- 1Daniel P. Haerther Center for Conservation and Research, Shedd Aquarium, Chicago, IL, United States
- 2Biological Sciences, Humbolt State University, Arcata, CA, United States
Establishing marine reserves can increase biomass and diversity relative to surrounding areas. However, such changes presumably alter species compositions, interactions, and associated demographic processes such as predation. We investigated relative predation pressure by fish inside and outside of a well-established no-take reserve using standardized predation tether units: Squidpops. We deployed Squidpops (N = 990) monitored by cameras to examine predation, as estimated by consumption rates, between paired seagrass and patch reef habitat sites proximate to the Exuma Cays Land and Sea Park, a well-enforced no-take Bahamian reserve since 1986. Surprisingly, there was no significant park effect on consumption nor observed diversity. Significantly higher consumption rates were found at patch reef sites relative to seagrass sites, occurred in association with higher fish diversity and with longer soak times. Observed fish diversity was predicted by habitat and distance inshore with more species found on patch reefs and further from the deep water Exuma Sound. Recorded fish bites were positively associated with patch reef habitat, with reef dwelling fish, and increased as the species became more commonly encountered. Deployment-specific factors, including temperature, depth, and time of day had little impact on measured predation, an expected result as the experiments occurred in similar locations over a fine temporal scale. In our experiment, ecosystem-based factors outweighed the place-based management effects that we expected from being within the oldest well-enforced no-take marine reserve in the Caribbean versus the surrounding actively fished waters. Despite well-documented increases in abundances across trophic levels from primary consumers to apex predators within the reserve, predation rates were not significantly different than adjacent areas. Evaluating the efficacy of a marine reserve in protecting vital ecosystem processes requires studies beyond abundance and diversity surveys. By focusing on predation on two of the most ubiquitous habitats throughout the region and on common species, rather than on exceptional sites and charismatic species, we reveal a process that seems relatively unchanged by strict marine conservation management.
Introduction
Marine protected areas (MPAs) are established for the purposes of biodiversity conservation and the preservation of ecologically and economically important species. Globally, MPAs can increase the abundance, size, and diversity of fish relative to pre-MPA levels or nearby fished areas (Lester et al., 2009). These are vital results given worldwide dependency on fisheries for protein (Cabral et al., 2020) and the precipitous decline in biodiversity across the planet, including within coral reef ecosystems (Hughes et al., 2017). Biodiversity increases and anthropogenic impacts decrease fish biomass (Duffy et al., 2016), thus conservation management can yield direct benefits. The management components that create an overall effective MPA include good enforcement, old-age, large-size and remoteness (Edgar et al., 2014), and evidence is continually accumulating that MPAs enhance ocean conservation (Grorud-Colvert et al., 2021) making them familiar tools as the planet’s marine ecosystems are rebuilt (Duarte et al., 2020).
Determining which habitat features boost MPA benefits is an integral part of their design. Fish diversity in shallow marine habitats is associated with higher complexity (Gratwicke and Speight, 2005a). Similarly, the features of seagrass beds that provide better structure, such as canopy height and leaves per shoot, can enhance fish abundance and diversity (Jones et al., 2021). However, over the past few decades Caribbean structural complexity has been in decline (Alvarez-Filip et al., 2009) which has resulted in broad conservation concerns about phase shifts and biodiversity loss amplified by disease outbreaks and climate change (Hughes et al., 2007; but see Bruno et al., 2009). Processes by fish communities, such as grazing, can increase structure by enhancing the recruitment of ecosystem building corals, and a protected fish population can facilitate a trophic cascade towards higher ecosystem biodiversity (Mumby et al., 2006). Further, the distribution of key species responsible for herbivory on seagrass beds can guide spatial MPA planning (Henderson et al., 2019) which protects ecosystem processes in addition to biodiversity.
The effectiveness of MPAs may vary in preserving ecosystem processes that are not accounted for through standard surveys of species abundance, diversity and benthic coverage. Tropical marine ecosystems, such as coral reefs, are often a focus of marine conservation and a recent review identified eight interacting core processes that should guide management efforts (Brandl et al., 2019). Predation is one of these key processes, thus research that links predation, biodiversity, ecosystem function and conservation management is of high value (Brandl et al., 2019).
As no-take MPAs increase the biomass and diversity of marine life, they can cause trophic cascades as predator populations recover from outside fishing pressure. Top-down control is a primary driver in reef fish community structure (Terborgh and Estes, 2013), MPAs protect exploited higher-level predators, and the functional traits and trophic level of an ecosystem can track recovery better than abundance trends (Coleman et al., 2015; Hadj-Hammou et al., 2021). In areas with long-term overharvest of piscine predators, MPAs are more likely to have higher levels of predation and maintain top-down control of the ecosystem (Boada et al., 2015). Still, increases in biomass across multiple trophic levels have been observed inside MPAs (Loflen and Hovel, 2010; Soler et al., 2015) and the same species may occupy a higher trophic level within an MPA versus surrounding areas (Dell et al., 2015).
The feeding intensity and consumption by generalist fish predators is a measurable indicator of relative predation (Duffy et al., 2015) that should change with conservation management. Through a global synthesis, Cheng et al. (2019) documented an increase of consumption pressure arising from predator accumulation within marine reserves. Higher predation rates are found in healthier habitats in MPAs with a higher biomass of piscine predators (Barley et al., 2017; Rojo et al., 2021). Predator behavior may be significantly altered within marine reserves, and bold foraging behaviors are implicated in higher consumption rates (Rhoades et al., 2019). Beyond MPAs, the relative level of predation has been shown to vary across temporal (Ritter et al., 2021), seasonal (Yarnall and Fodrie, 2020), and spatial (Rodemann and Brandl, 2017; Duncan et al., 2019) scales from experiments using general piscine feeding assays.
We examined relative predation (i.e., consumption) on two common marine benthos, patch reef and seagrass, both inside and outside an MPA to determine the effects of protection and habitat type. To do so we utilized Squidpops, a method to measure the feeding intensity of generalist predators that is cost-effective, non-invasive, and easily replicable (Duffy et al., 2015). Additionally, we deployed and analyzed video surveys at each Squidpop array. These tools yielded three variables with which to test hypotheses: relative consumption, fish diversity, and fish interactions. By comparing predation intensity across protection levels and habitat types, we aimed to investigate two main hypotheses: (H1) locations within the longstanding MPA will have increased consumption rates of Squidpops, and (H2) patch reef habitats will have increased consumption rates of Squidpops relative to seagrass habitats. Video data tested a further three hypotheses: (H3) more fish species will be observed at patch reef sites relative to seagrass, (H4) the same species will be the primary consumers of Squidpops between the park and fished areas, and (H5) fish within the park will be bolder, resulting in increased investigation and consumption of Squidpops that they encounter. Our goal is to determine the efficacy of an MPA at preserving a vital ecological process: fish predation.
Methods
Squidpops
Standardized assays of generalist predator feeding intensity, Squidpops, were built and deployed following Duffy et al. (2015). A single Squidpop consisted of a 1 cm diameter disk of dried squid (i.e., bait) tied to a 1 – 2 cm length of low-test clear monofilament and attached to a 60 cm green fiberglass stake using electrical tape. A transect of 25 Squidpops was deployed with 1 – 2 m between individual units. An underwater camera was placed towards the center of the transect where it monitored several Squidpops to approximate fish communities and fish interactions. Transects were laid out in 2 – 5 m deep water via skin diving. Transects were checked after 1 and 24 hours to determine predation intensity based on squid-bait removal. The number of Squidpops that were completely missing the squid disk was tallied in each case and observer-subjective partial removals were not counted.
Study area
Squidpops were used to measure relative predation intensity inside and outside the boundaries of the Exuma Cays Land and Sea Park (ECLSP) in the Bahamas (Figure 1). The 442 km2 ECLSP was established as a no-take reserve in 1986, making it one of the oldest and largest MPAs in the Caribbean (Chiappone and Sullivan-Sealey, 2000). Prolonged exclusion from fishing pressure within the park has resulted in a higher biomass of predatory fishes, compared with other areas, for decades (Kough et al., 2022; Chiappone and Sullivan-Sealey, 2000; Mumby et al., 2007). The ECLSP is centrally located in the Exuma Cays, an island chain containing more than 365 islands and stretching for nearly 200 km in the central Bahamas. The Exuma Cays separate the deep water Exuma Sound (> 3000 m) from the shallow (< 10 m) Great Bahamas Bank. Deployment locations were within 200 m of exposed land to focus on fringing patch reef areas potentially accessible to fishers even in adverse conditions and to include two habitat types common throughout the archipelago.
