- 1Department of Pharmacy, University of Patras, Patras, Greece
- 2School of Science and Technology, Hellenic Open University, Patras, Greece
- 3Institute of Physiology II, Faculty of Medicine, University of Freiburg, Freiburg, Germany
- 4Faculty of Medicine, Imperial College London, Hammersmith Hospital Campus, Burlington Danes, London, United Kingdom
Single nucleotide polymorphisms (SNPs) are genetic variations which can play a vital role in the study of human health. SNP studies are often used to identify point mutations that are associated with diseases. Arkadia (RNF111) is an E3 ubiquitin ligase that enhances transforming growth factor-beta (TGF-β) signaling by targeting negative regulators for degradation. Dysregulation of the TGF-β pathway is implicated in cancer because it exhibits tumor suppressive activity in normal cells while in tumor cells it promotes invasiveness and metastasis. Τhe SNP CGT > TGT generated an amino-acid (aa) substitution of Arginine 957 to Cysteine on the enzymatic RING domain of Arkadia. This was more prevalent in a tumor than in a normal tissue sample of a patient with colorectal cancer. This prompted us to investigate the effect of this mutation in the structure and activity of Arkadia RING. We used nuclear magnetic resonance (NMR) to analyze at an atomic-level the structural and dynamic properties of the R957C Arkadia RING domain, while ubiquitination and luciferase assays provided information about its enzymatic functionality. Our study showed that the R957C mutation changed the electrostatic properties of the RING domain however, without significant effects on the structure of its core region. However, the functional studies revealed that the R957C Arkadia exhibits significantly increased enzymatic activity supporting literature data that Arkadia within tumor cells promotes aggressive and metastatic behavior.
Introduction
Ubiquitination is a three-step enzymatic reaction catalyzed by the action of three key-enzymes. Initially, ubiquitin is activated in an ATP-dependent manner by the E1 activating enzyme. Activated ubiquitin afterwards is transferred to E2 conjugating enzyme. In the last step of the enzymatic reaction ubiquitin is covalently attached to the target protein, through an isopeptide bond between its G76 and the ε-amino group of a lysine residue of the target protein. The attachment of ubiquitin to substrate is catalyzed by the E3 ubiquitin ligase. The E3 ubiquitin ligases are considered crucial partners of the ubiquitination machinery because they act as specific substrate recognition elements. The ubiquitin ligases are characterized by the presence of either a HECT (Homologous to E6-AP Carboxyl Terminus) or a RING (Really Interesting New Gene) domain (Hershkos et al., 1983; Pickart, 2001). RING-type E3 ligases constitutes the largest class of E3 ligases and they are characterized by the following amino acids sequence: Cys-X2-Cys-X9-39-Cys-X1-3-His-X2-Cys/His-X2-Cys-X4-48-Cys-X2-Cys, wherein X represents any amino acid. The RING domain motif is constructed by Cys2His2, Cys3His or Cys4 metal binding sites which coordinate two zinc ions in a “cross-brace” arrangement (Freemont, 1993; Saurin et al., 1996).
Ubiquitination is a signaling pathway that regulates a broad spectrum of biochemical processes, e.g., it enhances the tumor suppression activity of some proteins or the oncogenic and metastatic properties of others and has a significant role in the life cycle of cells. Thus, many studies have shown that mutations in E3 ligases are implicated in cancer (Shangary and Wang, 2008; Nelson and Holt, 2010; Sharma et al., 2011; Chan et al., 2013; Wang et al, 2017) and other diseases (Kumar et al., 2017). Two of the thoroughly studied and well characterized RING E3 ligases are BRCA1 and HDM2. Tumor suppressor BRCA1 is a RING E3 ligase whose mutations lead to a high predisposition of breast and ovarian cancers (Brzovic et al., 2001; Rosen, 2013). Mutations observed in the RING sequence are now considered as significant markers for these types of cancer. Similarly, Hdm2 is a RING E3 ligase whose overexpression associates with many human tumor types (breast, esophageal, lung carcinomas etc.,), whereas its binding and degradation of p53 tumor suppressor is inactivated in more than 50% of human cancers (Moll and Petrenko, 2003; Sun, 2006). Targeting specific E3 ligases, that play a significant role in cancer, may contribute to the development of therapeutic strategies (Lakshmanan et al., 2008).
Arkadia (RNF111) is a RING ubiquitin ligase that positively regulates the TGF-β pathway by targeting its negative regulator SKI and its close homologue SKIL (SNON), as well as the inhibitory SMAD7 for ubiquitin-dependent proteasomal degradation. Arkadia functions as E3 ligase through its C-terminal RING-H2 domain (amino acids 942–983) (Levy et al., 2007; Mavrakis et al., 2007; Nagano et al., 2007). Arkadia was shown to enhance and support TGF-β tumor suppressing function in colorectal cancer (CRC) (Sharma et al., 2011). Furthermore, Le Scolan, et al., 2008 and Briones-Orta et al., 2013, using tumor cell lines driven by the TGF-β pathway, demonstrated that Arkadia has a potent tumor-promoting activity. These data indicate that Arkadia supports both properties of the TGF-β pathway, i.e., tumor suppression in normal cells and metastasis in tumor cells. Moreover, deep sequencing studies of the mRNA from tumors of CRC patients (Bravou et al., 2009; Sharma et al., 2011), led to the identification of somatic mutations that diminish Arkadia’s function (Sharma et al., 2011). They were listed in the COSMIC database of somatic mutations in cancer (Bravou et al., 2009; Sharma et al., 2011).
In the present study the single nucleotide polymorphism (SNP) of arginine (R) 957 (rs780099637, CGT > TGT, allele frequency = 0.000004 in gnomAD) enriched in CRC was studied. SNPs are genetic variations that are associated with individual susceptibility to diseases (Shastry, 2007). The R957C SNP was identified both in CRC and adjacent normal tissue samples (Supplementary Table S1). Existence of mutations in normal tissues adjacent to tumors is frequently observed and their association with cancer is under investigation (Risques and Kennedy 2018; Fiala and Diamandis, 2020; Oh and Sung, 2020). SNP studies are crucial to identify amino acids substitutions; in the protein coding regions that potentially alter the function or structure of a protein (Bhattacharya et al., 2017). Herein, we present a thorough overview about the effects of the R957C point mutation. Replacement of the positively charged arginine with cysteine affects the electrostatic properties of the protein, which may lead to alterations of structure, stability, and function of the enzymatic RING domain of the E3 ubiquitin ligase Arkadia. To predict the effect of the R957C mutation on Arkadia protein, the SNPs and GO and I-Mutant pathogenicity prediction servers were used (Capriotti et al., 2005; Capriotti et al., 2013). In order to investigate the impact of this mutation on the conformational dynamics and the function of the Arkadia RING domain, we prepared the recombinant polypeptide bearing a cysteine residue instead of arginine in position 957 of the human sequence and we conducted a structural and functional study to obtain an atomic-level insight into its conformational dynamics and activity in comparison with the native Arkadia RING domain.
Materials and Methods
Prediction of Single Nucleotide Polymorphism Impact
The effect of the R957C substitution was analyzed using SNPs and GO and I-Mutant tools. SNPs and GO is a tool which predicts disease associated amino acid substitution at a single position in a specific protein including functional classifications with >82% prediction accuracy. I-Mutant predicts the effects of single point mutation with 80% accuracy. A probability score higher than 0.5 reveals a disease related effect of the mutation on protein function. The input given to the SNPs and GO and I-Mutant tools was the UniProt accession number (Q6ZNA4) of Arkadia isoform-1 protein, the sequence position of the wild type amino acid and the mutated amino acid.
