- 1Instituto de Acuicultura Torre de la Sal, Consejo Superior de Investigaciones Científicas (IATS-CSIC), Castellón, Spain
- 2Alfred Wegener Institute, Helmholtz Centre for Polar and Marine Research, Bremerhaven, Germany
The fast and remarkable growth of global aquaculture in recent years has created new challenges, such as guaranteeing a sustainable supply of raw materials used for aquafeed formulation. Gammarids are low-trophic crustaceans with an increasing interest in aquaculture due to their high nutritional profiles and their capacity to grow under high-density conditions. Moreover, gammarids have the ability to thrive on a wide range of sidestreams while accumulating relatively high levels of long-chain (≥C20) polyunsaturated fatty acids (LC-PUFA). In the present study, juveniles of the marine gammarid Gammarus locusta were cultured at four different temperatures (5°C, 10°C, 15°C, and 20°C) for 21 days and fed three diets, including the seaweed Fucus sp. as control, and carrot leaves and coconut flesh representing two agri-food industry sidestreams. Our results indicate that both the survival and biomass of G. locusta were highly affected by diet, with coconut showing the lowest growth performance. The temperature had no effect on biomass, although high temperature (20°C) resulted in a decrease in survival. The effects of temperature on the gammarid fatty acids were not evident, with diet being the main modulator of the profiles. Furthermore, the results also reveal that the Fucus sp. diet was associated with relatively high percentages of n-3 and n-6 LC-PUFA. Interestingly, essential LC-PUFA such as eicosapentaenoic (20:5n-3, EPA) and docosahexaenoic (22:6n-3, DHA) acids were detected in gammarids fed on either Fucus sp. or any of the sidestreams irrespectively of their presence in the diets. These results suggest an ability of G. locusta for LC-PUFA biosynthesis (trophic upgrading) and/or retention, making this species a promising candidate for the production of high-value ingredients for aquafeeds.
Introduction
Rapidly expanding aquaculture worldwide has created new economic and ecological challenges (FAO, 2020). With regard to fish farming, such challenges have been mostly linked to modifying the supply of raw materials used for feed formulation in order to reduce the current usage of finite resources such as the so-called marine ingredients fishmeal (FM) and fish oil (FO). FM and FO are regarded as major sources of essential nutrients for aquafeeds, including long-chain (C20-24) polyunsaturated fatty acids (LC-PUFA) (Tocher, 2015; Shepherd et al., 2017). The current production of FM and FO largely relies on feed-grade species fisheries and, with the expansion of aquaculture worldwide, pressure on these fisheries has grown to an extent that they may not sustainably fulfill the increasing demand (Naylor et al., 2009; FAO, 2020).
Several efforts have been made to find alternative feeding sources to alleviate the abovementioned pressure on fisheries and reduce the dependence upon FM and FO in finfish aquaculture (Naylor et al., 2009; Turchini et al., 2011b; Jannathulla et al., 2019). Up to the present, the use of raw materials derived from animals or plants has become a widely extended practice (Turchini et al., 2009; Jannathulla et al., 2019; Galkanda-Arachchige et al., 2020). However, the replacement of FM and FO with nonmarine ingredients has often been associated with decreased nutritional value of fish farming products, including reduced levels of the health-promoting n-3 LC-PUFA eicosapentaenoic acid (20:5n-3, EPA) and docosahexaenoic acid (22:6n-3, DHA), as well as suboptimal growth (Bell et al., 2001; Mourente and Bell, 2006; Turchini et al., 2011a; Yıldız et al., 2018; Romano et al., 2020). Therefore, high-quality alternative ingredients are needed in order to successfully replace traditional sources of FM and FO in aquafeed while maintaining growth performance and nutritional value (i.e., levels of n-3 LC-PUFA) of farmed fish (Alberts-Hubatsch et al., 2019). Indeed, the search for novel aquatic ingredients for aquafeed is a priority within the EU, as is their production through Integrative Multi-Trophic Aquaculture (IMTA) strategies (Guerra-García et al., 2016).
Ingredients derived from biomasses of low-trophic marine crustaceans such as gammarids, krill, and copepods have been regarded as promising candidates for aquafeed formulations due to their balanced profiles of essential nutrients, including n-3 LC-PUFA (McKinnon et al., 2003; Suontama et al., 2007; Naylor et al., 2009; Dhont et al., 2013; Harlıoğlu and Farhadi, 2018). While the exploitation of wild populations of marine crustaceans poses negative ecological impacts similar to those of wild-capture fisheries alluded to above, biomass production using intensive aquaculture systems arises as an interesting strategy. Gammarids are abundant in benthic communities and inhabit practically all aquatic environments, which is reflected in their high diversity of feeding habits (Costa and Costa, 2000; Harlıoğlu and Farhadi, 2018). Moreover, gammarids are fish’s natural prey, and their use as an alternative ingredient for fish feeding has been explored (Ashour et al., 2021). Gammarids can be grown and maintained in laboratory cultures and adapt well to different culture conditions and diets (Sexton, 1928; Costa and Costa, 2000; Neuparth et al., 2002; Ahyong and Hughes, 2016; Beermann et al., 2018; Alberts-Hubatsch et al., 2019). Interestingly, recent investigations have also demonstrated that gammarids can be fed on a wide range of sidestreams from bioindustries such as agriculture and aquaculture itself (Alberts-Hubatsch et al., 2019; Jiménez-Prada et al., 2020). Such sidestreams are characterized by being deprived or having low contents of LC-PUFA, but, intriguingly, gammarids fed on these sidestreams have shown relatively high levels of LC-PUFA, suggesting that gammarids have some capacity for trophic upgrading via endogenous lipid metabolism (Alberts-Hubatsch et al., 2019; Jiménez-Prada et al., 2020). Thus, applying circular bioeconomy strategies by which sidestreams derived from bioindustries are used for the production of high nutritional value gammarid biomasses as potential fish feed ingredients has been proposed (Jiménez-Prada et al., 2020). However, up to date, little is known about the optimal culture conditions for reliable large-scale cultures of marine gammarids (Alberts-Hubatsch et al., 2019; Jiménez-Prada et al., 2020). Several factors, including diet, temperature, and salinity can modulate LC-PUFA metabolism, growth, and survival of aquatic invertebrates (Neuparth et al., 2002; Monroig and Kabeya, 2018; Alberts-Hubatsch et al., 2019). Hence, it is necessary to fine tune the culture conditions for the production of LC-PUFA–rich gammarid biomass.
A previous study comparing the effects of several diets on two gammarid species, namely Gammarus locusta and Echinogammarus marinus, suggested that G. locusta is the best candidate regarding LC-PUFA composition, growth, and survival when fed sidestreams (Alberts-Hubatsch et al., 2019). However, to the best of our knowledge, information on the combined effects of diet and environmental factors, on survival, growth, and LC-PUFA content of marine gammarids is lacking. The main goal of this study was to elucidate the effects of three diets and four temperatures on the FA profile and growth performance of the marine gammarid G. locusta. For this purpose, cultures were carried out at 5°C, 10°C, 15°C, and 20°C, representing ambient temperatures in the wild, with G. locusta occurring in coastal areas of the North Atlantic (Costa and Costa, 2000). Fucus spp. was used as a natural marine diet, and carrot leaves and coconut flesh as two different nonmarine diets, mimicking agriculture sidestreams of different nutritional values.
Materials and methods
Animal collection and culture
All G. locusta specimens used in the experiments were obtained from a laboratory culture at the Alfred Wegener Institute, with stock cultures originating from the German Bight, North Sea. Specimens were reared in the laboratory prior to the experiment at temperatures of 18°C, a salinity of 33–34 ppt, and pH 8. Broodstock cultures and juvenile G. locusta were raised on a mixture of dried Fucus spp. and vegetable greens (mainly carrot greens and kale leaves). Juvenile G. locusta (28 days posthatch) were collected from the same batch culture, maintained at 10°C for 5 days, and starved for 24 h prior to the experiment.
