- 1CAS Key Laboratory of Coastal Environmental Processes and Ecological Remediation, Shandong Key Laboratory of Coastal Environmental Processes, Research Center for Coastal Environment Engineering Technology of Shandong Province, Yantai Institute of Coastal Zone Research, Chinese Academy of Sciences, Yantai, China
- 2College of Marine Sciences, University of Chinese Academy of Sciences, Beijing, China
The biogeochemical cycle of trace metals plays an important role in the coastal zone. The distribution, interaction, and biogeochemical behavior of dissolved trace metals in surface and bottom seawater are still unclear. This study analyzed the distribution and performed an ecological health risk assessment of dissolved trace metals (Zn, Cd, Co, Pb and Cr) in surface and bottom seawater in the summer (August) of 2019 and 2020 in the seawater of the Yantai offshore area, China. Zinc (Zn), cadmium (Cd), cobalt (Co), lead (Pb) and chromium (Cr) ranged from 23.76–117.47 μg/L (42.67 μg/L ± 15.15), 0.21–0.51 μg/L (0.30 μg/L ± 0.05), 0.90–2.94 μg/L (1.09 μg/L ± 0.31), 0.13–1.06 μg/L (0.39 μg/L ± 0.17), and 2.00–9.9 μg/L (5.38 μg/L ± 2.99) in the summer of the two years. The average metal (Zn and Co) concentrations were slightly higher than the other Chinese coastal areas, while the major contamination pressures were from Zn and Pb. The largest contaminants were in the following order: Zn > Pb > Cd > Cr > Co. Correlation analysis, principal component analysis and hierarchical cluster were used to determine the potential origins and cause the spatial distribution of the multiple dissolved trace metals. The major sources of the trace metal contamination in study area were identified as external inputs from natural human and atmospheric settling and the natural release of the trace metals in sediments under external disturbances. Riverine inputs, the Lubei coastal current and aquaculture significantly influenced the multi-parameter horizontal distributions of the dissolved trace metals and dissolved organic carbon. Although the growth of phytoplankton was strong in summer, the uptake of Zn, Cd, Co and Pb was negligible compared with the exogenous input. The concentrations of Zn, Cd, Co and Pb were higher in the surface than those in the bottom. Monsoons, tides and wind waves also influence the migration, transformation and distribution of dissolved trace metals and dissolved organic carbon. This study provides important information on the biogeochemical cycle process (migration and conversion) and distribution of dissolved trace metals at different depths in coastal and marine pasture areas.
1 Introduction
Semi-enclosed bays are important economic activities including fisheries, aquaculture and industrial and populated areas due to their superior natural conditions (Peng, 2015). Served as filters and buffer zones to capture pollutants from the mainland to the open ocean, their poor water exchange is not conducive to the dilution and dispersion of trace metals and other pollutants, and the environmental quality of these bays has accordingly become a critical issue (Wang et al., 2019).
Metal contamination and effects have been one of the most concerned topic in aquatic environments (Sun et al., 2019). Trace metals influence the geology, chemistry, and various marine biological processes, and form an important part of marine ecosystems. The trace metal geochemical cycles influence the structure and function of marine ecosystems (Robbins et al., 2016). The metals’ nutritional requirements differ substantially between species, and the optimal metal concentration range is usually narrow. In too high concentrations, metals can be potentially toxic. It can bioaccumulate and biomagnify, and be persistent in the environment and organisms, especially in human beings (Achary et al., 2016). Trace metals such as Zn, Co, and Cd, promote the growth of marine organisms in specific concentration ranges (Morel et al., 2003). Trace metals such as Pb and Cr are non-essential and toxic to many marine organisms (Gumpu et al., 2015; Chasapis et al., 2022). High Pb intake can lead to memory problems, kidney disease, decreased bone growth, gastrointestinal tract, and neuro-degenerative diseases (Gumpu et al., 2015; Gutierrez-Ravelo et al., 2020). Cr causes chronic renal failure, dermatitis, bronchitis and extensive lesions, kidney lesions by contaminated fish (Ali et al., 2020). Trace metal concentrations in seawater are usually extremely low, in the order of nanomoles or picomolars (Li et al., 2017). Marine geochemists, therefore, measure very rare substances, akin to isolating 1 gram of iron from 1,250 tons of salt. Marine trace metal elements research began in 1970 in the GEOSECS project. Before that, it was very difficult to detect trace metals due to the low sensitivity of instruments and cross-contamination during sampling and analysis (Li et al., 2017). The GEOSECS project, in which scientists from various countries participated, achieved fruitful scientific research results (Li et al., 2017). It is however still difficult to solve some of the existing problems in current research. The development of research in this field is relatively slow in China because of limited involvement in GEOTRACEs and a longstanding lack of sampling equipment for seawater trace metals. Marine geochemical studies rely heavily on very clean sampling and analytical tools, and the continued technological development of these tools. Chinese marine scientists have recently strengthened their research in these areas (Li et al., 2017).
Complex processes control the transportation of trace metals in dynamic coastal systems. These systems have been studied in detail, but are still not fully understood (Li et al., 2017). Limited temporal and spatial data, and clean sampling and analytical procedures are the major obstacles to better understanding the behavior of trace metals in coastal areas. Recent scholarly research has promoted the understanding of the distribution and the biogeochemical behaviors of trace metals in coastal waters (Jiann et al., 2009; Achary et al., 2016; Wang et al., 2018c; Zhao et al., 2018). Investigations on the Bay of Bengal coast in Bangladesh, for example, showed that As, Cr, Cd, and Pb have reached high pollution levels due to the impact of anthropogenic activities on the surface and subsurface waters and sediments during the winter and summer (Ali et al., 2022). Clean sampling and analysis techniques for trace metals have improved the technology for determining dissolved trace metals in the Chinese coastal seawaters, and more reliable data have therefore been obtained. Wang et al. (2018b) and Jiann et al. (2009) have studied the distribution and behavior of trace metals (Cd, Cu, and Ni) in the surface waters and sediments of the East China Sea (ECS). Zhao et al. (2018) reported on the occurrence and potential risks of seven dissolved trace metals (Hg, Cd, Pb, As, Cr, Cu, and Zn) in Xiangshan Bay, China. In the offshore area of Dalian Bay, Sun et al. (2019) examined the concentration and pollution characteristics of dissolved trace metals (Cu, Pb, Zn, Cd, Hg, and As). Lu et al. (2020) studied the distribution of four size fractions of dissolved Cu, Pb, and Cd and the related influence on surface waters of the Yellow Sea, while Wang et al. (2015) analyzed the spatial distribution of dissolved and particulate trace metals (Cu, Cd, Zn, and Pb) in Jiaozhou Bay, Yellow Sea. In the Dingzi Bay and Laoshan Bay, the spatial distribution of selected trace elements (Hg, As, Pb, Cu, Cd, Cr, and Zn) and their potential ecological risk have been investigated by Pan et al. (2014) and Wang et al. (2019). Li et al. (2015) studied the spatial and temporal distribution of dissolved trace metals (Ag, Cd, Co, Cu, Ni, Pb, and Zn) in the surface and bottom seawater of Bohai Bay and Laizhou Bay over two years. The concentrations of dissolved metals from the two years are very similar at the surface and bottom locations. Although many researchers have increasingly concerned about trace metals in coastal areas and estuaries around the globe, dissolved trace metal concentrations and spatial distribution were seldom reported in seawater of the coastal areas of China (Wang et al., 2018). Studies on dissolved trace metals in coastal waters mainly focused on the surface seawater, while few studies focused on the bottom seawater. Only a few studies focused on the difference in the distribution of dissolved trace metals from the surface and bottom seawater and how they interact. The coastal sea is the most physically and biogeochemically active area because of the high degree of boundary exchanges and processes (Dai et al., 2012). The distribution and biogeochemical behaviors of dissolved trace metals in the coastal areas, therefore, remain unclear.
Five trace metals (Zn, Co, Cr, Cd, and Pb) in the Yantai offshore area (YOA), North Yellow Sea (China) were detected in this study. In addition to the determination of physicochemical parameters, the ecological risks and potential sources of trace metals were also explored in detail. These data were generated from the surface and bottom seawater in the summer (August) of 2019 and 2020 using clean sampling and analysis techniques for trace metals. In summer, with the rise of the temperature, the content of organic matter is higher, and the biological activities become more frequently. (Hatje et al., 2003; Zhang et al., 2022). The aquaculture organisms in the study area are in the feeding and rapid growth period. At this time, the distribution of trace metals was relatively complex, which was also a significant health concern at this time. The overall study aimed to assess the potential ecological risk, explore the mutual influence of total dissolved trace metals from the surface and bottom water in the coastal sea, and improve the understanding of the distribution and biogeochemical behaviors of these trace metals in the YOA including the Yantai Sishili Bay Marine Ranch.
2 Methods
2.1 Study region
The Yellow Sea is a typical semi-enclosed continental shelf marginal sea, with abundant oil and gas fields, and supports economic activities including fisheries and aquaculture. The waves, tides, and ocean currents undergo seasonal changes (Wang et al., 2003; Chen and Yu, 2011; Wang et al., 2020). The Yellow Sea is in the temperate zone and belongs to the East Asian monsoon climate with obvious seasonal changes (Li D. et al., 2022). Strong winds of magnitude 6 or higher occur in the Yellow Sea area all year round. These winds are mainly distributed from the Bohai Strait to the sea area near Chengshan Jiao at the top of the Shandong Peninsula (including the study area). From June to August, the prevailing wind direction in the study area is south and southeast. Most of the tidal currents in the Yellow Sea are regular semi-diurnal currents, but irregular diurnal currents occur near the coast of Yantai (Mask et al., 1998).
Yantai city is located in the North Yellow Sea with a long coastline, rich ocean resources, and unique advantages. The Guangdang, Han, and Nian Rivers, etc. occur along the coastline (Figure 1A) (Wang et al., 2013). Runoff injection from land-based sources, industrial and agricultural wastewater, urban sewage, and mariculture discharge have contributed to the deterioration of the marine environment in the YOA (Li M. et al., 2022). In the past, sea cucumbers, prawns, oysters, scallops, kelp, flounder, and other seafood were mainly cultured in traditional rafts in the Yantai Sishili Bay. The marine aquaculture was the main factoraffecting environmental changes in the Yantai Sishili Bay. The deterioration of the marine environment is attributed to high-density aquaculture, the single aquaculture mode, and the direct discharge of a large number of aquaculture wastes into the sea. To improve the marine ecological environment and promote sustainable and green development of these fishery resources, Yantai has planned the construction of four marine ranching demonstration areas, which includes the sea area of the Yantai Sishili Bay. Figure 1 shows a schematic diagram of the spatial layout of the YOA, including the Yantai Sishili Bay Marine Ranch area.
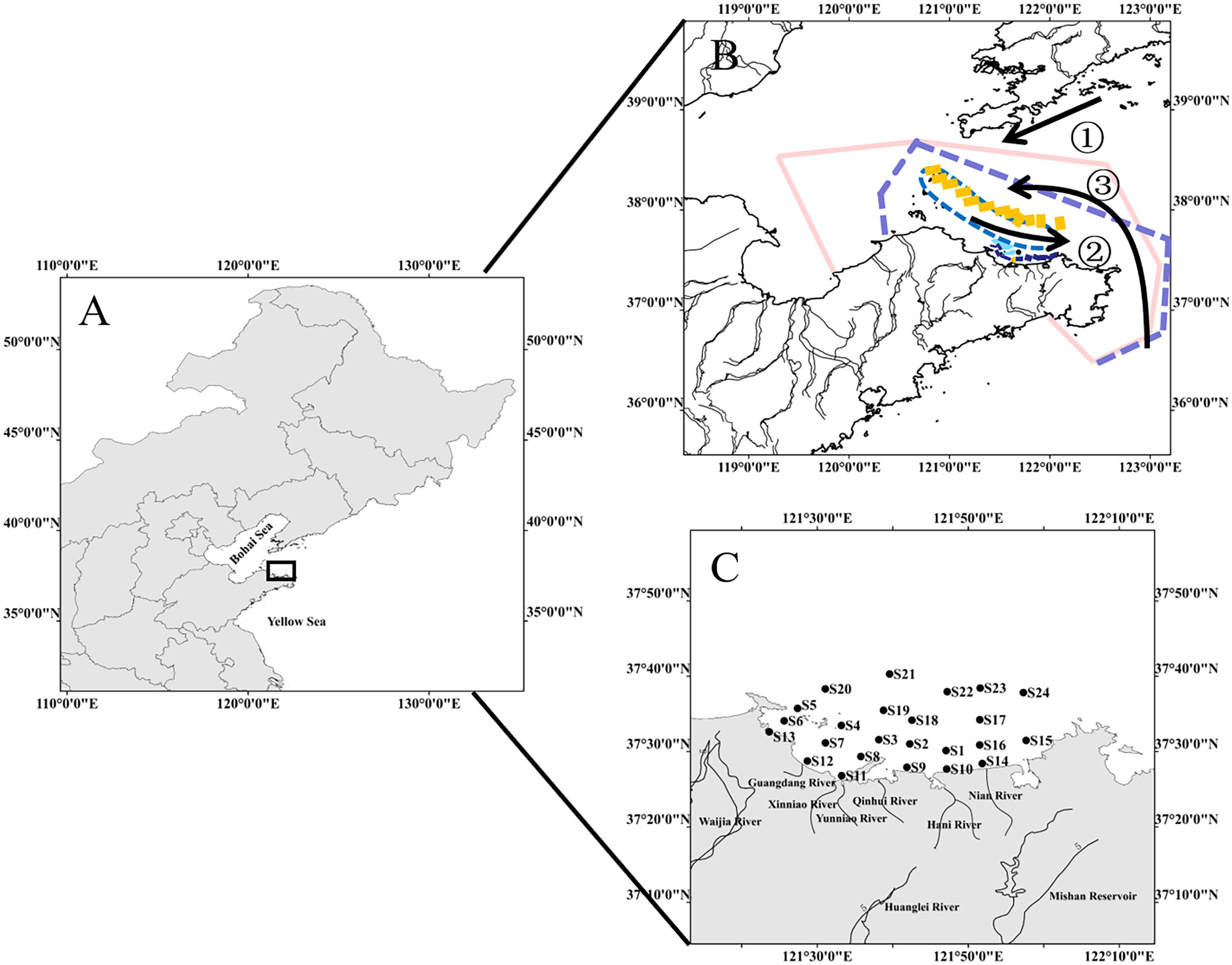
Figure 1 (A) Map of the study area, (B) Schematic diagram of the spatial layout of the Yantai Sishili Bay Marine Ranch and (C) Sampling sites in the YOA, China. In Figure B the colored lines represent a schematic diagram of the spatial layout of the Yantai Sishili Bay Marine Ranch and its surrounds: The orange line represents the offshore recreational sea fishing belt, the dark blue line indicates the coastal sightseeing fishery belt; the pink line represents the bottom sowing area of sea treasures in the northeastern peninsula; the purple dotted lines represent the main area of artificial reef construction in Jiaodong Peninsula; the blue dotted line represents the Yanwei offshore shellfish ecological park; the light blue line represents the marine ranch prohibited development zone. Marine dumping area in Yantai area in 2019: Dark spot represents the temporary dumping area; and the orange spot shows the formal dumping area. The major sea currents include ① Liaonan Coastal Current; ② Lubei Coastal Current; ③ Yellow Sea Warm Current.
