- 1Department of Integrative Biology, University of Windsor, Windsor, ON, Canada
- 2Fisheries and Oceans Canada, Arctic Aquatic Research Division, Winnipeg, MB, Canada
- 3Daniel P. Haerther Center for Conservation and Research, John G. Shedd Aquarium, Chicago, IL, United States
Variable movement strategies can complicate the conservation and management of mobile species. Given its extreme life history traits as a long-lived, deep-water species, the Greenland shark (Somniosus microcephalus) is vulnerable to fisheries bycatch, but little is known over its long-term movements across a spatially and seasonally variable Arctic environment. To address this knowledge gap, the movements of Greenland sharks in coastal fjords and offshore waters of Baffin Bay were examined using seven years of acoustic telemetry data. Seasonal patterns in broad-scale movements and inshore-offshore connectivity were compared among 155 sharks (101 males, 54 females [mean LT = 2.65 ± 0.48 m, range 0.93-3.5 m]) tagged in 6 discrete coastal locations spanning from Grise Fiord to Cumberland Sound (Nunavut). Sharks exhibited transient movements throughout coastal and offshore regions with some evidence of seasonally recurring hotspots revealed by repeat detections of individuals at sites over multiple years. Shark presence in coastal fjords occurred exclusively during the coastal ice-free period (July to November), regardless of the location of tagging or detection, while presence in the offshore was recorded during the period of ice re-formation and cover (November to July). Through multi-year telemetry, it was possible to reveal repetitive patterns in broad-scale habitat use for a complex marine predator with direct relevance for understanding the seasonal distribution of mobile Arctic consumers and informing regional fisheries management.
Introduction
Resource distribution and seasonality are important factors in regulating the movements of consumers across environmental landscapes (O’Neill et al., 1988). In seasonal environments, the occurrence of resource patches (primary productivity hotspots and associated prey aggregations) can vary substantially across time and space, producing patterns that are often reflected in the movements of mobile consumers (Boyd et al., 2002; Sims et al., 2003; Laidre et al., 2004). As a result, mobile consumers can drive food web stability by coupling parallel energy pathways from disparate sources of primary productivity (McCann et al., 2005; McMeans et al., 2013; Williams et al., 2018), and by adopting a flexible response to changing resource conditions to maximize energy flow (McCann et al., 2005). For example, Arctic marine environments are characterized by extreme seasonality in solar radiation and extent of sea-ice cover, generating spatial and temporal variability in pelagic and ice-associated primary production and in turn, the distribution of resource hotspots (Gradinger, 1995; Tremblay et al., 2012). In Baffin Bay, a deep-water ocean basin in the Eastern Canadian Arctic, this seasonal variability influences the movements of marine predators, producing patterns that predominantly coincide with the timing and location of sea-ice formation and retreat [e.g., Monodon monoceros − narwhal (Laidre et al., 2004; Shuert et al., 2022 In revision), Balaena mysticetus − bowhead whale (Dueck et al., 2006), Odobenus rosmarus − walrus, Erignathus barbatus – bearded seal, (Marcoux et al., 2017) and Reinhardtius hippoglossoides − Greenland halibut (Hussey et al., 2017; Barkley et al., 2018)]. Specifically, the presence of transient marine animals in the deep-water fjords of Baffin Island [e.g., R. hippoglossoides, (Barkley et al., 2018), M. monoceros, and B. mysticetus, (Marcoux et al., 2017)] corresponds tightly with the summer open water period when increased upwelling and nutrient inputs from glacial and terrestrial runoff stimulate primary productivity in these coastal systems (Gradinger, 1995; Tremblay et al., 2012). In the ice-covered winter months, offshore areas of significant upwelling and reduced ice cover such as the North Water (NOW) Polynya act as hotspots of late-season primary productivity that promote the aggregation of numerous marine mammals and birds (Melling et al., 2001; Heide-jørgensen et al., 2013). In this seasonally dynamic environment, a high degree of mobility and the semi-predictable spatial and temporal occurrence of primary productivity hotspots allows large-bodied consumers to exploit these episodic resource patches despite the landscape’s vast spatial scale.
Under sparse resource conditions, animals are required to operate at broader scales of habitat utilization in order to locate a maximal number of resource patches (O’Neill et al., 1988). Deep-sea habitats are highly nutrient-limited, leading to the evolution of highly efficient resource detection traits (e.g., chemoreception) and long-range mobility (Premke et al., 2003; Armstrong et al., 2012). Depending on the scale of habitat use, high levels of mobility can drive migratory marine species to move through waters managed by numerous jurisdictions and, in turn, to encounter various levels of protection throughout their individual lifespans (Lascelles et al., 2014; Heupel et al., 2015; Barkley et al., 2019). The movement of wide-ranging species through regions varying in levels of both risk and protection can complicate conservation and management efforts (Heupel et al., 2015). Importantly, migratory marine species often include large-bodied predators that play disproportionately important roles in ecosystem stability (McCann et al., 2005) and may act as indicators of ecosystem health (Zacharias and Roff, 2001).
As the largest fish species to inhabit the Arctic deep sea, the Greenland shark (Somniosus microcephalus) occupies a high trophic position as both scavenger and active predator and may provide stability to Arctic marine food webs (McMeans et al., 2013; Hussey et al., 2014). Despite the predicted high abundance of Greenland sharks throughout Baffin Bay (Devine et al., 2018) and their vulnerability to incidental capture by commercial and Inuit community fisheries (Idrobo and Berkes, 2012; Bryk et al., 2018; Madigan et al; in press), Greenland shark distribution and the timing of movements throughout the basin remain unknown. While exhibiting the slowest observed mean swim speed (0.34 ms-1) and tailbeat frequency (0.15 Hz) relative to body size for any fish species (Watanabe et al., 2012), Greenland sharks are capable of undertaking extensive horizontal movements (Campana et al., 2015a; Hussey et al., 2018) and maintain a broad distribution throughout the coastal and offshore waters of Baffin Bay (MacNeil et al., 2012). Given their current designation as the world’s longest-lived vertebrate (Nielsen et al., 2016), and the fact that they possess other K-selected life history traits such as low fecundity, slow growth (0.5 cm yr-1; Hansen, 1963), and an extremely low metabolic rate (Ste-Marie et al., 2020), appropriate management of this species is a priority (Davis et al., 2013; Edwards et al., 2019).
The goal of the current study was to provide a summarized overview of the movement patterns of Greenland sharks in coastal and offshore waters of Baffin Bay. Specifically, we aimed to determine: (i) multi-year trends in seasonal occurrence, (ii) evidence for recurrence of individuals at sites (i.e., return of individuals to a particular location over longer time periods; months-years) relative to spatial monitoring in the offshore and, (iii) transient or resident behaviors indicated by duration of detection days in different habitats. To achieve this, we analyzed the detection profiles of Greenland sharks recorded via static acoustic receivers deployed along the deep-water offshore banks of Baffin Bay (~3 y of monitoring, total area = ~34,458 km2) and in four coastal environments (~7 y of monitoring, total area range = ~348 - 2,152 km2). We hypothesized that the long lifespan, high degree of mobility, and opportunistic foraging strategy of the Greenland shark would drive it to adopt a temporally fluctuating distribution corresponding to the seasonal occurrence of resource patches throughout the basin, mirroring those reported for several other Arctic predators. Specifically, we expected sharks to concentrate in coastal fjords during periods of peak coastal productivity (spring and summer), in contrast to the less productive winter months, when sharks would disperse throughout the ice-covered ocean basin.
Methods
Study location
Baffin Bay is a large, semi-enclosed ocean basin situated between Baffin Island (Nunavut, Canada) and Northwestern Greenland with a maximum depth of approximately 2000 m (Figure 1). To the south, the bay is linked to the North Atlantic by a deep-water sill (640 m) in the Davis Strait, and in the north it connects to the Arctic Ocean via shallower sills located in Lancaster Sound (125 m depth), Jones Sound (190 m depth), and Nares Strait (220 m depth) (Münchow et al., 2015). Along its continental margins, Baffin Bay is ringed by wide, sloping shelf areas off Greenland, and more steeply sloping shelves off Baffin Island both of which are broken by a series of deep channels (~500 – 1000 m depth) connecting offshore waters to its coastal fjords (Münchow et al., 2015). Circulation patterns in Baffin Bay are driven by two major North Atlantic current systems known as the West Greenland and Baffin Island Currents. Warm and salty water (temperature > 0˚C, salinity > 34) from the North Atlantic enters the bay from the south through the eastern Davis Strait, moving northward along the west coast of Greenland, where it is met by inflows of Arctic water from the Smith, Lancaster, and Jones Sounds (Tang et al., 2004). Following this cyclonic flow, the Baffin Island Current moves southward down the eastern coast of Baffin Island, resulting in a prominent outflow through western Davis Strait (Tang et al., 2004). This deep-water basin is also characterized by seasonal, semi-complete coverage of sea-ice, with formation beginning in October, increasing in a southerly direction and reaching near-complete coverage in March (Tang et al., 2004). Predominantly ice-free periods occur only in August and September (Tang et al., 2004), however, a recurrent patch of open water known as the North Water (NOW) Polynya is typically found spanning the region between Smith and Lancaster Sounds (~76˚N to 79˚N and 70˚W to 80˚W) throughout the ice-covered months (Heide-jørgensen et al., 2013).
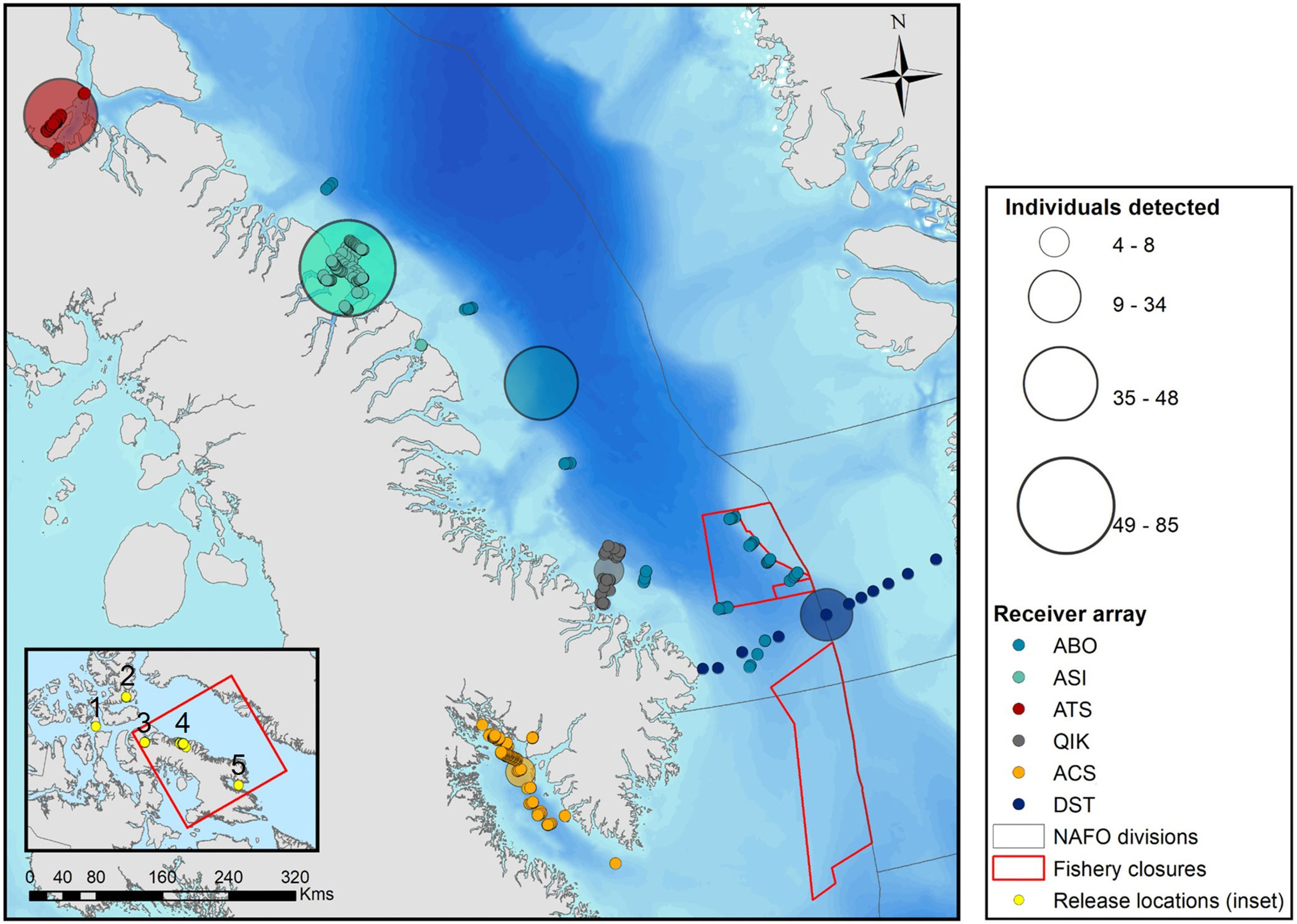
Figure 1 Locations of acoustic receivers used to monitor the presence of tagged Greenland sharks across four coastal regions of Nunavut (Eastern Canadian Arctic) and offshore Baffin Bay. Points are coloured by receiver array, where solid points represent the locations of individual receivers and semi-transparent points are scaled in size to represent the total number of individuals detected per array. Release locations of tagged Greenland sharks are depicted by yellow circles in the inset map and are numbered as follows: 1) Resolute Bay, 2) Grise Fjord, 3) Tremblay Sound, 4) Scott Inlet, 5) Cumberland Sound. [Colour online].
