- 1Department of Marine Biotechnology and Resources, National Sun Yat-sen University, Kaohsiung, Taiwan
- 2National Museum of Marine Biology & Aquarium, Pingtung, Taiwan
- 3Department of Post Modern Agriculture, Mingdao University, Changhua, Taiwan
- 4He Wei Precision Company Limited, Hsinchu, Taiwan
- 5Department of Applied Chemistry, National Chiayi University, Chiayi, Taiwan
- 6Graduate Institute of Marine Biology, National Dong Hwa University, Pingtung, Taiwan
Coral reefs worldwide are declining due to increasing concentrations of greenhouse gases, which, combined with local anthropogenic pressure, are exacerbating unprecedented mass coral bleaching. For corals to survive, restoring coral reefs through cryopreservation is crucial. The aim of this study was to vitrify and laser-warm Stylophora pistillata planulae to allow for feasible settlement, post-settlement survival, and the production of adult corals. The no-observed-effect concentrations were used to determine the best cryoprotective agents for S. pistillata. The larvae were then subjected to cooling and nanolaser warming (300 V, 10 ms pulse width, 2 mm beam diameter) by using two vitrification solutions (VSs; VS1: 2 M dimethyl sulfoxide and 1 M ethylene glycol and VS2: 2M EG and 1M DMSO and gold nanoparticles. The results revealed that VS1-treated larvae had a higher vitrification rate (65%), swimming rate (23.1%), settlement rate (11.54%), and post settlement survival rate (11.54%) than those treated with VS2. Seasonal variations also affected the cryopreservation of the planulae; VS1 was more favorable for the planulae in spring than in fall. Although laser-warmed larvae developed slower morphologically than their controlled counterparts, the production of adult S. pistillata corals from cryopreserved larvae was achieved. The proposed technique can improve the cryopreservation of corals and advance efforts to protect endangered coral species.
Introduction
Global warming poses a threat to the world’s coral reefs. Unprecedented declines in coral reefs manifest at the regional level, where the composition of coral assemblages are drastically changing (Hughes et al., 2018). Most reefs cannot maintain positive net carbonate production because of ocean warming and acidification (Cornwall et al., 2021). Ocean temperatures continue to rise, and corals cannot acclimate or adapt quickly. Thus, coral bleaching events continue to increase in frequency and intensity (Hoegh-Guldberg, 1999). The projected decline in coral reefs is being met with mitigation strategies that use new and radical interventions such as cryopreservation (Lin and Tsai, 2020). Cryopreservation is the process of preserving biological samples at extremely cold temperatures (Tsai and Lin, 2012). Cryobiology is a developing science that has a rudimentary theoretical framework (Gwo, 2011; Vanderzwalmen et al., 2020). Cryopreservation research is empirical and conducted through trial and error; thus, the protocol for cryopreservation continues to be optimized (Whaley et al., 2021).
The freezing protocols for coral samples such as sperm (Hagedorn et al., 2006; Hagedorn et al., 2017; Viyakarn et al., 2018; Zuchowicz et al., 2021), oocyte (Tsai et al., 2011; Tsai et al., 2015; Tsai et al., 2016a; Tsai et al., 2016b), tissue balls (Feuillassier et al., 2014), planula larvae (Daly et al., 2018; Cirino et al., 2019; Cirino et al., 2021a), and symbiotic algae of corals (Hagedorn et al., 2010; Chong et al., 2016; Lin et al., 2019) have been optimized to cryopreserve corals. The protocols involve a series of assessments of the suitability (Feuillassier et al., 2014), chilling sensitivity, cooling, and warming rates (Viyakarn et al., 2018; Zuchowicz et al., 2021) of cryoprotective agents (CPAs). These initial steps are crucial for developing cryobanks (Martínez-Páramo et al., 2017; di Genio et al., 2020; Toh et al., 2022) to mitigate the loss of genetic and species diversity (Mayfield et al., 2019; Lin CC et al., 2022) in coral reefs.
Cryopreservation techniques have evolved beyond the slow, fast, and ultrafast freezing techniques that use temperature control equipment (Narida et al., 2022b). Vitrification is gaining popularity in cryopreservation because it prevents ice crystal formation when the speed of temperature conduction is increased, which significantly increases cooling rates (Liebermann et al., 2002). Vitrification is the glass-like solidification of a solution (from approximately −80°C to −130°C) and differs from conventional freezing techniques (Bojic et al., 2021). In vitrification, the sample solidifies without ice crystals, and multiple (Tsai et al., 2016b; Jang et al., 2017; Magnotti et al., 2018) or no CPAs (Isachenko et al., 2003; Spis et al., 2019) are used for biological samples that require extremely high cooling rates (Bojic et al., 2021). Vitrification was first used in mouse (Mus musculus) embryos (Rall and Fahy, 1985) and then plants (Stiles, 1930), erythrocytes (Rapatz and Luyet, 1968), mammalian embryos (Rall and Fahy, 1985; Massip et al., 1986; Yuswiati and Holtz, 1990; Rall, 1993; Schiewe and Anderson, 2017), fish (Cuevas-Uribe et al., 2013; Figueroa et al., 2013; Godoy et al., 2013), invertebrates (Guo and Weng, 2020; Heres et al., 2021), and now corals (Tsai et al., 2015) and their unicellular dinoflagellate symbionts (di Genio et al., 2020; Kihika et al., 2022a; Kihika et al., 2022b). Researchers are striving to cryobank genetic materials from endangered coral species and save regional populations in decline.
Contemporary vitrification techniques are coupled with the use of lasers to rewarm the samples rather than applying conventional warming rates of 40°C/s to 200°C/s (Figueroa et al., 2013; Figueroa et al., 2015). Mouse oocytes and embryos were among the first samples that were successfully laser warmed (Jin et al., 2014; Jin and Mazur, 2015). One study applied droplet vitrification and laser warming to Danio rerio (zebra fish) embryos (>2mm) (Khosla et al., 2020). Vitrification and ultrarapid laser warming were also tested on Saccharomyces cerevisiae (Paredes, 2021). Another study used the gonadal tissues and oocytes from a cat to explore the effects of laser warming versus those of conventional thawing methods (Rowlison et al., 2022). The effectiveness of this technique has led to its popularity in coral cryopreservation. To date, the planula larvae from three species of corals, namely Lobactis [Fungia] scutaria (Daly et al., 2018), Pocillopora verrucosa, and Seriatopora caliendrum (Cirino et al., 2019), have successfully survived cryopreservation and resumed swimming after vitrification and nanolaser warming. Two of these species (P. verrucosa and S. caliendrum) (Cirino et al., 2019) were successfully settled.
Cryopreservation of coral oocytes and larvae poses many challenges due to their large sizes, high-fat content (Lin et al., 2013), high chilling sensitivities (Lin and Tsai, 2012; Cirino et al., 2022), and high lipid phase transition temperatures (Lin et al., 2014), Two ecologically distinct groups of corals exist from which biomaterials can be collected for cryopreservation. The first group, spawners, simultaneously release eggs and sperm, and fertilization occurs externally. Brooders, the second group, fertilize internally and release complex planulae that can metamorphose and settle within hours (Harrigan, 1972; Hughes et al., 1999). Spawners comprise 80% of scleractinian corals, whereas brooders only comprise 20%. Although brooder corals' planulae settle quickly, they are not as prevalent in reefs. Moreover, only brooders can acquire gonochorism, which is 100 times more likely to be lost than gained (Kerr et al., 2011). These characteristics make coral planula larvae excellent candidates for cryobanking. With the advent of cryopreservation techniques, novel devices for vitrification that enable long-term storage and cryobanking can be used to laser-warm coral samples; thus, planulae are a suitable coral biomaterial for cryopreservation (Narida et al., 2022a; Lin et al., 2023). The aim of this study was to vitrify and laser-warm S. pistillata planulae to allow for feasible settlement, post-settlement survival and the production of adult corals.
Materials and methods
Target coral species
S. pistillata (Esper, 1797) is a key scleractinian coral commonly found in reef fronts and submarine terraces of protected reefs around Taiwan and offshore islets (Dai, 1990). S. pistillata is an opportunistic and pioneering coral that can colonize new and/or undisturbed environments (Loya, 1976; Baird and Morse, 2004). It is a thermally intolerant (Cacciapaglia and van Woesik, 2016) and bleaching-sensitive (Loya et al., 2001; Marshall and Schuttenberg, 2006; van Woesik et al., 2011) species. A brooder that reproduces year-round (Atoda, 1947), exhibits lunar periodicity (Zakai et al., 2006) and does not require chemical cues to induce metamorphosis (Baird, 2001), which makes S. pistillata a good candidate for cryopreservation.
Coral collection
Colonies of S. pistillata (10–15 cm in diameter) were collected at a depth of 10 m from Houbihu Reef, Kenting National Park (KNP), Nanwan, Taiwan (GPS coordinates: N 21.931867°, E 120.744683°). An approved collection permit from KNP was received (permit number: 1090009719). The coral colonies were transported in a 60-L plastic crate with seawater. The individual colony was transferred and reared in 5-L flow-through tanks at the National Museum of Marine Biology. Handmade traps with a mesh size of 250 µm were deployed before sunset, and the planulae were collected at least 2 h after sunrise. The planulae were transported to the laboratory and washed thrice with filtered seawater (FSW) by using a 47-mm glass fiber filter with a mesh size of 0.2 µm (Pall, New York, NY, USA) attached to a vacuum pump (Rocker, Kaohsiung, Taiwan). Only planulae that were actively swimming and recently released were selected for the experiments.
Species identification and DNA analyses
The S. pistillata colonies were morphologically identified using Veron (2000) as a reference, and DNA analyses were performed to validate the identification of the species. The collected S. pistillata planulae were circular, approximately 640 µm in diameter, and had an elongated length of approximately 1000 µm. The planulae contained Symbiodiniaceae that were well distributed with a distinct dark-brown tail. Tissue samples were collected to verify the species of S. pistillata, and the DNA was extracted using a DNasy Blood & Tissue Kit (Qiagen, Hilden, Germany) according to the manufacturer’s instructions. The extracted DNA samples were amplified using a 10× AccuPrime polymerase chain reaction (PCR) buffer, 200 nM of each primer, and 5 U of AccuPrime Pfx (Invitrogen, Carlsbad, CA, USA) in a 50 μL reaction. The forward primer for coral nuclear ribosomal DNA was 5′-TAAAAGTCGTAACAAGGTTTCC-3′, and the reverse primer was 5′- CCTCCGCTTATTGATATGCTTAAAT-3′ (Forsman et al., 2009). The PCR and other sequencing procedures were described in a previous study (Wang et al., 2015).
Effects of cryoprotective agents
The collected planulae (five planulae per treatment) were exposed to five CPAs that varied in concentration (0.5, 1, and 2 M) and exposure time (5, 10, and 15 min). The five CPAs were dimethyl sulfoxide (DMSO; Sigma-Aldrich, St. Louis, MO, USA), methanol (MeOH; Merck, Darmstadt, Germany), glycerol (GLY; Merck, Darmstadt, Germany), propylene glycol (PG; JT Baker, Phillipsburg, NJ, USA), and ethylene glycol (EG; JT Baker). After CPA exposure, the planulae were washed thrice with FSW and kept in six-well petri dishes (Alpha Plus, Taipei, Taiwan) to observe viability (i.e., moving and swimming) and settlement. The number of planulae that moved and settled was used to calculate the no-observed-effect concentration (NOEC) and determine the highest concentration at which no effect on S. pistillata larvae was observed over a given time. Three replicates were performed for each CPA (Figure 1).
