- 1Ionic Liquid and Nano Materials Laboratory, Department of Physics, Faculty of Engineering and Technology, V. B. S. Purvanchal University, Jaunpur, India
- 2Department of Environmental Science, V. B. S. Purvanchal University, Jaunpur, India
- 3Centre for Nano Science and Technology, Rajju Bhaiya Institute of Physical Sciences for Study and Research, V. B. S. Purvanchal University, Jaunpur, India
- 4Quantum Computational Lab, Department of Physics, S. S. V. College, Hapur, India
- 5The Guo Photonics Laboratory, Changchun Institute of Optics, Fine Mechanics, and Physics, (CIOMP), Changchun, China
- 6Department of Biotechnology, V. B. S. Purvanchal University, Jaunpur, India
- 7Department of Physics, Graphic Era University, Dehradun, India
There is a significant amount of waste generated which creates a huge environmental issue for humanity/earth and a tremendous number of varieties of resources of a different kind are needed globally. In this context, nanoscience technology has shown its potential ability to solve the above issues and provides realistic applications and devices. The beauty of nanotechnology is its multidisciplinary approach, in which green nanotechnology has been translated to focus on waste materials. Waste materials are generally generated from biogenic (rice husk, dead leaves, waste food, etc.) and non-biogenic (several types of plastics waste, lard oil, etc.) materials produced from municipal or industrial waste. Currently, a large number of efforts have been made to utilize the waste materials for the synthesis of 2D materials in a greener way. This green synthetic approach has two advantages 1) it reduces the cost of synthesis and 2) includes minimal use of hazardous chemicals. Biogenic wastes (contains biomolecules) contain several significant constituents such as co-enzymes, enzymes, proteins, terpenoids, etc. These constituents or biomolecules are known to play an energetic role in the formation of a different variety of 2D materials and hence control the protocols of green synthesis of 2D materials. This review focuses on the exploration of the current understanding of 2D-layered material synthesis methods using waste material produce from biogenic and non-biogenic waste. It also investigates the applications of various 2D-layered materials in perspective with synthesis from waste and future challenges along with their limitations to industrial-scale synthesis.
Introduction
The exponential development and industrialization of the world broadly generates two kinds of waste. The first is related to municipal and industrial waste with tremendous amounts of construction and demolition debris, plastic waste, e-waste, biomedical waste, and other industrial hazardous and non-hazardous waste (Gabryszewska and Gworek, 2020). The second is related to the agricultural waste of villages. It generates bio-wastes containing crop residue, food waste, and other agro wastes. These kinds of waste materials are a big challenge for civilized society because the expected generation of annual waste in the next 30 years (by 2050) is 3.40 billion tons compared to 2.01 billion tons in the year 2018 (Kaza et al., 2018). Therefore, the world is looking toward developing and deploying various technologies to reduce, reuse, recycle, and produce new materials utilizing waste for industrial applications and renewable sources of energy. However, the waste management market (2.3 trillion U.S. dollars in 2027) is slowly growing because of the high cost of segregation, safety, and lack of training in the disposal of waste materials.
The growing human population and industrialization are the major factors for the increase in solid wastes on Earth (Glushkov et al., 2020). The massive amounts of solid wastes lead to public health and environment-related problems due to improper management of waste. Thus, there is an urgent need of ideas and research to recycle and reutilize wastes that could protect us from an unsustainable “tsunami of waste in (the) future.” One well-known and potential technique to solve various problems efficiently is nanoscience and nanotechnology (Bhardwaj et al., 2017a). Therefore, the modern scientific community of nanotechnology is focusing on a sustainable environment. Hence, they are evolving innovative and ground-breaking methods for the preparation of novel nano materials (Bhardwaj et al., 2018; Yang et al., 2020). There are a variety of nano materials from 0D, 1D, 2D, and 3D with their diverse potential in science and technology. These nano materials are generally synthesized by toxic, hazardous, and non-ecofriendly chemicals (Soufi and Iravani, 2020). Hence the concept of green and biogenic/non-biogenic methods has attracted the attention of chemists and materials scientists for the synthesis of new nano materials in a green, cheaper, and ecofriendly way. There are several green methods for synthesis of nano materials that have already been documented in literature (Verma et al., 2012; Hussain et al., 2016; Bhardwaj et al., 2017b). People have used enzymes, microorganisms, fungi, plants, fruits, and fruit peels for the synthesis of nano materials because of two main reasons: 1) avoiding toxic and hazardous chemicals and 2) utilization of waste materials. Alternatively, this type of synthesis reduces the cost of processing and has potential for large scale production. This is because of the availability of an enormous amount of raw materials including roots, stems, peels/pulp (waste), and poly(ethyleneterephthalate) plastic (PET), etc. (Maurya et al., 2016; Bhardwaj et al., 2017a).
The first two-dimensional (2D) nano material was discovered in 2004 Novoselov et al. (2004) and is commonly known as graphene. It is the pioneer member of the wide 2D family. Graphene is a two-dimensional sheet of carbon atoms arranged in a honeycomb structure in which carbon atoms are bonded with each other through sp2 hybridization. Graphene has peculiar properties, viz., high electrical conductivity, greater mechanical elasticity, high carrier transport mobility, high absorption in white light spectrum, high Young’s modulus, etc. (Novoselov et al., 2004). With these exotic properties, the thin flat surface of graphene shows high surface activity and high functionality, and hence graphene and its analogous materials (Rao et al., 2009) have found applications in diverse fields from energy storage and conversion, optical devices, DNA sequencing, hybrid materials, plasmonic sensors, etc. (Edwards and Coleman, 2013; Ren et al., 2018; Coros et al., 2019). Beyond graphene, there are many other 2D-layered materials which have exciting properties such as hexagonal boron nitride (h-BN), graphitic carbon nitrate (g-C3N4), transition metal dichalcogenides (TMDCS), metal nitrides/carbides (MXenes), black phosphorous (BP), monochalcogenides, monoelemental 2D semiconductors, etc. (Zhu et al., 2014; Qiu et al., 2017; Bertolazzi et al., 2018; Darkwah and Ao, 2018; Fu et al., 2019; Hu et al., 2020a; Rao 2020; Sarkar and Stratakis, 2020; Zhang et al., 2020; Gong et al., 2021). One of the key parameters of these 2D-layered materials is tunability of properties based on the number of layers and thickness. They have already shown their potential in LEDs, optoelectronics, FETs, spintronics, etc. A large number of synthesis methods has been demonstrated for the preparation of graphene (Avouris and Dimitrakopoulos, 2012; Madurani et al., 2020) and other analogous 2D-layered materials with different structure and properties (Zhan et al., 2019; Gutierrez, 2020). Although these methods have resulted in high-quality 2D-layered materials, their production still involves many chemicals which are not good for our environment. Therefore, the main objective is to design 2D-layered materials based on environmentally benign approaches for solving challenges related to environmental issues. Therefore, researchers are interested in developing the new ecofriendly synthesis of 2D-layered materials. These synthesis are based on the principles of green chemistry and utilizing waste materials. Accordingly, research in recent years has focused on the development of 2D-layered materials via ecofriendly, rapid, and cost-effective ways having advantages over other chemical methods.
To emphasize this alternative approach, recently researchers have focused on using biogenic and non-biogenic entities to synthesize 2D-layered materials. Biogenic and non-biogenic synthesis of graphene and other 2D-layered materials using agro waste, rice husk, coconut shell, plastics, scrap rubber/tyre, and industrial waste offer alternative ecofriendly approaches for producing graphene and other 2D-layered materials. Each of these biogenic and non-biogenic entities are used as stabilizing agents, surfactants, reducing agents, etc. to synthesize graphene and other graphene-like materials of high quality and sometimes better properties as compared to conventionally synthesized graphene and graphene-like carbon materials. There are few reviews Raghavan et al. (2017) and Kong et al. (2020) which discuss the synthesis of graphene and graphene-like carbon materials utilizing waste, biomass, and bioprecursors. Therein, discussions were mainly focused on the following points: 1) graphene sheets, doped graphene, inter-connected carbon nano sheets, 3D-strutted graphene, monolayer graphene, etc. derived from insects, chitosan, hemp, glucose, food/paper waste, etc., 2) graphene, porous graphene, N and B-doped graphene, and graphene-like porous and non-porous carbon synthesized from biomass like sucrose, gelatin, coconut coir and shell, bamboo char, soybean shell, pine bark, wheat straw, fungus, salvia splendens, etc. via pyrolysis via various methods using salts, templates, post exfoliation, chemical blowing, hydrothermal carbonization, etc., and 3) either applications were not discussed or related to 3D graphene, doped graphene, porous graphene-like carbon with few layers of graphene, and graphene-like carbon.
In the view of the above discussion the present review has following advantages: 1) It is strictly focused on 2D-layered materials, 2) it focuses on utilizing only waste either from biogenic or non-biogenic origin which includes domestic, agriculture, and industrial waste for the synthesis of 2D-layered materials graphene and beyond, and 3) it focuses on recent applications of biogenic or non-biogenic-derived 2D-layered materials (graphene, h-BN nano sheets, and g-C2N). It is of great significance to understand how 2D materials can be synthesized using biogenic and non-biogenic waste materials in a greener and ecofriendly way as well as the applications of derived materials in various areas of the science and engineering. This review, firstly discusses the evolution and requirement of using waste of biological and non-biological origins for the synthesis of 2D-layered materials. Secondly, it describes the recent highlights of the current understanding of the process of biogenically or non-biogenically synthesized 2D-layered materials. Followed by a brief discussion of applications of the concerned materials. Further, it also lists several key challenges and opportunities in this field. Moreover, suggestions are given for further enrichment, development, and additional enhancement of this important area of research. Finally, the conclusion and outlook with critical insight and prospective are given. Also, non-layered, doped, porous, and composite 2D materials did not fall within the scope of this review and hence are not discussed.
Why 2D-Layered Materials are Fascinating
Although extensive research progress has been achieved in the field of 2D-layered materials, such as the development of different synthetic methods, discovery of their structures and properties, and exploration of their potential applications, challenges still remain. Therefore, efforts on pursuing precise control on the structures and properties of 2D-layered materials are still on-going. Specifically, how to improve the existing synthetic approaches or develop new synthetic methods for scale-up production of 2D-layered materials still require great research efforts.
Two-dimensional (2D)-layered materials are a class of materials with sheet-like structures with transverse dimensions larger than 100 nm and thickness typically less than 5 nm. Change in thickness or number of layers are particularly important and key parameters for optimizing structural, optical, and electronic properties of many novel 2D-layered materials. In 2D-layered materials, atoms on the plane are connected by strong chemical bonds in each layer, while layers stack together to form bulk crystals through a weak van der Waals interaction. Further, 2D-layered materials possess a large surface area which makes them an ideal candidate for hybrid nano composites and sensors, etc. Moreover, the large lateral size and planar area of 2D-layered materials provide an excellent opportunity in surface active applications including electrochemistry in comparison to their bulk counterpart. Additionally, confinement of charge carriers along the thickness opens the door for tuning the band gap. Overall, 2D-layered materials (see Figure 1) are very significant materials in terms of composition, crystal structures, properties, multifunctionalities, low cost, and applications (Chen et al., 2017; Khan et al., 2017; Tyagi et al., 2020).
Biogenic and Non-Biogenic Materials for Synthesis of 2D-Layered Materials
Biogenic materials are primarily composed of carbon and hydrogen. These materials produce millions of tons of biomass waste every year. The huge amount of waste creates problems for farmers. Therefore, to avoid the accumulation of bio-waste, it is a customary practice to destroy these bio-wastes by direct combustion of the biomass. It releases huge amounts of CO2 into the atmosphere, expediting global warming (Bhardwaj et al., 2019). Waste material as a source of carbon can be found in biological and non-biological origins. Biological-origin or biogenic waste such as agro waste, rice husk, coconut shell, jatropha oil, banana peels, tea-tree extract, waste food, food products insects, and chicken fat, etc. have been employed to produce various nano materials and graphene-like materials. While non-biological-origin or non-biogenic waste including plastics, scrap rubber/tyre, industrial waste, etc. have also been reported for the fabrication of many nano materials including graphene. Non-biogenic waste includes solid and liquid wastes (leachate from municipal waste, glycerol, engine oil, and heavy oil residue, etc.) (Alfarisa et al., 2015). Researchers are hopefully looking into the utilization of waste materials in ecofriendly ways to develop an approach in order to solve problems related to waste management. Biogenic and non-biogenic (including wastes from industries) waste products are easily, cheaply, and widely available in a large amount which can be used as precursors for the synthesis of 2D-layered materials, and can makes the synthesis process environmentally benign. The advantages and disadvantages of biogenic, non-biogenic, and conventional synthesis of 2D materials are given in Figure 2. The synthesis of 2D-layered materials using biogenic and non-biogenic materials generally involves pretreatment of the waste materials followed by conventional and non-conventional methods as shown in Figure 3.
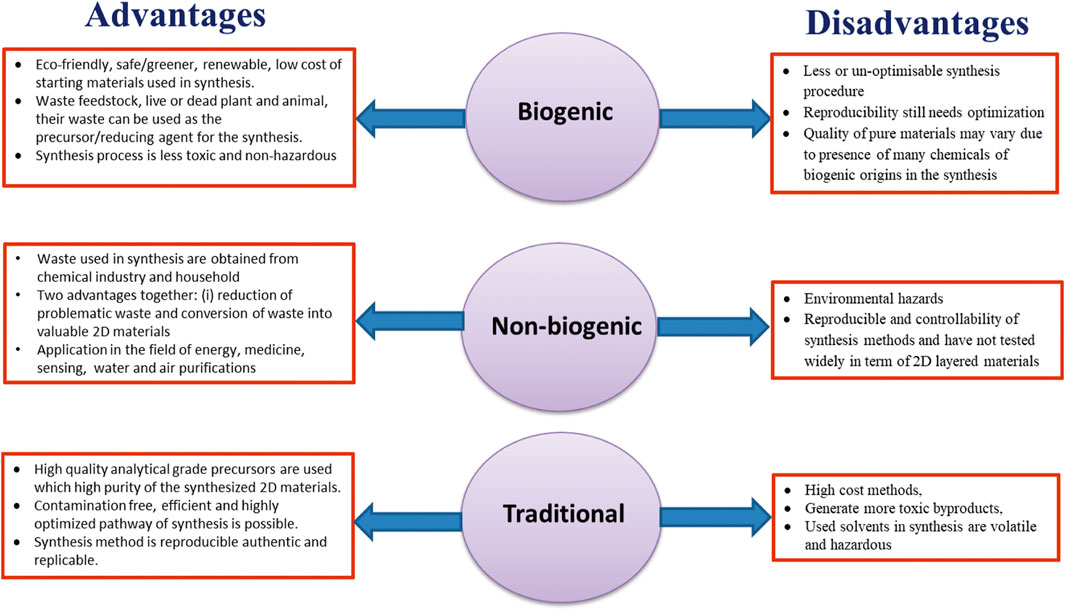
FIGURE 2. Advantage and disadvantage of biogenic, non-biogenic, and traditional fabrication of 2D-layered materials.
