- 1Division of Pathology, Department of Biomolecular Health Sciences, Faculty of Veterinary Medicine, Utrecht University, Utrecht, Netherlands
- 2Wageningen Marine Research, Wageningen University, Den Helder, Netherlands
- 3Institute for Terrestrial and Aquatic Wildlife Research, University of Veterinary Medicine Hannover, Foundation, Büsum, Germany
- 4Department of Bird Ecology, Bureau Waardenburg, Culemborg, Netherlands
- 5Department of Morphology and Pathology, Faculty of Veterinary Medicine, University of Liège, Liège, Belgium
- 6Department of Population Health Sciences, Faculty of Veterinary Medicine, Utrecht University, Utrecht, Netherlands
Humans impact natural systems at an unprecedented rate. The North Sea is one of the regions in the world with the highest levels of anthropogenic activity. Here, the harbor porpoise (Phocoena phocoena) is an abundant species and is often regarded as an ecosystem sentinel. A post-mortem surveillance program was established in the Netherlands aimed at increasing knowledge of the effects of human activities on harbor porpoises. In this study, we describe the pathological findings related to anthropogenic and natural causes of death categories in 612 harbor porpoises that stranded between 2008 and 2019, and assess their relations to age, sex, season, and location. The largest anthropogenic category was bycatch (17%), with mainly juveniles affected and peak periods in March and September–October. Other, infrequently diagnosed anthropogenic causes of death were trauma (4%), largely most likely due to ship collisions, and marine debris ingestion and entanglement (0.3%). The risk of dying from anthropogenic causes was highest for juveniles. Lesions compatible with noise-induced hearing loss were investigated in carcasses which were fresh enough to do so (n = 50), with lesions apparent in two porpoises. Non-direct human-induced threats included infectious diseases, which were by far the largest cause of death category (32%), and affected mainly adults. Also, gray seal (Halichoerus grypus) attacks were a frequently assigned cause of death category (24%). There were more acute predation cases in the earlier study years, while porpoises with lesions that suggested escape from gray seal attacks were diagnosed more recently, which could suggest that porpoises adapted to this threat. Our study contributes to understanding porpoise health in response to persisting, new, emerging, and cumulative threats. Building up such knowledge is crucial for conservation management of this protected species.
1 Introduction
Harbor porpoises (Phocoena phocoena) are charismatic and protected animals, with a broad societal, scientific, and political interest in their health and wellbeing (Evans and Hammond, 2004; Peltier et al., 2013; Evans, 2019). Harbor porpoise habitat includes both offshore and nearshore waters of the Northern Hemisphere (Evans and Hammond, 2004; Hammond et al., 2017; Nielsen et al., 2018). The harbor porpoise is currently listed as ‘least concern’ by the International Union for Conservation of Nature given their large global population size, which is likely well over a million individuals (Braulik et al., 2020). Nevertheless, for most geographical areas, population trends are unclear and there are numerous threats posing conservation concerns (Hammond et al., 2008). These concerns result from the frequent and emerging exposure of marine ecosystems to human activities (Aguirre and Tabor, 2004; Evans and Hammond, 2004; Halpern et al., 2008; Tyne et al., 2016; Wisniewska et al., 2018). As a result, harbor porpoises are key elements in many environmental monitoring programs (ASCOBANS, 1992; Evans and Hammond, 2004; Camphuysen and Siemensma, 2011; Bjørge and Tolley, 2018; Evans, 2019; LNV, 2020). These programs generally aim to increase knowledge of human-related activities impacting the environment and ultimately to protect and conserve species and their habitats.
The North Sea is one of the regions in the world with the highest level of anthropogenic activity and associated anthropogenic stressors (Halpern et al., 2008; Halpern et al., 2015; Nachtsheim et al., 2021). Harbor porpoise numbers in the Greater North Sea are estimated at around 350,000 individuals (Hammond et al., 2002; Hammond et al., 2013; Hammond et al., 2017). Porpoises are impacted by fishery activities through accidental bycatch, with underwater entanglement hampering them from air breathing, and competition for food (Kirkwood et al., 1997; Leeney et al., 2008; Dolman et al., 2016). Also, chemical pollution from persistent organic pollutants (Pierce et al., 2008; Weijs et al., 2009; Jepson et al., 2016; van den Heuvel-Greve et al., 2021), and underwater noise pollution from shipping (Wisniewska et al., 2018), seismic surveys, and unexploded ordnance detonations (von Benda-Beckmann et al., 2015; Aarts et al., 2016; Siebert et al., 2022) are known threats. More recent concerns and emerging threats include habitat loss due to the rapid growth of offshore activities related to the construction of wind farms (Madsen et al., 2006; Gilles et al., 2009; Teilmann and Carstensen, 2012) and the broadscale effects of climate change, including habitat degradation and changes in food quality and quantity (Simmonds and Isaac, 2007; Burge et al., 2014). These single and cumulative stressors threaten the direct survival of individual porpoises but may also induce nonlethal effects impacting population viability (Aguirre and Tabor, 2004; Evans and Hammond, 2004; Halpern et al., 2008; Tyne et al., 2016; Wisniewska et al., 2018).
Marine mammals are difficult to study at sea, but stranding records provide unique and cost-effective opportunities for surveillance purposes (Peltier et al., 2013; ten Doeschate et al., 2018). Stranding frequency in North Sea-bordering countries has increased since the 90s and has been particularly high in the southern part since 2005 (Camphuysen, 2004; Camphuysen et al., 2008; IJsseldijk et al., 2020a). Due to the statutory requirements of international legislation in combination with the high stranding numbers, stranding networks that record harbor porpoises and other cetaceans found ashore have been implemented decades ago in most North-Western European countries. Initiated post-mortem programs in order to sample stranded animals and monitor their causes of mortality and health status were initiated in association with these (Camphuysen and Siemensma, 2011; Peltier et al., 2013; ten Doeschate et al., 2018; LNV, 2020). In the Netherlands, post-mortem investigations have been conducted at the Faculty of Veterinary Medicine (Utrecht University) since 2008. The metrics routinely collected include pathological findings to investigate disease and causes of death, data on demographics, nutritional condition, and diet, and on marine debris ingestion. In addition, since 2010, special attention has been given to pathology indicative of hearing loss due to potential anthropogenic activities. Here we provide an overview of the causes of death of porpoises that were stranded in the Netherlands, as well as any pathology related directly to human activities. This knowledge is crucial for management, as it helps to understand how this protected species is vulnerable to certain human activities.
2 Materials and methods
2.1 Post-mortem examinations
Post-mortem examinations followed internationally standardized guidelines (IJsseldijk et al., 2019). In short, at the start of each post-mortem examination, external features were photographed. The data collection included date and location of stranding, sex, total body length (in cm), and mass (in kg). Animals were assigned to three age classes based on length: neonate (<90 cm), juvenile (90–130 cm), and adult (>130 cm), with the final differentiation between juveniles and adults made based on gonadal appearance. Nutritional status was assessed based on visual examination of the dorsal musculature, visceral fat, and blubber thickness measured immediately anterior to the dorsal fin at three locations (dorsal, lateral, and ventral, in mm). Nutritional condition was assessed on a six-point scale with one representing very fat and muscular animals and six representing emaciated animals. This was later grouped into three categories: good (NCC1–2), moderate (NCC3–4), and poor (NCC5–6). Additionally, all carcasses were given a decomposition condition code (DCC) at the time of necropsy, from DCC1 representing very fresh carcasses to DCC5, representing mummified carcasses or remains. Parasite loads were recorded for four major organ systems: lungs, liver, cranial sinuses (peribullar and pterygoid), and tympanic cavities, and stomachs. If parasites were present, the infection was categorized as (1) mild, with approx. 1–20 nematodes or <10% of the organ affected by trematodes, (2) moderate, with approx. 21–50 nematodes or 10%–30% of the organ affected by trematodes, or (3) severe, with approx. >50 nematodes or >30% of the organ affected by trematodes. The dates were later grouped by season, as winter: December–February, spring: March–May, summer: June–August, and autumn: September–November. Locations were grouped from south to north as: Zeeland; the mainland provinces of Noord-Holland and Zuid-Holland; the Wadden Sea islands (Texel, Vlieland, Terschelling, Ameland, and Schiermonnikoog); and Noord-Nederland (the mainland coasts of the provinces of Friesland and Groningen).
Live stranded harbor porpoises were submitted for rehabilitation at the SOS Dolfijn Foundation in the Netherlands until 2016. Porpoises that entered the rehabilitation center and (eventually) died there are further discussed elsewhere (van Elk et al., 2019). In the presented study, we referred to live-stranded individuals based on eyewitness reports of alive porpoises on the beach or on the presence of ante-mortem scavenging lesions. After the rehabilitation center closed in 2016, live stranded harbor porpoises that were euthanized based on welfare grounds were included in this study. None of the authors involved in this study took part in the decision-making around living with stranded individuals.
For this study, all cases in DCC1–3 for which the post-mortem investigation included gross- and histopathological assessment and for which external photographs were available were included (n = 612). Of these 612 porpoises, a range of tissue samples (for details see: IJsseldijk et al., 2019) was fixed in 10% neutral buffered formalin, embedded in paraffin, sectioned at 4–7 µm, stained with hematoxylin and eosin (HE) and microscopically examined. In the Results section, we give percentages of the number of cases out of the total number of cases assessed, since this could vary depending on carcass completeness. We refer to significant lesions when these are judged as multifocal mild, moderate, or severe, or focal moderate or severe. We present the main and most common findings and most likely causes of death, largely following the subdivision of Arbelo et al. (2013). In order to provide an overview, most likely causes of death were subdivided into categories. Each result section starts with a brief description of the category and the most relevant findings for animals that were placed in this category, where applicable, with associated references for diagnostic purposes.
2.2 Diet and marine debris investigation
Stomach walls and contents were visually inspected first, and stomach walls were then sampled, after which the complete remaining stomach with contents were temporarily stored in a sealed plastic bag at −20°C for later analyses. For analyses of the diet, contents were rinsed into a large glass beaker, placed under slowly running tap water so that light prey components such as tissue fragments and fluids were gently flushed out of the sample, while heavier prey remains could be collected from the bottom of the glass beaker (following van Franeker et al., 2018). Since 2010, the glass beaker has been placed on a metal 1 mm mesh sieve to make sure that no foreign items, especially small pieces of plastic, were overlooked. For full details on the methods as well as detailed results on plastic and other man-made litter in stomachs (2006–2013), see van Franeker et al. (2018). Prey remains were examined following earlier published methods (Leopold, 2015). Firstly, fish sagittal otoliths were used to identify fish species and to estimate fish length and weight. Prey mass was back-calculated following Leopold (2015) and Leopold et al. (2001). Detailed results of dietary analysis (2006–2015) are published in Leopold (2015). We included data on the presence or absence of marine debris in stomachs, and the presence or absence of prey in the stomach, number of prey items, and reconstructed prey mass (in grams) (Leopold, 2015; Leopold et al., 2015b).
2.3 Inner ear evaluation
The inner ears of 50 porpoises from 2010 to 2019, sampled and fixed within 24 h post-mortem, were collected following earlier published methods (Morell and André, 2009). Several complementary techniques were selected to analyze the sensory cells of the organ of Corti and type I afferent innervation using immunofluorescence (eleven samples) and/or ultrastructural methods: 36 inner ears were processed for scanning electron microscopy (SEM), and three for transmission electron microscopy (TEM). Protocols for processing the samples and results have been published previously (Morell et al., 2015; 2017a; 2017b; 2020; 2021; 2022). In short, for immunofluorescence, the sensory cells of the organ of Corti were labeled with phalloidin (FluoProbes® X5 505, FP-AZ0130, 1:100), anti-prestin (Santa Cruz, USA SC-22692, 1:200, courtesy of Dr. Zheng, 1:1,000), and/or anti-myosin VI (Proteus Biosciences 25-6791, 1:5,000) antibodies. Type I afferent innervation was labeled with anti-neurofilament 200KD (Sigma-Aldrich N0142, 1:400) or anti-neurofilament H (Millipore AB5539, 1:5,000) antibodies. Nuclei were counterstained with DAPI (4’, 6-diamidino-2’-phenylindole, dihydrochloride; Thermo Scientific 62247, 1:1,000). The inner ears were imaged with one or multiple of the following microscopes: 1) SEM (Hitachi S-3500N from the Institute of Marine Sciences, Spain, Hitachi S-4000 from CRIC, Montpellier, France, and Hitachi S-4700 from the University of British Columbia, UBC, Bioimaging Facility), TEM (Hitachi H-7100 TEM from CRIC, Hitachi H7600 from UBC Bioimaging Facility, and FEI Tecnai G2 Spirit from the Department of Cellular and Physiological Sciences, UBC), and 3) confocal microscopes (Olympus FV1000 at UBC Bioimaging Facility, and Zeiss LSM880 at Montpellier Resources Imagery).
2.4 Analyses
Age- and sex differences in causes of death categories were assessed. Analyses were carried out in Excel and R version 3.6.3 (R 2017) following Zuur et al. (2007; 2009). Following initial data exploration, Chi-square tests were conducted to assess the relationship with the categorical variable “cause of death” and variables: age class (three categories: neonate, juvenile, and adult) and sex (two categories: male and female). The significance threshold was set at p = 0.05. A Generalized Linear Model (GLM) with a binomial distribution and logit link was conducted in order to assess whether porpoises at a certain length have higher probabilities of dying of anthropogenic causes, using glm2 version 1.2.1 (Marschner, 2018) and ggplot2 for visualization (Wickham, 2016). Anthropogenic causes of death (indicated as 1 for bycatch and blunt forced and sharp trauma, and 0 for the following categories: infectious disease, insufficient food or feeding (non-neonates), and interspecific traumatic) were modeled as a function of body length (in cm, incomplete carcasses excluded) for all juvenile and adult porpoises. Model validation was done by evaluating calculated dispersion parameters and diagnostic plots using Pearson residuals.