The YOA is a semi-closed bay with poor water exchange, which is not conducive to the dilution and diffusion of trace metals and other pollutants (Pan et al., 2020). The major currents in the vicinity of the Yantai Sishili Bay Marine Ranch are the Liaonan Coastal Current (LnCC), the Lubei Coastal Current (LbCC), and the Yellow Sea Warm Current (YSWC) (Figure 1B) (Liu et al., 2017; Li et al., 2020). The flow direction of the LnCC remains unchanged throughout most of the year. But in summer, it has a large range at the surface sea and a small range at the bottom sea, along with a large flow velocity and narrow flow range (Chen et al., 2013). The low salinity LnCC provides abundant materials for the coastal waters (Xu et al., 2018), which may affect and control the ecological characteristics of the sea areas. The waters near the northern coast of the Shandong Peninsula are hydrodynamically active and are affected by the LbCC. This activity leads to the accumulation or outward transport of sediments from the Yellow River (Chen et al., 2013; Li et al., 2020). The LbCC and the LnCC formed a large counterclockwise vortex in the North Yellow Sea. This vortex transports offshore materials and deposits the Yellow River sediment in the middle of the North Yellow Sea. The YSWC, which is a branch of the Kuroshio Current, merges a stream of high temperature and high salinity water into the LbCC, after which it flows out of the strait (Mask et al., 1998; Xu et al., 2009). In summer, the more prominent bottom cold ocean water masses of the Yellow Sea weaken the YSWC (Dai et al., 2009). Typhoons and temperate storm surges affect the coastal area of Yantai, and nearshore red tides mainly occur in the Yantai Sishili Bay. The occurrence and flow of the ocean currents, typhoons, and storm surges would all affect the water quality (including trace metals) in the YOA area (Wang et al., 2020; Li D. et al., 2022). The marine ranch aims to build a “smart ocean” in the future. This will improve in-situ long-term monitoring and real-time dynamic monitoring of the distribution and characteristics of major environmental factors and how they change over time. Improving the monitoring methods for dissolved trace metals, understanding their spatial changes over time, and doing pollution assessments, are urgent in the major Yantai Sishili Bay marine ranch areas. (Dai et al., 2009).
2.2 Sample collection
Twenty-four survey stations were set up in the YOA in August 2019 and 2020 (Figure 1). Surface water samples (1-2 m deep) and bottom water samples (about 2 m above the water bottom) were collected. The contours corresponding to the depth of the station point were shown in Figure S1. The samples were analyzed for physicochemical parameters, including Dissolved oxygen (DO), Water temperature (T), pH, salinity (S), Chlorophyll a (Chl a), DOC (Dissolved organic carbon) and DIC (Dissolved inorganic carbon), and total dissolved trace metals (Zn, Co, Cr, Cd, and Pb). The sites collected in 2019 year (without S22) and 2020 year (without S14, S15, S19, S21, S23 and S24) were not the same because of the various cruises of our institute. Only the physicochemical parameters (DO, T, pH, S, and Chl a) on sites S1-S13 in 2019 year and 2020 year were provided from Research Station of our institute. These effects were however eliminated in subsequent explorations. Strict clean trace metal sampling procedures were maintained from water sampling to testing. Samples were collected with a 2.5 L pre-cleaned polypropylene sample collector that had been pre-rinsed three times by the seawater in the field and was then stored in pre-cleaned low-density polypropylene bottles (NalgeneTM, Thermo Fisher Scientific, Inc.). The samples were then kept at a temperature of 4 °C and brought back to the laboratory. At the laboratory, it was immediately filtered by a peristaltic pump (Cole-Parmer Inc.) through acid-treated 0.45 µm Millipore filters (Pall Corporation) into the pre-cleaned polyethylene bottles. Some samples for total dissolved metals were acidified to pH< 2 with high-purity HNO3 in a clean and low-temperature environment and stored until measurement. Other samples, earmarked for analyzing DOC, were stored at -20°C without acidification. The cleaning process by Lu et al. (2019) was used to clean the sample collector and sample bottles before sampling.
2.3 Dissolved metal analysis
The dissolved trace metal concentrations of selected metals (Zn, Co, Cr, Cd, and Pb) were determined after the samples had been collected using an inductively coupled plasma mass spectrometer (ICP-MS, ELAN DRC II, PerkinElmer® USA) (Lu et al., 2020). The ICP-MS method was verified to ensure the accuracy of the subsequent experimental methods. The ICP-MS method blanks were estimated by detecting diluted 0.5% HNO3 10 times with ultra-pure water. The detection limits (LOD) and Quantification limits (LOQ) for Zn, Co, Cr, Cd, and Pb were three times and ten times the standard deviation (3r or 10r) of the blanks, respectively. Table S1 shows the corresponding results and linear correlations (R2). The data presented high R2 and low LOD and LOQ, which verified the accuracy of the experimental detection method.
2.4 Determination of physicochemical parameters
Dissolved carbon concentrations (DOC and DIC) were measured with a TOC-VCPH total organic carbon analyzer (Shimadzu, Japan) (Lu et al., 2020; Yang et al., 2020). A CTD (Conductivity, Temperature, Depth) and a YSI sensor (Yang et al., 2022) were used to determine the DO, T, pH, S and Hitachi F-7000 fluorescence spectrophotometer was used to determine chlorophyll a (Chl a) (Yang et al., 2022). The detection precisions for S, T, DO, pH and Chl a were ±0.01‰, ± 0.1°C, ± 0.1mg/L, ± 0.01, and 0.01μg/L, respectively.
2.5 Correlation analysis, principal component analysis, and hierarchical cluster analysis
The Probability-Probability plot and the Kolmogorov-Smirnov test were used to check the normality of the data. Pearson correlation analysis was used to identify the correlations among the surface and bottom variables, respectively. The upper statistical analysis was performed using IBM SPSS Statistics 25.0 and presented through Origin 2021b.
Principle component analyses (PCA) were used to evaluate the possible origins of the dissolved trace metals in the surface and bottom seawater of the YOA (Poupard et al., 2005; Yano et al., 2019). The Keiser-Meyer-Olkin (KMO, 0.722) and Bartlett’s tests (<0.000) indicated that the PCA results were an effective method. The Hierarchical Cluster analysis (HCA) was used to evaluate the correlations between the concentration of dissolved trace metals and the sampling sites seawater of the YOA for the different years. HCA could be used to trace similarities and differences between variables, which could be used to further verify the results of the PCA analysis (Ma et al., 2016). PCA and HCA were created using Origin 2021b (Dang et al., 2021).
2.6 Water quality assessment method and principal component analysis method
This study evaluated seawater contamination by toxic substances by using contamination factors (Cƒ) and contamination degrees (Cd) (Hakanson, 1980; Lv et al., 2015; Wang et al., 2018). Cƒ and Cd represent the contamination of single elements and overall trace metals, respectively.
Cheavy metal equals the mean concentrations of trace metals, (i =Zn, Cd, Co, Pb, Cr); whereas Cbackgroundequals the background concentrations of the corresponding trace metals in the seawater. Background data for trace metals in seawater are lacking in the YOA. The grade-one seawater quality standard for China was therefore used as the background concentration (i.e.20 µg/L Zn, 1 µg/L Cd, 30 µg/L Co, 1 µg/L Pb and 5 µg/L Cr; GB 3097-1997) (AQSIQ, 1997). The Cƒ and Cd values were evaluated as recommended by Hakanson (i.e. Cƒ< 1 Low, 1≤ Cƒ< 3 Moderate, 3 ≤ Cƒ< 6 Considerable and Cƒ ≥ 6 Very high; Cd< 5 Low, 5 ≤Cd< 10 Moderate, 10≤Cd< 20 Considerable and Cd ≥ 20 Very high) (Hakanson, 1980).
3 Results and discussion
3.1 Investigation of hydrological parameters and dissolved carbon distribution
DO, Chl a, and S distribution were shown in Figure S2. The water temperature was high (T = 25.27 ± 0.81°C) (Table S2) and reached the optimum temperature for the growth of most phytoplankton (Wang et al., 2018a). The salinity (S = 31.05 ± 0.20 ‰) and pH value (7.93 ± 0.15) only varied to a limited extent (Table S2 and Figure S2). The distribution of salinity in the surface and bottom waters were almost similar to the results of Dai et al. (2009). The salinity distribution of the YOA surface water decreased from the inshore to the offshore, indicating that it was not strong freshwater signals input as shown in other regions, such as the Yangtze River and the Yellow River, etc (Li et al., 2015; Wei et al., 2016; Wei et al., 2016b). Compared to the surface water, bottom water had relatively higher salinity and lower temperature (Table S3 and Figure 5), which has been frequently observed in previous studies (Li et al., 2017).The salinity of the surface water was lower than the bottom water, indicating that it was also influenced by the external freshwater input from the River (Li et al., 2015). Theoretically, there is no direct correlation between DO and pH. DO and pH were positively correlated in this. Based on the DO and Chl a distribution in the YOA, the distribution trends of the content of both in the surface and bottom water were similar. The DO distribution of surface water was influenced by the Chl a, which was similar to the Liu et al. (2019b) study. The large variation in the spatial distribution of Chl a concentration was similar to the considerable spatial and temporal variability of phytoplankton blooms (especially in summer) previously studied by Fu et al. (2009).
DOC affects the chemical properties of the surrounding environment, including the behavior of trace metals and pollutants (Bhatt and Gardner, 2008; Birkel et al., 2017). A survey of the dynamics of DOC in water bodies will help in understanding its role in the variability of trace metals in the environment. The Joint Global Ocean Flux Study (JGOFS), which has been completed, provides a deeper understanding of the role of carbon in Marine biogeochemical processes. Scientific understanding of the role of DOC in the marine biogeochemical cycle of trace elements is however not perfect (Biller and Bruland, 2013). Figure 2 shows DOC and DIC in the YOA in August 2020. The DOC concentration of the surface and bottom of the main seawater is 0.72–1.08 mg/L and 0.41–0.54 mg/L, respectively (Hansell and Carlson, 1998). The average concentration of DOC in the estuary around the study area is about 5.28 mg/L. The DOC concentration in the estuary is usually higher than that of the open ocean due to the influence of the continental input in the coastal waters. The nearshore DOC concentration in this study is the highest in the estuary, followed by the nearshore and then the ocean (Hansell and Carlson, 1998; Dittmar et al., 2006). The mean DOC concentration of the bottom water was lower than the surface water in August. These results were similar to those of the northern Shandong Peninsula (Yang and Gao, 2019). Compared with the other areas in August, the DOC concentration is higher than that in the ECS (Zheng et al., 2018) but lower than the Canadian Shelf of the Beaufort Sea Green Bay (DeVilbiss et al., 2016).
