- 1Marine-Med: Marine Research, Epidemiology, and Veterinary Medicine, Bothell, WA, United States
- 2Cascadia Research Collective, Olympia, WA, United States
- 3Washington Department of Fish and Wildlife, Marine Mammal Investigations, Lakewood, WA, United States
- 4Northwest Fisheries Science Center, National Marine Fisheries Service, National Oceanic and Atmospheric Administration (NOAA), Seattle, WA, United States
- 5Northwest ZooPath, Monroe, WA, United States
- 6The SeaDoc Society, University of California (UC) Davis School of Veterinary Medicine, Karen C. Drayer Wildlife Health Center – Orcas Island Office, Eastsound, WA, United States
- 7The Whale Museum, Friday Harbor, WA, United States
- 8British Columbia Ministry of Agriculture, Animal Health Centre, Abbotsford, BC, Canada
Mucorales infections are increasing in frequency and are a One Health pathogen of concern. In humans and domestic animals, risk factors include being immunocompromised, elevated circulating serum iron, contaminated open wounds, or metabolic diseases such as ketoacidosis or uncontrolled diabetes. Mucormycosis was first identified in 2012 in Pacific Northwest marine mammals, predominantly in harbor porpoises. We performed an assessment to determine the overall qualitative risk, or risk score, of mucormycosis in harbor porpoises. Risk factors for this disease are unknown in aquatic mammals. In a separate risk factor analysis, potential risk factors such as pollutants, trace metals (e.g., iron), and co-infection with other pathogens (e.g., viruses and Brucella spp.) were examined in mucormycosis cases and noncases using a matched case-control study design, to determine the presence and strength of association of these factors with mucormycosis. Disease severity (gross and histopathology) and exposure scores were multiplied together to obtain the overall risk scores of 9 -16 which corresponded to moderate and severe, respectively. In the risk factor analysis, the factors most strongly associated with a mucormycosis case, relative to a control, were elevated liver iron, decreased blubber thickness, and the decreased ratio of the sum of PCB congeners/sum of PBDE congeners. The results of this study suggest that mucormycosis may pose an inordinately high risk to harbor porpoises (and potentially sympatric species in the Salish Sea such as southern resident killer whales) based on the detected prevalence and the severity of lesions observed at necropsy. However, the risk may be greater on an individual basis compared to the overall population, and is likely related to other factors such as increased POP and heavy metal burdens.
1 Introduction
Fungi of the order Mucorales are ubiquitous filamentous saprobionts and opportunistic organisms found in all climatic zones, containing approximately 50 genera and 300 species (Migaki and Jones, 1983; Petrikkos et al., 2012). These fungi are a One Health concern due to their ability to infect humans, other animals, plants, and persist in the environment in decaying organic matter such as leaves, soil, rotting wood and other vegetation, and dung (Migaki and Jones, 1983; American Society for Microbiology (ASM), 2019; Hassan and Voigt, 2019). Historically, mucormycosis has been called phycomycosis, and more recently zygomycosis, and is a disease caused by any species of the order Mucorales. Despite the pervasiveness of these fungi, the disease itself represents only 8-13% of all fungal infections in humans. Although Mucorales only represent a small portion of fungal infections, the mortality rate associated with them is striking (Roden et al., 2005) and increasing (Spellberg et al., 2005; Petrikkos et al., 2012). Infection in animals and humans is caused by inhalation of sporangiospores, direct inoculation into the skin, or ingestion, and is not considered zoonotic, nor does it transmit between individuals. In susceptible individuals the agent is aggressive and typically disseminates quickly throughout the body, invading blood vessel walls, resulting in thrombosis, infarcts (necrosis), and granulomatous inflammation.
Because mucormycosis is so aggressive, it is difficult to treat and often fatal (mortality >40%) (Roden et al., 2005). Though it occurs rarely in healthy individuals, mucormycosis is largely considered an opportunistic rather than primary pathogen in humans and animals. Infections may result from localized traumatic damage to the skin or generalized immune system suppression from chronic pre-existing disease or elevated contaminant levels (Ross, 2002; Desforges et al., 2016), such as humans with unregulated diabetes, HIV/AIDS, intravenous drug use, malnutrition, pregnancy, traumatic injuries and increased circulating levels of heavy metals such as iron or uptake of zinc, and/or coinfection with other infectious pathogens (Roden et al., 2005; Gomes et al., 2011; Petrikkos et al., 2012; Binder et al., 2014; Mouton et al., 2015; Wilson, 2015; Weiss and Carver, 2018). In humans, iron overload is a risk factor for mucormycosis (Petrikkos et al., 2012). A recent study in stranded false killer whales (Pseudorca crassidens) detected a strong link between opportunistic cutaneous fungal invaders and elevated levels of aluminum, selenium and zinc (Mouton et al., 2015). Although little is known about metal and trace element concentrations in marine mammals of the Pacific Northwest, a few studies have examined metals in harbor seals where regional differences in metal levels have been documented (Calambokidis et al., 1984; Calambokidis et al., 1991; Akmajian et al., 2014). Essential elements (e.g., zinc, copper, selenium) occur naturally in the environment and many have important biological functions, but excesses or deficiencies can have adverse health effects. Non-essential elements such as lead, cadmium and mercury can be toxic, depending on concentration and form, with evidence suggesting a possible link between long-term exposure to heavy metals such as mercury and infectious disease in harbor porpoises (Bennett et al., 2001; Wintle et al., 2011), immuno- and genotoxic effects in cetaceans (Kershaw and Hall, 2019), and more numerous lesions in dolphins with elevated cadmium and selenium levels (Monteiro et al., 2020). Underlying viral infections were also investigated considering herpesvirus was detected in harbor seals with mucormycosis and could be associated with the presence of the disease as well as a killer whale (Orcinus orca) under human care (Abdo et al., 2012b; Huggins et al., 2020).
In marine mammals, mucormycosis is reported less commonly than in humans, and is most frequently identified as those in human care, with a few isolated cases among wild, stranded individuals. It is unclear how marine mammals are exposed to Mucorales spp., but is suspected to be similar to humans with exposure to detritus containing the organism carried by winds or dispersal into the marine environment through flooding. The disease has been documented in delphinids, large whales, and pinnipeds (Kaplan et al., 1960; Best and McCully, 1979; Wünschmann et al., 1999; Robeck and Dalton, 2002; Naota et al., 2009; Morris et al., 2010; Barnett et al., 2011; Abdo et al., 2012a; Abdo et al., 2012b; Sosa et al., 2013; Isidoro-Ayza et al., 2014; Huckabone et al., 2015; Nakagun et al., 2018; Huggins et al., 2020).
Over the past 20 years, increasing numbers of harbor porpoise (Phocoena phocoena) have been documented in the northern Salish Sea (Washington State, USA), with expansion southward into Puget Sound proper (Evenson et al., 2016; Jefferson et al., 2016). Though significantly impacted by negative environmental factors such as pollution and entanglement in fishing gear, harbor porpoises are not currently considered a threatened or endangered species, and are relatively abundant throughout the Salish Sea and along the outer Pacific Northwest coast (Evenson et al., 2016; Jefferson et al., 2016; Carretta et al., 2017; Morin et al., 2021). Harbor porpoises are a sentinel species in other regions of the world such as the Baltic Sea (ASCOBANS, 2010) and Canada (COSEWIC, 2016). Investigations into the disease and health conditions of harbor porpoises are revealing diseases that are unusual, increasing, or not previously recorded in the region (Huggins et al., 2015). For example, mucormycosis cases were noted for the first time in 2012 in a harbor porpoise (Huggins et al., 2020). Between 2012 and 2019, 21 cases of mucormycosis were detected in marine mammals, 19 confirmed and 2 suspect; in 12 adults, 6 subadults, and 3 pups, including 15 harbor porpoises, 5 harbor seals (Phoca vitulina) and an endangered southern resident killer whale (SRKW), the latter following deployment of a LIMPET satellite tag into the dorsal fin (Department of Fisheries and Oceans Canada (DFO), 2016; National Marine Fisheries Service, 2016a; National Oceanic and Atmospheric Administration, 2016b; Huggins et al., 2020). Both sexes and all seasons were represented (Huggins et al., 2020). Cases increased over time and peaked in 2016 (n = 6), the year that coincided with the first documentation of the fungus in species other than harbor porpoise. Most of the cases in this study occurred in inland waters of Washington State and British Columbia, Canada (Figure 1).
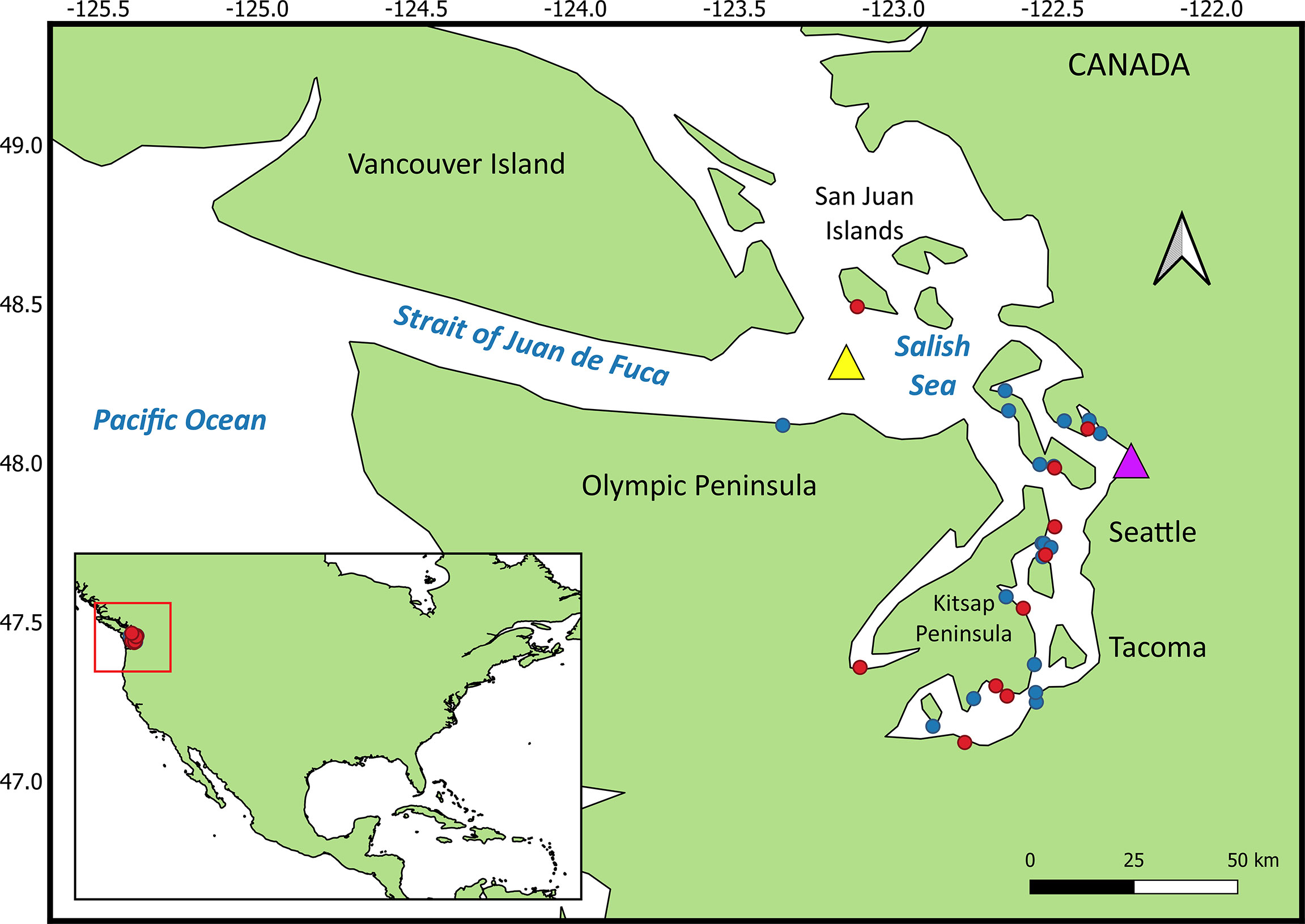
Figure 1 Mucormycosis cases (red dots) and controls (blue dots) in harbor porpoise. The yellow triangle is the location of the buoy that recorded mean yearly sea surface temperature. The purple triangle is the location where mean yearly precipitation was recorded.
Harbor porpoises in the Salish Sea are ideal indicator species for monitoring marine ecosystem health, including emerging pathogens such as Mucorales (Moore, 2008; Bossart, 2011). This is especially relevant for the endangered SRKWs within the Salish Sea, whose small population size, and thus vulnerability to disease, was identified as one of the important concerns for this population’s survival (NMFS, 2008; NMFS West Coast Region, 2016). SRKWs are a Vital Signs indicator for the Puget Sound Partnership to monitor the health of the Puget Sound ecosystem (Kershner et al., 2011). Given the prolonged timeframe, to acquire a sufficient sample size of tissues from killer whales for disease or contaminant analysis, along with the logistical challenges of studying their health, a more practical, efficient method is to monitor alternative surrogate species, such as harbor porpoises, that are easier to monitor and sample (Knap et al., 2002; Rabinowitz et al., 2005). Porpoises are sympatric with SRKWs, display inshore distribution, urban ecosystem residency, proximity to human populated areas, and greater abundance than the latter (Calambokidis et al., 2015; COSEWIC, 2016). Harbor porpoises and SRKWs eat high on the marine food web and display relatively strong regional site fidelity (Carretta et al., 2017). Thus, harbor porpoises are ideally suited to study for emerging pathogens that could affect SRKWs.
