- 1Departamento de Química, Universidad de Las Palmas de Gran Canaria (ULPGC), Las Palmas de Gran Canaria (Las Palmas de GC), Canary Islands, Spain
- 2Instituto Español de Oceanografía (IEO), Centro Oceanográfico de Canarias, Consejo Superior de Investigaciones Científicas (CSIC), Santa Cruz de Tenerife, Canary Islands, Spain
- 3Departamento de Química, Unidad Departamental de Química Analítica, Facultad de Ciencias, Universidad de La Laguna (ULL), La Laguna, Canary Islands, Spain
- 4Instituto Universitario de Enfermedades Tropicales y Salud Pública de Canarias, Universidad de La Laguna (ULL), La Laguna, Canary Islands, Spain
- 5Oceanografía Física y Geofísica Aplicada (OFYGA), IU-ECOAQUA, Universidad de Las Palmas de Gran Canaria, Las Palmas de Gran Canaria (Las Palmas de GC), Canary Islands, Spain
The presence of different types of microplastics (MPs) in the Atlantic Ocean has been well-documented, with data collected from the Canary Islands indicating high concentrations of MPs at four different beaches (hot spots). However, critical gaps persist regarding the abundance of MPs in diverse open ocean regions, at varying depths, and their transport behavior and origin. This study aims to evaluate, for the first time, the large-scale (>100 km) and mesoscale (10-100 km) ocean dynamic processes and the resulting transport of MPs in the region. On the one hand, this study investigates the surface velocity of currents between 27-32°N latitude range, establishing correlation with the accumulation of MPs on diverse beaches across several islands of the archipelago. This analysis goes beyond studying the predominant current by integrating data at a smaller geographical scale over a two-year period. Concurrently, previous and ongoing research suggests the presence of small MPs (SMPs,<1 mm) at high concentration at a depth of 1100 meters in this region. By integrating these vertical profiles of MPs with a deep understanding of the physical processes of the Canary region accumulated over decades of research, our study introduces an innovative model that outlines how SMPs are sink and transported in open oceans by water mases. This comprehensive approach not only enhances our understanding of the complex dynamics influencing the distribution of MPs but also provides crucial insights to address the widespread problem of MP pollution in the Atlantic.
1 Introduction
Nowadays, it is clear that plastic pollution is a worldwide hazard that affects every environmental compartment, marine environment being the one mostly studied up to now (Zhou et al., 2022). Despite the important efforts that have been developed over the last years, relevant issues still remain unclear, especially the ones concerning the movement in the open ocean or the existence of specific accumulation zones.
At a global scale, the ocean current systems are responsible for transporting marine debris -which is mostly composed of plastics (Barnes et al., 2009)- around the globe, reaching as far afield as Antarctica (Zhang et al., 2022) or the Artic Ocean (Cózar et al., 2017; Citterich et al., 2023). In coastal areas, the main factors affecting plastics transport are the wind, waves and tides, which mainly transport plastic particles according to their density, size and shape (Le Roux, 2005). In the open ocean, seabed, and water column, the behavior of plastic is influenced by internal factors, such as plastic composition and state (density, degradation state, size, among others), as well as external factors. The flow dynamics induced by the geostrophic balance, temperature, the Coriolis effect, the convective movement of water induced by its temperature difference and the wave action, are all external factors that influence the vertical and horizontal transport, and thus the dispersion of plastic debris (Kubota, 1994; Martinez et al., 2009; Browne et al., 2010; van Sebille et al., 2020). At surface, in the open ocean, floating marine plastic debris converges in several zones of accumulation of millions of km2 in areas known as “garbage patches” getting trapped for decades to centuries (van Sebille et al., 2012). These patches are generally formed as a consequence of the current systems of all five subtropical ocean gyres (Cózar et al., 2014; Eriksen et al., 2014).
Understanding the movement pattern of plastics in the open ocean, in particular microplastics (MPs, i.e., size between 1 µm and 5 mm, the most accepted definition) and their arrival to any part of the world, is useful for predicting the fate of these materials in the different environmental compartments (especially if they are originated elsewhere), their potential impact on marine biota and the ecosystems, and for assessing potential MPs removal techniques.
Worldwide, it is known that the concentration of marine litter on the ocean surface is growing exponentially (Borrelle et al., 2020), with an estimation of an increase of 7.2 trillion particles/year between 2011-2015 and 15.6 trillion particles/year between 2015-2019 (Eriksen et al., 2023). Additionally, there is enough experimental data to know that MPs not only appear on the surface or on the seafloor (i.e. in sediment samples), but also in the water column at different depths (Choy et al., 2019; Egger et al., 2020; Li et al., 2020; Pabortsava and Lampitt, 2020; Vega-Moreno et al., 2021), generally with sizes lower than 1 mm (Cincinelli et al., 2019; Simon-Sánchez et al., 2022).
The Canary Islands are an archipelago composed of eight main islands and several islets located within the North Atlantic Subtropical Gyre in the path of the Canary Current, and a zone of relatively high MPs arrival (Cardoso and Caldeira, 2021). Local oceanographic conditions significantly affect MPs concentrations (Adamopoulou et al., 2021). This is observed from the data compiled in previous research developed up to now in which MPs have been gathered in beaches of the region (Table 1). In this sense, and although there is not a clear definition of what a hotspot of MPs arrival is, several authors have already highlighted the existence of 4 of such spots in the Canaries (see Figure 1) (Baztan et al., 2014; Herrera et al., 2018; Álvarez-Hernández et al., 2019; Edo et al., 2019; González-Hernández et al., 2020; Rapp et al., 2020; Reinold et al., 2020; Hernández-Sánchez et al., 2021): located in Playa Grande (Tenerife Island) (Álvarez-Hernández et al., 2019), Playa de Famara (Lanzarote Island) (Baztan et al., 2014; Herrera et al., 2018), Playa Lambra (La Graciosa Island) (Baztan et al., 2014; Herrera et al., 2018) and Arenas Blancas (El Hierro Island) (Hernández-Sánchez et al., 2021), being the most recent. These beaches have in common that they have a north or north-east orientation with visual evidence of MPs contamination all over the year. Besides, more than 100 g/m2 of plastics or concentrations higher than 3,000 items/m2 have been reported in certain times of the year (Hernández-Sánchez et al., 2021). Although it is a common pattern, nowadays it is not fully understood why such places are more prone than others of the same islands to receive a higher arrival of MPs.
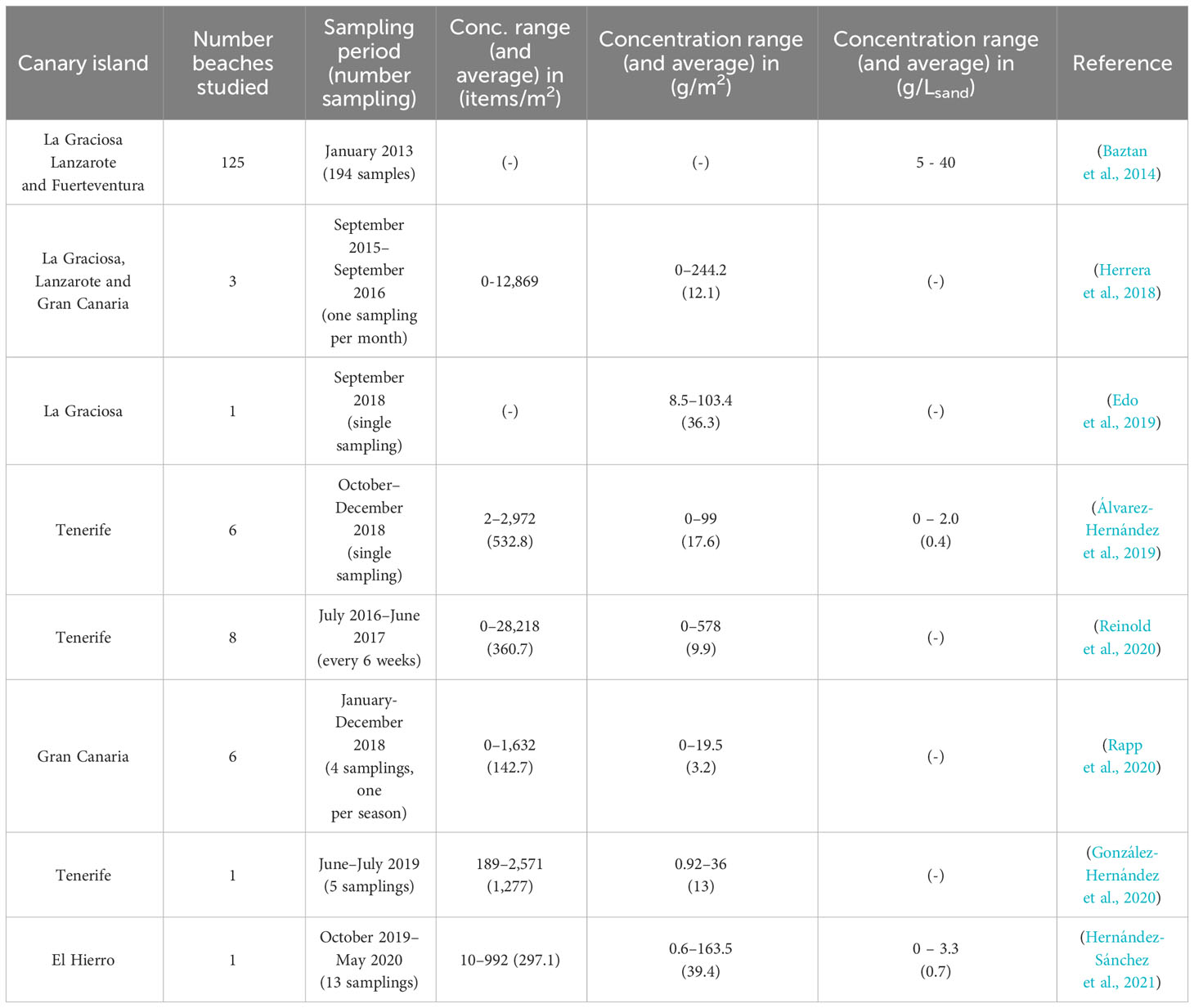
Table 1 Published literature (from less to more recent) where MPs (sizes in the range 1–5 mm) have been determined in beaches of the Canary Islands (Spain).
