- 1Institute of Marine Sciences – Okeanos and Institute of Marine Research - IMAR, University of the Azores, Horta, Portugal
- 2Faculty of Life and Environmental Sciences, University of Iceland, Reykjavik, Iceland
Disturbance from whale watching can induce a wide range of behavioral responses in cetaceans, some of which can affect their energetic balance and, ultimately, their long-term fitness. However, assessing disturbance effects on deep-diving cetaceans remains challenging, as the majority of their activities occurs underwater and are difficult to monitor from surface observations. To surpass this limitation, we attached high-resolution multi-sensor tags (DTAGs) on 24 sperm whales (Physeter macrocephalus) off the Azores, an area of intense whale watching, to evaluate changes in movement and acoustic parameters related to foraging effort and dives, locomotion effort, and non-foraging behavior. These parameters were compared between periods of exposure and non-exposure to whale watching vessels, using linear mixed-effect models to assess the presence of short-term effects, accounting for individual differences in behavior. We found no significant changes in foraging effort (number of buzzes, bottom phase depth, proportion of search and foraging phases) and overall dive behavior (dive duration, proportion of dive phases, and descending or ascending pitch) between non-exposure and exposure periods. In contrast, variation in body pitch during non-foraging periods increased during exposure but not variation in body roll. Taken together, these results suggest increased movements during resting activity, which may carry energetic costs to whales and impact their health. Vertical velocity during ascents was also significantly higher in response to vessel exposure, suggesting that sperm whales increased the frequency of fluke strokes, which was consistent with a higher Overall Dynamic Body Acceleration (ODBA) during ascents, incurring in higher energy expenditure. If the detected changes are repeated across multiple exposures, they might affect the individual’s energetic budget, and consequently their fitness. Our study contributes to a better understanding of the short-term behavioral responses of sperm whales to whale watching vessels and highlights the need for further studies examining the potential consequences from repeated disturbance to individuals.
Introduction
Effects of non-lethal disturbance from anthropogenic activities are increasingly recognized as a severe threat to marine wildlife, especially due to their potential for repercussions at the population level (Lima, 1998; Hazel et al., 2007; Christiansen et al., 2014; Avila et al., 2018; Pirotta et al., 2018; Montero-Quintana et al., 2020). Human-related disturbance stimuli (sensu Frid and Dill, 2002) may elicit costly avoidance behaviors (Kvadsheim et al., 2017; Onoufriou et al., 2021), reduce time for, or disrupt, critical activities such as foraging, resting, nursing or socializing (Visser et al., 2011; Christiansen et al., 2013; Wisniewska et al., 2018; Sprogis et al., 2020; Arranz et al., 2021), increase stress levels (Rolland et al., 2012), or induce temporary hearing loss (Finneran, 2015). The effects of such behavioral and physiological responses may accumulate with repeated exposure to disturbance, impacting the health and vital rates (survival, reproductive success) of individuals and, ultimately, their fitness (New et al., 2014; Pirotta et al., 2018; Wilson et al., 2020a). Depending on the proportion of individuals affected in the population, changes in individual fitness can scale up to influence population dynamics (Pirotta et al., 2018).
Despite being often hailed as a “green” or “sustainable” touristic activity, whale watching may disturb marine mammals through increased underwater noise and the physical presence of vessels near individuals (Bearzi, 2017). The presence of whale watching vessels often induce changes to cetacean behavioral budgets, with social, energetic and physiological consequences (Senigaglia et al., 2016). The observed behavior responses of cetaceans to whale watching vessels include alteration of blow rates, increased surface swim speed and frequency of aerial displays, and decreased dive durations, nursing and resting periods (Avila et al., 2015; Senigaglia et al., 2016; Schuler et al., 2019; Arranz et al., 2021; Currie et al., 2021). Studies have also reported disruption of foraging (Christiansen et al., 2013), shifts in acoustic behavior (Heiler et al., 2016; Burnham et al., 2021), and reduction of communication ranges (Erbe, 2002; Jensen et al., 2009). Although more difficult to document, an increasing body of evidence suggests long-term detrimental effects of whale watching activities on some cetacean populations, ranging from displacement to population decline (Bejder et al., 2006; Lusseau et al., 2006a; Bain et al., 2014; Christiansen and Lusseau, 2014). Thus, in order to implement management measures to ensure the sustainability of whale watching activity, it is essential to assess both short- and long-term effects on cetaceans (Lusseau and Bejder, 2007; Bearzi, 2017). This is even more critical, as these effects may interact and add up with effects from other anthropogenic stressors (e.g., global warming, marine debris and ocean noise) (Pirotta et al., 2022).
The sperm whale (Physeter macrocephalus) is classified as a vulnerable species according to the International Union for Conservation of Nature’s Red List of Threatened Species (Taylor et al., 2019). At lower latitudes, female and juvenile sperm whales live in social groups and spend about 70% of their time in foraging behavior (Amano and Yoshioka, 2003; Watwood et al., 2006). After each foraging dive, they remain about 8-10 minutes at the surface to recover and replenish their oxygen stores for the next foraging dive (Watwood et al., 2006). The remaining time is allocated to nursing, breeding, social interactions, resting and sleeping, often taking place at or near the surface (Whitehead, 2003; Miller et al., 2008). Sperm whales produce two types of echolocation sounds during foraging dives, thought to be involved in navigation and prey detection (usual clicks), and in prey capture attempts (buzzes) (Madsen et al., 2002b; Miller et al., 2004a).
In the presence of vessels, sperm whales often change behavior, either while foraging or when engaged in near-surface behaviors, which may be indirectly detrimental to their fitness, survival and reproductive success. For example, sperm whales produced less clicks while ships were approaching and after their passage compared to control periods (Azzara et al., 2013). During interactions with whale watching vessels, sperm whales changed their blow interval, surface period duration, heading and speed while at the surface, and number of aerial displays (Gordon et al., 1992; Magalhães et al., 2002; Richter et al., 2003). In addition, the presence of whale watching vessels increased the probability of sperm whales performing shallow dives (Cosentino, 2016). All these studies were based on surface observations or acoustic data collected from bottom moored hydrophones, and could not quantify the energetic costs of surface and near-surface responses of individual whales, or how such responses affected biologically important activities that occur underwater, such as feeding. Using hand-held hydrophones and towed arrays Isojunno et al. (2011) tracked individual whales through several dives before, during and after exposure to whale watching vessels. While there was no evidence that whale watching vessels significantly changed sperm whale foraging effort and feeding success, these authors stress that their results may have been affected by a small sample size (Isojunno et al., 2011). Hence, it remains unclear if and to what extent sperm whale responses to whale watching vessels impact their energy budget.
The aim of this study was to investigate if the presence of whale watching vessels affects sperm whale foraging effort and foraging dive behavior, locomotion activity, and non-foraging behavior. Whale watching vessels may indirectly affect dive and foraging effort by interfering with the individual’s recovery and oxygen replenishment from the previous dive, resulting in shorter or shallower dives, and/or in less time spent searching and foraging at the bottom phase, and ultimately reducing the number of prey capture attempts. Whale watching vessels may also elicit avoidance responses by sperm whales, leading to steeper or faster dive descents and increased locomotion activity (as measured by the overall dynamic body acceleration, ODBA), with potential implications for the remaining dive phases. As we expected short-term responses to whale watching vessels to be stronger immediately after the exposure, changes in parameters linked to foraging effort, dive behavior, and locomotion activity were investigated by comparing the first dive following exposure with non-exposure periods. Finally, we hypothesized that presence of whale watching vessels would interfere with the near-surface activities of sperm whales and elicit avoidance behavior, through increased movement and number of shallow dives. To address these hypotheses, we instrumented sperm whales with multi-sensor digital acoustic recording tags [DTAGs; Johnson and Tyack (2003)] in the Azores archipelago, where there is a seasonal whale watching activity targeting sperm whales, as one of the most important species (Oliveira et al., 2007).
Materials and methods
Field data collection
Fieldwork was conducted off the Azores archipelago (38.5°N, 28.5°W), between July 2017 and June 2019, where a regulated whale watching industry exists (Regional Law Decrees 10/2003/A and 13/2004/A and Ordinances 70/2005 and 17/2007). Within the study area, there are 10 whale watching companies that operate 23 vessels, all engine driven. The activity is seasonal (March to October) but during the summer peak each vessel can carry out 2-3 trips a day. Prior to the fieldwork season, whale watching companies working in the study area were informed about the project goals and protocol and they were asked to collaborate both on land and at sea.
Sperm whales were located by land-based spotters working for the whale watching operators or by hand-held directional hydrophone in the research boat. The intention was to work within a whale watching area and to tag individuals before the arrival of whale watching vessels. However, that was not always possible and, on several occasions, tagging occurred when whale watching vessels were already in the area, although we only approached whales for tagging when vessels were at >800 m from the target whale. Tagging was performed from either a 9 m or a 5 m rigid-hulled inflatable boat (RHIB), using a 11 m cantilevered pole or a 6 m hand pole. Audio and movement recording tags [DTAG-3 or DTAG-4; Johnson and Tyack (2003)] were attached to the whales using suction cups and programmed to release after 24 hours. At the surface, whales showed no reaction or only a mild behavioral response to tagging (e.g., typically a dorsal flex and occasional defecation with or without fluking). Size class of tagged whales was visually estimated by the research boat crew.
Whale watching vessels were informed about the tagging event over VHF radio, but there was no control on their approach to the animals, nor did we interfere in their maneuvers while observing the tagged whales. Although our aim was to let vessels behave “naturally”, we cannot assess if the way they operated was representative of their usual behavior towards sperm whales. Whenever possible, the number and distance of whale watching vessels to tagged individuals was also estimated by the research boat. However, as tagged whales were tracked (visually or using the VHF beacon on the tag) from a distance (i.e., >500 m, which corresponds to the limit of the observation area defined by the regional whale watching regulations), to avoid interfering with whale’s behavior (Isojunno and Miller, 2015), distances were only roughly estimated.
