Impact of the Construction of New Port Facilities on Primary Production of Plankton in the Neva Estuary (Baltic Sea)
- Zoological Institute of Russian Academy of Sciences, Saint-Petersburg, Russia
A significant increase in the human population on marine coast and steady growth of maritime water transport causes the construction of port infrastructure and the creation of new lands, which affects the ecosystems of coastal waters. Despite the widespread occurrence of such large-scale engineering projects in coastal areas, their impact on various components of aquatic ecosystems, including phytoplankton, is still poorly understood. The aim of the study was to assess the effect of the construction of ports and the alluvium of new lands in the Neva Estuary in 2000s on the productivity of phytoplankton. Digging and dredging of bottom sediments results in one order of magnitude elevation of suspended particulate matter (SM), which mostly consisted of sand and clayed deposits and in significant decrease water transparency, as compared to the average long-term values. Concentrations of total phosphorus in the estuarine waters during the works significantly positively correlated with the concentrations of SM. However, the multiple increase in nutrients was less important for phytoplankton development than expected. Analysis of variance and stepwise multiple regression analyses showed that the main predictor of the primary production of plankton in the periods of construction was water transparency. Gross primary production decreased significantly. In contrast to short-term effects caused by wind-induced events, which often stimulated phytoplankton development, long-term construction works of new port facilities negatively influenced phytoplankton productivity. Apart from pristine conditions when the phosphorus concentration was the main factor limiting the primary production in the estuary, the main limiting factor during long-term engineering projects became water transparency. Taking into account plans for further development of ports in coastal areas around the world, the influence of the large-scale engineering projects on the conditions for the development of phytoplankton may provide a new aspect of long-term regulation of algal blooms and ecosystem functioning in the coastal and estuarine zones.
Introduction
The most significant growth in human population and urbanization is predicted for coastal marine areas in the near future (Neumman et al., 2015). This causes the active development of coastal infrastructure, the construction of dams, the alluvium of new territories and digging fairways, which can affect the ecosystems of coastal zones (Bianchi, 2009; Wu et al., 2018). Coastal environments, in particular heavily populated semi-enclosed marginal seas and coasts like the Baltic Sea region, are strongly affected by human activities. A multitude of human impacts, including climate change, affects the different compartments of the environment, and these effects interact with each other (Reckermann et al., 2022). In recent decades, new lands, ports and fairways have been created at the mouth of the Neva River, which have become the infrastructure of the five million megapolis – St. Petersburg (Golubkov and Alimov, 2010).
Modern climate changes are causing a rise in the water level in the Baltic Sea, especially in its eastern part (Pindsoo and Soomere, 2020). Moreover, it has been shown that over the last 50 years the absolute (geocentric) mean sea level in the Baltic Sea has rising at a rate slightly higher than the world average, and this trend is likely to increase in the future (Weisse et al., 2021). The Flood Protective Facility that consists of 11 dams, has been protecting St. Petersburg from floods since the late 1980s (Ryabchuk et al., 2017), but future sea level rise is likely to lead to the need for their expansion and modernization. In the past, such works have already led to the appearance of a large amount of suspended matter in the waters of the Neva Estuary and affected it's ecosystem (Golubkov and Alimov, 2010).
The effect of suspended matter on phytoplankton has been studied in some detail in natural shallow water. In these ecosystems, significant amounts of suspended matter can rise from the bottom into the water column as a result of wind mixing (e.g.; Su et al., 2015; Kang et al., 2019) or turbulent tidal currents (e.g., de Jorge and van Beusekom, 1995; Guinder et al., 2009). In addition, according to climate models, coastal ecosystems will face an increased frequency of turbidity due to terrestrial sediment run-off induced by future increasing precipitation (Remy et al., 2017; Hoshiba et al., 2021).
A significant increase in the content of suspended particulate matter (SM) reduces light penetration in water and worsens conditions for the development of phytoplankton (Su et al., 2015; Horemans et al., 2020; Kumar et al., 2022). In opposite, the decrease in amount of SM with a concomitant increase in the penetration of solar radiation may be one of the main causes for the development of phytoplankton blooms (Guinder et al., 2009). Moreover, nutrients that have accumulated in the sediment over time can be recycled back into the water because of the intrusion of nutrient-rich pore water from sediments or bottom water layers into the whole water column (Su et al., 2015). This may release phytoplankton from nutrient limitation and stimulate plankton primary production (Su et al., 2015; He et al., 2022), but its response depends on the season and the species composition of the algae (Lagus et al., 2007; Su et al., 2015).
In some cases, the combined effect of both factors is important (Marzetz et al., 2020). Phytoplankton is likely to be light-limited at times of extreme turbidity because at lower turbidity light intensity is lower the threshold of light-limitation of phytoplankton species (Wang et al., 2019). Thus, the response of phytoplankton to resuspension of bottom sediments is rather complicated. In addition, it should be taken into account that resuspension events resulting from strong winds or tides usually last for several hours (e.g. de Jorge and van Beusekom, 1995; Martyanov and Ryabchenko, 2016). On the contrary, resuspension of bottom sediments can last for months or even years with the creation of new alluvial lands or port infrastructure. For example, the creation of new lands and the construction of ports at the mouth of the Neva River took several years (Ryabchuk et al., 2017).
Despite the widespread occurrence of mega-engineering works in coastal areas, their impact on various components of near-shore ecosystems, including phytoplankton, is still poorly understood. The aim of this study was to assess the effect of increased concentration of suspended particulate matter resulting from new port facilities construction on the primary production of plankton in the Neva Estuary. We tested the hypothesis that an increase in the concentration of nutrients in the water due to the resuspension of bottom sediments can compensate for the deterioration of light conditions for the development of phytoplankton in the Neva Estuary during the construction of port infrastructure.
Material and Methods
Study Sites
The Neva Estuary (Figure 1) is located in the northeastern part of the Baltic Sea. The Neva River, with an average discharge of 2,490 m3 s−1 (78.6 km3 yr−1), flows out of Lake Ladoga and goes into the Gulf of Finland. The catchment area of the estuary exceeds 280,000 km2 (Golubkov and Golubkov, 2020). It is located at the northern boundaries of the temperate zone and at the southern boundary of the subpolar zone (Meteoblue, 2021). Climate type in the region according to Köppen-Geiger climate classification (Kottek et al., 2006) is Dfc — Snowy climate, fully humid, with cool summers.