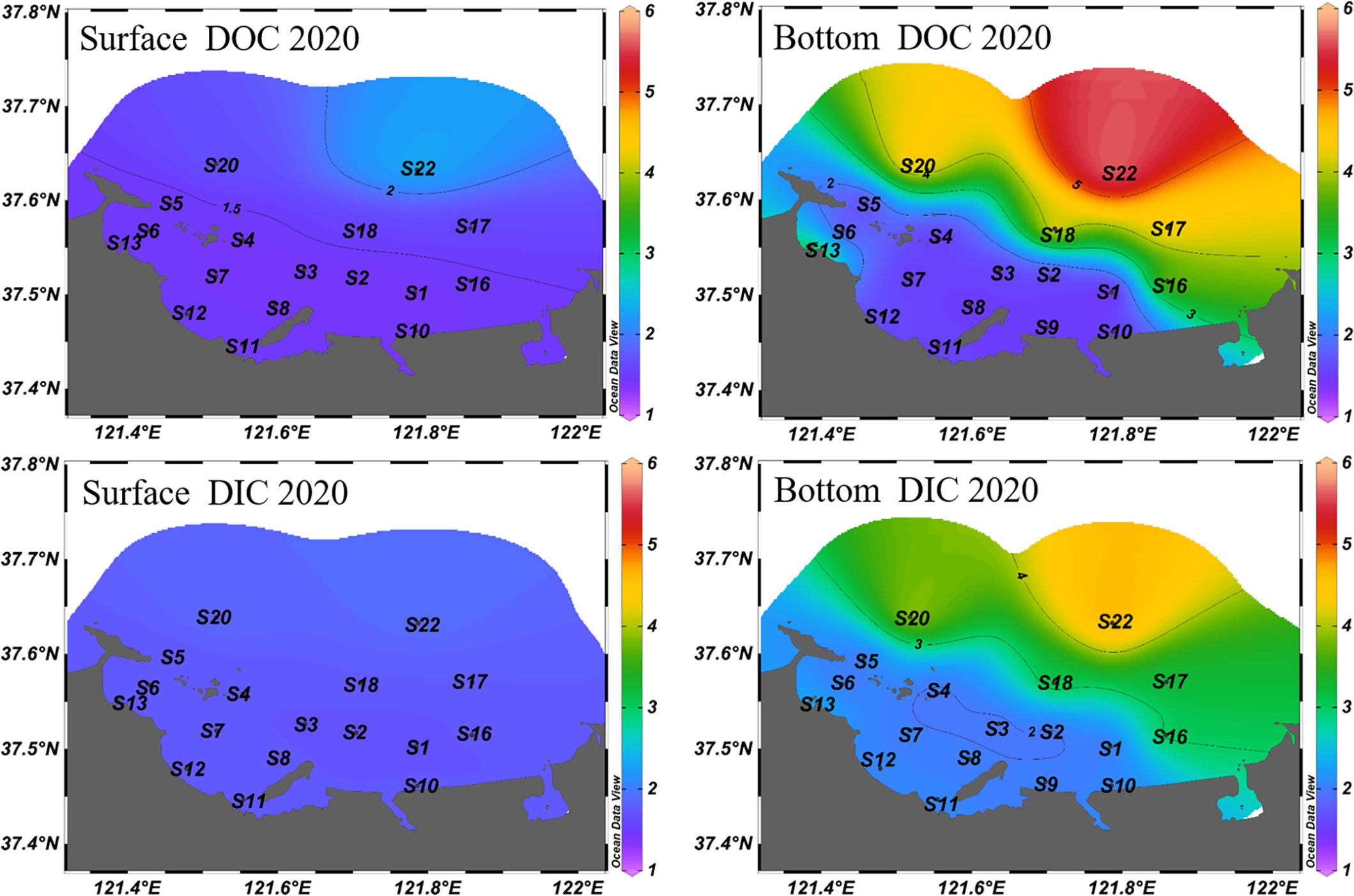
Figure 2 Spatial distribution of the DOC and DIC (unit: mg/L) in the YOA in August 2020 for surface seawater, and bottom seawater.
The dissolved carbon data showed a clear spatial distribution pattern. All the DOC and DIC values increased from the inshore to the offshore, from west to east, and from the surface to the bottom stations. Contrary to what was expected, the DOC value of the seawater was low in the nearshore and high in the far shore areas. This means that human activities and river input did not have a dominant influence on the DOC of the seawater. Previous studies have also shown that annual DOC discharged into the coastal ocean is relatively low compared to the total marine DOC pool (Dai et al., 2012). The concentration of DOC in the far shore area is higher than near shore the seawater. This is probably because this area is in or near the fish and shellfish breeding area (Figure 1), where phytoplankton grows easily and where the biomass of various organisms also increases. The high values of dissolved oxygen in the far shore area also prove this (Table S2). The Lubei Coastal Current flowing from west to east may influence the DOC concentrations, which gradually increase from west to east. The upper-ocean has a low DOC concentration. The DOC concentration might be affected by the low vertical stability of the water body and by the upwelling dilution of the low concentration of DOC in the bottom water due to the impact of cyclone in late July 2020. There is also a growing body of evidence for the discharge of submarine groundwater into the coastal ocean. This influence of this discharge is however currently difficult to estimate because the DOC data for the subsurface estuaries where the groundwater mixes with the seawater are very limited (Dai et al., 2012). The distribution of DIC in the study is similar to the results (Yang and Gao, 2019). The low DIC concentration in the surface seawater in August is caused by high primary productivity. The DIC concentration of the underlying layer is also significantly higher than the surface layer, which may mainly be due to the oxidation of the underlying organic matter.
3.2 Dissolved trace metal distribution
3.2.1 Zn, Cd, and Co
Figures 3, 4 show the distribution of dissolved Zn, Cd, Co, Pb, and Cr in the surface and bottom seawater of the YOA in August 2019 and 2020. Marine organisms accumulate trace metals such as Zn, Cd, and Co in their tissues and bones. These trace metal elements are essential for biological growth and are therefore often referred to as trace nutrients. Zn and Cd are absorbed by plankton in the upper water body and are vertically distributed in ocean waters (Bruland et al., 2013). When the organisms die, part of the biogenic material is recycled in the water body of the biosphere, while part moves to the middle and deep ocean water layers via particle sedimentation (Zhang et al., 2022). These trace metals, therefore, occur at low concentrations in surface water layers and high concentrations in the middle and deep ocean water layers. Contrary to the expected, Zn and Cd concentrations were high in the surface seawater layer and low in the bottom seawater layer (Table S2). The horizontal distributions of the Zn and Cd were similar in the surface and bottom layers for both sampling years. The Cd concentration of the S16 station was higher than other stations for both the surface and bottom layers in 2020, and the spatial distribution of Cd in the two layers was not completely consistent. The vertical distribution of Co was the same as those of Zn and Cd, and the concentration was higher in the surface seawater layer and lower in the deep seawater layer. The interannual variation of the Co content was the same as the Cd with unconspicuous spatial distribution difference of content, with the 2020 Co concentrations being slightly higher than the 2019 Co concentrations. The surface Zn concentrations decreased from the nearshore to the offshore stations in 2019. The results support the fact that the main transport pathway of Zn into the ocean is through continental runoff. The bottom seawater distribution of Zn was similar to that in the surface seawater for all stations except for stations S9, S21, and S24 (Li et al., 2015).
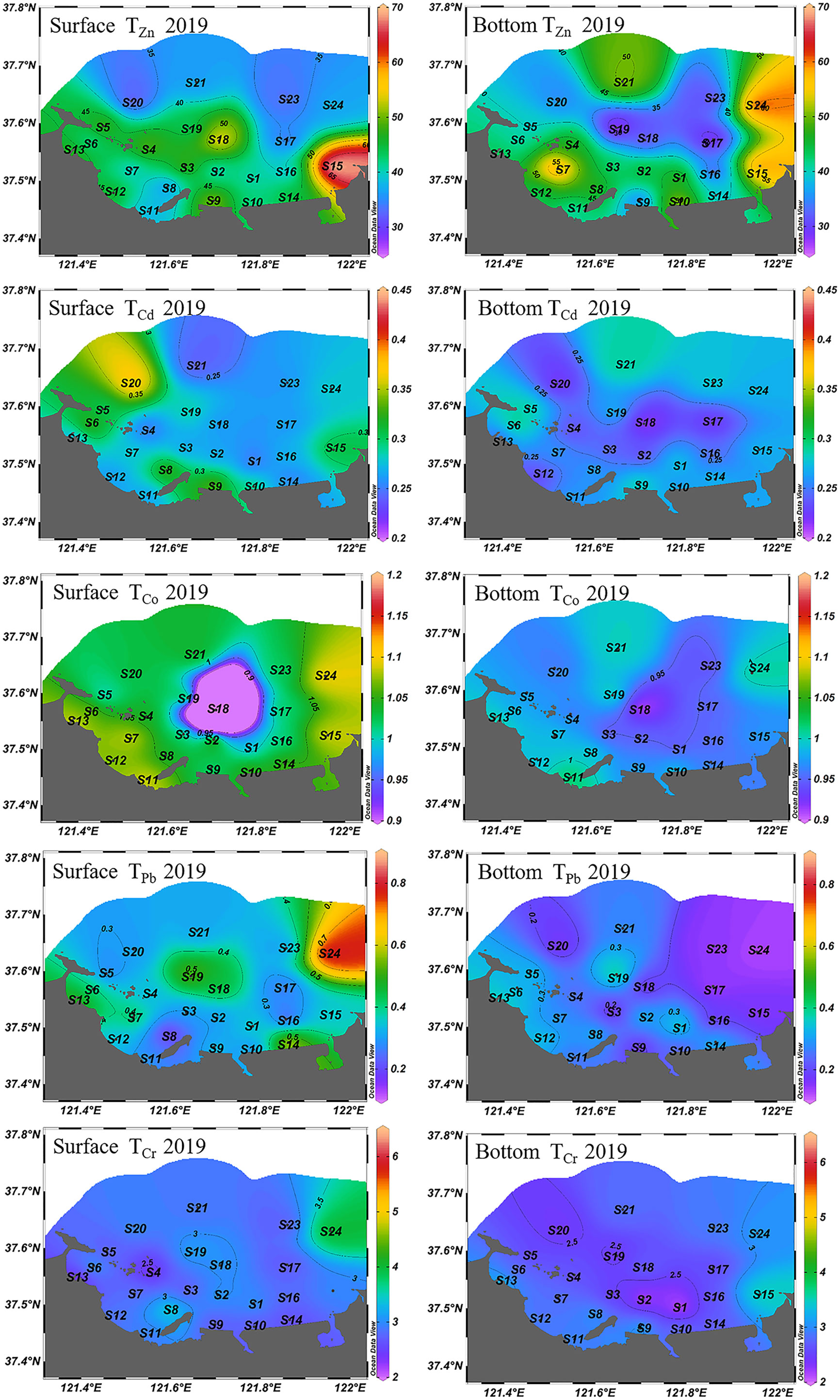
Figure 3 Spatial distribution of Zn, Cd, Co, Pb, and Cr (unit: μg/L) in the YOA in August 2019 for surface seawater and bottom seawater.
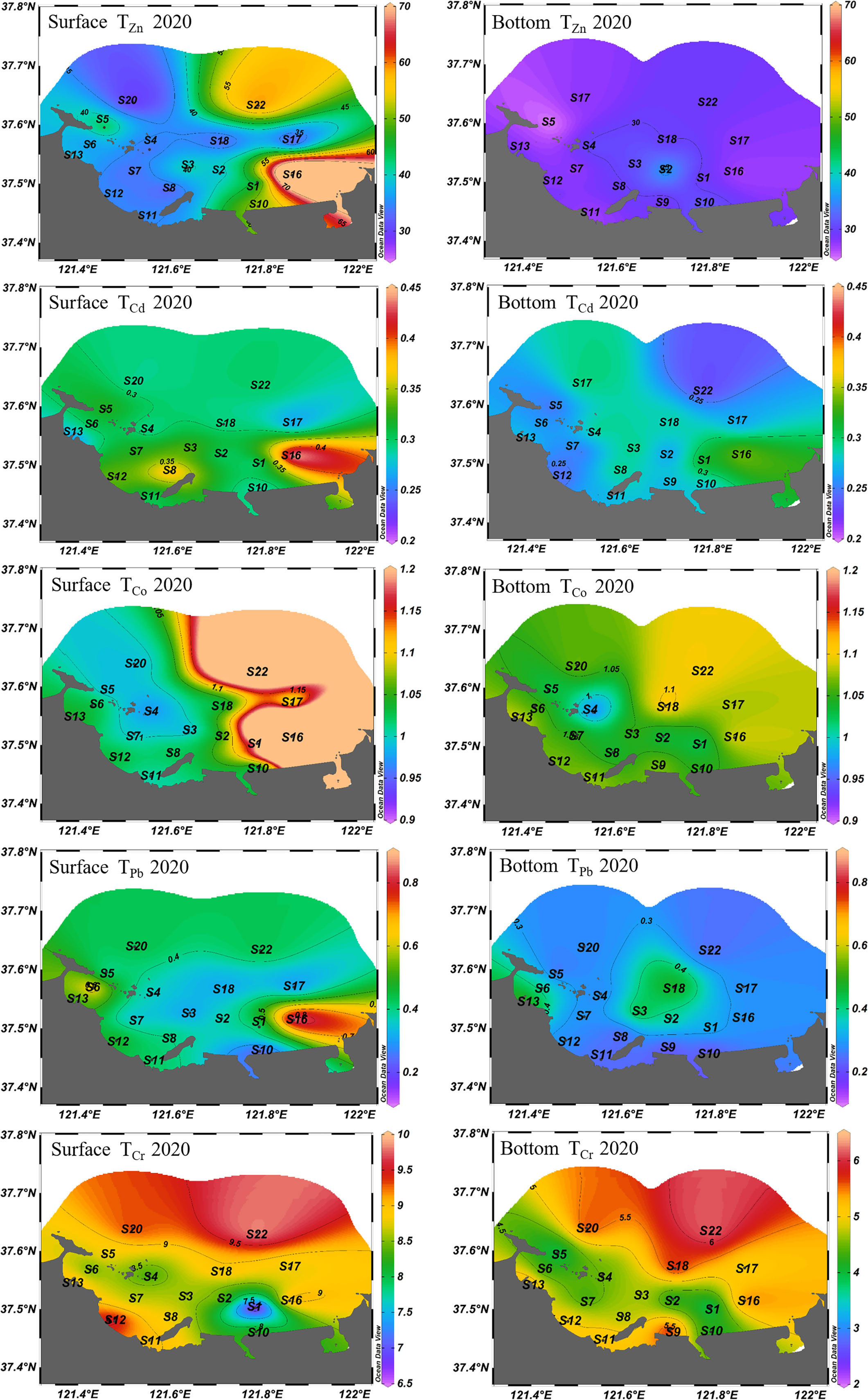
Figure 4 Spatial distribution of Zn, Cd, Co, Pb, and Cr (unit: μg/L) in the YOA in August 2020 for surface seawater and bottom seawater.