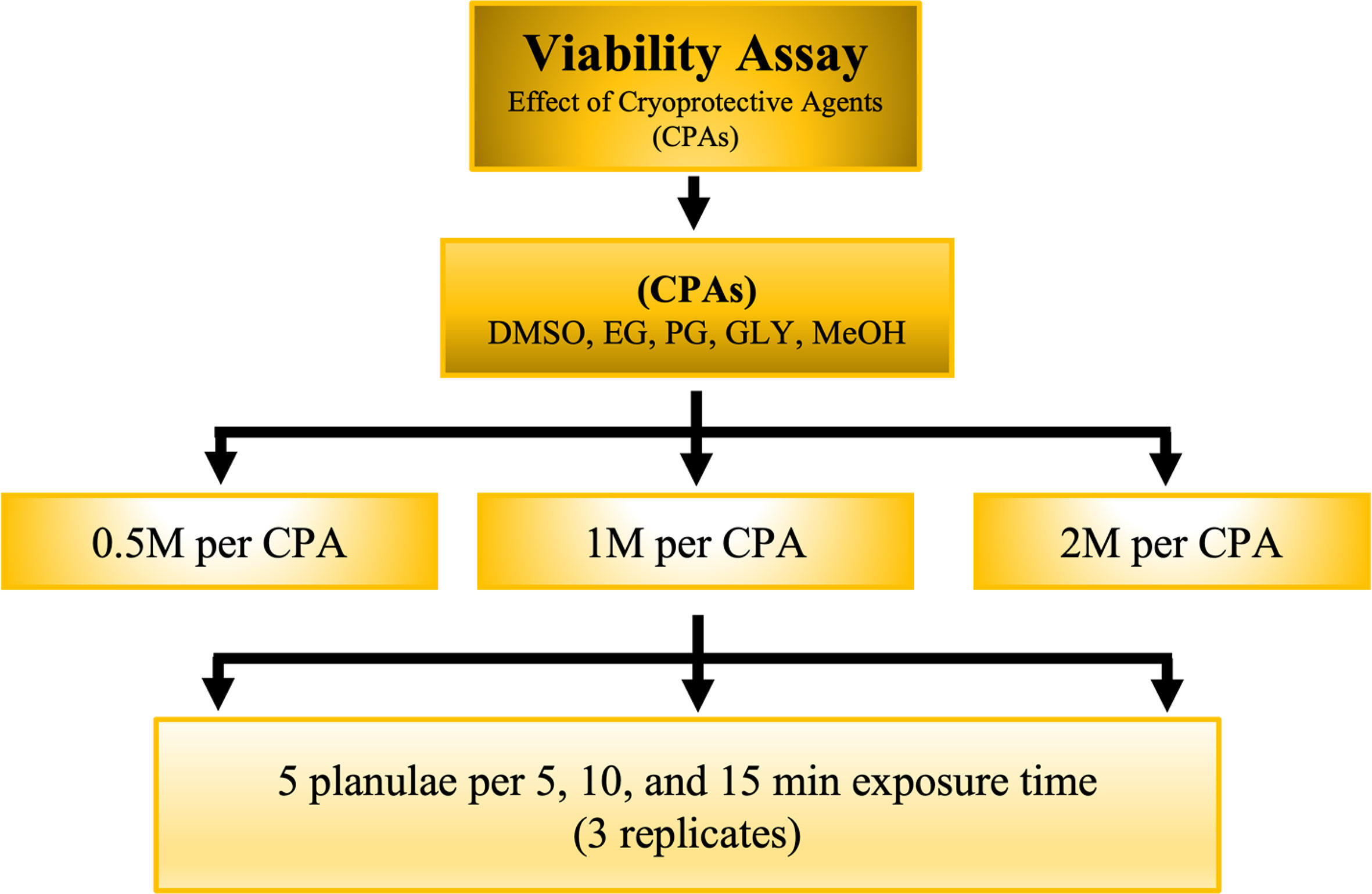
Figure 1 Schematic for evaluating the viability of S. pistillata exposure to different CPAs at different exposure times.
Gold nanoparticle synthesis
The synthesis of gold nanoparticles (AuNPs) in the absence of surfactants was based on the methods of Turkevich et al. (1951) and Frens (1973). All the solutions of HAuCl4 (Alfa Aeser, Haverhill, MA, USA) and Na3-citrate (Merck) were prepared using deionized water. First, 20 mL of 1 mM HAuCl was heated to boiling. Then, 0.6 mL of 10 mM Na3-citrate was added to the boiling solution. After approximately 30 s, the solution first turned dark blue (i.e., nucleation) and then changed to wine red, which indicated the formation of AuNPs. Digital images of the AuNPs were captured using a transmission electron microscope (TEM; JEM-2100, JEOL, Tokyo, Japan) connected to a camera system (Gatan digital micrograph; Gatan, Pleasanton, CA, USA) at an accelerating voltage of 200 kV (filament, 56 µA). Before being analyzed under the TEM, the AuNP colloid was dripped onto a carbon-coated copper grid and air-dried at room temperature.
Vitrification and laser warming
The vitrification solutions (VSs) were prepared according to the results of the CPA experiments. Two VSs were created. VS1 was composed of 2M DMSO and 1M EG and VS2 was composed of 2 M EG and 1M DMSO. Both VSs were mixed with 40% (w/v) Ficoll and 10% (v/v) AuNPs in FSW, the final concentration of which was 1.2 × 1018 particles/m3 with an optimized emission wavelength of 535 nm. Equilibration solutions (ESs) were prepared by serially diluting VS1 and VS2 at ratios of 1:3 (ES1) and 1:1 (ES2) to create quarter- and half-strength VSs. Each coral larva was initially exposed to a 10-µL drop of ES1 for 4 min and then a 10-µL drop of ES2 for 2 min. Finally, the coral larva was submerged in 10-µL VS for 1 min. After each solution was added to the planula, signs of irregularities and damage were recorded. Planulae that were damaged were discarded to preserve the larval quality before cryopreservation. After a series of solution exposures, a 0.80-µL drop (VS with planula) was placed on a self-made cryostick for vitrification; the cryostick was made using a bamboo stick (approximately 8 cm long) and a laminated thin sheet of acetate (160 µm; width: 1 mm; length: 4 cm). The planula was lowered into LN2 for at least 1 min by using a custom device and placed under a benchtop iWeld infrared Nd : YAG laser welder (980 Series; LaserStar Technologies, Riverside, RI, USA) at 60 joules for warming.
Assessment of larval vitrification, viability, settlement, and post settlement rates
A planula was successfully vitrified when it remained clear of ice crystals after being hit by a laser beam (as verified using a laser microscope). Vitrified planulae were rehydrated at 25°C by using 10 µL of ES2 for 2 min, followed by 10 µL of ES1 for 4 min. The planulae were then washed in FSW. Vitrification rates were computed using the following formula:
The S. pistillata planulae that were successfully laser-warmed were washed thrice with FSW and observed under a stereo-zoom microscope (Olympus, Tokyo, Japan). The planulae were transferred to a petri dish and left for 12 h. The untreated planulae were also kept inside a petri dish for comparisons between vitrified S. pistillata. Comparisons were made by dividing the number of settled laser-warmed planulae by the total number of settled untreated planulae and multiplying the result by 100. Successfully settled planulae were reared in 600-L fiberglass tanks with wavemakers (IceCap, Slidell, LA, USA) to maintain water circulation and a seawater temperature of 26°C. The petri dishes were cleaned at least thrice per week to avoid algal overgrowth. Digital images of S. pistillata spats were taken daily by using a SteREO Discovery V8 (Zeiss, White Plains, NY, USA) stereomicroscope. The swimming, settlement, and post settlement rates were computed using the following formulas:
Statistical analyses
Data analyses were performed using SPSS (version 17.0; Chicago, IL, USA). A one-sample Kolmogorov–Smirnov test was performed to test for normality, and Levene’s test was performed to test the equality of variance. A one-way analysis of variance (ANOVA) was conducted to determine the differences in NOEC within each CPA between the control and treatment groups.
Results
Species verification
The colonies were verified using 18s and internal transcribed spacer (ITS) sequencing to ensure that the planulae were taken from the same species regardless of differences in planula size or morphology (Figure 2A). The results of the 18s sequencing revealed 100% similarity, whereas the ITS sequences showed three base-pair differences between the colonies (see Figure S1 in the Supporting Information). Upon release, the planulae had a rod-shaped appearance (Figure 2B) and transitioned into a flat ball shape before settlement (Figure 2C). The planulae were released with Symbiodiniacea through direct inheritance from the parent (i.e., vertical transmission). According to the genetic analysis, the S. pistillata in this study was dominated by Cladocopium.
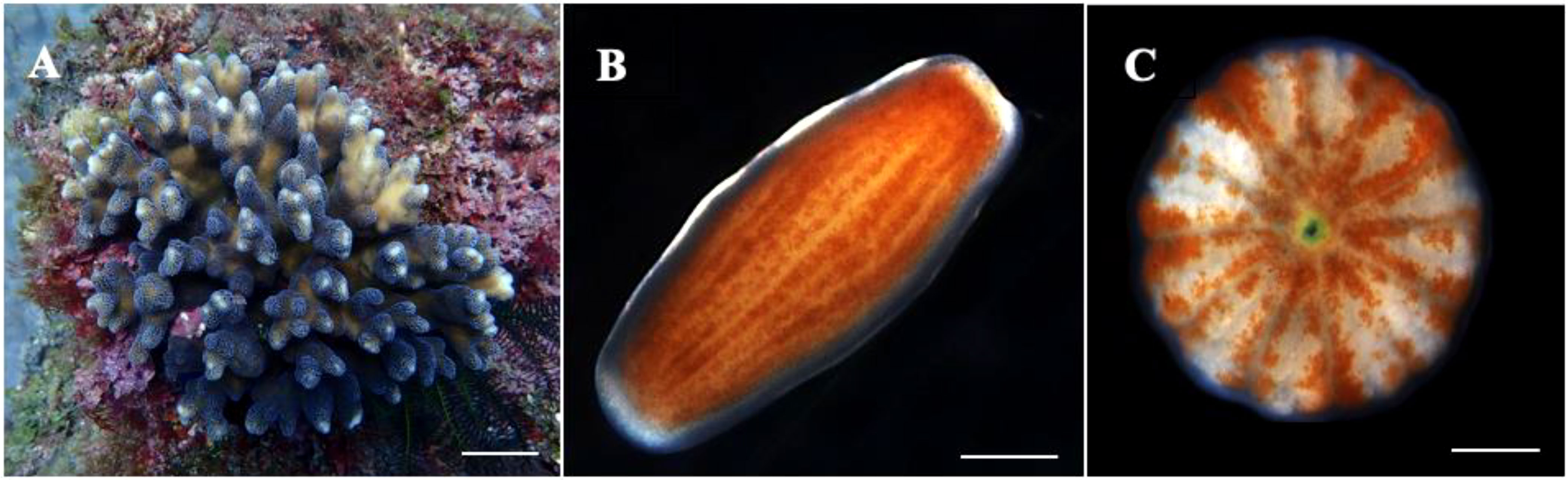
Figure 2 Sample photo of an S. pistillata colony (A). A rod-shaped swimming planula (B) was released from the colony and morphed into a circular flat planula shortly before settling (C). Scale bar: (A) 2.5 cm, (B) 250 µm, and (C) 250 µm.
Effects of cryoprotective agents
The effects of CPAs on S. pistillata were assessed before the experiment to identify the most suitable CPA for vitrification and laser warming. The results in Figure 3 and Table 1 show the NOECs of the CPAs at exposure times of 5, 10, and 15 min. As previously mentioned, the CPAs used in this study were DMSO, EG, PG, GLY, and MeOH, and the highest concentration was 2 M. The effects of the CPAs increased in the following order: EG< DMSO = MeOH< PG< GLY. Therefore, the most suitable CPA for S. pistillata was EG. The planulae could withstand EG at a concentration of 2 M for up to 10 min. According to the results of the NOECs, the planulae could be exposed to DMSO and MeOH at a concentration greater than 2 M for 5 min. GLY was the most toxic among the CPAs, even at concentrations of less than 0.5 M for 5 min. PG was the second most harmful, and the planulae could tolerate a concentration of up to 1 M regardless of exposure time. Therefore, the viability of the S. pistillata planula larvae in this study was not affected when treated with 2 M EG for 10 min and 2 M DMSO and MeOH for 5 min. The viabilities of the planulae after exposure to 2 M DMSO, EG, and MeOH for 5 min were 93% for DMSO and EG and 80% for MeOH. However, the viabilities when exposed to 1 M PG for 5 min and 0.5 M GLY for 5 min were 100% and 53%, respectively.
