- 1UMR SPE CNRS/UMS Stella Mare CNRS, Université de Corse, Corte, France
- 2Ifremer, Laboratoire Environnement Ressources Provence-Azur-Corse (LER/PAC), Bastia, France
- 3Environmental Agency of Corsica, Corte, France
- 4Corsican Regional Council, Service of the Biguglia lagoon Natural Reserve, Furiani, France
- 5Arctus Inc, Rimouski, QC, Canada
- 6MARBEC, Univ Montpellier, CNRS, Ifremer, IRD, Montpellier, France
Coastal lagoons are subjected to ever-increasing direct or indirect anthropic pressures and are inexorably deteriorating with serious issues regarding their resilience. In this paper, we assessed the functioning and evolution of the highly disturbed Biguglia coastal lagoon (Mediterranean Sea, Corsica) through an ecosystem-based approach (EBA), using multiple biotic and abiotic proxies (hydro-climatic context and eutrophication), considering its connectivity to sea and watershed and biological compartments (macrophytes, phytoplankton, and invasive species) and taking into account human influence (management actions and fishing activities). The aim of this work is firstly to provide a comprehensive analysis of its long-term (2000–2021) ecological evolution trajectory and then, based on these results, to anticipate management strategies for supporting its conservation and restoration, and the maintenance of ecosystem services it offers. Results revealed that while the lagoon showed these days a good capacity to recover after disturbance and absorb change, it recently exhibited considerable changes in its phytoplankton community composition, developed an increased susceptibility to biological invasion, and experienced a drastic reduction in fish stocks. The major interannual variations of the mean salinity, strongly dependent on management interventions beyond natural climatic variability, summarized this instability. In the future, the lagoon may no longer be able to cope with even small disturbances, which could then be sufficient to reach a breakpoint and tip the system permanently into undesired/degraded states. We demonstrated that local and punctual management actions are not always beneficial for the entire ecosystem or even detrimental in some instances. Such a retrospective ecosystem-based approach is fundamental for producing the holistic insights required to implement efficient integrated ecosystem management. This further helps enhance lagoon resilience and hence preserve its ecosystem services in the context of increasing global changes. Such lessons are useful anywhere for comparable ecosystems.
Introduction
Coastal systems are particularly fragile, as the development of human activities and climate change are increasingly causing a “coastal squeeze” phenomenon, meaning the subtraction or loss of available space for the natural functioning of these systems (Chacón Abarca et al., 2021), aquatic ecosystem degradation (Perrow and Davy, 2002; Lloret et al., 2008), reduction of biodiversity, and consequent loss of ecosystem services (Elmqvist et al., 2003; Nyström et al., 2012) with, ultimately, reduced resilience and resistance to climate change and other pressures (Defeo et al., 2021). Coastal lagoons are transitional systems, strictly dependent on their connection to adjacent systems (Tagliapietra et al., 2009). They are characterized by complex spatio-temporal diversity compared to other transitional systems, and this makes it difficult to distinguish human-driven impact from natural stress (Pérez-Ruzafa et al., 2019). Moreover, coastal lagoons are particularly subjected to more human-induced pressures than many other ecosystems (Elliott and Whitfield, 2011). Efficient management has become increasingly vital in order to sustain lagoons’ ecosystem services and avoid their loss (Jennerjahn and Mitchell, 2013).
Constant long-lasting (e.g., urbanization, exploitation, and temperature rise) or punctual (e.g., extreme events) disturbances bring about degradation in coastal lagoons, sometimes resulting in the shift of these systems into alternative stable states, different from the initial state, in which they can be locked due to higher stability of degraded states (Scheffer et al., 2001; Carpenter et al., 2012; Nyström et al., 2012; Perez-Ruzafa et al., 2018). The capacity of a natural system to experience disturbance without losing its essential function, structure, and identity is referred to as “resilience” (Walker et al., 2006). At first limited to the capacity of the system to return to equilibrium after disturbance, resilience is a concept that recently expanded to a human–environmental framework, since “human systems are a component of, and in turn shape, ecological ones” (Cote and Nightingale, 2012). Anthropic presence and growth impose a necessity for the sustainability of ecosystems, meaning the optimization of resource use and maintenance of ecosystem services provided (Cote and Nightingale, 2012; Pasqualini et al., 2020; Walker, 2020). Resilience is not linked to sustainability in a strict sense; however, a social component is inevitably present since the coexistence of “natural” ecosystem functioning and human activities leads to the aim to converge to a spectrum of “desired states”, in a dynamic context of constant (mostly of anthropogenic origin) change (Elmqvist et al., 2019; Walker, 2020). Resilience concept is hence now represented by a broader dynamic approach: it involves the social–ecological perception of natural environments and also anthropic activities as the ability of humans to maintain development in face of change (e.g., climatic, geopolitical, and anthropic context) and in a sustainable and durable way; it is tightly linked to management questions (Folke, 2016). That is why recently ecosystems are often considered as “social–ecological systems” constituted by ecological and human components and in which anthropic activities are integrated as part of their ecology. Hence, from a conservation point of view, resilience re-joins the idea of a “desired state” through the “resilience thinking” notion, which revolves around three main concepts: resilience, as persistence; adaptability, as the capacity of actors in a system to influence its resilience; and transformability, as the ability to transform the stable system into a different new one when it is no longer possible to sustain it with the preexisting ecological, economic, or social structures (Folke et al., 2010).
Changes are related to both temporal and spatial scales, as they are linked to local anthropic activities and broader scale processes such as climate change or geopolitical events and the interaction between these multiple factors (Folke et al., 2021). Hence, the present global changing and dynamic context results in the difficulty or even impossibility of the classic approach, based on historic reference state, to the conservation of natural ecosystems, and leads then to a wider approach of multi-disciplinary integrated management (Walker et al., 2006; Hobbs et al., 2009). However, integrated management is deeply intricate, especially in coastal lagoon systems, due to their complexity and natural variability. These environments show wide internal gradients of salinity, going from estuarine to hypersaline characters depending on variable freshwater influence and connection to the sea, exhibit different trophic regimes, and globally evolved in very contrasting anthropogenic contexts (Pérez-Ruzafa et al., 2011). These different contexts and functioning make it difficult to classify these systems and hence establish general rules or recommendations (Pérez-Ruzafa et al., 2011; García-Ayllón, 2017). In order to contemplate efficient integrative management, it is essential to understand what controls the resilience capacity of an ecosystem following disturbances and how human activities can alter this capacity, from a social–ecological point of view. This implies deep technical and scientific knowledge of the system’s functioning (Davoudi et al., 2016). To do so, it is particularly important to trace and analyze the trajectories of the system, in order to describe its evolution in marking events and highlighting its connectivity with other systems (Biggs et al., 2015; De Wit et al., 2020; Derolez et al., 2020; Pasqualini et al., 2020).
The main consequences of ecosystem degradation generally consist of a simplification of the biological community structure, with the loss of specialized species and the success of more generalist species, especially at the primary producers’ level, as these react more rapidly to environmental change (Pasqualini et al., 2017; Le Fur et al., 2019). In particular, different stages of primary producers shift along the degradation process of lagoon systems, mainly regulated by nutrient content and hydrological functioning, are well described in the literature (Schramm, 1999; Pergent-Martini et al., 2005; Pergent et al., 2006; Bec et al., 2011; De Wit et al., 2017). Basically, in pristine conditions characterized by low turbidity and dissolved inorganic nutrient concentrations, the community is dominated by slow-growing submerged angiosperms, like Ruppia and Zostera spp., with weak phytoplankton densities (Schramm, 1999; Pergent-Martini et al., 2005; Pergent et al., 2006). As nutrient loading increases, the system goes through successive stages: at first, light limitation occurs due to the development of epiphytes on angiosperms’ leaves; hence, angiosperms start decreasing and shift toward opportunistic macroalgae, such as Gracilaria, Ulva, and Cladophora spp., or phytoplankton starts to take place and may eventually flip the ecosystem into a phytoplankton-dominated and turbid state (Schramm, 1999; Bec et al., 2011; De Wit et al., 2017). Recently, phytoplankton biomass, community shifts, and proliferations of harmful species, known as harmful algal blooms (HABs), are increasing worldwide and especially in the Mediterranean region and coastal lagoon environment, linked to anthropogenic pressures (“cultural eutrophication”, Malone and Newton, 2020) and global change (Hallegraeff, 2010; Kudela et al., 2015; Fischer et al., 2020; Ligorini et al., 2022). Lately, shifts toward dinoflagellate dominance were increasingly observed (Xiao et al., 2018; Fischer et al., 2020). This class and especially some potentially toxic species, like Prorocentrum spp., can have the upper hand by taking advantage of rising temperatures, increasing eutrophication and nutrient inputs from terrestrial runoff in coastal environments or management actions (Aligizaki et al., 2009 and references herein; Collos et al., 2009; Xiao et al., 2018; Fischer et al., 2020). These events can lead to drawbacks on higher trophic levels, like biotic homogenization, decrease in zooplankton biomass, decrease in fish catch, toxin bioaccumulation, and risks to human health (Smayda, 1997; Fischer et al., 2020; Zingone et al., 2021).
Another cause of biotic homogenization and disturbance is the introduction (intentional or accidental) of non-native species. Such species may sometimes have the upper hand over native ones, usually by taking advantage of degradation, and successfully install themselves by becoming “invasive” and exacerbating homogenization and degradation, especially in lagoon environments (Boudouresque and Verlaque, 2012; Tordoni et al., 2019). Tracing and understanding biotic invasion are essential to preserve biological diversity and hence enhance the resistance capacity and resilience of ecosystems (Elmqvist et al., 2003). Invasions of the ecosystem engineer polychaete Ficopomatus enigmaticus (Fauvel, 1923) are long known in many lagoon environments worldwide (Argentina, Balearic Islands, Italy). This species is known to have negative impacts on macrophytes, fish nets, water and sediment circulation, inducing cascade effects along the trophic network and ecological and economic damages (Fornós et al., 1997; Bazterrica et al., 2012; Brundu and Magni, 2021). Eurasian carps Cyprinus carpio (Linnaeus, 1758) are the most widely introduced species in temperate aquatic systems and are drivers of ecological disruption by destructing seagrass beds and fueling phytoplankton blooms in lakes and reservoirs through their feeding behavior (Fischer et al., 2013; Qiu et al., 2019; Dalu et al., 2022). Other very recent and rapidly wide-spreading invaders in Mediterranean lagoons are the ctenophore Mnemiopsis leidyi (Agassiz, 1865) and the blue crab Callinectes sapidus (Rathbun, 1896). M. leidyi has colonized Mar Menor (Spain), S’Ena Arrubia (Italy), and eight Gulf of Lion French lagoons over the last 20 years, causing direct and indirect negative effects on fishing activities, through physical clogging of fish nets and predation on plankton (Marambio et al., 2013; Diciotti et al., 2016; Marchessaux and Belloni, 2021). C. sapidus invasions reported in Mediterranean lagoons are relatively recent and rapidly growing, probably linked to climate change, but they are extremely detrimental to the environment and fishing activities (Cilenti et al., 2015; Fuentes, 2019; Taybi and Mabrouki, 2020). This species colonized 17 out of 23 countries along the Mediterranean coasts and was found to negatively impact native wildlife and fisheries through its voracious behavior (Cilenti et al., 2015; Taybi and Mabrouki, 2020).
Integrative management of lagoon environments is therefore essential, especially with regard to the speeding deterioration of these systems over the last few decades, as highlighted by previous works. For instance, Badosa et al. (2007) demonstrated that some management actions may not have desired effects on the system if applied without taking into account the connectivity of the lagoon to surrounding systems. Nevertheless, this kind of study generally focuses on one or few biological compartments, like primary producers, plankton, or invasive species only, instead of describing the complex context of the ecological network and its connectivity (Badosa et al., 2007; Schallenberg et al., 2010; Delpy et al., 2012).
In this work, we explored the ecological trajectories of the Biguglia lagoon, which is a relevant example of Mediterranean coastal lagoons and can be considered a social–ecological system in its urbanized setting (Pasqualini et al., 2020). The originality of this work consists of the application of an ecosystem-based approach (EBA) to the lagoon socio-ecosystem over the last 20 years (2000–2021). We integrated multiple abiotic and biological compartments, including human activity, in the ecology of the system and also its connectivity to its watershed and exchanges with the sea. The aim of this study is to provide a large-scale comprehension of the lagoon system functioning through the tracing of long-term ecological evolution trajectories in order to provide support to the conservation or restoration of this environment and the maintaining of ecosystem services it offers, as well as improving useful scientific and methodological knowledge for future management of other similar environments. Three main questions are asked. What is the global ecological evolution context of the lagoon in the last 20 years (2000–2021; hydro-climatic context, connectivity of the lagoon and eutrophic situation, primary producers (macrophytes and phytoplankton), invasive species, and fishing activity)? In the particular eutrophication context of the Biguglia lagoon, how has the phytoplankton community evolved since 2010 observations? Is the system globally resilient and resistant, and which information can be provided to understand which factors influence these capacities and therefore help future management strategies?