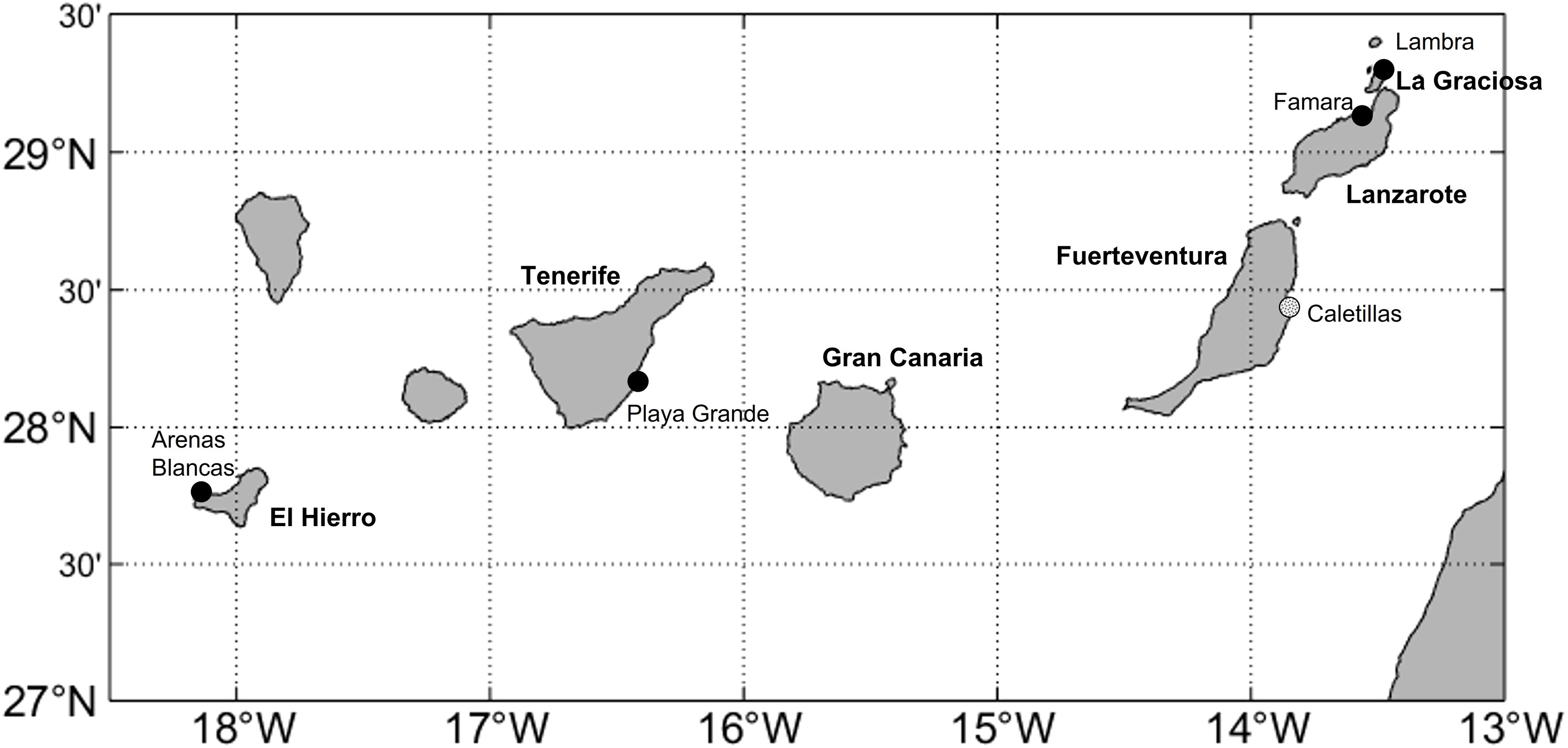
Figure 1 Map of the previously identified hotspots of MPs arrival in the Canary Islands (Spain) according to Table 1. Already published: ; not published yet:
.
Recently, Cardoso and Caldeira (2021) modelled the exposure of the Macaronesian islands to marine plastic pollution using Lagrangian simulations, finding that the North Atlantic Subtropical Gyre is the most conspicuous feature in particles and drifter trajectories. These authors also indicated that buoyant particles from the North Atlantic “garbage patch” are most likely to arrive to the archipelagos if they are significantly driven by the wind. To the best of our knowledge, this is the first publication regarding ocean surface modelling in this region. However, the authors focused on the characterization of the potential sources and pathways of floating marine plastic litter affecting such archipelagos at a macroscale level (>100 km), but not on the specific analysis and identification of high MPs arrival zones in the region.
Beyond the subtropical gyre, it is also well known that the Mediterranean outflow water at the Strait of Gibraltar (around 1300 km apart from the Canary Islands), upon coming in contact with the Atlantic water, sinks to the seafloor at this region producing the so called Mediterranean Water (MW) (Bashmachnikov et al., 2015). As a result of the high salinity of the MW, it sinks to the Atlantic basin following the topography until it reaches 1000-1200 m depth, where it finds its neutral density with the surrounding water near Cape San Vincent (Portugal). At this depth, MW extends across the Atlantic Ocean in different directions, predominantly northward but also westward and southward, thus reaching the Canary Islands region (Fraile-Nuez et al., 2010). The southern direction is favored by the presence of mesoscale Mediterranean water eddies (“meddies”) activity (Richardson et al., 2000; Bower et al., 2002), which are well-known structures of accumulation and conservation of water properties (Sangrà et al., 2009). Some of these meddies regularly reach the Canary Islands latitudes where their presence has been registered over several decades influencing the water characteristics at intermediate levels (Machín et al., 2006; Papiol et al., 2019).
Despite the distance travelled from its place of origin, MW still retains some properties present when exiting the Strait of Gibraltar. Such water mass has not only a salinity higher than the surrounding water, but may also have a higher concentration of MPs, as the Mediterranean Sea has high macro & MP pollution (Schmidt et al., 2018; Simon-Sánchez et al., 2022). In fact, it is also considered to be a hot spot of plastic pollution, receiving 5-10% of global plastic mass in a single semi-enclosed basin (Sharma et al., 2021; Tsiaras et al., 2022). However, the possible presence of MPs transported from the Mediterranean Sea into the Atlantic, in particular, to the Canary Islands region, has not been previously documented.
As all of the above evidences point out, addressing the surface and sub-surface ocean dynamics complexity is needed to understand the behavior of the transport of MPs in the open ocean not only at a macroscale level as subtropical gyres, but also at a mesoscale level (Brach et al., 2018; van Sebille et al., 2020). Moreover, it is necessary to work with model outputs to help to elucidate the fate and transport of MPs for a better understanding of the effect of MP presence in the environment (Cardoso and Caldeira, 2021; Phan and Luscombe, 2023). Thus, this work aims to (i) generate probability distribution maps for buoyant MP particles arriving from the North Atlantic Ocean to the Canary Islands’ coasts, enabling the identification of potential hotspots of MP arrival based on these trajectories and (ii) to assess the likely transport of MPs within the Middle Waters throughout the Atlantic Ocean at depths between 1000-1200 m. The main focus of this study is the construction of probability distribution maps to understand the observed spatial patterns of MP accumulation.
2 Materials and methods
With the aim of assessing the extent of the impact of plastic particles at the coasts and open ocean around the Canary Islands region, as well as determining their possible origin, the projects DeepPLAS and IMPLAMAC have been monitoring for the last few years the presence of MPs in the ocean as well as the arrival of marine litter, mainly MPs, to different beaches of the Macaronesian islands (archipelagos of the Canary Islands, Madeira, Azores and Cape Verde). The comprehension of the behavior of plastic waste in the sea is of vital importance to unveil aspects such as the degradation phenomena to which this marine waste is subjected: how long these particles remain in the sea and in the water column to know if the transport behavior is the same according to the range of MPs sizes and shapes; how long it can take plastic to travel a certain distance; or what are their most recurrent trajectories.
2.1 Lagrangian trajectories
The simulation of the Lagrangian trajectories of microparticles (simulated MPs) released at the sea surface located at the north of the Canary Islands archipelago was performed under Matlab. One thousand massless particles randomly distributed were daily deployed on the region between 30-31N and 19-10W. These particles were driven by the daily velocity field operationally provided by the Atlantic-Iberian Biscay Irish- Ocean Physics Reanalysis (IBI) product available at the Copernicus Marine Service (https://marine.copernicus.eu). The system is based on an application of the eddy-resolving NEMO model run at a horizontal resolution of 1/12 (~8-9 km) (Madec et al., 1998). The velocity data are distributed vertically over 50 z-levels with a resolution decreasing from about 1 m in the upper 10 m to more than 400 m in the deep ocean. The simulations were performed with the data set provided for the years 2020 and 2021 at a depth of 0.5 m. A total of 365000 trajectories per year were produced and each trajectory spanned 90 days. Finally, once a particle reaches a beach, its trajectory concludes, without accounting for potential effects of wave washing.
