- 1Institute of Marine Sciences, University of North Carolina at Chapel Hill, Morehead City, NC, United States
- 2National Centers for Coastal Ocean Science, National Ocean Service, National Oceanic and Atmospheric Administration, Beaufort, NC, United States
Epiphytic microalgae are important contributors to the carbon and nutrient cycles yet are often overlooked during ecological surveys. In reef habitats, epiphytes are often found living on host organisms, including seaweeds or corals, and can influence community composition of higher trophic level taxa. Hence, understanding how epiphytes respond to different reef substrate materials can help inform designs of substrates intentionally deployed to form artificial reefs which can encourage high biodiversity and ecological functioning. One such epiphyte, Gambierdiscus spp., is a harmful benthic dinoflagellate which produces toxins that bioaccumulate and cause ciguatera fish poisoning (CFP) when contaminated fish is consumed by humans. CFP is one of the most frequently reported seafood-associated illnesses around the world, occurring most often near tropical reefs. We surveyed the epiphytes amongst 13 natural and artificial reef sites located off the coast of North Carolina to determine the role of the reefs’ foundational substrate (e.g., natural marl, steel or concrete) on structuring the epiphyte community with an emphasis on Gambierdiscus spp. abundance. No Gambierdiscus spp. were detected among the sampled reefs, likely due to suboptimal water temperatures. An ex-situ substrate preference experiment for Gambierdiscus spp. was performed using marl to represent natural rocky reefs, and steel and concrete to represent artificial reefs. Experimental results indicated that Gambierdiscus spp. grew fastest in the presence of marl and density decreased significantly when exposed to steel. However, steel artificial reefs had the highest average epiphyte biomass and species richness amongst the sampled reefs. 18s rRNA gene sequence analysis revealed that natural reefs were more likely to be dominated by dinoflagellates, whereas steel and concrete reefs were dominated by diatoms. We found that epiphyte composition was related to material at a phylum level, but seaweed hosts played a more significant role at the species level. These findings suggest that CFP risk is relatively low on the reefs studied but natural reefs would likely be preferentially colonized by Gambierdiscus spp. should ambient conditions become appropriate.
Introduction
Efforts to evaluate the ecological function and significance of artificial reefs often focus on fish assemblages and secondary productivity (Paxton et al., 2020; Rouse et al., 2020). However, it is also important to consider the role of microbial organisms such as epiphytic microalgae which are found living on host organism such as macroalgae, seagrasses or corals. Epiphytes are important primary producers on reefs, attracting fish and other pelagic organisms (Thornber et al., 2016). These epiphytic organisms produce organic carbon via photosynthesis, play important roles in nutrient cycling and influence the composition of invertebrates and other higher trophic level taxa. Thus, understanding how epiphytes respond to different artificial reef materials is a potentially important, but largely overlooked consideration of artificial reef design.
A particular epiphyte of reef macroalgae, Gambierdiscus spp., is known for causing ciguatera fish poisoning (CFP) through the production of ciguatoxins and maitotoxins (Holland et al., 2013). These toxins do not directly affect fish but bioaccumulate via herbivory and result in severe symptoms when contaminated fish is consumed by humans. CFP is the most commonly reported seafood-related illness worldwide (Friedman et al., 2008), making it important to understand the conditions that encourage Gambierdiscus spp. presence. Gambierdiscus spp. are most prevalent in tropical regions as the maximum growth rates of most species occur at high water temperatures, with optimal temperature being 27°C (Xu et al., 2016). However, due to the proximity of the Gulf Stream to the coast of North Carolina, the warm temperatures have allowed tropical macroalgae and their associated Gambierdiscus spp. epiphytes to thrive south of Cape Hatteras (Mallin et al., 2000). The presence of Gambierdiscus spp. in North Carolina has been recorded several times (Litaker et al., 2009; Litaker et al., 2010) and a cluster of CFP cases occurred in the state in 2007 (CDC, 2009). Incidences of CFP are expected to increase as global warming extends the range and increases the abundance of Gambierdiscus spp. in North Carolina (Kibler et al., 2015). Community composition of epiphytes can be affected by a variety of factors, ranging from physical factors like temperature and depth, to biochemical conditions such as light and nutrient availability. Different artificial reef construction materials can alter the assemblage of microbial biofilms (Caruso, 2020; Guo et al., 2021), epibenthic invertebrate communities (Ushiama et al., 2016) and even fish communities (Lemoine et al., 2019). Substrate properties such as hydrophobicity, surface polarization and roughness may all affect patterns of bacterial adhesion (Caruso, 2020). Additionally, the materials chosen may affect the chemical composition of the surrounding water column as the structures succumb to corrosion. For example, steel structures (e.g. shipwrecks) may leach iron into the surrounding waters affecting productivity of microalage (Marchetti et al., 2006; MacLeod, 2016). Certain types of concrete, particularly those made of “green” or uncured cement may secrete calcium hydroxide and cause an increase in pH, making the surface of such substrates toxic to some invertebrates (Ballard et al., 2020). Therefore, different materials play both a physical and a chemical role in determining the formation and composition of biofilm communities. In turn, biofilms influence ecosystem functioning by affecting the settlement of macroalgal spores, coral larvae and other sessile marine organisms (Caruso, 2020).
Given that different artificial reef materials can affect the composition of other assemblages, it is reasonable to expect differential impacts on the composition and abundance of epiphytes, including Gambierdiscus spp. If certain materials encourage the growth of Gambierdiscus spp., it may result in a higher proportion of ciguateric fish around the artificial reef, thus increasing the likelihood of CFP due to the consumption of contaminated fish. This paper investigates the relationship between artificial reef material and the abundance of Gambierdiscus spp. on artificial reef macroalgae.
We hypothesized that Gambierdiscus spp. will be more abundant in macroalgae collected from steel structures (i.e., vessels), as the increased iron concentration will support the growth of macroalgae and the associated epiphytic microalgae (Marchetti et al., 2006; Rodriguez et al., 2016). To test this hypothesis, we sampled 13 artificial and natural reef sites off the coast of North Carolina, USA and examined the epiphyte communities using microscopy and molecular techniques. Additionally, we performed manipulative ex situ growth experiments on Gambierdiscus spp. to determine substrate preferences. We also assessed the abundance and species diversity of the total epiphyte community in order to describe the variations between different artificial reef materials. Ultimately, these results can be used to further assess the suitability of the materials currently being employed to construct artificial reefs.
Methods
Site selection
Macroalgae samples were collected from six natural and seven artificial reefs off the coast of North Carolina from 18th to 27th June 2021, 27th September 2021, and 6th to 19th June 2022 (Figure 1; Table S1). Natural reefs were composed of calcium carbonate rock whereas artificial reefs were composed of a variety of concrete or steel structures that were placed on the sea floor.
Qualitative survey of epiphyte population
In summer 2021, a survey was performed to fine tune sampling protocols and gain a general understanding of the epiphyte populations on North Carolina’s reefs. Single samples of macroalgae were collected from 3 concrete reefs, 2 steel reefs and 3 natural reefs, yielding 8 total samples. The samples were collected in gallon sized zipper locking bags along with ambient seawater. Epiphytes were extracted by shaking the bag vigorously to dislodge any attached cells. The resultant suspension was then transferred to a 40 mL scintillation vial and preserved with 1% Lugol solution. Upon return to the lab, the suspension was passed through a ~200 µm nylon mesh to remove pieces of macroalgae and debris. Subsequently, 1 mL of the suspension was pipetted onto a settling chamber and was left to settle for an hour. The 10 most common cell types from each sample were identified and enumerated using a Leica DMIRB inverted light microscope at 200X magnification. At least 400 cells or 100 fields of view were counted, and the resultant values were used to determine cell density (cells/mL).
