- 1Graduate School of Engineering and Science, University of the Ryukyus, Okinawa, Japan
- 2Sesoko Station, Tropical Biosphere Research Center, University of the Ryukyus, Okinawa, Japan
Mesophotic habitats are potential refugia for corals in the context of climate change. The seawater temperature in a mesophotic habitat is generally lower than in a shallow habitat. However, the susceptibility and threshold temperatures of mesophotic corals are not well understood. We compared 11 mesophotic and shallow species to understand their thermal stress thresholds using physiological parameters. Coral fragments were exposed to two thermal stress treatments, with temperatures set at ~30°C and ~31°C, and a low-temperature treatment set at ~28°C as the “no stress” condition for 14 days. We found that the threshold temperature of coral species at mesophotic depths is slightly lower or equal to that of corals in shallow depths. The results suggest that species in the mesophotic coral ecosystems can survive low (<4 degree heating weeks) thermal stress. However, mass bleaching and high mortality can be expected when temperatures rise above 4 degree heating weeks.
Introduction
Coral reefs are ecosystems threatened by anthropogenic activities and consequent extensive coral bleaching events (Hoegh-Guldberg et al., 2007; Spalding and Brown, 2015; Hughes et al., 2017). Shallow coral reefs are facing unprecedented bleaching events and subsequent degradation (Hughes et al., 2018b; Lough et al., 2018). The severity and frequency of the bleaching events may increase in the near future (McClanahan et al., 2015). Coral bleaching begins when a sea surface temperature anomaly exceeds 1°C above the maximum monthly mean recorded temperature (Glynn and D'Croz, 1990). The threshold temperature for coral bleaching substantially varies geographically based on the habitat temperature (Hughes et al., 2003; Jokiel, 2004; Hughes et al., 2017). The degree heating week (DHW) index is used to estimate the severity and duration of thermal stress (Kayanne, 2017; Skirving et al., 2020). DHWs indicate the accumulation of temperature anomalies exceeding the historical maximum monthly mean (MMM) temperature, and bleaching potentially occurs at more than 4 DHWs (Liu et al., 2013; Kayanne, 2017). Temperature stress strongly influences the physiological function of reef-building corals (Hoadley et al., 2016), and consequently can affect the integrity of the ecosystem (Baker et al., 2008). However, numerous studies have confirmed that thermally variable environments have led to more thermally tolerant species/colonies than those inhabiting moderate habitats (Oliver and Palumbi, 2011; Mayfield et al., 2013; Klepac and Barshis, 2020).
In contrast to shallow reefs, mesophotic coral ecosystems (MCEs; coral reefs below 30 m depth) are subjected to lower light intensity, lower wave disturbance, and exhibit variable temperature regimes that can range from moderate (Lesser et al., 2009; Kahng et al., 2010) to extreme variations (Schramek et al., 2019; Wyatt et al., 2020; Pérez-Rosales et al., 2021; Wyatt et al., 2023). While seawater temperatures in MCEs are generally considered cooler compared to their shallower counterparts (Lesser et al., 2009; Shlesinger et al., 2018; Turner et al., 2019; Tavakoli-Kolour et al., 2023), the extent of this thermal variability raises questions about the thermal tolerance of corals inhabiting these ecosystems. MCEs are considered potential refugia for coral reefs (Riegl and Piller, 2003; Bongaerts et al., 2010; Muir et al., 2017), and may safeguard reef biodiversity and ecological function in the face of climate change in the near future. Therefore, the “deep reef refugia” hypothesis (DRRH) has been postulated (Glynn, 1996; Bongaerts et al., 2010). However, there is limited empirical data thus far to support the DRRH (Bongaerts and Smith, 2019). Studies investigating the effectiveness of the DRRH have found that it is only applicable in certain cases (Bongaerts et al., 2017; Frade et al., 2018). Furthermore, it is unclear whether MCEs serve as refugia for both shallow and mesophotic species or only for shallow species.
Stress responses, such as bleaching, differ among species (Bieri et al., 2016; Traylor-Knowles, 2019). The thermal tolerance threshold of coral species depends on the temperature regime of their habitat and their acclimation/adaptation (Logan et al., 2014; Kleypas et al., 2016). Therefore, given the variability of the thermal regime in mesophotic ecosystems, it is important to consider the implications for the thermal tolerance of corals in these ecosystems. While there is growing interest in the study of the thermal tolerance of mesophotic corals (Nir et al., 2014; Smith et al., 2016; Schramek et al., 2018; Skutnik et al., 2020; Gould et al., 2021; Pérez-Rosales et al., 2021; Godefroid et al., 2023; Wyatt et al., 2023), there is still a significant gap in knowledge pertaining to MCEs.
Elucidating the physiological functions that respond to environmental stress can help understand the tolerance and acclimatization levels of corals in the unique ecological settings of MCEs. Temperature is a factor that affects the physiological functions of corals (Hoadley et al., 2016). For example, responses to thermal stress, which are involved in bleaching, have been examined in terms of physiological aspects, such as symbiotic algal density, algal chlorophyll content, and maximum photosynthetic efficiency (Nielsen et al., 2018; Ben-Zvi et al., 2020). Furthermore, mortality after bleaching and the degree of bleaching have been examined (Pratchett et al., 2020). These stress responses occur in genera- and species-specific manner (Abrego et al., 2008; Diaz et al., 2016; Dias et al., 2019), and the susceptibility of corals to thermal stress has been considered as a critical factor affecting the community structure of coral reef ecosystems (Marshall and Baird, 2000; Dias et al., 2018). While some studies have compared the thermal tolerance of corals from different depths (Skutnik et al., 2020; Gould et al., 2021), our current knowledge remains limited, providing an incomplete understanding of the physiological function and thermal thresholds across a wide range of species, from shallow coral ecosystems to MCEs. Published studies have found contradicting patterns of lower thresholds versus equal or potentially higher thresholds in mesophotic corals compared to shallow water corals. Consequently, further investigation is necessary to compare the thermal thresholds and physiological responses between corals from mesophotic and shallow depths.
In this study, we aimed to examine the response of eleven coral species from both mesophotic and shallow depths to thermal stress in terms of survivorship, bleaching, and photosynthetic activity. Furthermore, we discuss the acclimatization potential of these species to global warming.
Materials and methods
Coral collection and experimental design
This study was performed on five coral species, including Acropora valida, Galaxea fascicularis, Porites sp., Porites cylindrica, and Turbinaria mesenterina, from shallow depth (5–7 m) and five species, including Acropora cf. horrida, Galaxea cf. astreata, Porites sp., Seriatopora hystrix, and Leptoseris papyracea, from upper mesophotic depth (40 m), with one species, Pachyseris speciosa, collected at both depths (Figure 1). Three healthy colonies with roughly equal-sized (approximately 20-25 cm2) of each species were collected by SCUBA divers with hammer and chisel from shallow (26° 39’ N, 127° 52’ E) and mesophotic (26° 40’ N 127° 51’ E) depths north of Sesoko Island, Okinawa, Japan, in August 2018 and transferred to the Sesoko Station of the Tropical Biosphere Research Center of the University of the Ryukyus. The coral species were identified based on Wallace (1999) and Nishihira and Veron (1995). Twelve fragments of similar sizes were collected from each colony and mounted on plastic screws using super jelly glue. They were placed in seawater flow-through tanks at 28°C and maintained under experimental light conditions (as described below) for two weeks before starting the experiment to facilitate wound healing.
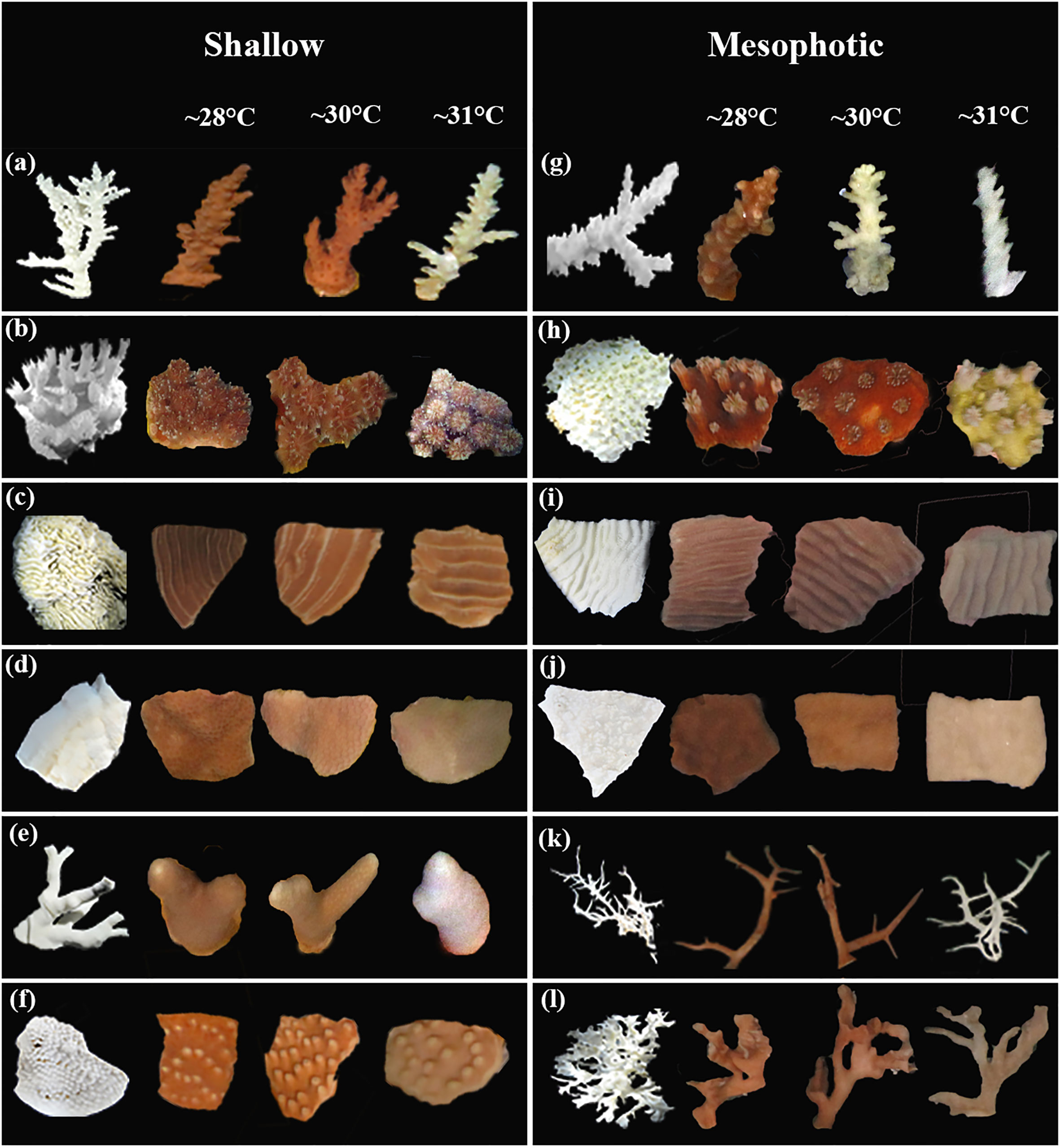
Figure 1 Images of 11 coral species fragments from shallow and mesophotic depths exposed to different temperature regimes to indicate interspecific responses of the species. The left column display the bleached skeleton of coral species. (A) Acropora valida; (B) Galaxea fascicularis; (C) Pachyseris speciosa; (D) Porites sp.; (E) Porites cylindrica; (F) Turbinaria mesenterina; (G) Acropora cf. horrida; (H) Galaxea cf. astreata; (I) Pachyseris speciosa; (J) Porites sp.; (K) Seriatopora hystrix; and (L) Leptoseris papyracea.
