- 1Department of Chemistry, School of Science, Gandhi Institute of Technology and Management (GITAM), Hyderabad, India
- 2Department of Chemistry, Koneru Lakshmaiah Education Foundation, Hyderabad, Telangana, India
This study presents the rational synthesis of Cu-supported doped-CeO2 catalysts designed for the oxidation of benzylamine, both in the absence and presence of 1,2-diaminobenzene. The catalysts were prepared using a two-step method and characterized by various techniques, including XRD, Raman spectroscopy, BET surface area analysis, NH3-TPD, pyridine-FTIR, H2-TPR, XPS, SEM, and TEM. Raman and XPS analyses confirmed the presence of oxygen vacancy sites, with CuO/CeO2-ZrO2 displaying the highest concentration of these sites. H2-TPR revealed strong metal-support interactions, while NH3-TPD indicated that CuO/CeO2-ZrO2 possessed the greatest number of acidic sites. The pyridine-FTIR results indicates both the acidic sites present on the catalyst surface. The Cu/CeZr sample exhibits the lowest Iu////ITotal ratio (0.0567) compared to the Cu/Ce (0.0843) and Cu/CeSi (0.0672) samples, indicating a higher number of Ce3+ species or a greater number of oxygen defect sites in the sample. The catalyst demonstrated excellent performance in converting benzylamine to imines and was also highly effective in the synthesis of benzimidazole from benzylamine and 1,2-diaminobenzene, broadening its application potential. The superior catalytic activity is attributed to the abundant oxygen vacancies, redox properties, strong metal-support interactions, and acidic sites. Furthermore, the CuO/CeO2-ZrO2 catalyst maintained its efficiency over five consecutive cycles, exhibiting robustness, high functional group tolerance, and reduced reaction times, making it a promising system for diverse catalytic applications.
1 Introduction
The oxidative coupling of benzyl amines to form N-benzylidenebenzyl amines and benzimidazoles is a highly significant reaction with extensive applications in both industrial and pharmaceutical chemistry (Dong et al., 2016; Zeng et al., 2021; He et al., 2022; Yamamoto et al., 2022). N-benzylidenebenzyl amines, along with their imine derivatives, serve as versatile intermediates in the synthesis of various essential compounds, including those used in inorganic, organic, pharmaceutical, polymer, and bio-fertilizer production (Oiye et al., 2019; Ay et al., 2020; Orr et al., 2021). In particular, imines are critical in the pharmaceutical industry for the creation of heterocyclic compounds, which are pivotal in drug discovery, as well as for synthesizing bioactive molecules, and dyes in various industrial chemical processes. Imines also play a key role as reactive intermediates in major reactions, including the Diels–Alder reaction, Umpolung reactions, Aza–Baylis–Hillman, and Amadori rearrangement, thereby expanding their utility in organic synthesis (Layer, 1963; Ravula et al., 2024) Benzimidazoles, which are formed in this process, are particularly important as a structural framework in numerous bioactive molecules. These nitrogen-containing hetero cycles exhibit diverse biological activities, making them valuable in pharmaceuticals, especially as anti-cancer, anti-viral, and anti-fungal agents (Brishty et al., 2021; Chung et al., 2023; Mahurkar et al., 2023; Ahmad G. et al., 2024; Kumari et al., 2024). In cancer treatment, benzimidazoles inhibit tumour growth, while their anti-viral properties help combat diseases like HIV and hepatitis by interfering with viral replication. Furthermore, they act as effective anti-fungal agents, protecting against fungal infections. In addition, benzimidazoles play key roles in agrochemicals, functioning as pesticides and fungicides to protect crops, and in material science, where they contribute to the development of polymers and dyes (Zubrod et al., 2019; Ahmad M. F. et al., 2024; Bai et al., 2024; Lewicka et al., 2024). Therefore, the sustainable synthesis of N-benzylidenebenzyl amines and benzimidazoles via oxidative coupling provides an eco-friendly pathway for producing these essential compounds, which are vital not only for pharmaceuticals but also for agriculture, materials science, and green chemistry initiatives.
In recent years, both homogeneous and heterogeneous catalysts have been extensively explored for the oxidation of benzyl amines and the synthesis of benzimidazoles (Chen et al., 2015; Lu et al., 2024) While homogeneous catalysts, such as KMnO4, K2Cr2O₇, two-iodoxybenzoic acid, and N-tert-butylphenylsulfinimidoyl chloride, have demonstrated high efficiency, their significant drawbacks include challenging catalyst separation, complex work-up procedures, and substantial waste produced (Matsuo et al., 2001; Nicolaou et al., 2003; 2004; Furukawa et al., 2011). For instance, Dong et al. reported a metal-free oxidative approach using salicylic acid derivatives under oxygen for the selective oxidation of benzyl amines to imines and the synthesis of nitrogen-containing hetero cycles, for example, benzimidazoles (Dong et al., 2016). Additionally, a sustainable alternative involves the dehydrogenative coupling of aromatic diamines with alcohols, yielding water and hydrogen gas as eco-friendly by-products. As a result, attention has shifted toward heterogeneous catalysts, which offer easy catalyst separation, reusability, and economic feasibility (Qian et al., 2018; Bera et al., 2019; Tian et al., 2024a; Wang et al., 2024b). Although noble metals like Pt, Pd, Ru, Ir, and Rh have shown effectiveness in oxidation reactions, their high cost and scarcity necessitate the development of more abundant non-noble metal alternatives (Tateyama et al., 2016; Slanina and Oberschmid, 2018). Recent advances highlight the potential of base metals viz., Fe, Cu, Co, and Mn in catalyzing the dehydrogenative coupling of alcohols with o-phenylenediamine for benzimidazole synthesis (Bauer and Knölker, 2015; Wang et al., 2015; Chakraborty et al., 2017; Sadhasivam et al., 2017; Huang et al., 2024; Tian et al., 2024b). Amongst, Cu-based catalysts stand out for their efficiency and environmental benefits, present a viable path toward scalable green methodologies for producing N-benzylidenebenzyl amines and benzimidazole derivatives. The attractiveness of copper as a catalyst stems from its excellent catalytic activity in facilitating oxidation reactions, which are essential for synthesizing various organic compounds. Copper’s redox flexibility, switching between Cu(I) and Cu(II) oxidation states, plays a critical role in promoting the oxidation reactions, making it highly effective for transformations like amine to imine conversion. This ability to facilitate electron transfer is crucial in reactions involving molecular oxygen as an oxidant, further enhancing copper’s catalytic efficiency in aerobic conditions. Recent efforts have focused on elucidating the strong metal–support interaction (SMSI) between CuO and support, as this interaction plays a crucial role in modulating catalytic behaviour. Therefore, the redox properties and catalytic performance of metal-oxide-supported catalysts are greatly influenced by several key factors, including SMSI, type of support, the method of catalyst preparation, dopants and the calcination temperature. These parameters collectively determine the dispersion of the active species, oxygen vacancy formation, acidic sites and the overall stability of the catalyst, all of which are crucial in enhancing catalytic efficiency.
Recent reports suggest that metal oxide catalysts were used in oxidation reactions because of their excellent acid and redox properties. There has been considerable interest in ceria-based material as catalysts for various oxidation reactions because of their significant properties such as oxygen storage/release capacity and redox properties and creates more reactive oxygen defects due to the redox nature of Ce3+/Ce4+. According to the literature, the crystalline structure of ceria (CeO2) is fluorite. However, pure CeO2 has less thermal resistance, and poor oxygen storage capacity. To account these limitations, suitable metal ions have been incorporated into the ceria lattice which creates high surface area, more oxygen defects there by enhances its unique structural and redox properties compared to parent Ceria (Rao et al., 2011). It is reported that the doping of suitable transition metal ions such as Si4+ and Zr4+ into the ceria lattice can introduce a strain in the ceria lattice attributed to the difference in the ionic radius of dopant and cerium. Furthermore, interaction of two metal cations in an oxide improves the properties such as formation of oxygen vacancies and improves the textural, redox, and acidic thus enhances the catalytic efficiency.
In this present study, we explored the rational design of CuO-supported doped-CeO2 catalysts for the selective oxidative coupling of benzyl amines to produce N-benzylidenebenzyl amines and benzimidazoles under ambient conditions. The role of acidic, redox, and defect sites, particularly oxygen vacancies, is highlighted as a key factor in improving the catalytic performance. Characterization techniques such as XRD, Raman spectroscopy, SEM, TEM, and XPS have been employed to confirm the structural and surface properties of the synthesized catalysts. Our findings provide a comprehensive understanding of how acidic, redox, and defect sites contribute to enhanced catalytic activity, offering valuable insights for developing efficient nano structured non-noble metal catalysts.
2 Experimental section
2.1 Catalyst preparation
The synthetic procedure for preparing the highly efficient ZrO2-doped CeO2 catalyst (Cu/CeZr) follows a two-step method, adapted from a recent publication by our group (Ravula et al., 2024). In the first step, ZrO2-doped CeO2 nanoparticles were synthesized via a coprecipitation method using dilute solutions. Specifically, an equimolar mixture (1:1) of Ce(NO3)3·6H2O and ZrO(NO3)2·xH2O (Merck) was added dropwise to a 500 mL three-neck round-bottom flask containing 250 mL of deionized water, with stirring maintained by a mechanical stirrer and a dropping funnel over a 30-min period at ambient temperature. Next, 20% aqueous ammonia solution was added carefully under constant stirring at room temperature until the pH of the reaction mixture reached 8.5. The formation of a white precipitate was observed as ammonia was introduced. This precipitate was then stirred for an additional 24 h, followed by aging for another 24 h at room temperature. Upon completion of the reaction, the precipitate was recovered by filtration, washed thoroughly with deionized water to remove anions and impurities, and then dried in a hot air oven at 383K for 12 h. Finally, the dried material was calcined at 773K for 5 h, with a heating rate of 5 K/min, under static atmosphere, yielding CeO2-ZrO2 nanoparticles. The synthesis of the CuO-promoted SiO2-doped CeO2 catalyst (Cu/CeSi) follows the same procedure.
In the second step, the CuO-promoted CeO2-ZrO2 catalyst was synthesized via a wet-impregnation method. First, an appropriate amount of Cu(NO3)2·3H2O was dissolved in deionized water. Simultaneously, the previously synthesized CeO2-ZrO2 nanoparticles were dispersed in deionized water and then added to the Cu(NO3)2·3H2O solution under constant stirring at room temperature for 20 min. Afterward, the mixture was dried in a hot air oven at 373K for 5 h, resulting in a brick-red colored fine powder, Cu(OH)x/CeO2-ZrO2. This powder was further dried at 383K for 12 h, followed by calcination at 773Kfor 5 h, with a heating rate of 5 K/min under a static atmosphere, producing CuO/CeO2-ZrO2 (Cu/CeZr) nanoparticles. The same procedure was used for the synthesis of the CuO-promoted SiO2-doped CeO2 catalyst (Cu/CeSi).
2.2 Catalyst characterization
The synthesized catalysts were characterized using X-ray diffraction (XRD), Brunauer–Emmett–Teller (BET) surface area analysis, X-ray photoelectron spectroscopy (XPS), and temperature-programmed desorption (TPD). All characterization techniques were performed using the same instruments and methodologies as outlined in my previous reports (Ravula et al., 2024). Further technical details are provided in the supplementary information.
2.3 Catalytic oxidative coupling of amines
The catalytic oxidative coupling of benzylamine was conducted over synthesized CeO2-based catalysts using oxygen as the oxidant, under base- and solvent-free conditions. The reactions were performed in a 10 mL three-necked round-bottom flask, where 0.5 mmol of substrate and 50 mg of catalyst were subjected to an O2 flow rate of 20 mL/min with constant stirring at 900 rpm. Upon completion, the catalyst was separated from the reaction mixture by centrifugation. The liquid products were characterized using a GC-MS system equipped with a DB-5 capillary column and a flame ionization detector (FID). Samples were taken at intervals and analyzed via GC, using an HP-5 capillary column with FID detection. These reactions were carried out under kinetic control, with conversion and selectivity of products calculated using standard equations (Ravula et al., 2024).
Where,
3 Results and discussions
The XRD profiles of CeO2, 50 wt% SiO2-doped CeO2 (CeSi), 50 wt% ZrO2-doped CeO2 (CeZr), and 10 wt% CuO-supported Ce, Ce-Si, and CeZr catalysts are shown in Figure 1. The diffraction peaks at approximately 29.3° (111), 32.78° (200), 47.88° (220), 56.33° (311), 59.14° (222), 69.52° (400), 76.36° (331), and 79.81° (420) correspond to the fluorite-type structure of ceria, consistent with previous reports (Potdar et al., 2011; Yashima et al., 1993; Sun and Sermon, 1996). The XRD patterns for the CeSi and CeZr samples did not exhibit any additional crystalline peaks for SiO2 or ZrO2, likely due to the amorphous nature of SiO2/ZrO2 or the complete incorporation of Si4⁺ and Zr4⁺ ions into the ceria lattice. Interestingly, the diffraction peaks for doped ceria were broadened, and 2θ values were shifted compared to pure ceria, indicating the presence of smaller crystallite sizes and lattice distortions. The shifts in peak positions for CeSi and CeZr relative to CeO2 can be explained by the difference in ionic radii between the dopant ions (Si4⁺ ∼0.040 nm, Zr4⁺ ∼0.084 nm) and Ce4⁺ (∼0.097 nm), resulting in lattice contraction due to the substitution of Ce4⁺ by smaller Si4⁺ and Zr4⁺ (Liang et al., 2008; Rao et al., 2011). The calculated lattice parameters corresponding to the highest-intensity XRD peak of CeO2 were determined to be 0.541, 0.540, and 0.539 nm, respectively. A noticeable reduction in lattice parameters was observed for the CeSi and CeZr supports compared to pristine CeO2. This reduction, coupled with peak broadening, lattice parameter variation, and peak position shifts, strongly suggests the incorporation of dopants such as SiO2 and ZrO2 into the CeO2 lattice. These findings confirm the successful formation of ceria-based solid solutions. These results were well agreement with earlier reports (Sudarsanam et al., 2014; Ravula et al., 2024).
