- 1Center for Ocean and Society, University of Kiel, Kiel, Germany
- 2Marine Geophysics and Hydroacoustics, Institute of Geosciences, University of Kiel, Kiel, Germany
- 3Department of Geography, Coastal Risks and Sea Level Rise, University of Kiel, Kiel, Germany
- 4Department of Geography, Landscape Ecology and Geoinformation, University of Kiel, Kiel, Germany
Coastal wetlands depend on vertical accretion to keep up with sea level rise in cases where embankment restricts accommodation space and landward migration. For coastal wetland survival, autogenic productivity (litter, root decay) as well as allogenic matter input are crucial. Beach wrack composed of seagrass and algae can serve as an important allogenic matter source, increase surface roughness, elevate the backshore, and influence the blue carbon budget. The objective of this study is to understand how human footpaths in a frequently accessed Baltic coastal wetland influence beach wrack transport and accumulation. Beach wrack monitoring during the winter storm season 2021/2022 was conducted in high spatial and temporal resolution with bi-weekly UAV flights. Object-based identification, segmentation, and classification of orthophotos with open-source software allowed the detection of beach wrack patches with a mean area of 0.6–2.7 m². Three major storm events occurred during the monitoring period (Arwen, Malik, Eunice). Regardless of wind speed or direction, the main accumulation zones remained stable. The east-west footpath that crosses the coastal wetland and connects the tourist hotspots served as a “highway” for water-mediated transport of beach wrack. Total area covered by beach wrack fluctuated between 1,793 and 2,378 m² with a peak after storm Malik in January 2022. The densely accumulated beach wrack along the main east-west footpath formed an elongated micro-cliff-like structure and limited landward transport. Additional aerial image analysis for the last 15 years showed that the position of the footpaths remained stable. This pioneering study offers first insights into the fate of beach wrack in an anthropogenically influenced Baltic coastal wetland where larger tidal channels that usually generate hydrological connectivity are missing. The identified transport patterns and accumulation hotspots are a starting point for further research on how beach wrack behaves in (waterlogged) coastal wetlands compared to decomposition on sandy beaches.
Introduction
Coastal wetlands, as the interface between aquatic and terrestrial ecosystems, are highly productive ecosystems that provide various habitats, sequester carbon, dissipate wave energy, or buffer nutrients (e.g., Reddy and DeLaune, 2008; Karstens et al., 2015; Jurasinski et al., 2018; Heckwolf et al., 2021; Buczko et al., 2022). However, coastal wetlands are at risk of submergence and thus loss of important ecosystem services if vertical growth cannot keep up with the rising sea level (Coleman et al., 2022). A main driver of wetland resilience is the availability of accommodation space, allowing wetlands to migrate landwards and compensate for seaward losses due to erosion or drowning (Schuerch et al., 2018). In case human-made constructions preclude landward migration, coastal wetland survival depends on vertical accretion, which is largely driven by suspended sediment concentrations in nearby coastal waters (Kirwan et al., 2016; FitzGerald et al., 2008; Howe et al., 2009; Reed, 1990; Morris et al., 2002). Although nature-based adaptation solutions receive growing attention (e.g., Thorslund et al., 2017; Kiesel et al., 2020; Leonardi and Dai, 2022), coastal protection in the Baltic region still relies most commonly on engineered solutions and large coastal strips are characterized by embankment. Under such conditions, coastal wetlands must act as bioengineers of their own environment with the feedback mechanisms of plant production and sediment trapping resulting in vertical accretion. For coastal wetland survival, autogenic productivity (litter, root decay) as well as allogenic matter input are crucial (Morris et al., 2002). In case sediment supply from land is suppressed by an embankment (Willemsen et al., 2016; Perillo et al., 2018), seagrass and macrophytes deposited on the shore as beach wrack could become an important source of allogenic matter. Beach wrack increases the surface roughness and has been identified as a key factor in trapping wind-blown sand (Nordstrom et al., 2011). Furthermore, beach wrack enhances plant colonization at more seaward-located positions because it elevates the backshore and provides essential nutrients (Dugan et al., 2011; Biel et al., 2018; Hyndes et al., 2021). For Baltic coast wetlands, Ward et al. (2016) have shown that already small alterations in elevation and thus inundation frequency can lead to substantial vegetation changes. Beach wrack transport and accumulation patterns and their impact on topography have thus direct implications with regard to ecological restoration efforts and target species defined in nature conservation plans (e.g., NATURA, 2000 management plans). In terms of a unit area, coastal vegetated ecosystems store much more carbon in both their biomass and sediments than most terrestrial ecosystems (e.g., Mcleod et al., 2011; Duarte et al., 2013). Seagrass in the Baltic Sea is also thought to have great potential for storing CO2. However, this refers to the submarine part with the storage of organic matter in the seafloor. Despite the ecological importance, so far, most studies have focused on sandy beaches (e.g., Dugan et al., 2011; Reimer et al., 2018; Hyndes et al., 2021; Pan et al., 2022) and the transport and fate of beach wrack in vegetated coastal ecosystems has not been studied yet.
The Baltic Sea represents one of the largest brackish waters of the Earth with strong lateral variations in the salt content from near marine in the western to near limnic conditions in the inner parts (Reusch et al., 2018). Over the course of the past century, Baltic Sea ecosystems have undergone drastic changes and anthropogenic pressures especially in the coastal zone remain high (HELCOM, 2010; Dietz et al., 2021). Coastal wetlands are particularly valuable as they provide essential coastal habitats for fish in the Baltic Sea (Kraufvelin et al., 2018), counteract eutrophication (Karstens et al., 2015), and provide coastal protection (Schernewski et al., 2018). Coastal wetland evolution is controlled by a variety of factors including biotic activities and biomass production, storm activities, subsidence, sediment supply, as well as changes in local hydrology (Boumans and Day, 1993; Cahoon et al., 2011). Tides play a minor role in the Baltic Sea and water level fluctuations are caused mainly by meteorological forcing, episodically leading to oscillating water levels (Keruss and Sennikovs, 1999; Niedermeyer et al., 2011). The tidal range limits channel depth in creek networks (Davidson-Arnott et al., 2019) and large tidal channel systems that generate hydrologically connectivity in North Sea salt marshes do not exist in Baltic coastal wetlands. However, many coastal wetlands along the Baltic coast are accessible and planned as well as unplanned walking footpaths develop. These footpaths create mosaics of vegetation patches and could influence the hydrological connectivity. In order to better assess the impact, it is important to understand if footpaths are seasonal structures emerging during the tourist season and potentially disappearing under fresh vegetation in the next year with new pathways opening up or whether the footpath persist over time at the same positions, impacting the study site on longer timescales. Comprehending the impact of such anthropogenic footprint is a prerequisite for the sustainable management of coastal wetlands.
The objective of this study is to understand how human footpaths in a frequently accessed coastal wetland influence the water-mediated transport of beach wrack. Baltic coastal wetlands remain largely understudied (Graversen et al., 2022) and the fate of beach wrack in these systems has not been explored yet. Bi-weekly UAV flights between December 2021 and April 2022 covering the winter storm season in combination with aerial image analysis of 2007–2019 enabled us to investigate the following research questions in high spatial and temporal resolution: (I) Are human footpaths hotspots for beach wrack accumulation? (II) How do those footpaths persist in wetlands over time? We anticipate that our results will help to better understand Baltic coastal wetland dynamics and effects of altered hydrological connectivity under intensifying climatic conditions (e.g., increased storm activity during winter months).
Methods and Material
Study Site
The coastal wetland Stein is situated in northern Germany at the outer Kiel Fjord, Baltic Sea (Figure 1A). As a result of the ongoing shore-parallel sediment transport, most of the bays between Kiel and the island Fehmarn are currently cutoff by spit formation. Bottsand (see Figure 1B) is the youngest spit growing to the west and has been advancing since 1880 (Niedermeyer et al., 2011). Bottsand has been a nature reserve since 1939 with various dune stages and salt marsh vegetation and access is completely forbidden (Diehl and Diehl, 1986). In contrast, the coastal wetland Stein south-west of Bottsand is a popular tourist area and frequently visited during all seasons. Accommodation space is limited as the wetland is squeezed by a dike in the hinterland and a marina in the east. The typical German beach chairs are placed each summer in the two sandy beach areas (see Figure 1C) and footpaths connect these areas with the walkway on the dike as well as with the marina. The coastal wetland offers a diverse species portfolio, but the seaward wetland edge is largely dominated by common reed (Phragmites australis) with a few patches of salt marsh bulrushes (Bolboschoenus maritimus). The composition of beach wrack is geographically highly diverse and overall the contribution of ephemeral and nutrient-opportunistic seaweeds increased along Schleswig-Holstein’s Baltic Sea coast (Weinberger et al., 2021). However, beach wrack at the study site Stein is dominated by seagrass (relative contribution of 89–95%, Weinberger et al., 2021), probably due to the large seagrass meadows north-west off the coast (Kuhwald et al., 2021; see Figure 1B). Aquatic vegetation is dominated by Zostera marina (Schubert et al., 2015) and to a lesser degree by Fucus vesiculosus and Fucus evanescens (Rohde et al., 2008).