We exposed shallow and mesophotic fragments to different light and temperature conditions for two weeks. Light conditions were set based on the light (photosynthetically active radiation, PAR) measured at shallow and mesophotic site (Prasetia et al., 2016). Due to the limitation of LED lamps, data from cloudy day were used as a reference for shallow reef (5m depth). The fragments were placed under a 12:12-h light/dark cycle, in two different light conditions corresponding to their original depths. LED lights (AI Hydra FiftyTwo HD LED, Aqua Illumination, USA) were used with shallow light irradiance (~200 µmol m−2 s−1) for the shallow coral fragments and mesophotic light irradiance (~25 µmol m−2 s−1) for the mesophotic coral fragments. To achieve this intensity for the mesophotic depth, the lamp was set to 20% of its maximum capacity.
Three temperature treatments were designated based on the determination of maximum monthly mean (MMM) temperatures at different depths. Due to temperature anomalies and coral bleaching observed at shallow depths of the study sites during 2016 and 2017, MMM values for the shallow depths at the study sites were obtained from the National Oceanic and Atmospheric Administration (NOAA) Coral Reef Watch (CRW) satellite products for the Northern Ryukyus Island 5 km Regional Virtual Station (Liu et al., 2014; Liu et al., 2017). The MMM for the 40m depth was calculated by averaging the highest monthly temperatures during the warmest months (June 21st to September 21st) of each year, based on in-situ water temperatures measured at hourly intervals from 2015 to 2018 at the mesophotic study site (Prasetia et al., 2022) using underwater temperature loggers (HOBO Water Temp Pro v2, Onset, USA) (Liu et al., 2003). The climatological MMM values were calculated for each depth: 28.4°C for the shallow depths and 27.9°C for the mesophotic depths. The solar calendar was used to accurately determine the corresponding seasons.
We conducted an experiment with three temperature treatments to induce moderate-duration thermal stress for 14 days. The temperature treatments were ~28°C, ~30°C, and ~31°C. The ~28°C temperature was set at 27.8 ± 0.9°C, which corresponds to the average maximum daily temperature at mesophotic depth during the experiment (Figures 2, S1). The thermal stress temperature of ~30°C was set at 29.8 ± 0.4°C, which corresponds to 4 > eDHWs in mesophotic and shallow depths for two weeks. The thermal stress temperature of ~31°C was set at 31.3 ± 0.5°C, which corresponds to 5~7 eDHWs in shallow and mesophotic depths. To achieve the desired temperatures and start the experiment, the temperature in the thermal stress conditions was ramped up by 0.5°C every 6 hours. Chillers were used to regulate the temperature conditions at ~28°C, while separate heaters controlled the thermal temperature conditions. Temperature loggers (HOBO Water Temp Pro v2) were used to monitor the temperatures (Figure S2A).
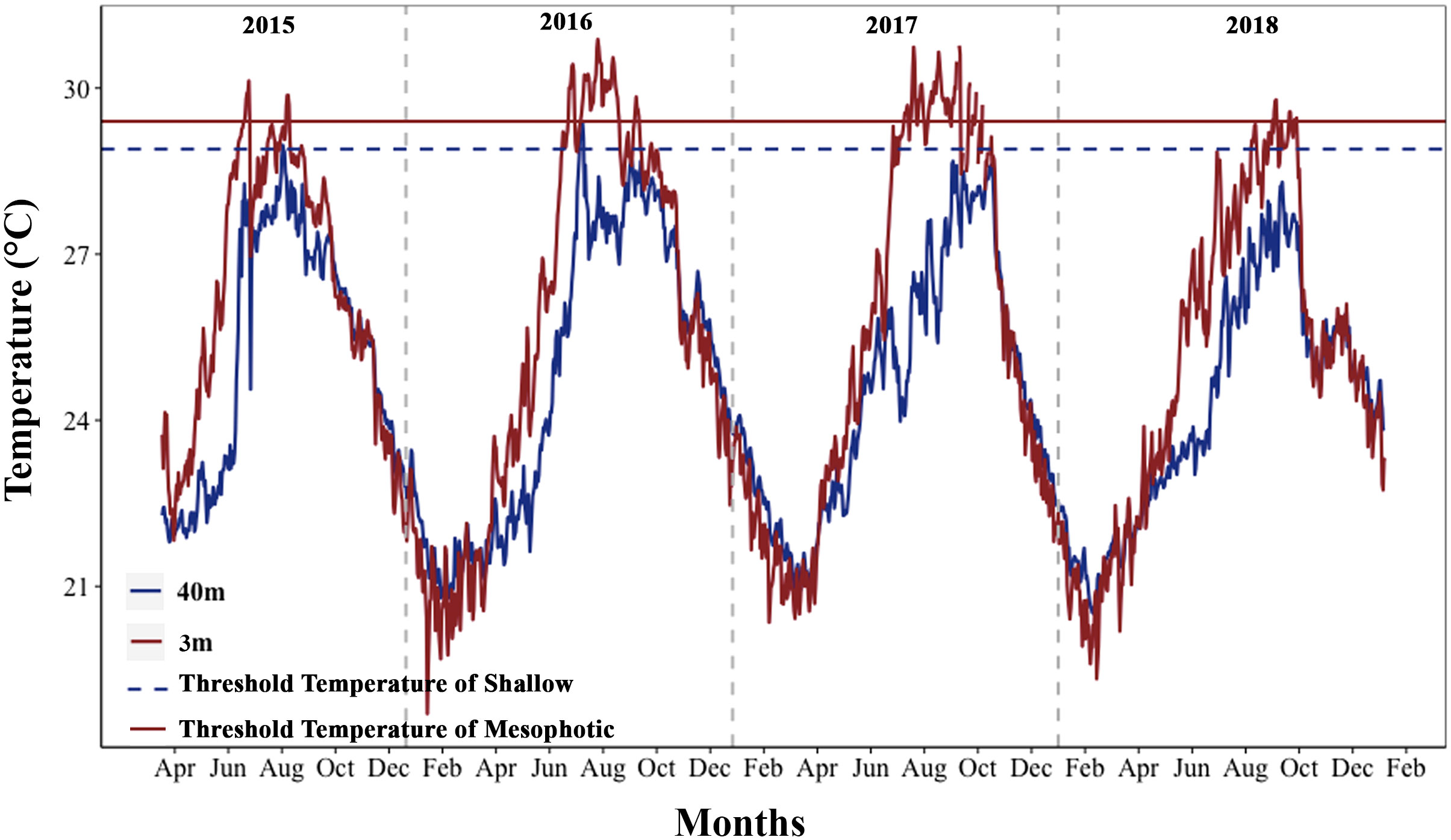
Figure 2 Annual temperature fluctuations in shallow and mesophotic depths of the study area (2015–2018). The horizontal dark red line (TTS) and the dark blue dashed line (TTM) represent the threshold temperature for shallow depth (29.4°C) and mesophotic depth (28.9°C), respectively. The vertical gray dashed lines separate the years.
A total of 18 aquariums were utilized in this study. Three replicates of 26-L flow-through (0.02 L s-1) aquaria were established for each temperature treatment under each light condition (Figure S2B). In each temperature treatment, twelve fragments of each species (with four replicates per colony) were randomly placed in three aquaria, resulting in a total of four fragments from each species per aquarium. At least one fragment of each colony was placed in an aquarium (Figure S2C).
Overall, each aquarium contained 24 fragments from six species, which were placed with a 4 cm distance between fragments. Finally, three fragments from each species were randomly collected from each temperature treatment and each species (one fragment per colony/aquarium) to measure physiological parameters on days seven and 14 of the experiment. The collected fragments were stored at -80°C until measurement.
Monitoring coral health and color
The health status of fragments (healthy and dead fragments with no live tissue) was visually assessed daily, and color was monitored every other day during the experiment. All the fragments in each aquarium were photographed using a digital camera (Canon Powershot G10; Canon, Japan) with constant white balance under identical illumination, and Coral Health Chart (Coral Watch) was used to assess bleaching intensity (Siebeck et al., 2006).
The coral images were analyzed using Adobe Photoshop CC 2015. The coral reflection color was recorded using the histogram function with the RGB channel and matched with the Coral Health Chart color category (Siebeck et al., 2006).
Photosynthetic efficiency
The maximum quantum yield of the photosystem II (Fv/Fm) was used as a sensitive indicator of symbiotic microalgal cells and monitored every other day for each fragment after 20 min of dark adaptation (after sunset) using a pulse-amplitude modulated fluorometer (Diving PAM; Walz, Effeltrich, Germany). Minimum fluorescence (Fo) was determined using 3μs pulses of a light emitting diode (blue LED, peak emission at 470 nm; MEAS-INT: 6, DAMP:1, GAIN:3), and the maximum fluorescence (Fm) of each dark-adapted sample was measured by a 0.8 s saturation light pulses (8000 μmol photons m−2s−1;SAT-INT: 12).
Symbiotic microalgal density and chlorophyll concentrations
To determine symbiotic microalgal density and chlorophyll content, samples stored at −80°C were used. Coral tissues were collected from each fragment (n = 3 per treatment) using an air pick and transferred to filtered (0.22 μm) seawater. Subsequently, the coral tissue slurry was homogenized using a Potter–Elvehjem tissue grinder and centrifuged at 5000 × g for 20 min at 4°C. Following this, the supernatant was discarded, and the pellet of symbiotic microalgae cells was resuspended in fresh filtered seawater. The cell density of the fragments (n = 3 for each species in each treatment) was counted with a hemocytometer under a light microscope.
One milliliter of the slurry was centrifuged for 20 min, and the supernatant was discarded for each fragment. The pellet was resuspended in 1 mL acetone and stored in darkness for 24 h at 4°C to extract chlorophyll. The absorbance of the samples was measured using a spectrophotometer (Shimadzu UV-1800 spectrophotometer; Shimadzu Inc., Kyoto, Japan), and chlorophyll a and c2 concentrations (n = 3 for each species in each treatment) were calculated using the equations of Jeffrey and Humphrey (1975). The symbiotic microalgae density and chlorophyll content were normalized and estimated to the surface area of each fragment by the wax dipping technique (Veal et al., 2010).
Assessment of thermal stress accumulation levels in treatments
The level of accumulated thermal stress within each treatment during the 14-day experiment was monitored using two metrics: experimental degree heating weeks (eDHW) (Leggat et al., 2022) and experimental degree heating days (eDHD) as described in Wyatt et al. (2020). These metrics were calculated based on the recorded temperature data in the treatments and provide an indication of the intensity and duration of thermal stress accumulation commonly associated with coral bleaching responses (Skirving et al., 2020; Wyatt et al., 2023). Briefly, to calculate the eDHW values, we sum of the recorded water temperatures anomalies that exceeded 1°C above the maximum monthly means (MMM; shallow treatment: 28.4°C; mesophotic treatment: 27.9°C) in each treatment over the 14-day period. We then divide this sum by seven to obtain weekly units. For the eDHD values, we sum of the recorded water temperature anomalies in 10-minute intervals that exceeded 1°C above the maximum monthly means (MMM) of each treatment. We then divide the sum by the number of recorded temperatures in each day (n=144, 10-minute intervals) to achieve daily units.