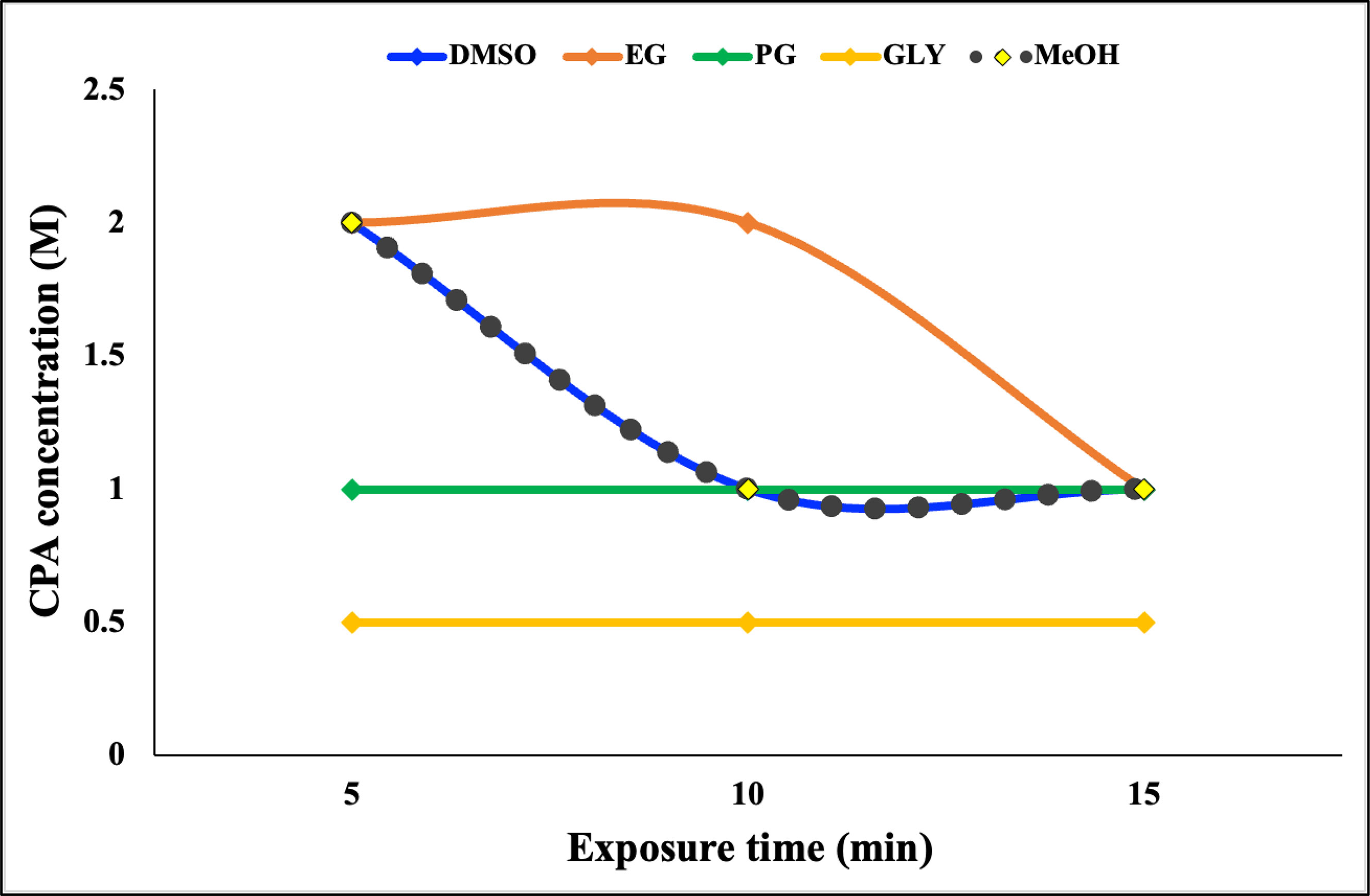
Figure 3 No-observed-effect concentrations of five cryoprotective agents in Stylophora pistillata planula larvae. Data are presented as the average triplicates; each replicate contained five coral planulae.
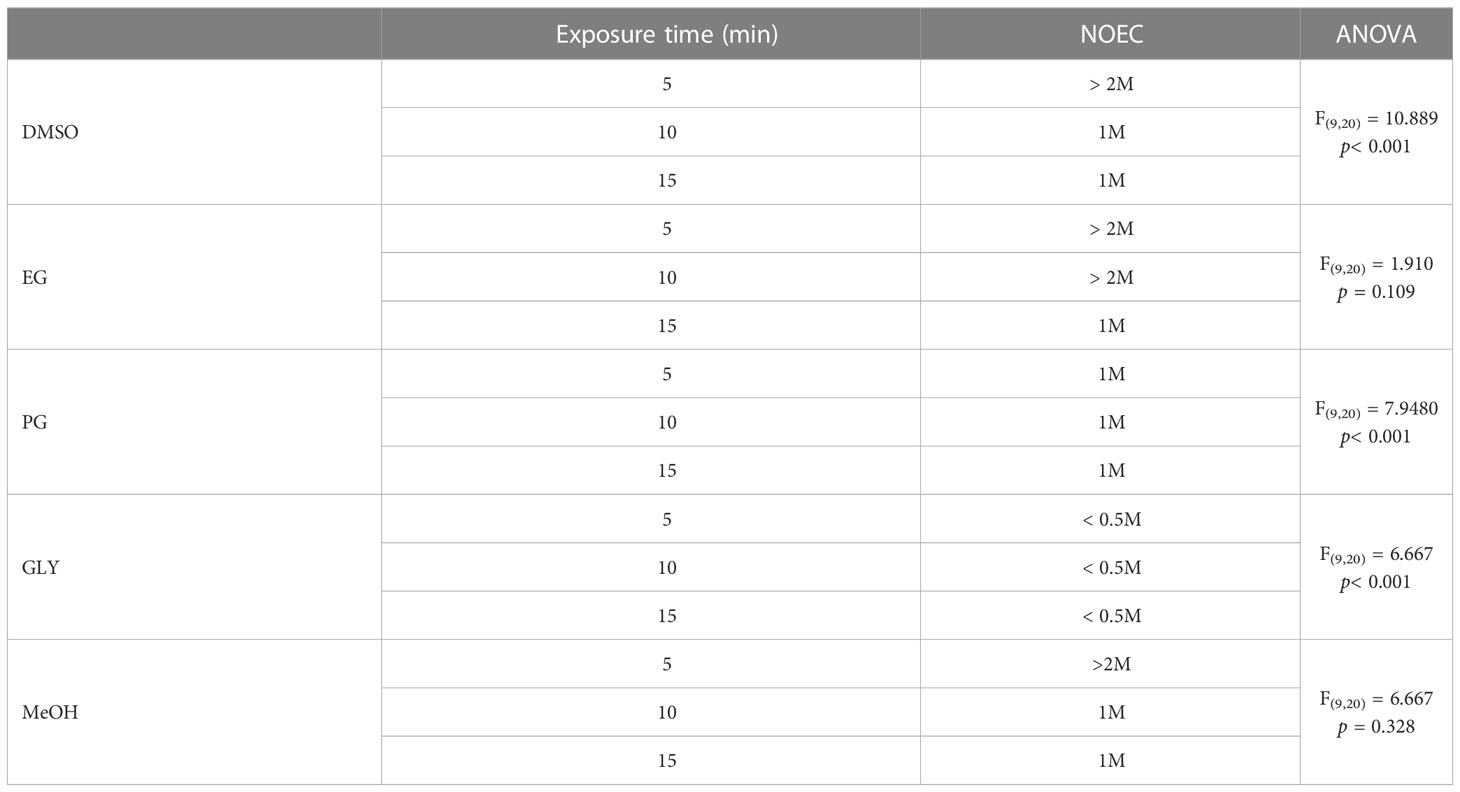
Table 1 No-observed-effect concentrations of cryoprotective agents used for Stylophora pistillata planulae.
Vitrification and nanolaser warming
The vitrification rates of S. pistillata were tested against two VSs after cooling and laser warming. The results in Figure 4 reveal that the vitrification rates after cooling (i.e., after VSs were applied to planulae and planulae were directly submerged into LN2) were 100% for both VSs. Experiments were halted when samples were devitrified after cooling. Thus, a 100% vitrification rate was required to laser warm the samples. After laser warming, the vitrification rates dropped significantly for both VSs; VS1 was associated with a slightly higher vitrification rate (65%) than VS2 (56.2%).
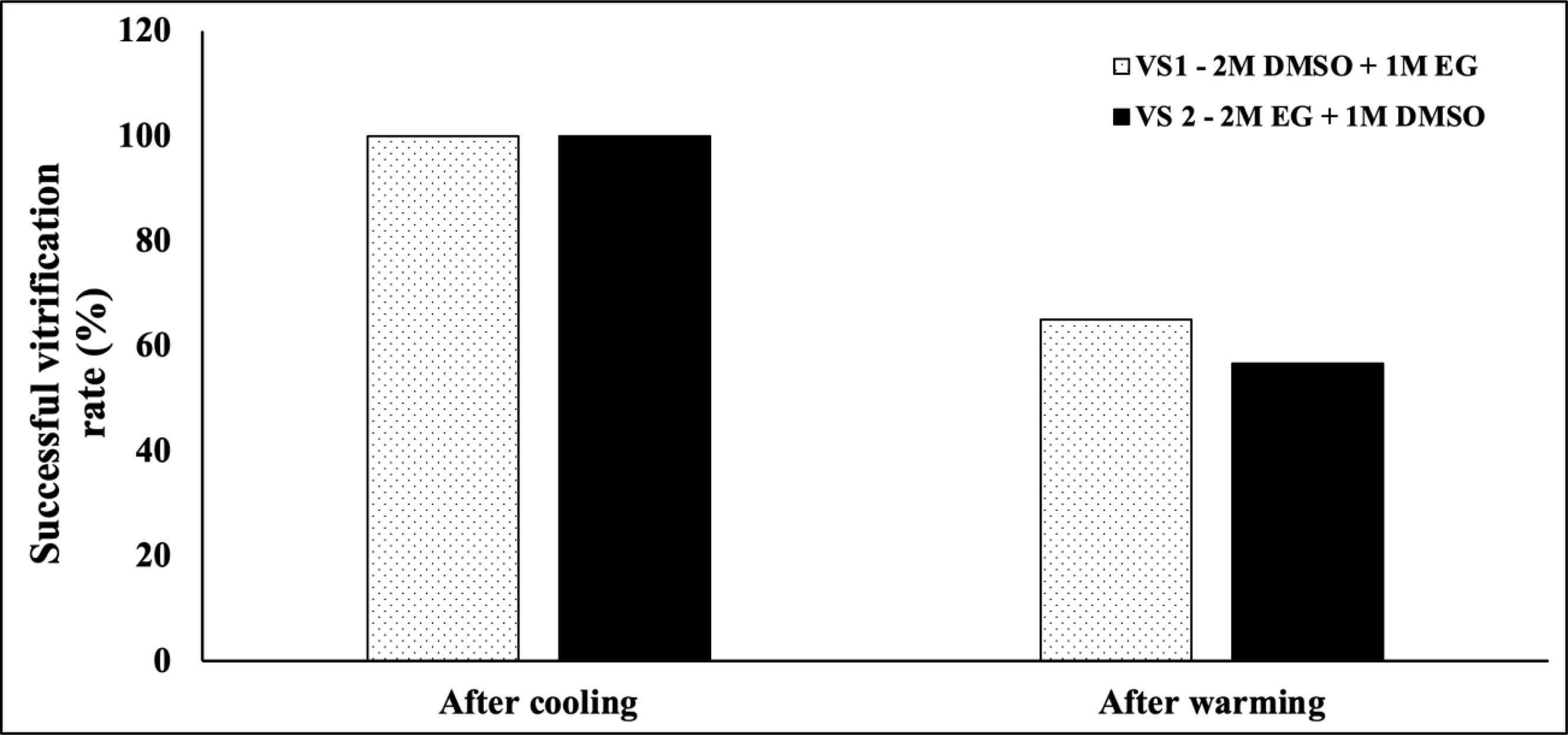
Figure 4 Vitrification rates of two vitrification solutions after cooling and laser warming (n = 333).
