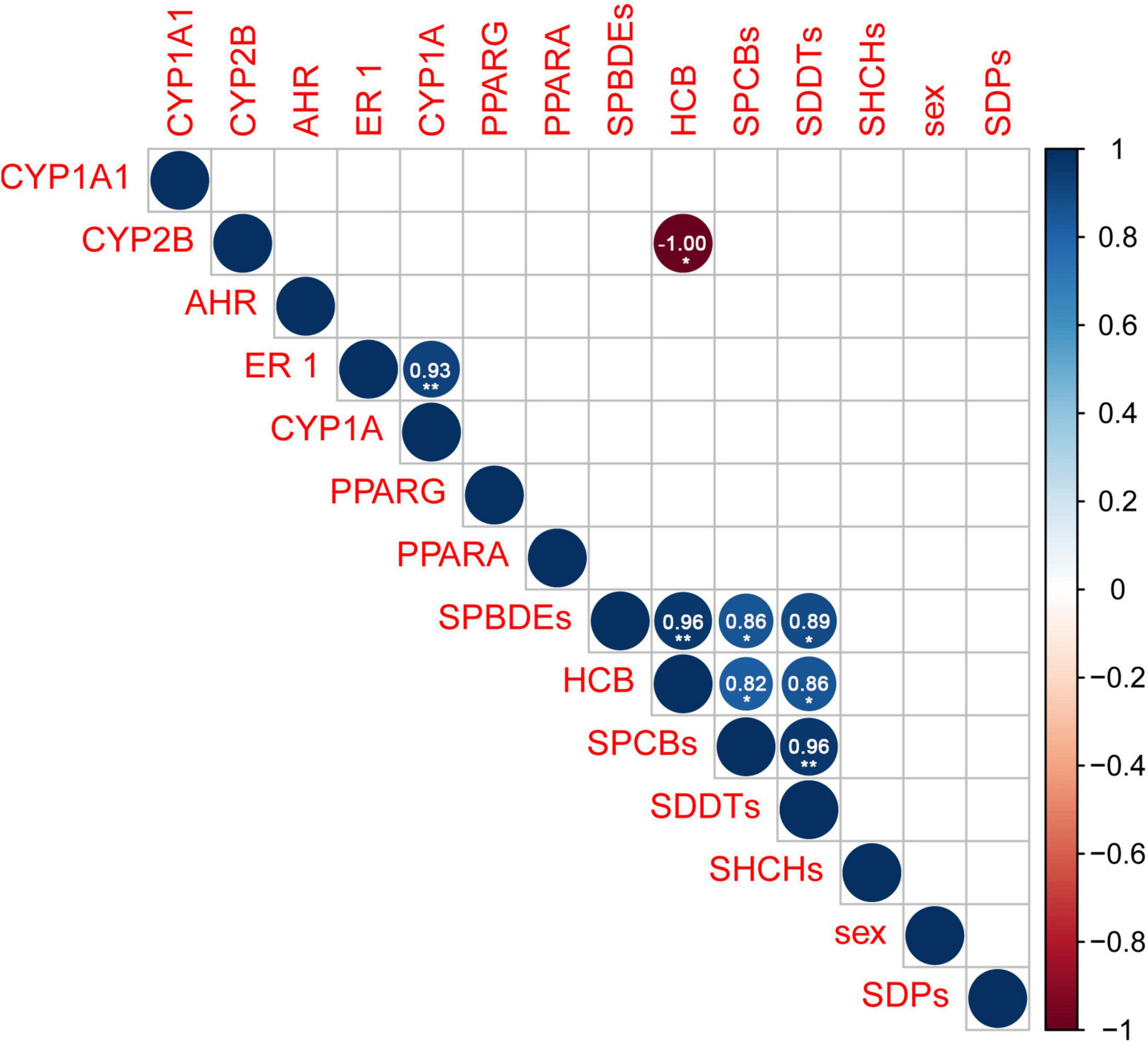
Figure 3. Correlation matrix of the variables analyzed in the RSKW samples. Blank square means no statistically significant correlation. Darker colors mean higher negative (red) or positive (blue) rho values. p Values: *p < 0.05; **p < 0.01, and rho values are displayed in the circles and reported in Supplementary Table 6.
The detection of cytochrome CYP450 protein expression by Western Blot technique was carried out for the first time on RSKWs. Cytochrome-like bands for the CYP1A1 (59 kDa) and CYP2B (56 kDa) were identified and quantified in the dermal part of five out of seven skin biopsies (Table 1). CYP1A1 and CYP2B protein expression have a broad range of expression among the samples, where CYP1A1 has higher values than CYP2B, as already shown in a previous study on the same species (Fossi et al., 2014) and other cetaceans (Hoydal et al., 2018; Baini et al., 2020).