Statistical analyses
The survival of species in different treatments was estimated using the log-rank test on the Kaplan–Meier curves (Kaplan and Meier, 1958) using the “survfit” function from the “survival” package on R (Therneau, 2015). Normality and homogeneity of variances were assessed using Shapiro–Wilk test and Levene’s test, respectively (Queen et al., 2002). Spearman correlation coefficients were used to analyze the relationships between the daily mean in situ seawater temperatures at depths of 3 and 40 meters during 2015 and 2018. Wilcoxon rank test was used to test the differences in maximum and mean daily temperatures between shallow and mesophotic depths. Generalized linear models (GLM) using the package “lme4” were used to evaluate the effect of temperature treatments on brightness and Fv/Fm for each species and depth. Temperature treatments and days were considered as fixed effect factors. A generalized linear model (GLM) was also used to test the effect of temperature on symbiotic algal density and chlorophyll concentration. Tukey’s test was performed using the “emmeans” package on R (Lenth, 2019).
To investigate inter-specific and depth-dependent variation in the thermal tolerance of corals to different treatments, the standardized mean differences of the data were obtained using Hedges’ G method (Hedges, 1981), and the effect size was calculated using the “metacont” function of “meta” package in R (Schwarzer et al., 2015). Forest plots were used to generate the effect size with 95% confidence intervals using the “ggplot” package. Statistical significance was set at p < 0.05. R v4.0.4 (R Core Team, 2021) was used for all statistical analyses.
Results
Temperatures in shallow and mesophotic reefs
Maximum monthly mean (MMM) climatological temperatures obtained were 28.4°C and 27.9°C in the shallow and mesophotic habitats, respectively, during three months of the high-temperature season. The thermal thresholds in the shallow and mesophotic habitats were predicted to be one degree higher than the MMM temperatures at each depth (shallow: 29.4, mesophotic: 28.9) (Figure 2). To obtain a comprehensive understanding of the thermal conditions at various depths, we analyzed the maximum and average daily temperatures at different depths using temperature data recorded over a five-year period from 2015 to 2020. Additionally, we examined the daily temperature fluctuations during both high-temperature seasons (summer: June 21st to September 21st) and low-temperature seasons (winter: December 21st to March 21st). The analysis of temperature data from different depths revealed that the maximum and mean daily temperatures in the mesophotic depth were lower than those in the shallow depth at the study sites (Wilcoxon rank test, p < 0.0001). However, temperature fluctuations increased with depth during the summer (GLM, p < 0.0001). In the winter, the mesophotic depth showed lower daily temperature fluctuations than the shallow depth (GLM, p < 0.0001; Figures 2, S1). Furthermore, a strong correlation was found between seawater temperatures at depths of 3 and 40 m (Spearman’s correlation, p < 0.0001, ρ = 0.908).
Survivorship of different species
All shallow and mesophotic species fragments survived in the ~28°C temperature until the end of the 14-day experiment. Among species from shallow depths, mortality was observed only at ~31°C temperatures (5.6 eDHWs in shallow), with the highest mortality rates observed in Porites cylindrica (22%), Porites sp. (11%), and Acropora valida (11%). In contrast, among species from mesophotic depths, high mortality was observed in ~30°C and ~31°C temperature treatments (3.7 and 6.7 eDHWs in mesophotic) for Acropora cf. horrida (100%) and ~ 31°C temperature treatments (6.7 eDHWs) for Seriatopora hystrix (100%), Galaxea cf. astreata (44%), and Porites sp. (11%).
Other species, such as Pachyseris speciosa, Turbinaria mesenterina, and Galaxea fascicularis from shallow depths and Pachyseris speciosa and Leptoseris papyracea from mesophotic depths, survived under all temperature conditions. The log-rank test showed significant differences in survival rates between colonies subjected to ~30°C and ~31°C temperatures compared to ~28°C, as well as between the depths (Figure 3; Table S1).
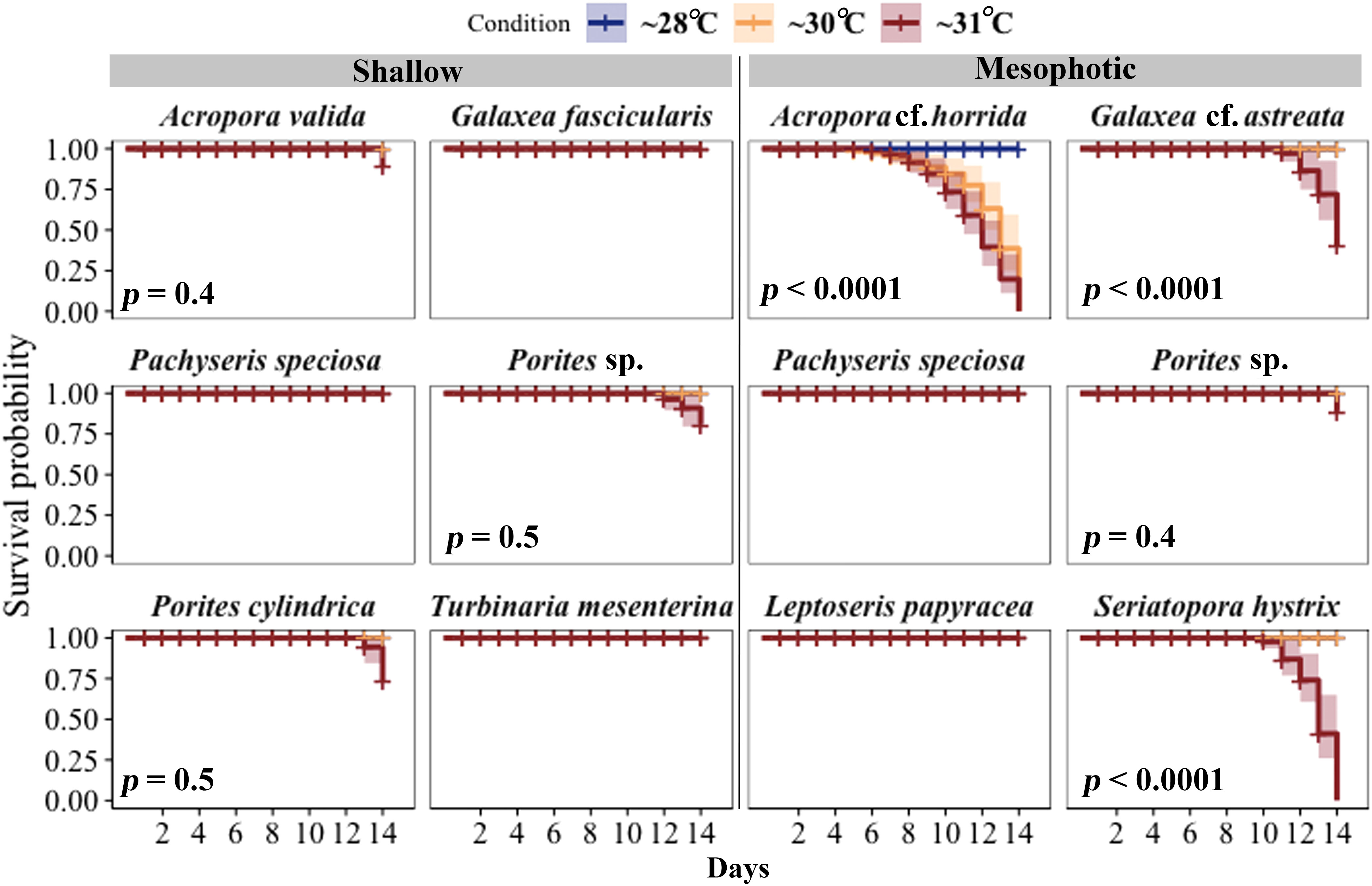
Figure 3 Survival probabilities of corals from mesophotic and shallow reefs over a period of 14 days under three different temperature conditions. Kaplan–Meier log-rank test p-values are shown for comparing survival curves in three different temperature treatments. All fragments of Pachyseris speciosa, Turbinaria mesenterina, and Galaxea fascicularis from shallow areas and P. speciosa and Leptoseris papyracea from mesophotic depths, exhibited 100% survivorship under all temperature conditions throughout the experimental period.
Coral brightness and bleaching
The color of shallow and mesophotic fragments exposed to higher temperatures turned pale (brighter color caused by bleaching) compared with those in the ~28°C treatment, and the brightness of the fragments gradually increased over the experimental period (Figure 4; Table S2). A significant increase in brightness was observed in all species at shallow and mesophotic depths at ~31°C treatment (GLM, p < 0.0001). In the ~30°C treatment, three out of six species showed a significant change in brightness: Acropora valida (GLM, p < 0.0001), Porites cylindrica (GLM, p < 0.0001), and Pachyseris speciosa (GLM, p = 0.0001). In contrast, four out of six mesophotic species exhibited a significant increase in brightness in the ~30°C treatment: Acropora cf. horrida (p < 0.0001), Pachyseris speciosa (p = 0.01), Leptoseris papyracea (p = 0.007), and Seriatopora hystrix (p = 0.003) (Figures 1, 4; Table S2).
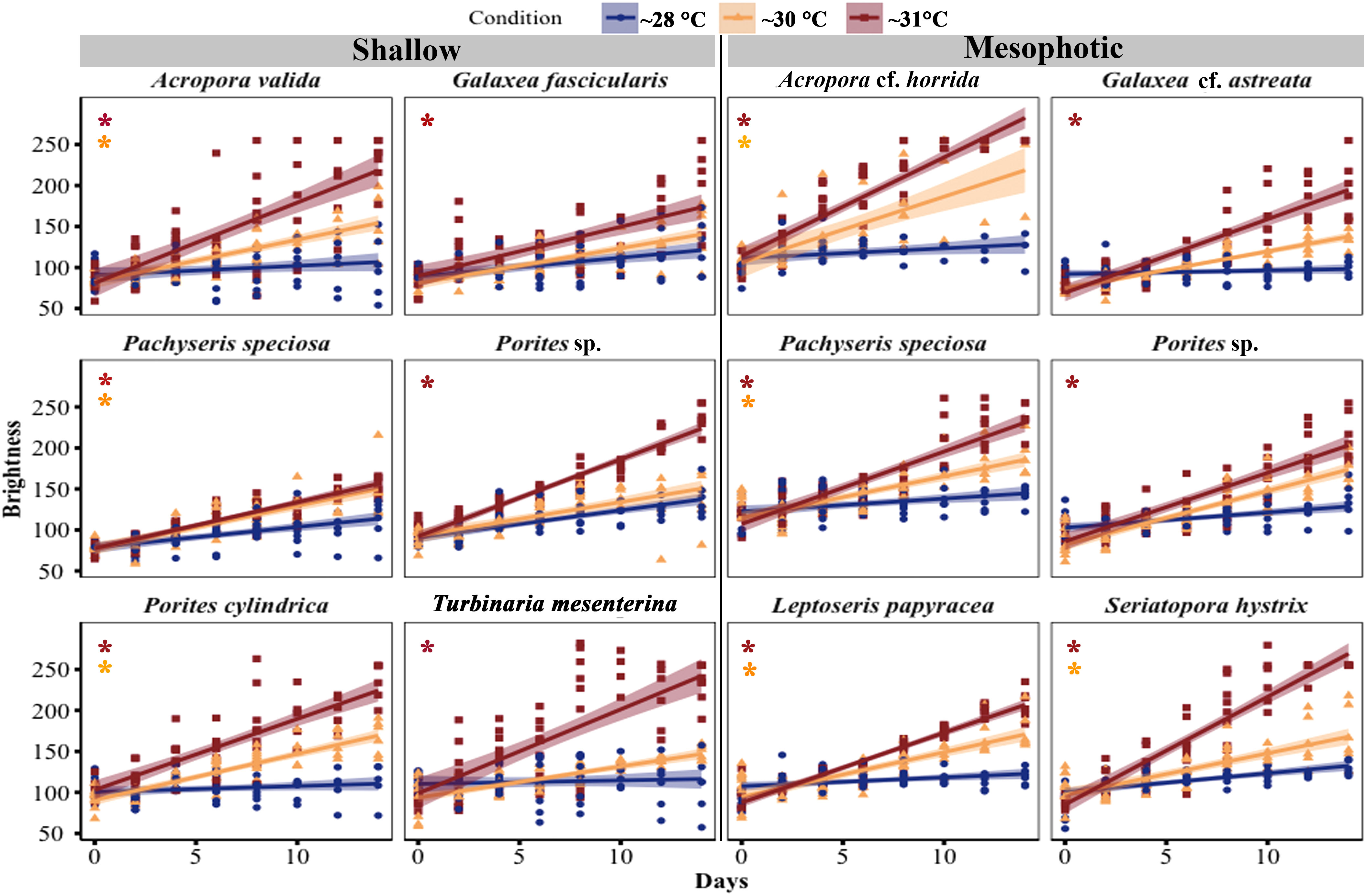
Figure 4 Changes in brightness of corals from mesophotic and shallow reefs under three different temperature treatments over a period of 14 days. The brightness was determined based on the hue value measured from photos. The brightness range varied from 55 to 255, with a value of 55 corresponding to healthy fragments, and a value of 255 indicating bleached fragments (Siebeck et al., 2006). An asterisk on the left side of the plots indicates a significant difference between treatments: orange represents a significant difference between ~28°C and ~30°C treatments, while red represents a significant difference between ~28°C and ~31°C treatments.
