- 1Department of Neurophysiology, Medical Faculty, Ruhr-University Bochum, Bochum, Germany
- 2International Graduate School of Neuroscience, Ruhr-University Bochum, Bochum, Germany
Psychosis is a clinical state that encompasses a range of abnormal conditions, including distortions in sensory information processing and the resultant delusional thinking, emotional discordance and cognitive impairments. Upon developing this condition, the rate at which cognitive and behavioral deteriorations progress steadily increases suggesting an active contribution of the first psychotic event to the progression of structural and functional abnormalities and disease establishment in diagnosed patients. Changes in GABAergic and glutamatergic function, or expression, in the hippocampus have been proposed as a key factor in the pathophysiology of psychosis. However, little is known as to the time-point of onset of putative changes, to what extent they are progressive, and their relation to disease stabilization. Here, we characterized the expression and distribution patterns of groups I and II metabotropic glutamate (mGlu) receptors and GABA receptors 1 week and 3 months after systemic treatment with an N-methyl-D-aspartate receptor (NMDAR) antagonist (MK801) that is used to model a psychosis-like state in adult rats. We found an early alteration in the expression of mGlu1, mGlu2/3, and GABAB receptors across the hippocampal dorsoventral and transverse axes. This expanded to include an up-regulation of mGlu5 levels across the entire CA1 region and a reduction in GABAB expression, as well as GAD67-positive interneurons particularly in the dorsal hippocampus that appeared 3 months after treatment. Our findings indicate that a reduction of excitability may occur in the hippocampus soon after first-episode psychosis. This changes, over time, into increased excitability. These hippocampus-specific alterations are likely to contribute to the pathophysiology and stabilization of psychosis.
Introduction
Schizophrenia is a chronic mental disorder, distinguished by a rich spectrum of symptoms that are clustered into the cognitive, negative, and psychotic domains (McGhie and Chapman, 1961; Kapur, 2003; Bowie and Harvey, 2005; Bürgy, 2008; Velligan and Alphs, 2008; Davis et al., 2014). Whilst a decline in learning and memory ability (cognitive symptoms) and social withdrawal (negative symptoms) become apparent early on, in the course of the disease (Keshavan et al., 2011; Keefe and Harvey, 2012), it is the manifestation of hallucinatory and delusionary experiences (psychotic symptoms) that lead to clinical diagnosis. Following the first major psychotic event, also known as the first-episode of psychosis (Larson et al., 2010; Keshavan et al., 2011; Ruby et al., 2014), the rate at which cognitive and behavioral deteriorations occur progressively increases (Häfner et al., 1994; Cheung et al., 2010; Alvarez-Jimenez et al., 2011). This suggests an active involvement of the first-episode of psychosis in the progression of structural and functional abnormalities in diagnosed patients (Borgwardt et al., 2008; Haijima et al., 2012; Guo et al., 2013).
It has been proposed that a dysfunction of the glutamatergic and GABAergic systems are key elements to the etiology of schizophrenia and psychosis (Belforte et al., 2010; Nakazawa et al., 2012; Snyder and Gao, 2013). In particular, it is believed that hypofunction of N-methyl-D-aspartate receptors (NMDAR) on GABAergic interneurons, particularly on parvalbumin-containing (PV) interneurons, leads to disinhibition of glutamatergic terminals and a hyperglutamatergic state (Marek et al., 2010; Riebe et al., 2016). This disinhibition further results in functional deficits of neurons, circuits and behavior that are characteristic of psychosis and can be emulated by NMDAR antagonists (Krystal et al., 1994; Lahti et al., 1995), and in transgenic animal models of NMDAR hypofunction (Belforte et al., 2010).
Importantly, reduced inhibitory and increased excitatory neurotransmission may, in turn, modulate the function of other glutamatergic and GABAergic receptors, as well as be modulated by them. Here, the (group I) metabotropic glutamate receptor 5 (mGlu5) is of prime interest as it shares a unique interrelationship, both in terms of physical coupling and functional modulation, with NMDAR (Tu et al., 1999; Marino and Conn, 2002; Alagarsamy et al., 2005). Another group I mGlu receptor, mGlu1, potentiates NMDAR currents (Benquet et al., 2002; Heidinger et al., 2002). Furthermore, mGlu1 and mGlu5 receptors both facilitate long-term potentiation (LTP) in the hippocampus (Mukherjee and Manahan-Vaughan, 2013), a brain region that is among the most affected in patients suffering from schizophrenia and psychosis (Heckers and Konradi, 2002; Kolomeets et al., 2007; Adriano et al., 2012; Yin et al., 2012). Group II mGlu receptors, mGlu2 and mGlu3, serve as autoreceptors at glutamatergic terminals (Shigemoto et al., 1997; Schoepp, 2001) and are critically required for persistent forms of hippocampal long-term depression (LTD) (Kobayashi et al., 1996; Manahan-Vaughan, 1998; Pöschel and Manahan-Vaughan, 2007; Altinbilek and Manahan-Vaughan, 2009). Thus, both group I and group II receptors play an intrinsic role in the regulation and support of NMDAR-dependent information processing and storage, as well as in the regulation of activity-dependent neural excitability.