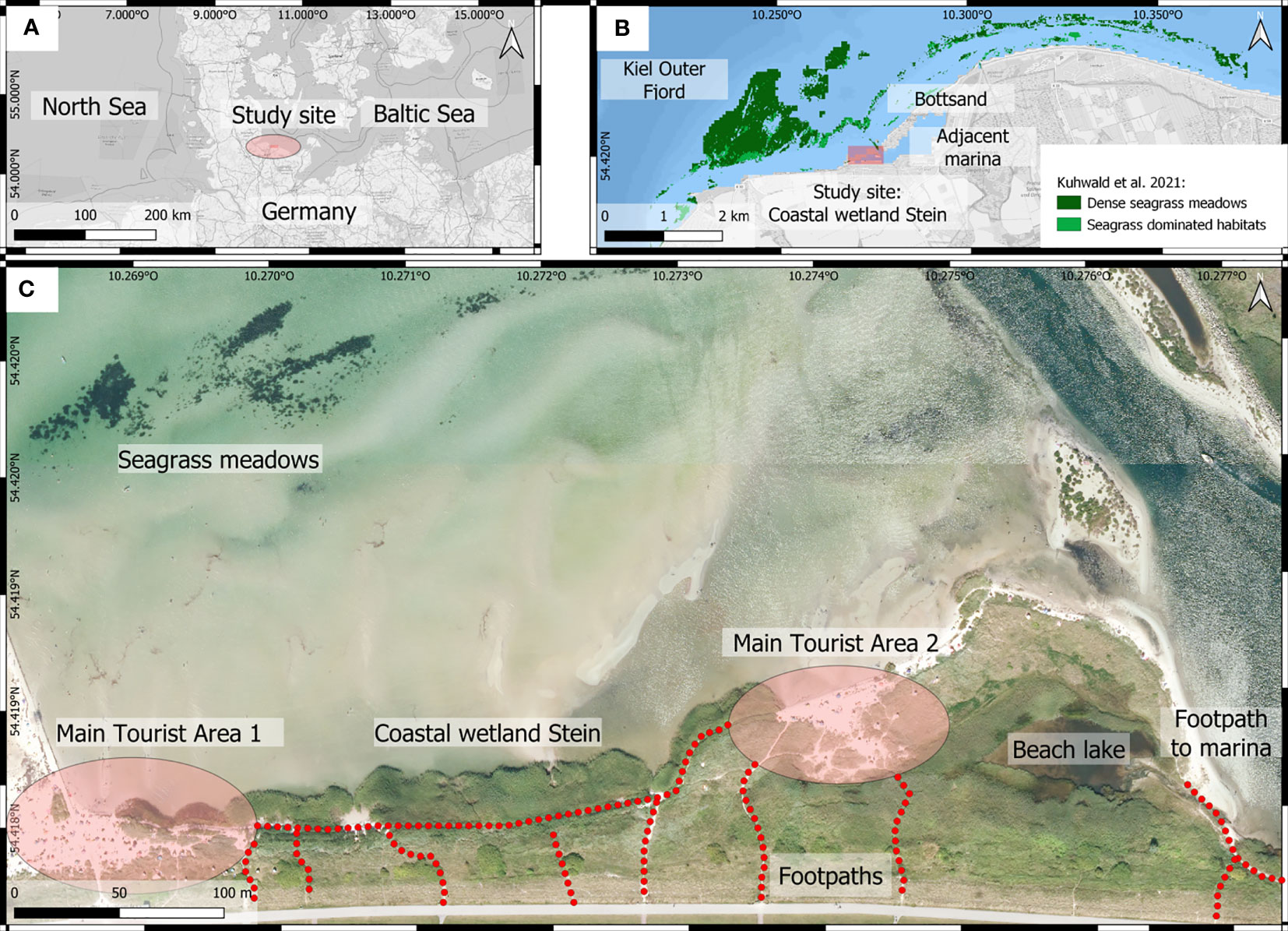
Figure 1 (A) The coastal wetland Stein is situated in northern Germany at the outer Kiel Fjord, Baltic Sea (Background map: Open Street Map). (B) Large seagrass meadows are located north-west of the study site (Data is derived from Sentinel satellite and cut off at 4 m water depth, provided by Kuhwald et al. (2021). Adjacent to the coastal wetland is the spit formation Bottsand, a nature protection area, as well as a marina. (C) Several footpaths cross the coastal wetland at Stein. The main footpath connects the tourist areas from east to west (The aerial image from 2019 was chosen as background image as it was taken during summer, showing the main tourist areas, data provided by the State Office for Surveying and Geoinformation Schleswig-Holstein).
Brackish reed wetlands dominated by P. australis are characteristic for the Baltic region, especially along the inner, protected coastal waters (Dijkema, 1990; Meriste et al., 2012). To access the water front, walking footpaths develop in these vegetated coastal ecosystems. Study sites from the work of Chubarenko et al. (2021) on beach wrack management around the Baltic Sea were used to check that our study site is not unique. Using Google Maps, we confirmed the following similar sites with footpaths and availability of beach wrack: Curonian Spit, Lithuania—55° 19′ 22.0″ N, 21° 02′ 21.8″ E; Vistula Spit, Poland—54° 25′ 49.2″ N, 19° 36′ 11.0″ E; Kalmar, Sweden—56° 38′ 51.7″ N, 16° 19′ 12.0″ E; Køge, Denmark—55° 24′ 01.5″ N, 12° 19′ 10.5″ E; Island Rügen, Germany—54° 17′ 12.3″ N, 13° 41′ 23.5″ E. P. australis is also a common species along other brackish coasts, e.g., along the Black Sea (Sangiorgio et al., 2008) and Chesapeake Bay (e.g., Rice et al., 2000; Chambers et al., 2008). Hence, studies on the interactions between reed and beach wrack are of global interest. The coordinates given are just examples for sites where footpaths in coastal vegetated ecosystems may impact the hydrological connectivity in systems with minor tidal influence, comparable to our study site Stein.
Data Acquisition and Processing
Beach wrack monitoring is challenging as its distribution is temporally and spatially variable. Uhl et al. (2022) developed classification ensembles for beach wrack detection at a spatial scale of 3–10 m with multispectral data of the sensors Sentinel-2 MSI and PlanetScope. However, the spatial resolution would not be suitable for beach wrack monitoring in coastal wetlands with footpaths sometimes not wider than 1 m. This can be achieved with high-resolution images acquired from sensors assembled on uncrewed aerial vehicles (UAVs). Pan et al. (2021) showed that UAV imagery and object-based image analysis (OBIA) are a cost- and labor-saving option to monitor beach wrack accumulation with a sub-decimeter spatial resolution. In this study, bi-weekly UAV surveys between December 2021 and April 2022 monitored and mapped beach wrack accumulation during the winter storm season. Flight height was 70 m (Figure 2A). A DJI ZenmuseX5S camera mounted on a DJI Inspire II UAV provided RGB imagery in sub-decimeter scale. Orthophotos and digital elevation models were generated based on structure-from-motion photogrammetry with the open-source software WebODM (Version 1.9.11, OpenDroneMap, 2022, see Mokrane et al., 2019; Vacca, 2020). WebODM uses multiple techniques and software libraries to process aerial image data. Orthophotos are generated by stitching individual, overlapping orthorectified aerial images producing a single file, that is then georeferenced and converted to a GeoTIFF (Vacca, 2020; Pell et al., 2022). A first step in this process is the generation of a textured 3D point cloud that represents the landscape which was captured by the UAV in several single images. WebODM uses a structure-from-motion software library called OpenSfM (OpenSfM, 2022) in combination with the Multi-View-Stereo (MVS 2022) technique (Vacca, 2020). Fifteen ground control points, taken with a Leica GNSS-RTK-Rover in the field, were provided for georeferencing. Georeferenced point cloud data was used for the processing of digital elevation models. WebODM extracts a surface model by using an inverse distance weighting interpolation method and the model is then smoothed using a media filter to remove noise (Vacca, 2020). Pell et al. (2022) evaluated the workflows and output products of different structure-from-motion photogrammetry software options. WebODM needed more processing time for larger datasets than for example Correlator3D and AgiSoft, but worked well for a variety of different ecosystems and had no problems to reconstruct underwater features in comparison with Pix4Dmapper. Aerial images from 2007 to 2019 were provided by the State Office for Surveying and Geoinformation Schleswig-Holstein (LVermGeo SH) and provided insights into past development of footpaths, vegetation, and shoreline evolution. Flight height was between 1,000 and 2,400 m and RGB image resolution between 10 and 15 cm. Segmentation and supervised classification procedures for all images from 2007 to 2022 were performed using the open-source Orfeo Toolbox (OTB Version 6.0, Grizonnet et al., 2017). Instead of classifying individual pixels, OBIA works with homogeneous and contiguous groups of pixels known as segments (Torres-Sánchez et al., 2015). For the segmentation, a spatial range of 50 and radial range of 7 was chosen, corresponding to the feasibility study by Pan et al. (2021). Support vector machine (SVM) as one option of machine learning methods was chosen, although Pan et al. (2021) showed that random forests (RF) and K-nearest neighbor (KNN) also performed well with an overall classification accuracy >75%. In supervised algorithms, a training phase is used to associate every segment with a pre-defined class (De Luca et al., 2019). On average, 120 training points for the following five classification classes were set-up manually for every orthophoto: (1) vegetation, (2) beach wrack, (3) sand, (4) water covered sand, and (5) water. Following De Luca et al. (2019), a regular square grid of points with dimensions of 20 × 20 m was placed over the study area for accuracy assessment (Figure 2B). Predicted values were compared with manual visual inspection. Confusion matrices were generated and producer’s and user’s accuracy were calculated with values >80% for all five classes (see Supplementary Material). The study area of approximately 58,000 m², which was surveyed by UAV, is also manageable by foot. Field observations along the footpaths were carried out simultaneously with the UAV fights and beach wrack composition was documented with photos. Visualization and area calculations were performed with open-source QGIS [Visualization and area calculations were performed with open-source QGIS (Figure 2C) Version 3.16 “Hannover”; QGIS, 2022].