Photosynthetic efficiency
In most species, the maximum quantum efficiency of photosystem II (Fv/Fm) was highest at ~28°C and decreased in corals exposed to temperature treatments at ~30°C and ~31°C (Figure 5; Table S3). Among the shallow species, four out of six species showed a significant decrease in Fv/Fm at ~31°C (Acropora valida, p < 0.0001; Porites sp., p = 0.01; Porites cylindrica, p < 0.0001; Turbinaria mesenterina, p < 0.0001), while no significant reduction was observed at ~30°C. Among the mesophotic species, all species exhibited a significant reduction in Fv/Fm at ~31°C (GLM, p < 0.05), and only Acropora cf. horrida showed a significant reduction at ~30°C (GLM, p = 0.0003).
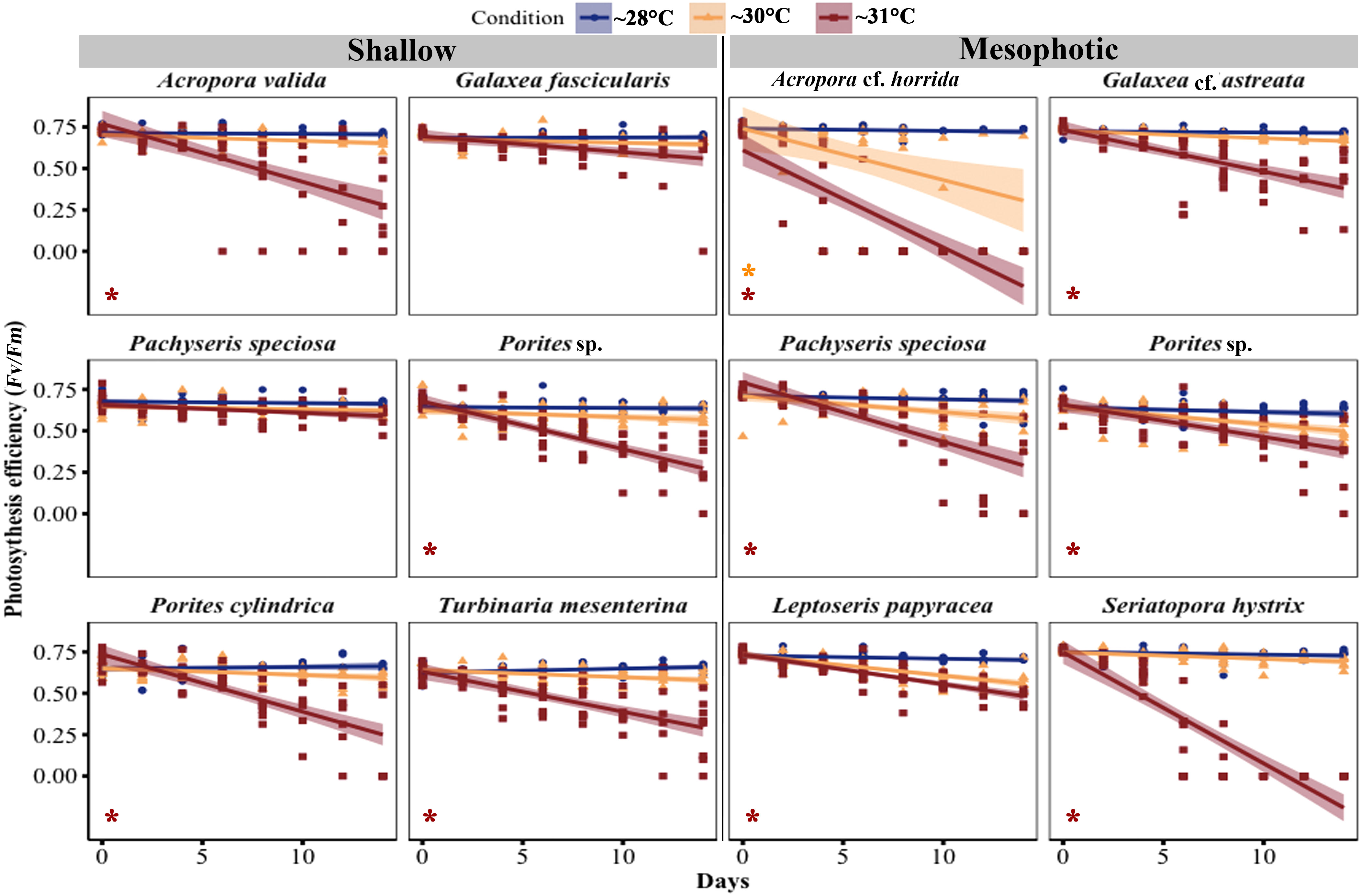
Figure 5 Changes in maximal quantum yield of photosystem II (Fv/Fm) in corals from mesophotic and shallow reefs under three temperature conditions over 14 days. An asterisk on the left side of the plots indicates a significant difference between treatments: orange represents a significant difference between ~28°C and ~30°C treatments, while red represents a significant difference between ~28°C and ~31°C treatments.
Pachyseris speciosa displayed different responses in shallow and mesophotic habitats. In mesophotic fragments, a significant reduction of 67.61% was observed at ~31°C (GLM, p < 0.0001), while at ~30°C, there was a non-significant reduction of 18.03% (GLM, p = 0.4) during the experimental period. In contrast, in the Fv/Fm of shallow Pachyseris speciosa fragments, there was no significant reduction (GLM, p > 0.5) at ~31°C, with a decrease of 14.7%, and at ~30°C, there was a 7.15% reduction during the experimental period.
Symbiotic algal density and chlorophyll
Throughout the experiment, the symbiont density in the fragments decreased in the ~30°C and ~31°C temperature treatments compared with the ~28°C treatments. A significant reduction in symbiotic algal density was observed in three out of six shallow species at ~31°C temperature treatments, including A. valida (GLM, p = 0.005), Porites cylindrica (GLM, p < 0.0001), and T. mesenterina (GLM, p = 0.0006), while there was no significant difference at ~30°C. In contrast, four out of six mesophotic species showed a significant decrease in symbiotic algal density in the 31-degree treatment, including Acropora cf. horrida (GLM, p = 0.0001), Pachyseris speciosa (GLM, p = 0.005), S. hystrix (GLM, p = 0.0001), and Galaxea cf. astreata (GLM, p = 0.01). Additionally, Acropora cf. horrida showed a significant reduction at ~30°C (GLM, p = 0.01) (Figure 6; Table S4).”
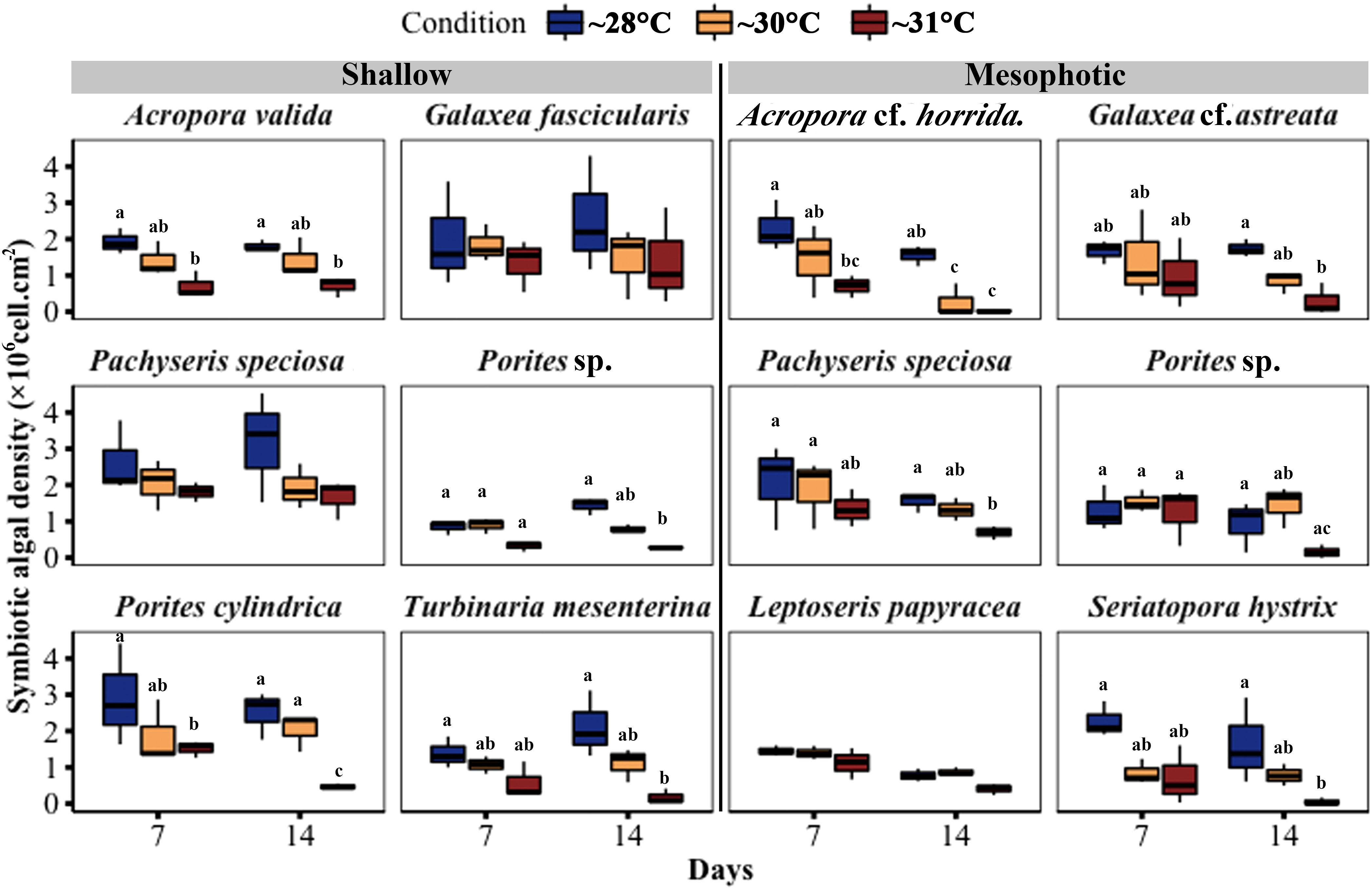
Figure 6 Changes in symbiotic algal density in corals from mesophotic and shallow reefs under three temperature conditions over 14 days. Boxes show the upper (75th percentile) and lower (25th percentile) quartiles, center lines represent medians, and whiskers extend to less than 1.5 × interquartile range measured data away from the first/third quartile. Letters indicate a significant difference between treatments each day and between days.
