- 1Institute for Marine Biological Resources and Biotechnology (IRBIM), National Research Council–CNR, Mazara del Vallo, Italy
- 2Department of Biological, Geological and Environmental Sciences (BiGeA) – Marine Biology and Fisheries Laboratory of Fano, University of Bologna, Fano, Italy
- 3Department of Integrative Marine Ecology, Stazione Zoologica Anton Dohrn, Rome, Italy
Holothurians or sea cucumbers are key organisms in marine ecosystems that, by ingesting large quantities of sediments, provide important ecosystem services. Among them, Parastichopus regalis (Cuvier, 1817) is one of the living sea cucumbers in the Mediterranean actively fished for human consumption mainly in Spain, where it is considered a gastronomic delicacy. In the Strait of Sicily (central Mediterranean Sea), this species is not exploited for commercial use even if it is used as bait by longline fishery. P. regalis is frequently caught by bottom trawling and discarded at sea by fishers after catch, and because of its capacity to resist air exposition (at least in cold months), it is reasonable to consider that it is not affected by fishing mortality. Having observed a significant decrease in abundance since 2018, the possible effects of some ecological factors related to current climate change (i.e., temperature and pH) were sought. Generalized additive models (GAMs) were applied to investigate the relationship among the abundance of P. regalis and environmental variables and fishing effort. Long time series of P. regalis densities (2008–2021) were extracted from the MEDITS bottom trawling survey and modeled as function of environmental parameters (i.e., salinity, dissolved oxygen, ammonium, pH, and chlorophyll α) and fishing effort (i.e., total number of fishing days per gross tonnage). Our results showed that this species prefers the soft bottoms (50–200 m) of the Adventure Bank and Malta Plateau, and its distribution changed over time with a slight deepening and a rarefaction of spatial distribution starting from 2011 and 2017, respectively. In addition, a positive relationship with pH concentration in surface waters during the larval dispersal phase (3-year lag before the survey) and nutrient concentration at sea bottom (1-year lag) has been found, suggesting that this species is sensitive to climate change and food availability. This study adds new knowledge about the population dynamics of an unexploited stock of P. regalis under fishing impact and environmental under climate change in fisheries management.
Introduction
The Anthropocene (sensu Crutzen and Stoermer, 2000) climate change has been impacting the marine environment and biodiversity since the early 20th century. Human activities mainly affect different processes such as the alteration of thermal regimes, water cycle, increase of ocean acidification and sea temperature (Huang et al., 2021), marked thermal stratification, and reduction of upwelling processes (Prakash, 2021).
The Mediterranean is a semi-enclosed sea, representing the boundary between the arid climate of North Africa and the temperate climate of Europe, and is characterized by a restricted hydrological exchange with the open ocean (Bethoux and Gentili, 1999; Diffenbaugh et al., 2007). For these reasons, it is particularly climate-vulnerable to even minor modifications, while it is warming at two to three times the rate for the global ocean (Vargas-Yáñez et al., 2010). Moreover, the Mediterranean Sea depicts a high imprint of anthropogenic CO2, which has already affected its pH by a decrease of 0.05–0.14 pH units since pre-industrial times (Touratier and Goyet, 2011). Both the rise in CO2 concentration and warming have directly and indirectly affected marine organisms that are vulnerable to changes in pH and temperature (González-Durán et al., 2021).
Holothurians, commonly called sea cucumbers, is an abundant and diverse group of worm-like and usually soft-bodied echinoderms. They are found almost everywhere in the marine environment ranging from the intertidal to the floor of the deepest oceans (Aydın and Erkan, 2015). Sea cucumbers are key organisms in the marine ecosystem because they provide different ecosystem services, such as recycling of nutrients and particulate organic matter, bioturbation of sediments with consequent oxygenation, bio-remediation of the bacterial component present in sediments and in the water column, and production of biomass (Ramón et al., 2010; MacTavish et al., 2012; Purcell et al., 2013; Costa et al., 2014; Purcell et al., 2016).
Similar to many other marine organisms, the holothurians are also affected by climate change (González-Durán et al., 2021). The combined effects of pH changes, water temperature, and salinity may trigger pathological responses by altering the anatomical characteristic of the sea cucumber organisms (Gullian, 2013), generating diverse biochemical and physiological adaptations (Zamora and Jeffs, 2012; Gullian, 2013; Wu et al., 2013; Gullian and Terrats, 2017), altering the concentration ratio of different inorganic carbon species CO2, HCO3−, and CO3−2, and reducing the saturation state of CaCO3 (Gao et al., 2019). These environmental variables can influence the population abundance of holothurians, and have been shown to negatively impact several biological aspects (e.g., the fertilization success, the larvae metamorphosis, the development time, the physiological performances, the respiratory rate as the temperature increases, and the larvae growth rate) (Asha and Muthiah, 2005; Hamel and Mercier, 2008; Pörtner, 2008; Yuan et al., 2015) and the recruitment efficiency (González-Durán et al., 2021). Furthermore, these environmental variables influence the growth and survival of juveniles and adults, although the effect exerted by the variation of pH and temperature is less than those observed in the larvae (Dong et al., 2006; Yuan et al., 2009). An indirect effect associated with sea temperature variation is related to an increase in the oxygen diffusion as well as oxygen demands to guarantee biological processes (Pörtner and Knust, 2007). To contrast hypo-metabolism, sea cucumbers most probably increase the defense mechanisms and physiological responses that generate dormancy and estivation by reducing the metabolic activity (Quiñones et al., 2002; Asha and Muthiah, 2005; Morgan, 2008; Yuan et al., 2009). Furthermore, few studies have suggested that food abundance is a key factor influencing sea cucumber larvae survival and development (Morgan, 2008; Brander, 2010).
Holothurians represent a fishery resource in many countries. Indeed, at least 66 species are fished from more than 40 countries, particularly in Asia and the Pacific regions where the catches amount to 20,000–40,000 tons/year, and most of the catches are processed locally and exported to Asian markets, where they are considered traditional medicine and gastronomic delicacy (Bruckner, 2006; Toral-Granda, 2007; Toral-Granda et al., 2008; Ramón et al., 2010; Purcell, 2010; Purcell et al., 2013; Maggi and González-Wangüemert, 2015). Sea cucumbers may be sold alive, fresh, frozen, and as “trepang” (gutted, boiled, and dried). Their edible part is usually the thick body tegument although other tissues may be consumed (Purcell et al., 2012). The rising demand in Asian markets has resulted in the decline of many sea cucumber populations (Toral-Granda et al., 2008; Purcell et al., 2012; Eriksson and Clarke, 2015) and more than 70% of their stocks around the world were overexploited or depleted (Purcell et al., 2013). To address the problem of increasing market demands, many aquaculture practices have been developed in different parts of the world recently (Han et al., 2016; González-Wangüemert et al., 2018).
Overexploitation is considered the main risk of extinction of the most commercially valuable species, with 16 species of sea cucumbers now classified as threatened with extinction on the International Union for Conservation of Nature (IUCN) red list (Conand et al., 2014; González-Wangüemert et al., 2014). The collapse of sea cucumber stocks has prompted governments to ban or regulate fishing or exports in several sea cucumber fisheries globally (Robinson and Lovatelli, 2015). In this regard, several studies have reported that overfished populations of sea cucumber can take up to half a century of no fishing activities to rebuild (Battaglene and Bell, 1999; Skewes et al., 2000; Bruckner et al., 2003).
According to Aydın and Erkan (2015), 37 holothurian species belonging to nine families and five orders live in the Mediterranean. Historically, sea cucumber fisheries have been of scarce importance in Mediterranean countries with the exception of the Provence region (France). Nowadays, large quantities of holothurians are caught in Greece, Turkey, and Spain (Meloni and Esposito, 2018). Turkey is the main Mediterranean country involved in the catch and export of frozen, dried, and salted sea cucumbers toward Asian countries, particularly the species belonging to the genus Holothuria (Aydin, 2008; González-Wangüemert et al., 2014; Gonzalez-Wangüemert et al., 2015; González-Wangüemert et al., 2018). In Greece, Holothuria (Holothuria) tubulosa (Gmelin, 1791) has been harvested as bait in long-line fisheries for more than a century (Antoniadou and Vafidis, 2011). Conversely, in Spain, the fishery is mainly focused on royal cucumber, Parastichopus regalis (Cuvier, 1817). Its spatial distribution includes the Mediterranean Sea, the eastern Atlantic Ocean from the south of the Canary Islands to the north of Ireland, and the Antilles and the Gulf of Mexico in the western Atlantic (Tortonese, 1965; Míguez-Rodríguez, 2009). It is a benthic species found at a wide range of depths (5–800 m according to Tortonese, 1965), being more abundant on sandy and bioclastic bottoms between 50 and 300 m in the Mediterranean (Pawson et al., 2009; Ramón et al., 2010). The species is fished by bottom trawl and considered a gastronomic delicacy in Spain (i.e., Catalonia, Valencia and Baleares; Ramón et al., 2010; González-Wangüemert et al., 2016). This sea cucumber is the most expensive sea product in the Catalan market, where auction prices ranged between 64 and 129€/kg in 2019 (Ramón et al., 2022).
P. regalis is routinely caught by bottom trawlers operating on the outer shelf-upper slope in the Strait of Sicily (central Mediterranean Sea), and despite the long tradition of consumption of marine food by Sicilian people, it is not consumed or commercialized in Sicily and is generally discarded by fishers after catch or rarely used as bait in long-line fisheries. Conversely, holothurian shallow water species are fished in Sardinia and Apulia (Italian seas), where the amount of sea cucumbers illegally harvested and destined for the Asiatic market in 2016 and 2017 was equal to 23.9 tons, while in 2015 alone, it was about 29 tons (Meloni and Esposito, 2018). According to a precautionary approach, specimens belonging to the Holothuroidea class cannot be fished, retained on board, landed, or sold in the Italian seas since 2018 (IMAFFP, 2018).
Considering the growing interest about holothurians as a fishable resource and given its important ecological role, exploring the effect of environmental and fishing variables on their standing stock allows to make a step forward in understanding the impacts of climate change on a Mediterranean sea cucumber and to inform future fishery management plans applied to these species. In this regard, the Strait of Sicily is a suitable site to investigate population dynamics of P. regalis under different anthropic and natural drivers. In particular, this study aims at exploring spatial distribution and abundance over time and the potential effect of environmental factors linked to the climate change and fishing pressure.
Materials and methods
The study area
The Geographical Sub-Area 16 (GSA 16, according to the General Fisheries Commission for the Mediterranean-GFCM) is located on the northern sector of the Strait of Sicily (south-central Mediterranean Sea), sited between the landmasses of the western and southern coast of Sicily and Tunisia to the south (Figure 1). This relatively shallow borderland has a complex configuration, currently affected by active tectonics and recent volcanism and swept by bottom currents (Colantoni et al., 1992), with two platform-like areas (the Adventure Bank westward and the Malta Platform eastward), two wide northern and southern epicontinental shelves, narrow interconnected NE–SW basins, several knolls and seamounts, and volcanic edifices (Colantoni et al., 1975; Calanchi et al., 1989).
