- 1Departamento de Biología Marina, Facultad de Ciencias del Mar, Universidad Católica del Norte, Coquimbo, Chile
- 2Oceana Chile, Santiago, Chile
- 3Laboratorio de Ecología Funcional, Instituto de la Patagonia, Universidad de Magallanes, Punta Arenas, Chile
Knowledge about the composition, diversity, and geographic distribution of marine species is important for successful conservation planning in the future. The ecological and zoogeographic patterns of benthic communities in Central Patagonia have been scarcely studied, due to the remoteness of the area combined with harsh weather conditions. During the past years, five scientific expeditions were executed in order to study the biodiversity, ecological, and biogeographical patterns of benthic invertebrates in the Katalalixar National Reserve (KNR) waters, Central Patagonia (~48°S). Our analyses comprised images from 26 video transects using a remotely operated vehicle, completed with biological sampling at four stations by means of SCUBA diving, covering a bathymetric range from 10 to 220 m depth. Stations covered the entire longitudinal range of the KNR, from inner channels to the Pacific Ocean. A total of 187 benthic invertebrate taxa were identified as OTUs (operational taxonomic units), with mollusks being the most conspicuous taxonomic group (18.7%), followed by sponges, echinoderms (16.6% each), and arthropods (14.4%). A higher OTU richness (42 to 51 OTUs) was observed in the central and western parts of the KNR waters. Analyses of the β-diversity indicated a similar level of species turnover between shallow, intermediate, and deep strata, as well as an important turnover between different locations. Dissimilitudes in the assemblage structure of invertebrates were explained mainly by changes in substrate types and longitude. Most of the species (49%) found in the KNR waters showed a wide latitudinal distribution range along the Eastern South Pacific Ocean (ESP) and the Chilean Patagonia of fjords and channels (CPFC) (~18°S and ~56°S), whereas 9.4% of the species have a wide distribution range between the CPFC and south of the Antarctic polar front (SAPF) (~42°S and ~65°S). Since only 16.7% of the species identified in the KNR are distributed exclusively in CPFC waters, it may be considered a transition area for marine invertebrates. It is distributed between northern ESP and SAPF. Knowledge of species composition and distribution patterns along spatial and environmental gradients is essential for any sustainable management, monitoring, and future conservation plans to protect the fragile and diverse marine ecosystems of Chilean Patagonia.
Introduction
Knowledge about the composition, diversity, and geographic distribution of marine species is a central issue to understanding the ecological patterns of marine ecosystems, especially considering that climate change already alters these patterns at the global scale (Cooley et al., 2022). In order to protect marine ecosystems and their functioning, efforts of the scientific community as well as of local governments and international non-governmental organizations have focused on the establishment of marine protected areas (MPAs) around the world. Currently, a total of 11 marine areas with different levels of protection (e.g., nature sanctuaries, marine reserves, marine protected areas, and marine parks) have been established inside the Chilean Patagonia fjord and channel region (CPFC), and four of them have been established during the last decade (Pitipalena-Añihue in northern Patagonia, Tortel in central Patagonia, and Seno Almirantazgo and Diego Ramírez-Drake passage in southern Patagonia; Tecklin et al., 2021). Despite these achievements in marine conservation in the CPFC, the structure of benthic communities and their ecological patterns have been poorly studied and show a comparable but unequal level of development.
The CPFC is subdivided into three major areas: North Patagonia (42°–46.5°S), Central Patagonia (46.5°–53°S), and South Patagonia (53°-56°S) (Silva and Prego, 2002; Aracena et al., 2011). Located in the central part of the CPFC, the Katalalixar National Reserve (KNR) is an archipelago surrounded mostly by brackish waters and interconnected by channels and fjords, representing a geographical puzzle of landscapes. The KNR waters could be considered one of the few remaining “pristine areas” of the CPFC; due to the remote access, the population density is still low, and the anthropogenic impacts associated with salmon farming or intensive fisheries are nearly absent. The KNR is nearly isolated from the central and southern areas of Patagonia, with limited access both from land and sea. The research of the KNR marine ecosystems is limited, and the sublittoral benthic communities and their ecological patterns are still almost unknown since only a few studies have been made in central Patagonia (e.g., Häussermann and Försterra, 2007; Quiroga et al., 2012; Försterrra et al., 2017; Friedlander et al., 2021). In contrast, most studies about the ecology of sublittoral benthic communities of the CPFC have been carried out mainly in the north (e.g., Häussermann et al., 2013; Zapata-Hernández et al., 2016; Betti et al., 2017; Betti et al., 2021; Villalobos et al., 2021) and south (e.g., Gerdes and Montiel, 1999; Montiel et al., 2005; Ríos et al., 2007; Montiel et al., 2011).
Worldwide research on benthic communities using remotely operated vehicles (ROVs) has increased recently in isolated areas, deep sea areas, or environments with harsh weather conditions that are inaccessible to diving or traditional benthic sampling methods (Costello et al., 2018; Tapia-Guerra et al., 2021). In the CPFC, studies based on underwater photography in northern and southern Patagonia were obtained by SCUBA diving (e.g., Cárdenas and Montiel, 2015; Betti et al., 2017; Villalobos et al., 2021), or by means of an underwater camera operated from a vessel (e.g., Gutt et al., 1999). Nevertheless, studies using ROV are lacking for central Patagonia, including the surrounding waters of KNR, and even more for benthic communities from the deeper areas of the channels and fjords.
The zoogeography of marine benthic species of the CPFC has been studied since the 19th century, with analysis of the distribution patterns achieved using two different approaches. Some studies comprise multiple taxonomic groups (e.g., Viviani, 1979; Brattström and Johanssen, 1983; Stuardo and Valdovinos, 1992; Lancellotti and Vásquez, 1999; Fernández et al., 2000; Camus, 2001), whereas other studies have been focused on selected taxonomic groups, such as Porifera (Pansini and Sarà, 1999), Anthozoa (Häussermann and Försterra, 2005; Häussermann, 2006), Mollusca (Linse, 1999), Polychaeta (Montiel et al., 2005), Peracarida (Brandt, 1999; De Broyer and Rauschert, 1999), Decapoda (Gorny, 1999), and Bryozoa (Moyano, 1999). However, all these studies show some divergences related to the biogeographical patterns of benthic species within the CPFC. In this sense, some studies analyzed the distribution patterns within the continental shelf of the Southern Pacific Coast (Viviani, 1979; Brattström and Johanssen, 1983; Lancellotti and Vásquez, 2000; Camus, 2001; Hernández et al., 2005), whereas others considered the extension of species distribution from the CPFC towards the continental shelf of the Antarctic continent (Gorny, 1999; Pansini and Sara, 1999; Moyano, 1999; De Broyer and Raushert 1999; Brandt, 1999; Montiel et al., 2005; Linse et al., 2006; Griffiths et al., 2009; Retamal and Moyano, 2010). However, emergent issues about how climate change and anthropogenic activities may affect species distribution have become more relevant since the biogeographical limits seem to have changed; for example, several species from cold temperate areas have been recorded lately to the south of the Antarctic Polar front and inside Antarctic waters (Thatje et al., 2005; Aronson et al., 2007; Cárdenas et al., 2020; Hughes et al., 2020) whereas others were found expanding southwards within Chilean Patagonia (Häussermann et al., 2021). On the other hand, how many Antarctic benthic species also inhabit the bottoms of the CPFC is still an open question.
In this context, the goals of this study include: 1) to determine the species composition, diversity (α and β), and structure of benthic invertebrate assemblages across the KNR waters, 2) to determine putative drivers affecting the spatial patterns of benthic invertebrate assemblages, and 3) to analyze the latitudinal distribution patterns of benthic taxa inhabiting the KNR waters. Since the KNR is in Central Patagonia, this study also provides the missing link to better understand the distribution patterns of benthic invertebrates within different regions across the CPFC and beyond the northern and southern limits of Patagonia, towards the Southeastern Pacific Ocean and Antarctic polar front.
Materials and methods
Study area
The KNR is in the fjord and channel region of Chilean Central Patagonia, between 47.5°S and 48.5°S, bordering with the Gulf of Penas in the north, and the Castillo Channel in the south. Across the longitudinal section, the KNR extends from the coastal lines of the islands fronting the Pacific Ocean in the west ~150 km towards the east in the Troya Channel. This reserve has a total surface area of 674,500 hectares (Gorny et al., 2020) (Figure 1). As in the entire CPFC region, most of the coastal borders are formed mainly by rocky reefs up to a depth of 40 m (Soto, 2009). The sea surface temperature fluctuates between 6.9 and 10.1°C, and the salinity of inner waters near effluents of rivers reaches 10 psu on average in the first few meters depth, whereas in areas further away from these bodies of freshwater, the salinity increases, reaching >32 psu towards the open ocean (Sievers et al., 2002). The waters around the inner islands are highly influenced by the Baker River, which provides organic matter, detritus, and nutrients derived from glaciers, influencing the composition of the macrobenthic communities in coastal zones (Quiroga et al., 2016). Recently, preliminary results on the benthic fauna of KNR waters were obtained by Gorny et al. (2020), who describe the different marine communities present in the area.
