- 1Department of Marine Plankton Research, Institute of Oceanography, University of Gdańsk, Gdynia, Poland
- 2Institute of Oceanology, Polish Academy of Sciences, Sopot, Poland
Oceanic fronts constitute boundaries between hydrologically distinct water masses and comprise one of the most productive regions of the world’s ocean. Fronts associated with density gradients (active fronts) profoundly structure planktonic communities in adjacent waters, but less is known about the impacts of density-compensated (passive) fronts. Two such fronts are found in the European Arctic, the Arctic Front (AF) and the Polar Front (PF), that both separate warmer and saltier, Atlantic water from the colder, but fresher Arctic water. As scrutinized research on the influence of passive fronts on zooplankton at large spatial and temporal scales had been lacking, we tackled the question of their role in maintaining distinct communities, employing globally unique, 12-year-long gelatinous zooplankton (GZ) and hydrological time series from the European Arctic. The GZ, owing to their fast reproductive cycles and passive dispersal, reflect particularly well the local environment. We therefore compared GZ communities between zones separated by the two fronts, disentangled their drivers, and analyzed community shifts occurring whenever front relocation occurred. We have identified fifteen GZ taxa, distributed among three distinct communities, specific for front-maintained zones, and selected the following taxa as indicators of each zone: W—west of the AF, within the Greenland Sea Gyre, Beroe spp.; C—central, in between the AF and the PF, Aglantha digitale; and E—east of the PF, in the West Spitsbergen Shelf Mertensia ovum. Taxonomic composition of these communities, and their specific abundance, persisted throughout time. We also showed that relocation of either front between the sampling years was subsequently followed by the restructuring of the GZ community. Our results indicate that passive oceanic fronts maintain distinct GZ communities, with probable limited exchange across a front, and provide a new perspective for the Arctic ecosystem evolution under progressing Atlantification.
Introduction
Hydrographic fronts form at the junction of distinct water masses, and hence are usually defined by a sharp gradient of water properties, like temperature, salinity, and/or density. The existence of a horizontal density gradient fuels cross-frontal, vertical circulation and surface convergence of water masses (Belkin et al., 2009), leading to the upwelling of nutrients (Allen et al., 2005) and sinking of organic matter (Stukel et al., 2017), both linked to the enhanced primary and secondary production at a front (le Fèvre, 1987; Russell et al., 1999). Density-related fronts also attract representatives of the upper trophic levels, as evidenced by records of local aggregations of schooling fish (e.g., Herron et al., 1989), seabirds, and whales (reviewed in Olson et al., 1994). Moreover, the presence of a geostrophic, along-front currents associated with the density fronts, plays a leading role in large-scale transport of heat, salt, and nutrients (Belkin, 2004), and in maintaining the boundary between the adjacent water masses (Belkin et al., 2009). Not all fronts are, however, associated with the strong horizontal gradients of density, and these so-called density-compensated or passive fronts thus do not exhibit enhanced primary or secondary production (Drinkwater and Tande, 2014). The extent to which such density-compensated fronts can structure adjacent ecosystems has yet to be explored.
Two such passive fronts are found in the European Arctic, where they bound the northward flow of the Atlantic water on both sides (Wassmann et al., 2015). In the Greenland Sea, the Arctic Front (AF), topographically steered by the Mohn and Knipovich Ridges (Swift and Aagaard, 1981; van Aken et al., 1995), separates the colder and fresher Arctic water of the Greenland Sea Gyre from the warmer and saltier Atlantic water in the western branch of the West Spitsbergen Current (Walczowski, 2013). The other passive front, the Polar Front (PF), crosses most of the Barents Sea (Oziel et al., 2016), where it marks the boundary between the inflowing Atlantic water, and that originating in the Arctic. In its western part, along the southern and western Spitsbergen shelf and near the Bear Island, the front location is largely controlled by the bottom topography (Loeng, 1991) and it borders the eastern branch of the West Spitsbergen Current and the Spitsbergen Polar Current (Strzelewicz et al., 2022). Although both the AF and the PF are topographically steered, the presence of topographic discontinuities (e.g., submarine canyons) that leads to the baroclinic and the barotropic instabilities (Teigen et al., 2010), promotes cross-front exchange and interleaving of the Atlantic and Arctic waters (Huthnance, 1995; Saloranta and Svendsen, 2001; Drinkwater and Tande, 2014). Such instabilities are associated with a higher concentration of particles and often with a larger primary production (Trudnowska et al., 2016), but whether these can propagate up the trophic chain remains unresolved.
The position of both the AF and the PF changes with the increasing inflow of the Atlantic water (e.g., Walczowski, 2013; Wassmann et al., 2015)—a manifestation of the Atlantification of the European Arctic (reviewed in Ingvaldsen et al., 2021). During warm years, when more of the warmer and saltier Atlantic water reaches the Arctic with the flow of the West Spitsbergen Current (Beszczynska-Möller et al., 2012; Walczowski et al., 2012), it pushes the AF westwards (Walczowski, 2013), while on the other side of the current, it increases the presence of the Atlantic water on the West Spitsbergen Shelf, weakening the PF in the northward direction, and shifting its southern part eastwards (Saloranta and Svendsen, 2001; Strzelewicz et al., 2022). Assuming that both fronts maintain, to some extent, the distinctiveness of adjacent ecosystems, shifts in their position, irrespective of the underlying mechanisms, should be traceable with the analysis of local biota distribution, provided the availability of the diversity baseline and a time-series dataset at the appropriate spatial scale.
Planktonic organisms, owing to their short generation times and rapid growth in response to favorable environmental conditions, have been advocated as sentinels of the climate change in marine ecosystems (Hays et al., 2005). Patterns of their distribution and diversity have already been linked with the locations of oceanic fronts (e.g., Basedow et al., 2014), though in the European Arctic, such studies have disproportionately focused on the PF (Basedow et al., 2014; Trudnowska et al., 2016; Balazy et al., 2018), seemingly omitting the AF. Overall, these works have identified the Atlantic-facing side of the fronts as more productive (Basedow et al., 2014), and harboring more abundant planktonic communities (Kwasniewski et al., 2010; Trudnowska et al., 2016). However, referenced data pertain to the well-studied planktonic groups, like hard-bodied copepods, or provide low taxonomic resolution, hampering interpolation of such results across the whole diversity of plankton.
One such group of animals, gelatinous zooplankton (GZ; here as pelagic cnidarians and ctenophores), has received surprisingly little attention in the front-position related studies (Luo et al., 2014; Haberlin et al., 2019), especially given that their passive and active mechanisms of aggregations at physical discontinuities in the ocean are well-recognized (Arai, 1992; Graham et al., 2001), as is the front-related partitioning of their diversity across adjacent water masses (Pagès and Gili, 1992; Haberlin et al., 2019). Although the majority of studies agree that the fronts act as an impermeable barrier for the GZ (e.g., Graham et al., 2001), contradictory evidence exists, which would suggest that they, in fact, allow for a continuous exchange between the adjacent GZ communities (e.g., Luo et al., 2014). These two opposing patterns could be related to the different nature of the active and passive fronts, but the paucity of data from the density-compensated fronts precludes further reasoning.
Additional support for using the GZ, as a model system to study the ecology at the passive fronts, comes with the existence of a solid baseline of their diversity in the North Atlantic (Licandro et al., 2015; Hosia et al., 2017) and the European Arctic (Mańko et al., 2015; Ronowicz et al., 2015; Mańko et al., 2020). Unfortunately, the GZ is either completely absent from the local zooplankton time series, or the taxonomic resolution of their records is insufficient (Long et al., 2021), due to their fragile body structure that often damages when sampled harshly. From a broader perspective, the recent recognition of the GZ’s trophic importance (Hays et al., 2018; Lüskow et al., 2021) as well as their diverse roles in the biogeochemical cycles (Wright et al., 2021) and the biological pump (Lebrato et al., 2019) renders understanding of the factors structuring their community pivotal for monitoring marine ecosystems and forecasting their climate-mediated evolution.
The Arctic Ocean is warming up at an unprecedented pace (IPCC, 2014) that is even more rapid in its European sector (Walczowski and Piechura, 2007), owing to the strengthening advection of Atlantic water flowing with the West Spitsbergen Current. Monitoring of the spatial extent of Atlantic water inflow is thus crucial for predicting the Arctic’s future. With that in mind, we designed a study that attempted to use the GZ to track the position of the AF and the PF, which flank the West Spitsbergen Current, and their role in maintaining distinctive pelagic communities across the European Arctic. For that purpose, we examined the 12-year-long (2003–2014) zooplankton time series, combined with detailed hydrographic measurements spanning Greenland, Norwegian, and Barents Seas, the so-called European Arctic. We hypothesize that (1) the GZ found on the side of the AF and PF that faces the West Spitsbergen Current will be more abundant but less diverse, and that (2) the two passive fronts would constitute a semi-impermeable barrier, with only a small proportion of the shared GZ taxa on either side of each front, thus justifying the use of GZ to monitor the shifting position of the fronts.
Materials and Methods
Data Collection
Zooplankton was sampled at 17 sites scattered throughout the European Arctic (Figure 1), from 2003 to 2014, onboard the R/V Oceania as part of the Institute of Oceanology of the Polish Academy of Sciences annual monitoring campaign—AREX (Arctic Research Expedition). Sampling took place from June to July, so that each site was visited within a 2-week time window each year. Noteworthy, the presence of the sea ice or rough sea has sometimes precluded accessing all planned sites in a given year; thus, data only from the sites sampled more than five times over the study period were included in the analyses.