Quantitative survey of epiphyte population
In 2022, the survey was repeated with improved sampling protocols to ensure better standardization of samples. Triplicate macroalgae samples were collected from 3 reefs of each material, resulting in 27 samples from 9 distinct sites. The samples were placed in quart zipper locking plastic bags along with ambient seawater and sealed for return to the dive vessel. Upon return to the lab, the macroalgae samples were transferred into a 1 L bottle filled with 0.2 µm filtered seawater. Replacing ambient seawater with filtered seawater ensured that any phytoplankton in the ambient seawater was not incorporated into the sample. The samples were then shaken vigorously to dislodge epiphytes including Gambierdiscus spp. Between 100 to 250 mL of the resultant suspension was passed through a 0.45 µm polyethersulfone (PES) filter via vacuum filtration. The filters were then stored at -80 °C pending DNA extraction. An additional 40 mL of the suspension samples were filtered through a ~200 µm nylon mesh to remove pieces of macroalgae and debris before being transferred into a sample preservation vial and fixed with 1% Lugol. The preserved samples were stored in the dark at room temperature until enumeration. Each macroalgae sample was patted dry and weighed before being identified to the genus level according to Schneider and Searles (1991).
Microscopic analysis of samples collected in 2022 was performed as for the 2021 samples described above. However, cell density was normalized by macroalgae biomass, resulting in units of cells/mL/g. This ensured that reported cell densities are not affected by differences in the amounts of macroalgae biomass collected between sites. Species diversity was calculated using the Shannon-Wiener diversity index. Because a fixed number of cells (n = 10) were counted in each sample, the richness is constant across all materials and the resultant value is better interpreted as species evenness. A one-way analysis of variance (ANOVA) was used to determine any significant difference of microalgae abundance and species diversity between the materials tested.
The V4 region of the 18S rRNA gene was sequenced to obtain taxonomic identification of epiphyte composition. DNA was extracted from the filters using the DNeasy Plant Mini Kit (QIAGEN, Hilden, Germany). Cells collected on the 0.45 µm PES filter were lysed using 0.2 g of sterile glass beads and 400 µl of buffer AP1 (QIAGEN) placed in a mini-beadbeater at 4800 rpm for 1 minute. Subsequent extraction and purification were performed according to manufacturer’s instructions. 18S gene fragments were amplified via polymerase chain reaction (PCR) using the following V4 primer sets: 18S forward (5’-CAGCASCYGCGGTAATTCC-3′) and reverse (5′-ACTTTCGTTCTTGAT-3′). These primers were modified according to Lin et al. (2017) to improve sequencing quality. PCR reactions were prepared using half reactions of the TaKaRa Ex Taq® DNA Polymerase Hot-Start Version kit to obtain a final reaction volume of 25 µl. 10 µM of primer was added to each reaction mixture. PCR was conducted with an initial activation step at 98°C for 1 minute, followed by 4 three-step cycles of 94°C for 30s, 45°C for 45s, 72°C for 60s, another 30 three-step cycles of 94˚C for 30s, 57°C for 45s, 72°C for 60s, and a final extension step of 72°C for 2 minutes. PCR was performed in triplicates for each sample and checked using 1% agarose gel electrophoresis to ensure a clear band was obtained. The PCR products were purified using the QIAquick PCR Purification kit and quantified using a Qubit dsDNA assay. Amplicons from each sample site were pooled in equimolar amounts and submitted to UNC’s High Throughput Sequencing Facility for sequencing using the Illumina MiSeq platform.
Demultiplexing was carried out using tools from QIIME 1.9.1 and Cutadapt 1.18. The averaged read count was 83206 after demultiplexing. The DADA2 tool in the software pipeline QIIME 2.0 was used to denoise and join paired ends, and remove chimeras. Resultant sequences were then clustered at a 97% similarity to obtain operational taxonomic units (OTUs). Taxonomic assignment of OTUs was performed using the PR2 database (version 4.14.0). OTUs classified as Metazoans or macroalgae were removed from the analyses.
Analysis of species composition was performed using R version 4.1.2 (R Core Team, 2021). The vegan package (Oksanen et al., 2022) was used to perform a non-metric multidimensional scaling analysis on the taxonomic data to understand differences in epiphyte composition. The distance between two points on an NMDS ordination plot is proportional to the dissimilarity of species composition of the respective samples. Analysis of similarities (ANOSIM, 9999 permutations) test was subsequently used to evaluate dissimilarity of species composition based on reef material and algae type. The ANOSIM R value describes the dissimilarity between groups, with a value close to 1 indicating high dissimilarity and a value close to 0 suggesting even distributions.
Ex-situ Gambierdiscus spp. substrate preference experiment
Gambierdiscus spp. preferences for different artificial reef materials were experimentally determined using a modified version of the procedure performed by Mustapa et al. (2019) and Rains and Parsons (2015) to establish Gambierdiscus spp.’s preferred macroalgal hosts.
A Gambierdiscus culture was provided by Mark Vandersea from NOAA’s National Center of Coastal Ocean Science (NCCOS). The culture contained Gambierdiscus carolinianus and Gambierdiscus caribaeus, two species that are most commonly found in NC’s offshore waters. Twelve mL of L1 growth media (Guillard and Hargraves, 1993) was pipetted into each well of two 6-well culture plates. To each well, 200 µL of Gambierdiscus culture was added, resulting in 812-1113 cells/well (average 984 cells/well). The culture plates were placed in an incubator at 26˚C, under a 12:12h light:dark photocycle for 5 days. Prior to start of the experiment, the number of Gambierdiscus cells were enumerated using inverted microscopy.
Because the experiment aimed to differentiate between materials, the macroalgae host was standardized across the various treatments. Padina spp. was collected from a rocky surface on Radio Island (34.7074°N, -76.6802°W, Morehead City, NC) along with ambient seawater in an 800 mL glass jar. Upon return to the lab, the sample was shaken vigorously for at least one minute to remove any epiphytes. Then, algal thalli were cut into fragments between 85 mg to 95 mg net weight.
The reef substrate materials selected were concrete, steel and marl. Concrete and steel are the two most popular materials deployed as artificial reefs in North Carolina (Comer and Love-Adrick, 2016). Marl was chosen to represent natural rocky reefs as it is a sedimentary carbonate-based rock. The materials were cut into pieces with similar surface areas that could fit into the wells of the culture plates. The mass and surface area of each material were recorded (Table 1). Each piece was placed in a separate well and topped up with 12 mL of 0.2 µL-filtered seawater. The Padina spp, fragments were carefully placed on top of each material. The culture plates were then covered and placed in the incubator at the same culture settings as mentioned above. The fragments were acclimatized with the different materials for 5 days, with daily water changes to remove any harmful excretions released from the cutting of algal thalli before the addition of Gambierdiscus cells (Rains and Parsons, 2015).
The reef substrate materials and Padina samples were transferred from culture well plates containing filtered seawater to wells containing growth media and Gambierdiscus cells. The experiment was conducted in triplicates, with three reef substrate treatments and a control containing only the macroalgae fragment and no substrate. The incubation was conducted at 26°C, under a 12:12 h light:dark photocycle for a total of 27 days. Gambierdiscus cell counts were performed daily for the first 5 days and twice a week subsequently (Days 10, 13, 17, 20, 24 and 27). Changes of fresh media were performed weekly by carefully removing 6 mL media with a transfer pipette from the surface of each well to prevent disturbing Gambierdiscus cells on the bottom and replacing it with 6mL of fresh media. This was done on days when cell counts were not being done, to prevent counts from being affected by the media change.