3 Results
A total of 612 harbor porpoises were included in this study: 84 neonates (14%), 343 juveniles (56%), and 185 adults (30%). Overall, there were more males than females: 333 compared to 277 (55% versus 45%; the sex of the two individuals remained unknown due to carcass incompleteness). Males dominated the immature age classes, but there were more females in the adult age class. Most animals were found in spring (30%), followed by summer (26%), winter (25%), and autumn (19%). These age class, sex, and seasonal differences are consistent with the stranding patterns of harbor porpoises along the southern North Sea (IJsseldijk et al., 2020a). In terms of spatial distribution, slightly more individuals came from the southern part of the country (Zeeland 25% and Zuid-Holland 30%) compared to the northern parts (Noord-Holland 20%, Wadden Sea islands 23%, and Noord-Nederland 2%) (Supplementary Table 1).
3.1 Pathological findings related to anthropogenic causes
3.1.1 Pathology consistent with accidental bycatch in fisheries
This category consists of harbor porpoises that died acutely, most likely as a result of underwater entrapment following accidental capture in fishing nets, referred to as bycatch. The diagnosis of this cause of death category was based on methods outlined in Kuiken (1996) and IJsseldijk et al. (2021c). The presence of superficial incisions and/or encircling imprints was judged as the most informative feature for placement in this category. However, the exclusion of another cause of death (based on other significant pathological findings), the presence of prey in the stomach, and the presence of pulmonary edema and/or emphysema were additionally considered. One hundred-three harbor porpoises (17%, 103/612) were classified in this category, of which 41 were female and 62 were males. There were mainly juveniles (73%), followed by adults (21%), and an additional six neonates most likely died due to bycatch (6%) as well. Bycaught animals were mainly found in spring (39%), and mostly on the Dutch mainland (Zuid-Holland 38% and Noord-Holland 25%) (Supplementary Table 1). Nutritional conditions were judged as good for 55% porpoises (NCC1–2: 57/103), moderate for 35% others (NCC3–4: 36/103), and poor for the remaining 10% of animals (NCC5–6: 10/103) (Supplementary Table 1).
Externally, 86% of the cases had clear and distinct lesions consistent with net entanglement, including encircling imprints (Figure 1A), or superficial cutaneous lesions on the rostrum or on fins and flukes (Figure 1B), while for the other animals this was less apparent or could not be diagnosed with confidence due to significant external defects. The diagnosis of underwater entrapment due to bycatch was based on a combination of internal findings that consisted of an acute and traumatic cause of death. Among all porpoises in the bycatch category, 51% of the cases (52/102) had subcutaneous bruising, hemorrhage in (parts of) the central nervous system, or acute skull or mandible fractures. Pulmonary edema was present in 89% (92/103) and multiple organ congestion in 29% of the cases (28/98) (Supplementary Table 2). Excluding cases without stomachs (e.g., due to scavenging), prey remains were present in 82% of the porpoises, with a median number of reconstructed prey of 30 and a median reconstructed summed prey mass of 276 g (Supplementary Table 3). Six porpoises in this cause of death category had non-natural material in their stomachs (see below), but no netting or other direct fishery-related material was found in these individuals.
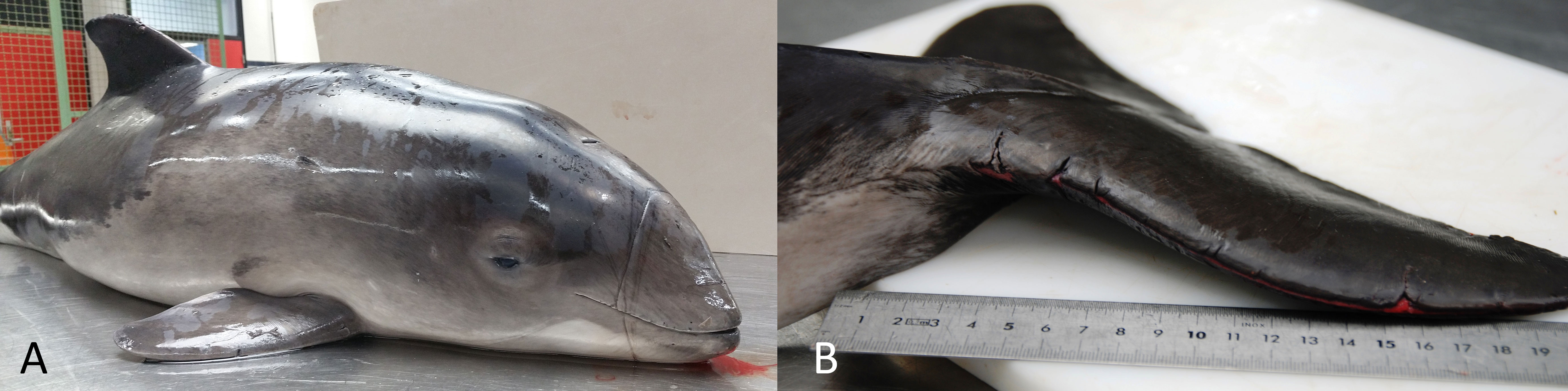
Figure 1 (A) Prominent net imprint encircling the rostrum. (B) Several incisions in the edges of the fluke.
The most frequently diagnosed conditions were moderate to severe lesions in the respiratory tract (53%), moderate to severe lesions in the liver in 29% of the cases, and ulceration of the alimentary tract in 14% of the porpoises in which this could be assessed. Eight percent of the animals had inflammatory lesions in three or more organs. An overview of pathological findings can be found in Supplementary Table 2. Parasites were present mainly in mild to moderate numbers in the respiratory tract and tympanic cavities, pterygoid, and peribullar sinuses (Supplementary Table 4). There were no significant macroscopic or microscopic lesions or signs of poor nutritional status in only 30% of all individuals in the bycatch category. These animals were considered “healthy” at the time of bycatch (31/103). This means that the majority of the porpoises had a compromised health status prior to the suspected bycatch.
3.1.2 Pathology due to man-made foreign bodies
This category represents animals with lesions associated with physical evidence of anthropogenic origin, including animals chronically entangled and animals that have ingested foreign bodies with associated pathological changes. There were two harbor porpoises which most likely died as a result of foreign bodies (0.3%, 2/612): one adult male and one juvenile male. Both animals were in good nutritional condition. The adult male had an encircling lesion just cranial to the pectoral fins (Figure 2A), which resulted in a subacute to chronic ulcerative dermatitis following entanglement. No monofilament or other man-made material was detected in the lesion, so the type of entanglement (e.g., plastic or netting) could not be determined. The other porpoise had an orange nylon fishing line wired around the epiglottis (Figure 2B), with a fishing hook attached to one end of the line. The fishing hook, used for angling, was not ingested but instead protruding outside the oral cavity. It was penetrating the blubber cranial to the pectoral fins. The hook and fishing line caused moderate necropurulent and ulcerative chronic dermatitis and necrotic laryngitis, and the animal additionally suffered from multifocal bronchopneumonia.
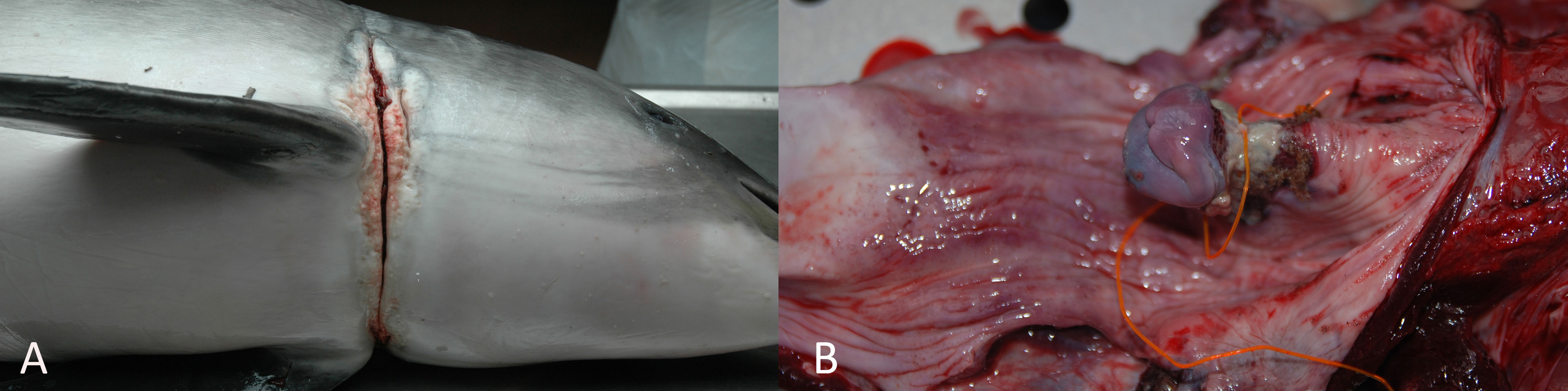
Figure 2 (A) Adult male harbor porpoise with sign of encircling, chronic lesion indicative of chronic entanglement. (B) Juvenile male harbor porpoise with a fishing line wired around the larynx, causing a necrotic laryngitis.
Marine debris was detected in 7% (39/597) of all stomachs assessed, in 25 juveniles and 14 adults. There was one notable case with a fishing hook in the forestomach. The fishing hook was in the center of a 5 cm in diameter ball of algae, mostly Sargassum muticum. The hook is a straight J-shaped (37 × 15.4 mm) fishing hook used for angling with a 6 × 3.3 × 1 mm bait stopper and a single barb. The hook was rusty, but the tip was sharp. Attached to the hook was a 45 mm long, 0.55 mm wide, piece of white nylon fishing line. This juvenile male was in poor nutritional condition and the surface of the stomach was affected by multifocal 1 mm in diameter ulcerations. There was a focal moderate to chronic granulomatous gastritis with intralesional trematodes, morphologically consistent with Pholeter gastrophilus. Other pathological findings included multiple small longitudinal ulcerations in the larynx and esophagus, and a small amount of black content in the third and fourth stomach and intestines (presumably digested blood). Since upon necropsy, the fishing hook was centrally placed in the ball of algae and there was no sign of perforation of the stomach wall, a causal relationship between the fishing hook and the gastric lesions could not be established. The cause of death of this animal was categorized as “infectious disease”. Harbor porpoises that ingest marine debris died due to a variety of causes (Supplementary Table 3), but most likely not as a direct result of the ingestion of this debris. In most cases, ingested materials were small pieces of rope or netting, or small fragments or sheets of plastic, all smaller than the average whiting otolith found in the stomachs, and these were not considered directly detrimental to porpoise health (for details see: van Franeker et al., 2018; Bravo Rebolledo, 2020).
3.1.3 Trauma consistent with anthropogenic origin
This category refers to those cases that suffered either severe sharp trauma resulting in rapid loss of life or severe blunt force trauma, or a combination of both. Twenty-five porpoises were placed in this category (25/612; 4%). Eleven of these had sharp-edged mutilations consistent with a vessel-propeller collision; 13 others presented solely with severe blunt force trauma, possibly as a result of a ship hull collision; and a final case had presumable knife-induced injuries. There were 20 juveniles and five adults, 14 males and 11 females. Most individuals were found in spring (52%) in Zeeland (36%). Nutritional conditions were judged as good for most animals (NCC1–2: 52%, NCC3–4: 20%, NCC5–6: 28%) (Supplementary Table 1). Prey remains were present in 78% of the porpoises, with a median number of prey of 50 and a median reconstructed summed prey mass of 326 g (Supplementary Table 3). Porpoises dying of trauma were infected with parasites in comparable percentages to porpoises dying due to other causes, although there were more porpoises with moderate parasitic infestation of the tympanic cavities, pterygoid, and peribullar sinuses (Supplementary Table 4).
Of the 11 animals with lesions consistent with vessel propeller collision (sharp trauma), ten had underlying fractures of the vertebral column (n = 7) (Figure 3), ribs (n = 2), or head (n = 1). Two porpoises had amputated tail flukes and one had an amputated rostrum. The presence of net-marks as a result of bycatch could not be ruled out in nine of the 11 cases, and possible seal-induced wounds could not be ruled out in two of the 11 cases due to extensive external defects. This highlights the difficulty in differential diagnosis for these causes of acute mortality.
