- 1School of Molecular and Life Sciences, Curtin University, WA, Bentley, Australia
- 2Negaunee Integrative Research Center, Field Museum of Natural History, Crawley, Chicago, IL, United States
- 3CSIRO Oceans and Atmosphere, Indian Ocean Marine Research Centre, The University of Western Australia, WA, Australia
- 4Department of Biological and Environmental Sciences, University of Gothenburg, Gothenburg, Sweden
Introduction: The west coast of Western Australia (WA) is a global hotspot for increasing sea surface temperatures and marine heatwaves.
Methods: We used visual survey transects to compare mollusc and echinoderm populations on three coastal intertidal platform reefs on the Perth shoreline with two intertidal platforms at the west end of Rottnest Island (32°S) which are under the influence of the southward flowing Leeuwin Current.
Results: In 1983, temperate species dominated Perth coastal molluscan diversity, but the tropical mussel Brachidontes sculptus dominated density. Species richness on coastal platforms remained constant in 2007 and 2021, but total densities were lower in 2007 as B. sculptus declined; partial recovery occurred on coastal platforms in 2021. Tropical species were a significant component of mollusc diversity and density at Rottnest Island in 1982 and 2007. Total mollusc density declined by 98% at the island sites of Radar Reef and 86% at Cape Vlamingh and total echinoderm density by 52% and 88% respectively from 2007 to 2021; species diversity also declined sharply.
Discussion: Tropical species have moved southward in WA subtidal environments, but tropical, temperate and WA endemic species all suffered losses of biodiversity and catastrophic declines in density of 90% or more on the two Rottnest Island intertidal platforms. Data presented here provide a sound basis for exploring the possible causes of the catastrophic mortality at the west end of Rottnest Island and monitoring for recovery.
Introduction
Global warming is recognized as one of the critical environmental issues in marine ecosystems, and the pace of change is increasing (O’Hara et al., 2021). Ocean circulation patterns and water chemistry are being disrupted, including changes in marine temperatures, stratification, nutrient input, oxygen content and ocean acidification (Harley et al., 2006; Doney et al., 2012).
Warming ocean temperatures are occurring globally, but the phenomenon is uneven; some areas are warming more rapidly than others. Hobday and Pecl (2013) analyzed monthly sea surface temperatures (SST) for the five decades of 1950 to 1999, reporting 24 global hotspots of increasing SST either in warming rate or being in the top 10% of warming. A related phenomenon is marine heatwaves (Pearce et al., 2011) where SST increase markedly for short periods in a local area then return to more normal levels (Hobday et al., 2016; Oliver et al., 2021). Marine heatwaves increased in frequency by 34% from 2005 to 2016 and their duration by 17% (Straub et al., 2019), with further increases in frequency and temperature spikes predicted (Oliver et al., 2019). The physical and chemical changes in marine systems modify biological parameters such as calcification rates, community structures, larval transport patterns and species ranges (Harley et al., 2006; Doney et al., 2012; Poloczanska et al., 2016).
A key impact of ocean warming is that the ranges of tropical species are increasing towards the poles (Wernberg et al., 2011; Yamano et al., 2011; Baird et al., 2012; Hyndes et al., 2016; Hastings et al., 2020; Melbourne-Thomas et al., 2021). In Australia, of the 198 range shifts recorded, 87% were poleward (Gervais et al., 2021). Babcock et al. (2019) collated the effects of extreme climatic events over 45% of the Australian coastline in the short period of 2011 to 2017: coral bleaching over much of northern Australia; loss of seagrasses on both the east and west coasts through excessive rainfall and marine heatwaves; loss of kelp forests on the west coast through marine heatwaves; and loss of mangroves during droughts and unusually low sea levels during the 2016 El Niño event.
Studies on the west coast of Western Australia (WA), extending over 12° of latitude from North West Cape south to Cape Leeuwin, have been particularly important in elucidating the effects of climate change on the marine environment. The region has the only poleward current on the west coast of a continent, the Leeuwin Current (Cresswell and Golding, 1980; Pearce and Walker, 1991; Pearce, 2007) which has naturally dispersed tropical species farther southward than they would otherwise occur. The lower west coast of WA is a global hotspot in warming SST which have risen ∼0.6°C since 1951 (Pearce and Feng, 2007; Hobday and Pecl, 2013). The region has also recently experienced several marine heatwaves, the largest of which was in the summer of 2011 when temperatures were up to 3°C above average for about three months and inshore temperatures increased by up to 5°C for shorter periods (Pearce and Feng, 2013). The heatwave caused considerable changes in the subtidal marine flora and fauna along the entire west coast. Extensive temperate kelp beds contracted 100km to the south and were replaced by tropical and subtropical marine algae, invertebrates, corals and fishes (Pearce and Feng, 2013). The changed community structure altered key ecological processes, suppressing the potential recovery of kelp (Wernberg et al., 2011; Wernberg et al., 2013; Hyndes et al., 2016; Wernberg et al., 2016). Coral bleaching occurred along the entire west coast (Thomson et al., 2011; Abdo et al., 2012; Moore et al., 2012; Smale and Wernberg, 2012). Valuable scallop, crab, abalone, prawn and rock lobster fisheries were severely affected. Some, but not all, fisheries have since partially recovered (Caputi et al., 2019).
Most of the known adverse effects of the 2011 marine heatwave in WA occurred in subtidal environments. The exception was a small commercial fishery of the temperate abalone Haliotis roei which was wiped out on intertidal platforms at Kalbarri (27.7°S) near the northern limit of its range; it has not recovered (Hart et al., 2018; Caputi et al., 2019; Strain et al., 2020).
With increasing SST in the Perth region on the lower west coast of Western Australia (Pearce and Feng, 2007; Hobday and Pecl, 2013) and the southward expansion of subtidal species in the region as a result of marine heatwaves (Wernberg et al., 2011; Wernberg et al., 2013; Hyndes et al., 2016; Wernberg et al., 2016) we hypothesize that there would have been an increase in tropical invertebrates on intertidal platform reefs in the Perth region in recent decades. To test this hypothesis, we used visual transect surveys on molluscs and echinoderms collected over the 39 years from 1982 to 2021.
Materials and methods
Platform reef habitat
There is a series of rocky ridges in the shallow water marine environment of the Perth region; some are emergent, reaching heights of up to 60m above sea level while others remain subsurface. Limestone platforms along the continental shoreline and on islands range from a few metres to over 100m wide at Cape Vlamingh at Rottnest Island. The region is microtidal, a mixture of diurnal and semidiurnal tides; tidal ranges vary from a few centimetres to up to 1m. Rock platforms occur from tidal datum to about 1m above; many are at about 0.3m. The platform surface is generally flat, but highly dissected by channels and pools on some platforms. There is usually a low ridge, or rim, up to 0.2m high and 2m wide on the seaward margin or along channels that traps water in a shallow pool on the platform. As seawater drains from the platform at low tide it is replaced by waves crashing over the seaward rim or water emerging from fissures and blowholes in the rock surface (Wells and Walker, 1993).
Molluscs and echinoderms were investigated on five platforms in the present study: Cape Vlamingh and Radar Reef at the western end of Rottnest Island and three platforms along the Perth coastline (Cottesloe, Trigg Point and Waterman) (Figure 1).