Zn, Cd, and Co were essential elements of the biogeochemical cycle (Morel and Price, 2003), as evidenced by the negative correlation of Chl a with them (Table S3 and Figure 5). The metabolic requirements for these elements were however low relative to the available metal concentrations in the coastal seawater and did not impact their spatial distribution appreciably. Their seawater distribution may be the result of several processes: (1) The water moves eastward along the Shandong Peninsula with the LbCC (Li et al., 2017). This hypothesis must however be confirmed by appropriate trace metal concentrations in the easterly or westerly seas, but no relevant water samples were collected for testing. (2) Elevated metal concentrations were found at stations S15, S18, S21, and S24 in 2019 which correspond to the S16 and S22 stations of 2020, which may be caused by local point source emissions. Many rivers empty into the YOA, including the Guangdang River, the Han River, and the Nian River, etc. (Figure 1B). In addition, the water depth is shallow and the exchange capacity is poor in the YOA. The water body is relatively aged, and desorption or remineralization can resuspend sediments and cause the metals to migrate from the solid phase into the water body (Santschi et al., 1990; Cantwell et al., 2008; Kalnejais et al., 2010; Lue et al., 2010). This resuspension has caused an increase in metal concentrations around this area. The high metal concentrations occur in both the surface and bottom seawater layers, which supports this hypothesis (Figure 3). (3) The spatial difference in the metal content is small and may be affected by marine disasters. On August 11, 2019, the storm surge of Typhoon Lekima caused the highest tidal level station in the YOA to reach blue status (Paul et al., 2018). In late July 2020, cyclone 200722 caused a wave with an effective wave height of 4.0 meters in the Yellow Sea. Tides and windblown waves are the main hydrodynamic factors that control the motion and mixing of seawater. They may also play the important role in the biogeochemical behavior of dissolved trace metals in the YOA (Lu et al., 2021).
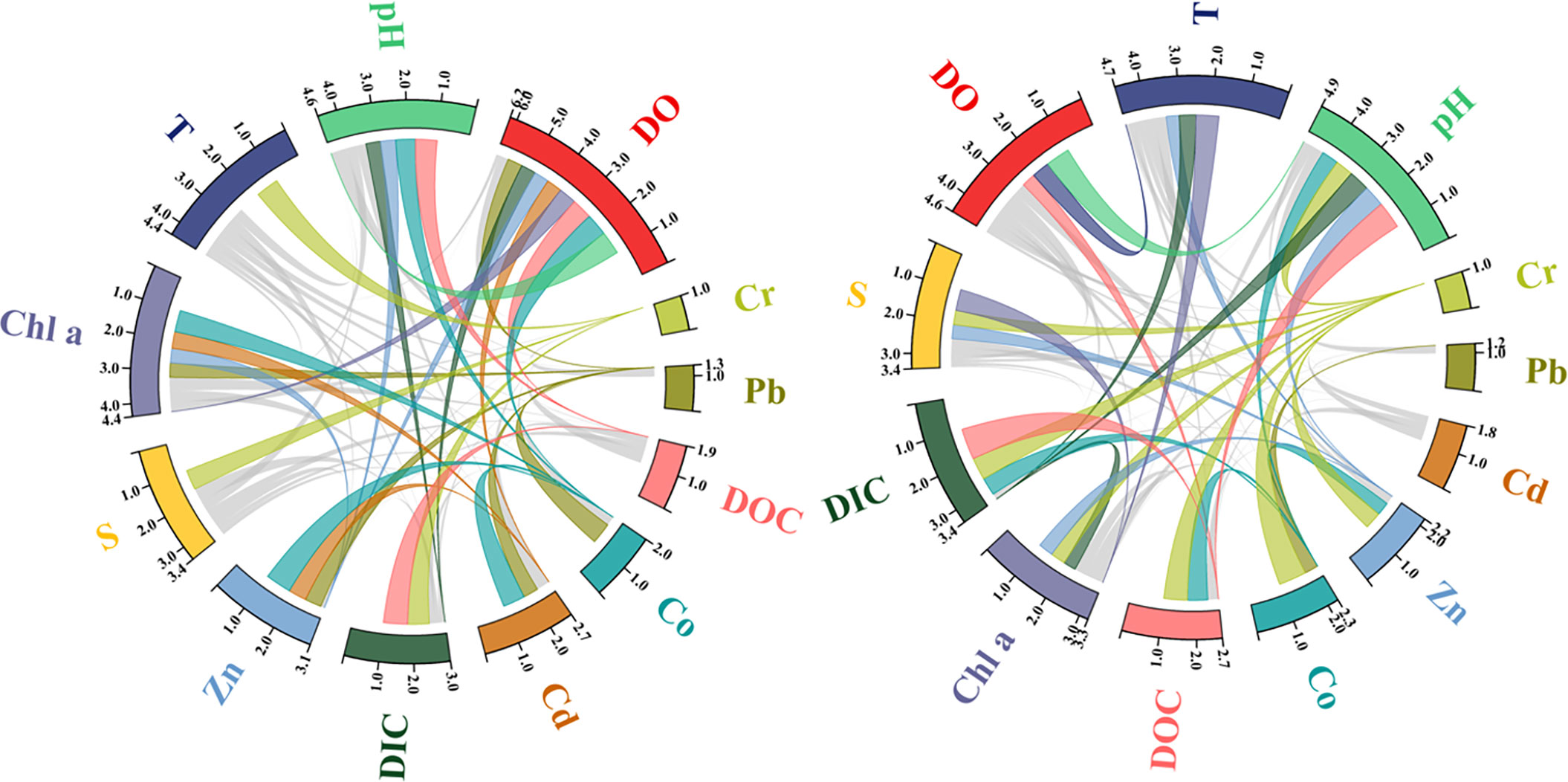
Figure 5 Pearson correlation diagram for the physicochemical parameters and dissolved trace metal concentrations in the study area (Left: surface layers correlation diagram; Right: bottom layers correlation diagram).
3.2.2 Pb and Cr
Pb and Cr are considered the most biologically toxic heavy metal pollutants. These two trace metals accumulate in organisms through multiple channels, which may be toxic and pose health risks to animals and humans (Xu et al., 2009; Zhao et al., 2018). The vertical distribution of Pb and Cr in seawater belongs to the surface enrichment type. Pb is mainly released into the atmosphere through mining mineral resources or fossil fuels. The Pb is then absorbed by atmospheric aerosol particles, and transported to the surface oceans via rainfall (Li et al., 2010; Bruland et al., 2013). Biologically induced oxidation-reduction reactions cause high Cr(III) concentrations in the surface seawater layer. The metal concentrations of Pb and Cr decreased from the surface to the bottom seawater layers (Table S2 and Figure 4). The Pb concentrations do not decrease from the coastal to the offshore areas, as observed in Bohai Bay (Li et al., 2015). The Pb concentration at station S13 is higher than in the surrounding sea area and may be due to input from the dumping area (Figure 1B). In 2020, the Pb concentration in the surface seawater layer at site S16 was abnormally high, similar to the other four trace metals (Zn, Cd, Co, and Cr), and may be caused by input from the Nian River (Figure 1C). The surface layer may be affected by the LbCC, atmospheric input, the influx of many rivers, and typhoons and storms, which may all cause the uneven distribution of the metals in the bottom seawater layer (Li et al., 2017; Pan et al., 2020). The variation of Cr in the bottom and surface seawater layers was relatively consistent, but its inter-annual variation was large. The Cr distribution decreased and then increased from the nearshore to the offshore areas in 2020. This was probably because the main source of Cr in this sea area is the input to the coastal water (Figure 1C). The coastal sightseeing fishery belt is the intermediate transition zone (with stations S1–S5, S16, S17) (Figure 1B), and Cr easily became enriched in the fish (Lin et al., 2021), which led to the decrease of Cr concentrations in the seawater. The Cr concentrations in the offshore seawater were higher and increased from the northwest to the northeast. The Yanwei offshore shellfish ecological park is also located northwest of the study area (Figure 1B), and the shellfish also became easily enriched by Cr. The LbCC however also affected the seawater Cr concentrations from west to east (Figure 1B). Studies that show serious Cr pollution of aquatic products along the Yellow Sea support this hypothesis (Lin et al., 2021). Preliminary conclusions from the above analysis are that the Pb and Cr concentrations may be the result of the complex combination of the following main processes. The main sources of Pb and Cr are the atmosphere and coastal water. The LbCC, aquaculture water bodies, and natural disasters all affect their distribution (Li et al., 2017; Pan et al., 2020). To investigate the possible sources, sinks, interrelationships, and the water quality of the trace metals in the YOA, a more detailed study was carried out in section 3.3.
3.3 Comparison of dissolved trace metals in the YOA with other coastal areas
The mean dissolved trace metals in the surface and bottom seawater Zn, followed by Cr, Co, Pb, and Cd. These results agree with most other research on dissolved trace metals (Table S2) (Wang et al., 2019). The dissolved trace metal concentrations in the YOA were compared with those in other bays and seas (Table 1). The Zn concentrations in the YOA are higher than in the other bays and seas but are comparable with the Zn concentrations in the Kaohsiung Harbour and on the Southwest coast of the Bay of Bengal (Lin et al., 2013; Achary et al., 2016). The Cd levels in the YOA are higher than in Bohai Bay and Laizhou Bay but lower than in the Yellow River Estuary in China (Li et al., 2015; Wang et al., 2019). The Cd concentrations are higher than the Cd levels in Port Jackson, Australia, the Mersey estuary, UK, and San Francisco Bay, USA, while they are comparable with or lower than the Cd levels in the other world bays and seas (Flegal et al., 1991; Comber et al., 1995; Hatje et al., 2003). The Co concentrations in the region are higher than in other sea areas but lower than in the Macrotidal Ennore creek, India (Jayaprakash et al., 2015). The Pb levels are lower than in other bays and seas, but higher than the Pb levels in Bohai Bay and Laizhou Bay, China (Li et al., 2015). The average Cr concentrations compare well with those of the other sea areas.
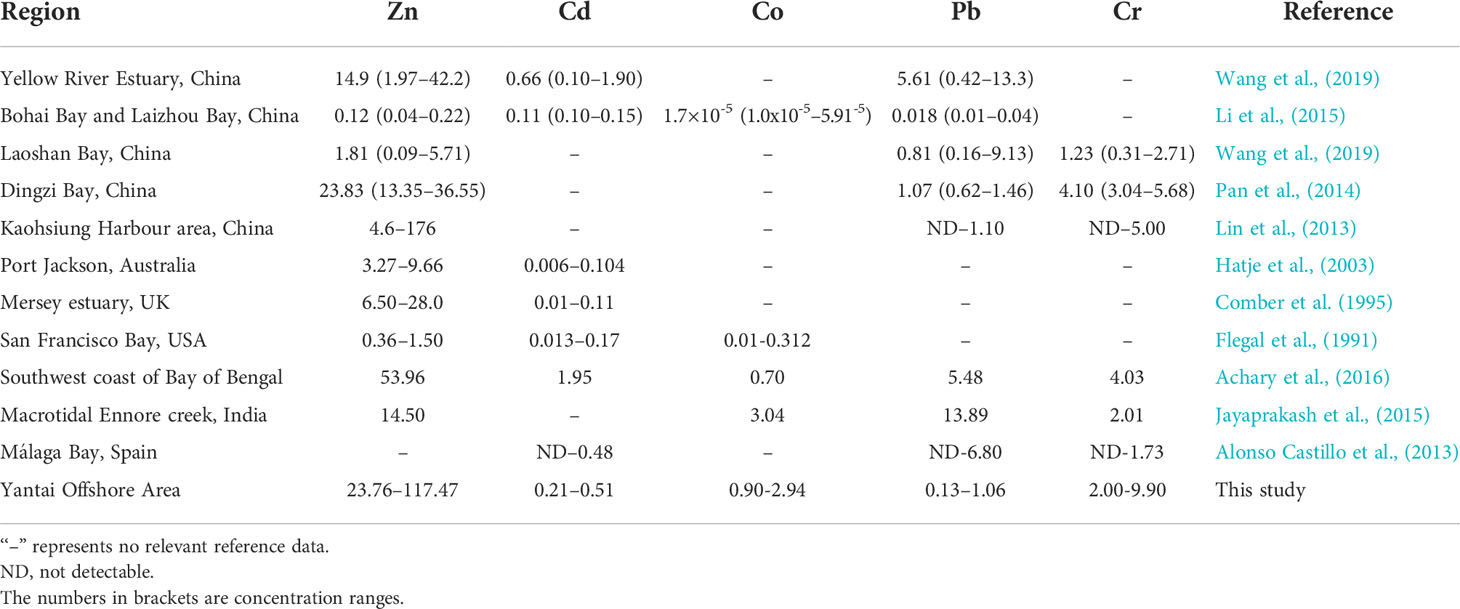
Table 1 Dissolved trace metal concentrations in seawater compared to other regions in the world (ug/L).
