- 1Van Hall Larenstein University of Applied Sciences, Leeuwarden, Netherlands
- 2Groningen Institute for Evolutionary Life Sciences, University of Groningen, Groningen, Netherlands
- 3Marine Animal Ecology Group, Wageningen University & Research, Wageningen, Netherlands
- 4Department of the Built Environment, Eindhoven University of Technology, Eindhoven, Netherlands
Caribbean coral reefs are in rapid decline and artificial reefs are increasingly often deployed to restore lost three-dimensional structure. The majority of artificial reefs and other marine infrastructure is built from concrete, with Ordinary Portland Cement (CEM I) as the most important ingredient. However, the production of CEM I results in substantial CO2 emissions. In addition, there are indications that the material is colonized by different benthic assemblages compared to natural reefs. To make artificial reefs more sustainable and ecologically optimal, research into alternative materials is required. For this study, CEM I was compared with five alternative substrates: a mixture of CEM III cement with recycled CEM I fines (CEM III), Calcium Sulfoaluminate cement (CSA), geopolymer-sediment tiles (GS), lime-sediment tiles (LS), and Xiriton (E0). Settlement of the long-spined sea urchin Diadema antillarum on the different materials was tested under marine laboratory conditions. Competent D. antillarum larvae were added to beakers with a tile made from one of the substrates and monitored for settlement after two days. Half of the tiles of each material were covered with a four-week old biofilm, the other half had no biofilm. Results show that substrate type and the presence of a biofilm affected settlement rates significantly. After 48h, highest settlement rates were found on CEM III with biofilm (30% settlement), CSA with biofilm (26% settlement) and E0 with biofilm (20% settlement). Without biofilm, the same substrates yielded only 4 to 10% settlement. CEM I, GS, and LS had overall low settlement rates (<5%) irrespective of biofilm. Post-settlement morphology was not affected by substrate type or biofilm, with juveniles having a mean test diameter of 593 ± 12 µm and a mean spine length of 487 ± 27 µm. This study provides alternative choices for regular concrete that enhance the larval settlement of the key herbivore D. antillarum. We recommend studying these alternative materials in the field to obtain a better understanding of the effects of substrate on the ecological community development over larger time- and spatial scales.
1 Introduction
Worldwide, coral reefs face many global and local threats (Andrello et al., 2022; Good and Bahr, 2021; Hughes et al., 2018; Putnam et al., 2017). Caribbean coral reefs are no exception, with a region-wide reduction in hard coral cover up to 80% since 1975 (Gardner et al., 2003; Jackson et al., 2014) due to global warming, diseases, fishing, land-based sedimentation, and pollution from agriculture and coastal development (Cramer et al., 2021; Jackson et al., 2014). Reefs have shifted from coral-dominance to algae-dominance (Hughes et al., 2010). A main contributor to this shift is the 1983–1984 mass die-off of the long spined sea urchin Diadema antillarum, a key herbivore on Caribbean coral reefs (Lessios, 1988). In 2015, population densities had recovered to 12% of pre die-off densities (Lessios, 2016) and have again decreased sharply due to a new Caribbean-wide die-off that took place in 2022 (Hylkema et al., 2023). Without this key herbivore, algal mats covering the reefs inhibit coral recruitment (Mumby et al., 2006). This results in flattening of coral reefs, with serious consequences for reef biodiversity, ecosystem functioning, and ecosystem services (Alvarez-Filip et al., 2009).
One way to increase three-dimensional structure on coral reefs is deploying artificial reefs (Baine, 2001): man-made structures that are purposely placed on the seabed (Seaman, 2007). In the Caribbean more than 200 artificial structures have been introduced, but only very few have been monitored for their ecological success (Hylkema et al., 2021). Artificial reefs provide a new substrate for reef-building species to settle on and enhance food resources for higher trophic levels (Cresson et al., 2014). The complexity of the artificial structure allows for different niches that can shelter numerous species. Artificial reefs can perform equally compared to natural reefs, although this is strongly dependent on the geographic setting, the artificial reef material, and the structural design (reviewed by Paxton et al., 2020). The more heterogenous the structural design, the larger the habitat complexity, resulting in a more abundant and diverse community attracted to the reefs (Bulleri and Chapman, 2010; Chapman and Underwood, 2011; Loke and Todd, 2016; Hylkema et al., 2020). Less is known about the effects of artificial reef material choice on its success (Davis et al., 2017; McManus et al., 2017).
Concrete is one of the materials used most often for artificial reefs (Hylkema et al., 2021; Lima et al., 2019), because it is strong, durable, available worldwide, relatively cheap, and easy to work with (Meyer, 2009). On the other hand, the cement industry is responsible for 5-8% of the global CO2 footprint (Kajaste and Hurme, 2016; Meyer, 2009) and accounting for 26% of the total industrial emissions (Guo et al., 2024). Moreover, concrete is different in chemical composition from natural hard substrates, one of the reasons being the high pH-value of its pore solution (pH ~13) and alkalinity of its surface (Jain and Neithalath, 2009; Sagüés et al., 1997). Studies have shown that artificial structures from concrete harbor different assemblages compared to natural reefs (Carvalho et al., 2013; Connell and Glasby, 1999). Therefore, multiple attempts have been made to enhance the bioreceptivity for the settlement of marine life (Bone et al., 2022) and to reduce the CO2 footprint of concrete (Lippiatt et al., 2020).
Concrete usually consists of cement (the binder), complemented with sand, gravel, water, and sometimes additives to increase strength or workability. Ordinary Portland cement (CEM I) is by far the most commonly used cement and therefore the focus of attention. Previous studies replaced CEM I by alternatives such as CEM III (Hayek et al., 2020; Natanzi et al., 2021; Ly et al., 2021) and sulfoaluminate cement (Chen et al., 2015; Xu et al., 2019). These alternatives show promising results in their workability and mechanical properties but are ecologically still largely understudied. Since CEM III and sulfoaluminate cement have a lower CO2 footprint than CEM I, they could be interesting alternatives for CEM I in ecological engineering (Chen et al., 2015; Xu et al., 2019). Other ways to reduce the CO2 footprint in building materials is using more natural ingredients, such as organic fibers (Merta and Tschegg, 2013) and dredged sediments (el Mahdi Safhi et al., 2019). It is unknown yet how marine life will respond to these materials.
Larval settlement of reef-builders and associated key species, such as D. antillarum, is known to depend on settlement cues (Bak, 1985; Hylkema et al., 2022). These can be biological, chemical, and/or physical cues, such as the presence of a biofilm, crustose coralline algae (CCA) and other calcifying macroalgae such as Halimeda sp (Bak, 1985; Pilnick et al., 2023; Wijers et al., 2024). Furthermore, a synergistic relationship between structural and biochemical cues appears to be present for D. antillarum (Pilnick et al., 2023). Regarding the role of pH, studies on other sea urchin species highlighted that settlement rates decreased when Centrostephanus rodgersii larvae were exposed to a higher pH (up to pH 8.3) (Mos et al., 2020). However, juvenile sea urchins had more abnormalities, fewer spines and shorter spines when exposed to a lower pH (pH 7.6 - 7.8) (Mos et al., 2020).
Currently it is unknown how larval settlement of D. antillarum is affected by artificial hard substrates, and whether settlement behavior can be explained by the pH of the substrates surface. We hypothesize that alternative materials with lower surface pH levels than CEM I provide a more suitable settlement substrate and higher settlement rates. Since it is unknown whether D. antillarum are likely to settle on bare artificial substrates, we included a natural biofilm as a verified settlement cue for this species (Wijers et al., 2024). This study investigated the effects of artificial hard substrate type and biofilm presence on larval settlement and post-settlement morphology of D. antillarum.
2 Methods
2.1 Production of materials
A total of six artificial hard substrate types were used in this study, including CEM I, the industry standard, as a reference material. Five alternative hard substrates with a lower CO2 footprint than CEM I were produced. The composition of each substrate is detailed in Table 1. The first alternative is CEM III*, a binder consisting of 75% CEM III/B cement and 25% recycled CEM I cement fines. The recycled fines were sourced from previous concrete crushing and sieved to ensure fineness compatible with the cementitious matrix. CEM III/B is a blast furnace cement, containing 66–80% ground granulated blast furnace slag (GGBFS), blended with 20–34% clinker. In the rest of this paper, we will refer to CEM III* as CEM III. The second alternative is Calcium Sulfoaluminate cement (CSA), a low-carbon alternative binder based on ye’elimite (C4A3Ŝ) and other aluminates. The three binder types (CEM I, CEM III, and CSA) were produced by Eindhoven University of Technology. Three additional composite substrate mixes made with natural aggregates and alternative binders were tested: geopolymer-sediment tiles (GS) and lime-sediment tiles (LS) were produced with dredged sediments as core ingredient. The last tested material is Xiriton, specifically type XM5S-E0 (E0). This material is composed of trass lime, Miscanthus gigantheus fibres, shell gravel, sea sand and sea water, produced by Acroniq (https://acroniq.nl/) (Table 1). All alternative hard substrates included in this study were selected based on their lower CO2 footprint than CEM I and their potential for applications in the marine environment. CEM III and CSA require significantly less energy during production compared to CEM I (Chen et al., 2015; Xu et al., 2019). GS, LS, and E0 were produced from locally available sustainable materials. GS and LS have dredged sediments as a key ingredient, similar to el Mahdi Safhi et al. (2019). Geopolymer was included as a binder for one of these mixes because of its low CO2 footprint (Hassan et al., 2019). For E0, organic fibers (Miscanthus gigantheus) are a key ingredient, making the material more CO2-neutral.
