- 1 Développement, Evolution et Plasticité du Système Nerveux, Centre National de la Recherche Scientifique, Gif-sur-Yvette, France
- 2 Laboratoire Physiologie de l’Insecte: Signalisation et Communication, Institut National de la Recherche Agronomique, Unité Mixte de Recherche 17, Versailles, France
- 3 Laboratoire Evolution, Génomes et Spéciation, Unité Propre de Recherche 9034, Centre National de la Recherche Scientifique, Université Paris-Sud 11 and Institut de Recherche pour le Développement, Gif-sur-Yvette, France
Memory performance depends not only on effective learning and storage of information, but also on its efficient retrieval. In Drosophila, aversive olfactory conditioning generates qualitatively different forms of memory depending on the number and spacing of conditioning trials. However, it is not known how these differences are reflected at the retrieval level, in the behavior of individual flies during testing. We analyzed conditioned behaviors after one conditioning trial and after massed and spaced repeated trials. The single conditioning produces an early memory that was tested at 1.5 h. Tested at 24 h after training, the spaced and the massed protocols generate two different forms of consolidated memory, dependent, or independent of de novo protein-synthesis. We found clearly distinct patterns of locomotor activity in flies trained with either spaced or massed conditioning protocols. Spaced-trained flies exhibited immediate and dynamic choices between punished and unpunished odors during the test, whereas massed-trained flies made a delayed choice and showed earlier disappearance of the conditioned response. Flies trained with single and spaced trials responded to the punished odor by decreasing their resting time, but not massed-trained flies. These findings demonstrate that genetically and pharmacologically distinct forms of memory drive characteristically different forms of locomotor behavior during retrieval, and they may shed light on our previous observation that memory retrieval in massed-trained flies is socially facilitated. Social interactions would enhance exploratory activity, and then reduce the latency of their conditioned choice and delay its extinction.
Introduction
Investigations of memory aim to understand how individuals adapt decision-making and other forms of behavior to environmental circumstances, as a function of their previous experience. Memory processing involves three steps: learning and acquisition of memory, memory storage, and memory retrieval. The respective contributions of these steps to memory performance are hard to separate, even in controlled laboratory studies, because measurement of memory performance is accessible mainly through expression of the conditioned behavior during retrieval. Consequently, an indispensable approach for studying memory is to precisely describe the conditioned response.
In Drosophila, olfactory aversive conditioning has been thoroughly studied for more than 30 years (Berry et al., 2008), with several operant or Pavlovian versions of the paradigm (Quinn et al., 1974; Tully and Quinn, 1985; Pascual and Preat, 2001; Mery and Kawecki, 2005; Claridge-Chang et al., 2009). The most widely used Pavlovian discriminatory set up involves the presentation of two odors: one associated with electric shocks and the other with no shocks (Tully and Quinn, 1985; Pascual and Preat, 2001). Memory performance is measured in groups of flies using a T-maze, in which flies have a set time to choose between both odors. At the end of the test, a mean performance index is calculated by counting the number of flies that made the correct and the incorrect choice. This paradigm provides a relatively easy and rapid assessment of learning and memory capacities, allowing screening, and characterization of mutants (for a review, see Waddell and Quinn, 2001). It can induce different forms of memory: labile short-term and middle-term memory (STM and MTM) after single or repeated learning trials, and two forms of consolidated memory that depend on the spacing of repeated learning trials (Tully et al., 1994; Isabel et al., 2004). Long-term memory (LTM), formed after spaced trials, requires de novo protein-synthesis, in contrast to anesthesia-resistant memory (ARM), which is formed after single or massed trials (Tully et al., 1994). It is still not clear if these two forms of memory coexist after spaced conditioning (Tully et al., 1994) or if only LTM is expressed after spaced conditioning (Isabel et al., 2004). Importantly, the olfactory conditioned response has been estimated mainly by the presence of flies in the punished or unpunished odor at the end of the test. However, the response has never been precisely characterized, either for individuals or groups of flies, by the different behavioral events that can influence the position of flies during the test.
The vast majority of studies using this protocol measure the responses of groups of flies during memory retrieval. Consequently, our understanding of memory phase dynamics in this system does not take into account potential differences between collective group behavior and decision-making by individual flies. However, a new version of the operant olfactory aversive conditioning paradigm has recently been set up to test shock-trained odorant avoidance of single flies (Claridge-Chang et al., 2009). Furthermore, using the classical paradigm we recently demonstrated that for the two long-lasting memories, group performances are influenced by interactions between flies during the test, with specific effects on memory performance (Chabaud et al., 2009). In contrast to LTM, ARM retrieval is impaired when tested individually but facilitated when tested in a group of conditioned flies. We show that the social interactions involved in the facilitation of retrieval are specific to conditioned flies, suggesting that they may use stress-like signals that enhance their attention or motivation to respond to the punished odor. The ARM conditioned flies tested individually might also be in a state of perceived social isolation, which is known to contribute to poorer overall cognitive performance, as reviewed elsewhere (Cacioppo and Hawkley, 2009). The social context of the test can be seen as one of a number of physiological states known to modulate memory performance, such as the effect of sleep deprivation upon aversive learning (Seugnet et al., 2008) or the effect of food motivation state upon appetitive learning and memory retrieval in Drosophila (Colomb et al., 2009; Krashes et al., 2009), and the gustatory context of the test of olfactory memory for Drosophila larvae (Gerber and Hendel, 2006).
To understand which component of the decision-making process is deficient in single flies tested for ARM, we decided to characterize, in detail, the conditioned responses of individual flies during the test. We compared the conditioned responses of individuals trained for ARM (24 h after massed conditioning) with those of individuals trained for early memory (EM 1.5 h after a single conditioning) and for LTM (24 h after spaced conditioning). For each memory type, we measured several behavioral parameters potentially related to memory retrieval, such as the time spent in each odor, walking, or resting states, and levels of exploratory activity between and within odors. In doing so, we analyzed the conditioned response over time during the test.
Our data reveal the behavioral features that sustain the difference in memory performance between massed- and spaced-trained flies. In particular we show that, when tested individually, spaced-trained flies make dynamic choices, with repeated avoidance of the punished odor from the beginning of the test. In contrast, conditioned avoidance in massed-trained flies is both delayed and less persistent than in spaced-trained flies, suggesting memory extinction occurs during the test. The behavior of individual massed-trained flies suggest that the social facilitation of ARM retrieval acts by decreasing the latency and/or retarding the extinction of the conditioned response during testing within a group.
Materials And Methods
Biological Material
The subjects used were adult Drosophila melanogaster from the wild-type strain Canton-Special. Flies were raised at 18°C on standard Drosophila medium, then trained and tested under red light at 25°C and at 80% relative humidity.
Olfactory Classical Conditioning
Discriminatory olfactory aversive conditioning was performed on samples of 30–40 flies between 2 and 3 days old. One conditioning trial consists of pairing a first odor with electric shocks (twelve 1.2 s pulses of 60 V over 1 min), followed by presentation of the second odor without electric shocks. Odors are referred to as punished and unpunished. Each odor presentation is always followed by 45 s of airflow without odor. 4-methylcyclohexanol and 3-octanol were used alternately as punished odor and unpunished odor, for every other set of flies (Tully and Quinn, 1985; Pascual and Preat, 2001). Flies trained with this paired procedure were compared with control flies trained with an unpaired protocol, in which electric shocks are delivered 2 min before the first odor. With this schedule, flies are not expected to learn any backward association between electric shocks and odor (Tanimoto et al., 2004).
Early memory, which we measured 1.5 h after a single conditioning trial, corresponds both to MTM and early ARM (Tully et al., 1990). Flies trained with the single conditioning protocol were compared to control flies that were trained with one trial of the unpaired procedure.
The massed conditioning protocol consists of five consecutive trials, without inter-trial intervals. ARM formed after massed conditioning was measured 24 h after training and was compared with control performance obtained with five repetitions of the unpaired procedure.