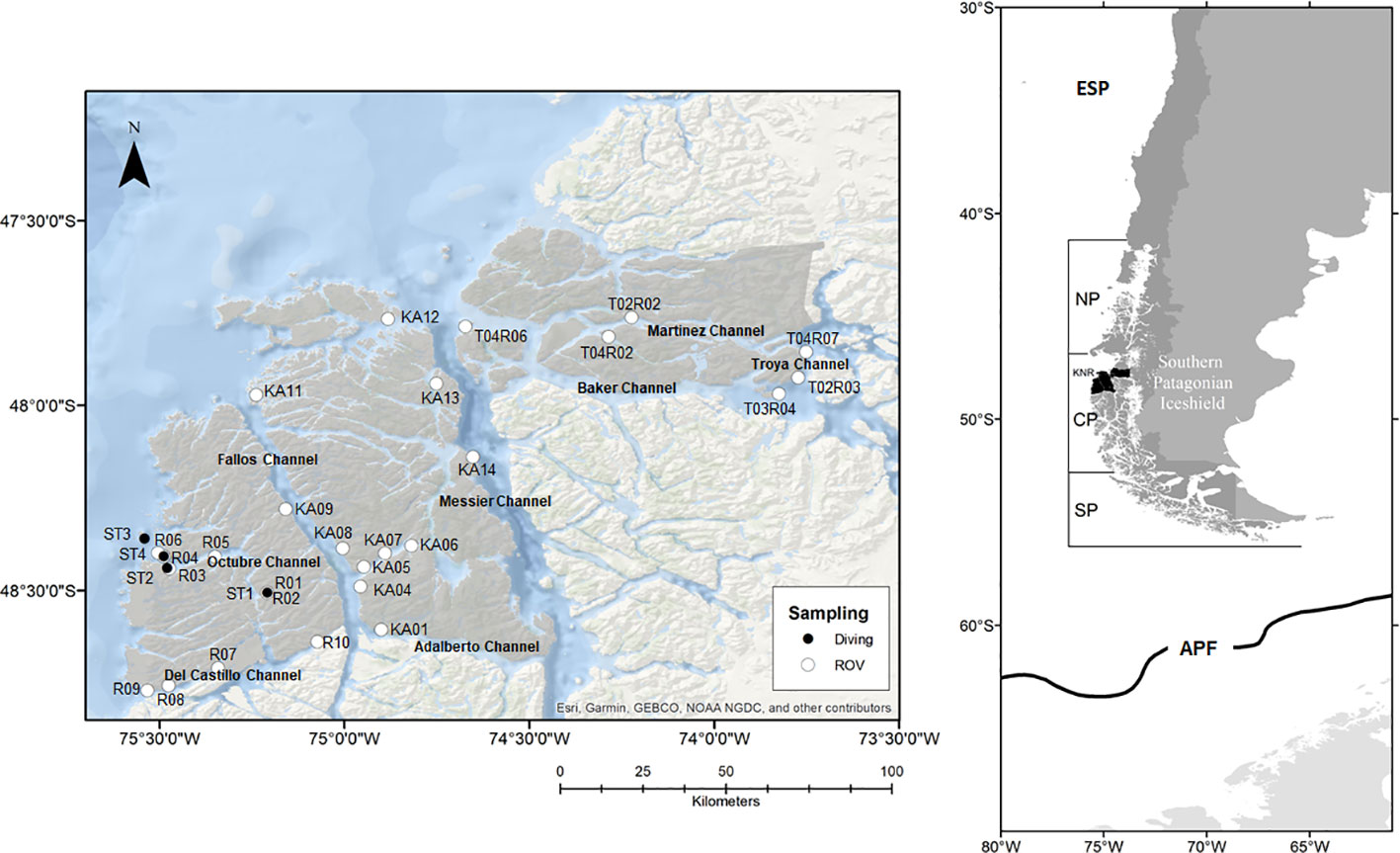
Figure 1 Map indicating the positions of the ROV and diving stations across the fjords and channels in the KNR waters. The gray landscape represents the area of the KNR.
Underwater video with a remotely operated vehicle
Video images of 26 transects using a remotely operated vehicle (ROV) were analyzed in this study, performed between austral spring 2008 and winter 2009 (six transects), austral summer 2017 (nine transects), and austral winter 2018 (11 transects) (Figure 1, Table 1). Except for the transects from 2008 and 2009, all videos were filmed using the ROV Commander MK II (Mariscope Meerestechnik, Kiel, Germany), equipped with an HD camcorder (Panasonic SD 909, resolution 1,920 × 1,080, 30 fps), a GoPro Hero camera (resolution 2,704 × 1,520, 60 fps), and two laser pointers (mounted 10 cm apart between the front cameras). The videos from 2008 and 2009 were obtained using a smaller ROV (FO, Mariscope) and filmed in HD (1,440 × 1,080, 30 fps). Front lights of 500 W each (four on the Commander and two on the FO) illuminated the areas where images were recorded. Both ROVs were equipped with an electronic compass and a depth sensor. These data were presented as video text on the surface monitor and were recorded separately and in real time on a laptop connected directly to the ROV remote control. Transects from 2008 and 2009 were included to complete the study using unpublished data from the easternmost part of the KNR waters. Altogether, the video transects covered a depth range between 10 and 220 m (Table 1).
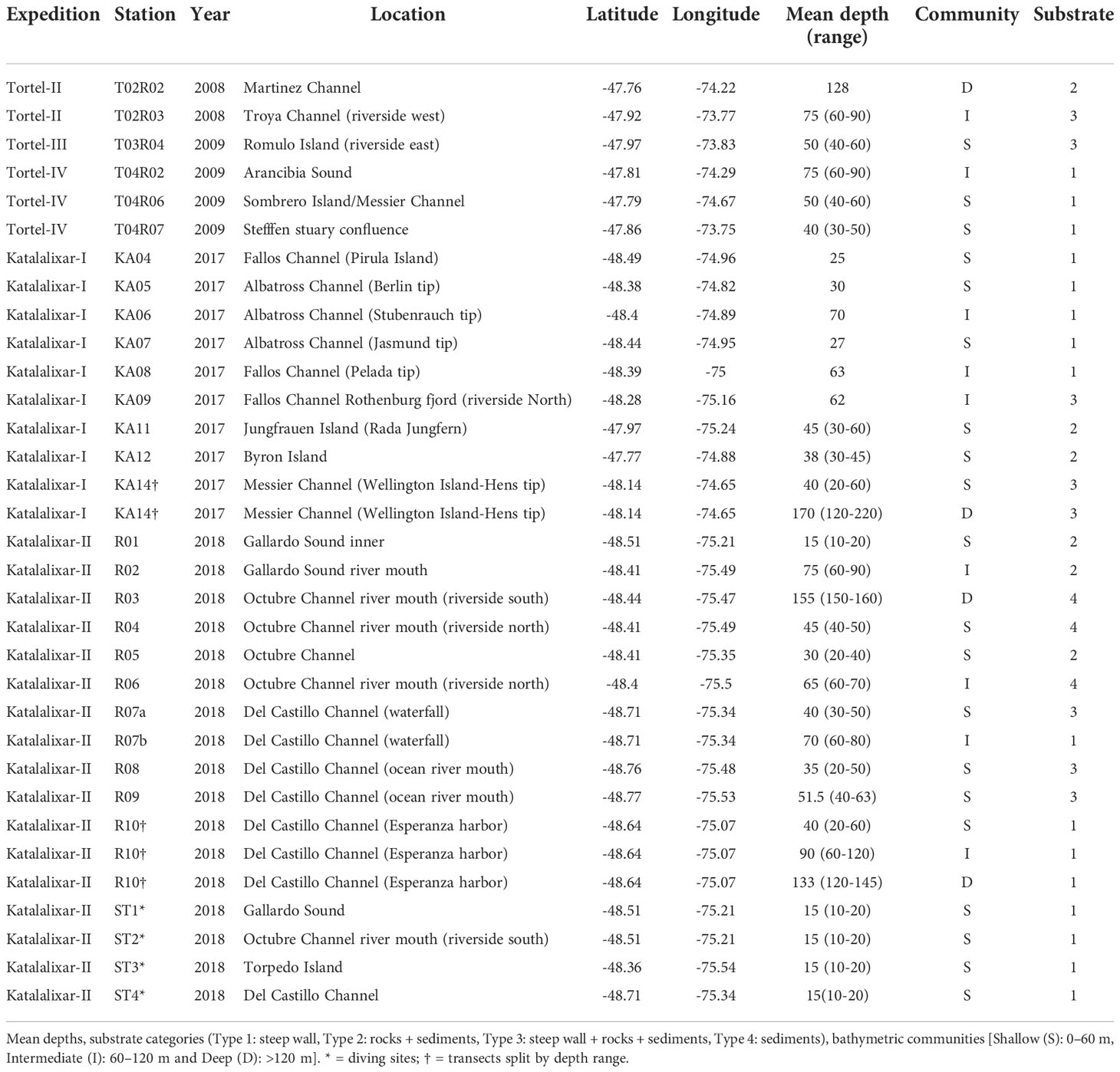
Table 1 Summary of expeditions; station list of sampling locations (latitude and longitude) during austral summer (2008, 2009, and 2018) and during austral winter (2009 and 2017).
Transects on hard substrates were conducted by maintaining the vessel parallel to the coast and lowering the ROV approximately to the grounding line between the rock wall and the plain seafloor of the commonly U-shaped channels. Then, the ROV was driven upward along the steep walls or terraces formed by rocks and boulders toward the surface. In some locations, the ROV was stopped near the surface (~20 m); in other places, the geomorphology, weather conditions, or strong currents allowed for observing only a limited depth range. On soft bottoms, a fixed water depth was filmed, maintaining the vessel directly above the ROV and following it when moving forward. No underwater GPS or sensor was mounted on the ROVs, but since the channel walls were comparably steep, the geographical position registered onboard the vessel was used, considering that the deviation between the ship and ROV was always less than 50 m, as measured by the length of the submarine tether connected to the ROV. On the flat ground, the deviation between vessel and ROV was even less, keeping the ship directly above the ROV. Each immersion of the ROV lasted between 15 and 30 min and a maximum of 1 h was achieved on the few very deep stations. The ROVs were operated as slowly as possible, most commonly with a speed of less than 0.2 knots, and occasionally the machines were landed on the ground to observe objects of specific interest. This technique allowed for close examination of small invertebrates and physical habitat features. The cameras of both ROVs were inclined vertically at an angle of ~10° to the ground, and, as estimated from calibrations, the observed areas varied between 0.7 m2 (ground) and 1.7 m2 when the ROV was about 1 m above the seafloor or away from the wall. All underwater videos were analyzed at half their normal speed or framewise in GOM Player Plus 2.3.55 (GOM and Company; https://www.gomlab.com/), and digital frames with a resolution of 200 pixel per inch were extracted to identify all taxa observed on the seafloor with a minimum size of 1 cm length or diameter.
SCUBA diving sampling
To complete the inventory of benthic invertebrates and to improve taxonomic identifications, additional sampling by SCUBA diving was performed in 2018 at four stations in the western area (Figure 1), where representative invertebrates were collected randomly by two divers through transects between 10 and 20 m water depth. The organisms were stored in diving bags and on board, fixed in 10% formol/seawater solutions buffered with borax for further taxonomic analyses at the Functional Ecology Laboratory (Universidad de Magallanes).
Taxonomic analysis
The species identification, either from ROV images or from specimens collected by diving, was carried out until the lowest possible taxonomic level or assigned to morphospecies categories due to external morphological traits, also known as OTUs (operational taxonomic units). The taxonomic identifications were made using the illustrated guide to the marine benthic fauna of Chilean Patagonia (Häussermann and Försterra, 2009) and were completed by further specialized taxonomic literature for selected taxonomic groups (e.g., Porifera: Hajdu et al., 2013; Decapoda: Ceseña et al., 2016; Mollusks: Forcelli, 2000), and when necessary, expert consultations were carried out.
Statistical analyses
In order to comprehend the similarity of invertebrate assemblages between ROV stations, hierarchical clustering was made using presence–absence matrices (binary) of species composition using the Ward algorithm as an agglomerative technique (option = “ward.D”), the distance method “binary” (presence–absence matrix), and using the pvclust function incorporated in the “pvclust” package (Suzuki and Chimodaira, 2006). P-values (expressed as % probability) were estimated for each cluster via multiscale bootstrap resampling (10,000).
In addition, similarity among ROVs and diving stations was represented through non-metric multidimensional scaling analyses (NMDS) based on the Jaccard dissimilarity metrics and using the metaMDS function (Oksanen et al., 2018). The relation between stations and categorical variables such as substrate types (Type 1: steep wall, Type 2: rocks + sediments, Type 3: steep wall + rocks + sediments, and Type 4: sediments) was represented by the position of the centroids for each category on the ordination. Subsequently, different numerical spatial variables, such as latitude, longitude, and mean depth, were tested as predictors of variation between stations (using 10,000 permutations), and only significant predictors were incorporated into the ordination as vectors using the envfit function (Oksanen et al., 2018). The proportion of variation explained and its significance were reported through r2 and P-values, respectively.