Post warming viability
The viability of S. pistillata planulae after laser warming is presented in Figure 5. A total of 333 pooled planulae were used in this study. The viabilities were based on the planulae’s ability to swim, settle, and survive post settlement when using two VSs. The results revealed that the planulae's swimming rate after laser warming was generally higher than the settlement rate and post settlement survival rate. Additionally, larval cryopreservation with VS1 led to a significantly higher swimming rate among planulae (14%) than treatment with VS2 (3.2%). After swimming, the planulae were monitored for calcification and settlement, and not all planulae that swam settled. Thus, the settlement rate and post settlement survival rate for VS1 were 2%, whereas those for VS2 were 1.6%.
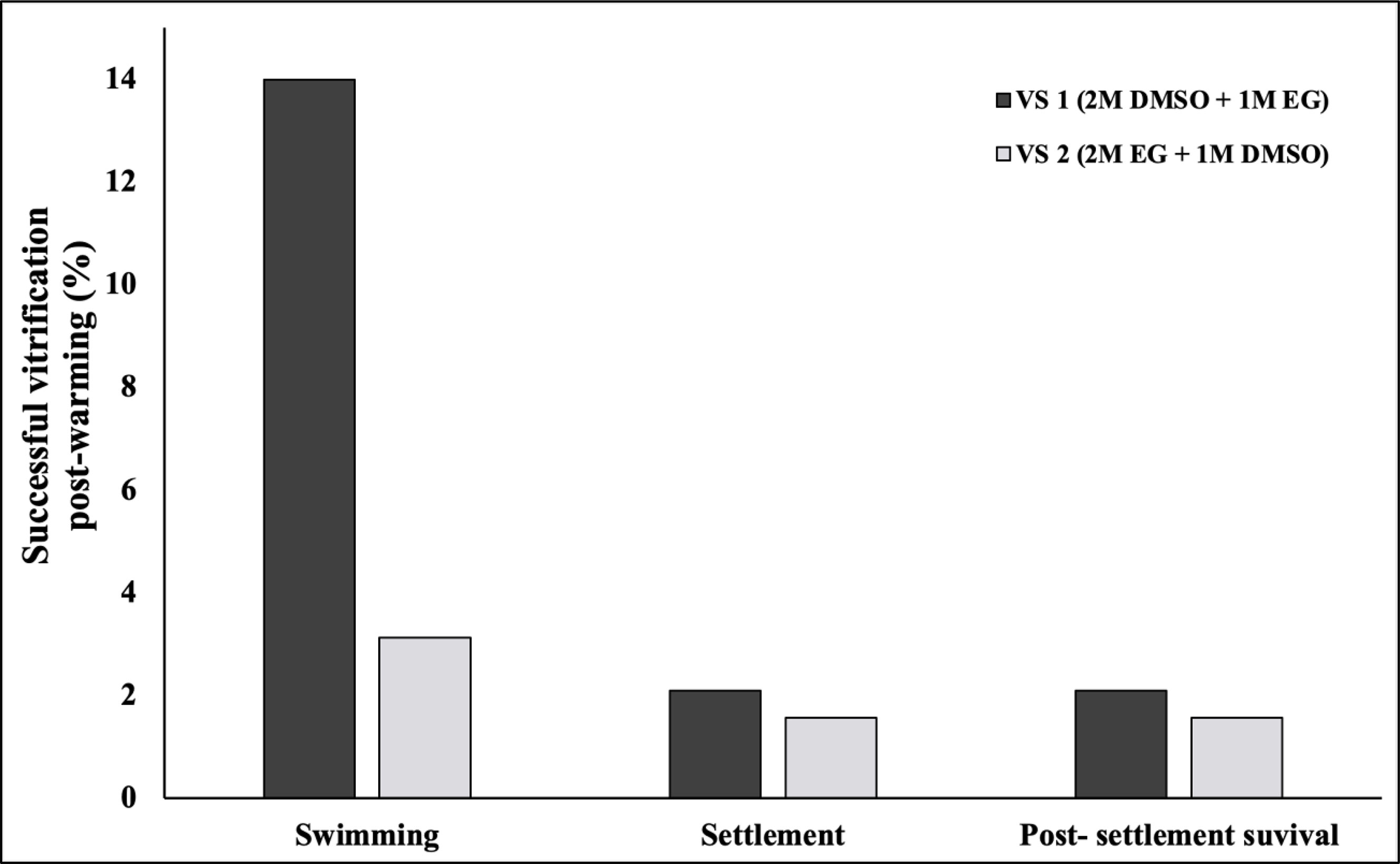
Figure 5 Swimming rate, settlement rate, and post settlement survival rate for two vitrification solutions.
Seasonal variability
The graphs in Figure 6 demonstrate the seasonal variations between Fall (Sep–Nov) and Spring (Mar–May) when using the two VSs. The results indicate that S. pistillata planulae had a higher swimming rate, settlement rate, and post settlement survival rate during the fall than the spring (Figure 6A). When using VS1, the swimming rate during the fall was 23.08%, which was higher than that during the spring. The high swimming rate also led to a significantly high settlement rate and post settlement survival rate (both 11.54%). The swimming rate remained at 11.54% during the spring, but no settlement or post settlement survival was observed when using VS1. When using VS2 during the fall, no swimming, settlement, or post settlement survival of planulae was observed; however, a relatively low rate of swimming, settlement, and post settlement survival (all 3.1%) was observed when using VS2 during the spring (Figure 6B).
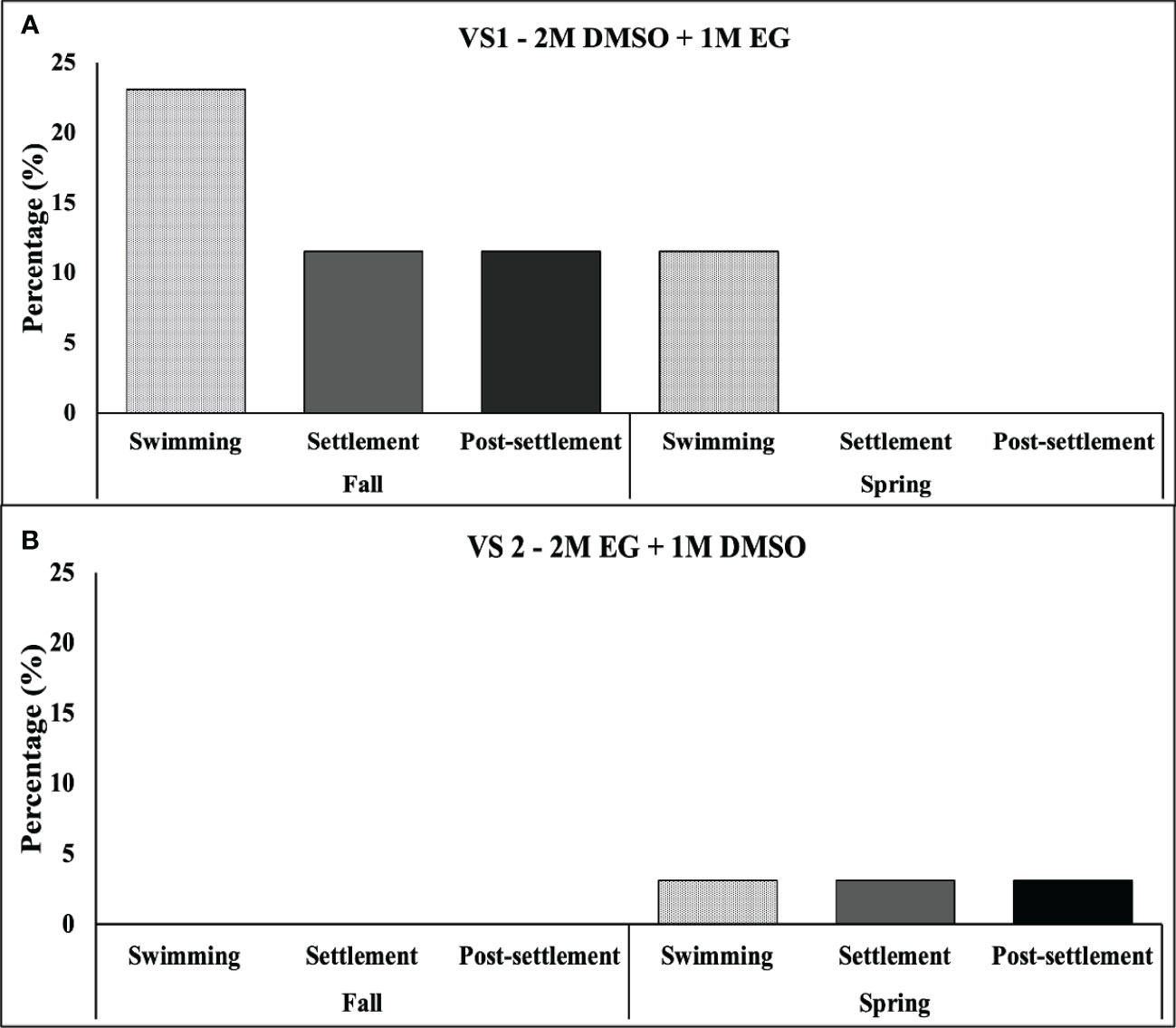
Figure 6 Rates (in %) of swimming, settlement, and post settlement survival of S. pistillata planulae during spring and fall when using VS1 (A) and VS2 (B). (n = 333).
Developmental differences between untreated and laser-warmed S. pistillata spat
The S. pistillata planulae (approximately 1000 µm in diameter) were spherical upon shedding and changed to pyriform, disk-like, and rod-like shapes during the presettlement stage. The planulae exhibited a light-brown color due to the presence of Symbiodiniacea. Upon release, the ball-shaped planulae could elongate to a 1-to-2-mm-long rod-like shape with smooth margins. After laser warming and cryopreservation, the ball-shaped planulae moved, swam, and gradually transitioned into a rod-like shape. During the presettlement stage, the planulae sank to the bottom of the tank and flattened into a disk-like shape. The basal plate started to form and attached to the polystyrene substrate on day 1. The laser-warmed planulae developed similarly to untreated planulae, except for a delay in the emergence of the secondary corallite (Figure 7), which budded off as early as the first week (Figure 7A). For the laser-warmed planulae, the corallite emerged 3 weeks after settlement (Figure 7B). No physical differences or defects were observed in the skeletal development of the laser-warmed coral spats.
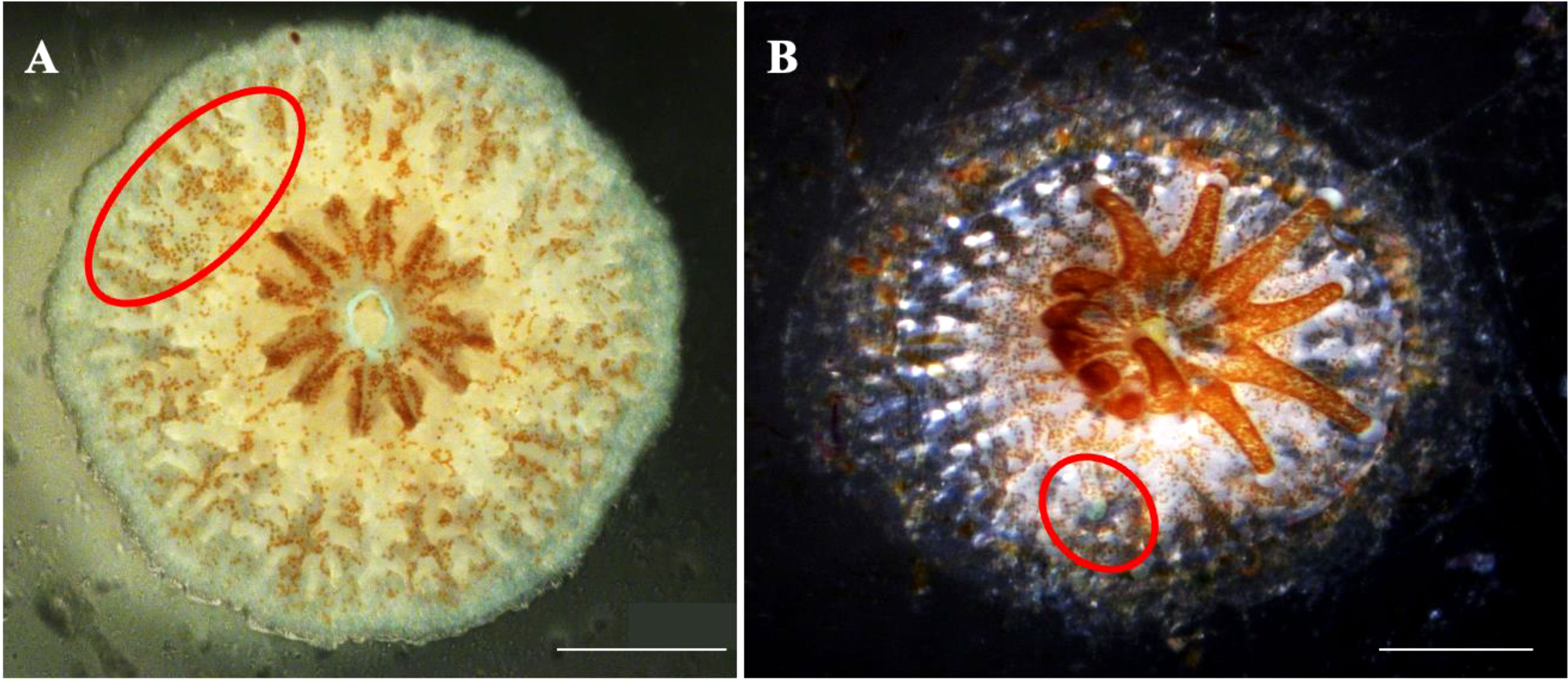
Figure 7 Differences between the untreated (A) and laser-warmed (B) Stylophora pistillata planulae. The secondary corallite (red circle) budded 1 week after settlement for the untreated planulae but 3 weeks after settlement for the laser-warmed planulae (B; red circle). Scale bar: 500 µm.
