- 1CAS Key Laboratory of Tropical Marine Bio-Resources and Ecology, South China Sea Institute of Oceanology, Chinese Academy of Sciences, Guangzhou, China
- 2University of Chinese Academy of Sciences, Beijing, China
- 3Innovation Academy of South China Sea Ecology and Environmental Engineering, Chinese Academy of Sciences, Guangzhou, China
- 4Department of Ecology and Institute of Hydrobiology, Jinan University, Guangzhou, China
Reef-building corals are invertebrate animals that associate with diverse microorganisms, including Symbiodiniaceae, bacteria, fungi, and archaea. This symbiotic consortium, called the holobiont, is a dynamic system and rapidly responds to environmental temperatures. At present, the effects of temperature on bacteria-bacteria interactions in the coral-associated bacterial community are not clear. Antagonism is considered one of the potential structuring forces in coral microbial communities. Here, we examined the antagonistic interactions among 32 coral-associated bacteria and the physiological and biochemical characteristics of these isolates at different temperatures. The results showed that the antagonism breadth (i.e. the total number of antagonistic interactions) increased but antagonism intensity (i.e. the size of the inhibition zone) decreased at 32°C. The antagonistic interaction network was nested and sender-determined both at 25°C and 32°C, suggesting that the competition networks of coral-associated bacteria were more influenced by the antagonist strains than sensitive strains. Furthermore, we found that the elevated temperature increased the complexity of the antagonistic network. By evaluating the correlations between antagonism and the phylogenetic and phenotypic distances, we demonstrated that the antagonism probability correlated with the phylogenetic distance rather than phenotypic distance. Moreover, the antagonist strains have a wider metabolic niche space, i.e., grew on more carbon sources, than the antagonized strains at 25°C, while there was no difference at 32°C, suggesting the trade-off between antagonism and resource exploitation shifted in the antagonistic interactions under the higher temperature. These findings will be helpful for understanding the bacterial interactions in coral holobionts and the assembly of bacterial community in altered environments, especially under heat stress.
Introduction
Reef-building corals associate with dinoflagellates within Symbiodiniaceae and other groups of microorganisms, such as fungi, bacteria, archaea, and viruses, that inhabit the coral tissue, mucus, gastro-vascular cavity, and skeleton (Rohwer et al., 2002; van Oppen and Blackall, 2019). The coral-associated microbiome is highly dynamic, the structure and composition of which can rapidly change with environmental conditions, such as temperature, pH, and eutrophication (Meron et al., 2012; Jessen et al., 2013; Pootakham et al., 2018). Previous studies have shown that coral-associated bacteria play an essential role in coral health, including translocating fixed nitrogen (Olson et al., 2009), preventing infection with pathogens (Nissimov et al., 2009; Rypien et al., 2010), and inducing coral larval settlement and metamorphosis (Negri et al., 2001; Tebben et al., 2011). Coral-associated bacteria could act as a defense barrier against pathogenic microbes to facilitate homeostasis and contribute to the survival of the coral holobiont (Glasl et al., 2016) through antibiotics production, living space occupation, and nutrients competition (Rohwer and Kelley, 2004).
Global coral reef ecosystems are threatened by climate change (e.g., increasing seawater temperatures, ocean acidification, and more frequent tropical storms) and direct anthropogenic pressure (e.g., pollution, over-exploitation, and eutrophication). According to records, the scale, frequency and intensity of coral bleaching events are increased caused by anthropogenic global warming, which leads to coral morbidity and mortality and has substantially decimated coral reefs (Hughes et al., 2017; Hughes et al., 2018; Sully et al., 2019). Notably, heat stress changes the community structures of coral-associated bacteria (Ainsworth and Hoegh-Guldberg, 2009; Littman et al., 2010; Littman et al., 2011; Lee et al., 2015; Tout et al., 2015; Ziegler et al., 2017; Grottoli et al., 2018; Li et al., 2021), and the abundances of potential pathogens subsequently increase (Littman et al., 2010; Littman et al., 2011; Tout et al., 2015). In addition, coral-associated bacterial communities may contribute to the thermal resilience of the coral host (Littman et al., 2010; Ziegler et al., 2017; Grottoli et al., 2018; Epstein et al., 2019; Li et al., 2021), suggesting that adaptation through changes in bacterial communities may constitute different possible mechanisms for counteracting adverse environmental effects (Ziegler et al., 2017). However, the mechanism of coral-associated bacterial assembly has not been well elucidated (Zhang et al., 2021). Antagonism among bacteria has been considered one of the potential structuring forces in microbial communities (Vetsigian et al., 2011; Perez-Gutierrez et al., 2013; Garcia-Bayona and Comstock, 2018), while it has rarely been investigated in coral holobionts, especially through experimental tests based on pure cultures (Long et al., 2005; Rypien et al., 2010). Additionally, the correlation between antagonism and phenotypical properties of coral-associated bacteria has not been studied.
The competition-relatedness hypothesis proposed by Darwin over 150 years ago (Darwin, 1859) is that phylogenetically closely related species are more likely to compete strongly than distantly associated species due to their functional similarity. This hypothesis has been proven (e.g., in oak, trees, and bacteria) (Cavender-Bares et al., 2004; Kunstler et al., 2016; Russel et al., 2017) or denied (e.g., in vascular plants and green algae) (Cahill et al., 2008; Venail et al., 2014; Alexandrou et al., 2015) according to researches on various subjects. Case and Gilpin (1974) hypothesized a trade-off between interference competition (i.e., antagonism) and resource exploitation efficiency. Russel et al. (2017) further speculated that antagonists were generalists, and there is a trade-off between specializing in exploiting few resources efficiently or growing on many resources and antagonizing the specialists. To test whether the antagonistic interactions occurred among the coral-associated bacteria satisfy these hypotheses, we investigated the antagonisms among 30 bacteria isolated from the tissue of coral Pocillopora damicornis and two pathogenic vibrions and the physiological and biochemical characteristics of these isolates at different temperatures. On the basis of these results, we analyzed the correlations between antagonism and phenotypic and phylogenetic characteristics, the trade-off between antagonism and resource exploitation, and the effects of temperature on the antagonistic interaction network. This study will help understand the bacterial interactions in coral holobionts and the assembly of bacterial community under altered environments.
Materials and Methods
Antagonistic Interaction Assays
Bacterial strains used in this study (Table S1) were mainly isolated from the tissue of the healthy coral P. damicornis, with strains Vibrio mediterranei DSM 13774 and Vibrio coralliilyticus DSM 19607 isolated from the bleached corals Oculina patagonica (Kushmaro et al., 1996) and P. damicornis (Ben-Haim and Rosenberg, 2002), respectively. Strains DSM 13774 and DSM 19607 were provided by DSMZ (German Collection of Microorganisms and Cell Cultures). Antagonistic interactions were tested on marine agar 2216 (MA; BD; Becton, Dickinson and Company) using Burkholder diffusion assays (Burkholder et al., 1966). Each isolate was streaked from the -80°C glycerol stock on MA, and a single colony was then transferred to 6 ml of marine broth 2216 (MB; Becton, Dickinson and Co.) and shaken at 25°C for 24 h. The OD600 was measured for each isolate before starting the assay using spectrophotometer (TU-1810, Persee General Instrument co., LTD, Beijing). Ten milliliters of top lawn bacteria (1 ml of bacterial culture added to 100 ml MA with 0.6% [w/v] agar, gently mixed) was poured on a sterile MA (with 1.5% agar) petri dish plate. The lawn was allowed to cool, and then 10 μl of each producer isolate was spotted onto the agar in petri dish plates (12 cm×12 cm). Control producer spots consisted of 10 μl of marine broth. Plates were incubated at 25°C and 32°C for 48 h, respectively, and then imaged. Antagonism was considered to occur when the semidiameter of the zone of inhibition was at least 0.4 mm greater than the semidiameter of the colony formed by the potential producer. This size of inhibition was chosen because it represents a spatial scale relevant to bacterial interactions (Long and Azam, 2001). The semidiameters of the inhibition halo and colony were measured by digital imaging software (ImageJ, NIH), and the decimal places were set to 3 in Set Measurements of ImageJ software. We tested the antagonistic interactions of 32 isolates in pairs, and the experiment was 32×32 (1024) times. Each experiment was carried out in 3 replicates. There are fourteen antagonistic interactions with ambiguous results that were re-verified with 3 replicates.
