- 1Istituto Zooprofilattico Sperimentale del Mezzogiorno, Portici (Naples), Italy
- 2Università degli Studi di Napoli Federico II, Dipartimento di Medicina Veterinaria e Produzioni Animali, Naples, Italy
- 3Centro di Referenza Nazionale per l’analisi e studio di correlazione ambiente, animale, uomo, Portici (Naples), Italy
Caretta Caretta are endangered sea turtles leaving in the Mediterranean Sea. We carried out a study on 195 sea turtles stranded along the coast of Southern Italy, evaluating anthropogenic and opportunistic factors affecting animal health status and probably threatening the species. More than 60% of the animals showed body injuries, mainly caused by marine traffic and fishing. Ingestion of marine litter of various origins was observed in the digestive tract of 38.5% of the turtles investigated. Chelonid herpesvirus 5 was detected (for the first time in this basin) in 10.8% of the turtles, which were however free from fibropapillomatosis. Analyses on trace elements showed a time-dependent reduction in the mean concentrations of Cd and Hg, a decrease in the levels of Pb, and a time-dependent increase of As. Particularly, a site-dependent accumulation of As was detected in turtles from the coasts of Calabria. There is a significant correlation between the levels of As in the kidney of Campania turtles and their straight-line carapace length, indicating a size-dependent accumulation of this metal. Overall, besides dangerous debris items, high levels of trace elements may weaken the immune system of Caretta caretta, the most common turtle of the Mediterranean Sea, making it more likely vulnerable to viruses.
Introduction
The loggerhead sea turtle, Caretta caretta (C. caretta), is the most abundant sea turtle species living and reproducing in the Mediterranean Sea (Pagano et al., 2019; Esposito et al., 2020; Oriá et al., 2020). This species is classified as vulnerable to endangered in the International Union for Conservation of Nature’s Red List of Threatened Species (https://www.iucnredlist.org ). Factors threatening C. caretta extinction are mainly linked to human activities (www.iucn.org): coastal areas anthropisation, high level of anthropisation in the coastal areas, marine traffic, sea macroscopic pollution (ingestion of plastic and other debris), chemical pollution, fisheries (entanglement in longlines, trawls and gill nets as well as ingestion of hooks and lines) (Tomas et al., 2001; Camiñas et al., 2006; Báez et al., 2010; Echwikhi et al., 2012; Blasi and Mattei, 2017; Caracappa et al., 2018; Pagano et al., 2019).
Chemical pollution, caused by human and industrial activities (use of fertilisers and pesticides, plastic, oil, and gas production), determines the release in the marine environment of large quantities of nonessential toxic metals, such as arsenic (As), cadmium (Cd), mercury (Hg), and lead (Pb) (Valavanidis and Vlachogianni, 2010; Varol, 2011; Esposito et al., 2020; Lambiase et al., 2021b), which are accumulated by sea turtles mainly through the ingestion of contaminated prey, but also through marine sediment and seawater (Storelli et al., 2011; Bucchia et al., 2015; Cammilleri et al., 2017; Lambiase et al., 2021a). The accumulation of these chemicals, as already reported for other marine pollutants (Gonzalez-Fernandez and Cuesta, 2022), could weaken the immune system of sea turtles (Chaousis et al., 2023), making them more vulnerable to bacterial or viral infections (Fiorito et al., 2019; Fiorito et al., 2021). In this regard, they are susceptible to an alphaherpesvirus belonging to the Herpesviridae family, named chelonid herpesvirus 5 (ChHV5). The virus can infect several species of aquatic turtles and is considered the causative agent of the formation and development of fibropapillomas (Aguirre et al., 1994; Quackenbush et al., 1998). Even though fibropapillomatosis (FP) mostly affects green sea turtles (Chelonia mydas), all marine turtles can contract the disease (Lawrance et al., 2018). FP is characterised by the presence of cutaneous lesions growing on the external soft tissue of the animal body, on the carapace, plastron, and cornea (Jones et al., 2016). The tumours, although not malignant, can hinder crucial functions (Rodenbusch et al., 2014), like the animal’s ability to see, breathe, swim, dive, forage, and avoid predators (Milton and Lutz, 2003; Perrault et al., 2021), thus indirectly causing its death. The lesions can also be observed (with reported prevalence varying from 17% to 41%, Work et al., 2004) in visceral organs; they mainly occur in the lungs and kidneys, but also, to a lesser extent, in the heart, gastrointestinal tract, and liver (Herbst and Klein, 1995; Alfaro-Nunez et al., 2016; Jones et al., 2016). ChHV5 has been consistently associated with FP, with fibropapillomas containing high concentrations of ChHV5 DNA (Alfaro-Nunez et al., 2016). However, recent PCR-based studies have detected high levels of ChHV5 DNA also in apparently healthy tissues without fibropapillomas (Page-Karjian et al., 2012), demonstrating that large proportions of asymptomatic sea turtles can also be virus carriers (Alfaro-Nunez et al., 2016) and suggesting the probable ability of ChHV5 to latently persist in their organism (Alfaro-Nunez et al., 2014, Alfaro-Nunez, 2016). In this regard, it is conceivable that, if particular conditions compromise the immune system of animals, like critical water temperature, coinfection with other pathogens, or the presence of pollutants (Jones et al., 2016; Lawrance et al., 2018; Chaousis et al., 2023), the virus could be reactivated and trigger the onset of a disease, not necessarily FP.
The present study was carried out (from 2015 to 2020) on specimens of sea turtles stranded along the coasts of Southern Italy overlooking the Mediterranean Sea. Animals underwent viral, bacterial, histological, and chemical investigations with the aim of carrying out a comprehensive analysis of the causes of turtle stranding and, more generally, to trace a picture of the anthropogenic and opportunistic factors involved in the animal health status and in the endangerment of the species.
Materials and methods
Sample collection
Following regional plans (Executive Decree No. 231 of 14 July 2015 for Campania region and DPGR-CA No.104 of 29 July 2013 for Calabria region), from 2015 to 2020, a total of 195 specimens of loggerhead sea turtles (C. caretta) were collected among those beached along the coasts of the Southern Tyrrhenian Sea in Southern Italy. Of these, 150 were stranded along the Campania region coasts (75 in the province of Naples, 39 in the province of Salerno, 18 in the province of Caserta, and 18 in the islands area among Procida, Ischia, and Capri); 45 were stranded along the Calabria region coasts (five in the province of Cosenza, 25 in the province of Reggio Calabria, two in the province of Vibo Valentia, and 13 in the province of Catanzaro) (Figure 1). A detailed indication of the animals examined per year in each region is reported in Table 1.
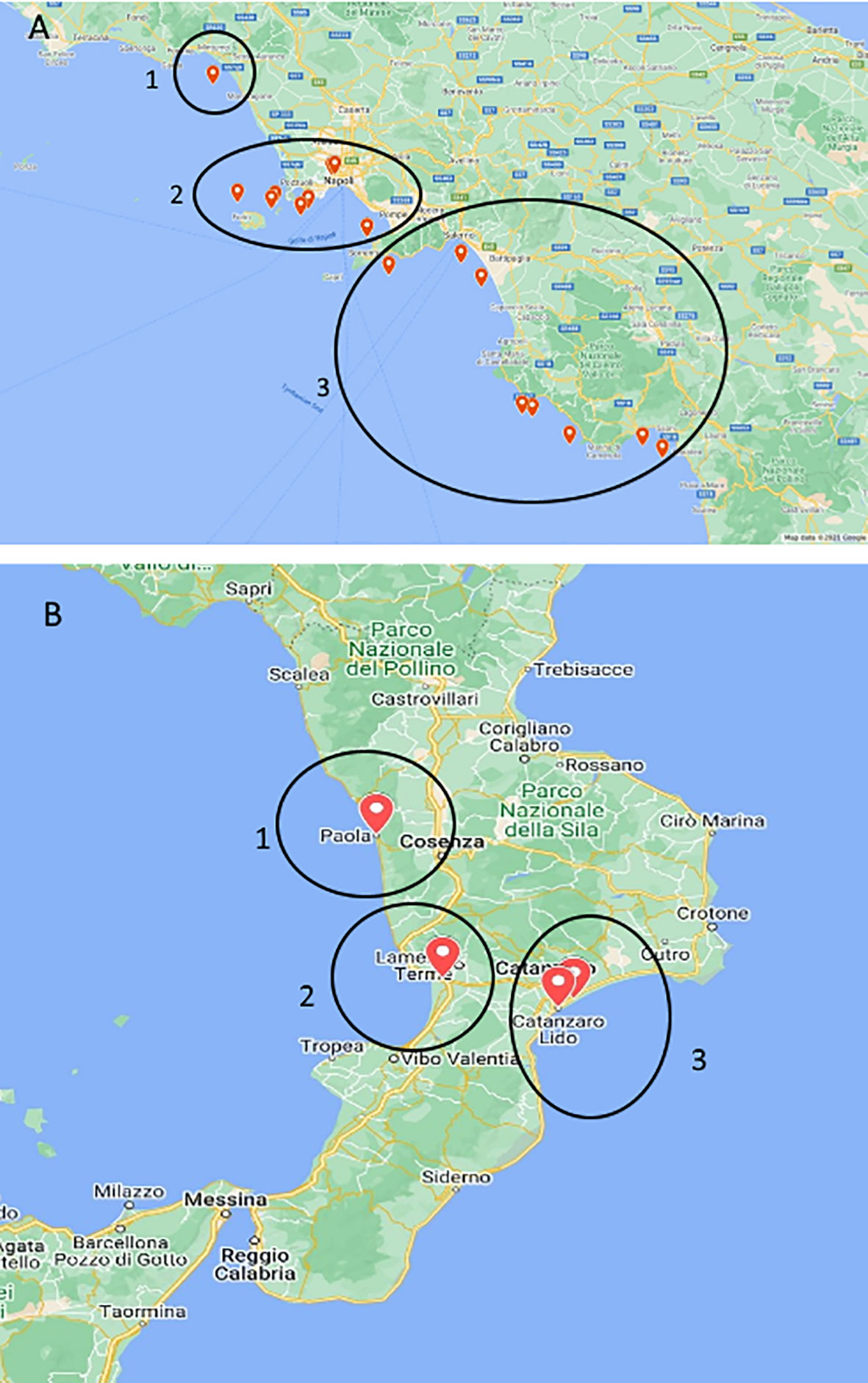
Figure 1 Maps of stranded Caretta caretta turtle (n = 195) sampling sites. In the circle, ChHV5-positive animal collection sites. (A) Campania region: 1, Caserta province; 2, Naples and islands; and 3, Salerno province. (B) Calabria region: 1, Cosenza province; 2, Vibo Valentia area; and 3, Catanzaro province.
Post-mortem investigations
Dead loggerhead sea turtles undergo necropsy as soon as possible, and if possible, do not alter the conservation state of the carcasses. The condition of the carcasses was determined following the “standard protocol for post-mortem examination on sea turtles” (https://www.plavi-svijet.org/bw/wp-content/uploads/2017/05/NETCET_Standard-protocols-for-post-mortem-examination-of-sea-turtles.pdf), and a code was assigned accordingly. Measurement of the following morphological parameters was performed: body weight (BW), curved carapace length (CCL), straight-line carapace length (SCL), curved carapace width (CCW), straight-line carapace width (SCW), head length (HL), and head width (HW). The CCL, measured from the mid-point of the nuchal scute to the posteriormost tip of the carapace (Wyneken, 2001) by a flexible meter tape, was used to determine the age of the animal. The sex of turtles is determined by a visual examination of the gonads (Wyneken, 2001). The nutritional condition score of each animal was appreciated by the ventral deposition of the fat under the plastron (Solomon et al., 1986), observed after its removal (https://www.plavi-svijet.org/bw/wp-content/uploads/2017/05/NETCET_Standard-protocols-for-post-mortem-examination-of-sea-turtles.pdf). During macroscopic investigations, the animal’s body was carefully inspected to detect the presence of any wounds and abrasions on the skin, fins, tail, carapace, and head, as well as to check for the presence of external neoplastic lesions like FP. The state of the eyes was also checked, and conjunctival hyperaemia was evaluated. Furthermore, the presence of fishing hooks or objects of plastic causing obstruction in the buccal cavity was ascertained. After macroscopic inspection, turtles underwent necropsy: tissues and organs were removed with sterile scalpels, and pieces of them were sent to the laboratories for bacterial, virological, histological, and chemical analyses.
Viral nucleic acid extraction procedures
Samples from the liver, heart, intestine, lungs, cloaca, skin, kidneys, and brain were individually homogenised by Tissue Lyser (Qiagen GmbH, Hilden, Germany). In brief, 25 mg of tissue were inserted in 2 ml of Eppendorf safe-lock tubes containing 1 ml of phosphate-buffered saline (PBS) solution and a 4.8-mm stainless-steel bead to allow mechanical lysis (30 Hz for 5 min). Homogenates were centrifuged at 2,000–4,000 rpm for 15 min to pellet the debris. Nucleic acid extraction was carried out on 200 µl of homogenate by using the QIAsymphony automated extraction system (Qiagen GmbH, Hilden, Germany) with the DSP Virus/Pathogen Mini kit (Qiagen GmbH, Hilden, Germany) according to the manufacturer’s instructions. Nucleic acids were eluted in 80 µl of elution buffer. Instead of homogenate, a sample made with 200 µl of PBS was used as a process control. PCR inhibition was evaluated using an external amplification control, murine norovirus, added to the samples prior to extraction, as indicated by Cioffi et al. (2020).
Real-time PCR identification of ChHV5
Detection of ChHV5 was carried out by a real-time PCR protocol targeting the glycoprotein B (gB) gene (Ackermann et al., 2012) with some modifications. In detail, the reaction was carried out in a total volume of 25 µl containing the following: 5 µl of template, 12.5 µl of TaqMan® Universal PCR Master Mix (Thermo Fisher Scientific, Waltham, MA, USA), 0.5 µl of primer (5 µM) 5′-pol-For (5′-ACTGGCTGGCACTCAGGAAA-3′), 0.5 µl of primer (5 µM) 3′-pol-Rev (5′-CAGCTGCTGCTTGTCCAAAA-3′), and 0.5 µl of probe (20 µM) pol-P (FAM-5′-CGATGAAAACCGCACCGAGCGA-3′-TAMRA). The thermal profile was the following: initial denaturation for 15 min at 95°C, followed by 45 cycles of 15 s at 95°C and 60 s at 60°C.
Samples were analysed in duplicate, and results were considered positive when the mean threshold cycle (Ct) was < 40. A nuclease-free water sample was included in the PCR as a negative control. Positive turtle tissues given by Dr. Alonzo Alfaro-Núñez (Department of Virus and Microbiological Special Diagnostics, Statens Serum Institut, Artillerivej 5, 2300 Copenhagen S, Denmark) were used as positive controls.