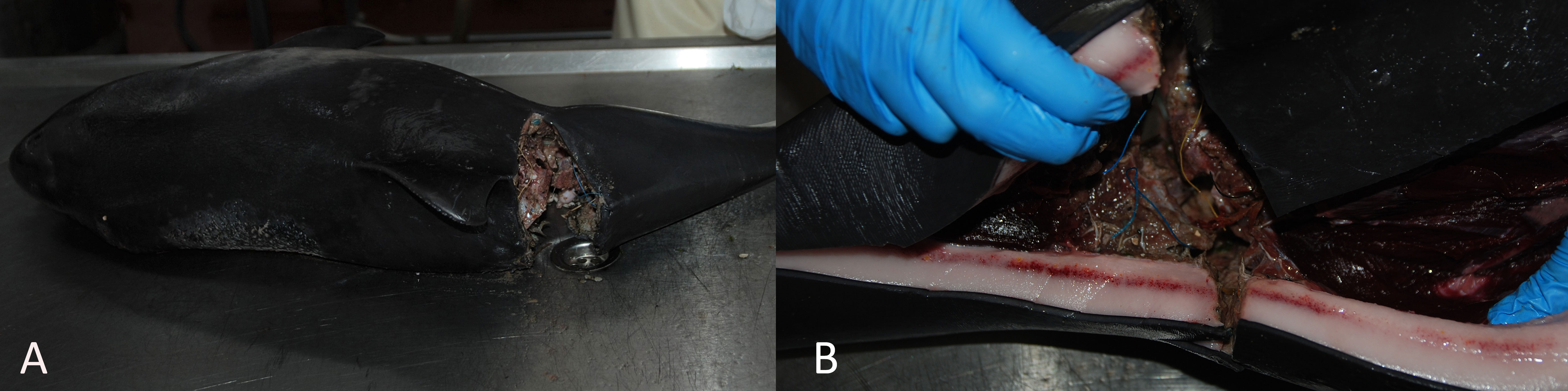
Figure 3 (A) Harbor porpoise with severe sharp trauma to the vertebral column, just caudal to the dorsal fin. (B) Associated hemorrhage in the blubber layer around the trauma.
There were 13 cases with severe blunt force trauma as the main diagnosis. Fractures of the mandible were most common (46%, 6/13). One porpoise with a mandible fracture also had a complete skull and cochlea fracture. One other porpoise had a skull fracture and additional fractures in the vertebral column. There was hemorrhage around fractures in all cases, demonstrating ante-mortem injury. Porpoises without fractures had extensive subcutaneous hemorrhage and hemorrhage surrounding the spinal cord (n = 1) or hemorrhage in the brain (n = 3). Two porpoises live stranded. One of them had a mandible fracture as well as hemorrhage in the spinal cord and cerebrum and additional pneumonia. The other had extensive subcutaneous hemorrhage as well as severe ulcerations of the upper alimentary tract (Supplementary Table 3). The causes of these blunt-forced traumas remain unclear. None of these porpoises had external evidence of sharp trauma or obvious external lesions consistent with bycatch. Collisions with ship hulls are possibilities, especially in cases with fractures.
Finally, one emaciated juvenile male had small but deep lacerations on the left lateral flank and caudal to the right pectoral fin. These presumable knife-induced injuries penetrated the abdominal cavity and ruptured the diaphragm and liver after which this animal most likely died because of hypovolemia.
3.1.4 Pathological findings compatible with noise-induced hearing loss
From the 50 ears analyzed in the period 2010–2019, we found six cases with focal hemorrhages in the cochlea. Nevertheless, there was no conclusive evidence of hearing loss in the majority of these cases, either because there were no lesions detected, or because the organ of Corti was not in a condition good enough for diagnosis due to post-mortem artifacts. However, there were four cases with missing sensory cells in the apical region of the cochlea. While the pattern of sensory cell loss in two cases suggested individual apical variability instead of lesions (Morell et al., 2022), the other two showed evidence of sensory cell loss that is compatible with noise exposure. More details on these cases have been published elsewhere (Morell et al., 2015; Morell et al., 2021).
3.2 Pathological findings related to non-anthropogenic causes
3.2.1 Peri-neonatal pathology
Of the 84 neonates necropsied in total, 63 neonatal harbor porpoises had morphological and etiological characteristics, most likely related to problems in gestation, birth, or nursing, and are therefore listed under this category (10%, 63/612). The vast majority was found in summer (79%) and mainly in the southern part of the Netherlands (Zeeland 37%; Zuid-Holland 38%) (Supplementary Table 1). Twenty-two porpoises had as their most significant findings general, internal, diffuse congestive-hemorrhagic lesions, and their deaths likely occurred perinatally. The other neonates had as their most significant finding hepatic and/or renal lipidosis and therefore most likely died due to starvation. There was one porpoise with complete fetal atelectasis. This was most likely a stillborn animal. Twelve neonates live stranded (21%). Ancillary findings were ulcers in the alimentary tract in 23%, lesions in the respiratory tract of 7%, and in the central nervous system of 4% of these neonates (Supplementary Table 2). Only 8% of the neonates (n = 5) had hard prey items in their stomachs, mainly gobies (Gobiidae) and invertebrates. These five porpoises had a median number of prey of two and a median summed reconstructed prey mass of 2.5 g. There were no parasites detected in the gastrointestinal-, respiratory tracts, and hepatic ducts, but there were two neonates with a mild nematode infestation in the tympanic complex (Supplementary Table 4). The mean length of animals in the perinatal category was 76.1 cm ( ± SD: 4.9 cm), consistent with the reported length at birth for porpoises in the North Sea (Lockyer, 2003). The mean length of animals dying due to starvation was 83.4 cm ( ± SD: 5 cm), suggesting that most likely these animals lived for a short period of time but had insufficient resources or additional problems related to nursing which led to their deaths.
3.2.2 Infectious diseases
This category is defined as being associated with the presence of subacute and/or chronic pathological findings that were sufficiently severe to be considered, ultimately responsible, alone or in combination with other factors, for the death and/or stranding of the animal. A total of 198 harbor porpoises (32%, 198/612) were classified in this category, of which 103 were male and 95 females. In contrast to other categories, there were mainly adults (55%, 109/198), followed by juveniles (40%, 80/198), and an additional nine neonates. These porpoises were found more often in winter compared to the anthropogenic categories (30% versus 16% trauma and 18% bycatch). Animals were mostly found on the Wadden Sea islands and in Zuid-Holland (both 27%) (Supplementary Table 1). Despite the fact that these animals most likely died from infectious diseases, 71% had some prey in their stomachs, with a median number of prey of 4 and a median reconstructed summed prey mass of 26 g (Supplementary Table 3).
Fifty porpoises presented a good nutritional condition (NCC1–2) and most likely died of an acute but significant infectious disease (50/198). Of these 50 cases, 21 live stranded (42%). Despite the good nutritional conditions of these porpoises, significant pathological changes were present. Most commonly diagnosed were moderate to severe lesions in the respiratory tract (88%, 43/49) and moderate to severe lesions in the liver (53%, 25/47). Seventeen porpoises had inflammatory infiltrates or other lesions in their central nervous system (36%, 17/47). Reported pathological findings in the other 148 porpoises, which most likely died of significant infectious diseases with signs of subacute to chronic deterioration based on their moderate to very poor nutritional conditions (NCC3–6), were very similar to the animals in good nutritional condition. The number of live strandings was high (33%). Moderate to severe lesions of the respiratory tract were most commonly diagnosed (86%, 125/146), followed by moderate to severe lesions in the liver (50%, 70/139). Inflammatory infiltrates and other lesions were detected in the central nervous system in 31% of these cases (40/130). An overview of all pathological findings for harbor porpoises in the infectious diseases category is provided in Supplementary Table 2. Most striking is the large percentage of animals suffering from inflammation in three or more organs (59%), likely also associated with the high parasite burden in the assessed organ systems (Supplementary Table 4). The high rate of multiple organ inflammation demonstrates the extensiveness of lesions in most porpoises within this category. The lesions found were caused by various micro-organisms, but the ancillary assessment of other biological agents other than parasites varied widely between cases. This hampers the calculation of the frequency of occurrence for other etiologic agents than parasites.
3.2.3 Insufficient food or feeding (non-neonates)
This category is comprised of 53 harbor porpoises and refers to non-neonatal harbor porpoises for which the most significant pathological finding pointed towards insufficient food intake as a cause of mortality (9%, 53/612). Hepatic lipidosis was the most significant pathological finding in eight young juveniles (length ranging from 93 to 113 cm). The additional 45 porpoises, both juveniles (87%, 39/45) and adults (13%, 6/45), were in poor to very poor nutritional condition (NCC5–6). In terms of causation, there was one notable case: a juvenile porpoise that passed through the harbor locks and swam up a canal and thus was found far inland. There were no prey remains in its stomach and it was severely emaciated and had extensive dermatitis. The latter was considered most likely as a result of the prolonged period (approximately 11 days) of residing in fresh water. There was substrate (mud) in the lungs and associated acute pneumonia. The underlying causes of the poor nutritional status remained unclear for the other porpoises in this category. Alimentary pathology was, however, diagnosed in half of all cases listed under this category (50%), which could have resulted in emaciation or vice versa, a lack of sufficient nutrition could have induced alimentary ulcerations. Other common pathological findings were moderate to severe lesions in the respiratory tract (40%), and moderate to severe lesions in the liver (31%). Additional pathological findings in these cases (see Supplementary Table 2) were deemed unrelated or not severe enough to explain the emaciation or starvation of these individuals. The porpoises under this category were mostly found in summer (32%) and autumn (26%), and mainly on the Wadden Sea islands (32%) (Supplementary Table 1). Despite the fact that these animals most likely died as a result of insufficient feeding, 67% had some prey in their stomachs, with a median number of prey of four and a low median reconstructed summed prey mass of 14 g (Supplementary Table 3). A previous and more detailed diet study, using partly the same individuals as in the current study, already showed that the stomachs of emaciated porpoises are not necessarily empty, but lack energy-rich prey (Leopold, 2015). Parasites were detected in slightly lower numbers compared to animals from other death categories (Supplementary Table 4), which could be explained by the many young animals in this category.
3.2.4 Interspecific traumatic interactions
This category refers to harbor porpoises with lesions consistent with gray seal (Halichoerus grypus) attacks. A total of 144 harbor porpoises (24%, 144/612) were classified within this category, of which 80 were male and 62 females (sex of two animals was not determinable). Similar to the bycatch category, there were mainly juveniles (74%), followed by adults (22%), with the remaining cases being neonates (3%). Porpoises were found mainly in spring (40%) and in winter (36%). In terms of spatial distribution, there was no clear hotspot area, as animals within this category were found in relative numbers similar to the geographical distribution of all animals (Supplementary Table 1). Most porpoises had prey in their stomachs (86%), with a median number of prey of 49 and a median reconstructed summed prey mass of 391 g (Supplementary Table 3). These findings correspond to what was reported by Leopold et al. (2015a), since cases from 2008 to 2015 were overlapping. Parasites were mainly present in mild-moderate numbers in the respiratory tract, but numbers in the other organ systems were equally high compared to the other death categories (Supplementary Table 4).
In terms of pathological findings, we further divided them into two subcategories: pathology associated with acute interactions, with the presence of large, sharp-edged mutilations; and pathology associated with interactions that did not immediately result in death. The first subcategory includes harbor porpoises that presented acute, ante-mortem, extensive mutilations, which were considered to be the most likely cause of death. Claw- and bite marks, inflicted by gray seals in a predatory attack, were often found associated with the extensive mutilations, conforming to the diagnosis outlined in Leopold et al. (2015a). Seventy-eight porpoises had these typical lesions. The majority of these predated porpoises were in good nutritional condition (63%, 49/78), or in moderate nutritional condition (33%, 26/78), and the final three porpoises were in poor nutritional condition (4%, 3/78). None of these porpoises live stranded. Despite the good nutritional conditions of the majority of these porpoises, additional pathological changes were detected. Pulmonary edema was a common finding (79%, 61/77). Over half of these porpoises suffered from moderate to severe lesions in the respiratory tract (57%, 37/65), and a third presented moderate to severe lesions in the liver (32%, 19/59), mostly associated with parasitic infestations. Other, less frequent recorded pathological changes are listed in Supplementary Table 2. Of these 78 harbor porpoises, six porpoises had additional healed lesions from previous bites. Another six animals had some suspicious skin lesions which could also have been from previous bites (8%–15%, 6–12/78), demonstrating multiple traumatic interactions between gray seals and porpoises.
Sixty-six harbor porpoises presented solely subacute to chronic skin lesions, which were morphologically most consistent with bite trauma induced by gray seals in a predatory attempt (Leopold et al., 2015a; Foster et al., 2019; Gilbert et al., 2020). However, these animals lacked the large, sharp-edged mutilation typically seen in gray seal victims. All the bite lesions of porpoises in this category had inflamed appearances. Unlike the porpoises that had died acutely from the attack, good nutritional conditions were seen in fewer animals with chronic lesions from previous bites (26%, 18/66 in NCC1–2; 35%, 24/66 in NCC3–4; 35%, 24/66 in NCC5–6). Eleven of these porpoises live stranded (16%). The percentage of cases with inflammation in multiple organs (three or more organs) was high: 45% (29/64). Alimentary pathology was apparent in 32% of the cases (21/66); moderate to severe lesions to the respiratory tract in 68% of the cases (44/65) and moderate to severe lesions to the liver in 38% of the cases (24/64). Other significant findings included lesions in the central nervous system (14%, 9/64) and the heart (10%, 6/62).
3.2.5 Miscellaneous
Five adult females had acutely died from dystocia (n = 3) or intestinal volvulus (n = 2) (1%, 5/612). The three cases of dystocia stranded in May, corresponding to the calving season of porpoises in the North Sea (Lockyer, 2003). One animal had a fetus partly protruding from the genital opening, which apparently got stuck at the height of the pectoral fins (Figure 4A). This adult was in good nutritional condition, had prey remains in the stomach, and no significant other pathological findings, and thus appeared healthy at the time of dystocia/death. The second porpoise, a fresh adult in poor nutritional condition without any prey remains in its stomach, had a moderately decomposed fetus located in the left uterine horn. There was extensive transmural uterine necrosis with additional peritonitis. The third porpoise had extensive, ante-mortem external and internal injuries as a result of the ‘breech’ position of the fetus, which was located with its dorsal fin in the genital opening. This had caused an ante-mortem uterus- and abdominal wall rupture and eventual death (Figure 4B) (Supplementary Tables 1–4).