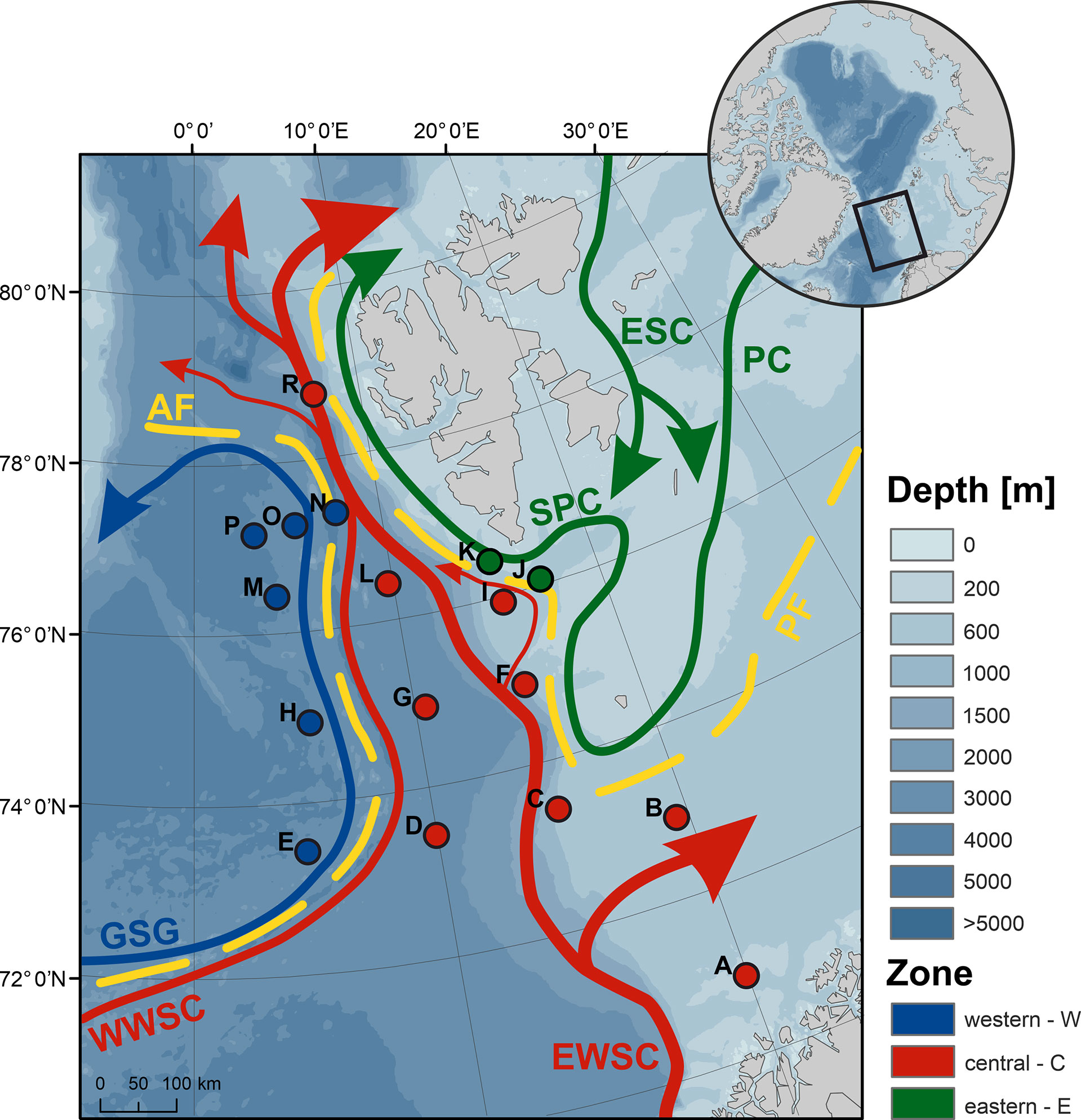
Figure 1 Location of sampling sites (A to R) with a general circulation pattern (ESC, East Spitsbergen Current; EWSC and WWSC, east and west branch of the West Spitsbergen Current; GSG, Greenland Sea Gyre; PC, Persey Current and SPC, Spitsbergen Polar Current), and with the two oceanic fronts (AF, Arctic Front and PF, Polar Front) marked in yellow. Both the oceanic currents and sites are colored according to the frontal zone classification (western W, blue; central C, red and eastern E, green), which, in the case of sites, reflects their prevailing classification (>70% of years falling within a given zone). Bathymetry data were derived from the International Bathymetric Chart of the Arctic Ocean (Jakobsson et al., 2012), while ocean currents were mapped after Beszczynska-Möller et al. (2012) and Lien et al. (2017). Inset map depicts the Arctic Ocean with the investigated area bordered by the black rectangle.
When on site, the Sea-Bird Electronics CTD (SBE 911plus) probe was first lowered down to the seabed, to record the vertical profiles of temperature and salinity. Then, the zooplankton was vertically sampled from the epipelagic zone (down to 200 m, or less at shallower, shelf sites) with the standard WP-2 net fitted with 180-µm filtering gauze, and then fixed with a borax-buffered 4% solution of formaldehyde in seawater. Noteworthy, no clogging of the net or any other issue that could impact calculations of the volume of water filtered by the net was reported for either of the sampling events. In all subsequent analyses, temperature and salinity were averaged over 0–200 m to match the zooplankton data.
Gelatinous animals were identified to the lowest taxonomic level possible, based on the key taxonomic references and species lists from the region (see Ronowicz et al., 2015, and references therein), and enumerated in each sample using a NIKON SMZ800 stereomicroscope. The abundance of GZ was expressed as the number of individuals per cubic meter [ind. m−3]. In the case of calycophoran siphonophores, separate numbers were given for the eudoxids and the polygastric colonies, with the latter corresponding to the numbers of anterior nectophores encountered, while the former equaled the number of eudoxid bracts. The number of physonect colonies was approximated, based on the nectophore counts, with the threshold of ten nectophores per colony (Guerrero et al., 2018) applied uniformly to all species found.
Position of the Fronts
The position of the AF was assumed to follow the 3°C isotherm at 100 m ± 5 m in the vicinity of the Knipovich Ridge (Walczowski et al., 2017). The position of the PF in Storfjorden Trough was evaluated based on the salinity averaged over 0–100 m, with the salinity of 34.86 taken as the threshold of the Atlantic water (Strzelewicz et al., 2022). The location of the PF along the West Spitsbergen Shelf was inferred from Strzelewicz et al. (2022) for years 2007–2014, and estimated from temperature and salinity distribution maps for 2003–2006. To facilitate comparison of the GZ community on either side of the fronts, a classification of sites, hereafter referred to as the frontal zone or zone for short, was introduced: sites located to the west of the AF, within the Greenland Sea Gyre—frontal zone W; sites to the east of the PF, on the West Spitsbergen Shelf—frontal zone E; and sites positioned centrally, between the two fronts—zone C (Figure 1). To analyze the consequences of the shifting biogeographic domains, an additional classification was used, which grouped the W and E frontal zones as the Arctic domain and referred to zone C as the Atlantic domain.
Interannual variation in the water mass distribution and position of the AF and PF was visualized based on the temperature and salinity measured at 100 m ( ± 5 m buffer), interpolated with the Data-Interpolating Variational Analysis (DIVA) and plotted in the Ocean Data View 4. A depth of 100 m was chosen as it corresponds to the upper part of the Atlantic water core in the European Arctic (Walczowski et al., 2012).
Data Preprocessing and Analysis
Analyzed data included GZ abundance and site-associated environmental data: temperature (averaged over 0–200 m; [°C]), salinity (averaged over 0–200 m), depth [m], latitude [DD], longitude [DD], and the frontal zone classification. The distribution of some data deviated significantly from the normal distribution (see the Supplementary Material for the results of Shapiro–Wilk tests); hence, non-parametric methods were used for testing differences between the frontal zones, and summaries were given as median ± interquartile range, unless otherwise stated. Exact values of test statistics and p were given in all cases, while the threshold for significance was set at p ≤ 0.05. To control the family-wise error rate, Holm–Bonferroni correction was used whenever multiple comparisons were run. Prior to further analyses, to reduce the weight of dominant taxa, GZ abundance was square root transformed, and the Bray–Curtis dissimilarity matrix was calculated.
First, the total abundance of GZ was compared between the frontal zones, between years and within each zone on an interannual scale with a series of Kruskal–Wallis tests, each followed by the Dunn’s post-hoc test for pairwise comparisons. Then, a permutational multivariate analysis of variance (PERMANOVA) (Anderson, 2001) was run with the adonis function from the vegan package (Oksanen et al., 2020) in R (v. 4.0.4) to test whether frontal zones differed in the GZ community composition, and, if so, then whether these differences were independent of time. Prior to running PERMANOVA, the assumption of homogeneity of group dispersion was tested with the permutest.betadisper function from the same package. Each Monte Carlo permutation was run in 999 replications. Full results of PERMANOVA are available in the Supplementary Material, while only pseudo-F and p-values are reported here.
Next, the community of the GZ in each frontal zone was analyzed, first with the comparative description of the percentage contribution of each species to the GZ community. Then, the median percentage contribution of the most abundant, Atlantic water-related species, Aglantha digitale (O. F. Müller, 1776), to the total abundance of GZ was compared between each frontal zone. Last, the data on the GZ community were scanned in the search of the taxa indicatory of the frontal zones, through the IndVal method using the strassoc function from the indicspecies package (de Cáceres and Legendre, 2009). Permutation p-values of the associations between species and frontal zones were then calculated with the signassoc function implemented in the same package (de Cáceres and Legendre, 2009).
In order to evaluate which environmental variables were the most influential in shaping the GZ community structure across the frontal zones, distance-based linear models (DistLM) were built, aided by a visual representation with the distance-based redundancy analysis (dbRDA), all run in PRIMER 7 with PERMANOVA+ add-on (Anderson et al., 2008). First, marginal effects were assessed separately for each explanatory variable (temperature, salinity, depth, longitude, and latitude). Because the GZ community remained under the joint influence of all variables, they were incorporated into the target model through the forward selection based on the adjusted R2 criterion (Legendre and Anderson, 1999).
Finally, to test whether position shifts of the AF and the PF were followed by changes in the GZ community, a series of tests were run. First, a subset of sites was chosen, which, throughout the study period, shifted from the Arctic domain (zone E or W) to the Atlantic domain, at least once. These were sites E, H, J, K, M, and N. Then, the GZ abundance and the median proportion of A. digitale were compared between the Arctic and the Atlantic community at a given site with the Wilcoxon signed-rank test. Additionally, another PERMANOVA with the test of multivariate homogeneity of group dispersion (permutest.betadisper) was run, to test whether shifting frontal association was followed by the change in the taxonomic composition of the GZ community.