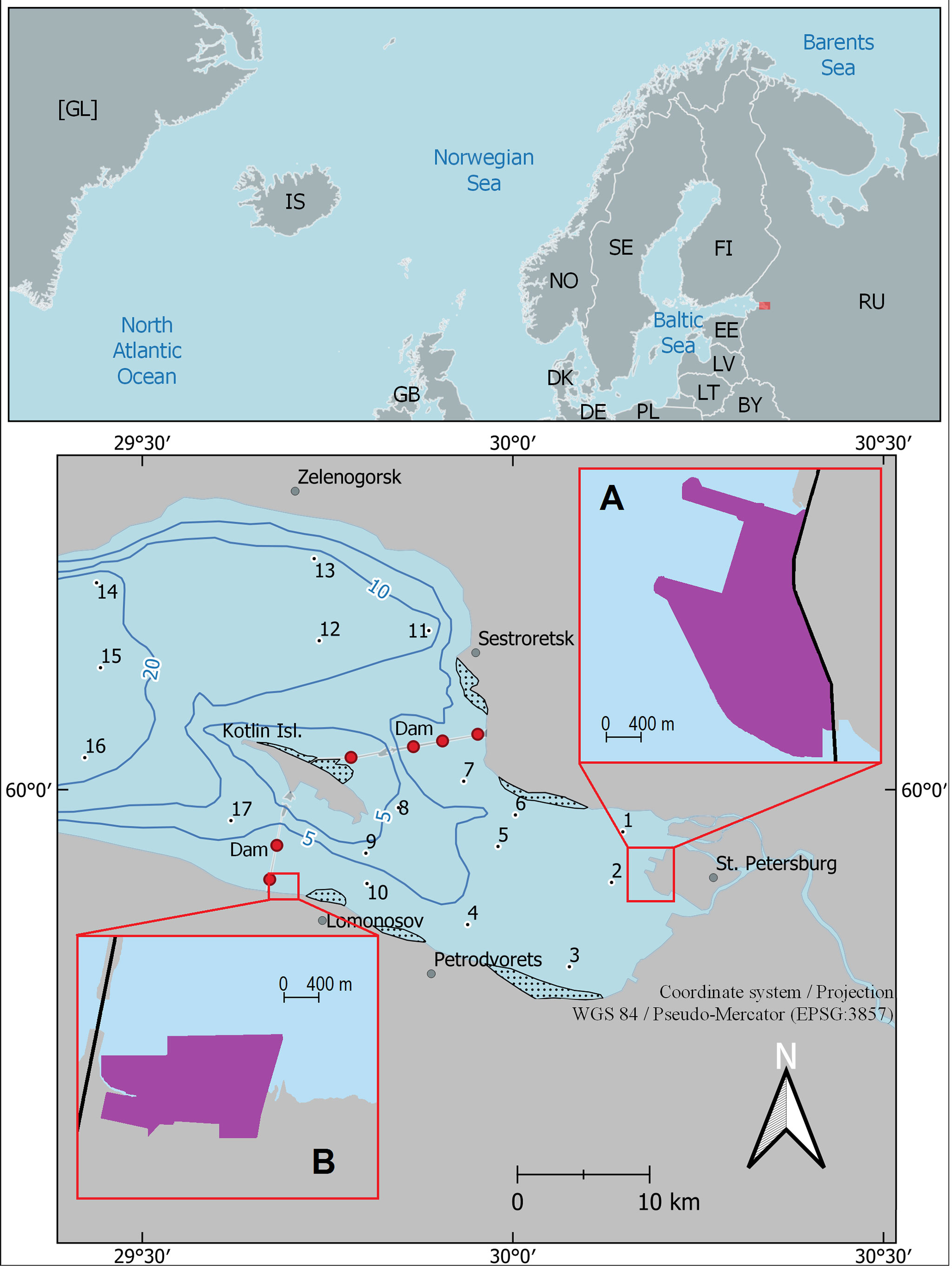
Figure 1 The upper and middle reaches of the Neva Estuary with indication of sampling stations (1–17) and new lands (violet) created in 2006 –2007 (A) and 2014 – 2015 (B). Blue lines: isobaths of 5, 10, and 20 m. Areas with dots indicate dense reeds. Dam — the St. Petersburg Flood Protection Facility. Red circles - waters gates in the Dam. Red rectangle in the top block of the map – the location of the Neva Estuary. Two-letter country codes are given according to ISO 3166-1 alpha-2 (International Organization for Standardization, 2022).
The Neva Estuary is shallow, non-tidal and with a smooth salinity gradient from fresh water in the upper part to slightly saline in the lower part (Golubkov and Golubkov, 2020). Since the five millionth megalopolis of St. Petersburg is located on the coast of the estuary, its ecosystem is subject to strong anthropogenic impact (Golubkov et al., 2019). The Flood Protective Facility that consists of 11 dams have separated the upper reach (UR) of the estuary from its middle reach (MR) since the end of 1980s (Figure 1). Temperature stratification is absent in the UR of the estuary, and low water transparency and rather strong currents prevent the development of benthic aquatic vegetation. The MR of the estuary is located between Kotlin Island and the conditional boundary of 29°10` E. In summer, there is temperature stratification there. A detailed description of both parts of the estuary was given earlier in (Golubkov and Golubkov, 2020, 2021). The main abiotic and biotic characteristics of the estuary are presented in Table 1.
In 2006–2007, 476.7 hectares of new territory were created in the UR of the estuary for the new Passenger Harbor of St. Petersburg (Figure 1). At the same time, the shipping channel was deepened to 14 meters for the passage of large ferries. The construction of the new multifunctional maritime shipping complex «Bronka» was carried out in 2014–2015 in the southwestern part of the UR (Figure 1). During these mega engineering works, large amounts of bottom sediment were dredged, removed and transferred from one part of the estuary to another (Ryabchuk et al., 2017). This led to the appearance of large amounts of suspended matter in the water.
Sampling
Samples were taken at 17 stations (Figure 1) in mid-summer (late July – early August) 2003–2020. Secchi-disk depth (Sec), salinity (S) and temperature (T) were measured at each station. T and S were measured by the CTD90m probe (Sea&Sun Tech., Germany) every 20 cm from the surface to the bottom in the water column. Since, according to these measurements, the whole water column in the shallow UR was mixed, samples were collected from the entire water column. Five water samples (2 l each) were taken at each station: from the surface, half a meter from the bottom and from three equal depths between them. Samples from different depths were taken in order to avoid errors associated with the vertical distribution of different phytoplankton species in the water column. These samples were composited and mixed to make up a pooled sample (10 l). Samples of total phosphorus, chlorophyll a, suspended particulate matter and suspended particulate organic matter (three replicates of water collection) were taken from these pooled samples.
In the MR, pooled water samples were taken from the layer above the thermocline (LAT). Five water samples (2 l each) were taken from LAT: from the surface, the thermocline and from three equal depths between them. These samples were mixed to create a pooled sample (10 l). The samples for chlorophyll a, total phosphorus, suspended particulate matter and suspended particulate organic matter analysis (three replicates) were taken from these pooled samples.
Sample Analysis
Three hundred millilitres of water were filtered through 0.85 µm membrane filters (Millipore AAWP) to determine the chlorophyll a (CHL) concentration, which was followed by 90% acetone extraction and spectrophotometric determination (Grasshoff et al., 1999). Total phosphorus (TP) was determined after acid hydrolysis with the molybdate blue method (Grasshoff et al., 1999). Suspended particulate matter (SM) samples were obtained by filtering water over preweighed and precombusted filters (Whatman GF/F). SM concentration was determined with a standard gravimetric technique (Grasshoff et al., 1999). Suspended particulate organic matter (SOM) was determined after filtration (Whatman GF/F filters) with the dichromate acid oxidation (Grasshoff et al., 1999). Suspended particulate mineral matter (SMM) was calculated as a difference between SM and SOM. After that, percentage of SMM in SM was calculated.
The primary production of plankton (PP) in the water column were measured by the oxygen method of light and dark bottles (Hall et al., 2007; Vernet and Smith, 2007). Since the depth of the LAT practically coincided with the depth of euphotic zone in mid-summer, 900 ml of water from the LAT pooled samples in the MR, and from the whole water column in the UR were used to determine PP. Three 100 ml light and three dark bottles were filled with the water from each sampling station and exposed in an aquarium on the ship’s deck in shadow during 6 h at a surface water temperature to estimate PP. Three 100 ml bottles (control bottles) were filled with the water from each sampling station to determine the oxygen contents in water at the beginning of the experiment. The Winkler method was used to determine the oxygen contents in the control, the light and the dark bottles (Hall et al., 2007). A more detailed description of the method and experimental design is given in Golubkov et al. (2017) and Golubkov and Golubkov (2020).