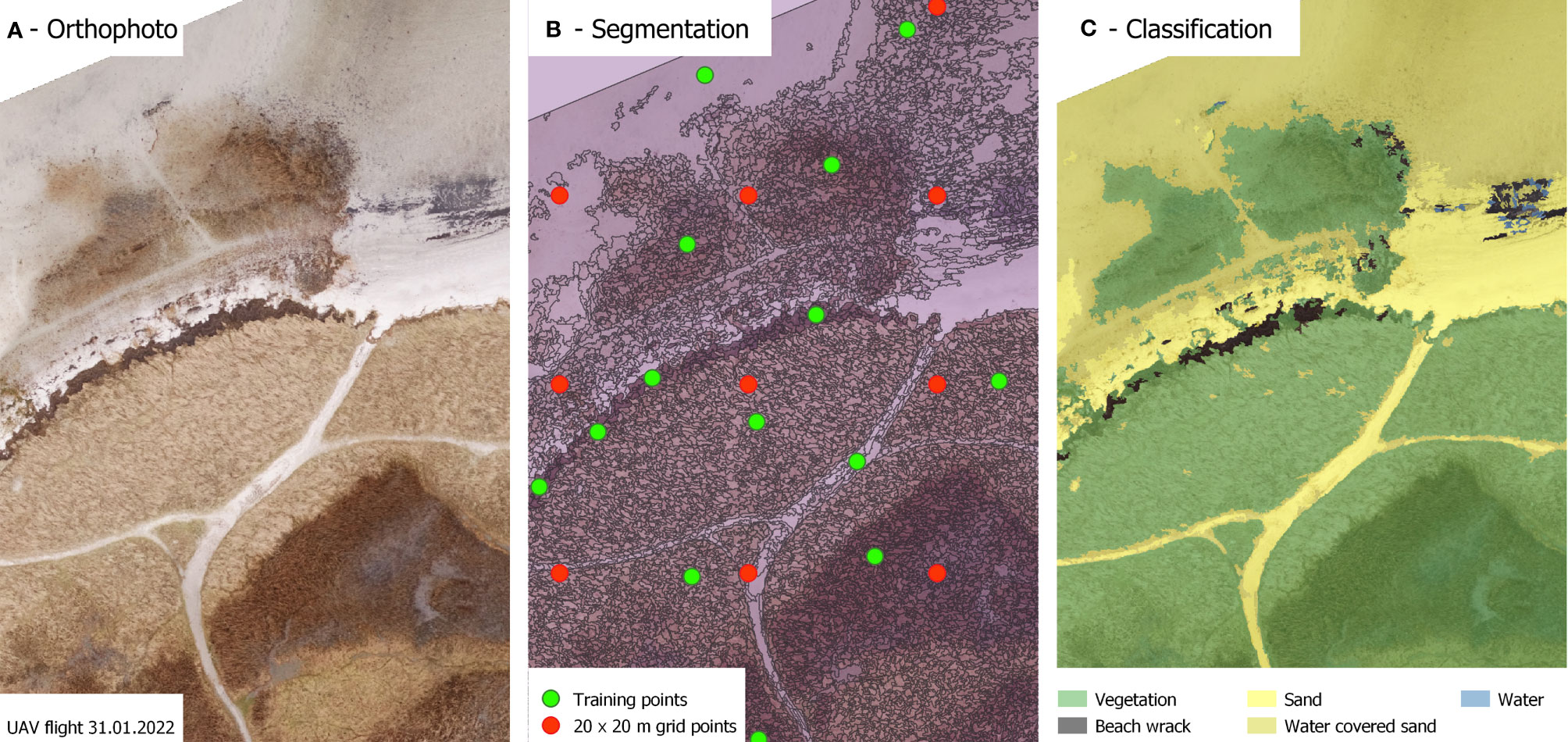
Figure 2 Data from UAV overflight in 70 m height. (A) Orthophoto showing unvegetated and vegetated beach with some beach wrack in the transition zone. (B) OTB segmentation results including training points for 5 classes as well as 20 × 20 m regular square grid of points for accuracy assessment. (C) Final classification results.
Weather data for the monitoring period was provided by the German Weather Service (DWD) and water level at the nearest station (Kiel lighthouse) was recorded by the Federal Waterways and Shipping Administration (WSV) and provided by the Federal Institute for Hydrology (BfG). Major events were storm Arwen (known as Hannelore in Germany), storm Malik (known as Nadia in Germany), and storm Eunice (known as Zeynep in Germany, see Figure 3). Storms Malik and Eunice caused serious damage in Europe with wind speeds of up to 141 km/h in Kiel.
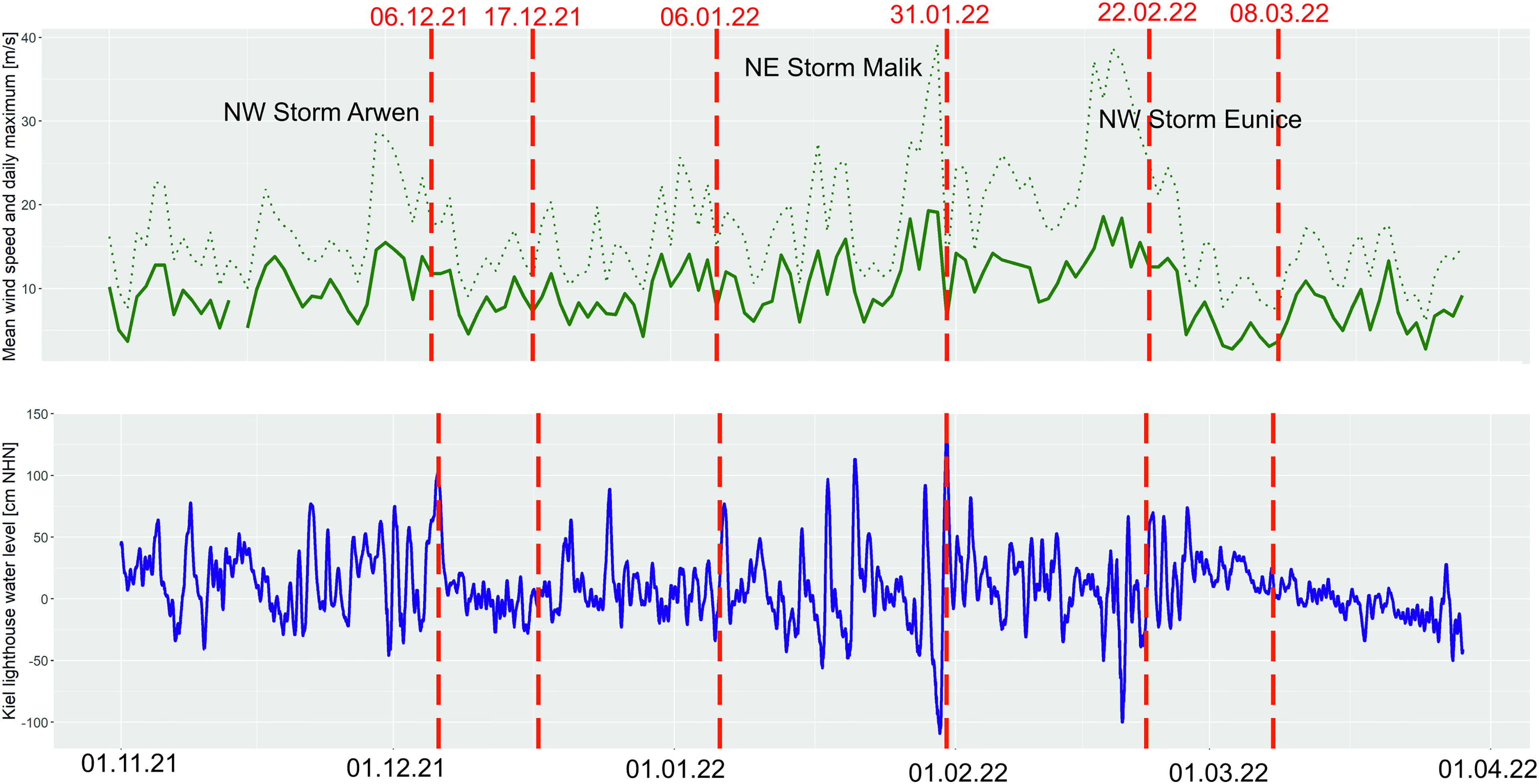
Figure 3 The monitoring period covered the major storm events Arwen, Malik, and Eunice. Green line displays mean wind speed [m/s] and green dotted line displays maximum wind speed [m/s]. Blue line shows water level fluctuations [cm NHN]. Dates for UAV flights are displayed as red dotted vertical lines (06.12.21, 17.12.21, 06.01.22, 31.01.22, 22.02.22, 08.03.22). Weather data for the monitoring period was provided by the German Weather Service (DWD) and water level was recorded by the Federal Waterways and Shipping Administration (WSV) and provided by the Federal Institute for Hydrology (BfG).
Results
Beach Wrack Accumulation During Winter Storm Season 2021/2022
Total area classified as beach wrack shows fluctuation between 1,793 and 2,378 m² during the monitoring period, with a peak after storm Malik in January (Table 1). An area of 492 m² was permanently covered by beach wrack. Overlapping beach wrack zones in between UAV surveys are listed in Table 1. Accumulation patterns were similar in all winter months with two distinct hotspot areas: (1) the east-west footpath connecting the main tourist areas and (2) the area between the beach lake outlet and the footpath coming from the marina in the east (see Figure 4). Beach wrack passed the wetland edge at open spaces between the dense Phragmites stands and accumulated along the main footpath (Figure 5). It formed a long and narrow cliff-like structure (see photo impressions in Table 1 and Figure 6). Beach wrack was rarely mapped along the small footpaths crossing the study area at various locations along the north-south direction, although these footpaths were flooded up to 75 m landwards from the seaward edge during some events (e.g., 06.01.2022).
