- 1Red Sea Research Centre (RSRC), King Abdullah University of Science and Technology, Thuwal, Saudi Arabia
- 2Centre of Marine Sciences (CCMAR), University of Algarve, Faro, Portugal
- 3Computational Bioscience Research Center (CBRC), King Abdullah University of Science and Technology, Thuwal, Saudi Arabia
A soundscape is the recording of all sounds present in an area, creating a holistic view of the acoustic profile in an ecosystem. Studying acoustic parameters of marine soundscapes as a whole has been shown to give an indication of the health status of the location, as well as correlate to which species may be present and using the area. With the rapid innovation of technology, especially data storage and declining cost of equipment, marine soundscape research is fast increasing, and these previous limitations have been switched for computing capacity for data analysis. Here, we perform a systematic assessment of literature of marine soundscape studies, from 1978, when the first soundscape study was reported, until 2021. We identified 200 primary research studies that recorded soundscapes and captured their geographical location, depth, habitat, duration of the study, and number of sites in each study. Using this data, we summarize the state of play in marine soundscapes studies, and identify knowledge gaps in the spatial coverage, depth profiles, habitat representation and study duration. Spatially, studies are biased towards the northern hemisphere. They are also more prevalent in more easily accessible ecosystems, in order from most to least studied, in coastal (38%), pelagic (20%), tropical coral reef (17%), rocky reef (7%), polar (5.5%), seagrass meadows, oyster reef and kelp/algal forest (<5% each) areas, with zones of cold-water coral the least studied (0.3%). Continuing the trend of accessibility, studies also tended to focus on shallow ecosystems. Most recordings (68%) were conducted in the upper 50 m, with 13% in 50-200 m depths, and only 0.6% at a depth >4000 m. With anthropogenic noise and other pollution sources increasing globally, these gaps in research should be further addressed, especially as they pertain to vulnerable ecosystems, many of which are affected by global climate change and anthropogenic influences. It is crucial that marine soundscape studies continue to be developed and pursued, to establish baselines for healthy ecosystems and/or document recovery following management actions.
Introduction
A soundscape is the result of a holistic recording of all acoustic activities present within an ecosystem or area of biological, geological, or man-made (anthropological) origin (Southworth, 1967; Schafer, 1969). Soundscapes, therefore, contain a fingerprint of biological, human and geological activities and dynamics in the ecosystem, which can be retrieved from analyses of their acoustic patterns and spectral characteristics to provide an integrative metric of the status, dynamics and health of an ecosystem (Pijanowski et al., 2011). Terrestrial soundscapes have been a focus of environmental study for over 40 years (Truax, 1978) as proxies for ecosystem health, however it is only recently that acoustic soundscape studies have moved underwater in earnest, where similar indices have been seen to discover parallel trends (Bertucci et al., 2016).
Owing in great part to the vast distances potentially travelled by underwater sound compared to any other cue, chemical or optical, soundscapes studies are particularly useful in marine ecosystems, providing the important role of sound on the ecology of marine organisms (Harris and Radford, 2014; Duarte et al., 2021). Advances in acoustic-recording technology have allowed scientists to characterize soundscapes for longer periods of time and at greater depths than previously possible (Howe et al., 2019). In parallel, new tools for data-rich soundscape analysis have emerged, facilitating both species-specific call recognition and summative approaches to analyze the entire acoustic spectrum, yielding important insights. For example, coastal habitats often have distinct acoustic signatures (Radford et al., 2010) that are used by a range of larval organisms to locate and select suitable settlement habitats (Simpson et al., 2005; Montgomery et al., 2006; Vermeij et al., 2010). Though methods for soundscape analyses continue to be improved to address their inherent complexities (Mckenna et al., 2021), underwater sound recording is an easily obtained metric that complements other ecosystem health measurements (Staaterman et al., 2017 Peck et al., 2021). Soundscape monitoring allows for long-term detection of change in a habitat, offering potential to contribute to future ecosystem monitoring and conservation research (Mooney et al., 2020).
Underwater, sound propagates approximately five times faster than in air, and is integral to marine species’ activities such as communication, navigation, and foraging. Larval fish have been found to use the sound created on reefs to navigate after pelagic development back to their home reef (Simpson et al., 2005). Other marine ecosystems in which soundscape studies have been conducted include rocky reefs, seagrass meadows, kelp/algal forests, oyster reefs, estuarine zones, mangrove forests and deep-sea zones such as canyons and seamounts. Marine soundscapes in the Anthropocene are now unavoidably punctuated with man-made noise such as pile-driving, deep-sea mining, seismic testing, and shipping noise (Miksis-Olds and Nichols, 2016; Howe et al., 2019; Duarte et al., 2021) which have been shown to have detrimental effects on marine life (Popper et al., 2003; Williams et al., 2015; Duarte et al., 2021). The low frequency nature of these sounds enables them to travel long distances underwater, rendering them prevalent components of contemporary marine soundscapes.
Some common acoustic indices to reduce the complexity of soundscape data, (candidates to provide proxy for ecosystem health and biodiversity), are the Acoustic Complexity Index (Pieretti et al., 2011), Broadband or overall sound pressure levels, Third Octave Levels, and distinguishing characteristic frequencies and spectra of specific marine species (Au and Hastings, 2008). For example, higher sound-pressure levels in low frequencies in coral reef ecosystems have correlated positively with visual measures of fish diversity, coral cover, and invertebrate abundance (Kennedy et al., 2010; Bertucci et al., 2016; Staaterman et al., 2017; Peck et al., 2021). However, these indices also require a degree of prior knowledge on the sound origins and characteristics, and are therefore complementary to traditional monitoring methods (Staaterman et al., 2017). Indeed, degraded marine ecosystems have been shown to exhibit simplified soundscapes (Butler et al., 2016; Gordon et al., 2018). A successful acoustic index of marine ecosystem health should be positively correlated with traditional measurements of species’ assemblages, be robust to changes in spectral and temporal resolution, and cope with potential interference by both natural (e.g., wind) and anthropogenic noise (Bohnenstiehl et al., 2018 Howe et al., 2019). Robust acoustic indices derived from ocean soundscapes may serve multiple purposes, such as provide targets for acoustic restoration of healthy soundscapes (Gordon et al., 2019), monitor illegal fishing where satellite-based methods fail (e.g., at night-time, by small vessels, or in remote locations), and monitor impacts of human activities such as climate change, habitat loss, overfishing and pollution events.
Because of the unique nature of sound propagation underwater, sound generated at the surface can travel to the deepest part of the ocean (Dziak et al., 2017). Studying ecosystems at extreme depths is complex, and benthic assessment of these areas using sound is an invaluable tool with no impact on the surrounding environment (Dziak et al., 2017). However, although sounds may propagate to the deep sea, the study of biological sounds originating in surface waters is not effective from deep sea recording, with the sound levels (decibels, or dB) greatly reduced, and therefore recordings offer a much lower spectral resolution for analysis than recordings closer to the source.
It is crucial that marine soundscape studies continue to be developed and pursued, in order to establish baselines for healthy ecosystems and/or document recovery following management actions (Lamont et al., 2021). With the rapid innovation of technology, especially data storage and declining cost of equipment, marine soundscape research is rapidly increasing. Soundscapes are typically recorded using underwater microphones, i.e. hydrophones, which are becoming more available for personal not only commercial use. It has also allowed the duration of recordings to greatly increase, delivering huge volumes of data. The duration of studies has also increased with higher connectivity between sites and availability of equipment, allowing patterns to be observed over years to months. The baselines of these soundscapes, and continued survey are vital in determining changes caused by anthropogenic influence.
Here we present an assessment of published marine soundscape studies, the habitats surveyed and their depth distribution, to assess the growth of marine soundscape assessments and detect research gaps that need to be targeted to derive a more comprehensive understanding of marine soundscapes. We do so based on a systematic assessment of literature, from 1978, when the first soundscape study was reported, to 2021.
Methods
We systematically searched the records of the Web of Science to retrieve the studies focusing on marine soundscapes, using the PRISMA method (Figure 1) (Moher et al., 2015). To detect those studies, we used a topic string on February 8th 2021: (Ocean* AND soundscape* OR marine AND soundscape* OR marine AND chorus* OR marine AND biodiversity AND bioacoustic* OR marine AND sound AND production AND pattern*). The string resulted in 492 articles published between 1900 and 2021.
