- 1Laboratory of Genetics and Genomics of Marine Resources and Environment, Department of Biological, Geological and Environmental Sciences, Alma Mater Studiorum University of Bologna Ravenna, Ravenna, Italy
- 2Department of Life and Environmental Sciences, University of Cagliari, Cagliari, Italy
- 3Institut de Ciències del Mar, Barcelona, Spain
- 4Marine Laboratory, Marine Scotland Science, Aberdeen, United Kingdom
- 5Instituto Español de Oceanografıa, Centro Oceanografico de Malaga, Biodiversity-Sustainability resources East-Central Atlantic Research Group, Malaga, Spain
- 6Institute for Marine Biological Resources and Biotechnology, National Research Council, Messina, Italy
- 7Instituto Español de Oceanografıa, Centre Oceanogràfic de les Balears, Ecosystem Oceanography Group, Palma de Mallorca, Spain
- 8Fisheries Research Institute, Hellenic Agricultural Organization Dimitra, Kavala, Greece
- 9Department of Biology, University of Padua, Padua, Italy
- 10Institute for Marine Biological Resources and Biotechnology, National Research Council, Mazara Del Vallo, Italy
- 11Portuguese Institute for the Ocean and Atmosphere, Division of Modelling and Management of Fishery Resources, Alges, Portugal
- 12Interuniversity Centre of Marine Biology and Applied Ecology, Livorno, Italy
- 13Italian Institute for Environmental Protection and Research, Rome, Italy
- 14Marine Biology and Fisheries Lab, Department of Biological, Geological and Environmental Sciences, Alma Mater Studiorum University of Bologna, Fano, Italy
Among the main measures adopted to reduce anthropogenic impacts on elasmobranch communities, understanding the ecology of deep-sea sharks is of paramount importance, especially for potentially vulnerable species highly represented in the bycatch composition of commercial fisheries such as the blackmouth catshark Galeus melastomus. In the present work, we unravelled the first indication of population genetic structure of G. melastomus by using a novel and effective panel of nuclear, and polymorphic DNA markers and compared our results with previous findings supporting high genetic connectivity at large spatial scales. Given the lack of species-specific nuclear markers, a total of 129 microsatellite loci (Simple Sequence Repeats, SSRs) were cross-amplified on blackmouth catshark specimens collected in eight geographically distant areas in the Mediterranean Sea and North-eastern Atlantic Ocean. A total of 13 SSRs were finally selected for genotyping, based on which the species exhibited signs of weak, but tangible genetic structure. The clearcut evidence of genetic differentiation of G. melastomus from Scottish waters from the rest of the population samples was defined, indicating that the species is genetically structured in the Mediterranean Sea and adjacent North-eastern Atlantic. Both individual and frequency-based analyses identified a genetic unit formed by the individuals collected in the Tyrrhenian Sea and the Strait of Sicily, distinguished from the rest of the Mediterranean and Portuguese samples. In addition, Bayesian analyses resolved a certain degree of separation of the easternmost Aegean sample and the admixed nature of the other Mediterranean and the Portuguese samples. Here, our results supported the hypothesis that the interaction between the ecology and biology of the species and abiotic drivers such as water circulations, temperature and bathymetry may affect the dispersion of G. melastomus, adding new information to the current knowledge of the connectivity of this deep-water species and providing powerful tools for estimating its response to anthropogenic impacts.
Introduction
Sharks are among the most vulnerable species inhabiting the marine realm, and although they are characterised by a long lifespan, they exhibit slow growth, late maturity and low fecundity, and other biological traits that may pose a significant threat to their populations (Stevens et al., 2000; Kousteni and Megalofonou, 2019). Habitat selection (DiGiacomo et al., 2020) and complex behaviours such as philopatry or site fidelity (Pillans et al., 2021) can additionally limit their capacity to recover from population declines related to overexploitation (Dell’Apa et al., 2012) and habitat fragmentation and depletion (Barausse et al., 2014). In deep waters, these intrinsic features may be exacerbated, increasing their vulnerability to human impacts and particularly exploitation (Rigby and Simpfendorfer, 2015). Concerns about the overexploitation of the deep-sea waters, lead to the identification, assessment and management of stock units of several shark species in the Atlantic Ocean (Veríssimo et al., 2011a; Daley et al., 2019; ICES, 2021), where bottom trawling has been banned below 800 metres (European Parliament, Council of the European Union, 2016). Within the Mediterranean Sea, the General Fisheries Commission for the Mediterranean (GFCM) banned bottom trawling below 1,000 m depth, allowing a possible refuge zone for mature individuals of those species showing depth-dependent size segregation (Gouraguine et al., 2011; Ramírez-Amaro et al., 2020). However, such measures may have likely shifted the trawling effort towards deeper waters (Tserpes et al., 2011; Farriols et al., 2019). On top of that, the effects of bycatch on already poorly investigated deep-water species may become even more dangerous, hindering the quantification of indirect impacts such as cascade effects on trophic interactions and loss of biomass (Myers et al., 2007; Cashion et al., 2019; Serena, 2021).
The blackmouth catshark Galeus melastomus Rafinesque, 1810 is a common benthic shark whose nominal family is still debated (Iglésias et al., 2005; Musick and Ellis, 2005; Dymek et al., 2021). Currently the species belongs to the family Pentachidae (Naylor et al., 2012; Kousteni et al., 2021) and it is widely distributed on the outer continental shelves and upper slopes in the North-eastern Atlantic Ocean and the Mediterranean Sea (Kallianiotis et al., 2000; Follesa et al., 2011; Abella et al., 2017; Froese and Pauly, 2019). The species shows a wide bathymetric range (from 55 to 2000 m depth), dwelling between 200 and 500 m depth (Ebert et al., 2021). The distribution, feeding habits and biological features of G. melastomus are well-studied and many authors have been focussing on the size at first maturity, sex ratio, sexual dimorphism, and egg deposition (Costa et al., 2005; Ragonese et al., 2009; Anastasopoulou et al., 2013; Metochis et al., 2018; D’Iglio et al., 2021a; Scacco et al., 2022). The species has shown signs of population differentiation across its distribution range regarding the maximum body-size, the length at maturity (Massutí and Moranta, 2003; Barría et al., 2015) and sex-related body weight (D’Iglio et al., 2021b). Data reported in literature showed differences in the maximum total length (TL) in individuals caught in the Atlantic and in the Mediterranean areas (TL 90 versus 70 cm, respectively; Massutí and Moranta, 2003; Barría et al., 2015), with the Mediterranean Sea individuals reaching the first maturity more rapidly (Massutí and Moranta, 2003; Moore et al., 2013; Metochis et al., 2018). In general, both sexes increase in size and abundance with depth (Rey et al., 2004), with females growing larger than males and reaching sexual maturity at a larger size (Moore et al., 2013; Metochis et al., 2018). Reproduction through spawning was observed all over the year (Capapé et al., 2008), and peaks were recorded in late spring-summer (Ragonese et al., 2009).
Little information is available about blackmouth catshark genetic diversity and population structure (Ferrari et al., 2018; Ramírez-Amaro et al., 2018), being the molecular method previously applied for integrated taxonomy (Castilho et al., 2007) and food traceability (Clarke et al., 2006). With the refinement of genetic and genomic techniques, deepening the knowledge about species genetic diversity and structuring is becoming one of the pillars of elasmobranchs’ conservation (Ovenden et al., 2019; Domingues et al., 2021; Delaval et al., 2022). Finding population structure across wide geographical or global scales failed in pelagic sharks (Hoelzel et al., 2006; Veríssimo et al., 2017) although some exceptions are reported (Kraft et al., 2020), while the identification of isolated populations on smaller regional scales has been documented in multiple coastal species (Portnoy et al., 2015; Catalano et al., 2022).
The above-mentioned population dynamics and habitat use of the blackmouth catshark may influence gene flow, being the latter limited by hydrographic barriers (e.g., currents and frontal systems and water masses), and progressively leading to genetic differentiation (Shaw et al., 2004). Currently, estimating population fragmentation is of primary interest for the conservation of cartilaginous fish species, whose population structure must be carefully assessed. Despite a high discard rate of the species (Ferretti et al., 2005), the International Union for the Conservation of Nature (IUCN) suggested that populations of blackmouth catshark in the Alboran, Balearic, Ligurian and Tyrrhenian Seas can be considered as stable (Ferretti et al., 2005; Ramírez-Amaro et al., 2020), even if the current fishing mortality does not ensure stock sustainability (Abella et al., 2017). Among the main threats, bycatch has been demonstrated to be the heaviest, leading to a steady decrease in mature individuals. According to the IUCN Red List Status, the species is characterised as Least Concern globally (Finucci et al., 2021) and in the Mediterranean Sea (Abella et al., 2016). Nevertheless, the North-eastern Atlantic Ocean and the Mediterranean Sea have been subjected to extensive and intensive deep-water fishing across much of the species’ known depth and spatial distribution (Finucci et al., 2021). Despite the management measures adopted to reduce deep-water shark fishing mortality, the species is still captured in demersal fisheries (e.g., in deep water shrimp trawl fishery), with a bycatch rate steadily increasing in some locations (Pauly and Zeller, 2015; Moura et al., 2018). Discard mortality of G. melastomus is unknown, but is likely to be high based on severe injuries obtained when individuals are captured (Finucci et al., 2021).
The present study aims at assessing the population genetic structure of G. melastomus within a large part of its range, from the North-eastern Atlantic Ocean and from the Western to the Eastern Mediterranean Sea, surveying the genetic diversity of 194 specimens collected from eight geographically distant areas at 13 cross-amplified microsatellite loci. Our findings, in conjunction with the results from previous studies that analysed the species’ genetic diversity by using mitochondrial DNA markers, will advance current knowledge about the species’ population units and contribute to future species assessment in the region.
Materials and methods
Sampling
A total of 194 tissue samples (i.e., skeletal muscle or fin clips) and associated information (i.e., biological and sampling data, pictures, and size measurements) of G. melastomus were collected during scientific surveys from eight sampling areas across the North-eastern Atlantic Ocean and the Mediterranean Sea: Scotland (SCO), Portugal (POR), Spain (SPA), Italy (LIG, TYR, SIC, ADR) and Greece (AEG) (Table 1; Figure 1; SM1). Samples were collected in 2018 and 2019 except for the AEG sample that was collected in 2011. Data points were collected at depths ranging from 116 to 1060 m. Tissue samples were stored in 96% ethanol at -20°C until laboratory analysis.