The spaced conditioning consists of five consecutive trials, with 15 min inter-trial intervals. It is still not clear if memory measured 24 h after training is composite, with the induction of both ARM and LTM (Tully et al., 1994), or if only LTM is present (Isabel et al., 2004). We showed, however, that if memory is composite, LTM expression appears to be dominant during retrieval (Chabaud et al., 2009). To facilitate the reading of this paper, we thus used the term LTM for the memory measured 24 h after spaced conditioning. Flies trained with the spaced conditioning protocol were compared to control flies that were trained with similar repetitions of the unpaired procedure.
Memory Tests
Tests were performed in a T-Maze set on a homogenous-lighting table (Waldmann, 50 Hz) covered by three transparent red sheets, which allowed diffusion of a red light that illuminated the T-Maze sufficiently to observe flies with the naked eye. For individual memory assays, single flies were collected without anesthesia from trained groups, and introduced alone in a T-maze apparatus (Tully and Quinn, 1985) to choose between octanol or methylcyclohexanol odor over a period of 3 min. Six flies per group (three males and three females) were tested individually. The remaining flies of a group (about 30) were then tested together in the T-maze for 3 min to provide a control, and to verify the positive effect of the group on ARM scores (Chabaud et al., 2009).
Data Recording
Data were recorded with The Observer® software (Noldus). We recorded the position of flies in the different parts of the T-maze, during the 3-min memory test, i.e., the arm carrying the unpunished odor (correct choice), the central part where flies were introduced, and the arm carrying the previously punished odor (incorrect choice). We also noted their position within each arm, dividing the arms into three zones (Figure 1). Time spent walking or resting was also recorded.
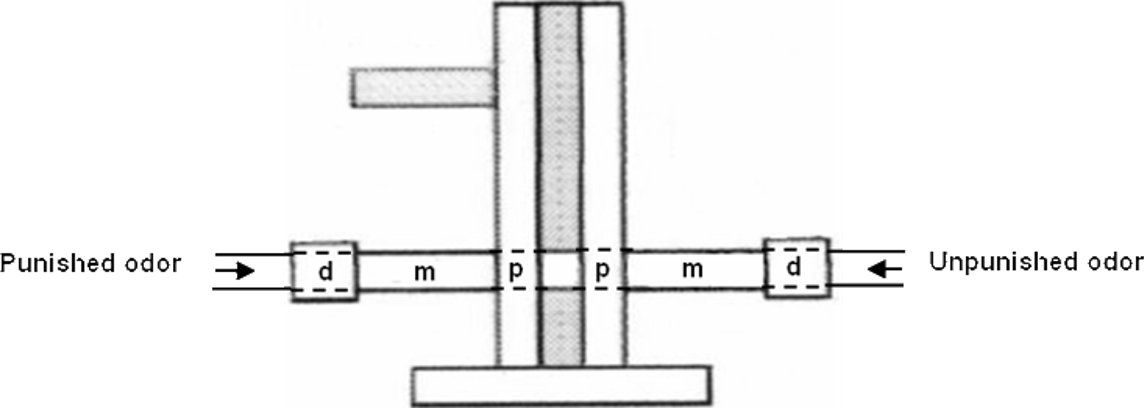
Figure 1. Scheme of the virtual division in three zones on each of the two arms of the T-maze. The proximal zone (p), measuring 1.8 cm long, is located at the entrance of the arm and near the central part of the T-maze, where flies are introduced at the beginning of the test and where both odors are extracted. The median part of the arm (m) measures 5.5 cm long. The distal part of the arm (d), measuring 2.2 cm, is located at the end of the arm, fitted inside a small plexiglas connector that insures the junction with the airflow tubing. The internal diameter is constant and equal to 1.5 cm.
Individual memory performance was estimated in two ways. First we calculated a “time score” for each fly, equal to the time spent by the fly in the unpunished odor minus the time spent in the punished odor, divided by the time spent in both odors during the 3-min test. The mean time score and its SE were then calculated for each conditioning protocol. Secondly, the evolution of odor choice during the test was analyzed using the position of the fly at different time points (at the first choice, 30 s, 1, 2, and 3 min). At these different time points, flies were either in the punished odor, in the unpunished odor, or in the central part of the T-maze. We calculated a global “position score” at each time point of the test, on all individual data of the different treatments, equal to the sum of flies in the unpunished odor minus the sum of flies in the punished odor, divided by the sum of both numbers.
To verify memory performance in groups and the positive effect of the group on ARM scores, we had to compare the memory score of individuals with that of the group from which they came. When flies are tested in groups, their memory is estimated using the position score, defined above. To estimate individual memory with a memory score similar in essence to that of the group score, we pooled individual positions (12 flies per pool, 6 conditioned to 3-octanol and 6 to 4-methylcyclohexanol). A position score was calculated as for the group score, for each pool of 12 flies. Mean individual position score of a given sample was the mean of the scores of the pools. In all cases, memory scores were multiplied by 100 and thus evaluated on a scale from −100 to 100. For the unpaired control procedure, “memory” scores were calculated as for the paired procedure, with the first odor, delivered 2 min after electric shocks, being considered as the “punished” odor.
Statistical Analyses
The chi-square test (χ2) was used to analyze (i) the distributions of duration scores (Figure 3B), (ii) the position scores (Figures 4 and 5), and (iii) the distribution of flies as a function of the number of odor changes (Figure 6). The Haber correction was applied for 1 df chi-square tests (Zar, 1999).
After normalization (arcsine transformation for proportions), and after checking assumptions of normality (Shapiro–Wilk test) and homogeneity of variances (Levene test), mean scores of conditioned group vs control group, and conditioned group vs conditioned individuals (Figure 2) were compared with a two-tailed Student’s t-test at the Dunn–Sidak corrected significance level α = 0.025 for the use of data in two comparisons (Zar, 1999). For all the other data, the assumption of normality of data was not satisfied even after normalization (arcsine transformation for proportions, logarithmic and square root transformations for latencies, and number of odor changes). Therefore, we used non-parametric tests. The median test (Z) was used to characterize the symmetry of the distribution of duration scores around the median for control flies (Figure 3B). The normal approximation to the Mann–Whitney test corrected for continuity (Z) was used to compare two independent samples (conditioned vs control groups) when minimum and maximum sample sizes exceeded 20 and 40, respectively (Zar, 1999; Tables 1 and 3; Figure 7). The Wilcoxon sign and rank test (V) was used to compare dependent data (Table 2). Correlations between the time memory score and the proportion of time spent resting or spent in the proximal zone of the punished odor, were analyzed using the Spearman rank correlation. Results of all statistical analyses refer to the significance level α = 0.05, and were calculated with Statistica 9 or Xlstat softwares.
