- 1Department of Observation and Study of the Land, the Atmosphere and the Ocean, Consejo Nacional de Humanidades, Ciencias y Tecnologías-El Colegio de la Frontera Sur (CONAHCYT-ECOSUR), Chetumal, Mexico
- 2Department of Sustainability Sciences, El Colegio de la Frontera Sur, Chetumal, Mexico
- 3Department of Observation and Study of the Land, the Atmosphere and the Ocean, El Colegio de la Frontera Sur, Chetumal, Mexico
Coastal karst structures have been recently explored and documented in Chetumal Bay, Mexico, at the southeast of the Yucatan Peninsula. These structures, recognized as blue holes, stand out for their remarkable dimensions within a shallow estuarine environment. Particularly the Taam Ja’ Blue Hole (TJBH), revealed a depth of ~274 mbsl based on echo sounder mapping, momentarily positioning it as the world's second-deepest blue hole. However, echo sounding methods face challenges in complex environments like blue holes or inland sinkholes arising from frequency-dependent detection and range limitations due to water density vertical gradients, cross-sectional depth variations, or morphometric deviations in non-strictly vertical caves. Initial exploration could not reach the bottom and confirm its position, prompting ongoing investigation into the geomorphological features of TJBH. Recent CTD profiler records in TJBH surpassed 420 mbsl with no bottom yet reached, establishing the TJBH as the deepest-known blue hole globally. Hydrographic data delineated multiple water layers within TJBH. Comparison with Caribbean water conditions at the Mesoamerican Barrier Reef System, reef lagoons, and estuaries suggests potential subterranean connections. Further research and implementation of underwater navigation technologies are essential to decipher its maximum depth and the possibilities of forming part of an interconnected system of caves and tunnels.
Introduction
Anchialine systems stand out as impressive and exciting environments to be explored across different disciplines. These systems provide a vast research field, from microbiology (Benítez et al., 2019; Little et al., 2021; Sha et al., 2021), to sea-level dynamics or paleoclimate (van Hengstum et al., 2011; Husson et al., 2018; van Hengstum et al., 2020; Wallace et al., 2021), stratigraphy (Vimpere, 2017), physicochemical water properties (Perry et al., 2002, Perry et al., 2009), as well as groundwater hydrology (Gondwe et al., 2010; Björnerås et al., 2020). However, a common basis across all disciplines is the need to understand the geomorphology and dimensions of the karst structures.
The Yucatan Peninsula, part of Central America's Maya block, lacks Paleozoic folds (Weber et al., 2012). With dynamic diagenesis and gradual Pliocene emergence, it exhibits significant geological structures in vadose (Perry et al., 2003, Perry et al., 2009) and phreatic settings (van Hengstum et al., 2010, van Hengstum et al., 2011), as well as in coastal submarine environments (Bauer-Gottwein et al., 2011a). Moreover, the Yucatan Peninsula's northern side hosts the Ring of Cenotes Fault, a regional-scale structure formed by sinkholes, related to the Chicxulub meteorite impact 65 million years ago (Bauer-Gottwein et al., 2011a). Simultaneously, the world's most extensive subterranean cave system, shaped by glacio-eustatic sea-level changes, is found on the western side (Supper et al., 2009; Kambesis and Coke, 2013). Across the eastern margin, parallel to the Caribbean coast, the Yucatan Peninsula features two regional fracture zones—the Holbox Fracture Zone to the north and the Rio Hondo Fault Zone to the south (Bauer-Gottwein et al., 2011a) with possible intersections and water exchange (Gondwe et al., 2011). To the southeast, inland sinkholes and lagoons aligned with the Rio Hondo Fault Zone have been extensively studied (e.g. Gischler et al., 2011; Perry et al., 2021). Also, recent exploration in Chetumal Bay reported large coastal karstic formations recognized as blue holes (Carrillo et al., 2009b; Alcérreca-Huerta et al., 2023; Flórez-Franco et al., 2023). These blue holes represented an outstanding revelation, particularly that of the Taam-ja’ Blue Hole (TJBH), preliminarily recognized as the world's second-deepest, surpassing the depths of the Dean’s Blue Hole in the Bahamas (~202 mbsl) (Vimpere, 2017), the Dahab Blue Hole in Egypt (~130 mbsl) (Li et al., 2018), or the Great Blue Hole in Belize (~125 mbsl) (Schmitt et al., 2021).
The TJBH, first documented by Alcérreca-Huerta et al. (2023), stands as a noteworthy geological feature. Bathymetric mapping employing echo sounder technology indicated an impressive maximum depth of 274.4 meters below sea level (mbsl). Echo sounding, serving as an indirect method, allowed a comprehensive 3D spatial coverage of the TJBH morphology. However, this method could grapple with constraints arising from frequency-dependent detection and range limitations (Colbo et al., 2014). These challenges are usually accentuated in blue holes and inland sinkholes due to fluctuations in water density (Cejudo et al., 2022) and cross-sectional variations in depth (Li et al., 2018), particularly in non-strictly vertical caves where the blue hole structure deviates from their entrance position. Direct methods for depth measurement employed in TJBH relied on CTD profiling but encountered limitations with measurements being restricted to a maximum depth of 200 mbsl to safeguard against potential instrument damage (Alcérreca-Huerta et al., 2023; Flórez-Franco et al., 2023). Notably, the measurements could not reach the bottom and confirm its position, leaving the depths of TJBH and the vertical thermohaline structure partially unresolved.
Therefore, recent direct methods for water depth measurement gathered with a SWiFT CTD Profiler reveal water depths within the TJBH that surpassed the previous reported records, but also the maximum water depth record held by the Sansha Yongle Blue Hole (SYBH) at ~301 mbsl in the South China Sea (Li et al., 2018). This groundbreaking finding establishes the TJBH as the recently confirmed deepest-known blue hole globally. Additionally, the hydrographic data collected is also described to delineate the water temperature and salinity variations along the recent depths reached, the formation of previously unknown pycnoclines, and comparison of the thermohaline conditions in TJBH with those found in the literature for waters in the Caribbean at the Mesoamerican Barrier Reef System and coastal reef lagoons, as a proxy of possible hydraulic connectivity between them and the blue hole.