Materials and methods
Study site
The Biguglia lagoon is the largest coastal lagoon on Corsica island, covering a surface of 14.5 km2 with a watershed of 180 km2 (42°36′N, 09°28′E), at the south of Bastia town (Figure 1). This brackish lagoon is characterized by shallow waters (maximum depth of 1.8 m). Exchanges with the sea take place through a long (1.5 km), narrow, natural sea channel located in the northern part. The sea channel mouth, allowing communication with the marine environment, is known as grau (Figure 1). Marine water inputs are estimated to reach ≈13 Mm3 per year (Mouillot et al., 2000) and are limited due to the tendency of the grau to naturally close through sand accumulation. Artificial opening interventions are frequently required to re-establish the connection with the sea, even though these take place only when necessary under the administration of a service within the Collectivity of Corsica regional institution, without a regular management pattern. Freshwater inputs dominate the water budget (≈90 Mm3 per year compared to the total volume of the lagoon, 18 Mm3, Dufresne et al., 2017) and are insured by numerous rivers and streams, pumping stations of the agricultural plain, sewage plants, and direct runoff. Major inputs come from the Bevinco river in the north (Figure 1). Freshwater inputs also take place in the southern part through the artificial channel connecting the Golo river to the lagoon at its southern end (Fossone canal; Figure 1); in particular, when Golo river’s discharge is limited by meteorological factors (i.e., low rainfall, winds, and currents) and, hence, its outlet tends to close, freshwater cannot flow completely in the sea and is then deviated to the lagoon through the Fossone canal (Figure 1). The hydrological functioning of the Biguglia lagoon is however more complex than this since exchanges with the sea can take place both in the extreme north of the lagoon, through the natural grau, and in the southern part, as when meteorological conditions (i.e. wind direction and velocity,and currents) are favourable sea water can reach the lagoon from the Golo outlet through the Fossone canal (Figure 1). Global historical knowledge of the lagoon report that, in addition to huge seasonal temperature variations (3°C–28°C), the water basin experienced historically a wide range of salinity conditions (4–37) at various temporal and spatial scales (Garrido et al., 2016), according to its high dependence on sea and freshwater inputs and the major impacts of water flux management performed under the government of the Collectivity of Corsica’s service.
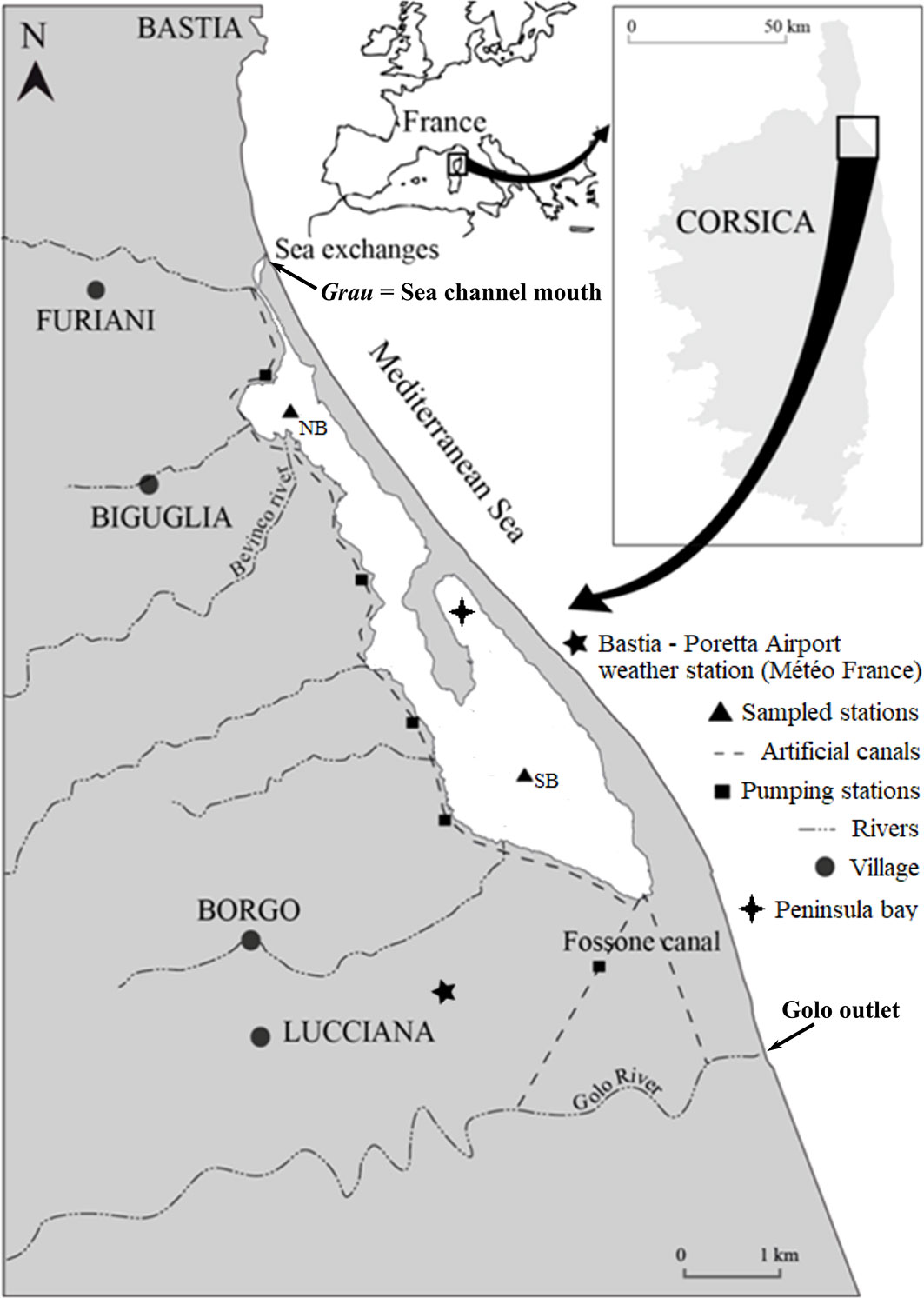
Figure 1 Study site localization: main elements of the Biguglia lagoon watershed, sampled stations, and Bastia-Poretta Airport meteorological station (Météo-France) are represented (figure from Pasqualini et al., 2017—modified).
Due to its patrimonial biological and ecological interest, the Biguglia lagoon has been recognized as RAMSAR wetland conservation site since 1991 and is a Natural Reserve (Natural Reserve of the Biguglia Lagoon, RNEB) since 1994. It offers numerous ecosystem services of social and economic interest, like fish exploitation and provision of drinking water, as its aquifer (40 Mm3) is the main source of drinking water for the urban area of Bastia (72,000 inhabitants) (Erostate et al., 2022). The lagoon is set in a highly urbanized context with growing population and increasing artificialization of its watershed and is subjected to regular management efforts under the Water Framework Directive (WFD). Ecological status was classed as “mediocre” in 2018, with phytoplankton, macrophytes, and benthic invertebrates as declassing parameters. The objective of “good status” imposed by the WFD has been postponed twice for the lagoon since 2010 (in 2015 and 2021) and is now set in 2027, indicating a need for the development of new and effective management strategies. The lagoon historically experienced major disturbances and alterations of its natural functioning and even underwent two major dystrophic crises in the past associated with low salinity conditions (in 1991 and 2007; (Cecchi et al., 2016; Garrido et al., 2016; Pasqualini et al., 2017; Erostate et al., 2022). It is currently subjected to ongoing eutrophication, due to increasing urbanization and agricultural exploitation of its watershed, and alterations of its connectivity to the adjacent marine environment (Pasqualini et al., 2020; Erostate et al., 2022).
Long-term data collection
To describe the long-term evolution of the lagoon, historical data on different environmental and biological parameters were collected from multiple sources. For meteorological data, daily rainfall and mean air temperature data were collected from Météo-France at the Bastia-Poretta Airport meteorological station (42°32′26″ N; 9°29′07″E; Figure 1). In order to characterize climatic long-term trends, an annual dataset of cumulative rainfall and mean air temperature from 1950 to 2021 was constructed. Potential multiannual monotonic trends were investigated through the Mann–Kendall (MK) trend test (modifiedmk package in R, (Hamed and Rao, 1998; Patakamuri and O’Brien, 2020), applied to annual cumulative rainfall and mean air temperature means. The Theil–Sen slope estimator was provided in the case of significant MK (i.e., p-value<0.05). Then, an analysis that focused on the 2000–2021 period was performed on cumulative annual rainfall and mean annual air temperature to characterize meteorological conditions over the study period. This was compared to the standard reference value over the reference period established for France (1991–2020) according to the World Meteorological Organization guidelines (WMO-No. 1203 © World Meteorological Organization, 2017). Over the same period, a monthly database was also constructed to discriminate and compare the extension of dry periods between different years.
In order to describe the global context of the study site, monthly cumulative water discharge and nutrient (nitrate, ) contents of the two main rivers of the watershed (Bevinco and Golo rivers) were obtained from publicly accessible data websites (http://www.naiades.eaufrance.fr/, https://www.hydro.eaufrance.fr/) at several available sampling stations from 2000 to 2021, located at 9 km (Bevinco) and 13 km (Golo) upstream the lagoon. Annual mean values of discharge were then obtained based on monthly available data, by multiplying the nutrient concentration by water discharge. Values obtained were then expressed as tonnes of per year.
A monthly database for salinity (PSU), water temperature (°C), ammonium (, µM), nitrate (, µM), nitrite (, µM), phosphates (DIP, µM), silicates (SiOH, µM), and chlorophyll a (µg L−1) data for the lagoon was constructed based on different sources (Garrido et al., 2013; Garrido et al., 2016; REPHY, 2019 (SEANOE https://doi.org/10.17882/47248); RNEB data; this work). This covers the 2000–2020 period, at two main stations, representing distinct basins inside the lagoon: North Basin (NB) and South Basin (SB) stations (Figure 1). When possible, mean values for the entire basin were calculated. Potential relationships between nutrient concentrations in the lagoon, salinity of the lagoon, inputs and flow from main rivers (Bevinco for the northern and Golo for the southern part), and monthly cumulative rainfall were assessed through the non-parametric Spearman’s rank-order correlation tests applied on available monthly data.
Qualitative information on the opening of the grau and the outlet of the Golo river were obtained by visual analysis of available satellite pictures from Landsat 4/5 (30-m resolution), Landsat 7 (15-m resolution), and Sentinel2 (10-m resolution) systems (https://glovis.usgs.gov/). Sixteen-day cycle data were available from January 2000 to October 2021. For each image, the outlets were assigned a status of “opened” (1), “closed” (0), or “partially opened” (0.5). To represent dominant trends over the study period, a mean value was then calculated per year. All the years considered within our study presented at least 10 observations seasonally distributed along the year. Furthermore, information on human-mediated openings of the grau was collected from the Natural Reserve of the Biguglia Lagoon (RNEB data) and expressed as the declared number of days of artificial openings per year.
In order to analyze biological compartments and evolution over time of different trophic levels, multiple sources were exploited. Data on fish captures (t), fish species evolution, and major invasive species were obtained from professional fishermen, managers of the RNEB, and literature (Bouchoucha, 2010; Etourneau, 2012). Macrophytes (angiosperms and macroalgae) mean percentage coverage over the basin and dominant species information were obtained from the Observatoire des Lagunes (OBSLAG) network data (monthly samples during the summer period from 2000 to 2021 at 15 stations in the Biguglia lagoon) and following the method described in Pasqualini et al. (2017). Finally, monthly phytoplankton cellular abundances (cell L−1) and community composition data were collected to analyze relative composition at the class level and identify dominant phytoplankton species and bloom events, defined as cellular proliferation over 100,000 cell L−1 threshold (Cecchi et al., 2016; Belin and Neaud-Masson, 2017). Data were issued from literature (Cecchi et al., 2016; Garrido et al., 2016; Leruste et al., 2019) and field sampling in 2020. All analyzed data came from optical microscopy identification (inverted microscope CKX31, OLYMPUS) and quantification through the Utermöhl method, applied on samples fixed with formaldehyde solution at 2.5% final concentration (Utermöhl, 1958; AFNOR, 2006). Available monthly data at the two stations covered the years 2010, 2012 (from May to December), 2013, 2014, 2019 (uniquely November), and 2020. Some missing months are due to the impossibility of sample analysis performance or technical problems impeding field sampling (2020). Data were treated for the two main sampling stations separately, NB and SB. For class-level analysis, original data were grouped into five main classes: “Bacillariophyceae” (or diatoms), “Dinophyceae” (or dinoflagellates), “Cyanophyceae”, “Cryptophyceae”, and “Chlorophyta”. All individuals that could not be assigned to one of these five classes were grouped in the class “Others”. To explore the evolution of the two dominant classes, diatoms and dinoflagellates, we calculated their ratio as the abundance of dinoflagellates over the sum of diatoms and dinoflagellates. A finer qualitative analysis of dominant and blooming (cellular density >100,000 cell L−1) taxonomic units was also performed.
Results
Environmental variables
The global meteorological context analyzed over the last 70 years (1950–2021) revealed that mean air temperature and cumulative rainfall were highly variable according to the years (Figure S1). The MK test highlighted a significant increasing trend in annual mean air temperature over the overall period (1950–2021) (p-value<0.001, Sen’s slope = 0.025), indicating a temperature rise of more than 1.7°C since 1950. From 2000 to 2021, annual mean air temperature ranged from 15.12°C (2005) to 16.96°C (2019) (Figure 2A). Until 2010, annual mean air temperatures rarely exceeded the standard reference normal value (16.14°C), except for a peak in 2003 (Figure 2A). On the contrary, since 2014, they were systematically higher than this value (Figure 2A). Conversely, cumulative annual rainfall did not show any significant monotonic trend over the period (MK p-value = 0.32). Over the 2000–2021 period, annual cumulative rainfall showed a minimum in 2021 (560.4 mm, Figure 2A). Until 2010, the standard reference value (816.87 mm) was exceeded five times (2000, 2005 2007, 2008, and 2010), culminating at 1,375.2 mm in 2008. After 2010, only 2018 resulted in a particularly wet year, reaching a cumulative rainfall of 1,132.4 mm (Figure 2A). Looking at the monthly scale, high mean air temperatures and low cumulative rainfall tend to last for several consecutive months in recent years, progressively increasing drought periods, while high cumulative rainfall tends to concentrate in few months, mainly in autumn (Figure S2).
