- 1Philips Research, Eindhoven, Netherlands
- 2Philips Molecular Pathway Dx, Eindhoven, Netherlands
Introduction: Sepsis is a life-threatening complication of a bacterial infection. It is hard to predict which patients with a bacterial infection will develop sepsis, and accurate and timely diagnosis as well as assessment of prognosis is difficult. Aside from antibiotics-based treatment of the causative infection and supportive measures, treatment options have remained limited. Better understanding of the immuno-pathophysiology of sepsis is expected to lead to improved diagnostic and therapeutic solutions.
Functional activity of the innate (inflammatory) and adaptive immune response is controlled by a dedicated set of cellular signal transduction pathways, that are active in the various immune cell types. To develop an immune response-based diagnostic assay for sepsis and provide novel therapeutic targets, signal transduction pathway activities have been analyzed in whole blood samples from patients with sepsis.
Methods: A validated and previously published set of signal transduction pathway (STP) assays, enabling determination of immune cell function, was used to analyze public Affymetrix expression microarray data from clinical studies containing data from pediatric and adult patients with sepsis. STP assays enable quantitative measurement of STP activity on individual patient sample data, and were used to calculate activity of androgen receptor (AR), estrogen receptor (ER), JAK-STAT1/2, JAK-STAT3, Notch, Hedgehog, TGFβ, FOXO-PI3K, MAPK-AP1, and NFκB signal transduction pathways.
Results: Activity of AR and TGFβ pathways was increased in children and adults with sepsis. Using the mean plus two standard deviations of normal pathway activity (in healthy individuals) as threshold for abnormal STP activity, diagnostic assay parameters were determined. For diagnosis of pediatric sepsis, the AR pathway assay showed high sensitivity (77%) and specificity (97%), with a positive prediction value (PPV) of 99% and negative prediction value (NPV) of 50%. For prediction of favorable prognosis (survival), PPV was 95%, NPV was 21%. The TGFβ pathway activity assay performed slightly less for diagnosing sepsis, with a sensitivity of 64% and specificity of 98% (PPV 99%, NPV 39%).
Conclusion: The AR and TGFβ pathways have an immunosuppressive role, suggesting a causal relation between increased pathway activity and sepsis immunopathology. STP assays have been converted to qPCR assays for further evaluation of clinical utility for sepsis diagnosis and prediction of prognosis, as well as for prediction of risk at developing sepsis in patients with a bacterial infection. STPs may present novel therapeutic targets in sepsis.
Introduction
Sepsis is a life-threatening infection in which the immune response is dysregulated resulting in multi-organ dysfunction or failure (1). Sepsis is generally a complication of severe bacterial infection and characterized by a systemic inflammatory response leading to septic shock. Mortality rates range between 25 and 30% for sepsis and 40% to 70% for septic shock (2–4).
Aside from antibiotics and supportive measures to maintain blood circulation of internal organs, no treatments have proven to be effective, although it cannot be excluded that some treatments may benefit a subset of patients who so far cannot be identified (1). One reason for failure to develop effective treatments is the heterogeneity among sepsis patients, that is, variation in underlying medical conditions and use of drugs, and genetic variations influencing the immune response in an individual patient.
Detailed assessment of the functional immune response in a patient with sepsis may enable a personalized treatment approach and improve treatment efficacy. Diagnostic assessment of immune function is currently limited to routine blood measurements, such as numbers of immune cells and inflammation markers (e.g., C-reactive protein), but is not informative on the functional activity state of the various types of immune cells, responsible for the abnormal immune response in a sepsis patient.
The functional state of immune cells is determined by a small number of so-called cellular signal transduction pathways (STPs) (5–9). Recently, novel assays have been developed to quantitatively measure activity of STPs in cell and tissue samples, including blood samples (10–13). Measuring combined activity of these STPs in blood cells is expected to enable quantitative assessment of the innate and adaptive immune response in an individual patient (8, 14).
In this study, STP analysis was performed on publicly available gene expression data from multiple clinical sepsis studies. Measurement of activity of the androgen receptor (AR) pathway, and to a lesser extent the TGFβ pathway, in a whole blood sample is shown to have value for sepsis diagnosis and prediction of prognosis in a sepsis patient, and may lead to novel personalized treatment options. Measurement of STP activity to identify patients with a bacterial infection who are at high risk to develop sepsis is discussed.
Methods
STP Assays to Determine Activity of AR, Estrogen Receptor (ER), FOXO-PI3K, JAK-STAT1/2, JAK-STAT3, Notch, Hedgehog (HH), TGFβ, and NFκB Pathways in Blood Cells
Development and validation of assays to quantify STP activity have been described before (10–13). In brief, target genes of transcription factors of the respective signal transduction pathways were identified, and a Bayesian network computational model was created for interpretation of measured mRNA levels of the pathway target genes to generate a quantitative pathway activity score (PAS). PAS are presented on a log2 odds scale as described (15, 16).
Affymetrix Expression Microarray Data Analysis
For analysis of clinical studies, STP assays were performed on public Affymetrix HG-U133 Plus2.0 microarray expression datasets from previously published clinical studies [deposited in the Gene Expression Omnibus (GEO) database (17)]. Quality control (QC) was performed on Affymetrix data of each individual sample prior to STP analysis, as described before (11), using available R packages (18, 19). Samples that failed QC were removed prior to data analysis.
A summary of clinical datasets used in this study is shown in Supplementary Table 1. All studies provided Affymetrix data from whole blood samples. Duplicate sample data between datasets of different studies were removed when such datasets were combined into one analysis or calculation, such as for determining normal pathway activity range thresholds and for sensitivity and specificity calculations. For these purposes, 8 duplicates from GSE26640, 28 duplicates from GSE8121, 10 duplicates from GSE9692, 48 duplicates from GSE13904, and 1 duplicate from GSE26378 were removed (specified in Supplementary Table 1).
Interpretation of Signal Transduction Pathway Activity Scores
An important and unique advantage of the STP assays is that they can in principle be performed on all cell types. A few considerations to bear in mind when interpretating log2 odds PAS, as described before (11), are:
(1) On the same sample, log2 odds PAS cannot be compared between different signaling pathways, since each of the signaling pathways has its own range in log2 odds activity scores (11).
(2) The log2 odds range for pathway activity (minimum to maximum activity) may vary depending on cell type. Once the range has been defined using samples with known pathway activity, on each new sample the absolute value can be directly interpreted against that reference. If the range has not been defined, only differences in log2 odds activity score between samples can be interpreted.
(3) PAS are highly quantitative, and even small differences in log2 odds PAS can be reproducible and meaningful.
(4) A negative log2 odds ratio does not necessarily mean that the pathway is inactive.
Statistics
Boxplots were made using the Python data visualization library function seaborn. Statistical annotations were created using the Python package statannot (20, 21). Two sided Mann-Whitney-Wilcoxon testing was used to compare PAS across groups. For paired testing (dataset GSE95233) a one-sided t-test was used. P-values are indicated in the figures. In accordance with current consensus regarding the use of statistical parameters, a p-value of 0.01 (indicated as double asterisk in the figures) was considered significant (22). Receiver Operating Characteristics (ROC) curves and Area Under Curve (AUC) was calculated in R. Normal range thresholds for diagnosis and prognosis classification were based on the normal range for pathway activity in healthy individuals, as determined by mean ±2 standard deviations (SD) of pathway activity of healthy individuals. Based on these thresholds, sensitivity, specificity, positive predictive value (PPV) and negative predictive value (NPV) were determined, and Fisher exact tests were used to compare groups.
