- 1Scottish Association for Marine Science (SAMS), Department of Science, University of the Highlands and Islands, Oban, United Kingdom
- 2Xi Engineering Consultants, Edinburgh, United Kingdom
- 3Department of Ecoscience – Marine Mammal Research, Aarhus University, Roskilde, Denmark
- 4SAMS Enterprise, Oban, United Kingdom
- 5Ocean Science Consulting Ltd., Dunbar, United Kingdom
- 6Institute of Sound and Vibration Research, University of Southampton, Southampton, United Kingdom
Continued development of offshore renewable energy is urgently needed to support the goal of achieving global Net Zero targets; however, concerns persist about impacts of construction noise on acoustically sensitive species such as the harbour porpoise (Phocoena phocoena). Population impact modelling frameworks have been proposed as a quantitative way to evaluate whether disturbance, as experienced by individual animals, might result in negative population-level consequences, and suggested as useful tools in support of Environmental Impact Assessments. However, questions remain regarding model applicability to projects of different spatial scales. This study applied two such models (iPCoD and DEPONS) to assess potential population-level impacts of construction of the East Anglia ONE wind farm on North Sea harbour porpoises. Both models were parameterised using project-specific input values underpinned by static passive acoustic monitoring data. The various iPCoD and DEPONS scenario simulations did not predict any long-term population-level impacts in response to pin-piling activities. This is likely due to discrepancy in scale between localised, short-term impacts of constructing a single wind farm in comparison to the range of the North Sea porpoise population. DEPONS modelling also revealed no long-term negative impact of pin-piling on predicted porpoise numbers within the boundary of the Southern North Sea Special Area of Conservation. Current results highlight the importance of scale when using either modelling framework to evaluate the likelihood of long-term population-level impacts on abundant, wide-ranging species from individual offshore renewables developments. Cumulative population effects from human activities, including other construction projects, commercial shipping, hydrocarbon exploitation, and fisheries bycatch, are not well understood but can be approached with the modelling frameworks explored here. However, such effects will not be apparent when applying these models to individual construction projects and in isolation from coexisting pressures. There is a risk that ignoring co-occurring pressures may result in an incomplete picture of simulated population trajectories that should be considered carefully when these models are proposed to evaluate impacts of offshore renewable construction projects. We advocate that long-term population impact modelling should be applied on a regional basis and take additional wind farm developments as well as other cumulative pressures into consideration.
1 Introduction
Expansion of the global offshore wind sector continues to accelerate in response to increasing demands for green energy to achieve challenging (inter-)national Net Zero emission targets (UN FCCC, 2015; GWEC, 2022). In many jurisdictions, offshore wind farm (OWF) sites are proliferating (UK Government, 2022; Musial et al., 2022), resulting in increases in renewable energy production capacity, but also raising concerns about potential impacts on surrounding marine environments. Underwater anthropogenic noise emissions associated with OWF construction have previously been identified as a potential concern for acoustically sensitive species such as marine mammals, whose distributions often overlap spatially with OWF sites (Lucke et al., 2006; Madsen et al., 2006). Whilst piling is the most important source of construction-related noise disturbance, other noise sources include high-order detonation of unexploded ordnance (UXO), geophysical and geotechnical surveys, and subsea cable installation. In addition, acoustic deterrent devices (ADDs) are often used to mitigate direct physical impacts to sensitive species, which together with support vessel activity also contribute to the soundscape around OWF sites.
Potential effects of OWF construction on marine mammals have been studied for over a decade, with most research focusing on construction-related impacts on sensitive species in European waters, especially the harbour porpoise (Phocoena phocoena; e.g. Brandt et al., 2016; Carstensen et al., 2006; Teilmann and Carstensen, 2012). In addition to at-sea construction activities, the species is vulnerable to several other anthropogenic pressures across its range, including fisheries bycatch, chemical pollution, and other anthropogenic noise sources (review by IAMMWG et al., 2015).
Underwater noise has been identified as an important driver of porpoise response to OWF construction (Tougaard et al., 2009; Brandt et al., 2011; Dähne et al., 2013; Kastelein et al., 2013), potentially resulting in impacts such as auditory injury, habitat exclusion, behavioural disturbance, and acoustic masking. This has driven calls for more detailed assessments of how localised disturbances might impact porpoise populations across different spatial and temporal scales, as well as highlighted the need to consider and mitigate potential cumulative effects from other concurrent activities (e.g. nearby OWF construction, shipping, oil and gas exploitation). Predictive population impact modelling approaches to resolve crucial questions about long-term population-level effects of (acoustic) disturbance as experienced by individual animals have been developed in response (NRC, 2005; Nabe-Nielsen et al., 2014; New et al., 2014; NASEM, 2017; Villegas-Amtmann et al., 2017; Cervin et al., 2020; Pirotta et al., 2022). In contrast to rule-based methods, where projected numbers of deaths are compared against a set threshold for mortalities that a population can sustain, predictive modelling approaches are particularly suitable to assess potential long-term population effects of sub-lethal anthropogenic disturbances (Sparling et al., 2017). Two main types of modelling approaches to simulate population responses are available: ‘top-down’ models that use information on, for example, average mortality and population carrying capacity, to simulate population responses (using e.g. matrix or differential equation models), and ‘bottom-up’ models, where these characteristics emerge from the actions of simulated individual animals (e.g. individual-based models; Table 1) (Grimm and Railsback, 2005).
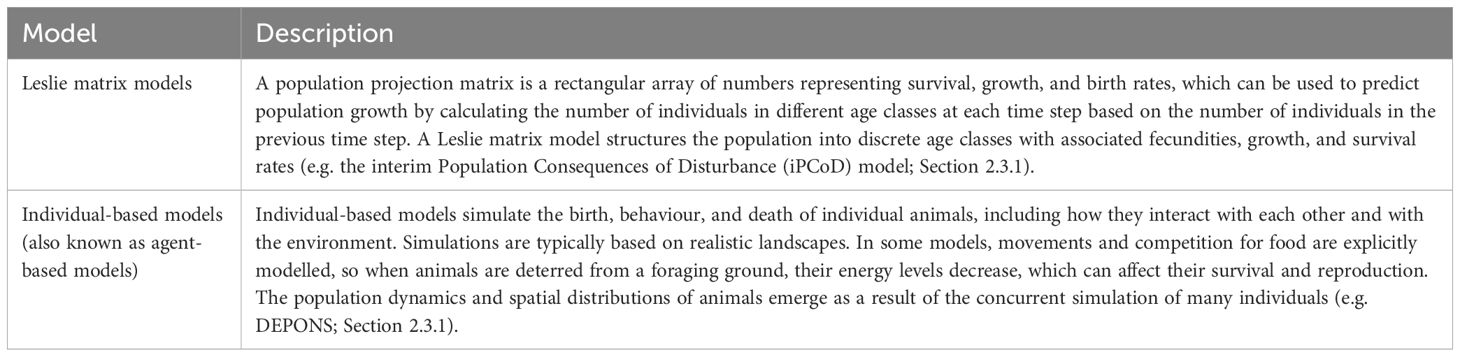
Table 1. Summary of models used in the prediction of population dynamics relevant to the current study.
Population impact models can provide guidance to regulators and other stakeholders as to the projected long-term impact of OWF construction or other anthropogenic activities. At present, two models have been developed that can be applied to assess long-term population-level impacts of marine renewable construction on the porpoise population in the North Sea, namely the interim Population Consequences of Disturbance (iPCoD) model and DEPONS. These models can be used to inform OWF construction planning, or as part of the consenting process (Nabe-Nielsen et al., 2018; Heinis et al., 2019; Sinclair et al., 2019; Mortensen et al., 2021).
Porpoises have long been protected under a range of European (inter)national regulations, notably the European Habitats Directive (referred to as the Habitats Regulations in a UK context), which requires that the Favourable Conservation Status of listed species (in the UK referred to as ‘European Protected Species’, or EPS) be maintained or restored through appropriate conservation measures. Inclusion of harbour porpoise under Annex II of the Habitats Directive (HD) additionally mandates establishment of a network of Special Areas of Conservation (SACs) for the species. In the UK, the Southern North Sea (SNS) SAC is one such designated site (JNCC, 2019a) which overlaps with numerous existing and proposed OWFs. Porpoises found within this SAC are considered part of the North Sea Management Unit (NS MU; ICES, 2014; IAMMWG, 2015 & 2023), hereafter also referred to as the ‘North Sea population’. The fact that the species is also listed in Annex IV of the HD requires conservation on the population level, and OWFs cannot be built if they harm the population.
The UK consenting process for an OWF license application involves developers producing an Environmental Statement (ES) as part of the Environmental Impact Assessment (EIA) to enable regulators to determine the potential for likely significant effects of the proposed OWF on the surrounding marine environment. In cases involving EPS such as harbour porpoise, a successful consenting application also requires one or more additional EPS licenses, which are only issued if ‘the actions authorised will not be detrimental to the maintenance of the population of the species concerned at favourable conservation status in their natural range’ (JNCC et al., 2010; Scottish Government, 2018). This phrasing illustrates that potential impacts are assessed against the scale of the entire relevant population of the species that overlaps with the proposed OWF development, rather than at the scale of individual animals impacted by specific OWF activities (Maclean et al., 2014; Sparling et al., 2015). Consequently, in the UK regulatory framework, OWF developers are encouraged to principally consider population-level impacts on EPS of their proposed developments. However, a lack of regulatory guidance currently exists on the actual implementation of the relevant EIA legislation (Maclean et al., 2014), including regarding advice on assessment of noise-related impacts on marine mammals, such that ‘…there are no rules or guidance as to which model should be used for impact assessments (project alone, cumulative or different sound sources) and nor is there guidance on when a population model should be used in an impact assessment’ (Sinclair et al., 2023).
Considering these ‘open policy’ questions, the uncertainty surrounding use of population impact models for evaluating potential impacts of single OWF developments needs to be addressed, especially when impacts are assessed in isolation from other concurrent pressures known to impact porpoises.
The present study sought to apply both iPCoD and DEPONS population impact modelling frameworks to a single OWF (East Anglia ONE) to assess the impact of pin-piling on the North Sea porpoise population. Additionally, this study aimed to evaluate results in the wider context of cumulative impacts and current UK regulations and management frameworks.
2 Methodology
2.1 Site description
The ScottishPower Renewables (SPR) East Anglia ONE (EA1) OWF is located in the southern North Sea, 43 km off the English coast. The site (300 km2, average water depth 45 m) lies within the SNS harbour porpoise SAC boundary. It contains 102 turbines (Siemens Gamesa) with a combined capacity of 714 MW. Construction started in 2018, and the OWF started operating in July 2020 (Figure 1). Each turbine has a three-legged jacket foundation, all of which required pin-piling with 2.5 m diameter piles for installation. Collectively, this resulted in 310 individual pin-piling events (including the offshore substation), which occurred intermittently between 25 April 2018 and 30 January 2019. Other noise sources associated with EA1 construction included support vessel presence, clearance of UXOs by high-order detonation, and use of Lofitech ADDs to exclude marine mammals from both construction and UXO detonation sites. Full EA1 pin-piling, ADD activation, and UXO detonation schedules and applied hammer energy (kJ) information were provided by SPR; pin-piling occurred only at single piles at any given moment.