Discussion
Cryopreservation in coral reef conservation is both challenging and promising. This proof-of-concept experiment verified that coral planulae can be cryopreserved, nanolaser warmed, and settled and ultimately survive under husbandry conditions. For aquatic species, sperm is the most common sample for cryopreservation when breeding and managing fish such as salmonids, cyprids, silurids, and fish of the family Acipesiridae (Tsvetkova et al., 1996; Barun, 2015). Most sperm research has focused on optimizing protocols for managing oyster sperm to improve hatchery operations, even in the absence of broodstock (Hassan et al., 2015). However, scaling up aquaculture operations from research to commercial production remains challenging. Studies in larval cryopreservation were not common until the early 1990s. More current studies on aquatic larval cryopreservation have been limited to shrimp (Alfaro et al., 2000; Diwan and Kandasami, 1997; Dong et al., 2004; Subramoniam and Arun, 1999; Subramoniam and Newton, 1993), clams (Anjos et al., 2022; Chao et al., 1997; Choi et al., 2008; Heres et al., 2021), mussels (Heres et al., 2019; Liu et al., 2020; Paredes et al., 2013, Paredes et al., 2012; Rusk et al., 2020; Wang et al., 2011), oysters (Anjos et al., 2022; Chao et al., 1997; Choi et al., 2007; Choi and Nam, 2014; Paniagua-Chávez and Tiersch, 2001; Paniagua-Chávez et al., 2011; Paniagua-Chávez et al., 1998; Paredes et al., 2013; Suneja et al., 2014; Usuki et al., 2002), barnacles (Khin Maung et al., 1998; Piazza et al., 2022), worms (Olive and Wang, 2002), and scleractinian corals (Daly et al., 2018; Cirino et al., 2019; Cirino et al., 2021a). Nonetheless, studies have only performed slow freezing until more recent studies on scleractinian coral. The advent of such technology has successfully been used to successfully cryopreserve coral larvae.
S. pistillata is a brooder; thus, the planulae develop within the coelenteron of the adult coral (Veron and Stafford-Smith, 2000). Our study demonstrated that the cryopreserved and laser-warmed planulae of S. pistillata could grow into adult corals when using a VS composed of DMSO and EG. One study reported that L. scutaria could survive laser warming and the use of DMSO, PG, and trehalose as a CPA; however, no settlement was observed (Daly et al., 2018). Another study found that S. caliendrum could be vitrified and warmed with EG and PG as VSs, settled, and then survive for 1 week (Cirino et al., 2019). Supplementing linoleic acid and phosphatidylethanolamine with both DMSO and EG as the VSs resulted in a higher settlement rate for P. verrucosa (Cirino et al., 2021a). Thus, the choice of VS is species-specific and an essential factor for achieving successful cryopreservation and laser warming.
Our findings revealed that larval seasonality was a key component in effective cryopreservation. We hypothesized that the variation in lipid content and the composition of S. pistillata planulae affect the swimming rate, settlement rate, and post settlement survival rate more during the fall than during the spring. According to Kim et al. (2001) and Seidel (2006), lipids provide essential functions during freezing and can alter cell viability. However, the lipid concentration and the composition of coral change with the seasons (Oku et al., 2003). The planula larvae are released from the parent colony and are comprised of 70% total lipid (TL) content, aiding in positive buoyancy and dispersal (Richmond, 1987; Harii et al., 2007). Some studies have investigated the TL content of scleractinian (Harii et al., 2007; Cirino et al., 2021b; Harii et al., 2002; Rivest et al., 2017) and nonscleractinian (Harii et al., 2002) corals. Harii et al. (2007) examined the TL content of newly released larvae from two brooder scleractinian corals, A. brueggemanni and P. damicornis, which demonstrated a TL content of 58% and 68%, respectively. Another scleractinian coral, a spawner coral, A. tenuis, and its 5-day-old planulae were examined, and exhibited a TL content of 61%. Additionally, the nonscleractinian coral Heliopora coerulea had a TL content of 41%. The TL content from these corals contained wax esters (WEs), triacylglycerol (TG) (except for A. tenuis), and phospholipids (PLs). Another study on P. damicornis from Taiwan (Rivest et al., 2017) revealed that the planulae contained an average TL content of 20.47 µg. The TL content included WEs (39%), TG (18%), and PLs (6%). Cirino et al. (2021b) also examined the TL content of S. caliendrum and P. verrucosa to determine how seasonality affects the lipid content of planulae. Analogous to the reported lipid content of coral planulae, intracellular WEs and TG were the predominant lipids of both S. caliendrum and P. verrucosa, primarily during fall. Higher TL concentrations can also lead to higher cellular membrane fluidity at low temperatures (Lin et al., 2013). Although the TL content and composition were not examined in the present study, the lipid composition of corals in the same genera or family may be similar and have similar metabolic patterns (Harii et al., 2007). S caliendrum and P. verrucosa are from the same family as S. pistillata and presumably share similar seasonal lipid patterns. Additionally, the planulae have specific lipid phase transition (LPT) temperatures—specific low temperatures—at which the physical state of lipids changes from a gel to a disordered liquid crystalline (Lin et al., 2014). Thus, the significant seasonal viability after vitrification and laser warming may be caused by the lipid content and composition, changes in the lipid profile, and the LPT of the planulae, all of which lead to changes in the lipid profile with the season and temperature (Oku et al., 2003). Such lipid storage patterns in coral from the same family may be worth exploring to improve vitrification for S. pistillata. Under the same circumstances, modifying the VS can increase planula vitality after laser warming.
Studies on coral skeletogenesis from settlement to post settlement have employed scanning electron microscopy (Vandermeulen and Watabe, 1973; Jell, 1979; Wallace, 1999; Stolarski, 2003; Sun et al., 2020) and microcomputed tomography systems (Janiszewska et al., 2013; Perrin et al., 2015) and involved killing (i.e., fixing) the sample to describe the complex processes. This study observed the skeletal ontogeny and biomineralization from coral settlement to adulthood after successfully cooling and laser-warming S. pistillata planulae. The skeletogenesis of the live broadcast spawning corals Galaxea fascicularis (12 months) and Mycedium elephantotus (14 months), the brooder coral S. caliendrum (2.5 months), and P. verrucosa (approximately 1 month) were described by Lin C. et al. (2022). At the macro level, the skeleton of the laser-warmed S. pistillata is composed of corallites (i.e., the building unit of the skeleton) with basic skeletal elements such as the septa, costae, septocostae, coenosteum, and the wall. The skeletal development was verified using the studies of Baird (2001) and Baird and Babcock (2000). No physical deformation was observed when compared with the control. S. pistillata possesses a tissue-over-skeleton growth mechanism, a typical characteristic for brooding Pocilloporid corals such as P. verrucosa and S. caliendrum (Lin C. et al., 2022). The development of S. pistillata was initially described by Atoda (1947) in Japan, Rinkevich and Loya (1979) in the Red Sea, and Baird and Babcock (2000) in Australia. The differences in the onset of development can be attributed to the location and environmental conditions of the species. However, the skeletal development of the laser-warmed planulae was similar to that of typical coral. Nonetheless, a delay in the emergence of the secondary corallite and a possible stagnation in growth were observed. Rinkevich and Loya (1979) found that a 2-year-old S. pistillata had an average size of 4–5 cm. However, due to the weedy nature of S. pistillata (Loya, 1976), the 8-month-old laser-warmed coral was smaller in diameter and possessed 16 fully developed corallites. Further experiments can be conducted to better understand the effects of cooling and warming on S. pistillata planula larvae. Nonetheless, no physical injuries were incurred from cooling or warming. Coral planulae have been studied to understand the skeletogenesis in scleractinians (le Tissier, 1988) and the effects of factors such as pollution (Rinkevich and Loya, 1979). Studies have also tested new biotechnology for coral reef research and restoration (Randall et al., 2019).
Successful cooling and warming are crucial for the post settlement survival of planulae. More research is necessary on open flow-through systems to ensure the survival of coral spats. The water conditions in the rearing tank post settlement were maintained at 26 °C, and the settlement plates were cleaned 3 times per week or as needed to prevent algal overgrowth. The algae compete for space and can even outgrow coral recruitment during husbandry setup, leading to coral mortality (Diaz-Pulido et al., 2009). Slow growth and the demise of juvenile coral in the present study were not directly related to possible damage during cryopreservation but to the postrearing state. The noncryopreserved larvae developed similarly to the cryopreserved planula larvae. Thus, the husbandry conditions should be closely monitored during the early stage of development to successfully produce viable coral recruits from the cryopreserved and laser-warmed planulae. Cryopreservation can be successful when the planulae swim and settle after laser warming. Growing and maintaining coral under husbandry conditions is a significant achievement.
In this study, successful cooling and laser warming were affected by various factors, namely the CPA, droplet volume, supplementation of GNP, and mastery of the technique. DMSO, EG, Ficoll, and GNP were the primary components of the VSs. The use of multiple CPAs necessitates lowering the CPA concentration and making it less toxic than when using a single CPA (Kader et al., 2009); therefore, this was performed in our VSs. Incorporating macromolecules such as Ficoll into the VS also reduces the osmotic shock of the sample (Liebermann et al., 2002). Studies have shown that adding Ficoll to a VS protected the samples from cryoinjury (Dumoulin et al., 1994), lowered the toxicity of the VS, and increased the effectiveness of the VS in the vitrification of mouse embryos (Kasai et al., 1990). In our study, the media or droplet’s volume and shape were carefully controlled when the planulae with VS were loaded onto the cryostick. The smaller the CPA droplet is, the less likely that ice crystals form. To increase the survival of vitrified embryos, this technique follows the minimum volume cooling concept, which involves reducing the volume of the VS with the sample (Hamawaki et al., 1999). The addition of GNP into the VS evens the distribution of heat when the laser hits the droplet to lessen or eliminate membrane damage to the planula (Daly et al., 2018; Cirino et al., 2019). The high VS concentrations of glass-promoting solutes during cooling allows for planulae to be suspended. A high cooling rate is crucial for achieving a high degree of vitrification and survival (Liebermann et al., 2002); therefore, direct-contact vitrification was applied in our study. The survival of vitrified samples depends on the warming conditions (Paredes, 2021). Ultrafast laser warming is gaining popularity as an alternative to slow, convective warming. Ultrafast warming can block ice recrystallization and retain cells (Seki and Mazur, 2009; Seki and Mazur, 2012), and the results of ultrafast warming are 100 times higher than conventional warming (Kleinhans and Mazur, 2015). Laser warming was used to prevent ice crystal formation and rewarm the S. pistillata planulae rapidly and uniformly. Finally, successful vitrification is dependent on the technician, who must decide on the CPAs, sample loading, and machine settings. Thus, technicians must possess a particular skillset that requires training and mastery.
