- 1Wetland Ecosystem Research Station of Hangzhou Bay, Research Institute of Subtropical Forestry, Chinese Academy of Forestry, Hangzhou, China
- 2Ministry of Education Key Laboratory for Biodiversity Science and Ecological Engineering, Coastal Ecosystems Research Station of Yangtze River Estuary, Institute of Biodiversity Science and Institute of Eco-Chongming, School of Life Sciences, Fudan University, Shanghai, China
Grazing prohibition has been used for some conservation purposes in salt marshes. However, the impact of this measure on microbe-mediated key nitrogen removal processes remains poorly understood. Therefore, this study assessed the impact of grazing prohibition on potential rates of nitrification and denitrification under short- and long-term grazing prohibition in high and middle elevation of the Dongtan salt marsh on Chongming Island, China. Compared with short-term grazing prohibition, we found that long-term grazing prohibition significantly increased nitrification and denitrification (except for nitrification in the high marsh), which indicates that the nitrogen removal ability of the salt marsh was improved along with the grazing prohibition time. Furthermore, we found that nitrification and denitrification in the high marsh were largely affected by NH4+ and soil moisture, respectively. Nitrification and denitrification in the middle marsh were mainly controlled by soil organic carbon (SOC) and nirS gene abundances, respectively. Our results indicate that the implementation of scientific and reasonable grazing prohibition policies in salt marshes has great potential to restore their ecosystem functions, maintain their ecosystem balance and realize their sustainable development.
Introduction
Salt marshes are located at the transition zone between terrestrial and marine ecosystems, providing various ecosystem services (Costanza et al., 1997; Valiela and Cole, 2002). Salt marshes, acting as filters, are of interest because they can remove large amounts of terrestrial N inputs from marine ecosystems, as such inputs adversely affect human and ecosystem health (Mitsch et al., 2001; Yang et al., 2015). However, these environments are under increasing anthropogenic pressure; for example, livestock grazing decreases aboveground plant material, potentially impairs coastal protection, and reduces invertebrate richness (Eldridge and Delgado-Baquerizo, 2017; Huang et al., 2021). Many regions of the world where livestock grazing is permitted have experienced great salt marsh erosion (Pagès et al., 2019). Thus, to eliminate the negative effects of continuous grazing, grazing prohibition has been extensively employed around the world and has been used as a management tool for protecting and restoring degraded salt marshes (Law et al., 2014).
Most of the world’s research on grazing prohibition has focused on grassland and forest ecosystems and has concentrated on studies related to plant community composition, primary production, soil properties and carbon cycling (Chen et al., 2012; Chen et al., 2016; Hu et al., 2016; Väisänen et al., 2021). For example, Hu et al. (2016) found that grazing prohibition increased vegetation biomass and soil carbon content by synthesizing the results of 51 sites in grasslands in China; Olofsson et al. (2006) revealed that herbivore exclusion increased primary production in the long term; Moradi et al. (2021) showed that soil physicochemical properties had a significant improvement after livestock exclusion. However, in contrast to the above research, there are fewer studies on the effects of grazing exclusion on nitrogen cycling processes, especially in salt marsh ecosystem. Although studies in grassland ecosystems had demonstrated that grazing prohibition could have a significant effect on the nitrogen cycle through changes in soil physicochemical properties (Li et al., 2021a), microbial community composition (Song et al., 2019) and soil nutrient content (Walker et al., 2009 ; Li et al., 2017), and some of these effects are positive and some are negative. However, salt marshes have distinct environmental characteristics that vary from those of terrestrial grasslands, such as high salinity, low redox potential, and the occurrence of periodic tidal inundation (Zhang et al., 2021 ; Martin and Reddy, 1997). These discrepancies may result in different responses of salt marshes to grazing prohibition, and the regulator of grazing prohibition impacts may differ between these ecosystems.
Nitrification and denitrification are two key nitrogen removal processes occurring in salt marshes (Valiela and Teal, 1979; Eriksson et al., 2003). Nitrification can affect the internal circulation of nitrogen, while denitrification combined with tidal water exchange results in nitrogen removal from salt marshes. Several studies have demonstrated the effects of grazing prohibition practices on nitrogen cycling processes (Walker et al., 2009; Wang et al., 2010; Liao et al., 2019). However, these studies have tended to focus on grasslands (Cheng et al., 2016; Che et al., 2018; Pan et al., 2016), alpine meadows (Yin et al., 2019), and riparian zones (Walker et al., 2009) and have ignored this issue in salt marshes. Given the essential roles of nitrification and denitrification in nitrogen cycling, discerning their responses to grazing prohibition, especially in salt marshes, is important for improving our understanding of the mechanisms of salt marsh restoration. Such knowledge can also enhance our capacity to forecast the responses of salt marsh ecosystems to anthropogenic effects.
In this study, we selected the Dongtan salt marsh on Chongming Island, China, as the study area. This region has long suffered from high nitrogen loading (Huang et al., 2006). This area has also been experiencing intensive human activities, including livestock grazing, which has reduced plant biomass, negatively affected soil decomposers, altered carbon resources, and disturbed the habitats of birds (Yang et al., 2017). The Chinese government imposed a policy of grazing prohibition, but it is largely unknown how grazing affects key nitrogen removal processes. To address these knowledge gaps, we conducted a field experiment in the Dongtan salt marsh on Chongming Island to investigate the effects of grazing prohibition on nitrogen removal processes. Our specific objectives were to (i) analyze how nitrification and denitrification respond to grazing prohibition in the high and middle marsh after short- and long-term grazing prohibition and (ii) identify the major drivers of nitrification and denitrification.
Materials and Methods
Study Site and Soil Sampling
This study was conducted in the Dongtan salt marsh on Chongming Island, China (31°69′N, 121°65′E) (Figure 1). The Dongtan salt marsh covers an area of approximately 4000 km2, is located at the mouth of the Yangtze Estuary in eastern China, and is an important stopover and wintering site for migratory birds. It is representative of salt marsh landscapes in China with high sediment deposition rates (Tang et al., 2020). This region has a subtropical monsoon climate, and the annual precipitation and temperature are 1145 mm and 15.3°C, respectively (Yang et al., 2017). Tidal fluctuations in the vicinity of Dongtan salt marsh are regular and semidiurnal. Ebb and flood tides are the two obvious diurnal tidal periods. The average elevations of the high and middle marsh are 380 cm and 330 cm above sea level, respectively, resulting in average monthly inundation frequencies of 17 and 39, respectively. The grazing history of Dongtan can be traced back to 1949 before the founding of the People’s Republic of China. From the beginning of April to the end of October, cattle were grazed in this region with a stocking density of one head of cattle per ha (Yang et al., 2017). Grazing by cattle disturbs the habitats of birds, changes the plant community composition and structure, and damages the environment in this area (Yang et al., 2017). To relieve these problems, the local government implemented a policy of grazing prohibition in 2011. Given the interests and acceptance of herders, grazing activities were not completely banned and were restricted to the southern portion of the Dongtan salt marsh (Figures 1C, D). In 2017, total comprehensive grazing prohibition was achieved. Salt marshes undergoing different durations of grazing prohibition time were selected in August 2018. Grazing has been prohibited at these sites since 2011 (Figures 1A, B) or 2017 (Figures 1C, D), corresponding to grazing prohibition for 7 years (long-term grazing prohibition, LGA) and 1 year (short-term grazing prohibition, SGA), respectively (Figure 1). Four soil cores (3.8 cm diameter, 15 cm depth) were randomly taken from each plot (15 m × 15 m) in August 2018 and mixed to obtain one composite sample. A total of 24 composite soil samples (6 replicates × 2 marsh zones × 2 grazing prohibition treatments) were collected and transported to the laboratory in an ice box.