Statistical Analysis of Persistent Organic Pollutants and Molecular Endpoints: Toxicological Implications
All the data obtained in the seven RSKWs were further analyzed to investigate if any endpoint or contaminant has the role as the main driver for the comprehensive ecotoxicological status evaluation in this species. The analysis was performed to highlight whether gene transcripts and protein concentrations are correlated with POP concentration or whether the alteration of gene and protein expression might be related to other potential stressors.
According to the PCA analysis, male and females did not cluster separately, probably due to the low number of samples, even if a trend of aggregation appears, mainly due to the contribution of POPs on dimension 2 and transcript values on dimension 1 (Figure 4), which contributes to explain more than 50% of the variance in the dataset.
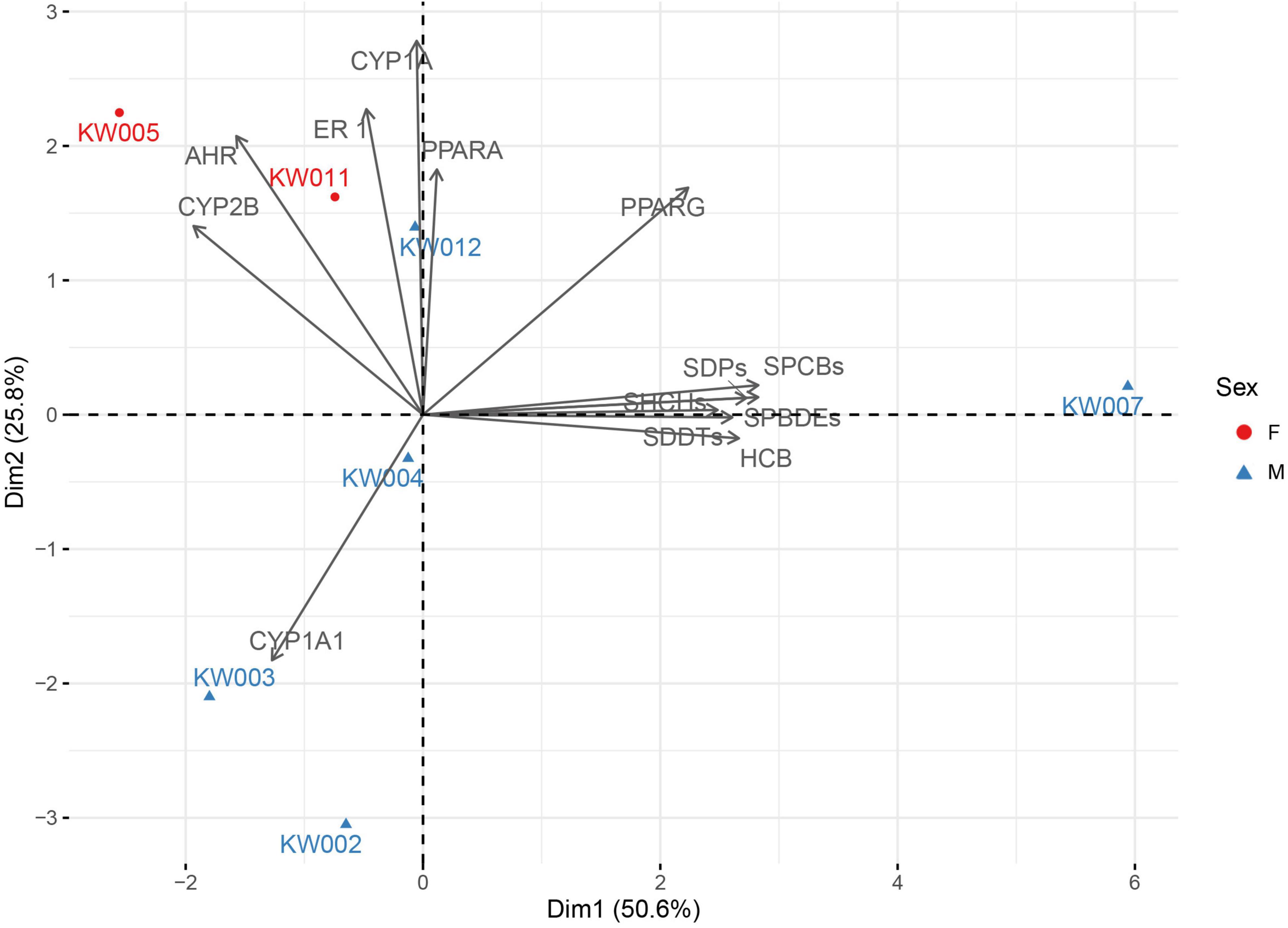
Figure 4. Principal component analysis (PCA) plot of mRNA level of the five target genes (PPARγ, PPARα, ERα, Cyp1a, and AhR), protein expression (CYP1A1 and 2B), and POPs (sum values of each class of compounds: PCBs, PBDEs, HCHs, HCB, and DP) in the seven individuals. Males are indicated by blue triangles and females by red circles. The total variance explained by the first two principal axes is 76.4%.
