- 1Istituto per lo studio degli Impatti Antropici e Sostenibilità in ambiente marino, Consiglio Nazionale delle Ricerche (IAS-CNR), Rome, Italy
- 2Institute of Geological Sciences, Freie Universität Berlin, Berlin, Germany
- 3Faculty of Geosciences, Utrecht University, Utrecht, Netherlands
- 4Ocean BioGeoscience, National Oceanography Centre, Southampton, United Kingdom
- 5Institut de Ciències del Mar, Spanish National Research Council (CSIC), Barcelona, Spain
- 6Dipartimento di Scienze della Terra, Sapienza Università di Roma, Rome, Italy
- 7School of Marine Science and Engineering, University of Plymouth, Plymouth, United Kingdom
- 8School of Marine and Environmental Science, University of Gibraltar, Gibraltar, Gibraltar
- 9School of Earth and Environmental Sciences, University of Manchester, Manchester, United Kingdom
- 10GRID-Arendal, Arendal, Norway
Marine litter is one of the most pervasive and fast-growing aspects of contamination in the global ocean, and has been observed in every environmental setting, including the deep seafloor where little is known about the magnitude and consequences of the problem. Submarine canyons, the main conduits for the transport of sediment, organic matter and water masses from shallow to abyssal depths, have been claimed to be preferential pathways for litter transport and accumulation in the deep sea. This is supported by ongoing evidence of large litter piles at great water depths, highlighting efficient transfer via canyons. The aim of this article is to present an overview of the current knowledge about marine litter in submarine canyons, taking a geological, process-based point of view. We evaluate sources, transport mechanisms and deposition of litter within canyons to assess the main factors responsible for its transport and accumulation in the deep sea. Few studies relate litter distribution to transport and depositional processes; nevertheless, results from available literature show that canyons represent accumulation areas for both land-based and maritime-based litter. Particularly, accumulation of fishing-related debris is mainly observed at the canyon heads and walls and is related to fishing activities carried out in and adjacent to canyons, while transport and accumulation of general waste and plastic along canyon axes can be related to different mechanisms, encompassing enhanced bottom currents, dense water cascading and turbidity currents, and is related to the proximity of canyons to shore. Global assessment of canyons exposure to riverine plastic inputs and fishing-related debris indicates varying susceptibility of canyons to litter, also highlighting that most of the canyons prone to receive large amounts of anthropogenic debris have not yet been surveyed. Considering that litter research in canyons is still in its infancy, several knowledge gaps need to be filled before the role of canyons as litter traps and the implication for benthic ecosystems can be fully understood.
Introduction
The input of solid waste into the Global Ocean has become a topic of worldwide concern, due to its negative consequences on marine ecosystems, the economy and potentially human health (Beaumont et al., 2019). As a result of the dramatic increase in waste production and mismanaged disposal over the last half century (Geyer et al., 2017), large quantities of litter have entered the ocean, either from terrestrial sources or directly from ships or other maritime infrastructures (UNEP, 2009). Approximately 10 million tons of land-derived plastic debris ended up in the oceans in 2010 alone (Jambeck et al., 2015); a scenario that is forecast to rise to 53 million metric tons per year by 2030 under a business-as-usual scenario (Borrelle et al., 2020).
Marine litter, defined as ‘any persistent, manufactured or processed solid material discarded, disposed or abandoned in the marine and coastal environment’ (UNEP, 2005), consists of a great variety of materials, among which plastic predominates, accounting for 60–80% of marine litter worldwide (Derraik, 2002). A significant proportion of the plastic lost in the marine environment consists of microplastics, small (<5 mm) fragments and fibres derived from the breakdown of larger plastic debris and synthetic textiles or originated as manufactured particles (Lebreton et al., 2017; Pabortsava and Lampitt, 2020). Both macro- and microparticles can have adverse impacts on species across trophic levels and threaten habitat integrity in different ways and at different spatial and temporal scales. These pollutants can be ingested by organisms, affecting individual fitness or resulting in direct mortality (Gall and Thompson, 2015). Litter, especially fishing-related debris, can entangle biota, hindering its ability to move, feed and breathe (Kühn et al., 2015; Bruemmer et al., 2023, this issue). Furthermore, plastic debris can act as a dispersal vector for alien species and pathogens (Barnes, 2002; Zettler et al., 2013) or as a source of persistent organic pollutants and toxic compounds (Hartmann et al., 2017), and is also able to alter the physical characteristics of the environment (Carson et al., 2011).
Marine litter and microplastics have now been reported from all environmental settings across the Global Ocean, from beaches and coastal surface waters (Willis et al., 2017; Schmidt et al., 2018) to the most remote environments such as the polar regions (Tekman et al., 2017), oceanic islands (Lavers and Bond, 2017) and the deep seafloor (Ramirez-Llodra et al., 2013; Peng et al., 2018), which is considered the final depositional sink for the majority of anthropogenic litter entering the ocean (Thompson et al., 2004; Pham et al., 2014; Woodall et al., 2015).
The spatial variability of litter abundance and composition in the ocean is driven by the complex interplay of anthropogenic factors linked to litter sources (such as coastal population densities and industrialization, fishing pressure, maritime traffic and tourism) and natural factors including hydrological, geomorphological and sedimentary processes, responsible for its transport and deposition (Ramirez-Llodra et al., 2013; Pham et al., 2014; Lopez-Lopez et al., 2017). Once at sea, buoyant litter can be transported by surface currents and winds and can be stranded on the coastlines or transferred offshore and concentrated by converging surface currents into the so-called floating “garbage patches” (Eriksen et al., 2014; Lebreton et al., 2017). However, estimation of floating plastic represent only a small percentage of global input to the ocean (Van Sebille et al., 2015), while a significant proportion of litter is thought to sink to the deep seafloor, where little is known about the extent, magnitude and impacts of this issue. Despite the increasing number of publications discussing seafloor litter over the last decades (Hernandez et al., 2022), papers on seafloor litter represent less than 15% of all studies on marine litter (Canals et al., 2021).
Furthermore, the physical processes governing the horizontal and vertical transport of anthropogenic debris and its fate on the seafloor, especially in deep-sea environments, are still poorly understood (Kane and Clare, 2019; Waldschläger et al., 2022). Notwithstanding the patchy survey coverage of the deep seafloor, current knowledge highlights an uneven distribution of benthic litter and microplastics (Harris, 2020; Canals et al., 2021; Galgani et al., 2022), which cannot be explained solely by vertical settling from concentrated surface accumulations. Oceanographic and sedimentary processes play a fundamental role in transporting and redistributing litter coming from land across continental margins, focusing it in specific physiographic settings, such as abyssal trenches, submarine canyons, bottom current drift deposits and on deep-sea fans located at the mouths of submarine canyons and channels (Pham et al., 2014; Peng et al., 2018; Kane and Clare, 2019; Kane et al., 2020). On the other hand, the rough topography characterizing geomorphological features such as seamounts and banks may favor entanglement and local accumulation of lost or abandoned fishing gears (Angiolillo et al., 2015; Angiolillo et al., 2021).
Submarine canyons are common erosive features found on all the world’s continental margins (Harris and Whiteway, 2011) connecting continental shelves to deep ocean basins and acting as preferential transport routes for sediment, organic matter and water masses (Shepard, 1981; Vetter and Dayton, 1999; Masson et al., 2010; Hage et al., 2022; Pope et al., 2022; Post et al., 2022). Canyons are considered to be important conduits for the transfer and accumulation of litter from land to the deep sea (Galgani et al., 1996; Tubau et al., 2015; Pierdomenico et al., 2019; Zhong and Peng, 2021). At the same time, due to their role in focusing currents and transporting sediment and nutrients and their complex topography, submarine canyons are sites of enhanced biodiversity and productivity (De Leo et al., 2010; Fernandez-Arcaya et al., 2017). As such, submarine canyons are often preferential targets for fishing activities (Puig et al., 2012), and susceptible to the accumulation of fishing-related debris along their course (Mordecai et al., 2011; Cau et al., 2017).
Although submarine canyons may feature among the largest hotspots of litter in the deep sea, research on this topic remains limited, and the processes that control the inputs, distribution and ultimate fate of litter within canyons are poorly understood (Kane and Clare, 2019; Harris, 2020; Canals et al., 2021; Zhong and Peng, 2021). Similarly, it is not clear to what degree canyons may act as sinks or conduits for litter transfer toward deeper areas, which type of canyons may be more susceptible to funnel and/or accumulate litter and on which timescales, as well as what is their potential for litter burial and sequestration in the sedimentary record (Zhong and Peng, 2021).
The overarching aim of this paper is therefore to assess the occurrence and distribution of macro- and microlitter in submarine canyons based on a review of the existing literature. Given that litter, and particularly plastics, is rapidly becoming a key indicator for the Anthropocene in the sedimentary record (Bancone et al., 2020), this review is presented from a geoscience perspective. We evaluate source, transport mechanism and deposition of litter as a type of anthropogenic ‘sediment’, and discuss the predisposing factors that may be responsible for their accumulation in and around submarine canyons.
Therefore, we estimate the potential exposure of individual canyons to riverine and maritime-sourced litter on a global scale and discuss how modeling of physical sediment transport processes combined with flume experiments can constrain the spatial variability and abundance of marine litter occurrences in submarine canyon systems. Finally, we identify knowledge gaps and perspectives on future research that could help to better understand the role of canyons as potential litter repositories and conduits to the deep-sea.
Methods
Review of litter assessment and transport processes in submarine canyons
To assess the current state of knowledge on litter in submarine canyons, a literature review was carried out using Google Scholar and the ISI Web of Knowledge. The keywords “litter”, “plastic” and “microplastics” in combination with “canyons”, were used to generate a list of peer-reviewed papers from 1996 till 2022. All papers dealing with litter in surface waters and the water column were excluded. From the selected paper we extracted information about: the geographical area and specific canyon studied; the method used to assess the presence of litter or microplastics; the number of surveyed sites and the depth range explored; litter abundance within the canyons, its spatial distribution and the dominant litter type. In parallel, selected literature on submarine canyons and their transport processes were used to discuss and infer the role of sedimentary and hydrodynamic processes in litter transfer and distribution.
Estimating the exposure of submarine canyons to plastics delivered by river systems
To identify the regions where submarine canyon heads are exposed to the highest input of river-derived litter, we combined a global model of river macroplastic input into the ocean (Meijer et al., 2021) with a global database of seafloor morphology (Harris et al., 2014; Bernhardt and Schwanghart, 2021a; Bernhardt and Schwanghart, 2021b). We calculated the distance from each submarine canyon head to the adjacent river outlets along the world’s coastlines. Then, we divided the modeled floating macroplastic emissions of the closest river outlet (in million metric tons per year (MT/yr)) of Meijer et al. (2021) by the distance from each canyon head to the closest river outlet.
This index is, of course, a simplified metric. It does not account for litter other than macroplastic (e.g., microplastic), the buoyancy of plastic litter and other pathways of litter introduction into the ocean system including coastal inputs, atmospheric deposition, and direct inputs from ships. It omits any oceanographic processes. Moreover, this approach refers to submarine canyons that were mapped on global low-resolution bathymetric data and hence only features large submarine canyons (see details in Harris et al., 2014). Additionally, we restricted this analysis to canyons between 50°N and 50°S and to the main continents including the major islands of the West-Pacific, where data coverage is best. However, we argue that this approach helps to depict highly vulnerable submarine canyons with potential high litter input, canyons with low potential input that may be declared as sanctuaries, and to design future targeted scientific surveys.
Estimating the exposure of submarine canyons to marine fishing debris
To estimate canyon exposure to fishing activity, we used the global compilation of commercial fishing activity (any type) of the NGO “Global Fishing Watch” (available here https://services7.arcgis.com/IyvyFk20mB7Wpc95/arcgis/rest/services/SDG_14_Global_Fishing_Activity_1/FeatureServer). We calculated the mean fishing activity per canyon per year for 4398 canyons of the global canyon database (Harris et al., 2014). All remaining canyons were not covered by fishing activity data. Commercial fishing activity is measured in hours per area (area = grid size = 0.1 x 0.1 degrees) and refers to the annual average of the pre-pandemic year 2019.
Differences in exposure to riverine plastic and to fishing activity between geographical regions have been tested by the non-parametric Kruskal-Wallis test and post-hoc pair-wise comparisons with the Wilcoxon method and Bonferroni correction, using the software R (R Core Team, 2016).
To compare observed litter abundances (Supplementary Material S1) with canyon exposure to riverine plastic and to fishing activity, these indices have been estimated in higher detail for selected canyons where litter data were available. Canyon head locations and canyon areas have been remapped using Emodnet bathymetry for European seas (https://emodnet.ec.europa.eu/) and Gebco 2020 bathymetry (https://www.gebco.net) elsewhere, as these bathymetric data sets are of higher resolution (100 m resolution for Emodnet dataset, 15-arc resolution for Gebco 2020 bathymetry) than the SRTM30_PLUS 30-arc second database (Becker et al., 2009) used in the canyon mapping of Harris et al. (2014).
Canyons hosting a prevalence of plastic litter have been compared to the riverine-plastic input exposure, whereas canyon with dominant fishing-related debris have been compared to the exposure factor to fishing pressure.
Research efforts on the study of seafloor litter and microplastics in canyons
Submarine canyons have been the subject of research for a long time. Due to the difficulty of surveying such complex deep-sea environments, our knowledge of canyons has come primarily from remote sensing and sampling, with contributions from various oceanographic disciplines (Inman et al., 1976; Shepard, 1981). Over the last few decades, the advances in marine robotics for mapping, imaging and sampling, coupled with long term time-series from submarine observatories and moorings has shed light on the diverse and complex hydrodynamics and geomorphic processes acting along canyons (e.g., Xu, 2011; Amaro et al., 2016; Chaytor et al., 2016; Carter et al., 2018), showing that active sediment transport during the present sea level highstand may be considerably higher than previously predicted (Puig et al., 2014; Heijnen et al., 2022a) and revealing the widespread occurrence of anthropogenic debris along their course. However, while a handful of individual canyon systems have received considerable attention, most canyons around the world have not yet been studied, or only to a very limited extent. Eleven canyons account for almost 50% of the body of submarine canyon literature (Matos et al., 2018) and these are mostly located along the North American and European continental margins. A similar geographical bias also characterizes the research on litter in canyons, as shown below.
We found 46 studies reporting the occurrence of macrolitter in submarine canyons (Supplementary Material S1). From the selected publications, litter was reported and/or quantified in more than 120 canyons around the world (Figure 1). Over half of the studies featured seafloor litter distribution as the primary research topic, while a large proportion (~40%) addressed the study of canyon habitats, mostly focusing on ecologically relevant communities such as cold-water corals (CWCs), and reported the presence of litter or its relative impacts as secondary aims. In two studies, litter accidentally collected in canyons during sampling for other purposes was analyzed to assess colonization of benthic organisms (Aymà et al., 2019; Santín et al., 2020).
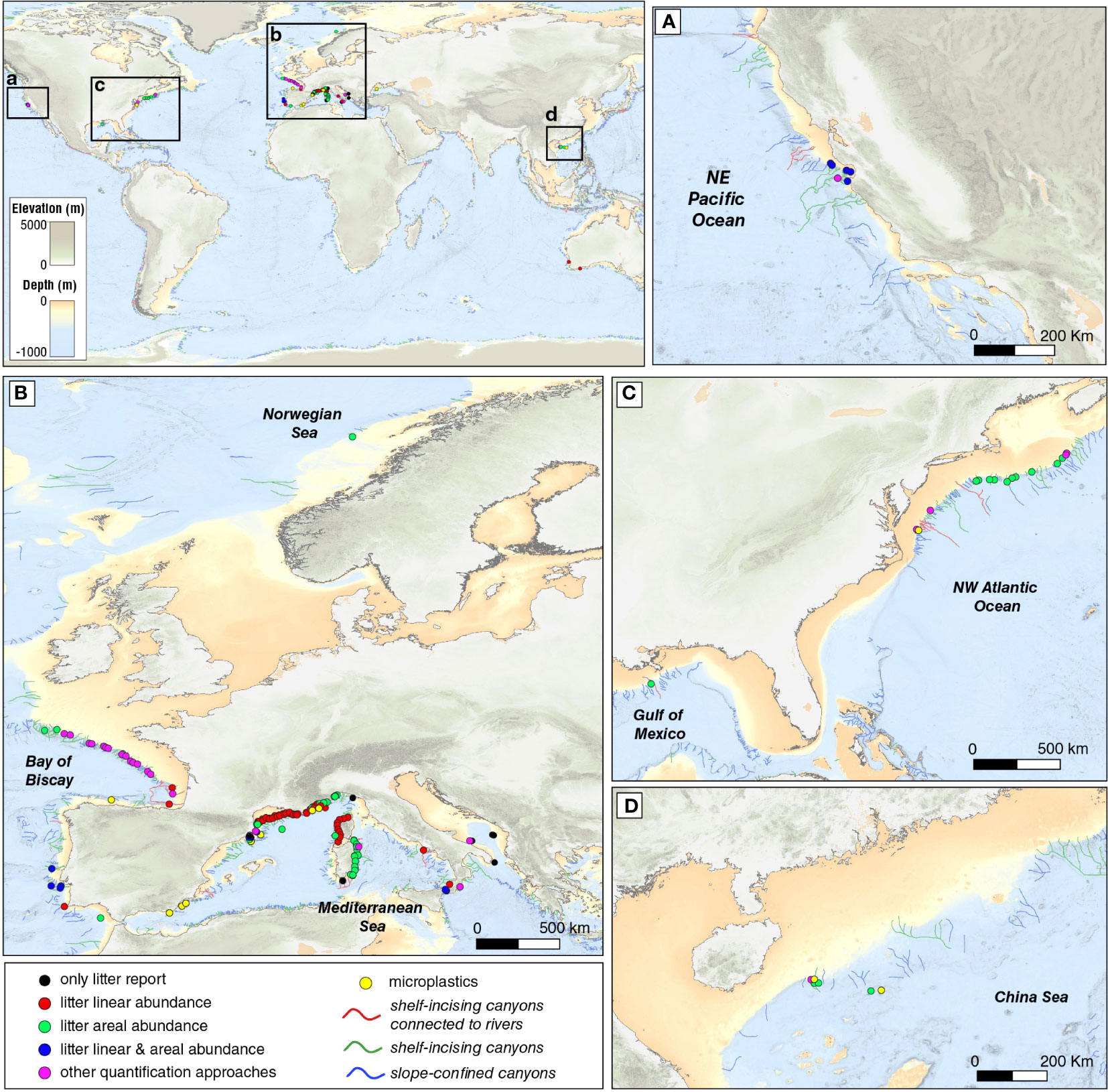
Figure 1 Global distribution of studies which quantified or reported litter and microplastics within submarine canyons. a-d. Zoom of geographical areas shown in the inset on the upper left. Bathymetric map has been obtained from GEBCO relief data (https://www.gebco.net). Canyons distribution and classification after Harris and Whiteway, 2011.