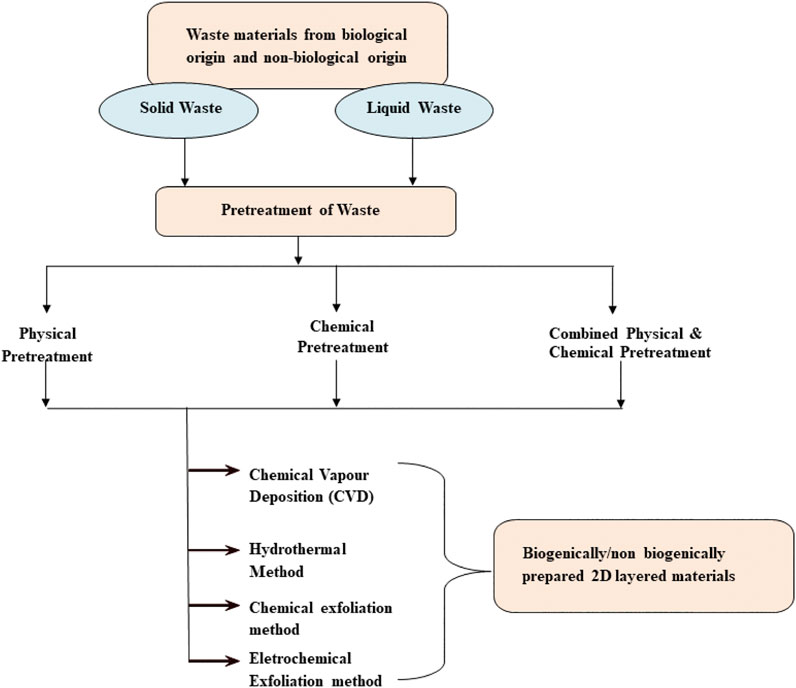
FIGURE 3. Protocol for synthesis of 2D-layered nano materials using biogenic and non-biogenic waste.
Pretreatment Processes
The synthesis of 2D-layered materials from wastes generated from biogenic and non-biogenic materials essentially involves pretreatment (Samaddar et al., 2018). Pretreatment methods can vary with the composition of the waste materials and the technique used for the synthesis of the layered 2D materials. The variety of pretreatment techniques are broadly divided into three categories, 1) physical, 2) chemical, and 3) a combination of physical and chemical techniques. For the synthesis of 2D-layered materials, the pretreatment of waste is quite crucial for efficient and high-quality synthesized material.
Physical Pretreatment
In this method, grinding and milling are the two most basic techniques that are used. In the process of grinding, the mortar and pestle provide mechanical energy to the reactant without any chemicals. Another important term in this section is milling; this process takes place in the presence of ball mills. The most important parameters for ball milling are time and energy which largely influence the expected reproducibility of results (Stolle et al., 2011). The importance of physical pretreatment was shown in a recent report by Pandey et al. (2019) during the preparation of graphene sheets using plastic waste. Initially, the plastic waste was chopped into smaller pieces. Then disk structure bentonite nano clay was mixed with the chopped plastic and slow pyrolysis was performed at 400°C in a nitrogen atmosphere. The slow pyrolysis led to removal of the oil’s hydrocarbon, and gases in the range of C1–C4 were removed from the plastic waste mixture which finally gave a black char used in graphene synthesis.
Chemical Pretreatment
Chemical pretreatment removes soluble contamination or undesirable things present from the waste before 2D-layered material synthesis. The solubility can be increased with increasing temperature or treating with suitable chemicals. Commonly, concentrated strong acids (HNO3, HCl, and H2SO4 etc.), alkalis, and other organic solvents are used to hydrolyze waste materials. However, the very corrosive nature of organic solvent always needs to be neutralized, and acids should be recycled due to minimization of the toxic effect to the environment (Bhardwaj et al., 2019). For instance, Sankar et al. (2018) pretreated a graphite rod (mosquito repellent refill) using (20%) diluted HCl. Then, the graphite rod was boiled in Millipore water for 10 min to remove any remaining impurities. The chemically treated graphite rod was ready for electrochemical exfoliation to prepare a graphene nano sheet.
Combination of Physical and Chemical Pretreatment
In various cases, the single pretreatment method is not particularly useful, therefore the combined method is important. The combination of chemical and physical processes simultaneously or one after another plays a significant role in the pretreatment. For instance, peanut shells are first crushed into a powder and dried in an ambient atmosphere. A few grams of peanut shell precursor powder are pyrolyzed at 800°C in the presence of argon atmosphere for 2 h to get carbonized material. The carbonized material is then washed using isopropanol to eliminate unwanted organic deposition. The process of activation is performed with carbonized material mixed with porogen (KOH) using a mortar and pestle to get a homogeneous mixture. The resulting material is kept for 2 h in a tubular furnace in an inert atmosphere of argon at 800°C to obtain porosity. Finally, functionalized activated carbon materials are obtained for graphene synthesis (Purkait et al., 2017) as shown in Figure 4. In a similar fashion, Nanaji et al. (2018) also reported the pretreatment of jute, which was activated by heat and KOH treatment to get 3D nano flakes. This was suitable for graphene synthesis.
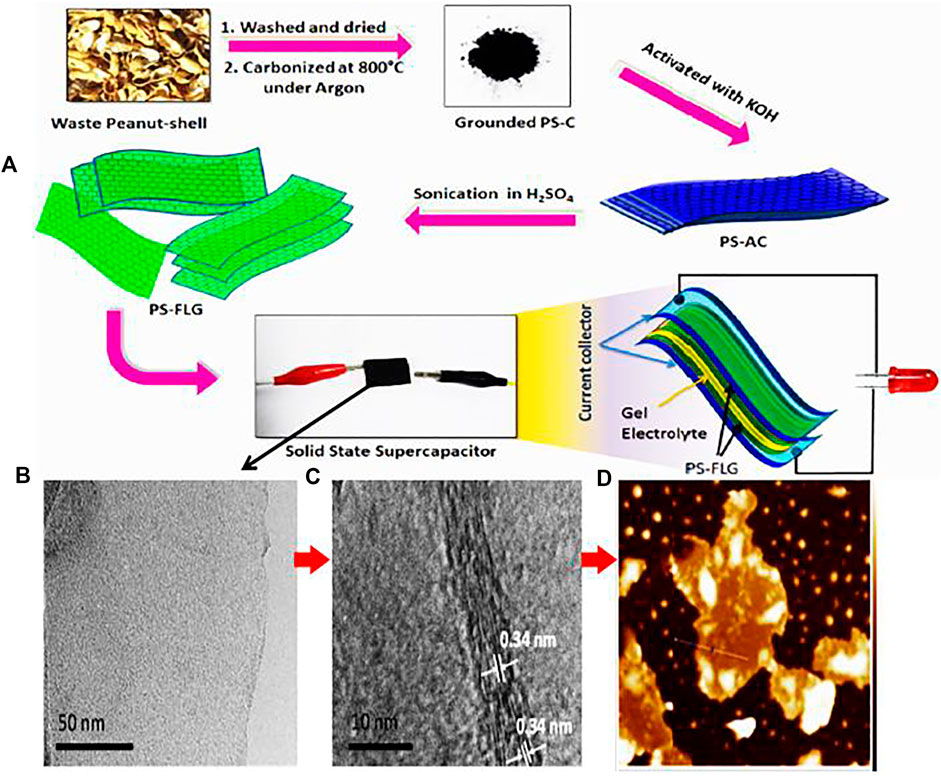
FIGURE 4. (A) Schematic representation of synthesis of few-layer graphene using peanut shell and its subsequent integration into a solid-state device, (B,C) HRTEM images showing crystallite size of about 4.2 nm, (D) tapping-mode AFM images representing 2D mapping. The figure is reprinted with permission from Purkait et al., 2017, Copyright 2017, Springer Nature.
Conventional and Non-Conventional Methods for Synthesis of 2D-Layered Materials Using Biogenic and Non-Biogenic Materials
Synthesis methods for the preparation of 2D-layered materials are well established and can be easily found in literature. However, there are two broad categories, namely top-down and bottom-up approaches for the synthesis of 2D-layered materials. In a top-down approach, nano materials are formed by reducing the size of the atomic plane or by removing the building blocks. In the bottom-up approach, 2D-layered materials are fabricated by building upon single atoms or molecules. In this technique, controlled segregation of atoms or molecules occurs as they are assembled into a desired nano structure. There are many processes in top-down and bottom-up approaches but only a few methods have been applied so far for the synthesis of 2D-layered materials using biogenic and non-biogenic waste materials. These methods are discussed below.
Hydrothermal Synthesis Technique (Bottom-Up Approach)
Hydrothermal synthesis is one of the most commonly used methods for preparation of 2D-layered materials in liquid phase. The meaning of the word is within the name itself, hydro means water and thermal means heat. In other words, it is a wet thermal technique. Hydrothermal synthesis of 2D-layered materials requires an extended range of temperature (room temperature to 1,000°C). To control the morphology of the 2D-layered materials, we need either low or high pressure depending on the vapor pressure of the solution. Through the accelerated dialysis process and physical control, improved 2D-layered nano materials can be obtained. The obtained 2D-layered materials must follow filtration, washing, and drying processes to obtain high-quality 2D-layered materials. Water acts as a solvent and as a mineralizer as well as a reaction medium in the reaction environment. It can also be used as a medium for pressure transfer, so that usually insoluble substances can be dissolved, and the reaction can also be recrystallized. This synthesis technique can be further modified by combining with other processes/techniques or devices such as microwave, sonication, etc.
Chemical Vapor Deposition Technique (Bottom-Up Approach)
Chemical vapor deposition (CVD) is the gas phase method that is popularly used for the synthesis of a thin sheet of 2D-layered materials. In the CVD technique, volatile metal halides, hydrides, or organic metal components are used as raw materials. It is the most tempting method to synthesize 2D-layered materials. As science and technology progress, the CVD technique has been continuously innovated, including laser CVD, plasma CVD, high frequency CVD, and so on. Some of the advantages of CVD are the production of a uniform film with low porosity, high purity and stability, homogeneous particles, small size, good diversity, high chemical reaction activity, and a controllable and continuous process. Normally, CVD involves two or more types of gaseous reactants on the surface of the matrix, and the products are deposited on the substrate. The mechanism of CVD includes the transport of reactant species to the substrate, followed by diffusion of the volatile molecule in the vicinity of the growth site, and the precursor gets adsorbed to the substrate followed by a chemical reaction, by-product desorption, and transport of by-products away from the substrate.
Electrochemical and Chemical Exfoliation Technique (Top-Down Approach)
Electrochemical synthesis is a technique that has been widely used in the synthesis of graphene and its analogous materials. In this method, an applied voltage drives ionic species to intercalate into layers of bulk materials where they form gaseous species that expand and exfoliate individual layers of 2D materials. The scope of electrochemical synthesis can be divided into inorganic and organic synthesis, and large- and small-scale synthesis. The reaction is controlled by the electrode potential and reaction conditions which depend on the cell and system design.
Chemical exfoliation is a process consisting of two steps, 1) by decreasing the van der Waals forces between the layers of bulk material, the interlayer spacing enlarges. Thus, it forms intercalated materials and 2) it exfoliates the layered materials with a single to few layers by rapid heating or sonication.
Family of 2D-Layered Materials Synthesized Biogenically and Non-Biogenically
2D-layered materials attracted much attention just after the birth of graphene. The excellent mechanical and coupling properties of 2D-layered materials play a very important role to optimize the performance and life of devices based on these materials. In the family of 2D-layered materials, only graphene is extensively synthesized either biogenically or non-biogenically. The other members of the 2D-layered materials family are much less studied in terms of synthesis utilizing waste derived from biogenic or non-biogenic materials. The following sections systematically discuss the synthesis of graphene, h-BN, and g-C3N4 utilizing waste of biogenic or non-biogenic origin.
Graphene
Graphene is considered to be the parent of all graphitic materials like carbon nano tubes (CNTs), fullerenes, etc. (Geim et al., 2010). This material is established as the world’s strongest, stiffest, and thinnest material which has surprising characteristics of extraordinary mechanical, thermal, and electrical properties. There are several synthetic routes that were shown in the recent past to obtain mono and multi-layer graphene based on either bottom-up or top-down approaches. However, it is also true that the community of science and technology is always looking for new techniques for easy, simple, commercially, and environmentally useful protocols for the synthesis of graphene. The biogenic and non-biogenic precursors utilized for the synthesis of graphene are mentioned in Table 1. In the coming sections, the discussion will focus on the synthesis of graphene using biogenic and non-biogenic waste materials.
Cellulose Acetate (Main Component of Cigarette Butts)
Cellulose acetate (CA) is a common photodegradable polymer and can be obtained from simple acetylation of cellulose (an abundant biomass). CA is the principle component in cigarette butts (used as filters). Cigarette butts are the most common litter or waste which are frequently available all over the world. The estimated number of waste cigarette butts is 4.5 trillion every year and could be 9.0 trillion by the year 2025 (Mackay et al., 2006; Murugan et al., 2018). This waste material has a serious impact on our ecosystem and living things as it takes a very long time to biodegrade. Therefore, its recycling/recovering or utilization is essential for waste management as well as to solve many environmental issues. Very recently, Chen et al. (2021) proposed a growth setup to prepare single-layer graphene using CA as shown in Figure 5. They also studied the optimization of the effect of growth parameters like hydrogen concentration, temperature, time, condition, and roll of Cu grain boundaries during synthesis of monolayer graphene in terms of quality and size. For the synthesis of monolayer graphene using CA, the authors modified the growth setup. In this modification, half of the Cu substrate was coated with CA whereas the other half remained uncoated. By doing this they resolved two main problems associated with the synthesis of monolayer graphene, 1) the formation of intermediate reduced graphene (r-GO), and 2) insufficient active sites on Cu to act as catalyst. The Cu substrate was prepared (as discussed) and transferred into a custom-made CVD system with pressure approximating 0.5 Pa and temperature increased to 1,050°C with a heating rate of ∼17°C/min. The mixture of Ar and H2 gas was pursed into the CVD system under a constant pressure of 1,600 Pa. With all these experimental conditions, growth took 20 min and the CVD system was cooled very rapidly (∼200°C/min) to room temperature. The growth mechanisms mainly included transfer of mass and the chemical reactions. The H2 molecules were transferred from the H2/Ar mixture to the surface of the CA-coated Cu substrate (mass transfer) and reacted with CA (chemical reactions) along with carbonization and graphitization to produce r-GO, water vapor, etc. As a result of this, r-GO covered the whole area of the CA-coated Cu substrate. Therefore, it stopped the further formation of r-GO by inactivating the Cu catalyst. The low molecular weight polymers (LMWPs) formed during the pyrolysis of CA were disorganized on the remaining half of the uncoated surface of the Cu substrate and formed carbon clusters, followed by the processes of carbonization and graphitization. Consequently, islands of r-GO were formed. These r-GO islands reacted with H2 under the presence of the Cu catalyst and eliminated functional groups with oxygen, followed by recrystallization and hexagonization to obtained monolayer graphene.
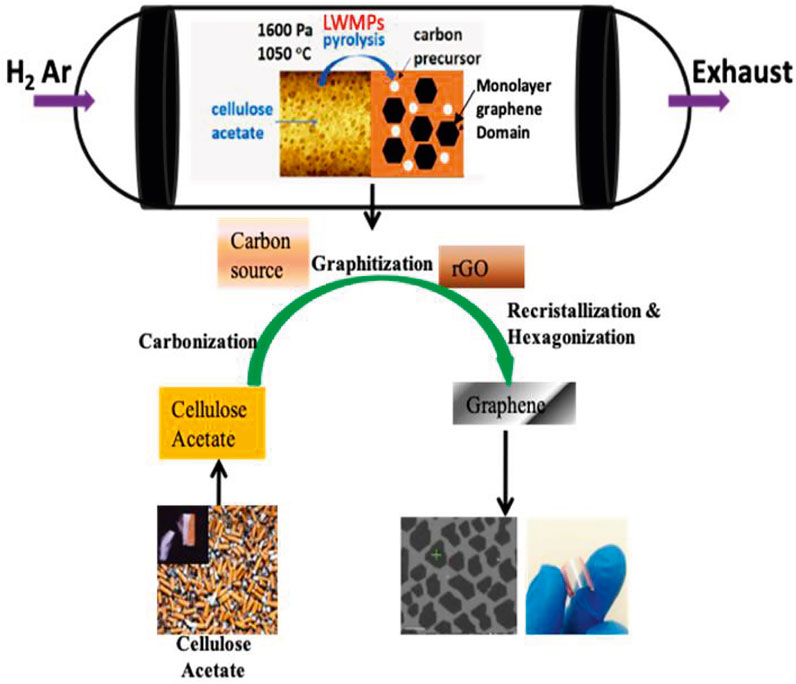
FIGURE 5. Schematic of the growth of monolayer graphene using cellulose acetate. Right bottom corner shows an optical image of the graphene/PET flexible heater. The device dimension was 1 cm × 1 cm. The figure is reprinted with permission from Chen et al., 2021, Copyright 2021, Elsevier.
