- 1Red Sea Research Center (RSRC) and Computational Bioscience Research Center (CBRC), King Abdullah University of Science and Technology (KAUST), Thuwal, Saudi Arabia
- 2Red Sea Research Center (RSRC), King Abdullah University of Science and Technology (KAUST), Thuwal, Saudi Arabia
Immobilization of marine invertebrates for research purposes has been commonly used and is often necessary to obtain high-quality findings. Despite these approaches being standard procedures, they can affect the specimens, and their responses as well. The effectiveness of magnesium chloride (MgCl2) to “narcotize” marine invertebrates has been recognized since 1946. Here, we determine the concentration of MgCl2 that immobilizes Cassiopea sp., at jellyfish stage, effectively and quickly, without causing mortality. The specimens were exposed to different concentrations of MgCl2, until the range was narrowed, and the most effective concentration was found (0.092M). In the final experiment, the jellyfish (~ 8.68 cm ± 1.70 SD of diameter) were exposed to a 0.092M MgCl2 solution for 2 and 24 hours. Before, during, and after the exposure we monitored the following biological responses: (1) cessation of bell pulsations, (2) responsiveness to bell-tap stimulus, (3) photochemical efficiency, (4) bell pulsation, (5) recovery time, and (6) survival after 24 and 48 hours from exposure. Environmental conditions (pH and dissolved oxygen, dO2) were monitored as well. Our findings indicate that, despite being an effective concentration for immobilization of Cassiopea sp. at jellyfish stage, their bell pulsations were significantly affected both after 2 and 24 hours when comparing the values measured in the “pre-incubation” and “immediate post-incubation” phases, whereas bell size was significantly affected only in the 24 hours incubation. In conclusion, we suggest that this concentration can be used to immobilize Cassiopea sp.at jellyfish stage for short-term analyses. However, the limitations related to the use of this chemical should be reported, as it could lead scientists to misleading conclusions.
Introduction
Marine invertebrates represent a versatile model system used across many research disciplines, ranging from marine ecology to biochemical engineering (Tsien, 1998) and human aging research (Bodnar, 2009). Advanced visualization systems and fiberoptic microsensors are now commonplace techniques used to study marine invertebrates. However, high-quality research outcomes from these techniques often require the subject specimens to be immobilized, (Perez and Weis, 2006), anesthetized, or even euthanatized. Despite the invasiveness of these approaches, the impacts of these protocols on the data obtained is not commonly assessed or reported. The development of protocols enabling immobilization, anesthesia, or euthanasia for marine invertebrates has accelerated in the last 10 years, presumably owing to the rising demand for such studies (Lewbart et al., 2012). These protocols usually involve the treatment of specimens with chemicals, including menthol (Steedman, 1976), chloral hydrate (Pantin, 1946), ethanol (Pizzi, 2012), magnesium sulphate (Brusca, 1980), potassium chloride (Battison et al., 2000), and magnesium chloride (Pantin, 1946).
Magnesium chloride (MgCl2) was first used in 1946 (Pantin, 1946) to “narcotize” marine invertebrates. Since then, it has been used extensively to anesthetize and euthanatize echinoderms, cephalopods, and coelenterates (Brusca, 1980; Messenger et al., 1985; Arai, 1997; Lewbart et al., 2012). Owing to the absence of side-effects at low MgCl2 concentrations (Messenger et al., 1985), this method has found additional applicability in aquaculture and the transportation of organisms to reduce stress (Arafa et al., 2007). Indeed, MgCl2 acts as muscle relaxant and immobilization agent and is known to prevent stress-induced spawning in bivalves (Heasman et al., 1995) and sea urchins (Arafa et al., 2007), and induces relaxation of cephalopods (Andrews and Tansey, 1981).
In cnidarians, MgCl2 is mostly used to anesthetize specimens prior to their fixation in formalin (e.g., Acuña et al., 2012; Amiel and Röttinger, 2021; Di Camillo et al., 2021). Experiments on cnidarians, such as the moon jellyfish Aurelia aurita, have also revealed that MgCl2 can be used to euthanize specimens without distress (i.e., death defined as cessation of bell pulsations and responsiveness to external stimulus; Doerr and Stoskopf, 2019) for nuclear magnetic resonance (NMR) applications. However, a recent study revealed that long-term exposure to magnesium excess or deficiency can induce negative side-effects (Evans et al., 2021), including death (Doerr and Stoskopf, 2019), impairment of nematocyst function (Ozacmak et al., 2001), several growth-related issues (Dabrowska et al., 1991; Lim and Klesius, 2003), and an increase of bell pulsations as reported for A. aurita (Abrams et al., 2015). This is likely due to the essential role of magnesium in cellular osmosis and catecholamine interference (Fawcett et al., 1999).
Hence, no reliable method is yet available to immobilize cnidarians for experimental purposes. In this study we focus on the jellyfish stage of Cassiopea sp., commonly known as the upside-down jellyfish, which is often used as a model system in climate change and symbiosis-related studies (e.g., Mellas et al., 2014; Aljbour et al., 2017; Aljbour et al., 2019; Banha et al., 2020). Cassiopea spp. are also well known for their bell pulsation activity (Arai, 1997; Hamlet et al., 2011) and for releasing mucus when stressed (Pitt et al., 2009). Movements of the body were previously observed to interfere with specific experimental approaches involving the use of fiberoptic microsensors (e.g., breaking of microsensors tips, noisy measurements, etc.; Arossa et al., 2021) and needed to be minimized as much as possible. Additionally, obtaining high quality photos using confocal microscopy can be affected by this factor. For this purpose, we tested the efficiency and the effects of the use of MgCl2 as an immobilization agent on individuals of Cassiopea sp. at jellyfish stage. The following biological responses were analyzed: bell pulsation frequency, photochemical efficiency (Fv/Fm), bell diameter, time to unresponsiveness, responsiveness to physical stimulus, and recovery.
Materials and Methods
Experimental Approach and Preliminary Tests
Twenty-three individuals at jellyfish stage of Cassiopea sp. (bell diameter average: 8.68 cm ± SD 1.70 cm), similar in condition and without abnormalities, were collected from the mangroves surrounding the KAUST Monument (22.340905°N, 39.09024°E), at 0.20 – 1 m depth, in the Central Red Sea in June 2021. Seawater temperature, salinity and light conditions, measured in situ, were 28.0°C ± 0.01, 42 ppt, and ~1246.2 µmol photons m-2 s-1 (photosynthetic active radiation [PAR]), respectively. The jellyfish were immediately transported to the Coastal and Marine Resources Core Labs (CMR) aquaria facilities at the King Abdullah University of Science and Technology (KAUST) for acclimation. Acclimation was performed in an outdoor tank (200 L) under natural light conditions (approximately 12:12-hr light: dark cycle, maximum 2130.00 µmol photons m-2 s-1 ± 101.70 during day and night) for 2 weeks. The tank was supplemented by a continuous flow of raw seawater (i.e. unfiltered) at a rate of ~300 L h-1 and mimicked conditions of the field collection site (at 28.8°C ± 0.23 and salinity of 42 ppt). The aquarium was equipped with a thermometer, temperature controller, and PAR sensor. The specimens were fed daily with freshly hatched Artemia naupilii, except during the exposure to MgCl2 and recovery time.