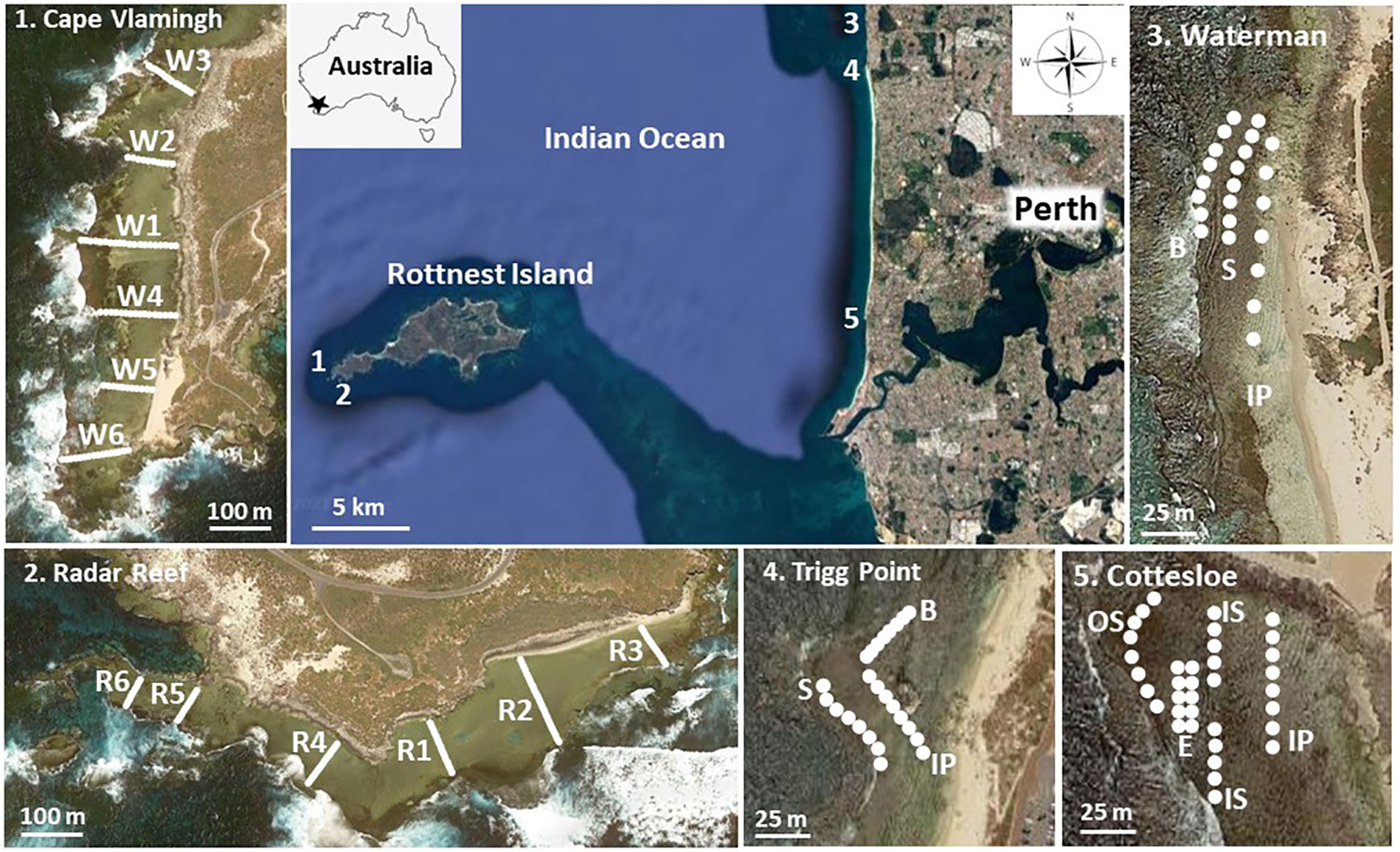
Figure 1 Map of the location of the study sites and transects at Rottnest Island and along the coastline of Perth, Western Australia. For the coastal platforms, habitat codes are IP inshore platform; S, Sargassum; B, bare; IS, inner Sargassum; OS, outer Sargassum; E, Ecklonia; Redrawn from Google (n.d.).
Transect surveys
Visual surveys were made along transects 100m apart from the base of the inshore cliff to the seaward edge of the intertidal platforms at Cape Vlamingh and Radar Reef in 1982. Every 5m all molluscs ≥5mm in their greatest dimension in a 1m2 quadrat were identified and counted. There were six transects with 97 quadrats at Cape Vlamingh and six transects with 65 quadrats at Radar Reef (Figure 1). A similar series of transects from the shoreline to the seaward edge of the platform was made at the coastal sites of Cottesloe, Trigg Point and Waterman in the austral summer of 1982. Results of the 1982 surveys were summarised from Wells (1985).
In 1983 a major investigation was initiated on molluscs present on the coastal platforms to assess possible overexploitation, particularly of the abalone Haliotis roei. From 1983 to 1987 an intensive summer survey (January to March) was made of molluscs (and in 1986 echinoderms) on the platforms at Cottesloe, Trigg Point and Waterman. Each platform was divided into habitats based on macroalgal assemblages present (Figure 1). Four zones were defined at Cottesloe: inshore platform; inner Sargassum on the middle of the platform; outer Sargassum at the seaward margin of the platform; and an Ecklonia zone in slightly deeper water (approximately 1m; 32m2). Three habitats were defined at Trigg Point and Waterman: an inshore platform with a diverse assemblage of macroalgae; Sargassum zone; and a seaward bare zone with only sparse macroalgal coverage (24m2 each at Trigg Point and Waterman). Transects commenced at the northern end of each zone (Figure 1) and were sampled parallel to shore at 5-10m intervals depending on the size of the platform. Quadrats were searched in detail for all molluscs ≥5mm. Data for each habitat were converted to average density for the overall platforms using estimates of the area of each habitat on each platform.
Molluscs and echinoderms on the Rottnest Island and coastal platforms were resurveyed in the summers of 2007 and 2021 using the same methodology, except that quadrats were reduced from 1m2 to 0.25m2 to minimise the workload. As far as possible the same transects were used for each platform. The number of quadrats in each habitat on coastal platforms was increased from 8 to 10, so there were 40 quadrats (10m2) at Cottesloe and 30 quadrats (7.5m2) each at Waterman and Trigg Point. Statistical analysis indicated that the reduced quadrat size would possibly reduce the numbers of species recorded on each platform but should not affect estimates of density (Irvine et al., 2008).
Taxonomic and biogeographic determinations
All molluscs collected in the 1982-1983 surveys were taken to the Western Australian Museum, Perth, WA where they were sorted to species, identified using the museum collections and published literature and counted. Representative specimens of each species were deposited in the museum. As far as possible identifications were made in the field in subsequent years and the animals returned to the platform to minimize study impacts.
Biogeographic relationships (tropical, temperate and species endemic to WA) are based on published distributions and/or collections of the WA Museum. The dominant mussel on inshore platforms was previously identified as a WA endemic species, Brachidontes ustulatus (Wells and Bryce, 1986; Wilson, 2002), but it is now considered to be the tropical species, B. sculptus (Huber, 2010). The species names presented in Supplementary Tables 1 and 2 have been updated using the World List of Marine Species (WoRMS Editorial Board, 2022).
Data analysis
Data analysis was performed using R statistical software, version 4.0. Non-metric multidimensional analysis (nMDS) using Bray-Curtis distance to describe differences in mollusc and echinoderm community assemblages was performed using the Vegan R package (Dixon, 2003). ANOSIM was used to establish significance levels (p<0.05) for changes in community assemblages between years, and between coastal versus Rottnest Island sampling sites. Heatmaps displaying mollusc density were restricted to the 16 most numerous species, with each individual species representing >1% of all individuals observed at any site for any given year. Echinoderm densities were restricted to the 9 most abundant species, with specimens with very low occurrence (<1%) or unidentified to species level presented as class aggregates. Hierarchical cluster analysis was performed using the Vegan R package (Dixon, 2003). Community diversity was estimated as species richness.
Sea surface temperature data (annual mean temperatures for southwestern Australia) were obtained from the Australian Bureau of Meteorology (available at: http://www.bom.gov.au/climate/change/#tabs=Tracker&tracker=timeseries&tQ=graph%3Dsst%26area%3Dsw%26season%3D0112%26ave_yr%3D10).
Results
Molluscs
Rottnest Island
In 1982, 53 mollusc species were collected and identified at two sites on the western end of Rottnest Island: 32 at Radar Reef and 44 at Cape Vlamingh (Table 1). Isolated individuals, mostly small juveniles, of 5 species at Radar Reef and 3 at Cape Vlamingh could not be identified. The total density of molluscs was 130.8 ± 17.9 ind/m2 at Radar Reef and 135.0 ± 14.8 ind/m2 at Cape Vlamingh. The number of tropical (12) and temperate (12) species identified at Radar Reef was equal and there were 8 WA endemic species. Tropical species (83.8 ± 17.0 ind/m2) dominated density, almost all of which were the mytilid Septifer bilocularis. The 8 WA endemic species had a density of 36.3 ± 7.2 ind/m2, and were primarily the small (1cm) gastropod Euplica bidentata. Temperate species contributed only 10.7 ± 1.6 ind/m2. A similar diversity pattern occurred at Cape Vlamingh: tropical (18) and temperate (16) species had the greatest species richness, with only 10 WA endemic species. However, mollusc density at Cape Vlamingh was dominated by WA endemic species (89.7 ± 11.9 ind/m2), primarily E. bidentata. Tropical species were 35.1 ± 7.3 ind/m2, again dominated by S. bilocularis. Temperate species (10.5 ± 1.4 ind/m2) were only a small proportion of total mollusc density.