Rice Husk
Rice is the third most cultivated cereal crop whose global production reached 497.7 million metric tons in the 2019–2020 crop year. Therefore, it is producing tons of rice husk. Rice husk is the external cover of the rice grain which is generally separated as waste in large amounts during the processing of rice in the milling machine. Rice husk is composed of mostly cellulose (∼38%), hemicellulose (∼20%), lignin (∼22%), and silica SiO2 (∼16–20%) (Bazargan et al., 2014; Sankar et al., 2017). Such high availability of worldwide carbon-rich materials are attractive to the scientific community. Muramatsu et al. (2014) reported the synthesis of graphene from rice husk by the KOH activation method. In a typical synthesis process, dried rice husk was converted to rice husk ash (RHA) by simple combustion in air. The activation process is as follow: The RHA (5 g) and KOH (25 g) were mixed and compactly transferred to a mullite crucible and covered with ceramic wool. The whole setup was put in a bigger-sized SiC crucible. This bigger crucible was again covered with ceramic wool and carbon powder (for protection against oxidation in air at higher temperature), and transferred to a furnace. The sample was annealed at 850°C for 2 h in air and an activated sample was obtained. This activated sample was further washed with distilled water and dried at 100°C for 24 h. The final yield was 10 wt%. The synthesized sample was characterized by aberration-corrected HRTEM and it was observed that the sample often contained crystalline mono or multi-layer graphene with atomically smooth edges as shown in Figure 6A and bi-layer graphene as shown in Figure 6B. They also observed a stacked well-defined atomically smooth zigzag edge (Figure 6C). The inset of Figure 6B indicates the hexagonal patterns of atoms in mono or bi-layer graphene. Similarly, Sankar et al. (2017) prepared crystalline ultrathin multi (five) layer graphene nano sheets with a low defect density using brown rice husk. They used the same synthesis protocol as Muramastu et al. (2014). The main differences are 1) brown rice husk instead of white rice husk, 2) lower combustion temperature (500°C) to obtain brown rice husk ash, 3) lower annealing temperature, and 4) avoidance of the use of carbon powder. The appearance of a 2D band clearly demonstrates the formation of 2D multi-layer graphene. In another report, Singh et al. (2017) followed the same synthesis procedure (as Muramatsu et al., 2014) to obtain few-layered graphene from rice husk as shown in Supplementary Figure S1. The key point in this synthesis was the replacement of carbon black powder with rice husk itself. From this discussion, it is clear that rice husk ash has the potential to become an excellent waste material for preparation of 2D-layered graphene. This is because of easy synthesis processes and huge availability across the world. However, a biogenically synthesized graphene sample has a porous structure, agglomerated silica, etc. Therefore, it is recommended that new researchers should develop a new strategy for obtaining high-quality and scalable biogenically synthesized graphene.
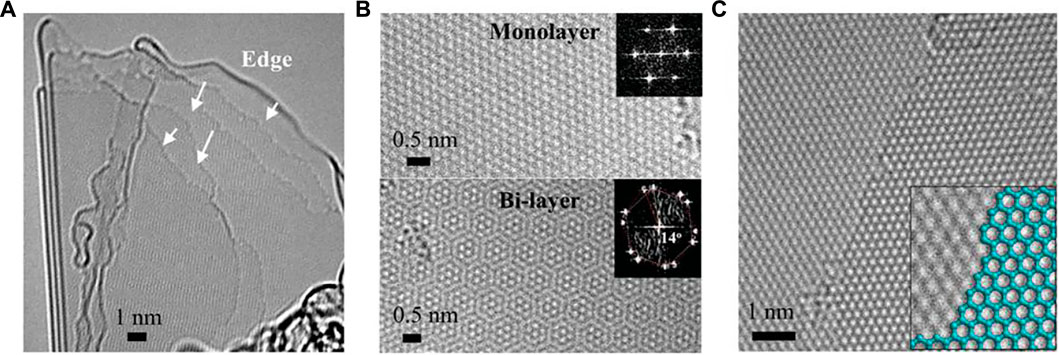
FIGURE 6. Atomic-scale TEM images of crystalline micro-sized graphene: (A) Crystalline-graphene with an exposed edge-rich structure; (B) monolayer graphene (upper) and bilayer graphene exhibiting 14° stacking rotation (bottom); (C) AA stacking exhibiting zigzag edges. The figure is reprinted with permission from Muramatsu et al., 2014, Copyright 2014, John Wiley and Sons.
Graphene From Paper Cups
Disposal paper cups are widely used for serving tea, coffee, water, and other beverages. The chief constituent of a paper cup is made up of cellulose, hemicelluloses and lignin from plants and tree products. Generally, the cups are discarded after a single use, which are then spread by animals, and end up choking the drainage/sewerage system (Durairaj et al., 2019). However, several workers have tried to prepare different graphene derivatives using biodegradable waste material paper/pulp-based waste materials and paper cups (Raghavan et al., 2017; Singu et al., 2018; Shukla et al., 2020). High in quality, crystallinity, and yield with little defects, few and multi-layer 2D graphene sheets have been reported by Zhao and Zhao (2013). They used a waste material (disposable wastepaper cups) and activated it with KOH to synthesize few/multi-layer 2D graphene sheets with the added advantage of obtaining Pt/graphene and Fe/graphene sheets. Figure 7A illustrates the proposed mechanism for the formation of layered graphene with Pt/graphene and Fe/graphene sheets. The synthesis process involved the treatment of the paper cup (composed of lignin, hemicellulose, wood etc.) wastes with KOH solution to form pulp. During the activation process, the metal ion (K+) form complexes with the main constituents of the paper cup (lignin and hemicellulose) through the hydroxy functional groups (alcohol, carboxylic acid etc.); cations were also incorporated to the –OH and –C=O functional groups of the cellulose and lignin structures. After the completion of the activation process, the metal ion-adsorbed paper pulps were passed through an ion exchange process to replace the K+ cations with Fe2+ in the pulp. It was graphitized at high temperature during which multiple graphene layers were produced leading to the formation of Fe/graphene sheets. Removal of Fe was done from these sheets through HCl treatment. Moreover, Pt/graphene sheets were easily obtained by exchanging Fe2+ with Pt2+. After the successful synthesis of the graphene sheets, the quality of the graphene sheets was examined. It was found that the synthesized graphene sheets were 1) hexagonally crystalline and present over a large area (see TEM image in Figure 7B), 2) few-layer to multi-layer (see HRTEM in Figure 7C), and 3) ∼1 µm in lateral size (as evident from AFM analysis, see Figure 7D).
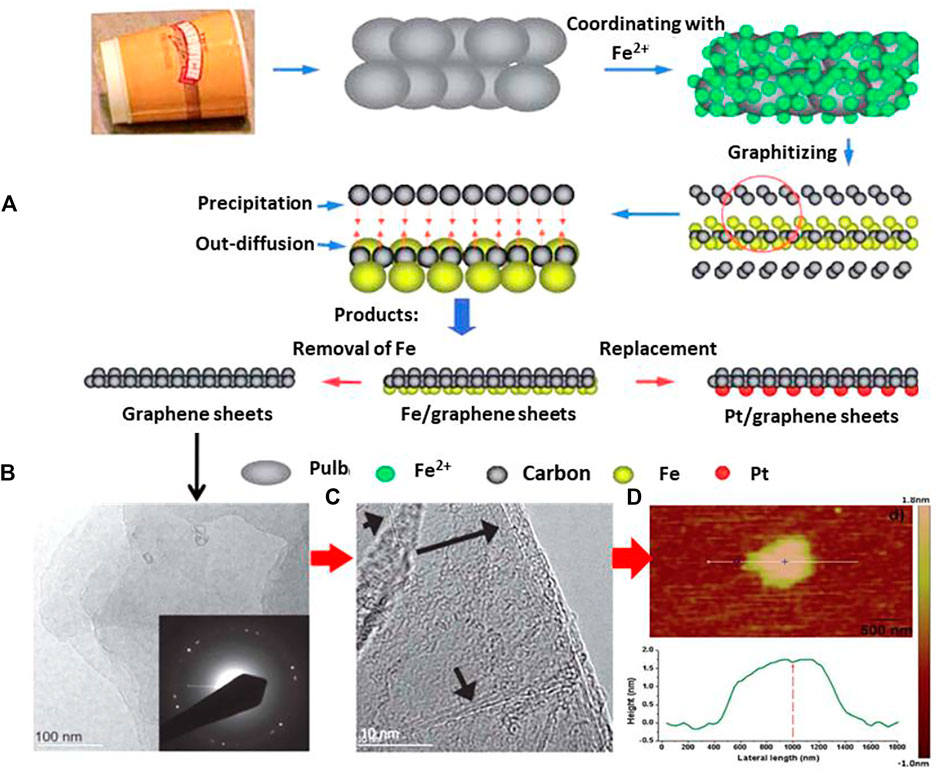
FIGURE 7. (A) Synthesis of Fe/graphene sheets, graphene sheets, and Pt/graphene sheets using disposable paper cups, (B) TEM image of graphene sheets with the SAED pattern in inset, (C) the graphene sheets mainly composed of few-layer and multilayer graphene as observed in the HRTEM image, (D) the AFM image and the corresponding height profile of the as-prepared graphene sheets. The figure is reprinted with permission from Zhao and Zhao 2013, Copyright 2013, Royal Society of Chemistry.
Black Liquor Obtained From Pulping
Pulp black liquor is a major by-product generated by paper and ethanol production industries. It is considered as the second most abundant naturally occurring biomass. Black liquor (BL) is extracted mainly from the wood cooking (kraft) process during the production of wood pulp and then into paper. The major constituent of aqueous black liquor is lignin along with other extractives. The lignin is composed of complex organic natural material, and is widely available all over the world. Therefore, it is believed that lignin is a very attractive material that can be utilized for the synthesis of high-value products like carbon-based nano materials, bio-oil, phenolic resin, etc. (Bajwa et al., 2019; Liu et al., 2015; Schlee et al., 2019). Recently, Ding et al. (2020) reported the synthesis method of mono to few-layer graphene sheets using biogenically derived waste of black liquor via hydrothermal reforming. Their approach was quite interesting in terms of ease of doing, green, potentially scalable, and use of waste material. This synthesis was started with making pulping black liquor from a grass, commonly known as elephant grass (hybrid Pennisetum grass) in a ratio of 1:10:0.24 (elephant grass: water: NaOH). This alkaline mixture was heated to obtain cooked BL followed by filtration, sealing, and storage for the next 30 days at an ambient temperature to get 15 wt% solid content per 100 ml of BL. This solid-containing BL was dissolved in deionized water and transferred into an autoclave (Teflon-lined) and heated at 180°C for various timespans. The resulting solution was filtered, washed, and dried in air. This was followed by centrifugation and dialysis to obtain graphene sheets. The synthesized graphene was examined by TEM, HRTEM, AFM, etc. The obtained graphene sheets were of a controllable average lateral size of 2.5 µm and thickness of 1 nm (Figure 8A). Interestingly, TEM and HRTEM analysis of the graphene sheets showed clear evidence of lamellar characteristics (Figure 8B) and folded edges (mono, bi, and tri-layers) as shown in Figure 8C. It was also observed that the synthesized few-layer graphene sheets had a six-ring carbon structure (left inset of Figure 8D) with lattice spacing of 0.21 nm and a well-ordered hexagonally crystalline structure (right inset of Figure 8D). The mechanism of conversion of pulping BL to 2D-layered graphene consists of aromatic rebounding facilitated by p-hydroxycinnamic acid units which are segments of lignin present in BL followed by re-fusion and finally deoxygenation reaction during activation in an alkaline atmosphere resulting in the formation of atomic carbon layers of sp2 hybridization. These authors have also demonstrated that the longer the reaction time, the smaller the defect in graphene sheets. Similar to elephant grass, another grass named Ophiopogon, collected from Rice University, United States, was used to synthesized a high-quality mono-layer graphene sheet via the CVD method (Ruan et al., 2011).
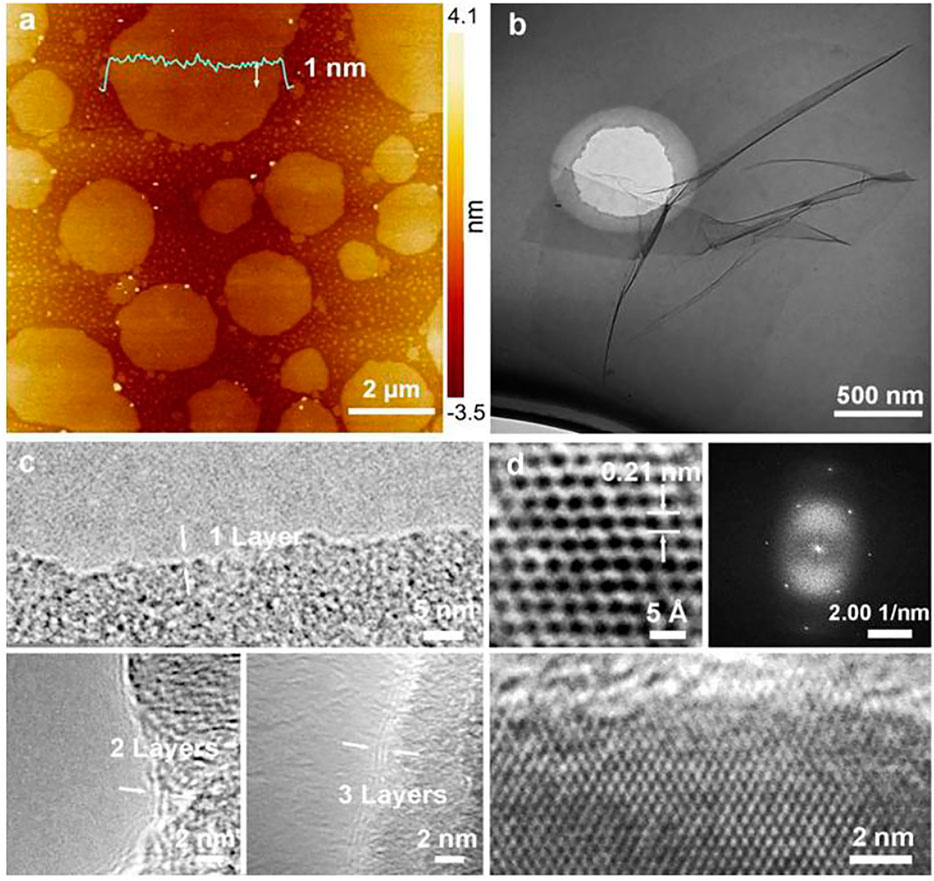
FIGURE 8. (A) and (B) The AFM and TEM images of black liquor-converted graphene (BL-G) sheets, (C) the HRTEM image of BL-G sheets with 1–3 layers, (D)top: The atomically resolved HR-TEM image (left) and selected area electron diffraction pattern (right) to show the well-ordered honeycomb lattice structure with lattice spacing of 0.21 nm. Bottom: The magnified HRTEM images indicated a large area well-ordered graphene lattice. The figure is reprinted with permission from Ding et al., 2020, Copyright 2020, Elsevier.