Methods
Study area
Cenotes, underground springs, freshwater inlets, and a complex lagoon and anchialine system develop at the southeastern region of the Yucatán Peninsula (Figure 1A). The system connects with Chetumal Bay, a semi-closed mesohaline tropical estuary developed over carbonated sedimentary deposits of the Miocene, Mio-Pliocene and Holocene (Gondwe et al., 2010; Domínguez-Herrera et al., 2023), which hydrographic conditions are described in Carrillo et al (2009a), Carrillo et al (2009b) and Ruíz-Pineda et al. (2016).
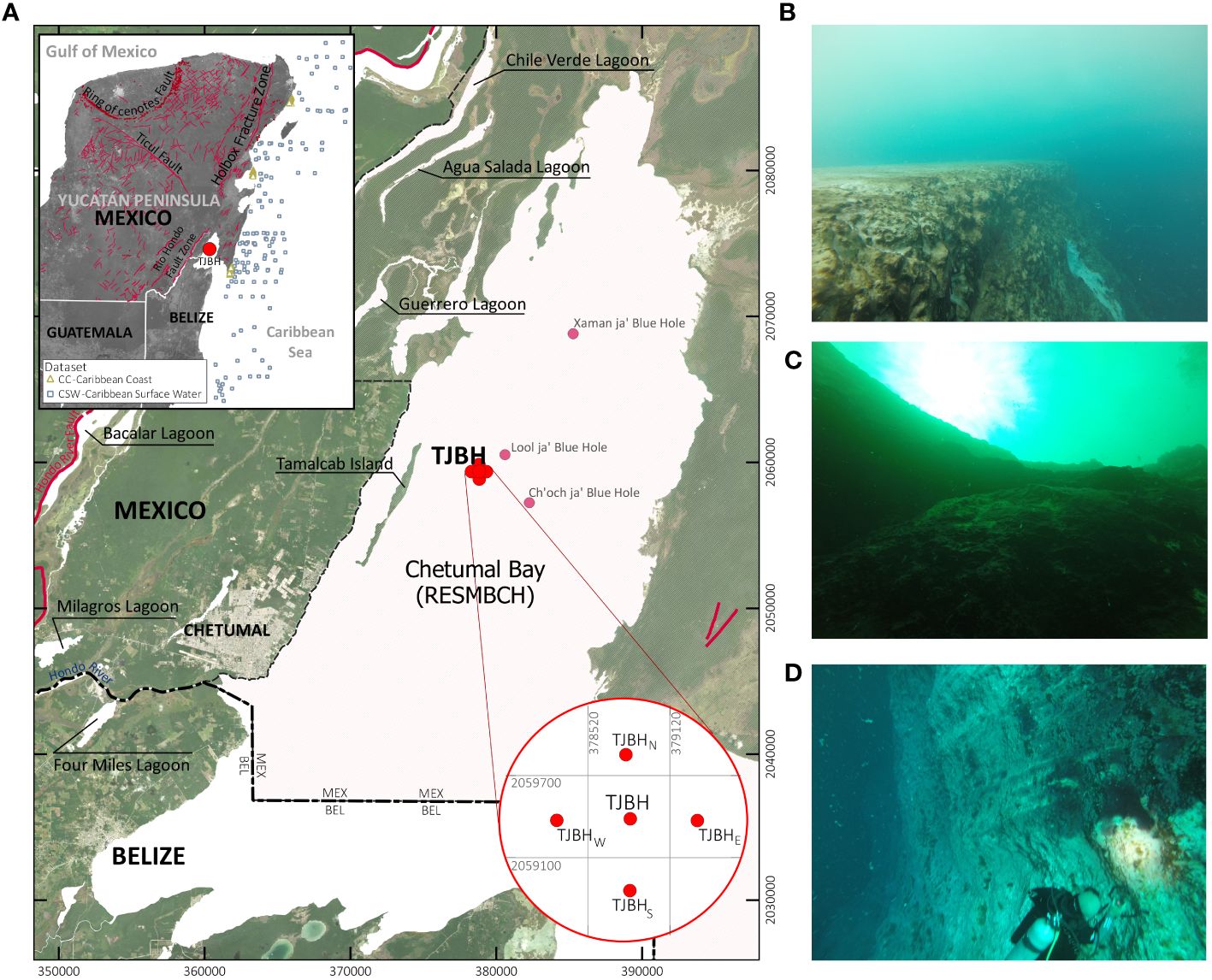
Figure 1 (A) Location of the Taam ja’ Blue Hole (TJBH) in Chetumal Bay, Mexico, is presented alongside the CC and CSW data regions for further comparison of water temperature and salinity conditions. Regional fracture zones and geological faults in the Yucatán Peninsula are indicated (INEGI, 2002), along with the locations of documented blue holes within Chetumal Bay. CB data was measured at sampling stations positioned at cardinal positions ~500 m apart of the TJBH (TJBHN, TJBHS, TJBHE and TJBHW). Images from scuba explorations of the TJBH at depths (B) 5.0 mbsl, (C) 20 mbsl, and (D) 30 mbsl are also presented.
The TJBH (378823 m E, 2059390 m N, UTM 16Q) is located in the central portion of Chetumal Bay, within the Mexican State Reserve “Chetumal Bay-Manatee Sanctuary” (RESMBCH). It is ~4.5 km from Tamalcab island, and ~19.2 km from Chetumal, the most urbanized area. TJBH, Lool ja’ Blue Hole (LJBH), and Ch’och-ja’ Blue Hole (CJBH) are among the blue holes recently documented in Chetumal Bay (Carrillo et al., 2009b; Alcérreca-Huerta et al., 2023; Flórez-Franco et al., 2023) (Figure 1A), for which preliminary insights into their geomorphological features, and temporal variability of physicochemical properties have been provided.
Field work and data analysis
On December 6th, 2023, a scuba diving expedition was conducted to identify the environmental conditions prevailing at the TJBH and related to factors such as visibility, substrate characteristics, and wall coverage within a depth range extending from 0 to 30 mbsl. Additionally, on December 6th and 13th, 2023, measurement of new CTD profiles was conducted within the TJBH aiming to reach its bottom and confirm the echo-sounding results described in Alcérreca-Huerta et al. (2023). Employing a SWiFT CTD Profiler (Valeport UK), single profiles at each campaign with simultaneous measurements of water pressure, temperature, and conductivity were acquired throughout the water column of TJBH. The coordinates for the CTD profiles were 378830.7 m E and 2059383.6 m N (UTM 16Q), selected based on preliminary echo sounding measurements that indicated water depths surpassing 250 mbsl. The vessel was anchored to prevent drifting caused by waves and currents. In this specific location, the CTD instrument was lowered, utilizing ~500 m of cable down to the bottom, adhering to the maximum depth supported by the instrument.