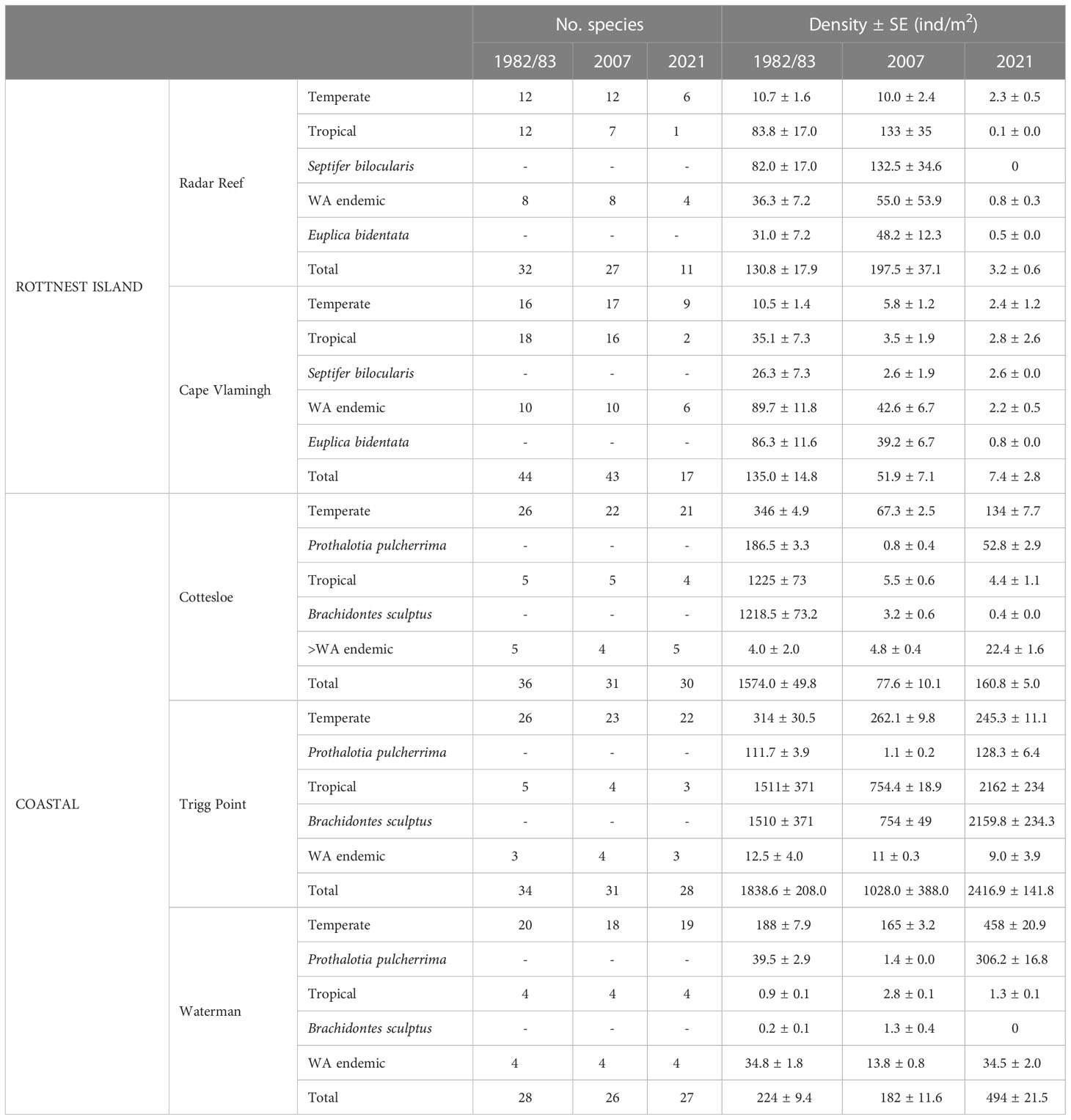
Table 1 Distribution patterns of mollusc species identified on intertidal platforms at Rottnest Island and the Perth coastline, Western Australia.
Fifty-four species of molluscs were found in 2007 at Rottnest Island: 27 at Radar Reef and 43 at Cape Vlamingh (Figures 2A, 3A; Table 1). Of the 50 identified species on these two platforms, 21 were temperate, 17 tropical and 12 WA endemics. The numbers of temperate (12) and WA endemic species (8) at Radar Reef was the same in 2007 as in 1982, but only 7 tropical species were found in 2007 (12 in 1982). Species composition at Cape Vlamingh in 2007 was very similar to 1982: 17 temperate, 16 tropical and 10 WA endemic. Density of molluscs at Radar Reef and Cape Vlamingh were similar in 1982 but diverged in 2007: increasing to 197.5 ± 37.1 ind/m2 at Radar Reef and decreasing at Cape Vlamingh (51.9 ± 7.1 ind/m2). Tropical species (67%), primarily S. bilocularis, again dominated density at Radar Reef followed by WA endemics (24%), primarily E. bidentata. As in 1982, the situation was reversed at Cape Vlamingh, where E. bidentata had a density of 39.2 ± 6.7 ind/m2 and S. bilocularis was only 2.6 ± 1.9 ind/m2. Total density at Cape Vlamingh was dominated by WA endemic species (82%), primarily E. bidentata. Temperate species were 11% of total density at Cape Vlamingh and tropical were 7% of the total mollusc density.
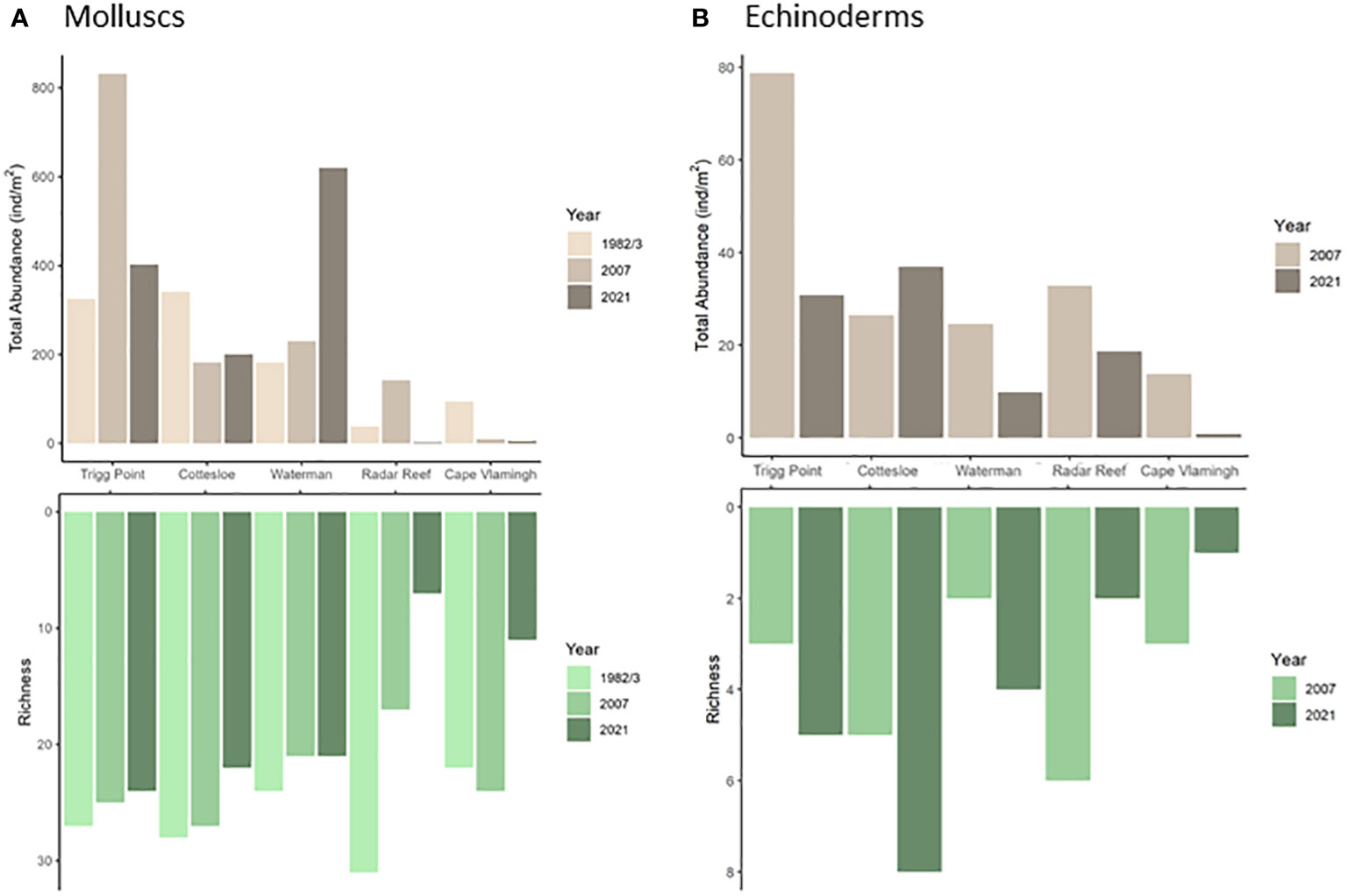
Figure 2 Density and diversity (as richness) of platform molluscs (A) and echinoderms (B) at five sites in 1982/3, 2007 and 2021.