Statistical Analyses
Concentration of SM and percentage of SMM in SM was averaged for each station for periods 2006 –2007, 2014 – 2015 and for 2003 – 2020 excluding the previous periods, and visualized using SURFER 8.0.
The pairwise Pearson correlation coefficients between concentrations of SM, SOM, SMM, Sec, TP and phytoplankton productivity indicators were calculated using Microsoft Excel. Before calculating correlation coefficients, the data were logarithm. Linear regression analysis was produced using Microsoft Excel, the original data were logarithm.
Statistical analysis was performed using R software (version 4.0.5) (R Development Core Team, 2022). Analysis of variance (ANOVA) was performed using the “anova” command of the R Stats package (R Development Core Team, 2022). Stepwise multiple linear regression was performed to determine the set of factors most influencing plankton primary production. Models “model.null” and “model.full” were built. The “step” function (direction = “both”) was then used to add and remove factors from the model and find the model with the lowest Akaike Information Criteria (AIC). AIC was used as an estimator of out-of-sample prediction error and thereby relative quality of statistical models for a given set of data. The lower the value, the better for the AIC (Mangiafico, 2022). When the model with lowest AIC was found it called final.model. In this way, combination of factors most influencing PP was found. The residual standard error (RSE) for the resulting model was calculated. RSE is a measure of the inconsistency of the model with the available data. If the RSE value is very close to the actual outcome value, then model fits the data well (James et al., 2013). After that, the adjusted R2 (Adj R2) was calculated to avoid the error of overestimating the quality of the models, arising from the addition of each additional variable to the models. Adj R2 is a version of R2, which is calculated to take into account the number of variables in the multiple regressions model. Adj R2 is always lower than the R-squared. The Fisher distribution for the model was also checked and reliable F-values were obtained, taking into account the degrees of freedom (df) of the model. In addition, the model was tested for unbiasedness and homoscedacity.
Results
Abiotic Environmental Factors
The most intensive construction of new port facilities in the Neva Estuary was carried out in 2006–2007. Because of these works, the concentration of suspended particulate matter sharply increased in all parts of the estuary, especially in its upper reach (Figure 2A). Suspended particulate matter concentrations during the works in the UR and MR of the estuary were about 20 times higher than the long-term average summer values (Figures 2A, C). The construction of the new multifunctional maritime shipping complex «Bronka» in 2014–2015 were carried out in the near-dam zone (Figure 1) and increased turbidity only in the western part of the UR (Figure 2B). The suspended particulate matter mainly came in the southern part of the MR, which led to an increase in its concentration (up to 30 g m-3) in the area of station 17 (Figure 2B). Concentrations of SM in this part of the Neva Estuary in 2014–2015 were approximately 6–7 times higher than the average long-term values (Figures 2B, C).
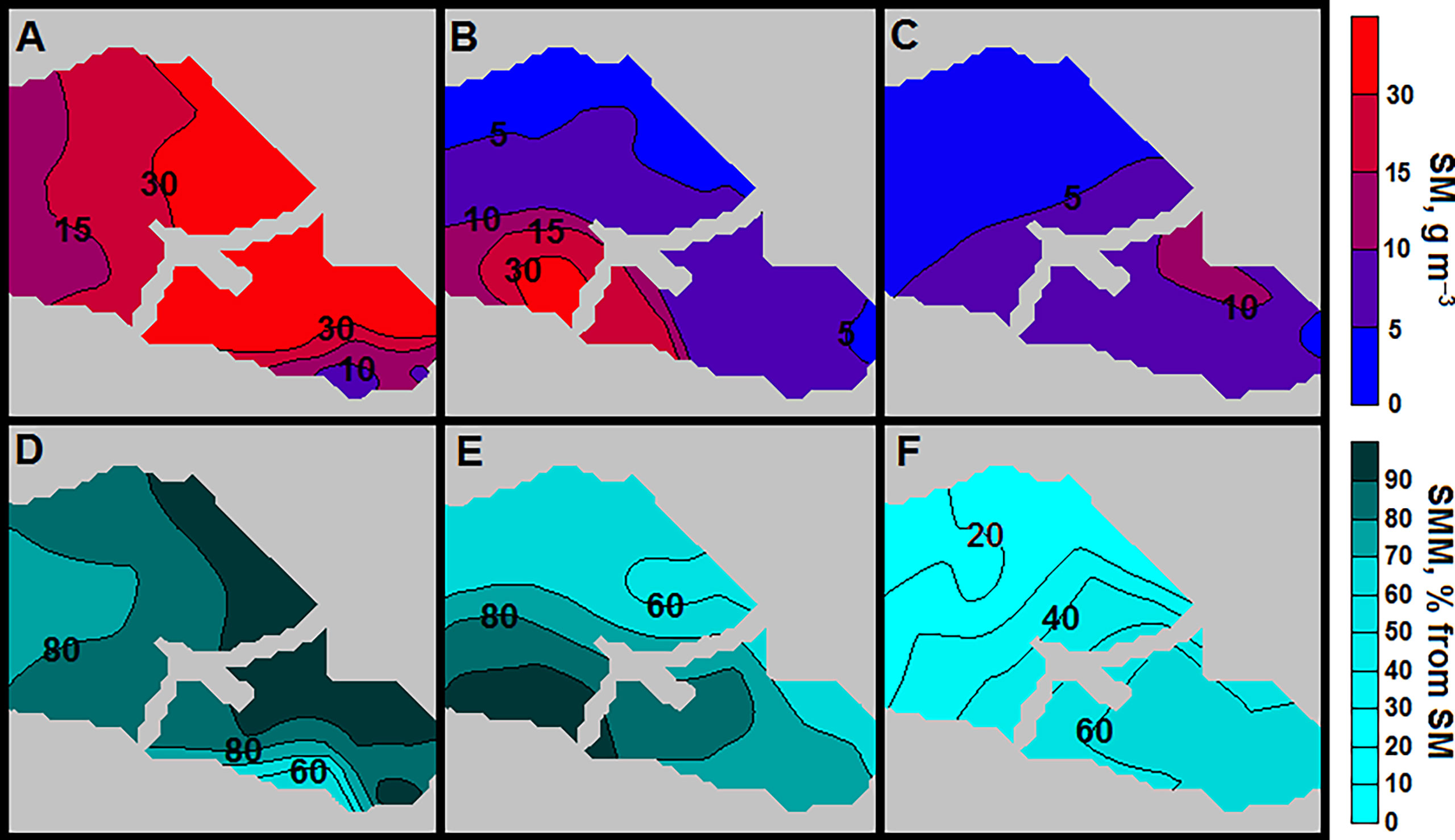
Figure 2 Average concentration of suspended matter during the periods of construction of new port facilities in 2006–2007 (A) and in 2014–2015 (B), and on average in the 2000s, without data for 2006–2007 and 2014–2015 (C), the percentage of mineral fraction in suspended matter in 2006–2007 (D) and in 2014–2015 (E), and on average for the 2000s, without data for 2006–2007 and 2014–2015 (F) in the Neva Estuary in midsummer. SM, concentration of total suspended particulate matter; SMM, concentration of suspended particulate mineral matter.
The analysis of SM showed that both in 2006–2007 and in 2014–2015 it mainly consisted of the mineral fraction (Figures 2D, E). In 2006–2007, the share of SMM in SM exceeded 90% in almost the entire UR and in a significant part of the MR of the estuary (Figure 2D). In 2014–2015, the area of increased concentration of suspended particulate matter was significantly smaller compared to 2006–2007 (Figure 2B), but in the southwestern zone near the dam, the share of the mineral fraction was also higher than 90% (Figure 2E). For comparison, when no mega-engineering works were carried out, the share of the mineral fraction in the SM was about 60% in the UR of the estuary, and less than 20% in its MR (Figure 2F).
