- Lighthouse Field Station, School of Biological Sciences, University of Aberdeen, Cromarty, United Kingdom
With increasing numbers of offshore structures being installed and decommissioned, a better understanding of their effect on marine predators is timely. There is some evidence that oil and gas platforms may attract marine mammals, acting as artificial reefs. However, it is unclear whether different man-made structure designs have similar effects or whether artificial structures modify the diel patterns of occurrence and foraging of marine mammals. Here, we used passive acoustics to investigate the occurrence and foraging activity of harbour porpoises (Phocoena phocoena) around four artificial structures of different age and complexity. We deployed an array of echolocation click detectors (CPODs) in 2021, along a gradient of distances to these structures and assessed the extent to which porpoises were attracted to them and their effect on porpoises’ diel patterns of occurrence and foraging activity. The probability of porpoise occurrence and foraging activity decreased with distance from offshore structures. A significant increase in porpoise occurrence and foraging was detected during night-time compared to daytime around all four offshore structures (< 200 m). Comparing pre- and post-installation porpoise detections, the daily patterns of occurrence and foraging activity shifted from a weak diel pattern before the structure was installed, to a strong nocturnal pattern when the structure was present. These findings provide evidence that marine mammals are attracted to man-made structures and that porpoises modify their diel patterns of occurrence and foraging activity around them. This research suggests that offshore structures play an important role as foraging areas for some marine mammals and provides key information for decommissioning considerations and the planning of decommissioning activities.
Introduction
Increasing numbers of oil and gas (O&G) structures are coming to the end of their operational life, and there is ongoing debate about the best ecological approaches to their removal or re-use (Bull and Love, 2019; Fortune and Paterson, 2020; Lemasson et al., 2021). In parallel, the rapid growth of offshore wind energy is leading to the installation of many new fixed artificial structures in shelf seas. Assessments of the biological costs and benefits of installing and decommissioning these structures are complicated by uncertainties over the importance of offshore artificial structures for many mobile species (Fortune and Paterson, 2020). In some regions, this constrains policy decisions over re-use of O&G structures as artificial reefs (Bull and Love, 2019). In others, policy decisions already require removal of structures (Lemasson et al., 2021), but the required EIAs are not mandated to consider effects on marine biota adhering to or dependent on the structure (Fortune and Paterson, 2020).
It is recognised that marine mammals may be attracted to artificial offshore structures in certain situations (Russell et al., 2014; Clausen et al., 2021), but the generality of these findings is less clear. This is partly because studies have focused on a limited number of the many designs of structure currently installed offshore, but also because research has been conducted only in a few ecological regions. In common with studies of many aspects of artificial structures, the lack of baseline data can hinder the assessment of decommissioning effects (Fortune and Paterson, 2020).
Harbour porpoises are abundant and widely distributed across the North Sea (Hammond et al., 2013). Given their known sensitivity to anthropogenic disturbance, they are considered key receptors within EIAs underpinning extensive offshore energy activities across this region (Thomsen et al., 2011). Future assessments will need, first, to consider the extent to which attraction to redundant structures may affect local densities during decommissioning activities. Second, mitigation measures will require an understanding of how local densities vary in time to identify periods when these receptors may be more, or less, sensitive to disturbance.
To date, just one study has demonstrated that harbour porpoises are locally attracted to O&G structures. In this case, within the Danish sector of the North Sea, porpoise echolocation activity was up to twofold higher within 800m of an operational O&G platform compared to reference sites (Clausen et al., 2021). As demonstrated in earlier work from a jack-up barge around a gas platform in the German sector of the North Sea, porpoise echolocation activity was also highest during the night (Todd et al., 2009). Similar diel patterns of activity in prey (Fujii and Jamieson, 2016) and avian predators (Ronconi et al., 2015) have been observed around active O&G platforms. However, it is not known whether such diel patterns in predator-prey interactions are driven by the physical presence of artificial structures or through attraction of prey to lights and flares on operational platforms (Todd et al., 2009; Clausen et al., 2021).
Here, using passive acoustic monitoring (PAM), we studied the occurrence and foraging activity of harbour porpoises around a cluster of redundant artificial structures within Scottish shelf waters. First, we assessed the extent to which porpoises were attracted to structures of different age and complexity. Secondly, we explored whether observed increased levels of nocturnal occurrence and foraging were also evident around platforms that have been abandoned, with lighting reduced to levels required for navigational safety. Finally, we used pre-installation baseline data at one site (Thompson et al., 2010) to provide a direct assessment of how the presence of structures affected diel patterns of occurrence and foraging activity of porpoises.
Material and methods
The study was carried out around the Smith Bank, within the Moray Firth, NE Scotland. The area has been subject to O&G exploration and production for several decades (Addy, 1987), and has more recently seen the development of demonstration (Thompson et al., 2010) and commercial (Graham et al., 2019) offshore wind energy. Several marine mammal species occur across the area, but harbour porpoises are the most abundant and widespread of these, providing a model species for understanding interactions between wildlife and offshore energy activities (Thompson et al., 2010; Thompson et al., 2013; Benhemma-Le Gall et al., 2021).
Acoustic deployments
In August 2021, an array of 23 click detectors (V.0 and V.1 CPODs; www.chelonia.co.uk) was deployed along a gradient of distances to four offshore structures on the Smith Bank: Jacky Wellhead platform, Beatrice Bravo O&G platform and the two Beatrice Demonstrator turbines (Figure 1 and detailed information on these offshore structures in Supplementary Material Table S1).