Apart from a few cases where litter is collected by trawling (i.e., Wei et al., 2012; Ramirez-Llodra et al., 2013; Pham et al., 2014), the assessment of seafloor litter within submarine canyons is mainly carried out using seafloor imagery, acquired by remotely operated vehicles (ROVs) (e.g., Mordecai et al., 2011; Tubau et al., 2015; Gerigny et al., 2019; Pierdomenico et al., 2019; Angiolillo et al., 2021), towed cameras (e.g., Buhl-Mortensen and Buhl-Mortensen, 2018), or submersibles (e.g., Watters et al., 2010; Peng et al., 2019). These instruments are more appropriate for studies in complex and rugged terrains, such as those occurring in canyons, which are usually inaccessible to bottom trawls. However, these methods do not give information about volumes or weight of litter, which are necessary for estimates of fluxes and mass balance models (Canals et al., 2021). Presence of litter is therefore primarily quantified as abundance (i.e., number of items), although there is a high variability in the units of measure adopted to report its density (see also Hernandez et al., 2022). Both abundance of litter per unit of area and/or abundance per linear distance are commonly used (Figure 1), along with other quantification approaches, such as abundance per time of observation (Schlining et al., 2013), per image frames (van den Beld et al., 2017; Zhong and Peng, 2021), frequency of occurrence (Moccia et al., 2019) or simple enumeration (Jones et al., 2022). The only attempt at mass estimates from video images was performed by Buhl-Mortensen and Buhl-Mortensen (2017), who assumed approximate weights for different items based on their composition.
The presence of microplastics in canyons is much less investigated than that of macrolitter, with only 4 studies reporting microplastics in seafloor sediments collected from 12 canyons around the world (Figure 1; Supplementary Material S1). Although all studies use density-based extraction methods, where lighter plastic particles are separated from sediment by mixing the sample with saturated solutions (Hidalgo-Ruz et al., 2012), the units of measure adopted to report microplastic concentrations are highly variable, encompassing the number of microparticles per unit of weight (Chen et al., 2020; Angiolillo et al., 2021), per unit of volume (Sanchez-Vidal et al., 2018) or per unit of area (Jones et al., 2022).
Distribution of studies: geographical and sampling biases
There is a strong geographic bias to the studies that have been performed to date. Most of the research on seafloor litter and microplastics has focused on Mediterranean canyons (accounting for more than 50% of the canyons where anthropogenic debris has been reported), followed by the northeast and northwest Atlantic canyons (Figure 2A). In the Pacific Ocean, few canyons have been studied for litter assessment, mostly offshore California (Watters et al., 2010; Schlining et al., 2013) and in the South China Sea (Peng et al., 2019; Chen et al., 2020; Zhong and Peng, 2021). Canyons in the Indian Ocean are almost absent from published reports, except for a recent study of two canyon systems on the SW Australian margin (Taviani et al., 2023). The striking differences in coverage by studies are also evident when analyzing the sampling effort (i.e., the number of stations surveyed) for individual canyons (Figure 2B). On average, studies on litter distribution in Mediterranean canyons have analyzed a larger number of samples than canyons in other regions (Figure 2B), especially those in the NW Mediterranean. La Fonera, Cap de Creus and Blanes Canyons on the Catalan margin (Orejas et al., 2009; Ramirez-Llodra et al., 2013; Tubau et al., 2015; Mecho et al., 2018; Dominguez-Carrió et al., 2020) and some canyons of the Gulf of Lyons have been extensively studied (Galgani et al., 1996; Fabri et al., 2014; Fabri et al., 2019; Gerigny et al., 2019), with tens of stations explored per single canyon. Similarly, for a few canyons in the Atlantic Ocean, namely Dangeard, Explorer and Whittard Canyons on the Celtic margin (Pham et al., 2014), Nazaré Canyon off the west coast of Portugal (Mordecai et al., 2011), and Baltimore and Norfolk Canyons off the US coast (Jones et al., 2022) a large number of stations (>10) have been surveyed. However, the assessment of seafloor litter is based in most cases on exploration of only one or two sites of the entire canyon system. This is also true for microplastics assessment, which has been performed mostly based on a single sediment sample, except for a few individual canyons such as Norfolk (Jones et al., 2022) and Blanes Canyons (Sanchez-Vidal et al., 2018) where several samples have been collected. Papers on litter in the Monterey Canyon, one of the most studied canyon systems in the world (Matos et al., 2018), do not report the exact number of sampled sites, although the large number of surveys covering decadal time spans and the availability of a wide database (332 stations for shelf and canyons of Central California as reported in Watters et al. (2010) and 1149 videos in Monterey Bay analyzed by Schlining et al. (2013)) indicate that the sampling effort is likely much higher than all other canyons.
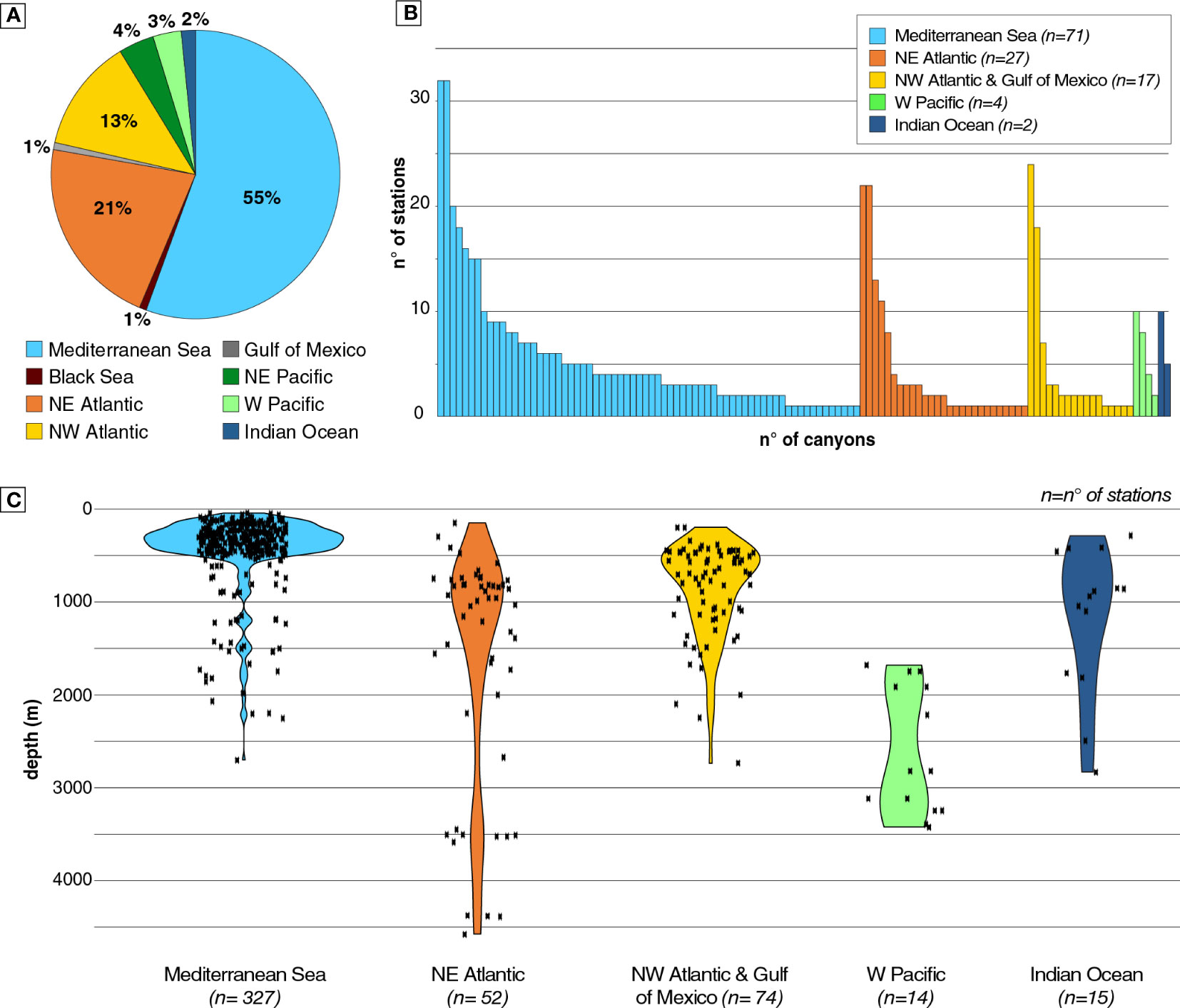
Figure 2 Research effort on seafloor litter and microplastics in canyons. (A) Pie chart showing the geographical location of publications reporting litter and microplastic in canyons. (B) Bar plot of sampling effort (number of stations) per submarine canyon carried out in each of the main geographical regions. (C) Violin plots showing the depth ranges covered by surveys in canyons in each of the five main geographical regions. For each available station, the mean depth of ROV transects/trawl hauls and the depth of sediment sampling for microplastic assessment were plotted.
Bathymetric ranges of observations are also highly variable (Figure 2C; Supplementary Material S1). Overall, Mediterranean canyons have been mainly surveyed in their heads or along their upper reaches, and only a few canyons offshore the Spanish and French coasts have been surveyed in their middle or lower reaches at depths >1500 m (Galgani et al., 2000; Ramirez-Llodra et al., 2013; Tubau et al., 2015; Dominguez-Carrió et al., 2020; Angiolillo et al., 2021). Conversely, in the Atlantic Ocean, wider depth ranges have been covered. Canyons of the Bay of Biscay have been surveyed from 300 to 2300 m depth (Galgani et al., 2000; van den Beld et al., 2017), similar to the canyons of the continental margin off the Northeastern US, with observations from 300 to 2100 m depth (Quattrini et al., 2015; Jones et al., 2022). Explorations at greater depths have been carried out in the canyons offshore of Portugal, from 1600 down to 4500 m depth (Mordecai et al., 2011), as well as in the Whittard (Pham et al., 2014) and Mississippi Canyons (Wei et al., 2012), down to 2600 and 2700 m depth, respectively. In the Pacific Ocean, litter and microplastics have been reported from the tributary canyons of the Xisha Trough at around 2000 m depth, with deeper exploration in the trough down to 3300 m depth (Peng et al., 2019; Chen et al., 2020; Zhong and Peng, 2021). Canyons off SW Australia have been surveyed from 180 m down to 3300 m depth (Taviani et al., 2023) (Figure 2C).
Submarine canyons are hotspots for litter accumulation
Overall, reviews of published data (Galgani et al., 2015; Angiolillo, 2018; Canals et al., 2021; Galgani et al., 2022; Hernandez et al., 2022) and studies covering wide geographic areas indicate that canyons are preferential accumulation areas for anthropogenic debris, often showing higher concentrations compared to the surrounding areas. Large-scale assessments of seafloor litter in European waters found higher litter abundance in canyons than in shelf and slope areas (Galgani et al., 2000), and also compared to other physiographic settings such as seamounts, mounds, ocean ridges and deep basins (Pham et al., 2014). Using a wide dataset of over 1700 video transects collected along the Norwegian margin in the national scientific program Mareano, Buhl-Mortensen and Buhl-Mortensen (2017) observed that litter densities in canyons were more than twice the densities of shelf and slope areas, and lower only than fjords. Trawl surveys in the northern Gulf of Mexico, from the outer continental shelf to the abyssal plain, showed that Mississippi Canyon was a focal point for litter accumulation (Wei et al., 2012). Even on a more local scale and with much limited sampling on the margin, several studies in the Mediterranean Sea and NE Atlantic report increased abundances of litter in canyons compared to the adjacent sectors (e.g., Galgani et al., 1996; Cau et al., 2017; van den Beld et al., 2017; Mecho et al., 2018; Gerigny et al., 2019; Dominguez-Carrió et al., 2020; Pierdomenico et al., 2020).
Canyons have also been shown to represent main hotspots for microplastics contamination, with higher abundances compared to other physiographic settings (Kane and Clare, 2019). This has been further supported by results from a large-scale survey of seafloor sediments in European seas from 42 m down to 3500 m depth, which revealed microplastic abundances in canyons almost twice those of adjacent open slopes (Sanchez-Vidal et al., 2018).
Slope-confined canyons are much less extensively investigated than shelf-indenting canyons, as their transport activity has been traditionally considered reduced during the present highstand of sea level, despite there is increasing evidence for intermittent sediment transport even in these systems (Yin et al., 2019; Post et al., 2022). Consequently, their role in litter accumulation remains practically unknown. Litter assessment in a slope-confined canyon and comparison with other physiographic domains in the Gulf of Cadiz showed higher abundance of litter within the canyon, with plastic concentrations more than twice than those observed on the adjacent continental slope; litter abundance was only higher in the nearby contouritic channels (Mecho et al., 2020).
Litter supply to canyons: the influence of natural and anthropogenic factors on global abundance and composition
The abundance and composition of litter reported are extremely variable, with spatial densities spanning several orders of magnitude (Figure 3) and no obviously discernible patterns related to geographical location and depth range (Hernandez et al., 2022). Such large variability has been observed between canyons of different geographical areas, as well as between nearby canyons along the same margin (e.g., van den Beld et al., 2017; Gerigny et al., 2019; Quattrini et al., 2014). This is linked to the fact that the abundance and composition of litter in canyons is the result of a combination of anthropogenic factors (e.g., sources and litter buoyancy), geomorphological factors and physical transport processes, which may differ between canyons as well as along different reaches of an individual canyon. In addition, the uneven geographical distribution, sampling effort and bathymetric range of studies in canyons, combined with the general lack of standardization of data collection and reporting in the literature (Canals et al., 2021; Hernandez et al., 2022), hinder comparisons between studies and estimates of absolute pollution magnitude. This information is essential to better assess the role of canyons in focusing, distributing and trapping litter and to evaluate the influence of natural and anthropogenic predisposing factors.
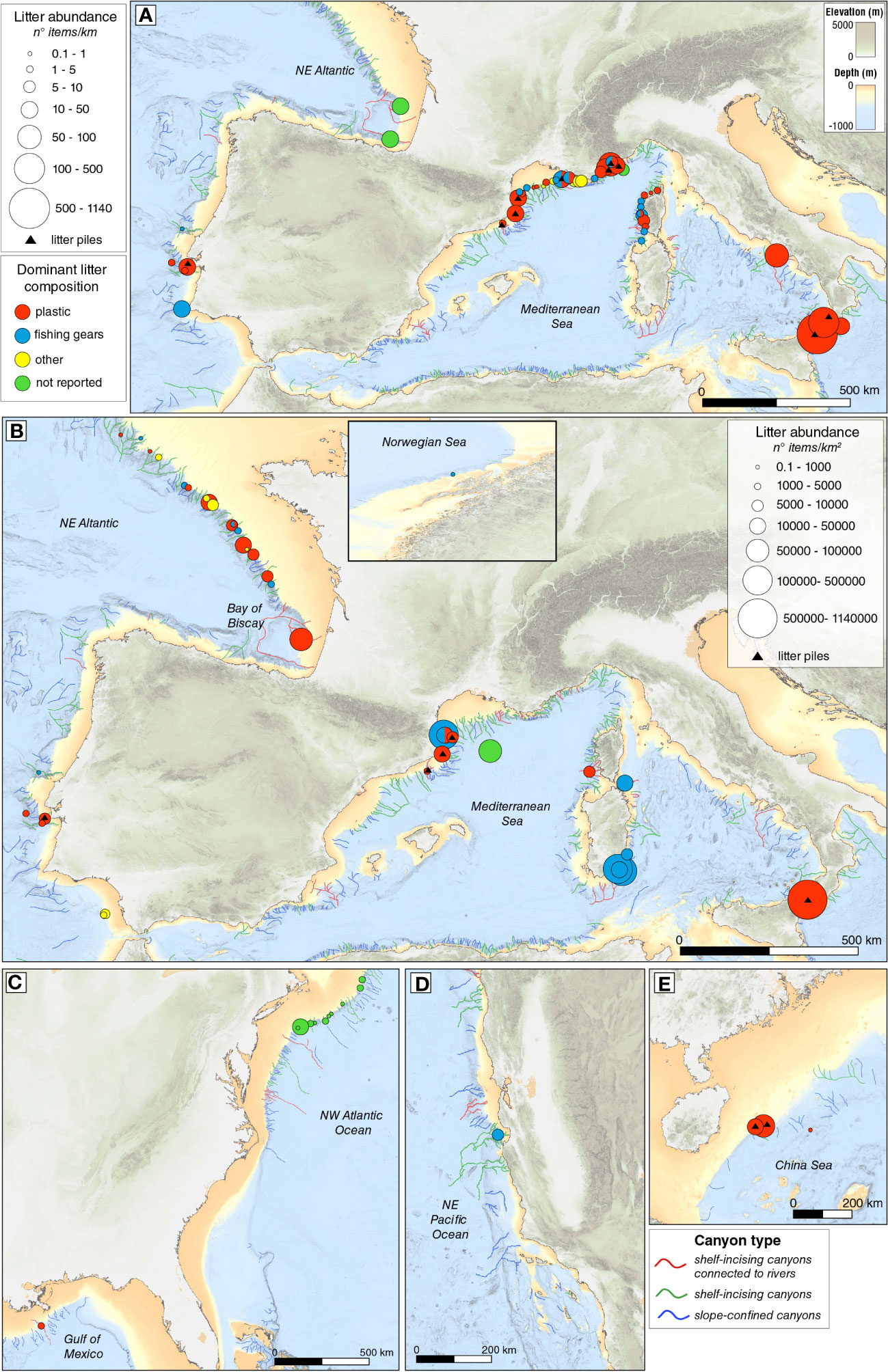
Figure 3 Global Abundance and composition of litter in canyons. (A) Bubble plot showing the abundance of litter reported as n° items per linear km of video track. (B-E) Bubble plots showing the abundance of litter reported as n° items per square km in different geographical areas. The plotted values refer to the mean abundance estimated per each canyon, while the colors of bubbles refer to the dominant type of litter. The black triangles indicate the occurrence of litter accumulation piles. For canyons included in more than one study all the mean abundances reported are plotted in the map.