Results
Oceanographic Data
The three front-related zones differed significantly in terms of hydrological conditions (Figure 2). The central zone C, located between the AF and the PF, was characterized by the highest median temperature (5.13°C ± 1.45°C) and salinity (35.11 ± 0.06) compared to the W and E zones (Figures 2A, B). The lowest median salinity was typical of zone E, which was located east of the PF (34.87 ± 0.07), while the lowest median temperature was recorded in zone W, west from the AF (1.40°C ± 1.54°C; Figures 2A, B). Uniformly shallow depths characterized the sites located within the eastern zone E, on the West Spitsbergen Shelf (166.5 m ± 126.0 m), while those scattered across zone C exemplified the largest variation in depth, with a median of 1,110.0 m ± 1,954.0 m. The deepest sampling sites were found in the western zone W, within the Greenland Sea Gyre (2,815.0 m ± 343.0 m). Overall, no significant differences in salinity or temperature were found between the zones belonging to the Arctic domain (zones W and E), but they did differ in terms of the average depth (Figure 2).
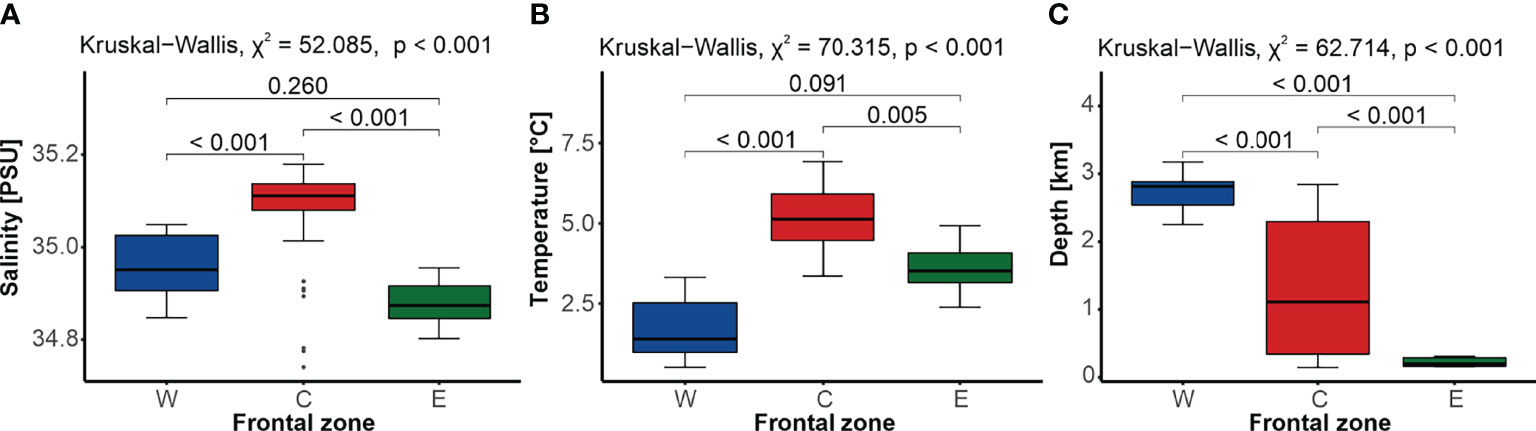
Figure 2 Comparison of water salinity (A), temperature (B), and depth (C) between sites located in the three frontal zones (western W, blue; central C, red; and eastern E, green), with the results of the Kruskal–Wallis test and the p-value of the Dunn’s post-hoc pairwise comparisons. Gray dots represent outliers.
Considerable temporal variations of both the temperature and the salinity were detected during the studied period (Figure 3). Interannual differences in temperature and salinity were observed during the two anomalously Atlantic-influenced periods (2004–2006 and 2011-2014), when the warm water masses (>6°C) occupied the largest part of the investigated area and reached the furthest north. Noteworthy, comparatively elevated temperatures were also detected in 2009. The presence of the colder, less saline waters over the West Spitsbergen Shelf also varied in time, with their largest extent onto the shelf during years 2003 and 2010 (Figure 3). Notable interannual differences were also observed regarding the Atlantic water penetration of the Storfjorden Trough, with years 2005, 2008, and 2010 characterized by the weakest presence of the warmer water in the trough. The varying position of the isotherm 3°C, indicatory of the AF, showcased maximal eastward displacement of the front in years 2003, 2008, and 2011. The spatial distributions of salinity and temperature corresponded well with each other, corroborating that in the anomalously warm years, more saline waters reached further north, as exemplified by the extremely high salinity in the southern region, exceeding even 35.3 (years: 2006, 2009, 2010, and 2013; Figure 3). Salinity distribution was also a good indicator of the extent of fresher, Arctic waters on the shelf, and these results agreed with the distribution of temperature. Additionally, the presence of the warm, but relatively fresh waters associated with the Norwegian Coastal Current could be inferred from Figure 3, as well as from Figure 2A, as evidenced by the presence of outliers.
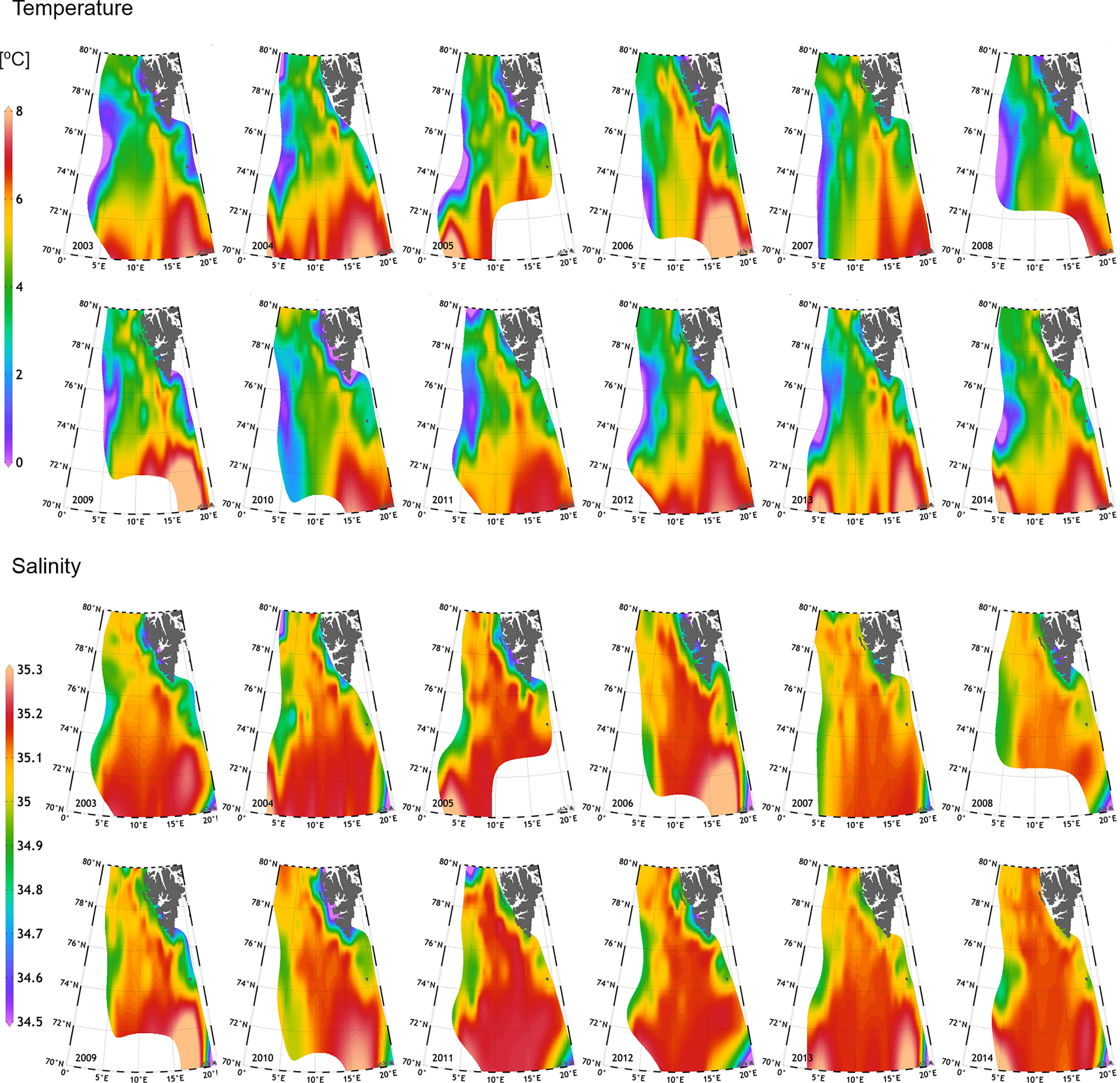
Figure 3 Interannual (2003–2014) variation in temperature (two upper rows) and salinity (two lower rows) at 100 m ± 5 m, within the European Arctic.
Gelatinous Zooplankton Abundance
GZ were present in all of the 120 examined samples. Their abundance was relatively low, with a median of 0.176 ind. m−3 ± 1.133 ind. m−3 and varied significantly between the frontal zones (Kruskal–Wallis, χ2 = 37.846, p < 0.001), though upon further examination, significant difference was identified only between the frontal zones W and C (Figure 4A). Consistently small numbers of GZ were typical of the zone W, with a median abundance of 0.059 ind. m−3 ± 0.056 ind. m−3 (Figure 4A). A slightly higher abundance of gelatinous animals was found in zone E (0.235 ind. m−3 ± 0.558 ind. m−3), while the most abundant community characterized the area between the fronts, in zone C (0.392 ind. m−3 ± 2.458 ind. m−3). Although based on the visual examination, the abundance of GZ seemed to vary interannually (Figure 4B), statistical analysis failed to uncover significant differences between years (Kruskal–Wallis, χ2 = 17.808, p = 0.086). However, when the frontal zones were analyzed separately, significant variation was found in the temporal variation of the GZ abundance within zone C (Kruskal–Wallis, χ2 = 23.044, p = 0.018; Supplementary Material).