Specific growth rate [µ (d-1)] was calculated after each cell count across the 27 days using the following equation:
where Nt and Nt-1 are the number of Gambierdiscus cells at the end and beginning of each growth period spanning Δt amount of time. A repeated measured ANOVA followed by post-hoc Tukey test was used to determine significant differences of Gambierdiscus cell counts over the 27-day incubation period. One-way ANOVA and Tukey test were performed on average growth rates. α was set at 0.05 for all hypothesis tests.
Results
Qualitative sampling results
In 2021, two major classes – diatoms and dinoflagellates – were observed within the samples. Diatoms were further classified into centric diatoms, which have radial symmetry, and pennate diatoms, which have bilateral symmetry. Dinoflagellates were further classified into desmokonts, a cell type with two flagella emerging from the anterior of the cell, and dinokonts, in which the two flagella are inserted ventrally; one is transverse and the other is longitudinal (Tomas, 1997).
No Gambierdiscus spp. were detected in the artificial and rocky reef samples. Epiphyte assemblages were dominated by centric diatoms at all sites. Notably, one genus Leptocylindrus accounted for most centric diatoms observed. Pennate diatoms were the second most abundant group observed and were significantly more diverse, consisting of Navicula spp., Cylindrotheca spp., Licmophora spp., Thalassionema sp., Gyrosigma spp., Pleurosigma spp., Striatella sp. and other unidentified pennate diatoms. Dinoflagellates (Prorocentrum sp. and Warnowia sp.) were only observed in a few sites and made up a very small proportion of the total microalgae cells. The two steel sites (AR-386 and AR-345) were the only sites that had both dinokont and desmokont dinoflagellates. Average epiphyte abundance was highest at steel sites and lowest at concrete sites.
Quantitative sampling results
Microscopy
In 2022, Gambierdiscus spp. were again not detected in the artificial and rocky reef samples. Three classes of epiphytes – diatoms, dinoflagellates and cyanobacteria – were observed within the samples (Figure 2A). Pennate diatoms dominated both the relative proportion of cells and the percentage of identified taxa within all samples, followed by centric diatoms, then dinoflagellates and sparingly observed cyanobacteria. Pennate diatoms showed much more diversity across the samples and made up about 75% of the identified taxa (Figure 2B). No significant differences in cell density (Figure 2C) or species evenness (Figure 2D) were observed across the three materials. However, steel had the highest median value, closely followed by concrete and then natural materials. In terms of species evenness, steel once again had the highest median, followed by concrete and then natural materials.
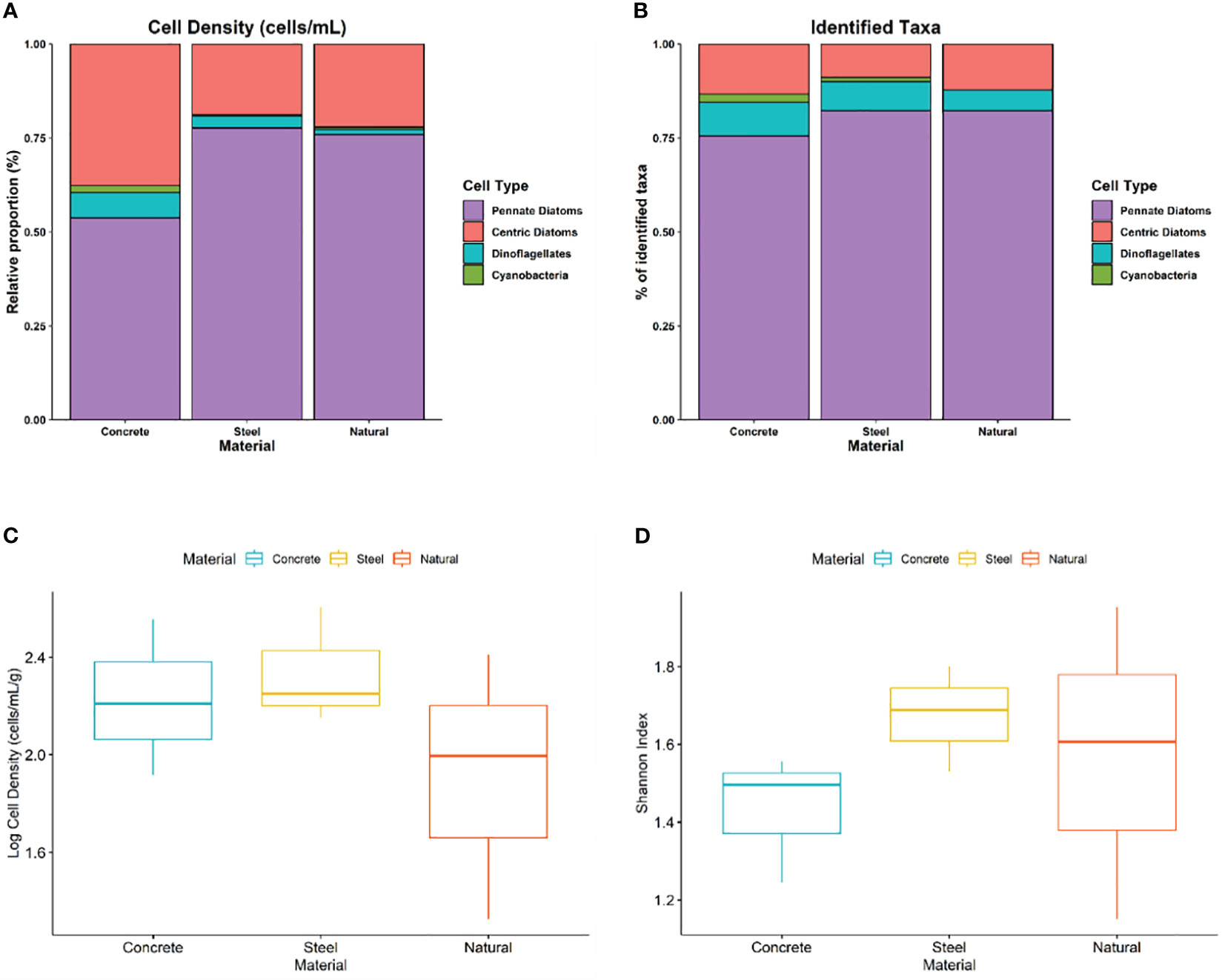
Figure 2 (A) Relative proportion of cell type observed across each material. (B) Percentage of identified taxa across each material. (C) Distribution of epiphyte abundance (cells/mL/g) based on material of each reef (F = 0.96, df = 2, p = 0.435). (D) Distribution of Shannon diversity index based on material of each reef (F = 0.63, df =2, p = 0.565).
18s rRNA analysis
As with microscopy, Gambierdiscus spp. were not detected via 18S rRNA gene analysis. Taxonomic identification via molecular techniques is a valuable complement to microscopy as it provides a much higher taxonomic resolution. 18s rRNA gene analysis ensures that all cells within the samples are accounted for, providing a more complete picture of variations in species composition across sampled sites compared to microscopy.
Epiphyte composition was very comparable across the reef sites, dominated either by phylum Ochrophyta or Pyrrophyta (Figure 3A). However, certain trends were observed across the different reef materials. The concrete reefs tend to be dominated by phylum Ochrophyta which includes diatoms, whereas natural reefs appear to favor phylum Pyrrophyta (dinoflagellates) over Ochrophyta. Steel reefs displayed more variability than concrete reefs, as steel reef number 1 (S1) was dominated by phylum Pyrrophyta but S2 and S3 had a higher proportion of phylum Ocrophyta.