Regarding litter composition, canyons can represent accumulation areas for both land-sourced and maritime-sourced litter, which is reflected in the overall types of litter found. A wide variety of objects has been reported from canyons, with plastic items and fishing gears featuring as the most common types of waste (Figure 3). Despite fishing materials currently being largely made of artificial polymers, fishing-related debris is often classified as a separate category because of its origin (Hernandez et al., 2022). It is not easy to ascertain the exact origin for many types of litter, except for specific categories such as fishing gears; however, the majority of plastic, one of the main components of urban waste, is thought to derive from terrestrial and coastal sources, at least on a global basis (Jambeck et al., 2015). Among plastics, single-use items such as bags, packaging and bottles are often the most common categories (e.g., Schlining et al., 2013; Tubau et al., 2015; Peng et al., 2019; Pierdomenico et al., 2019; Angiolillo et al., 2021; Grinyó et al., 2021), which reflects the large contribution of single-use items to the global marine plastic pollution (Schnurr et al., 2018) and highlights the high mobility of these light-weight objects.
The abundance and distribution of litter of terrestrial and maritime origins in canyons may differ due to the different entry points, transport and depositional mechanisms of these types of litter (Galgani et al., 2022). It has been proposed that the efficiency of litter transfer from onshore to the deep sea via canyons is dependent on the physiographic configuration of the continental margin and the connection of the canyon heads to terrestrial sources of sediment (Kane and Clare, 2019), together with the magnitude of litter inputs from inland. In the following paragraphs the main factors determining the connectivity of submarine canyons to a source of sediment are described, together with other maritime sources that may influence the supply of litter to canyons.
Rivers
Canyons which indent the shelf and are connected directly to river systems may be highly efficient at transferring sediment (and potentially litter) directly to the slope, bypassing the shelf. Dense sediment-laden water generated at the river mouth may plunge to form a hyperpycnal flow that can evolve into turbidity currents and supply sediment directly to the canyon (e.g., Var Canyon, NW Mediterranean; Mulder et al., 2003; Gaoping Canyon, Taiwan; Khripounoff et al., 2009; Carter et al., 2012; La Jolla Fan, Romans et al., 2016; Messina Canyons; Pierdomenico et al., 2019). Alternatively, high discharge from these river mouths may lead to rapid sediment accumulation near the river mouth or at the canyon head; these deposits may become flushed offshore as a buoyant river plume before sinking to the seabed (Tubau et al., 2015; van den Beld et al., 2017), or may collapse, to trigger turbidity currents (Carter et al., 2012; Pope et al., 2017; Hizzett et al., 2018; Hage et al., 2019; Talling et al., 2022).
Rivers, globally recognized as the main conveyers and transporting agents of land-based litter (Rech et al., 2014; Lebreton et al., 2017; Meijer et al., 2021), can be responsible for significant inputs of mismanaged anthropogenic waste to the sea, although many uncertainties still exist about the proportion of litter rapidly sinking close to the river mouth versus being transported offshore, and consequently about how riverine outflows may enter and interact with the sedimentary regime in canyons. While the largest discharging rivers have been traditionally considered to contribute almost entirely to the global river emissions (Lebreton et al., 2017; Schmidt et al., 2017), the important role of small (low discharge), heavily polluted urban rivers has been recently reconsidered (Meijer et al., 2021). Direct input from a small river affected by flash-floods and draining a heavily populated area explains the high concentration of litter in the Gioia-Petrace Canyon system, where up to 560 items per linear km of ROV track were observed (Pierdomenico et al., 2020). Even higher densities have been reported from the Messina Strait, ranging from 121,000 to up to 1.3 million items/km2, making it the most litter-affected canyon and deep-marine environment recorded to date globally (Figure 3, Pierdomenico et al., 2019). These canyons are connected to subaerial drainage networks and are frequently affected by sedimentary gravity flows triggered by river flash-floods, which are able to funnel huge amounts of anthropogenic waste into adjacent canyons. In this area, the high coastal population densities coupled with poor disposal practices likely contribute to the extreme density values observed (Pierdomenico et al., 2019).
The presence of large rivers may influence litter abundance even in canyons separated from land by wide continental shelves, such as observed in the Bay of Biscay, where the higher litter abundances found in the Belle-île and Arcachon Canyons (with maximum densities up to 59,000 items/km2, comparable to those observed in other Mediterranean coastal canyons), with respect to the other canyons of the margin, have been attributed to the influence of the Loire and Gironde rivers, respectively (van den Beld et al., 2017). In the Gulf of Lyon, despite low litter densities being observed off the mouth of Rhône River (Galgani et al., 1996) and in the upper reach of the Petit Rhône Canyon (Fabri et al., 2014; Gerigny et al., 2019), trawling on the deep-water Rhône fan at 2200 m depth, more than 150 km from the river mouth, revealed litter concentrations of 52,000 items/km2 (Galgani et al., 2000), suggesting efficient transfer of river-derived waste.
Distance of canyon head from land and coastal urbanization
Submarine canyons that indent the shelf, but that do not have a direct connection to a river mouth, are fed by along-shelf currents and wave and tide action, which redistributes and disperses sediments and litter (Mulder et al., 2012; Schlining et al., 2013; Eidam et al., 2019; Pierdomenico et al., 2020). Natural and anthropogenic debris may be transported along the shelf, until the load is diminished through wave and storm action, or until it meets an intersecting canyon head (e.g., La Jolla or Monterey Canyons, California; Xu et al., 2002; Covault et al., 2007). Particularly, high energy seasonal events that might enhance cross- and along-shelf transport such as storms, dense water shelf cascading, hurricanes or typhoons (e.g., Flemming, 1980; Canals et al., 2006; Harris and Heap, 2009), can be responsible for the transfer of heavy litter from the shelf to canyons (Wei et al., 2012; Tubau et al., 2015; Zhong and Peng, 2021).
Submarine canyons deeply incising the shelf offshore densely populated areas were observed to accumulate substantial amounts of litter, such as the Paillon Canyon offshore Nice (>80 items/km, Galgani et al., 1996), La Fonera and Cap de Creus Canyons (>25,000 items/km2 Tubau et al., 2015; Dominguez-Carrió et al., 2020) and the Dohrn Canyon, offshore Naples (50 items/km, Taviani et al., 2019) (Figure 3A). Reduced distances between the canyon head and the coastline may also in part explain the higher abundance of anthropogenic waste observed in the Ligurian and Corsica canyons with respect to the offshore Gulf of Lyon canyons (Gerigny et al., 2019). The higher litter abundance reported from the Lisbon Canyon compared to other canyons of the Portuguese margin was also interpreted as due to the proximity to a large population center (Mordecai et al., 2011). On the other hand, the low litter density observed in the canyon systems off southwestern Australia (1 to 1.7 items/km), has been attributed to reduced coastal urbanization in this region compared to other regions worldwide, combined with the national status as marine parks which further limits human activities in these areas (Taviani et al., 2023). Exploration of north-western Atlantic canyons, which are separated from land by a wide continental shelf, revealed an overall low litter abundance, despite anthropogenic debris being observed in most dives (Quattrini et al., 2015; Jones et al., 2022) (Figure 3). Surprisingly, the highest litter densities >10,000 items/km2 were found in an un-named slope-confined canyon at 1000-1100 m depth (Quattrini et al., 2015).
While the proximity of a canyon’s head to land may facilitate the transfer of litter of terrestrial origin from the coast and shelf environments into the canyons, one of the most littered canyons observed to date is the SY82 Canyon, a tributary of the Xisha Trough in the southern China Sea (Peng et al., 2019). This canyon has its head at 350 m depth, being located ~150 km from the coast. Here, large litter accumulations reaching densities >50,000 items/km2 were found in the middle course of the canyon at 1700-1800 m depth, indicating that the massive transfer of anthropogenic debris to the deep sea via canyons does not necessarily require close proximity of the canyon head to land. Even though it has been proposed that most of the debris came from fishery and navigation activities, the uneven and focused distribution of the litter accumulations indicates subsequent reworking and down-canyon transport of litter (Zhong and Peng, 2021), showing that even canyons indenting wide shelves may become hotspots of litter accumulation.
Global canyon exposure to river-derived plastic
The global maps in Figure 4 and the boxplots in Figure 5A show a highly spatially-variable exposure of canyons to river-supplied plastic fluxes, as confirmed by Kruskal-Wallis test (p<0.001) and Wilcoxon pair-wise comparisons (Supplementary Material S2). Overall, the Mediterranean Sea, the North Pacific Ocean and the South Atlantic Ocean show a significantly higher proportion of submarine canyons that are most exposed to plastic contamination when compared to other ocean basins (Figure 5A). Specifically, we infer high exposure of canyons to litter in areas where high macroplastic emission from rivers (Meijer et al., 2021) coincides with short distances between the river outlets and submarine canyon heads. For instance, in the Philippines and India, which are recognized as the largest contributing countries to global plastic emissions (Meijer et al., 2021), tectonics and high rainfall result in high sediment supply (and therefore litter export) to the oceans (Milliman and Meade, 1983). Narrow shelves and the higher percentage of shelf-indenting canyons compared to other margins (Harris and Whiteway, 2011) makes these areas more prone to transfer plastic from land to the deep sea via canyons. Similarly, canyons off West Africa, Central America and Brazil as well as those in the Mediterranean and Black seas are also susceptible to receiving large amounts of river-derived litter, mostly because of rivers draining highly populated coastal areas. They are therefore identified as hotspots for plastic emissions (Meijer et al., 2021).
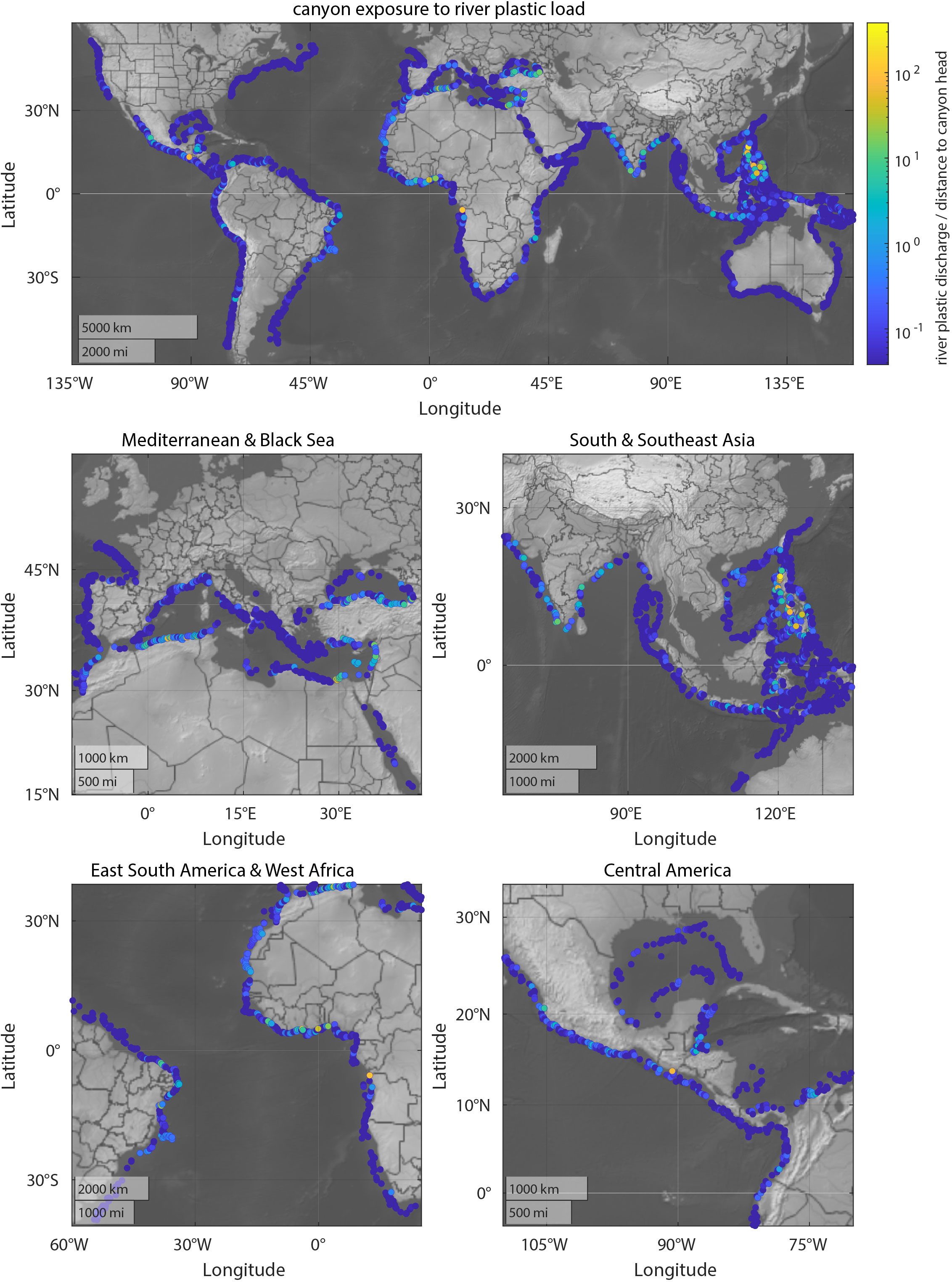
Figure 4 Global map showing the canyon head exposure to plastic input from rivers and insets of the regions with canyons most susceptible to receive substantial amount of land-derived plastic.
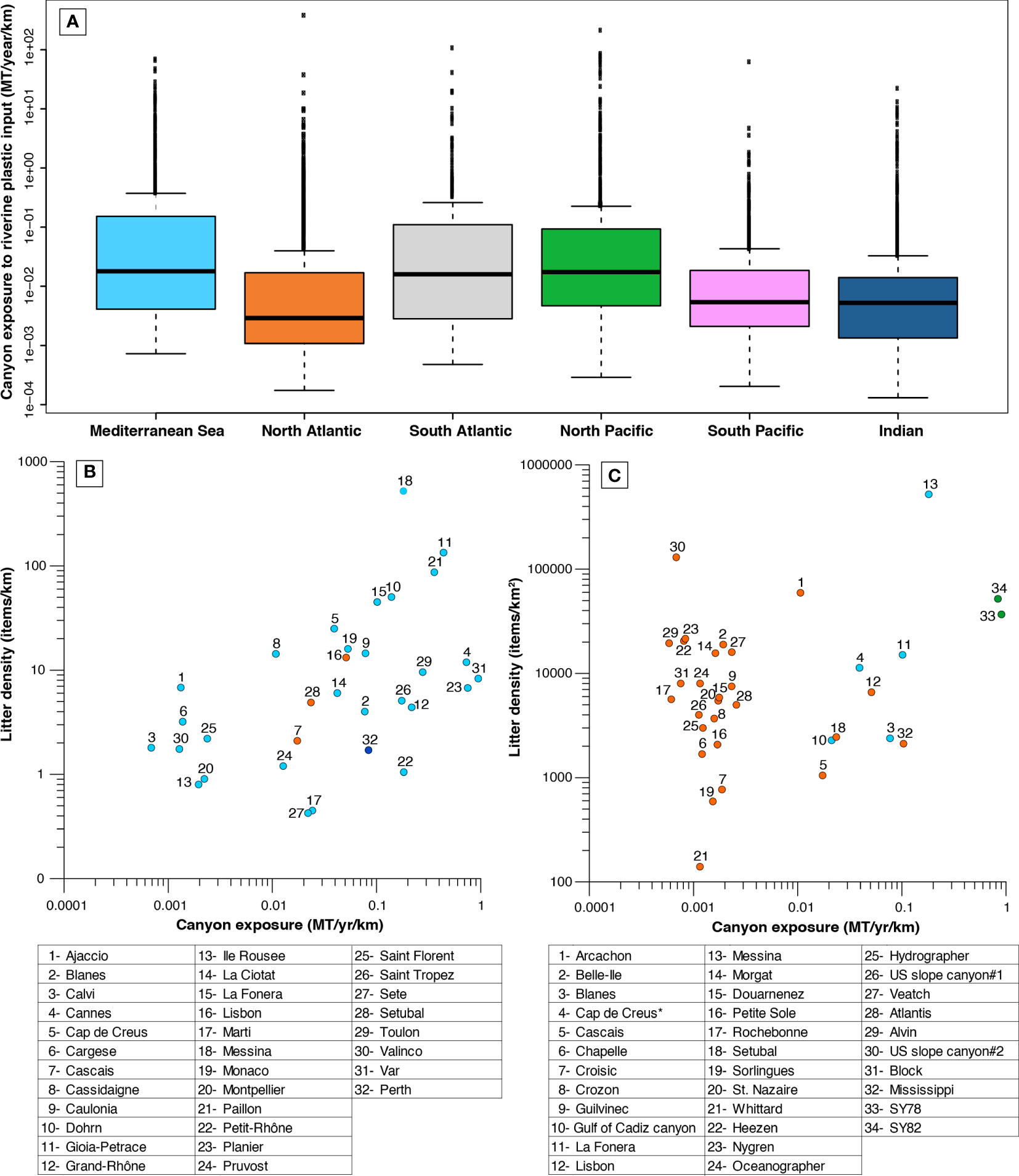
Figure 5 (A) Box plots of canyon head exposure to plastic input from rivers across different basins. (B, C) Scatter plots of litter density reported in canyons and corresponding canyon head exposure to plastic input from rivers. Canyons are colored according to their location, using the same colors of the geographical areas in (A). (B) Canyons with litter density reported as items/km. (C) Canyons with litter density reported as items/km2.