During the experiment, the jellyfish were kept inside 2L glass aquaria in autoclaved seawater (121°C for 20 min) maintained at 28°C and salinity of 43 ppt. The aquaria were kept inside biological incubators (Percival Scientific, Boone, IA) set to control temperature at 28°C and light at a day: night 12:12-hr cycle of ~200 µmol photons m-2 s-1 (PAR).
A preliminary test was performed across a range of MgCl2 concentrations: 0.37 M, 0.18 M, 0.09 M and 0.04 M, with the highest concentration having previously been reported to be effective in the immobilization of Aiptasia diaphana (Franchini and Steinke, 2017). These concentrations were used to narrow down the range of concentrations that provide the best results. This first test was performed without adequate replication (4 individuals), to minimize the number of specimens used. A concentration was considered effective when (a) immobilization was observed within a maximum of 20 minutes (Doerr and Stoskopf, 2019), (b) recovery was observed in maximum 10 minute from the seawater change (i.e., when autoclaved seawater with MgCl2 was replaced with autoclaved seawater without MgCl2), (c) jellyfish were still alive and displaying normal behavior after 24 and 48 hours. The terms “recovery” and “complete recovery” will be used from now on to indicate the start of pulsations after MgCl2 exposure and recovery to a normal/initial bell pulsations rate, respectively. A second pilot test was then performed using MgCl2 levels ranging from the effective concentration obtained from the first test (i.e., 0.092 M) to 0.07 M (i.e., 0.081 M and 0.07 M). Three jellyfish for each concentration were exposed to MgCl2 and maintained in the solution for 2 and 24 hours after immobilization (i.e., no bell pulsations and unresponsive to bell-tap stimulus). Specimens were then removed and immersed in autoclaved seawater without MgCl2. Immobilization time, recovery time after 2 hours of exposure, recovery time after 24 hours, and time of death (if applicable) were recorded through observation of the specimens. The most effective concentration obtained from these preliminary tests was used to design the main experiment presented in this study and described below.
Although higher replication would have been ideal, the chosen number of replicates (n = 3) represents a compromise due to the necessity to avoid overexploitation of the local population of Cassiopea. Additionally, previously published studies were used as a guide to decide the number of medusae considered adequate replication (e.g. n = 3 in Wangpraseurt et al., 2012; Wangpraseurt et al., 2014; Arossa et al., 2021; etc.) or did not clearly report replication (Shashar and Stambler, 1992; Al-Horani et al., 2003; Kühl et al., 1995; etc.).
Pre-Incubation Phase
Nine jellyfish were collected from the aquarium, transferred to the experimental aquaria, and allowed to acclimatize for 15 minutes. After acclimation, bell pulsations min-1, size (cm), photochemical efficiency (Fv/Fm), dissolved oxygen (dO2), and pH were recorded. We used these measurements as an indication of jellyfish health prior to MgCl2 exposure (termed henceforth as the “pre-incubation” phase), as dO2 and pH fluctuations can be driven by the responses of the holobiont (e.g., respiration, photosynthesis of the symbionts, bell pulsations, etc.; Arossa et al., 2021). Bell pulsations were used as a proxy for jellyfish behavior (sensu Nath et al., 2017) and measured by counting the number of bell contractions in two minutes, which were then averaged and presented as the number of bell pulsations min-1 (units). Jellyfish size was measured to the nearest millimeter using a bendable measuring tape so that measurements could be made through the glass and avoid disturbances prior the start of the incubation. Photochemical efficiency (Fv/Fm) was measured on three randomly selected oral arms per jellyfish using a mini-pulse amplitude modulator (miniPAM-R, Waltz GmbH, Germany). dO2 and pH were measured using a calibrated pH/dissolved oxygen meter (Mettler Toledo International USA, SevenGo Duo pro). The pH probe was calibrated using commercial pH standard solutions (pH 4.0, 7.0, and 10.0), whereas the dO2 sensor was calibrated following manufacturer instructions.
Incubation Phase
Jellyfish were randomly assigned to two treatments: (1) three jellyfish were exposed to a solution of 0.092 M MgCl2 for 2 hours and (2) three jellyfish were exposed to a solution of 0.092 M MgCl2 for 24 hours. Three jellyfish were used as controls (i.e., in seawater without MgCl2). Cessation of bell pulsations (i.e., time passed between the immersion in seawater with MgCl2 and the last bell pulsation) and unresponsiveness to bell-tap stimulus (i.e., when there’s a lack of “a single strong, synchronous pulse immediately after receiving a light tap to the top of the bell with a gloved finger”; Doerr and Stoskopf, 2019) were recorded at the start of the exposure. Unresponsiveness to bell-tap stimulus was tested right after the cessation of bell pulsations until absence of responses was observed (i.e., approximately every 5 seconds). When cessation of bell pulsations and unresponsiveness to bell-tap stimulus were observed, the jellyfish was considered immobilized. At the midpoint of the incubation pH, dO2, and photochemical efficiency (Fv/Fm) were recorded. Jellyfish were also monitored during the treatment time for potential pulsations and to assess the efficiency of the immobilization procedure using both visual observation and a camera positioned inside the incubator. This step will be called from now on “mid-incubation”
Post-Incubation Phase
At the end of the exposure time (i.e., called from now on “immediate post-incubation), jellyfish were removed and immersed in autoclaved seawater at 28.0°C without MgCl2 to evaluate their recovery, complete recovery, survival processes, and size. This seawater change was also performed on the jellyfish assigned to the control treatment to account for effects of the handling procedures. Recovery time (i.e., time between the immersion of jellyfish in seawater without MgCl2 and the first bell pulsation), responsiveness to bell-tap stimulus, pH, dO2, number of bell pulsations min-1, and photochemical efficiency (Fv/Fm) were recorded. At 24 and 48 hours from the end of the exposure, photochemical efficiency (Fv/Fm), number of bell pulsations min-1, bell size, and mortality was recorded. These steps will be called from now on “post-incubation 24 hours” and “post-incubation 48 hours”.
