- 1State Key Laboratory of Tropical Oceanography, South China Sea Institute of Oceanology, Chinese Academy of Sciences, Guangzhou, China
- 2Daya Bay Marine Biology Research Station, Chinese Academy of Sciences, Shenzhen, China
- 3Southern Marine Science and Engineering Guangdong Laboratory (Guangzhou), Guangzhou, China
- 4Sanya Institute of Oceanology, South China Sea Institute of Oceanology, Sanya, China
- 5College of Life Sciences, Linyi University, Linyi, China
Bacterial degradation of dimethylsulfoniopropionate (DMSP) plays a significant role in ecosystem productivity and global climate. In this study, the abundance and diversity of Roseobacter group DMSP degradation genes were explored in spatial scale of the South China Sea (SCS). Quantitative PCR showed that a higher abundance of dmdA (DMSP demethylase) and dddP (DMSP lyase) genes was detected above 75 m than deep water, especially in surface water. A high ratio of dmdA/dddP existed in all sites and increased with water depth, indicating that demethylation was the main degradation pathway in the Roseobacter group. High-throughput sequencing analysis showed that distribution of dmdA gene had a significant layering structure in the northern SCS, and high taxonomic diversity of dmdA gene was observed in near-surface waters (25 and 50 m). DmdA gene in the Roseobacter group, such as Leisingera, Nioella, Roseobacter, Roseovarius, Donghicola, Phaeobacter, and Tateyamaria, had remarkable specificity due to the effect of different sites and water depths. Different ecological strategies of DMSP degradation may be used by members of the bacterial community harboring demethylation genes. In addition, many dmdA sequences were affiliated with unidentified bacteria, indicating that the SCS reserved high diversity of DMSP-degrading bacteria. Canonical correspondence analysis (CCA) suggested that temperature and depth were the most important factors to determine the taxonomic distribution of DMSP degradation genes in the Roseobacter group, as well as their abundance. This study highlighted the understanding of the role of Roseobacter group in DMSP degradation in the tropical ocean.
Introduction
Dimethylsulfoniopropionate (DMSP), an important sulfur compound, is mainly produced by marine phytoplankton in marine water (Howard et al., 2006; Michaud et al., 2007; Levine et al., 2012; Moran and Durham, 2019). Association with phytoplankton aggregates may provide many ecophysiological advantages to the marine Roseobacter group, including easy access to DMSP and other algal products (González et al., 1999; Amin et al., 2015). When released from phytoplankton, DMSP is mainly assimilated and degraded by members of bacteria via a demethylation or cleavage pathway (Malmstrom et al., 2004; Curson et al., 2011; Burkhardt et al., 2017; Raina et al., 2017). The demethylation pathway transforms the majority of DMSP to 3-methiolpropionate, which is then incorporated into the cell biomass (Kiene et al., 1999; Reisch C. R. et al., 2011). By contrast, the cleavage pathway converts DMSP to produce dimethylsulfide (DMS) through various DMSP lyases (Johnston et al., 2016). DMS represents the largest volatile sulfide in the ocean, and its oxidation production can form cloud nucleation and affect global climate (Andreae and Crutzen, 1997; Simó, 2001).
The Roseobacter group is affiliated with Alphaproteobacteria, and the majority is of marine origin, constituting a large proportion of the total bacterial community (Brinkhoff et al., 2008; Simon et al., 2017). Members of the Roseobacter group can establish symbiotic relationships with phytoplankton, partly through the exchange of DMSP (Liu et al., 2018; Nowinski et al., 2019; O’Brien et al., 2022). Roseobacter are often dominant with functional genes, which can encode the capabilities of oxidation sulfur compounds and methylated amines, and catabolism of various carbohydrates (Zhang et al., 2016). The Roseobacter group is considered to be a key participant in DMSP metabolism, and nearly 1/3 of DMSP assimilation process is performed through the Roseobacter group in the coastal area (Malmstrom et al., 2004). At least 80% of Roseobacter group cells contain dmdA in the Sargasso Sea (Howard et al., 2006; Howard et al., 2008). Of the seven ddd genes identified (dddD, dddK, dddL, dddP, dddQ, dddY, and dddW), dddP and dddQ genes are the most frequently detected in marine bacteria and mainly found in the Roseobacter group (Howard et al., 2008; Todd et al., 2009; Todd et al., 2011; Sun et al., 2016). To date, more and more the whole genomes of marine Roseobacter strains have been sequenced (Newton et al., 2010; Luo et al., 2012; Voget et al., 2015; Billerbeck et al., 2016; Bakenhus et al., 2018). According to the above studies, dmdA and dddP genes are the most important genes for DMSP degradation in the Roseobacter group.
The northern South China Sea (nSCS) is a marginal sea characterized by tropical and subtropical climates and representing typical oligotrophic characteristics with significant environmental gradients. High concentrations of DMSP and DMS are detected in surface water, especially between 20 and 75 m (Yang, 2000; Yang et al., 2008). Previous studies reported that the abundance of DMSP degradation genes had spatial variability in the seawater (Howard et al., 2011; Levine et al., 2012; Varaljay et al., 2012; Choi et al., 2015; Cui et al., 2015), indicating that DMSP degradation genes are strongly impacted by primary production, UV radiation, DMSP and DMS concentrations, and Chl a concentration. To date, DMSP degradation genes have been little reported in the SCS, and biogeography of DMSP degradation genes in spatial-vertical distribution of marine water needs further study. In this study, dmdA and dddP genes were targeted and collected from the surface to 200 m depth during a cruise across the SCS. The aims of this study were to describe the spatial–vertical distribution and abundance of Roseobacter-like DMSP degradation genes, and to explore the diversity shift of DMSP degradation genes related to water environment in the SCS.
Materials and Methods
Sample Collection
The cruise was carried out in the South China Sea with the Shiyan 3 from August to September 2011 (Figure 1). A conductivity-temperature-depth (CTD) system (SeaBird SBE-911 Plus, US) was deployed to acquire hydrographic parameters. Seawater samples were collected at different depths (0, 25, 50, 75, 100, 150, and 200 m) with CTD 12-L Niskin bottles (General Oceanics, Inc., Miami, FL). Once collected, the samples were immediately filtered using polycarbonate membranes (EMD Millipore, US) with a pore size of 0.22 μm. The filter was immediately placed in a 1.5-ml sterile centrifuge tube and stored in liquid nitrogen for further DNA extraction. Sample DNA were extracted using the DNA Extraction Kit (OMEGA, US) according to the protocol instructions. Nitrate (NO3), phosphate (PO4), silicate (SiO3), and chlorophyll (Chl a) concentrations were measured according to the protocols of “the specialties for oceanography survey” (GB17378.4-2007, China).