The absence of a significant correlation between transcript profiles and POPs may indicate that levels of contaminants measured in the blubber of RSKWs might not exert a contaminant-related biological response at these concentrations or that other variables both endogenous and exogenous, as different anthropogenic stressors or other classes of contaminants, are responsible for the alterations of the biological responses investigated. The levels of PCBs, for instance, are about 20-fold lower (1,060 ng/g l.b.) than the levels in Norwegian fish-eating killer whales (429 mg/kg l.b.), hundred-fold lower than mixed-diet killer whales (Remili et al., 2021), and well below the lowest value (9.0 mg/kg l.b.) proposed as the toxicity threshold concentration for the onset of physiologic effects in marine mammals (Kannan et al., 2000; Jepson et al., 2016).
Considering the link between biochemical endpoints and concentration of POPs, the most interesting result, apart from the correlation between Cyp1a and ERα transcripts, which has also been previously demonstrated due to the crosstalk between these two genes, is the negative correlation between HCB and CYP2B protein (rho = 1; p = 0.017; Figure 3).
The PCBs, PBDEs, DDTs, and HCB are positively correlated confirming the same behavior and the rate of accumulation in the adipose tissue of marine organisms (Figure 3 and Supplementary Table 6).
Conclusion
This work highlights and confirms the usefulness of a multidisciplinary approach successfully applied to bind together feeding ecology, foraging behavior, and ecotoxicological data (from both molecular biomarkers and several classes of POPs) in a poorly studied killer whale ecotype, frequenting an area with a high ecological value. The outstanding value of the Ross Sea Region, recognized by the Commission on the Conservation of Antarctic Marine Living Resources (CCAMLR) through the establishment of the Ross Sea Region Marine Protected Area (RSA MP) (CCAMLR, 2016), has also been the driving force for the expansion of the scientific infrastructure in the area, which may have brought about an increase in the anthropogenic pressure in the area (Lauriano et al., 2020), in addition to the cumulative stress to which the polar regions are subjected.
It should be highlighted the heightened contribution in this species of an often overlooked BDE congener in marine foodwebs, such as BDE-209. In that sense, this study’s results could contribute to spur the systematic investigation of this as well as other higher brominated congeners while studying the occurrence of PBDEs in marine ecosystems. Furthermore, the low rate of DP biomagnification found in RSKWs in comparison with data reported for other Antarctic marine mammals should be investigated to decipher the metabolic or other causes behind this behavior. Moreover, PCBs in RSKWs far below the toxicity threshold concentration seem to not affect molecular responses of target genes and proteins, which underline the current low risk of exposure to organic contaminants.
Overall, and within the RSA MP context, this work contributes to the establishment of baseline data for the fish-eating killer whale as a sentinel in this fragile Antarctic ecosystem. The recent predicted global killer whale collapse due to pollution (in particular PCBs; Desforges et al., 2018) makes this ecotype, which has been shown to be exposed to low levels of POPs, as a reservoir for the recovery of this species and to be considered as a “control” population to set baselines for biological and toxicological levels of this species worldwide.
Data Availability Statement
The datasets presented in this study can be found in online repositories. The names of the repository/repositories and accession number(s) can be found in the article/Supplementary Material.
Ethics Statement
Biopsies were obtained in accordance with the relevant guidelines and regulations imposed by the Italian Ministry and the Italian National Institute for Environmental Protection and Research. The research permits also included the necessary ethical approval in terms of sample collection, analysis, and use for scientific studies.
Author Contributions
CP performed molecular sex determination and biomarkers analysis, analyzed the data, and coordinated the writing, revision, and editing of the manuscript. JM-A performed contaminant analysis (Methodology, Validation, Data curation) and contributed significantly to the writing, revision, and editing of the manuscript. LM assisted with conceptualization of the study and revised and edited the manuscript. SP participated in the sampling and data collection, writing, revision, and editing of the manuscript. MB performed the WB analysis, analyzed the data, and contributed to the writing, revision, and editing of the manuscript. BJ supervised and provided financial support for the contaminant analysis and contributed to the revision and editing of the manuscript. MF assisted with conceptualisation of the study, data interpretation, and writing and provided a general overview. GL performed the conceptualization of the study, obtained funding, and participated in the sampling and data collection, writing, revision, and editing. All authors, read, and approved the submitted version.