Graphene From Food Waste
Developed countries contribute more food wastage than developing countries in general, with an average of 280–300 kg per capita per year of food loss in Europe and North America and an average of 120–170 kg per capita per year of food loss in Sub-Saharan Africa and South and Southeast Asia (Papargyropoulou et al., 2014). Ruan et al. (2011) gained popularity when they synthesized single-layered graphene from different solid carbon sources of cookies (the Girl Scouts of America Troop 25,080 from Houston, Texas) and chocolate (Chocolate Kennedy Half Dollar Gold Coins). These raw carbon containing materials (can also be treated as wastes if left or thrown in bin) were loaded on carbon-coated Cu foil in a steeper-curved quartz boat and converted into graphene sheets by the CVD technique at 1,050°C for 15 min under a vacuum of 9.3 torr and with an Ar flow at 500 cm3 STP/min and H2 flow at 100 cm3 STP/min, resulting in the formation of mono-layer graphene sheets of high quality. The experimental synthesis setup is shown in Supplementary Figure S2. The cookies and chocolate-derived graphene sheets were subjected to XPS analysis and found only a sharp peak at 284.5 eV for the C1s peak (Figure 9). This single peak in XPS spectra clearly indicated a graphic peak of sp2 type. Also, the quality of the derived graphene sheet was excellent with no impurity elements present. Further, the graphene sheet was mono-layer with hexagonal crystalline behavior. Recently, Panahi-Kalamuei et al. (2020) worked with an innovative approach for recycling leftover waste bread into valuable round-shape mono/few-layer graphene using simple hydrothermal synthesis. The group obtained a gel by mixing bread waste with water at 70–80°C. The gel was subjected to hydrothermal treatment in a Teflon-lined steel autoclave at 180°C under different conditions of pH and reaction time. Then, the suspension was filtered to recover the precipitate formed. The obtained material was characterized by TEM, SEM, and XRD. They have also investigated the effect of precursor (waste bread) concentrations, temperature, and pH value. It was observed that the mono-layer graphene sheet of round shape formed with 0.1 g of precursor concentration in natural pH at 180°C. All other investigated precursor concentrations, pH values, and temperatures resulted in either porous, mono and porous mixed graphene, or graphene quantum dots.
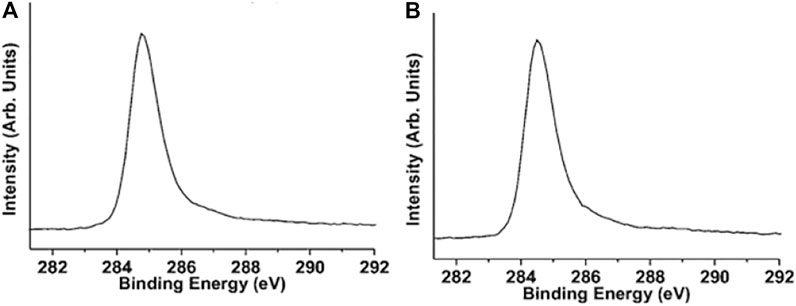
FIGURE 9. XPS spectra of graphene from two carbon sources. The C1s XPS spectra of the randomly selected detection spots on graphene derived from the (A) girl scout cookies and (B) chocolate. The figure is reprinted with permission from Raun et al., 2011, Copyright 2011, American Chemical Society.
Oil
Lard is a fat extracted from the fattiest portions of swine. After extraction, lard undergoes more or less advanced refining processes, depending on the end use of the products. It is used in high-heat cooking due to its relatively high smoke point. Lard is popular in British, central European, Chinese, and Mexican cuisines. It can easily be converted into colorless oil by pressing pure lard. The lard oil is significantly used in various parts of the world, therefore waste lard oil can be obtained from daily cooking and oil manufacturers. Waste lard oil is a naturally abundant, non-toxic, green, and low price source of carbon. Utilizing this waste, Wu et al. (2017) reported a novel single-step synthesis of interweaved few-layer vertical graphene via inductively coupled plasma (ICP)-enhanced chemical vapor deposition (PECVD). In a typical synthesis, nickel foam was uniformly smeared by 1 mg of waste lard oil obtained from a restaurant. This foam was transferred in a radio frequency ICP-PECVD system as depicted in Figure 10. A mixture of Ar and H2 carrier gas was used to maintain a reducing atmosphere at 800°C for 15 min. After the completion of synthesis, the silvery white nickel foam was completely converted into the black foam indicating the deposition of graphene sheets on foam. The obtained graphene was interweaved into a few-layer mesh-like vertical graphene sheet. It is also interesting to note that the synthesis temperature is very crucial as a relatively low temperature (600 and 700°C) resulted in chopped carbon sheets with homogeneous granular particles and spherical carbon particles with a relatively regular nano structure. The graphene sheets that were synthesized at a relatively high temperature of 900°C produced very low quality. The authors also tried to explain the formation of vertical graphene sheets using waste lard oil by giving the mechanism of this complex electrochemical process. In this process, ICP and high temperature jointly played significant roles. The initiated electrons in ICP collided with Ar and H2 which produced active species like radicals and excited the molecule responsible for radical reactions. At the same time, decomposition of waste lord oil in volatile components along with carbon-containing species occurred and reaction pressure was increased in the vessel. With this increased pressure and optimized temperature, the carbon-containing species started generating nucleation sites as well as amorphous carbon on the nickel surface. This was then followed by a reaction between active species and H-atoms which formed vertical graphene sheets on the Ni substrate. These steps are schematically shown in Figure 11.
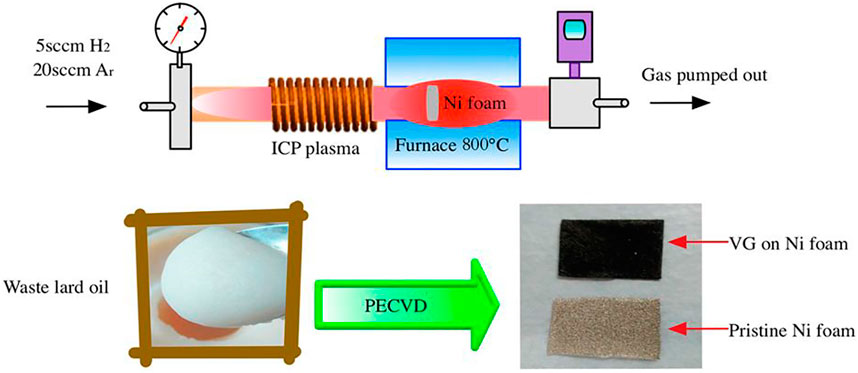
FIGURE 10. Schematic diagram of inductively coupled plasma-enhanced chemical vapor deposition (ICP-PECVD) applied for the synthesis of waste lard oil-based vertical graphene (Wu et al., 2017).
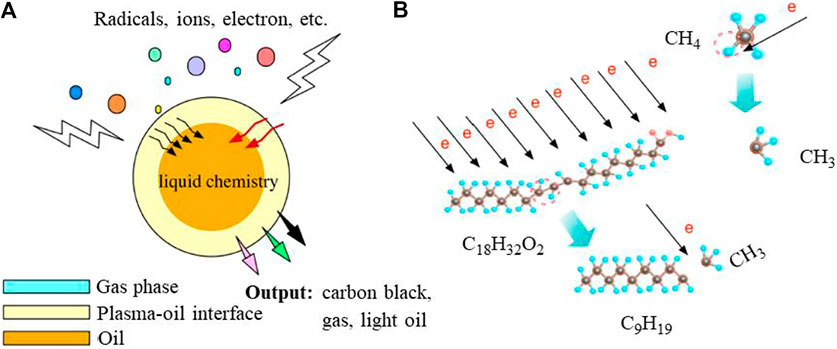
FIGURE 11. (A) Decomposition of waste lard oil in PECVD and the effect of electron collisions, (B) breakdown of fatty acid chains induced by electron collisions (Wu et al., 2017).
Mosquito-Repellent Graphite Rod
Mosquito repellents are widely used in the Asian Pacific, Africa, Middle East, Latin America, and other parts of the world like North America, Europe etc. There is a verity of mosquito repellents (plug-in vaporizer, spare, cream, oil, coil etc.) used over the world. The plug-in vaporizer is the second most popular segment in the global market of mosquito repellents. The mosquito repellent market is estimated at $4.1 billion in the year 2020 as per the latest report of Allied Market Research. The bigger market is expected to produce large waste material by plug-in mosquito repellents. This type of waste plug-in mosquito repellent vaporizer consists of an empty plastic bottle (refill) with a rod. Upcycling of these is quite important in terms of waste management. Recently, Sankar et al. (2018) prepared few-layered graphene via an electrochemical exfoliation method using graphite rods (Lai, 2014; Kumar, 2016) obtained from empty mosquito repellent refills (Good Knight from Godrej Consumer Products Ltd., Mumbai, India). These graphite rods were used as the source of graphene during synthesis. In this electrochemical exfoliation process of graphene, two cleaned rods (with diluted HCl of 20% and boiled Millipore water) of empty mosquito repellent refills were used as electrodes. In these two electrodes, one electrode worked as the anode and the other acted as the cathode with each one separated by 1.5 cm. The complete setup was kept under sonication in the presence of 0.02 (M) of sodium dodecyl benzene sulfonate aqueous solution and a DC voltage of 4.75 V for 4 h. The obtained solution was filtered and extracted to obtain layered graphene. The per hour yield obtained was 0.037 g at a DC voltage of 4.75 V. It was observed that increasing the DC or exfoliation voltage did not increase the yield. This is because of the separation of bigger particles. The quality of synthesized graphene is defective because it has different functional groups on the surface. Therefore, further treatment with sodium citrate is suggested to obtain a higher quality of graphene. However, a higher intensity of the 2D band in Raman spectra is a clear indication of a few-layer graphene sheet.
Dead Camphor Leaves and Tea Tree Leaves
The scientific name of the camphor tree is Cinnamomum Camphora and it belongs to the family of Lauraceae. Initially, it was found in the sub-tropics of Eastern Asia but currently it is found in most continents. The size of the camphor tree is generally big with bark and leaves colored a pale brown and dark green to yellowish, respectively. Wood and bark of this tree are used to prepare camphor whereas leaves have medical and cosmetic uses. However no significant use of dead leaves was reported until Shams et al. (2015) proposed an idea to utilize the dead camphor leaf as a precursor for high-valued product. In this synthesis, graphene was obtained from camphor leaves by single-step pyrolysis under an N2 atmosphere. The dead leaves were pretreated, dried, and chopped into smaller pieces for pyrolysis under an N2 atmosphere at 1,200°C at 10°C/min for 400 min. Then the sample was left to cool at room temperature. After the completion of the pyrolysis process, the obtained sample was mixed with D-tyrosine and tri chloroethane and ultrasonicated for 15 min in an ice bath. Followed by centrifugation, which was applied to obtain few-layer graphene (due to π-π interaction graphene and D-Tyrosine). However, the yield was quite low (0.08 mg of graphene per 100 mg of dead leaves). The synthesized graphene had a layered structure with seven estimated layers. The quality of the synthesized few-layer graphene was not very good due to the presence of other functional groups on the surface of the graphene. This was also evident by the less prominent D, G, and 2D bands of Raman spectra.
Melaleuca Alternifolia commonly known as tea tree is a native Australian plant and is found easily in Australia, Europe, and North America. This is widely used to make essential oils for traditional medicine and as an active component in an array of products. Leaves of tea tree are used to extract oil using a steam distillation process. Jacob et al. (2015) synthesized high-quality graphene using tea tree extract oil using a radio frequency (RF) capacitively coupled plasma-enhanced CVD process at about 800°C. This synthesis was very rapid (seconds to a few minutes), without catalyst, and suitable for many substrates. Despite of its high quality, large area, and environmentally-sustainable green synthesis, the obtained graphene had a 3D structure. Actually, the growth started in 2D along with a small increase in the vertical z-dimension of the graphene film. This is possibly due to the strain produced in the graphene film during the rapid growth process.
Plastic Wastes
Plastic products (polymeric materials) have become an integral part of our daily life as a result of which they are now produced at a massive scale worldwide after the first industrial scale production in the 1940s. In 2019, the global production of plastics reached 368 million metric tons due to continued growth in production year after year (Tiseo, 2021). Once plastic is discarded after its utility is over, it is known as plastic waste. Plastics are not inherently bad but plastic waste does not degrade. Therefore, it remains in the landscape for several hundred years. This causes serious problems to the soil, ecosystem, biodiversity, and ecosystem of the ocean. The most obvious solution of this problem is reuse or recycling of plastic materials. According to a recent report by the government of India, only ∼20% of waste plastic materials was recycled globally, while 25% was incinerated, and ∼55% of global plastic waste was discarded either in landfill or ended up polluting streams/groundwater resources in 2019 (www.mohua.gov.in, 2019). Scientists are constantly working on developing recycling technologies to reduce plastic waste, and transform them into materials that have a smaller environmental impact and are economically profitable. Previously, carbon nano tubes were prepared by pyrolysis of granular polyethylene at 420–450°C in a helium atmosphere using Ni plates as catalyst (Kukovitskii et al., 1997). Recently, Pandey et al. (2019) reported an invincible synthesis method of few-layered graphene from plastic solid waste via a two-step pyrolysis process (first at 400°C and second at 750°C). Initially, in the presence of disk structure bentonite nano clay mixed with shredded plastic, slow pyrolysis was performed at 400°C in a nitrogen atmosphere with a heating rate of 5°C/min. The slow pyrolysis led to removal of hydrocarbons from oil, and gases in the range of C1–C4 removed from the plastic waste mixture. After the completion of the first step, the obtained black charred residue was converted into a fine powder using a ball mill. Furthermore, the obtained ultrafine powder was heated at 750°C at the rate of 10°C/min in an inert N2 atmosphere which consequently formed a black-colored graphene sheet. The obtained final product also contained residual nano clay, therefore washing (distilled water) and acidic treatment (5% HCl) was performed to get high-quality few-layer graphene. This method has great potential for recycling waste plastic and producing graphene due to the high efficiency of conversion (35 kg of waste plastic converted to 5.25 kg of graphene). The synthesized material has been investigated experimentally and computationally [Gaussian09 and QuantumWise-Atomistix Toolkit (ATK) packages]. Experimental and computational data of the Raman investigation of D and G bands show close agreement. However, the 2D band in the experimental Raman spectra is broad (much smaller than D and G bands) indicating the presence of few-layer graphene nano sheets. This is also evident in TEM despite of a wrinkled morphology with arched boundaries. Further, it was found that the nucleation density must be controlled via pyrolysis temperature rate to gain higher-quality graphene nano sheets. Moreover, the synthesized graphene showed the appearance of oxygen (21.1%) in the sample indicating defective graphene. A similar synthesis method was also used by Karakoti et al. (2020) for production of graphene nano sheets using plastic waste. However, their second stage pyrolysis temperature was 900°C instead of 750°C to ensure the removal of oxygen present in the sample. Therefore, the graphene sheets worked according to the need of the supercapacitor. In another report, postconsumer plastic bottles made up of poly(ethyleneterephthalate) (PET) were used to synthesize graphene (El-Essawy et al., 2017). However, the synthesized graphene had a porous nature. In a recent study of Hu et al. (2019), they demonstrated that the preparation of few-layered graphene sheets via a two-step cross-linking strategy using waste expanded polystyrene (EPS) as a source of carbon precursor. This method is quite important for (Plastic wastemanagement issues, 2019) EPS has a large production (∼15.4 million tons per annum globally), low cycling rate (10–30%), and is the most difficult biodegradable class of plastic (Feng et al., 2011; Chaukuraet al., 2016). In a typical synthesis producer, first of all a pre-cross-linking strategy (using glacial acetic acid, formaldehyde dimethylacetal, and 0.3 g of clean EPS particles for 4 h) and subsequent hyper-cross-linking reaction (with 3 g of FeCl3 as catalyst) are carried out in 1,2- dichloroethane (DCE) (as a cross-linker and solvent) to get a good amount of yield. Followed by carbonization (at 600, 700, 800, and 900°C of FeCl2-loaded hyper-crosslinked EPS at 2°C min−1 and held for 2 h in a nitrogen flow resulting in few-layer graphene sheets after acid washing. Glacial acetic acid is used as a solvent to prevent EPS from dissolving in the second step of cross-linking to retain the framework structure of EPS. It is quite interesting that 70 wt% waste EPS is converted to a few-layer graphene sheet. Therefore, showing potential to scale up the production of few-layer graphene sheets using EPS. Alternatively, it could be an effective way for waste management of EPS. The size of few-layer graphene sheets did become smaller and isolated with more defects on the edges as the synthesized temperature increased from 700 to 900°C (Figures 12A–F). However, there was no change in the crystallinity (Figure 12C) but the intensity ratio of D and G bands of Raman spectra increased with temperature (Figure 12D). The carbon atoms in the sheets showed sp2 hybridization and long range ordering in a 2D hexagonal lattice of few-layer graphene by HRTEM (Figure 12G) and selected area electron diffraction (SAED) pattern (Figure 12H). In a another very recent study, Gu et al. (2021) reported the synthesis of high-quality bi-layer graphene using polyethylene (PE) plastic bag wastes as a precursor by ball milling and graphitization. In the typical synthesis, the waste PE plastic bags were first ultrasonically cleaned, chopped, and subjected to ball mill for 6 h to obtain a powdered sample. The small amount of sample (∼2 g) was sieved and carbonized at 1,300°C for 2 h in a vacuum oven to get a carbonized block. The obtained sample was ball-milled, washed, purified, and microwave-sintered for 6 min to obtain final black products. Graphene was observed after purification and vacuum-drying of black products. The as-prepared graphene exhibited distinct thin layers and different sizes. It is interesting to observe a 2D lattice structure of mono and bi-layers of atomically thin carbon atoms.