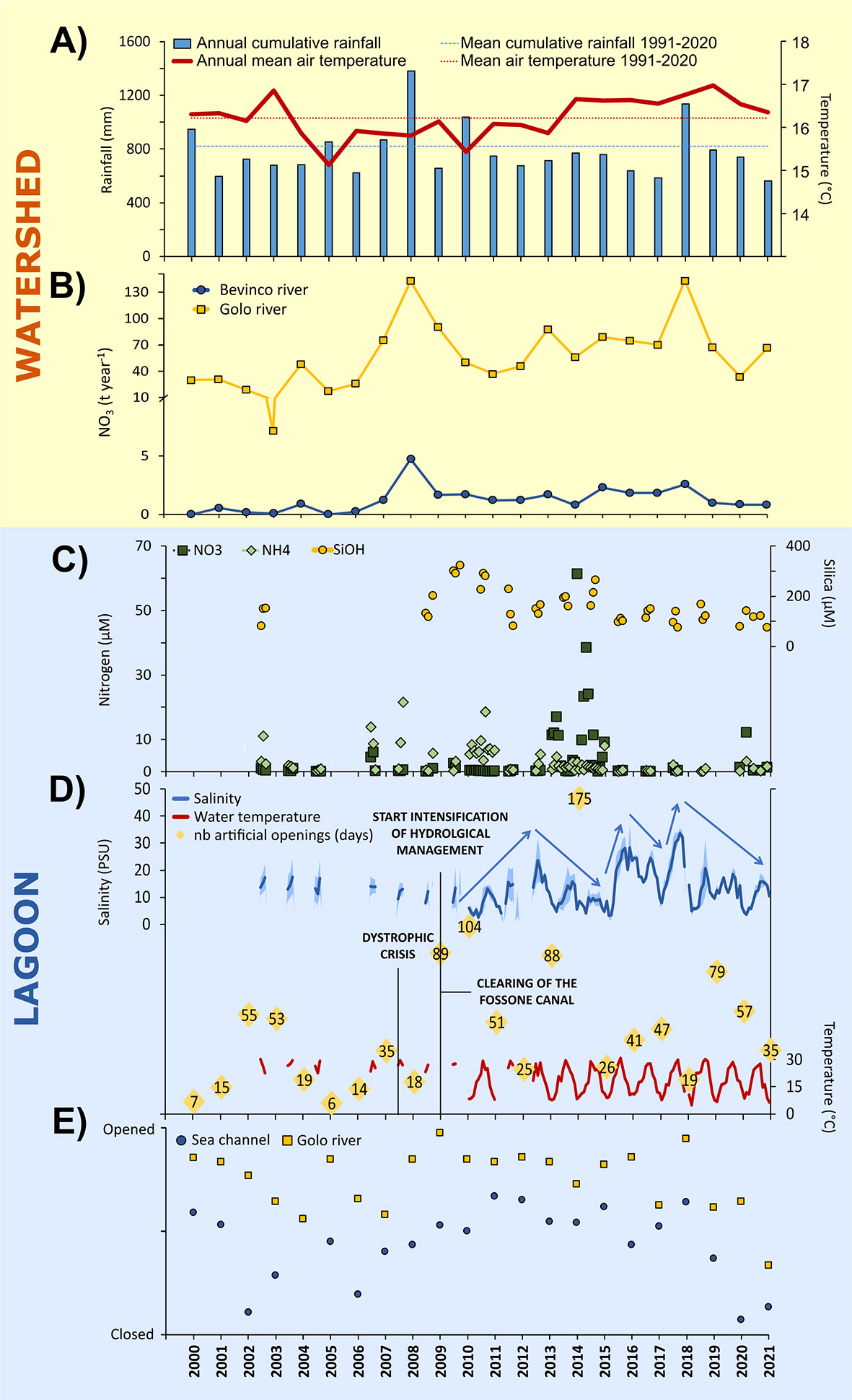
Figure 2 Multi-parameter evolutions over the 2000–2021 period for watershed and lagoon characterization over time. (A) Meteorological context is shown by annual cumulative rainfall (mm) and mean air temperature (°C), together with respective means over the standard reference period (1991–2020; WMO-No. 1203 © World Meteorological Organization, 2017. (B) Annual mean nitrate loads (t year−1) for the Bevinco and Golo rivers. (C) Nutrients monthly mean concentrations (, , SiOH; µM) for the water basin. (D) Mean monthly patterns of salinity (PSU), with light blue halo representing standard deviation of sampling stations and water temperature (°C), together with annual management effort on sea channel opening (expressed as days spent on artificial intervention) and major remarkable events. (E) Mean annual open/close status of the sea channel and Golo river outlet.
For characterizing the watershed, the water discharge and load of the two main tributaries (the Bevinco and the Golo rivers) were analyzed. Mean annual fluxes of the Bevinco and the Golo rivers were estimated at 0.66 and 14.2 m3 s−1, respectively (data not shown). The average annual load showed mostly parallel trajectories for both rivers and broadly followed the general pattern of rainfall (Figure 2B), with peaks in 2008, 2013, and 2018. For both rivers, these peaks were mainly due to monthly inputs peaks in January, May, and November in 2008; in March and November 2013; and from February to May and October 2018 (data not shown). The highest mean annual values were found in 2008 at 4.76 and 142.83 t year−1 for Bevinco and Golo rivers, respectively (Figure 2B).
Nutrient concentration data in the lagoon were only available for some months and mostly for the summer season. DIP concentration in the lagoon was low, ranging from 0.02 µM (June 2002) to 0.62 µM (August 2003) (data not shown). Silicate content reached a maximum in September 2009 (322.15 µM), while it dropped to 74.42 µM in August 2017 (Figure 2C). A gradual decrease was observed during the last 7 years, finally falling to 74.45 µM in November 2020 (Figure 2C). Nitrogen compounds showed high variability. concentrations ranged from 0.00 to 3.13 µM (June and July 2006) (data not shown). concentration culminated at 21.42 µM in August 2007 and remained below 5.5 µM from 2010 except for a peak at 8.04 µM in December 2014. concentrations varied during the period, and the highest values were observed in 2013–2014 when the maximum of 61.23 µM was reached in December 2013 (Figure 2C). Global trend showed rainfall was positively correlated with rivers’ flow and inputs from the watershed (Spearman’s rank-order correlation coefficients, 0.31 and 0.09, respectively, p< 0.05 for both) and negatively with the salinity of the lagoon (Spearman’s rank-order correlation coefficient, −0.34, p< 0.001). A significant positive correlation was found between and concentrations in the lagoon with inputs from the watershed (Spearman’s rank-order correlation coefficients, 0.31 and 0.28, p< 0.01 and 0.001, respectively) and Bevinco and Golo rivers’ water discharge (Spearman’s rank-order correlation coefficients, 0.47 and 0.42, respectively, p< 0.001 for both). All nutrient concentrations within the lagoon were significantly negatively correlated with the salinity of the lagoon (Spearman’s rank-order correlation coefficients, −0.50 (), −0.49 (), −0.30 (), and −0.58 (silicates), p< 0.001 for all).
Mean monthly water temperature followed seasonal variations and did not show any important interannual variability, fluctuating from a minimum of 4.83°C (February 2018) to a maximum of 30.88°C (August 2015) (Figure 2D). Salinity experienced high variability over time, ranging between 2.66 PSU (Mai 2010) to 33.67 PSU (September 2017), and significant trends could be identified. Progressive desalinization was observed until 2010 (Figure 2D). Starting from 2009, an intensification of management efforts to keep the grau open (e.g., up to 104 days of artificial intervention in 2010) resulted in gradual salinization until 2012, followed by another desalinization period (Figure 2D). Then, after a steep re-salinization during 2015, wide variations took place, and a gradual decrease went on until 2020, with a marked shoot in 2018 to 4.97 PSU (Figure 2D).
Parallel to these variations, some trends could be identified in the status of grau and outlet of the Golo river. From 2000 to 2006, both outlets tended to close, except in 2005 (Figure 2E). Then, an opening tendency went on until 2010, followed by a gradual closing trend that is still ongoing these days (Figure 2E).
Ecological parameters
Ecological trajectories were described through the multiple biotic compartment approach. Mean monthly chlorophyll a experienced high variability over the period, with a mean value of 11.57 µg L−1 over the whole period and a peak at 272.70 µg L−1 in 2007 during a dystrophic crisis (Figure 3A). Other lower peaks were reached in July 2010 (19.48 µg L−1), November 2012 (24.45 µg L−1), August 2017 (19.17 µg L−1), and February 2020 (16.83 µg L−1) (Figure 3A).
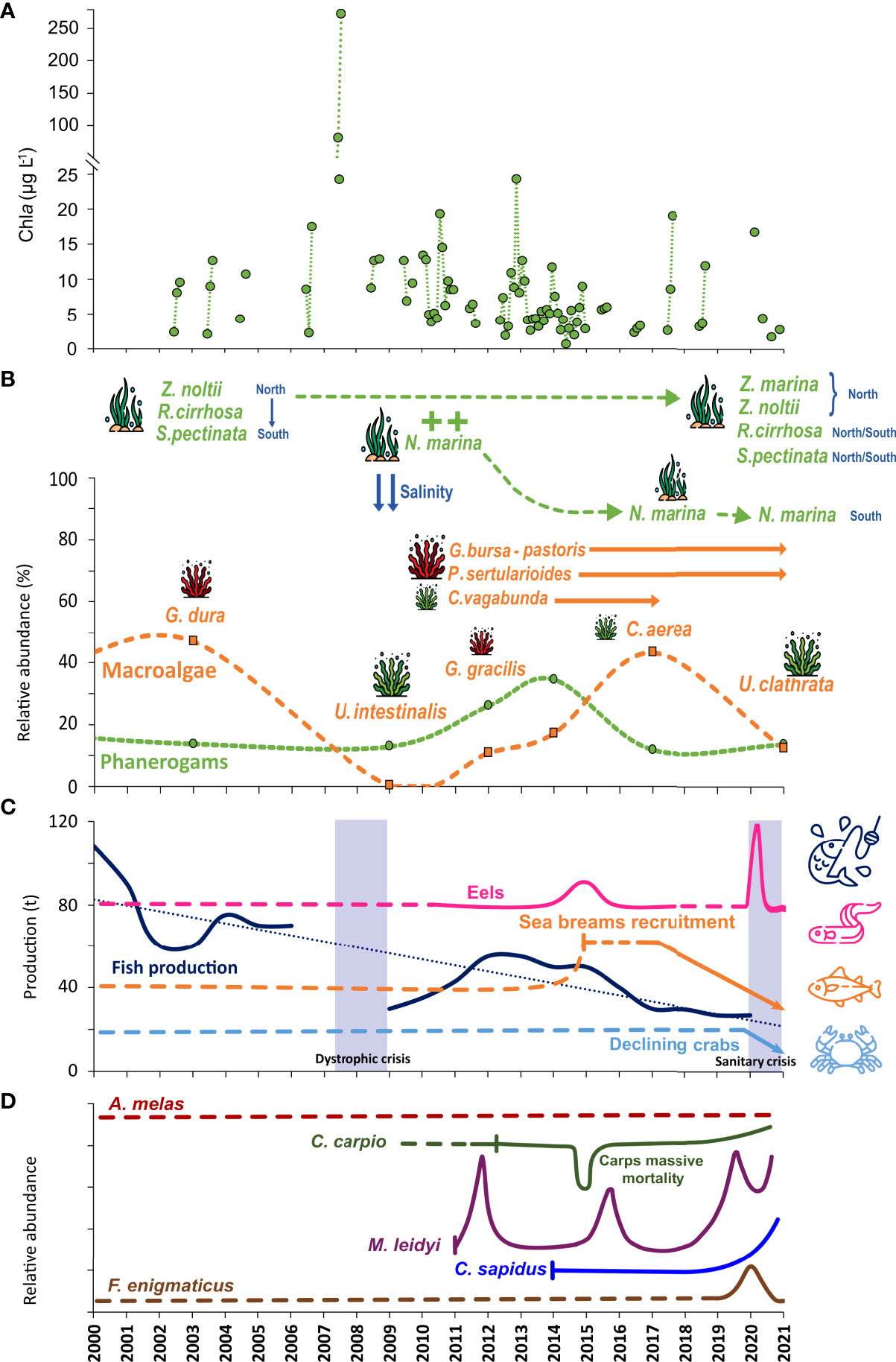
Figure 3 Ecological trajectories description of main biotic variables considered, over the 2000–2021 period. (A) Chlorophyll a (Chl a, µg L−1). (B) Macrophytes (for the genera or species: C.: Cladophora vagabunda, Chaetomorpha aerea; G.: Gracilaria; N.: Najas; P.: Polysiphonia; R.: Ruppia, S.: Stuckenia, U.: Ulva; Z.: Zostera) main trends in relative abundance and summary description of main dominant species presence and spatial distribution variations. (C) Fish production trends (2007 dystrophic crisis and 2020 COVID-19 sanitary crisis are indicated, as fishing activity was suspended due to these events) and major trajectories for interesting commercial fish based on fishermen interviews. (D) Invasive species major trends based on stakeholders’ and managers’ interviews and observations, literature, and recent observations during this work. Created with pictograms from https://www.freepik.com and https://www.flaticon.com/authors/nhor-phai.
The macrophyte compartment was dominated by macroalgae for most of the period (Figure 3B). Red macroalgae of the Gracilaria genus were usually dominant and systematically present, while green algae dominated sporadically the macroalgal community, notably of the Ulva genus (Ulva intestinalis (Linnaeus, 1753) in 2009 and Ulva clathrata [(Roth) C. Agardh, 1811] in 2021) and Cladophora vagabunda [(Linnaeus) Hoek 1963] and Chaetomorpha aerea [(Dillwyn) Kützing, 1849] in 2012 and 2017, respectively (Figure 3B). Angiosperm community experienced a decrease in percentage coverage until 2009, then increased until 2014, and finally showed degradation in the last few years (Figure 3B). Remarkable events were the expansion of Stuckenia pectinata [(Linnaeus) Börner, 1912], usually confined to the southern basin, to the northern basin from 2017 (Figure 3B). Apparition and important development of the freshwater species Najas marina (Linnaeus, 1753) was noticed in 2010 and 2018, and it expanded to the northern basin as well (Figure 3B). This species was then confined to the southern basin; still it was sporadically observed in the peninsula bay (Figure 1) in 2021. A decrease in Ruppia cirrhosa [(Petagna) Grande, 1918] was also observed between 2015 and 2017.