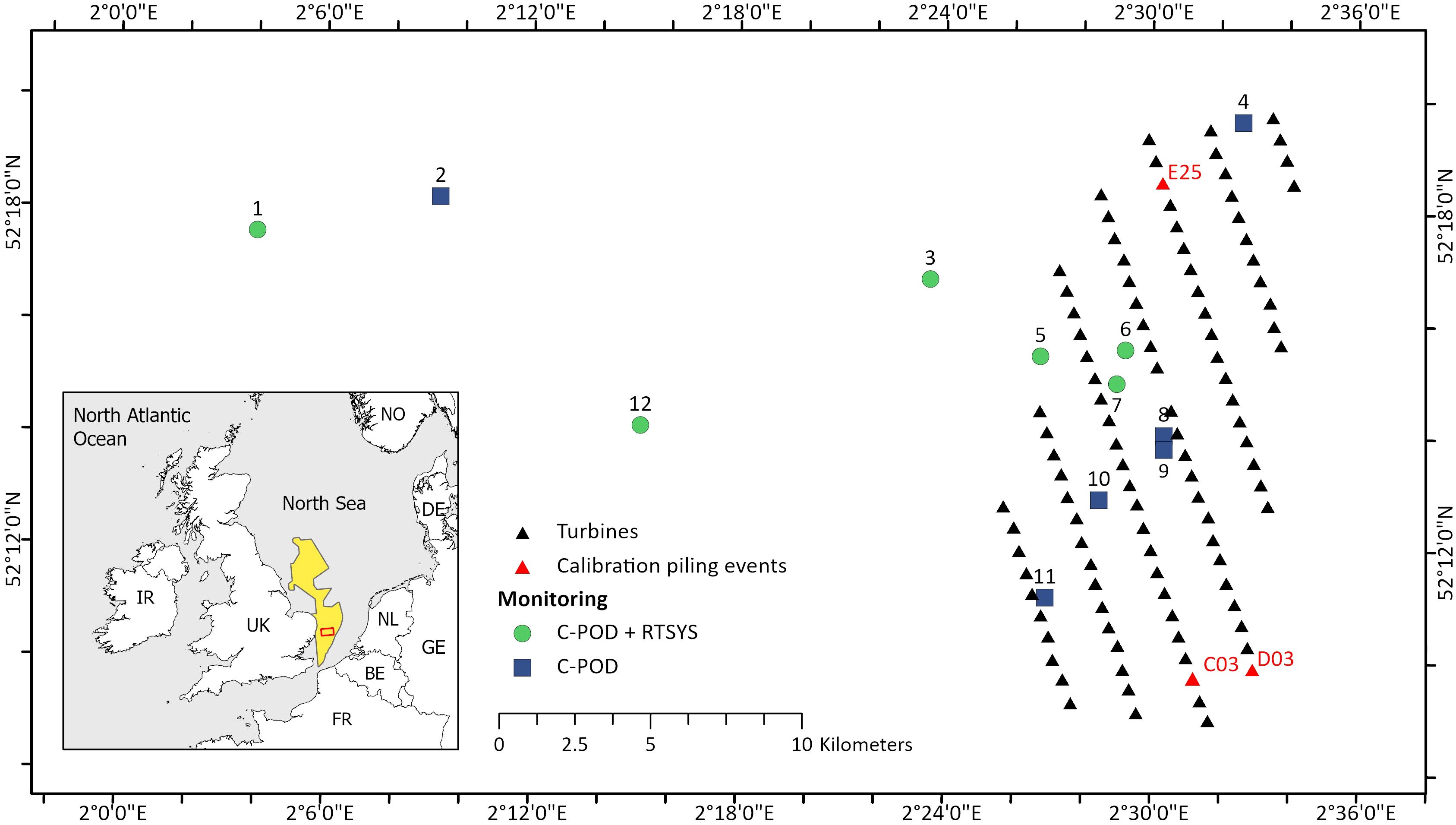
Figure 1. Location of the ScottishPower Renewables East Anglia ONE offshore wind farm off south-east England (red rectangle in inset) in relation to the Southern North Sea Special Area of Conservation (yellow area in inset), with positions of individual turbines (black triangles) and acoustic monitoring stations collecting C-POD porpoise echolocation data only (blue squares), or both C-POD and RTSYS recorder full bandwidth data (green circles). Data for calibration of the propagation model were collected during construction of turbines C03, D03, and E25 (red triangles). UK, United Kingdom; IR, Ireland; NO, Norway; DE, Denmark; NL, the Netherlands; BE, Belgium; GE, Germany; and FR, France. European country shapefile data were obtained from European Commission; Eurostat, GISCO @EuroGeographics and UN-FAO (https://ec.europa.eu/eurostat/web/gisco/geodata/reference-data/administrative-units-statistical-units/countries). Coordinates in World Geodetic System 1984.
2.2 Data collection
An array of twelve acoustic moorings was deployed (Locations 1 – 12; Figure 1) at varying distances from the piling locations over eight consecutive deployment periods (Deployments 1 – 8) from 11 March 2018 until 21 June 2019. Water depths of individual deployments varied between 32 – 56 m. All twelve moorings were equipped with C-POD porpoise click detectors (version 1; Chelonia Ltd., UK) deployed approximately 2 m above the seabed to detect the presence of echolocating porpoises. C-PODs were set to record continuously at all angles relative to vertical, and were programmed using default settings, including a limit of 4,096 clicks per minute. Six of these moorings were also equipped with EA-SDA14 full bandwidth (FBW) recorders (RTSYS, France), connected to a SPARTON PHOD-1 or a RESON TC4014 hydrophone (mean system sensitivity -180.6 dB re 1 V/μPa), to collect acoustic data for calibration of the transmission loss model, as well as quantification of ambient sound levels. These recorders operated at a sampling rate of 156 kHz (24 bit), initially on a 10/14 hour on/off duty cycle (during Deployment 1), and subsequently on a 6-hour on/off duty cycle during Deployments 2 – 8.
2.3 Population impact modelling
2.3.1 Model descriptions
2.3.1.1 iPCoD
The iPCoD framework was developed to investigate population-level consequences of the effects of individual marine mammals’ exposure to noise, primarily from piling activity during OWF construction (Harwood et al., 2014; King et al., 2015). Given current limited understanding of how changes in behaviour and hearing sensitivity affect survival and reproductive rates (i.e. fitness) of individual marine mammals, the iPCoD approach incorporates a statistical distribution of the predicted effects of 1) disturbance and 2) a permanent threshold shift (PTS) in hearing sensitivity on individual survival and reproductive rates, derived through expert elicitation (Booth and Heinis, 2018; Booth et al., 2019). Subsequently, population impact simulations use randomly selected values from these distributions to parameterise a Leslie matrix population model, as defined in Table 1. The interim framework thus enables assessment of population-level consequences from disturbance and/or injury, despite a lack of robust data regarding relationships between noise impact levels and resulting behavioural and physiological changes affecting individual fitness.
The iPCoD simulations involve a two-stage process. The first stage comprises a day-by-day simulation for a limited number of individuals (the number of which is determined by the size of the population; maximum is 1,000) throughout the period of predicted impact. It identifies the predicted number of individuals that experience disturbance and PTS, as well as the amount of impact experienced by each individual on an annual basis. This is based on user-defined estimates of 1) the number of individuals predicted to be impacted by exposure to a single day of construction, 2) the piling schedule, and 3) an estimate of the proportion of the population considered vulnerable to impacts. What constitutes the vulnerable section of the population is a judgement based on individual animal’s movements in relation to the extent of impact and construction duration. Whilst the model allows for identification of, and comparison between, different vulnerable sub-populations, it is not otherwise spatially explicit (i.e. when simulating population trajectories, the modelling processes do not take spatial locations into consideration).
The second stage extrapolates the results from the first stage to the total population size to create a Leslie Matrix model that is subsequently used to assess the population dynamics of the impacted population. This is done by modifying survival and birth rates based on the expected impacts of disturbance and PTS. At the same time, baseline survival and birth rates are applied to project the future trajectory of an un-impacted reference population in the absence of the anthropogenic pressure.
This process is repeated 1,000 times, with each simulation drawing parameter values from statistical distributions describing uncertainties in the parameters. Differences between these 1,000 simulations are determined by 1) environmental stochasticity (variation in survival and fertility rates resulting from changes in environmental conditions), 2) demographic stochasticity (i.e. individual variation in realised vital rates as a result of random processes), and 3) random selection from statistical distributions of the effect of disturbance and PTS on vital rates, as obtained through the expert elicitation process. This process results in outputs of trajectory distributions for both the reference and impacted populations, which can then be compared to assess any predicted long-term population effects, as well as the uncertainty surrounding these predictions. For further details, see Harwood et al. (2014); King et al. (2015), and Sinclair et al. (2019).
2.3.1.2 DEPONS
The DEPONS modelling framework enables a cumulative assessment of population-level impacts of offshore construction activities, bycatch, and shipping on porpoises (Nabe-Nielsen et al., 2018, 2021). DEPONS is an individual-based model that simulates porpoise movements and energetics in spatially realistic landscapes. Population dynamics arise from the individuals’ competition for food and altered movements in response to anthropogenic noise. Animals that are exposed to aversive noise are deterred from that location. Displacement from their foraging grounds reduces the individuals’ energetic status and eventually their survival. Animal movements have been calibrated based on telemetry data collected in the North Sea (van Beest et al., 2018; Nabe-Nielsen et al., 2018).
In the North Sea region, which contains patchy, limited, and seasonally fluctuating food resources, the fine-scale movements of simulated porpoises (i.e. porpoise agents) are dominated by correlated random walk behaviour (i.e. direction of movement at one time is correlated with direction of movement at the next time), as long as the energy intake is high. When local resources become depleted, individuals move towards known food patches, guided by a spatial memory of food patch locations and previous foraging success (Nabe-Nielsen et al., 2013). When food acquisition rate is persistently low and individuals cannot sustain their energy levels, fine-scale movements are abandoned, and animals start to make large-scale movements towards areas where food intake rates have previously been high. When a simulated porpoise encounters food, the animal’s energy level increases (but levels off as the porpoise becomes satiated), whilst energy levels decrease when an animal moves. Additionally, time-dependent variability in energy expenditure is incorporated into the energy costs depending on season and reproductive state (i.e. lactation in females). Survival of adults and juveniles is directly determined by their energetic status: the lower an individual’s energy levels are, the higher the risk of mortality. The survival of dependent calves relates to the energy budget of their mothers, as lactating females experiencing food shortages will not die immediately, but abandon their calves instead.
As simulated porpoises move around, individuals may become exposed to piling and (construction-related) shipping noise, with received noise levels determining how strongly animals react. These behavioural responses, in turn, affect the energy balances of porpoises by affecting foraging and travel behaviour, with potential consequences for mortality. During a simulation, the model updates the status of various entities such as porpoise movements, noise exposure, energetic status in half-hourly time intervals, and once a day records the number of animals, their energy levels, and the total amount of food. At the end of each day, life history processes, such as death, mating, birth and weaning, have a certain probability of occurring, whilst food distribution maps change seasonally. Each simulated ‘porpoise agent’ is considered a ‘super individual’ and represents several real-world female porpoises. Independent (i.e. weaned) males are not modelled explicitly; assuming an equal gender ratio, the population size is doubled at the end of a simulation. For additional DEPONS information, see Nabe-Nielsen et al. (2018, 2021). A comparison describing the similarities and differences between both modelling frameworks is provided by Nabe-Nielsen and Harwood (2016).
2.3.2 Acoustic data availability
Between March 2018 and June 2019, a total of 3,760 and 640 days of C-POD and full-bandwidth (FBW) acoustic data, respectively, were collected across the array (including both complete and partial days monitored). An overview of C-POD and FBW acoustic monitoring effort suitable for analysis is provided in Figure 2.
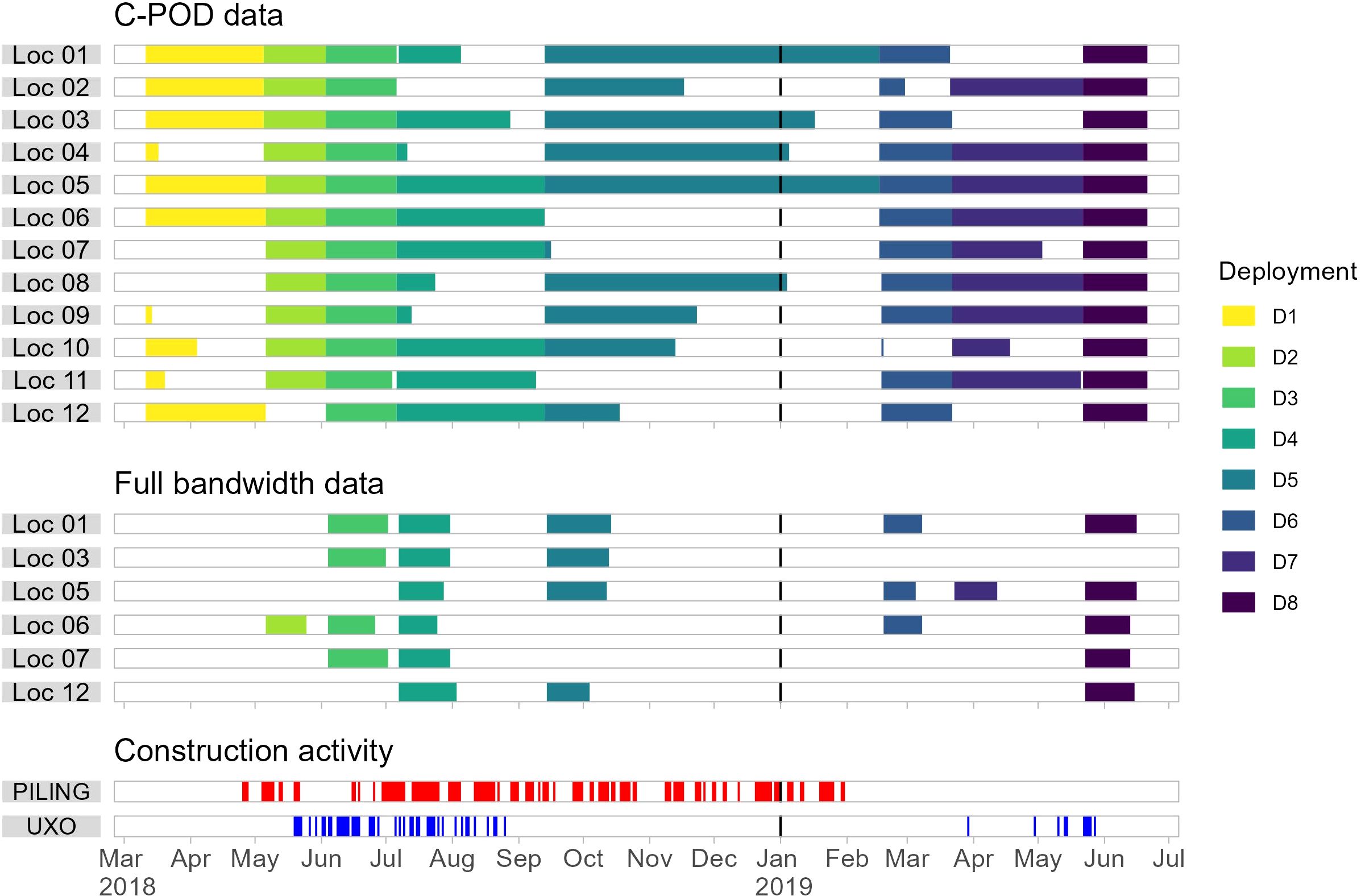
Figure 2. Summary of C-POD and FBW data suitable for subsequent analysis at deployment level for each monitoring location, in relation to realised EA1 piling and Unexploded Ordnance (UXO) detonation activity.