Assessment of Phenotypic Fingerprint
The phenotypic fingerprint of each isolate, including 71 carbon source utilization assays and 23 chemical sensitivity assays (the detailed assays were presented in Table S2), was tested by using BIOLOG GEN III MicroPlate (Biolog Inc.). Isolates were cultured for three days on MA at 25°C, a single fresh colony was then picked and dispersed in the modified inoculating fluid A (Biolog Inc.) with increasing salinity to 30‰ for maintaining the osmotic pressure as the salinity of culture medium MA was approximately 30 ‰, and the turbidity of the suspension was 92-95%T. The cell suspension was inoculated into the GENIII MicroPlate (100 μl per well) and incubated at 25°C and 32°C respectively. Color reactions in the microplate wells were determined using the Biolog Microbial Identification System, MicroStation Reader (Biolog Inc., ELx808BLG, USA) with absorbance measured at 590 nm every 24 h for a total duration of 7 days. The “borderline” value was scored as positive if the value of absorbance was higher than that of the negative control.
Phylogenetical and Statistical Analyses
For each interaction pair of coral-associated bacteria, one antagonistic pair was defined by the inhibition value in three statuses: x=1 if isolate A inhibited B, y=1 if B inhibited A or if both x and y were 1, which means reciprocal inhibition. If both x and y were zero, which means no inhibition occurred. A phylogenetic tree was constructed based on 16S rRNA gene sequences using the neighbor-joining method with MEGA X (Kumar et al., 2018), and the best DNA model Tamura-Nei was used. Topologies of the phylogenetic trees were evaluated using the bootstrap method with 1000 resamplings. Pairwise phylogenetic distances were calculated using the Tamura-Nei model with MEGA X (Kumar et al., 2018). Phenotypic dissimilarity was quantified by calculating the Jaccard distance according to the results of the GEN III MicroPlate tests. Correlations between antagonism and the phylogenetic and phenotypic distances were evaluated with logistic regression (glm, binomial family). To infer whether the correlation between antagonism and the phenotypic distances was confounded by the correlation between the phylogenetic and phenotypic distances, linear regression for phenotypic distance with phylogenetic distance was performed. The residuals (the variation was not explained by phylogenetic distance) were then used as independent variables in the logistic regressions (Russel et al., 2017). These analyses were performed using R version 4.0.1 (R Core Team, 2020).
Network Analysis
The interaction frequency was calculated as the number of antagonistic interactions observed divided by the number of total possible antagonistic interactions determined by the number of strains tested (Vetsigian et al., 2011). Sender-receiver asymmetry, which represents whether the network is more determined by the sender or receiver strains on average, was evaluated by comparing the variance of the sender and receiver degrees (Vetsigian et al., 2011; Perez-Gutierrez et al., 2013). The sender degree of one isolate means the number of isolates it inhibits, and the receiver degree represents the number of isolates that inhibit the isolate (Perez-Gutierrez et al., 2013). The network diameter, the longest path connecting any two strains (Newman, 2003), was calculated using the network analyzer tool implemented in Cytoscape v3.8.0 (Shannon et al., 2003). Nestedness, a property of the interaction network, was calculated as defined by Bascompte and colleagues (Bascompte et al., 2003) using the algorithm implemented in BINMATNEST (binary matrix nestedness temperature calculator) (Rodriguez-Girones and Santamaria, 2006). Antagonistic interaction networks among coral-associated bacteria were visualized with yFiles, a hierarchical layout option of Cytoscape v3.8.0 (Shannon et al., 2003). The “nodes” represent strains in the networks, and the “edges” depict directed antagonistic interactions. The connectivity of a network is the total number of interactions (Landi et al., 2018), represented by the total number of edges in this study. The width of the “edges” was multiple enlarged based on the intensity value (i.e., the size of the inhibition zone) among the antagonism interactions. The size of the “nodes” was enlarged according to the total number of sender and receiver degrees.
Results
Alterations in Physiological and Biochemical Characteristics at Different Temperatures
Comparing the phenotypic fingerprints of each isolate at 25°C and 32°C, we found that the physiological and biochemical characteristics of most strains varied at different temperatures except Marimonas sp. SCSIO 12655 and Halioglobus sp. SCSIO 12614 (Figure 1). Microbacterium sp. SCSIO 12466 showed the highest number of variations with 20 tested characteristics due to increased temperature (Figure 1 and Table S1). In contrast, Erythrobacter sp. SCSIO 12564 showed only one varied characteristic (i.e. resistance to minocycline), and the tested phenotypical characteristics of strains Halioglobus sp. SCSIO 12614 and Marimonas sp. SCSIO 12655 were not affected by the increasing temperature (Figure 1 and Table S1). According to the total number of positive tests, more kinds of sugar and amino acids could be utilized at the higher temperature (Figure S1). α-D-glucose and L-glutamic acid were commonly utilized at low temperature (Figure S1), while α-D-glucose, L-alanine and L-glutamic acid were commonly utilized at high temperature (Figure S1). In addition, the resistance of five antibiotics (Troleandomycin, Rifamycin SV, Minocycline, Lincomycin, Vancomycin) had no change under the evaluated temperature (Figure S1).
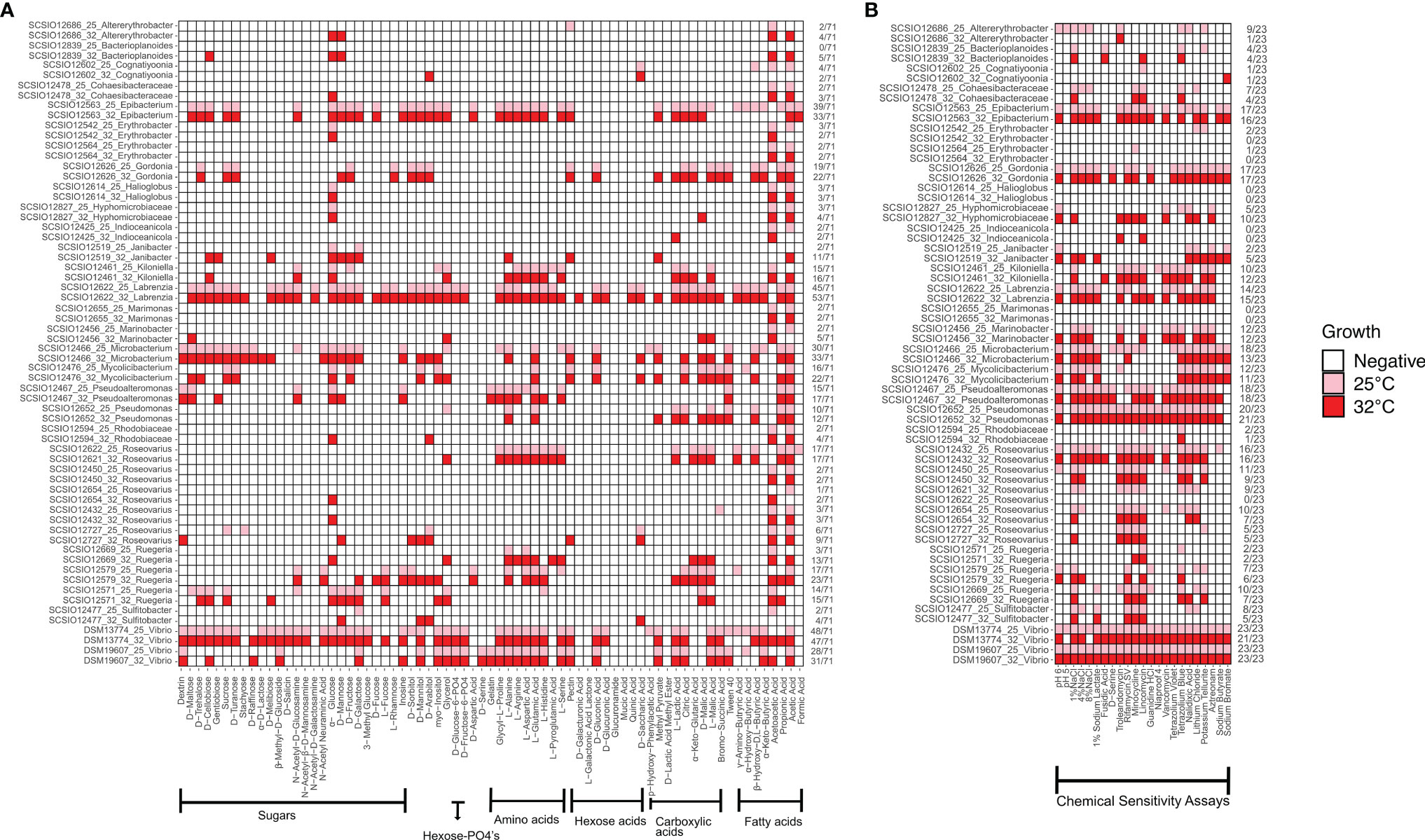
Figure 1 Phenotypic fingerprints of the coral-associated isolates at 25°C and 32°C. The pink (red) square of the heat map indicates that the phenotypic test is positive at 25°C (32°C). The white square of the heat map indicates that the phenotypic test is negative at 25°C and 32°C. Seventy-one carbon sources (A) plus 23 chemical sensitivity assays (B) were listed on the top of the heatmap. Isolates listed on the left side were ordered by taxa name. The categories of physiological and biochemical characteristics are shown at the bottom. The number of positive tests and the sum for each row were presented on the right side of the heatmap.