Data processing
The DTAG-3 recorded two channels of acoustic data at a sampling rate of 120 kHz, and sampled pressure, temperature, and tri-axial accelerometers and magnetometers at 20 Hz or 25 Hz. DTAG-4 recorded one acoustic channel at 192 kHz and sampled all other data at 25 Hz. DTAG data were calibrated and analyzed in Matlab 2007b, 2016b (Mathworks ©) with custom tools developed specifically for these data-loggers (https://www.soundtags.org/dtags/dtag-toolbox/). Whale orientation was determined from the accelerometer and magnetometer data, and whale depth from pressure and temperature, using established methods (Johnson and Tyack, 2003). An audit tool was used to analyze the audio files to identify and mark the periods with clicks associated with foraging, i.e., long-range echolocation search clicks (hereafter “usual clicks”), and foraging buzzes, i.e., short-range fast echolocation click trains which likely indicate prey capture attempts (Miller et al., 2004a). Clicks were assigned to the tagged or other non-tagged whales based on their angle-of-arrival [only for DTAGs-3; (Johnson et al., 2006)], their received levels and the depth of the tagged individual (for all DTAGs). The audit tool was also used to identify vessel noise of the research boat and whale watching vessels in the tag data.
Foraging dives were defined as dives with usual click and buzz production (Isojunno and Miller, 2015) and were divided into descent, bottom, ascent and surface phases [Figure 1; Miller et al. (2004b); Watwood et al. (2006)]. The descent phase refers to the period from the surface until the pitch of the whale exceeded 0 degrees. The ascent phase started when the pitch was consistently greater than 0 degrees until surfacing (Miller et al., 2004b). The bottom phase was the period between descent and ascent phases, and the surface phase was the interval between consecutive foraging dives (Watwood et al., 2006). To distinguish surfacing (i.e., oxygen replenishment and physiological recovery at the surface; Isojunno et al., 2016) from longer periods spent at and near the surface (hereafter termed “non-foraging”) we used an upper threshold of 18.2-minute duration, which corresponded to the 97.5th percentile of the durations between consecutive foraging dives (Supplementary Figure 1). Non-foraging periods likely include socializing and resting but may also include travelling. This category also contained periods of vertical resting and sleep underwater, when whales vertically drifted with their heads up or down (Miller et al., 2008). Finally, the search phase was defined as the period between the first and last usual clicks within a dive and the foraging phase as the period between the first and last buzzes (Watwood et al., 2006).
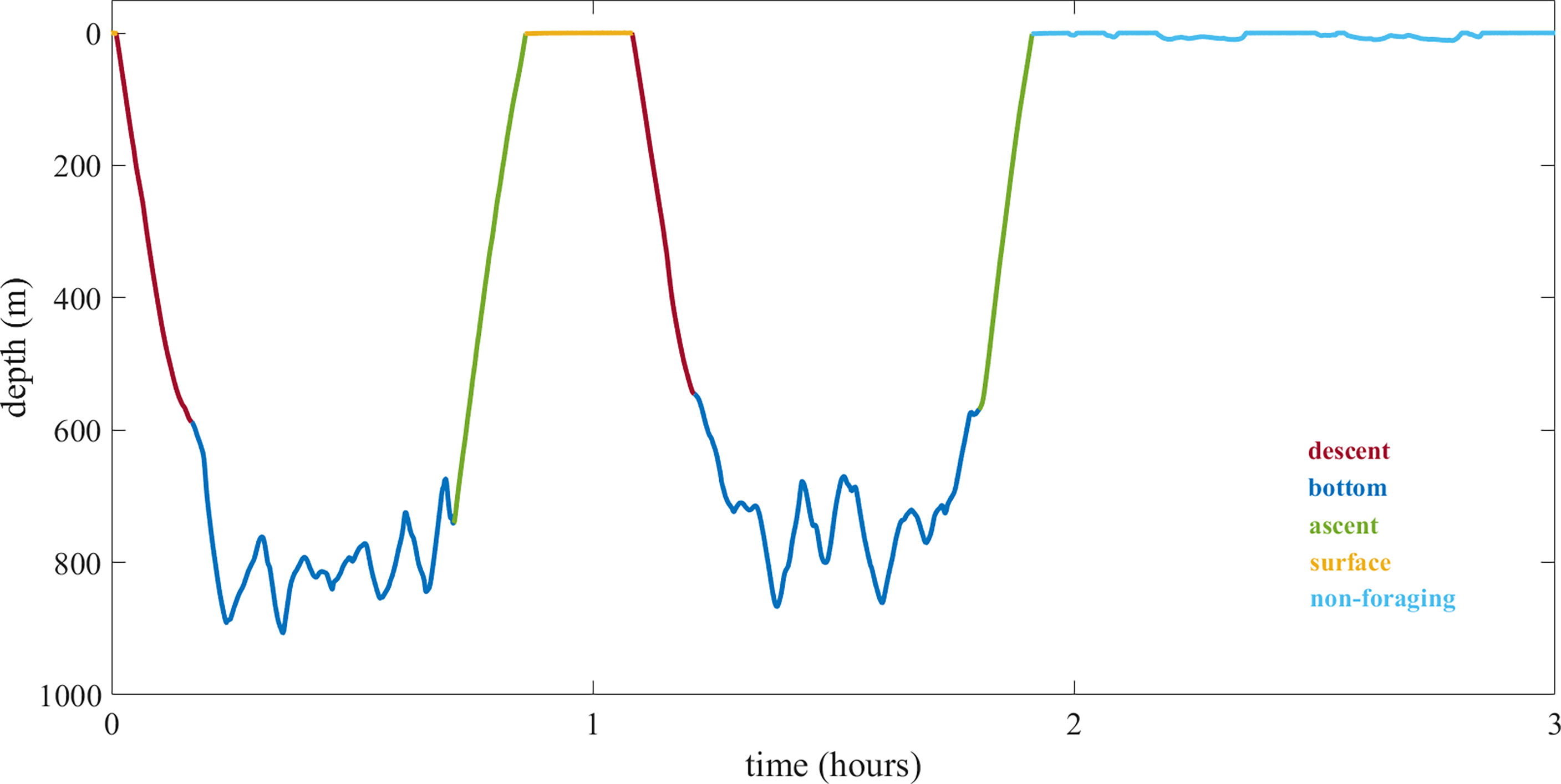
Figure 1 Example of foraging dive phases and a non-foraging period: descent (dark red), bottom (dark blue), ascent (green) and surface (orange) phases, and a non-foraging period (light blue).
Data collected during night time (calculated from the sunrise and sunset times NOAA Solar Calculator; https://www.esrl.noaa.gov/gmd/grad/solcalc/) were removed from the analyses because there was no whale watching activity at night and there is some evidence that sperm whale behavior exhibits diel variation (Aoki et al., 2007; André et al., 2017; Isojunno et al., 2020). To minimize potential effects of tagging on sperm whale behavior, the first foraging dive cycle (i.e., descent, bottom, ascent, and surface phases) after tagging was removed (Miller et al., 2009). For whales that remained at the surface after being tagged, we excluded the first 1 hour of data (i.e., approximate duration of a dive cycle). In addition, all dives and near-surface periods that had vessel noise that could have been produced by the research boat were removed. Vessel noise was attributed to the research boat whenever the boat was close to tagged individuals and there were no other vessels nearby. To determine the distance at which noise from the research boat could be detected on the tag, we used the GPS positions of the research boat and of 4 individuals that had a FastLoc GPS incorporated on the tag (sw18_170a, sw18_172a, sw18_173a and sw18_177a). The research boat was acoustically detected in 3% of the times it was < 500 m from the whale, in 1% of the times it was 501-1000 m from the tagged whale, and there were no detections at distances > 1000 m.
According to the whale watching regulations in the Azores, whale watching vessels cannot be at less than 50 m from a sperm whale (100 m for individuals/groups with calves) and a maximum of three vessels is allowed simultaneously within a 50-500 m radius of a whale. Each vessel cannot stay more than 15 minutes within this radius. Vessels need to approach whales from behind, navigate with a constant speed of a maximum 2 knots above the animal’s speed, and are not allowed to overtake the animal (Law Decrees 10/2003/A and 13/2004/A). While observing sperm whales at the surface, whale watching vessels typically remain in engine neutral mode, with occasional gear shifts to adjust the distance to the animals. When sperm whales start foraging, as indicated by a common fluke up, whale watching vessels usually wait for a minute or so and then gradually accelerate to move away from the diving whale. Based on this, we expected to detect whale watching vessel noise in tag sound recordings when vessels were observed near the tagged individuals. Indeed, a type I and II error analysis showed very good accordance between visual and acoustic detections of whale watching vessels during whale dives (0% of probability of making a type I error for all dive phases, and 50%, 28% and 37% of probability of making a type II error for surface, bottom and ascent phases, respectively). However, agreement between visual and acoustic detections of whale watching vessels was lower during non-foraging periods (43% of probability of making a type I error and 33% of probability of making a type II error), when whales were at or close to the surface (see Supplementary Material and Supplementary Table 1). Based on this analysis, the typical behavior of whale watching vessels around sperm whales, and the distances set by the whale watching regulations, we defined exposure and non-exposure as follows. Exposed foraging dives were the dives that followed the observation of one or more whale watching vessels within 500 m from the tagged whale while it was at the surface and/or the dives with vessel noise in the first 200 m of the descent phase. Thus, by definition, only the first dive following detection of whale watching vessels was considered to be exposed, when short-term responses of sperm whales are expected to be stronger (Miller et al., 2009). Non-exposed foraging dives were all dives where whale watching vessels were not observed or acoustically detected during the first 200 m of the descent phase. Exposed non-foraging periods were the ones where whale watching vessels were visually observed within 500 m from the surfacing tagged whale (Figure 2), while the remaining ones corresponded to non-exposed non-foraging periods. Both foraging and non-foraging non-exposure periods could have been preceded by exposure periods. Therefore, this study focuses on the immediate, short-term effects of whale watching vessels on sperm whale behavior and does not account for cumulative effects. Surfacing periods between foraging dives were excluded from subsequent analyses due to the insufficient number of exposures visually confirmed by the research boat.