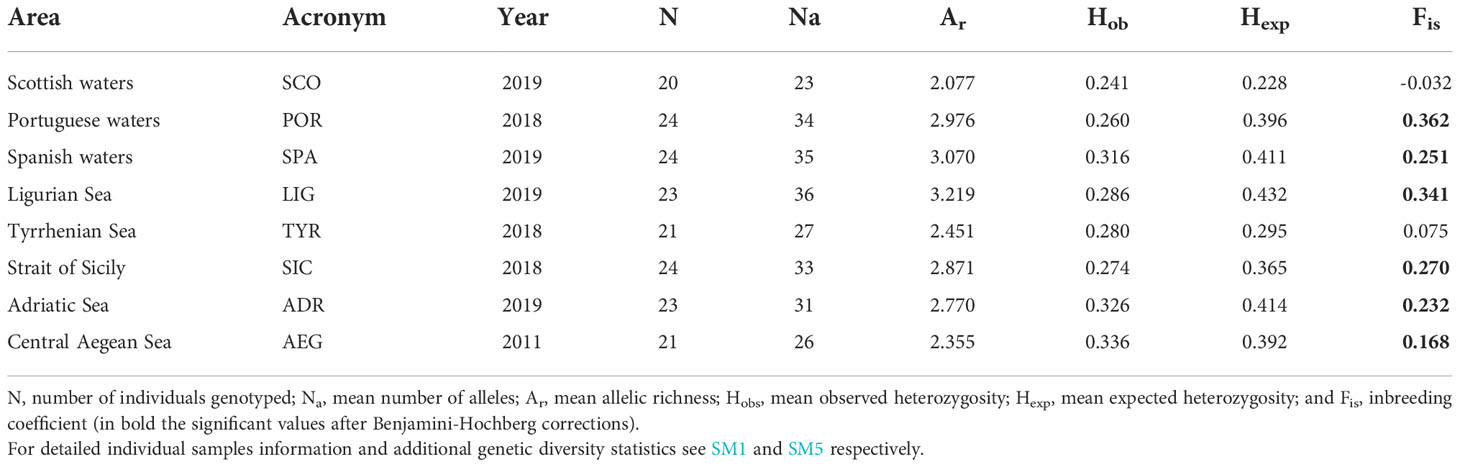
Table 1 Sampling summary and main genetic diversity statistics of Galeus melastomus geographical samples.
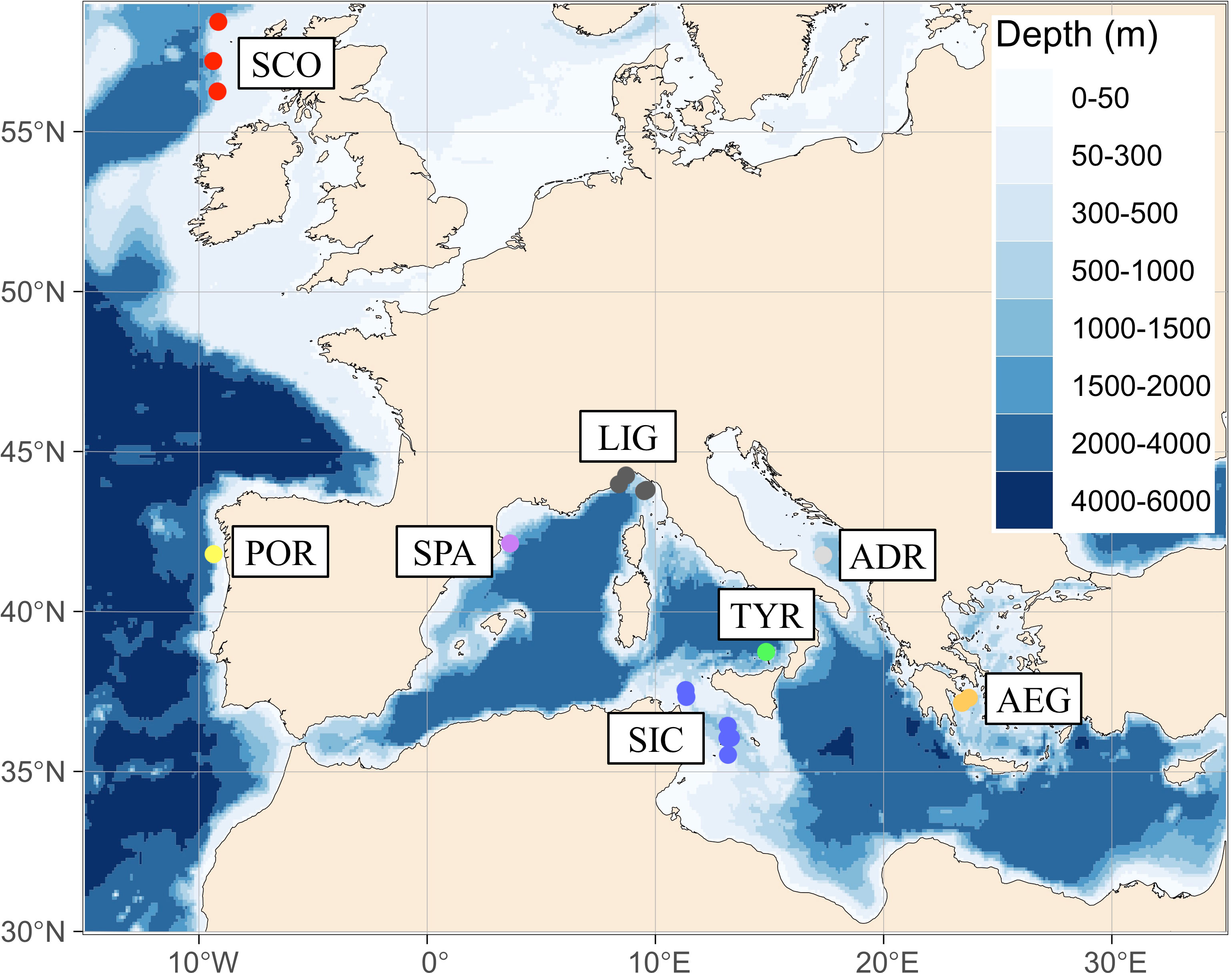
Figure 1 Sampling locations of Galeus melastomus. Acronyms of geographical samples are given as in Table 1. Data points are coloured according to the DAPC scatterplot of Figure 2.
Specimen identification followed field marks proposed by Rey et al. (2006) and confirmed by Castilho et al. (2007). When specimen identification between G. melastomus and Galeus atlanticus Vaillant, 1888 was ambiguous, molecular identification was performed using the DNA barcoding approach (Cariani et al., 2017). Results were cross-referenced with sequences available on the Barcode of Life Data Systems (Ratnasingham and Hebert, 2007).
DNA extraction and genotyping
Total genomic DNA (gDNA) was extracted from approximately 20 mg of tissue using the Wizard® SV Genomic DNA Purification System by Promega, according to the manufacturer’s instructions. The quality of the extracted gDNA was assessed on a 0.8% agarose gel electrophoresis.
A total of 129 heterospecific microsatellite loci (SM2) were selected and cross-amplified on 24 individuals of G. melastomus collected from the North Atlantic, Western and Eastern Mediterranean. Thirteen loci were associated with polymorphic amplicons in individuals from all three areas and were thus selected for the genotyping of the 194 individuals. In particular, the loci tested here were initially developed for Centroscymnus crepidater Barbosa du Bocage & de Brito Capello, 1864, Galeorhinus galeus Linnaeus, 1758, Hexanchus griseus Bonnaterre, 1788, Mustelus antarcticus Günther, 1870, Mustelus canis Mitchill, 1815, Mustelus mustelus Linnaeus, 1758 and Scyliorhinus canicula Linnaeus, 1758 (SM2).
The selected loci were amplified in three multiplexed PCR reactions (SM2; SM3). When necessary, single-locus PCR reactions were carried out following the same protocol described in SM3 with a total volume of 15 μL. Amplicons were further sized at Macrogen Korea using the GS-500LIZ internal size standard.
Data analysis
Alleles were scored using GENEMARKER® (SOFTGENETICS®, LLC). The presence of null alleles, stuttering, and allele drop-out was tested using MICRO-CHECKER 2.2.3 (Van Oosterhout et al., 2004) using 1,000 randomisations on Bonferroni correction. The statistical power of microsatellite loci to detect population homogeneity was verified with POWSIM 4.1 by using the allele frequencies obtained from the samples in this study (Ryman and Palm, 2006). Both Chi-square and Fisher’s exact tests were carried out by employing 1,000 iterations, 5,000 simulation runs, effective population (Ne) 1,000, and pairwise differentiation index (Fst) between 0.025 and 0.050. Furthermore, Type I α error, representing the probability of rejecting the null hypothesis, was inferred setting t generations’ drift to zero.
Allelic frequency, measures of heterozygosity, polymorphism index and the average number of alleles per locus were calculated using the software GENETIX 4.05. (Belkhir et al., 2004). The software FSTAT 2.9.3.2 (Goudet, 2001) was used to compute allelic richness, gene diversity and inbreeding coefficient (Fis). The Hardy-Weinberg equilibrium was assessed using the software GENEPOP 4.7.3 (Rousset, 2008), applying the Fisher’s (1935) exact test. The Markov Chain Monte Carlo (MCMC) approximation involved 10,000 dememorization steps, 1,000 batches and 10,000 iterations per batch. Probability tests were also performed, and the relative values were corrected for multiple testing at alpha 0.05 using the Bonferroni correction.
Pairwise Fst values were calculated with ARLEQUIN v.3.5 (Excoffier and Lischer, 2010) with 5,000 permutations and alpha value equal to 0.05. The same software was used for the analysis of molecular variance (AMOVA; Excoffier et al., 1992), grouping the samples based on a geographical structure on three hierarchical levels: among geographical areas, among populations within geographical areas and within populations. The statistical significance of the resulting values of the pairwise fixation index was estimated by comparing the observed distribution with a null distribution generated by 5,000 permutations and the Benjamini-Hochberg method was applied for multiple test correction (Benjamini and Hochberg, 1995).
The discriminant analysis of principal components (DAPC), implemented in the adegenet R package following Jombart et al. (2010), was used to investigate the genetic structure identified by AMOVA.
We investigated the underlying population genetic structure using a Bayesian clustering algorithm implemented in STRUCTURE 2.3.4 (Hubisz et al., 2009), incorporating the Admixture Model, Alleles Frequencies Correlated and sampling location as a LOCPRIOR. We performed 25 independent runs for each K (1-15) with 1,000,000 iterations and a burn-in of 100,000 generations. The optimal number of genetic clusters was inferred from the mean estimated log probability of the data and its second-order rate of change (ΔK) as calculated in STRUCTURE HARVESTER according to Earl and vonHoldt (2012). Cluster matching and permutation were performed using CLUMPAK (Kopelman et al., 2015). In addition, we estimated the best K using four alternative statistics (medmedk, medmeak, maxmedk and maxmeak) carried out using STRUCTURESELECTOR (Li and Liu, 2018).