Salinity and density values from CTD casts are computed employing the Chen and Millero/UNESCO international algorithm (Chen and Millero, 1977; Fofonoff and Millard, 1983), leading to an accuracy of ±0.01 PSU and ±0.01 kg/m³, respectively. Temperature data from SWiFT CTD Profiler measurements has an accuracy of ±0.01 °C. Data was resampled to achieve a fixed depth resolution of 0.5 m for the calculation of temperature (∂T/∂z), salinity (∂S/∂z), and density (∂ρ/∂z) vertical gradients, to delineate variations in these parameters with depth. The vertical gradient resulted from the absolute difference in a variable quantity over the vertical distance between their resampled measurement locations. Pycnoclines, indicative of density variations, were estimated by considering the maximum vertical density gradient surpassing a defined threshold of δ1 = 0.5 kg·m4 (Read et al., 2011; Flórez-Franco et al., 2023). Building upon the findings by Flórez-Franco et al. (2023), density transition zones are identified assuming a density gradient of δ2 ≥ 0.05 kg·m4.
A temperature-salinity diagram was also devised to identify a potential relationship between the waters of the TJBH and those in coastal and open-sea waters in the Caribbean. For this purpose, existing hydrographic data from the Caribbean Surface Water (CSW data) at the Mesoamerican Barrier Reef (0-150 mbsl) delineated in Carrillo et al. (2016) was employed. Insights derived from data detailed in Tovar et al. (2009), encompassing coastal reef lagoons within the Mexican Caribbean, were considered (CC data). Additionally, existing quarterly data measurements at stations ~500 m apart from the TJBH (i.e., TJBHN, TJBHS, TJBHE, TJBHW) between March 2021 to December 2023, were used to describe the observed conditions within Chetumal Bay and in the vicinity of the TJBH (CB data). Location of the different comparative study areas (CB, CSW and CC) is depicted in Figure 1A.
Results
The boundary of TJBH, clearly defined around 5.0 mbsl, features a soft substrate covered by biofilms, which extends across the upper walls of the blue hole (Figure 1B). The turbidity of Chetumal Bay's waters conceals this border from being visible at the surface. However, the border becomes clearly seen after a depth >4.0 mbsl The TJBH wall exhibits speleothem-like formations covered by biofilms, yet they are soft, fragile, and prone to collapse (Figure 1C). Beyond 25-30 mbsl, the wall steepens and develops a firm substrate. This substrate occasionally forms a tilted roof largely free of biofilms (i.e. 0-20% coverage), possibly due to limited natural light penetration (Figure 1D).
Profiles and vertical gradients of water temperature, salinity, and density are depicted in Figure 2. The depths attained from CTD casts on both December 6th and 13th, 2023, recorded 416.0 and 423.6 mbsl, respectively. Consequently, these new findings unequivocally establish the Taam Ja’ Blue Hole (TJBH) as the world's deepest known blue hole, with its bottom still not reached.
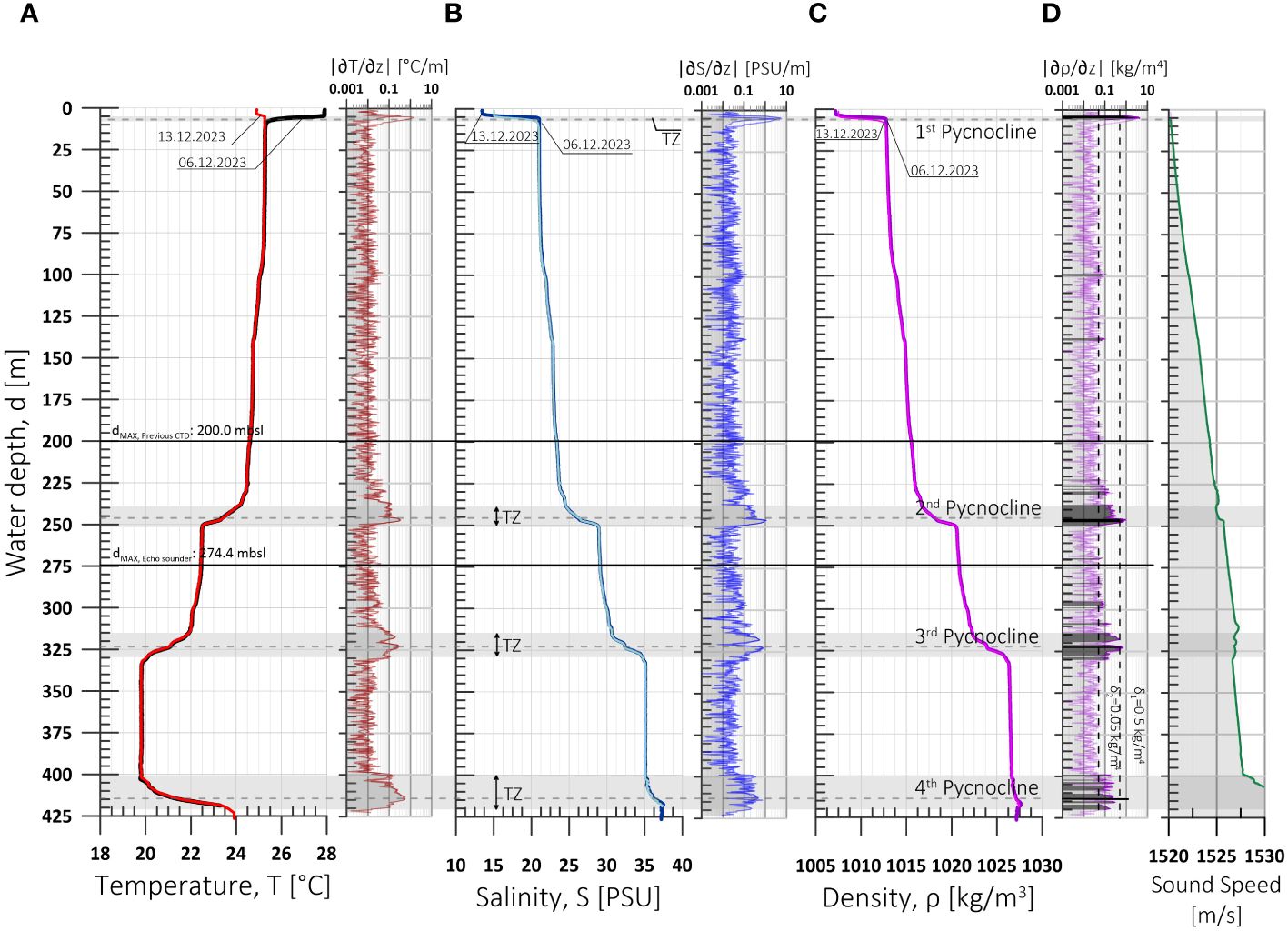
Figure 2 Vertical profiles and gradients of (A) water temperature, (B) salinity, (C) density, and (D) sound speed measured on 06.12.2023 and 13.12.2023 in TJBH with a CTD profiler. Pycnoclines are given by the maximum density gradient above a threshold δ1=0.5 kg/m4. Regions next to the pycnoclines location with a density gradient δ2>0.05 kg/m4 (TZ) are also shown.