2.3.3 Model parametrisation
Acoustic data on porpoise presence (C-PODs) and construction noise (RTSYS broadband recorders) collected at the EA1 OWF were analysed and combined with sound propagation and statistical modelling to generate site-specific input parameters required for application of the iPCoD and DEPONS frameworks. Specifically, measurements of piling noise were used in sound propagation modelling to determine the piling source levels and resulting received noise levels frequency-weighted for harbour porpoise hearing sensitivity for the locations of the recorders, as well as the frequency-weighted PTS range. The spreading loss factor (a constant influencing sound transmission loss; input for DEPONS) was inferred from analysing the frequency-weighted received levels against distance from piling. Generalized Additive Modelling was undertaken to investigate the relationship between harbour porpoise presence from the C-POD data and distance to piling to obtain the impact range (i.e. the distance until which the probability of detecting porpoise presence was lower during pin-piling activity than in the absence of piling). Subsequently, the associated frequency-weighted response threshold (the received sound level above which porpoises are starting to get deterred; input for DEPONS) was derived for this obtained impact range using the relationship between frequency-weighted received level and distance from piling as resulting from the propagation modelling. In addition to this response threshold, additional input values for this parameter were identified based on the results by Graham et al. (2019). The PTS and impact ranges were multiplied with local, season-specific porpoise densities to obtain the number of individuals experiencing disturbance or auditory injury (inputs for iPCoD). Detailed descriptions of analytical approaches used to derive input values for specific parameters are provided in Supplementary Material Sections 1 and 2, with results presented in Supplementary Material Section 3. Assessments for additional scenario-specific parameter input values are outlined in Supplementary Material Section 4. Full overviews of all iPCoD and DEPONS model parameter input values and parameter descriptions are included in Supplementary Material Section 5.
2.3.4 Model scenarios
To assess population-level consequences of piling activity during construction of EA1 on the North Sea porpoise population, a range of iPCoD and DEPONS scenarios were considered. These scenarios incorporated a range of fixed parameter input values that were consistently applied across all model-specific scenarios, while input values for a selected number of model parameters differed across scenarios - see the Supplementary Material for a comprehensive description of the parametrisation process.
2.3.4.1 iPCoD
For iPCoD simulations, effects of variability in input values of the following parameters were explored: population size, vulnerable sub-population, residual disturbance, and site-specific density (affecting the number of disturbed individuals and the number of individuals experiencing PTS) (Table 2; Supplementary Table SM5.1 in Supplementary Material Section 5.1.1). This resulted in a total of 60 possible iPCoD scenarios. To maximise efficiency, modelling started with a ‘conservative’ scenario, the scenario most likely to result in a population-level impact based on the combinations of parameter input values considered in this study. This scenario applied the smallest population size (i.e. 87,440; the lower 95% CL of the regional southern North Sea group), the smallest proportion of vulnerable sub-population (i.e. 0.5; representing the ‘local’ abundance estimate compared to the estimate for the ‘regional’ southern North Sea), a 1-day residual disturbance, and the highest seasonal local density estimations to obtain the number of individuals experiencing 1) disturbance and 2) PTS (2,502 and 4 individuals, respectively, in spring, summer and autumn; 3,754 and 6 individuals, respectively, in winter).
Subsequent scenarios were based on single-parameter modifications of the initial ‘conservative’ scenario, with each parameter scaled down (one step at a time), until no long-term difference could be identified between the modelled un-impacted reference population and the impacted population. Modelling was undertaken using iPCoD Version 5.2.
2.3.4.2 DEPONS
DEPONS simulations incorporated variation in the probability that animals became pregnant in a given year (0.68 or 0.34), the deterrence coefficient (a constant describing the linear relationship between received sound levels and the strength of an individual’s reaction) input values (0.035, 0.07, or 0.14), the response threshold (103.0, 124.6, 132.3, or 140.0 dB re 1 μPa2 SEL), as well as the specific food map applied for the winter period (autumn or spring food map). Combining these input value options resulted in the formulation of 16 modelling scenarios, summarised in Table 3 (see Supplementary Table SM5.3 in Supplementary Material Section 5.2.1 for more details). Five replicate simulations were completed for each scenario, with outputs assessed on three spatial levels: the North Sea modelling domain, the area of the SNS SAC, as well as a 20 km buffer zone around the outermost turbines of the EA1 OWF. Modelling was undertaken in DEPONS Version 2.2.
2.3.5 Long-term population-level impacts
The modelled iPCoD population size outcomes were assessed by determining the probability of an annual average population decline of >0.5% and >1% (i.e. the population size in 2040 being <88.67% and <78.57% of the pre-piling baseline, respectively). For DEPONS, the proportional population size outputs (i.e. the population size at the end of the 25-year simulation relative to the population size for the 2017 pre-piling baseline) were similarly compared against those associated with an average annual population decline thresholds of >0.5% and >1% (i.e. a proportion <0.8867 is indicative of a >0.5% decline, and <0.7857 of a decline >1%). For this study, the relevant population was the NS MU (ICES, 2014).
These model assessments were based on current EU and UK policies. A population decline of >1% per year is considered to signify Unfavourable conservation status under the EU Habitat’s Directive (Evans and Arvela, 2012). As a Habitat’s Directive Annex II listed priority species, porpoises are also specifically included under Descriptor 1 (Biological Diversity) of the EU Marine Strategy Framework Directive (MSFD; transposed into UK legislation by the Marine Strategy Regulations 2010). The MSFD requires Member States to achieve Good Environmental Status for listed species and habitats. In the UK, a ~0.5% annual reduction in population size is presently considered to indicate a significant decrease in abundance (DEFRA, 2019).
3 Results
3.1 Modelling results: iPCoD
Application of the ‘conservative’ modelling scenario did not reveal a long-term impact in mean population size resulting from EA1 piling activity (Figure 3). Despite very subtle differences when comparing the modelled impacted and reference populations, mean trajectories and 95% Confidence Intervals were almost identical over the 25-year simulation (Figure 3A). The distributions of modelled population sizes were also similar (Figure 3B). In the end, the mean size of the impacted population stabilised at ~99.61% of the mean size of the reference population (i.e. the predicted mean decline over the entire assessment period was ~0.39%).
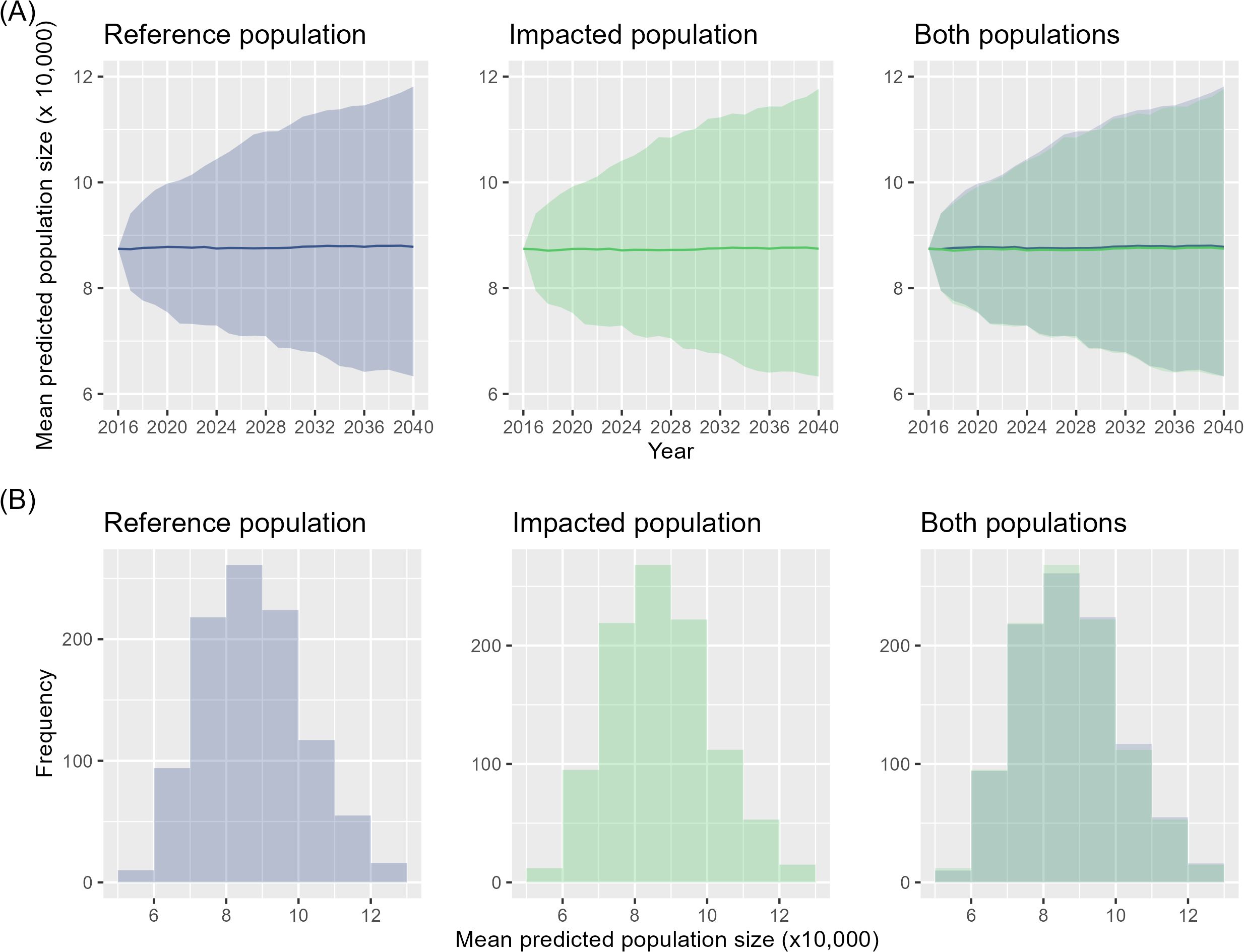
Figure 3. (A) Visualisation of the mean reference and impacted population trajectories (solid line) and associated 95% Confidence Intervals (shaded area) for the ‘conservative’ iPCoD scenario. (B) Histogram of predicted population size at the end of the 25-year model simulation for the ‘conservative’ scenario.
Compared to the reference population, the impacted population showed a slightly increased probability of an annual population decline (>0.5%: 256 vs. 249, and >1%: 83 vs. 80 out of 1,000 iterations for the impacted vs. reference populations, respectively).
Since this ‘conservative’ scenario did not generate a significant difference between impacted and reference populations, it was concluded that testing less conservative scenarios, would also not result in an impact. Consequently, no additional scenarios were assessed.