Experimental setup
The juvenile gammarids (5.327 ± 1.54 mm SD, n = 960) were randomly transferred into white 1-L buckets, filled with 500 ml of freshly filtered seawater (5µm tube filter) at 33–34 ppt and equipped with a mesh (70 × 70 mm, 5 mm mesh size) and an oyster shell as substrate. Each container was stocked with 20 specimens at 10°C and randomly allocated to the respective temperatures (5°C, 10°C, 15°C, and 20°C) and diet in quadruplicates. The temperature was slowly adjusted by transferring the containers into water baths at the desired temperature. Three different diets were prepared: one natural marine food source, thalli of Fucus spp. containing LC-PUFA (hereafter referred to as Fucus), and two nonmarine diets: carrot leaves (hereafter referred to as “Carrot”), an agricultural sidestream, (high shorter-chain (<C20) PUFA content), and a diet that mimics a potential sidestream rich in saturated fatty acids (SFA) consisting on coconut flesh (hereafter referred to as “Coco”). All diets were rinsed in fresh water and dried at 55°C for 24 h. The temperature in the experimental containers was recorded twice a day, and water exchange with fresh seawater adjusted to the respective temperature was done every second day. Feeding was done ad libitum with remaining food items removed and replaced during water exchange. Dead individuals were removed on a daily basis. Gammarids were cultured at the corresponding diet vs. temperature combination for 21 days, until sexual maturity was reached in the higher temperature treatments.
Growth and survival
Initially, a subsample of 100 juvenile gammarids (average 5 mm) was analyzed. Total body lengths of gammarids were measured at the beginning and end of the experiment by analyzing pictures of each replicate taken on scale paper using Fiji ImageJ (vers. 1.53q, Schneider et al., 2012). Total lengths were measured from the basal point of the antennae to the third urosome segment. Specific growth rate (SGR, in % day−1) was calculated as length gain per experimental duration for each pool as follows:
SGR = 100 × [ln (final length) − ln (initial length)/time interval]. Initial individual weights could not be taken at the beginning of the experiment due to the vulnerability of the early life stages. Therefore, at the end of the experiment, all remaining specimens were counted and wet biomass per replicate was recorded using an analytical scale (Sartorius Practum 213-S1, d = 0.001 g). The gammarids were rinsed in Milli-Q water twice, placed into Eppendorf tubes, and immediately frozen at −80°C. The frozen samples were then freeze-dried at −52°C within 2 weeks after the experiment and thereafter stored at −80°C until further analyses.
Fatty acid analysis
Total lipids and FA were analyzed from gammarid samples as well as from experimental diets. Briefly, total lipids were extracted from the homogenized samples using the Folch method (Folch et al., 1957). Subsequently, total lipids were used to prepare fatty acid methyl esters (FAME), which were analyzed using a Thermo Trace GC Ultra Gas Chromatograph (Thermo Electron Corporation, Waltham, MA, USA), equipped with a fused silica 30 m × 0.25 mm open tubular column (Tracer, TR-WAX, film thickness: 0.25 μm, Teknokroma, Sant-Cugat del Vallés, Spain), fitted with an on-column injection system, using helium as a carrier gas, and a flame ionization detector (FID). The analytical temperature was programmed from 50°C to 220°C. Chromatograms were integrated and analyzed with Azur Datlys (St Martin d’Heres, France) software. FAs were identified by comparison of retention times of each peak with those of well-characterized standards.
Statistical analysis
All data were subjected to statistical analyses using PAST (vers. 4.09) (Hammer et al., 2001). Prior to the analyses, data were tested for normality (Shapiro–Wilk) and homogeneity (Levene’s test). After assuring that normality and homogeneity criteria were met, data were analyzed using analysis of variance (fixed effects two-way ANOVA) with Tukey’s post-hoc test for multiple comparisons. Principal component analysis (PCA) was used to analyze and visualize the relationship between diet and FA profiles of gammarids. Differences in FA levels among treatments were tested by using a two-way permutational multivariate analysis of variance (PERMANOVA) with factors: “Temperature,” four different conditions (5°C, 10°C, 15°C, and 20°C), and “Diet,” three different treatments (Fucus, Carrot, Coco). One-way PERMANOVA was further used to compare the scores of the dietary groups. Ellipses were fitted to the scores at 95% confidence. Additionally, the percentage of similarity analysis (SIMPER) was used to determine the FA responsible for dissimilarities between conditions within the dietary groups. Unless otherwise stated, statistical significance was tested at 95% confidence level (p ≤ 0.05). The Unsaturation Index (UI) was used to establish a relationship between the FA levels of diets used and the FA levels of gammarids fed on the corresponding diets in order to determine how diet can affect the gammarids’ FA profiles and to ascertain previous retention. The UI was calculated according to the following formula: ∑ [area of fatty acid * number of unsaturations].
Results
Growth and survival
The two-way ANOVA revealed that both temperature (F (3, 36) = 12.43, p < 0.0001) and diet (F (2, 36) = 18.08, p < 0.0001) had a significant effect on the survival of G. locusta, although no interacting effects of temperature and diet on survival were observed (F (6, 36) = 0.38, p = 0.89). Pairwise comparisons of the survival of gammarids at different temperatures revealed that the highest temperature (20°C) resulted in significantly lower survival rates, whereas no differences were observed between the temperatures (Table 1). Regarding the diets, Coco resulted in significantly lower survival of gammarids, whereas no differences between the effects of Carrot and Fucus were found. The highest mortality was obtained with the Coco diet at 20°C (Table 1).
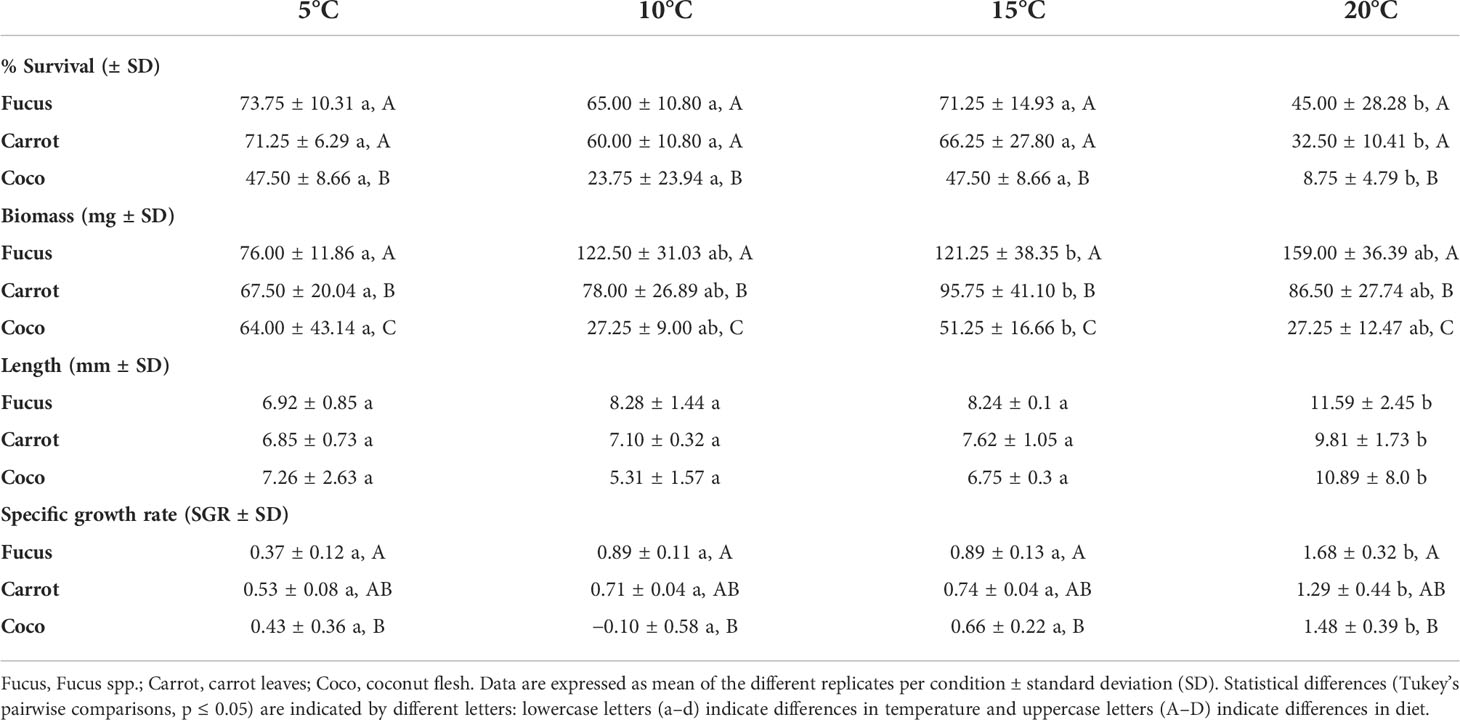
Table 1 Survival, total biomass, and total length of G. locusta in response to different diets and temperatures after the 21-day feeding trial.