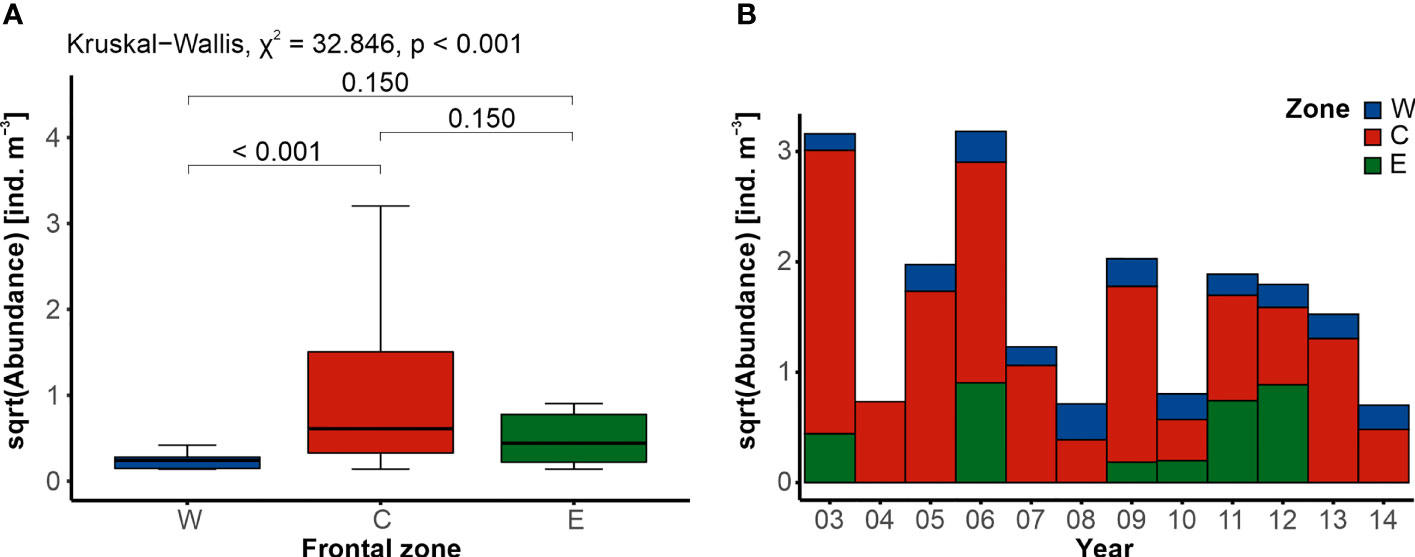
Figure 4 Differences in the abundance of gelatinous zooplankton. (A) Comparison of the average abundance between the three frontal zones (western W, blue; central C, red; and eastern E, green), with the results of statistical tests (Kruskal–Wallis followed by Dunn’s post hoc). (B) Interannual trend in the abundance within the frontal zones.
Gelatinous Zooplankton Diversity
Overall, fifteen taxa of the GZ were recorded throughout the study period (Figure 5C). The majority of these taxa were observed within the central zone C; however, these were mostly sporadic observations, with the most abundant species, A. digitale, comprising about 96% of all records. This species was found in all zones, with an average contribution to GZ abundance of ~67% in the frontal zone W, and ~56% in zone E (Figure 5A). The other taxa present in all zones were a siphonophore Dimophyes arctica (Chun, 1897) and a ctenophore Beroe spp. Interestingly, life-cycle stages (eudoxids and polygastric colonies) of D. arctica varied in their distribution between the zones, with no eudoxids found in the frontal zone W, and their numerical dominance (~5× times more abundant) over polygastric colonies in zones C and E. All the other siphonophore species identified in this study were restricted to the central zone C, while the other ctenophore species, Mertensia ovum (Fabricius, 1780), appeared both in frontal zones C and E. There were also some unidentified cydippid larvae, which occurred solely between the investigated oceanic fronts, in zone C. The only Narcomedusae species identified, Aeginopsis laurentii Brandt, 1838, was found exclusively in the frontal zone E, while no Anthomedusae were present there. Four species of Leptomedusae were identified, which, except for Melicertum octocostatum (M. Sars, 1835), were distributed only in the frontal zone C.
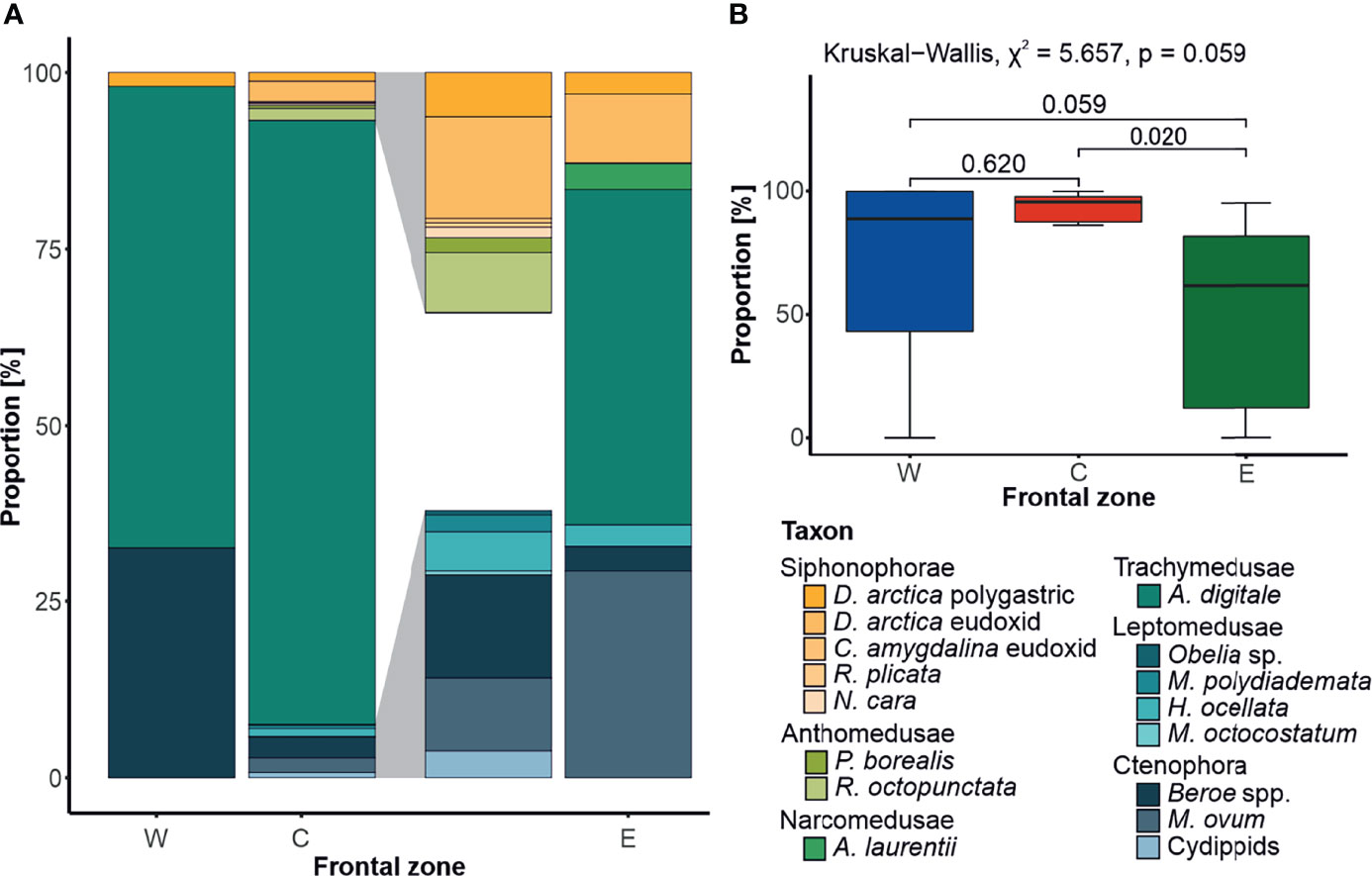
Figure 5 Front-related diversity of gelatinous zooplankton. (A) Species contribution to the gelatinous zooplankton diversity in the investigated frontal zones (W, western; C, central; and E, eastern), calculated based on the square root transformed data, with the 5× magnification of the portions of the middle bar highlighted in gray. (B) Comparison of Aglantha digitale median contribution [%] to the abundance of gelatinous zooplankton community in frontal zones, with the results of statistical tests (Kruskal–Wallis followed by Dunn’s post hoc).
The emerging picture of species/life-cycle stage affinity to a particular frontal zone was corroborated with the analysis of association. M. ovum (IndVal.g = 0.661, p = 0.001) was found to be indicatory of the eastern frontal zone E, alongside A. laurentii (IndVal.g = 0.354, p = 0.065) and eudoxids of D. arctica (IndVal.g = 0.369, p = 0.081). Beroe spp. exemplified strong affinity to zone W, located west of the AF (IndVal.g = 0.487, p = 0.044), while A. digitale was strongly associated with the central frontal zone C (IndVal.g = 0.833, p = 0.001).
Species composition of the GZ varied significantly between frontal zones (PERMANOVA, pseudo-F = 11.139, p = 0.003), and on the interannual scale (PERMANOVA, pseudo-F = 1.843, p = 0.003). Surprisingly, significant variation in the GZ community was found also on an interannual scale, within each zone (two-way PERMANOVA, pseudo-F = 2.429, p = 0.003). The analysis of multivariate homogeneity of group dispersion (pseudo-F = 1.515, p = 0.076) indicated, however, that the significance of the two-way PERMANOVA might have resulted from the variation in data dispersion within the two-factorial (Year × Frontal zone) groups.
Drivers of Gelatinous Zooplankton Community
A modelling approach revealed that species composition of GZ was tightly coupled with temperature and depth gradients and reflected the frontal zonation resulting from the presence of the AF and PF (Figure 6). Marginal tests within the DistLM framework revealed statistically significant influence on the community structure of the following variables: latitude (pseudo-F = 6.750, p = 0.001), longitude (pseudo-F = 16.831, p = 0.001), depth (pseudo-F = 13.661, p = 0.001), temperature (pseudo-F = 24.630, p = 0.001), and salinity (pseudo-F = 5.481, p = 0.002). However, when simultaneously incorporated to the model in the sequential test approach, only gradients of temperature (adjusted R2 = 0.166, p = 0.001) and depth (adjusted R2 = 0.176, p = 0.038) were found to significantly shape the community structure, with the explanatory power of 19% (Figure 6). Noteworthy, the addition of the remaining explanatory variables increased the explanatory power of the model to 21.1%, but this increment was not statistically significant.