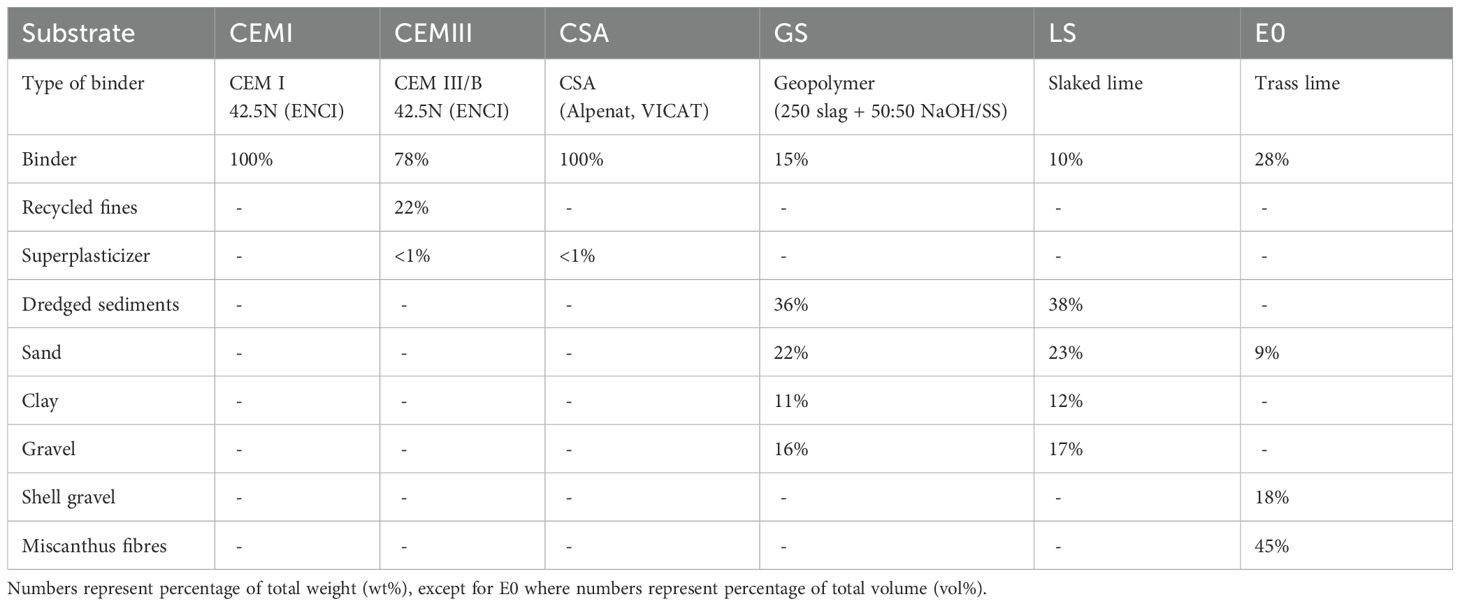
Table 1. Composition of the materials used as artificial hard substrate settlement tiles in this study.
The ingredients for each material were mixed according to the EN 196-1. A constant water-to-cement ratio of 0.4 was used for CEM I, CEM III, and CSA. For GS and LS, a water-to-solid ratio of 0.1 was applied, while E0 was prepared with a sea water-to-solid ratio of 0.2. Subsequently, the fresh mixture was cast in molds to make settlement tiles measuring 40x40x10 mm. The tiles were demolded at 24 h, then cured at 100% humidity and room temperature until 28 days after demolding. After this, the tiles were stored in dry boxes at the marine laboratory facilities of Van Hall Larenstein University of Applied Sciences in the Netherlands until the experiments started.
2.2 D. antillarum larval production
D. antillarum larvae were produced using the shaker bottle method as described by Wijers et al. (2023). Larvae were the offspring of 12 adult D. antillarum, 4 females and 8 males, cultured in the marine laboratory facilities of Van Hall Larenstein University of Applied Sciences (the Netherlands) in December 2020 and kept as brood stock. The adults were divided over two recirculation systems filled with 450 L artificial seawater (ASW) (Tropic Marin® REEF-MIX sea salt, Wartenburg, Germany) of 35‰ at 25°C with a light/dark period of 12/12 h with artificial lighting. A commercially available herbivore diet (Vitalis Marine Grazer Mini, Thorne, Great Britain) was fed to the adults 5 times per week. To induce spawning, all adults were transferred to a tank containing water 5°C above ambient holding conditions (Pilnick et al., 2021). Within 15 minutes, adults started to release gametes. Eggs and sperm were collected directly after release of the gametes with a 10 mL pipette and combined in a 1L bottle with screw cap. Two hours after spawning, eggs were checked for fertilization and divided over 1L bottles containing 500 mL ASW. Bottles were placed on a shaking table to keep the larvae in suspension during culture (Wijers et al., 2023). Larval densities started at 15 larvae mL-1 right after fertilization and were brought back to 1 larva mL-1 the day after. Larvae were fed twice a week with microalgae Rhodomonas salina at a concentration of 15000 cells mL-1 during the first 14 days post fertilization (DPF). Once the larvae reached 15 DPF, they were fed 30000 cells mL-1 and this increased to 45000 cells mL-1 for larvae >20 DPF. After 35 DPF larval densities were ~0.4 larvae mL-1 and 70%-80% of the larvae had reached competency. Competent larvae can be recognized by a clearly developed external and/or internal rudiment, visible as white, instead of transparent tissue, with internal or external tube feet and are assumed to be ready for settlement and metamorphosis (Pilnick et al., 2023). At 35 DPF, 600 competent larvae were selected for the settlement assays.
2.3 Settlement assays
2.3.1 Experimental set-up
To test the effect of substrate type on the settlement of D. antillarum, a settlement experiment was conducted. For each substrate type, ten settlement tiles (40 × 40 × 10 mm) were inoculated with biofilm-forming organisms by placing them into tanks filled with artificial seawater (ASW) and porous reef rocks. The tiles were kept under artificial light with a 12/12 h light/dark cycle for a period of four weeks prior to the settlement experiment. To be able to test the effect of biofilm presence, the biofilm on half the tiles of each substrate was removed by using a toothbrush and blade, after which the tiles were placed in an autoclave (105°C for 20 min) to kill the remaining organisms. For each of the six substrates, 5 tiles were now available with biofilm and 5 tiles without biofilm, resulting in 12 treatments (Figure 1). Each settlement tile was placed in a 250 mL-beaker and filled up to 100 mL with autoclaved ASW of 35‰, leading to a solid:liquid ratio of ~1:5 between the tile and the surrounding water. Using 12 treatments and 5 replicates, this resulted in 60 beakers in total. Beakers were placed on a table under artificial light using a light/dark period of 12/12h. In each beaker, ten competent larvae were added by using a 10 mL pipette to place the larvae on the tile. Fifty percent of the autoclaved ASW was refreshed three times per week. The temperature in the room was kept at 22.1 ± 0.4°C.