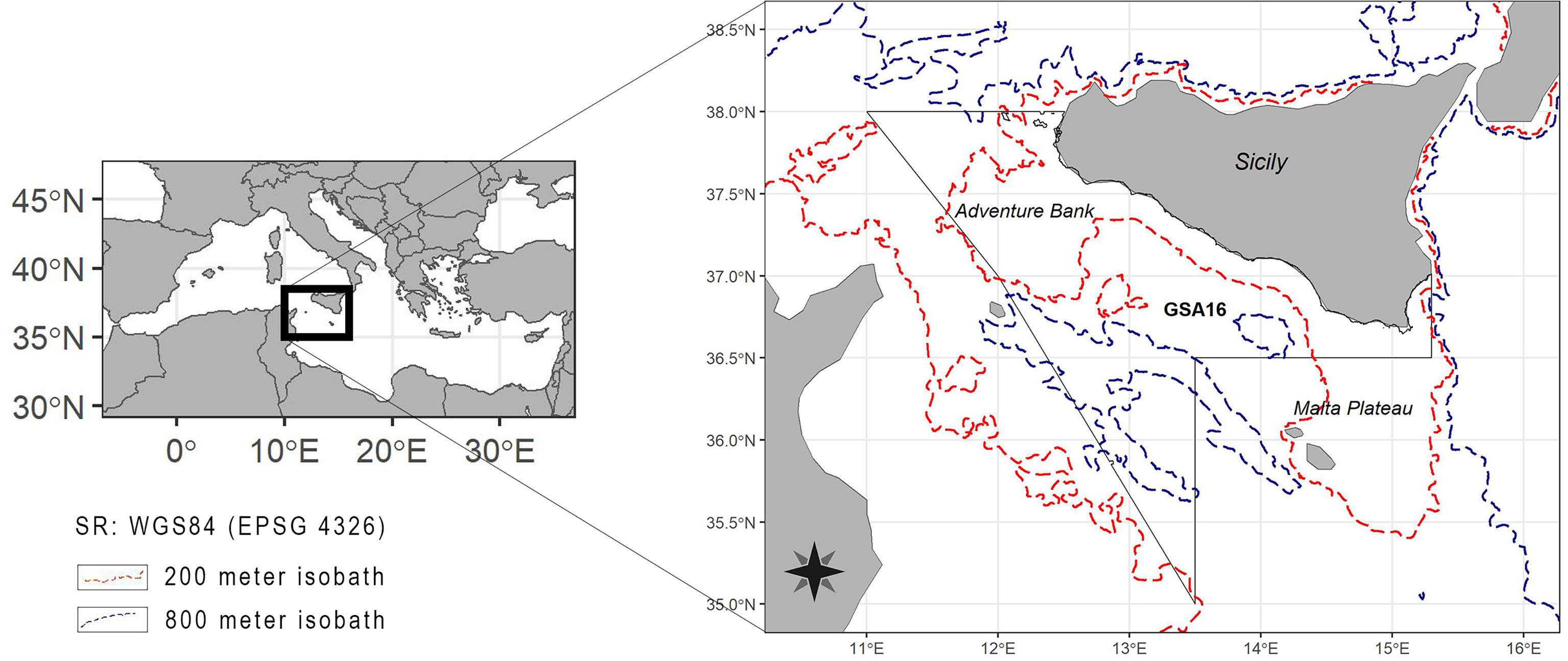
Figure 1 Map of the Strait of Sicily showing the location of the study area where the data of the P. regalis were collected: Geographical Sub-Area 16, south of Sicily. Red and blue dashed lines indicate the 200- and 800-m isobaths respectively.
In this area, the sedimentary facies and the depositional processes are heavily influenced by the physiographic structures and coastal erosion, river runoff and/or biogenic sedimentation. The western and eastern platforms (Adventure Bank and Malta Plateau) show exposed bedrock, coarse carbonate gravels and sands, and volcano-clastic sediments. Shelf modern sediments are strongly influenced by the local production of calcareous algal and shelly materials in shallower water and by clastic supply from coastal erosion and river drainage (Reeder et al., 2002). This seabed patchwork is highlighted by the high heterogeneity in benthic communities along the continental shelf (Di Lorenzo et al., 2018).
The currents in this region are mainly dictated by the Modified Atlantic Water (MAW) flowing eastward, and the Levantine Intermediate Water (LIW) flowing westward along the Sicilian slope in the 200–500 m depth range. Surface waters in the GSA derived from the Atlantic Ocean (Atlantic Ionian Stream, AIS) flow eastwards along the south Sicilian coast (Pinardi and Masetti, 2000; Béranger et al., 2004). The AIS shows a strong seasonal variability, being more intense during the spring–summer period in the 15–30 m depth range (Pinardi and Masetti, 2000; Sorgente et al., 2003; Sorgente et al., 2011). This surface circulation promotes the establishment of “permanent” upwellings towards the left side of the AIS in certain places, which are reinforced by westerly winds (Piccioni et al., 1988; Bonanno et al., 2014). The main source of nutrients in the area is associated with coastal upwellings and the doming of intermediate waters inside the cyclonic gyres, lying over the Adventure Bank and over the Malta shelf (Garcia Lafuente et al., 2002; Béranger et al., 2004). Primary production in the Strait of Sicily is seasonally controlled: a deep summer thermocline induces low biomass in late spring/summer; higher productivity is observed in late fall/winter, due to winter convention (D’Ortenzio and Ribera d’Alcalà, 2009; Di Donato et al., 2022).
Biological data
Spatial distribution and abundance data of P. regalis relative to the period 2008–2021 were collected from the bottom trawl surveys MEDITS (MEDiterranean International Bottom Trawl-Surveys) that have been carried out in the GSA16 annually in spring/summer since 1994 (Bertrand et al., 2002; Spedicato et al., 2019), except for 2013, 2014, 2017, and 2020, which were carried out in autumn due to administrative constraints. The bottom trawl surveys covered an area of about 31,400 km2 within the 10–800 m water depth range, and a total of 1,589 hauls were sampled (2008–2021). The survey follows a stratified random sampling with the allocation of hauls proportional to strata extension (depth strata: 10–50 m, 51–100 m, 101–200 m, 201–500 m, and 501–800 m). In particular, 120 hauls were carried out each year except for 2014 (55 hauls) and 2020 (94 hauls). Hauls were carried out by a standard bottom trawl net (GOC 73) with a 20-mm opening mesh size in the cod end (Fiorentini et al., 1999). The area swept in each haul was calculated according to the formula proposed by Fiorentini et al. (1999). For each haul, P. regalis abundance was standardized to the haul swept area (km2) and expressed as density index (herein DI), namely, the number of individuals per km2 (N/km2). The distribution curve of DI per haul as a function of depth was inspected to identify the preferred depth range of the species, which was found to be 20–200 m. The annual abundances of the species over this bathymetric range were then estimated. To account for the different numbers of hauls carried out during the time series considered in this study, DI was corrected by a common resampling without replacement procedure by reporting the number of hauls for each stratum to the minimum number observed during the time period considered (N of bootstrap samples = 99).
Environmental and fishery data sources
Concerning the climate-related drivers, four environmental predictors, i.e., temperature (T), salinity (S), oxygen (O2), and pH, were considered, given that several studies have shown they can influence the population density of holothurians (Widicombe and Spicer, 2008; Brierley and Kingsford, 2009; Doney et al., 2009; González-Durán et al., 2021). Similarly, chlorophyll α (Chl) and two nutrient concentrations (i.e., ammonium, NH4+ and phosphate, PO4−) were used as a proxy for primary productivity and food availability (Huot et al., 2007).
All environmental variables used for model construction were obtained from the Copernicus marine environment monitoring service (http://marine.copernicus.eu). Specifically, for each year, mean monthly layers of all environmental predictors were extracted with a spatial resolution of 1/24° × 1/24° (about 4 × 4 km) at two different depths: surface and 100 m of depth (Escudier et al., 2020; Cossarini et al., 2021). The rationale behind the choice of using two different depths is to evaluate the possible effect of the climatic variables on the larval as well as on the juvenile–adult phase of the royal sea cucumber. The values obtained at sea surface level have been averaged over the study area (GSA16) for each year between June and September (summer season), in order to investigate the possible effect of climatic conditions during the early life larvae stages, whereas the values obtained at 100 m depth (i.e., the bathymetry where the bulk of the species inhabits, Figure 2) have firstly been spatially clipped using the extent of a polygon representing the continental shelf within the study area (GSA16) and then averaged by year.
To investigate potential time lag effects of the environmental factors on the P. regalis larvae, the mean values obtained at sea surface level have been lagged of 3 years prior to the year of the survey in order to consider the temporal window running from the spawning until reaching the sizes of the specimen collected in this study. This choice was supported by the most representative size class sampled by the survey (approximately 19 cm total, Supplementary Figure 1) and, in the absence of growth studies of P. regalis, the corresponding mean length age proposed by Glockner-Fagetti et al. (2016) and Ramírez-González et al. (2020) for the similar sea cucumber Isostichopus fuscus (Ludwig, 1875) in the Pacific Ocean. Instead, the time lag relationship between the abundance of P. regalis and the 100-m environmental variables as well as the fishing effort (Fe) were lagged 1 year prior to the year of the survey.
Since fishery pressure has been shown to have negative effects on the populations of sea cucumbers in the Mediterranean and NE Atlantic waters (i.e., decrease in abundance, reduction of genetic diversity, loss of the biggest individuals, increase of diseases, and even “local extinction” in some sites; González-Wangüemert et al., 2016), data on the Fe of bottom trawling operating in the GSA16 were used as an independent variable affecting P. regalis density. The trawl fleet segment mainly operating on continental shelf bottoms of the study area is composed of vessels with a length overall less of 24 m (LOA < 24) (Scannella et al., 2020; Di Maio et al., 2022). Differently, the large fishing fleet is engaged in deep-sea fishing far away from the coast on sea bottoms reaching 700–800 m (Milisenda et al., 2017; Russo et al., 2019). Annual Fe was retrieved by Fisheries Dependent Information (FDI, https://stecf.jrc.ec.europa.eu/dd/fdi) database provided by the EU Member States (DCF, Data Collection Framework context) and expressed as the total number of fishing days per gross tonnage (DayAtSea*GT) of trawl fishing vessels with LOA < 24 m operating in the GSA16.
The variable acronyms, unit measurement, and some descriptive statistics are shown in Table 1.