3.4 Statistical analysis
The distribution and transport of trace metals were controlled by the physicochemical processes. Table S3 and Figure 5 show the Pearson correlation coefficients between the concentrations of the dissolved trace metals and the physicochemical parameters. Based on the Pearson correlation test there were positive correlations between Zn, Cd, Co, and Pb in the surface seawater layers (Pan et al., 2014; Sun et al., 2019). These results suggest that the sources of those trace metals sources are similar. The sources may be associated with surface fluvial inputs (see sections 3.2.1 and 3.2.2). Cr however only correlated positively with Cd and Co. DO and Chl a concentration correlated negatively with Zn, Cd, Co, and Pb concentrations, suggesting that phytoplankton growth produced more dissolved oxygen and consumed these metals (Liu et al., 2019b). This paper treats Pb as a toxic metal (Gumpu et al., 2015; Gutierrez-Ravelo et al., 2020). The T concentrations were positive correlations with Cr concentrations. These results were similar to the study of Wang et al. (2019). In the bottom seawater layers, DIC and DOC correlated positively with Co and Cr (Pan et al., 2014), but not with other dissolved trace metals. DIC and DOC showed concentration distributions similar to Co and Cr at the bottom seawater (Figures 2, 4). Dissolved organic compounds in water contain complex trace metals (Dyrssen et al., 1988). This study does however not show any significant or systematic correlation between the bottom-layer total dissolved metal concentration and the DOC concentrations. These results are similar to the results of Jiann et al. (2013). Natural organic matter complexation is therefore not the main factor controlling the trace metal concentrations. Follow-up PCA results confirmed this observation. DOC has no obvious contribution to trace metals compared with other factors, and accounts for only 4.5% of PC2. The Zn concentrations were strongly negatively correlated with Co and Cr, and the Co concentrations showed a strong positive correlation Pb and Cr. The S concentrations were also positive correlations with Cr and not correlated with any of the dissolved trace metals (Wang et al., 2018c). There were also positive correlations between DIC and DOC and DO and pH, and negative correlations between T and S (Wang et al., 2018c; Li et al., 2017) in the water body. These results suggest that, unlike for surface trace metal sources, the dominant source of the bottom metal distribution was not just river input.
PCA was used to correlate the different measured variables. This technique captures most of the information from the raw data and can transform a set of correlated variables into a smaller set of uncorrelated variables as principal components (PCs) (Li et al., 2000; Poupard et al., 2005).
The correlations and identified potential origins of multiple dissolved trace metals in the surface and bottom seawater were analyzed via PCA and the data was obtained via interannual monitoring. To gain a deeper understanding of the drivers and to identify correlations between raw variables, two principal components (PCs) with eigenvalues > 1 were extracted from the raw data via PCA. These two PCs explain 99.3% of the total dissolved trace metals. The first principal component (PC1) explained 94.8% of the variance and PC2 explained 4.5% of the total variance. PC1 explains as much of the variability in the data, and the subsequent components explain as much of the remaining variability as possible (Figure 6). Except for the Cr (B) and Cr (D), the other 6 dissolved trace metals showed negative loading on PC1(-0.506 - 0.557). Zn (B) and Zn (D) displayed high positive loadings (1.757- 2.006) (see Table S4 and Figure 6). A combination of natural and anthropogenic sources may therefore contribute to the variable distributions (Rajaram et al., 2020). The trace metals in the coastal seawater mainly come from atmospheric deposition and have external fluvial input (via weathering of rocks). Internal regeneration from the decomposition of particulate organisms and diffusion of the seafloor sediment from interstitial water to overlying water bodies also contribute to the heavy metal distributions. Previous studies show that Zn-containing products are widely used in fertilizers and aquaculture (including marine aquaculture) and galvanizing technology (Liu et al., 2019a; Pan et al., 2020). Many rivers, including the Guangdang River, the Han River, and the Nian River, etc. empty into the YOA (Figure 1C). These rivers may introduce anthropogenic pollution, discharged effluent, and industrial and aquaculture pollution to the ocean water (Yuan et al., 2012; Pan et al., 2020; Rajaram et al., 2020). Sea cucumbers, prawns, oysters, scallops, and other marine products are also cultivated in this region. Marine aquaculture industries often use chemical drugs to control pests. The drug residues can also cause trace metal contamination, including Zn (Wang et al., 2013). Previous studies show increased atmospheric fluxes of trace metals into the Chinese continental shelf, which may even be more important than the river inputs (Gao et al., 1992; Zhang et al., 1992). In the early summer, cold air currents can transport crustal aerosols from the northwestern desert region to the eastern coastal region. This has a significant impact on the atmospheric quality over the Yellow Sea (Gao et al., 1992). South and southeast winds are also predominant in the study area during summer (see section 2.1). This means that Pb, Cd, and Zn concentrations are much higher at the coastal stations than in the desert area (Liu, 2002). In summer, the rainfall may be more frequently scavenged from the atmosphere to the ocean. Pb was mainly released into the atmospheric aerosol particles and transported to the ocean surface through rainfall. PC1, therefore, indicates that the external input is a natural source of human and atmospheric settling. The dissolved trace metals with high PC1 scores exhibited Zn (B) and Zn (D), where the higher concentrations of Zn (B) correspond to the higher concentrations of Zn (D), and vice versa.
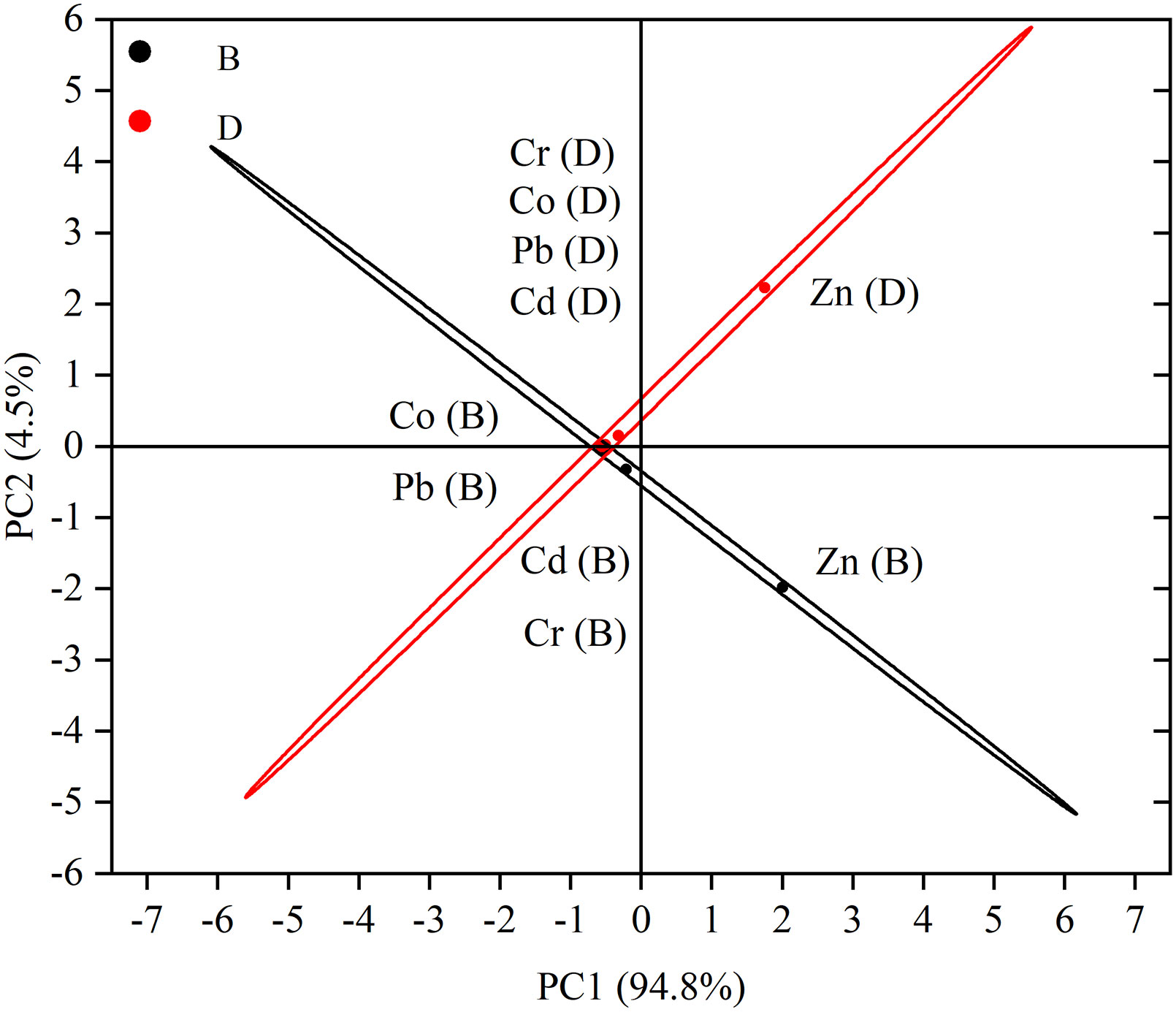
Figure 6 The principal component analysis loading plot of the dissolved trace metals in the B) surface seawater and D) bottom seawater.
PC2 explains almost all the remaining component variability (Figure 6). The dissolved trace metals Zn (B), Cr (B), Zn (D), and Cr (D) for PC2 showed strong loadings compared to the other metals. Table S4 and Figure 6 show that Zn (B) and Cr (B) showed negative loading, while Zn (D) and Cr (D) indicated high positive loadings. The load on the surface and the bottom seawater layers are opposite, which means that anthropogenic sources as a natural source can be excluded. Even though the river discharge solids and dissolved substances into the oceans, the emissions from sediments are generally limited to the coastal and estuarine areas. Trace metals associated with fine-grained suspended sediments are introduced into the estuarine and coastal environments and are eventually deposited with the marine sediments. In the offshore area, sediment plays an essential environmental role in the deposition and release of different compounds in the water and may act as a large reservoir for dissolved trace metals (Pang et al., 2015; Lu et al., 2021). Hydrodynamic factors such as the coastal ocean currents, runoff, and tides, play an important role in dispersing and transporting pollutants, including trace metals, offshore. A particle tracer release off the coast of Yantai has shown that the pathway roughly coincides with the movement of the prevailing ocean currents (Sun et al., 2011). As described in section 2.1, two currents play a key role in the study area. One is the hydrodynamically active LbCC, which causes the accumulation or outward transport of sediments from the Yellow River. The LbCC and the LnCC form a large counterclockwise vortex in the sea area, which causes the deposition of the Yellow River sediment. The trace metals in these sediments may however be released back into the water when environmental conditions (redox potential, pH, and salinity) change (Sundelin and Eriksson, 2001) (i.e., the solubility of the trace metals was increased in lower pH value).
The physical disturbance of typhoons and temperate storm surges, diagenesis, and changes in sediment thickness also affect the study area and may accelerate resuspension, redistribution, and the release of sediment-bound metals into the overlying water. (Petersen et al., 1995; Tsai et al., 2015; Wang et al., 2017; Li et al., 2018; Paul et al., 2018). PC2 may therefore represent a natural source for the release of trace metals in sediments under the influence of external disturbances. This conjecture is consistent with the negative correlation between the related trace metals in the bottom and surface seawater layers.