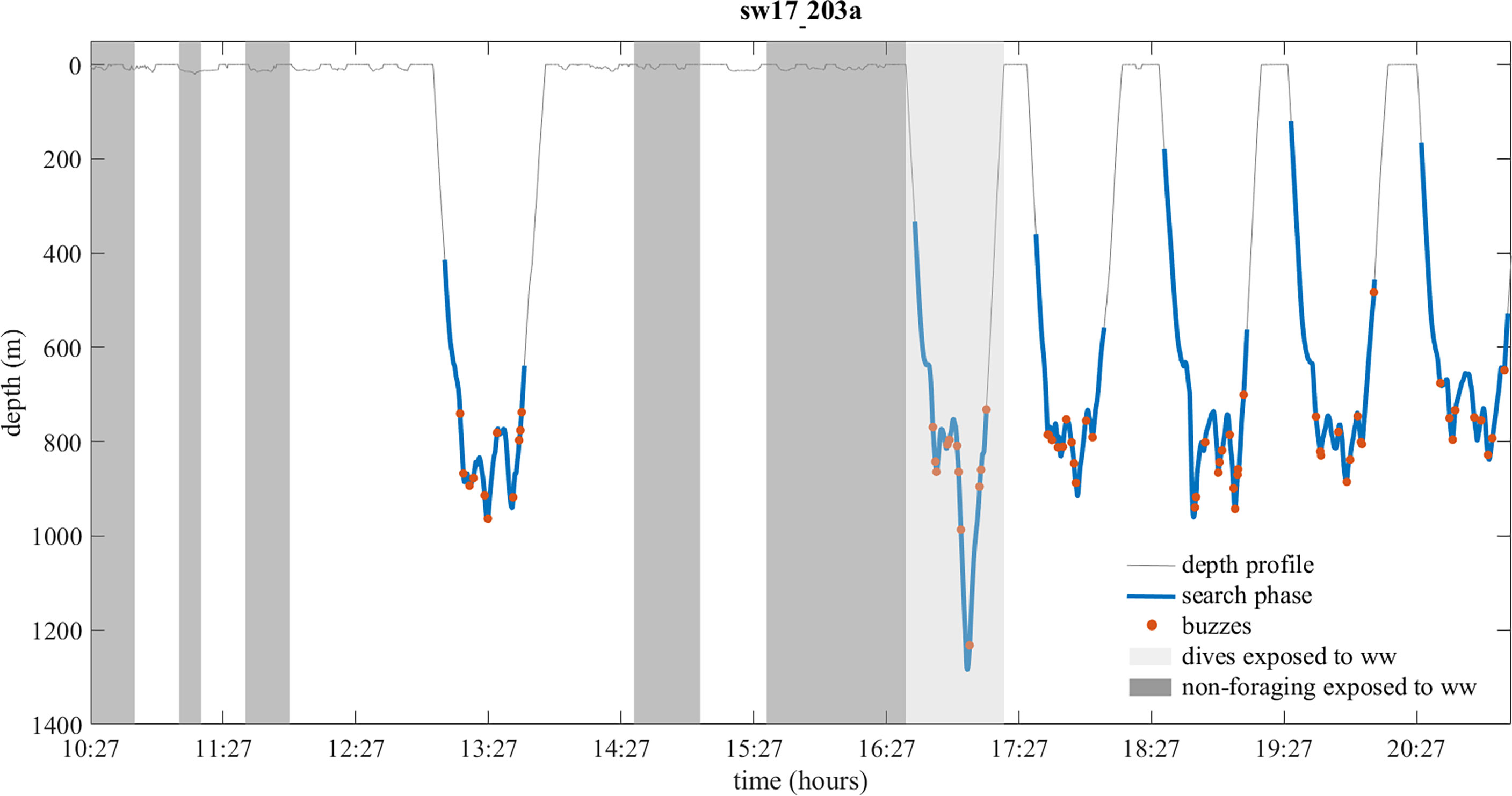
Figure 2 Dive profile (thin grey line) of tagged whale sw17_203a with its echolocation behavior (search phase – blue thick segments; buzzes – red circles) superimposed. Dives (light grey shadowing) and non-foraging periods (darker grey shadowing) exposed to whale watching (ww) vessels are indicated.
Behavioral indicator variables and hypotheses
We hypothesized that exposure to whale watching vessels influenced the foraging effort, foraging dive behavior, locomotion effort, and non-foraging periods in sperm whales. To investigate these hypotheses, we selected a set of variables describing the underwater movement and acoustic behavior of sperm whales. An exploratory analysis showed that several of these variables were correlated (see Supplementary Table 2) and did not differ significantly between exposure and non-exposure periods. Therefore, to reduce the number of multiple tests based on the same data (see below), we used only those variables that more adequately described the expected changes in sperm whale behavior during exposure to whale watching vessels (Table 1). Variables related to foraging effort included the median depth of the bottom phase (reflecting the prey depth layer targeted by the whale), the number of buzzes produced per minute within the foraging phase (i.e., buzzes/minute), and the proportion of time in the search and foraging phases within a dive (i.e., search and foraging ratios). Variables used to characterize the foraging dives were the dive duration, the duration of descent, bottom and ascent phases relative to the total dive duration, and the pitch angle in the descent and ascent phases. Variables related to locomotion activity were the vertical velocity (i.e., depth rate in meters per second) of descent and ascent phases, and the overall dynamic body acceleration (ODBA; Wilson et al., 2006; Halsey et al., 2009) of descent, bottom and ascent phases. ODBA is defined as the sum over a reference interval of the magnitude of the triaxial acceleration after the removal of a running mean, and is considered a proxy of energy expenditure (Wilson et al., 2006). While at the surface (i.e., during surface phases and non-foraging periods), there is the potential of ODBA estimations being influenced by sea state (Wilson et al., 2020b). For this reason, we did not calculate ODBA during non-foraging periods. Instead, we used the standard deviation (SD) of body pitch and roll to characterize changes in body orientation and examine whale maneuvers during non-foraging periods in response to presence of whale watching vessels (Table 1). To avoid the potential confounding effects of orientation shifts by whales while vertically resting on the values of SD pitch, we identified and removed from the analysis all these periods, i.e., the apparent 180° (3.14 radians) shifts in vertical position (Miller et al., 2008). These shifts were first detected through a visual inspection of the dive profile and the pitch signal and, later, an automatic detection was defined with shifts occurring in intervals of 2-3 minutes in a range of 103-114° (1.8-2 radians). Additionally, we also calculated the number of shallow dives (depth>2 m below the surface) per minute during non-foraging periods. Vertical velocity and ODBA were calculated using the previously referred tag tools. The mean of vertical velocity and ODBA, and the standard deviation of pitch and roll were calculated over 1-minute periods. To account for potential effects of variable tag position on ODBA calculations, ODBA values were standardized across in 1-minute intervals, by dividing by the median value of that individual and then multiplied by the median ODBA across all individuals (Isojunno and Miller, 2015). DTAG-4 had problems in the magnetometer data, thus, to avoid the use of distinct methods to calculate ODBA for the two types of tags used, ODBA was calculated using accelerometer data. For the same reason, fluke strokes could not be calculated for DTAG-4 tags, precluding the calculation of fluking and gliding periods in this study.