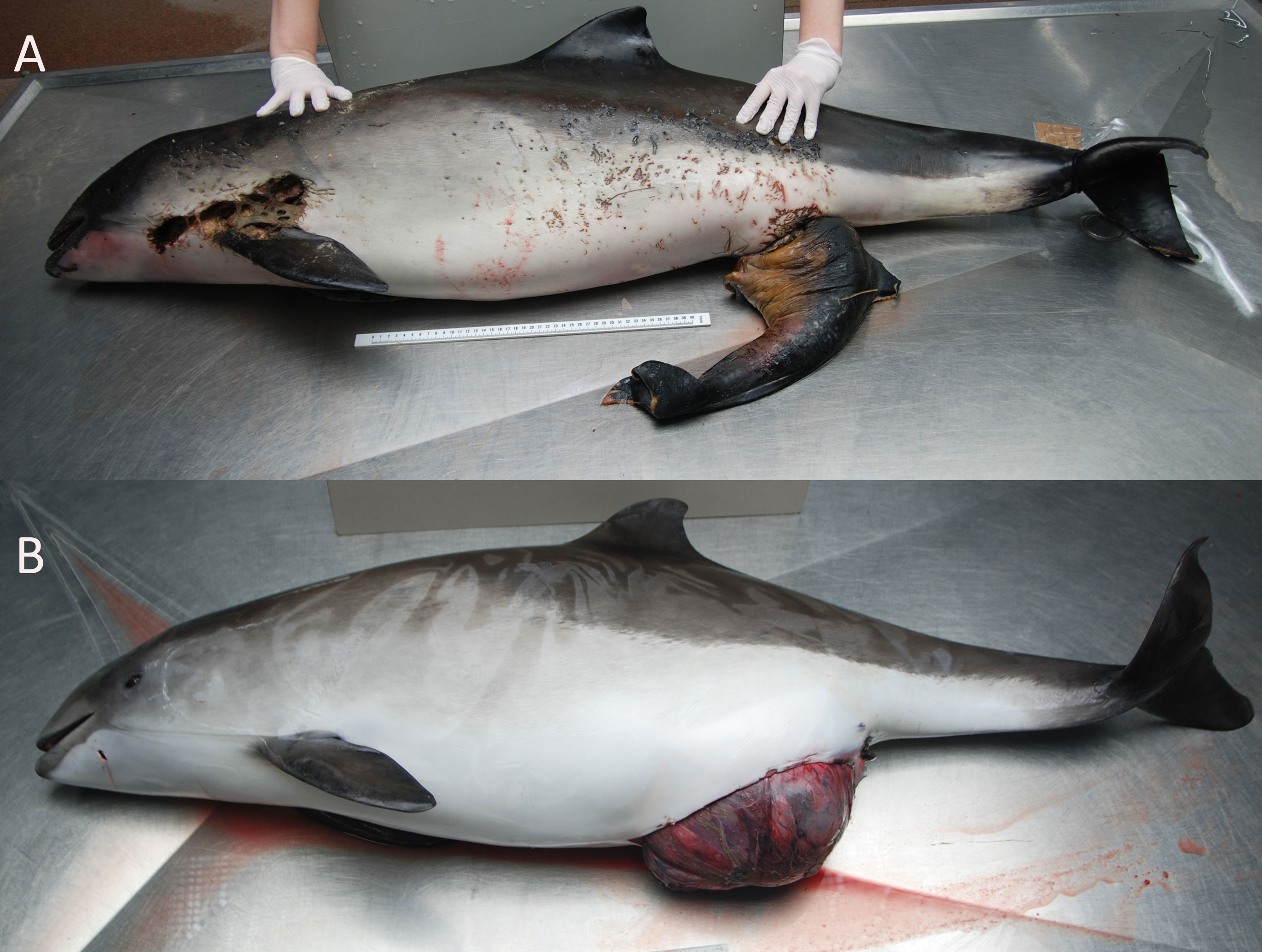
Figure 4 (A) Dystocia in an adult female harbor porpoises. There is a protruding fetus from the genital opening, which was stuck at the height of the pectoral fins. (B) Dystocia in an adult female harbor porpoises. The abdominal wall is ruptured as a result of a fetus in a “breech” position, with the dorsal fin protruding from the genital opening.
One live-stranded porpoise had an intestinal volvulus, with findings previously published (Begeman et al., 2013). No possible predisposing factors were noted for this particular case. The animal was in good nutritional condition, although the stomach was empty. The other animal with an intestinal volvulus stranded in May was pregnant. This was a relatively small female to be pregnant, measuring 139 cm. The fetus was just under half the size of the mother (63 cm). The pregnancy could have been a predisposing factor for the intestinal twist to occur. The adult was in good nutritional condition and had prey remains in its stomach. It had additional parasitic pneumonia and moderate amounts of trematodes in its liver (Supplementary Tables 1–4). Finally, there was one adult female that was resting (neither pregnant nor lactating) with an intestinal volvulus, but that porpoise is listed under the infectious disease category since it suffered from severe multiple organ inflammation.
3.2.6 Unestablished cause of death or stranding
For 19 harbor porpoises, there was no clear cause of death or, in the case of live stranding, there was no clear cause of stranding established (3%, 19/612). These were five adults and 14 juveniles. Five porpoises live-stranded, three of which died shortly after stranding, while two were euthanized based on clinical signs of disease and poor survival prognosis. These porpoises were mainly found in spring (42%) and winter (37%), and mostly in Zeeland (37%) and on the Wadden Sea islands (32%). Most of these harbor porpoises were in good nutritional condition (NCC1–2: 63%, 12/19) or in moderate nutritional condition (NCC3–4: 37%, 7/19) (Supplementary Table 1). Approximately 95% had prey in their stomachs, with a median number of prey of 20 and a median summed reconstructed prey mass of 137 g (Supplementary Table 3). No signs of significant infectious disease or clear signs of anthropogenic pathological changes could be detected in any of the 19 porpoises (Supplementary Table 2). The nutritional conditions, combined with dietary and pathological results, point towards an acute cause of mortality or stranding as most likely for these animals.
3.3 Age class- and sex-related difference in causes of death
Data exploration and analyses indicated a clear age class-related difference in cause of death (X2 (df = 18, n = 612) = 451.1, p <0.001). Neonates were, logically, predominantly listed under the peri-neonatal and starvation categories. Juveniles were largely present in the gray seal-related causes of death (both chronic lesions from previous bites and acute predation) as well as in trauma, bycatch, and emaciation categories. Adults dominated the infectious diseases category (Figure 5; Supplementary Figure 1). When we group the anthropogenic causes of mortality (bycatch and trauma) and compare these to other categories among the juveniles and adults, the probability of dying due to direct anthropogenic causes decreased with increasing total length (Figure 6, GLM: slope = −0.014, p <0.05). There was no significant relationship between sex and cause of death (X2 (df = 9, n = 612) = 7.2, p >0.05). Males were overrepresented in all but one (emaciation of unknown origin) of the cause of death categories (Supplementary Table 1; Supplementary Figure 2). Bycatch was the predominant cause of mortality among the juvenile porpoises until up to around 2017, when cumulative numbers of infectious diseases overtook (Figure 7; Supplementary Figure 1). One notable spike in the category of emaciation of unknown origin was detected in the year 2011 (Figure 7; Supplementary Figure 3). This was especially apparent in the absolute stranding numbers (Figure 7). In terms of season, animals in the bycatch category were predominantly found in March and September–October (Figure 8). Acute predation cases, and to a lesser extent, porpoises with chronic lesions from previous bites, were mainly seen throughout late winter and early spring (January–March) (Figure 8). Emaciation seemed to be a late-summer phenomenon (August–September) (Figure 8). Peri- and neonatal porpoises were mainly seen from May into June, corresponding to the calving season, and cases of starvation followed that period, peaking in July. These findings were apparent in terms of absolute numbers (Figure 8) and relative numbers (Supplementary Figure 4).
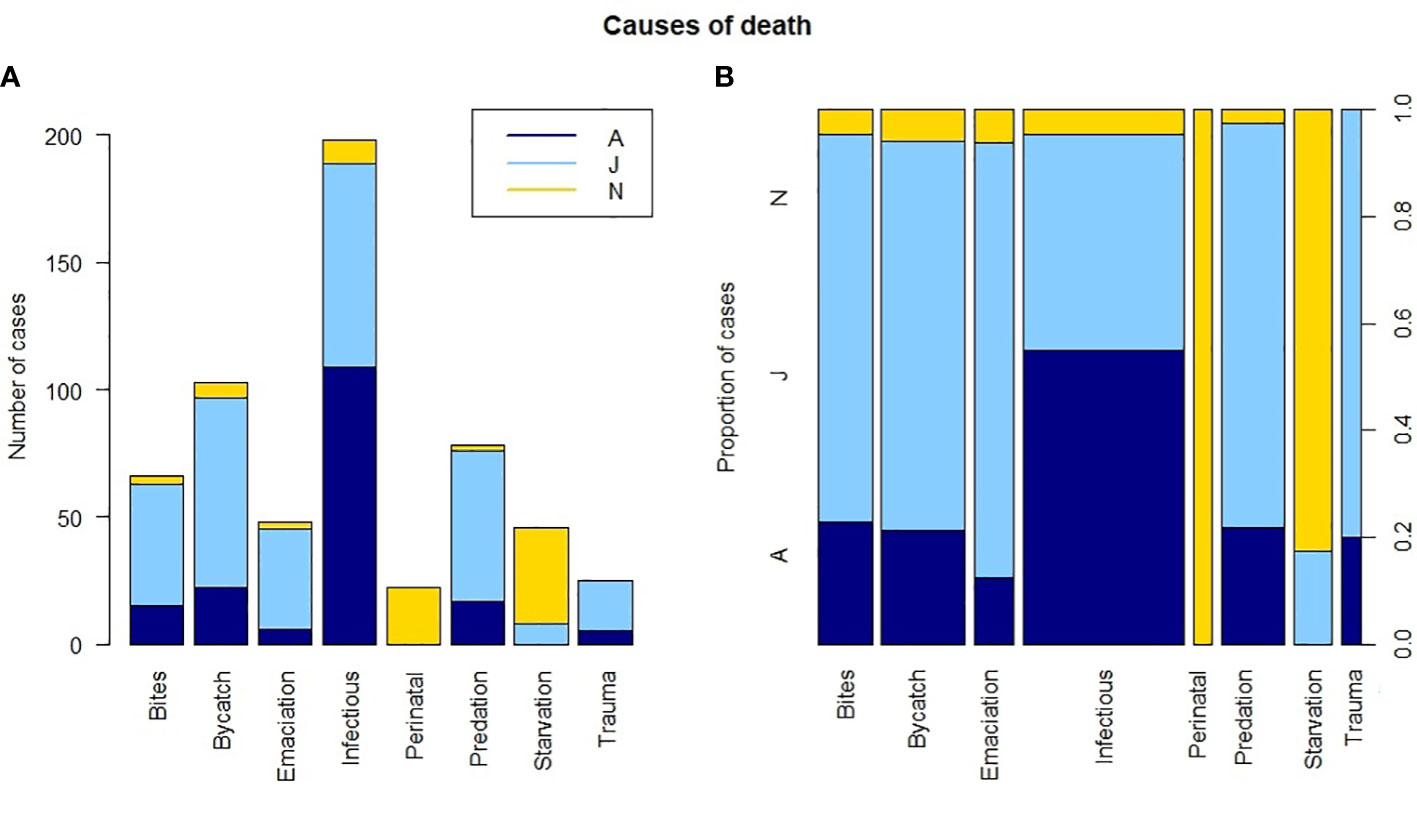
Figure 5 Age class per cause of death category. (A) Stacked absolute number of cases. (B) Proportion of cases with the width of the bars representative of sample size. Dark blue is adult (A), light blue is juvenile (J), and yellow is neonate (N).
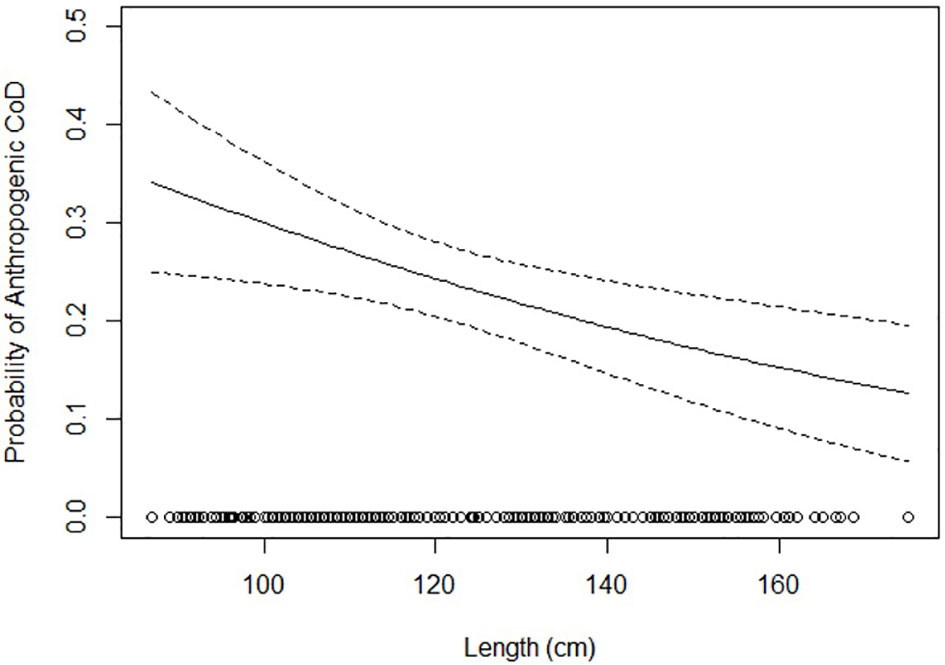
Figure 6 Probability of an anthropogenic cause of death based on harbor porpoise total length (in cm).
