- 1Laboratoire des sciences de l’Environnement Marin (LEMAR), Université de Brest, Institut Universitaire Européen de la Mer (IUEM), Centre National de la Recherche Scientifique (CNRS), Place Nicolas Copernic, Plouzané, France
- 2Institut des Sciences de la Mer, Université du Québec à Rimouski, Rimouski, QC, Canada
- 3Applied Ocean Physics and Engineering Department, Woods Hole Oceanographic Institution, Woods Hole, MA, United States
- 4Laboratoire de Biologie des Organismes et Ecosystèmes Aquatiques (BOREA), Unité Mixte de Recherche (UMR) 8067, Muséum National d'Histoire Naturelle (MNHN), Centre National de la Recherche Scientifique (CNRS), Sorbonne Université (SU), Institut de Recherche et de Développement (IRD) 207, Université de Caen Normandie (UCN), Université des Antilles (UA), Paris, France
Noise is now recognized as a new form of pollution in marine coastal habitats. The development of marine renewable energies has introduced new sonorous perturbations, as the wind farm installation requires pile driving and drilling operations producing low frequency sounds at high sound pressure levels. Exponential expansion of offshore wind farms is occurring worldwide, making impact studies, particularly on benthic species highly abundant and diverse in the coastal area used for wind farming, a necessity. As larval recruitment is the basis for establishing a population, we conducted an experimental study to assess the interactive effects of pile driving or drilling sounds and larval rearing temperature on the endobenthic bivalve Venus verrucosa. In ectothermic animals, temperature modifies the organism’s physiology, resulting in performance variability. We hypothesize that temperature modulation could change larval responses to noise and explore the potential interacting effects of temperature and noise. Using two distinct rearing temperatures, physiologically different batches of larvae were produced with contrasting fatty acid content and composition in the neutral and polar lipid fractions. Without defining any absolute audition threshold for the larvae, we demonstrate that the effects of temperature and noise were ontogenic-dependent and modulated larval performance at the peri-metamorphic stage, acting on the metamorphosis dynamic. At the pediveligers stage, a strong interaction between both factors indicated that the response to noise was highly related to the physiological condition of the larvae. Finally, we suggest that underwater noise reduces the compensatory mechanisms established to balance the temperature increase.
1 Introduction
Thermal tolerance is species specific (Rayssac et al., 2010), with each species occupying a particular thermal niche of optimal functioning outside which it may fail to survive. Within a thermal range, temperature controls various essential features of an ectothermic organism’s physiology, as it alters chemical and enzymatic reactions, rates of diffusion, membrane fluidity, and protein structure (reviewed in Sokolova, 2021), resulting in performance variability. The present study focused on an ectothermic infaunal bivalve species, the warty venus Venus verrucosa, which lives on seagrass habitats, detrital sandy, or coralline rhodolith bottoms to a depth down to 30 m and has a great commercial interest (Arneri et al., 1998). As other filter-feeding bivalve, it provides ecosystem services as reviewed in Vaughn and Hoellein (2018) and Smaal et al. (2019). V. verrucosa has broad thermal tolerance that explains its large distribution in the Atlantic from Norway to South Africa, and in the Mediterranean Sea (Poppe and Goto, 1993). Recently, Forêt et al. (2020) showed that rearing temperature modulates the fatty acid profile of V. verrucosa, as juveniles reared at 20°C contained largely less energetic (neutral) lipids than those reared at 15°C. As the main energetic reserve in marine bivalve larvae are the neutral lipids (Holland and Spencer, 1973; Gallager et al., 1986; Whyte et al., 1991), they positively correlate with their survival (Rayssac et al., 2010). Thus, temperature modulation could have long-term impacts on fitness. Moreover, energy metabolism modulates the responses to multiple stressors (Sokolova, 2021), and temperature is known to interact with many other factors. For example, Cherkasov et al. (2007) showed that temperature amplifies the toxicity of cadmium, leading to elevated oxidative stress in mitochondria, which may have important implications for the survival of Magallana gigas. Reciprocally, cadmium pollution reduces the thermal tolerance of M. gigas (Lannig et al., 2006).
Aquatic anthropogenic noise was recently recognized as a new form of pollution (Barber et al., 2010; Slabbekoorn et al., 2010) and, as it increases annually (Chapman and Price, 2011; Tournadre, 2014), several authors have emphasized its impact on adult marine organisms, including behavior (Fewtrell and McCauley, 2012), oxygen intake (Regnault and Lagardere, 1983; Wale et al., 2013a), food uptake (Wale et al., 2013b; Charifi et al., 2017), growth (Lagardère, 1982), and gene expression (Peng et al., 2016), and could even induce severe injuries (André et al., 2011). Noise also impacts larval development of invertebrates, as some studies have revealed significantly deep effects, particularly on growth, survival, and settlement success (Branscomb and Rittschof, 1984; de Soto et al., 2013; Gigot et al., in revision; Wilkens et al., 2012; Lillis et al., 2015; Jolivet et al., 2016). While many authors agree that the main sensory organ involved in sound perception in bivalve larvae are statocysts (ciliated cells containing statolith or statoconia; Budelmann, 1992), that are observed at the pediveliger stage (Cragg and Nott, 1997; but see extensive review on invertebrates in Solé et al., 2023), their audition thresholds or sensitivity to particular frequencies remains largely unknown. Such research is particularly pertinent in the context of renewable energy device installations, such as wind farms, which usually settle in shallow coastal water overlapping areas of rich biodiversity (Ramirez et al., 2020). Offshore wind farms are growing in size and number, with a global capacity that could increase 7-fold by 2030 (Lee and Zhao, 2021) and involves drilling and pile driving operations that generate high levels of anthropophony (Norro et al., 2013). Pile driving noise results in short impulses with high sound pressure and broadband spectrum below 1 kHz (SPLp−p = 205 dB re 1 μPa @ 100 m) (Robinson et al., 2013). Drilling is characterized by a continuous broadband sound, with maximum energy between 100 Hz and 10 kHz (SPLrms =184 dB re 1 μPa @ 1m) (Kyhn et al., 2014).
Within this context, we tested whether temperature could modulate the response of V. verrucosa larvae to anthropogenic noise. Most bivalve species display a biphasic life-cycle with early swimming pelagic veliger larvae developing into a competent pediveliger stage able of settling and metamorphosing into a benthic post-larva (Figure 1). Pediveliger larvae select their benthic habitat upon several environmental biotic and abiotic variables (Toupoint et al., 2012) including soundscape (Lillis et al., 2013). We decided to study this particular transient phase and exposed larvae at pre-metamorphic veliger and peri-metamorphic pediveliger stages.
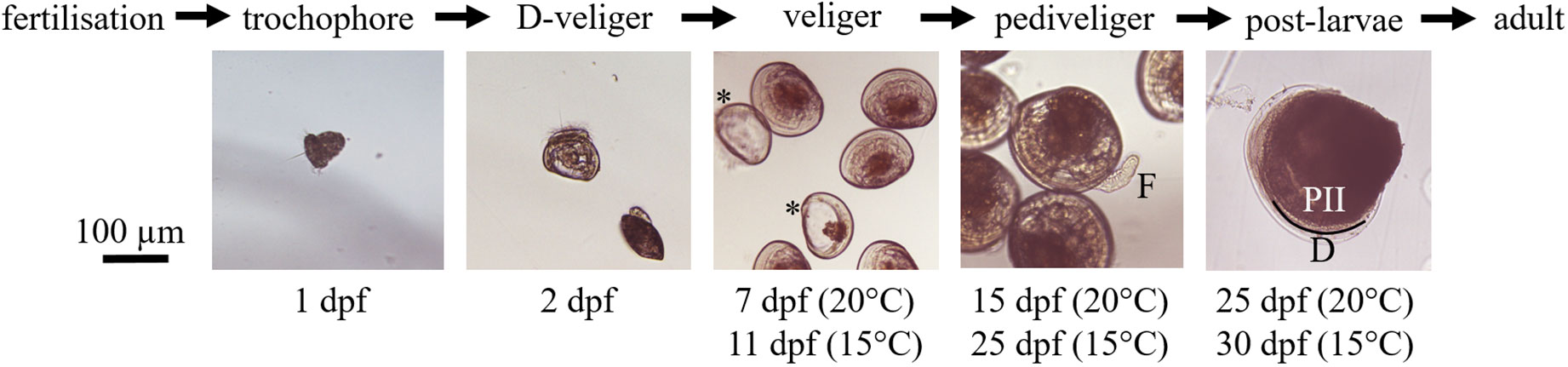
Figure 1 Larval development of V. verrucosa during the experiment. Corresponding days post-fertilization (dpf) are indicated below each picture for the two rearing temperatures. Stars (*) highlight two empty shells considered as dead veliger larvae. On pediveligers picture we can distinguish the ‘foot’ specific to this stage, indicated by (F). The demarcation between prodissoconch II (PII) and the dissoconch (D) shells, which is a criterion of metamorphosis, is materialized by a black line.
As sound impact on V. verrucosa has never been investigated, we hypothesize that drilling and pile driving playback modifies the settlement dynamics of competent pediveliger (Eggleston et al., 2016), as observed in epifauna species, such as blue mussel Mytilus edulis (Jolivet et al., 2016) and great scallop Pecten maximus (Gigot et al., 2023). Temperature mainly influences the physiological state of bivalve larvae by modifying the lipid composition (Pernet et al., 2007; Rayssac et al., 2010; Barret et al., 2016) and thereby could impact larval development, particularly the success of metamorphosis. Lipid accumulation (neutral) and membrane fatty acid (polar) composition acting on membrane fluidity are modified by temperature exposure and modulate the responses to stressors. We tested the hypothesis that response of warty venus to anthropogenic noise highly relates on their physiological state, both in terms of energetic reserves (neutral lipids) and fatty acid tissue composition (polar lipids). Thus, two larval batches were produced at different rearing temperature in the thermal niche of the warty venus to obtain contrasting total fatty acid (TFA) content and profiles, as already observed by Forêt et al. (2020) in young juveniles of V. verrucosa, before their exposure to pile driving and drilling noises.