3.2 Modelling results: DEPONS
3.2.1 Long-term population-level impacts across the North Sea MU
The North Sea MU results, averaged over the five simulations per scenario while applying the default pregnancy rate of 0.68 (S1-S8; Table 3), are presented in Table 4 (yearly population sizes). After the 15-year burn-in period (see Supplementary Material Section 4.2), the population stabilised around 8,000 of the 10,000 original simulated porpoise agents. For the North Sea porpoise MU, no annual rates of population decline >0.5% (relative to 2017 pre-piling levels) were observed when averaging within scenarios. Only one out of five non-piling reference (S1) simulations illustrated such a decline. The largest decline in average population size (5.37% overall reduction over 25 years across the five simulations) was observed in the non-piling reference scenario. Both reference and piling scenarios (S2-S8) revealed substantial inter-simulation variation, with population size at the end of the modelling period fluctuating between approximately +4.3% and -5.4% of 2017 pre-piling reference population size (up to approximately +6.6% and -12.1% for individual simulations) (Table 4).
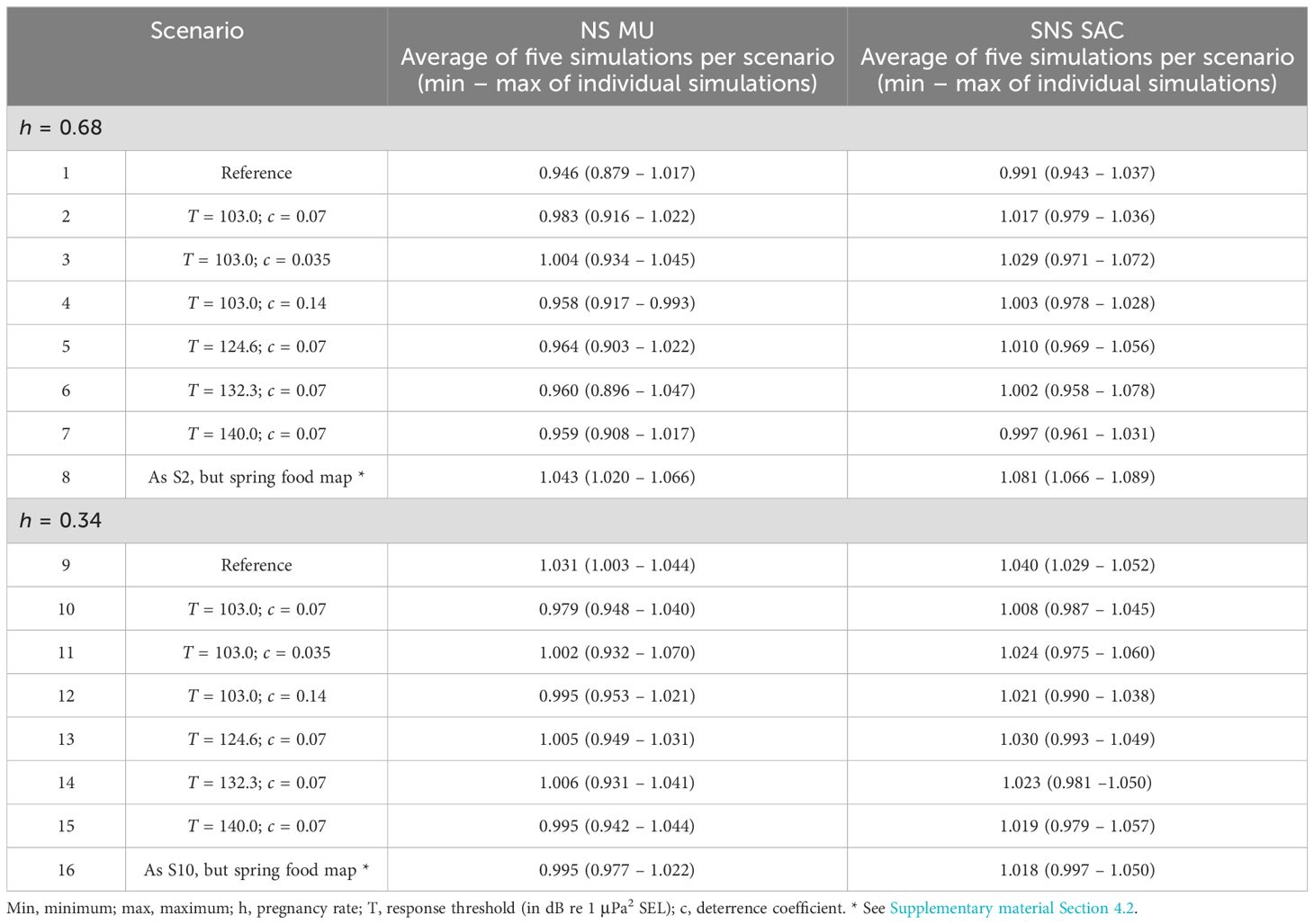
Table 4. Modelled mean annual harbour porpoise population sizes (North Sea Management Unit – NS MU) and number of porpoises within the boundary of the Southern North Sea Special Area of Conservation (SNS SAC) at the end of the DEPONS simulations relative to the pre-piling reference in 2017 (i.e. a value <1.0 indicates a decrease in the number of porpoises across the 25-year modelling period).
Decreasing the pregnancy rate from 0.68 to 0.34 (S9-S16; Table 3) resulted in very similar outcomes (Table 4). Following the 25-year burn-in period, the population stabilised around 8,500 of the 10,000 original porpoise agents. Based on the modelled results, construction of EA1 appeared to have no substantial long-term impacts on population size in any of the individual piling scenarios, with scenario averages at the end of simulations being similar to the pre-piling size of the North Sea MU. Changing the pregnancy rate also resulted in inter-simulation variability in population size; variability at the end of the modelling period ranged between approximately +3.1% and -2.1% for the averaged scenarios (and between +7.0% and -6.9% for individual simulations) (Table 4).
3.2.2 Impacts at Southern North Sea SAC scale
Projected changes to porpoise abundance within the SNS SAC boundary across different scenarios at the end of the simulation period are also presented in Table 4 (S1-S8: pregnancy rate = 0.68; S9-S16: pregnancy rate = 0.34). No substantial long-term negative impacts on the predicted numbers of porpoises present in the SNS SAC were indicated by any of the modelling scenarios. Instead, most scenarios predicted slightly increased numbers of individuals to occur within the boundary of the SNS SAC compared to 2017 levels (up to +8.1% for h=0.68, and +4.0% for h=0.34), with only the S1 reference scenario revealing an average 0.9% decrease in porpoise abundance after the 25-year modelling period. Individual simulations were 8.9% above or 6.7% below the pre-piling reference at the end of the simulations for S1-S8 and varied between +6.0% and -2.5% for S9-S16 (Table 4).
When scenario outputs were assessed at daily temporal resolution rather than annual averages, predicted porpoise numbers were observed to fluctuate through the year, with increases reflecting weaning of calves and decreases resulting from deaths through competition for food (Nabe-Nielsen, 2021). Predicted porpoise agent numbers within the SAC decreased during part of the piling period, but not more than during the same months in the years before or after construction. This trend was the same across all scenarios, including the non-piling reference scenario (Figures 4A, B).
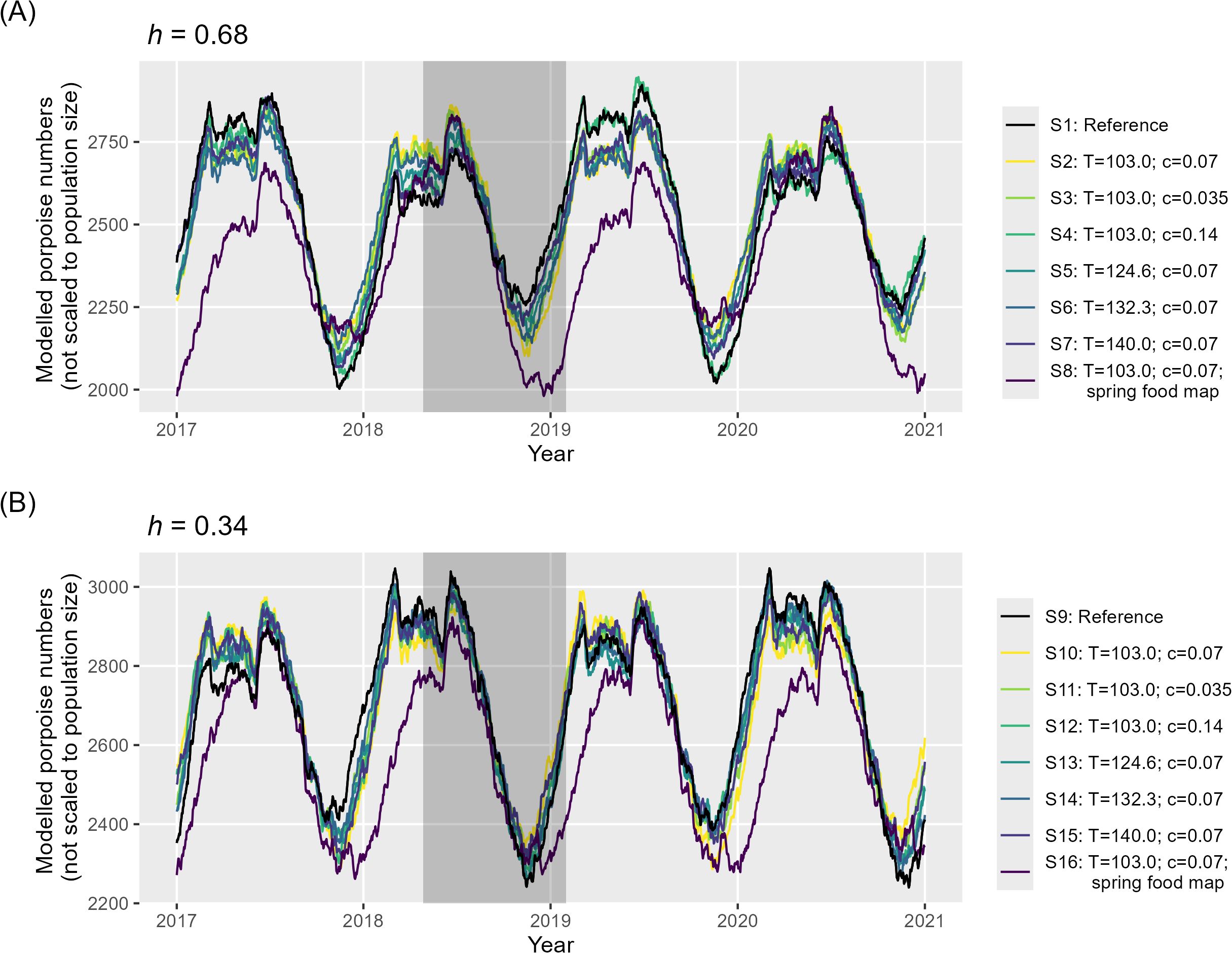
Figure 4. Predicted daily average number of harbour porpoise agents (not scaled to population size) within the Southern North Sea SAC for 2017-2020, based on a pregnancy rate of 0.68 (A) and 0.34 (B), and averaged over five simulations per DEPONS scenario (Table 3). The EA1 construction period is indicated by the dark grey colour. h = pregnancy rate; T = response threshold (in dB re 1 μPa2 SEL); c = deterrence coefficient.
For S1-S8 (h=0.68), the predicted porpoise abundance fluctuated interannually for most scenarios (i.e. S1, S4, S5, S7, and S8) suggesting a biennial pattern. However, whilst predicted numbers were highest in 2017 & 2019 for S1, S4, S5, and S7, an opposite pattern was present for S8 (i.e. highest modelled numbers for 2018 & 2020). Consequently, in alternate years, S8 (incorporating increased local food supply at the EA1 site for December – February), suggested an overall decrease in the number of modelled porpoises in the wider SAC compared to the other scenarios.
Results of S9-S16 (h=0.34) were very similar to each other when assessed at a daily temporal resolution; however S16 (which incorporated increased food availability at the EA1 wind farm during winter), revealed a decrease in predicted number of porpoises within the SAC for the winter and following spring months. Some biennial fluctuation in modelled porpoise abundance was also noted in several scenario outputs. This biennial periodicity was not as pronounced as for those scenarios applying the higher pregnancy rate, with the exception of S9 (non-piling reference).
3.2.3 Impacts at the EA1 scale
Whilst predicted porpoise numbers were much lower in the small 20 km buffer zone around the EA1 turbine locations compared to those in the much larger SNS SAC, the overall pattern of porpoise presence was broadly similar (Figure 5; only results based on h=0.68 are presented, as differences with simulations using h=0.34 were minimal), with numbers declining annually during part of the piling period. This negative trend in predicted numbers from spring into summer was mirrored in all scenarios, including the non-piling reference scenario, and also recurred in the same months in non-piling years.