Results
A total of 13 out of the 129 tested SSRs (10.1%) provided amplicons within the reference size and in both Atlantic and Mediterranean individuals (SM2). Considering a call rate threshold of 90%, a total of 180 individuals constituted the final dataset. Loci Gg11 and McaB28 were discarded since their amplification patterns suggested the presence of multiple genic regions. The most polymorphic locus was MaD2X (Na = 9), while the less polymorphic ones were Cc21 and MaND5 (both with Na = 2). The presence of null alleles (SM4) contributed to a moderate heterozygosity that was observed at the population level (Table 1). The population sample from the Ligurian Sea showed the highest number of alleles (Na = 36) and the highest allelic richness (Ar = 3.219), while the sample from Scottish waters showed the lowest values (Na = 23, Ar = 2.077). In general, genetic diversity estimators showed samples from the Ligurian Sea and Scottish waters as the putative populations with the highest and lowest genetic variability, respectively (SM4).
The pattern and the success of SSRs cross-amplification followed the levels of taxonomic relatedness between species since most of the loci effectively amplified in G. melastomus were those previously developed for species of the closest family Triakidae (Galeorhinus galeus and Mustelus spp.; extensive details and analyses are reported in SM5).
The simulation obtained with POWSIM highlighted the high-resolution power of the dataset. Both Chi-square and Fisher’s tests revealed genetic differentiation in most runs and were able to detect Fst values of as low as 0.025 in 100% of the runs (t100: χ2 = 1.000, F = 1.000, Pvalue < 0.05).
The mean pairwise Fst value among samples was 0.159 (Pvalue < 0.001) while the pairwise Fst values ranged from 0.037 (POR-ADR) to 0.348 (TYR-AEG) (SM6). All the pairwise Fst values were significant (Pvalue < 0.001) except for the comparison SPA-ADR. The AMOVA (Table 2 and Table 3) with the highest percentage of molecular variation among groups (13.62%) and the lowest value of molecular variation among populations within group (3.99%) was the one performed with the subdivision of geographical samples into five groups (SCO; POR; SPA-LIG-ADR; SIC-TYR; AEG).
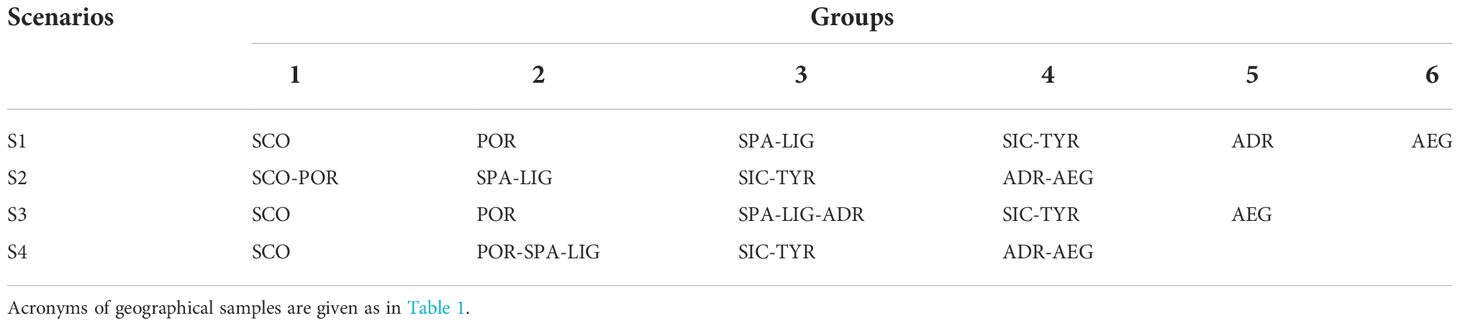
Table 2 Summary of a priori groupings tested by AMOVA analysis according to the geographic origin of the individuals.
The DAPC scatter plot showed a separation into three main groups (Figure 2). The SCO group was clearly separated from the rest of the samples along axis 2, the TYR-SIC group separated along axis 1, while the remaining samples only partially separated (e.g., POR, SPA, LIG, ADR and AEG).
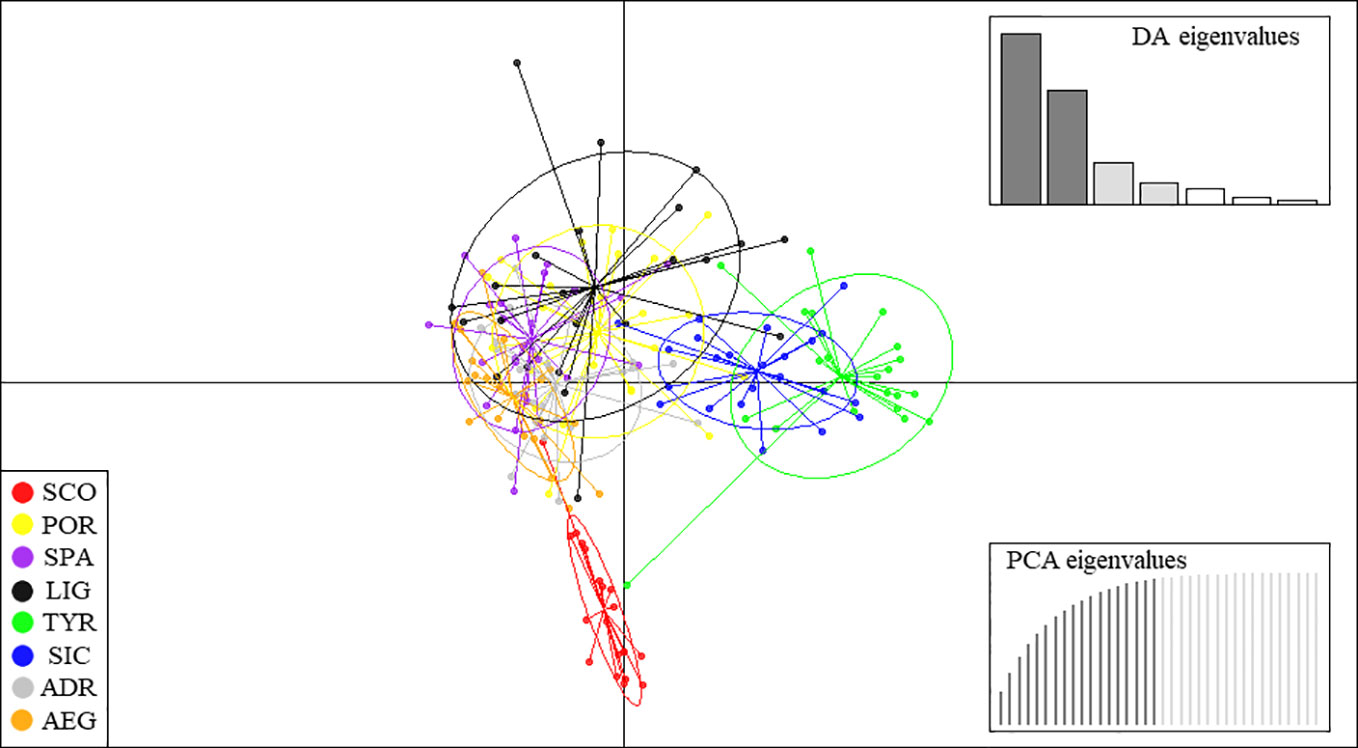
Figure 2 DAPC scatterplot of the Galeus melastomus samples. DAPC cluster ellipses were set to contain 95% of genotypes. Discriminant analysis (DA) eigenvalues and principal component analysis (PCA) eigenvalues were selected as displayed to avoid overfitting. Sample acronyms are given as in Table 1.
The STRUCTURE analyses inferred K = 2 and K = 3 as the optimal number of genetic clusters that best fitted the data with STRUCTURE HARVESTER (ΔK) and STRUCTURESELECTOR (K), respectively (Figure 3, Figure 4, SM7 and SM8). In Figure 3, the barplot of K = 2 showed all individuals except one from the Mediterranean TYR and SIC assigned to a genetic cluster different with respect to that of SCO and AEG individuals. The rest of the samples (POR, SPA, LIG and ADR) were genetically admixed with different proportions of individuals assigned to the two genetic clusters. The barplot of K = 3 improved the separation of SCO from AEG, as well as the admixed composition of POR, SPA, LIG and ADR with individuals associated to the three identified clusters in different proportions.
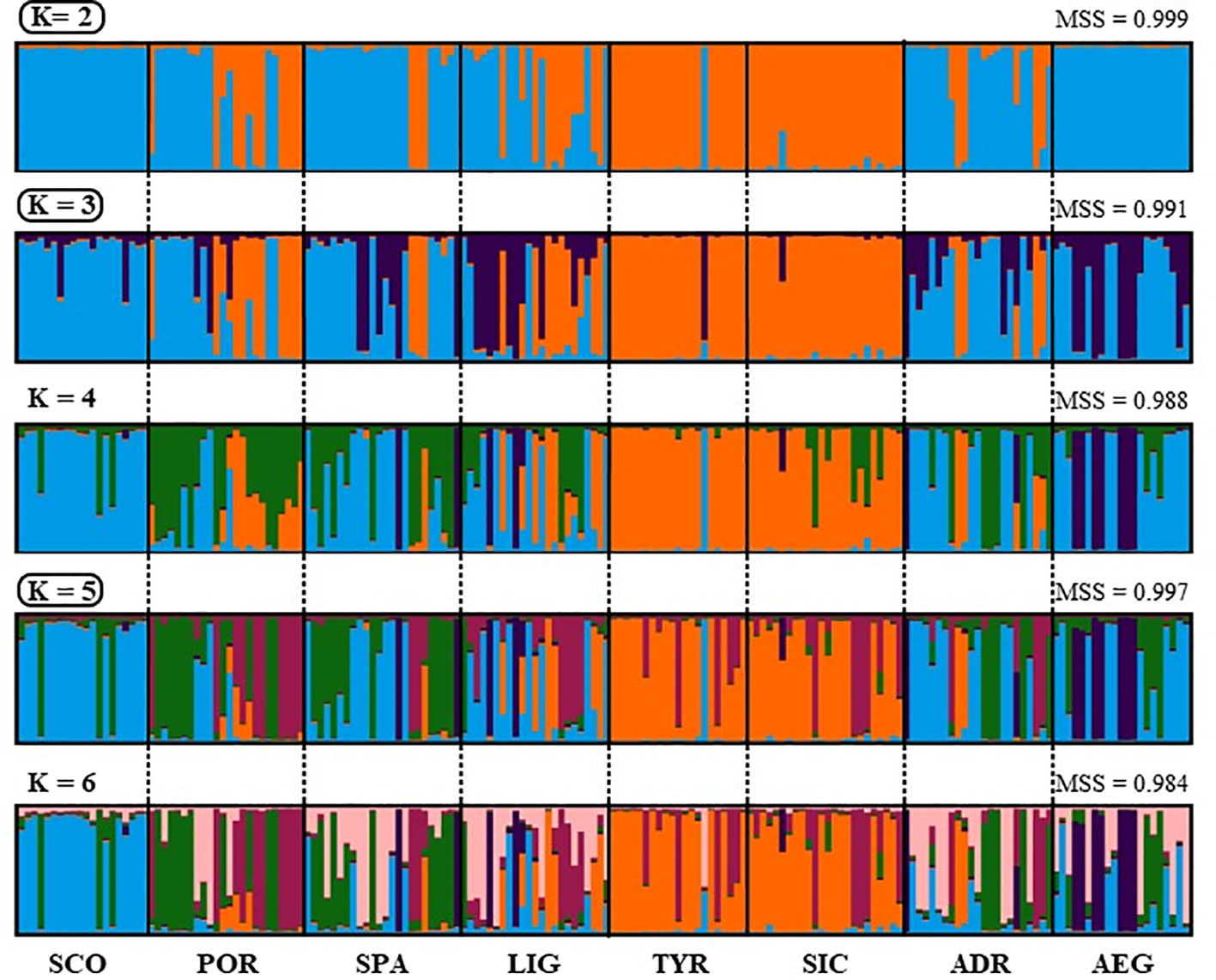
Figure 3 Bayesian clustering barplot showing membership probabilities (q) for each individual after CLUMPAK analysis, see Table 1 for geographical location codes. For each K analysed the mean similarity score (MSS) is reported.