Results
Measuring STP Activity in Whole Blood Samples of Pediatric and Adult Patients With Sepsis
PAS scores for the AR, ER, MAPK-AP1, FOXO-PI3K, JAK-STAT1/2, JAK-STAT3, Notch, Hedgehog, TGFβ, and NFκB pathways were measured using target gene expression data of whole blood samples from clinical studies on children (Supplementary Figures 1–6) and adults with sepsis and septic shock (Supplementary Figures 7, 8). An overview of included datasets is available in Supplementary Table 1.
AR and TGFβ Signal Transduction Pathway Activity Scores are Higher in Patients With Sepsis/Septic Shock
Activity of the AR pathway, and with exception of clinical study GSE57065, also of the TGFβ pathway, were consistently and significantly increased in pediatric and adult patients with sepsis and septic shock (Figures 1–4). No consistent differences in AR and TGFβ pathway activity scores were observed between men and women (Figures 1F, 2F, and Supplementary Figure 6), between patients categorized as “sepsis” vs. “septic shock” (Figures 1D, 2D, and Supplementary Figure 4), nor between patients categorized as “SAPSII-low” and “SAPSII-high” (Figures 3A, 4A, and Supplementary Figure 7). No difference was found between patients with gram-negative and gram-positive bacterial infections (clinical study datasets GSE4607 and GSE9692; data not shown).
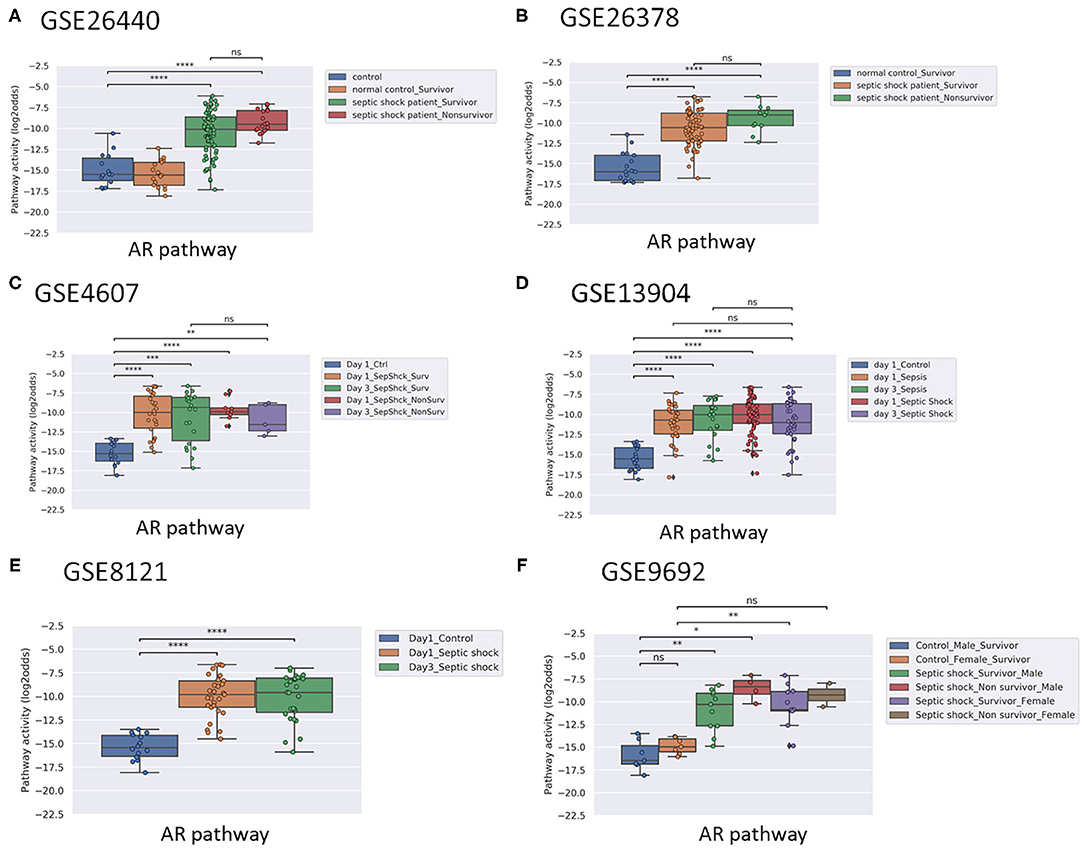
Figure 1. AR pathway activity scores (PAS) of Affymetrix data of whole blood samples of children with sepsis (<10 years of age). Samples were obtained within 24 h of initial presentation to the pediatric intensive care unit (PICU) (all datasets). Indicated when applicable: survivors and non-survivors (A–F); time (Day) on PICU (C); and gender (F). Control patients were recruited using the following exclusion criteria: a recent febrile illness (within 2 weeks), recent use of anti-inflammatory medications (within 2 weeks), or history of chronic or acute disease associated with inflammation. (A) Dataset GSE26440 (23). (B) GSE26378 (23). (C) Dataset GSE4607 (24). (D) Dataset GSE13904 (25). (E) Dataset GSE8121 (26). (F) Dataset GSE9692 (27). AR PAS on Y-axis on a log2 odds scale. Two sided Mann–Whitney–Wilcoxon statistical tests were performed; p-values are indicated in the figures as *p < 0.5, **p < 0.01, ***p < 0.001, ****p < 0.0001 or ns (not significant).
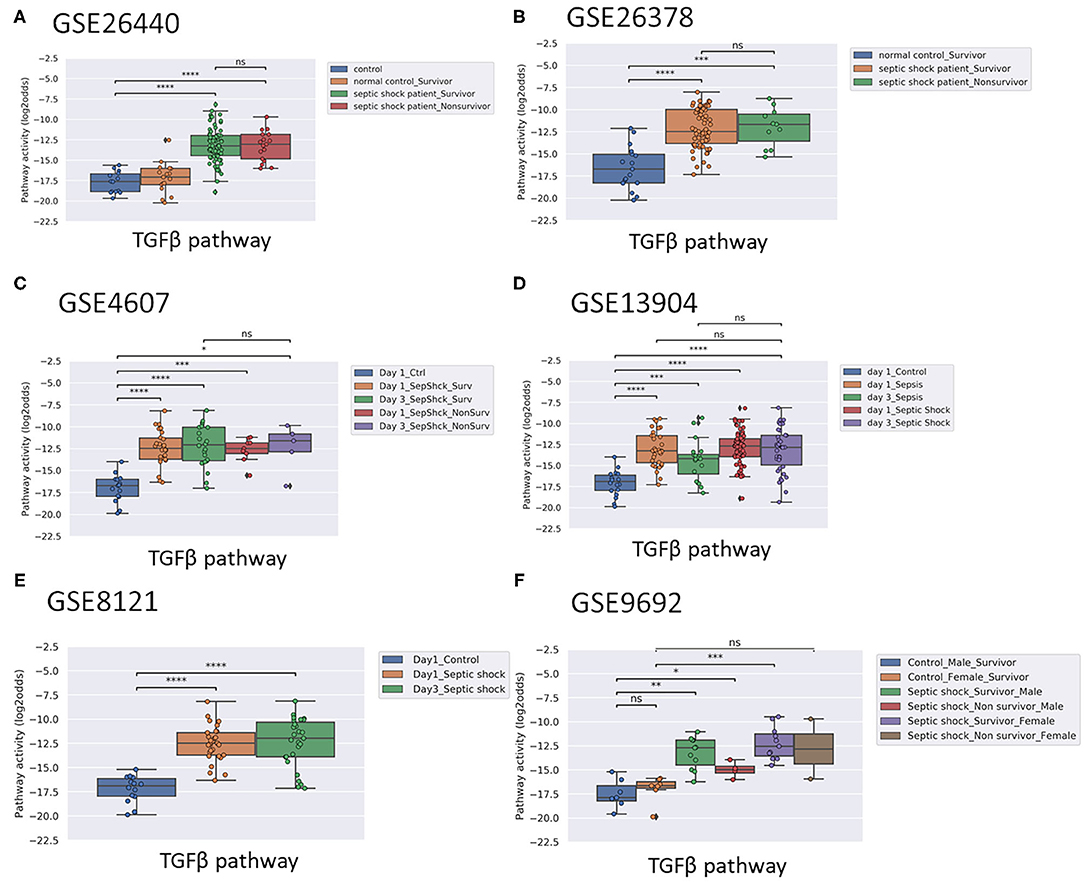
Figure 2. TGFβ PAS, same datasets as in Figure 1. Two sided Mann–Whitney–Wilcoxon statistical tests were performed; p-values are indicated in the figures as *p < 0.5, **p < 0.01, ***p < 0.001, ****p < 0.0001 or ns (not significant). (For explanation see Figure 1 caption).