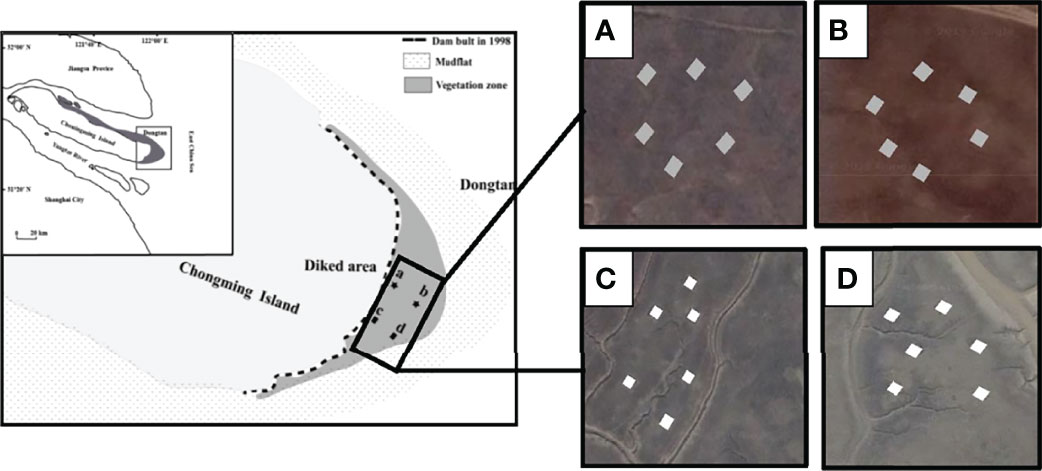
Figure 1 Locations of experimental site. (A) LGA (long-term grazing prohibition) in the high marsh; (B) LGA (long-term grazing prohibition) in the middle marsh; (C) SGA (short-term grazing prohibition) in the high marsh; (D) SGA (short-term grazing prohibition) in the middle marsh.
Soil Characteristics and Biomass Measurements
Soil temperature and redox potential (Eh) were measured in situ with a multiple digital meter (IQ Scientific Instruments, CA, USA) (Li et al, 2020). The pH and salinity of the samples were determined from soil suspensions at the soil water ratio was 1:5 (w/w) by using a conductivity meter (SevenExcellence S479-uMix, Mettler-Toledo, Switzerland) (Li et al., 2009). Soil moisture was determined by oven drying 50 g of fresh soil to a constant weight at 105°C (Cui et al., 2017). The total carbon (TC) and nitrogen (TN) concentrations were determined by using a N/C Soil Analyzer (Flash EA 11121 Series; Thermo Finnigan, Milan, Italy) (Nie et al., 2009). Ammonium (NH4+) and nitrate (NO3-) were extracted from fresh soil with 2 M KCl at in situ temperature for 1 h and measured using a continuous flow analyzer (SAN++, Skalar, the Netherlands) (Deng et al., 2015; Lin and Lin, 2022). Soil organic matter content (SOM) was determined as loss on ignition at 550°C for 5 h (Zhang et al., 2019). Aboveground living plant tissues were collected by clipping the entire contents of three randomly positioned 25 × 25 cm quadrats in each plot, and roots were collected from the same quadrats using a PVC tube (corer of 15 cm diameter, 20 cm depth) (Wei et al., 2020). The roots were immediately washed in clean water to remove all soil, and then both the aboveground living plant tissues and roots were oven dried at 80°C to a constant weight.
Nitrification and Denitrification
The nitrification potential was measured according to a modified shaken soil-slurry method (Hart et al., 1994; Li et al., 2021b). In brief, 15 g of sieved fresh sediment samples was placed in a 250 mL Erlenmeyer flask. Then, 100 mL of 1.0 mM phosphate buffer (pH=7.2) containing 1.0 mM NH4+ was added to the Erlenmeyer flask and mixed well. The soil slurry was shaken on an orbital shaker at 200 rpm in the dark for 24 h, and aeration was maintained. After shaking began, 10 mL of the soil slurry was collected at 2, 4, 12, 22, and 24 h. The collected slurry samples were centrifuged at 8000 ×g for 8 min, and then the supernatant was filtered and stored at -20°C until analysis. Nitrite (NO2-) and NO3- concentrations were measured by continuous-flow injection analysis (Skalar Analytical SAN++, the Netherlands). The calculation of potential rates of nitrification was based on a linear regression of (NO2-+NO3-) concentration over time.
The 15N tracer technique was used to measure the potential rates of denitrification (Hou et al., 2013; Lin et al., 2017). Briefly, soil slurries were prepared by mixing fresh sediment and helium-purged tidal water (30 min) at a sediment: water ratio of 1:7 (w/w). Then, the resulting slurries were transferred into 12-mL glass vials (Exetainer; Labco, High Wycombe, Buckinghamshire, UK) and sealed with butyl rubber stoppers. A 36-h preincubation step at the prevailing in situ temperature (25°C for August) was performed to consume the residual oxygen, NO3- and NO2- (Hou et al., 2015; Lin et al., 2016). After the preincubation period was complete, 100 µL of helium-purged stock solutions of 15NO3- (99% 15N) at a concentration of 100 µmol L-1 was spiked into each vial. Then, one-half of the vials were injected with 200 μL of 50% ZnCl2 solution and regarded as the initial samples. The remaining vials were regarded as the final samples, and these vials were again incubated for another 8 h and fixed with 200 μL of 50% ZnCl2 solution at the end of the incubation period. The 15N atom% (FN) was calculated by considering 15N atom% of stock solutions and any residual ambient NOx− (2.04–7.53 μM) as determined by difference, ranging from 0.92 to 0.99. The abundance of 29N2 and 30N2 in each vial was measured with membrane inlet mass spectrometry (MIMS) (Hou et al., 2013). The potential rates of denitrification and anammox were calculated using the following equation (Thamdrup and Dalsgaard, 2002):
Where P29 (nmol N g-1h-1) is the total 29N2 production rate; A29 and D29 (nmol N g-1h-1) represent the rates of 29N2 production from anammox and denitrification, respectively. 28N2, 29N2, and 30N2 generated from denitrification following random isotope pairing, D29, can be estimated by using equation (2):
Where P30 (nmol N g-1h-1) denotes the total 30N2 production rate, FN (%) is the fraction of 15N in the total NO3-, which can be calculated according to the concentrations of NO3- before and after the addition of 15NO3- to the incubated slurry; Hence, denitrification and anammox were calculated using equations (3) and (4), respectively, as follows:
where Dtotal is potential rate of denitrification, A29 (nmol N g-1h-1) denotes the potential rate of anammox.