Variation of Antagonistic Interactions at Different Temperatures
Twenty out of the 32 isolates inhibited at least one isolate (Table 1 and Figure 2). Ten of the twelve strains belonging to Rhodobacteraceae showed antagonistic activities (Table 1). Among them, strain SCSIO 12563 presented the strongest antagonistic activity that inhibited 19 (25°C) or 18 (32°C) strains belonging to various taxonomic groups (Table 1 and Figure 2). Seven isolates (Epibacterium sp. SCSIO 12563, Bacterioplanoides sp. SCSIO 12839, Ruegeria sp. SCSIO 12669, Roseovarius sp. SCSIO 12450, Roseovarius sp. SCSIO 12626, Mycolicibacterium sp. SCSIO 12476, Gordonia sp. SCSIO 12652) were not inhibited by any isolates at either 25°C or 32°C (Figure 2). In addition, strain Microbacterium sp. SCSIO 12466 showed autoinhibition (Figure 2).
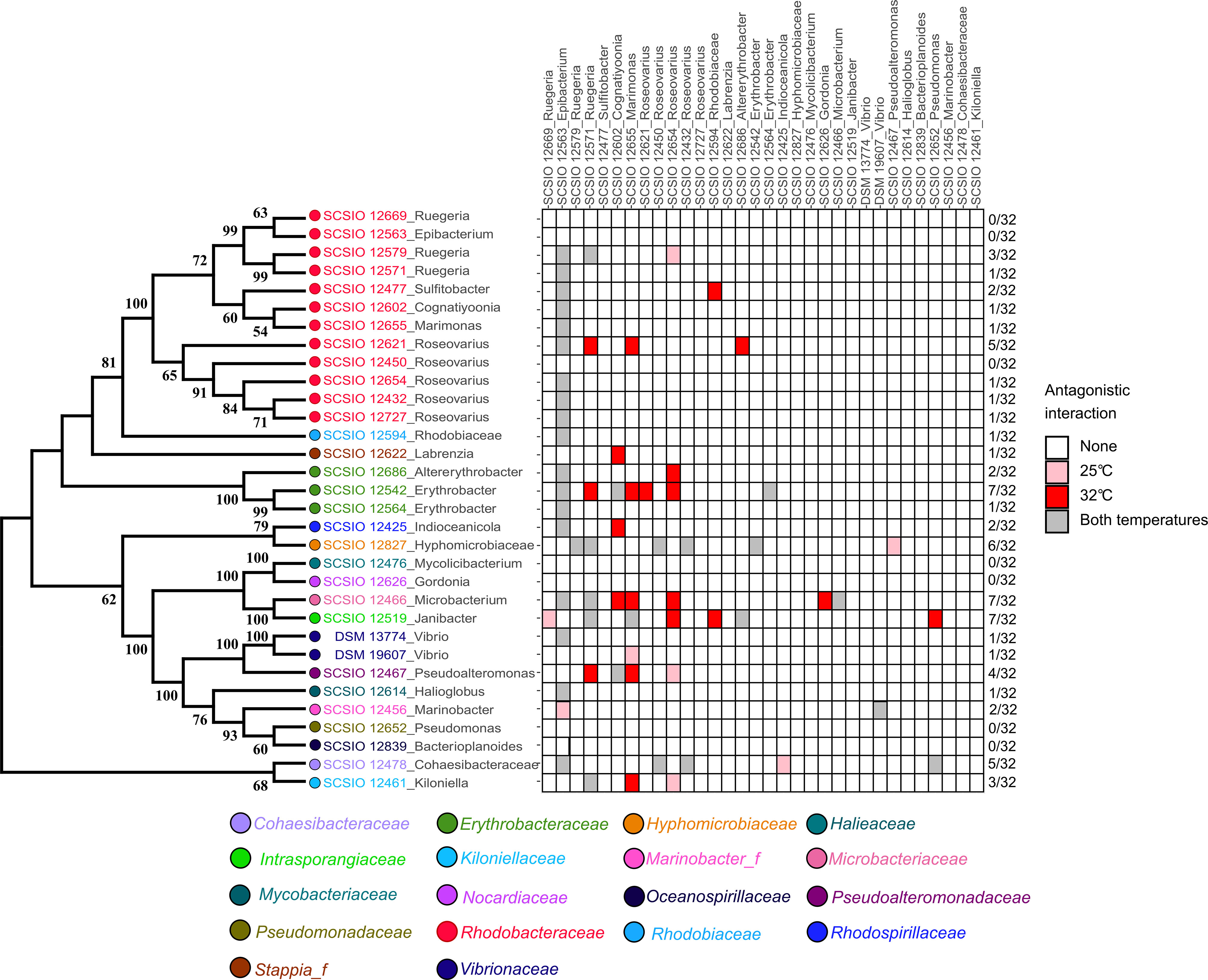
Figure 2 Heat map of the antagonistic interactions among the coral-associated bacteria integrated with a phylogenetic tree. Isolates listed on the top of the heatmap are producers, and those listed on the left side are inhibited. Colors of the strains numbers represent the taxonomic information at the family level. The number of antagonistic interactions and the sum for each row were presented on the right side of the heatmap. Pink and red blocks indicate antagonism at 25°C and 32°C, respectively. The gray block represents antagonism at both temperatures.
V. mediterranei DSM 13774 was inhibited by strain Epibacterium sp. SCSIO 12563 at both 25°C and 32°C (Tables 1, S3 and Figure 2). The growth of V. coralliilyticus DSM 19607 was inhibited by strain Marimonas sp. SCSIO 12655 at 25°C (Tables 1, S4 and Figure 2). V. mediterranei DSM 13774 did not inhibit any isolate growth, while V. coralliilyticus DSM 19607 inhibited Marinobacter sp. SCSIO 12456 at both 25°C and 32°C (Tables 1, S5 and Figure 2).
The total number of antagonistic interactions at 32°C was approximately 20% higher than that at 25°C (Figure S2). Twenty-one antagonistic interactions occurred only at higher temperature (32°C), while 8 occurred only at 25°C (Figure 2 and Table S4). At the level of order or class, the mean number of inhibited isolates increased at higher temperature for producers belonging to Rhodobacterales, Sphingomonadales, Rhizobiales, and Actinobacteria (Figure S3). At the level of strain, Ruegeria sp. SCSIO 12571, Cognatiyoonia sp. SCSIO 12602, Roseovarius sp. SCSIO 12654, Marimonas sp. SCSIO 12655, Altererythrobacter sp. SCSIO 12686, and Janibacter sp. SCSIO 12652 was able to inhibit more isolates with increasing temperature (Figure 2). These results indicated that more antagonistic interactions occurred at the higher temperature. Additionally, among the antagonistic interactions that occurred at both 25 and 32°C (Tables S3 and S5), most of them (62.2%) showed compromised inhibition activities at 32°C, while three strains (Epibacterium sp. SCSIO 12563, Marimonas sp. SCSIO 12655, and Microbacterium sp. SCSIO 12466) showed significantly increased inhibition activities (Table S5).
Effects of Elevated Temperature on Antagonistic Interactions Network
We further compared the structures of the antagonistic interaction networks at different temperatures (Figure 3), and found that the number of nodes increased by 1, and the number of edges increased by 28% at higher temperature (Figure 3 and Table S6). Additionally, the interaction frequency increased at 32°C (25°C, 0.04 and 32°C, 0.06) (Table 2). The value of sender-receiver asymmetry was negative at 25°C (-0.22) and 32°C (-0.15), indicating that the interaction matrix was sender-determined and temperature independent (Table 2). The values of nestedness, a vital descriptor of ecological network architecture (Landi et al., 2018), showed that the antagonistic network was significantly nested at both temperatures (Table 2; P < 0.00001 both at 25°C and 32°C). To test the relation between sender degree and receiver degree at different temperatures, we depicted their antagonistic interaction using a heatmap (Figure S4). The nested heatmap results showed that the antagonists were highly resistant, while the inhibited strains were unlikely to antagonize other strains (Figure S4).