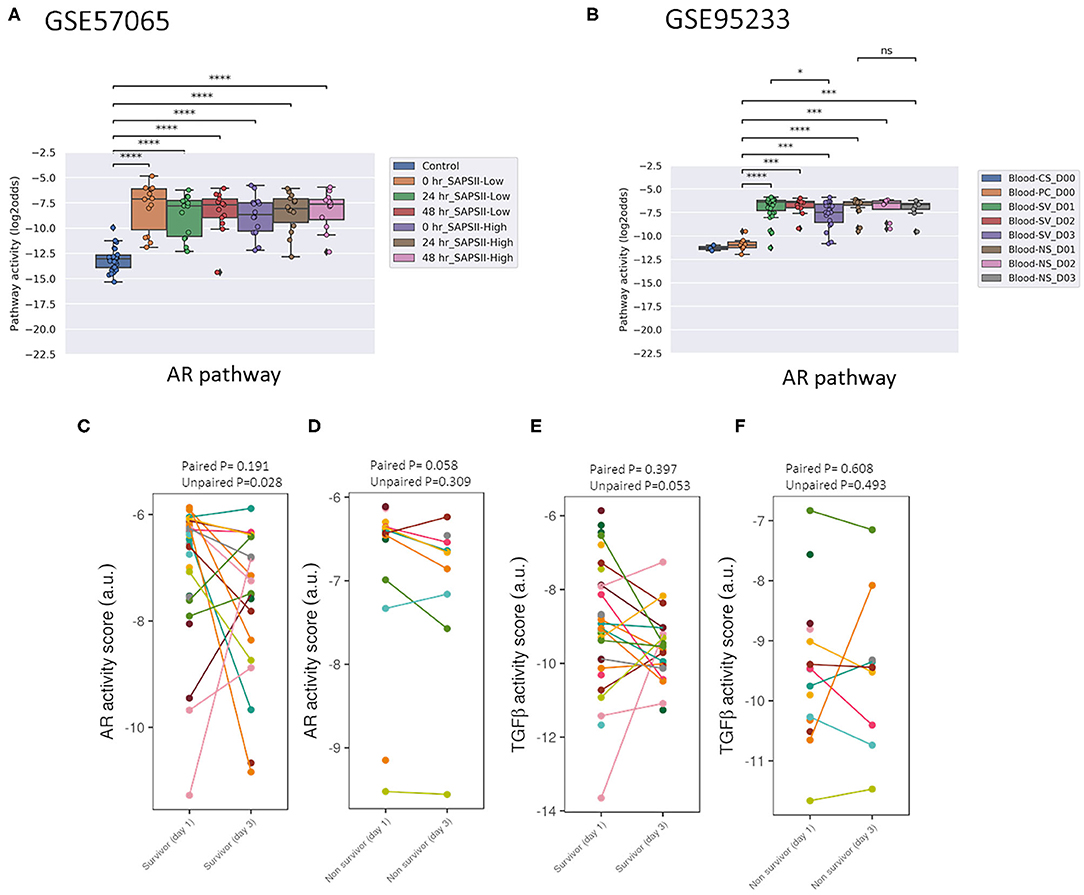
Figure 3. AR PAS of datasets of whole blood samples from adults with sepsis and healthy individuals. (A) Dataset GSE57065 (28), containing ICU patients included at the onset of septic shock. Blood samples were collected within 30 min, and 24 and 48 h after diagnosis of sepsis. Sepsis patients were divided into SAPSII-low and SAPSII-high groups according to the median of SAPSII score (SAPSII scores of <45 and >45, respectively). (B) Dataset GSE95233 (29), containing ICU patients included at diagnosis of septic shock. Blood samples were collected at admission, and at day 2 (D2) or day 3 (D3). SV indicates survivor, NS indicates non-survivor sepsis patient, CS indicates control healthy individual, PC indicates patient control. (C-E) Dataset GSE95233 (29). AR (C,D) and TGFβ (E,F) PAS for sepsis survivor vs. non-survivor patients, at day 1 and day 3 after diagnosis. Patient samples have been connected by lines. AR PAS on Y-axis on log2 odds scale. (A,B) two sided Mann–Whitney–Wilcoxon statistical tests. (C,D) one sided paired and unpaired t-test. p-values are indicated as *p < 0.5, ***p < 0.001, ****p < 0.0001 or ns (not significant).
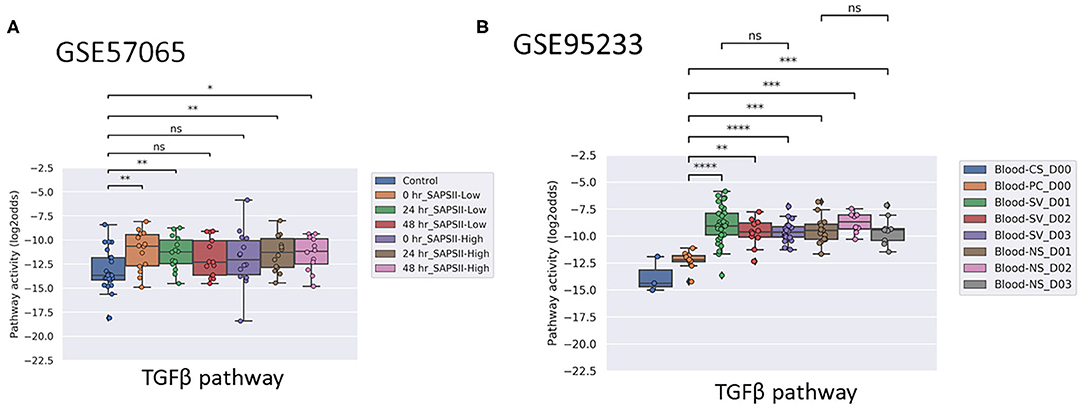
Figure 4. TGFβ PAS, same datasets as in Figures 3A,B. Two sided Mann–Whitney–Wilcoxon statistical tests were performed; p-values are indicated in the figures as *p < 0.5, **p < 0.01, ***p < 0.001, ****p < 0.0001 or ns (not significant). (For explanation see Figures 3A,B caption).
Two adult sepsis clinical studies [GSE57065 (28); GSE95233 (29)] allowed investigation of STP activity at several time points after disease onset. Blood samples had been collected within 30 min, and at 24 and 48 h after septic shock onset (Figures 3A, 4A, and Supplementary Figure 7) or at Day 1, Day 2, or Day 3 (Figure 3B and Supplementary Figures 8, 11). AR PAS were found to be significantly increased at first measurement and remained increased during subsequent days.
