Anti-nucleolin aptamer AS1411: an advancing therapeutic
- Rosalind Franklin University of Medicine and Science, North Chicago, IL, United States
Targeted therapy is highly desirable, as it allows for selective cytotoxicity on diseased cells without off-target side effects. Nucleolin is a remarkable target for cancer therapy given its high abundance, selective presence on the plasma membrane, and multifaceted influence on the initiation and progression of cancer. Nucleolin is a protein overexpressed on the cell membrane in many tumors and serves as a binding protein for several ligands implicated in angiogenesis and tumorigenesis. Nucleolin is present in the cytoplasm, nucleoplasm, and nucleolus and is used by selected pathogens for cell entry. AS1411 is a guanosine-rich oligonucleotide aptamer that binds nucleolin and is internalized in the tumor cells. AS1411 is well tolerated at therapeutic doses and localizes to tumor cells overexpressing nucleolin. AS1411 has a good safety profile with efficacy in relapsed acute myeloid leukemia and renal cell carcinoma producing mild or moderate side effects. The promising potential of AS1411 is its ability to be conjugated to drugs and nanoparticles. When a drug is bound to AS1411, the drug will localize to tumor cells leading to targeted therapy with fewer systemic side effects than traditional practices. AS1411 can also be bound to nanoparticles capable of detecting nucleolin at concentrations far lower than lab techniques used today for cancer diagnosis. AS1411 has a promising potential to change cancer diagnoses and treatment.
1 Introduction
Aptamers are a class of oligonucleotides with unique three-dimensional folding allowing them to be assembled into supramolecular multi-component structures to recognize and bind specific proteins (Keefe et al., 2010; He et al., 2020). As aptamers are generally formed from short single-stranded oligonucleotides (DNA or RNA), they can more easily enter and localize to their targets than immunoglobulins due to their smaller size (10–100 nucleotides) and diminished induction of the immune response (Kumar et al., 2020). Aptamers are produced chemically in a readily scalable process, and their production is not prone to viral or bacterial contamination. Aptamers are small, non-immunogenic, high-binding affinity molecules that can efficiently enter biological compartments. One of the strengths of aptamers is specificity and selection for specific cell-surface targets (Kelly et al., 2021). Some aptamers with guanosine (G)-rich sequences can adopt, in conjunction with metal ions, peculiar secondary structures named G-quadruplexes (G4s) (Tong et al., 2022). The methodology known as Systematic Evolution of Ligands by Exponential enrichment (SELEX), invented around 1990, is a technique used to identify specific oligonucleotides with high affinity and selectivity for their target (Ellington and Szostak, 1990; Tuerk and Gold, 1990). The advances of SELEX technology has led to more efficient and cost-effective development of specific and stable aptamers (Darmostuk et al., 2015; Guo et al., 2008; Catuogno and Esposito, 2017; Kohlberger and Gadermaier, 2022; Chen et al., 2023). The first aptamer for therapeutic use in humans, pegaptanib, was approved in 2004 by the FDA for use in wet age-related macular degeneration (Takeda et al., 2007). There is an excellent potential for use in healthcare treating diseases and research communities of aptamers, which are therefore investigated with numerous goals. The small size of aptamers sometimes makes them susceptible to renal filtration, leading to a shorter half-life. Conjugation chemistries for the attachment of dyes or functional groups can readily be introduced during aptamer synthesis (Healy et al., 2004). Unmodified aptamers are highly susceptible to degradation in serum but conjugated (polyethylene glycol or cholesterol) aptamers rather have increased the circulating half-life and nuclease resistance (Kang et al., 2007). Aptamer technologies have been optimized for activity and persistence under physiological conditions during selection (Guo et al., 2008; Catuogno and Esposito, 2017; Kohlberger and Gadermaier, 2022; Chen et al., 2023). Advancements in aptamer research have allowed sensitive pH responses to enhance control over molecular devices, and improve their diagnostic and therapeutic efficacy (Wilson et al., 2019; Thompson et al., 2020). Aptamers have been, and are currently being, studied in numerous clinical trials. Table 1 shows a selection of results from clinical trials involving aptamers. One of the most promising aptamers is AS1411, formerly AGRO100, now also known as ACT-GRO-777 (Bates et al., 2017). AS1411 is a natural 26-mer (G)-rich DNA [5′-d (GGT GGT GGT GGT TGT GGT GGT GGT GG)-3′]. It is among the aptamers most extensively studied, and is used as an anti-nucleolin drug (Wu et al., 2013).
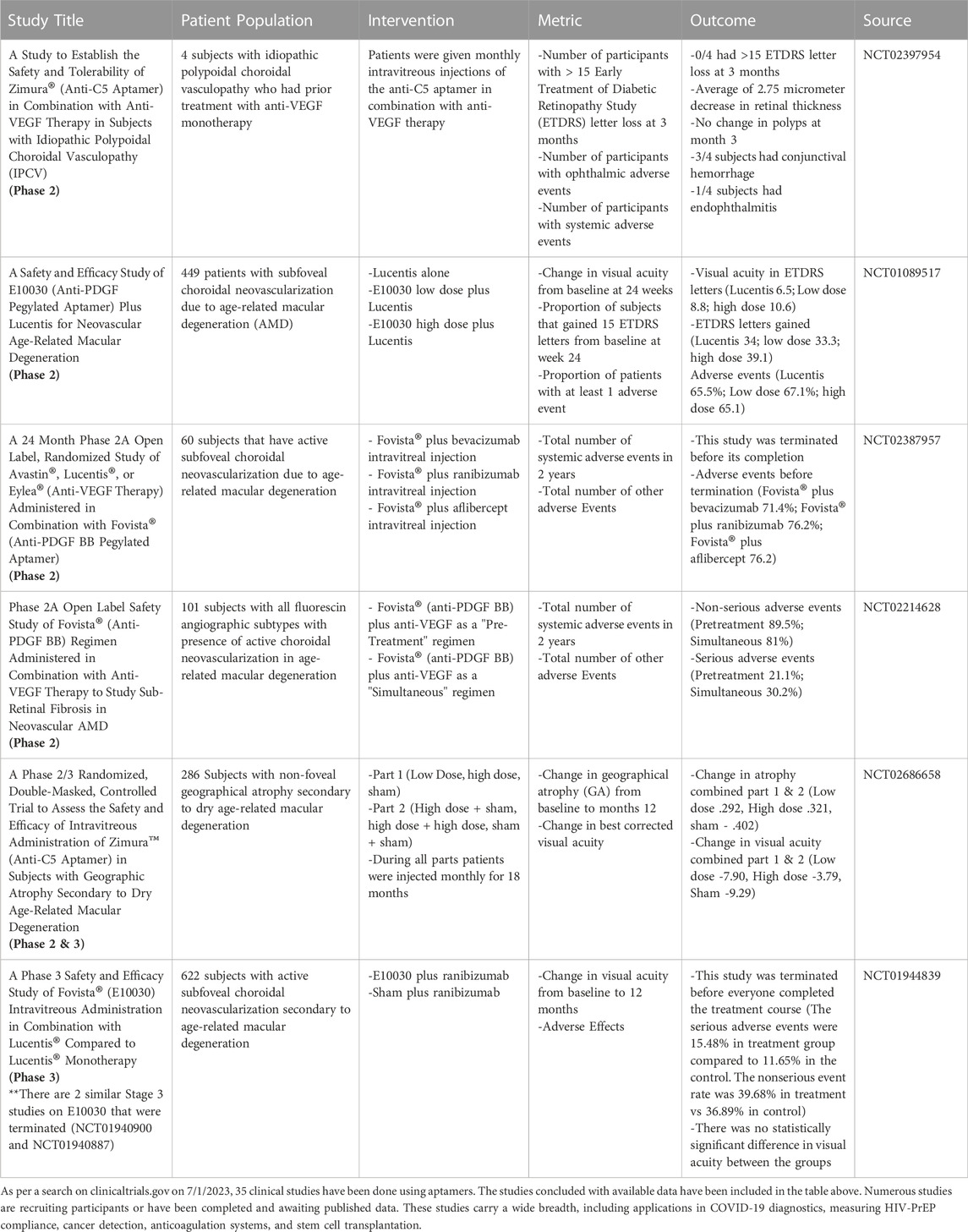
TABLE 1. As per a search on clinicaltrials.gov on 7/1/2023, 35 clinical studies have been done using aptamers. The studies concluded with available data have been included in the table above. Numerous studies are recruiting participants or have been completed and are awaiting published data. These studies carry a wide breadth, including applications in COVID-19 diagnostics, measuring HIV-PrEP compliance, cancer detection, anticoagulation systems, and stem cell transplantation.
1.1 AS1411 structure
AS1411 is a 26-base guanine-rich oligodeoxyribonucleotide aptamer that forms a G-quadruplex structure with many beneficial characteristics, notably its ability to bind to nucleolin. AS1411 was not developed by SELEX but discovered by chance (Bates et al., 2009). AS1411 targets cells with higher concentrations of nucleolin on the cell membrane and in the cytoplasm–a characteristic of many cancer cells and pathogen-infected cells. When in the proper pH, with available cationic metals to stabilize the structure, the guanines form Hoogsteen bonds forming G-tetrads; stacking two or more G-tetrads gives G-quadruplex structures that can assume several conformations. Depending on various factors, including the metal ion available, the DNA strands configure into parallel, anti-parallel, or mixed structures (Tong et al., 2022). The names of the G4 structures come from the orientation of the strands. Potassium provides the most stable and common G-quadruplex structures in the parallel conformation (Kosiol et al., 2021). There are 26 possible looping topologies and eight possible tetrad arrangements of AS1411; this means that a single AS1411 G4 complex can theoretically have 208 unique unimolecular conformations (Dailey et al., 2010).