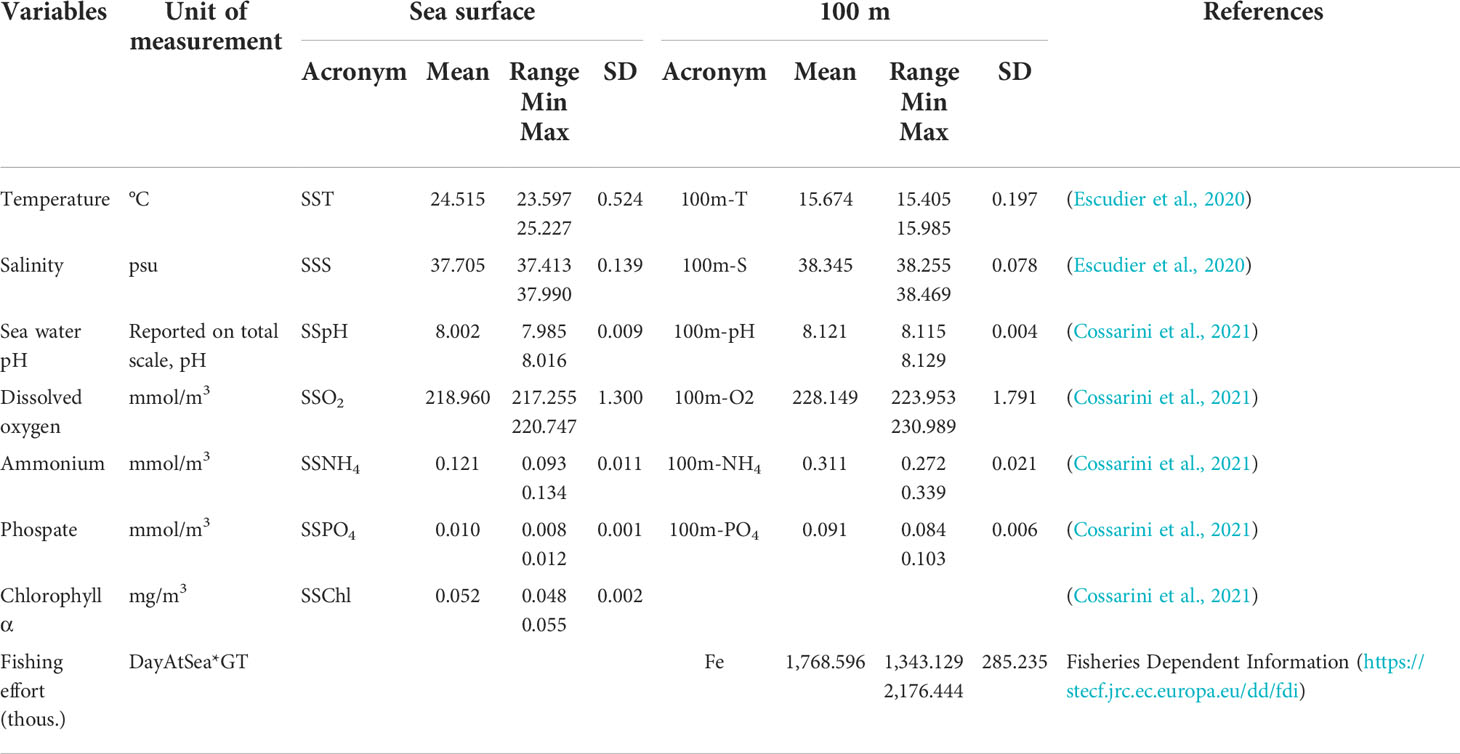
Table 1 Descriptive statistics [mean, range, and standard deviation (SD)] for selected predictor variables.
Temporal trend and spatial analysis
Segmented regression analysis was applied to log-transformed DI to objectively define the time point in which the trend significantly changes during the time frame considered in this study, using the R “segmented” package (Muggeo, 2008).
The DI of each haul was geo-referenced to spatially identify higher-density areas (spots) of the species and qualitatively assess the variation of the spatial pattern during different time periods. The distribution maps were obtained by applying the inverse distance-weighted (IDW) interpolation algorithm on the logarithm of the DI plus 1 using Quantum GIS software (QGIS Development Team, 2020).
To evaluate the possible deepening of the royal sea cucumber distribution in the study area in response to the change in oceanographical variables, the center of gravity (CoG) of the species (Stefanescu et al., 1992; Cartes et al., 2011) was computed, for the period 2008–2021, by the formula:
where DIi is the standardized density of the species at depth i and zi is the mean depth of the sampling station. Correlation among CoG and years were assessed by computing the Spearman’s rho (ρ) statistic and tested via t-test using a p-value of 0.05 to establish statistical significance.
As an exploratory analysis to evaluate the degree of association among environmental and fishery variables was conducted, the most related variables were highlighted using a matrix of Spearman’s rho (ρ) rank correlation coefficients for all possible pair combinations. This was graphically displayed by means of a correlogram plot using the “corrplot” R package (Wei and Simko, 2021). Classical linear regressions were also used to analyze linear trends of explanatory variables.
Modeling
A generalized additive model (GAM) with a Gaussian distribution with the canonical (identity) link function was used to investigate the shape and the strength of the relationship of royal sea cucumber abundance with the explanatory variables considered (environmental and fishing). GAMs are flexible non-linear regression models that allow one response variable being fitted by several predictors additively (Wood, 2006; Hastie and Tibshirani, 2017). The use of smoothing functions for the regressors gives GAMs greater flexibility over linear (or other parametric) types of models (Wood, 2006). Given the sample size of the observations, to control the degree of smoothing and avoid overfitting the data, a penalty term was added in the regression, setting the gamma argument equal to 1.4 (Kim and Gu, 2004). GAMs were fitted using the R “mgcv” package (Wood, 2001).
Model construction
Because the predictor variables are measured at different scales, they were firstly re-scaled by applying the z-score standardization by subtracting the mean of each variable from the raw data and then dividing it by the standard deviation of the original variable. In addition, the sea royal cucumber DI were log-transformed.
All variables were tested for collinearity using the Variance Inflation Factor (VIF). Variables showing a VIF value that exceeds 5, indicating a problematic amount of collinearity (James et al., 2013), were not considered for modeling, since the information that these variables provide about the response is redundant in the presence of the other variables with lower VIF (James et al., 2013). In particular, a VIF analysis has been performed in order to assess multicollinearity among all 100 m of depth explanatory variables (T, S, pH, O2, NH4+, and PO4−), Fe, and all sea surface variables (SST, SSS, SSpH, SSO2, SSNH4, SSPO4, and SSChl), 3 years lagged.
Model selection
Modeling selection was carried out in the framework of an information-theoretic approach (Burnham and Anderson, 2002). More specifically, starting from the full model that included all explanatory variables, the most parsimonious model has been selected based on the lowest Akaike’s Information criterion (AIC) (Grueber et al., 2011; Aho et al., 2014), corrected for small sample size (AICc; Burnham and Anderson, 2002). Candidate models were ranked using the difference in AICc (ΔAICc) calculated as the difference in AIC between a model i and the first-ranked model (Buckland et al., 1997; Burnham and Anderson, 2002) and by calculating the AICc weights (total amount of predictive power provided by the models). Competing models of the best supported model were selected when having their AICc within 2 of the minimum (Burnham and Anderson, 2002). Also, the relative importance of the predictor variables was estimated by summing the AICc weights of each model where the variable appears (Symonds and Moussalli, 2011). The variable sum of weights (SW) varies from 0 to 1, and high values indicate strong importance for a given predictor. Model performance was measured as the proportion of variation explained by the estimated regression using adjusted R2.
Graph plotting and statistical analysis were performed using the R v.4.1.2 software (R Core Team, 2021).
Results
P. regalis has been collected in a wide bathymetric range, from 20 to 500 m, although the largest abundances were recorded in the infralittoral and circalittoral zones, within the bathymetry of 200 m (Figure 2).
The mean DI over the shelf (10–200 m) showed a significant linear negative (r2 = 0.31, p = 0.03) trend over time with the lowest value of 6 N/km2 recorded in 2020 and 2021, while the highest value of 138.5 N/km2 was recorded in 2015. The trend of the log-transformed DI is shown in Figure 3. The segmented regression analysis on log-transformed DI data estimated a break point in the year 2017, separating clearly the temporal DI trend in two parts: a steady-state phase of the species between 2008 and 2017, in which the density oscillated around a mean value of about 4.3 N/km2 (log DI) and a decline phase reaching the lowest values of the entire time series in 2020 and 2021 (Figure 3).
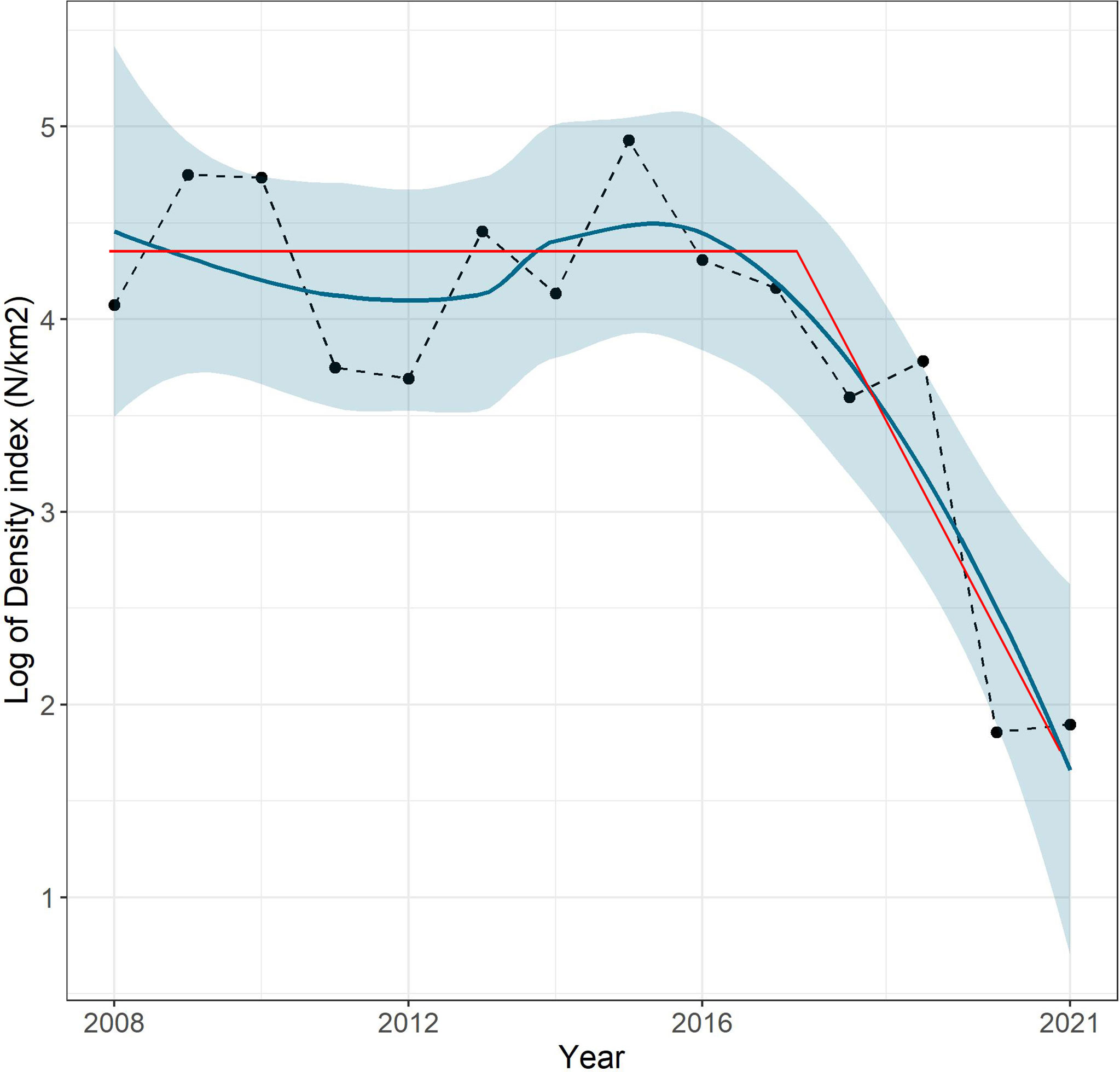
Figure 3 Trend of the density index logarithm (N/km2) for P. regalis in the GSA16 during time series 2008–2021. The blue line represents the smooth curve obtained using the LOESS interpolation method; the blue area indicates the 95% confidence interval. The red lines visualize the segmented regression model.