Normal Range AR Pathway Activity in Patients With Sepsis May be Indicative for Survival
In pediatric sepsis patients who survived, AR (but not TGFβ) pathway activity showed a trend toward lower PAS, within the PAS range of healthy controls (Figures 1A–C,F and Figures 2A–C). Only one adult sepsis study allowed investigation of the relation between survival and STP activity [GSE95233 (29), Figures 3B-F and Supplementary Figures 8, 11]. AR pathway activity tended to be lower for sepsis survivors at day three after diagnosis, decreased between Day 1 and Day 3 of sepsis in survivor patients and (Figures 3B-D and Supplementary Figure 11). For the TGFβ pathway this was not found (Figures 4B, 3E,F, and Supplementary Figure 11). Although not significant, results from these independent clinical studies (children and adults) suggest that AR pathway activity within the normal range may be favorable in patients with sepsis.
Activity of Other Signaling Pathways (Supplementary Figures 1–8)
ER pathway PAS were low and increased slightly in patients with sepsis, but the increase was not significant in dataset GSE95233 (pediatrics) and GSE9692 (adults). MAPK-AP1, FOXO-PI3K, JAK-STAT1/2, JAK-STAT3, NFκB and Notch signaling pathway PAS were either not significantly increased in sepsis patients, or not consistently increased across multiple independent clinical studies. Activity of the Hedgehog pathway tended to be lower in sepsis patients, only meeting statistical requirements in the adult sepsis studies (GSE57065 and GSE95233) (Supplementary Figures 7, 8).
Defining an Upper Threshold for Normal AR and TGFβ Signal Transduction Pathway Activity in Whole Blood Samples
To enable determination of STP assay performance parameters for diagnostic use in sepsis patients, normal STP PAS ranges in whole blood samples were determined. Eight datasets contained samples of healthy individuals [GSE26440 (22), GSE26378 (23), GSE4607 (24), GSE13904 (25), GSE8121(26), and GSE9692 (27)] (Supplementary Table 1).
Mean AR PAS in healthy individuals was −15.37 (log2 odds scale, SD 1.52) for children (n = 93) and −12.44 (log2 odds scale, SD 1.44) for adults (n = 37) (Supplementary Table 2). Combining healthy pediatric and adult sample data (n = 130), mean AR PAS was −14.5 (log2 odds scale, SD 2.0). No significant differences between men and women were found. Using this information, normal range (healthy) STP PAS thresholds were determined for each STP, based on the mean STP PAS score in healthy controls ±2 SD for combined datasets (Supplementary Table 2) and for individual datasets (Supplementary Table 4). The upper AR PAS threshold for normal AR pathway activity was calculated as the mean +2SD, resulting in a threshold for pediatric patients of −12.33 (log2 odds scale), for adults −9.57 (log2 odds scale), and for pediatric and adults combined −10.54 (log2 odds scale). For the TGFβ pathway mean PAS in healthy individuals was −17.05 (log2 odds scale, SD 1.63) for children and −13.01 (log2 odds scale, SD 1.83) for adults (Supplementary Table 2). Combining healthy pediatric and adult sample data, mean TGFβ PAS was −15.9 (log2 odds scale, SD 2.49).
Applying the pediatric upper threshold for normal AR PAS on the pediatric study data showed that only 3 out of 45 (7%) children in the non-survivor groups had an AR pathway activity in the normal range, while 53 out of 210 (25%) survivor children had AR pathway activity in the normal range (Fisher exact test p = 0.005). For the TGFβ pathway the percentages were respectively 31 and 32. Thus, AR PAS in the normal range was associated with sepsis survival, at least in children (Figures 1A-C,F and Figures 2A-C, F). We proceeded with calculating AR and TGFβ pathway assay performance parameters.
Sensitivity and Specificity of AR and TGFβ Pathway Activity Assays for Pediatric Sepsis Diagnosis and Prognosis Prediction
Using the pediatric upper threshold of the normal range of AR and TGFβ PAS, sensitivity, specificity, PPV, and NPV for diagnosis and prediction of survival in a pediatric sepsis patient were calculated (Tables 1, 2, and Supplementary Tables 5, 6), and a ROC curve was generated (Figure 5). For the same parameters of the other STPs, see Supplementary Figures 9, 10. For sepsis diagnosis, the AR pathway assay showed both a high sensitivity (77%) and specificity (97%), with a PPV of 99% and a NPV of 50%. AUC in the ROC curve was 0.94 for sepsis diagnosis. For prediction of favorable (survivor) prognosis, the PPV was 95%, indicating that the assay was highly specific (93%) in identifying survivor patients. The NPV was 21%, indicative of low sensitivity (25%) in predicting survivor patients. The TGFβ assay performed less, with a sensitivity for diagnosing sepsis of respectively 64% and specificity of 98%; all other STP assays performed less well (Supplementary Figure 9).
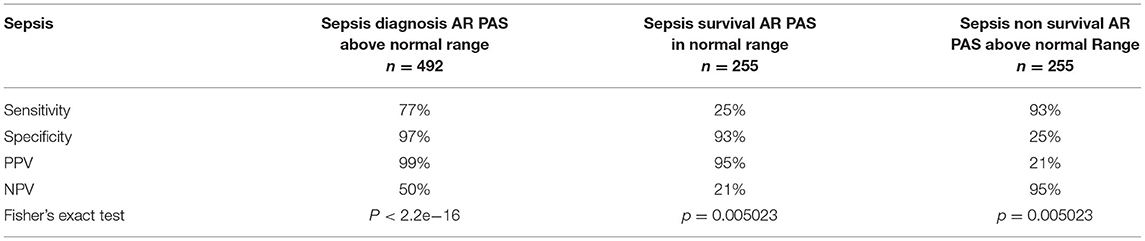
Table 1. Performance parameters of the AR pathway assay for diagnosis of pediatric sepsis and prediction of survival.
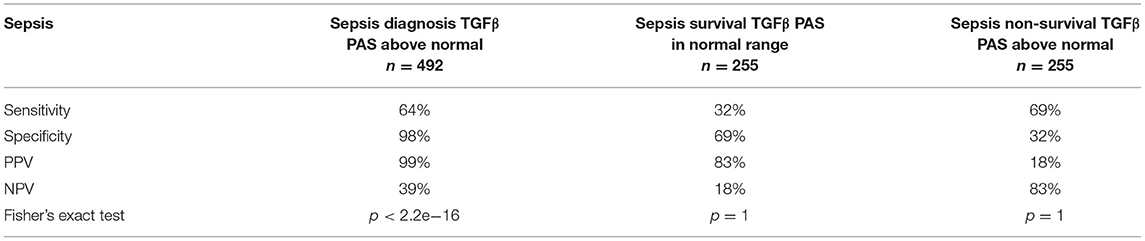
Table 2. Performance parameters of the TGFβ pathway assay for diagnosis of pediatric sepsis and prediction of survival.
