- 1Flanders Research Institute for Agriculture, Fisheries and Food (ILVO), Oostende, Belgium
- 2Marine Biology Research Group, Faculty of Sciences, Ghent University, Gent, Belgium
- 3Marine Ecology and Management, Operational Directorate Natural Environment, Royal Belgian Institute of Natural Sciences, Brussels, Belgium
Marine aggregates have been intensively extracted in the North-East Atlantic over the past decades. This study aimed to assess the effect of sand extraction on benthic ecosystem functioning using a combination of biological traits and functional indices (the bioturbation (BPc) and irrigation potential (IPc) and secondary production (SPc) of the macrobenthic community). Data on macrobenthos, sediment properties and extraction intensity were collected over a time period of ten years (2010 – 2019) for three coarse sediment extraction areas in the Belgian Part of the North Sea, each with a different extraction regime. Sediment parameters such as the medium sand fraction (250 – 500 µm) and median grain size showed a significant effect on all functional indices. Whilst sand extraction variables only significantly affected secondary production estimates. The secondary production of the macrobenthic community decreased following a high yearly extraction intensity, whereas a high cumulative (10-year period) extraction intensity resulted in a slightly increased secondary production. Species-specific responses revealed that these high cumulative extraction volumes increased the abundance of opportunistic species, which could have contributed to the higher SPc values observed in cumulative disturbed areas. Response traits such as tube-living and sessile individuals with a pelagic egg development were positively influenced by a long-term disturbance, an indication of a more disturbance-tolerant community. A short-term disturbance rather seemed to favor a macrobenthic community characterized by a higher burrowing capability. In terms of effect traits, both short- and long-term extraction clearly favored deposit feeders, which can structure organic matter distribution and thus indirectly influence nutrient and oxygen fluxes as well. Future in situ measurements in sand extraction areas could help to unravel and strengthen our understanding of the ecosystem processes linked to these trait-based observations.
1 Introduction
Next to petroleum, sand and gravel are among the most extracted mineral resources worldwide, but mining sites on land are getting scarce (Peduzzi, 2014; Torres et al., 2017). The extraction of marine aggregates has become an important alternative since the second half of the 20th century. Over the past 50 years, the yearly extraction of marine aggregates in the North Sea has increased from a few hundred thousand m3 to tens of millions m3 of sand and gravel in recent years (ICES, 2019). It has been proven that marine sand extraction activities exert significant direct and indirect impacts on the physical and biological properties of the seabed (Desprez, 2000; Robinson et al., 2005; Cooper et al., 2007; Krause et al., 2010; Cooper et al., 2011; Waye-Barker et al., 2015; Wyns et al., 2021). Habitat modifications and the removal of sediment and benthic fauna are the main direct and near-field impacts of sand extraction by trailing suction hopper dredgers (De Backer et al., 2014). Resuspension and redeposition of sediments are the main indirect impacts affecting both near- and far-field sediment characteristics which leads to the smothering of benthic organisms (Desprez et al., 2010; Le Bot et al., 2010). Several studies have shown substantial decreases in macrobenthos density, biomass and diversity in response to sand extraction (Desprez, 2000; Krause et al., 2010; Barrio Froján et al., 2011), while other studies observed local increases in density and diversity (Newell et al., 1999; De Backer et al., 2014; Wyns et al., 2021; Desprez et al., 2022). It is clear that the impact on the structural properties of macrobenthic communities (e.g. density, biomass, diversity measures) depends on the site-specific habitat characteristics (sedimentology) and extraction pressure (frequency, spatial extent and intensity of aggregate extraction) (ICES, 2016; Desprez et al., 2022).
Although the structural attributes of macrobenthic communities are good indicators to assess benthic ecosystem health (Dauer, 1993; Borja et al., 2000), they do not fully represent the functional role of macrobenthos in the ecosystem. Macrobenthic organisms can change the oxygen dynamics in the sediment through their particle reworking and burrow ventilation activities, thereby influencing mineralization processes and nutrient cycling (Kristensen et al., 2012; Volkenborn et al., 2019). In addition, macrobenthos also plays a significant role in the transfer of energy to higher trophic levels (Cusson & Bourget, 2005).
Because direct measurements of faunal activities are time-consuming and logistically demanding (Queirós et al., 2013; Van Der Meer et al., 2013), a number of trait-based proxies to approximate the functioning of macrobenthic communities have been developed over the past years (Solan et al., 2004; Bremner et al., 2006b; Brey, 2012; Beauchard et al., 2017; Renz et al., 2018; Wrede et al., 2018; Lam-Gordillo et al., 2020). One such proxy is the bioturbation potential (BPc). It is a functional index representing the sediment reworking capacity (particle transport) of a macrobenthic community based on each taxon’s mobility, feeding mode, biomass and abundance (Solan et al., 2004; Queirós et al., 2013). Another proxy is the irrigation potential of the community (IPc), which is an index for the burrow ventilation capacity of the macrobenthic community (solute exchange at the sediment–water interface), based on multiple traits such as feeding mode, burrowing capacity and injection depth as well as the taxon’s biomass and abundance (Wrede et al., 2018). Both BPc and IPc are indices representing bio-transport activities that can influence the ecosystem functioning by modifying the distribution of oxygen, organic matter (OM) and chemical elements in the sediment (Kristensen et al., 2012; Shull, 2019). Hence, several studies have identified BPc and IPc as important explanatory variables for benthic biogeochemical cycling and oxygen dynamics under different environmental conditions (Braeckman et al., 2014; Wrede et al., 2017; Wrede et al., 2019; Neumann et al., 2021; Toussaint et al., 2021). Furthermore, bioturbation and irrigation activities depend on sedimentary properties such as the median grain size where lower BPc and IPc are expected in areas with larger median grain sizes (Bernard et al., 2019; Gogina et al., 2020; Gogina et al., 2022). The secondary production of the macrobenthic community (SPc) (Brey, 2001; Brey, 2012) is a quantitative measure of the incorporation of energy or organic matter per unit of time and area (Cusson & Bourget, 2005). By integrating population dynamics (e.g. recruitment, mortality, life span, body mass, growth), biotic interactions (e.g. predation) and environmental conditions (temperature, depth), the secondary production can be a useful index for ecosystem health and functioning (Dolbeth et al., 2012).
Another widely used approach to describe changes in ecosystem functioning, besides the functional indices, is the biological trait analysis (BTA) (Bremner et al., 2006a; Bremner et al., 2006b; Bremner, 2008). BTA considers the distribution of species-specific physiological, morphological, behavioral and other relevant biological attributes in a community (Bremner et al., 2006b). To make inferences on how changes in the biological traits community may influence ecosystem functioning, a distinction has to be made between traits that exert an impact on the ecosystem properties (effect traits) and traits that define the response of a species (response traits) to a changing environment or disturbance event (Violle et al., 2007).
These proxies for ecosystem functioning have already been individually applied to assess the impact of anthropogenic activities such as demersal fish trawling (Fleddum et al., 2013; Van Denderen et al., 2015; Hinz et al., 2021; Tsikopoulou et al., 2022), dredge disposal (Birchenough et al., 2012; Bolam et al., 2016; Bolam et al., 2021), sewage discharge (Nasi et al., 2023), noise pollution (Wang et al., 2022) and sand extraction (Barrio Froján et al., 2011; Wan Hussin et al., 2012; Festjens et al., 2023) on ecosystem functioning. However, no proxy represents all processes within the ecosystem. We, therefore, used a combination of both functional indices (BPc, IPc, SPc) and BTA to achieve a more thorough understanding of how sand extraction potentially affects the functioning of the ecosystem.
In the Belgian Part of the North Sea (BPNS), sand extraction is restricted to dedicated concession areas with a long history of benthic environmental monitoring, rendering suitable datasets on sedimentology, sand extraction and biological parameters of the present macrobenthic communities (De Backer et al., 2014; Wyns et al., 2021). Wyns et al. (2021) examined the impact of marine sand extraction over a ten-year period on the structural patterns of the benthic communities in the BPNS. The current study builds further upon those results and the overall aim was to understand the impact of sand extraction on benthic ecosystem functioning by using a combination of trait-based proxies (functional indices and biological traits approach). Hence, following research questions were posed: (I) how does sand extraction intensity affect the three functional indices, BPc, IPc and SPc, (II) how are the effect and response traits affected by changes in both extraction and sediment variables, and (III) which macrobenthic species drive the response of the ecosystem functioning proxies to sand extraction?
2 Materials and methods
2.1 Study area and extraction activity
The Belgian Part of the North Sea, located in the southern North Sea, is characterized by subtidal sandbanks (habitat type 1110 under the EU Habitat Directive). Sand extraction activities are located in specific zones on the crests of certain sandbanks. We focused on three active sand extraction areas (EAs) each with a different extraction regime (Figure 1). In EA1 (Extraction Area 1 on Thorntonbank) and EA2od (Extraction Area 2 on Oostdyck) sand is extracted on a regular basis for industrial applications, while in EA4c (Extraction Area 4c on Oosthinder) sand is extracted irregularly for coastal protection measures. Extraction regimes and volumes are deduced from the Electronic Monitoring System (EMS) present on the extraction vessels operating in the BPNS since 2003. EA1 has been open for extraction since 2003 (Roche et al., 2017), although not much sand was extracted up till 2014 and part of the area (where no extraction has taken place) was closed in October 2010 to serve as a monitoring reference area. EA1 can be defined as a high intensity and high frequency area, with ca 1.5 to 2 million m³ sand extracted per year on an almost daily basis year-round since 2014. EA2od is a smaller extraction area that already opened in 1977, with sand extracted year-round at much lower intensities (yearly extracted volumes since 2010 ca 300,000 m³) (Wyns et al., 2021). EA4c is located furthest offshore and opened in 2012, where sand is extracted at high intensity, but irregularly during short time periods (generally from January to April) in certain years (Wyns et al., 2021). The cumulative volume extracted in EA4c (6.7 million m³ since 2012) is intermediate between those of EA1 (14.2 million m3) and EA2od (2.8 million m3) (Supplementary Material, Figure A1). Previous studies have shown that the natural reference areas of all three EAs are dominated by medium sands (250 – 500 µm) (Verfaillie et al., 2006) and represent a similar habitat type to permeable sediments, characterized by the functionally diverse Hesionura elongata macrobenthic community (Breine et al., 2018). Characteristic species of this reference community exhibit biological traits such as egg brooding, planktotrophic or direct larval development, small maximum sizes (<10 to 100 mm) and relatively short lifespans (Breine et al., 2018). Macrobenthic abundance and biomass in undisturbed permeable sediments are generally low (Wyns et al., 2021), resulting in a low bioturbation and irrigation potential and a low secondary production of the macrobenthic community (Bolam et al., 2014; Braeckman et al., 2014; Breine et al., 2018; Toussaint et al., 2021).