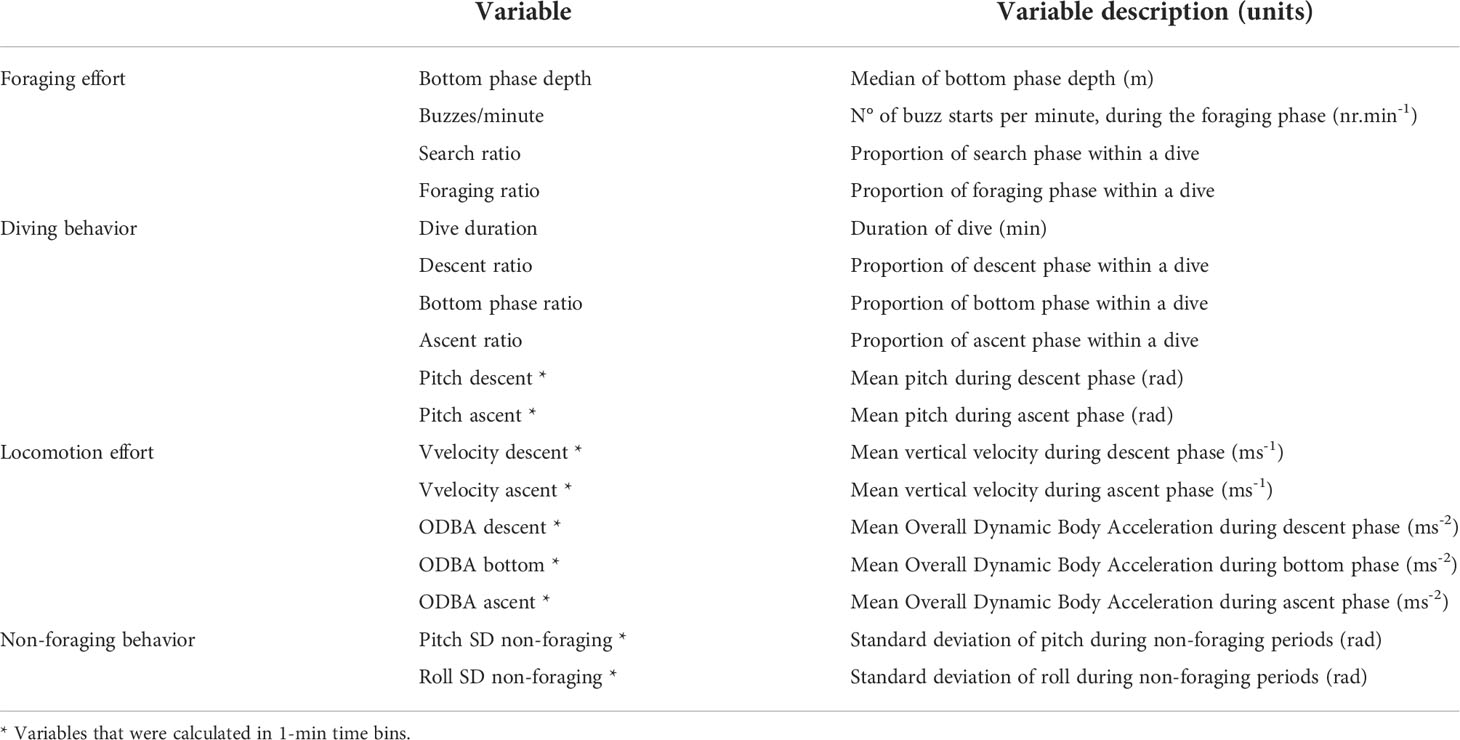
Table 1 Hypotheses, variable names and variable descriptions used in the analysis of the effects of whale watching vessels on sperm whales.
Statistical analysis
Linear Mixed-Effects Models were used to test the effects of whale watching vessel presence on all the behavioral variables (Table 1). Separate models were built for each variable, always including individual ID as a random effect to account for within-individual correlation. A log or squared root transformation was applied to variables when model assumptions were not met (Table 2). Model assumptions were verified by plotting normalized residuals versus fitted values to check for heterogeneity of variance, and histograms and Q-Q plots to check for normality (Zuur and Ieno, 2016). Additionally, autocorrelation plots (ACF and PACF) were visually inspected for the temporal dependency of the residuals (Zuur and Ieno, 2016). AR1 or ARMA correlation structures were included in the model, if needed, to account for the temporal autocorrelation. Finally, a correction controlling for false discovery rate (FDR) was applied to p-values obtained from the models to adjust for multiple comparisons using the same dataset (Jafari and Ansari-Pour, 2019). All analyses were performed using the R software (R Core Team, 2016) and the package “mgcv” (Wood, 2017). All values reported below are mean values unless otherwise stated.
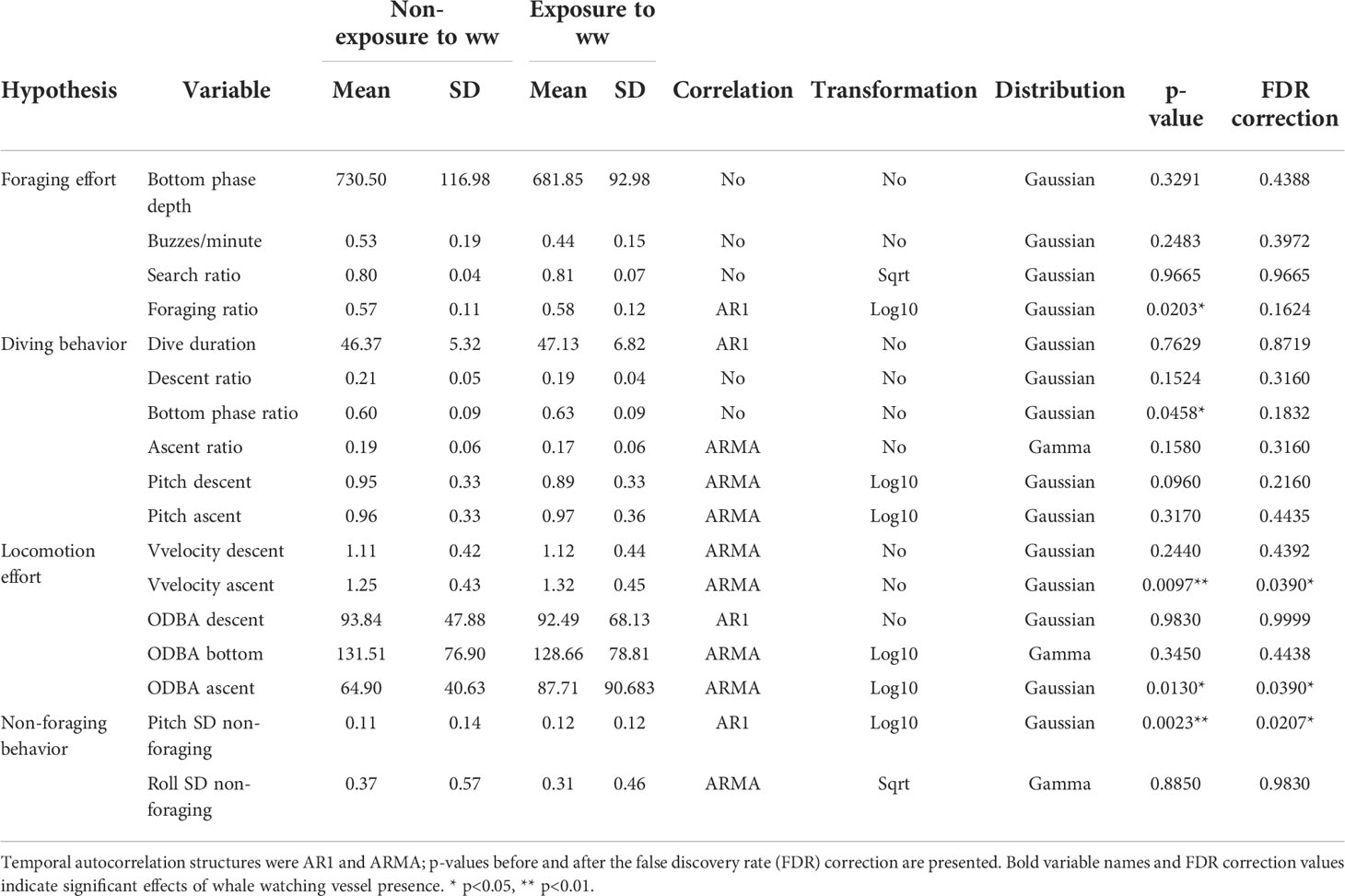
Table 2 Mean and standard deviation (SD) of variables used during non-exposure and exposure to whale watching (ww) periods and summary of results from the Linear Mixed-Effect Models testing the effects of ww vessel presence on the four sets of variables.
Results
Between 2017 and 2019, 24 sperm whales were tagged with either the DTAG-3 (n=16 whales) or DTAG-4 (n=8 whales). Tagged whales ranged approximately 9-11 m in length and all were found in social groups. A total of 93 dives and 1135 minutes of non-foraging periods were analyzed (Table 3). Sixteen out of the 24 tagged sperm whales were exposed to whale watching vessels. Overall, tagged individuals were exposed to whale watching vessels in 34 (37%) dives and 504 (44%) minutes of non-foraging periods (Table 3). In total, 13 premature detachments of DTAGs occurred due to breaches and only two individuals had deployment durations near the pre-programmed period of 24 hours (sw19_088a and sw19_160a). About 42% of tagged individuals were exposed to whale watching vessels prior tagging, 37% may have been exposed because there were whale watching vessels in the area, and 21% were not exposed.
Sperm whales exhibited higher vertical velocity (p=0.0390) and ODBA (p=0.0390) on the ascent phase of dives exposed to whale watching vessels (predicted vertical velocities: 1.35 ms-1 (SE=0.045) and 1.23 ms-1 (SE=0.044) for exposed and non-exposed dives, respectively; predicted ODBA: 1.79 ms-2 (SE=0.028) and 1.72 ms-2 (SE=0.044) for exposed and non-exposed dives, respectively) (Table 2 and Figures 3A, B). Dives exposed to whale watching vessels showed slightly shallower bottom phase depth (non-exposure=730.50 m; exposure=681.85 m) and fewer buzzes/minute (non-exposure=0.53; exposure=0.44) than non-exposed dives, but these differences were not statistically significant. The remaining variables related to foraging effort and dive behavior also did not reveal any statistical significance between exposed and non-exposed dives (Table 2).