The relationship between environmental and demographic variables in harbor porpoise and the occurrence of mucormycosis is currently not well understood. Understanding how these variables influence development of this fungal disease in porpoise is key to identifying ways to attenuate the number of new cases that develop each year and to help mitigate exposure or development of the disease in endangered species that inhabit the same waters as porpoise, such as SRKWs. Our preliminary data identify an unusually large number of mucormycosis cases in harbor porpoises compared to other species (Huggins et al., 2020). Among the porpoise cases, animals have been emaciated, lactating (n = 2), affected with concurrent disease or heavy parasitism, pregnant (n = 1), or free of any obvious underlying disease.
Based on the varied presentation of cases, the contributors to mucormycosis appear to be multifactorial and likely similar to those in humans; therefore, we set out to investigate whether any of the following potential risk factors are associated with infection caused by mucormycosis: elevated pollutants and trace metals (e.g., iron), co-infection with other pathogens (e.g., virus, bacteria), emaciation, or pregnancy and lactation, as well as environmental variables such as heavy precipitation in the Salish Sea region (Nnadi and Carter, 2021). Environmental disruptions due to climate change, such as more severe flooding and other natural disasters, can readily aerosolize and disperse fungal spores, which are small, throughout the environment. This is particularly true when the spores encounter favorable climatic conditions such as lower rainfall and higher temperatures (Richardson, 2009).
Qualitative risk assessments serve as initial steps in assessing the risk of a specific hazard such as acquiring a pathogen (Jakob-Hoff et al., 2014). Risk assessments help contribute to risk management by breaking down threats into categories that are identifiable, and define the impact of each risk or risk factor (Graves, 2000). Quantitative risk assessments provide more objective information and accurate data than qualitative risk assessments because the former are based on data that are measurable and often more realistic than the latter and are, thus, often preferred. However, quantitative risk assessments are often impractical or impossible to perform due to a lack of measurable data on which to obtain a valid, numerical health risk assessment (Graves, 2000; Leighton, 2002). Because there is a lack of accurate numerical information on mucormycosis in marine mammals, much less harbor porpoise, to perform a quantitative assessment, a qualitative risk assessment can be performed instead. It is easily performed, quick to implement, and practical due to the lack of statistical/numerical dependence (Leighton, 2002). Thus, our aims for this project were two-fold: to evaluate risk factors that might be associated with mucormycosis in harbor porpoise, with the intent of improving our ability to identify marine mammals at risk for the disease; and second, perform a qualitative risk assessment for mucormycosis in harbor porpoises within the Salish Sea.
2 Methods
2.1 Risk factor analysis
2.1.1 Study population and case/control selection
Study subjects were identified from the records of the National Oceanic and Atmospheric Administration (NOAA Fisheries), West Coast Region marine mammal stranding network, over the study period (2012-2019). Marine mammal stranding response groups collect dead beach cast animals, conduct examinations, and in many cases, complete necropsies and tissue sampling. The focus of this study was on harbor porpoise given the relatively high number of annual strandings and the overrepresentation of mucormycosis in this species (Huggins et al., 2020). Records of stranded harbor porpoises within the Salish Sea were thus extracted, from which cases and controls were selected. A confirmed case was defined as a harbor porpoise that stranded within the Salish Sea, presented with suspected findings at necropsy (e.g., marked swollen lymph nodes and/or presence of masses within organs), the pathogen detected at histopathological examination, with confirmation by culture and/or molecular sequencing. Controls were defined as a harbor porpoise that stranded within inland Washington State waters, and was negative for any histopathological or diagnostic findings as described for cases. In addition, the cause of death in controls must have been unrelated to fungal disease to avoid selection bias. Analyses were completed for potential risk factors using samples collected from fresh, dead-stranded harbor porpoise mucormycosis cases and controls within the Salish Sea.
Analyses of the following potential risk factors for mucormycosis were completed in cases of mucormycosis (n = 10) and controls (n = 20), (n = 30 total for study): persistent organic pollutants (POPs), and trace metals concentrations, and pathogen (viral and bacterial) screening. For all study samples, one-gram sections of blubber were collected from fresh stranded carcasses and frozen until analyzed for POPs and lipid content at NOAA’s Northwest Fisheries Science Center (Seattle, WA). The POPs included the sums of chlordanes (CHLD), dichloro-diphenyl-trichloroethane (DDT), hexachlorocyclohexanes (HCH), 46 polychlorinated biphenyl (PCB) congeners (as 40 chromatographic peaks), a subset of the PCB congeners (105, 118, 156) for which toxic equivalency factors were available to us, and 15 polybrominated diphenyl ether congeners (PBDEs). In addition, the following POP ratios were evaluated as potential risk factors: the sum of the 46 PCB congeners as a ratio to each of the sums of CHLDs, DDTs, HCHs, and PBDEs, respectively. Blocks of frozen liver samples from all cases and controls were submitted to ALS Environmental (Kelso, WA) and the California Animal Health and Food Safety Laboratory at University of California (Davis, CA) for the following trace metals/elements that serve important roles in the survival and the pathogenesis of invasive fungal disease (Gerwien et al., 2018): aluminum (Al), arsenic (As), copper (Cu), cadmium (Cd), iron (Fe), nickel (Ni), silver (Ag), lead (Pb), mercury (Hg), selenium (Se), and zinc (Zn).
To determine the presence of co-morbidity with infectious/noninfectious diseases, detailed necropsies were conducted on the fresh harbor porpoise carcasses following standard techniques (Geraci and Lounsbury, 2005). Histological examination was completed on all cases and controls at Northwest Zoopath (Monroe, WA), and Animal Health Center (Abbotsford, BC). Histochemical (Grocott methenamine silver, acid fast, and Gram) and immunohistochemical stains were used as needed and previously described in harbor porpoise mucormycosis cases (Huggins et al., 2020). Frozen tissues were submitted to the One Heath Institute Laboratory at the University of California (Davis, CA) for screening for morbilli- and herpesviruses by reverse transcriptase and standard polymerase chain reaction (PCR), respectively, based on established methods (Van Devanter et al., 1996; Tong et al., 2008). PCR for Brucella was conducted by the Veterinary Diagnostic Laboratory at the University of Illinois (Urbana, IL).
2.1.2 Specification of levels of potential risk factors
Descriptive data with no numeric value or order (nominal risk factor) included sex; age class (subadult or adult); and season of stranding - winter (December-February), spring (March-May), summer (June-August), and autumn (September-November). The binary data were recorded as yes versus no, unless otherwise indicated (e.g., sex) and included comorbid lesions present; and age class. Continuous variables included lateral blubber depth at maximum girth (cm); the POPs as listed under section 2.2; location, in decimal degrees latitude, of the stranding north or south relative to the approximate lateral midpoint of the Salish Sea (latitude 48°N; roughly at Everett, WA); and environmentally acquired metals that are deemed of importance to homeostatic regulation in pathogenic fungi, for which a sufficiently large body of literature exists, such as iron, zinc, and copper (Gerwien et al., 2018). Decimal degrees relative to latitude 48°N was selected as a variable because past evidence along the outer Washington coast suggested ratios of select contaminants, such as PCB and DDT in harbor porpoises, are associated with latitude (location), indicating this species’ movements may be restricted in some areas, helping to identify the geographic region from which the porpoise originated (Calambokidis and Barlow, 1991), as has been done in SRKWs (Krahn et al., 2007). Mean yearly sea surface temperature data for the Salish Sea were obtained for a buoy moored in the eastern Strait of Juan de Fuca (48.332° N, -123.179° W) (yellow triangle, Figure 1) for 2012-2019 and were reported as °C (National Oceanic and Atmospheric Administration, 2020a). Mean annual precipitation (cm), for the year preceding each mucormycosis case was reported, was obtained from a precipitation monitoring station approximately mid-Salish Sea, at Everett, Washington (purple triangle, Figure 1) (National Oceanic and Atmospheric Administration, 2020b). The outcome (dependent) variable for the condition regression model was case/control status.
2.1.3 Molecular identification
Molecular genotyping was performed in eight of the 10 cases and four of the control porpoises (retrospectively) at NOAA Fisheries’ Northwest Fisheries Science Center (Seattle, WA) to confirm (or rule out) the fungal family and/or species, and has been recently described in more detailed elsewhere (Huggins et al., 2020). Not all cases and controls were molecularly tested due to confirmation by other methods (e.g., histopathology). Targeted tissue samples from suspected case animals, that included those stored in RNAlater®, in paraffin blocks, or frozen in Whirl-Pak bags, were analyzed using polymerase chain reaction and sequencing.
2.1.4 Statistical analyses
To determine the presence and strength of association of the independent potential risk factors with confirmed mucormycosis, a matched case-control study was implemented using conditional logistic regression with two controls matched by age class to each case (Pearce, 2016). Matching was used to reduce confounding by the independent variables and to gain greater statistical efficiency and precision (Rose and van der Laan, 2009; Pearce, 2016). Conditional univariate logistic regression was performed to identify those independent variables associated with the dependent outcome variable (disease status) to determine if the variable would be included in the initial full model. Risk factors with P < 0.20 on univariate analysis were eligible for inclusion as were any potential 2-way interaction terms that were deemed biologically plausible (e.g., interaction between sex and levels of total CHLD, DDT, HCH, PBDE, and PCB, as well as degrees latitude from mid-Salish Sea and POPs). A threshold of P < 0.20 was chosen to prevent exclusion of a potentially marginally significant risk factor that may only become significant when in the presence of other risk factors during the multivariable analysis (Dohoo et al., 2003). Collinearity between related variables, such as POPs and POPs with degrees latitude of stranding relative to mid-Salish Sea, was examined via the Pearson correlation coefficient (r). When variables were strongly correlated (i.e., r > 0.70), the one with the greatest odds ratio (OR) on univariate analysis was kept for modeling purposes and the other(s) were excluded.
We used a manual, stepwise approach to model building. Independent variables at P < 0.20 were kept, and the effect of adding or subtracting each variable was examined for evidence of confounding, represented by a change in other covariate coefficients by more than 20%. Akaike information criterion (AIC) values were used to select the final model out of all candidate models (Akaike, 1974). The Hosmer-Lemeshow test assessed final model goodness-of-fit. Results of conditional logistic regression analysis were reported as ORs and 95% CIs. The OR represents the relative change in odds for mucormycosis associated with the presence of a given factor(s) or, for continuous variables, for each (single) unit change in that factor (e.g., precipitation). Odds ratios are used to quantify the magnitude of association between a potential risk factor and the outcome (disease). Therefore, when an OR = 1, there is no association; an OR > 1 indicates the risk factor is associated with an increased in odds of the outcome and the reverse for OR < 1.
A secondary regression analysis, using multiple linear regression (MLR), was used to evaluate the association of levels of various contaminants with independent risk factors (sex, length, age class, blubber thickness, mucormycosis status, degrees latitude distance relative to 48°N, season, and lipid percent). The dependent outcome variables were lipid-normalized sums of concentrations for each POP class (PBDE, CHLD, DDT, HCH, PCB). We explored a number of contaminant ratios, including the ratios of summed PCBs to those of PBDE, CHLD, DDT, and HCH. The resulting beta coefficients were standardized to evaluate which of the independent variables have a greater effect on the dependent variable since the independent variables are given in different units of measurement. The beta coefficients are essentially considered a general measure of effect size, or magnitude of the effect of one variable on another.
2.2 Qualitative risk assessment
Due to limited data on mucormycosis in harbor porpoise, a qualitative, versus quantitative, risk assessment approach was selected to determine the probability of risk to harbor porpoises of acquiring mucormycosis. We utilized a qualitative risk assessment based on the World Organisation for Animal Health (OIE) and Food and Agriculture Organization-World Health Organisation (FAO-WHO) (Food and Agriculture Organization of the United Nations/World Health Organization, 2011) assessment frameworks, incorporating available data from published and unpublished sources. This type of assessment consists of identifying the probability of a risk event (i.e., transmission of the disease agent), and the impact the risk will have if said event does occur (i.e., recipient of disease agent becomes infected). A framework for this approach has already been developed and used by multiple agencies for assessing animal diseases (Palmer et al., 2005; Heller et al., 2010; Food and Agriculture Organization of the United Nations/World Health Organization, 2011; Sharma et al., 2018). The two principal components of a qualitative risk assessment framework (Figure 2) include: (1) hazard identification (blue box) and (2) risk assessment (green box). The latter further consists of hazard characterization (pink boxes), exposure assessment (gold boxes), and risk characterization (purple boxes). All risks have both probability and impact. Once the hazard is identified, in this case mucormycosis in harbor porpoise, the overall risk can be assessed as a function of: a) the probability that a porpoise will be exposed to the fungi (exposure assessment); and b) the magnitude of the consequences of such a hazard (hazard characterization). The resulting probabilities (exposure and consequence) are then combined to give an overall qualitative estimation of risk using a matrix approach (risk characterization).
