- 1College of Fisheries, Guangdong Ocean University, Zhanjiang, Guangdong, China
- 2Guangdong Provincial Key Laboratory of Pathogenic Biology and Epidemiology for Aquatic Economic Animals, Zhanjiang, Guangdong, China
- 3Southern Marine Science and Engineering Guangdong Laboratory, Zhanjiang, Guangdong, China
In this study, the inhibitory effect of different doses of hydrogen peroxide nano-silver ion composite disinfectant (HPS) on DIV1 and bacteria was analyzed, and the antibacterial activity of three disinfectants, HPS, potassium monopersulfate (KMPS) and calcium hypochlorite (Ca(ClO)2), was compared. In addition, 16S rDNA amplification sequencing technology was used to analyze the effects of these three disinfectants on the intestinal microflora of Litopenaeus vannamei and the structure and composition of water microflora. The results showed that HPS did not positively affect the survival rate of prawns infected with DIV1, which needs to be verified in future studies. In the experimental design range, the higher the dosage of HPS, the more obvious the killing effect on the number of Vibrio and total bacteria in rearing water, and the two showed a negative correlation. 5,104 OTUs were obtained based on 16S rDNA high-throughput sequencing, of which 3,012 (59.01%) and 1,475 (28.90%) OUTs were annotated at the phylum and genus levels, respectively. Proteobacteria, Firmicutes, Actinobacteriota, Bacteroidetes, and Cyanobacteria dominated the water samples at the phylum level. At the genus level, the dominant bacterial genera in the intestinal bacterial community of shrimp were Photobacterium, Vibrio, and Ruegeria. The most dominant bacteria genera in water samples were Vibrio, Ruegeria, Pseudoalteromonas, and Nautella. In the water samples, the composition and structure of the Ca(ClO)2 microbial community were relatively simple, and the species richness and diversity of Ca(ClO)2 were significantly lower than those of HPS and KMPS disinfectant groups at 12 h and 24 h (p < 0.05). In terms of inhibiting the diversity and richness of the microbial community, Ca(ClO)2 had a significant effect (p < 0.05), but the intestinal microbial community diversity of shrimp treated with HPS was higher than that of the other two groups. It is worth noting that, compared with the three disinfectants, HPS has the strongest killing effect on Vibrio and total bacteria, and has a certain positive significance for maintaining the stable state of the microbial community. This study provides a scientific basis for applying HPS in aquaculture and broadens the application range of HPS.
1 Introduction
Aquaculture is one of the world’s fastest-growing food production areas (Bayliss et al., 2017; FAO, 2022). Currently, half of the world’s aquatic products come from aquaculture. With the pollution of the marine aquatic environment and the decline of the wild fishing industry, aquaculture is playing an increasingly important role in the sustainable food supply and has become an important supplement to ensure the world’s food security. According to the statistics of the Food and Agriculture Organization of the United Nations (FAO) (FAO, 2022), the world’s aquaculture output in 2020 reached 122,578.5 thousand tons. As the world’s largest aquaculture country, China’s aquaculture output accounted for 57.5% of the world’s total, providing a large amount of high-quality animal protein for people. Prawn is the backbone industry of aquaculture. In 2020, the total production of crustacean aquaculture in the world was 11,237.0 thousand tons, while the total production of Litopenaeus vannamei and Penaeus monodon in the world was 5812.2 thousand tons and 717.1 thousand tons, accounting for 51.7% and 6.4% of the total production of crustacean aquaculture, respectively, creating huge economic benefits.
In recent years, in pursuit of higher economic benefits, prawn farmers have been promoting high-density aquaculture, which has induced a series of ecological problems such as the deterioration of aquaculture waters and the imbalance of the ecological environment in offshore waters, which in turn has led to the frequent occurrence of prawn farming diseases, especially viral and bacterial diseases, seriously threatening the sustainable development of the s prawn farming industry.
The effective treatment of aquaculture water has become a major technical difficulty. Traditional aquaculture water treatment mainly uses physical filtration and chemical disinfection to eliminate and reduce pathogens, purify water, and control disease outbreaks. The main chemical disinfectants commonly used in aquaculture are potassium permanganate (KMnO4) (Abed et al., 2019), calcium hypochlorite (Ca(ClO)2) (Lewis, 2010), povidone-iodine (PVP-I) (Zhang et al., 2023), hydrogen peroxide (H2O2) (Pedersen and Pedersen, 2012), and potassium monopersulfate (KMPS) (Jin et al., 2018). These chemical disinfectants are strongly bactericidal and are active in farm epidemic prevention, wastewater decontamination, and medical and environmental disinfection. However, more and more evidence shows that most chemical disinfectants contain harmful byproducts in the disinfection process, such as trihalomethane (THM) and haloacetic acid (HAA), which are byproducts of chlorinated disinfectants and potassium persulfate composite disinfectants, and even residues caused by incomplete reactions of the disinfectants themselves, which may lead to chemical pollution and adverse health side effects, and there are safety risks (Smith et al., 2010; Ao et al., 2016; Kali et al., 2021).
Hydrogen peroxide (H2O2), in line with the principles of green chemistry, is an environmentally friendly, highly active, and safe disinfectant that does not produce harmful byproducts (Pedersen and Pedersen, 2012) and can be widely used in health care and veterinary environments and is licensed by the European Commission (SCENIHR, 2009). H2O2, which exerts its disinfection efficacy through the efficient oxidation of hydroxyl radicals directly destroying microbial cell membranes (Acosta et al., 2021), is commonly used in aquaculture to treat some gill and skin infections (Adams et al., 2012; Tkachenko et al., 2014), fulfilling the requirement to be a bactericidal disinfection alternative in aquaculture (Schmidt et al., 2006).
As a promising broad-spectrum antimicrobial agent, silver increasingly appears in various types of sterile antimicrobial materials, making a splash in the food and medical fields and becoming a focal point for antimicrobial material development. A biomaterial coating based on silver ion implantation coating with silicone has been reported to have a sustained antimicrobial effect that effectively reduces the risk of infection in implantable procedures (Heno et al., 2021). Silver implanted into polyethylene food packaging film inhibited the ability of bacteria to adhere to the surface and exert bactericidal effects as a potential protector of food quality and safety (Lu et al., 2021). Tambur et al. successfully decorated nano silver particles on functionalized multi-walled carbon nanotubes, and the study confirmed that this novel material (Ag-MWCNTs) has better antibacterial activity and effectively inhibited the activity of Bacillus subtilis, Staphylococcus aureus, Escherichia coli, and Pseudomonas aeruginosa (Tambur et al., 2020). Studies have shown that the antibacterial mechanisms of silver nanoparticles (AgNPs) and Ag+ are highly similar. On the one hand, AgNPs depend on electrostatic adsorption on bacterial cell membranes, disrupting membrane integrity and inhibiting protein activity, ultimately leading to bacterial lysis and death (Antoine et al., 2006; Dai et al., 2016; Hamad et al., 2020). On the other hand, the mechanism of Ag+ inhibition is through uptake by bacterial cell membranes, which results in a series of reactions that inhibit cellular production of adenosine triphosphate (ATP), block DNA replication channels, and generate reactive oxygen species (ROS) (Nikolaj et al., 2005).
Putting aside the fact that silver has good antibacterial properties, many people still question the safety of silver as an antibacterial agent, and the toxic effects of silver have attracted increasing attention from the scientific and public communities. Trace amounts of silver are harmless to humans, and the guidelines for drinking water quality published by the World Health Organization stipulate that the concentration of silver ions in drinking water is less than 0.1 mg/L and does not threaten human health (WHO, 2004). By analyzing the dermal toxicity and in vitro cytotoxicity of AgNPs, as well as the biosafety exposure of AgNPs and nano-silver materials, scholars showed that nano-silver ion skin disinfectants exhibit good killing effects on both bacteria and fungi without causing skin irritation, ensuring the safety of the application in skin management (Yan and Wang, 2020).
Based on the excellent bactericidal properties of H2O2 and silver, a new composite disinfectant, hydrogen peroxide nano-silver ion (HPS), has attracted wide interest from scholars. It was concluded that H2O2 and Ag+ have a synergistic antimicrobial effect (Pedahzur et al., 1997; Orta De Velasquez et al., 2008; Martin et al., 2015), and the implantation of silver helps stabilize H2O2, slow down the decomposition, and enhance the disinfection effect of H2O2. Martin et al. (Martin et al., 2015) compared the antimicrobial activity and mechanism of action of hydrogen peroxide nano-silver ion and H2O2 and found that the former has stronger bactericidal activity than the latter, confirming that the intervention of Ag+ increases the interaction of HSP with the bacterial cell surface. Girolamini et al. determined that related parameters of Legionella, Pseudomonas aeruginosa, and the heterotopic plate count (HPC) were analyzed, suggesting that the new formulation of H2O2 and silver salt (WTP828) was safe and effective as a potential alternative to conventional disinfection methods (Girolamini et al., 2019). Because of the high efficiency, stability, safety, and environmental friendliness achieved by the HPS, it is increasingly used in the disinfection and sterilization treatment of wastewater treatment, medical equipment, and drinking water (Mahnel and Schmidt, 1986; Liang, 2016). Notably, no studies have applied this compound disinfectant in aquaculture water disinfection.
This experiment aimed to investigate the antiviral effect of HPS in shrimp and the inhibition of Vibrio and total bacteria in the rearing water. The 16S rDNA amplicon sequencing technology was applied to analyze and compare the differences of HPS, potassium monopersulfate (KMPS), and Ca(ClO)2 on the microbiota of shrimp intestine and microbiota of rearing water, the interactions between them, and the regulatory pathways. This study is the first to introduce a new hydrogen peroxide nano-silver ion composite disinfectant into shrimp aquaculture, providing a scientific basis for further research of HPS in aquaculture in the future.
2 Materials and methods
2.1 Experimental disinfectants and shrimp feeding
The HPS used in the experiments was developed by Roam Technology, Belgium, and the active ingredients and their contents were H2O2 (7.5-7.9%) and Ag+ (0.042-0.053‰). KMPS and Ca(ClO)2 (32% active chlorine) were obtained from the East Island Marine Biology Research Laboratory, Guangdong Ocean University (Zhanjiang, China). The L. vannamei and Marsupenaeus japonicus used in the experiment were both obtained from the East Island Marine Biology Research Base, with the average body weight of 7.43 ± 0.51 g and 7.82 ± 0.73 g, respectively. Before the experiment, shrimp with intact appendages and no damage were selected and domesticated in a pre-oxygenated 0.3 m3 experimental bucket for 5 days. The artificial compound feed (Guangdong Yuequn Biotechnology Co., Ltd., China) was fed thrice daily at 10% of the shrimp’s body weight during this period. Water was changed once a day, with a water change of approximately 100%, and water quality parameters were checked and recorded, maintaining water temperature at 28.0 ± 0.5 °C, salinity at 35.0 ± 0.6, pH 8.0 ± 0.1.