Protein Expression and Purification
A human RNF111 gene encoding amino acids 927–994 of the full length Arkadia was sub-cloned in a pGEX-4T-1 vector and transformed in Escherichia coli (E. cloni®) EXPRESS BL21 (DE3) cells (Lucigen). Cells were grown at 37°C in minimal medium (M9) supplemented with 1 g/L15N ammonium chloride, 4 g/L glucose or 13C-glucose and 1 ml/L15N or 15N/13C Bioxpress™ (CIL) for single or double labeled samples, respectively. Cell cultures were induced at an optical absorption of 0.6–0.9 with 1 mM IPTG (final concentration). After 4 h incubation at 37°C cells were harvested and the resulting pellet was lysed by sonication in PBS (Phosphate Buffered Saline) pH 7.4 ± 0.2 containing a protease inhibitor cocktail (Sigma) and DNAase I. The cell lysate was cleared by centrifugation at 20.000rpm (rounds per minute) for 30 min. For protein purification the supernatant was loaded onto a GST-trap 5 ml column (GE Healthcare). The GST-tag and Arkadia927-994 were separated after overnight incubation with the protease thrombin (Merck Millipore) at 4°C. Arkadia927-994 was eluted in PBS pH 7.4 ± 0.2 and concentrated using Amicon® Ultra centrifugal filter units (3 kDa cutoff) (Merck Millipore). The protein was further purified by size exclusion chromatography on a Superdex75 column (GE Healthcare).
The E2 enzyme UBCH5B (UBE2D2) was expressed and purified as described elsewhere (Birkou et al., 2017) and ubiquitin was expressed in E. coli (E. cloni®) EXPRESS BL21 (DE3) cells (Lucigen). Cells were grown at 37°C in minimal medium (M9) supplemented with 4 g/L glucose and 1 g/L NH4Cl and induced with 1 mM (final concentration) IPTG. After 4 h of incubation at 37°C cells were harvested and lysed by sonication in PBS pH 7.4. The cell lysate was cleared by centrifugation at 20.000 rpm for 30 min. The supernatant was heated at 85°C for 15 min and centrifuged at 10.000 rpm for 20 min. For further purification ubiquitin was passed through a Superdex75 column (GE Healthcare).
Nuclear Magnetic Resonance Data and Structure Calculation
NMR spectra were recorded on a Bruker Avance 600 MHz spectrometer equipped with a TXI cryoprobe and Bruker Avance III 700 MHz spectrometer equipped with a four-channel 5 mm cryogenically cooled TCI gradient probe. Protein samples were prepared in a mixed solvent of 90% H20 (50 mM K2HPO4, 50 mM KH2PO4 pH 7), 10% D20, 1 mM NaN3 and 0.25 mM DSS (4,4-dimethyl-4-silapentane-1-sulfonic acid) as internal chemical shift standard. All NMR experiments are included in the Bruker pulse program library. Data were processed with Topspin 3.5 pl5 software and analyzed with CARA (Keller, 2004) and XEASY (Bartels et al., 1995). The sequence specific assignment of R957C Arkadia927−994 domain was obtained using conventional backbone and side chain assignment methods (Wüthrich, 1986; Ferentz and Wagner, 2000) and were deposited in the BioMagResBank (BMRB; accession no: 50,985). The NMR solution structure of the R957C mutant was determined with DYANA (Güntert et al., 1997) and the ensemble of 30 models with the lowest RMSD and target function values was deposited in the ProteinDataBank after energy minimization with AMBER (Pearlman et al., 1995) (PDB, accession no: 7P2K; statistical analysis is reported in Supplementary Table S2). The assignment of the backbone 1H-15N resonances of all non-proline residues of E2 enzyme UBCH5B was obtained from the BMRB database (accession no: 6,277).
15N Relaxation Data
The backbone mobility of the R957C Arkadia927-994 mutant on the ps-ns time scale was investigated through 15N relaxation measurements (15N T1 and T2, {1HN}-15N NOE at 298 K) on Bruker Avance 600 and Avance III 700 spectrometers (experiments are reported in Supplementary Table S3). Standard pulse programs of the Bruker library were used. The overall delays between scans were 1.8 s for the T1 and T2 measurements and 4 s for the interleaved heteronuclear NOE experiment, respectively. The delays used for the T1 experiments were 7, 18, 40, 85, 150, 230, 350, 500, 680, and 900 ms, whereas delays of 17, 34, 51, 68, 85, 102, 136, 187, and 221 ms were used for the T2 experiments. 15N relaxation data were analyzed according to the model-free approach as implemented in the Tensor 2 program (Dosset et al., 2000) (R1, R2 and (1H) 15N NOE diagrams are shown in Supplementary Figure S2).
Atomic Absorption Spectroscopy
To identify whether two zinc ions are bound to the R957C mutant, atomic absorption spectroscopy was performed (Perkin Elmer AAnalyst 700). For the zinc concentration determination proteins were purified as described above. Zinc ions standards of 0.1–1 ppm (Zinc Pure Standard, 1000 μg/ml, 2% HNO3, Perkin Elmer) were used and an appropriate calibration curve was constructed (Supplementary Figure S1). The concentration of zinc in the protein samples was determined dividing the intercept by the slope of calibration curve.
Metal-Chelation Experiments
To evaluate the stability of the R957C Arkadia927–994 domain metal-chelation experiments were performed with the ethylenediaminetetraacetic acid (EDTA). 1H-15N HSQC spectrums were recorded after each EDTA addition at a ratio 1:0.5, 1:1, 1:1.5 and 1:2 (Zn: EDTA).
Titration Experiments Monitored by Nuclear Magnetic Resonance
In order to identify the interaction interface between E3 R957C Arkadia927–994 and E2 UBCH5B, titration experiments were monitored by 1H–15N HSQC spectra of labeled 15N R957C Arkadia927–994 or 15N UBCH5B after each addition of the unlabeled protein partner. The unlabeled protein was added in eight steps in order to reach the following ratios and saturation of labeled/unlabeled protein: 1:0.25, 1:0.5, 1:0.75, 1:1, 1:1.25, 1:1.5, 1:1.75, 1:2. Combined chemical shift perturbations (CSPs) after binding were calculated using the equation:
Ubiquitination Assay
For the ubiquitination assays the Arkadia876–994 polypeptide bearing the R957C mutation was used. Briefly, ubiquitination assay was performed by incubating 0.5 μM hUbe1 enzyme (Boston Biochem), 5 μM UBCH5B, 100–150 μM ubiquitin and 15 μM wt/R957C Arkadia876-994 in 20 mM Tris-HCl pH 7.5, 50 mM NaCl, 5 mM ATP, 2 mM MgCl2 and 2 mM DTT at 37°C for 0–60 min. Time points were collected after the addition of ATP and the reactions were stopped by addition of SDS loading buffer. Samples were resolved by 15% SDS-PAGE and visualized by western blotting for ubiquitin using the anti-Ub antibody P4D1 (Santa Cruz Biotechnologies, SCBT) and the goat anti-mouse m-IgGk BP-HRP sc-516102 (SCBT).
Luciferase Assays
Luciferase assays were conducted in HEK293T cells (Cancer Research UK). using the Dual Luciferase reporter system (Promega), as previously described by Levy at al. 2007. Briefly, cells were transfected with the appropriate combination of promoter-reporter constructs and expression plasmids using FuGENE transfection reagent (Promega) and were cultured for 24 h after transfection. Luciferase activities in the cell lysates were measured following the manufacturer’s protocol. The experiments were repeated at least three times.