DNA Isolation and Quantitative PCR
Soil DNA was extracted from 0.25 g of frozen soil using Powersoil™ DNA Isolation Kits according to the manufacturer’s protocol (MOBIO, USA). The quality and quantity of the extracted DNA were checked with a NanoDrop spectrophotometer (NanoDrop Technologies, Wilmington, DE). The extracted soil DNA was stored at -20°C for further use. Gene abundances of nitrifiers (ammonia-oxidizing archaea (AOA) amoA and ammonia-oxidizing bacteria (AOB) amoA) and denitrifiers (nirK and nirS) were determined using real-time PCR (q-PCR). The extracted DNA samples were diluted 10-fold prior to q-PCR. All q-PCRs were performed in triplicate with an ABI 7500 Detection System (Applied Biosystems, Canada) using the SYBR Green method. The primers Arch-amoAF (STAATGGTCTGGCTTAGACG) and Arch-amoAR (GCGGCCATCCATCTGTATGT) were used to quantify the abundance of the AOA amoA gene (Gao et al., 2018), the primers amoA-1F (GGGGTTTCTACTGGTGGT) and amoA-2R (CCCCTCKGSAAAGCCTTCTTC) were used to quantify the abundance of the AOB amoA gene (Rotthauwe et al., 1997), the primers F1aCu (ATCATGGTSCTGCCGCG) and R3Cu (GCCTCGATCAGRTTGTGGTT) were used to quantify the abundance of the nirK gene (Hallin and Lindgren, 1999) and the primers cd3aF(GTSAACGTSAAGGARACSGG) and R3cd (GASTTCGGRTGSGTCTTGA) were used to quantify the abundance of the nirS gene (Throbäck et al., 2004). Details regarding the q-PCR conditions for these genes are provided in Table S1. In each amplification, the absence of DNA template was used as a negative control. The standard curves for these genes were created using a 10-fold dilution series (102–109 copies) of the standard plasmid DNA. Only results with efficiency and correlation coefficients above 95% and 98% were used. The abundances of the four genes were calculated based on the constructed standard curves.
Data Analyses and Statistical Tests
All data analysis in this research were done with SPSS Statistics version 19.0 (SPSS, Inc., Chicago, IL, USA) and RStudio software version 3.5.1 (RStudio, Inc., Boston, MA, USA). We used two-way ANOVA to determine the effects of grazing prohibition and marsh zone on plant community characteristics, soil physicochemical properties, the potential rates of nitrification and denitrification, and nitrifier and denitrifier gene abundances. Tukey’s HSD test was used to determine the significance level (p< 0.05). Values were the mean (n=6) ± standard error (SE). Pearson correlation heatmaps were applied to analyze potential rates of nitrification and denitrification with soil physicochemical properties and abundances of key nitrogen removal communities. Stepwise multiple regression analyses were used to identify which factors can best explain the variations in potential rates of denitrification. All statistical analyses were performed with R software.
Results
Effects of Grazing Prohibition on Nitrification and Denitrification
Potential rates of nitrification changed significantly with marsh zones and grazing prohibition time (Table S2, p< 0.05). Nitrification rates were higher in the high marsh than these in the middle marsh. In the high marsh, there was no significant difference in nitrification rates between short-term grazing prohibition and long-term grazing prohibition (Figure 2). In the middle marsh, the nitrification rates under long-term grazing prohibition were 25.70% higher than those under short-term grazing prohibition (Figure 2A).
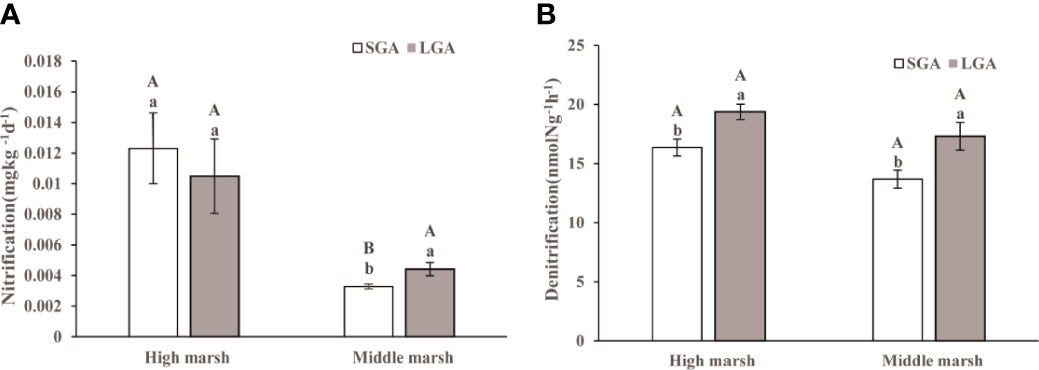
Figure 2 Nitrification and denitrification under different treatments in the middle and high marsh. (A) Nitrification and (B) Denitrification. SGA, Short-term grazing prohibition; LGA, Long-term grazing prohibition. Error bars indicate standard errors. Different uppercase letters indicate significant differences (p < 0.05) between elevations under the same treatment, and different lowercase letters indicate significant differences (p < 0.05) between different treatments.
Potential rates of denitrification were significantly affected by grazing prohibition time, but not affected by marsh zones (Table S2, p< 0.05). In both the middle marsh and the high marsh, long-term grazing prohibition dramatically increased the denitrification rate compared with that under short-term grazing prohibition (Figure 2B). Compared with that in the short-term grazing prohibition treatment, long-term grazing prohibition increased the denitrification rates in the high marsh and middle marsh by 15.56% and 21.00%, respectively (Figure 2B).
Effects of Grazing Prohibition on the Abundances of Nitrifiers and Denitrifiers
Grazing prohibition significantly influenced the abundances of nitrifiers. The abundances of the AOA amoA gene and AOB amoA gene under long-term grazing prohibition were 47.53% and 76.34% higher than those under short-term grazing prohibition, respectively (Figures 3A, B). There were no significant differences in the abundances of the nirK gene among the different grazing prohibition treatments (Figure 3C). However, compared with that under short-term grazing prohibition, the abundances of the nirS gene under long-term grazing prohibition in the high marsh and middle marsh increased by 51.12% and 73.13%, respectively (Figure 3D). In addition, marsh zones also had great influences on the abundances of the AOA amoA gene and nirK gene (Figure 3 and Table S3).
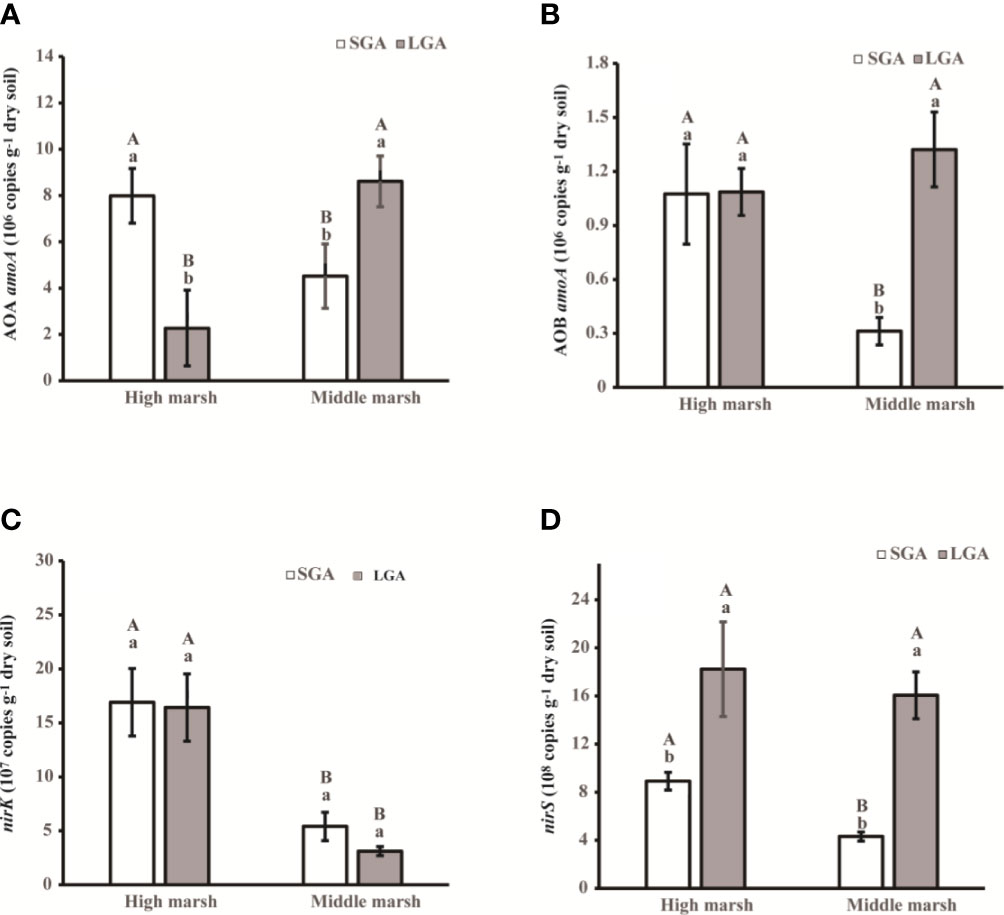
Figure 3 Abundance of amoA sequences from AOA and AOB and nirK and nirS sequences from soil in the different treatments. SGA, Short-term grazing prohibition; LGA, Long-term grazing prohibition. Error bars indicate standard errors. Different uppercase letters indicate significant differences (p < 0.05) between elevations under the same treatment, and different lowercase letters indicate significant differences (p < 0.05) between different treatments.