GABA is the primary inhibitory neurotransmitter in the mammalian brain. The ionotropic GABAA receptor is a ligand-gated chloride channel that is responsible for tonic inhibition in the brain. Because of their high affinity for GABA, along with their relatively slow desensitization rates, it has been proposed that GABAA receptors sense both the ambient concentration of GABA, as well as the activity- dependent spill-over of GABA from the synaptic cleft (Wlodarczyk et al., 2013). The GABAB receptor is a metabotropic receptor that serves as an autoreceptor (Waldmeier et al., 2008), but also can modulate GABAergic responses of neurons (Shen et al., 2017). The efficacy of both receptors is regulated by synaptic activity: activation of calcium calmodulin kinase II (CAMKII), a downstream target of NMDAR activation, results in phosphorylation of GABAB receptors (Zemoura et al., 2018). Furthermore, both protein kinase A (PKA) and protein kinase C (PKC) can mediate phosphorylation of GABAA receptors (Connelly et al., 2013). GABAA and GABAB receptors serve to keep local cellular and network excitation within a functional physiological range and enable the precise spatiotemporal conditions that are required for information encoding through synaptic plasticity (Kaila, 1994; Paulsen and Moser, 1998; Bettler et al., 2004; Kullmann et al., 2005). A disruption of GABAergic inhibitory control can, thus, be expected not only to have a potent effect at the level of tonic inhibition, but also at the level of excitation-inhibition balance. The effectivity of information processing related to cognition, learning and memory will consequently be compromised.
The hippocampus is a critical processing hub for a wide range of sensory and cognitive information (Kesner and Rolls, 2015) and is a primary site for activity-dependent synaptic plasticity that in turn enables both short- and long-term declarative memory (Kemp and Manahan-Vaughan, 2007). Patients with schizophrenia and psychosis exhibit a wide range of sensory and cognitive abnormalities (McGhie and Chapman, 1961; Kapur, 2003; Bowie and Harvey, 2005; Bürgy, 2008) that have been ascribed, at least in part, to dysfunctions in sensory information processing by the hippocampus (Arnold, 1999; Bast and Feldon, 2003; Behrendt, 2010; Bak et al., 2014; Kalweit et al., 2017). Not only is the trisynaptic circuit [dentate gyrus (DG) and CA regions (CA3 and CA1)] of the hippocampus responsible for the formation of multimodal memory representations (Hasselmo, 2005; Kesner, 2013a,b; Kesner and Rolls, 2015), but its longitudinal (dorso-ventral) axis is pivotal to the processing of visuo-spatial and socio-emotional elements of cognition (Bast et al., 2009; Fanselow and Dong, 2010; Strange et al., 2014). Strikingly, changes in GABAergic and groups I and II mGlu receptor function or expression in the hippocampus have been proposed to form a key factor in psychotic events in schizophrenia (Linden et al., 2006; Behrendt, 2010; Matosin et al., 2015; Zurawek et al., 2018). Nonetheless, little is known as to the time-point of onset of putative changes on these receptor systems, or to what extent changes are progressive during the course of chronification of the disease. Evidence for early changes in receptor function, soon after the first-episode of psychosis could offer novel possibilities for therapy and disease intervention.
In the present study our goal was to study putative changes in the abovementioned receptor systems by examining receptor expression in a rodent model of first-episode psychosis. Here, an acute systemic injection of MK801, an NMDAR antagonist, was used to mimic the first-episode of psychosis (Manahan-Vaughan et al., 2008a; Wiescholleck and Manahan-Vaughan, 2013a,b). Rodent features of a psychosis-like episode comprise significant deficits in prepulse inhibition of the acoustic startle response and startle inhibition, increased stereotypy and hyperactivity (Manahan-Vaughan et al., 2008a; Wiescholleck and Manahan-Vaughan, 2013a) that parallel symptoms exhibited by patients during the first episode of psychosis (Ludewig et al., 2003; Kim et al., 2011; Compton et al., 2015).
