- 1Gastroenterology Department, Institut d’Investigacions Biomèdiques August Pi i Sunyer (IDIBAPS), Centro de Investigación Biomédica en Red de Enfermedades Hepáticas y Digestivas (CIBERehd), Hospital Clínic, Barcelona, Spain
- 2Department of Cellular and Molecular Medicine and Department of Bioengineering and Moores Cancer Center, UC San Diego, La Jolla, CA, United States
- 3Inflammatory Bowel Disease Unit, Gastroenterology Department, Institut d'Investigacions Biomèdiques August Pi i Sunyer (IDIBAPS) Centro de Investigación Biomédica en Red de Enfermedades Hepáticas y Digestivas (CIBERehd), Hospital Clínic, Barcelona, Spain
- 4Pathology Department, Institut d’Investigacions Biomèdiques August Pi i Sunyer (IDIBAPS), Centro de Investigación Biomédica en Red de Enfermedades Hepáticas y Digestivas (CIBERehd) and Tumor Bank-Biobank, Hospital Clínic, Barcelona, Spain
Serrated polyposis syndrome (SPS) is one of the most frequent polyposis syndromes characterized by an increased risk for developing colorectal cancer (CRC). Although SPS etiology has been mainly associated with environmental factors, germline predisposition to SPS could also be relevant for cases with familial aggregation or a family history of SPS/CRC. After whole-exome sequencing of 39 SPS patients from 16 families, we identified a heterozygous germline frameshift variant in the POLD1 gene (c.1941delG, p.(Lys648fs*46)) in a patient with SPS and CRC. Tumor presented an ultra-hypermutated phenotype and microsatellite instability. The POLD1 germline variant segregated in three additional SPS-affected family members. We attempted to create yeast and cellular models for this variant but were no viable. Alternatively, we generated patient-derived organoids (PDOs) from healthy rectal tissue of the index case, as well as from a control donor. Then, we challenged PDOs with a DNA-damaging agent to induce replication stress. No significant differences were observed in the DNA damage response between control and POLD1-Lys648fs PDOs, nor specific mutational signatures were observed. Our results do not support the pathogenicity of the analyzed POLD1 frameshift variant. One possible explanation is that haplosufficiency of the wild-type allele may be compensating for the absence of expression of the frameshift allele. Overall, future work is required to elucidate if functional consequences could be derived from POLD1 alterations different from missense variants in their proofreading domain. To our knowledge, our study presents the first organoid model for germline POLD1 variants and establishes the basis for its use as a model for disease in SPS, CRC and other malignancies.
1 Introduction
Serrated polyposis syndrome (SPS) is a clinically defined syndrome characterized by multiple serrated polyps in the colon and rectum as well as an increased risk for developing colorectal cancer (CRC) (Carballal et al., 2022; Muller et al., 2022). Traditionally, serrated polyps were often missed during colonoscopy, and there was a lack of awareness regarding their malignancy potential as precursor lesions of CRC. Currently, SPS is the most common colorectal polyposis syndrome, although its underlying causes are still unclear. SPS has been mainly associated with environmental factors such as smoking and alcohol consumption (Bailie et al., 2017). However, it has also been hypothesized that SPS likely represents a spectrum of disease influenced by genetic and environmental factors (Mankaney et al., 2020). Germline predisposition to SPS could be relevant for those cases with familial aggregation or a family history of either SPS or CRC.
Studies attempting to identify the genetic basis of SPS have provided inconsistent data so far. RNF43 is the gene with more robust evidence of being involved in SPS predisposition (Gala et al., 2014; Taupin et al., 2015; Yan et al., 2017; Mikaeel et al., 2022; Murphy et al., 2022), although its association is quite controversial and is now believed to be a minor germline cause of SPS (Buchanan et al., 2017; Quintana et al., 2018). Polyposis-related genes are not commonly altered in SPS patients (Clendenning et al., 2013); but some isolated SPS cases harbor putatively pathogenic variants in MUTYH (Chow et al., 2006; Boparai et al., 2008; Murphy et al., 2022), SMAD4, CHEK2 and POLD1 (Murphy et al., 2022). Our research group has conducted germline sequencing analyses and has proposed new potential high-penetrance genes (Toma et al., 2020; Soares de Lima et al., 2021; 2022) and low-penetrance variants (Arnau-Collell et al., 2020) that could be associated with SPS susceptibility.
The high-fidelity polymerases epsilon (POLE) and delta (POLD1) have a selective polymerase active site coupled with a proofreading exonuclease domain, essential for an effective DNA repair activity during DNA replication. The alteration of their proofreading activity results in the accumulation of mutations throughout the genome, which leads to a high mutational burden and specific mutational signatures (termed, COSMIC signatures SBS10a-d) that can be identified by whole-exome or whole-genome sequencing (Alexandrov et al., 2020; Robinson et al., 2021). Germline mutations in the proofreading domains of POLE and POLD1 predispose to colorectal adenomas and adenocarcinomas (Palles et al., 2013) but also to extra-intestinal neoplasia such as ovarian, endometrial and brain tumors (Valle et al., 2019; Magrin et al., 2021). POLE and POLD1 can also be somatically mutated in colorectal tumors and, sometimes, mutations in these polymerases can appear in combination with deficiencies in DNA mismatch repair (Haradhvala et al., 2018). Other pathogenic POLE/POLD1 germline variants, either affecting the catalytic domain or intronic regions, have been associated with growth restriction and multisystem disorders such as IMAGe (intrauterine growth restriction, metaphyseal dysplasia, adrenal hypoplasia congenita and genitourinary abnormalities), FILS (facial dysmorphism, immunodeficiency, livedo and short stature), MDP (mandibular hypoplasia, deafness, progeroid features), or Werner syndrome (Schmit and Bielinsky, 2021).
Organoid technology has revolutionized cancer modeling, and it corresponds to a huge step forward for the study of tumor initiation and cancer progression (Lau et al., 2020). Both patient-derived organoids (PDO), which retain the original mutational background of the patient, or genetically-engineered organoids, hold great promise for hereditary cancers and comprehension of syndromes such as SPS. For instance, pathogenicity for variants in BRAF (Fessler et al., 2016) and RNF43 (Yan et al., 2017; Fang et al., 2022; Yamamoto et al., 2022) has been functionally assessed in these in vitro models. Also, the serrated pathway of carcinogenesis has been reproduced in organoids by sequential editing approaches (Lannagan et al., 2018; Kawasaki et al., 2020).
In this study, we have combined whole-exome sequencing with organoid modeling to assess the impact of a germline heterozygous POLD1 frameshift variant detected in a family with SPS.
2 Materials and methods
2.1 Patients and clinical samples
The SPS cohort comprised 16 families including 39 patients (≥2 patients per family) diagnosed with SPS and fulfilling the 2010 World Health Organization (WHO) criteria (Snover et al., 2010), as the new WHO guidelines released in 2019 (Rosty et al., 2019) were not available when this study was developed. The complete clinical and somatic characterization of this cohort is available at (Soares de Lima et al., 2021). The presence of germline alterations in APC, MUTYH and DNA mismatch repair (MMR) genes was discarded for all probands.
One patient (AA3531, family SPS.7, Figure 1) presented a loss-of-function variant in the POLD1 gene (c.1941delG; p.Lys648fs*46). The variant co-segregated in other six family members, three of them affected with SPS (Figure 1; Supplementary Figure S1). A summary of clinical characteristics of family SPS.7 is shown in Table 1.