The CTD measurements revealed a depth shorter than the cable length (~500 m) employed to lower the CTD profiler, indicating an oblique descent of the instrument at an angle of approximately 32.1-33.7° from the vertical. This deviation in orientation could be ascribed to either the specific geomorphology of the Taam Ja’ Blue Hole (TJBH) or the influence of prevailing underwater currents. Moreover, echo sounding data from prior investigations (Alcérreca-Huerta et al., 2023) had reported a maximum depth of 274.4 mbsl, with the deeper regions of the TJBH concentrated predominantly on the northern side, where depths were in average 250 mbsl. This depth coincides with the location of a pycnocline, positioned at a depth of 246.1 mbsl. Consequently, it can be inferred that the echo sounding results reported by Alcérreca-Huerta et al. (2023) might have been affected by a possibly non-strictly vertical morphology of the TJBH or acoustic scattering given by fluctuations in water density (Figure 2C, D).
The development of four primary clines with density gradients exceeding 0.5 kg/m4 is also shown in Figure 2A–C. Pycnoclines were delineated on average at 4.6-5.3 mbsl for the 1st pycnocline, 246.1 mbsl for the 2nd pycnocline, 323.3 mbsl for the 3rd pycnocline, and 414.5 mbsl for the 4th pycnocline. Transition zones (TZ) between layers above and below the pycnoclines are defined by gradients ∂ρ/∂z > 0.05 kg/m4.
The surface water layer (~0-4 mbsl) above the 1st pycnocline exhibits substantial variability in temperature (ranging from 24.9 to 27.9°C) and salinity (13.5-15.0 PSU) across measurements. Temperature and salinity variabilities decrease within the layers below the 1st pycnocline within the TJBH. On average, the layer between pycnoclines 1-2 describes an average temperature of 24.9±0.30 °C and salinity of 22.2±1.02 PSU within a depth range of 8 to 236 mbsl. In the layer encompassing depths of 249-313 mbsl (between pycnoclines 2-3), the average temperature decreases, while salinity increases, with values of 22.3±0.18 °C and 29.5±0.53 PSU, respectively. The layer below, spanning depths of 332-399 mbsl, registers an average salinity of 35.1±0.01 PSU and the lowest average temperature (19.8±0.01 °C). Beyond 400 mbsl, there is a significant increase in temperature within the transition zone, rising from 19.8 to 23.9 °C, accompanied by a salinity increase of up to 37.5 PSU and an average water density of 1027 kg/m3.
Possible hydrographic relationships across the TJBH, Chetumal Bay (CB), the Caribbean Surface Water (CSW) and Mexican Caribbean reef lagoons (CC) are explored in the temperature-salinity diagram in Figure 3. The CB data presents a wide variability of temperature (>25°C) and salinity (<17 PSU) with water densities below 1010 kg/m3, similar to those observed in the surface layer above the entrance of TJBH. This reflects the influence of the estuarine Chetumal Bay water atop the TJBH entrance.
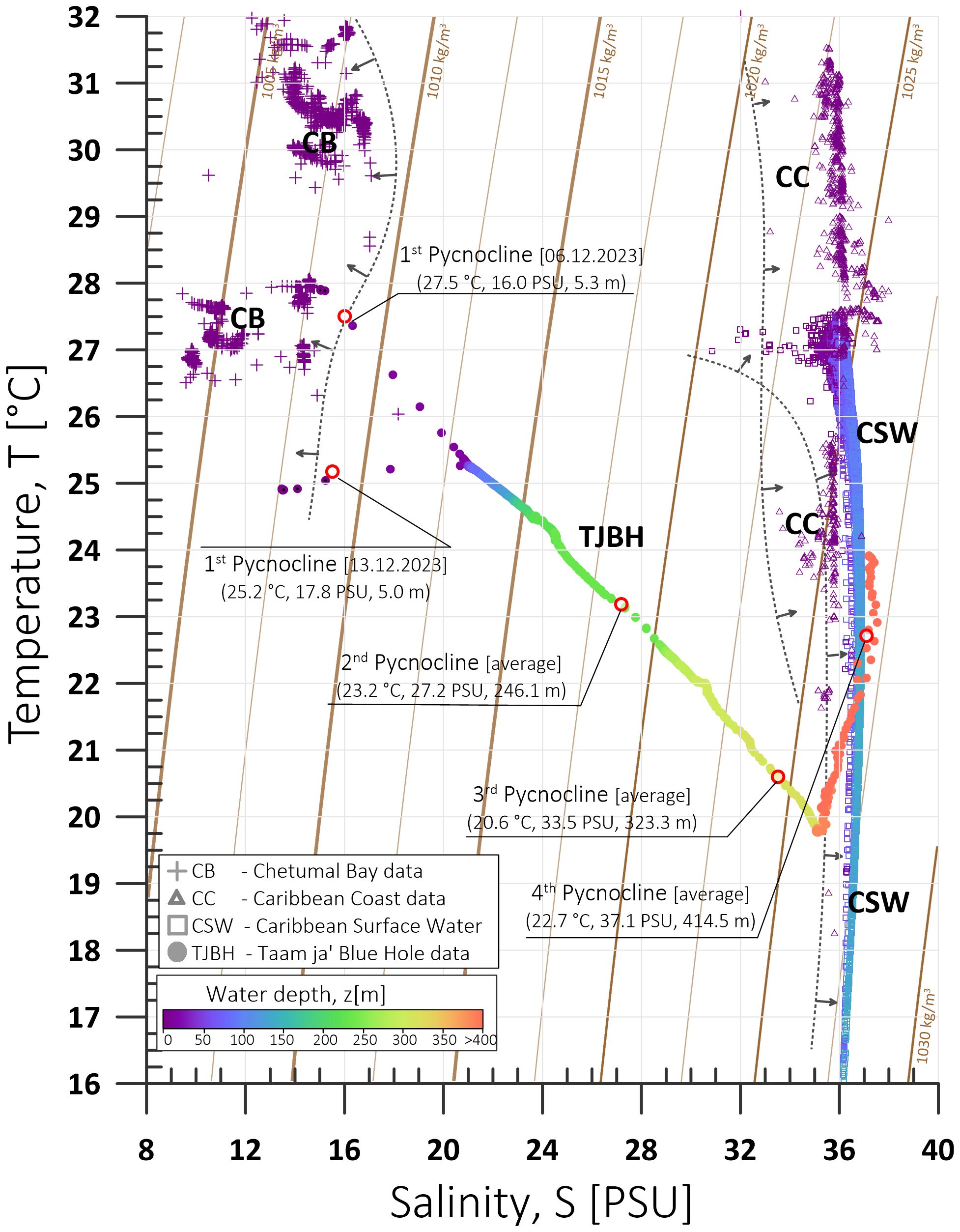
Figure 3 Temperature-salinity diagram for the water features corresponding to the TJBH. Water temperature and salinity from measured data in Chetumal Bay (CB) between 2021-2023 is also depicted together with data corresponding to the Caribbean Surface Water (CSW) for water depths 2-150 m (Carrillo et al., 2016) and to reef lagoons in the Caribbean Coast (CC) (Tovar et al., 2009). Curves show density in kilograms per cubic meter. Color bar refers to water depth in meters.