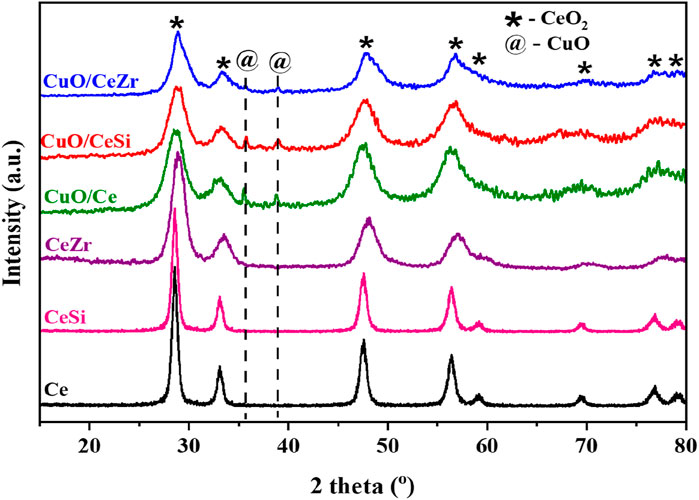
Figure 1. Powder X-ray diffraction pattern of the Ce (CeO2), CeSi (CeO2-SiO2), CeZr (CeO2-ZrO2), Cu/CeSi (CuO/CeO2-SiO2), and Cu/CeZr (CuO/CeO2-ZrO2) catalysts calcined at 773K.
For the CuO-added samples, CuO XRD peaks were observed at 35.5° (002) and 39.8° (200), in agreement with previously reported CuO particles (Rao et al., 2011; Sudarsanam et al., 2014). Peak broadening was also noted, attributed to changes in the crystallite size of ceria. To further investigate, the influence of dopants on the structural properties of ceria, the average crystallite size and specific surface area were determined (Table 1). The addition of dopants resulted in a reduction in crystallite size, indicating their role in inhibiting crystal growth, even under high thermal conditions. The average crystallite size was calculated using the Scherrer equation:
where λ is the X-ray wavelength, β is the full width at half maximum (FWHM), and d is the average crystallite size.
Raman spectroscopy is highly effective for investigating the structural characteristics of ceria-based materials due to its sensitivity to variations in M–O bond arrangements and lattice defects. Figure 2 Represents the Raman spectra of CeO2, SiO2-doped CeO2, ZrO2-doped CeO2, and CuO-supported on Ce, CeSi, and CeZr catalysts. The spectrum of pure CeO2 exhibits a prominent peak near 460 cm⁻1, attributed to the F2g Raman-active mode characteristic of the fluorite structure, in agreement with XRD results (Figure 1) and addition of dopants (SiO2, ZrO2) to the pure ceria shifts the peak around 457 cm-1 (F2g) attributed to the changes in M-O vibration frequencies, which are influenced by differences in the ionic radii of Ce4⁺ and the dopants (Reddy et al., 2005). The broadening of the F2g vibration mode observed may be due to the lattice defects, lattice strain, phonon confinement associated with the variation in the particle size. No Raman peaks associated with the SiO2, ZrO2 were observed confirming the formation of ceria solid solutions, which are correlating with the XRD results (Figure 1). An additional shoulder peak around 600 cm-1 was observed in CeSi, CeZr, Cu/Ce, Cu/CeSi and Cu/CeZr catalysts due to the formation of oxygen vacancies. The incorporation of dopants enhances the concentration of oxygen vacancies (Ov) which is evident from Figure 2. Interestingly, a strong enhancement in this peak was observed in Cu/CeZr due to formation of more oxygen vacancies and there by enhances the acidic properties of CuO doped into the Ce, CeSi and CeZr, respectively.
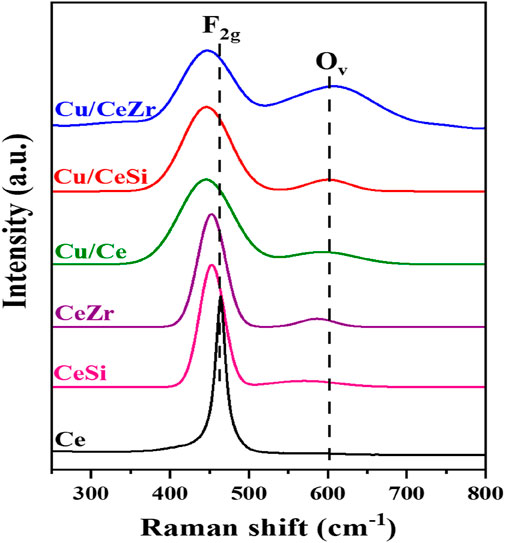
Figure 2. Raman spectra of the Ce (CeO2), CeSi (CeO2-SiO2), CeZr (CeO2-ZrO2), Cu/CeSi (CuO/CeO2-SiO2), and Cu/CeZr (CuO/CeO2-ZrO2) catalysts calcined at 773K.
The presence of defect sites, specifically oxygen vacancies (Ov), can be determined through the analysis of Raman spectra (Figure 2). As illustrated in Figure 2, all the synthesized samples exhibited a prominent band at ∼460 cm-1corresponding to F2g mode of vibration in the CeO2, which confirms the fluorite structure of Ceria. The broadening of the F2g vibration mode was observed when SiO2, ZrO2 and CuO were doped into the CeO2 lattice. This significant finding could be attributed to several factors, including lattice defects, lattice strain, phonon confinement, and variations in phonon relaxation associated with particle size. These results are in co-relation with the XRD results (Figure 1). An additional shoulder band around 600 cm-1 was observed in CeSi, CeZr, Cu/Ce, Cu/CeSi and Cu/CeZr catalysts due to the formation of oxygen vacancies (Sudarsanam et al., 2014; Ravula et al., 2024). The formation of oxygen vacancies is due to either the incorporation of Si4+ and Zr4+ into the CeO2 lattice or the interaction between the oxides of copper and Ce, CeSi and CeZr support matrix. Interestingly, a strong enhancement in this peak was observed in Cu/CeZr due to formation of more oxygen vacancies and there by enhances the acidic properties of CuO doped into the Ce, CeSi and CeZr, respectively.
XPS analysis was conducted to determine the elemental valence states and investigate changes in surface composition. The Ce 3d XP spectra of ceria-based mixed oxide-supported copper catalysts are depicted in Figure 3. The spectra, ranging from 870 to 920 eV, are notably complex due to the hybridization of the O 2p valence band with the Ce 4f level during the final state of photo ionization. The spin-orbit components 3d3/2 and 3d5/2 are labelled as u and v, respectively and these notations are same as that of literature. Peaks identified as u0, v0, u', and v' are characteristic of Ce3+ ions, featuring one main line and one satellite. In contrast, peaks marked as v, u, v'', u'', v''', and u''' correspond to Ce4+ ions, each displaying one main line and two satellites (the peaks v'' and v''' are the satellites arising from the ionization of Ce 3d5/2, whereas the signals u'' and u''' account for the ionization of Ce 3d3/2). Specifically, the u'''/v''' doublet is due to primary photoemission from Ce4+. In contrast, the u/v and u''/v'' doublets are shakedown features. These shakedown features result from the transfer of one or two electrons from a filled O 2p orbital to an empty Ce 4f orbital. Furthermore, the u0, v0, and u'/v' signals are attributed to photoemission from Ce3+ cations. As shown in Figure, the Ce 3d spectrum is integrated into subsequent peaks at about 881.38 eV (v0), 882.68 eV (v), 885.60 eV (v'), 888.81 eV (v''), 897.45 eV (v'''), 899.69 eV (u0), 901.76 eV (u), 904.08 eV (u'), 907.68 eV (u''), and 916.72 eV (u'''). The principal peaks of 3d5/2 and 3d3/2 are located at 882.68eV and 901.76 eV, respectively which are inconsistent with the previously reported results (Bêche et al., 2008; Rao et al., 2011; Isaacs et al., 2023). This indicates that all samples contain both Ce4+ and Ce3+ ions. The Ce 3d XP Spectra of Cu/CeZr sample shows signal splitting around 915–920 eV, which results in less concentration of Ce4+ ions in the sample. From Figure 3, the ratio of Ce3⁺ intensity Iu////ITotal to the total intensity provides insight into the proportion of Ce3⁺ ions in the sample (Reddy et al., 2005; Sudarsanam et al., 2014; Ravula et al., 2024). This ratio is significant because Ce3⁺ ions are closely associated with oxygen vacancies, which are critical defect sites in the material. The reduction of Ce4⁺ to Ce3⁺ often compensates for the creation of these oxygen vacancies, making the Iu////ITotal ratio a reliable indicator of defect density. The Cu/CeZr sample exhibits the lowest Iu////ITotal ratio (0.0567) compared to the Cu/Ce (0.0843) and Cu/CeSi (0.0672) samples, indicating a higher number of Ce3+ species or a greater number of oxygen defect sites in the sample. By analysing this ratio, XPS enables a deeper understanding of the material’s structural and electronic properties. This indicates a greater number of oxygen vacancies in the ceria lattice, which are in line with the Raman spectra results.
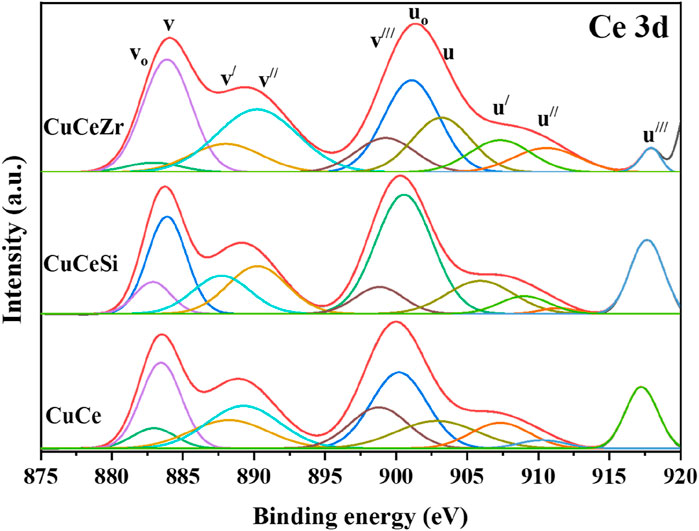
Figure 3. XPS patterns of the Ce 3d profiles for the Cu/Ce (CuO/CeO2), Cu/CeSi (CuO/CeO2-SiO2), and Cu/CeZr (CuO/CeO2-ZrO2) catalysts calcined at 773K.
Figure 4A–D presents the XPS of Cu 2p, O 1s, Si 2p, and Zr 3d spectra for all three samples. Figure 4A presents the XPS of Cu 2p spectra for all three samples, highlighting the characteristic spin-orbit splitting into the Cu 2p3/2 and Cu 2p1/2 peaks. The Cu 2p3/2 peak, a key feature for identifying the oxidation state of copper, appears prominently at approximately 932.8 eV across the samples. This binding energy is typically associated with Cu2⁺ species, confirming the presence of divalent copper ions (Rao et al., 2011; Mallesham et al., 2016). Additionally, a satellite peak observed around 941.9 eV further supports this assignment to Cu2⁺, as such satellite structures are known to arise from shake-up processes unique to high-spin Cu2⁺ species. However, the range of Cu 2p3/2 binding energies across the samples, spanning from 930.6 to 936.9 eV, suggests the co-existence of multiple copper species (Mallesham et al., 2016). This range indicates the possible presence of both Cu2⁺ and Cu⁺ species in the catalysts, as Cu⁺ typically exhibits binding energies lower than that of Cu2⁺. The calculated full width at half maximum (FWHM) of around 3 eV provides further evidence for this dual copper state, implying a mixture of oxidation states, which could influence catalytic performance through redox cycling between Cu⁺ and Cu2⁺ (Velu et al., 2005; Mallesham et al., 2016; Scherzer et al., 2019).
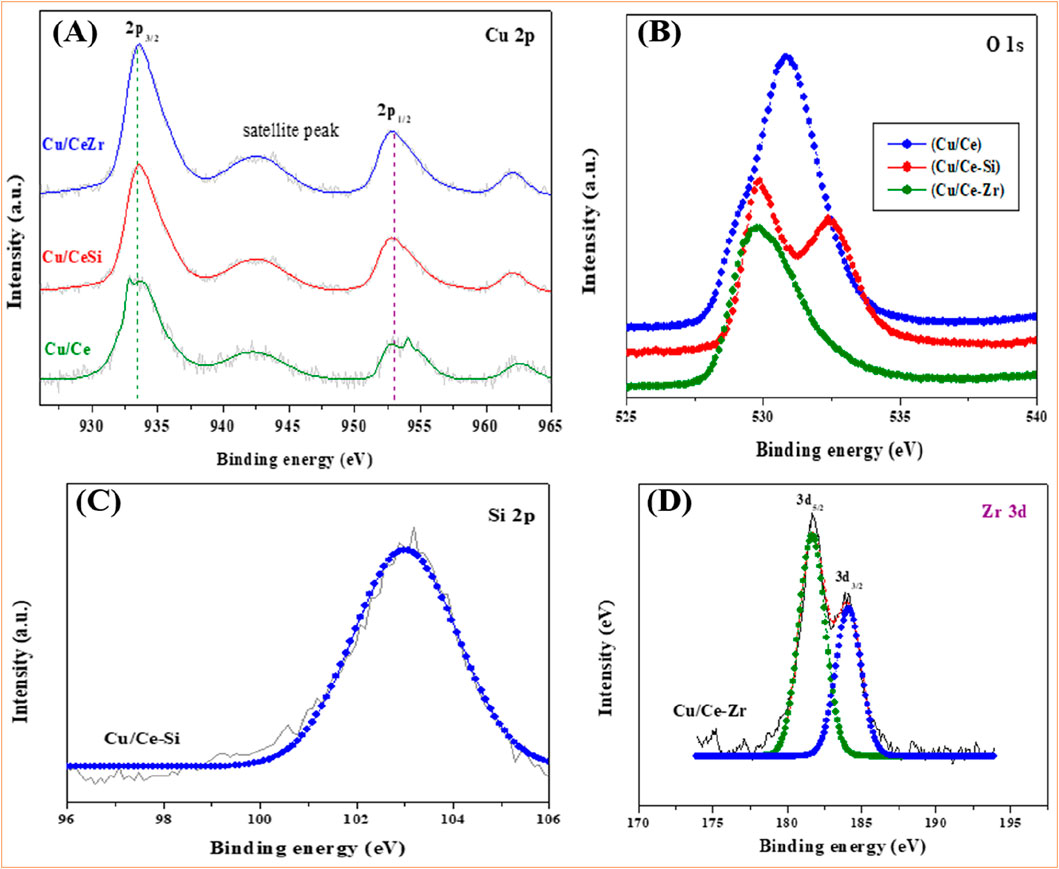
Figure 4. XPS spectra of the (A) Cu 2p, (B) O 1s, (C) Si 2p and (D) Zr 3d of Cu/CeZr (CuO/CeO2-ZrO2) catalyst calcined at 773K.