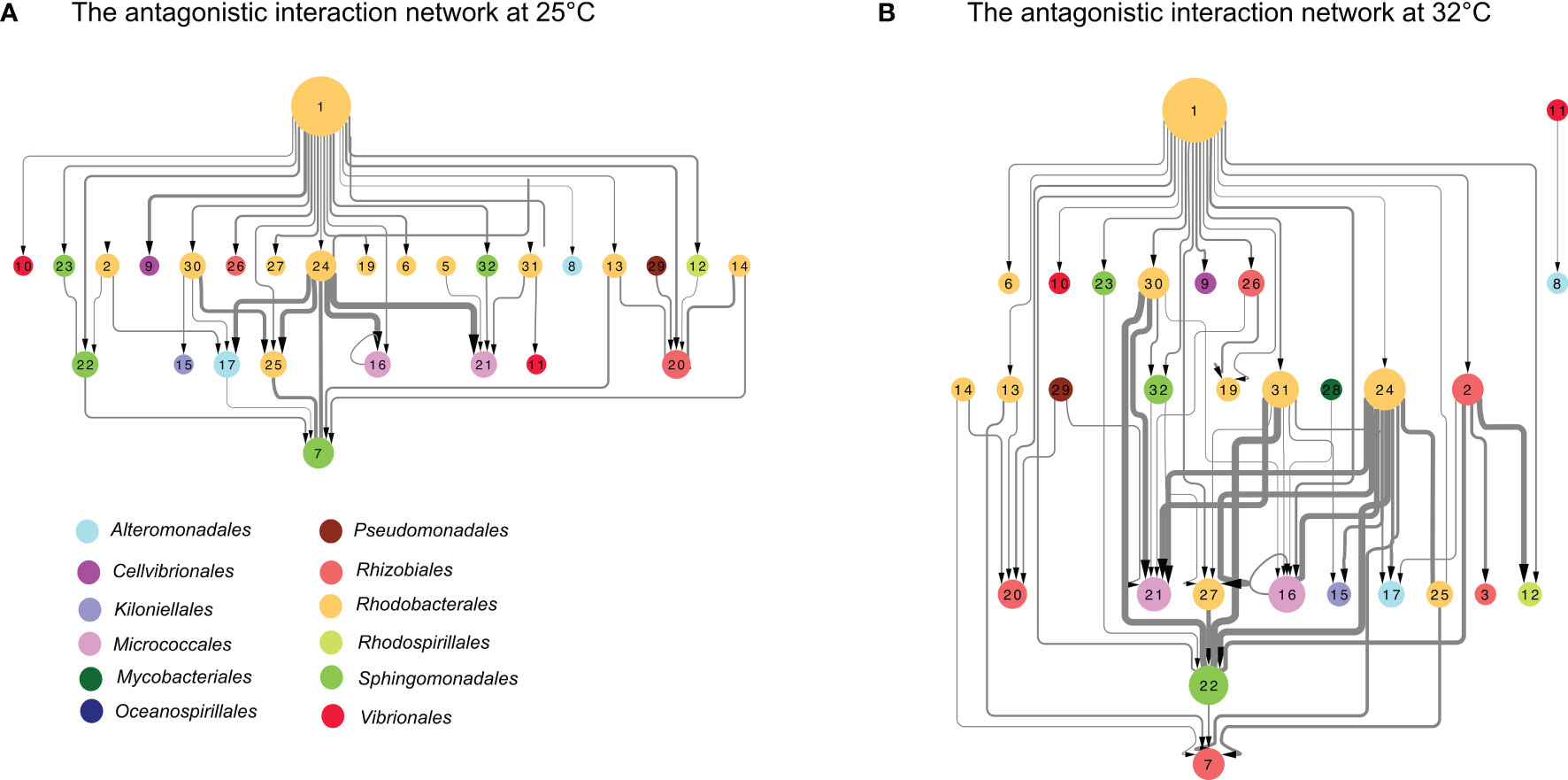
Figure 3 The antagonistic interaction network included antagonistic pairs and intensity at 25°C (A) and 32°C (B). The “edges” depict directed inhibitory interactions, and the “nodes” represent strains. The size of the “edges” shows the intensity of the interactions. The size of the “nodes” represents the total number of degrees, including sender degrees and receiver degrees. The code of each isolate was included in Table S1. Colors of the nodes represent the taxonomic information of isolates at the order level.
The Correlation Between Antagonism Probability and Phylogenetic and Phenotypic Distance
Among the 496 antagonistic test pairs (pairs of tested strains), we found that the antagonistic probability increased with decreasing 16S rRNA gene phylogenetic distance (Figures 4A, B). Furthermore, the correlation between antagonistic probability and phylogenetic distance was stronger at higher temperature (25°C, P = 0.028, pseudo-R2 = 0.021; 32°C, P = 0.020, pseudo-R2 = 0.021; logistic regression used throughout unless otherwise noted) (Figures 4A, B).
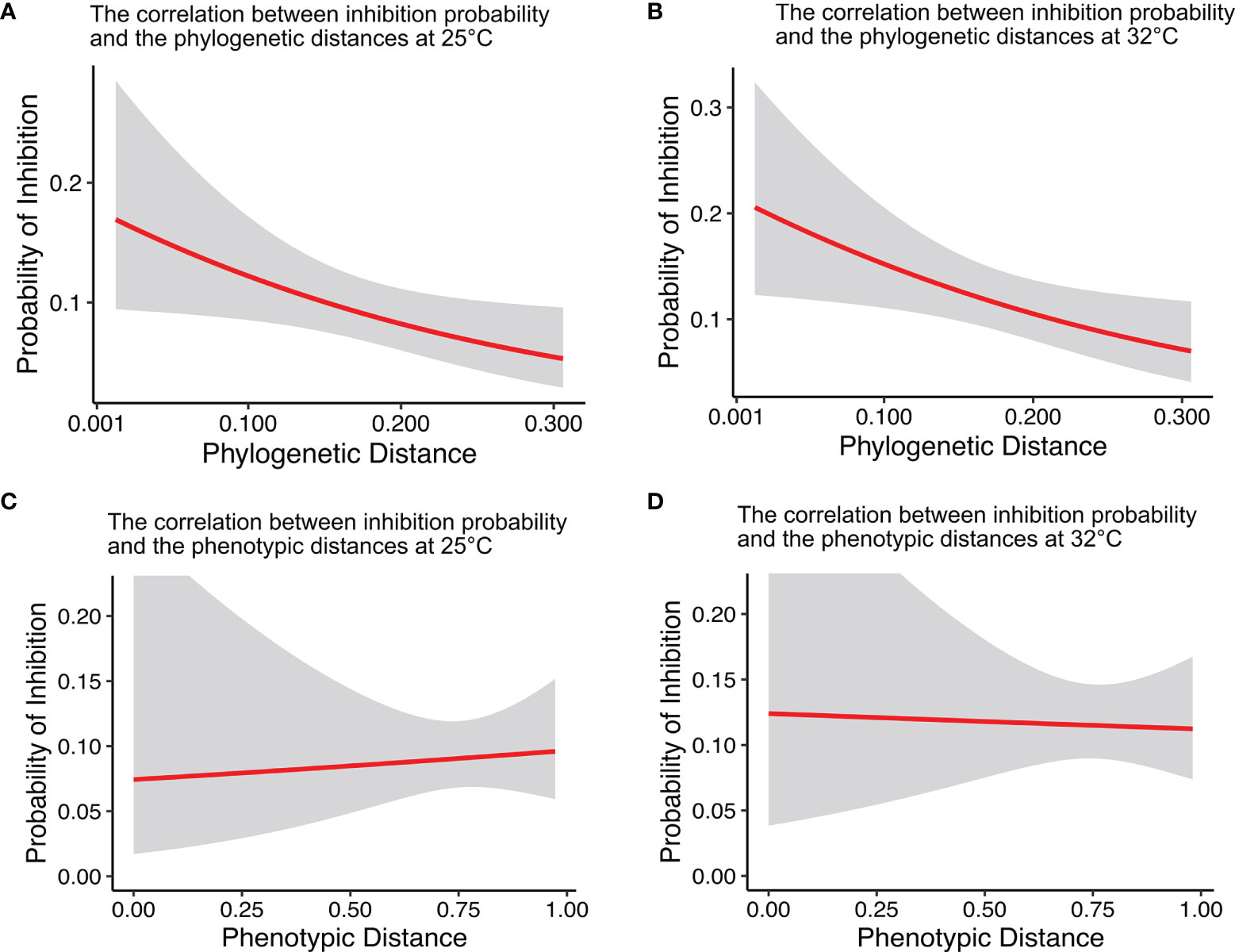
Figure 4 The correlation between inhibition probability and the phylogenetic (A, B) and phenotypic distances (C, D) at 25°C (A, C) and 32°C (B, D). The probability of inhibition increased with decreasing phylogenetic distance. The red line is a logistic regression, and the gray shaded area denotes the 95% confidence interval (A, 25°C, P = 0.0284, pseudo-R2 = 0.021; B, 32°C, P = 0.020; pseudo-R2 = 0.021; C, 25°C, P = 0.891; D, 32°C, P = 0.775, n = 496).