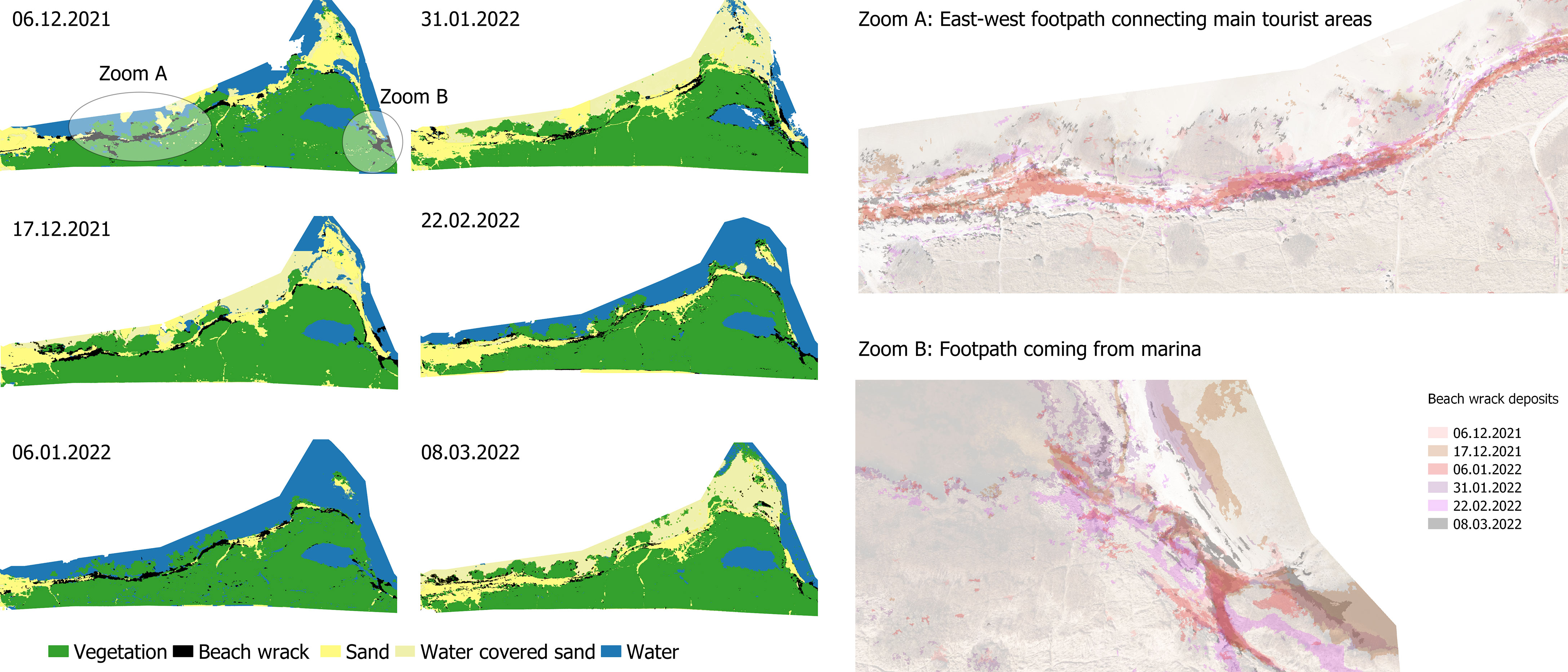
Figure 4 Classification results for the UAV imagery from December 2021 to April 2022. Regardless of weather or water level conditions, beach wrack accumulations could always be mapped in two hotspot areas: along the east-west footpath connecting the main tourist areas (Zoom A) and within the area between the beach lake outlet and the footpath coming from the marina in the east (Zoom B).
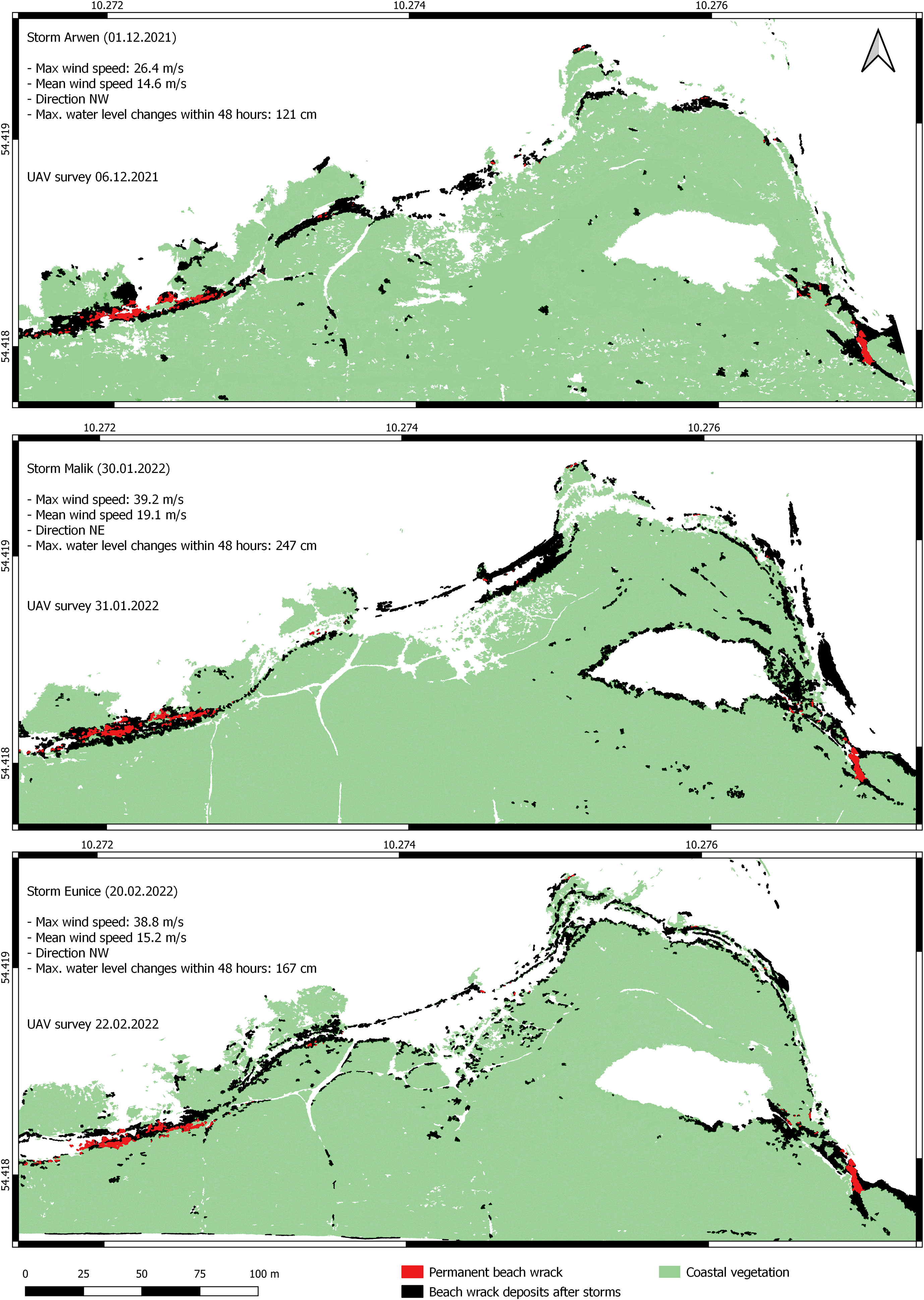
Figure 5 Beach wrack deposits after Storm Arwen in December 2021, Storm Malik in January 2022, and Storm Eunice in February 2022. Marked in red are the areas with permanent beach wrack during the winter storm season 2021/2022.
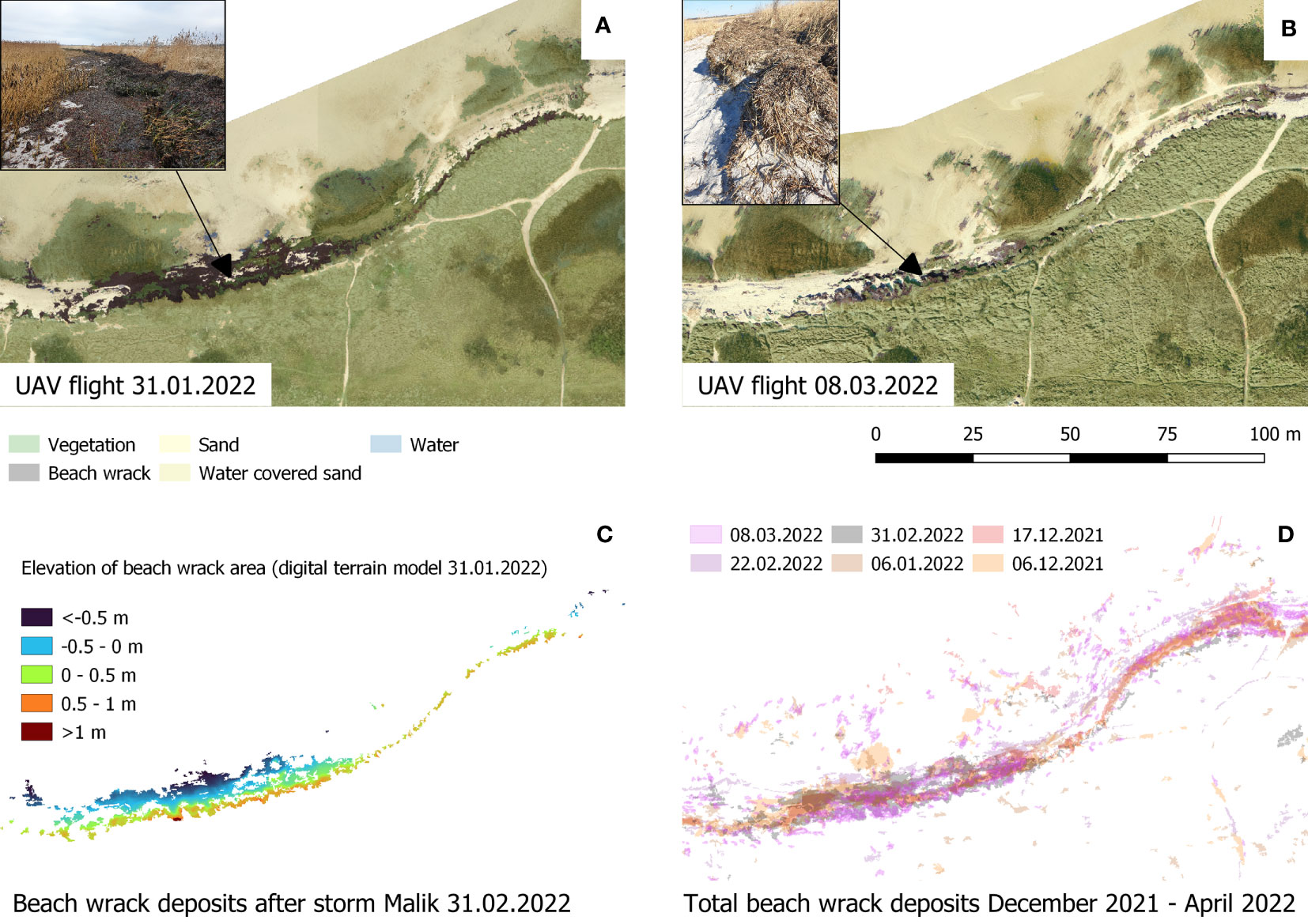
Figure 6 Zoom into the “beach wrack highway” along the east-west footpath. While fresh sea grass leaves were visible in the beach wrack during December and January (A), the beach wrack started to get covered by sand and mixed with Phragmites litter and broken stems in February and March (B). This underlines the importance of event-based monitoring. The elongated cliff-like structure becomes also obvious looking at the digital elevation model based on UAV photogrammetry with the open-source software WebODM for the 31.01.2022 after storm Malik (C). Beach wrack accumulated behind “the first line of defense” (mainly Phragmites patches) along the east-west footpath connecting the two sandy areas where most tourists reside. (D) Almost no beach wrack accumulated along the north-south footpaths.