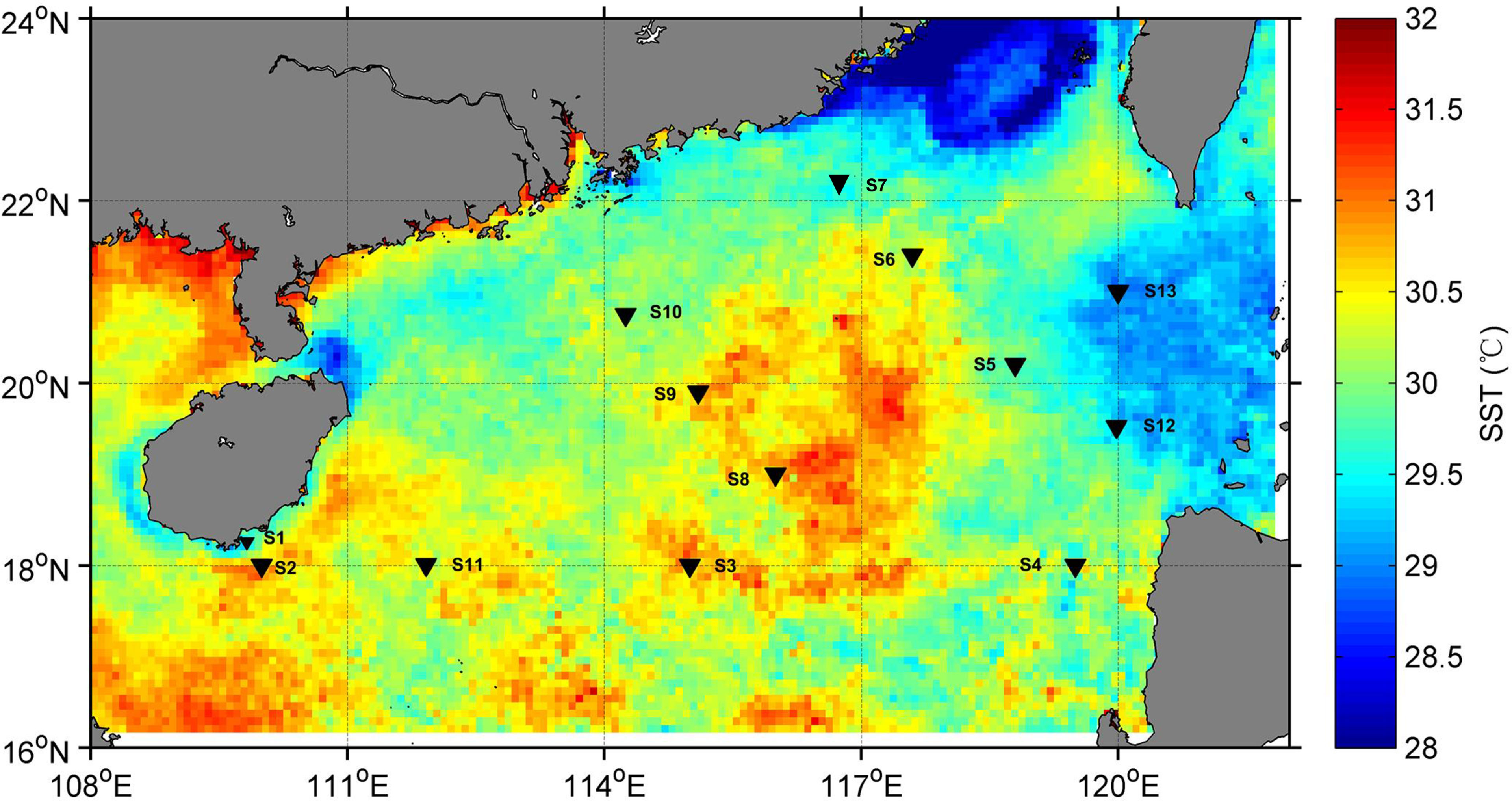
Figure 1 Map of sampling stations in the South China Sea. Sea surface temperature (SST) during investigation time was shown in the base map that was obtained from NOAA.
Quantitative PCR of dmdA and dddP Genes Abundance in the Roseobacter Group
Two dmdA primers were used to amplify the different Roseobacter subclades (A/1 and A/2) (Varaljay et al., 2010) for the DMSP demethylase gene, and dddP primers targeting the Roseobacter group were used to detect the DMSP lyase genes (Levine et al., 2012). The reactions were performed in the iQ5 Real-Time PCR Detection System (Bio-Rad, US). Quantification was based on the increasing fluorescence intensity of the SYBR green dye during amplification. QPCR standards were made from PCR products amplified from environmental samples using the pMD19-T cloning kit (TaKaRa, Japan). The real-time PCR assay was performed in a 20-μL reaction volume with SYBR Premix Ex Taq II (TaKaRa, Japan). All qPCRs were run in triplicate for each sample. QPCR conditions were as follows: predenaturation at 95°C for 30 s, 35 cycles at 95°C for 5 s, and annealing at 60°C for 30 s. Tenfold serially diluted standard and no-template controls were run in triplicate for each reaction.
Illumina MiSeq Sequencing of dmdA Genes in the Roseobacter Group
The primer pairs, dmdA282F (5′-TGCTSTSAACGAYCCSGT-3′) and dmdA591R (5′-ACRTAGAYYTCRAAVCCBCCYT-3′) (Zeng et al., 2016), were used for Roseobacter-like dmdA gene amplification. PCR conditions were as follows: 94°C for 3 min, 35 cycles at 94°C for 1 min, 54°C for 30 s, extension at 72°C for 30 s, and a final extension at 72°C for 10 min. The PCR comprised a 20-μL reaction volume containing 4 μL of 5× FastPfu Buffer, 2 μL of 2.5 mM dNTPs, 0.5 μL of FastPfu polymerase, 1.0 μL of primers (5 μM), and 10 ng of template DNA. A positive control and non-template control samples were run to validate PCR. PCR products were purified using the AxyPrep DNA Gel Extraction Kit (Axygen Biosciences, US), and quantified using QuantiFluor™-ST (Promega, US). An Illumina MiSeq platform (Illumina, US) was used for paired-end sequencing (2 × 300) according to the standard protocols.
For pair-ended reads obtained by Illumina sequencing, barcodes and primers were trimmed and then assembled using FLASH (V1.2.7). Reads that contained Ns were shorter than 50 bp or had primer mismatches which were also excluded. Sequences were compared with RDP reference database using VSEARCH (1.9.6) to detect chimeric sequences. Then sequences were grouped into OTUs (operational taxonomic units) using UPARSE (v7.0.1001), and pre-clustered at 97% sequence identity. The highest OTU frequencies were selected as representative OTU sequences. The taxonomy of each dmdA gene sequence was analyzed by RDP classifier algorithm against the NCBI non-redundant (nr) database using a confidence threshold of 70%.