To further assess whether the correlation between antagonism and phylogeny was driven by the metabolic niche space of individual isolates, the correlation between antagonism probability and phenotypic distance (calculated on the basis of the dissimilarity of the phenotypic fingerprints) was evaluated. There was no correlation between antagonism and phenotypic distances (25°C, P = 0.885; 32°C, P = 0.891) (Figures 4C, D). As the phylogenetic distance correlated with the phenotypic distance at both 25°C and 32°C (25°C, P < 0.01; 32°C, P < 0.05) (Figure S5), we also analyzed the correlation between antagonism probability and phenotypic distance by using the residuals from the linear regression for phenotypic distance with the phylogenetic distances. The results showed that antagonism did not correlate with phenotypic distances at either 25°C or 32°C (25°C, P = 0.510; 32°C, P = 0.908) (Figure S6).
The Trade-Off Between Antagonism and Resource Exploitation at Different Temperatures
In order to investigate the trade-off between antagonism and resource exploitation, we tested whether the antagonist had a wider metabolic niche space (growth on the 71 carbon sources of the Biolog GENIII MicroPlate) for each antagonistic interaction, and compared it to the non-antagonistic interactions. We found that the antagonists tended to have a wider metabolic niche space, i.e., grew on more carbon sources than the antagonized strains at 25°C (Fisher’s exact test, odds ratio = 2.064, P = 0.028), but not at 32°C (odds ratio = 0.825, P = 0.498) (Figure 5).
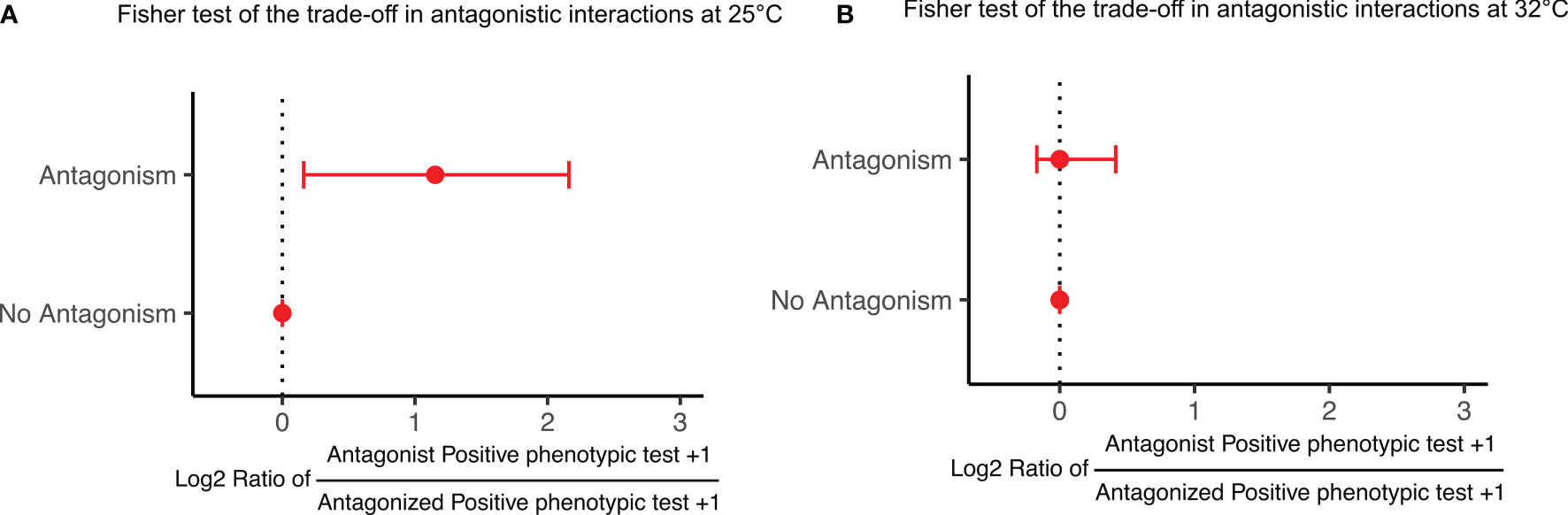
Figure 5 Fisher test of the trade-off in antagonistic interactions at 25°C (A) and 32°C (B). The log2 ratios between the number of carbon source utilized by the antagonists compared with the antagonized are larger than 0 when antagonism was observed (P = 0.052, n = 44), but not different from 0 for the non-antagonistic interactions (P = 0.999, n = 948) at 25°C (A); however, no differences from 0 for all interactions at 32°C (B) (antagonism: P = 0.881, n = 57; no antagonism: P = 0.981, n = 935). One autoinhibition pair was removed. P values are one-tailed tests of whether medians are larger than 0 estimated from 1,000 bootstrap realizations. Points are medians, and error bars are 90% bootstrapped confidence limits of the medians (equivalent to a 5% one-tailed test of the median).
Discussion
It is generally recognized that bacterial communities associated with corals alter under heat stress (Ziegler et al., 2017; Grottoli et al., 2018; Li et al., 2021), while the biotic interactions and their role in community assembly have not been elucidated. Here, through investigating the antagonism among coral-associated bacteria under different temperatures, we uncovered the alterations of antagonistic interactions due to the increased temperature and the correlation with genetic relationships and metabolic capabilities and hypothesized an association between bacterial antagonism interactions and community assembly in the coral holobiont.
Temperature Affects Antagonistic Interactions of Coral-Associated Bacteria
The results obtained in this study and reported previously indicated that antagonism was a common phenomenon in the coral-associated bacterial community, and temperature distinctly altered the breadth and intensity of antagonistic interactions (Rypien et al., 2010; Aguirre-von-Wobeser et al., 2014; Tang et al., 2019). The intensity of most antagonistic interactions decreased at higher temperature, which is consistent with previous results (Rypien et al., 2010). In contrast to the findings of Rypien et al. (2010), more antagonistic interactions occurred at higher temperature in this study, suggesting that the breadth of antagonism increased with increasing temperature. Previous studies showed that 6% or 12% of tested isolates were able to inhibit V. mediterranei (Nissimov et al., 2009; Rypien et al., 2010), and 3% or 10% were able to inhibit V. coralliilyticus (Rypien et al., 2010; Miura et al., 2019; Tang et al., 2019). Results of this study showed that strain Epibacterium sp. SCSIO 12563 inhibited the growth of V. mediterranei DSM 13774, and Marimonas sp. SCSIO 12655 inhibited V. coralliilyticus DSM 19607. Those two isolates (SCSIO 12563 and SCSIO 12655) were affiliated to different species in comparison to the antagonists observed in the previous studies (Nissimov et al., 2009; Rypien et al., 2010; Miura et al., 2019; Tang et al., 2019). These results suggest that these two opportunistic vibrion might face a challenging growth environment due to antagonistic interactions in the coral holobiont. Moreover, the growth of V. coralliilyticus DSM 19607 was only inhibited by strain Marimonas sp. SCSIO 12655 at 25°C, while the inhibition was removed at 32°C. The removal of inhibition might contribute to the enrichment of vibrion at higher temperature (Bourne et al., 2008; Frydenborg et al., 2014; Tout et al., 2015; Rajeev et al., 2021) and allow compromising the health of coral host (Gavish et al., 2021).