At a higher trophic level, a global decrease in fish production was observed (Figure 3C). Caught fish are constituted for the most part by mullets, European bass, Atherina, and eels. The latter experienced high recruitment in 2015 and an important fishing proportion in 2020 (Figure 3C). Great recruitment through the sea channel of the highly commercially important species Sparus aurata (Linnaeus, 1758) took place in 2015; then the fish stock was gradually consumed for the next 5 years (Figure 3C). Eurasian carps (C. carpio), introduced for recreational fishing in the surrounding streams around 2013, colonized the lagoon and became invasive (Figure 3D). This species is not commercialized in the area and always presents high abundance in the basin. In 2015, massive mortality of this species was observed following the salinization of the lagoon, with around 5 t of carcasses found in the canals around the lagoon (Figure 3D). Other invasive species are established in the lagoon, notably Ameiurus melas (Rafinesque, 1820), a non-commercial catfish species; the polychaete F. enigmaticus, which showed a worrying development in 2020 (especially in the northern basin) but then regressed in 2021 (Figure 3D); and the ctenophore M. leidyi and the crab C. sapidus, both introduced very recently (Figure 3D). M. leidyi was observed for the first time in autumn 2011 and was strongly present since its appearance, with sporadic developments at very high densities (Figure 3D). C. sapidus was firstly identified in the lagoon in February 2014. The fast rise observed in the last few years culminated with the capture of egg-bearing females in 2021 (Figure 3D). Parallel to the increase of invasive crab C. sapidus, a decrease of native crabs, usually inhabiting the northern part of the lagoon, was observed by fishermen exploiting the lagoon (Figure 3C).
Phytoplankton community
The phytoplankton community experienced some changes over the past 20 years, and remarkable shifts and blooms could be identified in the 2010–2020 period. Globally, total phytoplankton abundances showed wide interannual and monthly fluctuations, as it was the case for Chl a concentration, and without a clear trend (data not shown). In summer 2010, cyanobacteria were dominant at both northern and southern stations, with the genera Anabaena sp., Lyngbya sp., and Oscillatoria sp. (Figure 4A). The third genus also showed high contribution during summer 2012 at SB station, where a bloom of Cyanophyceae was also recorded in April 2014 (Figures 4A and 5). Chlorophyta group dominated only sporadically the community (Figure 4A). They were mostly present during summer 2010 at NB, in December 2014 at SB (Chlorophyceae), from July to December 2014 at SB, and in February 2020 (Trebouxiophyceae) at both stations, associated with very low salinity (mean 3.74 PSU). Globally, diatoms dominated the community between 2010 and 2012, and then dinoflagellate contribution began to increase starting from 2013 (Figure 4A). This is supported by the variations observed in Dinoflagellates/Diatoms+Dinoflagellates ratio: an increasing trend of the ratio was observed at both stations, meaning dinoflagellates rose to diatom detriment and with steeper slope at NB than SB (Figure 4B). Compared to 2010, annual mean and median values gradually increased, almost reaching the ratio = 1 plateau of total dinoflagellate dominance, especially at NB, and with a parallel decrease in interannual variability, meaning the dominance was more and more homogeneous over the recent years and independent from the season (Figure 4B). The year 2020 was almost completely dominated by dinoflagellates at both stations (Figures 4A, B). The most remarkable fact is that in 2020 the community was nearly systematically dominated by one single dinoflagellate species, producing a monospecific bloom, but not necessarily the same species each time. In the overall period, the majority of dominant and bloom-forming species belonged to the dinoflagellate class (Figure 5). The most frequent blooming species belonged to the Prorocentrum sp. genus (Figure 5). In particular, the first record of Prorocentrum cordatum ((Ostenfeld) J.D. Dodge, 1976) high bloom was in 2010, reaching a maximum of 5.93 × 105 cell L−1 at SB in November (Figure 5). Since then, this species provoked multiple persistent blooms, especially long-lasting in 2020, when it persisted from January to June with a slight time-lag between the two stations, a minimum density of 2.74 × 105 cell L−1 (May, NB) and a maximum of 5.42 × 106 cell L−1 (February, SB). During the same year, another Prorocentrum species (Prorocentrum micans (Ehrenberg 1834)) produced persistent blooms at both stations from August to December (Figure 5), touching the lowest and highest densities at SB of 1.18 × 105 cell L−1 (December) and 1.79 × 106 cell L−1 (October), respectively.
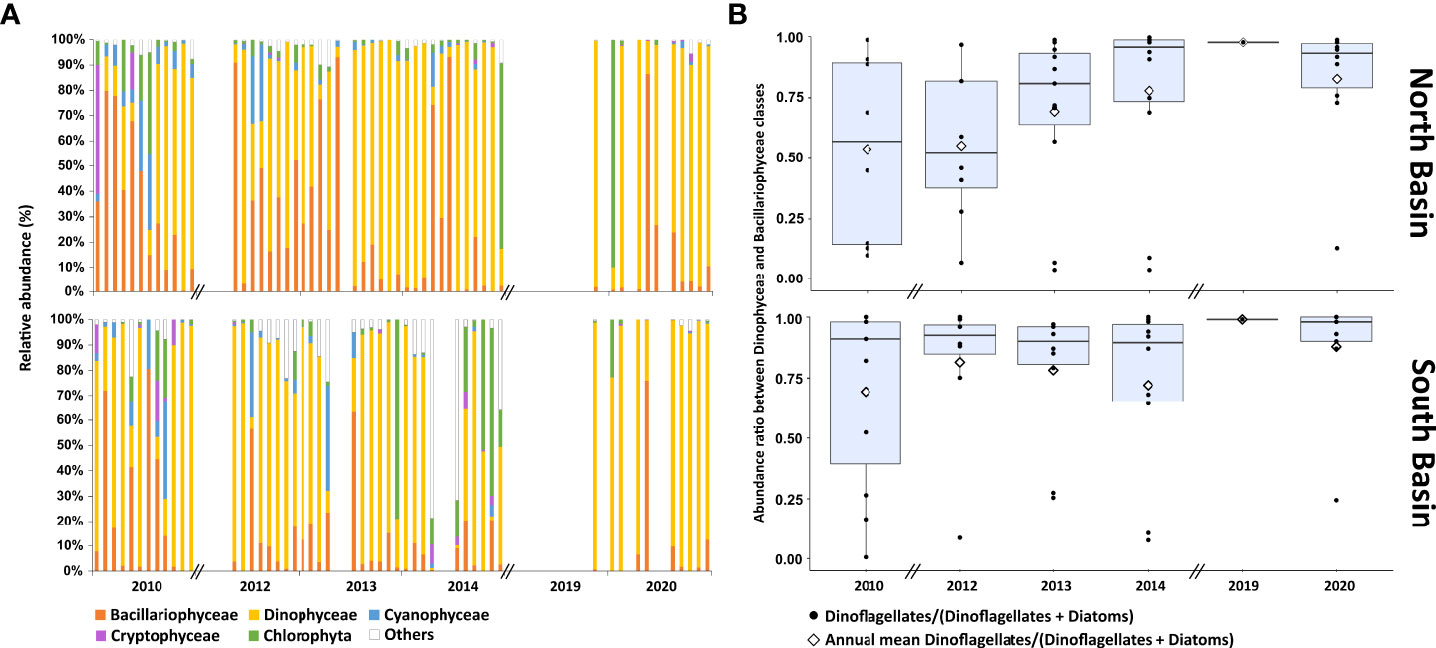
Figure 4 Phytoplankton community description over the study period considered (2010–2020). Class relative percentage composition (A) and Dinoflagellates/Diatoms+Dinoflagellates ratio (B) are represented for the two stations Northern Basin (NB), Southern Basin (SB). (B) Available monthly data on Dinoflagellates/Diatoms+Dinoflagellates ratio are represented by black points, while boxplots represent annual ratios with median (dark horizontal line) and mean (white lozenge) values.
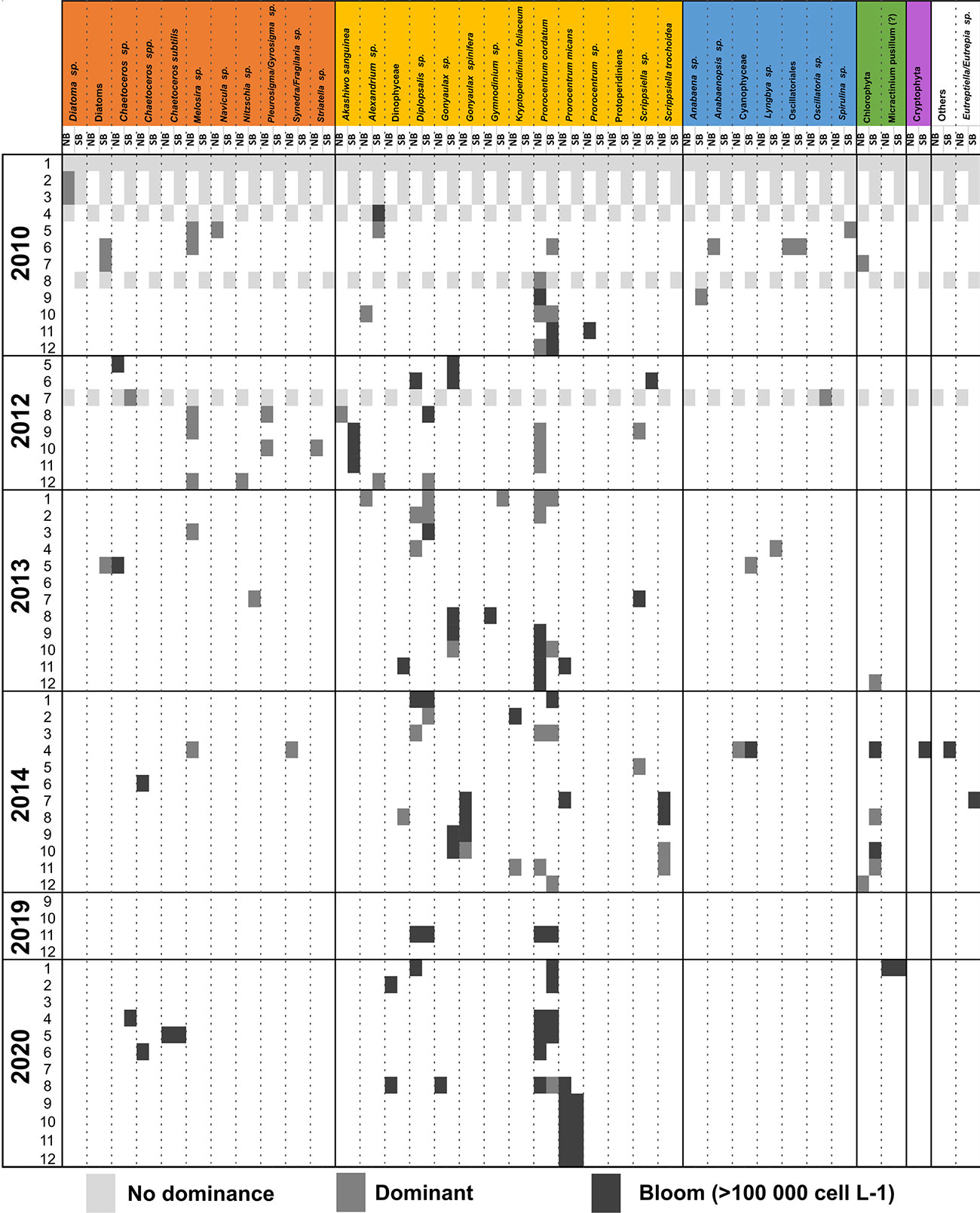
Figure 5 Bloom events and relative phytoplankton species (or taxonomic unit) were recorded in the lagoon over the 2010–2020 period for North Basin (NB) and South Basin (SB) stations. Taxonomic units are color-coded by class (for color legend see Figure 4A). White cases indicate unavailable samples; lightest colors indicate that samples were available but no dominance was highlighted; dark colors designate the dominance of the corresponding taxonomic unit; darkest colors indicate a bloom (>100,000 cell L−1) of the corresponding taxonomic unit.
P. cordatum is an invasive potentially toxic species. Blooms of other potentially toxic genera were observed in the lagoon. Akashiwo sanguinea ((K. Hirasaka) Gert Hansen & Moestrup 2000) produced a bloom lasting from September to November 2012 (Figure 5), reaching its peak at 4.06 × 105 cell L−1 in October. Blooms of Gonyaulax sp. were recorded sporadically in 2012, 2013, and 2014 (Figure 5), with a maximum in November 2013 (3.56 × 105 cell L−1). Scrippsiella sp. bloomed occasionally and mainly during the summer season (Figure 5), with a peak at 1.21 × 106 cell L−1 in July 2012. It is worthy to mention that a massive bloom of Dinophysis sp. occurred in November 2019 and expanded to the entire basin, with maximum density reached at NB (1.60 × 106 cell L−1) and associated with P. cordatum bloom too (maximum cellular density 1.37 × 106 cell L−1, NB) (Figure 5).