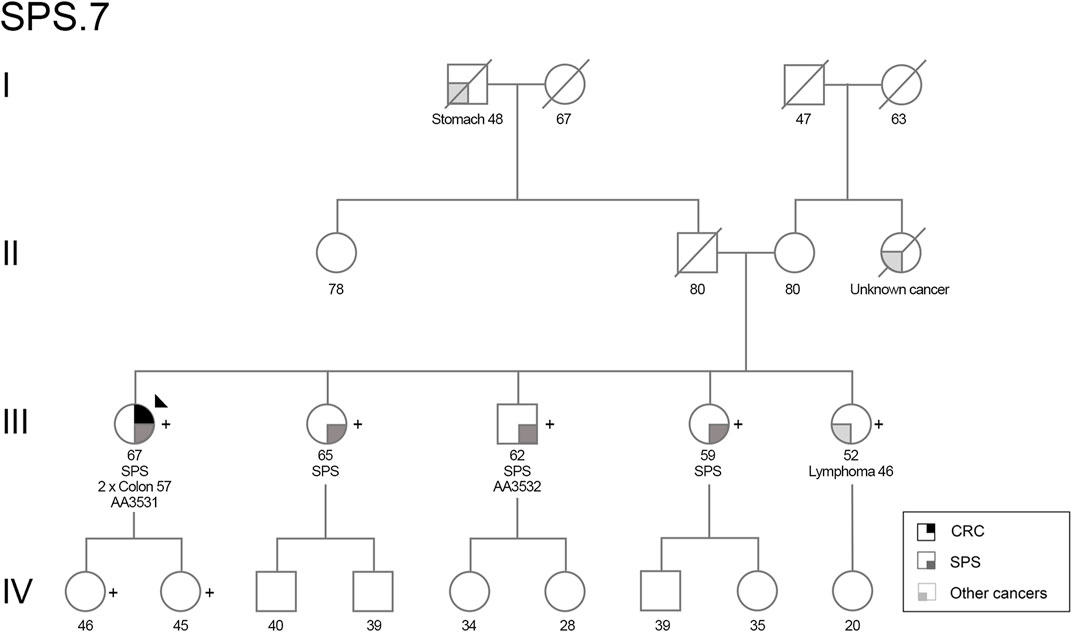
FIGURE 1. Pedigree of family SPS.7. Filled symbol indicates affected for CRC (upper right quarter), SPS (lower right quarter) or other types of cancer (lower left quarter). Stomach, colon and lymphoma refer to the type of cancer. The proband is indicated by an arrow, and POLD1 p.Lys648fs*46 variant carriers are indicated by (+). Ages at diagnosis are depicted. CRC, colorectal cancer; SPS, serrated polyposis syndrome.
The index case (III.1) was affected with 2 synchronous CRC at age 57 as well as more than 100 serrated polyps, and important proportion of them having a large size (>20 mm). Individual III.5 was diagnosed at 46 y. o. with MALT lymphoma (affecting gastrointestinal tract, breast and lung). A familial history of cancer was present with a paternal grandfather affected with stomach cancer at 48 y. o. and a maternal aunt diagnosed with cancer of unknown origin at 78 y. o.
Regarding the phenotype in the affected siblings, III.2 presented 11 polyps at 56–65 y. o., corresponding to one hyperplastic polyp in the rectum (3 mm), five sessile serrated lesions proximal to the rectum (5–8 mm) and 5 T/LGD (tubular, low-grade dysplasia) adenomas (4–8 mm) distributed all over her colon. III.3 presented 20 polyps <1 cm at 53–60 y. o., including 5 serrated polyps proximal to the rectum (5–6 mm), being four hyperplastic polyps and one sessile serrated lesion without dysplasia, and 15 T/LGD adenomas (<1 cm). III.4 presented 11 polyps at 49–57 y. o., comprising four serrated polyps being two sessile serrated lesions proximal to the rectum (>5 mm, <1 cm), and 7 T/LGD adenomas (one 1 cm at rectum, the rest <1 cm).
The study received the approval of Hospital Clínic de Barcelona Clinical Research Ethics committee (registration number 2013/8286). Written informed consent was obtained in all cases.
2.2 Whole-exome sequencing, variant identification and prioritization
Details on germline and tumoral whole-exome sequencing, quality control and alignment, variant calling and variant annotation have been already described in (Soares de Lima et al., 2021).
2.3 DNA extraction and amplification
Germline DNA and cell lines’ DNA was extracted using the QIAamp DNA Blood kit (Qiagen, Redwood City, CA, United States). Somatic DNA was obtained from formalin-fixed paraffin-embedded tissue using the QIAamp Tissue kit (Qiagen, Redwood City, CA, United States). POLD1 variant validation was performed by PCR amplification using the GC-Rich PCR System (Roche, Basel, Switzerland) followed by Sanger sequencing (Eurofins Genomics).
2.4 Somatic characterization
2.4.1 Microsatellite instability
Tumor MMR deficiency (MMRd) was evaluated by immunohistochemical staining of the four mismatch repair proteins (MLH1, MSH2, MSH6 and PMS2). MSI (microsatellite instability) status was assessed by analyzing five nearly monomorphic mononucleotide microsatellite loci (BAT-25, BAT-26, NR-21, NR-24, and MONO-27; Promega, Madison, WI).
DNA methylation status of the MLH1 promoter region was established by bisulfite genomic sequencing, as previously described (Moreira et al., 2015).
2.4.2 POLD1 immunohistochemistry
Immunostainings were performed on histological 2-μm sections. After deparaffination, antigen retrieval was performed with citrate buffer 10 mM, and tissue was permeabilized with 1% Triton X-100. Peroxidase activity was blocked with 3% hydrogen peroxide. Sections were treated for 1 h with Dako serum-free protein blocker (Agilent, Santa Clara, CA), incubated for 16 h with anti-POLD1 antibody (EPR15118, #ab186407, Abcam) diluted 1:500, and for 1 h with goat anti-rabbit secondary antibody at 37°C (Dako REAL EnVision HRP Rabbit; Agilent). Sections were revealed with diaminobenzidine for 10 s (Agilent), counterstained with hematoxylin and mounted. An Olympus BX41 microscope (Olympus, Tokyo, Japan) was used to visualize the immunostainings.
2.4.3 Loss of heterozygosity
Loss of heterozygosity (LOH) was tested by comparing germline-tumoral Sanger sequencing results of the same individual. Additionally, microsatellites mapping close to POLD1 (D19S866, D19S904, D19S246, D19S907) were assessed by PCR. Primer details are listed in Supplementary Table S1.
2.4.4 Mutational signatures
Assignment of reference mutational signatures was performed using our bioinformatics tool SigProfilerAssignment v0.0.14 (https://github.com/AlexandrovLab/SigProfilerAssignment/) (Islam et al., 2022) based on COSMIC mutational signatures v3.3 (GRCh37 genome build) (Tate et al., 2019).
2.5 RNA extraction from blood
Whole blood was collected into PAXgene Blood RNA tubes (PreAnalytiX, Hombrechtikon, Switzerland), and automated purification of total RNA was performed using the QIAcube and the PAXgene Blood RNA Kit (Qiagen), according to the manufacturer’s protocol.
Total RNA from cultured cells was isolated with the RNeasy Mini Kit, according to the manufacturer’s instructions (Qiagen, Hilden, Germany).
2.6 PBMCs isolation
Peripheral Blood Mononuclear Cells (PBMCs) were isolated from whole blood by density gradient centrifugation (Ficoll® Paque, GE Healthcare Life Sciences). Ten ml of whole blood were mixed 1:1 with PBS, layered on top of 3 ml of density gradient media, and separated by centrifugation at 1800 rpm for 25 min at room temperature with the brake turned off. After recovering the buffy coat, PBMCs were washed three times with cold PBS. Pelleted PBMCs were finally cryopreserved for subsequent protein expression testing.
2.7 Quantitative real time-PCR
RNA reverse transcription was performed with the High-Capacity cDNA reverse Transcription kit (Applied Biosystems). Quantitative PCR was run on a QuantStudio1 System (Applied Biosystems) by using Taqman® Gene Expression probes against POLD1-FAM (Hs01100821_m1) and GAPDH-VIC (4326317E), the latter for normalization purposes. Relative quantification was performed with the –∆∆Ct method.
2.8 Protein extraction and immunoblotting
Pelleted PBMCs or cultured cells were lysed with RIPA buffer solution (Sigma-Aldrich, MA, United States) supplemented with cOmplete Protease Inhibitor Cocktail and PhosSTOP (Roche, Basel, Switzerland).
Protein extracts were run in NuPAGE™ gels according to the manufacturer’s protocol (ThermoFisher, Waltham, MA) and transferred into PVDF membranes (Millipore, Bedford, MA). Blots were probed with anti-POLD1 (EPR15118, #ab186407, Abcam) or anti-GAPDH (clone 14C10, #2118, Cell Signaling) primary antibodies diluted 1:5000, followed by the incubation with the fluorescent Dylight 800 anti-rabbit secondary antibody (SA5-10036). Protein detection was carried out using Odyssey Imaging System (LI-COR, Lincoln, NE).
2.9 Cell lines
Human colorectal SW837 cells (diploid and MMR-proficient, Cat No. 91031104, ECACC, Sigma Aldrich) were cultured in RPMI-1640 medium (Gibco, Waltham, MA) supplemented with 10% fetal bovine serum. Human HEK293T cells (Cat No. CRL-3216, ATCC) were cultured in DMEM medium (Gibco, Waltham, MA) supplemented with 10% fetal bovine serum. Both cell lines were maintained under standard growth conditions (37°C, 5% CO2) and routinely tested for mycoplasma contamination using the Mycoplasma Gel Detection kit from Biotools (Madrid, Spain).