Beyond the depth of 400 mbsl within the TJBH, the water conditions gradually converge with those of in the Caribbean Sea (CSW and CC, Figure 3). Salinity levels in the Caribbean Surface Water reach up to 36.9 PSU, particularly at depths ranging from 115 to 150 mbsl, where the water densities are in average 1023±0.1 kg/m³ and reach up to 1026 kg/m³. These marine hydrographic values resemble the results obtained from CTD casts within TJBH at depths exceeding 400 mbsl with average salinity of 36.0±0.74 PSU and density of 1027±0.3 kg/m³. Similarly, data from the coastal reef lagoons of the Mexican Caribbean describe an average salinity value of 36.0±0.53 PSU, accompanied by water temperatures surpassing 18.3 °C and averaging approximately 27.9±2.48 °C. Coastal reef hydrographic data represents shallow areas (less than 9.5 mbsl) showing a wider range of density values between 1020 and 1026 kg/m³ with a mean value of 1023±0.8 kg/m³. This data alignment suggests a potential subterranean connection between these water bodies and the TJBH.
Discussion and concluding remarks
Hydrogeology and geomorphology of karst systems such as blue holes are highly valuable with implications for water resources, biodiversity, or physicochemical and geological processes. The initial results in Alcérreca-Huerta et al. (2023) yielded preliminary insights into the geomorphology, depths, and water properties of TJBH. Confirmation of the maximum depth was not possible due to instrumental limitations during the scientific expeditions in 2021, prompting the need for further exploration and analysis.
The recent records from CTD profiling in 2023 conclusively verifies that the TJBH is now the deepest blue hole discovered to date, exhibiting water depths surpassing 420 mbsl, with its bottom yet to be reached. In line with the approach undertaken by Li et al. (2018), further investigations should incorporate advanced underwater navigation technologies in conjunction with CTD profilers. This integrated methodology would allow an accurate three-dimensional spatial representation of the TJBH leading to a detailed analysis on its geomorphological features and water depths.
CTD measurements provided valuable results into the temperature–salinity stratification of the TJBH, contributing to a more comprehensive understanding of its hydrographical characteristics. Variations in temperature and salinity within the water layers of the TJBH and the pycnoclines development offered insights of TJBH in relation to surrounding marine environments. In this regard, the CTD measurements hint potential yet undiscovered connections with the seawater of either the coastal reef lagoons or deeper coastal zones of the Mesoamerican Barrier Reef System. The notable increase of temperature (~ΔT>4.0 °C) and salinity (up to 37.5 PSU) at depths beyond 400 mbsl could probably be related to these connections. The increase in salinity may stem from various mechanisms, as delineated by Fleury et al. (2007). These mechanisms could include salinization processes triggered by the inflow of marine water through a Venturi effect, water density differences (Mijatovic, 1962; Fleury et al., 2007), or the difference in hydraulic head as long as that of the seawater is higher than that of the freshwater (Whitaker and Smart, 1997). Thermal specific features could also be related to geological, volcanic or tectonic processes in relation to water circulation (Šušmelj et al., 2024). The increase in water temperature at depths >400 mbsl in TJBH could be hypothesized to resemble that observed in the Floridian aquifer (Meyer, 1989; Fleury et al., 2007), where geothermal activity warms cold seawater at deep layers, prompting its upward movement through existing sinkholes or factures at confining units. Subsequent interaction with the aquifer and the presence of further hydraulic connections with seawater could occur at upper layers, resulting in a reduction of the water temperature. This geothermal activity and the recharging areas from seawater have been related with fracture and fault zones in Florida (Whitaker and Smart, 1997) and the Northern Adriatic Sea (Šušmelj et al., 2024).
Research on blue holes encompasses a series of ambitious and exploration projects, often spanning several years or even decades, as occurred for the SYBH (e.g. Li et al., 2018; He et al., 2019; Xie et al., 2019; He et al., 2020; Jinwei et al., 2022; Chen et al., 2023) or the Bahamian blue holes (e.g. Bottrell et al., 1991; Mylroie, 2008; Gonzalez et al., 2011; Vimpere, 2017; van Hengstum et al., 2020; Sha et al., 2021). Moreover, the exploration and research of inland vertical caves, such as the Krubera–Voronya, the world's deepest known cave with a depth of 2191 meters, has continually set successive new depth records since 1960s (Klimchouk et al., 2009; Klimchouk, 2019). This evinces the needs of continuous exploration of these karst geological structures, their intricate geomorphology, and the development of cave branches. Delving into the underwater spatial geomorphology of TJBH, the focus is on deciphering its maximum depth and the possibilities of forming part of an underwater intricate and potentially interconnected system of caves and tunnels.