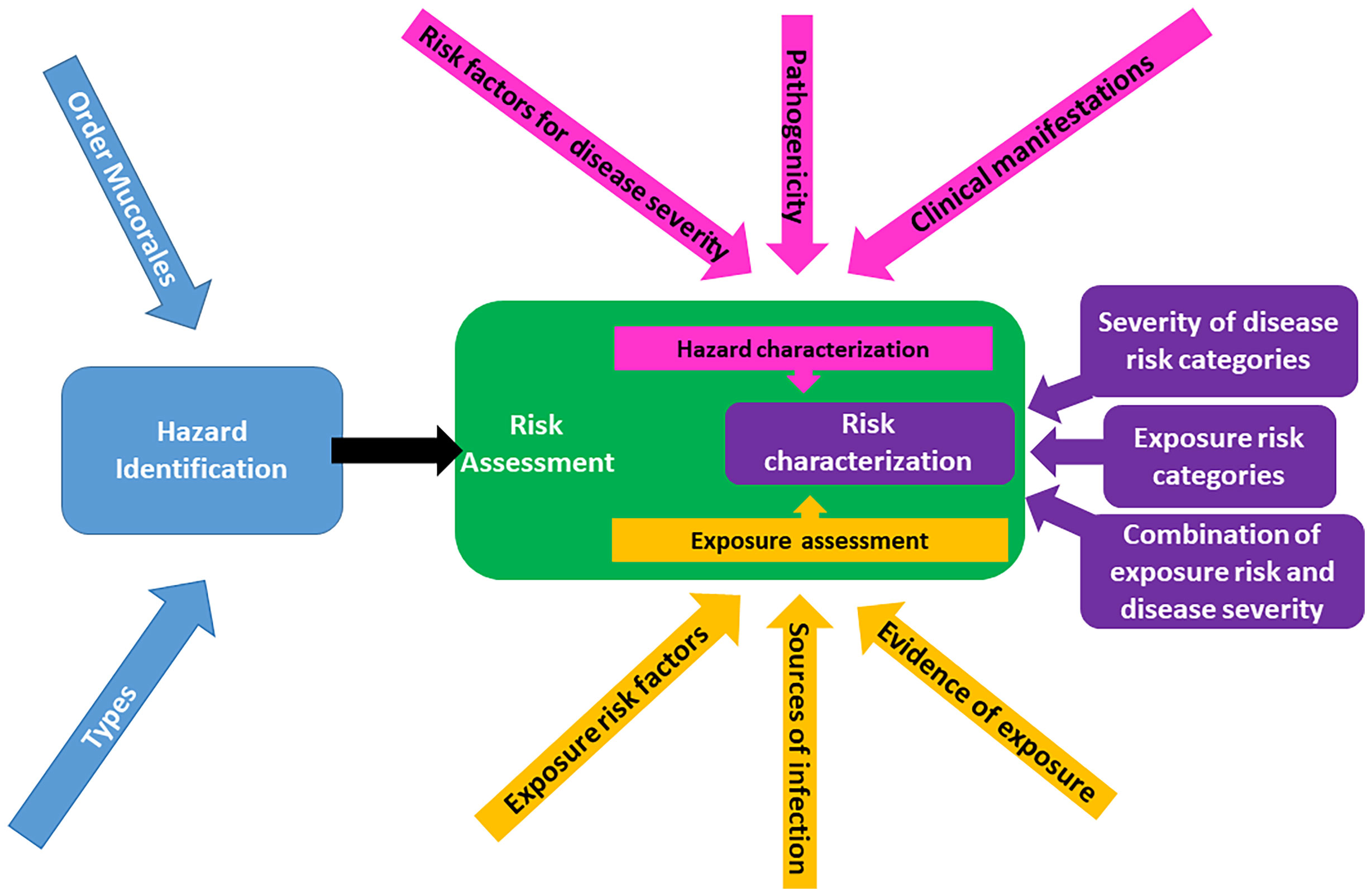
Figure 2 Structural framework for a qualitative risk assessment of mucormycosis in harbor porpoises, demonstrating the three major steps of the assessment: hazard identification (blue), risk assessment (green), and risk characterization (purple). Adapted from Food and Agriculture Organization of the United Nations/World Health Organization (2011) and Sharma et al. (2018).
Because relatively little information on fungal organisms in marine mammals exists, compared to terrestrial animal species or humans, data used to assess the risk were collected from published and unpublished sources. Information on health effects from, and exposure levels to, mucormycosis were extrapolated from the marine mammal literature where available, and otherwise from terrestrial species such as domestic animals and humans. Key words including ‘marine mammals’, ‘porpoise’, ‘dolphins’, ‘cetacean’, ‘seal’, ‘sea lion’, ‘pinniped’, ‘fungi’, ‘mucormycosis’, ‘zygomycosis’, ‘terrestrial’, ‘transmission’, and others were searched using PubMed and Web of Science in November 2019. Each publication was screened for its relevance to transmission, lesions, mortality, and clinical manifestation of mucormycosis. Health effects and exposure levels from other marine mammals and humans were extracted from the publications for guidance in developing the current risk assessment framework.
The categories of disease severity and probabilities of exposure were qualitatively assessed using a descriptive scale adapted from Zepeda-Sein (2002), according to four different levels of risk. The qualitative levels were determined subjectively according to the following definitions: negligible (the probability of occurrence of the event is sufficiently low to be ignored, or to be considered only in exceptional circumstances; low (the occurrence of an event is a possibility in a minority of cases); moderate (the occurrence of the event is a possibility in the majority of cases); and high (the occurrence of the event is probable), and were assigned disease severity scores of one, two, three, and four, respectively. The categories of exposure to Mucorales were expressed as prevalences (%) on the following scale: negligible; low; moderate; and high, likewise assigned to one through four, respectively. Lastly, the risk categories for disease severity and exposure risk were subsequently combined with each other to get a total combined qualitative estimation of risk of mucormycosis specific to harbor porpoise. The assignment of each total estimated risk value was also subjective and completed by the first author (SAN) only. The assignment of scores were derived from “clinical” manifestations of mucormycosis, of which the closest we could obtain were gross and histopathologic lesions and information from the few published clinical cases in marine mammals under human care. Baseline levels of iron and other heavy metals are unknown in this species, so could not be used as part of the hazard characterization. Hence, we ran the separate potential risk factor evaluation (regression) to identify possible factors to focus on in the future that could be used in future assessments.
3 Results
Cases (and controls) were represented by five males, five females (10 males, 10 females) and eight adults, two subadults (14 adults, six subadults), respectively (Supplementary Table 1). Mucormycosis was determined to be the primary cause of mortality in eight of the ten cases in which it was detected, and a secondary contributory to mortality in two of the ten. Many of these animals had other illnesses and/or poor body condition. Three adult females were lactating, but not pregnant, whereas another was pregnant, but not lactating. Multisystemic parasitism was common, and one porpoise was co-infected with encephalitis due to Toxoplasma gondii. One infected harbor porpoise was collected after researchers witnessed it being attacked and partially consumed by transient killer whales; the attack itself was the cause of mortality, but we were unable to determine the extent of the fungal infection from the remains.
3.1 Risk factor analysis
3.1.1 Matched case-control study
Assessment for collinearity left the following potential risk factors available for univariate analyses: sex, iron (Fe), copper (Cu), zinc (Zn), season, mean annual precipitation the year preceding the stranding, mean annual sea surface temperature the year of the stranding, year of stranding, body condition, maximum blubber thickness at necropsy (maxblub), sum of DDTs (sumDDT), sum of the PCB congeners 105, 118, 156 (sumCB105118156), the ratio of sum PCB/sum HCH (ratioPCB/HCH), the ratio of the sum PCB/sum PBDE (ratioPCBPBDE), presence of co-morbidities, the degrees of latitude north or south relative to mid-Salish Sea (latitude 48°N), straight length, and lipid percent in the blubber. Following univariate analyses, the full conditional logistic model contained the independent variables Fe, Cu, Zn, blubthick, and ratioPCBPBDE (Table 1). No interaction terms were deemed influential during model development. The best model fit based on AIC was the model with the variables Fe, blubber thickness, and the ratio of the sum of PCB/sum PBDE.
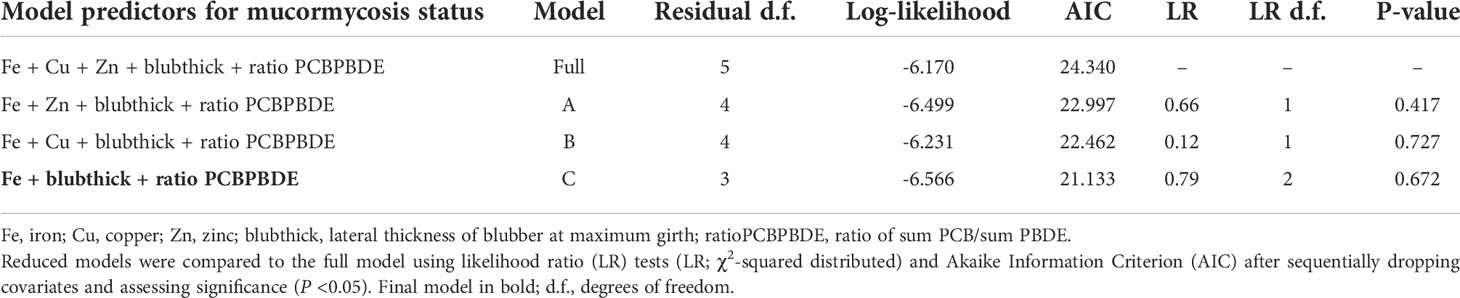
Table 1 Evaluation of conditional logistic regression models of mucormycosis case status (case or control).
The ORs serve as an approximation of “relative risk”. For example, an increase in Fe by a unit increases by two percentage points the probability that a harbor porpoise had mucormycosis (Table 2). Though a small percentage increase in probability, the small sample size and other unknown covariates may dampen the strength of the association between Fe levels and mucormycosis. The variable blubber thickness was marginally significant and may represent a biological surrogate for depletion of stored contaminants from the blubber as it is utilized that are released into the blood stream, along with the possibility of metabolism of these contaminants into compounds with higher toxicity, such as hyrdroxy-PCBs and -PBDEs.

Table 2 Maximum-likelihood estimates and odds ratio (OR) from fitting final conditional logistic regression model for a case-control study, matched on age class, to evaluate variation in mucormycosis status (case or control) associated with iron (Fe), blubber thickness (cm) at necropsy (blubthick), and the ratio of the sum PCB/sum PBDE (ratioPCBPBDE) in harbor porpoise (Phocoena phocoena) in the Salish Sea.
3.1.2 Evaluation of contaminants (multiple linear regression)
Ten models of MLR were created that evaluated the relationship between the various types of POPs and the independent variables: sex, length, age class, season, blubber thickness, mucormycosis status, year of stranding, latitude north or south relative to mid-Salish Sea (latitude 48°N), and lipid percent of the blubber sample (Supplementary Table 2). The association between POP type and the independent variables was significant multiple times for sex, age class, and blubber thickness. Although we explored a number of contaminant sums and ratios the only one that showed any significant association with mucormycosis was for the sum of the PCB congeners 105, 118, 156 (P < 0.001). When the POP was a ratio of two contaminants, sex was still often significant, but the latitude relative to mid-Salish Sea was significant for three of the four ratios.
3.2 Qualitative risk assessment
The results from the assessment follow the flow diagram depicted in Figure 2 with each section below referring to the corresponding section in the diagram.
3.2.1 Hazard identification
The resulting risk analysis framework (Figure 2) was based only on cases of mucormycosis in harbor porpoise, due to the paucity of data in other affected species, such as harbor seals and killer whales. In the present assessment, the hazard is identified as mucormycosis (Figure 2, blue boxes).
3.2.1.1 Types
Fungal speciation was determined in five cases and has been described previously (Huggins et al., 2020). The results of PCR sequencing of frozen tissues detected three cases of Rhizomucor pusillus and one of Lichtheimia corymbifera. Culture of tissues from one porpoise resulted in scant growth of the species Cunninghamella bertholletiae, which was identified by phenotypic characterization, and DNA sequencing.
3.2.1.2 Transmission modes, sources, and seasonality
Although the major mode of transmission in many fungi is aerosolization of their sporangiophores, the mode(s) of transmission and seasonality for harbor porpoises remains undetermined. The modes were assumed to include inhalation of airborne spores, ingestion, or disruption of the cutaneous skin or mucosal barrier.