2.2 Preparation of DIV1 inoculant and challenge experiment
The DIV1 inoculant was prepared and quantified using a method previously published by scholars (Chen et al., 2019), and the DIV1 disease material was obtained from our laboratory. Eighty healthy M. japonicus were randomly selected and divided into four groups for the attack experiment (20 shrimp per group), three of which were injected with 50 μl of 107 copies/ng DNA of DIV1 inoculant at their third abdominal segment muscle, and the other group was injected with 50 μl of phosphate-buffered saline (PBS, pH 7.4). Immediately after that, injected 50 μl of 0 g/m3, 5 g/m3, and 10 g/m3 of HPS to the three groups with DIV1-infected shrimp, respectively, and the group that had been injected with PBS was not injected HPS as a negative control. The injection method of HPS is the same as that of DIV1 inoculant. After the injection, mortality was observed and recorded every 6 h for 72 h, during which no water exchange was performed. The dying M. japonicus were isolated in time to avoid secondary infection.
2.3 Test of the inhibitory effects of different doses of HPS and different disinfectants on Vibrio and total bacteria
To study the effect of different doses of HPS on the inhibition of Vibrio and total bacteria in the rearing water, a dose gradient of 0 g/m3, 0.5 g/m3, 1.0 g/m3, 2.0 g/m3, and 5.0 g/m3 was set. Healthy L.vannamei were randomly selected and put into clean experimental bucket, with 50 prawns in each bucket (the actual experimental water volume was 0.25 m3). The experimental water was filtered seawater, and three replicates were set for each dose group. After 24 h of rearing, HPS was added to the rearing water in five dose gradients, and the rearing water was counted for Vibrio and total bacteria every 24 h for up to 120 h, during which no water exchange was performed.
Based on the dose experiment, three disinfectants, HPS, KMPS, and Ca(ClO)2, were selected to compare their differences in bacterial inhibition ability. Three disinfectants were used at a dose of 1 g/m3, with Ca(ClO)2 calculated as effective chlorine. Refer to the above HPS dose experiment for the experimental operation process.
Bacterial counts of water samples referred to the standard procedure (APHA, 2005), where water samples (10 mL) were collected from each repeat of disinfection treatment every 24 h. It was done in a special chamber. Serial dilutions were performed with sterilized PBS buffer solution in 10-fold orders of magnitude. After dilution to the appropriate order of magnitude, 0.1 mL of subsamples were uniformly coated on the prepared sterile thiosulfate citrate bile salt sucrose agar medium (TCBS) and 2216E agar medium (Qingdao Hope Bio-Technology Co. Ltd., China), respectively, for Vibrio count and total bacterial count. The media were placed in a constant temperature incubator at 36 ± 1 °C and incubated for 24 h and 48 h, respectively. The colonies were counted and expressed as colony-forming units (CFU/mL) per mL of the water sample (John O. Rawlings et al., 1998).
2.4 Analysis of intestinal and water microflora structure of L. vannamei treated with different disinfectants
The experimental disinfectants were HPS, KMPS, and Ca(ClO)2, all at 1 g/m3, with Ca(ClO)2 calculated as effective chlorine. Three disinfectant treatment groups and one control group were set up, with three replicates in each group. L. vannamei with intact and healthy appendages were placed in a pre-oxygenated 0.3 m3 bucket (the actual volume of the experimental water was 0.25 m3) with thirty shrimp in each replicate, and different disinfectants were added to the rearing water respectively after 24 h of feeding, while the control group was not added. In the microbiota results, the three disinfectant treatment groups of HPS, KMPS, and Ca(ClO)2 were denoted as group H, K, and Cl, respectively, while the control group was denoted as group B.
2.4.1 Microbial community sample collection
Microbiological samples from the intestine of the shrimp and microbiological samples from the rearing water were collected at the 12th and 24th h of the disinfection treatment test. Five L. vannamei were randomly retrieved from each replicate, and after washing and wiping the shrimp body surface with distilled water and alcohol disinfection tablets, the intestine and its contents of shrimp were extracted and placed in a 1.5 mL frozen storage tube, quickly placed in liquid nitrogen, and then transferred to the -80 °C refrigerator for storage until DNA extraction. 1 L of water was randomly taken from each experimental bucket and filtered through a 0.45 μm microporous filter membrane, with each filter membrane filtering 250-300 mL of experimental water, collected the membranes in a 50 mL sterile freezer-storage tube and stored quickly in a refrigerator at -80 °C until DNA extraction (He et al., 2020).
2.4.2 Total DNA extraction, amplification, and high-throughput sequencing
Soil DNA Kit (OMEGA, US) was applied to extract total DNA from shrimp intestines and rearing water samples. The concentration and purity of total DNA were determined, and total DNA quality was assessed by 1% agarose gel electrophoresis. The primer pairs 341F (5’-CCTAYGGGRBGCASCAG-3’) and 806R (5’-GGACTACNNGGGTATCTAAT-3’) were used to amplify the V3-V4 high variant region of the 16S rRNA gene. After the PCR products were qualified with 2% agar gel, the small fragment libraries were constructed according to the characteristics of the amplified 16S region, and the libraries were sequenced by the Illumina NovaSeq sequencing platform (Beijing Novozymes Technology Co., Ltd., China). The obtained sequences are available in the NCBI Sequence Read Archive (SRA) database (accession number: PRJNA945210).
2.4.3 Bioinformatic analysis
The original data of intestinal and water samples of L. vannamei were obtained by sequencing. Quantitative Insights Into Microbial Ecology (QIIME, http://qiime.org/index.html) was carried out on the original data splicing and filter to obtain high-quality tags data obtained (Clean Tags). Use Usparse (Version 7.0.1001, http://drive5.com/uparse/), and based on 97% similarity, OTUs (operational taxonomic units) clustering was performed. Based on the OTUs clustering results, on the one hand, species annotation was done for each OTU representative sequence to obtain the corresponding species information and species-based abundance distribution. Meanwhile, the abundance and Alpha diversity were calculated for OTUs to obtain the sample’s species richness and evenness information. On the other hand, multiple sequence comparisons were performed on OTUs, and phylogenetic trees were constructed to explore the differences in community structure among different samples or groups by Beta diversity analysis. The Tax4Fun software was selected to perform functional prediction analysis of microbial communities in the samples.
2.5 Statistical analysis
Data were organized and statistically analyzed using Excel 2019 software and SPSS 26.0 software (SPSS Inc., Chicago, IL, USA), and experimental data were expressed as mean ± standard deviation (SD). All results were analyzed using one-way analysis of variance (ANOVA) with a significance level of p < 0.05, and Duncan’s method was used for multiple comparisons when significant differences existed.
3 Results
3.1 Effects of different doses of HPS on the cumulative survival rate of M. japonicus infected with DIV1
Overall comparison of survival curves showed that no shrimp died in the negative control group injected only with PBS during the experiment, while all three groups died successively. There was no significant difference in the survival rate of M. japonicus infected with DIV1 at different doses of hydrogen peroxide nano-silver ion composite disinfectant (Logrank=0.085, p > 0.05) (Figure 1).
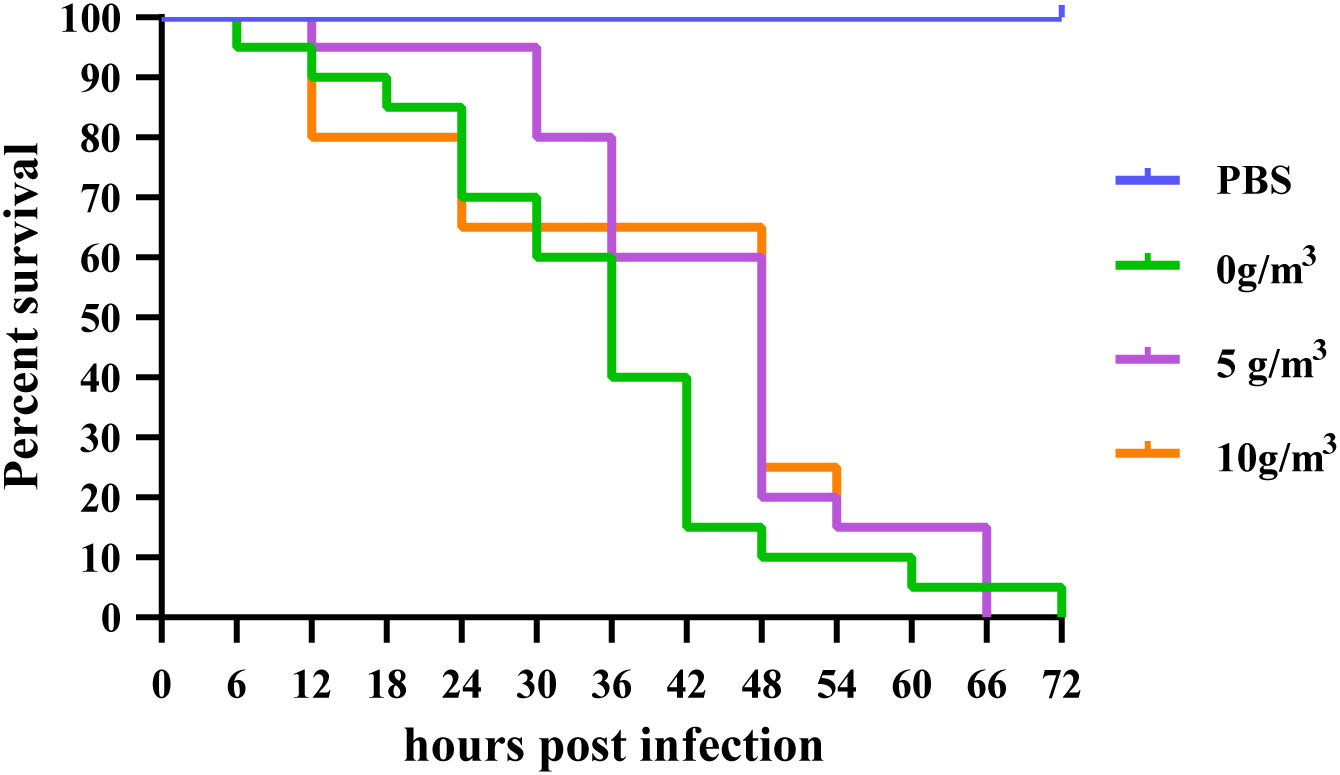
Figure 1 Effect of different doses of HPS on the cumulative survival of M. japonicus after infection with DIV1.