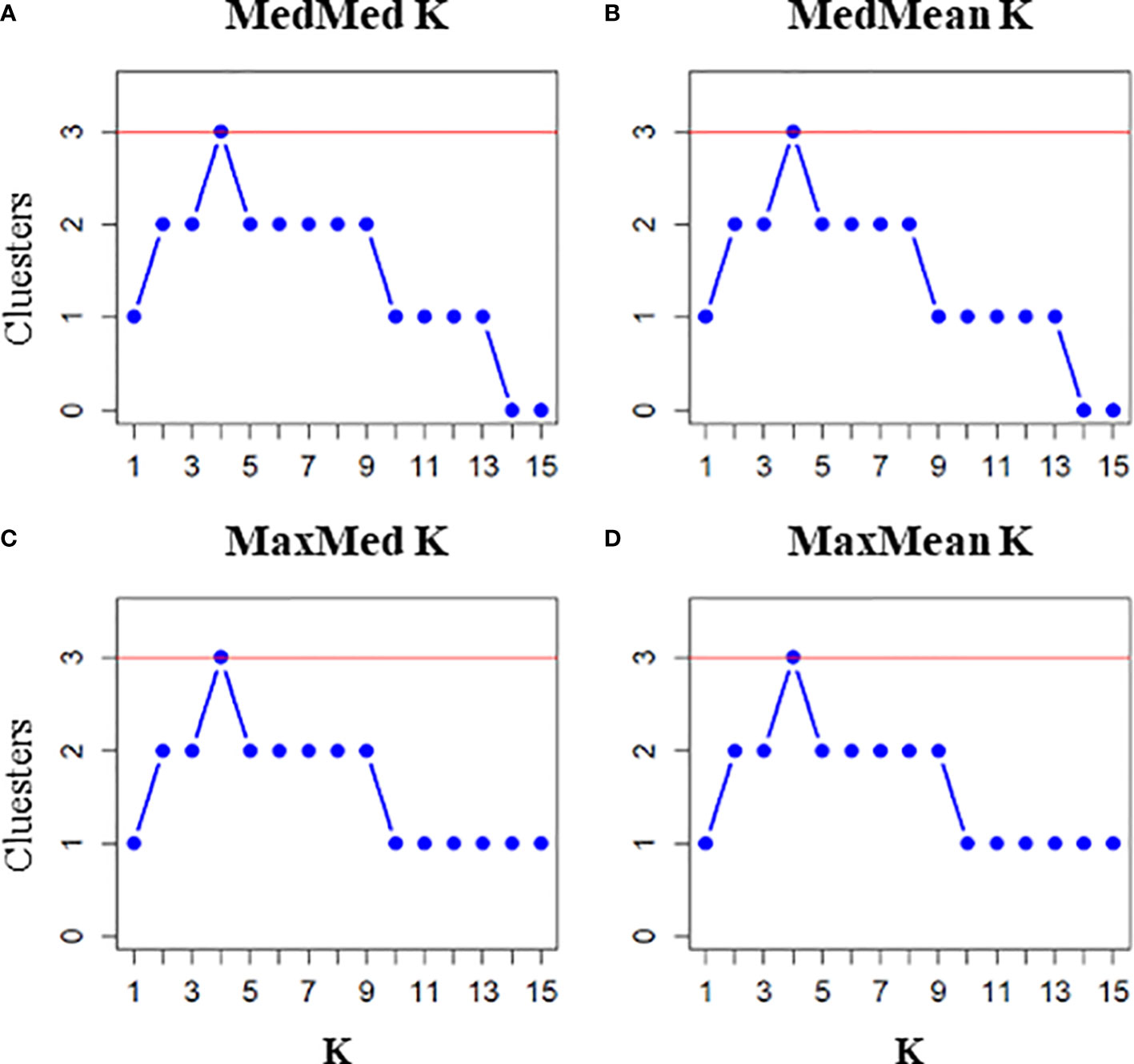
Figure 4 Graphical representation of results inferred with STRUCTURESELECTOR for each k and statistics (A: medmedk; B: medmeak; C: maxmedk; D: maxmeak).
Discussion
This research work revealed the first evidence of a detectable genetic structure of the necto-benthic, deep-water G. melastomus populations in the Mediterranean Sea and neighbouring North-East Atlantic areas, whose stocks are potentially affected and impacted by the demersal fishery (Tiralongo et al., 2021). Here, we described the first evidence of a weak albeit detectable genetic structure and the development of a technological tool represented by a panel of cross-specific microsatellite loci which were successful in detecting and measuring species genetic diversity and differentiation of blackmouth catshark over a large, regional geographic scale and a significant number of samples and individuals.
Our results are partially in contrast with previous findings obtained using multiple mitochondrial DNA markers and assessing genetic differentiation based on phylogeographic signals at a similar geographical scale, that suggested high connectivity with past population expansion of the species (Ferrari et al., 2018; Ramírez-Amaro et al., 2018). Indeed, our study newly unravels the genetic complexity of G. melastomus populations on a large geographical scale by using nuclear, and polymorphic DNA markers. Such recorded genetic complexity can be attributable to i) a detectable but weak level of genetic differentiation and population structure that ii) speaks in favour of the occurrence of groups of subpopulations and environmental and/or ecological barriers to gene flow and iii) a great amount of genetic diversity represented by a high number of alleles per locus, high allelic richness and moderately high proportion of heterozygous multilocus genotypes. Given the clearcut evidence of genetic differentiation of the blackmouth sharks from Scottish waters emerged from all the tests and analyses performed, the rest of the population samples clustered depending mostly by the test and the analysis performed but, all in all, indicating that the species is genetically structured in the Mediterranean Sea and in the adjacent North-eastern Atlantic. Both the DAPC and STRUCTURE analyses identified a genetic unit formed by the individuals collected in the Tyrrhenian Sea and the Strait of Sicily, which resulted quite differentiated from the rest of the Mediterranean and Portuguese samples. In addition, the STRUCTURE analyses resolved a certain degree of separation of the easternmost Aegean sample and the admixed nature of the other Mediterranean and the Portuguese samples. The separation of the Central Southern Mediterranean samples could be a combination of different factors, related on one hand to specific environmental conditions in this area as hydrodynamic regimes and front systems limiting the species migration or, on the other hand, related to the modifications induced by the fishing pressure. Although intensive fishing currently involves the whole Mediterranean basin, the Strait of Sicily has been suffering from intense overfishing for decades (Fiorentino and Vitale, 2021). Such impact has been found to contribute to the modification of relevant biological traits of G. melastomus as the decrease of size at first maturity (D’Iglio et al., 2021b). Although the species seems to be resilient and adaptable to human exploitation, mitigation measures based on population dynamics or mating success should not be excluded.
When looking at the interaction between some biological traits of G. melastomus and physical barriers of the marine realm, knowledge about the use of nursery areas (Capapé et al., 2008; Metochis et al., 2018) and sex-based migratory behaviour should be encouraged, since this species might be an important key-taxon for understanding deep-sea ecosystems (Rey et al., 2004). Moreover, if the large geographic distance between sampling locations is not sufficient to explain the lack of connectivity in deep-sea species (Veríssimo et al., 2011b; Catarino et al., 2015; Kousteni et al., 2015a; Kousteni et al., 2015b; Gubili et al., 2016), factors as bathymetry or geomorphology (e.g., the presence of canyons; Fernandez-Arcaya et al., 2017) and temperature may be influencing the genetic structuring of the species. As a matter of fact, large adults of G. melastomus appear to undertake periodical migration from deeper waters toward shallow environments for mating (D’Iglio et al., 2021b). It is reasonable to suppose that abiotic factors such as water circulations and bathymetry might be decreasing the contact potential between populations, contributing to the differentiation of samples from the Tyrrhenian Sea and the adjacent Strait of Sicily and to the homogenization of their genetic variability against other Mediterranean areas (Spanish, Ligurian, Adriatic and Aegean waters). As a matter of fact, within the Mediterranean Sea, the Levantine Intermediate Water and the Deep-water circulations (El-Geziry and Bryden, 2010) might be contributing to the differentiation of samples from the Tyrrhenian Sea and the adjacent Strait of Sicily and the homogenization of genetic variability between other sectors of the Basin (Spanish, Ligurian, Adriatic and Aegean waters). In addition, the important depth of the Strait of Sicily (over 1300 m deep) and the Ionian Sea (average depth near to 4000 m) can explain the very low similarity between samples from Tyrrhenian Sea and Strait of Sicily and the other groups. Specific dispersal potential related to species’ habits and life-history traits, such as the reproductive strategy, the use of mating and nursery areas and sex-based migratory behaviour, may contribute to population structuring (Hirschfeld et al., 2021; Catalano et al., 2022). Migration events or site fidelity may be strongly related to the optimal temperature at which the species is able to maximise growth rate and the bioenergetic efficiency, as already described in other sharks like S. canicula (Sims et al., 2006). The search for optimal temperature by G. melastomus is consistent with its reproduction hot-spot discovered in the deep waters 200 miles off the western Irish coast (O’Sullivan et al., 2018) and agrees with similar observations for other sharks, like Negaprion brevirostris Poey, 1868 (Kessel et al., 2014). High dispersal potential and panmixia have been inferred for some migratory shark species, including Squalus acanthias Linnaeus, 1758 (Veríssimo et al., 2010), Squalus blainville Risso, 1827 (Kousteni et al., 2015a), Centroscymnus coelolepis Barbosa du Bocage & de Brito Capello, 1864 and Centrophorus squamosus Bonnaterre, 1788 (Veríssimo et al., 2011a; Veríssimo et al., 2012). On the contrary, strong genetic differentiation has been described for the less vagile S. canicula when comparing samples from the Ionian and Aegean Seas with Atlantic, Spanish, and Algerian ones (Kousteni et al., 2015b) and within the Alboran Sea and Western Mediterranean (Ramírez-Amaro et al., 2018). According to Ramírez-Amaro et al. (2018), results for G. melastomus collected in the same areas did show genetic connectivity, being the latter well supported by a high number of migrants per generation crossing the Alboran and Balearic Seas.
