- College of Chemistry and Environmental Science, Guangdong Ocean University, Zhanjiang, China
Nitrogen (N) plays an important role in marine ecosystems as a biogenic element for phytoplankton. The tidal cycle had major influence on various biogeochemical parameters of the bay and changed nutrients input with the ebb and flow of the tide. In this study, we collected samples from Shuidong Bay (SDB) in China, in August 2021, to explore the periodic variations in coastal waters during spring and neap tides. The effects of spring and neap tides on different speciation of nitrogen in the SDB and the exchange fluxes between the SDB and the South China Sea (SCS) were investigated. The results indicated that the concentrations of particulate nitrogen (PN) and N-NO2- were significantly different between the spring and neap tides (P < 0.05). The total nitrogen (TN) concentrations in SDB during the spring and neap tides were 258.12 ± 89.49 μmol/L and 231.77 ± 56.86 μmol/L. During the spring and neap tides, total dissolved nitrogen (TDN) accounted for 54.1% and 52.2% of TN, respectively. In addition, dissolved organic nitrogen (DON) accounted for 81.4% and 69.9% of the TDN during the spring and neap tides, respectively. Furthermore, the net exchange fluxes of different speciation of nitrogen showed that the net exchange fluxes of TN were transported from SDB to SCS during the spring and neap tide, with the net exchange fluxes of 37.7 t and 8.8 t, respectively. The net exchange flux of TN during spring tide was 4.3 times higher than that of neap tide. In addition, a significant negative correlation was observed between dissolved inorganic nitrogen (DIN) and salinity in SDB during the spring and neap tides (P < 0.001), indicated that DIN was mainly influenced by terrestrial sources inputs. A significant positive correlation (P < 0.01) was observed between PN and Chlorophyll-a in SDB during the spring and neap tides, implied that the assimilation of a large number of planktonic organisms promoted PN formation. The present results revealed that tidal variation played an important role in regulating N speciation and exchange flux in coastal waters, which had great implications for N biogeochemistry and water quality improvement in SDB.
1 Introduction
Nitrogen is an essential nutrient for the growth and reproduction of marine organisms (Tian, 1990; Dittmar and Lara, 2001; Xia et al., 2007; Fang et al., 2008; Gao et al., 2009; Cheng et al., 2020). Nitrogen is the basis of marine primary productivity and food chain and play an important role in marine biogeochemistry, which affects the biomass of marine ecosystems and community structure, causing the eutrophication of water bodies (Loh, 2000; Berman et al., 2003; Han et al., 2003; Zhou et al., 2003; Xin et al., 2010; Lin et al., 2013; Sui et al., 2016). The nitrogen cycle is a crucial part of biogeochemical processes that control the availability of nitrogenous nutrients and productivity levels of organisms in marine ecosystems (Galloway, 2005; Zhong and Li, 2014; Huang, et al., 2021; Lin and Lin, 2022). The major sources of nitrogen in the ocean are terrestrial runoff, surface water and groundwater input, sewage discharge, atmospheric deposition, and nitrogen fixation (Watson et al., 1993; Howarth, 2008; White et al., 2013; Guo et al., 2019; Du et al., 2020). Extremely high nutrient content caused the eutrophication of water bodies and even disasters, such as red tides (Sun et al., 2005; Zheng, 2010; Carey et al., 2011; Liu et at., 2011; Fatemi et al., 2012; Guo et al., 2012; Zhang et al., 2020c). In contrast, extremely low nutrient content may cause dysfunctional nutrient structures, preventing the normal growth of phytoplankton, and affecting the stability of marine ecosystems (Bricker et al., 2003; Geeraert et al., 2021; Niu et al., 2016; Xu et al., 2020; Zhou, 2021). In the water column, nitrogen is characterized by variable speciation. DIN is involved in the speciation of N-NO3-, N-NO2-, and N-NH4+. The changes in DIN speciation affecting the primary production, changing competitiveness among species, and altering biodiversity in water column (Collos, 1998; Feng et al., 2012; Tang et al., 2013 Kang et al., 2020; Li et al., 2020). Phytoplankton constitutes an indispensable link in the aquatic food chain, and they grow by absorbing nutrients from the water column in a certain proportion (Eppley and Peterson, 1979; Falkowski, 1997; Joseph et al., 2008; Li et al., 2008; Liu et al., 2011; Li et al., 2017; Xiu et al., 2019). DIN is taken up by phytoplankton and then released in the DON forms, which primarily contains humic substances, urea, free amino acids, amides, and vitamins. Particulate organic nitrogen included organic nitrogen debris, bacteria, and phytoplankton components ( Yang et al., 1990; Moneta et al., 2014; Kanuri et al., 2018; Xiu et al., 2019). PN is an important speciation of nitrogen in the ocean and plays an important role in the transport of various elements of the ocean and in the supply of nutrients and biogeochemical processes. Changes in the ratio and concentration of PN plays an important role in the maintenance of primary productivity in the offshore (Fernandes, 2011; Yu et al., 2012; Zhou et al., 2019). The multiple species of nitrogen make its circulation in the marine environment relatively complex.
The tidal cycle has major influence on various physicochemical parameters of the bay and changes nutrients and organic matter input with the ebb and flow of the tide. The coastal zone is a key area for marine biogeochemical reactions, and the physical processes has a significant impact on biogeochemical reactions (Yin and Harrison, 2000; Qu et al., 2007; Fang et al., 2012; Li, 2021). Tidal forces are important factors controlling water–sediment dynamics in estuarine and coastal systems, including regulated water stratification and influenced the transport of freshwater and sediment from rivers into estuaries, further affecting the dynamics of organic and inorganic components (Tian, 1990; Dittmar and Lara, 2001; Xia et al., 2007; Fang et al., 2008; Gao et al., 2009; Cheng et al., 2020). The high intensity human activities had caused the ecological degradation of the bay, which were unique nearshore weak exchange hydrodynamic environments, were among most eutrophic water environments in the ocean under the influence of high-intensity human activities (Bricker et al., 2003; Daniel et al., 2009; Liu et al., 2011; Wang, 2019; Cui et al., 2020; Li et al., 2020). In recent years, nutrients in bay water have been influenced primarily by anthropogenic activities, resulting in the increase of terrestrial nutrients into coastal water (Zhang 2007b; Santos et al., 2008; Li et al., 2013; Amato et al., 2020). The distribution of nitrogen in coastal waters was influenced by tides, winds, convective diffusion, biological activities, and water-sediment interface exchange (Li, 2021; Liu et al., 2017; Zhang et al., 2016; Zhang et al., 2022). Therefore, nutrients had different biogeochemical processes and showed different spatial patterns under the influence of tidal variations, which further affect primary production and thus the nitrogen cycle in coastal waters (Zhou et al., 2003; Sun, 2008; Guo et al., 2014; Yuan et al., 2016).
Shuidong Bay (SDB) is a semi-enclosed bay, formed by the recent slight rise in the Earth’s crust (Li et al., 2015). The bay is slightly curved, with its wide mouth facing south, and surrounded by a large sand dam. The tides in SDB were characterized by semi-diurnal tidal variations (Qin et al., 2014; Li et al., 2015; Feng et al., 2017). Semi-diurnal tidal variations may have a considerable influence on the dynamics of nutrients in SDB (Peng, 1987; Yang et al., 2011; Qin et al., 2014; Li et al., 2015; Feng et al., 2017). In addition, the input and output of nutrients and hydrological conditions of coastal bays determined the residence time of nutrients, and the combined influence of adjacent waters further complicates the biogeochemical processes of the nutrients involved (Hopkins et al., 1993; Li, 2021). As different speciation of nitrogen flow between SDB and SCS with seawater, some nutrients may be consumed or produced during transport. That would result in high or low nutrient concentrations during tidal changes (Li, 2021). However, with the rapid development of mariculture in recent years, it had caused a decrease in the exchange capacity of SDB waters and an increase in water eutrophication (Qin et al., 2014; Li et al., 2015; Li et al., 2016; Feng et al., 2017). At present, studies had focused on the mechanisms of tides and their effects on hydrodynamics (Song et al., 2011; Wu et al., 2011; Cheng et al., 2020) and distribution patterns of nutrients in estuarine bays (Guo, 2020; Lu et al., 2020; Liu et al., 2021; Zhang et al., 2021; Ke et al., 2022). However, previous studies on SDB had primarily focused on the natural environment and dynamic conditions; previous studies lack real-time observations, particularly the simultaneous changes in nutrients during the spring and neap tidal cycles. In addition, most previous studies had focused on DIN for nitrogen speciation (Wang et al., 2016; Jiang et al., 2019; Yang et al., 2020; Zhang et al., 2020; Zhang et al., 2020b). However, the effects of tidal cycling on nitrogen species variations, particularly on PN and DON, were still scarce.
Therefore, to better understand the tidal effects on nitrogen speciation, we analyzed continuous observation data from four stations (S1, S2, S3, S4) in SDB to reveal the effects of tidal cycles on the concentrations of different nitrogen species and to analyze the causes of tidal variations affecting them. Thus, the main research objectives of this study were to explore (1) the effects of spring and neap tides on different nitrogen species, (2) exchange fluxes between SDB and SCS, and (3) caused of tidal variations in different nitrogen species. In this study, the effects of spring and neap tides on nitrogen speciation in SDB were assessed for the first time. Our results provided new insights into the analysis of the distribution and transport of different nitrogen species in coastal waters, which had great implications for nitrogen biogeochemical and water quality improvement in eutrophic SDB.
2 Materials and methods
2.1 Study areas
SDB is a semi-enclosed bay (Figure 1). It is connected to the SCS through a tidal channel that is 12.7 km long, 500–800 m wide, and 5–15 m deep (Li et al., 2015). The surface area of SDB is approximately 32 km2 narrow at the mouth. SDB had a wide range of water, topography of the seafloor in SDB was complex, distribution of deep troughs was remarkable, and mudflat area was large (Feng et al., 2017). The rivers flowing into SDB include the Xijian, Danchang, Zaitou, and Nanhai rivers (Li et al., 2015). The marine dynamic environment of SDB was dominated by tidal action, and the tidal type was irregular semi-diurnal tide, with a maximum average flow velocity of 0.3–0.5 m/s and maximum flow velocity of 1.0 m/s in the tidal channel (Qin et al., 2014). The development of coastal aquaculture and increasing terrestrial pollutants in SDB had recently caused the gradual shrinking of the water area in SDB and deterioration of water quality conditions, even directly changing the tidal pattern in SDB (Qin et al., 2014; Li et al., 2015; Feng et al., 2017). Therefore, in this study, we collected data on spring and neap tides from four stations in SDB, and water sampling was conducted according to the tidal characteristics and hydrology of SDB. The coastal water monitoring stations in SDB were selected according to the method specified in the Specification of Oceanographic Survey (General Administration of Quality Supervision, Inspection, and Quarantine of the People’s Republic of China).