3.2 Vibrio and total bacteria counts in the rearing water of L.vannamei after treatment with different doses of HPS and different disinfectants
After treatment with five different doses of HPS, the number of Vibrio in each group showed that the number of Vibrio in the group without disinfectant addition was always the largest during the experimental period ((26.00 ± 3.61) ×10² CFU/mL ~ (27.67 ± 2.08) ×10² CFU/mL) and did not show any inhibitory effect on Vibrio. In the four disinfectant addition groups, the number of Vibrio decreased with increasing disinfectant dose, and the number of Vibrio in the experimental group with 5.0 g/m3 addition was always the lowest (lowest (5.33 ± 0.58) ×10² CFU/mL) and always significantly lower (p < 0.05) than in the non-addition and low concentration addition groups (0.5 g/m3) (Figure 2A). The trend in the number of total bacteria was similar to that of Vibrio, with the inhibitory effect on bacteria increasing with the amount of disinfectant additive (Figure 2B), where the number of total bacteria was significantly higher (p < 0.05) in the unspiked and 0.5 g/m3-spiked groups than in the 5.0 g/m3-spiked group. From the 0th h to the 24th h, the number of Vibrio in the disinfectant-added group decreased sharply, and the higher the dose, the faster the bacterial abundance decreased, followed by a plateau and rebound period.
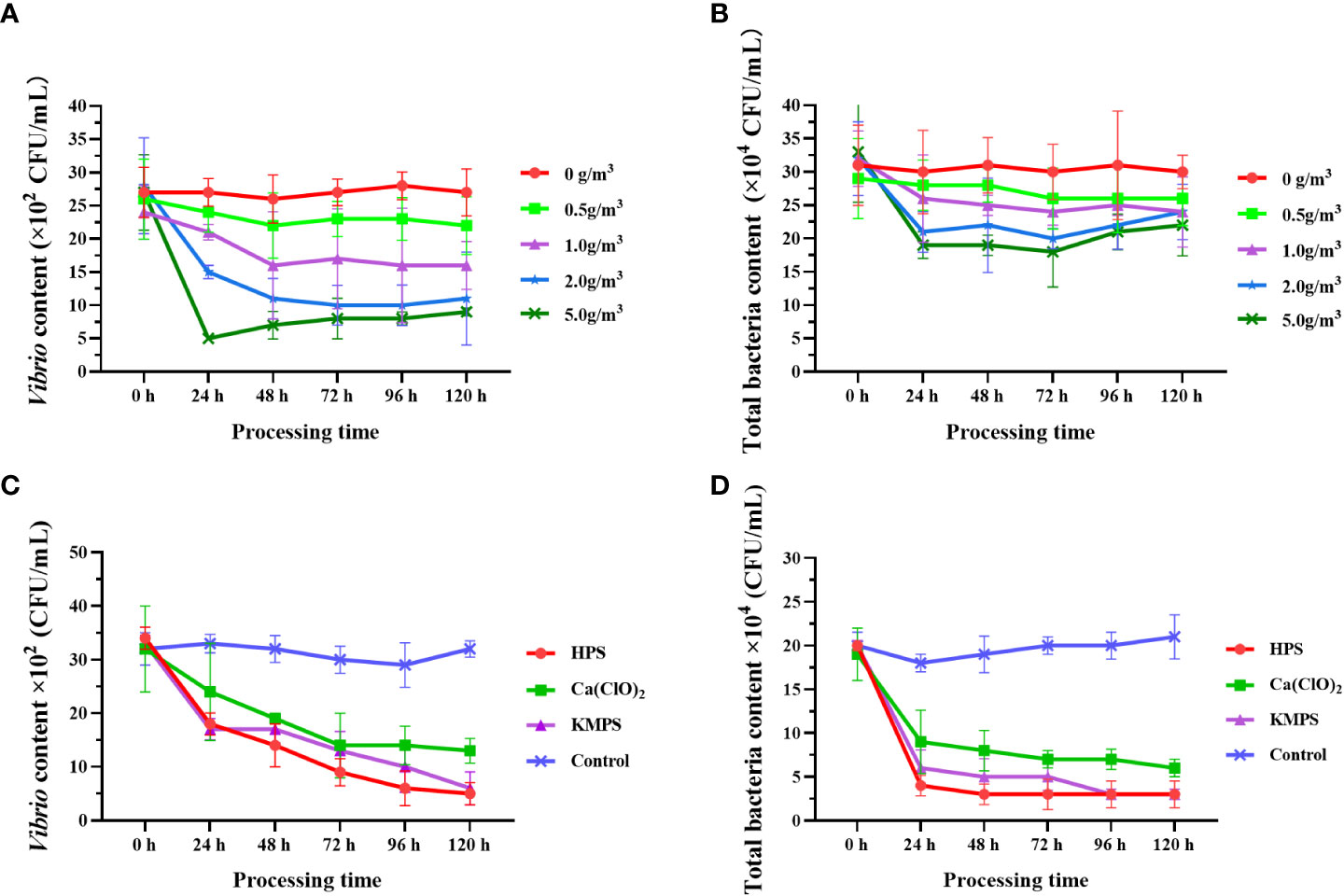
Figure 2 Quantity changes of Vibrio and total bacteria after treatment with different doses of HPS (A, B) and different disinfectants (C, D). Data are expressed as mean ± SD.
Comparison of the bacterial inhibition ability of different disinfectants showed that all three disinfectants showed significant inhibition of Vibrio and total bacteria, and the numbers of both Vibrio and total bacteria were significantly lower than the control group (p < 0.05), specifically the best bacterial inhibition ability of HPS, followed by KMPS (Figures 2C, D). Similarly, the number of bacteria decreased fastest within 24 h after the addition of disinfectants and then decreased slowly (Vibrio) or remained stable (total bacteria).
3.3 Overview of Illumina sequencing and OUT analysis
Sequencing was performed based on the Illumina Nova platform, and the reads were spliced. An average of 88,225 tags were measured per sample, the effective tags were obtained 80,351 effective sequences after quality control, the effective data volume reached 55,295, and the effective rate of quality control reached 62.70%. Sequences were clustered into OTUs using 97% identity as the criterion, and a total of 5,104 OTUs were obtained. Species annotation of OTUs sequences showed that the percentage of annotation to the phylum level was 59.01%; the percentage of annotation to the genus level was 28.90%.
Compared with 12 h, after 24 h treatment, except for the Cl group, the number of OTUs in intestinal microflora samples of the other three groups increased, and the B group (Control group) had the largest increase in OTUs (Table 1). Compared with the water samples treated with disinfectant for 12 h, the number of OTUs increased after treatment for 24 h, and the increasing amount of Cl group was the smallest, and the increasing amount of H group was the largest. In intestinal microflora samples, the number of annotations in K, H, and B groups at different levels (phylum, class, order, family, and genus) increased from 12 h to 24 h after treatment, but the number of annotations in the Cl group showed a downward trend. In the water microflora samples, the number of annotations at different levels (phylum, class, order, family and genus) in the disinfectant addition groups and the control group showed an increasing trend after 24 h of treatment compared with 12 h of treatment.
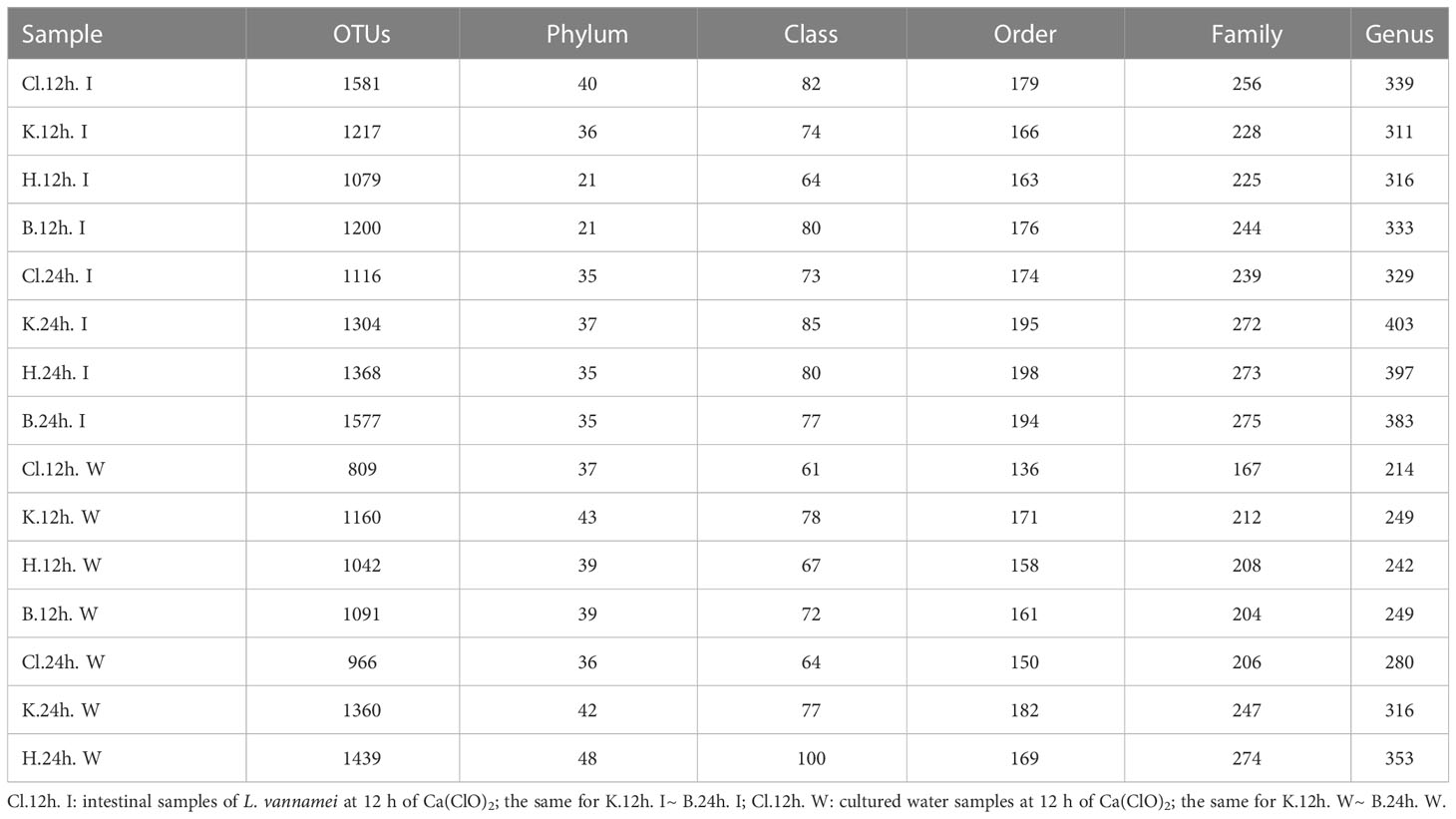
Table 1 Annotation overview of the number of OTUs and levels (phylum, class, order, family, and genus).