Therefore, the new findings and the discovered challenging depths of TJBH entails a multifaceted inquiry encompassing various scientific dimensions. Efforts should extend to unravel the hydrogeology, stratification, and mixing processes within TJBH, delineating their relationship with regional water bodies, hydraulic connections, water quality dynamics, and water residence times. Within the depths of TJBH could also lie a biodiversity to be explored and linked to physicochemical and geomorphological processes, forming a unique biotope. Geological studies should extend to understanding TJBH's relationship with the fault and fracture system of the region (i.e. the Rio Hondo Fault Zone), with implications for its origin. Analyses are needed to describe the stratigraphic sequence within TJBH and potential connections between TJBH, other blue holes and cenotes in or nearby Chetumal Bay. Thus, uncovering the challenges and mysteries concealed in TJBH urges further exploration, monitoring, and scientific inquiry.
Data availability statement
The datasets presented in this article are not readily available because the data belong to a project funded by the authors. Once published, data eventually will be shared on the institutional data reservoir. Requests to access the datasets should be directed to Laura Carrillo,bGNhcnJpbGxvQGVjb3N1ci5teA==.
Author contributions
JA: Conceptualization, Data curation, Formal analysis, Funding acquisition, Investigation, Methodology, Project administration, Supervision, Validation, Software, Visualization, Writing – original draft. OR: Investigation, Methodology, Writing – review & editing, Writing – original draft. JS: Investigation, Methodology, Writing – review & editing. TÁ: Investigation, Methodology, Writing – review & editing, Conceptualization, Funding acquisition, Project administration, Resources, Supervision, Validation. LC: Conceptualization, Funding acquisition, Investigation, Methodology, Project administration, Resources, Supervision, Validation, Writing – review & editing, Data curation, Formal analysis.
Funding
The author(s) declare that financial support was received for the research, authorship, and/or publication of this article. The first author personally funded fieldwork expenses during the survey. The fifth author funded the acquisition of the SWiFT CTD Profiler for hydrographic measurements. APCs funded by El Colegio de la Frontera Sur.
Acknowledgments
The support of Mr. Jesús Artemio Poot Villa (COBIA Team) for their navigation services and support during field surveys is gratefully acknowledged. Technical support of Johnny Omar Valdez from UNAM-UMDI during the fieldwork and scuba-explorations in TJBH is highly appreciated and recognized. Permissions and collaboration with IBANQROO (Institute of Biodiversity and Protected Areas of the State of Quintana Roo) are accredited. Recognition is given to the CONAHCYT (Mexican National Council of Humanities, Sciences and Technologies) program ‘Investigadoras e Investigadores por México’ (Project 761).
Conflict of interest
The authors declare that the research was conducted in the absence of any commercial or financial relationships that could be construed as a potential conflict of interest.
Publisher’s note
All claims expressed in this article are solely those of the authors and do not necessarily represent those of their affiliated organizations, or those of the publisher, the editors and the reviewers. Any product that may be evaluated in this article, or claim that may be made by its manufacturer, is not guaranteed or endorsed by the publisher.
References
Alcérreca-Huerta J. C., Álvarez-Legorreta T., Carrillo L., Flórez-Franco L. M., Reyes-Mendoza O. F., Sánchez-Sánchez J. A. (2023). First insights into an exceptionally deep blue hole in the Western Caribbean: The Taam ja’ Blue Hole. Front. Mar. Sci. 10. doi: 10.3389/fmars.2023.1141160
Bauer-Gottwein P., Gondwe B. R. N., Charvet G., Marín L. E., Rebolledo-Vieyra M., Merediz-Alonzo G. (2011a). Review : The Yucatán Peninsula karst aquifer , Mexico. Hydrogeol J. 19, 507–524. doi: 10.1007/s10040-010-0699-5
Benítez S., Iliffe T. M., Quiroz-Martínez B., Alvarez F. (2019). How is the anchialine fauna distributed within a cave? A study of the Ox Bel Ha System, Yucatan Peninsula, Mexico. Subterr Biol. 31, 15–28. doi: 10.3897/subtbiol.31.34347
Björnerås C., Škerlep M., Gollnisch R., Herzog S. D., Ekelund Ugge G., Hegg A., et al. (2020). Inland blue holes of The Bahamas – chemistry and biology in a unique aquatic environment. Fundam. Appl. Limnology 194, 95–106. doi: 10.1127/fal/2020/1330
Bottrell S. H., Smart P. L., Whitaker F., Raiswell R. (1991). Geochemistry and isotope systematics of sulphur in the mixing zone of Bahamian blue holes. Appl. Geochemistry 6, 97–103. doi: 10.1016/0883-2927(91)90066-X
Carrillo L., Johns E. M., Smith R. H., Lamkin J. T., Largier J. L. (2016). Pathways and hydrography in the Mesoamerican Barrier Reef System Part 2: Water masses and thermohaline structure. Cont Shelf Res. 120, 41–58. doi: 10.1016/j.csr.2016.03.014
Carrillo L., Palacios-Hernández E., Ramírez A. M., Morales-Vela B. (2009a). “Características hidrometeorológicas y batimétricas,” in El sistema ecológico de la bahía de Chetumal / Corozal: costa occidental del Mar Caribe. Eds. Espinoza-Avalos J., Islebe G., Hernández-Arana H. A. (ECOSUR, Chetumal, Mexico), 12–20.