The O 1s spectra of the catalysts reveal broad signals that represent overlapping contributions from oxygen species in both copper oxide and the ceria-based supports (Figure 4B). The binding energies associated with oxygen in the Cu/CeZr catalyst are observed at 529.7 eV and 530.8 eV. These peaks can be attributed to lattice oxygen in the CuO and CeO2 phases, as well as oxygen vacancies within the ceria-Zirconia matrix (Zhang et al., 2014; Mallesham et al., 2016; Yang et al., 2021). The formation of oxygen vacancies is a well-known characteristic of ceria-based materials, enhancing their redox properties and catalytic activity, particularly in oxidation reactions. For the Cu/CeSi catalyst, the O 1s binding energies at 529.7 eV and 532.4 eV suggest an interaction between silica and hydroxyl groups (Sudarsanam et al., 2014; Dong et al., 2016; Mallesham et al., 2016). The higher binding energy peak at 532.4 eV likely corresponds to surface hydroxyls, which may play a role in modulating the catalytic environment by influencing the dispersion and reducibility of the copper species. The accessibility of these hydroxyl groups, as suggested by the XPS data, indicates that silica enhances the interaction with copper species, potentially improving catalytic performance.
The Si 2p spectrum, with a prominent peak at 103.02 eV, indicates the presence of Si4⁺ in the Cu/CeSi catalyst. This result is consistent with the incorporation of silica into the catalyst framework, potentially affecting the distribution and electronic properties of the active copper sites. The presence of Si4⁺ may also contribute to the formation of stronger Cu-O-Si interactions, further stabilizing the catalyst. Similarly, the Zr 3d XPS spectra for the Cu/CeZr catalyst exhibit two distinct spin-orbit-split peaks, with Zr 3d5/2 at 181.7 eV and Zr 3d3/2 at 184.1 eV, indicative of Zr4⁺ (Zhang et al., 2005; Sudarsanam et al., 2014). The incorporation of Zirconia into the ceria support is expected to enhance the oxygen mobility and defect formation, which can facilitate redox reactions and improve the overall catalytic performance in oxidation processes.
Figure 5 represents the H2-TPR profiles of the Cu/Ce, Cu/CeSi, and Cu/CeZr samples reveal distinct reduction behaviour due to variations in copper oxide dispersion and interaction with the supports (Dou et al., 2021). The Cu/Ce sample shows multiple reduction peaks, indicating the presence of highly dispersed copper species (∼396.96K), weakly interacting copper (∼433.56K), small CuO/Cu2O clusters (∼470.15K), and crystalline CuO (∼508.21K), reflecting strong interactions with CeO2 (Rao et al., 2011; Sudarsanam et al., 2014). In contrast, the Cu/CeSi profile shifts towards higher temperatures, suggesting weaker interactions with the inert SiO2, leading to the presence of more crystalline copper oxide species and subsurface formations. These differences highlight the significant role of the support material in influencing the reduction behaviour and thermal stability of copper oxide species.
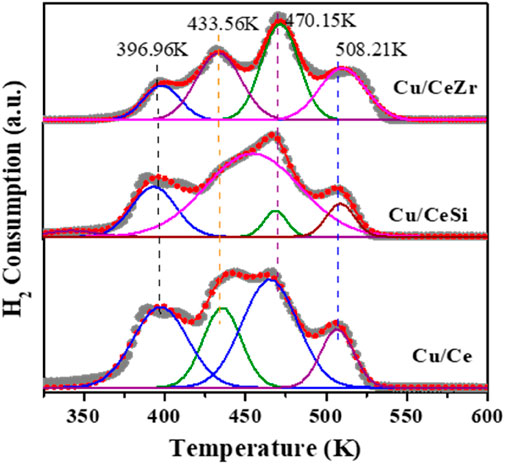
Figure 5. TPR patterns of the Cu/Ce(CuO/CeO2), Cu/CeSi (CuO/CeO2-SiO2), and Cu/CeZr (CuO/CeO2-ZrO2) catalysts calcined at 773K.
The H2-TPR profiles and XPS results together provide a comprehensive understanding of the metal-support interaction (MSI) in Cu/Ce, Cu/CeSi, and Cu/CeZr systems. In the Cu/Ce sample, the strong interaction between CuO and CeO2 is evident from multiple reduction peaks, indicating the presence of various copper species—ranging from highly dispersed (∼396.96K) to weakly interacting copper (∼433.56K), small clusters of CuO/Cu2O (∼470.15K), and crystalline CuO (∼508.21K). This broad range reflects a high degree of MSI, where CeO2, known for its oxygen storage capacity, creates oxygen vacancies that facilitate the reduction of dispersed copper at lower temperatures (Layer, 1963; Rao et al., 2011; Mallesham et al., 2016) The strong MSI in Cu/Ce stabilizes smaller copper species, promoting a higher degree of reduction. In contrast, Cu/CeSi displays a shift to higher reduction temperatures, which suggests weaker MSI due to the inert nature of SiO2. This weak interaction leads to the presence of more crystalline copper oxide species and subsurface formations, requiring more energy for reduction and resulting in less dispersed copper. XPS data corroborate these observations, as Cu/Ce likely shows a higher proportion of Cu⁺/Cu2⁺ species due to the stabilization of copper by CeO2. In contrast, Cu/CeSi would exhibit a larger proportion of Cu2⁺, indicative of less reduction and the presence of more bulk-like CuO. The TPR profiles, combined with the oxidation states revealed by XPS, underline how the support material significantly influences the dispersion, reduction behavior, and electronic properties of copper oxide species, with stronger MSI facilitating more efficient reduction and stabilization of reduced copper species in the Cu/CeZr.
The temperature-programmed desorption of ammonia (NH3-TPD) is a widely used technique for examining the quantitative and qualitative analysis of amount of acidic sites. NH3-TPD is the important method to understand the acidic sites of the Cu/Ce, Cu/CeSi and Cu/CeZr. In general, the distribution of acidic sites follows: weak acidic sites (<423K), medium acidic sites (423–623K) and strong acidic sites (>623K) (Boréave et al., 1997; Mohan et al., 2014). NH3-TPD profiles for the synthesized catalysts of Cu/Ce, Cu/CeSi and Cu/CeZr were shown in Figure 6. It is observed that the CuO promoted CeO2 doped catalysts show all the three types of acidic sites. The quantification of the acidic sites was also performed and the number of weak sites for Cu/Ce, Cu/CeSi and Cu/CeZr are ∼24, 35, 51 μmol/g, respectively. The number of medium sites follows ∼10, 17, and 18 μmol/g, respectively. Similarly, the number of strong acidic sites is ∼9, 10, and 14 μmol/g, respectively. When Zr4⁺ ions are incorporated into the CeO2 lattice in the CuO/CeO2-ZrO2 system, they induce structural distortions due to the smaller ionic radius of Zr4⁺ compared to Ce4⁺. These distortions generate oxygen vacancies, which play a critical role in enhancing the material’s catalytic properties (Oh et al., 2024). The creation of oxygen vacancies modifies the local electronic environment, leading to an increase in Lewis acidic sites. In the CuO/CeO2-ZrO2 catalyst, these oxygen vacancies and the enhanced Lewis acidity improve the dispersion and redox activity of CuO species (Zhang et al., 2019). This synergistic interaction between CuO and the modified CeO2-ZrO2 support contributes to superior catalytic performance, particularly in reactions requiring efficient oxygen transfer and strong metal-support interactions.
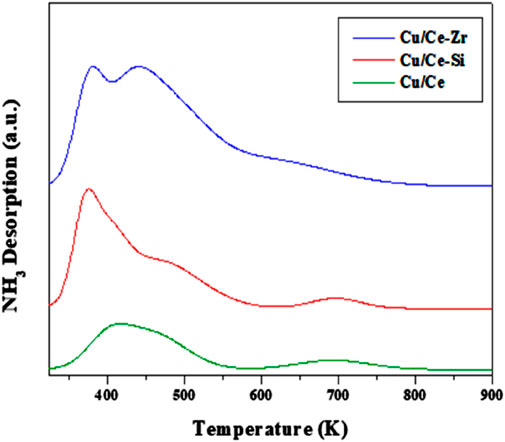
Figure 6. TPD patterns of the Cu/Ce(CuO/CeO2), Cu/CeSi (CuO/CeO2-SiO2), and Cu/CeZr (CuO/CeO2-ZrO2) catalysts calcined at 773K.
The FTIR spectra of pyridine-adsorbed samples, as depicted in Figure 7, reveal significant insights into the nature of acid sites present in the catalyst. The observed peaks around 1,545 cm⁻1, 1,490 cm⁻1, and 1,445 cm⁻1 can be ascribed to specific types of acid sites: the peak at 1,545 cm⁻1 corresponds to Brønsted acid sites, the one at 1,490 cm⁻1 indicates the presence of both Brønsted and Lewis acid sites, and the peak at 1,445 cm⁻1 is characteristic of Lewis acid sites [(Baithy et al., 2022; Mohan et al., 2014; Mallesham et al., 2020; Nagu et al., 2023)]. Additionally, distinct peaks around 1,590 cm⁻1 and 1,635 cm⁻1 further confirm the presence of Brønsted acid sites. These observations suggest that the catalyst samples exhibit a combination of Brønsted and Lewis acid sites. This duality is likely a key factor contributing to the samples’ exceptional catalytic activity, as both types of acid sites play critical roles in facilitating catalytic processes. The interplay between these sites enhances the catalyst’s overall performance, making it highly effective for the intended reactions.
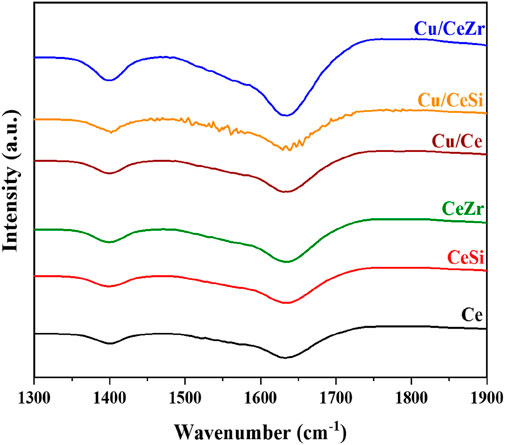
Figure 7. Pyridine adsorbed FTIR spectra of Ce (CeO2), CeSi (CeO2-SiO2), CeZr (CeO2-ZrO2), Cu/CeSi (CuO/CeO2-SiO2), and Cu/CeZr (CuO/CeO2-ZrO2) catalysts calcined at 773K.
The SEM and TEM analyses of the CuO/CeO2-ZrO2 catalyst provide critical insights into its morphology and structure (Figures 8A–D). The SEM images at resolutions of 100 nm and 200 nm reveal spherical CuO particles dispersed on the irregular surface of the CeO2 support, indicating a relatively homogeneous surface that enhances the catalyst’s surface area. TEM images at higher resolutions (20 nm and 2 nm) show that the CuO nanoparticles are uniformly distributed across the zirconia-doped CeO2 support, with lattice fringes confirming a well-defined cubic crystal structure aligned with the (111) plane. The measured particle size of ∼6.2 nm highlights the nanoscale precision, while the intimate contact between CuO and CeO2 observed in TEM suggests strong interfacial interactions, which are crucial for boosting catalytic performance. Together, the SEM and TEM results demonstrate a catalyst with well-dispersed, crystalline CuO nanoparticles that can significantly enhance catalytic activity.
3.1 Catalytic activity measurement
The catalytic performance of benzyl amine oxidation was investigated using all the synthesized CeO2-based heterogeneous catalysts under batch reaction conditions (benzyl amine = 5 mmol, temperature = 403 K, O2 flow rate = 20 mL/min, stirring speed = 900 rpm) for 3 h (Scheme 1). The oxidation of benzyl amine produced three key products is observed in the GC analysis: dibenzylimine (DBI), benzaldehyde (BA), and benzyl nitrile (BN), with varying percentage of selectivity (Figure 9). The pure CeO2 demonstrated a lower conversion rate of 20%, showing a selectivity of 78% towards DBI. Doping CeO2 with 50% SiO2 led to an improvement in both conversion and selectivity, achieving a 40% conversion and 80% selectivity towards DBI. A further enhancement has observed with the introducing of 50% ZrO2-doped CeO2, which increased the conversion to 45% while maintaining 80% selectivity towards DBI. The incorporation of 10 wt% CuO onto the doped-ceria catalysts further enhanced the conversion and selectivity. Among all the catalysts, the Cu/CeZr catalyst exhibited the highest performance, achieving 85% conversion and 90% selectivity for DBI. This improvement can be attributed to the greater number of oxygen vacancies and the presence of acidic sites in the Cu/CeZr catalyst, which are critical in promoting the oxidation reaction. The Raman results indicates that the enhancement of oxygen vacancies for the Cu/CeZr catalyst in comparison to the rest of the catalysts, which provides more active sites for oxygen activation, facilitating the easy oxidation process. These oxygen vacancies play a crucial role in enhancing the redox properties of the catalyst, making it more effective for oxidative processes (Wang et al., 2024a; Zeng et al., 2024). Additionally, CuO’s redox flexibility (Cu(I) and Cu(II)) balances the oxygen vacancy formation, facilitating electron transfer during the oxidation reaction, which contributes to higher conversion and selectivity, while the enhanced acidity supports better interaction with the benzyl amine substrate (Al-Hmoud and Jones, 2013; Gawande et al., 2016). These findings are consistent with the Raman, XPS and TPD analyses, which confirm the formation of additional oxygen vacancies and acidic sites in the CuO/CeO2-ZrO2 catalyst.