2.10 Development of a POLD1+/− cellular model by CRISPR/Cas9 gene editing
The Benchling (http://benchling.com) CRISPR tool was used to design suitable single guide RNAs (sgRNA) and homology-directed repair (HDR) templates flanking the POLD1 p.Lys648 region. Additionally, as a positive control, we designed a sgRNA and HDR template to model the POLD1 p.Leu474Pro mutation, a pathogenic founder mutation present in Spanish population (Accession ClinVar: VCV000144003.5) (Valle et al., 2014; Ferrer-Avargues et al., 2017).
sgRNA top and bottom strands were purchased from IDT (Coralville, IA) and cloned into the Esp3I site of the lentiCRISPRv2-Puro plasmid (#98290, Addgene), which also packages the Cas9 coding sequence. Each lentiCRISPRv2-POLD1 encoding vector was packaged into lentivirus by using the host cell line HEK293T and the CalPhos Mammalian Transfection kit (TakaraBio, Kusatsu, Japan). HEK293T cells were plated and co-transfected with at a 3:2:1 lentiCRISPRv2:psPAX2:pVSVG2 DNA ratio. Supernatants were harvested at two different time points (24 and 48 h after transfection), pooled, concentrated by centrifugation (15,000xg, 3 h, 4°C) and used for cell transduction in the presence of 8 μg/ml of polybrene. Infected cells were enriched by puromycin selection (1 μg/ml).
Cells with a stable expression of the sgRNA and the Cas9 protein were plated at a ratio of 400,000 cells per well in 12-well plates. Cells were transiently transfected in two consecutive rounds with a mixture of 10 pmol (500 ng) of the HDR template and 125 ng of an episomal vector for p53DD (#25989, Addgene) by using Lipofectamine 3000 reagent (ThermoFisher, Waltham, MA). The dominant negative p53DD inhibits the P53 double-strand break (DSB) response and was used to boost the DNA engineering process (Ihry et al., 2018). Cells were seeded into 96-well plates at a density of 1 cell per well and after 3 weeks, the obtained clones were screened for POLD1 gene editing by Sanger sequencing (Eurofins Genomics).
2.11 Cell viability assessment
Cell viability was determined using the colorimetric CellTiter 96® AQueous One Solution Cell Proliferation kit (Promega, Madison, WI). Either SW837 or SW837-POLD1+/− cells were seeded in 96-well plates at a density of 2500 cells per well, in triplicate. After 4 days, 20 μl of CellTiter reagent was added to each well. Plates were incubated at 37°C for 3 h and absorbance was read at 490 nm wavelength using an Epoch Microplate Spectrophotometer (BioTek, Winooski, VT).
2.12 Clonogenic assay
Single-cell suspensions were seeded at low density (400 cells per well into 12-well plates). After 16 days, colonies were fixed in cold methanol for 10 min and stained with a 0.5% crystal violet solution (Sigma Aldrich). After drying, plates were imaged on an EliSpot Reader System (AID GmbH, Strassberg, Germany) and analyzed with ImageJ (National Institutes of Health, Bethesda, MD).
2.13 Organoid culture establishment
The organoid cultures used in this study were established from normal rectum endoscopic biopsies derived from patient AA3531:III-1 (Figure 1) and a healthy donor, following already published procedures (Dotti et al., 2022).
2.14 γH2AX immunofluorescence assessment
Organoids were expanded in µ-Slide 8-well ibiTreat chambers (Ibidi, Fitchburg, WI) and treated with 200 nM camptothecin (CPT) for 24 h. As a control, some wells were left untreated. Phosphorylation of the Ser-139 residue of the histone variant H2AX (γH2AX) was assessed by immunofluorescence staining following already published protocols (Mayorgas et al., 2021), with some modifications. After the fixation step, organoids were stored in PBS overnight at 4°C, and the next day the permeabilization and blocking steps were performed as indicated. Samples were then incubated with anti-γH2AX (#ab81299, 1:750, Abcam) and EpCAM (#M0804, 1:150, Dako) primary antibodies overnight at 4°C. The next day, samples were incubated with the secondary antibodies anti-rabbit Alexa Fluor® 594 and anti-mouse Alexa Fluor® 488 (ThermoFisher, Waltham, MA), both diluted 1:500. After DAPI nuclear staining, samples were overlaid with Ibidi Mounting medium (#50001, Ibidi, Fitchburg, WI) and stored at 4°C for subsequent fluorescent microscope observation in a Zeiss LSM 880 confocal laser scanning microscope (CCiTUB optical microscopy facility, Universitat de Barcelona, Spain). Positive nuclei (γH2AX–DAPI colocalization) were counted by using the CellCounter plug-in in ImageJ software. The ratio of γH2AX-positive nuclei versus the total number of nuclei per organoid was calculated.
2.15 Organoid somatic mutational profile
Organoids were expanded in 48-well plates and treated with 200 nM CPT for 24 h. After the genotoxic challenge, organoids were cultured during a 5-day resting period to allow cells to accumulate mutations. Organoids were recovered, and DNA extraction was performed in order to assess changes in their mutational profile, as already mentioned in section 2.4.4. Somatic variant calling was performed using MuTect2 (McKenna et al., 2010) and Strelka2 (Kim et al., 2018), by only considering those variants shared by both computational tools and showing an allelic frequency above 0.20.
3 Results
3.1 Characterization of somatic mutations
Tumor MMRd testing was performed in both tumor samples from the proband. Immunostaining for the four MMR proteins confirmed loss of MLH1 and PMS2 (Figure 2A), and MSI molecular testing revealed that the tumor was indeed MSI-H, due to the alteration of three mononucleotide markers (BAT25, BAT26, Mono-27) (Figure 2B). One tumor also presented MLH1 promoter hypermethylation (25%), a likely cause for the observed MMR deficiency.
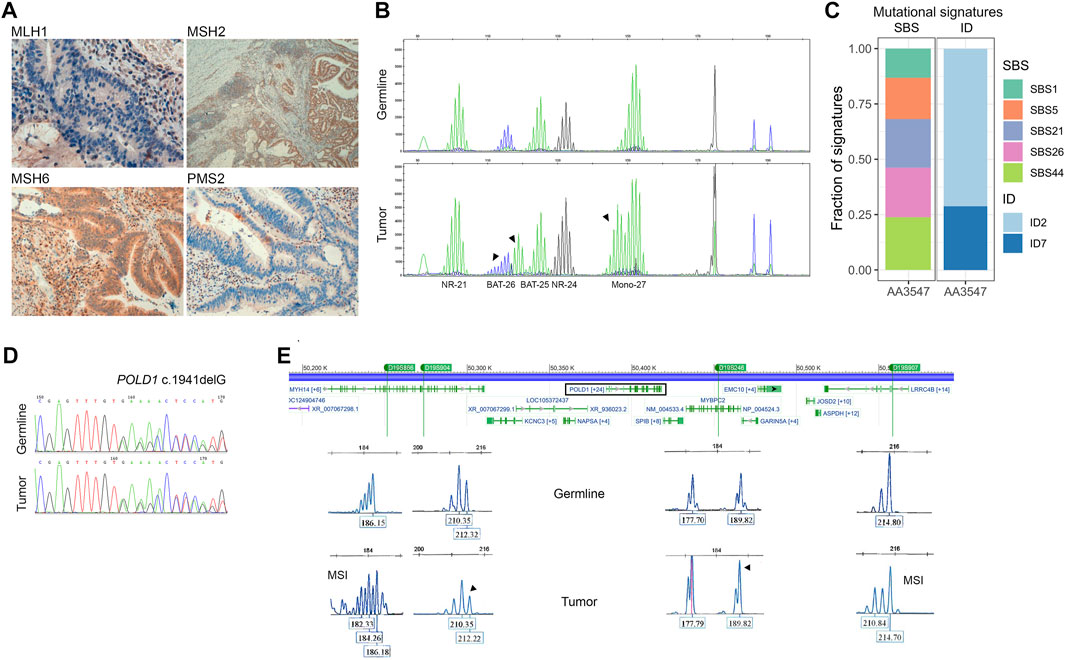
FIGURE 2. Somatic characterization of the index case. (A) Immunohistochemistry for MMR proteins showing loss of MLH1 and PMS2 expression. (B) Microsatellite instability analysis in germline DNA (upper panel) and tumor DNA (lower panel). All markers showing instability are marked with an arrow. (C) Contribution of single base substitution (SBS) and small insertions and deletions (ID) mutational signatures on a tumoral DNA sample from the proband (AA3547). (D) Loss-of-heterozygosity assessment by Sanger sequencing and by (E) PCR-amplification of four microsatellite markers close to POLD1 (rectangle). The four loci D19S866, D19S904, D19S246 and D19S907 are depicted in green. LOH is indicated by an arrow. MSI, microsatellite instability.