Our global assessment highlights that most of the canyons highly vulnerable to plastic input are located in areas that have not been yet surveyed for litter (see Figure 1 for comparison). Hence, we are likely far from a comprehensive knowledge of the real magnitude of this worldwide impact. Moreover, it also highlights areas with canyons that are potentially less affected by riverine plastic emissions, such as those located in the North Atlantic Ocean, on the Australian coasts and in the NE Pacific (Figure 4). These canyons, theoretically less impacted by plastic inputs along their course, may host well-preserved and relatively pristine ecosystems, and could be considered as priority areas to concentrate conservation efforts (Fernandez-Arcaya et al., 2017).
Notwithstanding the limitations of this approach (as explained in the methods section), the comparison of the estimated exposure values with litter densities observed in canyons shows an overall increase in litter abundance with increasing exposure values (Figures 5B, C). This supports the validity of the global assessment and stresses the strong role of rivers as main inputs of litter to the Global Ocean (Rech et al., 2014; Jambeck et al., 2015). The increase is more evident for Mediterranean canyons (Figure 5B), which have been more extensively surveyed, especially in their upper reaches, than other canyons worldwide. In canyon systems developed on the edge of wide continental shelves, as in the case of the North Atlantic passive margin, that show highly variable litter abundance for similar exposure values (Figure 5C), complex hydrographic regimes acting over large areas may concur to generate more complex dispersal patterns of litter. Departures from the overall trend could also be related to the fact that river plastic discharge from Meijer et al. (2021) may be not representative of local scenarios. The mismanagement of litter dumped in small torrential rivers may for instance explain the unpredicted higher abundances in the Messina Canyons with respect to other ones with comparable exposure (Pierdomenico et al., 2019).
Fishing and other maritime activities
Although overlooked by many global studies on plastic transport to the ocean, fishing activities can represent an important source of litter in canyons, which can be the dominant source of plastic pollution in some systems. Many canyons are the sites of intense commercial and artisanal fishery activities (due to the rich commercially-important fauna they host), including bottom trawling along the rims and long-line fisheries on the rocky substrates not accessible to trawling (Puig et al., 2012; Fernandez-Arcaya et al., 2017). Small-scale artisanal fishing activities may have a deep impact on littering in canyons, as indicated by the very high litter densities of 280,000 items/km2 observed in the East Sardinian canyons (Cau et al., 2017), with abandoned or discarded fishing gears comprising 84 to 100% of litter, approx. three times higher than other geomorphological settings (Cau et al., 2017). Similarly, concentrations of benthic long-line fishing gears of up to 220,000 items/km2 were observed on rocky outcrops along the flanks of Cap de Creus Canyon (Orejas et al., 2009). During submersible explorations off the Californian margin, hotspots of litter, with densities up to 380 items per linear km of ROV track, were found in Monterey Canyon and the south-western edge of Soquel Canyon at places that are traditionally fished (Watters et al., 2010).
Apart from fishing gear, other maritime activities are also attributed as the source for heavy items found in canyons such as glass, clinker or large metal objects, whose distribution has been tentatively linked to direct dumping from ships and therefore associated with marine traffic and shipping routes (e.g., Ramirez-Llodra et al., 2013; van den Beld et al., 2017). Other local factors may determine the accumulation of specific litter types, such as the presence of naval bases or military dumping sites, which are thought to be responsible for the large proportion of metal objects observed in some canyons of the Gulf of Lyon (Fabri et al., 2014; Gerigny et al., 2019) and the Gulf of Cadiz (Mecho et al., 2020).
Global canyon exposure to fishing-related debris
The exposure of global canyons to commercial fishing activity was calculated for 4398 large submarine canyons of the 9477 canyons mapped by Harris et al. (2014) (Figure 6). The remaining canyons do not overlap with fishing activity data as such data do not exist for large regions of the global ocean, such as the South and Southern Asia, the Central and South-Eastern America and the Southern Mediterranean Sea (Supplementary Material S3). However, the available results show that the canyons of the Mediterranean Sea are more heavily affected by fishing pressure along their course than canyons in other regions in the world (p<0.001). No striking differences in canyon exposure to fishing pressure were observed across the main oceans, except for a slightly higher exposure of canyons in the northern hemisphere, which is significant only for the Mediterranean Sea and the North Atlantic Ocean (Figure 7A; Supplementary Material S2).
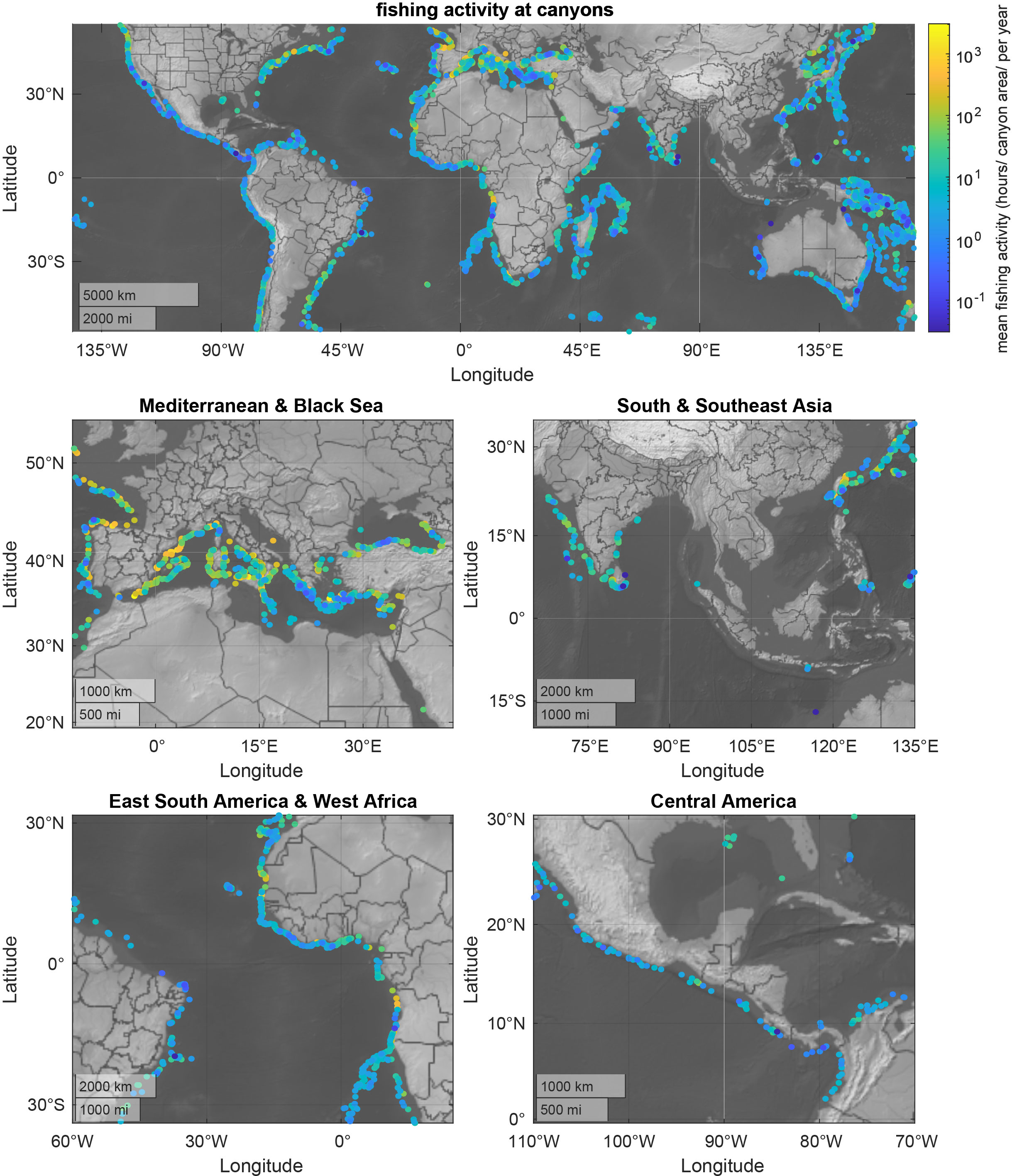
Figure 6 Global map showing the canyon exposure to commercial fishing activity and insets of the regions shown in Figure 4 for comparison. Data coverage is inhomogeneous and very sparse around Southeast Asia and the southern Mediterranean Sea where the fishing activity per submarine canyon could not be computed.
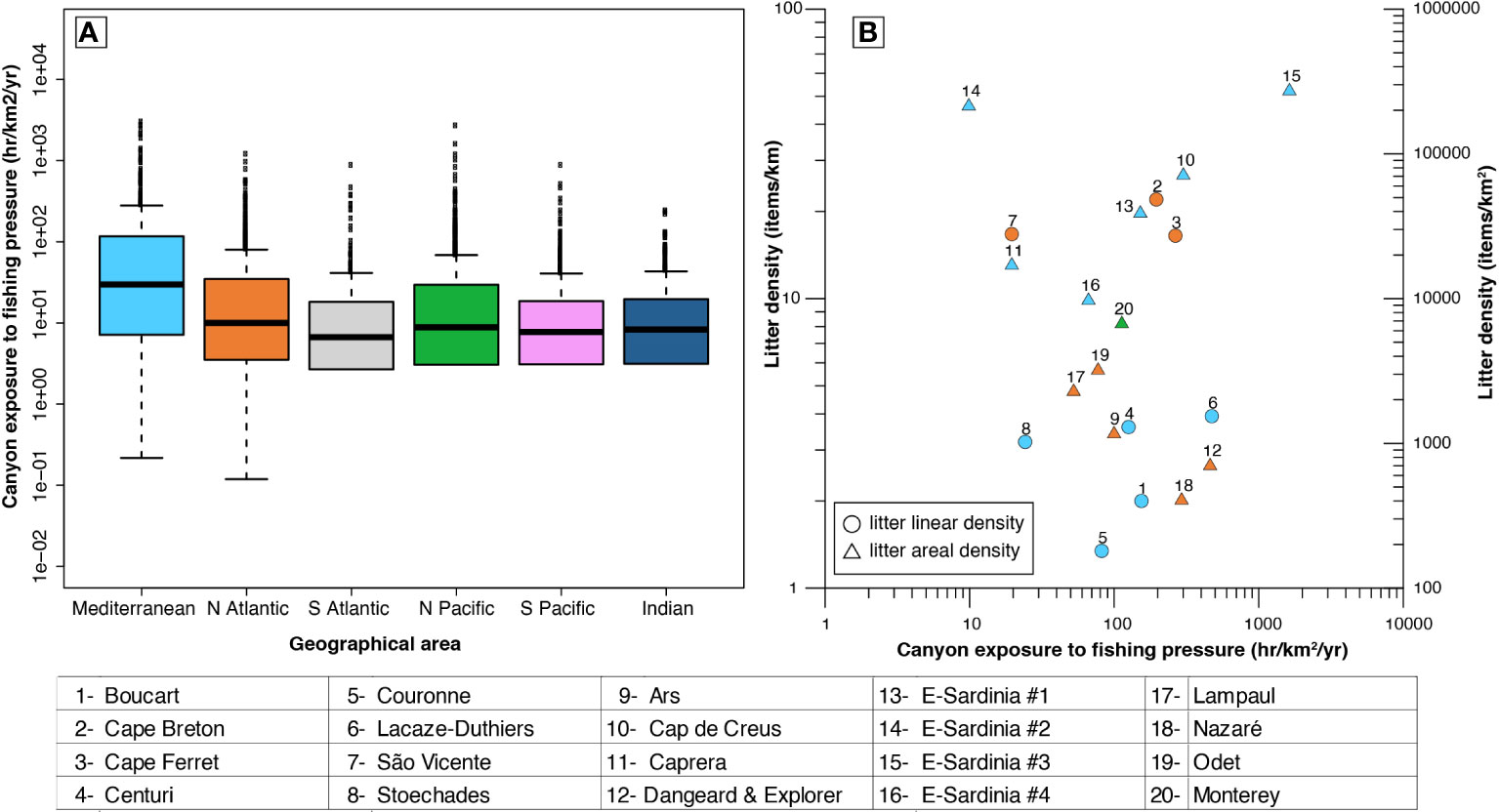
Figure 7 (A) Box plots of canyon exposure to fishing activity across different basins. (B) Scatter plot of the abundance of fishing-related debris reported in canyons and corresponding canyon exposure to commercial fishing activity. Canyons are colored according to their location, using the same colors of the geographical areas in (A).
Comparison of the observed litter densities with mean commercial fishing activities at submarine canyons, carried out for canyons where the dominant litter type was fishing-related debris, does not show clear trends (Figure 7B). However, along with the data gaps, it has to be considered that the calculated mean commercial fishing activity may not recognize the artisanal fishery activities, which are regarded as an important source of litter in specific cases (Cau et al., 2017). This may explain, for instance, the high densities of fishing gears in Sardinian canyons (Mediterranean Sea) despite variable fishing activity (Figure 7B). In addition, as the distribution of fishing debris may be largely controlled by the substrate topography and the occurrence of rocky outcrops that favor entanglement, the estimation of fishing-debris concentration may be strongly influenced by the sampling location along the canyon course.
Litter distribution within canyons: transport and deposition
Processes controlling the distribution of terrestrial litter
Plastic and other land-based litter that is funneled into canyon heads can be remobilized and redistributed downslope toward deep-sea areas by a variety of oceanographic and sedimentary processes, including enhanced bottom currents, dense water cascading and sediment gravity flows (Schlining et al., 2013; Tubau et al., 2015; Dominguez-Carrió et al., 2020; Pierdomenico et al., 2019; Pierdomenico et al., 2020; Zhong and Peng, 2021). These processes act at different temporal and spatial scales from the continental margin down to individual canyons and on to small-scale features along the canyon course and can be responsible for the uneven distribution of litter among and within individual canyons. Furthermore, various sediment-transport mechanisms (and by extension litter transport mechanisms) often coexist in a given submarine canyon and along-canyon transport is not a constant or unidirectional process (Puig et al., 2014; Amaro et al., 2016). This means that understanding the magnitude of transport activity and the role of canyons in funneling and accumulating litter in the deep sea is not a trivial task, as it is driven by a complex interplay of natural and anthropogenic factors linked to sources, transport, and depositional mechanisms (Ramirez-Llodra et al., 2013; Maier et al., 2019; Pearman et al., 2020; Pierdomenico et al., 2020).
Local topography and spatial variations in flow energy seem to play an important role in the deposition of litter in canyons. Litter has been observed frequently within the troughs of furrows, behind rock slabs, tree trunks or other obstacles (Figures 8A, B) (Galgani et al., 1996; Tubau et al., 2015; Pierdomenico et al., 2020). Specific types of litter, such as fishing gear, or cables and pipelines lying on the seafloor, can nucleate the formation of small litter accumulation points, where mostly plastic items get trapped (Figure 8C) (Mordecai et al., 2011; Tubau et al., 2015; Biede et al., 2022). On a larger scale, morphological features along the canyon axis such as scours, knickpoints, or changes in thalweg slope, which may interact with flows promoting deposition, can represent important accumulation areas for litter (Pierdomenico et al., 2019; Angiolillo et al., 2021; Zhong and Peng, 2021).
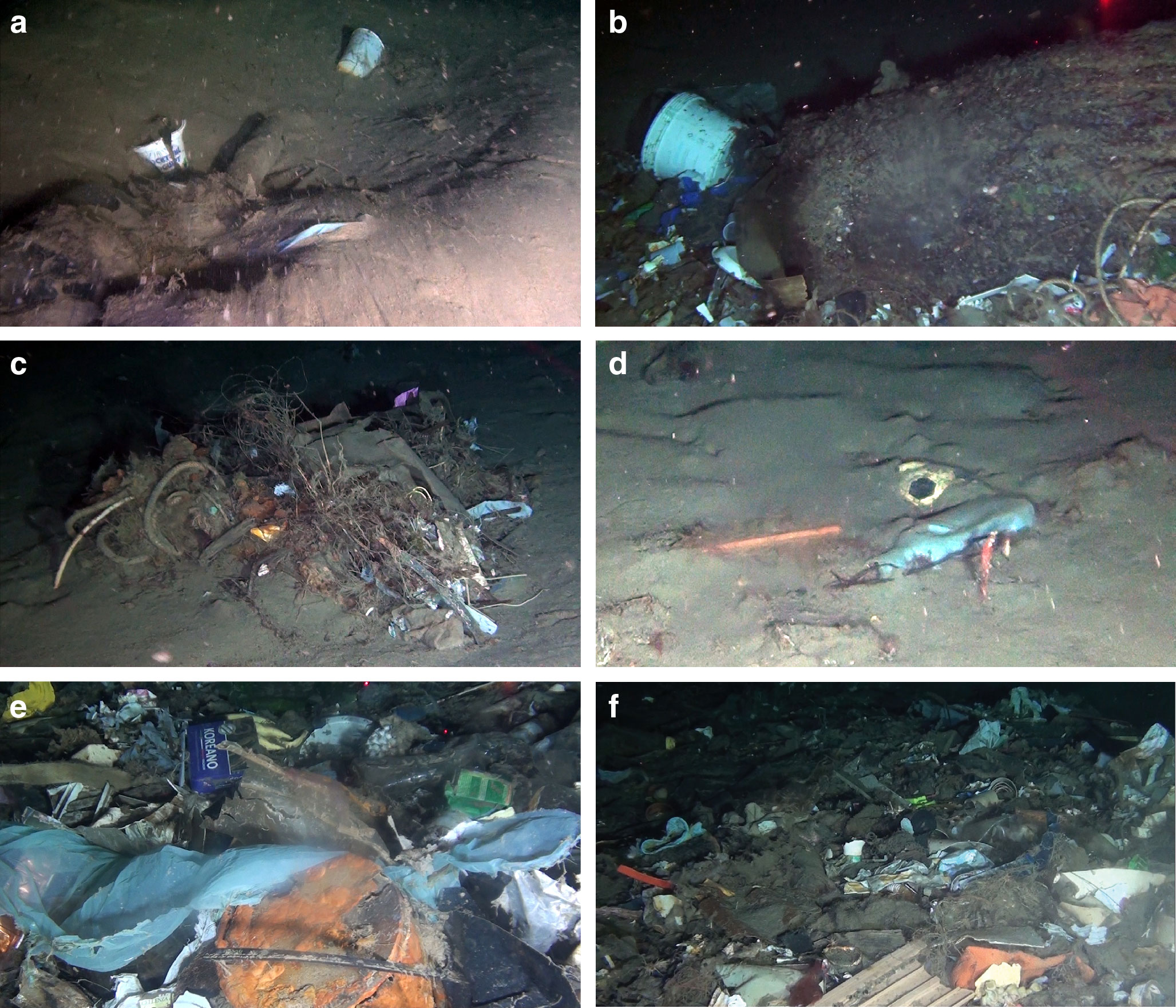
Figure 8 Examples of litter on the floor of submarine canyons. (A) plastic accumulating within a depression along the thalweg of Gioia Canyon (400 m depth). (B-F) accumulation hotspots in the Messina Strait Canyons (200-600 m depth).