The analysis of Pearson`s pairwise correlations showed that the concentrations of SM and SMM during mega-engineering works exhibit a high statistically significant positive correlation between each other (r=0.95, р<0.001, n=62) (Figure 3). The relationship between the SOM and SM was weaker (r = 0.55, p<0.001, n = 62) than between SM and SMM. Such a strong increase in SM led to a significant decrease in water transparency (Sec) during the years of construction of new port facilities, as evidenced by the analysis of Pearson’s pairwise correlations (Figure 3) and the regression dependence of Sec on SM (Figure 4A). The concentration of SOM also significantly negatively correlated with Sec, but the strength and reliability of their relationship were weaker (Figure 3). Mega-engineering works also influenced the concentration of total phosphorus (TP) in the estuary. Concentrations of TP in the waters of the UR and MR of the estuary during the works was significantly positively correlated with the concentrations of SM and SMM (Figure 3). Although the Pearson`s correlation coefficient between TP and SM concentrations was not very high and there was a noticeable scatter of points, the relationship was statistically significant (Figure 4B). TP concentrations also showed a weak but significant correlation with SOM concentrations (Figure 3).
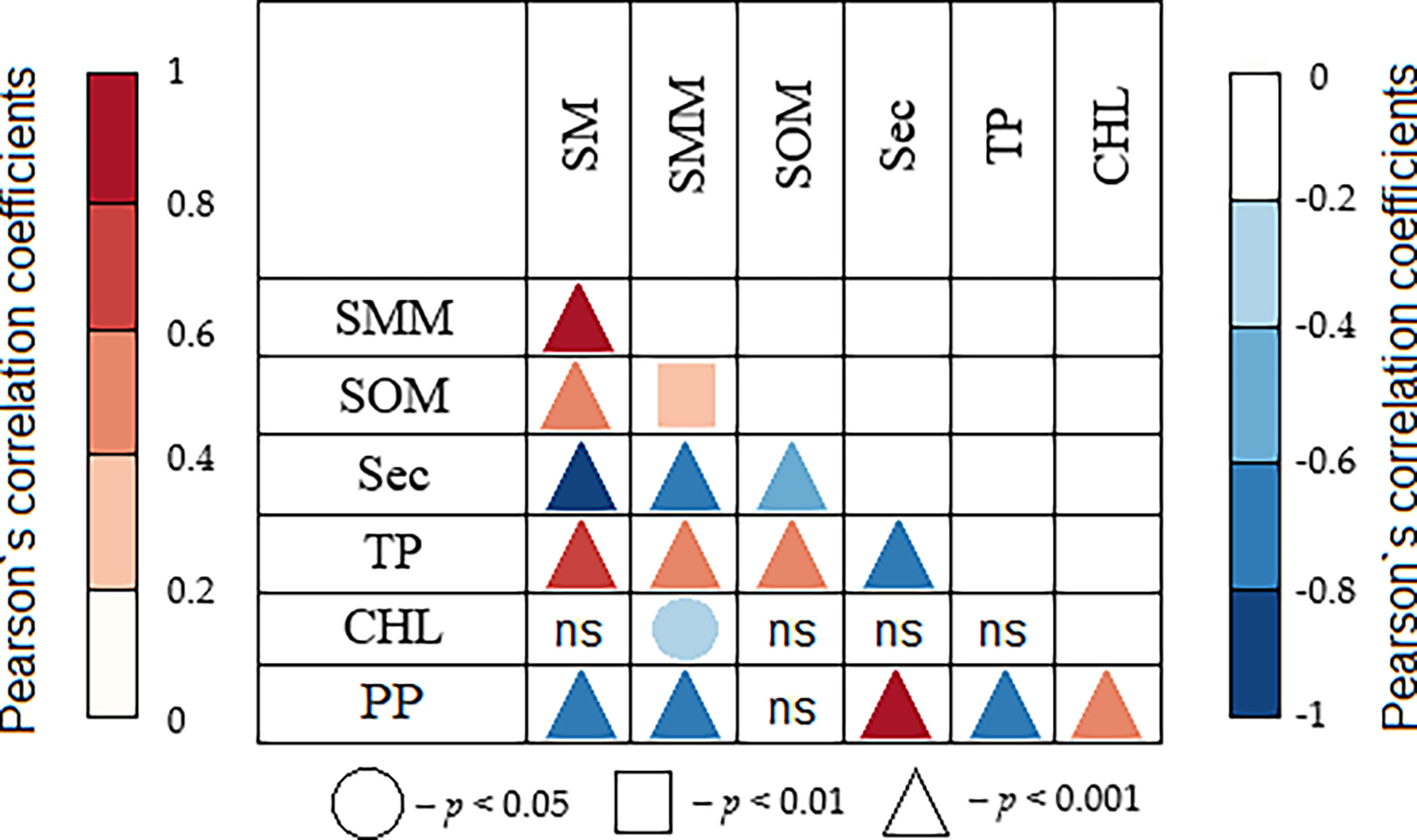
Figure 3 Pearson`s correlation coefficients between the studied variables during the construction of new port facilities in 2006–2007 and 2014–2015. SM, concentration of suspended particulate matter; SMM, concentration of suspended particulate mineral matter; SOM, concentration of suspended particulate organic matter; Sec, Secchi-disk depth; TP, concentration of total phosphorus; CHL, concentration of chlorophyll a; PP, plankton primary production; ns, not significant.
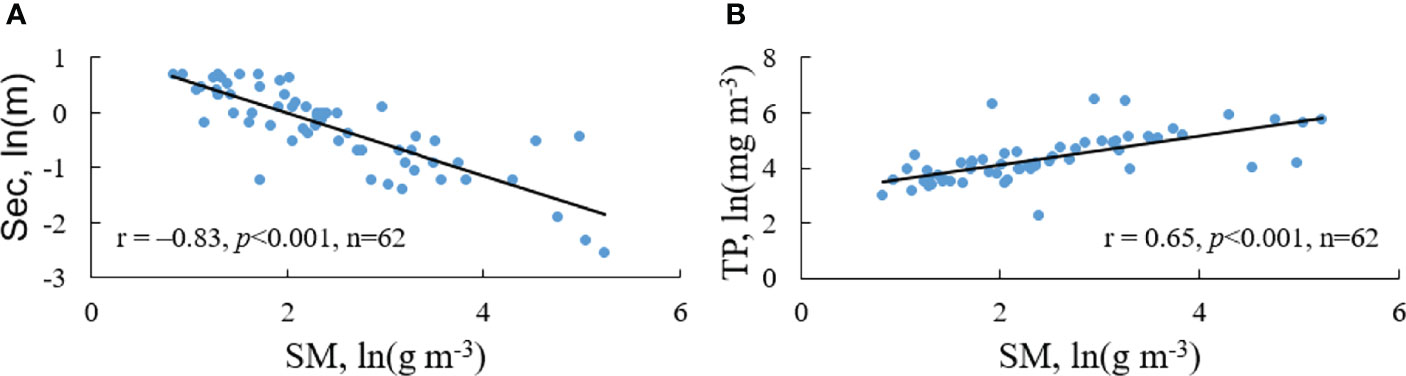
Figure 4 Dependence of the Secchi-disk depth (A) and the concentration of total phosphorus (B) on the concentration of suspended matter in the water during the periods of the construction of new port facilities in the Neva Estuary.