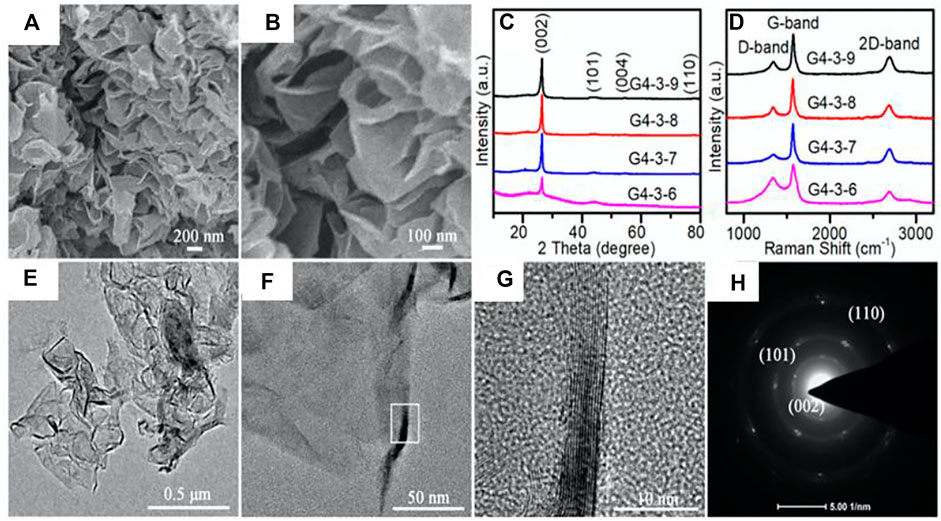
FIGURE 12. (A,B) Field emission scanning electron microscopy (FESEM) images of the as-synthesized G4-3-9. (C) XRD patterns and (D) Raman spectra of the as-synthesized samples at different carbonization temperatures. (E,F) TEM images, (G) high-resolution TEM (HRTEM), and (H) selected area electron diffraction (SAED) pattern of the sheets (G4-3-9). Graphene sheets prepared using pre-cross-linked samples after 4 h, and 3 g of FeCl3 at 900°C is labeled as G4-3-9. The figure is reprinted with permission from Hu et al., 2020b, Copyright 2020, American Chemical Society.
Recently, a new step changing approach was introduced for synthesis of high-quality graphene without use of a furnace, solvents, or reactive gases in less than a second (Luong et al., 2020). This method is known as direct current flash Joule heating (DC-FJH) and is much more cost effective compared to bottom-up CVD deposition or top-down exfoliation methods. This process has great potential for industrial scale production of high-quality graphene and improves the access of the graphene industry to users. In the process, carbon precursor material is placed inside a tube between two electrodes and treated with a high DC voltage electrical discharge. This discharge produces excess temperature (more than 2,727°C) to heat the sample for a hundred milliseconds, which is then converted into graphene. All other chemicals escape as volatile material. Algogeeb et al. (2020) used this method with a slight modification for direct conversion of waste plastics (pre-treatment not required) to flash graphene without any catalyst. They used AC-FJH followed by DC-FJH. In this synthesis, plastic wastes of various types [carbonated beverage bottles, milk jugs or Polywize (Jacksonville, TX), plumbing pipes, single-use plastics bags, disposable straws, food packaging plastic, disposable coffee cups] were powered and mixed with carbon black to get a conductive mixture during the FJH process Figure 13A. This mixture was put in a reaction tube of quartz and subjected to the AC-FJH process for ∼8 s and then the DC-FJH process was applied for 500 milliseconds to get very high quality of FG. It was found that the flash graphene was turbostratic, i.e., one layer of graphene can be rotated with respect to the next layer due to weaker interactions between layers compared to normal graphene (A-B or A-B-C-stacked layers). It was found that the sequential AC and DC FJH method is more effective for producing high-quality turbostratic flash graphene either utilizing PW (mixed plastic) or single plastic. Further, AC-FJH is also able to convert PW into turbostratic flash graphene (Figure 13B) but has lesser quality compared to the sequential AC and DC FJH method. However, the DC FJH method is not sufficient enough to produce high-quality flash graphene. Turbostratic flash graphene obtained either by only AC or the sequential AC and DC-FJH process clearly demonstrates highly graphitic sheets with an average number/size of four layers/16 nm Supplementary Figures S3A,B and six layers/27 nm Supplementary Figures S3C,D, respectively. Wyss et al. (2021) obtained turbostratic flash graphene sheets with 1–3 layers and a size of ∼20 nm using pyrolysis ash of plastic waste by the same process of DC-FJH.
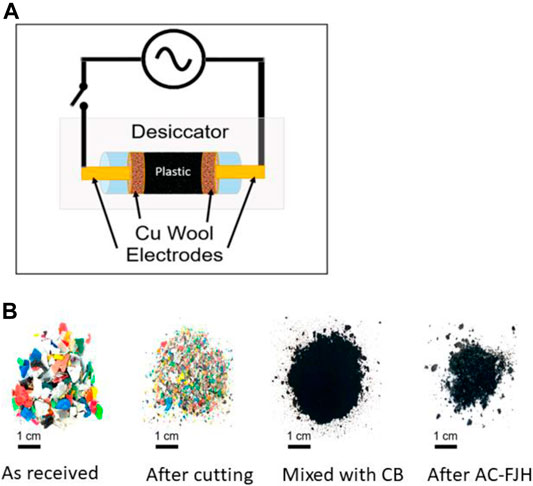
FIGURE 13. (A) Schematic of the 120 V AC circuit, (B) Pictures of (left to right) postconsumer plastic as received from a recycler; after cutting using a commercial cutter; after mixing with 5 wt % CB; and further conversion to FG using AC-FJH.The figure is reprinted with permission from Algogeeb et al., 2020, Copyright 2020, American Chemical Society
2D Nano Materials Beyond Graphene
The wide field of 2D-layered materials opened with the discovery of graphene in 2004 Novoselov et al. (2004). The 2D family of materials has increased their members quite rapidly because of exotic properties and significant advancement of synthesis methods. These new 2D-layered materials are transition-metal dichalcogenides, graphitic carbon nitride (g-C3N4) hexagonal boron nitride, black phosphorous, etc. Similar or some times better performances have been given by these new 2D-layered materials compared to graphene. The origin of better performance of newly discovered 2D-layered materials is due to 1) the effect of dimensionality and 2) a modified band structure. The hunt for 2D-layered materials using biogenic and non-biogenic waste beyond graphene has started with graphitic carbon nitride (g-C2N) and hexagonal boron nitride (h-BN). These materials are discussed below.
Biogenic Synthesis of Graphitic Carbon Nitride (g-C2N Structure)
Aloe Barbadensis is a species of the Liliaceae family and commonly known as Aloe vera or Barbados or Curacao Aloe. The Aloe vera plant is native to northern parts of Africa but currently it is available across the world. It has turgid green leaves joined at the stem in a rosette pattern which contain a transparent mucilaginous jelly. This jelly is known as Aloe vera gel. The gel offers several therapeutic benefits based on the high content of amino acids, sugars, enzymes, hormones, vitamins, minerals, etc. Tiwari and Ram (2019) used a simple biogenic approach to synthesize graphitic-carbon nitride using Aloe vera gel through a self-combustion process. In this synthesis, a fresh and filtered Aloe vera gel was taken from the plant of Aloe vera from the campus of IIT Kharagpur, India. The gel was then dried and burnt (using camphor) in a vacuum furnace at 250°C for 10 min to obtain a powdered sample. The obtained sample was pulverized and heated again at 600°C in a vacuum furnace for 2 h for removal of impurities, and finally a hybrid-phase g-C2N was obtained. Synthesized g-C2N has been investigated by FESEM, TEM, HRTEM, XPS, FTIR, and Raman spectroscopic studies. It was found that exfoliated graphitized sheets have a layered structure Figure 14A and are in the form of a transparent film of nanometer-thickness grafted with carbon nitride nano dots Figure 14B. The dispersed micro structure is also observed for carbon plate-embedding nano dots of g-C2N Figure 14C). HRTEM analysis showed that the amorphous graphite sheet was clearly separated with carbon nitride crystallites Figure 14D.
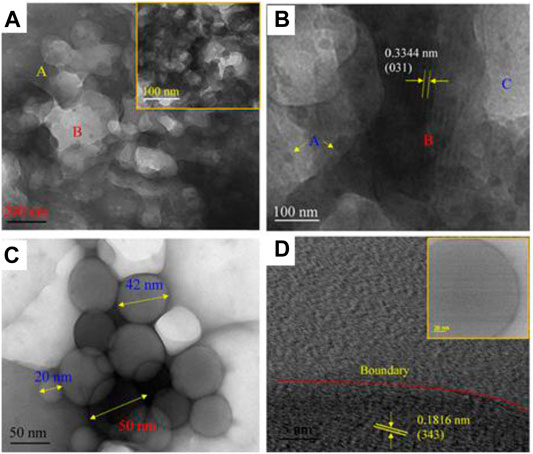
FIGURE 14. (A-D) HRTEM images of (A) layers of graphite sheets are more in region ‘A (in yellow colour) as compared to region B (in red colour) while inset shows magnified view of the graphitized carbon bonded to surface carbon nitride nanodots, (B,C) the carbon plate-embedding nanodots of graphitic carbon nitrate (g-C2N) and, (D) the lattice image of g-C2N where a boundary separates the amorphous graphite sheet with carbon nitride crystallites. The figure is reprinted with permission from Tiwari and Ram, 2019 Copyright 2019, American Chemical Society.
Biogenic Exfoliation of Bulk Hexagonal Boron Nitride to Nano Sheets
h-BN is known as white graphene. It has demonstrated many excellent and unique properties, for example it has a very wide direct band gap (5.97 eV) (Xu et al., 2013) which makes it a superior class of insulator. A variety of synthesis processes have already been shown to prepare h-BN nano sheets. However, these methods do not include a environmentally benign approach, and face problems related to scalable and industrial-level production. Recently, Deshmukh et al. (2019) developed a simple and environmentally benign process for the production of h-BN nano sheets from bulk h-BN using extracts of 17 plant (ranging from Panax ginseng, Hovenia dulcis, Morous nigra, Cercis chinensis, etc.) leaves/roots/stems cells/foliage/needles/cocklebur/seaweed followed by application of ultrasound. Figure 15 shows the schematic representation of various steps involved in the biogenic synthesis of h-BN nano sheets. This approach has significant advantages over other chemical methods as it is greener, energy saving, and cost effective. All the plant extracts have a different ability in the exfoliation process from h-BN to h-BN nano sheets depending upon the phytochemicals present in the plant extract. The mechanism of the exfoliation process is quite interesting and simple. It involves two steps. The first step is the acoustic cavitation produced by ultrasound in the mixture of bulk h-BN and plant extract solution which results in weaker van der Waals force between the adjacent layers. As a consequence of this, h-BN break into nano sheets. The second step is the adsorption of biomolecule moieties (from phytochemicals) on the surface of bulk h-BN. The adsorption of biomolecules helps in the exfoliation process as well as acts as a barrier in the aggregation of nano sheets. The TEM and AFM images of h-BN nano sheets exfoliated from bulk h-BN using plant extracts show (see Supplementary Figure S4) high-quality h-BN nano sheets, i.e., transparent, multi-layer, and average lateral dimension of 77 nm compared to pristine or bulk h-BN. More importantly, the yield in this biogenic exfoliation synthesis protocol is good (∼23%) compared to other exfoliation methods and has potential for large scale production.
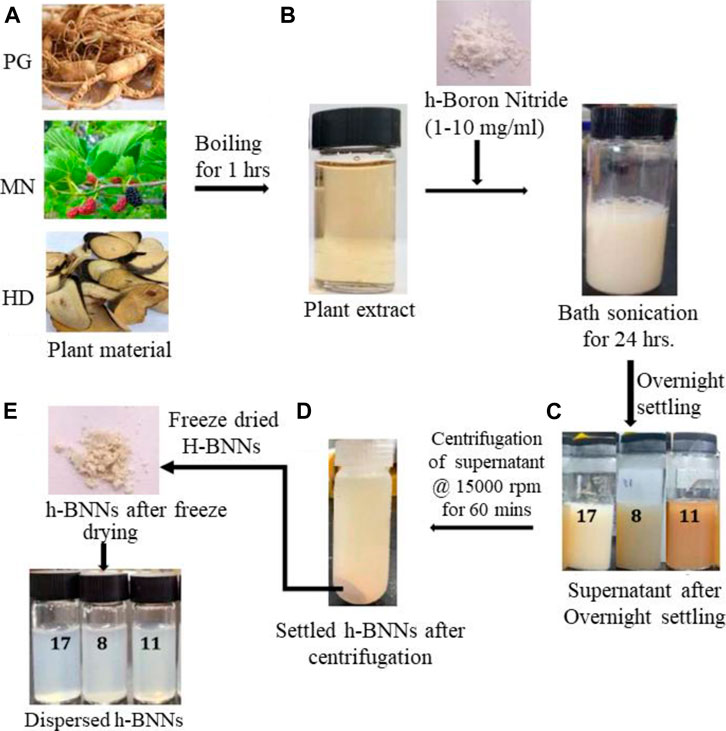
FIGURE 15. Schematic diagram of the biogenic exfoliation of bulk h-BN to h-BN nano sheets using plant material extract of Panax ginseng (PG), Morusnigra (MN) and, Hoveniadulcis (HD). The figure is reprinted with permission from Deshmukh et al., 2019, Copyright 2019, American Chemical Society.