2 Materials and methods
2.1 Thermal modulation of physiological state
Larvae were obtained following a modified protocol (Buestel et al., 1982) detailed by Forêt et al. (2020). Adults were collected by dredging in the Bay of Brest in January 2021 and fed continuously during gametogenesis at the ‘Ecloserie du Tinduff’ (Plougastel-Daoulas, France) with a DTCS diet (Diacronema lutheri, Tisochrysis luthea, Chaetoceros neogracilis, Skeletonema marinoi; ⅔ DT, ⅓ CS). Spawning was induced by thermal shock in 30 adults and cross-fertilization performed as described by Beaumont and Budd (1983). The resulting eggs were incubated for 48 h at 18°C in cylindro-conical tanks filled with 1-µm filtered, UV-treated seawater treated with 9 ppm erythromycin to avoid bacterial development (salinity = 33 psu; temperature = 19.5°C). Use of erythromycin treatment on scallop larvae has been demonstrated do not impact the long-term P. maximus larval performance (Holbach et al., 2015). Two days after fertilization (2 dpf), trochophore larvae were sieved and transferred to two larval tanks at a temperature of either 15°C or 20°C (Figure 2), and at 40 larvae/ml. Each day, the water was renewed and dead individuals counted and removed by sieving. Larvae were fed daily with a 3:3:2:2 ratio of DTCN diet (N for Nannochloropsis occulata) at 40 cells/µl adjusted to the biovolume of T. luthea (Helm and Bourne, 2006). At the pediveliger stage, larvae were fed with a DTCSN diet (1:1:1:1:1). We conducted two experiments, one at the veliger stage and the second at the pediveliger stage (Figure 2). During the first day (d0) of each experiment, larval samples were fixed in 4% formaldehyde until further abundance counting. Sampled larvae were counted and measured under a microscope (Zeiss Axioscope A1, x40 magnification) equipped with a digital camera (Moticam 3.0 10+). To assess the mortality rate at d0 in each tank sample (Figure 2 Section 1), we calculated the ratio between empty shells (Figure 1) and alive larvae. Mean shell length, from the umbo to the most distant part of the shell, was measured using Motic Images plus 3.0 software for 100 individuals in each tank. Growth rates were then calculated separately for veliger/pediveliger and 15/20°C batches by dividing the mean size deducted from the length at d0 by the number of days since fertilization (dpf).
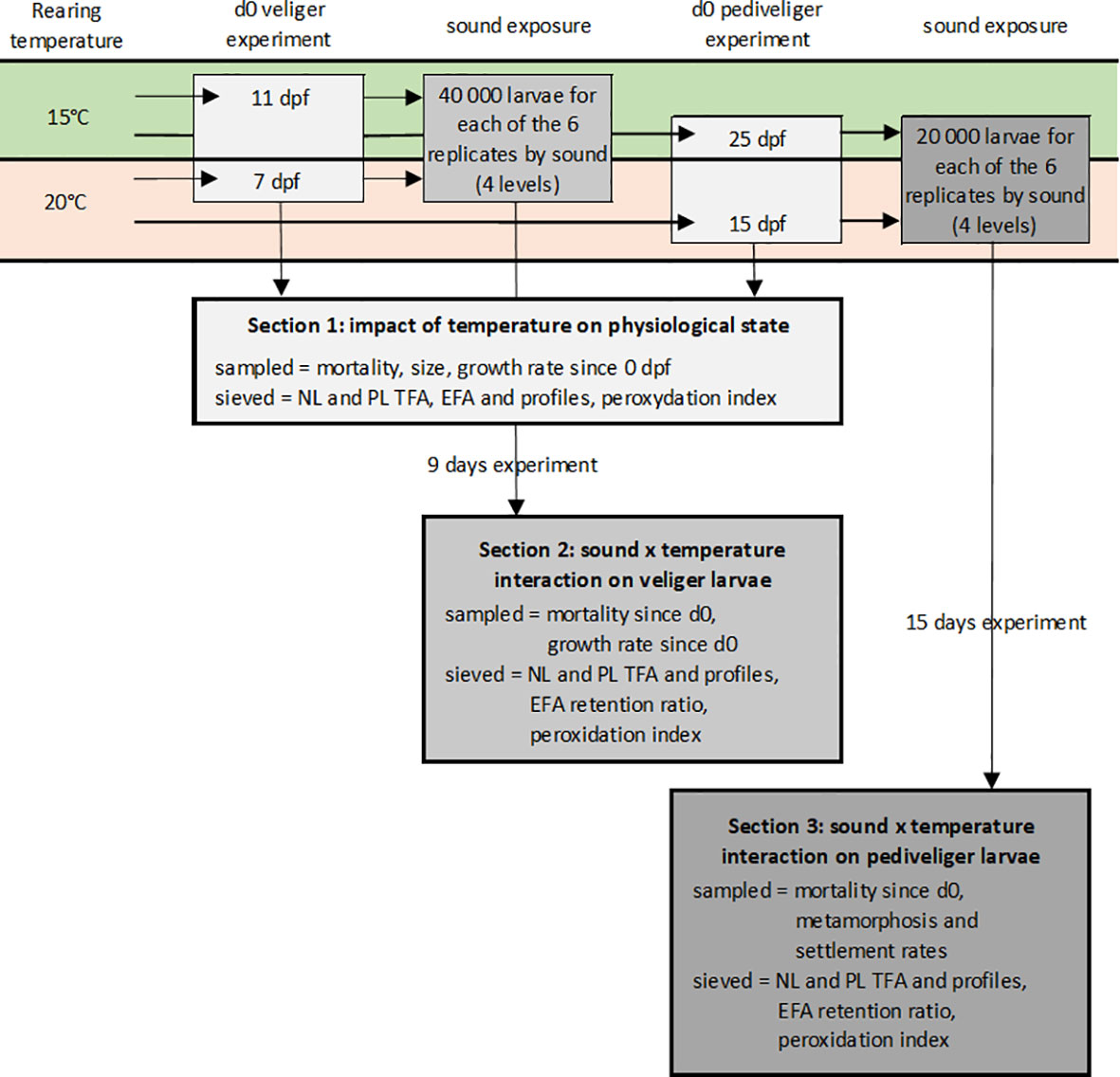
Figure 2 Schematic representation of the experimental protocol. Two batches of Venus verrucosa larvae were reared at 15 and 20°C. After 11 or 7 dpf and 25 or 15 dpf, depending on the batch, veliger and pediveliger larvae replicates were sampled and sieved to determine the impact of temperature on their physiological state. The veliger and pediveliger replicates were separately exposed to two temperatures (15 and 20°C) and four sound levels (control, low and high pile driving, and high drilling) for 9 and 15 days. At the end of the experiments, larvae were sampled and sieved to determine the potential interaction between the two factors.
At d0 of the veliger and pediveliger experiments (Figure 2 Section 1), batches of 40 000 and 20 000 larvae, respectively, from each of the 15 and 20°C populations, and DTCSN and DTCN diets (4 replicates of each) were sieved on pre-burned glass microfiber filters (GF/F) and stored at -80°C until fatty acid analyses. The GF/F filters were first lyophilized, weighed, and lipids extracted following the procedure in Folch et al. (1957) using dichloromethane–methanol instead of chloroform as modified by Parrish (1987). Extracts were separated into neutral and polar fractions by chromatography on silica gel micro-columns (30×5 mm i.d., packed with Kieselgel 60, 70–230 mesh; Merck, Darmstadt, Germany) (Marty et al., 1992). Neutral lipids represent energetic lipids mainly Triacylglycerids (TAG) and polar lipids are structural lipids mainly Phospholipids (PL). Each fraction was methylated in fatty acid methyl esters (FAMEs) following the modified method from Lepage and Roy (1984), and the NL samples were purified on an activated silica gel with 1 mL of hexane:ethyl acetate (v/v) to eliminate free sterols. FAMEs were analyzed in the full scan mode (ionic range: 50–650 m/z) on a Polaris Q ion trap coupled multichannel gas chromatograph (Trace GC ultra, Thermo Scientific, MA, USA) equipped with an autosampler (model Triplus), PTV injector, and mass detector (model ITQ900, Thermo Scientific, MA, USA). Separation was performed through a Supelco Omegawax 250 capillary column (30 m × 250 µm × 0.25 µm film thickness). The initial oven temperature was 100°C for 2 min, then 140°C for 1 min, and was increased at a rate of 10°C/min until it reached 270°C, where it was held for 15 min. The injector temperature was 90°C and a constant helium flow of 1.0 ml/min was used. A volume of 1 µl was injected. Fatty acids were identified and quantified by comparing retention times and mass spectra with a calibration curve of known standards with concentrations ranging from 0.5 to 20 μg/ml (Supelco 37 Component FAME Mix Supelco Inc., Belfonte, PA, USA) using Xcalibur v.2.1 software (Thermo Scientific, Mississauga, ON, CA). Fatty acids are designated as X:YwZ, where X is the number of carbons, Y the number of double bonds, and Z the position of the ultimate double bond from the terminal methyl group. We report the values of each fatty acid as absolute concentrations (mg/g) or percentage of TFA (for profile composition comparisons) separately for NL and PL fractions. We present the concentrations of three essential fatty acids (EFAs): arachidonic acid (ARA, 20:4w6), eicosapentoic acid (EPA, 20:5w3), and docosahexaenoic acid (DHA, 22:6w3).