Regarding total biomass, there was a significant interacting effect of temperature and diet as revealed by the two-way ANOVA (F (6, 36) = 3.17, p = 0.01). Here, our analysis shows no significance of temperature (F (3, 36) = 1.63, p = 0.20) but a highly significant effect of diet (F (2, 36) = 29.07, p < 0.001). Pairwise comparisons of the biomass output revealed highly significant differences (p < 0.005) between all diet groups, with the Fucus diet resulting in the highest (Table 1). No significant differences were found in the gammarid biomasses when comparing temperature groups.
The final length was not affected by the interaction of temperature and diet (F (6,35) = 1.47, p = 0.22). In this case, the diet had no effect on final length (F (2, 35) = 3.03, p = 0.06), in contrast to temperature, which strongly affected final length (F (3, 35) = 20, 73, p < 0.001). When looking at pairwise comparisons, it becomes evident that this effect was caused by the 20°C treatment, which resulted in significantly higher final lengths compared to all other temperature groups (p < 0.001), whereas no differences were found between the other groups (Table 1).
The SGR followed a similar pattern as the final length data, with temperature having a highly significant effect on SGR (F (3, 35) = 31.83, p < 0.001). This was also explained by the higher SGR in the 20°C treatment, which was significantly different from all other temperature treatments (p < 0.001) while the other temperatures were not different (Table 1). Diet had a significant effect on SGR (F (2, 35) = 5.26, p = 0.01), which was caused by the low SGR when regarding pairwise comparisons, in which Coco was significantly different from Fucus but not from Carrot (Table 1). This was biased by the very high mortality in this treatment group (see above). Similarly, a significant interaction effect of diet and temperature on SGR (F (6, 35) = 3.44, p = 0.008) was observed.
Fatty acid profiles of gammarids
The FA analysis of the diets revealed that Fucus was rich in oleic acid (18:1n-9) (29.8%), whereas α-linolenic acid (ALA, 18:3n-3) was the most abundant FA in carrot leaves (26.5%). On the other hand, the Coco diet showed high levels of SFA (90.4%), such as lauric acid (12:0) (48.7%) and myristic acid (14:0) (19.9%) (Table 2). Low levels of DHA (22:6n-3) were detected only in the Fucus diet (1.1%), whereas EPA (20:5n-3) was also present in Fucus (4.8%) and only in trace amounts (0.2%) in Carrot. Moreover, the Coco diet had the lowest UI (6.2) whereas the Fucus and Carrot diets showed similar levels (150.4 and 138.3, respectively), reflecting that the former is very poor in PUFA and MUFA and rich in SFA, as compared to the other treatments.
FA analysis of cultured G. locusta showed similar profiles when different temperature treatments were compared (Table 3). However, the two-way ANOVA of the FA analyses only showed significant differences when comparing the different dietary treatments (Table 4). One-way ANOVA of gammarids fed on the different diets (Table 5) did not show significant differences in EPA and DHA levels. The two-way PERMANOVA results did not show differences in FA among different temperatures (F (3, 28) = 1.20, p = 0.29), or the interaction temperature and diet (F (6, 28) = 0.73, p = 0.74). Moreover, the two-way PERMANOVA revealed diet as the main modulator of FA profiles (F (2, 28) = 8.97, p < 0.001). PCA revealed that the first component (PC1) accounted for the 60.81% of variance of this dataset, whereas the PC2 accounted for the 19.19% of variance (Figure 1). The PCA loading plot showed saturated fatty acids such as lauric acid (12:0) and myristic acid (16:0) are separated on the negative side of PC1 from unsaturated and polyunsaturated fatty acids including LC-PUFA such as arachidonic acid (20:4n-6, ARA), EPA (20:5n-3), docosapentaenoic acid (22:5n-3, DPA), and DHA (22:6n-3), which load on the positive side (Figure 1). On the other hand, 16:1n-7, LA (18:2n-6), and ALA (18:3n-3) load on the negative side of PC2. This variable distribution drives the scores segregation (Figure 1). The FA profiles of G. locusta fed on Coco are correlated with SFA (Figure 1). Those fed carrots are associated with LA and ALA, whereas those fed the Fucus diet are associated with LC-PUFA, among others. It is also interesting to note that the scores of the FA profiles of gammarids fed on the Fucus diet showed less dispersion than those fed the carrot leaves and coconut diets (95% ellipses, Figure 1). One-way PERMANOVA (F = 9.38, p < 0.0001), however, showed that the three dietary groups were significantly different (Supplementary Table S1). No significant differences were found for PC2. Additional SIMPER analysis (Supplementary Tables S2-S4) revealed that oleic acid (18:1n-9, OA) was the FA with more dissimilarity between the Fucus diet and the other diet groups (Carrot and Coco). On the other hand, the SIMPER analysis comparing Carrot vs. Coco showed that the SFA were the FA characteristic of the Coco diet.
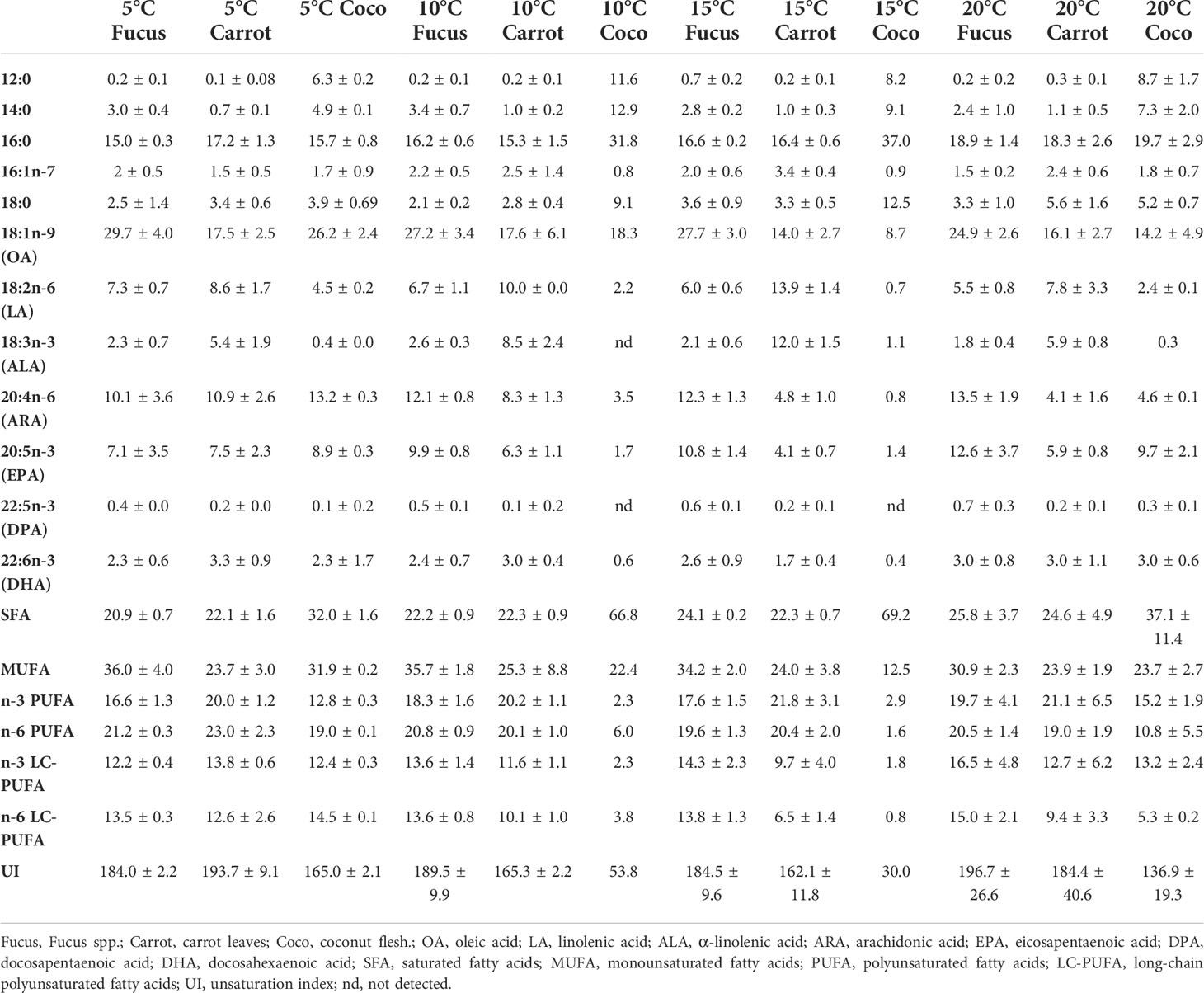
Table 3 Relative fatty acid (FA) composition (% of total FA) of samples of G. locusta fed different diets at four temperatures.