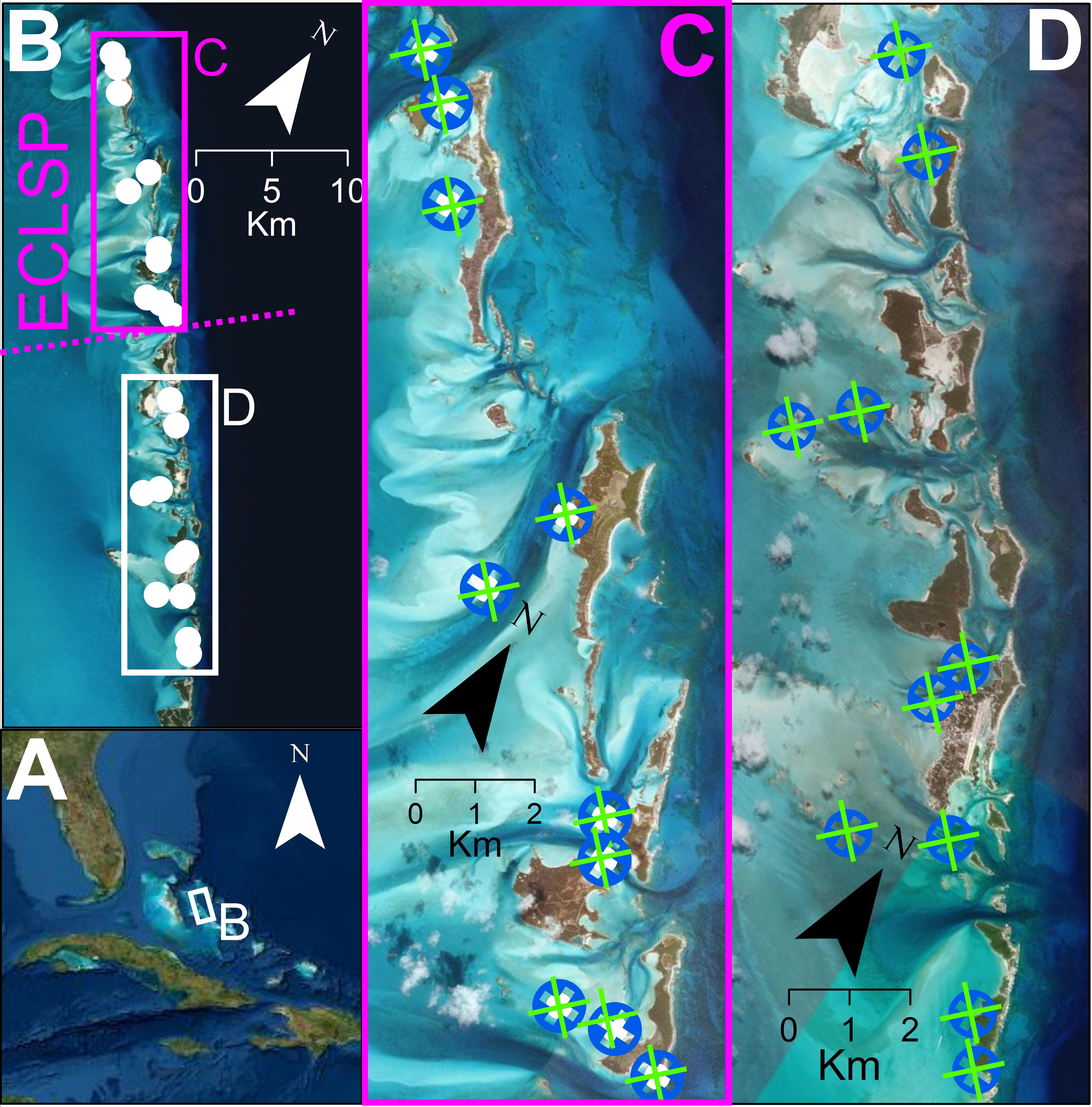
Figure 1 Study sites for Squidpop transects were in The Bahamas (A) within the Exuma Cays. The Exuma Cays Land and Sea Park (ECLSP) is a well-enforced, no-take marine reserve in the central Bahamas (B). Paired transects of 25 Squidpops were placed on seagrass (green) and patch reef (blue) sites at 10 locations within the ECLSP (C, purple) and 10 locations south of the ECLSP (D) to test the influence of management and habitat on piscine consumption.
Habitats
Predation in seagrass and patch reef habitat was assessed within the Exumas. Seagrass habitat featured stands of Thalassia testudinum as the dominant, climax biota. Occasionally, study sites included sparse specimens of Halodule wrightii and/or Syringodium filiforme. Substrate was typically sand or course gravel. To qualify as seagrass habitat, a site had living seagrass occurring within 1 m along a 25 m length of sediment that could be easily penetrated by the Squidpop stake to a depth of no less than 10 cm. Reef habitat featured live hard corals and/or gorgonians on hard bottom that fringed softer sediment that could be easily penetrated by the Squidpop stake to a depth of no less than 10 cm. Discontinuous patches adjacent to one another were focused on, rather than continuous stands of live coral. To qualify as reef habitat, a site had live coral occurring within 5 m along the 25 m transect of the benthos.
Spatial design
The experiment was divided by two scales: location and site. Locations were separated by approximately 1 km (mean 1160 m ± SD 395 m) and spanned a 40 km range (Figure 1). An equal number of locations were within the ECLSP (N = 10) and south of the ECLSP boundary (N = 10). Each location contained a pair of habitat sites, one seagrass and one patch reef, that each received a transect of 25 Squidpops. In cases where less than 25 Squidpops were recovered, likely as a result of wildlife interactions, the consumption rate was calculated relative to the number recovered (mean recovered = 24.8 ± SD 0.49). The two sites within each location were placed within approximately 50 m of each other (mean 51.4 m ± SD 40.5 m). Transects varied in length depending on the distance between subsequent Squidpops on each transect (1 – 2 m). Unsafe conditions (e.g., high winds, waves, and currents) and unique sediment and habitat configurations precluded precisely standardized transects but having transects of a constant length or shape is not critical towards Squidpop function (Duffy et al., 2015). A GPS (eTrex 10, Garmin, Switzerland) point taken midway along the transect helped relocate each site and maintain the spatial arrangement.
Fish diversity and frequency of approaches and bites
Video footage of approximately the first hour (mean 66 ± SD 28 min) of soak time during each Squidpop transect (N = 40) was viewed to determine the number of fish morphotypes passing in front of the camera. Fish were identified to the lowest taxonomic level possible. A screenshot and video time were cataloged for each unique morphotype at each site. Cataloged morphotypes were reviewed and verified by three individuals, including an author. Indistinguishable fish were given a separate category. The same video was viewed to quantify interactions between fish and Squidpops. Two fish interactions were quantified: approaches and bites. An approach was defined as a change in behavior to inspect or avoid a Squidpop that did not result in contact. A bite was defined as direct contact between the front of the fish and either the disk of squid, the tether, or the top of the stake. Approaches and bites were tallied and pooled for each morphotype observed in the video. For statistical analysis, fish diversity and interactions were standardized by the number of Squidpops clearly visible in each video and the amount of time monitored at a site (i.e., interactions/visible Squidpops/minute). The number of observed morphotypes and fish interactions yielded data on relative fish diversity and likely consumers.
Statistical analysis
The statistical approach of Duffy et al. (2015) was followed with the addition of further explanatory factors specific to the hypotheses and environment of this study. Depth and temperature were taken while doing the one-hour check using a SCUBA dive computer. Fish behavior changes over the course of the day, so Squidpop initial deployments were restricted to approximately midday but with an opportunistic range of 11:00 thru 18:12 (mean 14:10 ± 1´50῞). An evenly split categorical factor for deployment time (levels: “Early” and “Late”, N = 20 each) was used instead of a circular factor given that deployments did not occur around the clock. The distance from each location to pelagic water defined by Google Earth’s ocean layer, travelling around islands, was included (factor “inshore”) as this has been previously shown to shape benthic populations in the area (Kough et al., 2017). A within location factor was examined to account for effects of fish traveling potentially unequal distances between the patch reef and nearby seagrass among locations. This Separation factor was the distance between the two GPS points for the deployments, although the transects were variable in shape and length. The response variable, Consumption, was calculated as the proportion of Squidpops missing at a time point (i.e., after 1 or 24 hours) versus how many were recovered after 24 hours. A factor for morphotypes observed during video recordings was included after standardizing the footage by the number of Squidpops within the visible frame and the recording time.