Scheme 1. Synthesis of dibenzylimine from various benzyl aminederivatives over the CuO/CeO2-ZrO2 catalyst.
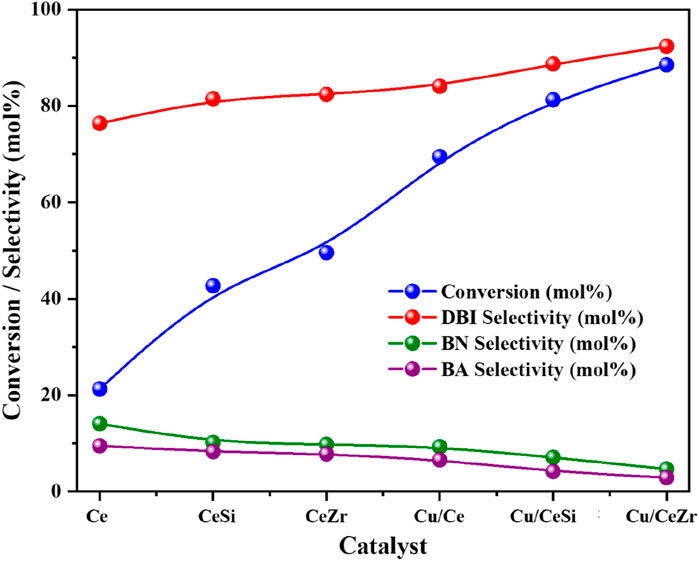
Figure 9. Catalytic oxidative coupling of benzyl amine over Ce (CeO2), CeSi (CeO2-SiO2), CeZr (CeO2-ZrO2), Cu/CeSi (CuO/CeO2-SiO2), and Cu/CeZr (CuO/CeO2-ZrO2) catalysts under solvent-free and base-free conditions. Reaction conditions: benzyl amine = 5 mmol, catalyst = 50 mg, temperature = 403 K, O2 flow rate = 20 mL/min, stirring speed = 900 rpm, time = 3 h.
The reaction kinetics for the oxidation of benzyl amine was studied over a reaction time range from 0.5 to 5 h (Figure 10). During this period, the conversion of benzyl amine steadily increased up to the 3 h of reaction time, with the catalyst demonstrating excellent activity during this initial phase. At the 3 h of reaction time, the conversion of benzyl amine approached to its maximum (85%) with selectivity of DBI (90%), indicates that the catalyst had significantly converted the benzyl amine. Notably, after the 3 h, both the conversion of benzyl amine and selectivity towards dibenzylimine (DBI) reached a plateau, as shown in the graph, with no significant change observed even at the 4 h. This behaviour suggests that the reaction has reached equilibrium at the 3 h. The selectivity remained consistently high throughout the reaction, indicating the catalyst’s effectiveness in selectively producing DBI, even when the conversion rate ceased to increase. Finally, it is clear that the kinetic insight helps optimize the catalytic process by avoiding unnecessary prolongation, which would result in wasted time and resources without improving yields.
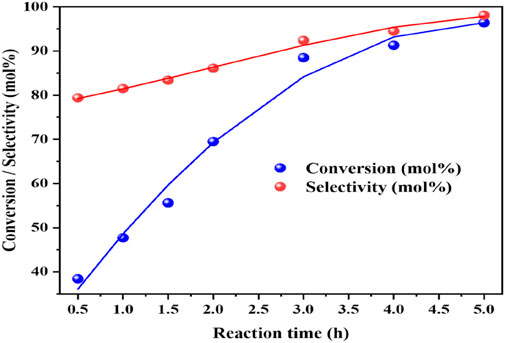
Figure 10. The effect of reaction time on the catalytic oxidative coupling of benzyl amine over the high performance CuO/CeO2-ZrO2 catalyst under solvent-free and base-free conditions. Reaction conditions: benzyl amine = 5 mmol, catalyst = 50 mg, temperature = 403 K, O2 flow rate = 20 mL/min, stirring speed = 900 rpm.
The effect of reaction temperature on the oxidative coupling of benzyl amine provides crucial insights into the reaction kinetics when using the Cu/CeZr catalyst (Figure 10). This study shows that as the temperature increases, both the conversion of benzyl amine and selectivity towards dibenzylimine (DBI) improve. By increasing temperature from 323 to 383 K increases the benzyl amine conversion and selectivity of the DBI. As seen clearly from Figure 11, the reaction temperature of 403 K is found to be optimal, as it provides a significant improvement to the catalytic activity, resulting in high conversion (85%) and selectivity (90%). Upon further increasing the temperature to 423 K, there is no substantial improvement in the conversion or selectivity is observed, indicating that the reaction has reached its kinetic optimum at 403 K. Therefore, 403 K of reaction temperature is identified as the optimal reaction temperature, providing the most efficient conversion and product selectivity, without any need for higher thermal response.
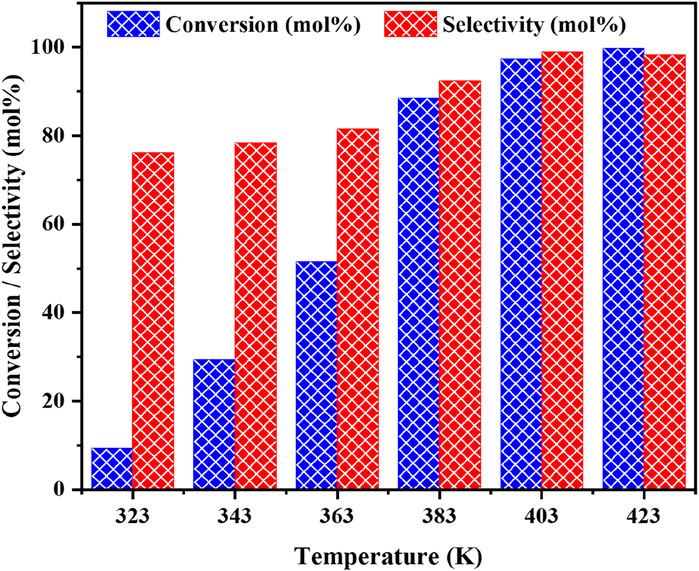
Figure 11. The effect of reaction temperature on the catalytic oxidative coupling of benzyl amine over the high performance CuO/CeO2-ZrO2 catalyst under solvent-free and base-free conditions. Reaction conditions: benzyl amine = 5 mmol, catalyst = 50 mg, O2 flow = 20 mL/min, stirring speed = 900 rpm and time = 3 h.
We also investigated the effect of benzylamine concentration on the performance of the Cu/CeZr catalyst (Figure 12). At an initial concentration of 0.5 mmol, the reaction achieved a conversion of 99.9% and a selectivity of 99.7% towards dibenzylimine (DBI), indicating both a high conversion rate and excellent selectivity. This can be attributed to the lower concentration of reactant, which allows for easier activation at the catalyst’s active sites. As the concentration of benzylamine was increased from 0.5 mmol to 5 mmol, a slight decrease in conversion was observed, although the selectivity towards DBI remained unchanged. However, when the concentration was further increased to 7 mmol, the conversion of benzylamine dropped significantly. From this, we conclude that the optimal reactant concentration for the reaction is 5 mmol, balancing conversion efficiency and high selectivity.
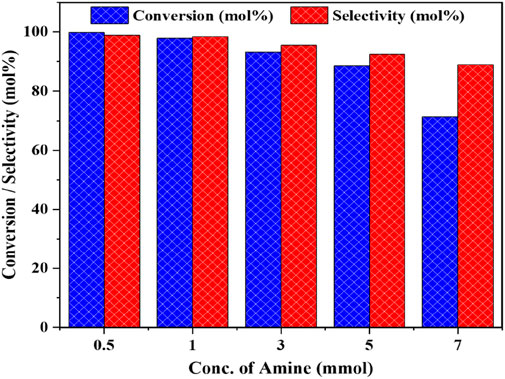
Figure 12. The influence of concentration on the catalytic oxidative coupling of benzyl amine over the high performance CuO/CeO2-ZrO2 catalyst under solvent-free and base-free conditions. Reaction conditions: catalyst = 50 mg, temperature = 403 K, O2 flow = 20 mL/min, stirring speed = 900 rpm, time = 3 h.
We explored the influence of concentration of the catalyst over reaction parameters. Under optimum reaction conditions, the oxidation of benzyl amine over Cu/CeZr catalyst was investigated at different concentrations of catalyst. From the above Figure 13, we can observe that on increasing the catalyst loading from 10mg to 50 mg the conversion of benzyl amine and selectivity of DBI increases significantly, i.e., from 10% to 88% conversion, respectively. Upon further increase in catalyst concentration from 50 mg to 70 mg increases the conversion to 99%, which can be due to the number of active sites (oxygen vacancies and acidic sites) increases on the catalyst surface with increased the catalysts loading. Further increase in catalyst loading up to 90 mg, it does not influence the conversion of the benzyl amine, but the selectivity of the DBI slightly decreases due to the further participated in the reaction to form by products.
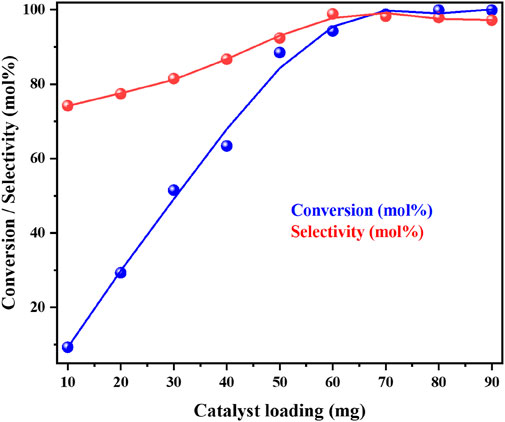
Figure 13. The influence of catalyst loading on the catalytic oxidative coupling of benzyl amine over the high performance CuO/CeO2-ZrO2 catalyst under solvent-free and base-free conditions. Reaction conditions: benzyl amine = 5 mmol, temperature = 403 K, O2 flow = 20 mL/min, stirring speed = 900 rpm and time = 3 h.
Under optimum reaction conditions, the recovery and recyclability of catalyst over oxidative coupling of benzyl amine was investigated (Figure 14). After the completion of the first cycle of the catalytic oxidative coupling reaction, the catalyst was meticulously separated from the reaction mixture. To ensure its purity and effectiveness for subsequent use, it was recovered through the process of centrifugation. This technique allowed for the efficient separation of the catalyst from the reaction by products. Once isolated, the catalyst underwent a thorough washing procedure, utilizing both deionised water and ethanol. This dual washing process was essential to effectively remove any adsorbed species or impurities that had accumulated on the catalyst’s surface during the reaction.
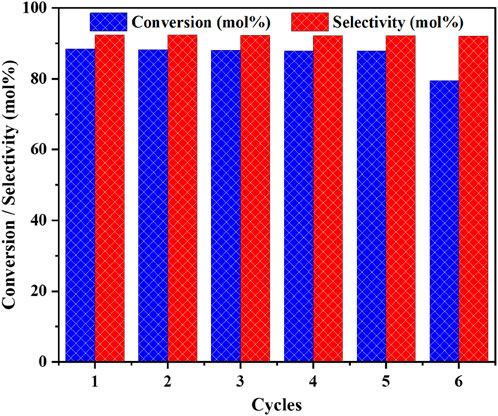
Figure 14. The reusability study on the catalytic oxidative coupling of benzyl amine over the high performance CuO/CeO2-ZrO2 catalyst under solvent-free and base-free conditions. Reaction conditions: benzyl amine = 5 mmol, temperature = 403 K, catalyst loading = 50 mg, O2 flow = 20 mL/min, stirring speed = 900 rpm and time = 3 h.
After the washing step, the catalyst was subjected to a drying process. It was placed in an oven and dried at a temperature of 423 K for duration of 4 h. This drying phase was crucial to eliminate any residual moisture and solvents, ensuring that the catalyst would be in an optimal state for reuse. Once dried, the catalyst was ready to be employed in the next cycle of the catalytic oxidative coupling reaction, demonstrating its reusability and stability under the specified conditions. Figure 14 illustrates that the Cu/CeZr catalyst exhibited substantial robustness and was capable of being recycled up to the fifth run without any observable alterations in amine conversion and imine selectivity. However, following the fifth cycle, a marked decline in imine selectivity was detected, indicating a significant change in the catalyst’s performance.
The proposed reaction mechanism for the formation of N-Benzylidenebenzylamine and benzimidazole over the CuO/CeO2-ZrO2 catalyst is schematically illustrated (Schemes 2, 4). The reaction starts with benzylamine and molecular oxygen interaction with the acidic and redox-active sites (oxygen vacancy site) of the CuO/CeO2-ZrO2 catalyst (Rao et al., 2016; Purohit et al., 2023). This interaction facilitates the oxidation of benzylamine, leading to the formation of an imine intermediate. Subsequently, the imine intermediate reacts with another molecule of benzylamine, resulting in the production of N-Benzylidenebenzylamine, as shown in Scheme 2. Additionally, the imine intermediate can undergo hydrolysis, generating benzaldehyde. The benzaldehyde further reacts with benzylamine through a condensation reaction at the acidic sites, also yielding N-Benzylidenebenzylamine [(Rao et al., 2016)]. This dual pathway highlights the versatility of the catalytic system in promoting multiple reaction routes for imine formation over the CuO/CeO2-ZrO2 catalyst.