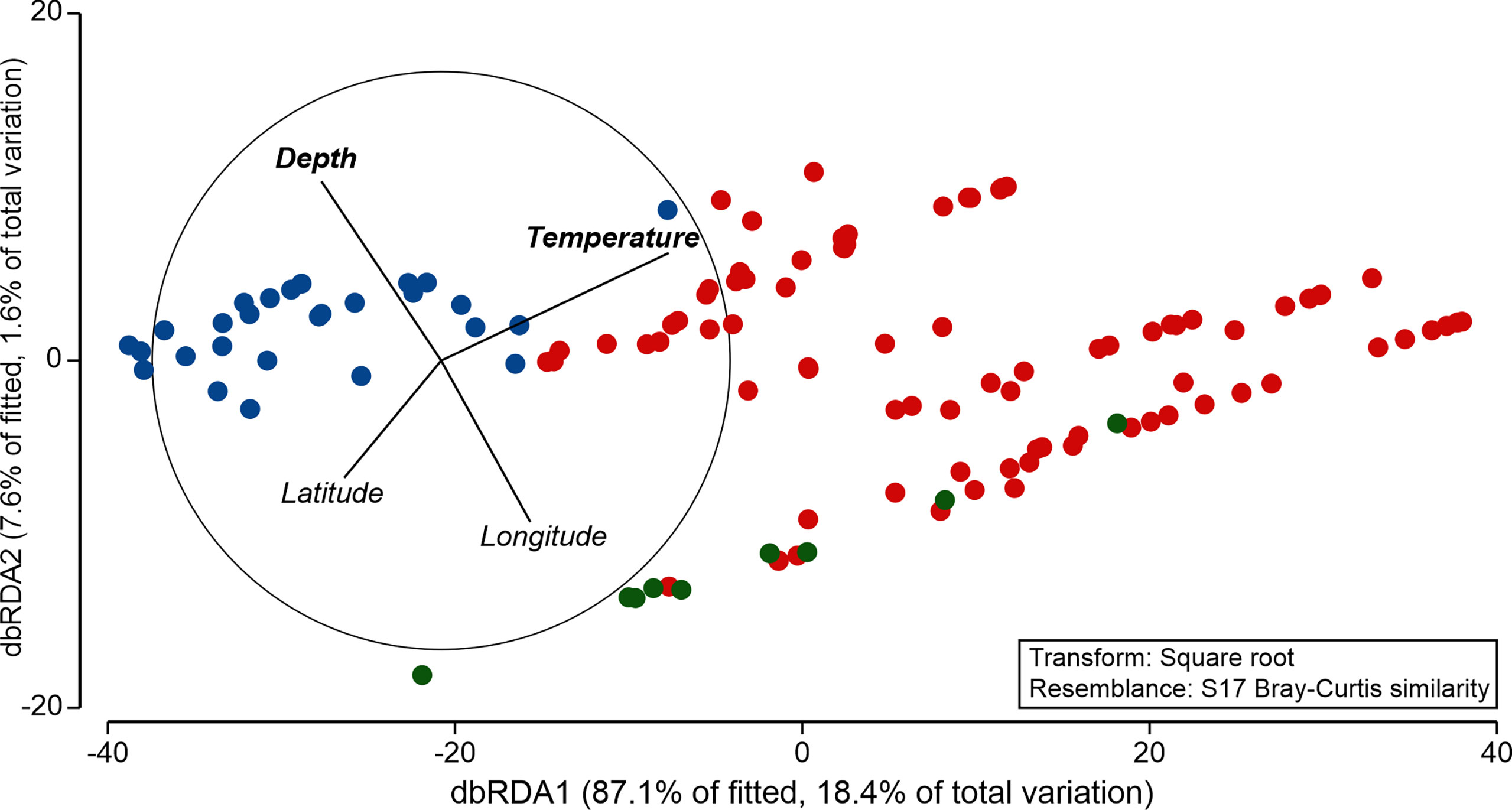
Figure 6 Similarity of the gelatinous zooplankton community in the frontal zones (W—blue, C—red, and E—green) along environmental gradients resolved by distance-based redundancy analysis (dbRDA). Variables in bold were statistically significant according to the DistLM model.
Impact of Shifting Fronts on GZ
Changes in the positions of oceanic fronts were accompanied by thorough restructuring of the local GZ community. Whenever a site went from being within the Arctic domain (zone W or E) to the Atlantic domain (zone C) or vice versa, a significant shift in the taxonomic composition was detected (PERMANOVA, pseudo-F = 2.303, p = 0.043). Moreover, when the front relocated, the site previously found in the Arctic domain ended up within the Atlantic domain (zone C), then the more abundant community was typically found at that site (Figure 7A). Interestingly, the shift in the domain was concomitant with the increase of the proportion of A. digitale in the abundance of the GZ community (Figure 7B).
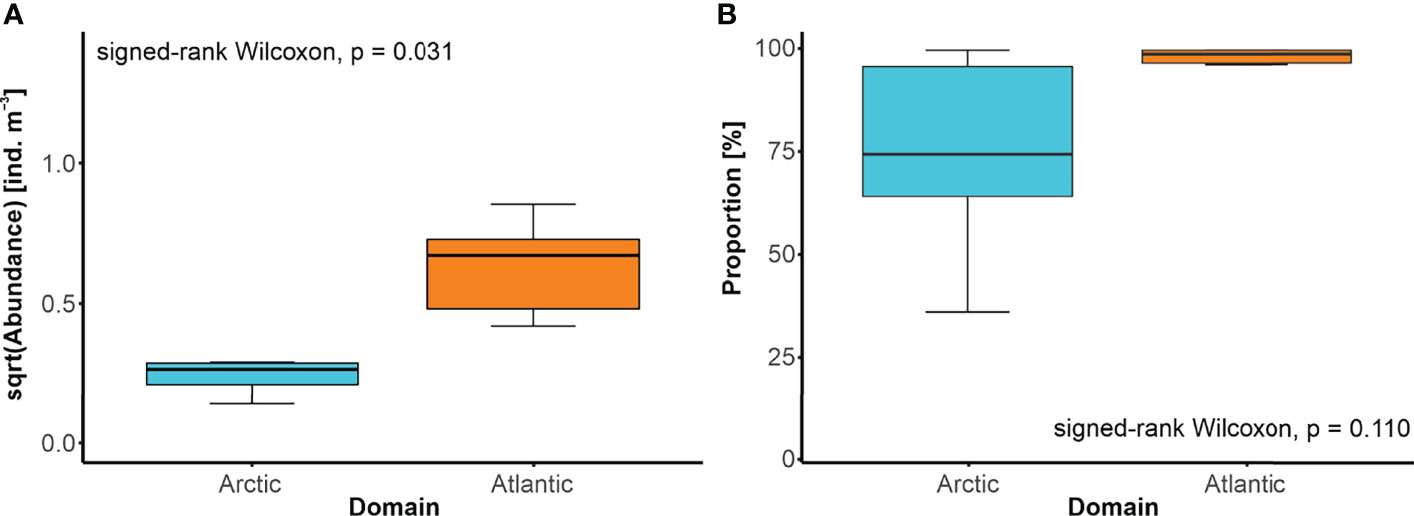
Figure 7 Modifications of the GZ community related to the shift in the front position. (A) Abundance of the GZ and (B) median proportion of Aglantha digitale [%] in the GZ abundance, on sites before and after the change in their affinity from the Arctic domain to the Atlantic domain, and vice versa, with the results of the Wilcoxon signed-rank test.
Discussion
Taking advantage of the unique, 12-year-long, polar planktonic time series, we showed that the two passive fronts of the European Arctic, the AF and the PF, maintained a certain degree of distinctiveness of the GZ communities between the adjacent water masses. We have documented that changes in the front position, mediated by the varying strength of the Atlantic water advection, were followed by a shift in the GZ community composition and abundance, thus further supporting the notion of oceanic fronts acting as impermeable barriers for GZ exchange. In a broader context, such shifts were found to reflect the clear-cut differences between the GZ in the Atlantic and Arctic domains, adding to the growing body of literature on the possible ecological impacts of the Atlantification (reviewed in Csapó et al., 2021).
The generally low abundance of GZ (0.176 ind. m−3 ± 1.133 ind. m−3; Figure 4) detected in this study, with the higher GZ numbers documented in the warmer, Atlantic water side of the AF and PF, is consistent with other records from the European Arctic (Mańko et al., 2020) and elsewhere in the world (e.g., Haberlin et al., 2019). When the front separates the dynamic shelf water from a warmer offshore water, more abundant GZ is usually found on the offshore-facing side of the front (Pagès et al., 1992). A plausible explanation is that the more stable, stratified oceanic waters provide a favorable condition for GZ to thrive (Pagès et al., 1992; Haberlin et al., 2019). However, epipelagic waters in the European Arctic, contrary to the remaining parts of the Arctic Ocean, are weakly stratified due to the increasing Atlantic water inflow (Polyakov et al., 2017; Polyakov et al., 2020), hence probably another mechanism underlies the GZ abundance pattern. Interestingly, an opposing GZ abundance distribution was described for the Mediterranean Sea, where the offshore water was numerically impoverished in GZ (Guerrero et al., 2016). Shelf waters are usually inhabited by the neritic taxa that exemplify a biphasic (polyp-medusae) life cycle, known to strongly relate to seasonality and local productivity (Gili et al., 1991), which could explain the atypical pattern of abundance found there. This points to the diversity of species as an important perspective for analyzing mesoscale patterns of GZ abundance distribution.
The two passive fronts of the investigated area have maintained distinct communities of the GZ (Figures 5, 6), in that a portion of taxa were unique for a particular frontal zone, but the few, most abundant ones, were common everywhere. Previous studies from the region arrived at a similar conclusion, like Descôteaux et al. (2021) who found that only one-third of meroplankton were shared among both sides of the PF. In any other place in the world’s ocean, the presence of the same taxa on both sides of the front would lead to the conclusion of the GZ community exchange across the passive fronts. However, in the European Arctic, an alternative explanation exists that accounts for an intricate pattern of the Atlantic water circulation. North of 76°N, a considerable portion of Atlantic water from the western branch of the West Spitsbergen Current recirculates towards the west and south as a Return Atlantic Current, which eventually combines with Polar water of the East Greenland Current, to form Arctic water flowing within the Greenland Sea Gyre (Walczowski, 2014; Raj et al., 2019). Similarly, the branch of the West Spitsbergen Current that wraps around the northern coast of Svalbard branches off and enters the Barents Sea, where it feeds the East Spitsbergen Current (Lind and Ingvaldsen, 2012) that reaches the Arctic-facing side of the PF. The presence of Atlantic-origin water in the eastern (E) and western (W) zones could thus plausibly justify the appearance of a typically boreal or boreo-Arctic species, like A. digitale, within the Arctic domain, simultaneously supporting barrier effects of the fronts (Figure 5). Alternatively, the presence of transient, shallow eddies, known to occur along the AF (van Aken et al., 1995), could provide some support for the notion of partial GZ community exchange between zones W and C.