Figure 7 shows the two-way hierarchical cluster results of the trace metals as a dendrogram. HCA was used to sort the data by creating a dual dendrogram. The vertical dendrogram showed the trace metal clustering of the sampling sites (S1–S24) for the different years (2019 - 2020). The horizontal dendrogram shows the clustering of the trace metals in the surface and bottom seawater layers based on their similarities. The vertical dendrogram displayed 4 clusters: Cluster 1 contained S9 (2020), and Cluster 2 comprised S16 (2020). Sites S7, S9, S11, S18, S19, S20, and S24 (2019) and sites S3, S5, S10, and S22 (2020) were aggregated as cluster 3. The remaining sites were sorted into cluster 4. Cluster 1 exhibited higher Cr concentrations at the S9 site, while other trace metal concentrations were relatively low. Compared with other points, cluster 2 exposed relatively high concentrations of all the trace metals at the S16 site, which was similar to the previous analysis and conclusions. Cluster 3 displayed a similar distribution and concentration of trace metals at sites of the corresponding surface and the bottom seawater layers, where the higher concentration of the surface layer corresponds to the higher concentration of the bottom layer. HCA clustered all the trace metals in the horizontal dendrogram into 2 different clusters. Cluster 1 contained Zn (B) and Zn (D). Cluster 2 comprised the remaining trace metals in the surface and bottom layers. The HCA analysis results were the same as the PCA results.
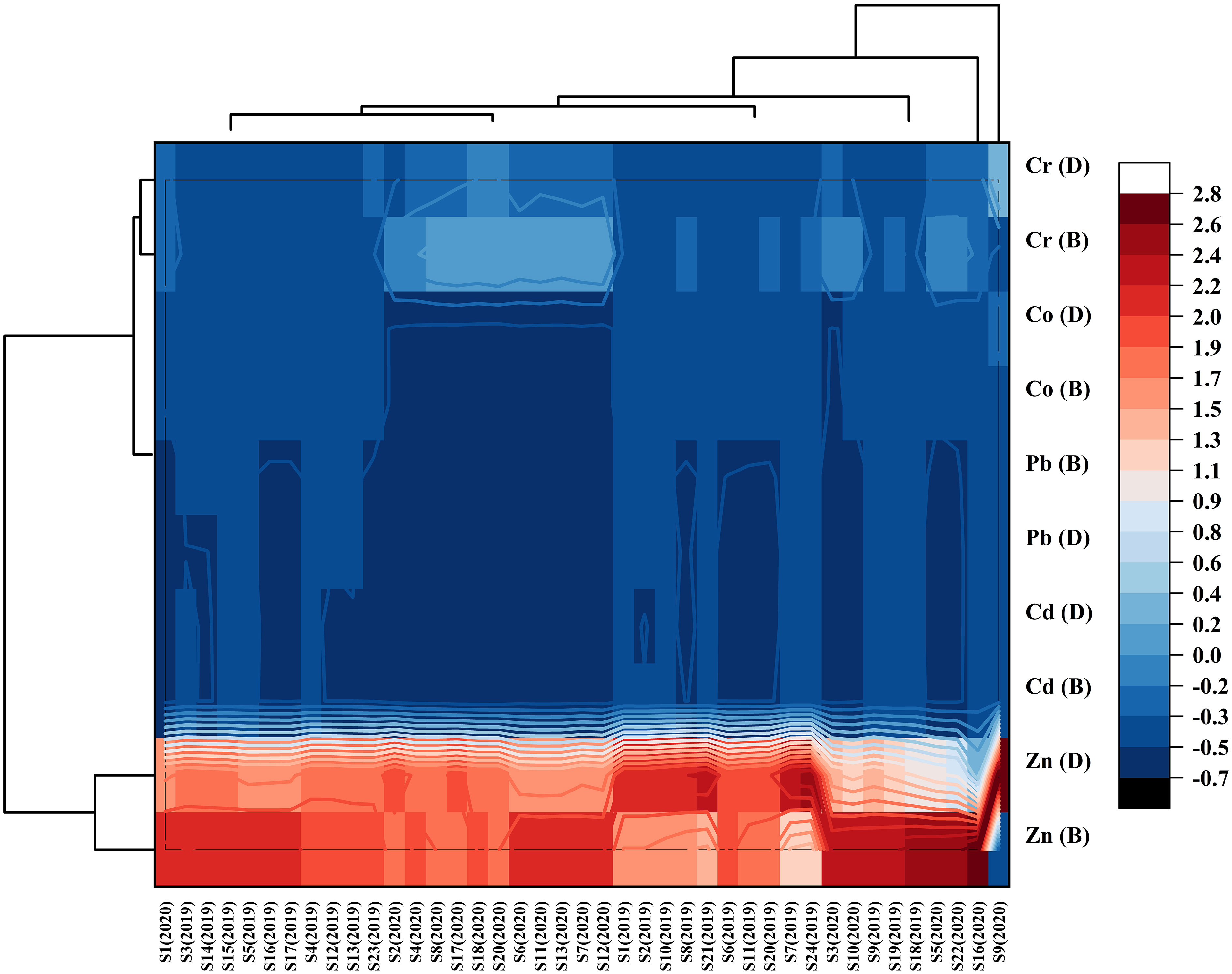
Figure 7 Two-way hierarchical cluster analysis for the dissolved trace metal in the B) surface seawater and D) bottom seawater and sampling sites.
3.5 Water quality assessment
The seawater quality standards of China were used to assess the concentrations of dissolved trace metals in the surface and bottom seawater of YOA (Table 2). The Cd, Co, and Cr concentrations in both the surface and bottom seawater layers were below the primary seawater quality standard of China. In August 2020, 5.88% of the Pb levels however exceeded this standard in the surface seawater layer. The Zn concentrations in all locations exceeded the primary water quality standard of China over the research period. Only in August 2020, the Zn concentration of the bottom seawater layer did not exceed the Grade 2 seawater quality standard, while over the rest of the research period, the Zn of some sites even worsened to a Grade 3 seawater quality.
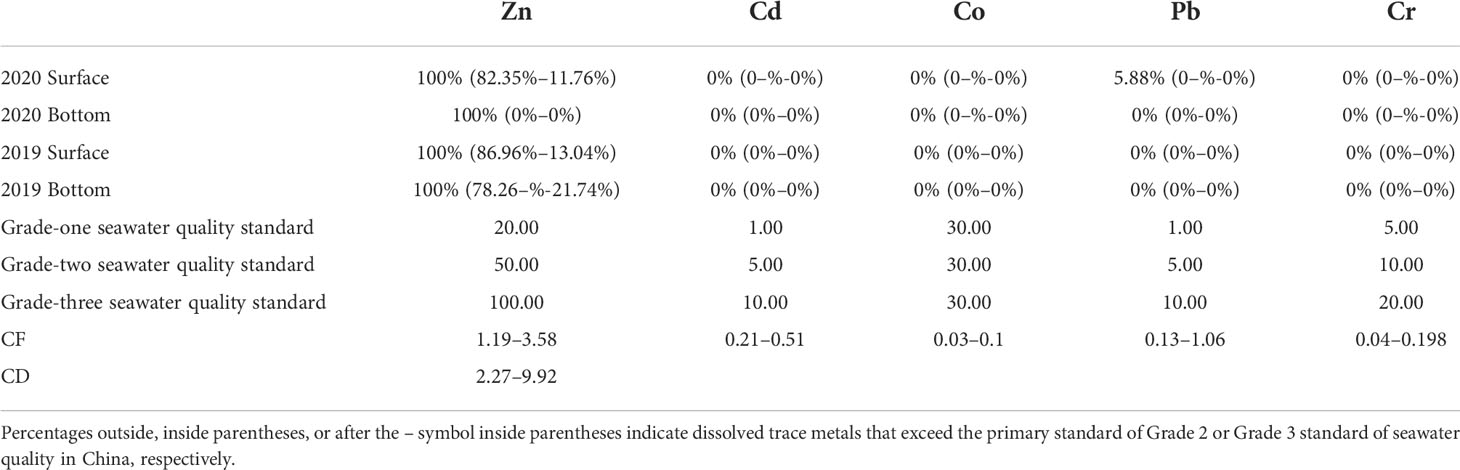
Table 2 Rates of dissolved trace metal concentrations at sampling locations exceeding the Chinese standards.
CF and CD calculations were done by using mean concentrations of dissolved trace metals over the study period (Table 2 and Figure 8). Zn had the highest CF value, followed by Pb, Cd, Cr, and Co. The CF of the Cd, Co, and Cr were low. In contrast, the CF of the Pb was low to moderate (Only at surface station S16 in 2020), whereas the CF of Zn was from moderate to considerable. This result indicated the significant contamination pressures from Zn and Pb in the YOA, which is similar to the results in the Yantai Sishili Bay (Pan et al., 2020). The CF values for Zn Cr, Pb, Cd, and Co were consistent in the surface and bottom seawater layers. The CF value of the surface layer was generally higher than that of the bottom layer, indicating an anthropogenic influence on the surface seawaters of the YOA. The CD of the YOA ranged from 2.27–9.92, indicating low to considerable levels of contamination. The contamination levels may rank differently when using the Grade 1 seawater quality standard as background data. In this case, the realistic pollution levels would be higher than in the present study.
4 Conclusion
This study collected water samples from the YOA to assess the spatial and annual distribution of dissolved trace metals (Zn, Cr, Co, Pb, and Cd) and dissolved organic carbon (DIC and DOC) in the surface and bottom seawater. Combined influences from physical, chemical, and biological processes, as well as local sources and processes, such as coastal currents, monsoons, tides, and wind waves all, influenced the spatial distribution of trace metals and dissolved carbon. Seafood farming in marine pastures and sediment resuspension played an important role in trace element migration and conversion. The average Zn and Co concentrations in the YOA were slightly higher than in other coastal areas of China. The average Pb and Cd concentrations in the YOA were however lower than in other Chinese bays and seas. There were strong positive correlations between Zn, Cd, Co, and Pb in the surface seawater layers. This means that these metals had a similar source, which was most likely surface fluvial inputs. Overall, the CF value of the metals from the surface seawater layer was higher than that from the bottom seawater layer, confirming the anthropogenic influence on the quality of surface seawaters in the study area. This study confirmed major contamination pressures presented by Zn and Pb in the YOA.
This is the first study that has tried to elucidate the biogeochemical behaviors of these dissolved trace metals in the YOA, China. To do this, the study investigated the differences in the distribution of dissolved trace metals in the surface and bottom seawater layers. Overall, the spatial distribution of trace metals in this semi-enclosed bay was different with that in the ocean during the biologically active summer months. Contrary to the expected, the concentrations of trace Zn and Cd were higher in the surface seawater layer and lower in the bottom seawater layer. We suspect that this phenomenon was caused by the relatively low metal uptake rate of phytoplankton, which also reflected the higher external influx rate. The external input rate was generally smaller than the metal uptake rate in the ocean. In nearshore and marine transitional waters, metal uptake rates and external input rates may be almost close, which might maintain similar concentrations of trace metals in the surface and bottom seawater. At this time, the behavior of nutrient trace metals might be similar to conservative elements. Additionally, the main pollution pressure component was the Zn anomaly, which was suspected to be caused by lower phytoplankton and animal uptake and sorption rates than the external input rates in the aquaculture area. Different cultured organisms had different requirements for feed and their ability to absorb and sorption metals. In the nearshore aquaculture area, if reducing the Zn concentration in the feed, Zn would not be the pollution pressure component or Zn concentration limitation. But further researches are still needed to improve our understanding on the mechanism of those phenomenon. Trace metal data analyses in water will become more reliable and comprehensive in the future as increasing attention is focused on the development of clean sampling and analysis protocols for trace metals. This study provides important information on the biogeochemical cycling process and the distribution of trace metals at different depths in the marine pasture areas of China. The assessment of the contamination of trace metals will aid in the development of appropriate strategies and approaches for pollution control.
Data availability statement
The original contributions presented in the study are included in the article/Supplementary Material. Further inquiries can be directed to the corresponding author.
Author contributions
YLi: Investigation, Formal analysis, Conceptualization, Methodology, Validation, Writing - original draft preparation. DP: Funding acquisition, Resources, Conceptualization, Methodology, Validation, Supervision, Writing - review & editing. CW and YLu: Investigation, characterization and draft modification. XF: characterization and draft modification. All authors contributed to the article and approved the submitted version.
Funding
This work was financially supported by the Strategic Priority Research Program (XDB42000000) and the Original Innovation Project (ZDBS-LY-DQC009) of Chinese Academy of Sciences, and the Taishan Scholar Project of Shandong Province (tsqn202103133).
Acknowledgments
The authors thank Muping Coastal Environment Research Station, Yantai Institute of Coastal Zone Research, Chinese Academy of Sciences, for providing part of physicochemical parameters.
Conflict of interest
The authors declare that the research was conducted in the absence of any commercial or financial relationships that could be construed as a potential conflict of interest.
Publisher’s note
All claims expressed in this article are solely those of the authors and do not necessarily represent those of their affiliated organizations, or those of the publisher, the editors and the reviewers. Any product that may be evaluated in this article, or claim that may be made by its manufacturer, is not guaranteed or endorsed by the publisher.