Shark capture and tagging
Greenland sharks were tagged during the summer/fall (July-Oct) from 2012 to 2018 at seven inshore sites along the Eastern coast of Baffin Island (see Table 1). Sharks were captured using bottom longlines (100-1500 m in length) set at depths between 100 and 1000 m for periods ranging from 3-12 h. In most cases, longlines were set with size 16, 18, and 20 circle hooks attached to 50 x 1.5 m steel leader gangions spaced 10 m apart and baited with either frozen squid, char, narwhal, or seal meat. At certain shallower inshore sites (Tremblay Sound, Resolute Bay, and Grise Fiord), reduced longlines were set consisting of 6-12 steel leader gangions as detailed above. Acoustic tagging was conducted using the following methods. Upon retrieval of the fishing gear, each shark was held alongside an inflatable zodiac using tail and body straps and a 69 kHz acoustic transmitter (V16-6x, V16-TP-4x; VEMCO) was surgically inserted through a small incision on the animal’s ventral side (anterior to the pelvic fins and just off the midline). The incision was then closed using 3-4 interrupted sutures. Acoustic tags were programmed to transmit at a random interval between 200 and 240 seconds. Tagging procedures, including measurement (total length [LT; cm]), sex determination, and biological sampling (blood extraction, fin clips) were conducted within ~10 min, after which time the shark was released at the capture location and was monitored for normal swimming behavior. Animal capture and handling were conducted in accordance with CCAC guidelines under permit through the Animal Care Committee at the University of Windsor and a Fisheries and Oceans License to Fish.
Acoustic arrays
The movements of tagged Greenland sharks were monitored throughout the coastal and offshore regions of Baffin Bay (Eastern Canadian Arctic) via static acoustic telemetry. Static receiver stations designed for the detection of tagged sharks were constructed using a nylon rope riser anchored to the seafloor by a 200 lb cast iron disc anchor and connected to an acoustic release mechanism (PORT MFE; EdgeTech, West Wareham, MA, USA) positioned approximately 10 m above the anchor. At each station, an acoustic receiver (VR2W; Innovasea, Bedford, Nova Scotia) was attached to a second nylon rope riser using zip ties and a security line and was suspended above the release mechanism and ~2 m below a subsurface float. The length of the upper riser (connecting the float and the release) was selected relative to the bottom depth at the deployment location, with longer risers (187 m) deployed at depths >800 m and shorter risers (12 m) on shallower deployments (<800 m depth). Equipment retrieval was facilitated by an acoustic release mechanism which, upon receipt of an acoustic command signal, released from the anchor riser allowing the upper riser along with its associated acoustic receiver and environmental sensor, to float to the surface where it was recovered. Station servicing and data collection were conducted annually from late September to early October, during which time stations were retrieved and subsequently redeployed in their original locations within a 24 h period. Across all study years and locations, stations were deployed at bottom depths ranging from 397 to 1150 m with a mean deployment depth of 535 ± 312.47 m. Receiver stations referred to in this study formed the basis of six separate arrays deployed across four coastal systems and in offshore waters along the western continental margin of Baffin Bay (Table 1). The maximum detection range of acoustic receivers was estimated at ~802 m based on range tests previously conducted at comparable depths (~1000 m) and under similar environmental conditions in Cumberland Sound, Nunavut (Hussey et al., 2017, Appendix S1).
Data analysis
Greenland shark detections were summarized using the statistical software R v.3.5.3 (R Core team, 2019) and the packages, tidyverse (Wickham et al., 2019) and glatos (Holbrook et al., 2020). All maps were produced using ArcMAP (ESRI, 2019) and additional figures were produced using the R package, ggplot2 (Wickham, 2016).
Inshore and offshore acoustic arrays
Offshore stations were classified as those deployed along the continental slope across a depth gradient between 600 and 1100 m while inshore stations were classified as all those deployed on the continental shelf between the coast and the shelf margins. Coastal arrays were deployed in Cumberland Sound (ACS), Tremblay Sound (ATS), Scott Inlet (ASI), and Qikiqtarjuaq (QIK), while offshore arrays included receivers deployed in southeastern Baffin Bay (DST and ABO) and along the western continental shelf (ABO) (Figures 1, 2).
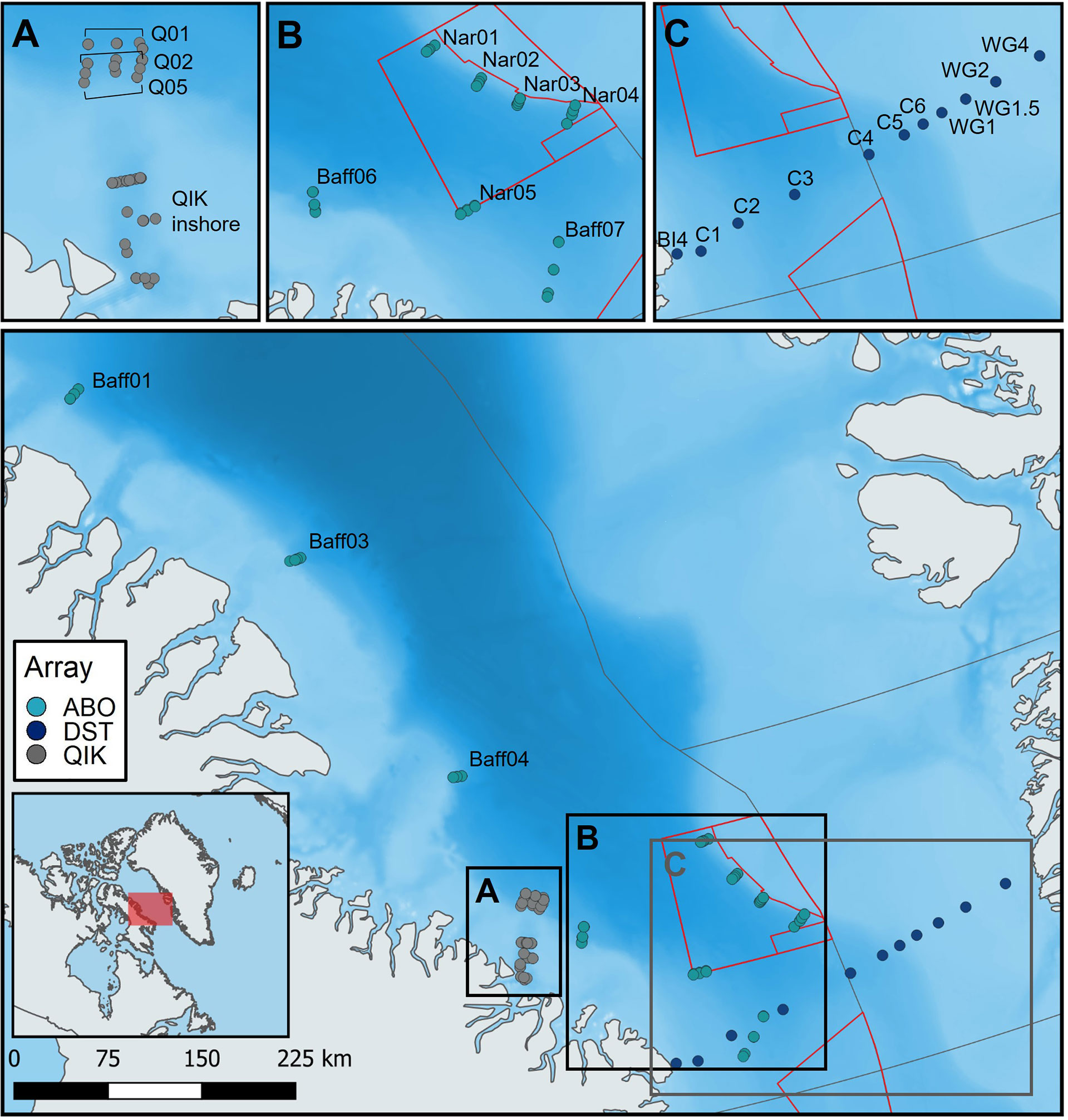
Figure 2 Locations of acoustic receivers used to monitor the presence of tagged Greenland sharks in offshore Baffin Bay. Points are coloured by array ID for each of the three offshore arrays (QIK, ABO, DST). Receiver gates in each array are labelled and depicted independently in panels (A) (QIK), (B) (ABO), and (C) (DST). [Colour online].
Coastal-offshore seasonality
Detections recorded across all sites were used to determine the seasonal abundance of tagged sharks present in each array between August 8, 2011 and September 27, 2018, hereby referred to as the study period. For each discrete receiver array, the active deployment period and the number of receivers that formed each array were summarized over this 7-y period (Table 1). The total number of detections recorded per array, the number of tagged individuals detected, and the proportion of receivers that detected the presence of tagged sharks were then calculated for the entire study period (Table 1, Figure 1). For both coastal and offshore arrays, the monthly abundance of detected individuals was compared to detect seasonal occurrence across the two habitat types. This acknowledges that the number of sharks detected in coastal vs. offshore regions is inherently biased by the fact that all tagged sharks were captured and released in coastal waters.
To define temporal patterns in the spatial distribution of Greenland sharks across inshore vs. offshore environments over a 12-month period, summarized detections from all arrays and study years were plotted by month and receiver latitude. This visualization method was then repeated using only the coastal array that was most connected to the offshore (QIK) to provide a fine-scale analysis of spatiotemporal coastal-offshore movements (see Supplementary Material).
Site fidelity in the offshore environment
Detection summaries categorized by discrete receiver array and by offshore receiver gate and station (i.e., array subcomponents) were used to identify locations of interannual recurrence for individual Greenland sharks within the offshore receiver network. Specifically, to detect multi-year recurrences of individual sharks at unique offshore locations, detection summaries were grouped by shark ID and by the year at liberty (i.e., the sequential year following tagging) during which the individual was detected (i.e., year 1 detections were recorded during the first year at liberty). These data were used as a metric to infer potential inter-annual site fidelity to specific regions. This analysis accepts that the combined offshore arrays provided limited spatial coverage relative to the scale of the open water environment and that a comparatively low number of detections were recorded per receiver (with high zero inflation) limiting statistical comparisons.
Transience vs. resident movement behavior in the offshore environment
To categorize the movement behaviors exhibited by Greenland sharks in offshore waters, (i.e. transiting vs. temporarily resident), the number of detections predicted for a Greenland shark moving in a straight line past a receiver at a known swimming speed was used as a proxy for transient movement. This predicted value was then compared to the observed number of sequential detections recorded for individual sharks by offshore receivers. The expected number of detections for a transiting shark - based on an individual moving in a linear path across the widest diameter of the receiver detection range - was calculated based on:
i. A receiver detection radius of 802 m at 60% detection efficiency – as reported by Hussey et al. (2017).
ii. An average swim speed of 0.34 ms-1 (range <0.17- 0.73 ms-1) for Greenland sharks (Watanabe et al., 2012).
iii. A minimum nominal tag delay of 200 sec (for a V16TP-4x tag).
Detections of tagged individuals recorded by each offshore receiver were classified into detection events – defined as clusters of sequential detections of a unique tag (i.e., tagged shark) at a single receiver station – using the ‘detection_events’ function in the glatos R package (Holbrook et al., 2020) with a time separation interval value of seven days (604800 sec). The latter value determines the maximum duration used to delineate individual detection events and was chosen as a conservative estimate of the average duration of detection events. To identify transient or temporarily resident behavior, the average number of detections and event duration for all unique offshore receivers were then calculated and compared to predicted values for an animal transiting past a receiver.
Coastal-offshore connectivity
A subset of sharks that were detected by both coastal and offshore receivers during the 3-y period of offshore array deployments (n = 48) was used to examine the frequency and timing of individual-level movements between the two environments. Specifically, individuals were classified into two groups based on the frequency of redetection by coastal and offshore arrays wherein individuals were either: (i) never detected by a coastal array (despite having been tagged and released in the coastal environment) or were detected in coastal waters during a single detection year, or (ii) were detected by coastal arrays in multiple detection years, indicating repeated coastal-offshore transitions.