Mixed-effect generalized linear models (GLMM) with a binomial distribution were used to examine Consumption at each site relative to continuous factors depth, temperature, inshore, and fish morphotype diversity and the categorical factors protection (levels: “MPA” and “fished”), start time (levels: “early” and “late”), soak time (levels: “1 hr” and “24 hr”) and habitat (levels: “seagrass” and “reef”). Continuous factors were centered and scaled prior to modeling. Sampling location was included as a random effect to account for the unique weather, oceanographic, and benthic characteristics at the scale of location. Categorical interactions between Habitat and Species, Habitat and Soak Time, and Species and Soak Time were also considered. The best fit GLMM from all possible combinations was determined using corrected Aikakes Information Criterion (AICc).
A set of GLMM with a Poisson distribution were used to examine variable Morphotypes relative to continuous factors monitored Squidpops, video time length, depth, temperature, and inshore, and the categorical factors protection (levels: “MPA” and “fished”), start time (levels: “early” and “late”), and habitat (levels: “seagrass” and “reef”). A Poisson distribution was used given that the response variable was non-zero counts. Continuous factors were centered and scaled prior to modeling. Categorical interactions were also considered. Sampling location was included as a random effect to account for the unique weather, oceanographic, and benthic characteristics at the scale of location. The best fit GLMM from all possible combinations was determined using AICc and the top ten models were retained to assemble a group of top candidate models.
Two sets of negative binomial generalized linear models (GLM) examined variable fish interactions (separate for Approaches and Bites) relative to continuous factors Squidpops monitored, minutes recorded and morphotype rarity, and categorical factors protection, habitat, and fish type. The factor rarity was the ratio of at how many sites a morphotype was recorded versus all sites, and the factor increased as a fish morphotype was more common. The categorical factor fish type had two levels: reef associated for morphotypes that only appeared at reef habitat sites, and generalist for morphotypes that appeared at both seagrass and reef sites. The two morphotypes that were likely seagrass specialists (Acanthostracion quadricornis and Bothidae sp.) did not have any interactions with the Squidpops and were assigned to the generalist level to enable fitting. No random effect was included so that the impact of rarity could be investigated without singularity.
Results
Squidpop consumption
The best-fit GLMM for Squidpop consumption included the fixed-effects of soak time, habitat and morphotypes (Figure 2; Table 1). Soak time explained the greatest amount of variance in the model, with a logical increase in consumption associated with the second time point attesting to the importance of using two temporal checkpoints. Habitat had a significant impact on consumption and, relative to seagrass, there was more than two orders of magnitude higher predation at reef sites. A continuous factor for the number of morphotypes observed at each site, relative to observed Squidpops and recording time, had a significant positive effect on consumption. All three terms in the best-fit model were present in all the top ten models as ranked by AICc. The random effect of Location was not significant in the top model.
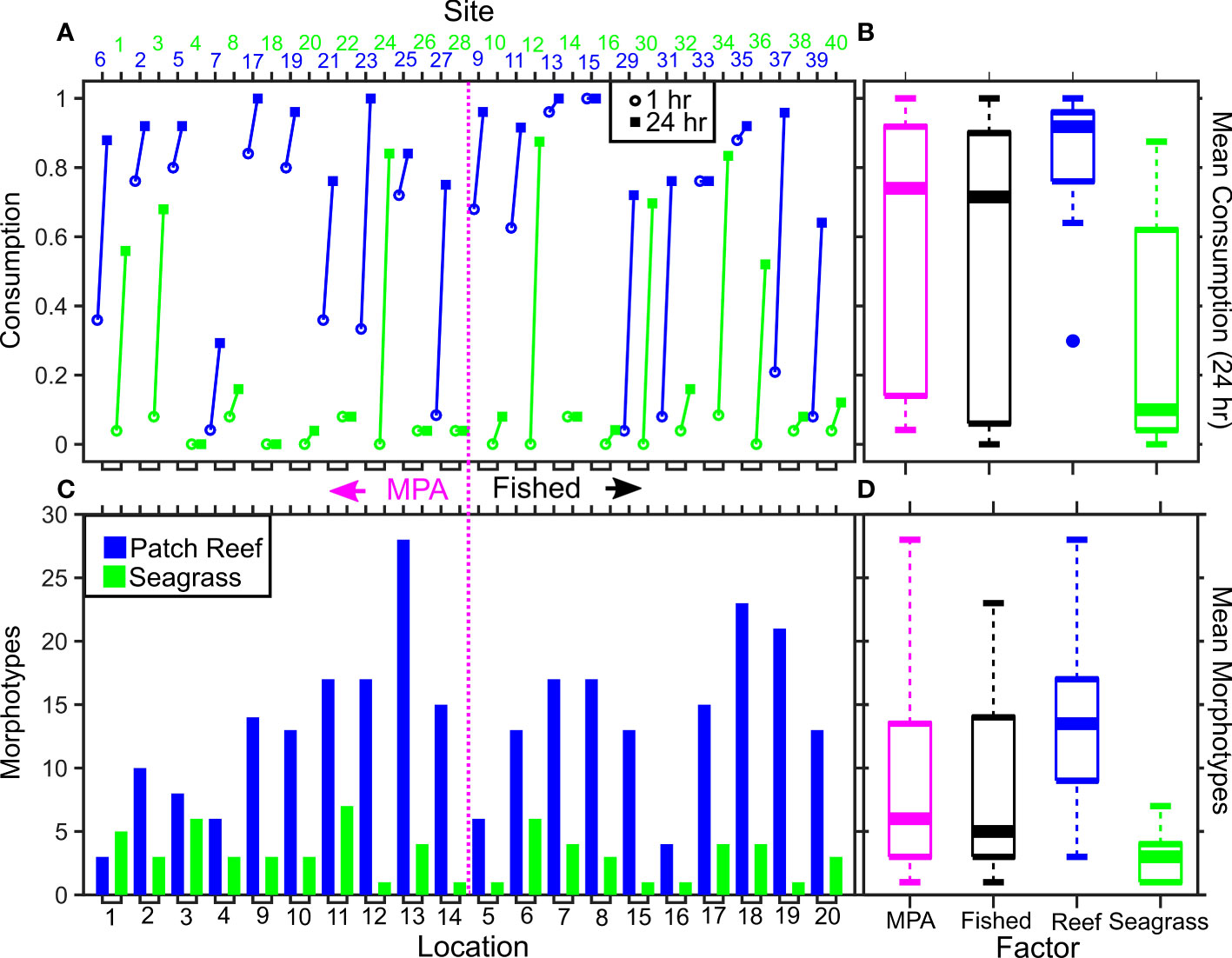
Figure 2 |Consumption and morphotypes varying by MPA, location, and habitat. The highest-ranked GLMM for predicting variable Squidpop consumption rate included soak time, habitat, and observed species with a random effect of location. Sites are ordered so that those within a well-enforced, old MPA are to the left of the dashed pink line, and fished sites are on the right. Sites are colored by habitat (blue = patch reef; green = seagrass). For each pair of Squidpop sites per location the consumption rate after 1 hour (open circle) and 24 hours (closed square) is shown (A). The observed count of unique morphotypes at each site is shown (C). The mean consumption rate after 24 hours (B) and mean number of morphotypes observed (D) are also presented with sites divided by MPA (pink) or Fished (black) and by habitat. MPA effects were not included in top-ranked models and had no discernable impact on consumption, nor morphotypes.
In the top-ten ranked models, further terms were present and may influence Squidpop consumption (Table 1). The categorical factor for being within an MPA was a focus of the experiment, was only present in one model and had a positive but not significant effect. Distance to the deep water Exuma Sound (“inshore”) was present but not significant in three top models and had a positive effect. The time that the experiment started (early or late) was present in three models and had a not significant positive effect on consumption. Temperature was not present in any top models, although differences throughout the deployments were likely masked by unknown tidal signals as the mean temperature was 28.0˚C ± SD 1.1. Similarly, Depth was not present in any top models. No interactions were significant when present in the top-ranked models.