Effects of Grazing Prohibition on Plant Community Characteristics and Soil Physicochemical Properties
Plant community characteristics was significantly affected by grazing prohibition time, but not affected by marsh zone (Table S4). Compared with that under short-term grazing prohibition, long-term grazing prohibition increased the aboveground and belowground biomass and plant community height, decreased the plant community density, changed the plant community composition, and caused Phragmites australis to become the single dominant species (Table 1).
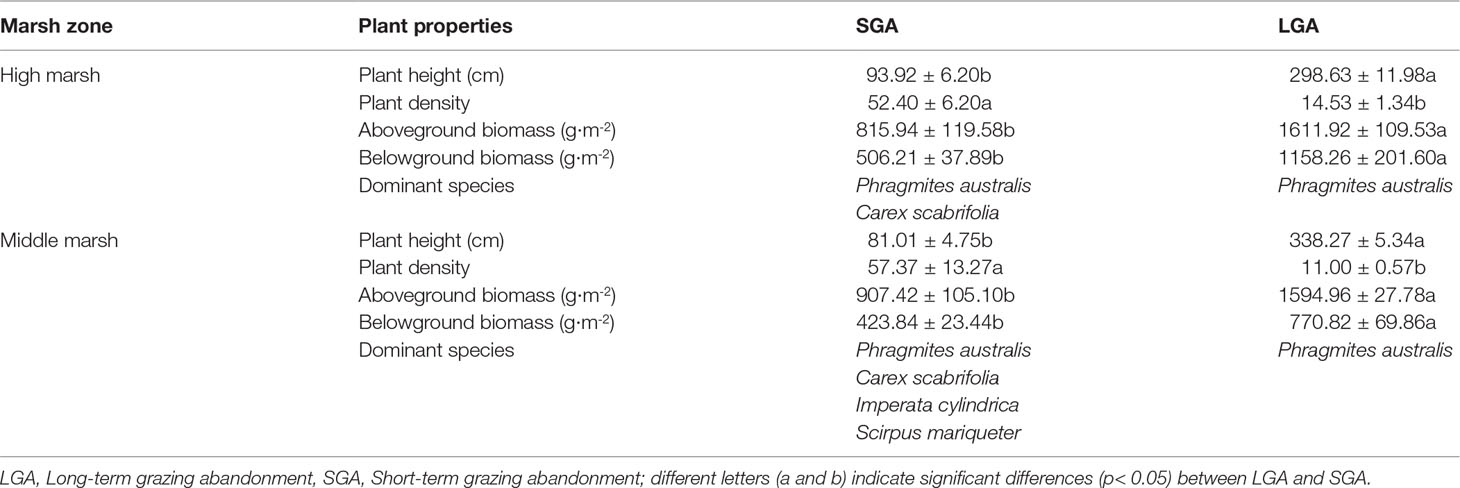
Table 1 Influence of grazing abandonment on plant averaged height, averaged density, biomass and community compositions (Mean ± SE).
Soil physicochemical properties changed significantly with marsh zones and grazing prohibition time (Table 2 and Table S5). Temperature, soil moisture, bulk density, SOC, TN and TC in the high marsh were higher than these in the middle marsh. In the high marsh, compared with that under short-term grazing prohibition, long-term grazing prohibition reduced the soil bulk density and temperature and significantly increased the soil Eh, salinity, soil organic carbon (SOC), NH4+, NO3−, TC, TN and moisture (p< 0.05) (Table 2). In the middle marsh, long-term grazing significantly increased the soil salinity, SOC, NH4+, NO3−, TN, and moisture(p< 0.05) (Table 2).
Factors Controlling Nitrification and Denitrification
Pearson correlation heatmap shown that potential rates of nitrification and denitrification in the middle marsh were significantly positive related to AOA amoA gene abundance and nirS gene abundance, respectively (Figure 4A). Whereas, in the high marsh, potential rates of nitrification and denitrification were affected not only by the abundance of functional microorganisms, but also by SOC and TN contents, respectively (Figure 4B).
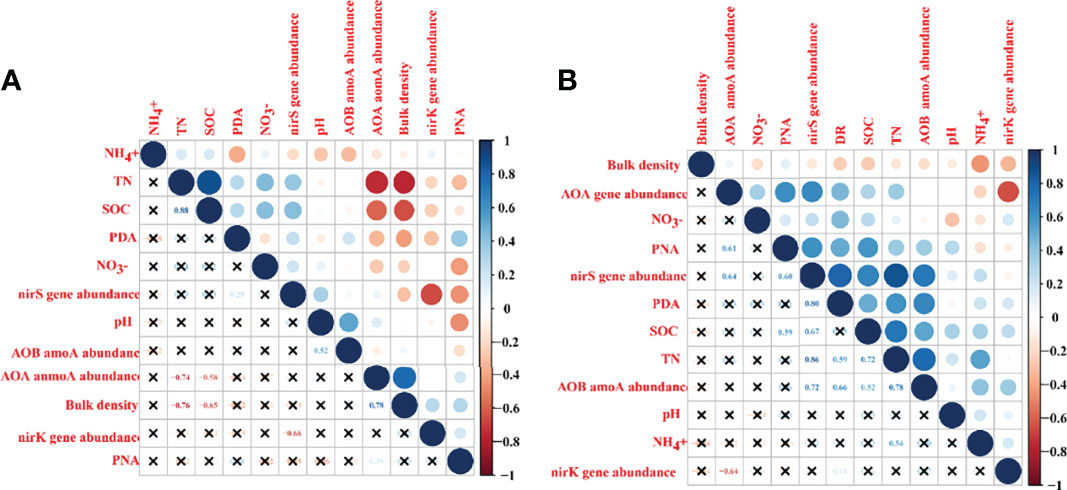
Figure 4 Pearson correlation heatmap of potential rates of nitrification and denitrification with soil physicochemical properties and abundances of key nitrogen removal communities. (A) in the middle marsh; (B) in the high marsh. The colors show in the figure represent the correlation between two variables. The darker the blue, the stronger the positive correlation, and the deeper the red, the stronger the negative correlation. The larger the circle, the greater the absolute value of the correlation coefficient, the cross in the figure indicates that there is no correlation between the two variables. PNA, potential rates of nitrification; PDA, potential rates of denitrification.
For different marsh zones, we executed multiple linear regression analysis to point out which linear combination of independent variables best to explain changes of nitrification and denitrification after grazing prohibition in salt marsh (Table 3). In the high marsh, the main factors affecting nitrification were belowground biomass and NH4+, while the main factor affecting denitrification was soil moisture. In the middle marsh, nitrification was mainly affected by SOC, while denitrification was largely explained by the nirS gene abundance (Table S6). The denitrification rate was also affected by soil salinity, temperature, clay content and nirK gene abundance.