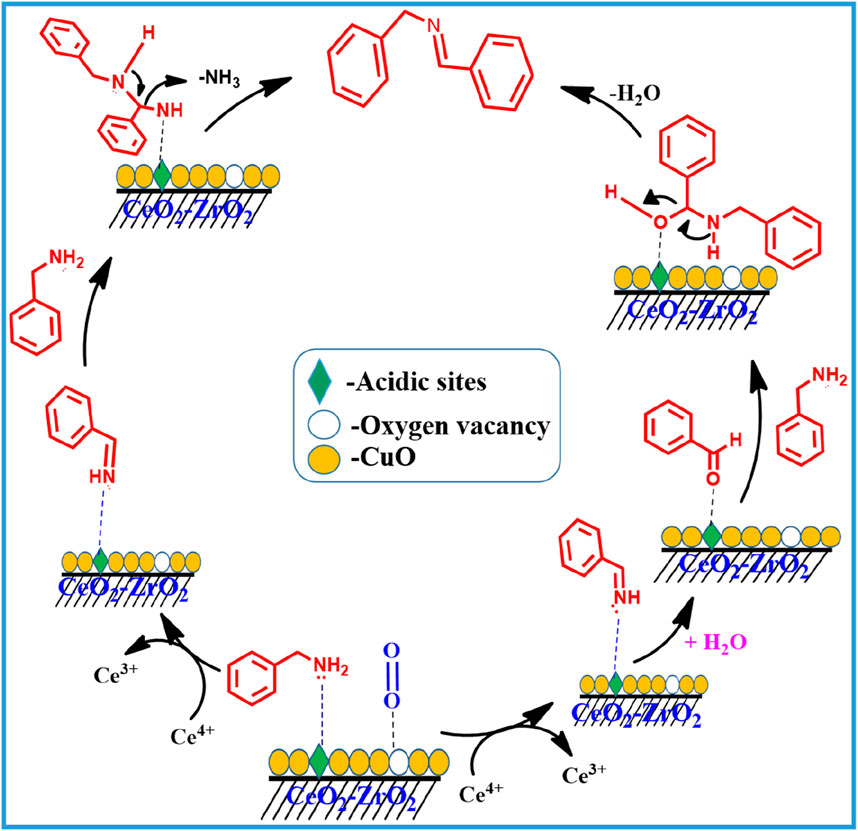
Scheme 2. Plausible reaction mechanism for the catalytic oxidative coupling of the benzyl amine to imine over the CuO/CeO2-ZrO2 catalyst.
The formation of benzimidazole over the CuO/CeO2-ZrO2 catalyst follows a well-defined parallel mechanism with oxidative coupling of the benzyl amine reaction (Scheme 3). Initially, benzylamine and molecular oxygen adsorb onto the acidic and vacancy sites of the catalyst. This interaction facilitates the oxidation of benzylamine, leading to the generation of an imine intermediate (Rao et al., 2016; Chung et al., 2023; Chai et al., 2024). The imine undergoes hydrolysis, producing benzaldehyde, a key intermediate in the process. The benzaldehyde then reacts with 1,2-diaminobenzene through a condensation reaction to form an imine derivative, which subsequently rearranges to yield the final product (Chai et al., 2024), benzimidazole, as illustrated in Scheme 4. The CuO/CeO2-ZrO2 catalyst’s unique redox properties and ability to stabilize reactive intermediates are pivotal in driving these transformations. The dual mechanistic pathways not only highlight the catalyst’s versatility but also demonstrate its capability to selectively and efficiently synthesize both imines and benzimidazole derivatives under controlled reaction conditions. These features make the catalyst an excellent choice for facilitating complex oxidation and condensation reactions, with mechanisms similar to those observed in other advanced redox-active catalytic systems reported in recent studies.
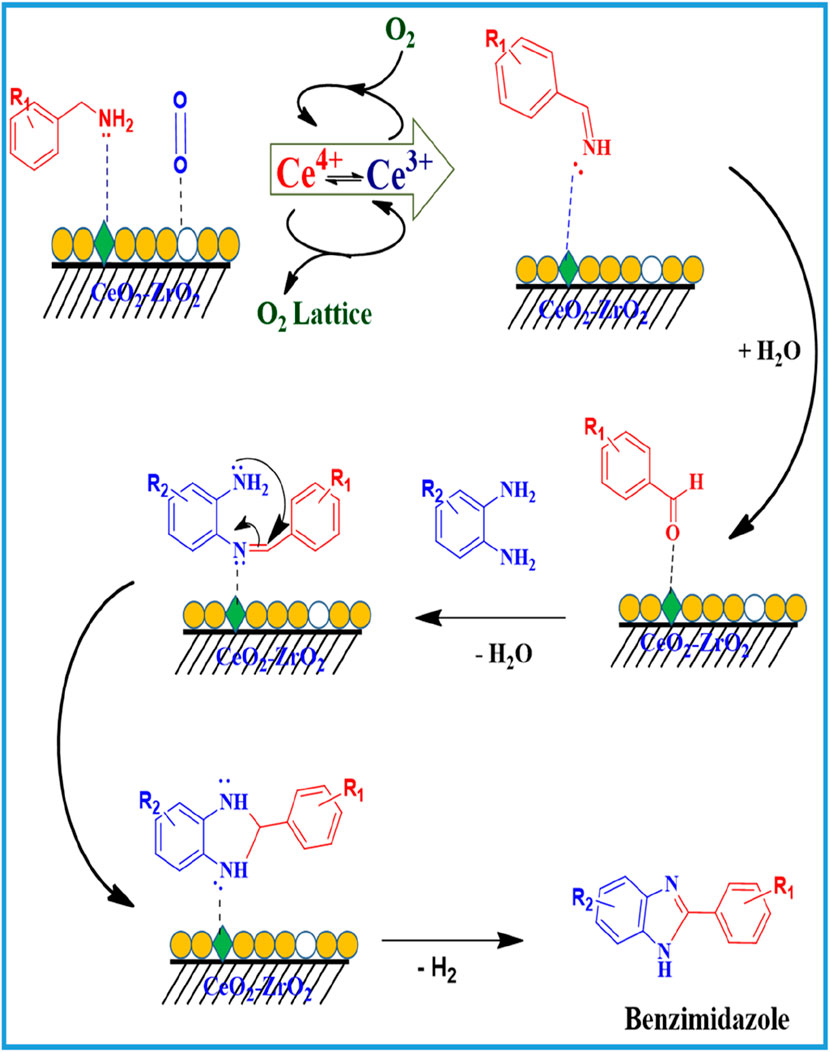
Scheme 4. Plausible reaction mechanism for the catalytic oxidative coupling of benzyl amine to benzimidazole over the CuO/CeO2-ZrO2 catalyst.
The CuO/CeO2-ZrO2 catalyst demonstrated high efficiency in the oxidative coupling of benzyl amine derivatives with various substituents, maintaining excellent conversion and selectivity across a wide range of substrates. For unsubstituted benzyl amine, the catalyst achieved near-complete conversion and high selectivity, serving as a baseline for the reactions (entries 1–12 in Table 2). Substrates with electron-donating groups, such as methyl and methoxy, displayed similarly high conversions, particularly in the ortho and para positions, where steric effects were minimal in comparison to meta-substituted benzyl amines. Ortho-substituted amines, while slightly hindered by steric factors, generally retained high conversion rates with selectivity close to or above 95%, indicating that the CuO/CeO2-ZrO2 catalyst efficiently manages steric hindrance. Interestingly, para-substituted amines, which are less sterically hindered, yielded higher conversions, further highlighting the catalyst’s ability to facilitate efficient oxidative coupling when substituents are positioned to minimize steric interference. Electron-withdrawing substituents, such as chlorine and nitro groups, introduced notable variations in conversion rates depending on their position on the phenyl ring. For example, para-chlorobenzyl amine and para-nitrobenzyl amine showed significantly reduced conversions, which can be attributed to the electron-withdrawing nature of these groups, decreasing the nucleophilicity of the amine. However, even in these cases, selectivity remained impressively high, suggesting that the catalyst retains strong control over product formation despite lower conversion rates. Meta-substituted amines generally exhibited the lowest conversions across all substituents, reflecting the combined effects of electronic deactivation and possible steric hindrance. Nonetheless, the catalyst consistently maintained selectivity for imine products between 95% and 99%, indicating its robustness and versatility across a variety of benzyl amine derivatives, regardless of the electronic properties of the substituents.
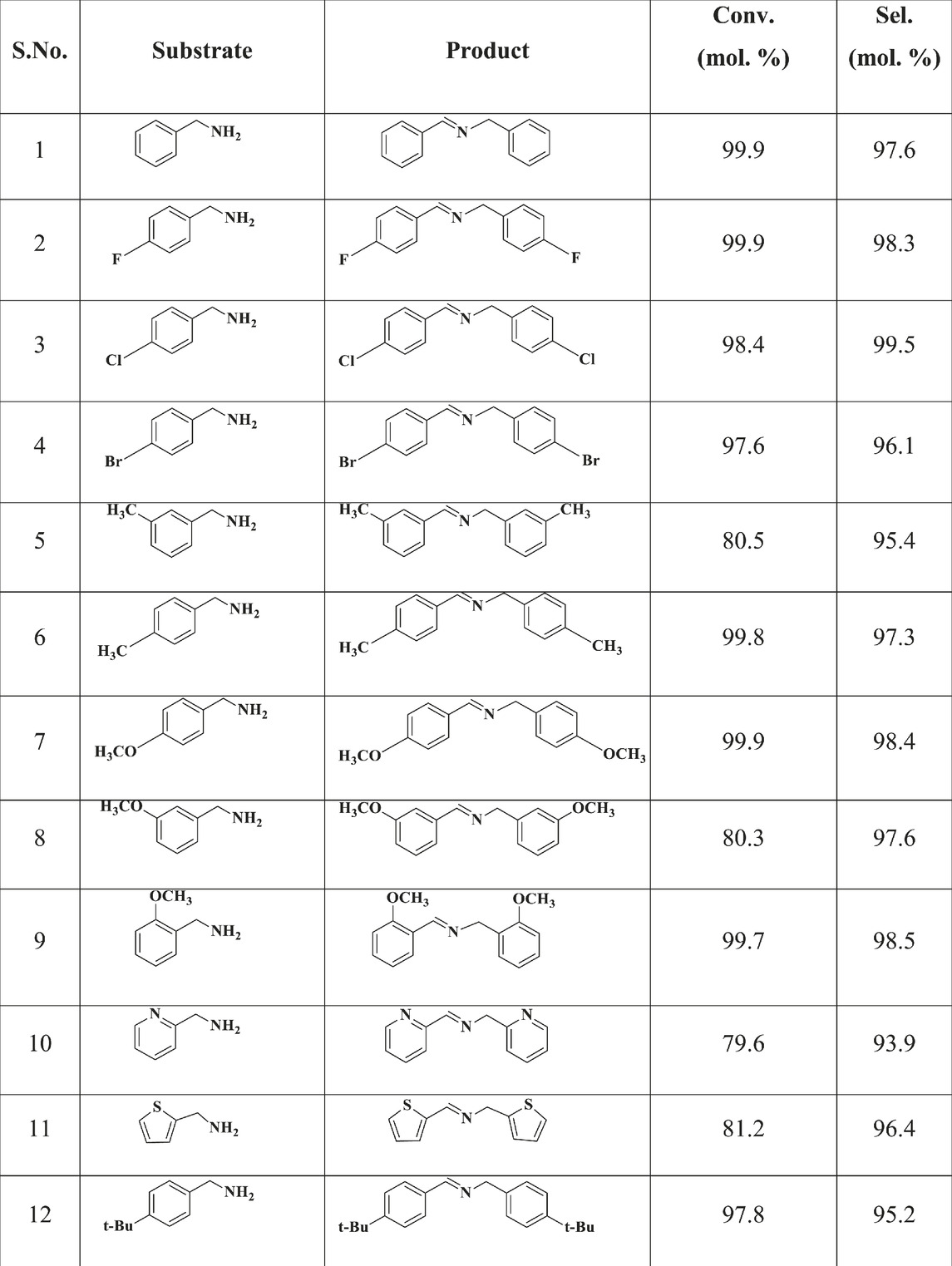
Table 2. The catalytic selective oxidative coupling of different substituted benzyl amine over CuO/CeO2-ZrO2 catalyst under solvent- and base-free conditions. Reaction conditions: benzyl amine = 5 mmol, temperature = 423 K, catalyst loading = 70 mg,O2 flow = 20 mL/min, stirring speed = 900 rpm and time = 5 h.
The above Table 3 compares the catalytic activity for the oxidation of benzylamine to imines using various catalysts. The Ce-doped MnOx catalyst synthesized via redox-precipitation (CeMn-RO) demonstrated excellent catalytic activity for imine synthesis through the oxidation of benzylamine under relatively mild conditions (353 K, 8 h, air atmosphere), achieving a benzylamine conversion rate of 92% and a good selectivity of 80%. The remarkable oxidative performance of this catalyst can be attributed to its elevated levels of surface Ce3⁺, Mn3⁺, and oxygen vacancies, which enhance the generation of reactive surface oxygen species critical to driving the reaction (Entry 1, Table 3) (Wu et al., 2022). A mesoporous mixed manganese and cobalt oxides (MMA-A) catalyst, prepared using an anionic surfactant template, was utilized for the homo-coupling of benzylamine to form imines. The incorporation of the anionic surfactant promotes micelle formation, aiding in structuring the catalyst. A higher Co2⁺:Co3⁺ ratio within the catalyst has been identified as a key factor enhancing its activity in the oxidation of benzylamine to imines, with a conversion of 76% and a selectivity of >99% (Entry 2, Table 3) (Khanna et al., 2020). The PdxCu1−x catalyst showed efficient catalytic performance toward imine synthesis from benzylamine oxidation. The enhanced catalytic performance of the PdCu intermetallic catalyst, achieving a benzylamine conversion of 94.0% and a dibenzylimine selectivity of 92.2%, can be attributed to its atomic ordering (Entry 3, Table 3) (Marakatti et al., 2017). Cd-MOFs with bpy ligands exhibit excellent light-harvesting and photo catalytic properties, enabling efficient, selective, and green photo-oxidation of benzylamine to N-benzylbenzaldimine with a conversion of 99.1% and a selectivity of >99% under mild conditions without additional sensitizers or co-catalysts (Entry 4, Table 3) (Shi et al., 2019).