To test homogeneity of multivariate dispersion between substrate categories (Types 1, 2, 3, and 4) and gear methods (ROV and diving), permutational homogeneity of multivariate dispersion analyses were independently estimated based on the Jaccard dissimilarity metrics and using the betadisper function (Anderson, 2006). Statistical differences for multivariate homogeneity dispersion were tested through variance analyses (ANOVA) and a posterior pairwise comparison test using the permutest function. A permutational multivariate analysis of variance (PERMANOVA) was used to test the null hypotheses of no significant differences in the position of centroids between substrate types (Anderson, 2017). Differences in species composition between different gear methods were tested using a no-parametric multivariate analysis of similarity (ANOSIM); the differences and their significance were reported through statistical R and P-values (Clarke, 1993).
Subsequently, a three-way permutational multivariate analysis of variance (PERMANOVA) was used to determine the individual and interactive effects of categorical (substrates: Types 1, 2, and 3) and significant numerical predictors (longitude and mean depths) on the dissimilarity of benthic communities analyzed through ROV methods (model: dissimilarity ~ substrates ∗ longitude ∗ mean depth) using the adonis function (Anderson, 2017).
Alpha and beta diversity analyses
The α-diversity was evaluated by estimating the OTU richness for each station using the specnumber function implemented in the “vegan” package (Oksanen et al., 2018). In order to understand the species turnover between depth strata, homogeneity of multivariate dispersion analysis was used as a measure of β-diversity for different depth range categories: shallow (0–60 m), intermediate (60–120 m), and deep (>120 m), using the Jaccard dissimilarity metrics created by the vegdist function and multivariate dispersion of groups estimated using betadisper function (Anderson et al., 2006; Oksanen et al., 2018). Statistical differences were also tested through ANOVA tests and using a significance level of α = 0.05 (Anderson et al., 2006). The functions metaMDS, envfit, adonis, specnumber, betadisper, and permutest are incorporated in the “vegan” package (Oksanen et al., 2018). In addition, to understand the spatial patterns of β-diversity between ROV stations, the contribution of turnover (βjtu) and nestedness (βjne) components relative to the pairwise Jaccard’s dissimilarity index (βjac), using the “betapart” package (Baselga and Orme, 2012), were calculated. βjac values close to 0 suggest that species composition is identical between locations, whereas values close to 1 indicate complete dissimilarity. The turnover component indicates species replacing between locations (measured as turnover fraction of Jaccard dissimilarity), whereas nestedness indicates that the extension of that group is a subset of another (measured as nestedness-resultant fraction of Jaccard dissimilarity) (Baselga and Orme, 2012). All analyses were performed in RStudio (RStudio Team, 2021).
Latitudinal distribution analysis
The taxonomic status of each determined species and their respective distribution ranges integrate information reported by Häussermann and Försterra (2009); Häussermann et al. (2021), and four databases: the World Register of Marine Species (WoRMS; Horton et al., 2022), the Ocean Biogeographic Information System (OBIS, 2021), the Register of Antarctic Marine Species (RAMS; De Broyer et al., 2022), and the biological collection of the Universidad Católica del Norte (SCBUCN; Sellanes, 2018).
According to the geographic distribution range, the presence of each species was assigned to three ecoregions in Chilean Patagonia following the zoogeographic scheme proposed by Försterra (2009). This classification considers that the CPFC is subdivided into three major provinces: North Patagonia (42°–46.5°S), Central Patagonia (46.5°–53°S), and South Patagonia (53°–56°S). This categorization was based on oceanographic (Silva and Prego, 2002; Aracena et al., 2011) and geological information, considering that the CPFC extends over a latitude of about 14° (42° to 56°S) and reaches up to three degrees of longitude in its widest part. The CPFC is only interrupted by two major geographical features, the Gulf of Penas and the Strait of Magellan, which may produce a zoogeographical subdivision (Rovira and Herreros, 2016). For species with a distribution range beyond the zoogeographic borders of the CPFC, we used two categories: species with a distribution range north of 42°S were assigned to occur also in the Eastern South Pacific Ocean (ESP) area, and those species with a distribution range towards the south of ~65°S were assigned as present in the southern part of the Antarctic Polar Front (SAPF) (Freeman and Lovenduski, 2016).
Results
Species composition
The images obtained from 26 ROV stations allowed for the identification of 140 OTUs (or morphospecies), with 76 OTUs assigned to species level (Table S1). Sponges contributed notably to the assemblage structure (30 OTUs), representing 21.4% of the total OTUs recorded, followed by echinoderms with 27 OTUs (19.3%), cnidarians, and mollusks with 24 OTUs each (17.1%; Figure 2A). Echinoderms were prevalent in the Messier Channel, with 13 OTUs recorded per transect (KA14); 14 sponge OTUs were recorded in the Del Castillo Channel (R07b); and a similar number of cnidarians was found in the Albatross Channel (14 OTUs, KA05) and the Fallos Channel (13 OTUs, KA09) (Figure 2A). The most frequent taxa between all analyzed stations were the sea urchin Arbacia dufresnii (19 transects), followed by the sea star Cosmasterias lurida (18 transects), the polychaete Apomatus sp. (17 transects), the cold-water coral Desmophyllum dianthus, the sea star Odontaster penicillatus, and the ascidiacea Didemnum sp. (16 transects) (Table S1).
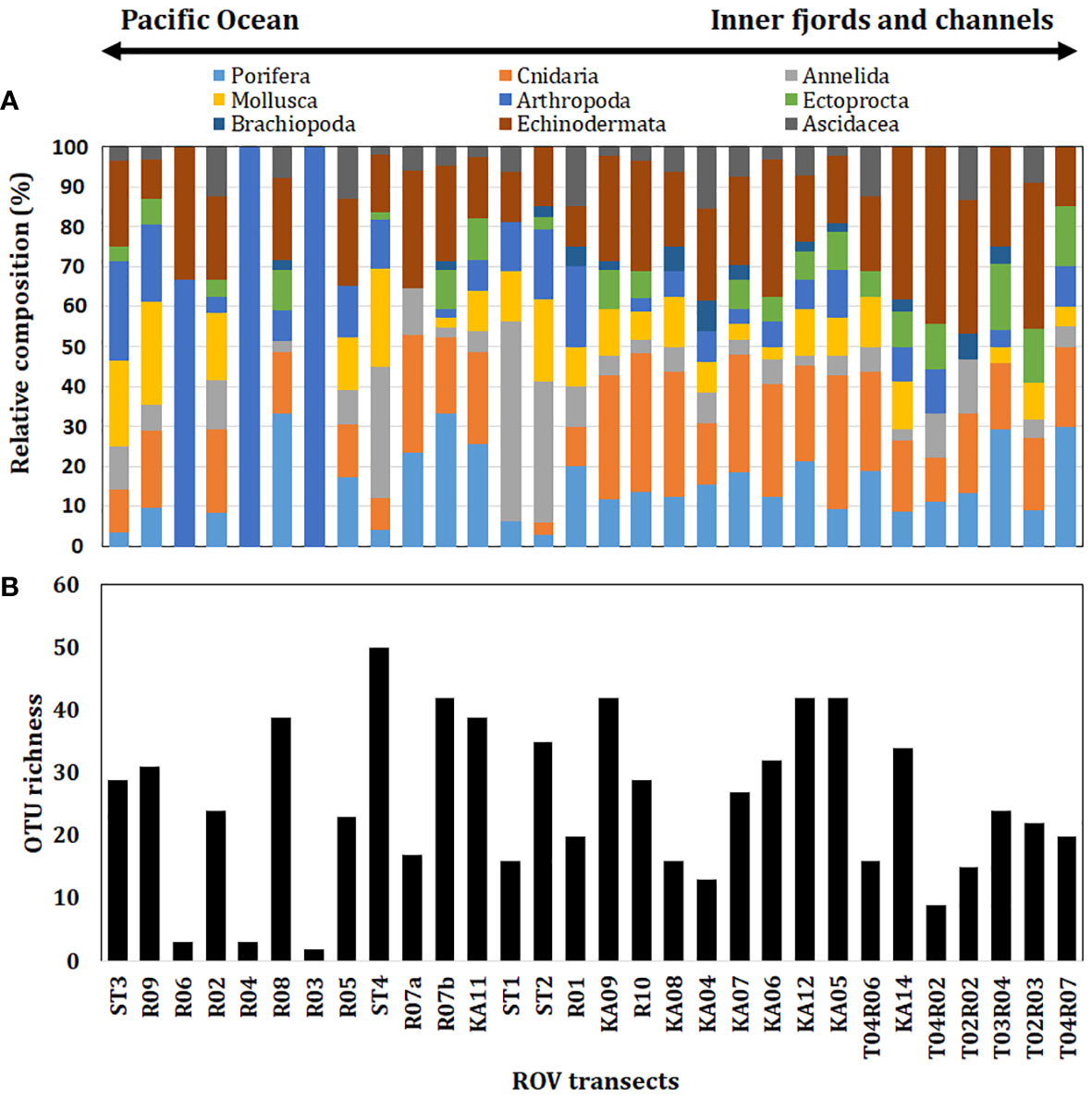
Figure 2 (A) Histogram of relative composition and (B) OTU richness of sublittoral benthic invertebrate assemblages in different ROV transects and SCUBA diving sampling across the KNR waters. Stations were ordered longitudinally.
Samples obtained through SCUBA diving from four sites allowed us to identify a total of 71 OTUs and incorporate 47 additional taxa into our records. Under this methodology, the most represented groups were Polychaeta, with 20 OTUs (29.0%), followed by Mollusca, with 17 OTUs (24.6%), and Arthropoda, with 11 OTUs (15.9%) (Figure 2A). Two Polychates species (e.g., Perkinsiana magalhaensis and Platynereis australis), amphipods (Amphipoda spp.), and sponges (Demospongiae) were common in the four stations sampled (Table S1). Therefore, including both approaches, a total of 187 OTUs were identified (Table S1), with mollusks being the most conspicuous taxonomic group (18.7%), followed by porifera, echinoderms (16.6% each), and arthropods (14.4%).
Diversity patterns
Based on ROV transects, OTU richness tended to be heterogeneous across different channels, but generally a higher OTU richness was observed in the Del Castillo and Fallos channels, that are linked directly to the Pacific Ocean (Figure 2B). Compared to the total area of this study, the highest values of OTU richness were observed at the Del Castillo, Fallos, and Albatross channels (each with 42 OTUs; stations R07b, K09, and K05, respectively), and with similar values at Byron Island (station KA12; Figure 2B), all in the vicinity of the Gulf of Penas. In contrast, lower OTU richness was reported at the confluence of the Octubre Channel with the Pacific Ocean (two OTUs at station R03 and three OTUs at station R04, respectively) and Sombrero Island (three OTUs at station R06; Figure 2B). For diving stations, higher OTU richness was also reported in the Del Castillo Channel (station-04: 50 OTUs), followed by the Octubre Channel confluence (station-02: 34 OTUs), and Torpedo Island (station-03: 30 OTUs); the lowest OTU richness was found in the Gallardo Sound (station-01: 16 OTUs) (Figure 2B).
