- 1Taronga Institute of Science and Learning, Taronga Conservation Society Australia, Mosman, NSW, Australia
- 2School of Biological, Earth, and Environmental Sciences, University of New South Wales, Sydney, NSW, Australia
- 3Australian Institute of Marine Science, Townsville, QLD, Australia
- 4Bush Heritage Australia, Melbourne, VIC, Australia
- 5Hawaii Institute of Marine Biology, University of Hawaii, Kaneohe, HI, United States
- 6Minderoo Foundation and University of Western Australia - Oceans Institute, University of Western Australia, Perth, WA, Australia
- 7School of BioSciences, Melbourne University, Parkville, VIC, Australia
- 8Center for Species Survival, Smithsonian Conservation Biology Institute, Smithsonian Institution, Front Royal, VA, United States
Active restoration or intervention programs will be required in the future to support the resilience and adaptation of coral reef ecosystems in the face of climate change. Selective propagation of corals ex situ can help conserve keystone species and the ecosystems they underpin; cross-disciplinary research and communication between science and industry are essential to this success. Zoos and aquaria have a long history of managing ex situ breed-for-release programs and have led the establishment of wildlife biobanks (collections of cryopreserved living cells) along with the development of associated reproductive technologies for their application to wildlife conservation. Taronga Conservation Society Australia’s CryoDiversity Bank includes cryopreserved coral sperm from the Great Barrier Reef, which represents the largest repository from any reef system around the globe. This paper presents results from an inventory review of the current collection. The review highlighted the skew toward five Acropora species and the necessity to increase the taxonomic diversity of the collection. It also highlighted the need to increase geographic representation, even for the most well represented species. The inventory data will inform Taronga’s future research focus and sampling strategy to maximize genetic variation and biodiversity within the biobank and provide a test case for other practitioners implementing biobanking strategies for coral conservation around the world. Through co-investment and collaboration with research partners over the next decade, Taronga will prioritize and resource critical applied research and expand biobanking efforts to assist interventions for reef recovery and restoration.
Introduction
The effects of climate change on our oceans imperil the health and resilience of coral reef systems around the globe. Addressing drivers of global climate change, while concurrently implementing restoration interventions at a large scale, provides the best chance for the continued existence of these ecosystems and their essential function and services (Anthony et al., 2017; Hoegh-Guldberg et al., 2019; Knowlton et al., 2021; Vardi et al., 2021). In Australia, the Great Barrier Reef (GBR) has experienced an increase in the frequency of climate-change-driven mass bleaching events over the last decade, including in its previously untouched southern range (Hughes et al., 2018; Australian Institute of Marine Science, 2021; Great Barrier Reef Marine Park Authority et al., 2022). These unprecedented heat stress events have caused a decline in coral cover and have reduced reproductive output (Hagedorn et al., 2016; Howells et al., 2016; Daly et al., 2022), consequently depressing larval supply (Cheung et al., 2021) and recruitment (Hughes et al., 2019).
In addition to action on climate change and improved local management practices, active reef restoration approaches to repair and re-seed reefs will likely be required for many sites to maintain or restore biodiversity and support or accelerate adaptation (Anthony et al., 2017; van Oppen et al., 2017; Suggett and van Oppen, 2022). Current restoration efforts primarily use asexual propagation methods (colony fragmentation) to generate new material for out-planting (Young et al., 2012; Boström-Einarsson et al., 2020); however, this approach has limitations for upscaling to a system as large as the GBR and it only generates clonal material. There is increasing interest in the sexual production of coral juveniles for out-planting (e.g., Randall et al., 2020), which is more amenable to upscaling, has the added advantage of helping to maintain population genetic diversity, and has the ability to strengthen or introduce adaptive traits to populations through selective breeding (Quigley et al., 2020; Hagedorn et al., 2021).
The sexual reproduction of corals ex situ is commonly achieved either by allowing individual colonies to naturally spawn in the same system or by controlling pairwise matches by selectively mixing freshly washed oocytes with optimized concentrations of fresh sperm (commonly termed ‘in-vitro fertilization’, or IVF). The relatively recent development of sperm cryopreservation technologies for corals (Hagedorn et al., 2006) enables selective breeding to also occur using sperm samples that are spatially and temporally removed from fresh oocyte supply. Cryopreservation refers to the controlled cooling of living cells and tissues to ultra-low temperatures using techniques that maintain the structure and function of cells when samples are brought back to physiological temperatures, allowing them to be stored for decades, possibly centuries, if maintained correctly. Biobanking of cryopreserved samples, when combined with assisted reproductive technologies such as IVF, can support species conservation by preserving genetically distinct specimens over long time periods. The potential application of biobanking to wildlife conservation was recognized in the 1970s (Watson, 1978; Benirschke, 1984) and has progressed over ensuing decades with zoos emerging as global leaders in the establishment of wildlife biobanks (Comizzoli and Wildt, 2017; Hagedorn et al., 2019; Hobbs et al., 2019; Hagedorn et al., 2021).
In 2011, scientists from the Taronga Conservation Society and Zoo (Sydney, Australia) and the Smithsonian Institution (United States of America) initiated a program with collaborators from the Australian Institute of Marine Science (AIMS) to apply coral sperm cryopreservation methods to species from the Great Barrier Reef. Cryopreservation techniques proved directly transferable to Australian Acropora species (Acropora tenuis, A. millepora), leading to the establishment of Australia’s first frozen coral biorepository (Hagedorn et al., 2012a; Hagedorn et al., 2012b). Moderate upscaling of IVF methods for the generation of live offspring (larvae and juveniles) using cryopreserved material was successfully demonstrated over the first three years of the program (Hagedorn et al., 2017). Subsequent improvements to the specifically designed cryopreservation apparatus (Zuchowicz et al., 2021) have aided in the up-scaling of time-sensitive sperm sample processing. Biobanking of gametes from GBR corals by the Taronga and Smithsonian teams has continued annually during spawning, utilizing wild coral colonies held temporarily at the AIMS National Sea Simulator (Cape Ferguson, Queensland, Australia). These achievements have resulted in the integration of coral biobanking via cryopreservation as an enabling technology within the largest research and development program for coral reefs globally, the Reef Restoration and Adaptation Program (RRAP1). A decade from its conception, we conducted a review and quality assurance check of Taronga’s CryoDiversity Bank coral inventory. This review aimed to generate census data on the existing collection to understand the quantity and scope of sampling from bioregions and species, and to identify gaps in quality assessments and metadata that need to be addressed. As the Taronga CryoDiversity Bank transitions from a relatively small-scale opportunistic sperm sample collection to a more targeted approach, we will use this information to direct future resource investment, inform collection management and ensure that the collection remains relevant to the needs of the coral conservation and restoration communities. It is hoped that the information and experiences of the Taronga CryoDiversity Bank will be useful to other coral biorepositories around the world that will likely face similar considerations as they seek to support coral conservation and restoration efforts.
Methods
Data associated with Taronga’s CryoDiversity Bank, in Sydney on Cammeraigal Country and in Dubbo on Wiradjuri Country, are managed using Microsoft Excel and stored on a user-restricted server. Prior to conducting the inventory review, a quality assurance check of the metadata was performed. An example of the metrics and metadata collected from each colony, and the evolution of these data fields over the 10+ year program, are included in Supplementary Material (Figure S1). New metadata fields were added as new technologies (e.g. computer assisted sperm analysis; CASA) were incorporated into best practices and metadata needs for future restoration were refined by collaborators (e.g. GIS data).
An inventory review was conducted of the data associated with physical sperm samples collected from corals of the GBR and cryopreserved between November 2011 and February 2022.
To obtain estimates of colony numbers and quantity of material from species, reefs, and regions, data were handled as follows:
• Colonies collected from the Far North and Cairns/Cooktown management areas (MAs) were combined into one group, referred to throughout as North MA.
• Database entries for 30 colonies (Central MA) were missing data on specific reef of origin. These entries were allocated to a general grouping “Unidentified Location Central”.
• During each spawning period, a colony may be sampled as an individual genetic unit and/or combined with samples from other individuals of the same species and reef to form a multi-colony pooled sperm sample. Pooled samples were created when individual sperm samples were either low in volume, or when batch spawning of corals within one container was conducted. Sperm samples were only pooled within species and source MA. When determining the number of colonies from each species, colonies were:
a. only counted once per year even if the colony was sampled on more than one spawning night each season;
b. counted towards the cumulative total of colonies sampled as individuals regardless of whether they also contributed to a pooled sperm sample that year, and;
c. counted towards the cumulative total of colonies contributing to pooled sperm samples if they were identified by colony name and not also sampled as individuals.