Results and Discussion
Characteristics of the Abundance of dmdA and dddP Gene in the South China Sea
Two subclades (A/1 and A/2) of dmdA genes and dddP genes were quantified using qPCR (Figure 2). The copy numbers of dmdA and dddP genes and their distribution in the northwestern SCS varied greatly among sampling sites and depths. This result was similar with the study in the Pacific Ocean (Cui et al., 2015), indicating that the abundance of DMSP degradation genes had great variability of abundance in the ocean. Overall, the abundance of dmdA and dddP genes located in the northwest SCS was higher than that located in the northeastern SCS. As seen from a spatial scale, sites (S1, S2, S3, S8, and S9) showed higher copy numbers of dmdA and dddP genes than other sites (S5, S6, S12, and S13). In these five stations, two sites (S1 and S2) are close to the shore, and the remaining three (S3, S8, S9) are in the middle of the survey area. Similar to previous studies in the SCS (Ling et al., 2012; Sun et al., 2015), bacterial community in the northwestern SCS had higher diversity than that in the northeastern SCS. The abundance difference is likely due to water temperature. As seen in Figure 1, the SST of the sites to the left of longitude 117°E is higher than that of the sites to the right. This result was similar to other reports that temperature is an important factor in determining the abundance of dmdA and dddP in surface water (Levine et al., 2012; Varaljay et al., 2012; Cui et al., 2015).
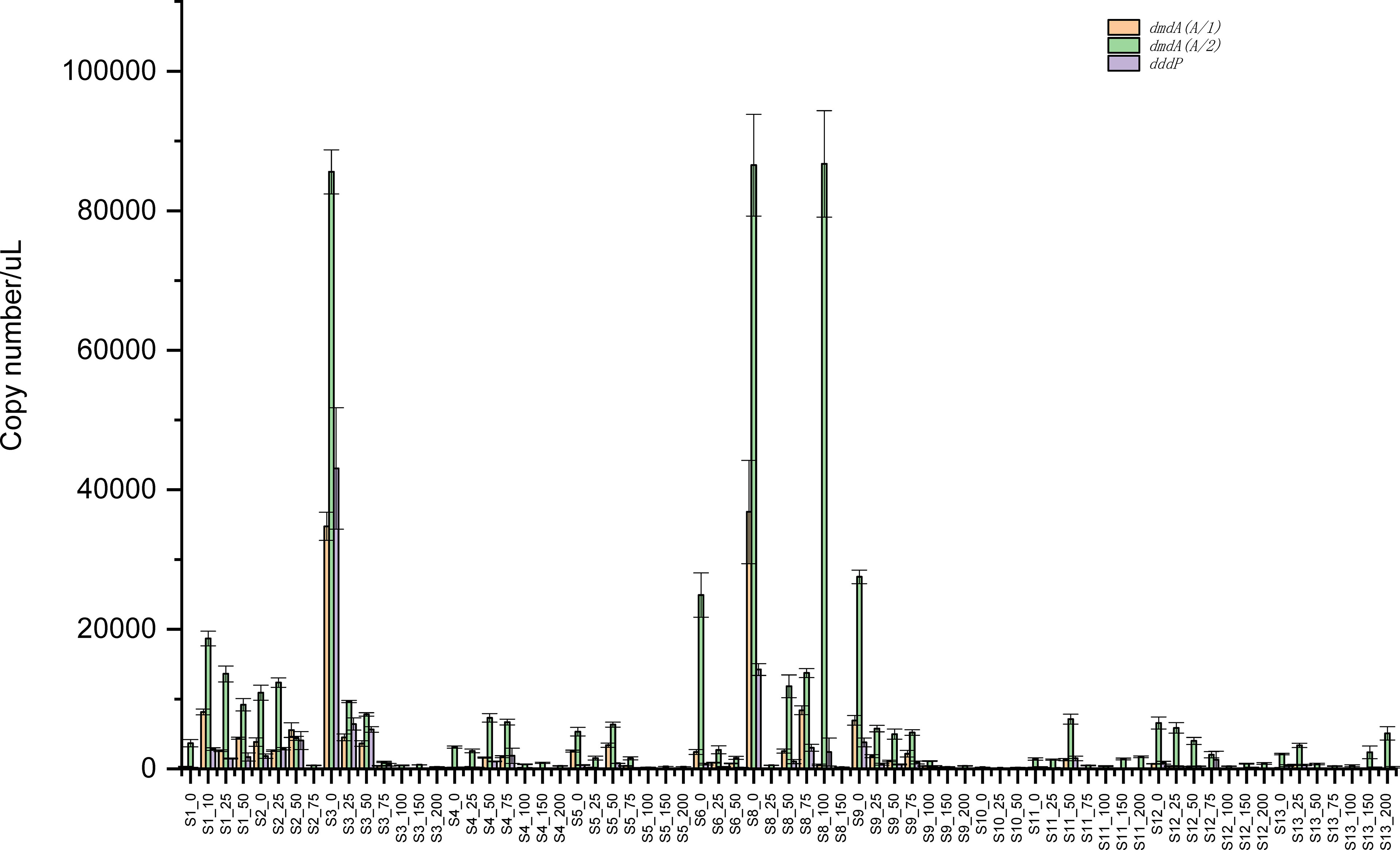
Figure 2 Abundance of dmdA and dddP in the SCS from surface water to 200 m depth based on quantitative PCR.
DmdA and dddP genes from the Roseobacter group were particularly enriched in surface waters with an order of magnitude difference in their abundance relative to deep waters (Figure 2), which indicated that the abundance of dmdA and dddP genes was strongly separated by water depth. Copy numbers of dmdA and dddP genes were higher above 75 m water than those below 100 m water, and the highest abundance of these genes was observed in the surface layer. Variation of gene abundance of dmdA and dddP may be closely related to the DMSP concentration in vertical depth. Previous studies reported that DMSP and DMS concentrations in SCS were markedly high in the surface seawater, and decreased gradually with increasing depth (Yang, 2000; Zhai et al., 2020). Other studies also reported that distribution patterns of dmdA and dddP were roughly consistent with the distribution characteristics of DMSP concentration, and were mainly influenced by the Chl a concentrations, depth, salinity, and temperature (Howard et al., 2011; Varaljay et al., 2012; Cui et al., 2015).