Two maps of the spatial distribution of the DIs in the steady-state phase (Figure 4A) and the declining phase have been produced (Figure 4B). In particular, Figure 4A shows the DIs of the year 2015 characterized by the highest abundance in the steady-state phase (2008–2017) while Figure 4B shows those of the last year (2021) of the declining phase (2018–2021). Our results show that P. regalis is prevalently distributed on the continental shelf (Figures 2 and 4A, B) of the study area. The species became more abundant in the northwestern Strait of Sicily and, in particular, along the southwestern Adventure Bank, showing density indices from 900 to 3,000 N/km2. Catches at depths over 200 m were occasional, except for two areas located in the northwestern sectors of the Adventure Bank where, however, low abundances (1–300 N/Km2) have been recorded.
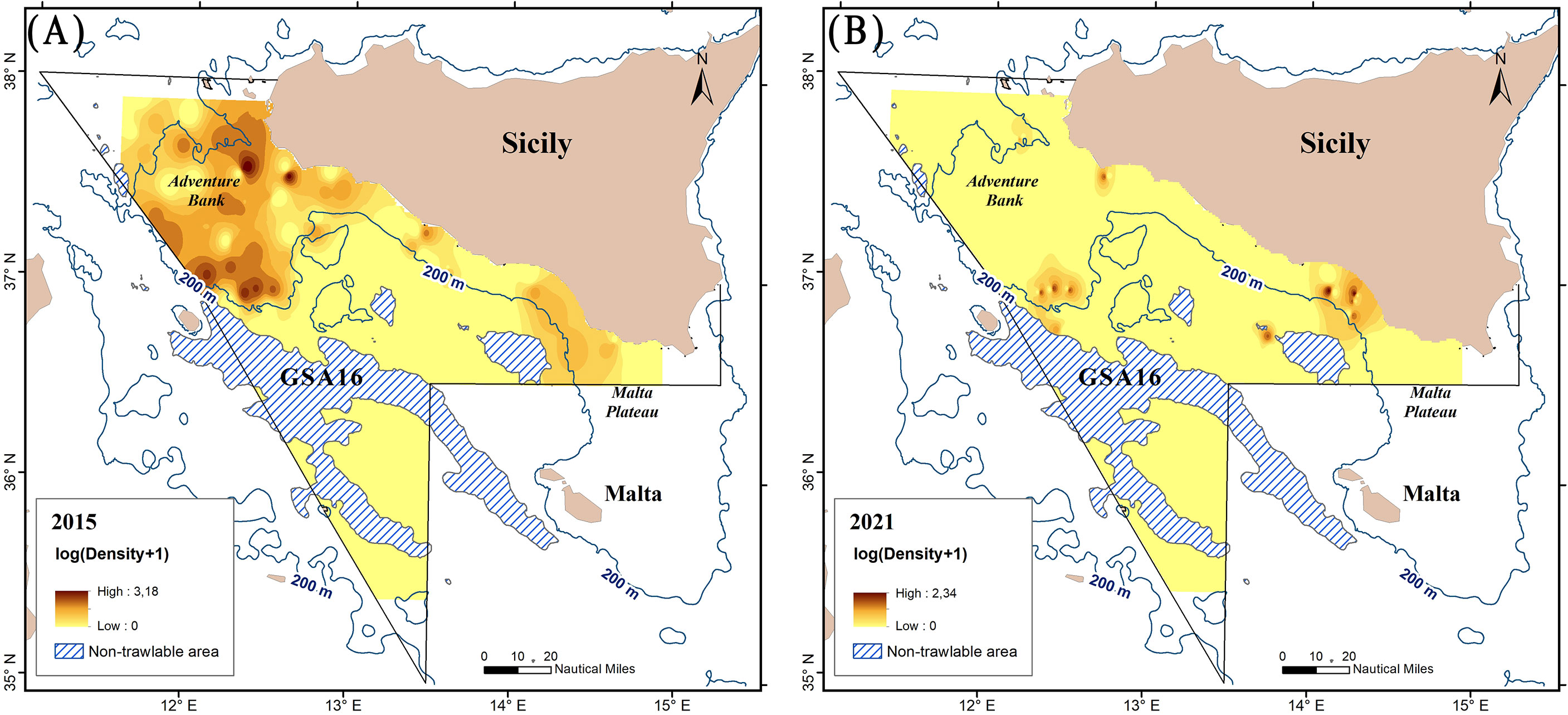
Figure 4 Spatial distribution, in terms of the logarithm of density index [(N+1)/km2], of P. regalis in the study area (GSA 16) (A) of the year (2015) with the highest density index in the steady-state phase (2008–2017) and (B) the last year (2021) of the declining phase (2018–2021) according to the temporal trend of the abundance of the species. The dashed areas depict water depths of more than 800 m not investigated during the MEDITS survey.
A marked difference in terms of the spatial pattern between the two phases is observed (Figures 4A, B). In particular, it is quite evident that the greatest loss of abundance occurred in correspondence of the Adventure Bank area, where the density spots are reduced substantially, and in some cases, as in the central part of the Bank, even disappear. It appears that there is also a drastic abundance reduction in the Malta Plateau area. According to the map in Figure 4B, to date, two little hot spots of the species seem to remain, one in the southernmost sector of the Adventure Bank and the other one in the eastern part of the GSA16.
During the entire time series considered (2008–2021), the shift of the CoG’s royal sea cucumber towards deeper bathymetry starting from 2011 seems to occur. However, this trend was not significant according to the Spearmen correlation (ρ = −0.41) (Figure 5).
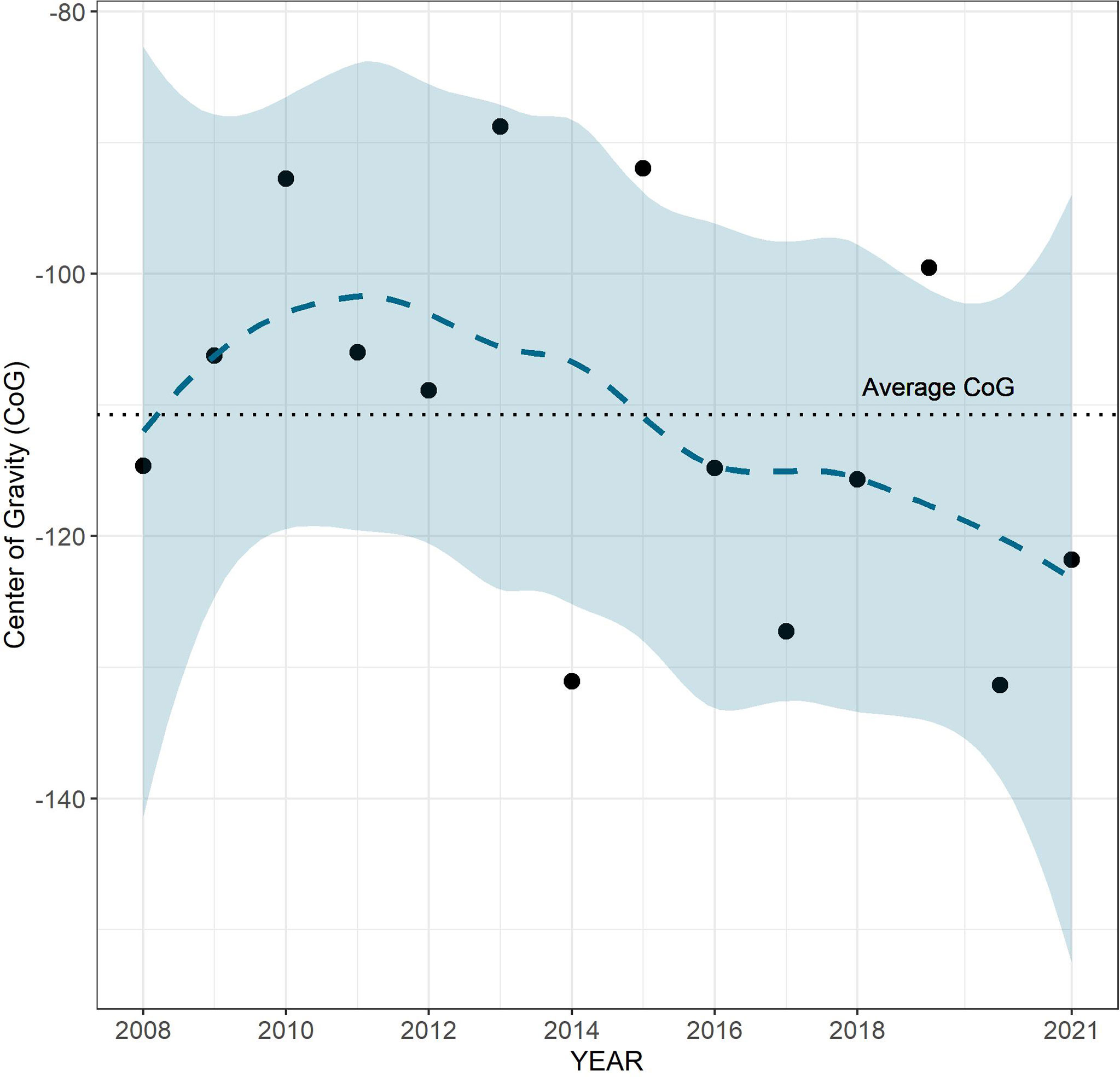
Figure 5 Trend of optimal average distribution (Center of Gravity, CoG) during the 2008–2021 period. The blue dotted line represents the smooth curve obtained using the LOESS interpolation method, the blue band indicates the 95% confidence interval.
During the period 2008–2020, climate variables varied considerably in the GSA16 (Supplementary Figures 2, 3). The sea surface salinity (SSS) in summer increased significantly (p = 0.03) at a rate of 0.014 psu year−1, as shown by linear regression (Supplementary Figure 2), while on the contrary, the mean sea surface pH (SSpH) decreased significantly (p = 0.04) at a rate of −0.0017 year−1, leading to a rise in its acidity. The other six sea surface variables did not show statistically significant trends (p > 0.05) although SSNH4, SSPO4, and SST moderately increased over the years, while SSChl and SSO2 decreased. The mean yearly temperature and salinity at 100 m depth (100m-T) in the continental shelf of the study area progressively increased in a statistically significant manner, at a rate of 0.037°C (p = 0.012) and 0.016 psu year−1 (p < 0.001), respectively, while 100m-pH decreased (−0.00086 units of pH year−1), leading to progressive sea acidification also in deeper layers (p < 0.001) (Supplementary Figure 3). Conversely, 100m-NH4, 100m-PO4, and 100m-O2 did not show significant trends (p > 0.05) although the nutrients seemed slightly to decrease while the oxygen moderately increased. Finally, the annual average of Fe showed a significant decreasing trend (p = 0.007) at a rate of −58,000 DayAtSea*GT year−1.