A similar approach was followed at intermediate levels to produce Lagrangian trajectories related to the spreading of Mediterranean Water in the eastern North Atlantic focusing on the region around the Canary Islands. In this case, the velocity data were also provided by Copernicus though their product GLORYS12V1, which has a regular grid of 1/12 with 50 vertical levels at standard depths. Stokes drift and small-scale turbulence are not included in the models used to produce the Lagrangian trajectories. In this case, 60501 particles were released at 1062 m depth in the vicinity of Cape Sant Vincent and followed for 4 years (2017-2020). In both cases, surface and intermediate levels, the Lagrangian trajectories are defined by the ODE , with the initial condition x(t0)=x0 (van Sebille et al., 2018; Nordam and Duran, 2020). An explicit Runge-Kutta scheme of order 4-5 in both space and time was then used to solve the ODE (Tyranowski and Desbrun, 2019; Komen et al., 2020). Windage data has not been considered on this model as MPs do not significantly protrude above the water’s surface (Onink et al., 2019).
2.2 Climatological variables data
World Ocean Atlas data (Reagan, 2023) are used to feature the climatological distribution of salinity and temperature, extracted from the Climate Normal Fields Database. In particular, their objectively analyzed mean on 1/4° and 1° grid of all available decades (1991-2020) was used. Horizontal contours and diagrams were generated with Matlab R2022a.
2.3 Water column microplastics sampling
MPs samples were collected during the oceanographic cruise VULCANA-III-0421 in the southern region of El Hierro island (Canary Islands, Spain). The sampling was conducted onboard R/V Ángeles Alvariño, which belongs to the Spanish Institute of Oceanography (IEO-CSIC). The collection samples were taken at five different stations between April 4th and 5th, 2021, specifically at coordinates 18°20’ W and between 26°40’-27°40’ N (see the stations map at Supplementary Figure 1.
To obtain discrete water samples for MPs analysis, a rosette of 24-12-L Niskin bottles was used to cover the water column from 0 to 1200 m depth. Seawater samples were collected and passed through filters with a mesh size of 100 µm. Each sample consisted of 60-L of seawater, obtained from 5 Niskin bottles (12-L per Niskin). This filter was subsequently washed with Milli-Q water, and the concentrated material was retained on a 47 mm Whatmann GF/F filter for MPs evaluation. The samples were stored at -20°C for later visual examination and counting. Additionally, a blank sample was processed to account for any contamination during the filtration procedure, and it was analyzed onboard.
An Euromex® Nexius Zoom EVO stereo-microscope with a digital camera (model NZ1703S) was used for MPs visual identification, counting and classification.
MPs were analyzed by micro-Fourier Transform Infrared Spectroscopy (µ-FTIR), using a Nicolet™ iN10 infrared microscope (Thermo Fisher Scientific, USA) for a preliminary study to identify the composition of MPs, limited to particles larger than 100 µm. A total of 146 fibers and 114 fragments were analyzed. To carry out the analysis, items were randomly selected and handpicked using a fine stainless-steel tweezer. Then, they were placed in a numbered spot of a reflection slide made of low-e glass slide. The analysis was performed using the OMNIC Picta software (Thermo Fisher Scientific, USA) in reflection mode with a LN-cooled mercury-cadmium-telluride (MCT) detector. Spectral resolution of 4 cm-1 and 16 scans (5 s of acquisition time) were chosen as working conditions in a spectral range from 4000 to 650 cm-1.
The spectral libraries ‘Biblioteque Particules’, ‘HR Sprouse Polymers by Transmission’ and ‘Polimeri’ supplied by Thermo Scientific, as well as ‘FLOPP’ and ‘FLOPP-e’ open libraries were used as polymer reference libraries (De Frond et al., 2021). The identification was considered satisfactory if the Pearson’s correlation coefficient between reference spectrum and acquired spectrum was ≥70%. Additionally, if the correlation score for a suspected MP was within the range of 60- 70%, the analysis of that particle was carefully to ensure its identification. Moreover, a double-check of the identification was accomplished using Open Specy databases (Cowger et al., 2021).
3 Results and discussion
3.1 Shoreline arrival of microplastics
The arrival of MPs at different beaches and coastal regions in the Canary Islands is not homogeneous, as previous results have shown (Table 1). A high variability is observed between the different islands, which are located less than 100 km from each other, and also within each island (Baztan et al., 2014; Álvarez-Hernández et al., 2019). A similar pattern has also been observed in the Azores, with large variations in MPs concentrations from one island to another, despite having similar orientations and closer distances (Pham et al., 2020).
Several authors have reported that MPs arrive to the Canary Islands coasts as a result of their position under the influence of the North Atlantic Subtropical Gyre (Herrera et al., 2018; Reinold et al., 2020), so MPs concentration is higher at beaches facing north aligned with the predominant current (Baztan et al., 2014; Herrera et al., 2018). However, field data reveal that some of the main MPs hot spots in the Canary Islands face somewhere else (Figure 1) (González-Hernández et al., 2020), which suggest that mesoscale processes as eddies (scales between 10-100 km) might play a dominant role on the transport of buoyant MPs at the ocean’s surface, distorting the classical pattern of MPs being found at facing north beaches.
In this regard, the lagrangian trajectories obtained for 1,000 virtual particles launched between 30-31°N along 2020 are presented in Figure 2. This same analysis was repeated for each day of 2020 and 2021, producing 730,000 trajectories lasting 90 days from the release date. All these trajectories are integrated according to their frequency of occurrence, hence producing areas of potential accumulation of particles. These integrations cover 3 months of data, obtaining four 2D histograms per year: January-March, April-June, July-September, October-December (Figure 3).
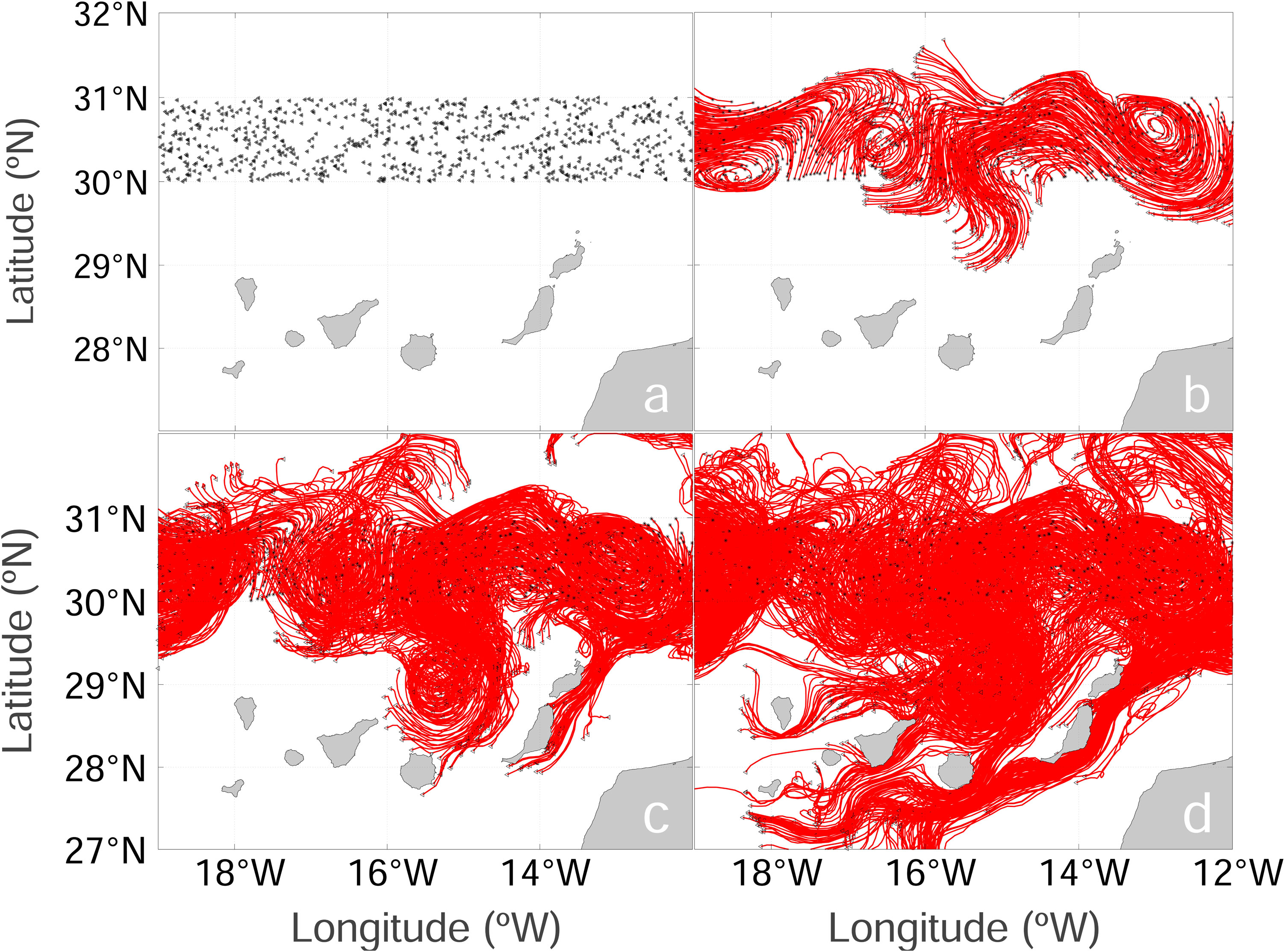
Figure 2 Lagrangian trajectories followed by 1,000 virtual particles along 2020 after (A) 0 days, (B) 12 days, (C) 38 days and (D) 76 days. The complete simulation can be found in the Supplementary Material.