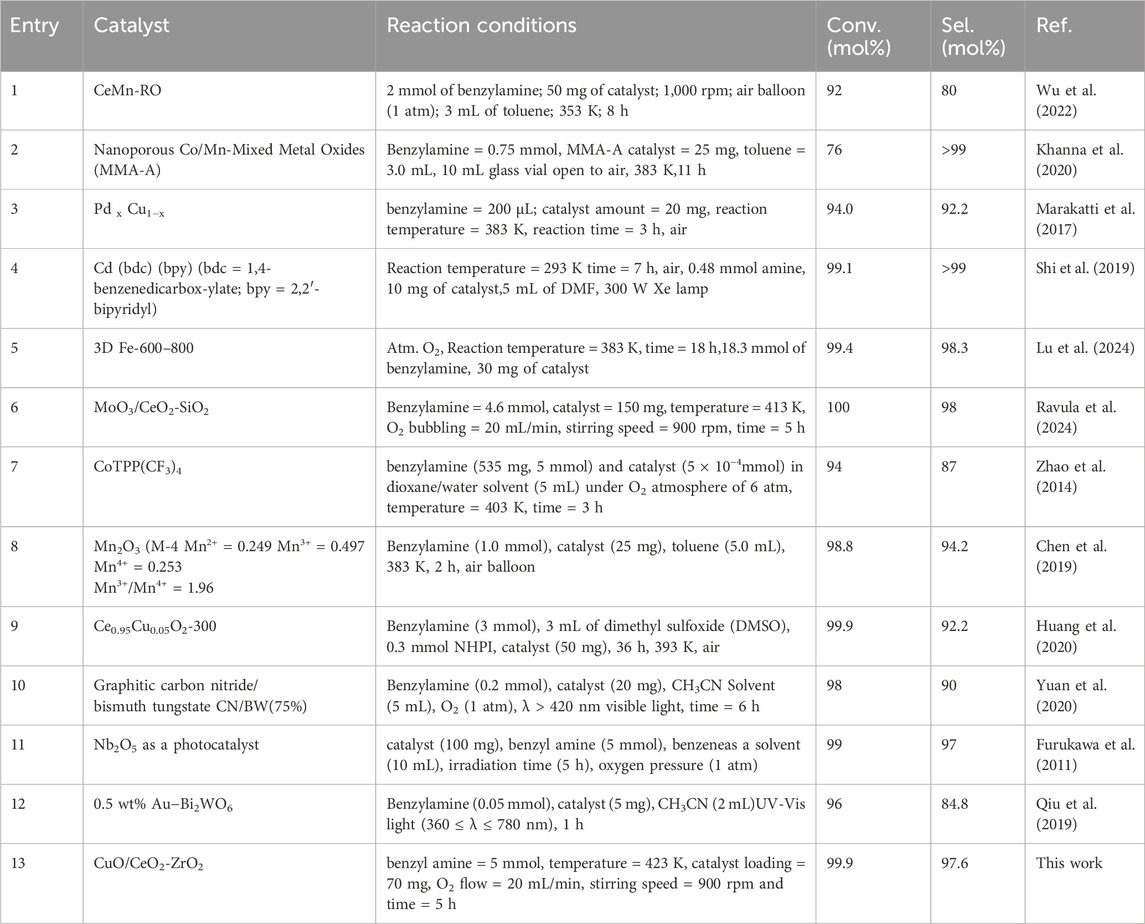
Table 3. Comparative study of catalytic oxidation of benzylamine to imine with various reported catalysts.
The 3D Fe-600–800 catalyst demonstrates superior catalytic performance, achieving a remarkable 97.7% yield of the target imines with 98.3% selectivity. This efficiency is attributed to the Fe−Nx active sites and the pivotal role of oxygen in the oxidation of benzylamine to imines (Entry 5, Table 3) (Lu et al., 2024). The MoO3/CeO2-SiO2 catalyst excelled in the oxidative coupling of benzylamine, delivering superior conversion rates and selectivity for the corresponding imines due to enhanced acidic properties and a higher ratio of Ce3⁺ ions (Iu/ITotal), reflecting an increased presence of oxygen vacancies (Entry 6, Table 3) (Ravula et al., 2024). The CoTPP(CF3)4 catalyst exhibits high conversion (94%) and selectivity (87%) in the oxidative coupling of benzylamine using molecular oxygen as an oxidant. The enhanced catalytic performance is due to the effect of four β-CF3 groups. The reaction is easily scaled up and may provide a convenient way to prepare many imines on a large scale (Entry 7, Table 3) (Zhao et al., 2014). The Mn2O3 (M-4, Mn2⁺ = 0.249, Mn3⁺ = 0.497, Mn4⁺ = 0.253, Mn3⁺/Mn4⁺ = 1.96) catalyst shows 96.2% selectivity for imines at 100% conversion of benzylamine. The high selectivity of imines was influenced by the Mn3⁺/Mn4⁺ ratio (Entry 8, Table 3) (Chen et al., 2019). Cu-doped CeO2 nano crystals were synthesized using a hydrothermal method. The catalyst demonstrates high catalytic activity (selectivity 92%) for imine synthesis from amines and maintains excellent stability, sustaining at least four reaction cycles under optimized reaction conditions. The high performance is attributed to the well-dispersed copper species and the presence of abundant oxygen vacancies (Entry 9, Table 3) (Huang et al., 2020).
The optimized CN/BW hetero junction with 75% BW content presents high conversion (∼98%) and selectivity (∼98%) in a 3 h oxidative coupling reaction of benzylamine. The good catalytic performance can be attributed to the transfer pathway of photo-excited electrons and holes in the hetero junction photo catalyst (Entry 10, Table 3) (Yuan et al., 2020). Nb2O5 exhibits catalytic activity with high selectivity (97%) for imines under visible light (λ > 390 nm) irradiation, due to the formation of excited electrons in the conduction band and positive holes in the valence band (Entry 11, Table 3) (Furukawa et al., 2011). The Au−Bi2WO6 hybrid photo catalyst with optimal Au content shows superior activity and selectivity (84.8%) for the selective oxidation of aromatic amines under visible light, outperforming pure Bi2WO6. The enhanced photo catalysis is due to the combined effects of hot charge carriers from Au nanoparticles, photo-excited electron−hole pairs from Bi2WO6, and oxygen vacancies that promote efficient O2 activation (Entry 12, Table 3) (Qiu et al., 2019). The CuO/CeO2-ZrO2 catalyst demonstrated high efficiency in the oxidative coupling of benzylamine to imine using molecular oxygen as an oxidant, with high conversion (99.9%) and high selectivity (97.6%). The catalytic efficiency is due to the increased number of acidic sites and the presence of abundant oxygen vacancies (Entry 13, Table 3).
To expand the scope of the reaction, the model reaction of benzylamine with 1,2-diaminobenzene was further investigated using the CuO/CeO2-ZrO2 catalyst, yielding benzimidazole as the product. The product formation is attributed to the condensation of nascent benzaldehyde, generated in situ via the oxidation of benzylamine followed by hydrolysis, with 1, 2-diaminobenzene and exhibits higher reactivity compared to pure benzaldehyde, as previously elucidated in earlier work (Dong et al., 2016; Nguyen and Largeron, 2016). To validate this mechanistic insight, an additional experiment was performed using pure benzaldehyde and 1,2-diaminobenzene in the presence of the CuO/CeO2-ZrO2 catalyst. The reaction achieved a conversion of 95.6% and a selectivity of 91.3% toward the desired product, benzimidazole, correlating the enhanced reactivity of nascent benzaldehyde. In contrast, a control experiment conducted without the CuO/CeO2-ZrO2 catalyst resulted in negligible conversion (<2%) and selectivity for the target product.
Furthermore, we screened the performance of the CuO/CeO2-ZrO2 catalyst in the oxidative coupling of various benzyl amine with different substituent on the phenyl ring and with different substituent on 1,2-diaminebenzene as detailed in Table 4. Generally, the oxidative couplings of amines (Entries 2–18, Table 4), which include both electron-withdrawing and electron-donating groups) reacts efficiently under the specified conditions, yielding excellent conversions and high selectivity for the corresponding benzimidazole (Table 4). This finding indicates that the CuO/CeO2-ZrO2 catalyst, designed with careful consideration, plays a crucial role in promoting the oxidative transformation reaction to a significant degree. The nature of the substituent on the benzyl amine ring significantly influenced both the amine conversion and the selectivity of the benzimidazole formed. Specifically, the oxidative coupling of ortho-, meta-, and para-substituted benzyl amine resulted in overall higher selectivity’s compared to substituted 1,2-diaminobenzene (Entries 3–9, Table 4). The selectivity for benzimidazole formation remained consistently high, ranging from approximately 91%–99% for all substituted benzyl amine. Notably, the selectivity for benzimidazole decreased approximately to 85% when the substituent on 1,2-diaminobenzene were electron-donating, whereas the introduction of electron-withdrawing substituent enhanced the selectivity to 96% (Entries 10–15, Table 4) with higher conversion rates. In contrast, heterocyclic analogues of amines exhibited lower conversion rates (78%–81%), while maintaining high selectivity in the range of 87%–91% (Entries 16–18, Table 4). These results demonstrate that the rationally synthesized CuO/CeO2-ZrO2 catalyst plays an important role in facilitating the oxidative transformation with high efficiency.
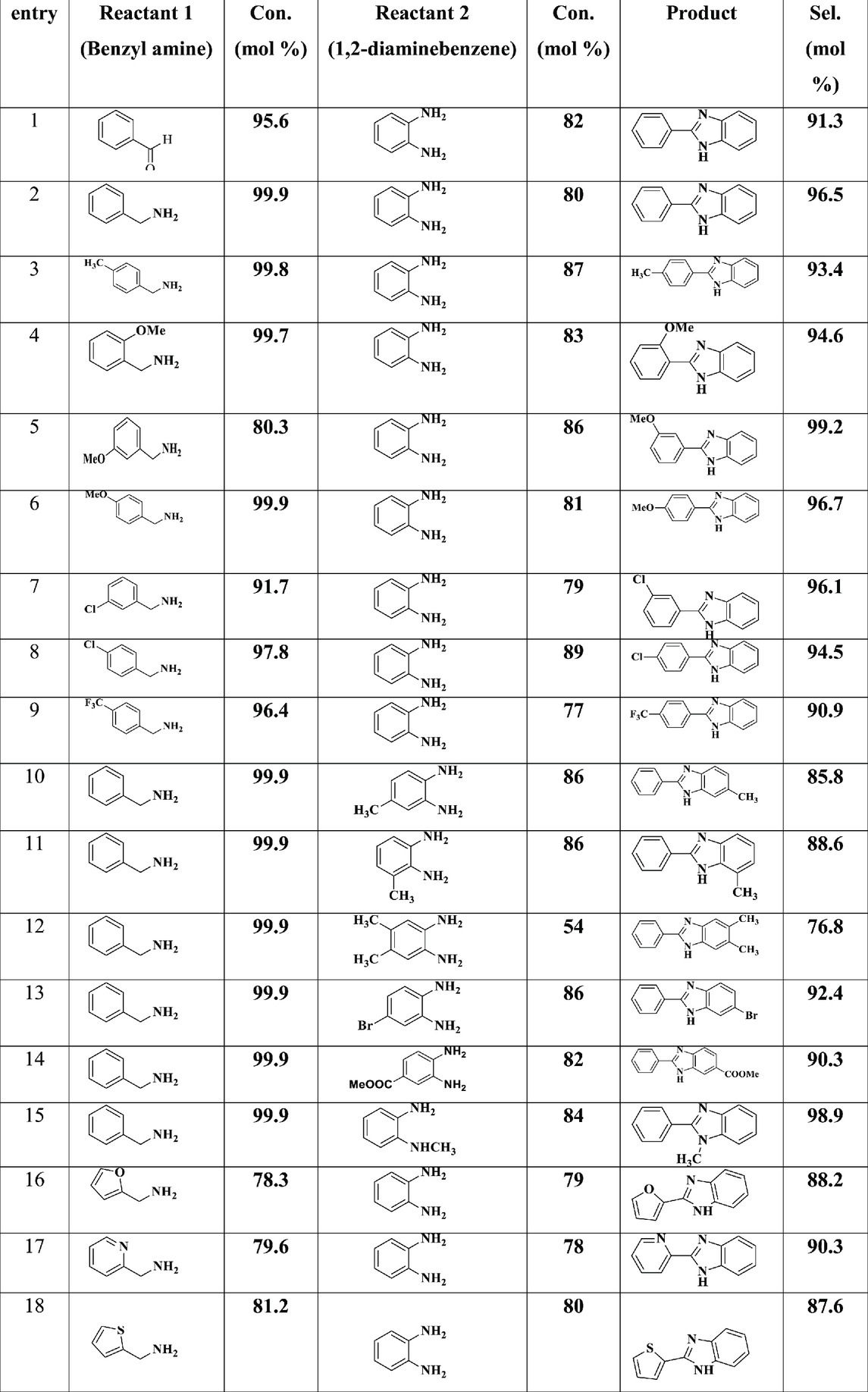
Table 4. The catalytic selective oxidative coupling of different substituted benzyl amine with substituted 1,2- diaminebenzene over CuO/CeO2-ZrO2 catalyst under solvent- and base-free conditions. Reaction conditions: benzyl amine = 5 mmol, substituted 1,2- diaminebenzene = 5 mmol, temperature = 403 K, catalyst loading = 50 mg, O2 flow = 20 mL/min, stirring speed = 900 rpm and time = 5 h.
The above Table 5 gives the comparison of various heterogeneous catalysts reported for the oxidation of benzylamine to benzimidazole. Dong et al., demonstrated that, under optimized conditions, the cyclization reaction between benzyl amine and 1,2-diaminobenzene, catalyzed by a salicylic acid derivative, was successfully carried out, resulting in the formation of benzimidazoles with a high yield of 93%. (Entry 1, Table 5) (Dong et al., 2016). Naresh et al., reported that under the optimized reaction conditions, the molecular iodine catalyzed cyclization of benzyl amine with 1,2-diaminobenzene was studied and the corresponding benzimidazole were obtained in good yield (80%) (Entry 2, Table 5) (Naresh et al., 2014). In the present study, we carried out the oxidative coupling of benzyl amine with 1,2-diaminobenzene using a CuO/CeO2-ZrO2 catalyst, achieving an excellent yield of 96.4% for the corresponding benzimidazole (Entry 3, Table 5).