At temperatures near or exceeding the Tm of 67°C, the aptamer can change its conformation to reach an equilibrium among different species. At lower temperatures, the concentration of the unfolded AS1411 is infinitesimal, thus leading to an equilibrium that may differ from the expected (Dailey et al., 2010). These properties create the need to quantify the structural makeup of an AS1411-containing solution. In 2010, Lane and Trent’s group deeply investigated AS1411 and discovered its high polymorphism in complex mixtures of different G4 structures. The behavior of AS1411 is not unique, and techniques such as circular dichroism, electrophoresis, UV melting, CD melting, and analytical ultracentrifugation are insufficient in fully characterizing the different conformations of AS1411 fully. If the exact structure of AS1411 is needed, high-resolution techniques like NMR are essential (Dailey et al., 2010). The G4 structures are very stable to heat and serum nucleases; they also preferentially enter cancer cells without permeating the cell membrane or causing damage to normal cells (Ogloblina et al., 2020; Saravanakumar et al., 2020).
AS1411 is the first anticancer “G-4 forming GRO” with good biological activity, reaching phase II clinical trials for acute myeloid leukemia and renal cell carcinoma (Bates et al., 2009; Rosenberg et al., 2014). Antiproliferative biological effects of AS1411 are due to its interaction with nucleolin and various nucleolin-independent effects (Bates et al., 2017; Ogloblina et al., 2018). The highlight of the clinical development of AS1411 was not only its anticancer and antiproliferative effects, but its ability to be loaded with several drugs that allow for the delivery of that drug to a specific target cell. AS1411 can also be linked with biomarkers that allow identification of cells with a high level of nucleolin (Bie et al., 2022; Tong et al., 2022). These advancements will be discussed in much detail throughout this review.
In 2021, the Miranda group developed a derivative of AS1411, termed AS1411-N6 (Miranda et al., 2021). AS1411-N6 had 6 nucleotides added at the 5′ end of the sequence of AS1411, forcing the aptamer to fold into a stem-loop structure. This new conformation decreases the structural polymorphism of the G4-forming aptamer; when AS1411-N6 aptamer is in the presence of potassium ions, it forms a unique duplex/G4 hybrid structure. The appeal of AS1411-N6 is that when loaded with a fluorescence marker, a 3-fold increase in the fluorescence at saturation nucleolin concentrations is detected (Miranda et al., 2021).
Other AS1411 derivatives include analogues 5′-conjugated with lipophilic tails (Riccardi et al., 2018). These lipid-conjugated aptamers have applications in liposomal formulations and lipid coated-nanoparticles for targeted cancer therapies, and these aptamers fold into stable, parallel unimolecular G-quadruplex structures, forming large aggregates like micelles (Riccardi et al., 2018). AS1411 has also been used as a supramolecular carrier for the cancer-selective delivery of acridine orange derivatives (C3, C5, and C8), especially in cervical cancer (Figueiredo et al., 2019). This delivery strategy relied on the non-covalent association of the acridine derivatives and the G4 structures (Figueiredo et al., 2019).
1.2 Nucleolin
Nucleolin is a ubiquitous multifunctional protein discovered in 1973 and was initially called C23. As its name suggests, nucleolin is a protein predominately found in the nucleolus, nucleoplasm, cytoplasm, and cell membrane (Orrick et al., 1973; Jing et al., 2020). Nucleolin consists of three main structural domains: the N-terminal domain, the central domain, and the C-terminal domain. The N-terminal domain is rich in acidic amino acids and is the predominant site of phosphorylation during the cell cycle (Peter et al., 1990; Ginisty et al., 1999); it participates in the transcription of rRNA and interacts with the components of the pre-rRNA processing complex. Extensive phosphorylation by casein kinase 2 (CK2) occurs at interphase and by cell division control protein 2 homolog (CDC2) during mitosis; the regulated phosphorylation of nucleolin regulates its role during the cell cycle (Khurts et al., 2004). The central domain contains four RNA-binding domains (RBD) (also called RNA-recognition domains) that predominately aid in producing rRNA by acting as a chaperone and a pre-ribosome component. The central domain also aids in the biological packaging of RNA, pre-mRNA splicing, and poly-A tail synthesis. The RBDs also interact with telomerase and are believed to be the localization mechanism to the nucleolus (Khurts et al., 2004). The C-terminal domain is rich in Arg-Gly-Gly repeats that lead to the unique structure of the C-terminus; this leads to interactions with mRNA and facilitates the interactions of RBDs and large RNA. The C-terminus is also essential for assembling the ribosome and importing ribosomal proteins into the nucleus (Jia et al., 2017). Although nucleolin lacks a transmembrane domain or signal sequence, nucleolin is nevertheless present on the surface of various cell types (Chen et al., 2008).
To function correctly, nucleolin has to travel between the nucleus, cytoplasm, and plasma membrane. Nucleolin can be modified to facilitate its function and shuttling; it can be phosphorylated, methylated, and ADP-ribosylated (Kang et al., 1975; Lischwe et al., 1982; Leitinger and Wesierska-Gadek, 1993). While nucleolin’s function is well-documented and summarized above, its RBD domain is found to be reductive. The knockout of nucleolin’s central functional unit, RBD, does not affect the transcription, rRNA maturation, and shape in chicken B-lymphocytes (Storck et al., 2009). They did find that nucleolin is required for the proliferation and survival of cancer cells (Storck et al., 2009). These data are an excellent example of why nucleolin is a promising target for cancer treatment, its inhibition in cancer cells limits the ability to grow and spread while not impacting the normal function of non-cancer cells.
Nucleolin is overexpressed in non-small cell lung, gastric, ovarian, breast, and kidney cancers (Masiuk et al., 2007; Cicchillitti et al., 2009; Qiu et al., 2013; Rosenberg et al., 2014; Xu et al., 2016). It can be found on the cell surface of HeLa cells, lymphoblastoid T-cells, breast carcinoma, hepatocarcinoma, laryngeal epithelial cells, and endothelial cells of angiogenic blood vessels (Fogal et al., 2009; Fujiki et al., 2014; Koutsioumpa and Papadimitriou, 2014; Niu et al., 2015). Viruses such as Human immunodeficiency virus-1 (HIV-1), Respiratory syncytial virus (RSV), Influenza A, Parainfluenza virus 3, and Enterovirus 71 have all been shown to use nucleolin to enter a human host cell (Bose et al., 2004; Su et al., 2015; Chan et al., 2016; Perrone et al., 2016; Mastrangelo et al., 2021). HSV-1, Rabies virus, Epstein-Barr virus (EBV), hepatitis C virus (HCV), Dengue virus, and bacterial infection F.tularensis have shown to use nucleolin in part of their life cycles (Shimakami et al., 2006; Barel et al., 2008; Callé et al., 2008; Balinsky et al., 2013; Oksayan et al., 2015; Lista et al., 2017). Numerous studies cited above have shown that inhibition of nucleolin leads to decreased vitality of corresponding cancers and viral infections. Nucleolin has a promising potential as a therapeutic target that can be used in diagnosis and treatment.
There is the promise that AS1411 might have potential in other viruses based on work done on other G4 structures. The Verma lab showed that G4 structures inhibit Kaposi’s sarcoma herpesvirus latency-associated nuclear antigen (KSHV-LANA) mRNA translation, a major latency-associated gene (Dabral et al., 2020). Our lab conducts an IACUC-approved in vivo study on AS1411 to treat KSHV+ Primary Effusion Lymphoma (PEL) in NOD/SCID mice. The mice are injected with 5 × 106 KSHV + PEL cell line BCBL-1 cells to induce tumorigenesis. They are then either injected with AS1411 or the control [5′-d (CCT CCT CCT CCT TCT CCT CCT CCT CC)-3′] and monitored for survival and tumor growth; after the mice have humanely euthanized, their tumors, spleens, and ascites are collected for further lab evaluations. This is one example of how AS1411 can be used experimentally in oncogenic viral infected-cell tumors. Numerous other in vivo studies and their findings are highlighted in Table 2.