• If multiple pooled sperm samples were collected from the same source colonies across more than one night in the same year, but individual colonies contributing to that pool were not specifically identified, only the pool with the highest number of individuals was counted towards the pooled colony total for each species (Table 1).
• Raw sample motility data were calculated as the average of percent total motility, whether data were collected by manual counting (2011-2017), or CASA (≥2018; Table 1). From 2019 onwards, raw motility data were derived from a sub-sample activated with a standardized concentration of caffeine and bovine serum albumen (refer to methods in Daly et al., 2022). Thus, any motility values of ⪅ 50% observed for samples collected prior to 2019 may be underestimated due to absent or incomplete activation.
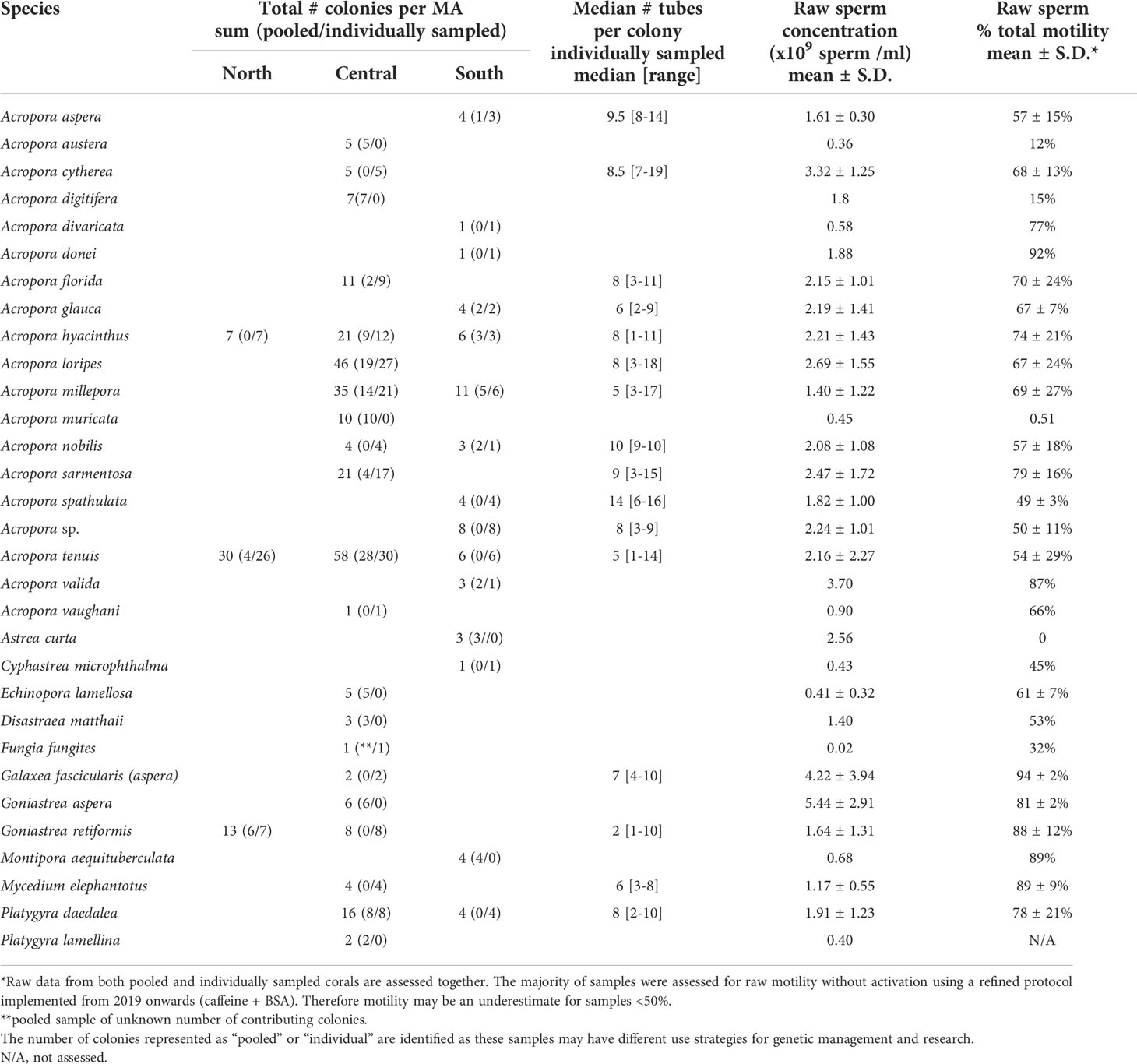
Table 1 Summary of sample quantity and type (pooled or individual), sample quality (motility and concentration), and the number and distribution of coral colonies across local management areas (origin) represented in Taronga’s CryoDiversity Bank.
Results
As of March 2022, Taronga’s CryoDiversity Bank contains 2614 cryovials of cryopreserved sperm sourced from:
• 381 coral colonies. Of these colonies, 230 (60%) are represented by single genotypes (i.e., sampled as individuals); the remaining 151 colonies only contributed to pooled sperm samples, comprising two or more colonies.
• 30 identified species of hard coral and one additional species of Acropora of unknown taxonomy (referred to throughout as Acropora sp.).
• Four MAs of the GBR Marine Park; North MA (combining Far North and Cairns/Cooktown MAs), Central MA (Townsville and Whitsunday) and South MA (Mackay/Capricorn).
Between 2011 and 2014 a total of 446 cryovials from 7 species were cryopreserved and banked (Figure 1A) consisting of pooled sperm samples only (Figure 1B). In 2016, the first medium-scale cryopreservation of coral sperm specifically for conservation biobanking was undertaken at the National Sea Simulator (AIMS) adjacent to the central GBR. This single collection event increased the total number of cryovials preserved, the number of colonies from which sperm were sampled, and the number of species represented by approximately 100%, to a cumulative total of 1088 cryovials from 123 colonies across 11 species. This was also the first year that sperm samples were collected and cryopreserved from single colonies, creating samples of individual genetic units (Figure 1B). Between 2016 and 2020, an average of 415 cryovials of cryopreserved sperm from 5 species and 60 new colonies were added to the bank each year.
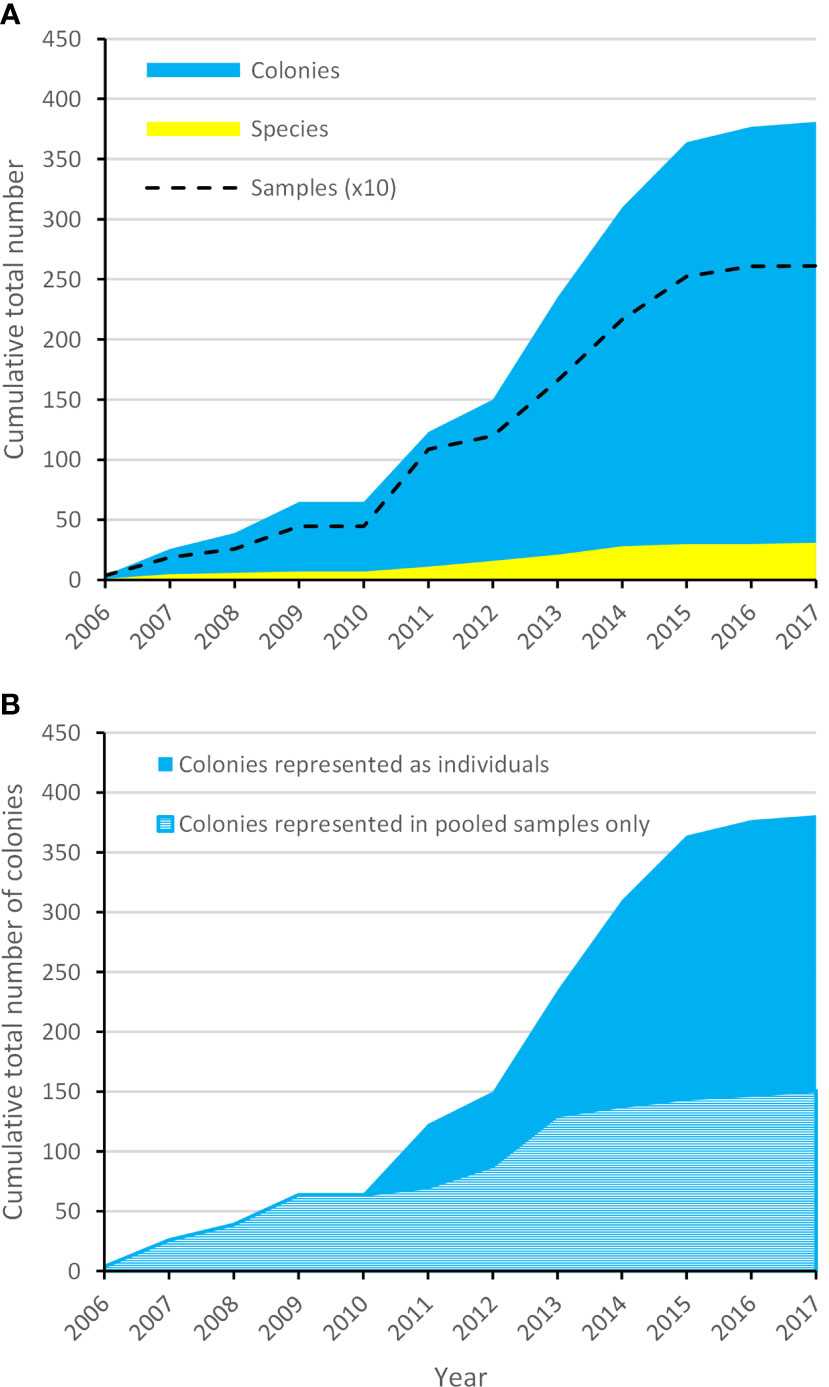
Figure 1 Growth (A) and diversification of sampling strategy (B) of Taronga’s CryoDiversity Bank coral collection between 2011 and 2022.