Results
The SNP and GO and I-Mutant pathogenicity prediction tools indicated, with high probability, that the R957C mutation in the Arkadia RING domain (Figure 1) is a disease-causing mutation (Supplementary Table S1). To determine the effect of the R957C mutation on Arkadia’s RING domain structure, stability and interaction properties NMR studies were performed. In addition, auto-ubiquitination and luciferase assays were carried out to investigate the biochemical activity of the R957C mutant.
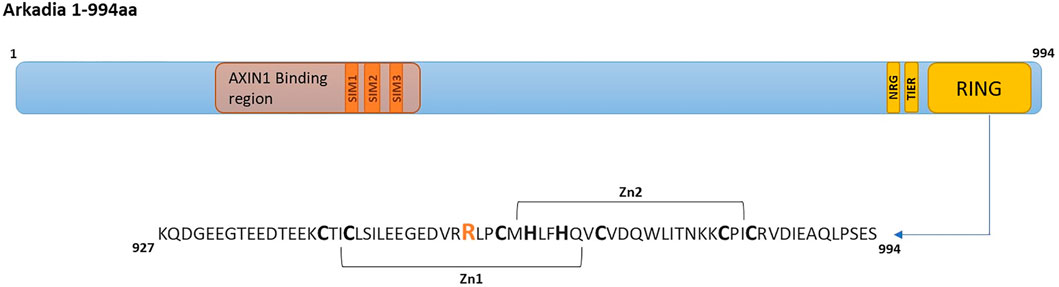
FIGURE 1. Arkadia E3 ubiquitin ligase domain organization and RING mutated sequence. SIMs (SUMO interacting motifs) (Poulsen et al., 2013) colored in orange, NRG, TIER segments and RING domain are colored in yellow.
Determination of R957C Arkadia927–994 Zinc Ions Content and Stability Upon Addition of Ethylenediaminetetraacetic Acid.
Atomic absorption spectroscopy was performed on R957C mutant to measure the Zn (II) content. A protein sample of 0.3 mM gave zinc concentration of 0.71 mM. These concentrations correspond to two zinc ions per protein molecule.
According to the structural role of the Zn (II) in RING domains, addition of the metal-chelating agent EDTA to a final ratio 1:2 (Zn: EDTA) led to the complete unfolding of the protein after 48 h as indicated by the loss of chemical shift dispersion in the 1H-15N HSQC spectra (Figure 2A). Similarly, addition of EDTA to the wt RING domain caused complete unfolding of the protein after 48 h of incubation with the chelating agent (Figure 2B). These results suggest that the addition of one more Cys residue in the RING domain of Arkadia has not affected the zinc ions binding and the stability of the mutated protein. According to the Cα (56 ppm) and Cβ (27 ppm) chemical shifts Cys957 in reduced state and does not participate in zinc ions binding or in disulfide bond formations (Sharma and Rajarathnam, 2000).
Solution Structure and 15N Relaxation Studies of the R957C Arkadia927–994 RING Domain
The NMR solution structure of the R957C mutant was determined based on a total of 906 NOEs distance constraints. The final family energy minimized NMR models contains two β-strands, namely β1 (Val955-Leu958) and β2 (His962-His965) forming an antiparallel β-sheet, two zinc binding loops and a 3-turn α-helix encompassing the residues Gln966-Thr975 (Figure 3). The two β-strands of the R957C mutant are one amino acid longer compared to wt RING Val955-Arg957 and Leu963-His965 β-strands. The a-helix observed in the R957C mutant is identical to the wt Arkadia RING helix in length and sequence position (Chasapis et al., 2012). The secondary structure elements of the R957C RING domain exhibit a ββα topology as observed in the wt Arkadia, RNF24 (PDB: 2EP4) and RNF168 RING domains (Zhang et al., 2013). The average distance between the two metal centers in the final 30 NMR models is 13.8 ± 0.4 Å (Figure 3). A detailed view of the coordination of the two Zn (II) ions is shown in Figure 4. The overall backbone and heavy atom RMSDs of the 30 energy-minimized models of R957C are 1.22 ± 0.48 Å and 2.08 ± 0.45 Å, respectively, for the core region between Glu939 and Ile986. Quality assessment of the final models reveals that 100% of the non-glycine/non-proline residues fall into favorable or allowed regions of the φ/ψ dihedral angle space in the Ramachandran plot (Supplementary Table S2).
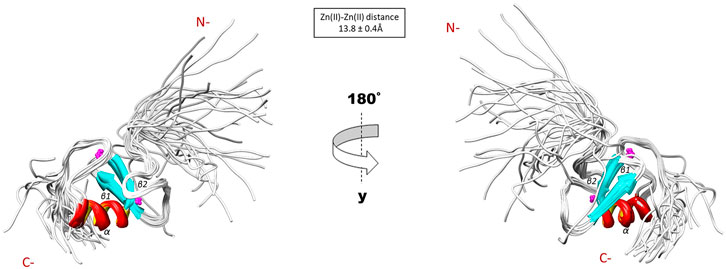
FIGURE 3. Solution structure of the R957C RING domain as represented by the final family of 30 energy minimized models.
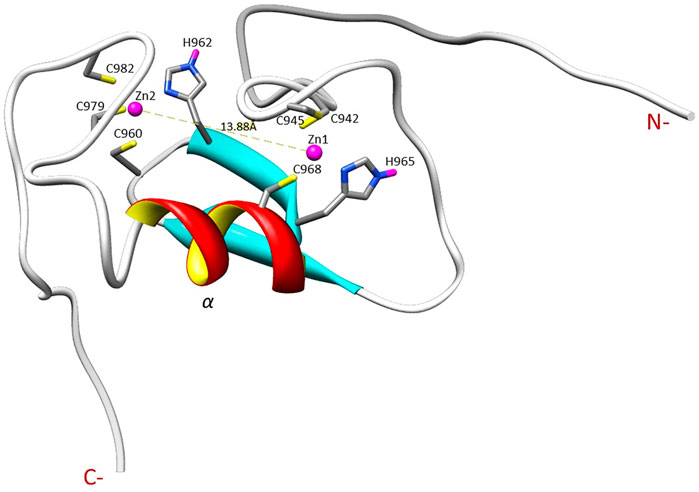
FIGURE 4. Illustration of the zinc ions binding sites and the Zn ions distance in the R957C RING domain as appeared in the first model of the family.
15N relaxation studies of R957C Arkadia927–994 revealed that mutant is a monomer in solution since the correlation time for isotropic tumbling measured based on the R2/R1 ratio is 4.69 ns corresponding to a MW of ∼7.81 kDa (theoretical MW of R957C RING is 7767 Da). In addition, model-free analysis of 15N relaxation data as implemented in the Tensor2 program showed that the core exhibited a rather rigid structure (Figure 5). The order parameters of the region Lys941-Ile986 (average S2 = 0.77) are higher than those of the N- and C-terminal residues (Lys927-Glu940 and Glu987-Ser994, respectively) closely resembling the 15N-relaxation properties of the wt Arkadia RING (Chasapis et al., 2012; Birkou et al., 2017) (Figure 5). The above data clearly support that there are no significant differences in the structure and dynamic properties between wt and R957C Arkadia RING domain.
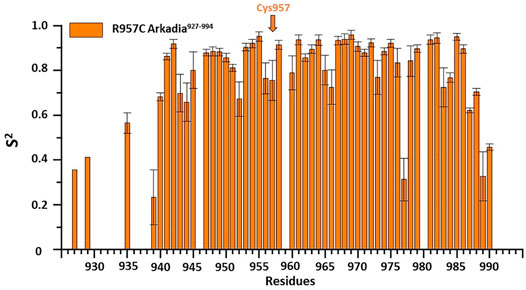
FIGURE 5. Order parameters (S2) derived from 15N-relaxation measurements [R1, R2 and heteronuclear (1HN)– 15N NOE] for R957C Arkadia927–994 domain at 600 MHz and 298 K.