Observing fish diversity
Video records revealed the presence of potential piscine consumers at every site selected for a Squidpop transect. Image quality was sufficient to discern 78 different morphotypes: 15 to the level of Family, 62 to the level of Species and a remaining bin for distant fish at the level of Class (Actinopterygii). Video data was insufficient to calculate abundance but was able to confirm the presence of morphotypes at each site and estimate relative diversity (i.e., species seen on camera proximate to Squidpops). A database of 337 site-unique records of morphotypes was assembled with 6 records to the level of class, 33 to the level of family and 298 to the level of species. The majority of the morphotypes occurred multiple times (N repeats = 45) in the study and the majority of the morphotypes interacted with the Squidpops at least once (either an approach or a bite; N = 40). Green turtles, Chelonia mydas (N = 3), were considered a species of fish for statistical analysis after one investigated an arrangement of Squidpops, and the camera observing them, and thus could have had an impact upon the study.
Predicting fish diversity
The best-fit GLMM predicting the count of observed species at each site contained significant factors of habitat and distance from sound, factor “Inshore” (Table 2). Sites in seagrass habitat had fewer species relative to reef sites. As the Inshore factor increased and sites became further removed from the Exuma Sound, the number of observed morphotypes increased. The factor Park was not in the top ten GLMM (Table 2). In addition, treating each location (i.e., each pair of reef and seagrass transects grouped by location) independently, there was no significant difference in the number of species between locations inside versus outside the park (Welch Two Sample t = 0.30043, df = 18, p-value = 0.7673). Finally, the co-presence of species (i.e., the same species was seen on video at both the patch reef site and the seagrass site at the same location) was not significantly related to how far apart two sites within a location were (Kendall’s τ = -0.24, N = 20, p = 0.183).
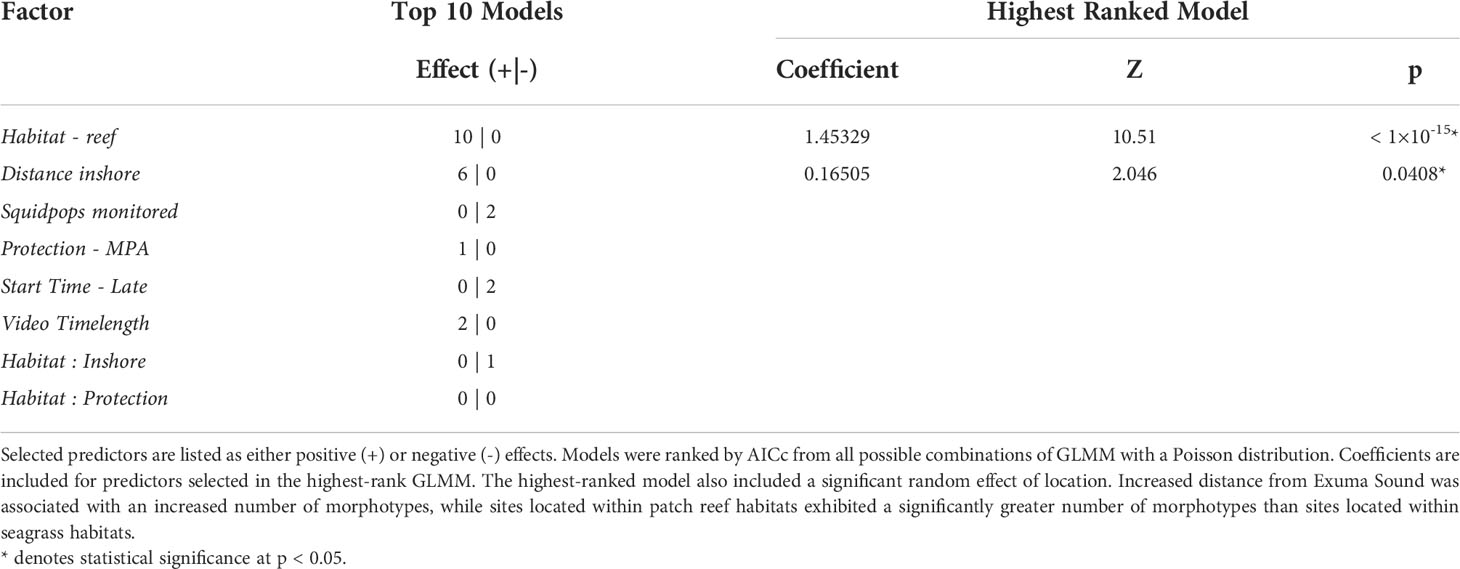
Table 2 Fixed factors included in top 10 models predicting observed fish morphotype diversity at monitored sites in the Exuma Cays.
Fish interactions
To examine the influence of fish rarity on Squidpop interactions, separate negative binomial GLM were run for variable Approaches and for Bites with site-unique records of morphotypes as samples. The best-fit GLM for Approaches contained an insignificant intercept and an insignificant but negative influence of the categorical factor park (Table 3). The best-fit GLM for Bites contained significant effects of Habitat, fish type, and rarity (Table 3). Fewer bites occurred in seagrass habitats relative to reef habitats. In addition, there was a positive effect associated with reef fish relative to generalist fish, but no interaction between the habitat and fish type. There was a significant, positive effect of fish rarity on Bites, and more common fish in the study were more likely to take a bite out of a Squidpop. The consumption rate of Squidpops over the first hour, when video monitoring occurred, is correlated to both Bites/Squidpop/Minute (Pearson’s r = 0.585, t = 4.448, df = 38, p = 0.733 × 10-5) and to Approaches/Squidpop/Minute (Pearson’s r = 0.463, t = 3.222, df = 38, p = 0.261 × 10-3).
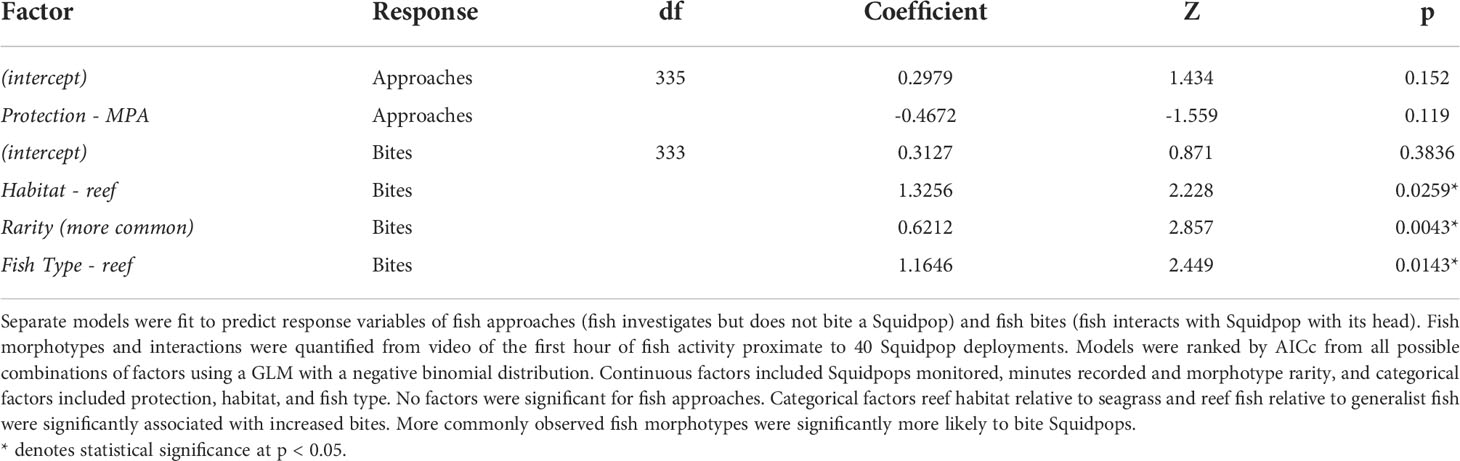
Table 3 Factors in best-fit models predicting observed fish interactions at monitored sites in the Exuma Cays.
Discussion
Habitat over MPA effects
Habitat, soak time and fish diversity determined relative predation rates. Our Squidpops experiment found higher consumption rates at patch reef sites relative to seagrass sites and higher consumption rates occurring in association with higher fish diversity. Similarly, reef-associated fish were more likely to bite Squidpops relative to generalist fish, bites were more likely at reef sites, and more bites were associated with the more common fish in the study. Finally, more fish species were found at patch reef sites and at sites further away from the Exuma Sound. Habitat type had a profound effect on all our measured variables, and we accept our hypotheses of increased consumption (H2) and increased fish diversity (H3) at patch reef sites. This agrees with other Caribbean works using the Squidpops methodology that suggested consumption rates varied the most by habitat classification and that results were consistent temporally (Ritter et al., 2021). We did not directly measure rugosity nor benthic coverage but by definition our two habitat classes contrasted in both metrics. Patch reefs sites had higher rugosity and a faunal composition including live corals while seagrass sites were flat and dominated by seagrass, thus higher abundance and biodiversity of fishes was expected at patch reef sites (Gratwicke and Speight, 2005a; Hall and Kingsford, 2021) as observed in our study.