Sediment gravity flows
Sediment gravity flows, principally turbidity currents, are the main agents for sediment transport down canyons and consequently for litter transfer to the deep sea (Pierdomenico et al., 2019; Zhong and Peng, 2021). There are several processes able to trigger sediment gravity flows within canyons (Piper and Normark, 2009), including the evolution of mass-failures along canyon walls (Paull et al., 2010), advection of resuspended shelf sediments by oceanographic currents or storm waves (Xu et al., 2010; Martín et al., 2011) and hyperpycnal flows during pulses of river discharge or ice melting (Mulder et al., 2003; Khripounoff et al., 2012; Hizzett et al., 2018; Bailey et al., 2021; Talling et al., 2022). Sediment resuspension induced by bottom trawling can be an additional trigger for the development of turbidity currents along canyons (Puig et al., 2012). However, it is increasingly apparent that major external triggers are not always required for the inception of gravity flows; the key driver appears to be the availability and supply of sediment, which may be sustained or ephemeral in its delivery to canyons (Bailey et al., 2021). While it has been long assumed that during the modern high-stand of sea level, the level of activity of canyons whose heads lie at large distances from terrestrial sediment sources is significantly reduced, recent studies have shown that turbidity flows with high frequencies (i.e., sub-annual) and velocities comparable with highly active coastal canyons, may also affect such land-detached canyons (Heijnen et al., 2022a), with potential implication for the transfer of litter and microplastics in the deep-sea realm via submarine canyons.
Due to the high flow velocities, transport capacity and the potential to run out to significant distances, turbidity currents are able to carry and redistribute significant amount of litter at high depths. In Monterey Canyon, which is frequently affected by powerful turbidity currents (Paull et al., 2010), surveys for litter from 25 to 4000 m depth found the greatest abundance of plastic between 2000 and 4000 m depth (Schlining et al., 2013). The activity of turbidity flows can also lead to the deposition of large litter accumulation hotspots, such as those found in the upper reach of the Messina Strait Canyons (200-600 m depth, Figures 8E, F) (Pierdomenico et al., 2019), in the middle reach of the SY82 Canyon at 1800-1900 m depth (Zhong and Peng, 2021) and at the base of the Monaco Canyon at 2100 m depth (Angiolillo et al., 2021). These accumulation hotspots are a few to tens of meters wide and formed by a mixture of items, whose chaotic arrangement evidences remobilization and emplacement by sediment transport processes (Pierdomenico et al., 2019; Zhong and Peng, 2021). Their composition, dominated by litter of urban origin, primarily plastic, the presence of terrestrial plant debris and the paucity of fishing-related debris further support their link with the action of turbidity flows carrying litter from shallower depths (Angiolillo et al., 2021). Furthermore, the occurrence of large rocky boulders and heavy litter items, such as pieces of furniture, metals sheets and cars, within litter piles testifies to the high transport competence of turbidity currents (Pierdomenico et al., 2019).
Even though it might be expected that turbidity flows (that can travel for hundreds of kilometers), should enable transport of anthropogenic debris to the lowest reaches of canyons, very little is known about potential accumulation at the base of canyons and on deep-sea fans, as these zones have not been extensively surveyed for litter. However, the high concentration of litter found in the Rhône deep-sea fan (Galgani et al., 2000) suggests that litter can be transferred throughout the entire canyon course in systems actively carrying sediment toward deep-sea fans, where it can eventually be prone to entrainment by bottom currents.
Sediment-gravity flows may also bury litter within the sediment, as indicated by reports of partially buried items in canyon sediments (Figure 8D) (Pierdomenico et al., 2019; Angiolillo et al., 2021). The ability of gravity flows to transport and bury litter is largely unstudied, although flume experiments evidenced that microplastic can be transported and buried by turbidity currents (Pohl et al., 2020). Furthermore, macroplastics enclosed within coarse grained turbidite deposits buried 2.5 m below the seafloor have been recovered from a sediment core in a prodelta channel, further demonstrating the potential of gravity flows in burying litter deeply beneath the sea floor (Pierdomenico et al., 2022).
Oceanographic processes
Oceanographic processes can also be involved in the transport of sediment and litter within canyons. The accumulation of plastic below 1000 m depth in the Cap de Creus Canyon has been linked to the action of dense shelf water cascading that generates strong bottom flows able to erode and entrain seafloor sediment in the upper reach of the canyon (Canals et al., 2006). These flows transport litter from the continental shelf and upper canyon, favoring its settling once the current speed has slowed down (Tubau et al., 2015; Dominguez-Carrió et al., 2020).
Bottom currents are recognized as important agents for the dispersal of microplastics in the deep sea (Kane et al., 2020) and can be steered down- and up-slope through canyons, where they can also transport larger macrodebris. In the Gioia Canyon, almost 40% of total macroplastic found between 50 and 490 m depth was observed drifting above the seafloor, demonstrating the important role of bottom currents in the downward flux of plastic (Pierdomenico et al., 2020). The decrease in the amount of drifting litter downslope suggests trapping of debris in the upper parts of canyons for some period of time.
Internal waves and tidal currents, which are usually amplified by the topographic constraint of submarine canyons, can contribute to sediment resuspension and advection along the canyon axis, sometimes favoring sediment and litter accumulation in specific regions (Hotchkiss and Wunsch, 1982; de Stigter et al., 2011; Pearman et al., 2020). Changes in strength and direction of currents driven by internal tides (Mulder et al., 2012), combined with the occurrence of biologically and geologically complex habitats promoting trapping of litter, are thought to be responsible for the higher abundances observed in specific mid-depth ranges (800-1100 m) in the Bay of Biscay canyons (van den Beld et al., 2017).
Processes controlling the distribution of fishing-related debris
Because of the different entry points and transport mechanisms, litter of terrestrial and maritime origins often shows a different distribution in the different morphological zones of canyons, as observed in the Cap de Creus Canyon (Dominguez-Carrió et al., 2020), the Dohrn Canyon (Taviani et al., 2019; Angiolillo et al., 2023) and the Gioia Canyon (Pierdomenico et al., 2020). In these canyons, fishing-related debris was observed mainly on rocky substrates at the canyon head or along the walls as opposed to general plastic items, which were concentrated within the thalweg. This may be related to the fact that accumulation of fishing debris is linked to the spatial distribution of fishing activities, which are often focused in specific sectors of canyons such as the canyon head and rims (Puig et al., 2012; Tubau et al., 2015). In addition, fishing gear may be too large or heavy to be easily displaced by bottom currents, compared to plastics (van den Beld et al., 2017), and due to their shape can be easily entangled in complex terrains such as rocky outcrops, coral frameworks or other biogenic structures that are common in canyons (Angiolillo et al., 2015). A dominance of fishing gear, especially lines and trammel nets, has been reported in several studies that surveyed the rocky habitats at the canyon head or flanks in the upper canyon reach (e.g., Watters et al., 2010; Oliveira et al., 2015; Cau et al., 2017; Enrichetti et al., 2020), often as additional observations within the assessment of coral communities that thrive in these habitats (Orejas et al., 2009; Cau et al., 2017, Giusti et al., 2019; Fabri et al., 2019; Angiolillo et al., 2023). The presence of fishing gear within the thalweg in the Nazaré Canyon down to 4300 m depth (Mordecai et al., 2011) suggests that fishing-related debris can be also funneled to the canyon thalweg and transported to great depths, although direct dumping by fishermen cannot be excluded. However, the greater abundance of fishing debris at shallower depths, compared to general waste (e.g., Schlining et al., 2013; Tubau et al., 2015; Angiolillo et al., 2021; Taviani et al., 2017; Dominguez-Carrió et al., 2020) and the paucity of fishing gear within the large litter piles found in the middle and lower reach of canyons (Angiolillo et al., 2021; Zhong and Peng, 2021), suggest a relatively lower mobility for this type of litter compared to general plastic items.
Physical transport processes of microplastics
Recent literature addresses transport and burial of microplastics by sediment gravity flows (Ballent et al., 2013; Pohl et al., 2020; Bell et al., 2021), with some attention being paid to interaction with bottom current systems (Kane et al., 2020). The resemblance of microplastics size to the natural sediment present in gravity-driven flows, has motivated application of existing sediment transport hydrodynamics of sediment gravity flows to microplastics transport, specifically the Rouse perspective (Kane and Clare, 2019; Waldschläger et al., 2022). Suspension of dense particles in turbulent environmental fluid flow is understood to result from a balance between downward settling due to gravity and upward mixing due to turbulence. This process was resolved by Rouse nearly a century ago for rivers (Rouse, 1937). In rivers, turbulence is exclusively generated through friction with the floor. Mixing of air into the top of rivers can be neglected. The non-dimensional Rouse number characterizes the ability of river-like flows to suspend particles of different size and density. It can be used to calculate vertical distributions of each particle type suspended in a flow (the Rouse equations). There are fundamental differences between rivers and turbidity currents due to the presence of ambient sea water above turbidity currents rather than air above rivers: a) the density difference between the flow and its surroundings is 1 to 2 orders of magnitude smaller; b) there is no viscosity discontinuity at the top of turbidity currents, and this generates friction and turbulence at the top of the flow as well as at the bottom; c) as a result of a) and b) ambient water can be mixed into the top of turbidity currents, which dilutes the particle concentration in the top of the flow. These fundamental differences from rivers are widely acknowledged to be problematic for the application of the Rouse equations to turbidity currents, yet Rouse still dominates quantification of particle suspension in turbidity currents (Hiscott, 1994; Hiscott et al., 1997; Straub and Mohrig, 2008; Bolla Pittaluga and Imran, 2014; Jobe et al., 2017; Bolla Pittaluga et al., 2018; Eggenhuisen et al., 2020). Indeed, Rouse equations satisfy observations for dense mineral sand with a Rouse number as low as ≈1/2 in turbidity currents (Eggenhuisen et al., 2020). However, here we test the Rouse equations with the experiments with plastic microfibers of Pohl et al. (2020) to reveal a critical shortcoming when applied to sediment gravity flows: the Rouse number of the microfibers is ≈1/50; yet the observed vertical distribution in the turbidity currents is identical to that of dense mineral sand with a Rouse number of ≈1/2 (Figure 9). This violation of the Rouse equations is a critical barrier to using the Rousean perspective to quantify fluxes of low-density particles such as plastics in sediment gravity flows. Plastic particles that are virtually neutrally buoyant should hardly settle downwards due to gravity, should be easily mixed homogenously throughout the water column, and are therefore unlikely to ever be deposited with dense mineral sand at the base of the flow. Observations of plastic floating in the water column above the seabed (Pierdomenico et al., 2020) are indeed intuitively explained by their approximately neutral buoyancy in sea water. However, the burial of litter beneath the seafloor together with dense mineral sand in both observations (Pierdomenico et al., 2022) and experiments (Pohl et al., 2020; Bell et al., 2021) is not. It appears that a conventional buoyancy and turbulent diffusion treatment does not suffice to treat transport and burial of plastic litter in sediment gravity flows in submarine canyons. Pohl et al. (2020) explain the preferential burial of microplastic fibres relative to microplastic fragments with shape effects. Burial of the fibres is promoted by their larger surface area and length. As one section of the fibre is pushed downwards by settling sediment particles it gets trapped in the seabed, and the rest of the fibre is subsequently buried, despite it still sticking upwards partly above the bed into the ongoing flow. This shape effect does explain predominance of burial of plastic fibres over plastic fragments, and this could extend to explain preferential burial of macrolitter films, sheets, nets, and wires. However, it cannot explain the similarity in transport to natural dense sediment with Rouse numbers of ≈1/2. Furthermore, biological processes such as fouling, consumption and incorporation in fecal material (Choy et al., 2019) could increase density and settling velocities of microparticles. Though further research is needed, provisional treatment of plastic litter in similarity to natural sediment of Ro ≈ ½ is advocated here based on the empiric evidence of our analyses (Figure 9) of the experiments by Pohl et al. (2020).
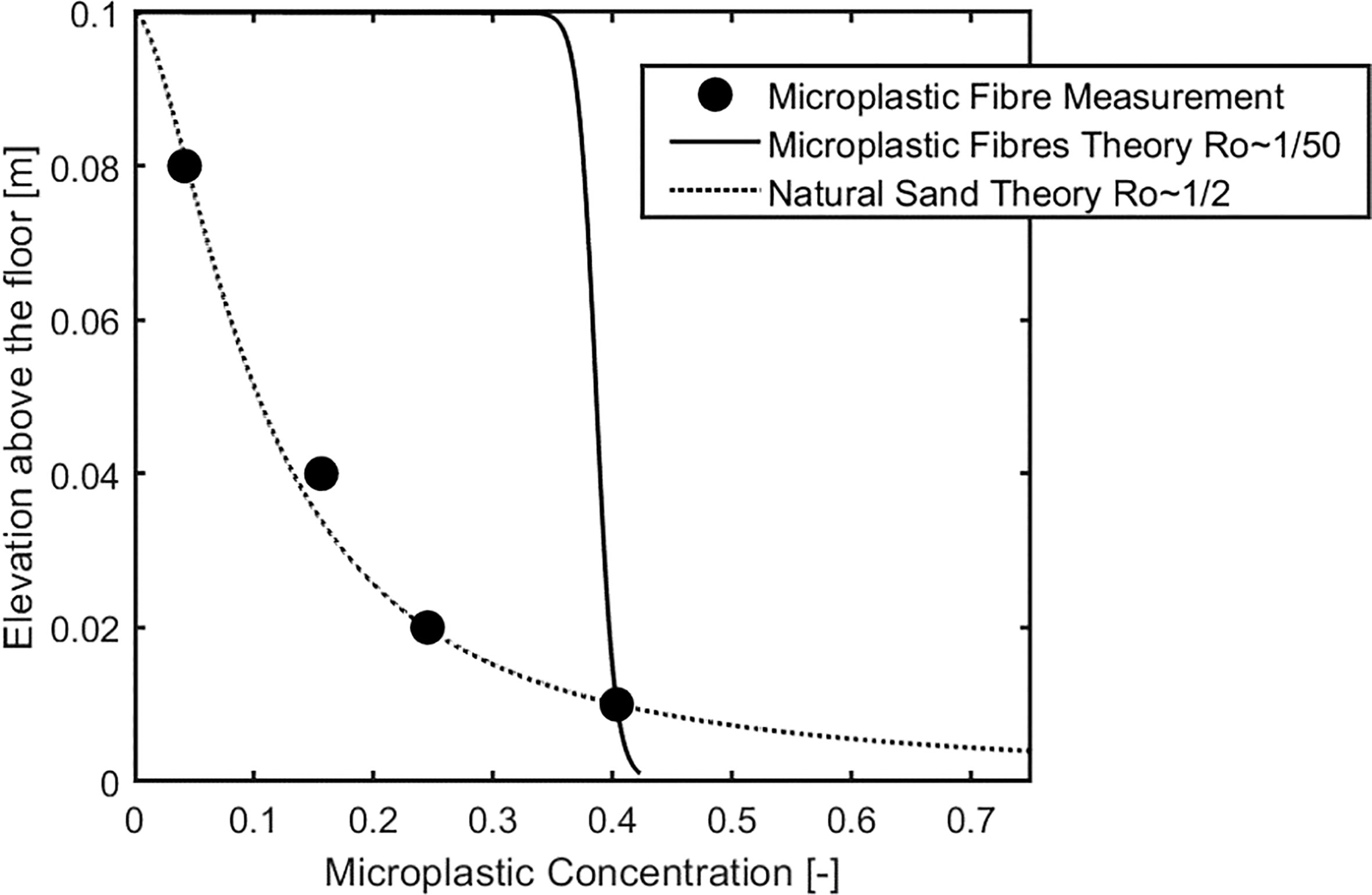
Figure 9 The Rouse equation for fine grained quartz sand also predicts the measured concentration of microplastic fibres in an experimental turbidity current. The equations yield incorrect results when calculated with the density and size of the fibres.