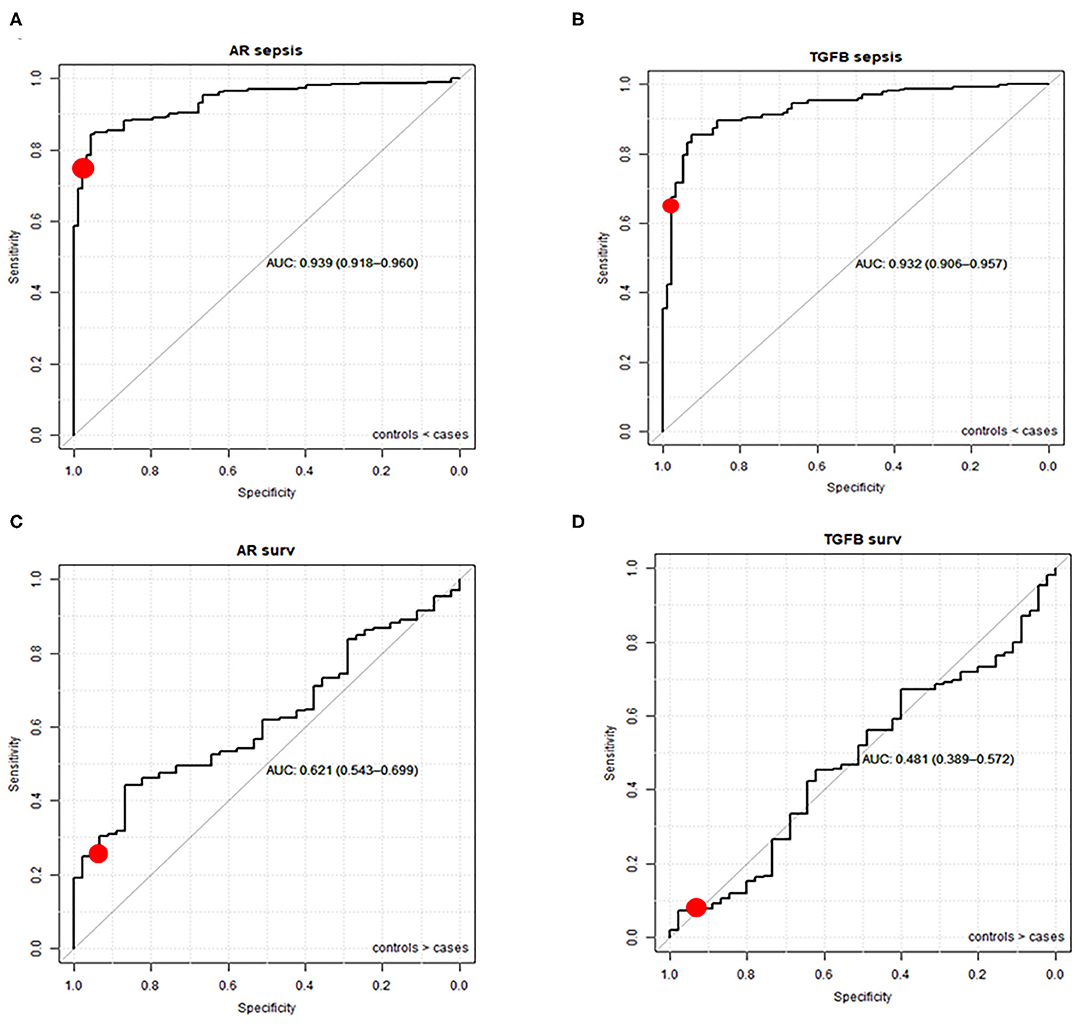
Figure 5. ROC curves (and AUC) of AR and TGFβ pathway activity assays for sepsis diagnosis (A,B) and survival prediction (C,D). Healthy controls and sepsis patients (sepsis and sepsis shock groups combined) from datasets GSE26440 (23), GSE26378 (23), GSE4607 (24), GSE13904 (25), GSE8121 (26), and GSE9692 (27) are included (duplicate samples excluded). The red dot represents sensitivity and specificity when the upper threshold is mean plus 2 SD of normal pathway activity.
Comparison of Affymetrix Data Analysis Results, Described in the Original Publication Associated With the Dataset, With Results of STP Analysis
For each Affymetrix dataset, bioinformatics tools used for data analysis as reported in the associated publication were listed, together with reported functional gene annotations and/or identified “pathways” as defined by the used tools (Ingenuity, PANTHER, D.A.V.I.D., ToppGene) (see Supplementary Information). In all analyses, groups of patient samples had been compared with respect to differential gene expression. In contrast to the current study, individual samples had not been analyzed. An association between sepsis/septic shock and signal transduction pathways had been identified using Ingenuity Pathways Analysis and PANTHER. The NFκB pathway (termed NFκB or TLR) (23, 24, 26, 27), the MAPK pathway (termed “p380MAPK, PDGF”) (24, 26–28), the JAK-STAT3 pathway (termed “IL6, IL10”) (24, 26–28), and the PI3K-FOXO pathway (termed “Integrin signaling/Insulin signaling/IGF1 signaling”) (26), were identified as associated with sepsis, but without information on activity state of these pathways. Hormonal AR and ER pathways were not mentioned and neither was the TGFβ pathway.
Discussion
Using a previously reported STP assay platform, we quantified activity of the most important signal transduction pathways that determine immune cell function, using RNA expression data of whole blood samples from previously published clinical pediatric and adult sepsis studies (9–13, 16).
Activity of Several Signaling Pathways is Increased in Whole Blood From Patients With Sepsis: Potential for Diagnostic Use
Activity of the AR pathway, and to a lesser extent of the TGFβ signaling pathway, was increased in sepsis, while a trend toward higher activity was observed for MAPK-AP1, ER, NFκB and JAK-STAT3 pathways, toward lower activity for the Hedgehog pathway, while activity of the JAK-STAT1/2 pathway did not differ between healthy controls and sepsis patients. Only the AR pathway assay could to some extent identify sepsis patients with a favorable prognosis. This assay showed high sensitivity and specificity for sepsis diagnosis, and high specificity for prediction of favorable (survival) prognosis in children with sepsis.
In sepsis, the immune system plays a crucial role, with both pro-inflammatory and anti-inflammatory mechanisms. A subset of patients with sepsis has been described to rapidly display signs of immunosuppression and inflammation, which is associated with worse prognosis (30, 31).
In line with our observations, for AR, ER, TGFβ, NFκB, and JAK-STAT3 pathways a number of functional roles in the immune response have been described, with a distinct immunosuppressive role for AR and TGFβ pathways and an inflammatory role for NFκB and JAK-STAT3 pathways (30–39).
The lack of increase in JAK-STAT1/2 pathway activity in sepsis patients is in line with our earlier findings that PAS scores of this signaling pathway only increase in virally, but not bacterially, infected patients, at least when measured in whole blood and Peripheral Blood Mononuclear Cell (PBMC) samples (9).
For the AR, ER, TGFβ, and NFκB pathways putative roles in sepsis have been described, generally in relation to specific immune cell types (40–44). This may explain the lack of diagnostic power of some of the measured STPs when measured in whole blood samples, since whole blood samples consist of a mix of multiple immune cell types.
Is There a Causal Relation Between AR Pathway Activity and Sepsis?
Despite the disadvantage of measuring in whole blood samples, the diagnostic performance of AR and TGFβ assays for sepsis was good. With respect to the TGFβ pathway, either decreased or elevated levels of various TGFβ pathway ligands have been reported in sepsis patients, while uncertainty remains with respect to the causal role of this pathway (44).
Multiple findings support a causal role for the AR pathway in sepsis. The AR signaling pathway is in principle activated by the presence of testosterone ligand, binding to a pocket in the AR transcription factor protein to induce RNA transcription of target genes (7). The AR protein is expressed in a wide variety of innate and adaptive immune cells including neutrophils, macrophages, mast cells, monocytes, megakaryocytes, B cells, and T cells, indicating that the AR signaling pathway can be activated in these blood cell types (42). Monocytes are one of the key cell types that play a pathogenic role in sepsis, and make up around 10% of whole blood cells (45, 46). Interestingly and in line, men have shown to be more susceptible to developing sepsis than women (47). Some studies suggest that the AR pathway may be a useful drug target in sepsis: testosterone blockade in patients with hemorrhage improved the prognosis if subsequently sepsis developed (48). Also AR pathway blockade reduced mortality in a preclinical mouse model for sepsis (38, 40). While suggestive of a role for the AR pathway in the pathophysiology of sepsis, we are not aware of any clinical studies investigating AR pathway targeted drugs in human sepsis patients.