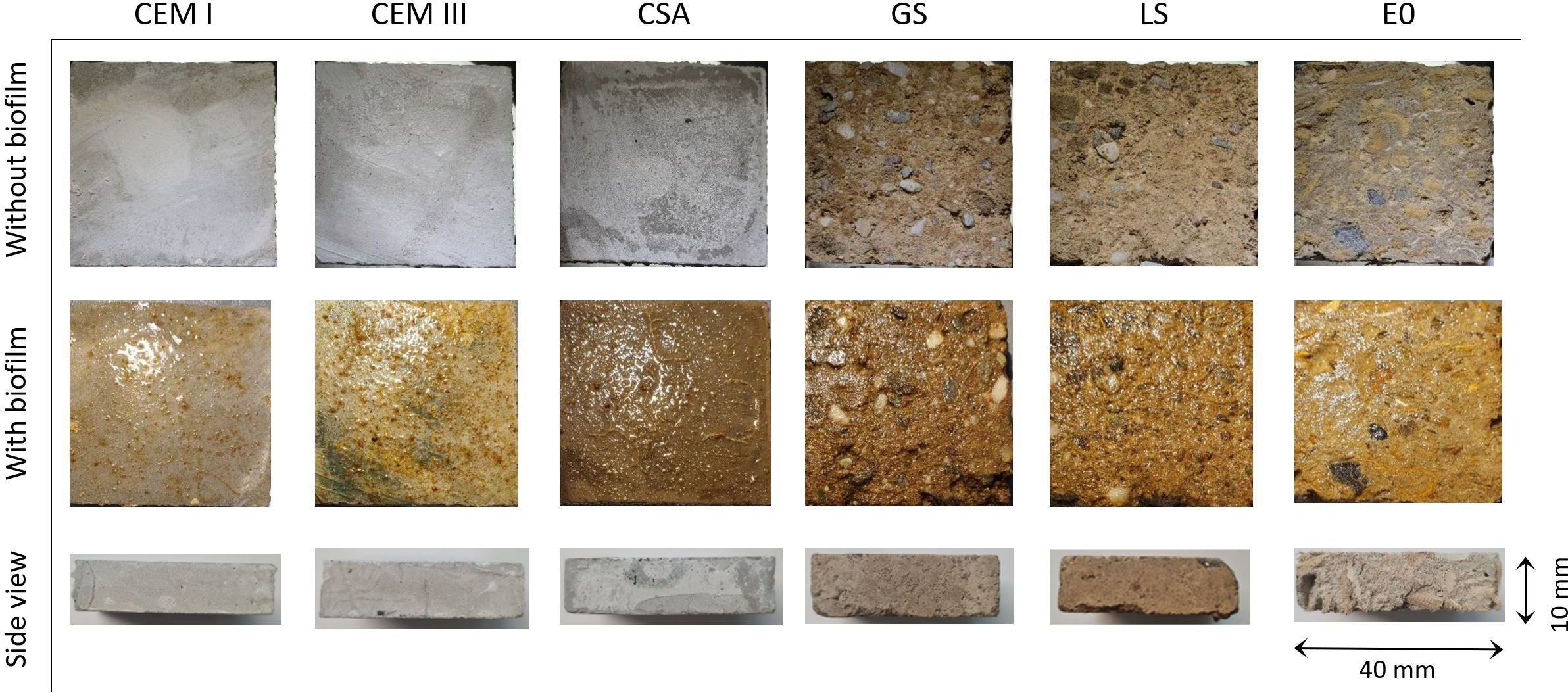
Figure 1. Overview of substrate types (CEM I, CEM III, CSA, GS, LS, and E0) with and without a four-week old biofilm at the start of the settlement experiment with D. antillarum. The first two rows show the top view of the tiles, the last row shows the side view (without biofilm).
2.3.2 Settlement measurements
Settlement was measured after 48h. All beakers were inspected thoroughly using a stereo microscope (Nikon SMZ745T). The following data were collected per beaker: number of alive, settled, and dead larvae. From these counts, we calculated the percentage of settled larvae per beaker. For example, if 3 out of the 10 larvae introduced in a beaker had settled on the substrate, this resulted in 30% settlement. We then averaged these percentages across the five replicate beakers to obtain the mean settlement for each treatment. The larvae were defined as settled when they were clearly attached to a substrate with their tube feet, retained a holdfast when a gentle water current was created with a 1 mL pipette, and had clear spine formation. Dead larvae were pale and brownish in color and often partly dissolving (Wijers et al., 2024) and were removed from the beakers. Temperature and oxygen levels of the ASW in the beakers were measured as control variables using a HQ40d Portable Multi Meter (Hach) after 48h. Salinity was measured using a hand-held refractometer (Atago).
2.3.3 pH measurements
At the start of the settlement experiment, before tiles were put in the water, the pH of the ASW was 8.57. The pH values of the water layer around the settlement tile of each beaker were measured with a HQ40d Portable Multi Meter (Hac) after 48h. The sensor of the Multi Meter was held in the water layer just above the settlement tile. The tiles were at this stage already 4–5 months old and had been in ASW for four weeks prior to the experiment (see 2.3.1).
2.3.4 Post-settlement morphology
To measure post-settlement morphology after one week, all settled juvenile D. antillarum were removed from the beaker, placed in a 1 mL-counting chamber, and photographed using the camera of a Nikon SMZ745T microscope. The test diameter (TD) and maximum spine length were assessed from the photographs using image analysis software (IC Measure, The Imaging Source). TD was determined by averaging two perpendicular measures across the test at the longest axes. Maximum spine length was calculated as the average of the five longest spines from each juvenile, following Mos et al. (2019).
2.4 Statistical analysis
Statistical analyses were performed with R (R Core Team, 2022) using R studio version 4.3.0. The effects of substrate type and biofilm presence on the number of settled larvae per treatment were analyzed using Generalized Linear Models (GLMs) with a Poisson distribution, which is the standard distribution for counts (Zuur et al., 2009). Model validation revealed no overdispersion. pH data also showed a Poisson shaped distribution, but since these were not count data we instead fitted a GLM with a Gamma distribution (with a log link function), to test the effect of substrate type and biofilm presence on the pH of the water. We compared models that included a full factorial combination of substrate type and biofilm, with additive models that excluded the interaction between the two factors; selecting the best-fitting models based on the lowest Akaike’s Information Criterion (AIC) (Zuur et al., 2009). The best-fitting models both for the number of settled larvae and pH included substrate type and biofilm presence, without their interaction (Supplementary Tables S1; S2).The significance of fixed effects in the GLMs was evaluated using likelihood ratio tests via the drop1() function in R and corresponding χ² values were reported. For the post-settlement morphology, test diameter and spine length data were analyzed using a 2-Factor ANOVA, with substrate type and biofilm presence as fixed factors. To test for post-hoc differences between specific treatments, pairwise comparisons were performed using estimated marginal means (EMMs) from the package emmeans (version 1.11.0) (Lenth et al., 2019), with Benjamini-Hochberg correction for multiple testing (Benjamini and Hochberg, 1995).
3 Results
3.1 Effect of substrate type and biofilm on larval settlement
A total number of 54 out of 600 D. antillarum larvae (9%) settled on the experimental tiles. Substrate type (χ² = 38.6, df = 5, p < 0.001) and the presence of a biofilm (χ² = 20.3, df = 1, p < 0.001) affected the settlement of D. antillarum significantly (Figure 2). All substrates with a biofilm had a significantly higher settlement than the same substrates without a biofilm. The highest settlement was found on the following substrates with biofilm: CEM III (30 ± 6%), followed by CSA (26 ± 10%), and E0 (20 ± 6%). Pairwise comparisons of substrates with biofilm revealed that CEM III, CSA and E0 differed significantly (p<0.05 for all comparisons, Supplementary Table S3) from the substrates CEM I (4 ± 3%), LS (4 ± 3%) and GS (2 ± 2%). Settlement rates on substrates without biofilm were on average low, however a similar effect of substrate type became apparent here: CEM III (6 ± 4%), CSA (4% ± 3%) and E0 (10 ± 5%) again yielded significantly higher settlement rates (p<0.05 for all comparisons, Supplementary Table S3) than CEM I (0 ± 0%), LS (2 ± 2%) and GS (0 ± 0%).