Eleven of the 31 species represented in Taronga’s CryoDiversity Bank had sperm samples collected and cryopreserved across more than one year (Figure 2), five of which were sampled across six or more years. Eighteen species have been sampled only on one occasion.
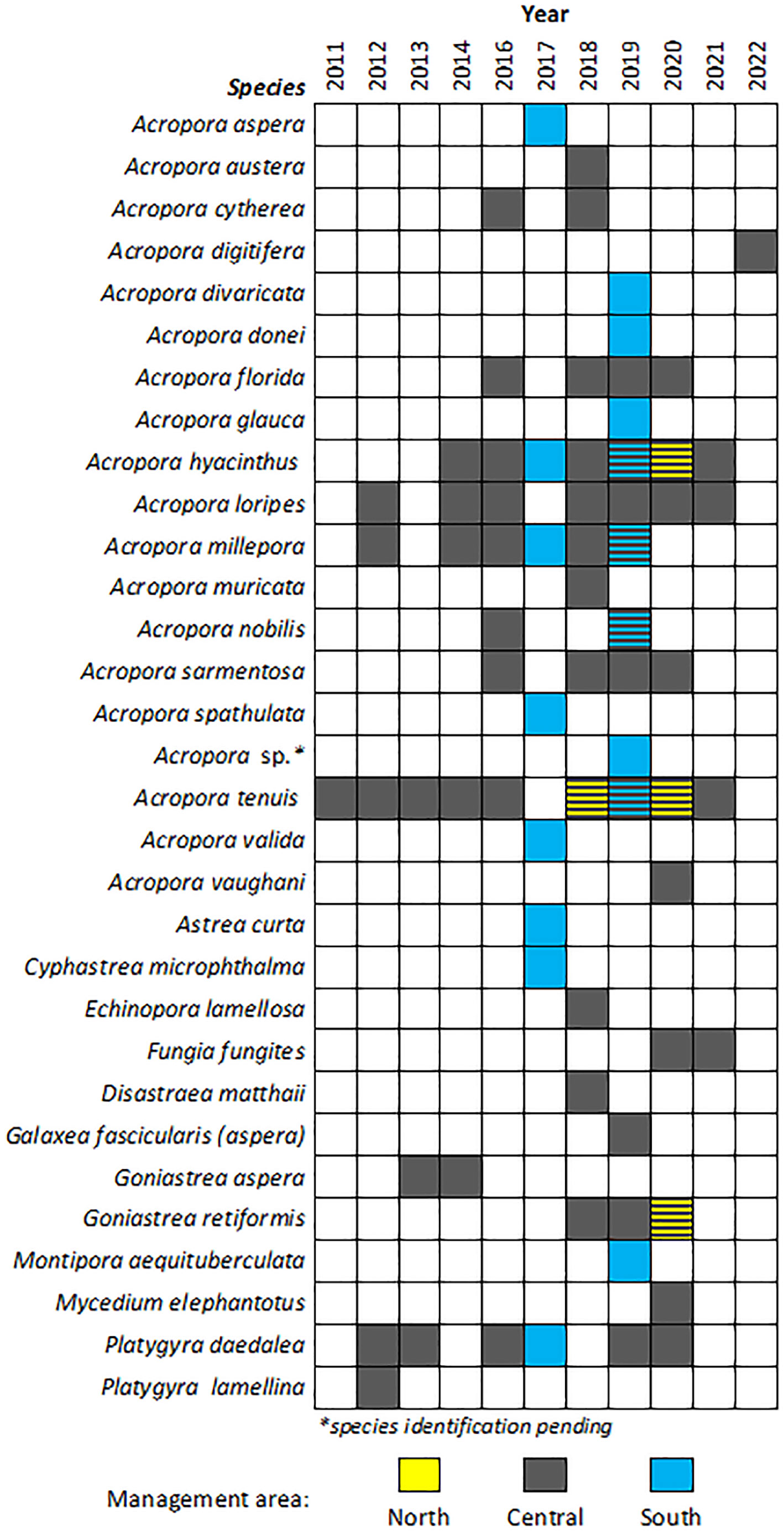
Figure 2 Species sampling events by year and local management area. Striped boxes indicate sampling from two MAs in a single year.
Geographic diversity
Between 2011 and 2022, sperm samples were collected and cryopreserved from corals originating from 22 reefs across the GBR Marine Park (Figure 3).
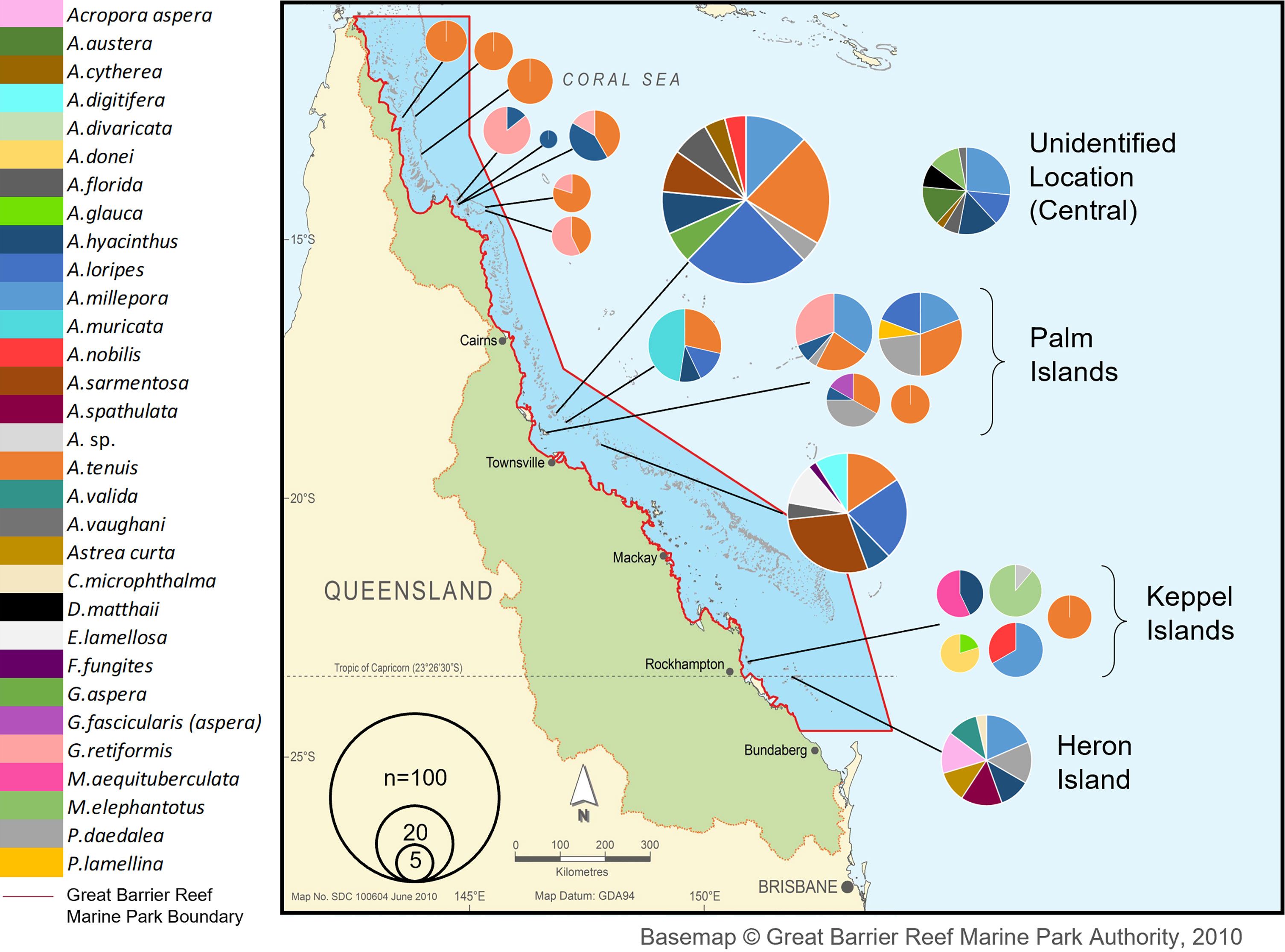
Figure 3 Species represented within Taronga’s CryoDiversity Bank mapped by reef location sampled. The 22 reefs sampled (starting top left to right and down) were: North MA: Curd, Long Sandy, Sand Bank No.7, South Warden, Munro, Switzer, Jewell, Parke; Central MA: Trunk, Backnumbers, Palm Islands (Falcon, Esk, West Pelorus, Mundy), Davies; and South MA: Keppel Islands (Humpy, Great Keppel, Outer Rocks, Pleasant (Conical) Island, Halfway Island), Heron Island. The size of each circle represents the total number of coral colonies collected from the location; each slice represents the proportion of colonies sampled by species. See Table 1 for full species names.