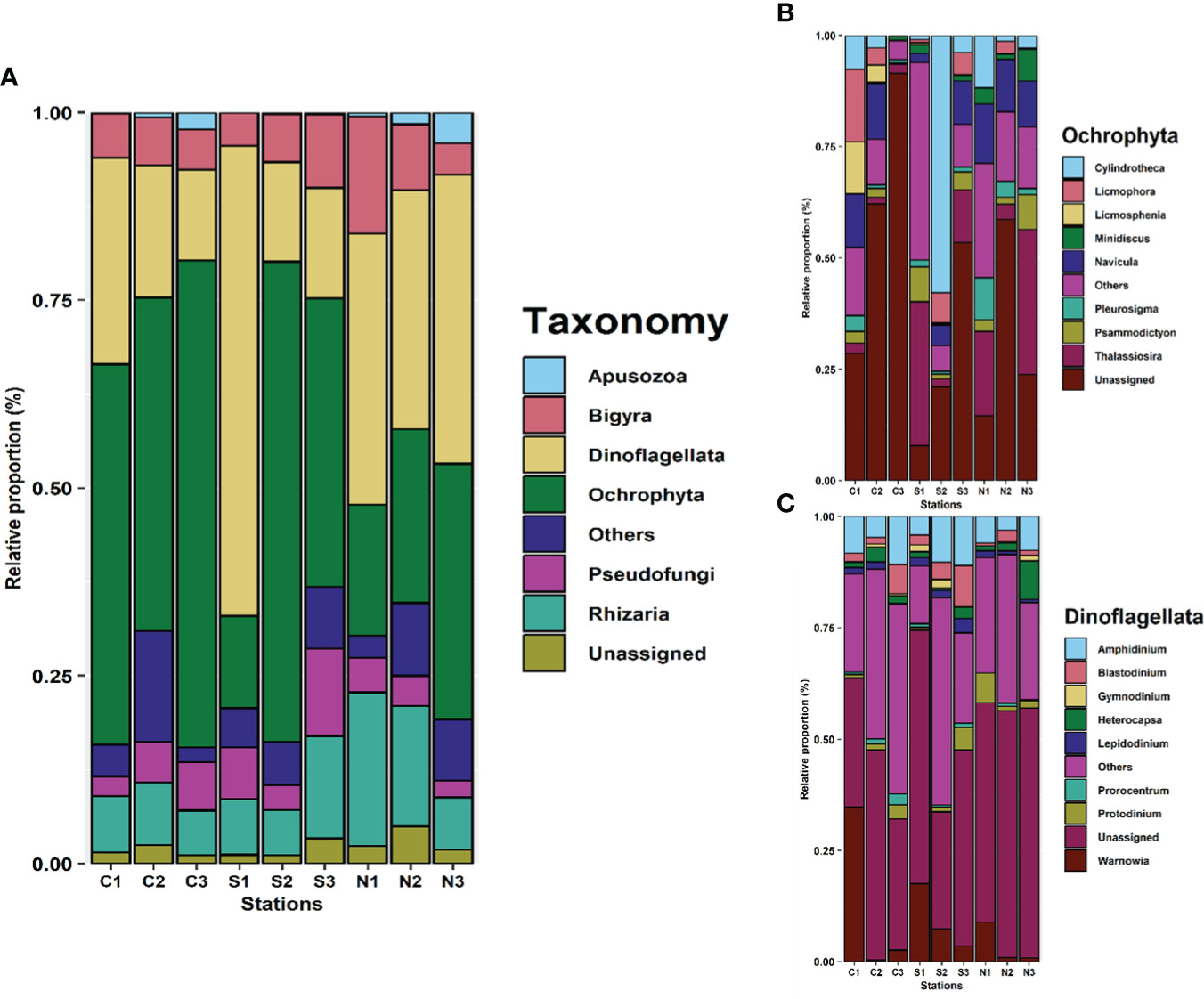
Figure 3 (A) Taxonomy bar plot showing relative proportions of major phyla identified. Triplicates were collected at each site but are presented in aggregate for simplicity. C1, C2 and C3 are the three concrete reefs, S1, S2 and S3 are the three steel reefs and N1, N2 and N3 are the three natural reefs. (B) Taxonomic breakdown of diatoms (Ocrophyta). (C) Taxonomic breakdown of dinoflagellates (Pyrophyta).
Pyrrophyta and Ochrophyta were further broken down into genera. Ochrophyta composition varied significantly across the sites, but frequently observed genera included Cylindrotheca, Licmophora, Navicula and Thalassiosira (Figure 3B). These genera were also observed via microscopy, which corroborates with the 18s rRNA analysis. Pyrrophyta composition was more consistent across sites with Warnowia dominating most samples (Figure 3C).
Although triplicate samples were collected at each site, constraints in seaweed distributions resulted in certain sites with different macroalgae types across the triplicates. As such, both seaweed type and reef material were analyzed. ANOSIM results indicated that there was no significant influence of reef material on epiphyte species composition. Seaweed type, however, resulted in significant differences, with the epiphyte composition of brown alga Sargassum sp. and Dictyota sp. clearly separating from red algae and other brown alga species (Figure 4).
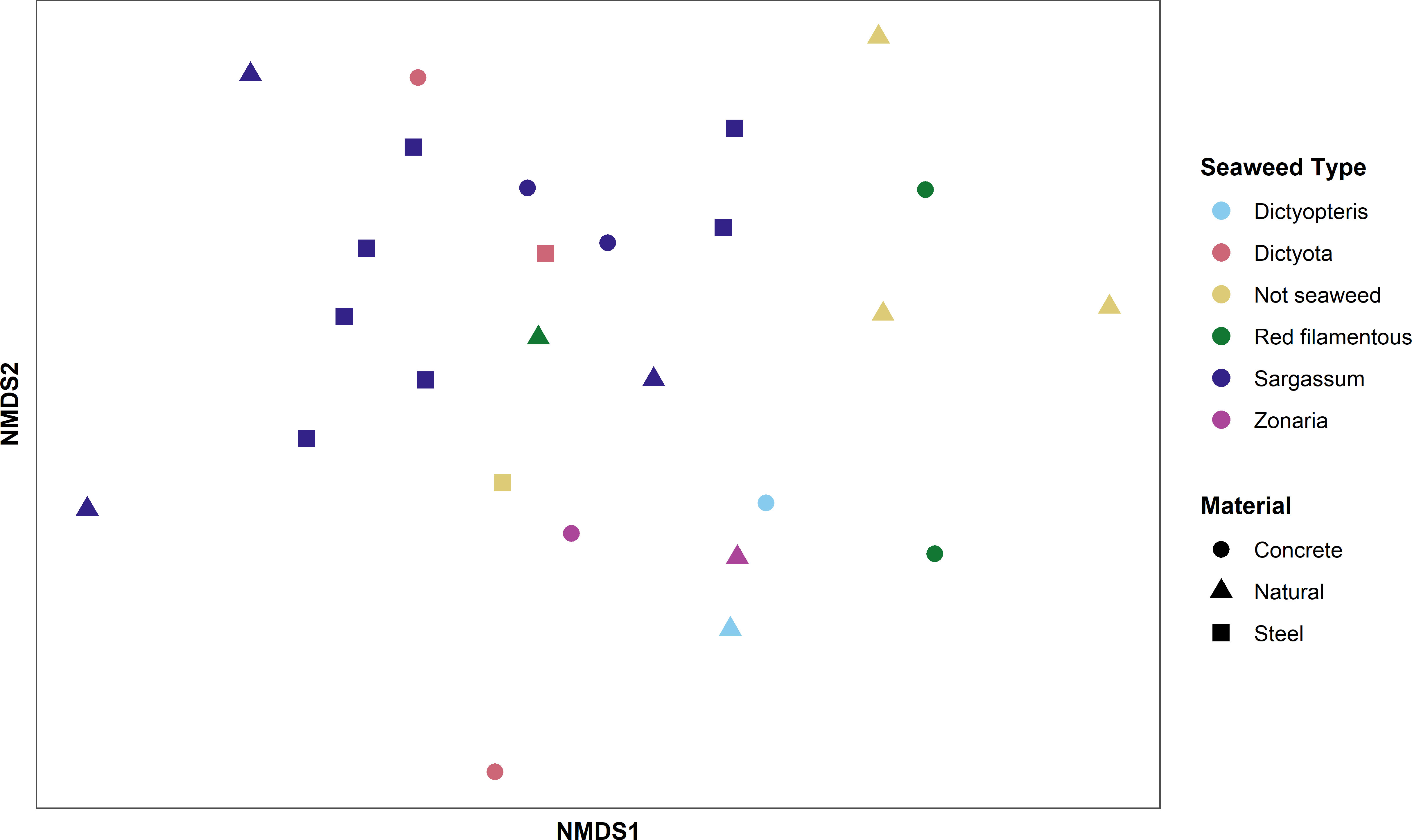
Figure 4 Non-metric multidimensional scaling (NMDS) plot by material and seaweed type. The shape of the points corresponds to the material of the reef the samples were collected from. Stress: 0.237, ANOSIM R = 0.067, significance = 0.103. The color of the points corresponds to the seaweed type the samples were collected from. Stress: 0.219, ANOSIM R = 0.407, significance = 0.002.