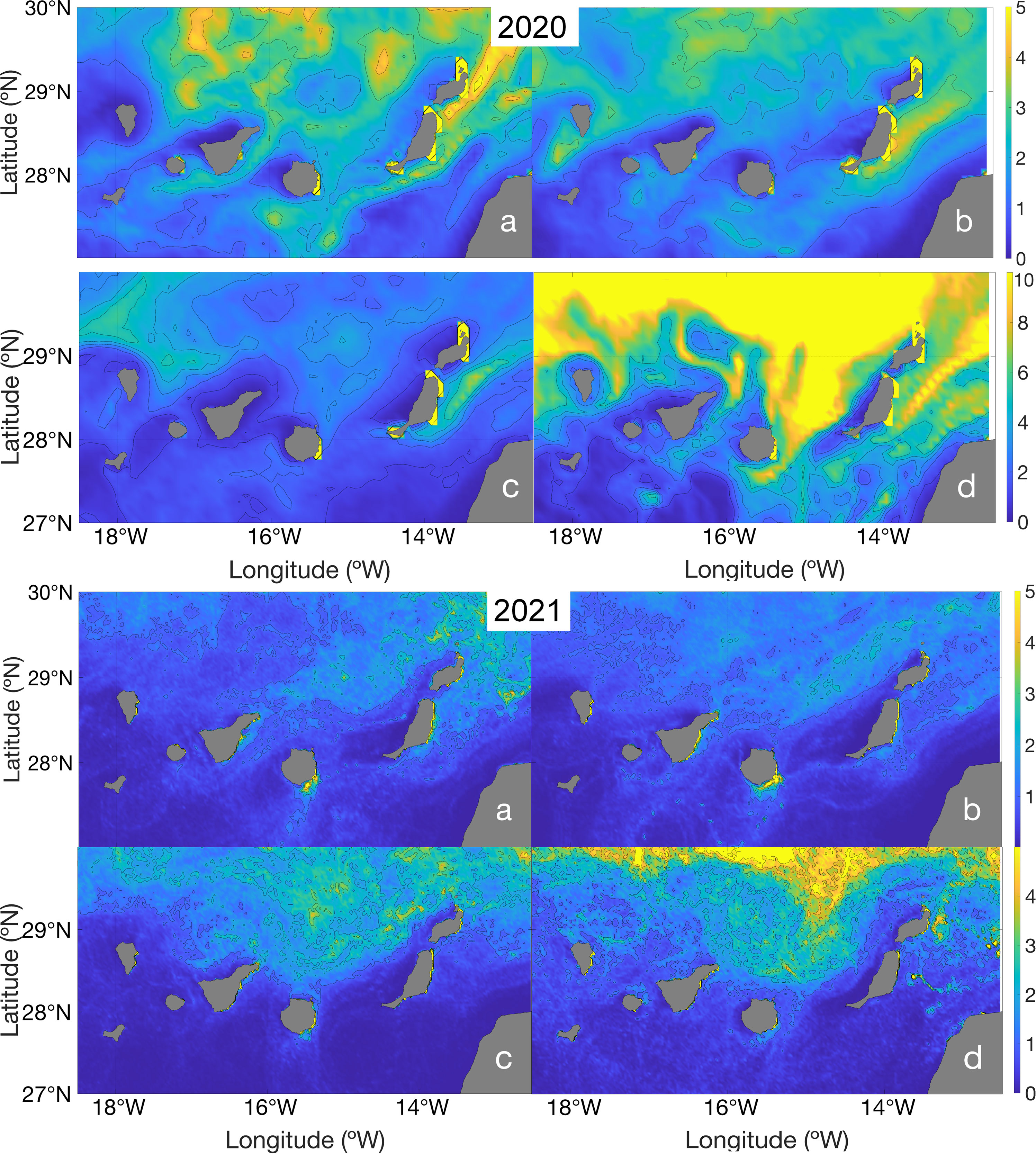
Figure 3 Lagrangian trajectories integrated according to their frequency of occurrence. Trajectories are grouped by months: (A) January-March; (B) April-June, (C) July-September, (D) October-December, for 2020 & 2021.
The areas with a higher potential for the accumulation of particles are highlighted in yellow, while the areas with a lower potential to accumulate particles are indicated in blue. These distribution maps are useful to highlight the preferred trajectories in the open ocean but also to reveal the locations in the coast with higher potential to receive and accumulate MPs. These locations with highest potential present indeed a good agreement with four of the previously reported “hot spots” of MPs arrival in the Canary Islands (Figure 1): Famara (Lanzarote Island), Lambra (La Graciosa Island), Playa Grande (Tenerife Island) and Caletillas (Fuerteventura Island). This last hot spot was recently discovered as part of the IMPLAMAC project and the Marine Litter Observatory (IMPLAMAC, 2022; OBAM, 2022). However, according to the model, no apparent accumulation of MPs take place in the westernmost hot spot located at El Hierro island. In this case, we note that it might be insightful to expand the release of particles further west on future simulations to elucidate whether another location with higher potential for the accumulation of MPs is also revealed.
Large-scale features such as the North Atlantic Subtropical Gyre and the eastern boundary represented by the Canary Current play a significant role in the overall transport of MP particles across the major oceans. However, our findings highlight the important role played by mesoscale structures in the vicinity of the Canary Islands, as there seems to be a direct correlation between surface currents at the mesoscale level and the areas with high potential for MPs accumulation. These hot spots exhibit a complex distribution pattern that is not simply oriented from north to south or northeast to southwest. Instead, they display considerable temporal and spatial variability influenced by the presence of eddies, which make these trajectories much more complex than the previously described. In order to accurately predict MP pollution in oceanic island regions like the Canary Islands, it is essential to consider these mesoscale phenomena.
3.2 Microplastic sampled at different depths near the Canary Islands
Marine MPs were sampled in the same region up to 1,200 m depth during an oceanographic cruise in 2021. Every single sample presented MPs, as it was also reported previously in the same region with samples from 2019 (Vega-Moreno et al., 2021). Both fibers and fragments were found, with an average concentration at all depths between 1 and 6 items/L per fibers, and between 3 to 220 items/L, being small fragments (<200 µm) the most abundant. This data agrees with the previous data analyzed in 2019.
We note that, in future studies, it would be recommended to expand our knowledge regarding the specific composition of these MPs. Nonetheless, a preliminary assessment of sample composition has been conducted using µ-FTIR, revealing that around 45% of the analyzed fibers are of synthetic origin (67 fibers) and 55% cellulosic (79 fibers). Interestingly, all the analyzed fragments (114 MPs) are of synthetic origin with diverse compositions, such as polyethylene, polypropylene and polyvinyl, among others.
MPs concentration (fibers and fragments) is not homogeneous in the water column. As can be seen in Figure 4, which shows MPs concentrations with depth, the concentration of small MPs (<200 µm) is much higher (30-220 items/L) than the bigger ones (3-20 items/L); this observation agrees with previous results that indicate that MPs concentration increases as the size decreases (Poulain et al., 2019). Furthermore, MPs concentrations usually present a maximum value around 200 m for both fragments and fibers. Third, a consistent minimum is found for MPs concentrations sampled at 600 m. Finally, a striking second maximum for small MPs fragments is generally observed at 1,100 m.
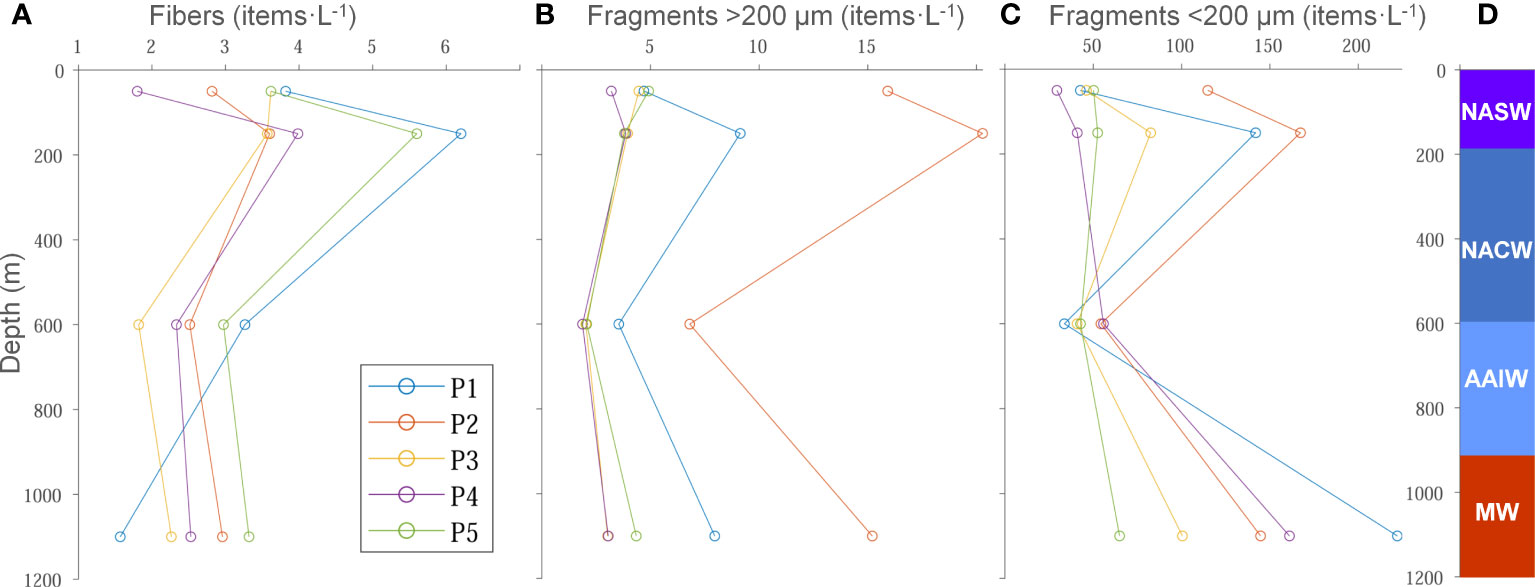
Figure 4 MPs concentration (items/L) at different depths (between 50 and 1100 m) for 5 stations in the same area. (A) Fibers; (B) Fragments with a size >200 µm, (C) Fragments with a size<200 µm; (D) Water masses profile at the Canary Islands region (NASW, North Atlantic Surface Water; NACW, North Atlantic Central Water; AAIW, Antarctic Intermediate Water; MW, Mediterranean Water).