Germline DNA (AA3531) and somatic DNA (AA3547) from one MMRd tumor of the proband underwent whole-exome sequencing for the assessment of mutational signatures, tumor substitution mutational burden (TMB), and tumor indel mutational burden (IDB). The sample displayed the COSMIC clock-like signatures SBS1 and SBS5, and a high contribution of the already described MMRd-associated signatures SBS21, SBS26, SBS44, ID2, and ID7 (Figure 2C), in concordance with the molecular MMRd characterization of the tumor. Neither the mutational signatures associated with a defective POLD1 proofreading (SBS10c, SBS10d) nor that associated with concurrent POLD1 mutations and defective DNA mismatch repair (SBS20) were detected. However, the sample appeared to be ultra-hypermutated (TMB = 117.46 mut/Mb, IDB = 100.6 mut/Mb), which is characteristic from combined mismatch-repair deficiency and polymerase alterations.
Additionally, LOH analysis was performed by Sanger sequencing and the detection of microsatellite markers flanking POLD1. The analysis revealed a LOH of the wild-type allele (Figures 2D,E).
3.2 POLD1 variant segregation and expression analysis in the SPS.7 family
The segregation analysis revealed that the POLD1 variant c.1941delG, p.(Lys648fs*46) segregated in six additional members of the family beside the index case: four siblings (three fulfilling SPS 2010’s criteria and one diagnosed with lymphoma at age of 46) and her two healthy daughters (Figure 1; Supplementary Figure S1). The variant is located in the polymerase domain of POLD1 and it causes a frameshift, which changes a Lysine to an Arginine at codon 648, and a premature stop codon is predicted at position 46 of the new reading frame (Supplementary Figure S2A). We evaluated the germline expression of this gene at both RNA and protein levels in the identified variant carriers. We observed a decrease on POLD1 levels (Figures 3A,B), suggesting that the altered mRNA was being degraded by the non-sense mediated decay pathway rather than producing a truncated protein. Nevertheless, although the germline protein expression pattern was markedly reduced in the Western Blot, the nuclear detection of POLD1 in the proband’s tumor revealed that POLD1 expression from the wild-type allele was still evident (Figure 3C).
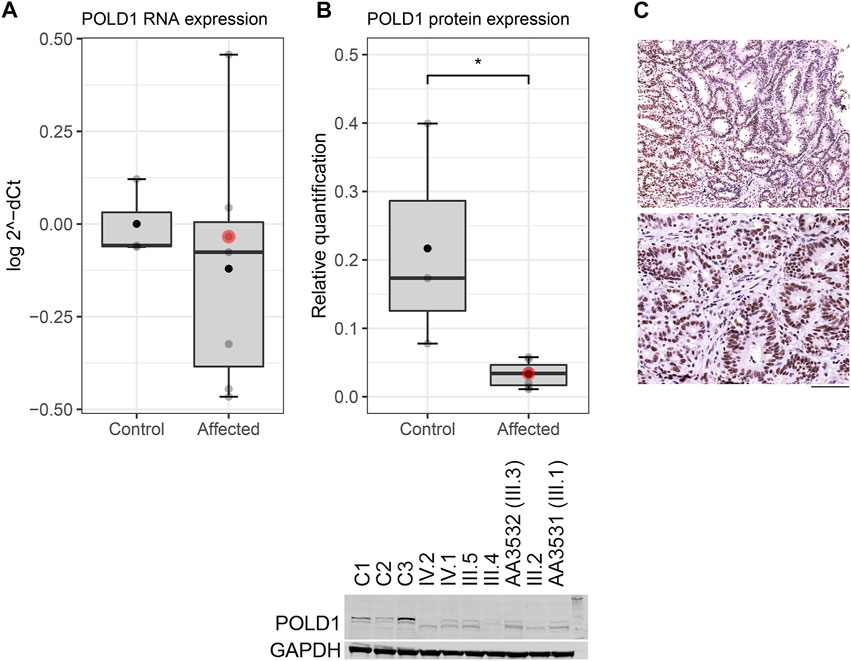
FIGURE 3. POLD1 expression in POLD1 p.Lys648fs*46 variant carriers at both (A) RNA and (B) protein levels. A significant difference of POLD1 protein expression was detected in POLD1 p.Lys648fs*46 carriers (affected) versus healthy controls (Wilcoxon test, p < 0.05). The horizontal line marks the median value, and the black dot indicates the mean value. The proband is indicated by a red dot. (C) Nuclear detection of POLD1 in two different adenocarcinoma sections from a proband’s tumor sample. Scale bars, 50 µm.
3.3 CRISPR/Cas9 generation of a POLD1+/− model
We planned to establish a POLD1 p.(Lys648fs*46) model in SW837 cells using CRISPR/Cas9 via homologous recombination. At the same time, we also intended to introduce the founder pathogenic mutation POLD1 p.Leu474Pro as a positive control. We tested three different CRISPR strategies: the transient transfection of the sgRNA and Cas9 cloned into a plasmid together with the HDR template; the transient nucleofection of the sgRNA and Cas9 as a ribonucleoprotein complex together with the HDR template; and the generation of a cellular model constitutively expressing the sgRNA and Cas9, in which the HDR template was transiently transfected. The high on-target efficiency of Cas9 and the high mortality observed in all the attempts confirmed POLD1 as an essential gene. After several targeting rounds, we failed to generate a heterozygous model encoding the POLD1 p.(Lys648fs*46) variant. However, one of the attempts with the last strategy randomly produced a clone with a different frameshift mutation and a premature stop codon (Supplementary Figure S2A). Although far from ideal, we pursued to functionally characterize this clone, termed hereafter POLD1+/− for convenience.
POLD1+/− cells showed POLD1 downregulation at both RNA and protein levels, similar to what was observed in POLD1 p.(Lys648fs*46) carriers (Supplementary Figures S2B, 2C). We next assessed whether a reduced amount of wild-type POLD1 could be important for cell viability. Cell proliferation and cell survival were not affected in POLD1+/− cells, indicating that reduced POLD1 levels did not affect cellular growth (Supplementary Figures S2D, 2E). We also challenged POLD1+/− cells with different concentrations of the DNA-damaging agent CPT, but no significant differences were observed between wild-type SW837 cells and POLD1+/− cells, suggesting that a single POLD1 functional copy allele could maintain normal function (Supplementary Figure S2F).
3.4 Patient-derived organoids
To study the specific functional consequences of POLD1 p.(Lys648fs*46) variant, we generated patient-derived organoids (PDOs) from normal rectum biopsies from the proband, which maintained the genetic background of the original tissue and, therefore, already had the loss-of-function POLD1 variant in their genome (POLD1K648fs). At the same time, PDOs from a control individual were also generated. PDOs were stimulated with CPT to evaluate the effect of POLD1 haplosufficiency in both DNA replication and DNA damage repair (Figure 4A). After the genotoxic stress challenge, we aimed to assess the amount of DNA damage by γH2AX immunofluorescence staining, as it has emerged as a highly specific and sensitive molecular marker for monitoring DNA damage initiation and resolution (Mah et al., 2010). After a 24-h treatment, organoid growth and shape were unaffected (Figure 4B). The increase in γH2AX phosphorylation upon CPT treatment was evident in both control and POLD1K648fs organoids, but no differences in the amount of γH2AX positive nuclei per organoid were observed between them (Figure 4C), indicating that reduced POLD1 expression did not alter the organoid sensitivity to CPT. We next assessed whether the CPT-induced DNA damage repair could be impaired in organoids lacking a functional copy of POLD1. After a 5-day resting period, the organoid growth arrest was evident in both control and POLD1K648fs organoids (Figure 4B). The DNA from CPT-treated organoids and their untreated counterparts was collected, and whole-exome sequencing was performed. The number of substitutions detected in the samples was very low, and the mutational profiles did not indicate any accumulation of drug-induced mutations (Figure 4D).