The remaining part of the similarity in the GZ community composition between the frontal zones can be attributed to a puzzling taxonomy of the Arctic ctenophores. Their significant abundance detected here (Figure 5) is in line with a common assumption of their numerical dominance in the epipelagic GZ community of the Arctic (Raskoff et al., 2005; Purcell et al., 2010). In spite of that, the diversity of the Arctic ctenophores remains poorly resolved (e.g., Majaneva & Majaneva, 2013), mostly due to the inability to preserve their delicate bodies in the regular plankton samples. Therefore, our genus level records of Beroe spp. likely corresponded to more than one species, probably to the epipelagic B. cucumis and a deeper water species B. abyssicola (Raskoff et al., 2010; Licandro et al., 2015), hence justifying the presence of Beroe records in both zones W and E.
The overall number of species detected here is in line with previous works from the region (Zelickman, 1972; Mańko et al., 2015; Mańko et al., 2020). The fact that the central zone C harbored the most diverse GZ community could potentially be attributed to the large spatial extent of this zone, which encompassed not only purely oceanic ecosystems, but also a shallow shelf region under the influence of the Norwegian Coastal Current (Figures 1, 3). Therefore, the detection of meroplanktonic species (with benthic polyp phase) there is unsurprising, as they are known to numerically prevail in the shallower, coastal areas (Gili et al., 1991; Mańko et al., 2020). Additionally, some authors argued that, in general, GZ communities are more diverse with the increasing oceanic influence (see Haberlin et al., 2019 and references therein), but this assumption does not align with the paucity of GZ taxa found in the frontal zone W (Figure 5).
The most abundant species, A. digitale, was found in all zones, but constituted the largest proportion of the GZ in zone C. This species has previously been attributed to waters of Atlantic origin (Mańko et al., 2020), and was found to be a key driver of dissimilarity between neritic and oceanic GZ communities in the Celtic Sea (Haberlin et al., 2019). Likewise, we also found this species to be indicatory of zone C (IndVal.g = 0.833, p = 0.001). As for the remaining zones, the strongly associated species belong to Ctenophora, with Beroe spp. typical for the western zone W (IndVal.g = 0.487, p = 0.044) and M. ovum for the eastern zone E (IndVal.g = 0.661, p = 0.001). Interestingly, a similar set of indicatory species were found in previous studies of the local GZ community (Mańko et al., 2015; Mańko et al., 2020), hence strengthening the notion that spatial distribution of these three taxa should suffice to approximate the location of the polar fronts.
The community of GZ across the whole investigated area was structured mainly by depth and temperature (Figure 6). Proximity to the seabed is a key factor shaping the relative contribution of meroplanktonic to holoplanktonic cnidarian species, as the former are more common in shallower regions. Temperature, in turn, was advocated as a major driver of the GZ diversity (Guerrero et al., 2018), and probably also their abundance (Purcell, 2005). Having found the same set of GZ community drivers, Luo et al. (2014) suggested that these drivers may have a controlling role in shaping the GZ community, with only a regulating effect of the front position. However, it must be noted that the work of Luo et al. (2014) pertained to a transient, salinity-related, mesoscale front; hence, their finding cannot be adequately extrapolated to the permanent, but spatially variable fronts of the European Arctic.
Temporal trends of the Atlantic water advection depicted in Figure 3 confirm that waters of the European Arctic are becoming noticeably warmer and more saline. The increase in Atlantic water inflow, in terms of both the heat content and the volume transported northwards (Ingvaldsen et al., 2021), is inevitably followed by shifts in the position of the AF and PF that flank the Atlantic water (Figure 3). Their position may also vary with the tidal currents (the PF; Saloranta & Svendsen, 2001), and large-scale atmospheric forcing, like the North Atlantic Oscillation (the AF; Schlichtholz & Goszczko, 2006; Walczowski, 2013), though conflicting results of the lack of the atmospheric forcing impact on the AF position also exist (Raj et al., 2019).
The results of our analysis on the consequences of shifting position of the fronts for the GZ community (Figure 7) provided additional support for the barrier effect at the front. When a particular site switched its position relative to the front, a restructuring of the GZ community followed, which reflected the distinct abundance and diversity patterns associated with a given domain (Figure 7). In agreement with literature data (Drinkwater and Tande, 2014; Wassmann et al., 2015; Mańko et al., 2020), we also showed that the Atlantic zooplankton community is far more abundant than that in the Arctic domain. An important observation is also that the extremely substantial proportion of A. digitale in the GZ community is a typical feature of the Atlantic-facing side of either front (Figures 7B, 5B). The expansion of the Atlantic domain (Csapó et al., 2021), which could even reach the Arctic fjords (Weydmann-Zwolicka et al., 2021), through increasing the abundance of A. digitale, and that of the other small-bodied zooplankton in the Arctic (Balazy et al., 2018), may thus accelerate the restructuring of the local ecosystems (Ingvaldsen et al., 2021). Atlantification-related shifts in the plankton composition have already been linked to the diet alterations of the planktivorous seabirds (Vihtakari et al., 2018) and their foraging strategies (Jakubas et al., 2017; Stempniewicz et al., 2021). These shifts may also explain why the distribution ranges of more and more boreal fish species expand northwards (Haug et al., 2017) or why the North Atlantic population of the right whale struggles to recover (Meyer-Gutbrod and Greene, 2018).
Although our main conclusion on the role of passive fronts in maintaining distinct GZ communities appears to be supported by the presented results and their discussion, certain limitations of this study must be acknowledged. First, the relatively coarse spatial and vertical resolution of zooplankton sampling (Figure 1; Luo et al., 2014) might have concealed some of the more intricate, fine patterns of the GZ distribution. For example, their patchiness (Trudnowska et al., 2016) at the mesoscale oceanographic features like the AF-associated eddies (diameter 40-60 km; van Aken et al., 1995). Moreover, since both the AF and the PF undergo seasonal variation, confined to their surface layer (van Aken et al., 1995; Raj et al., 2019), we might have failed to capture the more dynamic situation of the GZ there. However, since the seasonal variation (at least in the case of the PF; Strzelewicz et al., 2022) often leads to the formation of a strong density gradient in the upper 50 m of the water column (Strzelewicz et al., 2022), then this should only reinforce the barrier effect of the front. Despite these two limitations, our sampling framework was detailed enough to capture the large-scale pattern of the front-related structuring of the GZ diversity.
Conclusions
The two passive fronts of the European Arctic, the AF and the PF, maintained distinct GZ communities, which differed not only in their abundance, but also in their taxonomic composition. The community associated with the warmer and saltier Atlantic water was characterized by the overall higher abundance and diversity, but with a clear numerical dominance of a single species, A. digitale. In contrast, the colder Arctic water harbored less abundant and less diverse communities, which were readily identifiable by the largest proportion of ctenophores, with Beroe spp. for the Greenland Sea Gyre, west of the AF, and M. ovum for the West Spitsbergen Shelf, east of the PF. This adds to the growing body of literature that advocates the usage of GZ as hydrological indicators, but more importantly points to the potential direction of the Arctic ecosystems evolution, in the progressing Atlantification scenario.
Interannual shifts in the position of the fronts were coupled with the dynamics of Atlantic water inflow, which is flanked on both sides by the fronts. GZ was found to tightly follow the front relocations, in that if a particular site shifted its position relative to the front, from the Atlantic-facing side to the Arctic-facing side or vice versa, then a GZ community specific for a given domain appeared. Taking the intricate pattern of water circulation in the European Arctic into account, e.g., recirculating/return currents, the two passive fronts, the AF and the PF, appeared to provide a semi-impermeable barrier for the GZ community, with only a minor cross-frontal exchange.
Data Availability Statement
The raw data supporting the conclusions of this article will be made available by the authors, without undue reservation.
Author Contributions
MKM and AW-Z contributed to the conception and design of the study. MKM conducted laboratory work, statistical analyses, data visualization and wrote the first draft of the manuscripts. AW-Z supervised the project and participated in the statistical analysis. MM analyzed hydrological data. SK coordinated field work and curated sample collection. MKM, MM, and AWZ acquired funding for this research. All authors contributed to the review and editing of the first draft, and have read, and approved the submitted version.
Funding
This work was supported by the following grants: MKM received funding from the Polish Ministry of Science and Higher Education through a Diamond Grant program (no. DI2014 020344); AW-Z was supported by the grant HIDEA—Hidden diversity of plankton in the European Arctic no. 2017/27/B/NZ8/01056 from the National Science Centre, Poland; MM’s work was financed by the National Science Centre, Poland through the DWINS Project (2016/21/N/ST10/02920).
Conflict of Interest
The authors declare that the research was conducted in the absence of any commercial or financial relationships that could be construed as a potential conflict of interest.
Publisher’s Note
All claims expressed in this article are solely those of the authors and do not necessarily represent those of their affiliated organizations, or those of the publisher, the editors and the reviewers. Any product that may be evaluated in this article, or claim that may be made by its manufacturer, is not guaranteed or endorsed by the publisher.
Acknowledgments
We want to thank all the crew members of the R/V Oceania and those who participated in the AREX expeditions for their demanding work in often challenging conditions that allowed for this study to come into existence.
Supplementary Material
The Supplementary Material for this article can be found online at: https://www.frontiersin.org/articles/10.3389/fmars.2022.941025/full#supplementary-material
References
Allen J. T., Brown L., Sanders R., Moore C. M., Mustard A., Fielding S., et al. (2005). Diatom Carbon Export Enhanced by Silicate Upwelling in the Northeast Atlantic. Nature 437, 728–732. doi: 10.1038/nature03948
Anderson M. J. (2001). Permutation Tests for Univariate or Multivariate Analysis of Variance and Regression. Can. J. Fish. Aquat. Sci. 58, 626–639. doi: 10.1139/cjfas-58-3-626
Anderson M. J., Gorley R. N., Clarke K. R. (2008). PERMANOVA+ for PRIMER: Guide to Software and Statistical Methods. (Plymouth, UK: Primer-E Ltd.)