All data analyses were performed in PRIMER7 software using the PERMANOVA+ package. We performed analysis of variance for univariate or multivariate data on resemblance matrices using 10 000 permutations (Legendre and Legendre, 2012) under a reduced model. Similarity matrices were produced using Bray-Curtis or Euclidean distance on fatty acid profiles and all other data, respectively. For both veliger and pediveliger stages, univariate 1-factor PERMANOVA were performed on d0 data, including size, growth rate, and TFA and EFA concentrations separately in NL and PL fractions to test the impact of rearing temperature (15°C and 20°C). We also conducted a multivariate PERMANOVA on the whole fatty acid profile for both lipid fractions (NL/PL) and both larval stages (veliger/pediveliger). When a significant difference was detected in the fatty acid profiles (p-perm < 0.05), a similarity percentage breakdown (SIMPER) (Clarke, 1993) was computed to determine which fatty acid contributes the most to the difference.
2.2 Sound × temperature interaction on veliger larvae
Exposure to drilling and pile driving sounds was realized using the Larvosonic system (Olivier et al., 2023), which includes a main 800-l tank, and a Clark Synthesis AQ339 Diluvio™ underwater speaker (https://clarksynthesis.com/aq339/) connected to a power amplifier allows sound emission to six 5-l cylinders (independent replication units above the speaker) half-immersed in this water bath. Because invertebrates lack gas-filled organs classically used to sense the pressure component of sound, they are sensitive to the motion of water particles via statocysts (Mooney et al., 2012; Popper and Hawkins, 2018). However, in the Larvosonic system, we demonstrate experimentally that when the sound level decreases, both acoustic pressure and particle motion decrease by exactly the same level (Olivier et al., 2023). Audition thresholds of the larvae are unknown and our experiment is not intended to define them but to explore initially the potential responses of the larvae to anthropic sound at levels comparable to those emitted in the natural environment. Emission levels were calculated by recording 30 s of sound at the center of each tank using an RTSYS EA-SDA14 (https://rtsys.eu/) underwater acoustic recorder (sampling frequency 78 kHz, 32-bit resolution) equipped with an HTI-96-min hydrophone (sensitivity = -165 dB re 1 V/µPa). Then emission levels were adjusted to match our experimental design. The pile driving sound sequence was recorded during the building phase of an offshore marine wind farm in the North Sea (depth ~30 m, SOMME database), and the drilling sound sequence corresponds to a recording of geotechnical drilling made in June 2018 at a distance of 200 m from the boat (SOMME database). Both sounds were the same than those characterized in Olivier et al. (2023). Pile driving is an impulsive sound (one 200-ms impulse every 3 s) dominated by low frequencies (40 - 800 Hz) (Supplementary Material S1). Drilling is continuous, and its spectrum is characterized by a high level in the 150 - 600 Hz and 4000 - 7000 Hz frequency ranges (Supplementary Material S1). Different Larvosonic tanks (n=8) with non-filtered seawater were deployed equitably in two controlled rooms (15°C and 20°C) under a 12:12h photoperiod. In each room, we generated drilling at high intensity (called D) in one tank (SPLrms = 175.4 ± 2.3 dB re 1 µPa-1), and two increasing levels of pile driving (P and P+) in two other experimental tanks (SPLpp = 147.6 ± 2.5 and 187.6 ± 2.4 dB re 1 µPa-1). As no sound was emitted in the fourth control tank, it characterized the ambient sound of the experimental room. The frequency content was maximum under 1000 Hz (low-frequencies) and levels (SPLrms = 98.8 ± 0.8 dB re 1 µPa-1) and spectrum were consistent with ambient sound levels recorded in temperate coastal environments of the western English Channel with contrasting wind conditions (Mathias et al., 2016) (Supplementary Material S1). However control condition do not reproduce natural acoustic conditions and the objective is to investigate the effect of the addition of anthropic sounds.
Veliger experiments started when mean larval length reached 124 µm (i.e., 7 or 11 dpf for larvae reared at 20 or 15°C, respectively). On the first day of the experiment, cylinders were filled with 5 l of 1-µm filtered, UV-treated seawater and 9 ppm erythromycin. Approximately 40 000 veliger larvae were introduced into each of the 48 cylinders. Drilling and pile driving sounds were emitted following 19:5 h and 6:6 h on:off cycles, matching the on-site work conditions (Ailes Marines pers. com.) for 9 days (Figure 2 Section 2). Larvae were fed once a day with mix algae at a concentration of 40 cells/µl as already described. Every 3 days, dead and alive larvae were sieved and the water renewed. At the end of veliger exposure (day 9), three larval samples were taken in each cylinder and fixed with 4% formaldehyde. Mortality and growth rates were assessed as the difference between the means on day 9 (N = 48) and d0. The daily growth rate was measured on 33 individuals by replicate, then divided by the number of days (i.e., 9 days). The remaining larvae were sieved on GF/F filters and stored at -80°C until further analysis of the fatty acids as described previously. We calculated absolute concentrations of TFA, fatty acid profiles (%), and the EFA selective retention ratio (ratio between PL fatty acids contained in larvae and the concentration of the total fraction of the same fatty acid in diet to investigate potential selective retention) separately for the NL and PL fractions. If the relative proportion of a fatty acid in the larvae/diet was >1, it was selectively incorporated and could suggest potential dietary deficiency under this rearing condition.
Separately for each parameter (mortality and daily growth rates, TFA concentrations, fatty acid profiles, EFA ratios, and peroxidation index), two-way PERMANOVA was performed to assess the impact and potential interaction between temperature (15°C and 20°C) and sound (C, D, P, and P+) treatments. Significant differences were analyzed by multiple comparison pairwise tests, and fatty acids that contribute the most to the significant difference between fatty acid profiles were assessed by similarity percentage breakdown (SIMPER).
2.3 Sound × temperature interaction on pediveliger larvae
Pediveligers were exposed to similar sound and temperature treatments as veligers except for the following points. The experiment was started when pediveligers reached a mean length of 190 µm at 15 and 25 dpf for 20°C and 15°C batches, respectively. Approximately 20 000 pediveligers were introduced in each replicate cylinder and exposed to sound treatments for 15 days (Figure 2 Section 3). At each seawater renewal (days 3, 6, 9, 12, or 15), each cylinder was gently rinsed over a 60-µm square mesh sieve to collect swimming larvae. Crawling larvae were detached from the walls and bottom of each cylinder by a gentle water jet and set apart. Three samples were taken in both the swimming and crawling larval fraction for further counting. On days 9 and 12, the remaining crawlers were sieved on GF/F filters and swimmers were put back into the cylinders. On day 15, both fractions were sieved on GF/F filters and stored at -80°C. The NL and PL fatty acid content of larvae collected on day 15 in each replicate cylinder were assessed on pooled swimmer and crawler fractions using previously described methods.
Mortality rates were assessed for each of the samples (N = 48) on days 9, 12, and 15 by subtracting the d0 mortality rate. We used the presence of demarcation between prodissoconch II and the dissoconch shells as a criterion of metamorphosis (Martel et al., 1995) to determine metamorphosis rates. As on days 9 and 12, the crawler fraction was removed from the cylinders, and we integrated the mortality and metamorphosis rates of those fractions into the mortality rate of the following samples. is the number of metamorphosed larvae in the crawler fraction on day 9, is the number of metamorphosed larvae in the crawler fraction on day 12, is the number of metamorphosed larvae in the crawler and swimmer fractions on day 15, is the number of alive larvae in the crawler fraction on day 9, is the number of alive larvae in the crawler fraction on day 12, and is the number of alive larvae in the crawler and swimmer fractions on day 15. The cumulative metamorphosed rate on day 15 ( )as defined as:
Calculation of the mortality rate followed the same pattern, with the number of dead larvae instead of metamorphosed and total larvae instead of alive.
Separately for each parameter (mortality, metamorphosis and settlement rate, TFA concentrations, fatty acid profiles, EFA ratios, and peroxidation index), two-way PERMANOVA was performed to assess the impact and potential interaction between temperature (15°C and 20°C) and sound (C, D, P, P+). Significant differences were analyzed by multiple comparison pairwise tests and SIMPER analyses.
3 Results
3.1 Thermal modulation of physiological state
Daily growth rates varied according to rearing temperature at both the veliger and pediveliger stage (Table 1B). Growth was 55% and 67% higher for veliger and pediveliger larvae reared at 20°C compared to 15°C (Table 1A). To avoid a length difference between thermal batches at the start of both the veliger and pediveliger experiments (Table 1B), larvae were collected at different rearing times (7 and 11 dpf at 20 and 15°C, respectively).
In veligers, the fatty acid profiles in NL and PL fractions varied according to temperature (Table 1B, see SIMPER analyses S1(a) and S1(b) for fatty acid contributing to the differences). At 15°C, veligers accumulated 1.9- and 1.5-fold more 20:5w3 and 22:6w3 than rearing at 20°C (Table 1A); this difference was close to significant for 20:4w6 (Table 1B), but only in the NL fraction. These results reflect the accumulation of TFAs observed only in the NL fraction, with 57% more TFA at 15°C than 20°C (Tables 1A, B). Without changes to the fatty acid composition in the PL fraction, the membrane peroxidation index showed no difference between rearing temperatures.
At the pediveliger stage, the fatty acid profile varied according to temperature only in the NL fraction (Table 1B, see SIMPER analysis S1(c)) without changes in TFA or EFA concentrations. In the PL fraction, no differences were observed according to rearing temperature (Tables 1A, B).