2.2 Field sampling and pre-treatment
The current state of SDB environmental quality were surveyed and monitored under the hydrodynamic conditions of spring tide (August 22-23, 2021) and neap tide (August 29-30, 2021) in the summer. Four hydrodynamic monitoring stations and water quality monitoring stations (S1, S2, S3, S4) were deployed at sea on this simultaneous survey cruise for 24-h continuous monitoring, with hydrodynamic conditions monitored every 1 h and surface seawater sampling performed every 3 h. T1 was a tide gauge station for monitoring the tidal height at different times. Considering the shallow the coastal water in the SDB, samples were collected from the depth of 0.5m in the surface layer. All samples were collected, processed, and preserved until analysis, according to the methods specified in the Marine Monitoring Code (GB17378-2007). Seawater samples were collected through a CTD-Rosette system using Teflon-coated bottles (10 L, Sea Bird Inc., USA) equipped with temperature, salinity, and fluorescence sensors. The collected water samples were packed in 100 ml acid-washed HDPE bottles and stored at -20°C. Approximately 1 L of seawater was filtered through pre-acid-washed and pre-assembled (held at 450°C for 4 h) 45 mm diameter Whatman GF/F glass fibre filters. Filtrate samples for TDN, N-NH4+, N-NO3-, and N-NO2- determination were collected in acid-washed HDPE bottles and stored at -20°C until laboratory analysis. Water environmental factors (temperature, salinity, and pH) were determined in situ using a multiparameter water quality tester (Aquaprobe AP-7000).
2.3 Chemical analysis in the laboratory
Owing to the complexity of DON and PN, it was difficult to perform single component determination. Therefore, in this experiment, we used the potassium persulfate oxidation method to determine the concentration of TN and TDN in water bodies; the detection limit of this method was 2.64 μmol/L. The DON and PN concentrations were then calculated. The DON concentration was determined as the difference between TDN and DIN, and PN concentration was determined as the difference between TN and TDN. DIN was the sum of the N-NO3-, N-NH4+, and N-NO2- concentrations. The N-NH4+, N-NO3-, and N-NO2- concentrations in the water samples were determined via the hypobromite oxidation, zinc–cadmium reduction, and diazo–azo methods, respectively, and the detection limits were 0.03 μmol/L, 0.05 μmol/L, and 0.02 μmol/L, respectively. All of the above methods were measured at 543 nm using a UV-visible spectrophotometer (Shimadzu UV2600i). Suspended particulate matter (SPM) was measured by weight method. Chlorophyll-a was determined by spectrophotometric method. The relative standard deviation of the above methods was <5% for repeatability, reproducibility and precision. All samples were collected, pretreated, and analyzed in strict accordance with the Marine Monitoring Code (GB17378-2007), and full monitoring quality control was implemented (AQSIQ, 2007a).
2.4 Net exchange flux of nitrogen calculation during tidal cycle
The fluxes of different nitrogen species exchanged between the SCS and SDB in the positive direction of the designated flow to SDB were estimated using the method described next.
SDB was connected to the SCS by only a narrow bay channel, and sites S1, S2, and S3 were away from the bay mouth. Therefore, the fluxes of different nitrogen species from the bay mouth of SDB to the SCS were estimated from the S4 site of SDB, which represented the fluxes of different nitrogen species from the bay mouth of SDB. The flow velocity and direction of seawater in the surface layer of S4 were measured continuously with an electromagnetic current meter (AME-USB, JFE, Japan). When the flow direction was 45°-225° it showed the flow from SDB to SCS. When the flow direction was 225°-360° and 0°-45° it showed the flow from SCS to SDB. Calculated the flux of water through S4 at different times by equation (1). Then, the net exchange flux of different nitrogen species between SDB and SCS was estimated by multiplying the water flux by the concentrations of different nitrogen species in the net coastal inflow water column by equation (2). The net exchange fluxes of different speciation of nitrogen between SDB and SCS at other moments were estimated on the transects of both sides of the bay according to the temporal variation of the flow velocity, and then the net exchange fluxes of different speciation of nitrogen between SDB and SCS in one day were calculated.
Q = W × D × V (1)
Where Q was the net water flux at the mouth of SDB per unit time (L/h) in the vector, W was the width of S4 (m), D was the depth of S4 (m), V was the flow velocity at different times of S4 (cm/s).
FN = CN × Q × 10-6 × MN (2)
Where FN was the net exchange of different nitrogen species at the mouth of SDB (t/h), CN was the concentration of different nitrogen species flowing through the mouth of SDB per second (μmol/L), and MN was the relative atomic mass of nitrogen.
2.5 Statistical analysis
Student’s t test was used to test the normality of the concentrations of different speciation of nitrogen during spring and neap tides, but the data did not show a normal distribution. Then a non-parametric Mann-Whitney U test was used for analysis to find significant differences between different speciation of nitrogen during spring and neap tides (Sánchez-Carrillo et al., 2009). Spearman correlation analysis was used to determine the correlation between the variables by environmental factors and the concentration of different speciation of nitrogen (Zhang et al., 2020). In addition, a linear regression analysis was performed to analyze the relationship between fluxes and flow velocity of different speciaton of nitrogen in spring and neap tides. If P < 0.05, it indicates a significant difference between the variables.
3 Results
3.1 Hydrographic conditions in SDB
The environmental factors for the different stations of the SDB were presented in Table 1. SDB was an irregular semi-diurnal tide, with two high tides and two low tides in one day. During the SDB spring tide, two high tides occurred at 10:00 and 23:00, with tide heights of 382 cm and 254 cm respectively and two low tides occurred at 17:00 and 4:00, with tide heights of 47 cm and 130 cm respectively, and the tidal difference during the spring tide was 335 cm. During the neap tide in SDB, two high tides occurred at 14:00 and 3:00, with tide heights of 230 cm and 280 cm, and two low tides occurred at 20:00 and 9:00, with tide heights of 140 cm and 153 cm, respectively, and the tidal difference during the neap tide was 140 cm. During the spring and neap tides, the average flow velocity of all four stations in SDB were S3 > S4 > S2 > S1. During the spring and neap tides, the maximum flow velocity of S3 was 42.22 ± 24.64 cm/s and 25.38 ± 12.07 cm/s, respectively. Furthermore, the average salinity of all four stations in SDB were S4 > S3 > S2 > S1 during the spring and neap tides. The salinity of S4 was maximum during spring and neap tides with 27.91 ± 0.49‰ and 30.51 ± 0.80‰, respectively. During the SDB spring tide, the maximum tidal exchange from the SDB to the SCS occurred at 15:00 with a tidal exchange of 1.0×1010 t/h. The maximum tidal exchange from the SCS to the SDB occurred at 22:00 with a tidal exchange of 8.2×109 t/h. During the SDB neap tide, the maximum tidal exchange from the SDB to the SCS occurred at 16:00 with a tidal exchange of 6.1×109 t/h. The maximum tidal exchange from SCS to SDB occurred at 22:00, with a tidal exchange of 5.9×109 t/h. During the SDB spring and neap tides, the net tidal exchange was from SCS to SDB, the net tidal exchange is 4.4×109 t and 2.3×109 t respectively.
3.2 Dynamics of TN in SDB during the spring and neap tides
3.2.1 Dynamics of TN in SDB during the spring and neap tides
The TN concentrations did not differ between the spring and neap tides (P > 0.05), but the PN concentrations differed between the spring and neap tides (P > 0.05). The concentrations of different nitrogen species were presented in Table 2. The range of the TN concentration during the spring (Figure 2A) and neap tides (Figure 2B) was 77.79–421.79 μmol/L and 113.62–350.55 μmol/L, respectively, with averages of 258.12 ± 89.49 μmol/L and 231.77 ± 56.86 μmol/L, respectively; thus, the range of the average concentration during the spring and neap tides was small. The maximum value of the TN concentration occurred at 23:00 in S1, and the minimum value occurred at 23:00 in S4 during the spring tide. The averages of TN during the spring and neap tides in SDB indicated that S1 >S2 >S3 >S4 and S4 >S3 >S2 >S1, respectively; these results indicated that the TN concentration in SDB was influenced by the tide. The TN concentration in SDB during the spring tide was higher than that in the bay mouth, whereas the opposite was true during the neap tide. The TN concentrations of S1, S2, and S3 during the spring tide were higher than those during the neap tide; however, the TN concentration of S4 during the spring tide was lower than that during the neap tide. TDN accounted for 54.1% and 52.2% of TN during the spring and neap tides, respectively. This indicated that TDN was the dominant species of TN. The range of the PN concentration during the spring and neap tides was 8.51–304.66 μmol/L and 12.76–246.71 μmol/L, respectively, and the average concentrations were 118.51 ± 84.57 μmol/L and 110.74 ± 63.18 μmol/L, respectively; the average PN concentration of S1 was higher during the spring tide than during the neap tide, and the average PN concentration of S4 was lower during the spring tide than during the neap tide.