Results
Of a total of 193 Greenland sharks tagged at 6 coastal locations, 155 individuals (101 males, 54 females [mean LT = 2.65 ± 0.48 m, range = 0.93-3.5 m]) were detected between 65° and 72° latitude by 153 acoustic receiver stations deployed across 6 distinct coastal and offshore receiver arrays (Figure 1; Table 1). Of the 155 detected sharks, 138 were detected within coastal arrays (91 males, 47 females [mean LT = 2.62 ± 0.48 m]; Aug 2011 – Sept 2018): Resolute Bay (40% of tagged sharks detected, n = 15); Tremblay Sound (87% detected, n = 62); Scott Inlet (96% detected, n = 81); Cumberland Sound (39% detected, n = 23); and Home Bay (100% detected, n = 1). The three offshore arrays (ABO, DST, and QIK) recorded the presence of 55 unique individuals (33 males, 22 females [mean LT = 2.66 ± 0.49 m]) (Nov 2015 – Sept 2018; Figure 3). Individuals detected offshore included those tagged at 5 coastal locations, with large variation in the proportion of sharks detected in the offshore relative to the total number of animals tagged at each coastal location: Cumberland Sound (4% of total tagged, n = 23), Grise Fiord (58%, n = 12), Resolute Bay (40%, n = 15), Scott Inlet (33%, n = 81), and Tremblay Sound (23%, n = 62). In total, 88,009 shark detections were recorded across all arrays during the study period, with more detections recorded by inshore receivers (mean = 622.0 ± 1,353.0 detections/receiver) (range = 94-33,046) relative to offshore receivers (mean = 152.0 ± 296.0 detections/receiver) (range = 2-1,754). The minimum time at liberty prior to first being detected in the offshore was 61.61 d for a shark tagged in Scott Inlet in 2015, compared to a maximum time at liberty prior to offshore detection of ~6.37 y for one individual tagged in Cumberland Sound in 2011 (Figure 3).
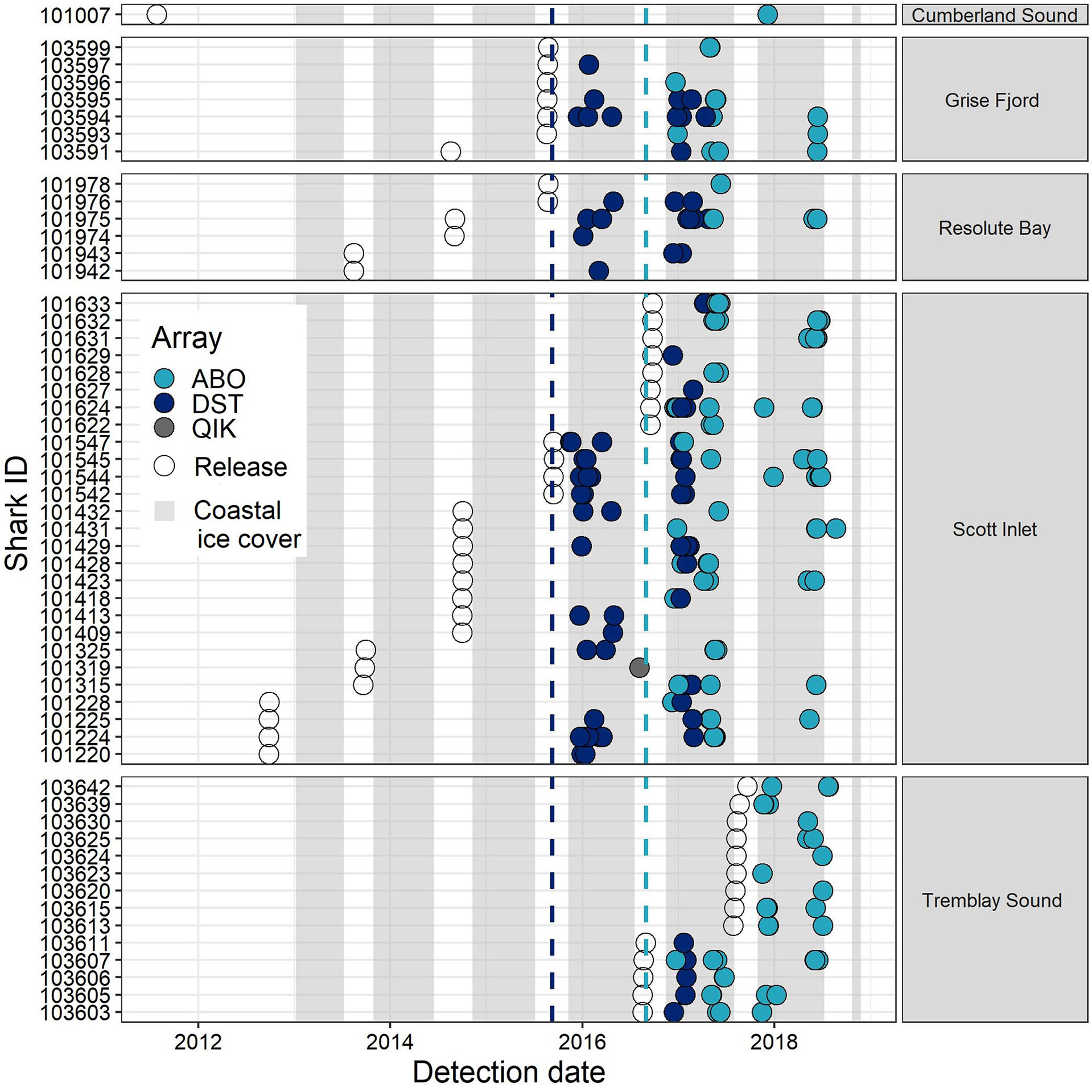
Figure 3 Temporal distribution of Greenland shark detections recorded by acoustic receiver stations in offshore Baffin Bay, listed by shark ID and coloured by array ID. Panels indicate the capture locations of individual sharks and open dots indicate the date of initial tagging and release for each individual. Vertical lines denote the date of first deployment for DST and ABO arrays, respectively, as indicated by line colour. Shaded bars denote the annual periods of coastal ice cover between the timing of formation (>75% sustained cover) and breakup (<75% sustained cover) in Scott Inlet (Nunavut) throughout the study period (data available at: https://ice-glaces.ec.gc.ca/). [Colour online].
Coastal-offshore seasonality
Apparent segregation in the timing of shark presence was observed between coastal and offshore environments. While sharks were detected across 10 months of the year by offshore receiver stations (ABO, DST, and QIK arrays; Figure 4), shark presence in coastal habitats was strongly tied to the summer ice-free months between July and November (Figure 5). This trend was consistent for all 4 coastal receiver arrays (ATS, ASI, QIK, and ACS arrays) across the 7-y study period. The distinct seasonal pattern in coastal waters was matched by a simultaneous decrease in the abundance of sharks detected by offshore receivers throughout the same 4-month period over the 3 consecutive years of monitoring (Figure 4).
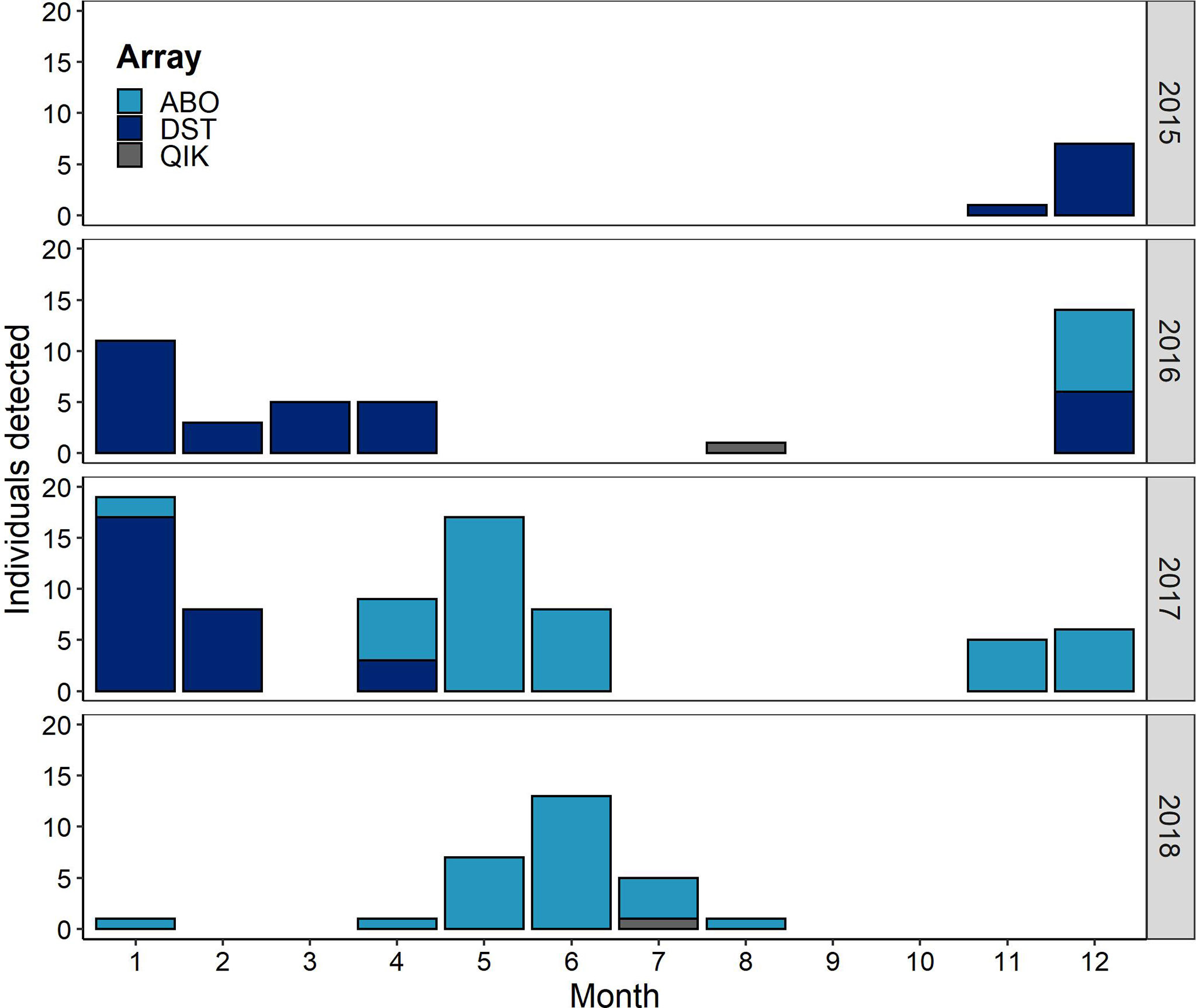
Figure 4 Total number of tagged Greenland sharks detected by acoustic receiver stations in offshore Baffin Bay (ABO, DST, and QIK arrays) listed by month and year and coloured by array ID. It should be noted that initial array deployment dates limit the number of detections observed before September 2016 (ABO array first deployed 2016-08-31, DST deployed 2015-09-09, and QIK deployed 2015-10-07). [Colour online].