In general, β-diversity estimations (measured as multivariate dispersion) were similarly higher for shallow, intermediate, and deep categories (average distance to centroid = 0.560, 0.558, and 0.576, respectively), being statistically not significant between depth categories (ANOVA, F = 0.0827, P = 0.921; Figures 3A, B). In this sense, these values suggest that around half of the species are exchanged between stations for each bathymetric category or depth strata. In addition, the Jaccard’s index of β-diversity indicates a high dissimilarity of benthic assemblages between ROV locations (βjac = 0.952), and taxonomic compositional differences were associated mainly with a high spatial turnover (βjtu = 0.922), rather than nestedness (βjne = 0.023).
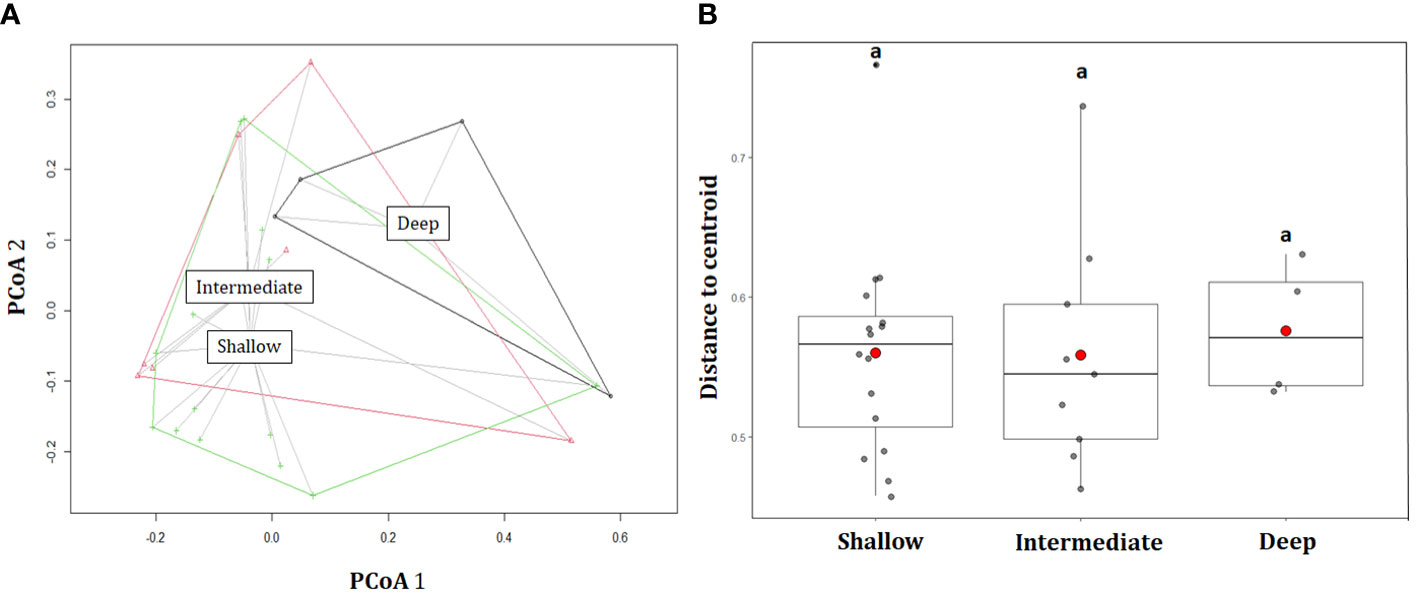
Figure 3 (A) Principal Coordinate Analysis (PCoA) and (B) boxplot of centroid distance for ROV stations (only “hard bottom” habitats) estimated for different bathymetric categories: shallow (0–60 m), intermediate (60–120 m), and deep (>120 m), as a measure of β-diversity. Mean values are represented by blue triangles. Same lowercase letters indicate not significant differences (P=>0.05).
Spatial patterns of invertebrate assemblages
The dendrogram of the hierarchical clustering and ordination (NMDS) analyses based on the species composition (presence–absence matrix) showed a clear relation among stations with combinations of different hard substrates (Types 1, 2, and 3), with clear dissimilitude relative to transects on sedimentary habitats (Type 4) (Figures S1, S2). In addition, other observed patterns showed that assemblages from shallow and intermediate depths were notably associated with the ordination (Figure 4). In this context, dissimilitude among assemblage compositions and their relationship with different categorical predictors suggest an important correlation with substrate types (r2 = 0.34; P ≤0.001) and sampling methods (ROV and diving) (r2 = 0.24; P ≤0.001). Furthermore, the relation with numerical variables (i.e., latitude, longitude, and mean depth) suggests only a positive and significant correlation with mean depth (r2 = 0.37, P = 0.001) and longitude (r2 = 0.24, P = 0.01; Figure 4).
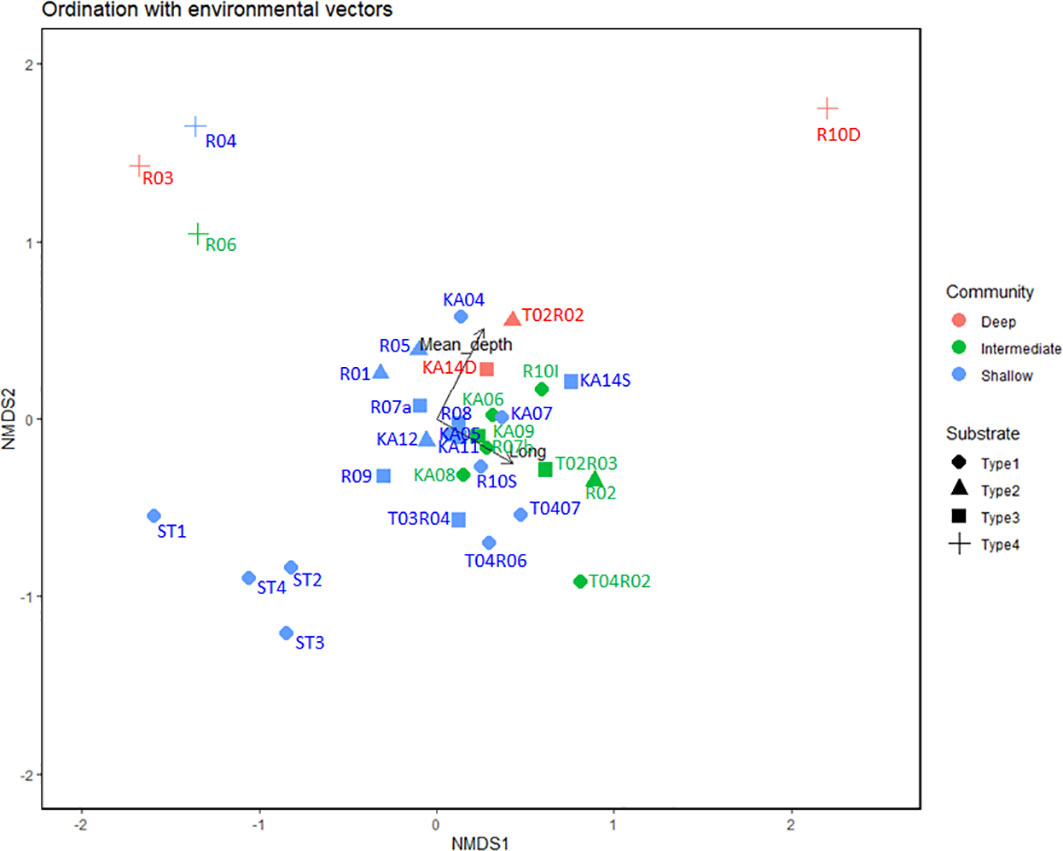
Figure 4 NMDS ordination plot based on presence–absence data of sublittoral benthic assemblages in the KNR waters (only ROV stations). Category of substrate types and vectors as longitude and mean depth were included in the ordination due to their significant correlation. Length of vectors are proportional to importance of each predictor variable (Stress = 0.141).
The multivariate homogeneity dispersion analyses revealed no significant differences between substrate categories (ANOVA, F = 1.18, P = 0.32; Figure S3). However, significant differences were observed between categories (PERMANOVA, F = 2.02, P ≤0.001); mainly between the sediments (Type 4) and “hard bottoms” categories Type 1 (P = 0.006), Type 2 (P = 0.036), and Type 3 (P = 0.024). On the other hand, sampling methods (ROV and diving) showed significant differences in multivariate dispersion (ANOVA, F = 7.37.98, P = 0.009; Figure S4); thus, when similitude analyses between assemblages were tested, these showed significant differences (ANOSIM, R = 0.543, P = 0.006); hence diving data were removed from the analysis.
Subsequent three-way PERMANOVA analyses using only ROV data and assemblages from different combinations of hard bottom habitats (Types 1, 2, and 3), corroborated that substrate types (R2 = 0.11, P = 0.003) and longitude (R2 = 0.068, P ≤0.001) may be significant predictors of dissimilitude, and their interaction (R2 = 0.095, P = 0.043) may, in part, explain the dissimilitude in the structure of sublittoral benthic invertebrate assemblages (Table 2).
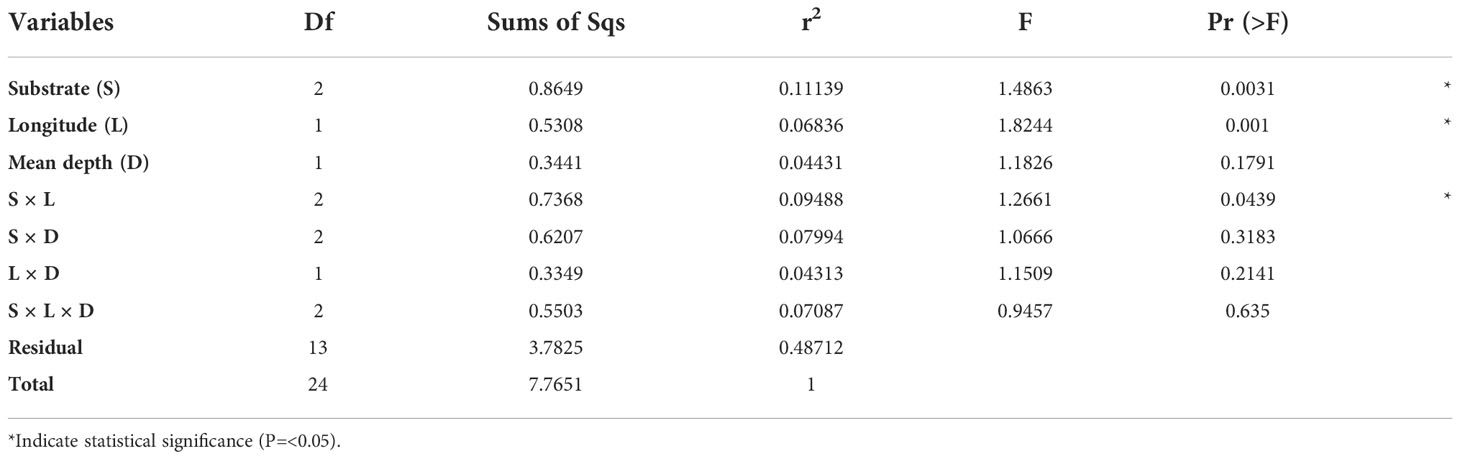
Table 2 Results of three way PERMANOVA modelling, including qualitative (substrate) and numerical variables (longitude and mean depth).