The antagonistic networks of coral-associated bacteria were sender-determined both at 25 and 32°C, which means that the structures of the networks were more influenced by antagonists than sensitive strains. Furthermore, our results showed that the antagonistic interaction network was significantly nested, with nestedness scores of 0.984 (25°C) and 0.969 (32°C). The highly nested and sender-determined network suggests that the antagonistic interaction network of the coral-associated bacteria community is more determined by antagonists. The nested antagonistic interaction networks were also respectively observed among Gammaproteobacteria in the oligotrophic water environment (Aguirre-von-Wobeser et al., 2014) and Carnobacterium maltaromaticum strains isolated from diverse habitats (e.g., dairy products, diseased fish, and meat products) (Ramia et al., 2020). These results suggested that the nested network is a common feature among bacteria antagonism interactions.
Coral-associated bacterial communities are known to alter in response to increasing seawater temperature (Tout et al., 2015; McDevitt-Irwin et al., 2017; Grottoli et al., 2018; Pootakham et al., 2018; Li et al., 2021). In this study, the antagonistic interaction network was found to be more hierarchical and complex at 32°C than 25°C (Figure 3). The study of Welsh et al. (2016) showed that heat stress decreased the complexity (network edges) of the coral mucus associated bacterial network, and the number of mutual exclusion was decreased in the network at the higher temperature (29-30°C). We should mentioned that the strains used in this study were isolated from coral tissue, while Welsh et al. (2016) investigated the network of bacteria associated with corals mucus. The microbial communities in different compartments of coral holobiont might show distinct networks. Even so, the inconsistent results obtained in this and previous studies highlight the necessity of interpretation of the interactions among coral-associated bacteria on the basis of both wet lab and dry lab results. The interactions among culturable strains could be experimentally investigated, while these results may not be enough for comprehensively understanding the interactions in the whole coral-associated bacterial community. On the other side, although the co-occurrence network constructed based on the relative abundances acquired through culture-independent analyses provides information for the whole bacterial community, it needs more cautions in interpreting the interactions presented in the co-occurrence network (Blanchet et al., 2020).
Competition (including antagonism) is considered one of the factors in community assembly (Perez-Gutierrez et al., 2013; Rocha et al., 2015; Zapien-Campos et al., 2015; Garcia-Bayona and Comstock, 2018). We hypothesize accordingly that the alteration of antagonistic interactions due to increased temperature is one of the processes that governs the assembly of coral-associated bacterial community, and encourage verifying it in combination with studies on natural communities.
Antagonism Correlates With Phylogenetic Rather Than Phenotypic Distance
Our results showed that closely related bacteria have a higher probability of antagonism than more distantly related bacteria, consistent with previous reports (Russel et al., 2017; Gonzalo et al., 2020). Moreover, we found that the correlation between antagonism and phylogenetic distance was stronger at higher temperature (32°C), implying that phylogenetically similar bacteria are likely to compete more fiercely under heat stress in coral holobionts. In contrast to the results of Russel et al. (2017), antagonism did not significantly correlate with phenotypic distances among coral-associated bacteria in this study, while this was similar to the finding in isolates of Pseudomonas aeruginosa from household drains (Mojesky and Remold, 2020). In the previous studies of both Russel et al. (2017) and Mojesky and Remold (2020), the utilization of 31 carbon sources were tested. These results suggest differential associations between antagonism and physiological and biochemical properties occur in distinct habitats, and phylogenetic similarity might predict the inhibition among bacteria associated with corals. Considering that the limitation in the range of phenotypic characteristics that could be screened using BIOLOG GEN III MicroPlate, expanding the tested index is necessary in the future.
Temperature Affects the Trade-Off Strategy
We investigated whether the antagonizing strains are generalists growing on many resources, and found that antagonists were indeed generalists at 25°C (Figure 5A), growing on ~20-130% more carbon sources than the inhibited strains. This result supports the hypothesis, i.e., the antagonist is also generalist, proposed by Russel et al. (2017). Meanwhile, the findings obtained at 32°C did not support this hypothesis, as the numbers of carbon sources utilized by antagonizing and antagonized strains were similar (Figure 5B). Additionally, more antagonistic interactions occurred due to the increased temperature (Figure S2 and Table S4). These results suggest that temperature significantly affects the trade-off between antagonism and resource exploitation in the antagonistic interactions of coral-associated bacteria. We further speculated that the shift of trade-off is an adaptation to nutrient reduction in bleached coral under thermal stress (Morris et al., 2019; Rädecker et al., 2021).
Conclusion
In this study, we investigated phenotypic fingerprints and antagonistic interactions of 32 coral-associated bacteria at different temperatures. We found that most of the tested strains showed alterations in the phenotypic fingerprints. Additionally, the elevated temperature increased the breadth of antagonism but decreased antagonism intensity among coral-associated bacteria. The complexity of the antagonistic network increased at the higher temperature. Our results showed that antagonism between coral-associated bacteria correlates with their genetic relationship rather than metabolic similarity. Moreover, increased temperature shifted the trade-off between antagonism and resource exploitation in the antagonistic interactions of coral-associated bacteria. The results of this study will be helpful in understanding of coral-associated bacterial interactions, and imply that the antagonistic interactions are involved in the assembly of coral-associated bacterial community.
Data Availability Statement
The datasets presented in this study can be found in online repositories. The names of the repository/repositories and accession number(s) can be found in the article/Supplementary Material.
Author Contributions
AG, JL, HJ, and QL performed the analyses. JL and SZ conceived this study. AG, JL, LW, LR, and SZ drafted the manuscript, and all authors commented on the manuscript and made suggestions.
Funding
The work was supported by the National Natural Science Foundation of China (42122045 and 41890853), K. C. Wong Education Foundation (GJTD-2020-12), Key Science and Technology Project of Hainan Province (ZDKJ202018), and Institution of South China Sea Ecology and Environmental Engineering, Chinese Academy of Sciences (ISEE2021ZD03).
Conflict of Interest
The authors declare that the research was conducted in the absence of any commercial or financial relationships that could be construed as a potential conflict of interest.
Publisher’s Note
All claims expressed in this article are solely those of the authors and do not necessarily represent those of their affiliated organizations, or those of the publisher, the editors and the reviewers. Any product that may be evaluated in this article, or claim that may be made by its manufacturer, is not guaranteed or endorsed by the publisher.
Acknowledgments
We thank Yiyang Zou and Yicong Zheng for their assistance in the experiment.