Ex-situ Gambierdiscus spp. substrate preference experiment
Gambierdiscus cells incubated with marl and the control experienced a net increase over the 27-day incubation (Figure 5A). Cells incubated with concrete increased up to day 20 before decreasing, resulting in a near zero growth rate over the full 27 d experimental period. The steel treatment caused a steady decrease in Gambierdiscsus cells over the incubation, with no cells being detected after day 24. Repeated measures ANOVA and Tukey test indicated significant differences between steel and marl (p = 0.027) and between steel and control (p = 0.045).
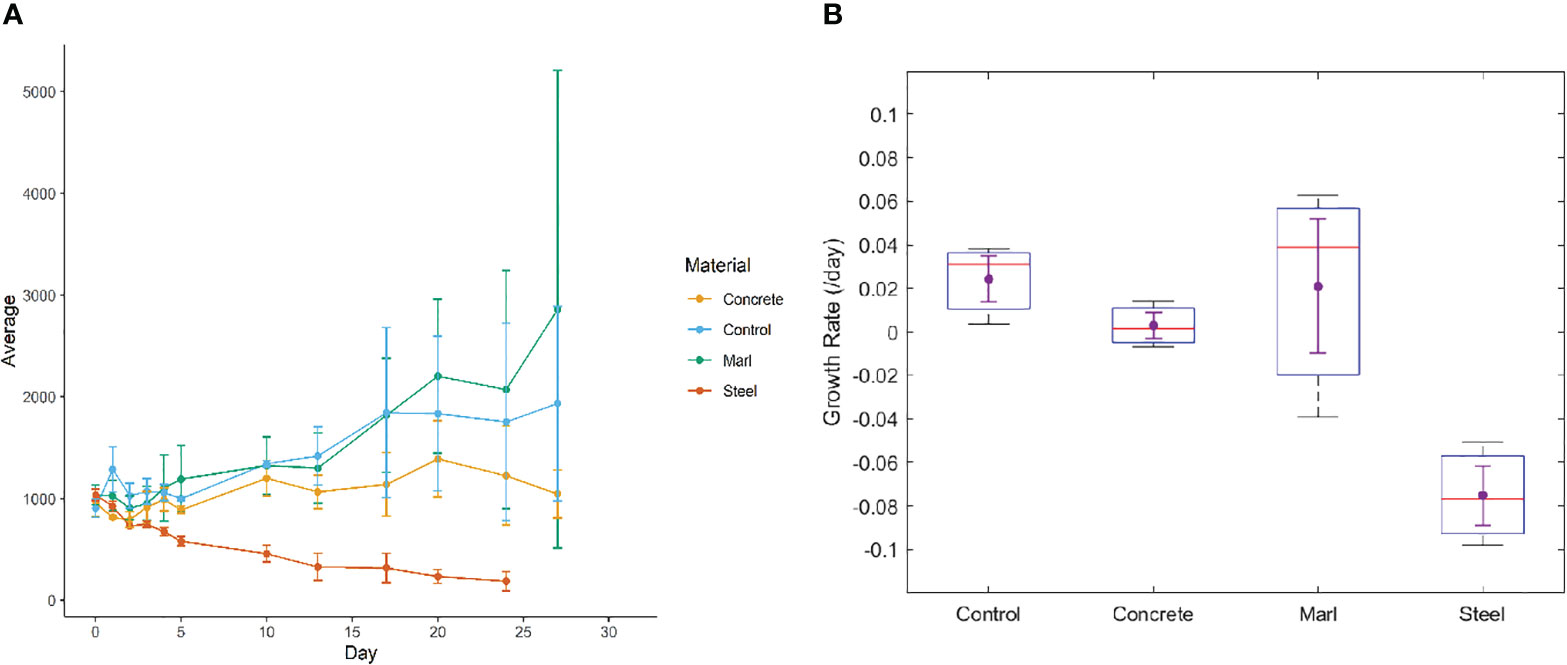
Figure 5 (A) Changes in mean number of Gambierdiscus cells across triplicate control and material treatment over the 27 days of incubation. Error bars shown are standard deviation. (B) Growth rate (day-1) of Gambierdiscus spp. across different treatments, as represented by the x-axis labels. The boxplot represents the distribution of growth rate data, with the whiskers indicating minimum and maximum growth rates, the box indicating the interquartile range and the red line indicating the median growth rate. Dots represent means with standard error bars.
Apart from marl, all treatments resulted in growth rates lower than the control (Figure 5B). Average growth rate of Gambierdiscus spp. ranged from -0.075 day-1 to 0.024 day-1. The highest growth rate was observed in the marl treatment (0.062 day-1) and the lowest growth rate was observed in the steel treatment (-0.098 day-1). As with the raw cell counts, there were significant differences between steel and marl (p = 0.022) and between steel and control (p = 0.018). Marl and control treatments resulted in very similar average growth rates (p = 0.999).
Discussion
Prevalence of Gambierdiscus spp.
The lack of Gambierdiscus spp. within the samples collected can likely be attributed to water temperatures at the sampled sites. Throughout the sampling periods, sea surface temperature at the sampled sites did not reach 27°C (Figure S1). Temperatures at depth ranged from 25-26°C (Figure S2). As a result, water temperatures at the sampled sites may not be sufficiently warm to support the proliferation of Gambierdsicus spp., resulting in no detection of the toxic dinoflagellate. Notably, sampling was only carried out during the summertime and does not represent community composition throughout the year. It is possible that monthly sampling across a year would result in detection of Gambierdiscus spp. That being said, artificial and natural reefs located further offshore experience warmer temperatures as they are closer to the Gulf Stream and may harbor higher abundances of Gambierdiscus spp. In fact, Gambierdiscus spp. have been identified from a number of offshore sites by Litaker et al. (Litaker et al., 2009; Litaker et al., 2010). It appears that the prevalence of Gambierdiscus spp. is currently limited to offshore sites closer to the Gulfstream due to warmer water temperatures compared to shallower, nearshore sites. Artificial reefs are often created to provide fishing sites and consequently, many of these sites are popular recreational fishing spots. Thus, the detection of Gambierdiscus spp. on artificial reefs will have serious public health implications. With rising ocean temperatures, the range of Gambierdiscus spp. is expected to expand and allow them to spread further inshore (Kibler et al., 2015). Long-term monitoring of artificial and natural reefs will be necessary to understand the distribution of Gambierdiscus spp. and manage the risk of CFP.