Productivity of Phytoplankton
Analysis of pairwise correlations showed that plankton primary production was significantly negatively correlated with the concentrations of SM and SMM (Figure 3). Regression analysis showed a statistically significant dependence of PP on Sec during the periods of construction of ports and alluvium of new lands (Figure 5A). In other words, PP in the water column decreased at stations where the highest SM were observed during the works, and PP increased where there was a low concentration of SM. At the same time, PP was statistically significantly negatively correlated with the concentration of TP (Figure 5B), which, apparently, was a consequence of the positive correlation of TP with SM and SMM (Figure 3).
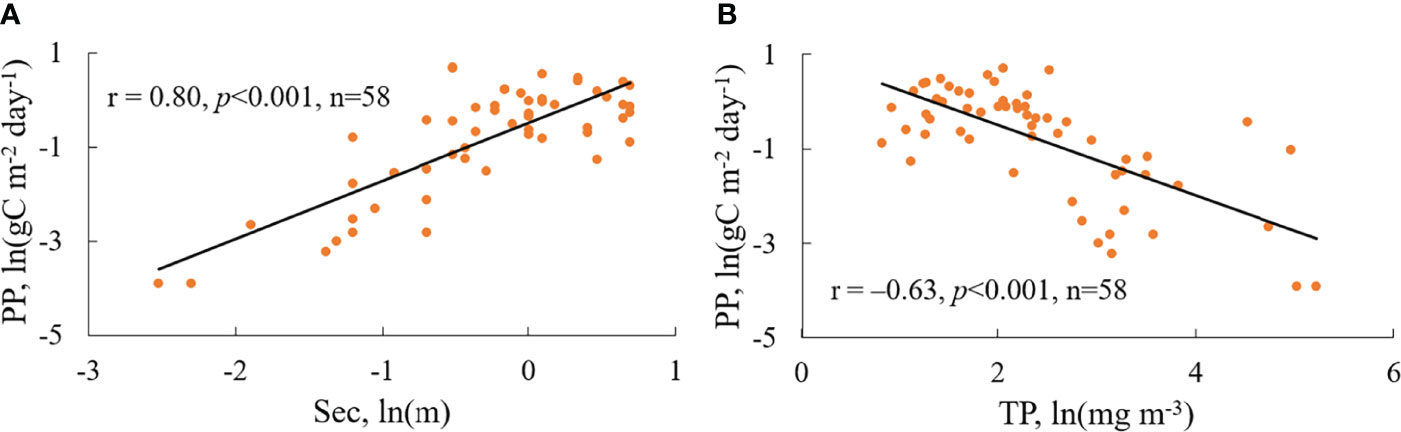
Figure 5 Dependence of the primary production of plankton on Secchi-disk depth (A) and the concentration of total phosphorus (B) during the periods of construction of new port facilities in the Neva Estuary.
Analysis of variance showed that water transparency had the greatest impact on the primary production of phytoplankton in the Neva Estuary during the periods of port infrastructure construction (Table 2). Salinity and temperature of the water, as well as the concentration of total phosphorus also affected the PP, but much weaker. Factors not taken into account in the study also had a significant influence (29.7%), but their role was less than that of water transparency (Table 2).
Stepwise multiple regression analyses showed that of all the studied factors, the primary production of plankton was influenced by a combination of four factors: transparency, salinity, water temperature and total phosphorus concentration (Table 3). The resulting multi-regression model has a high degree of reliability, because adjusted for the number of measurements, R2 is high, p-value<0.001. In addition, Fischer’s test for the model showed that F-value is high and statistically reliable at these degrees of freedom. Residual standard error also has a low value, which means that the simulation results describe the initial empirical data well (Table 3). The resulting model is homoscedastic and unbiased.
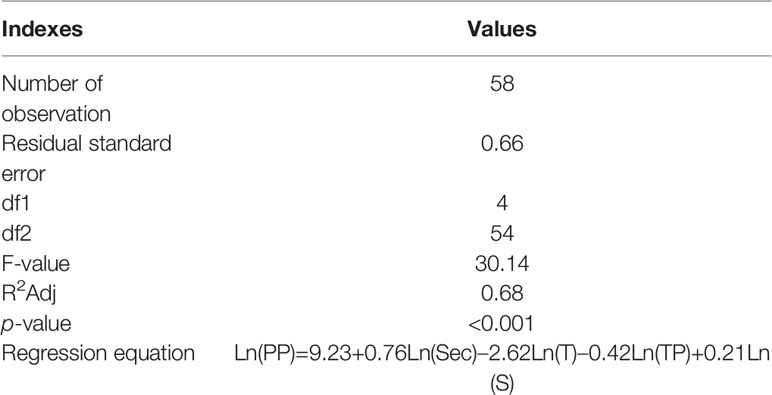
Table 3 Results of stepwise multiple regression analysis between the plankton primary production (PP, variable Y, predictand) and environmental variables (variables X, predictors).
The resulting multiregression equation shows that plankton primary production was positively related to water transparency, i.e. with its increase, the PP increased and vice versa. An increase in water salinity had a similar effect. In other words, PP was higher in clearer and more saline waters. However, this was most likely due to an increase in water salinity with distance from the epicenter of the port and alluvial works that took place in the mouth of the river and the upper reach of the estuary. Conversely, primary production was negatively related to phosphorus concentration and water temperature (Table 3). This is consistent with the results of the regression analysis, which also showed that phosphorus concentration was strongly positively related to suspended matter concentration (Figure 4B) and negatively to PP (Figure 5B).
Discussion
Concentrations of Suspended Matter and Total Phosphorus, Water Transparency
Periodic high increases in the concentration of suspended matter are common for shallow water, including estuaries (de Jorge and van Beusekom, 1995; Guinder et al., 2009; Su et al., 2015). Periodic rather high concentrations of SM and low Sec are also common for the Neva Estuary, especially for its shallow upper reach, even in the absence of mega-engineering works (Martyanov and Ryabchenko, 2016). Hydrobiological studies in the upper reach of the Neva Estuary, carried out at the beginning of the 20th century (1911–1912), showed that the concentration of suspended particulate matter in the summer period was within 0.9–75 g m-3, with an average concentration about 5 g m-3 and average Secchi-disk depth was 1.4 m (Zalessky and Wulf, 1913). The concentration of SM in 1959–1961 was 5–8 g m-3 in calm weather, but with a wind of 9–11 m s-1, it rapidly increased to 20–34 g m-3 (Ryabinina, 1965). The maximum concentration of SM (89 g m-3) in those years was recorded along the southern coast of UR with a long east wind and a wave height of 1 meter. At the same time, the Secchi-disk depth in summer was in the range of 0.3–2.1 m (Ryabinina, 1965). In the 2000s, when no mega-engineering works were carried out, the concentration of SM in the upper reach was in the range of 5–20 g m-3, and in the middle reach, it decreased below 5 g m-3 (Figure 2). The average Secchi-disk depth in the 2000s, when no works were carried out, was 1.3 meters.
Mega-engineering works also led to an increase in turbidity of water in various regions of the Baltic Sea. For instance, the SM concentration reached 126 g m-3, and the water transparency decreased to 0.2 meters along the Estonian coast during the dredging period (Liblik and Lips, 2011). The Neva Estuary has been under strong anthropogenic impact for over a hundred years (Golubkov et al., 2003; Golubkov et al., 2019). Dredging and digging of bottom sediments in the Neva Estuary were carried out periodically and before 2000s (Ryabchuk et al., 2017), which led to increased turbidity. For example, the concentration of SM at some stations in the upper reach of the estuary exceeded 110 g m-3 during the construction of the Flood Protective Facility of St. Petersburg in 1982–1984 (Umnova, 1987). However, the maximum concentration of suspended particulate matter recorded at station 7 (186 g m-3) in 2006–2007 was one and a half times higher than its maximum value in the 1980s.