Data Analysis
Data was tested for normality and homoscedasticity using Q–Q plots and standardized residual plots. Levene’s tests and Q-Q plots were performed to test for homogeneity of variance and normality, respectively. If not meeting normality assumptions, data was either ln or ln (x+1) transformed, if required. Biological responses (bell pulsations (min-1) and photochemical efficiency Fv/Fm) were analyzed using two-way linear mixed models (LMMs) in SPSS (IBM, version 27), with a repeated measures design. Two separate LMMs were used to analyze each response variable for the 2h and 24h incubations. Treatment (with two levels: control and MgCl2) and time (with five levels: pre-incubation, incubation, immediate post-incubation, 24h post-incubation, and 48h post-incubation) were considered as fixed factors. Time was also our repeated measure. Different covariance structures (e.g. Compound Symmetry, AR(1), and AR(1) Heterogenous) were examined to assess the model of best fit by comparing several goodness-of-fit statistics (e.g. -2 restricted log likelihood, Akaike’s information criterion (AIC) and Bayesian information criterion (BIC)). When no significant effect of one of the factors was revealed, this factor was removed, and the analysis rerun. If significant effects were detected, the Bonferroni post-hoc tests (for significant main effects) was performed to determine which means differed.
Independent t-tests were used to compare jellyfish size (% change - calculated from before/after measurements) between the control and incubation treatments in the 2h and 24h incubations.
Environmental parameters were reported as Mean ± SD, whereas all the other results were reported as Mean ± SE.
Results
Response Variables
The first pilot test showed that the most effective concentration was ~0.09 M, whereas in the second test the best results were obtained with a concentration of 0.92 M (Supplementary Materials 1). Lower concentrations didn’t induce immobilization (i.e., cessation of bell pulsations and unresponsiveness to bell-tap stimulus together) and higher concentrations induced signs of distress (e.g., unusual orientation). This concentration was subsequently chosen for the immobilization of Cassiopea jellyfish in our main experiment.
In the 2-hour incubation experiment jellyfish were completely immobilized (i.e., cessation of bell pulsations and unresponsiveness to bell-tap stimulus together) after 36.0 ± 11.0 seconds, whereas in the 24-hour incubation experiment, complete pulse cessation was observed after 68.0 ± 11.1 seconds (Table 1). Overall, immobilization was observed after ~ 52.0 ± 10.0 seconds, while unresponsiveness was reached after 71.0 ± 16.0 seconds in the jellyfish incubated for 2 hours and after 58.0 ± 27.0 seconds in the jellyfish incubated for 24 hours (Table 1). An average of ~64.0 ± 14.0 seconds was needed to obtain complete unresponsiveness. In the 2 hours incubation experiment, recovery was observed after 10.0 ± 5.0 seconds from the immersion in seawater without MgCl2. In the 24-hour incubation experiment, recovery time was 6.0 ± 2.0 seconds. Overall, recovery required 8.0 ± 2.0 seconds (see Video in Supplementary Materials). Mortality was not observed during these experiments and all jellyfish were still alive at 48 hours post-incubation.
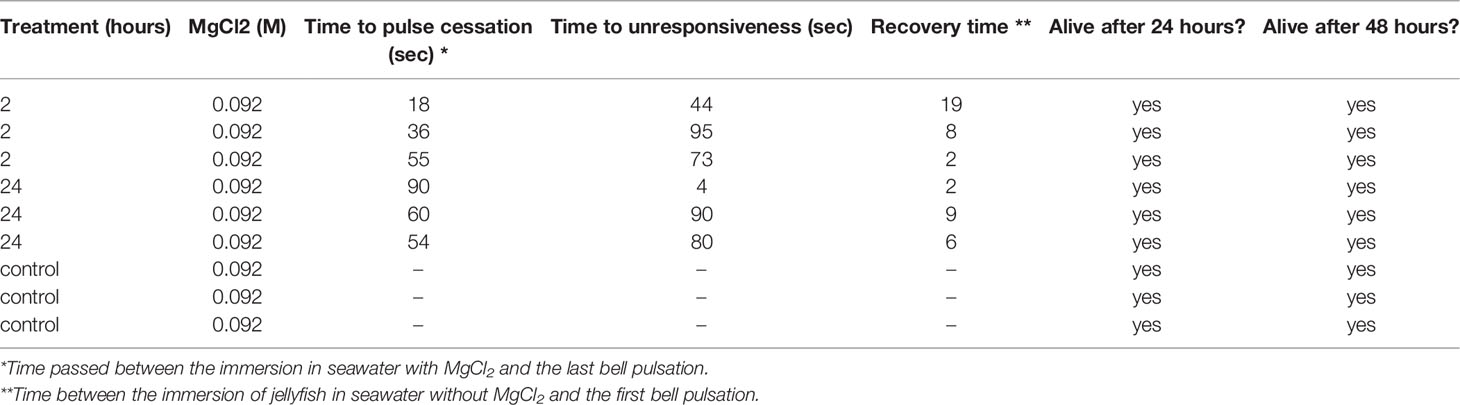
Table 1 Summary of the main responses of Cassiopea sp.jellyfish to the MgCl2 treatment (e.g., time to pulse cessation, time to unresponsiveness, recovery time, and survival).
Photochemical efficiency (Fv/Fm) was not affected by time or treatment (two-way LMMs, p > 0.05, Table 2) in neither the 2 hour or 24-hour incubation experiments (Figures 1A, B). Average bell pulsations min-1 were significantly affected by the treatment in both the 2 hour (one-way LMMs, p=0.010, Table 2; Figures 1C, D) and 24-hour incubation (one-way LMMs, p=0.001, Table 2). No effect of time was observed. Figure S2 in Supplementary Materials 2 shows average bell pulsations min-1 in treatment versus controls.
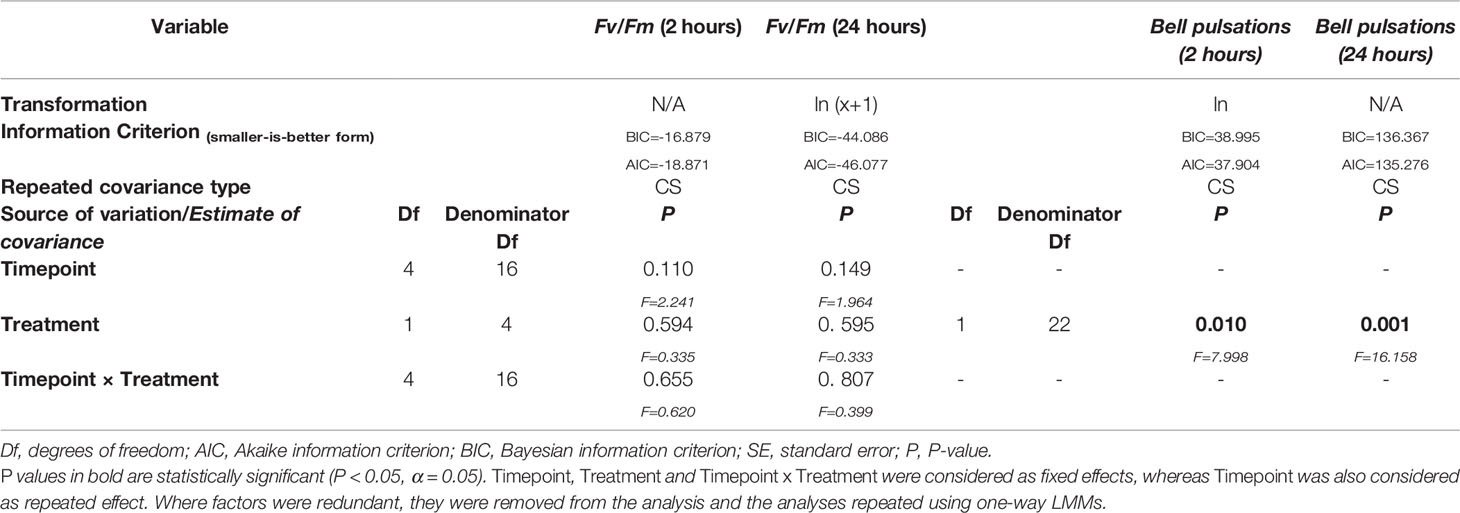
Table 2 Summary of results for Linear Mixed Models (LMMs) conducted on the investigated biological responses of Cassiopea jellyfish during the exposure to Magnesium Chloride (MgCl2) (i.e., photochemical efficiency Fv/Fm and bell pulsations min-1).