Testing a sediment process-based model to infer plastic transport in a submarine canyon: the case of the Congo Canyon
Modeling and quantification of physical transport processes of litter in submarine canyons can substantiate extrapolations of spatial occurrence and abundance of litter from sparse observations, and even provide justifications for predictions in systems that are designated as highly vulnerable to litter input, but that have not been surveyed yet. As an example of the potential of integrated studies in inferring litter distribution in canyons we apply the Sediment Budget Estimator (SBE) process model (Eggenhuisen et al., 2022) to the Congo Canyon, which we identified as a potential hotspot for plastic litter based on the canyon head exposure parameter to land-derived plastic litter (Figure 4). We assume that plastic is transported in association with natural sediment (Figure 9) in sediment gravity flows. Unfortunately, no direct survey information is available to quantitatively inform this association yet. However, such quantifications are available for the association between natural sediment and Particulate Organic Carbon (POC; Azpiroz-Zabala et al., 2017; Simmons et al., 2020). POC overlaps in density, sizes, and shapes with at least some plastic litter. Furthermore, we suggest that there is a physical similarity between transport of this litter and POC that can be leveraged. The potential for successful process modeling of litter fluxes is illustrated by populating the process model with input conditions (Supplementary Material S4) based on published monitoring studies at 2000 m water depth in the Congo Canyon (Cooper et al., 2013; Azpiroz-Zabala et al., 2017; Simmons et al., 2020), and comparing the simulated POC budgets to the estimates of those studies (Figure 10). The flow-velocity and sediment-concentration profiles of the sediment gravity flows compare well to the ADCP results. More importantly, resulting sediment fluxes closely match the variability observed in the 4 months monitoring deployment (Figure 10C). The simulated estimates for sediment-associated POC fluxes cover the range estimated from ADCP monitoring, though the histogram reveals an overestimation of the top end of the range reported by Azpiroz-Zabala et al. (2017). This success highlights that, when the association between litter and natural sediment is clarified, existing knowledge of sediment gravity flows suffices to quantitatively simulate fluxes and budgets of litter transport and burial down submarine canyons. Various approaches exist in process modeling of sediment gravity flows. The SBE demonstrated here uses a simplified stochastic approach. The benefits of this approach make it uniquely suited to integration with global estimation of canyon-head exposure to river-derived litter (Figure 4) and sparse modeling: input conditions can be based on robust estimates from bathymetric surveys and sparse monitoring results; and ensemble simulations can rapidly be performed for tens of thousands of events in one or more canyons. The modeling results are still critically dependent on direct flow monitoring results, specifically sediment gravity flow thickness and natural sediment concentration. This means that at present, process modeling is only feasible in parallel to continued efforts in sea going research. Specifically, there is an urgent need to establish the association of litter and natural sediment in sediment gravity flows and buried deposits.
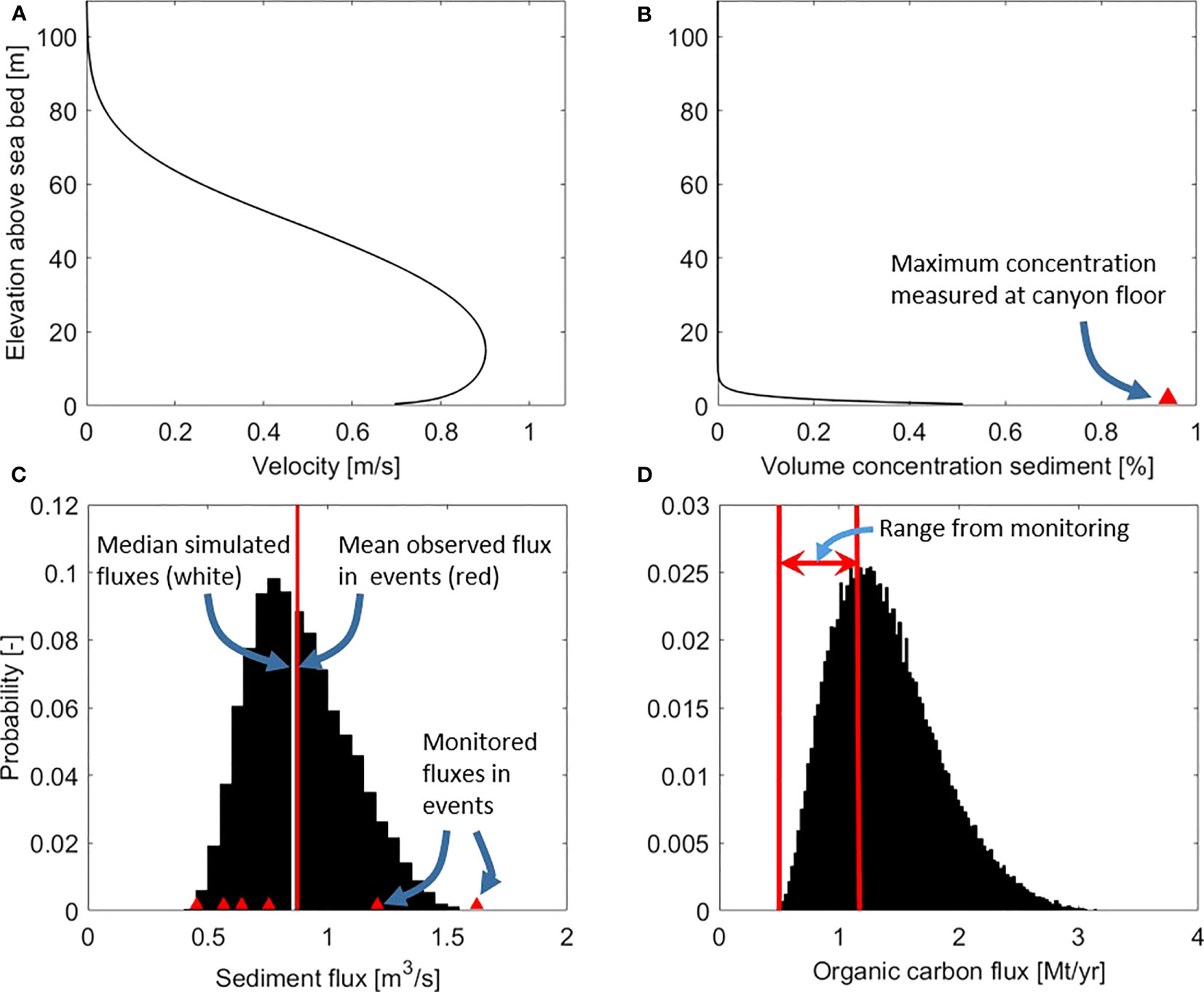
Figure 10 (A, B) Velocity and concentration profiles of simulated sediment gravity flow #5 of Azpiroz-Zabala et al. (2017). Maximum concentration at the seabed is from Simmons et al. (2020) whose ADCP backscatter inversion leads to 50% overestimation of concentration relative to the Azpiroz-Zabala et al. (2017) conditions simulated here. (C) Histogram of all simulated sediment fluxes, compared to sediment fluxes estimated from ADCP measurements in Azpiroz-Zabala et al. (2017). (D) Histogram of simulated POC-flux estimates compared to the range reported by Azpiroz-Zabala et al. (2017).
Final remarks
Although litter research in canyons is still in its infancy, it is clear that these geomorphic features represent preferential area for litter accumulation and that the distribution of litter within canyons may be greatly influenced by the diverse transport routes and processes characterizing them. However, several knowledge gaps exist about the pathways that bring litter and microplastics from their entry-points to the seafloor and into canyons as well as about the interplay of depositional and erosional processes controlling their dispersal, deposition and potential burial. This is not surprising, considering that less than 10% of submarine canyons worldwide have been surveyed so far (Matos et al., 2018), and, out of this estimate, no more than 1% canyons have been studied with focused efforts on marine litter (Hernandez et al., 2022). Particularly, information is missing for most of the canyons that our model highlighted as prone to receiving large litter inputs from land sources. On top of that, our ability to depict distribution patterns is constrained by the sampling strategy, frequently originally planned to address other topics than litter assessment and often targeting specific morphological zones or depth ranges. Beside the difficulties in comparisons between studies linked to the lack of standardized approaches in litter classification and quantification (Canals et al, 2021), the variability of geological settings and of spatio-temporal scales of natural processes within canyons makes local estimates less consistent and representative of the entire source to sink system.
Our work highlights the importance of the connection of canyon heads to terrestrial and maritime sources of litter. However, hydrographic processes, which play a crucial role in litter supply to canyons, are not taken into account in these estimations, due to their high temporal and spatial variability and to the lack of globally available datasets of coastal and shelf currents and sediment transport. Little is also known about the environmental residence times of litter and their final depositional sinks in the deep sea. Canyons are highly dynamic environments where sediment and litter storage is often transient, as also suggested by the shift of microplastic depocentres from the continental shelf to the canyons and then to the lower reach of the Xisha Trough observed within a 40-year time span from 1980s to 2018 (Chen et al., 2020). Gravity-driven flows are generally assumed to decrease in energy both spatially and temporally, although the relationships between event magnitude and frequency and its variation within distance, as well as the resulting depositional/erosional patterns, are not fully clarified (Heijnen et al., 2022a). Litter and sediment transported by high-frequency low-magnitude events could be confined and stored in the upper and middle reach of submarine canyons for decades or centuries, before being flushed further down canyon by high-magnitude events that can deliver material to the basin seafloor (Normark and Piper, 1991; Mas et al., 2010). Repeated burial and excavation over shorter timescales, with stepwise sediment transport and fragmentation by a range of flows with variable run-out has also been proposed (Stacey et al., 2019; Heijnen et al., 2022b). The lower reach of canyons would theoretically represent main depositional areas for litter, as also suggested by the report of large piles at thousands of meters depth, although these zones are largely unexplored for most canyons.
In summary, the geological processes-based approach highlighted in this work helps to elucidate land-to-sea transport and pathways by which litter is transferred and moved throughout canyons in deep-sea areas, but we are aware that a realistic assessment of this issue is still far to be reached. This is due to multiple problems, such as i) the paucity of systematic studies targeted to assess litter distribution in different morpho-sedimentary zones of canyons, ii) the lack of common standards in classification and quantification of litter, as well as of contextual information useful to constrain the depositional environment iii) the paucity of studies dealing with physical transport of litter (except for a few flume experiments targeting microplastics) and the fact that physical (e.g.: dimension, density) and chemical properties (e.g.: composition) of these pollutants can fundamentally differ from those of natural sediment, influencing their behavior and pathways. Considering the paramount role of canyons in funneling litter to the deep sea and the potential threat on deep-sea ecosystems, this issue should be carefully addressed by research communities together with monitoring agencies and policy-makers, to better assess global plastic budgets and to help delineate future mitigation strategies.
Author contributions
Conceptualization, MP, AB, MC, VH, JD, and PH; methodology, MP, AB, and JE; validation, MP, AB, and JD; formal analysis and investigation, MP, AB, and JE; data curation, MP, AB, and JD; writing original draft preparation, MP, AB, and JE; writing review and editing, MC, CLI, DC, JD, IK, VH, and PH. All authors contributed to the article and approved the submitted version.
Funding
MP acknowledges funding from CNR-DSSTTA “SNAPSHOT” Project (CIG Z503B9C139 - CUP B22F20000270001). DC acknowledges funding provided by “Progetto di Ateneo 2021” of University of Rome Sapienza “Morpho-sedimentary characterization of mass-wasting processes along Italian continental margins and insular volcanoes”. VH and MC acknowledge funding from the Natural Environment Research Council (NERC) National Capability Programme (NE/R015953/1) “Climate Linked Atlantic Sector Science”. VH was also funded through the EU H2020 project iAtlantic (Project No 818123) and enjoyed a Fellowship from the Hanse Wissenschaftskolleg Institute for Advanced Study during the final stages of this manuscript. CLI acknowledges funding from the EU-H2020 Marie Sklodowska Curie single action "Eco-hydrodynamics of cold water coral habitats across integrated spatial scales - HABISS" (GA 890815).
Acknowledgments
This work has been carried out in the framework of INCISE network and it has benefited from fruitful discussion of the Marine litter Working group during the 2021 INCISE Conference. The authors are grateful to Pia Urban (FU Berlin) for the global estimation of commercial fishing activity per canyon.
Conflict of interest
The authors declare that the research was conducted in the absence of any commercial or financial relationships that could be construed as a potential conflict of interest.
Publisher’s note
All claims expressed in this article are solely those of the authors and do not necessarily represent those of their affiliated organizations, or those of the publisher, the editors and the reviewers. Any product that may be evaluated in this article, or claim that may be made by its manufacturer, is not guaranteed or endorsed by the publisher.
Supplementary material
The Supplementary Material for this article can be found online at: https://www.frontiersin.org/articles/10.3389/fmars.2023.1224859/full#supplementary-material
References
Amaro T., Huvenne V. A. I., Allcock A. L., Aslam T., Davies J. S., Danovaro R., et al. (2016). The whittard canyon–a case study of submarine canyon processes. Prog. Oceanogr. 146, 38–57. doi: 10.1016/j.pocean.2016.06.003
Angiolillo M. (2018). “Debris in deep water,” in World seas: an environmental evaluation, 2nd ed. (Cambridge, MA: Academic Press), 251–268. doi: 10.1016/j.dsr.2023.103963
Angiolillo M., Bo M., Toma M., Giusti M., Salvati E., Giova A., et al. (2023). A baseline for the monitoring of Mediterranean upper bathyal biogenic reefs within the marine strategy framework directive objectives. Deep Sea Res. Part I: Oceanographic Res. Papers 194, 103963.
Angiolillo M., di Lorenzo B., Farcomeni A., Bo M., Bavestrello G., Santangelo G., et al. (2015). Distribution and assessment of marine debris in the deep tyrrhenian Sea (NW Mediterranean Sea, Italy). Mar. pollut. Bull. 92 (1-2), 149–159. doi: 10.1016/j.marpolbul.2014.12.044
Angiolillo M., Gérigny O., Valente T., Fabri M. C., Tambute E., Rouanet E., et al. (2021). Distribution of seafloor litter and its interaction with benthic organisms in deep waters of the ligurian Sea (Northwestern Mediterranean). Sci. Total Environ. 788, 147745. doi: 10.1016/j.scitotenv.2021.147745
Aymà A., Aguzzi J., Canals M., Lastras G., Mecho A., Lo Iacono C. (2019). “Occurrence of living cold-water corals at Large depths within submarine canyons of the northwestern Mediterranean Sea,” in Mediterranean Cold-water corals: past, present and future (Cham: Springer), 271–284.
Azpiroz-Zabala M., Cartigny M. J., Talling P. J., Parsons D. R., Sumner E. J., Clare M. A., et al. (2017). Newly recognized turbidity current structure can explain prolonged flushing of submarine canyons. Sci. Adv. 3 (10), e1700200. doi: 10.1126/sciadv.1700200
Bailey L. P., Clare M. A., Rosenberger K. J., Cartigny M. J., Talling P. J., Paull C. K., et al. (2021). Preconditioning by sediment accumulation can produce powerful turbidity currents without major external triggers. Earth Planetary Sci. Lett. 562, 116845. doi: 10.1016/j.epsl.2021.116845
Ballent A., Pando S., Purser A., Juliano M. F., Thomsen L. (2013). Modelled transport of benthic marine microplastic pollution in the nazaré canyon. Biogeosciences 10 (12), 7957–7970. doi: 10.5194/bg-10-7957-2013
Bancone C. E., Turner S. D., Ivar do Sul J. A., Rose N. L. (2020). The paleoecology of microplastic contamination. Front. Environ. Sci. 8, 574008. doi: 10.3389/fenvs.2020.574008
Barnes D. K. A. (2002). Invasions by marine life on plastic debris. Nature 416, 808–809. doi: 10.1038/416808a
Beaumont N. J., Aanesen M., Austen M. C., Börger T., Clark J. R., Cole M., et al. (2019). Global ecological, social and economic impacts of marine plastic. Mar. pollut. Bull. 142, 189–195. doi: 10.1016/j.marpolbul.2019.03.022
Becker J. J., Sandwell D. T., Smith W. H. F., Braud J., Binder B., Depner J. L., et al. (2009). Global bathymetry and elevation data at 30 arc seconds resolution: SRTM30_PLUS. Mar. Geodesy 32 (4), 355–371. doi: 10.1080/01490410903297766
Bell D., Soutter E. L., Cumberpatch Z. A., Ferguson R. A., Spychala Y. T., Kane I. A., et al. (2021). Flow-process controls on grain type distribution in an experimental turbidity current deposit: implications for detrital signal preservation and microplastic distribution in submarine fans. Depositional Rec. 7 (3), 392–415. doi: 10.1002/dep2.153
Bernhardt A., Schwanghart W. (2021a). Where and why do submarine canyons remain connected to the shore during sea-level rise? insights from global topographic analysis and Bayesian regression. Geophysical Res. Lett. 48 (10), e2020GL092234. doi: 10.1029/2020GL092234
Bernhardt A., Schwanghart W. (2021b). Global dataset of submarine canyon heads combined with terrestrial and marine topographic and oceanographic parameters. GFZ Data Serv. doi: 10.5880/fidgeo.2021.008
Biede V., Gates A. R., Pfeifer S., Collins J. E., Santos C., Jones D. O. (2022). Short-term response of deep-water benthic megafauna to installation of a pipeline over a depth gradient on the Angolan slope. Front. Mar. Sci. 9, 917. doi: 10.3389/fmars.2022.880453
Bolla Pittaluga M., Frascati A., Falivene O. (2018). A gradually varied approach to model turbidity currents in submarine channels. J. Geophysical Research: Earth Surface 123 (1), 80–96. doi: 10.1002/2017JF004331
Bolla Pittaluga M., Imran J. (2014). A simple model for vertical profiles of velocity and suspended sediment concentration in straight and curved submarine channels. J. Geophysical Research: Earth Surface 119 (3), 483–503. doi: 10.1002/2013JF002812
Borrelle S. B., Ringma J., Law K. L., Monnahan C. C., Lebreton L., McGivern A., et al. (2020). Predicted growth in plastic waste exceeds efforts to mitigate plastic pollution. Science 369 (6510), 1515–1518. doi: 10.1126/science.aba3656
Bruemmer A. L., Dissanayake A., Davies J. S. (2023). Marine litter-fauna interactions in submarine canyons: a standardised reporting framework and critical review of the current status of research. Front. Mar. Sci.