In addition, the copy numbers of the dddP gene were far lower than those of the dmdA gene at almost all sites and depths (Figure 2), even if only the dmdA A/2 clade was considered. The copy number ratios (dmdA/dddP) of these genes ranged from 2 to 156 times. The copy numbers of the dmdA A/2 gene were higher than those of the dmdA A/1 gene at almost all sites and depths, which suggested that subclade A2 was the main group of Roseobacter group in the demethylation pathway. The other study reported that two Roseobacter group dmdA gene subclades (A/1 and A/2) showed opposite depth distributions in the summer (Levine et al., 2012). Interestingly, high ratios of dmdA/dddP and dmdA2/dmdA1 were mainly observed in deep waters (below 75 m), even if these three genes had relatively low abundance, indicating that demethylation is the main pathway of DMSP degradation in the water. Previous studies suggested that 80% of DMSP degradation is processed through the demethylation pathway, and only 20% is cleaved to DMS (Kiene et al., 1999). Marine bacteria keep the ability of DMSP demethylation to a suitable evolutionary pressure, which can explain the consistently stable and high dmdA gene frequencies in the ocean (Varaljay et al., 2012). In marine waters, nutrients and organic sulfur such as DMSP are important because cells need increased sulfur demand for growth, causing more sulfur to be incorporated into cell protein (Kiene et al., 1999).
Diversity Distribution of dmdA Gene in the Roseobacter Group
A total of 231,246 valid reads and 688 OTUs were obtained from the 19 samples through Illumina MiSeq sequencing analysis. Each of the samples contained 9155 to 17,955 reads, with OTUs ranging from 12 to 55. The coverage was more than 0.999, which suggested that sequencing data had favorable coverage for dmdA diversity. Diversity indices, including Shannon, Chao, Ace, and Simpson, are demonstrated in Supplementary Table S1. The result indicated that the dmdA gene had higher community diversity above 50 m than in deep waters (100 and 200 m).
The study revealed that the composition of the dmdA gene varied significantly among the sites and depths (ANOVA, p < 0.01). Overall, the dmdA gene was mainly affiliated with Alphaproteobacteria and Gammaproteobacteria (Figure 3). In most sites, the dmdA gene was dominated by Alphaproteobacteria, whereas a few of the samples (S2_0, S6_0, S6_50, and S9_25) were dominated by Gammaproteobacteria. In addition, low abundance of Acidithiobacillia-like dmdA gene was detected (1.37%). High abundance of dmdA (2.64%–87.95%), which had very low similarity with the amino acid identity of uncultured bacteria, was found in some samples. Moreover, 21.78% of all sequences had 73%–79% amino acid similarity with Gammaproteobacteria. This result provided further evidence that ocean water contains a high diversity of dmdA genes, which has not yet been unearthed in the tropical ocean.
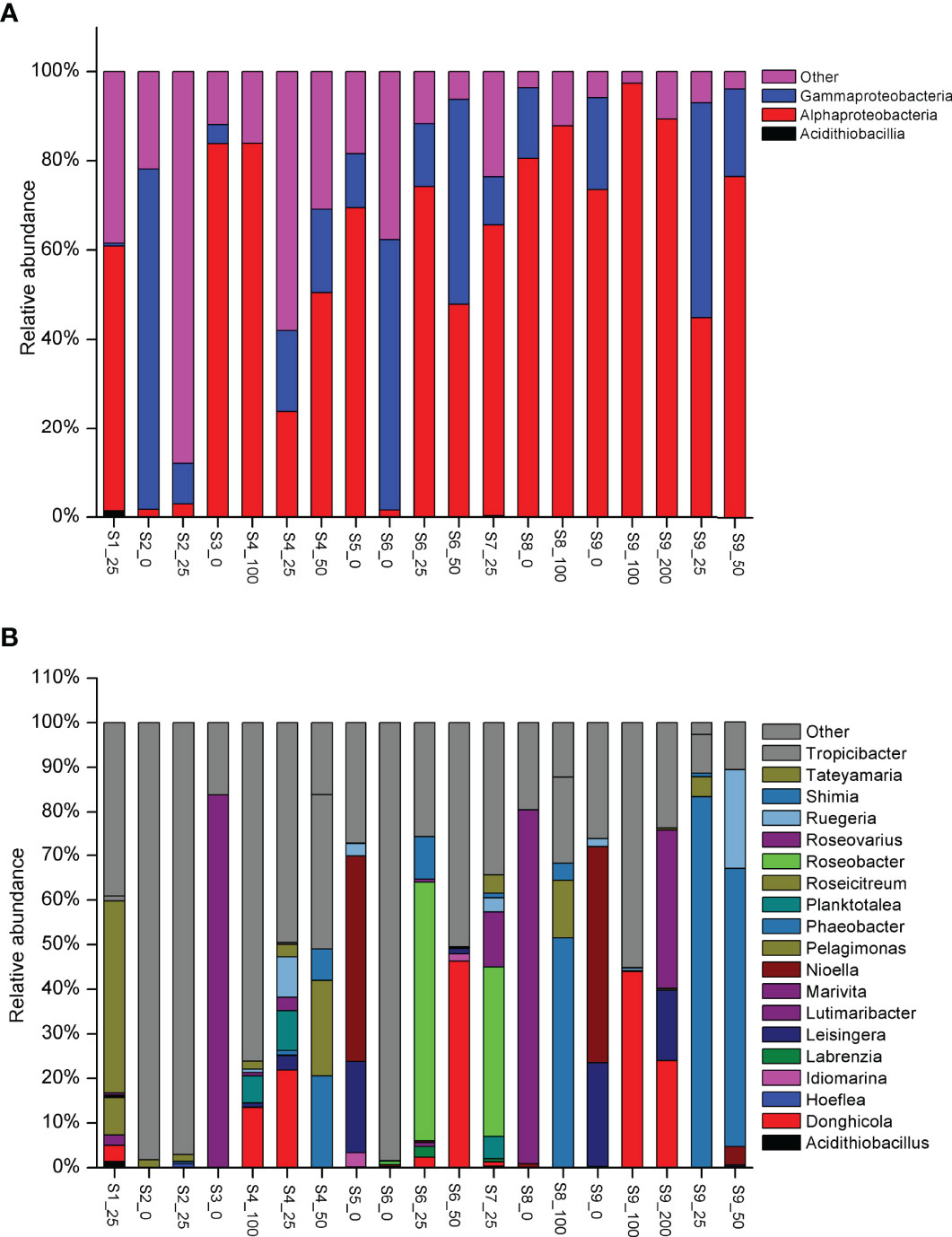
Figure 3 Taxonomic distribution of samples through High-throughput sequencing analysis in class level (A) and genus level (B).
Overall, dmdA genes in the nSCS showed greater variation near surface water than in deep waters, which is related to the vertical structure of water (Figure 3). At the genus level, high abundance of dmdA genes below 100 m belonged to Phaeobacter, Roseicitreum, Ruegeria, Tropicibacter, and Shimia. The upwelling site (S1-25) was characterized by Tateyamaria (43.11%) and Pelagimonas (8.40%). These findings indicated that coastal upwelling had different dmdA taxonomy of the Roseobacter group with open water. S5_0 and S9_0 sites were dominated by Leisingera (>20%) and Nioella (>46%). S3_0 and S8_0 sites were characterized by highly abundant Roseovarius (>80%). Site S6_25 was mainly composed of Roseobacter (58%) and Shimia (9.56%). Site S7_25 was mainly composed of Roseobacter (38%), Roseovarius (12.32%), and Planktotalea (4.97%). The dmdA gene of Donghicola mainly dominated in the 25- and 50-m water layer. Moreover, dmdA genes of Leisingera, Planktotalea, Roseovarius, and Ruegeria were dominant in the 25- or 50-m water layer.