3.2.2 Risk assessment
The risk assessment (Figure 2 – green box) component of the assessment framework was composed of three parts: 1) hazard characterization; 2) exposure assessment; and 3) risk characterization, the results of which follow this order.
3.2.2.1 Hazard characterization
Characterizing the hazard (Figure 2 – pink boxes) resulted a summary of the known effects of mucormycosis in marine mammals based on published reports, to determine the pathogenicity and risk factors for mucormycosis disease severity. The most common gross lesions noted in the present porpoise cases were associated with respiratory disease and associated lymph nodes, and if disseminated, included masses in other organs such as brain, kidney, and spleen among other organs. Histologically, vascular disease was noted in the present harbor porpoise cases. These lesions were similar to those noted in the marine mammal literature. However, there were relatively few reports of mucormycosis in marine mammals, many of which occurred in stranded animals such as the present cases, which are often affected with multiple contributors to mortality. This resulted in potentially confounding results of disease interpretation and uncertainties as to the contribution of Mucorales species to specific lesions.
3.2.2.1.1 Clinical manifestations
Clinical signs of infection vary depending on affected organs and the severity of infection. Given the inability to clinically observe free-ranging harbor porpoises affected with mucormycosis antemortem, we report lesions noted on necropsy and histological examinations. Of the ten mucormycosis cases in our study, three were in poor and 5 in fair body condition, respectively (Supplementary Table 1). In addition, the cases presented with fungal lesions in the brain (8/10), lymph nodes of various locations (5/10), and the lungs (7/10), and if observed alive, would have likely manifested clinical signs related to these organ pathologies. All 30 study animals tested negative for Brucella spp. and herpes-/morbilliviruses. The two most common co-morbidities detected in the study animals (n = 10 cases/20 controls) were parasitism, most frequently liver flukes and/or lungworms, as a contributory cause of death (n = 5/13) and infectious disease due to bacteria or viruses other than Brucella or herpes-/morbilliviruses (n = 1/7) (Supplementary Table 1). Protozoal organisms were contributory causes of death in one case (morphologic features most consistent with Toxoplasma gondii) and one control (unspecified protozoa).
Microscopically, lesions may vary depending on location and chronicity. Acute lesions are associated with necrosis of small vessel walls, thrombosis, and fungal elements within the thrombi, vessel walls and adjacent tissue, with associated hemorrhage, tissue necrosis and neutrophilic or eosinophilic inflammation. Inflammation becomes pyogranulomatous with lesion chronicity (see Isidoro-Ayza et al., 2014; Huggins et al., 2020). In the case that was co-infected with suspected T. gondii, at least three separate infectious disease processes were present, including pulmonary nematodiasis, a mycotic abscess in the brain, and meningoencephalitis associated with the protozoa. Another case presented with at least two separate infectious processes. Most of the pulmonary lesions were attributed to nematodiasis, but a large pulmonary abscess and the intraabdominal abscess were due to zygomycosis. Freeze artifact was substantial in this specimen and, in some areas, impeded microscopic interpretation. Though it was difficult to definitively determine the porpoise’s nutritional status due to freeze artifact, the animal may have been in suboptimal nutritional status likely due to the underlying infectious processes. Many of the microscopic lesions in the cases confirmed mucormycosis and the grossly observed parasitism.
3.2.2.1.2 Risk factors for disease severity
In marine mammals, risk factors for mucormycosis may include underlying illness such as co-infection with viruses, malnutrition, elevated chemical contaminant loads, pregnancy, traumatic injuries, and excess iron. In harbor porpoises, detailed information is lacking on current POP loads and elemental metal accumulation, as well as the relation of these factors to the occurrence of mucormycosis in this species. Immunosuppressive or tissue damaging viral infections such as herpes- and morbillivirus can predispose a marine mammal to other pathogens such as fungi (Barrett et al., 1993; Arbelo et al., 2010; Van Bressem et al., 2014). Neither of these viruses was detected in the harbor porpoise cases of the present study. Given that normal ranges of metal and trace elements are unknown in most marine mammal species, including those of the Pacific Northwest, regional differences have been noted in a few studies in harbor seals (Calambokidis et al., 1984; Calambokidis et al., 1991; Akmajian et al., 2014), and may be the case with harbor porpoises.
3.2.2.2 Exposure assessment
This portion of the qualitative risk assessment (Figure 2 – gold boxes) evaluated the likelihood that the susceptible porpoise(s) would come into contact with the hazard (e.g., Mucorales fungi) that could potentially facilitate transmission; in other words, estimate the likelihood of susceptible animals being exposed to the fungi. The following sections list the relevant biological, ecological and geographical factors and the associated assumptions.
3.2.2.2.1 Exposure risk factors
None of the published case reports of mucormycosis included in this assessment directly studied risk factors for exposure to Mucorales in harbor porpoise. Therefore, we extrapolated from risk factors identified in other marine mammal species, other porpoise infectious diseases, and humans, and assumed they likely represented risk factors in harbor porpoise. Without numerical values to quantify exposure risk, the results of exposure risk are presented in terms of qualitative values. For example, the general likelihood of exposure for Mucorales as with most fungal agents was considered moderate to high due to the ubiquitous presence of these pathogens in the environment. Fungi in general are not considered to be transmitted between individuals, so close physical interaction with other marine mammal species in the Salish Sea would not be a risk factor for exposure. Chronic exposure to heavy metals such as mercury, selenium, and zinc may predispose to immunosuppression and thus be associated with increased susceptibility to infectious disease, so the risk may be considered at least moderate. The POP levels measured in this study’s porpoises are not unexpected for the Salish Sea at the trophic level that porpoises feed. However, depending on their site fidelity and prey migratory patterns, porpoises may be spending more time or feeding on prey in more urbanized areas in the southern part of Puget Sound. Therefore, these individuals will be exposed to higher levels of POPs, on average compared to those spending more time in northern Puget Sound. Exposure may only require a single respiration or ingestion of the organism in an immunosuppressed or otherwise stressed animal; therefore, mitigating exposure will be very challenging.
3.2.2.2.2 Sources of exposure
Routes of exposure for Mucorales in harbor porpoise and other marine mammals is not completely known, but the fungi could be introduced into the marine ecosystem from terrestrial sources (Richardson, 2009). With the predominant mode of acquisition of these fungal infections presumed to be inhalation, this route was assumed to be the case for the present cases. However, we could not rule out the possibility the fungi alternatively entered through trauma to the skin or mucosal barrier from injuries such as interspecific interactions or predatory trauma. Although it is also possible a marine mammal could become infected by ingestion of prey containing sporangiospores, ingestion is more likely to occur from direct incidental swallowing of marine water containing sporangiospores during acquisition or ingestion of prey, and could have occurred in our case animals.
A monthly survey of ~80 sites throughout Puget Sound over a seven-month period (April through October) of pelagic seawater at a six-meter depth detected only ten positives out of 547 samples using a dual detection quantitative PCR specifically for Rhizomucor and Lichtheimia (Salehi et al., 2016; LDR, unpublished data). Positive sites were distributed across the geographic extent of Puget Sound, and the majority occurred in May and August. Although these fungal genera may have low frequency in the pelagic water column of harbor porpoise habitat, surface water may have higher levels of airborne spores deposited as fallout. As previously hypothesized for porpoises, disturbance of soil or detritus by environmental or climatic events, may introduce spores into the marine environment by winds or water runoff from terrestrial sources (Huggins et al., 2020).
3.2.2.2.3 Evidence of exposure
Few studies have documented exposure or clinical disease in live, free-ranging marine mammals. Cunninghamella bertholletiae was cultured from the blowhole and stomach of a presumed healthy, wild bottlenose dolphin (Tursiops truncatus) sampled during a microbial culture survey in the waters off Charleston, South Carolina (USA) (Morris et al., 2010). Two cases of nasal mucormycosis were documented in harbor seal pups presenting to rehabilitation, with concurrent herpesvirus infection, in Vancouver, British Columbia (Canada) (Huggins et al., 2020).
3.2.2.3 Risk characterization
The next three subsections collectively formed the risk characterization (Figure 2 – purple boxes). The first two subsections, severity of exposure to the agent Mucorales and severity of mucormycosis, which were approximated, were combined to calculate an overall estimate of risk associated with mucormycosis, which essentially estimates the consequences of the hazard (i.e., mucormycosis) occurring in Salish Sea harbor porpoise.
3.2.2.3.1 Risk categories for exposure (exposure assessment)
Though the incidence of mucormycosis is increasing worldwide, its prevalence and incidence are difficult to estimate since it is not a reportable disease, is currently considered rare, and the risk varies widely in different populations of humans and animals (Gomes et al., 2011). We assumed similar low incidences as observed in humans. Seroprevalence data on exposure of marine mammals to Mucorales are poorly defined. For this study, we extracted prevalence data from those reported in other marine mammal species, and used those values in the assessment as part of characterizing the overall risk of mucormycosis. Values ranged from < 0.025% in California (Huckabone et al., 2015) and 0.23% in the Baltic Sea (Wünschmann et al., 1999) to 1.16% in the Salish Sea (Huggins et al., 2020). Thus, the categories of exposure to Mucorales were expressed as prevalences (%) on the following scale: negligible (0-0.1%); low (0.2-0.5%); moderate (0.6-0.9%); and high (> 0.9%) (Table 3). Based on these published prevalences, we assumed a moderate to high incidence, and thus prevalence, in harbor porpoises, and considered exposure risk to be moderate (prevalence 0.6-0.9%; Score 3) or high (prevalence > 0.9%; Score 4).
3.2.2.3.2 Risk categories for disease severity (hazard characterization)
Categorizing mucormycosis disease severity, based on the presence of gross and microscopic lesions, resulted in four categories: negligible (1), low (2), moderate (3), or high (4) (Table 3). Though clinical signs have not been documented in harbor porpoises, they have been observed in a few captive animals, so these categories assumed similar clinical signs would also be present in porpoises if ante-mortem observation was possible. Associated disease, without overt clinical disease, has been occasionally reported in Salish Sea harbor porpoises (Huggins et al., 2015). The severity of lesions observed in Salish Sea cases is such that it is assumed clinical signs were present, but not observed in the field; therefore, a risk category of (4) is assigned.
3.2.2.3.3 Overall risk characterization (combination of disease severity and risk of exposure scores)
Overall risk characterization scores were calculated by multiplying the disease risk scores by the exposure scores (from Table 3). The resulting overall risk scores could range from 1 to 16, with 16 being the worst possible outcome (Table 4 and Figure 3). Insufficient data are available to determine any finer scale risk scores. For Salish Sea harbor porpoise, a final risk score of 6-8 (Table 4), or low-moderate risk, was calculated because of the range of presentations of disease severity in this species. For SRKWs, calculated a final risk score of eight (Table 4), or moderate risk. However, based on information from mucormycosis in humans and other animals, if these two species have elevated iron levels and/or POP levels, and are immunocompromised by other disease processes, are likely at higher risk for developing moderate to severe disease from mucormycosis. There are data gaps that give this risk assessment a relatively high level of uncertainty such as poor knowledge of mucormycosis prevalence in Salish Sea cetaceans.
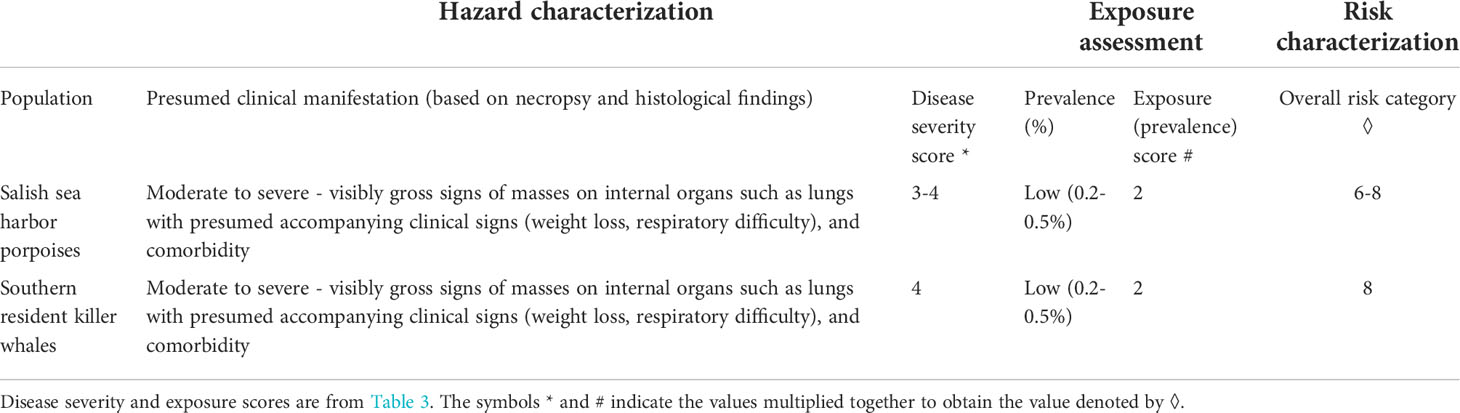
Table 4 Overall risk characterization score for harbor porpoise in the Salish Sea and southern resident killer whales, for comparison.