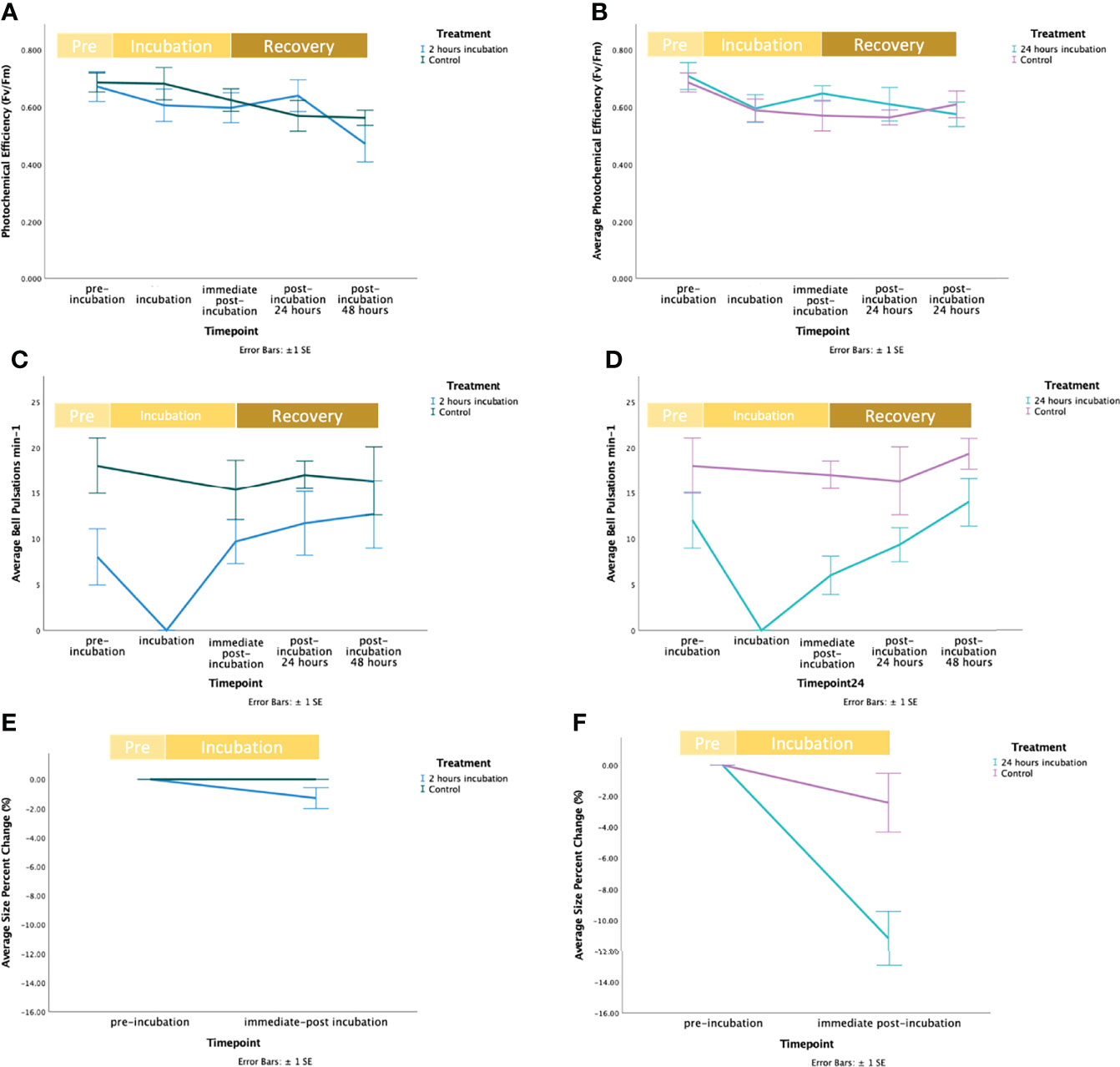
Figure 1 Fluctuation of response variables during the exposure of Cassiopea sp. jellyfish to Magnesium Chloride (MgCl2), including the recovery time. In figures a, c, and e the blue line represents the control, and the green line represents the treatment. In figures b, d, and f the purple line represents the control, and the mint line represents the treatment. (A) Fluctuations of average photochemical efficiency (Fv/Fm) at the start (“pre-incubation”), middle (“incubation”) and end (“immediate post-incubation”) of the 2 hours exposure to MgCl2 and during the recovery period (i.e., “post-incubation 24 hours” and “post-incubation 48 hours” indicates the recovery period of 24 and 48 hours after the end of the exposure to MgCl2). Bars represent standard error (± SE). (B) Fluctuations of average photochemical efficiency (Fv/Fm) at the start (“pre-incubation”), middle (“incubation”) and end (“immediate post-incubation”) of the 24-hour exposure to MgCl2 and during recovery period (i.e., “post-incubation 24 hours” and “post-incubation 48 hours” after the end of the exposure to MgCl2). Bars represent standard error (± SE). (C) Fluctuations of the average number of bell pulsations min-1 at the start (“pre-incubation”), middle (“incubation”) and end (“immediate post-incubation”) of the 2-hour exposure to MgCl2 and during the recovery period (i.e., “post-incubation 24 hours” and “post-incubation 48 hours” indicates the recovery period of 24 and 48 hours after the end of the exposure to MgCl2). Bars represent standard error (± 1SE). (D) Fluctuations of the average number of bell pulsations min-1 at the start (“pre-incubation”) and end (“immediate post-incubation”) of the 24-hour exposure to MgCl2 and during the recovery period (i.e., “post-incubation 24 hours” and “post-incubation 48 hours” indicates the recovery period of 24 and 48 hours after the end of the exposure to MgCl2). Bars represent standard error (± SE). (E) Fluctuations of the average size percent change (%) at the start (“pre-incubation”), and end (“immediate post-incubation”) of the 2-hour exposure to MgCl2. Bars represent standard error (± 1SE). (F) Fluctuations of the average size percent change (%) at the start (“pre-incubation”), and end (“immediate post-incubation”) of the 24-hour exposure to MgCl2. Bars represent standard error (± 1SE).