Ecosystem-based habitat factors outweighed the place-based management effects that we expected from being within the oldest well-enforced no-take marine reserve in the Caribbean versus the surrounding and actively fished waters. We reject our primary hypothesis (H1) and conclude that there was no discernable impact of a marine reserve on a vital ecosystem process: predation. In addition, the number of unique morphotypes observed at each site was not significantly affected by the MPA, thus we accept our hypothesis (H4). Further, there was no significant effect of the MPA on fish feeding behavior, bites, and a not significant but negative effect of MPA on fish approaches, thus we reject our hypothesis (H5). These surprising results could be caused by many factors which we did not quantify. Across smaller spatial scales than our study, isotopic analysis demonstrated changes in the ecological roles of the same species of fish inside versus outside of reserves (Dell et al., 2015). Indeed, a marine reserve can influence an area’s ecological function as inferred by analysis of the functional traits associated with fish assemblages (Coleman et al., 2015) and invertebrate communities (Gillepsie and Vincent, 2019). A deeper quantification of the fish community could reveal changes in predation within the MPA especially at the higher trophic levels likely to have the most benefit from a harvest ban. It took decades for an effect of protection to emerge in a trait-based analysis of small to medium sized fish like those that dominated our experiment (Hadj-Hammou et al., 2021). However, other work suggested an increase in similar size classes as a weak compensatory response from decreased predation as larger fish are harvested over shorter timescales (Dulvy et al., 2004). Inferred changes based on fish traits may exist, but by directly measuring consumption our data strongly suggest that habitats within the reserve and outside of it are experiencing similar levels of relative predation, despite long standing changes from protection that have resulted in greater abundances of fished species (Chiappone and Sullivan-Sealey, 2000; Lipcius et al., 2001; Mumby et al., 2007; Kough et al., 2019), apex predators (Gallagher et al., 2021) and higher quality habitat (Mumby et al., 2006) inside the ECLSP.
Observed fish assemblages
Video monitoring revealed that a diverse fish assemblage visited experimental sites and interacted with Squidpops (Figure 3). Previous works have captured far fewer species as part of Squidpop experiments. For example, Ritter et al. (2021) reported a total of only 12 species at four habitat types (fore reef, patch reef, seagrass and mangroves) that bit Squidpops in Belize. Relative to this past work (Ritter et al., 2021), we recorded greater than six times as many species present in our videos and nearly four times the diversity in species that interacted with the Squidpops (Supplementary Materials). A synthetic report (Harborne, 2017) combined three databases of fishery-independent SCUBA surveys in The Bahamas and described 97 commonly identifiable species of reef fish. Therefore, the Squidpops video successfully identified the majority of the fish (N = 62 to species and 15 to family) that would have likely been observed by divers in the area using standardized methods. Further, most of the observed morphotypes that we categorized interacted with the Squidpops on video at least once, suggesting that these were not random observations but were instead animals drawn to the experiment. These results strengthen the case that Squidpops are an appropriate tool for assessing relative predation at an ecosystem level by measuring the consumption of many species.
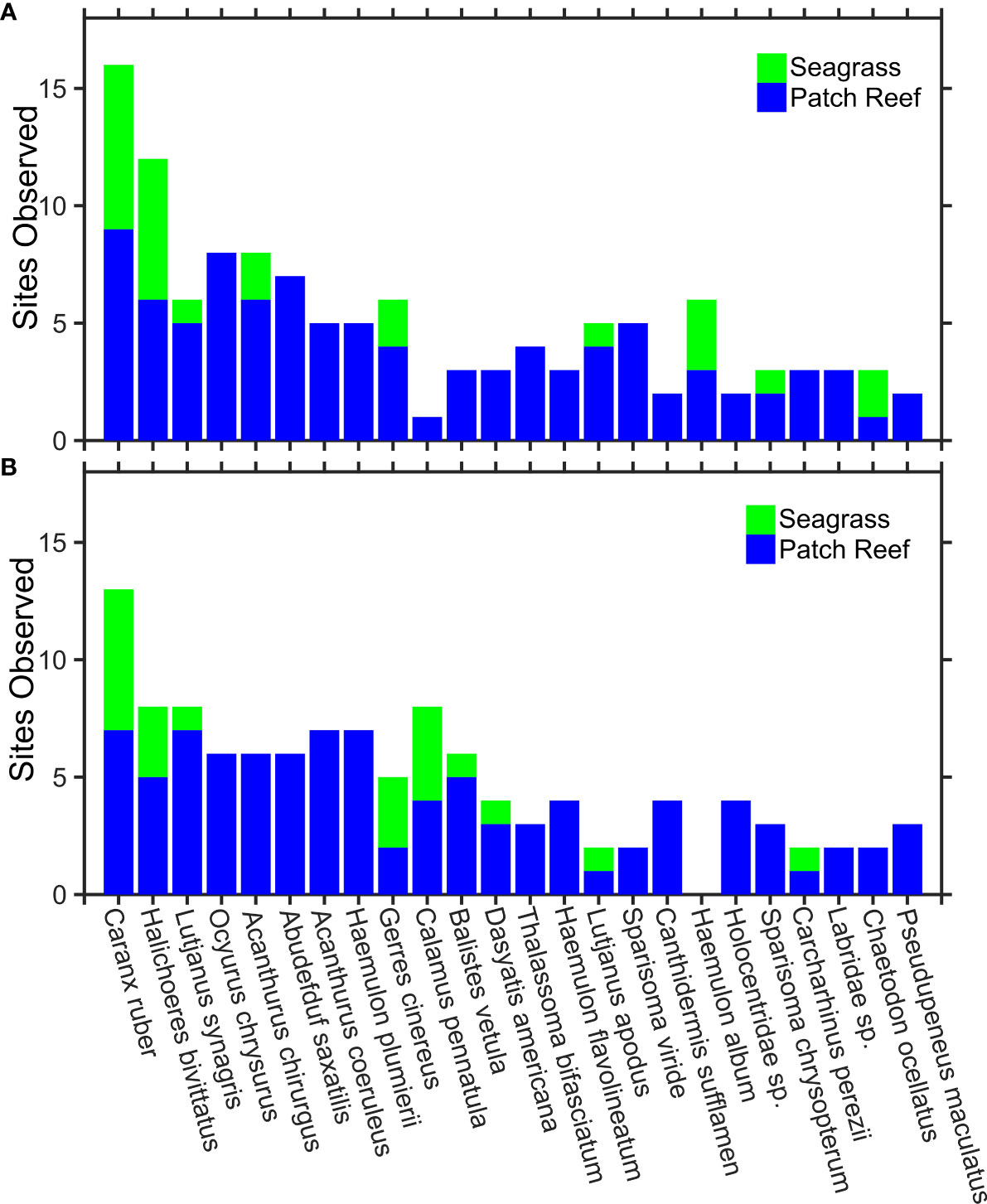
Figure 3 Top 24 observed species and families from Squidpop video. Stacked histograms show the number of sites within an MPA (A) and fished areas (B) where each morphotype was observed, colored by habitat (blue = reef, green = seagrass). The factor MPA was not included in the top-ranked GLMM predicting the number of morphotype observations, nor was there a significant difference between morphotype totals at locations inside and outside of the park. The full database of observed morphotypes, interactions and voucher images is available in the Supplementary Materials.
The size and feeding behavior of fish determines their interactions with Squidpops and our ability to describe them. Some families, like squirrelfish (Holocentridae spp.), never got close enough to the camera to make a positive species identification. Damselfish (Pomacentridae spp.) occasionally did, but typically remained outside of identifiable acuity except for remarkably identifiable species such as the sergeant majors (Abudefduf saxatilis) that were prevalent throughout the study. Smaller species like these may play an important ecosystem role that is not manifested in Squidpop results given that their size widely prevents their predation attempts from having success, although they did persistently bite and 18% of all recorded bites were from Pomacentridae, mainly Abudefduf saxatilis. Small wrasses were another ubiquitous Squidpop consumer, with greater bait-removal success, that often unleashed a fury of bites even after the bait was removed. As a group, Labridae spp. were responsible for 28% of all recorded bites and a school of Thalassoma bifasciatum attacked a series of three Squidpops 109 times within a 75-minute recording. It is unlikely that the larger-sized fish in the area that are prized by fishers and benefit from MPA protections and harvest restrictions (Kough et al., 2022), such as grouper (Epinephelinae spp.), would be directly impacting Squidpop consumption. Grouper, of at least two species (Mycteroperca bonaci and Epinephelus striatus), were observed in 18% of videos but never approached nor bit the Squidpops. However, snappers (Lutjanidae spp.) and grunts (Haemulidae spp.) are the most fished groups in the area (Davis, 2008) and they contributed 16% and 26% of all recorded bites, respectively, on our Squidpop videos. Bar jacks (Caranx ruber) were the most commonly observed fish in our study and occurred in 73% of the video recordings yet they accounted for less than 1% of all observed bites.