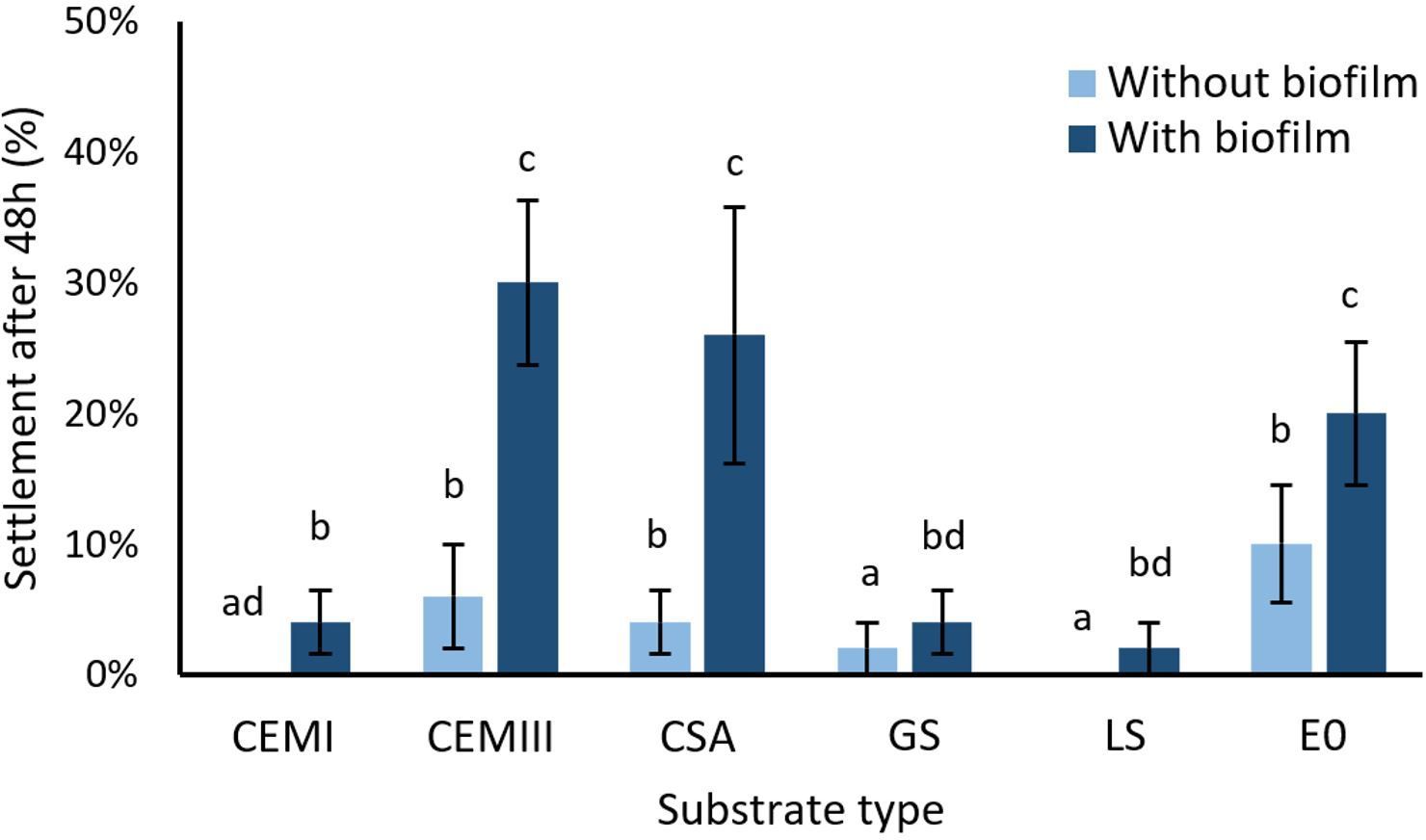
Figure 2. The effect of substrate type and biofilm on the settlement success of D. antillarum after 48h. The tested substrates are CEM I, CEM III, CSA, GS, LS, and E0. Each substrate was tested without biofilm (light bars) and with biofilm (dark bars), n=5 per treatment. Bars represent means ± S.E. Treatments with the same letter do not significantly differ (p > 0.05).
3.2 Effect of substrate type and biofilm on pH
The pH values of the ASW in the experimental beakers ranged from 8.00 to 8.85, 48h after the start of the experiment (Figure 3, Supplementary Table S4). Substrate type significantly affected the pH (χ² = 264.8, df = 5, p < 0.001) whereas the presence of a biofilm had no effect (χ² = 1.7, df = 1, p = 0.186). Pairwise comparisons revealed that CEM I, CEM III, and LS all had a significantly higher pH (p < 0.01) than CSA, E0, and GS (Figure 3, Supplementary Table S5). CEM I without biofilm had the highest pH (8.81 ± 0.02), significantly higher than all the other materials.
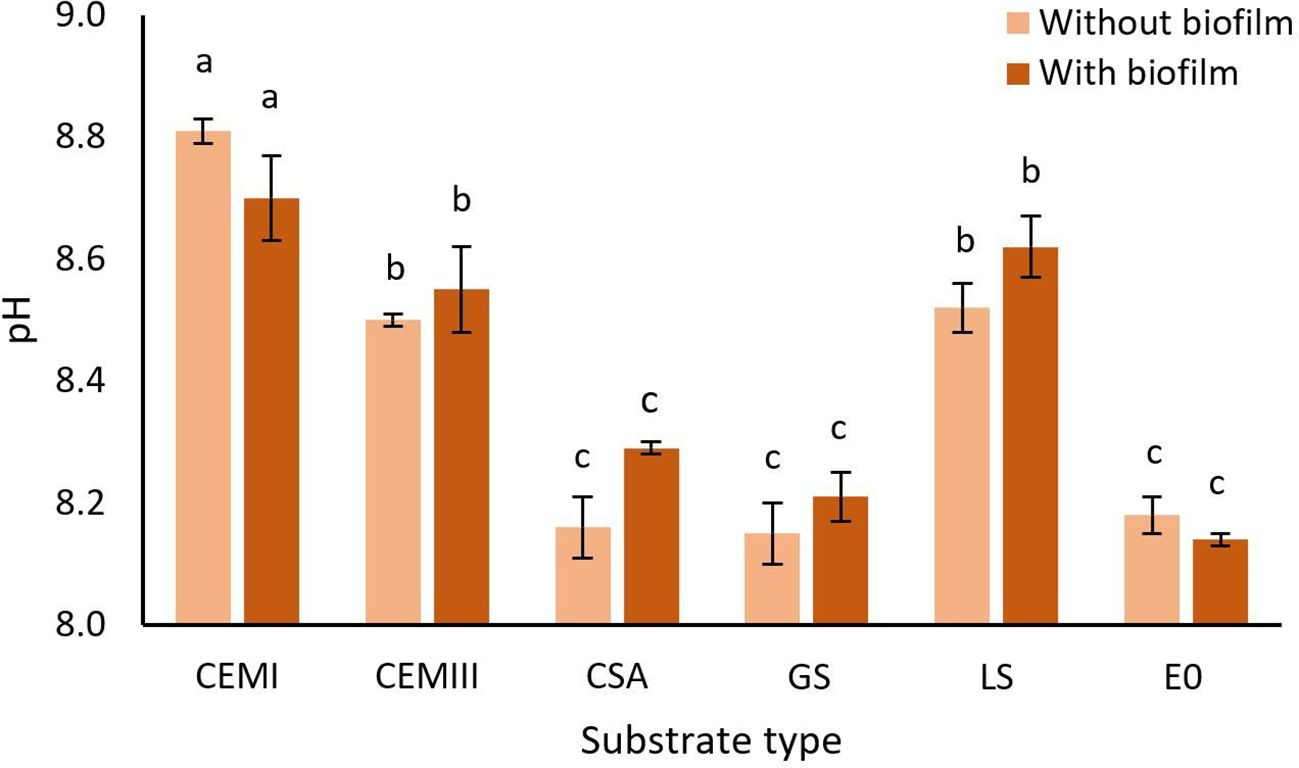
Figure 3. The effect of substrate type and biofilm on the pH in the D. antillarum settlement experiment. The pH values represent the pH of the ASW in the beaker with experimental tile and D. antillarum larvae, 48h after the start of the experiment. The tested substrates are CEM I, CEM III, CSA, GS, LS, and E0. Each substrate was tested without biofilm (light bars) and with biofilm (dark bars). Bars represent means ± S.E., n=5. Treatments with the same letter do not significantly differ (p > 0.05).
3.3 Effect of substrate type on post-settlement morphology
The average test diameter of the settled D. antillarum (Figure 4) one week after competent larvae were added to the beakers ranged between 566 and 617 µm. There was no effect of substrate type (F = 0.566, p = 0.716) or biofilm treatment (F = 2.13, p = 0.150) on the test diameter. The spine length of the larvae did not statistically differ between substrates (F = 1.986, p = 0.094) or biofilm treatment (F = 0.834, p = 0.365) (Table 2) but was generally lowest on CEM I (315 ± 39 µm) compared to other substrates.