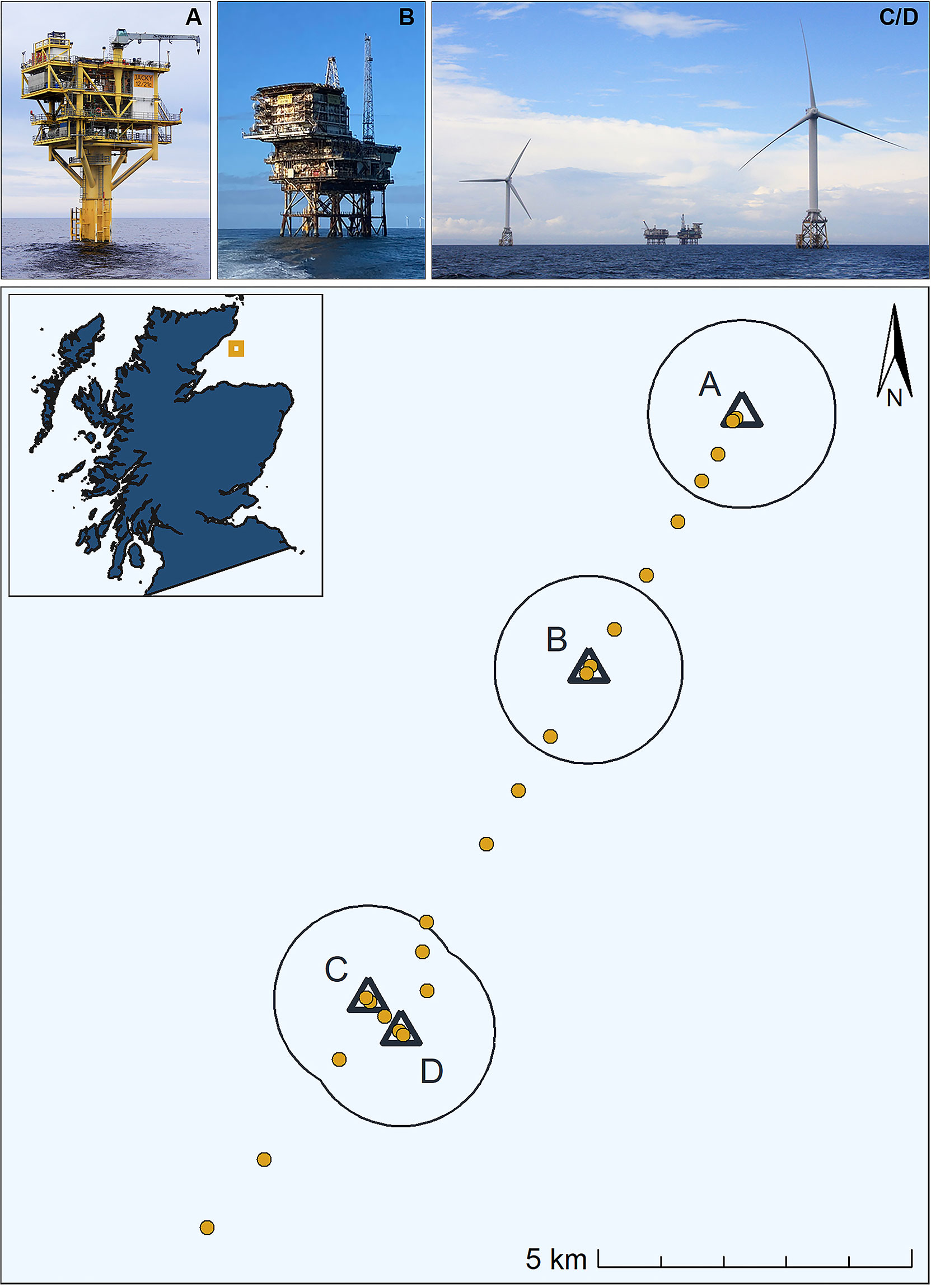
Figure 1 CPOD deployments (yellow circles) and offshore structures (black triangles) with a 1500 m buffer around them (black empty circles). (A) Jacky Wellhead O&G platform (© Stephen Hurrel), (B) Beatrice Bravo O&G platform (© Repsol Sinopec Resources UK LTD), (C, D) Beatrice Demonstrator turbines (© Stephen Hurrel).
Jacky Wellhead O&G platform, installed in 2008, is a monopile structure with three suction piles (jacket weight: 596 t). Beatrice Bravo O&G platform was installed in 1983 and has 10 leg piles and 4 skirt-piles (total jacket weight: 2,946 t). Oil production from the Beatrice field began in 1981 and ceased in 2015. Jacky started production in 2009 and stopped in 2014. The Beatrice Demonstrator turbines were installed in 2007 on a 4 skirt-pile jacket design (jacket weight: 804 t each). All four structures are un-manned, and their lighting is reduced to the minimum required to comply with national and international regulations on aviation and shipping navigation.
Two CPODs were located in close proximity to each of the structures (< 200 m), hereafter Structure CPODs. The remaining 15 CPODs were deployed at distances between 373 and 2700 m from the structures, hereafter Non-structure CPODs (detailed information in Supplementary Material Table S2). All CPODs were set to record continuously, with a 20 kHz High pass filter.
Data from these recent studies were compared with historic baseline data from one of the contemporary sites. In August 2005, two TPODs (v.4 TPODs; www.chelonia.co.uk) were deployed between the locations where the Beatrice Demonstrator turbines were planned to be installed to collect baseline data from the pre-installation period (Thompson et al., 2010). TPODs were configured to detect the presence of echolocation clicks from harbour porpoises following the set up and analysis methods described by Bailey et al. (2010). TPODs were the analogue predecessors of CPODs and, although TPODs had a less sophisticated detection algorithm than their successors, they were a well-established tool to study variation in the occurrence of harbour porpoises (Carlstrom, 2005; Thomsen et al., 2005; Todd et al., 2009).
Data processing
CPOD data were downloaded and processed using CPOD custom software (cpod.exe v. 2.044). Following the manufacturer’s manual, only echolocation clicks classified as high or moderate quality by the built-in “KERNO” classifier were included in the analyses.