Principal component analysis (PCA) illustrated that the dmdA gene of the bacterial community had distinct differences among different sites and depths (Figure 4). The results indicated that the dmdA gene of the Roseobacter group in shallow water (0 m, 25 m, and 50 m) and deep layer (100 and 200 m) had clustered together separately. The dmdA gene of the deep water was separated by a large distance from the shallow layer. Overall, the results indicated the clearly distinct structure among sampling sites and depths (ANOVA, p < 0.01). Veen analysis showed that dmdA gene diversity had 14 and 11 common OTUs between the surface water and 25-m depth of the horizontal scale. Considering the vertical depths, no common OTU was found from the surface to 200 m water depths (Figure 5).
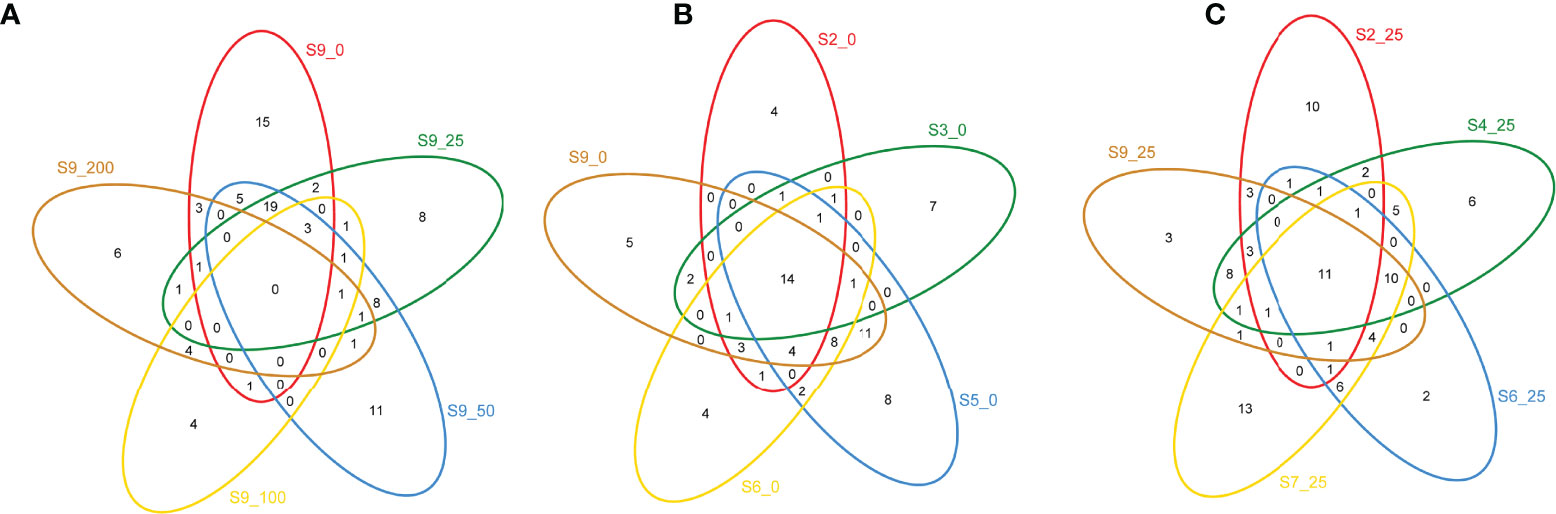
Figure 5 Veen map analysis of dmdA gene diversity in spatial and vertical scale. (A) common OTUs in the vertical layers; (B) common OTUs in the surface water; (C) common OTUs in the 25-m water layers.
Environmental Factors Affected the Distribution of the dmdA Gene
The CCA analysis constructed a correlation between environmental factors and dmdA gene diversity (Figure 6A). The first ordination axis accounted for 32.1% of the cumulative percentage variance in the matrix, while the second axis accounted for 31.2%. DmdA gene in the upwelling (S1-25) was mainly positively related to Chl a and silicate concentration (p<0.05). DmdA gene below 100-m depth was negatively defined by water depth (p<0.01), while shallow water samples (0 m and 25 m) were strongly determined by water temperature (p<0.01). Other environmental factors, such as salinity, nitrate, and phosphate, had no obvious effects on the diversity of dmdA genes.
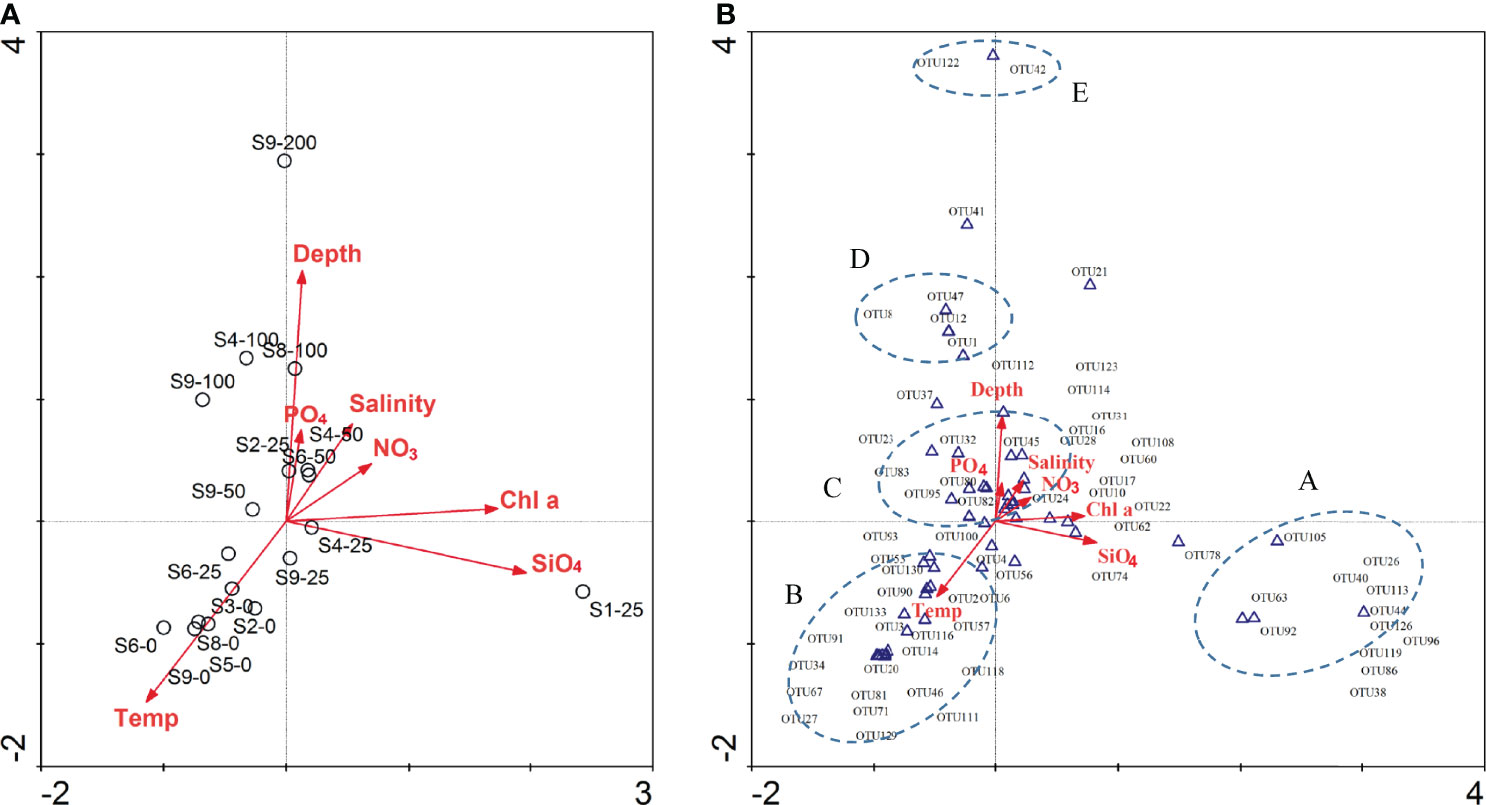
Figure 6 Canonical correspondence analysis (CCA) of the relationship of dmdA gene diversity (A) and OTU level (B) in the Roseobacter group, with environmental factors. Areas surrounded by the blue dashed lines represented the dominant OTUs of different genera.
In the CCA map (Figure 6B), cluster A, mainly composed by Tateyamaria, Pelagimonas, and Marivita, had significant correlation (p < 0.05) with Chl a and silicate concentration, which was influenced by the upwelling. High abundance OTUs of cluster B, which was afflicted with Leisingera, Nioella, Roseovarius, and Roseobacter, were mainly positively related to temperature. High abundance of OTUs (cluster C), which was afflicted with Roseobacter, Shimia, Planktotalea, and Donghicola, were mainly related to depth, phosphate, and salinity. Cluster D and E, mainly composed by Phaeobacter, Roseicitreum, Ruegeria, and Tropicibacter, were mainly positively related to depth, indicating that these Roseobacter OTUs were suitable to environment change with deep water. As described above, a high ratio of dmdA/dddP