3.4 Microbial community composition of L. vannamei intestine and rearing water
The microflora structure was similar between the intestinal tract of L. vannamei and the water samples, and the composition ratio was somewhat different. According to the results of species annotation, the top 10 microbial colonies with maximum abundance at phylum and genus levels were selected for analysis. At the phylum level, it is found that Proteobacteria, Firmicutes, Actinobacteria, Bacteroidetes, and Cyanobacteria occupy the dominant position (Figure 3A). In the intestinal microflora samples, after 12 h treatment, the relative abundance of Proteobacteria in the H group was the highest (80.48%), followed by Cl group (76.88%) and K group (60.24%), while the relative abundance of the control group (B group, 58.48%) was the lowest. Nevertheless, there was no significant difference between groups (p > 0.05) (Figure 3B). There was no significant difference in the relative abundance of Proteobacteria among all groups after 24 h treatment compared with 12 h treatment (p > 0.05). In addition, there were no significant differences in the relative abundance of Firmicutes, Actinobacteria, and Bacteroidetes among the four groups and between the two time periods (p > 0.05) (Figures 3C–E).
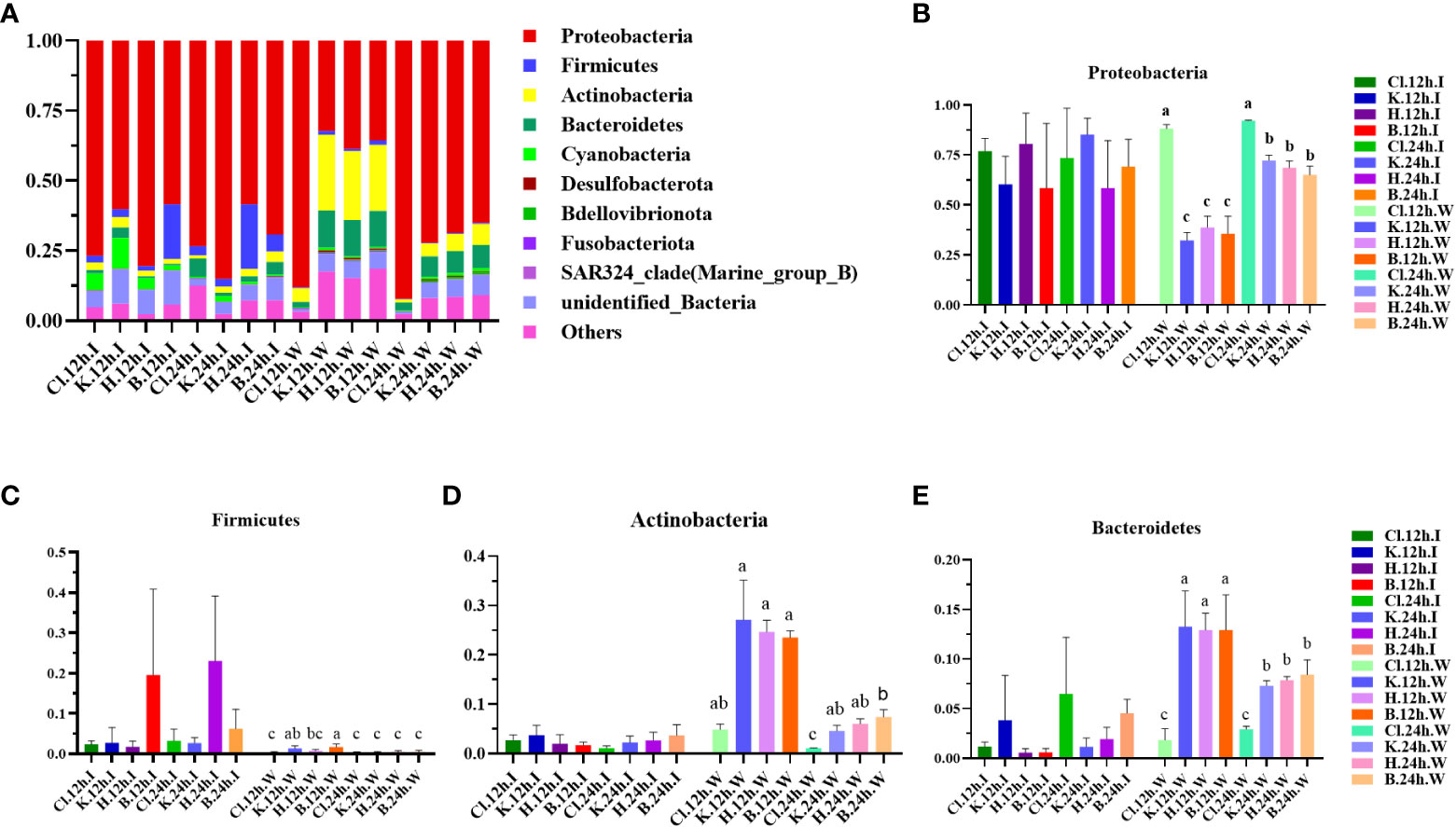
Figure 3 Structure and composition of intestinal dominant microflora and water dominant microflora of shrimp after different disinfectants at the phylum level. (A) shows the relative abundance of the top 10 dominant phyla, and (B–E) indicate the abundance changes of Proteobacteria, Firmicutes, Actinobacteria, and Bacteroidetes, respectively. Data are expressed as mean ± SD, with different letters indicating a significant difference (p < 0.05).
Among the water samples, the species composition of the Cl group was relatively simple, and the relative abundance of Proteobacteria in the Cl group was the highest (88.11%) after 12 h treatment. The relative abundance of Proteobacteria in the K, H, and B groups was 32.26%, 38.73%, and 35.62%, significantly lower than those in the Cl group (p < 0.05). After comparison for 12 h, the relative abundance of Proteobacteria in the other three groups, except the Cl group, was significantly up-regulated at 24 h (p < 0.05). After 12 h of treatment, there were no significant differences in the relative abundance of Actinobacteria and Bacteroidetes in the K, H, and B groups (p > 0.05). After 24 h treatment, the relative abundance of 2 dominant bacteria in the K and B groups was significantly lower than in groups treated for 12 h (p < 0.05).
At the genus level, the dominant bacterial genera in the intestinal microbial community of L. vannamei were Photobacterium, Vibrio, and Ruegeria (Figure 4A). The relative abundance of Photobacterium in the B group was lower than that in the disinfectant groups, and the relative abundance of Vibrio was the highest (Figures 4B, C). The most dominant bacteria genera in the water samples were Vibrio, Ruegeria, Pseudoalteromonas, and Nutella. The relative abundance of Vibrio in the Cl group was significantly higher than in the other three groups at 12 h and decreased significantly after 24 h (p < 0.05). Compared with 12 h, the relative abundance of Ruegeria in water samples of disinfectant groups and control group was significantly increased at 24 h (p < 0.05). The relative abundance of Pseudoalteromonas in each group increased after 24 h treatment compared with 12 h treatment (Figures 4D, E). According to the phylogenetic tree of species at the genus level, the main dominant bacteria genera (Photobacterium, Vibrio, Ruegeria, Pseudoalteromonas, and Nautella) belong to Proteobacteria (Figure 5).
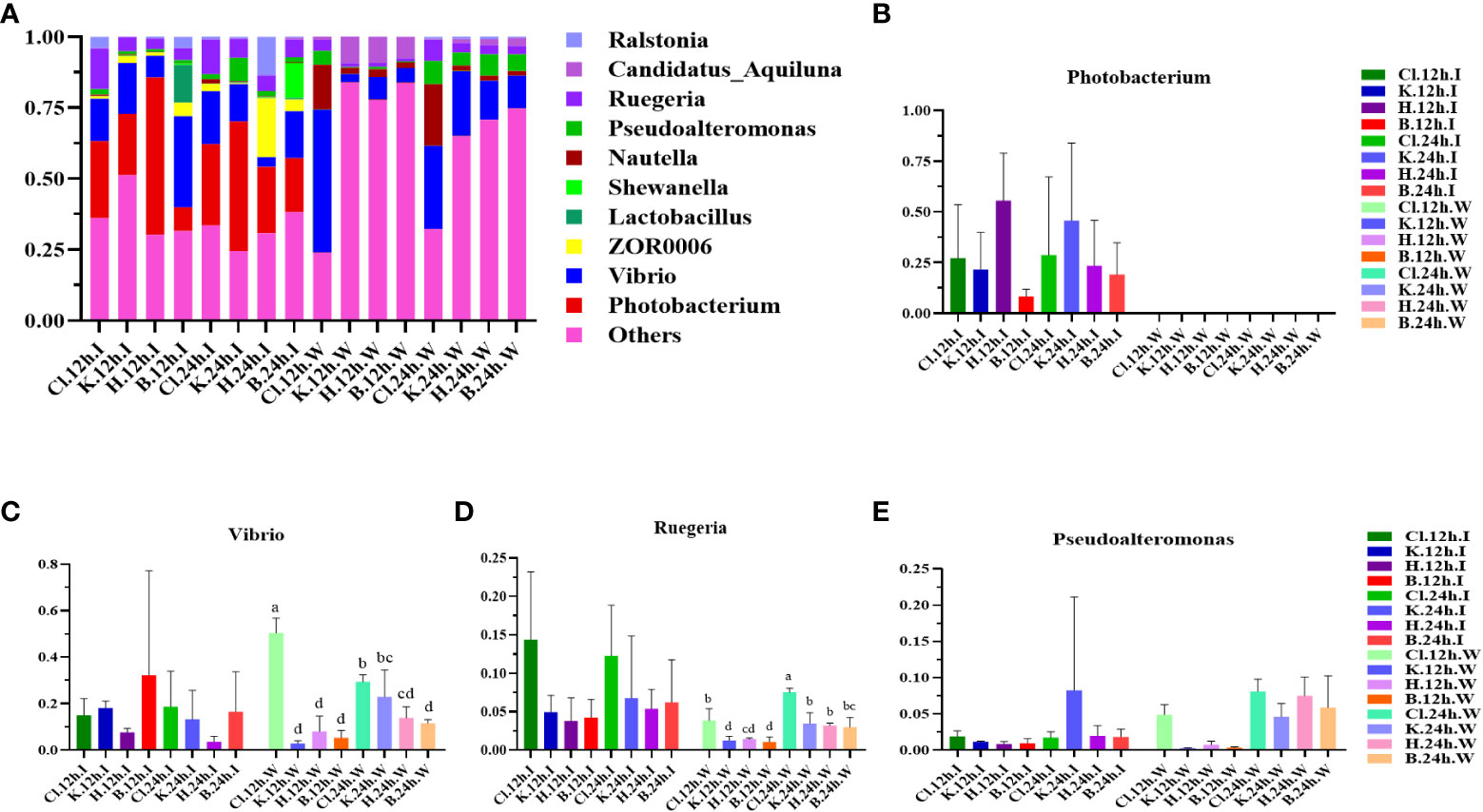
Figure 4 The structure and composition of intestinal dominant microflora and water dominant microflora of L. vannamei treated with different disinfectants at the genus level. (A) shows the relative abundance of the top 10 dominant genera, and (B–E) indicate the abundance changes of the Photobacterium, Vibrio, Ruegeria, and Pseudoalteromonas, respectively. Data were expressed as mean ± SD, with different letters indicating significant differences (p < 0.05).