Interaction Studies of R957C Arkadia927–994 Really Interesting New Gene Domain and UBCH5B
To determine whether the R957C mutation affects the interaction of the RING with E2 we used NMR driven interaction studies of 15N R957C with the E2 enzyme UBCH5B, which functions as a partner of Arkadia in vitro (Mavrakis et al., 2007). The analysis showed that the resonances exhibit either fast or intermediate exchange behavior, suggesting a moderate affinity for the two proteins. The largest chemical shift changes were observed for the sequential stretches Cys942–Thr943, Cys945-Ile947, Val955-Leu958, Phe964-His965, Val967-Asp970 and Trp972-Asn976 and for residue Ile981 (Figure 6A). The first three sequential stretches comprise the first and the third Zn(II)-binding motif, while the fourth and fifth are located at the C-end of the α-helix Gln966-Thr975. The above regions are essentially identical with those exhibiting the largest chemical shift changes upon interaction with UBCH5B in the native RING (Chasapis et al., 2012; Birkou et al., 2017).
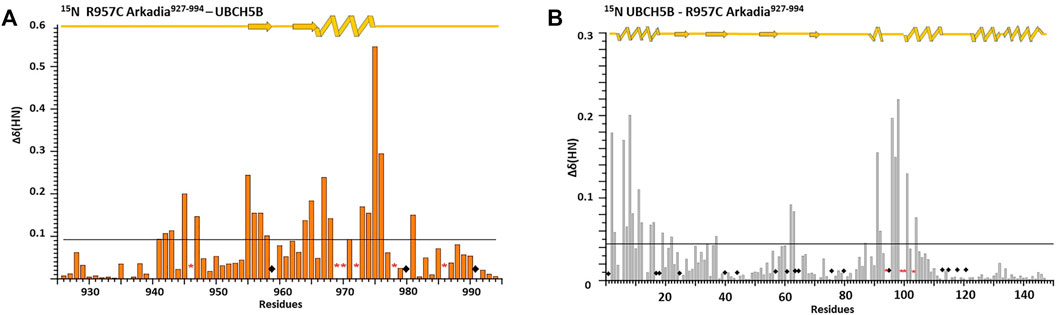
FIGURE 6. Interaction of E3 Ub ligase Arkadia RING with the E2 UBCH5B enzyme monitored by 1H-15N HSQC spectra. Diagram (A) illustrates the total CSPs measured at 1:2 M ratio for 15N R957C Arkadia/14N UBCH5B (left), while diagram (B) illustrates the CSPs for 15N UBCH5B/14N R957C Arkadia RING. Straight lines indicate the applied thresholds of CSPs representing the respective average CSP (Kornhaber et al., 2006). ◆represents proline residues and residues with no information.
The addition of unlabeled R957C RING to 15N-labeled UBCH5B resulted in the loss of four UBCH5B amide resonances (Ser94, Ile99, Ser100 and Leu103) in the 1H–15N HSQC spectra during the titration. The largest CSPs in UBCH5B were observed for residues Ala2-Leu3, Ile6-Glu9, Asn11-Asp12, Arg15-Asp16 (N-terminal helix α1) Ala19, Ser22 (β1 strand), Ile37 (β2 strand), Phe62-Lys63 (loop L1 between the β3 and β4 strands), Ser91-Glu92, Ala96-Thr98 (loop L2b), Lys101, and Leu104 (the first half of helix α2) (Figure 6B). All these residues were also found to participate in wt Arkadia RING–UBCH5B interactions (Chasapis et al., 2012; Birkou et al., 2017), strongly suggesting that UBCH5B utilizes the same interface for its interaction with the Arkadia RING mutant R957C.
The above data show that R957C Arkadia mutant and UBCH5B E2 enzyme interact via the surfaces that are expected to be ivolved in a canonical E2-E3 RING interaction (Chasapis et al., 2012; Gundogdu and Walden, 2019). However, it seems that the CSPs observed for the mutant are somewhat larger compared with those observed for the wt-E2 interaction [0.09/0.06 ppm for the R957C and wt RING, respectively and 0.042/0.038 ppm for the interacting UBCH5B with R957C and wt RING, respectively (Figure 5 and Birkou et al., 2017)].
Effect of the R957C Mutation on Arkadia’s Function
To examine the actual activity of the mutant protein we developed an in vitro ubiquitination assay. For the auto-ubiquitination assays, wt or mutated polypeptides containing NRG and TIER segments along with the RING domain (Arkadia876–994) were used, because the RING domain alone does not auto-ubiquitinate. We performed the assay in the presence of E2 UBCH5B and ubiquitin. Immunoblot analysis of these reactions, with anti-ubiquitin antibodies, showed that both wt and R957C Arkadia 876–994 were functional in this in vitro auto-ubiquitination assay, as indicated from the ladder-like bands that appeared after addition of ATP to the reaction mixture (Figure 7) due to the formation of a polyubiquitin chain on the substrates wt or R957C Arkadia 876–994. We found that the R957C Arkadia876–994 exhibit significantly rapid and enhanced autoubiquitination (Figure 7), indicating a gain of function and not loss of function.
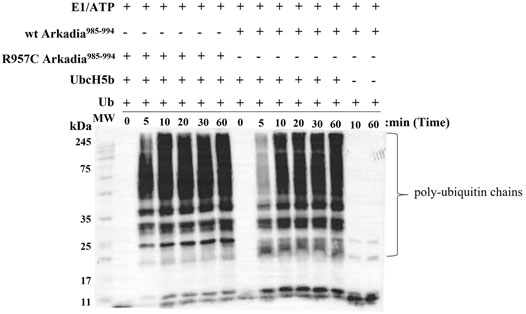
FIGURE 7. Western blot of in vitro ubiquitination assays of R957C Arkadia985–994 (left) and wt Arkadia985–994 (right).
Moreover, R957C Arkadia’s functionality was tested in vivo by luciferase assays. Luciferase experiments were carried out in HEK293T cells using the TGF-β SMAD-dependent reporter CAGA12-Luc, empty plasmid, the full length wt, C937A and R957C Arkadia proteins as described in Birkou et al., 2017. Overexpression of the R957C Arkadia protein enhanced reporter expression a slightly higher than that of the wt Arkadia, however, this was not statistical significant in this assay (Figure 8). These in vitro and in vivo results show that the R957C mutation on the RING domain does not reduce the activity of Arkadia as E3 ubiquitin ligase.
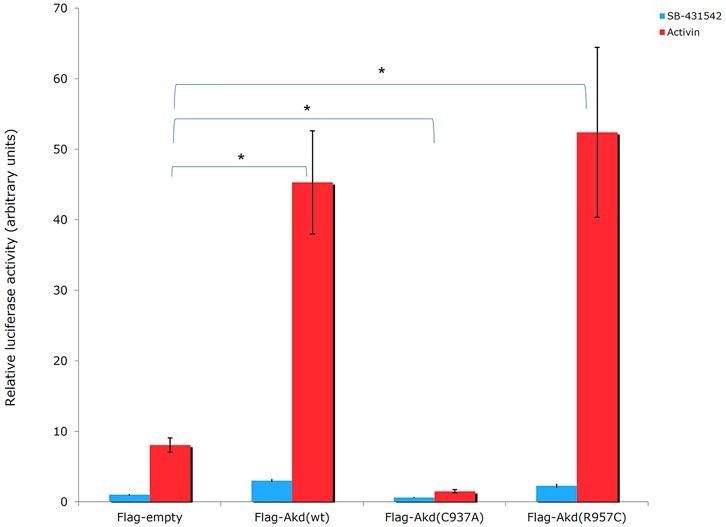
FIGURE 8. Luciferase CAGA12 reporter assay values. Activin: activator of the TGF-β pathway. SB-431542: inhibitor of TGF-β superfamily type I activin receptor-like kinase receptors. C937A is a ligase defective mutant, which forms mixed dimers (Erker et al., 2013) with the endogenous wt protein leading on suppression of wt function in HEK293T cells (Sharma et al., 2011).