Cultural methods
Organs positive for ChHV5 were further investigated by bacterial culture methods to ascertain the possible presence of co-infecting bacterial pathogens. Briefly, tissues were inoculated either into trypticase soy agar (TSA) supplemented with 5% sheep blood (Oxoid Ltd., Basingstoke, Hampshire, England) or into TSA supplemented with 2% NaCl (Oxoid Ltd., Basingstoke, Hampshire, England), and then aerobically incubated at 25°C for 24–72 h. Intestine samples were instead inoculated into TSA supplemented with 5% sheep blood (Oxoid Ltd., Basingstoke, Hampshire, England) and anaerobically incubated at 25°C for 24–72 h. All the suspected colonies underwent biochemical characterisation by API® 20E and API® 20NE (Biomerieux, Marcy-l’Etoile, France) with modified incubation temperature (25°C for 24–48 h), as indicated by Buller (2014) and by Vitek 2® (Biomerieux Marcy-l’Etoile, France). Identified species were confirmed by taxonomic identification through the MicroSEQ® rapid microbial identification system (Thermo Fisher Scientific, Waltham, MA, USA), based on sequencing of the 16s rRNA.
Histopathological analysis
Tissues and possible lesions from ChHV5-positive animals (21) were maintained in 10% neutral phosphate-buffered formalin to block the phenomena of autolysis and to preserve the samples. Afterwards, they were processed by sequential washing steps in ethanol to dehydrate the samples. Tissues were then included in paraffin blocks, which were cut into 3–4-µm-thick sections. Each section was stained with haematoxylin and eosin to be analysed under an optical microscope.
Chemical analysis
The determination of toxic elements, Hg, Pb, Cd, and As, was performed in the liver, muscle, and kidney of 94 turtles, specifically, 52 animals from Campania region and 42 from Calabria region. Mummified turtles were not considered for the chemical analysis. For the analysis, the samples were homogenised, then 0.75 g were weighted and subjected to acid mineralisation with nitric acid (69%) and hydrogen peroxide (30% v/v), using a microwave digestion system Ethos One (Milestone, Bergamo, Italy). Concentrations were measured by an atomic absorption spectrophotometer with a transversely heated graphite furnace (GF-AAS) with the Zeeman effect for electrothermal atomisation and equipped with an autosampler (Analyst 800, Perkin-Elmer, Germany). Hollow cathode lamps were used as line sources for both elements. The absorption wavelengths were 228.8, 283.3, and 193.7 nm for cadmium, lead, and arsenic, respectively. Total mercury was determined by an atomic absorption spectrophotometer (mod. 5010, Perkin-Elmer, Germany) after the reduction of mercury in volatile hydrides by sodium tetrahydroborate. Each sample was analysed in duplicates, and the mean results were expressed in milligrammes per kilogramme of wet weight (ww). The limits of quantification (LOQs) were 0.030, 0.005, 0.020, and 0.17 mg kg−1 for Hg, Cd, Pb, and As, respectively. The methods used were validated by means of an in-house quality control procedure and by using certified reference materials (NIST-SRM 2976 (mussel tissue—Community Bureau of Reference) to assess precision and accuracy. The accuracy of the method was assessed also through participation in several proficiency tests; the obtained z-score values were within ±2, indicating satisfactory results.
Statistical analysis
Statistical analysis was performed using Minitab v18 (Minitab, LLC, State College, Pennsylvania, USA). For the calculations, the results of metal concentrations below the LOQ values were considered equal to one-half LOQ, according to ISTISAN (Report 04/15). The correlations between contaminants found in turtle tissues and their morphometric parameters, as well as the ChHV5-positive samples, were assessed by applying the Spearman’s correlation test. The correlations greater than 0.500 with p < 0.05 were analysed using a linear regression approach.
Results
Animal classification based on morphological characters
The 195 specimens collected were made up of 103 (52.8%) females (75 from Campania and 28 from Calabria), 65 (33.3%) males (52 from Campania and 13 from Calabria), and 27 sea turtles (13.8%) whose sexes were undistinguishable due to the bad condition of the carcasses (animals defined as decomposed or mummified). The body weight and the CCL were measured in 178 of 195 marine turtles (see Table 2) (17 animals were considered “unknown” since they were not measurable due to their poor conservation conditions). On the basis of the CCL, the animals were divided into three groups (Piovano et al., 2011; Casale and Mariani, 2014; Solomando et al., 2022): the “early juveniles” (ej; CCL < 40 cm) to which belonged 12 sea turtles from each region, the “large juveniles” (lj; 40 cm ≤ CCL ≤ 70 cm) to which belonged 112 animals (83 from Campania and 29 from Calabria), and the “adults” (A; CCL > 70 cm), including 42 sea turtles, all from the Campania region. Furthermore, the age of the animals was estimated by the curved length of the carapace measurement using the equation of von Bertalanffy adapted to sea turtles (Piovano et al., 2011). Results showed that the early juveniles belonged to turtles with a calculated age of ≤7 years, the large juvenile group included animals with a calculated age from 8 to 23 years, while the adult group included ≥24-year-old turtles.
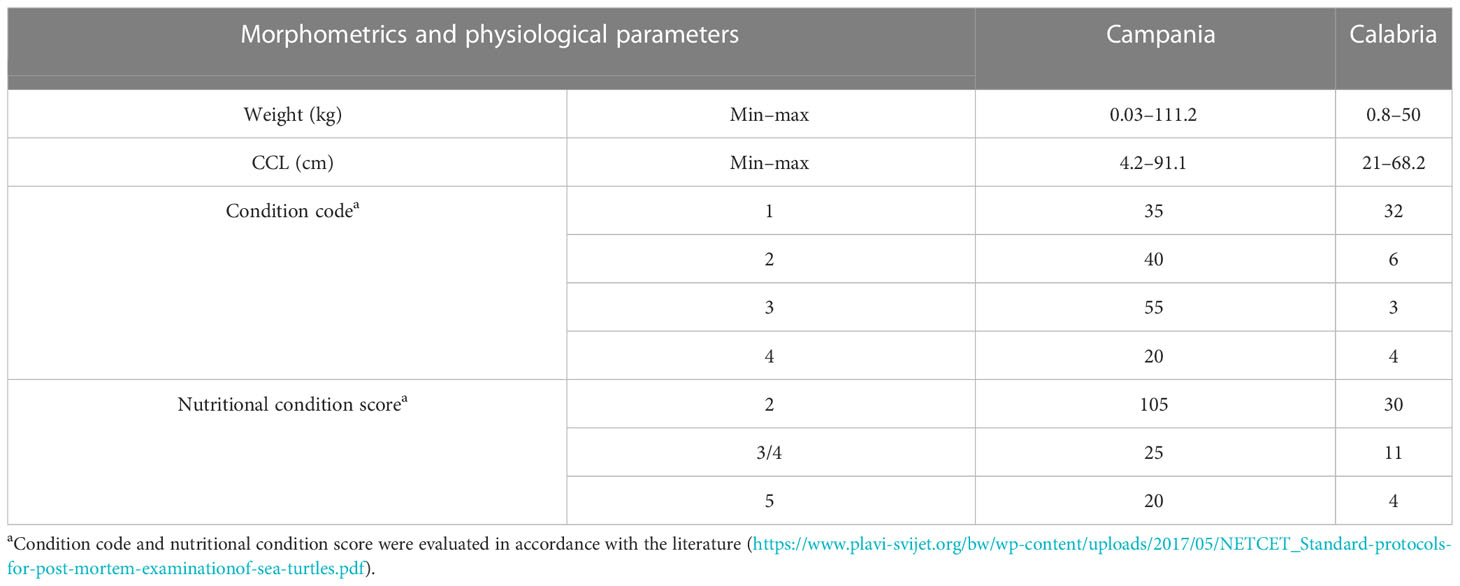
Table 2 Morphometrics and physiological parameters evaluated during necropsy in the Campania and Calabria regions.
Necropsy evaluation of sea turtles’ carcasses condition
Evaluation of carcass condition gave the following results: of the 195 carcasses examined, 67 (34.3%) were fresh (code 1), 46 (23.6%) were moderately decomposed (code 2), 58 (29.7%) were in the advanced decomposition state (code 3), and 24 (12.3%) were in the state of mummification (code 4). The nutritional condition was considered very good (score 2) for 135 turtles (69.2%), fair (score 3) or scarce (score 4) for 36/195 animals (18.5%), and not evaluable (score 5) for the mummified animals (24) (Table 2). In inspecting the digestive tract of the animals, the presence of hooks, lines, plastic debris, and other marine litter of unknown origin was found in 75/195 (22 from Calabria and 53 from Campania) of the specimens analysed (38.5%). In detail, in the Campania region, 56.6% (30/53) of the turtles ingested plastic debris, and the remaining 43.4% (23/53) ingested hooks and/or lines. In the Calabria region, 63.6% (14/22) of the sea turtles ingested unknown material, 31.8% hooks and/or lines (7/22), and 4.5% (1/22) plastic debris. Hooks mainly caused oesophagus laceration and, more rarely, pericardium laceration, while ingestion of lines determined intestinal plication, intussusception, and laceration (Figures 2, 3D). In 27 animals, the internal lesions observed were considered noncompatible with life. In particular, the ingestion of fishing gears was estimated to be fatal in six of seven turtles from Calabria (85.7%) and in 21/23 from Campania (91.3%) (see Table 4).
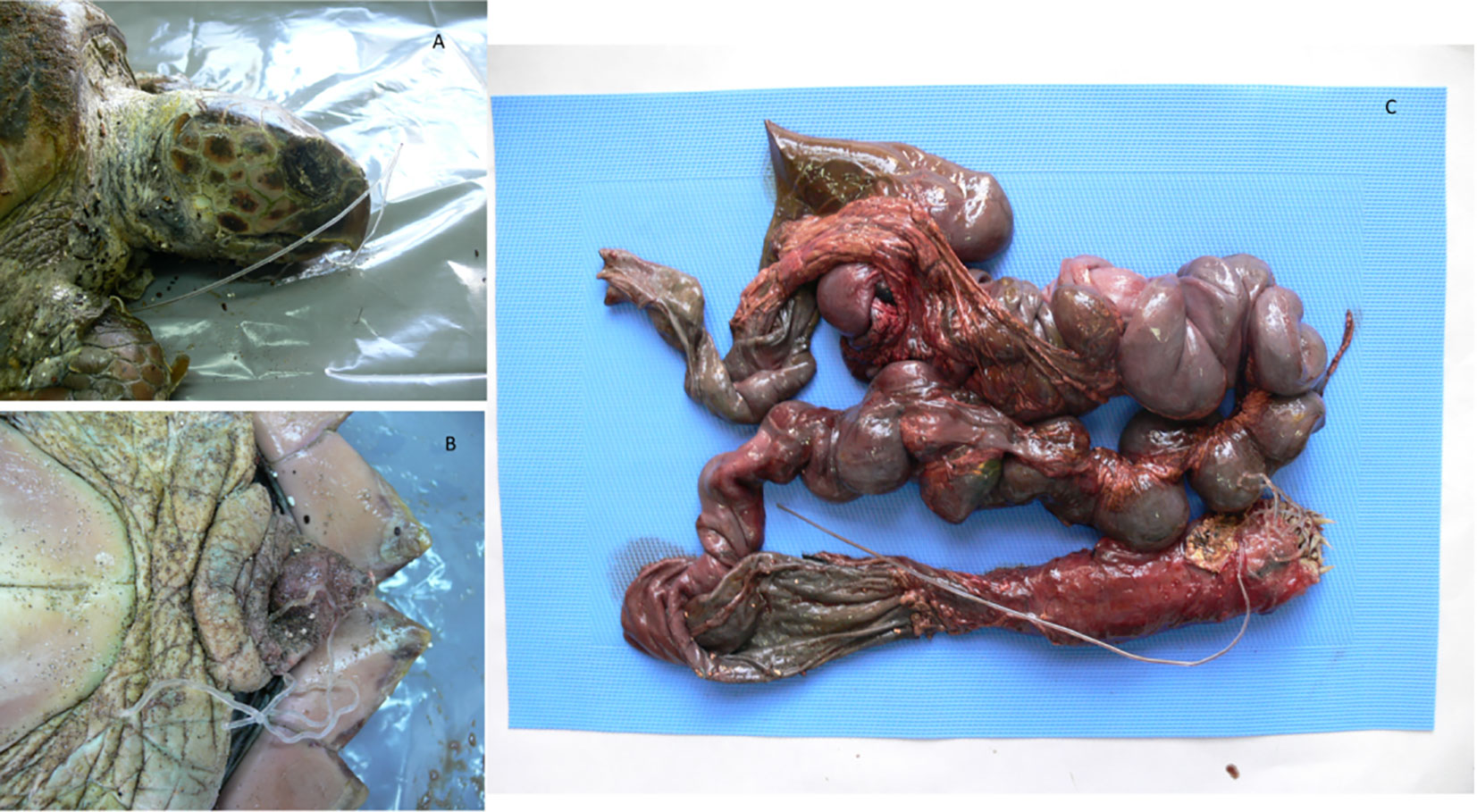
Figure 2 Loggerhead sea turtle that ingested a line. The object entered the rhamphotheca (A) and exited the cloaca, (B) causing plication and intussusception in both small and large tracts of the intestine (C).