Discussion
Global ecological evolution context
In its Mediterranean meteorological context, we demonstrated that the lagoon has been subjected to an increasing trend in mean air temperatures in the last 70 years, in accordance with universally established temperature rise due to climate change (Giorgi and Lionello, 2008). Moreover, during our 20-year study period, the first 10 years was rainier and colder, while the last few years was dryer and hotter, with impacts on the hydrological functioning of the lagoon. Due to its urban setting, the lagoon is exposed to increasing anthropic pressure, as urbanization has increased over fivefold during the last century (Pasqualini et al., 2020; Erostate et al., 2022). Historically, the lagoon experienced a wide range of environmental conditions and trophic states (Bec et al., 2011; Cecchi et al., 2016; Garrido et al., 2016; Pasqualini et al., 2017). The main fluctuations documented on the lagoon are linked to modifications of its hydrological regime, which were shaped in relation to human activities and the development of its watershed over time. A steep decrease in salinity was observed from 2000, mostly due to the gradual closing of the natural grau and to the Golo outlet’s closed status for a longer time. This led to the accumulation of nutrients (from runoff and reduced exchanges with seawater). Parallel to the desalinization, observed until 2010, an increase in nitrates concentration was reported, linked to agricultural activities, and still ongoing, despite management measures taken in the past few years for nitrogen reduction through improved sanitation (Pasqualini et al., 2017; Leruste et al., 2019). This inertial pollution was found to be also due to the accumulation of nitrate in the groundwater (Erostate et al., 2018; Erostate et al., 2019; Jaunat et al., 2019).
Until the 1970s, the lagoon showed a good status, characterized by macrophytes dominance; then, it went through multiple statuses and sometimes experienced shifts to phytoplankton and opportunistic macroalgae dominance (Pasqualini et al., 2017; Erostate et al., 2022). The most remarkable event in the ecological history of the lagoon was the dystrophic crisis in 2007. On this occasion, the observed peak in chlorophyll a concentration was due to the proliferation of the potentially toxic cyanobacteria Anabaenopsis circularis (Pasqualini et al., 2017), probably favored by salinity decrease (as already mentioned in Cabras Lagoon by Pulina et al., 2011) and loss of connection to the sea, together with an increase in temperature during summer. Management efforts, like the clearing of the Fossone canal and the management of water sewage discharges, were made to ameliorate water circulation in the lagoon and decrease eutrophication. Results seemed to actually improve the situation, as water quality was enhanced and submerged macrophytes started to regain coverage (Pasqualini et al., 2017). However, the operation resulted in a massive input of freshwater in the system, which was later linked to the appearance and proliferation of the invasive potentially toxic dinoflagellate P. cordatum in 2010 (Cecchi et al., 2016; Garrido et al., 2016). Moreover, especially in 2020 and 2021, we assisted in a novel drastic decline in submerged angiosperm coverage, together with the modification of species distribution patterns, like the expansion of freshwater species S. pectinata to the northern part of the lagoon (linked to decreased salinity) and the proliferation of opportunistic green macroalgae, like U. clathrata and C. aerea.
The present and last few years’ situations, in fact, present several alarming signs. Notably, the frequent and high proliferation of phytoplankton might be an early sign of a trajectory toward a regime shift, according to Schramm’s scheme (Schramm, 1999). We observed a shift toward dinoflagellates to the detriment of diatoms. This phenomenon was already observed in multiple environments after disturbance, as an indication of degradation (Xiao et al., 2018; Fischer et al., 2020). The success of dinoflagellates, and potentially toxic species like Gonyaulax spp., A. sanguinea, P. cordatum, and Dinophysis sp., could be linked to decreasing salinity of the basin, increasing nutrient input, especially nitrogen, and increasing temperature (Heil et al., 2005; Dhib et al., 2013; Xiao et al., 2018; Skarlato et al., 2018a; Fischer et al., 2020; Bouquet et al., 2022). These organisms, thanks to their mixotrophic capacity and motility, can take advantage of freshwater inputs and consequent short-term stratification induced so that blooms are easily fueled in these conditions and are expected to increase with forthcoming climate change (Aligizaki et al., 2009; Skarlato et al., 2018b; Fischer et al., 2020). Potentially, the shift toward a dinoflagellate-dominated phytoplankton community and homogenization of the composition, as monospecific dinoflagellate blooms were frequently observed during the study and particularly in 2020, may lead to cascade consequences on higher levels. For instance, these shifts have already been related to a decrease in zooplankton biomass and consequently in commercial fish, a phenomenon that was indeed highlighted during this study (Fischer et al., 2020). Despite the need for further investigations on fish catch decline observed in the Biguglia lagoon during the study, one of its causes might be associated with changes in the phytoplankton community: these can depend on factors linked to the alteration of salinity natural gradients and cause a decrease in fish catch, together with many other factors, such as decreased marine recruitment after the loss of sea connectivity but also the colonization of new species (Perez-Ruzafa et al., 2018).
One of the main issues of the global disruption of the ecosystems is that it enhances vulnerability to biological invasion, as invasive species show a wide range of growth conditions, making it difficult to link their establishment to one single factor, but can take advantage of disturbed conditions (Boudouresque and Verlaque, 2012; Delpy et al., 2012; Dalu et al., 2022). This was the case for Berre lagoon’s invasion by the ctenophore M. leidyi, where it was not possible to simply trace back this event to hydrological modification or salinity variations (Delpy et al., 2012). This widely recognized invader is rapidly expanding in multiple different lagoon environments, like French Palavasian complex lagoons and Spanish Mar Menor lagoon (Marambio et al., 2013; Marchessaux and Belloni, 2021), and is also well established in the Biguglia lagoon. However, M. leidyi is not the only non-native species in the Biguglia lagoon, as its degraded status in the past probably favored the successful establishment of multiple invasive species in the lagoon. The recent first-time capture of egg-bearing females of the non-native crab C. sapidus in 2021, since its first official record in 2014, is particularly concerning, as it is an indication of its establishment in the Biguglia lagoon, and this species is well known for being rapidly expanding in the Mediterranean and already becoming invasive in lagoon environments (Cilenti et al., 2015; Fuentes, 2019; Culurgioni, 2020; Kampouris et al., 2020; Taybi and Mabrouki, 2020; Kara and Chaoui, 2021; Mancinelli et al., 2021).
Once established, these invasive species can cause ecological damage. Moreover, the simultaneous degradation of the ecosystem and presence of invasive species can contribute to the self-sustain of the “new system” and also have cascade effects on human activities and economic drawbacks. For instance, the success of the dinoflagellate P. cordatum, which is also an invasive species itself, might be at the basis of the establishment and feedback sustaining mechanism of M. leidyi in the Biguglia lagoon, since it is a fundamental component of larval and post-larval stage diet of this species (Sullivan, 2004). M. leidyi could have played a role in fish stock reduction observed over the study, as it is known to negatively affect fishing activity directly and indirectly in other Mediterranean lagoons (Sullivan, 2004; Marambio et al., 2013; Diciotti et al., 2016). C. sapidus constitutes a direct threat to fishing activity, as fish gear damage and attacks on trapped fish have already been attributed to this species (Culurgioni, 2020; Taybi and Mabrouki, 2020). Moreover, a decrease in native crabs revealed during the study may also be linked to the C. sapidus invasion, as it was already observed in Italian and Spanish lagoons (Cilenti et al., 2015; Fuentes, 2019). Another concerning point is the presence of the invasive Eurasian carp C. carpio. This species is a really disruptive invader, as it can bring about physical, chemical, and biological alterations through its feeding behavior, by increasing turbidity and resuspending sediments. It is well known for boosting phytoplankton blooms and deteriorating seagrass meadows (Fischer et al., 2013; Qiu et al., 2019; Dalu et al., 2022). F. enigmaticus and A. melas seem to be less concerning in the Biguglia lagoon case, as they were long-established and seem to have reached an equilibrium in the system.
In general, biological invasion tends to lead to native species loss and homogenization. The Biguglia lagoon seems to show early signals of this situation; thus, it is important to control and maintain a good status of its native assemblages and protect biological diversity and redundancy (“response diversity”) (Elmqvist et al., 2003; Biggs et al., 2015). Cascade effects of homogenization can bring about socioeconomic drawbacks, by negatively impacting fishing activities for instance, or even influencing bird behavior and migrations (Bruschetti et al., 2009; Pejchar and Mooney, 2009; Maceda-Veiga et al., 2017). Hence, since the Biguglia lagoon system seems already altered on the ecological level, potential socioeconomic downsides, mainly on fisheries and tourism (avifauna), are not to be neglected for future sustainable development, stressing once again that global ecosystem approach results are essential for this purpose.
Resilience and future management
The scientific contribution of this work and previous studies led, hence, to the necessity of defining efficient management directions and establishing a “desired state” to which converge. The long-term scheme proposed in this work is intended to provide a holistic image of the Biguglia lagoon socio-ecosystem: we here discuss how based on indicators of “good” ecological status coupled with other parameters both human and environmentally linked, enhancing the resilience of “good functioning” status of the lagoon is the best way to preserve it and guarantee the convergence to this “desired state” previously mentioned. The aim is to avoid falling into non-desired states, which can in turn be very resilient too. The fact that we do not clearly quantify resilience or breakpoint threshold does not prevent coming to these conclusions: first of all, clear information on the reaching of tipping points is rarely provided (Perez-Ruzafa et al., 2018); secondly, one of the key points of resilience (in the sense of the guiding toward a “desired state” or, even better, a spectrum of “desired states”) is to avoid crossing dangerous thresholds, even still unknown or unpredicted ones, by promoting the functioning of the attributes that confer this resilience to the system, in general (Walker, 2020). In a “future management” set of mind, we here try to explore these attributes, such as response diversity, exposure to disturbance, and ability to quickly react to disturbance, thinking, planning, and managing across scales, through the description of different biotic, abiotic, and anthropic elements’ patterns and trends over ~20 years.
This study highlighted that many disruption signs are simultaneously emerging in the Biguglia lagoon, potentially affecting its resilience capacity. If nothing is done, in the near future, the risk is to let the system fall into a degraded stable state in case of any forthcoming new disturbance (Figure 6). Since now, the system proved itself resilient, meaning despite acting pressures, it was able to absorb disturbances without shifting definitively toward a new state (Walker et al., 2006; Folke, 2016; Pasqualini et al., 2017). However, the trajectories of degradation we highlighted should be considered alarming. The more the system is degraded, the more it would be easy to reach the breakpoint. If the Biguglia lagoon resilience properties are not protected, it will be gradually eroded, meaning that even a small disturbance might be sufficient to easily trigger a regime shift (Figure 6).
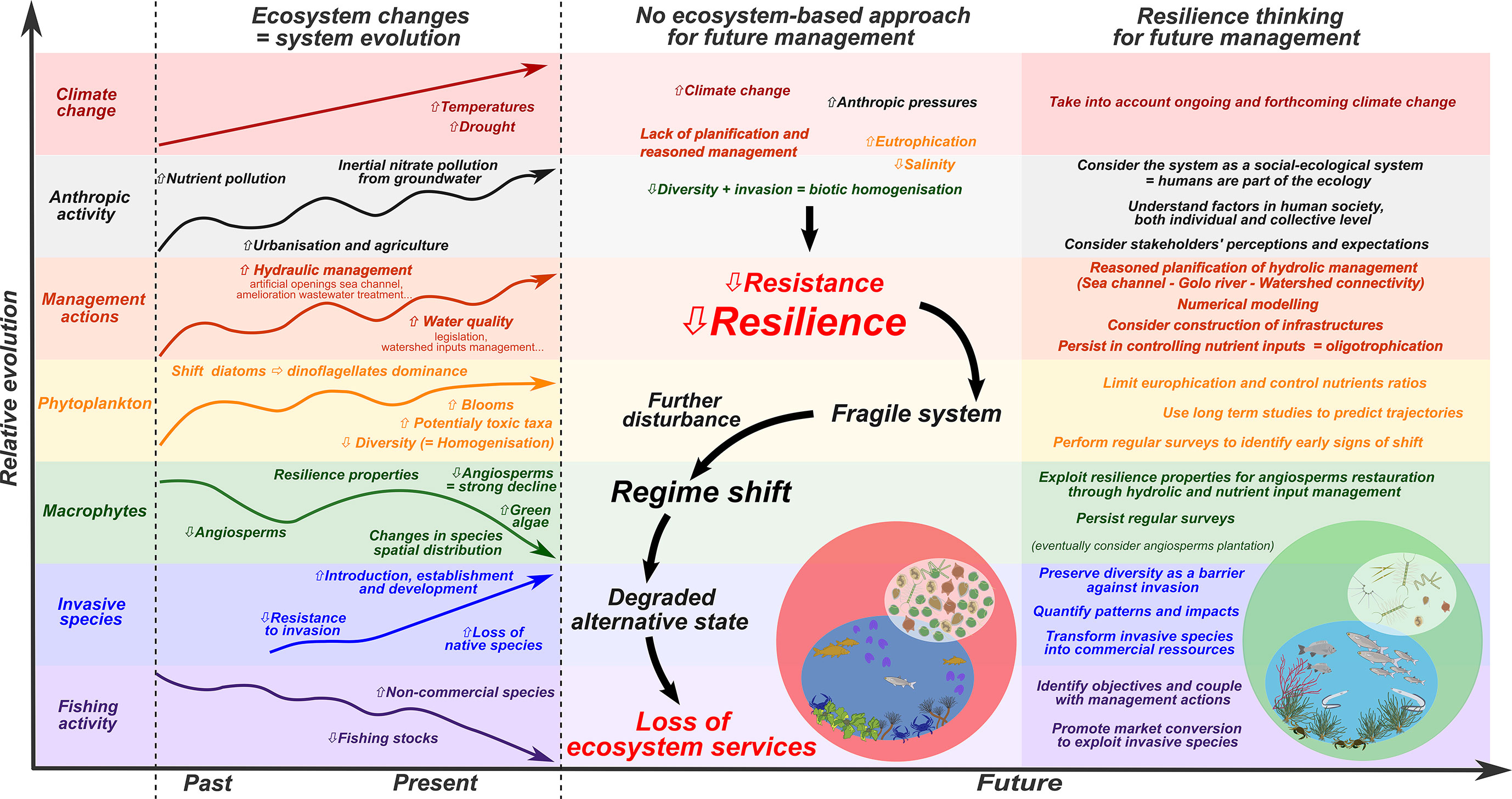
Figure 6 Scheme of functioning dynamics of the Biguglia lagoon: past and present evolutions of main compartment considered in the study, future alternative scenarios, and suggestions in the case of non-application or application of “resilience thinking” concept for future management. Created with pictograms from the Integration and Application Network, University of Maryland Center for Environmental Science (ian.umces.edu/media-library).