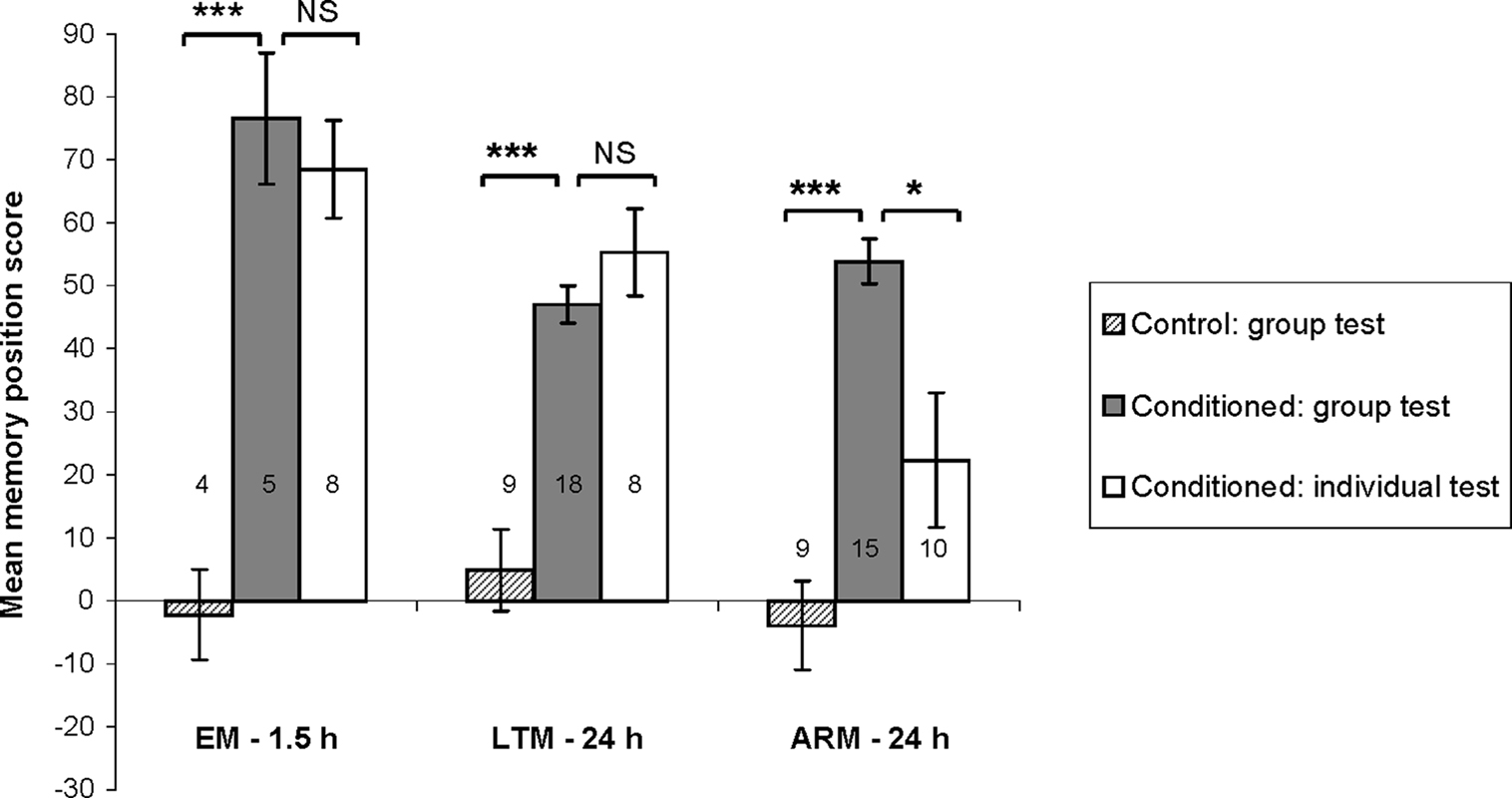
Figure 2. Confirming the social effect on memory performance. Bars represent mean values of memory position score ± SE. For each conditioning procedure (EM, early memory; LTM, long-term memory; ARM, anesthesia-resistant memory), position scores are compared between control and conditioned flies tested in group, and between conditioned flies tested in group or individually, using Student’s t-test. P-values are compared to α′ = 0.025. ***P < 0.001; *P < 0.05; NS: P ≥ 0.05. Numbers of groups tested and pools of flies tested individually are reported on the graph.
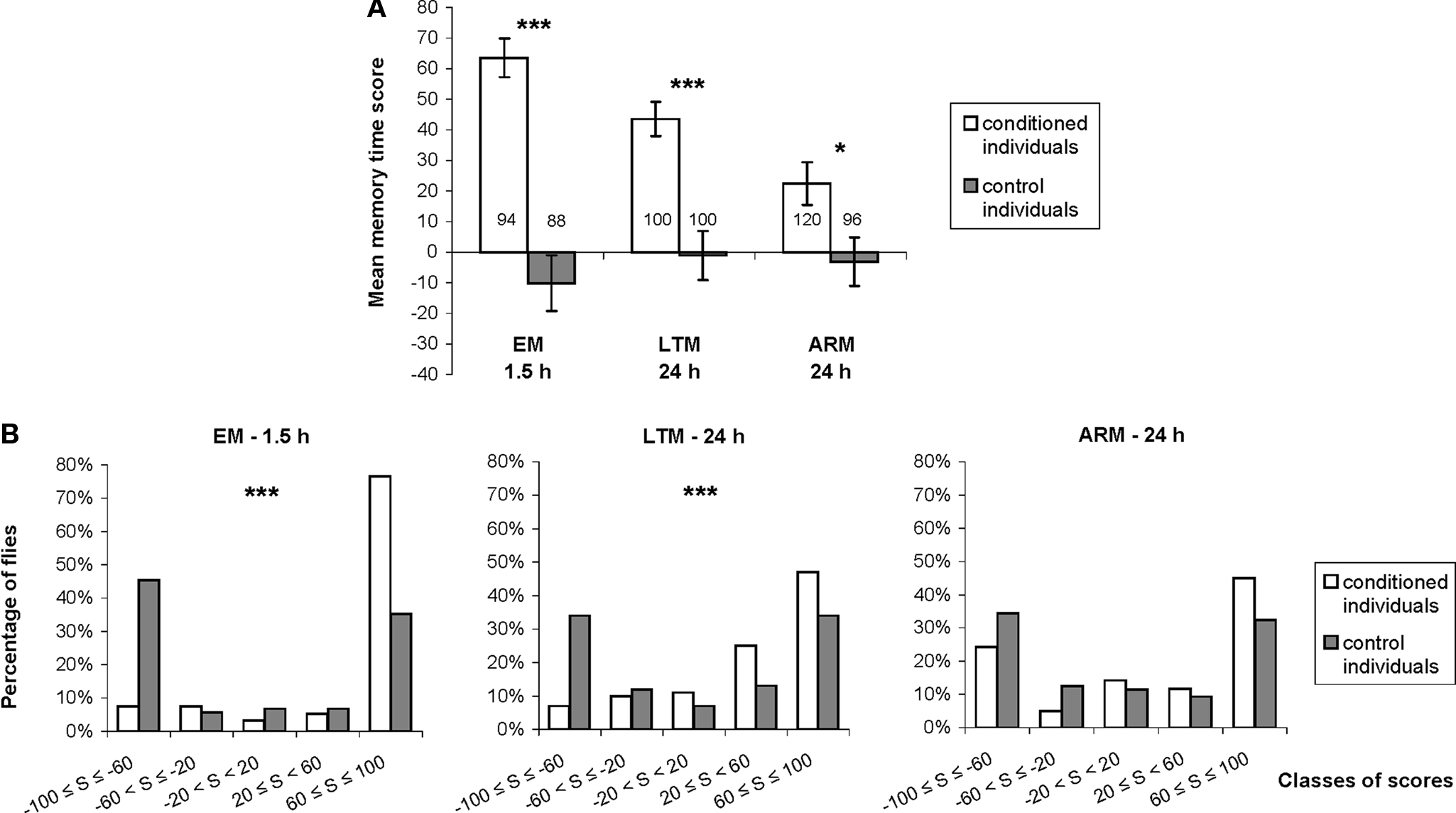
Figure 3. Distribution of individual memory scores. (A) We compared mean individual time scores between flies trained with associative (conditioned individuals) and unpaired (control individuals) protocols, using single cycle conditioning (EM), spaced conditioning (LTM), and massed conditioning (ARM). See Section “Materials and Methods” for the calculation of time score. Data represent the mean ± SE of the mean. Numbers of flies tested are reported on the graph. Mann–Whitney test: stars indicate significant differences between conditioned and control individuals, at P-values inferior to 0.05 (*), 0.01 (**), or 0.001 (***). (B) Graphs showing the distribution of individual time scores in five classes ranging from −100 to 100, represented by the percentage of flies in each class of scores for conditioned and control flies, trained and tested for EM, LTM, and ARM. Chi-square: stars indicate significant differences between scores distribution of conditioned and control individuals, at P-values inferior to 0.05 (*), 0.01 (**), or 0.001 (***).
Results
Confirming the Social Effect on Memory Performance
We first verified that, for the three conditioning procedures, conditioned groups show higher scores than their specific unpaired control groups following 3 min tests (Figure 2; t-test : t ≥ 6.38, P < 0.0001. We also confirmed the existence of social facilitation of retrieval when ARM-trained flies were tested in groups (Chabaud et al., 2009), with a higher ARM score when tested in groups than when tested as individuals (t = 2.91, P < 0.008). No group effect was observed in EM and LTM performance (t ≤ 1.35, P ≥ 0.19).