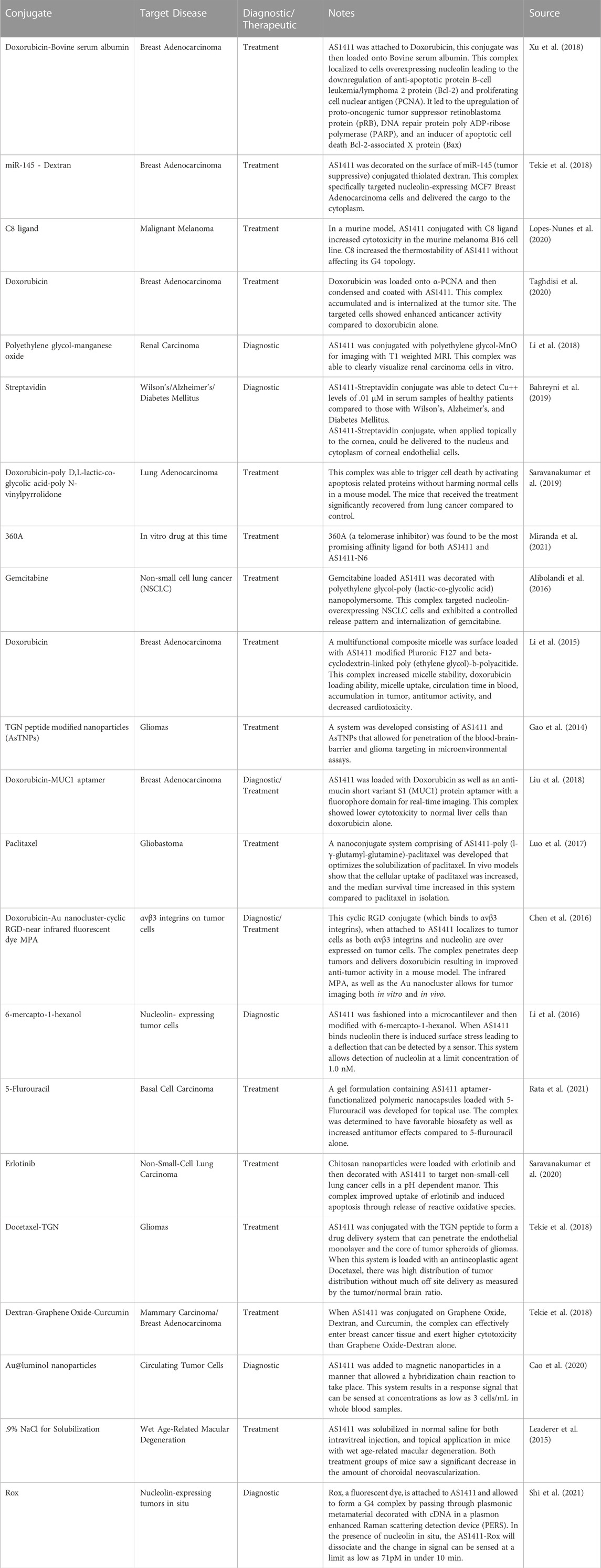
TABLE 2. A selection of publications citing non-clinical experiments using AS1411 conjugated to (a) nanoparticle(s). Each of these studies has an application in treatment, or detection of a disease state in humans..
2 Binding and uptake of AS1411
2.1 Binding of AS1411 to nucleolin
AS1411 can bind to nucleolin and subsequently enter the cytosol, leading AS1411 to deliver drugs and dyes to cancer cells. Whether inside the cell or on the plasma membrane, AS1411 finds and binds to nucleolin. The central region of nucleolin consists of 4 RBDs. The exact binding mechanism is not fully understood, but Bie et al. (2022) proposed a model in 2022. They proposed that AS1411 undergoes capping at the 5′ end, while the folding of AS1411 into a G4 structure leads to the Thymidine residues facing a groove of nucleolin. The 5′ cap forms the bond with the RBD1 of nucleolin; this binding is stabilized by AS1411 interacting with RBD2 via hydrogen bonds, salt bridges, and a water-mediated network. The binding of AS1411 downregulates nucleolin phosphorylation by blocking the phosphorylation sites near the N-terminus (Iturriaga-Goyon et al., 2021). Loss of the N-terminal phosphorylation leads to the inhibition of transcription of rRNA and interactions with the components of the pre-rRNA processing complex (Jia et al., 2017).
2.2 Role of nucleolin in endocytosis
Endocytosis is the broad term for the entry of a substrate into a cell enclosed by its cell membrane resulting in a vesicle. Endocytosis evolutionarily allows cells to accumulate nutrients and resources necessary for survival and remove foreign material from the aqueous environment. Pathogens can hijack this process for their replication and transmission; it can also be used therapeutically for drug delivery and signal transduction (Doherty and McMahon, 2009).
There are four mechanisms by which a cell achieves endocytosis: Phagocytosis, Caveolae, Pinocytosis, and Receptor-mediated endocytosis (also known as clathrin-mediated endocytosis). Phagocytosis is the process of internalizing particles larger than 0.5 μm in diameter; it is a crucial function of many cells in the immune system. Caveolae are non-clathrin-coated invaginations that consist of cholesterol, caveolin, and glycoproteins; they are prominent in smooth muscle, fibroblasts, adipocytes, type I pneumocytes, and endothelial cells (Doherty and McMahon, 2009). In 2010, Bates and Luo’s groups showed that caveolae are not involved in AS1411 nor nucleolin-associated viral uptake in prostate cancer cells, endothelial cells, or skin fibroblasts (Reyes-Reyes et al., 2010; Song et al., 2012).
Receptor-mediated endocytosis is the primary route for endocytosis in most cells. The cell can take up a known substrate bound to a specific extracellular domain. When the extracellular domain of a clathrin-associated receptor is activated, a conformational change of the cytoplasmic domain is initiated. The conformational change results in clathrin aggregating in the cytoplasm along the plasma membrane. Clathrin has a tri-skeleton structure allowing self-interaction and forming a clathrin cage. The clathrin cage results in a vesicle that contains the receptor, its bound substrate, and a small amount of extracellular fluid. This vesicle will be trafficked to an endosome to deliver its contents before recycling the receptor back to the plasma membrane (Doherty and McMahon, 2009). This process is summarized in Figure 1. One such receptor that utilizes clathrin-mediated endocytosis is nucleolin. Several viruses have utilized this receptor as a means of entry. HIV-1, RSV, Influenza A, Parainfluenza virus 3, and Enterovirus 71 have all been shown to use nucleolin to enter human host cell (Bose et al., 2004; Su et al., 2015; Chan et al., 2016; Perrone et al., 2016; Mastrangelo et al., 2021). Several viruses in non-human hosts also use this mechanism. Studies have also shown that decreased nucleolin levels achieved with siRNA and AS1411 have decreased viral titers in HIV-1, RSV, Influenza A, Parainfluenza virus 3, and Enterovirus 71 (Bose et al., 2004; Su et al., 2015; Chan et al., 2016; Perrone et al., 2016; Mastrangelo et al., 2021).
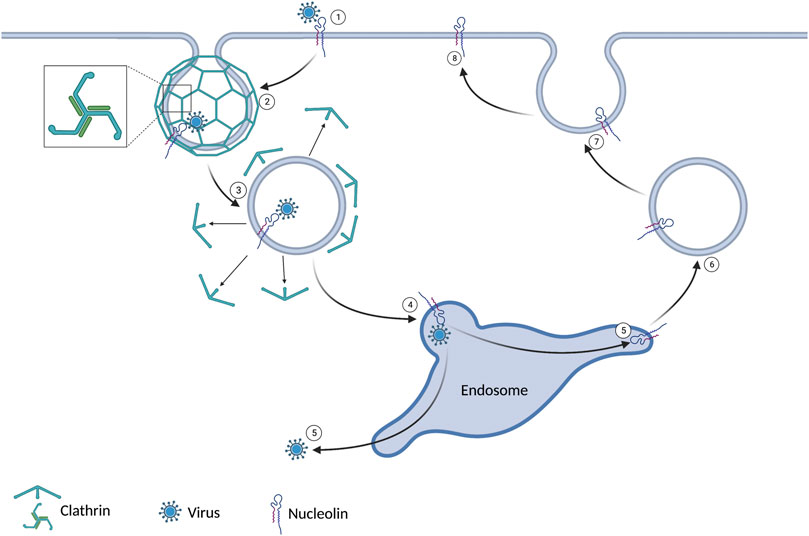
FIGURE 1. 1) The virus binds to nucleolin expressed on the cell surface. 2) Clathrin coats the intracellular plasma membrane around the virus-bound receptor. This leads to an invagination and ultimately a vesicle. 3) After the vesicle gets internalized, the clathrin dissociates. 4) The vesicle joins the endosome. 5) The virus evades the endosome and enters the cytoplasm. 6/7/8) The nucleolin is recycled to the extracellular domain.
As nucleolin can act as a receptor for receptor-mediated endocytosis, it can also act as a receptor for phagocytes. Phagocytosis is the process of internalizing particles larger than 0.5 mm in diameter. The first phase of phagocytosis is recognizing the particle to be injected (Uribe-Querol and Rosales, 2020). This can be done with various receptors, one of which is nucleolin. Nucleolin is used in microglia recognition of amyloid beta peptide 1–42 (Ozawa et al., 2013), macrophage recognition of maleyated bovine serum albumin, and acetylated low-density lipoprotein (Miki et al., 2015; Miki et al., 2015), to name a few. After recognition, there is the process of internalization through cytoskeleton rearrangement and, ultimately, degradation (Uribe-Querol and Rosales, 2020).
2.3 Proposed mechanism of endocytosis and localization of AS1411
Pinocytosis, coming from the Greek pino-meaning “to drink,” is the process by which a cell forms a vesicle containing solutes in the extracellular fluid. Pinocytosis can be divided into non-specific adsorptive pinocytosis and macropinocytosis. Non-specific adsorptive pinocytosis is a clathrin-mediated process. It differs from receptor-mediated endocytosis in that the clathrin dissociates from the vesicle immediately upon forming. The vesicles commonly fuse with early endosomes to digest their contents (Doherty and McMahon, 2009). Macropinocytosis is a clathrin-independent process that utilizes actin to induce “membrane ruffling,” resulting in the formation of vesicles. This actin remodeling is under tight control via several pathways, including the Ras-related C3 botulinum toxin substrate 1 (Rac1) pathway (Koronakis et al., 2011). Rac1 is a member of the Rho family of GTPases. Positive regulation of Rac1 is obtained through guanine exchange factors (GEF); when a GEF activates Rac1, the result is Rac1GTP. Endogenous activators of Rac1 include serine/threonine p21-activating kinase 1 (PAK1), Ras, phosphoinositide 3-kinase (PI3K), and SRC Proto-oncogene non-receptor tyrosine kinase (Src). Negative regulation of Rac1 results in Rac1GDP, the inactive form of Rac1; nucleolin is a negative regulator of Rac1 (Reyes-Reyes et al., 2015).