Conclusion
This study was the first proof-of-concept experiment on cryopreserved and laser-warmed planulae, which successfully settled and grew into adult corals. Coral planulae can be cryopreserved, stored, warmed, and grown ex situ by optimizing the nanolaser warming technology and the CPAs used in vitrification. Moreover, raising coral spats under controlled and well-monitored husbandry conditions can enhance the survival of laser-warmed planula larvae. This study was also the first attempt to describe coral skeletogenesis in successfully settled planulae through coral spat development after cooling and laser warming but without fixing the sample. This technological breakthrough can revolutionize the study, banking, and conservation of coral species. The technology can also address the need to preserve the genetic materials of threatened and endangered coral species.
Data availability statement
The original contributions presented in the study are included in the article/Supplementary Material. Further inquiries can be directed to the corresponding author.
Author contributions
Conceptualization, CL and ST. Methodology, WCH, LHW, CLH, and AN. Validation, CL and ST. Formal analyses, WCH, CLH, and AN. Resources, CL, ZHW and ST. Writing—original draft preparation, AN. Writing—;review and editing, CL, ST and AN. Visualization, CL and ST. Supervision, CL, ZHW and ST. Funding acquisition, CL and ZHW.
Funding
This work was funded by Taiwan's Ministry of Science and Technology (MOST 110-2313-B-291-001-MY3).
Conflict of interest
Author WCH is the owner of He Wei Precision Company Limited.
The remaining authors declare that the research was conducted in the absence of any commercial or financial relationships that could be construed as a potential conflict of interest.
Publisher’s note
All claims expressed in this article are solely those of the authors and do not necessarily represent those of their affiliated organizations, or those of the publisher, the editors and the reviewers. Any product that may be evaluated in this article, or claim that may be made by its manufacturer, is not guaranteed or endorsed by the publisher.
Supplementary material
The Supplementary Material for this article can be found online at: https://www.frontiersin.org/articles/10.3389/fmars.2023.1172102/full#supplementary-material
Supplementary Figure 1 | 18s (A) and internal transcribed spacer (ITS) (B) sequencing results for Stylophora pistillata showing no base-pair differences for 18s and three base-pair differences (highlighted in yellow) by using ITS.
References
Alfaro J., Komen J., Huisman E. A. (2000). Cooling, cryoprotectant and hypersaline sensitivity of penaeid shrimp embryos and nauplius larvae. Aquaculture 195 (2001), 353–366. doi: 10.1016/S0044-8486(00)00557-3
Anjos C., Duarte D., Diogo P., Matias D., Cabrita E. (2022). Assessment of larval quality of two bivalve species, Crassostrea angulata and Chamelea gallina, exposed and cryopreserved with different cryoprotectant solutions. Cryobiology 106, 24–31. doi: 10.1016/j.cryobiol.2022.04.007
Atoda K. (1947). The larva and postlarval development of some reef-building corals. II. Stylophora pistillata (Esper). Sci. Rep. Tohoku Univ. 18, 48–64.
Baird A. H., Babcock R. C. (2000). Morphological differences among three species of newly settled pocilloporid coral recruits. Coral Reefs. 19 (2), 179–183. doi: 10.1007/PL00006955
Baird A. H. (2001). The ecology of coral larvae: settlement patterns, habitat selection and the length of the larval phase (Doctoral dissertation, James Cook University).
Baird A. H., Morse A. N. C. (2004). Induction of metamorphosis in larvae of the brooding corals Acropora palifera and Stylophora pistillata. Mar. Freshw. Res. 55, 469–472. doi: 10.1071/MF03121
Barun S. (2015). A review on applications & advantages of cryopreservation in different fields of science. Beats Nat. Sci. 2, 1–6.
Bojic S., Murray A., Bentley B. L., Spindler R., Pawlik P., Cordeiro J. L., et al. (2021). Winter is coming: the future of cryopreservation. BMC Biol. 19, 1–20. doi: 10.1186/s12915-021-00976-8
Cacciapaglia C., van Woesik R. (2016). Climate-change refugia: shading reef corals by turbidity. Glob Chang Biol. 22, 1145–1154. doi: 10.1111/gcb.13166
Chao N. H., Lin T., Chen Y. J., Hsu H. W., Liao I. C. (1997). Cryopreservation of late embryos and early larvae in the oyster and hard clam. Aquaculture 155, 31–44. doi: 10.1016/S0044-8486(97)00107-5
Choi Y. H., Jo P. G., Kim T., Bai S. C., Chang Y. J. (2007). The effects of cryopreservation on fine structures of pearl oyster (Pinctada fucata martensii) larvae. Dev. Reprod. 11 (2), 79–84.
Choi Y. H., Lee J. Y., Chang Y. J. (2008). The influence of developmental stages and protective additives on cryopreservation of surf clam (Spisula sachalinensis) larvae. J. Environ. Biol. 29 (4), 461–463.
Choi Y. H., Nam T. J. (2014). Influence of the toxicity of cryoprotective agents on the involvement of insulin-like growth factor-I receptor in surf clam (Spisula sachalinensis) larvae. Cryo Letters. 35 (6), 537–543.
Chong G., Tsai S., Wang L. H., Huang C. Y., Lin C. (2016). Cryopreservation of the gorgonian endosymbiont Symbiodinium. Sci. Rep. 6, 1–9. doi: 10.1038/srep18816
Cirino L., Tsai S., Wang L. H., Chen C. S., Hsieh W. C., Huang C. L., et al. (2021a). Supplementation of exogenous lipids via liposomes improves coral larvae settlement post-cryopreservation and nano-laser warming. Cryobiology 98, 80–86. doi: 10.1016/j.cryobiol.2020.12.004
Cirino L., Tsai S., Wang L. H., Hsieh W. C., Huang C. L., Wen Z. H., et al. (2022). Effects of cryopreservation on the ultrastructure of coral larvae. Coral Reefs. 41, 131–147. doi: 10.1007/s00338-021-02209-4
Cirino L., Tsai S., Wen Z. H., Wang L. H., Chen H. K., Cheng J. O., et al. (2021b). Lipid profiling in chilled coral larvae. Cryobiology 102, 56–67. doi: 10.1016/j.cryobiol.2021.07.012
Cirino L., Wen Z. H., Hsieh K., Huang C. L., Leong Q. L., Wang L. H., et al. (2019). First instance of settlement by cryopreserved coral larvae in symbiotic association with dinoflagellates. Sci. Rep. 9, 18851. doi: 10.1038/s41598-019-55374-6
Cornwall C. E., Comeau S., Kornder N. A., Perry C. T., van Hooidonk R., DeCarlo T. M., et al. (2021). Global declines in coral reef calcium carbonate production under ocean acidification and warming. Proc. Natl. Acad. Sci. U.S.A. 118, 1–10. doi: 10.1073/pnas.2015265118
Cuervas-Uribe R., Chesney E. J., Daly J., Tiersch T. (2013). Vitrification of sperm from marine fish: effect on motility and membrane integrity. Aquac Res. 47 (7), 1–15. doi: 10.1111/are.12337
Dai C. F. (1990). Scleractinian fauna in the coastal waters of Kenting National Park. Natl. Park J. 2:1, 1–22.
Daly J., Zuchowicz N., Nuñez C. I. L., Khosla K., Lager C., Henley E. M., et al. (2018). Successful cryopreservation of coral larvae using vitrification and laser warming. Sci. Rep. 8, 15714. doi: 10.1038/s41598-018-34035-0
Diaz-Pulido G., McCook L. J., Dove S., Berkelmans R., Roff G., Kline D. I., et al. (2009). Doom and boom on a resilient reef: climate change, algal overgrowth and coral recovery. PloS One 4, e5239. doi: 10.1371/journal.pone.0005239
Diwan A. D., Kandasami K. (1997). Freezing of viable embryos and larvae of marine shrimp, Penaeus semisulcatus de haan. Aquac. Res. 28 (12), 947–950. doi: 10.1111/j.1365-2109.1997.tb01020.x
Dong Q., Huang C., Lin J. (2004). Effects of cryoprotectant toxicity on the embryos and larvae of pacific white shrimp Litopenaeus vannamei. Aquaculture 242, 655–670.
Dumoulin J. C. M., Bergers-Janssen J. M., Pieters M. H. E. C., Enginsu M. E., Geraedts J. P. M., Evers J. L. H. (1994). The protective effects of polymers in the cryopreservation of human and mouse zonae pellucidae and embryos. Fertil. Steril. 62 (4), 793–798. doi: 10.1016/S0015-0282(16)57006-X
di Genio S., Wang L. H., Meng P. J., Tsai S., Lin C. (2020). “Symbio-cryobank”: toward the development of a cryogenic archive for the coral reef dinoflagellate symbiont symbiodiniaceae. Biopreserv Biobank 19, 91–93. doi: 10.1089/bio.2020.0071
Esper E. J. C. (1797). Die pflanzenthiere in abbildungen nach der natur mit farben erleuchtet nebst beschreibungen Vol. 3 (Nuremberg: Raspischen Buchhandlung). Available at: https://www.marinespecies.org/aphia.php?p=sourcedetails&id=35915.
Feuillassier L., Martinez L., Romans P., Engelmann-Sylvestre I., Masanet P., Barthélémy D., et al. (2014). Survival of tissue balls from the coral Pocillopora damicornis L. exposed to cryoprotectant solutions. Cryobiology 69, 376–385. doi: 10.1016/j.cryobiol.2014.08.009
Figueroa E., Risopatrón J., Sánchez R., Isachenko E., Merino O., Isachenko V., et al. (2013). Spermatozoa vitrification of sex-reversed rainbow trout (Oncorhynchus mykiss): Effect of seminal plasma on physiological parameters. Aquaculture 372–375, 119–126. doi: 10.1016/j.aquaculture.2012.10.019
Figueroa E., Valdebenito I., Zepeda A. B., Figueroa C. A., Dumorné K., Castillo R. L., et al. (2015). Effects of cryopreservation on mitochondria of fish spermatozoa. Rev. Aquac 0, 1–12. doi: 10.1111/raq.12105
Forsman Z. H., Barshis D. J., Hunter C. L., Toonen R. J. (2009). Shape-shifting corals: molecular markers show morphology is evolutionarily plastic in Porites. BMC Evol. Biol. 9 (1), 1–9. doi: 10.1186/1471-2148-9-45
Frens G. (1973). Controlled nucleation for the regulation of the particle size in monodisperse gold suspensions. Nat. Phys. Sci. 241, 20–22. doi: 10.1038/physci241020a0
Godoy L. C., Streit D. P., Zampolla T., Bos-Mikich A., Zhang T. (2013). A study on the vitrification of stage III zebrafish (Danio rerio) ovarian follicles. Cryobiology 67, 347–354. doi: 10.1016/j.cryobiol.2013.10.002
Guo J. H., Weng C. F. (2020). Current status and prospects of cryopreservation in aquatic crustaceans and other invertebrates. J. Crustac Biol. 40, 343–350. doi: 10.1093/jcbiol/ruaa034
Gwo J. (2011). “Cryopreservation in aquatic species,” in Conservation in aquatic species. Eds. Tiersch T. R., Green C. C. (Baton Rouge, Louisiana, USA: World Aquaculture Society), 459–481.