Trends observed using microscopy
Epiphyte assemblages were dominated by pennate diatoms at all sites. Pennate diatoms were also the most diverse group observed, consisting of Navicula spp., Cylindrotheca spp., Licmophora spp., Thalassionema sp., Gyrosigma spp., Pleurosigma spp., Striatella sp. and other unidentified pennate diatoms. Dinoflagellates (Prorocentrum sp. and Warnowia sp.) and cyanobacteria (Oscillatoria sp.) were only observed in a few sites and made up a very small proportion of the total microalgae cells. The low abundance of dinoflagellates may indicate that these sites are not optimal environments for epiphytic dinoflagellates and further explain the lack of Gambierdiscus spp.
Overall, average epiphyte abundance was very similar between the concrete and the natural sites, whereas the steel sites had the highest median cell density and diversity of epiphytes. This indicates that concrete may be better at mimicking a natural assemblage whereas steel can promote increased biomass. Intriguingly, this trend was also observed within fish assemblages by Lemoine et al. (2019) on some of the same sites that we sampled here. They noted that concrete artificial reefs supported similar fish assemblages to natural reefs while steel artificial reefs had distinct assemblages but higher biomass and diversity. The similar trends in both epiphyte and fish composition suggests a potential relationship between epiphyte community composition and the resulting fish populations. On the other hand, both trends could have occurred independently of each other in response to the characteristics of the artificial reef materials. However, due to the small sample size examined, further research and observations are necessary to understand the mechanisms that drive the trends observed in this study.
Species composition based on 18S rRNA gene analysis
Evaluating epiphyte composition using microscopy tends to favor cells such as diatoms and heterotrophic dinoflagellates as their large size makes them more easily discernable while smaller cells tend to be overlooked during this process (Gong et al., 2020). Molecular analysis of species composition yields a better understanding of epiphyte assemblages observed at each site.
DNA samples yielded similar results to cell counts with diatoms constituting the largest proportion of epiphytes detected, but dinoflagellates also contributed to a significant fraction. Dinoflagellates tend to have larger genome sizes and thus high 18S gene copy numbers (Gong and Marchetti, 2019), which would account for the higher representation of dinoflagellates via 18S sequencing compared to cell counts. Although epiphyte composition was relatively consistent across the sampled sites, notable trends were observed with natural reefs being dominated by dinoflagellates, while concrete reefs were dominated by diatoms (Figure 3A).
The NMDS analysis (Figure 4) indicated that seaweed type plays a more important role on determining epiphyte composition compared to reef material. Consequently, it is reasonable to infer that seaweed type might also affect Gambierdiscus spp. abundance. However, prior research examining the interactions between host seaweeds and Gambierdiscus spp. present conflicting levels of host preference. Lee et al. (2020) found that Gambierdiscus spp. were more common in turf algae and some fleshy macroalgae habitats whereas Mustapa et al. (2019) observed no specific preference for macroalgal types. With this in mind, it is difficult to predict which macroalgae may be more favorable to Gambierdiscus spp., despite the observed influence of seaweed type on epiphyte composition.
Additionally, reef material and seaweed type do not sufficiently explain the dissimilarities observed in epiphyte communities. The outlier point, station C-1-B was collected from concrete reef AR465. All three macroalgae samples collected from this site were Sargassum algae, yet C-1-B had a drastically different species composition compared to the other two replicates. This indicates that there are other factors which drive epiphyte diversity which have not been considered in this study.
Gambierdiscus spp. substrate preference
Gambierdiscus cells in the marl treatment had a similar average growth rate to the control but experienced larger variations. Both steel and concrete treatments resulted in an average growth rate lower than that of the control. None of the treatments resulted in a substantial increase in Gambierdiscus cells, but Gambierdiscus spp. displayed a slight preference for marl over both concrete and steel. This implies that natural rocky reefs may harbor more Gambierdiscus spp. and have a higher risk of CFP. Interestingly, this aligns with the molecular data showing that natural reefs were more likely to be dominated by dinoflagellates compared to the steel and concrete reefs. The experimental results also suggest that the presence of an artificial substrate may not drive the proliferation of Gambierdiscus spp., yet additional research is needed to further explore whether steel and concrete can be utilized as artificial reefs without concern for increased risk of CFP.
The use of steel to construct artificial reefs may be beneficial in reducing the abundance of Gambierdiscus spp. should it spread further inshore with rising ocean temperatures. Given that the steel treatment resulted in a steady decrease in Gambierdiscus cells over time, increasing the number of steel artificial reefs may be a potential solution to limiting the prevalence of the toxic dinoflagellate. That said, there are some limitations to the current experimental design that may interfere with the reliability of the results. Rusting of the steel materials resulted in a significant production of rust particles within the steel treatment wells (Figure 6). Gambierdiscus cells could generally be distinguished from the rust particles, however it is possible that some cells were obscured, creating the appearance of fewer cells than were actually present.
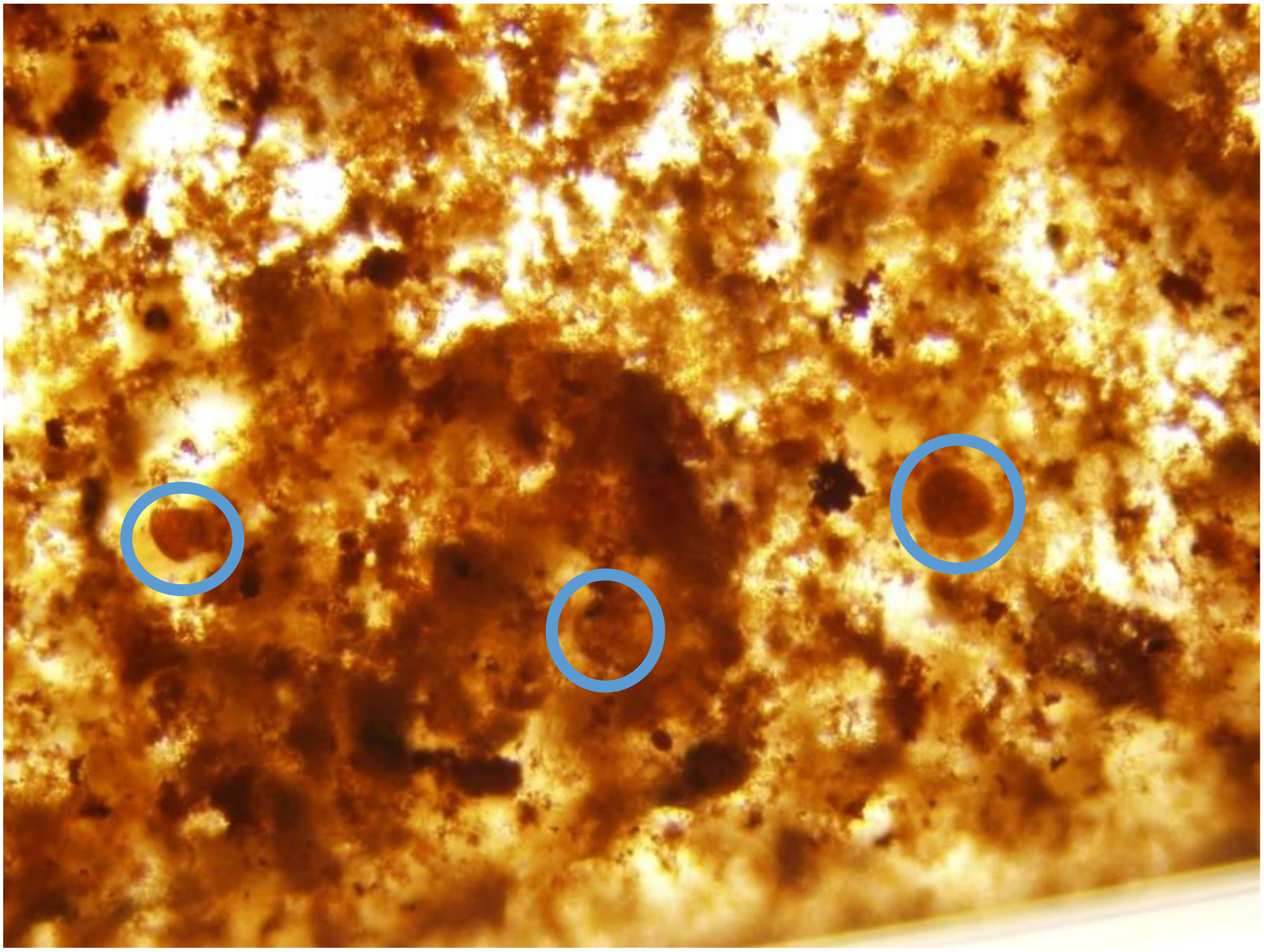
Figure 6 Image of rust particles occurring in steel treatment over the course of the experiment. Gambierdiscus cells are circled in blue.