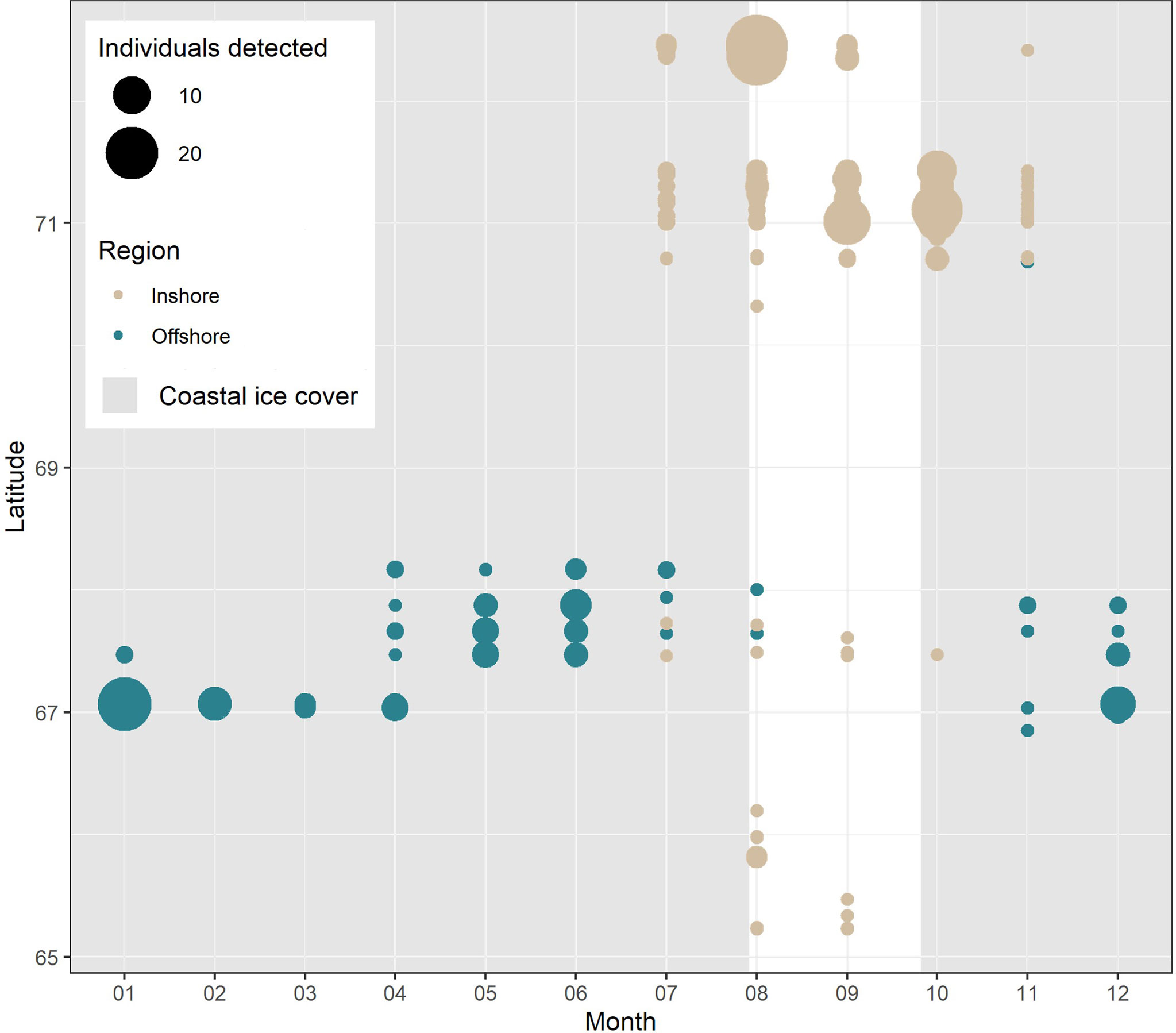
Figure 5 Greenland shark detections arranged by month and latitude. Points are scaled by the number of unique individuals recorded at each time and location and are coloured by the region in which the detections were recorded (i.e., inshore vs. offshore). Shaded bars denote the average annual period of ice cover between the timing of formation (>75% sustained cover) and breakup (<75% sustained cover) in Scott Inlet (Nunavut) throughout the study period (data available at: https://ice-glaces.ec.gc.ca/). [Colour online].
Sharks were detected in coastal arrays primarily during and just after active tagging periods with low detection rates in subsequent months and years. In Scott Inlet, annual peaks in detected shark abundance were recorded in September and October in the years 2012−2016 when active tagging took place (mean ± SD = 12.6 ± 6.29 individuals/month; ASI array). In contrast, fewer sharks were detected outside this period (mean of 2.86 ± 1.66 individuals/month) in July, August, and November across all years. A similar trend was observed in Tremblay Sound (ATS array), where a peak abundance of 22 ± 8.45 tagged individuals/month was observed in August (2017-2018) compared to a mean of 2.4 ± 1.20 individuals/month recorded during July, September, and November.
When considering the temporal trend of sharks detected in the offshore, no individuals were detected in either September or October (combined data from 2015-2018 and accepting different offshore array configurations; Figure 3). This absence of detections was bounded by low numbers of detected individuals in July, August, and November (mean = 0.9 ± 1.61 individuals/month detected across the entire offshore array for the 3-y deployment period). Over the remaining seven months, a mean of 6 ± 5.74 individuals was detected per month, with non-recurrent peaks in abundance recorded in December 2016 (n = 14), January 2016 and 2017 (n = 11, n = 17, resp.), May 2017 (n = 17) and June 2018 (n = 13).
Of the 56 receivers comprising the 3 offshore arrays, 21 recorded the presence of tagged sharks. Individuals were typically detected by a small number of offshore receivers per year (mean = 1.67 ± 0.98 receivers/y) and by a mean of 2.6 ± 1.71 receiver stations across the entire 3 y study period. Relative to offshore monitoring effort, the highest number of tagged sharks were detected in the ABO array (southeastern Baffin Bay) near the Disko Fan Conservation Area, ~213 km off the coast of Sisimiut, Greenland (DFO, 2008b). Specifically, most sharks were detected on four gates in the ABO array (Nar01, Nar02, Nar03, Nar04), located along the eastern border of the fishery closure (Figure 2). In 2017, sharks from 5 coastal tagging locations were detected by gates in the ABO array, with most individuals recorded on southernmost gate, Nar04 (n = 15) over a period of 5 months (April-June and Dec-Jan). Detections in this region (Nar01-04) occurred over two distinct periods – one in spring and early summer from April to July and another in winter from November to January (Figure 4). In spring, a maximum of 17 individuals was detected in May 2017 with peaks of 6-7 individuals in May and June 2017-2018. In winter, most individuals were detected in December but at a much lower abundance (n = 3 in both 2016 and 2017). During these peak periods, many individuals were also detected near the easternmost stations in the DST array, located south of the closure and spanning the mouth of a deep-water channel connecting southern Baffin Bay to the Davis Strait (Figures 2, 6). For example, in 2017, 23 individuals were detected on a single receiver in this region (C6) over the months of December (n = 7), January (n = 16), and April (n = 3) (Figure 6). The remaining gates in the ABO array, situated along the western continental slope of Baffin Bay (Figure 2), recorded only 3 sharks on 2 receivers (of 17 total receivers) over the 3-y period (Figure 6). Similarly, only 2 individuals were detected by the offshore QIK array (Figure 6).
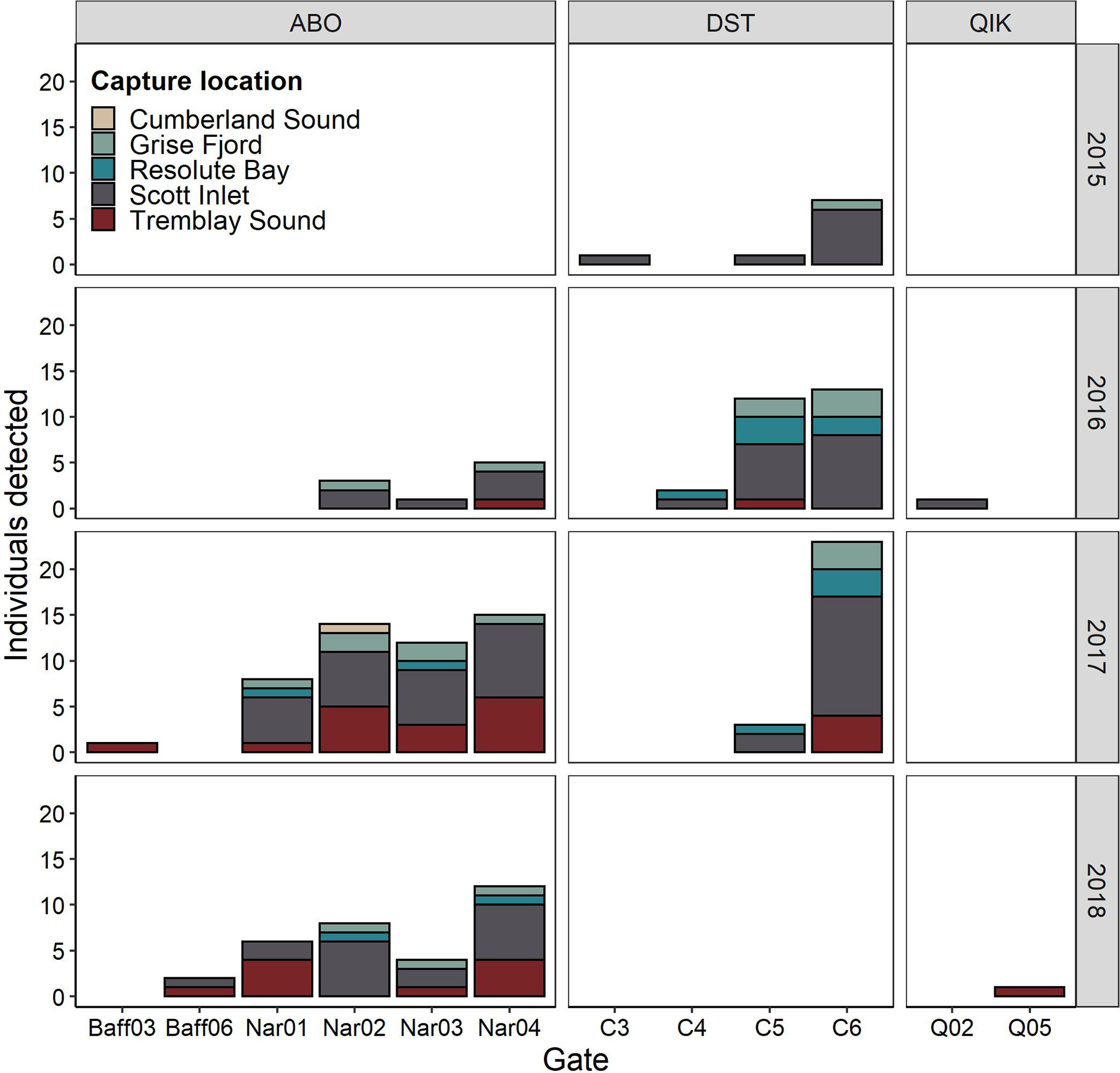
Figure 6 Total number of tagged Greenland sharks detected by offshore acoustic receiver stations listed by receiver gate and grouped by array (ABO, DST, and QIK). Bar colour denotes the capture locations of detected individuals. [Colour online].
More than half of the sharks detected in the offshore (n = 55) were redetected in subsequent years (20 sharks were detected over 2 y [n = 176 sharks tagged by end 2017], 9 were detected over 3 y [n = 145 sharks tagged by end 2016], and 2 were detected over 4 y [n = 121 sharks tagged by end 2015]), resulting in an average of 1.8 ± 0.85 years per individual. A total of 24 individuals were recorded only once by offshore arrays, representing 14% of the total tagged sharks available for detection in the offshore across 2 study years (i.e., those tagged by end 2017; n = 176).
Interannual recurrence in the offshore environment
Sharks detected over multiple years (n = 31) demonstrated a high degree of overlap in the offshore locations visited interannually, with 19 sharks visiting receivers in the same gate in multiple years (Figure S2, Supplementary). Gates C6 (DST array) and Nar04 (ABO array) showed the highest frequency of individual recurrence (n = 9 and n = 8 individuals, respectively) (Figure S1A, Supplementary). Although the remaining sharks (n = 12) were not detected by precisely the same gates across years, these individuals were largely detected within the same offshore region as the aforementioned group (gates C5-C6 and Nar01-Nar04) throughout the study period (Figure S2B, Supplementary).
Transience vs. resident movement behavior in the offshore environment
All offshore were categorized into 190 detection events within a maximum interval of 7 days (604,800 sec) between subsequent detections of an individual shark on a given receiver. Among the detection events calculated, the average number of detections recorded was 19 ± 32.37 per event, with a mean event duration of 31,231 ± 103,830.8 seconds (~8.68 h).
The mean duration of detection events varied by receiver gate, with the longest event durations recorded by gates in southeastern Baffin Bay (Nar01-Nar04, C5-C6; Table S1, Supplementary). Receiver gates located along Baffin Bay’s western shelf had detection events that were considerably shorter by comparison and recorded fewer overall detections and detection events (Baff03, Baff06, C3, C4, Q02, Q05).
Given the nominal delay of our acoustic tags and the average swimming speed of Greenland sharks (0.34 ms-1, range = <0.17 - 0.73 ms-1; Watanabe et al., 2012), the predicted time for an individual to transit on a linear path through the widest point of the detection radius of a receiver was calculated to be 4,717.65 sec (~1.31 h, range = 2,167.57 - 9,435.29 sec) with an expected 14.15 detections at detection efficiency of 60%. This estimated transit time suggests that sharks detected by gates C3 and C4 were transiting past receivers at the time of detection. Sharks detected by gates Baff06, C5, Nar01, and Nar03 were also likely to be exhibiting transient movements when considering the range of swim speeds recorded by Watanabe et al. (2012) and the additional time required for sharks to undertake vertical displacements while transiting through the detection radius. For 3 receiver gates (Nar04, Nar02, and C6) located in the two high activity offshore regions, detection events were much longer in duration than our minimum estimate, indicating that sharks were temporarily resident at those sites.