Latitudinal distribution patterns of benthic invertebrates
Based on 96 OTUs identified at the species level, 26% of the total species (25 taxa) recorded in the KNR waters have a distribution range exclusive to the CPFC. The remaining 74% (71 taxa) have a wide distribution range outside the CPFC (see below). The first group can be divided into one with a distribution range only inside the CPFC (16.7%) and the other with a distribution between the CPFC and to the south of the Antarctic Polar Front (SAPF) (9.4%) (Figures 5, S5). Among the second species group with wide distribution ranges, 49% was distributed between the Eastern South Pacific (ESP) and the CPFC, whereas the remaining 25% have also a wider distribution range between the ESP and SAPF (Figures 5, S5).
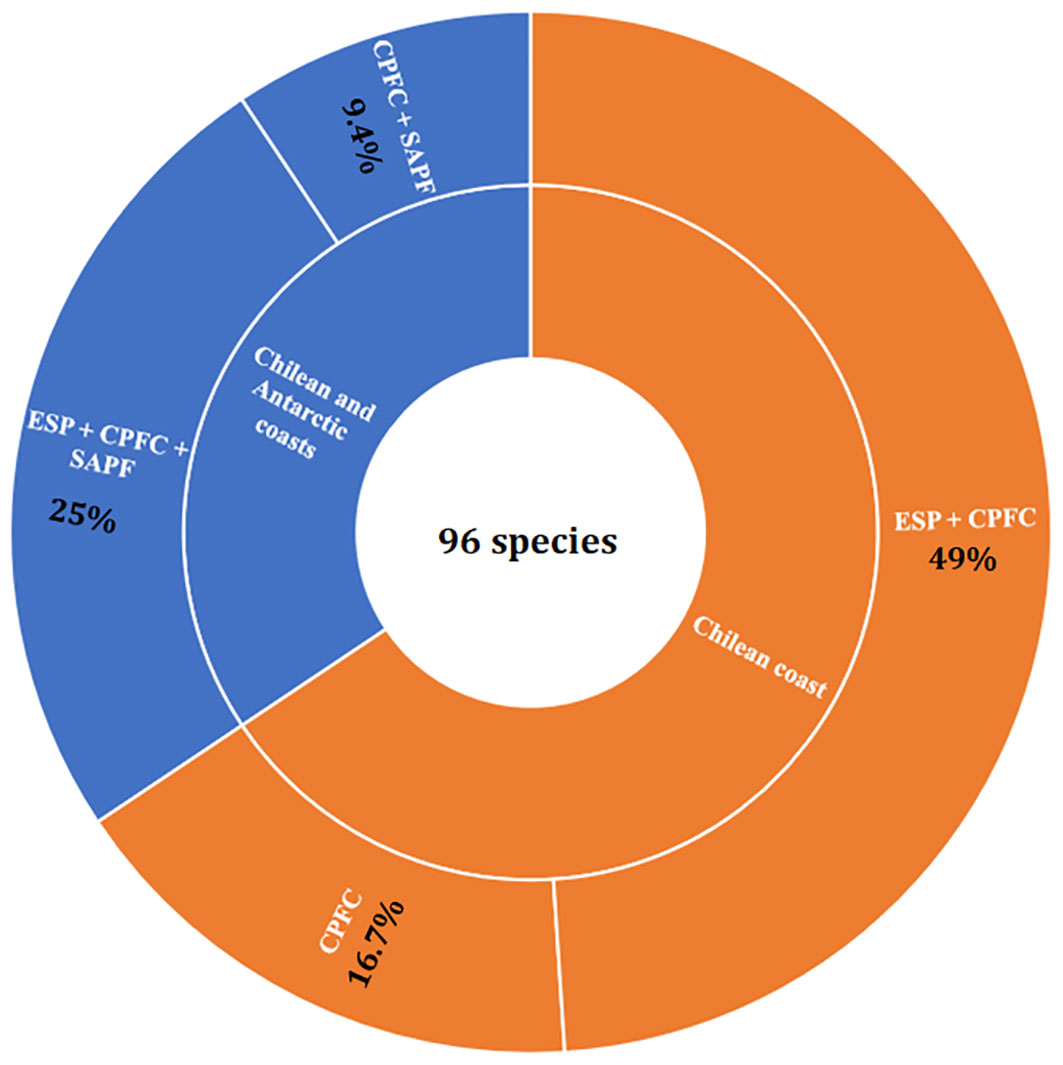
Figure 5 Latitudinal distribution ranges of the species recorded in the KNR waters. Species with distribution ranges north of 42°S are named as Eastern South Pacific (ESP); species distributed between 42° and 56°S are named as CPFC; species with a distribution range south of 65°S are classified as south of Antarctic polar front (SAPF).
Recent new records expanding the geographical distribution range of species into KNR waters have been reported by Gorny et al. (2020) and are completed in the current study. Together, the extension of latitudinal distribution ranges within the CPFC and towards 48°S has been established for cnidarians (e.g., Paranthus niveus), gastropods (e.g., Buchanania onchidioides), Polychaeta (e.g., Ampharete kerguelensis and Eunice pennata), and crustaceans (e.g., Pinnixa bahamondei). In contrast, records within the KNR waters extended for five species, with known distributions from further south towards 48°S, including the sponge Mycale magellanica, the polychaete Notaulax phaeotaenia, and the echinoderms Odontaster meridionalis and Ophiosabine rosea (Figure S5).
Discussion
Structure of invertebrate assemblages
All assemblages of benthic invertebrates in the present study were dominated mainly by sponges, echinoderms, cnidarians, and mollusks, which were also the most diverse groups, representing altogether nearly two-thirds (65.2%) of all OTUs reported (187 OTUs, including ROV and diving). Similar results from benthic communities in the shallow waters of the inner fjords in North Patagonia showed that sponges, followed by mollusks and echinoderms, constitute important groups in terms of species richness (Betti et al., 2021). Other studies from the channels and fjords of Central and South Patagonia highlighted the importance of suspension feeders (comprised of sponges, ascidians, and bivalves) as important components of benthic communities (e.g., Gutt et al., 1999; Friedlander et al., 2021), as well as echinoderms, which have been recognized as the most diverse group in samples obtained between the Penas Gulf and the Magellan Strait (Mutschke, 2008). The importance of suspension feeders in Patagonia may be related mainly to the important contribution of local phytoplankton production and allochthonous terrestrial organic matter reaching the bottoms, supporting the secondary production and benthic food webs (Zapata-Hernández et al., 2014; Zapata-Hernández et al., 2016; Cari et al., 2020; Ortiz et al., 2021).
The echinoderms C. lurida and A. dufresnii were the most common taxa in the ROV transects (19 sites), followed by the polychaete Apomatus sp. (17 sites), the cold-water coral D. dianthus, the ascidian Didemnum sp., and the sea star O. penicillatus (16 sites). Of these species, the sea star C. lurida is recognized as an important generalist predator in shallow waters (>25 m depth) across the CPFC ecosystems (Garrido et al., 2021), as well as being common in these latitudes between 5 and 460 m depth (Häussermann et al., 2021). On the other hand, the sea urchin A. dufresnii is an important grazer that could contribute to the incorporation of different organic matter sources (e.g., micro- and macro-algae as well as marine and terrestrial detritus), and its transfer into upper trophic levels through predator–prey interactions in fjord food webs (Zapata-Hernández et al., 2014). Indeed, stable isotope analyses of benthic invertebrates from the inner fjords in Central Patagonia indicate that echinoderms are a wide isotopic niche associated with the consumption of multiple food sources (Cari et al., 2020).
The benthic invertebrate inventory of KNR waters reached a total of 187 OTUs, of which 140 were determined from underwater image analyses obtained by ROV; 47 additional taxa were collected by SCUBA diving. These results indicate that the sampling strategy used in the present study was adequate because both sampling methods were complementary and also because the species collected by SCUBA diving were small; most of them were vagile fauna, whereas the underwater images presented most taxa classified as megafauna and sessile. These results are the highest diversity values for megafaunal taxa obtained using underwater imaging in the CPFC (Table 3). For instance, studies of fjords in northern Patagonia (using photo-quadrants) showed values between 60 and 114 taxa (Betti et al., 2017; Villalobos et al., 2021, respectively), whereas in southern Patagonia, between 71 and 116 taxa have been recorded (Gutt et al., 1999; Cárdenas and Montiel, 2015, respectively). Recently, Friedlander et al. (2021) recorded a total of 147 taxa in the meridional sector of central Patagonia through qualitative benthic surveys of fjord slopes (<40 m depth) (Table 3). However, the wide bathymetry range, covering about 200 m in our study, may complicate the comparison with these results. Despite this, our study covers an area that is poorly studied and could represent one of the most extensive surveys of benthic biodiversity performed across central Patagonia.
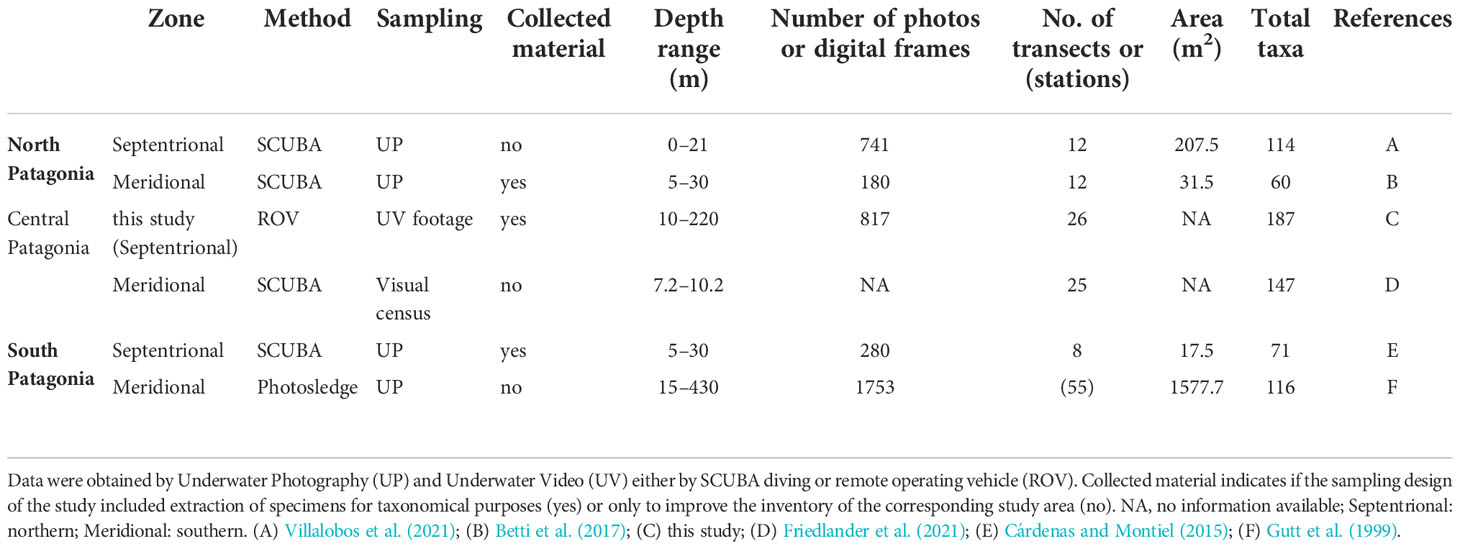
Table 3 Species richness (number of taxa) of selected investigations on benthic communities in the three main areas of the fjord and channel region in Chilean Patagonia, sorted by septentrional and meridional zones for each area.