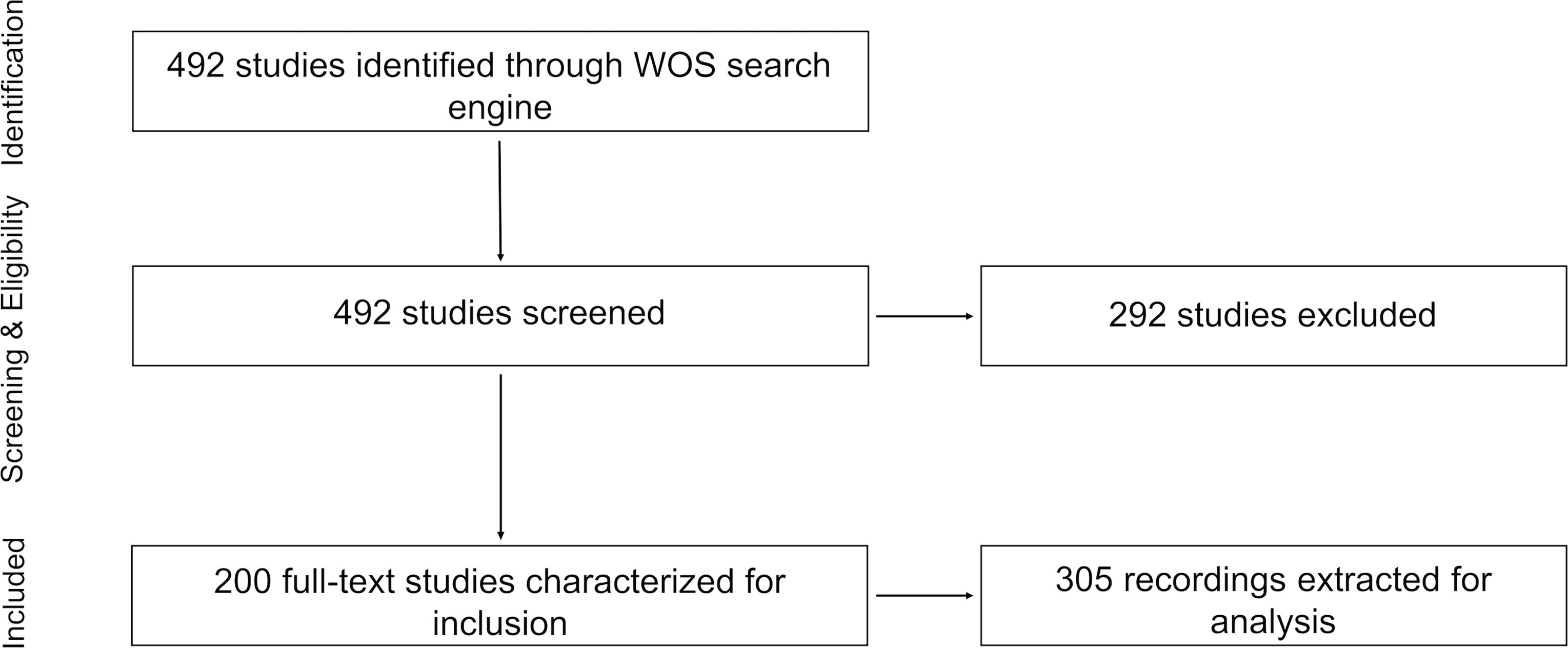
Figure 1 PRISMA diagram (Moher et al., 2015) of meta-analysis process.
Identifying Relevant Papers
Studies were only screened if they were full-text, original and peer-reviewed, and focused on marine soundscapes. We define a marine soundscape study, as that which records all sonic aspects of a marine ecosystem or area. All studies with ambient in-situ marine recordings were included. However, if a study focused solely on a specific sonic quality (e.g. the call of a single species), or on sound propagation modelling, without taking into account the entire soundscape, it was excluded. Ex-situ experiments, recordings from rivers upstream of an estuary and terrestrial recordings above water near a marine ecosystem, were also not included. A large number of studies captured by our search string were not relevant, since they focused on acoustic telemetry. Following this criteria 200 papers in total were eligible for assessment, and 292 were excluded (Moher et al., 2015).
Information Extraction and Categorization
From each eligible study, the following information categories were extracted: location (latitude and longitude), depth of sound measurements, habitat type, duration of recordings, and number of sites. If the exact geographic coordinates were not specified in the paper, the approximate location was used. The depth of sound measurements was divided into five categories: 0-50 m, 50-200 m, 200-1000 m, 1000-4000 m, >4000 m, based on ecosystem characteristics. Habitat type was divided into eleven different categories including: tropical coral reef, rocky reef, oyster reef, cold water coral reef, mangrove, seagrass meadow, kelp/algal forest, estuary, polar (ice cover), pelagic, and coastal. Habitats were selected as the area where the recordings were taken (Table 1). The duration of recordings was defined as the length of the entire study and divided into five categories: minutes, hours, days, months and years (e.g. a study recording one hour per day for several months in the same location was classified as “months”). When studies contained multiple geographic locations, and/or depth ranges, and/or habitat types, each category was documented separately and called a recording. This means that each study can have more than one recording. If a recording performed in a certain habitat type and at a certain depth had several different locations of soundscape measures (i.e. the front and the back of a reef), the number of those locations were captured and called sites. Following this procedure, from 200 studies, we extracted 305 recordings and 991 sites in total (Figure 1).
Results
The first study focusing on underwater soundscapes was published in 1978, describing marine animals’ choruses in the tropical waters of Australia (Cato, 1978). Twenty-eight years later, in 2006, a study focusing on a seagrass meadow in northern Sicily, including its soundscape, was published (Hermand, 2006). Between the years 2010 and 2012 one to two soundscape studies were published annually, increasing to six publications in 2013, and since then, the number of soundscape studies increased rapidly peaking at 30 papers in 2016 (Figure 2A).
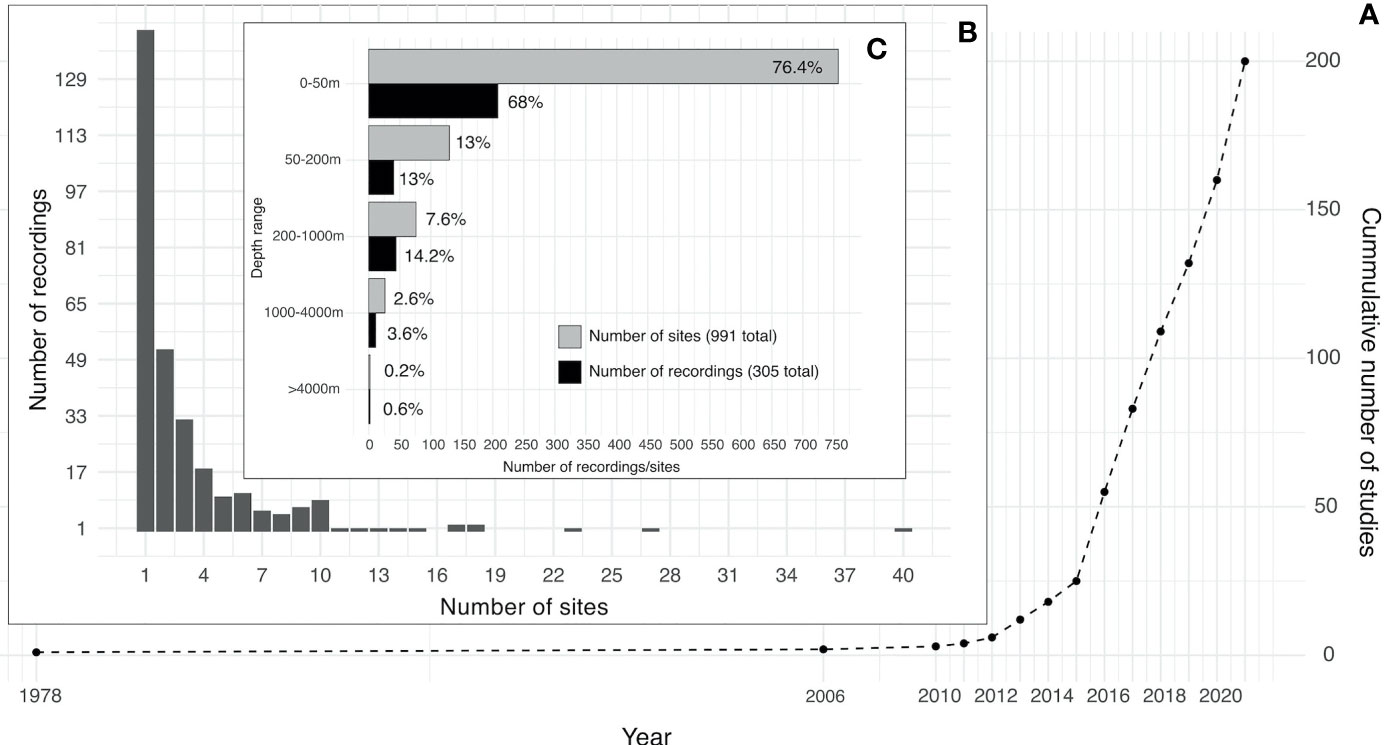
Figure 2 (A) Cumulative number of studies published on ocean soundscapes through time (see Supplementary Material for data sources). (B) Number of recordings extracted from all the studies versus their number of sites. (C) Number of recordings and their sites in different depth ranges.
Number of Sites
Most recordings had only one site per recording (140, Figure 2B). The number of recordings with between two and ten sites ranged between two and 36 recordings, and recordings with more than ten sites were very few and sporadic. Most recordings (68%) were conducted in the upper 50 m, with 13% in 50-200 m depths, followed by 14.2% in the mesopelagic region (200-1000 m), 3.6% in the bathypelagic zone (1000-4000 m) and only 0.6% (two recordings) at a depth >4000 m. In total, there were 757 sites out of 991 (76.4%) in the upper 50 m of depth, 130 sites (13%) in 50-200 m depths, 76 sites in the mesopelagic region (200-1000 m), 26 sites in the bathypelagic zone (1000-4000 m), and two sites at depth >4000 m (Figure 2C).
Spatial distribution of soundscape recordings in the upper 50 m of the ocean shows that the most represented regions were the East coast of the United States, the Caribbean and most of Western and Southern European countries (Figure 3). Accordingly, these regions also had the highest number of sites, with the maximum number of sites (40) on the West coast of Florida. Less represented, but still with significant effort were Canada, the West coast of the United States, the Pacific Islands, Brazil, Australia, New Zealand, and Southeast Asia. The regions with very low research effort were the Arctic, Antarctica, Africa, South America (except Brazil), and the Middle East (Figure 3). Similar distribution of effort was found on depths lower than 50 m, but with much less spatial coverage and lower number of sites. The maximum number of sites (17) was recorded on the Canadian Arctic Archipelago at depths between 200 m and 1000 m (Figure 4). Two sites measured soundscapes at depths deeper than 4000 m, one in the abyssal plain around the Minamitorishima Island in Japan (5500 m), and the other one in one of the deepest parts of the ocean, the Mariana Trench (10,829.7 m) (Figure 4).