Discussion
Mutations within the RING domain of E3 ligases such as BRCA1 are correlated with high risk of cancer (Ruffner et al., 2001). Usually, most of the SNPs cause missense mutations that are neutral or deleterious and unclassified variants. Deleterious SNPs/mutations cause phenotypic differences leading to various types of cancer (Ramensky et al., 2002). Here, we study the structural and functional consequences of the R957C SNP in the RING domain of Arkadia that was identified in normal and tumor tissues of a colorectal cancer patient.
Does the R957C mutation affect the solution structure and dynamics of Arkadia?
The NMR structure of the R957C mutant of Arkadia closely agrees with the NMR structure of the native RING domain (PDB: 2KIZ). Overlay of the first model of R957C RING domain with the first model of wt RING domain shows that there is no significant difference in the overall topology (Figure 9). Both contain a 3-turn α-helix, although in the final family of the R957C mutant 10 out of 30 models show a shightly distorted third turn of the α-helix due to the lack of helix-diagnostic NOEs. Replacement of Arg957 with Cys results in a slight bending of the β1-strand which is slightly bended, affecting the conformation of a loop that comprises the acidic residues Glu936 and Asp937. In wt, the positively charged NH1 and NH2 atoms of Arg957 interact electrostatically with the carboxyl groups of Glu936 and Asp937, respectively, leading to a compact and less flexible N-terminus (Figure 10). This electrostatic interaction is abolished in the R957C mutant resulting in an increased flexibility of the N-terminus as indicated by the backbone mobility data (Figure 5) and by the distances between the carboxyl groups of Glu936 and Asp937 and the sulfur atom of Cys957. As far as the mobility of the RING core is concerned, the 15N relaxation measurements show that the replacement of Arg957 with Cys has essentially no effects on the ps–ns time scale (Supplementary Figure S2).
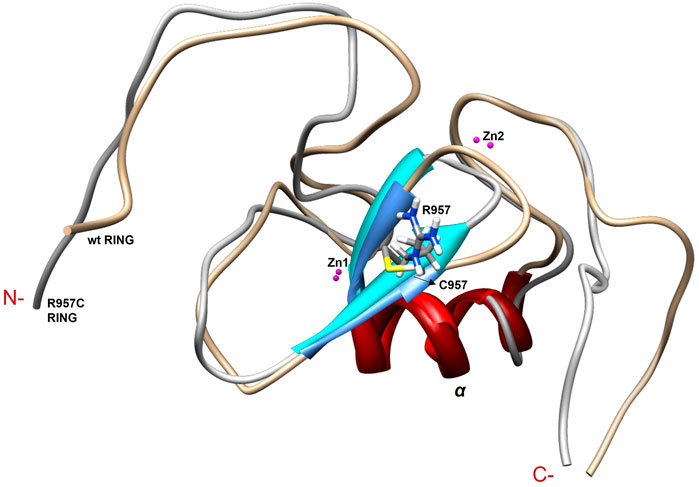
FIGURE 9. Overlay of the first model of the NMR family models of wt and R957C mutant of Arkadia RING.
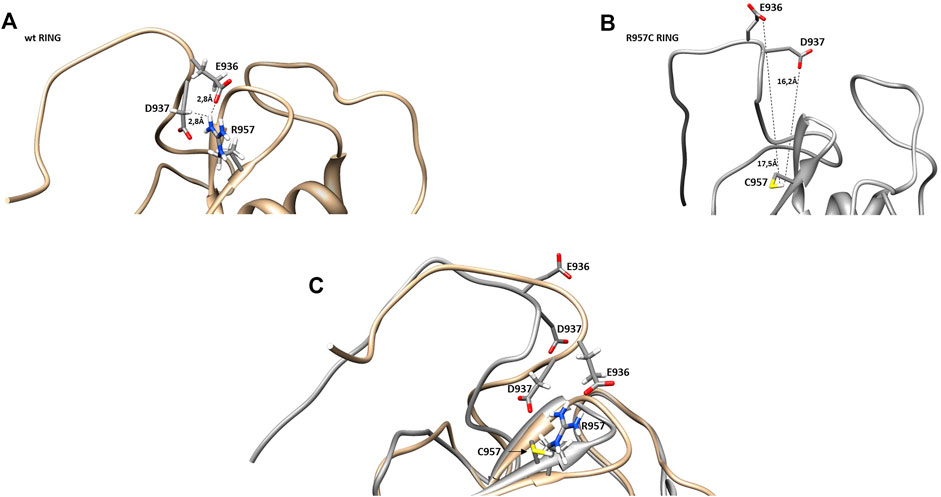
FIGURE 10. Representation of β-sheets region in (A) wt and (B) R957C RING domain. (C) Overlay of the β-sheet region of wt (gold) and R957C mutant (white).
Comparison of the NMR structure of the R957C mutant with the RING domains of RNF24 (PDB: 2EP4) and RNF168 (PDB: 4GB0) that exhibit a ββα topology reveals the similarity of the Arkadia mutant with the RING domain of RNF24 (Figure 11). Interestingly, both domains have β-strands, that are four residues long and adopt the same orientation (Figures 11A,B). Moreover, their β-strands do not exhibit sequence homology but rather physicochemical similarity in their amino acids composition (Figure 12).
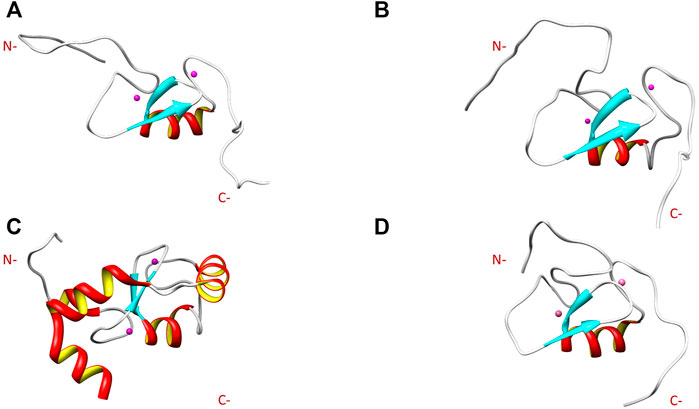
FIGURE 11. NMR solution structure of (A) RNF24 (PDB: 2EP4), (B) R957C Arkadia, (C) RNF168 (PDB: 4GB0) and (D) wt Arkadia (PDB: 2KIZ) RING domains.

FIGURE 12. Alignment of the RING domains of R957C Arkadia, RNF24 and RNF168 E3 ubiquitin ligases. Coloring by residue hydrophobicity and sequence conservation.
How does the R957C mutation affects Arkadia’s interaction properties and function?
The R957C Arkadia mutant is capable to interact with the E2 enzyme UBCH5B as shown by titration experiments monitored through 1H-15N HSQC spectra and auto-ubiquitination assays (Figure 6 and Figure 7). Interestingly, the observed CSPs suggest a native-like interaction, involving the two metal-binding loops and parts of the α-helix of the mutated RING (Figure 6). A similar surface, formed by the same RING structural elements participates in the E2-E3 interaction of BRCA1 (Brzovik et al., 2003), c-Cbl (Zheng et al., 2000) and RNF38 (Buetow et al., 2015) RING domains. Additionally, the UBCH5B regions that participate in the E2-E3 interaction are the same as those observed in the vast majority of E2-E3 pairs (Gundogdu and Walden, 2019).