Coastal-offshore connectivity
Of the total 155 sharks detected, 48 individuals exhibited movements between multiple receiver arrays (i.e., ≥ 2 coastal arrays, ≥ 2 offshore arrays, or on both coastal and offshore arrays) (Figure 7). Based on the number of years in which an individual was detected in coastal waters, two distinct groups of sharks were identified.
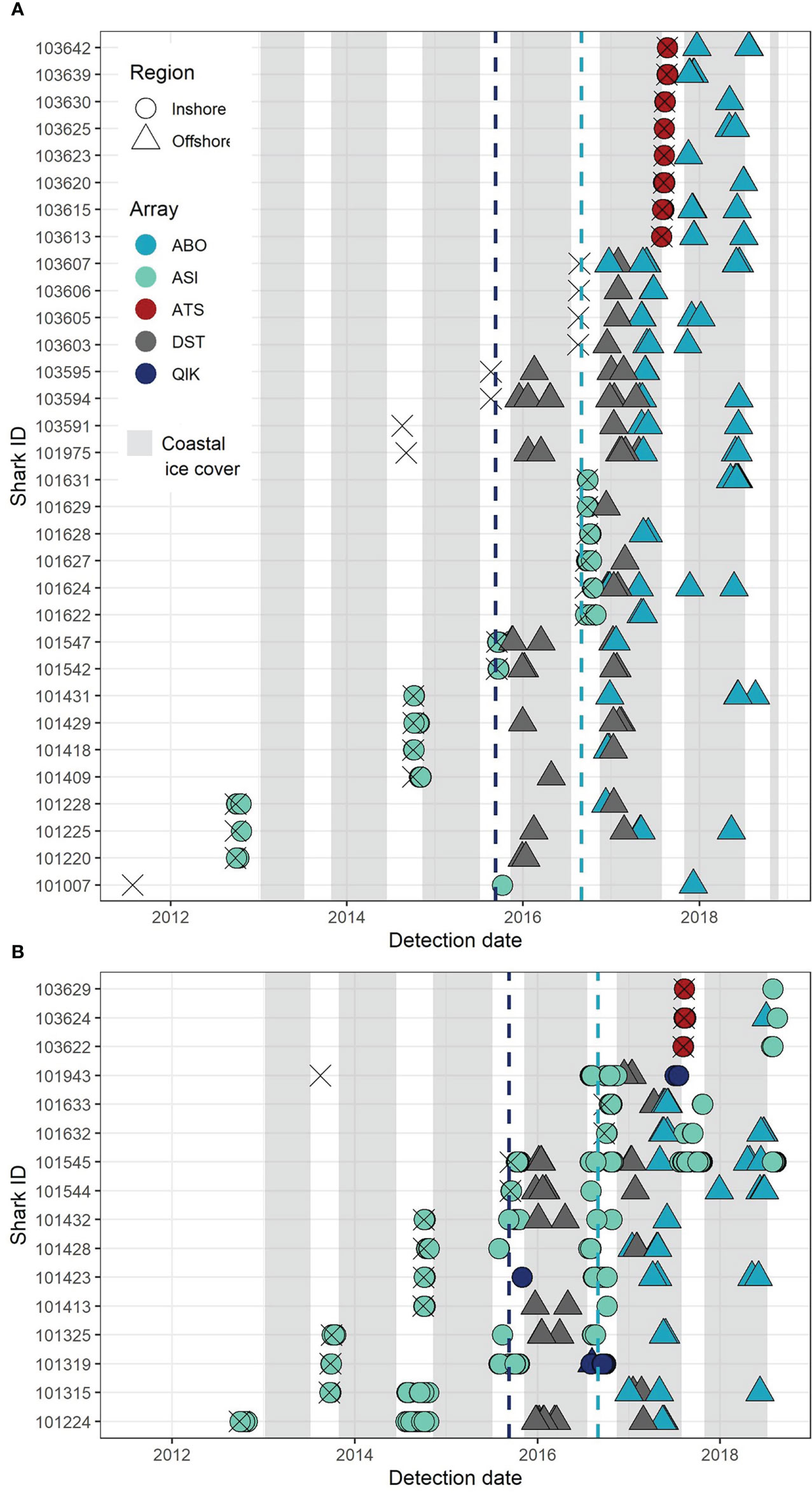
Figure 7 Greenland shark detections recorded by acoustic receiver stations in multiple arrays in coastal and offshore Baffin Bay, listed by the identification number of tagged sharks and the time of detection. (A) Sharks that were detected by more than one array but were detected in coastal waters in only one year. (B) Sharks detected by more than one array and in coastal waters over multiple years. Point colour denotes the array on which detections were recorded and point shape indicates the location of the detection as either inshore or offshore. Dashed lines indicate the deployment date of arrays by corresponding colour. The date of tagging and release for each animal is marked by an ‘X’. Shaded bars denote the annual periods of ice cover between the timing of formation (>75% sustained cover) and breakup (<75% sustained cover) in Scott Inlet (Nunavut) throughout the study period (data available at: https://ice-glaces.ec.gc.ca/). [Colour online.].
Group 1 contained sharks that were either never detected in coastal waters or that were detected in coastal arrays only once (i.e., in 1 y and principally when they were tagged; one exception, shark ID: 101007; Figure 7A). This group included 66.7% of the total sharks considered (n = 32), with 8 sharks (17% of total) detected only in the offshore (Figure 7). Despite being undetected by coastal arrays, sharks detected only in the offshore were, nonetheless, tagged in coastal regions and therefore demonstrated the same transitional movement exhibited by the remaining cohort of sharks in this subset (Figure 7A). Time at liberty between tagging/release and the first recorded detection ranged from 1-1,534 d (~4.2 y) for sharks in group 1, however, longer durations were driven by delays between the date on which sharks were tagged and the later deployment of offshore arrays. The majority of these sharks were tagged in coastal locations without active arrays present at the time of tagging/release (Grise Fiord [n = 2 individuals], Resolute Bay [n = 1 individual], Tremblay Sound [n = 4 individuals], and Cumberland Sound [n = 1 individual]; Figure 7A). Sharks that exhibited the longest periods prior to detection were those that were only detected in the offshore (apart from shark ID: 101007).
Group 2 contained 16 individuals (33% of total) that were detected in coastal regions across multiple years, including 2 sharks that were detected only in coastal waters and 6 that were detected by more than one distinct coastal array (Figure 7B). Across these 16 individuals, 17 coastal absence periods were identified (i.e., periods of time between consecutive events of temporary coastal residency) (Figure 7B). During these coastal absence periods, tagged sharks were detected by offshore arrays 76% of the time (n = 17 events) (Figure 7B). In contrast, only 4 coastal absence periods (24%, n = 17 events) were identified wherein sharks were not detected by offshore receivers (representing 4 individuals; Figure 7B).
A subset of individuals in group 2 demonstrated predictable coastal-offshore transitional movements which were repeated for up to a maximum of 4 years (shark ID: 101632, 101545, 101432; Figure 7B). One individual that demonstrated a similar pattern remained undetected in coastal waters in 2017, resulting in a longer perceived duration of offshore residence between 2016 and 2018 (shark ID: 101544; Figure 7B). Several sharks demonstrated movement patterns similar to those in group 1, where individuals were detected exclusively in coastal waters for the first 2-3 years after tagging (before the deployment of the offshore arrays), after which time they were detected in the offshore over the remainder of the study period (shark IDs: 101428, 101423, 101315, 101224; Figure 7B). Nearly all sharks in this group were tagged in Scott Inlet, where they were detected within the first 3 d following release (69%, n = 16 individuals; Figure 7B). Pre-detection periods for the remaining individuals ranged from 9-22 d (25%, n = 16), with one exception - a shark tagged in Resolute Bay with a pre-detection period of 1,081 d (~3 y) (shark ID: 101943; Figure 7B).
Discussion
Knowledge of the large-scale movements of Greenland sharks, particularly over long durations, is limited. To date, data have primarily been derived from short-term tracking studies lasting hours to months, providing insight into fine-scale horizontal (Skomal and Benz, 2004) and vertical movement behaviors (Stokesbury et al., 2005; Campana et al., 2015a; Gallant et al., 2016) and evidence of large-scale displacements (Fisk et al., 2012; Campana et al., 2015a; Hussey et al., 2018). The few studies examining the movements of Greenland sharks in offshore waters have used pop-off satellite archival tags (PSATs) that are limited to straight-line trajectories interpolated between the location of the tagged animal’s release and the location of the satellite tag’s first successful transmission to ARGOS satellites (Fisk et al., 2012; Campana et al., 2015a). Reconstruction of coarse-scale movements through attachment and sequential release of multiple mrPAT tags have provided continuous tracks of individual sharks, but these are still limited to months in duration (Hussey et al., 2018). The current study using static acoustic telemetry provides the longest period of continuous monitoring of Greenland shark movements (up to 7 y). To our knowledge, it is the first to identify repeated seasonal transitions among inshore and offshore habitats and to document the interannual recurrence of individual sharks to specific regions of offshore Baffin Bay. While these long-term data identify clear seasonal transitions in the movements of Greenland sharks, the capture of animals as bycatch during summer commercial offshore fisheries operations raises questions over the potential for distinct sub-population movement behaviors based on the season of tagging and/or short offshore excursions during the open water period when traversing between inshore habitats. Derived data will inform spatial planning and potential interaction rates for both community inshore fisheries development and the offshore commercial fishing industry given the vulnerability of this species as a result of its highly K-selected life history parameters.
Coastal-offshore connectivity
There is currently limited understanding of seasonal Greenland shark distribution and population abundance throughout its range. Primary records are largely based on fisheries-dependent methods (e.g., commercial and historical bycatch records, exploratory fisheries surveys and commercial stock assessments for Greenland halibut (R. hippoglossoides) and Northern shrimp (Pandalus borealis)) that are likely biased by fishing gear type, set duration, and the timing of fishing efforts which can affect the relative frequency and abundance of Greenland shark bycatch (Bryk et al., 2018). Nevertheless, telemetry data reported here confirm the presence of Greenland sharks in the inshore regions of Baffin Bay during the ice-free summer period (July to November) reported by fisheries dependent data. For example, inshore multi-species surveys conducted from high latitude coastal sites (Pond Inlet, Nunavut) to lower latitudes (Cumberland Sound) between 2010 and 2017 reported the incidental capture of a median 11 Greenland sharks per year (range = 0-47) over a median of 29 annual sets (range = 5-43) (Bryk et al., 2018). Equally, catch data from exploratory fisheries conducted between July 1st and Nov 10th in deep-water (>500 m) coastal areas near Qikiqtarjuaq (Hathaway, 1993) and Davis Strait, Resolution Island, and Cumberland Sound (Northlands Consulting, 1994) reported high numbers of Greenland sharks caught by a variety of gear types (52 sharks in 11 gillnet sets in 1993) (Treble and Stewart, 2010). Similar trends were shown by longline fisheries and fishery training courses conducted in Cumberland Sound in 2003 (12 sharks caught over 10 longline sets; Walsh, 2003) and 2009 (570 sharks caught in 55 sets; Treble and Stewart, 2010) as well as commercial bycatch records from 1987 to 2006, whereby annual reported captures ranged from 4 to 220 individuals (median = 60) over a median of 601 longline sets (DFO, 2008a). The importance of inshore regions for Greenland shark summer distribution in northern Baffin Bay (NAFO subarea 0) was also proposed following exploratory longline fisheries conducted in late September near Grise Fiord, Qikiqtarjuaq, Arctic Bay, and Resolute (Wheeland and Devine, 2018).
Similar to fisheries catch data, evidence of Greenland shark distribution collected by video surveys and telemetry methodologies have primarily been collected in coastal waters during the ice-free period. Baited Remote Underwater Video (BRUV) surveys identified shark presence in coastal systems during the open-water period with estimated relative abundances ranging from 0.4 (Resolute) to 15.5 (Scott Inlet) sharks/km2 (Devine et al., 2018). Furthermore, the use of multiple pop-off archival satellite tags deployed on individual Greenland sharks identified the use of coastal fjords in the summer, temporary coastal residency, and large-scale migrations among inshore systems in northeast Devon Island (Canada) and northwest Greenland (Hussey et al., 2018), while acoustic telemetry data showed repeated use of an inshore fjord system across years (Edwards et al., 2021). To date, only one telemetry study has followed the movements of tagged Greenland sharks in coastal waters under ice (Skomal and Benz, 2004). The paucity of information on the use of ice-covered coastal waters by Greenland sharks likely reflects logistical challenges associated with conducting fieldwork in Arctic winters, rather than a seasonal absence of Greenland sharks.