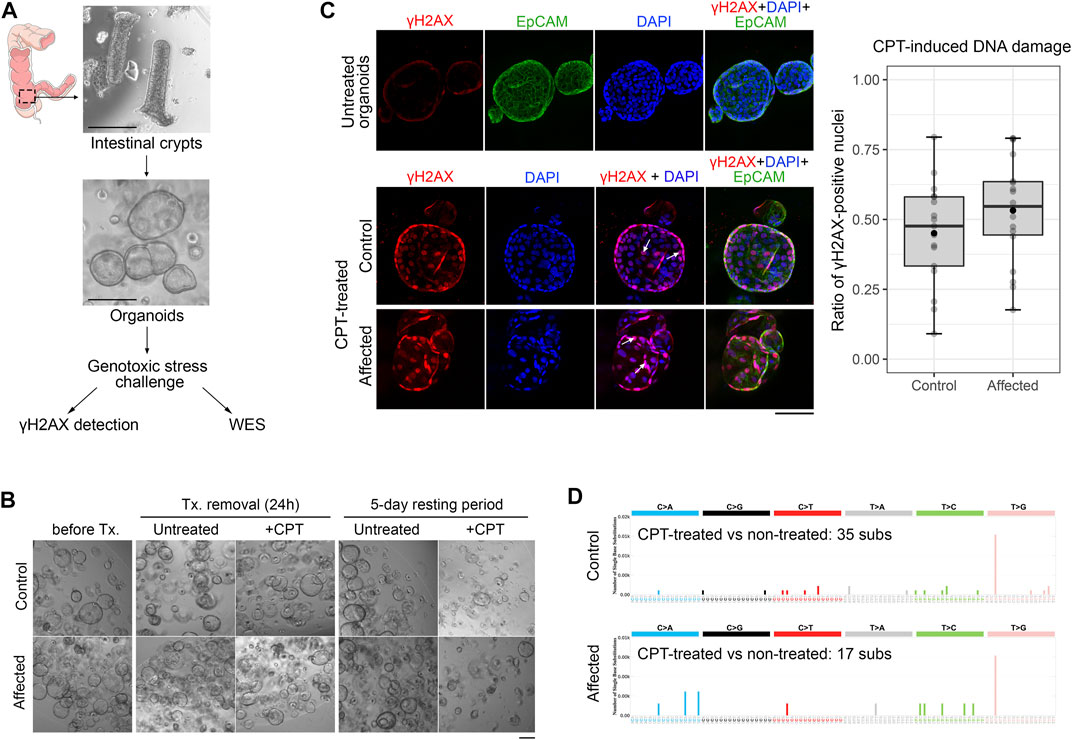
FIGURE 4. Generation of PDO and DNA damage and repair functional assessment in a POLD1 p.Lys648fs*46 carrier. (A) Overview of the PDO generation and functional characterization workflow. Endoscopic samples from the index case (POLD1 p.Lys648fs*46 carrier, Affected) and a healthy control were used to generate PDO. Organoids were subsequently exposed to CPT in order to induce genotoxic stress and evaluated for DNA damage (γH2AX) and DNA repair (WES) after a 5-day resting period. Scale bars, 200 µm. (B) Representative images of PDO from control and POLD1 p.Lys648fs*46 carrier (Affected) at different stages of the experiment. Panel scale bar, 200 µm. (C) DNA damage assessment after CPT treatment by γH2AX immunofluorescence staining. γH2AX-DAPI colocalization (white arrows) was assessed and γH2AX-positive nuclei were quantified. EpCAM counterstaining was also performed. Untreated organoids showed negligible γH2AX signal (representative image of control organoids is displayed). Panel scale bar, 100 µm. (D) DNA repair assessment by WES after CPT-treatment and a 5-day resting period. The profiles of the mutational signatures are depicted. CPT, camptothecin; Tx, treatment; WES, whole-exome sequencing.
4 Discussion
Currently, it is still controversial whether SPS’s origin could have an underlying genetic predisposition. Although smoking and other environmental exposures have been associated with SPS development, germline predisposition factors could still be relevant, especially in cases with familial aggregation.
In this study, we assessed the impact of the POLD1 p.(Lys648fs*46) variant detected by whole-exome sequencing in a proband with familiar history of SPS and cancer. Although one of the tumors from the proband displayed a MMRd profile, it also showed LOH and ultra-hypermutation, which is usually correlated with POLE/POLD1 alterations. At the same time, the detected POLD1 frameshift variant segregated in three additional family members with serrated polyposis. Therefore, the role of POLD1 p.(Lys648fs*46) alteration in the germline predisposition to SPS in this family remained to be assessed.
Several studies have focused on the effect of POLE/POLD1 germline variants located in the exonuclease domain (Elsayed et al., 2015; Bellido et al., 2016; Buchanan et al., 2018; Mur et al., 2020a; Siraj et al., 2020), but limited efforts have been made to determine the functional impact of those variants identified in the polymerase domain. Their potential pathogenicity is usually based on rarity of these variants in the general population and loss-of-function intolerance scores. Nevertheless, functionally relevant mutations could also occur outside the POLD1 exonuclease domain, leading to defects in nucleotide selectivity and error-prone polymerase activity (Daee et al., 2010; Mertza et al., 2015; Barbari and Shcherbakova, 2017). Also, a decrease in POLD1 protein levels seems to reduce the efficiency of both replicative DNA synthesis and DNA synthesis associated with DNA repair, probably due to an increased frequency of DNA polymerase slippage (Kokoska et al., 2000; Lemoine et al., 2008; Tumini et al., 2016). Since both POLD1 quantity and quality seem important for genome stability, frameshift/non-sense heterozygous variants’ functional implications could also be hypothesized.
In order to determine the functional consequences of the POLD1 p.(Lys648fs*46) variant, we proceeded to generate a suitable cellular model. Traditionally, yeast-based models have been used to assess the pathogenicity of POLD1 variants due to the high homology between the human and yeast polymerase delta catalytic subunit. We first intended to model POLD1 p.(Lys648fs*46) in Schizosaccharomyces pombe using ade6-485 reversion, a strategy we and others have previously used to validate missense variants of POLE and POLD1 (Palles et al., 2013; Esteban-Jurado et al., 2017; Mur et al., 2020a). However, POLD1 is an essential gene, and S. pombe strains are haploid, which hampers the modeling of non-sense/frameshift variants in this model. Therefore, we pursued editing S. pombe in its diploid state. After several attempts and high mortality rates, the model could not be established, as diploid strains are unstable and difficult to propagate in the laboratory.
The CRISPR/Cas9 technique offers a new approach to potentially edit any desired genome region. Nevertheless, the engineering of POLE and POLD1 in mammalian cells has been scarce and mainly performed in MMRd cell lines (Hodel et al., 2020; Job et al., 2020). In our case, the CRISPR-driven POLD1 editing was a major challenge in the MMR-proficient SW837 cell line and could not be accomplished for POLD1 p.(Lys648fs*46). The randomly obtained POLD1+/− model allowed us to corroborate that cells expressing a single POLD1 copy had reduced expression levels of POLD1 at both RNA and protein levels, similar to what was observed in POLD1 p.(Lys648fs*46) carriers. Although POLD1 reduction or depletion had been previously linked to cell cycle arrest and increased sensitivity to the DNA damaging agents CPT, hydroxyurea (Tumini et al., 2016) and methyl methane sulfonate (Kokoska et al., 2000), cellular growth and sensitivity to CPT were not altered in our cellular model.
The generation of PDOs from normal tissue of the proband allowed us to characterize the effect of the POLD1 frameshift variant on DNA damage and repair responses. POLD1K648fs and control organoids showed an equivalent DNA damage sensitivity to CPT treatment, in line with what was observed in the POLD1+/− cellular model. Also, we did not detect specific mutational signatures associated with DNA repair malfunction after a 5-day resting period. However, it should be considered that we did not perform clonal organoid culturing and expansion for a long time period, which could influence these results. Organoids have proven to be a suitable model in which mutational signatures can be associated with genetic defects. Using CRISPR-edited colon organoids, it was possible to confirm the mutational signatures associated with NTHL1 and MLH1 loss-of-function (Drost et al., 2017). Also, the mutational signatures associated with POLE/POLD1 exonuclease domain defects have been reproduced in vitro in tumor PDOs with a POLE hotspot mutation (Yan et al., 2020), POLE CRISPR-edited cells (Hodel et al., 2020) and POLD1 patient-derived fibroblasts (Andrianova et al., 2022). To our knowledge, this is the first time that POLD1 variants are functionally assessed by organoid modeling.
Altogether, our results do not support the pathogenicity of POLD1 heterozygous non-synonymous/frameshift variants. It could be hypothesized that haplosufficiency of the POLD1 wild-type allele compensates for the altered allele, a mechanism already reported in heterozygous pol3-exo− yeast mutants (Zhou et al., 2021) and heterozygous germline POLD1 p.Leu474Pro carriers (Andrianova et al., 2022). However, the origin of the ultra-hypermutated TMB detected in the proband remains elusive since only a hypermutated profile will be expected from MMRd. Neither the tumoral sample nor CPT-challenged POLD1K648fs organoids displayed signatures SBS10c-d or SBS20, linked to POLD1 exonuclease malfunction alone or in conjunction with MMRd. In a similar reported case, in which a patient with a POLE frameshift germline variant presented mainly somatic MMRd-associated signatures, it could not be concluded whether the identified variant increased colorectal cancer predisposition (Yamaguchi et al., 2019; Mur et al., 2020b; Yamaguchi and Furukawa, 2020). It has also been speculated that POLD1 pathogenic variants cause hypermutation only with concurrent MMRd, with the latter as an early event (Schamschula et al., 2022). However, it should also be considered whether mutational signatures associated with POLD1 exonuclease domain malfunction could differ from those arising from loss-of-function alterations.