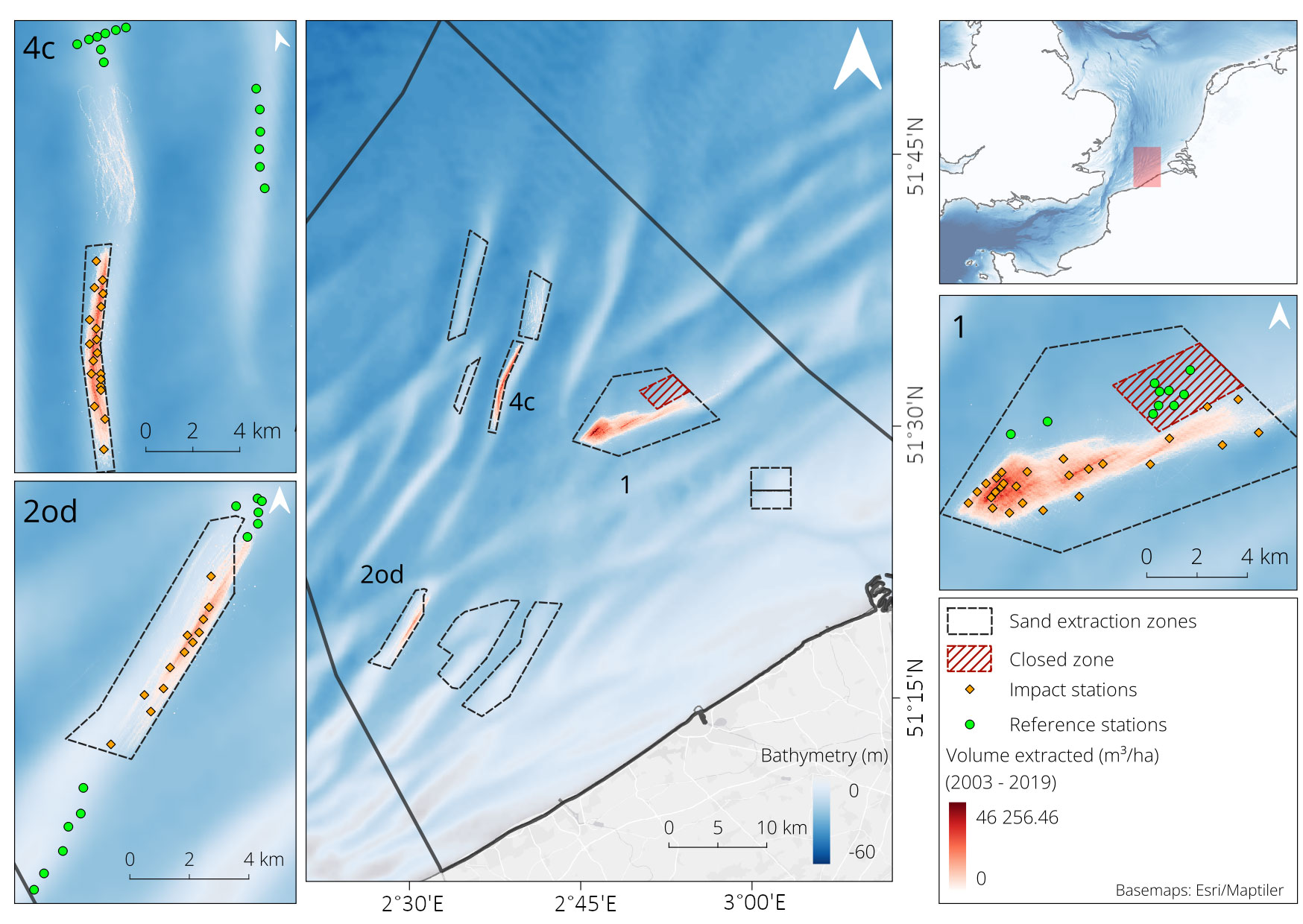
Figure 1 Sand extraction areas in the Belgian Part of the North Sea (BPNS) according to the marine spatial plan of 2014-2019. The three sand extraction areas used for this study (Thorntonbank – EA1, Oostdyck – EA2od, Oosthinder – EA4c) are visualized in more detail with the cumulative extracted volume (m3 ha-1) from 2003 to 2019, and the corresponding impact (orange) and reference (green) macrobenthos sampling locations. The red shaded zone depicts an area which is closed for extraction activities within the sand extraction area. EMS data from Continental Shelf Service – FPS Economy.
2.2 Data collection, availability and processing
2.2.1 Sand extraction data
Extracted volumes of sand were estimated based on the EMS (Electronic Monitoring System) data recorded on board of each trailing suction hopper dredger active in the BPNS. The automatic registration system registers date, time, position and speed of the ship as well as the pump activity. A complete EMS-dataset for the BPNS is available from 2003 onwards (Van Den Branden et al., 2017) and allows to quantify both yearly and cumulative sand extraction intensities (m3). The yearly sand extraction intensity represents the short-term and direct impact, while the latter focuses on the cumulative impact over the years. Around each sampling location (see 2.2.2), an area with a radius of 50 m was drawn in ArcGIS and the yearly extraction volumes within that area were calculated for the year preceding the macrobenthos sampling. The cumulative sand extraction intensity for the same areas was calculated per sampling location over the period 2003 – 2019.
2.2.2 Grab sample data
The dataset for this study covers 10 years (2010-2019) and was collected during environmental impact monitoring in the framework of sand extraction. For each EA, both impact and reference locations were sampled in autumn (September – October) using a Van Veen grab (surface area 0.1 m²). Sampling locations in the impact zones were defined based on the mapped extraction intensity derived from EMS (Continental Shelf Service – FPS Economy). Reference locations were located at similar depths and similar environmental conditions as the impact locations but without recent or past extraction activities. The number of locations per EA varied over the years based on changes in the extent of sand extraction activities or adaptations to the sampling design of the impact monitoring. Almost all locations were sampled on a yearly basis (Supplementary Material, Table A1), with a total of 454 sampling events at 93 locations distributed over the three extraction areas (Figure 1).
A small subsample was taken from each Van Veen Grab for granulometric sediment analyses, performed with laser diffraction on a Malvern Mastersizer 2000G hydro version 5.40). Grain size fractions between 1 and 2000 µm were defined according to the Wentworth scale. The shell fraction represents the sediment fractions larger than 1600 µm. Additionally, the median grain size of the sand fraction (63 – 2000 µm), hereafter called median grain size (MGS), was calculated.
The remaining of each Van Veen grab sample was sieved over a 1 mm sieve on board the research vessel and preserved in an 8% formaldehyde-seawater solution stained with eosin to facilitate further sorting. All macrobenthic organisms were identified to the lowest taxonomic level possible, and wet-weight measurements were obtained for surface-dried individuals (dried on blotting paper). Macrobenthos identifications were conducted in an ISO-regulated lab environment (NBN EN ISO/IEC 17025), holder of a BELAC T-315 certificate for macrobenthos analyses. Before data analysis, taxa which were not sampled representatively (e.g. meiobenthic and hyperbenthic taxa) or taxa for which insufficient information was available (e.g. mass-to-energy conversion factor for Phoronis sp.) were discarded from the dataset. In case of inconsistent identification to species level, species were lumped to the next higher taxon level. All taxon names were verified using the WoRMS taxon match tool (WoRMS Editorial Board, 2023).
For a more detailed overview and additional background information concerning the data collection procedures for the sand extraction variables and the sediment and biological parameters, we refer to Wyns et al. (2021).
2.2.3 Functional indices
The bioturbation potential of the macrobenthic community (BPc), an index of how benthic taxa affect ecosystem functioning (e.g. oxygen flow, mineralization processes and nutrient cycling at the sediment-water interface) through their bioturbation activity, was calculated for each sample as first described in Solan et al. (2004):
With Bi and Ai representing the biomass (gWW m-2) and abundance (ind. m-2) of taxon i, respectively. Two traits are further used in this formula: a mobility score (Mi) and a particle reworking score (Ri). Both traits are divided into subcategories to make sure all mobility modes (from 1: organisms living in fixed tubes to 4: organisms with free movement through a burrow system) and all reworking modes (1: epifaunal organisms to 5: regenerators) are represented. Species-specific scores for both traits were adopted from Queirós et al. (2013). If no score was available for a certain species, a score from the adjacent higher taxonomic level or its closest relative was adopted. The index was calculated at the community level (BPc), but also for the main individual taxa (BPi). The scores used to calculate the bioturbation potential can be found in the Supplementary Material (Supplementary Material, Table B1).
The irrigation potential of the macrobenthic community (IPc), an index representing the burrow ventilating capacity of the macrobenthic community, was calculated for every sample according to the formula described in Wrede et al. (2018):
Bi and Ai are as in the formula (1). Three functional traits are included in the formula, describing the irrigation behavior and impact of this behavior on the environment. Each taxon was assigned a specific score for burrow type (BTi; from 1: epifauna and internal irrigation to 3: blind-ended irrigation), feeding type (FTi; from 1: surface filter feeder to 4: subsurface filter feeder) and injection depth (IDi; from 1: 0-2 cm to 4: >10 cm). To obtain scores for the different traits per species (Supplementary Material, Table B1), literature and databases were consulted. Also this index was calculated on the community level (IPc) as on the taxon level (IPi).
Secondary production estimates (SP; kJ m-2 year-1) at the macrobenthic community (SPc) and taxon level (SPi) were derived from the standing biomass by means of production-to-biomass ratios (P/B). The P/B was estimated for every species in each sample according to the empirical model by Brey (2012). The model predicts annual P/B based on mean body mass (Joule), mean annual bottom water temperature (°C), depth of sampling (m) and information on taxonomy, sediment position, mobility, feeding mode, biotope and exploitation status of each species or taxon. The measured wet weights (gWW) were first converted to ash-free dry mass (mg AFDM) using conversion factors defined at family or higher level. Subsequently, the AFDM biomass was converted to energy values (Joule). All conversion factors were adopted from Brey et al. (2010).The depth of each station was defined using recent bathymetric maps of the sample locations (Supplementary Material, Table B2) (Wyns et al., 2021). The CORA database was consulted to extract the mean annual temperature for the BPNS during the study period (Supplementary Material, Table A2) (Szekely et al., 2023). Exploitation status was set at 0 for every taxon as macrobenthos is not commercially exploited in the BPNS. Information on the remaining life-history traits was adopted from traits databases published in Breine et al. (2018) and Clare et al. (2022). Scores for each species and parameter can be consulted in the Supplementary Material (Supplementary Material, Table B3). From the modeled P/B scores, the secondary production value was calculated following the formula:
Bi and (P/B)i represent the biomass and production-to-biomass ratio, respectively, for taxon i in a sample. An R script constructed by Saulnier et al. (2019) was used to estimate and predict the P/B and SPc values.