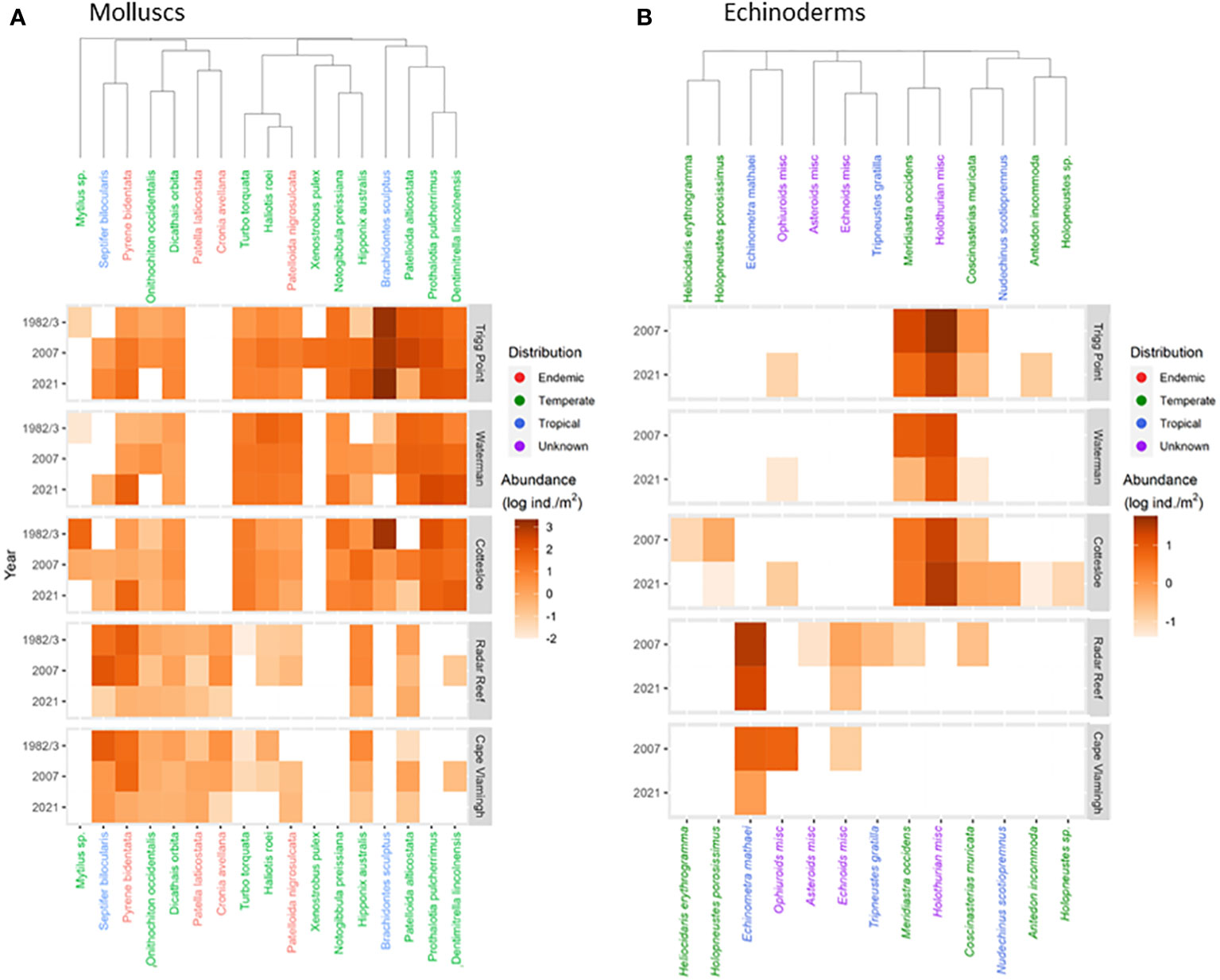
Figure 3 Heatmaps of population densities for molluscs (A) and echinoderms (B) for five sites in 1982/3, 2007 and 2021.
A catastrophic decline was recorded in 2021 for diversity (27 species down to 11; 59% decline) for molluscs at Radar Reef compared to 2007 (Figures 2A, 3A; Table 1) where all distribution patterns (tropical, temperate and WA endemic) declined sharply at Radar Reef, particularly tropical species, with only a single species being recorded in 2021. Similarly, the number of species declined from 43 to 17 at Cape Vlamingh (60% decline). Species richness in all three distributional patterns was reduced, again led by tropical species which declined from 16 to 2. Total mollusc density declined catastrophically between 2007 and 2021 at both platforms: a decline of 98.4% at Radar Reef and of 85.6% at Cape Vlamingh.
The decline in mollusc populations between 2007 and 2021 was not uniform across the platforms. The “limpet zone” at the seaward margins of the platforms receives incoming wave action which dissipates as it travels inshore across the platform. Large species: the giant limpet (Scutellastra laticostata), chitons (WA endemic Liolophura hirtosa and temperate Onithochiton quercinus) and abalone (temperate Haliotis roei) occur near the seaward margin. Total mollusc density on the general platform surface declined markedly in 2021 at Radar Reef (-99%) (Figures 2A, 3A; Table 2), but the declines in the seaward limpet zone were much lower (-54%). A similar pattern occurred to a lesser extent at Cape Vlamingh; the overall decline between 2007 and 2021 was 85.6% of density (Table 2). Density on the general platform surface declined by 99% but increased slightly (14%) in the wave swept limpet zone.
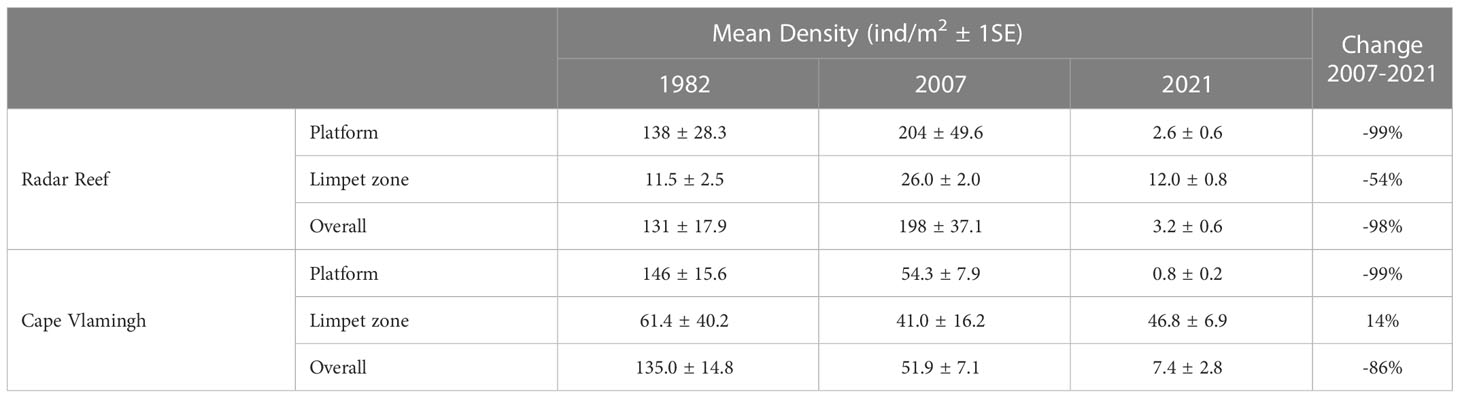
Table 2 Density of molluscs on intertidal platforms at Rottnest Island, Western Australia in 1982, 2007 and 2021.
Coastal platforms
Species diversity on the coastal platforms was remarkedly consistent in the three years surveyed: the total numbers of species at Cottesloe ranged from 30 to 36; 28-34 at Trigg Point and 26-28 at Waterman. Temperate species dominated in all years on all three platforms, ranging from 69% of mollusc species at Waterman in 2007 to 79% at Trigg Point in 2021 (mean 72.4 ± 1.1%) (Table 1). The percentages of WA endemic species (mean 13.6 ± 0.9%) were similar to tropical species (mean 14.2 ± 0.6).
In contrast to the relative stability of species richness, total mollusc density varied considerably on the coastal platforms (Table 1). Trigg Point had the greatest total mollusc density in all years. Cottesloe was second in 1983, but had lower total mollusc density than Waterman in 2007 and 2021.
Total mollusc density declined sharply (-95.1%) at Cottesloe from 1574.0 ± 49.8 ind/m2 in 1983 to only 77.6 ± 10.1 ind/m2 in 2007. This was largely due to reductions in the small (≤1cm) tropical mussel Brachidontes sculptus. Total mollusc density at Cottesloe recovered slightly to 160.8 ± 5.0 ind/m2 in 2021, primarily due to the increase in P. pulcherrima to 52.8 ± 2.9 ind/m2. The pattern was somewhat different at Trigg Point. B. sculptus formed continuous carpets on the rock surface in some areas of the Sargassum zone; individual quadrats had densities of B. sculptus of up to 10,000 ind/m2. Total mollusc density on the overall platform in 1983 was 1838.6 ind/m2 but declined to 1028 ind/m2 in 2007, driven primarily by a decline in B. sculptus. Total mollusc density at Trigg Point in 2021 was 2416.9 ± 141.8 ind/m2, 32% higher than in 1983, with the increased density of B. sculptus. In contrast to Cottesloe and Trigg Point, B. sculptus did not form dense aggregations at Waterman; the highest density measured at Waterman was 1.3 ± 0.4 ind/m2 in 2007. Total mollusc density at Waterman was driven by P. pulcherrima, which declined from 39.5 ± 2.9 ind/m2 in 1983 to 1.4 ± 0.0 ind/m2 in 2007, but increased to 306.2 ± 16.8 ind/m2 in 2021.