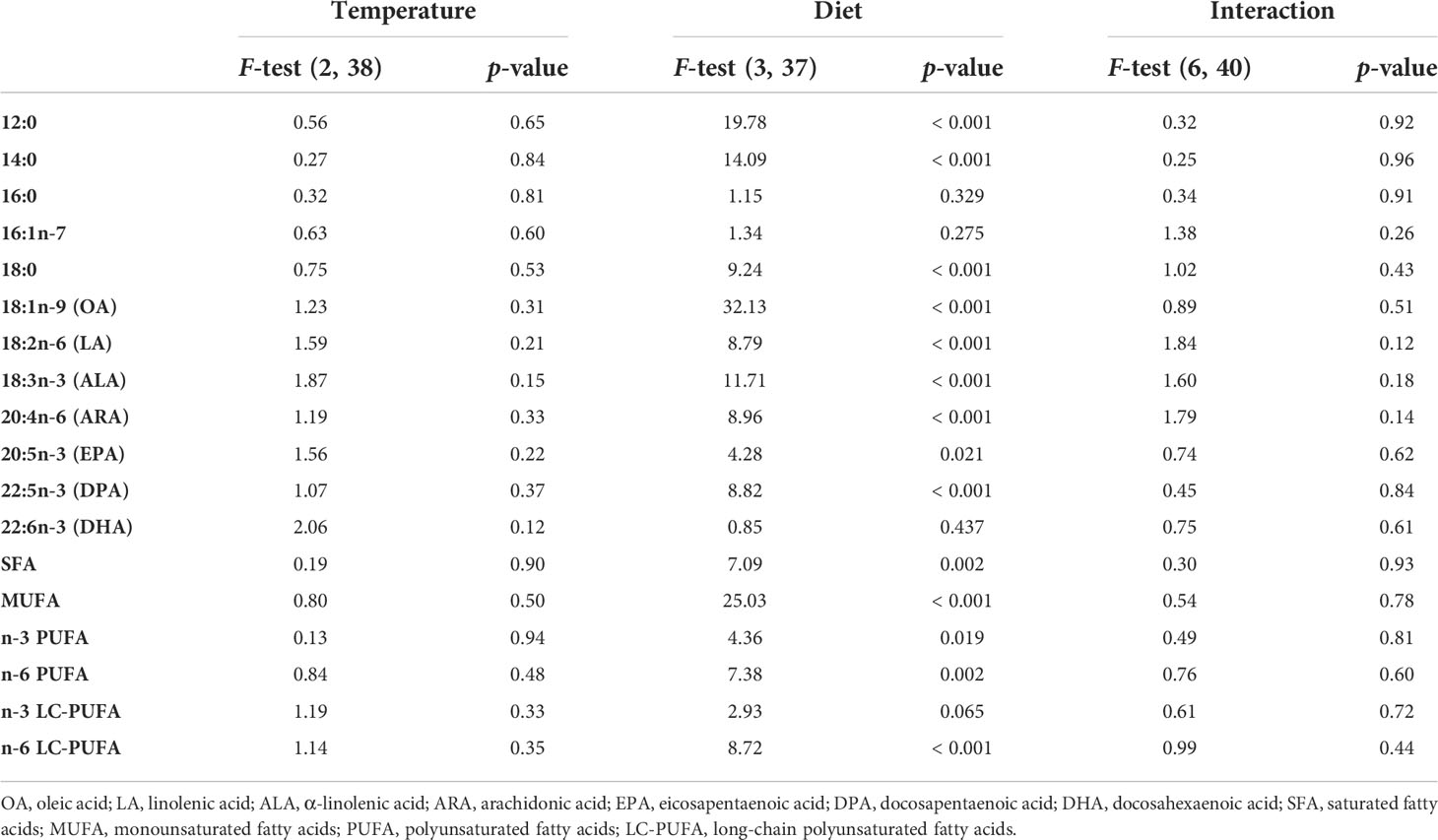
Table 4 Two-way ANOVA results of the fatty acid composition of samples of G. locusta fed different diets at four temperatures.
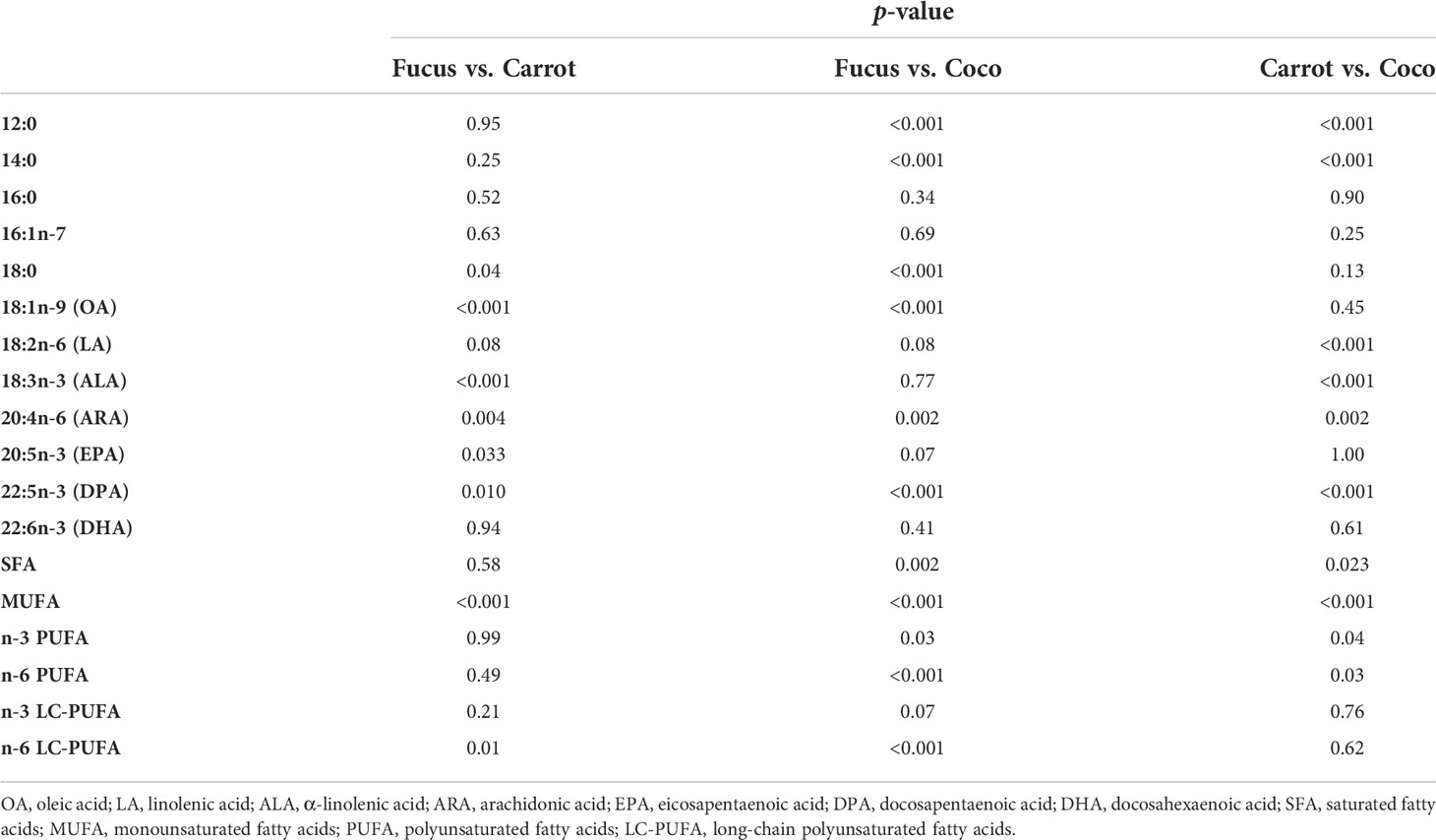
Table 5 Tukey’s post-hoc test after ANOVA of the fatty acid composition of G. locusta fed different diets.