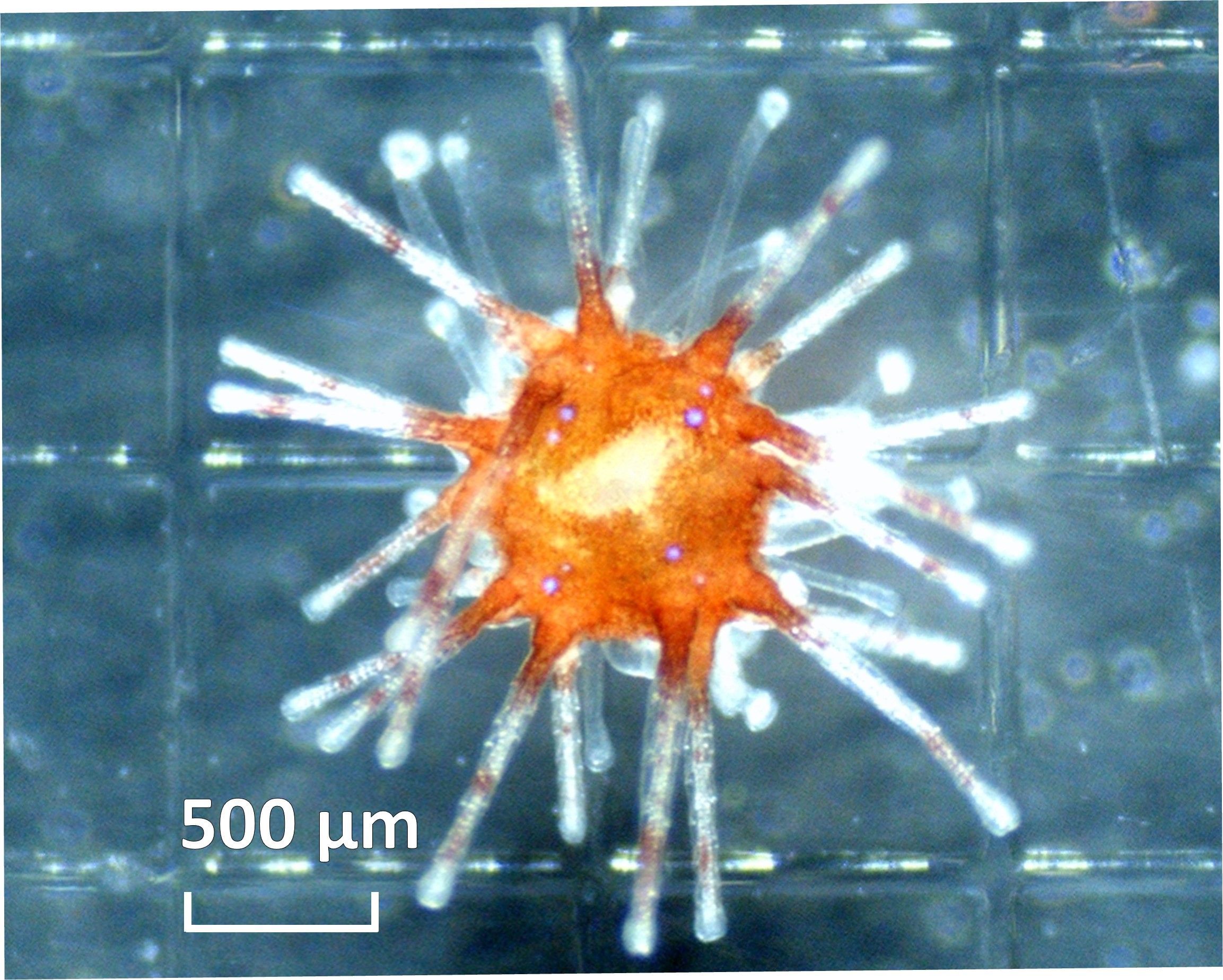
Figure 4. Post-settlement morphology of D. antillarum, one week after the start of the settlement experiment. This juvenile settled on CEM III with biofilm.
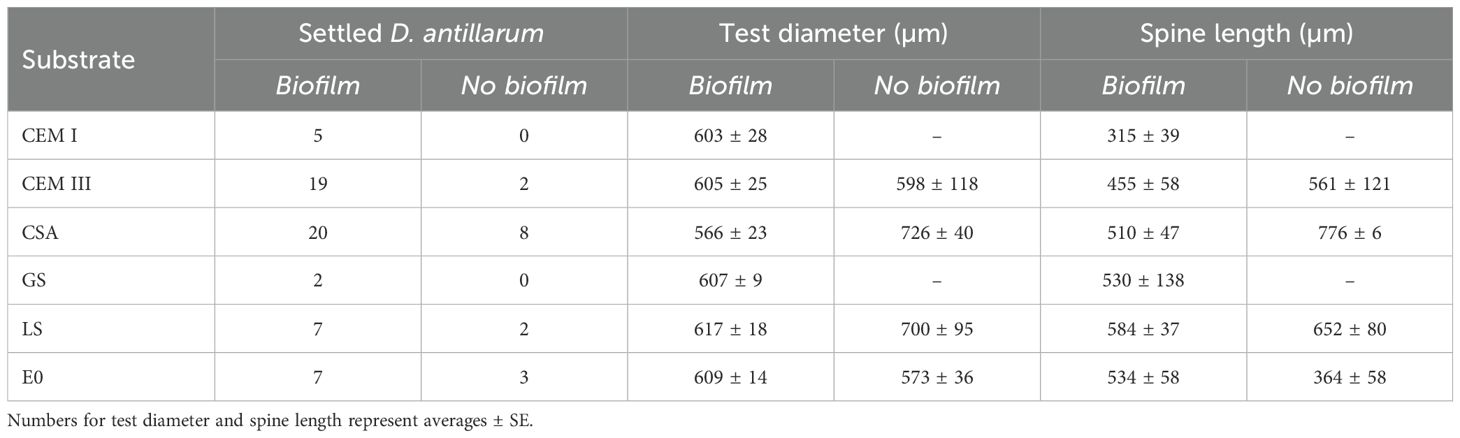
Table 2. Post-settlement morphology of juvenile D. antillarum, one week after the start of the experiment.
4 Discussion
Our results demonstrate that larval settlement rates of Diadema antillarum vary depending on the type of artificial hard substrate. Settlement rates after 48h were up to eight times higher on CEM III compared to CEM I. CSA, and E0 also performed significantly better than CEM I. These differences are strongly reinforced by the presence of a biofilm. This is corroborated by other studies on D. antillarum (Hylkema et al., 2022; Wijers et al., 2024) and different other marine invertebrates (Dworjanyn and Pirozzi, 2008; Hadfield, 2011; Hayek et al., 2020, 2021; Mos et al., 2011).
The differences in settlement rates between materials can likely be explained by the characteristics of the substrate, which can cause differences in biofilm formation and consequently in available settlement cues (Wijers et al., 2024). Hayek et al. (2021) showed that bacterial biofilm formation after one month was significantly greater on CEM III mortars than on CEM I. Although biofilm formation was not quantified in the current study, variations in biofilm presence were visible (Figure 1) and increased colonization of CEM III, CSA, and E0 by biofilm-forming organisms might have benefited the larval settlement of D. antillarum. A more detailed analysis of biofilm composition across substrate types is recommended to better understand these patterns. Furthermore, CEM I has the strongest alkaline effect of the three binders compared in this study (CEM I > CEM III > CSA) (Chen et al., 2015; Hayek et al., 2020, 2021; Xu et al., 2019). This high pH in the water layer around CEM I could have inhibited colonization of biofilm forming micro-organisms (Hayek et al., 2020) and therefore reduce settlement of D. antillarum.
However, the pH values of the tested artificial hard substrates do not fully explain the difference in D. antillarum larval settlement rates. The three materials that performed best in terms of settlement (CEM III, CSA and E0) had pH values in the higher and lower end of the measured range. From the pH values of the substrates with biofilm, it becomes clear that CEM III (pH=8.6 ± 0.1) is more similar to CEM I (pH=8.7 ± 0.1), than to CSA (pH=8.3 ± 0.0) or E0 (pH=8.1 ± 0.0). Therefore, in this study, pH does not appear to be the main driver of differences in D. antillarum settlement. This is in contrast with other studies emphasizing the importance of pH with regard to settlement and post-settlement development of another sea urchin, Centrostephanus rodgersii (Mos et al., 2019, 2020). In this study, settlement rates were reduced up to 26% in high pH treatments (pH=8.2-8.3) compared to ambient and low pH treatments (pH=7.6-8.1). Other attempts of reducing the pH of concrete to enhance its bioreceptivity were often not focused specifically on settlement of a key species such as D. antillarum, but more on increasing overall cover of marine life and/or biodiversity (Hsiung et al., 2020; Perkol-Finkel and Sella, 2014). Lowering initial substrate pH only increased species richness in field experiments at early time points (3–6 months), possibly related to greater biofilm development. These differences did not persist over time, emphasizing the temporally limited benefit of lowering the substrate pH (Bone et al., 2022; Hsiung et al., 2020).