Comparison of molluscs at Rottnest Island with coastal platforms
Differences in mollusc communities between coastal platforms and Rottnest Island sites, and between sampling years are illustrated by an unambiguous separation within the ordination space of the nMDS plot (Figure 4). Coastal sites were dominated by predominantly temperate species in all years, whereas offshore sites were influenced mostly by endemic and tropical species in 1982 and 2007, but not in 2021.
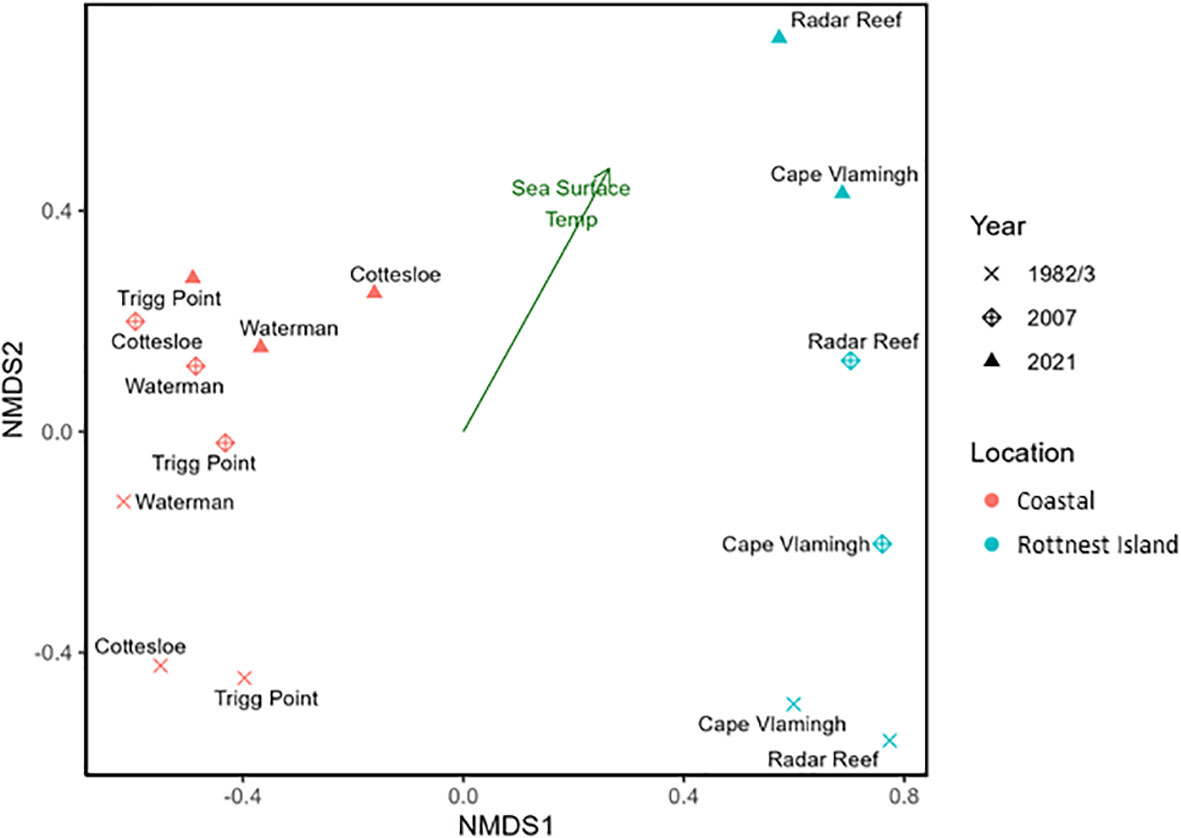
Figure 4 nMDS plot of mollusc assemblages in 1982/3, 2007 and 2021 for Perth coastal platforms and Rottnest Island sites.
Assemblages at coastal sites were significantly different from those at Rottnest Island (ANOSIM, R =0.26; p = 0.04), hence changes in mollusc communities over the decades in both coastal and Rottnest Island habitats were statistically analysed independently of each other. Regardless, changes in assemblages from 1982/3, 2007 and 2021 were not significantly different for either coastal (ANOSIM, R = -0.07; p = 0.45) or Rottnest Island sites (ANOSIM, R = 0.50; p = 0.13).
The increase in annual mean sea surface temperatures was congruent with the progression of sampling years within the ordination space.
Echinoderms
Nine species of echinoderms with a total density of 39.2 ± 5.7 ind/m2 (Figure 2B; Table 3) were recorded at Radar Reef in 2007. The tropical urchin Echinometra mathaei dominated, accounting for 80% of total echinoderm density. Two additional tropical species, the ophiuroid Ophiocomella sexradia and the echinoid Tripneustes gratilla, contributed a further 17% of echinoderm density; 3 temperate species represented only 3% of density. Only 4 species of echinoderms were recorded in 2021. Total echinoderm density declined by 52% to 18.7 ± 2.8 ind/m2 in 2021. Echinometra mathaei was 99% of the remaining echinoderm density and biomass at Radar Reef in 2021.
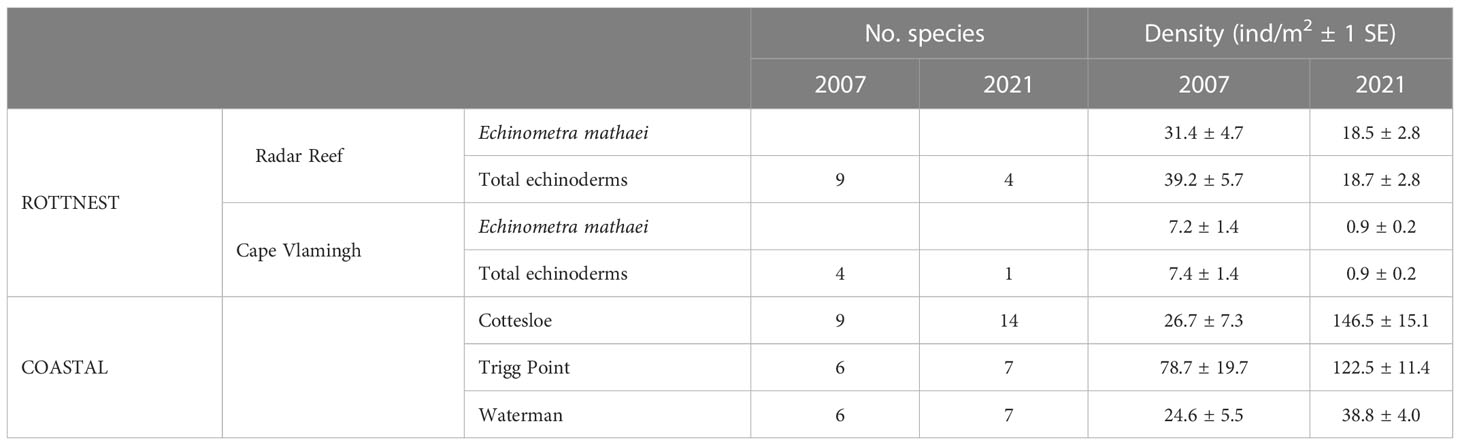
Table 3 Diversity and density of echinoderm species identified on intertidal platforms at Rottnest Island and the Perth coastline, Western Australia.
Four species of echinoderms were recorded at Cape Vlamingh in 2007 with a total density of 7.4 ± 1.4 ind/m2 (Table 3); E. mathaei contributed over 97% of total density. Only E. mathaei was recorded in 2021; its density had declined by 88%.
Diversity of echinoderms was also low on coastal platforms in 2007 (Figures 2B, 3B; Table 3); 6 species each at Trigg Point and Waterman and 9 at Cottesloe were recorded in 2007. Waterman (24.6 ± 5.5 ind/m2) and Cottesloe (26.7 ± 7.3 ind/m2) had much lower total echinoderm density than Trigg Point (78.7 ± 19.7 ind/m2). Diversity and density of echinoderms on coastal platforms increased in 2021, relative to the 2007 sampling (Table 3). The increased diversity at Trigg Point and Waterman (7 species versus 6) was negligible, but the increases in density (+56% at Trigg Point to 449% at Waterman) were substantial, partly because of reduced sand coverage of the coastal platform in 2021. Three species of small (≤2cm) sea cucumbers (WA endemic Psolidiella maculosa, tropical Psolidium spinuliferus and temperate Apsolidium handrecki) living attached to the underlying limestone rock on the coastal platform together constituted 83% of total density of echinoderms.