Buhl-Mortensen L., Buhl-Mortensen P. (2017). Marine litter in the Nordic seas: distribution composition and abundance. Mar. pollut. Bull. 125 (1-2), 260–270. doi: 10.1016/j.marpolbul.2017.08.048
Buhl-Mortensen P., Buhl-Mortensen L. (2018). Impacts of bottom trawling and litter on the seabed in Norwegian waters. Front. Mar. Sci. 5, 42. doi: 10.3389/fmars.2018.00042
Canals M., Pham C. K., Bergmann M., Gutow L., Hanke G., Van Sebille E., et al. (2021). The quest for seafloor macrolitter: a critical review of background knowledge, current methods and future prospects. Environ. Res. Lett. 16 (2), 023001. doi: 10.1088/1748-9326/abc6d4
Canals M., Puig P., De Madron X. D., Heussner S., Palanques A., Fabres J. (2006). Flushing submarine canyons. Nature 444, 354–357. doi: 10.1038/nature05271
Carson H. S., Colbert S. L., Kaylor M. J., McDermid K. J. (2011). Small plastic debris changes water movement and heat transfer through beach sediments. Mar. pollut. Bull. 62 (8), 1708–1713. doi: 10.1016/j.marpolbul.2011.05.032
Carter G. D., Huvenne V. A., Gales J. A., Iacono C. L., Marsh L., Ougier-Simonin A., et al. (2018). Ongoing evolution of submarine canyon rockwalls; examples from the whittard canyon, celtic margin (NE Atlantic). Prog. Oceanogr. 169, 79–88. doi: 10.1016/j.pocean.2018.02.001
Carter L., Milliman J. D., Talling P. J., Gavey R., Wynn R. B. (2012). Near-synchronous and delayed initiation of long run-out submarine sediment flows from a record-breaking river flood, offshore Taiwan. Geophysical Res. Lett. 39 (12), L12603. doi: 10.1029/2012GL051172
Cau A., Alvito A., Moccia D., Canese S., Pusceddu A., Rita C., et al. (2017). Submarine canyons along the upper sardinian slope (Central Western Mediterranean) as repositories for derelict fishing gears. Mar. pollut. Bull. 123 (1-2), 357–364. doi: 10.1016/j.marpolbul.2017.09.010
Chaytor J. D., Demopoulos A. W., ten Brink U. S., Baxter C., Quattrini A. M., Brothers D. S. (2016). “Assessment of canyon wall failure process from multibeam bathymetry and remotely operated vehicle (ROV) observations, US Atlantic continental margin,” in Submarine mass movements and their consequences: 7th international symposium (Switzerland: Springer International Publishing), 103–113.
Chen M., Du M., Jin A., Chen S., Dasgupta S., Li J., et al. (2020). Forty-year pollution history of microplastics in the largest marginal sea of the western pacific. Geochemical Perspective Lett. 13, 42–47. doi: 10.7185/geochemlet.2012
Choy C. A., Robison B. H., Gagne T. O., Erwin B., Firl E., Halden R. U., et al. (2019). The vertical distribution and biological transport of marine microplastics across the epipelagic and mesopelagic water column. Sci. Rep. 9 (1), 7843. doi: 10.1038/s41598-019-44117-2
Cooper C., Wood J., Andrieux O. (2013). Turbidity current measurements in the Congo canyon. In Offshore Technol. Conf. (Vol. 23992 pp, 6–9). doi: 10.4043/23992-MS
Covault J. A., Normark W. R., Romans B. W., Graham S. A. (2007). Highstand fans in the California borderland: the overlooked deep-water depositional systems. Geology 35 (9), 783–786. doi: 10.1130/G23800A.1
De Leo F. C., Smith C. R., Rowden A. A., Bowden D. A., Clark M. R. (2010). Submarine canyons: hotspots of benthic biomass and productivity in the deep sea. Proc. R. Soc. B: Biol. Sci. 277 (1695), 2783–2792. doi: 10.1098/rspb.2010.0462
de Stigter H. C., Jesus C. C., Boer W., Richter T. O., Costa A., van Weering T. C. E. (2011). Recent sediment transport and deposition in the Lisbon–setúbal and cascais submarine canyons, Portuguese continental margin. Deep Sea Res. Part II 58, 2321–2344. doi: 10.1016/j.dsr2.2011.04.001
Derraik J. G. (2002). The pollution of the marine environment by plastic debris: a review. Mar. Pollut. Bull. 44 (9), 842–852.
Dominguez-Carrió C., Sanchez-Vidal A., Estournel C., Corbera G., Riera J. L., Orejas C., et al. (2020). Seafloor litter sorting in different domains of cap de creus continental shelf and submarine canyon (NW Mediterranean Sea). Mar. pollut. Bull. 161, 111744. doi: 10.1016/j.marpolbul.2020.111744
Eggenhuisen J. T., Tilston M. C., de Leeuw J., Pohl F., Cartigny M. J. (2020). Turbulent diffusion modelling of sediment in turbidity currents: An experimental validation of the rouse approach. Depositional Rec. 6 (1), 203–216.
Eggenhuisen J. T., Tilston M. C., Stevenson C. J., Hubbard S. M., Cartigny M. J., Heijnen M. S., et al. (2022). The sediment budget estimator (SBE): a process model for the stochastic estimation of fluxes and budgets of sediment through submarine channel systems. J. Sedimentary Res. 92 (12), 1093–1115. doi: 10.2110/jsr.2021.037
Eidam E. F., Ogston A. S., Nittrouer C. A. (2019). Formation and removal of a coastal flood deposit. J. Geophysical Research: Oceans 124 (2), 1045–1062. doi: 10.1029/2018JC014360
Enrichetti F., Dominguez-Carrió C., Toma M., Bavestrello G., Canese S., Bo M. (2020). Assessment and distribution of seafloor litter on the deep ligurian continental shelf and shelf break (NW Mediterranean Sea). Mar. pollut. Bull. 151, 110872.
Eriksen M., Lebreton L. C., Carson H. S., Thiel M., Moore C. J., Borerro J. C., et al. (2014). Plastic pollution in the world's oceans: more than 5 trillion plastic pieces weighing over 250,000 tons afloat at sea. PLoS One 9 (12), e111913. doi: 10.1371/journal.pone.0111913
Fabri M. C., Pedel L., Beuck L., Galgani F., Hebbeln D., Freiwald A. (2014). Megafauna of vulnerable marine ecosystems in French mediterranean submarine canyons: spatial distribution and anthropogenic impacts. Deep Sea Res. Part II 104, 184–207. doi: 10.1016/j.dsr2.2013.06.016
Fabri M. C., Vinha B., Allais A. G., Bouhier M. E., Dugornay O., Gaillot A., et al. (2019). Evaluating the ecological status of cold-water coral habitats using non-invasive methods: an example from cassidaigne canyon, northwestern Mediterranean Sea. Prog. Oceanogr. 178, 102172. doi: 10.1016/j.pocean.2019.102172
Fernandez-Arcaya U., Ramirez-Llodra E., Aguzzi J., Allcock A. L., Davies J. S., Dissanayake A., et al. (2017). Ecological role of submarine canyons and need for canyon conservation: a review. Front. Mar. Sci. 4, 5. doi: 10.3389/fmars.2017.00005
Flemming B. W. (1980). Sand transport and bedform patterns on the continental shelf between Durban and port Elizabeth (south-east African continental margin). Sedimentary Geology 26 (1-3), 179–205. doi: 10.1016/0037-0738(80)90011-1
Galgani F., Hanke G., Maes T. (2015). “Global distribution, composition and abundance of marine litter,” in Marine anthropogenic litter. Eds. Bergmann M., Gutow L., Klages M. (Cham: Springer International Publishing), 29–56.
Galgani F., Leaute J. P., Moguedet P., Souplet A., Verin Y., Carpentier A., et al. (2000). Litter on the sea floor along European coasts. Mar. pollut. Bull. 40 (6), 516–527. doi: 10.1016/S0025-326X(99)00234-9
Galgani F., Michela A., Gérigny O., Maes T., Tambutté E., Harris P. T. (2022). “Marine litter, plastic, and microplastics on the seafloor,” in Plastics and the ocean: origin, characterization, fate, and impacts. Andrady A.L Ed. (John Wiley & Sons), 151–197.
Galgani F., Souplet A., Cadiou Y. (1996). Accumulation of debris on the deep sea floor off the French Mediterranean coast. Mar. Ecol. Prog. Ser. 142, 225–234. doi: 10.3354/meps142225
Gall S. C., Thompson R. C. (2015). The impact of debris on marine life. Mar. pollut. Bull. 92 (1-2), 170–179. doi: 10.1016/j.marpolbul.2014.12.041
Gerigny O., Brun M., Fabri M. C., Tomasino C., Le Moigne M., Jadaud A., et al. (2019). Seafloor litter from the continental shelf and canyons in French Mediterranean water: distribution, typologies and trends. Mar. pollut. Bull. 146, 653–666. doi: 10.1016/j.marpolbul.2019.07.030
Geyer R., Jambeck J. R., Law K. L. (2017). Production, use, and fate of all plastics ever made. Sci. Adv. 3 (7), e1700782. doi: 10.1126/sciadv.1700782
Giusti M., Canese S., Fourt M., Bo M., Innocenti C., Goujard A., et al. (2019). Coral forests and derelict fishing gears in submarine canyon systems of the ligurian Sea. Prog. Oceanography 178, 102186.
Grinyó J., Chevaldonné P., Schohn T., Le Bris N. (2021). Megabenthic assemblages on bathyal escarpments off the west Corsican margin (Western Mediterranean). Deep Sea Res. Part I: Oceanographic Res. Papers 171, 103475.
Hage S., Cartigny M. J., Sumner E. J., Clare M. A., Hughes Clarke J. E., Talling P. J., et al. (2019). Direct monitoring reveals initiation of turbidity currents from extremely dilute river plumes. Geophysical Res. Lett. 46 (20), 11310–11320. doi: 10.1029/2019GL084526
Hage S., Galy V. V., Cartigny M. J., Heerema C., Heijnen M. S., Acikalin S., et al. (2022). Turbidity currents can dictate organic carbon fluxes across river-fed fjords: an example from Bute inlet (BC, Canada). J. Geophysical Research: Biogeosciences 127 (6), e2022JG006824. doi: 10.1029/2022JG006824
Harris P. T. (2020). The fate of microplastic in marine sedimentary environments: a review and synthesis. Mar. pollut. Bull. 158, 111398. doi: 10.1016/j.marpolbul.2020.111398
Harris P. T., Heap A. (2009). Cyclone-induced net sediment transport pathway on the continental shelf of tropical Australia inferred from reef talus deposits. Continental Shelf Res. 29 (16), 2011–2019. doi: 10.1016/j.csr.2008.12.006
Harris P. T., Macmillan-Lawler M., Rupp J., Baker E. K. (2014). Geomorphology of the oceans. Mar. Geology 352, 4–24. doi: 10.1016/j.margeo.2014.01.011
Harris P. T., Whiteway T. (2011). Global distribution of large submarine canyons: geomorphic differences between active and passive continental margins. Mar. Geology 285, 69–86. doi: 10.1016/j.margeo.2011.05.008
Hartmann N. B., Rist S., Bodin J., Jensen L. H., Schmidt S. N., Mayer P., et al. (2017). Microplastics as vectors for environmental contaminants: exploring sorption, desorption, and transfer to biota. Integrated Environ. Assess. Manage. 13 (3), 488–493. doi: 10.1002/ieam.1904
Heijnen M. S., Clare M. A., Cartigny M. J., Talling P. J., Hage S., Pope E. L., et al. (2022b). Fill, flush or shuffle: how is sediment carried through submarine channels to build lobes? Earth Planetary Sci. Lett. 584, 117481. doi: 10.1016/j.epsl.2022.117481
Heijnen M. S., Mienis F., Gates A. R., Bett B. J., Hall R. A., Hunt J., et al. (2022a). Challenging the highstand-dormant paradigm for land-detached submarine canyons. Nat. Commun. 13 (1), 3448. doi: 10.1038/s41467-022-31114-9
Hernandez I., Davies J. S., Huvenne V. A., Dissanayake A. (2022). Marine litter in submarine canyons: a systematic review and critical synthesis. Front. Mar. Sci. 1615. doi: 10.3389/fmars.2022.965612
Hidalgo-Ruz V., Gutow L., Thompson R. C., Thiel M. (2012). Microplastics in the marine environment: a review of the methods used for identification and quantification. Environ. Sci. Technol. 46 (6), 3060–3075. doi: 10.1021/es2031505
Hiscott R. N. (1994). Loss of capacity, not competence, as the fundamental process governing deposition from turbidity currents. J. Sedimentary Res. 64, 209–214. doi: 10.2110/jsr.64.209
Hiscott R. N., Hall F. R., Pirmez C. (1997). “Turbidity-current overspill from the Amazon channel: texture of the silt/sand load, paleoflow from anisotropy of magnetic susceptibility, and implications for flow processes,” in Proceedings-ocean drilling program scientific results (Texas: National Science Foundation), 53–78.
Hizzett J. L., Hughes Clarke J. E., Sumner E. J., Cartigny M. J. B., Talling P. J., Clare M. A. (2018). Which triggers produce the most erosive, frequent, and longest runout turbidity currents on deltas? Geophysical Res. Lett. 45 (2), 855–863. doi: 10.1002/2017GL075751
Hotchkiss F. S., Wunsch C. (1982). Internal waves in Hudson canyon with possible geological implications. Deep Sea Res. Part I 29, 415–442. doi: 10.1016/0198-0149(82)90068-1
Inman D. L., Nordstrom C. E., Flick R. E. (1976). Currents in submarine canyons: an air-sea-land interaction. Annu. Rev. fluid mechanics 8 (1), 275–310. doi: 10.1146/annurev.fl.08.010176.001423
Jambeck J. R., Geyer R., Wilcox C., Siegler T. R., Perryman M., Andrady A., et al. (2015). Plastic waste inputs from land into the ocean. Science 347 (6223), 768–771. doi: 10.1126/science.1260352
Jobe Z., Sylvester Z., Bolla Pittaluga M., Frascati A., Pirmez C., Minisini D., et al. (2017). Facies architecture of submarine channel deposits on the western Niger delta slope: implications for grain-size and density stratification in turbidity currents. J. Geophysical Research: Earth Surface 122 (2), 473–491. doi: 10.1002/2016JF003903
Jones E. S., Ross S. W., Robertson C. M., Young C. M. (2022). Distributions of microplastics and larger anthropogenic debris in Norfolk canyon, Baltimore canyon, and the adjacent continental slope (Western north Atlantic margin, U.S.A.). Mar. pollut. Bull. 174, 113047. doi: 10.1016/j.marpolbul.2021.113047
Kane I. A., Clare M. A. (2019). Dispersion, accumulation, and the ultimate fate of microplastics in deep-marine environments: a review and future directions. Front. Earth Sci. 7, 80. doi: 10.3389/feart.2019.00080
Kane I. A., Clare M. A., Miramontes E., Wogelius R., Rothwell J. J., Garreau P., et al. (2020). Seafloor microplastic hotspots controlled by deep-sea circulation. Science 368 (6495), 1140–1145. doi: 10.1126/science.aba5899
Khripounoff A., Crassous P., Bue N., Dennielou B., Jacinto R. S. (2012). Different types of sediment gravity flows detected in the var submarine canyon (northwestern Mediterranean Sea). Prog. Oceanography 106, 138–153. doi: 10.1016/j.pocean.2012.09.001
Khripounoff A., Vangriesheim A., Crassous P., Etoubleau J. (2009). High frequency of sediment gravity flow events in the var submarine canyon (Mediterranean Sea). Mar. Geology 263 (1-4), 1–6. doi: 10.1016/j.margeo.2009.03.014
Kühn S., Bravo Rebolledo E. L., van Franeker J. A. (2015). “Deleterious effects of litter on marine life,” in Marine anthropogenic litter. Bergmann, Lutow, Klages (Eds) (Berlin: Springer Nature), 75–116.
Lavers J. L., Bond A. L. (2017). Exceptional and rapid accumulation of anthropogenic debris on one of the world’s most remote and pristine islands. Proc. Natl. Acad. Sci. 114 (23), 6052–6055. doi: 10.1073/pnas.1619818114
Lebreton L. C. M., van der Zwet J., Damsteeg J. W., Slat B., Andrady A., Reisser J. (2017). River plastic emissions to the world’s oceans. Nat. Communication 8, 1–10. doi: 10.1038/ncomms15611
Lopez-Lopez L., González-Irusta J. M., Punzón A., Serrano A. (2017). Benthic litter distribution on circalittoral and deep sea bottoms of the southern bay of Biscay: analysis of potential drivers. Continental. Shelf Res. 144, 112–119. doi: 10.1016/j.csr.2017.07.003
Maier K. L., Rosenberger K. J., Paull C. K., Gwiazda R., Gales J., Lorenson T., et al. (2019). Sediment and organic carbon transport and deposition driven by internal tides along Monterey canyon, offshore California. Deep Sea Res. Part I 153, 103108. doi: 10.1016/j.dsr.2019.103108
Martín J., Palanques A., Vitorino J., Oliveira A., De Stigter H. C. (2011). Near-bottom particulate matter dynamics in the nazaré submarine canyon under calm and stormy conditions. Deep Sea Res. Part II 58, 2388–2400. doi: 10.1016/j.dsr2.2011.04.004
Mas V., Mulder T., Dennielou B., Schmidt S., Khripounoff A., Savoye B. (2010). Multiscale spatio-temporal variability of sedimentary deposits in the var turbidite system (North-Western Mediterranean Sea). Mar. Geology 275, 37–52. doi: 10.1016/j.margeo.2010.04.006
Masson D. G., Huvenne V. A. I., De Stigter H. C., Wolff G. A., Kiriakoulakis K., Arzola R. G., et al. (2010). Efficient burial of carbon in a submarine canyon. Geology 38 (9), 831–834. doi: 10.1130/G30895.1
Matos F. L., Ross S. W., Huvenne V. A. I. I., Davies J. S., Cunha M. R. (2018). Canyons pride and prejudice: exploring the submarine canyon research landscape, a history of geographic and thematic bias. Prog. Oceanogr. 169, 6–19. doi: 10.1016/j.pocean.2018.04.010
Mecho A., Aguzzi J., De Mol B., Lastras G., Ramirez-Llodra E., Bahamon N., et al. (2018). Visual faunistic exploration of geomorphological human-impacted deep-sea areas of the north-western Mediterranean Sea. J. Mar. Biol. Assoc. United Kingdom 98 (6), 1241–1252. doi: 10.1017/S0025315417000431
Mecho A., Francescangeli M., Ercilla G., Fanelli E., Estrada F., Valencia J., et al. (2020). Deep-sea litter in the gulf of cadiz (Northeastern Atlantic, Spain). Mar. pollut. Bull. 153, 110969.