Both GS and LS had relatively low settlement rates. Contrary to LS, the performance of geopolymers (GP) in the marine environment has been tested before, but results varied per study: A year after deployment in the sea, 3D printed GP-based modules were less colonized by marine life compared to CEM III-based modules (Boukhelf et al., 2022). Yoris-Nobile et al. (2023) found the opposite: GP mortars showed higher marine biomass accumulation than cement mortars after three months. Bone et al. (2024) emphasize that primary chemical bioreceptivity of mortar mixes (ground granulated blast furnace slag and a natural, single source cement) seems to be inconsistent between ecological metrics and study sites. Therefore, other factors, such as the sustainability of materials, should be one of the priorities (Bone et al., 2024).
The relatively low settlement rates observed on GS and LS may be related to the leachates, liquids that seep from the substrates into the surrounding water. Santos et al. (2023) found that geopolymers leachates contain higher levels of trace elements than cement-based leachates, which may explain their lower success rate in embryo-larval development of the sea urchin Paracentrotus lividus (Santos et al., 2023). Since sea urchins are known for their sensitivity to a variety of marine pollutants, they are often used as an ecological indicator (Morroni et al., 2018). It might well be the case that this sensitivity also plays a role in the settlement of D. antillarum on the various artificial hard substrates. In addition to trace elements, leachates may also contain ions such as Ca2+, Mg2+, Sr2+. These ions can act as chemical cues for larval settlement, as shown for larvae of the Caribbean corals Colpophyllia natans and Orbicella faveolate (Deshpande et al., 2025). Yus et al. (2024) further demonstrated that larval settlement of these corals is influenced by both the base material composition (e.g. CaCO3) and the presence of inorganic additives (e.g. SrCO3), even without a biofilm. While leachate composition was not analyzed in the current study, GS and LS appeared to have a higher porosity, potentially leading to an accelerated release of (trace) elements into the water. This could in turn affect both biofilm development and the settlement success of D. antillarum.
Post-settlement morphology did not differ between treatments, as settler test diameter and spine length were not affected by substrate type or biofilm presence. Although this was not tested for D. antillarum before, a study on another sea urchin species found similar results, with no effect of substrate type on the spine length of Tripneustes gratilla two weeks post-settlement (Mos et al., 2019). Mos et al. (2019) did however find an effect of the interaction between pH and substrate on test diameter (TD). At pH 7.9 the TD was 12% greater in the concrete treatment than the greywacke and granite treatments, while at pH 8.1 this effect was opposite with TD being 15% greater in the greywacke treatment than the granite (no difference between concrete and greywacke). This interaction became apparent two weeks post-settlement, whereas in our study morphology was only examined one week after the start of the experiment. Survival of juveniles could not be measured in detail in the current study, since settlers were not separated from non-settled larvae and could therefore not individually be monitored. Only the total number of settled larvae per beaker at each time frame could be assessed, in line with the design of Mos et al. (2019). For a more detailed post-settlement survival analysis, a design similar to Mos et al. (2020) is suggested, where settled larvae are removed, placed in new containers, and maintained under the same experimental conditions.
One limitation in comparing the artificial substrates in this study is that the addition of dredged sediments and natural fibers in the mixes GS, LS, and E0 resulted in a rougher surface texture than the binders (CEM I, CEM III and CSA) (Figure 1). Surface microtopography is known to positively affect the settlement of coral reef-associated invertebrates (Levenstein et al., 2022a) and seems to play a role for D. antillarum as well (Pilnick et al., 2023). However, our results show that the highest settlement rates were both on smooth surfaces (CEM III, CSA) and a rougher surface (E0). This may be explained by the high degree of specificity with which larvae select their settlement substrate. Levenstein et al. (2022b) demonstrated that Caribbean coral larvae can detect soluble inorganic ions in the water column, are drawn to their source, and subsequently select settlement sites based on surface microtopography and/or composition – emphasizing the complex ecological processes that underlie larval recruitment. For future comparison of substrate types, surface roughness should therefore be quantified or standardized between treatments.
Our study shows that CEM III, CSA, and E0 support higher D. antillarum settlement than the industry-standard CEM I. These results were reinforced if the materials were allowed to develop a four-week old biofilm, underscoring the importance of biofilm-forming organisms as a settlement cue. Although pH differed significantly between treatments and may have affected the biofilm formation - and thereby indirectly D. antillarum settlement - no direct link between pH and D. antillarum settlement was observed. This study provides insights into alternative materials with increased bioreceptivity and a lower carbon footprint that could enhance the ecological performance of artificial reefs and other marine infrastructure. Whether the observed effects of substrate type remain significant over a larger temporal and spatial scale, for multiple key species, and combined with other engineering effects, should be the focus of future studies. This knowledge will help us to restore degraded ecosystems such as coral reefs more effectively while at the same time minimizing greenhouse gas emissions.
Data availability statement
The raw data supporting the conclusions of this article will be made available by the authors, without undue reservation.
Author contributions
JCR: Writing – original draft, Conceptualization, Investigation, Formal Analysis, Visualization, Methodology. TW: Writing – review & editing, Methodology, Conceptualization. CGEB: Writing – review & editing, Investigation. MA: Writing – review & editing. BKE: Supervision, Writing – review & editing, Formal Analysis. AH: Methodology, Supervision, Conceptualization, Writing – review & editing.
Funding
The author(s) declare that financial support was received for the research and/or publication of this article. This study was conducted within the scope of the RAAK Publiek ECODAMI project (project#RAAK.PUB07.014), partially funded by SIA, part of the Dutch Research Council (NWO); JCR was funded by the NWO Doctoral Grant for Teachers Programme (#023.017.101).
Acknowledgments
The authors would like to thank all ECODAMI project partners for their valuable contributions, and in particular Acroniq and TUE for providing the test materials for the project. We greatly appreciate the help in the lab with D. antillarum provided by Annemieke Borsch, Roel Fiselier, Stef Figee, Mia Großmann, and Heather Boback. We would also like to thank Katrin Schollbach and Charles Wesemann for their insightful discussions that helped shape the manuscript. Finally, we thank the two reviewers for their constructive feedback, which improved the quality of this work.
Conflict of interest
The authors declare that the research was conducted in the absence of any commercial or financial relationships that could be construed as a potential conflict of interest.
Generative AI statement
The author(s) declare that no Generative AI was used in the creation of this manuscript.
Publisher’s note
All claims expressed in this article are solely those of the authors and do not necessarily represent those of their affiliated organizations, or those of the publisher, the editors and the reviewers. Any product that may be evaluated in this article, or claim that may be made by its manufacturer, is not guaranteed or endorsed by the publisher.
Supplementary material
The Supplementary Material for this article can be found online at: https://www.frontiersin.org/articles/10.3389/fmars.2025.1596378/full#supplementary-material
References
Alvarez-Filip L., Dulvy N. K., Gill J. A., Côté I. M., and Watkinson A. R. (2009). Flattening of Caribbean coral reefs: Region-wide declines in architectural complexity. Proc. R. Soc. B: Biol. Sci. 276, 3019–3025. doi: 10.1098/rspb.2009.0339
Andrello M., Darling E. S., Wenger A., Suárez-Castro A. F., Gelfand S., and Ahmadia G. N. (2022). “A global map of human pressures on tropical coral reefs,” in Conservation letters, vol. 15.1. (John Wiley and Sons Inc). doi: 10.1111/conl.12858
Baine M. (2001). Artificial reefs: a review of their design, application, management and performance. Ocean Coast. Manage. 44, 19. doi: 10.1016/S0964-5691(01)00048-5
Bak R. P. M. (1985). “Recruitment patterns and mass mortalities in the Sea Urchin Diadema antillarum.,” in Proceedings of 5th inter-national coral reef congress, vol. 5. , 267–272.