Supplementary material
The Supplementary Material for this article can be found online at: https://www.frontiersin.org/articles/10.3389/fmars.2022.993965/full#supplementary-material
References
Achary M. S., Panigrahi S., Satpathy K. K., Prabhu R. K., Panigrahy R. C. (2016). Health risk assessment and seasonal distribution of dissolved trace metals in surface waters of kalpakkam, southwest coast of bay of Bengal. Reg. Stud. Mar. Sci. 6, 96–108. doi: 10.1016/j.rsma.2016.03.017
Ali M. M., Ali M. L., Proshad R., Islam S., Rahman Z., Tusher T. R., et al. (2020). Heavy metal concentrations in commercially valuable fishes with health hazard inference from karnaphuli river, Bangladesh. Hum. Ecol. Risk Assess. 26 (10), 2646–2662. doi: 10.3390/ijerph17197212
Ali M. M., Islam M. S., Islam A., Bhuyan M. S., Ahmed A. S. S., Rahman M. Z., et al. (2022). Toxic metal pollution and ecological risk assessment in water and sediment at ship breaking sites in the bay of Bengal coast, Bangladesh. Mar. pollut. Bull. 175, 113274. doi: 10.1016/j.marpolbul.2021.113274
Alonso Castillo M. L., Sanchez Trujillo I., Vereda Alonso E., Garcia de Torres A., Cano Pavon J. M. (2013). Bioavailability of heavy metals in water and sediments from a typical Mediterranean bay (Malaga bay, region of andalucia, southern Spain). Mar. pollut. Bull. 76 (1-2), 427–434. doi: 10.1016/j.marpolbul.2013.08.031
AQSIQ (1997). Sea Water quality standard (GB 3097-1997) (Beijing: Administration of Quality Supervision, Inspection and Quarantine. Standard Press of China).
Bhatt M. P., Gardner K. H. (2008). Variation in DOC and trace metal concentration along the heavily urbanized basin in Kathmandu valley, Nepal. Environ. Geol 58 (4), 867–876. doi: 10.1007/s00254-008-1562-z
Biller D. V., Bruland K. W. (2013). Sources and distributions of Mn, fe, Co, Ni, Cu, zn, and cd relative to macronutrients along the central California coast during the spring and summer upwelling season. Mar. Chem. 155, 50–70. doi: 10.1016/j.marchem.2013.06.003
Birkel C., Broder T., Biester H. (2017). Nonlinear and threshold-dominated runoff generation controls DOC export in a small peat catchment. J. Geophys. Res-Biogeo. 122 (3), 498–513. doi: 10.1002/2016jg003621
Bruland K. W., Middag R., Lohan M. C. (2013). “Controls of trace metals in seawater,” in: Treatise on Geochemistry Second Edition. Saunders: Elsevier Inc., pp.19–51. doi: 10.1016/B978-0-08-095975-7.00602-1
Cantwell M. G., Burgess R. M., King J. W. (2008). Resuspension of contaminated field and formulated reference sediments part I: Evaluation of metal release under controlled laboratory conditions. Chemosphere 73 (11), 1824–1831. doi: 10.1016/j.chemosphere.2008.08.007
Chasapis C. T., Peana M., Bekiari V. (2022). Structural identification of metalloproteomes in marine diatoms, an efficient algae model in toxic metals bioremediation. Molecules 27 (2), 378. doi: 10.3390/molecules27020378
Chen X., Li T., Zhang X., Li R. (2013). A Holocene yalu river-derived fine-grained deposit in the southeast coastal area of the liaodong peninsula. Chin. J. Oceanol. Limnol. 31 (3), 636–647. doi: 10.1007/s00343-013-2087-1
Chen T., Yu K. (2011). P/Ca in coral skeleton as a geochemical proxy for seawater phosphorus variation in daya bay, northern south China Sea. Mar. pollut. Bull. 62 (10), 2114–2121. doi: 10.1016/j.marpolbul.2011.07.014
Comber S., Gunn A. M., Whalley C. (1995). Comparison of the partitioning of trace metals in the humber and mersey estuaries. Mar. pollut. Bull. 30 (12), 851–860. doi: 10.1016/0025-326X(95)00092-2
Dai M., Peng S., Xu J., Liu C., Jin X., Zhan S. (2009). Decennary variations of dissolved heavy metals in seawater of bohai bay, north China. Bull. Environ. Contam. Toxicol. 83 (6), 907–912. doi: 10.1007/s00128-009-9842-1
Dai M., Yin Z., Meng F., Liu Q., Cai W. J. (2012). Spatial distribution of riverine DOC inputs to the ocean: An updated global synthesis. Curr. Opin. Env. Sust. 4 (2), 170–178. doi: 10.1016/j.cosust.2012.03.003
Dang P., Gu X., Lin C., Xin M., Zhang H., Ouyang W., et al. (2021). Distribution, sources, and ecological risks of potentially toxic elements in the laizhou bay, bohai Sea: Under the long-term impact of the yellow river input. J. Hazard. Mater. 413, 125429. doi: 10.1016/j.jhazmat.2021.125429
DeVilbiss S. E., Zhou Z., Klump J. V., Guo L. (2016). Spatiotemporal variations in the abundance and composition of bulk and chromophoric dissolved organic matter in seasonally hypoxia-influenced green bay, lake Michigan, USA. Sci. Total. Environ. 565, 742–757. doi: 10.1016/j.scitotenv.2016.05.015
Dittmar T., Hertkorn N., Kattner G., Lara R. J. (2006). Mangroves, a major source of dissolved organic carbon to the oceans. Global. Biogeochem. Cycles 20 (1), GB1012. doi: 10.1029/2005gb002570
Dyrssen D. (1988). Complexation reactions in aquatic systems—An analytical approach: by J. Buffle. Ellis Harwood Series in Analytical Chemistry, Chichester.
Flegal A. R., Smith G. J., Gill G. A., Sa Udo-Wilhelmy S., Anderson L. (1991). Dissolved trace element cycles in the san francisco bay estuary. Mar. Chem. 36 (1-4), 329–363. doi: 10.1016/S0304-4203(09)90070-6
Fu M., Wang Z., Li Y., Li R., Sun P., Wei X., et al. (2009). Phytoplankton biomass size structure and its regulation in the southern yellow Sea (China): Seasonal variability. Cont. Shelf Res. 29 (18), 2178–2194. doi: 10.1016/j.csr.2009.08.010
Gao Y., Arimoto R., Duce R. A., Lee D. S., Zhou M. Y. (1992). Input of atmospheric trace elements and mineral matter to the yellow sea during the spring of a low-dust year. J. Geophys. Re. 97 (1), 3767–3777. doi: 10.1029/91JD02686
Gumpu M. B., Sethuraman S., Krishnan U. M., Rayappan J. B. B. (2015). A review on detection of heavy metal ions in water – an electrochemical approach. Sens. Actuators B 213, 515–533. doi: 10.1016/j.snb.2015.02.122
Gutierrez-Ravelo A., Gutierrez A. J., Paz S., Carrascosa-Iruzubieta C., Gonzalez-Weller D., Caballero J. M., et al. (2020). Toxic metals (Al, cd, Pb) and trace element (B, ba, Co, Cu, cr, fe, Li, Mn, Mo, Ni C.OMMASr., V, zn) levels in sarpa salpa from the north-Eastern Atlantic ocean region. Int. J. Environ. Res. Public Health 17 (19), 7212. doi: 10.3390/ijerph17197212
Hakanson L. (1980). An ecological risk index for aquatic pollution control. a sedimentological approach. Water Res. 14, 975–1001. doi: 10.1016/0043-1354(80)90143-8
Hansell D. A., Carlson C. A. (1998). Net community production of dissolved organic carbon. Global Biogeochem. Cycles 12 (3), 443–453. doi: 10.1029/98gb01928
Hatje V., Apte S. C., Hales L. T., Birch G. F. (2003). Dissolved trace metal distributions in port Jackson estuary (Sydney harbour), Australia. Mar. pollut. Bull. 46 (6), 719–730. doi: 10.1016/s0025-326x(03)00061-4
Jayaprakash M., Kumar R. S., Giridharan L., Sujitha S. B., Sarkar S. K., Jonathan M. P. (2015). Bioaccumulation of metals in fish species from water and sediments in macrotidal ennore creek, chennai, SE coast of India: A metropolitan city effect. Ecotoxicol. Environ. Saf. 120, 243–255. doi: 10.1016/j.ecoenv.2015.05.042
Jiann K. T., Santschi P. H., Presley B. J. (2013). Relationships between geochemical parameters (pH, DOC, SPM, EDTA concentrations) and trace metal (Cd, Co, Cu, fe, Mn, Ni, Pb, zn) concentrations in river waters of Texas (USA). Aquat. Geochem. 19 (2), 173–193. doi: 10.1007/s10498-013-9187-6
Jiann K. T., Wen L. S., Gong G. C. (2009). Distribution and behaviors of cd, Cu, and Ni in the East China Sea surface water off the changjiang estuary. Bull. Geoghys. 20 (2), 4. doi: 10.3319/TAO.2008.05.09.01(Oc
Kalnejais L. H., Martin W. R., Bothner M. H. (2010). The release of dissolved nutrients and metals from coastal sediments due to resuspension. Mar. Chem. 121 (1-4), 224–235. doi: 10.1016/j.marchem.2010.05.002
Li D., Feng J., Zhu Y., Staneva J., Qi J., Behrens A., et al. (2022). Dynamical projections of the mean and extreme wave climate in the bohai Sea, yellow Sea and East China Sea. Front. Mar. Sci. 9. doi: 10.3389/fmars.2022.844113
Li M., He H., Mi T., Zhen Y. (2022). Spatiotemporal dynamics of ammonia-oxidizing archaea and bacteria contributing to nitrification in sediments from bohai Sea and south yellow Sea, China. Sci. Total Environ. 825, 153972. doi: 10.1016/j.scitotenv.2022.153972
Li Y., Lin Y., Wang L. (2018). Distribution of heavy metals in seafloor sediments on the East China Sea inner shelf: Seasonal variations and typhoon impact. Mar. pollut. Bull. 129 (2), 534–544. doi: 10.1016/j.marpolbul.2017.10.027
Li L., Liu J., Wang X., Shi X. (2015). Dissolved trace metal distributions and Cu speciation in the southern bohai Sea, China. Mar. Chem. 172, 34–45. doi: 10.1016/j.marchem.2015.03.002
Lin Y. C., Chang Chien G. P., Chiang P. C., Chen W. H., Lin Y. C. (2013). Multivariate analysis of heavy metal contaminations in seawater and sediments from a heavily industrialized harbor in southern Taiwan. Mar. pollut. Bull. 76 (1-2), 266–275. doi: 10.1016/j.marpolbul.2013.08.027
Lin Y., Lu J., Wu J. (2021). Heavy metals pollution and health risk assessment in farmed scallops: Low level of cd in coastal water could lead to high risk of seafood. Ecotoxicol. Environ. Saf. 208, 111768. doi: 10.1016/j.ecoenv.2020.11176
Li L., Pala F., Jiang M., Krahforst C., Wallace G. T. (2010). Three-dimensional modeling of Cu and Pb distributions in Boston harbor, Massachusetts and cape cod bays. Estuar. Coast. Shelf S. 88 (4), 450–463. doi: 10.1016/j.ecss.2010.05.003
Liu C. L. (2002). Spatial and temporal variability of trace metals in aerosol from the desert region of China and the yellow Sea. J. Geophys. Res. 107 (D14), 4215. doi: 10.1029/2001jd000635
Liu S. M., Altabet M. A., Zhao L., Larkum J., Song G. D., Zhang G. L., et al. (2017). Tracing nitrogen biogeochemistry during the beginning of a spring phytoplankton bloom in the yellow Sea using coupled nitrate nitrogen and oxygen isotope ratios. J. Geophys. Res-Biogeo 122 (10), 2490–2508. doi: 10.1002/2016jg003752
Liu R., Guo L., Men C., Wang Q., Miao Y., Shen Z. (2019a). Spatial-temporal variation of heavy metals' sources in the surface sediments of the Yangtze river estuary. Mar. pollut. Bull. 138, 526–533. doi: 10.1016/j.marpolbul.2018.12.010
Liu G., Lao Q., Su Q., Shen Y., Chen F., Qing S., et al. (2019b). Spatial and seasonal characteristics of dissolved heavy metals in the aquaculture areas of beibu gulf, south China. Hum. Ecol. Risk Assess. 26 (7), 1957–19691-13. doi: 10.1080/10807039.2019.1629273
Li X., Wai O., Li Y., Coles B., Ramsey M., Thornton I. (2000). Heavy metal distribution in sediment profiles of the pearl river estuary, south china. Appl. Geochem. 15 (5), 567–581. doi: 10.1016/S0883-2927(99)00072-4
Li W., Wang Z., Huang H. (2020). Indication of size distribution of suspended particulate matter for sediment transport in the south yellow Sea. Estuar. Coast. Shelf S. 235, 106619. doi: 10.1016/j.ecss.2020.106619
Li L., Xiaojing W., Jihua L., Xuefa S. (2017). Dissolved trace metal (Cu, cd, Co, Ni, and Ag) distribution and Cu speciation in the southern yellow Sea and bohai Sea, China. J. Geophys. Res- Oceans. 122 (2), 1190–1205. doi: 10.1002/2016jc012500
Lue X., Qiao F., Xia C., Wang G., Yuan Y. (2010). Upwelling and surface cold patches in the yellow Sea in summer: Effects of tidal mixing on the vertical circulation. Cont. Shelf Res. 30 (6), 620–632. doi: 10.1016/j.csr.2009.09.002
Lu Y., Gao X., Chen C. T. A. (2019). Separation and determination of colloidal trace metals in seawater by cross-flow ultrafiltration, liquid-liquid extraction and ICP-MS. Mar. Chem. 215, 103685. doi: 10.1016/j.marchem.2019.103685
Lu Y., Gao X., Song J., Chen C. A., Chu J. (2020). Colloidal toxic trace metals in urban riverine and estuarine waters of yantai city, southern coast of north yellow Sea. Sci. Total. Environ. 717, 135265. doi: 10.1016/j.scitotenv.2019.135265
Lu J., Li A., Dong J., Pei W., Feng X., Wang H. (2021). The effect of typhoon talim on the distribution of heavy metals on the inner shelf of the East China sea. Cont. Shelf Res. 229, 104547. doi: 10.1016/j.csr.2021.104547
Lv D., Zheng B., Fang Y., Shen G., Liu H. (2015). Distribution and pollution assessment of trace metals in seawater and sediment in laizhou bay. Chin. J. Oceanol. Limn. 33 (4), 1053–1061. doi: 10.1007/s00343-015-4226-3
Mask A. C., O'Brien J. J., Preller R. (1998). Wind-driven effects on the yellow Sea warm current. J. Geophys. Res-Oceans. 103 (C13), 30713–30729. doi: 10.1029/1998jc900007
Ma X., Zuo H., Tian M., Zhang L., Meng J., Zhou X., et al. (2016). Assessment of heavy metals contamination in sediments from three adjacent regions of the yellow river using metal chemical fractions and multivariate analysis techniques. Chemosphere 144, 264–272. doi: 10.1016/j.chemosphere.2015.08.026
Morel F. M. M., Milligan A. J., Saito. M. A. (2003). “Marine bioinorganic chemistry: The role of trace metals in the oceanic cycles of major nutrients,” in Treatise geochemistry. Eds. Elderfifield H., Holland H. D., Turekian K. K. (Cambridge, U. K: Elsevier Sci.), 113–143.