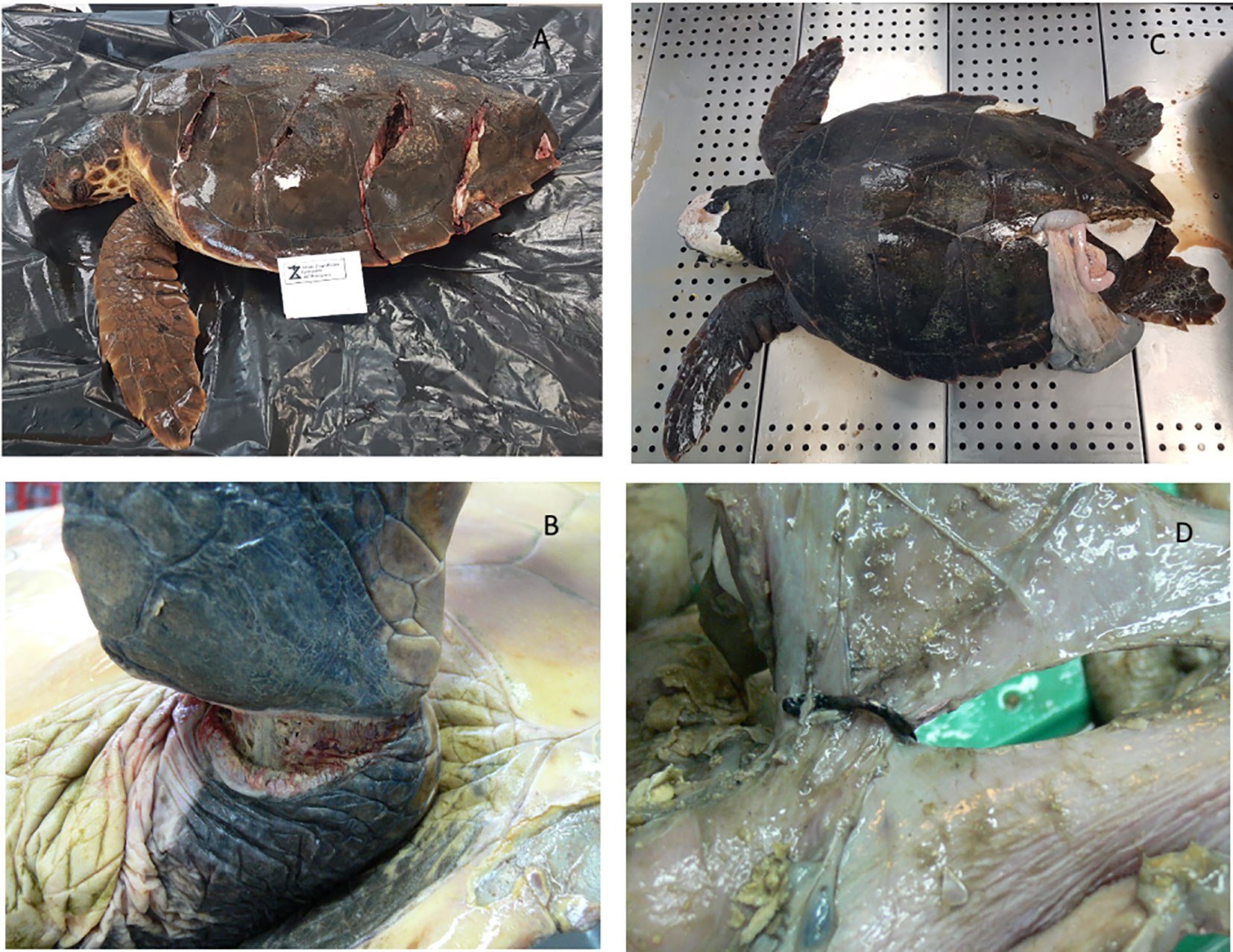
Figure 3 Animals with fatal wounds caused by anthropic impact. (A) Carapace fracture probably caused by the propeller of a boat. (B) Laceration at the basis of the fin likely determined by the entanglement of the animal in a net or a line. (C) Carapace fracture (with organ leakage) probably caused by a collision with a boat. (D) Laceration of the intestine caused by hook ingestion.
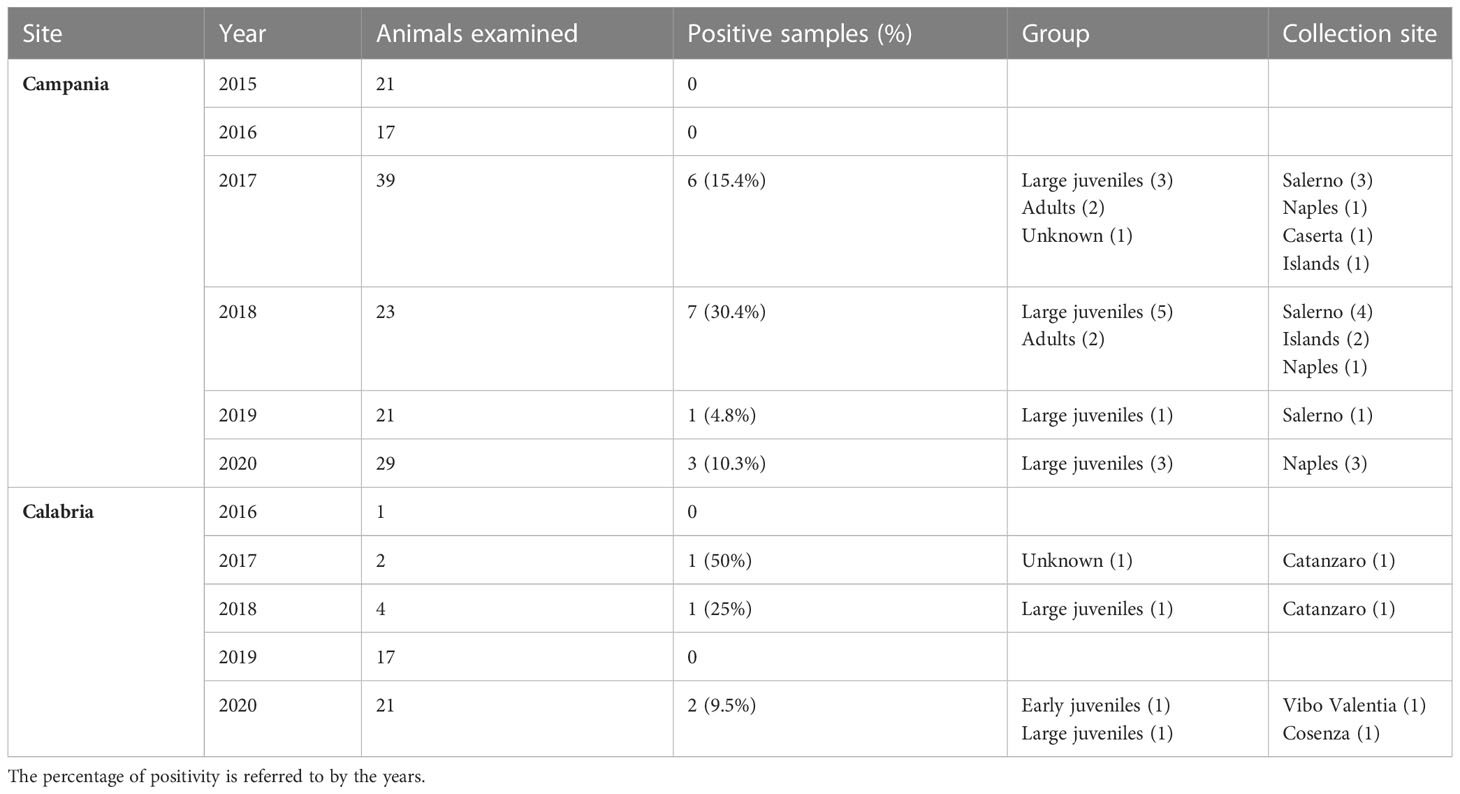
Table 4 Sea turtles examined from 2015 to 2020, the prevalence of positivity to ChHV5, age group, and collection sites of positive turtles.
Of the animals analysed, 21.5% (42/195), all from Campania (almost one-third of the animals analysed in this region), presented injuries like fractures of carapace, fins, and plastron (Figure 3), caused by collision with boats or by entanglement in nets and lines. As indicated in Table 3, more than one-half of these injuries (an example is the amputation of the head or important body fractures determining the leakage of the internal organs) were considered fatal (26/42, 61.9%). For 64 animals (Table 3), the mortality was not clearly attributed to a specific event but probably due to a mix of anthropogenic factors (ingestion of litter, injuries by collisions, etc.). In 54 sea turtles, no visible anthropogenic signs were found, and the cause of death was defined as unknown. No internal or external tumours, typically associated with FP, were detected.
Identification of pathogens and heavy metals
Positivity to ChHV5 virus
ChHV5 DNA was identified in 21 of the 195 sea turtles analysed (10.8%), 17/150 (11.3%) in Campania and 4/45 (8.9%) in Calabria, with the prevalence of detection varying in the years under study (Table 4). As reported in Figure 1 and Table 4, positive animals were distributed all along the coast of Campania, being found in the Salerno area, between the coasts of Naples in the islands, and in Caserta province. The four cases detected in Calabria were from the provinces of Catanzaro, Cosenza, and Vibo Valentia (Figure 1, Table 4). Of the 21 ChHV5-positive turtles, 14 (66.7%) belonged to the large juvenile group, four (19.0%) were adults, one (4.8%) belonged to early juvenile group, and two (9.5%) were not classifiable. The prevalence of the virus in the age groups was as follows: 12.5% in lj, 9.5% in A, and 4.2% in ej (see Figure 4). With respect to sex, no significant difference in virus prevalence was observed between females and males (11.65% and 12.3%, respectively).
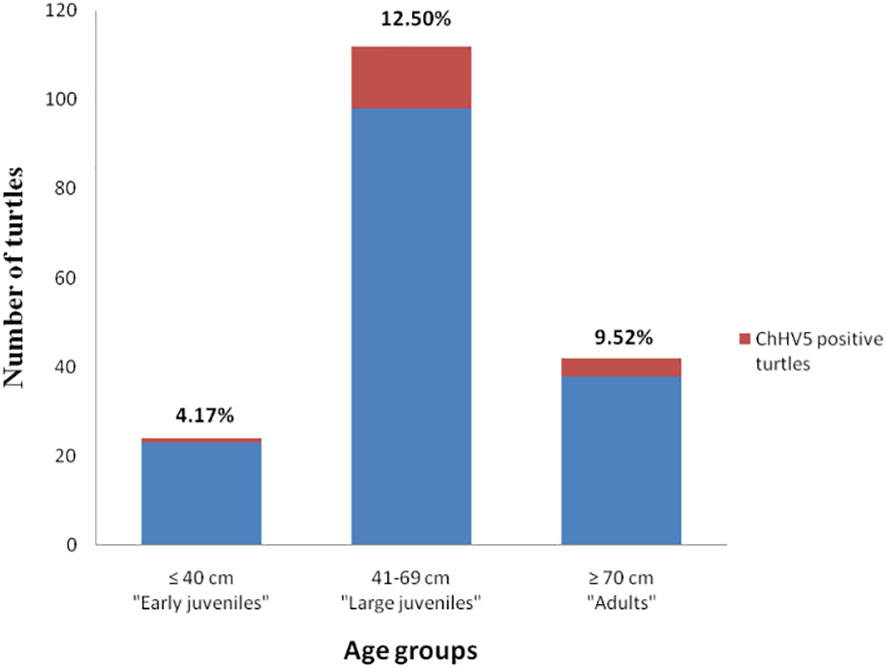
Figure 4 Prevalence of ChHV5 in the different age groups of sea turtles analysed. Histogram indicates the total number of turtles belonging to the age group, while the red part of it indicates the number of turtles positive for the virus.
ChHV5 was identified in all the types of organs analysed. With respect to the 21 positive animals, the prevalence was as follows: intestine, 12/21 (57.1%); lung, nine of 21 (42.8%); brain, eight of 21 (38.1%); kidney, eight of 21 (38.1%); liver, seven of 21 (33.3%); heart, six of 21 (28.5%); and cloaca, four of 21 (19.0%) (Figure 5). The spleen was only collected from three animals, and two of these samples were positive for the virus. Skin tissue always resulted negative. In eight turtles, the virus was detected only in one organ; in 10 animals, it was detected in two organs; in seven turtles, ChHV5 was simultaneously found in three organs; in one animal (from Calabria), it was detected in four (heart, spleen, intestine, and lungs) of the six organs investigated.
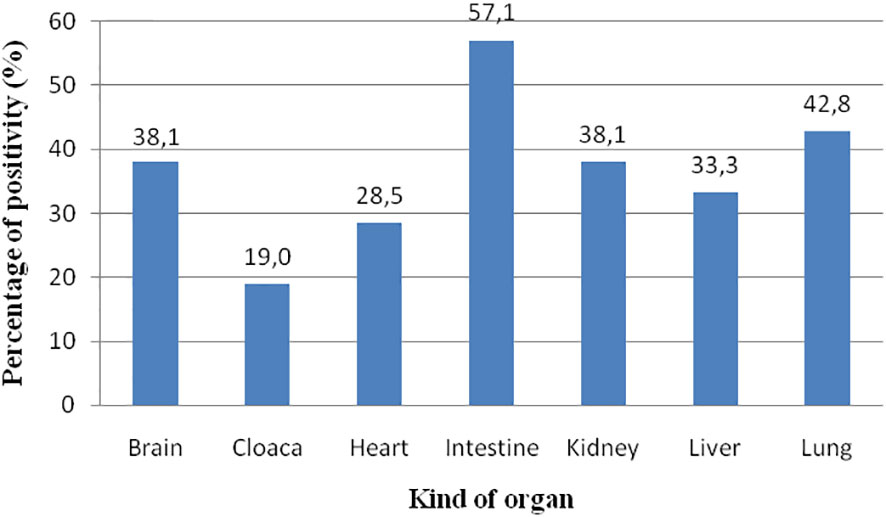
Figure 5 Prevalence of ChHV5 in the different organs analysed. The spleen is not considered since only three samples were analysed.
Macro- and microscopical observation of CHhV5-positive lungs
In ChHV5-positive animals, organs were more thoroughly investigated to observe any pathological condition connectable to the virus presence, as well as to point out the likely presence of co-infecting bacterial pathogens. In two of the 21 ChHV5-positive turtles, a macroscopical frame of acute-haemorrhagic bilateral bronchopneumonia was found (Figure 6). Those animals were both females belonging to the lj group, and viral DNA was found in the lungs and at least in another organ, the heart for one animal and the intestine and liver for the other. For histology, only animals positive to ChHV5 with tissues/organs in good conservation state (10 animals) were investigated.
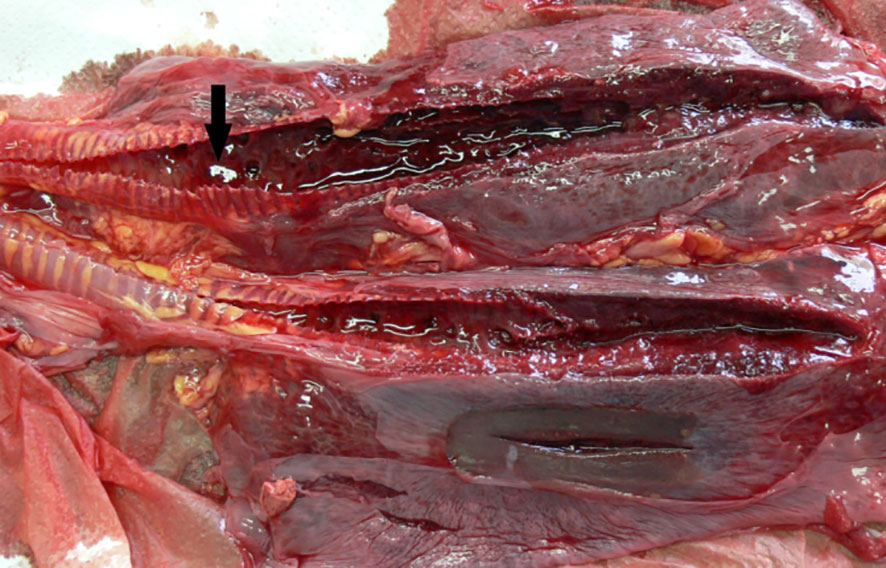
Figure 6 ChHV5-positive lungs with bilateral severe haemorrhagic congestion in the airways and in the parenchyma. Arrow indicates blood clots in the lumen of the airways.