Rac1GTP activates the WASP family verprolin homologous protein (WAVE) regulatory complex (WRC). The WRC is active when phosphorylated, activating Arp2/3, leading to actin polymerization, membrane ruffling, and, thus, endocytosis (Koronakis et al., 2011; Reyes-Reyes et al., 2015). The importance of highlighting this specific pathway is to show nucleolin’s role in cellular uptake. This is represented visually in Figure 2. It is hypothesized that one of the ways AS1411 gets localized to cancer cells lies in the increased level of macropinocytosis found in many cancer lines. This initial increase in extracellular fluid uptake leads to a basilary level of AS1411 entering the cytoplasm. The AS1411 then inhibits nucleolin, leading to an increase in Rac1GTP and, thus, further macropinocytosis in a feed-forward loop in which more AS1411 is taken up by the cell (Reyes-Reyes et al., 2015).
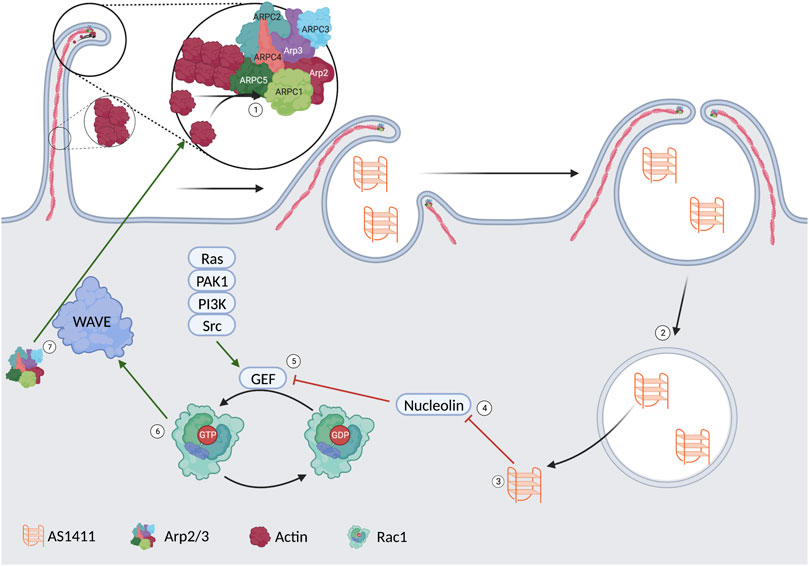
FIGURE 2. 1) Arp2/3 induces actin polymerization leading to membrane ruffling and the formation of a vesicle. 2/3) The AS1411 is released from the vesicle into the cytoplasm. 4) AS1411 leads to the inhibition of nucleolin. 5) The inhibited nucleolin leads to an upregulation of a guanosine exchange factor. 6) The activated GEF leads to the conversion of Rac1GDP to Rac1GTP. 7) rac1GTP leads to activation of the WAVE complex. 7) The WAVE complex upregulates Arp2/3 and more membrane ruffling.
3 AS1411 as an anti-nucleolin aptamer
AS1411 began being studied because of its ability to bind nucleolin. It was soon discovered that AS1411 significantly decreased the survivability of cancer cells expressing nucleolin. The specific role that AS1411 (and by effect nucleolin) plays in these pathways is yet to be clarified for many cancer-cell lines. For example, the Cheng et al. (2016) explored the expression level of several factors in glioma cells. They found that when glioma cells were treated with AS1411, there was an upregulation of tumor suppressor gene p53 and a downregulation of oncogenes Bcl-2 and Akt1. These findings were discovered when nucleolin was silenced, suggesting that these results were the effect of AS1411 directly inhibiting nucleolin. These authors cited the 2010 work of the Ishimaru group to propose a complete mechanism of action. Without AS1411, nucleolin’s RBD will bind to the 5′ UTR of B-cell Leukemia/lymphoma Protein 1 (Bcl-1) mRNA and the 5′ UTR of p53 mRNA. Binding to Bcl-1 promotes expression, while binding to p53 decreases expression. The decreased concentration of Akt1 is attributed to it being downstream of a Bcl-1 pathway (Ishimaru et al., 2010). AS1411, 5′ UTR of Bcl1 mRNA, and 5′ UTR of p53 mRNA all bind to the exact location; it is hypothesized that AS1411 acts as a competitive inhibitor for these targets.
The above mechanism was the basis for the hypothesis of using AS1411 alone as an anti-cancer drug. Using AS1411 alone to treat solid tumors has been investigated. In 2007, a phase I clinical trial was completed on 30 adult patients with advanced solid tumors to determine the maximum tolerated dose. In 2010, a phase II clinical trial was started, studying 35 patients with metastatic, clear-cell, renal cell carcinoma after only 2.9% reported a response (Rosenberg et al., 2014). No other studies in humans use AS1411 alone as of May 2023.
In addition to nucleolin’s role in ribosome maturation and interacting with the cell cycle intermediaries CK2 and CDC2, nucleolin also exists on the extracellular side of the plasma membrane. HIV-1 has a low-level affinity to the extracellular domain of nucleolin. Perrone et al., 2016 demonstrated that treatment with AS1411 can limit the entry of HIV-1 into the host cell in vitro (Figure 3) (Perrone et al., 2016). Respiratory Syncytial Virus (RSV) has been shown to use nucleolin as a functional receptor that passively interacts with fusion proteins (F proteins) (Figure 3). F proteins are glycoproteins on the virion surface and are crucial in the initial phase of infection (McLellan, Ray, and Peeples, 2013). Mastrangelo et al. (2021), in 2021 demonstrated that AS1411 could inhibit endocytosis and decrease the number of RSV (+) cells in vitro and mouse and rat models at a non-toxic level. The hemagglutinin glycoprotein found on the surface of Influenza A has been shown to bind to nucleolin in addition to sialic acid (Figure 3).
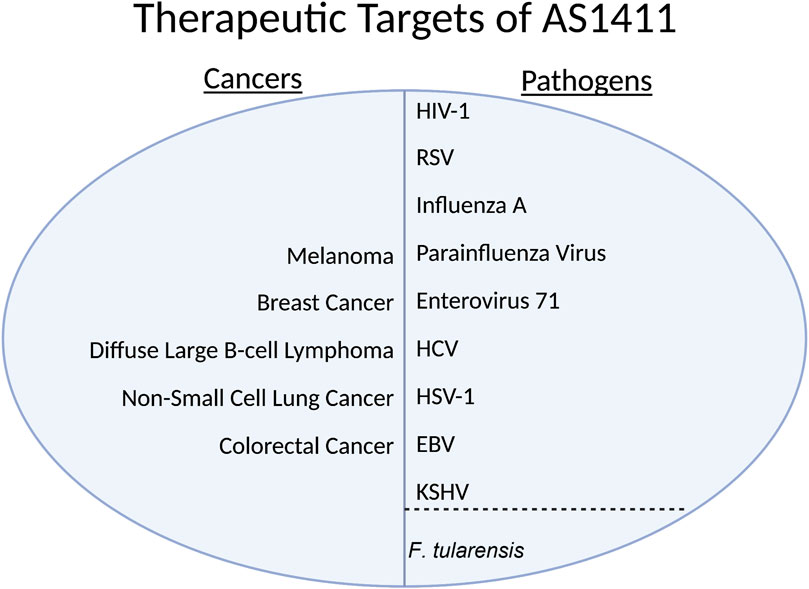
FIGURE 3. HIV-1, Human Immunodeficiency Virus 1; RSV, Respiratory Syncytial Virus; HCV, Hepatitis C Virus; HSV-1, Herpes Simplex Virus 1; EBV, Epstein-Barr Virus; KSHV, Kaposi’s sarcoma-associated herpesvirus.
Chan et al. (2016), in 2016 showed that inhibiting nucleolin with a specific nucleolin antibody, blocking the virus with purified protein, and siRNA knockdown all substantially decreased the internalization of several virus strains in lung endothelial cells in vitro (Figure 3). Bose, Basu, and Banerjee (2004) in 2004 showed that Parainfluenza virus type 3 requires nucleolin expression on the cell surface for efficient cellular entry into lung epithelial cells in vitro; they accomplished the knockdown via treatment with an anti-nucleolin antibody and preincubation with nucleolin (Figure 3). Enterovirus-71’s (EV71) capsid protein VP1 interacts directly with nucleolin expressed on the cell surface. Su et al., 2015 showed that cells treated with an anti-nucleolin antibody reduced the binding of EV71 to human cells while knockdown decreased binding, infection, and virus production (Figure 3). They also conferred the nucleolin gene in the mouse model; mice with human-nucleolin expressed on their cells conferred EV71 infection and production (Figure 3) (Su et al., 2015). In HSV-1, nucleolin is required for an efficient infection through its suspected involvement in the HSV-1 DNA replication process via ICP4 (Figure 3) (Callé et al., 2008).