In addition, ecological traits, such as feeding habits, may also contribute to population structuring. The relationship between predator abundance and prey density has been discussed in Barría et al. (2018); Ramírez-Amaro et al. (2020) and more recently in Sbrana et al. (2022) when describing marine predators’ foraging maximisation. In their works, these authors indicated the possibility that population structure and dynamics may depend on the adoption of opportunistic habits and a highly diverse diet, a trait that may enhance their susceptibility to spatial-temporal changes in prey abundance (Campana and Joyce, 2004; Anastasopoulou et al., 2013).
For some deep-sea elasmobranch species such as C. coelolepis (Catarino et al., 2015), Etmopterus spinax Linnaeus, 1758 (Gubili et al., 2016) and hypothetically also for G. atlanticus (Rey et al., 2010), the Strait of Gibraltar represents a breakpoint in the connectivity of the species. Differently, our results did not highlight such a strong barrier to the gene flow between Mediterranean and adjacent Atlantic, since samples collected along the Portuguese coasts were strongly different from the northernmost Scottish sample but were similar to those collected in the western Mediterranean (Spanish and Ligurian) waters. These results appear discordant with the identification of water circulation assumption as a physical barrier (see Roque et al., 2019 for Atlantic currents), but not with the bathymetry since these areas are divided by the Bay of Biscay characterised by an average depth of 1744 m, much deeper than the common depths inhabited by G. melastomus and reached only occasionally (Ebert et al., 2021). In addition, in the Atlantic Ocean temperature decreases with latitude, likely limiting the migration of individuals along the Atlantic coast and influencing their spatial dispersion (Hemmer-Hansen et al., 2007). Strong genetic differences were also detected by outlier SNPs between Northern and Southern North-eastern Atlantic samples of the European hake Merluccius merluccius Linnaeus, 1758 (Milano et al., 2014), a species with several ecological traits similar to the blackmouth shark.
The relationship existing between sensitive ecosystems (e.g., canyons and cold-water coral habitats, nurseries and refugia) and inhabiting species, should be preserved with effective conservation strategies (Carluccio et al., 2021). In this perspective, further in-depth research focusing on the monitoring of natural populations of G. melastomus and on the comparison of the genetic variability and differentiation of populations over multiple generations are required. Over temporal replicates, any difference in allelic richness and allele frequencies could reveal the existence of a founder effect or past bottleneck events. Additionally, including individuals of G. melastomus from other areas in the Mediterranean Sea as the North African coasts, Western Mediterranean waters and the Levantine Sea could be useful to describe any further differentiation in the Basin, especially in those areas with canyon systems and other essential fish habitats. Similarly, targeting the Atlantic Iberian coasts, the Bay of Biscay, the West of Ireland and the United Kingdom and Norway waters would pinpoint the species’ connectivity in the North-eastern Atlantic Ocean.
Maximising the monitoring of Mediterranean and Atlantic populations of blackmouth catshark both in its temporal and spatial coverage is advisable and it could be achieved by capitalising established scientific surveys where G. melastomus represents one of the target species (i.e., MEDITS and PNAB; Spedicato et al., 2020; Vieira et al., 2020). In addition, the employment of non-invasive sampling protocols (i.e., environmental DNA; Aglieri et al., 2021) represent a valuable approach to obtain data without sacrificing individuals for research purposes, even though such techniques have not been extensively applied to threatened and deep water species (Dugal et al., 2022; Merten et al., 2021). The improvement of the genotyping approach (i.e., the application of an increased number of polymorphic microsatellite loci or the development of panels of Single Nucleotide Polymorphisms, SNPs) will provide more statistical power for the robust delineation of the genetic structure of the species. The application of high throughput sequencing techniques would likely enhance the detection of randomly distributed genomic markers. In species where large numbers of SNPs have been screened, even a small fraction of these markers has been demonstrated to be very informative for population structure analysis, sometimes outperforming highly polymorphic microsatellites (Liu et al., 2005; Lao et al., 2006).
Within the technological framework, this research work advanced by providing a new panel of 13 heterospecific microsatellite markers that were deeply verified to cross-amplify genetic loci in G. melastomus. Very positive results of cross-amplification tests confirmed that the microsatellite loci investigated in G. melastomus are particularly well-conserved over time in related families (i.e., Triakidae with G. galeus and Mustelus spp.), but also in phylogenetically more distant families and species as Hexanchus griseus, within the family Hexanchidae, and C. crepidater, within the family Somniosidae, which are the most distant taxa from Pentachidae and G. melastomus (Naylor et al., 2012). Overall, similar rates of success in cross-amplification were reached here compared to the literature (Griffiths et al., 2011; Helyar et al., 2011; Veríssimo et al., 2011b; see SM9 for comparison of cross-amplification results on G. melastomus). Most importantly, since these loci resulted largely polymorphic at the pan-European scale, this novel panel of microsatellites could be considered an effective analytical tool for assessing and monitoring genetic diversity and differentiation among populations and stocks either over the whole species range and at the subregional level.
With this research work we investigated and assessed the first evidence of genetic structure over a large, regional geographic scale of G. melastomus, a potentially vulnerable species highly represented in the bycatch composition of demersal fisheries. Understanding the intrinsic characteristics of both Mediterranean basin (e.g., bathymetry, water currents and deep-water temperature) and the species life-history traits and ecology will likely disclose the drivers influencing species differentiation, as well as the potential decline in species abundance, meaning the loss of biodiversity at a local scale with important long-term effects leading to local depletion (Dell’Apa et al., 2012). For these reasons, the effective panel of cross-specific and polymorphic microsatellite loci developed here will enhance the monitoring of natural populations of G. melastomus. The results described enhance the refining and integration of the current knowledge on the diversity and connectivity of a key species for deep-sea ecosystems (D’Iglio et al., 2021b). As a mesopelagic predator with a diverse diet, the blackmouth catshark is becoming one of the most important sentinels of the health of deep habitats and an indicator of human impacts on the marine environment that should be effectively managed (Sbrana et al., 2022). The improvement and employment of genetic and genomic tools coupled with wide research actions (e.g., integrating data on biology, reproductive strategies, feeding and migratory behaviours) should be directed at gathering information on spawning and nursery areas, pursuing scientific surveys at sea to detect discrete groups of populations (Domingues et al., 2018). Robust data mining along with strengthened international networks of collaborations maximising the monitoring effort, will likely fill the gaps in our knowledge of this benthic shark, and encourage effective management and conservation plans for the key species and its habitat.
Data availability statement
The data presented in this study are included in the supplementary material (SM1; SM10), further inquiries can be directed to the corresponding authors.
Ethics statement
Ethical review and approval was not required for the animal study because the following information was supplied relating to ethical approvals (i.e., approving body and any reference numbers): samples of shark individuals analyzed in the present work were obtained from commercial and scientific fisheries. The activity was conducted with the observation of the Regulation of the European Parliament and the Council for fishing in the General Fisheries Commission for the Mediterranean (GFCM) Agreement area and amending Council Regulation (EC) No. 1967/2006. This Regulation is de facto the unique authorization needed to conduct this type of activity.
Author contributions
SC, AF, AC, and FT conceived and designed the study. SC performed the molecular and data analyses. RC and IM contributed to statistical revision. SC and AF wrote the first draft of the manuscript. CB, JD, LF-P, DG, MH, VK, DM, TM, JR, PS, US, FS, and MS provided the samples used in this work. All authors contributed to the article and approved the submitted version.
Funding
This research was funded by RFO and Canziani grants given to FT and AC, and by the RFO grant of the University of Bologna for the research fellowships of AF. The funders had no role in study design, data collection and analysis, decision to publish, or preparation of the manuscript.
Acknowledgments
We thank all contributors to this research. In particular, we are grateful to all the participants in the scientific surveys, as well as the crew of each research vessel for their sampling effort.
Conflict of interest
The authors declare that the research was conducted in the absence of any commercial or financial relationships that could be construed as a potential conflict of interest.
The reviewer FT is currently organizing a Research Topic with the author CB.
Publisher’s note
All claims expressed in this article are solely those of the authors and do not necessarily represent those of their affiliated organizations, or those of the publisher, the editors and the reviewers. Any product that may be evaluated in this article, or claim that may be made by its manufacturer, is not guaranteed or endorsed by the publisher.
Supplementary material
The Supplementary Material for this article can be found online at: https://www.frontiersin.org/articles/10.3389/fmars.2022.953895/full#supplementary-material
References
Abella A. J., Mancusi C., Mannini A., Serena F. (2017). “Galeus melastomus” in Synthesis of the knowledge on biology, ecology and fishery of the halieutic resources of the Italian seas. Eds Sartor P., Mannini A., Carlucci R., Massaro E., Queirolo S., Sabatini A., et al Mediterranean Marine Biology 24 (Suppl. 1), 136–143.
Abella A., Serena F., Mancusi C., Ungaro N., Hareide N. R., Guallart J., et al. (2016) Galeus melastomus (The IUCN Red List of Threatened Species) (Accessed 09 April 2022). e.T161398A16527793.