Funding
This study was funded by the Italian Antarctic Research Programme (PNRA) granted to Giancarlo Lauriano (award n° 2013/AZ1.08).
Conflict of Interest
The authors declare that the research was conducted in the absence of any commercial or financial relationships that could be construed as a potential conflict of interest.
Publisher’s Note
All claims expressed in this article are solely those of the authors and do not necessarily represent those of their affiliated organizations, or those of the publisher, the editors and the reviewers. Any product that may be evaluated in this article, or claim that may be made by its manufacturer, is not guaranteed or endorsed by the publisher.
Acknowledgments
This research was part of POLARCSIC activities. JM-A thanks CSIC and MAGRAMA for his contracts under Projects 15CAES004 and 17CAES004.
Supplementary Material
The Supplementary Material for this article can be found online at: https://www.frontiersin.org/articles/10.3389/fmars.2022.818370/full#supplementary-material
References
Ainley, D. G., Lindke, K., Ballard, G., Lyver, P. O., Jennings, S., Toniolo, V., et al. (2017). Spatio-temporal occurrence patterns of cetaceans near Ross Island, Antarctica, 2002–2015: implications for food web dynamics. Polar Biol. 40, 1761–1775. doi: 10.1007/s00300-017-2100-9
Alava, J. J., Ross, P. S., and Gobas, F. A. P. C. (2016). Food web bioaccumulation model for resident killer whales from the Northeastern Pacific ocean as a tool for the derivation of PBDE-sediment quality guidelines. Arch. Environ. Contam. Toxicol. 70, 155–168. doi: 10.1007/s00244-015-0215-y
Andvik, C., Jourdain, E., Lyche, J. L., Karoliussen, R., and Borgå, K. (2021). High levels of legacy and emerging contaminants in killer whales (Orcinus orca) from Norway, 2015 to 2017. Environ. Toxicol. Chem. 40, 1848–1858. doi: 10.1002/etc.5064
Andvik, C., Jourdain, E., Ruus, A., Lyche, J. L., Karoliussen, R., and Borgå, K. (2020). Preying on seals pushes killer whales from Norway above pollution effects thresholds. Sci. Rep. 10:11888. doi: 10.1038/s41598-020-68659-y
Atkinson, S., Branson, M., Burdin, A., Boyd, D., and Ylitalo, G. M. (2019). Persistent organic pollutants in killer whales (Orcinus orca) of the Russian Far East. Mar. Pollut. Bull. 149:110593.
Baini, M., Panti, C., Fossi, M. C., Tepsich, P., Jiménez, B., Coomber, F., et al. (2020). First assessment of POPs and cytochrome P450 expression in Cuvier’s beaked whales (Ziphius cavirostris) skin biopsies from the Mediterranean Sea. Sci. Rep. 10, 1–13.
Ballard, G., Jongsomjit, D., Veloz, S. D., and Ainley, D. G. (2012). Coexistence of mesopredators in an intact polar ocean ecosystem: the basis for defining a Ross Sea marine protected area. Biol. Conserv. 156, 72–82. doi: 10.1016/j.biocon.2011.11.017
Berube, M., and Palsbøll, P. (1996). Identification of sex in Cetaceans by multiplexing with three ZFX and ZFY specific primers. Mol. Ecol. 5, 283–287. doi: 10.1111/j.1365-294X.1996.tb00315.x
Borja, J., Taleon, D. M., Auresenia, J., and Gallardo, S. (2005). Polychlorinated biphenyls and their biodegradation. Process Biochem. 40, 1999–2013.
Buckman, A. H., Veldhoen, N., Ellis, G., Ford, J. K. B., Helbing, C. C., and Ross, P. S. (2011). PCB-associated changes in mRNA expression in killer whales (Orcinus orca) from the NE Pacific Ocean. Environ. Sci. Technol. 45, 10194–10202. doi: 10.1021/es201541j
Castelli, M. G., Rusten, M., Goksøyr, A., and Routti, H. (2014). mRNA expression of genes regulating lipid metabolism in ringed seals (Pusa hispida) from differently polluted areas. Aquat. Toxicol. 146, 239–246. doi: 10.1016/j.aquatox.2013.11.015
CCAMLR (2016). Report of the Thirty-Fifth Meeting of the Commission. Hobart: Commission for the Conservation of Antarctic Marine Living Resources.