Benjamini Y. and Hochberg Y. (1995). Controlling the false discovery rate- A practical and powerful approach to multiple testing. J. R. Stat. Society: Ser. B (Methodological) 57, 289–300. doi: 10.1111/j.2517-6161.1995.tb02031.x
Bone J. R., Hall A. E., Stafford R., and Herbert R. J. H. (2024). Inconsistent bioreceptivity of three mortar mixes in subtidal sites. Ecol. Eng. 204. doi: 10.1016/j.ecoleng.2024.107265
Bone J. R., Stafford R., Hall A. E., and Herbert R. J. H. (2022). The intrinsic primary bioreceptivity of concrete in the coastal environment – A review. Developments Built Environ. 10, 100078. doi: 10.1016/j.dibe.2022.100078
Boukhelf F., Sebaibi N., Boutouil M., Yoris-nobile A. I., Blanco-fernandez E., Castro-fresno D., et al. (2022). On the properties evolution of eco-material dedicated to manufacturing artificial reef via 3D printing : long-term interactions of cementitious materials in the marine environment. Sustainability 14, 9353. doi: 10.3390/su14159353
Bulleri F. and Chapman M. G. (2010). The introduction of coastal infrastructure as a driver of change in marine environments. J. Appl. Ecol. 47, 26–35. doi: 10.1111/j.1365-2664.2009.01751
Carvalho S., Moura A., Cúrdia J., Cancela da Fonseca L., and Santos M. N. (2013). How complementary are epibenthic assemblages in artificial and nearby natural rocky reefs? Mar. Environ. Res. 92, 170–177. doi: 10.1016/j.marenvres.2013.09.013
Chapman M. G. and Underwood A. J. (2011). Evaluation of ecological engineering of “armoured” shorelines to improve their value as habitat. J. Exp. Mar. Biol. Ecol. 400, 302–313. doi: 10.1016/j.jembe.2011.02.025
Chen C., Ji T., Zhuang Y., and Lin X. (2015). Workability, mechanical properties and affinity of artificial reef concrete. Construction Building Materials 98, 227–236. doi: 10.1016/j.conbuildmat.2015.05.109
Connell S. D. and Glasby T. M. (1999). Do urban structures influence local abundance and diversity of subtidal epibiota? A case study from Sydney Harbour, Australia. Mar. Environ. Res. 47, 373–387. doi: 10.1016/S0141-1136(98)00126-3
Cramer K. L., Donovan M. K., Jackson J. B. C., Greenstein B. J., Korpanty C. A., Cook G. M., et al. (2021). The transformation of Caribbean coral communities since humans. Ecol. Evol. 11, 10098–10118. doi: 10.1002/ece3.7808
Cresson P., Ruitton S., and Harmelin-Vivien M. (2014). Artificial reefs do increase secondary biomass production: Mechanisms evidenced by stable isotopes. Marine Ecology Progress Series. 509, 15–26. doi: 10.3354/meps10866
Davis K. L., Coleman M. A., Connell S. D., Russell B. D., Gillanders B. M., and Kelaher B. P. (2017). Ecological performance of construction materials subject to ocean climate change. Mar. Environ. Res. 131, 177–182. doi: 10.1016/j.marenvres.2017.09.011
Deshpande K., Gysbers D., Yus J., van Bendegom D., Nixon E., McClintock R., et al. (2025). Direct observation and quantitative characterization of chemotactic behaviors in Caribbean coral larvae exposed to organic and inorganic settlement cues. Sci. Rep. 15, 10173. doi: 10.1038/s41598-025-93194-z
Dworjanyn S. A. and Pirozzi I. (2008). Induction of settlement in the sea urchin Tripneustes gratilla by macroalgae, biofilms and conspecifics : A role for bacteria ? Aquaculture 274, 268–274. doi: 10.1016/j.aquaculture.2007.11.030
el Mahdi Safhi A., Benzerzour M., Rivard P., and Abriak N. E. (2019). Feasibility of using marine sediments in SCC pastes as supplementary cementitious materials. Powder Technol. 344, 730–740. doi: 10.1016/j.powtec.2018.12.060
Gardner T. A., Côté I. M., Gill J. A., Grant A., and Watkinson A. R. (2003). Long-term region-wide declines in Caribbean corals. Science 301, 958–960. doi: 10.1126/science.os-2.57.341-a
Good A. M. and Bahr K. D. (2021). “The coral conservation crisis: interacting local and global stressors reduce reef resiliency and create challenges for conservation solutions,” in SN applied sciences, vol. 3.3. (Springer Nature). doi: 10.1007/s42452-021-04319-8
Guo Y., Luo L., Liu T., Hao L., Li Y., Liu P., et al. (2024). A review of low-carbon technologies and projects for the global cement industry. In J. Environ. Sci. (China) 136, 682–697). doi: 10.1016/j.jes.2023.01.021
Hadfield M. G. (2011). Biofilms and marine invertebrate larvae : what bacteria produce that larvae use to choose settlement sites. Annu. Rev. Mar. Sci. 3, 453–470. doi: 10.1146/annurev-marine-120709-142753
Hassan A., Arif M., and Shariq M. (2019). Use of geopolymer concrete for a cleaner and sustainable environment – A review of mechanical properties and microstructure. In J. Cleaner Production 223, 704–728). doi: 10.1016/j.jclepro.2019.03.051
Hayek M., Salgues M., Habouzit F., Bayle S., Souche J.-C., De Weerdt K., et al. (2020). In vitro and in situ tests to evaluate the bacterial colonization of cementitious materials in the marine environment. Cement Concrete Composites 113, 103748. doi: 10.1016/j.cemconcomp.2020.103748
Hayek M., Salgues M., Souche J., Cunge E., Giraudel C., and Paireau O. (2021). Influence of the intrinsic characteristics of cementitious materials on biofouling in the marine environment. Sustainability 13, 2625. doi: 10.3390/su13052625
Hsiung A. R., Tan W. T., Loke L. H. L., Firth L. B., Heery E. C., Ducker J., et al. (2020). Little evidence that lowering the pH of concrete supports greater biodiversity on tropical and temperate seawalls. Mar. Ecol. Prog. Ser. 656, 193–205. doi: 10.3354/meps13365
Hughes T. P., Graham N. A. J., Jackson J. B. C., Mumby P. J., and Steneck R. S. (2010). Rising to the challenge of sustaining coral reef resilience. Trends Ecol. Evol. 25, 633–642. doi: 10.1016/j.tree.2010.07.011
Hughes T. P., Kerry J. T., Baird A. H., Connolly S. R., Dietzel A., Mark Eakin C., et al. (2018). Global warming transforms coral reef assemblages. Nature 556, 492–496. doi: 10.1038/s41586-018-0041-2
Hylkema A., Debrot A. O., Osinga R., Bron P. S., Heesink D. B., Izioka A. K., et al. (2020). Fish assemblages of three common artificial reef designs during early colonization. Ecol. Eng. 157, 105994. doi: 10.1016/j.ecoleng.2020.105994
Hylkema A., Debrot A. O., Pistor M., Postma E., and Williams Stacey Kitson-Walters M.K. (2022). High peak settlement of Diadema antillarum on different artificial collectors in the 2 Eastern Caribbean. J. Exp. Mar. Biol. Ecol. 549 , 151693. doi: 10.1016/j.jembe.2022.151693
Hylkema A., Hakkaart Q. C. A., Reid C. B., Osinga R., Murk A. J., and Debrot A. O. (2021). Artificial reefs in the Caribbean: A need for comprehensive monitoring and integration into marine management plans. Ocean Coast. Manage. 209, 105672. doi: 10.1016/j.ocecoaman.2021.105672
Hylkema A., Kitson-Walters K., Kramer P. R., Patterson J. T., Roth L., Sevier M. L. B., et al. (2023). The 2022 Diadema antillarum die-off event: Comparisons with the 1983–1984 mass mortality. Front. Mar. Sci. 9. doi: 10.3389/fmars.2022.1067449
Jackson J., Donovan M., Cramer K., and Lam V. (2014). Status and trends of Caribbean coral reefs: 1970-2012 (Gland, Switzerland: Global Coral Reef Monitoring Network; International Union for the Conservation of Nature (IUCN).
Jain J. and Neithalath N. (2009). Analysis of calcium leaching behavior of plain and modified cement pastes in pure water. Cement Concrete Composites 31, 176–185. doi: 10.1016/j.cemconcomp.2009.01.003
Kajaste R. and Hurme M. (2016). Cement industry greenhouse gas emissions - Management options and abatement cost. J. Cleaner Production 112, 4041–4052. doi: 10.1016/j.jclepro.2015.07.055
Lenth R., Singmann H., Love J., Buerkner P., and Herve M. (2019). Emmeans: estimated marginal means, aka least-squares means (Version 1.3. 4). Emmeans Estim Marg Means Aka Least-Sq Means 4. Available online at: https://cran.r-project.org/package=emmeans.