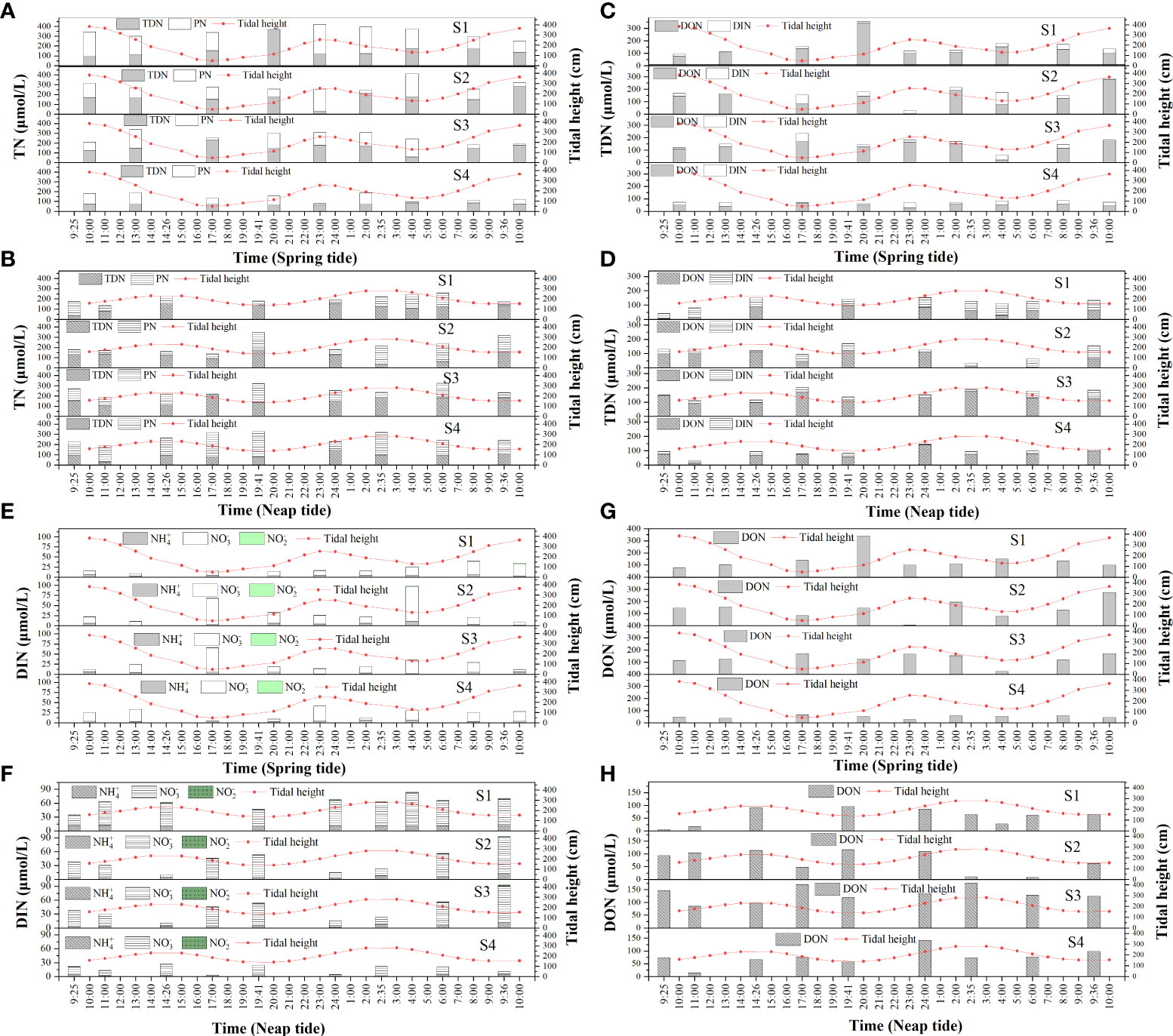
Figure 2 Dynamics of TN (A), TDN (C), DIN (E) and DON (G) during the spring tide in SDB and dynamics of TN (B), TDN (D), DIN (F) and DON (H) during the neap tide in SDB.
3.2.2 Dynamics of TDN in SDB during the spring and neap tides
The TDN concentrations did not differ between the spring and neap tides (P > 0.05). The range of the TDN concentration during the spring (Figure 2C) and neap tides (Figure 2D) was 31.53–356.93 μmol/L and 27.81–204.86 μmol/L, respectively, and the average concentrations were 139.61 ± 66.09 μmol/L and 121.03 ± 41.77 μmol/L, respectively. The average TDN concentration during the spring and neap tides changed little; however, the variations in the TDN concentration during the spring tide was more evident, and the maximum TDN concentration occurred at 20:00 in S1. In addition, DON accounted for 81.4% and 69.9% of the TDN during the spring and neap tides, respectively. This indicates that DON was the dominant species of TDN. The average concentrations of TDN in SDB during the spring and neap tides were S2>S1>S3>S4 and S3>S1>S2>S4, respectively. S4 was less affected by tide, and the TDN concentration in SDB was higher than that at the mouth of the bay.
3.3 Dynamics of DIN in SDB during the spring and neap tides
There were no significant differences in the concentrations of DIN, N-NO3- and N-NH4+ during the spring and neap tides (P > 0.05), but the N-NO2- concentrations were significantly different during the spring and neap tides (P < 0.05). The ranges of DIN during the spring (Figure 2E) and neap tides (Figure 2F) were 4.67–97.78 μmol/L and 3.68–92.70 μmol/L, respectively, with averages of 26.03 ± 18.49 μmol/L and 36.49 ± 23.81 μmol/L. The DIN concentration during the spring tide exceeded the seawater quality index category II standard, whereas the DIN concentration during the neap tide exceeded the seawater quality index category IV standard. Only S2 exceeded the second category of seawater quality index during the spring tide, while S1 and S2 exceeded the fifth category of seawater quality index during the neap tide, and the DIN concentration of S1 was considerably higher than that of S2. During spring tide, N-NH4+, N-NO3-, and N-NO2- accounted for 17.6%, 80.9%, and 1.5% of DIN, respectively. During the neap tide, N-NH4+, N-NO3-, and N-NO2- accounted for 16.5%, 81.1%, and 2.4% of DIN, respectively. DIN was primarily dominated by N-NO3-, followed by N-NH4+, and N-NO2-. The N-NO3- concentration ranged from 2.38 to 84.93 μmol/L and from 1.00 to 78.95 μmol/L during the spring and neap tides, respectively, with averages of 21.06 ± 17.37 μmol/L and 29.60 ± 20.37 μmol/L, respectively, and the N-NO3- concentrations were higher in the neap tide than in the spring tide. The N-NO3- concentrations were lower during the S1 spring tide than during the neap tide, whereas the N-NO3- concentrations were higher during the S4 spring tide than during the neap tide. The ranges of N-NH4+ concentrations during the spring and neap tides were 1.17–10.94 μmol/L and 1.95–11.65 μmol/L, respectively, with averages of 4.58 ± 1.86 μmol/L and 6.03 ± 3.48 μmol/L, respectively. The ranges of N-NO2- concentrations during the spring and neap tides were 0.02–1.91 μmol/L and 0.02–2.25 μmol/L, respectively, with averages of 0.40 ± 0.38 μmol/L and 0.86 ± 0.71 μmol/L, respectively. Although N-NH4+ and N-NO2- only accounted for a small part of DIN, the average concentration of N-NH4+ was still considerably larger than that of N-NO2-, and both N-NH4+ and N-NO2- had higher concentrations in the neap tide than in the spring tide. The averages of DIN, N-NO3-, and N-NH4+ during the spring and neap tides in SDB were S2 >S1 >S3 >S4 and S1> S2> S3> S4, respectively. The average concentrations of N-NO2- during the spring and neap tides in SDB were S2 >S3 >S1 >S4 and S1 >S2 >S3 >S4, respectively. The DIN concentrations (including N-NO3-, N-NO2-, and N-NH4+) all exhibited higher concentrations in the bay than at the mouth of the bay.
3.4 Dynamics of DON in SDB during the spring and neap tides
There were no significant differences in the concentrations of DON during the spring and neap tides (P > 0.05). The ranges of DON concentrations during the spring (Figure 2G) and neap tides (Figure 2H) were 5.08–342.37 μmol/L and 5.87–175.54 μmol/L, respectively, with averages of 113.58 ± 67.38 μmol/L and 84.54 ± 43.51 μmol/L, respectively. The concentration and range of DON at spring tide were higher than neap tide. The average DON concentrations in SDB during the spring and neap tides were S1 >S2 >S3 >S4 and S3 >S4 >S2 >S1, respectively, which indicated that the tide had a great influence on DON concentration. The DON concentration at stations S1 and S2 was higher during the spring tide than during the neap tide, and DON concentration at S3 and S4 was slightly lower during the spring tide than during the neap tide.
3.5 The net exchange TN flux with SCS during the spring and neap tides in SDB
During spring and neap tides, the net exchange fluxes between SDB and SCS were different. During the SDB spring tide (Figure 3A) and neap tide (Figure 3B), the daily net exchange fluxes of TN and PN flowed from SDB to SCS. However, the daily net exchange fluxes of TDN (including DIN and DON) flowed from SCS to SDB during the SDB spring tide (Figure 3C) and neap tide (Figure 3D). In addition, the daily net exchange fluxes of TN and PN during spring tide were 37.7 t and 44.3 t, respectively, and the daily net exchange fluxes of DIN and DON were 1.9 t and 4.7 t, respectively. Furthermore, the daily net exchange fluxes of TN and PN during neap tide in SDB were 8.8 t and 15.0 t, respectively, and the daily net exchange fluxes of DIN and DON were 0.8 t and 5.4 t, respectively. The net exchange flux of TN in spring tide was 4.3 times higher than that of neap tide. Therefore, SDB was a source of TN for SCS.