Concentration of suspended particulate matter can affect phytoplankton in different ways (de Jorge and van Beusekom, 1995). For instance, SM resuspended from the bottom was an important source of nitrogen and phosphorus in the shallow estuary in the Gulf of Bothnia (Nilsson and Jansson, 2002). At the same time, an increase in turbidity has a negative effect on water transparency, the second most important factor in the development of phytoplankton (Kirk, 2011). An increase in the SM in the Neva Estuary during the works related to the creation of a new port infrastructure and the alluvium of new lands also correlated with these two environmental factors. On the one hand, this led to an increase in the concentration of TP (Figures 3, 4B), the main element limiting the development of algae in the estuary (Golubkov and Golubkov, 2020). It was also shown that the bulk of nutrients entering the Neva Estuary through various mechanisms are associated with inorganic substances in the suspended particulate matter (Lehtoranta et al., 2004). On the other hand, the increase in concentration of suspended matter due to these works negatively affected the transparency of water in the estuary (Figures 3, 4A).
Primary Production of Plankton
The large-scale construction of mega-engineering facilities can have a greater impact on estuarine ecosystems than the facility itself (He et al., 2022). This usually leads to an increase in turbidity and nutrient concentration, which affects phytoplankton (Wang et al., 2019).
In the Nevа Estuary, an increase in the concentration of phosphorus as a result of the resuspension of a large amount of suspended particulate matter into the water during the periods of mega-engineering construction should have resulted in the intensive development of phytoplankton, but this did not happen. The concentration of chlorophyll a did not increase, and the primary production of plankton during the years of construction was the lowest in the entire 2000s (Golubkov and Golubkov, 2021). This was obviously a consequence of a decrease in water transparency due to the high concentration of SM (Figure 4A), which, in turn, led to a decrease in phytoplankton productivity because of a lack of light (Figure 5A and Table 3). Light limitation of plankton primary production in the Neva Estuary during the periods of construction is confirmed by the negative correlation between PP and SM in those years (Figure 3).
The composition of substances resuspended from the bottom can also affect the productivity of phytoplankton. For example, the use of active clay as a technique to combat the mass development of toxic algal species in coastal waters is now gaining popularity (de Magalhães et al., 2017; Yu et al., 2017). This method is quite simple and cheap (Song et al., 2021). The addition of clay particles to the water causes sedimentation of joint aggregates of clay and algae cells, resulting in a decrease in the number of algae in the water column (de Magalhães et al., 2019; Cao et al., 2022). Moreover, clay particles absorb nutrients from the water and thus become inaccessible to algae (de Magalhães et al., 2019). Suspended material during the period of mega-engineering projects in the Neva Estuary mainly contained fine sand, consisting of silica, and clayey Quaternary deposits, which make up the underlying bedrock of the bottom of the upper reach of the estuary (Ryabchuk et al., 2017). Sandy material was excavated from sand pits in the lower part of the estuary and delivered by barges to its upper part, where this material was poured out to create new territories, and the clayey deposits fell into the water column when new deep fairways were dug. It is likely that a significant positive relationship between total phosphorus and the concentration of suspended matter (Figure 4B) and a negative relationship with primary production (Figure 3) observed during construction periods is due to the appearance of a large amount of clay particles in estuary water.
Salinity and water temperature also influenced the primary production of plankton in the estuary (Table 2, 3). However, the influence of these predictors was more likely to be associated with the action of other factors. Previously, it was shown that in the middle reaches of the Neva Estuary, primary production is higher than in the upper reaches (Golubkov et al., 2017). In the upper part of the estuary, the runoff is faster, and the residence time of water is therefore shorter than in the middle part of the estuary (Golubkov and Golubkov, 2020). Due to the short residence time of water, developing algae are washed out from the upper to the middle reaches of the estuary, where they accumulate in large biomasses. The salinity of water in the middle reaches is higher than in the upper reaches, and therefore, salinity appears in the multi-regression as a positive predictor (Table 3). In addition, it has been shown that the biomass of algae is higher in less flowing and saline parts of the estuary (Golubkov et al., 2021). In addition, the upper shallow part of the estuary is usually warmer in summer compared to its middle part. As a result, water temperature is also a negative predictor in the multi-regression equation for the dependence of PP on environmental factors (Table 3).
Thus, our study showed that light was the main factor limiting the primary production of plankton in the Neva Estuary during the periods of mega-engineering construction. This factor limited the primary production despite the increase in the phosphorus concentration in the water during these periods. Similar limitation of primary production with an excess of nutrients by light was observed in some other estuaries under natural conditions as a results of shallowness and high resuspension of bottom sediments or overeutrophication (Yoshiyama and Sharp, 2006; Guinder et al., 2009; Horemans et al., 2020). The Neva Estuary is one of the most eutrophic water areas in the Baltic Sea; the primary production and biomass of phytoplankton are quite high at the peak of summer phytoplankton development (Golubkov et al., 2017; Golubkov et al., 2021). However, under natural conditions, the light intensity is not a factor limiting the development of phytoplankton in the estuary. This factor became limiting during the construction of port infrastructure due to the resuspension of a large amount of suspended matter into the water column. An increase in the concentration of nutrients during these periods did not have a stimulating effect on the development of phytoplankton in the estuary under conditions of light limitation.
Conclusions
Large-scale engineering projects carried out in the Neva Estuary are a reflection of the general global trend towards the rapid development of coastal territories and port infrastructure at the mouths of large rivers. This trend is closely related to the steady growth of international trade and the large role of maritime water transport in it. An example of the construction of a new port infrastructure and the creation of new lands in the Neva Estuary shows that the implementation of such mega-projects is associated with an intense impact on the estuary ecosystem, including its primary productivity. The conducted study shows that construction work in the estuary water area caused increased concentrations of suspended particulate matter in the estuary water, which significantly exceeded the natural background. This led, on the one hand, to a significant increase in the content of nutrients in the water, and, on the other hand, to a multiple increase in turbidity. As a result, there was a change in the factor limiting the productivity of phytoplankton. The multiple increase in nutrients turned out to be less important than expected. Against the background of high concentrations of phosphorus, the main factor limiting the productivity of phytoplankton became transparency of water. In the case of the Neva Estuary, this led to a decrease in plankton primary production. Taking into account plans for further development of port areas in the Neva Estuary and other coastal areas around the world, the influence of the large-scale engineering projects on the conditions for the development of phytoplankton may provide a new aspect of long-term regulation of algal blooms and ecosystem functioning in the coastal and estuarine zones.
Data Availability Statement
The original contributions presented in the study are included in the article/Supplementary Material. Further inquiries can be directed to the corresponding author.
Author Contributions
Both authors drafted, contributed to, and approved the manuscript.
Funding
The study was supported by Zoological Institute RAS (project 122031100274-7).
Conflict of Interest
The authors declare that the research was conducted in the absence of any commercial or financial relationships that could be construed as a potential conflict of interest.
Publisher’s Note
All claims expressed in this article are solely those of the authors and do not necessarily represent those of their affiliated organizations, or those of the publisher, the editors and the reviewers. Any product that may be evaluated in this article, or claim that may be made by its manufacturer, is not guaranteed or endorsed by the publisher.