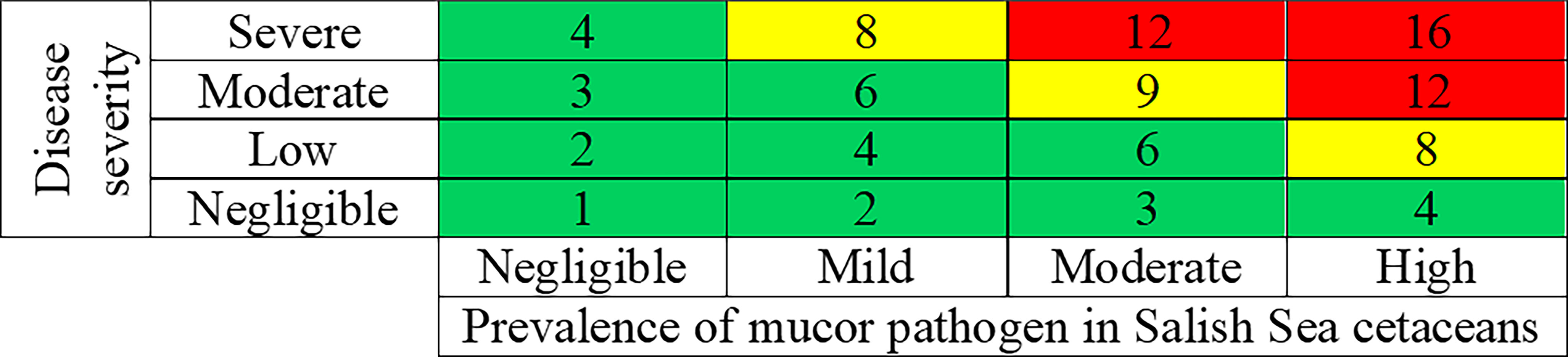
Figure 3 Risk matrix for combining the results of the disease severity scores (from Table 3) and the exposure scores (from Table 3), resulting in overall risk characterizations scores for mucormycosis in harbor porpoise. Green, yellow and red, respectively, represent negligible to low (combined together), moderate, and high risk.
4 Discussion
We conducted a qualitative assessment of the risk of acquisition of mucormycosis in harbor porpoises between 2012-2019 in the Salish Sea. Results of the risk factor analysis indicated that elevated levels of iron in the tissues (and possibly blood), decreasing blubber thickness and decreasing ratio of total PCBs/PBDEs may be associated with mucormycosis and might serve as factors to include in future qualitative risk assessments for this disease. Based on ‘clinical’ (i.e., gross and histopathologic lesions) signs, and likely prevalences of exposure to the fungal agents, harbor porpoise, and thus killer whales, are currently at low to moderate risk for mucormycosis. The study provided an opportunity to obtain estimates of the potential overall risk of mucormycosis in harbor porpoises, and more importantly, to attribute qualitative risk rankings to the various components of the assessment such as disease severity and exposure risk. Our findings indicated that Salish Sea harbor porpoise may currently be at increased risk; however, there are a very limited amount of published data available so perceived risk may change in the future as more data are gathered on the ecology of this disease in harbor porpoise and their urban environment. However, seals and killer whales may be as susceptible to fungal disease as harbor porpoises and should not be ignored when assessing mucormycosis risk in the future. The study identifies data gaps that require attention and prioritization to increase the likelihood of future quantitative risk assessments.
Assessing the risks of mucormycosis in marine mammals is important from a health and conservation perspective. Given their coastal inland water home range and prey preferences, harbor porpoises in the Pacific Northwest are a good model to investigate pollutants and potential links to primary or opportunistic diseases, including mucormycosis. The emergence of Mucorales as a source of marine mammal mortality is of particular concern for nearby endangered cetacean populations, such as southern resident killer whales. We identified risk factors (Fe overload and potentially contaminants) that should be more thoroughly investigated for future monitoring. Baseline porpoise and environmental prevalence data will be helpful to detect changes in the presence of this fungal organism in the Salish Sea ecosystem linked to anthropogenic or environmental forces. The presence of fungal species in marine waters does not appear to be high (∼1,100 fungal species retrieved exclusively from the marine environment), with a relatively small percentage of described species associated with marine environments (Amend et al., 2019). Conspicuously little is known about the diversity and ecological functions of fungi in marine ecosystems, compared to their bacterial counterparts. If the pressure from fungi is not large, susceptibility to potentially pathogenic fungi in the host may be greater compared to bacteria because the host is not exposed to the former as often as the latter, and thus may be naïve to infections from fungi. Interactions among fungi and other marine biota likely have considerable implications extending beyond the individual host or local community (e.g., influencing the ocean geochemistry) (Amend et al., 2019).
Comprehensive health profiles demonstrate that the vulnerability of these animals to infectious diseases such as mucormycosis may be influenced by susceptibility to pathogens and the ability to cope with the infection (Hall et al., 2006; Egan and Gardiner, 2016; Hodges and Tomcej, 2016; Raverty et al., 2017). Pollutant loads in higher trophic level feeders such as harbor porpoise can reflect environmental burdens. Harbor porpoises and other regional marine mammals have accumulated POPs, such as PCBs and PBDEs (Calambokidis and Barlow, 1991; Ross et al., 2013). Though key viruses such as herpes- and morbillivirus were not detected in any of the study animals, two harbor seals were reported with nasal mucormycosis were co-infected with phocid herpesvirus (Huggins et al., 2020). A killer whale was co-infected with disseminated mucormycosis and a herpesvirus, distinct to phocine herpesvirus (Abdo et al., 2012b). Co-infection with bacterial microbes has been documented in animals affected with mucormycosis and may play a role in susceptibility to or disease severity (Wünschmann et al., 1999; Robeck and Dalton, 2002; Abdo et al., 2012a; Isidoro-Ayza et al., 2014; Huggins et al., 2020). In cases of disseminated mucormycosis lesions may also be seen in other organs such as the kidney (Sosa et al., 2013; Huggins et al., 2020), skeletal or cardiac muscle (Best and McCully, 1979; Wünschmann et al., 1999; Robeck and Dalton, 2002; Naota et al., 2009; Barnett et al., 2011; Abdo et al., 2012b; Huggins et al., 2020), central nervous system (brain) (Wünschmann et al., 1999; Robeck and Dalton, 2002; Barnett et al., 2014; Isidoro-Ayza et al., 2014; Huggins et al., 2020), and skin (Robeck and Dalton, 2002; Huggins et al., 2020).
The risk assessment identified several knowledge gaps which should be addressed and are similar to those identified for Toxoplasma gondii infection in Arctic beluga whales (Delphinapterus leucas) in Canada (Sharma et al., 2018). The gaps include major transmission routes of the various Mucorales species to marine mammals, risk factors that promote infection and establishment of the fungi in the animal, the role of terrestrial species, including humans, in contributing to the environmental distribution of the fungi, and variation in Mucorales genotypes within harbor porpoises compared to other marine mammal species including southern resident killer whales (Huggins et al., 2020). Mucorales spores are easily aerosolized and dispersed by the air and insects, and are taken up by the human or animal via inhalation, ingestion, or through disruption of the cutaneous skin or mucosal barrier, with the number of airborne spores dependent on the presence of climatic conditions that favor their dispersal and growth (Richardson, 2009). Mucormycosis agents are typically found in soil and dust, composting vegetation, on rotting fruit, during heavy excavation and construction, and in air conditioning filters. The increased temperatures present in composting organic substrates are selective for thermophilic species, such as some species of Absidia, Mucor, Rhizopus, and Rhizomucor. Other potential sources of Mucorales spores include dog skin, bird feathers (e.g., poultry), rodent feces and natural disasters such as storms (Cabañes et al., 1996; Saidi et al., 2000; Lim, 2005; Stejskal et al., 2005; Richardson, 2009). Spatiotemporal investigations of the role of anthropogenic disturbance of soil and land use changes around the Salish Sea region are also needed to understand the ecology of these fungi. Monitoring mycobiomes along the coastline can help determine if clinically relevant fungal species are present and understand their community and environmental dynamics (Nilsson et al., 2019; Wunderlich, 2020). Though environmental sampling specific to fungi has not been conducted in the Puget Sound region, studies in other geographic regions revealed the following mucor genera: Mucor, Rhizopus, Lichtheimia, and Cunninghamella as being some of the most commonly isolated (Calvo et al., 1980; Mousavi, 2018). As currently observed and projected global temperatures are expected to rise, and shifts in precipitation patterns and extreme climatic events increase in frequency globally (IPCC, 2014) possible shifts in fungal risk for wildlife, including marine mammals may increase.
Mucormycosis has not previously been regarded as having a seasonal occurrence. For some molds in multiple United States geographical locations, atmospheric concentrations of spores were highest in the summer and autumn, while lowest in winter and spring (Shelton et al., 2002). In addition, results from other studies that specifically targeted mucormycosis suggest the organisms, and thus cases, follow the same general seasonal pattern (Talmi et al., 2002; Al-Ajam et al., 2006; Sivagnanam et al., 2017). Furthermore, a study of dog skin biota revealed a peak spore prevalence of Rhizopus and Mucor species in the summer and autumn, respectively (Cabañes et al., 1996). The predominant mode of acquisition of these fungal infections is presumed to be via the respiratory tract (Gomes et al., 2011). A few air sampling studies have detected Mucorales species, but little testing for Mucorales in marine ecosystem waters has been done (Raverty et al., 2017). Environmental air samples, obtained outdoors throughout an aquarium with cases of Aspergillus fumigatus in their collection of beluga whales were positive for Mucor spp. (Young et al., 1999). The ubiquitous nature and small size of Mucorales sporangiospores allows them to remain airborne for prolonged periods, which can increase the exposure risk. Though exposure to Mucorales sporangiospores is considered likely from the air during inhalation at the sea surface microlayer (Raverty et al., 2017), the fungi can also enter through trauma to the skin or mucosal barrier from injuries such as interspecific interactions, predatory trauma, or injections (Vainrub et al., 1988; Lim, 2005; Nakagun et al., 2018; Huggins et al., 2020). Ingestion of Mucorales fungi in the infected tissues of prey items can be another possible mode of exposure for carnivorous marine mammals. Gastritis associated with a nematode infection in the first stomach compartment of a harbor porpoise was suspected to be the origin of entry for systemic mucormycosis due to a Rhizopus spp. (Wünschmann et al., 1999). Cases of mucormycosis have been reported in immunosuppressed humans who ingested food or medicine contaminated with sporangiospores (Oliver et al., 1996).
Clinical signs attributed to mucormycosis have been reported in marine mammals (Robeck and Dalton, 2002; Naota et al., 2009; Barnett et al., 2011; Abdo et al., 2012a; Abdo et al., 2012b; Sosa et al., 2013; Barnett et al., 2014; Nakagun et al., 2018); however, clinical mucormycosis has not been reported in harbor porpoise. This is due to the fact that few harbor porpoise are kept in captivity or are rehabilitated as lesions associated with the fungi have been observed in dead stranded animals within the Salish Sea. It is likely that species of Mucorales would be detected in live, free-ranging Salish Sea harbor porpoise if their respiratory and gastrointestinal systems were sampled (Morris et al., 2010), and based on the presentation of live-stranded harbor seal pups with mucormycosis in a rehabilitation setting (Huggins et al., 2020). Grossly, mucormycosis lesions appear similar to other types of systemic fungal infections and consisted of variably sized often cavitating masses with a pulmonary predilection as noted in case reports of other affected marine mammal species (Kaplan et al., 1960; Best and McCully, 1979; Wünschmann et al., 1999; Barnett et al., 2011; Abdo et al., 2012a; Huggins et al., 2020). Clinical signs observed in marine mammals in managed care are nonspecific and include inappetence (Robeck and Dalton, 2002; Naota et al., 2009; Abdo et al., 2012a; Abdo et al., 2012b; Barnett et al., 2014), respiratory difficulties (Abdo et al., 2012a; Nakagun et al., 2018), renal failure (Sosa et al., 2013), dermatological (Robeck and Dalton, 2002; Barnett et al., 2011) and neurological abnormalities that include seizures, abnormal motor function, nystagmus, or dull mentation (Barnett et al., 2011; Nakagun et al., 2018). These neurological presentations may render harbor porpoise, and other marine mammals, susceptible to predation, stranding, or trauma from vessel or propeller strikes. Furthermore, inter- or intraspecific trauma to cutaneous or mucosal barriers to animals, such as commonly encountered in the daily lives of marine mammals, could serve as a portal for entry of Mucorales species, of which several have a propensity for cutaneous or subcutaneous tissues as well as from inhalation of the organism at the sea surface microlayer (Robeck and Dalton, 2002; Barnett et al., 2011; Gomes et al., 2011; Raverty et al., 2017; Huggins et al., 2020). Such dermal infections by mucormycosis can be a risk to marine mammal species in the Salish Sea, especially those under pressure from other sources of mortality and reproductive stressors such as the SRKW (Raverty et al., 2017).