Echinoderm community assemblages differed between coastal and Rottnest Island sites, but not between sampling years, as demonstrated by a distinct separation within the nMDS ordination plot (Figure S1).
Discussion
Intertidal limestone platform reefs at the western end of Rottnest Island have long been known to have a relatively large component of tropical species compared to similar platforms along the Perth coast (Hodgkin and Marsh, 1957; Hodgkin et al., 1959; Black and Johnson, 1984; Wells, 1985; Veron and Marsh, 1988; Jones and Morgan, 1993). Description of the southward flowing Leeuwin Current (Cresswell and Golding, 1980) provided a mechanism for the distribution of tropical species to the west end of Rottnest Island, where Radar Reef and Cape Vlamingh are located. The current reaches the west end of Rottnest Island but has limited influence along the continental coastline in the Perth region. Wells (1985) and this paper demonstrated that in 1982/3 tropical species were a significant component of mollusc diversity and density at both Cape Vlamingh and Radar Reef at Rottnest Island. In contrast, diversity of molluscs on coastal platforms was dominated by temperate species and density by the tropical mussel on the coastal platforms, tropical species were limited in species richness. A similar pattern was found in 2007 (Wells et al., 2007). While there were differences in species composition and densities, essentially the same patterns were found at Rottnest Island and coastal sites in 2007.
A follow-up survey in 2021 unexpectedly showed a catastrophic decline in both molluscs and echinoderms at the west end of Rottnest Island in species diversity and density. Compared to the 2007 data, mollusc diversity at Radar Reef in 2021 decreased by 59% and density by 98%. Corresponding figures for Cape Vlamingh were 60% and 86% decline in diversity. Echinoderm diversity decreased by 56% and density by 52% at Radar Reef. Three of the four echinoderm species recorded at Cape Vlamingh in 2007 were not found in 2021. Virtually the total density of echinoderms in 2007 was due to the tropical urchin Echinometra mathaei which had decreased by 88% at Cape Vlamingh in 2021.
What caused the catastrophic declines of molluscs and echinoderms at Radar Reef and Cape Vlamingh between 2007 and 2021? There are several unlikely possibilities. The platforms are at the western end of the island, furthest away from Perth, the Western Australian capital. While the island is a key short-term holiday hotspot, it is a Class A reserve, the highest level of protection in WA. Visitors congregate in Thompson Bay and Geordie Bay in eastern side of Rottnest Island. There are no public access routes to the two platforms. Radar Reef and Cape Vlamingh are in sanctuary zones and removal of benthic marine species is totally prohibited. There have been no reports of eutrophication and there are no artificial structures on the platforms. The only pollutant recorded at the western end of Rottnest Island was low levels of tributyltin (TBT) (Burt and Ebell, 1995), an antifoulant added to vessel paints that came into widespread use worldwide in the early 1970s. The first detection of imposex, a reproductive abnormality caused by TBT, in Western Australia was at Rottnest Island in 1991 (Kohn and Almasi, 1993), primarily from specimens collected at the western end of the island. Restrictions on the use of TBT were imposed, and imposex is now virtually absent in snail populations at Rottnest Island, including both Cape Vlamingh and Radar Reef (Wells et al., 2017; Wells and Gagnon, 2020). It is unlikely the above causes impacted diversity and densities of molluscs and echinoderms at the two study sites on Rottnest Island.
Marine heat waves are another possible cause of the declines recorded at Radar Reef and Cape Vlamingh. Over the five months from late December 2010 to late May 2011 SST off Rottnest Island were up to 4°C higher than the long-term monthly average of 21.5–21.9°C, with the increased temperatures extending down to >50m (Thomson et al., 2011). The 2011 marine heatwave during a La Niña event caused extensive coral bleaching at Rottnest Island (Thomson et al., 2011; Moore et al., 2012). Nearly 30% of the Rottnest Island corals were bleached five years later (2016) during a record El Niño event (Le Nohaïc et al., 2017). In another effect of the 2011 marine heatwave, two tropical species of fish increased in abundance (Pearce et al., 2016).
Most of the adverse effects of the 2011 marine heatwave in WA occurred in subtidal environments. The known exception was a small commercial fishery of the temperate abalone Haliotis roei which was wiped out during the 2011 marine heatwave on intertidal platforms at Kalbarri (27.7°S) near the northern limit of its range. There has been virtually no natural recovery of the Kalbarri population (Caputi et al., 2019; Strain et al., 2020), paralleling the situation at Rottnest Island. The catastrophic mortality recorded here was apparently restricted to Radar Reef and Cape Vlamingh. Rifai et al. (2020) used haphazardly scattered quadrats to survey invertebrates on four intertidal platforms at Rottnest Island in the summer of 2018; three in Strickland Bay on the south coast of the island 2.7-4km east of Radar Reef and one at North Point 8km to the northeast of Radar Reef. Forty species were recorded, including 23 species of molluscs. Echinometra mathaei, the population of which crashed at Radar Reef and Cape Vlamingh, was abundant at all four sites surveyed by Rifai et al. (2020) in 2018, ranging from 26.5% of total invertebrates at Strickland West to 71.2% at Strickland Far East. Pyrene bidentata, another species that crashed at the west end was recorded in small numbers at two sites but was abundant at Strickland West (22.3% of total invertebrates) and North Point (32.3%). Similarly, Wells et al. (2021) found the heterobranch gastropod Bursatella hirsuta to be abundant on the platform at Little Armstrong Bay, adjacent to North Point, with an estimated population of >600,000 individuals over the ∼300m long platform (Wells et al., 2021). The Western Australian Museum conducted a detailed survey of Roe Reef, 1 km offshore of the northeastern end of Rottnest Island in 2013 and 2014, recording 287 marine species, including 40 species of molluscs and 16 echinoderms (Richards et al., 2016). The ranges of 28 mollusc species from Roe Reef could be determined: 19 were temperate, 4 tropical and 5 endemic to WA. These results suggest the catastrophic mortality reported here was restricted to Radar Reef and Cape Vlamingh at the western tip of the island, where the influence of the Leeuwin Current is greatest.
The presence of viable populations of invertebrates on adjacent platform reefs at Rottnest Island suggests there would be ample planktonic larvae available to repopulate the platforms at Radar Reef and Cape Vlamingh. In addition, there are numerous islands and subtidal reefs in the area which could be sources of local species for replenishment (Kendrick and Rule, 2014). Further north, the Houtman Abrolhos Islands provide a source of tropical species that can be brought south to Rottnest Island by the Leeuwin Current (Wells, 1997).
In contrast to the catastrophic declines in mollusc and echinoderm populations at the western end of Rottnest Island, viable populations of both groups remained on the Perth coastal platforms. Echinoderms increased modestly in species richness between 2007 and 2021 but substantially in density. Wells et al. (in prep.) combined the data in this paper into a temporal study of mollusc populations on all three coastal platform reefs (Cottesloe, Trigg Point and Waterman) and also reformatted data collected annually from 1984 to 1986 conducted (Wells and Keesing, 1986; Wells et al., 1986). The full dataset was conducted annually in summer from 1983 to 1987, and in 2007 and 2021. Total mollusc densities varied considerably between years at Cottesloe and Trigg Point, primarily because of changes in the populations of the short-lived mussel Brachidontes sculptus (as B. ustulatus) and the small gastropod Prothalotia pulcherrima, which has a one year life cycle (Wells and Keesing, 1987; Wells et al., in prep.). Mollusc density at the Waterman platform, which lacked the mussel populations, was relatively stable between 1983 and 2021. Despite the reduced sampling effort, the total number of species recorded on the three platforms in 2007 (52) and 2021 (44) was consistent with the results in the 1980s. The 44 species recorded in 2021 was a single species less than the 45 recorded in 1987. The 52 species identified in 2007 was within the 1980s range of 45-58. The eight most commonly recorded species in the 1980s were also common in 2021.
Increasing SST typically cause temperate biota to be replaced by tropical species (Yamano et al., 2011; Gervais et al., 2021), a phenomenon reported for subtidal environments off the west coast of Western Australia (Wernberg et al., 2011; Baird et al., 2012; Wernberg et al., 2013; Hyndes et al., 2016; Wernberg et al., 2016); however the responses of intertidal invertebrates to rising sea surface temperatures did not translate into population replenishments on the intertidal platform reefs at Rottnest Island. All three marine biogeographic components of molluscs and echinoderms (tropical, temperate and WA endemic species) suffered extensive population declines; temperate species were not replaced by tropical ones. The reason(s) for the catastrophic decline in tropical, temperate and WA endemic mollusc and echinoderm species and their failure to repopulate the intertidal rock platforms at Cape Vlamingh and Radar Reef are unknown, but the data presented here provide a sound basis for exploring the possible causes and monitoring for recovery.
Data availability statement
The raw data supporting the conclusions of this article will be made available by the authors, without undue reservation.