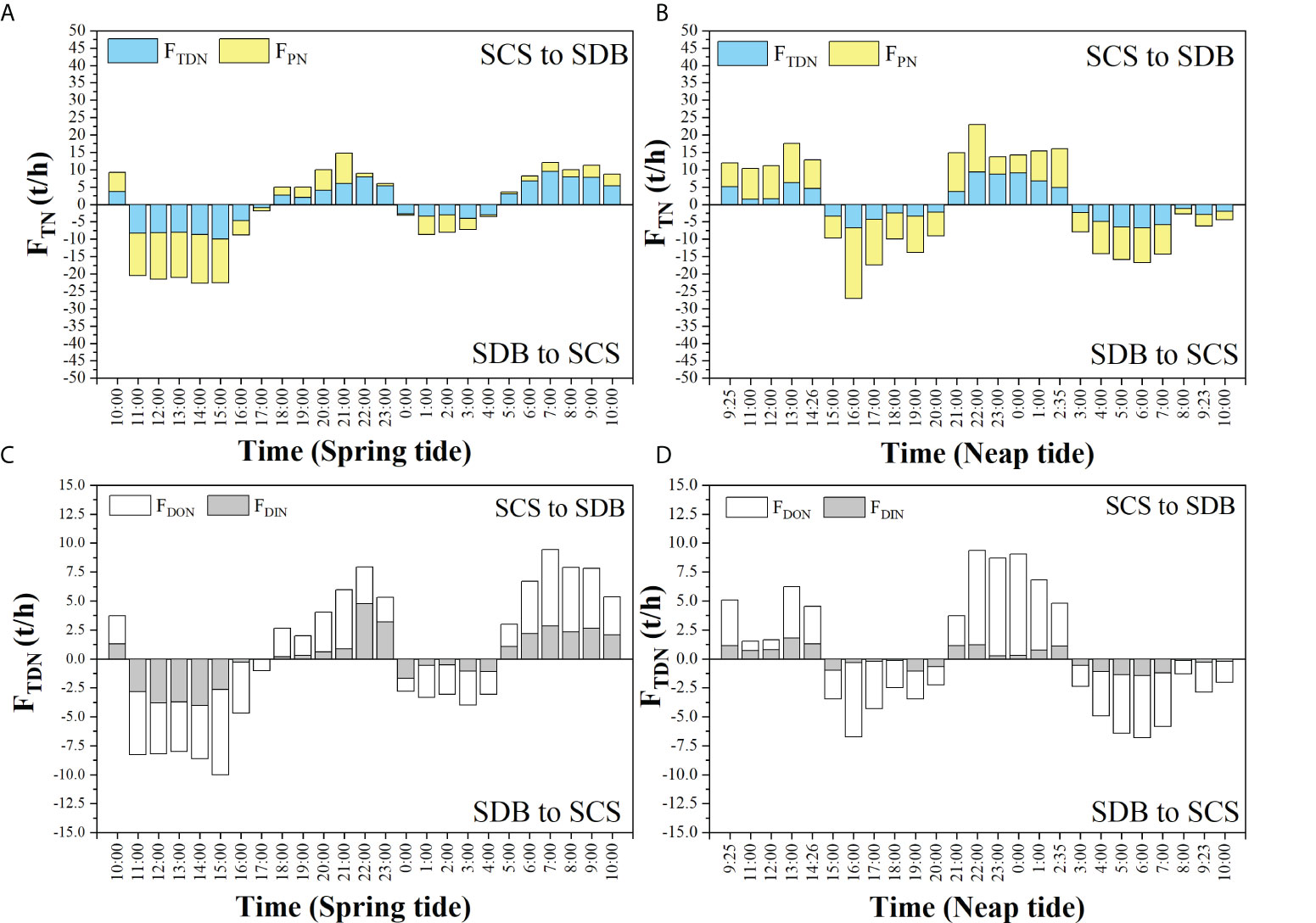
Figure 3 During the spring tide, TN (A) and TDN (C) in the exchange flux between SDB and SCS. During the neap tide, TN (B) and TDN (D) in the exchange flux between SDB and SCS.
4 Discussion
4.1 Comparison of the TN levels in estuaries and coastal waters worldwide
A comparison of the nitrogen concentrations in SDB with other semi-enclosed bays in China and the world was presented in Table 3. SDB had the highest TN concentration, which was higher than estuaries and bays with human influence such as Liusha Bay, Chesapeake Bay, Yangtze Estuary and Mississippi Estuary (Turner et al., 2007; Cao et al., 2011; Harding et al., 2016; Ge et al., 2021). In addition, TDN/TN in SDB was only 53.2%, which was considerably lower than the corresponding values in Daya bay and Yangtze Estuary (Zhou et al., 2019; Ge et al., 2021). The highest PN concentration was found in SDB, which was much higher than that in Daya Bay and Yangtze River Estuary (Zhou et al., 2019; Ge et al., 2021). By comparing the Chlorophyll-a of SDB with that of Jiaozhou Bay and Zhanjiang Bay (Li et al., 2005; Zhou et al., 2021), the highest Chlorophyll-a was found in SDB, indicated the high biomass of SDB. The high concentration of PN in SDB may be due to assimilation of DIN during the photosynthesis by phytoplankton. Furthermore, the DIN concentration in SDB was lower than that in Mississippi Estuary and Pearl River Estuary (Turner et al., 2007; Ke et al., 2022), but higher than that in Jiaozhou Bay, Persian Gulf and Daya Bay (Lu et al., 2016; Zhou et al., 2019; Maryam et al., 2021). The N-NO3- content in SDB was second only to that in the Pearl River Estuary (Zhao et al., 2000; Wu, 2014; Zhou et al., 2019; Ge et al., 2021; Maryam et al., 2021; Ke et al., 2022), and N-NO3- was the dominant component of DIN in SDB. With the increase of population and development of mariculture in SDB (Qin et al., 2014; Li et al., 2015; Li et al., 2016; Feng et al., 2017), containing higher concentrations of N-NH4+ was discharged into the SDB with the river and will be oxidized with the shoreline to N-NO3- (Archana et al., 2018). The high nitrogen load in SDB may be caused by the untreated urban polluted wastewater and large amount of farming wastewater discharged into SDB. Furthermore, the TDN/TN ratios were 53.3%, 55.8%, 61.3% and 44.15% for S1, S2, S3 and S4 during the spring and neap tides in SDB, respectively. This indicated that TDN was the dominant component in S1, S2 and S3, but PN was the dominant component in S4. During spring and neap tides, the net exchange fluxes of different speciation of nitrogen in SDB showed that TN and PN flowed from SDB to SCS, while TDN (including DIN and DON) flowed from SCS to SDB, which resulted in larger TDN/TN ratios in S1, S2 and S3 than in S4.
4.2 Hydrodynamics induced by tidal variations on nitrogen species dynamics
The correlation between different nitrogen species and environmental factors during the spring and neap tides were shown in Figure 4A and Figure 4B, respectively. A significant positive correlation existed between PN and Chlorophyll-a during the spring and neap tides (P < 0.01), which was related to the strong biological action of particulate matter (Lin et al., 2013). The assimilation of a large number of planktonic organisms promoted PN formation. Moreover, the living fraction of PN influenced DIN by producing secretions (Chowdhury et al., 2016), detritus, and excretions (Belcher et al., 2016), whereas the non-living fractions buffer DIN via processes such as adsorption–desorption (Lebo et al., 1991). The quality and source of PN determines its impact on coastal water quality. DON can be microbially converted to DIN, which can adversely affect the water quality of the bay (Stedmon et al., 2007; Mesfioui et al., 2012; Seitzinger and Sanders, 1997). Coastal waters were mainly influenced by ocean currents, as SDB and SCS exchange tidal currents through the mouth of the bay, which facilitates the dilute mixing of SDB seawater (Bejaoui et al., 2016; Bancon-Montigny et al., 2019). When the flow velocity slowed down, the particulate organic matter being settled down will be decomposed by bacteria (Yin et al., 2000). Simultaneously, sediment resuspension caused strong changes in the chemical composition of the overlying water column, released nutrients that may be available to pelagic species (Bancon-Montigny et al., 2019). With the rapid development of mariculture in the SDB, had caused a large amount of pollutants containing DON to enter the SDB (Qin et al., 2014; Li et al., 2015; Feng et al., 2017). Since DON and PN were likely to be absorbed by microorganisms and converted into DIN, coupled with the rich microbial population in the bay, the impact of DON and PN on the water quality of the bay should be taken seriously (Lu et al., 2020).

Figure 4 Correlation analysis of nitrogen species with environmental factors during spring tide (A) and neap tide (B) in SDB.
During spring and neap tides, TDN and DON showed a significant positive correlation (P < 0.001), and DON, as the main substance of TDN, influenced the concentration of TDN through the migration transformation of DON. During SDB spring tide, DIN showed a significant negative correlation with tidal height (P < 0.05), indicating that DIN was tidally influenced during spring tide, with DIN concentration decreasing with high tide and increasing with low tide. However, there was a significant negative correlation between DIN and salinity during spring and neap tides (P < 0.0001). Nutrient was higher in waters with low salinity, which was consistent with the findings in Chongming Dongtan and Hongwan (Wang, 1999; Qu et al., 2007; Li et al., 2012; Fu et al., 2020; Zhou et al., 2022). This suggested that terrestrial input may be responsible for influencing the variation of DIN. In addition, a significant positive correlation (P < 0.001) existed between N-NO3-, the dominant species of DIN, and DIN during the spring and neap tides, indicating a similarity in the transformation and transport between N-NO3- and DIN. A significant negative correlation (P < 0.01) was found between N-NH4+ and salinity during neap tide, indicating that N-NH4+ was mainly influenced by terrestrial inputs, and with the development of terrestrial aquaculture in the SDB, effluents containing high concentrations of N-NH4+ was discharged into the SDB (Chen et al., 2011; Liu et al., 2011). Furthermore, there was a negative correlation between N-NH4+ and DON during neap tides (P < 0.05). This demonstrated that N-NH4+ and DON transformed their concentrations through mutual migration. In addition, DON can be converted to N-NH4+ via microbial activity (Lu et al., 2016; Pisam, et al., 2017). Additionally, the respiratory activity of marine organisms was a key process in nutrient regeneration.
4.3 Impacts of the exchange of TN speciation flux between SDB and SCS
Figure 5A and Figure 5B showed the TN and TDN flux as a function of the flow velocity during the spring and neap tides, respectively. When the flow velocity was greater than 0, it indicated that the exchange flux flowed from SCS to SDB, and the flow velocity was less than 0 indicated that the exchange flux flowed from SDB to SCS. The results indicated that there were significant relationships between the flow velocity and nitrogen flux. During the spring tide and neap tide in SDB, FTN was significantly negatively correlated (P < 0.01) with flow velocity when the net exchange flux of TN flowed from SDB to SCS. Furthermore, FTN was significantly positively correlated with flow velocity, when the net exchange flux of TN flowed from SCS to SDB during the spring tide and neap tide in SDB (P < 0.05). The high TN in the SDB was discharged from the terrestrial pollutants and flowed from the SDB to the SCS. The high flow velocity tended to greatly increase the exchange flux of different nitrogen species between SDB and SCS. Therefore, the hydrological conditions of the sea area predominated the transport of different species of nitrogen in coastal waters (Zhao et al., 1998; Passeri et al., 2015; Chen et al., 2019). Thus, the flow velocity of coastal water between the open ocean and bay was influenced by hydrodynamic conditions, resulting in the net exchange fluxes of different nitrogen species both into and out of SDB during the day.