Size change (%) in treatments during the 2 hours incubations was -1.290 ± 0.722%, but did not significantly differ from that of controls (independent t-test, p = 0.185; Table 2; Figures 1E, F). On the contrary, size change (%) in treatments in the 24-hour incubation was equal to -11.179 ± 1.744% and a significant effect of the treatment was found (independent t-test, p = 0.027; Table 1 in Supplementary Materials; Figures E, F).
Environmental Parameters
pH and dO2 fluctuated during the incubation with MgCl2 (Figures 2 and S1 in Supplementary Materials 2). In the 2 hours incubation experiment, pH slightly increased by approximately 0.071 ± 0.008 SD in controls (from 8.166 ± 0.032 SD to 8.237 ± 0.036 SD) and 0.090 ± 0.023 SD in treatments (from 7.945 ± 0.013 SD to 8.035 ± 0.013 SD), but largely remained unchanged. In the 24-hour incubation experiment, variations of pH and dO2 followed a day/night cycle. pH increased by 0.208 ± 0.033 SD units in controls (from 8.166 ± 0.032 SD to 8.375 ± 0.003 SD) and similarly, it increased by 0.437 ± 0.059 SD in treatments (from 7.955 ± 0.010 SD to 8.375 ± 0.003 SD). Overall, dO2 increased over time in both treatments and controls. In the 2-hour incubation experiment, dO2 increased by 2.030 ± 0.095 SD mg/L in controls (from 8.807 ± 0.185 SD mg/L to 10.837 ± 0.090 SD mg/L) and 2.587 ± 0.424 SD mg/L in treatments (from 9.360 ± 0.183 SD mg/L to 11.947 ± 0.511 SD mg/L). In the 24-hour incubation experiment, it increased by 7.047 ± 0.490 SD mg/L in controls (from 8.807 ± 0.185 SD mg/L to 15.853 ± 0.660 SD mg/L) and 11.050 ± 1.355 SD mg/L in treatments (from 9.620 ± 0.230 SD mg/L to 20.670 ± 1.546 SD mg/L) (Figures 1C, D, 2C and d in Supplementary Materials 2). However, during the 2-hour exposure the rise in dO2 followed a linear trend, whereas during the 24 hour incubation dO2 reached the lowest value mid-incubation equal to 3.353 ± 0.854 SD mg/L (4.724 ± 0.690 SD mg/L in controls and 3.354 ± 0.854 SD in treatments).
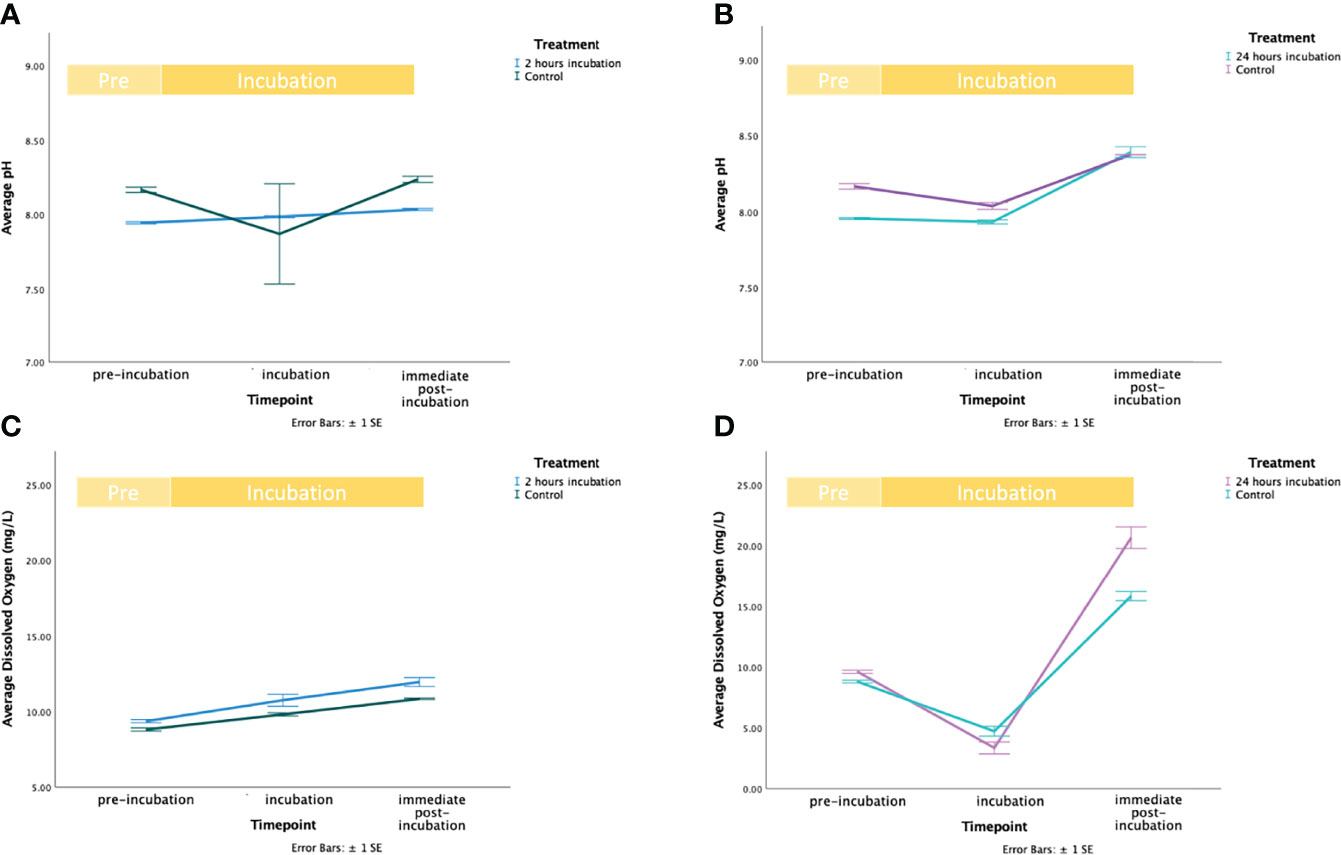
Figure 2 Environmental parameters variation during the exposure of Cassiopea jellyfish to Magnesium Chloride (MgCl2). In figures a, and c the green line represents the control, and the blue line represents the treatment. In figures b, and d, the mint line represents the control, and the purple line represents the treatment. (A) Fluctuations of average pH levels before the start (“pre-incubation”), middle (“incubation”) and end (“immediate post-incubation”) of the 2 hours exposure to MgCl2. Bars represent standard error (± 1SE). (B) Fluctuations of average pH levels before the start (“pre-incubation”), middle (“incubation”) and end (“immediate post-incubation”) of the 24 hours exposure to MgCl2. Bars represent standard error (± 1SE). (C) Fluctuations of average dO2 levels before the start (“pre-incubation”), middle (“incubation”) and end (“immediate post-incubation”) of the 2 hours exposure to MgCl2. Bars represent standard error (± 1SE). (D) Fluctuations of average dO2 levels before the start (“pre-incubation”), middle (“incubation”) and end (“immediate post-incubation”) of the 24 hours exposure to MgCl2. Bars represent standard error (± 1SE).