In summary, our results do not support the pathogenicity of POLD1 frameshift variants, and we hypothesize that POLD1 could be an essential gene that exhibits haplosufficiency. To our knowledge, our study presents the first organoid model for germline POLD1 variants. Overall, it is still unclear if functional consequences could be derived from POLD1 alterations different from missense variants in their proofreading domain.
Data availability statement
The original contributions presented in the study are included in the article/Supplementary Material, further inquiries can be directed to the corresponding authors.
Ethics statement
The studies involving human participants were reviewed and approved by Hospital Clínic de Barcelona Clinical Research Ethics committee (registration number 2013/8286). The patients/participants provided their written informed consent to participate in this study.
Author contributions
Conceptualization: LB, YS, MD-G, and SC-B; funding acquisition: AC and SC-B; investigation: LB, YS, MD-G, ID, JM, LM, SC, TO, MC, OO, AC, MP, FB, AS, LA, and SC-B; resources: LM, SC, TO, MC, OO, AC, MP, FB, and SC-B; supervision: LB and SC-B; visualisation: LB, YS, MD-G, ID, JM, MC, and SC-B; guarantor: SC-B; writing–original draft: LB and SC-B; writing–review and editing: all authors.
Funding
YS was awarded an INPhINIT fellowship (LCF/BQ/DI18/11660058) from “la Caixa” Foundation (ID100010434 funded by the EU Horizon 2020 Programme Marie Skłodowska-Curie grant agreement no. 713673). MD-G was supported by a contract from Agència de Gestió d’Ajuts Universitaris i de Recerca -AGAUR- (Generalitat de Catalunya, 2019FI_B2_00203). JM was supported by a contract from CIBEREHD. LB was supported by a Juan de la Cierva postdoctoral contract (FJCI-2017-32593). CIBEREHD is funded by the Instituto de Salud Carlos III. This research was supported by grants from Fundació La Marató de TV3 (2019-202008-10), Fondo de Investigación Sanitaria/FEDER (17/00878, 20/00113), Fundación Científica de la Asociación Española contra el Cáncer (PRYGN211085CAST), PERIS (SLT002/16/00398, Generalitat de Catalunya), CERCA Program (Generalitat de Catalunya), Xarxa de Bancs de Tumors de Catalunya (XBTC, Pla Director d’Oncologia de Catalunya) and Agència de Gestió d'Ajuts Universitaris i de Recerca (Generalitat de Catalunya, GRPRE 2017SGR21, GRC 2017SGR653). Research at the LA lab at UC San Diego was supported by a Packard Fellowship for Science and Engineering and by NIH grants R01ES030993-01A1, R01ES032547, and R01CA269919. This article is based upon work from COST Action CA17118, supported by COST (European Cooperation in Science and Technology). www.cost.eu.
Acknowledgments
We are sincerely grateful to the patients, and Biobanks of Hospital Clínic–IDIBAPS, IDIBELL and Biobanco Vasco. The work was carried out (in part) at the Esther Koplowitz Centre.
Conflict of interest
LA is a compensated consultant and has equity interest in io9, LLC. His spouse is an employee of Biotheranostics, Inc. LA is an inventor of a U.S. Patent 10,776,718 and he also declares U.S. provisional patent applications with serial numbers: 63/289,601, 63/269,033, 63/412,835, 63/366,392, and 63/367,846.
The remaining authors declare that the research was conducted in the absence of any commercial or financial relationships that could be construed as a potential conflict of interest.
Publisher’s note
All claims expressed in this article are solely those of the authors and do not necessarily represent those of their affiliated organizations, or those of the publisher, the editors and the reviewers. Any product that may be evaluated in this article, or claim that may be made by its manufacturer, is not guaranteed or endorsed by the publisher.
Supplementary material
The Supplementary Material for this article can be found online at: https://www.frontiersin.org/articles/10.3389/fmolb.2023.1119900/full#supplementary-material
References
Alexandrov, L. B., Kim, J., Haradhvala, N. J., Huang, M. N., Tian Ng, A. W., Wu, Y., et al. (2020). The repertoire of mutational signatures in human cancer. Nature 578, 94–101. doi:10.1038/s41586-020-1943-3
Andrianova, M. A., Seplyarskiy, V. B., Terradas, M., Sánchez-Heras, A. B., Mur, P., Soto, J. L., et al. (2022). Extended family with germline pathogenic variant in polymerase delta provides strong evidence for recessive effect of proofreading inactivation. bioRxiv Prepr. Available at:Accessed November 14, 2022). doi:10.1101/2022.07.20.500591
Arnau-Collell, C., Soares de Lima, Y., Díaz-Gay, M., Muñoz, J., Carballal, S., Bonjoch, L., et al. (2020). Colorectal cancer genetic variants are also associated with serrated polyposis syndrome susceptibility. J. Med. Genet. 57, 677–682. doi:10.1136/jmedgenet-2019-106374
Bailie, L., Loughrey, M. B., and Coleman, H. G. (2017). Lifestyle risk factors for serrated colorectal polyps: A systematic review and meta-analysis. Gastroenterology 152, 92–104. doi:10.1053/j.gastro.2016.09.003
Barbari, S. R., and Shcherbakova, P. V. (2017). Replicative DNA polymerase defects in human cancers: Consequences, mechanisms, and implications for therapy. DNA Repair (Amst) 56, 16–25. doi:10.1016/j.dnarep.2017.06.003
Bellido, F., Pineda, M., Aiza, G., Valdés-Mas, R., Navarro, M., Puente, D. A., et al. (2016). POLE and POLD1 mutations in 529 kindred with familial colorectal cancer and/or polyposis: Review of reported cases and recommendations for genetic testing and surveillance. Genet. Med. 18, 325–332. doi:10.1038/gim.2015.75
Boparai, K. S., Dekker, E., Van Eeden, S., Polak, M. M., Bartelsman, J. F. W. M., Mathus-Vliegen, E. M. H., et al. (2008). Hyperplastic polyps and sessile serrated adenomas as a phenotypic expression of MYH-associated polyposis. Gastroenterology 135, 2014–2018. doi:10.1053/j.gastro.2008.09.020
Buchanan, D. D., Clendenning, M., Zhuoer, L., Stewart, J. R., Joseland, S., Woodall, S., et al. (2017). Lack of evidence for germline RNF43 mutations in patients with serrated polyposis syndrome from a large multinational study. Gut 66, 1170–1172. doi:10.1136/gutjnl-2016-312773
Buchanan, D. D., Stewart, J. R., Clendenning, M., Rosty, C., Mahmood, K., Pope, B. J., et al. (2018). Risk of colorectal cancer for carriers of a germ-line mutation in POLE or POLD1. Genet. Med. 20, 890–895. doi:10.1038/gim.2017.185
Carballal, S., Balaguer, F., and Ijspeert, J. E. G. (2022). Serrated polyposis syndrome; epidemiology and management. Best. Pract. Res. Clin. Gastroenterol. 58–59, 101791. doi:10.1016/j.bpg.2022.101791
Chow, E., Lipton, L., Lynch, E., D’Souza, R., Aragona, C., Hodgkin, L., et al. (2006). Hyperplastic polyposis syndrome: Phenotypic presentations and the role of MBD4 and MYH. Gastroenterology 131, 30–39. doi:10.1053/j.gastro.2006.03.046
Clendenning, M., Young, J. P., Walsh, M. D., Woodall, S., Arnold, J., Jenkins, M., et al. (2013). Germline mutations in the polyposis-associated genes BMPR1A, SMAD4, PTEN, MUTYH and GREM1 are not common in individuals with serrated polyposis syndrome. PLoS One 8, e66705. doi:10.1371/journal.pone.0066705
Daee, D. L., Mertz, T. M., and Shcherbakova, P. v. (2010). A cancer-associated DNA polymerase δ variant modeled in yeast causes a catastrophic increase in genomic instability. Proc. Natl. Acad. Sci. U. S. A. 107, 157–162. doi:10.1073/pnas.0907526106
Dotti, I., Mayorgas, A., and Salas, A. (2022). Generation of human colon organoids from healthy and inflammatory bowel disease mucosa. PLoS One 17, e0276195. doi:10.1371/journal.pone.0276195
Drost, J., van Boxtel, R., Blokzijl, F., Mizutani, T., Sasaki, N., Sasselli, V., et al. (2017). Use of CRISPR-modified human stem cell organoids to study the origin of mutational signatures in cancer. Science 358, 234–238. doi:10.1126/science.aao3130
Elsayed, F. A., Kets, C. M., Ruano, D., van den Akker, B., Mensenkamp, A. R., Schrumpf, M., et al. (2015). Germline variants in POLE are associated with early onset mismatch repair deficient colorectal cancer. Eur. J. Hum. Genet. 23, 1080–1084. doi:10.1038/ejhg.2014.242