In the Rabies virus, nucleolin interacts with isoform P3 (Figure 3). Depletion of nucleolin leads to decreased protein expression and lower viral production in infected cells (Oksayan et al., 2015). In the Dengue virus, nucleolin plays a role in viral morphogenesis by interacting with viral capsid protein before spread (Figure 3) (Balinsky et al., 2013). In HCV, nucleolin is required to interact with NS5B for RNA-dependent RNA polymerase to function (Figure 3) (Shimakami et al., 2006). The Lista group in 2017 showed that in EBV, nucleolin expression levels are unique, as the virus uses nucleolin to evade the immune response (Figure 3). The viral protein Epstein-Barr virus nuclear antigen 1 (EBNA1) is a soluble protein crucial for the virus to establish and maintain infection; it is only functional in its free form. It is highly antigenic to the human immune system. The viral mRNA for EBNA1 binds to nucleolin, limiting the immune response to the viral mRNA; however, the mRNA bound to nucleolin cannot be translated.
Thus, the virus must exist in an environment with a nucleolin concentration rather similar to the standard concentration of a normal cell. Too little nucleolin and the immune system will mount a response; too much nucleolin and there will be an insufficient amount of EBNA1 protein. This use of nucleolin has yet to be seen in other viruses that infect humans (Lista et al., 2017). In the bacteria F.tularensis, it has been shown that nucleolin binds to bacterial ligand elongation factor-thermo-unstable (EF-Tu), aiding its adhesion and entry process. EF-Tu, a vital protein in F.tularensis translates bacterial proteins (Figure 3) (Barel et al., 2008).
4 AS1411s ability to bind modified particles
The functional aspect of the G4-forming AS1411 lies in the central region. This allows the 5′ and 3′ tails of AS1411 to be modified without resulting in a decreased affinity for nucleolin. One such modification is a thiol modification that allows AS1411 to be linked to drugs/biomarkers via a covalent sulfide bond at either the 5′ or 3′ end (Pon and Yu, 2004). Riccardi et al. (2018) studied three derivatives of AS1411 with different lipophilic tails attached to the 5′ domain of the aptamer. Their studies in pseudo-physiological buffers that mimic extra- and intracellular environments showed that the ability of AS1411 to maintain its G4 motif is unaffected by lipophilic tails. This study showed that these lipophilic tails decreased the cytotoxicity of AS1411, so it is unlikely to be used in cancer therapeutics; however, it demonstrates the ability of AS1411 to maintain its G4 complex (and thus its nucleolin binding ability) while conjugated. Like lipid tails, AS1411 has also been conjugated to niosomes for targeted delivery to cancer cells. Niosomes are vesicles made of non-ionic surfactants that can be conjugated with lipids to deliver hydrophilic and lipophilic drugs (Riccardi et al., 2018). The size of the groups attached to AS1411 can vary significantly and, in many cases, dwarf AS1411 without affecting its ability to bind nucleolin. The consequence of this capability is that the nanoparticle attached to AS1411 is preferentially delivered to where nucleolin is, with limited off-target binding, a valuable feature in cancer/virus therapeutics and diagnosis (Wandtke, Woźniak, and Kopiński, 2015; Tong et al., 2022).
Some of the major complications of traditional chemotherapeutics are the systemic effects of off-target delivery. Significant advancements have been made utilizing the increased extracellular expression of nucleolin in cancer cells. When AS1411 is loaded with an anti-cancer drug, the medication will be preferentially found in upregulated nucleolin-expressing cells. Most particles conjugated to AS1411, per a thorough PubMed review, consist of established drugs/markers often linked in series. An example is the delivery of orange derivative (C8) and Imiquimod to gynecological carcinoma cells from the Lopez-Nunes group. They could use gold nanoparticles to covalently conjugate these particles to AS1411, intracellularly allowing on-target dissociation. This conjugate was delivered using a topical gel comprised of polyethylene glycol that could be applied directly to the female genital tract. Their study showed decreased viability of the cancer cells in their porcine model (Lopes-Nunes et al., 2020). The Carvalho group demonstrated that AS1411 can be valuable therapeutically when bound to lipids. These AS1411-lipid conjugates form nano aggregates suitable for pharmaceutical applications due to their small size, negative charge, and drug release capabilities. They demonstrated these findings by seeing a decrease in HeLa cell proliferation and increased cell uptake (Carvalho et al., 2021). The Riccardi et al., 2018 group showed that when AS1411 is conjugated to a niosome it can deliver ruthenium (III) complexes to HeLa cells. This model demonstrated increased antiproliferative activity when the niosomes were decorated with AS1411 than with the control.
A similar idea can be used if AS1411 is loaded with a biomarker and exposed to a sample suspected of cancer; a greater level of AS1411 bound can be highly suggestive of a positive result (Tong et al., 2022). In viral infections, similar patterns might be expected for treatment and diagnosis, with the added potential of preventing infection. For example, the anti-gag RNA aptamer DP6-12 has been shown experimentally to lower HIV-1 infection (Wandtke et al., 2015). Table 2 summarizes several nanoparticles that can be attached to AS1411 and the conjugates target.
5 Conclusion
Nucleolin is a protein overexpressed in many cancers and is used by pathogens to invade host cells and propagate. This allows for the design of targeted therapies and diagnostic tools using the anti-nucleolin aptamer AS1411. AS1411 has been shown to localize and internalize in numerous tumor cells. Once in the cell, it can inhibit nucleolin function and deliver a drug it is conjugated to, allowing for targeted therapy with few off-target effects. AS1411 could also be conjugated to a nanoparticle that allows for the diagnosis of cancer cells at lower concentrations than existing techniques. AS1411 has the potential to do more than deliver drugs or aid in diagnostics. In 2023, the Puzzo lab published their work using AS1411 in gene therapy. They could conjugate AS1411 to an Adeno-associated virus that led to ligand-specific transduction without off-target transduction in peripheral organs (Puzzo et al., 2023). As gene therapy continues to be explored, aptamers such as AS1411 could help to ensure that the therapy limits off-target effects. As research continues, AS1411 has a promising potential to aid in the targeted diagnosis and treatment of a myriad of pathologies.
Author contributions
AV wrote the manuscript and created the images and tables. In addition, NS-W and AV revised the manuscript. All images were created with BioRender.com. All authors contributed to the article and approved the submitted version.
Funding
We gratefully acknowledge the funding from NIH-CA192970 and NIH-CA278641. We gratefully acknowledge the publishing cost support from Chicago Medical School and the Center for Cancer Cell Biology, Immunology, and Infection, RFUMS.
Acknowledgments
We are deeply appreciative of Miroslava Repak and Mehnaz Patwa Madraswalla for introducing AS1411 research in viral cancers in the lab and proofreading the manuscript, respectively.
Conflict of interest
The authors declare that the research was conducted in the absence of any commercial or financial relationships that could be construed as a potential conflict of interest.
Publisher’s note
All claims expressed in this article are solely those of the authors and do not necessarily represent those of their affiliated organizations, or those of the publisher, the editors and the reviewers. Any product that may be evaluated in this article, or claim that may be made by its manufacturer, is not guaranteed or endorsed by the publisher.