was found in deep water, which indicated that the Roseobacter group had more nutrition demand and greater degree of demethylation than DMSP cleavage. Different ecological strategies of DMSP degradation may be used by members of the bacterial community harboring demethylation and/or cleavage genes (Reisch C. et al., 2011). In previous studies, the Roseobacter group is the dominant microbial taxa in the offshore, upwelling, and mesoscale eddy of the South China Sea (Zhang et al., 2016; Sun et al., 2020; Sun et al., 2022), and diversity of DMSP degradation potential has obvious significance for sulfur material cycling and transformation.
Conclusion
This study showed that DMSP degradation genes varied significantly in spatial scale of the South China Sea. The current study found that temperature and water depth mainly induced variations in taxonomic affiliations of Roseobacter group DMSP degradation genes over space. These findings further implied that additional factors including light, salinity, and temperature, which are caused by an increase in depth, may play important roles in regulating the DMSP degradation genes. These factors play an important role in regulating the switch process between bacterial DMSP demethylation and DMSP cleavage. This study highlighted the understanding of the role of Roseobacter group in sulfur cycling and transformation conversion in the tropical ocean.
Data Availability Statement
The datasets presented in this study can be found in online repositories. The names of the repository/repositories and accession number(s) can be found below: NCBI [accession: SRP133746].
Author Contributions
All authors contributed to the article and approved the submitted version.
Funding
The present study was supported financially by Hainan Province Science and Technology Special Fund (ZDYF2021XDNY131), the Strategic Priority Research Program of the Chinese Academy of Sciences (XDA19060204), the Key Special Project for Introduced Talents Team of Southern Marine Science and Engineering Guangdong Laboratory (Guangzhou) (GML2019ZD0303), the National Natural Science Foundation of China (31971480, 41406130 and 42073078), the National Key Research and Development Plan (2017FY100700), and the Project of Guangdong Science and Technology Department (2017A020216008).
Conflict of Interest
The authors declare that the research was conducted in the absence of any commercial or financial relationships that could be construed as a potential conflict of interest.
Publisher’s Note
All claims expressed in this article are solely those of the authors and do not necessarily represent those of their affiliated organizations, or those of the publisher, the editors and the reviewers. Any product that may be evaluated in this article, or claim that may be made by its manufacturer, is not guaranteed or endorsed by the publisher.