Storm Arwen in the beginning of December 2021 with NW wind speeds of up to 26.4 m/s and a water level increase of 121 cm within 48 h pushed fresh sea grass leaves along the footpath oriented parallel to the shoreline that connects the two main tourist areas as well as along the footpath between the beach lake and adjacent marina (Figure 5). NE storm Malik in January with wind speeds of up to 39.2 m/s caused massive water level fluctuations, rising from -100 cm below mean sea level to 150 cm above mean sea level (Figure 3). Beach wrack was pushed further into the beach lake. Large amounts of fresh sea grass leaves were also deposited against the wetland edge in the north-east of the study site. However, only the beach wrack trapped along the footpaths embedded with vegetation along both sides remained trapped and permanently covered the ground throughout the monitoring period (Figure 5). After storm Eunice in February, beach wrack started to get covered by sand, making mapping via UAV RGB imagery more difficult and potentially resulting into an underestimation of beach wrack area. However, fresh green sea grass leaves were visible in the beach wrack after storm Arwen (December) and after storm Malik (January), but not after storm Eunice (February). Starting in January, the beach wrack got mixed with Phragmites litter and broken stems (Table 1). Storm Eunice resembled storm Arwen with NW winds, but higher wind speeds of up to 38.8 m/s and a more pronounced water level increase of 167 cm within 48 h. In contrast to storm Malik with one peak, wind speeds above 30 m/s remained for five consecutive days during storm Eunice (Figure 3). Highest water level during that time was 67 cm above mean sea level but when water level dropped to -38 cm below mean sea level on the 20th of February, maximum wind speed was still 28.2 m/s, explaining why beach wrack was covered by sand being blown from the main beach area in the west.
Temporal and Spatial Evolution of Footpaths and Wetland Edge
The width of the east-west footpath connecting the main tourist areas varied during the last 15 years. Mean width at location I (see Figure 6A) was 14 m in March 2007, 6 m in March 2013, and 13 m in March 2019. During vegetation peak in summer, the width of the footpaths can decrease to <3 m (see images 2010 and 2016 in Figure 7A). The width of the various north-south footpaths (location II) never exceeded 3 m throughout the years and seasons. The position of those footpaths remained stable since 2013. This was also confirmed during the winter storm season 2021/2022, where the east-west footpath oriented parallel to the shoreline increased in width, while the north-south footpaths remained stable. The width of the footpath coming from the marina (location III) decreased from 5 m in 2007 to 3 m in 2022. Since 2007, the coastal wetland did not propagate seaward and the vegetation at the wetland edge remained relatively stable. Spaces between the Phragmites patches remain open throughout the years (Figure 6B).
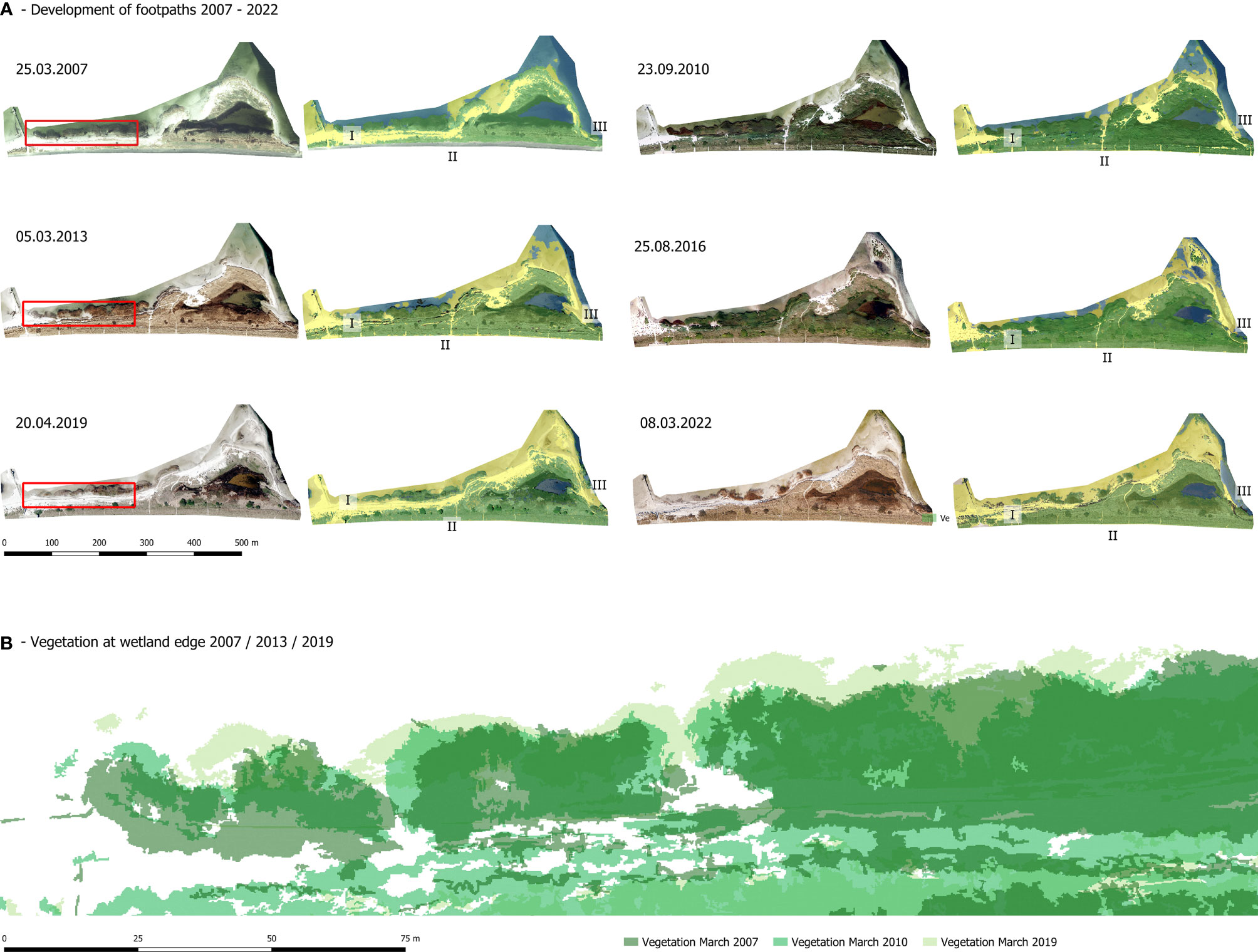
Figure 7 (A) Coastal wetland dynamic between 2007 and 2022. Classification results of the aerial images show that the positions of footpaths remained stable, whereas the widths varied. Locations I–III where the widths of the footpaths were measured are shown in the map. (B) Zoom into the wetland edge for March 2007, March 2013, and March 2019. The picture section is marked in red in (A). Aerial mages from 2007 to 2019 were provided by the State Office for Surveying and Geoinformation Schleswig-Holstein (LVermGeo SH).
Discussion
Beach wrack at the study site Stein is accumulated along the main footpaths that connect the tourist hotspots (the two main sandy beach areas as well as the adjacent marina, see Figure 1). In the Odense Fjord (Denmark), tidal amplitude has the largest impact on beach wrack deposition, with onshore transport during high tide (Pan et al., 2022). Tides play a minor role at our study site but massive water level fluctuations were caused by winter storms Arwen, Malik, and Eunice with amplitudes of 121 cm, 247 cm, and 114 cm, respectively. Large amounts of beach wrack were deposited along the wetland edge and pushed into the footpath oriented parallel to the shoreline. This east-west footpath appears to be a “highway” for beach wrack transport (Figure 4). Seagrass and macrophyte depositions at beaches are highly dynamic (Chubarenko et al., 2021; Pan et al., 2022) and beach wrack can be washed up onshore and then back into the sea within several hours (Pan et al., 2022). This dynamic was also observed in the Western Baltic Sea despite the lack of tides (Hammann and Zimmer, 2014). Overlapping areas at our study site Stein where beach wrack remained between UAV surveys decreased throughout time (Table 1) and beach wrack deposits at the outer wetland edge and in the sandy areas of our study site are also spatially and temporally dynamic. However, beach wrack trapped along east-west footpaths oriented parallel to the shoreline stayed permanently on the ground throughout the monitoring period (Figure 5). Although the north-south footpaths were flooded during the monitoring period and the hydrological connectivity of the system was visible, their role regarding beach wrack transport is negligible. Mean size of monitored beach wrack patches was 0.6–2.7 m², thus identifiable via UAV imagery, but not detectable by methods for beach wrack monitoring applied to global scale data products such as Sentinel-2 MSI or PlanetScope (Uhl et al., 2022). No large beach wrack accumulations along the north-south footpaths were observed during field inspections. Rather than the smaller width of the footpaths, it seems reasonable that the accumulated beach wrack along the east-west footpath itself prevented the transport landwards. The beach wrack formed an elongated micro-cliff-like structure parallel to the shoreline and persisted throughout the winter storm season 2021/2022 (Figures 5, 6). Wave attenuation is enhanced at micro-cliff sites compared to smoother transition sites (Möller and Spencer, 2002). The “beach-wrack-micro-cliff” provides an effective protection landwards. The footpaths have existed for more than 15 years and create mosaics of vegetation patches. They are not seasonal features that disappear with the end of the tourist season, but influence biological and hydrological connectivity at the study site on longer timescales. In cases where wetland restoration projects take place in accessible areas welcoming visitors, path orientation that might impact water-mediated transport and allogenic material trapping should be taken into consideration.