Chlorophyll (a and c2) content per surface area showed a significant decrease in three out of six species from shallow depths, namely Pachyseris speciosa, Porites cylindrica, and T. mesenterina, at ~31°C temperature treatments. Additionally, Pachyseris speciosa exhibited a significant reduction in chlorophyll content at ~30°C treatment. In contrast, among coral fragments from mesophotic depth, five out of six species, including Acropora cf. horrida, Galaxea cf. astreata, Pachyseris speciosa, Porites sp., and S. hystrix, showed a significant decrease in chlorophyll content at ~31°C temperature treatments. Among these mesophotic species, only Pachyseris speciosa also displayed a significant reduction in chlorophyll content at ~30°C temperature treatments. It is worth noting that the total chlorophyll content in the other species did not differ between the temperature treatments (see Figure 7; Table S5).
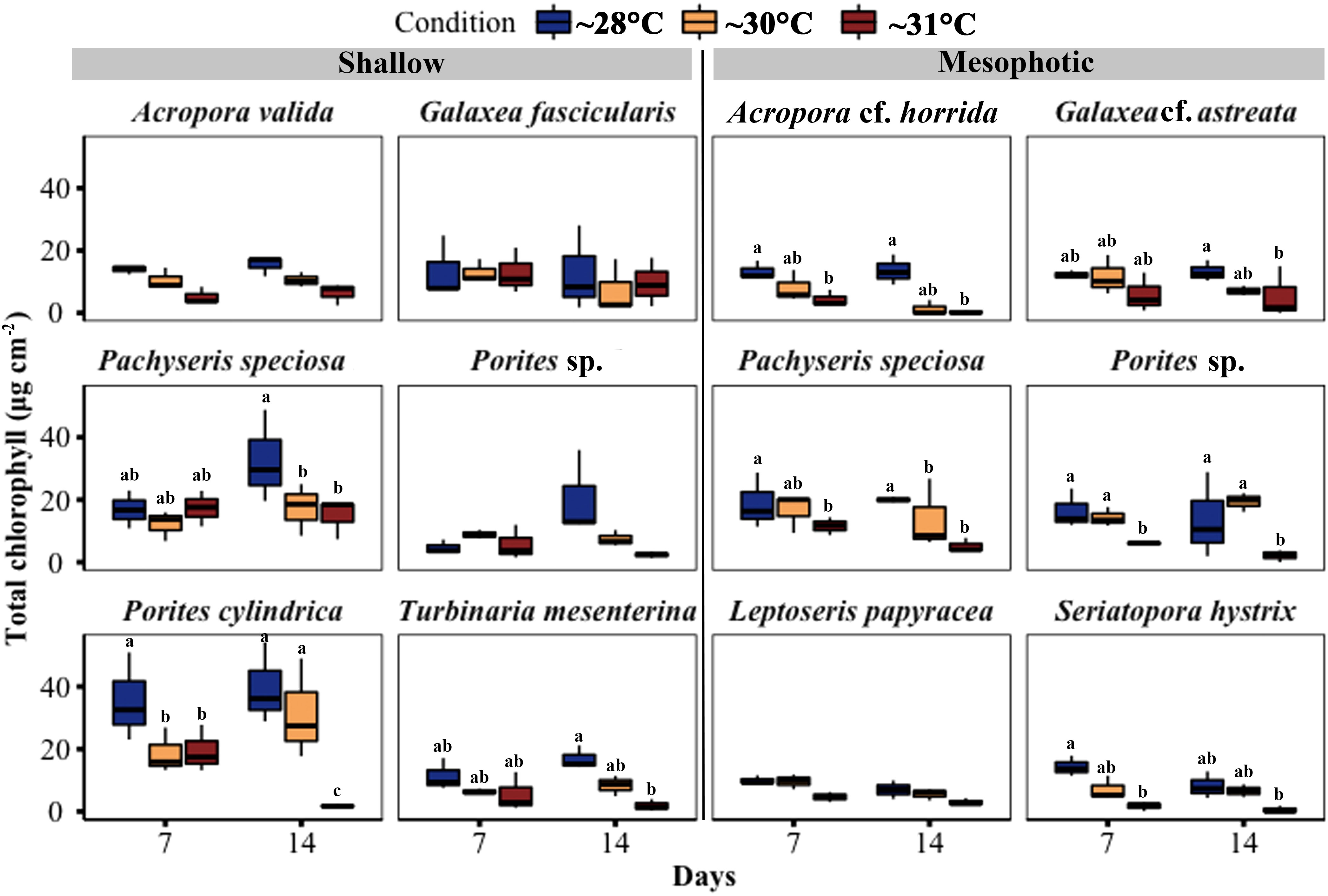
Figure 7 Changes in total chlorophyll (Chl a + c2) concentration per cm2 in corals from mesophotic and shallow reefs under three temperature conditions over 14 days. Boxes show the upper (75th percentile) and lower (25th percentile) quartile, center lines represent medians, and whiskers extend to less than 1.5×interquartile range measured data away from the first/third quartile. Letters indicate a significant difference between treatments each day and between days.
Thermal responses, eDHWs and eDHD
We examined coral fragments from shallow and mesophotic depths in response to water temperature using two metrics: eDHW and eDHD. The accumulation of thermal stress exposure over the 14-day experimental period reached an eDHW (Degree Heating Weeks) of 2.6°C-weeks for shallow fragments and 3.7°C-weeks for mesophotic fragments under moderate conditions (~30°C treatments). Under higher stress conditions (~31°C treatments), the eDHW was 5.6°C-weeks for shallow fragments and 6.7°C-weeks for mesophotic fragments. The accumulation of thermal stress exposure in terms of Degree Heating Days (eDHD) over the experimental period was 18.8°C-days for shallow fragments and 26.5°C-days for mesophotic fragments under moderate conditions. Under higher stress conditions, the eDHD was 39.6°C-days for shallow fragments and 47.3°C-days for mesophotic fragments.
The results indicated variable reactions (Table 1). In terms of brightness, bleaching was observed in Acropora cf. horrida among mesophotic species, while among shallow species, slight bleaching was observed in a few fragments of Acropora valida, Porites sp., and Galaxea fascicularis. Additionally, at 5 < eDHWs, the brightness in mesophotic fragments of Pachyseris speciosa was significantly higher than in shallow fragments (LMM, p < 0.0001). In terms of survival, among the mesophotic species, only Acropora cf. horrida showed low survivorship at 5 < eDHWs and all other species survived through the treatment, while among the shallow species slight mortality was observed in Acropora valida, Porites sp. and Porites cylindrica at 4DHWs although it was not significant (Figure 3, Table 1). Interestingly, Fv/Fm for Pachyseris speciosa was not significantly different between the mesophotic and shallow depths (LMM, p = 0.29) but was different in brightness (LMM, p < 0.0001).
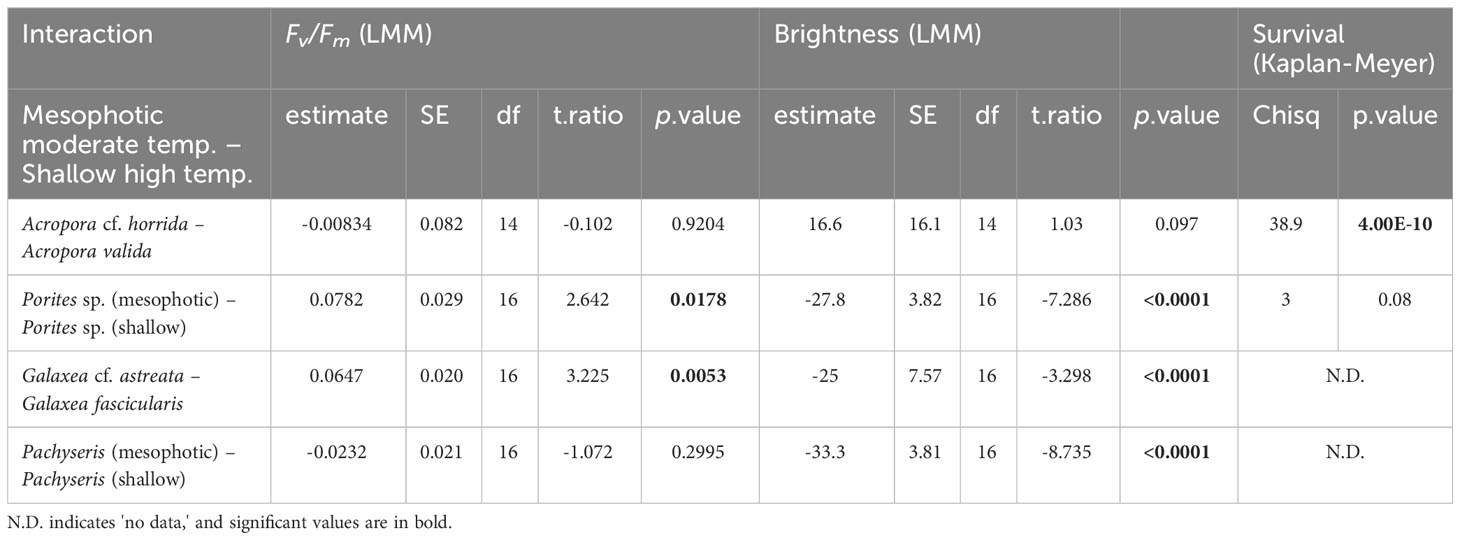
Table 1 Comparison between +4DHWs in moderate temperature for mesophotic- and higher temperature for shallow species.
Discussion
The present study indicates that the thermal threshold of mesophotic corals is slightly lower or equal to that of corals distributed in shallow water. Organisms in colder environments show lower thermal tolerance and higher sensitivity than those in warmer climates (Jokiel and Coles, 1990; Jokiel, 2004). Since the thermal tolerance of corals may be linked to the temperature of their habitats (Oliver and Palumbi, 2011; Schoepf et al., 2015; Safaie et al., 2018), it is reasonable to expect depth-dependent differences in their thermal tolerance. Species inhabiting highly variable shallow habitats often possess higher thermal tolerance and better resistance to bleaching compared to those inhabiting stable habitats (Schoepf et al., 2015; Smith et al., 2016; Schoepf et al., 2019).