Spatial patterns of biodiversity
Our analyses indicated that benthic communities change their species composition between different substrate categories, and spatially along a longitudinal gradient. The relation between species composition and substrate morphology has been recognized by Försterra (2009). In addition, Betti et al. (2021) found comparable results for shallow water benthic communities (5 to 30 m depth) in North Patagonian fjords, with a similar relation between species composition and predictor variables, such as distance from the mouth of the fjord (longitudinally oriented) and depth. On the other hand, the KNR waters cover an extensive area, with important freshwater discharges in the eastern area of inner waters, fed by large lakes and glacial melting, with important contributions of terrestrial material (Rebolledo et al., 2019). On the other hand, in the western and north-western areas, the islands tend to be exposed directly to the open Pacific Ocean waters and are strongly influenced by wave exposure, generally higher salinities, and minor sediment stress (Försterra, 2009). In this sense, OTU richness of the megafauna was higher (>40 OTUs) in the large channels, including the Messier, Fallos, and Del Castillo channels, and are likely affected by only a moderate freshwater influence and moderate levels of terrestrial organic carbon (Försterra, 2009; Rebolledo et al., 2019). Furthermore, β-diversity measures (centroid distances) indicated that for each bathymetric range analyzed (shallow, intermediate, and deep), the species turnover between transects were similar, with no differences between depth strata. In this way, the β-diversity between ROV stations was high (βjac = 0.952), suggesting an important species turnover between locations and highlighting the importance of spatial heterogeneity of benthic assemblages from inner fjords and channels towards the Pacific Ocean waters.
Bathymetric patterns of benthic communities in CPFC systems have been established for shallow benthic communities influenced by freshwater inputs, where barnacles and mussels cover the hard bottoms at protected and semi-protected rocky shores (Försterra, 2009). However, it is recognized that below the surface layer, at lower salinities, subtidal benthic communities change their spatial organization from stratified to patchy patterns (Försterra, 2009). In this way, the effect of depth driving changes in the community structure was minor, which, in part, could be related to the vertical structure of the water masses; where subantarctic surface waters penetrate into the fjords below the freshwaters, forming a mixture of estuarine waters and modified subantarctic waters that reach ~150 m depth, thus homogenizing the structure of benthic invertebrate assemblages. Below these depths the equatorial subsurface water, containing a lower dissolved oxygen content, can penetrate the deeper strata of fjords and channels and cover the bottoms between ~200 and 300 m depth (Sobarzo, 2009), likely affecting the structure of benthic communities at deeper zones. On the other hand, studies on deep-water emergence processes across the Patagonian waters indicate that temperature may be the most important environmental driver affecting the bathymetric distribution of benthic invertebrates to <50 m depth, whereas dissolved oxygen could be more relevant for benthic communities >200 m depth (Häussermann et al., 2021).
Latitudinal distribution patterns among zoogeographical regions
The Taitao Peninsula (46°S) on the north of the Penas Gulf was proposed as an important biogeographic barrier (Pickard, 1973; Viviani, 1979; Stuardo and Valdovinos, 1992). Lancellotti and Vásquez (2000) suggested that this area represents the southern boundary of the transitional zone where western wind drift reaches the South American continent and creates the Cape Horn current that drives the template-cold region. Analysis of the geographical distribution of sea anemones has shown important changes in species composition in this area (Häussermann, 2006). Based on current knowledge on the geographical distribution of benthic invertebrates identified across the KNR waters (48°S), our results indicate that invertebrates recorded in the KNR waters were dominated by species distributed between the ESP and CPFC, followed by those distributed latitudinally towards the SAPF (Figure S5). However, an important number of species in the KNR waters are distributed exclusively in the CPFC (16.7%) and a minor fraction (9.4%) distributed from the CPFC to the APF. Therefore, it is suggested that KNR waters correspond to a transition area between the northern and southern fauna of the CPFC. These complex patterns of latitudinal ranges found in the benthic fauna of KNR waters likely reflect the glaciological history of the CPFC. This region, with an extension of more than 14 degrees of latitude, is the result of complex erosional processes caused by glacial advances and retreats during the last Pleistocene glaciation era (Glasser et al., 2008; Hein et al., 2017). Therefore, the benthic fauna of Patagonia originated from species inhabiting the proto-continental shelf margins prior to the Cretaceous era, when the South American and Antarctic continents drifted apart about 30 million years ago (Arntz, 1999). Then, the inner fjords and channels of Patagonia were colonized successively by fauna after the retreat of the glaciers about 10–20 thousand years ago (Gordillo, 1999; Arntz, 1999; McCulloch and Davis, 2001; Gordillo et al., 2010).
Conclusion and future perspectives
The effects of climate change, combined with increasing human activities such as aquaculture in the southernmost areas of the South American continent, may drastically alter marine ecosystems in the near future. This study represents a baseline of shallow and sublittoral benthic invertebrate assemblages across the KNR waters (Central Patagonia), whose structures can change and be highly heterogeneous along a longitudinal axis. Due to the transitional features of species distribution, the waters of the reserve could be considered a reservoir for species distributed in the northern and southern parts of Patagonia, even for some species distributed south of the Antarctic Polar Front. However, due to the great proportion of taxa identified through ROV photographs, these results should be considered with caution because of potential bias associated with taxonomic identification based on images. In addition, more detailed analyses of the relationship between biodiversity patterns and local environmental variables (e.g., primary productivity, salinity, oxygen, and suspended organic material) still need to be explored in detail, as well as changes in the trophic structure and food webs along the environmental gradients between the inner waters and the Pacific Ocean. Moreover, the paucity of sampling in the area and the absence of temporal monitoring of marine communities could complicate effective management of natural resources within this remote area. Therefore, a detailed knowledge of species composition and distribution patterns along spatial and environmental gradients in the southern tip of South America is essential for any sustainable management, monitoring, and future conservation plans to protect the fragile and highly diverse ecosystems of the Chilean fjords and channels.
Data availability statement
The original contributions presented in the study are included in the article/Supplementary Material; further inquiries can be directed to the corresponding author.
Author contributions
GZ-H supported ROV operations and SCUBA diving sampling, analyzed images of ROV video transects, determined species on video transects, performed statistical analyses, conceived the idea, and wrote the manuscript. MG generated funding and obtained permits for the expeditions, piloted the ROV during expeditions, identified decapods from ROV images, analyzed the zoogeographical data, conceived the idea, and wrote the manuscript. AM participated in SCUBA diving sampling, determined specimens collected by SCUBA diving, identified polychaetes from ROV images, checked the species identified on video transects, analyzed zoogeographical data towards the south polar front, and conceived the idea and wrote the manuscript. All authors listed have made a substantial, direct, and intellectual contribution to the work and approved it for publication.
Funding
Oceana financed the expedition to obtain the data and the exchange between the authors. Oceana complied with all authorizations to realize sampling and video transects during the expedition, including authorization to realize the investigation in the Katalalixar National Reserve. GZ-H was partially funded by Beca de Postdoctorado MINEDUC UCN-19101 N°02.
Acknowledgments
We would like to thank the crew of the L/M Patagonia Explorer. This study formed part of a cooperation between the Laboratory of Functional Ecology (UMAG) and OCEANA-Chile, and we wish to thank the directors of both institutions. OCEANA financed the expeditions, and the institute provided laboratory facilities. We thank J.C.C. Fernández (TaxPo) for his help in determining Porifera taxa and L. Moreno for revising the English grammar in the last version of this manuscript. This article is dedicated to Eduardo Quiroga (RIP), who dedicated part of his life to study the benthic marine ecosystems in Chilean Patagonia.
Conflict of interest
The authors declare that this research was conducted in the absence of any commercial or financial relationships that could be interpreted as a potential conflict of interests.
Publisher’s note
All claims expressed in this article are solely those of the authors and do not necessarily represent those of their affiliated organizations, or those of the publisher, the editors and the reviewers. Any product that may be evaluated in this article, or claim that may be made by its manufacturer, is not guaranteed or endorsed by the publisher.