AR Pathway Assay as a Diagnostic Assay for Sepsis
Current diagnosis of sepsis is based on assessment of multiple clinical symptoms and biomarkers, but timely diagnosis and even more so, prediction of prognosis of a sepsis patient, remain a clinical challenge. Despite the limitation of measuring in whole blood samples, determination of AR PAS showed very good performance to diagnose sepsis, while as a prognostic assay, results suggest potential clinical utility to identify good prognosis patients.
The normal range in AR pathway activity differed between healthy adults and children. This may be a “real” difference, for example caused by differences in testosterone levels between adults and children. However, the normal range in AR pathway activity did not differ between men and women. Alternatively, differences may have been caused by different handling of samples between clinical centers involved in pediatric vs. adult studies (22–26, 26–28, 28, 29).
Since Affymetrix analysis of sample data takes too long to be useful in a clinical setting, STP assays have currently been adapted to qPCR for future diagnostic use (16). The time-to-result of the qPCR-based STP-assays is a few hours, and clinical implementation of such assays can be fast, following determination of normal ranges of STP activities. In general, correlation between STP activity determined by Affymetrix-based STP analysis and qPCR-based analysis is very good (15).
Thus, while we show feasibility of determining a normal range of AR pathway activity in whole blood samples based on Affymetrix data analysis, clinical adoption of the AR pathway activity assay for diagnostic use in sepsis will require determination of normal AR pathway activity ranges for both adults and children, using the qPCR-based AR pathway assay, followed by further confirmation of clinical utility.
In addition to diagnosing sepsis, and prediction of prognosis, we hypothesize that the AR pathway activity assay may find clinical utility in prediction of risk at serious infectious complications in the period after surviving sepsis. This is of relevance since patients who survive sepsis often remain immune-compromised for longer periods of time, which is associated with increased risk at secondary infections with poor clinical outcome (3, 49). In view of the immunosuppressive role of the AR pathway, we hypothesize that this may be causally related to persistent high AR pathway activity (50).
Similarly, the assay may have value in predicting risk at developing sepsis in patients with a bacterial infection. To illustrate this clinical use case, AR pathway activity was measured in RNAseq data [GSE161731, (51)] from samples of patients presenting either with Community Acquired bacterial Pneumonia (CAP) or with viral influenza infection at the emergency ward, using an RNAseq-converted AR pathway assay (Supplementary Figure 12). In whole blood of CAP patients mean AR pathway activity was increased, compared to healthy individuals or patients with an influenza infection, while around one third of CAP patients had AR pathway activity in the normal range. Within the perspective of the current study, we hypothesize that the latter group with normal AR pathway activity did not have sepsis at admission and was at lower risk to develop sepsis, possibly not needing hospital admission. This is in line with data showing that at least a third of patients presenting with CAP have or develop sepsis (52, 53). Future clinical studies are necessary to further investigate these clinical use cases.
STP Assays to Guide Therapy Choices
STP activity measurements may be used to develop novel therapies for sepsis, on the premise of a causal relation with sepsis. Targeted drugs are available against the AR, TGFβ, MAPK-AP1, NFκB, and JAK-STAT3 pathways, all involved in immune responses, offering a new perspective on treating sepsis (42). It is expected that a specific drug will only be effective if the causal signaling pathway(s) is (are) abnormally active. Variation in STP PAS was high in sepsis patients, suggesting that only a subpopulation of patients may benefit from pathway-targeted therapy. Thus, investigation of potential benefit of for example androgen antagonists will require a personalized treatment approach based on measuring signal transduction pathway activity.
Interestingly, the AR pathway has been suggested to play a role in severe COVID-19 infections, and an AR inhibitor (proxalutamide) has been reported to reduce disease severity, overall mortality, and length of hospitalization (54, 55). Secondary bacterial infections frequently complicate severe COVID-19 pneumonia and we hypothesize that increased AR pathway activity may be indicative of immunosuppression and high risk at sepsis, explaining the benefit of anti-androgen therapy in this setting.
Comparison of Our Data Analysis Results With the Original Data Analysis Performed in the Clinical Studies
Since we frequently get questions regarding the difference between our STP analysis and other bioinformatics tools for Pathway Analysis, such as GSEA and Ingenuity, we compared our STP analysis results with data analysis results performed by the investigators who generated the Affymetrix data. Using a variety of bioanalytical biomarker discovery tools (Ingenuity, PANTHER, D.A.V.I.D., and ToppGene) associations between sepsis and the NFκB, MAPK, FOXO-PI3K and JAK-STAT3 pathways had been found, in agreement with our STP analysis results, but no associations with the AR, ER, and TGFβ pathways. Identification of “pathways” using these bioinformatics tools is not suited for analysis of individual sample data and is not informative on the actual (and quantitative) activation state of the signaling pathway. To our best knowledge the sepsis-associated gene signatures discovered by earlier bioinformatics analysis of these clinical studies have not been implemented in clinical practice.
STP assays were developed for diagnostic purposes and have been analytically validated for measuring signaling pathway activity prior to use on the current data. STP results are potentially clinically actionable, since many targeted drugs inhibit activity of the signal transduction pathways that were found to be overactive in sepsis and septic shock.
In summary, our current results show initial results supporting clinical validity and potential clinical utility of measuring activity of the AR and TGFβ signal transduction pathways in patients with sepsis, fulfilling the basic requirements for biomarker assays (56). In addition, good reproducibility between the different clinical studies suggests that defining a normal (“healthy”) range of pathway activity in whole blood samples will be feasible. This is important for future clinical implementation. STP assays have been converted to qPCR assays, which can be easily performed in a routine hospital lab, and provide a pathway activity score within a few hours, enabling timely clinical decisions (16).
Limitations and Future Studies
Clinical studies are needed to confirm the clinical utility of measuring AR (and TGFβ) pathway activity in patients with, or at risk for developing, sepsis or septic shock. Only two studies with adult patients were available for STP analysis. Although results were comparable, causes of sepsis are far more variable in adult patients and in in future studies it would be important to include more adult patient populations.
Data Availability Statement
The original contributions presented in the study are included in the article/Supplementary Material, further inquiries can be directed to the corresponding author.
Author Contributions
WB: data, results analysis, and concept and writing. WV: pathway model development and results analysis. AS: pathway model development, results analysis, and writing. All authors contributed to the article and approved the submitted version.
Conflict of Interest
All authors are employees of Philips.
Publisher's Note
All claims expressed in this article are solely those of the authors and do not necessarily represent those of their affiliated organizations, or those of the publisher, the editors and the reviewers. Any product that may be evaluated in this article, or claim that may be made by its manufacturer, is not guaranteed or endorsed by the publisher.
Acknowledgments
We acknowledge Yvonne Wesseling-Rozendaal for analyzing dataset GSE161731 and preparing Supplementary Figure 12; and Paul van de Wiel, Diederick Keizer, and Yvonne Wesseling-Rozendaal for critically reviewing the manuscript. We also wish to acknowledge all investigators who generated the GEO datasets used in the current analysis.