2.2.4 Biological traits data
For the fourth-corner analysis, biological traits were assigned to only a selected set of taxa (see 2.3.2. for selection procedure). Ten biological traits containing several modalities, encompassing a wide variety of physiological, morphological and behavioral characteristics of each taxon, were chosen for this study (Table 1). Five traits were classified as effect traits, while the other five were labeled as response traits, as proposed by Bolam et al. (2016). The biological traits dataset originally published by Bolam et al. (2016) and slightly adapted for the BPNS by Breine et al. (2018) was consulted. In these studies, each taxon was assigned to one or more trait modalities based on the fuzzy coding approach (Chevenet et al., 1994). However, the fourth-corner can only fit one value per taxon and trait, hence we chose the modality with the highest fuzzy code for each particular trait and taxon. For example, fuzzy coding for the mobility trait of the polychaete Lanice conchilega yields a 0.75 score for the sessile modality and 0.25 for the crawler modality. Consequently, in our trait matrix the sessile trait modality was assigned to L. conchilega. In case of equal scoring or in absence of trait information, additional datasets were explored (Shojaei et al., 2015; Beauchard et al., 2022; Clare et al., 2022). The trait value reported in the majority of the consulted datasets or sources was chosen. The biological traits dataset used in this study, including references, is available as Supplementary Material (Supplementary Material, Table B4).
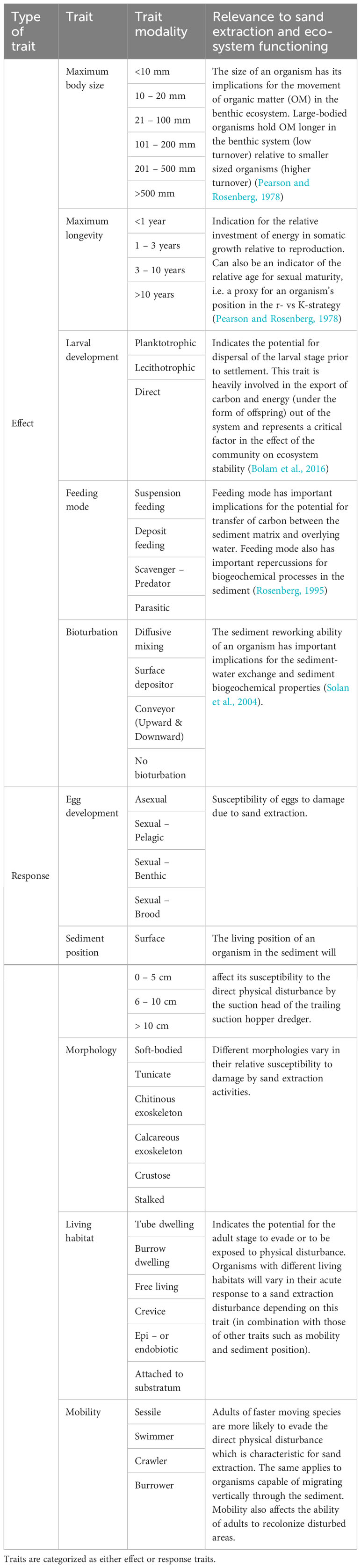
Table 1 Overview of the selected traits and their modalities in this study adopted and modified from Bolam et al. (2016).
2.3 Statistical analyses
2.3.1 Environmental effects on the functional proxies
The final dataset for the univariate analyses consisted of the sand extraction data, grab sample data on sedimentology and macrobenthos and functional proxies for the macrobenthic community (BPc, IPc, SPc). To determine the effects of sand extraction and various sediment descriptors on the proxies for ecosystem functioning (BPc, IPc, SPc), we adopted explanatory (generalized) linear mixed models ((G)LMM). Prior, a thorough data exploration was conducted following the protocol described in Zuur et al. (2010). To exclude collinearity between explanatory variables, predictors were plotted against each other by means of pairwise scatterplots, and the Pearson correlation coefficient and variance inflation factor (VIF) were calculated (Supplementary Material, Figure A2). Explanatory variables with a correlation >0.7 or VIF values >3 were considered collinear, in which case the variable with the highest biological relevance (based on local ecological knowledge) was kept in the analyses. Six explanatory variables were retained in the analysis: yearly sand extraction intensity, cumulative sand extraction intensity, very fine sand fraction (63 – 125 µm), shell fraction (>1600 µm), medium sand fraction (250 – 500 µm) and median grain size of the sand fraction (MGS). The sand extraction intensity variables were rescaled to 103 m3 in order to better fit the model. The presence of outliers was visually inspected using Cleveland dotplots. One influential outlier was identified for very fine sand and left out of the dataset after a sensitivity analysis with BPc as response variable (Supplementary Material, Figure A3). To account for the possible variation caused by the position of the sampling location on the asymmetrical sand waves (Cheng et al., 2020) and the fact that repeated measures are involved, the variable year, and a nested structure including sampling location, sampling position on the sand bank and sand extraction area were included in the model as random factors. The sample position on the sand bank (crest, gentle and steep slope) was determined using bathymetric maps from Wyns et al. (2021).For BPc and SPc, GLMMs were run using a Gamma distribution with a log-link. The IPc was subjected to a box-cox transformation and was therefore modelled using a linear mixed model (LMM). Model assumptions were visually inspected by using the DHARMa package (Hartig, 2022) (Supplementary Material, Figure A4).
2.3.2 Environmental impacts on biological trait composition of the macrobenthos
Model-based multivariate analyses for abundance data were performed using the mvabund package (Wang et al., 2012) to elucidate which taxa and traits drive the responses of the ecosystem functioning proxies to changes or differences in sediment characteristics and sand extraction regimes. This model-based approach differs from classic distance-based multivariate analyses, by taking into account the mean-variance relationship of the response variable. Preliminary multivariate analyses on the entire dataset with 219 taxa were overly complex, so only a subset of taxa was retained for this analysis, based on three selection criteria according to Gogina et al. (2020). Firstly, the common heart urchin Echinocardium cordatum was excluded from the selection procedure, because of its very high biomass compared to the other species in the dataset. Then a subset was created by selecting those taxa that contributed at least 0.5% to the sum of a functional index over all samples, and those taxa that accounted for at least 60% of a functional index at a particular location. Finally, the dataset was further reduced, retaining only those taxa that were present in more than 2.5% of the samples. Afterwards, E. cordatum was included in the final dataset, as it met all selection criteria. The biological traits analyses were equally performed on this reduced dataset (Supplementary Material, Table A3).
The impact of the explanatory environmental variables (related to extraction regime and sediment granulometry) on the community composition, based on the reduced dataset of 38 selected macrobenthos species, was investigated using the manyglm function from the mvabund package (Wang et al., 2012). The manyglm function fits separate generalized linear models (GLM), including a series of explanatory variables, to each taxon in the multivariate abundance dataset. A quadratic mean-variance relationship of the abundance data suggested a negative binomial distribution for the manyglm models (Supplementary Material, Figure A5). The models were built by means of a manual forward stepwise regression. The level of significance of the model was estimated with a PIT-trap resampling method using 999 bootstrap iterations (Wang et al., 2012). The final model was selected by using AICsum, which sums the AIC scores over all variables in the model (Akaike, 1974). After the final model selection, the Dunn-Smyth residuals were visually inspected to assess the probability distribution of the model (Supplementary Material, Figure A6).
The traitglm function was chosen to identify the biological traits associated with different species responses to the extraction and sediment variables. A traitglm fits a fourth-corner model on multivariate abundance data to test for environment-by-trait interactions (Brown et al., 2014). Three different dataframes were used for the fourth-corner analysis: a dataset containing the abundances for each of the 38 selected taxa across all locations, a dataset of the sediment and extraction variables across all locations, and a dataset containing either effect or response traits across all 38 taxa. Separate traitglm’s were fitted on the effect and response trait sets. Models were fit with a LASSO (least absolute shrinkage and selection operator) penalty to reduce any trait-environment interaction to zero if it did not contribute to lowering the Bayesian Information Criteria (BIC) score of the model (Supplementary Material, Figure A7).
All analyses were conducted in R Studio using the R software (v4.2.3; R Core Team, 2023). The significance level was set at 0.05 for all analyses.
3 Results
3.1 Environmental effects on functional proxies
The variation in all three functional indices (BPc, IPc and SPc) was explained by the proportion of the medium sand fraction (%) in the sample and the median grain size (MGS, µm). An increase in both MGS and the presence of the medium sand fraction lead to significant decreases in BPc (PMGS = < 2 × 10-16, P250-500µm = 8.5 × 10-13), IPc (PMGS = < 2 × 10-16, P250-500µm = 5.71 × 10-9) and SPc (PMGS = < 2 × 10-16, P250-500µm = 2 × 10-11). For IPc, also a slightly significant relation with the very fine sand fraction was found (P63-125µm = 0.041), with highest IPc values at lower very fine sand fractions. Only SPc was significantly affected by sand extraction as such, with a slight decrease in SPc at increasing yearly extracted volumes (103 m3) (PYearly extraction= 0.026) and increasing SPc when cumulative extracted volume increased (103 m3) (PCumulative extraction = 0.011) (Table 2). Plots between the response and significant explanatory variables may be found in the Supplementary Material (Supplementary Material, Figure A8-A10)
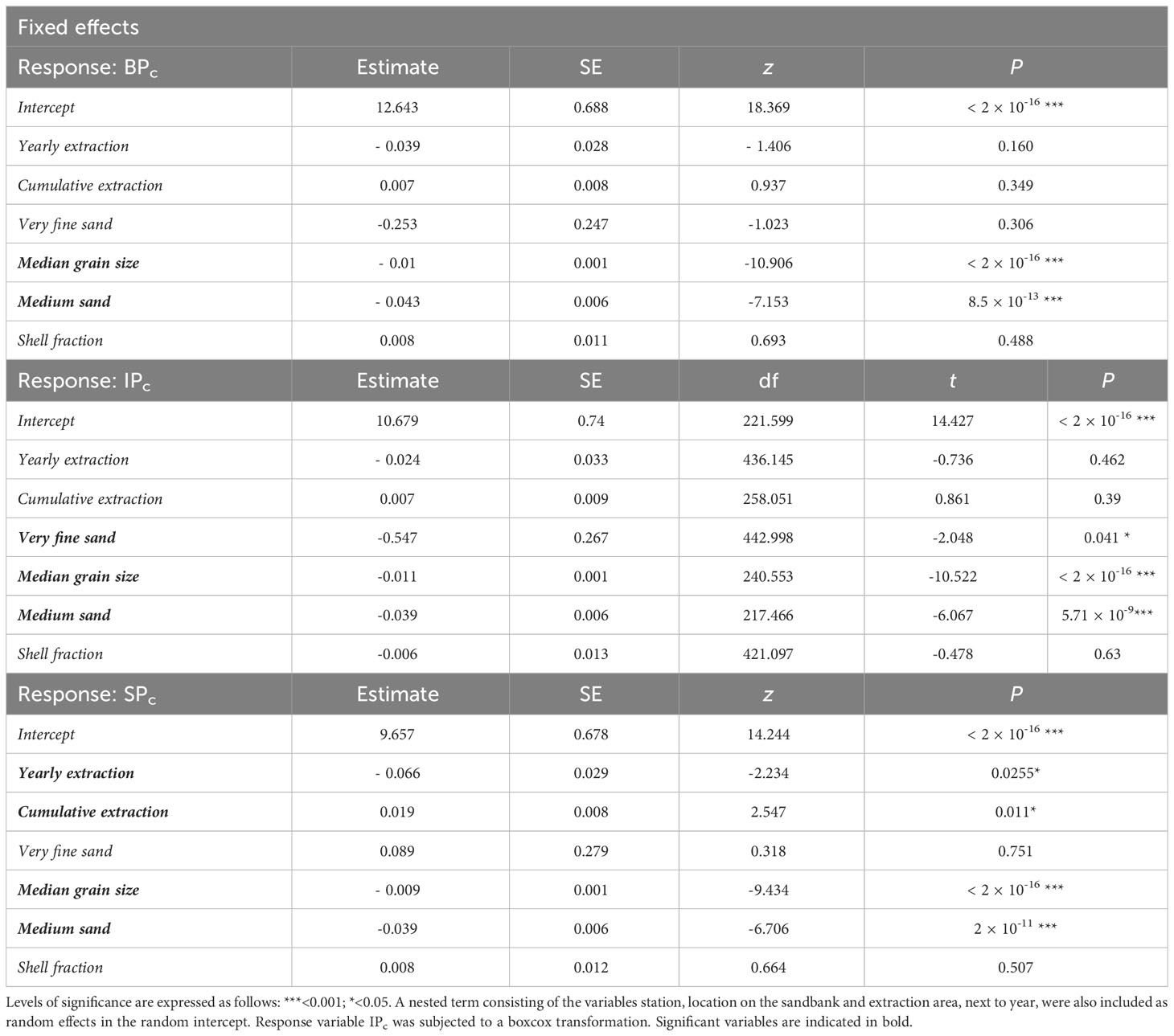
Table 2 Results for the fixed effects of the (generalized) linear mixed models for BPc, IPc and SPc.