The area covered by beach wrack peaked after storm Malik in January 2022. Although storm Eunice in February lasted longer, the beach wrack area did not increase and no fresh sea grass leaves were visible. One seagrass shoot litters 6–30 leaves per year (Mateo et al., 2006; Weinberger et al., 2021) and the seagrass meadows offshore (see Kuhwald et al., 2021) provide a large source. Still, it seems as if most cast material was already transported onshore by storm Malik, thus beach wrack accumulation might depend more on timing and source availability than on storm strength. Statements on the importance of storm direction cannot be derived as the study site is exposed to NW, N, and NE to more or less the same degree and other storm directions did not occur during the study period. Similar observations were made by Hamann and Zimmer (2014), where no significant correlation between weekly beach wrack depositions and mean wind speed existed. The authors state that spatial and temporal dynamics are less predictable in tide-free coastal systems and might depend on the abruptness of changes in wind speed. Changes in water level and wind speed were most extreme during storm Malik, resulting also in the largest beach wrack deposition during our monitoring period. Both wind and wave climate that influence the biogeographic patterns of beach wrack (Reimer et al., 2014; Hyndes et al., 2021) will change in the next decades and alter the beach wrack depositions patterns. Wind and wave projections for the Baltic Sea are highly uncertain due to large natural variability; but in areas no longer covered by sea ice, wind fetch and wind speeds could increase (Ahola et al., 2021).
The wetland edge is less densely vegetated in winter and open, unvegetated spaces remained throughout the last 15 years (Figure 6). Bending stiffness of P. australis varies seasonally with lowest values in February and highest in August (Das and Tanaka, 2007), thus beach wrack accumulation patterns might differ in summer. Less beach wrack might break through the “first line of defense” in summer. Furthermore, broken Phragmites stems contributed to the beach wrack composition in late winter (see Table 1). Still the Phragmites stems show lower susceptibility to breaking under mechanical stress than inter alia Schoenoplectus lacustris (Das and Tanaka, 2007) and also contribute to wave attenuation, water flow reduction, and trapping of beach wrack behind the wetland edge along the footpath during the winter months. P. australis is not only a characteristic species in the Baltic region, but also common along other brackish basins such as the Black Sea (Sangiorgio et al., 2008) and Chesapeake Bay (e.g., Rice et al., 2000; Chambers et al., 2008), thus studies on the interactions between reed and beach wrack are of global interest.
Accumulation of organic material from allochthonous sources is a key component that influences coastal wetland sediments (Bianchi et al., 2011). Beach wrack increases the surface roughness and elevates the backshore (Nordstrom et al., 2011; Biel et al., 2018). At our study site, beach wrack formed an elongated micro-cliff-like structure at the interface of the footpath and the next vegetated area. Due to the strong elevation–vegetation relationship in Baltic coastal wetlands (Ward et al., 2016), small eco-hydrological changes can impact vegetation characteristics and structural as well as functional connectivity within the wetland. B. maritimus and S. lacustris can outcompete P. australis in less elevated and thus more frequently inundated areas (Ward et al., 2016). These vegetation changes could then result in altered resilience to storm surge conditions, due to the species-specific control of wave attenuation. Flexible low-growing species are more resilient compared to rigid and all canopies (Rupprecht et al., 2017). At the time of this study Phragmites patches are dominant at our study site compared to Bolboschoenus or Schoenoplectus patches. Beach wrack deposition influences not only elevation changes but also nutrient content and oxygen conditions in the soil, further important factors that influence vegetation patterns. Nutrient remineralization from beach wrackplays a large role (Dugan et al., 2011) and seagrass buried by sand (as happened after storm Eunice) decomposes almost twice as fast as on top of the sand (Hamann and Zimmer 2014). Long-term monitoring would be needed to estimate how beach wrack accumulation impacts sediment composition and thereby vegetation changes.
Wetland width is another key parameter that impacts the effectiveness of flood risk reduction (Kiesel et al., 2022). Over the past years, the wetland edge at Stein has been relatively stable (Figure 7). Thus, in order to thrive and function as nature-based solution, the wetland depends on vertical accretion that has to be equal or greater than the sum of total subsidence and local sea level rise (Cahoon, 2015). Landward migration as well as sediment supply from land is restricted and the fate of beach wrack in the area impacts the wetland evolution. In coastal environments where beaches are fringed with riparian vegetation, reciprocal matter exchange between submerged and emerged vegetation exists (Heerhartz et al., 2014). Beach wrack washed onshore after storm events and trapped along footpaths enhances wetland resilience. Beach wrack itself also provides a variety of ecosystem services such as stabilizing soft bottom substrates, providing food and shelter to different organisms or storing blue carbon (Defeo et al., 2009; Malm et al., 2004; Vanhooren et al., 2011; Gilburn, 2012; Weinberger et al., 2021; Pan et al., 2021). However, not only Baltic coastal wetlands are understudied with regard to carbon sequestration and storage (Graversen et al., 2022; Buczko et al., 2022), but also the role of beach wrack remains overlooked (Duarte et al., 2013). Blue carbon storage as well as the potential of greenhouse gas emissions due to decomposition should be considered (Pan et al., 2021). This study offers first insights into the fate of beach wrack in coastal wetlands. Further research on how beach wrack behaves in (waterlogged) coastal wetlands compared to decomposition on sandy beaches is needed to better understand system dynamics.
Conclusion
This study offers first insights into the fate of beach wrack in an anthropogenically influenced Baltic coastal wetland where larger tidal channels that usually generate hydrological connectivity are missing. Total area covered by beach wrack fluctuated between 1,793 and 2,378 m² with a peak after storm Malik in January 2022. The smaller north-south footpaths played a minor role. However, it seems as if not the width of footpaths but the position is crucial. Footpaths oriented parallel to the shoreline turned out to act as major beach wrack trap and “highway” for water-mediated transport. If this is a generic feature, path orientation could be designed in a way to enhance entrapping allogenic material. The densely accumulated beach wrack formed an elongated micro-cliff-like structure and limited landward transport. The existing footpaths remained stable in their position for the last 15 years and future dynamics will continue to be influenced by these human footprints. Repeated, event-based UAV surveys not only offer great resolution to identify vegetation patches, but also disclose tempo-spatial dynamics of shape and color of detected wrack segments. This could help to improve the interpretation of satellite-derived beach wrack analyses. The identified transport patterns and accumulation hotspots are a starting point for further research on how beach wrack behaves, decomposes, and impacts sediment composition inter alia in the beach lake with continuously standing water, within vegetated patches or deposited in sandy, unvegetated areas.
Data Availability Statement
The datasets generated for this study (e.g. orthophotos) can be found in the KüNO data portal, a joint data portal for national North Sea - Baltic Sea research that combines metadata from previous and current KüNO projects as well as corresponding metadata from MDI-DE (Marine Data Infrastructure Germany) https://deutsche-kuestenforschung.de.
Author Contributions
SK developed the article concept, took care of the data collection and analyses, and did most of the article writing. LP and KE supported the data collection, data analyses, and commented on the paper. AV and JS supported the analysis and commented on the paper. JK and FG supported the article concept development, the writing, and the analysis. All authors contributed to the article and approved the submitted version.
Funding
This research is part of following projects: (1) Multi-dimensional Ocean Hazard Risk Assessment (FON-2020-04, allocation of funds from the state of Schleswig-Holstein for the measure ensuring top-level interdisciplinary and transdisciplinary research in the marine sciences in Kiel), (2) ECAS-BALTIC: Strategies of ecosystem-friendly coastal protection and ecosystem-supporting coastal adaptation for the German Baltic Sea Coast (BMBF funded, 03F0860H), (3) BONUS ECOMAP project, supported by BONUS (Art 185), funded jointly by the EU and the Federal Ministry of Education and Research of Germany (BMBF), the National Centre for Research and Development of Poland (NCBR), and the Innovation Fund Denmark (Innovationsfonden). We acknowledge financial support by DFG within the funding programme Open Access-Publikationskosten.
Conflict of Interest
The authors declare that the research was conducted in the absence of any commercial or financial relationships that could be construed as a potential conflict of interest.
Publisher’s Note
All claims expressed in this article are solely those of the authors and do not necessarily represent those of their affiliated organizations, or those of the publisher, the editors and the reviewers. Any product that may be evaluated in this article, or claim that may be made by its manufacturer, is not guaranteed or endorsed by the publisher.
Acknowledgments
We would like to thank the Center for Ocean and Society, a Kiel Marine Science platform that supports interdisciplinary research and involve societal actors in transdisciplinary projects.
Supplementary Material
The Supplementary Material for this article can be found online at: https://www.frontiersin.org/articles/10.3389/fmars.2022.929274/full#supplementary-material
References
Ahola M., Bergström L., Blomqvist M. (2021). Climate Change in the Baltic Sea. 2021 Fact Sheet. Helsinki Commission – HELCOM 2021. https://helcom.fi/media/publications/Baltic-Sea-Climate-Change-Fact-Sheet-2021.pdf
(2022) Open Drone Map [Computer Software]. Available at: https://github.com/OpenDroneMap/OpenDroneMap.
(2022) Open Structure From Motion – OpenSfM. Available at: https://github.com/mapillary/OpenSfM/.
Multi-View Environment 2022. Available at: https://github.com/simonfuhrmann/mve.
Bianchi T. S., Wysocki L. A., Schreiner K. M., Filley T. R., Corbett D. R., Kolker A. S. (2011). Sources of Terrestrial Organic Carbon in the Mississippi Plume Region: Evidence for the Importance of Coastal Marsh Inputs. Aquat. Geochem. 17 (4), 431–456. doi: 10.1007/s10498-010-9110-3
Biel R., Moore L. J., Goldstein E. B., Itzkin M. (2018). “Influence of Wrack on Coastal Foredune Development and Dune Habitat Complexity,” in AGU Fall Meeting Abstracts, vol. Vol. 2018. , EP11A–EP107.