Lessons and caveats with Squidpops
Site selection was successful and approximately equivalent habitats were able to be found across a wide spatial scale. The effects of temperature, depth, distance from the sound, and time of day had little impact on measured consumption, an expected result if the experiments occurred in similar habitats, as intended, over a fine temporal scale. Within a location, shared species were either very mobile (i.e., bar jack, snappers, barracudas) or very common (i.e., slippery dick), and the separation between two sites was not related to the number of shared species.
Low-effort video monitoring increased our ability to explain consumption and replaced diver surveys. Each video captured at least one morphotype which suggests that the piscine community was present and actively feeding at the sites we selected. We did not survey biomass or independently use visual surveys to gauge community makeup, thus we relied on video to make inferences about what was consuming the Squidpops. However, previous works suggest that fish biomass is unrelated to consumption rates measured by Squidpops (Ritter et al., 2021). The majority of the site-specific records were simply of a morphotype present on video and did not contain bites or approaches to the Squidpops. However, the video recorded approximately the first hour of soak time and consumption rates increased with soak time, thus the fish may have been acclimating to the experiment while the video was active and feeding later. In addition, nocturnal predators would not have been captured on exclusively diurnal videos. For example, grunts were exclusively seen on patch reefs, but they leave structure and forage on nearby seagrass nocturnally (Beets et al., 2003). Around-the-clock monitoring using far red light and long-lasting cameras could capture a wider range of piscine behavior and better explain the communities adjacent to and responsible for Squidpop consumption.
Video monitoring suggested that Squidpops were likely capturing predominately fish consumption. Outside of a green turtle blundering through the transect, other observed genera (i.e., cephalopods, crustaceans) did not interact with the experiment. A reef squid (Sepioteuthis sepioidea) positioned itself directly on top of the camera for one deployment, and its tentacles partially obscured the lens, but this was more comedic than impactful (haiku: Oddly pulsating/This obstruction, not algae/Reef squid pass on by). It is plausible, but inconclusive from our methods, that nocturnal communities of invertebrates such as crustaceans or swimming polychaetae worms also fed on the Squidpops.
Conservation implications
Quantifying how far ecological processes extend away from a patch reef has practical considerations for conservation planning in small island systems. We found that consumption rates varied with habitat differences over a relatively small spatial scale. An average of 50 m (± 40 m SD) separated the habitat transects between our paired sites at each location, yet habitat differences exerted the strongest effect on all our measured variables. The connectivity and compositions of fish communities among these habitats can be distinct with far greater diversity and abundance in reef communities that decreases as habitat shifts to seagrasses (Campbell et al., 2011). The landscape impacts of grazing around patch reefs creates a signal visible from satellite imagery (Madin et al., 2011) which is more pronounced in MPAs (Bilodeau et al., 2022). Predation risk for smaller fish is a strong driver of movement patterns between seagrass and patch reef habitats (Rooker et al., 2018), and fish occupy and forage within different habitats across the diel cycle (Nagelkerken et al., 2000). Our results suggest that consumption rates may vary more strongly with habitat type than time of day over a similar spatial scale within a location. Estimating a gradient in predation from a reef was beyond the constrains of our experiment, but merits investigation. For example, Squidpops have shown greater predation intensity closer to a reef (Gusmao et al., 2018). However, few patch reefs can sustain larger predators, making it likely that pressure from top predators in our experiment was transitory versus localized. High-resolution habitat maps (< 4 m) are now available throughout the Caribbean (Schill et al., 2021) making informed conservation planning possible even on small islands. For example, once effect ranges are quantified, development plans for channels or docks could be adjusted on the scales of 10s of m to decrease disruptions to patch reefs and the aquatic communities they sustain.
Our study adds to long-standing evidence that habitat structure shapes tropical fish ecosystems. Structure within complex and protected reefs determines predation pressure and maintains a diverse and productive ecosystem, but this structure is under threat (Rogers et al., 2014). Ecosystem shifts that remove critical differences at patch reefs, such as the ongoing surge in stony coral tissue loss disease (Muller et al., 2020), may remove structure building species and smooth over the habitat differences. Some activities, such as scuba diving by tourists, are often focused on high quality habitat where they can increase susceptibility to disease (Lamb et al., 2014). Certain community members, such as herbivores, have been previously shown to enhance recruitment and drive community structure across the ecosystem (Mumby et al., 2006; Ruttenberg et al., 2019) yet are facing increased threats from humans (Bellwood et al., 2011; Callwood, 2021) and can be repelled by tourist disturbances (Albuquerque et al., 2014). Future work that quantifies predation across a range of habitat degradation would be useful to forecast the implications in a changing environment, and patch reefs may offer the optimal experimental set-up.
Patch reefs are a ubiquitous feature of the wider Caribbean and an easy to monitor sentinel of change. Our finding of increased diversity at patch reefs that were further removed from the deep water sound, and more continuous spur and grove reef areas, was non-intuitional yet speaks to the importance of these habitats as refuges for animals dispersed over the expansive Bahamian bank. On a local scale relative to nearby seagrass beds, the shelter offered by a patch reef determines fish abundance (Gratwicke and Speight, 2005b). A recent review shows a myriad of techniques are being applied to restore and conserve coral reefs and the majority of these efforts occur over short-temporal scales and restore areas on average of 100 m2 (Boström-Einarsson et al., 2020). The experimental tractability of patch reefs enables researchers to investigate new methods before scaling them upwards. For example, artificial enhancement of invertebrate herbivores yielded a cascade of positive impacts on patch reefs including increased fish and coral recruitment (Spadaro and Butler, 2020). Fish diversity and abundance are shaped by habitat complexity and predation, although the effects of both are modulated by the fish life-stage (Almany, 2004). Consumption rates are another simple to measure metric of ecosystem function that can be easily added to restoration work to disentangle interactions between structure and function. We suggest including process-based monitoring as an addendum to abundance and diversity surveys that is achievable by a wide range of scientists, students and amateur naturalists.
Data availability statement
The original contributions presented in the study are included in the article/Supplementary Material. Further inquiries can be directed to the corresponding author.
Author contributions
AK: conceived, collected, analyzed, wrote, coordinated; CB: conceived, collected, edited. All authors contributed to the article and approved the submitted version.
Acknowledgments
Funded by the John G. Shedd Aquarium Society. Aquarium volunteers Tim Cain and Alexandra Brainerd spent hours scrutinizing videos of fish bites. Small vessel mechanical support from Shedd Aquarium Captains Kip and James. Logistic support and encouragement provided by the Bahamas National Trust, especially Joe and Nicole Ierna. Research permitted under MAMR/FIS/17 from Bahamas Department of Marine Resources and from the Bahamas National Trust.
Conflict of interest
The authors declare that the research was conducted in the absence of any commercial or financial relationships that could be construed as a potential conflict of interest.
Publisher’s note
All claims expressed in this article are solely those of the authors and do not necessarily represent those of their affiliated organizations, or those of the publisher, the editors and the reviewers. Any product that may be evaluated in this article, or claim that may be made by its manufacturer, is not guaranteed or endorsed by the publisher.
Supplementary material
The Supplementary Material for this article can be found online at: https://www.frontiersin.org/articles/10.3389/fmars.2022.921463/full#supplementary-material
Data Sheet 1 | Excel workbook containing the code and data used for analysis in the manuscript.
Data Sheet 2 | Excel workbook containing video times and frame vouchers for each of our morphotypes.