Histological examination (Figure 7) revealed similar findings, showing the multifocal inflammatory reaction of the airways with severe and diffuse inflammatory changes in all sections of the lungs. Airways contained a variably abundant luminal accumulation of numerous sloughed necrotic epithelial cells, erythrocytes, and heterophils. The pulmonary interstitium was diffusely expanded by hyperemia and numerous heterophils and lymphocytes. The interfoveolar interstitium was characterised by congestion and inflammation foci with the presence of lymphocytes and heterophils, a frame consistent with subacute focal interstitial bronchopneumonia. Furthermore, the organs of these animals, when analysed for bacterial pathogen co-infection, were negative.
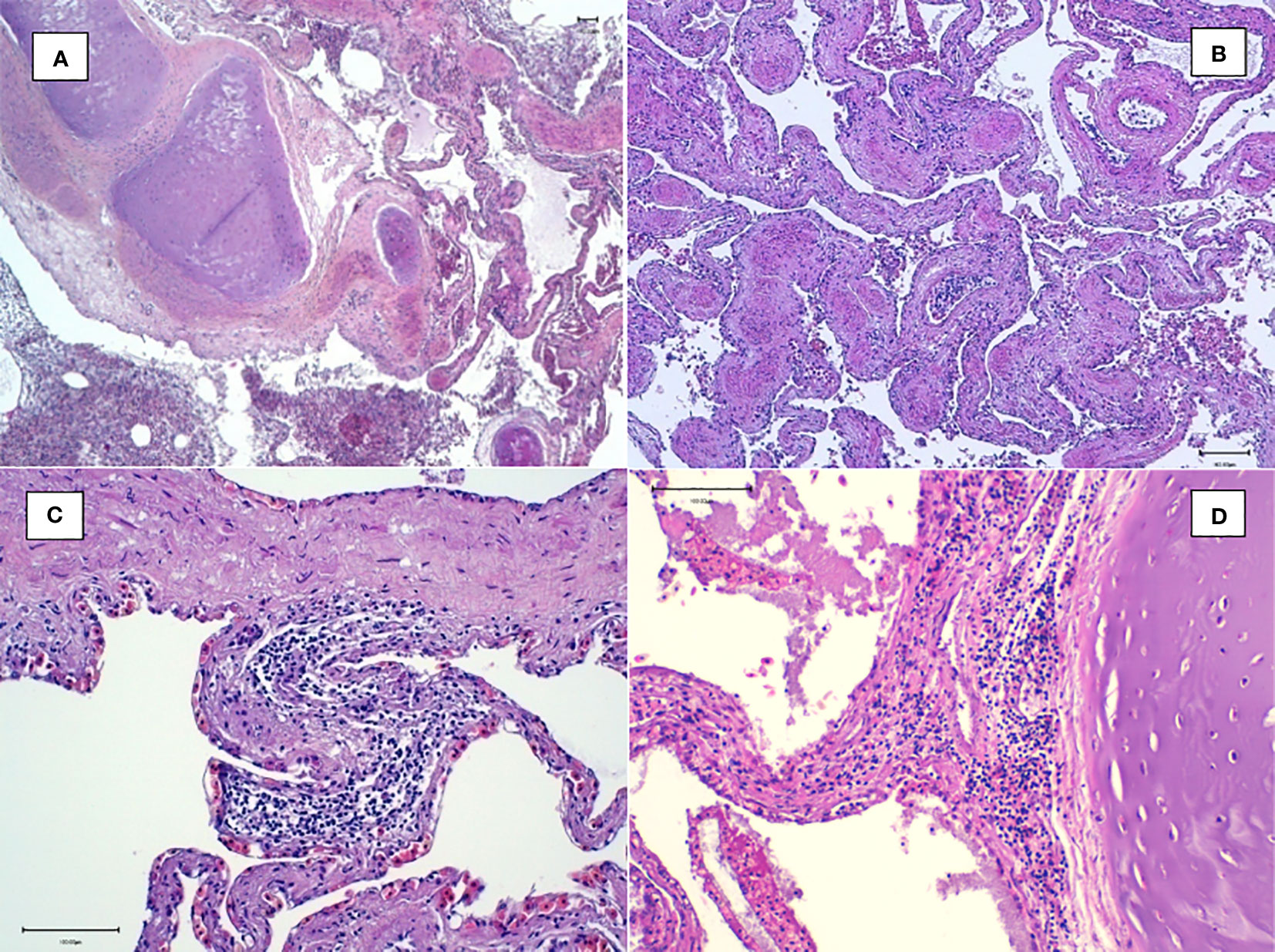
Figure 7 Histological examination of the lungs. (A) Magnification at ×4: multifocal inflammatory reaction of the airways containing sloughed epithelial cells. (B) Magnification at ×10: congestion and inflammation foci in the interfoveolar interstitium. (C, D) Magnification at ×20: heterophilic inflammatory peribronchial foci with the presence of lymphocytes and heterophils in the interfoveolar interstitium.
Analysis of toxic metals
The levels of nonessential toxic elements like As, Cd, Hg, and Pb were determined in the liver, kidney, and muscle of 94 sea turtles, 52 from the Campania region and 42 from the Calabria region (Figure 8, Table 5).
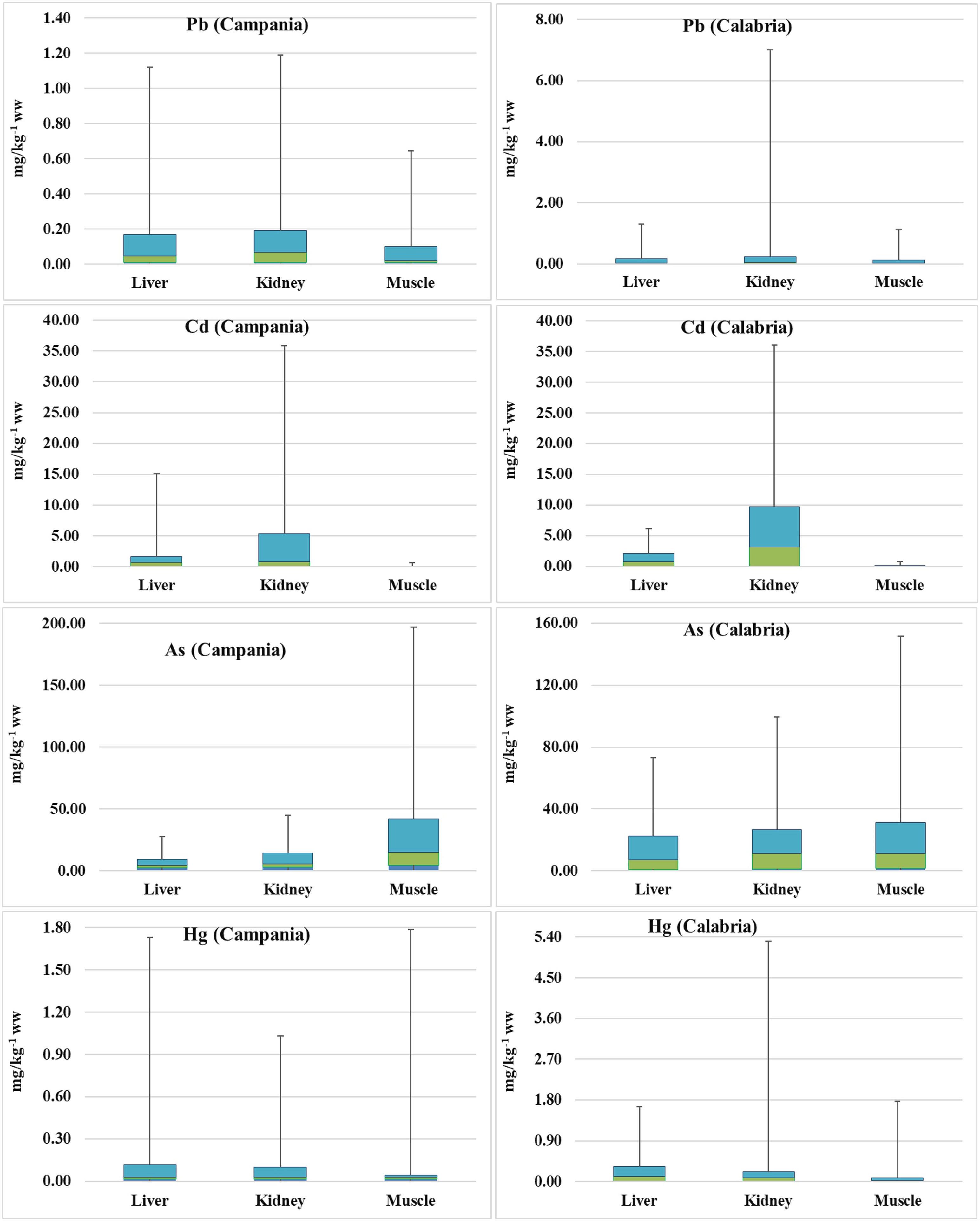
Figure 8 Box plots of As, Hg, Pb, Cd, and Pb concentrations (mg kg−1 ww) in turtles. The lines in the middle of the box plots represent the medians.
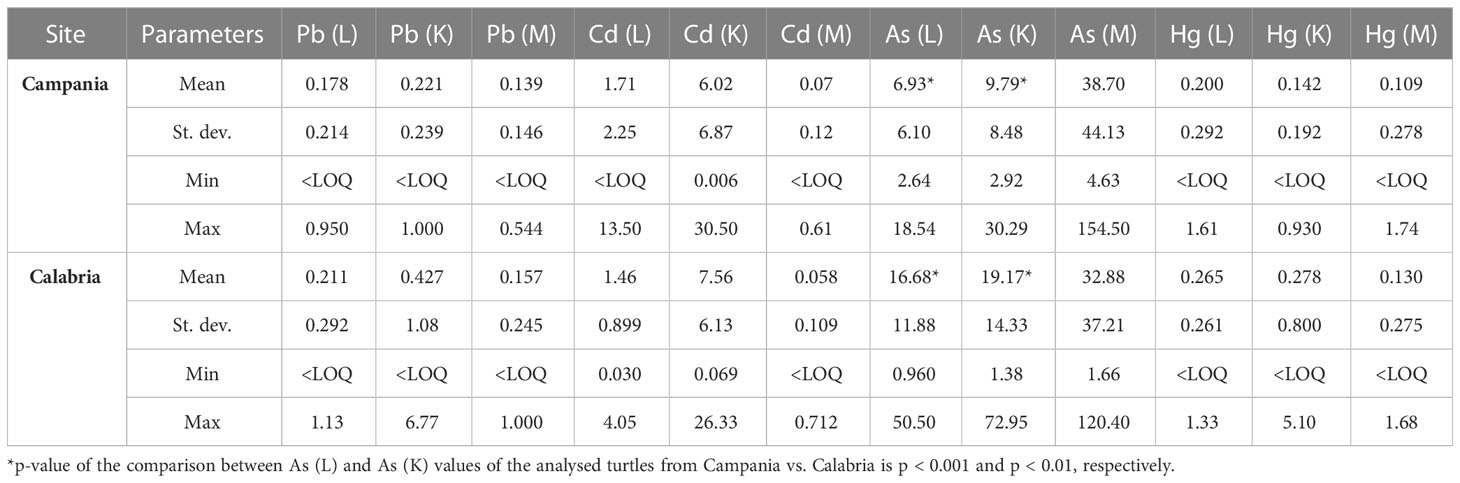
Table 5 Range, mean, and standard deviation of As, Cd, Hg, and Pb (mg kg−1 wet weight) in the liver (L), kidney (K), and muscle (M) of analysed turtles according to the collection site.
Preliminary results were already demonstrated (see Esposito et al., 2020; Canzanella et al., 2021). When As concentrations found in the animals from the Campania region were measured, they varied in the range from 2.64 to 18.54 mg kg−1 (n = 30) in the liver, 2.92 to 30.29 mg kg−1 (n = 23) in the kidney, and 4.63 to 154.50 mg kg−1 (n = 30) in the muscle. In turtles from the Calabria region, the concentrations of As in the liver varied in the range of 0.960–50.50 mg kg−1 (n = 41), in the kidney between 1.38 and 72.95 mg kg−1 (n = 39), and in the muscle in the range of 1.66–120.40 mg kg−1 (n = 41). Comparing the data from the two regions, the values of As in the liver and kidney were higher in turtles from the Calabria region than in the Campania region (p < 0.001 and p < 0.01, respectively).
In turtles from the Campania region, Hg in the liver showed levels ranging between <LOQ and 1.61 mg kg−1 (n = 49); in the kidney, the levels were <LOQ–0.930 mg kg−1 (n = 41); and in the muscle, Hg levels ranged between <LOQ and 1.74 mg kg−1 (n = 50). The concentration of Hg in animals from the Calabria region was as follows: in the liver, it ranged between <LOQ and 1.33 mg kg−1 (n = 42); in the kidney, it varied in the range of <LOQ–5.10 mg kg−1 (n = 40); and in the muscle, it varied in the range of <LOQ–1.68 mg kg−1 (n = 42). With respect to Pb, in turtles from the Campania region, its concentrations in the liver varied in the range of <LOQ–0.950 mg kg−1 (n = 50), in the kidney between <LOQ and 1.000 mg kg−1 (n = 40), and in the muscle varied in the range of <LOQ–0.544 mg kg−1 (n = 51). In the Calabria region, Pb in the liver ranged between <LOQ and 1.13 mg kg−1 (n = 42), whereas those in the kidney ranged between <LOQ and 6.77 mg kg−1 (n = 40), and those in the muscle varied from <LOQ to 1.000 mg kg−1 (n = 42). Levels of Cd in turtles from the Campania region were as follows: in the liver, they ranged from <LOQ to 13.50 mg kg−1 (n = 50); in the kidney, they ranged between 0.006 and 30.50 mg kg−1 (n = 40); and in the muscle, they ranged from <LOQ to 0.61 mg kg−1 (n = 50). In turtles from the Calabria region, Cd showed concentrations in the liver ranging from 0.030 to 4.05 mg kg−1 (n = 42), in the kidney from 0.069 to 26.33 mg kg−1 (n = 40), and in the muscle from <LOQ to 0.712 mg kg−1 (n = 42).