Supplementary material
The Supplementary Material for this article can be found online at: https://www.frontiersin.org/articles/10.3389/fmars.2022.951195/full#supplementary-material
References
Anderson M. J. (2006). Distance-based tests for homogeneity of multivariate dispersions. Biometrics 62 (1), 245–253. doi: 10.1111/j.1541-0420.2005.00440.x
Anderson M. J. (2017). “Permutational multivariate analysis of variance (PERMANOVA)”, in Wiley StatsRef: Statistics Reference Online Eds. Balakrishnan N., Colton T., Everitt B., Piegorsch W., Ruggeri F., Teugels J.L.. doi: 10.1002/9781118445112.stat07841
Anderson M. J., Ellingsen K. E., McArdle B. H. (2006). Multivariate dispersion as a measure of beta diversity. Ecol. Lett. 9, 683–693. doi: 10.1111/j.1461-0248.2006.00926.x
Aracena C., Lange C. B., Iriarte J. L., Rebolledo L., Pantoja S. (2011). Latitudinal patterns of export production recorded in surface sediments of the Chilean Patagonian fjords (41–55°S) as a response to water column productivity. Cont. Shelf Res. 31 (3–4), 340–355. doi: 10.1016/j.csr.2010.08.008
Arntz W. E. (1999). Magellan-Antarctic: Ecosystems that drifted apart. Summary review. Sci. Mar. 63 (S1), 503–511. doi: 10.3989/scimar.1999.63s1503
Aronson R. B., Thatje S., Clarke A., Peck L. S., Blake D. B., Wilga C. D., et al. (2007). Climate change and invasibility of the Antarctic benthos. Annu. Rev. Ecol. Evol. Syst. 38, 129–154. doi: 10.1146/annurev.ecolsys.38.091206.095525
Baselga A., Orme C. D. L. (2012). Betapart: An r package for the study of beta diversity. Methods Ecol. Evol. 3 (5), 808–812. doi: 10.1111/j.2041-210X.2012.00224.x
Betti F., Bavestrello G., Bo M., Enrichetti F., Loi A., Wanderlingh A., et al. (2017). Benthic biodiversity and ecological gradients in the seno Magdalena (Puyuhuapi fjord, Chile). Estuar. Coast. Shelf Sci. 198, 269–278. doi: 10.1016/j.ecss.2017.09.018
Betti F., Enrichetti F., Bavestrello G., Costa A., Moreni A., Bo M., et al. (2021). Hard-bottom megabenthic communities of a Chilean fjord system: Sentinels for climate change? Front. Mar. Sci. 8. doi: 10.3389/fmars.2021.635430
Brandt A. (1999). On the origin and evolution of Antarctic peracarida (Crustacea, malacostraca). Sci. Mar. 63 (S1), 261–274. doi: 10.3989/scimar.1999.63s1261
Brattström H., Johanssen A. (1983). Ecological and regional zoogeography of the marine benthic fauna of Chile: Report no. 49 of the Lund University Chile expedition 1948–49. Sarsia 68 (4), 289–339. doi: 10.1080/00364827.1983.10420583
Camus P. A. (2001). Biogeografía marina de Chile continental. Rev. Chil. Hist. Nat. 74 (3), 587–617. doi: 10.4067/S0716-078X2001000300008
Cárdenas L., Lecrerc J.-C., Bruning P., Garrido I., Détrée C., Figueroa A., et al. (2020). First mussel settlement observed in Antarctica reveals the potential for future invasions. Sci. Rep. 10, 5552. doi: 10.1038/s41598-020-62340-01
Cárdenas C. A., Montiel A. (2015). The influence of depth and substrate inclination on sessile assemblages in subantarctic rocky reefs (Magellan region). Polar Biol. 38 (10), 1631–1644. doi: 10.1007/s00300-015-1729-5
Cari I., Andrade C., Quiroga E., Mutschke E. (2020). Benthic trophic structure of a Patagonian fjord (47°S): The role of hydrographic conditions in the food supply in a glaciofluvial system. Estuar. Coast. Shelf Sci. 233, 106536. doi: 10.1016/j.ecss.2019.10653
Ceseña F., Meyer R., Mergl C. P., Häussermann V., Försterra G., McConnell K., et al. (2016). Decapoda of the huinay fiordos-expeditions to the Chilean fjords 2005-2014: Inventory, pictorial atlas and faunistic remarks (Crustacea, malacostraca). Spixiana 39 (2), 153–198.
Clarke K. R. (1993). Non-parametric multivariate analyses of changes in community structure. Aust. Ecol. 18, 117–143. doi: 10.1111/j.1442-9993.1993.tb00438.x
Cooley S., Schoeman D., Bopp L., Boyd P., Donner S., Ghebrehiwet D. Y., et al. (2022). “Ocean and coastal ecosystems and their services, in climate change 2022: Impacts, adaptation, and vulnerability,” in Contribution of working group II to the sixth assessment report of the intergovernmental panel on climate change. Eds. Pörtner H.-O., Roberts D. C., Tignor M., Poloczanska E. S., Mintenbeck K., Alegría A., Craig M., Langsdorf S., Löschke S., Möller V., Okem A., Rama B. (Cambridge, UK and New York, NY, USA: Cambridge University Press) pp. 379–550. doi: 10.1017/9781009325844.005
Costello M. J., Basher Z., Sayre R., Breyer S., Wright D. J. (2018). Stratifying ocean sampling globally and with depth to account for environmental variability. Sci. Rep. 8, 11259. doi: 10.1038/s41598-018-29419-1
De Broyer C., Clarke A., Koubbi P., Pakhomov E., Scott F., Vanden Berghe E., et al. (2022) Register of Antarctic marine species. Available at: https://www.marinespecies.org/rams%20on%202022-04-01.
De Broyer C., Rauschert M. (1999). Faunal diversity of the benthic amphipods (Crustacea) of the Magellan region as compared to the Antarctic (preliminary results). Sci. Mar. 63 (S1), 281–293. doi: 10.3989/scimar.1999.63s1281
Fernández M., Jaramillo E., Marquet P. A., Moreno C. A., Navarrete S. A., Ojeda F. P., et al. (2000). Diversity, dynamics and biogeography of Chilean benthic nearshore ecosystems: an overview and guidelines for conservation. Rev. Chil. Hist. Nat. 73 (4), 797–830. doi: 10.4067/S0716-078X2000000400021
Forcelli D. O. (2000). Moluscos magallánicos: guía de moluscos de Patagonia y sur de Chile. (Buenos Aires: Vásquez Mazzini Editores), 200 pp.
Försterra G. (2009). “Ecological and biogeographical aspects of the Chilean fjord region,” in Marine benthic fauna of Chilean Patagonia. Eds. Häussermann V., Försterra G. (Santiago, Chile: Nature In Focus), 61–76.
Försterra G., Häussermann V., Laudien J. (2017). “Animal forests in the Chilean fjords: discoveries, perspectives and threats in shallow and deep waters,” in Marine animal forests. Ed. Rossi S, Rossi S., Bramanti L., Gori A., Orejas C. (Switzerland: Springer) 35 p. doi: 10.1007/978-3-319-17001-5_3-1
Freeman N. M., Lovenduski N. S. (2016). Mapping the Antarctic polar front: weekly realizations from 2002 to 2014. Earth Syst. Sci. Data 8, 191–198. doi: 10.5194/essd-8-191-2016
Friedlander A. M., Ballesteros E., Goodell W., Hüne M., Muñoz A., Salinas-de-León P., et al. (2021). Marine communities of the newly created kawésqar national reserve, Chile: From glaciers to the pacific ocean. PloS One 16 (4), e0249413. doi: 10.1371/journal.pone.0249413
Garrido I., Pardo L. M., Johnson L. E., Schories D. (2021). Selective feeding by a predatory Sea star across a depth gradient in northern Patagonia, Chile. Front. Mar. Sci. 8. doi: 10.3389/fmars.2021.636208
Gerdes D., Montiel A. (1999). Distribution patterns of macrozoobenthos: A comparison between the Magellan region and the weddell Sea (Antarctica). Sci. Mar. 63, 149–154. doi: 10.3989/scimar.1999.63s1149
Glasser N. F., Jansson K. N., Harrison S., Kleman J. (2008). The glacial geomorphology and pleistocene history of south America between 38°S and 56°S. Quat. Sci. Rev. 27 (3–4), 365–390. doi: 10.1016/j.quascirev.2007.11.011
Gordillo S. (1999). Holocene Molluscan assemblages in the Magellan region. Sci. Mar. 63 (S1), 15–22. doi: 10.3989/scimar.1999.63s115
Gordillo S., Cusminsky G., Bernasconi E., Ponce J. F., Rabassa J. O., Pino M. (2010). Pleistocene marine calcareous macro-and-microfossils of navarino island (Chile) as environmental proxies during the last interglacial in southern South America. Quat. Int. 221 (1–2), 159–174. doi: 10.1016/j.quaint.2009.10.025
Gorny M. (1999). On the biogeography and ecology of the southern ocean decapod fauna. Sci. Mar. 3 (Supl. 1), 367–382. doi: 10.3989/scimar.1999.63s1367
Gorny M., Montiel A., Zapata-Hernández G., Pereda R. (2020). “Las comunidades marinas bentónicas de la reserva nacional katalalixar (Chile),” in Oceanografía. Ed. Saraiva M. (Curitiva, Brazil: Editora Artemis). doi: 10.37572/EdArt_1633110203
Griffiths H. J., Barnes D. K., Linse K. (2009). Towards a generalized biogeography of the southern ocean benthos. J. Biogeogr. 36 (1), 162–177. doi: 10.1111/j.1365-2699.2008.01979.x
Gutt J., Helsen E., Arntz W., Buschmann A. (1999). Biodiversity and community structure of the mega-epibenthos in the Magellan area (South America). Sci. Mar. 63 (S1), 155–170. doi: 10.3989/scimar.1999.63s1155
Hajdu E., Desqueyroux-Faúndez R., Carvalho M. D. S., Lôbo-Hajdu G., Willenz P. (2013). Twelve new demospongiae (Porifera) from Chilean fjords, with remarks upon sponge-derived biogeographic compartments in the SE pacific. Zootaxa 3744, 1–64. doi: 10.11646/zootaxa.3744.1.1
Häussermann V. (2006). Biodiversity of Chilean sea anemones (Cnidaria: Anthozoa): Distribution patterns and zoogeographic implications, including new records for the fjord region. Investig. Mar. 34 (2), 23–35. doi: 10.4067/S0717-71782006000200003
Häussermann V., Ballyram S. A., Försterra G., Cornejo C., Ibáñez C. M., Sellanes J., et al. (2021). Species that fly at a higher game: Patterns of deep–water emergence along the Chilean coast, including a global review of the phenomenon. Front. Mar. Sci. 8. doi: 10.3389/fmars.2021.688316
Häussermann V., Försterra G. (2005). “Distribution patterns of Chilean shallow-water sea anemones (Cnidaria: Anthozoa: Actiniaria, corallimorpharia), with a discussion of the taxonomic and zoogeographic relationships between the actinofauna of the south East pacific, the south West Atlantic and the Antarctic,” in The Magellan-Antarctic connection: links and frontiers at high southern latitudes, vol. 69 . Eds. Arntz W. E., Lovrichm G. A., Thatje S. Sci. Mar. 69, 91–102.
Häussermann V., Försterra G. (2007). “Large Assemblages of cold-water corals in Chile: a summary of recent findings and potential impacts,” in Conservation and adaptive management of seamount and deep-sea coral ecosystems. Eds. George R. Y., Cairns S. D. (Miami: University of Miami), 195–1207. Rosenstiel School of Marine and Atmospheric Science.
Häussermann V., Försterra G. (2009). “Marine benthic fauna of Chilean Patagonia,” in Nature in focus (Santiago, Chile), 1000.
Häussermann V., Försterra G., Melzer R. R., Meyer R. (2013). Gradual changes of benthic biodiversity in comau fjord, Chilean Patagonia–lateral observations over a decade of taxonomic research. Spixiana 36 (2), 161–171.
Hein A. S., Cogez A., Darvill C. M., Mendelova M., Kaplan M. R., Herman F., et al. (2017). Regional mid-pleistocene glaciation in central Patagonia. Quat. Sci. Rev. 164, 77–94. doi: 10.1016/j.quascirev.2017.03.023
Hernández C. E., Moreno R. A., Rozbaczylo N. (2005). Biogeographical patterns and rapoport’s rule in southeastern pacific benthic polychaetes of the Chilean coast. Ecography 28 (3), 363–373. doi: 10.1111/j.0906-7590.2005.04013.x
Horton T., Kroh A., Ahyong S., Bailly N., Bieler R., Boyko C. B., et al. (2022) World register of marine species. Available at: https://www.marinespecies.org.