To save CPOD memory in noisy environments, a maximum number of recorded clicks per minute (scan limit) can be set. When the scan limit is reached, CPODs stop recording for the rest of the minute and start again at the next one. We set 19 CPODs to record a maximum of 4096 clicks min-1 while the remaining 4 CPODs did not have any scan limit (Supplementary Material Table S2). To minimise false-negative detections, CPOD data days when the scan limit was reached in more than 1% of the total minutes were excluded from the analyses.
CPOD data were first used to assess variation in porpoise occurrence, with those hours containing echolocation clicks being defined as detection positive hours (Brookes et al., 2013; Williamson et al., 2016). We then identified the presence of buzzes within each of these hours by modelling the variation in harbour porpoise inter-click intervals (ICIs). To do so, we extracted high and moderate quality click details of porpoise origin and we fitted a Gaussian mixture-model to log transformed ICIs (Pirotta et al., 2014b). We set the number of component distributions k to three, dividing ICIs into three groups: inter-train, regular and buzzes. The first and second groups included ICIs between distinct click trains and ICIs within regular click trains, respectively. The third group included click trains with high repetition rate, known as buzzes. Porpoises use buzzes for both foraging activity and social communication (Clausen et al., 2011; Sørensen et al., 2018). Since it is not possible to distinguish between these two behaviours, in line with previous work (Pirotta et al., 2014a; Williamson L. et al., 2017; Benhemma-Le Gall et al., 2021) we assumed that all the identified buzzes could be used as a proxy for foraging.
Variation in harbour porpoise occurrence and foraging activity linked to offshore structures
To investigate the effect of offshore structures on harbour porpoise occurrence and foraging activity, we performed four generalized linear mixed-effects models (GLMM; Bolker et al., 2009).
First, to assess the extent to which porpoises were attracted to offshore structures we modelled the proportion of detection positive hours (DPH) and buzz positive hours (BPH) per day as a function of distance to the closest offshore structure. Proportion of detection positive hours per day was defined as the ratio between the number of hours when porpoises were detected and the total number of hours of the day (Brookes et al., 2013; Williamson et al., 2016). Proportion of buzz positive hours per day was defined as the ratio between the number of hours in which at least one buzz was detected and the number of hours in which porpoises were detected in that day (Pirotta et al., 2014b). For these models we considered the complete CPOD array.
Second, to investigate whether increased levels of nocturnal occurrence and foraging persisted around offshore structures with low levels of lighting, we divided the day into two diel phases (day/night) based on local sunrise and sunset times. We then summarised the proportion of DPH and BPH per diel phase and modelled them as a function of the interaction between the CPOD group (two levels: Structure/Non-structure CPODs), the closest offshore structure (three levels: Jacky/Beatrice Bravo/Beatrice Demonstrators) and the diel phase (two levels: day/night). Tukey Honestly Significant Difference tests (Tukey HSD; Tukey, 1991) were conducted as a post-hoc test to identify significant differences between group means. In this analysis, we only considered the CPODs deployed within 1500 m of an offshore structure.
All GLMMs were fitted with a binomial family distribution (probit link function) and included a unique identifier for CPOD, to account for variation in device sensitivity, and Julian day as random effects.
Comparison of harbour porpoise diel patterns of occurrence and foraging before and after installation of offshore structures
To assess whether the presence of structures affected diel patterns of occurrence and foraging activity of harbour porpoises, the hourly presence/absence of porpoise detections and buzzes were modelled as a function of the interaction between the hour of the day (0-24h) and the presence of the structure (two levels: Present/Absent). We fitted generalised additive models (GAMs; Wood, 2006) with a binomial distribution and a logit link. In this analysis, we only considered one site, located between the Beatrice Demonstrator turbines (< 375 m; Supplementary material Table S2), where pre-installation baseline data were available from a previous study (Thompson et al., 2010).
Results
Harbour porpoises were detected every day throughout the 31-day study period for an average of 17 hours day-1. The complete dataset comprised 636 data days from 22 CPOD deployments, with only 23 data days (< 4%) needing to be excluded from further analyses due to excessive background noise (Supplementary Material Table S2).
Variation in harbour porpoise occurrence and foraging activity linked to offshore structures
The probability of both porpoise occurrence (Figure 2A) and foraging activity (Figure 2B) decreased significantly with distance from offshore structures (porpoise occurrence: GLMM X2 = 16.01, df = 1, P < 0.001; porpoise foraging activity: GLMM X2 = 14.59, df = 1, P < 0.001). Porpoise occurrence decreased from 0.76 (95% CI: 0.73-0.79) around structures (< 200 m) to 0.63 (95% CI: 0.58-0.68) further away (2500 m). The probability of foraging activity decreased from 0.55 (95% CI: 0.49-0.60) around structures (< 200 m) to 0.36 (95% CI: 0.31-0.44) further away (2500 m).
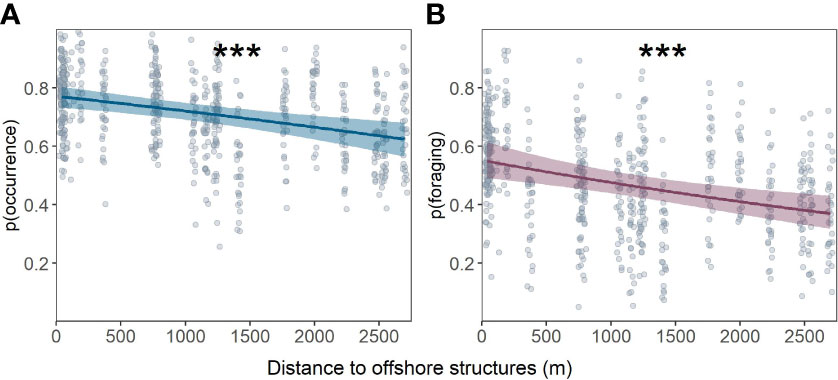
Figure 2 Predicted probability of harbour porpoise occurrence (A) and foraging activity (B) in relation to distance from offshore structures including raw data points (circles). Shaded areas are the 95% confidence intervals for the fixed effects only. Significance of the explanatory variable indicated at the top: ***P < 0.001.