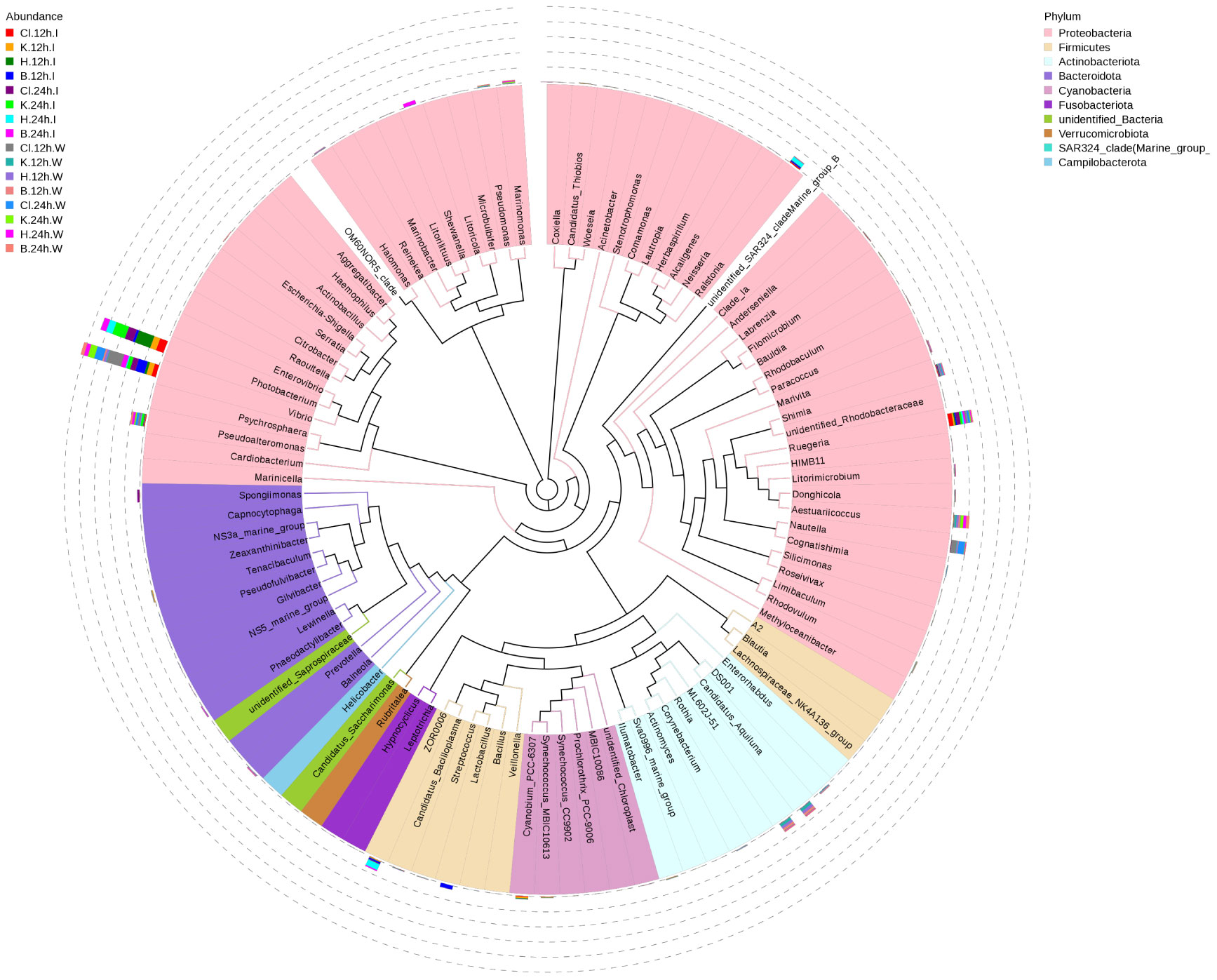
Figure 5 Species phylogeny at the genus level. The phylogenetic tree is constructed from the representative sequences of species at the genus level. The colors of the branches and sectors indicate their corresponding phyla, and the stacked column chart outside the sector circle indicates the abundance distribution information of the genus in different samples.
3.5 Alpha and beta diversity analysis
The Alpha analysis index (Shannon, Simpson, Chao, and ACE) of different samples under the consistency threshold of 97% is statistically analyzed. The results show that the diversity Shannon index and Simpson index of intestinal samples of prawn after 12 h and 24 h treatment with the three disinfectants ranged from 3.47 to 5.32 and 0.65 to 0.90, respectively. There was no significant difference among all groups (p > 0.05) (Figures 6A, B). The diversity index of the Cl and K groups decreased after 24 h treatment compared with 12 h treatment with disinfectant. The Chao index and ACE index of intestinal samples ranged from 779.83 to 1041.51 and 823.32 to 1097.35, respectively, with no significant difference among all groups (p > 0.05) (Figures 6C, D). Except for the Cl group, the richness index (Chao and ACE) of the other three groups at 24 h was higher than that at 12 h.
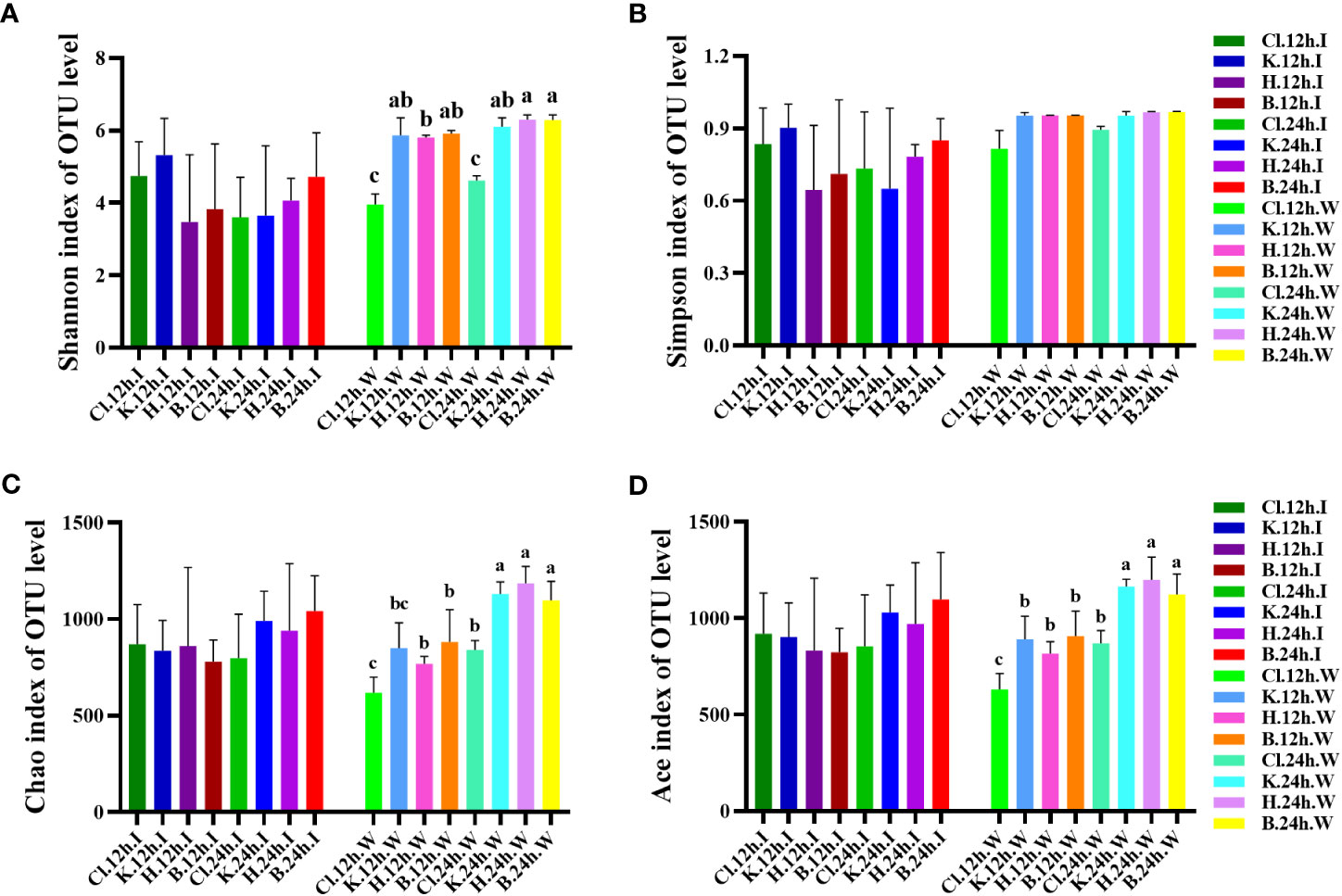
Figure 6 At the OTU level, the changes in bacterial species diversity index and richness index in different groups. Diversity is represented by (A) the Shannon index and (B) the Simpson index, and richness is represented by (C) the Chao index and (D) the Ace index. Data were expressed as mean ± SD, and different letters indicate a significant difference (p <0.05).
In the water samples, the Shannon index of diversity ranged from 3.958 to 6.294, and the Cl group was significantly lower than the other three groups after 12 h and 24 h treatment with disinfectant (p < 0.05). Compared with 12 h treatment with disinfectant, the Shannon index of water samples in the Cl and H groups after 24 h treatment was significantly increased (p < 0.05). The Simpson diversity index was between 0.816 and 0.968, with no significant difference among all groups (p > 0.05). The richness index (Chao and ACE) of water samples ranged from 618.726 to 1185.808 and 630.975 to 1198.368, respectively. The richness index of the three disinfectant addition groups and control group at 24 h was significantly higher than that at 12 h (p < 0.05). The Cl group was significantly lower than that in K, H, and B groups (p < 0.05).
The non-metric multidimensional calibration method (NMDS) can reflect samples’ inter-group and intra-group differences. In this experiment, Stress=0.107 (< 0.2) indicates that NMDS can accurately reflect the degree of difference between samples. There was no significant difference in the distance between the three disinfectant addition groups and the control group in intestinal samples. In the water samples, the points represented by the 12 h and 24 h samples treated with disinfectants clustered together, respectively, while the Cl group samples had a certain distance from the central aggregation point. Intestinal and water samples were distributed on two sides, far apart (Figure 7).