Application of Graphene in Supercapacitor
A supercapacitor is a popular energy storage device compared to traditional energy storage devices such as capacitors and rechargeable batteries because it delivers much higher capacitance than conventional capacitors and delivers the stored energy much faster than batteries. A conventional capacitor stores charge by polarization of the dielectric between two plates, but in supercapacitors the charge is stored at the electrode themselves. Supercapacitors have a wider operating temperature range, high specific energy, and long service ability in comparison with conventional energy storage devices. According to different energy storage mechanisms, supercapacitors are divided in two ways; one is an electric double-layered capacitor (ELDC: stores charge from ions adsorption and desorption) and the other is a pseudocapacitor (stores charge by Faradic reaction). To design high energy density supercapacitors, a layered material is required which combines a multilayer nano structure with high surface area to promote excellent electrolyte permeability and ion exchange. The high electrocatalytic property of graphene makes it a deserving material for electrochemical energy storage and many other applications as shown in Table 2. Graphene-based supercapacitors exhibit excellent reversibility, high power density, and long cycle life. Singh et al. (2017) synthesized few-layer graphene using RHA and investigated it as an active material (for electrode) for supercapacitors. This graphene in a 1 M Na2SO4 solution was investigated electrochemically with a three electrode system. The specific capacitance of RHA-derived graphene was found to be 86 F/g with a scan rate of 500 mV/s. Similarly, Muramatsu et al. (2014) observed a slightly lower value (80 F/g) of specific capacitance of the same active material but with a different solution of 1 M H2SO4. Recently, Karakoti et al. (2020) prepared low-cost electrode material graphene nano sheets from plastic waste for supercapacitor application. Electrochemical investigations were performed on four types of cells with different current collectors (CuT, ITO, GS, and AlS), PVA-H3PO4 polymer gel was the electrolyte, and graphene nano sheets were the active electrode material. The CV of the fabricated cells with different current collectors over the scan rate 5–500 mV/s showed the typical nature of supercapacitors. The increase in specific capacitance follows the order AlS > GS > ITO > CuT at 5 mV/s scan rate and maximum 19.52 F/g for AlS. Galvanic charge discharge (GCD) measurements at a current sweep of 0.1 mA up to a voltage range of 1 V have shown the same trend as for CV. Also, the EIS analysis showed that the highest specific capacitance was 36.78 F/g at 10 MHz for AlS. It is demonstrated that non-biogenically derived graphene nano sheets from plastic waste are better and quite compatible with the AlS current collector along with the highest specific capacitance value. Pandey et al. (2019) and Pandey et al. (2021) synthesized graphene nano sheets using plastic wastes. However, the derived graphene sheets had some defective surfaces. They compared the thermodynamic stability, electronic nature, and quantum capacitance of defective and pristine graphene sheets computationally using the QuantumWise-Atomistix Toolkit (ATK) package with density functional theory (DFT). It was found that graphene with the defective surface had lower stability but enhanced quantum capacitance when compared to the pristine one. It was also evident that quantum capacitance of the defective graphene increased with defect concentration (1.38–9.72%) over the potential range of −0.11–0.22 V. Thus, quantum capacitance is quite promising for energy storage devices based on defective graphene. Particularly, in graphene supercapacitors, the higher the value of quantum capacitance resulted in higher total capacitance and hence it can achieve higher energy density (Pandey et al., 2019). In a very recent report, Pandey et al. (2021) also observed that the defective graphene had a higher quantum capacitance compared to defective graphene oxide (GO) and pristine graphene. Following all this information, they fabricated a supercapacitor cell (graphite sheet|defective graphene sheets|1 M H3PO4|defective graphene sheets|graphite sheet) using defective graphene derived from waste plastic. The fabricated cell shows very good reversibility and an approximately rectangular shape of curve at different scan rates over the performed voltages, which is a typical supercapacitor characteristic as shown in Figure 16A. The fabricated cell has shown lower equivalent sheet resistance (ESR) and smaller charge transfer resistance as seen from the Nyquist plot (Figure 16B). Moreover, the triangular shape of the galvanostatic charging discharging (GCD) curves (Figure 16C) represents the capacitive nature of the fabricated cell. The highest specific capacitance of the cell via CV at 5 mV/s was evaluated to 398 F/g whereas highest specific capacitance using galvanic charge/discharge at 1A/g of current density was found to be 273.69 F/g. Further, the fabricated cell showed remarkable energy density and power density of 38 Wh/kg and 1,009.74 W/kg, respectively (Pandey et al., 2021).
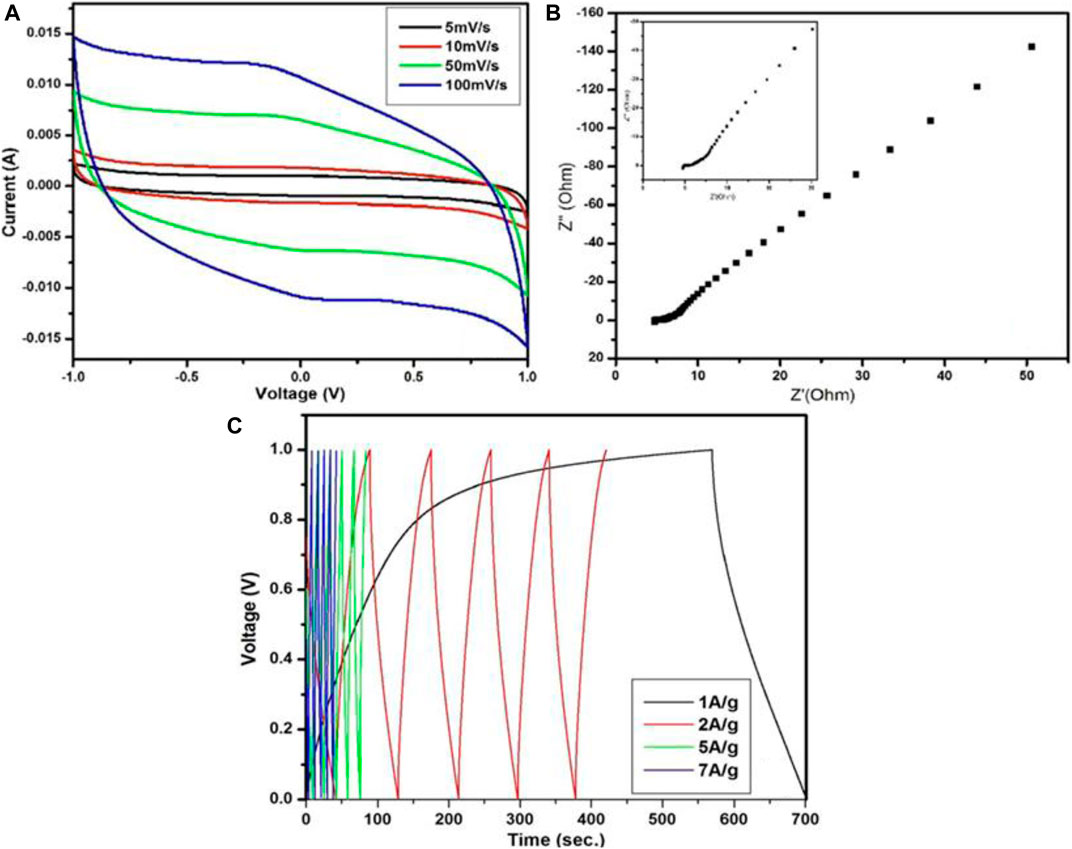
FIGURE 16. (A) Cyclic Voltammetry (CV) curve (B) Nyquist plot (C) GCD curve of the graphene sheets based cell in 1 M H3PO4 electrolyte (Pandey et al., 2021).
Memristor (Memory Resistance)
A memristor is a non-linear two-terminal electrical component whose resistance varies as a function of flux and charge. The memristor or RRAM (Resistive Random-Access Memory) is the next-generation stable memory device which can be designed in the presence of an external electric field with a low resistance state (LRS) or high resistance state (HRS). A tentative memristor cell can form with a sandwich configuration of conductor/semiconductor or insulator/conductor. For the application of graphene film in RRAM, Jacob et al. (2015) used a sustainable bottom-up approach for synthesis of high-quality graphene using a biogenic precursor. The precursor was obtained from an extract of leaves of Melaleuca alternifolia, commonly known as the tea tree plant. The prepared graphene film showed the lowest surface energy (33 mJ/m2). Low surface energy is potentially useful for stability of electronic devices. Keeping this in mind, Jacob et al. (2015) sandwiched a layer of p-type organic semiconductor between the biogenically derived graphene and aluminum electrode to form a typical memory device and found memristive behavior. The typical I-V characteristic shows (left-hand graph in Supplementary Figure S5A) a switching behavior at 1.8 V. They observed that after a 400 On/OFF cycle, there was no change in the magnitude of the current corresponding to HRS and LRS as shown in the right-hand graph of Supplementary Figure S5B.
Oxygen Reduction Reaction
The oxygen reduction reaction (ORR) is the most crucial reaction for energy conversion including fuel cells and biological respiration in life. ORR is a slow kinetics reaction which occurs in different ways for different solutions, for an aqueous solution it works in two ways; one includes two-electron reduction pathways from O2 to hydrogen peroxide (H2O2) and the other includes four-electron reduction pathways from O2 to H2O and for the non-aqueous solution/alkaline solution, a one-electron reduction pathway from O2 to superoxide (O2-). Zhao and Zhao (2013) synthesized a graphene sheet from the disposable cups. One of the added advantages of this synthesis was the easy formation of graphene sheet with Ag, Pd, and Pt by simple replacement reaction. The graphene sheets with Pt are used as catalyst for the application of oxygen reduction reaction in fuel cells, and compared with an industrial E-TEK catalyst (Pt/Vulcan). The characteristic curve as given in Supplementary Figure S6 for the ORR application recorded in 1.0 M HClO4 solution was saturated with pure oxygen. The Linear Sweep Voltammetry (LSV) curves of the commercial and as-prepared Pt/graphene catalyst clearly indicate that the as-prepared Pt/graphene catalyst showed a higher and more positive current density. The higher catalytic activity is attributed to better dispersion of Pt nano particles, a smaller number of defects, good crystallinity, and no stabilizers/surfactants. However, detail investigation is still required for better understanding and application of the prepared catalyst.
Application of Biogenically Exfoliated H-BN Nano Sheets
Extensive research in the area of 2D-layered h-BN has shown that it has many interesting features, viz., ideal dielectric substrate for graphene, dangling bond-free flat surface, etc. These features have proven that it is an ideal candidate for many applications. Recently, Deshmukh et al. (2019) reported the applications of a biogenically h-BN nano sheet in removal of dyes (cationic and anionic both), antioxidant activity, and as a nano filler of bio-based polyurethane.
Adsorbent
Recently, it was shown that the adsorption performance of biogenically synthesized h-BN nano sheets was much better than pristine h-BN and h-BN synthesized in isopropanol (control). It was found that more than 90% of cationic and anionic dyes were removed using biogenically synthesized h-BN nano sheets. Therefore, these nano sheets have demonstrated their potential adsorbent properties (Deshmukh et al., 2019).
Antioxidant Activity
DPPH (2,2-diphenyl-1-picryl-hydrazyl-hydrate) free radical scavenging is a commonly used technique for the evaluation of the antioxidant activity of natural products. It is a spectrophotometric technique based on quenching stable colored radicals like DPPH and shows the radical scavenging ability of antioxidants of plant extracts. Deshmukh et al. (2019) completed a DPPH assay for assessment of the antioxidant activity of biogenically derived h-BN nano sheets and compared it with pristine h-BN bulk material. It was observed that biogenically derived h-BN nano sheets (using PG, MN, and HD plant extracts) showed a scavenging activity while pristine h-BN materials did not show any activity. The scavenging activity of the PG-derived nano sheet was higher compared to the h-BN nano sheets synthesized using MN and HD plant extracts. DPPH free radical scavenging activity and color effect are shown in Supplementary Figure S7.
Nano Fillers for Biopolymer
Nano fillers have been known for many years and have shown significance in many applications ranging from the polymer industry to energy storage and conversation devices. Basically, they are used as an additive in solid state which is structurally and compositionally different from the host matrix. Inactive fillers increase the quantity and reduce the cost, whereas active fillers are used to improve mechanical or physical properties. Nano fillers for polymer composite applications are primarily categorized in 1D (nano graphene platelets), 2D (carbon nano tubes, h-BN), and 3D (nano silica) nano fillers (Singh et al., 2014; Verma et al., 2020). There are a variety of 2D nano fillers for many diverse applications in the area of energy (Wan et al., 2014), sensors (Shahjamali et al., 2014), catalysis (Rao et al., 2014), photocatalysts, nano containers, nano reactors (Pradhan et al., 2013), etc. In a recent report, h-BN nano sheets derived from biogenically waste plant extracts have been used as nano fillers for bio-based polymer nano composites (Deshmukh et al., 2019). The addition of 0.5% h-BN nano sheets in polyurethane (PU) film increased the ductility delays fracturing to 152% compared to 92% of pure polyurethane film (Supplementary Figure S8A). Also, the ultimate tensile strength and stress vs. strain curves (Supplementary Figure S8B) for pure polyurethane and the nano composite of polyurethane with h-BN nano sheets showed a significant enhancement in ultimate tensile strength from 7.33 to 10.1 MPa.
Application of Biogenically Derived g-C2N
Organic compounds are major chemical products used in the textile, food, and chemical industries, especially in synthetic dyes. Dyes are structurally complex materials which enter the environment due to various textile industry processes like dyeing and cloth completion processes. Methylene blue is a heterocyclic aromatic model cationic dye employed by industries such as the textile industry for a variety of purposes. Therefore, its degradation is highly demanded. There are many techniques for degradation but a cost-effective and environmental benign method is most preferred (Yuan et al., 2014; Zhang et al., 2014; Perreault et al., 2015). Photodegradation efficiency of biogenically synthesized graphitic carbon nitride was investigated by Tiwari and Ram (2019). For this, they used an experimental set up in which UV (24 W, intensity 400 lux, λ < 400 nm, and xenon lamp 900) and visible light bulbs were used and the solution was magnetically stirred in the dark for 30 min to establish adsorption-desorption equilibration. Biogenically synthesized graphitic carbon nitride catalyst (∼1.6 mg) was added to the methyl blue (MB) concentration of 2.6 × 10–5 M to get a final concentration of ∼5 × 10–5 M. The aqueous solution of MB concentration 5 × 10–5 M with photo catalyst (biogenically synthesized graphitic carbon nitride) had a loading of 1 g/L. A small amount of the sample from the suspension at 30 min intervals was taken and put into a quartz cell and the adsorption spectrum was measured with a UV-Vis spectroscopy. Comparative photodegradation efficiency of without and with biogenically synthesized graphitic carbon nitride is presented in Figure 17A. Figure 17B shows the concentration of MB at the irradiation time t, and C0 is the concentration in the absorption equilibrium of photocatalysts before irradiation. It was found that the photocatalytic activity of biogenically synthesized graphitic carbon nitride was about two times higher compared to the photocatalytic activity without the catalyst.
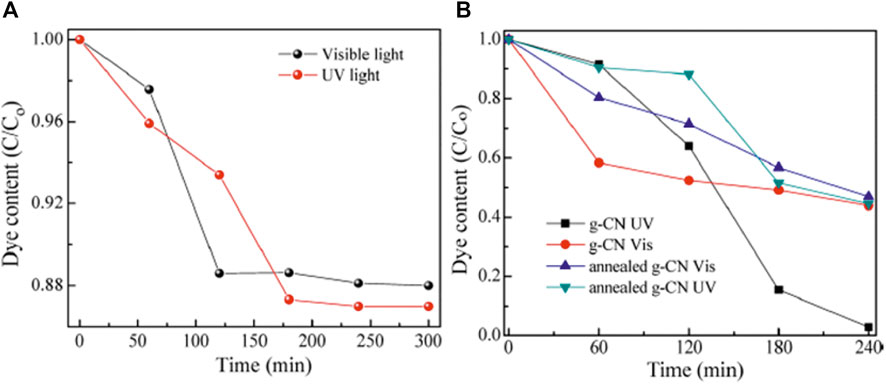
FIGURE 17. Phodegradation efficiency of MB without (A) and with (B) biogenically synthesized graphitic carbon nitride. The figure is reprinted with permission from Tiwari and Ram, 2019 Copyright 2019, American Chemical Society.