Thus, the R957 mutation which is located on the β1-strand, does not seem to have an impact on the RING domain interaction properties. Since R957 is not considered as a residue that is crucial for E2-E3 interaction (Chasapis et al., 2012) its mutation to cysteine is not expected to disrupt the interaction with the E2 enzyme UBCH5B. Generally, it is well established that mutations of conserved residues in RING domains disrupt E2 interaction and result in the loss of E3 ligase enzymatic activity (Deshaies and Joazeiro et al., 2009). For example, mutation of the conserved tryptophan (Trp) in the α-helix of most RING domains disrupts the recruitment of E2 and this is sufficient to abolish the ligase activity. A recent study on Arkadia’s RING domain revealed that mutation of Trp972 to arginine affected Arkadia’s interaction interface and abolished the E3 ligase function (Birkou et al., 2017). Another example is the mutation of Phe964 in the Arkadia RING domain. This phenylalanine is conserved in RING-H2 ligases and precedes the fifth zinc binding residue. Mutation of F964 to alanine (Ala, A) changed the folding of the RING domain (Figure 13A), which resulted in the disruption of E2-E3 interaction (Figure 13B).
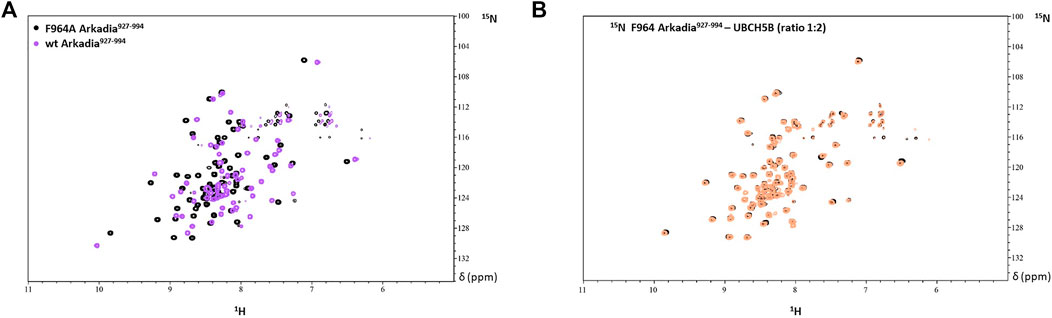
FIGURE 13. (A) Overlay of the 1H-15N HSQC spectrum of F964A and wt Arkadia RING domain (black and purple, respectively). (B) Overlay of the 1H-15N HSQC spectrum of F964A before (black) and after (orange) the addition of UBCH5B to a 1:2 ratio.
The auto-ubiquitination assay revealed R957C mutation is a gain of function mutation that enhances the enzymatic activity of the RING (Figure 7), and consistent to this, the luciferase assays shows that the R957C mutation exhibits slightly increased activity of Arkadia although it is not statistically significant here (Figure 8). The TGF-β pathway has tumor suppressive properties in normal tissues and promotes metastasis within tumors. Similarly, Arkadia enhances TGF-β signaling and exhibit the same bidiretional properties (Sharma et al., 2011; Briones-Orta et al., 2013). Indeed, Sharma et al., 2011 has shown that reduction of Arkadia in normal cells increases significantly the susceptibility to cancer. Furthermore, Le Scolan et al., 2008 and Briones-Orta et al., 2013 performing loss of function experiments in tumor cell lines have shown that Arkadia supports metastatic phenotypes via a TGF-β dependent manner. Our data presented here support the pro-tumorigenic functions of Arkadia in a context-cell type-dependent manner because it was found enriched in tumor compared to the adjacent normal tissue. However, it is possible that the presence of the R957C mutation in the adjacent normal cells represent infiltration of a metastatic tumor cells and not a polymorphism. More experiments are necessary to verify the role of this hyperactive mutant Arkadia within tumor cells.
Conclusion
Analysis of the consequences of SNPs on the 3D protein structure is beneficial in understanding both their function and their role in diseases. SNPs at the level of proteins usually affect their activity, binding/association, assembly, and rearrangement and promote their aggregation (Bhattacharya et al., 2017). Most studies analyzing the effects of SNPs on the 3D protein structure are restricted to specific diseases. The goal of this study is to provide experimental data about an SNP that was found in CRC and adjacent normal tissue samples of a patient and its effects on structure, stability, binding properties, and function of the Arkadia RING domain. Our NMR structural data show that the R957C mutation, in the β1 strand of this domain is located far away from the two Zn (II) binding motifs. It does not interfere with the metal binding and does not alter the geometry of the cross-brace metal coordination; moreover, it does not change the conformation of the E2 docking surface of the RING domain. The R957C mutation does not lead to conformational change in the β1−β2 surface, so no significant change of the overall conformational features of the RING domain is observed, and the same holds for the backbone dynamics of the RING core. Finally, the functional assays (Figures 7, 8) also showed that Arkadia does not lose its E3 ligase activity, despite the R957C mutation. It is worth mentioning that auto-ubiquitination assays indicated a gain of function effect of the R957C mutation of Arkadia, while the luciferase functional assay showed milder hyperactivity. The finding that this SNP was present in normal tissue and enriched within the tumor, suggests that it segrates with the tumor and possibly with infiltration of adjacent tissue by metastatic tumor cells.
Data Availability Statement
The datasets presented in this study can be found in online repositories. The names of the repository/repositories and accession number(s) can be found in the article/Supplementary Material.
Author Contributions
Conceptualization: VE, GS, Methodology: MB, KM, VR, and AT, Validation: MB, KM, KB, DB, VE, and GS, Writing-original draft preparation: MB, Writing-review and editing: KM, DB, VE, and GS, Resources: GS. All authors reviewed the results and approved the final version of the paper.
Funding
This research is co-financed by Greece and the European Union (European Social Fund—ESF) through the Operational Programme «Human Resources Development, Education and Lifelong Learning» in the context of the project “Reinforcement of Postdoctoral Researchers—2nd Cycle” (MIS5033021), implemented by the State Scholarships Foundation (ΙΚΥ). The work was also supported by the INSPIRED (MIS 5002550) which is implemented under the Action “Reinforcement of the Research and Innovation Infrastructure,” funded by the Operational Program “Competitiveness, Entrepreneurship and Innovation” (NSRF 2014–2020) and co-financed by Greece and the European Union (European Regional Development Fund).
Conflict of Interest
The authors declare that the research was conducted in the absence of any commercial or financial relationships that could be construed as a potential conflict of interest.
Publisher’s Note
All claims expressed in this article are solely those of the authors and do not necessarily represent those of their affiliated organizations, or those of the publisher, the editors and the reviewers. Any product that may be evaluated in this article, or claim that may be made by its manufacturer, is not guaranteed or endorsed by the publisher.
Acknowledgments
EU FP7 REGPOT CT-2011-285950—“SEE-DRUG” project is acknowledged for the purchase of UPAT’s 700 MHz NMR equipment. State Scholarships Foundation-MIS 5033021 and INSPIRED-MIS 5002550 are acknowledged for the financial support.