4.4 Implications for the effective improvement of water quality in SDB
Under the implementation of the 14th Five-Year Plan for Marine Ecological Environment Protection in Guangdong Province and the programme of key engineering measures for marine ecological protection and restoration in SDB (Department of Ecological Environment in Guangdong Province, 2022), terrestrial pollutant inputs should be reduced based on the characteristics of SDB to improve water quality (Li et al., 2018; Zhang et al., 2019; Zhang et al., 2019b; Zhou et al., 2020). In this study, we revealed that terrestrial sources and hydrodynamics jointly dominated the nitrogen under tidal variations. On the one hand, SDB and SCS should be considered as a single system to prevent nitrogen pollution. Owing to the high intensity of anthropogenic activities during the past decades, land reclamation decreasing seawater area, resulting in a sharp decline in water exchange and purification capacity (Feng, 2017). However, to achieve accurate terrestrial source reduction in SDB, there should be a shift from only control DIN to control TN in water. The discharge of land-source pollutants should be reduced based on environmental capacity, considering local urban sewage, industrial wastewater, and sewage from livestock breeding and aquaculture. However, there were inconsistencies in the current monitoring nitrogen indicators of river (TN) and coastal water quality (DIN). The total control of land-source pollution in polluted areas and management of river nutrient discharges into the sea should be strengthened (Strokal et al., 2017; Zhang et al., 2020), and the future coastal seawater quality should be linked to the management of the flux control of TN (Zhang et al., 2020). In addition, mangrove systems should be restored to reduce terrestrial nitrogen sources in SDB, particularly in marine aquaculture. Therefore, the long-term monitoring of nitrogen continued in SDB and SCS, as it will help us improve our understanding of the influence of tidal variations on the different nitrogen species in SDB. The establishment of a land–ocean space can effectively control and manage the water quality conditions of SDB.
5 Conclusions
In this study, we investigated the variations in nitrogen species in SDB during the spring and neap tides. The results indicated that the concentrations of PN and N-NO2- were significantly different between the spring and neap tides (P < 0.05). The TN concentrations in SDB during the spring and neap tides were 258.12 ± 89.49 μmol/L and 231.77 ± 56.86 μmol/L, respectively. During the spring and neap tides, TDN accounted for 54.1% and 52.2% of TN, respectively. DIN and DON exhibited semi-diurnal cyclic variation, indicating a period of high concentration at low tide and relatively low concentration in hight tide. In addition, the contribution of DON to TDN was the largest. A significant negative correlation was observed between the concentration of DIN and salinity (P < 0.001), and a significant positive correlation was observed between PN and Chlorophyll-a in SDB during the spring and neap tides (P < 0.01). This indicated that the concentration of different nitrogen species in SDB was not only controlled by tidal variations but also by the terrestrial source input. Moreover, the net exchange fluxes of different speciation of nitrogen between SDB and SCS showed that the TN were transported from SDB to SCS during the spring and neap tide, with the daily net exchange fluxes of 37.7 t and 8.8 t, respectively. The net exchange flux of TN in spring tide was 4.3 times higher than that of neap tide. Thus, SDB was a source of TN for SCS. Semi-diurnal tidal variations may drive the changes in the concentrations of different nitrogen species, thereby affecting the transport flux, biogeochemical behavior of nitrogen, and primary production. The present results provided scientific basis and key parameters for studying the tidal behaviors of different nitrogen species.
Data availability statement
The original contributions presented in the study are included in the article/supplementary material. Further inquiries can be directed to the corresponding author.
Author contributions
Conceptualization, PZ. Methodology, PZ and JZ. Software, WL, MF, MC, and JX. Validation, WL, MF, MC, and JX. Formal analysis, WL, MF, MC, and JX. Writing-original draft preparation, PZ and WL. Writing-review and editing, PZ and WL. Visualization, PZ and JZ. Supervision, PZ and JZ. Project management, PZ and JZ. Funding acquisition, PZ and JZ. All listed authors made substantial, direct, and intellectual contributions to the work and are approved for publication.
Funding
This research was financially supported by Research and Development Projects in Key Areas of Guangdong Province (2020B1111020004), Guangdong Basic and Applied Basic Research Foundation (2020A1515110483), Research on hydrodynamic changes and environmental remediation plan of Gemei Sea dike diversion (B21137). Guangdong Ocean University Fund Project (R18021), Science and Technology Special Project of Zhanjiang City (2019B01081), First-class Special Fund (231419018), Innovation Strong School Project (230420021) of Guangdong Ocean University.
Acknowledgments
The authors are grateful for the anonymous reviewers’ careful review and valuable suggestions to improve the manuscript.
Conflict of interest
The authors declare that the research was conducted in the absence of any commercial or financial relationships that could be construed as a potential conflict of interest.
Publisher’s note
All claims expressed in this article are solely those of the authors and do not necessarily represent those of their affiliated organizations, or those of the publisher, the editors and the reviewers. Any product that may be evaluated in this article, or claim that may be made by its manufacturer, is not guaranteed or endorsed by the publisher.
References
Amato H. K., Wong N. M., Pelc C. (2020). Effects of concentrated poultry operations and cropland manure application on anti- biotic resistant escherichia coli and nutrient pollution in Chesapeake bay watersheds. Sci. Total. Environ. 735 (15), 1–11. doi: 10.1016/j.scitotenv.2020.139401
Archana A., Thibodeau B., Geeraert N., Xu M. N., Kao S. J., Baker D. M. (2018). Nitrogen sources and cycling revealed by dual isotopes of nitrate in a complex urbanized environment. Water Res. 142 (oct.1), 459–470. doi: 10.1016/j.watres.2018.06.004
AQSIQ (2007a) Specifications for oceanographic surey-part 4: Survey of chemical parameters in sea water (GB/T 12764.4-2-7). (Beijing: Standards Press of China)
AQSIQ (2007b) The specification for marine monitoring-part 4: Seawater analysis (GB 17378.4-2007). (Beijing: Standards Press of China)
Bancon-Montigny C., Gonzalez C., Delpoux S., Avenzac M., Spinelli S., Mhadhbi T., et al. (2019). Seasonal changes of chemical contamination in coastal waters during sediment resuspension. CHEMOSPHERE. 235, 651–661. doi: 10.1016/j.chemosphere.2019.06.213
Béjaoui B. C. S., Harzallah A., Chevalier C., Chapelle A., Zaaboub N., Aleya L. 3D modeling of phytoplankton seasonal variation and nutrient budget in a southern Mediterranean lagoon. Mar. pollut. Bull. 114 (2), 962–976. doi: 10.1016/j.marpolbul.2016.11.001.
Belcher A., Iversen M., Manno C. (2016). The role of particle associated microbes in remineralization offecal pellets in the upper mesopelagic of the Scotia Sea, Antarctica. Limnol. Oceanogr. 61, 1049–1064. doi: 10.1002/lno.10269
Berman T., Bronk D. A. (2003). Dissolved organic nitrogen: a dynamic participant in aquatic ecosystems. Aquat. Microb. Ecol. 31, 279–305. doi: 10.3354/ame031279
Bricker S. B., Ferreira J. G., Simas T. (2003). An integrated methodology for assessment of estuarine trophic status. Ecol. Model. 169 (1), 39–60. doi: 10.1016/S0304-3800(03)00199-6
Cao Z. W., Wang D. P. (2011). Investigation and evaluation of water quality conditions in ganxi liushawan and yingpan pearl culture areas. Trans. Oceanology Limnology 4, 31–38. doi: CNKI:SUN:GXSC.0.2010-04-013
Carey R. O., Migliaccio K. W., Brown M. T. (2011). Nutrient discharges to Biscayne bay, Florida: trends, loads, and a pollutant index. Sci. Total. Environ. 409 (3), 530–539. doi: 10.1016/j.scitotenv.2010.10.029
Cheng P., Yu F., Chen N., Wang A. (2020). Observational study of tidal mixing asymmetry and eddy viscosity-shear covariance-induced residual flow in the jiulong river estuary. Cont. Shelf. Res. 193, 104035. doi: 10.1016/j.csr.2019.104035
Chen H. M., Sun C. X., Wu Y. Q. (2011). Analysis of trend of nutrient structure and influencing factors in changjiang estuary and its adjacent sea during 23 years. Mar. Environ. Sci. 30 (04), 551–553+563. doi: 10.3969/j.issn.1007-6336.2011.04.022
Chen B., Xu Z. X., Ya H. Z., Chen X. Y., Xu M. B. (2019). Impact of the water input from the eastern qiongzhou strait to the beibu gulf on guangxi coastal circulation. Acta Oceanol. Sin. 38 (9), 1–11. doi: 10.1007/s13131-019-1472-2
Chowdhury C., Majumder N., Jana T. K. (2016). Seasonal distribution and correlates of transparentexopolymer particles (TEP) in the waters surrounding mangroves in the sundarbans. J. Sea. Res. 112, 65–74. doi: 10.1016/j.seares.2016.01.004
Collos Y. (1998). Nitrate uptake, nitrite release and uptake, and new production estimates. Mar. Ecol. Prog. Ser. 171, 293–301. doi: 10.3354/meps171293
Cui X., Huang C., Wu J., Liu X., Hong Y. (2020). Temporal and spatial variations of net anthropogenic nitrogen inputs (NANI) in the pearl river basin of China from 1986 to 2015. PloS One 15 (2), 0228683. doi: 10.1371/journal.pone.0228683
D'Croz L., O'Dea A. (2007). Variability in upwelling along the pacific shelf of Panama and implications for the distribution of nutrients and chlorophyll. Estuar. Coast. Shelf. S. 73 (1–2), 325–340. doi: 10.1016/j.ecss.2007.01.013
Daniel J. C., Hans W. P., Rober W. H. (2009). Controlling eutrophication: nitrogen and phosphorus. SCIENCE. 323, 1014–1015. doi: 10.1126/science.1167755
Dan S. F., Liu S. M., Udoh E. C., Ding S. (2019). Nutrient biogeochemistry in the cross river estuary system and adjacent gulf of Guinea, south East Nigeria (West Africa). Cont. Shelf. Res. 179, 1–17. doi: 10.1016/j.csr.2019.04.001
Department of Ecological Environment in Guangdong Province (2022). In "14th five year plan" for marine ecological environment protection in guangdong province. (Guangdong Province: Department of ecological environment), 1–40.