3.2 Sound × temperature interaction on veliger larvae
In veliger larvae, no interaction between the two stressors was observed for each variable measured, but effects related to temperature were observed for the majority of variables and the effect of sound for fewer variables. Mortality, daily growth, and TFA concentration in the NL fraction were related to temperature change only without the impact of sound (Table 2B). Thus, mortality rates and daily growth rates at 20°C were 5.8- and 1.2-fold higher than in the 15°C batches, respectively (Table 2A; Figure 3C). Larvae reared at 15°C contained 35% more NL fatty acids. Rearing temperature also impacted the fatty acid composition of the NL fraction of larvae (Table 2B), with higher levels of 20:5w3 at 15°C (see SIMPER analyses S2(b) for fatty acids contributing to the difference). In this case, the sound treatment also showed a significant effect, with variation only between drilling (D) and high-level pile driving (P+) (p-perm pairwise = 0.0467). In the PL fraction, differences were related to temperature and sound treatment. Fatty acid profiles varied according to temperature and pile driving but not to drilling noise in the PL (p-perm = 0.0196 and 0.0021 for C vs. P and C vs. P+ pairwise test, respectively) The 20:5w3, 16:0, and 16:1w5 were higher in larvae reared at 15°C, but with a lower value for 22:6w3. The two pile driving treatments were associated with higher accumulation of 22:6w3, 20:5w3, and 16:1w5 than control larvae (see SIMPER analyses S2(c) and S2(d)). The TFA concentration varied according to temperature, with 14% higher fatty acid concentration in the PL fraction of 15°C larvae, and according to sound, as larvae exposed to pile driving concentrated 18% more TFA in their PL fraction (Tables 2A, B; p-perm = 0.0065 and 0.001 for C vs. P and C vs. P+ pairwise test, respectively). Consequently, these changes in TFA and fatty acid composition modified the peroxidation index in the PL fraction according to rearing temperature and sound exposure, with values 16.5% higher for larvae reared at 15°C and 23% higher for larvae exposed to pile driving (Table 2A; p-perm = 0.0034 and 0.0006 for C vs. P and C vs. P+ pairwise test, respectively).
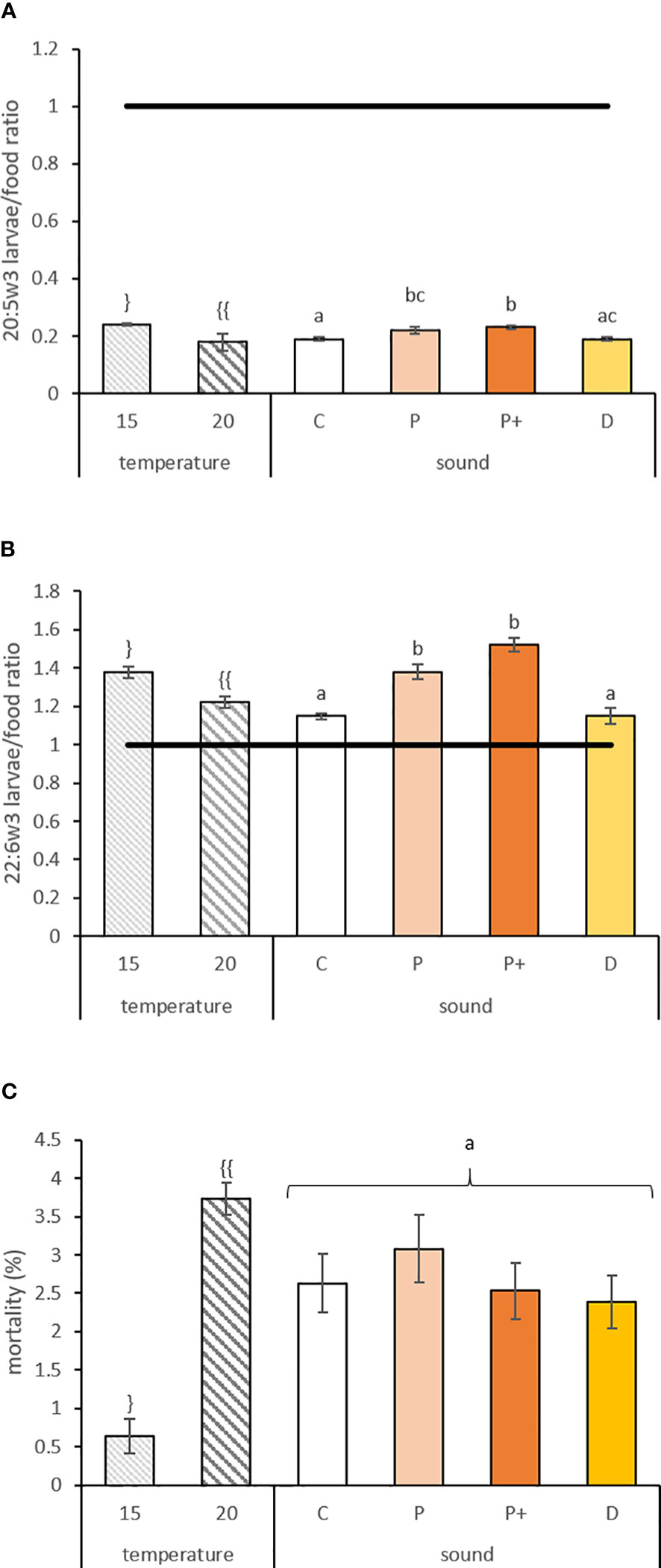
Figure 3 (A) 20:5w3 and (B) 22:6w3 larvae/food ratio and (C) mortality (%) ± standard error for veligers larvae reared at different temperatures (15 and 20°C) and exposed to sounds for 9 days (C, P, P+, and D represent control, low level pile driving, high level pile driving, and drilling, respectively). The line indicates equal amounts of fatty acids in the larvae and in the diet. Values annotated with different letters or symbols differed significantly at p-perm< 0.05. “}” and “{{“ represent significant difference of the values due to a temperature effect. “a”, “b” and “c” represent significant difference and similarity of the values due to sound effect.
The EFA selective retention ratios of 20:5w3 and 22:6w3 varied according to temperature and sound exposure, with levels 25% and 12% higher in larvae exposed to 15°C for 20:5w3 (df = 1; p-perm = 0.0001) and 22:6w3 (df = 1; p-perm = 0.0231), respectively (Figures 3A, B). These two fatty acids were also 18% and 26% higher in larvae exposed to pile driving sounds compared to the control (Table 2A) for 20:5w3 (df = 3; p-perm = 0.0026) and 22:6w3 (df = 3; p-perm = 0.0026), respectively (Figures 3A, B). However, all ratios were systemically less than or approximately 1. However, 20:4w6 showed no variation (p-perm = 0.3321 and 0.6703 for temperature and sound, respectively), with a mean ratio<1. Thus, a potential dietary deficiency of EFA was not observed for veliger larvae for any of the tested treatments.
3.3 Sound × temperature interaction on pediveliger larvae
In pediveliger larvae, interactions between temperature and sound were significantly observed only for some variables associated with fatty acids. Mortality rates varied according to rearing temperature and sound treatment (Table 3B); they were 9-fold higher when larvae were reared at 15°C (Table 3A) and reduced by 33% and 29% when larvae were exposed to drilling and pile driving sounds, respectively (Table 3A; Figure 4C). Metamorphosis and settlement rates varied only according to temperature, with higher values observed at 15°C compared to 20°C (Table 3B), with a 33% and 30% increase for metamorphosis and settlement, respectively (Table 3A). Settlement rates varied according to temperature; when reared at 20°C, larvae settled 30% lower than when reared at 15°C (Table 3A). The interaction between sound and temperature was near significant (p-perm=0.06; Table 3B), with settlement 29% lower in larvae reared at 20°C and exposed to pile driving sounds compared to the control condition (Table 3A).
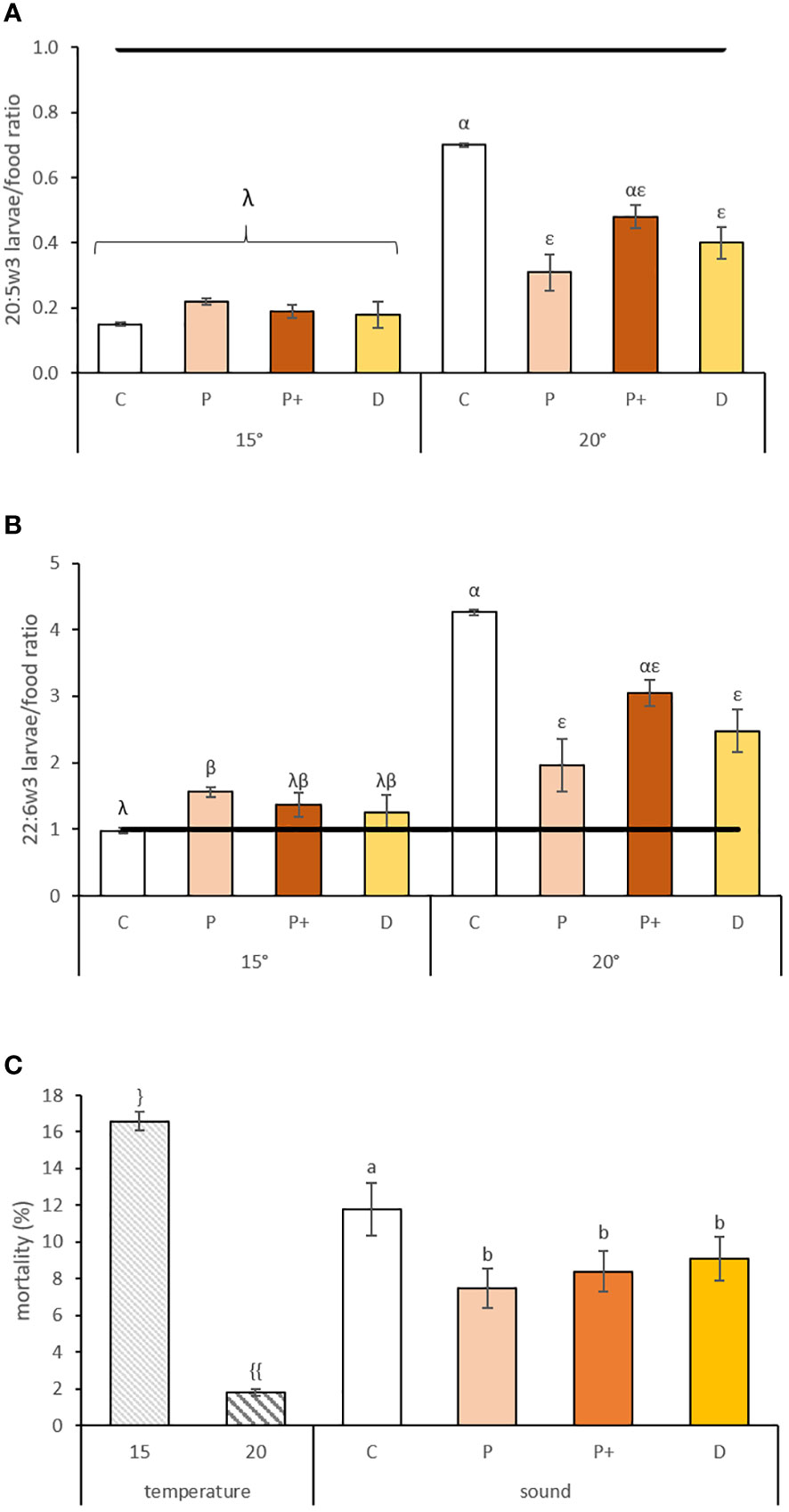
Figure 4 (A) 20:5w3 and (B) 22:6w3 larvae/food ratio and (C) mortality (%) ± standard error for pediveligers larvae reared at different temperatures (15 and 20°C) and exposed to sounds for 15 days (C, P, P+ and D represent control, low level pile driving, high level pile driving, and drilling, respectively). The line indicates equal amounts of fatty acids in the larvae and in the diet. Values annotated with different letters or symbols differed significantly at p-perm< 0.05. “}” and “{{“ represent significant difference of the values due to a temperature effect. “a” and “b” represent significant difference and similarity of the values due to sound effect.” Greek letters represent significant effect of temperature and sound interaction, with “λ” and “β” representing significant difference and similarity due to sound effect for the 15°C exposed larvae and “α” and “ε” representing significant difference and similarity due to sound effect for the 20°C exposed larvae.