The thermal threshold may be associated with species and temperature in their habitat; thus, the thresholds differ among species and locations. Previous studies have also indicated lower thermal tolerance among colonies from mesophotic depths compared to shallow depths (Smith et al., 2016; Skutnik et al., 2020; Frates et al., 2021). However, some regions have had contrasting results, such as the Great Barrier Reef (Frade et al., 2018) and Moorea in French Polynesia (Pérez-Rosales et al., 2021). In these areas, mesophotic coral communities have shown a decreased incidence of bleaching across depths, particularly from shallow to upper mesophotic depths (40 m). Furthermore, lower mesophotic communities (> 60 m) in Polynesia have been observed to escape the detrimental effects caused by thermal bleaching events (Pérez-Rosales et al., 2021). This could be attributed to the relatively stable and constant temperatures experienced by shallow and upper mesophotic depths in Polynesia during the high-temperature season (Pérez-Rosales et al., 2021), which might affect the thermal tolerance of corals in upper mesophotic depths. Nevertheless, in the present study site in Okinawa, mesophotic depths generally experienced lower temperatures than shallow depths. Additionally, the high-temperature fluctuations caused by short-term cooling events induced by internal waves further contribute to maintaining a cooler environment in deeper coral ecosystems (Wyatt et al., 2020; Godefroid et al., 2023; Wyatt et al., 2023). Consequently, this leads to a lower thermal threshold for mesophotic corals than their shallow counterparts.
Bleaching responses were highly variable but more severe in most of the examined species from mesophotic depths. The bleaching responses, represented as a decrease in algal density and an increase in color brightness in the studied species, were observed in both shallow and mesophotic areas, but their rates of change were not consistent (Figures 3, 6). Bleaching was prominently observed in the mesophotic species Acropora cf. horrida, Seriatopora hystrix, and Galaxea cf. astreata (Figures 3, 6). The variable responses in the examined species were congruent with those in previous reports; susceptibility to thermal stress varied among genera, species, and populations (Loya et al., 2001; Hongo and Yamano, 2013; Palumbi et al., 2014). The corals with high bleaching prevalence were physiologically damaged and suffered increased mortality (Glynn, 1984; Berkelmans et al., 2004; Hughes et al., 2018a).
Low thermal tolerance of S. hystrix has been documented in shallow reefs (Marshall and Baird, 2000; Loya et al., 2001; van Woesik et al., 2011; Seveso et al., 2014; Hoey et al., 2016). Notably, the disappearance of S. hystrix in shallow reefs around Sesoko Island followed a mass coral bleaching event in 1998 (van Woesik et al., 2011). Subsequently, a dense population of the species was found in the upper mesophotic depth north of Sesoko Island (Sinniger et al., 2013). This surviving population was likely protected from the extreme heat stress on shallow reefs because of the depth, underscoring the relationship between their habitat and susceptibility to environmental stressors. However, considering rising seawater temperature (0.2–1°C per decade) and the predicted annual coral bleaching phenomenon in the next decades (Donner et al., 2005), the mesophotic coral population may not be able to persist in the future due to the strong correlation with depth-dependent temperatures (Figures 3, 4, 8). Given the rising temperature, the duration, and magnitude of heatwaves, they will surpass the threshold temperature of the coral species, particularly at mesophotic depths where the corals live in cooler habitats and experience more stable thermal regimes. Indeed, coral bleaching due to high temperatures has been documented in some MCEs (Nir et al., 2014; Smith et al., 2016; Frade et al., 2018; Pérez-Rosales et al., 2021; Eyal et al., 2022). Owing to the discrepancy between a rapidly changing environment and phenotypic adaptation, the acclimatization/adaptation of corals to changing environments can be considered a major challenge (Putnam, 2021). Furthermore, even if the mesophotic population has the potential to replenish its shallow counterpart for a relatively long period as has been hypothesized in DRRH, the rising temperature and lower thermal threshold of this population limit the success of such regeneration.
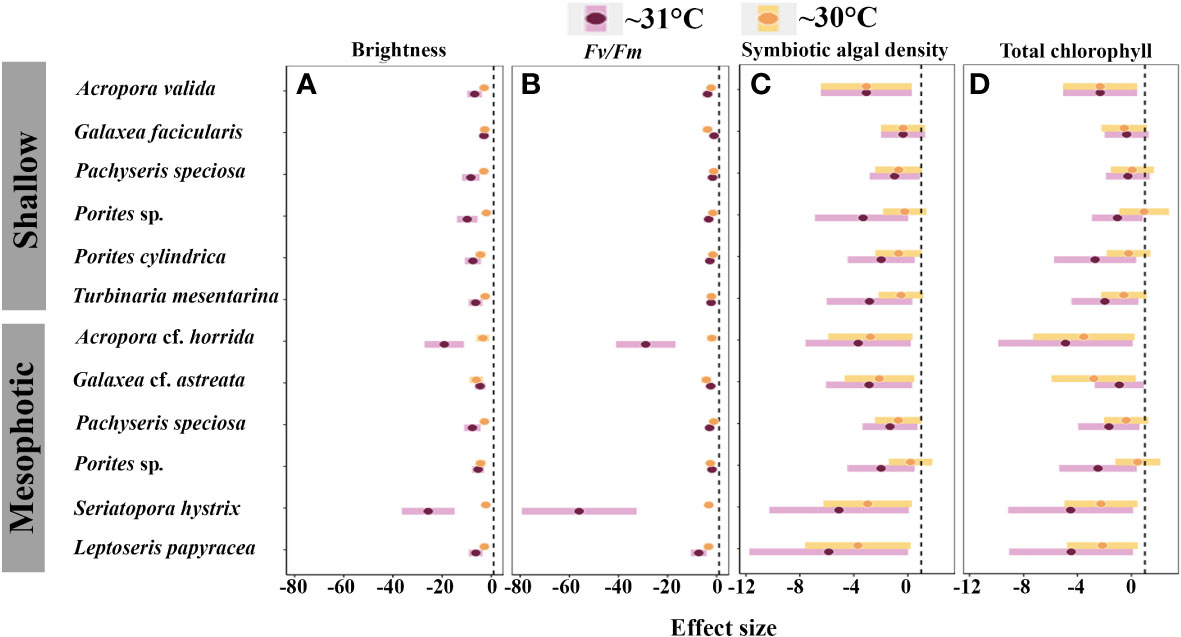
Figure 8 Inter-specific effect of medium- and high-temperature treatments on: (A) brightness; (B) Fv/Fm; (C) symbiotic algal density; and (D) total chlorophyll. The black dashed line indicates zero effect, and negative points indicate the negative impact of thermal treatments on the physiological function of species.
The heat tolerance of the species from both mesophotic and shallow reefs is essential for reef persistence. For example, despite differences in the thermal tolerance between A. cf. horrida from mesophotic depths and A. valida from shallow depths, both species were found to be susceptible to and physiologically damaged by high water temperature. In contrast, Porites sp. from both depth ranges and L. papyracea exhibited lower susceptibility to high water temperatures. Moreover, the laminar species Pachyseris speciosa demonstrated the highest heat tolerance (Figures 7, 8). However, mesophotic fragments of this species displayed significantly higher brightness levels than those shallow fragments at > 5 eDHWs. Previous studies have also shown that other laminar species exhibit higher heat tolerance than branching species (Loya et al., 2001; van Woesik et al., 2011). Pachyseris speciosa is widely distributed in mesophotic depths, including the Ryukyu archipelago (Sinniger and Harii, 2018). If water temperature increases by 2°C, heat-tolerant species such as Pachyseris speciosa may dominate the reef ecosystem. The acclimatization and adaptation potential of other species need to be clarified.
The responses to heat stress were highly variable among the examined species. In terms of DHWs, up to 4 eDHWs did not cause a critical negative effect on the survivorship of most species, except for Acropora cf. horrida, from mesophotic depths (Figure 3). In terms of brightness, bleaching was observed in only one fragments of Pachyseris speciosa and S. hystrix (Figures 4–7). In corals from shallow depths, a value of 5.71 eDHW, indicating a state of high-stress conditions, significantly impacted most of the examined parameters (Figures 3–7). However, the impact of temperature on species from mesophotic depths was most severe, and most of the examined species from this depth in the high thermal stress treatment (6.81 eDHWs) showed high levels of brightness and mortality. Regarding the DHWs index, DHWs from +4 to 8, considered moderate thermal stress, were expected to cause medium bleaching (Liu et al., 2018).
In this study, except for Acropora cf. horrida from mesophotic depth, other coral species from shallow and mesophotic habitats showed heat tolerance up to 4 eDHW. Therefore, we conclude that the upper-mesophotic habitats could act as refugia for coral species if the accumulation of thermal stress in mesophotic depth does not exceed 4 DHW. Some mesophotic ecosystems are protected from temperature anomalies because of the thermocline depth (Bridge et al., 2013), which could serve as potential thermal refugia. Otherwise, corals in mesophotic habitats should tolerate a similar temperature threshold as their shallow counterparts to withstand rising temperatures (Gould et al., 2021). Similar responses have been proposed for mesophotic and shallow colonies of Montastraea cavernosa (Skutnik et al., 2020) and Orbicella faveolata (Frates et al., 2021) against thermal stress. However, the temperature in mesophotic depth in the present study was considerably correlated with shallow depth temperature. Changes in shallow-depth temperature alter mesophotic depth temperature. Owing to rising sea surface temperature and annual consecutive thermal stress, which have been predicted (van Hooidonk et al., 2013), the water temperature in the mesophotic reef will eventually rise. Therefore, considering our findings, the mesophotic reef could act as a refugium for the species which could tolerate heat stress up to 4 DHW. However, the corals that inhabit mesophotic depths have significantly limited capacity for thermal tolerance when faced with high levels of thermal stress (>6 DHWs), which could have serious implications for the health and survival of these important ecosystems. On the other hand, the thresholds of the thermal tolerance in the corals are diverse and not essentially different in several species inhabiting mesophotic to shallow areas.
Data availability statement
The raw data supporting the conclusions of this article will be made available by the authors, without undue reservation.
Author contributions
PT-K, FS, and SH conceived and designed the experiments and conducted fieldwork. PT-K performed laboratory experiments. TN supported photo-physiological measurements. PT-K and MM analyzed the data and wrote the original draft of the manuscript. All authors contributed to the article and approved the submitted version.
Funding
This study was supported by Grants-in-Aid for Scientific Research (KAKENHI) from Japan Society for the Promotion of Science to SH (grant numbers 16H02490, 16KK0164, and 21H04943), FS (20K06210), and MM (21H05304 and 22H02369).
Acknowledgments
We thank Dr. H. Rouzé, E. D. S. Sitorus, S. Kadena, and M. Jinza for field assistance and Dr. S. Hazraty-Kari for laboratory assistance. The English language in this document has been checked by at least two professional editors, both native speakers of English.
Conflict of interest
The authors declare that the research was conducted in the absence of any commercial or financial relationships that could be construed as a potential conflict of interest.
Publisher’s note
All claims expressed in this article are solely those of the authors and do not necessarily represent those of their affiliated organizations, or those of the publisher, the editors and the reviewers. Any product that may be evaluated in this article, or claim that may be made by its manufacturer, is not guaranteed or endorsed by the publisher.