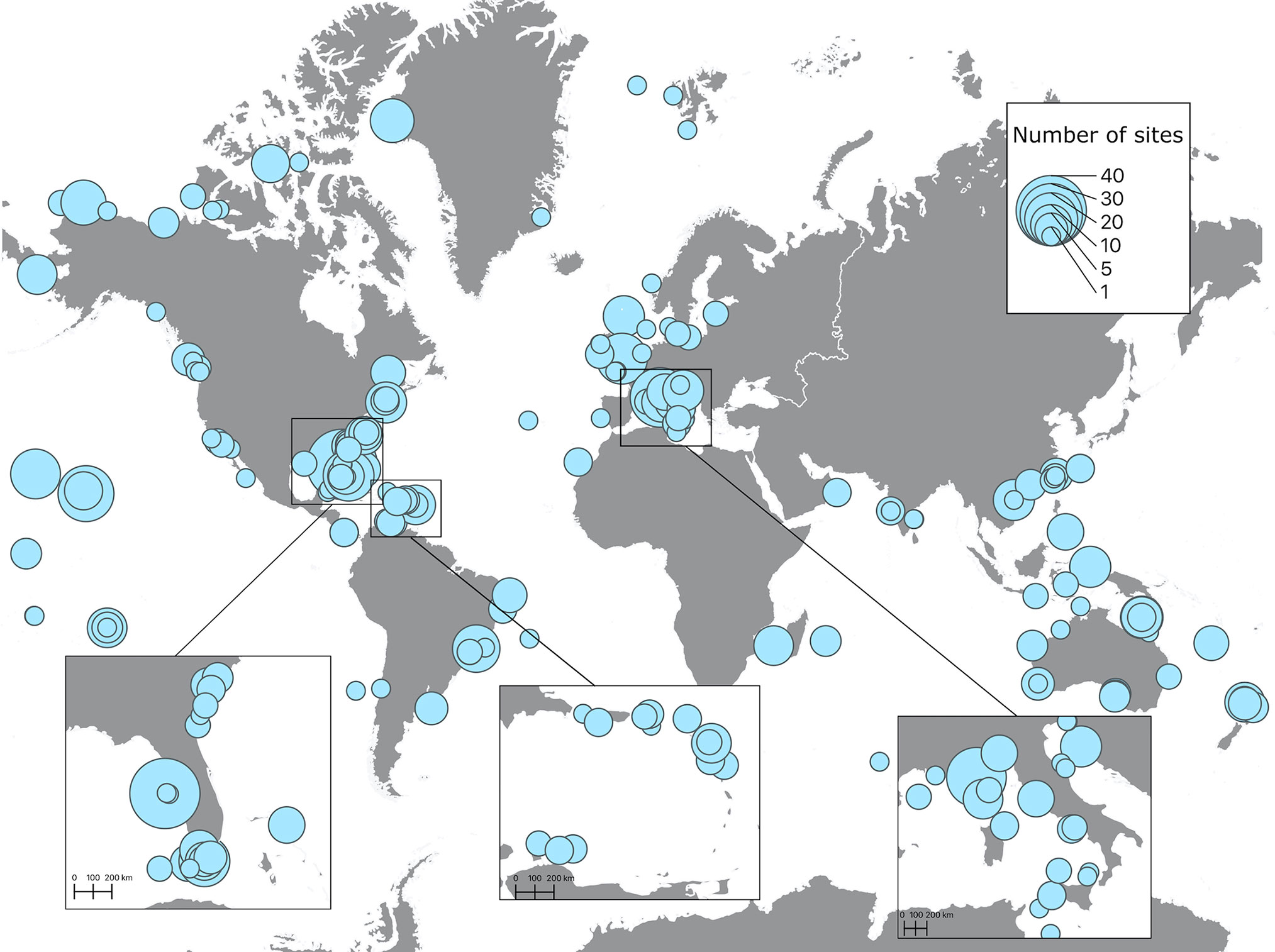
Figure 3 Spatial distributions of recordings extracted from published studies on ocean soundscapes (see Supplementary Material for data sources) recorded between 0 and 50 m depth. Points on the map represent areas where underwater recordings were made, with the size of the point being proportional to the number of sites of each recording.
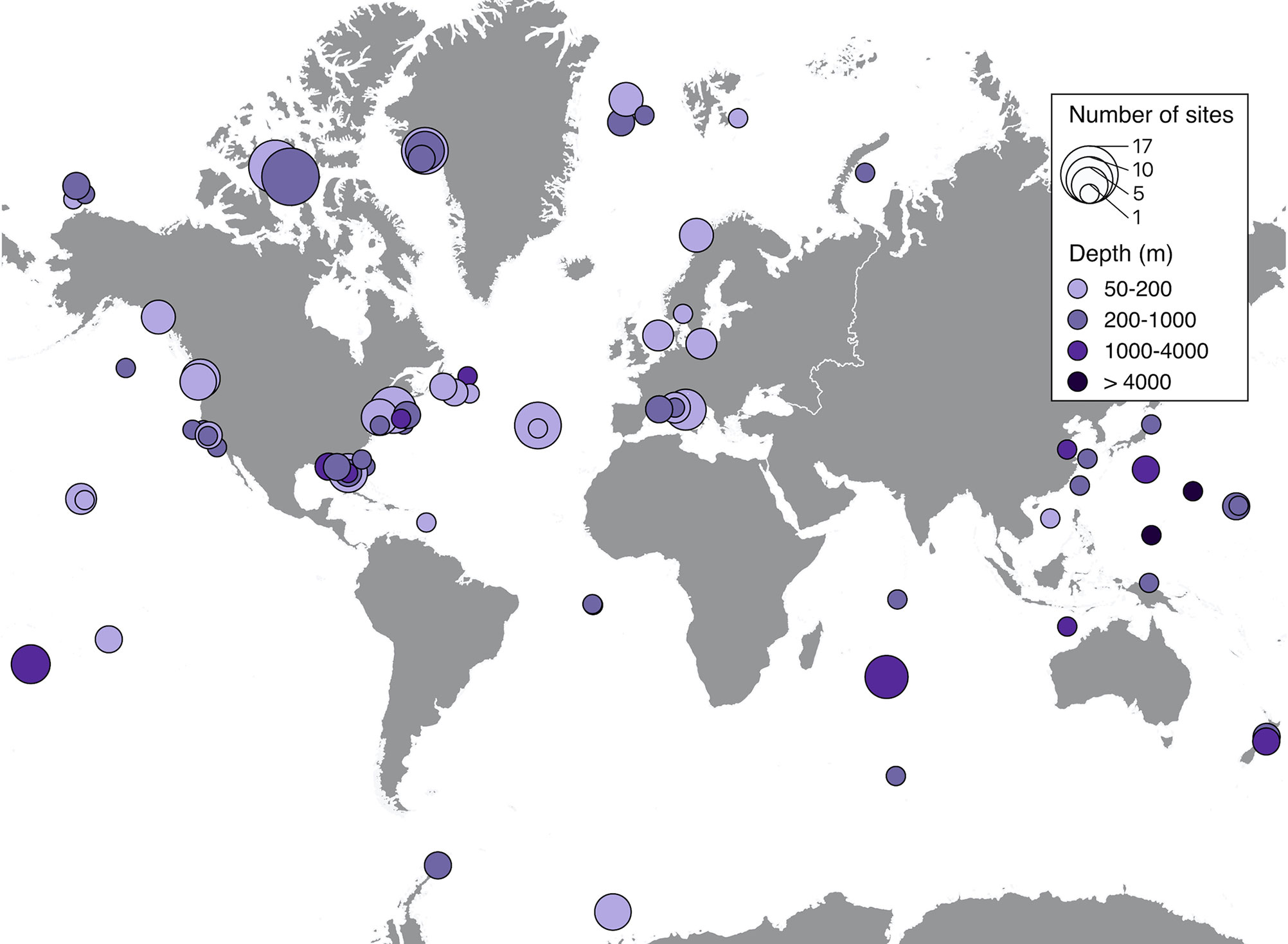
Figure 4 Spatial distributions of recordings extracted from published studies on ocean soundscapes (see Supplementary Material for data sources) recorded at depths greater than 50 m. Closed circles on the map represent areas where soundscape recordings were obtained, with the size of the symbol being proportional to the number of sites of each recording, and the color indicating the depth range of the recording.
Out of the 305 recordings we extracted from 200 studies, most (38%) were conducted in coastal habitats (Figure 5, cf. Table 1). Recordings focusing on pelagic habitats and tropical coral reefs received a similar effort, 20% and 17% of the total volume, respectively. This contrasts with underrepresented habitats including rocky reefs (7%), polar (5.5%), and seagrass meadow, oyster reef, and kelp/algal forest habitats (<5% of effort, Figure 5). Cold-water coral reef soundscapes were measured only in one location, a marine protected area that connects Norway and Sweden (De Clippele and Risch, 2021), accounting for 0.3% of the total volume (Figure 5).