While combined data indicate a peak in the presence of Greenland sharks in coastal systems in the summer months relative to the winter period (Idrobo, 2008; Walsh, 2018), the reported catch of Greenland sharks in winter contrasts the lack of winter detections of sharks in coastal regions shown in the current study. Winter fisheries for Greenland halibut in Cumberland Sound (DFO, 2008a) and Scott Inlet (Walsh, 2018) reported catching high numbers of Greenland sharks using deep-water longlines set through the ice. However, a comparison of historical catch records from summer and winter fisheries in Cumberland Sound support a relative increase in the coastal presence of Greenland sharks during the summer months. An open water summer longline fishery conducted in 2009 reported an average catch rate of 6.3 sharks per 1000 hooks (570 total individuals) (Young, 2010; Madigan et al. In press) while only 1.1 sharks per 1000 hooks (ranging between 0.4 and 2.9 sharks/1,000 hooks) were reported as bycatch in the Pangnirtung winter Greenland halibut fishery between 1987 and 2006 (DFO, 2008a; Bryk et al., 2018). Despite reporting relatively stable catches of Greenland halibut throughout the winter fishing season (late January/early February to the end of April/early May), local fishermen also suggested that Greenland shark abundance varies cyclically (Idrobo, 2008). Higher numbers of Greenland are observed early in the season, followed by a no-shark period lasting until the end of March, and terminated by a sudden resurgence lasting until the end of the fishing season (Idrobo, 2008).
Exploratory fishing in the Scott Inlet/Sam Ford fjord system also reported significant Greenland shark bycatch, with a total of 29 Greenland sharks (mean TL = 2.94 ± 0.13 m) caught over 42 longline sets in late May of 2007 (Walsh, 2018). This contradicts the pattern observed for tagged Greenland sharks in Scott Inlet that were detected between July and November (Figure 4). In addition, tagged sharks were absent from all four inshore arrays (ATS, ASI, QIK, ACS) throughout the ice-covered period from December to June (Figure 4). These contrasting findings raise two key questions: (i) If a subpopulation of Greenland sharks displays year-round coastal residency, why was this behavior not exhibited by any of our tagged individuals? Alternatively, (ii) do Greenland sharks exhibit sub-population behavior whereby individuals temporally segregate their use of coastal and offshore habitats? While we are currently unable to directly answer these questions, we propose the following explanations.
The short-term residence displayed by tagged individuals in all four coastal systems, their absence throughout the winter months, and movements between inshore and offshore arrays indicate that Greenland sharks are unlikely to reside permanently (i.e., your-round) in coastal systems. This is further supported by our large sample size, tagging efforts across multiple years, and tagging at several locations that did not reveal residency. Alternatively, these and other telemetry data indicate that Greenland sharks are highly transient, but display some evidence of site fidelity to specific coastal systems (e.g., Scott Inlet; Edwards et al., 2021) and offshore regions. In addition, Greenland sharks alternated visitations to different coastal systems over multiple years, further supporting evidence of their transitory behavior.
A likely explanation for the disparity between coastal bycatch reports and the lack of coastal detections during the winter months is the existence of a subcomponent of the population that is not represented in our tagged population. While sex and size class are known to drive differences in movement behavior and habitat use among sharks (see Supplementary for further detail), we propose the possibility that a behavioral dichotomy exists within the studied Greenland shark population, wherein individuals are segregated into subpopulations that are present in coastal waters exclusively during the summer or winter (Edwards et al., 2021). We might thereby attribute the absence of temporary winter residents in our tagged population to a lack of winter tagging efforts in coastal waters. Focused tagging of Greenland sharks in winter, building on the work of Skomal and Benz (2004), will provide a solution to determine whether sub-population behaviors exist with a component of the population temporarily resident in coastal regions under ice. Targeted tagging of sharks in the offshore would also reveal the nature of population-wide seasonal movements and consistency in observed patterns.
Occurrence and movements in the offshore environment
In ~3 y of monitoring and accepting the limited spatial extent of offshore receivers relative to total area, we detected 55 unique sharks, all tagged and released in coastal systems across a wide range of latitudes. These data revealed consistent patterns in behavior and habitat use among sharks tagged throughout the Eastern Canadian Arctic, highlighting the significance of offshore habitat for this species. While sharks were detected across 38% of offshore stations, notable temporal, and spatial variation in the number of individuals detected throughout the offshore was observed. Interannual variation in the monthly detection rates of sharks reflected changes in the number of arrays present across each study year and the number of sharks available for detection (see Table 1 for array deployment and retrieval dates). As expected, months with the highest recorded shark abundance corresponded to years when multiple offshore arrays were deployed (39 sharks were detected when ABO, DST, and QIK were deployed simultaneously in 2017; Figure 4). During this period of high receiver coverage, tagged sharks were also detected throughout more months of the year relative to periods of time when fewer arrays were present. Similarly, spatial differences in shark abundance were biased by the number of stations deployed in each array, where the arrays with the greatest spatial coverage showed a higher efficiency for detecting tagged individuals (Figure 6).
Nevertheless, when considering the spatial distribution of shark detections, we observed a region of high shark activity towards the southeastern portion of Baffin Bay in the vicinity of a fishery closure, the Disko Fan Conservation Area (DFO, 2008b), during the winter. This area was designated an Ecologically and Biologically Significant Area (EBSA) in 2011, based on its diverse and well established deep-water coral communities, bathymetric and oceanographic complexity, and sea-ice characteristics that permit the overwintering of marine mammals (i.e., persistent leads through winter pack ice) (DFO, 2008b). This habitat hosts several prey species potentially targeted by Greenland sharks, including marine mammals whose seasonal presence in the region overlaps with that of monitored Greenland sharks (Hiltz et al., 2019). Throughout Baffin Bay and the Canadian Arctic, areas of high seasonal diversity and abundance typically occur in locations where favorable environmental and oceanographic conditions lead to spikes in primary productivity, resulting in increased benthic and pelagic biomass (Yurkowski et al., 2018). These trends likely explain the disproportionately high number of Greenland sharks detected in Disko Fan compared to other offshore stations and high repeated visitation rates of individuals across multiple years.
Our results also indicate that a small subset of Greenland sharks exhibited temporarily resident behavior in the Disko Fan region. While mark-recapture and genetics studies have revealed natal and sex-specific reproductive philopatry in a number of shark species (Sims et al., 2001; Tillett et al., 2012; Mourier and Planes, 2013; Feldheim et al., 2014), evidence of individual-level site fidelity (return to a study region) obtained via electronic tagging methods are more limited (Hueter et al., 2005; Jorgensen et al., 2010; Kessel et al., 2014). Previous studies on elasmobranchs have identified site fidelity associated with mating (Pratt and Carrier, 2001; Feldheim et al., 2002), birthing (Kessel et al., 2014), and feeding (Lowe et al., 2006; Espinoza et al., 2011; Driggers et al., 2014). For example, white sharks (Carcharodon carcharias) in the Eastern Pacific and North Atlantic undertake highly predictable movements with individuals showing annual fidelity to specific locations during residency periods (Jorgensen et al., 2010; Franks et al., 2021). Based on our findings, we propose that Greenland sharks use the Disko Fan region to some degree as a winter foraging ground and that individuals likely exhibit similar levels of site fidelity to other high-productivity/biomass marine regions across offshore Baffin Bay, similar to previously reported recurrent summer visitations to a productive coastal fjord system (Edwards et al., 2021). Alternatively, the predictable occurrence of sharks in the southern region of Baffin Bay across years could indicate a north-south seasonal transition during winter. Interannual variability in the return of individuals to specific coastal and offshore regions and the detection of unique individuals by multiple coastal arrays (Figure 7) suggests that Greenland sharks undertake periods of temporary residency in a number of foraging areas. Fidelity tied to mating or birthing is unlikely given the majority of the tagged sharks were immature.
In contrast to southeastern Baffin Bay, tagged sharks were detected by only 4 receiver stations among the 9 gates deployed along ~760 km of the basin’s western continental shelf (ABO and offshore QIK arrays). This outcome was unexpected, given the high numbers of detections recorded in adjacent coastal fjords along the same stretch of coastline. These data indicate that Greenland sharks are either transiting close to shore, are entering coastal systems directly from offshore waters, or that our array design was ineffective at detecting sharks. This behavior contrasts that of Greenland halibut (Reinhardtius hippoglossoides), wherein fish are detected and targeted by commercial fisheries as they move along the shelf edge (DFO, 2013). Previous work has shown distinct behaviors among pelagic sharks, with some preferentially avoiding shallow waters (e.g., common thresher sharks, Alopias vulpinus; Cartamil et al., 2010) while others use shallow waters while moving along the continental shelf (e.g., porbeagle, Lamna nasus, and school shark, Galeorhinus galeus; West and Stevens, 2001; Pade et al., 2009). Given the frequent use of offshore waters and seasonally alternating pattern in coastal and offshore detections observed in the current study, we speculate that Greenland sharks transit through offshore regions when entering coastal fjords (i.e., use of deep-water channels; Edwards et al., 2021), but likely transit either in coastal waters or along banks when moving between distinct coastal fjords along the western border of Baffin Bay. Bycatch of Greenland sharks in offshore commercial fisheries focused on these banks further indicate Greenland shark presence, however, shark numbers relative to other offshore regions have not yet been characterized. Further work is required to understand transitional movements between inshore fjords during the summer period when commercial offshore fisheries are operating.
While a large number of tagged sharks were detected in offshore waters over multiple years, individuals were only detected by a few offshore receiver stations on average and with low annual numbers of detections outside of the southeastern Baffin Bay array (DST and southeastern ABO). This observation suggests that Greenland sharks undertake largely transient movements in those regions when travelling in offshore waters where arrays were present. Fine-scale behaviors exhibited during large-scale displacements of marine fishes are, in general, difficult to study and are therefore not well understood, especially when using static telemetry (Comeau et al., 2002). However, tracking studies have shown large, mobile marine species exhibiting directed movements over vast distances in relatively short periods of time (Domeier and Nasby-Lucas, 2008; Matthews et al., 2011; Hearn et al., 2016). In agreement, previous work has reported Greenland sharks travelling several hundred kilometers through open-water regions over a few months (Campana et al., 2015b; Hussey et al., 2018).
Conclusion
The multi-year movement records examined in this study provide insights on Greenland shark movements at an unprecedent spatio-temporal scale. Three years of detections in the offshore waters of Baffin Bay identify the predominant use of deep, offshore waters during the ice-covered winter period between November and June, with fewer offshore detections recorded in July and August. Tagged sharks exhibited notable site fidelity to specific offshore areas, with contrasting transient movements throughout the remainder of monitored offshore areas. Greenland sharks were also detected in four coastal habitats across a seven-year period; however, these inshore detections were tightly restricted to the ice-free summer months between July and November.
Bycatch records from commercial fisheries, exploratory fisheries surveys and commercial stock assessments, as well as abundance estimates from BRUV surveys and satellite tracking data, substantiate the high numbers of tagged Greenland sharks present in coastal arrays during the summer months. However, tagged sharks were not detected by any of our coastal receiver arrays in the winter months, contrasting Greenland shark bycatch records from winter fisheries in Cumberland Sound (DFO, 2008a) and Scott Inlet (Walsh, 2018), and the mobile (active) acoustic tracking of sharks under ice in the fjords of northern Baffin Island. To explain this trend, we suggest that Greenland sharks may demonstrate a behavioral dichotomy wherein individuals belong to one of two subpopulations that are present in inshore waters exclusively in the winter or summer periods. We thereby attribute a lack of winter tagging efforts in coastal systems to the absence of temporary winter residents in our tagging population and propose further tagging efforts are required.
Seasonality in the inshore-offshore movements of Greenland sharks throughout Baffin Bay likely reduces interaction rates with seasonally restricted commercial fisheries in NAFO Division 0A by minimizing regional spatiotemporal overlap. Potential north-south transitions during winter, however, could expose sharks to offshore commercial fisheries in the Davis Strait (i.e. NAFO Division 0B). Nevertheless, given the likely expansion of Arctic fisheries (Christiansen et al., 2014) and the continued development of community-led coastal fisheries in both summer and winter months (Hussey et al., 2017; Walsh, 2018; Young, 2010), these periods of overlap will extend and the movement behaviors of Greenland sharks may modify with loss of sea ice. Furthermore, the potential existence of subpopulations with divergent seasonal movement patterns suggests that, at the population-level, Greenland sharks inhabiting Baffin Bay are vulnerable to fisheries capture year-round in both coastal and offshore environments.
Data availability statement
The datasets presented in this study can be found in online repositories. The names of the repository/repositories and accession number(s) can be found below: https://members.oceantrack.org/OTN/.
Ethics statement
The animal study was reviewed and approved by Animal Care Committee, University of Windsor (Windsor, ON, Canada).