Dittmar T., Lara R. J. (2001). Driving forces behind nutrient and organic matter dynamics in a mangrove tidal creek in north Brazil. Estuar. Coast. Shelf. S, 52(2). doi: 10.1006/ecss.2000.0743
Du E., Terrer C., Pellegrini A. F. A., Ahlström A., Lissa C. J., Zhao X., et al. (2020). Global patterns of terrestrial nitrogen and phosphorus limitation. Nat. Geosci. 13 (3), 221–226. doi: 10.1038/s41561-019-0530-4
Eppley R. W., Peterson B. J. (1979). Particulate or-ganic-matter flux and planktonic new production in the deep ocean. NATURE, 282. doi: 10.1038/282677a0
Falkowski P. G. (1997). Evolution of the nitrogen cycle and its influence on the biological sequestration of CO2 in the ocean. Nature.: Int. weekly. J. Sci. 387, 272–275. doi: 10.1038/387272a0
Fang T., Feng Z. H., Gao L. (2012). Tidal cycle variation of nutrient salts and chlorophyll a in the south branch waters of the Yangtze river estuary. Trans. Oceanology. Limnology. 03), 58–65.
Fang T., Li D. J., Kong D. J., Yu L. H., Li Y., Gao L., et al. (2008). Distribution of n and p nutrients and their tidal variations in the Yangtze river estuary and adjacent waters in summer and autumn. Mar. Environ. Sci. 05), 437–442.
Fatemi S. M. R., Nabavi S. M. B., Vosoghi G. (2012). The relation between environmental parameters of hormuzgan coastline in Persian gulf and occurrence of the first harmful algal bloom of cochlodinium polykrikoides (Gymnodiniaceae). Iran. J. Fish. Sci. 11 (3), 475–489.
Feng S. J. (2017). Characterization and 3D numerical simulation of tidal currents in shuidong bay. J. Appl. Oceanogr. 36 (03), 333–340.
Feng L. L., Li Z. K., Zhou T. (2012). Temporal and spatial distributions of phytoplankton and various forms of inorganic nitrogen in lake taihu. J. Lake. Sci. 24 (5), 739–745.
Fernandes L. (2011). Origin and biochemical cycling of particulate nitrogen in the mandovi estuary. Estuar. Coast. Shelf. S. 94, 291–298. doi: 10.1016/j.ecss.2011.07.007
Fu D., Zhong Y., Chen F., Yu G., Zhang X. (2020). Analysis of dissolved oxygen and nutrients in zhanjiang bay and the adjacent sea area in spring. Sustainability-Basel. 12 (3), 899. doi: 10.3390/su12030889
Galloway J. N. (2005). The global nitrogen cycle: past, present and future. Sci. China. Ser. C. Life Sci. S2), 669–677. doi: 10.1007/BF03187108
Gao L., Li D. J., Ding P. X. (2009). Quasi-simultaneous observation of currents, salinity and nutrients in the changjiang (Yangtze river) plume on the tidal timescale. J. Mar. Systems.: J. Mar. Sci. Tech-Japan. 75 (1-2), 265–279. doi: 10.1016/j.jmarsys.2008.10.006.
Geeraert N., Archana A., Xu M. N., Kao S. J., Baker D. M., Thibodeau B. (2021). Investigating the link between pearl river-induced eutrophication and hypoxia in Hong Kong shallow coastal waters. Sci. Total. Environ. 772, 145007. doi: 10.1016/j.scitotenv.2021.145007
Ge T. T., Wang X. Y., Tan L. J., Li T. (2021). Influence of water stratification and tidal action on the transport of particulate nitrogen and phosphorus in the Yangtze estuary in summer. Mar. Sci. 45 (08), 1–9.
Guo J. (2020). Nitrogen biogeochemical processes and nutrient deposition records in the nearshore waters of beibu gulf, guangxi (Nanning: Guangxi University).
Guo J., Wang Y., Lai J., Pan C., Wang S., Fu H., et al. (2019). Spatiotemporal distribution of nitrogen biogeochemical processes in the coastal regions of northern beibu gulf, south China Sea. CHEMOSPHERE 239, 124803. doi: 10.1016/j.chemosphere.2019.124803
Guo Z. H., Wang C., Yan L., Wang J. K. (2012). Spatial and temporal variation characteristics of major pollutants in the jiulong river estuary. China Environ. Sci. 32 (04), 679–686. doi: 10.3969/j.issn.1000-6923.2012.04.017
Guo W. D., Yang L. Y., Zhai W. D., Chen W. Z., Osburn C. L., Huang X., et al. (2014). Runoff-mediated seasonal oscillation in the dynamics of dissolved organic matter in different branches of a large bifurcated estuary–the changjiang estuary. J. Geophys. Res. 119 (5). doi: 10.1002/2013JG002540
Han X. Y., Wang X. L., Sun X., Shi X. Y., Zhu C. J., Zhang C. S., et al. (2003). Nutrient distribution and its relationship with occurrence of red tide in coastal area of East China Sea. J. Appl. Ecol. 14 (7), 1097–1101.
Harding L. W., Gallegos C. L., Perry E. S., Miller W. D., Adolf J. E., Mallonee M. E., et al. (2016). Long-term trends of nutrients and phytoplankton in chesapeake bay. Estuaries. Coasts. 39 (3), 664–681. doi: 10.1007/s12237-015-0023-7
Herbeck L. S., Unger D., Wu Y., Jennerjahn T. C. (2013). Effluent, nutrient and organic matter export from shrimp and fish ponds causing eutrophication in coastal and back-reef waters of NE hainan, tropical China. Cont. Shelf. Res. 57, 92–104. doi: 10.1016/j.csr.2012.05.006
Hopkins J. S., Hamilton R. D. II, Sandifer P. A., Browdy C. L., Stokes A. D. (1993). Effect of water exchange rate on production, water quality, effluent characteristics and nitrogen budgets of intensive shrimp ponds. J. World Aquacult. Soc 24, 304–320. doi: 10.1111/j.1749-7345.1993.tb00162.x
Howarth R. W. (2008). Coastal nitrogen pollution: A review of sources and trends globally and regionally. Harmful. Algae. 8 (1), 0–20. doi: 10.1016/j.hal.2008.08.015
Huang F. J., Lin X. B., Yin K. D. (2021). Effects of marine produced organic matter on the potential estuarine capacity of NOx(-) removal. Sci. Total. Environ. 812, 151471. doi: 10.1016/j.scitotenv.2021.151471
Jiang S., Müller M., Jin J., Wu Y., Zhu K., Zhang G. S., et al. (2019). Dissolved inorganic nitrogen in a tropical estuary in Malaysia: transport and transformation. BIOGEOSCIENCES. 16, 2821–2836. doi: 10.5194/bg-16-2821-2019
Joseph N., Boyer C. R., Kelble P. B., David T. R. (2008). Phytoplankton bloom status: Chl-a biomass as an indicator of water quality condition in the southern estuaries of Florida. U.S.A. Ecol. Indic. 9 (6). doi: 10.1016/j.ecolind.2008.11.013
Kang J. H., Lin Y. L., Wang Y., Lan W. L. (2020). Evaluation of the eutrophication level of marine environment in qinzhou bay and its effect on phytoplankton chlorophyll a. Ocean. Dev. Management. 37 (11), 67–74.
Kanuri V. V., Muduli P. R., Robin R. S., Charan K. B., Lova R. A., Sivaji P., et al. (2018). Bioavailable dis- solved organic matter and its spatio-temporal variation in a river dominated tropical brackish water lagoon, India. Mar. Pollut. Bull. 131, 460–467. doi: 10.1016/j.marpolbul.2018.04.059
Ke S., Zhang P., Ou S. J., Zhang J. X., Chen J. Y., Zhang J. B. (2022). Spatiotemporal nutrient patterns, composition, and implications for eutrophication mitigation in the pearl river estuary, China. Estuarine. Estuar. Coast. Shelf. S. 226, 107749. doi: 10.1016/j.ecss.2022.107749
Lebo M. E. (1991). Particle-bound phosphorus along an urbanized coastal plain estuary. Mar. Chem. 34, 225–246. doi: 10.1016/0304-4203(91)90005-H
Li J. C. (2021). Influence of tidal action, typhoon and human activities on the circulation of biogenic elements in zhanjiang bay. (Zhanjiang: Master's dissertation Guangdong Ocean University.).
Li K. Q., He J., Li J. L., Guo Q., Liang S. K., Li Y. B., et al. (2018). Linking water quality with the total pollutant load control management for nitrogen in jiaozhou bay. China. Ecol. Indicat. 85, 57–66. doi: 10.1016/j.ecolind.2017.10.019
Li S., Huang Z. R., Chen Z. Z. (2015). Structural characteristics of phytoplankton communities in spring and autumn in shuidong bay. South. Aquat. Science. 11 (04), 27–33. doi: 10.3969/j.issn.2095-0780.2015.04.004
Li Y., Li D. J., Fang T., Wang Y. M. (2008). Sunday Variation of chlorophyll a concentration in the Yangtze river estuary. Mar. Bull. 05), 30–34. doi: CNKI:SUN:HUTB.0.2008-05-007
Li B. S., Li C. X., Jin Y. X., Ji P., Zhao X. L., He S. (2020). Evaluation of spatial and temporal distribution of nutrient salts and eutrophication in guanghai bay sea. Mar. Environ. Sci. 39 (05), 657–663.
Li R. H., Liu S. M., Zhang G. L., Ren J. L., Zhang J. (2013). Biogeochemistry of nutrients in an estuary affected by human activities: The wanquan river estuary, eastern hainan island. China. Cont. Shelf. Res. 57 (1), 18–3. doi: 10.1016/j.csr.2012.02.013
Li K. Q., Ma Y. P., Dai A. Q., Wang X. L. (2017). Degradation dynamics and bioavailability of land-based dissolved organic nitrogen in the bohai Sea: Linking experiment with modeling. Mar. Pollut. Bull. 124 (2). doi: 10.1016/j.marpolbul.2017.02.033
Li G. J., Ma Y. L., Wei Li, Wang J. L., Wei H. (2012). Evaluation of nutrient distribution and potential eutrophication in bohai bay during spring. J. Tianjin. Univ. Sci. Technology. 27 (05), 22–27. doi: CNKI:SUN:TQYX.0.2012-05-006
Lin P., Guo L. D., Chen M. (2013). Distribution, partitioning and mixingbehavior of phosphorus species in the jiulong river estuary. Mar. Chem. 157, 93–105. doi: 10.1016/j.marchem.2013.09.002
Lin G. M., Lin X. B. (2022). Bait input altered microbial community structure and increased greenhouse gases production in coastal wetland sediment. Water Res. 218, 118520. doi: 10.1016/j.watres.2022.118520
Liu S. (2011). Diatom to dinoflagellate shift in the summer phytoplankton community in a bay impacted by nuclear power plant thermal effluent. Mar. Ecol. Prog. Ser. 424, 75–85. doi: 10.3354/meps08974
Liu X. F., Duan X. Y., Tian Y., Cao K., Gao F., Yin P., et al. (2021). Nutrient changes in the water column of sanmen bay and its response to human activities. Front. Mar. Geol. 37 (05), 46–56. doi: 10.16028/j.1009-2722.2020.052
Liu Y. L., Gao L., Chen W. Q., Wang T., Li D. J. (2017). Dynamics of nutrients in the northeastern east China sea in summer. Oceanol. Limnol. Sin. 48, 734–744.