Carrillo L., Palacios-Hernández E., Yescas M., Ramírez-Manguilar A. M. (2009b). Spatial and seasonal patterns of salinity in a large and shallow tropical estuary of the western caribbean. Estuaries Coasts 32, 906–916. doi: 10.1007/s12237-009-9196-2
Cejudo E., Ortega-Almazán P. J., Ortega-Camacho D., Acosta-González G. (2022). Hydrochemistry and water isotopes of a deep sinkhole in north Quintana Roo, Mexico. J. South Am. Earth Sci. 116, 103846. doi: 10.1016/j.jsames.2022.103846
Chen C.-T., Millero F. J. (1977). Speed of sound in seawater at high pressures. J. Acoust Soc. Am. 62, 1129–1135. doi: 10.1121/1.381646
Chen L., Yao P., Yang Z., Fu L. (2023). Seasonal and vertical variations of nutrient cycling in the world’s deepest blue hole. Front. Mar. Sci. 10, 1172475. doi: 10.3389/fmars.2023.1172475
Colbo K., Ross T., Brown C., Weber T. (2014). A review of oceanographic applications of water column data from multibeam echosounders. Estuarine, Coastal Shelf Sci. 145, 41–56. doi: 10.1016/j.ecss.2014.04.002
Domínguez-Herrera E., Luna-gonzález L., Velázquez-Torres D. (2023). Mapa de distribución de geodiversidad de Quintana Roo, México, escala 1:800,000. Terra Digitalis 7 (1), 1–17. doi: 10.22201/igg.25940694e.2023.1.99
Fleury P., Bakalowicz M., de Marsily G. (2007). Submarine springs and coastal karst aquifers: a review. J. Hydrol (Amst) 339, 79–92. doi: 10.1016/j.jhydrol.2007.03.009
Flórez-Franco L. M., Alcérreca-Huerta J. C., Reyes-Mendoza O. F., Sánchez-Sánchez J. A., Álvarez-Legorreta T., Carrillo L. (2023). Coastal blue holes in a large and shallow tropical estuary: geomorphometry and temporal variability of the physicochemical properties. Estuaries Coasts. 47, 686–700. doi: 10.1007/s12237-023-01304-9
Fofonoff N. P., Millard J. R.C. (1983). Algorithms for computation of fundamental properties of seawate). UNESCO Tech. papers Mar. science. 44, 53. doi: 10.25607/OBP-1450
Gischler E., Golubic S., Gibson M. A., Oschmann W., Hudson J. H. (2011). “Microbial mats and microbialites in the freshwater laguna bacalar, yucatan peninsula, Mexico,” in Advances in stromatolite geobiology. Lecture notes in earth sciences, vol. 131. (Springer, Berlin, Heidelberg), 187–205. doi: 10.1007/978-3-642-10415-2_13
Gondwe B. R. N., Lerer S., Stisen S., Marín L., Rebolledo-Vieyra M., Merediz-Alonso G., et al. (2010). Hydrogeology of the south-eastern Yucatan Peninsula: New insights from water level measurements, geochemistry, geophysics and remote sensing. J. Hydrol (Amst) 389, 1–17. doi: 10.1016/j.jhydrol.2010.04.044
Gondwe B. R. N., Merediz-Alonso G., Bauer-Gottwein P. (2011). The influence of conceptual model uncertainty on management decisions for a groundwater-dependent ecosystem in karst. J. Hydrol (Amst) 400, 24–40. doi: 10.1016/j.jhydrol.2011.01.023
Gonzalez B. C., Iliffe T. M., Macalady J. L., Schaperdoth I., Kakuk B. (2011). Microbial hotspots in anchialine blue holes: initial discoveries from the Bahamas. Hydrobiologia 677, 149–156. doi: 10.1007/s10750-011-0932-9
He H., Fu L., Liu Q., Fu L., Bi N., Yang Z., et al. (2019). Community structure, abundance and potential functions of bacteria and archaea in the sansha yongle blue hole, xisha, south China sea. Front. Microbiol. 10. doi: 10.3389/fmicb.2019.02404
He P., Xie L., Zhang X., Li J., Lin X., Pu X., et al. (2020). Microbial diversity and metabolic potential in the stratified sansha yongle blue hole in the south China sea. Sci. Rep. 10, 5949. doi: 10.1038/s41598-020-62411-2
Husson L., Pastier A., Pedoja K., Elliot M., Paillard D., Authemayou C., et al. (2018). Reef carbonate productivity during quaternary sea level oscillations. Geochemistry, geophysics. Geosystems 19, 1148–1164. doi: 10.1002/2017GC007335
INEGI (2002). Conjunto de datos vectoriales geológicos, continuo nacional. Fallas-fracturas Vol. 1 (Mexico: Instituto Nacional de Estadística y Geografía), 1000000.
Jinwei G., Tengfei F., Minghui Z., Hanyu Z., Liyan T. (2022). Preliminary study on formation process of Sansha Yongle Blue Hole. J. Trop. Oceanography 41, 171–183. doi: 10.11978/2021077
Kambesis P. N., Coke VI, J. G. (2013). “Overview of the controls on eogenetic cave and karst development in quintana roo, Mexico,” in Coastal karst landforms. Eds. Lace M. J., Mylroie J. E. (Springer, New York, London), 347–373. doi: 10.1007/978-94-007-5016-6_16
Klimchouk A. (2019). “Krubera (Voronja) cave,” in Encyclopedia of caves (Elsevier), 627–634. doi: 10.1016/B978-0-12-814124-3.00074-1
Klimchouk A., Samokhin G. V., Kasian Y. M. (2009). “The deepest cave in the world in the Arabika massif (Western Caucasus) and its hydrogeological and paleogeographic significance,” in ICS Proceedings, 15th International Congress of Speleology. 898–905 (USA: Kerrville).
Li T., Feng A., Liu Y., Li Z., Guo K., Jiang W., et al. (2018). Three-dimensional (3D) morphology of Sansha Yongle Blue Hole in the South China Sea revealed by underwater remotely operated vehicle. Sci. Rep. 8, 17122. doi: 10.1038/s41598-018-35220-x
Little S. N., van Hengstum P. J., Beddows P. A., Donnelly J. P., Winkler T. S., Albury N. A. (2021). Unique habitat for benthic foraminifera in subtidal blue holes on carbonate platforms. Front. Ecol. Evol. 9. doi: 10.3389/fevo.2021.794728
Meyer F. W. (1989). Hydrogeology, ground-water movement, and subsurface storage in the Floridian aquifer system in Southern Florida. US Geological Survey Prof. Paper 1403-G, 64.
Mijatovic B. F. (1962). “Contribution a la solution qualitative du problème de l’èquilibre hydraulique de l’eau douce et salèe dans les collecteurs du karst littoral,” in Association Internationnale des Hydrogèologues Publ., Rèunion d’Athènes (Greek Institute for Geology and Subsurface Research, Athènes), 184–193.