Author contributions
JE contributed to the data curation, conducted formal analyses and data visualizations, and wrote the original manuscript. KH contributed to the study design, funding and resource acquisition, field investigations, and review of this manuscript. SK contributed to the study design, field investigations, and review of this manuscript. NH contributed to the study design, funding acquisition, process of investigation, acquisition of resources, and review and editing of this manuscript, in addition to providing supervision and acting as the administrator of the project.
Acknowledgments
The authors are extremely grateful for the many supporters of this project whose assistance and funding were essential for the completion of this research. Firstly, we would like to acknowledge the invaluable contributions of the community and HTO members of Kangiqtugaapik (Clyde River), Mittimatalik (Pond Inlet), and Qikiqtarjuaq, Nunavut, and the crew of the fishing vessels Kiviuq 1 and Nuliajuk whose individual efforts and hard work in the field made this study possible. We would like to thank the Government of Nunavut, CanNor, and the Nunavut Fisheries Association who provided essential funding to NH, and KH. We acknowledge additional contributions from the Molson Foundation, ArcticNet, and the Natural Sciences and Engineering Research Council of Canada (NSERC) Discovery grant awarded to NH, as well as the W. Garfield Weston Foundation and NSERC for financial support provided to JE. We are also grateful for equipment and support services provided by the Ocean Tracking Network over the duration of this project and original project support awarded to Aaron Fisk. Finally, the authors would like to acknowledge A. Barkley, S. Popov, and E. Davidson (University of Windsor) who provided invaluable advice and support during data organization and analysis. We also thank the anonymous reviewers for their role in refining this manuscript.
Conflict of interest
The authors declare that the research was conducted in the absence of any commercial or financial relationships that could be construed as a potential conflict of interest.
Publisher’s note
All claims expressed in this article are solely those of the authors and do not necessarily represent those of their affiliated organizations, or those of the publisher, the editors and the reviewers. Any product that may be evaluated in this article, or claim that may be made by its manufacturer, is not guaranteed or endorsed by the publisher.
Supplementary material
The Supplementary Material for this article can be found online at: https://www.frontiersin.org/articles/10.3389/fmars.2022.902854/full#supplementary-material
References
Armstrong C. W., Foley N. S., Tinch R., van den Hove S. (2012). Services from the deep: Steps towards valuation of deep sea goods and services. Ecosyst. Serv. 2, 2–13. doi: 10.1016/j.ecoser.2012.07.001
Barkley A. N., Gollock M., Samoilys M., Llewellyn F., Shivji M., Wetherbee B., et al. (2019). Complex transboundary movements of marine megafauna in the Western Indian ocean. Anim. Conserv. 22 (5), 420–431. doi: 10.1111/acv.12493
Barkley A. N., Hussey N. E., Fisk A. T., Hedges K. J., Treble M. A. (2018). Transient movements of a deep- water flatfish in coastal waters : Implications of inshore-offshore connectivity for fisheries management. Journal of applied ecology 55 (3), 1071–1081. doi: 10.1111/1365-2664.13079
Boyd I. L., Staniland I. J., Martin A. R. (2002). Distribution of foraging by female Antarctic fur seals. Mar. Ecol. Prog. Ser. 242 (Boyd 1998), 285–294. doi: 10.3354/meps242285
Bryk J. L., Hedges K. J., Treble M. A. (2018). Summary of Greenland shark (Somniosus microcephalus) catch in Greenland halibut (Reinhardtius hippoglossoides) fisheries and scientific surveys conducted in NAFO subarea 0.
Campana S. E., Fisk A. T., Peter Klimley A. (2015a). Movements of Arctic and northwest Atlantic Greenland sharks (Somniosus microcephalus) monitored with archival satellite pop-up tags suggest long-range migrations. Deep-Sea Res. Part II: Topical Stud. Oceanogr. 115, 109–115. doi: 10.1016/j.dsr2.2013.11.001
Campana S. E., Fisk A. T., Peter Klimley A. (2015b). Movements of Arctic and northwest Atlantic Greenland sharks (Somniosus microcephalus) monitored with archival satellite pop-up tags suggest long-range migrations. Deep-Sea Res. Part II: Topical Stud. Oceanogr. 115, 109–115. doi: 10.1016/j.dsr2.2013.11.001
Cartamil D., Wegner N. C., Aalbers S., Sepulveda C. A., Baquero A., Graham J. B. (2010). Diel movement patterns and habitat preferences of the common thresher shark (Alopias vulpinus) in the southern California bight. Mar. Freshw. Res. 61, 596–604. doi: 10.1071/MF09153
Christiansen J. S., Mecklenburg C. W., Karamushko O. V. (2014). Arctic marine fishes and their fisheries in light of global change. Global change biology 20 (2), 352–359.
Comeau L. A., Campana S. E., Castonguay M. (2002). Automated monitoring of a large-scale cod (Gadus morhua) migration in the open sea. Can. J. Fish. Aquat. Sci. 59, 1845–1850. doi: 10.1139/f02-152
Davis B., VanderZwaag D. L., Cosandey-Godin A., Hussey N. E., Kessel S. T., Worm B. (2013). The conservation of the Greenland shark (Somniosus microcephalus): Setting scientific, law, and policy coordinates for avoiding a species at risk. J. Int. Wildlife Law Policy 16 (4), 300–330. doi: 10.1080/13880292.2013.805073
Devine B. M., Wheeland L. J., Fisher J. A. D. (2018). First estimates of Greenland shark (Somniosus microcephalus) local abundances in Arctic waters. Sci. Rep. 8 (1), 1–10. doi: 10.1038/s41598-017-19115-x
DFO (2008a). Cumberland Sound Greenland halibut (Turbot) inshore fishery. Can. Sci. Advisory Sec. Sci. Advisory Rep. 40 (August), 1–20.
DFO (2008b). Development of a closed area in NAFO 0A to protect narwhal over-wintering grounds, including deep-sea corals. CSAS Sci. Response 2007/002 (July 2007), 16.
DFO (2013). Integrated fisheries management plan: Greenland halibut (Reinhardtius hippoglossoides) Northwest Atlantic fisheries organization subarea 0, effective 2013. Fish. Oceans Canada Cent. Arctic Region, 111.
Domeier M. L., Nasby-Lucas N. (2008). Migration patterns of white sharks carcharodon carcharias tagged at Guadalupe island, Mexico, and identification of an eastern pacific shared offshore foraging area. Mar. Ecol. Prog. Ser. 370, 221–237. doi: 10.3354/meps07628
Driggers W. B., Frazier B. S., Adams D. H., Ulrich G. F., Jones C. M., Hoffmayer E. R., et al. (2014). Site fidelity of migratory bonnethead sharks sphyrna tiburo (L. 1758) to specific estuaries in south Carolina, USA. J. Exp. Mar. Biol. Ecol. 459, 61–69. doi: 10.1016/j.jembe.2014.05.006
Dueck L. P., Hiede-Jørgensen M. P., Jensen M. V., Postma L. D. (2006). Update on investigations of bowhead whale (Balaena mysticetus) movements in the eastern arcti- 2005, based on satellite-linked telemetry. Can. Sci. Advisory Sec. Res. Document, 57.
Edwards J. E., Hedges K. J., Hussey N. E. (2021). Seasonal residency, activity space, and use of deep-water channels by Greenland sharks (Somniosus microcephalus) in an Arctic fjord system. Can. J. Fish. Aquat. Sci 79 (2), 314–330. doi: 10.1139/cjfas-2021-0009
Edwards J. E., Hiltz E., Broell F., Bushnell P. G., Campana S. E., Christiansen J. S., et al. (2019). Advancing research for the management of long-lived species: A case study on the Greenland shark. Front. Mar. Sci. 6, 87. doi: 10.3389/fmars.2019.00087
Espinoza M., Farrugia T. J., Lowe C. G. (2011). Habitat use, movements and site fidelity of the gray smooth-hound shark (Mustelus californicus gill 1863) in a newly restored southern California estuary. J. Exp. Mar. Biol. Ecol. 401 (1–2), 63–74. doi: 10.1016/j.jembe.2011.03.001
ESRI. (2019). ArcMAP Release 10.2.2). Environmental Systems Research Institute. (Redlands, CA: Esri Inc).
Feldheim K. A., Gruber S. H., Ashley M. V. (2002). The breeding biology of lemon sharks at a tropical nursery lagoon. Proc. R. Soc. B: Biol. Sci. 269 (1501), 1655–1661. doi: 10.1098/rspb.2002.2051
Feldheim K. A., Gruber S. H., Dibattista J. D., Babcock E. A., Kessel S. T., Hendry A. P., et al. (2014). Two decades of genetic profiling yields first evidence of natal philopatry and long-term fidelity to parturition sites in sharks. Mol. Ecol. 23 (1), 110–117. doi: 10.1111/mec.12583
Fisk A. T., Lydersen C., Kovacs K. M. (2012). Archival pop-off tag tracking of Greenland sharks somniosus microcephalus in the high Arctic waters of Svalbard, Norway. Mar. Ecol. Prog. Ser. 468 (September 2017), 255–265. doi: 10.3354/meps09962
Franks B. R., Tyminski J. P., Hussey N. E., Braun C. D., Newton A. L., Thorrold S. R., et al. (2021). Spatio-temporal variability in white shark (Carcharodon carcharias) movement ecology during residency and migration phases in the western north Atlantic. Front. Mar. Sci. 8, 744202. doi: 10.3389/fmars.2021.744202
Gallant J. J., Rodriguez M. A., Stokesbury M. J. W., Harvey-Clark C. (2016). Influence of environmental variables on the diel movements of the Greenland shark (Somniosus microcephalus) in the st. Lawrence estuary. Can. Field-Naturalist 130 (1), 1. doi: 10.22621/cfn.v130i1.1784
Gradinger R. (1995). Climate change and biological oceanography of the Arctic ocean. Philos. Trans. - R. Soc. London A 352 (1699), 277–286. doi: 10.1098/rsta.1995.0070
Hansen P. M. (1963). Tagging experiments with the Greenland shark (Somniosus microcephalus (Block and schneider)) in subarea 1. In: International Commission of Northwest Atlantic Fisheries Special Publication 4, North Atlantic Fish Marking Symposium. 172–175.
Hathaway B. L. (1993). Exploratory fishing in Davis Strait/Broughton island area (Summer 1993). Unpublished report to Baffin Region Sub-Committee for Fisheries. 29.
Hearn A. R., Green J., Román M. H., Acuña-Marrero D., Espinoza E., Klimley A. P. (2016). Adult female whale sharks make long-distance movements past Darwin island (Galapagos, Ecuador) in the Eastern tropical pacific. Mar. Biol. 163 (10), 214. doi: 10.1007/s00227-016-2991-y
Heide-jørgensen M. P., Burt L. M., Hansen R. G., Nielsen N. H., Rasmussen M., Fossette S., et al. (2013). The significance of the north water polynya to Arctic top predators. AMBIO. 42, 596–610. doi: 10.1007/s13280-012-0357-3
Heupel M. R., Simpfendorfer C. A., Espinoza M., Smoothey A. F., Tobin A., Peddemors V. (2015). Conservation challenges of sharks with continental scale migrations. Front. Mar. Sci. 2 (FEB). doi: 10.3389/fmars.2015.00012
Hiltz E., Fuller S. D., Mitchell J. (2019). Disko fan conservation Area : A Canadian case study. PARKS 24 (June 2018), 1–17. doi: 10.2305/IUCN.CH.2018.PARKS-24-SIEH.en
Holbrook C., Hayden T., Binder T., Pye J. (2020) Glatos: A package for the great lakes acoustic telemetry observation system. r package version 0.4.1. Available at: https://gitlab.oceantrack.org/GreatLakes/glatos.