Supplementary Material
The Supplementary Material for this article can be found online at: https://www.frontiersin.org/articles/10.3389/fmars.2022.895613/full#supplementary-material
References
Amin S. A., Hmelo L. R., Van Tol H. M., Durham B. P., Carlson L. T., Heal K. R., et al. (2015). Interaction and Signalling Between a Cosmopolitan Phytoplankton and Associated Bacteria. Nature 522 (7554), 98–101. doi: 10.1038/nature14488
Andreae M. O., Crutzen P. J. (1997). Atmospheric Aerosols - Biogeochemical Sources and Role in Atmospheric Chemistry. Science 276 (5315), 1052–1058. doi: 10.1126/science.276.5315.1052
Bakenhus I., Voget S., Poehlein A., Brinkhoff T., Daniel R., Simon M. (2018). Genome Sequence of Planktotalea Frisia Type Strain (SH6-1T), a Representative of the Roseobacter Group Isolated From the North Sea During a Phytoplankton Bloom. Stand. Genomic Sci. 13 (1), 7. doi: 10.1186/s40793-018-0311-5
Billerbeck S., Wemheuer B., Voget S., Poehlein A., Giebel H.-A., Brinkhoff T., et al. (2016). Biogeography and Environmental Genomics of the Roseobacter-Affiliated Pelagic CHAB-I-5 Lineage. Nat. Microbiol. 1, 16063. doi: 10.1038/nmicrobiol.2016.63
Brinkhoff T., Giebel H. A., Simon M. (2008). Diversity, Ecology, and Genomics of the Roseobacter Clade: A Short Overview. Arch. Microbiol. 189 (6), 531–539. doi: 10.1007/s00203-008-0353-y
Burkhardt I., Lauterbach L., Brock N. L., Dickschat J. S. (2017). Chemical Differentiation of Three DMSP Lyases From the Marine Roseobacter Group. Org. Biomol. Chem. 15 (20), 4432–4439. doi: 10.1039/C7OB00913E
Choi D. H., Park K.-T., An S. M., Lee K., Cho J.-C., Lee J.-H., et al. (2015). Pyrosequencing Revealed SAR116 Clade as Dominant dddP-Containing Bacteria in Oligotrophic NW Pacific Ocean. PloS One 10 (1), e0116271. doi: 10.1371/journal.pone.0116271
Cui Y., Suzuki S., Omori Y., Wong S.-K., Ijichi M., Kaneko R., et al. (2015). Abundance and Distribution of Dimethylsulfoniopropionate Degradation Genes and the Corresponding Bacterial Community Structure at Dimethyl Sulfide Hot Spots in the Tropical and Subtropical Pacific Ocean. Appl. Environ. Microbiol. 81 (12), 4184–4194. doi: 10.1128/aem.03873-14
Curson A. R. J., Todd J. D., Sullivan M. J., Johnston A. W. B. (2011). Catabolism of Dimethylsulphoniopropionate: Microorganisms, Enzymes and Genes. Nat. Rev. Microbiol. 9, 849. doi: 10.1038/nrmicro2653
González J. M., Kiene R. P., Moran M. A. (1999). Transformation of Sulfur Compounds by an Abundant Lineage of Marine Bacteria in the α-Subclass of the ClassProteobacteria. Appl. Environ. Microbiol. 65 (9), 3810–3819. doi: 10.1128/AEM.65.9.3810-3819.1999
Howard E. C., Henriksen J. R., Buchan A., Reisch C. R., Bürgmann H., Welsh R., et al. (2006). Bacterial Taxa That Limit Sulfur Flux From the Ocean. Science 314 (5799), 649–652. doi: 10.1126/science.1130657
Howard E. C., Sun S., Biers E. J., Moran M. A. (2008). Abundant and Diverse Bacteria Involved in DMSP Degradation in Marine Surface Waters. Environ. Microbiol. 10 (9), 2397–2410. doi: 10.1111/j.1462-2920.2008.01665.x
Howard E. C., Sun S., Reisch C. R., del Valle D. A., Bürgmann H., Kiene R. P., et al. (2011). Changes in Dimethylsulfoniopropionate Demethylase Gene Assemblages in Response to an Induced Phytoplankton Bloom. Appl. Environ. Microbiol. 77 (2), 524–531. doi: 10.1128/AEM.01457-10
Johnston A. W. B., Green R. T., Todd J. D. (2016). Enzymatic Breakage of Dimethylsulfoniopropionate—A Signature Molecule for Life at Sea. Curr. Opin. Chem. Biol. 31, 58–65. doi: 10.1016/j.cbpa.2016.01.011
Kiene R. P., Linn L. J., González J., Moran M. A., Bruton J. A. (1999). Dimethylsulfoniopropionate and Methanethiol Are Important Precursors of Methionine and Protein-Sulfur in Marine Bacterioplankton. Appl. Environ. Microbiol. 65 (10), 4549–4558. doi: 10.1128/AEM.65.10.4549-4558.1999
Levine N. M., Varaljay V. A., Toole D. A., Dacey J. W. H., Doney S. C., Moran M. A. (2012). Environmental, Biochemical and Genetic Drivers of DMSP Degradation and DMS Production in the Sargasso Sea. Environ. Microbiol. 14 (5), 1210–1223. doi: 10.1111/j.1462-2920.2012.02700.x
Ling J., Dong J.-D., Wang Y.-S., Zhang Y.-Y., Deng C., Lin L., et al. (2012). Spatial Variation of Bacterial Community Structure of the Northern South China Sea in Relation to Water Chemistry. Ecotoxicology 21 (6), 1669–1679. doi: 10.1007/s10646-012-0941-0
Liu J., Liu J., Zhang S.-H., Liang J., Lin H., Song D., et al. (2018). Novel Insights Into Bacterial Dimethylsulfoniopropionate Catabolism in the East China Sea. Front. Microbiol. 9. doi: 10.3389/fmicb.2018.03206
Luo H., Löytynoja A., Moran M. A. (2012). Genome Content of Uncultivated Marine Roseobacters in the Surface Ocean. Environ. Microbiol. 14 (1), 41–51. doi: 10.1111/j.1462-2920.2011.02528.x
Malmstrom R. R., Kiene R. P., Kirchman D. L. (2004). Identification and Enumeration of Bacteria Assimilating Dimethylsulfoniopropionate (DMSP) in the North Atlantic and Gulf of Mexico. Limnol. Oceanogr. 49 (2), 597–606. doi: 10.4319/lo.2004.49.2.0597
Michaud S., Levasseur M., Cantin G. (2007). Seasonal Variations in Dimethylsulfoniopropionate and Dimethylsulfide Concentrations in Relation to the Plankton Community in the St. Lawrence Estuary. Estuar. Coast Shelf Sci. 71 (3-4), 741–750. doi: 10.1016/j.ecss.2006.09.020
Moran M. A., Durham B. P. (2019). Sulfur Metabolites in the Pelagic Ocean. Nat. Rev. Microbiol. 17 (11), 665–678. doi: 10.1038/s41579-019-0250-1
Newton R. J., Griffin L. E., Bowles K. M., Meile C., Gifford S., Givens C. E., et al. (2010). Genome Characteristics of a Generalist Marine Bacterial Lineage. ISME J. 4 (6), 784–798. doi: 10.1038/ismej.2009.150
Nowinski B., Motard-Côté J., Landa M., Preston C. M., Scholin C. A., Birch J. M., et al. (2019). Microdiversity and Temporal Dynamics of Marine Bacterial Dimethylsulfoniopropionate Genes. Environ. Microbiol. 21 (5), 1687–1701. doi: 10.1111/1462-2920.14560