The MA with the highest diversity of species represented in the bank is the Central MA surrounding Townsville, with 21 species sourced from at least seven reefs (Figure 3). Almost as diverse is representation from the South MA, where sperm have been cryopreserved from 15 species across five reefs in the Keppel Islands and around Heron Island. Ten of the 15 species from this MA have not had sperm samples preserved from any other region, and only 20% of all colonies represented within the bank are from this MA. Only three species from eight reefs of the North MAs are represented in the CryoDiversity Bank (A. tenuis, A. hyacinthus and Goniastrea retiformis).
Six species from which sperm have been sampled originated from more than one MA. Acropora tenuis and A. hyacinthus are the only species with sperm samples cryopreserved across all MAs, originating from 14 and 11 reefs, respectively. Eight species were sampled from between two and six reefs and the remaining 21 species have been sampled from one reef only.
Biodiversity and representation
The most highly represented species in the collection, based on the total number of colonies sampled, are A. tenuis (25% of all colonies sampled), A. loripes (12%), A. millepora (12%), A. hyacinthus (9%), and A. sarmentosa (6%). Combined, these five species make up 63% of all colonies sampled and 71% of all cryovials in the bank. Four species (A. divaricata, A. donei, A. vaughani, Cyphastrea microphthalma) have had sperm samples cryopreserved from only one colony. Fourteen species have had two to five colonies sampled; five species have had between five and 19 colonies sampled; and seven species have had 20 or more colonies sampled (Table 1).
The most represented species in the collection is A. tenuis with 94 colonies represented, 62 of which have been sampled as individuals. On average, sperm samples from 6.7 (median 6.0; range 3-21) A. tenuis colonies have been cryopreserved from each of the 14 source reefs. Acropora hyacinthus is the second most geographically diverse species in the collection (34 colonies), with an average of 3.1 (median 3.0; range 1-11) colonies sourced from 11 different reefs, although both A. millepora and A. loripes exceed it in number of colonies represented, at 46 colonies per species (Table 1).
Approximately half the species (15 species: 8 Central, 7 South) represented in the bank have 20 or fewer cryovials banked in total. Sperm from nine species are represented as pooled samples only (A. austera, A. digitifera, A. muricata, Astrea curta, Echinopora lamellosa, Dipsastraea matthaii, Goniastrea aspera, Montipora aequituberculata, Platygyra lamellina). Nine species were sampled only as individual genotypes and the remaining 12 species were sampled in both formats (Table 1; Figure S2).
Sample quality metadata
Assessment of sperm quality metrics is essential in biobanking, both to determine the suitability of material for banking and to select frozen samples for end-use. Fresh and post-thaw sperm metrics are collected to assess cryopreservation efficacy. Average sperm motility of fresh samples was highly variable both within and between species (Table 1) and across years. The average fresh sperm concentration in 22 of 31 species was above 1 billion sperm cells per mL. Samples from the gonochoric species Fungia fungites recorded the lowest sperm concentration, at 20 million cells/mL.
Discussion
Zoos are global leaders in the establishment of wildlife biobanks both for fundamental research and as a tool to support genetic management of ex situ breeding programs (Comizzoli and Wildt, 2017; Hagedorn et al., 2019; Hobbs et al., 2019; Traylor-Holzer et al., 2019; Holt & Comizzoli, 2021). Alongside biobanking, development of reproductive technologies across a range of taxa (Comizzoli, 2015; Herrick, 2019) has seen these tools more frequently integrated into breed-for-release programs and species recovery plans in recent years (Swanson et al., 2007; Howard et al., 2016; O'Brien et al., 2016; Della Togna et al., 2020; Hagedorn et al., 2021; Hobbs et al., 2021). Biobanking has been championed by these organisations for decades as a viable conservation tool that does not detract from, and should not be to the exclusion of, other conservation strategies.
Through collaborative programs over the last decade, Taronga’s CryoDiversity Bank coral collection has become the largest single biorepository of cryopreserved coral sperm2,3, in both number of species represented and quantity of material. Crucially, this collection includes sperm samples that pre-date the recent mass-bleaching events on the GBR (e.g., 2016, 2017, 2020 and 2022) along with samples from colonies sourced from targeted resilient populations that have the demonstrated ability to produce heat-tolerant offspring (Quigley and van Oppen, 2022). The CryoDiversity Bank therefore provides an important reservoir of genotypic and phenotypic biodiversity that could become a unique resource for reef restoration practices, especially those that incorporate adaptation and adaptive management into their strategies (Quigley et al., 2022; Shaver et al., 2022). Our recent review showed that the Bank contains sperm samples from over 380 individual colonies of 30 identified species of scleractinian corals collected from a wide geographic area of the GBR Marine Park. Over the lifetime of Taronga’s Reef Recovery Project3, most sperm samples have been collected opportunistically from colonies prioritized and sourced by our collaborators conducting their own research on a small number of coral species year-to-year. Hence, the collection is dominated (70% of samples) by five species (A. hyacinthus, A. loripes, A. millepora, A. sarmentosa and A. tenuis) and by reefs in close proximity to the National Sea Simulator on the Central GBR.
Sampling strategies have been suggested to support ex situ propagation of genetically diverse coral populations (e.g., Shearer et al., 2009; Baums et al., 2019; Quigley et al., 2019), which are directly applicable to the sampling of coral donors for sperm biobanking. One such target is the sampling of ≥30 genets per species, which captures approximately 95% of allelic diversity of an average population of unknown genetic heritage (Shearer et al., 2009). It is important to undertake genotyping of all banked samples, particularly for those species with less resolved population genetics, to confirm genetic diversity prior to potential future use of cryopreserved sperm for conservation breeding and to inform ongoing collection targets. To this end, we recommend that a fragment, or voucher sample, be taken from each coral colony and banked alongside cryopreserved sperm samples to permit future genotyping and species identification (Voolstra et al., 2021). The most highly represented species in Taronga’s biobank, A. tenuis, easily surpasses this target with sperm samples preserved from 94 colonies. However, only six colonies have been sampled from the southern GBR where A. tenuis is known to have higher genetic diversity compared to northern populations (Lukoschek et al., 2016) so future effort will be focussed on bolstering genets collected from this region. For other species with fewer samples, a target of 30 genets per species and population will prioritized. Sourcing rare and poorly represented species will remain a challenge on the GBR as the majority of its reefs are tens of kilometres from the mainland; to reach them requires significant resourcing. Engagement with Traditional Owner and other community groups to prioritize culturally significant and biodiverse sites and species will help to ensure that limited resources have the most impact for people and communities.