4 Discussion
In this study, we provide an estimation of the occurrence of anthropogenic and natural causes of mortality of stranded harbor porpoises in the Netherlands over a twelve-year period (2008–2019). The largest anthropogenic cause of death category was bycatch (17%), with mainly juveniles affected and peak periods in March and September–October. This seasonal variance warrants further investigation in relation to fishing efforts in the southern North Sea. Other anthropogenic causes of mortality were infrequently diagnosed: trauma (4%), largely most likely due to ship collisions, and marine debris ingestion and entanglement (0.3%). Lesions compatible with noise-induced hearing loss could only be investigated for a small subset of cases but were apparent in two harbor porpoises. Natural causes of mortality included infectious diseases, which were by far the most common cause of death category (32%), mainly for adults, and were rather constant over time. Also, lesions consistent with gray seal attacks were found in almost a quarter of all stranded porpoises (24%), with more acute predation cases in the earlier years of the study, while porpoises with pathology associated with interactions that did not immediately result in death were diagnosed more recently. Two-thirds of all stranded neonates had morphological and etiological characteristics most likely related to problems in gestation, birth, or nursing, while others died most likely due to infectious diseases, interspecific interactions, or bycatch. Signs of food shortage (starvation and/or emaciation) were the most significant finding in 9% of porpoises (non-neonates). There was one notable spike in the category of emaciation of unknown origin in 2011, especially for juveniles. In a previous study, it already became apparent that overall harbor porpoise stranding numbers in 2011 were excessively high in the Netherlands, especially in summer, which was declared an unusual mortality event (UME) (IJsseldijk et al., 2021a). The post-mortem results presented here suggest that food shortages, or an inability to feed or hunt, were the probable cause of this UME.
4.1 Accidental bycatch in fisheries
The impact of fishing activities on different species of cetaceans is considered one of the main threats to small cetaceans globally (Jefferson and Curry, 1994; Moore, 2014; Dolman et al., 2016). The overall proportion of harbor porpoises in our study that most likely died from bycatch was 17%. In other geographic areas in northwestern EU waters, reported bycatch proportions varied widely. For the United Kingdom, several studies overlapping in time and space report bycatch percentages ranging from 11% to as much as 38% (Baker, 1994; Kuiken et al., 1994; Kirkwood et al., 1997). For the southernmost parts of the North Sea, specifically Belgium and Northern France, bycatch proportions were similar to those we reported for the Netherlands (15%, Jauniaux et al., 2002), although mass bycatch mortality events have also been reported from Belgium (Haelters and Camphuysen, 2009) before recreational set-netting was banned there. In Sweden, 31% of all post-mortem examined porpoises between 2006 and 2020 most likely died following bycatch (Neimanis et al., 2022). Bycatch numbers probably run into the thousands of animals per year in the Danish North Sea (Vinther and Larsen, 2004). Harbor porpoise mortality related to bycatch in the German North and Baltic Seas was not provided; instead, the authors gave the number of stranded porpoises with net-marks. This was 42% and 20%, respectively (Siebert et al., 2020). Stranded porpoises suspected to be bycaught were often deemed too decomposed for extensive necropsy, hampering the establishment of a bycatch prevalence (Siebert et al., 2020). This is likely also true for other areas, including the Netherlands. Here, only approximately 10%–15% of all stranded porpoises are necropsied annually due to financial constraints. This has unknown effects on the prevalence of all cause of death categories over time. Bycatch numbers as presented here might only reflect a minimum number of cases that die annually following entanglement. However, our study and post-mortem studies from bordering countries indicate that bycatch-related mortality persists, and might still exceed the acceptable percentage of human-induced mortality (1.7%) as agreed upon in the Agreement on the Conservation of Small Cetaceans of the Baltic and North Seas (ASCOBANS).
4.2 Marine debris ingestion and entanglement
The wide distribution and abundance of man-made litter, in particular plastics, affects a broad range of marine organisms through entanglement and ingestion (Kühn et al., 2015; Unger et al., 2016; van Franeker et al., 2018; Kühn, 2020). A previous study on harbor porpoises was conducted with individuals overlapping our study (van Franeker et al., 2018). The incidence of marine debris in stomachs was between 7% and 15%, depending on the methodology used. These pieces of debris can be expected to pass through the gastrointestinal tract in a similar way to food items, and hence are indicative of pollution of the marine environment. Ingestion of marine debris occurs more frequently than entanglement in debris, and fishery-related debris is of particular concern (van Franeker et al., 2018; Bravo Rebolledo, 2020; this study). We presented one case of fatal debris ingestion (wired epiglottis), and another porpoise with signs of severe and prolonged external entanglement. Although this marks the number of porpoises dying as a direct result of marine debris as very low (0.3%, 2/612), the welfare state of both individuals was severely compromised as a result of the entanglement. This demonstrates the harmful effects that marine debris, specifically macroscopic items, can have on individual marine animals. The detection of small particles ingested by cetaceans is hampered by protocols and methodologies, and the prevalence of microplastics (<1 mm) in porpoises is likely higher (van Franeker et al., 2018; Philipp et al., 2021).
4.3 Sharp and blunt force trauma
Twenty-five porpoises had trauma as the most significant pathological finding (25/612, 4%). Blunt force injuries may result from collisions with non-rotating features, such as the bow of a ship or slowly rotating tidal energy turbines, with common pathological findings being well-defined focal areas of subcutaneous hemorrhage and edema, physically disrupted muscle, fractured bones, and disruption of organ systems (Martinez and Stockin, 2013; McLellan et al., 2013; IJsseldijk et al., 2014; this study). Collisions with ship propellers are often associated with major injuries such as repetitive incised wounds and lacerations, and amputations, inflicted by heavy metal blades rotating at high speed (Lightsey et al., 2006; Byard et al., 2012; Costidis et al., 2013; IJsseldijk et al., 2020b; this study). However, necropsy findings in the blunt force trauma category were sometimes also consistent with bycatch criteria, such as the presence of bruising and fractures (Cox et al., 1998; Jepson et al., 2013; Moore et al., 2013). Although the most reliable indicator of bycatch is the presence of net-marks or encircling imprints (Kuiken, 1996; Jepson et al., 2013; IJsseldijk et al., 2021c), post-mortem and stranding-related degradation of the skin may hamper the detection of such external lesions. This highlights the diagnostic difficulties among these anthropogenic categories of acute death.
4.4 Infectious diseases
Bronchopneumonia was the most commonly detected disease, not only in the category of infectious diseases but also among the porpoises dying of acute causes (both anthropogenic and natural, Supplementary Table 2). Often, bronchopneumonia was associated with a high prevalence of nematodes (Supplementary Table 4). A high rate of bronchopneumonia with an associated high parasitic infestation was also seen in porpoises stranded in the Netherlands from 1983–1986 (van Nie, 1989) and 1970–1994 (Addink et al., 1995). Generally, parasite occurrence increases with total body length as a proxy of age (ten Doeschate et al., 2017). The relative pathogenicity of the different parasite species is uncertain and the role of parasites as a cause of stranding or death has widely been discussed in the scientific literature (Jepson et al., 2000; Arbelo et al., 2013; Siebert et al., 2020). Structural microbiological screening was beyond the scope of our study. The investigation into other infectious agents is hampered by finances, logistics, and tissue quality, and an overview and estimated prevalence of pathogens is lacking. Several infectious agents have, however, been targeted and published separately, including on the prevalence of Brucella spp. (Maio et al., 2014), mycoses, specifically Aspergillus fumigatus (Kapetanou et al., 2020), herpesvirus (van Beurden et al., 2015), and Toxoplasma gondii (van de Velde et al., 2016; Morell et al., 2021).
4.5 Gray seal interaction
When studying deceased marine mammals and determining causes of death, the interpretations of lesions are limited by the knowledge and experience of those conducting these investigations. A key example of this is the identification of the gray seal as a major predator of harbor porpoises a decade ago. Severely wounded porpoises were initially thought to have died from bycatch, as the large mutilations were judged to be “man-made cuts” and the bite lesions interpreted as “rope-marks” (Camphuysen and Oosterbaan, 2009). Once the gray seal was suggested as a predator of porpoises by Haelters et al. (2012), the detection of gray seal DNA in attack wounds on harbor porpoises revealed the fatal interaction (for porpoises) between these two marine mammals (Jauniaux et al., 2014; van Bleijswijk et al., 2014). A retrospective analysis of post-mortem findings and subsequent re-diagnosis of their causes of death demonstrated the large scale of gray seal predation on harbor porpoises in the southern North Sea (Leopold et al., 2015a) and significantly reduced suspected bycatch numbers in the Netherlands.
Predation events of gray seals on porpoises have been reported for a wide geographical range (Bouveroux et al., 2014; Jauniaux et al., 2014; Foster et al., 2019; van Neer et al., 2020). Acute predation cases are, however, just the tip of the iceberg, with potentially many more porpoises suffering from bite injuries with occasionally deadly outcomes (Leopold et al., 2015a; Foster et al., 2019; Gilbert et al., 2020). Several of our affected porpoises had chronic lesions from earlier bites in addition to the final, acute mutilations, suggesting previous failed predatory attempts. This demonstrates frequent interactions between gray seals and porpoises in the southern North Sea. Knowing the frequency and consequences of lethal and non-lethal interactions between predators and their prey is important when trying to understand the behavior of prey within an ecosystem (Lima, 2002). Increasing predator pressure could result in hunger-dependent risk-taking and influence abundance, habitat use, and energy intake of prey. Gray seal numbers have been restored after a successful rehabilitation program aiming at their conservation when numbers were at their lowest (Brasseur, 2017). With increasing numbers of gray seals as well as an increase in harbor porpoise numbers in the southern North Sea, their interactions have undoubtedly become more common in the last decades. The direct and indirect effects of these interactions warrant further investigation, but it is conceivable that the current large number of gray seals may negatively affect harbor porpoise health, behavior, and fitness. This may particularly be true in areas such as the Eastern Scheldt, with a small resident population of porpoises, from which seal victims are frequently observed (Podt and IJsseldijk, 2018) and demonstrates that conserving one species might have detrimental effects on another.
4.6 Peri- and neonatal mortality
Perinatal mortality, diagnosed in 26% of necropsied neonates in this study, may be related to asphyxiation due to aspiration of meconium or amniotic fluid and subcutaneous hemorrhages (Arbelo et al., 2013). Larger neonates more often died with signs of acute starvation and/or emaciation. Hepatic lipidosis was a common finding. Nursing calves have a high intake of carbohydrates, which results in the accumulation of excessive triglycerides in the hepatocytes, leading to pale and waxy livers (Jaber et al., 2004; St. Leger et al., 2018). The significance of neonatal hepatic lipidosis is deemed unclear (Siebert et al., 2001; Hiemstra et al., 2015). In harbor porpoises stranded in Germany, diffuse hepatocellular lipidosis was found in 13 of 29 (44%) calves (Siebert et al., 2001), and neonatal mortality attributed to starvation was also reported to be high among calves stranded in the United Kingdom (Kirkwood et al., 1997). Neonatal death may be a result of maternal separation or nursing difficulties, but in the majority of neonates, it is impossible to further pinpoint exact causes. It is possible that maternal inexperience or failure played a role in some of our cases, although another explanation could be disturbance from natural or anthropogenic threats, causing e.g., mother-calf separation.
4.7 Management implications
There is an increasing need to understand marine mammal health and how this changes over time to determine the effects of conservation measures but also of persisting, new, emerging, and cumulative threats affecting wild populations (Bossart, 2011; NAS, 2017; IJsseldijk et al., 2018; Siebert et al., 2020). Knowledge of the changes in health as well as mortality is crucial for the management of species or subgroups within species, which are particularly vulnerable to human interaction, like the harbor porpoise. Accidental bycatch in fisheries has historically been a focal topic within cetacean conservation and management programs (ASCOBANS, 1992; Camphuysen and Siemensma, 2011; Dolman et al., 2016). Despite binding legal requirements to monitor and reduce bycatch (Dolman et al., 2021), the high incidence of mortality most likely related to bycatch in our study (17%), as well as in similar studies from neighboring countries (Jauniaux et al., 2002; Siebert et al., 2020), shows that bycatch is a persisting issue for harbor porpoises in the North Sea. However, besides this persisting threat, temporal trends in all cause of death categories should be investigated.
A more emerging anthropogenic threat, both in terms of scale and focus, is over-exposure to anthropogenic noise. Establishing if a porpoise suffered from over-exposure to underwater noise, by examining the sensory cells of the organ of Corti, can usually only be done within 24–30 h after death (Morell et al., 2017b). Injuries in the auditory system following detonations of unexploded ordnance (UXO) can, however, be studied in porpoises in more advanced states of decomposition (Siebert et al., 2022). Despite frequent UXO detonations in the southern North Sea (von Benda-Beckmann et al., 2015), (micro)CT-scans of the auditory system are not routinely conducted in the Netherlands. This would be a recommended addition to monitoring schemes aimed at determining the effects of anthropogenic pressures on stranded marine mammals. High intensity sonars, seismic surveys, pile-driving for offshore windfarm construction, and shipping, among other activities, can also result in temporary hearing loss, masking, behavioral and other physiological changes. It is not possible to investigate or detect this by solely conducting inner ear analysis (Morell et al., 2017b; Wisniewska et al., 2018). There is clearly a need for a better understanding and investigation of the many consequences of exposure to various anthropogenic sounds and the effects of noise pollution on marine mammals. Continuation of a comprehensive research program on harbor porpoises with targeted studies on the auditory system is a means to increase our knowledge of the anatomy of and pathologies in the auditory pathway, which could ultimately help to distinguish lesions attributable to noise pollution from other causes of hearing loss such as aging or infectious disease.