Supplementary material
The Supplementary Material for this article can be found online at: https://www.frontiersin.org/articles/10.3389/fmolb.2023.1217769/full#supplementary-material
References
Alibolandi, M., Ramezani, M., Abnous, K., and Hadizadeh, F. (2016). AS1411 aptamer-decorated biodegradable polyethylene glycol-poly(lactic-co-glycolic acid) nanopolymersomes for the targeted delivery of gemcitabine to non-small cell lung cancer in vitro. J. Pharm. Sci. 105 (5), 1741–1750. doi:10.1016/j.xphs.2016.02.021
Bahreyni, A., Ramezani, M., Alibolandi, M., Hassanzadeh, P., Abnous, K., and Seyed, M. T. (2019). High affinity of AS1411 toward copper; its application in a sensitive aptasensor for copper detection. Anal. Biochem. 575, 1–9. doi:10.1016/j.ab.2019.03.016
Balinsky, C. A., Schmeisser, H., Ganesan, S., Singh, K., Pierson, T. C., and Zoon, K. C. (2013). Nucleolin interacts with the Dengue virus capsid protein and plays a role in formation of infectious virus particles. J. Virology 87 (24), 13094–13106. doi:10.1128/JVI.00704-13
Barel, M., Hovanessian, A. G., Meibom, K., Briand, J-P., Dupuis, M., and Charbit, A. (2008). A novel receptor - ligand pathway for entry of francisella tularensis in monocyte-like THP-1 cells: interaction between surface nucleolin and bacterial elongation factor tu. BMC Microbiol. 8 (9), 145. doi:10.1186/1471-2180-8-145
Bates, P. J., Laber, D. A., Miller, D. M., D Thomas, S., and John, O. T. (2009). Discovery and development of the G-rich oligonucleotide AS1411 as a novel treatment for cancer. Exp. Mol. Pathology 86 (3), 151–164. doi:10.1016/j.yexmp.2009.01.004
Bates, P. J., Reyes-Reyes, E. M., Malik, M. T., Murphy, E. M., O’Toole, M. G., and John, O. T. (2017). G-quadruplex oligonucleotide AS1411 as a cancer-targeting agent: uses and mechanisms. Biochimica Biophysica Acta General Subj. 1861 (5 Pt B), 1414–1428. doi:10.1016/j.bbagen.2016.12.015
Bie, L., Wang, Y., Jiang, F., Xiao, Z., Zhang, L., and Wang, J. (2022). Insights into the binding mode of AS1411 aptamer to nucleolin. Front. Mol. Biosci. 9, 1025313. doi:10.3389/fmolb.2022.1025313
Bose, S., Basu, M., and Banerjee, A. K. (2004). Role of nucleolin in human Parainfluenza virus type 3 infection of human lung epithelial cells. J. Virology 78 (15), 8146–8158. doi:10.1128/JVI.78.15.8146-8158.2004
Callé, A., Ugrinova, I., Epstein, A. L., Bouvet, P., Diaz, J-J., and Greco, A. (2008). Nucleolin is required for an efficient Herpes Simplex virus type 1 infection. J. Virology 82 (10), 4762–4773. doi:10.1128/JVI.00077-08
Cao, H-X., Liu, P-F., Wang, L., Liu, Z-J., Ye, S-Y., and Liang, G-X. (2020). Nonenzymatic chemiluminescence detection of circulating tumor cells in blood based on au@luminol nanoparticles, hybridization chain reaction and magnetic isolation. Sensors Actuators B Chem. 318, 128287. doi:10.1016/j.snb.2020.128287
Carvalho, J., Lopes-Nunes, J., Vialet, B., Rosado, T., Gallardo, E., Vale, J., et al. (2021). Nanoaggregate-forming lipid-conjugated AS1411 aptamer as a promising tumor-targeted delivery system of anticancer agents in vitro. Nanomedicine Nanotechnol. Biol. Med. 36 (8), 102429. doi:10.1016/j.nano.2021.102429
Catuogno, S., and Lucia Esposito, C. (2017). Aptamer cell-based selection: overview and advances. Biomedicines 5 (4), 49. doi:10.3390/biomedicines5030049
Chan, C-M., Chu, H., Zhang, A. J., Leung, L-H., Kao, Y-T., KennChik, K-H., et al. (2016). Hemagglutinin of Influenza A virus binds specifically to cell surface nucleolin and plays a role in virus internalization. Virology 494 (July), 78–88. doi:10.1016/j.virol.2016.04.008
Chen, L., Zhang, B., Wu, Z., Liu, G., Li, Weihua, and Tang, Y. (2023). In silico discovery of aptamers with an enhanced library design strategy. Comput. Struct. Biotechnol. J. 21, 1005–1013. doi:10.1016/j.csbj.2023.01.002
Chen, X., DianneKube, M., Cooper, M. J., and Davis, P. B. (2008). Cell surface nucleolin serves as receptor for DNA nanoparticles composed of pegylated polylysine and DNA. Mol. Ther. J. Am. Soc. Gene Ther. 16 (2), 333–342. doi:10.1038/sj.mt.6300365
Cheng, Y., Zhao, G., Zhang, S., Nigim, F., Zhou, G., Yu, Z., et al. (2016). AS1411-Induced growth inhibition of glioma cells by up-regulation of P53 and down-regulation of bcl-2 and Akt1 via nucleolin. PloS One 11 (12), e0167094. doi:10.1371/journal.pone.0167094
Cicchillitti, L., Michela Di, M., Urbani, A., Ferlini, C., Benedetta Donat, M., Scambia, G., et al. (2009). Comparative proteomic analysis of paclitaxel sensitive A2780 epithelial ovarian cancer cell line and its resistant counterpart A2780TC1 by 2D-DIGE: the role of ERp57. J. Proteome Res. 8 (4), 1902–1912. doi:10.1021/pr800856b
Dabral, P., Babu, J., Zareie, A., and Verma, S. C. (2020). LANA and HnRNP A1 regulate the translation of LANA MRNA through G-quadruplexes. J. Virology 94 (3), e01508-19. doi:10.1128/JVI.01508-19
Dailey, M. M., Miller, M. C., Bates, P. J., Lane, A. N., and John, O. T. (2010). Resolution and characterization of the structural polymorphism of a single quadruplex-forming sequence. Nucleic Acids Res. 38 (14), 4877–4888. doi:10.1093/nar/gkq166
Darmostuk, M., Rimpelova, S., Gbelcova, H., and Ruml, T. (2015). Current approaches in SELEX: an update to aptamer selection technology. Biotechnol. Adv. 33 (6 Pt 2), 1141–1161. doi:10.1016/j.biotechadv.2015.02.008
Doherty, G. J., and McMahon, H. T. (2009). Mechanisms of endocytosis. Annu. Rev. Biochem. 78, 857–902. doi:10.1146/annurev.biochem.78.081307.110540
Ellington, A. D., and Szostak, J. W. (1990). In vitro selection of RNA molecules that bind specific ligands. Nature 346 (6287), 818–822. doi:10.1038/346818a0
Figueiredo, J., Lopes-Nunes, J., Carvalho, J., Antunes, F., Ribeiro, M., Campello, M. P. C., et al. (2019). AS1411 derivatives as carriers of G-quadruplex ligands for cervical cancer cells. Int. J. Pharm. 568 (9), 118511. doi:10.1016/j.ijpharm.2019.118511
Fogal, V., Sugahara, K. N., Ruoslahti, E., and Christian, S. (2009). Cell surface nucleolin antagonist causes endothelial cell apoptosis and normalization of tumor vasculature. Angiogenesis 12 (1), 91–100. doi:10.1007/s10456-009-9137-5
Fujiki, H., Watanabe, T., and Suganuma, M. (2014). Cell-surface nucleolin acts as a central mediator for carcinogenic, anti-carcinogenic, and disease-related ligands. J. Cancer Res. Clin. Oncol. 140 (5), 689–699. doi:10.1007/s00432-014-1587-5
Gao, H., Yang, Z., Zhang, S., Pang, Z., Liu, Q., and Jiang, X. (2014). Study and evaluation of mechanisms of dual targeting drug delivery system with tumor microenvironment assays compared with normal assays. Acta Biomater. 10 (2), 858–867. doi:10.1016/j.actbio.2013.11.003
Ginisty, H., Sicard, H., Roger, B., and Bouvet, P. (1999). Structure and functions of nucleolin. J. Cell. Sci. 112(6), 761–772. doi:10.1242/jcs.112.6.761
Guo, K-T., Ziemer, G., Paul, A., and Wendel, H. P. (2008). CELL-SELEX: novel perspectives of aptamer-based therapeutics. Int. J. Mol. Sci. 9 (4), 668–678. doi:10.3390/ijms9040668
He, F., Wen, N., Xiao, D., Yan, J., Xiong, H., Cai, S., et al. (2020). Aptamer-based targeted drug delivery systems: current potential and challenges. Curr. Med. Chem. 27 (13), 2189–2219. doi:10.2174/0929867325666181008142831
Healy, J. M., ScottLewis, D., Kurz, M., Boomer, R. M., Thompson, K. M., Wilson, C., et al. (2004). Pharmacokinetics and biodistribution of novel aptamer compositions. Pharm. Res. 21 (12), 2234–2246. doi:10.1007/s11095-004-7676-4
Ishimaru, D., Zuraw, L., Ramalingam, S., TapasSengupta, K., Bandyopadhyay, S., Reuben, A., et al. (2010). Mechanism of regulation of bcl-2 MRNA by nucleolin and A+U-rich element-binding factor 1 (AUF1). J. Biol. Chem. 285 (35), 27182–27191. doi:10.1074/jbc.M109.098830
Iturriaga-Goyon, E., Vivanco-Rojas, O., Magaña-Guerrero, F. S., Buentello-Volante, B., Castro-Salas, I., Aguayo-Flores, J. E., et al. (2021). AS1411 nucleolin-specific binding aptamers reduce pathological angiogenesis through inhibition of nucleolin phosphorylation. Int. J. Mol. Sci. 22 (23), 13150. doi:10.3390/ijms222313150
Jia, W., Yao, Z., Zhao, J., Guan, Q., and Gao, L. (2017). New perspectives of physiological and pathological functions of nucleolin (NCL). Life Sci. 186 (10), 1–10. doi:10.1016/j.lfs.2017.07.025
Jing, Y., Cai, M., Zhou, L., Jiang, J., Gao, J., and Wang, H. (2020). Aptamer AS1411 utilized for super-resolution imaging of nucleolin. Talanta 217 (9), 121037. doi:10.1016/j.talanta.2020.121037
Kang, J., Lee, M. S., Watowich, S. J., and Gorenstein, D. G. (2007). Combinatorial selection of a RNA thioaptamer that binds to Venezuelan equine encephalitis virus capsid protein. FEBS Lett. 581 (13), 2497–2502. doi:10.1016/j.febslet.2007.04.072
Kang, Y. J., Olson, M. O., Jones, C., and Busch, H. (1975). Nucleolar phosphoproteins of normal rat liver and novikoff hepatoma ascites cells. Cancer Res. 35 (6), 1470–1475.