At present the most effective cryopreservation methodology for coral is sperm preservation, as cryopreservation technologies for other coral cell sample types are not yet sufficiently developed for biobanking. Taronga’s CryoDiversity Bank and similar groups working in Hawaii, Florida, Mexico, the Red Sea, and the Caribbean have therefore focused their acquisitions on cryopreservation of the male germline (Hagedorn et al., 2006; Grosso-Becerra et al., 2021; Zuchowicz et al., 2021). Approximately 63% of coral species surveyed are broadcast spawners (Baird et al., 2009) so the capture of sperm for most species should be possible, albeit with some notable challenges such as gonochores (e.g., Porites species) and species for which reproductive timing remains unknown (Baird et al., 2021). Coral sperm cryopreservation can help diversify shrinking populations through selective breeding, but currently relies on availability of fresh oocytes; we also need additional strategies to mitigate species extinctions by preserving whole organisms, or somatic cells. Cryopreservation and laser-warming of coral larvae (Daly et al., 2018) is a technology with promise but may be too complex for large-scale or field application. Newer, simpler cryopreservation technologies may provide additional opportunities for coral; for example, cryo-grid technology can easily be used to cryopreserve large numbers of Drosophila embryos and pancreatic cells from various species (Zhan et al., 2021; Zhan et al., 2022). Taronga’s CryoDiversity Bank coral acquisitions have only recently expanded to include experimental preservation of other cell types (e.g., embryonic cells: Hagedorn et al., 2012b), life history stages (e.g., larvae: Daly et al., 2018; Cirino et al., 2019), and the microalgal endosymbionts of corals (Symbiodiniaceae: Hagedorn et al., 2010; Hagedorn and Carter, 2015; Lin et al., 2019; Kihika et al., 2022). While cryopreservation of sperm should remain a priority in the near term, the inclusion of new sample types into existing biorepositories and restoration programs will be rapidly adopted as the technologies mature and become ready for broader application.
The most efficient and effective use of cryopreserved sperm samples for reef restoration will likely be for genetic management of brood-stock within ex situ coral aquaculture systems, where sexually produced juveniles are generated under controlled conditions (Hagedorn et al., 2017; Randall et al., 2020) and microbiome manipulations are possible (Buerger et al., 2020; Santoro et al., 2021; Maire and van Oppen, 2022) prior to deployment onto selected reefs (e.g., Quigley et al., 2021; Randall et al., 2021). The use of cryopreserved sperm is a cost-effective way to increase the effective breeding population (e.g., Howell et al., 2021), providing greater control over brood-stock and genetic management for trait selection via selective crosses among individual colonies. Alternatively, pooled samples enable “batch” multi-colony fertilisation mixtures to maximise the number and diversity of larvae produced, and fit with the planned automation of ex situ coral spawning activities to generate restoration-scale quantities of material. Although the Taronga CryoDiversity Bank has tended towards cryopreservation of sperm from individual colonies in recent years, future collections should aim to achieve a mix of both individual and pooled samples.
Cryopreserved sperm could also be used to overcome temporal differences in spawning between colonies. Importantly, cryopreserved sperm can easily be moved between institutions and populations to facilitate assisted gene flow (e.g., Hagedorn et al., 2021; Daly et al., 2022), reducing the cost and risk of disease transfer associated with moving coral colonies. An example of where this approach may be important is the Florida Coral Rescue program4, which has brought over 2300 colonies from 20 species of coral into the care of 27 facilities managed by 28 partners (including zoos and aquaria) in 15 states across the USA in response to extensive coral mortality in the wild from stony coral tissue loss disease (SCTLD). To manage population genetics within this ex situ breeding program and permit genetic crosses amongst institutions, cryopreserved sperm could be utilized to produce new corals for reef restoration and for biobanking to ensure that founder population genetics are retained (B. Firchau, pers. comm.).
Depending on the scale to which fertilization using cryopreserved sperm can be expanded, it may be possible to generate larvae directly for settlement and deployment using biobanked sperm samples. In vitro fertilization methods for some coral species are well established and typically involve a standard fertilization ratio of 10 eggs per ml exposed to 1×106 sperm/ml, corresponding to approximately 1×105 sperm per egg (Pollock et al., 2017; dela Cruz and Harrison, 2020). In the case of bulk fertilization of freshly collected gametes, fertilization volumes can reach up to 200 L for large mixed-batch cultures, generating hundreds of thousands of embryos (Negri and Heyward, 2000). Cryopreservation methods were developed to preserve 1 mL aliquots of sperm at a 1×109 sperm/mL, with a predicted efficiency of 50% sperm survival and consequently a reduction in overall motility (Hagedorn et al., 2017). Existing IVF production methods using cryopreserved coral sperm (Hagedorn et al., 2021; Daly et al., 2022) are capable of producing tens of thousands of coral larvae, suitable for the genetic maintenance of brood-stock or for small-scale restoration activities (e.g. Grosso-Becerra et al., 2021), especially if combined with advanced microfragmentation and husbandry techniques (Page et al., 2022). Current protocols for sperm cryopreservation and use may therefore already be suitable for many restoration applications; however, to support the production of millions of coral larvae, different approaches to sperm cryopreservation, sample packaging, and fertilisation methods, such as those used in the agriculture and aquaculture industries, may be required.
Reef recovery programs developing and applying reef restoration practices at the scale required to help large reef ecosystems (e.g., Bay et al., 2019; National Academies of Sciences, Engineering, and Medicine, 2019) will necessitate a prioritization of species and populations; this is especially true for the GBR, which encompasses hundreds of species across thousands of individual reefs along thousands of kilometres of coastline. Taronga’s coral cryo-collection will, in part, support the production needs of an established aquaculture program (Gibbs et al., 2019; Worley Parsons Services and Australian Institute of Marine Science, 2019) whose species priorities will be informed by restoration modelling and species selection tools (Madin et al., 2021). Large-scale aquaculture of this type will likely target a smaller number of species to produce large quantities of coral recruits for direct deployment onto reefs, potentially leading to a further increase in sampling from species that are already well represented in the bank (e.g., A. tenuis and A. hyacinthus). Of equal importance, however, will be the need to establish a more diverse collection that provides a reservoir of biodiversity from which to draw once initial coral cover is restored. This strategy will warrant continued collection from lesser represented species and regions, which will be supported by competitive funding streams and philanthropy in partnership with Traditional Owners and various community groups. Striking the balance between securing broad biodiversity and storing large volumes of prioritized species to support aquaculture will be an important logistical challenge for all coral biobanking programs going forward. Modelling efforts aimed at prioritizing the array of suggested interventions (e.g., Condie et al., 2021) will also be required before we can fully understand the scale at which cryopreservation will integrate with coral production in Australia and therefore the quantities of cryopreserved material that will be required.
Conclusions and future work
Coral reefs are complex ecosystems to conserve; ongoing applied research and modelling will be needed to improve our understanding of the necessary interventions, their scale, and their design (Sivapalan and Bowen, 2020). Based on our experience, biobanking activities should ensure that: each colony is uniquely identified and either genotyped or vouchered; collection and cryopreservation of sperm is prioritized and new sample types are introduced as technologies become available; and collections target a mix of individual and pooled samples matching production strategy and restoration goals. To achieve this will require increased resourcing for infrastructure to support the required biobanking capacity, along with continued refinement of metadata and database management to ensure that these collections can be maintained in perpetuity.
Aquaculture and biobanking on the scale required for coral conservation worldwide will require strategic partnerships between science, industry, restoration specialists, government, and community. Taronga scientists, along with scientists from other institutions utilizing coral cryopreservation strategies globally, are collaborating to refine coral cryopreservation technologies and build capacity through groups such as the Coral Restoration Consortium (CRC) Cryopreservation and Biobanking Working Group and the Coral Biobank Alliance. It is hoped that these initiatives will help to achieve the shared goal of minimizing the loss of biodiversity on coral reefs globally.