References
Albuquerque T., Loiola M., José de Anchieta C. C., Reis-Filho J. A., Sampaio C. L., Leduc A. O. (2014). In situ effects of human disturbances on coral reef-fish assemblage structure: temporary and persisting changes are reflected as a result of intensive tourism. Mar. Freshw. Res 66 (1), 23–32. doi: 10.1071/MF13185
Almany G. R. (2004). Differential effects of habitat complexity, predators and competitors on abundance of juvenile and adult coral reef fishes. Oecologia 141, 105–113. doi: 10.1007/s00442-004-1617-0
Alvarez-Filip L., Dulvy N. K., Gill J. A., Côté I. M., Watkinson A. R. (2009). Flattening of Caribbean coral reefs: region-wide declines in architectural complexity. Proc. R. Soc. B. 276, 3019–3025. doi: 10.1098/rspb.2009.0339
Barley S. C., Meekan M. G., Meeuwig J. J. (2017). Species diversity, abundance, biomass, size and trophic structure of fish on coral reefs in relation to shark abundance. Mar. Ecol. Prog. Ser. 565, 163–179. doi: 10.3354/meps11981
Beets J., Muehlstein L., Haught K., Schmitges H. (2003). Habitat connectivity in coastal environments: Patterns and movements of Caribbean coral reef fishes with emphasis on bluestriped grunt, Haemulon sciurus. gulf carib. Res. 14 (2), 29–42. doi: 10.18785/gcr.1402.03
Bellwood D. R., Hoey A. S., Hughes T. P. (2011). Human activity selectively impacts the ecosystem roles of parrotfishes on coral reefs. Proc. R. Soc B. 279, 1621–1629 doi: 10.1098/rspb.2011.1906
Bilodeau S. M., Schwartz A. W. H., Xu B., Paul Pauca V., Silman M. R. (2022). A low-cost, long-term underwater camera trap network coupled with deep residual learning image analysis. PloS One 17 (2), e0263377. doi: 10.1371/journal.pone.0263377
Boada J., Arthur R., Farina S., Santana Y., Mascaro O., Romero J., et al. (2015). Hotspots of predation persist outside marine reserves in the historically fished Mediterranean Sea. Biol. Cons. 191, 67–74. doi: 10.1016/j.biocon.2015.06.017
Boström-Einarsson L., Babcock R. C., Bayraktarov E., Ceccarelli D., Cook N., Ferse S. C. A., et al. (2020). Coral restoration – a systematic review of current methods, successes, failures and future directions. PloS One 15 (1), e0226631. doi: 10.1371/journal.pone.0226631
Brandl S. J., Rasher D. B., Côté I. M., Casey J. M., Darling E. S., Lefcheck J. S., et al. (2019). Coral reef ecosystem functioning: eight core processes and the role of biodiversity. Front. Ecol. Environ. 17 (8), 445–454. doi: 10.1002/fee.2088
Bruno J. F., Sweatman H., Precht W. F., Selig E. R., Schutte V. G. W. (2009). Assessing evidence of phase shifts from coral to macroalgal dominance on coral reefs. Ecology. 90 (6), 1478–1484. doi: 10.1890/08-1781.1
Cabral R. B., Bradley D., Mayorga J., Goodell W., Friedlander A. M., Sala E., et al. (2020). A global network of marine protected areas for food. Proc. Nat. Acad. Sci. 117 (45), 28134–28139 doi: 10.1073/pnas.2000174117
Callwood K. A. (2021). Examining the development of a parrotfish fishery in the Bahamas: Social considerations & management implications. Glob. Ecol. Cons. 28, e01677. doi: 10.1016/j.gecco.2021.e01677
Campbell S. J., Kartawijaya T., Sabarini E. K. (2011). Connectivity in reef fish assemblages between seagrass and coral reef habitats. Aquat. Biol. 13, 65–77. doi: 10.3354/ab00352
Cheng B. S., Altieri A. H., Torchin M. E., Ruiz G. M. (2019). Can marine reserves restore lost ecosystem functioning? A Global synthesis. Ecol. 100 (4), 1–13. doi: 10.1002/ecy.2617
Chiappone M., Sullivan-Sealey K. M. (2000). Marine reserve design criteria and measures of success: lessons learned from the exuma cays land and Sea park, Bahamas. Bull. Mar. Sci. 66 (3), 691–705.
Coleman M. A., Bates A. E., Stuart-Smith R. D., Malcolm H. A., Harasti D., Jordan A., et al. (2015). Functional traits reveal early responses in marine reserves following protection from fishing. Div. Distrib. 21, 876–887. doi: 10.1111/ddi.12309
Davis M. (2008). Do boaters overfish and under spend? a case study in the exumas, Bahamas (UC San Diego: Center for Marine Biodiversity and Conservation). Available at: https://escholarship.org/uc/item/3b826731.
Dell C., Montoya J. P., Hay M. E. (2015). Effect of marine protected areas (MPAs) on consumer diet: MPA fish feed higher in the food chain. Mar. Ecol. Prog. Ser. 540, 227–234. doi: 10.3354/meps11487
Duarte C. M., Agusti S., Barbier E., Britten G.L., Castilla J.C., Gattuso J.P.. (2020). Rebuilding marine life. Nature 580, 39–51. doi: 10.1038/s41586-020-2146-7
Duffy J. E., Lefcheck J. S., Stuart-Smith R. D., Navarrete S. A., Edgar G. J. (2016). Biodiversity enhances reef fish biomass and resistance to climate change. Proc. Nat. Acad. Sci. 113, (22) 6230–6235. doi: 10.1073/pnas.1524465113
Duffy J. E., Ziegler S. L., Campbell J. E., Bippus P. M., Lefcheck J. S. (2015). Squidpops: A simple tool to crowdsource a global map of marine predation intensity. PloS One 10 (11), e0142994. doi: 10.1371/journal.pone.0142994
Dulvy N., Polunin N. V., Mill A., Graham N. A. (2004). Size structural change in lightly exploited coral reef fish communities: Evidence for weak indirect effects. Can. J. Fish. Aqu. Sci. 61, 466–475. doi: 10.1139/f03-169
Duncan C. K., Gilby B., Olds A. D., Connolly R. M., Ortodossi N. L., Henderson C. J., et al. (2019). Landscape context modifies the rate and distribution of predation around habitat restoration sites. Biol. Conserv. 237, 97–104. doi: 10.1016/j.biocon.2019.06.028
Edgar G., Stuart-Smith R., Willis T., Kininmonth S., Baker S.C., Banks S., et al. (2014). Global conservation outcomes depend on marine protected areas with five key features. Nature 506, 216–220. doi: 10.1038/nature13022
Gallagher A. J., Shipley O. N., van Zinnicq Bergmann M. P., Brownscombe J. W., Dahlgren C. P., Frisk M. G., et al. (2021). Spatial connectivity and drivers of shark habitat use within a Large marine protected area in the Caribbean, the Bahamas shark sanctuary. Front. Mar. Sci. 7 doi: 10.3389/fmars.2020.608848
Gillepsie K. M., Vincent A. C. J. (2019). Marine reserves drive both taxonomic and functional change in coral reef invertebrate communities. Biol. Conserv. 28, 921–938 doi: 10.1007/s10531-019-01702-1
Gratwicke B., Speight M. R. (2005a). The relationship between fish species richness, abundance and habitat complexity in a range of shallow tropical marine habitats. J. Fish Biol. 66 (3), 650–667. doi: 10.1111/j.0022-1112.2005.00629.x
Gratwicke B., Speight M. R. (2005b). Effects of habitat complexity on Caribbean marine fish assemblages. Mar. Ecol. Prog. Ser. 292, 301–310. doi: 10.3354/meps292301
Grorud-Colvert K., Sullivan-Stack J., Roberts C. M., Lubchenco J. (2021). The MPA guide: A framework to achieve global goals for the ocean. Science 373 (6560). doi: 10.1126/science.abf0861
Gusmao J. B., Lee M. R., MacDonald I., Ory N. C., Sellanes J., Watling L., et al. (2018). No reef-associated gradient in the infaunal communities of rapa nui (Easter island) – are oceanic waves more important than reef predators? Estuar. Coast. Shelf Sci. 210, 123–131. doi: 10.1016/j.ecss.2018.06.019
Hadj-Hammou J., McClanahan T. R., Graham N. A. J. (2021). Decadal shifts in traits of reef fish communities in marine reserves. Sci. Rep. 11, 23470. doi: 10.1038/s41598-021-03038-9
Hall A. E., Kingsford M. J. (2021). Habitat type and complexity drive fish assemblages in a tropical seascape. J. Fish Biol. 99 (4), 1364–1379. doi: 10.1111/jfb.14843
Harborne A. (2017) Modelling and mapping fishing impact and the current and potential standing stock of coral-reef fishing in the bahamas. supporting marine reserve design report to the nature conservancy. Available at: https://marineplanning.org/wp-content/uploads/2019/07/Bahamas-Protected_Mapping-Coral-Fisheries_Final-Report_2017.pdf.