Hagedorn M., Carter V., Henley E., van Oppen M., Hobbs R., Spindler R. (2017). Producing coral offspring with cryopreserved sperm: A tool for coral reef restoration. Sci. Rep. 7, 1–9. doi: 10.1038/s41598-017-14644-x
Hagedorn M., Carter V. L., Leong J. C., Kleinhans F. W. (2010). Physiology and cryosensitivity of coral endosymbiotic algae (Symbiodinium). Cryobiology 60, 147–158. doi: 10.1016/j.cryobiol.2009.10.005
Hagedorn M., Carter V., Steyn R., Krupp D., Leong J., Lang R., et al. (2006). Preliminary studies of sperm cryopreservation in the mushroom coral, Fungia scutaria. Cryobiology 52, 454–458. doi: 10.1016/j.cryobiol.2006.03.001
Hamawaki A., Kuwayama M., Hamano A. (1999). Minimum volume cooling method for bovine blastocyst vitrification. Theriogenology 51, 165. doi: 10.1016/S0093-691X(99)91724-7
Harii S., Kayanne H., Takigawa H., Hayashibara T., Yamamoto M. (2002). Larval survivorship, competency periods and settlement of two brooding corals, Heliopora coerulea and Pocillopora damicornis. Mar. Biol. 141 (1), 39–46. doi: 10.1007/s00227-002-0812-y
Harii S., Nadaoka K., Yamamoto M., Iwao K. (2007). Temporal changes in settlement, lipid content and lipid composition of larvae of the spawning hermatypic coral Acropora tenuis. Mar. Ecol. Prog. Ser. 346, 89–96. doi: 10.3354/meps07114
Harrigan J. F. (1972). The planula larva of Pocillopora damicornis: Lunar periodicity of swarming and substratum selection behavior. (University of Hawaii).
Hassan Md M., Qin J. G., Li X. (2015). Sperm cryopreservation in oysters: a review of its current status and potentials for future application in aquaculture. Aquaculture 438, 24–32. doi: 10.1016/j.aquaculture.2014.12.037
Heres P., Rodriguez-Riveiro R., Troncoso J., Paredes E. (2019). Toxicity tests of cryoprotecting agents for Mytilus galloprovincialis (Lamark, 1819) early developmental stages. Cryobiology 86, 40–46. doi: 10.1016/j.cryobiol.2019.01.001
Heres P., Troncoso J., Paredes E. (2021). Larval cryopreservation as new management tool for threatened clam fisheries. Sci. Rep. 11, 1–15. doi: 10.1038/s41598-021-94197-2
Hoegh-Guldberg O. (1999). Climate change, coral bleaching and the future of the world's coral reefs. Mar. freshw.res. 50 (8), 839–866.
Hughes T. P., Baird A. H., Dinsdale E. A., Moltschaniwskyj N. A., Pratchett M. S., Tanner J. E., et al. (1999). Patterns of recruitment and abundance of corals along the great barrier reef. Nature 397, 59–63. doi: 10.1038/16237
Hughes T. P., Kerry J. T., Baird A. H., Connolly S. R., Dietzel A., Eakin C. M., et al. (2018). Global warming transforms coral reef assemblages. Nature 556, 492–496. doi: 10.1038/s41586-018-0041-2
Isachenko E., Isachenko V., Katkov I. I., Dessole S., Nawroth F. (2003). Vitrification of mammalian spermatozoa in the absence of cryoprotectants: From past practical difficulties to present success. Reprod. BioMed. Online 6, 191–200. doi: 10.1016/S1472-6483(10)61710-5
Jang T. H., Park S. C., Yang J. H., Kim J. Y., Seok J. H., Park U. S., et al. (2017). Corneal cryopreservation and its clinical application. Integr. Med. Res. 6, 12–18. doi: 10.1016/j.imr.2016.12.001
Janiszewska K., Jaroszewicz J., Stolarski J. (2013). Skeletal ontogeny in basal scleractinian micrabaciid corals. J. Morphol 274, 243–257. doi: 10.1002/jmor.20085
Jell J. S. (1979). Skeletogenesis of newly settled planulae of the hermatypic coral Porites lutea. Acta Palaeontol 25, 311–320.
Jin B., Kleinhans F., Mazur P. (2014). Survivals of mouse oocytes approach 100% after vitrification in 3-fold diluted media and ultra-rapid warming by an IR laser pulse. Cryobiology 68, 419–430. doi: 10.1016/j.cryobiol.2014.03.005
Jin B., Mazur P. (2015). High survival of mouse oocytes/embryos after vitrification without permeating cryoprotectants followed by ultra-rapid warming with an IR laser pulse. Sci. Rep. 5, 1–6. doi: 10.1038/srep09271
Kader A. A., Choi A., Orief Y., Agarwal A. (2009). Factors affecting the outcome of human blastocyst vitrification. Reprod. Biol. Endocrinol. 7, 99. doi: 10.1186/1477-7827-7-99
Kasai M., Komi J. H., Takakamo A., Tsudera H., Sakurai T., Machida T. (1990). A simple method for mouse embryo cryopreservation in a low toxicity vitrification solution, without appreciable loss of viability. J. Reprod. Fertil. 89 (1), 91–97. doi: 10.1530/jrf.0.0890091
Kerr A. M., Baird A. H., Hughes T. P. (2011). Correlated evolution of sex and reproductive mode in corals (Anthozoa: Scleractinia). Proc. R Soc. Lond B Biol. Sci. 278, 75–81. doi: 10.1098/rspb.2010.1196
Khin Maung O., Kurokura H., Iwano T., Okamoto K., Kado R., Hino A. (1998). Cryopreservation of nauplius larvae of the barnacle, Balanus amphitrite Darwin. Fish. Sci. 64 (6), 857–860.
Khosla K., Kangas J., Liu Y., Zhan L., Daly J., Hagedorn M., et al. (2020). Cryopreservation and laser nanowarming of zebrafish embryos followed by hatching and spawning. Adv. Biosyst. 4, 1–11. doi: 10.1002/adbi.202000138
Kihika J. K., Wood S. A., Rhodes L., Smith K. F., Miller M. R., Pochon X., et al. (2022a). Cryopreservation of six symbiodiniaceae genera and assessment of fatty acid profiles in response to increased salinity treatments. Sci. Rep. 12, 1–15. doi: 10.1038/s41598-022-16735-w
Kihika J. K., Wood S. A., Rhodes L., Smith K. F., Thompson L., Challenger S., et al. (2022b). Cryoprotectant treatment tests on three morphologically diverse marine dinoflagellates and the cryopreservation of Breviolum sp. (Symbiodiniaceae). Sci. Rep. 12, 1–12. doi: 10.1038/s41598-021-04227-2
Kim J. Y., Kinoshita M., Ohnishi M., Fukui Y. (2001). Lipid and fatty acid analysis of fresh and frozen-thawed immature and in vitro matured bovine oocytes. Reproduction 122 (1), 131–138. doi: 10.1530/rep.0.1220131
Kleinhans F., Mazur P. (2015). Physical parameters, modeling, and methodological details in using IR laser pulses to warm frozen or vitrified cells ultra-rapidly. Cryobiology 70, 195–203. doi: 10.1016/j.cryobiol.2015.02.003
le Tissier M. D. A. A. (1988). Patterns of formation and the ultrastructure of the larval skeleton of Pocillopora damicornis. Mar. Biol. 98, 493–501. doi: 10.1007/BF00391540
Liebermann J., Nawroth F., Isachenko V., Isachenko E., Rahimi G., Tucker M. J. (2002). Potential importance of vitrification in reproductive medicine. Biol. Reprod. 67, 1671–1680. doi: 10.1095/biolreprod.102.006833
Lin C., Kang C. M., Huang C. Y., Li H. H., Tsai S. (2022). Study on the development and growth of coral larvae. Appl. Sci. 12, 1–19. doi: 10.3390/app12105255
Lin C., Kuo F. W., Chavanich S., Viyakarn V. (2014). Membrane lipid phase transition behavior of oocytes from three gorgonian corals in relation to chilling injury. PloS One 9, 1–6. doi: 10.1371/journal.pone.0092812
Lin C. C., Li H. H., Tsai S., Lin C. (2022). Tissue cryopreservation and cryobanking: establishment of a cryogenic resource for coral reefs. Biopreserv Biobank 20, 409–411. doi: 10.1089/bio.2021.0089
Lin C., Thongpoo P., Juri C., Wang L.H., Meng P.J., Kuo F.W., et al. (2019). Cryopreservation of a thermotolerant lineage of the coral reef dinoflagellate Symbiodinium. Biopreserv Biobank 17, 520–529. doi: 10.1089/bio.2019.0019
Lin C., Tsai S. (2012). The effect of chilling and cryoprotectants on hard coral (Echinopora spp.) oocytes during short-term low temperature preservation. Theriogenology 77, 1257–1261. doi: 10.1016/j.theriogenology.2011.09.021
Lin C., Tsai S. (2020). Fifteen years of coral cryopreservation. Platax 17, 53–76. doi: 10.29926/platax.202012_17.0004
Lin C., Wang L. H., Meng P. J., Chen C. S., Tsai S. (2013). Lipid content and composition of oocytes from five coral species: Potential implications for future cryopreservation efforts. PloS One 8, 2–7. doi: 10.1371/journal.pone.0057823
Lin C., Hsieh W. C., Loeslakwiboon K., Huang C. L., Chen T. C., Tsai S. (2023). Refined Techniques for Enabling Long-Term Cryo-Repository Using Vitrification and Laser Warming. Bioengineering, 10 (5), 605. doi: 10.3390/bioengineering10050605
Loya Y. (1976). The red Sea coral Stylophora pistillata is a n r strategist. Nature 259, 478–480. doi: 10.1038/259478a0
Loya Y., Sakai K., Nakano Y., van Woesik R. (2001). Coral bleaching: the winners and the losers. Ecol. Lett. 4, 122–131. doi: 10.1046/j.1461-0248.2001.00203.x
Liu Y., Gluis M., Miller-Ezzy P., Qin J., Han J., Zhan X., et al. (2020). Development of a programmable freezing technique on larval cryopreservation in Mytilus galloprovincialis. Aquaculture 516, 1–6. doi: 10.1016/j.aquaculture.2019.734554
Magnotti C., Cerqueira V., Lee-Estevez M., Farias J. G., Valdebenito I., Figueroa E. (2018). Cryopreservation and vitrification of fish semen: A review with special emphasis on marine species. Rev. Aquac 10, 15–25. doi: 10.1111/raq.12145
Marshall P., Schuttenberg H. (2006). A reef manager’s guide to coral bleaching (Townsville, Australia: Great Barrier Reef Marine Park Authority).
Martínez-Páramo S., Horváth Á., Labbé C., Zhang T., Robles V., Herráez P., et al. (2017). Cryobanking of aquatic species. Aquaculture 472, 156–177. doi: 10.1016/j.aquaculture.2016.05.042
Massip A., van der Zwalmen P., Scheffen B., Ectors F. (1986). Pregnancies following transfer of cattle embryos preserved by vitrification. Cryo Lett. 7, 270–273.
Mayfield A. B., Tsai S., Lin C. (2019). The coral hospital. Biopreserv Biobank 17, 355–369. doi: 10.1089/bio.2018.0137
Narida A., Hsieh W., Huang C., Wen Z. (2022a). Novel long-term cryo-storage using vitrification and laser warming techniques. Biopreserv Biobank 00, 1–6. doi: 10.1089/bio.2022.0033
Narida A., Tsai S., Huang C. Y., Wen Z.-H., Lin C. (2022b). The effects of cryopreservation on the cell ultrastructure in aquatic organisms. Biopreserv Biobank 00, 1–8. doi: 10.1089/bio.2021.0132
Oku H., Yamashiro H., Onaga K., Sakai K., Iwasaki H. (2003). Seasonal changes in the content and composition of lipids in the coral Goniastrea aspera. Coral Reefs 22 (1), 83–85. doi: 10.1007/s00338-003-0279-4
Olive P. J. W., Wang W. B. (2002). Cryopreservation of Nereis virens (Polychaeta, Annelida) larvae: The mechanism of cryopreservation of a differentiated metazoan. Cryobiology 34 (3), 284–294. doi: 10.1006/cryo.1997.2006
Paniagua-Chávez C. G., Buchanan J. T., Supan J. E., Tiersch T. R. (1998). Settlement and growth of Eastern oysters produced from cryopreserved larvae. Cryo Lett. 19 (5), 283–292.
Paniagua-Chávez C. G., Ortiz-Gallarza S. M., Aguilar-Juárez M. (2011). National subsystem for aquatic genetic resources: The use of cryopreservation for conservation of aquatic genetic resources in Mexico. Hidrobiologica 21 (3), 415–429.