The results of Spearman’s correlation among P. regalis density index (expressed as logarithmic) and the normalized predictor variables are shown in Supplementary Figure 4. The correlogram revealed that among all environmental factors analyzed, only two variables showed a significant relationship (p < 0.05) to species abundance. In particular, 100m-NH4 and SSpH seemed to act directly on the population dynamics of P. regalis having found a positive correlation equal to 0.76 and 0.60, respectively.
The best GAM model retained the 3-year lagged pH and NH4+ at 100 m as shown in Table 2, while the relative importance of predictor variables for the species is shown in Table 3. The overall model fit was good with 82.8% of the total deviance explained. In particular, the relationship with NH4+ at 100 m was almost linear, with a negative effect on the abundance of P. regalis until a value at ca. 0.315, above which the effect became positive. The effect of the 3-year lagged pH was non-linear with a local positive peak at ca 8.003, and negatively affecting the abundance of P. regalis at values lower than 8.00 and higher than ca. 8.012 as shown in Figure 6.

Table 3 Relative importance of predictor variables (calculated as the sum AIC weights from models that contain that variable within the 95% confidence interval) for P. regalis.
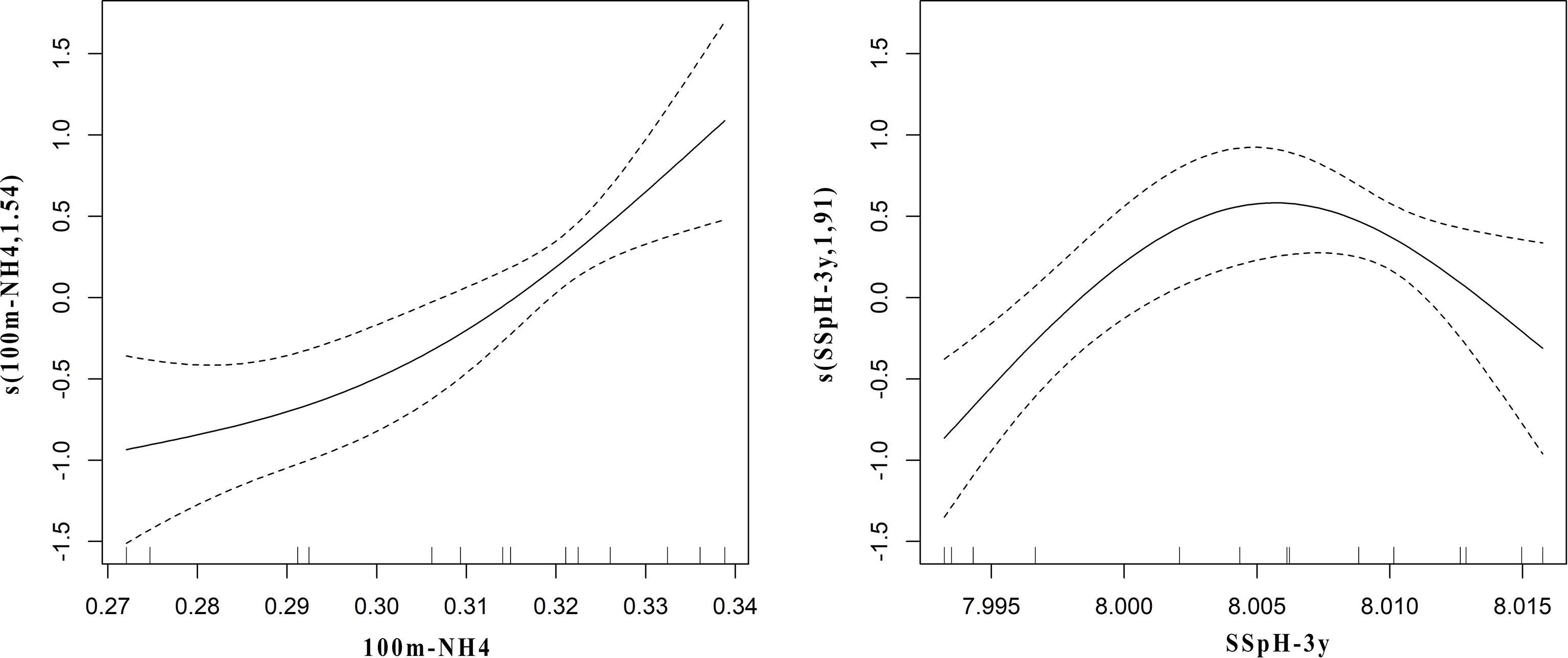
Figure 6 Partial GAM plots for the GAM model for P. regalis population in the Strait of Sicily. Each plot represents the estimated smoothing curves (solid line) obtained for 100m-NH4 (left panel) and SSph-3y (right panel). The environmental variables are represented on the x-axis, and the y-axis shows the contribution of the smoother to the fitted values. Untransformed values are provided on the x-axis for easier interpretation. Dotted lines indicate the 95% confidence intervals.
Discussion
This study suggests the potential negative effect of climate change on the abundance of P. regalis, a species of growing interest as a fishable resource in the central Mediterranean Sea. In addition, the positive influence of nutrient availability on this species was highlighted. Despite the fact that some information on the abundance and demography of P. regalis in the Mediterranean is available, according to our knowledge, nothing is known on the effect of environmental factors on this species in the wild and few information exists in experimental conditions (Galimany et al., 2018). On the other hand, some data on physiological responses to environmental variability of other sea cucumber species deriving from aquaculture are available (Asha and Muthiah, 2005; Dong et al., 2006; Yuan et al., 2009). Our results showed that the species mainly inhabits the soft bottoms (25–200 m) of the Adventure Bank and Malta Plateau, while also a rarefaction of its spatial distribution and a slight shift towards deeper depths (about −20 m) have been evidenced since 2018 and 2011, respectively. Previous research has shown that this species is found on a wide range of depths (5–800 m; Tortonese, 1965) even if more abundant between 100- and 300-m depths (Massutí and Renones, 2005; Ramón et al., 2010). Ramón et al. (2010), studying the population inhabiting the continental shelf and slope of Balearic Islands, found a strongly aggregated spatial distribution of the royal cucumber population, with the abundance being the highest between 100 and 300 m. Abad et al. (2007), describing the composition and abundance of the megabenthic fauna caught by the commercial trawl fleet in the Alboran Sea, reported the high abundance (4.99 ± 1.19 kg h−1) of P. regalis in the small seamount known as Chella Bank (310–360 m).
Concerning the role of environmental factors on the life cycle of P. regalis, little information is available. Galimany et al. (2018), comparing vital performances of specimens reared at seawater temperature higher than the preferred temperature (13°C) for aquaculture purposes, showed that 90% of the sea cucumbers exposed to 28°C died before 48 h, and at 23°C, they had 50% survivorship after 2 weeks of exposure.
Conversely, within the temperature range observed in the Strait of Sicily, namely, 15.4–25.2°C, Galimany et al. (2018) did not find significant effects on the species’ density. Our results exclude a direct effect of temperature on P. regalis population and agreed with Galimany et al (2018) findings on adult specimens. On the other hand, considering the knowledge about life cycles of other sea cucumbers (Al Rashdi et al., 2012; Qiu et al., 2015; Rakaj et al., 2018; Neelmani and D.T. Vanghela, 2018), the decreasing trend of the royal sea cucumber density in the investigated area might be influenced by the low tolerance of its larvae to the variation of sea pH during their pelagic phase.
This is in agreement with González-Durán et al. (2021), who reported that the most sensitive life stage of temperate and tropical holothurians to the adverse effects of temperature and pH was the larval dispersal and settlement phase. Conversely, Yuan et al. (2015) showed that a decrease in pH from 8.1 to 7.4 had relatively small effects on Apostichopus japonicus (Selenka, 1867) early life history compared to other echinoderms. On the other hand, Asha and Muthiah (2005), maintaining auricularia larvae of Holothuria (Theelothuria) spinifera (Théel, 1886) for 12 days at pH values ranging from 6.5 to 9.0, temperature between 28 and 32°C, and salinity at 35 psu, showed that, at pH 7.8, the larvae developed faster and grew better, showing better survival rates. All these contributions suggest that both larval development and survival rates are highly sensitive to pH than temperature and salinity. In particular, extreme alkaline values (pH 9.0) generated malformation and disintegration of individuals, while the reduction of 0.5 pH units from the optimum (pH 8.0) reduced survival rates by 49% (González-Durán et al., 2021).
Furthermore, exposing adult A. japonicus to different levels of pH, Yuan et al. (2016) highlighted that, the current increasing trend of acidification of the oceans may negatively influence the grazing capability and growth, thereby influencing its ecological functioning as an “ecosystem engineer” and potentially harming its culture output. More recently, Gullian and Terrats (2017) found that Isostichopus badionotus (Selenka, 1867) is a eurythermal organism capable of tolerating a wide range of temperatures from 16 to 34°C at pH 7.70. Environmental conditions for optimal physiological performance were found to be pH 8.17 and a temperature range of 24–28°C. The interaction between the two stressors (temperature and pH) activated antioxidant enzymes at various critical thermal limits to protect intracellular redox homeostasis.
Despite the high variability in responses observed, the effect exerted by the alteration of pH and temperature on the juveniles survival of sea cucumbers is less than those observed for the larvae. However, variations of pH and temperature from the optimal values can affect population abundance and density (Widicombe and Spicer, 2008; Brierley and Kingsford, 2009; Doney et al., 2009), impairing the success of the reproduction. Since holothurians are gonochoric and sedentary, their reproductive success depends largely on their gregarious behavior and their chemical communication, and spawning synchrony. Therefore, a reduction in density can affect fertilization, causing a further decrease in population size (Hutchings, 2014; Kuparinen and Hutchings, 2014; González-Durán et al., 2018).
However, other factors such as biotic factors could affect variation in P. regalis abundance. Although the available information on this species is scarce, it is well known that fishes, crustaceans, gastropods, and mainly seastars feed on holothurians, as young and adult (Francour, 1997), and they could have a relevant role as predators in regulating abundance of sea royal cucumbers. Therefore, it cannot be excluded that the effect of predation could contribute to the decreasing of the species in the Strait of Sicily.