Henderson C. J., Stevens T., Lee S. Y., Gilby B.L., Schlacher T.A., Connolly R.N.. (2019). Optimising seagrass conservation for ecological functions. Ecosystems 22, 1368–1380. doi: 10.1007/s10021-019-00343-3
Hughes T., Barnes M., Bellwood D., Cinner J.E., Cumming G.S., Jackson J.B.C., et al. (2017). Coral reefs in the anthropocene. Nature 546, 82–90. doi: 10.1038/nature22901
Hughes T. P., Rodrigues M. J., Bellwood D. R., Ceccarelli D., Hoegh-Guldberg O., McCook L., et al. (2007). Phase shifts, herbivory, and the resilience of coral reefs to climate change. Curr. Biol. 17, 360–365. doi: 10.1016/j.cub.2006.12.049
Jones B. L., Nordlund L. M., Unsworth R. K. F., Jiddawi N. S., Eklöf J. S. (2021). Seagrass structural traits drive fish assemblages in small-scale fisheries. Front. Mar. Sci. 8. doi: 10.3389/fmars.2021.640528
Kough A. S., Belak C. A., Paris C. B., Lundy A., Cronin H., Gnanalingam G., et al. (2019). Ecological spillover from a marine protected area replenishes an over-exploited population across an island chain. Conserv. Sci. Pract. 1 (3), e17 doi: 10.1111/csp2.17
Kough A. S., Cronin H., Skubel R., Belak C. A., Stoner A. W. (2017). Efficacy of an established marine protected area at sustaining a queen conch lobatus gigas population during three decades of monitoring. Mar. Ecol. Prog. Ser. 573, 177–189. doi: 10.3354/meps12163
Kough A. S., Gutzler B. C., Tuttle J. G., Palma N., Knowles L. C., Waterhouse L. (2022)Anthropause shows differential influence of tourism and a no-take reserve on the abundance and size of two fished species. Aquat. Conserv. Mar. Freshw. Eco. 32 (10), 1693–1709. doi: 10.1002/aqc.3856
Lamb J. B., True J. D., Piromvaragorn S., Willis B. L. (2014). Scuba diving damage and intensity of tourist activities increases coral disease prevalence. Biol. Conserv. 178, 88–96 doi: 10.1016/j.biocon.2014.06.027
Lester S. E., Halpern B. S., Grorud-Colvert K., Lubchenco J., Ruttenberg B. I., Gaines S. D., et al. (2009). Biological effects within no-take marine reserves: a global synthesis. Mar. Ecol. Prog. Ser. 384, 33–46 doi: 10.3354/meps08029
Lipcius R.N., Stockhausen W.T., Eggleston D.B. (2001). Marine reserves for Caribbean spiny lobster: empirical evaluation and theoretical metapopulation recruitment dynamics. Mar. Freshw. Res 52 (8), 1589–98. doi: 10.1071/MF01193
Loflen C. L., Hovel K. A. (2010). Behavioral responses to variable predation risk in the California spiny lobster Panulirus interruptus. Mar. Ecol. Prog. Ser. 420, 135–144. doi: 10.3354/meps08850
Madin E., Madin J., Booth D. (2011). Landscape of fear visible from space. Sci. Rep. 1, 14. doi: 10.1038/srep00014
Muller E. M., Sartor C., Alcaraz N. I., van Woesik R. (2020). Spatial epidemiology of the stony-Coral-Tissue-Loss disease in Florida. Front. Mar. Sci. 7. doi: 10.3389/fmars.2020.00163
Mumby P. J., Dahlgren C. P., Harborne A. R., Kappel C. V., Micheli F., Brumbaugh D. R., et al. (2006). Fishing, trophic cascades, and the process of grazing on coral reefs. Science. 311 (5757), 98–101. doi: 10.1126/science.1121129
Mumby P. J., Harborne A. R., Williams J., Kappel C. V., Brumbaugh D. R., Micheli F., et al. (2007). Trophic cascade facilitates coral recruitment in a marine reserve. Proc. Natl. Acad. Sci. 104, 8362–8367. doi: 10.1073/pnas.0702602104
Nagelkerken I., Dorenbosch M., Verberk W. C. E. P., Cocheret de la Morinière E., van der Velde G. (2000). Day-night shifts of fishes between shallow-water biotopes of a Caribbean bay, with emphasis on the nocturnal feeding of haemulidae and lutjanidae. Mar. Ecol. Prog. Ser. 194, 55–64. doi: 10.3354/meps194055
Rhoades O. K., Lonhart S. I., Stachowicz J. J. (2019). Human-induced reductions in fish predator boldness decrease their predation rates in kelp forests. Proc. R. Soc B. 286, 20182745 doi: 10.1098/rspb.2018.2745
Ritter C. J., Harper L. M., Lefcheck J. S., Paul V. J., Whippo R., Jones S., et al. (2021). Patterns of consumption across a Caribbean seascape: Roles of habitat and consumer species composition through time. Front. Mar. Sci. 8. doi: 10.3389/fmars.2021.742907
Rodemann J. R., Brandl S. J. (2017). Consumption pressure in coastal marine environments decreases with latitude and in artificial vs. natural habitats. Mar. Ecol. Prog. Ser. 574, 167–179. doi: 10.3354/meps12170
Rogers A., Blanchard J. L., Mumby P. J. (2014). Vulnerability of coral reef fisheries to a loss of structural complexity. Curr. Biol. 24, 1000–1005. doi: 10.1016/j.cub.2014.03.026
Rojo I., Anadón J. D., García-Charton J. A. (2021). Exceptionally high but still growing predatory reef fish biomass after 23 years of protection in a marine protected area. PloS One 16 (2), e0246335. doi: 10.1371/journal.pone.0246335
Rooker J. R., Dance M. A., Wells R. J. D., Quigg A., Hill R. L., Appeldoorn R. S., et al. (2018). Seascape connectivity and the influence of predation risk on the movement of fishes inhabiting a back-reef ecosystem. Ecosphere 9 (4), e02200. doi: 10.1002/ecs2.2200
Ruttenberg B. I., Adam T. C., Duran A., Burkepile D. E. (2019). Identity of coral reef herbivores drives variation in ecological processes over multiple spatial scales. Ecol. Appl. 29, e01893. doi: 10.1002/eap.1893
Schill S. R., McNulty V. P., Pollock F. J., Lüthje F., Li J., Knapp D. E., et al. (2021). Regional high-resolution benthic habitat data from planet dove imagery for conservation decision-making and marine planning. Remote Sens. 13 (21), 4215. doi: 10.3390/rs13214215
Soler G. A., Edgar G. J., Thomson R. J., Kininmonth S., Campbell S. J., Dawson T. P., et al. (2015). Reef fishes at all trophic levels respond positively to effective marine protected areas. PloS One 10 (10), e0140270. doi: 10.1371/journal.pone.0140270
Spadaro A. J., Butler M. J. I. V. (2020). Herbivorous crabs reverse the seaweed dilemma on coral reefs. Curr. Biol. 31 (4), 853–859. doi: 10.1016/j.cub.2020.10.097
Terborgh J., Estes J. A. (2013). Trophic cascades: predators, prey, and the changing dynamics of nature (Chicago: Island Press).
Keywords: Squidpops, consumption, MPA, habitat complexity, coral reef, seagrass
Citation: Kough AS and Belak CA (2022) Piscine predation rates vary relative to habitat, but not protected status, in an island chain with an established marine reserve. Front. Mar. Sci. 9:921463. doi: 10.3389/fmars.2022.921463
Received: 15 April 2022; Accepted: 28 September 2022;
Published: 17 October 2022.
Edited by:
Bernardo Antonio Perez Da Gama, Fluminense Federal University, BrazilReviewed by:
Miguel-Ángel Ruiz-Zárate, El Colegio de la Frontera Sur, MexicoHocein Bazairi, Mohammed V University, Morocco
Copyright © 2022 Kough and Belak. This is an open-access article distributed under the terms of the Creative Commons Attribution License (CC BY). The use, distribution or reproduction in other forums is permitted, provided the original author(s) and the copyright owner(s) are credited and that the original publication in this journal is cited, in accordance with accepted academic practice. No use, distribution or reproduction is permitted which does not comply with these terms.
*Correspondence: Andrew S. Kough, YW5kcmV3LmtvdWdoQGdtYWlsLmNvbQ==