3.2 Species-specific responses
Medium sand fraction and median grain size significantly explained the changes in community composition of the selected species (n = 38, resp. P250-500µm = 0.001 and PMGS = 0.028), while cumulative extracted volume was nearly significant (PCumulative extraction volume = 0.058, Table 3). The majority (n = 27) showed a significant negative association with the medium sand fraction, with the strongest negative response being observed for the polychaetes Lagis koreni and Poecilochaetus serpens and the small sea urchin Echinocyamus pusillus. Only three taxa (the amphipod Eurydice sp., mysid Gastrosaccus spinifer and polychaete Ophelia borealis) showed a significant positive relationship with the medium sand fraction (Figure 2A). Similarly, 28 taxa exhibited a significant relationship with MGS. Seven taxa, including Eurydice sp., G. spinifer, the bristleworms O. borealis, Glycera sp. (juvenile) and Hesionura elongata, the bivalve Asbjornsenia pygmaea and Oligochaeta, increased in abundance in response to a higher median grain size, while taxa such as the amphipods Urothoe brevicornis and Urothoe sp. (juvenile) and the bristleworm L. koreni became less abundant with increasing MGS (Figure 2B). Cumulative extraction volume significantly affected the abundances of 22 taxa, in most cases with higher abundances in locations with higher cumulative extraction volumes, in particular the amphipod Corophium sp., the polychaetes P. serpens and Lanice conchilega and Anthozoa. On the contrary, Oligochaeta, H. elongata and the amphipod Bathyporeia elegans abundances decreased with increasing cumulative extraction (Figure 2C) (Supplementary Material, Table A4).
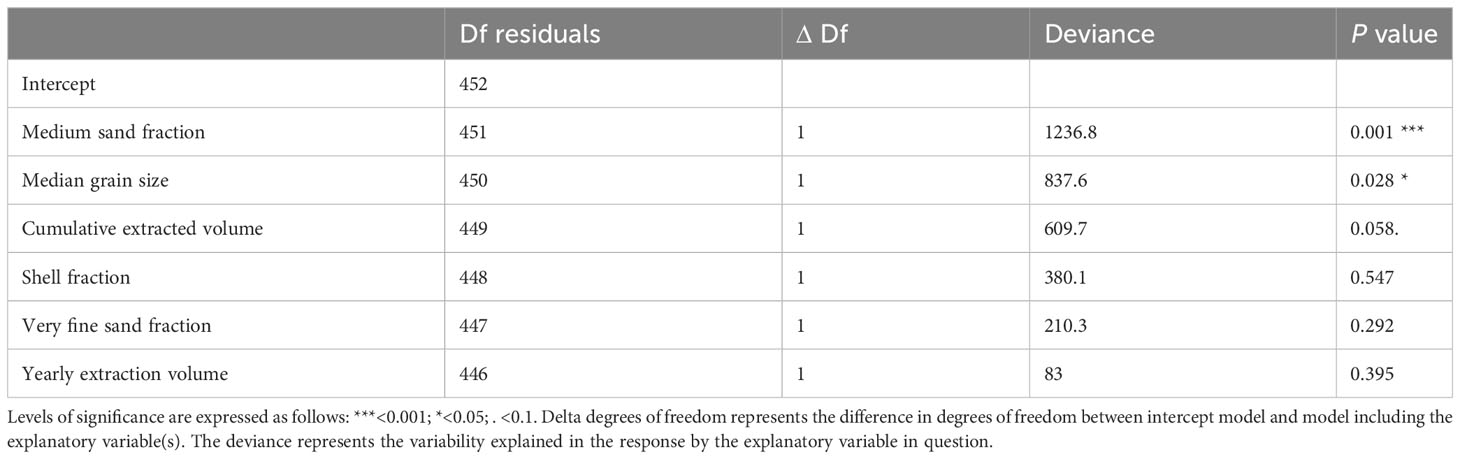
Table 3 Results of the final multivariate generalized linear model fitted on the reduced species matrix.
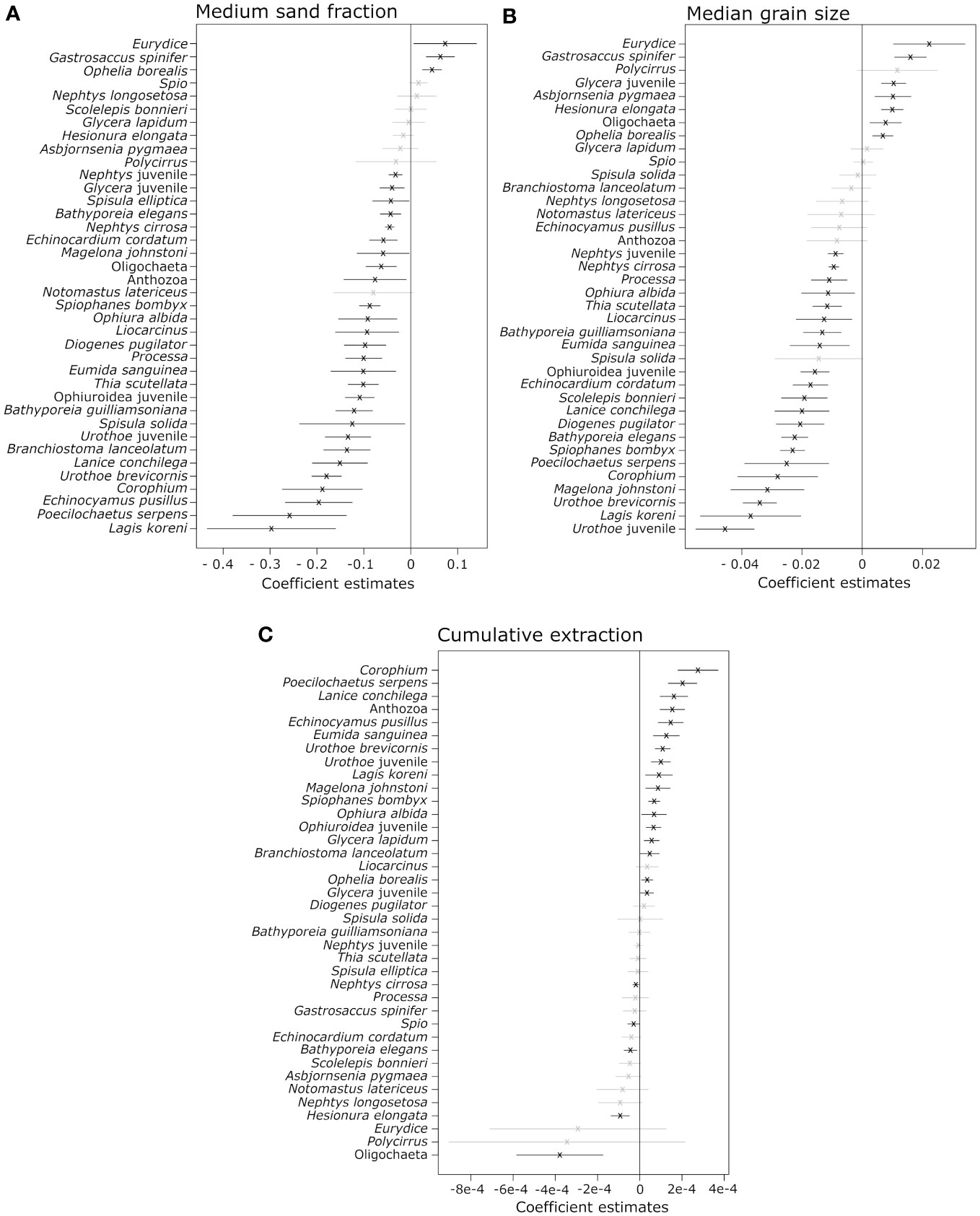
Figure 2 Coefficient plots for the sediment variables, (A) proportion of the medium sand fraction (250 – 500 µm) in a sample (%) and (B) median grain size (MGS, µm) that significantly explain the variability in species abundances in the multivariate generalized model and (C) cumulative extracted sand volume (m³). Values on the X axis present the values for the coefficient estimates of every species in the regression model. Crosses indicate the corresponding coefficient estimate for each species per explanatory variable. The horizontal lines represent the 95% confidence interval for each estimate for each species. Estimates left from the vertical line show negative associations, right of the line positive associations. If the confidence interval overlaps with zero, the association is non-significant (grey).
3.3 Trait-specific responses
Only 35 of the 45 different possible trait modalities were present in the selected subset of 38 taxa. For the response traits, 4 to 9 associations were found between the trait modalities and the different sediment variables, while 4 to 7 associations were observed for the extraction variables (Figure 3). For the effect traits, also 4 to 8 associations were found for the different sediment variables, but only 2 to 5 associations for the extraction variables. More trait-interactions were observed with the cumulative extraction variable compared to the yearly extracted volume in both response and effect traits. With the exception of a common positive association between the deposit feeding mode and both extraction variables, the yearly extraction variable and cumulative extraction variable each affected different response and effect traits. Areas with high yearly extraction volumes contained fewer crawlers and species with a benthic egg development, while burrowing species were favored in terms of abundance by high yearly extraction volumes. Species with a soft exoskeleton (chitinous), a pelagic egg development or tube-dwelling and sessile species, increased in abundance with higher cumulative extraction volumes, while the abundance of free-living, soft-bodied and swimming species decreased when cumulative extraction volumes were higher (Figure 3A). The effect trait modalities maximum longevity between 3-10 years and deposit feeders were positively associated with yearly sand extraction. Deposit feeders were also favored by higher cumulative extraction volumes. This also holds for species with a planktotrophic larval development and a maximum length between 201 and 500 mm. On the other hand, the abundance of smaller species measuring between 101 and 200 mm and species with a lecithotrophic larval development decreased with increasing cumulative extraction volumes (Figure 3B). Several response and effect traits that were associated with one or both the extraction variables, such as deposit feeding, maximum length between 201 and 500 mm, tube-dwellers and fauna with an exoskeleton, showed an opposite relationship with median grain size.