Hughes K. A., Pescott O. L., Peyton J., Adrieans T., Cottier-Cook E. J., Key G., et al. (2020). Invasive non-native species likely to threaten biodiversity and ecosystems in the Antarctic peninsula region. Glob. Change Biol. 26, 2702–2716. doi: 10.1111/gcb.14938
Lancellotti D. A., Vásquez J. A. (1999). Biogeographical patterns of benthic macroinvertebrates in the southeastern pacific littoral. J. Biogeogr. 26 (5), 1001–1006. doi: 10.1046/j.1365-2699.1999.00344.x
Lancellotti D. A., Vásquez J. A. (2000). Zoogeografía de macroinvertebrados bentónicos de la costa de Chile: Contribución para la conservación marina. Rev. Chil. Hist. Nat. 73 (1), 99–129. doi: 10.4067/S0716-078X2000000100011
Linse K. (1999). Mollusca Of the Magellan region. a checklist of the species and their distribution. Sci. Mar. 63 (S1), 399–407. doi: 10.3989/scimar.1999.63s1399
Linse K., Griffiths H. J., Barnes D. K., Clarke A. (2006). Biodiversity and biogeography of Antarctic and sub-Antarctic mollusca. Deep-Sea Res. II: Top. Stud. Oceanogr. 53 (8-10), 985–1008. doi: 10.1016/j.dsr2.2006.05.003
McCulloch R. D., Davies S. J. (2001). Late-glacial and Holocene palaeoenvironmental change in the central strait of Magellan, Southern Patagonia. Palaeogeogr. Palaeoclimatol. Palaeoecol. 173 (3–4), 143–173. doi: 10.1016/S0031-0182(01)00316-9
Montiel A., Gerdes D., Arntz W. E. (2005). Distributional patterns of shallow-water polychaetes in the Magellan region: A zoogeographical and ecological synopsis. Sci. Mar. 69 (S2), 123–133. doi: 10.3989/scimar.2005.69s2123
Montiel A., Quiroga E., Gerdes D. (2011). Diversity and spatial distribution patterns of polychaete assemblages in the paso ancho, straits of Magellan. Chile. Cont. Shelf Res. 31 (3–4), 304–314. doi: 10.1016/j.csr.2010.11.010
Moyano H. I. (1999). Magellan Bryozoa: a review of the diversity and of the Sub-Antarctic and Antarctic zoogeographical links. Sci. Mar. 63 (S1), 219–226. doi: 10.3989/scimar.1999.63s1219
Mutschke E. (2008). “Macrobenthic biodiversity and community structure in austral Chilean channels and fjords,” in Progress in the oceanographic knowledge of Chilean interior waters, from Puerto montt to cape horn. Eds. Silva N., Palma S. (Chile: Comité Oceanográfico Nacional - Pontificia Universidad Católica de Valparaíso, Valparaíso), 133–141.
OBIS (2021) Ocean biodiversity information system. intergovernmental oceanographic commission of UNESCO. Available at: https://obis.org/.
Oksanen J., Blanchet F. G., Friendly M., Kindt R., Legendre P., McGlinn D., et al. (2018) Vegan: Community ecology package. r package version 2.5-1. Available at: https://CRAN.R-project.org/package=vegan.
Ortiz P., Quiroga E., Montero P., Hamame M., Betti F. (2021). Trophic structure of benthic communities in a Chilean fjord (45°S) influenced by salmon aquaculture: Insights from stable isotopic signatures. Mar. Pollut. Bull. 173, 113149. doi: 10.1016/j.marpolbul.2021.113149
Pansini M., Sarà M. (1999). Taxonomical and biogeographical notes on the sponges of the straits of Magellan. Sci. Mar. 63 (S1), 203–208. doi: 10.3989/scimar.1999.63s1203
Pickard G. L. (1973). “Water structure in Chilean fjords,” in Oceanography of the south pacific. Ed. Fraser R. (Wellington: New Zealand National Commission for UNESCO), 95–104.
Quiroga E., Ortiz P., Gerdes D., Reid B., Villagran S., Quiñones R. (2012). Organic enrichment and structure of macrobenthic communities in the glacial baker fjord, northern Patagonia. Chile. J. Mar. Biolog. Assoc. U.K. 92 (1), 73–83. doi: 10.1017/S0025315411000385
Quiroga E., Ortiz P., González-Saldías R., Reid B., Tapia F. J., Pérez-Santos I., et al. (2016). Seasonal benthic patterns in a glacial Patagonian fjord: The role of suspended sediment and terrestrial organic matter. Mar. Ecol. Prog. Ser. 561, 31–50. doi: 10.3354/meps11903
Rebolledo L., Bertrand S., Lange C. B., Tapia F. J., Quiroga E., Troch M., et al. (2019). Compositional and biogeochemical variations of sediments across the terrestrial-marine continuum of the baker-martínez fjord system (Chile, 48°S). Prog. Oceanogr. 174, 89–104. doi: 10.1016/j.pocean.2018.12.004
Retamal M. A., Moyano H. I. (2010). Zoogeografía de los crustáceos decápodos chilenos marinos y dulceacuícolas. Lat. Am. J. Aquat. Res. 38 (3), 302–328. doi: 10.3856/vol38-issue3-fulltext-1
Ríos C., Arntz W. E., Gerdes D., Mutschke E., Montiel A. (2007). Spatial and temporal variability of the benthic assemblages associated to the holdfasts of the kelp macrocystis pyrifera in the straits of Magellan, Chile. Polar Biol. 31 (1), 89–100. doi: 10.1007/s00300-007-0337-4
Rovira J., Herreros J. (2016). Clasificación de ecosistemas marinos chilenos de la zona económica exclusiva. Ministerio del Medio Ambiente. 48 pp Available at: https://mma.gob.cl/wp-content/uploads/2018/03/%20Clasificacion-ecosistemas-marinos-deChile.pdf..
RStudio Team (2021). RStudio: Integrated development for r. RStudio (Boston, MA: PBC). Available at: http://www.rstudio.com/.
Sellanes J. (2018). Base de datos de la Sala de Colecciones Biológicas de la Universidad Católica del Norte (SCBUCN) Chile, Version 1.2. (Chile: Universidad Católica del Norte). Occurrence dataset, doi: 10.15468/d3auf9
Sievers H., Calvete C., Silva N. (2002). Distribución de características físicas, masas de agua y circulación general para algunos canales australes entre el golfo de penas y el estrecho de magallanes (Crucero CIMAR-2 fiordos), Chile Cienca Tecnol. Mar. 25 (2), 17–43.
Silva N., Prego R. (2002). Carbon and nitrogen spatial segregation and stoichiometry in the surface sediments of southern Chilean inlets (41–56°S). Estuar. Coast. Shelf Sci. 55 (5), 763–775. doi: 10.1006/ecss.2001.0938
Sobarzo M. (2009). “La región de los fiordos de la zona sur de Chile: aspectos oceanográficos,” in Fauna marina bentónica de la Patagonia chilena. Eds. Häusssermann V., Försterra G. (Santiago: Nature in Focus), 53–60.
Soto M. (2009). “Geography of the Chilean fjord region,” in Marine benthic fauna of Chilean Patagonia. Eds. Häussermann V., Försterra G. (Santiago: Nature in Focus), 43–52.
Stuardo J., Valdovinos C. (1992). “Barreras, límites faunísticos y provincias biogeoquímicas en sudamérica austral,” in Oceanografía en antártida. Eds. Gallardo V. A., Ferretti O., Moyano H. I. (Concepción: Centro EULA, Universidad de Concepción), 443–451.
Suzuki R., Shimodaira H. (2006). Pvclust: an r package for assessing the uncertainty in hierarchical clustering. Bioinformatics 22 (12), 1540–1542. doi: 10.1093/bioinformatics/btl117
Tapia-Guerra J. M., Mecho A., Easton E. E., Gallardo M.L.Á., Gorny M., Sellanes J. (2021). First description of deep benthic habitats and communities of oceanic islands and seamounts of the nazca desventuradas marine park, Chile. Sci. Rep. 11 (1), 6209. doi: 10.1038/s41598-021-85516-8
Tecklin D., Farias A., Peña M. A., Gélvez X., Castilla J. C., Sepúlveda A., et al. (2021). “Coastal-marine protection in Chilean Patagonia: Historical progress, current situation, and challenges,” in Conservación en la Patagonia chilena: evaluación del conocimiento, oportunidades y desafios. Eds. Castilla J. C., Armesto J. J., Martínez-Harms M. J. (Santiago, Chile: Ediciones Universidad Católica), 129–166.
Thatje S., Anger K., Calcagno J. A., Lovrich G. A., Pörtne H. O., Arntz W. E. (2005). Challenging the cold: Crabs reconquer the Antarctic. Ecology 86 (3), 619–625. doi: 10.1890/04-0620
Villalobos V. I., Valdivia N., Försterra G., Ballyram S., Espinoza J. P., Burgos-Andrade K., et al. (2021). Depth-dependent diversity patterns of rocky subtidal macrobenthic communities along a temperate fjord in northern Chilean Patagonia. Front. Mar. Sci. 8, 438. doi: 10.3389/fmars.2021.635855
Viviani C. A. (1979). Ecogeografía del litoral chileno. Stud. neotrop. fauna Environ. 14 (2–3), 65–123. doi: 10.1080/01650527909360548
Zapata-Hernández G., Sellanes J., Mayr C., Muñoz P. (2014). Benthic food web structure in the comau fjord, Chile (~ 42°S): preliminary assessment including a site with chemosynthetic activity. Prog. Oceanogr. 129, Part A, 149–158. doi: 10.1016/j.pocean.2014.03.005
Keywords: marine biodiversity, sublittoral benthos, zoogeography, channels and fjords, chilean patagonia, remotely operate vehicle, species turnover
Citation: Zapata-Hernández G, Gorny M and Montiel A (2022) Filling ecological gaps in Chilean Central Patagonia: Patterns of biodiversity and distribution of sublittoral benthic invertebrates from the Katalalixar National Reserve waters (~48°S). Front. Mar. Sci. 9:951195. doi: 10.3389/fmars.2022.951195
Received: 23 May 2022; Accepted: 14 November 2022;
Published: 01 December 2022.
Edited by:
Juan Armando Sanchez, University of Los Andes, ColombiaReviewed by:
Cristian Lagger, CCT CONICET Córdoba, ArgentinaVerena Häussermann, San Ignacio del Huinay Foundation, Chile
Copyright © 2022 Zapata-Hernández, Gorny and Montiel. This is an open-access article distributed under the terms of the Creative Commons Attribution License (CC BY). The use, distribution or reproduction in other forums is permitted, provided the original author(s) and the copyright owner(s) are credited and that the original publication in this journal is cited, in accordance with accepted academic practice. No use, distribution or reproduction is permitted which does not comply with these terms.
*Correspondence: Germán Zapata-Hernández, emFwYXRhLmJtQGdtYWlsLmNvbQ==