Variation in both occurrence (Figure 3A) and foraging activity (Figure 3B) were best explained by the interaction between CPOD group, closest structure and diel phase (porpoise occurrence: GLMM X2 = 24.3, df = 2, P < 0.001; porpoise foraging activity: GLMM X2 = 45.8, df = 2, P < 0.001). The nature and extent of changes in occurrence varied slightly between structures. However, there was a stronger and more consistent pattern in variation in foraging activity across all three structure levels (Figure 3).
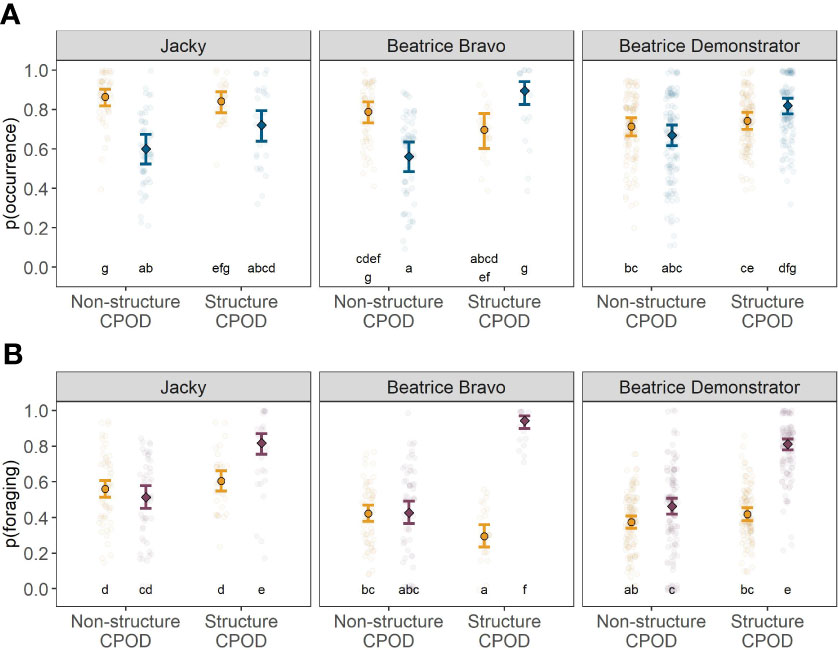
Figure 3 Predicted probability of harbour porpoise occurrence (A) and foraging activity (B) around Structure and Non-structure CPODs at each of the structures during the day (error bars with yellow circles) and during the night (error bars with diamonds) including raw data points (translucid circles). Unlike letters denote groups that differed statistically from each other in Tukey post-hoc test (e.g. a and b: P < 0.05; a and ab: P > 0.05).
Around structures (< 200 m), harbour porpoise occurrence was significantly higher during night-time compared to daytime at both Beatrice Bravo and Beatrice Demonstrator turbines (Tukey HSD: P < 0.001), while no significant variation in diel occurrence was observed at Jacky (Tukey HSD: P > 0.05; Figure 3A and Supplementary Table S3). At Beatrice Bravo, the probability of occurrence around structures increased from 0.69 during daytime (95% CI: 0.60-0.77) to 0.87 during night-time (95% CI: 0.78-0.92). At Beatrice Demonstrator turbines, the probability of occurrence around structures increased from 0.74 during daytime (95% CI: 0.69-0.78) to 0.83 during night-time (95% CI: 0.79-0.86). In contrast, away from structures (200-1500 m), porpoise occurrence was significantly lower during night-time compared to daytime around Jacky and Beatrice Bravo (Tukey HSD: P < 0.001), while no significant variation in diel occurrence was detected around the Beatrice Demonstrator turbines (Tukey HSD: P > 0.05).
An increase in foraging activity during night-time compared to daytime was observed around all offshore structures (< 200 m; Figure 3B and Supplementary Table S4). At Jacky, the probability of foraging activity increased from 0.52 during daytime (95% CI: 0.47-0.58) to 0.78 during night-time (95% CI: 0.71-0.83). At Beatrice Bravo, foraging activity increased from 0.29 during daytime (95% CI: 0.23-0.35) to 0.94 during night-time (95% CI: 0.90-0.97). At the Beatrice Demonstrator turbines, foraging activity increased from 0.39 during daytime (95% CI: 0.36-0.43) to 0.79 at night (95% CI:0.75-0.82). During night-time, the foraging activity was significantly higher around all structures (< 200 m) compared to distances further away (200-1500 m; Figure 3B and Supplementary Table S4).
Comparison of harbour porpoise diel patterns of occurrence and foraging before and after installation of offshore structures
There was a marked change in diel patterns of occurrence and foraging activity when comparing our 2021 data around the Beatrice demonstrators with baseline data collected in 2005 prior to the installation of these structures (Figure 4).