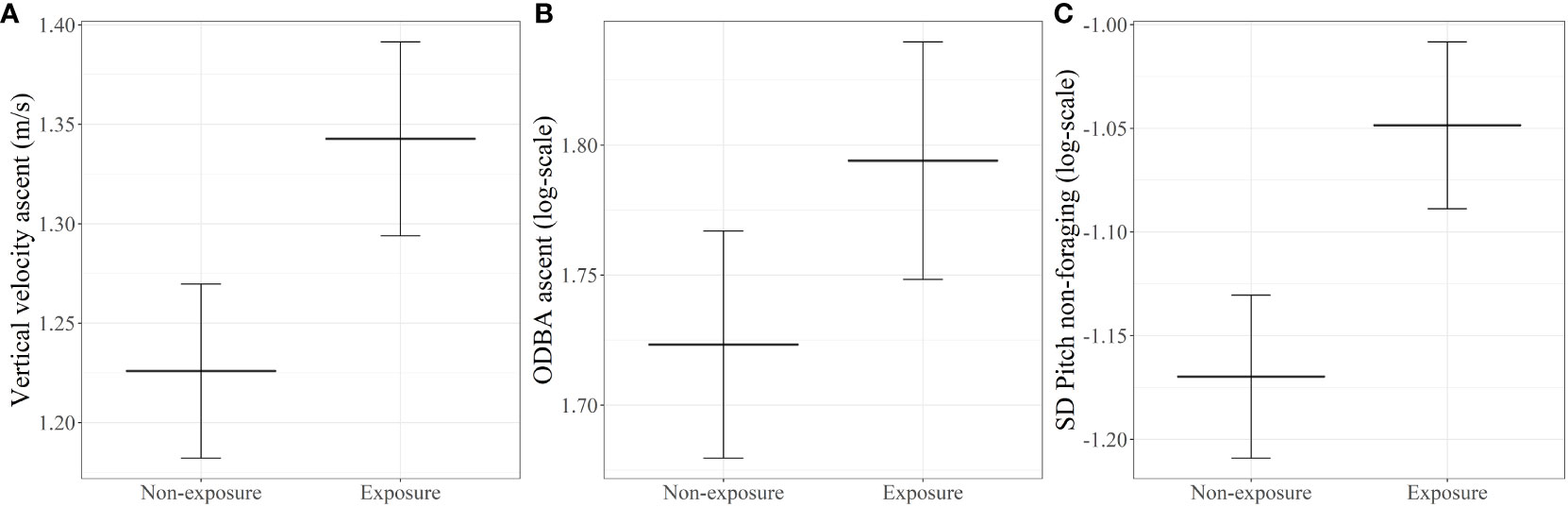
Figure 3 Model-predicted effects on the vertical velocity during ascent periods (A), ODBA during ascent periods (B), and on the SD of pitch (on the log scale) during non-foraging periods (C), for non-exposure and exposure periods to whale watching vessels. Error bars correspond to standard errors.
SD of body pitch during non-foraging periods exposed to whale watching vessels was significantly higher with -1.05 radians (SE: 0.040) compared to -1.17 radians (SE: 0.039) during non-exposure periods (p=0.0207) (Table 2, Figure 3C and Supplementary Figure 2). On the other hand, the roll SD during non-foraging periods was not significantly different between exposure and non-exposure periods (non-exposure=0.37 radians; exposure=0.31 radians). In addition, sperm whales made slightly more shallow dives per minute, when exposed to whale watching vessels (median ± SD of non-exposure=0.05 ± 0.29; median ± SD of exposure=0.06 ± 0.08), but differences were not statistically significant (H(1)=0.22, p=0.6413).
Discussion
Previous studies have assessed the impact of whale watching vessels on sperm whale behavior mostly based on their near-surface responses (Gordon et al., 1992; Magalhães et al., 2002; Richter et al., 2003; Richter et al., 2006; Isojunno et al., 2011; Cosentino, 2016); Isojunno et al., 2011; Cosentino, 2016) and they found changes in sperm whale ventilation patterns and surface heading, duration of surface periods between foraging dives, occurrence of aerial displays, increased number of shallow dives and delayed start of first long-range click. To the best of our knowledge, this is the first study investigating changes on the fine-scale underwater movements and acoustic behavior of sperm whales exposed to whale watching vessels, by instrumenting 24 whales with DTAGs in a region with relatively high whale watching pressure. Here, we found that, in the presence of whale watching vessels, sperm whales perform fastest vertical ascents from foraging dives, expend more energy during those ascents, and have more disturbed non-foraging periods.
Sperm whale underwater behavior during whale watching vessel exposure
We did not detect significant differences in the number of prey capture attempts per minute (buzzes/minute) between non-exposed and exposed dives. In New Zealand, in a study performed with towed-array hydrophones, there were also no changes in the parameters related to prey encounter rates that could be attributed to whale watching vessels (Isojunno et al., 2011). Additionally, changes in other variables associated to foraging effort and dive behavior such as dive duration, search, foraging and bottom phase ratios, and body pitch during dive descents or ascents, were also small and not significant. Hence, there was no evidence for short-term changes in energy intake during exposure to whale watching vessels. Likewise, differences between exposed and non-exposed dives in descent phase and bottom phase ODBA, a proxy for locomotion effort, were not statistically significant.
Sperm whale’s vertical velocity during ascent was significantly higher in dives exposed to whale watching vessels than in non-exposed dives. In marine mammals, energy expenditure and oxygen consumption increase rapidly with speed during active swimming (Kooyman, 1989; Hind and Gurney, 1997; Otani et al., 2001). To maximize oxygen available to foraging at depth, diving animals should conserve energy during descent and ascent transits by adopting optimal speeds during active stroking, and glide passively whenever buoyancy assists movement in the right direction (Williams et al., 2000; Sato et al., 2003; Miller et al., 2004b). The predominant swimming style in sperm whales is steady fluking during descent, and stroke-and-glide during ascent, with prolonged gliding during the terminal part of the ascent (Miller et al., 2004b; Oliveira, 2014). While buoyancy and drag forces influence gliding behavior and optimal swim speed both across dives and individuals (Miller et al., 2004b; Aoki et al., 2017), it is unlikely that these forces changed between exposure and non-exposure conditions in this study. Hence, even though we were unable to calculate fluke rates and duration of gliding, the higher vertical velocity during ascents in exposed dives without any changes in pitch angle, suggests that sperm whales increased the stroke frequency. An increase in active stroking is consistent with the increased ODBA during ascents exposed to whale watching vessels relative to non-exposed ascents, which indicates a higher energetic cost during exposed dives, when compared to non-exposed dives.
In the Azores, whale watching vessels attempt to observe several fluke-ups in the same trip, often transiting at high speed across the sperm whale foraging area to reach surfacing whales before they dive. The behavior by whale watching vessels combined with higher sperm whale vertical velocities while ascending from foraging dives, can expose whales to risk of collisions with vessels, as this species shows difficulties in performing escape maneuvers during approaches from fast vessels (Gannier and Marty, 2015). Studies assessing the risk of vessel collisions with sperm whales will be necessary to understand the magnitude of the problem.
Sperm whale near-surface behavior during whale watching vessel exposure
While exposed to whale watching vessels during non-foraging periods, sperm whales exhibited a significant increase in the SD of body pitch. Increased variability in pitch did not arise from orientation shifts while whales were vertically resting (Miller et al., 2008), as these were removed prior to the analysis. This higher variability could reflect a greater frequency of shallow evading dives (Lundquist et al., 2008), as was the case of mature males off Northern Norway (Cosentino, 2016). However, in our study the number of shallow dives was not significantly different between exposure and non-exposure non-foraging periods. Another possible explanation for the change in pitch variation would be an increase in social interactions. However, this activity is generally associated with higher variability in body roll movements (Gordon, 1998) and, in the present study, SD body roll did not differ between exposure and non-exposure periods. On the other hand, if presence of whale watching vessels disrupted social interactions, we would expect to see a decrease in SD roll. A third and, more likely explanation, is that the increased pitch variability reflects increased movement during resting periods when sperm whales are supposed to remain with a more less constant pitch (horizontal resting with pitch~0° (0 radians) and vertical resting in drifting position [pitch near ±90° ( ± 1.57 radians); Miller et al. (2008)]. Altogether, these results suggest that presence of whale watching vessels disrupts resting activity in sperm whales.
The most consisting finding across studies examining changes in cetacean activity budgets in response to presence of whale watching vessels was an increase in time spent travelling, and consequently in energy expenditure, and reduction in time allocated to resting, as well as to foraging (Senigaglia et al., 2016). Although we haven’t measured activity budgets of sperm whales, increased movements during resting and social periods also suggests increased energetic costs for whales exposed to whale watching vessels. These costs were not compensated by an increase in prey capture attempts, or any other proxy for foraging effort, suggesting an imbalance between energy intake and energy expenditure at least in the short-term. Additionally, sleep is a critical behavior, believed to play a key role in support of the central nervous system of animals (Rattenborg et al., 2017), and sleep disturbance has been shown to results in a range of negative health effects (Shaw et al., 2002; Andersen et al., 2009). If repeated over time, disruption of resting could impact the individual’s health and energetic balance, and affect other vital activities such as feeding, nursing or mating (Bejder et al., 2006; Lusseau et al., 2006b; Christiansen and Lusseau, 2014).
Limitations of this study
The power to detect significant effects in our analyses was likely constrained by the small sample of exposure periods, the unbalanced sample between exposure and non-exposure, as well as the high amounts of unexplained variation in sperm whale behavior.
Although we collected data from a healthy number of DTAG deployments (n=24), this study was based on opportunistic observations, which implies that we had no control on the number of exposures and non-exposures. Moreover, premature tag detachments due to breaching events and occasionally imperfect suction cup attachments, contributed to short tagging durations, thereby reducing the likelihood of whale exposures to whale watching vessels. In several deployments the tag slid to the whale’s flank, reducing the efficiency of VHF tracking and making it difficult to focal-follow the tagged individuals and visually detect interactions with whale watching vessels. To overcome this limitation and increase sample size, we used the data recorded by DTAG hydrophones to detect noise from whale watching vessels. Although acoustic detection may not have been 100% accurate, and some exposure periods could have been missed, the approach proved to be effective at detecting presence of whale watching vessels when tagged whales descended to their foraging dives (Supplementary Table 1). However, the accuracy of acoustic detections dropped when whales were at or near the surface, during the surface phases and non-foraging periods, and we had to rely only on data from visual observations. As a result, we could not compare exposure and non-exposure during surface phases, and the comparison during non-foraging periods, albeit possible, suffered from low sample size.