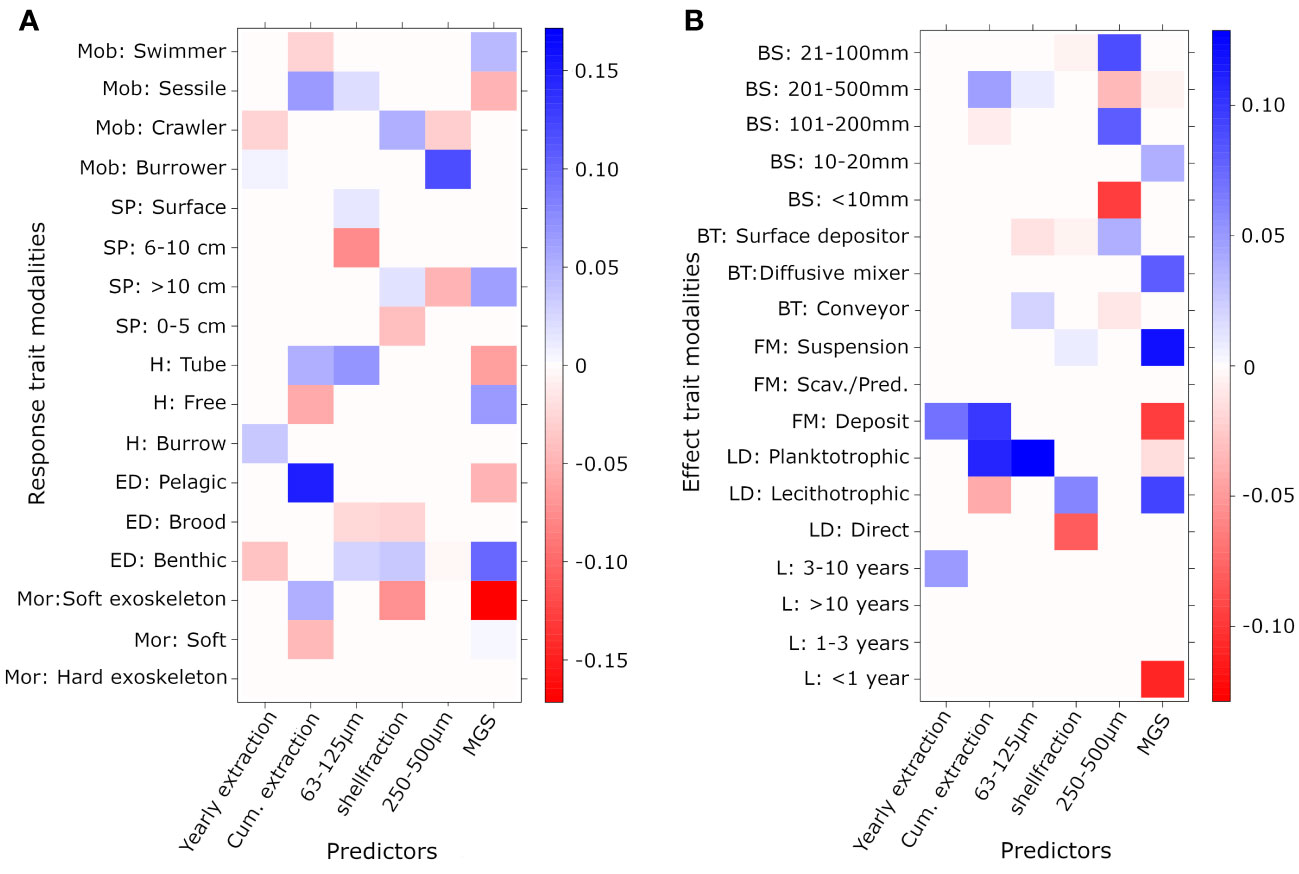
Figure 3 Fourth-corner plots showing (A) response and (B) effect traits and their relationships with sediment and extraction variables. Blue shades represent significant negative associations and red shades show significant positive associations.
4 Discussion
The aim of this study was to gain insight in how sand extraction activities affect benthic ecosystem functioning by using functional indices (BPc, IPc and SPc) and a biological traits analysis (BTA) as proxies for ecosystem functioning. Our results indicate that mainly sediment variables, and more specifically the proportion of medium sand (250 – 500 µm) and median grain size, explain the variability in the functional indices and biological traits composition, compared to the direct sand extraction variables. This likely corresponds to the highly dynamic nature of the permeable sediments in the study area, which are usually characterized by low BPc and IPc values. On the other hand, SPc was significantly impacted by sand extraction, with antagonistic effects of both the yearly and cumulative extraction intensity variables. Furthermore, several response and effect trait modalities were associated with both sand extraction variables, although again sediment variables usually showed a higher number of associations with the traits.
4.1 Sediment parameters explain variability in proxies for ecosystem functioning
Several studies have demonstrated the importance of sediment parameters in structuring the distribution of benthic communities (Snelgrove and Butman, 1994; Kröncke et al., 2004; Van Hoey et al., 2004; Breine et al., 2018). According to our results, this holds true as well for both the functional indices BPc, IPc and SPc and the biological traits. A coarsening of sandbank sediments led to a general decrease in the BPc, IPc and SPc values, which is in line with other studies that also reported lower values in coarse permeable sediments compared to more cohesive sediments (Bolam et al., 2014; Braeckman et al., 2014; Breine et al., 2018; Gogina et al., 2020; Toussaint et al., 2021). Permeable, well-oxygenated sediments, such as found in our reference areas, have a high mineralization capacity and therefore naturally harbor low concentrations of organic matter (Huettel and Rusch, 2000). This relatively low food availability in turn limits the secondary production of heterotrophic macrobenthic communities, leading to lower SPc values with increasing grain size (median grain size and proportion of medium sand fraction). Moreover, these undisturbed permeable coarse sand habitats, such as the reference zones on the offshore sandbanks in the BPNS, harbor a distinctive macrobenthic community that has adapted to the prevailing dynamic conditions and is characterized by small, free-living and interstitial polychaete species such as Hesionura elongata, Spio spp., Nephtys cirrosa and oligochaetes (Hall, 1994; Van Hoey et al., 2004; Ruddick and Lacroix, 2006; Breine et al., 2018). This was corroborated by our biological traits analysis where we observed an increase in the abundance of free-living, mobile and small-sized individuals with increasing median grain size, as well as increased abundances in suspension feeders and diffusive mixing bioturbators. These traits relate well with the species that were positively associated with median grain size, such as the amphipod Eurydice sp., the mysid shrimp Gastrosaccus spinifer, the polychaetes Ophelia borealis and Hesionura elongata and oligochaetes, which all contribute little to the bioturbation and irrigation potential of the community resulting in overall lower values for both BPc and IPc.
Although, sediment characteristics are of major importance in explaining the variability in functional proxies, they cannot be considered as exclusively environmental or natural parameters. Instead, they rather reflect a combination of natural and anthropogenic influences. Sand extraction activities do not only affect benthic communities, but also impact sediment properties through extraction, screening and overflow processes (Cooper et al., 2011; Wyns et al., 2021). In most cases, the extraction industry targets specific sand fractions, depending on whether sand is meant for construction or coastal protection. In some cases, the extracted aggregates are sieved on board the trailing suction hopper dredgers and unwanted grain sizes are deposited back to the seafloor (screening). Additionally, excess water together with low density sediment particles (mud and smallest sand fraction) are released to optimize storage capacity and consequently deposited on the seabed (overflow). These processes can cause a coarsening or fining of the sediment, or a mixture of both. As such, sand extraction might indirectly affect the different proxies for ecosystem functioning by changing the sedimentary environment.
4.2 Variable effects of sand extraction on functional indices and biological traits
Secondary production of the macrobenthic community was the only functional index that revealed significant changes in response to both extraction variables, which suggests that both short- and long-term sand extraction activities affect the macrobenthos secondary production. However, these were antagonistic effects: while cumulative sand extraction activities caused higher SPc values compared to the reference situation, a significant decrease in SPc was observed in response to high yearly extraction volumes. A decrease in SPc after a short-term disturbance can be attributed to the overall removal of benthic organisms from the upper sediment layers during extraction activities (Desprez et al., 2022). In addition, a high disturbance frequency might prevent the recovery or recolonization of large and slow growing species, which translates in a decrease in the production of the macrobenthic community (Reiss et al., 2009).
The biological response traits clearly showed how the community adapted to the direct short-term disturbance of sand extraction. Increased abundances of burrowers and burrow-dwelling organisms were associated with a high extraction intensity over a period of one year. The burrowing capacity of macrobenthic species allow them to escape the physical disturbances until a certain degree. On the contrary, organisms that release their eggs on the seafloor and thus more susceptible to physical disturbances, were negatively affected by increased yearly extraction volumes. A possible explanation for the negative impact of short-term sand extraction on crawling organisms might be that highly mobile species, such as swimmers, are better at escaping sand extraction disturbances compared to crawlers who are more limited in their mobility.
Sustained sand extraction, on the other hand, enhanced macrobenthic production. A number of processes could have driven this subtle increase in the total macrobenthic community production. First, over time, sand extraction introduces fine sediments in naturally coarse sediments due to overflow (Cooper et al., 2011). These fine sands can consequently trap more organic matter from the overlying seawater and enrich the seabed. This increased food source can either stimulate the production of the naturally occurring benthic species or attract new opportunistic species (Pearson and Rosenberg, 1978; Dolbeth et al., 2015). Secondly, sand extraction is known to resuspend the sediment, causing a displacement of organic matter to the surrounding sediments and a nutrient release to the water column. As a result of this new available OM, benthic production is stimulated in the direct vicinity of the extracted locations (Newell et al., 1998; Newell et al., 1999). As organic matter serves as an important food source for deposit feeders, an increase in the abundance of deposit feeders was observed for both sand extraction variables. Deposit feeders are known to structure the vertical distribution of OM through their feeding activities, and may as such indirectly regulate the nutrient and oxygen fluxes in the sediment (Thrush et al., 2006).