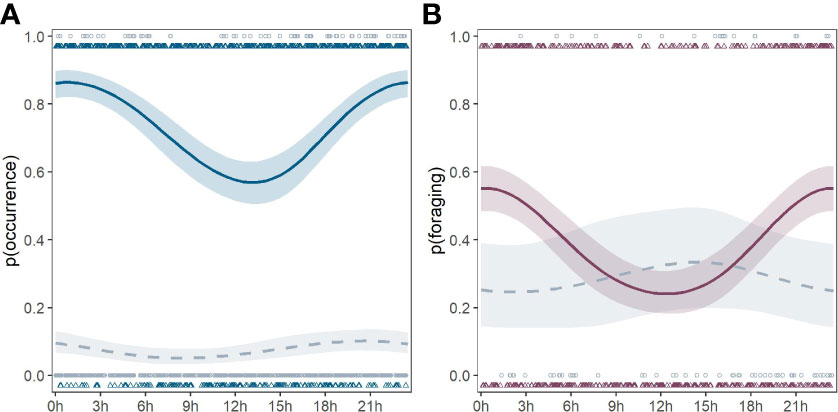
Figure 4 Predicted probability of harbour porpoise occurrence (A) and foraging activity (B) per hour when Beatrice demonstrator turbines were absent (dashed line) and present (solid line) including raw data points (circles: structure absent; triangles: structure present). Shaded areas are the 95% CI.
GAM model results confirmed that the presence of the structure had a significant effect on the diel patterns of both harbour porpoise occurrence (Figure 4A) and foraging activity (Figure 4B). In the baseline year, neither porpoise occurrence nor foraging activity changed with the diel cycle. However, in 2021, the probability of both harbour porpoise occurrence and foraging activity increased significantly during night-time.
Discussion
An extensive PAM array around four offshore structures revealed that harbour porpoise occurrence and foraging activity was higher around structures and that this effect was explained by an increase in occurrence and foraging at night. Further, we showed a change in the diel pattern of porpoise occurrence linked to the presence of an offshore structure.
Consistent with previous work (Russell et al., 2014; Clausen et al., 2021), our results provide further evidence that offshore structures attract some species of marine mammals, which use these structures to forage. A previous study found an increase in harbour porpoise activity within an operating windfarm compared to reference sites further away but raised uncertainty about the drivers of attraction as the windfarm was also subject to restricted fishing and vessel activity (Scheidat et al., 2011). Here, we also found a significant increase in harbour porpoise occurrence and foraging activity near isolated offshore structures compared to locations further away. Similarly, Clausen et al. (2021) detected an increase in porpoise occurrence within 800 m of an O&G platform compared to more distant areas. Marine artificial structures create artificial reefs that are colonised by epifaunal communities which, in turn, cause an increase in shallow- and mid-water pelagic species (Stanley and Wilson, 2000; Degraer et al., 2020). O&G platforms have been described as highly productive areas that support high fish density (Claisse et al., 2014; Love et al., 2019a). Therefore, one possible explanation for the observed increase in porpoise occurrence and foraging activity closer to the structures is that they provide more foraging opportunities. Alternatively, porpoises may perceive offshore structures as more complex areas to navigate and, since they use echolocation both to navigate and communicate (Clausen et al., 2011; Sørensen et al., 2018), the increase in occurrence and foraging activity detected in this study could partly be explained by changes in echolocation behaviour around structures. However, the attraction to man-made structures has also been detected in seals, which also use these areas to forage (Russell et al., 2014; Arnould et al., 2015). Furthermore, the increase in bentho-pelagic communities around man-made structures is well documented in the literature (Gates et al., 2019; Perry and Heyman, 2020). Therefore, we suggest that the enhanced foraging opportunities around offshore structures is the most plausible explanation for the observed increase in porpoise detections.
Our analyses showed strong diel patterns in the occurrence and foraging activity of harbour porpoises around offshore structures (< 200 m). Porpoise occurrence was significantly higher at night compared to daytime around three of four structures investigated here, while foraging activity was significantly higher at night around all four of them. Similar nocturnal increases in porpoise occurrence and foraging activity near man-made structures were found in previous studies (Todd et al., 2009; Brandt et al., 2014; Clausen et al., 2021). However, in those studies the authors highlighted that any influence of physical structures on predator-prey interactions could be confounded by the lighting on these structures attracting prey species at night (Todd et al., 2009; Clausen et al., 2021). In our study, lighting on all four structures had been reduced to minimum levels required to comply with national and international regulations on aviation and shipping navigation. Consequently, it is likely to be the physical presence of structures that shape these foraging patterns. The precise mechanisms underlying this nocturnal increase in foraging remain unclear, but it seems likely to be related to diel movements of prey or changes in their activity or schooling behaviour (Todd et al., 2009; Brandt et al., 2014; Clausen et al., 2021). Further research to directly investigate activity patterns of fish (Williamson B. J. et al., 2017) in relation to porpoise movements (e.g. Gillespie et al., 2020) are now required to better understand the drivers of porpoise activity around offshore structures.
Importantly, our study also provides direct evidence of a change in harbour porpoise diel patterns in relation to the introduction of an offshore structure. Although baseline data were available from only one of our sites, this analysis demonstrated a clear difference in nocturnal patterns of occurrence and foraging activity when the structure was present compared to the year before the structure was installed (Figure 4). It should be noted that, for this comparison, we used data sets obtained with different devices: CPODs, when the offshore structure was present, and their predecessors TPODs, when the structure was absent (Thompson et al., 2010). Current CPODs include a more sophisticated detection algorithm, which results in a lower false positive rate compared to TPODs. Therefore, the absolute differences in levels of porpoise occurrence and foraging activity cannot be directly compared. Nevertheless, the difference in the diel patterns within each of these data sets should be robust to device-specific differences in detection probability. Additionally, the larger contemporary dataset also demonstrated a clear increase in nocturnal occurrence and foraging around the structures (< 200 m) compared to locations further away (Figure 3). Together, these two datasets provide strong support for the hypothesis that the change in diel patterns of porpoise occurrence and foraging are linked to the presence of structures. Future studies using similar devices before and after the installation of a man-made structure could investigate this further.