O’Brien J., McParland E. L., Bramucci A. R., Siboni N., Ostrowski M., Kahlke T., et al. (2022). Biogeographical and Seasonal Dynamics of the Marine Roseobacter Community and Ecological Links to DMSP-Producing Phytoplankton. ISME Commun. 2 (1), 16. doi: 10.1038/s43705-022-00099-3
Raina J.-B., Clode P. L., Cheong S., Bougoure J., Kilburn M. R., Reeder A., et al. (2017). Subcellular Tracking Reveals the Location of Dimethylsulfoniopropionate in Microalgae and Visualises its Uptake by Marine Bacteria. eLife 6, e23008. doi: 10.7554/eLife.23008
Reisch C., Moran M., Whitman W. (2011). Bacterial Catabolism of Dimethylsulfoniopropionate (DMSP). Front. Microbiol. 2 (172). doi: 10.3389/fmicb.2011.00172
Reisch C. R., Stoudemayer M. J., Varaljay V. A., Amster I. J., Moran M. A., Whitman W. B. (2011). Novel Pathway for Assimilation of Dimethylsulphoniopropionate Widespread in Marine Bacteria. Nature 473, 208. doi: 10.1038/nature10078
Simó R. (2001). Production of Atmospheric Sulfur by Oceanic Plankton: Biogeochemical, Ecological and Evolutionary Links. Trends Ecol. Evol. 16 (6), 287–294. doi: 10.1016/S0169-5347(01)02152-8
Simon M., Scheuner C., Meier-Kolthoff J. P., Brinkhoff T., Wagner-Döbler I., Ulbrich M., et al. (2017). Phylogenomics of Rhodobacteraceae Reveals Evolutionary Adaptation to Marine and Non-Marine Habitats. ISME J. 11 (6), 1483–1499. doi: 10.1038/ismej.2016.198
Sun J., Todd J. D., Thrash J. C., Qian Y., Qian M. C., Temperton B., et al. (2016). The Abundant Marine Bacterium Pelagibacter Simultaneously Catabolizes Dimethylsulfoniopropionate to the Gases Dimethyl Sulfide and Methanethiol. Nat. Microbiol. 1 (8), 16065. doi: 10.1038/nmicrobiol.2016.65
Sun F.-L., Wang Y.-S., Wu M.-L., Jiang Z.-Y., Sun C.-C., Cheng H. (2015). Spatial and Vertical Distribution of Bacterial Community in the Northern South China Sea. Ecotoxicology 24 (7-8), 1478–1485. doi: 10.1007/s10646-015-1472-2
Sun F., Wu M., Wang Y., Sun C., Xu Z. (2020). Diversity and Potential Function of Bacterial Communities in Different Upwelling Systems. Estuar. Coast Shelf Sci. 237, 106698. doi: 10.1016/j.ecss.2020.106698
Sun F., Xia X., Simon M., Wang Y., Zhao H., Sun C., et al. (2022). Anticyclonic Eddy Driving Significant Changes in Prokaryotic and Eukaryotic Communities in the South China Sea. Front. Mar. Sci. 9. doi: 10.3389/fmars.2022.773548
Todd J. D., Curson A. R. J., Dupont C. L., Nicholson P., Johnston A. W. B. (2009). The dddP Gene, Encoding a Novel Enzyme That Converts Dimethylsulfoniopropionate Into Dimethyl Sulfide, Is Widespread in Ocean Metagenomes and Marine Bacteria and Also Occurs in Some Ascomycete Fungi. Environ. Microbiol. 11 (6), 1376–1385. doi: 10.1111/j.1462-2920.2009.01864.x
Todd J. D., Curson A. R., Kirkwood M., Sullivan M. J., Green R. T., Johnston A. W. (2011). DddQ, a Novel, Cupin-Containing, Dimethylsulfoniopropionate Lyase in Marine Roseobacters and in Uncultured Marine Bacteria. Environ. Microbiol. 13 (2), 427–438. doi: 10.1111/j.1462-2920.2010.02348.x
Varaljay V. A., Gifford S. M., Wilson S. T., Sharma S., Karl D. M., Moran M. A. (2012). Bacterial Dimethylsulfoniopropionate Degradation Genes in the Oligotrophic North Pacific Subtropical Gyre. Appl. Environ. Microbiol. 78 (8), 2775–2782. doi: 10.1128/AEM.07559-11
Varaljay V. A., Howard E. C., Sun S., Moran M. A. (2010). Deep Sequencing of a Dimethylsulfoniopropionate-Degrading Gene (Dmda) by Using PCR Primer Pairs Designed on the Basis of Marine Metagenomic Data. Appl. Environ. Microbiol. 76 (2), 609–617. doi: 10.1128/AEM.01258-09
Voget S., Billerbeck S., Simon M., Daniel R. (2015). Closed Genome Sequence of Octadecabacter Temperatus SB1, the First Mesophilic Species of the Genus Octadecabacter. Genome Announcements 3 (5), e01051–e01015. doi: 10.1128/genomeA.01051-15
Yang G.-P. (2000). Spatial Distributions of Dimethylsulfide in the South China Sea. Deep Sea Res. Pt I: Oceanogr. Res. Papers 47 (2), 177–192. doi: 10.1016/S0967-0637(99)00058-8
Yang G.-P., Jing W.-W., Kang Z.-Q., Zhang H.-H., Song G.-S. (2008). Spatial Variations of Dimethylsulfide and Dimethylsulfoniopropionate in the Surface Microlayer and in the Subsurface Waters of the South China Sea During Springtime. Mar. Environ. Res. 65 (1), 85–97. doi: 10.1016/j.marenvres.2007.09.002
Zeng Y.-X., Qiao Z.-Y., Yu Y., Li H.-R., Luo W. (2016). Diversity of Bacterial Dimethylsulfoniopropionate Degradation Genes in Surface Seawater of Arctic Kongsfjorden. Sci. Rep. 6, 33031. doi: 10.1038/srep33031
Zhai X., Song Y.-C., Li J.-L., Yang J., Zhang H.-H., Yang G.-P. (2020). Distribution Characteristics of Dimethylated Sulfur Compounds and Turnover of Dimethylsulfide in the Northern South China Sea During Summer. J. Geophys. Res.: Biogeosci. 125 (2), e2019JG005363. doi: 10.1029/2019JG005363
Keywords: Roseobacter group, dimethylsulfoniopropionate degradation genes, diversity, abundance, South China Sea
Citation: Sun F, Wang Y, Jiang Z, Sun C, Wang Y and Wu M (2022) Abundance and Diversity of Dimethylsulfoniopropionate Degradation Genes of Roseobacter Group in the Northern South China Sea. Front. Mar. Sci. 9:895613. doi: 10.3389/fmars.2022.895613
Received: 14 March 2022; Accepted: 01 April 2022;
Published: 03 May 2022.
Edited by:
Fajin Chen, Guangdong Ocean University, ChinaReviewed by:
Peng Wu, Chinese Academy of Fishery Sciences (CAFS), ChinaYanpei Zhuang, Jimei University, China
Yong Zhang, Fujian Normal University, China
Copyright © 2022 Sun, Wang, Jiang, Sun, Wang and Wu. This is an open-access article distributed under the terms of the Creative Commons Attribution License (CC BY). The use, distribution or reproduction in other forums is permitted, provided the original author(s) and the copyright owner(s) are credited and that the original publication in this journal is cited, in accordance with accepted academic practice. No use, distribution or reproduction is permitted which does not comply with these terms.
*Correspondence: Meilin Wu, bWx3dUBzY3Npby5hYy5jbg==