Discussion
This study demonstrates that MgCl2 can be used as an efficient chemical technique to immobilize Cassiopea sp. medusae (i.e., jellyfish stage). However, our results show that this procedure can significantly affect some key biological responses that may influence experimental results. The proposed effective concentration (0.092 M) was chosen because of the following observations: (1) it induces complete immobilization (i.e., cessation of bell pulsations and unresponsiveness to bell-tap stimulus together) after ~52 seconds in individuals with a diameter of ~ 8.68 cm ± 1.70 SD cm, (2) specimens are still alive after the treatment, after 24 and 48 hours. On the contrary, lower concentrations (i.e., 0.081 and 0.07 M) showed no immobilization of medusae and higher concentrations induced signs of distress (i.e., unusual behavior, such as upside-down orientation).
Numerous are the studies reporting the concentration of MgCl2 used for anesthesia prior preservation or fixation in Cnidarians (e.g., 0.36 M for polyps of Goniopora stockesi in Hawkridge et al., 2000; 0.63 M for Rhodactis in Chen et al., 1995; etc.) and, more specifically, of jellyfish (e.g., 0.37 M for Tripedialia cystophora in Petie et al., 2013; 0.75 M for the hydromedusa Aglantha digitale in Bickell-Page and Mackie, 1991). A concentration of 1.74 M was reported to cause effective euthanasia within ~32 seconds in the jellyfish Aurelia aurita (Doerr and Stoskopf, 2019). However, the actual immobilization (i.e., the cessation of bell pulsations and unresponsiveness to bell-tap) and recovery time of jellyfish was never reported.
This method has, however, some limitations as some biological responses, such as bell pulsations and bell size, can be affected by this procedure. We observed that bell pulsations were significantly affected by the MgCl2 treatment both after 2 and 24 hours when comparing the values measured in the “pre-incubation” and “immediate post-incubation” phases. Bell size was affected by the treatment, especially in the 24-hour incubation. This change, however, was not significantly different between controls and treatments, in the 2-hour incubation. Indeed, symbionts in Cassiopea are capable to provide up to 169% of carbon to support the respiration of the host (Verde and McCloskey, 1998). Whereas under longer immobilization time, the significant reduction of size observed in the treatments might be due to two factors: (1) no food was provided during the experiment, and (2) the lack of pulsation might have reduced the flux of seawater and compounds needed to support the symbiont’s photosynthetic activity.
Even though the main effect of MgCl2 as an immobilization chemical is the cessation of bell pulsations, this is unlikely to have caused major side effects to Cassiopea for this limited time. Although this is necessary to support a continuous flux of seawater in Cassiopea’s body and to allow heterotrophic feeding (Hamlet et al., 2011), Cassiopea commonly undergoes sleep-like behavior during nighttime (Nath et al., 2017) involving a reduction of bell pulsations. Hence, this is a naturally occurring status that doesn’t cause any harm to the holobiont.
Additionally, a recent study has demonstrated that magnesium, being an important component of the osmosis balancing and of the catecholamine interference systems (Fawcett et al., 1999), may lead to unexpected deleterious side-effects (Evans et al., 2021), especially if used for long time (~28 days). However, even though the effective concentration used in our study is significantly higher than those used by Evans and colleagues (2021) when reporting these effects, which ranged from 0.003 to 0.01 M, it might not lead to such deleterious effects if used for short-term experiments. Therefore, we suggest reporting limitations related to the use of MgCl2 in the study, avoiding the reuse of the specimens for further analyses, and to limit the use to short-term experiments only.
sp.During our experiment, significant fluctuations of pH and dO2 occurred. Addition of MgCl2 to seawater did not affect pH nor dO2, therefore the observed variations in environmental parameters were specifically driven by the metabolic activity of the specimens. We observed that these variations were more intense during the 24-hour incubation. This incubation included light-dark shifts (12h:12h), whereas the 2-hour incubation was performed in light only. As a consequence, we hypothesized that the fluctuations were driven by metabolic processes in the holobiont (i.e., photosynthesis during the day performed by the symbionts and respiration during the night performed by both symbionts and jellyfish; Arossa et al., 2021) despite being immobilized. Therefore, this variability followed a day/night cycle. This is particularly important and needs to be taken into account when conducting experiments with MgCl2 in closed-systems or preparing the specimens for microsensor measurements.
Conclusions
MgCl2 is commonly used for relaxation, anesthesia, and euthanasia of cnidarian species for many research purposes, including microsensor measurements and confocal microscopy. However, this chemical may have effects on the specimens, thus influencing scientists’ findings. Therefore, we suggest a specific concentration of MgCl2 (0.092 M) that can be used to quickly immobilize Cassiopea sp.jellyfish for short-term experiments or analyses. The use of this proposed concentration of MgCl2 would allow researchers to: (1) conduct experiments that require immobilization of individuals at jellyfish stage without causing their death, (2) use specimens for experimental techniques requiring immobilization (e.g., NMR spectroscopy; Doerr and Stoskopf, 2019), (3) obtain photos in confocal microscopy without limitations due to bell pulsations, and (4) avoid any damage to instruments analyzing the internal environment of the jellyfish or effects of motion on their measurements (e.g., fiberoptic microsensors). Also, studies have reported that the immobilization of cnidarians may reduce the mucus production, making easier their observation (Passano, 1965). However, the limitations related to the use of this chemical should be reported and further studies are needed to confirm the absence of physiological concerns which could affect the outcomes of the studies.
Data Availability Statement
The original contributions presented in the study are included in the article/Supplementary Material. Further inquiries can be directed to the corresponding author.
Author Contributions
CD and MA conceived the idea. SA and SK designed the experiments. SA wrote the first draft of the manuscript and prepared the display figures, tables and Supplementary Materials. SA and AP performed the pilot tests, and SA conducted the experiments. SK performed the statistical analyses. All the authors contributed to and approved the final version of the manuscript.
Funding
Financial support for this research was provided by the King Abdullah University of Science and Technology and the Tarek Ahmed Juffali Research Chair on Red Sea Ecology including the baseline research funds of CD.