Author contributions
FW and JK contributed to conception and design of the study. FW, JK, MG, TI, and CB participated in field surveys. FW and JK were primarily responsible for species identifications. FS performed the statistical analysis. FW wrote the first draft of the manuscript. All authors contributed to the article and approved the submitted version.
Funding
The 1983 survey was funded by the WA Fisheries Department, now part of DPIRD. The 2007 study was funded by the Swan Catchment Council (now the Perth region NRM Inc.). The 1982 and 2021 fieldwork did not receive any specific grant from funding agencies in the public, commercial, or not-for-profit sectors.
Acknowledgments
Survey permits were approved by the Rottnest Island Authority, Western Australian Department of Biodiversity Conservation and Attractions (DBCA) and WA Department of Primary Industry and Regional Development (DPIRD). Loisette Marsh identified the holothurians in 2007; John Keesing identified the other echinoderms.
Conflict of interest
The authors declare that the research was conducted in the absence of any commercial or financial relationships that could be construed as a potential conflict of interest.
Publisher’s note
All claims expressed in this article are solely those of the authors and do not necessarily represent those of their affiliated organizations, or those of the publisher, the editors and the reviewers. Any product that may be evaluated in this article, or claim that may be made by its manufacturer, is not guaranteed or endorsed by the publisher.
Supplementary material
The Supplementary Material for this article can be found online at: https://www.frontiersin.org/articles/10.3389/fmars.2023.1075228/full#supplementary-material
References
Abdo D. A., Bellchambers L. M., Evans S. N. (2012). Turning up the heat: Increasing temperature and coral bleaching at the high latitude coral reefs of the Houtman Abrolhos Islands. PloS One 7, e43878. doi: 10.1371/journal.pone.0043878
Babcock R. C., Bustamante R. H., Fulton E. A., Fulton D. J., Haywood M. D. E., Hobday A. J., et al. (2019). Severe continental-scale impacts of climate change are happening now: extreme climate events impact marine habitat forming communities along 45% of Australia’s coast. Front. Mar. Sci. 6. doi: 10.3389/fmars.2019.00411
Baird A. H., Sommer B., Madin J. S. (2012). Pole-ward range expansion of Acropora spp. along the east coast of Australia. Coral Reefs 31, 1063. doi: 10.1007/s00338-012-0928-6
Black R., Johnson M. S. (1984). Marine biological studies on Rottnest Island. J. R. Soc West. Aust. 66, 24–28.
Burt J. S., Ebell G. E. (1995). Organic pollutants in mussels and sediments of the inshore waters off Perth, Western Australia. Mar. Poll. Bull. 30, 723–732. doi: 10.1016/0025-326X(95)00063-S
Caputi N., Kangas M., Chandrapavan A., Hart A., Feng M., Marin M., et al. (2019). Factors affecting the recovery of invertebrate stocks from the 2011 Western Australian extreme marine heatwave. Front. Mar. Sci. 6(484). doi: 10.3389/fmars.2019.00484
Cresswell G. R., Golding T. J. (1980). Observations of a south-flowing current in the southeastern Indian Ocean. Deep-Sea Res. 27A, 449–466. doi: 10.1016/0198-0149(80)90055-2
Dixon P. (2003). VEGAN, a package of R functions for community ecology. J. Veg. Sci. 14, 927–930. doi: 10.1111/j.1654-1103.2003.tb02228.x
Doney S. C., Ruckelshaus M., Duffy J. E., Barry J. P., Chan F., English C. A., et al. (2012). Climate change impacts on marine ecosystems. Ann. Rev. Mar. Sci. 4, 11–37. doi: 10.1146/annurev-marine-041911-111611
Gervais C. R., Champion C., Pecl G. T. (2021). Species on the move around the Australian coastline: A continental-scale review of climate-driven species redistribution in marine systems. Glob. Change Biol. 27, 3200–3217. doi: 10.1111/gcb.15634
Google Maps (n.d.) [Perth metropolitan coastline Western Australia] Retrieved 12 Dec 2022 fromhttps://goo.gl/maps/TZ8ZGXkWkSAHWTb99
Harley C. D. G., Hughes A. R., Hultgren K. M., Miner B. G., Sorte C. J. B., Thornber C. S., et al. (2006). The impacts of climate change in coastal marine systems. Ecol. Lett. 9, 228–241. doi: 10.1111/j.1654-1103.2003.tb02228.x
Hart A. M., Strain L. W. S., Brown J. (2018). Regulation dynamics of exploited and protected populations of Haliotis roei, and their response to a marine heatwave. ICES J. Mar. Sci. 75, 1924–1939. doi: 10.1093/icesjms/fsy064
Hastings R. A., Rutterford L. A., Freer J. J., Collins R. A., Simpson S. D., Genner M. J. (2020). Climate change drives poleward increases and equatorward declines in marine species. Curr. Biol. 30, 1572–1577. doi: 10.1016/j.cub.2020.02.043
Hobday A. J., Alexander L. V., Perkins S. E., Smale D. A., Straub S. C., Oliver E. C., et al. (2016). A hierarchical approach to defining marine heatwaves. Progr. Oceanogr. 14, 227–238. doi: 10.1016/j.pocean.2015.12.014
Hobday A. J., Pecl G. T. (2013). Identification of global marine hotspots: sentinels for change and vanguards for adaptation action. Rev. Fish Biol. Fisheries 24, 415–425. doi: 10.1007/s11160-013-9326-6
Hodgkin E. P., Marsh L. M. (1957). On the significance of a tropical element in the littoral fauna of southwestern Australia. Abstract. CR 3° Congrès de la PIOSA, Transactions Section B, 61.
Hodgkin E. P., Marsh L. M., Smith G. G. (1959). The littoral environment of Rottnest Island. J. R. Soc West. Aust. 42, 85–88.
Huber M. (2010). Compendium of Bivalves. A full-color guide to 3,300 of the world's marine bivalves. A status on Bivalvia after 250 years of research. (Hackenheim, Germany: ConchBooks).
Hyndes G. A., Heck K. L. Jr., Vergés A., Harvey E. S., Kendrick G. A., Lavery P. S., et al. (2016). Accelerating tropicalization and the transformation of temperate seagrass meadows. BioScience 66, 938–948. doi: 10.1093/biosci/biw111
Irvine T. R., Keesing J. K., Wells F. E. (2008). Assessment of invertebrate populations on intertidal platforms at Rottnest Island, Western Australia (Perth, Western Australia: Report to Swan Catchment Council Inc by CSIRO Marine Research and the Department of Fisheries).
Jones D. S., Morgan G. J. (1993). “An annotated checklist of the Crustacea of Rottnest Island, Western Australia,” in The marine flora and fauna of Rottnest Island, Western Australia. Eds. Wells F. E., Walker D. I., Kirkman H., Lethbridge R. (Perth, Western Australia: Western Australian Museum), 135–162.
Kendrick A. J., Rule M. J. (2014). An annotated checklist of intertidal reef invertebrates from Marmion and Shoalwater Islands Marine Parks. Cons. Sci. West. Aust. 9, 201–213.
Kohn A. J., Almasi K. N. (1993). Imposex in Australian Conus. J. Mar. Biol. Assoc. U. K. 73, 241–244.
Le Nohaïc M., Ross C. L., Cornwall C. E., Comeau S., Lowe R., McCulloch M. T., et al. (2017). Marine heatwave causes unprecedented regional mass bleaching of thermally resistant corals in northwestern Australia. Nat. Sci. Rep. 7, 14999. doi: 10.1038/s41598-017-14794-y
Melbourne-Thomas J., Audzijonyte A., Brasier M. J., Cresswell K., Fogarty H. E., Haward M., et al. (2021). Poleward bound: adapting to climate-driven species redistribution. Rev. Fish Biol. Fisheries. 32, 231–251. doi: 10.1007/s11160-021-09641-3
Moore J. A. Y., Bellchambers L. M., Depczynski M. R., Evans R. D., Evans S. N., Field S. N., et al. (2012). Unprecedented mass bleaching and loss of coral across 12° of latitude in Western Australia in 2010–11. PloS One 7 (12), e51807. doi: 10.1371/journal.pone.0051807
O’Hara C. C., Frazier M., Halpern B. S. (2021). At-risk marine biodiversity faces extensive, expanding and intensify human impacts. Science 372, 84–87. doi: 10.1126/science.abe6731
Oliver E. C. J., Benthuysen J. A., Darmaraki S., Donat M. G., Hobday A. J., Holbrook N. J., et al. (2021). Marine heatwaves. Ann. Rev. Mar. Sci. 13, 313–342. doi: 10.1146/annurev-marine-032720-095144
Oliver E. C. J., Burrows M. T., Donat M. G., Sen Gupta. A., Alexander L. V., Perkins-Kirkpatrick S. E., et al. (2019). Projected marine heatwaves in the 21st century and the potential for ecological impact. Front. Mar. Sci. 6. doi: 10.3389/fmars.2019.00734
Pearce A., Feng M. (2007). Observations of warming on the Western Australian continental shelf. Mar. Freshw. Res. 58, 914–920. doi: 10.1071/MF07082
Pearce A. F., Feng M. (2013). The rise and fall of the “marine heat wave” off Western Australia during the summer of 2010/2011. J. Mar. Sys. 111–112, 139–156. doi: 10.1016/j.jmarsys.2012.10.009
Pearce A., Hutchins B., Hoschke A., Fearns P. (2016). Record high damselfish recruitment at Rottnest Island, Western Australia, and the potential for climate- induced range extension. Reg. Stud. Mar. Sci. 8, 77–88. doi: 10.1016/j.rsma.2016.09.009
Pearce A. F., Lenanton R., Jackson G., Moore J., Feng M., Gaughan D. (2011). The “marine heat wave” off Western Australia during the summer of 2010/11. Technical Report 222 (North Beach, Western Australia: Western Australian Fisheries and Marine Research Laboratory).