Taronga Conservation Society commits to working in a way that respects, recognizes and includes First Nations people. Taronga will consult with Traditional Owners to ensure that the proposed cryopreservation interventions are socially and culturally safe, including that: consent is obtained to collect material from sea Country; movement of cryopreserved material to Taronga’s biobank facilities is performed in collaboration with Traditional Owners of sea Country and Cammeraigal (North Sydney, NSW) and Wiradjuri (Dubbo, NSW) people; and access to biobanked material only occurs within the consent provided by the relevant Traditional Owner group. Moreover, Taronga recognizes the current and ongoing heritage and spiritual connection of Traditional Owners to their sea Country and will permanently track Traditional Custodianship within the CryoDiversity Bank electronic database. Recognition of the Traditional Custodians of living cells within the biobank into perpetuity will be vital to the long-term stewardship of these samples and harmonizes with the integrated and inclusive approach to species conservation being undertaken by Taronga and RRAP.
The prospects of the Taronga CryoDiversity Bank to support reef restoration are promising; however, the reality is that the causes of climate change progress unabated. Recent fine-scale modelling (Dixon et al., 2022; Kalmus et al., 2022) predicts that an elevated temperature of 1.5°C could see <1% of coral surviving by the mid-2030s. Securing biodiversity through continued sperm cryopreservation, and the development of simple, field-ready, technologies for coral embryo cryopreservation, will be crucial to stop-gap species loss in the coming years and decades. We hope the results of our work and efforts may give future generations options for healthier, more diverse reef ecosystems.
Data availability statement
The raw data supporting the conclusions of this article will be made available by the authors, without undue reservation.
Author contributions
RH compiled and analysed the data, wrote the manuscript and prepared the figures. JD did the main manuscript revision and editing. MH, RS and JO’B contributed significantly to the establishment and ongoing management of the Taronga CryoDiversity Bank coral collection and reviewed the manuscript. All other authors listed have made a substantial, direct, and intellectual contribution to the work over the life of Taronga’s reef recovery program, reviewed the manuscript and approved the manuscript for publication.
Funding
Taronga’s CryoDiversity Bank infrastructure and biobanking collection trips were co-funded by Taronga Conservation Society Australia, the Taronga Conservation Science Initiative and other philanthropists supporting the Taronga Foundation and we acknowledge the Australian Institute of Marine Science which provided laboratory space and facilities within the National Sea Simulator. Development, refinement and training in enabling technologies (MH, VC, NZ, JD) were co-funded through the Smithsonian Conservation Biology Institute, the Hawaii Institute of Marine Biology, the Australian Institute of Marine Science, the Paul M. Angell Family Foundation, the Smithsonian’s Women’s Committee, the Roddenberry Foundation, the Seaver Institute, the William H. Donner Foundation, the Barrett Family Foundation, the Skippy Frank Translational Medicine and Life Sciences Fund, the Compton Foundation, the Cedar Hill Foundation and the Anela Kolohe Foundation. From 2020, co-funding was provided by the Taronga Foundation and the Reef Restoration and Adaptation Program, a partnership between the Australian Government’s Reef Trust and the Great Barrier Reef Foundation.
Acknowledgments
The authors would like to acknowledge the Traditional Owner groups of the sea Country where coral colonies have been collected from. The authors thank those Traditional Owners who have given permission to preserve and store samples cryopreserved from their corals and commit to continuing consultation with all Traditional Owners regarding both historic and future collection of material for biobanking from sea Country. We would also like to acknowledge the support of The Australian Institute of Marine Science staff and scientists who facilitated the collection and spawning of colonies within the National Sea Simulator and the Great Barrier Reef Legacy who enabled access to corals from the North MA to be preserved. This work was undertaken as an activity of the Cryopreservation sub-program (RRAP-CP-01) for the Reef Restoration and Adaptation Program, a collaboration of leading experts working to create a suite of innovative measures to help preserve and restore the Great Barrier Reef. Funded by the Australian Government, partners include: the Australian Institute of Marine Science, CSIRO, the Great Barrier Reef Foundation, Southern Cross University, The University of Queensland, Queensland University of Technology and James Cook University.
Conflict of interest
The authors declare that the research was conducted in the absence of any commercial or financial relationships that could be construed as a potential conflict of interest.
Publisher’s note
All claims expressed in this article are solely those of the authors and do not necessarily represent those of their affiliated organizations, or those of the publisher, the editors and the reviewers. Any product that may be evaluated in this article, or claim that may be made by its manufacturer, is not guaranteed or endorsed by the publisher.
Supplementary material
The Supplementary Material for this article can be found online at: https://www.frontiersin.org/articles/10.3389/fmars.2022.960470/full#supplementary-material
Footnotes
- ^ https://gbrrestoration.org/program/cryopreservation/.
- ^ https://nationalzoo.si.edu/center-for-species-survival/coral-species-cryopreserved-global-collaborators
- ^ https://taronga.org.au/conservation-and-science/current-research/reef-recovery
- ^ https://myfwc.maps.arcgis.com/apps/dashboards/eba7dc2cabc64f60819e6d4b084d94cd
References
Anthony K., Bay L. K., Costanza R., Firn J., Gunn J., Harrison P., et al. (2017). New interventions are needed to save coral reefs. Nat. Ecol. Evol. 1, 1420–1422. doi: 10.1038/s41559-017-0313-5
Australian Institute of Marine Science (2021) Long-term monitoring program: Annual summary report of coral reef condition 2020/2021. Available at: https://www.aims.gov.au/reef-monitoring/gbr-condition-summary-2020-2021.
Baird A. H., Guest J. R., Edwards A. J., Bauman A. G., Bouwmeester J., Mera H., et al. (2021). An indo-pacific coral spawning database. Sci. Data 8, 35. doi: 10.1038/s41597-020-00793-8
Baird A. H., Guest J. R., Willis B. L. (2009). Systematic and biogeographical patterns in the reproductive biology of scleractinian corals. Ann. Rev. Ecol. Evol. Sys 40 (1), 551–571. doi: 10.1146/annurev.ecolsys.110308.120220
Baums I. B., Baker A. C., Davies S. W., Grottoli A. G., Kenkel C. D., Kitchen S. A., et al. (2019). Considerations for maximizing the adaptive potential of restored coral populations in the western Atlantic. Ecol. Appl. 29 (8), e01978. doi: 10.1002/eap.1978
Bay L., Rocker M., Bostroüm-Einarsson L., Babcock R., Buerger P., Cleves P., et al. (2019). Reef restoration and adaptation program: Intervention technical summary. a report provided to the Australian government by the reef restoration and adaptation program. 89pp. Australian Institute of Marine Science, Townsville.
Boström-Einarsson L., Babcock R. C., Bayraktarov E., Ceccarelli D., Cook N., Sebastian C. A., et al. (2020). Coral restoration – a systematic review of current methods, successes, failures and future directions. PloS One 15 (1), e0226631. doi: 10.1371/journal.pone.0226631
Buerger P., Alvarez-Roa C., Coppin C. W., Pearce S. L., Chakravarti L. J., Oakeshott J. G., et al. (2020). Heat-evolved microalgal symbionts increase coral bleaching tolerance. Sci. Adv. 6, eaba2498. doi: 10.1126/sciadv.aba2498
Cheung M. W. M., Hock K., Skirving W., Mumby P. J. (2021). Cumulative bleaching undermines systemic resilience of the great barrier reef. Curr. Biol. 31 (23), 5385–5392.e4. doi: 10.1016/j.cub.2021.09.078
Cirino L., Wen Z. H., Hsieh K., Huang C.-L., Lun Leong Q., Wang L.-H., et al. (2019). First instance of settlement by cryopreserved coral larvae in symbiotic association with dinoflagellates. Sci. Rep. 9, 18851. doi: 10.1038/s41598-019-55374-6
Comizzoli P. (2015). Biotechnologies for wildlife fertility preservation. Anim. Front. 5 (1), 73–78. doi: 10.2527/af.2015-0011
Comizzoli P., Wildt D. E. (2017). “Cryobanking biomaterials from wild animal species to conserve genes and biodiversity: Relevance to human biobanking and biomedical research,” in Biobanking of human biospecimens (Cham: Springer), 217–235.