High intra- and inter-individual variability in sperm whale behavior also limited our ability to detect significant exposure effects, albeit the inclusion of individual ID as a random term in the models. Part of this variability was likely driven by intrinsic individual differences in behavior, as well as changes in internal state, social context, or environmental conditions over time. However, differences in exposure conditions across observations were probably responsible for an important part of this variability. For example, the number and distance of whale watching vessels are known to influence the severity of behavioral responses in a number of cetaceans (Williams and Ashe, 2007; Stamation et al., 2010; Steckenreuter et al., 2011; Villagra et al., 2021). Here, because of the way observations were conducted and limitations in sample size, we could not account for either factor in the analysis. Converting the full range of exposure conditions into a binary variable (i.e., presence/absence of whale watching vessels) and contrasting the average response of whales, may have obscured real responses and weakened the detectability of significant effects (Williams and Ashe, 2007). Additionally, our ability to detect changes in behavior may have also been affected by potential cumulative effects of disturbance, if previous exposure to whale watching vessels conditioned whale’s response during observed exposure periods, or their behavior during periods of non-exposure. However, unless cumulative effects subsist only for hours, this problem is difficult to circumvent in studies conducted in whale watching areas.
Finally, our analyses involved multiple statistical tests on many behavioral variables, which is known to increase the probability of wrongly rejecting a null hypothesis. To account for this problem, we adjusted the p-values to control the false discovery rate (FDR), i.e., the rate at which null hypotheses are falsely rejected (Benjamini and Hochberg, 1995). Methods that control the FDR are becoming increasingly popular because they enable controlling the proportion of type I errors at or below a specified level and show greater power to detect true positives compared to more traditional approaches (e.g., Bonferroni corrections) (Benjamini and Hochberg, 1995). Nevertheless, more flexible modelling approaches such as generalized additive mixed models or multi-state hidden Markov models should be explored in the future to enable a better understanding of some of our results. Specifically, statistically significant differences prior FDR control in relative durations of bottom and foraging phases between exposed and non-exposed dives warrant further investigation.
Conclusions
Despite the caveats mentioned, this study provides novel insights on the short-term effects of whale watching vessels on sperm whale foraging and non-foraging behavior. Our findings indicate that exposure to whale watching vessels had no detectable effect on the individuals’ foraging effort and behavior, including on prey capture attempts. In contrast, sperm whales increased the vertical speed and body acceleration during dive ascents, and showed increased movements during resting, suggesting that sperm whale responses to disturbance from whale watching likely carry energetic costs.
In the Azores, most individually identified sperm whales are transients but several social units are known to remain months in the area and return nearly every year (Boys et al., 2019). Individuals within these social units may be subjected to repeated interactions with whale watching vessels and may be under cumulative effects of vessel disturbance for prolonged periods of time. Moreover, some of these social units also use the waters around Madeira and Canary Islands (Steiner et al., 2015) and may be exposed to whale watching activities there. Therefore, it is essential to understand how sperm whales respond to repeated disturbance from whale watching vessels and assess the potential long-term consequences of these responses.
Data availability statement
The datasets presented in this study can be found in online repositories. The names of the repository/repositories and accession number(s) can be found below: The datasets analyzed for this study can be found in the https://figshare.com/articles/dataset/Exposure_to_whale_watching_vessels_affects_dive_ascents_and_resting_behavior_in_sperm_whales/21355338.
Ethics statement
The animal study was reviewed and approved by Regional Secretariat of Science and Technology, Regional Directorate of Sea Affairs.
Author contributions
MAS, CO and RP conceived and designed the study, secured funding and managed the project. RP, CO, MAS, PJW, IC and SPJ collected the data. CO, IC, RP and MAS processed the data. CO and SPJ analyzed the data. CO wrote the first manuscript draft. CO, SPJ and MAS reviewed and wrote the revised manuscript. All authors commented on all previous versions of the manuscript and read and approved the final manuscript.
Funding
Research was supported by the Portuguese Science & Technology Foundation (FCT), the Azorean Science & Technology Fund (FRCT), and the EC through research projects WATCH IT (Acores-01-0145-FEDER-000057), FCTExploratory (IF/00943/2013/CP1199/CT0001), and META (FA_06_2017_017), co-funded by FEDER, COMPETE, QREN, POPH, ESF, PO AZORES 2020, Portuguese Ministry for Science and Education. CO was supported by WATCH IT (Acores-01-0145-FEDER-000057), tenders with SRMCT/DRAM under project RAGES (GA 110661/2018/794607/SUB/ENV.C.2) and INTERTAGUA (MAC2/1.1a/385), and with the University of St. Andrews under project ACCURATE (ONR-ID314_02-14-2019_0852-30), IC by FCT-IP Project UIDP/05634/2020, RP by FCT grant SFRH/BPD/108007/2015, PW by RANNÍS Icelandic Research Fund grant 207081. RP and MAS are co-financed by PO AZORES 2020 (Fund 01-0145-FEDER-000140) “MarAZ Researchers: Consolidate a body of researchers in Marine Sciences in the Azores” of the EU. Okeanos is funded by FCT (UIDB/05634/2020) and by the Regional Government of the Azores (M1.1.A/REEQ.CIENTÍFICO UI&D/2021/010).
Acknowledgments
We thank Peter Teglberg Madsen and Patrick J. O. Miller for providing DTAGs in some years. We are grateful to Peter Teglberg Madsen, Pernille Tønnesen, Per Henriksen, Bruno Castro and Renato Bettencourt for fieldwork participation and support. We also thank the whale watching companies and lookouts from the islands of Faial and Pico for all the support on land and at sea. We are also grateful to Pernille Tønnesen, Mark Johnson, Stacy L. DeRuiter, K. Alex Shorter, Joaquin Gabaldon, Saana Isojunno, Elliot Lee Hazen, Alec Burslem and Leslie New for advice on data analysis. We also thank Vicente Fernández Rodilla and Ricardo Medeiros for data analysis support. We are also grateful to the reviewers that helped us to significantly improve the manuscript.
Conflict of interest
The authors declare that the research was conducted in the absence of any commercial or financial relationships that could be construed as a potential conflict of interest.
Publisher’s note
All claims expressed in this article are solely those of the authors and do not necessarily represent those of their affiliated organizations, or those of the publisher, the editors and the reviewers. Any product that may be evaluated in this article, or claim that may be made by its manufacturer, is not guaranteed or endorsed by the publisher.
Supplementary material
The Supplementary Material for this article can be found online at: https://www.frontiersin.org/articles/10.3389/fmars.2022.914397/full#supplementary-material
References
Amano M., Yoshioka M. (2003). Sperm whale diving behavior monitored using a suction-cup attached TDR tag. Mar. Ecol. Prog. Ser. 258, 191–295. doi: 10.3354/meps258291
Andersen M. L., Ribeiro D. A., Bergamaschi C. T., Alvarenga T. A., Silva A., Zager A., et al. (2009). Distinct effects of acute and chronic sleep loss on DNA damage in rats. Prog. Neuropsychopharmacol. Biol. Psychiatry 33, 562–567. doi: 10.1016/j.pnpbp.2009.02.014
André M., Caballé A., van der Schaar M., Solsona A., Houégnigan L., Zaugg S., et al. (2017). Sperm whale long-range echolocation sounds revealed by ANTARES, a deep-sea neutrino telescope. Sci. Rep. 7, 45517. doi: 10.1038/srep45517
Aoki K., Amano M., Yoshioka M., Mori K., Tokuda D., Miyazaki N. (2007). Diel diving behavior of sperm whales off Japan. Mar. Ecol. Prog. Ser. 349, 277–287. doi: 10.3354/meps07068
Aoki K., Sato K., Isojunno S., Narazaki T., Miller P. J. O. (2017). High diving metabolic rate indicated by high-speed transit to depth in negatively buoyant long-finned pilot whales. J. Exp. Biol. 220, 3802–3811. doi: 10.1242/jeb.158287
Arranz P., Glarou M., Sprogis K. R. (2021). Decreased resting and nursing in short-finned pilot whales when exposed to louder petrol engine noise of a hybrid whale-watch vessel. Sci. Rep. 11, 21195. doi: 10.1038/s41598-021-00487-0
Avila I. C., Correa L. M., Parsons E. C. M. (2015). Whale-watching activity in bahía málaga, on the pacific coast of Colombia, and its effect on humpback whale (Megaptera novaeangliae) behavior. Tour. Mar. Environ. 11, 19–32. doi: 10.3727/154427315X14398263718394
Avila I. C., Kaschner K., Dormann C. F. (2018). Current global risks to marine mammals: Taking stock of the threats. Biol. Conserv. 221, 44–58. doi: 10.1016/j.biocon.2018.02.021
Azzara A. J., Zharen W. M., Newcomb J. J. (2013). Mixed-methods analytic approach for determining potential impacts of vessel noise on sperm whale click behavior. J. Acoust. Soc Am. 134, 4566–4574. doi: 10.1121/1.4828819