Keefe, A. D., Pai, S., and Ellington, A. (2010). Aptamers as therapeutics. Nat. Rev. Drug Discov. 9 (7), 537–550. doi:10.1038/nrd3141
Kelly, L., Maier, K. E., Yan, A., and Levy, M. (2021). A comparative analysis of cell surface targeting aptamers. Nat. Commun. 12 (1), 6275. doi:10.1038/s41467-021-26463-w
Khurts, S., Masutomi, K., Delgermaa, L., Arai, K., Oishi, N., Mizuno, H., et al. (2004). Nucleolin interacts with telomerase. J. Biol. Chem. 279 (49), 51508–51515. doi:10.1074/jbc.M407643200
Kohlberger, M., and Gadermaier, G. (2022). Selex: critical factors and optimization strategies for successful aptamer selection. Biotechnol. Appl. Biochem. 69 (5), 1771–1792. doi:10.1002/bab.2244
Koronakis, V., PeterHume, J., Humphreys, D., Liu, T., Hørning, O., Jensen, O. N., et al. (2011). WAVE regulatory complex activation by cooperating GTPases arf and Rac1. Proc. Natl. Acad. Sci. U. S. A. 108 (35), 14449–14454. doi:10.1073/pnas.1107666108
Kosiol, N., Juranek, S., Brossart, P., Heine, A., and Paeschke, K. (2021). G-quadruplexes: a promising target for cancer therapy. Mol. Cancer 20 (1), 40. doi:10.1186/s12943-021-01328-4
Koutsioumpa, M., and Papadimitriou, E. (2014). Cell surface nucleolin as a target for anti-cancer therapies. Recent Pat. Anti-Cancer Drug Discov. 9 (2), 137–152. doi:10.2174/1574892808666131119095953
Kumar, K., Hussain, B., and Yüce, M. (2020). Current perspectives on aptamers as diagnostic tools and therapeutic agents. Pharmaceutics 12 (7), 646. doi:10.3390/pharmaceutics12070646
Leaderer, D., Cashman, S. M., and Kumar-Singh, R. (2015). Topical application of a G-quartet aptamer targeting nucleolin attenuates choroidal neovascularization in a model of age-related macular degeneration. Exp. Eye Res. 140, 171–178. doi:10.1016/j.exer.2015.09.005
Leitinger, N., and Wesierska-Gadek, J. (1993). ADP-ribosylation of nucleolar proteins in HeLa tumor cells. J. Cell. Biochem. 52 (2), 153–158. doi:10.1002/jcb.240520207
Li, H., Bai, X., Wang, N., Chen, X., Jing, L., Zhang, Z., et al. (2016). Aptamer-based microcantilever biosensor for ultrasensitive detection of tumor marker nucleolin. Talanta 146, 727–731. doi:10.1016/j.talanta.2015.06.034
Li, J., Wu, C., Hou, P., Zhang, M., and Xu, K. (2018). One-pot preparation of hydrophilic manganese oxide nanoparticles as T1 nano-contrast agent for molecular magnetic resonance imaging of renal carcinoma in vitro and in vivo. Biosens. Bioelectron. 102, 1–8. doi:10.1016/j.bios.2017.10.047
Li, X., Yang, Y., Qian, J., and Qiu, L. (2015). Targeted delivery of anticancer drugs by aptamer AS1411 mediated pluronic F127/cyclodextrin-linked polymer composite micelles. Nanomedicine Nanotechnol. Biol. Med. 11 (1), 175–184. doi:10.1016/j.nano.2014.08.013
Lischwe, M. A., Roberts, K. D., Yeoman, L. C., and Busch, H. (1982). Nucleolar specific acidic phosphoprotein C23 is highly methylated. J. Biol. Chem. 257 (24), 14600–14602. doi:10.1016/s0021-9258(18)33317-9
Lista, M., José, M., Martins, R. P., Billant, O., Contesse, M-A., Findakly, S., et al. (2017). Nucleolin directly mediates epstein-barr virus immune evasion through binding to G-quadruplexes of EBNA1 MRNA. Nat. Commun. 8 (7), 16043. doi:10.1038/ncomms16043
Liu, X., Wu, L., Wang, L., and Jiang, W. (2018). A dual-targeting DNA tetrahedron nanocarrier for breast cancer cell imaging and drug delivery. Talanta 179, 356–363. doi:10.1016/j.talanta.2017.11.034
Lopes-Nunes, J., Lifante, J., Shen, Y., Ximendes, E. C., Jaque, D., Iglesias-de la Cruz, M. C., et al. (2020). Biological studies of an ICG-tagged aptamer as drug delivery system for malignant melanoma. Eur. J. Pharm. Biopharm. Official J. Arbeitsgemeinschaft Fur Pharmazeutische Verfahrenstechnik e.V 154 (9), 228–235. doi:10.1016/j.ejpb.2020.07.018
Luo, Z., Yan, Z., Jin, K., Pang, Q., Jiang, T., Lu, H., et al. (2017). Precise glioblastoma targeting by AS1411 aptamer-functionalized poly (l-γ-glutamylglutamine)-paclitaxel nanoconjugates. J. Colloid Interface Sci. 490, 783–796. doi:10.1016/j.jcis.2016.12.004
Masiuk, M., Urasinska, E., and Domagala, W. (2007). Intranuclear nucleolin distribution during cell cycle progression in human invasive ductal breast carcinomas in relation to estrogen receptor status. Anticancer Res. 27 (6B), 3957–3962.
Mastrangelo, P., Chin, A. A., Tan, S., AmyJeon, H., Ackerley, C. A., KarenSiu, K., et al. (2021). Identification of RSV fusion protein interaction domains on the virus receptor, nucleolin. Viruses 13 (2), 261. doi:10.3390/v13020261
McLellan, J. S., Ray, W. C., and Peeples, M. E. (2013). Structure and function of respiratory syncytial virus surface glycoproteins. Curr. Top. Microbiol. Immunol. 372, 83–104. doi:10.1007/978-3-642-38919-1_4
Miki, Y., Koyama, K., Kurusu, H., Hirano, K., Beppu, M., and Fujiwara, Y. (2015). Nucleolin is a receptor for maleylated-bovine serum albumin on macrophages. Biol. Pharm. Bull. 38 (1), 116–121. doi:10.1248/bpb.b14-00624
Miki, Y., Tachibana, Y., Ohminato, Y., and Fujiwara, Y. (2015). Nucleolin acts as a scavenger receptor for acetylated low-density lipoprotein on macrophages. Biol. Pharm. Bull. 38 (9), 1420–1424. doi:10.1248/bpb.b15-00260
Miranda, A., Santos, T., Largy, E., and Cruz, C. (2021). Locking up the AS1411 aptamer with a flanking duplex: towards an improved nucleolin-targeting. Pharm. (Basel, Switz. 14 (2), 121. doi:10.3390/ph14020121
Niu, H., Yang, X., Xu, Z., Tong, D., and Wang, R. (2015). Cell surface nucleolin interacts with CXCR4 receptor via the 212 C-terminal portion. Tumour Biol. J. Int. Soc. Oncodevelopmental Biol. Med. 36 (2), 1099–1104. doi:10.1007/s13277-014-2734-y
Ogloblina, A. M., Khristich, A. N., NataliaKarpechenko, Y., Semina, S. E., Belitsky, G. A., Dolinnaya, N. G., et al. (2018). Multi-targeted effects of G4-aptamers and their antiproliferative activity against cancer cells. Biochimie 145 (2), 163–173. doi:10.1016/j.biochi.2017.11.020
Ogloblina, A. M., Iaccarino, N., Capasso, D., Di Gaetano, S., Garzarella, E. U., Dolinnaya, N. G., et al. (2020). Toward G-quadruplex-based anticancer agents: biophysical and biological studies of novel AS1411 derivatives. Int. J. Mol. Sci. 21 (20), 7781. doi:10.3390/ijms21207781
Oksayan, S., Nikolic, J., David, C. T., Blondel, D., Jans, D. A., and Moseley, G. W. (2015). Identification of a role for nucleolin in Rabies virus infection. J. Virology 89 (3), 1939–1943. doi:10.1128/JVI.03320-14
Orrick, L. R., Olson, M. O., and Busch, H. (1973). Comparison of nucleolar proteins of normal rat liver and novikoff hepatoma ascites cells by two-dimensional polyacrylamide gel electrophoresis. Proc. Natl. Acad. Sci. U. S. A. 70 (5), 1316–1320. doi:10.1073/pnas.70.5.1316
Ozawa, D., Nakamura, T., Koike, M., Hirano, K., Miki, Y., and Beppu, M. (2013). Shuttling protein nucleolin is a microglia receptor for amyloid beta peptide 1-42. Biol. Pharm. Bull. 36 (10), 1587–1593. doi:10.1248/bpb.b13-00432
Perrone, R., Butovskaya, E., Lago, S., Garzino-Demo, A., Pannecouque, C., Palù, G., et al. (2016). The G-quadruplex-forming aptamer AS1411 potently inhibits HIV-1 attachment to the host cell. Int. J. Antimicrob. Agents 47 (4), 311–316. doi:10.1016/j.ijantimicag.2016.01.016
Peter, M., Nakagawa, J., Dorée, M., Labbé, J. C., and Nigg, E. A. (1990). Identification of major nucleolar proteins as candidate mitotic substrates of Cdc2 kinase. Cell. 60 (5), 791–801. doi:10.1016/0092-8674(90)90093-t
Pon, R. T., and Yu, S. (2004). Linker phosphoramidite reagents for the attachment of the first nucleoside to underivatized solid-phase supports. Nucleic Acids Res. 32 (2), 623–631. doi:10.1093/nar/gkh222