Conflict of Interest
The authors declare that the research was conducted in the absence of any commercial or financial relationships that could be construed as a potential conflict of interest.
Publisher’s Note
All claims expressed in this article are solely those of the authors and do not necessarily represent those of their affiliated organizations, or those of the publisher, the editors and the reviewers. Any product that may be evaluated in this article, or claim that may be made by its manufacturer, is not guaranteed or endorsed by the publisher.
Acknowledgments
We thank the team of KAUST Coastal and Marine Resources Core Labs (CMOR) for their support and assistance.
Supplementary Material
The Supplementary Material for this article can be found online at: https://www.frontiersin.org/articles/10.3389/fmars.2022.870832/full#supplementary-material
References
Abrams M. J., Basinger T., Yuan W., Guo C., Goentoro L. (2015). Self-Repairing Symmetry in Jellyfish Through Mechanically Driven Reorganization. Proc. Natl. Ac. Sci. U.S.A. 112 (26), E3365–E3373. doi: 10.1073/pnas.1502497112
Acuña F. H., Cortés J., Garese A. (2012). Ocurrence of the Sea Anemone Telmatactis Panamensis (Verrill 1869) (Cnidaria: Anthozoa: Actiniaria) at Isla Del Coco National Park, Costa Rica. Rev. Biol. Trop. 60, 201–205.
Al-Horani F. A., Al-Moghrabi S. M., de Beer D. (2003). Microsensor Study of Photosynthesis and Calcification in the Scleractinian Coral, Galaxea fascicularis: Active Internal Carbon Cycle. J. Experimental Mar. Biol. Ecol. 288 (1), 1–15. doi: 10.1016/S0022-0981(02)00578-6
Aljbour S. M., Zimmer M., Al-Horani F. A., Kunzmann A. (2019). Metabolic and Oxidative Stress Responses of the Jellyfish Cassiopea Sp. To Changes in Seawater Temperature. J. Sea. Res. 145, 1–7. doi: 10.1016/j.seares.2018.12.002
Aljbour S. M., Zimmer M., Kunzmann A. (2017). Cellular Respiration, Oxygen Consumption, and Trade-Offs of the Jellyfish Cassiopea Sp. In Response to Temperature Change. J. Sea. Res. 128, 92–97. doi: 10.1016/j.seares.2017.08.006
Amiel A. R., Röttinger E. (2021). “Experimental Tools to Study Regeneration in the Sea Anemone Nematostella Vectensis,” in In Developmental Biology of the Sea Urchin and Other Marine Invertebrates (New York, NY: Humana), 69–80. doi: 10.1007/978-1-0716-0974-3_
Andrews P. L. R., Tansey E. M. (1981). A Technique for Central Drug Administration in Octopus Vulgaris. J. Neurosci. Methods 4 (3), 243–247. doi: 10.1016/0165-0270(81)90035-2
Arafa S., Sadok S., Abed A. E. (2007). Assessment of Magnesium Chloride as an Anaesthetic for Adult Sea Urchins (Paracentrotus Lividus): Incidence on Mortality and Spawning. Aquac. Res. 38 (15), 1673–1678. doi: 10.1111/j.1365-2109.2007.01842.x
Arossa S., Barozzi A., Callegari M., Klein S. G., Parry A. J., Hung S. H., et al. (2021). The Internal Microenvironment of the Symbiotic Jellyfish Cassiopea Sp. From the Red Sea. Front. Mar. Sci. 8. doi: 10.3389/fmars.2021.705915
Banha T. N. S., Mies M., Güth A. Z., Pomory C. M., Sumida P. Y. (2020). Juvenile Cassiopea Andromeda Medusae are Resistant to Multiple Thermal Stress Events. Mar. Biol. 167, 173. doi: 10.1007/s00227-020-03792-w
Battison A., MacMillan R., MacKenzie A., Rose P., Cawthorn R., Horney B. (2000). Use of Injectable Potassium Chloride for Euthanasia of American Lobsters (Homarus Americanus). Comp. Med.; 50 (5), 545–550.
Bickell-Page L. R., Mackie G. O. (1991). Tentacle Autotomy in the Hydromedusa Aglantha Digitale (Cnidaria): An Ultrastructural and Neurophysiological Analysis. Philos. Trans. R. Soc Lond. B.: Biol. Sci. 331 (1260), 155–170. doi: 10.1098/rstb.1991.0005
Bodnar A. G. (2009). Marine Invertebrates as Models for Aging Research. Exp. Gerontol. 44 (8), 477–484. doi: 10.1016/j.exger.2009.05.001
Brusca R. C. (1980). “Cnidarians,” in Common Intertidal Invertebrates of the Gulf of California. Ed. Brusca R. C. (Tucson (AZ: University of Arizona Press).
Chen C. L. A., Chen C. P., Chen I. M. (1995). Sexual and Asexual Reproduction of the Tropical Corallimorpharian Rhodactis (= Discosoma) Indosinensis (Cnidaria: Corallimorpharia) in Taiwan. Zool. Stud. 34 (1), 29–40.
Dabrowska H., Meyer-Burgdorff K., Gunther K. D. (1991). Magnesium Status in Freshwater Fish, Common Carp and the Dietary Protein–Magnesium Interaction. Fish. Physiol. Biochem. 9, 165–172. doi: 10.1007/BF02265132
Di Camillo G. C., Arossa S., Pica D., Azzurra B., Torsani F., Cerrano C. (2021). Phenology of Anemonia Viridis and Exaiptasia Diaphana (Cnidaria: Anthozoa) From Marine Temperate Ecosystems. Mediterranean. Marine. Sci. 22 (1), 40–50. doi: 10.12681/mms.24600
Doerr M., Stoskopf M. K. (2019). Evaluation of Euthanasia of Moon Jellyfish (Aurelia Aurita) Using Simple Salt Solutions. J. Zoo Wildl. Med. 50 (1), 123–126. doi: 10.1638/2018-01510
Evans D., Millar Z., Wolvin S., Pham P. H., LePage V., Lumsden J. S. (2021). Magnesium Concentration Influences Size and Pulse Rate in the Upside-Down Jellyfish, Cassiopea Andromeda. Zoo Biol. 40 (5), 472–478. doi: 10.1002/zoo.21631
Fawcett W. J., Haxby E. J., Male D. A. (1999). Magnesium: Physiology and Pharmacology. Br. J. Anaesth. 83 (2), 302–320. doi: 10.1093/bja/83.2.302
Franchini F., Steinke M. (2017). Quantification of Dimethyl Sulfide (DMS) Production in the Sea Anemone Aiptasia Sp. To Simulate the Sea-To-Air Flux From Coral Reefs. Biogeosci 14 (24), 5765–5774. doi: 10.5194/bg-14-5765-201
Hamlet C., Santhanakrishnan A., Miller L. A. (2011). A Numerical Study of the Effects of Bell Pulsation Dynamics and Oral Arms on the Exchange Currents Generated by the Upside-Down Jellyfish Cassiopea Xamachana. J. Exp. Biol. 214 (11), 1911–1921. doi: 10.1242/jeb.052506
Hawkridge J. M., Pipe R. K., Brown B. E. (2000). Localisation of Antioxidant Enzymes in the Cnidarians Anemonia Viridis and Goniopora Stokesi. Mar. Biol. 137 (1), 1–9. doi: 10.1007/s002270000324
Heasman M. P., O’Connor W. A., Frazer A. W. (1995). Induction of Anaesthesia in the Commercial Scallop, Pecten Fumatus Reeve. Aquac 131 (3-4), 231–238. doi: 10.1016/0044-8486(94)00360-Z
Kühl M., Cohen Y., Dalsgaard T., Jørgensen B. B., Revsbech N. P. (1995). Microenvironment and Photosynthesis of Zooxanthellae in Scleractinian Corals Studied With Microsensors for O2, pH and Light. Mar. Ecology-Progress Series 117 (1–3), 159–172.