Correlation of toxic metals with age, gender, and stranding site
Values, including mean, range, and standard deviation, of As, Pb, Cd, and Hg with respect to gender and site of the collection are reported in Table 6. Of the 94 turtles analysed for metals, 40 were males, 47 were females, and seven were unknown. Especially in the liver and kidney, As was higher in Calabria than in Campania. Indeed, in the comparison between As (liver) and As (kidney) values of male turtles from Campania vs. Calabria, significant differences were found (p < 0.05 and p < 0.01, respectively). A similar trend was detected for As in liver and kidney in male turtles from Calabria vs. female turtles from the Campania region (p < 0.05). The comparison between As (liver) values of the male from Campania vs. female turtles from the Calabria region was significant (p < 0.05), as was the p-value of the comparison between As (liver) values of female turtles from Campania vs. female turtles from the Calabria region (p < 0.05). Differences almost significant were also detected between Cd values in the liver of male vs. female turtles from Calabria (p = 0.06). The values, including mean, range, and standard deviation, of As, Pb, Cd, and Hg with respect to animals’ age are reported in Table 7. Of the 52 turtles analysed from the Campania region, two were ej, 30 lj, and 13 were A. Whereas of the 42 turtles from the Calabria region, five were ej and 12 lj. Age-dependent bioaccumulation of Pb and As was observed in the liver and muscle. Indeed, significant differences were found in Campania region when Pb (liver) and As (muscle) values of turtles from the adult groups were compared vs. large juvenile groups (p < 0.001 and p < 0.05, respectively). The values of Pb in the muscle of adults from the Campania region were higher than those of early juveniles from the Calabria region (p < 0.05). A p-value of the comparison between Pb (liver) levels of turtles from the Campania region adults vs. Calabria early juveniles and large juveniles was significant (p < 0.01 and p < 0.01, respectively). Moreover, a site-dependent accumulation of As was observed from the Calabria region. Indeed, the levels of As in the liver of large juvenile turtles from the Campania region were lower than in early juvenile and large juvenile turtles from Calabria (p < 0.01 and p < 0.01, respectively). Of 94 analysed animals for metals, 15 were positive for ChHV5, 12 (9 males + 3 females) from Campania, and three (2 males + 1 female) from the Calabria region.
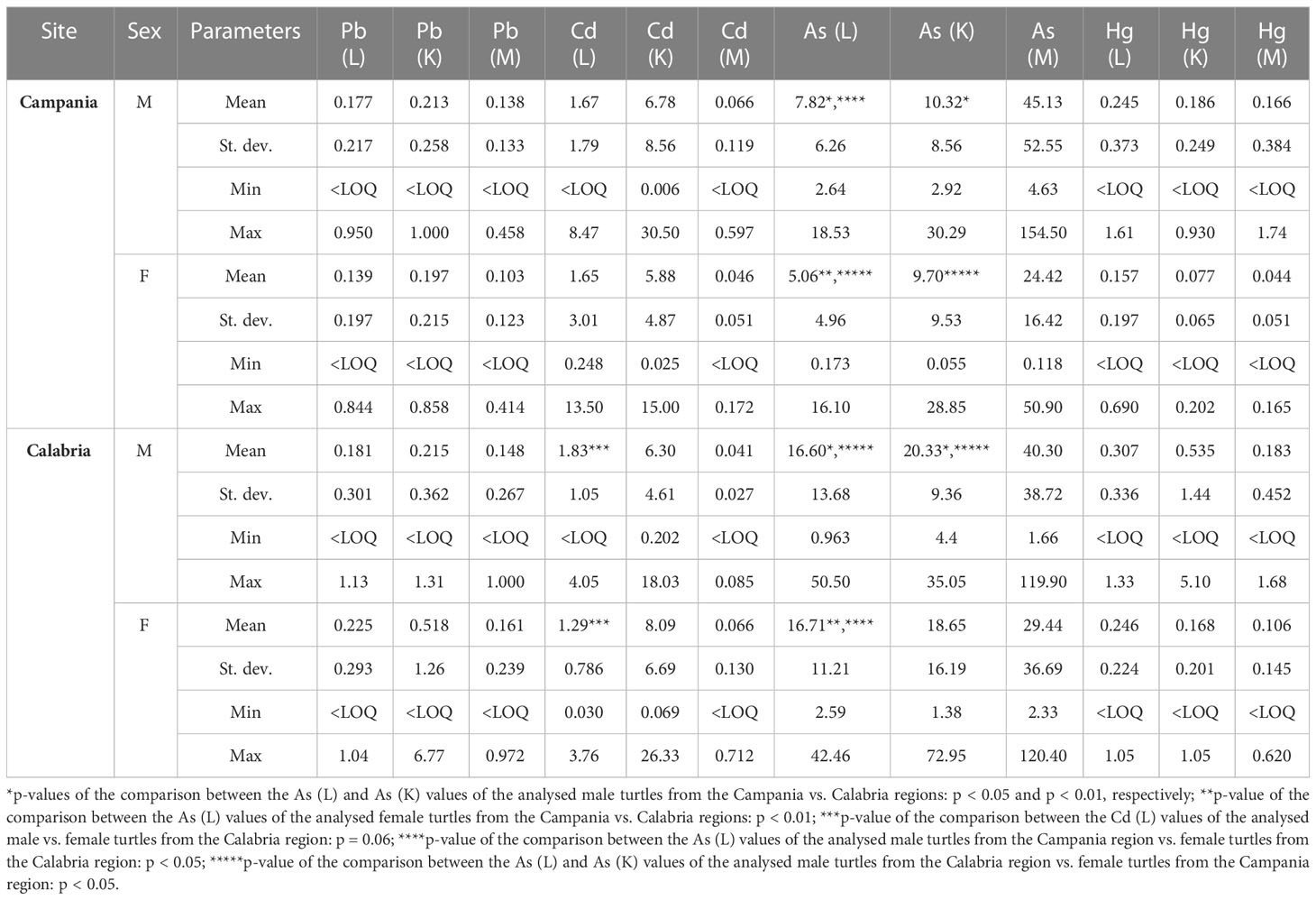
Table 6 Range, mean, and standard deviation of As, Cd, Hg, and Pb (mg kg−1 wet weight) in the liver (L), kidney (K), and muscle (M) of the analysed turtles according to sex in male (M) or female (F) and site.
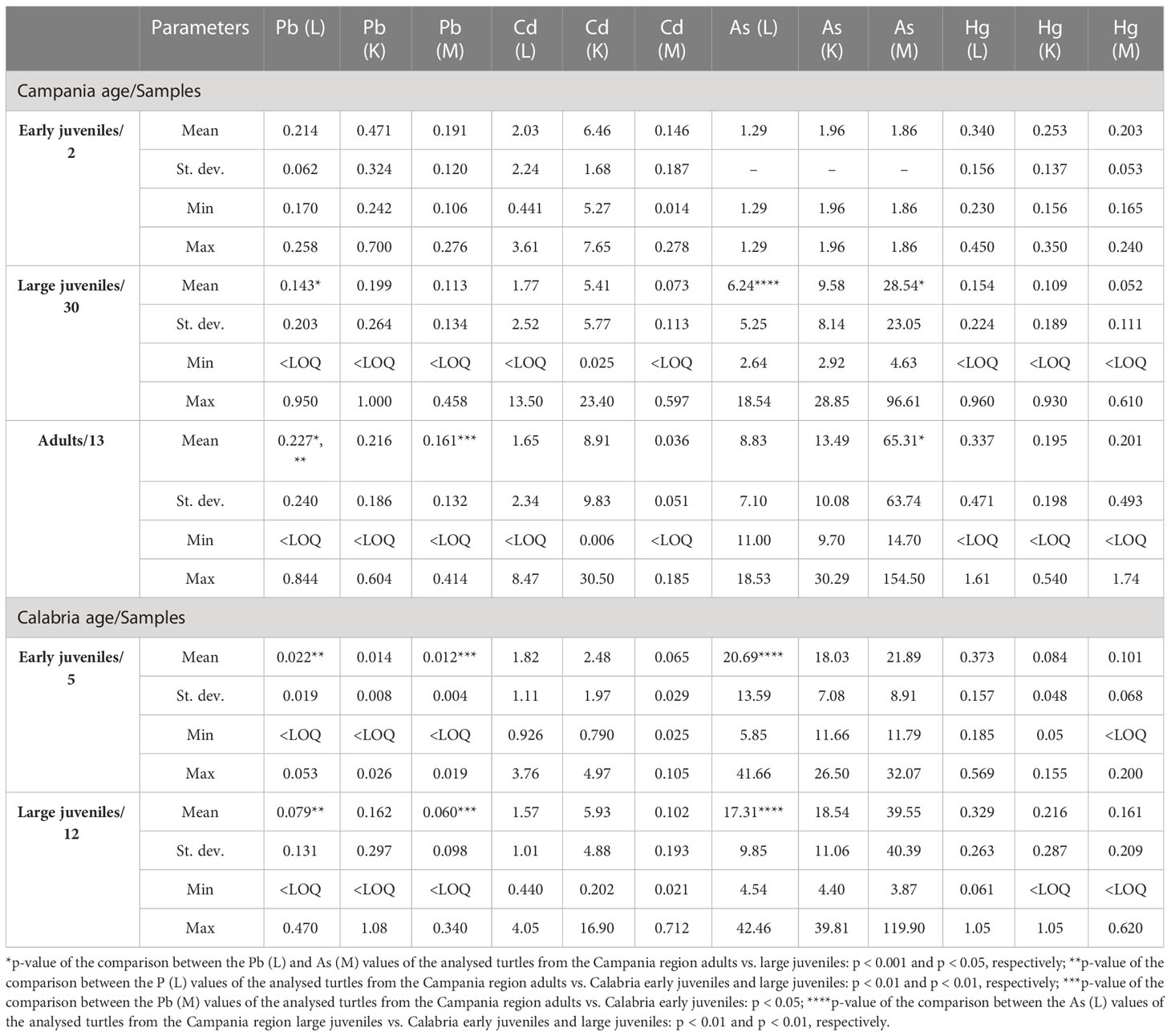
Table 7 Range, mean, and standard deviation of As, Cd, Hg, and Pb (mg kg−1 wet weight) in the liver (L), kidney (K), and muscle (M) of analysed turtles according to sampling region and age.
The correlation between the accumulation of the contaminants and the size of the turtles was studied by performing Spearman’s rank correlation. So, the contamination levels determined in the liver, kidney, and muscle were correlated with the BW, SCL, and SCW morphometric parameters of the turtles. The Spearman’s rank test revealed a statistically significant positive correlation (p < 0.01) between the levels of As found in the kidney of Campania turtles and their SCL, indicating that the accumulation of this metal is size-dependent (Supplementary Table S1).
Discussion
In the last few years, marine animal stranding has become frequent, and loggerhead sea turtles are involved in this phenomenon. Indeed, in our study, during the 6 years of observation, 195 sea turtles have been found dead along the coasts of the Campania and Calabria regions. Potential health risks for this species are mainly caused by increased anthropogenic pressure on the marine ecosystem (Monteiro et al., 2021), due to marine traffic, sea pollution, both macro- and microscopic, fishing activities (Pagano et al., 2019), and infectious diseases (Monteiro et al., 2021).
Results from our study have confirmed that anthropogenic factors are involved, to a different extent, in a large rate of turtle stranding/death, as already reported by other authors (Casale et al., 2010). Overall, our findings indicate that fishing activities are among the most important threats to sea turtles in our basin, in agreement with existing literature (Tomas et al., 2008; Casale et al., 2010; Caracappa et al., 2018). Necropsies have revealed that 117 sea turtles out of 195 (60%) suffered from anthropic impact. Indeed, we detected internal and/or external injuries caused by collision with boats, entanglement in fishing gears, and marine litter ingestion. Marine litter, a growing problem worldwide, impacts all marine organisms in different ways. Sea turtles usually ingest plastic debris and other litter, mistaking them for food (Campani et al., 2013). We found a high percentage (38.5%) of animals with objects in their digestive tract, similar to other studies (Casale et al., 2008; Lazar and Gračan, 2011), carried out on C. caretta in the Mediterranean Sea (37.9% and 48.1%, respectively), but lower than the rate reported by other authors (71.0% and 75.9%) in the same basin (respectively Tomás et al., 2002; Campani et al., 2013). Ingestion of plastic objects was found in 31/195 (15.9%) of the sea turtles analysed, but marine plastic ingestion has never been associated with direct mortality. However, it may cause sublethal effects, such as gastrointestinal lacerations, blockage, reduced feeding, and absorption of toxic compounds, as well as death from complete intestinal occlusion (Kühn et al., 2015; Matiddi et al., 2017; Sala et al., 2021).
Observing the injuries and the internal/external lesions has helped to clearly address the specific cause of mortality in 53 animals. In the other 64 sea turtles, the death could be ascribed to a mix of anthropic effects. Of the remaining 54 animals, 24 have been excluded from this analysis because they were mummified, and 30 have not been visibly damaged by anthropic factors, but various hypotheses about mortality were developed; for example, a lethal infection can be difficult to diagnose or die from embolism and/or drowning due to prolonged apnoea caused by fishing by-catch (trawling), which may not leave any visible traces on stranded carcasses (Casale et al., 2010).
In this study, a complete absence of both internal and external fibropapillomas in the animals tested was observed. The analysis included a substantial number of turtles (195) and covered an extended period of observation (6 years). Thus, so far, the Mediterranean Sea seems not to be affected by FP, a neoplastic disease whose development is likely linked to the presence of ChHV5 (James et al., 2021; Loganathan et al., 2021; Perrault et al., 2021), as well as too polluted and eutrophicated habitats (Dujon et al., 2021; Monteiro et al., 2021). Our results were quite surprising, considering that this disease is panzootic in green turtles (Williams et al., 1994; Jones et al., 2016), has been described in marine turtles worldwide (Alfaro-Nunez et al., 2014; Espinoza et al., 2020), and appears to be increasing in some parts of the world (Loganathan et al., 2021). It has been established that FP prevalence is related to marine pollution (Loganathan et al., 2021). In this regard, the Mediterranean Sea, a semi-closed sea mainly surrounded by industrialised countries, is considered a contaminated ecosystem (Gomez-Ramirez et al., 2020). Hence, the complete absence of FP in turtles from the Mediterranean Basin seems to indicate that other factors than pollution are involved in FP formation.