Distribution of Individual Memory Scores
Individual memory was estimated using the time score. For this parameter, we first checked that each associative training procedure induced a significant individual avoidance of the punished odor compared to corresponding control procedures (Figure 3A; Mann–Whitney test, Z ≥ 2.51, P ≤ 0.012), with similar memory performances for males and females (data not shown; EM♂ = 65 ± 9 and EM♀ = 62 ± 9, LTM♂ = 39 ± 8 and LTM♀ = 48 ± 8, ARM♂ = 20 ± 10 and ARM♀ = 25 ± 10; Mann–Whitney test, Z ≤ 1.17, P ≥ 0.24).
For all unpaired control procedures, time scores were close to 0, indicating that unpaired training induces neither avoidance nor preference for any odors (Figure 3A). Control individual scores were, however, not normally distributed and showed bipolar and symmetrical distributions around the means (Figure 3B; median test: z ≤ 1.96, P ≥ 0.23, 1 df). Thus, control individuals chose one of the two odors randomly during the test and tended to stay in this odor for most of the test period.
Following single or spaced conditioning protocols, time score distributions were significantly different from those of the corresponding controls (Figure 3B; χ2 ≥ 24.73, P ≤ 3.10−5, 4 df). The majority of EM-trained flies showed high scores, higher, or equal to 60. As expected, the LTM-trained flies performed more poorly, as shown by a significantly higher proportion of flies in the immediately inferior class (20–60) compared to EM-trained flies (χ2 = 12.89, P < 10−4, 1 df). In ARM-trained flies, low performance did not correspond to an increased in intermediate scores, but resulted from a subpopulation of flies with very low scores (inferior to −60); these flies were significantly more numerous than in EM and LTM-trained flies (χ2 = 18.03, P < 10−3, 2 df). The distribution of ARM individual scores was therefore more bipolar, and not significantly different from the distribution of ARM-control individuals (Figure 3B; χ2 = 6.57, P = 0.16, 4 df). This suggests that a fraction of individually tested, ARM-trained flies effectively make a random odor choice.
Evolution of Position Memory Scores Along the Test
To circumvent the cumulative nature of the time score, the evolution of the conditioned response was analyzed using position data. Individuals conditioned with the single-trial (EM) or the spaced-trial (LTM) protocols showed a significantly higher score from the beginning of the test compared to that of control individuals (Figures 4A,B; P < 0.005, 1 df). In contrast, ARM-trained individuals made their first choice at random, like control individuals (
P > 0.80, 1 df), and from 30 s they gained a significantly higher score than controls (
P < 0.05, 1 df). This demonstrates a delayed conditioned response compared to EM- or LTM-trained flies. Thus, the bipolar distribution of duration scores for control and ARM-trained flies (Figure 3B) might be linked to their random first choice. We therefore analyzed the effect of the first choice on the evolution of position scores during the test.
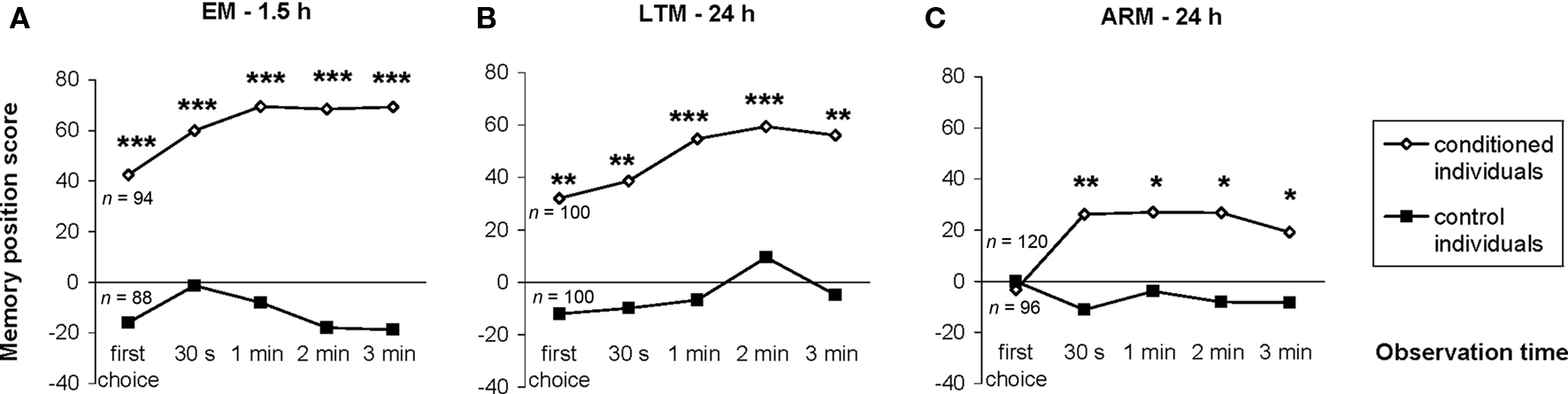
Figure 4. Evolution of individual memory scores during the test period. The memory position score of a given sample of individually tested flies was the difference between the numbers of flies in the punished and unpunished odors divided by the total number of flies. It was calculated at each observation time until the end of the 3-min test, for conditioned and control flies trained and tested for EM (A), LTM (B) and ARM (C). n corresponds to the number of flies tested. Chi-square test: stars indicate significant differences between conditioned and control individuals, at P-values inferior to 0.05 (*), 0.01 (**), or 0.001 (***). For the latency of the first choice, see Section “Latency of the First Choice does not Depend on the Odor Status.”
A Significant Effect of the First Choice on the Evolution of Individual ARM Scores
For all control procedures, the influence of the first choice disappeared progressively during the test; scores were not significantly different by the 1-min point of test (control for ARM and EM; Figures 5B,F; P > 0.05, 1 df) or 2 min (control for LTM; Figure 5D;
P = 0.10, 1 df). Control flies chose the first odor at random but did not necessarily stay in this odor during the entire test.