Aglieri G., Baillie C., Mariani S., Cattano C., Calò A., Turco G., et al. (2021). Environmental DNA effectively captures functional diversity of coastal fish communities. Mol. Ecol. 30 (13), 3127–3139. doi: 10.1111/mec.15661
Anastasopoulou A., Mytilineou C., Lefkaditou E., Dokos J., Smith C. J., Siapatis A., et al. (2013). Diet and feeding strategy of blackmouth catshark Galeus melastomus. J. Fish Biol. 83, 1637–1655. doi: 10.1111/jfb.12269
European Parliament, Council of the European Union. (2016). Regulation (EU) 2016/2336 of the European Parliament and of the Council of 14 December 2016 establishing specific conditions for fishing for deep-sea stocks in the north-east Atlantic and provisions for fishing in international waters of the north-east Atlantic and repealing Council Regulation (EC) No 2347/2002. OJ L 354, 23.12.2016, p. 1–19. Available at: http://data.europa.eu/eli/reg/2016/2336/oj
Barausse A., Correale V., Curkovic A., Finotto L., Riginella E., Visentin E., et al. (2014). The role of fisheries and the environment in driving the decline of elasmobranchs in the northern Adriatic Sea. ICES J. Mar. Sci. 71 (7), 1593–1603. doi: 10.1093/icesjms/fst222
Barría C., Navarro J., Coll M. (2018). Feeding habits of four sympatric sharks in two deep-water fishery areas of the western Mediterranean Sea. Deep Sea Res. Part I Oceanogr. Res. Pap. 142, 34–43. doi: 10.1016/j.dsr.2018.09.010
Barría C., Navarro J., Coll M., Fernandez-Arcaya U., Sáez-Liante R. (2015). Morphological parameters of abundant and threatened chondrichthyans of the northwestern Mediterranean Sea. J. Appl. Ichthyol. 31, 114–119. doi: 10.1111/jai.12499
Belkhir K., Borsa P., Chikhi L., Raufaste N., Bonhomme F. (2004). “GENETIX 4.05, logiciel sous windows TM pour la génétique des populations,” in Laboratoire génome, populations, interactions, CNRS UMR 5171 (Montpellier, France: Université de Montpellier II).
Benjamini Y., Hochberg Y. (1995). Controlling the false discovery rate: a practical and powerful approach to multiple testing. J. R. Stat. Soc Ser. B Stat. Methodol. . 57, 289–300. doi: 10.1111/j.2517-6161.1995.tb02031.x
Campana S. E., Joyce W. N. (2004). Temperature and depth associations of porbeagle shark (Lamna nasus) in the northwest Atlantic. Fish. Oceanogr. 13, 52–64. doi: 10.1111/J.1365-2419.2004.00236.X
Capapé C., Guélorget O., Vergne Y., Reynaud C. (2008). Reproductive biology of the blackmouth catshark, Galeus melastomus (Chondrichthyes: Scyliorhinidae) off the languedocian coast (southern France, northern Mediterranean). J. Mar. Biol. Assoc. UK 88, 415–421. doi: 10.1017/S002531540800060X
Cariani A., Messinetti S., Ferrari A., Arculeo M., Bonello J. J., Bonnici L., et al. (2017). Improving the conservation of Mediterranean chondrichthyans: The ELASMOMED DNA barcode reference library. PloS One 12 (1), e0170244. doi: 10.1371/journal.pone.0170244
Carluccio A., Capezzuto F., Maiorano P., Sion L., D’Onghia G. (2021). Deep-water cartilaginous fishes in the central Mediterranean Sea: Comparison between geographic areas with two low impact tools for sampling. J. Mar. Sci. Eng. 9 (7), 686. doi: 10.3390/jmse9070686
Cashion M. S., Bailly N., Pauly D. (2019). Official catch data underrepresent shark and ray taxa caught in Mediterranean and black Sea fisheries. Mar. Policy. 105, 1–9. doi: 10.1016/j.marpol.2019.02.041
Castilho R., Freitas M., Silva G., Fernandez-Carvalho J., Coelho R. (2007). Morphological and mitochondrial DNA divergence validates blackmouth, Galeus melastomus, and Atlantic sawtail catsharks, Galeus atlanticus, as separate species. J. Fish Biol. 70, 346–358. doi: 10.1111/j.1095-8649.2007.01455.x
Catalano G., Crobe V., Ferrari A., Baino R., Massi D., Titone A., et al. (2022). Strongly structured populations and reproductive habitat fragmentation increase the vulnerability of the Mediterranean starry ray Raja asterias (Elasmobranchii, rajidae). Aquat. Conserv. 32 (1), 66–84. doi: 10.1002/aqc.3739
Catarino D., Knutsen H., Veríssimo A., Olsen E. M., Jorde P. E., Menezes G., et al. (2015). The pillars of Hercules as a bathymetric barrier to gene flow promoting isolation in a global deep-sea shark (Centroscymnus coelolepis). Mol. Ecol. 24 (24), 6061–6079. doi: 10.1111/mec.13453
Clarke S. C., Magnussen J. E., Abercrombie D. L., McAllister M. K., Mahmood S., Shivji M. S. (2006). Identification of shark species composition and proportion in the Hong Kong shark fin market based on molecular genetics and trade records. Conserv. Biol. 20 (1), 201–211. doi: 10.1111/j.1523-1739.2005.00247.x
Costa M. E., Erzini K., Borges T. C. (2005). Reproductive biology of the blackmouth catshark, Galeus melastomus (Chondrichthyes: Scyliorhinidae) off the south coast of Portugal. Mar. Biol. Assoc. United Kingdom. J. Mar. Biolog. Assoc. UK. 85 (5), 1173. doi: 10.1017/S0025315405012270
Daley R. K., Hobday A. J., Semmens J. M. (2019). Simulation-based evaluation of reserve network performance for Centrophorus zeehaani (Centrophoridae): a protected deep-sea gulper shark. ICES J. Mar. Sci. 76 (7), 2318–2328. doi: 10.1093/icesjms/fsz087
Delaval A., Frost M., Bendall V., Hetherington S. J., Stirling D., Hoarau G., et al. (2022). Population and seascape genomics of a critically endangered benthic elasmobranch, the blue skate Dipturus batis. Evol. Appl. 15 (1), 78–94. doi: 10.1111/eva.13327
Dell’Apa A., Kimmel D. G., Clò S. (2012). Trends of fish and elasmobranch landings in Italy: associated management implications. ICES J. Mar. Sci. 69 (6), 1045–1052. doi: 10.1093/icesjms/fss067
DiGiacomo A. E., Harrison W. E., Johnston D. W., Ridge J. T. (2020). Elasmobranch use of nearshore estuarine habitats responds to fine-scale, intra-seasonal environmental variation: observing coastal shark density in a temperate estuary utilizing unoccupied aircraft systems (UAS). Drones 4 (4), 74. doi: 10.3390/drones4040074
D’Iglio C., Albano M., Tiralongo F., Famulari S., Rinelli P., Savoca S., et al. (2021b). Biological and ecological aspects of the blackmouth catshark (Galeus melastomus rafinesque 1810) in the southern tyrrhenian Sea. J. Mar. Sci. Eng. 9 (9), 967. doi: 10.3390/jmse9090967
D’Iglio C., Savoca S., Rinelli P., Spanò N., Capillo G. (2021a). Diet of the deep-Sea shark Galeus melastomus rafinesque 1810, in the Mediterranean Sea: What we know and what we should know. Sustainability 13 (7), 3962. doi: 10.3390/su13073962
Domingues R. R., Bunholi I. V., Pinhal D., Antunes A., Mendonça F. F. (2021). From molecule to conservation: DNA-based methods to overcome frontiers in the shark and ray fin trade. Conserv. Genet. Resour. 13 (2), 231–247. doi: 10.1007/s12686-021-01194-8
Domingues R. R., Hilsdorf A. W. S., Gadig O. B. F. (2018). The importance of considering genetic diversity in shark and ray conservation policies. Conserv. Genet. 19, 501–525. doi: 10.1007/s10592-017-1038-3
Dugal L., Thomas L., Jensen M. R., Sigsgaard E. E., Simpson T., Jarman S., et al. (2022). Individual haplotyping of whale sharks from seawater environmental DNA. Mol. Ecol. Resour. 22 (1), 56–65. doi: 10.1111/1755-0998.13451
Dymek J., Muñoz P., Mayo-Hernández E., Kuciel M., Żuwała K. (2021). Comparative analysis of the olfactory organs in selected species of marine sharks and freshwater batoids. Zool. Anz. 294, 50–61. doi: 10.1016/j.jcz.2021.07.013
Earl D. A., vonHoldt B. M. (2012). STRUCTURE HARVESTER: a website and program for visualizing STRUCTURE output and implementing the evanno method. Conserv. Genet. Resour. 4 (2), 359–361. doi: 10.1007/s12686-011-9548-7
Ebert D. A., Dando M., Fowler S. (2021). Sharks of the world: A complete guide (Princeton, New Jersey: WILDNATUREPRESS, Plymouth, UK and Princeton University Press).
El-Geziry T. M., Bryden I. G. (2010). The circulation pattern in the Mediterranean Sea: issues for modeller consideration. J. Oper. Oceanogr. 3 (2), 39–46. doi: 10.1080/1755876X.2010.11020116
Excoffier L., Lischer H. E. L. (2010). Arlequin suite ver 3.5: A new series of programs to perform population genetics analyses under Linux and windows. Mol. Ecol. Resour. 10 (3), 564–567. doi: 10.1111/j.1755-0998.2010.02847.x
Excoffier L., Smouse P. E., Quattro J. M. (1992). Analysis of molecular variance inferred from metric distances among DNA haplotypes: application to human mitochondrial DNA restriction data. Genetics 131 (2), 479–491. doi: 10.1093/genetics/131.2.479
Farriols M. T., Ordines F., Carbonara P., Casciaro L., Di Lorenzo M., Esteban A., et al. (2019). Spatio-temporal trends in diversity of demersal fish assemblages in the Mediterranean. Sci. Mar. 83 (S1), 189–206. doi: 10.3989/scimar
Fernandez-Arcaya U., Ramirez-Llodra E., Aguzzi J., Allcock A. L., Davies J. S., Dissanayake A., et al. (2017). Ecological role of submarine canyons and need for canyon conservation: A review. Front. Mar. Sci. 4. doi: 10.3389/fmars.2017.00005
Ferrari A., Tinti F., Bertucci Maresca V., Velonà A., Cannas R., Thasitis I., et al. (2018). Natural history and molecular evolution of demersal Mediterranean sharks and skates inferred by comparative phylogeographic and demographic analyses. PeerJ 6, e5560. doi: 10.7717/peerj.5560
Ferretti F., Myers R. A., Sartor P., Serena F. (2005). Long term dynamics of the chondrichthyan fish community in the upper tyrrhenian Sea. ICES CM. 25, 1–34. Available at: https://www.ices.dk/sites/pub/CM%20Doccuments/2005/N/N2505.pdf.
Finucci B., Derrick D., Neat F. C., Pacoureau N., Serena F., VanderWright W. J. (2021)Galeus melastomus. In: The IUCN red list of threatened species: e.T161398A124477972 (Accessed 09 April 2022).