The fatty acid profiles of pediveliger larvae varied according to temperature in both the NL and PL fractions without any interaction or effect of sound (Table 3B) and was mainly associated with higher accumulation of 22:5w3 and 16:1w5 and lower level of 22:6w3 in the NL fraction. In the PL fraction, we observed a higher accumulation of 22:6w3 and 16:0 in combination with lower levels of 18:0 at 20°C (see SIMPER analyses S3(a) and S3(b)). An interaction between both factors was observed in TFA concentration (Table 3B), but only for the NL fraction, with higher values in larvae reared at 20°C, particularly for the control, with nearly twice the TFA concentration than larvae exposed to anthropogenic sounds (Table 3A). In the PL fraction, only temperature affected the TFA concentration, with a value 2.2-fold higher at 20°C than at 15°C (Table 3A). The higher fatty acid concentration in the PL fraction of larvae reared at 20°C in combination with higher accumulation of 22:6w3, resulting in an increased peroxidation index (Table 3B).
The EFA selective retention ratio of 20:4w6 varied according to temperature (df = 1; p-perm = 0.0001) and was 1.4-fold lower for larvae reared at 20°C compared to 15°C (1.78 vs. 1.28, respectively). The selective retention ratio of 20:5w3 and 22:6w3 varied according to an interaction between sound and temperature (df = 3 and p-perm = 0.0056 for 20:5w3; df = 3 and p-perm = 0.0106 for 22:6w3). Larvae reared at 20°C had a 2.3- or 1.75-fold reduction of the 20:5w3 ratio when exposed to low level pile driving or drilling sounds compared to the control condition, but all ratios were<1 (Figure 4A). The retention ratio of 22:6w3 showed selective retention for all treatments and was 2.2-fold reduced by exposure to low-level pile driving sounds and 1.7-fold increased by exposure to drilling sounds, but only for larvae exposed to 20°C (Figure 4B). Thus, a potential dietary deficiency was observed for 22:6w3, mainly in pediveliger larvae reared at 20°C (control value >4), and anthropogenic sounds seem to decrease the level of selective retention and, thus, the dietary deficiency with ratio values ≤3.
4 Discussion
As expected, the present study showed that the physiological state of veliger and pediveliger stages of V. verrucosa was highly impacted by the temperature experienced by larvae during their development. In the veliger stage, larvae accumulated more neutral lipids when exposed at 15°C, and pediveliger showed a potential dietary deficiency of EFAs at 20°C, specifically 22:6n3. We show complex interactions between rearing temperature and anthropogenic sound exposure associated with the installation of offshore wind turbines that clearly impact larvae. The response of warty venus to noise appears to be highly dependent on both developmental stage and physiological state.
4.1 Impact of rearing temperature on larval physiology
The physiological state of bivalve larvae is based on their lipid content (Pernet et al., 2005), which relies on biotic and abiotic environmental factors, such as diet quality (Delaunay et al., 1993; Pernet and Tremblay, 2004) and temperature (Pernet et al., 2007). Lipids play a central role in supporting larval development (Glencross, 2009). By rearing larvae at two temperatures, we were able to produce two physiologically contrasting larval batches with distinct performances, fatty acid content, and profiles, mainly for NL fractions. As expected, larval growth was faster at 20°C (Bayne, 1965; Pechenik, 1990), which explains why veliger and pediveliger experiments with 15 and 20°C batches started at different times post-fertilization based on size criteria. This size threshold we adopted allows larval experiments to start at a similar developmental stage (Forêt et al., 2020). Lipids are essential for the development of bivalves, specifically polar lipids, which are mainly phospholipids incorporated in membranes and maintain cell membrane integrity in invertebrate species (Gallager et al., 1986). Because all fatty acids have different properties, the fatty acid composition of the PL fraction influences the membrane fluidity and peroxidation index, which is a proxy for the membrane susceptibility to peroxidation. For example, the membrane is prone to peroxidative damage as higher proportions of polyunsaturated fatty acids are found in the PL fraction (Hulbert et al., 2007). Conversely, a membrane with higher proportions of saturated and monounsaturated fatty acids will be more resistant to lipid peroxidation, which produces highly reactive molecules that can also cause damage to membrane DNA and proteins (Sukhotin et al., 2002). By modulating the rearing temperature from 15°C to 20°C, we modified the fatty acid composition of larval membrane in pediveligers, increasing lipid peroxidation 2.4-times and subsequent sensitivity to membrane lipids to potential oxidative damage. However, this pattern was not observed in veliger larvae.
The lipid content and composition of the NL fraction traduced the larval energetic storage (Gallager et al., 1986). Veligers reared at 20°C had lower energetic reserves (TFA in NL fraction) and concentrations of EFAs in the NL fraction, as well as a higher mortality rate at the end of the experiment. At the subsequent pediveliger stage, 20°C larvae accumulated more energetic reserves and no significant differences from larvae reared at 15°C were detected when starting pediveliger experiments. However, at the end of the experiment, pediveligers reared at 20°C had 2.6-fold more energetic lipids and 2.2-fold more membrane lipids than those reared at 15°C, which was coupled with a highly reduced mortality rate. However, lipid accumulation in the NL fraction was different between sound treatments, with higher values in the control. The positive correlation between higher temperature and increased lipid content at the pediveliger stage highly depends on the thermal optimum, which is species-specific (Rayssac et al., 2010; Pörtner et al., 2017). Fatty acid content is highly correlated with larval performance (Delaunay et al., 1992; Pernet and Tremblay, 2004), with a positive relationship between energy reserves and survival rates (Rayssac et al., 2010). Previous work on young stages of V. verrucosa showed that 60 dpf juveniles reared at 20°C accumulated 2 to 3 fewer lipids, as well as less lower triacylglycerols content, a main component of energetic storage, than those reared at 15°C (Forêt et al., 2020). Such thermal influence diverges from our data acquired on pediveligers but is in agreement with the veliger data affording for ontogenic variations (Pernet et al., 2007). High variation among larval stages has been reported in the literature; Marty et al. (1992) described 10-fold higher TFA content in great scallop pediveligers than in veligers. Previous studies suggest that the selective pressure of temperature is highly ontogenic (Pörtner et al., 2017) and predominant during early ontogeny (Rayssac et al., 2010), which is in accordance with the inverted effect of rearing temperature observed on veliger and pediveliger larvae. Thus, V. verrucosa seems to accumulate less energetic reserves and structural lipids at pre-metamorphic (our results) and post-metamorphic (Forêt et al., 2020) stages when reared at high temperature, whereas the peri-metamorphic stage (our results) stores more energetic and structural lipids. As increasing temperature usually raises the metabolism, this lipid accumulation at the pediveliger stage could be related to higher metabolic and energetic needs during metamorphosis. The costs associated with acclimatizing to thermal stress during metamorphosis seems to be offset by higher fatty acid accumulation (Zippay and Helmuth, 2012).