Animals in captive aquatic facilities may be at increased risk because fungi are known to contaminate wet environments even after disinfection, the animals live in close contact with each other and share husbandry staff, food preparation areas, and ambient air (Young et al., 1999; Barnett et al., 2011). In humans, patients with hematological malignancies, immunosuppression, diabetes, and chronic corticosteroid therapy are at risk for disseminated infection caused by mucormycosis. However, some Mucorales species can infect immunocompetent individuals, usually after traumatic injuries or injections are administered (Robeck and Dalton, 2002; Nakagun et al., 2018). Routes of exposure for Mucorales in harbor porpoise and other marine mammals is not completely known, but the fungi could be introduced into the marine ecosystem from terrestrial sources (Richardson, 2009).
The diagnosis of mucormycosis is difficult in free-ranging animals and is typically detected in moderate to severe infections identified at necropsy. Blow sampling is a potential way to detect pathogens in free-ranging cetaceans; however, smaller cetaceans such as porpoises might be more challenging to sample compared to larger species such as killer whales (Raverty et al., 2017). Blow sampling by drones offers a potential route to sample respiratory tracts for fungal organisms. This type of sampling helps with epidemiological studies to further investigate risk factors for mucormycosis. However, it is logistically and financially challenging to screen wild species for the purpose of obtaining high quality samples, so dead stranded animals will continue to be the prime source of tissues to study mucormycosis. Application of tools such as next-generation sequencing hold potential to provide more rapid and lower cost detection capabilities for more widespread environmental and tissue fungal screening around the Salish Sea (Zoll et al., 2016).
This matching case-control study results highlight the highly likely role that elevated liver Fe may contribute to or modulate mucormycosis in harbor porpoise as it does in human serum (Ibrahim et al., 2012; Kousser et al., 2019). In humans and animals that are considered healthy, limiting serum iron availability is a universal host defense mechanism against many microbes, but particularly fungi such as Mucorales because these fungi grow poorly in normal serum unless iron from exogenous sources are added (Artiss et al., 1982; Boelaert et al., 1993; Ibrahim et al., 2012). Though individually, Cu and Zn were significantly associated with mucormycosis status, this was not retained when other independent variables were considered in the modeling. The loss of significance of these metals may be due to confounding by other factors not considered in this study or from the small study sample size, despite matching two controls to one case to help with study efficiency. Given the strong association of mucormycosis with Fe (Tables 1, 2), it would be worthwhile to further investigate the effect of other metals on mucormycosis. The interpretation of iron levels relative to mucormycosis should be interpreted conservatively, as iron is sequestered following emaciation (a potentially immunosuppressive condition), and is also sequestered in various tissues during chronic infections, to limit iron metabolism by the infectious agent. Therefore, trace mineral levels and fungal infection may be associated through more than one process. Although increasing blubber thickness measured at maximum girth was significantly associated with the odds of being a mucormycosis case, this finding was likely confounded by other factors such as comorbidities, POP levels, or other undetermined factors. The ratio of PCB/PBDE as a risk factor for mucormycosis, although not significant in the final model, is more difficult to interpret because it is unknown whether a smaller or larger ratio of these POPs would be clinically or biological relevant to the animal.
In humans, mucormycosis is most commonly detected in individuals with compromised immune systems from organ transplants, chemotherapy, or chronic diseases such as unregulated diabetes (El-Herte et al., 2012; Petrikkos et al., 2012). While few of these conditions are ever observed in free-ranging cetaceans, potentially compromised immunity in stranded marine mammals has been most commonly related to elevated POP levels, presence of infectious or chronic diseases and starvation/emaciation/limited prey availability, so there may be some precedent for being predisposed to mucormycosis. The final conditional logistic regression model and the multiple linear regression models (MLR) suggest that contaminants may play a role in the pathogenesis of mucormycosis. However, caution must be exercised when interpreting the modeling results due to the small sample size and the fact that association does not equate to causation. There may be other confounding factors such as individual variability in contaminant loads, the animal’s innate immunity, physiological make-up, and other biological determinants that can influence the association between contaminant load and mucormycosis status. The MLRs in which the ratio of two classes of POPs was the dependent output variable, and was significantly associated with distance away from the latitude (48°N) at mid-Salish Sea, suggest potential regional patterns (or gradients) of POPs present in harbor porpoises within the Sea (north versus south of the Sea’s midpoint at 48° N). If this is the case, then latitude may simply be a proxy measure of the likelihood of exposure to POPs, particularly if these animals have a strong site fidelity to a relatively small range (Elliser et al., 2018; Elliser and Hall, 2021). A geographic association between POPs and latitude may also be influenced by urbanization and use patterns in the Salish Sea and should be further investigated (Ross et al., 2013), as mucormycosis has been associated with soil disturbances during anthropogenic land use alterations (Miller and Lodge, 2007; Richardson, 2009).
This qualitative risk assessment and risk factor analysis are a strong start to identifying the underlying environmental and anthropogenic perturbations that increase the risk of developing this aggressive disease in a marine sentinel species like harbor porpoise. Underlying contributors to infection are likely prevalent and geographically widespread in the marine ecosystem, suggesting locally resident endangered populations such as southern resident killer whales could also be at significant risk. Because Mucorales pose a health risk within animal, human, and environments, studying this group of pathogens benefits from a One Health approach in which contributions stem from all three health sectors.
5 Conclusion
Though Mucorales are considered relatively uncommon, their recent emergence as a recognized pathogen in select marine mammal populations signals the need to identify environmental and host features in susceptible animals that predispose them to infection. Qualitative risk assessments are tools that help summarize the best available information and identify knowledge gaps for a pathogen, and aid in highlighting future areas of research. Even with high uncertainty, risk assessments can help health agencies, conservation groups, and natural resource managers develop strategies to help prevent or mitigate pathogen occurrence. Qualitative risk assessments can be updated as needed to aid in efforts to conserve highly endangered species such as southern residents, as well as inform those in the environmental and public health sectors within the One Health framework. The results of this study suggest that mucormycosis may pose an inordinately high risk to harbor porpoises (and potentially sympatric species such as southern resident killer whales) based on the detected prevalence and the severity of lesions observed at necropsy. However, the risk may be greater on an individual basis compared to the population as a whole, and may be related to other factors such as POP and trace metal burdens. Further investigations should include periodic updating of the risk assessment input data and more detailed studies of metal and contaminant trends and occurrence in apparently healthy animals.
Data availability statement
The original contributions presented in the study are included in the article/supplementary material. Further inquiries can be directed to the corresponding author.
Ethics statement
Ethical review and approval was not required for the animal study because the animals included in this study were documented during routine stranding response activities conducted under the authorization of the Department of Fisheries and Oceans (Canada) and the National Oceanic and Atmospheric Administration Marine Mammal Health and Stranding Response Program (United States). The authors adhered to all guidelines for animal use provided by the governing agencies. No living animals were used for this study; samples were collected during routine necropsy of deceased animals only.
Author contributions
SN, JH, and JC conceived the project design and scope. SN, JH, DL, JG, and AS conducted data collection and submitted samples for diagnostic testing. MG and SR performed the histological examinations. LR completed fungal molecular analyses. JB conducted contaminant analyses. SN performed the risk assessment and statistical analyses. SN was responsible for the drafting of the text and received extensive comment and review by all other authors. All authors contributed to the article and approved the submitted version.
Acknowledgments
The authors thank the regional stranding networks for assistance with carcass recovery and sample collection; particularly Erin Keene (Cascadia Research Collective), Garry Heinrich, Susan Berta and Sandy Dubpernell (Central Puget Sound Marine Mammal Stranding Network), and the volunteers of the San Juan County Marine Mammal Stranding Network. Support for stranding response and sample collection was provided by grants to some of the stranding response groups from the John H. Prescott Marine Mammal Rescue Assistance Grant Program. Funding for this study was provided by a grant from the SeaDoc Society, a program of the University California Davis Karen C. Drayer Wildlife Health Center.
Conflict of interest
The authors declare that the research was conducted in the absence of any commercial or financial relationships that could be construed as a potential conflict of interest.
Publisher’s note
All claims expressed in this article are solely those of the authors and do not necessarily represent those of their affiliated organizations, or those of the publisher, the editors and the reviewers. Any product that may be evaluated in this article, or claim that may be made by its manufacturer, is not guaranteed or endorsed by the publisher.
Supplementary material
The Supplementary Material for this article can be found online at: https://www.frontiersin.org/articles/10.3389/fmars.2022.962857/full#supplementary-material.
References
Abdo W., Kakizoe Y., Ryono M., Dover S. R., Fukushi H., Okuda H., et al. (2012a). Pulmonary zygomycosis with Cunninghamella bertholletiae in a killer whale (Orcinus orca). J. Comp. Path. 147, 94–99. doi: 10.1016/j.jcpa.2011.08.012
Abdo W., Kawachi T., Sakai H., Fukushi H., Kano R., Shibahara T., et al. (2012b). Disseminated mycosis in a killer whale (Orcinus orca). J. Vet. Diagn. Invest. 24, 211–218. doi: 10.1177/1040638711416969
Akaike H. (1974). A new look at the statistical model identification. IEEE T. Automat. Contr. 19, 716–723. doi: 10.1109/TAC.1974.1100705
Akmajian A., Calambokidis J., Huggins J. L., Lambourn D. (2014). Age, region, and temporal patterns of trace elements measures in stranded harbor seals (Phoca vitulina richardii) from Washington inland waters. Northwest Nat. 95, 83–91. doi: 10.1898/NWN13-26.1
Al-Ajam M. R., Bizri A. R., Mokhbat J., Weedon J., Lutwick L. (2006). Mucormycosis in the eastern Mediterranean: a seasonal disease. Epidemiol. Infect. 134, 341–346. doi: 10.1017/S0950268805004930
Amend A., Burgaud C., Cunliffe M., Edgcomb V. P., Ettinger C. L., Gutiérrez M. H., et al. (2019). Fungi in the marine environment: open questions and unsolved problems. mBio 10 (2), e01189–e01118. doi: 10.1128/mBio.01189-18
American Society for Microbiology (ASM) (2019). “One health,” in Fungal pathogens of humans, animals, and plants (Washington, DC: Report on an American Academy of Microbiology Colloquium).
Arbelo M., Sierra E., Esperón F., Watanabe T. T. N., Bellière E. N., Espinosa de los Monteros A., et al. (2010). Herpesvirus infection with severe lymphoid necrosis affecting a beaked whale stranded in the canary islands. Dis. Aquat. Organ. 89, 261–264. doi: 10.3354/dao02208
Artiss W. M., Fountain J. A., Delcher H. K., Jones H. E. (1982). A mechanism of susceptibility to mucormycosis in diabetic ketoacidosis: transferrin and iron availability. Diabetes 31 (12), 1109–1114. doi: 10.2337/diacare.31.12.1109
ASCOBANS (2010). HELCOM indicator fact sheet: Decline of the harbour porpoise (Phocoena phocoena) in the southwestern Baltic Sea (UN Campus, Bonn, Germany: 17th ASCOBANS Advisory Committee Meeting), 13 pp. Available at: http://www.ascobans.org/es/document/helcom-indicator-fact-sheetdecline-harbour-porpoisephocoena-phocoena-southwestern-baltic. AC17/Doc.7-04 (C).
Barnett J. E. F., Davison N. J., Thornton S. M., Riley P., Cooper T., Wessels M. E. (2011). Systemic mucormycosis in a hooded seal (Cystophora cristata). J. Zoo Wildl. Med. 42, 338–341. doi: 10.1638/2010-0185.1
Barnett J. F., Riley P., Cooper T., Linton C., Wessels M. (2014). Mycotic encephalitis in a grey seal (Halichoerus grypus) pup associated with Rhizomucor pusillus infection. Vet. Rec. Case Rep. 2, e000115. doi: 10.1136/vetreccr-2014-000115
Barrett T., Visser I. K., Mamaev L., Goatley L., van Bressem M. F., Osterhaust A. D. (1993). Dolphin and porpoise morbilliviruses are genetically distinct from phocine distemper virus. Virology 193 (2), 1010–1012. doi: 10.1006/viro.1993.1217
Bennett P. M., Jepson P. D., Law R. J., Jones B. R., Kuiken T., Baker J. R., et al. (2001). Exposure to heavy metals and infectious disease mortality in harbour porpoises from England and Wales. Environ. pollut. 112, 33–40. doi: 10.1016/S0269-7491(00)00105-6
Best P. B., McCully R. M. (1979). Zygomycosis (phycomycosis) in a right whale (Eubalaena australis). J. Comp. Path. 89, 341–348. doi: 10.1016/0021-9975(79)90023-9
Binder U., Maurer E., Lass-Flörl C. (2014). Mucormycosis – from the pathogens to the disease. Clin. Microbiol. Infect. 20(6), 60–66. doi: 10.1111/1469-0691.12566
Boelaert J. R., de Locht M., Van Cutsem J., Kerrels V., Cantinieaux B., Verdonck A., et al. (1993). Mucormycosis during deferoxamine therapy is a siderophore-mediated infection: in vitro and in vivo animal studies. J. Clin. Invest. 91, 1979–1986. doi: 10.1172/JCI116419
Bossart G. D. (2011). Marine mammals as sentinel species for oceans and human health. Vet. Pathol. 48, 676–690. doi: 10.1177/2F0300985810388525
Cabañes F. J., Abarca M. L., Bragulat M. R., Castellá G. (1996). Seasonal study of the fungal biota of the fur of dogs. Mycopathologia 133, 1–7. doi: 10.1007/BF00437092
Calambokidis J., Barlow J. (1991)Chlorinated hydrocarbon concentrations and their use for describing population discreetness in harbor porpoises from Washington, Oregon, and California. In: Marine mammal strandings in the united states. proceedings of the second marine mammal stranding workshop (Miami, Florida: U. S. Dept. Commer. NOAA Tech. Rep. NMFS 98). Available at: https://repository.library.noaa.gov/view/noaa/5978 (Accessed January 14, 2020).