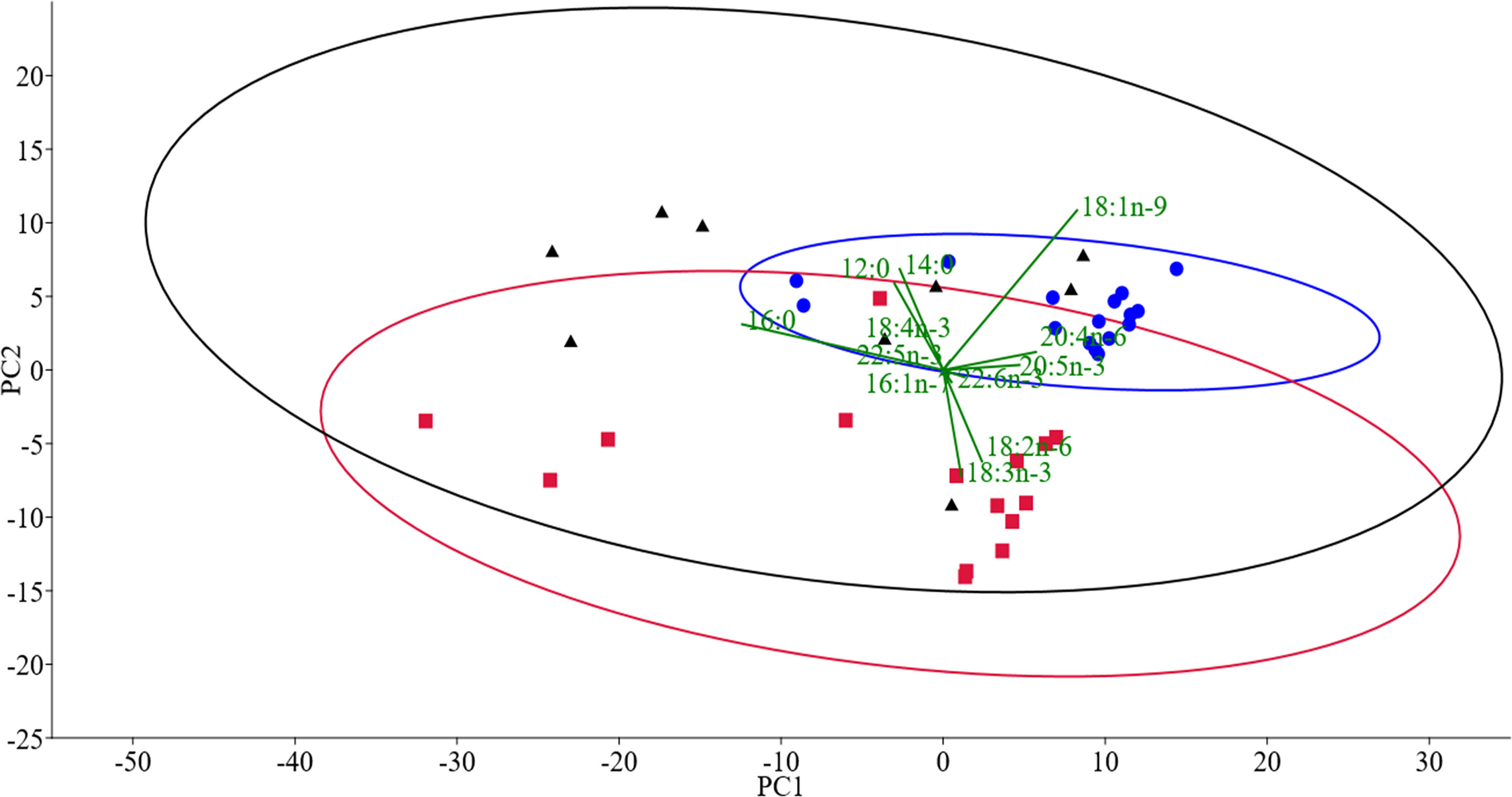
Figure 1 PCA of the fatty acid composition of G. locusta fed different diets and reared at different temperatures. Dots (blue), squares (red), and triangles (black) are the scores of G. locusta fed on Fucus spp., carrot leaves, and coconut, respectively. Fatty acids responsible for the grouping pattern are displayed in the biplot (green vectors); 95% confidence ellipses are shown. For the sake of clarity, and due to the lack of statistical significance, temperature labels are not shown.
Generally, G. locusta fed on Fucus showed FA profiles richer in LC-PUFA (C20-24) than those fed on Carrot or Coco, whereas G. locusta fed on Carrot showed the highest levels of PUFA (<C20) such as LA and ALA (Table 3). Notably, levels of EPA and DHA were detected in G. locusta fed on either Carrot or Coco regardless of these FA not being present in those diets. Regarding SFA, G. locusta fed on Coco showed higher percentages as compared to G. locusta fed on either Fucus or Carrot (Table 3). It is important to note as well that lower percentages of SFA were detected in cultured G. locusta fed on Coco in comparison with the Coco diet itself, while maintaining moderate LC-PUFA levels.
Discussion
The nutritional profiles of gammarids have been studied as alternative sustainable ingredients for aquafeeds (Kolanowski et al., 2007; Baeza-Rojano et al., 2010; Baeza-Rojano et al., 2013; Baeza-Rojano et al., 2014; Jiménez-Prada et al., 2018) due to the potential of these organisms to feed on a wide range of sidestreams (Harlıoğlu and Farhadi, 2018). However, only a few investigations have focused on culture strategies based on the use of aquaculture and/or agriculture waste as primary food (Alberts-Hubatsch et al., 2019; Jiménez-Prada et al., 2020), and up to date, there are no investigations reporting on the effect of food and different environmental conditions on the fatty acid profile, growth, and survival of these organisms. In the present study, juveniles of G. locusta survived and reached sexual maturity in all the conditions tested for both temperature and diet groups. Interestingly, the survival of G. locusta was significantly lower at 20°C than at the rest of the temperatures. This result supports the hypothesis that at 20°C there is an acceleration of growth and reduction of the life cycle of G. locusta, resulting in a faster length growth and therefore metabolism, which results in an increased mortality (Neuparth et al., 2002). Regarding the effect of different diets, survival was significantly lower in G. locusta fed on Coco, whereas no differences were found between those of gammarids fed on the Carrot and Fucus diets. The absence of physiologically essential compounds such as LC-PUFA in the coconut diet may explain this difference (Jump, 2002; Monroig et al., 2022). Indeed, it is tempting to hypothesize that the high content of SFA in the diet may be related to an impairment of the membrane composition and properties, ultimately resulting in an increase in mortality under the most stressful temperature conditions (Hazel and Eugene Williams, 1990; Hazel, 1995; Ernst et al., 2016; Laila, 2021; Zinoöcker et al., 2021). Noticeably, cannibalistic behavior was observed in gammarids fed on a coconut diet, suggesting a potential corrective mechanism towards the nutritional impairment. Although LC-PUFA was low in carrot leaves, the survival of gammarids fed on this diet was similar to that obtained with Fucus. As previously reported by Alberts-Hubatsch et al. (2019), this higher survival may be partially explained by the presence of other health-promoting nutrients like carotenoids that can thus compensate for suboptimal levels of LC-PUFA (Saini et al., 2015; González-Peña et al., 2021). Indeed, carotenoids are regarded as biomolecules that protect against unspecific and oxidant stress conditions such as suboptimal temperatures, which can ultimately cause increased mortality in gammarids (Agnew and Taylor, 1986; Neuparth et al., 2002; Wijnhoven et al., 2003). Significant differences in total gammarid biomass outputs were found when comparing different diets, and, regarding temperature, only significant differences were found between 5°C and 15°C, the latter having been reported as the optimal growth temperature for G. locusta (Neuparth et al., 2002). Interestingly, culturing gammarids at 20°C and fed on Fucus resulted in the highest output of biomass as well as final length and SGR. This may be due to the combined effects of the high nutritional value of the Fucus diet (Alberts-Hubatsch et al., 2019) as well as the increased growth performance of G. locusta when grown at 20°C (Neuparth et al., 2002) that compensates for the biomass loss associated with the abovementioned high mortality rates detected at 20°C.