The strong increase in lipid concentration and selective storage of dietary lipids 20:4w6 and 22:6w3 at the pediveliger stage compared to the veliger stage indicates a transition from endogenous to exogenous nutrition (Delaunay et al., 1992; Pernet et al., 2005). At the early veliger stage, the energetic content of larvae is mostly based on lipid reserves transferred from the mother to the egg (Yamamoto et al., 1999). We hypothesize that, at the young veliger stage, higher temperature causes a high metabolic and energetic demand that larvae cannot compensate through feeding with the selected diet, inducing higher mortality rates. As larvae age, feeding capacities and activity increase to compensate for the higher metabolic and energetic needs of the metamorphosis process. Larvae can selectively accumulate fatty acids (Pernet and Tremblay, 2004) and the ratio of EFA in the larva to the same fatty acid originating from the diet indicates whether larvae selectively incorporate a specific EFA from microalgae (Cabrol et al., 2015). A ratio > 1 means that the proportion of EFAs in the PL fraction is higher than in the diet, suggesting selective incorporation into membrane phospholipids. Higher selective retention highlights potential deficiencies in diet to meet the physiological needs. Our results at the pediveliger stage included higher DHA (22:6w3) retention ratios (> 3) at a rearing temperature of 20°C, suggesting that food quantity or quality seems too low to satisfy the metabolic needs at 20°C and that larvae could compensate by increasing feeding. Although there is no comparative study on filtration rate in V. verrucosa, Bayne (1965) showed an increase in the clearance rate of M. edulis with increasing temperature. Our data highlight that higher lipid content and EFA retention ratios are associated with a lower metamorphosis rate. The physiological status of competent larvae determines the active substrate prospection and selectivity during settlement (Pernet et al., 2005). Pediveligers accumulating more lipids would be more selective, potentially delaying their metamorphosis if the habitat for settlement is unsuitable (Tremblay et al., 2007). During the competence phase, the larva consumes its energetic reserves until reaching a threshold below which “the desperate larvae” can no longer delay metamorphosis and settle anywhere (Toonen and Pawlik, 2001). We hypothesize that higher temperature increases the larval selectivity capacity by increasing energetic lipid accumulation.
To prevent mortality in small experimental tanks with high biomass larvae (Holbach et al., 2015), antibiotics were used to avoid any bacterial contamination. However, antibiotics also prevented the development of a biofilm, which constitutes a positive settlement cue for bivalve larvae (Leyton and Riquelme, 2008), inducing a negative effect on their settlement (Pernet et al., 2006). Furthermore, in the absence of air injection and water agitation in experimental tanks to avoid sound perturbation for anthropogenic noise emission, no positive settlement cue was related to hydrodynamics/turbulence (Tremblay et al., 2020). Finally, with the absence of artificial collectors in the tanks, larvae could only settle on the smooth walls of the cylinders, which is less suitable than filamentous or rough surfaces (Le Tourneux and Bourget, 1988; Harvey et al., 1993). We suggest that these experimental conditions used to maintain better soundscape conditions were not optimal for larval settlement, stimulating metamorphosis delay and potential “desperate” conditions. However, such conditions are often encountered in the natural environment (Toonen and Pawlik, 2001). We conclude that higher metabolism and feeding at 20°C delayed metamorphosis and raised the selectivity of pediveliger larvae.
4.2 Sound reduces larvae settlement, mortality, and thermic compensatory mechanisms
The present study on an endobenthic bivalve demonstrated the ontogenic effect of anthropogenic sounds on larvae. Pile driving sounds slightly modified the energetic state of veliger larvae without inducing any effect on their mortality or growth. Under pile driving exposure, the veliger fatty acid profile changed in the sole PL fraction, particularly for EPA and DHA. The fatty acid content in the PL fraction and EFA retention ratio gradually increased with pile driving sound levels, suggesting that larvae accumulated more membranous fatty acids, particularly EFAs. Such results could relate to settler growth stimulation or traduce an increase in the metabolic level due to stress (Spiga et al., 2016), but further experiments are needed.
Our results highlight an ontogenic interaction between the physiological consequences of rearing temperature and the acoustic response of larvae, but only at the pediveliger stage. Both sounds reduce fatty acid content in the NL fraction of larvae reared at 20°C, but not at 15°C. Such observations concur with similar studies showing that temperature amplifies the effect of another stressor (Cherkasov et al., 2007) because physiological stress induced by one factor reduces the resistance of another (Zippay and Helmuth, 2012). For example, Lannig et al. (2006) concluded that cadmium pollution reduces the thermal tolerance of the oyster Crassostrea virginica. The present results also indicate that sound reduces the compensatory mechanisms established to balance the temperature increase.
However, the retention ratio indicated another pattern, as the DHA (22:6w3) retention ratio for larvae reared at 20°C decreased in response to sound (from > 4 to < 3) exposure. Thus, anthropogenic sounds could stimulate feeding or assimilation of pediveliger larvae at 20°C, decreasing the impact of the potential diet deficiency. However, this stimulation does not seem to be enough to compensate for the temperature impact in the context of sound exposure, as the TFA content in NL fractions was nearly 2-time less with sound treatments compared to control.
If energetic fatty acid accumulation (TFA in NL fraction) in response to increased temperature enhances larval selectivity and delays metamorphosis in the context of a non-optimal habitat for settlement, no acceleration of metamorphosis would be observed. Higher TFA content in the NL fraction was associated with a higher settlement rate but without changes in the success of metamorphosis. As described by Delaunay et al. (1992), there is not necessarily a direct relationship between lipids and larval growth. Despite the lower energetic content, larvae do not adopt a “desperate” behavior. Inversely, the settlement process was slowed down by pile driving sounds at 20°C, as indicated by the slightly lower proportion of larvae crawling on the cylinder surface. We then hypothesized that such anthropogenic noise is a negative settlement cue for V. verrucosa larvae. Our results agree with those from Balanus amphitrite, in which metamorphosis was delayed in response to low-frequency sounds (Branscomb and Rittschof, 1984). However, it also contrasts with other acoustic impact studies on bivalve species showing increased settlement in response to low-frequency anthropogenic sound for mytilids M. edulis (Jolivet et al., 2016) and P. canaliculus (Wilkens et al., 2012). Thus, the response to sound is highly species-specific. As it was demonstrated on adults bivalves (Zhao et al., 2021) further experiments are needed to determine if sound reduce attachment performances of larvae. In contrast to 20°C larvae, the fatty acid content of 15°C-reared larvae did not decrease with sound exposure, as it was already low. The effect of sound diverges between the 15 and 20°C rearing conditions, showing that the response to sound is highly dependent on the larval physiological state. Our study agrees with previous studies showing strong interactions between the physiological state of larvae and the response to an environmental stressor (Lannig et al., 2006; Freuchet et al., 2015; Torres et al., 2021). This study gives precursory results on the effects of sound on marine invertebrates larvae but it is important to keep in mind that this study carried out in the laboratory does not reproduce the real natural conditions. Its goal is to standardize as many parameters as possible to make only the factors tested vary and being reproducible. Therefore the results demonstrated here cannot be directly extrapolated to the natural environment but still give answers on the acoustic sensitivity of invertebrate larvae. Although, given that thermal variations in the marine environment can modulate the acoustic response of bivalve larvae, there is an urgent need to integrate multiple factor interactions into future anthropogenic noise studies.
Data availability statement
The raw data supporting the conclusions of this article will be made available by the authors, without undue reservation.
Author contributions
MG conducted the experiments, performed data analysis, and wrote the original draft. RT supervised fatty acid analysis, and contributed to results interpretation and manuscript review. JB supervised the acoustics analyses and reviewed the manuscript. LC conceived the study, led the project administration, and funding acquisition. FO conceived the experimental design and methods, supervised the experiments, and reviewed the manuscript. All authors contributed to the article and approved the submitted version.
Funding
The work reported in this paper was a part of the PhD of Mathilde Gigot, funded by the CNRS. Additional financial support was provided by the IMPAIC project (‘IMPacts Acoustiques sur les Invertébrés de la baie de Saint-BrieuC’) supported by Ailes Marines. This study is a contribution to the BeBEST2 International Research Project (CNRS INEE/LEMAR and UQAR/ISMER).
Acknowledgments
We deeply thank all of the Tinduff hatchery team for their technical support, their useful advice, and their remarkable skill in larval production. We thank Guillaume Bridier for conducting FA identification. We thank Joséphine Broussin for helping during the experimentations.
Conflict of interest
The authors declare that the research was conducted in the absence of any commercial or financial relationships that could be construed as a potential conflict of interest.
Publisher’s note
All claims expressed in this article are solely those of the authors and do not necessarily represent those of their affiliated organizations, or those of the publisher, the editors and the reviewers. Any product that may be evaluated in this article, or claim that may be made by its manufacturer, is not guaranteed or endorsed by the publisher.
Supplementary material
The Supplementary Material for this article can be found online at: https://www.frontiersin.org/articles/10.3389/fmars.2023.1117431/full#supplementary-material
References
André M., Solé M., Lenoir M., Durfort M., Quero C., Mas A., et al. (2011). Low-frequency sounds induce acoustic trauma in cephalopods. Front. Ecol. Environ. 9 (9), 489−493. doi: 10.1890/100124
Arneri E., Giannetti G., Antolini B. (1998). Age determination and growth of Venus verrucosa l. (Bivalvia : veneridae) in the southern Adriatic and the Aegean Sea. Fisheries. Res. 38 (2), 193−198. doi: 10.1016/S0165-7836(98)00146-5
Barber J. R., Crooks K. R., Fristrup K. M. (2010). The costs of chronic noise exposure for terrestrial organisms. Trends Ecol. Evol. 25 (3), 180−189. doi: 10.1016/j.tree.2009.08.002
Barret L., Miron G., Ouellet P., Tremblay R. (2016). Settlement behavior of American lobster (Homarus americanus) : effect of female origin and developmental temperature. Fisheries. Oceanogr. 26 (1), 69–8. doi: 10.1111/fog.12187
Bayne B. L. (1965). Growth and the delay of metamorphosis of the larvae of Mytilus edulis (L.). Ophelia 2 (1), 1−47. doi: 10.1080/00785326.1965.10409596
Beaumont A. R., Budd M. D. (1983). Effects of self-fertilisation and other factors on the early development of the scallop pecten maximus. Mar. Biol. 76 (3), 285−289. doi: 10.1007/BF00393030
Branscomb E. S., Rittschof D. (1984). An investigation of low frequency sound waves as a means of inhibiting barnacle settlement. J. Exp. Mar. Biol. Ecol. 79 (2), 149−154. doi: 10.1016/0022-0981(84)90215-6
Budelmann B. U. (1992). “Hearing in nonarthropod invertebrates,” in The evolutionary biology of hearing. Eds. Webster D. B., Popper A. N., Fay R.R. (Springer New York), 141−155. doi: 10.1007/978-1-4612-2784-7_10
Buestel D., Cochard J.-C., Dao J.-C., Gérard A. (1982). Production artificielle de naissain de coquilles saint-Jacques pecten maximus (L.). premiers résultats en rade de Brest. Vie. Mar. 4, 24−28.