Hence, what can be done to strengthen the system resistance and resilience and prevent further degradation? First, it is important to consider that multiple objectives are desired for the Biguglia lagoon: the alleviation of its eutrophication, the improvement of fishing activity, and the maintenance of other important ecological services (e.g., ornithological interest and tourism) (Figure 6).
This study allowed the identification of some specific needs, applicable to the Biguglia lagoon but also generally applicable to other Mediterranean lagoons in similar cases. Firstly, it is of fundamental importance to understand and monitor lagoon connections and exchanges with adjacent water systems (sea, rivers, watershed, etc.). Globally, correlations highlighted on available samples between lagoon nutrients, and inputs from watersheds, salinity, and rainfall suggest nutrient inputs are mainly driven by water discharge from major freshwater sources and linked to meteorological events. However, we were not able to identify a clear pattern between load from major tributaries and nutrient concentration in the lagoon. This is probably due to different sampling periods available and complex dynamics and multiple factors determining nutrient concentration in the water column. Furthermore, no data on connectivity to groundwater was considered, despite that its importance in the dynamics of the nitrates in the lagoon has already been demonstrated (Erostate et al., 2019). Based on our investigation, we might conclude that the Biguglia lagoon ecological functioning depends on its connection to the sea. The status of the grau and especially the Golo river outlet strictly depend on rainfall, as water flows prevent outlets’ closing through sediment accumulation. Low flow levels are not sufficient to open the Golo outlet but rather divert freshwater to the South part of the lagoon through the Fossone canal, enhancing desalinization and increasing nutrients and suspended matter inputs. Intensification of this phenomenon is expected with climate change, as decreased rainfall and flash flood events are predicted to increase (Giorgi and Lionello, 2008). In the last few years, the natural grau tended to close due to lower or less efficient management owing to stronger sand accumulation and/or insufficient digging depth of the channel. Despite management efforts, energies invested in the artificial openings of the grau in terms of days of intervention are often not reflected by significant evolution of the desired effect. The connectivity of the lagoon to the sea is of primary importance (Biggs et al., 2015), although, as suggested by other studies, parallel to hydrologic management, it is also necessary to proceed with the control and reduction of inputs from the watershed and its monitoring for long-term remediation (Schallenberg et al., 2010; Jones et al., 2018) (Figure 6). For the Biguglia lagoon, improving exchanges with the sea would mean to reduce confinement, mitigate ongoing desalinization and eutrophication through mixing with seawater, potentially decrease phytoplankton blooms and proliferation of opportunistic and invasive species, hopefully restore angiosperms, and enhance better fish recruitment to boost fisheries activity. Mitigation of desalinization would also allow to reduce intervention in mosquito control, which is currently often carried out since some species developing on the lagoon are vectors for illnesses and constitute a threat to human health. The most common mosquito species, Culex pipiens (Linnaeus, 1758) and Anopheles maculipennis (Meigen, 1818), are favored by desalinization of the basin; hence, the hydrologic management and salinization can result in a useful tool to prevent or control their development in the basin (Schaffner et al., 2001).
Our results suggest that management of the grau is required and can be supported by other studies, which demonstrated that artificial openings to the sea may prevent or even revert shifts to phytoplankton-dominated regimes in a lagoon environment (Lloret et al., 2008; Jones et al., 2018). This means that for future management, the openings of the Golo outlet might be also considered or at least regulation of the Golo flow to the lagoon through the Fossone canal should be considered (Figure 6), in parallel with the sea outlet control. Numerical modeling could be in this case a useful tool to build different scenarios and discuss potential management options (Pete et al., 2020). Management of connections should anyway be carefully considered according to local specificities (Tagliapietra et al., 2009; Pérez-Ruzafa et al., 2019). A further complexity resides in the potential shift into a state of brackish waters at higher salinity: this could potentially impact aquatic plants with freshwater affinity, like rush and Kosteletzkya pentacarpos (L. Ledeb., 1842), thus possibly affecting birds’ communities, for which Biguglia is also an important migratory site. For instance, one of the most emblematic wintering species on this site, the migratory Anatidae Aythya ferina (Linnaeus, 1758), showed a decline in France over the last 20 years according to the Wetlands International waterbirds’ survey counting. However, the Biguglia lagoon is at the top of French wintering sites, for this species and populations’ fluctuations seemed to follow salinity patterns in the lagoon. Nevertheless, it is difficult to link salinity variation to avifauna trajectories, since multiple factors are involved at both local and larger geographical scales, especially for migratory birds. We suggest that further efforts should be made to understand the complexity of these dynamics and maybe be artificial interventions or even the construction of infrastructures might be considered to control and manage exchanges at the Fossone canal level (Figure 6). Nevertheless, sometimes management actions result in unexpected drawbacks, as we highlighted in the Biguglia lagoon. One of the main reasons could be the scattering of decisional power over the lagoon and its surroundings: multiple institutions are charged with decisions and actions that directly impact the lagoon ecosystem, without the implication of management figures of the Natural Reserve. These institutional problems result in a dispersed and diluted management, where the expertise and global view of Natural Reserve actors are set aside. The fragmentation of management does not allow a coherent approach (De Wit et al., 2021); hence, to ameliorate and make effectively efficient management actions, we believe that stronger concertation between different actors should be foreseen, in order to centralize decisional power and coordinate actions in accordance with the global expected or desired vision of the system that can be provided by manager institution.
A useful tool to improve management is to combine scientific and technical expertise with stakeholders’ perception (Figure 6): this would help with political decisions for the allocation of financial resources and the identification of priorities and “desired state”, as well as help in the development of a roadmap to make the system converge to this state in a process of ecological restoration (De Wit et al., 2020). Ecological restoration raises multiple economic questions on costs and times required, as well as ecological questions on possible consequences of actions or passivity in the face of disturbance or degradation. For these reasons, multi-factorial modeling can be a helpful tool to design potential scenarios and reactions of ecosystems (Pete et al., 2020). These models can then be used to guide exchanges, transfer knowledge from different domains of expertise, and hence help concertation between actors involved in order to ameliorate and converge to adapted and reasoned management plans and decisions (Figure 6). In some cases, the restoration of angiosperms meadows through plantation was proposed to restore degraded environments (Schallenberg et al., 2010; Rodrigo et al., 2013). However, this operation is expensive, complex, and not necessarily efficient (Rodrigo et al., 2013). We consider that for the Biguglia lagoon, reasoned management of hydrological functioning and inputs from the watershed should be sufficient to restore seagrass meadows at present status (Duarte et al., 2015; Le Fur et al., 2019) (Figure 6).
An additional problem that should be addressed is a biological invasion. It is particularly important to better investigate invasive species: quantifying their patterns of introduction, distributions, expansion, individual and population sizes, and life cycle stages is essential to monitor—and partially mitigate—the invasion or prevent new invasion events, especially with regard to climate change (Aligizaki et al., 2009; Skarlato et al., 2018b; Radinger and García-Berthou, 2020) (Figure 6). Also, further knowledge of effective impacts of invasive species on local activities, like fisheries, is capital to decision-making and management (Mancinelli et al., 2017) (Figure 6).
Lastly, we suggest the application of adaptability in the resilience thinking concept (Figure 6). For example, introduced species are now successfully established in the Biguglia lagoon, meaning that they are now part of the “new system”. It has become more and more difficult to remove non-native species from ecosystems, but they can have potential in the “resilience thinking” optic, as they can constitute important elements in novel systems and therefore be essential in providing ecosystem services in the future (Hobbs et al., 2009). This should be carefully handled as often the non-native species can become invasive and contribute to loss in diversity and then potentially affect the resilience capacity of the system (Boudouresque and Verlaque, 2012). However, this could be taken into account as a temporary control measure of invasion, providing economic advantage. Some successful examples of this idea exist: for instance, the invasive species C. sapidus was transformed from a threat into a resource in some cases, like the Gargano lagoon (Italy) where a new market was created and the consequent transformation of fishing activity (Cilenti et al., 2015; Mancinelli et al., 2017). Nevertheless, the commercialization of invasive species as a form of management and control is still strongly debated, due to associated underhand drawbacks at both ecological and economic levels. The dependence of fisheries on invasive species as new food resources can lead to economic problems like overfishing and consequential loss of commercial value (Mancinelli et al., 2017). Hence, the commercialization of invasive species brings about socioeconomic challenges and should be carefully handled, considering all negative potential effects previously mentioned: it should better not be considered as long-term sustainable exploitation but rather be viewed as a temporary solution to decrease and control expansion, foreseeing an exit option afterward (Pasko and Goldberg, 2014).
In conclusion, this study represented a unique opportunity to stress the importance of EBA for shallow Mediterranean coastal lagoons conservation and management. In fact, the Biguglia lagoon is a relevant example of Mediterranean coastal lagoons, as it is a complex system, set in a changing context: it is subjected to increasing anthropic pressure and climate change and exhibits multiple signs of degradation. Our study revealed a degradation pattern and increased sensitivity to disturbance, and we demonstrated how management interventions may not always be beneficial for the entire ecosystem. This work is a good example of why a multiple compartment approach is fundamental in lagoon environments. The evolution scheme proposed, putting together all remarkable events and successions observed over 20 years, constitutes a holistic representation of the social–ecological system’s functioning. If the study can be perceived as very descriptive to a broad audience, it meets the perception and expertise of daily contact with the system of scientific researchers, managers, fishermen, and stakeholders in general, who are very aware of the need to cooperate to converge on a spectrum of “desired states” to promote. A global ecosystem approach is essential for the understanding of the functioning and resistance/resilience properties of the system, the planning, and the decision-making for a sustainable evolution in the lagoon environment. This is the basis for good integrative management of these systems in order to preserve them and maintain or eventually restore the ecosystem services they provide in a changing world, especially in the face of increasing anthropogenic disturbance and climate change. Our study is a good starting point in this direction and lays the first stone for a new way of foreseeing the future and constitutes a good foothold for actors involved in management. We suggest future investigations should integrate other ecological compartments, such as sediments’ analysis, benthic macro-, and meiofauna, entomofauna, and avifauna, in order to provide an even deeper insight into the system’s complex network and functioning.
Data availability statement
The datasets generated and/or analysed during the current study are available from the corresponding author on reasonable request. Part of the long-term datasets analysed during the current study are available in the REPHY repository, https://doi.org/10.17882/47248.
Author contributions
VL performed the field sampling, data collection, and analysis and wrote the original draft. NM and MG conceptualized the project, contributed to the data collection and analysis, and supervised and contributed to the revision and editing of the manuscript. BF contributed to the field sampling and revision and editing of the manuscript. SE provided the data from the RNEB and contributed to field sampling, revision, and editing of the manuscript. AL contributed to the data collection and revision and editing of the manuscript. CD contributed to the revision and editing of the manuscript. PC conceptualized the project, contributed to the data collection and analysis, and supervised and contributed to the revision and editing of the manuscript. VP conceptualized the project, contributed to the data collection and analysis, supervised and contributed to the revision and editing of the manuscript, and administrated the project and funding acquisition. All authors equally contributed to the draft preparation and its redaction and have read and agreed to the published version of the manuscript.
Funding
VL was awarded a grant from the Corsican Regional Council and the University of Corsica. This study was supported by funding from the French Government and the Corsican Regional Council (CPER Gerhyco project).
Acknowledgments
The authors are grateful to the Biguglia lagoon fish exploiters and the Natural Reserve team for their cooperation during field investigations.
Conflict of interest
Author CD is employed by Arctus Inc.
The remaining authors declare that the research was conducted in the absence of any commercial or financial relationships that could be construed as a potential conflict of interest.
Publisher’s note
All claims expressed in this article are solely those of the authors and do not necessarily represent those of their affiliated organizations, or those of the publisher, the editors and the reviewers. Any product that may be evaluated in this article, or claim that may be made by its manufacturer, is not guaranteed or endorsed by the publisher.
Supplementary material
The Supplementary Material for this article can be found online at: https://www.frontiersin.org/articles/10.3389/fmars.2022.937795/full#supplementary-material
References
AFNOR (2006). Norme guide pour le dénombrement du phytoplancton par microscopie inversée. Méthode Utermöhl NF EN 15204, 39 pp.
Aligizaki K., Nikolaidis G., Katikou P., Baxevanis A. D., Abatzopoulos T. J. (2009). Potentially toxic epiphytic Prorocentrum (Dinophyceae) species in Greek coastal waters. Harmful Algae 8, 299–311. doi: 10.1016/j.hal.2008.07.002
Badosa A., Boix D., Brucet S., López-Flores R., Quintana X. D. (2007). Short-term effects of changes in water management on the limnological characteristics and zooplankton of a eutrophic Mediterranean coastal lagoon (NE Iberian peninsula). Mar. Pollut. Bull. 54, 1273–1284. doi: 10.1016/j.marpolbul.2007.01.021
Bazterrica M. C., Botto F., Iribarne O. (2012). Effects of an invasive reef-building polychaete on the biomass and composition of estuarine macroalgal assemblages. Biol. Invasions 14, 765–777. doi: 10.1007/s10530-011-0115-7
Bec B., Collos Y., Souchu P., Vaquer A., Lautier J., Fiandrino A., et al. (2011). Distribution of picophytoplankton and nanophytoplankton along an anthropogenic eutrophication gradient in French Mediterranean coastal lagoons. Aquat. Microbial. Ecol. 63, 29–45. doi: 10.3354/ame01480
Belin C., Neaud-Masson N. (2017). Cahier de procédures REPHY. document de prescription. version 1 (Nantes: Ifremer). Available at: https://archimer.ifremer.fr/doc/00393/50389/.