For the first time, according to our knowledge, the presence of ChHV5 was detected in 21 specimens of C. caretta living in the Mediterranean Sea. The detection of the virus without FP association did not surprise us since the occurrence of this alphaherpesvirus in sea turtles without tumours is well documented (Alfaro-Nunez et al., 2014; Alfaro-Nunez et al., 2016). The prevalence of ChHV5 observed in this study (10.8%) agrees with previous researchers who reported a percentage of viral positivity of 11.5% in healthy turtles belonging to the same species (Alfaro-Nunez et al., 2014). Furthermore, in 19 of the 21 positive C. caretta, beyond FP, the presence of the virus could not be associated with any specific pathological condition of the animal.
As already supposed by other authors (Page-Karjian et al., 2017), our results suggest the presence of persistent subclinical and latent infection due to ChHV5 in apparently healthy sea turtles. Indeed, the virus is characterised by the common feature of establishing long latency to escape immune system detection, in line with other herpesviruses (Alfaro-Nunez et al., 2016; Oriá et al., 2020; Monteiro et al., 2021; Piret and Boivin, 2021; Esposito et al., 2022a). The presence of ChHV5 in all the organs analysed (heart, kidney, brain, spleen, liver) agrees with data reported by other authors (Page-Karjian et al., 2017), who have identified the virus in kidney, heart, and nerve from green turtles not affected by FP. In addition, the simultaneous identification of the virus in multiple anatomical sites of some turtles may be due to the capacity of alphaherpesviruses to infect a wide variety of host cell types (Page-Karjian et al., 2017). According to age, the rates of ChHV5 positivity in the large juvenile (12.5%) and adult (9.5%) groups were similar, while a lower prevalence was revealed in the early juvenile group (4.2%). These results could be explained by the age-dependent difference in habits of sea turtles, as they spend the first period of life in the open seas, while in their juvenile or adult phase, they live in coastal areas, not deep waters (Pagano et al., 2019), and are probably more exposed to viral contamination.
In chelonians (turtles and tortoises), herpetic infections have been related to different pathological states, including proliferative and ulcerative stomatitis, respiratory infections, dermatitis, conjunctivitis, hepatitis, and dermatitis (Jacobson et al., 1986; Pettan-Brewer et al., 1996; Hervas et al., 2002; Johnson et al., 2005). In C. caretta, herpesviruses have been associated not only with FP but also with oral, respiratory, cutaneous, and genital lesions (Stacy et al., 2008). Interestingly, our results showed in two animals real-time PCR positivity for ChHV5 (in the lungs), a clinical frame of bronchopneumonia observable both macroscopically and histologically (Figures 6, 7). Our histopathological findings are consistent with studies describing, in chelonians, herpesvirus infections associated with heterophilic bronchitis and pneumonia, with the presence of heterophilic inflammation and sloughed epithelial cells in the airways (Origgi et al., 2004; Stacy et al., 2008; Marschang et al., 2009; Marenzoni et al., 2018). Indeed, to date, ChHV5 has been associated, apart from FP, only with ocular pathological manifestations (Oriá et al., 2020), while its involvement in respiratory disorders has never been reported in the literature. Similarly, heterophilic inflammation in the lung could be due to any insult, and it is not pathognomonic for ChHV5. Nevertheless, even though no other pathogen associable with respiratory disease has been found in the lungs of these two specimens of C. caretta, the hypothetical implication of ChHV5 in bronchopneumonia does not fulfil all the criteria necessary to claim a causal relationship between a pathogen and a disease (Fredricks and Relman, 1996).
An important aspect of this research is the analysis of the levels of trace metals in various organs of the turtles. Climate changes and the consequent geochemical differences considerably affect the quality, temperatures, and biochemical parameters of seawater in Southern Europe Seas (https://ec.europa.eu/clima/climate-change; Mitchell et al., 2011; Iordache et al., 2022). The large-scale (6 years; 94 animals) survey provides a very clear overall picture of the current situation regarding trace element levels in loggerhead turtles from Southern Italy’s coasts. Thus, it makes it easier to evaluate accumulated concentrations as well as to compare the levels of time revealed among different surveys in these areas. Overall, a relevant time-dependent accumulation increase of As levels was detected in liver and muscle tissues (Storelli and Marcotrigiano, 2000; Esposito et al., 2020; Canzanella et al., 2021). In particular, the concentrations of As in the muscle were higher than in the kidney and liver. Metalloid levels, such as As, in the liver tend to decrease more than in other tissues, because of a detoxification process that occurs in the liver before going into other tissues (kidney and muscle) (da Silva et al., 2014; Sulato et al., 2022), as detected in the liver of C. mydas (Sulato et al., 2022). The levels of Hg, which are similar to Cd, appeared similar to or lower than those detected in other studies (Maffucci et al., 2005; Storelli et al., 2005; Esposito et al., 2020; Gomez-Ramirez et al., 2020; Canzanella et al., 2021) and were instead higher in the liver and kidney than in the muscle. Whereas the highest levels of Pb and Cd were found in the kidney, followed by the liver and muscle. A similar pattern of bioaccumulation was previously found in turtles stranded along the southwestern coasts of the Tyrrhenian Sea (Esposito et al., 2020; Canzanella et al., 2021). These findings suggest that the accumulation of each metal is tissue-dependent. Interestingly, the levels of As in the liver and kidney of turtles from Calabria were considerably higher than those from the Campania region. Herein, the values of As in loggerhead turtles were highest in the muscle, followed by the kidney and liver. This trend, probably due to their feeding habit rich in molluscs (generally containing high levels of this metalloid), was already described by us (Esposito et al., 2020; Canzanella et al., 2021; Lambiase et al., 2021b). It is well known indeed that the main route of turtles’ exposure to marine environment contaminants occurs through food, consequently, the levels of metals found in the organs depend on the specific dietary habits of the loggerhead turtles, which is directly correlated to the levels of contamination of the marine environment (Esposito et al., 2020; Canzanella et al., 2021; Lambiase et al., 2021a). In this regard, about one-fifth of the foraging areas in the Mediterranean resulted contaminated; therefore, they represent a potential risk of exposure for turtles (Almpanidou et al., 2022). Although a lowering in Pb levels was observed in our samples, likely due to reduced use of lead in gasoline in European Countries since early 1980 (https://www.eea.europa.eu), regrettably, climate warming affects both saltwater and freshwater, provoking metal toxic level perturbation (LeMonte et al., 2017; Iordache et al., 2022). The concentration of As in the Calabria region is affected by multiple hydrogeochemical processes, which determine variable levels in groundwater (Apollaro et al., 2022). In addition, sea level rise also induces arsenic release from contaminated coastal soils (LeMonte et al., 2017). Another plausible explanation for such high levels of As contamination in the loggerheads of the Calabria coast may be connected with harbours, ship traffic, and the oil and gas industry. Indeed, other studies have pointed out that high levels of As are closely related to industrial contamination, including the oil and gas industry in the case of the green sea turtles found stranded in Southeastern Brazil (Bruno et al., 2021). Important information, which could explain the higher contamination of turtles from Calabria than the animals from Campania, is described by Garofalo et al. (2013) in a study on the genetic characterisation of central Mediterranean stocks of the loggerhead turtles using mitochondrial and nuclear markers. Garofalo et al. (2013), using MSA analysis, found out that the Calabrian colony represented the only considerable contribution to the Tyrrhenian stock. Furthermore, it has been reported that loggerhead turtles are able to be active even in colder waters, and this could increase their exposure to environmental contaminants (Garofalo et al., 2013). Overall, higher concentrations of As were detected in the liver and kidney of turtles from Calabria. Moreover, in turtles from the Calabria region, males had almost significantly higher Cd levels in the liver than females. Probably, this variation could be explained by metabolic differences between males and females (Maffucci et al., 2005) and by their feeding habits. No significant differences between the sexes (p > 0.05) were found for Pb and Hg. Age-dependent bioaccumulation of Pb and As was detected in the liver and muscle, respectively. Indeed, an adult group from the Campania region showed Pb (L) and As (M) values significantly higher than the large juvenile group. Moreover, Pb in the muscle of adults from the Campania region was remarkably higher than those of early juvenile turtles from the Calabria region. The levels of Pb (L) in adults from the Campania region were significantly higher than those in early juvenile and large juvenile groups from Calabria. Moreover, a significant site-dependent accumulation of As was detected in the liver of both early juvenile and large juvenile turtles from the Calabria region vs. large juvenile turtles from Campania. This difference may be explained as reported above. The phenomenon of age-dependent bioaccumulation, as well as the size, may explain these differences (Adel et al., 2017). The relationship between the accumulation of the contaminants and the size of the turtles was studied by performing statistical tests such as Spearman’s rank correlation. For the statistical study, the contamination levels determined in the liver, kidney, and muscle were correlated with the BW, SCL, and SCW morphometric parameters of the turtles. The Spearman’s rank test showed a statistically significant positive correlation (p < 0.01) between the levels of As found in the kidneys of Campania turtles and their SCL, indicating that the accumulation of this metal is size-dependent (Supplementary Table S1). The increase in metal concentrations with size was also shown by a linear regression model (Supplementary Figure S1), in accordance with the results reported in other studies on the bioaccumulation of environmental contaminants in turtles (Lazar et al., 2011; Lambiase et al., 2021a). Considering that the size of the turtles can be related to their age, it is possible to assume that the older turtles had a higher body burden of metals due to longer exposure to contaminants (Lambiase et al., 2021a). The increase in the metal accumulation with size could also be explained by considering the feeding behaviour of the turtles, which depends on their developmental stage. In fact, during the oceanic stage (CCL < 40 cm), turtle feeding consists mostly of planktonic organisms, while in the neritic stage (CCL > 40 cm), their diet becomes mainly constituted by benthic organisms (Lazar et al., 2011; Nicolau et al., 2017; Lambiase et al., 2021a). Genetic characterisation of Mediterranean loggerhead turtles C. caretta indicates that the distribution of juveniles from different rookeries may not be homogeneous among the major Mediterranean foraging grounds because of a complex pattern of surface currents in the Mediterranean (Clusa et al., 2014; Garofalo et al., 2013; Caracappa et al., 2016). These findings might have possible implications for the burden of contaminants found in this population, indicating a special concern. For example, abnormalities in the number of scutes have already been found by Caracappa et al. (2016) through an epigenetic mechanism probably caused by the environmental context during embryonic growth. Despite the data reported in the literature as well as the results shown herein on the bioaccumulation of the metals in the turtles from the Campania region, which highlight the correlation between the contamination levels found in the animal tissues and their size, the statistical analysis did not show any significant correlation between the As, Pb, Cd, and Hg concentrations in the turtles from the Calabria region and their morphometric parameters (Supplementary Table S2). Moreover, the statistical analysis showed strong positive correlations (p < 0.001) between the Pb, As, and Hg levels found in the tissues of the turtles from both regions, indicating that there is a strong correlation between the bioaccumulation of contaminants in the different organs, despite the fact that it is tissue-dependent (Supplementary Table S1).
In the frame of anthropogenic threats, it is important to consider that other environmental contaminants, such as organic pollutants including polychlorinated dibenzo-p-dioxins (PCDDs), polychlorinated dibenzofurans (PCDFs), and poly-chlorinated biphenyls (PCBs), may also cause harmful effects in turtles (Lambiase et al., 2021b; Esposito et al., 2022b). Some chemicals may reduce the immune function of marine animals and increase susceptibility to microbes (Fiorito et al., 2019; Fiorito et al., 2021). Particularly, a recent study using nontargeted proteomics has revealed altered immune responses in geographically distinct populations of green sea turtles (Chelonia mydas) from the Queensland coast (Chaousis et al., 2023). Here, to establish a relationship between viruses and chemicals, we have examined this hypothetical correlation. Nevertheless, the statistical analysis did not show significant correlations between the presence of the virus and the metal concentrations. The lack of relationships among chemical and viral parameters was probably due to the too-small number of virus-positive tissues, which did not allow us to perform an accurate statistical analysis.
In conclusion, this study provided valuable information on various aspects related to loggerhead sea turtles living in the Mediterranean Sea, with a focus on the factors involved in the endangerment of the species. We identified for the first time the presence of chelonid herpesvirus 5 in C. caretta living in this ecosystem. We found relatively high levels of lead, mercury, arsenic, and cadmium in the organs of the sea turtles investigated, even though no correlation was found with animal health status, including viral infection. One-fifth of the sea turtles analysed presented injuries caused by collision with boats or caused by entanglement in nets and lines, and more than one-half of these injuries were probably fatal. In some cases, ingested debris such as plastic, filament line, and fishhooks were involved in the animals’ death. For some of the investigated turtles, the cause of death remained unknown and was considered probably due to a mix of not-determined factors. Moreover, in the last few years, maybe due to climate warming allowing the turtles to find new habitats previously too cold for embryos incubation, a notable increase in nesting activity of loggerhead turtles has been detected in the Western Mediterranean (Hochscheid et al., 2022). In 2021, from the Italian coasts, 244 nests were reported. Specifically, both Campania and Calabria coasts (53 and 77 nesting sites, respectively) have been chosen for turtle spawning (Pietroluongo et al., 2023). However, these beaches are characterised by a high flow of tourism that may threaten eggs and hatchlings, as well as the development of new rookeries. Hence, further studies are needed to monitor and identify risk parameters that might involve C. caretta, with the aim of implementing conservation measures to protect the species from extinction.
Data availability statement
The original contributions presented in the study are included in the article/Supplementary Material. Further inquiries can be directed to the corresponding authors.
Ethics statement
Ethical review and approval was not required for this study as our investigation was performed on stranded/dead turtles. Consequently, no ethical declarations were requested.
Author contributions
FF, ME, SL, and MA delineated the study. FS, DI, FF, FN, ME, SL, GF, and MA collected the data. FS, DI, FF, FN, ME, EE, CC, SL, BU, GL, and MA processed and analysed the data. FS, DI, FF, FN, ME, SL, BU, GL, EC, GF, and MA contributed resources and wrote the manuscript. All authors have read and agreed to the published version of the manuscript.
Funding
This work was partially funded by the Italian Ministry of Health, Project IZSME RC 07/22.
Conflict of interest
The authors declare that the research was conducted in the absence of any commercial or financial relationships that could be construed as a potential conflict of interest.
Publisher’s note
All claims expressed in this article are solely those of the authors and do not necessarily represent those of their affiliated organizations, or those of the publisher, the editors and the reviewers. Any product that may be evaluated in this article, or claim that may be made by its manufacturer, is not guaranteed or endorsed by the publisher.