Cabrol J., Winkler G., Tremblay R. (2015). Physiological condition and differential feeding behaviour in the cryptic species complex eurytemora affinis in the St Lawrence estuary. J. Plankton. Res. 37 (2), 372−387. doi: 10.1093/plankt/fbu111
Chapman N. R., Price A. (2011). Low frequency deep ocean ambient noise trend in the northeast pacific ocean. J. Acoustical. Soc. America 129 (5), EL161−EL165. doi: 10.1121/1.3567084
Charifi M., Sow M., Ciret P., Benomar S., Massabuau J.-C. (2017). The sense of hearing in the pacific oyster, magallana gigas. PloS One 12 (10), e0185353. doi: 10.1371/journal.pone.0185353
Cherkasov A. A., Overton R. A., Sokolov E. P., Sokolova I. M. (2007). Temperature-dependent effects of cadmium and purine nucleotides on mitochondrial aconitase from a marine ectotherm, crassostrea virginica: a role of temperature in oxidative stress and allosteric enzyme regulation. THE. J. Exp. Biol. 210 (1), 46–55. doi: 10.1242/jeb.02589
Clarke K. R. (1993). Non-parametric multivariate analyses of changes in community structure. Austral Ecol. 18 (1), 117−143. doi: 10.1111/j.1442-9993.1993.tb00438.x
Cragg S. M., Nott J. A. (1997). The ultrastructure of the statocysts in pediveliger larvae of Pecten maximus (L.) (Bivalvia). J. Exp. Mar. Biol. Ecol. 27, 23−36. doi: 10.1016/0022-0981(77)90051-X
Delaunay F., Marty Y., Moal J., Samain J.-F. (1992). Growth and lipid class composition of pecten maximus (L.) larvae grown under hatchery conditions. J. Exp. Mar. Biol. Ecol. 163 (2), 209−219. doi: 10.1016/0022-0981(92)90050-K
Delaunay F., Martyb Y., Moal J., Samain J.-F. (1993). The effect of monospecifk algal diets on growth and fatty acid composition of pecten maximus (L.) larvae. J. Exp. Mar. Biol. Ecol. 173 (2), 163−179. doi: 10.1016/0022-0981(93)90051-O
de Soto N. A., Delorme N., Atkins J., Howard S., Williams J., Johnson M. (2013). Anthropogenic noise causes body malformations and delays development in marine larvae. Sci. Rep. 3 (1), 2831. doi: 10.1038/srep02831
Eggleston D. B., Lillis A., Bohnenstiehl D. R. (2016). “Soundscapes and larval Settlement : larval bivalve responses to habitat-associated underwater sounds,” in The effects of noise on aquatic life II, vol. 875 . Eds. Popper A. N., Hawkins A. (Springer New York), 255−263. doi: 10.1007/978-1-4939-2981-8_30
Fewtrell J. L., McCauley R. D. (2012). Impact of air gun noise on the behaviour of marine fish and squid. Mar. pollut. Bull. 64 (5), 984−993. doi: 10.1016/j.marpolbul.2012.02.009
Folch J., Lees M., Sloane-Stanlez G. H. (1957). A simple method for the isolation and purification of total lipids from animal tissues. J. Biol. Chem. 226, 497−509.
Forêt M., Olivier F., Miner P., Winkler G., Nadalini J., Tremblay R. (2020). Influence of the physiological condition of bivalve recruits on their post-settlement dispersal potential. Mar. Ecol. Prog. Ser. 636, 77−89. doi: 10.3354/meps13223
Freuchet F., Tremblay R., Flores A. (2015). Interacting environmental stressors modulate reproductive output and larval performance in a tropical intertidal barnacle. Mar. Ecol. Prog. Ser. 532, 161−175. doi: 10.3354/meps11377
Gallager S. M., Mann R., Sasaki G. C. (1986). Lipid as an index of growth and viability in three species of bivalve larvae. Aquaculture 56 (2), 81−103. doi: 10.1016/0044-8486(86)90020-7
Gigot M., Olivier F., Cervello G., Tremblay R., Mathias D., Meziane T., et al. (2023). Pile driving and drilling underwater sounds impact the metamorphosis dynamics of pecten maximus (L) larvae. Mar. Poll. Bul. doi: 10.1016/j.marpolbul.2023.114969
Glencross B. D. (2009). Exploring the nutritional demand for essential fatty acids by aquaculture species. Rev. Aquacult. 1 (2), 71−124. doi: 10.1111/j.1753-5131.2009.01006.x
Harvey M., Bourget E., Miron G. (1993). Settlement of Iceland scallop chlamys islandica spat in response to hydroids and filamentous red algae : field observations and laboratory experiments. Mar. Ecol. Prog. Ser. 99, 283−292. doi: 10.3354/meps099283
Helm M., Bourne N. (2006). Hatchery culture of bivalves : a practical manual(Technical document) (Rome: FAO, Organisation de nations unies pour l’alimentation et l’agriculture). Available at: http://www.fao.org/3/y5720e/y5720e00.htm.
Holbach M., Robert R., Boudry P., Petton B., Archambault P., Tremblay R. (2015). Scallop larval survival from erythromycin treated broodstock after conditioning without sediment. Aquaculture 437, 312−317. doi: 10.1016/j.aquaculture.2014.12.003
Holland D. L., Spencer B. E. (1973). Biochemical changes in fed and starved oysters, Ostrea Edulis l. during larval development, metamorphosis and early spat growth. J. Mar. Biol. Assoc. United. Kingdom. 53 (2), 287−298. doi: 10.1017/S002531540002227X
Hulbert A. J., Pamplona R., Buffenstein R., Buttemer W. A. (2007). Life and Death : metabolic rate, membrane composition, and life span of animals. Physiol. Rev. 87 (4), 1175−1213. doi: 10.1152/physrev.00047.2006
Jolivet A., Tremblay R., Olivier F., Gervaise C., Sonier R., Genard B., et al. (2016). Validation of trophic and anthropic underwater noise as settlement trigger in blue mussels. Sci. Rep. 6 (1), 1−8. doi: 10.1038/srep33829
Kyhn L. A., Sveegaard S., Tougaard J. (2014). Underwater noise emissions from a drillship in the Arctic. Mar. pollut. Bull. 86 (1−2), 424−433. doi: 10.1016/j.marpolbul.2014.06.037
Lagardère J. P. (1982). Effects of noise on growth and reproduction of crangon crangon in rearing tanks. Mar. Biol. 71 (2), 177−185. doi: 10.1007/BF00394627
Lannig G., Flores J. F., Sokolova I. M. (2006). Temperature-dependent stress response in oysters, crassostrea virginica : pollution reduces temperature tolerance in oysters. Aquat. Toxicol. 79 (3), 278−287. doi: 10.1016/j.aquatox.2006.06.017
Lee J., Zhao F. (2021) Global offshore wind report (Global wind energy council). Available at: https://gwec.net/global-offshore-wind-report-2021/.
Lepage G., Roy C. C. (1984). Improved recovery of fatty acid through direct transesterification without prior extraction or purification. J. Lipid Res. 25 (12), 1391−1396. doi: 10.1016/S0022-2275(20)34457-6
Le Tourneux F., Bourget E. (1988). Importance of physical and biological settlement cues used at different spatial scales by the larvae of semibalanus balanoides. Mar. Biol. 97 (1), 57−66. doi: 10.1007/BF00391245
Leyton Y. E., Riquelme C. E. (2008). Use of specific bacterial-microalgal biofilms for improving the larval settlement of argopecten purpuratus (Lamarck 1819) on three types of artificial spat-collecting materials. Aquaculture 276 (1−4), 78−82. doi: 10.1016/j.aquaculture.2008.01.037
Lillis A., Bohnenstiehl D. R., Eggleston D. B. (2015). Soundscape manipulation enhances larval recruitment of a reef-building mollusk. PeerJ 3, e999. doi: 10.7717/peerj.999
Lillis A., Eggleston D. B., Bohnenstiehl D. R. (2013). Oyster larvae settle in response to habitat-associated underwater sounds. PloS One 8 (10). doi: 10.1371/journal.pone.0079337
Martel A., Hynes T. M., Buckland-Nicks J. (1995). Prodissoconch morphology, planktonic shell growth, and size at metamorphosis in Dreissena polymorpha. Can. J. Zool. 73 (10), 1835−1844. doi: 10.1139/z95-216
Marty Y., Delaunay F., Moal J., Samain J.-F. (1992). Changes in the fatty acid composition of pecten maximus (L.) during larval development. J. Exp. Mar. Biol. Ecol. 163 (2), 221−234. doi: 10.1016/0022-0981(92)90051-B
Mathias D., Gervaise C., Di Iorio L. (2016). Wind dependence of ambient noise in a biologically rich coastal area. J. Acoustical. Soc. America 139 (2), 839−850. doi: 10.1121/1.4941917
Mooney T. A., Hanlon R., Madsen P. T., Christensen-Dalsgaard J., Ketten D. R., Nachtigall P. E. (2012). “Potential for sound sensitivity in cephalopods,” in The effects of noise on aquatic life, vol. 730 . Eds. Popper A. N., Hawkins A. (Springer New York), 125−128. doi: 10.1007/978-1-4419-7311-5_28
Norro A., Botteldooren D., Dekoninck L., Haelters J., Rumes B. (2013). Qualifying and quantifying offshore wind farm-generated noise, Vol. 8.