3.6 Function prediction
According to the annotation information of microbial community functions in intestinal samples and water samples of shrimp, the functions with the highest abundance were selected for cluster analysis. The results showed that, at the KEGG Level 1, the functional characteristics of intestinal and cultured water samples were similar after being treated with three disinfectants. The top six predictive functions of relative abundance were “Metabolism” (relative abundance from 44.55% to 49.30%), “Genetic Information Processing” (from 17.86% to 22.22%), “Environment Information Processing” (from 12.27% to 17.23%), “Cellular Processes” (from 6.71% to 8.33%), “Human Diseases” (from 2.62% to 3.33%), and “Organismal Systems” (from 1.56% to 1.89%) (Figures 8A, B). Among the functions classified at KEGG Level 2, the abundance of metabolism-related pathways occupies the majority, including “Amino acid metabolism”, “Energy metabolism”, “Carbohydrate metabolism”, “Metabolism of cofactors and vitamins”, “Nucleotide metabolism” and “Lipid metabolism” (Figure 8C, D).
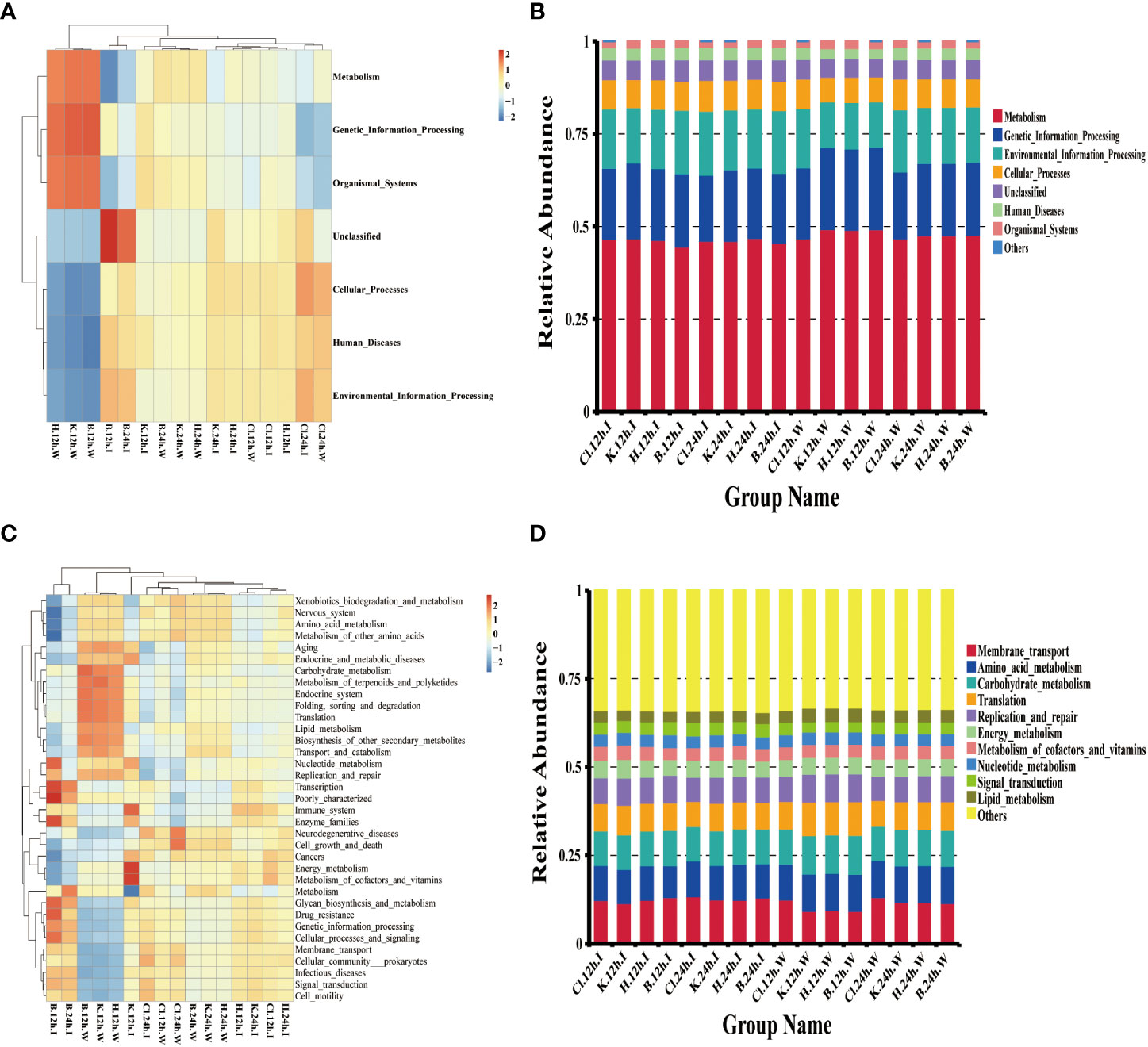
Figure 8 Microbial function prediction based on the Tax4Fun calculation method. (A, C) is the heatmap of the functional abundance of KEGG Level 1 and Level 2, respectively, (B) the histogram of the first 6 functional relative abundance of KEGG Level 1, and (D) the histogram of the top 10 functions of KEGG Level 2.
4 Discussion
Disinfectants can purify water quality, kill or control the viruses, harmful bacteria, and harmful algae in aquaculture water, stabilize the water microenvironment, and provide good growth conditions for cultured animals (Wang, 2015). As a new compound disinfectant with great potential, HPS has become the focus of the disinfection community due to its efficient sterilization and safe and toxic toxicity (Mahnel and Schmidt, 1986; Khazaei et al., 2008; De Giglio et al., 2014), and this study first introduced HPS to the aquaculture field to explore its sterilization effect in shrimp culture.
The research shows that the silver peroxide compound disinfectant has an effective inactivation effect on the influenza virus, parainfluenza virus, and Newcastle disease virus (Duan et al., 2020), and the inactivation effect of the disinfectant on the virus has a better persistence (Mahnel and Schmidt, 1986). However, the results of this experiment showed that the survival rate of M. japonicus infected with DIV1 was not significantly affected by different doses of HPS (p > 0.05). It is reported that DIV1 is highly pathogenic and can replicate and transcribe the virus by affecting the metabolism of shrimp blood cells (He et al., 2021). Once infected, it will cause extremely serious death (Qiu et al., 2017; Qiu et al., 2018; Liao et al., 2022). It is undoubtedly a great challenge to inject HPS into shrimp infected with DIV1, try to kill the virus in vivo, and improve the survival rate. The disinfection route of HPS in shrimp and the killing degree of the virus are not yet clear. In this respect, the experiment has some limitations, which need to be solved in future research.
This study used the 16S rDNA high-throughput sequencing method to analyze the effects of three disinfectants, including HPS, KMPS, and Ca(ClO)2, on intestinal microflora and water microflora of L. vannamei. Based on 16S rDNA high-throughput sequencing, 5,104 OTUs were obtained, of which 3,012 (59.01%) OTUs were annotated to the phylum level and 1,475 (28.90%) OTUs were annotated to the genus level. In the water samples, the number of OTUs in each group and the number of annotations in different levels (phylum, class, order, family, and genus) showed an increasing trend. The results showed that with the extension of disinfectant treatment time, the bactericidal effect weakened, and the number of bacteria rebounded. In the water microbial community, the diversity and richness of bacteria in the Ca(ClO)2 disinfectant group were lower than those in HPS and KMPS disinfectant groups at two treatment periods, and the Shannon index, Chao index, and Ace index were significantly different (P < 0.05). These results indicated that Ca(ClO)2 was superior to the other two disinfectants in controlling the diversity and richness of the microbial community in water.
After 12h treatment, the intestinal microbial community diversity of the HPS disinfectant group was lower than that of the Ca(ClO)2 disinfectant group and KMPS disinfectant group, which differed from the rule of the water microbial community. The studies showed that hydrogen peroxide and silver had synergistic antibacterial effects and strong bacteria-killing activity (Pedahzur et al., 1995; Pedahzur et al., 1997). Catalase (CAT) plays a vital role in enhancing the efficacy of this compound disinfectant, and the disinfection effect increases with the increase of catalase content (Martin et al., 2015). Catalase (CAT) is an important oxidoreductase in a multi-enzyme system, which exists widely in prokaryotes and eukaryotes (Kashiwagi et al., 1997; Klotz et al., 1997). In the study of Yang et al. (Yang et al., 2015), CAT was widely distributed in the heart, hepatopancreas, gills, stomach, intestine, and blood cells of shrimp, and it also played an important role in maintaining the homeostasis of intestinal microflora. Therefore, it is believed that the effect of HPS disinfectant on inhibiting the diversity of intestinal microflora of shrimp is better than that of rearing water because the rich CAT in the shrimp’s intestine promotes the killing ability of the disinfectant.
The comparative analysis of Beta diversity showed no significant difference between the intestinal microflora of L. vannamei treated with the three disinfectants, and the same was true between the water microbial community. The distance between the microbiota in the water and the intestine is far. Combined with the analysis of Alpha diversity and Beta diversity, it was concluded that the three disinfectants significantly affected the diversity and richness of the water microbial community when treated for 12 h and 24 h. However, due to the short-acting time of disinfectants, more time is needed to cause significant effects on the intestinal microbial community of shrimp.
The analysis of species’ relative abundance in the disinfectant groups and the control group showed that, at the phylum level, the dominant microflora were Proteobacteria, Firmicutes, Actinobacteria, Bacteroidetes, and Cyanobacteria, among which Proteobacteria had the largest relative abundance, which was similar to the results of previous studies (Fan et al., 2019; Cheng et al., 2021). At the genus level, the dominant bacterial genera in the intestinal bacterial community of L. vannamei were Photobacterium, Vibrio, and Ruegeria. In the water samples, the largest dominant bacteria genera are Vibrio, Ruegeria, Pseudoalteromonas, and Nautella, which is similar to the research results of (Zhang et al., 2021), which found that Flavobacterium, Vibrio, and Pseudoalteromonas in the water of control group and chlorine dioxide group are the main dominant bacteria genera, and their relative abundance is higher than that of other experimental groups. The phylogenetic tree of species at the genus level showed that the dominant bacteria genera annotated in Proteobacteria in this study were Photobacterium, Vibrio, Ruegeria, Pseudoalteromonas, and Nautella.