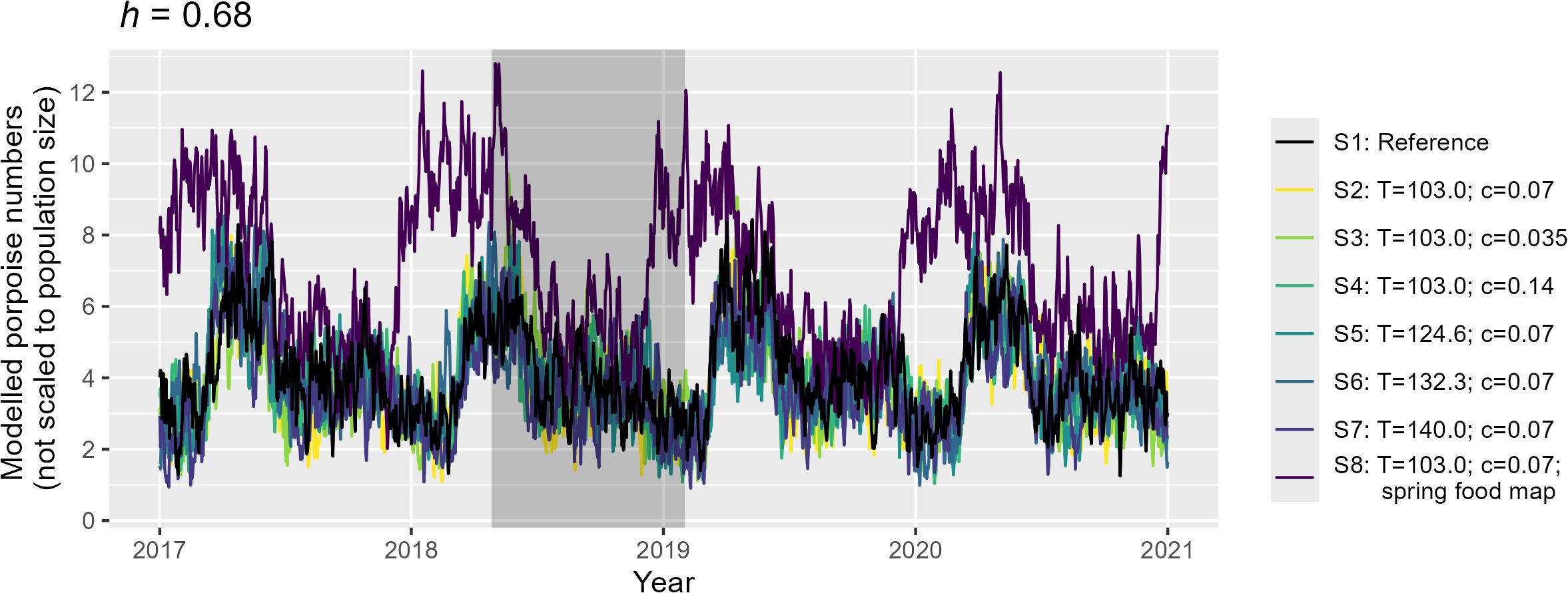
Figure 5. Daily average number of predicted harbour porpoise agents (not scaled to population size) within the 20 km buffer zone around the EA1 wind farm for 2017-2020, based on a pregnancy rate of 0.68, and averaged over five simulations per DEPONS scenario (Table 3). The EA1 construction period is indicated by the dark grey colour. h = pregnancy rate; c = deterrence coefficient.
In contrast to the SNS SAC results, the localised increased winter food availability modelled in S8 (& S16 not presented here) resulted in higher predicted porpoise numbers in the buffer zone during these months. Additionally, outcomes from these latter scenarios also predicted an overall higher number of porpoises present year-round within the 20 km buffer zone, when compared to S1-S7 and S9-S15.
4 Discussion
This study investigated potential impacts of pin-piling activity associated with the construction of the ScottishPower Renewables East Anglia ONE offshore wind farm on the North Sea porpoise population using iPCoD and DEPONS population impact modelling frameworks.
To the best of our knowledge, this study is the first to simultaneously apply both iPCoD and DEPONS to the same OWF development, and also the first time that project-specific data (i.e. EA1 passive acoustic porpoise monitoring and sound propagation modelling calibrated through pin-piling noise measurements) have been applied to both modelling frameworks to enable a project-specific post-construction impact assessment.
4.1 Evaluation of iPCoD and DEPONS population impact models
4.1.1 EA1 impact
When considering the entire North Sea porpoise population, neither impact modelling framework predicted long-term population-level impacts in response to EA1 pin-piling activity. Results of both frameworks showed that, following a 25-year simulation period, sizes of modelled populations exposed to pin-piling could not be distinguished from populations modelled under reference conditions without piling (Figure 4; Table 4). Both iPCoD and DEPONS results showed high average population sizes relative to the 2017 pre-construction baseline, with none of the scenarios indicating a strong probability of a long-term >0.5% annual decline in population abundance. Specifically, under the ‘conservative’ iPCoD scenario (applying a population size of 87,440 individuals), the increased probability of a >1% annual population decline due to piling (i.e. accounting for this occurring in the absence of piling) was 0.3%. For an annual >0.5% decline, this was 0.7%. Similarly, none of the DEPONS averaged scenarios predicted a long-term annual decline of >0.5%. Although an annual reduction in porpoise population size of >0.5%, caused by EA1 construction activity, cannot be ruled out completely based on these results, the probability of this occurring appears very low. In DEPONS, the population returns to carrying capacity after being disturbed for a short period of time, and thus long-term population-level impacts are not expected.
While no long-term population-level effects were indicated by either model, the spatially explicit nature of DEPONS allowed exploration of potential impacts on porpoise numbers at smaller spatial scales, specifically within 1) the Southern North Sea SAC, and 2) a 20 km buffer zone around the EA1 turbines. Due to underlying model differences (reviewed in Nabe-Nielsen and Harwood, 2016), the models were not applied across the same spatial resolutions. In particular, unlike DEPONS, iPCoD is not spatially explicit, and although assessments on smaller spatial scales could be addressed, predicted porpoise numbers could not be extracted for the SNS SAC and the 20-km EA1 buffer zone using the current setup. Conversely, DEPONS simulates the dynamics of the entire population, in this case the North Sea population. A hypothetical southern North Sea grouping cannot be forced, but emerges from the foraging patterns of individual animals. The consequences of such a grouping was tested using iPCoD.
DEPONS modelling outcomes at the scale of the SNS SAC revealed no long-term negative impacts of pin-piling on predicted absolute porpoise numbers within the SAC boundary, with pre-construction population sizes slightly lower or comparable to the means of the post-construction population sizes estimated by the various simulations across all scenarios (Table 4). The EA1 OWF is located within the ‘winter area’ (October – March) of the Southern North Sea SAC, which was designated based on seasonally persistent above-average porpoise densities (Heinänen and Skov, 2015; JNCC, 2019b). A general decrease in porpoise detections during the construction period (most of which occurred during the summer months) could thus be anticipated based on natural seasonal variation at the site. This seasonal variability was reflected in the DEPONS simulation results where daily modelled porpoise numbers were extracted for the area contained within the SAC boundary (Figure 4), as well as for the 20 km buffer zone around the wind turbines (Figure 5). For both spatial scales, predicted numbers of local porpoises decreased during the construction phase; this was, however, comparable to the decrease observed both in 2017 (pre-piling baseline), and in 2019-2020 (post-construction). Moreover, predicted numbers during construction periods were similar to those predicted for the non-piling reference scenario. Collectively, this suggests that observed decreases in local densities were therefore driven by larger-scale seasonal changes in porpoise distribution.
For both these smaller spatial scales, differences between scenarios in predicted porpoise numbers were mainly related to differences in applied local food availability during the winter months (Scenarios 8 & 16), with reduced numbers within the SAC and increased numbers in the buffer zone when applying the spring food map instead of the autumn food map for the winter months. This latter result is not surprising, considering that the alternative spring food distribution map specifically reflected increased food availability at the EA1 site.
An increased porpoise presence could be expected over time within the 20 km buffer zone, due to enhanced prey biomass around the OWF infrastructure driven by local fisheries exclusions, artificial reef effects, etc (Scheidat et al., 2011; Fernandez-Betelu et al., 2022). However, such effects were not investigated here.
4.1.2 Interpretation of ‘no-impact’ results
This study assessed the impact of the construction of 102 turbines and one offshore station (resulting in 310 pin-piling events) through application of an impact range of 14,0 km (Supplementary material Section 3.1), and did not demonstrate population-level impacts at the North Sea scale from EA1 pin-piling activity using iPCoD and DEPONS modelling frameworks. This, however, does not mean there was no local impact on porpoise presence (see van Geel et al., 2023a & 2023b), but instead suggests that the large North Sea population is inherently resilient to local impacts such as those assessed here. The population was unaffected by piling activities, irrespective of which input parameter values that were used in the scenarios. The lack of a population response predicted by the two modelling frameworks indicates that the population is not affected by the explored disturbances. This is not surprising given the relatively small spatial and temporal scale of the impact of a single development in relation to the abundance and geographic range of the population.
Even when considering cumulative impacts of multiple developments, Smith et al. (2019) did not find long-term cumulative population-level (i.e. North Sea scale) impacts of piling and blasting on porpoises when applying iPCoD to assess impacts of seven offshore wind construction developments and a harbour expansion project off eastern Scotland over a ~7-year construction period. Booth et al. (2017), investigating the impacts of 13 OWF developments constructed in English North Sea waters, reported a 0.3-5.2% increase in the risk of a >1% piling-associated decline in average annual porpoise population size 12 years after construction ended, despite using a range of conservative scenarios. The probability of increased risk depended strongly on a) specification of the vulnerable sub-population, b) which density estimates and noise impact ranges were applied (through a dose-response function), as well as c) which modelled (residual) disturbance days and associated impact ranges were used (all of which are poorly understood, as described in the Supplementary Material).
When Nabe-Nielsen et al. (2018) applied DEPONS to assess North Sea scale population-level impacts of different piling regimes related to numerous OWF construction developments across the southern North Sea (65 wind farms representing 3,900 individual turbines), resulting porpoise population dynamics were indistinguishable from a non-piling baseline when an 8.9 km impact range was incorporated. Finally, assessment of potential impacts of the construction of 1,650 wind turbines in German waters over a 20-year period, testing both 11.35 km and 25 km as maximum impact ranges, also did not reveal any localised short- or long-term impacts on porpoise population size (Nabe-Nielsen, 2021). The lack of longer-term impacts of pin-piling, especially at smaller spatial scales like those investigated in the current study, might result from simulated animals simply returning and continuing to forage after having been temporarily displaced by the piling noise (Nabe-Nielsen et al., 2018), and/or be driven by different, ‘naïve’ animals moving into the area.
4.1.3 Assessing effects of parameter uncertainty
Where possible, input parameters were either derived from analyses of the acoustic data to reflect project-specific conditions or obtained from the literature as relevant to the North Sea population. Uncertainty in input values for several of these parameters was incorporated into the stepwise approach for iPCoD and into the 16 fixed DEPONS scenarios. Application of a ‘conservative’ iPCoD scenario did not result in a long-term population-level impact, and no further scenarios were modelled. The lack of impact for this scenario also meant that no comprehensive assessment of the sensitivity of the model to a range of potential input values could be undertaken. The results of the different DEPONS scenarios were generally very similar, except for those where local winter food availability was increased when assessed at smaller spatial (SNS SAC; buffer zone) and temporal scales (daily average). Differences at finer spatio-temporal scales indicate the importance of carefully considering modelling requirements, especially in light of recent developments incorporating detailed energetic models (Section 4.2).
This work was underpinned by acoustic porpoise monitoring efforts (detailed in the Supplementary Material), which were unequally distributed in space and time. As in other acoustic monitoring programmes, this study relied on the detection of vocalising animals (Verfuss et al., 2018), which is affected by various factors influencing detectability (e.g. ambient noise levels, porpoises’ orientation relative to the receiver; Clausen et al., 2019; Macaulay et al., 2020, etc.). Moreover, changes in detection rates may reflect a change in animal presence, altered vocalisation behaviour, or both. In addition, tidal flow-related ‘buffering’ (i.e. reducing effective monitoring time) negatively affected C-PODs’ ability to detect porpoises, potentially confounding observed temporal patterns in porpoise presence across the array. To address this issue, considerable amounts of C-POD data (up to 38.7%) were excluded from specific monitoring locations, meaning that conclusions drawn based on such data are only valid during reduced tidal flow.
4.2 Management implications
The results of this study show that, when assessed on its own, the construction of an individual wind farm is unlikely to result in significant impacts on the North Sea harbour porpoise population. As previously mentioned, this outcome is unsurprising and likely results from the relatively local and brief impacts of EA1-associated construction activities.