Biggs R., Schlüter M., Schoon M. L. (Eds.) (2015). “Principles for building resilience,” in Sustaining ecosystem services in social-ecological systems (Cambridge: Cambridge University Press). doi: 10.1017/CBO9781316014240
Bouchoucha M. (2010) Inventaire des peuplements de poissons sur les milieux lagunaires corses. RST.DOP/LER-PAC/10-03. Available at: https://archimer.ifremer.fr/doc/00028/13919/.
Boudouresque C., Verlaque M. (2012). An overview of species introduction and invasion processes in marine and coastal lagoon habitats. Cahiers Biol. Mar. 53, 309–317.
Bouquet A., Laabir M., Rolland J. L., Chomérat N., Reynes C., Sabatier R., et al. (2022). Prediction of Alexandrium and Dinophysis algal blooms and shellfish contamination in French Mediterranean lagoons using decision trees and linear regression: a result of 10 years of sanitary monitoring. Harmful Algae 115, 102234. doi: 10.1016/j.hal.2022.102234
Brundu G., Magni P. (2021). Context-dependent effect of serpulid reefs on the variability of soft-bottom macrobenthic assemblages in three Mediterranean lagoons (Sardinia, Italy). Estuarine Coast. Shelf. Sci. 262, 107589. doi: 10.1016/j.ecss.2021.107589
Bruschetti M., Bazterrica C., Luppi T., Iribarne O. (2009). An invasive intertidal reef-forming polychaete affect habitat use and feeding behavior of migratory and locals birds in a SW Atlantic coastal lagoon. J. Exp. Mar. Biol. Ecol. 375, 76–83. doi: 10.1016/j.jembe.2009.05.008
Carpenter S. R., Arrow K. J., Barrett S., Biggs R., Brock W. A., Crépin A.-S., et al. (2012). General resilience to cope with extreme events. Sustainability 4, 3248–3259. doi: 10.3390/su4123248
Cecchi P., Garrido M., Collos Y., Pasqualini V. (2016). Water flux management and phytoplankton communities in a Mediterranean coastal lagoon. part II: Mixotrophy of dinoflagellates as an adaptive strategy? Mar. Pollut. Bull. 108, 120–133. doi: 10.1016/j.marpolbul.2016.04.041
Chacón Abarca S., Chávez V., Silva R., Martínez M. L., Anfuso G. (2021). Understanding the dynamics of a coastal lagoon: Drivers, exchanges, state of the environment, consequences and responses. Geosciences 11, 301. doi: 10.3390/geosciences11080301
Cilenti L., Pazienza G., Scirocco T., Fabbrocini A. (2015). First record of ovigerous Callinectes sapidus (Rathbun 1896) in the gargano lagoons (south-west Adriatic Sea). BIR 4, 281–287. doi: 10.3391/bir.2015.4.4.09
Collos Y., Bec B., Jauzein C., Abadie E., Laugier T., Lautier J., et al. (2009). Oligotrophication and emergence of picocyanobacteria and a toxic dinoflagellate in thau lagoon, southern France. J. Sea Res. 61, 68–75. doi: 10.1016/j.seares.2008.05.008
Cote M., Nightingale A. J. (2012). Resilience thinking meets social theory: Situating social change in socio-ecological systems (SES) research. Prog. Hum. Geogr. 36, 475–489. doi: 10.1177/0309132511425708
Culurgioni J. (2020). Distribution of the alien species Callinectes sapidus (Rathbun 1896) in sardinian waters (western Mediterranean). BIR 9, 65–73. doi: 10.3391/bir.2020.9.1.09
Dalu T., Cuthbert R. N., Moyo S., Wasserman R. J., Chari L. D., Weyl O. L. F., et al. (2022). Invasive carp alter trophic niches of consumers and basal resources in African reservoirs. Sci. Total Environ. 813, 152625. doi: 10.1016/j.scitotenv.2021.152625
Davoudi S., Zaucha J., Brooks E. (2016). Evolutionary resilience and complex lagoon systems. Integrated Environ. Assess. Manage. 12, 711–718. doi: 10.1002/ieam.1823
Defeo O., McLachlan A., Armitage D., Elliott M., Pittman J. (2021). Sandy beach social–ecological systems at risk: regime shifts, collapses, and governance challenges. Front. Ecol. Environ. 19, 564–573. doi: 10.1002/fee.2406
Delpy F., Pagano M., Blanchot J., Carlotti F., Thibault-Botha D. (2012). Man-induced hydrological changes, metazooplankton communities and invasive species in the berre lagoon (Mediterranean Sea, France). Mar. Pollut. Bull. 64, 1921–1932. doi: 10.1016/j.marpolbul.2012.06.020
Derolez V., Malet N., Fiandrino A., Lagarde F., Richard M., Ouisse V., et al. (2020). Fifty years of ecological changes: Regime shifts and drivers in a coastal Mediterranean lagoon during oligotrophication. Sci. Total Environ. 732, 139292. doi: 10.1016/j.scitotenv.2020.139292
De Wit R., Chaubron-Couturier P., Galletti F. (2021). Diversity of property regimes of Mediterranean coastal lagoons in s. france; implications for coastal zone management. Ocean Coast. Manage. 207, 105579. doi: 10.1016/j.ocecoaman.2021.105579
De Wit R., Leruste A., Le Fur I., Sy M. M., Bec B., Ouisse V., et al. (2020). A multidisciplinary approach for restoration ecology of shallow coastal lagoons, a case study in south France. Front. Ecol. Evol. 8. doi: 10.3389/fevo.2020.00108
De Wit R., Rey-Valette H., Balavoine J., Ouisse V., Lifran R. (2017). Restoration ecology of coastal lagoons: new methods for the prediction of ecological trajectories and economic valuation. Aquat. Conserv.: Mar. Freshw. Ecosyst. 27, 137–157. doi: 10.1002/aqc.2601
Dhib A., Frossard V., Turki S., Aleya L. (2013). Dynamics of harmful dinoflagellates driven by temperature and salinity in a northeastern Mediterranean lagoon. Environ. Monit. Assess. 185, 3369–3382. doi: 10.1007/s10661-012-2797-4
Diciotti R., Culurgioni J., Serra S., Trentadue M., Chessa G., Satta C. T., et al. (2016). First detection of Mnemiopsis leidyi (Ctenophora, bolinopsidae) in Sardinia (S’Ena arrubia lagoon, Western mediterranean): a threat for local fishery and species recruitment. Mediterr. Mar. Sci. 17, 714–719. doi: 10.12681/mms.1719
Duarte C. M., Borja A., Carstensen J., Elliott M., Krause-Jensen D., Marbà N. (2015). Paradigms in the recovery of estuarine and coastal ecosystems. Estuaries Coasts 38, 1202–1212. doi: 10.1007/s12237-013-9750-9
Dufresne C., Malet N., Coudray S., Fiandrino A. (2017)Fonctionnement hydrodynamique de la lagune de biguglia. partie 1. In: Mise en place de la modélisation numérique 3D. Available at: https://archimer.ifremer.fr/doc/00433/54453/ (Accessed February 18, 2022).
Elliott M., Whitfield A. K. (2011). Challenging paradigms in estuarine ecology and management. Estuarine Coast. Shelf. Sci. 94, 306–314. doi: 10.1016/j.ecss.2011.06.016
Elmqvist T., Andersson E., Frantzeskaki N., McPhearson T., Olsson P., Gaffney O., et al. (2019). Sustainability and resilience for transformation in the urban century. Nat. Sustain 2, 267–273. doi: 10.1038/s41893-019-0250-1
Elmqvist T., Folke C., Nyström M., Peterson G., Bengtsson J., Walker B., et al. (2003). Response diversity, ecosystem change, and resilience. Front. Ecol. Environ. 1, 488–494. doi: 10.1890/1540-9295(2003)001[0488:RDECAR]2.0.CO;2
Erostate M., Ghiotti S., Huneau F., Jouffroy D., Garel E., Garrido M., et al. (2022). The challenge of assessing the proper functioning conditions of coastal lagoons to improve their future management. Sci. Total Environ. 803, 150052. doi: 10.1016/j.scitotenv.2021.150052
Erostate M., Huneau F., Garel E., Lehmann M. F., Kuhn T., Aquilina L., et al. (2018). Delayed nitrate dispersion within a coastal aquifer provides constraints on land-use evolution and nitrate contamination in the past. Sci. Total Environ. 644, 928–940. doi: 10.1016/j.scitotenv.2018.06.375
Erostate M., Huneau F., Garel E., Vystavna Y., Santoni S., Pasqualini V. (2019). Coupling isotope hydrology, geochemical tracers and emerging compounds to evaluate mixing processes and groundwater dependence of a highly anthropized coastal hydrosystem. J. Hydrol. 578, 123979. doi: 10.1016/j.jhydrol.2019.123979
Etourneau M. S. (2012). Signalement du cténophore invasif mnemiopis leidyi (Agassiz 1865) sur la réserve naturelle de l’Étang de biguglia et premières approches bibliographiques de ses impacts sur la pêche. Département de la Haute-Corse, Service de la Réserve Naturelle de l’Etang de Biguglia, 29 pp.
Fischer A. D., Hayashi K., McGaraghan A., Kudela R. M. (2020). Return of the “age of dinoflagellates” in Monterey bay: Drivers of dinoflagellate dominance examined using automated imaging flow cytometry and long-term time series analysis. Limnol. Oceanography 65, 2125–2141. doi: 10.1002/lno.11443
Fischer J. R., Krogman R. M., Quist M. C. (2013). Influences of native and non-native benthivorous fishes on aquatic ecosystem degradation. Hydrobiologia 711, 187–199. doi: 10.1007/s10750-013-1483-z
Folke C., Carpenter S., Elmqvist T., Gunderson L., Walker B. (2021). Resilience: Now more than ever. Ambio 50, 1774–1777. doi: 10.1007/s13280-020-01487-6
Folke C., Carpenter S., Walker B., Scheffer M., Chapin T., Rockström J. (2010). Resilience thinking: Integrating resilience, adaptability and transformability. Ecol. Soc. 20, 15. doi: 10.5751/ES-03610-150420
Fornós J. J., Forteza V., Martínez-Taberner A. (1997). Modern polychaete reefs in Western Mediterranean lagoons: Ficopomatus enigmaticus (Fauvel) in the albufera of menorca, Balearic islands. Palaeogeography Palaeoclimatol. Palaeoecol. 128, 175–186. doi: 10.1016/S0031-0182(96)00045-4
Fuentes M. (2019). Rapid invasion of the American blue crab Callinectes sapidus rathbun 1896 in the north-East of the Iberian peninsula. BIR 8, 113–118. doi: 10.3391/bir.2019.8.1.12
García-Ayllón S. (2017). Integrated management in coastal lagoons of highly complexity environments: Resilience comparative analysis for three case-studies. Ocean Coast. Manage. 143, 16–25. doi: 10.1016/j.ocecoaman.2016.10.007
Garrido M., Cecchi P., Collos Y., Agostini S., Pasqualini V. (2016). Water flux management and phytoplankton communities in a Mediterranean coastal lagoon. part I: How to promote dinoflagellate dominance? Mar. Pollut. Bull. 104, 139–152. doi: 10.1016/j.marpolbul.2016.01.049
Garrido M., Cecchi P., Vaquer A., Pasqualini V. (2013). Effects of sample conservation on assessments of the photosynthetic efficiency of phytoplankton using PAM fluorometry. Deep Sea Res. Part I.: Oceanographic Res. Papers 71, 38–48. doi: 10.1016/j.dsr.2012.09.004
Giorgi F., Lionello P. (2008). Climate change projections for the Mediterranean region. Global Planetary Change 63, 90–104. doi: 10.1016/j.gloplacha.2007.09.005
Hallegraeff G. M. (2010). Ocean climate change, phytoplankton community responses, and harmful algal blooms: a formidable predictive challenge. J. Phycol. 46, 220–235. doi: 10.1111/j.1529-8817.2010.00815.x
Hamed K. H., Rao R. A. (1998). A modified Mann-Kendall trend test for autocorrelated data. J. Hydrol. 204, 182–196. doi: 10.1016/S0022-1694(97)00125-X
Heil C. A., Glibert P. M., Fan C. (2005). Prorocentrum minimum (Pavillard) Schiller. Harmful Algae 4, 449–470. doi: 10.1016/j.hal.2004.08.003
Hobbs R. J., Higgs E., Harris J. A. (2009). Novel ecosystems: implications for conservation and restoration. Trends Ecol. Evol. 24, 599–605. doi: 10.1016/j.tree.2009.05.012
Jaunat J., Garel E., Huneau F., Erostate M., Santoni S., Robert S., et al. (2019). Combinations of geoenvironmental data underline coastal aquifer anthropogenic nitrate legacy through groundwater vulnerability mapping methods. Sci. Total Environ. 658, 1390–1403. doi: 10.1016/j.scitotenv.2018.12.249
Jennerjahn T. C., Mitchell S. B. (2013). Pressures, stresses, shocks and trends in estuarine ecosystems – an introduction and synthesis. Estuarine Coast. Shelf. Sci. 130, 1–8. doi: 10.1016/j.ecss.2013.07.008
Jones H. F. E., Özkundakci D., McBride C. G., Pilditch C. A., Allan M. G., Hamilton D. P. (2018). Modelling interactive effects of multiple disturbances on a coastal lake ecosystem: Implications for management. J. Environ. Manage. 207, 444–455. doi: 10.1016/j.jenvman.2017.11.063
Kampouris T. E., Kouroupakis E., Batjakas I. E. (2020). Morphometric relationships of the global invader Callinectes sapidus rathbun 1896 (Decapoda, brachyura, portunidae) from papapouli lagoon, NW Aegean Sea, greece. with notes on its ecological preferences. Fishes 5, 5. doi: 10.3390/fishes5010005
Kara M. H., Chaoui L. (2021). Strong invasion of mellah lagoon (South-Western Mediterranean) by the American blue crab Callinectes sapidus rathbu. Mar. Pollut. Bull. 164, 112089. doi: 10.1016/j.marpolbul.2021.112089
Kudela R. M., Berdalet E., Bernard S., Burford M., Fernand L., Lu S., et al. (2015). “Harmful algal blooms,” in A scientific summary for policy makers (Paris: IOC/UNESCO). (IOC/INF-1320).