Supplementary Material
The Supplementary Material for this article can be found online at: https://www.frontiersin.org/articles/10.3389/fmed.2021.767145/full#supplementary-material
References
1. Cecconi M, Evans L, Levy M, Rhodes A. Sepsis and septic shock. Lancet. (2018) 392:75–87. doi: 10.1016/S0140-67361830696-2
2. Lever A, Mackenzie I. Sepsis: definition, epidemiology, and diagnosis. BMJ. (2007) 335:879–83. doi: 10.1136/bmj.39346.495880.AE
3. Hotchkiss RS, Moldawe LL, Opal SM, Reinhart K, Turnbull IR, Vincent JL. Sepsis and septic shock. Nat Rev Dis. (2016) 2:1–21. doi: 10.1038/nrdp.20216.45
4. Singer M, Deutschman CS, Seymour CW, Shankar-Hari M, Annane D, Bauer M, et al. The Third International Consensus Definitions for Sepsis and Septic Shock (Sepsis-3). JAMA. (2016) 315:801–10. doi: 10.1001/jama.2016.0287
5. Newton K, Dixit VM. Signaling in innate immunity and inflammation. Cold Spring Harb Perspect Biol. (2012) 4:1–20. doi: 10.1101/cshperspect.a006049
6. Cantrell D. Signaling in lymphocyte activation. Cold Spring Harb Perspect Biol. (2015) 7:1–13. doi: 10.1101/cshperspect.a018788
7. Cantley LC, Hunter T, Tony Sever R, Thorner JW. Signal Transduction : Principles, Pathways, and Processes. Cold Spring Harbor, NY : Cold Spring Harbor Laboratory Press (2014). Available online at: https://trove.nla.gov.au/version/203634108 (accessed Dec 2, 2018)
8. Wesseling-Rozendaal Y, van Doorn A, Willard-Gallo K, van de Stolpe A. Characterization of immunoactive and immunotolerant CD4+ T cells in breast cancer by measuring activity of signaling pathways that determine immune cell function. BioRxiv. (2020) p1–6. doi: 10.1101/2020.10.08.292557
9. Bouwman W, Verhaegh W, Holtzer L, van de Stolpe A. Measurement of Cellular Immune Response to Viral Infection and Vaccination. Front Immunol. (2020) 11:575074. doi: 10.3389/fimmu.2020.575074
10. Verhaegh W, Ooijen H, van Inda MA, Hatzis P, Versteeg R, Smid M, et al. Selection of personalized patient therapy through the use of knowledge-based computational models that identify tumor-driving signal transduction pathways. Cancer Res. (2014) 74:2936–45. doi: 10.1158/0008-5472.CAN-13-2515
11. van de Stolpe A, Holtzer L, van Ooijen H, Inda MA, de Verhaegh W. Enabling precision medicine by unravelling disease pathophysiology: quantifying signal transduction pathway activity across cell and tissue types. Sci Rep. (2019) 9:1603. doi: 10.1038/s41598-018-38179-x
12. van Ooijen H, Hornsveld M, Dam-de Veen C, Velter R, Dou M, Verhaegh W, et al. Assessment of functional phosphatidylinositol 3-kinase pathway activity in cancer tissue using forkhead box-o target gene expression in a knowledge-based computational model. Am J Pathol. (2018) 188:1956–72. doi: 10.1016/j.ajpath.2018.05.020
13. Canté-Barrett K, Holtzer L, van Ooijen H, Hagelaar R, Cordo' V, Verhaegh W, et al. A molecular test for quantifying functional notch signaling pathway activity in human cancer. Cancers (Basel). (2020) 12:3142. doi: 10.3390/cancers12113142
14. Bouwman W, Verhaegh W, Holtzer L, van de Stolpe A. Measurement of cellular immune response to viral infection and vaccination. Front Immunol. (2020) 11:575074. doi: 10.3389/fimmu.2020.5750744 eCollection 2020
15. Inda MA, Blok EJ, Kuppen PJK, Charehbili A, Biezen-Timmermans EC, den Brussel A, van, et al. Estrogen receptor pathway activity score to predict clinical response or resistance to neoadjuvant endocrine therapy in primary breast cancer. Mol Cancer Ther. (2020) 19:680–9. doi: 10.1158/1535-7163.MCT-19-0318
16. van de Stolpe A, Verhaegh W, Blay J-Y, Ma CX, Pauwels P, Pegram M, et al. RNA based approaches to profile oncogenic pathways from low quantity samples to drive precision oncology strategies. Front Genet. (2021) 11:598118. doi: 10.3389/fgene.2020.598118
17. NCBI. Home - GEO DataSets. (2019). Available online at: https://www.ncbi.nlm.nih.gov/gds/ (accessed Feb 24, 2021).
18. affyQCReport: QC Report Generation for affyBatch objects version 1,.68.0 from Bioconductor. (2020). Available online at: https://rdrr.io/bioc/affyQCReport/ (accessed Feb 24, 202)
19. Gautier L, Cope L, Bolstad BM, Irizarry RA. affy–analysis of Affymetrix GeneChip data at the probe level. Bioinformatics. (2004) 20:307–15. doi: 10.1093/bioinformatics/btg405
20. seaborn. Seaborn.Boxplot — Seaborn 0.11.1 Documentation. Available online at: https://seaborn.pydata.org/generated/seaborn.boxplot.html (accessed Feb 24, 2021).
21. Weber M. Webermarcolivier/Statannot. (2021). Available online at: https://github.com/webermarcolivier/statannot [accessed Feb 24, 2021].
22. Amrhein V, Greenland S, McShane B. Scientists rise up against statistical significance. Nature. (2019) 567:305–7. doi: 10.1038/d41586-019-00857-9
23. Wynn JL, Cvijanovich NZ, Allen GL, Thomas NJ, Freishtat RJ, Anas N, et al. The influence of developmental age on the early transcriptomic response of children with septic shock. Mol Med. (2011) 17:1146–56. doi: 10.2119/molmed.2011.00169
24. Wong HR, Shanley TP, Sakthivel B, Cvijanovich N, Lin R, Allen GL, et al. Genome-level expression profiles in pediatric septic shock indicate a role for altered zinc homeostasis in poor outcome. Physiol Genomics. (2007) 30:146–55. doi: 10.1152/physiolgenomics.00024.2007
25. Wong HR, Cvijanovich N, Allen GL, Lin R, Anas N, Meyer K, et al. Genomic expression profiling across the pediatric systemic inflammatory response syndrome, sepsis, and septic shock spectrum. Crit Care Med. (2009) 37:1558–66. doi: 10.1097/CCM.0b013e31819fcc08
26. Shanley TP, Cvijanovich N, Lin R, Allen GL, Thomas NJ, Doctor A, et al. Genome-level longitudinal expression of signaling pathways and gene networks in pediatric septic shock. Mol Med. (2007) 13:495–508. doi: 10.2119/2007-00065.Shanley
27. Cvijanovich N, Shanley TP, Lin R, Allen GL, Thomas NJ, Checchia P, et al. Validating the genomic signature of pediatric septic shock. Physiol Genomics. (2008) 34:127–34. doi: 10.1152/physiolgenomics.00025.2008
28. Cazalis M-A, Lepape A, Venet F, Frager F, Mougin B, Vallin H, et al. Early and dynamic changes in gene expression in septic shock patients: a genome-wide approach. Intensive Care Med Exp. (2014) 2:20. doi: 10.1186/s40635-014-0020-3
29. Venet F, Schilling J, Cazalis M-A, Demaret J, Poujol F, Girardot T, et al. Modulation of LILRB2 protein and mRNA expressions in septic shock patients and after ex vivo lipopolysaccharide stimulation. Hum Immunol. (2017) 78:441–50. doi: 10.1016/j.humimm.2017.03.010
30. Venet F, Monneret G. Advances in the understanding and treatment of sepsis-induced immunosuppression. Nat Rev Nephrol. (2018) 14:121–37. doi: 10.1038/nrneph.2017.165
31. Fattahi F, Ward PA. Understanding immunosuppression after sepsis. Immunity. (2017) 47:3–5. doi: 10.1016/j.immuni.2017.07.007
32. Flavell RA, Sanjabi S, Wrzesinski SH, Licona-Limón P. The polarization of immune cells in the tumour environment by TGFbeta. Nat Rev Immunol. (2010) 10:554–67. doi: 10.1038/nri2808
33. Kovats S. Estrogen receptors regulate innate immune cells and signaling pathways. Cell Immunol. (2015) 294:63–9. doi: 10.1016/j.cellimm.2015.01.018
34. Hillmer EJ, Zhang H, Li HS, Watowich SS. STAT3 signaling in immunity. Cytokine Growth Factor Rev. (2016) 31:1–15. doi: 10.1016/j.cytogfr.2016.05.001
35. Campbell JD, Cook G, Robertson SE, Fraser A, Boyd KS, Gracie JA, et al. Suppression of IL-2-induced T cell proliferation and phosphorylation of STAT3 and STAT5 by tumor-derived TGF beta is reversed by IL-15. J Immunol. (2001) 167:553–61. doi: 10.4049/jimmunol.167.1.553
36. Baldwin AS. The NF-kappa B and I kappa B proteins: new discoveries and insights. Annu Rev Immunol. (1996) 14:649–83. doi: 10.1146/annurev.immunol.14.1.649
37. Sun S-C. The non-canonical NF-κB pathway in immunity and inflammation. Nat Rev Immunol. (2017) 17:545–58. doi: 10.1038/nri.2017.52
38. Angele MK, Pratschke S, Hubbard WJ, Chaudry IH. Gender differences in sepsis. Virulence. (2014) 5:12–9. doi: 10.4161/viru.26982
39. Gubbels Bupp MR, Jorgensen TN. Androgen-Induced Immunosuppression. Front Immunol. (2018) 9:794. doi: 10.3389/fimmu.2018.00794
40. Roell D. Androgen-Regulation of Sepsis response: Beneficial Role of Androgen Receptor Antagonists. (2015). Available online at: /paper/Androgen-regulation-of-sepsis-response-%3A-beneficial-Roell/56789a32d9dc5d88b2324534b4eda12eeed9513f (accessed Feb 25, 2021).