Figure 5 compiles some photographs illustrating the different types of fibers and fragments observed in such oceanic samples, with predominance of small fragments.
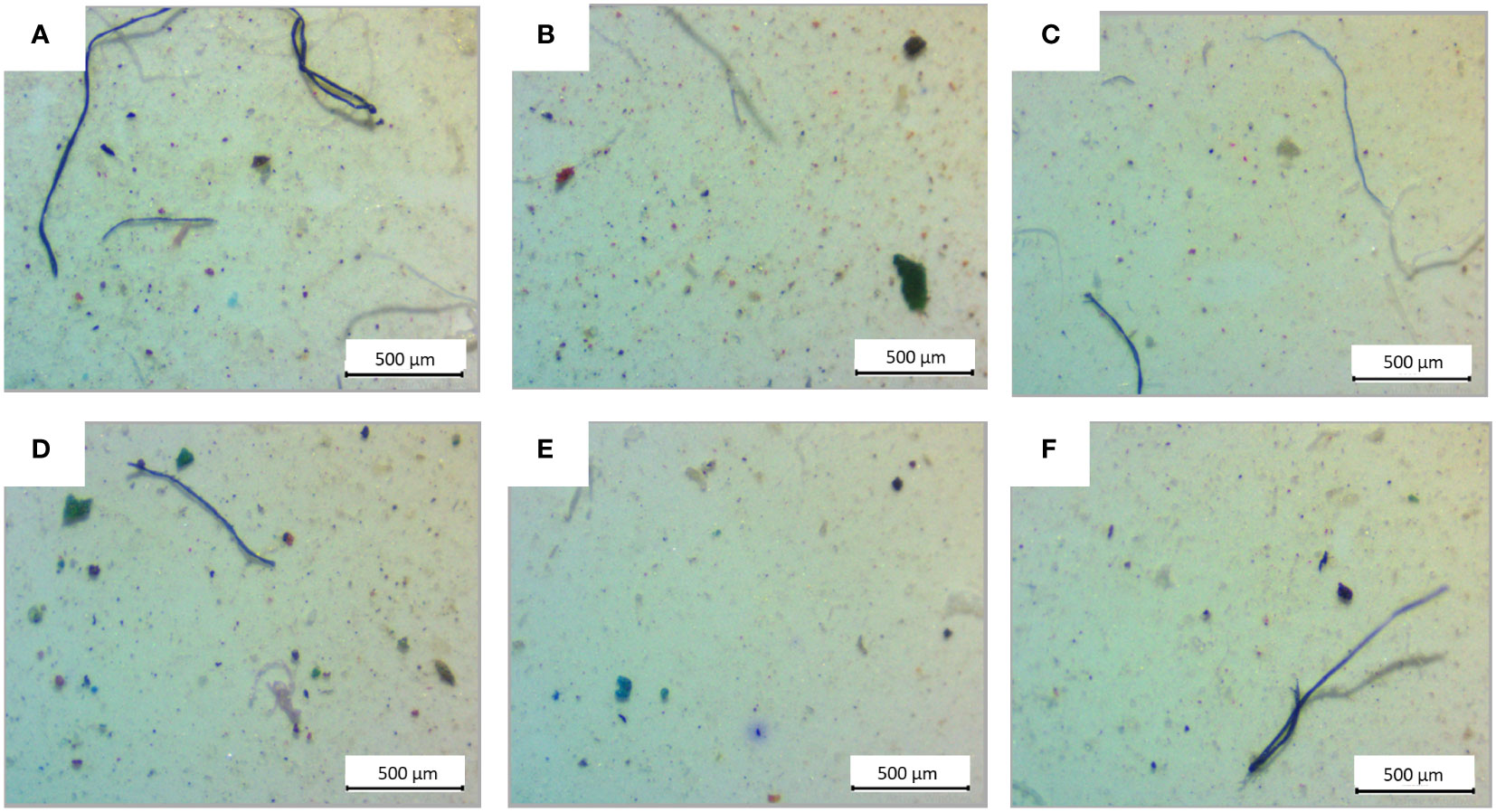
Figure 5 Stereomicroscope photographs of some of the MPs sampled at different depths in the Canary Islands region. In each case 60 L of seawater were filtered through a mesh size of 100 µm. (A) 50 m depth; (B) 150 m; (C) 50 m; (D) 1,100 m; (E) 600 m; (F) 1,100 m.
3.3 The role of Mediterranean water masses in the transport of microplastics in intermediate waters
As previously emphasized, there is a notable concentration of small MPs at a depth of 1100 m, which is surprisingly comparable to, or even exceeds, concentrations found at shallower levels at the same latitude (28°N). This leads us to suggest that these plastic fragments < 100-200 µm have not sunk directly from the surface to 1100 m at 28°N but are being transported to the Canary Islands domain and are finally resident at this depth. The proposed process would be as follows: MW leaves the Mediterranean Sea towards the Atlantic Ocean at 36°N through the Strait of Gibraltar (Iorga and Lozier, 1999). Its primary flow is westward and northward, sinking below the North Atlantic Central Water (NACW) down to 1000-1200 m where it stabilizes according to its density. However, MW is also present at latitudes further south around the Canary Islands (Machín et al., 2006; Fraile-Nuez et al., 2010). This near-horizontal distribution of MW in the eastern North Atlantic is summarized in Figure 6; Supplementary Figure 3 with a climatological approach, presenting the salinity on the Atlantic Ocean at 1100 m (data from the World Ocean Atlas). Salinity is chosen due to its higher value for this water mass compared to other surrounding water masses at this latitude [actually, the variable contoured in Figure 6 is the salinity anomaly, as defined by Richardson et al. (2000)].
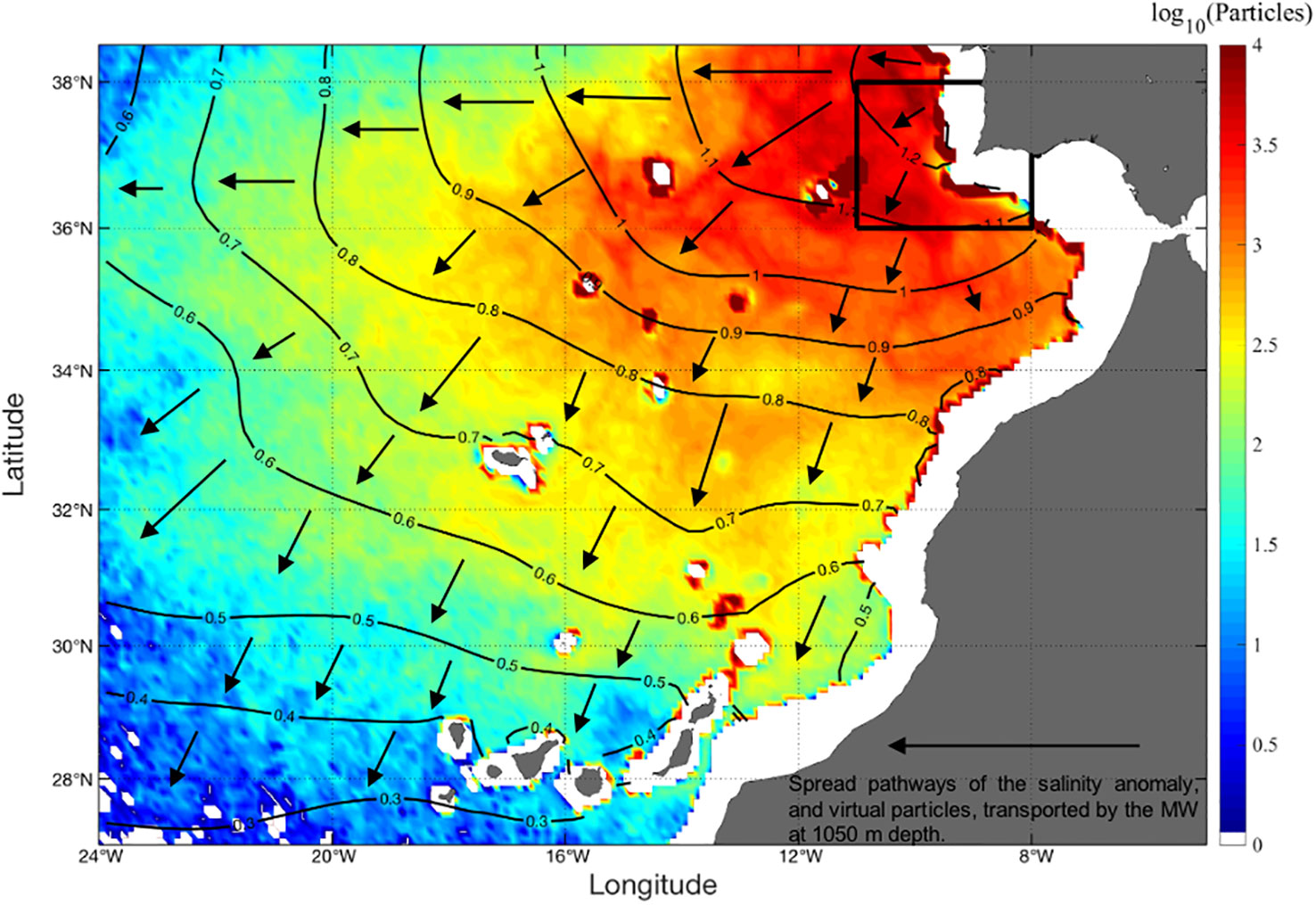
Figure 6 Horizontal distribution of the salinity anomaly (as contour lines) relative to 35.01, and transported by the Mediterranean Water while spreading across the Atlantic Ocean at 1050 m depth. This depiction was constructed using the annual mean of WOA23 (Boyer et al., 2018) and is based on a figure by Needler and Heath (1995) and shown by Joyce (1981). The black polygon indicates the particle releasing area of the modelling simulation. They represent the quantity of particles resulting from the cumulative accumulation of particles passing through a specific grid cell at each time step during a 4-year simulation (2017-2020) of forward trajectories. Warm (cold) colors indicate a high concentration of virtual particles. The resolution of the initial particle positions is 0.01°.