Supplementary Material
The Supplementary Material for this article can be found online at: https://www.frontiersin.org/articles/10.3389/fmars.2022.840384/full#supplementary-material
References
Aguirre-von-Wobeser E., Soberon-Chavez G., Eguiarte L. E., Ponce-Soto G. Y., Vazquez-Rosas-Landa M., Souza V. (2014). Two-Role Model of an Interaction Network of Free-Living Gamma-Proteobacteria From an Oligotrophic Environment. Environ. Microbiol. 16 (5), 1366–1377. doi: 10.1111/1462-2920.12305
Ainsworth T. D., Hoegh-Guldberg O. (2009). Bacterial Communities Closely Associated With Coral Tissues Vary Under Experimental and Natural Reef Conditions and Thermal Stress. Aquat. Biol. 4 (3), 289–296. doi: 10.3354/ab00102
Alexandrou M. A., Cardinale B. J., Hall J. D., Delwiche C. F., Fritschie K., Narwani A., et al. (2015). Evolutionary Relatedness Does Not Predict Competition and Co-Occurrence in Natural or Experimental Communities of Green Algae. Proc. R. Soc B-Biol. Sci. 282, 20141745. doi: 10.1098/rspb.2014.1745
Bascompte J., Jordano P., Melian C. J., Olesen J. M. (2003). The Nested Assembly of Plant-Animal Mutualistic Networks. Proc. Natl. Acad. Sci. U. S. A. 100 (16), 9383–9387. doi: 10.1073/pnas.1633576100
Ben-Haim Y., Rosenberg E. (2002). A Novel Vibrio Sp Pathogen of the Coral Pocillopora Damicornis. Mar. Biol. 141 (1), 47–55. doi: 10.1007/s00227-002-0797-6
Blanchet F. G., Cazelles K., Gravel D. (2020). Co-Occurrence Is Not Evidence of Ecological Interactions. Ecol. Lett. 23 (7), 1050–1063. doi: 10.1111/ele.13525
Bourne D., Iida Y., Uthicke S., Smith-Keune C. (2008). Changes in Coral-Associated Microbial Communities During a Bleaching Event. ISME J. 2 (4), 350–363. doi: 10.1038/ismej.2007.112
Burkholder P. R., Pfister R. M., Leitz F. H. (1966). Production of a Pyrrole Antibiotic by a Marine Bacterium. Appl. Microbiol. 14 (4), 649–653. doi: 10.1128/aem.14.4.649-653.1966
Cahill J. F. Jr., Kembel S. W., Lamb E. G., Keddy P. A. (2008). Does Phylogenetic Relatedness Influence the Strength of Competition Among Vascular Plants? Perspect. Plant Ecol. 10 (1), 41–50. doi: 10.1016/j.ppees.2007.10.001
Case T. J., Gilpin M. E. (1974). Interference Competition and Niche Theory. Proc. Natl. Acad. Sci. U. S. A. 71 (8), 3073–3077. doi: 10.1073/pnas.71.8.3073
Cavender-Bares J., Ackerly D. D., Baum D. A., Bazzaz F. A. (2004). Phylogenetic Overdispersion in Floridian Oak Communities. Am. Nat. 163, 823–843, 6. doi: 10.1086/386375
Epstein H. E., Torda G., van Oppen M. J. H. (2019). Relative Stability of the Pocillopora Acuta Microbiome Throughout a Thermal Stress Event. Coral Reefs. 38, 373–386. doi: 10.1007/s00338-019-01783-y
Frydenborg B. R., Krediet C. J., Teplitski M., Ritchie K. B. (2014). Temperature-Dependent Inhibition of Opportunistic Vibrio Pathogens by Native Coral Commensal Bacteria. Microb. Ecol. 67, 392–401. doi: 10.1007/s00248-013-0334-9
Garcia-Bayona L., Comstock L. E. (2018). Bacterial Antagonism in Host-Associated Microbial Communities. Science 361, aat2456. doi: 10.1126/science.aat2456
Gavish A. R., Shapiro O. H., Kramarsky-Winter E., Vardi A. (2021). Microscale Tracking of Coral-Vibrio Interactions. ISME Commun. 1, 1–18. doi: 10.1038/s43705-021-00016-0
Glasl B., Herndl G. J., Frade P. R. (2016). The Microbiome of Coral Surface Mucus has a Key Role in Mediating Holobiont Health and Survival Upon Disturbance. ISME J. 10 (9), 2280–2292. doi: 10.1038/ismej.2016.9
Gonzalo M., Deveau A., Aigle B. (2020). Inhibitions Dominate But Stimulations and Growth Rescues Are Not Rare Among Bacterial Isolates From Grains of Forest Soil. Microb. Ecol. 80 (4), 872–884. doi: 10.1007/s00248-020-01579-6
Grottoli A. G., Martins P. D., Wilkins M. J., Johnston M. D., Warner M. E., Cai W.-J., et al. (2018). Coral Physiology and Microbiome Dynamics Under Combined Warming and Ocean Acidification. PloS One 13, 0191156. doi: 10.1371/journal.pone.0191156
Hughes T. P., Anderson K. D., Connolly S. R., Heron S. F., Kerry J. T., Lough J. M., et al. (2018). Spatial and Temporal Patterns of Mass Bleaching of Corals in the Anthropocene. Science 359 (6371), 80–83. doi: 10.1126/science.aan8048
Hughes T. P., Kerry J. T., Alvarez-Noriega M., Alvarez-Romero J. G., Anderson K. D., Baird A. H., et al. (2017). Global Warming and Recurrent Mass Bleaching of Corals. Nature 543, 373–377. doi: 10.1038/nature21707
Jessen C., Lizcano J. F. V., Bayer T., Roder C., Aranda M., Wild C., et al. (2013). In-Situ Effects of Eutrophication and Overfishing on Physiology and Bacterial Diversity of the Red Sea Coral Acropora Hemprichii. PloS One 8, e60291. doi: 10.1371/journal.pone.0062091
Kumar S., Stecher G., Li M., Knyaz C., Tamura K. (2018). MEGA X: Molecular Evolutionary Genetics Analysis Across Computing Platforms. Mol. Biol. Evol. 35 (6), 1547–1549. doi: 10.1093/molbev/msy096
Kunstler G., Falster D., Coomes D. A., Hui F., Kooyman R. M., Laughlin D. C., et al. (2016). Plant Functional Traits Have Globally Consistent Effects on Competition. Nature 529, 204–207. doi: 10.1038/nature16476
Kushmaro A., Loya Y., Fine M., Rosenberg E. (1996). Bacterial Infection and Coral Bleaching. Nature 380, 396–396. doi: 10.1038/380396a0
Landi P., Minoarivelo H. O., Brannstrom A., Hui C., Dieckmann U. (2018). Complexity and Stability of Ecological Networks: A Review of the Theory. Popul. Ecol. 60 (4), 319–345. doi: 10.1007/s10144-018-0628-3
Lee S. T. M., Davy S. K., Tang S.-L., Fan T.-Y., Kench P. S. (2015). Successive Shifts in the Microbial Community of the Surface Mucus Layer and Tissues of the Coral Acropora Muricata Under Thermal Stress. FEMS Microbiol. Ecol. 91, fiv142. doi: 10.1093/femsec/fiv142
Li J., Long L. J., Zou Y. Y., Zhang S. (2021). Microbial Community and Transcriptional Responses to Increased Temperatures in Coral Pocillopora Damicornis Holobiont. Environ. Microbiol. 23 (2), 826–843. doi: 10.1111/1462-2920.15168
Littman R. A., Bourne D. G., Willis B. L. (2010). Responses of Coral-Associated Bacterial Communities to Heat Stress Differ With Symbiodinium Type on the Same Coral Host. Mol. Ecol. 19 (9), 1978–1990. doi: 10.1111/j.1365-294X.2010.04620.x
Littman R., Willis B. L., Bourne D. G. (2011). Metagenomic Analysis of the Coral Holobiont During a Natural Bleaching Event on the Great Barrier Reef. Environ. Microbiol. Rep. 3 (6), 651–660. doi: 10.1111/j.1758-2229.2010.00234.x
Long R. A., Azam F. (2001). Microscale Patchiness of Bacterioplankton Assemblage Richness in Seawater. Aquat. Microb. Ecol. 26 (2), 103–113. doi: 10.3354/ame026103
Long R. A., Rowley D. C., Zamora E., Liu J. Y., Bartlett D. H., Azam F. (2005). Antagonistic Interactions Among Marine Bacteria Impede the Proliferation of Vibrio Cholerae. Appl. Environ. Microbiol. 71 (12), 8531–8536. doi: 10.1128/aem.71.12.8531-8536.2005
McDevitt-Irwin J. M., Baum J. K., Garren M., Vega Thurber R. L. (2017). Responses of Coral-Associated Bacterial Communities to Local and Global Stressors. Fron. Mar. Sci. 4, 262. doi: 10.3389/fmars.2017.00262
Meron D., Rodolfo-Metalpa R., Cunning R., Baker A. C., Fine M., Banin E. (2012). Changes in Coral Microbial Communities in Response to a Natural pH Gradient. ISME J. 6 (9), 1775–1785. doi: 10.1038/ismej.2012.19
Miura N., Motone K., Takagi T., Aburaya S., Watanabe S., Aoki W., et al. (2019). Ruegeria Sp. Strains Isolated From the Reef-Building Coral Galaxea Fascicularis Inhibit Growth of the Temperature-Dependent Pathogen Vibrio Coralliilyticus. Mar. Biotechnol. 21 (1), 1–8. doi: 10.1007/s10126-018-9853-1
Mojesky A. A., Remold S. K. (2020). Spatial Structure Maintains Diversity of Pyocin Inhibition in Household Pseudomonas Aeruginosa. Proc. R. Soc B-Biol. Sci. 287, 20201706. doi: 10.1098/rspb.2020.1706
Morris L. A., Voolstra C. R., Quigley K. M., Bourne D. G., Bay L. K. (2019). Nutrient Availability and Metabolism Affect the Stability of Coral–Symbiodiniaceae Symbioses. Trends Microbiol. 27 (8), 678–689. doi: 10.1016/j.tim.2019.03.004
Negri A. P., Webster N. S., Hill R. T., Heyward A. J. (2001). Metamorphosis of Broadcast Spawning Corals in Response to Bacteria Isolated From Crustose Algae. Mar. Ecol. Prog. Ser. 223, 121–131. doi: 10.3354/meps223121
Newman M. E. J. (2003). The Structure and Function of Complex Networks. SIAM Rev. 45 (2), 167–256. doi: 10.1137/s003614450342480
Nissimov J., Rosenberg E., Munn C. B. (2009). Antimicrobial Properties of Resident Coral Mucus Bacteria of Oculina Patagonica. FEMS Microbiol. Lett. 292 (2), 210–215. doi: 10.1111/j.1574-6968.2009.01490.x
Olson N. D., Ainsworth T. D., Gates R. D., Takabayashi M. (2009). Diazotrophic Bacteria Associated With Hawaiian Montipora Corals: Diversity and Abundance in Correlation With Symbiotic Dinoflagellates. J. Exp. Mar. Biol. Ecol. 371 (2), 140–146. doi: 10.1016/j.jembe.2009.01.012
Perez-Gutierrez R. A., Lopez-Ramirez V., Islas A., Alcaraz L. D., Hernandez-Gonzalez I., Olivera B. C., et al. (2013). Antagonism Influences Assembly of a Bacillus Guild in a Local Community and Is Depicted as a Food-Chain Network. ISME J. 7 (3), 487–497. doi: 10.1038/ismej.2012.119
Pootakham W., Mhuantong W., Putchim L., Yoocha T., Sonthirod C., Kongkachana W., et al. (2018). Dynamics of Coral-Associated Microbiomes During a Thermal Bleaching Event. MicrobiologyOpen 7 (5). doi: 10.1002/mbo3.604
Rädecker N., Pogoreutz C., Gegner H. M., Cárdenas A., Roth F., Bougoure J., et al. (2021). Heat Stress Destabilizes Symbiotic Nutrient Cycling in Corals. Proc. Natl. Acad. Sci. U. S. A. 118 (5), e2022653118. doi: 10.1073/pnas.2022653118
Rajeev M., Sushmitha T. J., Aravindraja C., Toleti S. R., Pandian S. K. (2021). Thermal Discharge-Induced Seawater Warming Alters Richness, Community Composition and Interactions of Bacterioplankton Assemblages in a Coastal Ecosystem. Sci. Rep. 11 (1), 17341. doi: 10.1038/s41598-021-96969-2
Ramia N. E., Mangavel C., Gaiani C., Muller-Gueudin A., Taha S., Revol-Junelles A.-M., et al. (2020). Nested Structure of Intraspecific Competition Network in Carnobacterium Maltaromaticum. Sci. Rep. 10 (11), 1–9. doi: 10.1038/s41598-020-63844-5
R Core Team (2020). R: A Language and Environment for Statistical Computing (Vienna, Austria: R Foundation for Statistical Computing).