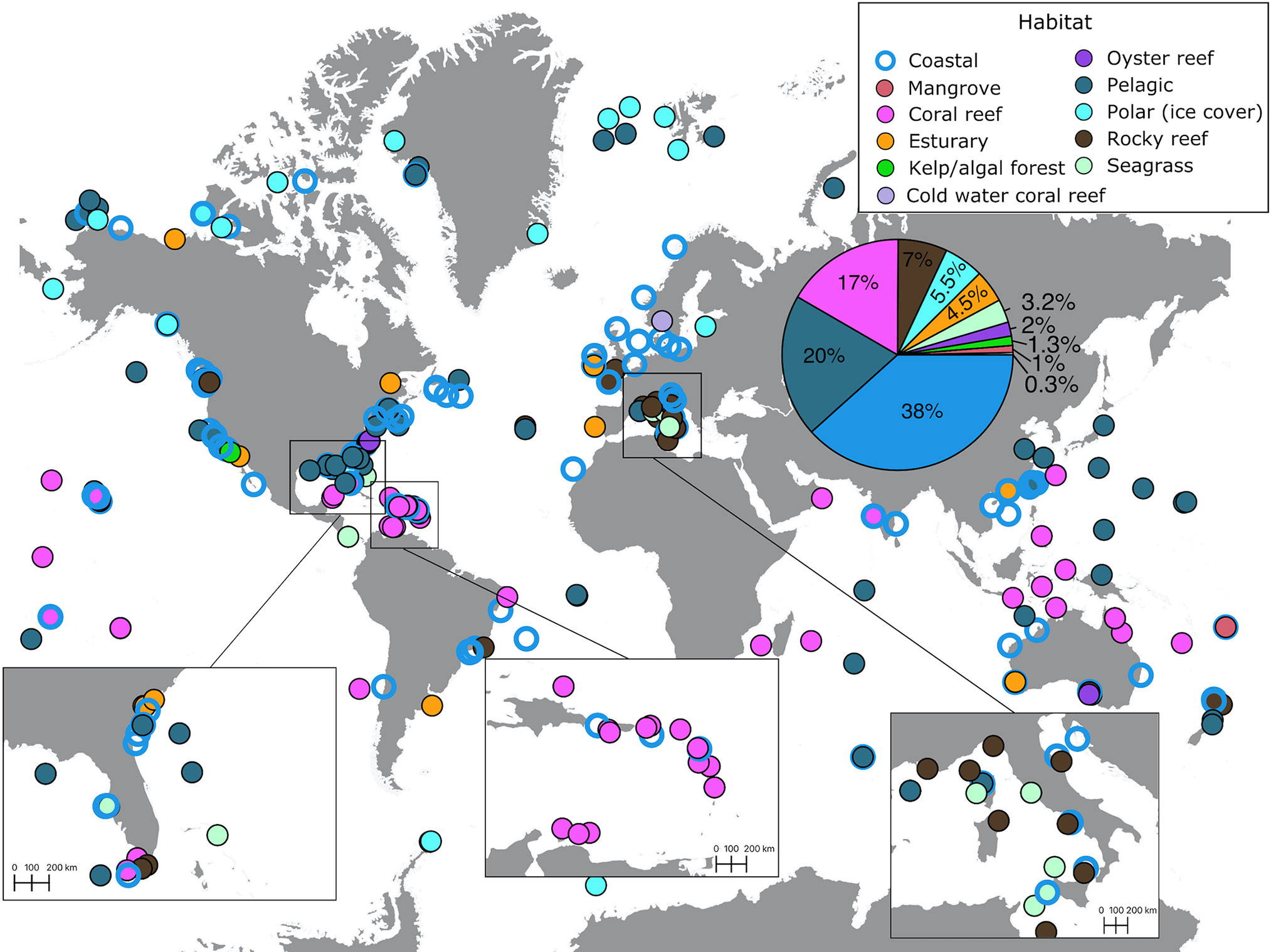
Figure 5 Distribution of habitats where soundscape recordings have been reported (see Supplementary Material for data sources). The pie chart in the insert indicates the percentage of recordings (out of 305 recordings) conducted in different habitats.
Most soundscapes were recorded during a span of a couple of months (40%, Figures 6, 7). Close to 30% of recordings were lasting several days, and around 25% were conducted over a period of several years (Figure 6). A small percentage of recordings were lasting several hours (7.2%) and minutes (1.3%). Longer recordings (days to years) were mostly concentrated in the United States, the Caribbean, Europe, Australia, and New Zealand. Shorter recordings (hours and minutes) were carried out across the world but tended to be the only recordings conducted in underrepresented regions, such as the West coast of South America, Africa, and the Arabian Peninsula (Figure 6). The duration of recordings increased throughout the years, especially in 2016 onwards, extending to months and years (Figure 7A).
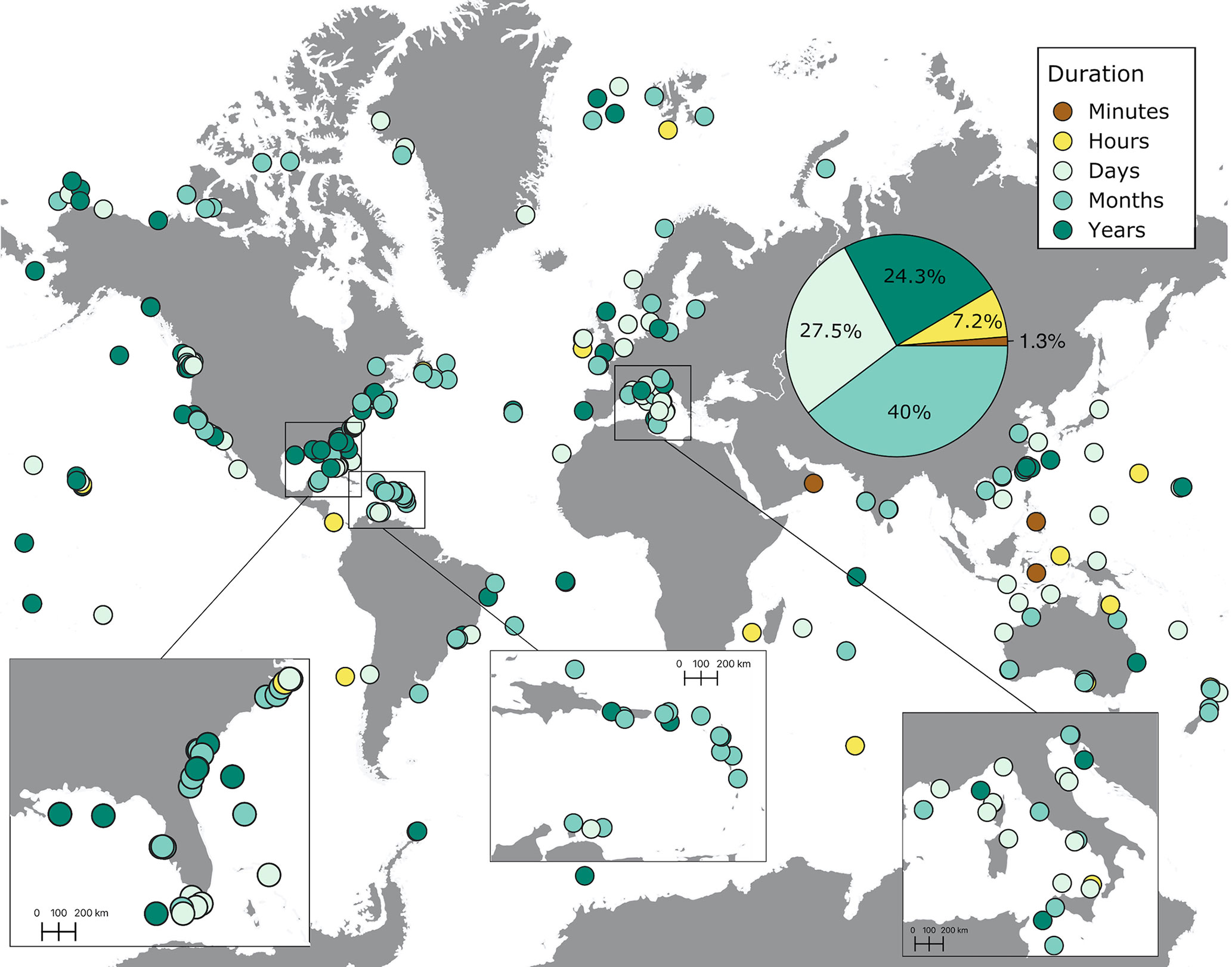
Figure 6 The distribution of the duration of soundscape recordings extracted from published studies on ocean soundscapes (see Supplementary Material for data sources). The pie chart shows the percentage of recordings (out of 305 recordings) conducted for different lengths of time. The duration represents the time elapsed from the first to the end of the last recording in each site, regardless of the frequency of recording within the time period covered.