Supplementary Material
The Supplementary Material for this article can be found online at: https://www.frontiersin.org/articles/10.3389/fmolb.2022.844129/full#supplementary-material
References
Baker, K. A., Hilty, C., Peti, W., Prince, A., Pfaffinger, P. J., Wider, G., et al. (2006). NMR-derived Dynamic Aspects of N-type Inactivation of a Kv Channel Suggest a Transient Interaction with the T1 Domain. Biochemistry 45, 1663–1672. doi:10.1021/bi0516430
Bartels, C., Xia, T.-h., Billeter, M., Güntert, P., and Wüthrich, K. (1995). The Program XEASY for Computer-Supported NMR Spectral Analysis of Biological Macromolecules. J. Biomol. NMR 6, 1–10. doi:10.1007/bf00417486
Bhattacharya, R., Rose, P. W., Burley, S. K., and Prlić, A. (2017). Impact of Genetic Variation on Three Dimensional Structure and Function of Proteins. PLoS One 12 (3), e0171355. doi:10.1371/journal.pone.0171355
Birkou, M., Chasapis, C. T., Marousis, K. D., Loutsidou, A. K., Bentrop, D., Lelli, M., et al. (2017). A Residue Specific Insight into the Arkadia E3 Ubiquitin Ligase Activity and Conformational Plasticity. J. Mol. Biol. 429, 2373–2386. doi:10.1016/j.jmb.2017.06.012
Bravou, V., Antonacopoulou, A., Papadaki, H., Floratou, K., Stavropoulos, M., Episkopou, V., et al. (2009). TGF-β Repressors SnoN and Ski Are Implicated in Human Colorectal Carcinogenesis. Anal. Cell Pathol. 31, 41–51. doi:10.3233/clo-2009-046010.1155/2009/975602
Briones-Orta, M. A., Levy, L., Madsen, C. D., Das, D., Erker, Y., Sahai, E., et al. (2013). Arkadia Regulates Tumor Metastasis by Modulation of the TGF-β Pathway. Cancer Res. 73, 1800–1810. doi:10.1158/0008-5472.CAN-12-1916
Brzovic, P. S., Keeffe, J. R., Nishikawa, H., Miyamoto, K., Fox, D., Fukuda, M., et al. (2003). Binding and Recognition in the Assembly of an Active BRCA1/BARD1 Ubiquitin-Ligase Complex. Proc. Natl. Acad. Sci. 100 (10), 5646–5651. doi:10.1073/pnas.0836054100
Brzovic, P. S., Rajagopal, P., Hoyt, D. W., King, M.-C., and Klevit, R. E. (2001). Structure of a BRCA1-BARD1 Heterodimeric RING-RING Complex. Nat. Struct. Biol. 8, 833–837. doi:10.1038/nsb1001-833
Buetow, L., Gabrielsen, M., Anthony, N. G., Dou, H., Patel, A., Aitkenhead, H., et al. (2015). Activation of a Primed RING E3-E2-Ubiquitin Complex by Non-covalent Ubiquitin. Mol. Cel 58 (2), 297–310. doi:10.1016/j.molcel.2015.02.017
Capriotti, E., Calabrese, R., Fariselli, P., Martelli, P., Altman, R. B., and Casadio, R. (2013). WS-SNPs&GO: a Web Server for Predicting the Deleterious Effect of Human Protein Variants Using Functional Annotation. BMC Genomics 14, S6–S7. doi:10.1186/1471-2164-14-S3-S6
Capriotti, E., Fariselli, P., and Casadio, R. (2005). I-Mutant2.0: Predicting Stability Changes upon Mutation from the Protein Sequence or Structure. Nucleic Acids Res. 33, W306–W310. doi:10.1093/nar/gki375
Chan, C.-H., Morrow, J. K., Li, C.-F., Gao, Y., Jin, G., Moten, A., et al. (2013). Pharmacological Inactivation of Skp2 SCF Ubiquitin Ligase Restricts Cancer Stem Cell Traits and Cancer Progression. Cell 154, 556–568. doi:10.1016/j.cell.2013.06.048
Chasapis, C. T., Kandias, N. G., Episkopou, V., Bentrop, D., and Spyroulias, G. A. (2012). NMR-based Insights into the Conformational and Interaction Properties of Arkadia RING-H2 E3 Ub Ligase. Proteins 80, 1484–1489. doi:10.1002/prot.24048
Deshaies, R. J., and Joazeiro, C. A. P. (2009). RING Domain E3 Ubiquitin Ligases. Annu. Rev. Biochem. 78, 399–434. doi:10.1146/annurev.biochem.78.101807.093809
Dosset, P., Hus, J.-C., Blackledge, M., and Marion, D. (2000). Efficient Analysis of Macromolecular Rotational Diffusion from Heteronuclear Relaxation Data. J. Biomol. NMR 16, 23–28. doi:10.1023/a:1008305808620
Erker, Y., Neyret-Kahn, H., Seeler, J. S., Dejean, A., Atfi, A., and Levy, L. (2013). Arkadia, a Novel SUMO-Targeted Ubiquitin Ligase Involved in PML Degradation. Mol. Cel Biol 33 (11), 2163–2177. doi:10.1128/MCB.01019-12
Ferentz, A. E., and Wagner, G. (2000). NMR Spectroscopy: A Multifaceted Approach to Macromolecular Structure. Quart. Rev. Biophys. 33, 29–65. doi:10.1017/s0033583500003589
Fiala, C., and Diamandis, E. P. (2020). Mutations in normal Tissues-Some Diagnostic and Clinical Implications. BMC Med. 18, 1–9. doi:10.1186/s12916-020-01763-y
Freemont, P. S. (1993). The RING Finger. Ann. NY Acad. Sci. 684, 174–192. doi:10.1111/j.1749-6632.1993.tb32280.x
Garrett, D. S., Seok, Y.-J., Peterkofsky, A., Clore, G. M., and Gronenborn, A. M. (1997). Identification by NMR of the Binding Surface for the Histidine-Containing Phosphocarrier Protein HPr on the N-Terminal Domain of Enzyme I of the Escherichia coli Phosphotransferase System. Biochemistry 36, 4393–4398. doi:10.1021/bi970221q
Gundogdu, M., and Walden, H. (2019). Structural Basis of Generic versus Specific E2-RING E3 Interactions in Protein Ubiquitination. Protein Sci. 28, 1758–1770. doi:10.1002/pro.3690
Güntert, P., Mumenthaler, C., and Wüthrich, K. (1997). Torsion Angle Dynamics for NMR Structure Calculation with the New Program DYANA. J. Mol. Biol. 273 (1), 283–298. doi:10.1006/jmbi.1997.1284
Hershko, A., Heller, H., Elias, S., and Ciechanover, A. (1983). Components of Ubiquitin-Protein Ligase System. Resolution, Affinity Purification, and Role in Protein Breakdown. J. Biol. Chem. 258 (13), 8206–8214. doi:10.1016/s0021-9258(20)82050-x
Keller, R. (2004). The Computer Aided Resonance Assignment Tutorial. 1st Edition. Goldau, Switzerland: CANTINA Verlag.