Table 3 Multiple linear regression equations reported for nitrification or denitrification versus several environmental factors and abundances of key nitrogen removal communities.
Discussion
Understanding the changes in nitrification and denitrification after grazing prohibition in salt marshes is of vital importance to assess the consequences of conversion measures for salt marsh ecosystem functions. In this study, we quantified grazing prohibition-induced changes in the potential microbial activities driving soil nitrogen dynamics in high and middle marshes. Our results show that long-term grazing prohibition increased nitrification and denitrification compared with those processes under short-term grazing prohibition, which indicates that the nitrogen removal ability of salt marshes improved after grazing prohibition after a certain time (Figure 5). Our results were consistent with previous findings in other systems (Song et al., 2019; Pan et al., 2015; Walker et al., 2009). Compared to other ecosystems around the world, salt marshes have a similar ability to recover soil N cycling processes after grazing prohibition. For example, a research conducted by Olofsson et al. (2006) found that excluding rabbits less than four years had no significant effect on N mineralization, whereas N mineralization level increased after herbivore exclusion for a long time. The report by Walker et al. (2009) showed that cattle exclusion increased N trace gas fluxes and denitrification rates in a restored riparian zone in the southern Appalachians. Song et al. (2019) found that potential rates of microbial N turnover is facilitated by grazing prohibition in a semiarid area in China.
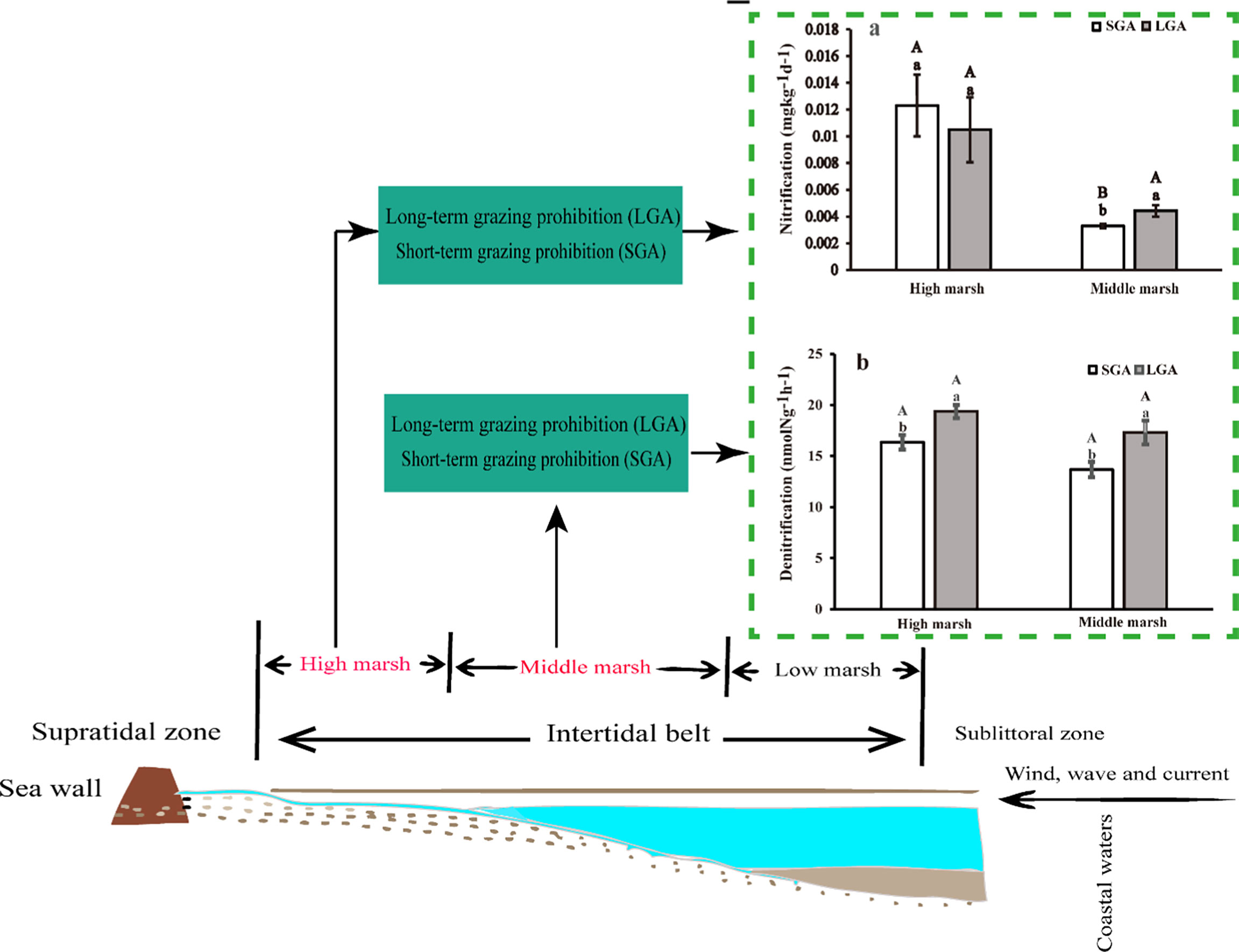
Figure 5 Graphical abstract of grazing prohibition on nitrification and denitrification in the different marsh zones in salt marshes.
In the high marsh, belowground biomass and NH4+ were key factors influencing nitrification. Previous studies have shown that grazing prohibition could reduce nutrient and have significantly impact on vegetation index, belowground biomass, and soil nutrients (Anderson et al., 2007; Chen et al., 2012; Li et al., 2022). Our results showed that belowground biomass increased with grazing prohibition time, which mainly due to the absence of eating and trampling by herbivores (Liu et al., 2019). Growing vegetation intercepts more inorganic nitrogen but also improves the absorption of NH4+ in the sediment with grazing prohibition time (Martin and Reddy, 1997; Berg et al., 1997). Moreover, nutrients from cattle manure and urine entering the soil were gradually diluted as the grazing prohibition time increased (Paul et al., 2013), which resulted in no difference in NH4+ between short- and long-term grazing prohibition. NH4+ provides a substrate for nitrification (McGilloway et al., 2003; Huang et al., 2022), which resulted in no changes in nitrification between the short- and long-term grazing prohibition treatments (Figure 2A). Soil moisture was a key factor influencing denitrification. There was no trampling by herbivores after grazing prohibition was implemented, the soil gradually became looser, the soil bulk density gradually decreased, and the soil porosity gradually increased. Therefore, the ability of water to penetrate and accumulate was improved, and the migration of NO3− from tidal water to the soil and its subsequent transformation were accelerated, which was conducive to the occurrence of denitrification (Reddy et al., 1989).
In the middle marsh, SOC and nirS gene abundance were the most important factors affecting nitrification and denitrification, respectively. As grazing prohibition proceeds, the vegetation community gradually recovers (Su et al., 2005), aboveground biomass significantly increases, and a large amount of litter is returned to the sediment (Belsky, 1992; Liu et al., 2019). The higher plant biomass caused nutrient accumulation, including the accumulation of SOC, NH4+ and NO3- (Table 2), which can provide energy and substrates for nitrifying and denitrifying bacteria (Pinay et al., 2003; Navada et al., 2020). Our study showed that in comparison with that under short-term grazing prohibition, the SOC content and the abundance of the nirS gene significantly increased after long-term grazing prohibition in the middle marsh (Table 2), which resulted in an increase in nitrification and denitrification under long-term grazing prohibition when compared to that under short-term grazing prohibition.