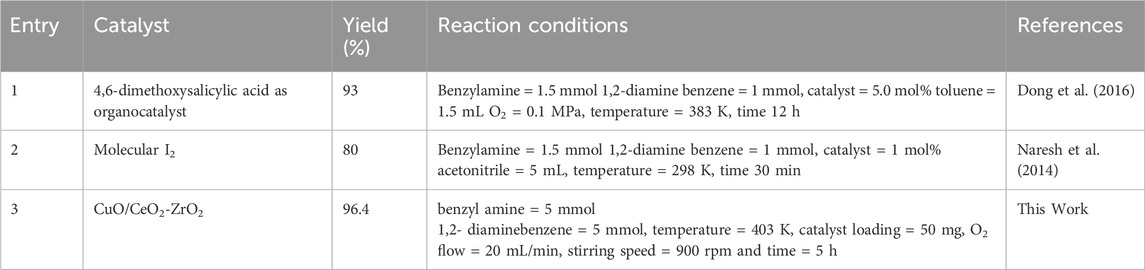
Table 5. Comparative study of catalytic oxidation of benzylamine to benzimidazole with various reported catalysts.
4 Conclusion
In summary, the CuO/CeO2-ZrO2 catalyst developed in this study offers a highly efficient, robust, and recyclable system for the selective oxidative coupling of benzylamines to imine (99.5%), benzimidazole (99.2%) and its derivatives under solvent-free conditions. The catalytic activity is obtained by the presence of oxygen vacancy sites, enhanced redox behavior, strong metal support interaction, and a high density of acidic sites, which collectively contribute to its superior performance. The catalyst also demonstrates excellent functional group tolerance, short reaction times, and durability over multiple cycles (upto fifth cycle). These features make the catalyst an attractive option for industrial applications in green and sustainable chemistry, particularly in the fields of pharmaceuticals and organic synthesis. The achievement of the CuO/CeO2-ZrO2 catalytic system covers the way for further exploration of doped ceria-based catalysts in oxidative processes.
Data availability statement
The raw data supporting the conclusions of this article will be made available by the authors, without undue reservation.
Author contributions
SS: Formal Analysis, Investigation, Methodology, Writing–original draft. NK: Formal Analysis, Investigation, Methodology, Software, Writing–original draft. SJ: Data curation, Formal Analysis, Investigation, Methodology, Writing–original draft. MV: Data curation, Formal Analysis, Validation, Visualization, Writing–review and editing. MB: Conceptualization, Funding acquisition, Project administration, Resources, Supervision, Writing–original draft, Writing–review and editing.
Funding
The author(s) declare that no financial support was received for the research, authorship, and/or publication of this article.
Acknowledgments
The authors thank the Department of Science and Technology (DST)-SERB, New Delhi, for the support under the SRG theme (SRG/2022/001201, dated: 9th September 2022). SS, NK, JS, and MB thank the GITAM University Hyderabad for providing the research specialities in the CIF lab.
Conflict of interest
The authors declare that the research was conducted without any commercial or financial relationships that could be interpreted as a potential conflict of interest.
Generative AI statement
The author(s) declare that no Generative AI was used in the creation of this manuscript.
Publisher’s note
All claims expressed in this article are solely those of the authors and do not necessarily represent those of their affiliated organizations, or those of the publisher, the editors and the reviewers. Any product that may be evaluated in this article, or claim that may be made by its manufacturer, is not guaranteed or endorsed by the publisher.
Supplementary material
The Supplementary Material for this article can be found online at: https://www.frontiersin.org/articles/10.3389/fnano.2024.1513783/full#supplementary-material
References
Ahmad, G., Sohail, M., Bilal, M., Rasool, N., Qamar, M. U., Ciurea, C., et al. (2024a). N-heterocycles as promising antiviral agents: a comprehensive overview. Molecules 29, 2232. doi:10.3390/molecules29102232
Ahmad, M. F., Ahmad, F. A., Alsayegh, A. A., Zeyaullah, M., AlShahrani, A. M., Muzammil, K., et al. (2024b). Pesticides impacts on human health and the environment with their mechanisms of action and possible countermeasures. Heliyon 10, e29128. doi:10.1016/j.heliyon.2024.e29128
Al-Hmoud, L., and Jones, C. W. (2013). Reaction pathways over copper and cerium oxide catalysts for direct synthesis of imines from amines under aerobic conditions. J. Catal. 301, 116–124. doi:10.1016/j.jcat.2013.01.027
Ay, B., Gönül, İ., Demir, B. S., Saygıdeğer, Y., and Kani, İ. (2020). Synthesis, structural characterization and in vitro anticancer activity of two new nickel complexes bearing imine bonds. Inorg. Chem. Commun. 114, 107824. doi:10.1016/j.inoche.2020.107824
Bai, S., Zhang, M., Tang, S., Li, M., Wu, R., Wan, S., et al. (2024). Research progress on benzimidazole fungicides: a review. Molecules 29, 1218. doi:10.3390/molecules29061218
Baithy, M., Mukherjee, D., Rangaswamy, A., and Reddy, B. M. (2022). Structure–activity relationships of WOx-promoted TiO2–ZrO2 solid acid catalyst for acetalization and ketalization of glycerol towards biofuel additives. Catal. Lett. 152, 1428–1440. doi:10.1007/s10562-021-03733-2
Bauer, I., and Knölker, H.-J. (2015). Iron catalysis in organic synthesis. Chem. Rev. 115, 3170–3387. doi:10.1021/cr500425u
Bêche, E., Charvin, P., Perarnau, D., Abanades, S., and Flamant, G. (2008). Ce 3d XPS investigation of cerium oxides and mixed cerium oxide (CexTiyOz). Surf. Interface Anal. 40, 264–267. doi:10.1002/sia.2686
Bera, A., Sk, M., Singh, K., and Banerjee, D. (2019). Nickel-catalysed dehydrogenative coupling of aromatic diamines with alcohols: selective synthesis of substituted benzimidazoles and quinoxalines. Chem. Commun. 55, 5958–5961. doi:10.1039/C9CC02319D
Boréave, A., Auroux, A., and Guimon, C. (1997). Nature and strength of acid sites in HY zeolites: a multitechnical approach. Microporous Mater 11, 275–291. doi:10.1016/S0927-6513(97)00047-3
Brishty, S. R., Hossain, M. J., Khandaker, M. U., Faruque, M. R. I., Osman, H., and Rahman, S. M. A. (2021). A comprehensive account on recent progress in pharmacological activities of benzimidazole derivatives. Front. Pharmacol. 12, 762807. doi:10.3389/fphar.2021.762807
Chai, K., Xia, W., Chen, H., Su, W., Xu, W., and Su, A. (2024). Mesoporous CuMn2O4 nanoframework as an efficient catalyst for the synthesis of benzimidazole derivatives through air oxidative coupling in water. Mol. Catal. 561, 114159. doi:10.1016/j.mcat.2024.114159
Chakraborty, S., Das, U. K., Ben-David, Y., and Milstein, D. (2017). Manganese catalyzed α-olefination of nitriles by primary alcohols. J. Am. Chem. Soc. 139, 11710–11713. doi:10.1021/jacs.7b06993
Chen, B., Wang, L., and Gao, S. (2015). Recent advances in aerobic oxidation of alcohols and amines to imines. ACS Catal. 5, 5851–5876. doi:10.1021/acscatal.5b01479
Chen, F., Yang, T., Zhao, S., Jiang, T., Yu, L., Xiong, H., et al. (2019). Highly selective oxidation of amines to imines by Mn2O3 catalyst under eco-friendly conditions. Chin. Chem. Lett. 30, 2282–2286. doi:10.1016/j.cclet.2019.09.007
Chung, N. T., Dung, V. C., and Duc, D. X. (2023). Recent achievements in the synthesis of benzimidazole derivatives. RSC Adv. 13, 32734–32771. doi:10.1039/D3RA05960J
Dong, C., Higashiura, Y., Marui, K., Kumazawa, S., Nomoto, A., Ueshima, M., et al. (2016). Metal-free oxidative coupling of benzylamines to imines under an oxygen atmosphere promoted using salicylic acid derivatives as organocatalysts. ACS Omega 1, 799–807. doi:10.1021/acsomega.6b00235
Dou, B., Yang, D., Kang, T., Xu, Y., Hao, Q., Bin, F., et al. (2021). Morphology effects of CeO2-ZrO2 on the catalytic performance of CuO/CeO2-ZrO2 for toluene oxidation. Carbon Resour. Convers. 4, 55–60. doi:10.1016/j.crcon.2021.01.007
Furukawa, S., Ohno, Y., Shishido, T., Teramura, K., and Tanaka, T. (2011). Selective amine oxidation using Nb2O5 photocatalyst and O2. ACS Catal. 1, 1150–1153. doi:10.1021/cs200318n
Gawande, M. B., Goswami, A., Felpin, F.-X., Asefa, T., Huang, X., Silva, R., et al. (2016). Cu and Cu-based nanoparticles: synthesis and applications in catalysis. Chem. Rev. 116, 3722–3811. doi:10.1021/acs.chemrev.5b00482
He, Y., Zhang, H., Wang, Z., Zheng, Z., Wang, P., Liu, Y., et al. (2022). Photoelectrochemical oxidation of amines to imines and production of hydrogen through Mo-doped BiVO 4 photoanode. ACS Omega 7, 12816–12824. doi:10.1021/acsomega.2c00048
Huang, X., Lu, G., Zhang, K., Liu, J., Zhang, H., Guo, Z., et al. (2020). Selective synthesis of imines by direct oxidative coupling of amines on Cu-doped CeO2 catalysts. Appl. Surf. Sci. 514, 145948. doi:10.1016/j.apsusc.2020.145948
Huang, Z.-S., Wang, Y.-F., Qi, M.-Y., Conte, M., Tang, Z.-R., and Xu, Y.-J. (2024). Interface synergy of exposed oxygen vacancy and Pd Lewis acid sites enabling superior cooperative photoredox synthesis. Angew. Chem. Int. Ed. n/a 63, e202412707. doi:10.1002/anie.202412707
Isaacs, M. A., Drivas, C., Lee, R., Palgrave, R., Parlett, C. M. A., and Morgan, D. J. (2023). XPS surface analysis of ceria-based materials: experimental methods and considerations. Appl. Surf. Sci. Adv. 18, 100469. doi:10.1016/j.apsadv.2023.100469
Khanna, H. S., Eddy, N. A., Dang, Y., Bhosale, T. S., Shubhashish, S., Suib, S. L., et al. (2020). Nanoporous Co/Mn-mixed metal oxides templated via polysulfones for amine oxidation. ACS Appl. Nano Mat. 3, 11923–11932. doi:10.1021/acsanm.0c02492
Kumari, G., Dhillon, S., Rani, P., Chahal, M., Aneja, D. K., and Kinger, M. (2024). Development in the synthesis of bioactive thiazole-based heterocyclic hybrids utilizing phenacyl bromide. ACS Omega 9, 18709–18746. doi:10.1021/acsomega.3c10299
Lewicka, K., Szymanek, I., Rogacz, D., Wrzalik, M., Łagiewka, J., Nowik-Zając, A., et al. (2024). Current trends of polymer materials’ application in agriculture. Sustainability 16, 8439. doi:10.3390/su16198439
Liang, Q., Wu, X., Weng, D., and Lu, Z. (2008). Selective oxidation of soot over Cu doped ceria/ceria–zirconia catalysts. Catal. Commun. 9, 202–206. doi:10.1016/j.catcom.2007.06.007
Lu, G., Schwiderowski, P., Shen, Z., Li, X., Schulwitz, J., Peng, B., et al. (2024). Macroporous carbon-supported Fe-based catalysts for the solvent-free oxidative coupling of benzylamine. Chem. Mat. 36, 2049–2060. doi:10.1021/acs.chemmater.3c03099
Mahurkar, N. D., Gawhale, N. D., Lokhande, M. N., Uke, S. J., and Kodape, M. M. (2023). Benzimidazole: a versatile scaffold for drug discovery and beyond – a comprehensive review of synthetic approaches and recent advancements in medicinal chemistry. Results Chem. 6, 101139. doi:10.1016/j.rechem.2023.101139
Mallesham, B., Rangaswamy, A., Rao, B. G., Rao, T. V., and Reddy, B. M. (2020). Solvent-free production of glycerol carbonate from bioglycerol with urea over nanostructured promoted SnO2 catalysts. Catal. Lett. 150, 3626–3641. doi:10.1007/s10562-020-03241-9
Mallesham, B., Sudarsanam, P., Reddy, B. V. S., and Reddy, B. M. (2016). Development of cerium promoted copper-magnesium catalysts for biomass valorization: selective hydrogenolysis of bioglycerol. Appl. Catal. B Environ. 181, 47–57. doi:10.1016/j.apcatb.2015.07.037
Marakatti, V. S., Sarma, S. C., Joseph, B., Banerjee, D., and Peter, S. C. (2017). Synthetically tuned atomic ordering in PdCu nanoparticles with enhanced catalytic activity toward solvent-free benzylamine oxidation. ACS Appl. Mat. Interfaces 9, 3602–3615. doi:10.1021/acsami.6b12253
Matsuo, J., Kawana, A., Fukuda, Y., and Mukaiyama, T. (2001). Oxidative deamination of various primary amines to the corresponding carbonyl compounds by using N-tert-Butylphenylsulfinimidoyl chloride. Chem. Lett. 2001, 712–713. doi:10.1246/cl.2001.712
Mohan, V., Venkateshwarlu, V., Pramod, C. V., Raju, B. D., and Rao, K. S. R. (2014). Vapour phase hydrocyclisation of levulinic acid to γ-valerolactone over supported Ni catalysts. Catal. Sci. Technol. 4, 1253–1259. doi:10.1039/C3CY01072D
Nagu, A., Raju, A., Vennela, A., Varkolu, M., Raju, B. D., and Rao, K. S. R. (2023). Single-step conversion of levulinic acid to valeric acid over bimetallic Ni-Co/H-ZSM-5 catalysts. ChemistrySelect 8, e202300509. doi:10.1002/slct.202300509