However, in reality, the construction of offshore wind projects does not happen in isolation and cumulative impacts from other renewable energy construction projects as well as from other industries (e.g. fisheries, shipping, oil- and gas exploration and extraction, pollution) frequently overlap in time and space. It is in relation to these larger cumulative human impact scenarios on marine mammals, that population effect models have been developed originally. Consequently, rather than assessing impacts of individual OWF sites, the strength of the iPCoD and DEPONS modelling frameworks lies in their ability to assess large-scale, aggregate impacts (i.e. underwater noise) across multiple offshore wind developments (e.g. Booth et al., 2017; Brandt et al., 2016, 2018; de Jong et al., 2019; Heinis et al., 2019, 2022; Nabe-Nielsen et al., 2018; Rumes and Bebosschere, 2018). For example, these models can contribute to marine spatial planning by enabling exploration of different piling scenarios (Nabe-Nielsen et al., 2018), by identifying noise thresholds for offshore wind developments (Heinis et al., 2019), by investigating effects of mitigation measures to reduce piling impacts (Rumes and Bebosschere, 2018; Verfuss et al., 2016), and assessing scenarios for mitigating porpoise bycatch (Lusseau et al., 2023; van Beest et al., 2017).
With OWF construction expected to continue in the southern North Sea throughout the next decade, assessing cumulative impacts of these developments, concurrently with other pressures (e.g. bycatch in fisheries, underwater noise from shipping, chemical pollution, vessel collisions, habitat degradation, prey depletion, biological interactions, and climate change) is crucial (e.g. IAMMWG et al., 2015, 2023; Murphy et al., 2019; Sarnocińska et al., 2020; Williams et al., 2020). Importantly, climate change will drive redistribution of porpoise prey and predators (Sadykova et al., 2020; Declerck et al., 2023), with potential for as-yet unknown population-level impacts on porpoises in this MU (but see Gallagher et al., 2021b). Ideally, population-level impact assessments should therefore include all natural and anthropogenic pressures experienced by the population of interest (Dutch Ministry of Agriculture, Nature and Food Quality, 2020). However, at present, population models are not yet capable of assessing cumulative impacts of all these stressors on the North Sea porpoise population. As such, cumulative effects of EA1 wind farm construction on long-term porpoise population trajectories remain largely unexplored.
Some progress towards cumulative impact assessments using the modelling frameworks explored here have been made in recent years. For example, both frameworks are already capable of incorporating bycatch, and developments are ongoing to expand their cumulative assessment capabilities, for example, through inclusion of detailed energetic models (Harwood et al., 2020 & 2023; Gallagher et al., 2021a & b). This would allow impacts of multiple stressors to be modelled more accurately and increase utility of both models for cumulative impact assessments. Since offshore construction adds to the cumulative impact on the porpoise population, modelling results suggesting a lack of long-term population-level impacts of individual offshore wind developments, as described here, should not be interpreted as implying that impacts of OWF development is necessarily negligible, but instead highlights the fact that model outputs should always be evaluated with these cumulative pressures in mind.
Given that the greatest utility of the models used in this study lies in large-scale marine spatial planning and impact evaluation, it is important to consider the appropriateness of using population modelling frameworks to contribute to current regulatory processes (e.g. Environmental Impact Assessments). If such models were to become an integral part of current processes (e.g. to satisfy conditions for a site development license), a transparent protocol would be required, describing how model results would be assessed (e.g. setting acceptable population decline thresholds, cross-industrial sector contribution specifications, clarifying what happens with concurrent and successive applications in the same general area and across international borders). Scenarios where adjacent wind farm operators each independently conclude (based on modelling frameworks such as those used here), that their developments do not pose a significant risk to the local porpoise population need to be avoided. In the UK, under existing Environmental Impact Assessment legislation, a cumulative impact assessment is undertaken by individual developers to assess whether multiple OWF projects, including their own, may have negative effects on sensitive species. To the best of our knowledge, there is no requirement for developers to make use of either model approach described above at this stage in the process, despite the fact that such models are designed to be used at larger spatial scales. Based on the results from this study and previous experience, a government coordinated application of these models at a regional scale seems more appropriate than running them at the level of individual developments.
Regional-scale assessments across multiple developments and integrating coexisting pressures, are specifically important in the context of SAC management, designated to protect key habitats. Concerns have been raised about the potential impact of OWF construction and operation on the continued functioning and integrity of the SNS SAC for harbour porpoises (BEIS, 2020). Rather than focusing on site-specific model-based risk assessments, several European countries regulate impact through piling noise thresholds and noise reduction measures e.g. via alternative installation methods (Potlock et al., 2023) or noise abatement (Dähne et al., 2017; Merchant, 2019; Merchant and Robinson, 2020; Verfuss et al., 2019). There are indications that similar regulation might be imposed in the UK, which would preclude the necessity to conduct assessments of population-level consequences by individual developers (Sinclair et al., 2023). However, even if such a threshold were to be applied in the future, it would remain important to undertake regional population impact assessments to address cumulative impacts.
In conclusion, neither iPCoD nor DEPONS models revealed long-term population-level impacts in response to the EA1 pin-piling activity. In particular, the results of both frameworks showed that modelled population sizes of populations exposed to pin-piling activity could not be differentiated from those under reference conditions following a 25-year simulation period. Based on the outcomes, and not incorporating all other pressures experienced by animals in this population (e.g. bycatch and vessel noise), a population-level impact of a single wind farm development is not a likely scenario. This is largely due to discrepancy in scale between the localised and relatively short-term impact of an individual development and the large-scale North Sea harbour porpoise population.
Results from this study raise the issue of whether use of these modelling frameworks is appropriate to address questions about population-level impact in the context of single-project Environmental Impacts Assessments for harbour porpoise in the North Sea. In addition to more localised impact assessments of individual wind farms, regional impact assessments will likely be more useful to evaluate impacts on a larger scale. These should encompass multiple renewable energy projects and take other stressors into account to realistically assess potential long-term population-level impacts. Various co-occurring pressures can currently not or only partially be captured by these models, which may result in an incomplete picture of long-term population trajectories. This should be considered carefully if these models are to be used more widely.
Data availability statement
The datasets presented in this article are not readily available because the data are owned by ScottishPower Renewables. Requests to access the datasets should be directed to https://www.scottishpowerrenewables.com/pages/contact_us.aspx.
Ethics statement
Data used in the current study were collected through non-intrusive passive acoustic monitoring. Approval was obtained from various UK regulatory authorities & Statutory Nature Conservation Bodies, incl. the Department of Energy and Climate Change (currently part of the Department for Business, Energy and Industrial Strategy (BEIS)), Natural England (NE), the Joint Nature Conservation Committee (JNCC), and the Marine Management Organisation (MMO), pertaining to documentation supporting the consenting application, and detailing the Habitats Regulation Assessment, the Appropriate Assessment, the Marine Mammal Mitigation Plan, and the European Protected Species License application. The study was conducted in accordance with the local legislation and institutional requirements.
Author contributions
NG: Data curation, Formal analysis, Funding acquisition, Methodology, Visualization, Writing – original draft, Writing – review & editing. SB: Formal analysis, Funding acquisition, Methodology, Writing – original draft, Writing – review & editing. BM: Formal analysis, Funding acquisition, Methodology, Visualization, Writing – review & editing. JN-N: Methodology, Software, Writing – review & editing. AW: Funding acquisition, Project administration, Writing – review & editing. DR: Formal analysis, Funding acquisition, Writing – review & editing. VT: Data curation, Writing – review & editing. BW: Funding acquisition, Supervision, Writing – review & editing.
Funding
The author(s) declare that financial support was received for the research and/or publication of this article. This work was funded by ScottishPower Renewables, and we wish to express our gratitude to SPR’s Offshore Environment Team for their support.
Acknowledgments
We would like to thank Dr Cormac Booth (SMRU Consulting, St Andrews) and Dr Carol Sparling (SMRU, University of St Andrews) for their assistance and advice during this project. Additionally, we would like to thank the representatives of the following organisations for their contributions to the Stakeholder Workshops: Natural England, JNCC, Marine Scotland Science, NatureScot, DEFRA, the Crown Estate, the MMO, and the Wildlife Trust. We thank Ian Todd, Sophie Cox, and Vilislav Velikov (Ocean Science Consulting) for collecting data. Megan Ryder (SMRU Consulting) and Dr Eiren Jacobson (Centre for Research into Ecological and Environmental Modelling – CREEM, University of St Andrews) provided helpful analytical advice on the GAM modelling process. Access to additional harbour porpoise density data was provided by Dr Anita Gilles (University of Veterinary Medicine Hannover).
Conflict of interest
Author BM was employed by Xi Engineering Consultants. Author AW was employed by SAMS Enterprise. Author VT was employed by Ocean Science Consulting Ltd.
The remaining authors declare that the research was conducted in the absence of any commercial or financial relationships that could be construed as a potential conflict of interest.
The authors declare that this study received funding from ScottishPower Renewables. The funder had the following involvement in the study: selection of acoustic monitoring locations (in discussion with UK regulatory bodies), encouraging application of both the iPCoD and DEPONS modelling frameworks should this be appropriate (in discussion with UK regulatory bodies), coordination of meetings between the authors and UK regulatory bodies, approving publication of this work, and reviewing the manuscript.
Generative AI statement
The author(s) declare that no Generative AI was used in the creation of this manuscript.
Publisher’s note
All claims expressed in this article are solely those of the authors and do not necessarily represent those of their affiliated organizations, or those of the publisher, the editors and the reviewers. Any product that may be evaluated in this article, or claim that may be made by its manufacturer, is not guaranteed or endorsed by the publisher.
Supplementary material
The Supplementary Material for this article can be found online at: https://www.frontiersin.org/articles/10.3389/fmars.2025.1539143/full#supplementary-material
References
BEIS (Department for Business, Energy & Industrial Strategy) (2020). Record of the Habitats Regulations Assessment undertaken under Regulation 65 of the Conservation of Habitats and Species 2017 and Regulation 33 of the Conservation of Offshore Marine Habitats and Species Regulations 2017. Review of Consented Offshore Wind Farms in the Southern North Sea Harbour Porpoise SAC. Available online at: https://www.gov.uk/government/publications/review-of-consented-offshore-wind-farms-in-the-southern-north-sea-harbour-porpoise-special-area-of-conservation (Accessed April 25, 2025).
Booth C., Harwood J., Plunkett R., Mendes S., and Walker R. (2017). Using the ‘Interim PcoD’ framework to assess the potential effects of planned offshore wind developments in eastern English waters on harbour porpoises in the North Sea. Natural England Joint Report Number 024. Available online at: http://publications.naturalengland.org.uk/publication/4813967957950464 (Accessed April 25, 2025).
Booth C. G. and Heinis F. (2018). Updating the Interim PCoD Model: Workshop Report – New transfer functions for the effects of permanent threshold shifts on vital rates in marine mammal species. Unpublished Report SMRUC-UOA-2018-006, submitted to the University of Aberdeen and Department for Business, Energy and Industrial Strategy (BEIS). Available online at: https://www.researchgate.net/publication/362172971_Updating_the_Interim_PCoD_Model_Workshop_Report_-New_transfer_functions_for_the_effects_of_permanent_threshold_shifts_on_vital_rates_in_marine_mammal_species (Accessed April 25, 2025).
Booth C. G., Heinis F., and Harwood J. (2019). Updating the Interim PcoD Model: Workshop Report – New transfer functions for the effects of disturbance on vital rates in marine mammal species. Report Code SMRUC-BEI-2018-011, submitted to the Department for Business, Energy and Industrial Strategy (BEIS), February 2019 (unpublished). Available online at: https://www.researchgate.net/publication/362172988_Updating_the_Interim_PCoD_Model_Workshop_Report_-New_transfer_functions_for_the_effects_of_disturbance_on_vital_rates_in_marine_mammal_species (Accessed April 25, 2025).
Brandt M. J., Diederichs A., Betke K., and Nehls G. (2011). Responses of harbour porpoises to pile driving at the Horns Rev II offshore wind farm in the Danish North Sea. Mar. Ecol. Prog. Ser. 421, 205–216. doi: 10.3354/meps08888
Brandt M. J., Dragon A.-C., Diederichs A., Bellmann M. A., Wahl V., Pipier W., et al. (2018). Disturbance of harbour porpoises during construction of the first seven offshore wind farms in Germany. Mar. Ecol. Prog. Ser. 596, 213–232. doi: 10.3354/meps12560
Brandt M. J., Dragon A.-C., Diederichs A., Schubert A., Kosarev V., Nehls G., et al. (2016). Effects of offshore pile driving on harbour porpoise abundance in the German Bight. Assessment of noise effects. Final report prepared for Offshore Forum Windenergy. Available online at: https://www.offshore-stiftung.de/sites/offshorelink.de/files/documents/Study_Effects_of_offshore_pile_driving_on_harbour_porpoise_abundance_in_the_German_Bight_0.pdf (Accessed April 25, 2025).