While a significant increase in nocturnal foraging was detected around all four offshore structures studied here, the highest increase was detected around Beatrice Bravo (from 0.29 during daytime to 0.94 during night-time; Figure 3B). More complex subsea structures exhibit higher fish density and greater species richness (Love et al., 2019b). Furthermore, species diversity also increases with the age of the artificial reef (Perkol-Finkel and Benayahu, 2005). Beatrice Bravo is both the oldest and the most complex structure among those investigated here, and, in line with those studies, our results suggest that higher foraging opportunities may exist around it compared to simpler structures, such as the Jacky monopile. Nevertheless, our findings are based only on individual (Beatrice Bravo & Jacky) or pairs (Beatrice Demonstrator) of similar offshore structures, where other factors could confound patterns of predator occurrence and foraging activity. Furthermore, all the structures that we studied had been in the water for at least 12 years, and we could not determine the age at which they started becoming attractive to porpoises. A recent study in the Southern North Sea found no evidence of attraction to a gas production platform in its first five years of operation (Todd et al., 2022), perhaps suggesting that it may take several years for prey communities to develop to levels at which they influence predator activity. Additionally, Clausen et al. (2021) found seasonal variability in porpoise activity around O&G platforms. Previous work in our study area has also shown seasonal changes in porpoise occurrence, with a peak in August, when the present study was conducted (Graham et al., 2019). Whether our observed attraction to structures during this month remains consistent throughout other seasons remains unclear. Further research of larger groups of similar man-made structures throughout their life cycle is now required to investigate how structure age, complexity and seasonality influence the occurrence and foraging activity of marine predators.
In conclusion, our analyses showed that harbour porpoises are attracted to isolated offshore structures and that porpoises use these structures to forage, especially at night. These findings suggest that offshore structures play an important role as foraging areas for some marine mammals, filling a key gap in the ecological understanding of offshore decommissioning in the North Sea (Fowler et al., 2020). These findings now provide important baseline to support the assessment and mitigation of future decommissioning projects.
Data availability statement
The datasets presented in this study can be found in online repositories. The names of the repository/repositories and accession number(s) can be found below: https://doi.org/10.5061/dryad.mpg4f4r2p
Ethics statement
Ethical review and approval was not required for the animal study because it was a non-invasive, acoustic observational study of harbour porpoise activity around offshore structures. No animals were captured or tagged during this study and no research or animal ethical assessments were required. Harbour porpoise activity was determined using remote passive acoustic devices on seabed moorings licensed for scientific use by Marine Scotland and consented by the Crown Estate. Moorings were deployed and recovered using vessels with appropriate certification, accreditation, and endorsements.
Author contributions
OF-B: Conceptualization, data curation, formal analysis, funding acquisition, methodology, visualization, writing – original draft. IG: Conceptualization, data curation, funding acquisition, methodology, supervision, writing – review & editing. PT: Conceptualization, funding acquisition, methodology, project administration, resources, supervision, writing – review & editing. All authors contributed to the article and approved the submitted version.
Funding
This study was funded by the UK Department for Business, Energy & Industrial Strategy (BEIS) Offshore Energy Strategic Environmental Assessment research programme under contracts OESEA-20-125 and OESEA-21-133, using equipment previously purchased by UK Department of Energy and Climate Change, Scottish Government, Oil and Gas UK, COWRIE and Moray Offshore Renewables Ltd. Funding for the 2005 baseline studies was provided by the EU DOWNVinD Project and Talisman Energy (UK) Ltd., who were not involved in the study design, collection, analysis, interpretation of data, the writing of this article, or the decision to submit it for publication.
Acknowledgments
We would like to thank Bill Ruck, Moray First Marine and colleagues from the University of Aberdeen for assistance with the data collection, and John Hartley for his support through the project and for his comments on the manuscript. We are very grateful to Ithaca Energy (UK) Limited, Repsol Sinopec Resources UK Limited and SSE Generation Limited for allowing access to the Jacky platform, Beatrice Bravo platform and Beatrice wind demonstrator turbine sites.
Conflict of interest
The authors declare that the research was conducted in the absence of any commercial or financial relationships that could be construed as a potential conflict of interest.
Publisher’s note
All claims expressed in this article are solely those of the authors and do not necessarily represent those of their affiliated organizations, or those of the publisher, the editors and the reviewers. Any product that may be evaluated in this article, or claim that may be made by its manufacturer, is not guaranteed or endorsed by the publisher.