The climatological MW footprint suggests that the Canary Islands region is clearly influenced by MW, retaining some of its properties from its origin at 36°N. In the vicinity of the Canary Islands, salinity clearly decreases latitudinally from approximately 32°N to 29°N at intermediate levels, revealing a reduction of the contribution from Mediterranean Water to the observations in the range from 900 down to some 1400 m (Supplementary Figure 5). To test the feasibility of this hypothesis, we performed a 4-year numerical modelling simulation (2017-2020), where virtual particles were released near Cape St. Vincent at 1062 m depth. The motivation behind releasing particles at this particular location is supported by the recurrent pathway followed by the MW entering into the Atlantic as displayed in Supplementary Figure 4 (see the spread of the high salinity signal); contouring the southwestern slope of the Iberian Peninsula and shedding meddies at Cape St. Vincent (Serra et al., 2002). Thus, the simulation results are also presented in Figure 6 to support the likelihood that marine pollution transported by MW is expected to be found across the Atlantic Ocean wherever MW mixes with other water masses. These results underscore the dynamic nature of the influence of MW in the Canary Islands region. The latitudinal reduction in salinity and the recurrent path of MW indicate a changing pattern, supported by the 4-year numerical simulation. The release of particles near Cape St. Vincent and their dispersion along the southwestern slope illustrate the dynamics related to the MW, highlighting the possibility of finding marine pollution in various areas of the Atlantic where this water mass is observed.
In this regard, the high content of MPs at 1100 m also suggests that MW would leave the Strait of Gibraltar with a large amount of MPs of various kinds and sizes; the larger MPs (LMPs: 1-5 mm) mostly remain close to the surface (if it is buoyant plastic) or sink to the seafloor (for non-buoyant MPs), but the smallest ones (small MPs, SMPs,< 1 mm) sink along within the water mass down to 1100 m and are then transported beyond 36°N, in particular to the latitude of the Canary Islands (28°N) at this depth.
The main reason to observe SMPs at intermediate waters is therefore not due to the sinking process of the plastic particles by themselves, nor to density equilibrium processes associated with the composition of the plastic in relation to the density of seawater (weight-thrust forces), nor it is even due to physical-chemical changes in the plastic by degradation, weathering, or biological processes as biofouling, among others. On the contrary, SMPs sink through the water column behaving like passive drifters. This is related to the drag by subduction processes of the water mass itself, forced by the ocean dynamics. Thus, the SMPs vertical transport (or sinking) is not dictated by the chemical or biological properties of the MPs particles, because their size is so small to counteract ocean transport processes governed by the physical properties of the water masses.
In summary, if MPs are somewhat larger in size (generally > 1 mm), particle density will be a determining factor and the weight-thrust forces will condition the driving of MPs (close to the surface in the case of a buoyant MPs). However, the SMPs cannot counteract the ocean dynamics regardless of particle composition or density and will subduct down to 1100 m where MW stabilizes. For intermediate sizes or near to the limit of this classification between LMPs & SMPs (sizes in the range 0.5 – 1 mm), it will be decisive for its behavior not only their composition, but also their shape, state of degradation, or biofouling. Therefore, the larger and more spherical particles can stay at the surface more easily (for buoyant MPs) (Kooi et al., 2017; Eo et al., 2021). Despite the sinking of the water mass containing them, the larger (0.5 – 1 mm) and spherical particles will persist at the surface, while those that are more flattened, degraded, and smaller will sink with the water mass.
4 Conclusions
The examination of surface currents and Lagrangian trajectories of marine floating virtual particles in the Canary Islands region provides compelling evidence of a direct correlation between coastal areas with high potential for MPs accumulation and the observed hot spots of MPs. Remarkably, four out of five identified hot spots, determined through regular in situ MP collection, align with the predicted trajectories of the virtual particles. These analyses prove particularly valuable for larger buoyant MPs, ranging in size from 1-5 mm. However, for smaller MPs (<1 mm), it becomes necessary to extend the exploration beyond 100 m depth, i.e., below the influence of the ocean-atmosphere interface and the surface mixed layer.
Through the water column, MPs present an irregular distribution. Typically, the largest values are usually found in the samples taken at depths of around 150-200 m, surpassing the values gathered at the shallowest location (~50 m). Conversely, the lowest MP values are obtained at the samples taken at 600 m, likely related to low inputs from both lateral water masses and from the upper levels. Interestingly, a noteworthy anomaly arises at intermediate depths, where the deepest level sampled demonstrates remarkably high MP concentrations comparable to those observed at 150-200 m depth.
Regarding the analysis of MPs transported at intermediate waters, we suggest that SMPs can be dragged by the subduction process of the Mediterranean Water mass at the Strait of Gibraltar down to 1100 m due to physical processes (MW is denser than North Atlantic Central Water). In this context, MW would transport SMPs across the Atlantic Ocean at 1100 m depth from the Strait of Gibraltar up to the Canary Basin among other locations. We consider that this hypothesis has the potential to be applied in areas where intermediate depths (in the range of 1000 m) or deep-water masses (below 2000 m) are forming, including polar regions.
Furthermore, considering the findings of our study, it would be worthwhile to generate Lagrangian trajectories using eddy-resolving models across the entire Macaronesian region. This approach would enable the prediction of areas with high potential for MPs accumulation based on the ocean dynamics associated with large and mesoscale processes. In order to validate those predictions, it is necessary to enhance the frequency of open-ocean sampling and intensify monitoring efforts on beaches identified as hot spots with a significant potential for MPs accumulation. By combining predictive modelling and comprehensive in situ monitoring, we can further improve our understanding of MP transport dynamics and effectively address the problem of MP pollution in the region.
Data availability statement
The raw data supporting the conclusions of this article will be made available by the authors, without undue reservation.
Author contributions
DV-M: Conceptualization, Funding acquisition, Investigation, Methodology, Project administration, Writing – original draft, Writing – review & editing. SS-G: Conceptualization, Data curation, Methodology, Software, Writing – original draft. CD-H: Data curation, Investigation, Writing – original draft. EM-G: Conceptualization, Data curation, Methodology, Software, Writing – original draft. BA-G: Conceptualization, Formal Analysis, Investigation, Methodology, Software, Validation, Writing – review & editing. JH-B: Investigation, Validation, Visualization, Writing – original draft, Writing – review & editing. EF-N: Conceptualization, Funding acquisition, Investigation, Methodology, Project administration, Resources, Writing – review & editing. FM: Conceptualization, Investigation, Methodology, Visualization, Writing – original draft, Writing – review & editing.
Funding
The author(s) declare financial support was received for the research, authorship, and/or publication of this article. DeepPLAS project: Microplastics evaluation at deep water at Canary region and their chemical pollutants associated, ProID2020010030. Funded by the Canary Government co-financed with FEDER funds (European Regional Development Fund). MICROAD project: Environmental microplastics adsorbed contaminants: Improvement of analytical methodologies with a green analytical chemistry approach, PID2020-112867GB-I00. Funded by Spanish Ministry of Science and Innovation. IMPLAMAC project: MAC2/1.1a/265. Funded by the Transnational Cooperation Program Azores-Madeira-Canary Islands. VULCANA project: VULcanología CAnaria SubmariNA, VULCANA-II, IEO-2019-2021. Funded by the Spanish Institute of Oceanography (IEO-CSIC).
Acknowledgments
Authors acknowledge financial support from DeepPLAS project funded by Canary Government co-financed with FEDER funds, MICROAD project (National Goberment) and IMPLAMAC project, funded by the Transnational Cooperation Program Azores-Madeira-Canary Islands financed with FEDER funds. Authors would also like to thank to the Spanish Institute of Oceanography (IEO-CSIC) for their support in the context of VULCANA project funded by IEO allowing our participation in the oceanographic cruise. High resolution Harmonie wind data were kindly provided by the Spanish Meteorological Agency (AEMET) in the collaboration framework with the Spanish Institute of Oceanography (IEO-CSIC).
Conflict of interest
The authors declare that the research was conducted in the absence of any commercial or financial relationships that could be construed as a potential conflict of interest.
The author(s) declared that they were an editorial board member of Frontiers, at the time of submission. This had no impact on the peer review process and the final decision.
Publisher’s note
All claims expressed in this article are solely those of the authors and do not necessarily represent those of their affiliated organizations, or those of the publisher, the editors and the reviewers. Any product that may be evaluated in this article, or claim that may be made by its manufacturer, is not guaranteed or endorsed by the publisher.