Supplementary Material
The Supplementary Material for this article can be found online at: https://www.frontiersin.org/articles/10.3389/fmars.2022.851043/full#supplementary-material
References
Bianchi T. S., Allison M.A.(2009).Large-River Delta-Front Estuaries as Natural ‘‘Recorders’’ of Global Environmental Change. PNAS 66 (20), 88085–88092. doi: 10.1073/pnas.0812878106
Cao S., Liu Z., Zhou B., Jiang Y., Xu M., Wang Y. (2022). Post-Ecological Effect and Risk Assessment of Using Modified Clay in Harmful Algal Bloom Mitigation: An Attempt Based on the Responses of Zooplankton Brachionus Plicatilis and Bivalve Mytilus Edulis. Ecotoxicology Environ. Saf. 230, 113134. doi: 10.1016/j.ecoenv.2021.113134
de Jorge V. N., van Beusekom J. E. E. (1995). Wind- and Tide-Induced Resuspension of Sediment and Microphytobenthos From Tidal Flats in the Ems Estuary. Limnol. Oceanogr. 40, 766–778. doi: 10.4319/lo.1995.40.4.0776
de Magalhães L., Noyma N. P., Furtado L. L., Leite E., Furtado V. B. G., Mucci M., et al. (2019). Managing Eutrophication in a Tropical Brackish Water Lagoon: Testing Lanthanum-Modified Clay and Coagulant for Internal Load Reduction and Cyanobacteria Bloom Removal. Estuaries Coasts 42, 390–402. doi: 10.1007/s12237-018-0474-8
de Magalhães L., Noyma N. P., Furtado L. L., Mucci M., van Oosterhout F., Huszar V. L. M., et al. (2017). Efficacy of Coagulants and Ballast Compounds in Removal of Cyanobacteria (Microcystis) From Water of the Tropical Lagoon Jacarepaguá (Rio De Janeiro, Brazil). Estuaries Coasts 40, 121–133. doi: 10.1007/s12237-016-0125-x
Golubkov S., Alimov A. (2010). Ecosystem Changes in the Neva Estuary (Baltic Sea): Natural Dynamics or Response to Anthropogenic Impacts? Mar. pollut. Bull. 61, 198–204. doi: 10.1016/J.MARPOLBUL.2010.02.014
Golubkov S. M., Alimov A. F., Telesh I. V., Anokhina L. E., Maximov A. A., Nikulina V. N., et al (2003). Functional Response of Midsummer Planktonic and Benthic Communities in the Neva Estuary (Eastern Gulf of Finland) to Anthropogenic Stress. Oceanologia 45, 53–66.
Golubkov M., Golubkov S. (2020). Eutrophication in the Neva Estuary (Baltic Sea): Response to Temperature and Precipitation Patterns. Mar. Freshw. Res. 71 (6), 583–595. doi: 10.1071/MF18422
Golubkov M., Golubkov S. (2021). Relationships Between Northern Hemisphere Teleconnection Patterns and Phytoplankton Productivity in the Neva Estuary (Northeastern Baltic Sea). Front. Mar. Sci. 8. doi: 10.3389/fmars.2021.735790
Golubkov S. M., Golubkov M. S., Tiunov A. V. (2019). Anthropogenic Carbon as a Basal Resource in the Benthic Food Webs in the Neva Estuary (Baltic Sea). Mar. pollut. Bull. 146, 190–200. doi: 10.1016/j.marpolbul.2019.06.037
Golubkov S., Golubkov M., Tiunov A., Nikulina L. (2017). Long-Term Changes in Primary Production and Mineralization of Organic Matter in the Neva Estuary (Baltic Sea). J. Mar. Syst. 171, 73–80. doi: 10.1016/j.jmarsys.2016.12.009
Golubkov M. S., Nikulina V. N., Golubkov S. M. (2021). Species-Level Associations of Phytoplankton With Environmental Variability in the Neva Estuary (Baltic Sea). Oceanologia 63 (1), 149–162. doi: 10.1016/j.oceano.2020.11.002
Grasshoff K., Ehrhardt M., Kremling K. (1999). Methods of Seawater Analysis, 3rd (New York: Wiley-VCH).
Guinder V. A., Popovich C. A., Perillo G. M. E. (2009). Particulate Suspended Matter Concentrations in the Bahıra Blanca Estuary, Argentina: Implication for the Development of Phytoplankton Blooms. Estuar. Coast. Shelf S. 85, 157–165. doi: 10.1016/j.ecss.2009.05.022
Hall R., Thomas S., Gaiser E. E. (2007). “Measuring Freshwater Primary Production and Respiration,” in Principles and Standards for Measuring Primary Production. Eds. Fahey T. J., Knapp A. K. (Oxford: Oxford University Press), 175–203. doi: 10.1093/acprof:oso/9780195168662.003.0010
He Y., Wang Y., Wu H. (2022). Regulation of Algal Bloom Hotspots Under Mega Estuarine Constructions in the Changjiang River Estuary. Front. Mar. Sci. 8. doi: 10.3389/fmars.2021.791956
Horemans D. M. L., Meire P., Cox T. J. S. (2020). The Impact of Temporal Variability in Light-Climate on Time-Averaged Primary Production and a Phytoplankton Bloom in a Well-Mixed Estuary. Ecol. Model. 436, 109287. doi: 10.1016/j.ecolmodel.2020.109287
Hoshiba Y., Hasumi H., Itoh S., Matsumura Y., Nakada S.. (2021). Biogeochemical Impacts of Flooding Discharge With High Suspended Sediment on Coastal Seas: A Modeling Study for a Microtidal Open Bay. Sci. Rep. 11, 21322. doi: 10.1038/s41598-021-00633-8
International Organization for Standardization (ISO) (2022) Country Codes –ISO 3166. Available at: https://www.iso.org/iso-3166-country-codes.html (Accessed March 7, 2022).
James G., Witten D., Hastie T., Tibshirani R. (2013). An Introduction to Statistical Learning With Applications (R. New York: Springer).
Kang L., He Y., Dai L., He Q., Ai H., Yang G., et al. (2019). Interactions Between Suspended Particulate Matter and Algal Cells Contributed to the Reconstruction of Phytoplankton Communities in Turbulent Waters. Water Res. 149, 251–262. doi: 10.1016/j.watres.2018.11.003
Kirk J. T. (2011). Light and Photosynthesis in Aquatic Ecosystems. 3rd Edition (Cambridge: Cambridge University Press).
Kottek M., Grieser J., Beck C., Rudolf B., Rubel F. (2006). World Map of the Köppen-Geiger Climate Classification Updated. Meteorol. Z. 15, 259–263. doi: 10.1127/0941-2948/2006/0130
Kumar P. S., Venkatnarayanan S., Pandey V., Ratnam K., Jha D. K., Rajaguru S., et al. (2022). Multivariate Approach to Evaluate the Factors Controlling the Phytoplankton Abundance and Diversity Along the Coastal Waters of Diu, Northeastern Arabian Sea. Oceanologia. 64 (2), 267–275. doi: 10.1016/j.oceano.2021.11.005
Lagus A., Suomela J., Helminen H., Sipura J. (2007). Impacts of Nutrient Enrichment and Sediment on Phytoplankton Community Structure in the Northern Baltic Sea. Hydrobiologia 579, 351–368. doi: 10.1007/s10750-006-0491-7
Lehtoranta J., Heiskanen A.-S., Pitkänen H. (2004). Particulate N and P Characterizing the Fate of Nutrients Along the Estuarine Gradient of the River Neva (Baltic Sea). Estuar. Coast. Shelf S. 61, 275–287. doi: 10.1016/j.ecss.2004.04.016
Liblik T., Lips U. (2011). Spreading Suspended Matter Shallow Sea Area Influenced by Dredging Activities Variable Atmospheric Forcing: Results In-Situ Measurements. J. Coast. Res. SI 64, 561–566.