Future Prospective and Challenges of 2D Materials Derived From Biogenic and Non-Biogenic Waste Materials
The first member (graphene) of 2D-layered materials was born by mechanical exfoliation of bulk graphite into a graphene nano sheet with the help of Scotch tape (Novoselov et al., 2004). Since then, the family of 2D-layered materials has extended rapidly and attracted much attention of physicists, chemists, materials scientists, and engineers for various applications. However, synthesis of 2D-layered materials is not straight forward and there are issues which need to be addressed. One of the major challenges is to develop a synthesis protocol for 2D-layered materials derived from biogenic and non-biogenic waste materials. The hurdles in the synthesis protocol are reproducibility, quality, size and morphology of yield, inconsistent properties of starting waste materials, etc. After the first paper on monolayer graphene derived from waste materials in 2011 (Ruan et al., 2011), there have been many interesting approaches used to derive 2D-layered materials using biogenic and non-biogenic waste materials. However, to date there are no commercially available 2D-layered materials based on waste materials. We believe that there are good opportunities for developing industrial-scale production of waste-derived 2D-layered materials. The biogenic and non-biogenic synthesis of 2D-layered materials using waste materials will help researchers not only to design 2D-layered materials but also promote the understanding of greener and safer concerns of 2D-layered materials. In future, more research is required in the area of biogenic and non-biogenic waste-derived 2D-layered materials to explore other members of the 2D family and their applications. Many other new biogenic and non-biogenic waste materials should be explored and utilized for sustainable applications. More technological innovations are necessary to be developed from the perspective of quality and morphology of waste-derived 2D-layered materials. Considerable efforts should be devoted for critical evaluation of waste-derived 2D-layered materials. The parameters of critical assessment of waste-derived 2D-layered materials are fast but simple synthesis, a cheaper as well as controllable process, and ecofriendly. Therefore, the resulting waste-derived 2D-layered materials should have high quality and monolayer stability with fascinating physicochemical properties for emerging valuable applications. Overall, it is demonstrated that the use of biogenic and non-biogenic waste synthesis of 2D-layered materials is an emerging and exciting area of nanotechnology and may have a significant impact on further advances in nanoscience and technology.
Conclusion and Outlook
The utilization of waste derived from biogenic and non-biogenic materials in the synthesis of 2D-layered materials is still very much in its early stage. However, in the last few years it has attracted much attention of materials scientists and engineers. This is because of exceptional properties of 2D materials which make them highly desirable for a wide range of applications in sensing, purification, energy, catalysis electronics, etc. However, the synthesis of 2D materials is expensive and time and energy consuming. Therefore, 2D materials derived from biogenic and non-biogenic waste are an excellent alternative approach to reduce the cost, speed up the process, and be effective in the management of waste. The studies discussed in the present review highlight recent advances in the synthesis of 2D-layered materials (graphene, h-BN, g-C2N) using biogenic and non-biogenic waste materials. Graphene is mostly synthesized compared to h-BN and g-C2N. The work related to biogenic and non-biogenic synthesis of h-BN and g-C2N are very limited and lots of work is required in this specific research area. In this type of synthesis approach, the most used methods are pyrolysis, CVD, FJH, hydrothermal, and electrochemical exfoliation. The quality and characteristics of the synthesized graphene, h-BN, and g-C2N strongly depend on the starting materials, exfoliation solvent, and technique. Also at present, the quality of waste-derived graphene or other 2D materials might not compete with the quality of graphene derived from conventional methods. However, graphene or other 2D materials derived in this way will certainly provide next steps toward new technology of high-value products with the added advantage of effective waste material management. Despite the issues related to the quality (small lateral dimension sheet, defected surface, etc.) of 2D materials obtained from biogenic and non-biogenic waste, they have shown potential to be great substitutes for current graphene, h-BN, and g-C2N-based applications in supercapacitors, memristors (memory device), oxygen reduction reaction in fuel cells, as a nano filler for bio-polymer, photocatalytic activity, etc. Also, this review offers some basic idea of biogenic and non-biogenic waste materials such as agro waste, rice husk, dead leaves, tea tree extract, waste food, food products, disposable paper cups, black liquor, plastic waste, and industrial waste etc. having the capacity to provide a new road map of using waste materials. This type of ecofriendly procedure has the potential to offer an unconventional approach over conventional routes that utilize non ecofriendly chemicals and solvents. Most work to date on 2D materials (graphene, h-BN, and g-C2N) derived from biogenic or non-biogenic waste materials have suffered from dedicated studies on the optical and polycrystalline properties which have a direct influence on electronic applications. Further, the recent research based on waste plastics and FJH have shown a new hope to solve the problems related to scalability, energy, and cost of derived 2D materials (particularly graphene). Finally, one keeps in mind that this area of research is still less explored and hence offering new opportunities for further development of green synthesis to produce 2D-layered materials utilizing biogenic and non-biogenic waste material is beneficial. Finally, it is believed that this inclusive review will encourage researchers in this relatively new and unexplored field.
Author Contributions
MPS designed and wrote the manuscript. Also supervised this work and revised manuscript. AKB, KB and RaPS wrote some sections. KV, SKC, SK, AS, RiPS and RN Discussion and corrections in manuscript. All authors contributed to the article and agreed on the submitted version.
Conflict of Interest
The authors declare that the research was conducted in the absence of any commercial or financial relationships that could be construed as a potential conflict of interest.
Publisher’s Note
All claims expressed in this article are solely those of the authors and do not necessarily represent those of their affiliated organizations, or those of the publisher, the editors and the reviewers. Any product that may be evaluated in this article, or claim that may be made by its manufacturer, is not guaranteed or endorsed by the publisher.
Acknowledgments
AB is highly thankful to V.B.S. Purvanchal University, Jaunpur, (U.P.), India for providing their Purvanchal University Postdoctoral Fellowship (PUPDF) (Grant No. 01 PUPDF/EVS). KB is thankful to University Grant Commission, New Delhi, India for providing the Junior Research Fellowship (JRF).
Supplementary Material
The Supplementary Material for this article can be found online at: https://www.frontiersin.org/articles/10.3389/fnano.2021.685427/full#supplementary-material
References
Alfarisa, S., Abu Bakar, S., Mohamed, A., Hashim, N., Kamari, A., Md Isa, I., et al. (2015). Carbon Nanostructures Production from Waste Materials: A Review. Amr 1109, 50–54. doi:10.4028/www.scientific.net/amr.1109.50
Algozeeb, W. A., Savas, P. E., Luong, D. X., Chen, W., Kittrell, C., Bhat, M., et al. (2020). Flash Graphene from Plastic Waste. ACS Nano 14, 15595–15604. doi:10.1021/acsnano.0c06328
Avouris, P., and Dimitrakopoulos, C. (2012). Graphene: Synthesis and Applications. Mater. Today 15, 86–97. doi:10.1016/s1369-7021(12)70044-5
Bajwa, D. S., Pourhashem, G., Ullah, A. H., and Bajwa, S. G. (2019). A Concise Review of Current Lignin Production, Applications, Products and Their Environmental Impact. Ind. Crops Prod. 139, 111526. doi:10.1016/j.indcrop.2019.111526
Bazargan, A., Tan, J., Hui, C. W., and McKay, G. (2014). Utilization of rice Husks for the Production of Oil Sorbent Materials. Cellulose 21, 1679–1688. doi:10.1007/s10570-014-0203-9
Bertolazzi, S., Gobbi, M., Zhao, Y., Backes, C., and Samorì, P. (2018). Molecular Chemistry Approaches for Tuning the Properties of Two-Dimensional Transition Metal Dichalcogenides. Chem. Soc. Rev. 47, 6845–6888. doi:10.1039/c8cs00169c
Bhardwaj, A. K., Gupta, M. K., Naraian, R., and Naraian, R. (2019). “Myco-Nanotechnological Approach for Improved Degradation of Lignocellulosic Waste: Its Future Aspect,” in Myco-Nanotechnological Approach for Improved Degradation of Lignocellulosic Waste: Its Future aspect Mycodegradation of Lignocelluloses (Springer), 227–245. doi:10.1007/978-3-030-23834-6_12
Bhardwaj, A. K., Shukla, A., Maurya, S., Singh, S. C., Uttam, K. N., Sundaram, S., et al. (2018). Direct Sunlight Enabled Photo-Biochemical Synthesis of Silver Nanoparticles and Their Bactericidal Efficacy: Photon Energy as Key for Size and Distribution Control. J. Photochem. Photobiol. B: Biol. 188, 42–49. doi:10.1016/j.jphotobiol.2018.08.019
Bhardwaj, A. K., Shukla, A., Mishra, R. K., Singh, S. C., Mishra, V., Uttam, K. N., et al. (2017a). Power and Time Dependent Microwave Assisted Fabrication of Silver Nanoparticles Decorated Cotton (SNDC) Fibers for Bacterial Decontamination. Front. Microbiol. 08, 330. doi:10.3389/fmicb.2017.00330
Bhardwaj, A. K., Shukla, A., Singh, S., Uttam, K. N., Nath, G., and Gopal, R. (2017b). Green Synthesis of Cu2O Hollow Microspheres. Amp 2, 132–138. doi:10.5185/amp.2017/215
Chaukura, N., Gwenzi, W., Bunhu, T., Ruziwa, D. T., and Pumure, I. (2016). Potential Uses and Value-Added Products Derived from Waste Polystyrene in Developing Countries: A Review. Resour. Conservation Recycling 107, 157–165. doi:10.1016/j.resconrec.2015.10.031
Chen, M., Li, J., Zhang, J., Ma, Y., Dong, H., Li, W., et al. (2021). Evolution of Cellulose Acetate to Monolayer Graphene. Carbon 174, 24–35. doi:10.1016/j.carbon.2020.11.084
Chen, Y., Yang, K., Jiang, B., Li, J., Zeng, M., and Fu, L. (2017). Emerging Two-Dimensional Nanomaterials for Electrochemical Hydrogen Evolution. J. Mater. Chem. A. 5, 8187–8208. doi:10.1039/c7ta00816c
Coros, M., PogaceanMagerusan, F. L., Socaci, C., and Pruneanu, S. (2019). A Brief Overview on Synthesis and Applications of Graphene and Graphene Based Nanomaterials. Front. Mater. Sci. 13, 23–32.
Darkwah, W. K., and Ao, Y. (2018). Mini Review on the Structure and Properties (Photocatalysis), and Preparation Techniques of Graphitic Carbon Nitride Nano-Based Particle, and its Applications. Nanoscale Res. Lett. 13, 388. doi:10.1186/s11671-018-2702-3
Deshmukh, A. R., Jeong, J. W., Lee, S. J., Park, G. U., and Kim, B. S. (2019). Ultrasound-Assisted Facile Green Synthesis of Hexagonal Boron Nitride Nanosheets and Their Applications. ACS Sustainable Chem. Eng. 7, 17114–17125. doi:10.1021/acssuschemeng.9b03387
Ding, Z., Yuan, T., Wen, J., Cao, X., Sun, S., Xiao, L.-P., et al. (2020). Green Synthesis of Chemical Converted Graphene Sheets Derived from Pulping Black Liquor. Carbon 158, 690–697. doi:10.1016/j.carbon.2019.11.041
Durairaj, A., Sakthivel, T., Ramanathan, S., Obadiah, A., and Vasanthkumar, S. (2019). Conversion of Laboratory Paper Waste into Useful Activated Carbon: a Potential Supercapacitor Material and a Good Adsorbent for Organic Pollutant and Heavy Metals. Cellulose 26, 3313–3324. doi:10.1007/s10570-019-02277-4
Edwards, R. S., and Coleman, K. S. (2013). Graphene Synthesis: Relationship to Applications. Nanoscale 5, 38–51. doi:10.1039/c2nr32629a
El Essawy, N. A., Ali, S. M., Farag, H. A., Konsowa, A. H., Elnouby, M., and Hamad, H. A. (2017). Green Synthesis of Graphene from Recycled PET Bottle Wastes for Use in the Adsorption of Dyes in Aqueous Solution. Ecotoxicology Environ. Saf. 145, 57–68. doi:10.1016/j.ecoenv.2017.07.014
Feng, H.-M., Zheng, J.-C., Lei, N.-Y., Yu, L., Kong, K. H.-K., Yu, H.-Q., et al. (2011). Photoassisted fenton Degradation of Polystyrene. Environ. Sci. Technol. 45, 744–750. doi:10.1021/es102182g
Fu, Z., Wang, N., Legut, D., Si, C., Zhang, Q., Du, S., et al. (2019). Rational Design of Flexible Two-Dimensional Mxenes with Multiple Functionalities. Chem. Rev. 119, 11980–12031. doi:10.1021/acs.chemrev.9b00348
Gabryszewska, M., and Gworek, B. (2020). Impact of Municipal and Industrial Waste Incinerators on PCBs Content in the Environment. PLoS ONE 15, e0242698. doi:10.1371/journal.pone.0242698
Geim, A. K., Novoselov, K. S., and Rodgers, P. (2010). Nanoscience and Technology: A Collection of Reviews from Nature Journals. World Scientific, 11–19. The Rise of Graphene.
Glushkov, D., Kuznetsov, G., and Paushkina, K. (2020). Switching Coal-Fired thermal Power Plant to Composite Fuel for Recovering Industrial and Municipal Waste: Combustion Characteristics, Emissions, and Economic Effect. Energies 13, 259. doi:10.3390/en13010259
Gong, Y., Xu, Z.-Q., Li, D., Zhang, J., Aharonovich, I., and Zhang, Y. (2021). Two-dimensional Hexagonal boron Nitride for Building Next-Generation Energy-Efficient Devices. ACS Energ. Lett. 6, 985–996. doi:10.1021/acsenergylett.0c02427
Gu, J., Pang, A., Guo, X., Li, L., Huang, D., and Li, F. (2021). Green Preparation of High-Quality and Low-Cost Graphene from Discarded Polyethylene Plastic Bags. Chem. Commun. 57, 129–132. doi:10.1039/d0cc06999j
Gutiérrez, H. R. (2020). Two-dimensional Layered Materials Offering Expanded Applications in Flatland. ACS Appl. Nano Mater. 3, 6134–6139. doi:10.1021/acsanm.0c01763
Hu, R., Liao, G. C., Huang, Z., Qiao, H., Liu, H., Shu, Y., et al. (2020a). Recent Advances of Monoelemental 2D Materials for Photoctalytic Applications. J. Hazard. Mater. 405, 124179. doi:10.1016/j.jhazmat.2020.124179
Hu, T., Chen, J., Lu, X., Chen, J., Chen, Z., Fu, J., et al. (2020b). Synthesis of Few-Layer Graphene Sheets from Waste Expanded Polystyrene by Dense Fe Cluster Catalysis. ACS Omega 5, 4075–4082. doi:10.1021/acsomega.9b03743
Hu, T., Meia, X., Wang, Y., Weng, X., Liang, R., and Wei, M. (2019). Two-dimensional Nanomaterials: Fascinating Materials in Biomedical Field. Sci. Bull. 64, 1707–1727. doi:10.1016/j.scib.2019.09.021
Hussain, I., Singh, N. B., Singh, A., Singh, H., and Singh, S. C. (2016). Green Synthesis of Nanoparticles and its Potential Application. Biotechnol. Lett. 38, 545–560. doi:10.1007/s10529-015-2026-7
Jacob, M., Rawat, R. S., Ouyang, B., Bazaka, K., Kumar, D. S., Taguchi, D., et al. (2015). Catalyst Free Plasma Enhanced Growth of Graphene from Sustainable Sources. Nano Lett. 15, 5702–5708. doi:10.1021/acs.nanolett.5b01363
Karakoti, M., Pandey, S., Jangra, R., Dhapola, P. S., Singh, P. K., Mahendia, S., et al. (2020). Waste Plastics Derived Graphene Nanosheets for Supercapacitor Application. Mater. Manuf. Process. 36, 171–177. doi:10.1080/10426914.2020.1832680
Kaza, S., Yao, L. C., Bhada-Tata, P., and Van Woerden, F. (2018). What a Waste 2.0: A Global Snapshot of Solid Waste Management to 2050. Washington, DC: Urban developmentWorld Bank.
Khan, A. H., Ghosh, S., Pradhan, B., Dalui, A., Shrestha, L. K., Acharya, S., et al. (2017). Two-dimensional (2D) Nanomaterials towards Electrochemical Nano Architectonics in Energy-Related Applications. Bull. Chem. Soc. Jpn. 90, 627–648. doi:10.1246/bcsj.20170043
Kong, X., Zhu, Y., Lei, H., Wang, C., Zhao, Y., Huo, E., et al. (2020). Synthesis of Graphene-like Carbon from Biomass Pyrolysis and its Applications. Chem. Eng. J. 399, 125808. doi:10.1016/j.cej.2020.125808
Kukovitskii, E., Chernozatonskii, L., Lvov, S., and MelNik, N. (1997). Carbon Nanotubes of Polyethylene. Chem. Phys. Lett. 266, 323–328. doi:10.1016/s0009-2614(97)00020-1
Kumar, L. (2016). World Intellectual Property Organization No.WO2014199388 A1. Geneva, Switzerland: Muktha Laboratories private limited.