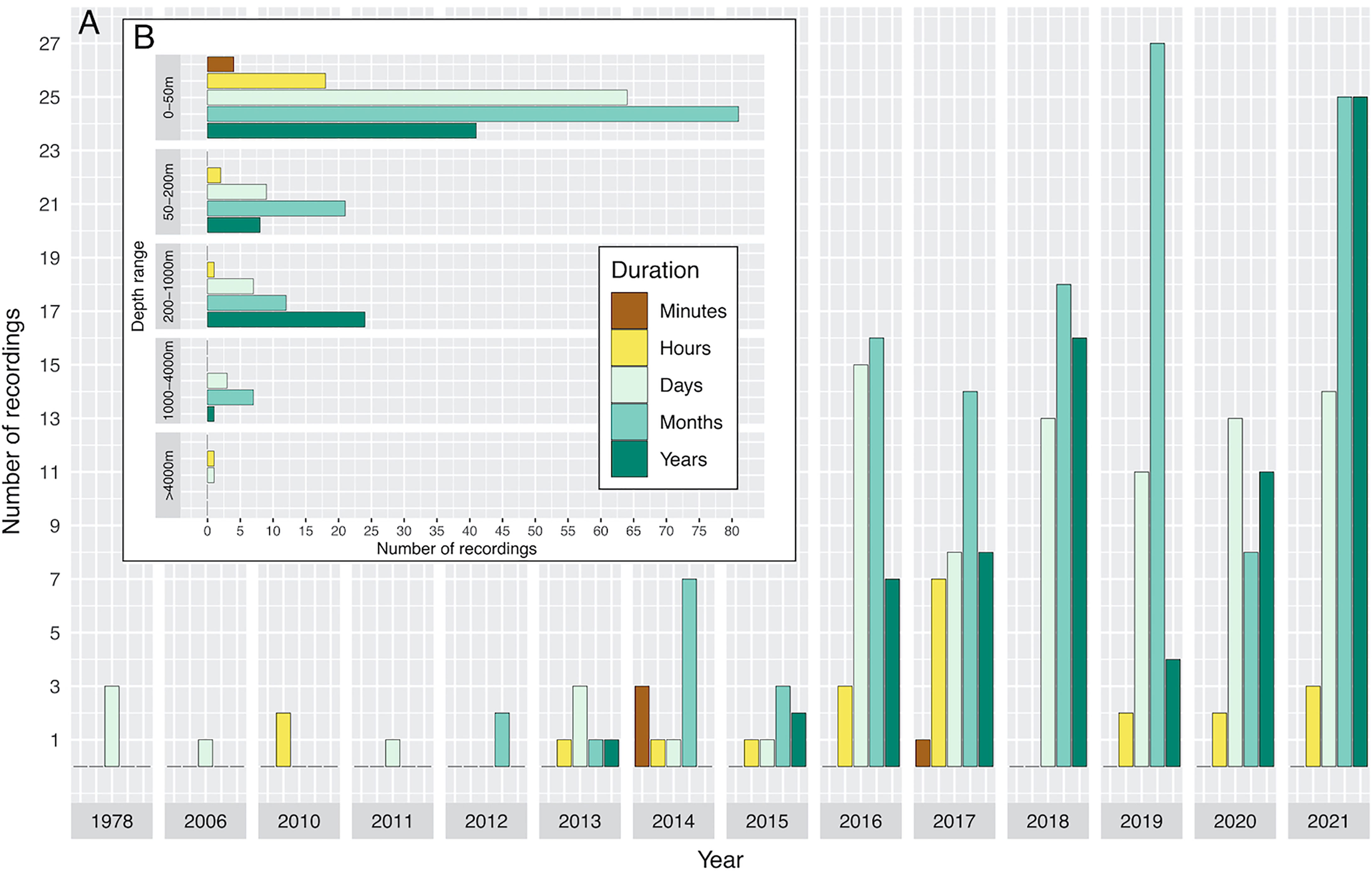
Figure 7 Temporal distribution of the duration of recordings extracted from published studies on ocean soundscapes (see Supplementary Material for data sources) indicating the year in which the study was carried out, and how long the study was undertaken for (A). (B) shows the depth range of recordings versus the length of time studied.
An overall decrease of published recordings was evident in 2020 with publication effort raising again by February 2021 (where this metanalysis dataset ends) (Figure 7A). In the upper 200 m depth, the most frequent durations of measurements were months, followed by days, years, hours, and minutes respectively (Figure 7B). On depths between 200 and 1000 m, most recordings lasted several years, followed by months, days, and hours. Between 1000 and 4000 m several recordings were carried out for several months, and few over the course of several days and years. The abyssal ocean (>4000 m) was recorded in only two studies, lasting several hours and days (Figure 7B).
Discussion
Research efforts to record and analyze entire soundscapes have increased over the past 50 years, with an exponential rise beginning at the turn of the century (Figure 2), in line with research on anthropogenic noise in the ocean (Duarte et al., 2021). The first marine soundscape study was undertaken in 1978 (Cato, 1978), followed by a large gap until 2006, attributed to the lack of affordable and accessible technology at the time. An increase occurred in earnest after 2010, with a peak in studies occurring with 30 papers in 2016, accompanied with technological and methodological advancements. The discovery that soundscapes can represent integrative proxies of ecosystem health and human pressures (Pijanowski et al., 2011; Harris and Radford, 2014; Bohnenstiehl et al., 2018), in great part contributed to this increase.
Learning From Disruption
The decrease of reported soundscapes in 2020 corresponds with the disruptions on field studies derived from confinement measures during the beginning of the COVID pandemic, which has greatly impacted on field research (Bates et al., 2020), but also provided opportunities to learn on the impacts of human activity on ocean soundscapes (Rutz et al., 2020). This decline is unfortunate, as previously unimaginable in an ever increasingly loud ocean, with levels rising by 3.3 dB since 1950 (Frisk, 2012), the mass pause in human activity and marine traffic in 2020-2021 gave an unprecedented look into how the ocean would sound without anthropogenic influence (Bates et al., 2021). Soundscape recordings that continued into this period reported an overall reduction in noise, especially in low-frequencies associated with vessel noise (Basan et al., 2021; De Clippele and Risch, 2021; Gabriele et al., 2021; Ryan et al., 2021) with one study showing a reduction in noise from terrestrial traffic influence (Leon-Lopez et al., 2021), owing mainly to the widespread border closures and lock-downs put in place during the pandemic. In one case, low-frequency sound pressure decreased by up to 13% (1.2 dB) in an area of usual high traffic (Basan et al., 2021). These insights support the notion that hydrophone recordings should be part of monitoring systems, and should be designed to gain in autonomy, so they can continue to operate unassisted when disruptive events, such as the pandemic, impacts on human availability to operate the systems.
Proximity Means More Studies
The most studied ecosystem soundscapes are sandy-bottom coastal, pelagic, and tropical coral reef ecosystems (between 17-38% of studies each). Coastal ecosystems, due to their proximity to humanity, including research labs, are both easier to study and rank amongst the most influenced by anthropogenic noise. Furthermore, increasing intensity of storms in coastal ecosystems, will require acoustic baseline indices to aid in restoration efforts (Michener et al., 1997).
As one of the most visually drastic changing ecosystems, coral reefs also garner a significant amount of studies (17%, Figure 5). Impoverished reefs have been paired with quieter, less complex soundscapes (Gordon et al., 2018), so this monitoring tool is an essential key ecosystem variable, which can provide a collective metric of habitat simplification with increasing impacts of climate change, including acidification and ocean warming.
Vulnerable Shallow Soundscapes
Forming similar sized gaps in research, in order from least to most studied, are the ecosystems of cold-water coral reefs, kelp/algal forests, mangroves, seagrass meadows, polar (ice-cover), estuaries, and rocky reefs (between 0.3-7% of studies each). This is a surprising paucity of records provided the important role of all these (mostly benthic) habitats in supporting biodiversity. Tropical coral reef soundscape studies have shown that in addition to reflecting ecosystem health (Gordon et al., 2018; Peck et al., 2021) soundscapes play a key role in guiding recruitment of larvae and juvenile organisms to these habitats where they will spend their adult lives (Simpson et al., 2005; Montgomery et al., 2006; Vermeij et al., 2010), suggesting that exploring the soundscapes of these and other neglected coastal habitats will greatly accelerate our understanding of their heath and restoration potential.
Mangroves, kelp and seagrasses are all considered ecosystem engineers, biogenic habitat providers, are important in coastal processes and are pivotal to carbon sequestration (Alongi, 2012; Fourqurean et al., 2012; Krause-Jensen et al., 2018), yet only 1%, 1.3% and 3.2% respectively of all soundscape studies focus on them. Acoustic monitoring could provide a solution to the observation of the remote and inaccessible areas where these habitats commonly thrive.
Kelps are some of the most diverse and productive habitats globally (Teagle et al., 2017), amounting, in global area and productivity, to the Amazonian forest [Duarte et al., 2022 (in press)]. Kelp forests create biogenic habitat structures supporting secondary productivity, and provide billions of dollars of essential ecosystem services to humanity (Beaumont et al., 2008). They are key players in blue carbon capture and climate control (Krause-Jensen et al., 2018). The current limited studies on algal/kelp forest soundscapes indicate correlations between acoustic parameters and the state of the ecosystem (Gottesman et al., 2020; Butler et al., 2021), as well as any regime shifts occurring (Rossi et al., 2017).
Seagrass ecosystems, stretching from Tropical-Polar regions, support a major global blue carbon stock (Fourqurean et al., 2012),and can be considered “coastal canaries” (Krause-Jensen et al., 2018), being one of the first to show decline in deteriorating coastal conditions. They are in serious threat by deleterious effects of climate change, such as regression of surface area (Chefaoui et al., 2018), making it imperative to monitor changes. Seagrass habitats often co-occur with other habitats such as mangroves, tropical coral and rocky reefs (Butler et al., 2016; Staaterman et al., 2017; Lyon et al., 2019). In the few studies available on seagrass meadow soundscapes, it is clear that acoustics play a large role in their ecological importance. Certain fish may dominate seagrass meadow soundscapes during calling (Staaterman et al., 2017; Boyd et al., 2021) potentially spanning months, such as in Posidonia oceanica meadows in the Mediterranean (Di Iorio et al., 2018), and complexity indexes have correlated with biodiversity of fish (La Manna et al., 2021). In other locations and/or seasons, sound pressure levels in seagrass meadows can be lowered, potentially due to the dominant presence of juvenile fish, which are seen to vocalize less frequently (Lyon et al., 2019). Seagrass meadows can also produce lower complexity of soundscape than other adjacent habitats, such as mangrove (Butler et al., 2016) but have higher complexity than sandy bottom habitats (Ceraulo et al., 2018). The bubbles on the foliage created by photosynthesis can also attenuate sounds (Hermand, 2006), creating a potential acoustic refuge for fish from detection by the echolocation of marine mammals (Wilson et al., 2013).
Like seagrass, mangrove ecosystems also represent a significant percentage of tropical coastlines, protecting land from wave pressure and providing high bio-complexity in ecological assemblages (Feller et al., 2010). With 20-25% of the world’s mangroves destroyed in the past 50 years by anthropogenic pressures such as coastal development and sea-level rise (Polidoro et al., 2010) monitoring ongoing changes in pristine and restored areas is of utmost importance to conservation. The three mangrove soundscape studies indicate that like seagrass, mangroves could provide acoustic refuges for certain species with potentially higher attenuation of sounds than adjacent ecosystems (Staaterman et al., 2017), but have alternatively also shown higher sound pressure levels than adjacent areas such as seagrass, due to presence of fish and alpheid shrimp (Butler et al., 2016).