Supplementary material
The Supplementary Material for this article can be found online at: https://www.frontiersin.org/articles/10.3389/fmars.2024.1314754/full#supplementary-material
References
Adamopoulou A., Zeri C., Garaventa F., Gambardella C., Ioakeimidis C., Pitta E., et al. (2021). Distribution patterns of floating microplastics in open and coastal waters of the eastern mediterranean sea (Ionian, aegean, and levantine seas). Front. Mar. Sci. 8, 699000. doi: 10.3389/fmars.2021.699000
Álvarez-Hernández C., Cairós C., López-Darias J., Mazzetti E., Hernández-Sánchez C., González-Sálamo J., et al. (2019). Microplastic debris in beaches of Tenerife (Canary Islands, Spain). Mar. pollut. Bull. 146, 26–32. doi: 10.1016/j.marpolbul.2019.05.064
Barnes D. K. A., Galgani F., Thompson R. C., Barlaz M. (2009). Accumulation and fragmentation of plastic debris in global environments. Philos. Trans. R. Soc B Biol. Sci. 364, 1985–1998. doi: 10.1098/rstb.2008.0205
Bashmachnikov I., Nascimento, Neves F., Menezes T., Koldunov N. V. (2015). Distribution of intermediate water masses in the subtropical northeast Atlantic. Ocean Sci. 11, 803–827. doi: 10.5194/os-11-803-2015
Baztan J., Carrasco A., Chouinard O., Cleaud M., Gabaldon J. E., Huck T., et al. (2014). Protected areas in the Atlantic facing the hazards of micro-plastic pollution: First diagnosis of three islands in the Canary Current. Mar. pollut. Bull. 80, 302–311. doi: 10.1016/j.marpolbul.2013.12.052
Borrelle S. B., Ringma J., Law K. L., Monnahan C. C., Lebreton L., McGivern A., et al. (2020). Mitigate plastic pollution. Science 1518, 1515–1518. doi: 10.1126/science.aba3656
Bower A. S., Serra N., Ambar I. (2002). Structure of the Mediterranean Undercurrent and Mediterranean Water spreading around the southwestern Iberian Peninsula. J. Geophys. Res. Ocean. 107, 1–19. doi: 10.1029/2001jc001007
Boyer T. P., García H. E., Locarnini R. A., Zweng M. M., Mishonov A. V., Reagan J. R., et al. (2018). World Ocean Atlas 2018 (NOAA National Centers for Environmental Information). Available at: https://www.ncei.noaa.gov/archive/accession/NCEI-WOA18.
Brach L., Deixonne P., Bernard M. F., Durand E., Desjean M. C., Perez E., et al. (2018). Anticyclonic eddies increase accumulation of microplastic in the North Atlantic subtropical gyre. Mar. pollut. Bull. 126, 191–196. doi: 10.1016/j.marpolbul.2017.10.077
Browne M. A., Galloway T. S., Thompson R. C. (2010). Spatial patterns of plastic debris along estuarine shorelines. Environ. Sci. Technol. 44, 3404–3409. doi: 10.1021/es903784e
Cardoso C., Caldeira R. M. A. (2021). Modeling the exposure of the macaronesia islands (NE atlantic) to marine plastic pollution. Front. Mar. Sci. 8. doi: 10.3389/fmars.2021.653502
Choy C. A., Robison B. H., Gagne T. O., Erwin B., Firl E., Halden R. U., et al. (2019). The vertical distribution and biological transport of marine microplastics across the epipelagic and mesopelagic water column. Sci. Rep. 9, 7843. doi: 10.1038/s41598-019-44117-2
Cincinelli A., Martellini T., Guerranti C., Scopetani C., Chelazzi D., Giarrizzo T. (2019). A potpourri of microplastics in the sea surface and water column of the Mediterranean Sea. TrAC - Trends Anal. Chem. 110, 321–326. doi: 10.1016/j.trac.2018.10.026
Citterich F., Giudice A., Azzaro M. (2023). A plastic world : A review of microplastic pollution in the freshwaters of the Earth’s poles. Sci. Total Environ. 869, 161847. doi: 10.1016/j.scitotenv.2023.161847
Cowger W., Steinmetz Z., Gray A., Munno K., Lynch J., Hapich H., et al. (2021). Microplastic spectral classification needs an open source community: open specy to the rescue! Anal. Chem. 93, 7543–7548. doi: 10.1021/acs.analchem.1c00123
Cózar A., Echevarría F., González-Gordillo J. I., Irigoien X., Úbeda B., Hernández-León S., et al. (2014). Plastic debris in the open ocean. Proc. Natl. Acad. Sci. U. S. A. 111, 10239–10244. doi: 10.1073/pnas.ments
Cózar A., Martí E., Duarte C. M., García-de-Lomas J., Van Sebille E., Ballatore T. J., et al. (2017). The Arctic Ocean as a dead end for floating plastics in the North Atlantic branch of the Thermohaline Circulation. Sci. Adv. 3, 1–9. doi: 10.1126/sciadv.1600582
De Frond H., Rubinovitz R., Rochman C. M. (2021). μATR-FTIR spectral libraries of plastic particles (FLOPP and FLOPP-e) for the analysis of microplastics. Anal. Chem. 93, 15878–15885. doi: 10.1021/acs.analchem.1c02549
Edo C., Tamayo-Belda M., Martínez-Campos S., Martín-Betancor K., González-Pleiter M., Pulido-Reyes G., et al. (2019). Occurrence and identification of microplastics along a beach in the Biosphere Reserve of Lanzarote. Mar. pollut. Bull. 143, 220–227. doi: 10.1016/j.marpolbul.2019.04.061
Egger M., Sulu-Gambari F., Lebreton L. (2020). First evidence of plastic fallout from the Great Pacific Garbage Patch. Sci. Rep. 10, 7495. doi: 10.1038/s41598-020-64465-8
Eo S., Hong S. H., Song Y. K., Han G. M., Seo S., Shim W. J. (2021). Prevalence of small high-density microplastics in the continental shelf and deep sea waters of East Asia. Water Res. 200, 117238. doi: 10.1016/j.watres.2021.117238
Eriksen M., Cowger W., Erdle L. M., Coffin S., Villarrubia-Gómez P., Moore C. J., et al. (2023). A growing plastic smog, now estimated to be over 170 trillion plastic particles afloat in the world’s oceans—Urgent solutions required. PloS One 18, 1–12. doi: 10.1371/journal.pone.0281596
Eriksen M., Lebreton L. C. M., Carson H. S., Thiel M., Moore C. J., Borerro J. C., et al. (2014). Plastic Pollution in the World’s Oceans: More than 5 Trillion Plastic Pieces Weighing over 250,000 Tons Afloat at Sea. PloS One 9, 1–15. doi: 10.1371/journal.pone.0111913
Fraile-Nuez E., MacHín F., Vélez-Belchí P., López-Laatzen F., Borges R., Benítez-Barrios V., et al. (2010). Nine years of mass transport data in the eastern boundary of the North Atlantic Subtropical Gyre. J. Geophys. Res. Ocean. 115 (C9). doi: 10.1029/2010JC006161
González-Hernández M., Hernández-Sánchez C., González-Sálamo J., López-Darias J., Hernández-Borges J. (2020). Monitoring of meso and microplastic debris in Playa Grande beach (Tenerife, Canary Islands, Spain) during a moon cycle. Mar. pollut. Bull. 150, 110757. doi: 10.1016/j.marpolbul.2019.110757
Hernández-Sánchez C., González-Sálamo J., Díaz-Peña F. J., Fraile-Nuez E., Hernández-Borges J. (2021). Arenas Blancas (El Hierro island), a new hotspot of plastic debris in the Canary Islands (Spain). Mar. pollut. Bull. 169, 112548. doi: 10.1016/j.marpolbul.2021.112548
Herrera A., Asensio M., Martínez I., Santana A., Packard T., Gómez M. (2018). Microplastic and tar pollution on three Canary Islands beaches: An annual study. Mar. pollut. Bull. 129, 494–502. doi: 10.1016/j.marpolbul.2017.10.020
IMPLAMAC (2022). IMPLAMAC Project. Evaluación del impacto de microplásticos y contaminantes emergentes en las costas de la Macaronesia. Transnatl. Coop. Progr. Azores-Madeira-Canary Islands. Available at: https://implamac.com/
Iorga M. C., Lozier M. S. (1999). Signatures of the Mediterranean outflow from a North Atlantic climatology 1. Salinity and density fields. J. Geophys. Res. Ocean. 104, 25985–26009. doi: 10.1029/1999jc900115
Joyce T. M. (1981). Influence of the mid-Atlantic Ridge upon the circulation and the properties of the Mediterranean Water southwest of the Azores. J. Mar. Res. 39, 31–52. doi: 10.1575/1912/1488
Komen E. M. J., Frederix E. M. A., Coppen T. H. J., D’Alessandro V., Kuerten J. G. M. (2020). Analysis of the numerical dissipation rate of different Runge–Kutta and velocity interpolation methods in an unstructured collocated finite volume method in OpenFOAM®. Comput. Phys. Commun. 253, 107145. doi: 10.1016/j.cpc.2020.107145
Kooi M., Van Nes E. H., Scheffer M., Koelmans A. A. (2017). Ups and downs in the ocean: effects of biofouling on vertical transport of microplastics. Environ. Sci. Technol. 51, 7963–7971. doi: 10.1021/acs.est.6b04702
Kubota M. (1994). A Mechanism for the accumulation of floating marine debris North of Hawaii. J. Phys. Oceanogr. 24, 1059–1064. doi: 10.1175/1520-0485(1994)024<1059:AMFTAO>2.0.CO;2
Le Roux J. P. (2005). Grains in motion: A review. Sediment. Geol. 178, 285–313. doi: 10.1016/j.sedgeo.2005.05.009
Li D., Liu K., Li C., Peng G., Andrady A. L., Wu T., et al. (2020). Profiling the vertical transport of microplastics in the west pacific ocean and the east Indian ocean with a novel in situ filtration technique. Environ. Sci. Technol. 54, 12979–12988. doi: 10.1021/acs.est.0c02374
Machín F., Hernández-Guerra A., Pelegrí J. L. (2006). Mass fluxes in the canary basin. Prog. Oceanogr. 70, 416–447. doi: 10.1016/j.pocean.2006.03.019
Madec G., Delecluse P., Imbard M., Lévy C. (1998). OPA 8.1 Ocean General Circulation Model reference manual. Note du Pole Model., 91. Available at: https://www.nemo-ocean.eu/wp-content/uploads/Doc_OPA8.1.pdf
Martinez E., Maamaatuaiahutapu K., Taillandier V. (2009). Floating marine debris surface drift: Convergence and accumulation toward the South Pacific subtropical gyre. Mar. pollut. Bull. 58, 1347–1355. doi: 10.1016/j.marpolbul.2009.04.022
Needler G. T., Heath R. A. (1995). Diffusion coefficients calculated from the Mediterranean salinity anomaly in the North Atlantic Ocean. J. Phys. Oceanogr. 5, 173–182. doi: 10.1175/1520-0485(1975)005<0173:DCCFTM>2.0.CO;2
Nordam T., Duran R. (2020). Numerical integrators for Lagrangian oceanography. Geosci. Model. Dev. 13, 5935–5957. doi: 10.5194/gmd-13-5935-2020
OBAM (2022) Fuerteventura Marine Litter Observatory (OBAM). Available at: https://www.programapleamar.es/proyectos/obam-ii-observatorio-de-basura-marina-ii.