Rocha J., Pinto M. P., Boubli J. P., Grelle C. E. V. (2015). The Role of Competition in Structuring Primate Communities Under Different Productivity Regimes in the Amazon. PloS One 10 (12), e0145699–e0145699. doi: 10.1371/journal.pone.0145699
Rodriguez-Girones M. A., Santamaria L. (2006). A New Algorithm to Calculate the Nestedness Temperature of Presence-Absence Matrices. J. Biogeogr. 33 (5), 924–935. doi: 10.1111/j.1365-2699.2006.01444.x
Rohwer F., Kelley S. (2004). “Culture-Independent Analyses of Coral-Associated Microbes,” in Coral Health and Disease. Eds. Rosenberg E., Loya Y. (Berlin, Heidelberg: Springer).
Rohwer F., Seguritan V., Azam F., Knowlton N. (2002). Diversity and Distribution of Coral-Associated Bacteria. Mar. Ecol.: Prog. Ser. 243, 1–10. doi: 10.3354/meps243001
Russel J., Roder H. L., Madsen J. S., Burmolle M., Sorensen S. J. (2017). Antagonism Correlates With Metabolic Similarity in Diverse Bacteria. Proc. Natl. Acad. Sci. U. S. A. 114 (40), 10684–10688. doi: 10.1073/pnas.1706016114
Rypien K. L., Ward J. R., Azam F. (2010). Antagonistic Interactions Among Coral-Associated Bacteria. Environ. Microbiol. 12 (1), 28–39. doi: 10.1111/j.1462-2920.2009.02027.x
Shannon P., Markiel A., Ozier O., Baliga N. S., Wang J. T., Ramage D., et al. (2003). Cytoscape: A Software Environment for Integrated Models of Biomolecular Interaction Networks. Genome Res. 13 (11), 2498–2504. doi: 10.1101/gr.1239303
Sully S., Burkepile D. E., Donovan M. K., Hodgson G., van Woesik R. (2019). A Global Analysis of Coral Bleaching Over the Past Two Decades. Nat. Commun. 10 (1), 1264. doi: 10.1038/s41467-019-09238-2
Tang K., Zhan W., Zhou Y., Xu T., Chen X., Wang W., et al. (2019). Antagonism Between Coral Pathogen Vibrio Coralliilyticus and Other Bacteria in the Gastric Cavity of Scleractinian Coral Galaxea Fascicularis. Sci. China Earth Sci. 63 (1), 157–166. doi: 10.1007/s11430-019-9388-3
Tebben J., Tapiolas D. M., Motti C. A., Abrego D., Negri A. P., Blackall L. L., et al. (2011). Induction of Larval Metamorphosis of the Coral Acropora Millepora by Tetrabromopyrrole Isolated From a Pseudoalteromonas Bacterium. PloS One 6 (4), e19082. doi: 10.1371/journal.pone.0019082
Tout J., Siboni N., Messer L. F., Garren M., Stocker R., Webster N. S., et al. (2015). Increased Seawater Temperature Increases the Abundance and Alters the Structure of Natural Vibrio Populations Associated With the Coral Pocillopora Damicomis. Front. Microbiol. 6. doi: 10.3389/fmicb.2015.00432
van Oppen M. J. H., Blackall L. L. (2019). Coral Microbiome Dynamics, Functions and Design in a Changing World. Nat. Rev. Microbiol. 17 (9), 557–567. doi: 10.1038/s41579-019-0223-4
Venail P. A., Narwani A., Fritschie K., Alexandrou M. A., Oakley T. H., Cardinale B. J. (2014). The Influence of Phylogenetic Relatedness on Species Interactions Among Freshwater Green Algae in a Mesocosm Experiment. J. Ecol. 102 (5), 1288–1299. doi: 10.1111/1365-2745.12271
Vetsigian K., Jajoo R., Kishony R. (2011). Structure and Evolution of Streptomyces Interaction Networks in Soil and In Silico. PloS Biol. 9 (10), e1001184. doi: 10.1371/journal.pbio.1001184
Welsh R. M., Zaneveld J. R., Rosales S. M., Payet J. P., Burkepile D. E., Thurber R. V. (2016). Bacterial Predation in a Marine Host-Associated Microbiome. ISME J. 10 (6), 1540–1544. doi: 10.1038/ismej.2015.219
Zapien-Campos R., Olmedo-Alvarez G., Santillan M. (2015). Antagonistic Interactions are Sufficient to Explain Self-Assemblage of Bacterial Communities in a Homogeneous Environment: A Computational Modeling Approach. Front. Microbiol. 6. doi: 10.3389/fmicr.2015.00489
Zhang J., Hu A., Sun Y., Yang Q., Dong J., Long L., et al. (2021). Dispersal Limitation Expands the Diversity of Coral Microbiome Metacommunity in the South China Sea. Front. Mar. Sci. 8. doi: 10.3389/fmars.2021.658708
Keywords: antagonistic interactions, coral-associated bacteria, phenotypic pattern, community assembly, competition-relatedness hypothesis
Citation: Guo A, Li J, Wang L, Ju H, Li Q, Ren L and Zhang S (2022) Temperature Affects Antagonism Among Coral-Associated Bacteria. Front. Mar. Sci. 9:840384. doi: 10.3389/fmars.2022.840384
Received: 21 December 2021; Accepted: 08 March 2022;
Published: 28 March 2022.
Edited by:
Anderson B. Mayfield, Atlantic Oceanographic and Meteorological Laboratory (NOAA), United StatesReviewed by:
Jean Lim, University of Miami, United StatesStephanie M. Rosales, Oregon State University, United States
Copyright © 2022 Guo, Li, Wang, Ju, Li, Ren and Zhang. This is an open-access article distributed under the terms of the Creative Commons Attribution License (CC BY). The use, distribution or reproduction in other forums is permitted, provided the original author(s) and the copyright owner(s) are credited and that the original publication in this journal is cited, in accordance with accepted academic practice. No use, distribution or reproduction is permitted which does not comply with these terms.
*Correspondence: Jie Li, bGlqaWV0YXJlbkBzY3Npby5hYy5jbg==