As a response to a high cumulative sand extraction intensity, the traits analyses indicated a shift of the naturally occurring community towards an even more disturbance-tolerant community, while attracting new and productive opportunistic species (van Dalfsen et al., 2000; Dolbeth et al., 2015). Though the multivariate analyses showed a borderline non-significant effect on the community composition, at species level we saw an increased abundance of opportunistic species, such as the amphipod genus Corophium, the polychaetes Poecilochaetus serpens and Magelona johnstoni, the small sea urchin Echinocyamus pusillus, and juvenile brittlestars Ophiuroidea. The cumulative extraction variable showed, at the same time, significant positive associations with a number of biological response traits and modalities that are characteristic for disturbance-tolerant species. For example, tube-dwelling organisms (inherently linked with a sessile mobility) are known to persist in heavily disturbed areas by optimizing their recruitment (Beauchard et al., 2022). Also, species that shed their eggs in the water column (pelagic egg development) are considered to be relatively more robust against a continuous disturbance which explains their increased abundance with higher cumulative extraction volumes (Bolam et al., 2014). The negative associations between the cumulative sand extraction variable and free-living and swimming organisms might be explained by the fact that highly mobile species are more successful in evading the physical disturbance of the suction head, and therefore leave the continuously extracted area more easily. Surprisingly, our biological traits analysis showed a positive relationship between the sand extraction variables and individuals with a medium-to-long lifespan (3-10 years) and large body size (201-500 mm). This can be explained by at least two reasons. One, the biological traits analysis uses the maximum age and body size of organisms, which do not always reflect the age and size at time of sampling. To circumvent this problem, the use of length-frequency distributions might give more insight into this aspect. Secondly, these patterns may be driven by other traits of the organisms (Beauchard et al., 2022). For example, many species in our dataset with a maximum lifespan of 3-10 years have a planktotrophic larval development, which is a characteristic trait for both disturbance-tolerant and opportunistic species.
Sand extraction activities did not exert a direct significant impact on the bioturbation and bioirrigation potential of the community in this study. As previously mentioned, macrobenthic species inhabiting the coarse sediments naturally contribute little to the BPc and IPc. This was also true for the two species which were positively influenced by sand extraction intensity, such as the amphipod genus Corophium and the polychaete Poecilochaetus serpens. Both are primarily surficial modifiers with a relatively shallow injection depth (max. 5 cm) (Queirós et al., 2013; Wrede et al., 2018). Similar findings have been observed for the effect of demersal fish trawling. Sciberras et al. (2016) reported an absence of trawling impact on the bioturbation potential, while Hinz et al. (2021) only observed an impact on BPc of communities consisting of vulnerable and moderately sensitive species, whereas the BPc of communities harboring resilient species remained unaffected.
Though functional indices and biological traits are a useful tool to assess the impact of an anthropogenic disturbance on ecosystem functioning, especially in absence of in situ measurements, these approaches also hold some disadvantages. The use of biological traits does not fully account for the behavioral plasticity under different environmental conditions and anthropogenic pressures. Similarly, one trait can be both an effect and response trait at the same time (Beauchard et al., 2017), which was not accounted for in this study. Also, BPc and IPc do not account for interspecific interactions (Solan et al., 2004), while species might change their feeding or burrowing activity in relation to changed local environmental conditions or the presence of anthropogenic pressures or other species (Mermillod-Blondin et al., 2005; Queirós et al., 2013). The inclusion of more detailed information on the different life-stages might improve the BPc and IPc estimations and the biological traits analyses. Despite known seasonal variations in the biomass of benthic species, the total annual production of macrobenthic communities is often calculated based on one sampling event per year. However, this might result in significant under- or overestimations of the secondary production, depending on the sampling period (Beukema and Dekker, 2013; Bolam and Eggleton, 2014; Saulnier et al., 2019). In this study, biomass values were used from late-summer, early-fall sampling events, leading to an overestimation of the secondary production. Therefore, one has to be careful when comparing secondary production estimates to these values, especially when data originates from different seasons or sites (Saulnier et al., 2019). Despite the drawbacks, empirical models have proven to perform at least as good as or better than cohort- or size-based methods in predicting secondary production or productivity (Cusson and Bourget, 2005; Dolbeth et al., 2005; Petracco et al., 2012).
5 Conclusion
This study revealed that sediment parameters, such as the proportion of medium sand fraction and median grain size, played an important role in influencing the different proxies of ecosystem functioning. Unlike the sand extraction variables, they significantly influenced all functional indices and revealed more associations with the biological traits of the community compared to the yearly and cumulative sand extraction variables. Yet this does not imply that sand extraction had no impact on the functional proxies and biological traits composition. Sand extraction activities could indirectly influence the proxies for ecosystem functioning by changing the sediment composition. Moreover, the total macrobenthic production was affected by sand extraction, although with an antagonistic effect of the two sand extraction variables. While the extraction of large sand volumes within one year led to a decrease in the secondary production, a slight increase was observed following high cumulative extracted volumes. The cumulative sand extraction variable also revealed more associations with the biological response and effect traits and exhibited a larger impact on the selected taxa. These results suggest that the persistence of sand extraction has a larger impact compared to the impact of the initial disturbance on benthic ecosystem functioning, at least in dynamic and permeable sandy habitats. Beside this antagonistic effect on secondary production, both yearly and cumulative extraction variables were correlated with an increased abundance of deposit feeders, which could have implications on the vertical distribution of OM in the sediment and thus indirectly affect nutrient exchange and oxygen availability in the sediment. However, to further increase our understanding on how the proxies really relate to the ecosystem functioning under the pressure of sand extraction, in-situ measurements of the main ecosystem processes are needed. A direct link between the ecosystem functioning (e.g. oxygen consumption of the benthic community, nutrient and carbon fluxes, OM content) and the estimated functional proxies should be assessed to strengthen the trait-based studies and to make more accurate predictions on how ecosystem functioning changes, both directly under variable sand extraction intensities and indirectly linked to changes in the sediment properties due to sand extraction.
Data availability statement
The data analyzed in this study is subject to the following licenses/restrictions: Data will be made available upon request. Requests to access these datasets should be directed to bmFub3UuZ29lZGVmcm9vQGlsdm8udmxhYW5kZXJlbi5iZQ==.
Ethics statement
The manuscript presents research on animals that do not require ethical approval for their study.
Author contributions
NG: Conceptualization, Data curation, Formal Analysis, Investigation, Methodology, Visualization, Writing – original draft. UB: Methodology, Supervision, Writing – review & editing, Conceptualization. KH: Methodology, Writing – review & editing, Conceptualization. JV: Methodology, Writing – review & editing, Conceptualization. TM: Methodology, Writing – review & editing, Conceptualization. AD: Conceptualization, Methodology, Supervision, Writing – review & editing.
Funding
The author(s) declare financial support was received for the research, authorship, and/or publication of this article. Data were gathered in the framework of the environmental monitoring program on sand extraction of ILVO, financially supported through the revenues of marine aggregate extraction in the BPNS. This study contributes to the BELSPO FED-tWIN METRIC project: Marine EcosysTem Responses In a multiple pressures Context.
Acknowledgments
The Measurement Service Ostend of the RBINS is acknowledged for the management of the EMS system on board the trailing hopper suction dredgers and the colleagues of FPS Economy are thanked for processing the EMS data. We acknowledge the crew of the RV Belgica for the excellent cooperation during the biological impact monitoring, as well as the Belgian Science Policy (Belspo) and RBINS - OD Nature for providing ship time on R.V. Belgica. Also, the crew of the R.V. Simon Stevin and VLIZ are acknowledged for providing ship time, and the organization and smooth execution of a number of sampling campaigns. ILVO colleagues from the macrobenthos lab are thanked for analyzing the macrobenthos samples.
Conflict of interest
The authors declare that the research was conducted in the absence of any commercial or financial relationships that could be construed as a potential conflict of interest.
The author(s) declared that they were an editorial board member of Frontiers, at the time of submission. This had no impact on the peer review process and the final decision.
Publisher’s note
All claims expressed in this article are solely those of the authors and do not necessarily represent those of their affiliated organizations, or those of the publisher, the editors and the reviewers. Any product that may be evaluated in this article, or claim that may be made by its manufacturer, is not guaranteed or endorsed by the publisher.
Supplementary material
The Supplementary Material for this article can be found online at: https://www.frontiersin.org/articles/10.3389/fmars.2023.1268999/full#supplementary-material
References
Akaike H. (1974). A new look at the statistical model identification. IEEE Trans. Automat Contr 19, 716–723. doi: 10.1109/TAC.1974.1100705
Barrio Froján C. R. S., Cooper K. M., Bremner J., Defew E. C., Wan Hussin W. M. R., Paterson D. M. (2011). Assessing the recovery of functional diversity after sustained sediment screening at an aggregate dredging site in the North Sea. Estuar. Coast. Shelf Sci. 92, 358–366. doi: 10.1016/j.ecss.2011.01.006
Beauchard O., Mestdagh S., Koop L., Ysebaert T., Herman P. M. J. (2022). Benthic synecology in a soft sediment shelf: habitat contrasts and assembly rules of life strategies. Mar. Ecol. Prog. Ser. 682, 31–50. doi: 10.3354/meps13928
Beauchard O., Veríssimo H., Queirós A. M., and Herman P. M. J. (2017). The use of multiple biological traits in marine community ecology and its potential in ecological indicator development. Ecol. Indic 76, 81–96. doi: 10.1016/j.ecolind.2017.01.011
Bernard G., Gammal J., Järnström M., Norkko J., Norkko A. (2019). Quantifying bioturbation across coastal seascapes: Habitat characteristics modify effects of macrofaunal communities. J. Sea Res. 152, 101766. doi: 10.1016/j.seares.2019.101766
Beukema J. J., Dekker R. (2013). Evaluation of Brey’s production/biomass model on the basis of a long-term data set on a clam population. Mar. Ecol. Prog. Ser. 489, 163–175. doi: 10.3354/meps10409
Birchenough S. N. R., Parker R. E., McManus E., Barry J. (2012). Combining bioturbation and redox metrics: Potential tools for assessing seabed function. Ecol. Indic. 12 (1), 8–16. doi: 10.1016/j.ecolind.2011.03.015
Bolam S. G., Coggan R. C., Eggleton J., Diesing M., Stephens D. (2014). Sensitivity of macrobenthic secondary production to trawling in the English sector of the Greater North Sea: A biological trait approach. J. Sea Res. 85, 162–177. doi: 10.1016/j.seares.2013.05.003
Bolam S. G., Eggleton J. D. (2014). Macrofaunal production and biological traits: Spatial relationships along the UK continental shelf. J. Sea Res. 88, 47–58. doi: 10.1016/j.seares.2014.01.001
Bolam S. G., McIlwaine P. S. O., Garcia C. (2016). Application of biological traits to further our understanding of the impacts of dredged material disposal on benthic assemblages. Mar. pollut. Bull. 105, 180–192. doi: 10.1016/j.marpolbul.2016.02.031
Bolam S. G., McIlwaine P., Garcia C. (2021). Marine macrofaunal traits responses to dredged material disposal. Mar. pollut. Bull. 168, 112412. doi: 10.1016/j.marpolbul.2021.112412
Borja A., Franco J., Erez V. P. (2000). A marine biotic index to establish the ecological quality of soft-bottom benthos within european estuarine and coastal environments. Mar. pollut. Bull. 40, 1100–1114. doi: 10.1016/S0025-326X(00)00061-8
Braeckman U., Foshtomi M. Y., Van Gansbeke D., Meysman F., Soetaert K., Vincx M., et al. (2014). Variable importance of macrofaunal functional biodiversity for biogeochemical cycling in temperate coastal sediments. Ecosystems 17, 720–737. doi: 10.1007/s10021-014-9755-7
Breine N. T., De Backer A., Van Colen C., Moens T., Hostens K., Van Hoey G. (2018). Structural and functional diversity of soft-bottom macrobenthic communities in the Southern North Sea. Estuar. Coast. Shelf Sci. 214, 173–184. doi: 10.1016/j.ecss.2018.09.012
Bremner J. (2008). Species’ traits and ecological functioning in marine conservation and management. J. Exp. Mar. Biol. Ecol. 366, 37–47. doi: 10.1016/j.jembe.2008.07.007
Bremner J., Rogers S. I., Frid C. L. J. (2006a). Matching biological traits to environmental conditions in marine benthic ecosystems. J. Mar. Syst. 60, 302–316. doi: 10.1016/j.jmarsys.2006.02.004
Bremner J., Rogers S. I., Frid C. L. J. (2006b). Methods for describing ecological functioning of marine benthic assemblages using biological traits analysis (BTA). Ecol. Indic 6, 609–622. doi: 10.1016/j.ecolind.2005.08.026
Brey T. (2001) Population dynamics in benthic invertebrates. A virtual handbook. Version 01.2. Available at: http://www.thomas-brey.de/science/virtualhandbook/ (Accessed 2023 October 3).