The assessment of age classes within the causes of death categories indicated several critical periods for immature harbor porpoises. Peri-neonatal porpoises face problems related to gestation, birth, or nursing, with maternal nutrition and care deemed vital to sustain pregnancy and for the survival of newborns, respectively (IJsseldijk et al., 2021b; this study). Neonates and juveniles are prone to acute starvation and/or emaciation due to their high metabolic requirements. In this study, there were indications that, especially in summer, resources might not be sufficiently available for juvenile harbor porpoises. Immature animals that seem to be in a better state of nutritional condition are not safe and are at risk from bycatch and predation. The individuals that do reach sexual maturity are exposed to a range of infectious diseases. This suggests that porpoises face different risks during their lifetimes. However, more robust analyses are needed, especially incorporating multiple variables that, in combination, might influence results. This was outside the scope of the current study, but would be a recommended next step.
Globally, reported infectious disease outbreaks in marine mammals have been on the rise, with factors causing these still being mostly unclear (Gulland and Hall, 2007; Sanderson and Alexander, 2020). There are, however, signs that especially chemical pollutants may be involved, since the persisting organic pollutants in the marine environment have been associated with disrupted endocrine and immune function in cetaceans (Jepson et al., 1999; Jepson et al., 2005; Jepson et al., 2016). Also, climate change, another emerging conservation issue, may lead to new indirect and thus more nebulous health effects, likely in terms of habitat degradation, changes in resource quantity and quality, and increasing infectious disease outbreaks (Learmonth et al., 2006; Simmonds and Isaac, 2007; Bossart, 2011; Sanderson and; Alexander, 2020). There therefore is a pressing need to expand the monitoring efforts to contaminant- and infectious agent screening, which would be an important way to investigate the effects of those human-related threats that do not leave distinct lesions on animals but rather have indirect effects on their immune system, behavior, health status, or reproduction.
Data availability statement
The original contributions presented in the study are included in the article/Supplementary Material. Further inquiries can be directed to the corresponding author.
Ethics statement
Ethical review and approval was not required for the animal study because the animals described in this study were free-living harbor porpoises which died of natural causes or were euthanized on welfare grounds and not for the purpose of this, or other, studies. No consent from an Animal Use Committee is required, as the animals described in this study were not used for scientific or commercial testing. Consequently, animal ethics committee approval was not applicable to this work.
Author contributions
LIJ, ML, LB, LW, HH and AG contributed to the conception and design of the post-mortem program and/or this specific study. LIJ, MK, LB, LW, TJ and AG conducted the post-mortem examinations. ML conducted the diet analyses. EBR conducted the marine debris analyses. MM conducted the inner ear analysis. LIJ organized the database, conducted the data analyses and drafted the manuscript. ML, HH and AG supervised the study. All authors contributed to the article and approved the submitted version.
Funding
Necropsies of harbor porpoises in the Netherlands are commissioned by the Ministry of Agriculture, Nature and Food Quality, and since 2016 embedded under the Legal Research Tasks Nature & Environment unit of Wageningen UR (project reference numbers 140000353, WOT-04-009-045).The ears were analyzed at the Technical University of Catalonia, Spain (UPC-Barcelona Tech) from 2008 to 2013, at the University of British Columbia (UBC), Canada from 2013 to 2017 and to the Institute for Neurosciences of Montpellier, France from 2017 to 2019 with appropriate CITES permits. The inner ear collection, preservation and analysis were funded by Rijkswaterstaat (WoZEP 2016), the Natural Sciences and Engineering Research Council (NSERC) of Canada Discovery and Accelerator grants RGPAS 446012-13 and RGPAN 312039-13, the Spanish Ministry of the Environment (grant number: 083/SDGTB/2007) and Marie Skłodowska-Curie Individual Post-doctoral Fellowship (grant number: 751284-H2020-MSCA-IF-2016).
Acknowledgments
We sincerely thank the stranding network volunteers and organizations for reporting and retrieving deceased harbor porpoises for our research. The post-mortem investigations and stomach analyses were conducted with the help of many colleagues, students and volunteers, for which we specifically want to acknowledge Johan van Amerongen, Louis van den Boom, Kees Camphuysen, Natashja Ennen-Buijs, Sjoukje Hiemstra, Guido Keijl, Darryl Leydekkers, Manon Lock, Liliane Solé, Mariel ten Doeschate, Linde van Schalkwijk en Ruby Wagensveld. In addition, we are thankful for the efforts of Folchert van Dijken, Jeroen Vis, Anne-Marie Svoboda, and Steve Geelhoed in funding acquisition and project management.
Conflict of interest
The authors declare that the research was conducted in the absence of any commercial or financial relationships that could be construed as a potential conflict of interest.
Publisher’s note
All claims expressed in this article are solely those of the authors and do not necessarily represent those of their affiliated organizations, or those of the publisher, the editors and the reviewers. Any product that may be evaluated in this article, or claim that may be made by its manufacturer, is not guaranteed or endorsed by the publisher.
Supplementary material
The Supplementary Material for this article can be found online at: https://www.frontiersin.org/articles/10.3389/fmars.2022.997388/full#supplementary-material
Supplementary Figure 1 | Cumulative number of causes of death per year, framed per age class.
Supplementary Figure 2 | Sex per cause of death category. Left: stacked absolute numbers. Right: proportions with the width of the bars representative of sample size and dotted horizontal line at 0.5. Blue = males. Pink = females.
Supplementary Figure 3 | Radar plots of causes of death categories per year (2009-2019) corrected for n/year.
Supplementary Figure 4 | Radar plots of causes of death categories per month corrected for n/month.
References
Aarts G., von Benda-Beckmann A. M., Lucke K., Sertlek H. Ö., Van Bemmelen R., Geelhoed S. C., et al. (2016). Harbour porpoise movement strategy affects cumulative number of animals acoustically exposed to underwater explosions. Mar. Ecol. Prog. Ser. 557, 261–275. doi: 10.3354/meps11829
Addink M. J., García-Hartmann M., Smeenk C. (1995). “The harbour porpoise Phocoena phocoena in Dutch waters: Life history, pathology and historical records,” in International whaling commission scientific community document, IWC SC/47/SM5, Scientific Commission of the International Whaling Commission, Cambridgeshire, United Kingdom. 1–8.
Aguirre A. A., Tabor G. (2004). Introduction: Marine vertebrates as sentinels of marine ecosystem health. Ecohealth 1, 236–238. doi: 10.1007/s10393-004-0091-9
Arbelo M., de Los Monteros A. E., Herráez P., Andrada M., Sierra E., Rodríguez F., et al. (2013). Pathology and causes of death of stranded cetaceans in the Canary islands, (1999– 2005). Dis. Aquat. Org. 103 (2), 87–99. doi: 10.3354/dao02558
ASCOBANS (1992) Agreement on the conservation of small cetaceans of the Baltic, north-East Atlantic, Irish and North Seas. Available at: https://www.ascobans.org/en/documents/agreement-text.
Baker J. R. (1994). By-catches of cetaceans around the coast of Wales. in: Diagnosis of by-catch in cetaceans. proceedings of the 2nd ECS workshop on cetacean pathology. Eur. Cetacean Soc. Newslett. 26, 35–37.
Begeman L., St. Leger J. A., Blyde D. J., Jauniaux T. P., Lair S., Lovewell G., et al. (2013). Intestinal volvulus in cetaceans. Veterinary Pathol. 50 (4), 590–596. doi: 10.1177/0300985812465327
Bjørge A., Tolley K. A. (2018). “Harbor porpoise Phocoena phocoena,” in Encyclopedia of marine mammals third edition. Eds. Wursig B., Thewissen J. G. M., Kovacs K. M., 448–451. doi: 10.1016/C2015-0-00820-6
Bossart G. D. (2011). Marine mammals as sentinel species for oceans and human health. Oceanography 19 (2), 134–137. doi: 10.1177/0300985810388525
Bouveroux T., Kiszka J., Heithaus R., Jauniaux T., Pezeryl S. (2014). Direct evidence for gray seal (Halichoerus grypus) predation and scavenging on harbor porpoises (Phocoena phocoena). Mar. Mammal. Sci. 30, 1542–1548. doi: 10.1111/mms.12111
Brasseur S. M. J. M. (2017). Seals in motion: How movements drive population development of harbour seals and grey seals in the North Sea (The Netherlands: Wageningen University).
Braulik G., Mintor G., Amano M., Bjørge A. (2020). Harbor Porpoise. The IUCN red list of threatened species 2020. Available at: https://dx.doi.org/10.2305/IUCN.UK.2020-2.RLTS.T17027A50369903.en. (Accessed 25-03-2022).
Bravo Rebolledo E. L. (2020). Afval in bruinvismagen: Voor en na de MSC Zoe containerramp. Report of Bureau Waardenburg, Culemborg, The Netherlands.
Burge C. A., Mark Eakin C., Friedman C. S., Froelich B., Hershberger P. K., Hofmann E. E., et al. (2014). Climate change influences on marine infectious diseases: Implications for management and society. Annu. Rev. Mar. Sci. 6, 249–277. doi: 10.1146/annurev-marine-010213-135029
Byard R. W., Winskog C., MacHado A., Boardman W. (2012). The assessment of lethal propeller strike injuries in sea mammals. J. Forensic Legal Med. 19, 158–161. doi: 10.1016/j.jflm.2011.12.017
Camphuysen C. J. (2004). The return of the harbour porpoise (Phocoena phocoena) in Dutch coastal waters. Lutra 47, 113–122.
Camphuysen C. J., Oosterbaan A. (2009). Het raadsel van de bruinvismutilaties: Extreme verminking en frequente strandingen van bruinvissen in noord Nederland, winter 2008/2009. Sula 22, 25–35.
Camphuysen C. J., Siemensma M. L. (2011) Conservation plan for the harbour porpoise Phocoena phocoena in the Netherlands: Towards a favourable conservation status. Available at: https://edepot.wur.nl/187229.
Camphuysen C. J., Smeenk C., Addink M., van Grouw H., Jansen O. E. (2008). Cetaceans stranded in the Netherlands from 1998 to 2007. Lutra 51, 87–122.
Costidis A. M., Berman M., Cole T., Knowlton A., McLellan W. A., Neilson J., et al. (2013). Sharp trauma induced by vessel collision with pinnipeds and cetaceans. Dis. Aquat. Org. 103, 251–256. doi: 10.3354/dao02566
Cox T. M., Read A. J., Barco S., Evans J., Gannon D. P., Koopman H. N., et al. (1998). Documenting the bycatch of harbor porpoises, Phocoena phocoena, in coastal gillnet fisheries from stranded carcasses. Fish. Bull. 96, 727–734.
Dolman S., Baulch S., Evans P. G. H., Read F., Ritter F. (2016). Towards an EU action plan on cetacean bycatch. Mar. Policy 72, 67–75. doi: 10.1016/j.marpol.2016.06.020
Dolman S. J., Evans P. G. H., Ritter F., Simmonds M. P., Swabe J. (2021). Implications of new technical measures regulation for cetacean bycatch in European waters. Mar. Policy 124, 104320. doi: 10.1016/j.marpol.2020.104320
Evans P. G. H. (2019). “European Whales, dolphins, and porpoises,” in Marine mammal conservation in practice (Academic Press).
Evans P. G. H., Hammond P. S. (2004). Monitoring cetaceans in European waters. Mamm. Rev. 34, 131–156. doi: 10.1046/j.0305-1838.2003.00027.x
Foster G., Whatmore A. M., Dagleish M. P., Malnick H., Gilbert M. J., Begeman L., et al. (2019). Forensic microbiology reveals that Neisseria animaloris infections in harbour porpoises follow traumatic injuries by grey seals. Sci. Rep. 9 (1), 1–8. doi: 10.1038/s41598-019-50979-3
Gilbert M. J., IJsseldijk L. L., Rubio-García A., Gröne A., Duim B., Rossen J., et al. (2020). After the bite: bacterial transmission from grey seals (Halichoerus grypus) to harbour porpoises (Phocoena phocoena). R. Soc. Open Sci. 7 (5), 192079. doi: 10.1098/rsos.192079
Gilles A., Scheidat M., Siebert U. (2009). Seasonal distribution of harbour porpoises and possible interference of offshore wind farms in the German North Sea. Mar. Ecol. Prog. Ser. 383, 295–307. doi: 10.3354/meps08020
Gulland F. M., Hall A. J. (2007). Is marine mammal health deteriorating? trends in the global reporting of marine mammal disease. EcoHealth 4 (2), 135–150. doi: 10.1007/s10393-007-0097-1
Haelters J., Camphuysen C. J. (2009). The harbour porpoise (Phocoena phocoena l.) in the southern North Sea. abundance, threats, research and management proposals (Brussels: Royal Belgian Institute of Natural Sciences (RBINS), department Management Unit of the North Sea Mathematical Models (MUMM) & Royal Netherlands Institute for Sea Research (NIOZ). Project financed by IFAW (International Fund for Animal Welfare)-IFAW Internationaler Tierschutz-Fonds GmbH, IFAW).