Polar regions, although arguably at the forefront of climate change policy focus, have been largely neglected in holistic soundscape measurements. The soundscape of the polar regions is not only rich with the biophony of marine mammals, invertebrates and fish, found to correlate positively with biodiversity assessments (Roca and Van Opzeeland, 2020; Heimrich et al., 2021), but as ice melts, it produces a recognizable sound signature (Mahanty et al., 2020) allowing for acoustic observation of climate change. Calving of icebergs, and glaciers is also recordable and present in analyses. The melting of polar icecaps was predicted almost 50 years ago (Mercer, 1968), and since then the phenomena is accelerating (Melia et al., 2016; Parkinson, 2019). As the polar oceans rapidly warm and change (Schofield et al., 2010), it is imperative that monitoring continues. Soundscape monitoring is a particularly robust tool for polar regions, where access is cumbersome, particularly in winter. The predicted shift to more kelp dominated areas in polar regions (Krause-Jensen and Duarte, 2014) should also be reflected in soundscape recordings, which could provide an approach to identifying these areas of shift, due to difficulty of access.
Cold-water reefs, although they have been known to exist since the 18th century, have been recently revealed to cover a much larger spatial extent than previously thought (Roberts et al., 2006). Only one soundscape study exists (De Clippele and Risch, 2021) off the coast of Norway and Sweden. These long-lived (often thousands of years old), slow-growing, underwater oases can create 300 m high carbonate pinnacles, and be as wide as kilometers across (Freiwald et al., 2004). Existing at depths between 40 to several hundreds of meters, they are at threat to bottom trawling fishing practices. Although banned in the UK, the destructive practice of bottom trawling is still permitted in many places globally (Freiwald et al., 2004). Another pertinent stressor is rising sea temperatures, as many cold-water corals are currently existing at their thermal thresholds such as in the Mediterranean. Experiments using a 2°C rise, resulted in lower energy reserves and slower growth in cold water corals, and 4°C in death (Chapron et al., 2021).
Sounding Out the Deep to Protect It
Although advances in acoustic-recording technology has allowed scientists to characterize soundscapes for longer periods of time and at greater depths than previously possible, most of all recordings were taken in shallow depths of 0-50 m (68%, Figure 2C) and only 0.2% of soundscape studies represented depths lower than 4000m, and 3.6% of 1000-4000 m. The mesopelagic layer, 200-1000 m, or off the continental shelf is only represented in 14.2% of records, despite it containing the largest stock of fish in the ocean (Irigoien et al., 2014). Below 50 m lie understudied ecosystems, insofar blocked from in-depth research by technological limitation. Hence, available soundscape data is biased toward shallow, nearshore environments. Research on Mesophotic reefs so far show a hugely diverse, and fragile ecosystem (Lesser et al., 2009), that provide fascinating insight into corals adapted to thrive under colder and dimmer light conditions than their shallow counterparts.
Compared with the upper ocean, very limited data is available for the deep sea (Figure 4). Deep-sea ecosystems have traditionally been inaccessible, but as technology advances allowing deep-sea mining, exploration, and deep-sea fishing, they have become one of the ecosystems most vulnerable to change. Evidence has shown with their extremely slow ecosystem turnover and growth-rates, recovery capacity is greatly reduced, and degradation can be potentially irreversible (Clark et al., 2016). Soundscapes hold vital information to these cryptic environments, with larval recruitment and settlement thought to be driven potentially by sonic signatures of thermal vents (Lin et al., 2019). Making these geophonic and biophonic acoustic studies even more critical, is the increasing interference of anthropogenic factors such as shipping and seismic testing (Miksis-Olds and Nichols, 2016; Duarte et al., 2021), which can mask biological sound-cues. Monitoring deep sea soundscapes is likely to produce new insights into deep-sea ecology while also alerting of disruptive practices, such as deep-sea mining, trawling, and drilling.
Duration and Sites
A single site within a marine habitat can have great variation within it. Sound may propagate differently according to the topography and placement of the hydrophone, or biological components may differ affecting the spectral characteristics (Bertucci et al., 2016). Duration, we defined as the length of the study between the first recording in a site and the last. The specific timing of the recording can also change the spectral results, as seasonal and lunar cycles can influence the presence and propagation of biological sound. These cycles can be invaluable to understand for the management of an area and its surrounding waters, and assess future changes responding to altering ambient conditions (Radford, 2008; Staaterman et al., 2014; Ricci et al., 2016; Mcwilliam et al., 2017; Lillis and Mooney, 2018).
A main issue of extended soundscape studies of large areas is that they rely on collaboration, but rarely are protocols universally agreed upon (Howe et al., 2019) making data difficult to share, reproduce, integrate and use. Though preferable to determine changes over time, studies that last years are not as popular as months and days, and month-long studies seem to dominate efforts as technology improves. A push to publish data quickly may also contribute to studies lasting for years being less represented.
The global distribution of sites where soundscape analyses have been conducted is unsurprisingly spatially unbalanced, like most research efforts on oceans that are characteristically biased toward the north hemisphere, where most marine research institutions are located. There is a clear need to extend efforts to areas underrepresented, such as seagrass meadows, kelp/algal forests, polar and deep-sea ecosystems, and an increase in spatial replication and temporal coverage are also required.
Conclusion
With the advancements of the past 50 years, soundscape studies have demonstrated their worth in marine ecosystem characterization, impact detection, and restoration. Looking ahead, to take full advantage of the utility of soundscape studies, there must be more focus on seagrass, kelp and mangrove, polar, cold water coral and deep-sea ecosystems vulnerable to climate change and anthropogenic influence, especially due to their vital role in carbon drawdown [seagrass (Fourqurean et al., 2012), mangrove (Alongi, 2012), polar ecosystems (Schofield et al., 2010) and deep sea ecosystems (Clark et al., 2016)]. More integrative studies to link sound with biodiversity assessments, possibly using emerging technologies such as environmental DNA, for example, will allow sound to become a more efficient, accurate and simpler analytical tool.
On-going monitoring efforts must be made resilient to disruptions while new tools and concepts to analyze them and derive useful metrics and diagnostics are needed. Developing these tools further can draw from developments in big-data and artificial intelligence, such as speech recognition, for automatic classification and simulation of ocean soundscapes, thus enhancing the diagnostic value of ocean soundscapes. These advances in processing will also allow more sites per study. Finally, soundscapes are a non-invasive study that would generate a wealth of information on understudied areas and areas difficult to access, to better inform conservation decisions. In this post-COVID era where we have seen the ecological changes brought about in the absence of anthropogenic sound (Rutz et al., 2020; Bates et al., 2021) we have a unique opportunity to use these results to create adequate quiet zones (see International Quiet Ocean project).
Data Availability Statement
The original contributions presented in the study are included in the article/Supplementary Material. Further inquiries can be directed to the corresponding author.
Author Contributions
M-NH, MP, and CMD contributed to conception and design of the study. M-NH and MP organized the database. M-NH and MP performed the meta-analysis and wrote the first draft of the manuscript. All authors contributed to manuscript revision, read, and approved the submitted version. CMD secured the funding.
Funding
Baseline funding secured by CMD.
Conflict of Interest
The authors declare that the research was conducted in the absence of any commercial or financial relationships that could be construed as a potential conflict of interest.
Publisher’s Note
All claims expressed in this article are solely those of the authors and do not necessarily represent those of their affiliated organizations, or those of the publisher, the editors and the reviewers. Any product that may be evaluated in this article, or claim that may be made by its manufacturer, is not guaranteed or endorsed by the publisher.
Acknowledgments
Reny P. Devassy for help with initial data collection in meta-analysis.
Supplementary Material
The Supplementary Material for this article can be found online at: https://www.frontiersin.org/articles/10.3389/fmars.2022.919418/full#supplementary-material
Supplementary Data Sheet 1 | Data analysis sheet of selected publications.