Onink V., Wichmann D., Delandmeter P., van Sebille E. (2019). The role of ekman currents , geostrophy , and stokes drift in the accumulation of floating microplastic. J. Geophys. Res. Ocean. 124 (3), 1474–1490. doi: 10.1029/2018JC014547
Pabortsava K., Lampitt R. S. (2020). High concentrations of plastic hidden beneath the surface of the Atlantic Ocean. Nat. Commun. 11, 1–11. doi: 10.1038/s41467-020-17932-9
Papiol V., Cartes J. E., Vélez-Belchí P., Martín-Sosa P. (2019). Near-bottom zooplankton over three seamounts in the east Canary Islands: Influence of environmental variables on distribution and composition. Deep. Res. Part I Oceanogr. Res. Pap. 149, 103025. doi: 10.1016/j.dsr.2019.04.003
Pham C. K., Pereira J. M., Frias J. P. G. L., Ríos N., Carriço R., Juliano M., et al. (2020). Beaches of the Azores archipelago as transitory repositories for small plastic fragments floating in the North-East Atlantic. Environ. pollut. 263, 1–8. doi: 10.1016/j.envpol.2020.114494
Phan S., Luscombe C. K. (2023). Recent trends in marine microplastic modeling and machine learning tools : Potential for long-term microplastic monitoring. J. Appl. Phys. 133, 020701. doi: 10.1063/5.0126358
Poulain M., Mercier M. J., Brach L., Martignac M., Routaboul C., Perez E., et al. (2019). Small microplastics as a main contributor to plastic mass balance in the North Atlantic subtropical gyre. Environ. Sci. Technol. 53, acs.est.8b05458. doi: 10.1021/acs.est.8b05458
Rapp J., Herrera A., Martinez I., Raymond E., Santana Á., Gómez M. (2020). Study of plastic pollution and its potential sources on Gran Canaria Island beaches (Canary Islands, Spain). Mar. pollut. Bull. 153, 110967. doi: 10.1016/j.marpolbul.2020.110967
Reagan J. R., NOAA National Centers for Environmental Information. World Ocean Atlas 2023 - objectively analyzed in situ temperature and salinity climatologies for the 1991-2020 climate normal period (NCEI Accession 0270533). NOAA National Centers for Environmental Information. (2023) https://www.ncei.noaa.gov/archive/accession/0270533.
Reinold S., Herrera A., Hernández-González C., Gómez M. (2020). Plastic pollution on eight beaches of Tenerife (Canary Islands, Spain): An annual study. Mar. pollut. Bull. 151, 110847. doi: 10.1016/j.marpolbul.2019.110847
Richardson P. L., Bower A. S., Zenk W. (2000). A census of Meddies tracked by floats. Prog. Oceanogr. 45, 209–250. doi: 10.1016/S0079-6611(99)00053-1
Sangrà P., Pascual A., Rodríguez-Santana Á., Machín F., Mason E., McWilliams J. C., et al. (2009). The Canary Eddy Corridor: A major pathway for long-lived eddies in the subtropical North Atlantic. Deep. Res. Part I Oceanogr. Res. Pap. 56 (12), 2100–2114. doi: 10.1016/j.dsr.2009.08.008
Schmidt N., Thibault D., Galgani F., Paluselli A., Sempéré R. (2018). Occurrence of microplastics in surface waters of the Gulf of Lion ( NW Mediterranean Sea ). Prog. Oceanogr. 163, 214–220. doi: 10.1016/j.pocean.2017.11.010
Serra N., Sadoux S., Ambar I., Renouard D. (2002). Observations and laboratory modeling of meddy generation at Cape St. Vincent. J. Phys. Oceanogr. 32, 3–25. doi: 10.1175/1520-0485(2002)032<0003:OALMOM>2.0.CO;2
Sharma S., Sharma V., Chatterjee S. (2021). Microplastics in the mediterranean sea: sources, pollution intensity, sea health, and regulatory policies. Front. Mar. Sci. 8. doi: 10.3389/fmars.2021.634934
Simon-Sánchez L., Grelaud M., Franci M., Ziveri P. (2022). Are research methods shaping our understanding of microplastic pollution? A literature review on the seawater and sediment bodies of the Mediterranean Sea. Sci. Total Environ. 292, 118275. doi: 10.1016/j.envpol.2021.118275
Tsiaras K., Costa E., Morgana S., Gambardella C., Piazza V., Faimali M., et al. (2022). Microplastics in the mediterranean: variability from observations and model analysis. Front. Mar. Sci. 9. doi: 10.3389/fmars.2022.784937
Tyranowski T. M., Desbrun M. (2019). Variational partitioned runge–kutta methods for lagrangians linear in velocities. Mathematics 7, 1–31. doi: 10.3390/MATH7090861
van Sebille E., Aliani S., Law K. L., Maximenko N., Alsina J. M., Bagaev A., et al. (2020). The physical oceanography of the transport of floating marine debris. Environ. Res. Lett. 15 (2), 023003. doi: 10.1088/1748-9326/ab6d7d
van Sebille E., England M. H., Froyland G. (2012). Origin, dynamics and evolution of ocean garbage patches from observed surface drifters. Environ. Res. Lett. 7 (4), 044040. doi: 10.1088/1748-9326/7/4/044040
van Sebille E., Griffies S. M., Abernathey R., Adams T. P., Berloff P., Biastoch A., et al. (2018). Lagrangian ocean analysis: Fundamentals and practices. Ocean Model. 121, 49–75. doi: 10.1016/j.ocemod.2017.11.008
Vega-Moreno D., Abaroa-Pérez B., Rein-loring P. D., Presas-navarro C., Fraile-nuez E., Machín F. (2021). Distribution and transport of microplastics in the upper 1150 m of the water column at the Eastern North Atlantic Subtropical Gyre, Canary Islands, Spain. Sci. Total Environ. 788, 147802. doi: 10.1016/j.scitotenv.2021.147802
Zhang S., Zhang W., Ju M., Qu L., Chu X., Huo C., et al. (2022). Distribution characteristics of microplastics in surface and subsurface Antarctic seawater. Sci. Total Environ. 838, 156051. doi: 10.1016/j.scitotenv.2022.156051
Keywords: marine debris, transport, microplastics, surface and sub-surface ocean dynamics, passive drifters, deep ocean
Citation: Vega-Moreno D, Sicilia-González S, Domínguez-Hernández C, Moreira-García E, Aguiar-González B, Hernández-Borges J, Fraile-Nuez E and Machín F (2024) Exploring the origin and fate of surface and sub-surface marine microplastics in the Canary Islands region. Front. Mar. Sci. 11:1314754. doi: 10.3389/fmars.2024.1314754
Received: 10 October 2023; Accepted: 11 January 2024;
Published: 02 February 2024.
Edited by:
Kai Liu, East China Normal University, ChinaReviewed by:
Abilash Gangula, University of Missouri, United StatesAlessandro Stocchino, Hong Kong Polytechnic University, Hong Kong SAR, China
Copyright © 2024 Vega-Moreno, Sicilia-González, Domínguez-Hernández, Moreira-García, Aguiar-González, Hernández-Borges, Fraile-Nuez and Machín. This is an open-access article distributed under the terms of the Creative Commons Attribution License (CC BY). The use, distribution or reproduction in other forums is permitted, provided the original author(s) and the copyright owner(s) are credited and that the original publication in this journal is cited, in accordance with accepted academic practice. No use, distribution or reproduction is permitted which does not comply with these terms.
*Correspondence: Eugenio Fraile-Nuez, eugenio.fraile@ieo.csic.es
‡ORCID: Eugenio Fraile-Nuez, orcid.org/0000-0003-4250-4445