Olivier O., Gigot M., Mathias D., Jezequel Y., Meziane T., L’Her C., et al. (2023). Assessing the impacts of anthropogenic sounds on early stages of benthic invertebrates: the ‘Larvosonic system’. Limnol. Oceanogr.: Methods. 2021, 51–68. doi: 10.1002/lom3.10527
Parrish C. C. (1987). Separation of aquatic lipid classes by chromarod thin-layer chromatography with measurement by iatroscan flame ionization detection. Can. J. Fisheries. Aquat. Sci. 44 (4), 722−731. doi: 10.1139/f87-087
Pechenik J. A. (1990). Delayed metamorphosis by larvae of benthic marine invertebrates : does it occur? is there a price to pay? Ophelia 32 (1−2), 63−94. doi: 10.1080/00785236.1990.10422025
Peng C., Zhao X., Liu S., Shi W., Han Y., Guo C., et al. (2016). Effects of anthropogenic sound on digging behavior, metabolism, Ca 2+/Mg 2+ ATPase activity, and metabolism-related gene expression of the bivalve sinonovacula constricta. Sci. Rep. 6 (1), 1−12. doi: 10.1038/srep24266
Pernet F., Bricelj V. M., Cartier S. (2005). Lipid class dynamics during larval ontogeny of sea scallops, placopecten magellanicus, in relation to metamorphic success and response to antibiotics. J. Exp. Mar. Biol. Ecol. 329 (2), 265−280. doi: 10.1016/j.jembe.2005.09.008
Pernet F., Tremblay R. (2004). Effect of varying levels of dietary essential fatty acid during early ontogeny of the sea scallop placopecten magellanicus. J. Exp. Mar. Biol. Ecol. 310 (1), 73−86. doi: 10.1016/j.jembe.2004.04.001
Pernet F., Tremblay R., Comeau L., Guderley H. (2007). Temperature adaptation in two bivalve species from different thermal habitats : energetics and remodelling of membrane lipids. J. Exp. Biol. 210 (17), 2999−3014. doi: 10.1242/jeb.006007
Popper A. N., Hawkins A. D. (2018). The importance of particle motion to fishes and invertebrates. J. Acoustical. Soc. America 143 (1), 470−488. doi: 10.1121/1.5021594
Pörtner H.-O., Bock C., Mark F. C. (2017). Oxygen- and capacity-limited thermal tolerance : bridging ecology and physiology. J. Exp. Biol. 220 (15), 2685−2696. doi: 10.1242/jeb.134585
Ramirez L., Fraile D., Brindley (2020). Offshore wind in Europe (Wind Europe). Available at: https://windeurope.org/wp-content/uploads/files/about-wind/statistics/WindEurope-Annual-Offshore-Statistics-2019.pdf.
Rayssac N., Pernet F., Lacasse O., Tremblay R. (2010). Temperature effect on survival, growth, and triacylglycerol content during the early ontogeny of Mytilus edulis and M. Trossulus. Mar. Ecol. Prog. Ser. 417, 183−191. doi: 10.3354/meps08774
Regnault M., Lagardere J.-P. (1983). Effects of ambient noise on the metabolic level of crangon crangon(Decapoda, natantia). Mar. Ecol. Prog. Series. Oldendorf. 11 (1), 71−78. doi: 10.3354/meps011071
Robinson S. P., Theobald P. D., Lepper P. A. (2013). "Underwater noise generated from marine piling" in Proceedings of Meetings on Acoustics. 070080. doi: 10.1121/1.4790330
Slabbekoorn H., Bouton N., van Opzeeland I., Coers A., ten Cate C., Popper A. N. (2010). A noisy spring : the impact of globally rising underwater sound levels on fish. Trends Ecol. Evol. 25 (7), 419−427. doi: 10.1016/j.tree.2010.04.005
Smaal A. C., Ferreira J. G., Grant J., Petersen J. K., Strand Ø. (2019). Goods and services of marine bivalves (Springer), 1–591. doi: 10.1007/978-3-319-96776-9
Sokolova I. (2021). Bioenergetics in environmental adaptation and stress tolerance of aquatic ectotherms : linking physiology and ecology in a multi-stressor landscape. J. Exp. Biol. 224 (Suppl_1), jeb236802. doi: 10.1242/jeb.236802
Solé M., Kaifu K., Mooney T. A., Nedelec S. L., Olivier F., Radford A. N., et al. (2023). Marine invertebrates and noise. Front. Mar. Sci. 10. doi: 10.3389/fmars.2023.1129057
Spiga I., Caldwell G. S., Bruintjes R. (2016). Influence of pile driving on the clearance rate of the blue mussel, mytilus edulis (L.). Proc. Meetings. Acoustics. 27 (1), 040005. doi: 10.1121/2.0000277
Sukhotin A. A., Abele D., Portner H. O. (2002). “Growth, metabolism and lipid peroxidation in mytilus edulis: age and size effects. Mar. Ecol. Prog. Ser. 226, 223–234. doi: 10.3354/meps226223
Toonen R. J., Pawlik J. R. (2001). Settlement of the gregarious tube worm hydroides dianthus (Polychaeta: serpulidae) II: testing the desperate larvae hypothesis. Mar. Ecol. Prog. Ser. 224, 115–131. doi: 10.3354/meps224115
Torres G., Charmantier G., Wilcockson D., Harzsch S., Giménez L. (2021). Physiological basis of interactive responses to temperature and salinity in coastal marine invertebrate : implications for responses to warming. Ecol. Evol. 11 (11), 7042−7056. doi: 10.1002/ece3.7552
Toupoint N., Gilmore-Solomon L., Bourque F., Myrand B., Pernet F., Olivier F., et al. (2012). Match/mismatch between the Mytilus edulis larval supply and seston quality: effect on recruitment. Ecology 93 (8), 1922−1934. doi: 10.1890/11-1292.1
Tournadre J. (2014). Anthropogenic pressure on the open ocean : the growth of ship traffic revealed by altimeter data analysis. Geophys. Res. Lett. 41 (22), 7924−7932. doi: 10.1002/2014GL061786
Tremblay R., Christophersen G., Nadalini J.-B., Redjah I., Magnesen T., Andersen S. (2020). Improving scallop (Pecten maximus and placopecten magellanicus) spat production by initial larvae size and hydrodynamic cues used in nursery system. Aquaculture 516, 734650. doi: 10.1016/j.aquaculture.2019.734650
Tremblay R., Olivier F., Bourget E., Rittschof D. (2007). Physiological condition of balanus amphitrite cyprid larvae determines habitat selection success. Mar. Ecol. Prog. Ser. 340, 1−8. doi: 10.3354/meps340001
Vaughn C. C., Hoellein T. J. (2018). Bivalve impacts in freshwater and marine ecosystems. Annu. Rev. Ecol. Evol. Syst. 49 (1), 183–208. doi: 10.1146/annurev-ecolsys-110617-062703
Wale M. A., Simpson S. D., Radford A. N. (2013a). Size-dependent physiological responses of shore crabs to single and repeated playback of ship noise. Biol. Lett. 9 (2). doi: 10.1098/rsbl.2012.1194
Wale M. A., Simpson S. D., Radford A. N. (2013b). Noise negatively affects foraging and antipredator behaviour in shore crabs. Anim. Behav. 86 (1), 111−118. doi: 10.1016/j.anbehav.2013.05.001
Whyte J. N. C., Bourne N., Ginther N. G. (1991). Depletion of nutrient reserves during embryogenesis in the scallop patinopecten yessoensis (Jay). J. Exp. Mar. Biol. Ecol. 149 (1), 67−79. doi: 10.1016/0022-0981(91)90117-F
Wilkens S. L., Stanley J. A., Jeffs A. G. (2012). Induction of settlement in mussel (Perna canaliculus) larvae by vessel noise. Biofouling 28 (1), 65−72. doi: 10.1080/08927014.2011.651717
Yamamoto H., Shimizu K., Tachibana A., Fusetani N. (1999). Roles of dopamine and serotonin in larval attachment of the barnacle,Balanus amphitrite. J. Exp. Zool. 284 (7), 746−758. doi: 10.1002/(SICI)1097-010X(19991201)284:7<746::AID-JEZ4>3.0.CO;2-J
Zhao X., Sun S., Shi W., Sun X., Zhang Y., Zhu L., et al. (2021). Mussel byssal attachment weakened by anthropogenic noise. Front. Mar. Sci. 8. doi: 10.3389/fmars.2021.821019
Keywords: anthropophony, energetic metabolism, larval recruitment, metamorphosis trigger, fatty acids
Citation: Gigot M, Tremblay R, Bonnel J, Chauvaud L and Olivier F (2023) Physiological condition of the warty venus (Venus verrucosa L. 1758) larvae modulates response to pile driving and drilling underwater sounds. Front. Mar. Sci. 10:1117431. doi: 10.3389/fmars.2023.1117431
Received: 06 December 2022; Accepted: 10 April 2023;
Published: 19 June 2023.
Edited by:
Marta Solé, BarcelonaTech (UPC), SpainReviewed by:
Lucille Chapuis, University of Bristol, United KingdomXinguo Zhao, Chinese Academy of Fishery Sciences, China
Copyright © 2023 Gigot, Tremblay, Bonnel, Chauvaud and Olivier. This is an open-access article distributed under the terms of the Creative Commons Attribution License (CC BY). The use, distribution or reproduction in other forums is permitted, provided the original author(s) and the copyright owner(s) are credited and that the original publication in this journal is cited, in accordance with accepted academic practice. No use, distribution or reproduction is permitted which does not comply with these terms.
*Correspondence: Mathilde Gigot, bWF0aGlsZGUuZ2lnb3QucHJvQGdtYWlsLmNvbQ==