Liu F., Li X. Q., Dong G. C., Qin Y. G., Chen Y. G., Wang Z. Z., et al. (2011). Study of water quality pollutants in coastal wetlands of the yellow river estuary. China Environ. Sci. 31 (10), 1705–1710.
Liu S. M., Li R. H., Zhang G. L., Wang D. R., Du Z., Herbeck L. S., et al. (2011). The impact of anthropogenic activities on nutrient dynamics in the tropical wenchanghe and wenjiaohe estuary and lagoon system in east hainan, China. Mar. Chem. 125 (1-4), 49–68. doi: 10.1016/j.marchem.2011.02.003
Li C. L., Zhang F., Shen X., Yang B., Shen Z. L., Sun S. (2005). Concentration and distribution characteristics of chlorophyll in jiaozhou bay and its annual changes. Ocean. Lake. Marsh. 2005 (06), 21–28. doi: 10.3321/j.issn:0029-814X.2005.06.003
Li J. L., Zheng B. H., Zhang S. Z., Jin X. W., Hu X. P., Liu F., et al. (2016). Eutrophication characteristics and difference analysis of major estuarine bays in China. China Environ. Sci. 36 (02), 506–516. doi: 10.3969/j.issn.1000-6923.2016.02.030
Loh A. N., Bauer J. E. (2000). Distribution, partitioning and fluxes of dissolved and particulate organic c, n and p in the eastern north pacific and southern oceans. DEEP-SEA. Res. Pt. I. 47 (12), 0–2316. doi: 10.1016/S0967-0637(00)00027-3
Lu D. L., Kang Z. J., Yang B., Dan S. F., Zhang D., Zhang P., et al. (2020). Compositions and spatio-temporal distributions of different nitrogen species and lability of dissolved organic nitrogen from the dafengjiang river to the sanniang bay. China. Mar. Pollut. Bull. 156, 111205. doi: 10.1016/j.marpolbul.2020.111205
Lu D. L., Yang N. N., Liang S. K., Li K. Q., Wang X. L. (2016). Comparison of land-based sources with ambient estuarine concentrations of total dissolved nitrogen in jiaozhou bay (China). Estuar. Coast. Shelf. Sci. 180, 82–90. doi: 10.1016/j.ecss.2016.06.032
Maryam G., Behrooz A., Sara G. (2021). Spatial distribution of nutrients and chlorophyll a across the Persian gulf and the gulf of Oman. Ocean. Coast. Manage., 201, 105476. doi: 10.1016/j.ocecoaman.2020.105476.
Mesfioui R., Love N. G., Bronk D. A., Mulholland M. R., Hatcher P. G. (2012). Reactivit and chemical characterization of effluent organic nitrogen from wastewater treatment plants determined by Fourier transform ion cyclotron resonance mass spec-trometry. Water Res. 46, 622–634. doi: 10.1016/j.watres.2011.11.022
Moneta A., Veuger B., Van Rijswijk P., Meysman F. J. R., Soetaert K., Middelburg J. J. (2014). Dissolved inorganic and organic nitrogen uptake in the coastal north Sea: A seasonal study. Eestuar. Coast. Shelf. S. 147, 78–86. doi: 10.1016/j.ecss.2014.05.022
Niu L. X., Gelder P.H.A.J.M., Zhang C. K., Guan Y. Q., Vrijling J. K. (2016). Physical control of phytoplankton bloom development in the coastal waters of jiangsu (China). Ecol. Model. 321, 75–83. doi: 10.1016/j.ecolmodel.2015.10.008
Passeri D. L., Hagen S. C., Medeiros S. C., Bilskie M. V. (2015). Impacts of historic morphology and sea level rise on tidal hydrodynamics in a microtidal estuary (Grand bay, Mississippi). CONT. SHELF. Res. 111, 150–158. doi: 10.1016/j.csr.2015.08.001
Peng B. J. (1987). Asymmetry of flow velocity in the tidal channel of the eastern part of the western guangdong water and analysis of flow simulation. Mar. Sci. 06), 17–22.
Pisam O., Boyer J. N., Podgorski D. C., Thomas C. R., Coley T., Jafféet R. (2017). Molecular composition and bioavailability of dissolved organic nitrogen in a lake flow-influenced river in south Florida, USA. Aquatic. Sci. 79, 891–908. doi: 10.1007/s00027-017-0540-5
Qi X. H., Liu M. S., Zhang J., Ren J. L., Zhang G. L. (2011). Cycling of phosphorus in the jiaozhou bay. Acta Oceanologica. Sinica. 30 (2), 62–74. doi: 10.1007/s13131-011-0106-7
Qin F. S., Yang Z. G., Yao S. S., Li S. (2014). Simulation study on the effect of comprehensive improvement project on water and sand dynamics in shuidong bay of maoming city. China Harbour. Engineering. 3, 51–56. doi: 10.7640/zggwjs201403010
Qu F., Liu M., Hou L. J., Xu S. Y., Liu Q. M., Ou D. N. (2007). Changes of overlying water environmental factors and nitrogen nutrients under the influence of tidal cycle and their correlation. Resour. Environ. Yangtze. Basin. 3, 345–350. doi: 10.3969/j.issn.1004-8227.2007.03.016
Rabalais N. N., Turner R. E., JustiĆ D., Quay D., William J W., Sen Gupta B. K. (1996). Nutrient changes in the Mississippi river and system responses on the adjacent continental shelf. Estuaries. 19 (2), 386–407. doi: 10.2307/1352458
Sánchez-Carrillo S., Sánchez-Andrés R., Alatorre L. C., Angeler D. G., M Álvarez-Cobelas J. A., Arreola-Lizárraga (2009). Nutrient fluxes in a semi-arid microtidal mangrove wetland in the gulf of california. Estuar. Coast. Shelf. S. . 82 (4), 654–662. doi: 10.1016/j.ecss.2009.03.002
Santos I. R. S., Burnett W. C., Chanton J. (2008). Nutrient biogeochemistry in a gulf of Mexico subterranean estuary and groundwater - derived fluxes to the coastal ocean. Limnol. Oceanogr. 53 (2), 705–718. doi: 10.4319/lo.2008.53.2.0705
Seitzinger S. P., Sanders R. W. (1997). Contribution of dissolved organic nitrogen from rivers to estuarine eutrophication. Mar. Ecol. Prog. Ser. 159, 1–12. doi: 10.3354/meps159001
Shi Y. Z., Zhang C. X., Zhang J. B., Chen C. L., Zhao L. R., Sun S. L. (2017). Characteristics of tidal distribution of phytoplankton and its relationship with environmental factors in the sea of shuidong bay. J. Ecol. 37 (18), 5981–5992. doi: 10.5846/stxb201606291286
Song D. H., Wang X. H., Andrew E., Bao X. W. (2011). The contribution to tidal asymmetry by different combinations of tidal constituents. J. Geophys. Res-Oceans. 116 (C12), C12007. doi: 10.1029/2011JC007270
Stedmon C. A., Markager S., Tranvik L., Kronberg L., TSlätis T., Martinsen W. (2007). Photochemical production of ammonium and transformation of dissolved organic matter in the Baltic Sea. Mar. Chem. 104, 227–240. doi: 10.1016/j.marchem.2006.11.005
Strokal M., Kroeze C., Wang M., Ma L. (2017). Reducing future river export of nutrients to coastal waters of China in optimistic scenarios. Sci. Total. Environ. 579, 517–528. doi: 10.1016/j.scitotenv.2016.11.065
Sui Q., Xia B., Xie H. B., Cui Y., Chen B. J., Cui Z. G., et al. (2016). Study on temporal and spatial variation of nutrients and evaluation on eutrophication in the seawater of the bohai Sea in winter and spring of 2014. Prog. Fishery. Sci. 37 (02), 10–15. doi: 10.11758/yykxjz.20150309002
Sun S. (2008). Distribution of nutrients and exchange fluxes at the sediment-water interface in the East and yellow seas. China Ocean. Univ. doi: 10.7666/d.y1338095
Sun P. X., Wang Z. L., Zhan R., Xia B., Wang X. Q. (2005). Distribution and eutrophication of inorganic nitrogen in the seawater of jiaozhou bay. Adv. Mar. Sci. 04), 466–471.
Tang S. M., Yan Y., Chen B. (2013). Effects of warm water drainage from daya bay nuclear power plant on the structure of marine phytoplankton community in spring and summer. J. Oceanogr. 32 (03), 373–382. doi: 10.3969/J.ISSN.2095-4972.2013.03.010
Tian X. P. (1990). The role of jet diffusion of outflow water from tidal channels on the formation of barrage sand. J. Zhongshan. University. Nat. Sci. Ed. 03), 177–182. doi: CNKI:SUN:ZSDZ.0.1990-03-028
Turner R. E., Rabalais N. N., Alexander R. B., Mcisaac G., Howarth R. W. (2007). Characterization of nutrient, organic carbon, and sediment loads and concentrations from the Mississippi river into the northern gulf of Mexico. Estuar. Coast. 30 (5), 773–790. doi: 10.1007/BF02841333
Wang C. Y. (2019). Investigation of water quality conditions in the main bays of zhangzhou city and evaluation of eutrophication. Fish. Res. 41 (06), 526–531. doi: 10.14012/j.cnki.fjsc.2019.06.010
Wang X. P., Jia X. P., Lin Q., Li C. H., Gan J. L., Cai W. G., et al. (1999). Characteristics and interrelationships of dissolved oxygen, pH, salinity and nutrient distribution in honghai bay. Mar. Bull. 5, 35–40. doi: 10.3969/j.issn.1001-6392.1999.05.005
Wang J. N., Li X. Y., Yan W. J., Chen L., Zhang Z. (2016). Nitrogen balance and dissolved inorganic nitrogen fluxes in the Yangtze river basin based on MEA scenarios: Integrated watershed-estuary/bay nitrogen management. J. Environ. Sci. 36 (01), 38–46. doi: 10.13671/j.hjkxxb.2015.0449
Watson P. G., Frickers P. E., Howland R. J. M. (1993). Benthic fluxes of nutrients and some trace metals in the Tamar estuary, SW England. Neth. J. Aquat. Ecol. 27, 135–146. doi: 10.1007/BF02334776
White A. E., Foster R. A., Benitez-Nelson C. R., Masqué P., Verdeny E., Popp B. N., et al. (2013). Nitrogen fixation in the gulf of California and the Eastern tropical north pacific. Prog. Oceanogr. 109, 1–17. doi: 10.1016/j.pocean.2012.09.002
Wu M. L. (2014). Study on the distribution characteristics of nutrient salts and its impact on the ecosystem in the northern part of beibu bay (Xiamen: Xiamen Ocean University).