Calambokidis J., Peard J., Steiger G. H., Cubbage J. C., DeLong R. L. (1984) Chemical contaminants in marine mammals from Washington state (U.S. Dept. Commer. NOAA Tech. Memo. NOS OMS 6). Available at: https://repository.library.noaa.gov/view/noaa/2794 (Accessed January 17, 2020).
Calambokidis J., Steiger G. H., Curtice C., Harrison J., Ferguson M. C., Becker E., et al. (2015). 4. biologically important areas for selected cetaceans within U.S. waters –west coast region. in biologically important areas for cetaceans within U.S. waters. Aquat. Mamm. 4 (1), 39–53. doi: 10.1578/AM.41.1.2015.1
Calambokidis J., Steiger G. H., Lowenstine L. J., Becker D. S. (1991)Chemical contamination of harbor seal pups in puget sound. U.S env. protect. agen. In: Report EPA 910/9-91-032. Available at: https://www.cascadiaresearch.org/publications/chemical-contamination-harbor-seal-pups-puget-sound (Accessed January 17, 2020).
Calvo M. A., Guarro J., Suarez G., Ramírez C. (1980). Air-borne fungi in the air of Barcelona (Spain). IV. various isolated genera. Mycopathologia 71, 119–123. doi: 10.1007/BF00440618
Carretta J. V., Oleson E. M., Lang A. R., Weller D. W., Baker J. D., Muto M. M., et al. (2017)2016 U.S. pacific marine mammal stock assessments. In: (U.S. Dept. Commer. NOAA Tech. Memo., NOAA-TM-NMFS-SWFSC-561). Available at: http://doi.org/10.7289/V5/TM-SWFSC-577 (Accessed October 13, 2019).
COSEWIC (2016) COSEWIC assessment and status report on the harbour porpoise phocoena vomerina, pacific ocean population (Canada. Ottawa: Committee on the Status of Endangered Wildlife in Canada). Available at: https://species-registry.canada.ca/index-en.html#/documents?documentTypeId=18&taxonomies=5&sortBy=sortDate&sortDirection=asc¤tPage=2&pageSize=10 (Accessed January 24, 2020).
Department of Fisheries and Oceans Canada (DFO) (2016) Necropsy results: Southern resident killer whale L95, October 5, 2016. Available at: http://www.pac.dfo-mpo.gc.ca/fmgp/speciesespeces/mammals-mammiferes/srkw-eprs-l95-eng.html (Accessed January 24, 2020).
Desforges J.-P. W., Sonne C., Levin M., Siebert U., De Guise S., Dietz R. (2016). Immunotoxic effects of environmental pollutants in marine mammals. Environ.Int 86, 126–139. doi: 10.1016/j.envint.2015.10.007
Dohoo I., Martin W., Stryhn H. (2003). “Detecting highly correlated (collinear) variables,” in Veterinary epidemiologic research. Eds. Dohoo I., Martin W., Stryhn H. (Charlottetown, PEI: AVC Inc), 388–389.
Egan S., Gardiner M. (2016). Microbial dysbiosis: rethinking disease in marine ecosystems. Front. Microbiol. 7. doi: 10.3389/fmicb.2016.00991
El-Herte R. I., Baban T. A., Kanj S. S. (2012). Mucormycosis: a review on environmental fungal spores and seasonal variation of human disease. Adv. Infect. Dis. 2, 76–81. doi: 10.4236/aid.2012.23012
Elliser C. R., Hall A. (2021). Return of the salish Sea harbor porpoise, Phocoena phocoena: knowledge gaps, current research, and what we need to do to protect their future. Front. Mar. Sci. 8. doi: 10.3389/fmars.2021.618177
Elliser C. R., MacIver K. H., Green M. (2018). Group characteristics, site fidelity, and photo-identification of harbor porpoises, Phocoena phocoena, in burrows pass, fidalgo island, Washington. Mar. Mamm. Sci. 34 (2), 365–384. doi: 10.1111/mms.12459
Evenson J. R., Anderson D., Murphie B. L., Cyra T. A., Calambokidis J. (2016)Disappearance and return of harbor porpoise to puget sound: 20-year pattern revealed from winter aerial surveys. In: Technical report, Washington department of fish and wildlife (Olympia, WA: Wildlife Program and Cascadia Research Collective). Available at: https://wdfw.wa.gov/publications/01787 (Accessed January 16, 2020).
Food and Agriculture Organization of the United Nations/World Health Organization (2011) FAO/WHO guide for application of risk analysis during food safety emergencies. Available at: https://apps.who.int/iris/handle/10665/44739 (Accessed February 19, 2020).
Geraci J. R., Lounsbury V. J. (2005). “Marine mammals ashore,” in A field guide for strandings (Galveston: Texas A&M University Sea Grant College Program Publication).
Gerwien F., Skrahina V., Kasper L., Hube B., Brunke S. (2018). Metals in fungal virulence. FEMS Microbiol. Rev. 42 (1), 1–21. doi: 10.1093/femsre/fux050
Gomes M. Z. R., Lewis R. E., Kontoyiannis D. P. (2011). Mucormycosis caused by unusual mucormycetes, non-rhizopus, -mucor, and -lichtheimia species. Clin. Microbiol. Rev. 24, 411–445. doi: 10.1128/CMR.00056-10
Hall A. J., Hugunin K., Deaville R., Law R. J., Allchin C. R., Jepson P. D. (2006). The risk of infection from polychlorinated biphenyl exposure in the harbor porpoise (Phocoena phocoena): A case–control approach. Environ. Health Persp. 114, 704–711. doi: 10.1289/ehp.8222
Hassan M. I. A., Voigt K. (2019). Pathogenicity patterns of mucormycosis: epidemiology, interaction with immune cells and virulence factors. Med. Mycol. 57, S245–S256. doi: 10.1093/mmy/myz011
Heller J., Kelly L., Reid S. W. J., Mellor D. J. (2010). Qualitative risk assessment of the acquisition of meticillin-resistant Staphylococcus aureus in pet dogs. Risk Anal. 30, 458–472. doi: 10.1111/j.1539-6924.2009.01342.x
Hodges E., Tomcej V. (2016). Is there a link between pollutant exposure and emerging infectious disease? Can. Vet. J. 57, 535–537.
Huckabone S. E., Gulland F. M. D., Johnson S. M., Colegrove K. M., Dodd E. M., Pappagianas D., et al. (2015). Coccidioidomycosis and other system mycoses of marine mammals stranding along the central California, USA coast: 1998-2012. J. Wildl. Dis. 51, 295–308. doi: 10.7589/2014-06-143
Huggins J. L., Garner M. M., Raverty S. A., Lambourn D. M., Norman S. A., Rhodes L. D., et al. (2020). The emergence of mucormycosis in free-ranging marine mammals of the pacific Northwest. Fron. Mar. Sci. 7. doi: 10.3389/fmars.2020.00555
Huggins J. L., Raverty S. A., Norman S. A., Calambokidis J., Gaydos J. K., Duffield D. A., et al. (2015). Increased harbor porpoise mortality in the pacific Northwest, USA: understanding when higher levels may be normal. Dis. Aquat. Organ. 115, 93–102. doi: 10.3354/dao02887
Ibrahim A. S., Spellberg B., Walsh T. J., Kontoyiannis D. P. (2012). Pathogenesis of mucormycosis. Clin. Infect. Dis. 54, S16–S22. doi: 10.1093/cid/cir865
IPCC (2014). “Climate change 2014: synthesis report,” in Contribution of working groups I, II and III to the fifth assessment report of the intergovernmental panel on climate change. Eds. Team C. W., Pachauri R. K., Meyer L. A. (Geneva, Switzerland: IPCC), 151pp.
Isidoro-Ayza M., Pérez L., Cabañes F. J., Castellà G., Andrés M., Vidal E., et al. (2014). Central nervous system mucormycosis caused by Cunninghamella bertholletiae in a bottlenose dolphin (Tursiops truncatus). J. Wildl. Dis. 50, 634–638. doi: 10.7589/2013-10-284
Jakob-Hoff R. M., MacDiarmid S. C., Lees C., Miller P. S., Travis D., Kock R. (2014)Manual of procedures for wildlife disease risk analysis. In: World organisation for animal health (Paris: Published in association with the International Union for Conservation of Nature and the Species Survival Commission, 2014). Available at: https://portals.iucn.org/library/sites/library/files/documents/2014-007.pdf (Accessed January 23, 2020).
Jefferson T. A., Smultea M. A., Courbis S. S., Campbell G. S. (2016). Harbor porpoise (Phocoena phocoena) recovery in the inland waters of Washington: estimates of density and abundance from aerial surveys 2013–2015. Can. J. Zool. 94, 505–515. doi: 10.1139/cjz-2015-0236
Kaplan W., Goss L. J., Ajello L., Ivens M. S. (1960). Pulmonary mucormycosis in a harp seal caused by Mucor pusillus. Mycopathol. Mycol. Appl. 12, 101–102. doi: 10.1007/BF02051389
Kershaw J. L., Hall A. J. (2019). Mercury in cetaceans: exposure, bioaccumulation and toxicity. Sci. Total Environ. 694, 133683. doi: 10.1016/j.scitotenv.2019.133683
Kershner J., Samhouri J. F., James C. A., Levin P. S. (2011). Selecting indicator portfolios for marine species and food webs: a puget sound case study. PloS One 6, e25248. doi: 10.1371/journal.pone.0025248
Knap A., Dewailly E., Furgal C., Galvin J., Baden D., Bowen R. E., et al. (2002). Indicators of ocean health and human health: developing a research and monitoring framework. Environ. Health Persp. 110, 839–845. doi: 10.1289/ehp.02110839
Kousser C., Clark C., Sherrington S., Voelz K., Hall R. A. (2019). Pseudomonas aeruginosa inhibits Rhizopus microsporus germination through sequestration of free environmental iron. Sci. Rep. 9, 5714. doi: 10.1038/s41598-019-42175-0
Krahn M. M., Herman D. P., Matkin C. O., Durban J. W., Barrett-Lennard L., Burrows D. G., et al. (2007). Use of chemical tracers in assessing the diet and foraging regions of eastern north pacific killer whales. Mar. Environ. Res. 63 (2), 91–114. doi: 10.1016/j.marenvres.2006.07.002
Leighton F. A. (2002). Health risk assessment of the translocation of wild animals. Rev. Sci. tech. Off. Int. Epiz. 21 (1), 187–195.
Lim P. L. (2005). Wound infections in tsunami survivors: a commentary. Ann. Acad. Med. Singapore 34, 582–585.
Migaki G., Jones S. R. (1983). “Mycotic diseases,” in Pathobiology of marine mammal diseases, vol. Vol. II . Ed. Howard E. B. (Boca Raton, FL: CRC Press), 1–29.