Under the present experimental conditions, G. locusta showed similar FA profiles regardless of the rearing temperature. Only slight differences among temperatures within the same diet were evident, indicating either an apparent lack of temperature effect or a much more predominant dietary phenotypic impact. The FA dietary fingerprint was noticeable in the Fucus treatment in the LC-PUFA of the gammarid’s profiles, whereas Carrot treatment resulted in higher levels of LA and ALA, and Coco induced higher amounts of SFA such as lauric and myristic acids in the final biomass. Interestingly, G. locusta fed on either Carrot or Coco still showed EPA and DHA percentages similar to those of G. locusta fed on Fucus, regardless of the absence of these LC-PUFA in the diets. This finding is in agreement with the potential capacity of gammarids for trophic upgrading, as has been suggested by several authors (Baeza-Rojano et al., 2013; Baeza-Rojano et al., 2014; Jiménez-Prada et al., 2018; Alberts-Hubatsch et al., 2019; Jiménez-Prada et al., 2020), indicating that these animals could bioconvert the short-chain FA present in sidestreams into high nutritional value LC-PUFA via their biosynthetic pathway.
LC-PUFA biosynthesis relies especially upon the gene repertoire and in the coordinated action of two types of enzymes (Monroig and Kabeya, 2018; Monroig et al., 2022). These two enzymes, elongation of very long-chain-fatty acid proteins (commonly known as “elongases”), and front-end desaturases (also named “desaturases”), need to be present and active in the organism in order to efficiently biosynthesize LC-PUFA such as EPA and DHA from dietary FA (Castro et al., 2016; Monroig and Kabeya, 2018; Monroig et al., 2022). A recent report on the three different elongases in the marine gammarid E. marinus (Ribes-Navarro et al., 2021) indicates the potential capacity for endogenous production of LC-PUFA in marine gammarids, but the presence and activity of desaturases remain to be elucidated. The presence of EPA and DHA can be due to reasons other than endogenous biosynthesis, such as the accumulation and retention of these FA from sources other than the diet provided, like the occasional and practically unavoidable presence of prey organisms such as copepods and rotifers in the culture tanks during the experiment. These organisms, particularly copepods, are characterized by having high LC-PUFA contents (McKinnon et al., 2003; Miller et al., 2008; Naylor et al., 2009; Nielsen et al., 2019; Boyen et al., 2020; Kabeya et al., 2021). It should be noted, however, that during the experiment, it was evident that gammarids fed actively on the vegetal substrates, and although impossible to rule out, the possible contribution of the above-mentioned accompanying fauna entering the system as well as that of the rapid development of biofilm seems at most testimonial. Other factors, like the fungal contribution described in the digestive process for the freshwater G. pulex (Chamier and Willoughby, 1986), can also be invoked to explain the presence of unexpected components in the final profiles, but this can only be clarified in experiments run in controlled conditions, far beyond the scope of the present experimental design.
In summary, the present study demonstrates that gammarids can be fed on agricultural sidestreams (carrot leaves) without drastically affecting their growth performance and survival when reared at temperatures ranging from 5°C to 15°C. A culture at 20°C is not recommended due to the impairment between growth and survival. This study is the first study of this kind to show interactions between rearing temperatures and novel feed ingredients for this species, further steps (e.g., other under-used sidestreams from agriculture) need to be taken to optimize biomass gain in addition to survival. In particular, this study shows that G. locusta can be a potential candidate for applying circular economy principles by which sidestreams such as carrot leaves can be used as the main feed for gammarid culture and potential biomass generation. The aim of this study was also to get independent from marine resources, such as macroalgae, that can be costly or not sustainable to use for feeding gammarids. Thus, showing that at least carrot greens are providing sufficient nutrients for a successful culture of G. locusta gives important directions in terms of sustainability. Moreover, under the present experimental conditions tested herein, diet has been established as the main modulator of FA profiling in G. locusta. However, a combination of agricultural and aquaculture sidestreams, along with other modulating conditions such as salinity, should be considered in order to set the best environment for large-scale gammarid production. Aside from the strong phenotypic effect of diet on the final FA profile, the presence of LC-PUFA like EPA and DHA, detected on G. locusta fed on diets devoid of these compounds, points towards unknown mechanisms of trophic upgrading beyond the theoretical endogenous biosynthetic capacity of the species.
Data availability statement
The original contributions presented in the study are included in the article/Supplementary Material. Further inquiries can be directed to the corresponding author.
Ethics statement
All experiments were carried out under the European Directive 2010/63/EU (European Parliament, Council of the European Union, 2010) for the protection of animals used for experimentation and other scientific purposes, from which invertebrates other than cephalopods are excluded. The experiments were run bearing in mind the 3R guidelines.
Author contributions
Conceptualization: HA-H and JN. Methodology: AR-N, HA-H, JN, and ÓM. Formal analysis: AR-N, HA-H, JN, and ÓM. Investigation: AR-N, HA-H, JN, FH, and ÓM. Resources: HA-H. Writing—original draft preparation: AR-N, HA-H, and JN. Writing—review and editing: AR-N, FH, HA-H, JN, and ÓM. Project administration: FH, HA-H, JN, and ÓM. Funding acquisition: FH, HA-H, JN, and ÓM. All authors contributed to the article and approved the submitted version.
Funding
This research was supported by the ERA-NET BlueBio COFUND Project SIDESTREAM [Grant ID 68], cofunded through national funds provided by the Agencia Española de Investigación, Spain, grant no. PCI2020-111960/MCIN/AEI/10.13039/501100011033 and the EU NextGenerationEU/PRTR, and the Funding by the German Federal Ministry of Education and Research (BMBF), FKZ161B0950B. Additional funding was received through the project IMPROMEGA Agencia Española de Investigación, Spain, grant no. RTI2018-095119-B-100, MCIU/AEI/FEDER/UE/MCIN/AEI/10.13039/501100011033/and FEDER “A way to make Europe.”
Acknowledgments
This is a short text to acknowledge the contributions of specific colleagues, institutions, or agencies that aided the efforts of the authors.
Conflict of interest
The authors declare that the research was conducted in the absence of any commercial or financial relationships that could be construed as a potential conflict of interest.
Publisher’s note
All claims expressed in this article are solely those of the authors and do not necessarily represent those of their affiliated organizations, or those of the publisher, the editors and the reviewers. Any product that may be evaluated in this article, or claim that may be made by its manufacturer, is not guaranteed or endorsed by the publisher.
Supplementary material
The Supplementary Material for this article can be found online at: https://www.frontiersin.org/articles/10.3389/fmars.2022.931991/full#supplementary-material
Supplementary Table 1 | Calculated p values for the one-way PERMANOVA (Bonferroni corrected p values).