For the first time, we explored the effects of grazing prohibition on the nitrogen removal ability of salt marsh ecosystems. Our results show that grazing prohibition could improve the nitrogen removal ability of salt marshes, indicating that the ecosystem service ability of salt marshes was improved during the restoration process. The improvement of the nitrogen removal capacity of salt marshes after grazing prohibition helps to alleviate the environmental pressure exerted by excessive terrestrial N inputs on marine ecosystems.
Nitrogen removal capacity potential is facilitated by grazing prohibition, probably through enhanced biomass production via increases in nutrient and carbon sources into the soil. In addition, previous studies on the ecosystem multifunctionality of salt marshes have mainly focused on the variables related to the operating rates of ecosystems but often ignored variables related to ecosystem services (Jing et al., 2015; Zhang et al., 2021). Therefore, it is necessary to integrate indicators related to ecosystem function and ecosystem services when conducting research on salt marsh ecosystem multifunctionality.
Conclusions
Nitrogen removal is a vital ecosystem service provided by coastal salt marshes. Based on the findings of this study, we established that the nitrogen removal ability of salt marshes was significantly improved after grazing prohibition for a certain period in salt marshes. Nitrification and denitrification under long-term grazing prohibition were higher than those under short-term grazing prohibition, and this information is beneficial to better assess the ecosystem services provided by salt marshes after restoration, especially in terms of their nitrogen removal capacity. Changes in soil N cycling mechanisms following restoration of the salt marshes were primarily driven by nutrient and carbon sources into the soil due to the recovery of plant communities. Nevertheless, this study analyzed only the dynamic changes in nitrification and denitrification after the restoration of salt marshes over a certain period time. The restoration of the ecosystem functions of salt marshes after disturbance may occur different situations over time. Therefore, to provide more evidence for a comprehensive scientific assessment of grazing prohibition, continued monitoring should be carried out over a long-time scale in the future. In general, our findings are important for assessing the effects of grazing prohibition on the key nitrogen removal processes occurring in salt marshes.
Data Availability Statement
The original contributions presented in the study are included in the article/Supplementary Material. Further inquiries can be directed to the corresponding author.
Author Contributions
NL, methodology, investigation, software, formal analysis, and writing original draft. MN and MW, conceptualization, writing-review and editing, and funding acquisition. JW, conceptualization, resources, methodology, supervision, project administration, writing-review and editing, and funding acquisition. All authors contributed to the article and approved the submitted version.
Funding
This research was supported by the NSFC funding (32030067) and the Shanghai Science and Technology Committee (20DZ1204702).
Conflict of Interest
The authors declare that the research was conducted in the absence of any commercial or financial relationships that could be construed as a potential conflict of interest.
Publisher’s Note
All claims expressed in this article are solely those of the authors and do not necessarily represent those of their affiliated organizations, or those of the publisher, the editors and the reviewers. Any product that may be evaluated in this article, or claim that may be made by its manufacturer, is not guaranteed or endorsed by the publisher.
Acknowledgments
We thank J. Chai for help in field sampling. JW and NL designed the study. NL conducted the work and analyzed the data, and all authors wrote the manuscript.
Supplementary Material
The Supplementary Material for this article can be found online at: https://www.frontiersin.org/articles/10.3389/fmars.2022.958803/full#supplementary-material
References
Anderson T. M., Ritchie M. E., McNaughton S. (2007). Rainfall and Soils Modify Plant Community Response to Grazing in Serengeti National Park. Ecology 88, 1191–1201. doi: 10.1890/06-0399
Belsky A. J. (1992). Effects of Grazing, Competition, Disturbance and Fire on Species Composition and Diversity in Grassland Communities. J. Veg. Sci. 3, 187–200. doi: 10.2307/3235679
Berg W. A., Bradford J. A., Sims P. L. (1997). Long-Term Soil Nitrogen and Vegetation Change on Sandhill Rangeland. J. Range Manage. 50, 482–486. doi: 10.2307/4003702
Cheng J., Jing G., Wei L., Jing Z. (2016). Long-Term Grazing Exclusion Effects on Vegetation Characteristics, Soil Properties and Bacterial Communities in the Semi-Arid Grasslands of China. Ecol. Eng. 97, 170–178. doi: 10.1016/j.ecoleng.2016.09.003
Chen Y., Li Y., Zhao X., Awada T., Shang W., Han J. (2012). Effects of Grazing Exclusion on Soil Properties and on Ecosystem Carbon and Nitrogen Storage in a Sandy Rangeland of Inner Mongolia, Northern China. Environ. Manage. 50, 622–632. doi: 10.1007/s00267-012-9919-1
Chen J., Zhou X., Wang J., Hruska T., Shi W., Cao J., et al. (2016). Grazing Exclusion Reduced Soil Respiration But Increased its Temperature Sensitivity in a Meadow Grassland on the Tibetan Plateau. Ecol. Evol. 6, 675–687. doi: 10.1002/ece3.1867
Che R., Qin J., Tahmasbian I., Wang F., Zhou S., Xu Z., et al. (2018). Litter Amendment Rather Than Phosphorus can Dramatically Change Inorganic Nitrogen Pools in a Degraded Grassland Soil by Affecting Nitrogen-Cycling Microbes. Soil Biol. Biochem. 120, 145–152. doi: 10.1016/j.soilbio.2018.02.006
Costanza R., D’Arge R., de Groot R., Farber S., Grasso M., Hannon B., et al. (1997). The Value of the World’s Ecosystem Services and Natural Capital. Nature. 387, 253–260. doi: 10.1038/387253a0
Cui J., Chen X., Nie M., Fang S., Tang B., Quan Z., et al. (2017). Effects of Spartina Alterniflora Invasion on the Abundance, Diversity, and Community Structure of Sulfate Reducing Bacteria Along a Successional Gradient of Coastal Salt Marshes in China. Wetlands 37, 221–232. doi: 10.1007/s13157-016-0860-6
Deng F., Hou L., Liu M., Zheng Y., Yin G., Li X., et al. (2015). Dissimilatory Nitrate Reduction Processes and Associated Contribution to Nitrogen Removal in Sediments of the Yangtze Estuary. J. Geophys. Res. Biogeosci. 120, 1521–1531. doi: 10.1002/2015JG003007
Eldridge D. J., Delgado-Baquerizo M. (2017). Continental-Scale Impacts of Livestock Grazing on Ecosystem Supporting and Regulating Services. L. Degrad. Dev. 28, 1473–1481. doi: 10.1002/ldr.2668
Eriksson P. G., Svensson J. M., Carrer G. M. (2003). Temporal Changes and Spatial Variation of Soil Oxygen Consumption, Nitrification and Denitrification Rates in a Tidal Salt Marsh of the Lagoon of Venice, Italy. Estuar. Coast. Shelf. Sci. 58, 861–871. doi: 10.1016/j.ecss.2003.07.002
Gao J., Hou L., Zheng Y., Liu M., Yin G., Yu C., et al. (2018). Shifts in the Community Dynamics and Activity of Ammonia-Oxidizing Prokaryotes Along the Yangtze Estuarine Salinity Gradient. J. Geophys. Res. Biogeosci. 123, 3458–3469. doi: 10.1029/2017JG004182