Mangiafico S. S. (2022) An R Companion for the Handbook of Biological Statistics. Available at: https://rcompanion.org/rcompanion/e_05.html (Accessed March 7, 2022).
Martyanov S., Ryabchenko V. (2016). Bottom Sediment Resuspension in the Easternmost Gulf of Finland in the Baltic Sea: A Case Study Based on Three–Dimensional Modeling. Cont. Shelf Res. 117, 126–137. doi: 10.1016/j.csr.2016.02.011
Marzetz V., Spijkerman E., Striebel M., Wacker A. (2020). Phytoplankton Community Responses to Interactions Between Light Intensity, Light Variations, and Phosphorus Supply. Front. Environ. Sci. 8. doi: 10.3389/fenvs.2020.539733
Meteoblue (2021) Climate Zones. Available at: https://content.meteoblue.com/en/meteoscool/general-climate-zones (Accessed November 30, 2021).
Neumann B., Vafeidis A., Vafeidis A., Zimmermann J., Nicholls R. J. (2015). Future Coastal Population Growth and Exposure to Sea-Level Rise and Coastal Flooding - A Global Assessment. PloS One 10 (6), e0131375. doi: 10.1371/journal.pone.0118571
Nilsson P., Jansson M. (2002). Hydrodinamic Control of Nitrogen and Phosphorus Turnover in an Eutroficated Estuary in the Baltic. Water Res. 36, 4616–4626. doi: 10.1016/s0043-1354(02)00171-9
Pindsoo K., Soomere T. (2020). Basin-Wide Variations in Trends in Water Level Maxima in the Baltic Sea. Cont. Shelf Res. 193, 104029. doi: 10.1016/j.csr.2019.104029
R Development Core Team (2022) The R Project for Statistical Computing (Version 4.0.5). Available at: https://www.r-project.org (Accessed March 7, 2022).
Reckermann M., Omstedt A., Soomere T., Aigars J., Akhtar N., Bełdowska M., et al. (2022). Human Impacts and Their Interactions in the Baltic Sea Region. Earth System Dynamics 13 (1), 1–80. doi: 10.5194/esd-13-1-2022
Remy M., Helmut H., Flöder S. (2017). Stability of Marine Phytoplankton Communities Facing Stress Related to Global Change: Interactive Effects of Heat Waves and Turbidity. J. Exp. Mar. Biol. Ecol. 497, 219–229. doi: 10.1016/j.jembe.2017.10.002
Ryabchuk D., Zhamoida V., Orlova M., Sergeev A., Bublichenko J., Bublichenko A., et al. (2017). “Neva Bay: A Technogenic Lagoon of the Eastern Gulf of Finland (Baltic Sea),” in The Diversity of Russian Estuaries and Lagoons Exposed to Human Influence. Ed. Kosyan R. (Cham: Springer International Publishing), 191–221. doi: 10.1007/978-3-319-43392-9
Ryabinina N. V. (1965). “Nanosy I Zanosimost' Farvaterov I Kanalov Nevskoy Guby (Deposits and Drifts of the Fairways and Canals of the Neva Bay)”, in Gidrologiya Ust'yevoy Oblasti Nevy. (Moscow: Gidrometeoizdat), 223–240.
Song X., Zhang Y., Yu Z. (2021). An Eco-Environmental Assessment of Harmful Algal Bloom Mitigation Using Modified Clay. Harmful Algae 107, 102067. doi: 10.1016/j.hal.2021.102067
Su J., Tian T., Krasemann H., Schartau M., Wirtz K. (2015). Response Patterns of Phytoplankton Growth to Variations in Resuspension in the German Bight Revealed by Daily MERIS Data in 2003 and 2004. Oceanologia 57, 328—341. doi: 10.1016/j.oceano.2015.06.001
Umnova L. P. (1987). “Vzveshennoye Organicheskoye I Mineral'noye Veshchestvo I Khlorofill (Suspended Organic and Mineral Matter and Chlorophyll),” in Nevskaya Guba: Gidrobiologicheskiye Issledovaniya. Eds. Winberg G. G., Gutel'makher B. L. (Lenngrad: Nauka), 7–14.
Vernet M., Smith R. C. (2007). “Measuring and Modeling Primary Production in Marine Pelagic Ecosystems,” in Principles and Standards for Measuring Primary Production. Eds. Fahey T. J., Knapp A. K. (Oxford: Oxford University Press), 142–174. doi: 10.1093/acprof:oso/9780195168662.003.0009
Wang Y., Wu H., Lin J., Zhu J., Zhang W., Li C. (2019). Phytoplankton Blooms Off a High Turbidity Estuary: A Case Study in the Changjiang River Estuary. J. Geophysical Research: Oceans 124, 8036, 8059. doi: 10.1029/2019JC015343
Weisse R., Dailidiene I., Hünicke B., Kahma K., Madsen K., Omstedt A., et al. (2021). Sea Level Dynamics and Coastal Erosion in the Baltic Sea Region. Earth Syst. Dynam. 12, 871–898. doi: 10.5194/esd-12-871-2021
Wu H., Wu T., Bai M. (2018). Mega Estuarine Constructions Modulate the Changjiang River Plume Extension in Adjacent Seas. Estuaries Coasts 41, 1234–1252. doi: 10.1007/s12237-017-0357-4
Yoshiyama K., Sharp J. H. (2006). Phytoplankton Response to Nutrient Enrichment in an Urbanized Estuary: Apparent Inhibition of Primary Production by Over Eutrophication. Limnol. Oceanogr. 51, 424–434. doi: 10.4319/lo.2006.51.1_part_2.0424
Yu Z., Song X., Cao X., Liu Y. (2017). Mitigation of Harmful Algal Blooms Using Modified Clays: Theory, Mechanisms, and Applications. Harmful Algae 69, 48–64. doi: 10.1016/j.hal.2017.09.004
Zalessky I. A., Wulf G. F. (1913). Rezul'taty Fiziko-Khimicheskikh Issledovaniy Nevskoy Guby (Results of Physicochemical Studies of the Neva Bay),” in Materialy Po Issledovaniyu Vody Nevskoy Guby V Sanitarnom Otnoshenii (Materials on the Study of Water in the Neva Bay in a Sanitary Sense). Ed. G.V. Khlopin. (St. Petersburg), 8–14.
Keywords: primary production, chlorophyll, suspended matter, light conditions, euthrophication, nutrients, phosphorus, Gulf of Finland
Citation: Golubkov M and Golubkov S (2022) Impact of the Construction of New Port Facilities on Primary Production of Plankton in the Neva Estuary (Baltic Sea). Front. Mar. Sci. 9:851043. doi: 10.3389/fmars.2022.851043
Received: 08 January 2022; Accepted: 23 May 2022;
Published: 23 June 2022.
Edited by:
Anas Ghadouani, University of Western Australia, AustraliaReviewed by:
Jerónimo Pan, Consejo Nacional de Investigaciones Científicas y Técnicas (CONICET), ArgentinaAna Laura Delgado, CONICET Instituto Argentino de Oceanografía (IADO), Argentina
Copyright © 2022 Golubkov and Golubkov. This is an open-access article distributed under the terms of the Creative Commons Attribution License (CC BY). The use, distribution or reproduction in other forums is permitted, provided the original author(s) and the copyright owner(s) are credited and that the original publication in this journal is cited, in accordance with accepted academic practice. No use, distribution or reproduction is permitted which does not comply with these terms.
*Correspondence: Mikhail Golubkov, golubkov_ms@mail.ru