Kornhaber, G. J., Snyder, D., Moseley, H. N. B., and Montelione, G. T. (2006). Identification of Zinc-Ligated Cysteine Residues Based on 13Cα and 13Cβ Chemical Shift Data. J. Biomol. NMR 34, 259–269. doi:10.1007/s10858-006-0027-5
Kumar, A., Chaugule, V. K., Condos, T. E. C., Barber, K. R., Johnson, C., Toth, R., et al. (2017). Parkin-phosphoubiquitin Complex Reveals Cryptic Ubiquitin-Binding Site Required for RBR Ligase Activity. Nat. Struct. Mol. Biol. 24, 475–483. doi:10.1038/nsmb.3400
Lakshmanan, M., Bughani, U., Duraisamy, S., Diwan, M., Dastidar, S., and Ray, A. (2008). Molecular Targeting of E3 Ligases - a Therapeutic Approach for Cancer. Expert Opin. Ther. Targets 12 (7), 855–870. doi:10.1517/14728222.12.7.855
Le Scolan, E., Zhu, Q., Wang, L., Bandyopadhyay, A., Javelaud, D., and Mauviel, A. (2008). Transforming Growth Factor-Beta Suppresses the Ability of Ski to Inhibit Tumor Metastasis by Inducing its Degradation. Cancer Res. 68 (9), 3277–85. doi:10.1158/0008-5472.CAN-07-6793
Levy, L., Howell, M., Das, D., Harkin, S., Episkopou, V., and Hill, C. S. (2007). Arkadia Activates Smad3/Smad4-dependent Transcription by Triggering Signal-Induced SnoN Degradation. Mol. Cel. Biol. 27 (17), 6068–6083. doi:10.1128/MCB.00664-07
Marousis, K. D., Tsika, A. C., Birkou, M., Matsoukas, M.-T., and Spyroulias, G. A. (2018). Lead Identification through the Synergistic Action of Biomolecular NMR and In Silico Methodologies. Methods Mol. Biol. 1824, 299–316. doi:10.1007/978-1-4939-8630-9_18
Mavrakis, K. J., Andrew, R. L., Lee, K. L., Petropoulou, C., Dixon, J. E., Navaratnam, N., et al. (2007). Arkadia Enhances Nodal/TGF-β Signaling by Coupling Phospho-Smad2/3 Activity and Turnover. Plos Biol. 5, e67. doi:10.1371/journal.pbio.0050067
Nagano, Y., Mavrakis, K. J., Lee, K. L., Fujii, T., Koinuma, D., Sase, H., et al. (2007). Arkadia Induces Degradation of SnoN and C-Ski to Enhance Transforming Growth Factor-β Signaling. J. Biol. Chem. 282, 20492–20501. doi:10.1074/jbc.M701294200
Nelson, A. C., and Holt, J. T. (2010). Impact of RING and BRCT Domain Mutations on BRCA1 Protein Stability, Localization and Recruitment to DNA Damage. Radiat. Res. 174, 1–13. doi:10.1667/RR1290.1
Oh, J.-H., and Sung, C. O. (2020). Comprehensive Characteristics of Somatic Mutations in the normal Tissues of Patients with Cancer and Existence of Somatic Mutant Clones Linked to Cancer Development. J. Med. Genet. 58, 433–441. doi:10.1136/jmedgenet-2020-106905
Pearlman, D. A., Case, D. A., Caldwell, J. W., Ross, W. S., Cheatham, T. E., DeBolt, S., et al. (1995). AMBER, a Package of Computer Programs for Applying Molecular Mechanics, normal Mode Analysis, Molecular Dynamics and Free Energy Calculations to Simulate the Structural and Energetic Properties of Molecules. Comp. Phys. Commun. 91, 1–41. doi:10.1016/0010-4655(95)00041-d
Pickart, C. M. (2001). Mechanisms Underlying Ubiquitination. Annu. Rev. Biochem. 70, 503–533. doi:10.1146/annurev.biochem.70.1.503
Poulsen, S. L., Hansen, R. K., Wagner, S. A., van Cuijk, L., van Belle, G. J., Streicher, W., et al. (2013). RNF111/Arkadia Is a SUMO-Targeted Ubiquitin Ligase that Facilitates the DNA Damage Response. J. Cel Biol. 201 (6), 797–807. doi:10.1083/jcb.201212075
Ramensky, V., Bork, P., and Sunyaev, S. (2002). Human Non-synonymous SNPs: Server and Survey. Nucleic Acids Res. 30 (17), 3894–3900. doi:10.1093/nar/gkf493
Risques, R. A., and Kennedy, S. R. (2018). Aging and the Rise of Somatic Cancer-Associated Mutations in normal Tissues. Plos Genet. 14, e1007108. doi:10.1371/journal.pgen.1007108
Rosen, E. M. (2013). BRCA1 in the DNA Damage Response and at Telomeres. Front. Genet. 4, 1–14. doi:10.3389/fgene.2013.00085
Ruffner, H., Joazeiro, C. A. P., Hemmati, D., Hunter, T., and Verma, I. M. (2001). Cancer-predisposing Mutations within the RING Domain of BRCA1: Loss of Ubiquitin Protein Ligase Activity and protection from Radiation Hypersensitivity. Proc. Natl. Acad. Sci. 98 (9), 5134–5139. doi:10.1073/pnas.081068398
Saurin, A. J., Borden, K. L. B., Boddy, M. N., and Freemont, P. S. (1996). Does This Have a Familiar RING? Trends Biochem. Sci. 21 (6), 208–214. doi:10.1016/s0968-0004(96)80017-x
Shangary, S., and Wang, S. (2008). Targeting the MDM2-P53 Interaction for Cancer Therapy. Clin. Cancer Res. 14, 5318–5324. doi:10.1158/1078-0432.CCR-07-5136
Sharma, D., and Rajarathnam, K. (2000). 13C Nmr Chemical Shifts Can Predict Disulfide Bond Formation. J. Biomol. NMR 18, 165–171. doi:10.1023/a:1008398416292
Sharma, V., Antonacopoulou, A. G., Tanaka, S., Panoutsopoulos, A. A., Bravou, V., Kalofonos, H. P., et al. (2011). Enhancement of TGF-β Signaling Responses by the E3 Ubiquitin Ligase Arkadia Provides Tumor Suppression in Colorectal Cancer. Cancer Res. 71, 6438–6449. doi:10.1158/0008-5472.CAN-11-1645
Shastry, B. S. (2007). SNPs in Disease Gene Mapping, Medicinal Drug Development and Evolution. J. Hum. Genet. 52, 871–880. doi:10.1007/s10038-007-0200-z
Sun, Y. (2006). E3 Ubiquitin Ligases as Cancer Targets and Biomarkers. Neoplasia 8, 645–654. doi:10.1593/neo.06376
Wang, D., Ma, L., Wang, B., Liu, J., and Wei, W. (2017). E3 Ubiquitin Ligases in Cancer and Implications for Therapies. Cancer Metastasis Rev. 36, 683–702. doi:10.1007/s10555-017-9703-z
Zhang, X., Chen, J., Wu, M., Wu, H., Arokiaraj, A. W., Wang, C., et al. (2013). Structural Basis for Role of Ring finger Protein RNF168 RING Domain. Cell Cycle 12, 312–321. doi:10.416/Fcc.23104
Keywords: Arkadia, snps, NMR, RING, E3 ligase
Citation: Birkou M, Raptis V, Marousis KD, Tsevis A, Bourikas K, Bentrop D, Episkopou V and Spyroulias GA (2022) Impact of a Single Nucleotide Polymorphism on the 3D Protein Structure and Ubiquitination Activity of E3 Ubiquitin Ligase Arkadia. Front. Mol. Biosci. 9:844129. doi: 10.3389/fmolb.2022.844129
Received: 27 December 2021; Accepted: 31 January 2022;
Published: 23 February 2022.
Edited by:
Piero Andrea Temussi, University of Naples Federico II, ItalyReviewed by:
Christopher Berndsen, James Madison University, United StatesChi-Fon Chang, Academia Sinica, Taiwan
Copyright © 2022 Birkou, Raptis, Marousis, Tsevis, Bourikas, Bentrop, Episkopou and Spyroulias. This is an open-access article distributed under the terms of the Creative Commons Attribution License (CC BY). The use, distribution or reproduction in other forums is permitted, provided the original author(s) and the copyright owner(s) are credited and that the original publication in this journal is cited, in accordance with accepted academic practice. No use, distribution or reproduction is permitted which does not comply with these terms.
*Correspondence: Georgios A. Spyroulias, Ry5BLlNweXJvdWxpYXNAdXBhdHJhcy5ncg==; Vasso Episkopou, dmFzc28uZXBpc2tvcG91QGltcGVyaWFsLmFjLnVr
†These authors have contributed equally to this work