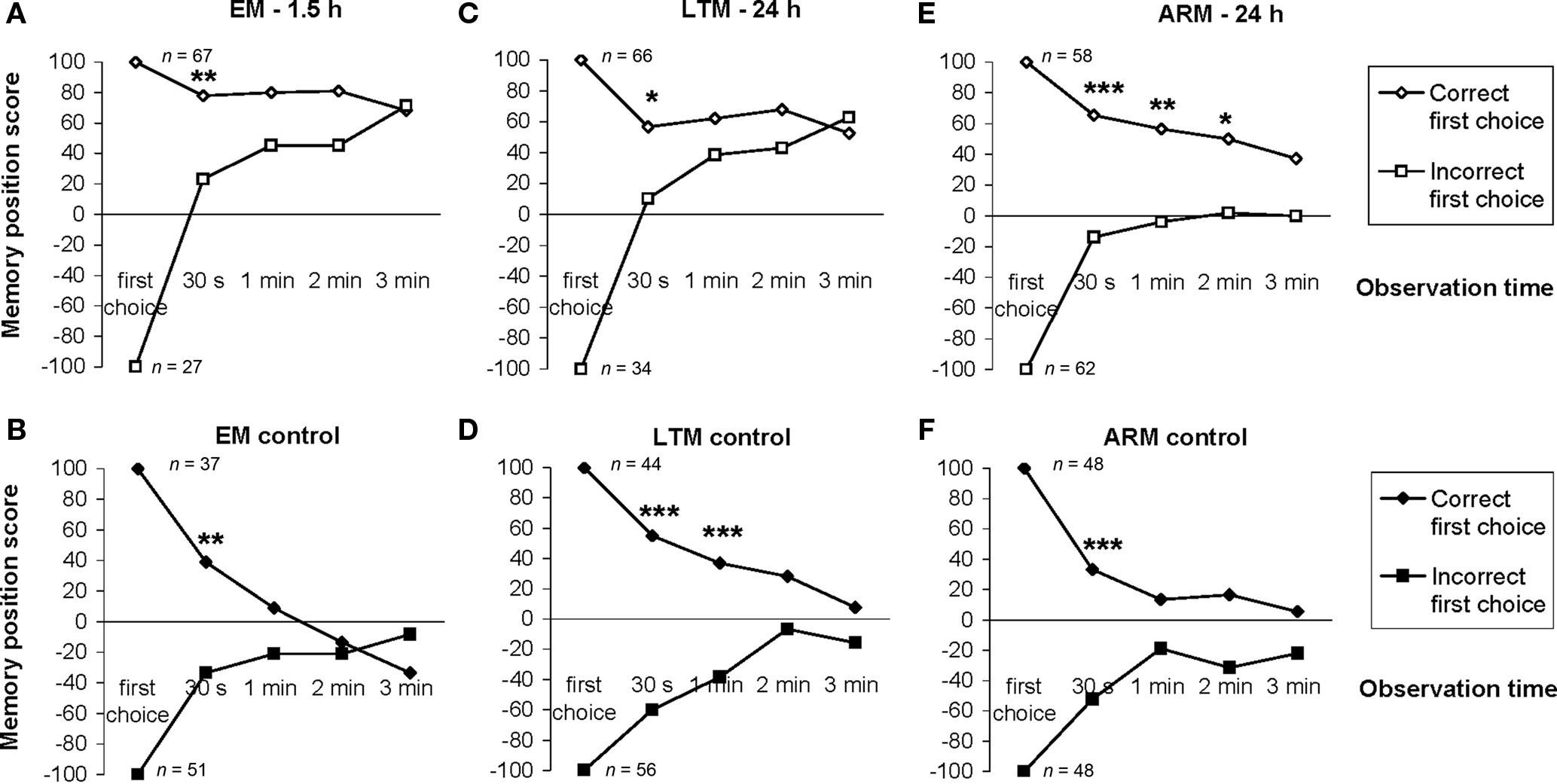
Figure 5. Effect of the first choice on the evolution of the individual memory score. Position scores were calculated for two categories of flies as a function of their first odor choice. As the test starts at the first choice, 100% of the flies making the correct choice are in the unpunished odor and this category has, therefore, a score equal to 100. Then part of the flies move from one odor to the other, so at the 30-s point the score of the category is less than 100. (A,C,E) EM-, LTM-, and ARM-trained flies respectively. (B,D,F) Control flies for EM, LTM, and ARM, respectively. n corresponds to the number of flies. Chi-square test: stars indicate significant differences between scores of flies making a correct or incorrect first choice, at P-values inferior to 0.05 (*), 0.01 (**), or 0.001 (***).
For the associative training procedures, EM- and LTM-trained flies that made the incorrect first choice had higher scores than control flies at 30 s (Figure 5A vs 5B, Figure 5C vs 5D; P < 0.05, 1 df), but this difference was only marginal in ARM-trained flies (Figure 5E vs 5F,
P = 0.051, 1 df). This suggests that the memories generated after the EM and LTM conditioning protocols allow flies to recognize and leave the punished odor significantly before 30 s of test, but to a lesser extent after the ARM conditioning protocol.
In EM- and LTM-trained flies, the effect of the incorrect first choice disappeared during the test; scores of “initially mistaken” flies approached those of “initially correct” flies, with no significant differences observed after 1 min of testing (Figures 5A,C; P > 0.05, 1 df). In contrast, for ARM-trained flies, an incorrect first choice had an irreversible effect on the score, which remained close to 0 from 30 s to the end of the test (Figure 5E; comparison with zero value:
P > 0.50, 1 df). Initially correct flies retained significantly higher scores than initially mistaken flies until 2 min of testing (Figure 5E;
P < 0.02, 1 df), after which their scores decreased; the difference was no longer significant at 3 min (Figure 5E;
P > 0.05, 1 df).
To better understand the mechanisms that sustain the evolution of memory scores, we then analyzed the exploratory behavior of flies during the test. In particular, we scrutinized the latency of the first choice, the frequency of odor changes as a function of the first choice, and locomotor activity in both odors.
Latency of the First Choice does not Depend on the Odor Status
Flies left the central part of the T-maze and made their first odor choice in a median time of 3.1–3.5 s, without significant differences between paired and control protocols (data not shown; Mann–Whitney test, Z ≤ 0.90, P ≥ 0.36). Moreover, the latency of the first choice did not depend on the odor (whether punished or not; data not shown; Z ≤ 1.79, P ≥ 0.07), except for EM-trained flies that took a little more time to enter the punished odor (i.e., median time of 4.1 vs 3.1 s to enter the unpunished odor; Z = 2.65, P = 0.008).
Different Patterns of Transition between the Odors are a Function of Memory Type
During the 3-min test, the mean number of changes between odors was significantly higher in LTM-trained flies than in LTM-control flies (Table 1. Mann–Whitney test: P = 3.10−5). The transition rates of EM- and ARM-trained flies did not differ significantly from their control flies.
The distribution of flies as a function of the number of changes between the odors is represented on Figure 6, comparing initially correct and initially mistaken flies. For all control procedures, the distributions did not depend on the first odor choice, suggesting that flies continued to randomly stop in one of the two odors until the end of the test (Figures 6B,D,F; χ2 ≤ 8.003, P ≥ 0.15, 5 df). One-third of flies stayed in the first odor (i.e., class 0 odor change) and nearly one-third more stayed in the second, opposite, odor (i.e., class 1 odor change). This explains why the position score of control flies tends toward 0 after the first choice (Figures 5B,D,F).
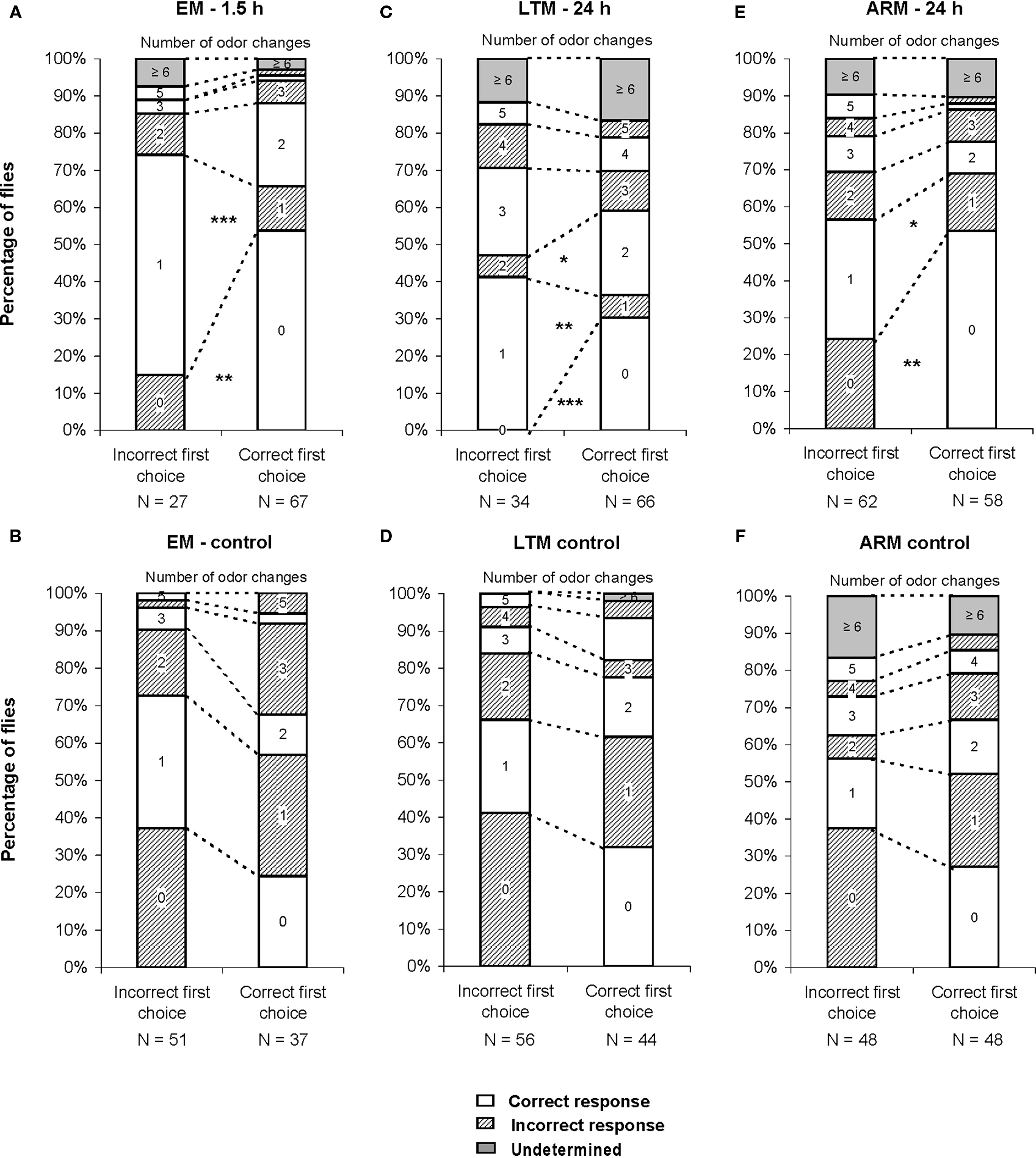
Figure 6. Number of odor changes as a function of the first choice. The graphs represent the distribution of flies as a function of the number of odor changes performed during the test, for each category of first choice (correct vs incorrect). (A,C,E) Conditioned flies, trained for EM, LTM, and ARM. (B,D,F) control flies, exposed to unpaired protocols for EM, LTM, and ARM. Flies of class 0 are those staying in the first chosen odor until the end of the test, flies of class 1 are those staying in the opposite odor after one change, etc. Chi-square test: stars indicate, for each class of odor change, significant differences between the number of flies in the unpunished odor (correct response) or in the punished odor (incorrect response) at the end of the test, at P-values inferior to 0.05 (*), 0.01 (**), or 0.001 (***). N is the sample size.