Corsolini, S. (2009). Industrial contaminants in Antarctic biota. J. Chromatogr. A 1216, 598–612. doi: 10.1016/j.chroma.2008.08.012
Corsolini, S., Ademollo, N., Martellini, T., Randazzo, D., Vacchi, M., and Cincinelli, A. (2017). Legacy persistent organic pollutants including PBDEs in the trophic web of the Ross Sea (Antarctica). Chemosphere 185, 699–708. doi: 10.1016/j.chemosphere.2017.07.054
Desforges, J.-P., Hall, A., McConnell, B., Rosing-Asvid, A., Barber, J. L., Brownlow, A., et al. (2018). Predicting global killer whale population collapse from PCB pollution. Science 361, 1373–1376. doi: 10.1126/science.aat1953
Desforges, J.-P. W., Sonne, C., Levin, M., Siebert, U., De Guise, S., and Dietz, R. (2016). Immunotoxic effects of environmental pollutants in marine mammals. Environ. Int. 86, 126–139. doi: 10.1016/j.envint.2015.10.007
Foote, A. D., Vijay, N., Ávila-Arcos, M. C., Baird, R. W., Durban, J. W., Fumagalli, M., et al. (2016). Genome-culture coevolution promotes rapid divergence of killer whale ecotypes. Nat. Commun. 7:11693. doi: 10.1038/ncomms11693
Fossi, M. C., Baini, M., Panti, C., Galli, M., Jiménez, B., Muñoz-Arnanz, J., et al. (2017). Are whale sharks exposed to persistent organic pollutants and plastic pollution in the Gulf of California (Mexico)? First ecotoxicological investigation using skin biopsies. Comp. Biochem. Physiol. C Toxicol. Pharmacol. 199, 48–58. doi: 10.1016/j.cbpc.2017.03.002
Fossi, M. C., Casini, S., Bucalossi, D., and Marsili, L. (2008). First detection of CYP1A1 and CYP2B induction in Mediterranean cetacean skin biopsies and cultured fibroblasts by Western blot analysis. Mar. Environ. Res. 66, 3–6. doi: 10.1016/j.marenvres.2008.02.006
Fossi, M. C., Panti, C., Baini, M., and Baulch, S. (2018). “Impacts of marine litter on cetaceans: a focus on plastic pollution,” in Marine Mammal Ecotoxicology: Impacts of Multiple Stressors on Population Health, eds M. C. Fossi and C. Panti (Cambridge, MA: Academic Press).
Fossi, M. C., Panti, C., Marsili, L., Maltese, S., Coppola, D., Jimenez, B., et al. (2014). Could feeding habit and migratory behaviour be the causes of different toxicological hazard to cetaceans of Gulf of California (Mexico)? Environ. Sci. Pollut. Res. 21, 13353–13366. doi: 10.1007/s11356-014-2574-8
Godard, C. A. J. (2004). Induction of cetacean cytochrome P4501A1 by -naphthoflavone exposure of skin biopsy slices. Toxicol. Sci. 80, 268–275. doi: 10.1093/toxsci/kfh124
Godard-Codding, C. A. J., and Fossi, M. C. (2018). “Field sampling techniques and ecotoxicologic biomarkers in cetaceans,” in Marine Mammal Ecotoxicology, eds M. C. Fossi and C. Panti (Amsterdam: Elsevier), 237–259.
Griffin, M. D., Pereira, S. R., DeBari, M. K., and Abbott, R. D. (2020). Mechanisms of action, chemical characteristics, and model systems of obesogens. BMC Biomed. Eng. 2:6. doi: 10.1186/s42490-020-00040-6
Hao, Y., Li, Y., Han, X., Wang, T., Yang, R., Wang, P., et al. (2019). Air monitoring of polychlorinated biphenyls, polybrominated diphenyl ethers and organochlorine pesticides in West Antarctica during 2011–2017: concentrations, temporal trends and potential sources. Environ. Pollut. 249, 381–389. doi: 10.1016/j.envpol.2019.03.039
Haraguchi, K., Hisamichi, Y., Kotaki, Y., Kato, Y., and Endo, T. (2009). Halogenated bipyrroles and methoxylated tetrabromodiphenyl ethers in tiger shark (Galeocerdo cuvier) from the Southern Coast of Japan. Environ. Sci. Technol. 43, 2288–2294. doi: 10.1021/es802999k
Hoydal, K. S., Jenssen, B. M., Letcher, R. J., Dam, M., and Arukwe, A. (2018). Hepatic phase I and II biotransformation responses and contaminant exposure in long-finned pilot whales from the Northeastern Atlantic. Mar. Environ. Res. 134, 44–54. doi: 10.1016/j.marenvres.2017.12.010
Hückstädt, L. A., McCarthy, M. D., Koch, P. L., and Costa, D. P. (2017). What difference does a century make? Shifts in the ecosystem structure of the Ross Sea, Antarctica, as evidenced from a sentinel species, the Weddell seal. Proc. R. Soc. B. 284:20170927. doi: 10.1098/rspb.2017.0927
Jepson, P. D., Deaville, R., Barber, J. L., Aguilar, À, Borrell, A., Murphy, S., et al. (2016). PCB pollution continues to impact populations of orcas and other dolphins in European waters. Sci. Rep. 6:18573.