In recent years, the harvesting of sea cucumbers in the Mediterranean Sea has been showing signs of systematic overexploitation being negative impacts observed such as decreased density, abundance, and genetic diversity; loss of larger individuals; increased incidence of some diseases; and even “local extinction” in some places (González-Wangüemert et al., 2018). Maggi and González-Wangüemert (2015), studying P. regalis Mediterranean populations from the Spanish coast, suggested the negative effects of overexploitation on populations living off the Catalan coast. Indeed, the population off Catalonia exhibited the highest occurrence of small individuals compared to other Spanish populations with a prevalence of mean or large-size specimens. Moreover, genetic data showed that Catalonia populations had the lowest number of haplotypes and haplotype diversity. According to the authors, the absence of shared haplotypes in Catalonia, which were present in other Spanish populations, may be due to the loss of haplotypes caused by fishing pressure.
Because of the high sensitivity to changes in environmental conditions (such as temperature and pH), the need to include the effects of pH and temperature into population models of sea cucumber has been suggested to inform the fishery management (González-Durán et al., 2021). Given the overall overexploitation of the Mediterranean resources (Colloca et al., 2017; Fiorentino and Vitale, 2021) and the growing interest of P. regalis as fishable species, it is necessary to improve the knowledge about its biological aspects (growth rate, longevity, reproductive aspects, thermal, and pH tolerance), abundance, and survival once hauled on deck.
In recent years, an uncontrolled fishing of sea cucumbers has been reported in Sardinia and Puglia waters (Italy) (Meloni and Esposito, 2018). Therefore, since 2018, the Italian authorities have prohibited fishing (target and/or accessory by catch), holding on board, transship, and landing of specimens belonging to the Holothuroidea class (IMAFFP, 2018).
Considering overfishing of some P. regalis stock in Mediterranean Sea, Ramón et al. (2022) have suggested some management measures to reach a more sustainable exploitation of the resources, namely, establishment of a fishing season and closure during spawning. Due to the high sensitivity of abundance dynamics of the species to the global change, when the impact of the fisheries seems to be weak, our results suggest that a precautionary approach in exploiting holothurians should be pursued.
The decreasing trend in abundance of P. regalis calls for an urgent conservation measure because its population reduction can compromise the functioning of the ecosystems with loss or reduction of the ecosystem services, such as enhanced nutrient cycling and local productivity in sediments, and trigger cascading effects on ecosystems that might diminish their ability to withstand other broad-scale stressors (Purcell et al., 2016; Ramón et al., 2019).
Data availability statement
The raw data supporting the conclusions of this article will be made available by the authors, without undue reservation.
Ethics statement
Ethical review and approval was not required for the animal study because no tests or experiments were performed on vertebrate animals.
Author contributions
DS, FFi, DM, VL, and MM: Conceptualization and design of the study. DS, VL, FQ, FFa, and GG: statistical analysis and data curation. AT, GS, VG, MLG, FD, and DM: data collection and validation. DS, FFi, and DM: writing—original draft. DS, GB, MDL, FFi, GG, MM, VL, and SV: writing—review and editing. GB and GG secured funding. All authors contributed to the article and approved the submitted version.
Funding
This work was supported by European Data Collection Framework (DCF)–Transversal Variables and MEDITS survey modules funded by the European Union and the Italian Ministry for Agricultural, Food and Forestry Policies.
Acknowledgments
This work is conducted thanks to the European Data Collection Framework (DCF)—Medits survey module—funded by the European Union and the Italian Ministry for Agricultural, Food and Forestry Policies. We are grateful to all staff of CNR-IRBIM of Mazara del Vallo, who collected and processed samples from the trawl surveys.
Conflict of interest
The authors declare that the research was conducted in the absence of any commercial or financial relationships that could be construed as a potential conflict of interest.
Publisher’s note
All claims expressed in this article are solely those of the authors and do not necessarily represent those of their affiliated organizations, or those of the publisher, the editors and the reviewers. Any product that may be evaluated in this article, or claim that may be made by its manufacturer, is not guaranteed or endorsed by the publisher.
Supplementary material
The Supplementary Material for this article can be found online at: https://www.frontiersin.org/articles/10.3389/fmars.2022.934556/full#supplementary-material
References
Abad E., Preciado I., Serrano A., Baro J. (2007). Demersal and epibenthic assemblages of trawlable grounds in the northern alboran Sea (western Mediterranean). Sci. Mar. 71, 513–524. doi: 10.3989/scimar.2007.71n3513
Aho K., Derryberry D., Peterson T. (2014). Model selection for ecologists: The worldviews of AIC and BIC. Ecology 95, 631–636. doi: 10.1890/13-1452.1
Al Rashdi K. M., Eeckhaut I., Claereboudt M. R. (2012). A manual on hatchery of sea cucumber holothuria scabra in the sultanate of Oman (Aquaculture Centre, Muscat: Ministry of Agriculture and Fisheries Wealth). Sultanate of Oman.
Antoniadou C., Vafidis D. (2011). Population structure of the traditionally exploited holothurian Holothuria tubulosa in the south Aegean Sea. Cah. Biol. Mar. 52, 171–175.
Asha P. S., Muthiah P. (2005). Effects of temperature, salinity and pH on larval growth, survival and development of the sea cucumber Holothuria spinifera theel. Aquaculture 250, 823–829. doi: 10.1016/j.aquaculture.2005.04.075
Aydin M. (2008). The commercial sea cucumber fishery in Turkey. SPC Beche-de-mer Infor Bull. 28, 40–41.
Aydın M., Erkan S. (2015). Identification and some biological characteristics of commercial sea cucumber in the Turkey coast waters. Int. J. Fish Aquat. 3, 260–265.
Battaglene S. C., Bell J. D. (1999). Potential of the tropical Indo-Pacific sea cucumber, Holothuria scabra, for stock enhancement. Proceedings First International Symposium on Stock Enhancement and Sea Ranching, 8–11 September 1997, (Bergen, Norway: Blackwell, Oxford), 478–90.
Béranger K., Mortier L., Gasparini G. P., Gervasio L., Astraldi M., Crépon M. (2004). The dynamics of the Sicily strait: a comprehensive study from observations and models. Deep-Sea Res. II: Top. Stud. Oceanogr. 51, 411–440. doi: 10.1016/j.dsr2.2003.08.004
Bertrand J. A., Gil de Sola L., Papaconstantinou C., Relini G., Souplet A. (2002). The general specifications of the MEDITS surveys. Sci. Mar. 66, 9–17. doi: 10.3989/scimar.2002.66s29
Bethoux J. P., Gentili B. (1999). Functioning of the Mediterranean Sea: past and present changes related to freshwater input and climate changes. J. Mar. Syst. 20, 33–47. doi: 10.1016/S0924-7963(98)00069-4
Bonanno A., Placenti F., Basilone G., Mifsud R., Genovese S., Patti B., et al (2014). Variability of water mass properties in the Strait of Sicily in summer period of 1998-2013. Ocean Sci. 10, 759–70. doi: 10.5194/os-10-759-2014
Brander K. (2010). Impacts of climate change on fisheries. J. Mar. Syst. 79, 389–402. doi: 10.1016/j.jmarsys.2008.12.015
Brierley A. S., Kingsford M. J. (2009). Impacts of climate change on marine organisms and ecosystems. Curr. Biol. 19, R602–R614. doi: 10.1016/j.cub.2009.05.046
Bruckner A. W. (2006). Proceedings of the CITES workshop on the conservation of sea cucumbers in the families Holothuriidae and Stichopodidae. NOAATechnical Memorandum NMFS-OPR (Silver Spring, MD) 34, 244.
Bruckner A. W., Johnson K. A., Field J. D. (2003). Conservation strategies for sea cucumbers. can a CITES appendix II listing promote sustainable international trade? SPC Beche-de-mer Infor Bull. 18, 24–33.
Buckland S. T., Burnham K. P., Augustin N. H. (1997). Model selection: An integral part of inference. Biometrics 53, 603–618. doi: 10.2307/2533961
Burnham K. P., Anderson D. R. (2002). Model selection and multimodel inference: A practical information-theoretic approach, 2nd edn (NewYork: Springer).
Calanchi N., Colantoni P., Rossi P. L., Saitta M., Serri G. (1989). The strait of Sicily continental rift systems: physiography and petrochemistry of the submarine volcanic centres. Mar. Geol. 87, 55–83. doi: 10.1016/0025-3227(89)90145-X
Cartes J. E., Maynou F., Abelló P., Emelianov M., de Sola L. G., Solé M. (2011). Long-term changes in the abundance and deepening of the deep-sea shrimp Aristaeomorpha foliacea in the Balearic basin: relationships with hydrographic changes at the levantine intermediate water. J. Marine. Syst. 88, 516–525. doi: 10.1016/j.jmarsys.2011.07.001
Colantoni P., Del Monte M., EFK Z. (1975). Il banco Graham: un vulcano recente del canale di sicilia. Giornale di Geologia. 40, 141–162. Bologna.
Colantoni P., Gennesseaux M., Vanney J. R., Ulzega A., Melegari G., Trombetta A. (1992). Processi dinamici del canyon sottomarino di gioia tauro (Mare tirreno). Giornale di Geologia. 54, 199–213.
Colloca F., Scarcella G., Libralato S. (2017). Recent trends and impacts of fisheries exploitation on Mediterranean stocks and ecosystems. Front. Mar. Sci. 4. doi: 10.3389/fmars.2017.00244
Conand C., Polidoro B., Mercier A., Gamboa R., Hamel J. F., Purcell S. (2014). The IUCN red list assessment of aspidochirotid sea cucumbers and its implications. SPC Beche-de-mer Infor. Bull. 34, 3–7.