The three disinfectants affected the relative abundance of intestinal microflora and the dominant microflora of the aquatic microflora of L. vannamei, but their effects were different. Vibrio is the dominant bacteria in the intestinal tract of prawns and marine water. Vibrio is mostly a pathogenic bacteria, and the overflow of pathogenic Vibrio will cause the outbreak of shrimp diseases (Xiong et al., 2017), resulting in serious economic losses. It is reported that pathogenic Vibrio is an important pathogen causing diseases such as luminescent vibriosis (Lavilla-Pitogo et al., 1990), acute hepatopancreatic necrosis disease (AHPND) (Soto-Rodriguez et al., 2015), bright-red syndrome (Soto Rodriguez et al., 2010), and is one of the greatest threats to the shrimp farming industry. In this experiment, the killing effect of Ca(ClO)2 on Proteobacteria (especially Vibrio and Ruegeria) was weak at 12 h and 24 h. After treatment with HPS and KMPS, Vibrio abundance was significantly lower than that of the Ca(ClO)2 group. This result was verified in the experiment comparing the number of Vibrio and total bacteria with three different disinfectants, indicating that HPS had a strong killing effect on total bacteria and Vibrio. In addition, the quantity of Vibrio and total bacteria in rearing water negatively correlated with the dose of HPS. That is, within a certain dose range, the higher the dose of HPS, the more obvious the inhibition effect on Vibrio and total bacteria.
Actinomyces are important in protecting the environment and material circulation and are often used in synthesizing antibiotics and antimicrobials (Zothanpuia et al., 2018; Han, 2021). In addition, Actinomyces are critical in maintaining intestinal homeostasis in animals (Binda et al., 2018; Fan et al., 2019). Bacteroides have a certain effect on the utilization of dissolved organic matter (Cottrell and Kirchman, 2000) and play a role in the degradation of cellulose, pectin, and chitin (Williams et al., 2013), and the abundance of Bacteroides in water increases with the accumulation of residual baited feces (Rossello-Mora et al., 1999). The results showed that Ca(ClO)2 disinfectant had a significant killing effect on Actinobacteria and Bacteroidetes (p < 0.05), and the relative abundance of both bacteria phyla was higher than that of Ca(ClO)2 group after HPS and KMPS disinfectants treatment. Therefore, it is believed that using HPS and KMPS disinfectants is more effective in controlling the number of Vibrio and inhibiting the outbreak of vibriosis in the process of culture, and can protect the abundance of beneficial bacteria while sterilization and maintain the balance of microenvironment to a certain extent.
5 Conclusion
In summary, this study described the effects of different doses of HPS on inhibiting DIV1, Vibrio, and total bacteria, compared the killing effects of HPS, KMPS, and Ca (ClO)2 disinfectants on Vibrio and total bacteria, and compared the characteristics of intestinal microflora and water microflora after treatment of aquaculture water with three disinfectants. The results showed that HPS did not play a positive role in improving the survival rate of shrimp infected with DIV1, and further research was needed to prove it. Ca (ClO)2 has more advantages than HPS in controlling the diversity and abundance of microbial communities. However, HPS plays a significant role in inhibiting Vibrio, and its inhibition of beneficial bacteria is weak, which helps maintain the microenvironment balance during sterilization. In aquaculture water, there is a negative correlation between the number of total bacteria and Vibrio and the dose of HPS in a certain range.
Data availability statement
The datasets presented in this study can be found in online repositories. The names of the repository/repositories and accession number(s) can be found in the article/supplementary material.
Ethics statement
Ethical review and approval was not required for the animal study because The experimental animal involved in this experiment is shrimp.
Author contributions
DH and CS contributed to the conception and design of this study. DH, ZL, JZ, and YX participated in the experimental operation and sample collection. DH wrote the first draft of the manuscript. ZL helps with data statistical analysis and chart drawing. CS conducted written review and editing, and made contributions to project management and fund acquisition. All authors contributed to the article and approved the submitted version.
Funding
This work was supported by the key research and development projects in Guangdong Province (Grant No.2020B0202010009), the project of the innovation team for the innovation and utilization of Economic Animal Germplasm in the South China Sea (Grant No.2021KCXTD026).
Acknowledgments
We thank all funders for this work.
Conflict of interest
The authors declare that the research was conducted in the absence of any commercial or financial relationships that could be construed as a potential conflict of interest.
Publisher’s note
All claims expressed in this article are solely those of the authors and do not necessarily represent those of their affiliated organizations, or those of the publisher, the editors and the reviewers. Any product that may be evaluated in this article, or claim that may be made by its manufacturer, is not guaranteed or endorsed by the publisher.
References
Abed A. R., Khudhair A. M., Hussein I. M. (2019). In vitro study of topical antiseptics used to treat mycological gill rot disease in Cyprinus carpio. J. Pure Appl. Microbiol. 13 (1), 537–544. doi: 10.22207/JPAM.13.1.60
Acosta F., Montero D., Izquierdo M., Galindo-Villegas J. (2021). High-level biocidal products effectively eradicate pathogenic gamma-proteobacteria biofilms from aquaculture facilities. Aquaculture 532. doi: 10.1016/j.aquaculture.2020.736004
Adams M. B., Crosbie P. B. B., Nowak B. F. (2012). Preliminary success using fihydrogen peroxide to treat Atlantic salmon, Salmo salar l., affected with experimentally induced amoebic gill disease (AGD). J. Fish Dis. 35 (11), 839–848. doi: 10.1111/j.1365-2761.2012.01422.x
American Public Health Association (APHA). (2005). Standard methods for the examination of water and wastewater. 21st edition (Washington, DC, USA: American Public Health Association).
Antoine T., Ophélie Z., Olivier S., Franck C., Jerôme R., Mélanie A., et al. (2006). Cytotoxicity of CeO2 nanoparticles for Escherichia coli. physico-chemical insight of the cytotoxicity mechanism. Environ. Sci. Technol. 40 (19), 6151–6. doi: 10.1021/ES060999b
Ao X.-W., Li H.-J., Liu W.-J., Yu J.-R. (2016). Characteristics of disinfection by-products and genotoxicity during drinking water disinfection with potassium monopersulfate compound powder. Environ. Sci. 37 (11), 4241–4246. doi: 10.13227/j.hjkx.201604116
Bayliss S. C., Verner.-Jeffreys D. W., Bartie K. L., Aanensen D. M., Sheppard S. K., Adams A., et al. (2017). The promise of whole genome pathogen sequencing for the molecular epidemiology of emerging aquaculture pathogens. Front. Microbiol. 8. doi: 10.3389/fmicb.2017.00121
Binda C., Lopetuso L. R., Rizzatti G., Gibiino G., Cennamo V., Gasbarrini A. (2018). Actinobacteria: a relevant minority for the maintenance of gut homeostasis. Digestive Liver Dis. 50 (5), 421–428. doi: 10.1016/j.dld.2018.02.012
Chen X., Qiu L., Wang H., Zou P., Dong X., Li F., et al. (2019). Susceptibility of exopalaemon carinicauda to the infection with shrimp hemocyte iridescent virus (SHIV 20141215), a strain of decapod iridescent virus 1 (DIV1). Viruses 11 (4), 387–387. doi: 10.3390/v11040387
Cheng Y., Ge C., Li W., Yao H. (2021). The intestinal bacterial community and functional potential of Litopenaeus vannamei in the coastal areas of China. Microorganisms 9 (9), 1793–1793. doi: 10.3390/microorganisms9091793
Cottrell M. T., Kirchman D. L. (2000). Natural assemblages of marine proteobacteria and members of the cytophaga-flavobacter cluster consuming low- and high-molecular-weight dissolved organic matter. Appl. Environ. Microbiol. 66 (4), 1692–1697. doi: 10.1128/AEM.66.4.1692-1697.2000
Dai X., Guo Q., Zhao Y., Zhang P., Zhang T., Zhang X., et al. (2016). Functional silver nanoparticle as a benign antimicrobial agent that eradicates antibiotic-resistant bacteria and promotes wound healing. ACS Appl. Materials Interfaces 8 (39), 25798–25807. doi: 10.1021/acsami.6b09267
De Giglio O., Coretti C., Lovero G., Barbuti G., Caggiano G. (2014). Pilot study on the antibacterial activity of hydrogen peroxide and silver ions in the hospital environment. Annali di igiene medicina preventiva e di comunita 26 (2), 181–185. doi: 10.7416/ai.2014.1974
Duan W., Deng H., Xiao X., Wang P., Jiang C., Zheng C. (2020). Study on the disinfection-related performance of a hydrogen peroxide silver ion compound disinfectant. Chin. J. Disinfection 37 (11), 812–815.
Fan L., Wang Z., Chen M., Qu Y., Li J., Zhou A., et al. (2019). Microbiota comparison of pacific white shrimp intestine and sediment at freshwater and marine cultured environment. Sci. Total Environ. 657, 1194–1204. doi: 10.1016/j.scitotenv.2018.12.069
FAO. (2022). The state of world fisheries and aquaculture 2022.Towards blue transformation (Rome: Food and Agriculture Organization).
Girolamini L., Dormi A., Pellati T., Somaroli P., Montanari D., Costa A., et al. (2019). Advances in Legionella control by a new formulation of hydrogen peroxide and silver salts in a hospital hot water network. Pathogens 8 (4), 209. doi: 10.3390/pathogens8040209
Hamad A., Khashan K. S., Hadi A. (2020). Silver nanoparticles and silver ions as potential antibacterial agents. J. Inorganic Organometallic Polymers Materials 30 (12), 4811–4828. doi: 10.1007/s10904-020-01744-x
Han X. (2021). Effect of actinomycetes biofertilizer on crop growth and regulation of rhizosphere microbial community (China: Northwest A&F University).
He Z., Chen X., Zhao J., Hou D., Fu Z., Zhong Y., et al. (2021). Establishment of infection mode and Penaeus monodon hemocytes transcriptomics analysis under decapod iridescent virus 1 (DIV1) challenge. Aquaculture 542, 17. doi: 10.1016/j.aquaculture.2021.736816
He Z., Zhao J., Liao X., Chen X., Fu Z., Sun C., et al. (2020). The secondary bacterial infection caused by WSSV outbreaks impacts shrimp Marsupenaeus japonicus' growth as well as its intestinal microbiota's composition and function. Israeli J. Aquaculture-Bamidgeh 17. doi: 10.46989/001c.21687
Heno F., Azoulay Z., Khalfin B., Craddock H. A., Silberstein E., Moran-Gilad J., et al. (2021). Comparing the antimicrobial effect of silver ion-coated silicone and gentamicin-irrigated silicone sheets from breast implant material. Aesthetic Plast. Surg. 45 (6), 2980–2989. doi: 10.1007/s00266-021-02348-7
Jin B., Niu J., Dai J., Li N., Zhou P., Niu J., et al. (2018). New insights into the enhancement of biochemical degradation potential from waste activated sludge with low organic content by potassium monopersulfate treatment. Bioresource Technol. 265, 8–16. doi: 10.1016/j.biortech.2018.05.032
Kali S., Khan M., Ghaffar M. S., Rasheed S., Waseem A., Iqbal M. M., et al. (2021). Occurrence, influencing factors, toxicity, regulations, and abatement approaches for disinfection by-products in chlorinated drinking water: a comprehensive review. Environ. pollut. 281, 116950–116950. doi: 10.1016/j.envpol.2021.116950
Kashiwagi A., Kashiwagi K., Takase M., Hanada H., Nakamura M. J. C. B., Biochemistry P. P. B., et al. (1997). Comparison of catalase in diploid and haploid Rana rugosa using heat and chemical inactivation techniques. Comp. Biochem. Physiol. Part B: Biochem. Mol. Biol. 118 (3), 499–503. doi: 10.1016/S0305-0491(97)00216-2
Khazaei M., NABIZADEH N. R., Yunesian M. (2008). Qom wastewater disinfection with hydrogen peroxide-silver ion complex. Qom Univ. Med. Sci. J. 1 (4), 31–36.