Arai M. N. (1992). Active and Passive Factors Affecting Aggregations of Hydromedusae: A Review. Sci. Mar. 56, 99–108.
Balazy K., Trudnowska E., Wichorowski M., Błachowiak-Samołyk K. (2018). Large Versus Small Zooplankton in Relation to Temperature in the Arctic Shelf Region. Polar. Res. 37, 1427409. doi: 10.1080/17518369.2018.1427409
Basedow S. L., Zhou M., Tande K. S. (2014). Secondary Production at the Polar Front, Barents Sea , August 2007. J. Mar. Syst. 130, 147–159. doi: 10.1016/j.jmarsys.2013.07.015
Belkin I. M. (2004). Propagation of the “Great Salinity Anomaly” of the 1990s Around the Northern North Atlantic. Geophys. Res. Lett. 31, L08306. doi: 10.1029/2003GL019334
Belkin I. M., Cornillon P. C., Sherman K. (2009). Fronts in Large Marine Ecosystems. Prog. Oceanogr. 81, 223–236. doi: 10.1016/j.pocean.2009.04.015
Beszczynska-Möller A., Fahrbach E., Shauer U., Hansen E. (2012). Variability in Atlantic Water Temperature and Transport at the Entrance to the Arctic Ocean 1997–2010. ICES. J. Mar. Sci. 69, 852–863. doi: 10.1093/icesjms/fss056
Csapó H. K., Grabowski M., Węsławski J. M. (2021). Coming Home - Boreal Ecosystem Claims Atlantic Sector of the Arctic. Sci. Total. Environ. 771, 144817. doi: 10.1016/j.scitotenv.2020.144817
de Cáceres M., Legendre P. (2009). Associations Between Species and Groups of Sites: Indices and Statistical Inference. Ecology 90, 3566–3574. doi: 10.1890/08-1823.1
Descôteaux R., Ershova E., Wangensteen O. S., Præbel K., Renaud P. E., Cottier F., et al. (2021). Meroplankton Diversity, Seasonality and Life-History Traits Across the Barents Sea Polar Front Revealed by High-Throughput DNA Barcoding. Front. Mar. Sci. 8. doi: 10.3389/fmars.2021.677732
Drinkwater K. F., Tande K. (2014). Biophysical Studies of the Polar Front in the Barents Sea and the Arctic Front in the Norwegian Sea: Results From the NESSAR Project. J. Mar. Syst. 130, 131–133. doi: 10.1016/j.jmarsys.2013.11.011
Gili J. M., Pagès F., Fusté X. (1991). Mesoscale Coupling Between Spatial Distribution of Planktonic Cnidarians and Hydrographic Features Along the Galician Coast (Northwestern Iberian Peninsula). Sci. Mar. 55, 419–426.
Graham W. M., Pagès F., Hamner W. M. (2001). A Physical Context for Gelatinous Zooplankton Aggregations: A Review. Hydrobiologia 451, 199–212. doi: 10.1023/A:1011876004427
Guerrero E., Gili J.-M., Grinyó J., Sabatés A. (2018). Long-Term Changes in the Planktonic Cnidarian Community in a Mesoscale Area of the NW Mediterranean. PLos One 13, e0196431. doi: 10.1371/journal.pone.0196431
Guerrero E., Marrodán A., Sabatés A., Orejas C., Gili J. M. (2016). High Spatial Heterogeneity of Two Planktonic Cnidarian Species Related to the Variability of a Shelf-Slope Front at Short Time Scales. Sci. Mar. 80, 487–497. doi: 10.3989/scimar.04452.03A
Haberlin D., Raine R., McAllen R., Doyle T. K. (2019). Distinct Gelatinous Zooplankton Communities Across a Dynamic Shelf Sea. Limnol. Ocean. 64, 1802–1818. doi: 10.1002/lno.11152
Haug T., Bogstad B., Chierici M., Gjøsæter H., Hallfredsson E. H., Høines Å.S., et al. (2017). Future Harvest of Living Resources in the Arctic Ocean North of the Nordic and Barents Seas: A Review of Possibilities and Constraints. Fish. Res. 188, 38–57. doi: 10.1016/j.fishres.2016.12.002
Hays G. C., Doyle T. K., Houghton J. D. R. (2018). A Paradigm Shift in the Trophic Importance of Jellyfish? Trends Ecol. Evol. 33, 874–884. doi: 10.1016/j.tree.2018.09.001
Hays G. C., Richardson A. J., Robinson C. (2005). Climate Change and Marine Plankton. Trends Ecol. Evol. 20, 337–344. doi: 10.1016/j.tree.2005.03.004
Herron R. C., Leming T. D., Li J. (1989). Satellite-Detected Fronts and Butterfish Aggregations in the Northeastern Gulf of Mexico. Cont. Shelf. Res. 9, 569–588. doi: 10.1016/0278-4343(89)90022-8
Hosia A., Falkenhaug T., Baxter E. J., Pagès F. (2017). Abundance, Distribution and Diversity of Gelatinous Predators Along the Northern Mid-Atlantic Ridge: A Comparison of Different Sampling Methodologies. PLos One 12, 1–18. doi: 10.1371/journal.pone.0187491
Huthnance J. M. (1995). Circulation, Exchange and Water Masses at the Ocean Margin: The Role of Physical Processes at the Shelf Edge. Prog. Oceanogr. 35, 353–431. doi: 10.1016/0079-6611(95)80003-C
Ingvaldsen R. B., Assmann K. M., Primicerio R., Fossheim M., Polyakov I. V., Dolgov A. V. (2021). Physical Manifestations and Ecological Implications of Arctic Atlantification. Nat. Rev. Earth Environ. 2, 874–889. doi: 10.1038/s43017-021-00228-x
IPCC Core Writing Team (2014). “Climate Change 2014: Synthesis Report,” in Contribution of Working Groups I, II and III to the Fifth Assessment Report of the Intergovernmental Panel on Climate Change. Eds. Pachauri R. K., Meyer L. A. (Geneva, Switzerland: IPCC).
Jakobsson M., Mayer L., Coakley B., Dowdeswell J. A., Forbes S., Fridman B., et al. (2012). The International Bathymetric Chart of the Arctic Ocean (IBCAO) Version 3.0. Geophys. Res. Lett. 39, L12609. doi: 10.1029/2012GL052219
Jakubas D., Wojczulanis-Jakubas K., Iliszko L. M., Strøm H., Stempniewicz L. (2017). Habitat Foraging Niche of a High Arctic Zooplanktivorous Seabird in a Changing Environment. Sci. Rep. 7, 16203. doi: 10.1038/s41598-017-16589-7
Kwasniewski S., Gluchowska M., Jakubas D., Wojczulanis-Jakubas K., Walkusz W., Karnovsky N., et al. (2010). The Impact of Different Hydrographic Conditions and Zooplankton Communities on Provisioning Little Auks Along the West Coast of Spitsbergen. Prog. Oceanogr. 87, 72–82. doi: 10.1016/j.pocean.2010.06.004
Lebrato M., Pahlow M., Frost J. R., Küter M., Mendes P. D. J., Molinero J. C., et al. (2019). Sinking of Gelatinous Zooplankton Biomass Increases Deep Carbon Transfer Efficiency Globally. Global Biogeochem. Cycles. 33, 1764–1783. doi: 10.1029/2019GB006265
le Fèvre J. (1987). Aspects of the Biology of Frontal Systems. Adv. Mar. Biol. 23, 163–299. doi: 10.1016/S0065-2881(08)60109-1
Legendre P., Anderson M. J. (1999). Distance-Based Redundancy Analysis: Testing Multispecies Responses in Multifactorial Ecological Experiments. Ecol. Monogr. 69, 1–24. doi: 10.1890/0012-9615(1999)069[0001:DBRATM]2.0.CO;2
Licandro P., Blackett M., Fischer A., Hosia A., Kennedy J., Kirby R. R., et al. (2015). Biogeography of Jellyfish in the North Atlantic, by Traditional and Genomic Methods. Earth Syst. Sci. Data 7, 173–191. doi: 10.5194/essd-7-173-2015
Lien V. S., Schlichtholz P., Skagseth Ø., Vikebø F. B. (2017). Wind-Driven Atlantic Water Flow as a Direct Mode for Reduced Barents Sea Ice Cover. J. Clim. 30, 803–812. doi: 10.1175/JCLI-D-16-0025.1
Lind S., Ingvaldsen R. B. (2012). Variability and Impacts of Atlantic Water Entering the Barents Sea From the North. Deep. Res. Part I. Oceanogr. Res. Pap. 62, 70–88. doi: 10.1016/j.dsr.2011.12.007
Loeng H. (1991). Features of the Physical Oceanographic Conditions of the Barents Sea. Polar. Res. 10, 5–18. doi: 10.3402/polar.v10i1.6723
Long A. P., Haberlin D., Lyashevska O., Brophy D., O’Hea B., O’Donnell C., et al. (2021). Interannual Variability of Gelatinous Mesozooplankton in a Temperate Shelf Sea: Greater Abundance Coincides With Cooler Sea Surface Temperatures. ICES. J. Mar. Sci. 78, 1372–1385. doi: 10.1093/icesjms/fsab030
Luo J. Y., Grassian B., Tang D., Irisson J. O., Greer A. T., Guigand C. M., et al. (2014). Environmental Drivers of the Fine-Scale Distribution of a Gelatinous Zooplankton Community Across a Mesoscale Front. Mar. Ecol. Prog. Ser. 510, 129–149. doi: 10.3354/meps10908
Lüskow F., Galbraith M. D., Hunt B., Perry R. I., Pakhomov E. A. (2021). Gelatinous and Soft-Bodied Zooplankton in the Northeast Pacific Ocean: Organic, Elemental, and Energy Contents. Mar. Ecol. Prog. Ser. 665, 19–35. doi: 10.3354/meps13663
Majaneva S., Majaneva M. (2013). Cydippid Ctenophores in the Coastal Waters of Svalbard: Is it Only Mertensia Ovum? Polar. Biol. 36, 1681–1686. doi: 10.1007/s00300-013-1377-6
Mańko M. K., G, ł, uchowska M., Weydmann-Zwolicka A. (2020). Footprints of Atlantification in the Vertical Distribution and Diversity of Gelatinous Zooplankton in the Fram Strait (Arctic Ocean). Prog. Oceanogr. 189, 102414. doi: 10.1016/j.pocean.2020.102414
Mańko M. K., Panasiuk-Chodnicka A., Żmijewska M. I. (2015). Pelagic Coelenterates in the Atlantic Sector of the Arctic Ocean − Species Diversity and Distribution as Water Mass Indicators. Oceanol. Hydrobiol. Stud. 44, 466–479. doi: 10.1515/ohs-2015-0044
Meyer-Gutbrod E. L., Greene C. H. (2018). Uncertain Recovery of the North Atlantic Right Whale in a Changing Ocean. Glob. Change Biol. 24, 455–464. doi: 10.1111/gcb.13929
Oksanen J., Simpson G. L., Blanchet F. G., Kindt R., Legendre P., Minchin P. R., et al (2020). vegan: Community Ecology Package. R package version 2.5-7
Olson D., Hitchcock G., Mariano A., Ashjian C., Peng G., Nero R., et al. (1994). Life on the Edge: Marine Life and Fronts. Oceanography 7, 52–60. doi: 10.5670/oceanog.1994.03
Oziel L, Sirven J, Gascard J.-C (2016). The Barents Sea frontal zones and water masses variability (1980-2011). Ocean Sci.12, 169–18410.5194/os-12-169-2016
Pagès F., Gili J. M. (1992). Influence of Agulhas Waters on the Population Structure of Planktonic Cnidarians in the Southern Benguela Region. Sci. Mar. 56, 109–123.