In the case of trained flies, for all training protocols the distributions depended on the first odor choice (Figures 6A,C,E; χ2 ≥ 13.2, P ≤ 0.033, 6 df). A higher percentage of flies stayed in the first and the second odor when it was the unpunished odor (Figures 6A,C,E, class 0 odor change; P < 0.01, 1 df; class 1 odor change;
P < 0.05, 1 df).
The fraction of mistaken flies that never left the punished odor during the test (Figures 6A,E, class 0 odor change) likely underlies the effect of incorrect first choice on the evolution of scores during the test, especially for ARM-trained flies (Figure 5E). Initially mistaken flies were more numerous in ARM-trained flies than in EM-trained and LTM-trained flies (Figures 6A,C,E, class 0 odor change; respectively 24, 15, and 0% initially mistaken flies).
Flies leaving the punished odor (Figures 6A,C,E, class 1 odor change) after an incorrect first choice, were responsible for the fast increase in scores observed for all three types of conditioning, though this was to a lesser extent for ARM flies (Figures 5A,C,E). In ARM-trained flies, no avoidance of the punished odor was observed following the second odor change (Figure 6E, class 2 odor changes; P ≥ 0.30, 1 df). This could explain the stagnation of the score close to 0 after an incorrect first choice, and the decrease of the score after a correct first choice (Figure 5E). Similarly, EM-trained flies did not avoid the punished odor from the second odor change (Figure 6A, class 2 odor changes;
P ≥ 0.20, 1 df). The conditioned response was present for a longer time in LTM-trained flies; it was only after the third odor change that flies no longer showed significant avoidance (Figure 6C, class 3 odor changes;
P ≥ 0.10, 1 df). In LTM-trained flies, avoidance of the punished odor is therefore a more dynamic choice than in EM- and ARM-trained flies.
Locomotor Activity During the Test
Locomotor activity decreased through the duration of the test, as indicated by the significant increase of resting time after 1 min of test, in both trained flies and their respective controls (Table 2; 1st minute vs 2nd + 3rd minute; Wilcoxon test, P < 10−4). This decrease in locomotor activity explains the limited mean number of odor changes (Table 1), and the fact that individual memory scores reach a plateau from 1 min of test (Figure 4).
When in the punished odor, EM- and LTM-trained flies spent less time resting than the corresponding control flies (Table 3). This difference was not observed in ARM-trained flies. This result shows that decreased resting in the punished odor is a component of the conditioned response of EM- and LTM-trained flies.
Control flies spent equivalent time resting in both odors. In contrast, flies of the three conditioned groups appeared to spend more time resting when in the unpunished odor than when in the punished one (this could not be statistically tested because only a portion of the flies visited both odors, thus data were either dependent or independent according to the fly’s behavior). This time spent in the unpunished odor also tended to be higher in EM-, LTM-, and ARM-trained flies than in their corresponding control, but this was not significant despite large sample sizes. Altogether, these data provide no clear evidence that aversive conditioning increases the time spent resting in the unpunished odor.
Exploration of the T-Maze
When looking at the percentage of time allocated to the three defined zones of the arms of the T-maze by control flies (Figure 7), it appears that they spent most time (around 50%) in the proximal zone. They spent about 30–40% of time in the median zone, and only 10% of time in the distal zone of the arm.
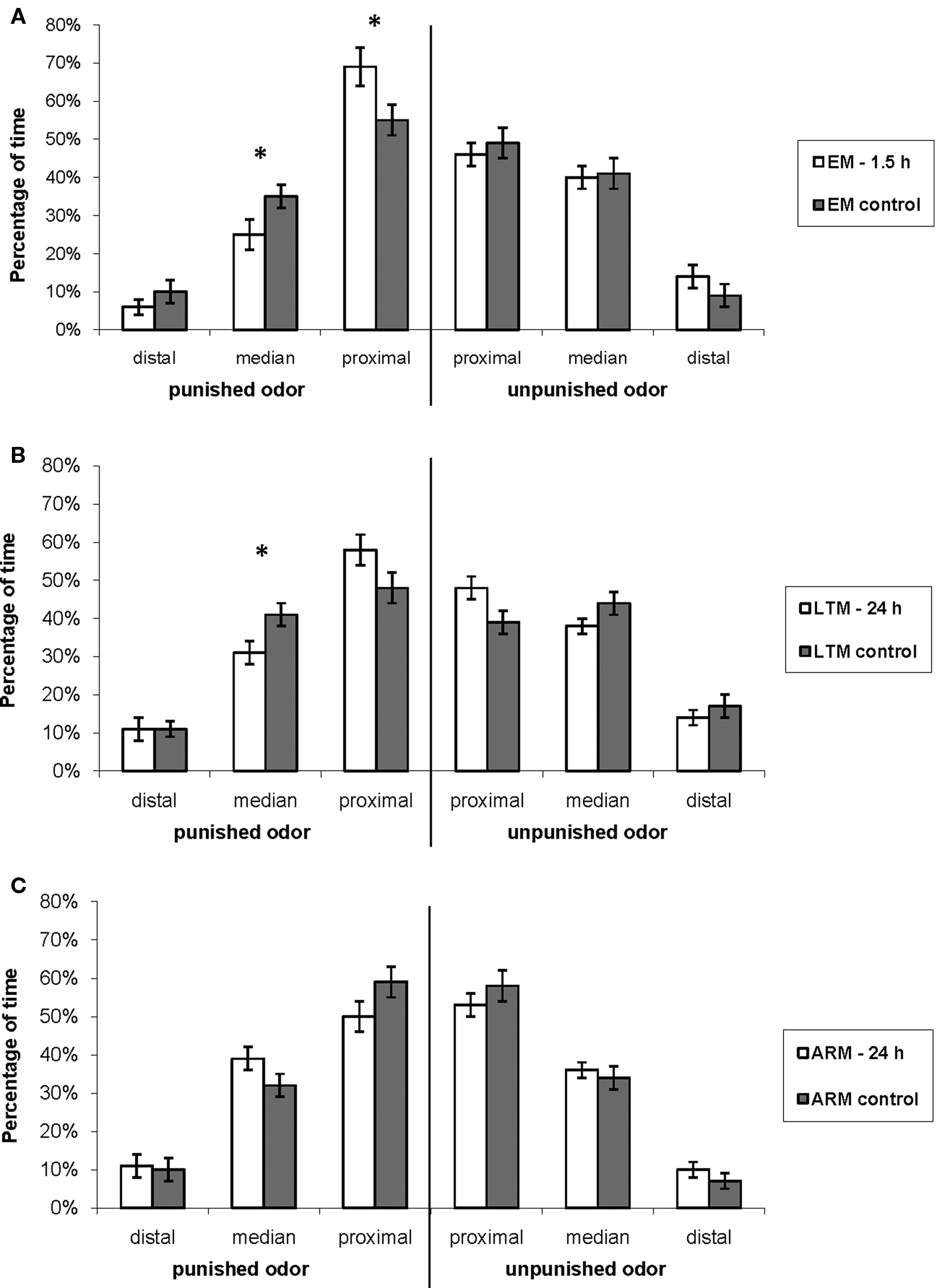
Figure 7. Exploration of the T-maze arm when flies are in the punished or in the unpunished odor. Mean percentage of time allocated to the distal, median, and proximal zones of the arm relative to the total time spent in each of the odor types during the 3-min test, for flies trained and tested (A) for EM, (B) LTM, and (C) ARM (blank bars), and their respective controls (gray bars). Data represent the mean ± SE of the mean. Stars indicate a significant difference between conditioned and control flies, tested with the Mann–Whitney Z-test (*P < 0.05). Sample sizes are those of Table 3.