Liu, W. L., Jiang, H., and Yu, H. Q. (2015). Thermochemical Conversion of Lignin to Functional Materials: a Review and Future Directions. Green. Chem. 17, 4888–4907. doi:10.1039/c5gc01054c
Luong, D. X., Bets, K. V., Algozeeb, W. A., Stanford, M. G., Kittrell, C., Chen, W., et al. (2020). Gram-scale Bottom-Up Flash Graphene Synthesis. Nature 577, 647–651. doi:10.1038/s41586-020-1938-0
Mackay, J., Eriksen, M., and Shafey, O. (2006). The Tobacco Atlas. 2nd edn. Atlanta: The American Cancer Society.
Madurani, K. A., Suprapto, S., Machrita, N. I., Bahar, S. L., Illiya, W., and Kurniawan, F. (2020). Progress in Graphene Synthesis and its Application: History, challenge and the Future Outlook for Research and Industry. ECS J. Solid State. Sci. Technol. 9, 093013. doi:10.1149/2162-8777/abbb6f
Maurya, S., Bhardwaj, A., Gupta, K., Agarwal, S., and Kushwaha, A. (2016). Green Synthesis of Silver Nanoparticles Using Pleurotus and its Bactericidal Activity. Cell.Mol. Biol. 62, 131.
Muramatsu, H., Kim, Y. A., Yang, K. S., Cruz‐Silva, R., Toda, I., Yamada, T., et al. (2014). Rice Husk‐derived Graphene with Nano‐sized Domains and Clean Edges. Small 10, 2766–2770. doi:10.1002/smll.201400017
Murugan, K., Suresh, U., Panneerselvam, C., Rajaganesh, R., RoniAziz, M. A. T., Hwang, , et al. (2018). Managing Wastes as green Resources: Cigarette Butt-Synthesized Pesticides Are Highly Toxic to Malaria Vectors with Little Impact on Predatory Copepods. Environ. Sci. Pollut. Res. 25, 10456–10470. doi:10.1007/s11356-017-0074-3
Nanaji, K., Upadhyayula, V., Rao, T. N., and Anandan, S. (2018). Robust, Environmentally Benign Synthesis of Nanoporous Graphene Sheets from Biowaste for Ultrafast Supercapacitor Application. ACS Sustain. Chem. Eng. 7, 2516–2529. doi:10.1021/acssuschemeng.8b05419
Novoselov, K., Geim, A., Morozov, S., Jiang, D., Zhang, Y., DubonosS, G., et al. (2004). Electric Field Effect in Atomically Thin Carbon Films. Science 306, 666–669. doi:10.1126/science.1102896
Panahi-Kalamuei, M., Amiri, O., and Salavati-Niasari, M. (2020). Green Hydrothermal Synthesis of High Quality Single and Few Layers Graphene Sheets by Bread Waste as Precursor. J. Mater. Res. Technol. 9, 2679–2690. doi:10.1016/j.jmrt.2020.01.001
Pandey, S., Karakoti, M., Dhali, S., Karki, N., SanthiBhushan, B., Tewari, C., et al. (2019). Bulk Synthesis of Graphene Nanosheets from Plastic Waste: an Invincible Method of Solid Waste Management for Better Tomorrow. Waste Manag. 88, 48–55. doi:10.1016/j.wasman.2019.03.023
Pandey, S., Karakoti, M., Surana, S., Dhapola, N., SanthiBhushan, B., Ganguli, S., et al. (2021). Graphene Nanosheets Derived from Plastic Waste for the Application of DSSCs and Supercapacitors. Sci. Repo. 11, 3916. doi:10.1038/s41598-021-83483-8
Papargyropoulou, E., Lozano, R., Steinberger, J. K., Wright, N., and Ujang, Z. bin. (2014). The Food Waste Hierarchy as a Framework for the Management of Food Surplus and Food Waste. J. Clean. Prod. 76, 106–115. doi:10.1016/j.jclepro.2014.04.020
Perreault, F., Fonseca de Fariaa, A., and Elimelech, M. (2015). Environmental Applications of Graphene-Based Nanomaterials. Chem. Soc. Rev. 44, 5861–5896. doi:10.1039/c5cs00021a
Plastic wastemanagement issues (2019). Plastic Wastemanagement Issues, Solutions and Case studiesMinistry of Housing and Urban Affairs. In March 2019 Available at: www.mohua.gov.
Pradhan, S., Lach, R., Le, H. H., Grellmann, W., Radusch, H-J., and Adhikari, R. (2013). Effect of Filler Dimensionality on Mechanical Properties of Nanofiller Reinforced Polyolefin Elastomers. ISRN Polym. Sci. 284504, 1–9. doi:10.1155/2013/284504
Purkait, T., Singh, G., Singh, M., Kumar, D., and Dey, R. S. (2017). Large Area Few-Layer Graphene with Scalable Preparation from Waste Biomass for High Performance Supercapacitor. Sci. Rep. 7, 15239. doi:10.1038/s41598-017-15463-w
Qiu, M., Sun, Z. T., Sang, D. K., Han, X. G., Zhang, H., and Niub, C. M. (2017). Current Progress in Black Phosphorus Materials and Their Applications in Electrochemical Energy Storage. Nanoscale 9, 13384–13403. doi:10.1039/c7nr03318d
Raghavan, N., Thangavel, S., and Venugopal, G. (2017). A Short Review on Preparation of Graphene from Waste and Bioprecursors. Appl. Mater. Today 7, 246–254. doi:10.1016/j.apmt.2017.04.005
Rao, C. N. R. (2020). Exciting Journey from the 0D to the 2D Nanoworld. Nano Lett. 20, 4061–4063. doi:10.1021/acs.nanolett.0c01077
Rao, C. N. R., Sood, A. K., Subrahmanyam, K. S., and Govindaraj, A. (2009). Graphene: the New Two-Dimensional Nanomaterial. Angew. Chem. Int. Ed. 48, 7752–7777. doi:10.1002/anie.200901678
Rao, K. S., Senthilnathan, J., Ting, J-M., and Yoshimura, M. (2014). Continuous Production of Nitrogen-Functionalized Graphene Nanosheets for Catalysis Applications. Nanoscale 6, 12758–12768. doi:10.1039/c4nr02824d
Ren, S., Rong, P., and Yu, Q. (2018). Preparations, Properties and Applications of Graphene in Functional Devices: A Concise Review. Ceram. Int. 44, 11940–11955. doi:10.1016/j.ceramint.2018.04.089
Ruan, G., Sun, Z., Peng, Z., and Tour, J. M. (2011). Growth of Graphene from Food, Insects, and Waste. ACS Nano 5, 7601–7607. doi:10.1021/nn202625c
Samaddar, P., Ok, Y. S., Kim, K., Kwon, E. E., and DanielTsang, C. W. D. C. W. (2018). Synthesis of Nanomaterials from Various Wastes and Their New Age Applications. J. Clean. Prod. 197, 1190–1209. doi:10.1016/j.jclepro.2018.06.262
Sankar, G. U., Moorthy, C. G., and RajKumar, G. (2018). Synthesizing Graphene from Wastemosquito Repellent Graphite Rod by Using Electrochemical Exfoliation Forbattery/supercapacitor Applications. Energ. Source Part. A. 40, 1209–1214. doi:10.1080/15567036.2018.1476609
Sankar, S., Lee, H., Jung, H., Kim, A., Ahmed, A. T. A., Inamdar, A. I., et al. (2017). Ultrathin Graphene Nanosheets Derived from rice Husks for Sustainable Supercapacitor Electrodes. New J. Chem. 41, 13792–13797. doi:10.1039/c7nj03136j
Schlee, P., Hosseinaei, O., Baker, D., Landmér, A., Tomani, P., Mostazo-Lopez, M. J., et al. (2019). From Waste to Wealth: from Kraft Lignin to Free-Standing Supercapacitors. Carbon 145, 470–480. doi:10.1016/j.carbon.2019.01.035
Shahjamali, M. M., Salvador, M., Bosman, M., Ginger, D. S., and Xue, C. (2014). Edge-Gold-Coated Silver Nanoprisms: Enhanced Stability and Applications in Organic Photovoltaics and Chemical Sensing. J. Phys. Chem. C 118, 12459–12468. doi:10.1021/jp501884s
Shams, S. S., Zhang, L. S., Hu, R., Zhang, R., and Zhu, J. (2015). Synthesis of Graphene from Biomass: a green Chemistry Approach. Mater. Lett. 161, 476–479. doi:10.1016/j.matlet.2015.09.022
Shukla, K., Verma, A., Verma, L., Rawat, S., and Singh, J. (2020). A Novel Approach to Utilize Used Disposable Paper Cups for the Development of Adsorbent and its Application for the Malachite green and Rhodamine-B Dyes Removal from Aqueous Solutions. Nat. Environ. Pollut. Technol. 19, 57–70.
Singh, M. P., Singh, R. K., and Chandra, S. (2014). Ionic Liquids Confined in Porous Matrices: Physicochemical Properties and Applications. Prog. Mater. Sci. 64, 73–120. doi:10.1016/j.pmatsci.2014.03.001
Singh, P., Bahadur, J., and Pal, K. (2017). One-step One Chemical Synthesis Process of Graphene from rice Husk for Energy Storage Applications. Graphene 6, 61–71. doi:10.4236/graphene.2017.63005
Singu, D. C., Joseph, B., Velmurugan, V., Ravuri, S., and Grace, A. N. (2018). Combustion Synthesis of Graphene from Waste Paper for High Performance Supercapacitor Electrodes. Int. J. Nanosci. 17, 1760023. doi:10.1142/s0219581x17600237
Soufi, G. J., and Iravani, S. (2020). Eco-friendly and Sustainable Synthesis of Biocompatible Nanomaterials for Diagnostic Imaging: Current Challenges and Future Perspectives. Green. Chem. 22, 2662–2687. doi:10.1039/d0gc00734j
Stolle, A., Szuppa, T., Leonhardt, S. E., and Ondruschka, B. (2011). Ball Milling in Organic Synthesis: Solutions and Challenges. Chem. Soc. Rev. 40, 2317–2329. doi:10.1039/c0cs00195c
Tiseo, I. (2021). Global Cumulative Production of Plastic 1950-2050. Available at: https://www.statista.com/statistics/1019758/plastics-production-volume-worldwide/.
Tiwari, B., and Ram, S. (2019). Biogenic Synthesis of Graphitic Carbon Nitride for Photocatalytic Degradation of Organic Dyes. ACS Omega 4, 10263–10272. doi:10.1021/acsomega.9b00989
Tyagi, D., Wang, H., Huang, W., Hu, L., Tang, Y., Guo, Z., et al. (2020). Recent Advances in Two-Dimensional-Material-Based Sensing Technology toward Health and Environmental Monitoring Applications. Nanoscale 12, 3535–3559. doi:10.1039/c9nr10178k
Verma, Y. L., Singh, M. P., Kumar, S., Dhar, R., and Singh, R. K. (2020). Behaviour of Ionic Liquid Adsorbed on the Surface of Nano Silica Particles and in Confined System of Silica Matrices. Sur. Sci. 701, 121701. doi:10.1016/j.susc.2020.121701
Wan, J., Kaplan, A. F., Zheng, J., Han, X., Chen, Y., Weadock, N. J., et al. (2014). Two Dimensional Silicon Nanowalls for Lithium Ion Batteries. J. Mater. Chem. A. 2, 6051–6057. doi:10.1039/c3ta13546b
Wu, A., Li, X., YangDuShen, J. S. W., and Yan, J. (2017). Upcycling Waste Lard Oil into Vertical Graphene Sheets by Inductively Coupled Plasma Assisted Chemical Vapor Deposition. Nanomaterials 7, 318. doi:10.3390/nano7100318
Wyss, K. M., Beckhem, J. L., Chen, W., Luong, D. X., Hundi, P., Raghuraman, S., et al. (2021). Converting Plastic Waste Pyrolysis Ash into Flash Graphene. Carbon 174, 430–438. doi:10.1016/j.carbon.2020.12.063
Xu, M., Liang, T., Shi, M., and Chen, H. (2013). Graphene like Two-Dimentional Materials. Chem. Rev. 113, 3766–3798. doi:10.1021/cr300263a
Yang, G., Zhang, Y. M., Cai, Y., Yang, B., Gu, C., and Zhang, S. X. A. (2020). Advances in Nanomaterials for Electrochromic Devices. Chem. Soc. Rev. 49, 8687–8720. doi:10.1039/d0cs00317d
Yuan, L., Yu, Q. Q., Zhang, Y. H., and Xu, Y.-J. (2014). Graphene-TiO2 Nanocomposite Photocatalysts for Selective Organic Synthesis in Water under Simulated Solar Light Irradiation. RSC Adv. 4, 15264–15270. doi:10.1039/c4ra01190b
Zhan, H., Guo, D., and Xie, G. (2019). Two-dimensional Layered Materials: from Mechanical and Coupling Properties towards Applications in Electronics. Nanoscale 11, 13181–13212. doi:10.1039/c9nr03611c
Zhang, J., Wang, P., Sun, J., and Jin, Y. (2014). High-efficiency Plasmon-Enhanced and Graphene-Supported Semiconductor/metal Coresatellite Hetero-Nanocrystal Photocatalysts for Visible-Light Dye Photodegradation and H2 Production from Water. ACS Appl. Mater. Inter. 6, 19905–19913. doi:10.1021/am505371g
Zhang, Y. Z., El-Demellawi, J. K., Jiang, Q., Ge, G., Liang, H., Lee, K., et al. (2020). MXene Hydrogels: Fundamentals and Applications. Chem. Soc. Rev. 49, 7229–7251. doi:10.1039/d0cs00022a
Zhao, H., and Zhao, T. S. (2013). Graphene Sheets Fabricated from Disposable Paper Cups as a Catalyst Support Material for Fuel Cells. J. Mater. Chem. A. 1, 183–187. doi:10.1039/c2ta00018k
Keywords: biogenic waste, green synthesis, non biogenic materials, biomolecules, 2D layered nanomaterials
Citation: Singh MP, Bhardwaj AK, Bharati K, Singh RP, Chaurasia SK, Kumar S, Singh RP, Shukla A, Naraian R and Vikram K (2021) Biogenic and Non-Biogenic Waste Utilization in the Synthesis of 2D Materials (Graphene, h-BN, g-C2N) and Their Applications. Front. Nanotechnol. 3:685427. doi: 10.3389/fnano.2021.685427
Received: 25 March 2021; Accepted: 28 June 2021;
Published: 30 September 2021.
Edited by:
Sibo Wang, Fuzhou University, ChinaReviewed by:
Min-Quan Yang, Fujian Normal University, ChinaXue Feng Lu, Nanyang Technological University, Singapore
Copyright © 2021 Singh, Bhardwaj, Bharati, Singh, Chaurasia, Kumar, Singh, Shukla, Naraian and Vikram. This is an open-access article distributed under the terms of the Creative Commons Attribution License (CC BY). The use, distribution or reproduction in other forums is permitted, provided the original author(s) and the copyright owner(s) are credited and that the original publication in this journal is cited, in accordance with accepted academic practice. No use, distribution or reproduction is permitted which does not comply with these terms.
*Correspondence: Manish Pratap Singh, ZHJtYW51OTlAZ21haWwuY29t; Abhishek Kumar Bhardwaj, YWtiaGFyZHdhakBnd2EuYW1pdHkuZWR1; Kunwar Vikram, dmlrYmh1QGdtYWlsLmNvbQ==
†Present Address: Abhishek Kumar Bhardwaj Amity School of Life Sciences, Department of Environmental Science, Amity University Gwalior, Gwalior, India