Supplementary material
The Supplementary Material for this article can be found online at: https://www.frontiersin.org/articles/10.3389/fmars.2023.1116804/full#supplementary-material
References
Ackermann M., Koriabine M., Hartmann-Fritsch F., de Jong P. J., Lewis T. D., Schetle N., et al. (2012). The genome of chelonid herpesvirus 5 harbors atypical genes. PLoS One 7, e46623. doi: 10.1371/journal.pone.0046623
Adel M., Cortés-Gómez A. A., Dadar M., Riyahi H., Girondot G. (2017). A comparative study of inorganic elements in the blood of Male and female Caspian pond turtles (Mauremys caspica) from the southern basin of the Caspian Sea. Environ. Sci. pollut. Res. Int. 24, 24965–24979. doi: 10.1007/s11356-017-0067-2
Aguirre A. A., Balazs G. H., Zimmerman B., Spraker T. R. (1994). Evaluation of Hawaiian green turtles (Chelonia mydas) for potential pathogens associated with fibropapillomas. J. Wildl. Dis. 30, 8–15. doi: 10.7589/0090-3558-30.1.8
Alfaro-Nunez A., Bojesen A. M., Bertelsen M. F., Wales N., Balazs G. H., Gilbert M. T. (2016). Further evidence of chelonid herpesvirus 5 (ChHV5) latency: high levels of ChHV5 DNA detected in clinically healthy marine turtles. Peer J. 4, e2274. doi: 10.7717/peerj.2274
Alfaro-Nunez A., Frost Bertelsen M., Bojesen A. M., Rasmussen I., Zepeda-Mendoza L., Tange Olsen M., et al. (2014). Global distribution of chelonid fibropapilloma-associated herpesvirus among clinically healthy Sea turtles. BMC Evol. Biol. 14, 206. doi: 10.1186/s12862-014-0206-z
Almpanidou V., Tsapalou V., Chatzimentor A., Cardona L., Claro F., Hostetter P., et al. (2022). Foraging grounds of adult loggerhead sea turtles across the Mediterranean Sea: key sites and hotspots of risk. Biodivers. Conserv. 31, 143–160. doi: 10.1007/s10531-021-02326-0
Apollaro C., Di Curzio D., Fuoco I., Buccianti A., Dinelli E., Vespasiano G., et al. (2022). A multivariate non-parametric approach for estimating probability of exceeding the local natural background level of arsenic in the aquifers of calabria region (Southern Italy). Sci. Total Environ. 806 (Pt 1), 150345. doi: 10.1016/j.scitotenv.2021.150345
International union for conservation of nature’s red list of threatened species. Available at: https://www.iucnredlist.org.
Báez J. C., Real R., Macías D., de la Serna J. M., Bellido J. J., Camiñas J. A. (2010). Swordfish Xiphias gladius Linnaeus 1758 and loggerhead Caretta caretta (Linnaeus 1758) captures associated with different combinations of bait in the Western Mediterranean surface longline fishery. J. App. Ichthyol. 26, 126–127. doi: 10.1111/j.1439-0426.2009.01342.x
Blasi M. F., Mattei D. (2017). Seasonal encounter rate, life stages and main threats to the loggerhead sea turtle (Caretta caretta) in the aeolian archipelago (southern thyrrenian Sea). Aquat. Conserv. Mar. Freshw. Ecosyst. 27, 617–630. doi: 10.1002/aqc.2723
Bruno D., Willmer I. Q., Rocha R. C. C., Saint’Pierre T. D., Baldassin P., Scarelli A. C. S., et al. (2021). Metal and metalloid contamination in green Sea turtles (Chelonia mydas) found stranded in southeastern Brazil. Front. Mar. Sci. 8. doi: 10.3389/fmars.2021.608253
Bucchia M., Camacho M., Santos M. R., Boada L. D., Roncada P., Mateo R., et al. (2015). Plasma levels of pollutants are much higher in loggerhead turtle populations from the Adriatic Sea than in those from open waters (Eastern Atlantic ocean). Sci. Total Environ. 523, 161–169. doi: 10.1016/j.scitotenv.2015.03.047
Buller N. B. (2014). Bacterial and fungi from fish and other acquatic animals. 2nd ed. (CABI). Available at: https://www.cabidigitallibrary.org
Camiñas J. A., Báez J. C., Valeiras X., Real R. (2006). Differential loggerhead by-catch and direct mortality due to surface longlines according to boat strata and gear type. Scientia Marina. 70, 661–665. doi: 10.3989/scimar.2006.70n4661
Cammilleri G., Calvaruso E., Pantano L., Cascio G. L., Randisi B., Macaluso A., et al. (2017). Survey on the presence of non-dioxine-like PCBs (NDL-PCBs) in loggerhead turtles (Caretta caretta) stranded in south Mediterranean coasts (Sicily, southern Italy). Environ. Toxicol. Chem. 36, 2997–3002. doi: 10.1002/etc.3866
Campani T., Baini M., Giannetti M., Cancelli F., Mancusi C., Serena F., et al. (2013). Presence of plastic debris in loggerhead turtle stranded along the Tuscany coasts of the pelagos sanctuary for Mediterranean marine mammals (Italy). Mar. pollut. Bull. 74, 225–230. doi: 10.1016/j.marpolbul.2013.06.053
Canzanella S., Danese A., Mandato M., Lucifora G., Riverso C., Federico G., et al. (2021). Concentrations of trace elements in tissues of loggerhead turtles (Caretta caretta) from the tyrrhenian and the Ionian coastlines (Calabria, Italy). Environ. Sci. pollut. Res. Int. 28, 26545–26557. doi: 10.1007/s11356-021-12499-4
Caracappa S., Persichetti M. F., Piazza A., Caracappa G., Gentile A., Marineo S., et al. (2018). Incidental catch of loggerhead sea turtles (Caretta caretta) along the Sicilian coasts by longline fishery. Peer J. 6, e5392. doi: 10.7717/peerj.5392
Caracappa S., Pisciotta A., Persichetti M. F., Caracappa G., Alduina R., Arculeo M. (2016). Nonmodal scutes patterns in the loggerhead Sea turtle (Caretta caretta): a possible epigenetic effect? Can. J. Zool. 94, 379–383. doi: 10.1139/cjz-2015-0248
Casale P., Abbate G., Freggi D., Conte N., Oliverio M., Argano R. (2008). Foraging ecology of loggerhead sea turtles caretta caretta in the central Mediterranean Sea: evidence for a relaxed life history model. Mar. Ecol. Prog. Ser. 372, 265–276. doi: 10.3354/meps07702
Casale P., Affronte M., Insacco G., Freggi D., Vallini C., D’Astore P. P., et al. (2010). Sea Turtle strandings reveal high antropogenic mortality in Italian waters. Aquat. Conserv: Mar. Freshw. Ecosyst. 20, 611–620. doi: 10.1002/aqc.1133
Casale P., Mariani P. (2014). The first "lost year" of Mediterranean sea turtles: dispersal patterns indicate subregional management units for conservation. Mar. Ecol. Prog. Ser. 498, 263–274. doi: 10.3354/meps10640
Chaousis S., Leusch F. D., Limpus C. J., Nouwens A., Weijs L. J., Weltmeyer A., et al. (2023). Non-targeted proteomics reveals altered immune response in geographically distinct populations of green sea turtles (Chelonia mydas). Environ. Res. 216, 114352. doi: 10.1016/j.envres.2022.114352
Cioffi B., Monini M., Salamone M., Pellicanò R., Di Bartolo I., Guida M., et al. (2020). Environmental surveillance of human enteric viruses in wastewaters, groundwater, surface water and sediments of campania region. Regional Stud. Mar. Science. 38. doi: 10.1016/j.rsma.2020.101368
Clusa M., Carreras C., Pascual M., Gaughran S. J., Piovano S., Giacoma C., et al. (2014). Fine-scale distribution of juvenile Atlantic and Mediterranean loggerhead turtles (Caretta caretta) in the Mediterranean Sea. Mar. Biol. 161, 509–519. doi: 10.1007/s00227-013-2353-y
da Silva C. C., Varela A. S., Barcarolli I. F., Bianchini A. (2014). Concentrations and distributions of metals in tissues of stranded green sea turtles (Chelonia mydas) from the southern Atlantic coast of Brazil. Sci. Total Environ. 466–467, 109–118. doi: 10.1016/j.scitotenv.2013.06.094
Dujon A. M., Schofield G., Venegas R. M., Thomas F., Ujvari B. (2021). Sea Turtles in the cancer risk landscape: a global meta-analysis of fibropapillomatosis prevalence and associated risk factors. Pathogens. 10 (10), 1295. doi: 10.3390/pathogens10101295
Echwikhi K., Jribib I., Bradaiaand M. N., Bouain A. (2012). Overview of loggerhead turtles coastal nets interactions in the Mediterranean Sea. Aquat. Conserv: Mar. Freshw. Ecosyst. 22, 827–835. doi: 10.1002/aqc.2270
Espinoza J., Hernandez E., Lara-Uc M. M., Resendiz E., Alfaro-Nunez A., Hori-Oshima S., et al. (2020). Genetic analysis of chelonid herpesvirus 5 in marine turtles from Baja California peninsula. Ecohealth. 17, 258–263. doi: 10.1007/s10393-020-01482-z
Esposito M., Canzanella S., Iaccarino D., Bruno T., Esposito E., Di Nocera F., et al. (2022b). Levels of non-dioxin-like PCBs (NDL-PCBs) in liver of loggerhead turtles (Caretta caretta) from the tyrrhenian Sea (Southern Italy). Chemosphere. 308, 136393. doi: 10.1016/j.chemosphere.2022.136393
Esposito M., De Roma A., Sansone D., Capozzo D., Iaccarino D., Di Nocera F., et al. (2020). Non-essential toxic element (Cd, as, Hg and Pb) levels in muscle, liver and kidney of loggerhead sea turtles (Caretta caretta) stranded along the southwestern coasts of tyrrhenian sea. Comp. Biochem. Physiol. C. Toxicol. Pharmacol. 231, 108725. doi: 10.1016/j.cbpc.2020.108725
Esposito C., Fiorito F., Miletti G., Serra F., Balestrieri A., Cioffi B., et al. (2022a). Involvement of herpesviruses in cases of abortion among water buffaloes in southern Italy. Vet. Res. Commun. 46, 719–729. doi: 10.1007/s11259-022-09887-7
Fiorito F., Amoroso M. G., Lambiase S., Serpe F. P., Bruno T., Scaramuzzo A., et al. (2019). A relationship between environmental pollutants and enteric viruses in mussels (Mytilus galloprovincialis). Environ. Res. 169, 156–162. doi: 10.1016/j.envres.2018.11.001
Fiorito F., Di Concilio D., Lambiase S., Amoroso M. G., Langellotti A. L., Martello A., et al. (2021). Crassostrea gigas, a good model for correlating viral and chemical contamination in the marine environment. Mar. pollut. Bull. 172, 112825. doi: 10.1016/j.marpolbul.2021.112825
Fredricks D., Relman D. A. (1996). Sequence-based identification of microbial pathogens: a reconsideration of koch’s postulates. Clin. Microbiol. Rev. 9 (1), 18–33. doi: 10.1128/CMR.9.1.18
Garofalo L., Mastrogiacomo A., Casale P., Carlini R., Eleni C., Freggi D., et al. (2013). Genetic characterization of central Mediterranean stocks of the loggerhead turtle (Caretta caretta) using mitochondrial and nuclear markers, and conservation implications. Aquat. Conserv. Mar. Freshw. Ecosyst. 23, 868–884. doi: 10.1002/aqc.2338
Gomez-Ramirez P., Espin S., Navas I., Martinez-Lopez E., Jimenez P., Maria-Mojica P., et al. (2020). Mercury and organochlorine pesticides in tissues of loggerhead Sea turtles (Caretta caretta) stranded along the southwestern Mediterranean coastline (Andalusia, Spain). Bull. Environ. Contam. Toxicol. 104, 559–567. doi: 10.1007/s00128-020-02822-z
Gonzalez-Fernandez C., Cuesta A. (2022). Nanoplastics increase fish susceptibility to nodavirus infection and reduce antiviral immune responses. Int. J. @ Mol. Sci. 23 (3), 1483. doi: 10.3390/ijms23031483
Herbst L. H., Klein P. A. (1995). Green turtle fibropapillomatosis: challenges to assessing the role of environmental cofactors. Environ. Health Perspect. 103 (Suppl 4), 27–30. doi: 10.1289/ehp.95103s427
Hervas J., Sanchez-Cordon P. J., Chacon de Lara F., Carrasco L., Gomez-Villamandos J. C. (2002). Hepatitis associated with herpes viral infection in the tortoise ( Testudo horsfieldii ). J. Vet. Med. B Infect. Dis. Vet. Public Health 49, 111–114. doi: 10.1046/j.1439-0450.2002.00522.x
Hochscheid S., Maffucci F., Abella E., Bradai M. N., Camedda A., Carreras C., et al. (2022). Nesting range expansion of loggerhead turtles in the Mediterranean: phenology, spatial distribution, and conservation implications. Global Ecol. Conserv. 38. doi: 10.1016/j.gecco.2022.e02194
Iordache A. M., Nechita C., Voica C., Pluháček T., Schug K. A. (2022). Climate change extreme and seasonal toxic metal occurrence in Romanian freshwaters in the last two decades–case study and critical review. NPJ Clean Water. 5, 2. doi: 10.1038/s41545-021-00147-w
Jacobson E. R., Gaskin J. M., Roelke M., Greiner E. C., Allen J. (1986). Conjunctivitis, tracheitis, and pneumonia associated with herpesvirus infection in green sea turale. J. Am. Vet. Med. Assoc. 189, 1020–1023.