References
Alongi D. M. (2012). Carbon Sequestration in Mangrove Forests. Carbon Manage. 3, 313–322. doi: 10.4155/cmt.12.20
Basan F., Fischer J.-G., Kühnel D. (2021). Soundscapes in the German Baltic Sea Before and During the Covid-19 Pandemic. Front. Mar. Sci. 8, 828. doi: 10.3389/fmars.2021.689860
Bates A. E., Primack R. B., Biggar B. S., Bird T. J., Clinton M. E., Command R. J., et al. (2021). Global COVID-19 Lockdown Highlights Humans as Both Threats and Custodians of the Environment. Biol. Conserv. 263, 109175. doi: 10.1016/j.biocon.2021.109175
Bates A. E., Primack R. B., Moraga P., Duarte C. M. (2020). COVID-19 Pandemic and Associated Lockdown as a “Global Human Confinement Experiment” to Investigate Biodiversity Conservation. Biol. Conserv. 248, 108665. doi: 10.1016/j.biocon.2020.108665
Beaumont N., Austen M., Mangi S., Townsend M. (2008). Economic Valuation for the Conservation of Marine Biodiversity. Mar. Pollut. Bull. 56, 386–396. doi: 10.1016/j.marpolbul.2007.11.013
Bertucci F., Parmentier E., Lecellier G., Hawkins A. D., Lecchini D. (2016). Acoustic Indices Provide Information on the Status of Coral Reefs: An Example From Moorea Island in the South Pacific. Sci. Rep. 6, 33326. doi: 10.1038/srep33326
Bohnenstiehl D. R., Lyon R. P., Caretti O. N., Ricci S. W., Eggleston D. B. (2018). Investigating the Utility of Ecoacoustic Metrics in Marine Soundscapes. J. Ecoacoustics 2, 1–1. doi: 10.22261/JEA.R1156L
Boyd A. D., Gowans S., Mann D. A., Simard P. (2021). Tropical Storm Debby: Soundscape and Fish Sound Production in Tampa Bay and the Gulf of Mexico. PLoS One 16, e0254614. doi: 10.1371/journal.pone.0254614
Butler J., Pagniello C. M., Jaffe J. S., Parnell P. E., Širović A. (2021). Diel and Seasonal Variability in Kelp Forest Soundscapes Off the Southern California Coast. Front. Mar. Sci. 8, 629643. doi: 10.3389/fmars.2021.629643
Butler J., Stanley J. A., Butler M. J. IV (2016). Underwater Soundscapes in Near-Shore Tropical Habitats and the Effects of Environmental Degradation and Habitat Restoration. J. Exp. Mar. Biol. Ecol. 479, 89–96. doi: 10.1016/j.jembe.2016.03.006
Cato D. H. (1978). Marine Biological Choruses Observed in Tropical Waters Near Australia. J. Acoustical Soc. America 64, 736–743. doi: 10.1121/1.382038
Ceraulo M., Papale E., Caruso F., Filiciotto F., Grammauta R., Parisi I., et al. (2018). Acoustic Comparison of a Patchy Mediterranean Shallow Water Seascape: Posidonia Oceanica Meadow and Sandy Bottom Habitats. Ecol. Indic. 85, 1030–1043. doi: 10.1016/j.ecolind.2017.08.066
Chapron L., Galand P. E., Pruski A. M., Peru E., Vétion G., Robin S., et al. (2021). Resilience of Cold-Water Coral Holobionts to Thermal Stress. Proc. R. Soc. B 288, 20212117. doi: 10.1098/rspb.2021.2117
Chefaoui R. M., Duarte C. M., Serrão E. A. (2018). Dramatic Loss of Seagrass Habitat Under Projected Climate Change in the Mediterranean Sea. Global Change Biol. 24, 4919–4928. doi: 10.1111/gcb.14401
Clark M. R., Althaus F., Schlacher T. A., Williams A., Bowden D. A., Rowden A. A. (2016). The Impacts of Deep-Sea Fisheries on Benthic Communities: A Review. ICES J. Mar. Sci. 73, i51–i69. doi: 10.1093/icesjms/fsv123
De Clippele L. H., Risch D. (2021). Measuring Sound at a Cold-Water Coral Reef to Assess the Impact of COVID-19 on Noise Pollution. Front. Mar. Sci. 712. doi: 10.3389/fmars.2021.674702
Di Iorio L., Raick X., Parmentier E., Boissery P., Valentini-Poirier C. A., Gervaise C. (2018). ‘Posidonia Meadows Calling’: A Ubiquitous Fish Sound With Monitoring Potential. Remote Sens. Ecol. Conserv. 4, 248–263. doi: 10.1002/rse2.72
Duarte C. M., Chapuis L., Collin S. P., Costa D. P., Devassy R. P., Eguiluz V. M., et al. (2021). The Soundscape of the Anthropocene Ocean. Science 371, eaba4658. doi: 10.1126/science.aba4658
Duarte C. M., Gattuso J.-P., Hancke K., Gundersen H., Filbee-Dexter K., Pedersen M. F., et al. (2022). Global Estimates of the Extent and Production of Macroalgal Forests. Global Ecol. Biogeography 32, 1–18. doi: 10.1111/geb.13515
Dziak R. P., Haxel J. H., Matsumoto H., Lau T.-K., Heimlich S., Nieukirk S., et al. (2017). Ambient Sound at Challenger Deep, Mariana Trench. Oceanography 30, 186–197. doi: 10.5670/oceanog.2017.240
Feller I. C., Lovelock C. E., Berger U., Mckee K. L., Joye S. B., Ball M. C. (2010). Biocomplexity in Mangrove Ecosystems. Annu. Rev. Mar. Sci. 2, 395–417. doi: 10.1146/annurev.marine.010908.163809
Fourqurean J. W., Duarte C. M., Kennedy H., Marbà N., Holmer M., Mateo M. A., et al. (2012). Seagrass Ecosystems as a Globally Significant Carbon Stock. Nat. Geosci. 5, 505–509. doi: 10.1038/ngeo1477
Freiwald A., Fossa J. H., Grehan A., Koslow T., Roberts J. M. (2004). Cold-Water Coral Reefs: Out of Sight-No Longer Out of Mind (Cambridge, UK: UNEP-WCMC).
Frisk G. V. (2012). Noiseonomics: The Relationship Between Ambient Noise Levels in the Sea and Global Economic Trends. Sci. Rep. 2, 1–4. doi: 10.1038/srep00437
Gabriele C. M., Ponirakis D. W., Klinck H. (2021). Underwater Sound Levels in Glacier Bay During Reduced Vessel Traffic Due to the COVID-19 Pandemic. Front. Mar. Sci. 736. doi: 10.3389/fmars.2021.674787
Gordon T. A., Harding H. R., Wong K. E., Merchant N. D., Meekan M. G., Mccormick M. I., et al. (2018). Habitat Degradation Negatively Affects Auditory Settlement Behavior of Coral Reef Fishes. Proc. Natl. Acad. Sci. 115, 5193–5198. doi: 10.1073/pnas.1719291115
Gordon T. A., Radford A. N., Davidson I. K., Barnes K., Mccloskey K., Nedelec S. L., et al. (2019). Acoustic Enrichment can Enhance Fish Community Development on Degraded Coral Reef Habitat. Nat. Commun. 10, 1–7. doi: 10.1038/s41467-019-13186-2
Gottesman B. L., Sprague J., Kushner D. J., Bellisario K., Savage D., Mckenna M. F., et al. (2020). Soundscapes Indicate Kelp Forest Condition. Mar. Ecol. Prog. Ser. 654, 35–52. doi: 10.3354/meps13512
Harris S. A., Radford C. A. (2014). “Marine Soundscape Ecology,” in INTER-NOISE and NOISE-CON Congress and Conference Proceedings (Melbourne, Australia: Institute of Noise Control Engineering), 5003–5011.
Heimrich A. F., Halliday W. D., Frouin-Mouy H., Pine M. K., Juanes F., Insley S. J. (2021). Vocalizations of Bearded Seals (Erignathus Barbatus) and Their Influence on the Soundscape of the Western Canadian Arctic. Mar. Mammal Sci. 37, 173–192. doi: 10.1111/mms.12732
Hermand J.-P. (2006). Continuous Acoustic Monitoring of Physiological and Environmental Processes in Seagrass Prairies With Focus on Photosynthesis. Acoustic Sens. Techniques Shallow Water Environ Springer, 183–196. doi: 10.1007/978-1-4020-4386-4_14
Howe B. M., Miksis-Olds J., Rehm E., Sagen H., Worcester P. F., Haralabus G. (2019). Observing the Oceans Acoustically. Front. Mar. Sci. 6, 1–2. doi: 10.3389/fmars.2019.00426
Irigoien X., Klevjer T. A., Røstad A., Martinez U., Boyra G., Acuña J. L., et al. (2014). Large Mesopelagic Fishes Biomass and Trophic Efficiency in the Open Ocean. Nat. Commun. 5, 1–10. doi: 10.1038/ncomms4271
Kennedy E. V., Holderied M. W., Mair J. M., Guzman H. M., SIMPSON S. D. (2010). Spatial Patterns in Reef-Generated Noise Relate to Habitats and Communities: Evidence From a Panamanian Case Study. J. Exp. Mar. Biol. Ecol. 395, 85–92. doi: 10.1016/j.jembe.2010.08.017
Krause-Jensen D., Duarte C. M. (2014). Expansion of Vegetated Coastal Ecosystems in the Future Arctic. Front. Mar. Sci. 1, 77. doi: 10.3389/fmars.2014.00077
Krause-Jensen D., Lavery P., Serrano O., Marbà N., Masque P., Duarte C. M. (2018). Sequestration of Macroalgal Carbon: The Elephant in the Blue Carbon Room. Biol. Lett. 14, 20180236. doi: 10.1098/rsbl.2018.0236
La Manna G., Picciulin M., Crobu A., Perretti F., Ronchetti F., Manghi M., et al. (2021). Marine Soundscape and Fish Biophony of a Mediterranean Marine Protected Area. PeerJ 9, e12551. doi: 10.7717/peerj.12551
Lamont T. A., Williams B., Chapuis L., Prasetya M. E., Seraphim M. J., Harding H. R., et al. (2021). The Sound of Recovery: Coral Reef Restoration Success Is Detectable in the Soundscape. J. Appl. Ecology 59, 743–756. doi: 10.1111/1365-2664.14089
Leon-Lopez B., Romero-Vivas E., Viloria-Gomora L. (2021). Reduction of Roadway Noise in a Coastal City Underwater Soundscape During COVID-19 Confinement. J. Acoustical Soc. America 149, 652–659. doi: 10.1121/10.0003354
Lesser M. P., Slattery M., Leichter J. J. (2009). Ecology of Mesophotic Coral Reefs. J. Exp. Mar. Biol. Ecol. 375, 1–8. doi: 10.1016/j.jembe.2009.05.009
Lillis A., Mooney T. A. (2018). Snapping Shrimp Sound Production Patterns on Caribbean Coral Reefs: Relationships With Celestial Cycles and Environmental Variables. Coral Reefs 37, 597–607. doi: 10.1007/s00338-018-1684-z
Lin T.-H., Chen C., Watanabe H. K., Kawagucci S., Yamamoto H., Akamatsu T. (2019). Using Soundscapes to Assess Deep-Sea Benthic Ecosystems. Trends Ecol. Evol. 34, 1066–1069. doi: 10.1016/j.tree.2019.09.006
Lyon R. P., Eggleston D. B., Bohnenstiehl D. R., Layman C. A., Ricci S. W., Allgeier J. E. (2019). Fish Community Structure, Habitat Complexity, and Soundscape Characteristics of Patch Reefs in a Tropical, Back-Reef System. Mar. Ecol. Prog. Ser. 609, 33–48. doi: 10.3354/meps12829
Mahanty M. M., Latha G., Venkatesan R., Ravichandran M., Atmanand M. A., Thirunavukarasu A., et al. (2020). Underwater Sound to Probe Sea Ice Melting in the Arctic During Winter. Sci. Rep. 10, 1–7. doi: 10.1038/s41598-020-72917-4
Mckenna M. F., Baumann-Pickering S., Kok A., Oestreich W. K., Adams J. D., Barkowski J., et al. (2021). Advancing the Interpretation of Shallow Water Marine Soundscapes. Front. Mar. Sci. 8 (719258), 1–17. doi: 10.3389/fmars.2021.719258
Mcwilliam J. N., Mccauley R. D., Erbe C., Parsons M. J. G. (2017). Patterns of Biophonic Periodicity on Coral Reefs in the Great Barrier Reef. Sci. Rep. 7, 1–13. doi: 10.1038/s41598-017-15838-z
Melia N., Haines K., Hawkins E. (2016). Sea Ice Decline and 21st Century Trans-Arctic Shipping Routes. Geophysical Res. Lett. 43, 9720–9728. doi: 10.1002/2016GL069315
Mercer J. H. (1968). Antarctic Ice and Sangamon Sea Level USA: Institute of Polar Studies, Ohio State University, USA.