Hallin S., Lindgren P. E. (1999). PCR Detection of Genes Encoding Nitrite Reductase in Denitrifying Bacteria. Appl. Environ. Microbiol. 65, 1652–1657. doi: 10.1128/AEM.65.4.1652-1657.1999
Hart S. C., Stark J. M., Davidson E. A., Firestone M. K. (1994). “Nitrogen Mineralization, Immobilization, and Nitrification,” In: Weaver RW (ed) Methods of Soil Analysis: Microbiological and Biochemical Properties, 3rd edn. Soil Science Society of America, Madison, pp 985 –1018
Hou L., Zheng Y., Liu M., Gong J., Zhang X., Yin G., et al. (2013). Anaerobic Ammonium Oxidation (Anammox) Bacterial Diversity, Abundance, and Activity in Marsh Sediments of the Yangtze Estuary. J. Geophys. Res. Biogeosci. 118, 1237–1246. doi: 10.1002/jgrg.20108
Hou L., Zheng Y., Liu M., Li X., Lin X., Yin G., et al. (2015). Anaerobic Ammonium Oxidation and its Contribution to Nitrogen Removal in China’s Coastal Wetlands. Sci. Rep. 5, 1–11. doi: 10.1038/srep15621
Huang F., Lin X., Hu W., Zeng F., He L., Yin K. (2021). Nitrogen Cycling Processes in Sediments of the Pearl River Estuary: Spatial Variations, Controlling Factors, and Environmental Implications. Catena. 206, 105545. doi: 10.1016/j.catena.2021.105545
Huang F., Lin X., Yin K. (2022). Effects of Marine Produced Organic Matter on the Potential Estuarine Capacity of NOx– Removal. Sci. Total Environ. 812, 151471. doi: 10.1016/j.scitotenv.2021.151471
Huang Q., Shen H., Wang Z., Liu X., Fu R. (2006). Influences of Natural and Anthropogenic Processes on the Nitrogen and Phosphorus Fluxes of the Yangtze Estuary, China. Reg. Environ. Change 6, 125–131. doi: 10.1007/s10113-005-0001-x
Hu Z., Li S., Guo Q., Niu S., He N., Li L., et al. (2016). A Synthesis of the Effect of Grazing Exclusion on Carbon Dynamics in Grasslands in China. Glob. Chang Biol. 22, 1385–1393. doi: 10.1111/gcb.13133
Jing X., Sanders N. J., Yu S., Chu H., Classen A. T., Zhao K., et al. (2015). The Links Between Ecosystem Multifunctionality and Above-and Belowground Biodiversity are Mediated by Climate. Nat. Commun. 6, 8159. doi: 10.1038/ncomms9159
Law A., Jones K. C., Willby N. J. (2014). Medium vs. Short Term Effects of Herbivory by Eurasian Beaver on Aquatic Vegetation. Aquat. Bot. 116, 27–34. doi: 10.1016/j.aquabot.2014.01.004
Liao L. R., Wang J., Zhang C., Liu G. B., Song Z. L. (2019). Effects of Grazing Exclusion on the Abundance of Functional Genes Involved in Soil Nitrogen Cycling and Nitrogen Storage in Semiarid Grassland. J. Appl. Ecol. 30, 3473–3481. doi: 10.13287/j.1001-9332.201910.002
Li W., Cao W., Wang J., Li X., Xu C., Shi S. (2017). Effects of Grazing Regime on Vegetation Structure, Productivity, Soil Quality, Carbon and Nitrogen Storage of Alpine Meadow on the Qinghai-Tibetan Plateau. Ecol. Eng. 98, 123–133. doi: 10.1016/j.ecoleng.2016.10.026
Li B., Liao C., Zhang X., Chen H., Wang Q., Chen Z., et al. (2009). Spartina Alterniflora Invasions in the Yangtze River Estuary, China: An Overview of Current Status and Ecosystem Effects. Ecol. Eng. 35, 511–520. doi: 10.1016/j.ecoleng.2008.05.013
Li N., Li B., Nie M., Wu J. (2020). Effects of Exotic Spartina Alterniflora on Saltmarsh Nitrogen Removal in the Yangtze River Estuary, China. J. Clean. Prod. 271, 122557. doi: 10.1016/j.jclepro.2020.122557
Lin X., Hou L., Liu M., Li X., Zheng Y., Yin G., et al. (2016). Nitrogen Mineralization and Immobilization in Sediments of the East China Sea: Spatiotemporal Variations and Environmental Implications. J. Geophys. Res. Biogeosci. 121, 2842–2855. doi: 10.1002/2016JG003499
Li N., Nie M., Li B., Wu J., Zhao J. (2021b). Contrasting Effects of the Aboveground Litter of Native Phragmites Australis and Invasive Spartina Alterniflora on Nitrification and Denitrification. Sci. Total Environ. 764, 144283. doi: 10.1016/j.scitotenv.2020.144283
Lin X., Li X., Gao D., Liu M., Cheng L. (2017). Ammonium Production and Removal in the Sediments of Shanghai River Networks: Spatiotemporal Variations, Controlling Factors, and Environmental Implications. J. Geophys. Res. Biogeosci. 122, 2461–2478. doi: 10.1002/2017JG003769
Lin G., Lin X. (2022). Bait Input Altered Microbial Community Structure and Increased Greenhouse Gases Production in Coastal Wetland Sediment. Water Res. 218, 118520. doi: 10.1016/j.watres.2022.118520
Liu J., Bian Z., Zhang K., Ahmad B., Khan A. (2019). Effects of Different Fencing Regimes on Community Structure of Degraded Desert Grasslands on Mu Us Desert, China. Ecol. Evol. 9, 3367–3377. doi: 10.1002/ece3.4958
Li M., Wang L., Li J., Peng Z., Wang L., Zhang X., et al. (2022). Grazing Exclusion had Greater Effects Than Nitrogen Addition on Soil and Plant Community in a Desert Steppe, Northwest of China. BMC Plant Biol. 22, 60. doi: 10.1186/s12870-021-03400-z
Li J., Zhao Y., Zhang A., Song B., Hill R. L. (2021a). Effect of Grazing Exclusion on Nitrous Oxide Emissions During Freeze-Thaw Cycles in a Typical Steppe of Inner Mongolia. Agric. Ecosyst. Environ. 307, 107217. doi: 10.1016/j.agee.2020.107217
Martin J. F., Reddy K. R. (1997). Interaction and Spatial Distribution of Wetland Nitrogen Processes. Ecol. Modell. 105, 1–21. doi: 10.1016/S0304-3800(97)00122-1
McGilloway R. L., Weaver R. W., Ming D. W., Gruener J. E. (2003). Nitrification in a Zeoponic Substrate. Plant Soil. 256, 371–378. doi: 10.1023/A:1026174026995
Mitsch W. J., Day J. W., Gilliam J. W., Groffman P. M., Hey D. L., Randall G. W., et al. (2001). Reducing Nitrogen Loading to the Gulf of Mexico From the Mississippi River Basin: Strategies to Counter a Persistent Ecological Problem. Bioscience. 51, 373–388. doi: 10.1641/0006-3568(2001)051[0373:RNLTTG]2.0.CO;2
Moradi M., Jorfi M. R., Basiri R., Yusef Naanaei S., Heydari M. (2021). Beneficial Effects of Livestock Exclusion on Tree Regeneration, Understory Plant Diversity, and Soil Properties in Semiarid Forests in Iran. Land Degrad. Dev. 33, 324–332. doi: 10.1002/ldr.4154
Navada S., Knutsen M. F., Bakke I., Vadstein O. (2020). Nitrifying Biofilms Deprived of Organic Carbon Show Higher Functional Resilience to Increases in Carbon Supply. Sci. Rep. 10, 1–11. doi: 10.1038/s41598-020-64027-y
Nie M., Zhang X. D., Wang J. Q., Jiang L. F., Yang J., Quan Z. X., et al. (2009). Rhizosphere Effects on Soil Bacterial Abundance and Diversity in the Yellow River Deltaic Ecosystem as Influenced by Petroleum Contamination and Soil Salinization. Soil Biol. Biochem. 41, 2535–2542. doi: 10.1016/j.soilbio.2009.09.012
Olofsson J., Mazancourt C. D., Crawley M. J. (2006). Contrasting Effects of Rabbit Exclusion on Nutrient Availability and Primary Production in Grasslands at Different Time Scales. Oecologia. 150, 582–589. doi: 10.1007/s00442-006-0555-4
Pagès J. F., Jenkins S. R., Bouma T. J., Sharps E., Skov M. W. (2019). Opposing Indirect Effects of Domestic Herbivores on Saltmarsh Erosion. Ecosystems. 22, 1055–1068. doi: 10.1007/s10021-018-0322-5
Pan H., Li Y., Guan X., Li J., Xu X., Liu J., et al. (2015). Management Practices Have a Major Impact on Nitrifier and Denitrifier Communities in a Semiarid Grassland Ecosystem. J. Soils Sediments. 16, 896–908. doi: 10.1007/s11368-015-1321-1
Pan H., Li Y., Guan X., Li J., Xu X., Liu J., et al. (2016). Management Practices Have a Major Impact on Nitrifier and Denitrifier Communities in a Semiarid Grassland Ecosystem. J. Soils Sediments. 16, 896–908.