Klotz M. G., Klassen G. R., Loewen P. C. (1997). Phylogenetic relationships among prokaryotic and eukaryotic catalases. Mol. Biol. Evol. 14 (9), 951–8. doi: 10.1093/oxfordjournals.molbev.a025838
Lavilla-Pitogo C. R., Baticados M. C. L., Cruz-Lacierda E. R., de la Pena L. D. (1990). Occurrence of luminous bacterial disease of Penaeus monodon larvae in the Philippines. Aquaculture 91 (1-2), 1–13. doi: 10.1016/0044-8486(90)90173-K
Lewis K. A. (2010). “Chapter 9: hypochlorination – sodium hypochlorite,” White’s Handbook of Chlorination and Alternative Disadvantages (USA: John Wiley & Sons Inc).
Liang R.-Z. (2016). Study on DUWLs microbial contamination monitoring by ATP biological fluorescence method and the disinfection effect of hydrogen peroxide silver ion (China: Doctoral Dissertation, Nanjing Medical University).
Liao X., He J., Li C. (2022). Decapod iridescent virus 1: an emerging viral pathogen in aquaculture. Rev. Aquaculture 14 (4), 1779–1789. doi: 10.1111/raq.12672
Lu N., Chen Z., Zhang W., Yang G., Liu Q., Boettger R., et al. (2021). Effect of silver ion implantation on antibacterial ability of polyethylene food packing films. Food Packaging Shelf Life 28. doi: 10.1016/j.fpsl.2021.100650
Mahnel H., Schmidt M. (1986). Effect of silver compounds on viruses in water. Zentralblatt fur Bakteriologie Mikrobiologie und Hygiene. Serie B Umwelthygiene Krankenhaushygiene Arbeitshygiene praventive Med. 182 (4), 381–392.
Martin N. L., Bass P., Liss S. N. (2015). Antibacterial properties and mechanism of activity of a novel silver-stabilized hydrogen peroxide. PloS One 10 (7), 131345. doi: 10.1371/journal.pone.0131345
Nikolaj L., Andersen O., Roge R., Larsen T., Petersen R., Riis J. (2005). Silver nanoparticles (Aalborg: Institute for Physics Nanotechnology, Aalborg University).
Orta De Velasquez M. T., Yanez-Noquez I., Jimenez-Cisneros B., Luna Pabello V. M. (2008). Adding silver and copper to hydrogen peroxide and peracetic acid in the disinfection of an advanced primary treatment effluent. Environ. Technol. 29 (11), 1209–1217. doi: 10.1080/09593330802270632
Pedahzur R., Lev O., Fattal B., Shuval H. I. (1995). The interaction of silver ions and hydrogen peroxide in the inactivation of E. coli: a preliminary evaluation of a new long acting residual drinking water disinfectant. Water Sci. Technol. 31 (5-6), 123–129. doi: 10.1016/0273-1223(95)00252-I
Pedahzur R., Shuval H. I., Ulitzur S. (1997). Silver and hydrogen peroxide as potential drinking water disinfectants: their bactericidal effects and possible modes of action. Water Sci. Technol. 35 (11-12), 87–93. doi: 10.1016/S0273-1223(97)00240-0
Pedersen L.-F., Pedersen P. B. (2012). Hydrogen peroxide application to a commercial recirculating aquaculture system. Aquacultural Eng. 46, 40–46. doi: 10.1016/j.aquaeng.2011.11.001
Qiu L., Chen M.-M., Wan X.-Y., Li C., Zhang Q.-L., Wang R.-Y., et al. (2017). Characterization of a new member of iridoviridae, shrimp hemocyte iridescent virus (SHIV), found in white leg shrimp (Litopenaeus vannamei). Sci. Rep. 7, 1–13. doi: 10.1038/s41598-017-10738-8
Qiu L., Chen M.-M., Wang R.-Y., Wan X.-Y., Li C., Zhang Q.-L., et al. (2018). Complete genome sequence of shrimp hemocyte iridescent virus (SHIV) isolated from white leg shrimp, Litopenaeus vannamei. Arch. Virol. 163 (3), 781–785. doi: 10.1007/s00705-017-3642-4
Rawlings J. O., Pantula S. G., Dickey D. A. (1998). Applied regression analysis (Springer, New York, NY: Springer New York).
Rossello-Mora R., Thamdrup B., Schafer H., Weller R., Amann R. (1999). The response of the microbial community of marine sediments to organic carbon input under anaerobic conditions. Systematic Appl. Microbiol. 22 (2), 237–248. doi: 10.1016/s0723-2020(99)80071-x
Schmidt L. J., Gaikowski M. P., Gingerich W. H. (2006). Environmental assessment for the use of hydrogen peroxide in aquaculture for treating external fungal and bacterial diseases of cultured fish and fish eggs. USGS Rep. 180.
Scientific Committee on Emerging and Newly Identified Health Risks (2009). Assessment of the antibiotic resistance effects of biocides (Belgium: European Commission, Directorate-General for Health & Consumers Brussels).
Smith E. M., Plewa M. J., Lindell C. L., Richardson S. D., Mitch W. A. (2010). Comparison of byproduct formation in waters treated with chlorine and iodine: relevance to point-of-Use treatment. Environ. Sci. Technol. 44 (22), 8446–8452. doi: 10.1021/es102746u
Soto Rodriguez S. A., Gomez Gil B., Lozano R., Roque A. (2010). Density of vibrios in hemolymph and hepatopancreas of diseased pacific white shrimp, Litopenaeus vannamei, from northwestern Mexico. J. World Aquaculture Soc. 41, 76–83. doi: 10.1111/j.1749-7345.2009.00335.x
Soto-Rodriguez S. A., Gomez-Gil B., Lozano-Olvera R., Betancourt-Lozano M., Morales-Covarrubias M. S. (2015). Field and experimental evidence of Vibrio parahaemolyticus as the causative agent of acute hepatopancreatic necrosis disease of cultured shrimp (Litopenaeus vannamei) in northwestern Mexico. Appl. Environ. Microbiol. 81 (5), 1689–1699. doi: 10.1128/aem.03610-14
Tambur P., Bhagawan D., Kumari B. S., Kasa R. R. (2020). A facile synthesis of implantation of silver nanoparticles on oxygen-functionalized multi-walled carbon nanotubes: structural and antibacterial activity. SN Appl. Sci. 2 (14), 56–58. doi: 10.1007/s42452-020-2797-x
Tkachenko H., Kurhaluk N., Grudniewska J. (2014). Oxidative stress biomarkers in different tissues of rainbow trout (Oncorhynchus mykiss) exposed to disinfectant-CIP formulated with peracetic acid and hydrogen peroxide. Arch. Polish Fisheries 22 (3), 207–219. doi: 10.2478/aopf-2014-0021
Wang Y.-T. (2015). Water disinfectants for aquaculture and their application technology (serial III). China Fisheries 03), 44–47.
Williams T. J., Wilkins D., Long E., Evans F., DeMaere M. Z., Raftery M. J., et al. (2013). The role of planktonic flavobacteria in processing algal organic matter in coastal East Antarctica revealed using metagenomics and metaproteomics. Environ. Microbiol. 15 (5), 1302–1317. doi: 10.1111/1462-2920.12017
Xiong J., Zhu J., Dai W., Dong C., Qiu Q., Li C. (2017). Integrating gut microbiota immaturity and disease-discriminatory taxa to diagnose the initiation and severity of shrimp disease. Environ. Microbiol. 19 (4), 1490–1501. doi: 10.1111/1462-2920.13701
Yan X., Wang X. (2020). Treatment and research of skin healthcare based on nanosilver ion material technology. Int. J. Nanotechnol 17 (2-6), 124–145. doi: 10.1504/ijnt.2020.110711
Yang H.-T., Yang M.-C., Sun J.-J., Guo F., Lan J.-F., Wang X.-W., et al. (2015). Catalase eliminates reactive oxygen species and influences the intestinal microbiota of shrimp. Fish Shellfish Immunol. 47 (1), 63–73. doi: 10.1016/j.fsi.2015.08.021
Zhang R., Wu G. T., Zhu J. Y., Wang X. W., Liu L. L., Li H. J., et al. (2023). Povidone iodine exposure alters the immune response and microbiota of the gill and skin in koi carp, Cyprinus carpio. Aquaculture 563. doi: 10.1016/j.aquaculture.2022.738926
Zhang W.-W., Huang Z., Duan Y.-F., et al. (2021). Effects of different inhibitors on water quality and microbial community structure of Litopenaeus vannamei. South China Fisheries Sci 17 (02), 1–10.
Keywords: hydrogen peroxide nano-silver ion, calcium hypochlorite, potassium monopersulfate, disinfectant, Vibrio, microbial community
Citation: Hou D, Lin Z, Zhou J, Xue Y and Sun C (2023) Germicidal effect of hydrogen peroxide nano-silver ion composite disinfectant and its effect on the microbial community of shrimp intestine and rearing water. Front. Mar. Sci. 10:1189013. doi: 10.3389/fmars.2023.1189013
Received: 18 March 2023; Accepted: 26 May 2023;
Published: 16 June 2023.
Edited by:
Bin Xia, Qingdao Agricultural University, ChinaReviewed by:
Yafei Duan, South China Sea Fisheries Research Institute, ChinaChanghong Cheng, South China Sea Fisheries Research Institute (CAFS), China
Copyright © 2023 Hou, Lin, Zhou, Xue and Sun. This is an open-access article distributed under the terms of the Creative Commons Attribution License (CC BY). The use, distribution or reproduction in other forums is permitted, provided the original author(s) and the copyright owner(s) are credited and that the original publication in this journal is cited, in accordance with accepted academic practice. No use, distribution or reproduction is permitted which does not comply with these terms.
*Correspondence: Chengbo Sun, c3VuY2JAZ2RvdS5lZHUuY24=