Michener W. K., Blood E. R., Bildstein K. L., Brinson M. M., Gardner L. R. (1997). Climate Change, Hurricanes and Tropical Storms, and Rising Sea Level in Coastal Wetlands. Ecol. Appl. 7, 770–801. doi: 10.1890/1051-0761(1997)007[0770:CCHATS]2.0.CO;2
Miksis-Olds J. L., Nichols S. M. (2016). Is Low Frequency Ocean Sound Increasing Globally? J. Acoustical Soc. America 139, 501–511. doi: 10.1121/1.4938237
Moher D., Shamseer L., Clarke M., Ghersi D., Liberati A., Petticrew M., et al. (2015). Preferred Reporting Items for Systematic Review and Meta-Analysis Protocols (PRISMA-P) 2015 Statement. Systematic Rev. 4, 1–9. doi: 10.1186/2046-4053-4-1
Montgomery J. C., Jeffs A., Simpson S. D., Meekan M., Tindle C. (2006). Sound as an Orientation Cue for the Pelagic Larvae of Reef Fishes and Decapod Crustaceans. Adv. Mar. Biol. 51, 143–196. doi: 10.1016/S0065-2881(06)51003-X
Mooney T. A., Di Iorio L., Lammers M., Lin ,. T.-H., Nedelec S. L., Parsons M., et al. (2020). Listening Forward: Approaching Marine Biodiversity Assessments Using Acoustic Methods. R. Soc. Open Sci. 7, 201287. doi: 10.1098/rsos.201287
Parkinson C. L. (2019). A 40-Y Record Reveals Gradual Antarctic Sea Ice Increases Followed by Decreases at Rates Far Exceeding the Rates Seen in the Arctic. Proc. Natl. Acad. Sci. 116, 14414–14423. doi: 10.1073/pnas.1906556116
Peck M., Tapilatu R. F., Kurniati E., Rosado C. (2021). Rapid Coral Reef Assessment Using 3D Modelling and Acoustics: Acoustic Indices Correlate to Fish Abundance, Diversity and Environmental Indicators in West Papua, Indonesia. PeerJ 9, e10761. doi: 10.7717/peerj.10761
Pieretti N., Farina A., Morri D. (2011). A New Methodology to Infer the Singing Activity of an Avian Community: The Acoustic Complexity Index (ACI). Ecol. Indic. 11, 868–873. doi: 10.1016/j.ecolind.2010.11.005
Pijanowski B. C., Farina A., Gage S. H., Dumyahn S. L., Krause B. L. (2011). What is Soundscape Ecology? An Introduction and Overview of an Emerging New Science. Landscape Ecol. 26, 1213–1232. doi: 10.1007/s10980-011-9600-8
Polidoro B. A., Carpenter K. E., Collins L., Duke N. C., Ellison A. M., Ellison J. C., et al. (2010). The Loss of Species: Mangrove Extinction Risk and Geographic Areas of Global Concern. PLoS One 5, e10095. doi: 10.1371/journal.pone.0010095
Popper A. N., Fewtrell J., Smith M. E., Mccauley R. D. (2003). Anthropogenic Sound: Effects on the Behavior and Physiology of Fishes. Mar. Technol. Soc. J. 37, 35–40. doi: 10.4031/002533203787537050
Radford C. (2008). Temporal Patterns in Ambient Noise of Biological Origin From a Shallow Water Temperate Reef. Oecologia 156, 921–929. doi: 10.1007/s00442-008-1041-y
Radford C., Stanley J., Tindle C., Montgomery J., Jeffs A. (2010). Localised Coastal Habitats Have Distinct Underwater Sound Signatures. Mar. Ecol. Prog. Ser. 401, 21–29. doi: 10.3354/meps08451
Ricci S., Eggleston D., Bohnenstiehl D., Lillis A. (2016). Temporal Soundscape Patterns and Processes in an Estuarine Reserve. Mar. Ecol. Prog. Ser. 550, 25–38. doi: 10.3354/meps11724
Roberts J. M., Wheeler A. J., Freiwald A. (2006). Reefs of the Deep: The Biology and Geology of Cold-Water Coral Ecosystems. Science 312, 543–547. doi: 10.1126/science.1119861
Roca I. T., Van Opzeeland I. (2020). Using Acoustic Metrics to Characterize Underwater Acoustic Biodiversity in the Southern Ocean. Remote Sens. Ecol. Conserv. 6, 262–273. doi: 10.1002/rse2.129
Rossi T., Connell S. D., Nagelkerken I. (2017). The Sounds of Silence: Regime Shifts Impoverish Marine Soundscapes. Landscape Ecol. 32, 239–248. doi: 10.1007/s10980-016-0439-x
Rutz C., Loretto M.-C., Bates A. E., Davidson S. C., Duarte C. M., Jetz W., et al. (2020). COVID-19 Lockdown Allows Researchers to Quantify the Effects of Human Activity on Wildlife. Nat. Ecol. Evol. 4, 1156–1159. doi: 10.1038/s41559-020-1237-z
Ryan J. P., Joseph J. E., Margolina T., Hatch L. T., Azzara A., Reyes A., et al. (2021). Reduction of Low-Frequency Vessel Noise in Monterey Bay National Marine Sanctuary During the COVID-19 Pandemic. Front. Mar. Sci. 8, 587. doi: 10.3389/fmars.2021.656566
Schafer R. M. (1969). The New Soundscape (BMI Canada Limited Don Mills: Associated Music Publishers, Inc., NY).
Schofield O., Ducklow H. W., Martinson D. G., Meredith M. P., Moline M. A., Fraser W. R. (2010). How do Polar Marine Ecosystems Respond to Rapid Climate Change? Science 328, 1520–1523. doi: 10.1126/science.1185779
Simpson S. D., Meekan M., Montgomery J., Mccauley R., Jeffs A. (2005). Homeward Sound. Science 308, 221–221. doi: 10.1126/science.1107406
Southworth M. F. (1967). The Sonic Environment of Cities (Cambridge, MA: Massachusetts Institute of Technology).
Staaterman E., Ogburn M., Altieri A., Brandl S., Whippo R., Seemann J., et al. (2017). Bioacoustic Measurements Complement Visual Biodiversity Surveys: Preliminary Evidence From Four Shallow Marine Habitats. Mar. Ecol. Prog. Ser. 575, 207–215. doi: 10.3354/meps12188
Staaterman E., Paris C., Deferrari H., Mann D., Rice A., D’Alessandro E. (2014). Celestial Patterns in Marine Soundscapes. Mar. Ecol. Prog. Ser. 508, 17–32. doi: 10.3354/meps10911
Teagle H., Hawkins S. J., Moore P. J., Smale D. A. (2017). The Role of Kelp Species as Biogenic Habitat Formers in Coastal Marine Ecosystems. J. Exp. Mar. Biol. Ecol. 492, 81–98. doi: 10.1016/j.jembe.2017.01.017
Vermeij M. J., Marhaver K. L., Huijbers C. M., Nagelkerken I., Simpson S. D. (2010). Coral Larvae Move Toward Reef Sounds. PLoS One 5, e10660. doi: 10.1371/journal.pone.0010660
Williams R., Wright A. J., Ashe E., Blight L., Bruintjes R., Canessa R., et al. (2015). Impacts of Anthropogenic Noise on Marine Life: Publication Patterns, New Discoveries, and Future Directions in Research and Management. Ocean Coast. Manage. 115, 17–24. doi: 10.1016/j.ocecoaman.2015.05.021
Keywords: soundscape, marine, bioacoustics, sound ecology, anthropogenic noise
Citation: Havlik M-N, Predragovic M and Duarte CM (2022) State of Play in Marine Soundscape Assessments. Front. Mar. Sci. 9:919418. doi: 10.3389/fmars.2022.919418
Received: 13 April 2022; Accepted: 30 May 2022;
Published: 29 June 2022.
Edited by:
Elva G. Escobar-Briones, National Autonomous University of Mexico, MexicoReviewed by:
Anna Maria Addamo, Joint Research Centre (JRC), ItalyMarco Marcelli, University of Tuscia, Italy
Copyright © 2022 Havlik, Predragovic and Duarte. This is an open-access article distributed under the terms of the Creative Commons Attribution License (CC BY). The use, distribution or reproduction in other forums is permitted, provided the original author(s) and the copyright owner(s) are credited and that the original publication in this journal is cited, in accordance with accepted academic practice. No use, distribution or reproduction is permitted which does not comply with these terms.
*Correspondence: Michelle-Nicole Havlik, bWljaGVsbGVuaWNvbGUuaGF2bGlrQGthdXN0LmVkdS5zYQ==
†These authors have contributed equally to this work and share first authorship