Lessios H. A. (1988). Mass mortality of Diadema antillarum in the Caribbean: what have we learned? Annu. Rev. Ecol. Systematics. Vol. 19, 371–393. doi: 10.1146/annurev.ecolsys.19.1.371
Lessios H. A. (2016). The great diadema antillarum die-off: 30 years later. Annu. Rev. Mar. Sci. 8, 267–283. doi: 10.1146/annurev-marine-122414-033857
Levenstein M. A., Gysbers D. J., Marhaver K. L., Kattom S., Tichy L., Quinlan Z., et al. (2022a). Millimeter-scale topography facilitates coral larval settlement in wave-driven oscillatory flow. PloS One 17. doi: 10.1371/journal.pone.0274088
Levenstein M. A., Marhaver K. L., Quinlan Z. A., Tholen H. M., Tichy L., Yus J., et al. (2022b). Composite substrates reveal inorganic material cues for coral larval settlement. ACS Sustain. Chem. Eng. 10, 3960–3971. doi: 10.1021/acssuschemeng.1c08313
Lima J. S., Zalmon I. R., and Love M. (2019). Overview and trends of ecological and socioeconomic research on artificial reefs. Mar. Environ. Res. 145, 81–96. doi: 10.1016/j.marenvres.2019.01.010
Lippiatt N., Ling T., and Pan S. (2020). Towards carbon-neutral construction materials : Carbonation of cement-based materials and the future perspective. J. Building Eng. 28, 101062. doi: 10.1016/j.jobe.2019.101062
Loke L. H. L. and Todd P. A. (2016). Structural Complexity and component type increase intertidal biodiversity independently of area. Ecology 97, 383–393. doi: 10.1890/15-0257.1
Ly O., Yoris-Nobile A. I., Sebaibi N., Blanco-Fernandez E., Boutouil M., Castro-Fresno D., et al. (2021). Optimisation of 3D printed concrete for artificial reefs: Biofouling and mechanical analysis. Construction and Building Materials 272, 121649. doi: 10.1016/j.conbuildmat.2020.121649
McManus R. S., Archibald N., Comber S., Knights A. M., Thompson R. C., and Firth L. B. (2017). Partial replacement of cement for waste aggregates in concrete coastal and marine infrastructure: A foundation for ecological enhancement? Ecol. Eng. 120, 655–667. doi: 10.1016/j.ecoleng.2017.06.062
Merta I. and Tschegg E. K. (2013). Fracture energy of natural fibre reinforced concrete. Construction Building Materials 40, 991–997. doi: 10.1016/j.conbuildmat.2012.11.060
Meyer C. (2009). The greening of the concrete industry. Cement Concrete Composites 31, 601–605. doi: 10.1016/j.cemconcomp.2008.12.010
Morroni L., Pinsino A., Pellegrini D., and Regoli F. (2018). Reversibility of trace metals effects on sea urchin embryonic development. Ecotoxicology Environ. Saf. 148, 923–929. doi: 10.1016/j.ecoenv.2017.11.013
Mos B., Byrne M., and Dworjanyn S. A. (2020). Effects of low and high pH on sea urchin settlement, implications for the use of alkali to counter the impacts of acidification. Aquaculture 528, 735618. doi: 10.1016/j.aquaculture.2020.735618
Mos B., Cowden K. L., Nielsen S. J., and Dworjanyn S. A. (2011). Do cues matter? Highly inductive settlement cues don’t ensure high post-settlement survival in sea urchin aquaculture. PloS One 6 (10), e28054. doi: 10.1371/journal.pone.0028054
Mos B., Dworjanyn S. A., Mamo L. T., and Kelaher B. P. (2019). Building global change resilience: Concrete has the potential to ameliorate the negative effects of climate-driven ocean change on a newly-settled calcifying invertebrate. Sci. Total Environ. 646, 1349–1358. doi: 10.1016/j.scitotenv.2018.07.379
Mumby P. J., Hedley J. D., Zychaluk K., Harborne A. R., and Blackwell P. G. (2006). Revisiting the catastrophic die-off of the urchin Diadema antillarum on Caribbean coral reefs: Fresh insights on resilience from a simulation model. Ecol. Model. 196, 131–148. doi: 10.1016/j.ecolmodel.2005.11.035
Natanzi A. S., Thompson B. J., Brooks P. R., Crowe T. P., and McNally C. (2021). Influence of concrete properties on the initial biological colonisation of marine artificial structures. Ecol. Engineering 159(December 2020), 106104. doi: 10.1016/j.ecoleng.2020.106104
Paxton A. B., Shertzer K. W., Bacheler N. M., Kellison G. T., Riley K. L., and Taylor J. C. (2020). Meta-analysis reveals artificial reefs can be effective tools for fish community enhancement but are not one-size-fits-all. Front. Mar. Sci. 7. doi: 10.3389/fmars.2020.00282
Perkol-Finkel S. and Sella I. (2014). Ecologically active concrete for coastal and marine infrastructure: innovative matrices and designs. From Sea to Shore - Meeting Challenges Sea, 1139–1149. doi: 10.1680/fsts597571139
Pilnick A. R., Neil K. L. O., Moe M., and Patterson J. T. (2021). A novel system for intensive Diadema antillarum propagation as a step towards population enhancement. Sci. Rep. 0123456789, 1–13. doi: 10.1038/s41598-021-90564-1
Pilnick A. R., Petrosino A., Hassan M. M., and Patterson J. T. (2023). Cue selection and ontogeny reveal larval settlement dynamics of the long-spined sea urchin Diadema antillarum, a keystone coral reef herbivore. Mar. Biol. 170 (11), 139. doi: 10.1007/s00227-023-04290-5
Putnam H. M., Barott K. L., Ainsworth T. D., and Gates R. D. (2017). The vulnerability and resilience of reef-building corals. Current biology, vol. 27.11. (Cell Press), R528–R540. doi: 10.1016/j.cub.2017.04.047
R Core Team (2022). R: a language and environment for statistical computing (Vienna: R Foundation for Statistical Computing).
Sagüés A. A., Moreno E. I., and Andrade C. (1997). Evolution of pH during in-situ leaching in small comcrete cavities. Cement Concrete Res. 27, 1747–1759. doi: 10.1016/S0008-8846(97)00177-4
Santos J., Cifrian E., Rodriguez-Romero A., Yoris-Nobile A. I., Blanco-Fernandez E., Castro-Fresno D., et al. (2023). Assessment of the environmental acceptability of potential artificial reef materials using two ecotoxicity tests: Luminescent bacteria and sea urchin embryogenesis. Chemosphere 310, 136773. doi: 10.1016/j.chemosphere.2022.136773
Seaman W. (2007). Artificial habitats and the restoration of degraded marine ecosystems and fisheries. Hydrobiologia 580, 143–155. doi: 10.1007/s10750-006-0457-9
Wijers T., Hylkema A., Pilnick A. R., and Patterson J. T. (2023). Novel cultivation method for the long spined sea urchin (Diadema antillarum: Philippi 2023) results in high larval survival and settlement rates. Aquaculture 562, 738855. doi: 10.1016/j.aquaculture.2022.738855
Wijers T., van Herpen B., Mattijssen D., Murk A. J., Patterson J. T., and Hylkema A. (2024). Implications of changing Caribbean coral reefs on Diadema antillarum larvae settlement. Mar. Biol. 171 (2), 48. doi: 10.1007/s00227-023-04368-0
Xu Q., Ji T., Yang Z., and Ye Y. (2019). Preliminary investigation of artificial reef concrete with sulphoaluminate cement, marine sand and sea water. Construction Building Materials 211, 837–846. doi: 10.1016/j.conbuildmat.2019.03.272
Yoris-Nobile A. I., Slebi-Acevedo C. J., Lizasoain-Arteaga E., Indacoechea-Vega I., Blanco-Fernandez E., Castro-Fresno D., et al. (2023). Artificial reefs built by 3D printing: Systematisation in the design, material selection and fabrication. Construction Building Materials 362, 129766. doi: 10.1016/j.conbuildmat.2022.129766
Yus J., Nixon E. N., Li J., Gimenez J. N., Bennett M. J., Flores D., et al. (2024). Composite substrates for coral larval settlement and reef restoration based on natural hydraulic lime and inorganic strontium and magnesium compounds. Ecol. Eng. 202, 107236. doi: 10.1016/j.ecoleng.2024.107236
Keywords: long-spined sea urchin, larval settlement, hard substrates, concrete, biofilm, coral reef
Citation: Rippen JC, Wijers T, Van Bruggen CGE, Antoun M, Eriksson BK and Hylkema A (2025) Material matters: artificial substrate composition and biofilm presence influence larval settlement of Diadema antillarum. Front. Mar. Sci. 12:1596378. doi: 10.3389/fmars.2025.1596378
Received: 19 March 2025; Accepted: 24 April 2025;
Published: 15 May 2025.
Edited by:
Lorenzo Mari, Polytechnic University of Milan, ItalyReviewed by:
Beverly Pl Goh, Nanyang Technological University, SingaporeJoaquin Yus, University of Illinois at Urbana–Champaign, United States
Copyright © 2025 Rippen, Wijers, Van Bruggen, Antoun, Eriksson and Hylkema. This is an open-access article distributed under the terms of the Creative Commons Attribution License (CC BY). The use, distribution or reproduction in other forums is permitted, provided the original author(s) and the copyright owner(s) are credited and that the original publication in this journal is cited, in accordance with accepted academic practice. No use, distribution or reproduction is permitted which does not comply with these terms.
*Correspondence: Johanna Catharina Rippen, am9yaWVuLnJpcHBlbkBodmhsLm5s