Supplementary material
The Supplementary Material for this article can be found online at: https://www.frontiersin.org/articles/10.3389/fmars.2022.980388/full#supplementary-material
References
Addy J. M. (1987). Environmental monitoring of the Beatrice oilfield development. Philos. Trans. R. Soc. London B Biol. Sci. 316 (1181), 655–668. doi: 10.1098/rstb.1987.0044
Arnould J. P., Monk J., Ierodiaconou D., Hindell M. A., Semmens J., Hoskins A. J., et al. (2015). Use of anthropogenic Sea floor structures by Australian fur seals: Potential positive ecological impacts of marine industrial development? PloS One 10 (7), e0130581. doi: 10.1371/journal.pone.0130581
Bailey H., Clay G., Coates E. A., Lusseau D., Senior B., Thompson P. M. (2010). Using T-PODs to assess variations in the occurrence of coastal bottlenose dolphins and harbour porpoises. Aquat. Conservation: Mar. Freshw. Ecosyst. 20, 150–158. doi: 10.3354/meps08789
Benhemma-Le Gall A., Graham I. M., Merchant N. D., Thompson P. M. (2021). Broad-scale responses of harbor porpoises to pile-driving and vessel activities during offshore windfarm construction. Front. Mar. Sci. 8, 735. doi: 10.3389/fmars.2021.664724
Bolker B. M., Brooks M. E., Clark C. J., Geange S. W., Poulsen J. R., Stevens M. H. H., et al. (2009). Generalized linear mixed models: a practical guide for ecology and evolution. Trends Ecol. Evol. 24 (3), 127–135. doi: 10.1016/j.tree.2008.10.008
Brandt M. J., Hansen S., Diederichs A., Nehls G. (2014). Do man-made structures and water depth affect the diel rhythms in click recordings of harbor porpoises (Phocoena phocoena)? Mar. Mammal Sci. 30 (3), 1109–1121. doi: 10.1111/mms.12112
Brookes K. L., Bailey H., Thompson P. M. (2013). Predictions from harbor porpoise habitat association models are confirmed by long-term passive acoustic monitoring. J. Acoust. Soc. America 134 (3), 2523–2523. doi: 10.1121/1.4816577
Bull A. S., Love M. S. (2019). Worldwide oil and gas platform decommissioning: A review of practices and reefing options. Ocean Coast. Manage. 168, 274–306. doi: 10.1016/j.ocecoaman.2018.10.024
Carlstrom J. (2005). Diel variation in echolocation behavior of wild harbor porpoises. Mar. Mammal Sci. 21 (1), 1–12. doi: 10.1111/j.1748-7692.2005.tb01204.x
Claisse J. T., Pondella D. J. 2nd, Love M., Zahn L. A., Williams C. M., Williams J. P., et al. (2014). Oil platforms off California are among the most productive marine fish habitats globally. Proc. Natl. Acad. Sci. 111 (43), 15462–15467. doi: 10.1073/pnas.1411477111
Clausen K. T., Teilmann J., Wisniewska D. M., Balle J. D., Delefosse M., Beest F. M. (2021). Echolocation activity of harbour porpoises, Phocoena phocoena, shows seasonal artificial reef attraction despite elevated noise levels close to oil and gas platforms. Ecol. Solutions Evid. 2 (1), e12055. doi: 10.1002/2688-8319.12055
Clausen K. T., Wahlberg M., Beedholm K., Deruiter S., Madsen P. T. (2011). Click communication in harbour porpoises Phocoena phocoena. Bioacoustics 20 (1), 1–28. doi: 10.1080/09524622.2011.9753630
Degraer S., Carey D. A., Coolen J. W. P., Hutchison Z. L., Kerckhof F., Rumes B., et al. (2020). Offshore wind farm artificial reefs affect ecosystem structure and functioning - a synthesis. Oceanography 33 (4), 48–57. doi: 10.5670/oceanog.2020.405
Fortune I. S., Paterson D. M. (2020). Ecological best practice in decommissioning: a review of scientific research. ICES J. Mar. Sci. 77 (3), 1079–1091. doi: 10.1093/icesjms/fsy130
Fowler A. M., Jørgensen A. M., Coolen J. W. P., Jones D. O. B., Svendsen J. C., Brabant R., et al. (2020). The ecology of infrastructure decommissioning in the north Sea: what we need to know and how to achieve it. ICES J. Mar. Sci. 77 (3), 1109–1126. doi: 10.1093/icesjms/fsz143
Fujii T., Jamieson A. J. (2016). Fine-scale monitoring of fish movements and multiple environmental parameters around a decommissioned offshore oil platform: A pilot study in the north sea. Ocean Eng. 126, 481–487. doi: 10.1016/j.oceaneng.2016.09.003
Gates A. R., Horton T., Serpell-Stevens A., Chandler C., Grange L. J., Robert K., et al. (2019). Ecological role of an offshore industry artificial structure. Front. Mar. Sci. 6. doi: 10.3389/fmars.2019.00675
Gillespie D., Palmer L., Macaulay J., Sparling C., Hastie G. (2020). Passive acoustic methods for tracking the 3D movements of small cetaceans around marine structures. PloS One 15 (5), e0229058. doi: 10.1371/journal.pone.0229058
Graham I. M., Merchant N. D., Farcas A., Barton T. R., Cheney B., Bono S., et al. (2019). Harbour porpoise responses to pile-driving diminish over time. R. Soc. Open Sci. 6 (6), 190335. doi: 10.1098/rsos.190335
Hammond P. S., Macleod K., Berggren P., Borchers D. L., Burt L., Cañadas A., et al. (2013). Cetacean abundance and distribution in European Atlantic shelf waters to inform conservation and management. Biol. Conserv. 164, 107–122. doi: 10.1016/j.biocon.2013.04.010
Lemasson A. J., Knights A. M., Thompson M., Lessin G., Beaumont N., Pascoe C., et al. (2021). Evidence for the effects of decommissioning man-made structures on marine ecosystems globally: a systematic map protocol. Environ. Evid. 10 (1), 4. doi: 10.1186/s13750-021-00218-y
Love M. S., Claisse J. T., Roeper A. (2019a). An analysis of the fish assemblages around 23 oil and gas platforms off California with comparisons with natural habitats. Bull. Mar. Sci. 95 (4), 477–514. doi: 10.5343/bms.2018.0061
Love M. S., Kui L., Claisse J. T. (2019b). The role of jacket complexity in structuring fish assemblages in the midwaters of two California oil and gas platforms. Bull. Mar. Sci. 95 (4), 597–616. doi: 10.5343/bms.2017.1131