Condie S. A., Anthony K. R. N., Babcock R. C., Baird M. E., Beeden R., Fletcher C. S., et al. (2021). Large-Scale interventions may delay decline of the great barrier reef. R. Soc Open Sci. 8 (4), 201296. doi: 10.1098/rsos.201296
Daly J., Hobbs R. J., Zuchowicz N., O’Brien J. K., Bouwmeester J., Bay L., et al. (2022). Cryopreservation can assist gene flow on the great barrier reef. Coral Reefs 41, 455–462. doi: 10.1007/s00338-021-02202-x
Daly J., Zuchowicz N., Nuñez Lendo C. I., Khosla K., Lager C., Henley E. M., et al. (2018). Successful cryopreservation of coral larvae using vitrification and laser warming. Sci. Rep. 8, 15714. doi: 10.1038/s41598-018-34035-0
dela Cruz D. W., Harrison P. L. (2020). Optimising conditions for in vitro fertilization success of acropora tenuis, a. millepora and favites colemani corals in northwestern Philippines. J. Exp. Mar. Biol. Ecol. 524, 151286. doi: 10.1016/j.jembe.2019.151286
Della Togna G., Howell L. G., Clulow J., Langhorne C. J., Marcec-Greaves R., Calatayud N. E. (2020). Evaluating amphibian biobanking and reproduction for captive breeding programs according to the amphibian conservation action plan objectives. Therio 1 (150), 412–431. doi: 10.1016/j.therio.2020.02.024
Dixon A. M., Forster P. M., Heron S. F., Stoner A. M. K., Beger M. (2022). Future loss of local-scale thermal refugia in coral reef ecosystems. PloS Clim 1, e0000004. doi: 10.1371/journal.pclm.0000004
Gibbs M., Mead D., Babcock R. C., Harrison D., Ristovski Z., Harrison P., et al. (2019) Reef restoration and adaptation program: Future deployment scenarios and costing. a report provided to the Australian government by the reef restoration and adaptation program. Available at: https://gbrrestoration.org/wp-content/uploads/2020/09/T5-Future-Deployment-Scenarios-and-Costing-V7.9-3.pdf.
Great Barrier Reef Marine Park Authority, Australian Institute of Marine Science, Commonwealth Scientific and Industrial Research Organisation (2022). Reef snapshot: Summer 2021-22 (Townsville: Great Barrier Reef Marine Park Authority).
Grosso-Becerra M. V., Mendoza-Quiroz S., Maldonado E., Banaszak A. T. (2021). Cryopreservation of sperm from the brain coral diploria labyrinthiformis as a strategy to face the loss of corals in the Caribbean. Coral Reefs 40, 937–950. doi: 10.1007/s00338-021-02098-7
Hagedorn M., Carter V. L. (2015). Seasonal preservation success of the marine dinoflagellate coral symbiont, symbiodinium sp. PloS One 10 (9), e0136358. doi: 10.1371/journal.pone.0136358
Hagedorn M., Carter V. L., Henley E. M., van Oppen M. J. H., Hobbs R. J., Spindler R. E. (2017). Producing coral offspring with cryopreserved sperm: A tool for coral reef restoration. Sci. Rep. 7, 14432. doi: 10.1038/s41598-017-14644-x
Hagedorn M., Carter V. L., Lager C., Camperio C. J. F., Dygert A. N., Schleiger R. D., et al. (2016). Potential bleaching effects on coral reproduction. Reprod. Fert. Dev. 28, 1061–1071. doi: 10.1071/RD15526
Hagedorn M., Carter V. L., Leong J. C., Kleinhans F. W. (2010). Physiology and cryosensitivity of coral endosymbiotic algae (Symbiodinium). Cryobiol 60 (2), 147–158. doi: 10.1016/j.cryobiol.2009.10.005
Hagedorn M., Carter V., Martorana K., Paresa M. K., Acker J., Baums I. B., et al. (2012b). Preserving and using germplasm and dissociated embryonic cells for conserving Caribbean and pacific coral. PloS One 7 (3), e33354. doi: 10.1371/journal.pone.0033354
Hagedorn M., Carter V. L., Steyn R. A., Krupp D., Leong J. C., Lang R. P., et al. (2006). Preliminary studies of sperm cryopreservation in the mushroom coral, fungia scutaria. Cryobiol 52 (3), 454–458. doi: 10.1016/j.cryobiol.2006.03.001
Hagedorn M., Page C. A., O’Neil K. L., Flores D. M., Tichy L., Conn T., et al. (2021). Assisted gene flow using cryopreserved sperm in critically endangered coral. PNAS 118 (38), e2110559118. doi: 10.1073/pnas.2110559118
Hagedorn M., Spindler R., Daly J. (2019). Cryopreservation as a tool for reef restoration. Adv. Exp. Med. Biol. 1200, 489–505. doi: 10.1007/978-3-030-23633-5_16
Hagedorn M., van Oppen M. J., Carter V., Henley M., Abrego D., Puill-Stephan E., et al. (2012a). First frozen repository for the great barrier reef coral created. Cryobiol 65 (2), 157–158. doi: 10.1016/j.cryobiol.2012.05.008
Herrick J. R. (2019). Assisted reproductive technologies for endangered species conservation: developing sophisticated protocols with limited access to animals with unique reproductive mechanisms. Biol. Reprod. 100 (5), 1158–1170. doi: 10.1093/biolre/ioz025
Hobbs R. J., O’Brien J., Calatayud N., Hunter D., Magrath M., Gilbert D., et al. (2021). “Integration of strategic biobanking into amphibian conservation translocation programs,” in ZAA Conference.
Hobbs R. J., Spindler R. E., O’Brien J. K. (2019). “Chapter 4: Strategic gene banking for conservation: The ins and outs of a living bank,” in Scientific foundations of zoos and aquariums: Their role in conservation and research. Eds. Kaufman A., Maple T., Bashaw M. (Cambridge:Cambridge University Press), Pp. 112–146. doi: 10.1017/9781108183147.005
Hoegh-Guldberg O., Pendleton L., Kaup A. (2019). People and the changing nature of coral reefs. Reg. Stud. Mar. Sci. 30, 100699. doi: 10.1016/j.rsma.2019.100699
Holt W. V., Comizzoli P. (2021). Genome resource banking for wildlife conservation: Promises and caveats. Cryoletters 42 (6), 309–320(12).
Howard J. G., Lynch C., Santymire R. M., Marinari P. E., Wildt D. E. (2016). Black-footed ferret gene restoration. Anim. Conserv. 19, 102–111. doi: 10.1111/acv.12229
Howell L. G., Frankham R., Rodger J. C., Rodger J. C., Witt R. R., Clulow S., et al. (2021). Integrating biobanking minimises inbreeding and produces significant cost benefits for a threatened frog captive breeding programme. Conserv. Lett. 14, e12776. doi: 10.1111/conl.12776
Howells E. J., Ketchum R. N., Bauman A. G., Mustafa Y., Watkins K. D., Burt. J. A. (2016). Species-specific trends in the reproductive output of corals across environmental gradients and bleaching histories. Mar. pollut. Bull. 105 (2), 532–539. doi: 10.1016/j.marpolbul.2015.11.034
Hughes T. P., Kerry J. T., Baird A. H., Connolly S. R., Chase T. J., Dietzel A., et al. (2019). Global warming impairs stock–recruitment dynamics of corals. Nature 568 (7752), 1–14. doi: 10.1038/s41586-019-1081-y
Hughes T. P., Kerry J. T., Simpson T. (2018). Large-Scale bleaching of corals on the great barrier reef. Ecology 99, 501–501. doi: 10.1002/ecy.2092
Kalmus P., Ekanayaka A., Kang E., Baird M., Gierach M. (2022). Past the precipice? projected coral habitability under global heating. Earth's Future 10, e2021EF002608. doi: 10.1029/2021EF002608
Kihika J. K., Wood S. A., Rhodes L., Smith K. F., Thompson L., Challenger S., et al. (2022). Cryoprotectant treatment tests on three morphologically diverse marine dinoflagellates and the cryopreservation of breviolum sp. (Symbiodiniaceae). Sci. Rep. 12, 646. doi: 10.1038/s41598-021-04227-2
Knowlton N., Grottoli A. G., Kleypas J., Obura D., Corcoran E., de Goeij J. M., et al. (2021). Rebuilding coral reefs: A decadal grand challenge. international coral reef. Soc. Future Earth Coasts, 56. doi: 10.53642/NRKY9386
Lin C., Thongpoo P., Juri C., Wang L.-H., Meng P.-J., Kuo F.-W., et al. (2019). Cryopreservation of a thermotolerant lineage of the coral reef dinoflagellate symbiodinium Biopreserv. Biobank 17 (6), 520–529. doi: 10.1089/bio.2019.0019
Lukoschek V., Riginos C., van Oppen M. J. (2016). Congruent patterns of connectivity can inform management for broadcast spawning corals on the great barrier reef. Mol. Ecol. 25 (13), 3065–3080. doi: 10.1111/mec.13649
Madin J. S., McWilliam M., Quigley K., Bay L. K., Bellwood D., Doropoulos C., et al. (2021). Selecting species for restoration in foundational assemblages. bioRxiv 2021. doi: 10.1101/2021.11.03.467181
Maire J., van Oppen M. J. H. (2022). A role for bacterial experimental evolution in coral bleaching mitigation? Trends. Microbiol. 30, 217–228. doi: 10.1016/j.tim.2021.07.006
National Academies of Sciences, Engineering, and Medicine (2019). A research review of interventions to increase the persistence and resilience of coral reefs (Washington, D.C: National Academies Press).