Lewbart G. A., Mosley C. (2012). Clinical Anesthesia and Analgesia in Invertebrates. J. of Exotic Pet. Med. 21 (1), 59–70. doi: 10.1053/j.jepm.2011.11.007
Lim C., Klesius P. H. (2003). Influence of Dietary Levels of Magnesium on Growth, Tissue Mineral Content, and Resistance of Channel Catfish Ictalurus Punctatuschallenged With Edwardsiella Ictaluri. J.World Aquac. Soc 34, 18–28. doi: 10.1111/j.1749-7345.2003.tb00035.x
Mellas R., McIlroy S., Fitt W., Coffroth M. (2014). Variation in Symbiont Uptake in the Early Ontogeny of the Upside-Down Jellyfish, Cassiopea Spp. J. Exp. Mar. Biol. Ecol. 459, 38–44. doi: 10.1016/j.jembe.2014.04.026
Messenger J. B., Nixon M., Ryan K. P. (1985). Magnesium Chloride as an Anaesthetic for Cephalopods. Comp. Biochem. Physiol. Part C. Comp. Pharmacol. 82 (1), 203–205. doi: 10.1016/0742-8413(85)90230-0
Nath R. D., Bedbrook C. N., Abrams M. J., Basinger T., Bois J. S., Prober D. A., et al. (2017). The Jellyfish Cassiopea Exhibits a Sleep-Like State. Cur. Biol. 27 (19), 2984–2990. doi: 10.1016/j.cub.2017.08.014
Ozacmak V. H., Thorington G. U., Fletcher W. H., Hessinger D. A. (2001). N-Acetylneuraminic Acid (NANA) Stimulates in Situ Cyclic AMP Production in Tentacles of Sea Anemone: (Aiptasia Pallida): Possible Role in Chemosensitization of Nematocyst Discharge. J. Exp.l Biol. 204, 2011–2020. doi: 10.1242/jeb.204.11.2011
Pantin C. F. A. (1946). Notes on Microscopical Technique for Zoologists (New York: University Press).
Passano L. M. (1965). Pacemakers and Activity Patterns in Medusae: Homage to Romanes. Am. Zool. 5 (3), 465–481. doi: 10.1093/icb/5.3.465
Perez S., Weis V. (2006). Nitric Oxide and Cnidarian Bleaching: An Eviction Notice Mediates Breakdown of a Symbiosis. J. Exp. Biol. 209 (14), 2804–2810. doi: 10.1242/jeb.02309
Petie R., Garm A., Nilsson D. E. (2013). Contrast and Rate of Light Intensity Decrease Control Directional Swimming in the Box Jellyfish Tripedalia Cystophora (Cnidaria, Cubomedusae). Hydrobiol 703 (1), 69–77. doi: 10.1007/s10750-012-1345-0
Pitt K. A., Welsh D. T., Condon R. H. (2009). Influence of Jellyfish Blooms on Carbon, Nitrogen and Phosphorus Cycling and Plankton Production. Hydrobiol 616 (1), 133–149. doi: 10.1007/s10750-008-9584-9
Pizzi R. (2012). Spiders. In: Invertebrate Medicine, (ed. Lewbart G. A., Ames I. A. Oxford: Blackwell Publishing), pp. 187–222.
Shashar N., Stambler N. (1992). Endolithic Algae Within Corals-Life in an Extreme Environment. J. Experimental Mar. Biol. Ecol. 163 (2), 277–286. doi: 10.1016/0022-0981(92)90055-F
Steedman H. R. (1976). Zooplankton Fixation and Preservation. UNESCO. Monogr. Oceanogr. Methodol. 4, 350. doi: 10.1139/f77-187
Tsien R. Y. (1998). The Green Fluorescent Protein. Annu. Rev. Biochem. 67 (1), 509–544. doi: 10.1146/annurev.biochem.67.1.509
Verde E. A., McCloskey L. R. (1998). Production, Respiration, and Photophysiology of the Mangrove Jellyfish Cassiopea Xamachana Symbiotic With Zooxanthellae: Effect of Jellyfish Size and Season. Mar. Ecol. Prog. Ser. 168, 147–162. doi: 10.3354/meps168147
Wangpraseurt D., Weber M., Røy H., Polerecky L., De Beer D., Nugues M. M. (2012). In Situ Oxygen Dynamics in Coralalgal Interactions. PloS One 2 (2), e31192. doi: 10.1371/journal.pone.0031192
Keywords: invertebrate, magnesium chloride, MgCl2, Cassiopea sp., cnidaria
Citation: Arossa S, Klein SG, Parry AJ, Aranda M and Duarte CM (2022) Assessing Magnesium Chloride as a Chemical for Immobilization of a Symbiotic Jellyfish (Cassiopea sp.). Front. Mar. Sci. 9:870832. doi: 10.3389/fmars.2022.870832
Received: 07 February 2022; Accepted: 06 April 2022;
Published: 29 April 2022.
Edited by:
Thanos Dailianis, Hellenic Centre for Marine Research, GreeceReviewed by:
Robyn J. Crook, San Francisco State University, United StatesShelley Templeman, James Cook University, Australia
Edgar Gamero-Mora, Consejo Nacional de Ciencia y Tecnología (CONACYT), Mexico
Copyright © 2022 Arossa, Klein, Parry, Aranda and Duarte. This is an open-access article distributed under the terms of the Creative Commons Attribution License (CC BY). The use, distribution or reproduction in other forums is permitted, provided the original author(s) and the copyright owner(s) are credited and that the original publication in this journal is cited, in accordance with accepted academic practice. No use, distribution or reproduction is permitted which does not comply with these terms.
*Correspondence: Silvia Arossa, c2lsdmlhLmFyb3NzYUBrYXVzdC5lZHUuc2E=