Brey T. (2012). A multi-parameter artificial neural network model to estimate macrobenthic invertebrate productivity and production. Limnol Oceanogr Methods 10, 581–589. doi: 10.4319/lom.2012.10.581
Brey T., Müller-Wiegmann C., Zittier Z. M. C., Hagen W. (2010). Body composition in aquatic organisms - A global data bank of relationships between mass, elemental composition and energy content. J. Sea Res. 64, 334–340. doi: 10.1016/j.seares.2010.05.002
Brown A. M., Warton D. I., Andrew N. R., Binns M., Cassis G., Gibb H. (2014). The fourth-corner solution - using predictive models to understand how species traits interact with the environment. Methods Ecol. Evol. 5, 344–352. doi: 10.1111/2041-210X.12163
Cheng C. H., Soetaert K., Borsje B. W. (2020). Sediment characteristics over asymmetrical tidal sand waves in the Dutch north sea. J. Mar. Sci. Eng. 8(6), 409. doi: 10.3390/JMSE8060409
Chevenet F., Dolédec S., Chessel D. (1994). A fuzzy coding approach for the analysis of long-term ecological data. Freshw. Biol. 31, 295–309. doi: 10.1111/j.1365-2427.1994.tb01742.x
Clare D. S., Bolam S. G., McIlwaine P. S. O., Garcia C., Murray J. M., Eggleton J. D. (2022). Biological traits of marine benthic invertebrates in Northwest Europe. Sci. Data 9, 339. doi: 10.1038/s41597-022-01442-y
Cooper K., Boyd S., Aldridge J., Rees H. (2007). Cumulative impacts of aggregate extraction on seabed macro-invertebrate communities in an area off the east coast of the United Kingdom. J. Sea Res. 57, 288–302. doi: 10.1016/j.seares.2006.11.001
Cooper K. M., Curtis M., Wan Hussin W. M. R., Barrio Froján C. R. S., Defew E. C., Nye V., et al. (2011). Implications of dredging induced changes in sediment particle size composition for the structure and function of marine benthic macrofaunal communities. Mar. pollut. Bull. 62, 2087–2094. doi: 10.1016/j.marpolbul.2011.07.021
Cusson M., Bourget E. (2005). Global patterns of macroinvertebrate production in marine benthic habitats. Mar. Ecol. Prog. Ser. 297, 1–14. doi: 10.3354/meps297001
Dauer D. M. (1993). Biological criteria, environmental health and estuarine macrobenthic community structure. Mar. pollut. Bull. 26, 249–257. doi: 10.1016/0025-326X(93)90063-P
De Backer A., Van Hoey G., Coates D., Vanaverbeke J., Hostens K. (2014). Similar diversity-disturbance responses to different physical impacts: Three cases of small-scale biodiversity increase in the Belgian part of the North Sea. Mar. pollut. Bull. 84, 251–262. doi: 10.1016/j.marpolbul.2014.05.006
Desprez M. (2000). Physical and biological impact of marine aggregate extraction along the French coast of the Eastern English Channel: Short-and long-term post-dredging restoration. ICES J. Mar. Sci. 57 (5), 1428–1438. doi: 10.1006/jmsc.2000.0926
Desprez M., Pearce B., Le Bot Desprez S., Pearce M., Bot L. (2010). The biological impact of overflowing sands around a marine aggregate extraction site: Dieppe (eastern English Channel). ICES J. Mar. Sci. 67, 270–277. doi: 10.1093/icesjms/fsp245
Desprez M., Stolk A., Cooper K. M. (2022). Marine aggregate extraction and the Marine Strategy Framework Directive: A review of existing research. ICES Cooperative Res. Rep. 354. doi: 10.17895/ices.pub.19248542
Dolbeth M., Lillebø A. I, Cardoso P. G., Ferreira S. M., Pardal M. A. (2005). Annual production of estuarine fauna in different environmental conditions: An evaluation of the estimation methods. J. Exp. Mar. Biol. Ecol. 326 (2), 115–127. doi: 10.1016/j.jembe.2005.05.010
Dolbeth M., Cusson M., Sousa R., Pardal M. A. (2012). Secondary production as a tool for better understanding of aquatic ecosystems. Can. J. Fisheries Aquat. Sci. 69, 1230–1253. doi: 10.1139/F2012-050
Dolbeth M., Dolédec S., Pardal M.Â. (2015). Relationship between functional diversity and benthic secondary production in a disturbed estuary. Mar. Ecol. Prog. Ser. 539, 33–46. doi: 10.3354/meps11473
Festjens F., Buyse J., De Backer A., Hostens K., Lefaible N., Vanaverbeke J., et al. (2023). Functional trait responses to different anthropogenic pressures. Ecol. Indic 146, 109854. doi: 10.1016/j.ecolind.2022.109854
Fleddum A., Atkinson L. J., Field J. G., Shin P. (2013). Changes in biological traits of macro-benthic communities subjected to different intensities of demersal trawling along the west coast of southern Africa. J. Mar. Biol. Assoc. United Kingdom 93 (8), 2027–2038. doi: 10.1017/S0025315413000647
Gogina M., Renz J. R., Forster S., Zettler M. L. (2022). Benthic macrofauna community bioirrigation potential (BIPc): regional map and utility validation for the south-western baltic sea. Biology 11 (7), 1085. doi: 10.3390/biology11071085
Gogina M., Zettler M. L., Vanaverbeke J., Dannheim J., Van Hoey G., Desroy N., et al. (2020). Interregional comparison of benthic ecosystem functioning: Community bioturbation potential in four regions along the NE Atlantic shelf. Ecol. Indic 110, 105945. doi: 10.1016/j.ecolind.2019.105945
Hall S. J. (1994). “Physical disturbance and marine benthic communities: life in unconsolidated sediments,” in Oceanography and marine biology: an annual review. Eds. Ansell A. D., Gibson R. N., Barnes M. (London: UCL Press), 179–239.
Hartig F. (2022) DHARMa: Residual Diagnostics for Hierarchical (Multi-Level/Mixed) Regression Models_. R package version 0.4.6. Available at: https://CRAN.R-project.org/package=DHARMa.
Hinz H., Törnroos A., de Juan S. (2021). Trait-based indices to assess benthic vulnerability to trawling and model loss of ecosystem functions. Ecol. Indic 126, 107692. doi: 10.1016/j.ecolind.2021.107692
Huettel M., Rusch A. (2000). Transport and degradation of phytoplankton in permeable sediment. Limnol Oceanogr 45, 534–549. doi: 10.4319/lo.2000.45.3.0534
ICES (2016). Effects of extraction of marine sediments on the marine environment 2005-2011 (International Council for the Exploration of the Sea).
ICES (2019). Working group on the effects of extraction of marine sediments on the marine ecosystem (WGEXT). doi: 10.17895/ices.pub.5733
Krause J. C., Diesing M., Arlt G. (2010). The physical and biological impact of sand extraction: a case study of the western baltic sea. J. Coast. Res. SI 51, 215–226. doi: 10.2112/SI51-020.1
Kristensen E., Penha-Lopes G., Delefosse M., Valdemarsen T., Quintana C. O., Banta G. T. (2012). What is bioturbation? The need for a precise definition for fauna in aquatic sciences. Mar. Ecol. Prog. Ser. 446, 285–302. doi: 10.3354/meps09506
Kröncke I., Stoeck T., Wieking G., Palojärvi A. (2004). Relationship between structural and functional aspects of microbial and macrofaunal communities in different areas of the North Sea. Mar. Ecol. Prog. Ser. 282, 13–31. doi: 10.3354/meps282013
Lam-Gordillo O., Baring R., Dittmann S. (2020). “Ecosystem functioning and functional approaches on marine macrobenthic fauna: A research synthesis towards a global consensus,” in Ecological indicators, vol. 115. (Elsevier B.V). doi: 10.1016/j.ecolind.2020.106379
Le Bot S., Lafite R., Fournier M., Baltzer A., Desprez M. (2010). Morphological and sedimentary impacts and recovery on a mixed sandy to pebbly seabed exposed to marine aggregate extraction (Eastern English Channel, France). Estuar. Coast. Shelf Sci. 89, 221–233. doi: 10.1016/j.ecss.2010.06.012
Mermillod-Blondin F., François-Carcaillet F., Rosenberg R. (2005). Biodiversity of benthic invertebrates and organic matter processing in shallow marine sediments: An experimental study. J. Exp. Mar. Biol. Ecol. 315, 187–209. doi: 10.1016/j.jembe.2004.09.013
Nasi F., Vesal S. E., Relitti F., Bazzaro M., Teixidó N., Auriemma R., et al. (2023). Taxonomic and functional macrofaunal diversity along a gradient of sewage contamination: A three-year study. Environ. pollut. 323, 121022. doi: 10.1016/j.envpol.2023.121022
Neumann A., van Beusekom J. E. E., Eisele A., Emeis K. C., Friedrich J., Kröncke I., et al. (2021). Macrofauna as a major driver of bentho-pelagic exchange in the southern North Sea. Limnol Oceanogr 66, 2203–2217. doi: 10.1002/lno.11748
Newell R. C., Hitchcock D. R., Seiderer L. J. (1999). Organic enrichment associated with outwash from marine aggregates dredging: A probable explanation for surface sheens and enhanced benthic production in the vicinity of dredging operations. Mar. pollut. Bull. 38, 809–818. doi: 10.1016/S0025-326X(99)00045-4
Newell R. C., Seiderer L. J., Hitchcock D. R. (1998). The impact of dredging works in coastal waters: a review of the sensitivity to disturbance and subsequent recovery of biological resources on the sea bed. Oceanography Mar. Biology: an Annu. Rev. 36, 127–178.
Pearson T. H., Rosenberg R. (1978). Macrobenthic succession in relation to organic enrichment and pollution of the marine environment. Oceanography Mar. Biol. 16, 229–311.