Perkol-Finkel S., Benayahu Y. (2005). Recruitment of benthic organisms onto a planned artificial reef: shifts in community structure one decade post-deployment. Mar. Environ. Res. 59 (2), 79–99. doi: 10.1016/j.marenvres.2004.03.122
Perry R. L., Heyman W. D. (2020). Considerations for offshore wind energy development effects on fish and fisheres in the united states. Oceanography 33 (4), 28–37. doi: 10.5670/oceanog.2020.403
Pirotta E., Brookes K. L., Graham I. M., Thompson P. M. (2014a). Variation in harbour porpoise activity in response to seismic survey noise. Biol. Lett. 10 (May), 5–5. doi: 10.1098/rsbl.2013.1090
Pirotta E., Thompson P. M., Miller P. I., Brookes K. L., Cheney B., Barton T. R., et al. (2014b). Scale-dependent foraging ecology of a marine top predator modelled using passive acoustic data. Funct. Ecol. 28 (1), 206–217. doi: 10.1111/1365-2435.12146
Ronconi R. A., Allard K. A., Taylor P. D. (2015). Bird interactions with offshore oil and gas platforms: Review of impacts and monitoring techniques. J. Environ. Manage. 147, 34–45. doi: 10.1016/j.jenvman.2014.07.031
Russell D. J. F., Brasseur S., Thompson D., Hastie G. D., Janik V. M., Aarts G., et al. (2014). Marine mammals trace anthropogenic structures at sea. Curr. Biol. 24 (14), R638–R639. doi: 10.1016/j.cub.2014.06.033
Sørensen P. M., Wisniewska D. M., Jensen F. H., Johnson M., Teilmann J., Madsen P. T. (2018). Click communication in wild harbour porpoises (Phocoena phocoena). Sci. Rep. 8 (1), 9702. doi: 10.1038/s41598-018-28022-8
Scheidat M., Tougaard J., Brasseur S., Carstensen J., van Polanen Petel T., Teilmann J., et al. (2011). Harbour porpoises (Phocoena phocoena) and wind farms: a case study in the Dutch north sea. Environ. Res. Lett. 6 (2), 025102. doi: 10.1088/1748-9326/6/2/025102
Stanley D. R., Wilson C. A. (2000). Variation in the density and species composition of fishes associated with three petroleum platforms using dual beam hydroacoustics. Fisheries Res. 47 (2-3), 161–172. doi: 10.1016/S0165-7836(00)00167-3
Thompson P. M., Brookes K. L., Graham I. M., Barton T. R., Needham K., Bradbury G., et al. (2013). Short-term disturbance by a commercial two-dimensional seismic survey does not lead to long-term displacement of harbour porpoises. Proc. R. Soc. B: Biol. Sci. 280 (1771), 20132001–20132001. doi: 10.1098/rspb.2013.2001
Thompson P. M., Lusseau D., Barton T., Simmons D., Rusin J., Bailey H. (2010). Assessing the responses of coastal cetaceans to the construction of offshore wind turbines. Mar. pollut. Bull. 60 (8), 1200–1208. doi: 10.1016/j.marpolbul.2010.03.030
Thomsen F., McCully S. R., Weiss L. R., Wood D. T., Warr K. J., Barry J., et al. (2011). Cetacean stock assessments in relation to exploration and production industry activity and other human pressures: Review and data needs. Aquat. Mammals 37 (1), 1–93. doi: 10.1578/AM.37.1.2011.1
Thomsen F., van Elk N., Brock V., Piper W. (2005). On the performance of automated porpoise-click-detectors in experiments with captive harbor porpoises (Phocoena phocoena). J. Acoust. Soc. Am. 118 (1), 37–40. doi: 10.1121/1.1937347
Todd V. L. G., Pearse W. D., Tregenza N. C., Lepper P. A., Todd I. B. (2009). Diel echolocation activity of harbour porpoises (Phocoena phocoena) around north Sea offshore gas installations. ICES J. Mar. Sci. 66 (4), 734–745. doi: 10.1093/icesjms/fsp035
Todd V. L. G., Williamson L. D., Couto A. S., Todd I. B., Clapham P. J. (2022). Effect of a new offshore gas platform on harbor porpoises in the dogger bank. Mar. Mammal Sci. 1–14 doi: 10.1111/mms.12949
Tukey J. W. (1991). The philosophy of multiple comparisons. Stat. Sci., 100–116. doi: 10.1214/ss/1177011945
Williamson L., Brookes K. L., Scott B. E., Graham I. M., Bradbury G., Hammond P. S., et al. (2016). Echolocation detections and digital video surveys provide reliable estimates of the relative density of harbour porpoises. Methods Ecol. Evol. 77, 762–769 doi: 10.1111/2041-210X.12538
Williamson L., Brookes K. L., Scott B. E., Graham I. M., Thompson P. M. (2017). Diurnal variation in harbour porpoise detection – potential implications for management. Mar. Ecol. Prog. Ser. 570, 223–232. doi: 10.3354/meps12118
Williamson B. J., Fraser S., Blondel P., Bell P. S., Waggitt J. J., Scott B. E. (2017). Multisensor acoustic tracking of fish and seabird behavior around tidal turbine structures in Scotland. IEEE J. Oceanic Eng., 1–18. doi: 10.1109/JOE.2016.2637179
Keywords: artificial reefs, oil and gas industry (O&G), offshore renewable energy installations, marine mammal, passive acoustic monitoring (PAM), diel patterns, foraging behaviour
Citation: Fernandez-Betelu O, Graham IM and Thompson PM (2022) Reef effect of offshore structures on the occurrence and foraging activity of harbour porpoises. Front. Mar. Sci. 9:980388. doi: 10.3389/fmars.2022.980388
Received: 28 June 2022; Accepted: 05 September 2022;
Published: 16 September 2022.
Edited by:
Victoria Louise Georgia Todd, Ocean Science Consulting Ltd., United KingdomReviewed by:
Magnus Wahlberg, University of Southern Denmark, DenmarkJonas Teilmann, Aarhus University, Denmark
Copyright © 2022 Fernandez-Betelu, Graham and Thompson. This is an open-access article distributed under the terms of the Creative Commons Attribution License (CC BY). The use, distribution or reproduction in other forums is permitted, provided the original author(s) and the copyright owner(s) are credited and that the original publication in this journal is cited, in accordance with accepted academic practice. No use, distribution or reproduction is permitted which does not comply with these terms.
*Correspondence: Oihane Fernandez-Betelu, b2loYW5lLmZlcm5hbmRlekBhYmRuLmFjLnVr