Miller R. M., Lodge D. J. (2007). “Fungal responses to disturbance: agriculture and forestry,” in Environmental and microbial relationships, vol. Vol. 4 . Eds. Kubicek C., Druzhinina I. (Berlin, Springer), 47–68. doi: 10.1007/978-3-540-71840-6_4
Monteiro S. S., Bozzetti M., Torres J., Tavares A. S., Ferreira M., Pereira A. T., et al. (2020). Striped dolphins as trace element biomonitoring tools in oceanic waters: accounting for health-related variables. Sci. Total Environ. 699, 134410. doi: 10.1016/j.scitotenv.2019
Moore S. E. (2008). Marine mammals as ecosystem sentinels. J. Mammal. 89, 534–540. doi: 10.1644/07-MAMM-S-312R1.1
Morin P. A., Forester B. R., Forney K. A., Crossman C. A., Hancock-Hanser B. L., Robertson K. M., et al. (2021). Population structure in a continuously distributed coastal marine species, the harbor porpoise, based on microhaplotypes derived from poor-quality samples. Mol. Ecol. 30 (6), 1457–1476. doi: 10.1111/mec.15827
Morris P. J., Johnson W. R., Pisani J., Bossart G. D., Adam J., Reif J. S., et al. (2010). Isolation of culturable microorganisms from free-ranging bottlenose dolphins (Tursiops truncatus) from the southeastern united states. Vet. Microbiol. 148, 440–447. doi: 10.1016/j.vetmic.2010.08.025
Mousavi B. (2018). Occurrence and species distribution of pathogenic mucorales in unselected soil samples from France. Med. Mycol. 56, 315–321. doi: 10.1016/j.mycmed.2015.06.044
Mouton M., Przybylowicz W., Mesjasz-Przybylowicz J., Postma F., Thornton M., Archer E., et al. (2015). Linking the occurrence of cutaneous opportunistic fungal invaders with elemental concentrations in false killer whale (Pseudorca crassidens) skin. Env. Microbiol. Rep. 7, 728–737. doi: 10.1111/1758-2229.12302
Nakagun S., Okazaki M., Toyotome T., Sugiyama N., Watanabe K., Horiuchi N., et al. (2018). Fatal pulmonary and cerebellar zygomycosis due to Rhizomucor pusillus in a ringed seal (Pusa hispida). Mycopathologia 183, 979–985. doi: 10.1007/s11046-018-0268-3
Naota M., Shimada A., Morita T., Kimura K., Ochiai K., Sano A. (2009). Granulomatous pericarditis associated with systemic mucormycosis in a finless porpoise (Neophocaena phocaenoides). J. Comp. Path. 140, 64–66. doi: 10.1016/j.jcpa.2008.09.006
National Oceanic and Atmospheric Administration (2020a) National data buoy center. Available at: https://www.ndbc.noaa.gov/station_page.php?station=46088 (Accessed February 13, 2020).
National Oceanic and Atmospheric Administration (2020b) National centers for environmental information. Available at: https://www.ncdc.noaa.gov/ (Accessed February 21, 2020).
Nilsson R. H., Anslan S., Bahram M., Wurzbacher C., Baldrian P., Tedersoo L. (2019). Mycobiome diversity: high-throughput sequencing and identification of fungi. Nat. Rev.Microbiol. 17 (2), 95–109. doi: 10.1093/clinids/22.3.521
NMFS (2008). Recovery plan for southern resident killer whales (Orcinus orca) (Seattle, WA: National Marine Fisheries Service).
NMFS (2016a) Southern resident killer whale (Orcinus orca) stranding event expert review summary, September 21, 2016. Available at: http://www.westcoast.fisheries.noaa.gov/publications/protected_species/marine_mammals/killer_whales/l95_expert_panel.pdf (Accessed January 18, 2020).
NMFS (2016b) Final report AHC case: 16-1760. Available at: http://www.westcoast.fisheries.noaa.gov/publications/protected_species/marine_mammals/killer_whales/l95necropsy.pdf (Accessed January 18, 2020).
NMFS West Coast Region (2016) Southern resident killer whales (Orcinus orca) 5-year review: summary and evaluation. Available at: https://repository.library.noaa.gov/view/noaa/17031 (Accessed April 3, 2022).
Nnadi N. E., Carter D. A. (2021). Climate change and the emergence of fungal pathogens. PloS Pathog. 17 (4), e1009503. doi: 10.1371/journal.ppat.1009503
Oliver M. R., Van Voorhis W. C., Boeckh M., Mattson D., Bowden R. A. (1996). Hepatic mucormycosis in a bone marrow transplant recipient who ingested naturopathic medicine. Clin. Infect. Dis 22, 521–524. doi: 10.1093/clinids/22.3.521
Palmer S., Brown D., Morgan D. (2005). Early qualitative risk assessment of the emerging zoonotic potential of animal diseases. BMJ 331, 1256–1260. doi: 10.1136/bmj.331.7527.1256
Petrikkos G., Skiada A., Lortholary O., Roilides E., Walsh T. J., Kontoyiannis D. P. (2012). Epidemiology and clinical manifestations of mucormycosis. Clin. Inf. Dis. 54 (S1), S23–S34. doi: 10.1093/cid/cir866
Rabinowitz P. M., Gardner H. S. Jr., Bantle J. A., De Rosa C. T., Finch R. A., Reif J. A., et al. (2005). Animals as sentinels of human environmental health hazards: an evidence-based analysis. Ecohealth 2, 26–37. doi: 10.1007/s10393-004-0151-1
Raverty S. A., Rhodes L. D., Zabek E., Eshghi A., Cameron C. E., Hanson M. B., et al. (2017). Respiratory microbiome of endangered southern resident killer whales and microbiota of surrounding sea surface microlayer in the eastern north pacific. Sci. Rep. 7, 394. doi: 10.1038/s41598-017-00457-5
Richardson M. (2009). The ecology of the zygomycetes and its impact on environmental exposure. Clin. Microbiol. Infect. 15, 2–9. doi: 10.1111/j.1469-0691.2009.02972.x
Robeck T. R., Dalton L. M. (2002). Saksenaea vasiformis and Apophysomyces elegans zygomycotic infections in bottlenose dolphins (Tursiops truncatus), a killer whale (Orcinus orca), and pacific white-sided dolphins (Lagenorhynchus obliquidens). J. Zoo Wildl. Med. 33, 356–366. doi: 10.1638/1042-7260(2002)033[0356:SVAAEZ]2.0.CO;2
Roden M. M., Zaoutis T. E., Buchanan W. L., Knudsen T. A., Sarkisova T. A., Schaufele R. L., et al. (2005). Epidemiology and outcome of zygomycosis: a review of 929 reported cases. Clin. Infect. Dis. 41, 634–653. doi: 10.1086/432579
Rose S., van der Laan T. J. (2009). Why match? Investigating matched case-control study designs with causal effect estimation. Int. J. Biostat. 5, 1. doi: 10.2202/1557-4679.1127
Ross P. (2002). The role of immunotoxic environmental contaminants in facilitating theemergence of infectious diseases in marine mammals. Hum. Ecol. Risk. Assess. 8, 277–292. doi: 10.1080/20028091056917
Ross P. S., Nöel M., Lambourn D., Dangerfield N., Calambokidis J., Jeffries S. (2013). Declining concentrations of persistent PCBs, PBDEs, PCDEs, and PCNs in harbor seals (Phoca vitulina) from the salish Sea. Prog. Oceanogr. 115, 160–170. doi: 10.1016/j.pocean.2013.05.027
Saidi S. A., Das P., Sikdar A. (2000). Keratinophilic fungi of poultry and their environment in India. Indian J. Comp. Microbiol. Immunol. Infect. Dis. 21, 49–55.
Salehi E., Hedayati M. T., Zoll J., Rafati H., Ghasemi M., Doroudinia A., et al. (2016). Discrimination of aspergillosis, mucormycosis, fusariosis, and scedosporiosis in formalin-fixed paraffin-embedded tissue specimens by use of multiple real-time quantitative PCR assays. J. Clin. Microbiol. 54, 2798–2803. doi: 10.1128/JCM.01185-16
Sharma R., Loseto L. L., Ostertag S. K., Tomaselli M., Bredtmann C. M., Crill C., et al. (2018). Qualitative risk assessment of impact of toxoplasma gondii on health of beluga whales, Delphinapterus leucas, from the Eastern Beaufort Sea, Northwest territories. Arctic Sci. 4, 321–337. doi: 10.1139/as-2017-0037
Shelton B. G., Kirkland K. H., Flanders W. D., Morris G. K. (2002). Profiles of airborne fungi in buildings and outdoor environments in the united states. Appl. Environ. Microbiol. 68, 1743–1753. doi: 10.1128/AEM.68.4.1743-1753.2002
Sivagnanam S., Sengupta D. J., Hoogestraat D., Jain R., Stednick R., Fredricks D. N., et al. (2017). Seasonal clustering of sinopulmonary mucormycosis in patients with hematologic malignancies at a large comprehensive cancer center. Antimicrob. Resist. Infect. Control 6, 123. doi: 10.1186/s13756-017-0282-0
Sosa M., Gamble K. C., Delaski K., Righton A. (2013). Clinical challenge: systemic Rhizopus microsporus infection with renal cavitation in a grey seal (Halichoerus grypus). J. Zoo Wildl. Med. 44, 1134–1138. doi: 10.1638/2013-0089R.1
Spellberg B., Edwards J. Jr., Ibrahim A. (2005). Novel perspectives on mucormycosis: pathophysiology, presentation, and management. Clin. Microbiol. Rev. 18, 556–569. doi: 10.1128/CMR.18.3.556-569.2005
Stejskal V., Hubert J., Kubátová A., Vánová M. (2005). Fungi associated with rodent feces in stored grain environment in the Czech republic. J. Plant Dis. Protect. 112, 98–102.
Talmi Y. P., Goldschmied-Reouven A., Bakon M., Barshack I., Wolf M., Horowitz Z., et al. (2002). Rhino-orbital and rhino-orbito-cerebral mucormycosis. Otolaryngol. Head Neck Surg. 127, 22–31. doi: 10.1067/2Fmhn.2002.126587
Tong S., Chern S.-W. W., Li Y., Pallansch M., Anderson L. J. (2008). Sensitive and broadly reactive reverse transcription-PCR assays to detect novel paramyxoviruses. J. Clin. Microbiol. 46, 2652–2658. doi: 10.1128/JCM.00192-08
Vainrub B., Macareno A., Mandel S., Musher D. M. (1988). Wound zygomycosis (mucormycosis) in otherwise healthy adults. Am. J. Med. 84, 546–548. doi: 10.1016/0002-9343(88)90282-3
Van Bressem M.–F., Duignan P. J., Banyard A., Barbieri M., Colegrove K. M., De Guise S., et al. (2014). Cetacean morbillivirus: current knowledge and future directions. Viruses 6, 5145–5181. doi: 10.3390/v6125145
Van Devanter D. R., Warrener P., Bennett L., Schultz E. R., Coulter S., Garber R. L., et al. (1996). Detection and analysis of diverse herpesviral species by consensus primer PCR. J. Clin. Microbiol. 34, 1666–1671. doi: 10.1007/s10344-018-1173-2
Weiss G., Carver P. L. (2018). Role of divalent metals in infectious disease susceptibility and outcome. Clin. Microbiol. Inf. 24, 16–23. doi: 10.1016/j.cmi.2017.01.018
Wilson D. (2015). An evolutionary perspective on zinc uptake by human fungal pathogens. Metallomics 7 (6), 979–985. doi: 10.1039/c4mt00331d
Wintle N. J. P., Duffield D. A., Barros N. B., Jones R. D., Rice J. M. (2011). Total mercury in stranded marine mammals from the Oregon and southern Washington coasts. Mar. Mammal Sci. 27, E268–E278. doi: 10.1111/j.1748-7692.2010.00461.x
Wunderlich G. (2020). Spatiotemporal characterization of the Sydney beach sand mycobiome using traditional and molecular techniques - a clinical connection? (Sydney, NSW: University of Sydney).
Wünschmann A., Siebert U., Weiss R. (1999). Rhizopusmycosis in a harbor porpoise from the Baltic Sea. J. Wildl. Dis. 35, 569–573. doi: 10.7589/0090-3558-35.3.569
Young S. J. F., Huff D. G., Stephen C. (1999). A risk-management approach to mycotic disease potential in captive beluga whale (Delphinapterus leucas). Zoo Biol. 18, 5–16. doi: 10.1002/(SICI)1098-2361(1999)18:1%3C5::AID-ZOO3%3E3.0.CO;2-W
Zepeda-Sein C. (2002)Risk analysis: a decision support tool for the control and prevention of animal diseases. In: Proc. 70th general session of the international committee, world organisation for animal health (OIE) (Paris). Available at: https://pdfs.semanticscholar.org/8c4a/dc5e856bfdf5561d85f6a0d2b1293278024f.pdf (Accessed February 3, 2020). Document 70 SG/10.
Keywords: harbor porpoise, fungi, killer whale, marine mammal, mucormycosis, One Health, qualitative risk assessment, Salish Sea
Citation: Norman SA, Huggins JL, Lambourn DM, Rhodes LD, Garner MM, Bolton JL, Gaydos JK, Scott A, Raverty S and Calambokidis J (2022) Risk factor determination and qualitative risk assessment of Mucormycosis in Harbor Porpoise, an emergent fungal disease in Salish Sea marine mammals. Front. Mar. Sci. 9:962857. doi: 10.3389/fmars.2022.962857
Received: 06 June 2022; Accepted: 11 July 2022;
Published: 29 July 2022.
Edited by:
Lorenzo Mari, Politecnico di Milano, ItalyReviewed by:
Sebastian Gnat, University of Life Sciences of Lublin, PolandCindy R Elliser, Pacific Mammal Research (PacMam), United States
Copyright © 2022 Norman, Huggins, Lambourn, Rhodes, Garner, Bolton, Gaydos, Scott, Raverty and Calambokidis. This is an open-access article distributed under the terms of the Creative Commons Attribution License (CC BY). The use, distribution or reproduction in other forums is permitted, provided the original author(s) and the copyright owner(s) are credited and that the original publication in this journal is cited, in accordance with accepted academic practice. No use, distribution or reproduction is permitted which does not comply with these terms.
*Correspondence: Stephanie A. Norman, c3RlcGhhbmllQG1hcmluZS1tZWQuY29t