Paul S., Meer H. V., Onduru D., Ebanyat P., Zake J., Wouters B., et al. (2013). Effects of Cattle and Manure Management on the Nutrient Economy of Mixed Farms in East Africa: A Scenario Study. Afr. J. Agric. Res. 8, 5129–5148. doi: 10.5897/AJAR10.009
Pinay G., O’Keefe T., Edwards R., Naiman R. J. (2003). Potential Denitrification Activity in the Landscape of a Western Alaska Drainage Basin. Ecosystems. 6, 336–343. doi: 10.1007/s10021-002-0169-6
Reddy K. R., Patrick W. H., Lindau C. W. (1989). Nitrification-Denitrification at the Plant Root-Sediment Interface in Wetlands. Limnol. Oceanogr. 34, 1004–1013. doi: 10.4319/LO.1989.34.6.1004
Rotthauwe J. H., Witzel K. P., Liesack W. (1997). The Ammonia Monooxygenase Structural Gene Amoa as a Functional Marker: Molecular Fine-Scale Analysis of Natural Ammonia-Oxidizing Populations. Appl. Environ. Microbiol. 63, 4704–4712. doi: 10.1128/aem.63.12.4704-4712.1997
Song Z., Wang J., Liu G., Zhang C. (2019). Changes in Nitrogen Functional Genes in Soil Profiles of Grassland Under Long-Term Grazing Prohibition in a Semiarid Area. Sci. Total Environ. 673, 92–101. doi: 10.1016/j.scitotenv.2019.04.026
Su Y. Z., Li Y. L., Cui J. Y., Zhao W. Z. (2005). Influences of Continuous Grazing and Livestock Exclusion on Soil Properties in a Degraded Sandy Grassland, Inner Mongolia, Northern China. Catena. 59, 267–278. doi: 10.1016/j.catena.2004.09.001
Tang H., Nolte S., Jensen K., Yang Z., Wu J., Mueller P. (2020). Grazing Mediates Soil Microbial Activity and Litter Decomposition in Salt Marshes. Sci. Total Environ. 720, 137559. doi: 10.1016/j.scitotenv.2020.137559
Thamdrup B., Dalsgaard T. (2002). Production of N2 Through Anaerobic Ammonium Oxidation Coupled to Nitrate Reduction in Marine Sediments. Appl. Environ. Microbiol. 68, 1312–1318. doi: 10.1128/AEM.68.3.1312-1318.2002
Throbäck I. N., Enwall K., Jarvis Å., Hallin S. (2004). Reassessing PCR Primers Targeting Nirs, nirK and nosZ Genes for Community Surveys of Denitrifying Bacteria With DGGE. FEMS Microbiol. Ecol. 49, 401–417. doi: 10.1016/j.femsec.2004.04.011
Väisänen M., Tuomi M. W., Bailey H. L., Welker J. M. (2021). Plant and Soil Nitrogen in Oligotrophic Boreal Forest Habitats With Varying Moss Depths: Does Exclusion of Large Grazers Matter? Oecologia. 196, 839–849. doi: 10.1007/s00442-021-04957-0
Valiela I., Cole M. L. (2002). Comparative Evidence That Salt Marshes and Mangroves may Protect Seagrass Meadows From Land-Derived Nitrogen Loads. Ecosystems. 5, 92–102. doi: 10.1007/s10021-001-0058-4
Valiela I., Teal J. M. (1979). The Nitrogen Budget of a Salt Marsh Ecosystem. Nature. 280, 652–656. doi: 10.1038/280652a0
Walker J. T., Vose J. M., Knoepp J., Geron C. D. (2009). Recovery of Nitrogen Pools and Processes in Degraded Riparian Zones in the Southern Appalachians. J. Environ. Qual. 38, 1391–1399. doi: 10.2134/jeq2008.0259
Wang C., Han X., Xing X. (2010). Effects of Grazing Exclusion on Soil Net Nitrogen Mineralization and Nitrogen Availability in a Temperate Steppe in Northern China. J. Arid. Environ. 74, 1287–1293. doi: 10.1016/j.jaridenv.2010.05.024
Wei H., Gao D., Liu Y., Lin X. (2020). Sediment Nitrate Reduction Processes in Response to Environmental Gradients Along an Urban River-Estuary-Sea Continuum. Sci. Total Environ. 718, 137185. doi: 10.1016/j.scitotenv.2020.137185
Yang Z., Nolte S., Wu J. (2017). Tidal Flooding Diminishes the Effects of Livestock Grazing on Soil Micro-Food Webs in a Coastal Saltmarsh. Agric. Ecosyst. Environ. 236, 177–186. doi: 10.1016/j.agee.2016.12.006
Yang W. H., Traut B. H., Silver W. L. (2015). Microbially Mediated Nitrogen Retention and Loss in a Salt Marsh Soil. Ecosphere. 6, 1–15. doi: 10.1890/ES14-00179.1
Yin Y., Wang Y., Li S., Liu Y., Zhao W., Ma Y., et al. (2019). Soil Microbial Character Response to Plant Community Variation After Grazing Prohibition for 10 Years in a Qinghai-Tibetan Alpine Meadow. Plant Soil. 458, 175–189. doi: 10.1007/s11104-019-04044-7
Zhang Y., Pennings S. C., Li B., Wu J. (2019). Biotic Homogenization of Wetland Nematode Communities by Exotic Spartina Alterniflora in China. Ecology. 100, 1–11. doi: 10.1002/ecy.2596
Keywords: grazing prohibition, nitrification, denitrification, salt marshes, Chongming Island
Citation: Li N, Nie M, Wu M and Wu J (2022) The Nitrogen Removal Ability of Salt Marsh Improved After Grazing Prohibition. Front. Mar. Sci. 9:958803. doi: 10.3389/fmars.2022.958803
Received: 01 June 2022; Accepted: 20 June 2022;
Published: 18 July 2022.
Edited by:
Xianbiao Lin, Ocean University of China, ChinaCopyright © 2022 Li, Nie, Wu and Wu. This is an open-access article distributed under the terms of the Creative Commons Attribution License (CC BY). The use, distribution or reproduction in other forums is permitted, provided the original author(s) and the copyright owner(s) are credited and that the original publication in this journal is cited, in accordance with accepted academic practice. No use, distribution or reproduction is permitted which does not comply with these terms.
*Correspondence: Jihua Wu, amlodWF3dUBmdWRhbi5lZHUuY24=