Fiorentino F., Vitale S. (2021). How can we reduce the overexploitation of the Mediterranean resources? Front. Mar. Sci. 8, 674633. doi: 10.3389/fmars.2021.674633
Follesa M. C., Porcu C., Cabiddu S., Mulas A., Deiana A. M., Cau A. (2011). Deep-water fish assemblages in the central-western Mediterranean (south sardinian deep-waters). J. Appl. Ichthyol. 27 (1), 129–135. doi: 10.1111/j.1439-0426.2010.01567.x
Froese R., Pauly D. (2019) FishBase (World Wide Web electronic publication). Available at: https://www.fishbase.org (Accessed February 15, 2022).
Goudet J. (2001). FSTAT, a program to estimate and test gene diversities and fixation indices (version 2.9.3; 2001). Updated from Goudet (1995). Available at http://www2.unil.ch/popgen/softwares/fstat.htm.
Gouraguine A., Hidalgo M., Moranta J., Bailey D. M., Ordines F., Guijarro B., et al. (2011). Elasmobranch spatial segregation in the western Mediterranean. Sci. Mar. 75 (4), 653–664. doi: 10.3989/scimar.2011.75n4653
Griffiths A. M., Casane D., McHugh M., Wearmouth V. J., Sims D. W., Genner M. J. (2011). Characterisation of polymorphic microsatellite loci in the small-spotted catshark (Scyliorhinus canicula l.). Conserv. Genet. Resour. 3 (4), 705–709. doi: 10.1007/s12686-011-9438-z
Gubili C., Macleod K., Perry W., Hanel P., Batzakas I., Farrell E. D., et al. (2016). Connectivity in the deep: Phylogeography of the velvet belly lanternshark. Deep Sea Res. Part I Oceanogr. Res. Pap. 115, 233–239. doi: 10.1016/j.dsr.2016.07.002
Helyar S., Coscia I., Sala-Bozano M., Mariani S. (2011). New microsatellite loci for the longnose velvet dogfish Centroselachus crepidater (Squaliformes: Somniosidae) and other deep sea sharks. Conserv. Genet. Resour. 3 (1), 173–176. doi: 10.1007/s12686-010-9316-0
Hemmer-Hansen J., Nielsen E. E., Grønkjaer P., Loeschcke V. (2007). Evolutionary mechanisms shaping the genetic population structure of marine fishes; lessons from the European flounder (Platichthys flesus l.): Population structure in European flounder. Mol. Ecol. 16 (15), 3104–3118. doi: 10.1111/j.1365-294X.2007.03367.x
Hirschfeld M., Dudgeon C., Sheaves M., Barnett A. (2021). Barriers in a sea of elasmobranchs: From fishing for populations to testing hypotheses in population genetics. Global Ecol. Biogeogr. 30 (11), 2147–2163. doi: 10.1111/geb.13379
Hoelzel A. R., Shivji M. S., Magnussen J., Francis M. P. (2006). Low worldwide genetic diversity in the basking shark (Cetorhinus maximus). Biol. Lett. 2 (4), 639–642. doi: 10.1098/rsbl.2006.0513
Hubisz M. J., Falush D., Stephens M., Pritchard J. K. (2009). Inferring weak population structure with the assistance of sample group information. Mol. Ecol. Resour. 9 (5), 1322–1332. doi: 10.1111/j.1755-0998.2009.02591.x
ICES (2021). Working group on elasmobranch fishes (WGEF). ICES Sci. Rep. 3 (59), 1–822. doi: 10.17895/ices.pub.8199
Iglésias S. P., Lecointre G., Sellos D. Y. (2005). Extensive paraphylies within sharks of the order carcharhiniformes inferred from nuclear and mitochondrial genes. Mol. Phylogenet. Evol. 34 (3), 569–583. doi: 10.1016/j.ympev.2004.10.022
Jombart T., Devillard S., Balloux F. (2010). Discriminant analysis of principal components: a new method for the analysis of genetically structured populations. BMC Genet. 11 (1), 94. doi: 10.1186/1471-2156-11-94
Kallianiotis A., Sophronidis K., Vidoris P., Tselepides A. (2000). Demersal fish and megafaunal assemblages on the Cretan continental shelf and slope (NE mediterranean): seasonal variation in species density, biomass and diversity. Prog. Oceanogr. 46 (2-4), 429–455. doi: 10.1016/S0079-6611(00)00028-8
Kessel S. T., Chapman D. D., Franks B. R., Gedamke T., Gruber S. H., Newman J. M., et al. (2014). Predictable temperature-regulated residency, movement and migration in a large, highly mobile marine predator (Negaprion brevirostris). Mar. Ecol. Prog. Ser. 514, 175–190. doi: 10.3354/meps10966
Kopelman N. M., Mayzel J., Jakobsson M., Rosenberg N. A., Mayrose I. (2015). Clumpak: a program for identifying clustering modes and packaging population structure inferences across K. Mol. Ecol. Resour. 15 (5), 1179–1191. doi: 10.1111/1755-0998.12387
Kousteni V., Kasapidis P., Kotoulas G., Megalofonou P. (2015a). Evidence of high genetic connectivity for the longnose spurdog Squalus blainville in the Mediterranean Sea. Mediterr. Mar. Sci. 17 (2), 371–383. doi: 10.12681/mms.1222
Kousteni V., Kasapidis P., Kotoulas G., Megalofonou P. (2015b). Strong population genetic structure and contrasting demographic histories for the small-spotted catshark (Scyliorhinus canicula) in the Mediterranean Sea. Heredity 114 (3), 333–343. doi: 10.1038/hdy.2014.107
Kousteni V., Mazzoleni S., Vasileiadou K., Rovatsos M. (2021). Complete mitochondrial DNA genome of nine species of sharks and rays and their phylogenetic placement among modern elasmobranchs. Genes 12 (3), 324. doi: 10.3390/genes12030324
Kousteni V., Megalofonou P. (2019). Reproductive strategy of Scyliorhinus canicula (L. 1758): a holistic approach based on macroscopic measurements and microscopic observations of the reproductive organs. Mar. Freshw. Res. 71 (6), 596–616. doi: 10.1071/MF18474
Kraft D. W., Conklin E. E., Barba E. W., Hutchinson M., Toonen R. J., Forsman Z. H., et al. (2020). Genomics versus mtDNA for resolving stock structure in the silky shark (Carcharhinus falciformis). PeerJ 8, e10186. doi: 10.7717/peerj.10186
Lao O., Van Duijn K., Kersbergen P., De Knijff P., Kayser M. (2006). Proportioning whole-genome single-nucleotide-polymorphism diversity for the identification of geographic population structure and genetic ancestry. Am. J. Hum. Genet. 78 (4), 680–690. doi: 10.1086/501531
Li Y., Liu J. (2018). STRUCTURESELECTOR: A web-based software to select and visualize the optimalnumber of clusters using multiple methods. Mol. Ecol. Resour. 18, 176–177. doi: 10.1111/1755-0998.12719LIANDLIU|177
Liu N., Chen L., Wang S., Oh C., Zhao H. (2005). Comparison of single-nucleotide polymorphisms and microsatellites in inference of population structure. BMC Genet. 6, S26. doi: 10.1186/1471-2156-6-S1-S26
Massutí E., Moranta J. (2003). Demersal assemblages and depth distribution of elasmobranchs from the continental shelf and slope trawling grounds off the Balearic islands (western Mediterranean). ICES J. Mar. Sci. 60 (4), 753–766. doi: 10.1016/S1054-3139(03)00089-4
Merten V., Bayer T., Reusch T. B., Puebla O., Fuss J., Stefanschitz J., et al. (2021). An integrative assessment combining deep-Sea net sampling, in situ observations and environmental DNA analysis identifies cabo Verde as a cephalopod biodiversity hotspot in the Atlantic ocean. Front. Mar. Sci. 9. doi: 10.3389/fmars.2021.760108
Metochis C. P., Carmona-Antoñanzas G., Kousteni V., Damalas D., Megalofonou P. (2018). Population structure and aspects of the reproductive biology of the blackmouth catshark, Galeus melastomus rafinesque 1810(Chondrichthyes: Scyliorhinidae) caught accidentally off the Greek coasts. J. Mar. Biol. Assoc. United Kingdom. 98 (4), 909–925. doi: 10.1017/S0025315416001764
Milano I., Babbucci M., Cariani A., Atanassova M., Bekkevold D., Carvalho G. R., et al. (2014). Outlier SNP markers reveal fine-scale genetic structuring across European hake populations (Merluccius merluccius). Mol. Ecol. 23 (1), 118–135. doi: 10.1111/mec.12568
Moore D. M., Neat F. C., McCarthy I. D. (2013). Population biology and ageing of the deep water sharks Galeus melastomus, Centroselachus crepidater and Apristurus aphyodes from the rockall trough, north-east Atlantic. J. Mar. Biol. Assoc. UK 93 (7), 1941–1950. doi: 10.1017/S0025315413000374
Moura T., Fernandes A., Figueiredo I., Alpoim R., Azevedo M. (2018). Management of deep-water sharks’ by-catch in the Portuguese anglerfish fishery: from EU regulations to practice. Mar. Policy. 90, 55–67. doi: 10.1016/j.marpol.2018.01.006
Musick J. A., Ellis J. K. (2005). “Reproductive evolution of chondrichthyans,” in Reproductive biology and phylogeny of Chondrichthyes: Sharks, batoids and chimaeras. Ed. Hamlett W. C. (Boca Raton, London, New York: CRC Press), 45–80.
Myers R. A., Baum J. K., Shepherd T. D., Powers S. P., Peterson C. H. (2007). Cascading effects of the loss of apex predatory sharks from a coastal ocean. Science 315 (5820), 1846–1850. doi: 10.1126/science.1138657
Naylor G. J., Caira J. N., Jensen K., Rosana K. A. M., Straube N., Lakner C. (2012). “Elasmobranch phylogeny: a mitochondrial estimate based on 595 species,” in Biology of sharks and their relatives. Ed. Evans D. H. (Boca Raton, FL: CRC Press), 31–56.
O’Sullivan D., Leahy Y., Healy L. (2018). EMFF Offshore Reef Survey, Sensitive Ecosystem Assessment and ROV Exploration of Reef - SeaRover 2018 Cruise Report. Cruise Report prepared by INFOMAR, the Marine Institute, Galway, Ireland and the National Parks and Wildlife Service for the Department of Agriculture, Food and the Marine, the European Maritime and Fisheries Fund and the Department of Culture, Heritage and the Gaeltacht. Available at http://hdl.handle.net/10793/1434.
Ovenden J. R., Dudgeon C., Feutry P., Feldheim K., Maes G. E. (2019). “Genetics and genomics for fundamental and applied research on elasmobranchs,” in Shark research: Emerging technologies and applications for the field and laboratory. Ed. Evans D. H. (Boca Raton, FL: CRC Press), 235–253.