This time allocation was modified in the punished odor in EM- and LTM-trained flies in comparison with the control flies. EM-trained flies showed a displacement from the median to the proximal zone (Figure 7A, Mann–Whitney test, proximal zone: Z = 2.40, P = 0.016; median zone: Z = 2.14, P = 0.032). LTM-trained flies spent also less time in the median zone, and more in the proximal zone, though this difference was not significant (Figure 7B. median zone: Z = 2.32, P = 0.020; proximal zone: Z = 1.79, P = 0.074).
This change in position may be a component of the conditioned response and it increases the probability that flies leave the punished odor. It was not observed in ARM-trained flies, which had the same exploratory behavior as the corresponding control flies (Figure 7C; Mann–Whitney test: Z ≤ 1.70, P ≥ 0.088).
In the unpunished odor, EM-, LTM-, and ARM- trained flies exhibited the same pattern of exploration as the respective control groups (Figures 7A–C; Mann–Whitney test: Z ≤ 1.64, P ≥ 0.10).
Correlation between the Memory Score and Behavioral Parameters
One question is whether the conditioned changes observed above are typical for EM, ARM, or LTM, or if they are dependent on the mean level of memory generated by the three training protocols, i.e., if individual flies’ behavior is linked to its score value regardless of the training protocol. To investigate this hypothesis, we looked for correlations between the memory score and conditioned behavioral variables. For the three memory types, not surprisingly, the percentage of time spent resting in the punished odor was negatively correlated with the memory score (Spearman coefficient of correlation: −56 ≤ R ≤ −61, P < 10−4), as well as the percentage of time spent in the median zone of the punished odor (−50 ≤ R ≤ −77; P < 10−4). These two conditioned changes in locomotor and exploratory activity were therefore quantitative and not typical for any one memory form.
Discussion
Characteristics of the Individual Conditioned Response as a Function of Memory Type
In our assay, memory performance is measured as the time allocated to the punished vs the unpunished odor. This response involves first recognizing the odor, recalling its conditioned character and then translating the information into a behavioral response. Only the latter can be observed, but our work suggests that detailed characterization of conditioned behavior during retrieval can provide us with information on earlier stages of the memory retrieval process.
EM-trained and LTM-trained flies retrieve memory efficiently, and do so from the beginning of the test. All stages of the recognition-retrieval-response process are completed in approximately 3–4 s. In contrast, the random first choice of ARM-trained flies (Figure 4C) suggests that they do not immediately recognize the conditioned repulsive character of the odor. Apparently, ARM is not efficient enough to prevent flies from entering and exploring the arm of the punished odor (Figures 6E and 7), but is sufficient to encourage some of these flies to leave (Figure 6E) suggesting that they remember the repulsive character of the odor, but with a degree of latency.
The first choice has a major impact on memory performance in ARM-trained flies, as it is definitive for half of the flies entering the unpunished odor, and for one-quarter of the flies entering the punished odor (Figure 6E). In contrast, no LTM-trained fly stayed in the punished odor after a first incorrect choice (Figure 6C). After two stays in the punished odor, ARM-trained flies did not avoid it anymore (Figure 6E). This low level of leaving the punished odor, initially or later during the test, can be explained by weaker conditioned reactions. Resting behavior and progression into the arm carrying the punished odor are less inhibited in ARM-trained flies than in EM- and LTM-trained flies (Table 3; Figure 7). Such deficiency in ARM recall is initially present, as shown by the random choice of odors in this group, and may well be aggravated by memory extinction. Memory can be rapidly extinguished by repeatedly exposing Drosophila to the punished odor in the absence of punishment (Tully and Quinn, 1985; Schwaerzel et al., 2002; Lagasse et al., 2009). In the bee Apis mellifera, appetitive memory extinction begins from the first presentation of the conditional stimulus without reinforcement (Bitterman et al., 1983; Sandoz and Pham-Delègue, 2004). This suggests that ARM-trained flies might be susceptible to memory extinction during the test. In contrast to the classical protocol of memory extinction in Drosophila, in which a single trial produces neither reconsolidation nor extinction (Lagasse et al., 2009), our set up may correspond to an operant form of memory extinction in individual flies, by allowing the flies to repeatedly experience the punished odor in the absence of negative reinforcement.
In the case of EM, which is thought to be a composite of early ARM and MTM (Tully et al., 1990), some characteristics of the conditioned response were similar to that of the ARM conditioned response. These characteristics included a similar low transition rate between the odors, the existence of a proportion of flies (albeit smaller than for ARM) that never correct their initially mistaken choice, and apparent memory extinction that occurs from the second odor change, earlier than for LTM (Figures 6A,C,E). The similarities between EM and ARM conditioned responses could be due to the fact that ARM is already formed 1.5 h after single conditioning, or could more simply be due to similar mechanisms of memory storage, without de novo protein-synthesis. However, EM-trained flies had a much higher score than ARM-trained flies, associated with a higher magnitude of behavioral changes including less time spent resting in the punished odor and less time spent in the median zone of the arm carrying the punished odor. These quantitative differences are likely due to less forgetting in EM-trained flies, because EM-trained flies are tested only 1.5 h after conditioning.
Long-term memory-trained flies shift more often between the odors than their control, which is not the case in EM-trained and ARM-trained flies (Table 1). However, such shifting does not hamper good individual LTM performance. LTM-trained flies leave, then re-enter the unpunished odor frequently and are able to avoid staying in the punished odor several times during the test (Figure 6C). In LTM-trained flies, avoidance of the punished odor therefore appears to be a dynamic process. After a certain number of odor changes, they may be sensitive to a mechanism of memory extinction, but this occurs less rapidly than in ARM-trained flies since random selection of odor in LTM flies occurs only after a higher number of odor changes than in ARM flies.
We observed that forgetting is more gradual for LTM-trained flies and more rapid for ARM-trained flies. This is shown by the distribution of LTM-trained flies in high and intermediate memory score classes. Some ARM-trained flies exhibit high memory scores but the remaining ones are distributed like the corresponding control flies (Figure 3B) due to the important deficit in memory recall (see Hypotheses for the Positive Effect of the Group on ARM Performance). In contrast with ARM, the clearly distinct and robust conditioned response 24 h after spaced conditioning would be due to the specific mechanism of protein-synthesis dependent LTM storage. Altogether, the differences found between ARM- and LTM-trained flies in the present study suggest that distinct memories are indeed expressed 24 h after massed and spaced procedures, favoring the model of exclusivity of ARM and LTM (Isabel et al., 2004), or at least that LTM expression is dominant over ARM expression if ARM and LTM coexist (Tully et al., 1994).