Bain D. E., Williams R., Trites A. W. (2014). “Energetic linkages between short-term and long-term effects of whale-watching disturbance on cetaceans.” In: Whale-watching, sustainable tourism and ecological management. pp.206–228. doi: 10.1017/CBO9781139018166.018
Bearzi M. (2017). “Impacts of marine mammal tourism”. In: Blumstein D. T., Geffroy B., Samia D. S. M., Bessa E. (eds). Ecotourism’s Promise and Peril: A Biological Evaluation. (Cham: Springer International Publishing), pp. 73–96. doi: 10.1007/978-3-319-58331-0_6
Bejder L., Samuels A., Whitehead H., Gales N., Mann J., Connor R., et al. (2006). Decline in relative abundance of bottlenose dolphins exposed to long-term disturbance. Conserv. Biol. 20, 1791–1798. doi: 10.1111/j.1523-1739.2006.00540.x
Benjamini Y., Hochberg Y. (1995). Controlling the false discovery rate: A practical and powerful approach to multiple testing. J. R. Stat. Soc Ser. B 57, 289–300. doi: 10.1111/j.2517-6161.1995.tb02031.x
Boys R. M., Oliveira C., Pérez-Jorge S., Prieto R., Steiner L., Silva M. A. (2019). Multi-state open robust design applied to opportunistic data reveals dynamics of wide-ranging taxa: the sperm whale case. Ecosphere 10, e02610. doi: 10.1002/ecs2.2610
Burnham R. E., Duffus D. A., Malcolm C. D. (2021). Towards an enhanced management of recreational whale watching: The use of ecological and behavioural data to support evidence-based management actions. Biol. Conserv. 255, 109009. doi: 10.1016/j.biocon.2021.109009
Christiansen F., Lusseau D. (2014). Understanding the ecological effects of whale-watching on cetaceans. Whale-watching, sustainable tourism and ecological management. (Cambridge, UK: Cambridge Univ. Press). pp. 177–192. doi: 10.1017/CBO9781139018166.016
Christiansen F., Rasmussen M., Lusseau D. (2013). Whale watching disrupts feeding activities of minke whales on a feeding ground. Mar. Ecol. Prog. Ser. 478, 239–251. doi: 10.3354/meps10163
Christiansen F., Rasmussen M. H., Lusseau D. (2014). Inferring energy expenditure from respiration rates in minke whales to measure the effects of whale watching boat interactions. J. Exp. Mar. Bio. Ecol. 459, 96–104. doi: 10.1016/j.jembe.2014.05.014
Cosentino A. M. (2016). Effects of whale-watching vessels on adult male sperm whales off andenes, Norway. Tour. Mar. Environ. 11, 215–227. doi: 10.3727/154427316X14580612748560
Currie J. J., McCordic J. A., Olson G. L., Machernis A. F., Stack S. H. (2021). The impact of vessels on humpback whale behavior: The benefit of added whale watching guidelines. Front. Mar. Sci. 8. doi: 10.3389/fmars.2021.601433
Erbe C. (2002). Underwater noise of whale-watching boats and potential effects on killer whales (Orcinus orca), based on an acoustic impact model. Mar. Mammal Sci. 18, 394–418. doi: 10.1111/j.1748-7692.2002.tb01045.x
Finneran J. J. (2015). Noise-induced hearing loss in marine mammals: A review of temporary threshold shift studies from 1996 to 2015. J. Acoust. Soc Am. 138, 1702–1726. doi: 10.1121/1.4927418
Frid A., Dill L. (2002). Human-caused disturbance stimuli as a form of predation risk. Conserv. Ecol. 6, 11. doi: 10.5751/ES-00404-060111
Gannier A., Marty G. (2015). Sperm whales ability to avoid approaching vessels is affected by sound reception in stratified waters. Mar. pollut. Bull. 95, 283–288. doi: 10.1016/j.marpolbul.2015.03.029
Gordon J., Leaper R., Hartley G. F., Chappell O. (1992). Effects of whale-watching vessels on the surface and underwater acoustic behaviour of sperm whales of kaikoura, new Zealand (Wellington, New Zealand: Department of Conservation).
Halsey L. G., Shepard E. L. C., Quintana F., Gomez Laich A., Green J. A., Wilson R. P. (2009). The relationship between oxygen consumption and body acceleration in a range of species. Comp. Biochem. Physiol. Part A Mol. Integr. Physiol. 152, 197–202. doi: 10.1016/j.cbpa.2008.09.021
Hazel J., Lawler I. R., Marsh H., Robson S. (2007). Vessel speed increases collision risk for the green turtle chelonia mydas. Endanger. Species Res. 3, 105–113. doi: 10.3354/esr003105
Heiler J., Elwen S. H., Kriesell H. J., Gridley T. (2016). Changes in bottlenose dolphin whistle parameters related to vessel presence, surface behaviour and group composition. Anim. Behav. 117, 167–177. doi: 10.1016/j.anbehav.2016.04.014
Hind A. T., Gurney W. S. C. (1997). The metabolic cost of swimming in marine homeotherms. J. Exp. Biol. 200, 531–542. doi: 10.1242/jeb.200.3.531
Isojunno S., Curé C., Kvadsheim P. H., Lam F.-P. A., Tyack P. L., Wensveen P. J., et al. (2016). Sperm whales reduce foraging effort during exposure to 1–2 kHz sonar and killer whale sounds. Ecol. Appl. 26, 77–93. doi: 10.1890/15-0040
Isojunno S., Fernandes M. C., Gordon J. (2011). “Effects of whale watching on underwater acoustic behaviour of sperm whales in the kaikoura canyon area,” in . eff. tour. behav. sperm whales inhabiting kaikōura canyon. Eds. Markowitz T. M., Richter C., Gordon J. (Rep. Dep. Conserv. NZ).
Isojunno S., Miller P. J. O. (2015). Sperm whale response to tag boat presence: biologically informed hidden state models quantify lost feeding opportunities. Ecosphere 6, art6. doi: 10.1890/ES14-00130.1
Isojunno S., Wensveen P. J., Lam F.-P. A., Kvadsheim P. H., von Benda-Beckmann A. M., Martín López L. M., et al. (2020). When the noise goes on: received sound energy predicts sperm whale responses to both intermittent and continuous navy sonar. J. Exp. Biol. 223, jeb219741. doi: 10.1242/jeb.219741
Jafari M., Ansari-Pour N. (2019). Why, when and how to adjust your p values? Cell J. 20, 604–607. doi: 10.22074/cellj.2019.5992
Jensen F. H., Bejder L., Wahlberg M., Aguilar Soto N., Johnson M., Madsen P. T. (2009). Vessel noise effects on delphinid communication. Mar. Ecol. Prog. Ser. 395, 161–175. doi: 10.3354/meps08204
Johnson M., Madsen P. T., Zimmer W. M. X., de Soto N. A., Tyack P. L. (2006). Foraging blainville’s beaked whales (Mesoplodon densirostris) produce distinct click types matched to different phases of echolocation. J. Exp. Biol. 209, 5038–5050. doi: 10.1242/jeb.02596
Johnson M. P., Tyack P. L. (2003). A digital acoustic recording tag for measuring the response of wild marine mammals to sound. IEEE J. Ocean. Eng. 28, 3–12. doi: 10.1109/JOE.2002.808212
Kooyman G. L. (1989). Diverse divers: physiology and behavior (Berlin: Springer Science & Business Media).
Kvadsheim P. H., DeRuiter S., Sivle L. D., Goldbogen J., Roland-Hansen R., Miller P. J. O., et al. (2017). Avoidance responses of minke whales to 1–4kHz naval sonar. Mar. pollut. Bull. 121, 60–68. doi: 10.1016/j.marpolbul.2017.05.037
Lima S. L. (1998). Nonlethal effects in the ecology of predator-prey interactions: What are the ecological effects of anti-predator decision-making? Bioscience 48, 25–34. doi: 10.2307/1313225
Lundquist D., Sironi M., Würsig B., Rowntree V. (2008). Changes in the movement patterns of southern right whales in response to simulated swim-with-whale tourism at península valdés, Argentina. J. Cetacean Res. Manage. 1–12.
Lusseau D., Bejder L. (2007). The long-term consequences of short-term responses to disturbance experiences from whalewatching impact assessment. Int. J. Comp. Psychol. 20, 228–236.
Lusseau D., Slooten L., Currey R. J. C.(2006a). Unsustainable dolphin-watching tourism in fiordland, new Zealand. Tour. Mar. Environ. 3, 173–178. doi: 10.3727/154427306779435184
Lusseau D., Slooten L., Currey R. J. C.(2006b). Unsustainable dolphin-watching tourism in fiorland, new Zealand. Tour. Mar. Environ. 3, 173–178. doi: 10.3727/154427306779435184
Madsen P. T., Payne R., Kristiansen N. U., Wahlberg M., Kerr I., Møhl B. (2002). Sperm whale sound production studied with ultrasound time/depth-recording. J. Exp. Biol. 205, 1899–1906. doi: 10.1242/jeb.205.13.1899
Magalhães S. M., Prieto R., Silva M. A., Gonçalves J. M., Afonso-Dias M., Santos R. S. (2002). Short-term reactions of sperm whales (Physeter macrocephalus) to whale-watching vessels in the Azores. Aquat. Mamm. 28, 267–274.
Miller P. J. O., Aoki K., Rendell L. E., Amano M. (2008). Stereotypical resting behavior of the sperm whale. Curr. Biol. 18, R21–R23. doi: 10.1016/j.cub.2007.11.003
Miller P. J. O., Johnson M. P., Madsen P. T., Biassoni N., Quero M., Tyack P. L. (2009). Using at-sea experiments to study the effects of airguns on the foraging behavior of sperm whales in the gulf of Mexico. Deep. Res. Part I 56, 1168–1181. doi: 10.1016/j.dsr.2009.02.008
Miller P. J. O., Johnson M. P., Tyack P. L. (2004a). Sperm whale behaviour indicates the use of echolocation click buzzes ‘creaks’ in prey capture. Proc. R. Soc London Ser. B-Biological Sci. 271, 2239–2247. doi: 10.1098/rspb.2004.2863
Miller P. J. O., Johnson M. P., Tyack P. L., Terray E. A. (2004b). Swimming gaits, passive drag and buoyancy of diving sperm whales physeter macrocephalus. J. Exp. Biol. 207, 1953–1967. doi: 10.1242/jeb.00993
Montero-Quintana A. N., Vázquez-Haikin J. A., Merkling T., Blanchard P., Osorio-Beristain M. (2020). Ecotourism impacts on the behaviour of whale sharks: an experimental approach. Oryx 54, 270–275. doi: 10.1017/S0030605318000017
New L., Clark J., Costa D., Fleishman E., Hindell M., Klanjšček T., et al. (2014). Using short-term measures of behaviour to estimate long-term fitness of southern elephant seals. Mar. Ecol. Prog. Ser. 496, 99–108. doi: 10.3354/meps10547
Oliveira C. (2014). Behavioural ecology of the sperm whale (Physeter macrocephalus) in the North Atlantic ocean. PhD thesis at the University of the Azores, 1–123.