To overcome these challenges, it would be ideal to set up mesocosm experiments with multiple algae hosts attached to structures of different materials. Addition of Gambierdiscus cells into such controlled environments will allow us to better understand the combined impact of reef substrate material and macroalgal hosts, while eliminating confounding factors that would occur in the natural environment.
Conclusion
The lack of Gambierdiscus spp. observed in the artificial and natural reef samples indicated these sites carry low risk of exposure to CFP, at the time of our sample collection.However, many popular fishing sites are located further offshore in deeper waters where warmer water temperatures may be more favorable to Gambierdiscus spp. Additionally, rising sea temperatures may result in range expansion of Gambierdiscus spp., increasing the occurrence of ciguatoxins in North Carolina waters. It is important to continue monitoring both artificial and natural reefs to understand how Gambierdiscus populations are shifting and to implement proper policies which reduce the risk and minimize occurrences of CFP in North Carolina.
This study also sheds light on how artificial reef materials relate to epiphyte composition. The trends in epiphyte assemblage align with trends observed in fish assemblages by Lemoine et al. (2019), with concrete artificial reefs supporting similar communities to natural reefs while steel artificial reefs led to increases in biomass and diversity. This demonstrates a potential relationship between epiphyte composition and fish populations, but more research is needed to better understand the interactions between epiphytic microalgae and pelagic fish populations, as well as how interactions may be mediated by reef characteristics, such as area and vertical relief.
Ultimately, it appears that the reef substrate material does not play as significant of a role in determining epiphyte composition compared to seaweed type, particularly within the shallow reefs sampled in this study. Thus, the optimal artificial reef construction material is dependent on the goal of resource managers. If the goal is to create habitats that mimic natural rocky reefs, then concrete or marl should be used. On the other hand, if the goal is to increase biomass and diversity of the resultant communities, then steel may be the better option. Steel materials may also be able to limit Gambierdiscus spp. growth and help manage the risk of CFP. While deeper reef sites were not sampled, it is reasonable to assume similar material-based trends would occur at those sites.
Data availability statement
This Targeted Locus Study project has been deposited at DDBJ/EMBL/GenBankunder the accession KIAW00000000. The version described in this paperis the first version, KIAW01000000.
Author contributions
PL conducted the experiments, data analysis and writing. AP and CT assisted in sample collection and editing. NH served as the Principal Investigator and supervised the entire project. All authors contributed to the article and approved the submitted version.
Acknowledgments
We would like to thank Dr Adrian Marchetti for providing sampling equipment and laboratory space required to perform molecular analyses, Dr. Mark Vandersea for providing the Gambierdiscus cell culture, Dr. Wilson Freshwater for assisting in macroalgae identification, Virginia Gilliland and the crew of the NOAA Ship Nancy Foster for their help with sample collection and processing. We thank Mark Vandersea, Alex Hounshell, and Tomma Barnes for thoughtful reviews of the manuscript. This project was supported by the North Carolina Coastal Recreational Fishing License Grant, the Knoble Family Honors Carolina Excellence Fund administered by Honors Carolina, the Sherry Reed Memorial Undergraduate Marine Conservation Scholarship sponsored by the family and friends of Women Divers Hall of Fame Member Sherry Reed, and the Explorers Club Rising Explorer Grant. The views and conclusions contained in this document are those of the authors and should not be interpreted as representing the opinions or policies of the US Government, nor does mention of trade names or commercial products constitute endorsement or recommendation for use.
Conflict of interest
The authors declare that the research was conducted in the absence of any commercial or financial relationships that could be construed as a potential conflict of interest.
Publisher’s note
All claims expressed in this article are solely those of the authors and do not necessarily represent those of their affiliated organizations, or those of the publisher, the editors and the reviewers. Any product that may be evaluated in this article, or claim that may be made by its manufacturer, is not guaranteed or endorsed by the publisher.
Supplementary material
The Supplementary Material for this article can be found online at: https://www.frontiersin.org/articles/10.3389/fmars.2023.1232524/full#supplementary-material
Supplementary Figure 1 | Sea surface temperatures overlaid with location of sampled sites on 27th September 2021 (NOAA GOES, n.d.)
Supplementary Figure 2 | Water temperatures on each sampling day recorded by dive computer during surveys. Red dashed line indicates 27°C, the optimal growing temperature for Gambierdiscus spp.
Supplementary Table 1 | Coordinates (decimal degrees) and reef substrate material of sampled sites. AR designated artificial reef. NR designates natural reef.
References
Caruso G. (2020). Microbial colonization in marine environments: Overview of current knowledge and emerging research topics. J. Mar. Sci. Eng. 8 (2), 78. doi: 10.3390/jmse8020078
CDC (2009) Cluster of Ciguatera Fish Poisoning. Available at: https://www.cdc.gov/Mmwr/preview/mmwrhtml/mm5811a3.htm.
Comer A. M., Love-Adrick R. (2016). North Carolina Division of Marine Fisheries Artificial Reef Guide.