Kannan, K., Blankenship, A. L., Jones, P. D., and Giesy, J. P. (2000). Toxicity reference values for the toxic effects of polychlorinated biphenyls to aquatic mammals. Hum. Ecol. Risk Assess. Int. J. 6, 181–201. doi: 10.1080/10807030091124491
Kester, M. H. A., Bulduk, S., Tibboel, D., Meinl, W., Glatt, H., Falany, C. N., et al. (2000). Potent inhibition of estrogen sulfotransferase by hydroxylated PCB metabolites: a novel pathway explaining the estrogenic activity of PCBs. Endocrinology 141, 1897–1900. doi: 10.1210/endo.141.5.7530
Kim, E.-Y., Iwata, H., Suda, T., Tanabe, S., Amano, M., Miyazaki, N., et al. (2005). Aryl hydrocarbon receptor (AHR) and AHR nuclear translocator (ARNT) expression in Baikal seal (Pusa sibirica) and association with 2,3,7,8-TCDD toxic equivalents and CYP1 expression levels. Comp. Biochem. Physiol. C Toxicol. Pharmacol. 141, 281–291. doi: 10.1016/j.cca.2005.07.007
Krahn, M. M., Pitman, R. L., Burrows, D. G., Herman, D. P., and Pearce, R. W. (2008). Use of chemical tracers to assess diet and persistent organic pollutants in Antarctic Type C killer whales. Mar. Mamm. Sci. 24, 643–663. doi: 10.1111/j.1748-7692.2008.00213.x
Kurtz, A. E., Reiner, J. L., West, K. L., and Jensen, B. A. (2019). Perfluorinated alkyl acids in hawaiian cetaceans and potential biomarkers of effect: peroxisome proliferator-activated receptor alpha and cytochrome P450 4A. Environ. Sci. Technol. 53, 2830–2839. doi: 10.1021/acs.est.8b05619
Lauriano, G., Fortuna, C. M., and Vacchi, M. (2011). Occurrence of killer whales (Orcinus orca) and other cetaceans in Terra Nova Bay, Ross Sea, Antarctica. Antartic Sci. 23, 139–143. doi: 10.1017/S0954102010000908
Lauriano, G., Pirotta, E., Joyce, T., Pitman, R. L., Borrell, A., and Panigada, S. (2020). Movements, diving behaviour and diet of type-C killer whales (Orcinus orca) in the Ross Sea, Antarctica. Aquat. Conserv. Mar. Freshw. Ecosyst. 30, 2428–2440. doi: 10.1002/aqc.3371
Lawson, T. M., Ylitalo, G. M., O’Neill, S. M., Dahlheim, M. E., Wade, P. R., Matkin, C. O., et al. (2020). Concentrations and profiles of organochlorine contaminants in North Pacific resident and transient killer whale (Orcinus orca) populations. Sci. Total Environ. 722:137776. doi: 10.1016/j.scitotenv.2020.137776
Livak, K. J., and Schmittgen, T. D. (2001). Analysis of relative gene expression data using real-time quantitative PCR and the 2-ΔΔCT method. Methods 25, 402–408. doi: 10.1006/meth.2001.1262
Lühmann, K., Lille-Langøy, R., Øygarden, L., Kovacs, K. M., Lydersen, C., Goksøyr, A., et al. (2020). Environmental pollutants modulate transcriptional activity of nuclear receptors of whales in vitro. Environ. Sci. Technol. 54, 5629–5639. doi: 10.1021/acs.est.9b06952
Matthews, J., Wihlén, B., Heldring, N., MacPherson, L., Helguero, L., Treuter, E., et al. (2007). Co-planar 3,3′,4,4′,5-pentachlorinated biphenyl and non-co-planar 2,2′,4,6,6′-pentachlorinated biphenyl differentially induce recruitment of oestrogen receptor α to aryl hydrocarbon receptor target genes. Biochem. J. 406, 343–353. doi: 10.1042/BJ20070585
McCormley, M. C., Noren, D. P., Ylitalo, G. M., and St. Leger, J. (2021). Partitioning of persistent organic pollutants between blubber and blood in killer whales (Orcinus orca). Mar. Mam. Sci. 37, 1531–1543. doi: 10.1111/mms.12819