Boumans R. M., Day J. W. (1993). High Precision Measurements of Sediment Elevation in Shallow Coastal Areas Using a Sedimentation-Erosion Table. Estuaries 16 (2), 375–380. doi: 10.2307/1352509
Buczko U., Jurasinski G., Glatzel S., Karstens S. (2022). Blue Carbon in Coastal Phragmites Wetlands Along the Southern Baltic Sea. Estuaries Coasts. 1–9. doi: 10.1007/s12237-022-01085-7
Cahoon D. R., Perez B. C., Segura B. D., Lynch J. C. (2011). Elevation Trends and Shrink–Swell Response of Wetland Soils to Flooding and Drying. Estuarine, Coastal and Shelf Science, 91 (4), 463–474. doi: 10.1016/j.ecss.2010.03.022
Cahoon D. R. (2015). Estimating Relative Sea-Level Rise and Submergence Potential Ata Coastal Wetland. Estuar. Coasts 38, 1077–1084. doi: 10.1007/s12237-014-9872-8
Chambers R. M., Havens K. J., Killeen S., Berman M. (2008). Common Reed Phragmites Australis Occurrence and Adjacent Land Use Along Estuarine Shoreline in Chesapeake Bay. Wetlands 28 (4), 1097–1103. doi: 10.1672/07-61.1
Chubarenko B., Woelfel J., Hofmann J., Aldag S., Beldowski J., Burlakovs J., et al. (2021). Converting Beach Wrack Into a Resource as a Challenge for the Baltic Sea (an Overview). Ocean. Coast. Manage. 200, 105413. doi: 10.1016/j.ocecoaman.2020.105413
Coleman D. J., Schuerch M., Temmerman S., Guntenspergen G., Smith C. G., Kirwan M. L. (2022). Reconciling Models and Measurements of Marsh Vulnerability to Sea Level Rise. Limnol. Oceanogr. Lett. 7 (2), 140-149 doi: 10.1002/lol2.10230
Das S. C., Tanaka N. (2007). The Effects of Breaking or Bending the Stems of Two Rhizomatous Plants, Phragmites Australis and Miscanthus Sacchariflorus, on Their Communities. Landscape Ecol. Eng. 3 (2), 131–141. doi: 10.1007/s11355-007-0028-x
Davidson-Arnott R., Bauer B., Houser C. (2019). Introduction to Coastal Processes and Geomorphology ( Cambridge university press).
Defeo O., Mclachlan A., , Schoeman D.S., Schlacher T. A., Dugan J., Jones A., Lastra M., Scapini F. (2009). Threats to Sandy Beach Ecosystems: A review. Estuarine, Coastal and Shelf Science81:1–12. doi: 10.1016/j.ecss.2008.09.022
De Luca G., Silva J. M. N., Cerasoli S., Araújo J., Campos J., et al. (2019). Object-Based Land Cover Classification of Cork Oak Woodlands Using UAV Imagery and Orfeo ToolBox. Remote Sens. 11 (10), 1238. doi: 10.3390/rs11101238
Diehl M., Diehl D. (1986). Naturschutzgebiete an der Ostseeküste Schleswig-Holsteins. Verein Natur und Heimat. Heft 19/20, Lübeck 1986, ISSN 0067-5806.
Dietz R., Sonne C., Jenssen B. M., Das K., de Wit C. A., Harding K. C., et al. (2021). The Baltic Sea: An Ecosystem With Multiple Stressors. Environ. Int. 147, 106324. doi: 10.1016/j.envint.2020.106324
Dijkema K. S. (1990). Salt and Brackish Marshes Around the Baltic Sea and Adjacent Parts of the North Sea: Their Vegetation and Management. Biol. Conserv. 51 (3), 191–209. doi: 10.1016/0006-3207(90)90151-E
Duarte C. M., Losada I. J., Hendriks I. E., Mazarrasa I., Marbà N. (2013). The Role of Coastal Plant Communities for Climate Change Mitigation and Adaptation. Nature Climate Change, 3 (11), 961–968. doi: 10.1038/nclimate1970
Dugan J. E., Hubbard D. M., Page H. M., Schimel J. P. (2011). Marine Macrophyte Wrack Inputs and Dissolved Nutrients in Beach Sands. Estuaries Coasts 34 (4), 839–850. doi: 10.1007/s12237-011-9375-9
FitzGerald D. M., Fenster M. S., Argow B. A., Buynevich I. V.(2008) Coastal Impacts Due to Sea-Level Rise. Annual Review of Earth and Planetary Sciences 36 (1), 601–647.doi: 10.1146/annurev.earth.35.031306.140139
Gilburn A. S. (2012). Mechanical Grooming and Beach Award Status are Associated With Low Strandline Biodiversity in Scotland. Estuarine Coast. Shelf Sci. 107, 81–88. doi: 10.1016/j.ecss.2012.05.004
Graversen A. E. L., Banta G. T., Masque P., Krause-Jensen D. (2022). Carbon Sequestration is Not Inhibited by Livestock Grazing in Danish Salt Marshes. Limnol. Oceanogr.1–17. doi: 10.1002/lno.12011
Grizonnet M., Michel J., Poughon V., Inglada J., Savinaud M., Cresson R. (2017). Orfeo ToolBox: Open Source Processing of Remote Sensing Images. Open Geospatial Data Software Standards 2 (1), 1–8. doi: 10.1186/s40965-017-0031-6
Hammann S., Zimmer M. (2014). Wind-Driven Dynamics of Beach-Cast Wrack in a Tide-Free System. Open J. Mar. Sci. 4:2014. doi: 10.4236/ojms.2014.42009
Heckwolf M. J., Peterson A., Jänes H., Horne P., Künne J., Liversage K., et al. (2021). From Ecosystems to Socio-Economic Benefits: A Systematic Review of Coastal Ecosystem Services in the Baltic Sea. Sci. Total Environ. 755, 142565. doi: 10.1016/j.scitotenv.2020.142565
Heerhartz S. M., Dethier M. N., Toft J. D., Cordell J. R., Ogston A. S. (2014). Effects of Shoreline Armoring on Beach Wrack Subsidies to the Nearshore Ecotone in an Estuarine Fjord. Estuaries Coasts 37 (5), 1256–1268. doi: 10.1007/s12237-013-9754-5
HELCOM (2010). Ecosystem Health of the Baltic Sea 2003- 2007: HELCOM Initial Holistic Assessment. In Baltic Sea Environment Proceedings (Vol. 122, No. 63, P. 2010) (Helsinki(Finnland: Baltic Marine Environment Protection Commission).
Howe A. J., Rodríguez J. F., Saco P. M., & (2009). Surface Evolution and Carbon Sequestration in Disturbed and Undisturbed Wetland Soils of the Hunter Estuary, Southeast Australia Estuarine, Coastal and Shelf Science, 84 (1), 75–83. doi: 10.1016/j.ecss.2009.06.006
Hyndes G. A., Berdan E., Duarte C., Dugan J. E., Emery K. A., Hambäck P. A., et al. (2021). Flotsam and Jetsam: A Global Review of the Role of Inputs of Marine Organic Matter in Sandy Beach Ecosystems. EcoEvoRxiv. doi:10.32942/osf.io/68as7
Jurasinski G., Janssen M., Voss M., Boettcher M. E., Brede M., Burchard H., et al. (2018). Understanding the Coastal Ecocline: Assessing Sea–Land Interactions at non-Tidal, Low-Lying Coasts Through Interdisciplinary Research. Front. Mar. Sci. 5, 342. doi: 10.3389/fmars.2018.00342
Karstens S., Buczko U., Glatzel S. (2015). Phosphorus Storage and Mobilization in Coastal Phragmites Wetlands: Influence of Local-Scale Hydrodynamics. Estuarine Coast. Shelf Sci. 164, 124–133. doi: 10.1016/j.ecss.2015.07.014
Keruss M., Sennikovs J. (1999). “Determination of Tides in Gulf of Riga and Baltic Sea. Proc,” in Modelling of Material Processing (Riga: International Scientific Colloqium), 28–29.