Pagès F., Gili J.-M., Bouillon J. (1992). Medusae (Hydrozoa, Scyphozoa, Cubozoa) of the Benguela Current (Southeastern Atlantic). Sci. Mar. 56, 1–64.
Polyakov I. V., Alkire M. B., Bluhm B. A., Brown K. A., Carmack E. C., Chierici M., et al. (2020). Borealization of the Arctic Ocean in Response to Anomalous Advection From Sub-Arctic Seas. Front. Mar. Sci. 7. doi: 10.3389/fmars.2020.00491
Polyakov I. V., Pnyushkov A. V., Alkire M. B., Ashik I. M., Baumann T. M., Carmack E. C., et al. (2017). Greater Role for Atlantic Inflows on Sea-Ice Loss in the Eurasian Basin of the Arctic Ocean. Science 356, 285–291. doi: 10.1126/science.aai8204
Purcell J. E. (2005). Climate Effects on Formation of Jellyfish and Ctenophore Blooms: A Review. J. Mar. Biol. Assoc. United. Kingdom. 85, 461–476. doi: 10.1017/S0025315405011409
Purcell J. E., Hopcroft R. R., Kosobokova K. N., Whitledge T. E. (2010). Distribution, Abundance, and Predation Effects of Epipelagic Ctenophores and Jellyfish in the Western Arctic Ocean. Deep. Res. Part II. Top. Stud. Oceanogr. 57, 127–135. doi: 10.1016/j.dsr2.2009.08.011
Raj R. P., Chatterjee S., Bertino L., Turiel A., Portabella M. (2019). The Arctic Front and its Variability in the Norwegian Sea. Ocean. Sci. 15, 1729–1744. doi: 10.5194/os-15-1729-2019
Raskoff K. A., Hopcroft R. R., Kosobokova K. N., Purcell J. E., Youngbluth M. (2010). Jellies Under Ice: ROV Observations From the Arctic 2005 Hidden Ocean Expedition. Deep. Res. Part II. Top. Stud. Oceanogr. 57, 111–126. doi: 10.1016/j.dsr2.2009.08.010
Raskoff K. A., Purcell J. E., Hopcroft R. R. (2005). Gelatinous Zooplankton of the Arctic Ocean: In Situ Observations Under the Ice. Polar. Biol. 28, 207–217. doi: 10.1007/s00300-004-0677-2
Ronowicz M., Kukliński P., Mapstone G. M. (2015). Trends in the Diversity, Distribution and Life History Strategy of Arctic Hydrozoa (Cnidaria). PLos One 10, 1–19. doi: 10.1371/journal.pone.0120204
Russell R. W., Harrison N. M., Hunt J. G.L. (1999). Foraging at a Front: Hydrography, Zooplankton, and Avian Planktivory in the Northern Bering Sea. Mar. Ecol. Prog. Ser. 182, 77–93. doi: 10.3354/meps182077
Saloranta T. M., Svendsen H. (2001). Across the Arctic Front West of Spitsbergen: High- Resolution CTD Sections From 1998 – 2000. Polar. Res. 20, 177–184. doi: 10.3402/polar.v20i2.6515
Schlichtholz P., Goszczko I. (2006). Interannual Variability of the Atlantic Water Layer in the West Spitsbergen Current at 76.5N in Summer 1991-2003. Deep. Res. Part I. Oceanogr. Res. Pap. 53, 608–626. doi: 10.1016/j.dsr.2006.01.001
Stempniewicz L., Weydmann-Zwolicka A., Strzelewicz A., Goc M., G, ł, uchowska M., Kidawa D., et al. (2021). Advection of Atlantic Water Masses Influences Seabird Community Foraging in a High-Arctic Fjord. Prog. Oceanogr. 193, 102549. doi: 10.1016/j.pocean.2021.102549
Strzelewicz A., Przyborska A., Walczowski W. (2022). Increased Presence of Atlantic Water on the Shelf South-West of Spitsbergen With Implications for the Arctic Fjord Hornsund. Prog. Oceanogr. 200, 102714. doi: 10.1016/j.pocean.2021.102714
Stukel M. R., Aluwihare L. I., Barbeau K. A., Chekalyuk A. M., Goericke R., Miller A. J., et al. (2017). Mesoscale Ocean Fronts Enhance Carbon Export Due to Gravitational Sinking and Subduction. Proc. Natl. Acad. Sci. 114, 1252–1257. doi: 10.1073/pnas.1609435114
Swift J. H., Aagaard K. (1981). Seasonal Transitions and Water Mass Formation in the Iceland and Greenland Seas. Deep. Res. 28A, 1107–1129. doi: 10.1016/0198-0149(81)90050-9
Teigen S. H., Nilsen F., Gjevik B. (2010). Barotropic Instability in the West Spitsbergen Current. J. Geophys. Res. Oceans. 115, C07016. doi: 10.1029/2009JC005996
Trudnowska E., Gluchowska M., Beszczynska-Möller A., Blachowiak-Samolyk K., Kwasniewski S. (2016). Plankton Patchiness in the Polar Front Region of the West Spitsbergen Shelf. Mar. Ecol. Prog. Ser. 560, 1–18. doi: 10.3354/meps11925
van Aken H. M., Budéus G., Hähnel M. (1995). The Anatomy of the Arctic Frontal Zone in the Greenland Sea. J. Geophys. Res. 100, 15999–16014. doi: 10.1029/95JC01176
Vihtakari M., Welcker J., Moe B., Chastel O., Tartu S., Hop H., et al. (2018). Black-Legged Kittiwakes as Messengers of Atlantification in the Arctic. Sci. Rep. 8, 1178. doi: 10.1038/s41598-017-19118-8
Walczowski W. (2013). Frontal Structures in the West Spitsbergen Current Margins. Ocean. Sci. 9, 957–975. doi: 10.5194/os-9-957-2013
Walczowski W. (2014). Atlantic Water in the Nordic Seas. Properties, Variability, Climatic Importance (Berlin Heidelberg: Springer). doi: 10.1007/978-3-319-01279-7
Walczowski W., Beszczynska-Möller A., Wieczorek P., Merchel M., Grynczel A. (2017). Oceanographic Observations in the Nordic Sea and Fram Strait in 2016 Under the IOPAN Long-Term Monitoring Program AREX. Oceanologia 59, 187–194. doi: 10.1016/j.oceano.2016.12.003
Walczowski W., Piechura J. (2007). Pathways of the Greenland Sea Warming. Geophys. Res. Lett. 34, L10608. doi: 10.1029/2007GL029974
Walczowski W., Piechura J., Goszczko I., Wieczorek P. (2012). Changes in Atlantic Water Properties: An Important Factor in the European Arctic Marine Climate. ICES. J. Mar. Sci. 69, 864–869. doi: 10.1093/icesjms/fss068Changes
Wassmann P., Kosobokova K. N., Slagstad D., Drinkwater K. F., Hopcroft R. R., Moore S. E., et al. (2015). The Contiguous Domains of Arctic Ocean Advection: Trails of Life and Death. Prog. Oceanogr. 139, 42–65. doi: 10.1016/j.pocean.2015.06.011
Weydmann-Zwolicka A., Prątnicka P., Łącka M., Majaneva S., Cottier F., Berge J. (2021). Zooplankton and Sediment Fluxes in Two Contrasting Fjords Reveal Atlantification of the Arctic. Sci. Total Environ. 773, 145599. doi: 10.1016/j.scitotenv.2021.145599
Wright R. M., le Quéré C., Buitenhuis E., Pitois S., Gibbons M. J. (2021). Role of Jellyfish in the Plankton Ecosystem Revealed Using a Global Ocean Biogeochemical Model. Biogeosciences 18, 1291–1320. doi: 10.5194/bg-18-1291-2021
Keywords: jellyfish, Atlantification, West Spitsbergen Current, Arctic Front, Polar Front, Climate Change
Citation: Mańko MK, Merchel M, Kwasniewski S and Weydmann-Zwolicka A (2022) Oceanic Fronts Shape Biodiversity of Gelatinous Zooplankton in the European Arctic. Front. Mar. Sci. 9:941025. doi: 10.3389/fmars.2022.941025
Received: 11 May 2022; Accepted: 06 June 2022;
Published: 14 July 2022.
Edited by:
Daniel J. Mayor, National Oceanography Centre, United KingdomReviewed by:
Cathy Lucas, University of Southampton, United KingdomClive Fox, Scottish Association For Marine Science, United Kingdom
Copyright © 2022 Mańko, Merchel, Kwasniewski and Weydmann-Zwolicka. This is an open-access article distributed under the terms of the Creative Commons Attribution License (CC BY). The use, distribution or reproduction in other forums is permitted, provided the original author(s) and the copyright owner(s) are credited and that the original publication in this journal is cited, in accordance with accepted academic practice. No use, distribution or reproduction is permitted which does not comply with these terms.
*Correspondence: Maciej K. Mańko, bWFjaWVqLm1hbmtvQHVnLmVkdS5wbA==