McHugh, B., Law, R. J., Allchin, C. R., Rogan, E., Murphy, S., Foley, M. B., et al. (2007). Bioaccumulation and enantiomeric profiling of organochlorine pesticides and persistent organic pollutants in the killer whale (Orcinus orca) from British and Irish waters. Mar. Pollut. Bull. 54, 1724–1731. doi: 10.1016/j.marpolbul.2007.07.004
Morales, P., Roscales, J. L., Muñoz-Arnanz, J., Barbosa, A., and Jiménez, B. (2022). Evaluation of PCDD/Fs, PCBs and PBDEs in two penguin species from Antarctica. Chemosphere 286:131871. doi: 10.1016/j.chemosphere.2021.131871
Morin, P. A., Parsons, K. M., Archer, F. I., Ávila-Arcos, M. C., Barrett-Lennard, L. G., Dalla Rosa, L., et al. (2015). Geographic and temporal dynamics of a global radiation and diversification in the killer whale. Mol. Ecol. 24, 3964–3979. doi: 10.1111/mec.13284
Muñoz-Arnanz, J., Chirife, A. D., Galletti Vernazzani, B., Cabrera, E., Sironi, M., Millán, J., et al. (2019). First assessment of persistent organic pollutant contamination in blubber of Chilean blue whales from Isla de Chiloé, southern Chile. Sci. Tot. Environ. 650, 1521–1528. doi: 10.1016/j.scitotenv.2018.09.070
Muñoz-Arnanz, J., Roscales, J. L., Ros, M., Vicente, A., and Jiménez, B. (2016). Towards the implementation of the stockholm convention in Spain: five-year monitoring (2008–2013) of POPs in air based on passive sampling. Environ. Pollut. 217, 107–113. doi: 10.1016/j.envpol.2016.01.052
Na, G., Yao, Y., Gao, H., Li, R., Ge, L., Titaley, I. A., et al. (2017). Trophic magnification of dechlorane plus in the marine food webs of fildes peninsula in antarctica. Mar. Pollut. Bull. 117, 456–461. doi: 10.1016/j.marpolbul.2017.01.049
Noël, M., Barrett-Lennard, L., Guinet, C., Dangerfield, N., and Ross, P. S. (2009). Persistent organic pollutants (POPs) in killer whales (Orcinus orca) from the Crozet Archipelago, southern Indian Ocean. Mar. Environ. Res. 68, 196–202.
Noël, M., Loseto, L. L., Helbing, C. C., Veldhoen, N., Dangerfield, N. J., and Ross, P. S. (2014). PCBs are associated with altered gene transcript profiles in arctic beluga whales (Delphinapterus leucas). Environ. Sci. Technol. 48, 2942–2951. doi: 10.1021/es403217r
Panti, C., Spinsanti, G., Marsili, L., Casini, S., Frati, F., and Fossi, M. C. (2011). Ecotoxicological diagnosis of striped dolphin (Stenella coeruleoalba) from the Mediterranean basin by skin biopsy and gene expression approach. Ecotoxicology 20, 1791–1800. doi: 10.1007/s10646-011-0713-2
Pedro, S., Dietz, R., Sonne, C., Rosing-Asvid, A., Hansen, M., and McKinney, M. A. (2019). Are vitamins A and E associated with persistent organic pollutants and fatty acids in the blubber of highly contaminated killer whales (Orcinus orca) from Greenland? Environ. Res. 177:108602. doi: 10.1016/j.envres.2019.108602
Pitman, R. L., and Ensor, P. (2003). Three forms of killer whales (Orcinus orca) in Antarctic waters. J. Cetacean Res. Manag. 5, 131–140.
Pitman, R. L., Fearnbach, H., and Durban, J. W. (2018). Abundance and population status of Ross Sea killer whales (Orcinus orca, type C) in McMurdo Sound, Antarctica: evidence for impact by commercial fishing? Polar Biol. 41, 781–792. doi: 10.1007/s00300-017-2239-4
R Core Team (2021). R: A Language and Environment for Statistical Computing. Vienna: R Foundation for Statistical Computing.
Rayne, S., Ikonomou, M. G., Ross, P. S., Ellis, G. M., and Barrett-Lennard, L. G. (2004). PBDEs, PBBs, and PCNs in three communities of free-ranging killer whales (Orcinus orca) from the northeastern Pacific Ocean. Environ. Sci. Technol. 38, 4293–4299.