Puzzo, F., Zhang, C., Gray, B. P., Zhang, F., Sullenger, B. A., and Kay, M. A. (2023). Aptamer-programmable adeno-associated viral vectors as a novel platform for cell-specific gene transfer. Mol. Ther. - Nucleic Acids 31 (March), 383–397. doi:10.1016/j.omtn.2023.01.007
Qiu, W., Zhou, F., Zhang, Q., Sun, X., Shi, X., Liang, Y., et al. (2013). Overexpression of nucleolin and different expression sites both related to the prognosis of gastric cancer. APMIS Acta Pathologica, Microbiol. Immunol. Scand. 121 (10), 919–925. doi:10.1111/apm.12131
Rata, D. M., Anca, N. C., Leonard, I. A., Popa, M., Cosmin-Teodor, M., Solcan, C., et al. (2021). Topical formulations containing aptamer-functionalized nanocapsules loaded with 5-fluorouracil - an innovative concept for the skin cancer therapy. Mater. Sci. Eng. C, Mater. Biol. Appl. 119, 111591. doi:10.1016/j.msec.2020.111591
Reyes-Reyes, E. M., Šalipur, F. R., Shams, M., Forsthoefel, M. K., and Bates, P. J. (2015). Mechanistic studies of anticancer aptamer AS1411 reveal a novel role for nucleolin in regulating Rac1 activation. Mol. Oncol. 9 (7), 1392–1405. doi:10.1016/j.molonc.2015.03.012
Reyes-Reyes, E. M., Teng, Y., and Bates, P. J. (2010). A new paradigm for aptamer therapeutic AS1411 action: uptake by macropinocytosis and its stimulation by a nucleolin-dependent mechanism. Cancer Res. 70 (21), 8617–8629. doi:10.1158/0008-5472.CAN-10-0920
Riccardi, C., Fàbrega, C., Grijalvo, S., Vitiello, G., D’Errico, G., Eritja, R., et al. (2018). AS1411-decorated niosomes as effective nanocarriers for Ru(iii)-based drugs in anticancer strategies. J. Mater. Chem. B 6 (33), 5368–5384. doi:10.1039/C8TB01563E
Riccardi, C., Musumeci, D., Piccolo, M., Irace, C., Paduano, L., and Montesarchio, D. (2018). Exploring the conformational behaviour and aggregation properties of lipid-conjugated AS1411 aptamers. Int. J. Biol. Macromol. 118 (October), 1384–1399. doi:10.1016/j.ijbiomac.2018.06.137
Rosenberg, J. E., Bambury, R. M., Allen, E. M. V., Drabkin, H. A., Lara, P. N., Harzstark, A. L., et al. (2014). A phase II trial of AS1411 (a novel nucleolin-targeted DNA aptamer) in metastatic renal cell carcinoma. Investig. New Drugs 32 (1), 178–187. doi:10.1007/s10637-013-0045-6
Saravanakumar, K., Hu, X., Shanmugam, S., Chelliah, R., Sekar, P., Oh, D. H., et al. (2019). Enhanced cancer therapy with PH-dependent and aptamer functionalized doxorubicin loaded polymeric (poly D, L-lactic-Co-glycolic acid) nanoparticles. Archives Biochem. Biophysics 671, 143–151. doi:10.1016/j.abb.2019.07.004
Saravanakumar, K., Sathiyaseelan, A., Jeevithan, E., Hu, X., Shin, S., and Wang, M-H. (2020). Dual stimuli-responsive release of aptamer AS1411 decorated erlotinib loaded chitosan nanoparticles for non-small-cell lung carcinoma therapy. Carbohydr. Polym. 245 (October), 116407. doi:10.1016/j.carbpol.2020.116407
Shi, C. F., Li, Z. Q., Wang, C., Li, J., and Xia, X. H. (2021). Ultrasensitive plasmon enhanced Raman scattering detection of nucleolin using nanochannels of 3D hybrid plasmonic metamaterial. Biosens. Bioelectron. 178, 113040. doi:10.1016/j.bios.2021.113040
Shimakami, T., Honda, M., Kusakawa, T., Murata, T., Shimotohno, K., Kaneko, S., et al. (2006). Effect of hepatitis C virus (HCV) NS5B-nucleolin interaction on HCV replication with HCV subgenomic replicon. J. Virology 80 (7), 3332–3340. doi:10.1128/JVI.80.7.3332-3340.2006
Song, N., Ding, Y., Zhuo, W., Ting, H., Fu, Z., Chen, Y., et al. (2012). The nuclear translocation of endostatin is mediated by its receptor nucleolin in endothelial cells. Angiogenesis 15 (4), 697–711. doi:10.1007/s10456-012-9284-y
Storck, S., Thiry, M., and Bouvet, P. (2009). Conditional knockout of nucleolin in DT40 cells reveals the functional redundancy of its RNA-binding domains. Biol. Cell. 101 (3), 153–167. doi:10.1042/BC20080054
Su, P-Y., Wang, Y-F., Huang, S-W., Lo, Y-C., Wang, Y-H., Wu, S-R., et al. (2015). Cell surface nucleolin facilitates Enterovirus 71 binding and infection. J. Virology 89 (8), 4527–4538. doi:10.1128/JVI.03498-14
Taghdisi, S. M., Noor, M. D., Morteza, A. N., Bahreyni, A., Ramezani, M., Alibolandi, M., et al. (2020). Co-delivery of doxorubicin and α-PCNA aptamer using AS1411-modified pH-responsive nanoparticles for cancer synergistic therapy. J. Drug Deliv. Sci. Technol. 58, 101816. doi:10.1016/j.jddst.2020.101816
Takeda, A. L., Colquitt, J., Clegg, A. J., and Jones, J. (2007). Pegaptanib and ranibizumab for neovascular age-related macular degeneration: a systematic review. Br. J. Ophthalmol. 91 (9), 1177–1182. doi:10.1136/bjo.2007.118562
Tekie, F. S. M., Soleimani, M., Zakerian, A., Dinarvand, M., Amini, M., Dinarvand, R., et al. (2018). Glutathione responsive chitosan-thiolated dextran conjugated MiR-145 nanoparticles targeted with AS1411 aptamer for cancer treatment. Carbohydr. Polym. 201, 131–140. doi:10.1016/j.carbpol.2018.08.060
Thompson, I. A. P., Zheng, L., Eisenstein, M., and Soh, H. T. (2020). Rational design of aptamer switches with programmable PH response. Nat. Commun. 11 (1), 2946. doi:10.1038/s41467-020-16808-2
Tong, X., Lu, G., Ai, J., and Wang, Y. (2022). Progress in cancer drug delivery based on AS1411 oriented nanomaterials. J. Nanobiotechnology 20 (1), 57. doi:10.1186/s12951-022-01240-z
Tuerk, C., and Gold, L. (1990). Systematic evolution of ligands by exponential enrichment: RNA ligands to bacteriophage T4 DNA polymerase. Sci. (New York, N.Y.) 249 (4968), 505–510. doi:10.1126/science.2200121
Uribe-Querol, E., and Rosales, C. (2020). Phagocytosis: our current understanding of a universal biological process. Front. Immunol. 11, 1066. doi:10.3389/fimmu.2020.01066
Wandtke, T., Woźniak, J., and Kopiński, P. (2015). Aptamers in diagnostics and treatment of viral infections. Viruses 7 (2), 751–780. doi:10.3390/v7020751
Wilson, B. D., Hariri, A. A., Thompson, I. A. P., Eisenstein, M., and Soh, H. T. (2019). Independent control of the thermodynamic and kinetic properties of aptamer switches. Nat. Commun. 10 (1), 5079. doi:10.1038/s41467-019-13137-x
Wu, J., Song, C., Jiang, C., Shen, Xin, Qiao, Q., and Hu, Y. (2013). Nucleolin targeting AS1411 modified protein nanoparticle for antitumor drugs delivery. Mol. Pharm. 10 (10), 3555–3563. doi:10.1021/mp300686g
Xu, J-Y., Lu, S., Xu, X-Y., Hu, S-L., Li, B., Li, W-X., et al. (2016). Prognostic significance of nuclear or cytoplasmic nucleolin expression in human non-small cell lung cancer and its relationship with DNA-PKcs. Tumour Biol. J. Int. Soc. Oncodevelopmental Biol. Med. 37 (8), 10349–10356. doi:10.1007/s13277-016-4920-6
Keywords: AS1411, nucleolin, targeted therapy, cancer, endocytosis, pathogens
Citation: Van den Avont A and Sharma-Walia N (2023) Anti-nucleolin aptamer AS1411: an advancing therapeutic. Front. Mol. Biosci. 10:1217769. doi: 10.3389/fmolb.2023.1217769
Received: 05 May 2023; Accepted: 01 August 2023;
Published: 21 September 2023.
Edited by:
Carla Lucia Esposito, National Research Council (CNR), ItalyReviewed by:
Domenica Musumeci, University of Naples Federico II, ItalySimona Camorani, National Research Council (CNR), Italy
Daniela Montesarchio, University of Naples Federico II, Italy
Copyright © 2023 Van den Avont and Sharma-Walia. This is an open-access article distributed under the terms of the Creative Commons Attribution License (CC BY). The use, distribution or reproduction in other forums is permitted, provided the original author(s) and the copyright owner(s) are credited and that the original publication in this journal is cited, in accordance with accepted academic practice. No use, distribution or reproduction is permitted which does not comply with these terms.
*Correspondence: Neelam Sharma-Walia, nsharmawalia@gmail.com, neelam.sharma-walia@rosalindfranklin.edu