Paniagua-Chavez C., Tiersch T. (2001). Laboratory studies of cryopreservation of sperm and trochophore larvae of the eastern oyster. Cryobiology 43 (3), 211–223. doi: 10.1006/cryo.2001.2346
Paredes E., Adams S. L., Tervit H. R., Smith J. F., McGowan L. T., Gale S. L., et al. (2012). Cryopreservation of GreenshellTM mussel (Perna canaliculus) trochophore larvae. Cryobiology 65 (3), 256–262. doi: 10.1016/j.cryobiol.2012.07.078
Paredes E., Bellas J., Adams S. L. (2013). Comparative cryopreservation study of trochophore larvae from two species of bivalves: Pacific oyster (Crassostrea gigas) and blue mussel (Mytilus galloprovincialis). Cryobiology 67 (3), 274–279. doi: 10.1016/j.cryobiol.2013.08.007
Paredes E. (2021). Vitrification and ultra-rapid laser warming of yeast Saccharomyces cerevisiae. Cryo Lett. 42, 19–24. doi: 10.1016/j.cryobiol.2016.09.090
Perrin J., Vielzeuf D., Ricolleau A., Dallaporta H., Valton S., Floquet N. (2015). Block-by-block and layer-by-layer growth modes in coral skeletons. Am. Mineralogist 100, 681–695. doi: 10.2138/am-2015-4990
Piazza V., Gambardella C., Costa E., Miroglio R., Faimali M., Garaventa F. (2022). Cold storage effects on lethal and sublethal responses of Amphibalanus amphitrite nauplii. Ecotoxicology. 31, 1078–1086. doi: 10.1007/s10646-022-02571-1
Rall W. (1993). “Cryobiology of gametes and embryos from nonhuman primates,” in In vitro fertilization and embryo transfer in primates. Eds. Wolf D. P., Stouffer R. L., Brenner R. M. (New York, NY: Springer New York), 223–245.
Rall W., Fahy G. (1985). Ice-free cryopreservation of mouse embryos at -196°C by vitrification. Nature 313, 573–575. doi: 10.1038/313573a0
Randall C. J., Giuliano C., Mead D., Heyward A. J., Negri A. P. (2019). Immobilisation of living coral embryos and larvae. Sci. Rep. 9, 1–7. doi: 10.1038/s41598-019-51072-5
Rapatz G., Luyet B. (1968). Electron microscope study of erythrocytes in rapidly cooled suspensions containing various concentrations of glycerol. Biodynamica 10, 193—210.
Richmond R. (1987). Energetics, competency, and long-distance dispersal of planula larvae of the coral Pocillopora damicornis. Mar. Biol. 93, 527–533. doi: 10.1038/093124a0
Rinkevich B., Loya Y. (1979). The reproduction of the red Sea coral Stylophora pistillata. II. Synchronizaton in breeding and seasonality of planulae shedding. Mar. Ecol. Prog. Ser. 1, 145–152. doi: 10.3354/meps001133
Rivest E. B., Chen C. S., Fan T. Y., Li H. H., Hofmann G. E. (2017). Lipid consumption in coral larvae differs among sites: A consideration of environmental history in a global ocean change scenario. Proc. R. Soc. B 284, 1–9. doi: 10.1098/rspb.2016.2825
Rowlison T., Nagashima J., Acker J. P., Ben R., Daly J., Hagedorn M., et al. (2022). First report of successful laser warming for frozen gonadal tissues and oocytes in the domestic cat model. Biopreserv Biobank. 0, 0:0. doi: 10.1089/bio.2022.0071
Rusk A. B., Alfaro A. C., Young T., Watts E., Adams S. L. (2020). Development stage of cryopreserved mussel (Perna canaliculus) larvae influences post-thaw impact on shell formation, organogenesis, neurogenesis, feeding ability and survival. Cryobiology. 93, 121–132. doi: 10.1016/j.cryobiol.2020.01.021
Schiewe M. C., Anderson R. E. (2017). Vitrification: The pioneering past to current trends and perspectives of cryopreserving human embryos, gametes and reproductive tissue. J. Biorepos Sci. Appl. Med. 5, 57–68. doi: 10.2147/bsam.s139376
Seidel G. E. (2006). Modifying oocytes and embryos to improve their cryopreservation. Theriogenology 65 (1), 228–235. doi: 10.1016/j.theriogenology.2005.09.025
Seki S., Mazur P. (2009). The dominance of warming rate over cooling rate in the survival of mouse oocytes subjected to a vitrification procedure. Cryobiology 59, 75–82. doi: 10.1016/j.cryobiol.2009.04.012
Seki S., Mazur P. (2012). Ultra-rapid warming yields high survival of mouse oocytes cooled to -196°C in dilutions of a standard vitrification solution. PloS One 7, 1–9. doi: 10.1371/journal.pone.0036058
Spis E., Bushkovskaia A., Isachenko E., Todorov P., Sanchez R., Skopets V., et al. (2019). Conventional freezing vs. cryoprotectant-free vitrification of epididymal (MESA) and testicular (TESE) spermatozoa: Three live births. Cryobiology 90, 100–102. doi: 10.1016/j.cryobiol.2019.08.003
Stiles W. (1930). On the cause of cold death of plants. Protoplasma 9 (1), 459–468. doi: 10.1007/BF01943364
Stolarski J. (2003). Three-dimensional micro- and nanostructural characteristics of the scleractinian coral skeleton: A biocalcification proxy. Acta Palaeontol Pol. 48, 497–530.
Subramoniam T., Arun R. (1999). Cryopreservation and aquaculture: A case study with penaeid shrimp larvae. Curr. Sci. 76 (3), 361–368.
Subramoniam T., Newton S. S. (1993). Cryopreservation of penaeid prawn embryos. Curr. Sci. 65 (2), 176–178.
Sun C. Y., Stifler C. A., Chopdekar R., Schmidt C. A., Parida G., Schoeppler V., et al. (2020). From particle attachment to space-filling coral skeletons. Proc. Natl. Acad. Sci. U.S.A. 117, 30159–30170. doi: 10.1073/pnas.2012025117
Suneja S., Alfaro A. C., Rusk A. B., Morrish J. R., Tervit H. R., McGowan L. T., et al. (2014). Multi-technique approach to characterise the effects of cryopreservation on larval development of the Pacific oyster (Crassostrea gigas). N. Z. J. Mar. Freshw. Res. 48 (3), 335–349. doi: 10.1080/00288330.2014.914545
Toh E. C., Liu K. L., Tsai S., Lin C. (2022). Cryopreservation and cryobanking of cells from 100 coral species. Cells 11, 2668. doi: 10.3390/cells11172668
Tsai S., Chang W. C., Chavanich S., Viyakarn V., Lin C. (2016a). Ultrastructural observation of oocytes in six types of stony corals. Tissue Cell 48, 349–355. doi: 10.1016/j.tice.2016.05.005
Tsai S., Lin C. (2012). Advantages and applications of cryopreservation in fisheries science. Braz. Arch. Biol. Technol. 55, 425–434. doi: 10.1590/S1516-89132012000300014
Tsai S., Spikings E., Huang I. C., Lin C. (2011). Study on the mitochondrial activity and membrane potential after exposing later stage oocytes of two Gorgonian corals (Junceella juncea and Junceella fragilis) to cryoprotectants. CryoLetters 32, 1–12.
Tsai S., Yang V., Lin C. (2016b). Comparison of the cryo-tolerance of vitrified Gorgonian oocytes. Sci. Rep. 6, 1–8. doi: 10.1038/srep23290
Tsai S., Yen W., Chavanich S., Viyakarn V., Lin C. (2015). Development of cryopreservation techniques for Gorgonian (Junceella juncea) oocytes through vitrification. PloS One 10, 1–14. doi: 10.1371/journal.pone.0123409
Tsvetkova L. I., Cosson J., Linhart O., Billard R. (1996). Motility and fertilizing capacity of fresh and frozen-thawed spermatozoa in sturgeons Acipenser baeri and A. ruthenus. J. Appl. Ichthyol 12;2, 107–112. doi: 10.1111/j.1439-0426.1996.tb00071.x
Turkevich J., Stevenson P. C., Hillier J. (1951). A study of the nucleation and growth processes in the synthesis of colloidal gold. Discuss Faraday Soc. 11, 55–75. doi: 10.1039/DF9511100055
Usuki H., Hamaguchi M., Ishioka H. (2002). Effects of developmental stage, seawater concentration and rearing temperature on cryopreservation of Pacific oyster Crassostrea gigas larvae. Fish. Sci. 68 (4), 757–762. doi: 10.1046/j.1444-2906.2002.00490.x
Vandermeulen J. H., Watabe N. (1973). Studies on reef corals. I. skeleton formation by newly settled planula larva of Pocillopora. Mar. Biol. 23, 47–57. doi: 10.1007/BF00394111
Vanderzwalmen P., Ectors F., Panagiotidis Y., Schu M., Murtinger M., Wirleitner B. (2020). The evolution of the cryopreservation techniques in reproductive medicine—exploring the character of the vitrified state intra- and extracellularly to better understand cell survival after cryopreservation. Reprod. Med. 1, 142–157. doi: 10.3390/reprodmed1020011
van Woesik R., Sakai K., Loya Y. (2011). Revisiting the winners and the losers a decade after coral bleaching. Mar. Ecol. Prog. Ser. 434, 67–76. doi: 10.3354/meps09203
Veron J. E. N., Stafford-Smith M. (2000). Corals of the world (Townsville, MC Qld, Australia: Australian Institute of Marine Science).
Viyakarn V., Chavanich S., Chong G., Tsai S., Lin C. (2018). Cryopreservation of sperm from the coral Acropora humilis. Cryobiology 80, 130–138. doi: 10.1016/j.cryobiol.2017.10.007
Wallace C. C. (1999). Staghorn corals of the world: A revision of the coral genus Acropora (Scleractinia; Astrocoeniina; Acroporidae) worldwide, with emphasis on morphology, phylogeny and biogeography. (Collingwood, Vic, Australia: CSIRO).
Wang H., Li X., Wang M., Clarke S., Gluis M., Zhang Z. (2011). Effects of larval cryopreservation on subsequent development of the blue mussels, Mytilus galloprovincialis lamarck. Aquac. Res. 42, 1816–1823. doi: 10.1111/j.1365-2109.2010.02782.x
Wang L. H., Chen H. K., Jhu C. S., Cheng J. O., Fang L. S., Chen C. S. (2015). Different strategies of energy storage in cultured and freshly isolated Symbiodinium sp. J. Phycol 51 (6), 1127–1136. doi: 10.1111/jpy.12349
Whaley D., Damyar K., Witek R. P., Mendoza A., Alexander M., Lakey J. R. (2021). Cryopreservation: An overview of principles and cell-specific considerations. Cell Transplant. 30, 1–12. doi: 10.1177/0963689721999617
Yuswiati E., Holtz W. (1990). Work in progress: Successful transfer of vitrified goat embryos. Theriogenology 34, 629–632. doi: 10.1016/0093-691X(90)90018-O
Zakai D., Dubinsky Z., Avishai A., Caaras T., Chadwick N. E. (2006). Lunar periodicity of planula release in the reef-building coral Stylophora pistillata. Mar. Ecol. Prog. Ser. 311, 93–102. doi: 10.3354/meps311093
Keywords: Stylophora pistillata, cryopreservation, laser warming, larvae, season
Citation: Narida A, Tsai S, Hsieh W-C, Wen Z-H, Wang L-H, Huang C-L and Lin C (2023) First successful production of adult corals derived from cryopreserved larvae. Front. Mar. Sci. 10:1172102. doi: 10.3389/fmars.2023.1172102
Received: 06 March 2023; Accepted: 08 May 2023;
Published: 25 May 2023.
Edited by:
Alaa El-Din Hamid Sayed, Assiut University, EgyptReviewed by:
Sebastian G. Gornik, Heidelberg University, GermanyYuehuan Zhang, Chinese Academy of Sciences (CAS), China
Copyright © 2023 Narida, Tsai, Hsieh, Wen, Wang, Huang and Lin. This is an open-access article distributed under the terms of the Creative Commons Attribution License (CC BY). The use, distribution or reproduction in other forums is permitted, provided the original author(s) and the copyright owner(s) are credited and that the original publication in this journal is cited, in accordance with accepted academic practice. No use, distribution or reproduction is permitted which does not comply with these terms.
*Correspondence: Chiahsin Lin, chiahsin@nmmba.gov.tw