Supplementary material
The Supplementary Material for this article can be found online at: https://www.frontiersin.org/articles/10.3389/fmars.2023.1210662/full#supplementary-material
References
Abrego D., Ulstrup K. E., Willis B. L., van Oppen M. J. H. (2008). Species-specific interactions between algal endosymbionts and coral hosts define their bleaching response to heat and light stress. Proc. R. Soc B 275, 2273–2282. doi: 10.1098/rspb.2008.0180
Baker A. C., Glynn P. W., Riegl B. (2008). Climate change and coral reef bleaching: An ecological assessment of long-term impacts, recovery trends and future outlook. Estuar. Coast. Shelf Sci. 80, 435–471. doi: 10.1016/j.ecss.2008.09.003
Ben-Zvi O., Tamir R., Keren N., Tchernov D., Berman-Frank I., Kolodny Y., et al. (2020). Photophysiology of a mesophotic coral 3 years after transplantation to a shallow environment. Coral Reefs 39, 903–913. doi: 10.1007/s00338-020-01910-0
Berkelmans R., De'ath G., Kininmonth S., Skirving W. J. (2004). A comparison of the 1998 and 2002 coral bleaching events on the Great Barrier Reef: spatial correlation, patterns, and predictions. Coral Reefs 23, 74–83. doi: 10.1007/s00338-003-0353-y
Bieri T., Onishi M., Xiang T. T., Grossman A. R., Pringle J. R. (2016). Relative contributions of various cellular mechanisms to loss of algae during cnidarian bleaching. PloS One 11. doi: 10.1371/journal.pone.0152693
Bongaerts P., Ridgway T., Sampayo E. M., Hoegh-Guldberg O. (2010). Assessing the 'deep reef refugia' hypothesis: focus on Caribbean reefs. Coral Reefs 29, 309–327. doi: 10.1007/s00338-009-0581-x
Bongaerts P., Riginos C., Brunner R., Englebert N., Smith S. R., Hoegh-Guldberg O. (2017). Deep reefs are not universal refuges: reseeding potential varies among coral species. Sci. Adv. 3, e1602373. doi: 10.1126/sciadv.1602373
Bongaerts P., Smith T. B. (2019). “Beyond the “Deep Reef Refuge” hypothesis: a conceptual framework to characterize persistence at depth,” in Mesophotic Coral Ecosystems (Springer Cham), 881–895.
Bridge T. C., Hoey A. S., Campbell S. J., Muttaqin E., Rudi E., Fadli N., Baird A. H. (2013). Depth-dependent mortality of reef corals following a severe bleaching event: implications for thermal refuges and population recovery. F1000Res 16(2), 187. doi: 10.12688/f1000research.2-187.v3
Dias M., Ferreira A., Gouveia R., Cereja R., Vinagre C. (2018). Mortality, growth and regeneration following fragmentation of reef-forming corals under thermal stress. J. Sea Res. 141, 71–82. doi: 10.1016/j.seares.2018.08.008
Dias M., Madeira C., Jogee N., Ferreira A., Gouveia R., Cabral H., et al. (2019). Oxidative stress on scleractinian coral fragments following exposure to high temperature and low salinity. Ecol. Indic. 107. doi: 10.1016/j.ecolind.2019.105586
Diaz J. M., Hansel C. M., Apprill A., Brighi C., Zhang T., Weber L., et al. (2016). Species-specific control of external superoxide levels by the coral holobiont during a natural bleaching event. Nat. commu 7. doi: 10.1038/ncomms13801
Donner S. D., Skirving W. J., Little C. M., Oppenheimer M., Hoegh-Guldberg O. (2005). Global assessment of coral bleaching and required rates of adaptation under climate change. Glob. Change Bio. 11, 2251–2265. doi: 10.1111/j.1365-2486.2005.01073.x
Eyal G., Laverick J. H., Ben-Zvi O., Brown K. T., Kramer N., Tamir R., et al. (2022). Selective deep water coral bleaching occurs through depth isolation. Sci. Total Environ. 844, 157180. doi: 10.1016/j.scitotenv.2022.157180
Frade P. R., Bongaerts P., Englebert N., Rogers A., Gonzalez-Rivero M., Hoegh-Guldberg O. (2018). Deep reefs of the Great Barrier Reef offer limited thermal refuge during mass coral bleaching. Nat. commu 9. doi: 10.1038/s41467-018-05741-0
Frates E., Hughes A., Randall A. (2021). Orbicella faveolata: Shallow versus Mesophotic Coral Responses to Temperature Change. Boston University 15.
Glynn P. W. (1984). Widespread coral mortality and the 1982–83 el niño warming event. Environ. Conserv. 11, 133–146. doi: 10.1017/S0376892900013825
Glynn P. W. (1996). Coral reef bleaching: Facts, hypotheses and implications. Glob. Change Bio. 2, 495–509. doi: 10.1111/j.1365-2486.1996.tb00063.x
Glynn P. W., D'Croz L. (1990). Experimental evidence for high temperature stress as the cause of El Niño-coincident coral mortality. Coral Reefs 8, 181–191. doi: 10.1007/BF00265009
Godefroid M., Dubois P., Under The Pole Consortium, Hédouin L. (2023). Thermal performance with depth: Comparison of a mesophotic scleractinian and an antipatharian species subjected to internal waves in Mo'orea, French Polynesia. Mar. Environ. Res. 184, 105851. doi: 10.1016/j.marenvres.2022.105851
Gould K., Bruno J. F., Ju R., Goodbody-Gringley G. (2021). Upper-mesophotic and shallow reef corals exhibit similar thermal tolerance, sensitivity and optima. Coral Reefs 40, 907–920. doi: 10.1007/s00338-021-02095-w
Hedges L. V. (1981). Distribution theory for Glass's estimator of effect size and related estimators. J. Educ. Stat. 6, 107–128. doi: 10.3102/10769986006002107
Hoadley K. D., Pettay D. T., Grottoli A. G., Cai W. J., Melman T. F., Levas S., et al. (2016). High-temperature acclimation strategies within the thermally tolerant endosymbiont Symbiodinium trenchii and its coral host, Turbinaria reniformis, differ with changing pCO(2) and nutrients. Mar. Biol. 163. doi: 10.1007/s00227-016-2909-8
Hoegh-Guldberg O., Mumby P. J., Hooten A. J., Steneck R. S., Greenfield P., Gomez E., et al. (2007). Coral reefs under rapid climate change and ocean acidification. Science 318, 1737–1742. doi: 10.1126/science.1152509
Hoey A. S., Howells E., Johansen J. L., Hobbs J. P. A., Messmer V., McCowan D. M., et al. (2016). Recent advances in understanding the effects of climate change on coral reefs. Diversity 8. doi: 10.3390/d8020012
Hongo C., Yamano H. (2013). Species-specific responses of corals to bleaching events on anthropogenically turbid reefs on okinawa island, Japan, over a 15-year period, (1995-2009). PloS One 8. doi: 10.1371/journal.pone.0060952
Hughes T. P., Anderson K. D., Connolly S. R., Heron S. F., Kerry J. T., Lough J. M., et al. (2018a). Spatial and temporal patterns of mass bleaching of corals in the Anthropocene. Science 359, 80–8+. doi: 10.1126/science.aan8048
Hughes T. P., Baird A. H., Bellwood D. R., Card M., Connolly S. R., Folke C., et al. (2003). Climate change, human impacts, and the resilience of coral reefs. Science 301, 929–933. doi: 10.1126/science.1085046
Hughes T. P., Barnes M. L., Bellwood D. R., Cinner J. E., Cumming G. S., Jackson J. B. C., et al. (2017). Coral reefs in the anthropocene. Nature 546, 82–90. doi: 10.1038/nature22901
Hughes T. P., Kerry J. T., Baird A. H., Connolly S. R., Dietzel A., Eakin C. M., et al. (2018b). Global warming transforms coral reef assemblages. Nature 556, 492–496. doi: 10.1038/s41586-018-0041-2
Jeffrey S. W., Humphrey G. F. (1975). ew spectrophotometric equations for determining chlorophylls a, b, c1 and c2 in higher plants, algae and natural phytoplankton. Biochem. Physiol. Pflanz. 167, 191–194
Jokiel P. L. (2004). “Temperature stress and coral bleaching, in: Rosenberg,” in Coral health and disease (Berlin, Germany: Springer), 401–425.
Jokiel P. L., Coles S. L. (1990). Response of Hawaiian and other Indo-Pacific reef corals to elevated temperature. Coral Reefs 8, 155–162. doi: 10.1007/BF00265006
Kahng S. E., Garcia-Sais J. R., Spalding H. L., Brokovich E., Wagner D., Weil E., et al. (2010). Community ecology of mesophotic coral reef ecosystems. Coral Reefs 29, 255–275. doi: 10.1007/s00338-010-0593-6
Kaplan E. L., Meier P. (1958). Nonparametric estimation from incomplete observations. J. Am. Stat. Assoc. 53, 457–481. doi: 10.1080/01621459.1958.10501452
Kayanne H. (2017). Validation of degree heating weeks as a coral bleaching index in the northwestern Pacific. Coral Reefs 36, 63–70. doi: 10.1007/s00338-016-1524-y
Klepac C. N., Barshis D. J. (2020). Reduced thermal tolerance of massive coral species in a highly variable environment. Proc. R. Soc B 287. doi: 10.1098/rspb.2020.1379
Kleypas J. A., Thompson D. M., Castruccio F. S., Curchitser E. N., Pinsky M., Watson J. R. (2016). Larval connectivity across temperature gradients and its potential effect on heat tolerance in coral populations. Glob. Change Bio. 22, 3539–3549. doi: 10.1111/gcb.13347
Leggat W., Heron S. F., Fordyce A., Suggett D. J., Ainsworth T. D. (2022). Experiment Degree Heating Week (eDHW) as a novel metric to reconcile and validate past and future global coral bleaching studies. J. Environ. Manage. 301, 113919. doi: 10.1016/j.jenvman.2021.113919
Lenth R. (2019). Emmeans: Estimated marginal means, aka least squares means. (R package version 1.4.1). Available online at: https://cran.r-project.org/package=emmeans
Lesser M. P., Slattery M., Leichter J. J. (2009). Ecology of mesophotic coral reefs. J. Exp. Mar. Biol. Ecol. 375, 1–8. doi: 10.1016/j.jembe.2009.05.009
Liu G., Eakin C. M., Chen M., Kumar A., de la Cour J. L., Heron S. F., et al. (2018). Predicting heat stress to inform reef management: NOAA coral reef watch's 4-month coral bleaching outlook. Front. Mar. Sci. 5, 57. doi: 10.3389/fmars.2018.00057
Liu G., Heron S., Eakin C., Muller-Karger F., Vega-Rodriguez M., Guild L., et al. (2014). Reef-scale thermal stress monitoring of coral ecosystems: New 5-km global products from NOAA coral reef watch. Remote Sens. 6 (11), 11,579–11,606. doi: 10.3390/rs61111579
Liu G., Rauenzahn J. L., Heron S. F., Eakin C. M., Skirving W. J., Christensen T., et al. (2013). NOAA coral reef watch 50 km satellite sea surface temperature-based decision support system for coral bleaching management. NOAA Tech. Rep. Nesdis 143:33.
Liu G., Skirving W. J., Geiger E. F., La J. L. D. (2017). NOAA coral reef watch’s 5-km satellite coral bleaching heat stress monitoring product suite version 3 and four-month outlook version 4, Vol. 32. 7.