Morel F. M. M., Price N. M. (2003). The biogeochemical cycles of trace metals in the oceans. Science 300 (5621), 944–947. doi: 10.1126/science.1083545
Pan D., Ding X., Han H., Zhang S., Wang C. (2020). Species, spatial-temporal distribution, and contamination assessment of trace metals in typical mariculture area of north China. Front. Mar. Sci. 7 552893. doi: 10.3389/fmars.2020.552893
Pang H. J., Lou Z. H., Jin A. M., Yan K. K., Jiang Y., Yang X. H., et al. (2015). Contamination, distribution, and sources of heavy metals in the sediments of andong tidal flat, hangzhoubay, China. Cont. Shelf Res. 110, 72–84. doi: 10.1016/j.csr.2015.10.002
Pan J., Pan J. F., Wang M. (2014). Trace elements distribution and ecological risk assessment of seawater and sediments from dingzi bay, Shandong peninsula, north China. Mar. pollut. Bull. 89 (1-2), 427–434. doi: 10.1016/j.marpolbul.2014.10.022
Paul S. A. L., Gaye B., Haeckel M., Kasten S., Koschinsky A. (2018). Biogeochemical regeneration of a nodule mining disturbance site: Trace metals, DOC and amino acids in deep-Sea sediments and pore waters. Front. Mar. Sci. 5. doi: 10.3389/fmars.2018.00117
Peng S. (2015). The nutrient, total petroleum hydrocarbon and heavy metal contents in the seawater of bohai bay, China: Temporal–spatial variations, sources, pollution statuses, and ecological risks. Mar. pollut. Bull. 95 (1), 445–451. doi: 10.1016/j.marpolbul.2015.03.032
Petersen W., Wallman K., Pinglin L., Schroeder F., Knauth H. D. (1995). Exchange of trace elements at the sediment-water interface during early diagenesis processes. Mar. Freshw. Res. 46 (1), 19–. doi: 10.1071/mf9950019
Poupard O., Blondeau P., Iordache V., Allard F. (2005). Statistical analysis of parameters influencing the relationship between outdoor and indoor air quality in schools. Atmos. Environ. 39 (11), 2071–2080. doi: 10.1016/j.atmosenv.2004.12.016
Rajaram R., Ganeshkumar A., Vinothkumar S., Arun G. (2020). Ecological risk assessment of toxic metals contamination in tuticorin coast of gulf of mannar, southern India. Chem. Ecol. 37 (2), 132–148. doi: 10.1080/02757540.2020.1819986
Robbins L. J., Lalonde S. V., Planavsky N. J., Partin C. A., Reinhard C. T., Kendall B., et al. (2016). Trace elements at the intersection of marine biological and geochemical evolution. Earth-Sci. Rev. 163, 323–348. doi: 10.1016/j.earscirev.2016.10.013
Santschi P., Hohener P., Benoit G., Buchholtz-ten Brink. M. (1990)., chemical processes at the sediment-water interface. Mar. Chem. 30, 269–315. doi: 10.1016/0304-4203(90)90076-O
Sundelin B., Eriksson A. K. (2001). Mobility and bioavailability of trace metals in sulfidic coastal sediments. Environ. Toxicol. Chem. 20 (4), 748–756. doi: 10.1002/etc.5620200408
Sun Q., Fan G., Chen Z., Yang W., Li D. (2019). The content and pollution evaluation of heavy metals in surface seawater in dalian bay. IOP Conf. Ser: Earth Environ. Sci. 227 (6), 62021. doi: 10.1088/1755-1315/227/6/062021
Sun Q., Liu D., Liu T., Di B., Wu F. (2011). Temporal and spatial distribution of trace metals in sediments from the northern yellow Sea coast, China: Implications for regional anthropogenic processes. Environ. Earth Sci. 66 (3), 697–705. doi: 10.1007/s12665-011-1277-4
Tsai Y. C., Chung C. Y., Chung C. C., Gau H. S., Lai W. L., Liao S. W. (2015). The impact of typhoon morakot on heavy metals of dapeng bay and pollution from neighboring rivers. Environ. Model. Assess. 21 (4), 479–487. doi: 10.1007/s10666-015-9474-2
Wang C., Liang S., Li Y., Li K., Wang X. (2015). The spatial distribution of dissolved and particulate heavy metals and their response to land-based inputs and tides in a semi-enclosed industrial embayment: Jiaozhou bay, China. Environ. Sci. pollut. Res. Int. 22 (14), 10480–10495. doi: 10.1007/s11356-015-4259-3
Wang Y., Ling M., Liu R. H., Yu P., Tang A. K., Luo X. X., et al. (2017). Distribution and source identification of trace metals in the sediment of yellow river estuary and the adjacent laizhou bay. Phys. Chem. Earth. Parts A/B/C 97, 62–70. doi: 10.1016/j.pce.2017.02.002
Wang Y., Liu D., Richard P., Li X. (2013). A geochemical record of environmental changes in sediments from sishili bay, northern yellow Sea, China: Anthropogenic influence on organic matter sources and composition over the last 100 years. Mar. pollut. Bull. 77 (1-2), 227–236. doi: 10.1016/j.marpolbul.2013.10.001
Wang X., Liu L., Zhao L., Xu H., Zhang X. (2019). Assessment of dissolved heavy metals in the laoshan bay, China. Mar. pollut. Bull. 149, 110608. doi: 10.1016/j.marpolbul.2019.110608
Wang C. C., Pan D. W., Han H. T., Hu X. P. (2018a). Distribution and contamination assessment of arsenic and mercury in surface sediments from the intertidal zone of yantai sishili bay, China. Hum. Ecol. Risk Assess. 24 (8), 2024–2035. doi: 10.1080/10807039.2018.1436435
Wang B. B., Wang X.-l., Zhan R. (2003). Nutrient conditions in the yellow Sea and the East China Sea. Estuar. Coast. Shelf S 58 (1), 127–136. doi: 10.1016/s0272-7714(03)00067-2
Wang J., Yu Z., Wei Q., Yang F., Dong M., Li D., et al. (2020). Intra- and inter-seasonal variations in the hydrological characteristics and nutrient conditions in the southwestern yellow Sea during spring to summer. Mar. pollut. Bull. 156, 111139. doi: 10.1016/j.marpolbul.2020.111139
Wang X., Zhao L., Xu H., Zhang X. (2018c). Spatial and seasonal characteristics of dissolved heavy metals in the surface seawater of the yellow river estuary, China. Mar. pollut. Bull. 137, 465–473. doi: 10.1016/j.marpolbul.2018.10.052
Wang C., Zou X., Feng Z., Hao Z., Gao J. (2018b). Distribution and transport of heavy metals in estuarine-inner shelf regions of the East China Sea. Sci. Total Environ. 644, 298–305. doi: 10.1016/j.scitotenv.2018.06.383
Wei Q. S., Yu Z. G., Wang B. D., Fu M. Z., Xia C. S., Liu L. U., et al. (2016). Coupling of the spatial–temporal distributions of nutrients and physical conditions in the southern yellow Sea. J. Mar. Syst. 156, 30–45. doi: 10.1016/j.jmarsys.2015.12.001
Wei Q. S., Yu Z. G., Wang B. D., Wu H., Sun J. C., Zhang X. L., et al. (2016b). Offshore detachment of the changjiang river plume and its ecological impacts in summer. J. Oceanogr 7, 277–294. doi: 10.1007/s10872-016-0402-0
Xu Y., Pan S., Gao J., Hou X., Ma Y., Hao Y. (2018). Sedimentary record of plutonium in the north yellow Sea and the response to catchment environmental changes of inflow rivers. Chemosphere 207, 130–138. doi: 10.1016/j.chemosphere.2018.05.082
Xu L. L., Wu D. X., Lin X. P., Ma C. (2009). The study of the yellow Sea warm current and its seasonal variability. J. Hydrodyn 21 (2), 159–165. doi: 10.1016/s1001-6058(08)60133-x
Yang B., Gao X. (2019). Chromophoric dissolved organic matter in summer in a coastal mariculture region of northern Shandong peninsula, north yellow Sea. Cont. Shelf Res. 176 19-35, 19–35. doi: 10.1016/j.csr.2019.02.006
Yang B., Gao X., Zhao J., Lu Y., Gao T. (2020). Biogeochemistry of dissolved inorganic nutrients in an oligotrophic coastal mariculture region of the northern Shandong peninsula, north yellow Sea. Mar. pollut. Bull. 150 110693. doi: 10.1016/j.marpolbul.2019.110693
Yang B., Gao X., Zhao J., Xie L., Liu Y., Lv X., et al. (2022). The impacts of intensive scallop farming on dissolved organic matter in the coastal waters adjacent to the yangma island, north yellow Sea. Sci. Total. Environ. 807 (Pt 3), 150989. doi: 10.1016/j.scitotenv.2021.150989
Yano K., Morinaka Y., Wang F., Huang P., Takehara S., Hirai T., et al. (2019). GWAS with principal component analysis identifies a gene comprehensively controlling rice architecture. Proc. Natl. Acad. Sci. U.S.A. 116 (42), 21262–21267. doi: 10.1073/pnas.1904964116
Yuan H., Song J., Li X., Li N., Duan L. (2012). Distribution and contamination of heavy metals in surface sediments of the south yellow Sea. Mar. pollut. Bull. 64 (10), 2151–2159. doi: 10.1016/j.marpolbul.2012.07.040
Zhang J., Huang W. W., Liu S. M., Liu M. G., Yu Q., Wang J. H. (1992). Transport of particulate heavy metals towards the china sea: a preliminary study and comparison. Mar. Chem. 40 (3-4), 161–178. doi: 10.1016/0304-4203(92)90021-2
Zhang X., Wang B., Pan F., Cai Y., Wu X., Liu H., et al. (2022). Potential pollution assessment of labile trace metals in xixi river estuary sediments in xiamen, China. J. Contam Hydrol. 250, 104055. doi: 10.1016/j.jconhyd.2022.104055
Zhao B., Wang X., Jin H., Feng H., Shen G., Cao Y., et al. (2018). Spatiotemporal variation and potential risks of seven heavy metals in seawater, sediment, and seafood in xiangshan bay, China, (2011-2016). Chemosphere 212, 1163–1171. doi: 10.1016/j.chemosphere.2018.09.020
Keywords: dissolved trace metals, geographical distribution, marine ranch, ecological risk assessment, dissolved organic carbon
Citation: Liang Y, Pan D, Wang C, Lu Y and Fan X (2022) Distribution and ecological health risk assessment of dissolved trace metals in surface and bottom seawater of Yantai offshore, China. Front. Mar. Sci. 9:993965. doi: 10.3389/fmars.2022.993965
Received: 14 July 2022; Accepted: 31 August 2022;
Published: 26 September 2022.
Edited by:
Jun Sun, China University of Geosciences Wuhan, ChinaReviewed by:
Liqiang Zhao, Guangdong Ocean University, ChinaRenjith K. R., Centre for Water Resources Development and Management, India
Copyright © 2022 Liang, Pan, Wang, Lu and Fan. This is an open-access article distributed under the terms of the Creative Commons Attribution License (CC BY). The use, distribution or reproduction in other forums is permitted, provided the original author(s) and the copyright owner(s) are credited and that the original publication in this journal is cited, in accordance with accepted academic practice. No use, distribution or reproduction is permitted which does not comply with these terms.
*Correspondence: Dawei Pan, ZHdwYW5AeWljLmFjLmNu