Haelters J., Kerckhof F., Jauniaux T., Degraer S. (2012). The grey seal (Halichoerus grypus) as a predator of harbour porpoises (Phocoena phocoena)? Aquat. Mammals 38, 343–353. doi: 10.1578/AM.38.4.2012.343
Halpern B. S., Frazier M., Potapenko J., Casey K. S., Koenig K., Longo C., et al. (2015). Spatial and temporal changes in cumulative human impacts on the world’s ocean. Nat. Commun. 6, 7615. doi: 10.1038/ncomms8615
Halpern B. S., Walbridge S., Selkoe K. A., Kappel C. V., Micheli F., D'agrosa C., et al. (2008). A global map of human impact on marine ecosystems. Science 319 (5865), 948–952. doi: 10.1126/science.1149345
Hammond P. S., Bearzi G., Bjørge A., Forney K., Karczmarski L., Kasuya T., et al. (2008). “Phocoena phocoena,” in The IUCN red list of threatened species. Available at: https://dx.doi.org/10.2305/IUCN.UK.2008.RLTS.T17027A6734992.en (Accessed 25-03-2022).
Hammond P. S., Berggren P., Benke H., Borchers D. L., Collet A., Heide-Jørgensen M. P., et al. (2002). Abundance of harbour porpoise and other cetaceans in the North Sea and adjacent waters. J. Appl. Ecol. 39 (2), 361–376. doi: 10.1046/j.1365-2664.2002.00713.x
Hammond P. S., Lacey C., Gilles A., Viquerat S., Boerjesson P., Herr H., et al. (2017) Estimates of cetacean abundance in European Atlantic waters in summer 2016 from the SCANS-III aerial and shipboard surveys. Available at: https://synergy.st-andrews.ac.uk/scans3/files/2017/05/SCANS-III-design-based-estimates-2017-05-12-final-revised.pdf.
Hammond P. S., Macleod K., Berggren P., Borchers D. L., Burt M. L., Cañadas A., et al. (2013). Cetacean abundance and distribution in European Atlantic shelf waters to inform conservation and management. Biol. Conserv. 164, 107–122. doi: 10.1016/j.biocon.2013.04.010
Hiemstra S., Harkema L., Wiersma L. C. M., Keesler R. I. (2015). Beyond parasitism: Hepatic lesions in stranded harbor porpoises (Phocoena phocoena) without trematode (Campula oblonga) infections. Veterinary Pathol. 52, 1243–1249. doi: 10.1177/0300985814560233
IJsseldijk L. L., Brownlow A. C., Mazzariol S. (Eds.) (2019). “Best practice on cetacean post mortem investigation and tissue sampling,” in Joint ACCOBAMS and ASCOBANS document. Available at: https://osf.io/zh4ra/ (Accessed 25-03-2022). doi: 10.31219/osf.io/zh4ra
IJsseldijk L. L., Camphuysen C. J., Keijl G. O., Troost G., Aarts G. (2021a). Predicting harbor porpoise strandings based on near-shore sightings indicates elevated temporal mortality rates. Front. Mar. Sci. 8, 668038. doi: 10.3389/fmars.2021.668038
IJsseldijk L. L., Scheidat M., Siemensma M., Couperus B., Leopold M. F., Morell M., et al. (2021c). Challenges in the assessment of bycatch: Post-mortem findings in harbor porpoises (Phocoena phocoena) retrieved from gillnets. Veterinary Pathol. 58 (2), 405–415. doi: 10.1177/0300985820972454
IJsseldijk L. L., Steenbergen J., Gröne A., Hiemstra S., Kik M. J. L., Begeman L. (2014). Apparent emergence of bow-caught fin whales (Balaenoptera physalus) found in the Netherlands. Aquat. Mammals 40 (4), 317. doi: 10.1578/AM.40.4.2014.317
IJsseldijk L. L., ten Doeschate M. T. I., Brownlow A. C., Davison N. J., Deaville R., Galatius A., et al. (2020a). Spatiotemporal mortality and demographic trends in a small cetacean: Strandings to inform conservation management. Biol. Conserv. 249, 108733. doi: 10.1016/j.biocon.2020.108733
IJsseldijk L. L., ten Doeschate M. T. I., Davison N. J., Gröne A., Brownlow A. C. (2018). Crossing boundaries for cetacean conservation: Setting research priorities to guide management of harbour porpoises. Mar. Policy 95, 77–84. doi: 10.1016/j.marpol.2018.07.006
IJsseldijk L. L., van Schalkwijk L., van den Berg A., ten Doeschate M. T. I., Everaarts E., Keijl G., et al. (2020b). Fatal attraction: The death of a solitary-sociable bottlenose dolphin due to anthropogenic trauma in the Netherlands. Lutra 63 (1-2), 17–32.
Jaber J. R., Pérez J., Arbelo M., Andrada M., Hidalgo M., Gómez-Villamandos J. C., et al. (2004). Hepatic lesions in cetaceans stranded in the Canary islands. Veterinary Pathol. 41 (2), 147–153. doi: 10.1354/vp.41-2-147
Jauniaux T., Garigliany M. M., Loos P., Bourgain J. L., Bouveroux T., Coignoul F., et al. (2014). Bite injuries of grey seals (Halichoerus grypus) on harbour porpoises (Phocoena phocoena). PloS One 9 (12), e108993. doi: 10.1371/journal.pone.0108993
Jauniaux T., Petitjean D., Brenez C., Borrens M., Brosens L., Haelters J., et al. (2002). Post-mortem findings and causes of death of harbour porpoises (Phocoena phocoena) stranded from 1990 to 2000 along the coastlines of Belgium and northern France. J. Comp. Pathol. 126 (4), 243–253. doi: 10.1053/jcpa.2001.0547
Jefferson T. A., Curry B. E. (1994). A global review of porpoise (Cetacea: Phocoenidae) mortality in gillnets. Biol. Conserv. 67 (2), 167–183. doi: 10.1016/0006-3207(94)90363-8
Jepson P. D., Baker J. R., Kuiken T., Simpson V. R., Kennedy S., Bennett P. M. (2000). Pulmonary pathology of harbour porpoises (Phocoena phocoena) stranded in England. Veterinary Rec. 146, 721–728. doi: 10.1136/vr.146.25.721
Jepson P. D., Barbieri M., Barco S. G., de Quiros Y. B., Bogomolni A., Danil K., et al. (2013). Peracute underwater entrapment of pinnipeds and cetaceans. Dis. Aquat. Org. 103, 229–264. doi: 10.3354/dao02566
Jepson P. D., Bennett P. M., Allchin C. R., Law R. J., Kuiken T., Baker J. R., et al. (1999). Investigating potential associations between chronic exposure to polychlorinated biphenyls and infectious disease mortality in harbour porpoises from England and Wales. Sci. Total Environ. 243, 339–348. doi: 10.1016/S0048-9697(99)00417-9
Jepson P. D., Bennett P. M., Deaville R., Allchin C. R., Baker J. R., Law R. J. (2005). Relationships between polychlorinated biphenyls and health status in harbor porpoises (Phocoena phocoena) stranded in the United Kingdom. Environ. Toxicol. Chem.: Int. J. 24 (1), 238–248. doi: 10.1897/03-663.1
Jepson P. D., Deaville R., Barber J. L., Aguilar À., Borrell A., Murphy S., et al. (2016). PCB Pollution continues to impact populations of orcas and other dolphins in European waters. Sci. Rep. 6, 18573. doi: 10.1038/srep18573
Kapetanou A., IJsseldijk L. L., Willems D. S., Broens E. M., Everaarts E., Buil J. B., et al. (2020). Mycotic infections in free-ranging harbor porpoises (Phocoena phocoena). Front. Mar. Sci. 7, 344. doi: 10.3389/fmars.2020.00344
Kirkwood J. K., Bennett P. M., Jepson P. D., Kuiken T., Simpson V. R., Baker J. R. (1997). Entanglement in fishing gear and other causes of death in cetaceans stranded on the coasts of England and Wales. Veterinary Rec. 141 (4), 94–98. doi: 10.1136/vr.141.4.94
Kühn S. (2020). Message in a belly: Plastic pathways in fulmars (The Netherlands: Wageningen University).
Kühn S., Bravo Rebolledo E. L., van Franeker J. A. (2015). Deleterious effects of litter on marine life. in: Marine anthropogenic litter. Eds. Bergmann M., Gutow L., Klages M. (Springer International Publishing), 75–116.
Kuiken T. (1996). “Review of the criteria for the diagnosis of by-catch in cetaceans,” in Diagnosis of by-catch in cetaceans: Proceedings of the second ECS workshop on cetacean pathology, montpellier, France, 2 march 1994, vol. 26, Special Issue . Ed. Kuiken T. (European Cetacean Society Newsletter).
Kuiken T., Bennett P. M., Allchin C. R., Kirkwood J. K., Baker J. R., Lockyer C. H., et al. (1994). PCBs, cause of death and body condition in harbour porpoises (Phocoena phocoena) from British waters. Aquat. Toxicol. 28 (1-2), 13–28. doi: 10.1016/0166-445X(94)90017-5
Learmonth J. A., MacLeod C. D., Santos M. B., Pierce G. J., Crick H. Q. P., Robinson R. A. (2006). Potential effects of climate change on marine mammals. Oceanography Mar. Biol. 44, 431. doi: 10.1201/9781420006391.ch8
Leeney R. H., Amies R., Broderick A. C., Witt M. J., Loveridge J., Doyle J., et al. (2008). Spatio-temporal analysis of cetacean strandings and bycatch in a UK fisheries hotspot. Biodiversity Conserv. 17 (10), 2323. doi: 10.1007/s10531-008-9377-5
Leopold M. F. (2015). Eat and be eaten: Porpoise diet studies (The Netherlands: Wageningen University).
Leopold M. F., Begeman L., van Bleijswijk J. D., IJsseldijk L. L., Witte H. J., Gröne A. (2015a). Exposing the grey seal as a major predator of harbour porpoises. Proc. R. Soc. B.: Biol. Sci. 282 (1798), 20142429. doi: 10.1098/rspb.2014.2429
Leopold M. F., Begeman L., Heße E., van der Hiele J., Hiemstra S., Keijl G. O., et al. (2015b). Porpoises: from predators to prey. J. Sea Res. 97, 14–23. doi: 10.1016/j.seares.2014.12.005
Leopold M. F., van Damme C. J. G., Philippart C. J. M., Winter C. J. N. (2001). Otoliths of North Sea fish: Interactive guide of identification of fish from the SE North Sea, wadden Sea and adjacent waters by means of otoliths and other hard parts (ETI, Amsterdam: CD-ROM).
Lightsey J. D., Rommel S. A., Costidis A. M., Pitchford T. D. (2006). Methods used during gross necropsy to determine watercraft-related mortality in the Florida manatee (Trichechus manatus latirostris). J. Zoo Wildlife Med. 37, 262–275. doi: 10.1638/04-095.1
Lima S. L. (2002). Putting predators back into behavioral predator–prey interactions. Trends Ecol. Evol. 17 (2), 70–75. doi: 10.1016/S0169-5347(01)02393-X
LNV (2020) Updated conservation plan for the harbour porpoise Phocoena phocoena in the Netherlands. Available at: https://open.overheid.nl/repository/ronl-dfa0b577-d562-4115-9fd5-110e8bd6eb02/1/pdf/bijlage-updated-conservation-plan-for-the-harbour-porpoise-phocoena-phocoenain-the-netherlands.pdf (Accessed 25-03-2022).
Lockyer C. (2003). “Harbour porpoises (Phocoena phocoena) in the North Atlantic: Biological parameters,” in Harbour porpoises in the North Atlantic, vol. 5 . Eds. Haug T., Desported G., Vikingsson G. A., Witting L. (NAMMCO Scientific Publications), 71–89.
Madsen P. T., Wahlberg M., Tougaard J., Lucke K., Tyack A. P. (2006). Wind turbine underwater noise and marine mammals: Implications of current knowledge and data needs. Mar. Ecol. Prog. Ser. 309, 279–295. doi: 10.3354/meps309279
Maio E., Begeman L., Bisselink Y., van Tulden P., Wiersma L., Hiemstra S., et al. (2014). Identification and typing of brucella spp. in stranded harbour porpoises (Phocoena phocoena) on the Dutch coast. Veterinary Microbiol. 173 (1-2), 118–124. doi: 10.1016/j.vetmic.2014.07.010
Marschner I. (2018). “glm2: Fitting generalized linear models,” in R package version 1.2.1. Available at: https://cran.r-project.org/web/packages/glm2/index.html. (Accessed 15-09-2022).
Martinez E., Stockin K. A. (2013). Blunt trauma observed in a common dolphin delphinus sp. likely caused by a vessel collision in the Hauraki Gulf, New Zealand. Pacific Conserv. Biol. 19, 19–27. doi: 10.1071/PC130019
McLellan W. A., Berman M., Cole T., Costidis A. M., Knowlton A., Neilson J. (2013). Blunt force trauma induced by vessel collisions with large whales. In: Criteria and case definitions for serious injury and death of pinnipeds and cetaceans caused by anthropogenic trauma. Dis. Aquat. Org. 103(3), 245–250. doi: 10.3354/dao02566
Moore M. J. (2014). How we all kill whales. ICES J. Mar. Sci. 71, 760–763. doi: 10.1093/icesjms/fsu008
Moore M. J., van der Hoop J., Barco S. G., Costidis A. M., Gulland F. M., Jepson P. D., et al. (2013). Criteria and case definitions for serious injury and death of pinnipeds and cetaceans caused by anthropogenic trauma. Dis. Aquat. Org. 103 (3), 229–264. doi: 10.3354/dao02566
Morell M., André M. (2009) Cetacean ear extraction and fixation protocol. Available at: http://www.zoology.ubc.ca/files/Ear_extraction_and_fixation_protocol_UBC.pdf.