Liu G., Strong A. E., Skirving W. (2003). Remote sensing of sea surface temperature during 2002 Barrier Reef coral bleaching. EOS 84 (15), 137–144. doi: 10.1029/2003EO150001
Logan C. A., Dunne J. P., Eakin C. M., Donner S. D. (2014). Incorporating adaptive responses into future projections of coral bleaching. Glob. Chang Biol. 20, 125–139. doi: 10.1111/gcb.12390
Lough J. M., Anderson K. D., Hughes T. P. (2018). Increasing thermal stress for tropical coral reefs: 1871-2017. Sci. Rep. 8, 6079. doi: 10.1038/s41598-018-24530-9
Loya Y., Sakai K., Yamazato K., Nakano Y., Sambali H., van Woesik R. (2001). Coral bleaching: the winners and the losers. Ecol. Lett. 4, 122–131. doi: 10.1046/j.1461-0248.2001.00203.x
Marshall P. A., Baird A. H. (2000). Bleaching of corals on the Great Barrier Reef: differential susceptibilities among taxa. Coral Reefs 19, 155–163. doi: 10.1007/s003380000086
Mayfield A. B., Chen M. N., Meng P. J., Lin H. J., Chen C. S., Liu P. J. (2013). The physiological response of the reef coral Pocillopora damicornis to elevated temperature: results from coral reef mesocosm experiments in Southern Taiwan. Mar. Environ. Res. 86, 1–11. doi: 10.1016/j.marenvres.2013.01.004
McClanahan T. R., Maina J., Ateweberhan M. (2015). Regional coral responses to climate disturbances and warming is predicted by multivariate stress model and not temperature threshold metrics. Clim. Change 131, 607–620. doi: 10.1007/s10584-015-1399-x
Muir P. R., Marshall P. A., Abdulla A., Aguirre J. D. (2017). Species identity and depth predict bleaching severity in reef-building corals: shall the deep inherit the reef? Proc. R. Soc B 284. doi: 10.1098/rspb.2017.1551
Nielsen D. A., Petrou K., Gates R. D. (2018). Coral bleaching from a single cell perspective. ISME J. 12, 1558–1567. doi: 10.1038/s41396-018-0080-6
Nir O., Gruber D. F., Shemesh E., Glasser E., Tchernov D. (2014). Seasonal mesophotic coral bleaching of Stylophora Pistillata in the Northern Red Sea. PloS One 9. doi: 10.1371/journal.pone.0084968
Oliver T. A., Palumbi S. R. (2011). Do fluctuating temperature environments elevate coral thermal tolerance? Coral Reefs 30, 429–440. doi: 10.1007/s00338-011-0721-y
Palumbi S. R., Barshis D. J., Traylor-Knowles N., Bay R. A. (2014). Mechanisms of reef coral resistance to future climate change. Science 344, 895–898. doi: 10.1126/science.1251336
Pérez-Rosales G., Rouze H., Torda G., Bongaerts P., Pichon M., Parravicini V., et al. (2021). Mesophotic coral communities escape thermal coral bleaching in French Polynesia. R. Soc Open Sci. 8. doi: 10.1098/rsos.210139
Prasetia R., Sinniger F., Harii S. (2016). Gametogenesis and fecundity of Acropora tenella (Brook 1892) in a mesophotic coral ecosystem in Okinawa, Japan. Coral Reefs 35, 53–62. doi: 10.1007/s00338-015-1348-1
Prasetia R., Sinniger F., Nakamura T., Harii S. (2022). Limited acclimation of early life stages of the coral Seriatopora hystrix from mesophotic depth to shallow reefs. Sci. Rep. 12, 12836. doi: 10.1038/s41598-022-16024-6
Pratchett M. S., Caballes C. F., Newman S. J., Wilson S. K., Messmer V., Pratchett D. J. (2020). Bleaching susceptibility of aquarium corals collected across northern Australia. Coral Reefs 39, 663–673. doi: 10.1007/s00338-020-01939-1
Putnam H. M. (2021). Avenues of reef-building coral acclimatization in response to rapid environmental change. J. Exp. Biol. 224. doi: 10.1242/jeb.239319
Queen J. P., Quinn G. P., Keough M. J. (2002). Experimental design and data analysis for biologists (Cambridge university press, United Kingdom).
R Core Team (2021). R: A Language and Environment for Statistical Computing (Vienna, Austria: R Foundation for Statistical Computing). Available at: https://www.R-project.org/.
Riegl B., Piller W. E. (2003). Possible refugia for reefs in times of environmental stress. Int. J. Earth Sci. 92, 520–531. doi: 10.1007/s00531-003-0328-9
Safaie A., Silbiger N. J., McClanahan T. R., Pawlak G., Barshis D. J., Hench J. L., et al. (2018). High frequency temperature variability reduces the risk of coral bleaching. Nat. commu. 9. doi: 10.1038/s41467-018-04074-2
Schoepf V., Carrion S. A., Pfeifer S. M., Naugle M., Dugal L., Bruyn J., et al. (2019). Stress-resistant corals may not acclimatize to ocean warming but maintain heat tolerance under cooler temperatures. Nat. commu 10, 4031. doi: 10.1038/s41467-019-12065-0
Schoepf V., Stat M., Falter J. L., McCulloch M. T. (2015). Limits to the thermal tolerance of corals adapted to a highly fluctuating, naturally extreme temperature environment. Sci. Rep. 5. doi: 10.1038/srep17639
Schramek T. A., Colin P. L., Merrifield M. A., Terrill E. J. (2018). Depth-dependent thermal stress around corals in the tropical Pacific Ocean. Geophys. Res. Lett. 45, 9739–9747. doi: 10.1029/2018GL078782
Schramek T. A., Cornuelle B. D., Gopalakrishnan G., Colin P. L., Rowley S. J., Merrifield M. A., et al. (2019). Tropical western Pacific thermal structure and its relationship to ocean surface variables: A numerical state estimate and forereef temperature records. Oceanography 32, 156–163. doi: 10.5670/oceanog.2019.421
Schwarzer G., Carpenter J. R., Rücker G. (2015). Meta-analysis with R (Springer Cham). doi: 10.1007/978-3-319-21416-0
Seveso D., Montano S., Strona G., Orlandi I., Galli P., Vai M. (2014). The susceptibility of corals to thermal stress by analyzing Hsp60 expression. Mar. Environ. Res. 99, 69–75. doi: 10.1016/j.marenvres.2014.06.008
Shlesinger T., Grinblat M., Rapuano H., Amit T., Loya Y. (2018). Can mesophotic reefs replenish shallow reefs? Reduced coral reproductive performance casts a doubt. Ecology 99, 421–437. doi: 10.1002/ecy.2098
Siebeck U. E., Marshall N. J., Kluter A., Hoegh-Guldberg O. (2006). Monitoring coral bleaching using a colour reference card. Coral Reefs 25, 453–460. doi: 10.1007/s00338-006-0123-8
Sinniger F., Harii S. (2018). “Studies on mesophotic coral ecosystems in Japan,” in Coral Reef Studies of Japan (Singapore: Springer), 149–162.
Sinniger F., Morita M., Harii S. (2013). "Locally extinct" coral species Seriatopora hystrix found at upper mesophotic depths in Okinawa. Coral Reefs 32, 153–153. doi: 10.1007/s00338-012-0973-1
Skirving W., Marsh B., de la Cour J., Liu G., Harris A., Maturi E., et al. (2020). CoralTemp and the coral reef watch coral bleaching heat stress product suite version 3.1. Remote Sens. 12, 3856. doi: 10.3390/rs12233856
Skutnik J. E., Otieno S., Khoo S. K., Strychar K. B. (2020). Examining the effect of heat stress on Montastraea cavernosa (Linnaeus 1767) from a mesophotic coral ecosystem (MCE). Water 12. doi: 10.3390/w12051303
Smith T. B., Gyory J., Brandt M. E., Miller W. J., Jossart J., Nemeth R. S. (2016). Caribbean mesophotic coral ecosystems are unlikely climate change refugia. Glob. Chang Biol. 22, 2756–2765. doi: 10.1111/gcb.13175
Spalding M. D., Brown B. E. (2015). Warm-water coral reefs and climate change. Science 350, 769–771. doi: 10.1126/science.aad0349
Tavakoli-Kolour P., Sinniger F., Morita M., Harii S. (2023). Acclimation potential of Acropora to mesophotic environment. Mar. Pollut. Bull. 188, 114698. doi: 10.1016/j.marpolbul.2023.114698
Therneau T. (2015). A package for survival analysis in S R package. Available online at: https://CRAN.R-project.org/package=survival
Traylor-Knowles N. (2019). Heat stress compromises epithelial integrity in the coral, Acropora hyacinthus. Peerj 7. doi: 10.7717/peerj.6510
Turner J. A., Andradi-Brown D. A., Gori A., Bongaerts P., Burdett H. L., Ferrier-Pagès C., et al. (2019). “Key questions for research and conservation of mesophotic coral ecosystems and temperate mesophotic ecosystems,” in Mesophotic coral ecosystems: Coral reefs of the world, vol. 12 . Eds. Loya Y., Puglise K., Bridge T. (Springer, Cham), 989–1003.
van Hooidonk R., Maynard J. A., Planes S. (2013). Temporary refugia for coral reefs in a warming world. Nat. Clim. Change 3, 508–511. doi: 10.1038/nclimate1829
van Woesik R., Sakai K., Ganase A., Loya Y. J. (2011). Revisiting the winners and the losers a decade after coral bleaching. Mar. Ecol. Prog. Ser. 434, 67–76. doi: 10.3354/meps09203
Veal C. J., Carmi M., Fine M., Hoegh-Guldberg O. (2010). Increasing the accuracy of surface area estimation using single wax dipping of coral fragments. Coral Reefs 29, 893–897. doi: 10.1007/s00338-010-0647-9
Wallace C. C. (1999). Staghorn Corals of the World: A revision of the genus Acropora (Collingwood, Australia: CSIRO Publishing).
Wyatt A. S. J., Leichter J. J., Toth L. T., Miyajima T., Aronson R. B., Nagata T. (2020). Heat accumulation on coral reefs mitigated by internal waves. Nat. Geosci. 13, 28–34. doi: 10.1038/s41561-019-0486-4
Keywords: mesophotic coral ecosystems (MCEs), stress response, resilience, threshold, temperatures, heat stress, bleaching
Citation: Tavakoli-Kolour P, Sinniger F, Morita M, Nakamura T and Harii S (2023) Variability in thermal stress thresholds of corals across depths. Front. Mar. Sci. 10:1210662. doi: 10.3389/fmars.2023.1210662
Received: 23 April 2023; Accepted: 21 August 2023;
Published: 14 September 2023.
Edited by:
Aldo Cróquer, The Nature Conservancy Dominican RepublicReviewed by:
Shashank Keshavmurthy, Academia Sinica, TaiwanDaniel J. Barshis, Old Dominion University, United States
Copyright © 2023 Tavakoli-Kolour, Sinniger, Morita, Nakamura and Harii. This is an open-access article distributed under the terms of the Creative Commons Attribution License (CC BY). The use, distribution or reproduction in other forums is permitted, provided the original author(s) and the copyright owner(s) are credited and that the original publication in this journal is cited, in accordance with accepted academic practice. No use, distribution or reproduction is permitted which does not comply with these terms.
*Correspondence: Saki Harii, c2hhcmlpQGxhYi51LXJ5dWt5dS5hYy5qcA==