Esteban-Jurado, C., Giménez-Zaragoza, D., Muñoz, J., Franch-Expósito, S., Álvarez-Barona, M., Ocaña, T., et al. (2017). POLE and POLD1 screening in 155 patients with multiple polyps and early-onset colorectal cancer. Oncotarget 8, 26732–26743. doi:10.18632/oncotarget.15810
Fang, L., Ford-Roshon, D., Russo, M., O’Brien, C., Xiong, X., Gurjao, C., et al. (2022). RNF43 G659fs is an oncogenic colorectal cancer mutation and sensitizes tumor cells to PI3K/mTOR inhibition. Nat. Commun. 13, 3181. doi:10.1038/S41467-022-30794-7
Ferrer-Avargues, R., Díez-Obrero, V., Martín-Tomás, E., Hernández-Illán, E., Castillejo, M. I., Codoñer-Alejos, A., et al. (2017). Characterization of a novel POLD1 missense founder mutation in a Spanish population. J. Gene Med. 19, e2951. doi:10.1002/jgm.2951
Fessler, E., Drost, J., van Hooff, S. R., Linnekamp, J. F., Wang, X., Jansen, M., et al. (2016). TGFβ signaling directs serrated adenomas to the mesenchymal colorectal cancer subtype. EMBO Mol. Med. 8, 745–760. doi:10.15252/emmm.201606184
Gala, M. K., Mizukami, Y., Le, L. P., Moriichi, K., Austin, T., Yamamoto, M., et al. (2014). Germline mutations in oncogene-induced senescence pathways are associated with multiple sessile serrated adenomas. Gastroenterology 146, 520–529. doi:10.1053/j.gastro.2013.10.045
Haradhvala, N. J., Kim, J., Maruvka, Y. E., Polak, P., Rosebrock, D., Livitz, D., et al. (2018). Distinct mutational signatures characterize concurrent loss of polymerase proofreading and mismatch repair. Nat. Commun. 9, 1746. doi:10.1038/S41467-018-04002-4
Hodel, K. P., Sun, M. J. S., Ungerleider, N., Park, V. S., Williams, L. G., Bauer, D. L., et al. (2020). POLE mutation spectra are shaped by the mutant allele identity, its abundance, and mismatch repair status. Mol. Cell 78, 1166–1177. e6. doi:10.1016/j.molcel.2020.05.012
Ihry, R. J., Worringer, K. A., Salick, M. R., Frias, E., Ho, D., Theriault, K., et al. (2018). p53 inhibits CRISPR–Cas9 engineering in human pluripotent stem cells. Nat. Med. 24, 939–946. doi:10.1038/S41591-018-0050-6
Islam, S. M. A., Díaz-Gay, M., Wu, Y., Barnes, M., Vangara, R., Bergstrom, E. N., et al. (2022). Uncovering novel mutational signatures by de novo extraction with SigProfilerExtractor. Cell Genomics 2, 100179. doi:10.1016/j.xgen.2022.100179
Job, A., Tatura, M., Schäfer, C., Lutz, V., Schneider, H., Lankat-Buttgereit, B., et al. (2020). The POLD1R689W variant increases the sensitivity of colorectal cancer cells to ATR and CHK1 inhibitors. Sci. Rep. 10, 18924. doi:10.1038/s41598-020-76033-1
Kawasaki, K., Fujii, M., Sugimoto, S., Ishikawa, K., Matano, M., Ohta, Y., et al. (2020). Chromosome engineering of human colon-derived organoids to develop a model of traditional serrated adenoma. Gastroenterology 158, 638–651. e8. doi:10.1053/j.gastro.2019.10.009
Kim, S., Scheffler, K., Halpern, A. L., Bekritsky, M. A., Noh, E., Källberg, M., et al. (2018). Strelka2: Fast and accurate calling of germline and somatic variants. Nat. Methods 15, 591–594. doi:10.1038/s41592-018-0051-x
Kokoska, R. J., Stefanovic, L., DeMai, J., and Petes, T. D. (2000). Increased rates of genomic deletions generated by mutations in the yeast gene encoding DNA polymerase delta or by decreases in the cellular levels of DNA polymerase delta. Mol. Cell Biol. 20, 7490–7504. doi:10.1128/mcb.20.20.7490-7504.2000
Lannagan, T. R. M., Lee, Y. K., Wang, T., Roper, J., Bettington, M. L., Fennell, L., et al. (2018). Genetic editing of colonic organoids provides a molecularly distinct and orthotopic preclinical model of serrated carcinogenesis. Gut 68, 684–692. doi:10.1136/gutjnl-2017-315920
Lau, H. C. H., Kranenburg, O., Xiao, H., and Yu, J. (2020). Organoid models of gastrointestinal cancers in basic and translational research. Nat. Rev. Gastroenterol. Hepatol. 17, 203–222. doi:10.1038/S41575-019-0255-2
Lemoine, F. J., Degtyareva, N. P., Kokoska, R. J., and Petes, T. D. (2008). Reduced levels of DNA polymerase delta induce chromosome fragile site instability in yeast. Mol. Cell Biol. 28, 5359–5368. doi:10.1128/mcb.02084-07
Magrin, L., Fanale, D., Brando, C., Fiorino, A., Corsini, L. R., Sciacchitano, R., et al. (2021). POLE, POLD1, and NTHL1: The last but not the least hereditary cancer-predisposing genes. Oncogene 40, 5893–5901. doi:10.1038/s41388-021-01984-2
Mah, L. J., El-Osta, A., and Karagiannis, T. C. (2010). gammaH2AX: a sensitive molecular marker of DNA damage and repair. Leukemia 24, 679–686. doi:10.1038/leu.2010.6
Mankaney, G., Rouphael, C., and Burke, C. A. (2020). Serrated polyposis syndrome. Clin. Gastroenterol. Hepatol. 18, 777–779. doi:10.1016/j.cgh.2019.09.006
Mayorgas, A., Dotti, I., Martínez-Picola, M., Esteller, M., Bonet-Rossinyol, Q., Ricart, E., et al. (2021). A novel strategy to study the invasive capability of adherent-invasive Escherichia coli by using human primary organoid-derived epithelial monolayers. Front. Immunol. 12, 646906. doi:10.3389/fimmu.2021.646906
McKenna, A., Hanna, M., Banks, E., Sivachenko, A., Cibulskis, K., Kernytsky, A., et al. (2010). The genome analysis toolkit: A MapReduce framework for analyzing next-generation DNA sequencing data. Genome Res. 20, 1297–1303. doi:10.1101/gr.107524.110
Mertza, T. M., Sharmab, S., Chabesb, A., and Shcherbakovaa, P. v. (2015). Colon cancer-associated mutator DNA polymerase δ variant causes expansion of dNTP pools increasing its own infidelity. Proc. Natl. Acad. Sci. U. S. A. 112, E2467–E2476. doi:10.1073/pnas.1422934112
Mikaeel, R. R., Young, J. P., Li, Y., Poplawski, N. K., Smith, E., Horsnell, M., et al. (2022). RNF43 pathogenic Germline variant in a family with colorectal cancer. Clin. Genet. 101, 122–126. doi:10.1111/cge.14064
Moreira, L., Muñoz, J., Cuatrecasas, M., Quintanilla, I., Leoz, M. L., Carballal, S., et al. (2015). Prevalence of somatic mutl homolog 1 promoter hypermethylation in Lynch syndrome colorectal cancer. Cancer 121, 1395–1404. doi:10.1002/cncr.29190
Muller, C., Yamada, A., Ikegami, S., Haider, H., Komaki, Y., Komaki, F., et al. (2022). Risk of colorectal cancer in serrated polyposis syndrome: A systematic review and meta-analysis. Clin. Gastroenterol. Hepatol. 20, 622–630.e7. e7. doi:10.1016/j.cgh.2021.05.057
Mur, P., García-Mulero, S., del Valle, J., Magraner-Pardo, L., Vidal, A., Pineda, M., et al. (2020a). Role of POLE and POLD1 in familial cancer. Genet. Med. 22, 2089–2100. doi:10.1038/S41436-020-0922-2
Mur, P., Palles, C., Tomlinson, I., and Valle, L. (2020b). Reply to: “Development of an MSI-positive colon tumor with aberrant DNA methylation in a PPAP patient. J. Hum. Genet. 65, 513–514. doi:10.1038/s10038-019-0701-6
Murphy, A., Solomons, J., Risby, P., Gabriel, J., Bedenham, T., Johnson, M., et al. (2022). Germline variant testing in serrated polyposis syndrome. J. Gastroenterol. Hepatol. 37, 861–869. doi:10.1111/jgh.15791
Palles, C., Cazier, J. B., Howarth, K. M., Domingo, E., Jones, A. M., Broderick, P., et al. (2013). Germline mutations affecting the proofreading domains of POLE and POLD1 predispose to colorectal adenomas and carcinomas. Nat. Genet. 45, 136–144. doi:10.1038/ng.2503
Quintana, I., Mejías-Luque, R., Terradas, M., Navarro, M., Piñol, V., Mur, P., et al. (2018). Evidence suggests that germline RNF43 mutations are a rare cause of serrated polyposis. Gut 67, 2230–2232. doi:10.1136/gutjnl-2017-315733
Robinson, P. S., Coorens, T. H. H., Palles, C., Mitchell, E., Abascal, F., Olafsson, S., et al. (2021). Increased somatic mutation burdens in normal human cells due to defective DNA polymerases. Nat. Genet. 53, 1434–1442. doi:10.1038/s41588-021-00930-y
Rosty, C., Brosens, L. A. A., Dekker, E., and Nagtegaal, I. D. (2019). Serrated polyposis” in WHO classification of tumours of the digestive system. 5th edition. Lyon, France: IARC, 532–534.