Hypotheses for the Positive Effect of the Group on ARM Performance
We showed recently that ARM-trained flies tested in groups have higher memory retrieval performance than when tested individually (Chabaud et al., 2009). A fly tested individually has not forgotten the learned odor but has a memory retrieval deficit. We know this because its memory score is good when it is tested in a group of trained flies, even when the group has been trained to the reverse odor combination (Chabaud et al., 2009). The data presented here clarify this hypothesis of retrieval deficit in individually tested flies, by pointing out an absence or a latency of memory retrieval, depending on the individuals. Individual ARM-trained flies tend to stay in the punished odor though they rest less in the punished odor than in the unpunished one, indicating that they may perceive the danger (Table 3). Their deficient exploratory behavior might be interpreted as a deficiency in decision-making associated with a physiological state of perceived social isolation (Cacioppo and Hawkley, 2009), a factor that has not yet been investigated in Drosophila.
The present analysis highlights the characteristics of the conditioned response that might be positively affected when flies are tested in groups. The presence of other trained flies could limit random first choice, or might reduce resting and exploration of the arm carrying the punished odor, and, thus, results in decreased time spent there. Interactions produced by ARM-trained flies in groups, possibly mediated by stress signals (Chabaud et al., 2009), would enhance their mates’ attention while making the initial choice, and would create a less favorable context for memory extinction. It would be interesting to record the behavior of a single ARM-trained fly during testing within a group. Such an analysis is, however, beyond the capability of our current laboratory set up.
Conclusion
This work is the first behavioral analysis of individual punished odor avoidance in the T-maze, a set up used for over 30 years for neurogenetic studies of memory, based on group memory scores in Drosophila. It documents how individual flies produce quantitatively and/or qualitatively distinct avoidance response as a function of the conditioning procedure used and the type of memory formed, and sheds light on the precise behaviors that are negatively affected by social isolation in the case of aversive memory retrieval. Our study raises new questions about the processes underlying memory retrieval and decision-making in the fly brain.
Conflict of Interest Statement
The authors declare that the research was conducted in the absence of any commercial or financial relationships that could be construed as a potential conflict of interest.
Acknowledgments
We thank members of our laboratories for helpful discussions, Niki Scaplehorn for linguistic editing of the manuscript, Vincent Denecheau for his help in data handling, and Philippe Vernier and Gérard Baud for their support. This work was supported by grants from the Agence Nationale pour la Recherche (Thomas Preat) and the Fondation pour la Recherche Médicale (Thomas Preat). Marie-Ange Chabaud was supported by a grant from the Université Paris 13, Ecole Doctorale Vivant et Sociétés (Ministry of Research).
References
Berry, J., Krause, W. C., and Davis, R. L. (2008). Olfactory memory traces in Drosophila. Prog. Brain Res. 169, 293–304.
Bitterman, M. E., Menzel, R., Fietz, A., and Schäfer, S. (1983). Classical conditioning of proboscis in honeybees (Apis mellifera). J. Comp. Psychol. 97, 107–119.
Cacioppo, J. T., and Hawkley, L. C. (2009). Perceived social isolation and cognition. Trends Cogn. Sci. 13, 447–454.
Chabaud, M. A., Isabel, G., Kaiser, L., and Preat, T. (2009). Social facilitation of long-lasting memory retrieval in Drosophila. Curr. Biol. 19, 1654–1659.
Claridge-Chang, A., Roorda, R. D., Vrontou, E., Sjulson, L., Li, H., Hirsch, J., and Miesenböck, G. (2009). Writing memories with light-addressable reinforcement circuitry. Cell 139, 405–415.
Colomb, J., Kaiser, L., Chabaud, M. A., and Preat, T. (2009). Parametric and genetic analysis of Drosophila appetitive long-term memory and sugar motivation. Genes Brain Behav. 8, 407–415.
Gerber, B., and Hendel, T. (2006). Outcome expectations drive learned behaviour in larval Drosophila. Proc. Biol. Sci. [B] 273, 2965–2968.
Isabel, G., Pascual, A., and Preat, T. (2004). Exclusive consolidated memory phases in Drosophila. Science 304, 1024–1027.
Krashes, M. J., DasGupta, S., Vreede, A., White, B., Armstrong, J. D., and Waddell, S. (2009). A neural circuit mechanism integrating motivational state with memory expression in Drosophila. Cell 139, 416–427.
Lagasse, F., Devaud, J. M., and Mery, F. (2009). A switch from cycloheximide-resistant consolidated memory to cycloheximide-sensitive reconsolidation and extinction in Drosophila. J. Neurosci. 29, 2225–2230.
Pascual, A., and Preat, T. (2001). Localization of long-term memory within the Drosophila mushroom body. Science 294, 1115–1117.
Quinn, W. G., Harris, W. A., and Benzer, S. (1974). Conditioned behaviour in Drosophila melanogaster. Proc. Natl. Acad. Sci. U.S.A. 71, 708–712.
Sandoz, J. C., and Pham-Delègue, M. H. (2004). Spontaneous recovery after extinction of the conditioned proboscis extension response in the Honeybee. Learn. Mem. 11, 586–597.
Schwaerzel, M., Heisenberg, M., and Troy, Z. (2002). Extinction antagonizes olfactory memory at the subcellular level. Neuron 35, 951–960.
Seugnet, L., Suzuki, Y., Vine, L., Gottschalk, L., and Shaw, P. J. (2008). D1 receptor activation in the mushroom bodies rescues sleep-loss-induced learning impairments in Drosophila. Curr. Biol. 18, 1110–1117.
Tanimoto, H., Heisenberg, M., and Gerber, B. (2004). Event timing turns punishment to reward. Nature 430, 983.
Tully, T., Boynton, S., Brandes, C., Dura, J. M., Mihalek, R., Preat, T., and Villella, A. (1990). Genetic dissection of memory formation in Drosophila melanogaster. Cold Spring Harbor. Symp. Quant. Biol. 55, 203–211.
Tully, T., Preat, T., Boynton, S. C., and Del Vecchio, M. (1994). Genetic dissection of consolidated memory in Drosophila. Cell 79, 35–47.
Tully, T., and Quinn, W. G. (1985). Classical conditioning and retention in normal and mutant Drosophila melanogaster. J. Comp. Physiol. [A] 157, 263–277.
Keywords: insect, aversive conditioning, memory retrieval, behavior, odor choice, locomotion
Citation: Chabaud M-A, Preat T and Kaiser L (2010) Behavioral characterization of individual olfactory memory retrieval in Drosophila melanogaster. Front. Behav. Neurosci. 4:192. doi: 10.3389/fnbeh.2010.00192
Received: 01 June 2010;
Accepted: 20 December 2010;
Published online: 31 December 2010.
Edited by:
Jean-Christophe Sandoz, Centre National de la Recherche Scientifique, FranceReviewed by:
Bertram Gerber, Universität Würzburg, GermanyAndreas S. Thum, University of Fribourg, Switzerland
Copyright: © 2010 Chabaud, Preat and Kaiser. This is an open-access article subject to an exclusive license agreement between the authors and the Frontiers Research Foundation, which permits unrestricted use, distribution, and reproduction in any medium, provided the original authors and source are credited.
*Correspondence: Laure Kaiser, Laboratoire Evolution, Génomes et Spéciation, Centre National de la Recherche Scientifique, 1 Avenue de la Terrasse, 91198 Gif-sur-Yvette Cedex, France. e-mail:bGF1cmUua2Fpc2VyLWFybmF1bGRAbGVncy5jbnJzLWdpZi5mcg==; Thomas Preat, Gènes et Dynamique des Systèmes de Mémoire, Ecole Supérieure de Physique et de Chimie Industrielles, 10 rue Vauquelin, 75005 Paris, France. e-mail:dGhvbWFzLnByZWF0QGVzcGNpLmZy