Le Fur I., Wit R. D., Plus M., Oheix J., Derolez V., Simier M., et al. (2019). Re-oligotrophication trajectories of macrophyte assemblages in Mediterranean coastal lagoons based on 17-year time-series. Mar. Ecol. Prog. Ser. 608, 13–32. doi: 10.3354/meps12814
Leruste A., Guilhaumon F., Wit R. D., Malet N., Collos Y., Bec B. (2019). Phytoplankton strategies to exploit nutrients in coastal lagoons with different eutrophication status during re-oligotrophication. Aquat. Microbial. Ecol. 83, 131–146. doi: 10.3354/ame01906
Ligorini V., Malet N., Garrido M., Derolez V., Amand M., Bec B., et al. (2022). Phytoplankton dynamics and bloom events in oligotrophic Mediterranean lagoons: seasonal patterns but hazardous trends. Hydrobiologia. 849, 2353–2375. doi: 10.1007/s10750-022-04874-0
Lloret J., Marín A., Marín-Guirao L. (2008). Is coastal lagoon eutrophication likely to be aggravated by global climate change? Estuarine Coast. Shelf. Sci. 78, 403–412. doi: 10.1016/j.ecss.2008.01.003
Maceda-Veiga A., López R., Green A. J. (2017). Dramatic impact of alien carp Cyprinus carpio on globally threatened diving ducks and other waterbirds in Mediterranean shallow lakes. Biol. Conserv. 212, 74–85. doi: 10.1016/j.biocon.2017.06.002
Malone T. C., Newton A. (2020). The globalization of cultural eutrophication in the coastal ocean: Causes and consequences. Front. Mar. Sci. 7. doi: 10.3389/fmars.2020.00670
Mancinelli G., Chainho P., Cilenti L., Falco S., Kapiris K., Katselis G., et al. (2017). On the Atlantic blue crab (Callinectes sapidus rathbun 1896) in southern European coastal waters: Time to turn a threat into a resource? Fish. Res. 194, 1–8. doi: 10.1016/j.fishres.2017.05.002
Mancinelli G., Bardelli R., Zenetos A. (2021). A global occurrence database of the Atlantic blue crab Callinectes sapidus. Sci. Data 8, 111. doi: 10.1038/s41597-021-00888-w
Marambio M., Franco I., Purcell J., Canepa A., Guerrero E., Fuentes V. (2013). Aggregations of the invasive ctenophore Mnemiopsis leidyi in a hypersaline environment, the mar menor lagoon (NW Mediterranean). AI 8, 243–248. doi: 10.3391/ai.2013.8.2.11
Marchessaux G., Belloni B. (2021). Expansion of Mnemiopsis leidyi in the French Mediterranean lagoons along the gulf of lion. J. Sea Res. 168, 101995. doi: 10.1016/j.seares.2021.101995
Mouillot D., Titeux A., Migon C., Sandroni V., Frodello J.-P., Viale D. (2000). Anthropogenic influences on a mediterranean nature reserve: modelling and forecasting. Environ. Model. Assess. 5, 185–192. doi: 10.1023/A:1011533811237
Nyström M., Norström A. V., Blenckner T., de la Torre-Castro M., Eklöf J. S., Folke C., et al. (2012). Confronting feedbacks of degraded marine ecosystems. Ecosystems 15, 695–710. doi: 10.1007/s10021-012-9530-6
Pasko S., Goldberg J. (2014). Review of harvest incentives to control invasive species. MBI 5, 263–277. doi: 10.3391/mbi.2014.5.3.10
Pasqualini V., Derolez V., Garrido M., Orsoni V., Baldi B., Etourneau S., et al. (2017). Spatiotemporal dynamics of submerged macrophyte status and watershed exploitation in a Mediterranean coastal lagoon: Understanding critical factors in ecosystem degradation and restoration. Ecol. Eng. 102, 1–14. doi: 10.1016/j.ecoleng.2017.01.027
Pasqualini V., Erostate M., Garel E., Garrido M., Huneau F., Robert S. (2020). An ecosystem-based approach to study the biguglia lagoon socio-ecosystem (Mediterranean Sea). Vie Milieu 70, 121–128.
Patakamuri S. K., O’Brien N. (2020) Modified versions of Mann Kendall and spearman’s rho trend tests. r-package ‘modifiedmk’. Available at: https://cran.r-project.org/web/packages/modifiedmk/modifiedmk.pdf.
Pejchar L., Mooney H. A. (2009). Invasive species, ecosystem services and human well-being. Trends Ecol. Evol. 24, 497–504. doi: 10.1016/j.tree.2009.03.016
Perez-Ruzafa A., Marcos C., Pérez-Ruzafa I. (2018). “When maintaining ecological integrity and complexity is the best restoring tool: The case of the mar menor lagoon”, in Management and restoration of Mediterranean coastal lagoons in Europe, vol. 10 . Eds. Quintana X., Boix D., Gascón S., Sala J. (Càtedra d’Ecosistemes Litorals), 67–95. Proyecto LIFE 13 NAT/ES/001001, Recerca y territori.
Pérez-Ruzafa A., Marcos C., Pérez-Ruzafa I. M., Pérez-Marcos M. (2011). Coastal lagoons: “transitional ecosystems” between transitional and coastal waters. J. Coast. Conserv. 15, 369–392. doi: 10.1007/s11852-010-0095-2
Pérez-Ruzafa A., Pérez-Ruzafa I. M., Newton A., Marcos C. (2019). “Coastal lagoons: Environmental variability, ecosystem complexity, and goods and services uniformity,” in Coasts and estuaries, the future. Eds. Wolanski E., Day J., Elliott M., Ramesh R. (Amsterdam: Elsevier), 253–276. doi: 10.1016/B978-0-12-814003-1.00015-0
Pergent-Martini C., Pasqualini V., Ferrat L., Pergent G., Fernandez C. (2005). Seasonal dynamics of Zostera noltii hornem. in two Mediterranean lagoons. Hydrobiologia 543, 233–243. doi: 10.1007/s10750-004-7454-7
Pergent G., Pasqualini V., Pergent-Martini C., Ferrat L., Fernandez C. (2006). Variability of ruppia cirrhosa in two coastal lagoons with differing anthropogenic stresses. Botanica Marina 49, 103–110. doi: 10.1515/BOT.2006.014
Perrow M. R., Davy A. J. (2002). Handbook of ecological restoration: Volume 1 principles of restoration. Ecol. Eng. 21, 205–206. doi: 10.1016/j.ecoleng.2004.02.001
Pete R., Guyondet T., Bec B., Derolez V., Cesmat L., Lagarde F., et al. (2020). A box-model of carrying capacity of the thau lagoon in the context of ecological status regulations and sustainable shellfish cultures. Ecol. Model. 426, 109049. doi: 10.1016/j.ecolmodel.2020.109049
Pulina S., Padedda B. M., Sechi N., Lugliè A. (2011). The dominance of cyanobacteria in Mediterranean hypereutrophic lagoons: a case study of cabras lagoon (Sardinia, Italy). Sci. Marina 75, 111–120. doi: 10.3989/scimar.2011.75n1111
Qiu X., Mei X., Razlutskij V., Rudstam L. G., Liu Z., Tong C., et al. (2019). Effects of common carp (Cyprinus carpio) on water quality in aquatic ecosystems dominated by submerged plants: a mesocosm study. Knowl. Manage. Aquat. Ecosyst. 420, 28. doi: 10.1051/kmae/2019017
Radinger J., García-Berthou E. (2020). The role of connectivity in the interplay between climate change and the spread of alien fish in a large Mediterranean river. Global Change Biol. 26, 6383–6398. doi: 10.1111/gcb.15320
REPHY (2019). French Observation and monitoring program for phytoplankton and hydrology in coastal waters. 1987-2018 metropolitan data (SEANOE). doi: 10.17882/47248
Rodrigo M. A., Rojo C., Alonso-Guillén J. L., Vera P. (2013). Restoration of two small Mediterranean lagoons: The dynamics of submerged macrophytes and factors that affect the success of revegetation. Ecol. Eng. 54, 1–15. doi: 10.1016/j.ecoleng.2013.01.022
Schaffner F., Angel G., Geoffroy B., Hervy J. P., Rhaiem A., Brunhes J. (2001). “Les Moustiques d’Europe / the mosquitoes of Europe,” (Montpellier, France: EID Méditerranée&IRD, CD-Rom Collection Didactiques IRD Editions).
Schallenberg M., Larned S. T., Hayward S., Arbuckle C. (2010). Contrasting effects of managed opening regimes on water quality in two intermittently closed and open coastal lakes. Estuarine Coast. Shelf. Sci. 86, 587–597. doi: 10.1016/j.ecss.2009.11.001
Scheffer M., Carpenter S., Foley J. A., Folke C., Walker B. (2001). Catastrophic shifts in ecosystems. Nature 413, 591–596. doi: 10.1038/35098000
Schramm W. (1999). Factors influencing seaweed responses to eutrophication: some results from EU-project EUMAC. J. Appl. Phycol. 11, 69. doi: 10.1023/A:1008076026792
Skarlato S., Filatova N., Knyazev N., Berdieva M., Telesh I. (2018a). Salinity stress response of the invasive dinoflagellate Prorocentrum minimum. Estuarine Coast. Shelf. Sci. 211, 199–207. doi: 10.1016/j.ecss.2017.07.007
Skarlato S. O., Telesh I. V., Matantseva O. V., Pozdnyakov I. A., Berdieva M. A., Schubert H., et al. (2018b). Studies of bloom-forming dinoflagellates Prorocentrum minimum in fluctuating environment: contribution to aquatic ecology, cell biology and invasion theory. Protistology 12, 113–157. doi: 10.21685/1680-0826-2018-12-3-1
Smayda T. J. (1997). Harmful algal blooms: Their ecophysiology and general relevance to phytoplankton blooms in the sea. Limnol. Oceanography 42, 1137–1153. doi: 10.4319/lo.1997.42.5_part_2.1137
Sullivan L. J. (2004). Diet of the larval ctenophore Mnemiopsis leidyi a. agassiz (Ctenophora, lobata). J. Plankton Res. 26, 417–431. doi: 10.1093/plankt/fbh033
Tagliapietra D., Sigovini M., Volpi Ghirardini A. (2009). A review of terms and definitions to categorise estuaries, lagoons and associated environments. Mar. Freshw. Res. 60, 497–509. doi: 10.1071/MF08088
Taybi A. F., Mabrouki Y. (2020). The American blue crab Callinectes sapidus rathbun 1896 (Crustacea: Decapoda: Portunidae) is rapidly expanding through the Mediterranean coast of Morocco. Thalassas 36, 267–271. doi: 10.1007/s41208-020-00204-0
Tordoni E., Petruzzellis F., Nardini A., Savi T., Bacaro G. (2019). Make it simpler: Alien species decrease functional diversity of coastal plant communities. J. Vegetation Sci. 30, 498–509. doi: 10.1111/jvs.12734
Utermöhl H. (1958). Zur vervollkommnung der quantitativen phytoplankton-methodik. Int. Ver. Theor. Angew. Limnol. 9, 1–38.
Walker B. (2020). Resilience: what it is and is not. Ecol. Soc. 25, 11. doi: 10.5751/ES-11647-250211
Walker B., Gunderson L., Kinzig A., Folke C., Carpenter S., Schultz L. (2006). A handful of heuristics and some propositions for understanding resilience in social-ecological systems. E&S 11, art13. doi: 10.5751/ES-01530-110113
Xiao W., Liu X., Irwin A. J., Laws E. A., Wang L., Chen B., et al. (2018). Warming and eutrophication combine to restructure diatoms and dinoflagellates. Water Res. 128, 206–216. doi: 10.1016/j.watres.2017.10.051
Keywords: mediterranean coastal lagoon, resilience, management, ecosystem-based approach (EBA), biological invasion, dinoflagellates, HABs
Citation: Ligorini V, Malet N, Garrido M, Four B, Etourneau S, Leoncini AS, Dufresne C, Cecchi P and Pasqualini V (2022) Long-term ecological trajectories of a disturbed Mediterranean coastal lagoon (Biguglia lagoon): Ecosystem-based approach and considering its resilience for conservation? Front. Mar. Sci. 9:937795. doi: 10.3389/fmars.2022.937795
Received: 06 May 2022; Accepted: 04 August 2022;
Published: 26 August 2022.
Edited by:
Stelios Katsanevakis, University of the Aegean, GreeceReviewed by:
Concepción Marcos, University of Murcia, SpainGeorg Umgiesser, Department of Earth System Sciences and Technologies for the Environment, (CNR), Italy
Copyright © 2022 Ligorini, Malet, Garrido, Four, Etourneau, Leoncini, Dufresne, Cecchi and Pasqualini. This is an open-access article distributed under the terms of the Creative Commons Attribution License (CC BY). The use, distribution or reproduction in other forums is permitted, provided the original author(s) and the copyright owner(s) are credited and that the original publication in this journal is cited, in accordance with accepted academic practice. No use, distribution or reproduction is permitted which does not comply with these terms.
*Correspondence: Viviana Ligorini, bGlnb3JpbmlfdkB1bml2LWNvcnNlLmZy
†ORCID: Viviana Ligorini, orcid.org/0000-0001-9846-568X