Naresh, G., Kant, R., and Narender, T. (2014). Molecular Iodine Promoted Divergent Synthesis of Benzimidazoles, Benzothiazoles, and 2-Benzyl-3-phenyl-3,4-dihydro-2H-benzo[e] [1,2,4]thiadiazines. J. Org. Chem. 79, 3821–3829. doi:10.1021/jo5000797
Nguyen, K. M. H., and Largeron, M. (2016). Catalytic oxidative coupling of primary amines under air: a flexible route to benzimidazole derivatives. Eur. J. Org. Chem. 2016, 1025–1032. doi:10.1002/ejoc.201501520
Nicolaou, K. C., Mathison, C. J. N., and Montagnon, T. (2003). New reactions of IBX: oxidation of nitrogen- and sulfur-containing substrates to afford useful synthetic intermediates. Angew. Chem. Int. Ed. 42, 4077–4082. doi:10.1002/anie.200352076
Nicolaou, K. C., Mathison, C. J. N., and Montagnon, T. (2004). o-Iodoxybenzoic acid (IBX) as a viable reagent in the manipulation of nitrogen- and sulfur-containing substrates: scope, generality, and mechanism of IBX-mediated amine oxidations and dithiane deprotections. J. Am. Chem. Soc. 126, 5192–5201. doi:10.1021/ja0400382
Oh, S. H., Kim, H.-K., Park, S.-Y., Kim, Y.-C., Kwon, D.-H., Yang, S., et al. (2024). Investigating the nano-scale structure and composition dynamics during the phase transition towards complete separation of CeO2–ZrO2 solid solutions. J. Mat. Chem. A 12, 21148–21155. doi:10.1039/D4TA02923B
Oiye, É. N., Ribeiro, M. F. M., Katayama, J. M. T., Tadini, M. C., Balbino, M. A., Eleotério, I. C., et al. (2019). Electrochemical sensors containing schiff bases and their transition metal complexes to detect analytes of forensic, pharmaceutical and environmental interest. A review. Crit. Rev. Anal. Chem. 49, 488–509. doi:10.1080/10408347.2018.1561242
Orr, S. A., Andrews, P. C., and Blair, V. L. (2021). Main group metal-mediated transformations of imines. Chem. – A Eur. J. 27, 2569–2588. doi:10.1002/chem.202003108
Potdar, H. S., Jeong, D.-W., Kim, K.-S., and Roh, H.-S. (2011). Synthesis of highly active nano-sized Pt/CeO2 catalyst via a cerium hydroxy carbonate precursor for water gas shift reaction. Catal. Lett. 141, 1268–1274. doi:10.1007/s10562-011-0656-5
Purohit, M., Kalla, S., and Jangir, R. (2023). A comprehensive review on Cu-catalysed aerobic oxidation of amines to imines. ChemistrySelect 8, e202300386. doi:10.1002/slct.202300386
Qian, X., Ren, Q., Wu, X., Sun, J., Wu, H., and Lei, J. (2018). Enhanced water stability in Zn-doped zeolitic imidazolate framework-67 (ZIF-67) for CO2 capture applications. ChemistrySelect 3, 657–661. doi:10.1002/slct.201702114
Qiu, G., Wang, R., Han, F., Tao, X., Xiao, Y., and Li, B. (2019). One-step synthesized Au–Bi2WO6 hybrid nanostructures: synergistic effects of Au nanoparticles and oxygen vacancies for promoting selective oxidation under visible light. Ind. Eng. Chem. Res. 58, 17389–17398. doi:10.1021/acs.iecr.9b03371
Rao, B. G., Sudarsanam, P., Mallesham, B., and Reddy, B. M. (2016). Highly efficient continuous-flow oxidative coupling of amines using promising nanoscale CeO 2 –M/SiO 2 (M = MoO 3 and WO 3) solid acid catalysts. RSC Adv. 6, 95252–95262. doi:10.1039/C6RA21218B
Rao, K. N., Venkataswamy, P., and Reddy, B. M. (2011). Structural characterization and catalytic evaluation of supported copper-ceria catalysts for soot oxidation. Ind. Eng. Chem. Res. 50, 11960–11969. doi:10.1021/ie201474p
Ravula, M., Dosarapu, V., Bandalla, S., Mavurapu, S., Varkolu, M., Rajeevan, A., et al. (2024). Development of molybdenum oxide promoted CeO 2 −SiO 2 mixed-oxide catalyst for efficient catalytic oxidation of benzylamine to N-benzylidenebenzylamine. ChemistrySelect 9, e202304534. doi:10.1002/slct.202304534
Reddy, B. M., Khan, A., Lakshmanan, P., Aouine, M., Loridant, S., and Volta, J.-C. (2005). Structural characterization of nanosized CeO2−SiO2, CeO2−TiO2, and CeO2−ZrO2 catalysts by XRD, Raman, and HREM techniques. J. Phys. Chem. B 109, 3355–3363. doi:10.1021/jp045193h
Sadhasivam, V., Balasaravanan, R., Chithiraikumar, C., and Siva, A. (2017). Incorporating Pd(OAc)2 on imine functionalized microporous covalent organic frameworks: a stable and efficient heterogeneous catalyst for suzuki-miyaura coupling in aqueous medium. ChemistrySelect 2, 1063–1070. doi:10.1002/slct.201601440
Scherzer, M., Girgsdies, F., Stotz, E., Willinger, M.-G., Frei, E., Schlögl, R., et al. (2019). Electrochemical surface oxidation of copper studied by in situ grazing incidence X-ray diffraction. J. Phys. Chem. C 123, 13253–13262. doi:10.1021/acs.jpcc.9b00282
Shi, J., Zhang, J., Liang, T., Tan, D., Tan, X., Wan, Q., et al. (2019). Bipyridyl-containing cadmium–organic frameworks for efficient photocatalytic oxidation of benzylamine. ACS Appl. Mat. Interfaces 11, 30953–30958. doi:10.1021/acsami.9b09879
Slanina, T., and Oberschmid, T. (2018). Rhodamine 6G radical: a spectro (fluoro) electrochemical and transient spectroscopic study. ChemCatChem 10, 4182–4190. doi:10.1002/cctc.201800971
Sudarsanam, P., Mallesham, B., Reddy, P. S., Großmann, D., Grünert, W., and Reddy, B. M. (2014). Nano-Au/CeO2 catalysts for CO oxidation: influence of dopants (Fe, La and Zr) on the physicochemical properties and catalytic activity. Appl. Catal. B Environ. 144, 900–908. doi:10.1016/j.apcatb.2013.08.035
Sun, Y., and Sermon, P. A. (1996). Surface reactivity and bulk properties of ZrO2. Part 2.—importance of homogeneity in the stabilisation of high surface area CeO2–ZrO2 aerogels. J. Mat. Chem. 6, 1025–1029. doi:10.1039/JM9960601025
Tateyama, K., Wada, K., Miura, H., Hosokawa, S., Abe, R., and Inoue, M. (2016). Dehydrogenative synthesis of benzimidazoles under mild conditions with supported iridium catalysts. Catal. Sci. Technol. 6, 1677–1684. doi:10.1039/C5CY01601K
Tian, B., Wu, H., Zhang, Y., Chen, C., Abdalla, K. K., Sendeku, M. G., et al. (2024a). Cu-induced interfacial water engineering of SnO 2 for durable and highly selective CO 2 electroreduction. ACS Catal. 14, 10904–10912. doi:10.1021/acscatal.4c01670
Tian, B., Wu, H., Zhang, Y., Chen, C., Abdalla, K. K., Sendeku, M. G., et al. (2024b). Cu-induced interfacial water engineering of SnO2 for durable and highly selective CO2 electroreduction. ACS Catal. 14, 10904–10912. doi:10.1021/acscatal.4c01670
Velu, S., Suzuki, K., Vijayaraj, M., Barman, S., and Gopinath, C. S. (2005). In situ XPS investigations of Cu1−xNixZnAl-mixed metal oxide catalysts used in the oxidative steam reforming of bio-ethanol. Appl. Catal. B Environ. 55, 287–299. doi:10.1016/j.apcatb.2004.09.007
Wang, X., Wang, X., and Guo, Z. (2015). Functionalization of platinum complexes for biomedical applications. Acc. Chem. Res. 48, 2622–2631. doi:10.1021/acs.accounts.5b00203
Wang, Y., Chan, Y. S., Zhang, R., and Yan, B. (2024a). Insights into the contribution of oxygen vacancies on CO2 activation for dry reforming of methane over ceria-based solid solutions. Chem. Eng. J. 481, 148360. doi:10.1016/j.cej.2023.148360
Wang, Y., Guo, Z., Li, Y., Black, L. E., MacDonald, D., Bao, S., et al. (2024b). Oxygen vacancy modulation of nanolayer TiOx to improve hole-selective passivating contacts for crystalline silicon solar cells. J. Mat. Chem. A 12, 29833–29842. doi:10.1039/D4TA05538A
Wu, C., Bu, J., Wang, W., Shen, H., Cao, Y., and Zhang, H. (2022). Imine synthesis by benzylamine self-coupling catalyzed by cerium-doped MnO2 under mild conditions. Ind. Eng. Chem. Res. 61, 5442–5452. doi:10.1021/acs.iecr.2c00311
Yamamoto, Y., Yamakawa, C., Nishimura, R., Dong, C.-P., Kodama, S., Nomoto, A., et al. (2022). Metal-free synthesis of 2-substituted quinazolines via green oxidation of o-aminobenzylamines: practical construction of N-containing heterocycles based on a salicylic acid-catalyzed oxidation system. Front. Chem. 9, 822841. doi:10.3389/fchem.2021.822841
Yang, M., Shen, G., Wang, Q., Deng, K., Liu, M., Chen, Y., et al. (2021). Roles of oxygen vacancies of CeO2 and Mn-doped CeO2 with the same morphology in benzene catalytic oxidation. Molecules 26, 6363. doi:10.3390/molecules26216363
Yashima, M., Ishizawa, N., and Yoshimura, M. (1993). High-temperature X-ray study of the cubic–tetragonal diffusionless phase transition in the ZrO2─ErO1.5System: II, temperature dependences of oxygen ion displacement and lattice parameter of compositionally homogeneous 12 mol% ErO1.5─ZrO2. J. Am. Ceram. Soc. 76, 649–656. doi:10.1111/j.1151-2916.1993.tb03655.x
Yuan, A., Lei, H., Wang, Z., and Dong, X. (2020). Improved photocatalytic performance for selective oxidation of amines to imines on graphitic carbon nitride/bismuth tungstate heterojunctions. J. Colloid Interface Sci. 560, 40–49. doi:10.1016/j.jcis.2019.10.060
Zeng, C., Tsui, L. S., Lam, F. L. Y., Wu, T., and Yip, A. C. K. (2024). Revisiting the crucial roles of oxygen vacancies in photo/electro-catalytic degradation of aqueous organic pollutants. Appl. Catal. O Open 190, 206930. doi:10.1016/j.apcato.2024.206930
Zeng, Y., Lyu, P., Cai, Y., Gao, F., Zhuo, O., Wu, Q., et al. (2021). Hierarchical carbon nanocages as efficient catalysts for oxidative coupling of benzylamine to N -benzylidene benzylamine. Acta Chim. Sin. 79, 539. doi:10.6023/a20110527
Zhang, Q., Xu, L., Ning, P., Gu, J., and Guan, Q. (2014). Surface characterization studies of CuO-CeO2-ZrO2 catalysts for selective catalytic reduction of NO with NH3. Appl. Surf. Sci. 317, 955–961. doi:10.1016/j.apsusc.2014.09.017
Zhang, S., Zhao, C., Liu, Y., Li, W., Wang, J., Wang, G., et al. (2019). Cu doping in CeO2 to form multiple oxygen vacancies for dramatically enhanced ambient N2 reduction performance. Chem. Commun. 55, 2952–2955. doi:10.1039/C9CC00123A
Zhang, X., Shi, H., and Xu, B.-Q. (2005). Catalysis by gold: isolated surface Au3+ ions are active sites for selective hydrogenation of 1,3-butadiene over Au/ZrO2 catalysts. Angew. Chem. Int. Ed. 44, 7132–7135. doi:10.1002/anie.200502101
Zhao, S., Liu, C., Guo, Y., Xiao, J.-C., and Chen, Q.-Y. (2014). Oxidative coupling of benzylamines to imines by molecular oxygen catalyzed by cobalt(II) β-Tetrakis(trifluoromethyl)-meso-tetraphenylporphyrin. J. Org. Chem. 79, 8926–8931. doi:10.1021/jo5017212
Keywords: Cu-supported catalysts, doped-CeO2, benzylamine oxidation, oxygen vacancies, benzimidazole synthesis, acidic sites and redox behavior
Citation: Sakinala S, Kothoori NPS, Jeedi S, Varkolu M and Baithy M (2025) Boosting catalytic efficiency of nanostructured CuO-supported doped-CeO2 in oxidative coupling of benzyl amines to N-benzylidenebenzyl amines and benzimidazoles: impact of acidic and defect sites. Front. Nanotechnol. 6:1513783. doi: 10.3389/fnano.2024.1513783
Received: 19 October 2024; Accepted: 18 December 2024;
Published: 15 January 2025.
Edited by:
Satyabrata Mohapatra, Guru Gobind Singh Indraprastha University, IndiaReviewed by:
Biswarup Chakraborty, Indian Institute of Technology Delhi, IndiaAnupam Singha Roy, Dev Bhoomi Uttarakhand University, India
Copyright © 2025 Sakinala, Kothoori, Jeedi, Varkolu and Baithy. This is an open-access article distributed under the terms of the Creative Commons Attribution License (CC BY). The use, distribution or reproduction in other forums is permitted, provided the original author(s) and the copyright owner(s) are credited and that the original publication in this journal is cited, in accordance with accepted academic practice. No use, distribution or reproduction is permitted which does not comply with these terms.
*Correspondence: Mallesham Baithy, bWJhaXRoeUBnaXRhbS5lZHU=, YmFpdGh5Lm1AZ21haWwuY29t