Carstensen J., Henriksen O., and Teilmann J. (2006). Impacts of offshore wind farm construction on harbour porpoises: Acoustic monitoring of echolocation activity using porpoise detectors (T-PODs). Mar. Ecol. Prog. Ser. 321, 295–308. doi: 10.3354/meps321295
Cervin L., Harkonen T., and Harding K. C. (2020). Multiple stressors and data deficient populations; A comparative life-history approach sheds new light on the extinction risk of the highly vulnerable Baltic harbour porpoises (Phocoena phocoena). Environ. Int. 144, 106076. doi: 10.1016/j.envint.2020.106076
Dähne M., Gilles A., Lucke K., Peschko V., Adler S., Krügel K., et al. (2013). Effects of pile-driving on harbour porpoises (Phocoena phocoena) at the first offshore wind farm in Germany. Environ. Res. Lett. 8, 25002. doi: 10.1088/1748-9326/8/2/025002
Dähne M., Tougaard J., Carstensen J., Rose A., and Nabe-Nielsen J. (2017). Bubble curtains attenuate noise from offshore wind farm construction and reduce temporary habitat loss for harbour porpoises. Mar. Ecol. Prog. Ser. 580, 221–237. doi: 10.3354/meps12257
Declerck M., Trifonova N., Hartley J., and Scott B. E. (2023). Cumualtive effects of offshore renewables: From pragmatic policies to holistic marine spatial planning tools. Environ. Impact Assess. Rev. 101, 107153. doi: 10.1016/j.eiar.2023.107153
DEFRA (Department for Environment Food & Rural Affairs) (2019). Marine Strategy Part One: UK updated assessment and Good Environmental Status. Available online at: https://www.gov.uk/government/publications/marine-strategy-part-one-uk-updated-assessment-and-good-environmental-status (Accessed April 25, 2025).
De Jong C. A. F., Heinis F., von Benda-Beckmann A. M., and Binnerts B. (2019). Testing CEAF in SEANSE case studies - Impact of piling for wind farms on North Sea harbour porpoise population. TNO Report 2019 R11563. Available online at: https://www.vliz.be/en/imis?module=ref&refid=322611&printversion=1&dropIMIStitle=1 (Accessed April 25, 2025).
Dutch Ministry of Agriculture, Nature and Food Quality (2020). Updated Conservation Plan for the harbour porpoise Phocoena phocoena in the Netherlands: Maintaining a Favourable Conservation Status. Available online at: https://open.overheid.nl/documenten/ronl-dfa0b577-d562-4115-9fd5-110e8bd6eb02/pdf (Accessed April 25, 2025).
Evans D. and Arvela M. (2012). Assessment and reporting under Article 17 of the Habitats Directive. Explanatory Notes & Guidelines for the period 2007-2012. Available online at: https://circabc.europa.eu/sd/a/2c12cea2-f827-4bdb-bb56-3731c9fd8b40/Art17-Guidelines-final.pdf (Accessed April 25, 2025).
Fernandez-Betelu O., Graham I. M., and Thompson P. M. (2022). Reef effect of offshore structures on the occurrence and foraging activity of harbour porpoises. Front. Marine Sci. 9. doi: 10.3389/fmars.2022.980388
Gallagher C. A., Chimienti M., Grimm V., and Nabe-Nielsen J. (2021b). Energy-mediated responses to changing prey size and distribution in marine top predator movements and population dynamics. J. Anim. Ecol. 91, 241–254. doi: 10.1111/1365-2656.13627
Gallagher C. A., Grimm V., Kyhn L. A., Kinze C. C., and Nabe-Nielsen J. (2021a). Movement and seasonal energetics mediate vulnerability to disturbance in marine mammal populations. Am. Nat. 197, 296–311. doi: 10.1086/712798
Graham I. M., Merchant N. D., Farcas A., Barton T. R., Cheney B., Bono S., et al. (2019). Harbour porpoise responses to pile-driving diminish over time. R. Soc. Open Sci. 6, 190335. doi: 10.1098/rsos.190335
Grimm V. and Railsback S. F. (2005). Individual-Based Modeling and Ecology (Princeton: Princeton University Press).
GWEC (Global Wind Energy Council) (2022). Global offshore wind report 2022. Available online at: https://www.gwec.net/reports/globaloffshorewindreport/2022v (Accessed April 25, 2025).
Harwood J., Booth C., Sinclair R., and Hague E. (2020). Developing marine mammal dynamic energy budget models and their potential for integration into the iPCoD framework. Scottish Marine Freshwater Sci. 11. Available at: https://data.marine.gov.scot/dataset/developing-marine-mammal-dynamic-energy-budget-models-and-their-potential-integration-0 (Accessed April 25, 2025).
Harwood J., Chudzinska M., and Booth C. (2023). Further development of marine mammal dynamic energy budgets models for application to environmental assessments and integration for application into the iPCoD framework. Report SMRUC-MSC-2021-015 provided to Marine Scotland. Available online at: https://www.gov.scot/publications/developing-marine-mammal-dynamic-energy-budget-models-potential-integration-ipcod-framework/documents/ (Accessed April 25, 2025).
Harwood J., King S. L., Schick R., Donovan C., and Booth C. (2014). A protocol for implementing the Interim Population Consequences of Disturbance (PcoD) approach: Quantifying and assessing the effects of UK offshore renewable energy developments on marine mammal populations. Report SMRUL-TCE-2013-014. Scottish Marine Freshwater Sci. 5. Available at: http://www.gov.scot/Resource/0044/00443360.pdf (Accessed April 25, 2025).
Heinänen S. and Skov H. (2015). The identification of discrete and persistent areas of relatively high harbour porpoise density in the wider UK marine area. Joint Nature Conservation Committee, JNCC Report No. 544. Available online at: https://hub.jncc.gov.uk/assets/f7450390-9a89-4986-8389-9bff5ea1978a (Accessed April 25, 2025).
Heinis F., de Jong C. A. F., and von Benda-Beckmann A. M. (2022). Framework for assessing Ecological and Cumulative Effects 2021 (KEC 4.0) – Marine mammals. TNO Report TNO 2021 R12503-UK. Available online at: https://repository.tno.nl/islandora/object/uuid%3A11e3bc5d-baec-4178-bada-56df2fdce9d8 (Accessed April 25, 2025).
Heinis F., de Jong C. A. F., von Benda-Beckmann S., and Binnerts B. (2019). Framework for Asssessing Ecological and Cumulative Effects – 2018: Cumulative effects of offshore wind farm construction on porpoises. Report 18.153RWS_KEC2018 by HWE and TNO on behalf of Rijkswaterstaat Sea and Delta. Available online at: https://www.noordzeeloket.nl/en/functions-and-use/offshore-wind-energy/ecology/accumulation-ecological-effects/framework-assessing-ecological-cumulative-effects/:~:text=The%20Framework%20for%20Assessing%20Ecological%20and%20Cumulative%20Effects,look%20at%20whether%20site-specific%20effects%20can%20be%20expected (Accessed April 25, 2025).
IAMMWG (Inter-Agency Marine Mammal Working Group), Camphuysen C. J., and Siemensma M. L. (2015). A conservation literature review for the harbour porpoise (Phocoena phocoena). JNCC Report No. 566. JNCC, Peterborough. Available online at: https://hub.jncc.gov.uk/assets/e3c85307-1294-4e2c-9864-f4dd0f195e1e (Accessed April 25, 2025).
IAMMWG (Inter-Agency Marine Mammal Working Group) (2015). Management Units for cetaceans in UK waters (January 2015). JNCC Report No. 547, JNCC, Peterborough. Available online at: https://hub.jncc.gov.uk/assets/f07fe770-e9a3-418d-af2c-44002a3f2872 (Accessed April 25, 2025).
IAMMWG (Inter-Agency Marine Mammal Working Group) (2023). Review of Management Unit boundaries for cetaceans in UK waters (2023). JNCC Report No. 734, JNCC, Peterborough. Available online at: https://hub.jncc.gov.uk/assets/b48b8332-349f-4358-b080-b4506384f4f7 (Accessed April 25, 2025).
ICES (International Council for the Exploration of the Sea) (2014). OSPAR request on implementation on MSFD for marine mammals. Special Request. Available online at: https://ices-library.figshare.com/articles/report/OSPAR_request_on_implementation_of_MSFD_for_marine_mammals/18687344 (Accessed April 25, 2025).
JNCC (Joint Nature Conservation Committee) (2019a). 1351 Harbour porpoise Phocoena phocoena. Available online at: https://sac.jncc.gov.uk/species/S1351/ (Accessed April 25, 2025).
JNCC (Joint Nature Conservation Committee) (2019b). Harbour porpoise (Phocoena phocoena) Special Area of Conservation: Southern North Sea. Conservation objectives and advice on operations (Aberdeen: JNCC). Available at: https://data.jncc.gov.uk/data/206f2222-5c2b-4312-99ba-d59dfd1dec1d/SouthernNorthSea-conservation-advice.pdf (Accessed April 25, 2025).
JNCC (Joint Nature Conservation Committee), NE (Natural England), and CCW (Countryside Council for Wales) (2010). The protection of marine European Protected Species from injury and disturbance – Guidance for the marine area in England and Wales and the UK offshore marine area (Draft). Available online at: https://assets.publishing.service.gov.uk/government/uploads/system/uploads/attachment_data/file/850708/Draft_Guidance_on_the_Protection_of_Marine_European_Protected_Species_from_Injurt_and_Disturbance.pdf (Accessed April 25, 2025).
Kastelein R. A., van Heerden D., Gransier R., and Hoek L. (2013). Behavioral responses of a harbor porpoise (Phocoena phocoena) to playbacks of broadband pile driving sounds. Marine Environ. Res. 92, 206–214. doi: 10.1016/j.marenvres.2013.09.020
King S. L., Schick R. S., Donovan C., Booth C. G., Burgman M., Thomas L., et al. (2015). An interim framework for assessing the population consequences of disturbance. Methods Ecol. Evol. 6, 1150–1158. doi: 10.1111/2041-210X.12411
Lucke K., Storch S., Cooke J., and Siebert U. (2006). “Literature review of offshore wind farms with regard to marine mammals,” in Ecological research on offshore wind farms: International exchange of experiences – Part B: Literature review of ecological impacts. Eds. Zucco C., Wende W., Merck T., Köchling I., and Köppel J. (Bundesamt für Naturschutz (BfN), Bonn, Germany), 199–284.
Lusseau D., Kindt-Larsen L., and van Beest F. M. (2023). Emergent interactions in the management of multiple threats to the conservation of harbour porpoises. Sci. Total Environ. 855, 158936. doi: 10.1016/j.scitotenv.2022.158936
Macaulay J. D., Malinka C. E., Gillespie D., and Madsen P. T. (2020). High resolution three-dimensional beam radiation pattern of harbour porpoise clicks with implications for passive acoustic monitoring. J. Acoustical Soc. America 147, 4175–4188. doi: 10.1121/10.0001376
Maclean I. M. D., Inger R., Benson D., Booth C. G., Embling C. B., Grecian W. J., et al. (2014). Resolving issues with environmental impact assessment of marine renewable energy installations. Front. Marine Sci. 1. doi: 10.3389/fmars.2014.00075
Madsen P. T., Wahlberg M., Tougaard J., Lucke K., and Tyack P. (2006). Wind turbine underwater noise and marine mammals: Implications of current knowledge and data needs. Mar. Ecol. Prog. Ser. 309, 279–295. doi: 10.3354/meps309279
Merchant N. D. (2019). Underwater noise abatement: Economic factors and policy options. Environ. Sci. Policy 92, 116–123. doi: 10.1016/j.envsci.2018.11.014
Merchant N. D. and Robinson S. P. (2020). Abatement of underwater noise pollution from pile-driving and explosions in UK waters (Report of the UKAN workshop held on 12 November 2019 at The Royal Society). doi: 10.6084/m9.figshare.11815449
Mortensen L. O., Chudzinska M. E., Slabbekoorn H., and Thomsen F. (2021). Agent-based models to investigate sound impact on marine animals: Bridging the gap between effects on individual behaviour and population level consequences. Oikos 130, 1074–1086. doi: 10.1111/oik.08078
Murphy S., Tougaard J., Evans P. G. H., Caurant F., and Hammond P. S. (2019). “Area status report North Sea,” in Report of joint IMR/NAMMCO international workshop on the status of harbour porpoises in the North Atlantic. NAMMCO (North Atlantic Marine Mammal Commission) and the IMR (Norwegian Institute of Marine Research, Tromsø, Norway), 150–192. Available at: https://nammco.no/wp-content/uploads/2020/03/final-report_hpws_2018_rev2020.pdf (Accessed April 25, 2025).