Cossarini G., Feudale L., Teruzzi A., Bolzon G., Coidessa G., Solidoro C., et al. (2021). High-resolution reanalysis of the Mediterranean Sea biogeochemistry, (1999–2019). Front. Mar. Sci. 1537. doi: 10.3389/fmars.2021.741486
Costa V., Mazzola A., Vizzini S. (2014). Holothuria tubulosa gmelin 1791 (Holothuroidea, Echinodermata) enhances organic matter recycling in Posidonia oceanica meadows. J. Exp. Mar. Biol. Ecol. 461, 226–232. doi: 10.1016/j.jembe.2014.08.008
Crutzen P. J., Stoermer E. F. (2000). “The ‘Anthropocene'’’ in The Future of Nature: Documents of Global Change, ed. Robin L. S., Warde SörlinP. (New Haven: Yale University Press). 2013, 479–90. doi: 10.12987/9780300188479-041
Di Donato V., Sgarrella F., Sprovieri R., Di Stefano E., Martín-Fernández J. A., Incarbona A. (2022). High-frequency modification of the central Mediterranean seafloor environment over the last 74 ka. Palaeogeogr. Palaeocl. 593, 110924. doi: 10.1016/j.palaeo.2022.110924
Diffenbaugh N. S., Pal J. S., Giorgi F., Gao X. (2007). Heat stress intensification in the Mediterranean climate change hotspot. Geophys Res. Lett. 34, L11706. doi: 10.1029/2007GL030000
Di Lorenzo M., Sinerchia M., Colloca F. (2018). The north sector of the strait of Sicily: a priority area for conservation in the Mediterranean Sea. Hydrobiologia 821, 235–253. doi: 10.1007/s10750-017-3389-7
Di Maio F., Geraci M. L., Scannella D., Russo T., Fiorentino F. (2022). Evaluation of the economic performance of coastal trawling off the southern coast of Sicily (Central Mediterranean Sea). Sustainability 14, 4743. doi: 10.3390/su14084743
Doney S., Fabry V., Feely R., Kleypas J. (2009). Ocean acidification: the other CO2 problem. Ann. Rev. Mar. Sci. 1, 169–192. doi: 10.1146/annurev.marine.010908.163834
Dong Y., Dong S., Tian X., Wang F., Zhang M. (2006). Effects of diel temperature fluctuations on growth, oxygen consumption and proximate body composition in the sea cucumber Apostichopus japonicas selenka. Aquaculture 255, 514–521. doi: 10.1016/j.aquaculture.2005.12.013
D’Ortenzio F., Ribera d’Alcalà M. (2009). On the trophic regimes of the Mediterranean Sea: a satellite analysis. Biogeosciences 6, 139–148. doi: 10.5194/bg-6-139-2009
Eriksson H., Clarke S. (2015). Chinese Market responses to overexploitation of sharks and sea cucumbers. Biol. Conserv. 184, 163–173. doi: 10.1016/j.biocon.2015.01.018
Escudier R., Clementi E., Omar M., Cipollone A., Pistoia J., Aydogdu A., et al. (2020). Mediterranean Sea Physical reanalysis (CMEMS MED-currents) (version 1)[Data set]. Copernicus Monit. Environ. Mar. Service (CMEMS). doi: 10.25423/CMCC/MEDSEA_MULTIYEAR_PHY_006_004_E3R1
Fiorentini L., Dremière P. Y., Leonori I., Sala A., Palumbo V. (1999). Efficiency of the bottom trawl used for the Mediterranean international trawl survey (MEDITS). Aquat Living Resour. 12, 187–205. doi: 10.1016/S0990-7440(00)88470-3
Fiorentino F., Vitale S. (2021). How can we reduce the overexploitation of the Mediterranean resources? Front. Mar. Sci 8, 74633. doi: 10.3389/fmars.2021.674633
Francour P. (1997). Predation on holothurians: a literature review. Invertebr. Biol. 116, 52–60. doi: 10.2307/3226924
Galimany E., Baeta M., Ramón M. (2018). Immune response of the sea cucumber Parastichopus regalis to different temperatures: Implications for aquaculture purposes. Aquaculture 497, 357–363. doi: 10.1016/j.aquaculture.2018.08.005
Gao K., Beardall J., Hä der D. P., Hall – Spencer J. M., Gao G., Hutchins D. A. (2019). Effects of ocean acidification on marine photosynthetic organisms under the current influences of warming, UV radiationm and deoxigenation. Front. Mar. Sci. 6. doi: 10.3389/fmars.2019.00322
Glockner-Fagetti A., Calderon-Aguilera L. E., Herrero-Pérezrul M. D. (2016). Density decrease in an exploited population of brown sea cucumber Isostichopus fuscus in a biosphere reserve from the Baja California peninsula, Mexico. Ocean Coast. Manage. 121, 49–59. doi: 10.1016/j.ocecoaman.2015.12.009
González-Durán E., Hernández-Flores Á., Headley M. D., Canul J. D. (2021). On the effects of temperature and pH on tropical and temperate holothurians. Conserv. Physiol. 9, coab092. doi: 10.1093/conphys/coab092
González-Durán E., Hernández-Flores A., Seijo J. C., Cuevas-Jiménez A., Moreno-Enriquez A. (2018). Bioeconomics of the allee effect in fisheries targeting sedentary resources. ICES J. Mar. Sci. 75, 1362–1373. doi: 10.1093/icesjms/fsy018
González-Wangüemert M., Aydin M., Conand C. (2014). Assessment of sea cucumber populations from the Aegean Sea (Turkey): First insights to sustainable management of new fisheries. Ocean Coast. Manage. 92, 87–94. doi: 10.1016/j.ocecoaman.2014.02.014
González-Wangüemert M., Domínguez-Godino J. A., Cánovas F. (2018). The fast development of sea cucumber fisheries in the Mediterranean and NE Atlantic waters: from a new marine resource to its over-exploitation. Ocean Coast. Manage 151, 165–177. doi: 10.1016/j.ocecoaman.2017.10.002
Gonzalez-Wangüemert M., Valente S., Aydin M. (2015). Effects of fishery protection on biometry and genetic structure of two target sea cucumber species from the Mediterranean Sea. Hydrobiologia 743, 65–74. doi: 10.1007/s10750-014-2006-2
González-Wangüemert M., Valente S., Henriques F., Domínguez-Godino J. A., Serrão E. A. (2016). Setting preliminary biometric baselines for new target sea cucumbers species of the NE Atlantic and Mediterranean fisheries. Fish. Res. 179, 57–66. doi: 10.1016/j.fishres.2016.02.008
Grueber C. E., Nakagawa S., Laws R. J., Jamieson I. G. (2011). Multimodel inference in ecology and evolution: challenges and solutions. J. Evol. Biol. 24, 699–711. doi: 10.1111/j.1420-9101.2010.02210.x
Gullian M. (2013). Physiological and immunological condition of the sea cucumber Isostichopus badionotus (Selenka, 1867) during dormancy. J. Exp. Mar. Biol. Ecol. 444, 31–37. doi: 10.1016/j.jembe.2013.03.008
Gullian K. M., Terrats P. M. (2017). Effect of pH on temperature controlled degradation of reactive oxygen species, heat shock protein expression, and mucosal immunity in the sea cucumber Isostichopus badionotus. PloS One 12, e0175812. doi: 10.1371/journal.pone.0175812
James G., Witten D., Hastie T., and Tibshirani R. (2013). An introduction to statistical learning (New York: Spinger). 426 p.
Hamel J. F., Mercier A. (2008). “Precautionary management of cucumaria frondosa in Newfoundland and Labrador, Canada,” in Sea Cucumbers. a global review of fisheries and trade. Eds. Toral-Granda V., Lovatelli A., Vasconcellos M. (Rome: FAO Fisheries and Aquaculture Technical Paper), 293–306. No. 516FAO.
Han Q., Keesing J. K., Liu D. (2016). A review of sea cucumber aquaculture, ranching, and stock enhancement in China. Rev. Fish. Sci. Aquac. 24, 326–341. doi: 10.1080/23308249.2016.1193472
Hastie T. J., Tibshirani R. J. (2017). Generalized additive models (New York: Routledge). doi: 10.1201/9780203753781
Huang B., Liu C., Freeman E., Graham G., Smith T., Zhang H. M. (2021). Assessment and intercomparison of NOAA daily optimum interpolation sea surface temperature (DOISST) version 2.1. J. Climate. 34, 7421–7441. doi: 10.1175/JCLI-D-20-0166.1
Huot Y., Babin M., Bruyant F., Grob C., Twardowski M. S., Claustre H. (2007). Does chlorophyll a provide the best index of phytoplankton biomass for primary productivity studies? Biogeosci. Discuss. 4, 707–745.
Hutchings J. (2014). Renaissance of a caveat: Allee effects in marine fish. contribution to the special issue: commemorating 100 years since hjort’s 1914 treatise on fluctuations in the great fisheries of northern Europe. ICES J. Mar. Sci. 71, 2152–2157. doi: 10.1093/icesjms/fst179
IMAFFP (2018). Italian Ministry of Agricultural, Food and Forestry Policies, ministerial decree 156/2018. In: Official Journal of the Italian Republic of February 27th, 2018. Divieto della Pesca delle Oloturie.
James G., Witten D., Hastie T., Tibshirani R. (2013). An introduction to statistical learning. (New York: Springer) 426.
Kim Y. J., Gu C. (2004). Smoothing spline Gaussian regression: more scalable computation via efficient approximation. J. R. Stat. Soc Ser. B. Methodol. 66, 337–356. doi: 10.1046/j.1369-7412.2003.05316.x
Kuparinen A., Hutchings J. (2014). Increased natural mortality at low abundance can generate allee effect in a marine fish. R. Soc Open Sci. 1, 140075. doi: 10.1098/rsos.140075.1–5
Lafuente J. G., Garcia A., Mazzola S., Quintanilla L., Delgado J., Cuttita A., et al. (2002). Hydrographic phenomena influencing early life stages of the Sicilian channel anchovy. Fish. Oceanogr. 11, 31–44. doi: 10.1046/j.1365-2419.2002.00186.x
MacTavish T., Stenton-Dozey J., Vopel K., Savage C. (2012). Deposit-feeding sea cucumbers enhance mineralization and nutrient cycling in organically-enriched coastal sediments. PloSE One 7, e50031. doi: 10.1371/journal.pone.0050031
Maggi C., González-Wangüemert M. (2015). Genetic differentiation among Parastichopus regalis populations from Western Mediterranean Sea: potential effects of its fishery and current connectivity. Med. Mar. Sci. 16, 489–501. doi: 10.12681/mms.1020
Massutí E., Renones O. (2005). Demersal resource assemblages in the trawl fishing grounds off the Balearic islands (western Mediterranean). Sci. Mar. 69, 167–181. doi: 10.3989/scimar.2005.69n1167
Meloni D., Esposito G. (2018). Hygienic and commercial issues related to the illegal fishing and processing of sea cucumbers in the Mediterranean: A case study on over-exploitation in Italy between 2015 and 2017. Reg. Stud. 19, 43–46. doi: 10.1016/j.rsma.2018.03.009
Míguez-Rodríguez L. J (2009). “Equinodermos (crinoideos, equinoideos y holothuroideos) litorales,” in Batiales y abisales de Galicia (Universidade de Santiago de Compostela). dissertation thesis doctoral.
Milisenda G., Vitale S., Massi D., Enea M., Gancitano V., Giusto G. B., et al. (2017). Discard composition associated with the deep water rose shrimp fisheries (Parapenaeus longirostris, Lucas 1846) in the south-central Mediterranean Sea. Mediterr. Mar. Sci. 18, 53–63. doi: 10.12681/mms.1787
Morgan A. (2008). The effect of food availability on phenotypic plasticity in larvae of the temperate sea cucumber Australostichopus mollis. J. Exp. Mar. Biol. Ecol. 363, 89–95. doi: 10.1016/j.jembe.2008.06.025
Muggeo V. M. (2008). Segmented: an r package to fit regression models with broken-line relationships. R News. 8, 20–25.
Neelmani, Vaghela D. T. (2018). “Reproduction and developmental biology of (Holothuria scabra) Sea cucumber,” in Research trends in agriculture sciences naresh, Ed. Naresh R. K. (AkiNik Publications), 97–108.
Pawson D., Vance D. J., Messing C., Solis-Marin A. F., Mah C. L. (2009). Echinodermata of the Gulf of Mexico. In Gulf of Mexico origin, waters, and biota. Eds. Felder D. L., Camp D. K. (Texas: A&M University Press), 1177–1204.
Piccioni A., Gabriele M., Salusti E., Zambianchi E. (1988). Wind-induced upwellings off the southern coast of Sicily. Oceanol. Acta 11, 309–314.