Friedman M. A., Fleming L. E., Fernandez M., Bienfang P., Schrank K., Dickey R., et al. (2008). Ciguatera fish poisoning: Treatment, prevention and management. Mar. Drugs 6 (3), 456–479. doi: 10.3390/md6030456
Gong W., Hall N., Paerl H., Marchetti A. (2020). Phytoplankton composition in a eutrophic estuary: Comparison of multiple taxonomic approaches and influence of environmental factors. Environ. Microbiol. 22 (11), 4718–4731. doi: 10.1111/1462-2920.15221
Gong W., Marchetti A. (2019). Estimation of 18S gene copy number in marine eukaryotic plankton using a next-generation sequencing approach. Front. Mar. Sci. 6. doi: 10.3389/fmars.2019.00219
Guillard R. R. L., Hargraves P. E. (1993). Stichochrysis immobilis is a diatom, not a chrysophyte. Phycologia 32 (3), 234–236. doi: 10.2216/i0031-8884-32-3-234.1
Guo Z., Wang L., Cong W., Jiang Z., Liang Z. (2021). Comparative analysis of the ecological succession of microbial communities on two artificial reef materials. Microorganisms 9 (1), 120. doi: 10.3390/microorganisms9010120
Holland W. C., Litaker R. W., Tomas C. R., Kibler S. R., Place A. R., Davenport E. D., et al. (2013). Differences in the toxicity of six Gambierdiscus (Dinophyceae) species measured using an in vitro human erythrocyte lysis assay. Toxicon 65, 15–33. doi: 10.1016/j.toxicon.2012.12.016
Kibler S. R., Tester P. A., Kunkel K. E., Moore S. K., Litaker R. W. (2015). Effects of ocean warming on growth and distribution of dinoflagellates associated with ciguatera fish poisoning in the Caribbean. Ecol. Model. 316, 194–210. doi: 10.1016/j.ecolmodel.2015.08.020
Lee L. K., Lim Z. F., Gu H., Chan L. L., Litaker R. W., Tester P. A., et al. (2020). Effects of substratum and depth on benthic harmful dinoflagellate assemblages. Sci. Rep. 10 (1), 11251. doi: 10.1038/s41598-020-68136-6
Lemoine H. R., Paxton A. B., Anisfeld S. C., Rosemond R. C., Peterson C. H. (2019). Selecting the optimal artificial reefs to achieve fish habitat enhancement goals. Biol. Conserv. 238, 108200. doi: 10.1016/j.biocon.2019.108200
Lin Y., Cassar N., Marchetti A., Moreno C., Ducklow H., Li Z. (2017). Specific eukaryotic plankton are good predictors of net community production in the Western Antarctic Peninsula. Sci. Rep. 7 (1), 14845. doi: 10.1038/s41598-017-14109-1
Litaker R. W., Vandersea M. W., Faust M. A., Kibler S. R., Chinain M., Holmes M. J., et al. (2009). Taxonomy of Gambierdiscus including four new species, Gambierdiscus caribaeus, Gambierdiscus carolinianus, Gambierdiscus carpenteri and Gambierdiscus ruetzleri (Gonyaulacales, Dinophyceae). Phycologia 48 (5), 344–390. doi: 10.2216/07-15.1
Litaker R. W., Vandersea M. W., Faust M. A., Kibler S. R., Nau A. W., Holland W. C., et al. (2010). Global distribution of ciguatera causing dinoflagellates in the genus Gambierdiscus. Toxicon 56 (5), 711–730. doi: 10.1016/j.toxicon.2010.05.017
MacLeod I. D. (2016). In-situ corrosion measurements of WWII shipwrecks in Chuuk Lagoon, quantification of decay mechanisms and rates of deterioration. Front. Mar. Sci. 3 (MAR). doi: 10.3389/fmars.2016.00038
Mallin M. A., Burkholder J. M., Cahoon L. B., Posey M. H. (2000). North and South Carolina coasts. Mar. pollut. Bull. 41 (1–6), 56–75. doi: 10.1016/S0025-326X(00)00102-8
Marchetti A., Maldonado M. T., Lane E. S., Harrison P. J. (2006). Iron requirements of the pennate diatom Pseudo-nitzschia: Comparison of oceanic (high-nitrate, low-chlorophyll waters) and coastal species. Limnol. Oceanogr. 51 (5), 2092–2101. doi: 10.4319/lo.2006.51.5.2092
Mustapa N. I., Yong H. L., Lee L. K., Lim Z. F., Lim H. C., Teng S. T., et al. (2019). Growth and epiphytic behavior of three Gambierdiscus species (Dinophyceae) associated with various macroalgal substrates. Harmful Algae 89, 101671. doi: 10.1016/j.hal.2019.101671
NOAA GOES Geostationary Satellite Server. Available at: https://www.goes.noaa.gov/.
Oksanen J., Simpson G. L., Blanchet F. G., Kindt R. (2022) vegan: Community Ecology Package. Available at: https://CRAN.R-project.org/package=vegan.
Paxton A. B., Shertzer K. W., Bacheler N. M., Kellison G. T., Riley K. L., Taylor J. C. (2020). Meta-analysis reveals artificial reefs can be effective tools for fish community enhancement but are not one-size-fits-all. Front. Mar. Sci. 7. doi: 10.3389/fmars.2020.00282
Rains L. K., Parsons M. L. (2015). Gambierdiscus species exhibit different epiphytic behaviors toward a variety of macroalgal hosts. Harmful Algae 49, 29–39. doi: 10.1016/j.hal.2015.08.005
R Core Team (2021) R: A Language and Environment for Statistical Computing. Available at: https://www.R-project.org/.
Rodriguez I. B., Lin S., Ho J., Ho T.-Y. (2016). Effects of trace metal concentrations on the growth of the coral endosymbiont symbiodinium kawagutii. Front. Microbiol. 7. doi: 10.3389/fmicb.2016.00082
Rouse S., Porter J. S., Wilding T. A. (2020). Artificial reef design affects benthic secondary productivity and provision of functional habitat. Ecol. Evol. 10 (4), 2122–2130. doi: 10.1002/ece3.6047
Schneider C. W., Searles R. B. (1991). “Seaweeds of the Southeastern United States,” in Seaweeds of the Southeastern United States (Duke University Press). doi: 10.2307/j.ctv1220hvb
Tomas C. R. (Ed.) (1997). “Identifying Marine Phytoplankton”. (Academic Press). doi: 10.1016/B978-0-12-693018-4.X5000-9
Thornber C., Jones E., Thomsen M. (2016). Epibiont-marine macrophyte assemblages. Mar. Macrophytes as Foundation Species, 43–75. doi: 10.4324/9781315370781-4
Ushiama S., Smith J. A., Suthers I. M., Lowry M., Johnston E. L. (2016). The effects of substratum material and surface orientation on the developing epibenthic community on a designed artificial reef. Biofouling 32 (9), 1049–1060. doi: 10.1080/08927014.2016.1224860
Keywords: Gambierdiscus, artificial reefs, epiphytes, ciguatera fish poisoning, community composition
Citation: Lim P, Paxton AB, Taylor JC and Hall NS (2023) Survey of epiphytic microalgae to evaluate risk of ciguatera fish poisoning across natural and artificial reefs in North Carolina. Front. Mar. Sci. 10:1232524. doi: 10.3389/fmars.2023.1232524
Received: 31 May 2023; Accepted: 16 August 2023;
Published: 11 September 2023.
Edited by:
Andrew Stanley Mount, Clemson University, United StatesReviewed by:
Renato Crespo Pereira, Fluminense Federal University, BrazilMohammad Reza Shokri, Shahid Beheshti University, Iran
Copyright © 2023 Lim, Paxton, Taylor and Hall. This is an open-access article distributed under the terms of the Creative Commons Attribution License (CC BY). The use, distribution or reproduction in other forums is permitted, provided the original author(s) and the copyright owner(s) are credited and that the original publication in this journal is cited, in accordance with accepted academic practice. No use, distribution or reproduction is permitted which does not comply with these terms.
*Correspondence: Prisca Lim, cHJpc2NhQGxpdmUudW5jLmVkdQ==
†ORCID: Avery B. Paxton, orcid.org/0000-0002-4871-9167