Pauly D., Zeller D. (2015) Sea Around us concepts, design and data. Available at: http://www.seaaroundus.org (Accessed 09 April 2022).
Pillans R., Rochester W. A., Babcock R. C., Thomson D. P., Haywood M. D., Vanderklift M. (2021). Long-term acoustic monitoring reveals site fidelity, reproductive migrations and sex specific differences in habitat use and migratory timing in a large coastal shark (Negaprion acutidens). Front. Mar. Sci. 8. doi: 10.3389/fmars.2021.616633
Portnoy D. S., Puritz J. B., Hollenbeck C. M., Gelsleichter J., Chapman D., Gold J. R. (2015). Selection and sex-biased dispersal in a coastal shark: The influence of philopatry on adaptive variation. Mol. Ecol. 24 (23), 5877–5885. doi: 10.1111/mec.13441
Ragonese S., Nardone G., Ottonello D., Gancitano S., Giusto G. B., Sinacori G. (2009). Distribution and biology of the blackmouth catshark Galeus melastomus in the strait of Sicily (Central Mediterranean Sea). Mediterr. Mar. Sci. 10 (1), 55–72. doi: 10.12681/mms.122
Ramírez-Amaro S., Ordines F., Esteban A., Garcia C., Guijarro B., Salmeròn F., et al. (2020). The diversity of recent trends for chondrichthyans in the Mediterranean reflects fishing exploitation and a potential evolutionary pressure towards early maturation. Sci. Rep. 10 (1), 1–18. doi: 10.1038/s41598-019-56818-9
Ramírez-Amaro S., Picornell A., Arenas M., Castro J. A., Massutí E., Ramon M. M., et al. (2018). Contrasting evolutionary patterns in populations of demersal sharks throughout the western Mediterranean. Mar. Biol. 165 (1), 3. doi: 10.1007/s00227-017-3254-2
Ratnasingham S., Hebert P. D. (2007). BOLD: The barcode of life data system (http://www.barcodinglife.org). Mol. Ecol. Notes. 7 (3), 355–364. doi: 10.1111/j.1471-8286.2007.01678.x
Rey J., Coelho R., Lloris D., Séret B., de Sola G. L. (2010). Distribution pattern of Galeus atlanticus in the alborán Sea (south western Mediterranean) and some sexual character comparison with Galeus melastomus. mar. Biol. Res. 6 (4), 364–372. doi: 10.1080/17451000903042487
Rey J., de Sola L. G., Massutí E. (2004). Distribution and biology of the blackmouth catshark Galeus melastomus in the alboran Sea (Southwestern Mediterranean). J. Northwest Atl. Fish. Sci. 35, 215–223. doi: 10.2960/J.v35.m484
Rey J., Séret B., Lloris D., Coelho R., Sola L. G. D. (2006). A new redescription of Galeus atlanticus (Vaillant 1888) (Chondrichthyes: Scyliorhinidae) based on field marks. Cybium 30 (4), 7–14. Available at http://hdl.handle.net/10400.1/8956
Rigby C., Simpfendorfer C. A. (2015). Patterns in life history traits of deep-water chondrichthyans. Deep-Sea Res. II: Top. Stud. Oceanogr. 115, 30–40. doi: 10.1016/j.dsr2.2013.09.004
Roque D., Parras-Berrocal I., Bruno M., Sánchez-Leal R., Hernández-Molina F. J. (2019). Seasonal variability of intermediate water masses in the gulf of cádiz: implications of the Antarctic and subarctic seesaw. Ocean Science. 15, 1381–1397. doi: 10.5194/os-15-1381-2019
Rousset F. (2008). Genepop’007: a complete re-implementation of the genepop software for windows and Linux. Mol. Ecol. Resour. 8 (1), 103–106. doi: 10.1111/j.1471-8286.2007.01931.x
Ryman N., Palm S. (2006). POWSIM: a computer program for assessing statistical power when testing for genetic differentiation. Mol. Ecol. Resour. 6 (3), 600–602. doi: 10.1111/j.1471-8286.2006.01378.x
Sbrana A., Cau A., Cicala D., Franceschini S., Giarrizzo T., Gravina M. F., et al. (2022). Ask the shark: Blackmouth catshark (Galeus melastomus) as a sentinel of plastic waste on the seabed. Mar. Biol. 169 (98). doi: 10.1007/s00227-022-04084-1
Scacco U., Mancini E., Marcucci F., Tiralongo F. (2022). Microplastics in the deep: comparing dietary and plastic ingestion data between two mediterranean bathyal opportunistic feeder species, Galeus melastomus, rafinesque 1810 and Coelorinchus caelorhincus (Risso 1810), through stomach content analysis. J. Mar. Sci. Eng. 10 (5), 624. doi: 10.3390/jmse10050624
Serena F. (2021). “Elasmobranchs,” in Incidental catch of vulnerable species in Mediterranean and black Sea fisheries - A review. Studies and Reviews No. 101 Eds Carpentieri P., Nastasi A., Sessa M., Srour A., General Fisheries Commission for the Mediterranean. Rome, FAO., 111–197. doi: 10.4060/cb5405en
Shaw P. W., Arkhipkin A. I., Al-Khairulla H. (2004). Genetic structuring of Patagonian toothfish populations in the southwest Atlantic ocean: the effect of the Antarctic polar front and deep-water troughs as barriers to genetic exchange. Mol. Ecol. 13, 3293–3303. doi: 10.1111/j.1365-294X.2004.02327.x
Sims D. W., Wearmouth V. J., Southall E. J., Hill J. M., Moore P., Rawlinson K., et al. (2006). Hunt warm, rest cool: bioenergetic strategy underlying diel vertical migration of a benthic shark. J. Anim. Ecol. 75 (1), 176–190. doi: 10.1111/j.1365-2656.2005.01033.x
Spedicato M. T., Massuti E., Merigot B., Tserpes G., Jadaud A., Relini G. (2020). The MEDITS trawl survey specifications in an ecosystem approach to fishery management. Sci. Mar. 84 (3), 309–309. doi: 10.3989/scimar.04915.11X
Stevens J. D., Bonfil R., Dulvy N. K., Walker P. A. (2000). The effects of fishing on sharks, rays, and chimaeras (chondrichthyans), and the implications for marine ecosystems. ICES J. Mar. Sci. 57 (3), 476–494. doi: 10.1006/jmsc.2000.0724
Tiralongo F., Mancini E., Ventura D., De Malherbe S., Paladini De Mendoza F., Sardone M., et al. (2021). Commercial catches and discards composition in the central tyrrhenian Sea: a multispecies quantitative and qualitative analysis from shallow and deep bottom trawling. Mediterr. Mar. Sci. 22 (3), 521–531. doi: 10.12681/mms.25753
Tserpes G., Tzanatos E., Peristeraki P. (2011). Spatial management of the Mediterranean bottom-trawl fisheries: the case of the southern Aegean Sea. Hydrobiologia 670 (1), 267–274. doi: 10.1007/s10750-011-0667-7
Van Oosterhout C., Hutchinson W. F., Wills D. P. M., Shipley P. (2004). Micro-checker: software for identifying and correcting genotyping errors in microsatellite data. Mol. Ecol. Notes. 4 (3), 535–538. doi: 10.1111/j.1471-8286.2004.00684.x
Verissimo A., Grubbs D., McDowell J., Musick J., Portnoy D. (2011a). Frequency of multiple paternity in the spiny dogfish Squalus acanthias in the Western north Atlantic. J. Hered. 102, 88–93. doi: 10.1093/jhered/esq084
Veríssimo A., Mcdowell J. R., Graves J. E. (2010). Global population structure of the spiny dogfish Squalus acanthias, a temperate shark with an antitropical distribution. Mol. Ecol. 19 (8), 1651–1662. doi: 10.1111/j.1365-294X.2010.04598.x
Veríssimo A., McDowell J. R., Graves J. E. (2011b). Population structure of a deep-water squaloid shark, the Portuguese dogfish (Centroscymnus coelolepis). ICES J. Mar. Sci. 68 (3), 555–563. doi: 10.1093/icesjms/fsr003
Veríssimo A., McDowell J. R., Graves J. E. (2012). Genetic population structure and connectivity in a commercially exploited and wide-ranging deepwater shark, the leafscale gulper (Centrophorus squamosus). Mar. Freshw. Res. 63 (6), 505–512. doi: 10.1071/MF11237
Veríssimo A., Zaera-Perez D., Leslie R., Iglesias S. P., Seter B., Grigoriou P., et al. (2017). Molecular diversity and distribution of eastern Atlantic and Mediterranean dogfishes squalus highlight taxonomic issue in the genus. Zool. Scr. 46 (4), 414–428. doi: 10.1111/zsc.12224
Keywords: conservation, cross-amplification, deep-sea, microsatellite loci, population differentiation, sharks, North-eastern Atlantic Ocean, Mediterranean Sea
Citation: Di Crescenzo S, Ferrari A, Barría C, Cannas R, Cariani A, Drewery J, Fernández-Peralta L, Giordano D, Hidalgo M, Kousteni V, Marino IAM, Massi D, Moura T, Rey J, Sartor P, Scacco U, Serena F, Stagioni M and Tinti F (2022) First evidence of population genetic structure of the deep-water blackmouth catshark Galeus melastomus Rafinesque, 1810. Front. Mar. Sci. 9:953895. doi: 10.3389/fmars.2022.953895
Received: 26 May 2022; Accepted: 08 November 2022;
Published: 30 November 2022.
Edited by:
Maria Lourdes D. Palomares, Institute for the Oceans and Fisheries, University of British Columbia, CanadaReviewed by:
Mauro Sinopoli, Stazione Zoologica Anton Dohrn Napoli, ItalyFrancesco Tiralongo, University of Catania, Italy
Maria Flavia Gravina, University of Rome “Tor Vergata”, Italy
Copyright © 2022 Di Crescenzo, Ferrari, Barría, Cannas, Cariani, Drewery, Fernández-Peralta, Giordano, Hidalgo, Kousteni, Marino, Massi, Moura, Rey, Sartor, Scacco, Serena, Stagioni and Tinti. This is an open-access article distributed under the terms of the Creative Commons Attribution License (CC BY). The use, distribution or reproduction in other forums is permitted, provided the original author(s) and the copyright owner(s) are credited and that the original publication in this journal is cited, in accordance with accepted academic practice. No use, distribution or reproduction is permitted which does not comply with these terms.
*Correspondence: Simone Di Crescenzo, c2ltb25lLmRpY3Jlc2NlbnpvQHVuaWNhLml0; Alessia Cariani, YWxlc3NpYS5jYXJpYW5pQHVuaWJvLml0
†These authors have contributed equally to this work