Supplementary Table 2 | SIMPER analysis of the dietary groups Fucus vs Carrot. All FA detected were included in the analysis and those above the cumulative cutoff of 70% were not shown in the table, except for the first one.
Supplementary Table 3 | SIMPER analysis of the dietary groups Fucus vs Coco. All FA detected were included in the analysis and those above the cumulative cut-off of 70% were not shown in the table, except for the first one.
Supplementary Table 4 | SIMPER analysis of the dietary groups Carrot vs Coco. All FA detected were included in the analysis and those above the cumulative cut-off of 70% were not shown in the table, except for the first one.
References
Agnew D. J., Taylor A. C. (1986). Effects of oxygen tension, temperature, salinity, and humidity on the survival of two intertidal gammarid amphipods. Mar. Ecol. Prog. Ser. 32, 27–33.
Ahyong S. T., Hughes L. E. (2016). Collecting and processing amphipods. J. Crustac. Biol. 36, 584–588. doi: 10.1163/1937240X-00002450
Alberts-Hubatsch H., Slater M. J., Beermann J. (2019). Effect of diet on growth, survival and fatty acid profile of marine amphipods: Implications for utilisation as a feed ingredient for sustainable aquaculture. Aquac. Environ. Interact. 11, 481–491. doi: 10.3354/aei00329
Ashour M., Abo-Taleb H. A., Hassan A. K. M., Abdelzaher O. F., Mabrouk M. M., Elokaby M. A., et al. (2021). Valorization use of amphipod meal, gammarus pulex, as a fishmeal substitute on growth performance, feed utilization, histological and histometric indices of the gut, and economic revenue of grey mullet. J. Mar. Sci. Eng. 9(12), 1336. doi: 10.3390/jmse9121336
Baeza-Rojano E., Domingues P., Guerra-García J. M., Capella S., Noreña-Barroso E., Caamal-Monsreal C., et al. (2013). Marine gammarids (Crustacea: Amphipoda): a new live prey to culture octopus maya hatchlings. Aquac. Res. 44, 1602–1612. doi: 10.1111/j.1365-2109.2012.03169.x
Baeza-Rojano E., García S., Garrido D., Guerra-García J. M., Domingues P. (2010). Use of amphipods as alternative prey to culture cuttlefish (Sepia officinalis) hatchlings. Aquaculture 300, 243–246. doi: 10.1016/j.aquaculture.2009.12.029
Baeza-Rojano E., Hachero-Cruzado I., Guerra-García J. M. (2014). Nutritional analysis of freshwater and marine amphipods from the strait of Gibraltar and potential aquaculture applications. J. Sea Res. 85, 29–36. doi: 10.1016/j.seares.2013.09.007
Beermann J., Boos K., Gutow L., Boersma M., Peralta A. C. (2018). Combined effects of predator cues and competition define habitat choice and food consumption of amphipod mesograzers. Oecologia 186, 645–654. doi: 10.1007/s00442-017-4056-4
Bell J. G., McEvoy J., Tocher D. R., McGhee F., Campbell P. J., Sargent J. R. (2001). Replacement of fish oil with rapeseed oil in diets of Atlantic salmon (Salmo salar) affects tissue lipid compositions and hepatocyte fatty acid metabolism. J. Nutr. 131, 1535–1543. doi: 10.1093/jn/131.5.1535
Boyen J., Fink P., Mensens C., Hablützel P. I., De Troch M. (2020). Fatty acid bioconversion in harpacticoid copepods in a changing environment: A transcriptomic approach. Philos. Trans. R. Soc B Biol. Sci. 375. doi: 10.1098/rstb.2019.0645
Castro L. F. C., Tocher D. R., Monroig O. (2016). Long-chain polyunsaturated fatty acid biosynthesis in chordates: Insights into the evolution of fads and elovl gene repertoire. Prog. Lipid Res. 62, 25–40. doi: 10.1016/j.plipres.2016.01.001
Chamier A., Willoughby L. G. (1986). The role of fungi in the diet of the amphipod gammarus pulex (L.): an enzymatic study. Freshw. Biol. 16, 197–208. doi: 10.1111/j.1365-2427.1986.tb00964.x
Costa F. O., Costa M. H. (2000). Review of the ecology of gammarus locusta (L.). Pol. Arch. Hydrobiol. 47, 541–559.
Dhont J., Dierckens K., Støttrup J., Van Stappen G., Wille M., Sorgeloos P. (2013). ““Rotifers, artemia and copepods as live feeds for fish larvae in aquaculture,”,” in Advances in aquaculture hatchery technology (Cambridge, UK: Woodhead Publishing Limited), 157–202. doi: 10.1533/9780857097460.1.157
Ernst R., Ejsing C. S., Antonny B. (2016). Homeoviscous adaptation and the regulation of membrane lipids. J. Mol. Biol. 428, 4776–4791. doi: 10.1016/j.jmb.2016.08.013
FAO (2020). The state of world fisheries and aquaculture 2020. sustainability in action. (Rome, Italy: FAO). doi: 10.4060/ca9229en
Folch J., Lees M., Sloane Stanley G. H. (1957). A simple method for the isolation and purification of total lipides from animal tissues. J. Biol. Chem. 226, 497–509. doi: 10.1016/S0021-9258(18)64849-5
Galkanda-Arachchige H. S. C., Wilson A. E., Davis D. A. (2020). Success of fishmeal replacement through poultry by-product meal in aquaculture feed formulations: a meta-analysis. Rev. Aquac. 12, 1624–1636. doi: 10.1111/raq.12401
González-Peña M. A., Lozada-Ramírez J. D., Ortega-Regules A. E. (2021). Carotenoids from mamey (Pouteria sapota) and carrot (Daucus carota) increase the oxidative stress resistance of caenorhabditis elegans. Biochem. Biophys. Rep. 26. doi: 10.1016/j.bbrep.2021.100989
Guerra-García J. M., Hachero-Cruzado I., González-Romero P., Jiménez-Prada P., Cassell C., Ros M. (2016). Towards integrated multi-trophic aquaculture: Lessons from caprellids (Crustacea: Amphipoda). PloS One 11, e0154776. doi: 10.1371/journal.pone.0154776
Hammer Ø., Harper D. A. T., Ryan P. D. (2001). PAST : Paleontological statistics software package for education and data analysis, Vol. 4. (California: Palaentologia Electronica, Coquina Press) 1–9.