Peduzzi P. (2014). Sand, rarer than one thinks. Environ. Dev. 11, 208–218. doi: 10.1016/j.envdev.2014.04.001
Petracco M., Cardoso R. S., Corbisier T. N., Turra A. (2012). Secondary production of sandy beach macrofauna: An evaluation of predictive models. Estuarine Coast. Shelf Sci. 115, 359–365. doi: 10.1016/j.ecss.2012.10.002
Queirós A. M., Birchenough S. N. R., Bremner J., Godbold J. A., Parker R. E., Romero-Ramirez A., et al. (2013). A bioturbation classification of European marine infaunal invertebrates. Ecol. Evol. 3, 3958–3985. doi: 10.1002/ece3.769
R Core Team (2023). R: A language and environment for statistical computing (Vienna, Austria: R Foundation for Statistical Computing). Available at: https://www.R-project.org/.
Reiss H., Greenstreet S. P. R., Sieben K., Ehrich S., Piet G. J., Quirijns F., et al. (2009). Effects of fishing disturbance on benthic communities and secondary production within an intensively fished area. Mar. Ecol. Prog. Ser. 394, 201–213. doi: 10.3354/meps08243
Renz J. R., Powilleit M., Gogina M., Zettler M. L., Morys C., Forster S. (2018). Community bioirrigation potential (BIPc), an index to quantify the potential for solute exchange at the sediment-water interface. Mar. Environ. Res. 141, 214–224. doi: 10.1016/j.marenvres.2018.09.013
Robinson J. E., Newell R. C., Seiderer L. J., Simpson N. M. (2005). Impacts of aggregate dredging on sediment composition and associated benthic fauna at an offshore dredge site in the southern North Sea. Mar. Environ. Res. 60, 51–68. doi: 10.1016/j.marenvres.2004.09.001
Roche M., Degrendele K., Vandenreyken H., Schotte P. (2017). Multi time and space scale monitoring of the sand extraction and its impact on the seabed by coupling EMS data and MBES measurements in Belgian marine sand: a scarce resource? Eds. Degrendele K., Vandenreyken H., 6–37.
Rosenberg R. (1995). Benthic marine fauna structured by hydrodynamic processes and food availability. Netherlands J. Sea Res. 34, 303–317. doi: 10.1016/0077-7579(95)90040-3
Ruddick K., Lacroix G. (2006). “Hydrodynamics and meteorology of the belgian coastal zone,” in Current status of euthrophication in the belgian coastal zone, (Brussels: Presses Universitaires de Bruxelles) 1–15.
Saulnier E., Brind’Amour A., Tableau A., Rufino M. M., Dauvin J. C., Luczak C., et al. (2019). Seasonality in coastal macrobenthic biomass and its implications for estimating secondary production using empirical models. Limnol Oceanogr 64, 935–949. doi: 10.1002/lno.11086
Sciberras M., Parker R., Powell C., Robertson C., Kröger S., Bolam S., et al. (2016). Impacts of bottom fishing on the sediment infaunal community and biogeochemistry of cohesive and non-cohesive sediments. Limnol Oceanogr 61, 2076–2089. doi: 10.1002/lno.10354
Shojaei M. G., Gutow L., Dannheim J., Pehlke H., Brey T. (2015). “Functional diversity and traits assembly patterns of benthic macrofaunal communities in the southern north sea,” in Towards an interdisciplinary approach in earth system science: advances of a helmholtz graduate research school (Heidelberg: Springer International Publishing), 183–195. doi: 10.1007/978-3-319-13865-7_20
Shull D. H. (2019). Bioturbation in Encyclopedia of ocean sciences, 3rd Edition vol. 2 Eds. Kirk C. J., Henry B. J., Patricia Y. L. (Elsevier), 671–676.
Snelgrove P. V. R., Butman C. A. (1994). Animal-sediment relationships revisited: cause versus effect. Oceanogrophy Mar. Biology: an Annu. Rev. 32, 111–177.
Solan M., Cardinale B. J., Downing A. L., Engelhardt K. A., Ruesink J. L., Srivastava D. S. (2004). Extinction and ecosystem function in the marine benthos. Sci. (1979) 306, 1177–1180. doi: 10.1126/science.1103960
Szekely T., Gourrion J., Pouliquen S., Reverdin G. (2023). CORA, coriolis ocean dataset for reanalysis (SEANOE). doi: 10.17882/46219
Thrush S. F., Hewitt J. E., Gibbs M., Lundquist C., Norkko A. (2006). Functional role of large organisms in intertidal communities: Community effects and ecosystem function. Ecosystems 9 (6), 1029–1040. doi: 10.1007/s10021-005-0068-8
Torres A., Brandt J., Lear K., Liu J. (2017). A looming tragedy of the sand commons. Sci. (1979) 357, 970–971. doi: 10.1126/science.aao0503
Toussaint E., De Borger E., Braeckman U., De Backer A., Soetaert K., Vanaverbeke J. (2021). Faunal and environmental drivers of carbon and nitrogen cycling along a permeability gradient in shallow North Sea sediments. Sci. Total Environ. 767, 144994. doi: 10.1016/j.scitotenv.2021.144994
Tsikopoulou I., Smith C. J., Papadopoulou K. N., Austen M. C. (2022). Linking species functional traits to specific biogeochemical processes under trawling pressure. Biol. (Basel) 11 (10), 1378. doi: 10.3390/biology11101378
van Dalfsen J. A., Essink K., Toxvig Madsen H., Birklund J., Romero J., Manzanera M. (2000). Differential response of macrozoobenthos to marine sand extraction in the North Sea and the Western Mediterranean. ICES J. Mar. Sci. 57 (5), 1439–1445. doi: 10.1006/jmsc.2000.0919
Van Den Branden R., De Schepper G., Naudts L. (2017). “The Electronic Monitoring System (EMS) as a minimum requirement for monitoring the extraction of an increasingly scarce raw material,” in Belgian marine sand: a scarce resource. Eds. Degrendele K., Vandenreyken H., 39–45.
Van Denderen P. D., Bolam S. G., Hiddink J. G., Jennings S., Kenny A., Rijnsdorp A. D., et al. (2015). Similar effects of bottom trawling and natural disturbance on composition and function of benthic communities across habitats. Mar. Ecol. Prog. Ser. 541, 31–43. doi: 10.3354/meps11550
Van Der Meer J., Brey T., Heip C., Herman P. M. J., Moens T., Van Oevelen D. (2013). “Measuring the flow of energy and matter in marine benthic animal populations,” in Methods for the study of marine benthos. Ed. Eleftheriou A. (John Wiley & Sons, Ltd).
Van Hoey G., Degraer S., Vincx M. (2004). Macrobenthic community structure of soft-bottom sediments at the Belgian Continental Shelf. Estuarine Coast. Shelf Sci. 59 (4), 599–613. doi: 10.1016/j.ecss.2003.11.005
Verfaillie E., Van Lancker V., Van Meirvenne M. (2006). Multivariate geostatistics for the predictive modelling of the surficial sand distribution in shelf seas. Cont Shelf Res. 26, 2454–2468. doi: 10.1016/j.csr.2006.07.028
Violle C., Navas M.-L., Vile D., Kazakou E., Fortunel C., Hummel I., et al. (2007). Let the concept of trait be functional! Oikos 116, 882–892. doi: 10.1111/j.2007.0030-1299.15559.x
Volkenborn N., Woodin S. A., Wethey D. S., Polerecky L. (2019). “Bioirrigation,” in Encyclopedia of ocean sciences (Elsevier), 663–670. doi: 10.1016/B978-0-12-409548-9.09525-7
Wang Y., Naumann U., Wright S. T., Warton D. I. (2012). Mvabund- an R package for model-based analysis of multivariate abundance data. Methods Ecol. Evol. 3, 471–474. doi: 10.1111/j.2041-210X.2012.00190.x
Wang S. V., Wrede A., Tremblay N., Beermann J. (2022). Low-frequency noise pollution impairs burrowing activities of marine benthic invertebrates. Environ. pollut. 310, 119899. doi: 10.1016/j.envpol.2022.119899
Wan Hussin W. M. R., Cooper K. M., Froján C. R. S. B., Defew E. C., Paterson D. M. (2012). Impacts of physical disturbance on the recovery of a macrofaunal community: A comparative analysis using traditional and novel approaches. Ecol. Indic 12, 37–45. doi: 10.1016/j.ecolind.2011.03.016
Waye-Barker G. A., McIlwaine P., Lozach S., Cooper K. M. (2015). The effects of marine sand and gravel extraction on the sediment composition and macrofaunal community of a commercial dredging site (15 years post-dredging). Mar. pollut. Bull. 99, 207–215. doi: 10.1016/j.marpolbul.2015.07.024
WoRMS Editorial Board (2023) World register of marine species (VLIZ). Available at: https://www.marinespecies.org (Accessed 2023-07-28).
Wrede A., Andresen H., Asmus R., Wiltshire K. H., Brey T. (2019). Macrofaunal irrigation traits enhance predictability of nutrient fluxes across the sediment-water interface. Mar. Ecol. Prog. Ser. 632, 27–42. doi: 10.3354/meps13165
Wrede A., Beermann J., Dannheim J., Gutow L., Brey T. (2018). Organism functional traits and ecosystem supporting services – A novel approach to predict bioirrigation. Ecol. Indic 91, 737–743. doi: 10.1016/j.ecolind.2018.04.026
Wrede A., Dannheim J., Gutow L., Brey T. (2017). Who really matters: Influence of German Bight key bioturbators on biogeochemical cycling and sediment turnover. J. Exp. Mar. Biol. Ecol. 488, 92–101. doi: 10.1016/j.jembe.2017.01.001
Wyns L., Roche M., Barette F., Van Lancker V., Degrendele K., Hostens K., et al. (2021). Near-field changes in the seabed and associated macrobenthic communities due to marine aggregate extraction on tidal sandbanks: A spatially explicit bio-physical approach considering geological context and extraction regimes. Cont Shelf Res. 229, 104546. doi: 10.1016/j.csr.2021.104546
Keywords: marine aggregate extraction, ecosystem functioning, macrobenthos, environmental impact, proxies
Citation: Goedefroo N, Braeckman U, Hostens K, Vanaverbeke J, Moens T and De Backer A (2023) Understanding the impact of sand extraction on benthic ecosystem functioning: a combination of functional indices and biological trait analysis. Front. Mar. Sci. 10:1268999. doi: 10.3389/fmars.2023.1268999
Received: 28 July 2023; Accepted: 16 October 2023;
Published: 31 October 2023.
Edited by:
Federica Nasi, National Institute of Oceanography and Applied Geophysics, ItalyReviewed by:
Seyed Ehsan Vesal, National Research Council (CNR), ItalyMonika Kędra, Polish Academy of Sciences, Poland
Mehdi Ghodrati Shojaei, Tarbiat Modares University, Iran
Copyright © 2023 Goedefroo, Braeckman, Hostens, Vanaverbeke, Moens and De Backer. This is an open-access article distributed under the terms of the Creative Commons Attribution License (CC BY). The use, distribution or reproduction in other forums is permitted, provided the original author(s) and the copyright owner(s) are credited and that the original publication in this journal is cited, in accordance with accepted academic practice. No use, distribution or reproduction is permitted which does not comply with these terms.
*Correspondence: Nanou Goedefroo, bmFub3UuZ29lZGVmcm9vQGlsdm8udmxhYW5kZXJlbi5iZQ==