Mylroie J. E. (2008). Late Quaternary sea-level position : Evidence from Bahamian carbonate deposition and dissolution cycles. Quaternary Int. 183, 61–75. doi: 10.1016/j.quaint.2007.06.030
Perry E., Leal-Bautista R. M., Velázquez-Olimán G., Sánchez-Sánchez J. A., Wagner N. (2021). Aspects of the hydrogeology of southern campeche and quintana roo, Mexico. Boletín la Sociedad Geológica Mexicana 73, A011020. doi: 10.18268/BSGM2021v73n1a011020
Perry E., Paytan A., Pedersen B., Velazquez-Oliman G. (2009). Groundwater geochemistry of the Yucatan Peninsula, Mexico: Constraints on stratigraphy and hydrogeology. J. Hydrol (Amst) 367, 27–40. doi: 10.1016/j.jhydrol.2008.12.026
Perry E., Velazquez-Oliman G., Marin L. (2002). ). The hydrogeochemistry of the karst aquifer system of the Northern Yucatan Peninsula, Mexico. Int. Geol Rev. 44, 191–221. doi: 10.2747/0020-6814.44.3.191
Perry E., Velazquez-Oliman G., Socki R. (2003). “Hydrogeology of the yucatán peninsula,” in The lowland Maya: three millennia at the human–wildland interface. Eds. Gomez-Pompa A. ,. M., Allen S., Fedick, Jimenez-Osornio J. (Food Products Press, London), 115–138.
Read J. S., Hamilton D. P., Jones I. D., Muraoka K., Winslow L. A., Kroiss R., et al. (2011). Derivation of lake mixing and stratification indices from high-resolution lake buoy data. Environ. Model. Software 26, 1325–1336. doi: 10.1016/j.envsoft.2011.05.006
Ruíz-Pineda C., Suárez-Morales E., Gasca R. (2016). Copépodos planctónicos de la Bahía de Chetumal, Caribe Mexicano: variaciones estacionales durante un ciclo anual. Rev. Biol. Mar. Oceanogr 51, 301–316. doi: 10.4067/S0718-19572016000200008
Schmitt D., Gischler E., Walkenfort D. (2021). Holocene sediments of an inundated sinkhole: facies analysis of the “Great Blue Hole”, Lighthouse Reef, Belize. Facies 67, 10. doi: 10.1007/s10347-020-00615-8
Sha Y., Zhang H., Lee M., Björnerås C., Škerlep M., Gollnisch R., et al. (2021). Diel vertical migration of copepods and its environmental drivers in subtropical Bahamian blue holes. Aquat Ecol. 55, 1157–1169. doi: 10.1007/s10452-020-09807-4
Supper R., Motschka K., Ahl A., Bauer-Gottwein P., Gondwe B., Alonso G. M., et al. (2009). Spatial mapping of submerged cave systems by means of airborne electromagnetics: an emerging technology to support protection of endangered karst aquifers. Near Surface Geophysics 7, 613–627. doi: 10.3997/1873-0604.2009008
Šušmelj K., Čenčur Curk B., Kanduč T., Rožič B., Verbovšek T., Vreča P., et al. (2024). Hydrogeochemical conditions of submarine and terrestrial karst sulfur springs in the Northern Adriatic. Environ. Earth Sci. 83, 214. doi: 10.1007/s12665-024-11476-7
Tovar E., Suárez-Morales E., Carrillo L. (2009). Multiscale variability of the Chaetognatha along a Caribbean reef lagoon system. Mar. Ecol. Prog. Ser. 375, 151–160. doi: 10.3354/meps07770
van Hengstum P. J., Reinhardt E. G., Beddows P. A., Gabriel J. J. (2010). Linkages between Holocene paleoclimate and paleohydrogeology preserved in a Yucatan underwater cave. Quaternary Sci. Reviews2 29, 2788–2798. doi: 10.1016/j.quascirev.2010.06.034
van Hengstum P. J., Scott D. B., Gröcke D. R., Charette M. A. (2011). Sea level controls sedimentation and environments in coastal caves and sinkholes. Mar. Geol 286, 35–50. doi: 10.1016/j.margeo.2011.05.004
van Hengstum P. J., Winkler T. S., Tamalavage A. E., Sullivan R. M., Little S. N., MacDonald D., et al. (2020). Holocene sedimentation in a blue hole surrounded by carbonate tidal flats in The Bahamas: Autogenic versus allogenic processes. Mar. Geol 419. doi: 10.1016/j.margeo.2019.106051
Vimpere L. (2017). Stratigraphy and sedimentology of Quaternary carbonate units around and whitin Deans’s Blue Hole, Long Island, Bahamas (Switzerland: University of Geneva, Faculty of Sciences).
Wallace E., Donnelly J., van Hengstum P., Winkler T., Dizon C., LaBella A., et al. (2021). Regional shifts in paleohurricane activity over the last 1500 years derived from blue hole sediments offshore of Middle Caicos Island. Quat Sci. Rev. 268, 107126. doi: 10.1016/j.quascirev.2021.107126
Weber B., Scherer E. E., Martens U. K., Mezger K. (2012). Where did the lower Paleozoic rocks of Yucatan come from? A U-Pb, Lu-Hf, and Sm-Nd isotope study. Chem. Geol 312–313, 1–17. doi: 10.1016/j.chemgeo.2012.04.010
Whitaker F. F., Smart P. L. (1997). Groundwater circulation and geochemistry of a karstified bank–marginal fracture system, South Andros Island, Bahamas. J. Hydrol (Amst) 197, 293–315. doi: 10.1016/S0022-1694(96)03274-X
Keywords: coastal karst structures, underwater geomorphology, blue holes, Yucatán Peninsula, Mexican Caribbean, cave system, anchialine system
Citation: Alcérreca-Huerta JC, Reyes-Mendoza OF, Sánchez-Sánchez JA, Álvarez-Legorreta T and Carrillo L (2024) Recent records of thermohaline profiles and water depth in the Taam ja’ Blue Hole (Chetumal Bay, Mexico). Front. Mar. Sci. 11:1387235. doi: 10.3389/fmars.2024.1387235
Received: 17 February 2024; Accepted: 15 April 2024;
Published: 29 April 2024.
Edited by:
Juan Jose Munoz-Perez, University of Cádiz, SpainReviewed by:
Henry Bokuniewicz, The State University of New York (SUNY), United StatesAntonio Contreras De Villar, University of Cádiz, Spain
Copyright © 2024 Alcérreca-Huerta, Reyes-Mendoza, Sánchez-Sánchez, Álvarez-Legorreta and Carrillo. This is an open-access article distributed under the terms of the Creative Commons Attribution License (CC BY). The use, distribution or reproduction in other forums is permitted, provided the original author(s) and the copyright owner(s) are credited and that the original publication in this journal is cited, in accordance with accepted academic practice. No use, distribution or reproduction is permitted which does not comply with these terms.
*Correspondence: Laura Carrillo, bGNhcnJpbGxvQGVjb3N1ci5teA==