Harlıoğlu M. M., Farhadi A. (2018). Importance of gammarus in aquaculture. Aquac. Int. 26, 1327–1338. doi: 10.1007/s10499-018-0287-6
Hazel J. R. (1995). Thermal adaptation in biological membranes: Is homeoviscous adaptation the explanation? Annu. Rev. Physiol. 57, 19–42. doi: 10.1146/annurev.ph.57.030195.000315
Hazel J. R., Eugene Williams E. (1990). The role of alterations in membrane lipid composition in enabling physiological adaptation of organisms to their physical environment. Prog. Lipid Res. 29, 167–227. doi: 10.1016/0163-7827(90)90002-3
Jannathulla R., Rajaram V., Kalanjiam R., Ambasankar K., Muralidhar M., Dayal J. S. (2019). Fishmeal availability in the scenarios of climate change: Inevitability of fishmeal replacement in aquafeeds and approaches for the utilization of plant protein sources. Aquac. Res. 50, 3493–3506. doi: 10.1111/are.14324
Jiménez-Prada P., Hachero-Cruzado I., Giráldez I., Fernández-Diaz C., Vilas C., Cañavate J. P., et al. (2018). Crustacean amphipods from marsh ponds: A nutritious feed resource with potential for application in integrated multi-trophic aquaculture. PeerJ 2018. doi: 10.7717/peerj.4194
Jiménez-Prada P., Hachero-Cruzado I., Guerra-García J. M. (2020). Aquaculture waste as food for amphipods: the case of gammarus insensibilis in marsh ponds from southern Spain. Aquac. Int. 139–153. doi: 10.1007/s10499-020-00615-z
Jump D. B. (2002). The biochemistry of n-3 polyunsaturated fatty acids. J. Biol. Chem. 277, 8755–8758. doi: 10.1074/jbc.R100062200
Kabeya N., Ogino M., Ushio H., Haga Y., Satoh S., Navarro J. C., et al. (2021). A complete enzymatic capacity for biosynthesis of docosahexaenoic acid (DHA, 22: 6n-3) exists in the marine harpacticoida copepod tigriopus californicus. Open Biol. 11. doi: 10.1098/rsob.200402
Kolanowski W., Stolyhwo A., Grabowski M. (2007). Fatty acid composition of selected freshwater gammarids (Amphipoda, crustacea): A potentially innovative source of omega-3 LC PUFA. JAOCS J. Am. Oil Chem. Soc 84, 827–833. doi: 10.1007/s11746-007-1116-7
Laila A. (2021). Limitations in the “homeoviscous adaptation to dietary lipids” model. Am. J. Clin. Nutr. 114, 822–823. doi: 10.1093/ajcn/nqab230
McKinnon A. D., Duggan S., Nichols P. D., Rimmer M. A., Semmens G., Robino B. (2003). The potential of tropical paracalanid copepods as live feeds in aquaculture. Aquaculture 223, 89–106. doi: 10.1016/S0044-8486(03)00161-3
Miller M. R., Nichols P. D., Carter C. G. (2008). n-3 oil sources for use in aquaculture alternatives to the unsustainable harvest of wild fish. Nutr. Res. Rev. 21, 85–96. doi: 10.1017/S0954422408102414
Monroig Ó., Kabeya N. (2018). Desaturases and elongases involved in polyunsaturated fatty acid biosynthesis in aquatic invertebrates: a comprehensive review. Fish. Sci. 84, 911–928. doi: 10.1007/s12562-018-1254-x
Monroig Ó, Shu-Chien A. C., Kabeya N., Tocher D. R., Castro L. F. C. (2022). Desaturases and elongases involved in long-chain polyunsaturated fatty acid biosynthesis in aquatic animals: From genes to functions. Prog. Lipid Res. 86, 101157. doi: 10.1016/j.plipres.2022.101157
Mourente G., Bell J. G. (2006). Partial replacement of dietary fish oil with blends of vegetable oils (rapeseed, linseed and palm oils) in diets for European sea bass (Dicentrarchus labrax l.) over a long term growth study: Effects on muscle and liver fatty acid composition and e. Comp. Biochem. Physiol. B Biochem. Mol. Biol. 145, 389–399. doi: 10.1016/j.cbpb.2006.08.012
Naylor R. L., Hardy R. W., Bureau D. P., Chiu A., Elliott M., Farrell A. P., et al. (2009). Feeding aquaculture in an era of finite resources. Proc. Natl. Acad. Sci. U. S. A. 106, 15103–15110. doi: 10.1073/pnas.0905235106
Neuparth T., Costa F. O., Costa M. H. (2002). ). effects of temperature and salinity on life history of the marine amphipod gammarus locusta. implications for ecotoxicological testing. Ecotoxicology 11, 61–73. doi: 10.1023/A:1013797130740
Nielsen B. L. H., Gøtterup L., Jørgensen T. S., Hansen B. W., Hansen L. H., Mortensen J., et al. (2019). N-3 PUFA biosynthesis by the copepod apocyclops royi documented using fatty acid profile analysis and gene expression analysis. Biol. Open 8, 1–12. doi: 10.1242/bio.038331
Ribes-Navarro A., Navarro J. C., Hontoria F., Kabeya N., Standal I. B., Evjemo J. O., et al. (2021). ). biosynthesis of long-chain polyunsaturated fatty acids in marine gammarids: Molecular cloning and functional characterisation of three fatty acyl elongases. Mar. Drugs 19. doi: 10.3390/MD19040226
Romano N., Kumar V., Yang G., Kajbaf K., Rubio M. B., Overturf K., et al. (2020). Bile acid metabolism in fish: disturbances caused by fishmeal alternatives and some mitigating effects from dietary bile inclusions. Rev. Aquac. 12, 1792–1817. doi: 10.1111/raq.12410
Saini R. K., Nile S. H., Park S. W. (2015). Carotenoids from fruits and vegetables: Chemistry, analysis, occurrence, bioavailability and biological activities. Food Res. Int. 76, 735–750. doi: 10.1016/j.foodres.2015.07.047
Schneider C. A., Rasband W. S., Eliceiri K. W. (2012). NIH Image to ImageJ: 25 years of image analysis. Nat. Methods 9, 671–675. doi: 10.1038/nmeth.2089
Sexton E. W. (1928). On the rearing and breeding of gammarus in laboratory conditions. J. Mar. Biol. Assoc. United Kingdom 15, 33–55. doi: 10.1017/S0025315400055521
Shepherd C. J., Monroig O., Tocher D. R. (2017). Future availability of raw materials for salmon feeds and supply chain implications: The case of Scottish farmed salmon. Aquaculture 467, 49–62. doi: 10.1016/j.aquaculture.2016.08.021
Suontama J., Kiessling A., Melle W., Waagbø R., Olsen R. E. (2007). Protein from northern krill (Thysanoessa inermis), Antarctic krill (Euphausia superba) and the Arctic amphipod (Themisto libellula) can partially replace fish meal in diets to Atlantic salmon (Salmo salar) without affecting pro. Aquac. Nutr. 13, 50–58. doi: 10.1111/j.1365-2095.2007.00453.x
Tocher D. R. (2015). Omega-3 long-chain polyunsaturated fatty acids and aquaculture in perspective. Aquaculture 449, 94–107. doi: 10.1016/j.aquaculture.2015.01.010
Turchini G. M., Francis D. S., Senadheera S. P. S. D., Thanuthong T., De Silva S. S. (2011a). Fish oil replacement with different vegetable oils in Murray cod: Evidence of an “omega-3 sparing effect” by other dietary fatty acids. Aquaculture 315, 250–259. doi: 10.1016/j.aquaculture.2011.02.016
Turchini G. M., Ng W., Tocher D. (2011b). Fish oil replacement and alternative lipid sources in aquaculture feeds (Boca Rat: CRC Press).
Turchini G. M., Torstensen B. E., Ng W. K. (2009). Fish oil replacement in finfish nutrition. Rev. Aquac. 1, 10–57. doi: 10.1111/j.1753-5131.2008.01001.x
Wijnhoven S., Van Riel M. C., van der Velde G. (2003). Exotic and indigenous freshwater gammarid species: Physiological tolerance to water temperature in relation to ionic content of the water. Aquat. Ecol. 37, 151–158. doi: 10.1023/A:1023982200529
Yıldız M., Eroldoğan T. O., Ofori-Mensah S., Engin K., Baltacı M. A. (2018). The effects of fish oil replacement by vegetable oils on growth performance and fatty acid profile of rainbow trout: Re-feeding with fish oil finishing diet improved the fatty acid composition. Aquaculture 488, 123–133. doi: 10.1016/j.aquaculture.2017.12.030
Keywords: agri-food sidestreams, circular economy, gammarus locusta, long-chain polyunsaturated fatty acids, novel marine ingredients
Citation: Ribes-Navarro A, Alberts-Hubatsch H, Monroig Ó, Hontoria F and Navarro JC (2022) Effects of diet and temperature on the fatty acid composition of the gammarid Gammarus locusta fed alternative terrestrial feeds. Front. Mar. Sci. 9:931991. doi: 10.3389/fmars.2022.931991
Received: 29 April 2022; Accepted: 13 July 2022;
Published: 04 August 2022.
Edited by:
Sílvia Lourenço, Instituto Politécnico de Leiria, PortugalReviewed by:
Renata Goncalves, Technical University of Denmark, DenmarkCatherine J. Stevens, University of Victoria, Canada
Copyright © 2022 Ribes-Navarro, Alberts-Hubatsch, Monroig, Hontoria and Navarro. This is an open-access article distributed under the terms of the Creative Commons Attribution License (CC BY). The use, distribution or reproduction in other forums is permitted, provided the original author(s) and the copyright owner(s) are credited and that the original publication in this journal is cited, in accordance with accepted academic practice. No use, distribution or reproduction is permitted which does not comply with these terms.
*Correspondence: Juan C. Navarro, amMubmF2YXJyb0Bjc2ljLmVz