Oliveira C., Filla G., Gonçalves J., Silva M. A., Prieto R., Magalhães S. M., et al. (2007). A social-economic perspective of the whale watching activity in the Azores (Anchorage: International Whaling Commission).
Onoufriou J., Russell D. J. F., Thompson D., Moss S. E., Hastie G. D. (2021). Quantifying the effects of tidal turbine array operations on the distribution of marine mammals: Implications for collision risk. Renew. Energy 180, 157–165. doi: 10.1016/j.renene.2021.08.052
Otani S., Naito Y., Kato A., Kawamura A. (2001). Oxygen consumption and swim speed of the harbor porpoise phocoena phocoena. Fish. Sci. 67, 894–898. doi: 10.1046/j.1444-2906.2001.00338.x
Pirotta E., Booth C. G., Costa D. P., Fleishman E., Kraus S. D., Lusseau D., et al. (2018). Understanding the population consequences of disturbance. Ecol. Evol. 8, 9934–9946. doi: 10.1002/ece3.4458
Pirotta E., Thomas L., Costa D. P., Hall A. J., Harris C. M., Harwood J., et al. (2022). Understanding the combined effects of multiple stressors: A new perspective on a longstanding challenge. Sci. Total Environ. 821, 153322. doi: 10.1016/j.scitotenv.2022.153322
Rattenborg N. C., de la Iglesia H. O., Kempenaers B., Lesku J. A., Meerlo P., Scriba M. F. (2017). Sleep research goes wild: new methods and approaches to investigate the ecology, evolution and functions of sleep. Philos. Trans. R. Soc B Biol. Sci. 372, 20160251. doi: 10.1098/rstb.2016.0251
Richter C. F., Dawson S. M., Slooten E. (2003). Sperm whale watching off kaikoura, new Zealand: effects of current activities on surfacing and vocalisation patterns. Sci. Conserv. 219, 1–78.
Richter C., Dawson S., Slooten E. (2006). Impacts of commercial whale watching on male sperm whales at kaikoura, new Zealand. Mar. Mammal Sci. 22, 46–63. doi: 10.1111/j.1748-7692.2006.00005.x
Rolland R. M., Parks S. E., Hunt K. E., Castellote M., Corkeron P. J., Nowacek D. P., et al. (2012). Evidence that ship noise increases stress in right whales. Proc. R. Soc B Biol. Sci. 279, 2363–2368. doi: 10.1098/rspb.2011.2429
Sato K., Mitani Y., Cameron M. F., Siniff D. B., Naito Y. (2003). Factors affecting stroking patterns and body angle in diving weddell seals under natural conditions. J. Exp. Biol. 206, 1461–1470. doi: 10.1242/jeb.00265
Schuler A. R., Piwetz S., Di Clemente J., Steckler D., Mueter F., Pearson H. C. (2019). Humpback whale movements and behavior in response to whale-watching vessels in Juneau, AK. Front. Mar. Sci. 6. doi: 10.3389/fmars.2019.00710
Senigaglia V., Christiansen F., Bejder L., Gendron D., Lundquist D., Noren D. P., et al. (2016). Meta-analyses of whale-watching impact studies: comparisons of cetacean responses to disturbance. Mar. Ecol. Prog. Ser. 542, 251–263. doi: 10.3354/meps11497
Shaw P. J., Tononi G., Greenspan R. J., Robinson D. F. (2002). Stress response genes protect against lethal effects of sleep deprivation in drosophila. Nature 417, 287–291. doi: 10.1038/417287a
Sprogis K. R., Videsen S., Madsen P. T. (2020). Vessel noise levels drive behavioural responses of humpback whales with implications for whale-watching. Elife 9, e56760. doi: 10.7554/eLife.56760
Stamation K. A., Croft D. B., Shaughnessy P. D., Waples K. A., Briggs S. V. (2010). Behavioral responses of humpback whales (Megaptera novaeangliae) to whale-watching vessels on the southeastern coast of Australia. Mar. Mammal Sci. 26, 98–122. doi: 10.1111/j.1748-7692.2009.00320.x
Steckenreuter A., Harcourt R., Möller L. (2011). Distance does matter: close approaches by boats impede feeding and resting behaviour of indo-pacific bottlenose dolphins. Wildl. Res. 38, 455–463. doi: 10.1071/WR11048
Steiner L., Pérez M., der Van Linde M., Freitas L., Santos R., Martins V., et al. (2015). “Long distance movements of female/immature sperm whales in the north Atlantic,” in: Poster, Proceedings of the 21st Biennial Conference of the Society for Marine Mammalogy, San Francisco, USA, December 13-18 2015.
Taylor B. L., Baird R., Barlow J., Dawson S. M., Ford J., Mead J. G., et al. (2019). Physeter macrocephalus (amended version of 2008 assessment). List Threat. Species 2019, e.T41755A160983555. doi: 10.2305/IUCN.UK.2008.RLTS.T41755A160983555.en
Villagra D., García-Cegarra A., Gallardo D. I., Pacheco A. S. (2021). Energetic effects of whale-watching boats on humpback whales on a breeding ground. Front. Mar. Sci. 7. doi: 10.3389/fmars.2020.600508
Visser F., Hartman K. L., Rood E. J. J., Hendriks A. J. E., Zult D. B., Wolff W. J., et al. (2011). Risso’s dolphins alter daily resting pattern in response to whale watching at the Azores. Mar. Mammal Sci. 27, 366–381. doi: 10.1111/j.1748-7692.2010.00398.x
Watwood S. L., Miller P. J. O., Johnson M., Madsen P. T., Tyack P. L. (2006). Deep-diving foraging behaviour of sperm whales (Physeter macrocephalus). J. Anim. Ecol. 75, 814–825. doi: 10.1111/j.1365-2656.2006.01101.x
Whitehead H. (2003). Sperm whales. social evolution in the ocean (Chicago; London: University of Chicago Press).
Williams R., Ashe E. (2007). Killer whale evasive tactics vary with boat number. J. Zool. 272, 390–397. doi: 10.1111/j.1469-7998.2006.00280.x
Williams T. M., Davis R. W., Fuiman L. A., Francis J., Le Boeuf B. J., Horning M., et al. (2000). Sink or swim: strategies for cost-efficient diving by marine mammals. Sci. (80-.). 288, 133–136. doi: 10.1126/science.288.5463.133
Wilson R. P., Börger L., Holton M. D., Scantlebury D. M., Gómez-Laich A., Quintana F., et al. (2020b). Estimates for energy expenditure in free-living animals using acceleration proxies: A reappraisal. J. Anim. Ecol. 89, 161–172. doi: 10.1111/1365-2656.13040
Wilson M. W., Ridlon A. D., Gaynor K. M., Gaines S. D., Stier A. C., Halpern B. S. (2020a). Ecological impacts of human-induced animal behaviour change. Ecol. Lett. 23, 1522–1536. doi: 10.1111/ele.13571
Wilson R. P., White C. R., Quintana F., Halsey L. G., Liebsch N., Martin G. R., et al. (2006). Moving towards acceleration for estimates of activity-specific metabolic rate in free-living animals: the case of the cormorant. J. Anim. Ecol. 75, 1081–1090. doi: 10.1111/j.1365-2656.2006.01127.x
Wisniewska D. M., Johnson M., Teilmann J., Siebert U., Galatius A., Dietz R., et al. (2018). High rates of vessel noise disrupt foraging in wild harbour porpoises (Phocoena phocoena). Proc. R. Soc B Biol. Sci. 285, 20172314. doi: 10.1098/rspb.2017.2314
Wood S. N. (2017). Generalized additive models: An introduction with r. 2nd ed (Boca Raton, FL, USA: Chapman and Hall/CRC). doi: 10.1201/9781315370279
Keywords: Physeter macrocephalus, disturbance, whale watching, foraging and diving effort, locomotion effort, resting and socializing
Citation: Oliveira C, Pérez-Jorge S, Prieto R, Cascão I, Wensveen PJ and Silva MA (2022) Exposure to whale watching vessels affects dive ascents and resting behavior in sperm whales. Front. Mar. Sci. 9:914397. doi: 10.3389/fmars.2022.914397
Received: 06 April 2022; Accepted: 12 October 2022;
Published: 02 November 2022.
Edited by:
Jorge M. Pereira, University of Coimbra, PortugalReviewed by:
Marta Guerra, University of Otago, New ZealandLadd M. Irvine, Oregon State University, United States
Fleur Visser, University of Amsterdam, Netherlands
Copyright © 2022 Oliveira, Pérez-Jorge, Prieto, Cascão, Wensveen and Silva. This is an open-access article distributed under the terms of the Creative Commons Attribution License (CC BY). The use, distribution or reproduction in other forums is permitted, provided the original author(s) and the copyright owner(s) are credited and that the original publication in this journal is cited, in accordance with accepted academic practice. No use, distribution or reproduction is permitted which does not comply with these terms.
*Correspondence: Cláudia Oliveira, Y2xhdWRpYS5pYi5vbGl2ZWlyYUB1YWMucHQ=
†These authors share first authorship