Morell M., Lehnert K., IJsseldijk L. L., Raverty S. A., Wohlsein P., Gröne A., et al. (2017a). Parasites in the inner ear of harbour porpoise: Cases from the North and Baltic Seas. Dis. Aquat. Org. 127 (1), 57–63. doi: 10.3354/dao03178
Morell M., Brownlow A., McGovern B., Raverty S. A., Shadwick R. E., André M. (2017b). Implementation of a method to visualize noise-induced hearing loss in mass stranded cetaceans. Sci. Rep. 7 (1), 1–8. doi: 10.1038/srep41848
Morell M., IJsseldijk L. L., Berends A. J., Gröne A., Siebert U., Raverty S. A., et al. (2021). Evidence of hearing loss and unrelated toxoplasmosis in a free-ranging harbour porpoise (Phocoena phocoena). Animals 11 (11), 3058. doi: 10.3390/ani11113058
Morell M., IJsseldijk L. L., Piscitelli-Doshkov M., Ostertag S., Estrade V., Haulena M., et al. (2022). Cochlear apical morphology in toothed whales: Using the pairing hair cell–deiters' cell as a marker to detect lesions. Anatomical Rec. 305, 622–642. doi: 10.1002/ar.24680
Morell M., Lenoir M., Shadwick R. E., Jauniaux T., Dabin W., Begeman L., et al. (2015). Ultrastructure of the odontocete organ of corti: Scanning and transmission electron microscopy. J. Comp. Neurol. 523 (3), 431–448. doi: 10.1002/cne.23688
Morell M., Vogl A. W., IJsseldijk L. L., Piscitelli-Doshkov M., Tong L., Ostertag S., et al. (2020). Echolocating whales and bats express the motor protein prestin in the inner ear: a potential marker for hearing loss. Front. Veterinary Sci. 7, 429. doi: 10.3389/fvets.2020.00429
Nachtsheim D. A., Viquerat S., Ramírez-Martínez N. C., Unger B., Siebert U., Gilles A. (2021). Small cetacean in a human high-use area: Trends in harbor porpoise abundance in the North Sea over two decades. Front. Mar. Sci. 7, 1135. doi: 10.3389/fmars.2020.606609
National Academies of Sciences, Engineering, and Medicine (NAS) (2017). Approaches to understanding the cumulative effects of stressors on marine mammals (Washington, DC: The National Academies Press).
Neimanis A., Stavenow J., Ågren E. O., Wikström-Lassa E., Roos A. M. (2022). Causes of death and pathological findings in stranded harbour porpoises (Phocoena phocoena) from Swedish waters. Animals 12 (3), 369. doi: 10.3390/ani12030369
Nielsen N. H., Teilmann J., Sveegaard S., Hansen R. G., Sinding M. H. S., Dietz R., et al. (2018). Oceanic movements, site fidelity and deep diving in harbour porpoises from Greenland show limited similarities to animals from the North Sea. Mar. Ecol. Prog. Ser. 597, 259–272. doi: 10.3354/meps12588
Peltier H., Baagøe H. J., Camphuysen C. J., Czeck R., Dabin W., Daniel P., et al. (2013). The stranding anomaly as population indicator: The case of harbour porpoise Phocoena phocoena in north-Western Europe. PloS One 8 (4), e62180. doi: 10.1371/journal.pone.0062180
Philipp C., Unger B., Ehlers S. M., Koop J. H. E., Siebert U. (2021). First evidence of retrospective findings of microplastics in harbour porpoises (Phocoena phocoena) from German waters. Front. Mar. Sci. 8, 682532. doi: 10.3389/fmars.2021.682532
Pierce G. J., Santos M. B., Murphy S., Learmonth J. A., Zuur A. F., Rogan E., et al. (2008). Bioaccumulation of persistent organic pollutants in female common dolphins (Delphinus delphis) and harbour porpoises (Phocoena phocoena) from western European seas: Geographical trends, causal factors and effects on reproduction and mortality. Environ. pollut. 153 (2), 401–415. doi: 10.1016/j.envpol.2007.08.019
Podt A. E., IJsseldijk L. L. (2018). Grey seal attacks on harbour porpoises in the Eastern scheldt. Lutra 60, 105–116.
Sanderson C. E., Alexander K. A. (2020). Unchartered waters: Climate change likely to intensify infectious disease outbreaks causing mass mortality events in marine mammals. Global Change Biol. 26, 4284–4301. doi: 10.1111/gcb.15163
Siebert U., Pawliczka I., Benke H., von Vietinghoff V., Wolf P., Pilāts V., et al. (2020). Health assessment of harbour porpoises (Phocoena phocoena) from Baltic area of Denmark, Germany, Poland and Latvia. Environ. Int. 143, 105904. doi: 10.1016/j.envint.2020.105904
Siebert U., Stürznickel J., Schaffeld T., Oheim R., Rolvien T., Prenger-Berninghoff E., et al. (2022). Blast injury on harbour porpoises (Phocoena phocoena) from the Baltic Sea after explosions of deposits of world war II ammunition. Environ. Int. 159, 107014. doi: 10.1016/j.envint.2021.107014
Siebert U., Wünschmann A., Weiss R., Frank H., Benke H., Frese K. (2001). Post-mortem findings in harbour porpoises (Phocoena phocoena) from the German North and Baltic Seas. J. Comp. Pathol. 124 (2-3), 102–114. doi: 10.1053/jcpa.2000.0436
Simmonds M. P., Isaac S. J. (2007). The impacts of climate change on marine mammals: Early signs of significant problems. Oryx 41, 19–26. doi: 10.1017/S0030605307001524
St. Leger J. A., Raverty S. A., Mena A. (2018). “Cetacea,” in Pathology of wildlife and zoo animals. Eds. Terio K. A., McAloose D., St. Leger J. A. (Elsevier, EAN: Andre Gerhard Wolff), 533–567.
Teilmann J., Carstensen J. (2012). Negative long term effects on harbour porpoises from a large scale offshore wind farm in the Baltic–evidence of slow recovery. Environ. Res. Lett. 7 (4), 045101. doi: 10.1088/1748-9326/7/4/045101
ten Doeschate M. T. I., Brownlow A. C., Davison N. J., Thompson P. M. (2018). Dead useful: Methods for quantifying baseline variability in stranding rates to improve the ecological value of the strandings record as a monitoring tool. J. Mar. Biol. Assoc. U. K. 98 (5), 1205–1209. doi: 10.1017/S0025315417000698
ten Doeschate M. T. I., IJsseldijk L. L., Hiemstra S., De Jong E. A., Strijkstra A., Gröne A., et al. (2017). Quantifying parasite presence in relation to biological parameters of harbour porpoises Phocoena phocoena stranded on the Dutch coast. Dis. Aquat. Org. 127 (1), 49–56. doi: 10.3354/dao03182
Tyne J. A., Loneragan N. R., Johnston D. W., Pollock K. H., Williams R., Bejder L. (2016). Evaluating monitoring methods for cetaceans. Biol. Conserv. 201, 252–260. doi: 10.1016/j.biocon.2016.07.024
Unger B., Bravo Rebolledo E. L., Deaville R., Gröne A., IJsseldijk L. L., Leopold M. F., et al. (2016). Large Amounts of marine debris found in sperm whales stranded along the North Sea coast in early 2016. Mar. pollut. Bull. 112 (1-2), 134–141. doi: 10.1016/j.marpolbul.2016.08.027
van Beurden S. J., IJsseldijk L. L., Ordonez S. R., Förster C., de Vrieze G., Gröne A., et al. (2015). Identification of a novel gammaherpesvirus associated with (muco)cutaneous lesions in harbour porpoises (Phocoena phocoena). Arch. Virol. 160 (12), 3115–3120. doi: 10.1007/s00705-015-2607-8
van Bleijswijk J. D., Begeman L., Witte H. J., IJsseldijk L. L., Brasseur S. M., Gröne A., et al. (2014). Detection of grey seal Halichoerus grypus DNA in attack wounds on stranded harbour porpoises Phocoena phocoena. Mar. Ecol. Prog. Ser. 513, 277–281. doi: 10.3354/meps11004
van den Heuvel-Greve M. J., van den Brink A. M., Kotterman M. J., Kwadijk C. J., Geelhoed S. C., Murphy S., et al. (2021). Polluted porpoises: Generational transfer of organic contaminants in harbour porpoises from the southern North Sea. Sci. Total Environ. 796, 148936. doi: 10.1016/j.scitotenv.2021.148936
van de Velde N., Devleesschauwer B., Leopold M., Begeman L., IJsseldijk L. L., Hiemstra S., et al. (2016). Toxoplasma gondii in stranded marine mammals from the North Sea and Eastern Atlantic Ocean: Findings and diagnostic difficulties. Veterinary Parasitol. 230, 25–32. doi: 10.1016/j.vetpar.2016.10.021
van Elk C. E., van de Bildt M. W., van Run P. R., Bunskoek P., Meerbeek J., Foster G., et al. (2019). Clinical, pathological, and laboratory diagnoses of diseases of harbour porpoises (Phocoena phocoena), live stranded on the Dutch and adjacent coasts from 2003 to 2016. Veterinary Res. 50 (1), 1–17. doi: 10.1186/s13567-019-0706-3
van Franeker J. A., Bravo Rebolledo E. L., Heße E., IJsseldijk L. L., Kühn S., Leopold M. F., et al. (2018). Plastic ingestion by harbour porpoises Phocoena phocoena in the Netherlands: Establishing a standardised method. Ambio 47 (4), 387–397. doi: 10.1007/s13280-017-1002-y
van Neer A., Gross S., Kesselring T., Grilo M. L., Ludes-Wehrmeister E., Roncon G., et al. (2020). Assessing harbour porpoise carcasses potentially subjected to grey seal predation. Sci. Rep. 10 (1), 1–9. doi: 10.1038/s41598-020-80737-9
van Nie C. J. (1989). Postmortem findings in stranded harbour porpoises (Phocoena phocoena, l. 1758) in the Netherlands from 23rd march 1983 to 25th June 1986. Aquat. Mammals 15 (2), 80–83.
Vinther M., Larsen F. I. N. N. (2004). Updated estimates of harbour porpoise (Phocoena phocoena) bycatch in the Danish North Sea bottom-set gillnet fishery. J. Cetacean Res. Manage. 6 (1), 19–24.
von Benda-Beckmann A. M., Aarts G., Sertlek H. Ö., Lucke K., Verboom W. C., Kastelein R. A., et al. (2015). Assessing the impact of underwater clearance of unexploded ordnance on harbour porpoises (Phocoena phocoena) in the southern North Sea. Aquat. Mammals 41 (4), 503. doi: 10.1578/AM.41.4.2015.503
Weijs L., Dirtu A. C., Das K., Gheorghe A., Reijnders P. J., Neels H., et al. (2009). Inter-species differences for polychlorinated biphenyls and polybrominated diphenyl ethers in marine top predators from the southern North Sea: Part 1. accumulation patterns in harbour seals and harbour porpoises. Environ. pollut. 157 (2), 437–444. doi: 10.1016/j.envpol.2008.09.024
Wisniewska D. M., Johnson M., Teilmann J., Siebert U., Galatius A., Dietz R., et al. (2018). High rates of vessel noise disrupt foraging in wild harbour porpoises (Phocoena phocoena). Proc. R. Soc. B.: Biol. Sci. 285 (1872), 20172314. doi: 10.1098/rspb.2017.2314
Zuur A. F., Ieno E. N., Smith G. M. (2007). Analyzing ecological data (New York, USA: Springer Science & Business Media). ISSN: 1431-8776.
Keywords: cetacean, post-mortem investigation, bycatch, hearing damage, infectious diseases, ship strike, marine debris
Citation: IJsseldijk LL, Leopold MF, Begeman L, Kik MJL, Wiersma L, Morell M, Bravo Rebolledo EL, Jauniaux T, Heesterbeek H and Gröne A (2022) Pathological findings in stranded harbor porpoises (Phocoena phocoena) with special focus on anthropogenic causes. Front. Mar. Sci. 9:997388. doi: 10.3389/fmars.2022.997388
Received: 18 July 2022; Accepted: 21 September 2022;
Published: 11 October 2022.
Edited by:
Juan José Alava, University of British Columbia, CanadaReviewed by:
Carlos Sacristán Yagüe, Centro de Investigación en Sanidad Animal (CISA), SpainJames Carretta, Southwest Fisheries Science Center (NOAA), United States
Joanna Kershaw, University of Aberdeen, United Kingdom
Copyright © 2022 IJsseldijk, Leopold, Begeman, Kik, Wiersma, Morell, Bravo Rebolledo, Jauniaux, Heesterbeek and Gröne. This is an open-access article distributed under the terms of the Creative Commons Attribution License (CC BY). The use, distribution or reproduction in other forums is permitted, provided the original author(s) and the copyright owner(s) are credited and that the original publication in this journal is cited, in accordance with accepted academic practice. No use, distribution or reproduction is permitted which does not comply with these terms.
*Correspondence: Lonneke L. IJsseldijk, TC5MLklKc3NlbGRpamtAdXUubmw=
†Present address: Lineke Begeman, Department of Viroscience, Erasmus University Medical Centre, Rotterdam, Netherlands;Lidewij Wiersma, European Board of Veterinary Specialisation, Utrecht, Netherlands