James A., Page-Karjian A., Charles K. E., Edwards J., Gregory C. R., Cheetham S., et al. (2021). Chelonid alphaherpesvirus 5 prevalence and first confirmed case of Sea turtle fibropapillomatosis in Grenada, West indies. Anim. (Basel). 11 (6), 1490. doi: 10.3390/ani11061490
Johnson A. J., Pessier A. P., Wellehan J. F., Brown R., Jacobson E. R. (2005). Identification of a novel herpesvirus from a California desert tortoise (Gopherus agassizii). Vet.Microbiol. 111, 107–116. doi: 10.1016/j.vetmic.2005.09.008
Jones K., Ariel E., Burgess G., Read M. (2016). A review of fibropapillomatosis in green turtles (Chelonia mydas). Vet. J. 212, 48–57. doi: 10.1016/j.tvjl.2015.10.041
Kühn S., Bravo Rebolledo E. L., van Franeker J. A. (2015). Deleterious Effects of Litter on Marine Life. In: Bergmann M., Gutow L., Klages M. (eds) Marine Anthropogenic Litter. Springer, Cham. doi: 10.1007/978-3-319-16510-3_4
Lambiase S., Ariano A., Serpe F. P., Scivicco M., Velotto S., Esposito M., et al. (2021b). Polycyclic aromatic hydrocarbons (PAHs), arsenic, chromium and lead in warty crab (Eriphia verrucosa): occurrence and risk assessment. Environ. Sci. pollut. Res. Int. 28, 35305–35315. doi: 10.1007/s11356-021-14824-3
Lambiase S., Serpe F. P., Pilia M., Fiorito F., Iaccarino D., Gallo P., et al. (2021a). Polychlorinated organic pollutants (PCDD/Fs and DL-PCBs) in loggerhead (Caretta caretta) and green (Chelonia mydas) turtles from central-southern tyrrhenian Sea. Chemosphere. 263, 128226. doi: 10.1016/j.chemosphere.2020.128226
Lawrance M. F., Mansfield K. L., Sutton E., Savage A. E. (2018). Molecular evolution of fibropapilloma-associated herpesviruses infecting juvenile green and loggerhead sea turtles. Virology. 521, 190–197. doi: 10.1016/j.virol.2018.06.012
Lazar B., Gračan R. (2011). Ingestion of marine debris by loggerhead sea turtles, caretta caretta, in the Adriatic Sea. Mar. pollut. Bull. 62, 43–47. doi: 10.1016/j.marpolbul.2010.09.013
Lazar B., Maslov L., Romanic S. H., Gracan R., Krauthacker B., Holcer D., et al. (2011). Accumulation of organochlorine contaminants in loggerhead sea turtles, caretta caretta, from the eastern Adriatic Sea. Chemosphere. 82, 121–129. doi: 10.1016/j.chemosphere.2010.09.015
LeMonte J. J., Stuckey J. W., Sanchez J. Z., Tappero R., Rinklebe J., Sparks D. L. (2017). Sea Level rise induced arsenic release from historically contaminated coastal soils. environ. Sci. Technol. 51, 5913–5922. doi: 10.1021/acs.est.6b06152
Loganathan A. L., Palaniappan P., Subbiah V. K. (2021). First evidence of chelonid herpesvirus 5 (ChHV5) infection in green turtles (Chelonia mydas) from sabah, Borneo. Pathogens. 10, 1404. doi: 10.3390/pathogens10111404
Maffucci F., Caurant F., Bustamante P., Bentivegna F. (2005). Trace element (Cd, Cu, Hg, Se, zn) accumulation and tissue distribution in loggerhead turtles (Caretta caretta) from the Western Mediterranean Sea (southern Italy). Chemosphere. 58, 535–542. doi: 10.1016/j.chemosphere.2004.09.032
Marenzoni M. L., Santoni L., Felici A., Maresca C., Stefanetti V., Sforna M., et al. (2018). Clinical, virological and epidemiological characterization of an outbreak of testudinid herpesvirus 3 in a chelonian captive breeding facility: lessons learned and first evidence of TeHV3 vertical transmission. PLoS One 13. doi: 10.1371/journal.pone.0197169
Marschang R. E., Papp T., Ferretti L., Hochscheid S., Bentivegna F. (2009). Detection and partial characterization of herpesviruses from Egyptian tortoises (Testudo kleinmanni) imported into Italy from Libya. J. Zoo Wildl Med. 40, 211–213. doi: 10.1638/2008-0045.1
Matiddi M., Hochsheid S., Camedda A., Baini M., Cocumelli C., Serena F., et al. (2017). Loggerhead sea turtles (Caretta caretta): a target species for monitoring litter ingested by marine organisms in the Mediterranean Sea. Environ. Pollution. 230, 199–209. doi: 10.1016/j.envpol.2017.06.054
Milton S. L., Lutz P. L. (2003). “Physiological and genetic responses to environmental stress,” in The biology of Sea turtles. Eds. Lutz P. L., Musick J. A., Wyneken J.(Boca Raton, FL, USA: CRC Press), 163–197.
Mitchell E., Frisbie S., Sarkar B. (2011). Exposure to multiple metals from groundwater-a global crisis: geology, climate change, health effects, testing, and mitigation. Metallomics. 3, 874–908. doi: 10.1039/c1mt00052g
Monteiro J., Duarte M., Amadou K., Barbosa C., El Bar N., Madeira F. M., et al. (2021). Fibropapillomatosis and the chelonid alphaherpesvirus 5 in green turtles from West Africa. Ecohealth. 18, 229–240. doi: 10.1007/s10393-021-01526-y
Nicolau N., Monteiro S. S., Pereira A. T., Marcalo A., Ferreira M., Torres J., et al. (2017). Trace elements in loggerhead turtles (Caretta caretta) stranded in mainland Portugal: bioaccumulation and tissue distribution. Chemosphere. 179, 120–126. doi: 10.1016/j.chemosphere.2017.03.108
Oriá A. P., Silva D. N., Raposo A. C., Estrela-Lima A., Pires T. T., Gattamorta M. A., et al. (2020). Atypical ocular chelonoid herpesvirus manifestations in a captive loggerhead turtle (Caretta caretta). Vet. Ophthalmol. 24, 97–102. doi: 10.1111/vop.12837
Origgi F. C., Romero C. H., Bloom D. C., Klein P. A., Gaskin J. M., Tucker S. J., et al. (2004). Experimental transmission of a herpesvirus in Greek tortoises (Testudo graeca). Vet. Pathol. 41, 50–61. doi: 10.1354/vp.41-1-50
Pagano M., Vazzana I., Gentile A., Caracappa G., Faggio C. (2019). Hematological and biochemical parameters in Sea turtles (Caretta caretta) after stranding. Regional Stud. Mar. Science. 32, 100832. doi: 10.1016/j.rsma.2019.100832
Page-Karjian A., Gottdenker N. L., Whitfield J., Herbst L., Norton T. M., Ritchie B. (2017). Potential noncutaneous sites of chelonid herpesvirus 5 persistence and shedding in green Sea turtles chelonia mydas. J. Aquat. Anim. Health 29 (3), 136–142. doi: 10.1080/08997659.2017.1321590
Page-Karjian A., Torres F., Zhang J., Rivera S., Diez C., Moore P., et al. (2012). Presence of chelonid fibropapilloma-associated herpesvirus in tumored and non-tumored green turtles, as detected by polymerase chain reaction, in endemic and non-endemic aggregations, Puerto Rico. Springerplus. 1, 35. doi: 10.1186/2193-1801-1-35
Perrault J. R., Levin M., Mott C. R., Bovery C. M., Bresette M. J., Chabot R. M., et al. (2021). Insights on immune function in free-ranging green Sea turtles (Chelonia mydas) with and without fibropapillomatosis. Anim. (Basel). 11 (3), 861. doi: 10.3390/ani11030861
Pettan-Brewer K. C., Drew M. L., Ramsay E., Mohr F. C., Lowenstine L. J. (1996). Herpesvirus particles associated with oral and respiratory lesions in a California desert tortoise (Gopherus agassizii). J. Wildl. Dis. 32, 521–526. doi: 10.7589/0090-3558-32.3.521
Pietroluongo G., Centelleghe C., Sciancalepore G., Ceolotto L., Danesi P., Pedrotti D., et al. (2023). Environmental and pathological factors affecting the hatching success of the two northernmost loggerhead sea turtle (Caretta caretta) nests. Sci. Rep. 13 (1), 2938. doi: 10.1038/s41598-023-30211-z
Piovano S., Clusa M., Carreras C., Giacoma C., Pascual M., Cardona L. (2011). Different growth rates between loggerhead sea turtles (Caretta caretta) of Mediterranean and Atlantic origin in the Mediterranean Sea. Mar. Biol. 158, 2577–2587. doi: 10.1007/s00227-011-1759-7
Piret J., Boivin G. (2021). DNA Polymerases of herpesviruses and their inhibitors. Enzymes. 50, 79–132. doi: 10.1016/bs.enz.2021.07.003
Quackenbush S. L., Work T. M., Balazs G. H., Casey R. N., Rovnak J., Chaves A., et al. (1998). Three closely related herpesviruses are associated with fibropapillomatosis in marine turtles. Virology. 246, 392–399. doi: 10.1006/viro.1998.9207
Rodenbusch C. R., Baptistotte C., Werneck M. R., Pires T. T., Melo M. T., de Ataide M. W., et al. (2014). Fibropapillomatosis in green turtles chelonia mydas in Brazil: characteristics of tumors and virus. Dis. Aquat. Organ. 111, 207–217. doi: 10.3354/dao02782
Sala B., Balasch A., Eljarrat E., Cardona L. (2021). First study on the presence of plastic additives in loggerhead sea turtles (Caretta caretta) from the Mediterranean Sea. Environ. pollut. 283, 117108. doi: 10.1016/j.envpol.2021.117108
Solomando A., Pujol F., Sureda A., Pinya S. (2022). Ingestion and characterization of plastic debris by loggerhead sea turtle, caretta caretta, in the Balearic islands. Sci. Total. Environ. 826, 154159. doi: 10.1016/j.scitotenv.2022.154159
Solomon S. E., Hendrickson J. R., Hendrickson L. P. (1986). The structure of the carapace and plastron of juvenile turtles, chelonia mydas (the green turtle) and caretta caretta (the loggerhead turtle). J. Anat. 145, 123–131.
Stacy B. A., Wellehan J. F. X., Foley A. M., Coberley S. S., Herbst L. H., Manire C. A., et al. (2008). Two herpesviruses associated with disease in wild Atlantic loggerhead sea turtles (Caretta caretta). Vet. Microbiol. 126, 63–73. doi: 10.1016/j.vetmic.2007.07.002
Storelli M. M., Barone G., Storelli A., Marcotrigiano G. O. (2011). Levels and congener profiles of PCBs and PCDD/Fs in blue shark (Prionace glauca) liver from the south-Eastern Mediterranean Sea (Italy). Chemosphere. 82, 37–42. doi: 10.1016/j.chemosphere.2010.10.014
Storelli M. M., Marcotrigiano G. O. (2000). Total organic and inorganic arsenic from marine turtles caretta caretta beached along the Italian coast (South Adriatic Sea). Bull. Environ-mental Contamination Toxicology. 65, 732–739. doi: 10.1007/s0012800184
Storelli M. M., Storelli A., D'Addabbo R., Marano C., Bruno R., Marcotrigiano G. O. (2005). Trace elements in loggerhead turtles (Caretta caretta) from the eastern Mediterranean Sea: overview and evaluation. Environ. pollut. 135 (1), 163–170. doi: 10.1016/j.envpol.2004.09.005
Sulato E. T., Luko-Sulato K., Pedrobom J. H., de Oliveira L. M. S., Lima G. D. S., Govone J. S., et al. (2022). Metals and metalloids in green turtle hepatic tissue (Chelonia mydas) from santos basin, Brazil. Environ. Res. 203, 111835. doi: 10.1016/j.envres.2021.111835
Tomas J., Aznar F. J., Raga J. A. (2001). Feeding ecology of the loggerhead turtle caretta caretta in the western Mediterranean. J. Zool. Lond. 255, 525–532. doi: 10.1017/S0952836901001613
Tomas J., Gozalbes P., Raga J. A., Godley B. J. (2008). Bycatch of loggerhead sea turtles: insights from 14 years of stranding data. Endangered Species Res. 5, 167–169. doi: 10.3354/esr00116
Tomás J., Guitart R., Mateo R., Raga J. A. (2002). Marine debris ingestion in loggerhead sea turtles, caretta caretta, from the Western Mediterranean. Mar. pollut. Bull. 44, 211–216. doi: 10.1016/s0025-326x(01)00236-3
Valavanidis A., Vlachogianni T. (2010). Metal pollution in ecosystems. ecotoxicology studies and risk assessment in the marine environment. Sci. Adv. Environment Toxicol. Ecotoxicology Issues. https://www.chem-tox-ecotox
Varol M. (2011). Assessment of heavy metal contamination in sediments of the Tigris river (Turkey) using pollution indices and multivariate statistical techniques. J. Hazard Mater. 195, 355–364. doi: 10.1016/j.jhazmat.2011.08.051
Williams E. H., Bunkley-Wlliams L., Peters E. C., Pinto-Rodriguez B., Matos-Morales R., Mignucci-Giannoni A. A., et al. (1994). An epizootic of cutaneous fibropapillomas in green turtles chelonia mydas of the Caribbean: part of a panzootic? Aquat. Anim. Health 6, 70–78. doi: 10.1577/1548-8667(1994)006<0070:AEOCFI>2.3.CO;2
Work T. M., Balazs G. H., Rameyer R. A., Morris R. A. (2004). Retrospective pathology survey of green turtles Chelonia mydas with fibropapillomatosis in the Hawaiian islands 1993-2003. Dis. Aquat. Org. 62, 163–176. doi: 10.3354/dao062163
Keywords: sea turtles, marine environment, chelonid herpesvirus 5, trace elements, intestinal plication, fracture of carapace
Citation: Serra F, Iaccarino D, Fiorito F, Di Nocera F, Esposito M, Cerracchio C, Esposito E, Lambiase S, Degli Uberti B, Lucifora G, De Carlo E, Fusco G and Amoroso MG (2023) Investigation on anthropogenic and opportunistic factors relevant to the incidence of stranded loggerhead sea turtle Caretta caretta along South Tyrrhenian coasts. Front. Mar. Sci. 10:1116804. doi: 10.3389/fmars.2023.1116804
Received: 05 December 2022; Accepted: 11 April 2023;
Published: 27 April 2023.
Edited by:
Julian Blasco, Spanish National Research Council (CSIC), SpainReviewed by:
Salvatore Siciliano, Fundação Oswaldo Cruz (Fiocruz), BrazilMontserrat Sole, Institute of Marine Sciences (CSIC), Spain
Copyright © 2023 Serra, Iaccarino, Fiorito, Di Nocera, Esposito, Cerracchio, Esposito, Lambiase, Degli Uberti, Lucifora, De Carlo, Fusco and Amoroso. This is an open-access article distributed under the terms of the Creative Commons Attribution License (CC BY). The use, distribution or reproduction in other forums is permitted, provided the original author(s) and the copyright owner(s) are credited and that the original publication in this journal is cited, in accordance with accepted academic practice. No use, distribution or reproduction is permitted which does not comply with these terms.
*Correspondence: Maria Grazia Amoroso, bWFyaWFncmF6aWEuYW1vcm9zb0BpenNtcG9ydGljaS5pdA==; Filomena Fiorito, Zmlsb21lbmEuZmlvcml0b0B1bmluYS5pdA==; Mauro Esposito, bWF1cm8uZXNwb3NpdG9AaXpzbXBvcnRpY2kuaXQ=
†These authors have contributed equally to this work and share first authorship