41. Liu SF, Malik AB. NF-κB activation as a pathological mechanism of septic shock and inflammation. Am J Physiol Lung Cell Mol Physiol. (2006) 290:L622–45. doi: 10.1152/ajplung.00477.2005
42. Ben-Batalla I, Vargas-Delgado ME, von Amsberg G, Janning M, Loges S. Influence of androgens on immunity to self and foreign: effects on immunity and cancer. Front Immunol. (2020) 11:1184. doi: 10.3389/fimmu.2020.01184
43. Straub RH. The complex role of estrogens in inflammation. Endocr Rev. (2007) 28:521–74. doi: 10.1210/er.2007-0001
44. Bergmann CB, Beckmann N, Salyer CE, Hanschen M, Crisologo PA, Caldwell CC. Potential targets to mitigate trauma- or sepsis-induced immune suppression. Front Immunol. 12:622601. doi: 10.3389/fimmu.2021.622601
45. Clinical Laboratory int. The Role of Monocytes in the Progression of Sepsis. (2020). Available online at: https://clinlabint.com/the-role-of-monocytes-in-the-progression-of-sepsis/ (accessed Feb 24, 2021).
46. Haveman JW, Muller Kobold AC, Tervaert JW, van den Berg AP, Tulleken JE, Kallenberg CG, et al. The central role of monocytes in the pathogenesis of sepsis: consequences for immunomonitoring and treatment. Neth J Med. (1999) 55:132–41. doi: 10.1016/S0300-2977(98)00156-9
47. Bauman KA, Han MK. Chapter 27 - Gender Differences in Susceptibility, Outcomes, and Pathophysiology of Sepsis. In: Legato MJ, editor. Principles of Gender-Specific Medicine. 2nd ed. San Diego: Academic Press (2010) p. 291–301. Available online at: https://www.sciencedirect.com/science/article/pii/B9780123742711000277 doi: 10.1016/B978-0-12-374271-1.00027-7 (accessed Feb 24, 2021).
48. Angele MK, Wichmann MW, Ayala A, Cioffi WG, Chaudry IH. Testosterone receptor blockade after hemorrhage in males. Restoration of the depressed immune functions and improved survival following subsequent sepsis. Arch Surg. (1997) 132:1207–14. doi: 10.1001/archsurg.1997.01430350057010
49. Cao C, Yu M, Chai Y. Pathological alteration and therapeutic implications of sepsis-induced immune cell apoptosis. Cell Death Dis. (2019) 10:1–14. doi: 10.1038/s41419-019-2015-1
50. Hotchkiss RS, Monneret G, Payen D. Sepsis-induced immunosuppression: from cellular dysfunctions to immunotherapy. Nat Rev Immunol. (2013) 13:862–74. doi: 10.1038/nri3552
51. McClain MT, Constantine FJ, Henao R, Liu Y, Tsalik EL, Burke TW, et al. Dysregulated transcriptional responses to SARS-CoV-2 in the periphery. Nat Commun. (2021) 12:1079. doi: 10.1038/s41467-021-21289-y
52. Montull B, Menéndez R, Torres A, Reyes S, Méndez R, Zalacaín R, et al. Predictors of severe sepsis among patients hospitalized for community-acquired pneumonia. PLoS ONE. (2016) 11:e0145929. doi: 10.1371/journal.pone.0145929
53. Cillóniz C, Dominedò C, Ielpo A, Ferrer M, Gabarrús A, Battaglini D, et al. Risk and prognostic factors in very old patients with sepsis secondary to community-acquired pneumonia. J Clin Med. (2019) 8:961. doi: 10.3390/jcm8070961
54. Pharmaceuticals K. Kintor Pharmaceutical Announces Results from Investigator-Initiated Brazil Trial Demonstrating 92% Reduction in Mortality in Hospitalized COVID-19 Patients. Available online at: https://www.prnewswire.com/news-releases/kintor-pharmaceutical-announces-results-from-investigator-initiated-brazil-trial-demonstrating-92-reduction-in-mortality-in-hospitalized-covid-19-patients-301245257.html (accessed Apr 12, 2021).
55. Cadegiani FA, McCoy J, Gustavo Wambier C, Vaño-Galván S, Shapiro J, Tosti A, et al. Proxalutamide Significantly Accelerates Viral Clearance and Reduces Time to Clinical Remission in Patients with Mild to Moderate COVID-19: Results from a Randomized, Double-Blinded, Placebo-Controlled Trial. Cureus. 13:e13492. doi: 10.7759/cureus.13492
Keywords: sepsis, pathway activity assay, host response, infectious diseases, diagnosis, prediction, signal transduction pathways, androgen receptor pathway
Citation: Bouwman W, Verhaegh W and Stolpe Avd (2021) Androgen Receptor Pathway Activity Assay for Sepsis Diagnosis and Prediction of Favorable Prognosis. Front. Med. 8:767145. doi: 10.3389/fmed.2021.767145
Received: 30 August 2021; Accepted: 01 November 2021;
Published: 23 November 2021.
Edited by:
Felipe Dal Pizzol, Universidade do Extremo Sul Catarinense, BrazilReviewed by:
Chaonan Jin, University of Bern, SwitzerlandJaqueline Generoso, Universidade do Extremo Sul Catarinense, Brazil
Copyright © 2021 Bouwman, Verhaegh and Stolpe. This is an open-access article distributed under the terms of the Creative Commons Attribution License (CC BY). The use, distribution or reproduction in other forums is permitted, provided the original author(s) and the copyright owner(s) are credited and that the original publication in this journal is cited, in accordance with accepted academic practice. No use, distribution or reproduction is permitted which does not comply with these terms.
*Correspondence: Anja van de Stolpe, YW5qYS52YW4uZGUuc3RvbHBlQHBoaWxpcHMuY29t