Wu H., Zhu J. R., Shen J., Wang H. (2011). Tidal modulation on the changjiang river plume in summer. J. Geophys. Res-Oceans. 116 (8), C08017. doi: 10.1029/2011JC007209
Xia H. Y., Lin D. Y., Niu Z. W. (2007). Prediction of effects of tidal and reclamation on the seabed erodion-accretions in the zhanjiang bay. Mar. Sci. Bull. 1, 61–66. doi: 10.3969/j.issn.1001-6392.2007.01.010
Xin M., Wang B. D., Sun X., Xie L. P. (2010). The distribution characteristics and seasonal variation of nutrients in guangxi coastal area. Mar. Sci. 34 (9), 5–9.
Xiu B., Liang S. K., He X. L., Wang X. K., Cui Z. G., Jiang Z. J. (2019). Bioavailability of dissolved organic nitrogen and its uptake by ulva prolifera: Implications in the outbreak of a green bloom off the coast of qingdao, China. Mar. Pollut. Bull. 140, 563–572. doi: 10.1016/j.marpolbul.2019.01.057
Xu H. Y., Wang B. D., Xin M., Sun X., Wang Z. X., Chen C., et al. (2020). Long-term changes of nutrient salinity in jiaozhou bay and its ecological effects. Adv. Mar. Science. 38 (02), 276–286. doi: 10.3969/j.issn.1671-6647.2020.02.008
Yang B., Gao X., Zhao J., Lu Y., Gao T.. (2020). Biogeochemistry of dissolved inorganic nutrients in an oligotrophic coastal mariculture region of the northern Shandong peninsula, north yellow Sea. Mar. Pollut. Bull. 50, 110693. doi: 10.1016/j.marpolbul.2019.110693
Yang L. Z., Liu H. K., Ren J., Yu F. H. (2011). Preliminary study on two-way jet system in the near-shore waters of shuidong bay. Acta Scientiarum. Naturalium. Universitatis. Sunyatseni. 50 (02), 116–119.
Yang X. L., Zhu M. Y. (1990). New progress of phytoplankton nutrient metabolism research. Adv. Mar. Science. 03), 65–74.
Yin K. D., Harrison P. (2000). Influences of flood and ebb tides on nutrient fluxes and chlorophyll on an intertidal flat. Mar. Ecol. Prog. Ser. 196 (3), 75–85. doi: 10.3354/meps196075
Yuan Q., Xu Z. Y., Peng H. Q., Lu J. M., Huang L. C., Liang X. J., et al. (2016). Study on the trends of nitrogen and phosphorus in seawater in zhanjiang bay and its nearby waters in the past 5 years. J. Green Sci. Technology. 24), 41–45. doi: CNKI:SUN:LVKJ.0.2016-24-016
Yu Y., Song J. M., Li X. G., Yan H. M., Li N. (2012). Distribution, sources and budgets of particulate phosphorus and nitrogen in the East China Sea. Cont. Shelf. Res. 43, 142–155. doi: 10.1016/j.csr.2012.05.018
Zhang P., Chen Y., Peng C. H., Dai P. D., Lai J. Y., Zhao L. R., et al. (2020b). Spatiotemporal variation, composition of DIN and its contribution to eutrophication in coastal waters adjacent to hainan island, China. Reg. Stud. Mar. Sci. 37, 101332. doi: 10.1016/j.rsma.2020.101332
Zhang P., Dai P. D., Zhang J. B., Li J. X., Zhao H., Song Z. G. (2021). Spatiotemporal variation, speciation, and transport flux of TDP in leizhou peninsula coastal waters, south China Sea. Mar. Pollut. Bull., 167. doi: 10.1016/j.marpolbul.2021.112284
Zhang P., Peng C., Zhang J., Zhang J., Chen J., Zhao H. (2022). Long-term harmful algal blooms and nutrients patterns affected by climate change and anthropogenic pressures in the zhanjiang bay, China. Front. Mar. Sci. 9, 849819. doi: 10.3389/fmars.2022.849819
Zhang P., Wei L. R., Lai J. Y., Dai P. D., Chen Y., Zhang J. B. (2019b). Concentration, composition and fluxes of land-based nitrogen and phosphorus source pollutants input into zhanjiang bay in summer. J. Guangdong. Ocean. U. 39 (4), 46–55. doi: 10.3969/j.issn.1673-9159.2019.04.000
Zhang P., Xu J. L., Zhang J. B., Li J. X., Zhang Y. C., Li Y., et al. (2020c). Spatiotemporal dissolved silicate variation, sources, and behavior in the eutrophic zhanjiang bay, China. Water-Sui. 12 (12), 3586–3586. doi: 10.3390/w12123586
Zhang J. B., Zhang P., Dai P. D., Lai J. Y., Chen Y. (2019). Spatial and temporal distribution of dissolved inorganic phosphorus and eutrophication in the nearshore waters of hainan island. J. Environ. Sci-china. 39 (06), 2541–2548. doi: CNKI:SUN:ZGHJ.0.2019-06-041
Zhang Y. Y., Zhang J., Wu Y., Zhu Z. Y. (2007b). Characteristics of dissolved oxygen and its affecting factors in the Yangtze estuary. Environ. Sci. 28 (8), 1649–1654. doi: 10.3321/j.issn:0250-3301.2007.08.001
Zhang J. B., Zhang Y. C., Zhang P., Li Y., Li J. X., Luo X. Q., et al. (2020). Seasonal phosphorus variation in coastal water affected by the land-based sources input in the eutrophic zhanjiang bay, China. Estuar. Coast. Shelf. S, 252. doi: 10.1016/J.ECSS.2021.107277
Zhang Y. J., Zhao R. D., Hu Y. C., Liang S. K., Wang X. L. (2016). The influence of typhoon- induced precipitation on biogenic element supplementary and distribution of jiaozhou bay. China. J. Ocean. U. China 46 (4), 79–88.
Zhao W. H., Jiao N. Z., Zhao Z. X. (2000). Distribution and dynamics of nutrient salts in the cultured waters of yantai shiliwan. Mar. Sci. 4, 31–34. doi: CNKI:SUN:HYKX.0.2000-04-010
Zhao X. D., Zhu C. J., Shi Z. L. (1998). The forms and distributions of nitrogen of seawater in eastern jiaozhou bay. Mar. Sci. 01), 40–44. doi: CNKI:SUN:HYKX.0.1998-01-016
Zheng X. H. (2010). Changes in nitrogen and phosphate and eutrophication character in minjiang estuary. J. Oceanogr. Taiwan. Strait. 29 (1), 42–46. doi: 10.1080/09500340.2010.529951
Zhong H. G., Li P. (2014). Study on the dynamics of total nitrogen and total phosphorus in coastal waters of sanya bay, dadonghai and yalong bay. Hebei. Fishery. 05), 12–14+26. doi: 10.3969/j.issn.1004-6755.2014.05.005
Zhou M. (2021). Study on the spatial and temporal distribution of chlorophyll a and nutrient salts and potential eutrophication in Zhanjiang Bay sea surface in autumn 2017-2020. Guangdong. Ocean. Univ. doi: 10.27788/d.cnki.ggdhy.2021.000178
Zhou W. H., Huo W. Y., Yuan X. C., Yin K. D. (2003). Distribution features of chlorophyll a and primary productivity in high frequency area of red tide in East China Sea during spring. J. Appl. Ecol. 14 (7), 1055–1059.
Zhou Y., Wang L. L., Zhou Y. Y., Mao X. Z. (2020). Eutrophication control strategies for highly anthropogenic influenced coastal waters. Sci. Total. Environ. 705, 135760. doi: 10.1016/j.scitotenv.2019.135760
Zhou C. F., Yuan G. W., Peng M., Zhang P. (2022). Characteristics, composition and nutrient level evaluation of nitrogen and phosphorus nutrient distribution in the sea adjacent to qidong, jiangsu. Environ. pollut. Control. 44 (01), 104–110. doi: 10.15985/j.cnki.1001-3865.2022.01.018
Zhou C. H., Zhang J. P., Huang X. P., Zhao C. Y., Wu Y. C. (2019). Spatial and temporal distribution, key influencing factors and potential ecological significance of particulate nitrogen and phosphorus in daya bay. Mar. Environ. Sci. 38 (05), 696–702+711. doi: CNKI:SUN:HYHJ.0.2019-05-008
Keywords: total nitrogen, speciation, exchange flux, tidal variation, Shuidong Bay
Citation: Zhang P, Luo W, Fu M, Zhang J, Cheng M and Xie J (2022) Effects of tidal variations on total nitrogen concentration, speciation, and exchange flux in the Shuidong Bay coastal water, South China Sea. Front. Mar. Sci. 9:961560. doi: 10.3389/fmars.2022.961560
Received: 04 June 2022; Accepted: 27 July 2022;
Published: 18 August 2022.
Edited by:
Xianbiao Lin, Ocean University of China, ChinaReviewed by:
Fangjuan Huang, Sun Yat-sen University, ChinaHongming Yao, Shenzhen Institutes of Advanced Technology (CAS), China
Copyright © 2022 Zhang, Luo, Fu, Zhang, Cheng and Xie. This is an open-access article distributed under the terms of the Creative Commons Attribution License (CC BY). The use, distribution or reproduction in other forums is permitted, provided the original author(s) and the copyright owner(s) are credited and that the original publication in this journal is cited, in accordance with accepted academic practice. No use, distribution or reproduction is permitted which does not comply with these terms.
*Correspondence: Jibiao Zhang, emhhbmdqYkBnZG91LmVkdS5jbg==