Musial W., Spitsen P., Duffy P., Beiter P., Marquis M., Hammond R., et al (2022). Offshore Wind Market Report: 2022 Edition. Report by the National Renewable Energy Laboratory for the U.S. Department of Energy. doi: 10.2172/1883382
Nabe-Nielsen J. (2021). Impacts of wind farm construction and the importance of piling order for harbour porpoises in the German Exclusive Economic Zone of the North Sea – Assessment based on DEPONS 2.2. Aarhus, Denmark: Aarhus University, DCE – Danish Centre for Environment and Energy. Available at: https://dce.au.dk/fileadmin/dce.au.dk/Udgivelser/Notater_2021/N2021_68.pdf (Accessed April 25, 2025).
Nabe-Nielsen J. and Harwood J. (2016). Comparison of the iPCoD and DEPONS models for modelling population consequences of noise on harbour porpoises. Aarhus, Denmark: Aarhus University, DCE – Danish Centre for Environment and Energy. Available at: http://dce2.au.dk/pub/SR186.pdf (Accessed April 25, 2025).
Nabe-Nielsen J., Sibly R. M., Tougaard J., Teilmann J., and Sveegaard S. (2014). Effects of noise and by-catch on a Danish harbour porpoise population. Ecol. Modelling 272, 242–251. doi: 10.1016/j.ecolmodel.2013.09.025
Nabe-Nielsen J., Tougaard J., Teilmann J., Lucke K., and Forchhammer M. C. (2013). How a simple adaptive foraging strategy can lead to emergent home ranges and increased food intake. Oikos 122, 1307–1316. doi: 10.1111/j.1600-0706.2013.00069.x
Nabe-Nielsen J., van Beest F. M., Grimm V., Sibly R. M., Teilmann J., and Thompson P. M. (2018). Predicting the impacts of anthropogenic disturbances on marine populations. Conserv. Lett. 11, e12563. doi: 10.1111/conl.12563
Nabe-Nielsen J., van Beest F. M., Grimm V., Sibly R. M., Teilmann J., and Thompson P. M. (2021). TRACE document DEPONS 2.2: Individual-based model for simulating impact of wind farm construction noise on the North Sea harbour porpoise population. Available online at: https://github.com/jacobnabe/DEPONS/blob/master/DEPONS%202.2%20%E2%80%93%20TRACE%202021-08-31.pdf (Accessed April 25, 2025).
NASEM (National Academies of Sciences, Engineering, and Medicine) (2017). Approaches to understanding the cumulative effects of stressors on marine mammals (Washington, DC: The National Academies Press). doi: 10.17226/23479
New L. F., Clark J. S., Costa D. P., Fleishman E., Hindell M. A., Klanjšček T., et al. (2014). Using short-term measures of behaviour to estimate long-term fitness of southern elephant seals. Mar. Ecol. Prog. Ser. 496, 99–108. doi: 10.3354/meps10547
NRC (National Research Council) (2005). Marine mammal populations and ocean noise: Determining when noise causes biologically significant effects. Washington, DC, U.S.A.: National Research Council; Division on Earth and Life Studies; Ocean Studies Board; Committee on Characterizing Biological Significant Marine Mammal Behavior. Available at: https://www.nrc.gov/docs/ML1434/ML14345A574.pdf (Accessed April 25, 2025).
Pirotta E., Booth C. G., Calambokidis J., Costa D. P., Fahlbusch J. A., Friedlaender A. S., et al. (2022). From individual responses to population effects: Integrating a decade of multidisciplinary research on blue whales and sonar. Anim. Conserv. 25, 796–810. doi: 10.1111/acv.12785
Potlock K. M., Temple A. J., and Berggren P. (2023). Offshore construction using gravity-base foundations indicates no long-term impacts on dolphins and porpoises. Marine Biol. 170, 92. doi: 10.1007/s00227-023-04240-1
Rumes B. and Bebosschere J. (2018). “Chapter 8 – Modelling the impact of pile driving on porpoise population in the Belgian part of the North Sea,” in Environmental impacts of offshore wind farms in the Belgian part of the North Sea: Assessing and managing effect spheres of influence. Eds. Degrear S., Brabant R., Rumes B., and Vigin L. (Royal Belgian Institute of Natural Sciences, OD Natural Environment, Marine Ecology and Management, Brussels), 117–128. Available at: https://odnature.naturalsciences.be/downloads/mumm/windfarms/winmon_report_2018_final.pdf (Accessed April 25, 2025).
Sadykova D., Scott B. E., De Dominics M., Wakelin S. L., Wolf J., and Sadykov A. (2020). Ecological cost of climate change on marine predator-prey population distributions by 2050. Ecol. Evol. 10, 1069–1086. doi: 10.1002/ece3.5973
Sarnocińska J., Teilmann J., Dalgaard Balle J., van Beest F. M., Delefosse M., and Tougaard J. (2020). Harbor porpoise (Phocoena phocoena) reaction to a 3D seismic airgun survey in the North Sea. Front. Marine Sci. 6. doi: 10.3389/fmars.2019.00824
Scheidat M., Tougaard J., Brasseur S., Carstensen J., van Polanen Petel. T., Teilmann J., et al. (2011). Harbour porpoises (Phocoena phocoena) and wind farms: A case study in the Dutch North Sea. Environ. Res. Lett. 6, 25102. doi: 10.1088/1748-9326/6/2/025102
Scottish Government (2018). Marine Scotland consenting and licensing guidance - For offshore wind, wave and tidal energy applications. Available online at: https://www.gov.scot/publications/marine-scotland-consenting-licensing-manual-offshore-wind-wave-tidal-energy-applications/documents/ (Accessed April 25, 2025).
Sinclair R., Booth C., Harwood J., and Sparling C. (2019). Helpfile for the interim PCoD v5 model. Available online at: https://www.researchgate.net/publication/362909970_Helpfile_for_the_interim_PCoD_v5_model (Accessed April 25, 2025).
Sinclair R. R., Kazer S., Ryder M., New P., and Verfuss U. K. (2023). Review and recommendations on assessment of noise disturbance for marine mammals. NRW Evidence Report No. 529. (Bangor: Natural Resources Wales). Available at: https://cdn.cyfoethnaturiol.cymru/media/696882/review-and-recommendations-on-assessment-of-noise-disturbance-for-marine-mammals-final.pdf (Accessed April 25, 2025).
Smith H., Carter C., and Manson F. (2019). Cumulative impact assessment of Scottish east coast offshore windfarm construction on key species of marine mammals using iPCoD. Scottish Natural Heritage Research Report No. 1081. Available online at: https://www.nature.scot/naturescot-research-report-1081-cumulative-impact-assessment-scottish-east-coast-offshore-windfarm (Accessed April 25, 2025).
Sparling C., Smith K., Benjamins S., Wilson B., Gordon J., Stringell T., et al. (2015). Guidance to inform marine mammal site characterisation requirements at wave and tidal steam energy sites in Wales. NRW Evidence Report Number 82. Natural Resources Wales. doi: 10.13140/RG.2.1.3483.8245
Sparling C. E., Thompson D., and Booth C. G. (2017). Guide to Population Models used in Marine Mammal Impact Assessments. JNCC Report No. 607. JNCC, Peterborough. Available online at: https://hub.jncc.gov.uk/assets/e47f17ec-30b0-4606-a774-cdcd90097e28.
Teilmann J. and Carstensen J. (2012). Negative long term effects on harbour porpoises from a large scale offshore wind farm in the Baltic – Evidence of slow recovery. Environ. Res. Lett. 7, 45101. doi: 10.1088/1748-9326/7/4/045101
Tougaard J., Henriksen O. D., and Miller L. A. (2009). Underwater noise from three offshore wind turbines: Estimation of impact zones for harbor porpoises and harbor seals. J. Acoust. Soc. America. 125, 3766–3773. doi: 10.1121/1.3117444
UK (United Kingdom) Government (2022). British energy security strategy – Secure, clean and affordable British energy for the long term. Available online at: https://www.gov.uk/government/publications/british-energy-security-strategy (Accessed April 25, 2025).
UN FCCC (United Nations Framework Convention on Climate Change) (2015). The Paris agreement. Available online at: http://unfccc.int/files/essential_background/convention/application/pdf/english_paris_agreement.pdf (Accessed April 25, 2025).
van Beest F. M., Kindt-Larsen L., Bastardie F., Bartolino V., and Nabe-Nielsen J. (2017). Predicting the population-level impact of mitigating harbor porpoise bycatch with pingers and time-area fishing closures. Ecosphere 8, e01785. doi: 10.1002/ecs2.1785
van Beest F. M., Teilmann J., Dietz R., Galatius A., Mikkelsen L., Stalder D., et al. (2018). Environmental drivers of harbour porpoise fine-scale movements. Marine Biol. 165, 95. doi: 10.1007/s00227-018-3346-7
van Geel N. C. F., Benjamins S., Risch D., Allen C., and Wittich A. (2023a). Southern North Sea harbour porpoise population modelling validation – Acoustic Processing Report. A report by SAMS Enterprise for ScottishPower Renewables. Available online at: https://www.scottishpowerrenewables.com/pages/east_anglia_one_document_library.aspx (Accessed April 25, 2025).
van Geel N. C. F., Benjamins S., Risch D., Wittich A., and Wilson B. (2023b). Southern North Sea harbour porpoise population modelling validation – Population Impact Modelling Report. A report by SAMS Enterprise for ScottishPower Renewables. Available online at: https://www.scottishpowerrenewables.com/pages/east_anglia_one_document_library.aspx (Accessed April 25, 2025).
Verfuss U. K., Gillespie D., Gordon J., Marques T. A., Miller B., Plunkett R., et al. (2018). Comparing methods suitable for monitoring marine mammals in low visibility conditions during seismic surveys. Mar. Pollut. Bull. 126, 1–18. doi: 10.1016/j.marpolbul.2017.10.034
Verfuss U. K., Plunkett R., Booth C. G., and Harwood J. (2016). Assessing the benefit of noise reduction measures during offshore wind farm construction on harbour porpoises. Report Number SMRUC-WWF-2016-008 provided to WWF UK. Available online at: https://www.wwf.org.uk/sites/default/files/2016-10/15_11_24_wwf:_finalreport%5B1%5D.pdf (Accessed April 25, 2025).
Verfuss U. K., Sinclair R. R., and Sparling C. E. (2019). A review of noise abatement systems for offshore wind farm construction noise, and the potential for their application in Scottish waters. NatureScot Research Report 1070. Available online at: https://www.nature.scot/doc/naturescot-research-report-1070-review-noise-abatement-systems-offshore-wind-farm-construction-noise (Accessed April 25, 2025).
Villegas-Amtmann S., Schwartz L. K., Gailey G., Sychenko O., and Costa D. P. (2017). East or west? The energetic cost of being a gray whale and the consequence of losing energy to disturbance. Endangered Species Res. 34, 167–183. doi: 10.3354/esr00843
Williams R., ten Doeschate M., Curnick D. J., Brownlow A., Barber J. L., Davison N. J., et al. (2020). Levels of polychlorinated biphenyls are still associated with toxic effects in harbor porpoises (Phocoena phocoena) despite having fallen below proposed toxicity thresholds. Environ. Sci. Technol. 54, 2277–2286. doi: 10.1021/acs.est.9b05453
Keywords: Interim Population Consequences of Disturbance (iPCoD), DEPONS agent-based population model, cumulative impact assessments, legislative context, conservation management, marine renewables, pin-piling, C-POD
Citation: van Geel NCF, Benjamins S, Marmo B, Nabe-Nielsen J, Wittich A, Risch D, Todd VLG and Wilson B (2025) Suitability of assessing population-level impacts from construction of a single wind farm – a case study on North Sea harbour porpoises. Front. Mar. Sci. 12:1539143. doi: 10.3389/fmars.2025.1539143
Received: 03 December 2024; Accepted: 14 April 2025;
Published: 15 May 2025.
Edited by:
Xuelei Zhang, Ministry of Natural Resources, ChinaReviewed by:
Zhongchang Song, Xiamen University, ChinaLiang Fang, Chinese Academy of Fishery Sciences (CAFS), China
Copyright © 2025 van Geel, Benjamins, Marmo, Nabe-Nielsen, Wittich, Risch, Todd and Wilson. This is an open-access article distributed under the terms of the Creative Commons Attribution License (CC BY). The use, distribution or reproduction in other forums is permitted, provided the original author(s) and the copyright owner(s) are credited and that the original publication in this journal is cited, in accordance with accepted academic practice. No use, distribution or reproduction is permitted which does not comply with these terms.
*Correspondence: N. C. F. van Geel, TmllbmtlLnZhbkdlZWxAc2Ftcy5hYy51aw==