Kiesel J., Schuerch M., Christie E. K., Möller I., Spencer T., Vafeidis A. T. (2020). Effective Design of Managed Realignment Schemes can Reduce Coastal Flood Risks. Estuarine Coast. Shelf Sci. 242, 106844. doi: 10.1016/j.ecss.2020.106844
Kiesel J., MacPherson L. R., Schuerch M., Vafeidis A. T., & (2022). Can managed realignment buffer extreme surges? The relationship between marsh width, vegetation cover and surge attenuation. Estuaries and Coasts, 45 (2), 345–362. doi: 10.1007/s12237-021-00984-5
Kirwan M. L., Temmerman S., Skeehan E. E., Guntenspergen G. R., Fagherazzi S. (2016). Overestimation of Marsh Vulnerability to Sea Level Rise. Nat. Climate Change 6 (3), 253–260. doi: 10.1038/nclimate2909
Kraufvelin P., Pekcan-Hekim Z., Bergström U., Florin A. B., Lehikoinen A., Mattila J., et al. (2018). Essential Coastal Habitats for Fish in the Baltic Sea. Estuarine Coast. Shelf Sci. 204, 14–30. doi: 10.1016/j.ecss.2018.02.014
Kuhwald K., Schneider von Deimling J., Schubert P., Oppelt N. (2021). How can Sentinel-2 Contribute to Seagrass Mapping in Shallow, Turbid Baltic Sea Waters? Remote Sens. Ecol. Conserv. 8 (3), 328–346. doi: 10.1002/rse2.246
Leonardi N., Dai Z. J. (2022). Coastal Wetlands Dynamics. Front. Mar. Sci. 317. doi: 10.3389/978-2-88974-849-5
Malm T., Råberg S., Fell S., Carlsson P. (2004). Effects of Beach Cast Cleaning on Beach Quality, Microbial Food Web, and Littoral Macrofaunal Biodiversity. Estuarine Coast. Shelf Sci. 60 (2), 339–347. doi: 10.1016/j.ecss.2004.01.008
Mateo M., Cebrián J., Dunton K., Mutchler T. (2006). Carbon Flux in Seagrass Ecosystems. Seagrasses: biol. Ecol. Conserv., 159–192. doi: 10.1007/1-4020-2983-7_7
Mcleod E., Chmura G. L., Bouillon S., Salm R., Björk M., Duarte C. M., Silliman B. R. (2011). A Blueprint for Blue Carbon: Toward an Improved Understanding of the Role of Vegetated Coastal Habitats in Sequestering CO2. Frontiers in Ecology and the Environment, 9 (10), 552–560. doi: 10.1890/110004
Meriste M., Kirsimäe K., Freiberg L. (2012). Relative Sea-Level Changes at Shallow Coasts Inferred From Reed Bed Distribution Over the Last 50 Years in Matsalu Bay, the Baltic Sea. J. Coast. Res. 28 (1), 1–10. doi: 10.2112/JCOASTRES-D-10-00049.1
Mokrane A., Choukchou-Braham A., Cherki B. (2019). “DEM Generation Based On UAV Photogrammetry,” in 2019 International Conference on Advanced Electrical Engineering (ICAEE). 1–5. IEEE. doi: 10.1109/ICAEE47123.2019.9014805
Möller I., Spencer T. (2002). Wave Dissipation Over Macro-Tidal Saltmarshes: Effectsof Marsh Edge Typology and Vegetation Change. J. Coast. Res. SI 36, 506–521. doi: 10.2112/1551-5036-36.sp1.506
Morris J. T., Sundareshwar P. V., Nietch C. T., Kjerfve B., Cahoon D. R. (2002). Responses of Coastal Wetlands to Rising Sea Level. Ecology 83 (10), 2869–2877. doi: 10.1890/0012-9658(2002)083[2869:ROCWTR]2.0.CO;2
NATURA (2000). Network of Nature Protection Areas in the Territory of the European Union. NATURA 2000. https://ec.europa.eu/environment/nature/natura2000/
Niedermeyer R. O., Lampe R., Janke W., Schwarzer K., Duphorn K., Kliewe H., et al. (2011). Die Deutsche Ostseeküste, ISBN: ISBN 978-3-443-15091-4. Schweizbart, 370
Nordstrom K. F., Jackson N. L., Korotky K. H. (2011). Aeolian Sediment Transport Across Beach Wrack. J. Coast. Res. 59), 211–217. doi: 10.2112/SI59-022.1
Pan Y., Flindt M., Schneider-Kamp P., Holmer M. (2021). Beach Wrack Mapping Using Unmanned Aerial Vehicles for Coastal Environmental Management. Ocean. Coast. Manage. 213, 105843. doi: 10.1016/j.ocecoaman.2021.105843
Pan Y., Ayoub N., Schneider-Kamp P., Flindt M., Holmer M. (2022). Beach Wrack Dynamics Using a Camera Trap as the Real-Time Monitoring Tool. Frontiers in Marine Science. doi: 10.3389/fmars.2022.813516
Pell T., Li J. Y., Joyce K. E. (2022). Demystifying the Differences Between Structure-From-MotionSoftware Packages for Pre-Processing Drone Data. Drones 6 (1), 24. doi: 10.3390/drones6010024
Perillo G., Wolanski E., Cahoon D. R., Hopkinson C. S. (Eds.) (2018). Coastal Wetlands: An Integrated Ecosystem Approach ( Elsevier). 1124
QGIS (2022). QGIS Geographic Information System ( QGIS Association). Available at: http://www.qgis.org.
Reddy K. R., DeLaune R. D. (2008). Biogeochemistry of Wetlands: Science and Applications (Boca Raton Fl: CRC Press).
Reed D. J. (1990). The Impact of Sea-level Rise on Coastal Salt Marshes. Progress in Physical Geography, 14 (4), 465–481. doi: 10.1177/2F030913339001400403
Reimer J. N., Hacker S. D., Menge B. A., Ruggiero P. (2018). Macrophyte Wrack on Sandy Beaches of the US Pacific Northwest is Linked to Proximity of Source Habitat, Ocean Upwelling, and Beach Morphology. Mar. Ecol. Prog. Ser. 594, 263–269. doi: 10.3354/meps12565
Reusch T. B., Dierking J., Andersson H. C., Bonsdorff E., Carstensen J., Casini M., et al. (2018). The Baltic Sea as a Time Machine for the Future Coastal Ocean. Sci. Adv. 4 (5), eaar8195. doi: 10.1126/sciadv.aar8195
Rice D., Rooth J., Stevenson J. (2000). Colonization and Expansion of Phragmites Australis in Upper Chesapeake Bay Tidal20, 2, 280–299. doi: 10.1672/0277-5212(2000)020[0280:CAEOPA]2.0.CO;2
Rohde S., Hiebenthal C., Wahl M., Karez R., Bischof K. (2008). Decreased Depth Distribution of Fucus vesiculosus (Phaeophyceae) in the Western Baltic: Effects of Light Deficiency and Epibionts on Growth and Photosynthesis. European Journal of Phycology, 43 (2), 143–150. doi: 10.1080/09670260801901018
Rupprecht F., Möller I., Paul M., Kudella M., Spencer T., Van Wesenbeeck B. K., et al. (2017). Vegetation-Wave Interactions in Salt Marshes Under Storm Surge Conditions. Ecol. Eng. 100, 301–315. doi: 10.1016/j.ecoleng.2016.12.030
Sangiorgio F., Basset A., Pinna M., Sabetta L., Abbiati M., Ponti M., et al. (2008). Environmental Factors Affecting Phragmites Australis Litter Decomposition in Mediterranean and Black Sea Transitional Waters. Aquat. Conserv.: Mar. Freshw. Ecosyst. 18 (S1), S16–S26. doi: 10.1002/aqc.955
Schernewski G., Schumacher J., Weisner E., Donges L. (2018). A Combined Coastal Protection, Realignment and Wetland Restoration Scheme in the Southern Baltic: Planning Process, Public Information and Participation. J. Coast. Conserv. 22 (3), 533–547. doi: 10.1007/s11852-017-0542-4
Schubert P. R., Hukriede W., Karez R., Reusch T. B. (2015). Mapping and Modeling Eelgrass Zostera Marina Distribution in the Western Baltic Sea. Mar. Ecol. Prog. Ser. 522, 79–95. doi: 10.3354/meps11133
Schuerch M., Spencer T., Temmerman S., Kirwan M. L., Wolff C., Lincke D., et al. (2018). Future Response of Global Coastal Wetlands to Sea-Level Rise. Nature 561 (7722), 231–234. doi: 10.1038/s41586-018-0476-5
Thorslund J., Jarsjo J., Jaramillo F., Jawitz J. W., Manzoni S., Basu N. B., et al. (2017). Wetlands as Large-Scale Nature-Based Solutions: Status and Challenges for Research, Engineering and Management. Ecol. Eng. 108, 489–497. doi: 10.1016/j.ecoleng.2017.07.012
Torres-Sánchez J., López-Granados F., Pena J. M. (2015). An Automatic Object-Based Method for Optimal Thresholding in UAV Images: Application for Vegetation Detection in Herbaceous Crops. Comput. Electron. Agric. 114, 43–52. doi: 10.1016/j.compag.2015.03.019
Uhl F., Græsdal Rasmussen T., Oppelt N. (2022). Classification Ensembles for Beach Cast and Drifting Vegetation Mapping With Sentinel-2 and PlanetScope. Geosciences 12 (1), 15. doi: 10.3390/geosciences12010015
Vacca G. (2020). “WEB Open Drone Map (WebODM) a Software Open Source to Photogrammetry Process,” in Fig Working Week Smart Surveyors for Land and Water Management(Amsterdam).
Vanhooren S., Maelfait H., Belpaeme K. (2011). Moving Towards an Ecological Management of the Beaches. J. Coast. Res. 61 (10061), 81–86. doi: 10.2112/SI61-001.70
Ward R. D., Burnside N. G., Joyce C. B., Sepp K. (2016). Importance of Microtopography in Determining Plant Community Distribution in Baltic Coastal Wetlands. J. Coast. Res. 32 (5), 1062–1070. doi: 10.2112/JCOASTRES-D-15-00065.1
Weinberger F., Sundt S., Staerck N., Merk C., Karez R., Rehdanz K. (2021). Shifting Beach Wrack Composition in the SW Baltic Sea and its Effect on Beach Use. Ecol. Soc. 26 (4) 43. doi: 10.5751/ES-12759-260443
Keywords: beach wrack, coastal wetland, storm season, Baltic Sea, UAV imagery, object-based image analysis
Citation: Karstens S, Kiesel J, Petersen L, Etter K, Schneider von Deimling J, Vafeidis AT and Gross F (2022) Human-Induced Hydrological Connectivity: Impacts of Footpaths on Beach Wrack Transport in a Frequently Visited Baltic Coastal Wetland. Front. Mar. Sci. 9:929274. doi: 10.3389/fmars.2022.929274
Received: 26 April 2022; Accepted: 23 June 2022;
Published: 26 July 2022.
Edited by:
Dongdong Shao, Beijing Normal University, ChinaReviewed by:
Simone Simeone, Istituto per l’Ambiente Marino Costiero di Oristano (CNR), ItalyWeiwei Sun, Ningbo University, China
Megan Dethier, University of Washington, United States
Copyright © 2022 Karstens, Kiesel, Petersen, Etter, Schneider von Deimling, Vafeidis and Gross. This is an open-access article distributed under the terms of the Creative Commons Attribution License (CC BY). The use, distribution or reproduction in other forums is permitted, provided the original author(s) and the copyright owner(s) are credited and that the original publication in this journal is cited, in accordance with accepted academic practice. No use, distribution or reproduction is permitted which does not comply with these terms.
*Correspondence: Svenja Karstens, c3ZlbmphLmthcnN0ZW5zQGlmZy51bmkta2llbC5kZQ==