Negri A. P., Heyward A. J. (2000). Inhibition of fertilization and larval metamorphosis of the coral acropora millepora (Ehrenberg 1834) by petroleum products. Mar. pollut. Bull. 417–12, 420–427. doi: 10.1016/S0025-326X(00)00139-9
O'Brien J. K., Montano G. A., Steinman K. J., Robeck T. R. (2016). Zoological research for understanding penguin reproductive biology and maximizing population genetic diversity (Cape Town, South Africa: 9th International Penguin Congress).
Page C., Perry R., Lager C., Daly J., Bouwmeester J., Henley E. M., et al. (2022). Tank fouling community enhances coral microfragment growth. bioRxiv 2022. doi: 10.1101/2022.07.04.498770
Pollock F. J., Katz S. M., van de Water J. A. J. M., Davies S. W., Hein M., Torda G., et al. (2017). Coral larvae for restoration and research: A large-scale method for rearing Acropora millepora larvae, inducing settlement, and establishing symbiosis. PeerJ 5, e3732. doi: 10.7717/peerj.3732
Quigley K. M., Bay L. K., van Oppen M. (2019). The active spread of adaptive variation for reef resilience. Ecol. Evol. 9 (19), 11122–11135. doi: 10.1002/ece3.5616
Quigley K. M., Bay L. K., van Oppen M. J. H. (2020). Genome-wide SNP analysis reveals an increase in adaptive genetic variation through selective breeding of coral. Mol. Ecol. 29, 2176–2188. doi: 10.1111/mec.15482
Quigley K. M., Hein M., Suggett D. J. (2022). Translating the 10 golden rules of reforestation for coral reef restoration. Conserv. Biol., 36:e13890. doi: 10.1111/cobi.13890
Quigley K. M., Marzonie M., Ramsby B., Abrego D., Milton G., van Oppen M. J. H., et al. (2021). Variability in fitness trade-offs amongst coral juveniles with mixed genetic backgrounds held in the wild. Front. Mar. Sci. 8. doi: 10.3389/fmars.2021.636177
Quigley K. M., van Oppen M. J. H. (2022). Predictive models for the selection of thermally tolerant corals based on offspring survival. Nat. Commun. 13, 1543. doi: 10.1038/s41467-022-28956-8
Randall C. J., Giuliano C., Heyward A. J., Negri A. P. (2021). Enhancing coral survival on deployment devices with microrefugia. Front. Mar. Sci. 8. doi: 10.3389/fmars.2021.662263
Randall C. J., Negri A. P., Quigley K. M., Foster T., Ricardo G. F., Webster N. S., et al. (2020). Sexual production of corals for reef restoration in the anthropocene. Mar. Ecol. Prog. Ser. 635, 203–232. doi: 10.3354/meps13206
Santoro E. P., Borges R. M., Espinoza J. L., Freire M., Messias C. S. M. A., Villela H. D. M., et al. (2021). Coral microbiome manipulation elicits metabolic and genetic restructuring to mitigate heat stress and evade mortality. Sci. Adv. 7, eabg3088. doi: 10.1126/sciadv.abg3088
Shaver E. C., McLeod E., Hein M. Y., Palumbi S. R., Quigley K., Vardi T., et al. (2022). A roadmap to integrating resilience into the practice of coral reef restoration. Glob. Change Biol. 00, 1–14. doi: 10.1111/gcb.16212
Shearer T., Porto I., Zubillaga L. (2009). Restoration of coral populations in light of genetic diversity estimates. NIH 28 (3), 727–733. doi: 10.1007/s00338-009-0520-x
Sivapalan M., Bowen J. (2020). Decision frameworks for restoration & adaptation investment–applying lessons from asset-intensive industries to the great barrier reef. PloS One 15 (11), e0240460. doi: 10.1371/journal.pone.0240460
Suggett D. J., van Oppen M. J. H. (2022). Horizon scan of rapidly advancing coral restoration approaches for 21st century reef management. Emerg. Top. Life Sci. 146 (1), 125–136. doi: 10.1042/ETLS20210240
Swanson W. F., Stoops M. A., Magarey G. M., Herrick J. R. (2007). Sperm cryopreservation in endangered felids: developing linkage of in situ-ex situ populations. Soc Reprod. Fertil. Suppl. 65, 417–432.
Traylor-Holzer K., Leus K., Bauman K. (2019). Integrated collection assessment and planning (ICAP) workshop: helping zoos move toward the One Plan Approach. Zoo biol. 38(1). 95–105. doi: 10.1002/zoo.21478
van Oppen M. J. H., Gates R. D., Blackall L. L., Cantin N., Chakravarti L. J., Chan W. Y., et al. (2017). Shifting paradigms in restoration of the world's coral reefs. Glob. Change Biol. 23, 3437–3448. doi: 10.1111/gcb.13647
Vardi T., Hoot W. C., Levy J., Shaver E., Winters R. S., Banaszak A. T., et al. (2021). Six priorities to advance the science and practice of coral reef restoration worldwide. Restor. Ecol. 29, e13498. doi: 10.1111/rec.13498
Voolstra C. R., Quigley K. M., Davies S. W., Parkinson J. E., Peixoto R. S., Aranda M., et al. (2021). Consensus guidelines for advancing coral holobiont genome and specimen voucher deposition. Front. Mar. Sci. 8. doi: 10.3389/fmars.2021.701784
Watson P. F. (1978). A review of techniques of semen collection in mammals. Symposia of the Zoological Society of London, Suppl No. 43: 97–126
Worley Parsons Services and Australian Institute of Marine Science (2019). Concept design & capital estimate, reef restoration and adaption programme. report no. 301012-02608 (Sydney:Worley Parsons Services Pty Ltd). Available at: https://gbrrestoration.org/wp-content/uploads/2020/09/T11-Automated-Aquaculture-Production-and-Deployment_21-3-19.pdf.
Young C. N., Schopmeyer S. A., Lirman D. (2012). A review of reef restoration and coral propagation using the threatened genus acropora in the Caribbean and Western Atlantic. Bull. Mar. Sci. 88 (4), 1075–1098. doi: 10.5343/bms.2011.1143
Zhan L., Li M.-G., Hays T., Bischof J. (2021). Cryopreservation method for drosophila melanogaster embryos. Nat. Commun. 12, 2412. doi: 10.1038/s41467-021-22694-z
Zhan L., Sushil Rao J., Sethia N., Slama M. Q., Han Z., Tobolt D., et al. (2022). Pancreatic islet cryopreservation by vitrification achieves high viability, function, recovery and clinical scalability for transplantation. Nat. Med. 28, 798–808. doi: 10.1038/s41591-022-01718-1
Keywords: reef restoration, cryopreservation, biobanking, coral spawning, coral
Citation: Hobbs RJ, O'Brien JK, Bay LK, Severati A, Spindler R, Henley EM, Quigley KM, Randall CJ, van Oppen MJH, Carter V, Zuchowicz N, Hagedorn M and Daly J (2022) A decade of coral biobanking science in Australia - transitioning into applied reef restoration. Front. Mar. Sci. 9:960470. doi: 10.3389/fmars.2022.960470
Received: 03 June 2022; Accepted: 26 July 2022;
Published: 16 September 2022.
Edited by:
Steven T. Kessel, Shedd Aquarium, United StatesReviewed by:
Erinn M. Muller, Mote Marine Laboratory and Aquarium, United StatesOlivia Williamson, University of Miami, United States
Copyright © 2022 Hobbs, O'Brien, Bay, Severati, Spindler, Henley, Quigley, Randall, van Oppen, Carter, Zuchowicz, Hagedorn and Daly. This is an open-access article distributed under the terms of the Creative Commons Attribution License (CC BY). The use, distribution or reproduction in other forums is permitted, provided the original author(s) and the copyright owner(s) are credited and that the original publication in this journal is cited, in accordance with accepted academic practice. No use, distribution or reproduction is permitted which does not comply with these terms.
*Correspondence: Rebecca J. Hobbs, Rhobbs@zoo.nsw.gov.au