Meijer L. J., van Emmerik T., van der Ent R., Schmidt C., Lebreton L. (2021). More than 1000 rivers account for 80% of global riverine plastic emissions into the ocean. Sci. Adv. 7 (18), eaaz5803. doi: 10.1126/sciadv.aaz5803
Milliman J. D., Meade R. H. (1983). World-wide delivery of river sediment to the oceans. J. Geology 91 (1), 1–21. doi: 10.1086/628741
Moccia D., Cau A., Alvito A., Canese S., Cannas R., Bo M., et al. (2019). New sites expanding the “Sardinian cold-water coral province” extension: a new potential cold-water coral network? Aquat. Conservation: Mar. Freshw. Ecosyst. 29 (1), 153–160. doi: 10.1002/aqc.2975
Mordecai G., Tyler P. A., Masson D. G., Huvenne V. A. I. (2011). Litter in submarine canyons off the west coast of Portugal. Deep Sea Res. Part II 58, 2489–2496. doi: 10.1016/j.dsr2.2011.08.009
Mulder T., Syvitski J. P. M., Migeon S., Faugères J. C., Savoye B. (2003). Marine hyperpycnal flows: initiation, behaviour and related deposits. a review. Mar. Petroleum Geology 20 (6-8), 861–882. doi: 10.1016/j.marpetgeo.2003.01.003
Mulder T., Zaragosi S., Garlan T., Mavel J., Cremer M., Sottolichio A., et al. (2012). Present deep-submarine canyons activity in the bay of Biscay (NE Atlantic). Mar. Geology 295, 113–127. doi: 10.1016/j.margeo.2011.12.005
Normark W. R., Piper D. J. W. (1991). “Initiation processes and flow evolution of turbidity currents: implications for the depositional record,” in From shoreline to abyss: contributions in marine geology in honor of Francis Parker Shepard (Tulsa, OK: SEPM), 207–230.
Orejas C., Gori A., Lo Iacono C., Puig P., Gili J. M., Dale M. R. T. (2009). Cold-water corals in the cap de creus canyon, northwestern Mediterranean: spatial distribution, density and anthropogenic impact. Mar. Ecol. Prog. Ser. 397, 37–51. doi: 10.3354/meps08314
Oliveira F., Monteiro P., Bentes L., Henriques N. S., Aguilar R., Gonçalves J. M. (2015). Marine litter in the upper são Vicente submarine canyon (SW portugal): Abundance, distribution, composition and fauna interactions. Mar. pollut. Bull. 97 (1-2), 401–407.
Pabortsava K., Lampitt R. S. (2020). High concentrations of plastic hidden beneath the surface of the Atlantic ocean. Nat. Commun. 11 (1), 1–11. doi: 10.1038/s41467-020-17932-9
Paull C. K., Ussler W. III, Caress D. W., Lundsten E., Covault J. A., Maier K. L., et al. (2010). Origins of large crescent-shaped bedforms within the axial channel of Monterey canyon, offshore California. Geology 6 (6), 755–774. doi: 10.1130/GES00527.1
Pearman T. R. R., Robert K., Callaway A., Hall R., Iacono C. L., Huvenne V. A. (2020). Improving the predictive capability of benthic species distribution models by incorporating oceanographic data–towards holistic ecological modelling of a submarine canyon. Prog. Oceanography 184, 102338. doi: 10.1016/j.pocean.2020.102338
Peng X., Chen M., Chen S., Dasgupta S., Xu H., Ta K., et al. (2018). Microplastics contaminate the deepest part of the world’s ocean. Geochemical Perspective Lett. 9, 1–5. doi: 10.7185/geochemlet.1829
Peng X., Dasgupta S., Zhong G., Du M., Xu H., Chen M., et al. (2019). Large Debris dumps in the northern south China Sea. Mar. pollut. Bull. 142, 164–168. doi: 10.1016/j.marpolbul.2019.03.041
Pham C. K., Ramirez-Llodra E., Alt C. H., Amaro T., Bergmann M., Canals M., et al. (2014). Marine litter distribution and density in European seas, from the shelves to deep basins. PLoS One 9 (4), e95839. doi: 10.1371/journal.pone.0095839
Pierdomenico M., Casalbore D., Chiocci F. L. (2019). Massive benthic litter funnelled to deep sea by flash-flood generated hyperpycnal flows. Sci. Rep. 9, 1–10. doi: 10.1038/s41598-019-41816-8
Pierdomenico M., Casalbore D., Chiocci F. L. (2020). The key role of canyons in funnelling litter to the deep sea: a study of the gioia canyon (Southern tyrrhenian Sea). Anthropocene 30, 100237. doi: 10.1016/j.ancene.2020.100237
Pierdomenico M., Ridente D., Casalbore D., Di Bella L., Milli S., Chiocci F. L. (2022). Plastic burial by flash-flood deposits in a prodelta environment (Gulf of patti, southern tyrrhenian Sea). Mar. pollut. Bull. 181, 113819. doi: 10.1016/j.marpolbul.2022.113819
Piper D. J. W., Normark W. R. (2009). Processes that initiate turbidity currents and their influence on turbidites: a marine geology perspective. J. Sedimentary Res. 79 (6), 347–362. doi: 10.2110/jsr.2009.046
Pohl F., Eggenhuisen J. T., Kane I. A., Clare M. A. (2020). Transport and burial of microplastics in deep-marine sediments by turbidity currents. Environ. Sci. Technol. 54, 4180–4189. doi: 10.1021/acs.est.9b07527
Pope E. L., Heijnen M. S., Talling P. J., Jacinto R. S., Gaillot A., Baker M. L., et al. (2022). Carbon and sediment fluxes inhibited in the submarine Congo canyon by landslide-damming. Nat. Geosci. 15 (10), 845–853. doi: 10.1038/s41561-022-01017-x
Pope E. L., Talling P. J., Carter L., Clare M. A., Hunt J. E. (2017). Damaging sediment density flows triggered by tropical cyclones. Earth Planetary Sci. Lett. 458, 161–169. doi: 10.1016/j.epsl.2016.10.046
Post A. L., Przeslawski R., Nanson R., Siwabessy J., Smith D., Kirkendale L. A., et al. (2022). Modern dynamics, morphology and habitats of slope-confined canyons on the northwest Australian margin. Mar. Geology 443, 106694. doi: 10.1016/j.margeo.2021.106694
Puig P., Canals M., Company J. B., Martín J., Amblas D., Lastras G., et al. (2012). Ploughing the deep sea floor. Nature 489 (7415), 286–289. doi: 10.1038/nature11410
Puig P., Palanques A., Martín J. (2014). Contemporary sediment-transport processes in submarine canyons. Annu. Rev. Mar. Sci. 6, 53–77. doi: 10.1146/annurev-marine-010213-135037
Quattrini A. M., Nizinski M. S., Chaytor J. D., Demopoulos A. W., Roark E. B., France S. C., et al. (2015). Exploration of the canyon-incised continental margin of the northeastern United States reveals dynamic habitats and diverse communities. PloS One 10 (10), e0139904. doi: 10.1371/journal.pone.0139904
Ramirez-Llodra E., De Mol B., Company J. B., Coll M., Sardà F. (2013). Effects of natural and anthropogenic processes in the distribution of marine litter in the deep Mediterranean Sea. Prog. Oceanogr. 118, 273–287. doi: 10.1016/j.pocean.2013.07.027
Rech S., Macaya-Caquilpán V., Pantoja J. F., Rivadeneira M. M., Madariaga D. J., Thiel M. (2014). Rivers as a source of marine litter–a study from the SE pacific. Mar. pollut. Bull. 82 (1-2), 66–75.
R Core Team (2016). R: a language and environment for statistical computing (Vienna, Austria: R Foundation for Statistical Computing). Available at: http://www.R-project.org/.
Romans B. W., Castelltort S., Covault J. A., Fildani A., Walsh J. P. (2016). Environmental signal propagation in sedimentary systems across timescales. Earth-Science Rev. 153, 7–29. doi: 10.1016/j.earscirev.2015.07.012
Rouse H. (1937). Modern conceptions of the mechanics of turbulence. Trans. Am. Soc. Civil Engineers 102 (1), 463–543. doi: 10.1061/TACEAT.0004872
Sanchez-Vidal A., Thompson R. C., Canals M., De Haan W. P. (2018). The imprint of microfibres in southern European deep seas. PloS One 13 (11), e0207033. doi: 10.1371/journal.pone.0207033
Santín A., Grinyó J., Bilan M., Ambroso S., Puig P. (2020). First report of the carnivorous sponge lycopodina hypogea (Cladorhizidae) associated with marine debris, and its possible implications on deep-sea connectivity. Mar. pollut. Bull. 159, 1–7. doi: 10.1016/j.marpolbul.2020.111501
Schlining K., Von Thun S., Kuhnz L., Schlining B., Lundsten L., Stout N. J., et al. (2013). Debris in the deep: using a 22-year video annotation database to survey marine litter in Monterey canyon, central California, USA. Deep Sea Res. Part I 79, 96–105. doi: 10.1016/j.dsr.2013.05.006
Schmidt C., Krauth T., Wagner S. (2017). Export of plastic debris by rivers into the sea. Environ. Sci. Technol. 51 (21), 12246–12253.
Schmidt N., Thibault D., Galgani F., Paluselli A., Sempéré R. (2018). Occurrence of microplastics in surface waters of the gulf of lion (NW Mediterranean Sea). Prog. Oceanography 163, 214–220. doi: 10.1016/j.pocean.2017.11.010
Schnurr R. E., Alboiu V., Chaudhary M., Corbett R. A., Quanz M. E., Sankar K., et al. (2018). Reducing marine pollution from single-use plastics (SUPs): A review. Mar. pollut. Bull. 137, 157–171.
Shepard F. P. (1981). Submarine canyons: multiple causes and long-time persistence. Aapg Bull. 65 (6), 1062–1077. doi: 10.1306/03B59459-16D1-11D7-8645000102C1865D
Simmons S. M., Azpiroz-Zabala M., Cartigny M. J. B., Clare M. A., Cooper C., Parsons D. R., et al. (2020). Novel acoustic method provides first detailed measurements of sediment concentration structure within submarine turbidity currents. J. Geophysical Research: Oceans 125 (5), e2019JC015904. doi: 10.1029/2019JC015904
Stacey C. D., Hill P. R., Talling P. J., Enkin R. J., Hughes Clarke J., Lintern D. G. (2019). How turbidity current frequency and character varies down a fjord-delta system: Combining direct monitoring, deposits and seismic data. Sedimentology 66 (1), 1–31.
Straub K. M., Mohrig D. (2008). Quantifying the morphology and growth of levees in aggrading submarine channels. J. Geophysical Research: Earth Surface 113, F03012. doi: 10.1029/2007JF000896
Talling P. J., Baker M. L., Pope E. L., Ruffell S. C., Jacinto R. S., Heijnen M. S., et al. (2022). Longest sediment flows yet measured show how major rivers connect efficiently to deep sea. Nat. Commun. 13 (1), 1–15. doi: 10.1038/s41467-022-31689-3
Taviani M., Angeletti L., Canese S., Cannas R. I.T.A., Cardone F., Cau A M., et al (2017). The “Sardinian cold-water coral province” in the context of the Mediterranean coral ecosystems. Deep Sea Res. Part II: Topical Studies Oceanography 145, 61–78.
Taviani M., Angeletti L., Cardone F., Montagna P., Danovaro R. (2019). A unique and threatened deep water coral-bivalve biotope new to the Mediterranean Sea offshore the Naples megalopolis. Sci. Rep. 9 (1), 3411. doi: 10.1038/s41598-019-39655-8
Taviani M., Foglini F., Castellan G., Montagna P., McCulloch M. T., Trotter J. A. (2023). First assessment of anthropogenic impacts in submarine canyon systems off southwestern Australia. Sci. Total Environ. 857, 159243. doi: 10.1016/j.scitotenv.2022.159243
Tekman M. B., Krumpen T., Bergmann M. (2017). Marine litter on deep Arctic seafloor continues to increase and spreads to the north at the HAUSGARTEN observatory. Deep Sea Res. Part I 120, 88–99. doi: 10.1016/j.dsr.2016.12.011
Thompson R. C., Olsen Y., Mitchell R. P., Davis A., Rowland S. J., John A. W., et al. (2004). Lost at sea: where is all the plastic? Science 304 (5672), 838–838. doi: 10.1126/science.1094559
Tubau X., Canals M., Lastras G., Rayo X., Rivera J., Amblas D. (2015). Marine litter on the floor of deep submarine canyons of the northwestern Mediterranean Sea: the role of hydrodynamic processes. Prog. Oceanography 134, 379–403. doi: 10.1016/j.pocean.2015.03.013
van den Beld I. M. J., Guillaumont B., Menot L., Bayle C., Arnaud-Haond S., Bourillet J. F. (2017). Marine litter in submarine canyons of the bay of Biscay. Deep Sea Res. Part II 145, 142–152. doi: 10.1016/j.dsr2.2016.04.013
Van Sebille E., Wilcox C., Lebreton L., Maximenko N., Hardesty B. D., Van Franeker J. A., et al. (2015). A global inventory of small floating plastic debris. Environ. Res. Lett. 10 (12), 124006. doi: 10.1088/1748-9326/10/12/124006
Vetter E. W., Dayton P. K. (1999). Organic enrichment by macrophyte detritus, and abundance patterns of megafaunal populations in submarine canyons. Mar. Ecol. Prog. Ser. 186, 137–148. doi: 10.3354/meps186137
Waldschläger K., Brückner M. Z., Almroth B. C., Hackney C. R., Adyel T. M., Alimi O. S., et al. (2022). Learning from natural sediments to tackle microplastics challenges: a multidisciplinary perspective. Earth-Science Rev. 228, 104021. doi: 10.1016/j.earscirev.2022.104021
Watters D. L., Yoklavich M. M., Love M. S., Schroeder D. M. (2010). Assessing marine debris in deep seafloor habitats off California. Mar. pollut. Bull. 60, 131–138. doi: 10.1016/j.marpolbul.2009.08.019
Wei C. L., Rowe G. T., Nunnally C. C., Wicksten M. K. (2012). Anthropogenic “Litter” and macrophyte detritus in the deep northern gulf of Mexico. Mar. pollut. Bull. 64, 966–973. doi: 10.1016/j.marpolbul.2012.02.015
Willis K., Denise Hardesty B., Kriwoken L., Wilcox C. (2017). Differentiating littering, urban runoff and marine transport as sources of marine debris in coastal and estuarine environments. Sci. Rep. 7, 1–9. doi: 10.1038/srep44479
Woodall L. C., Robinson L. F., Rogers A. D., Narayanaswamy B. E., Paterson G. L. J. (2015). Deep-sea litter: a comparison of seamounts, banks and a ridge in the Atlantic and Indian oceans reveals both environmental and anthropogenic factors impact accumulation and composition. Front. Mar. Sci. 2, 3. doi: 10.3389/fmars.2015.00003
Xu J. P. (2011). Measuring currents in submarine canyons: technological and scientific progress in the past 30 years. Geosphere 7, 868–876. doi: 10.1130/GES00640.1
Xu J. P., Noble M., Eittreim S. L., Rosenfeld L. K., Schwing F. B., Pilskaln C. H. (2002). Distribution and transport of suspended particulate matter in Monterey canyon, California. Mar. Geology 181 (1-3), 215–234. doi: 10.1016/S0025-3227(01)00268-7
Xu J. P., Swarzenski P. W., Noble M., Li A. C. (2010). Event-driven sediment flux in hueneme and mugu submarine canyons, southern California. Mar. Geology 269, 74–88. doi: 10.1016/j.margeo.2009.12.007
Yin S., Lin L., Pope E. L., Li J., Ding W., Wu Z., et al. (2019). Continental slope-confined canyons in the pearl river mouth basin in the south China Sea dominated by erosion 2004–2018. Geomorphology 344, 60–74. doi: 10.1016/j.geomorph.2019.07.016
Zettler E. R., Mincer T. J., Amaral-Zettler L. A. (2013). Life in the “plastisphere”: microbial communities on plastic marine debris. Environ. Sci. Technol. 47, 7137–7146. doi: 10.1021/es401288x
Keywords: submarine canyons, marine litter, microplastics, fishing-related debris, litter transport, sediment transport, deep-sea litter
Citation: Pierdomenico M, Bernhardt A, Eggenhuisen JT, Clare MA, Lo Iacono C, Casalbore D, Davies JS, Kane I, Huvenne VAI and Harris PT (2023) Transport and accumulation of litter in submarine canyons: a geoscience perspective. Front. Mar. Sci. 10:1224859. doi: 10.3389/fmars.2023.1224859
Received: 18 May 2023; Accepted: 22 June 2023;
Published: 21 July 2023.
Edited by:
Alessandro Cau, University of Cagliari, ItalyReviewed by:
Giorgio Castellan, Consiglio Nazionale delle Ricerche (CNR), ItalyMing Su, Sun Yat-sen University, China
Copyright © 2023 Pierdomenico, Bernhardt, Eggenhuisen, Clare, Lo Iacono, Casalbore, Davies, Kane, Huvenne and Harris. This is an open-access article distributed under the terms of the Creative Commons Attribution License (CC BY). The use, distribution or reproduction in other forums is permitted, provided the original author(s) and the copyright owner(s) are credited and that the original publication in this journal is cited, in accordance with accepted academic practice. No use, distribution or reproduction is permitted which does not comply with these terms.
*Correspondence: Martina Pierdomenico, bWFydGluYS5waWVyZG9tZW5pY29AaWFzLmNuci5pdA==