Remili, A., Letcher, R. J., Samarra, F. I. P., Dietz, R., Sonne, C., Desforges, J.-P., et al. (2021). Individual prey specialization drives PCBs in icelandic killer whales. Environ. Sci. Technol. 55, 4923–4931. doi: 10.1021/acs.est.0c08563
Rosen, E. D., Sarraf, P., Troy, A. E., Bradwin, G., Moore, K., Milstone, D. S., et al. (1999). PPARγ is required for the differentiation of adipose tissue in vivo and in vitro. Mol. Cell 4, 611–617. doi: 10.1016/S1097-2765(00)80211-7
Ross, P. S., Ellis, G. M., Ikonomou, M. G., Barrett-Lennard, L. G., and Addison, R. F. (2000). High PCB concentrations in free-ranging pacific killer whales, Orcinus orca: effects of age, sex and dietary preference. Mar. Pollut. Bull. 40, 504–515. doi: 10.1016/S0025-326X(99)00233-7
Routti, H., Diot, B., Panti, C., Duale, N., Fossi, M. C., Harju, M., et al. (2019). Contaminants in Atlantic walruses in Svalbard Part 2: relationships with endocrine and immune systems. Environ. Pollut. 246, 658–667. doi: 10.1016/j.envpol.2018.11.097
Routti, H., Lille-Langøy, R., Berg, M. K., Fink, T., Harju, M., Kristiansen, K., et al. (2016). Environmental chemicals modulate polar bear (Ursus maritimus) peroxisome proliferator-activated receptor gamma (PPARG) and adipogenesis in vitro. Environ. Sci. Technol. 50, 10708–10720. doi: 10.1021/acs.est.6b03020
Schlingermann, M., Berrow, S., Craig, D., McHugh, B., Marrinan, M., O’Brien, J., et al. (2020). High concentrations of persistent organic pollutants in adult killer whales (Orcinus orca) and a foetus stranded in Ireland. Mar. Pollut. Bull. 151:110699. doi: 10.1016/j.marpolbul.2019.110699
Schupp, M., and Lazar, M. A. (2010). Endogenous ligands for nuclear receptors: digging deeper. J. Biol. Chem. 285, 40409–40415. doi: 10.1074/jbc.R110.182451
Spinsanti, G., Panti, C., Lazzeri, E., Marsili, L., Casini, S., Frati, F., et al. (2006). Selection of reference genes for quantitative RT-PCR studies in striped dolphin (Stenella coeruleoalba) skin biopsies. BMC Mol. Biol. 7:32. doi: 10.1186/1471-2199-7-32
Tartu, S., Lille-Langøy, R., Størseth, T. R., Bourgeon, S., Brunsvik, A., Aars, J., et al. (2017). Multiple-stressor effects in an apex predator: combined influence of pollutants and sea ice decline on lipid metabolism in polar bears. Sci. Rep. 7:16487. doi: 10.1038/s41598-017-16820-5
Vorkamp, K., Rigét, F., Sanderson, H., Bossi, R., and Mantzius, K. (2019). POP/PBT Characterisation of Dechlorane Plus and Novel Brominated Flame Retardants and No: Scientific Report from DCE–Danish Centre for Environment and Energy. Denmark: Aarhus University.
Wilson, J. Y., Wells, R., Aguilar, A., Borrell, A., Tornero, V., Reijnders, P., et al. (2007). Correlates of cytochrome P450 1A1 expression in bottlenose dolphin (Tursiops truncatus) integument biopsies. Toxicol. Sci. 97, 111–119. doi: 10.1093/toxsci/kfm031
Keywords: cetaceans, persistent organic pollutants (POPs), dechlorane plus (DP), gene expression, qRT- PCR, protein expression, PPARs
Citation: Panti C, Muñoz-Arnanz J, Marsili L, Panigada S, Baini M, Jiménez B, Fossi MC and Lauriano G (2022) Ecotoxicological Characterization of Type C Killer Whales From Terra Nova Bay (Ross Sea, Antarctica): Molecular Biomarkers, Legacy, and Emerging Persistent Organic Contaminants. Front. Mar. Sci. 9:818370. doi: 10.3389/fmars.2022.818370
Received: 19 November 2021; Accepted: 27 January 2022;
Published: 10 March 2022.
Edited by:
Juan José Alava, University of British Columbia, CanadaReviewed by:
Annalisa Zaccaroni, University of Bologna, ItalyMassimiliano Rosso, CIMA Research Foundation, Italy
Ana Tirapé, ESPOL Polytechnic University, Ecuador
Copyright © 2022 Panti, Muñoz-Arnanz, Marsili, Panigada, Baini, Jiménez, Fossi and Lauriano. This is an open-access article distributed under the terms of the Creative Commons Attribution License (CC BY). The use, distribution or reproduction in other forums is permitted, provided the original author(s) and the copyright owner(s) are credited and that the original publication in this journal is cited, in accordance with accepted academic practice. No use, distribution or reproduction is permitted which does not comply with these terms.
*Correspondence: Cristina Panti, cGFudGk0QHVuaXNpLml0