Pearce A. F., Walker D.I. (1991). The Leeuwin Current: An influence on the coastal climate and marine life of Western Australia. J. R. Soc West. Aust. 74, 1–140.
Poloczanska E. S., Burrows M. T., Brown C. J., García Molinos J., Halpern B. S., Hoegh-Guldberg O., et al. (2016). Responses of marine organisms to climate change across Oceans. Front. Mar. Sci. 3. doi: 10.3389/fmars.2016.00062
Richards Z., Kirkendale L., Moore G., Hosie A., Huisman J., Bryce M., et al. (2016). Marine biodiversity in temperate Western Australia: multi-taxon surveys of Minden and Roe Reefs. Diversity 8 (7), 1–25. doi: 10.3390/d8020007
Rifai H., Perisha B., Zulpikar F., Renyaan J., Anggiani M., Rasyidin A. (2020). Comparison of intertidal invertebrate assemblages at four sites around Rottnest Island, Western Australia after seven years of marine heatwaves. Omni-Akuatika 16, 69–76. doi: 10.20884/1.oa.2020.16.1.803
Smale D. A., Wernberg T. (2012). Ecological observations associated with an anomalous warming event at the Houtman Abrolhos Islands, Western Australia. Coral Reefs 31, 441. doi: 10.1007/s00338-012-0873-4
Strain L., Brown J., Jones R. (2020). ““West coast Roe’s abalone resource status report 2020,” in Status reports of the fisheries and aquatic resources of Western Australia 2019/20: The state of the fisheries. Eds. Gaughan D. J., Santoro K. (Perth, Western Australia: Department of Primary Industries and Regional Development) 1, 37–42.
Straub S. C., Wernberg T., Thomsen M. S., Moore P. J., Burrows M. T., Harvey B. P., et al. (2019). Resistance, extinction, and everything in between – the diverse responses of seaweeds to marine heatwaves. Front. Mar. Sci. 6. doi: 10.3389/fmars.2019.00763
Thomson D. P., Bearham D., Graham F., Eagle J. V. (2011). High latitude, deeper water coral bleaching at Rottnest Island, Western Australia. Coral Reefs 30, 1107. doi: 10.1007/s00338-011-0811-x
Veron J. E. N., Marsh L. M. (1988). Hermatypic corals of Western Australia (Perth, Western Australia: Western Australian Museum).
Wells F. E. (1985). Zoogeographical importance of tropical marine mollusc species at Rottnest Island, Western Australia. West. Aust. Nat. 16, 40–45.
Wells F. E. (Ed.) (1997). The marine flora and fauna of the Houtman Abrolhos Islands, Western Australia Vol. 566 (Perth, Western Australia: Western Australian Museum).
Wells F. E., Bessey C., Gagnon M. M., Keesing J. K., Prince J. (2021). A recurring population of the sea hare Bursatella hirsuta (Gastropoda: Aplysiidae) at Rottnest Island, Western Australia. Moll. Res. 41, 285–288. doi: 10.1080/13235818.2021.2007750
Wells F. E., Bryce C. W. (1986). Seashells of Western Australia. (Perth: Western Australian Museum).
Wells F. E., Gagnon M. M. (2020). A quarter century of recovery of the whelk Thais orbita from tributyltin pollution off Perth, Western Australia. Mar. Poll. Bull. 158, 11408. doi: 10.1016/j.marpolbul.2020.111408
Wells F. E., Keesing J. K. (1986). An investigation of mollusc assemblages on intertidal beachrock platforms in the Perth metropolitan area, with particular emphasis on the abalone, Haliotis roei Vol. 2 volumes (Report to W.A. Department of Fisheries, Perth), 369 pages.
Wells F. E., Keesing J. K. (1987). Population characteristics of the gastropod Cantharidus pulcherrimus on intertidal platforms of the Perth area of Western Australia. J. Malac. Soc Aust. 8, 23–35. doi: 10.1080/00852988.1987.10673988
Wells F. E., Keesing J. K., Brearley A. (2017). Recovery of marine Conus (Mollusca: Caenogastropoda) from imposex at Rottnest Island, Western Australia, over a quarter of a century. Mar. Poll. Bull. 123, 182–187. doi: 10.1016/j.marpolbul.2017.08.064
Wells F. E., Keesing J. K., Irvine T. R. (2007). Assessment of invertebrate populations on intertidal platforms in the Perth metropolitan area. Report to the Swan Catchment Council by the Department of Fisheries (Perth, Western Australia).
Wells F. E., Keesing J. K., Sellers R. J. (1986). “The 1986 survey of mollusc and echinoderm assemblages on intertidal beachrock platforms in the Perth metropolitan area,” in Collected technical reports on the Marmion Marine Park., Perth, Western Australia. Ed. Moore E. 19, 125–161. Environmental Protection Authority, Perth, Technical Series.
Wells F. E., Walker D. I. (1993). “Introduction to the marine environment of Rottnest Island, Western Australia,” in The marine flora and fauna of Rottnest Island, Western Australia. Eds. Wells F. E., Walker D. I., Kirkman H., Lethbridge R. (Perth, Western Australia: Western Australian Museum), 1–10.
Wernberg T., Bennett S., Babcock R. C., de Bettignies T., Cure K., Depczynski M., et al. (2016). Climate-driven regime shift of a temperate marine ecosystem. Science 353, 169–172. doi: 10.1126/science.aad8745
Wernberg T., Smale D. A., Tuya F., Thomsen M. S., Langlois T. J., De Bettignies T., et al. (2013). An extreme climatic event alters marine ecosystem structure in a global biodiversity hotspot. Nat. Clim. Change 3, 78–82. doi: 10.1038/nclimate
Wernberg T., Thomsen M. S., Tuya F., Kendrick G. A. (2011). Biogenic habitat structure of seaweeds change along a latitudinal gradient in Ocean temperature. J. Exp. Mar. Biol. Ecol. 400, 264–271. doi: 10.1016/j.jembe.2011.02.017
Wilson B. (2002). A handbook to Australian seashells on seashores east to west and north to south. (Sydney: Reed New Holland).
WoRMS Editorial Board (2022) World register of marine species. Available at: https://www.marinespecies.org (Accessed 17 July 2022). VLIZ.
Keywords: Indian Ocean, climate change, marine heatwave, Leeuwin Current, sea surface temperatures, biogeography, molluscs, echinoderms
Citation: Wells FE, Keesing JK, Gagnon MM, Bessey C, Spilsbury F and Irvine TR (2023) Responses of intertidal invertebrates to rising sea surface temperatures in the southeastern Indian Ocean. Front. Mar. Sci. 10:1075228. doi: 10.3389/fmars.2023.1075228
Received: 20 October 2022; Accepted: 01 February 2023;
Published: 23 February 2023.
Edited by:
Punyasloke Bhadury, Indian Institute of Science Education and Research Kolkata, IndiaReviewed by:
Simon Morley, British Antarctic Survey (BAS), United KingdomPat Hutchings, Australian Museum, Australia
Copyright © 2023 Wells, Keesing, Gagnon, Bessey, Spilsbury and Irvine. This is an open-access article distributed under the terms of the Creative Commons Attribution License (CC BY). The use, distribution or reproduction in other forums is permitted, provided the original author(s) and the copyright owner(s) are credited and that the original publication in this journal is cited, in accordance with accepted academic practice. No use, distribution or reproduction is permitted which does not comply with these terms.
*Correspondence: Fred E. Wells, ZnJlZC53ZWxsc0BjdXJ0aW4uZWR1LmF1
†These authors share first authorship
‡ORCID: Fred E. Wells, orcid.org/0000-0002-0730-6614
John K. Keesing, orcid.org/0000-0002-0876-2144
Marthe Monique Gagnon, orcid.org/0000-0002-3190-5094
Cindy Bessey, orcid.org/0000-0002-4081-0214
Francis Spilsbury, orcid.org/0000-0002-8794-8528