Pinardi N., Masetti E. (2000). Variability of the large scale general circulation of the Mediterranean Sea from observations and modelling: a review. Palaeogeogr. Palaeocl. 158, 153–173. doi: 10.1016/S0031-0182(00)00048-1
Pörtner H. O. (2008). Ecosystem effects of ocean acidification in times of ocean warming: a physiologist’s view. Mar. Ecol. Prog. Ser. 373, 203–217. doi: 10.3354/meps07768
Pörtner H., Knust R. (2007). Climate change affects marine fishes through the oxygen limitation of thermal tolerance. Science 315, 95–97. doi: 10.1126/science.1135471
Prakash S. (2021). Impact of climate change on aquatic ecosystem and its biodiversity: An overview. Int. J. Biol. Innov. 3. doi: 10.46505/IJBI.2021.3210
Purcell S. W. (2010). Diel burying by the tropical sea cucumber Holothuria scabra: effects of environmental stimuli, handling and ontogeny. Mar. Biol. 157, 663–671. doi: 10.1007/s00227-009-1351-6
Purcell S. W., Mercier A., Conand C., Hamel J. F., Toral-Granda M. V., Lovatelli A., et al. (2013). Sea Cucumber fisheries: global analysis of stocks, management measures and drivers of overfishing. Fish 14, 34–59. doi: 10.1111/j.1467-2979.2011.00443.x
Purcell S. W., Piddocke T. P., Dalton S. J., Wang Y. G. (2016). Movement and growth of the coral reef holothuroids Bohadschia argus and Thelenota ananas. Mar. Ecol. Prog. Ser. 551, 201–214. doi: 10.3354/meps11720
Purcell S. W., Samyn Y., Conand C. (2012)Commercially important sea cucumbers of the world. FAO Species Catalogue for Fishery Purposes No. 6.
QGIS Development Team (2020). QGIS geographic information system (Open Source Geospatial Foundation Project). Available at: http://qgis.osgeo.org.
Qiu T., Zhang T., Hamel J. F., Mercier A., Elsevier (2015). “Development, settlement, and post-settlement growth,” in Developments in aquaculture and fisheries science, ed. Yang H., Hamel J-F, Mercier A. (Developments in Aquaculture and Fisheries Science, Elsevier) 111–131.
Quiñones J., Rosa R., Ruiz D., Garcia-Arrarás J. (2002). Extracellular matrix remodeling and metalloproteinase involvement during intestine regeneration in the sea cucumber Holothuria glaberrima. Dev. Biol. 250, 181–197. doi: 10.1006/dbio.2002.0778
Rakaj A., Fianchini A., Boncagni P., Lovatelli A., Scardi M., Cataudella S. (2018). Spawning and rearing of Holothuria tubulosa: A new candidate for aquaculture in the Mediterranean region. Aquac 49, 557–568. doi: 10.1111/are.13487
Ramírez-González J., Moity N., Andrade-Vera S., Mackliff H. R. (2020). Estimation of age and growth and mortality parameters of the sea cucumber Isostichopus fuscus (Ludwig 1875) and implications for the management of its fishery in the Galapagos marine reserve. Aquac. Fish. 5, 245–252. doi: 10.1016/j.aaf.2020.01.002
Ramón M., Amor M. J., Galimany E. (2022). Reproductive biology of the holothurian Parastichopus regalis in the Mediterranean Sea and its implications for fisheries management. Fish. Res. 247, 106191. doi: 10.1016/j.fishres.2021.106191
Ramón M., Lleonart J., Massutí E. (2010). Royal cucumber (Stichopus regalis) in the northwestern Mediterranean: distribution pattern and fishery. Fish. Res. 105, 21–27. doi: 10.1016/j.fishres.2010.02.006
Ramón M., Simarro G., Galimany E., Lleonart J. (2019). Evaluation of sediment particle size selection during feeding by the holothurian Parastichopus regalis (Cuvier 1817). Reg. Stud. Mar. Sci. 31, 100763. doi: 10.1016/j.rsma.2019.100763
R Core Team (2021). R: A language and environment for statistical computing (Vienna, Austria: R Foundation for Statistical Computing). Available at: https://www.R-project.org/.
Reeder M. S., Stow D. A., Rothwell R. G. (2002). Late quaternary turbidite input into the east Mediterranean basin: new radiocarbon constraints on climate and sea-level control. Geol. Soc Spec. Publ. London. 191, 267–278. doi: 10.1144/GSL.SP.2002.191.01.18
Robinson G., Lovatelli A. (2015). Lovatelli globalsea cucumber fisheries and aquaculture FAO’s inputs over the past few years. FAO Aquacult. Newsl. 53, 55–57.
Russo T., Carpentieri P., D’Andrea L., De Angelis P., Fiorentino F., Franceschini S., et al. (2019). Trends in effort and yield of trawl fisheries: a case study from the Mediterranean Sea. Front. Mar. Sci. 6. doi: 10.3389/fmars.2019.00153
Scannella D., Gancitano V., Falsone F., Geraci M. L., Vitale S., Colloca F., et al. (2020). Stock assessment form of red mullet (M. barbatus) in GSA 16. Zenodo. doi: 10.5281/zenodo.4382456
Skewes T., Dennis D., Burridge C. (2000). Survey of holothuria scabra (sandfish) on warrior reef, Torres strait (Queensland, Australia: Report to Queensland Fisheries Management Authority).
Sorgente R., Drago A. F., Ribotti A. (2003). Seasonal variability in the central Mediterranean Sea circulation. Ann. Geophys. 21, 299–322. doi: 10.5194/angeo-21-299-2003
Sorgente R., Olita A., Oddo P., Fazioli L., Ribotti A. (2011). Numerical simulation and decomposition of kinetic energy in the central Mediterranean: insight on mesoscale circulation and energy conversion. Ocean Sci. 7, 503–519. doi: 10.5194/os-7-503-2011
Spedicato M. T., Massutí E., Mérigot B., Tserpes G., Jadaud A., Relini G. (2019). The medits trawl survey specifications in an ecosystem approach to fishery management. Sci. Mar. 83, 9–20. doi: 10.3989/scimar.04915.11X
Stefanescu C., Rucabado J., Lloris D. (1992). Depth-size trends in western Mediterranean demersal deep-sea fishes. Mar. Ecol. Prog. Ser. 81, 205–2013. doi: 10.3354/meps081205
Symonds M. R., Moussalli A. (2011). A brief guide to model selection, multimodel inference and model averaging in behavioural ecology using akaike’s information criterion. Behav. Ecol. Sociobiol. 65, 13–21. doi: 10.1007/s00265-010-1037-6
Toral-Granda V. (2007). Facts on sea cucumber fisheries worldwide. SPC Beche-demer Inf. Bull. 25, 39–41.
Toral-Granda V., Lovatelli A., Vasconcellos M. (2008). Sea Cucumbers. A global review of fisheries and trade. FAO fish. FAO Fisheries and Aquaculture Technical Paper. No. 516. (Rome: FAO), 317.
Touratier F., Goyet C. (2011). Impact of the Eastern Mediterranean transient on the distribution of anthropogenic CO2 and first estimate of acidification for the Mediterranean Sea. Deep Sea Res. Part I Oceanogr. Res. Pap. 58, 1–15. doi: 10.1016/j.dsr.2010.10.002
Vargas-Yáñez M., Moya F., García-Martínez M. D. C., Tel E., Zunino P., Plaza F., et al. (2010). Climate change in the Western Mediterranean sea 1900–2008. J. Mar. Syst. 82, 171–176. doi: 10.1016/j.jmarsys.2010.04.013
Wei T., Simko V. (2021) R package ‘corrplot’: Visualization of a correlation matrix. (Version 0.92). Available at: https://github.com/taiyun/corrplot.
Widicombe S., Spicer J. I. (2008). Predicting the impact of ocean acidification on benthic biodiversity: what can animal physiology tell us? J. Exp. Mar. Biol. Ecol. 366, 187–197. doi: 10.1016/j.jembe.2008.07.024
Wood S. N. (2006). Generalized additive models: an introduction with r (New York: chapman and hall/CRC). doi: 10.1201/9781420010404
Wu H., Li D., Zhu B., Sun J., Zheng J., Wang F., et al. (2013). Proteolysis of noncollagenous proteins in sea cucumber, Stichopus japonicus, body wall: characterization and the effects of cysteine protease inhibitors. Food. Chem. 141, 1287–1294. doi: 10.1016/j.foodchem.2013.03.088
Yuan X., Shao S., Dupont S., Meng L., Liu Y., Wang L. (2015). Impact of CO2-driven acidification on the development of the sea cucumber Apostichopus japonicus (Selenka) (Echinodermata: Holothuroidea). Mar. pollut. Bull. 95, 195–199. doi: 10.1016/j.marpolbul.2015.04.021
Yuan X., Yang H., Wang L., Zhou Y., Gabr H. (2009). Bioenergetic responses of sub-adult sea cucumber Apostichopus japonicus (Selenka) (Echinodermata: Holothuroidea) to temperature with special discussion regarding its southernmost distribution limit in China. J. Therm Biol. 34, 315–319. doi: 10.1016/j.jtherbio.2009.05.001
Yuan X., Shao S., Yang X., Yang D., Xu Q., Zong H., et al. (2016). Bioenergetic trade-offs in the sea cucumber Apostichopus japonicus (Echinodermata: Holothuroidea) in response to CO2-driven ocean acidification. Environ. Sci. Pollut. Res.23, 8453–8461. doi: 10.1007/s11356-016-6071-0
Keywords: sea cucumbers, unexploited resources, environmental changes, acidification, fishing impact, GAM, ecosystem services, Strait of Sicily
Citation: Scannella D, Bono G, Di Lorenzo M, Di Maio F, Falsone F, Gancitano V, Garofalo G, Geraci ML, Lauria V, Mancuso M, Quattrocchi F, Sardo G, Titone A, Vitale S, Fiorentino F and Massi D (2022) How does climate change affect a fishable resource? The case of the royal sea cucumber (Parastichopus regalis) in the central Mediterranean Sea. Front. Mar. Sci. 9:934556. doi: 10.3389/fmars.2022.934556
Received: 02 May 2022; Accepted: 22 August 2022;
Published: 21 September 2022.
Edited by:
Gualtiero Basilone, National Research Council (CNR), ItalyReviewed by:
Francesco Tiralongo, University of Catania, ItalyEve Galimany, Institute of Marine Sciences, (CSIC), Spain
Copyright © 2022 Scannella, Bono, Di Lorenzo, Di Maio, Falsone, Gancitano, Garofalo, Geraci, Lauria, Mancuso, Quattrocchi, Sardo, Titone, Vitale, Fiorentino and Massi. This is an open-access article distributed under the terms of the Creative Commons Attribution License (CC BY). The use, distribution or reproduction in other forums is permitted, provided the original author(s) and the copyright owner(s) are credited and that the original publication in this journal is cited, in accordance with accepted academic practice. No use, distribution or reproduction is permitted which does not comply with these terms.
*Correspondence: Valentina Lauria, dmFsZW50aW5hLmxhdXJpYUBjbnIuaXQ=
†These authors have contributed equally to this work