Schamschula, E., Kinzel, M., Wernstedt, A., Oberhuber, K., Gottschling, H., Schnaiter, S., et al. (2022). Teenage-Onset colorectal cancers in a digenic cancer predisposition syndrome provide clues for the interaction between mismatch repair and polymerase δ proofreading deficiency in tumorigenesis. Biomolecules 12, 1350. doi:10.3390/biom12101350
Schmit, M., and Bielinsky, A. K. (2021). Congenital diseases of DNA replication: Clinical phenotypes and molecular mechanisms. Int. J. Mol. Sci. 22, 911–938. doi:10.3390/ijms22020911
Siraj, A. K., Bu, R., Iqbal, K., Parvathareddy, S. K., Masoodi, T., Siraj, N., et al. (2020). POLE and POLD1 germline exonuclease domain pathogenic variants, a rare event in colorectal cancer from the Middle East. Mol. Genet. Genomic Med. 8, e1368. doi:10.1002/mgg3.1368
Snover, D. C., Ahnen, D. J., Burt, R. W., and Odze, R. D. (2010). Serrated polyps of the colon and rectum and serrated polyposis” in WHO Classification of Tumours of the Digestive System. 4th edition. Lyon, France: IARC, 160–165.
Soares de Lima, Y., Arnau-Collell, C., Muñoz, J., Herrera-Pariente, C., Moreira, L., Ocaña, T., et al. (2022). Germline mutations in WNK2 could be associated with serrated polyposis syndrome. J. Med. Genet., 2022–108684. doi:10.1136/jmg-2022-108684
Soares de Lima, Y., Arnau-Collell, C., Díaz-Gay, M., Bonjoch, L., Franch-Expósito, S., Muñoz, J., et al. (2021). Germline and somatic whole-exome sequencing identifies new candidate genes involved in familial predisposition to serrated polyposis syndrome. Cancers (Basel) 13, 929. doi:10.3390/cancers13040929
Tate, J. G., Bamford, S., Jubb, H. C., Sondka, Z., Beare, D. M., Bindal, N., et al. (2019). Cosmic: The catalogue of somatic mutations in cancer. Nucleic Acids Res. 47, D941–D947. doi:10.1093/nar/gky1015
Taupin, D., Lam, W., Rangiah, D., McCallum, L., Whittle, B., Zhang, Y., et al. (2015). A deleterious RNF43 germline mutation in a severely affected serrated polyposis kindred. Hum. Genome Var. 2, 15013. doi:10.1038/hgv.2015.13
Toma, C., Díaz-Gay, M., Franch-Expósito, S., Arnau-Collell, C., Overs, B., Muñoz, J., et al. (2020). Using linkage studies combined with whole-exome sequencing to identify novel candidate genes for familial colorectal cancer. Int. J. Cancer 146, 1568–1577. doi:10.1002/ijc.32683
Tumini, E., Barroso, S., Pérez-Calero, C., and Aguilera, A. (2016). Roles of human POLD1 and POLD3 in genome stability. Sci. Rep. 6, 38873. doi:10.1038/srep38873
Valle, L., de Voer, R. M., Goldberg, Y., Sjursen, W., Försti, A., Ruiz-Ponte, C., et al. (2019). Update on genetic predisposition to colorectal cancer and polyposis. Mol. Asp. Med. 69, 10–26. doi:10.1016/j.mam.2019.03.001
Valle, L., Hernández-Illán, E., Bellido, F., Aiza, G., Castillejo, A., Castillejo, M. I., et al. (2014). New insights into POLE and POLD1 germline mutations in familial colorectal cancer and polyposis. Hum. Mol. Genet. 23, 3506–3512. doi:10.1093/hmg/ddu058
Yamaguchi, K., and Furukawa, Y. (2020). Response to the correspondence referring to our article “development of an MSI-positive colon tumor with aberrant DNA methylation in a PPAP patient” by pilar mur, claire palles, ian tomlinson, laura valle. J. Hum. Genet. 65, 515–516. doi:10.1038/s10038-020-0752-8
Yamaguchi, K., Shimizu, E., Yamaguchi, R., Imoto, S., Komura, M., Hatakeyama, S., et al. (2019). Development of an MSI-positive colon tumor with aberrant DNA methylation in a PPAP patient. J. Hum. Genet. 64, 729–740. doi:10.1038/S10038-019-0611-7
Yamamoto, D., Oshima, H., Wang, D., Takeda, H., Kita, K., Lei, X., et al. (2022). Characterization of RNF43 frameshift mutations that drive Wnt ligand- and R-spondin-dependent colon cancer. J. Pathol. 257, 39–52. doi:10.1002/path.5868
Yan, H. H. N., Lai, J. C. W., Ho, S. L., Leung, W. K., Law, W. L., Lee, J. F. Y., et al. (2017). RNF43 germline and somatic mutation in serrated neoplasia pathway and its association with BRAF mutation. Gut 66, 1645–1656. doi:10.1136/gutjnl-2016-311849
Keywords: serrated polyposis syndrome, POLD1, genetic predisposition to disease, gene editing, functional genomics
Citation: Bonjoch L, Soares de Lima Y, Díaz-Gay M, Dotti I, Muñoz J, Moreira L, Carballal S, Ocaña T, Cuatrecasas M, Ortiz O, Castells A, Pellisé M, Balaguer F, Salas A, Alexandrov LB and Castellví-Bel S (2023) Unraveling the impact of a germline heterozygous POLD1 frameshift variant in serrated polyposis syndrome. Front. Mol. Biosci. 10:1119900. doi: 10.3389/fmolb.2023.1119900
Received: 09 December 2022; Accepted: 10 January 2023;
Published: 23 January 2023.
Edited by:
Filipa Mendes, University of Lisbon, PortugalReviewed by:
Hassan Dastsooz, University of Turin, ItalyDaniele Fanale, Azienda Ospedaliera Universitaria Policlinico Paolo Giaccone, Italy
Copyright © 2023 Bonjoch, Soares de Lima, Díaz-Gay, Dotti, Muñoz, Moreira, Carballal, Ocaña, Cuatrecasas, Ortiz, Castells, Pellisé, Balaguer, Salas, Alexandrov and Castellví-Bel. This is an open-access article distributed under the terms of the Creative Commons Attribution License (CC BY). The use, distribution or reproduction in other forums is permitted, provided the original author(s) and the copyright owner(s) are credited and that the original publication in this journal is cited, in accordance with accepted academic practice. No use, distribution or reproduction is permitted which does not comply with these terms.
*Correspondence: Laia Bonjoch, Ym9uam9jaEByZWNlcmNhLmNsaW5pYy5jYXQ=; Sergi Castellví-Bel, c2JlbEByZWNlcmNhLmNsaW5pYy5jYXQ=