Hueter R. E., Heupel M. R., Heist E. J., Keeney D. B. (2005). Evidence of philopatry in sharks. and implications for the management of shark fisheries. J. Northwest Atlantic Fish. Sci. 35, 239–247. doi: 10.2960/j.v35.m493
Hussey N. E., Hedges K. J., Barkley A. N., Treble M. A., Peklova I., Webber D. M., et al. (2017). Movements of a deep-water fish: Establishing marine fisheries management boundaries in coastal Arctic waters. Ecol. Appl. 27 (3), 687–704. doi: 10.1002/eap.1485
Hussey N. E., Macneil M. A., Mcmeans B. C., Olin J. A., Dudley S. F. J., Cliff G., et al. (2014). Rescaling the trophic structure of marine food webs. Ecol. Lett. 17 (2), 239–250. doi: 10.1111/ele.12226
Hussey N. E., Orr J., Fisk A. T., Hedges K. J., Ferguson S. H., Barkley A. N. (2018). Mark report satellite tags (mrPATs) to detail large-scale horizontal movements of deep water species: First results for the Greenland shark (Somniosus microcephalus). Deep-Sea Res. Part I: Oceanogr. Res. Pap. 134 (March), 32–40. doi: 10.1016/j.dsr.2018.03.002
Idrobo C. J. (2008). The pangnirtung inuit and the greenland shark . Masters Thesis (Canada: University of Manitoba). Available at: http://www.umanitoba.ca/institutes/natural_resources/canadaresearchchair/thesis/Idrobo.Masters%20Thesis.Feb%2009.pdf
Idrobo C. J., Berkes F. (2012). Pangnirtung Inuit and the Greenland shark: Co-producing knowledge of a little discussed species. Hum. Ecol. 40 (3), 405–414. doi: 10.1007/s10745-012-9490-7
Jorgensen S. J., Reeb C. A., Chapple T. K., Anderson S., Perle C., Van Sommeran S. R., et al. (2010). Philopatry and migration of pacific white sharks. Proc. R. Soc. B: Biol. Sci. 277 (1682), 679–688. doi: 10.1098/rspb.2009.1155
Kessel S. T., Chapman D. D., Franks B. R., Gedamke T., Gruber S. H., Newman J. M., et al. (2014). Predictable temperature-regulated residency, movement and migration in a large, highly mobile marine predator (Negaprion brevirostris). Mar. Ecol. Prog. Ser. 514, 175–190. doi: 10.3354/meps10966
Laidre K. L., Heide-Jørgensen M. P., Logdson M. L., Hobbs R. C., Heagerty P., Dietz R., et al. (2004). Seasonal narwhal habitat associations in the high Arctic. Mar. Biol. 145 (4), 821–831. doi: 10.1007/s00227-004-1371-1
Lascelles B., Notarbartolo G., Sciara D., Agardy T., Cuttelod A., Eckert S., et al. (2014). Migratory marine species: Their status, threats and conservation management needs. Aquatic Conservation: Marine and Freshwater Ecosystems 24, 111–127. doi: 10.1002/aqc.2512
Lowe C. G., Wetherbee B. M., Meyer C. G. (2006). Using acoustic telemetry monitoring techniques to quantity movement patterns and site fidelity of sharks and giant trevally around French frigate shoals and midway atoll. Atoll Res. Bull. 543, 281–303.
MacNeil M. A., McMeans B. C., Hussey N. E., Vecsei P., Svavarsson J., Kovacs K. M., et al. (2012). Biology of the Greenland shark somniosus microcephalus. J. Fish Biol. 80 (5), 991–1018. doi: 10.1111/j.1095-8649.2012.03257.x
Marcoux M., Ferguson S. H., Roy N., Bedard J. M., Simard Y. (2017). Seasonal marine mammal occurrence detected from passive acoustic monitoring in Scott inlet, Nunavut, Canada. Polar Biol. 40 (5), 1127–1138. doi: 10.1007/s00300-016-2040-9
Matthews C. J. D., Luque S. P., Petersen S. D., Andrews R. D., Ferguson S. H. (2011). Satellite tracking of a killer whale (Orcinus orca) in the eastern Canadian Arctic documents ice avoidance and rapid, long-distance movement into the north Atlantic. Polar Biol. 34, 1091–1096. doi: 10.1007/s00300-010-0958-x
McCann K. S., Rasmussen J. B., Umbanhowar J. (2005). The dynamics of spatially coupled food webs. Ecol. Lett. 8 (5), 513–523. doi: 10.1111/j.1461-0248.2005.00742.x
McMeans B. C., Arts M. T., Lydersen C., Kovacs K. M., Hop H., Falk-Petersen S., et al. (2013). The role of Greenland sharks (Somniosus microcephalus) in an Arctic ecosystem: Assessed via stable isotopes and fatty acids. Mar. Biol. 160 (5), 1223–1238. doi: 10.1007/s00227-013-2174-z
Melling H., Gratton Y., Ingram G. (2001). Ocean circulation within the north water polynya of Baffin bay ocean circulation within the north water polynya of Baffin bay. Atmosphere-Ocean. 39 (3), 301–325. doi: 10.1080/07055900.2001.9649683
Mourier J., Planes S. (2013). Direct genetic evidence for reproductive philopatry and associated fine-scale migrations in female blacktip reef sharks (Carcharhinus melanopterus) in French Polynesia. Mol. Ecol. 22 (1), 201–214. doi: 10.1111/mec.12103
Münchow A., Falkner K. K., Melling H. (2015). Baffin island and West Greenland current systems in northern Baffin bay Progress in oceanography 132, 305–317. doi: 10.1016/j.pocean.2014.04.001
Nielsen J., Hedeholm R. B., Heinemeier J., Bushnell P. G., Christiansen J. S., Olsen J., et al. (2016). Eye lens radiocarbon reveals centuries of longevity in the Greenland shark (Somniosus microcephalus). Science 353 (6300), 702–704. doi: 10.1126/science.aaf1703
Northlands Consulting (1994). Exploratory Fishery: A report on exploratory fishing 10 for shrimp and groundfish in Cumberland Sound, Davis Strait and Resolution 11 Island survey areas. Unpublished report to Baffin Region Sub-Committee for Fisheries. 46.
O’Neill R. V., Milne B. T., Turner M. G., Gardner R. H. (1988). Resource utilization scales and landscape pattern. Landscape Ecol. 2 (1), 63–69. doi: 10.1007/BF00138908
Pade N. G., Queiroz N., Humphries N. E., Witt M. J., Jones C. S., Noble L. R., et al. (2009). First results from satellite-linked archival tagging of porbeagle shark, lamna nasus: Area fidelity, wider-scale movements and plasticity in diel depth changes. J. Exp. Mar. Biol. Ecol. 370 (1–2), 64–74. doi: 10.1016/j.jembe.2008.12.002
Pratt H. L., Carrier J. C. (2001). A review of elasmobranch reproductive behavior with a case study on the nurse shark, ginglymostoma cirratum. Environ. Biol. Fishes 60 (1–3), 157–188. doi: 10.1023/A:1007656126281
Premke K., Muyakshin S., Klages M., Wegner J. (2003). Evidence for long-range chemoreceptive tracking of food odour in deep-sea scavengers by scanning sonar data. J. Exp. Mar. Biol. Ecol 285, 283–294. doi: 10.1016/S0022-0981(02)00533-6
R Core Team (2019). R: A language and environment for statistical computing (Vienna, Austria: R Foundation for Statistical Computing). Available at: http://www.R-project.org/.
Shuert C., Marcoux M., Hussey N. E., Heidi-Jorgensen M. P., Auger-Methe M. (2022). Keeping pace with climate change? narwhal migration phenology shifts over the last three decades. Proc. Natl. Acad. Sci. United States America In revision.
Sims D., Nash J., Morritt D. (2001). Movements and activity of male and female dogfish in a tidal sea lough: Alternative behavioral strategies and apparent sexual segregation. Mar. Biol. 139, 1165–1175. doi: 10.1007/s002270100666
Sims D. W., Southall E. J., Richardson A. J., Reid P. C., Metcalfe J. D. (2003). Seasonal movements and behavior of basking sharks from archival tagging: No evidence of winter hibernation. Mar. Ecol. Prog. Ser. 248 (Priede 1984), 187–196. doi: 10.3354/meps248187
Skomal G. B., Benz G. W. (2004). Ultrasonic tracking of Greenland sharks, somniosus microcephalus, under Arctic ice. Mar. Biol. 145 (3), 489–498. doi: 10.1007/s00227-004-1332-8
Ste-Marie E., Watanabe Y. Y., Semmens J. M., Marcoux M., Hussey N. E. (2020). A first look at the metabolic rate of Greenland sharks (Somniosus microcephalus) in the Canadian Arctic. Scientific reports 10 (1), 1–8. doi: 10.1038/s41598-020-76371-0.
Stokesbury M. J.W., Harvey-Clark C., Gallant J., et al. (2005). Movement and environmental preferences of Greenland sharks (Somniosus microcephalus) electronically tagged in the St. Lawrence Estuary, Canada. Marine Bioly. 148, 159–165. doi: 10.1007/s00227-005-0061-y
Tang C. C. L., Ross C. K., Yao T., Petrie B., Detracey B. M., Dunlap E. (2004). The circulation, water masses and sea-ice of Baffin bay. Prog. Oceanogr. 63, 183–228. doi: 10.1016/j.pocean.2004.09.005
Tillett B. J., Meekan M. G., Field I. C., Thorburn D. C., Ovenden J. R. (2012). Evidence for reproductive philopatry in the bull shark carcharhinus leucas. J. Fish Biol. 80 (6), 2140–2158. doi: 10.1111/j.1095-8649.2012.03228.x
Treble M. A., Stewart R. E. A. (2010). Impacts and risks associated with a Greenland halibut (Reinhardtius hippoglossoides) gillnet fishery in inshore areas of NAFO subarea 0. In Canadian Science Advisory Secretatiat Research Document. Ottawa: Canadian Science Advisory Secretariat. 032. , 1–18.
Tremblay J. É., Robert D., Varela D. E., Lovejoy C., Darnis G., Nelson R. J., et al. (2012). Current state and trends in Canadian Arctic marine ecosystems: I. primary production. Climatic Change. doi: 10.1007/s10584-012-0496-3
Walsh P. J. (2003). Cumberland Sound resource assessment and fisheries training project (Memorial University, Kabva Marine Services and Pangnirtung Hunters and Trappers Organization: Centre for Sustainable Aquatic Resources), 25.
Walsh P. (2018). Winter longline fishing in Scott inlet/Sam fjord, Baffin island. technical report (Fisheries and Marine Institute of Memorial University of Newfoundland & Clyde River Hunters and Trappers Association.: Centre for Sustainable Aquatic Resources). doi: 10.13140/RG.2.2.26815.76966
Watanabe Y. Y., Lydersen C., Fisk A. T., Kovacs K. M. (2012). The slowest fish: Swim speed and tail-beat frequency of Greenland sharks. J. Exp. Mar. Biol. Ecol. 426, 5–11. doi: 10.1016/j.jembe.2012.04.021
West G. J., Stevens J. D. (2001). Archival tagging of school shark, galeorhinus galeus, in Australia: Initial results. In The behavior and sensory biology of elasmobranch fishes: an anthology in memory of Donald Richard Nelson (Dordrecht: Springer) 283–298. doi: 10.1023/A:1007697816642
Wheeland L., Devine B. (2018). Bycatch of Greenland shark (Somniosus microcephalus) from inshore exploratory fisheries adjacent to NAFO Division 0. 18, 044.
Wickham, et al. (2019). Welcome to the tidyverse. J. Open Source Software 4 (43), 1686. doi: 10.21105/joss.01686
Williams J. J., Papastamatiou Y. P., Caselle J. E., Bradley D., Jacoby D. M. P. (2018). Mobile marine predators: An understudied source of nutrients to coral reefs in an unfished atoll. Proc. R. Soc. B: Biol. Sci. 285 (1875), 20172456. doi: 10.1098/rspb.2017.2456
Young A. (2010). Development of the Cumberland sound inshore summer fishery (Department of Environment Fisheries and Sealing Division, Iqaluit: Government of Nunavut), 102.
Yurkowski D. J., Lunn N. J., Méthé M. A., Hussey N. E., Mallory M. L., Wong S. N. P., et al. (2018). Abundance and species diversity hotspots of tracked marine predators across the north American Arctic. Diversity Distributions 25 (3), 328–345. doi: 10.1111/ddi.12860
Keywords: acoustic telemetry, arctic marine ecosystem, movement ecology, seasonality, distribution, Somniosus microcephalus
Citation: Edwards JE, Hedges KJ, Kessel ST and Hussey NE (2022) Multi-year acoustic tracking reveals transient movements, recurring hotspots, and apparent seasonality in the coastal-offshore presence of Greenland sharks (Somniosus microcephalus). Front. Mar. Sci. 9:902854. doi: 10.3389/fmars.2022.902854
Received: 23 March 2022; Accepted: 02 September 2022;
Published: 21 September 2022.
Edited by:
Rowan Trebilco, Centre for Marine Socioecology, AustraliaReviewed by:
Ruth H. Carmichael, Dauphin Island Sea Lab, United StatesChristopher Mull, Dalhousie University, Canada
Copyright © 2022 Edwards, Hedges, Kessel and Hussey. This is an open-access article distributed under the terms of the Creative Commons Attribution License (CC BY). The use, distribution or reproduction in other forums is permitted, provided the original author(s) and the copyright owner(s) are credited and that the original publication in this journal is cited, in accordance with accepted academic practice. No use, distribution or reproduction is permitted which does not comply with these terms.
*Correspondence: Jena E. Edwards, ZWR3YXIxMW5AdXdpbmRzb3IuY2E=; ZWR3YXJkc2o2N0BnbWFpbC5jb20=