The first episode-like event in rodents is followed by a persistent loss of hippocampal LTP, deficits in hippocampus-dependent learning and memory (Manahan-Vaughan et al., 2008b; Wiescholleck and Manahan-Vaughan, 2013b; Wlodarczyk et al., 2013), changes in hippocampal neuronal oscillations (Kehrer et al., 2007; Kalweit et al., 2017), persistent elevations of neuronal excitability and somatic immediate early gene expression (Grüter et al., 2015). In patients, overt changes in brain function comprise decreases in hippocampal functional connectivity and deficits in relational memory (Samudra et al., 2015; Avery et al., 2018), increased cortical excitability (Díez et al., 2017), and abnormal neural oscillations (Uhlhaas and Singer, 2010). These changes form the fundament of the “disconnection hypothesis” (Friston, 1998; Díez et al., 2017) and are widely believed to be caused by a combination of focal brain changes and a disruption of synaptic plasticity that leads to impairments of functional integration at the cognitive level (Stephan et al., 2006, 2009). As mentioned above, changes in GABA and glutamate receptor function have been proposed to underlie these changes (Stephan et al., 2006, 2009; Corlett et al., 2011; Gonzalez-Burgos and Lewis, 2012). In line with this, changes in expression of NMDAR subunits along the dorsoventral axis of the hippocampus have been reported in a rodent model of psychosis (Dubovyk and Manahan-Vaughan, 2018a). Less is known about changes in GABA receptors, or subunits of mGlu receptors that may accompany disease progression.
We characterized the expression and distribution patterns of metabotropic glutamatergic and GABAergic receptors 1 week and 3 months after the emulation of the first psychotic event in adult rats. In particular, we looked for changes in the expression of mGlu1, mGlu2/3, mGlu5, GABAA, and GABAB receptors across the laminar structure of the trisynaptic circuit and along the longitudinal axis of the hippocampus. We detected time-dependent and neural subcompartment-specific changes in the expression of these receptors across the longitudinal axis of the hippocampus. Our findings indicate that an early adaptive reorganization of the hippocampus occurs after first-episode psychosis that contributes to a reduction of network excitation, and is superseded by a state of permanently increased network excitability and reduced interneuron number. These changes are likely to support loss of synaptic gain control that have been proposed by others (Adams et al., 2013; Díez et al., 2017) to contribute importantly to the pathophysiology and stabilization of psychosis.
Materials and Methods
Animals
All experiments were done with healthy male Wistar rats (Charles River Laboratories, Germany). Animals were housed in custom-made climatised and ventilated holding cupboards in an animal-housing room with a controlled 12-h light/dark cycle. No female rats were housed in the room. Animals had free access to food and water. The study was carried out in accordance with the European Communities Council Directive of September 22nd, 2010 (2010/63/EU) for care of laboratory animals. Prior permission was obtained from the ethics commission of the local government authority (Landesamt für Arbeitsschutz, Umwelt- und Naturschutz, Nordrhein-Westfalen).
Drug Treatment
Seven-to-eight-week-old Wistar rats were divided into two groups where each animal received a single intraperitoneal (i.p.) injection of the NMDAR antagonist [+]-5-methyl-10,11-dihydro-5Hdibenzo-[a,d]-cyclohepten-5,10-imine hydrogen maleate (MK801, Tocris, Germany). The compound was dissolved in 0.9% physiological saline and administered in a concentration of 5 mg/kg, in accordance with previous studies conducted by our group (Manahan-Vaughan et al., 2008a,b). This treatment protocol results in a first-episode-like state in rodents (Wöhrl et al., 2007; Manahan-Vaughan et al., 2008a,b, 2013a,b; Wiescholleck and Manahan-Vaughan, 2012). Two control animal groups of identical age and strain received a single i.p. injection of 0.9% physiological saline (10 ml/kg). Animals were sacrificed 1 week or 3 months after the injection. Each group included 5 animals, with the exception of the 3-month-group where 3 additional animals were added to confirm a strong statistical tendency in the original dataset. From each animal, one section of the DH, IH, or VH was analyzed with regard to a specific receptor. Both, right and left hippocampi were used for the analysis and considered as replicates.
Slice Preparation
Wistar rats were deeply anesthetized with sodium pentobarbital and transcardially perfused with cold Ringer’s solution + heparin (0.2%) followed by 4% paraformaldehyde (PFA) in phosphate buffered saline (PBS, 0.025 M). Brains were removed, fixed in 4% PFA for 24 h, and cryoprotected in 30% sucrose in 0.1 M PBS for at least 3 days. Serial 30-μm thick horizontal sections were collected with a freezing microtome. For each animal, three horizontal sections from the most dorsal (between 3.6 and 4.1 mm posterior to bregma), middle intermediate (around 5.6 mm posterior to bregma) and most ventral hippocampal parts (between 7.1 and 7.6 mm posterior to bregma) were simultaneously used for immunohistochemical staining (Figure 1A). Sections from the Vehicle- and MK801-treated animals were always analyzed in pairs.
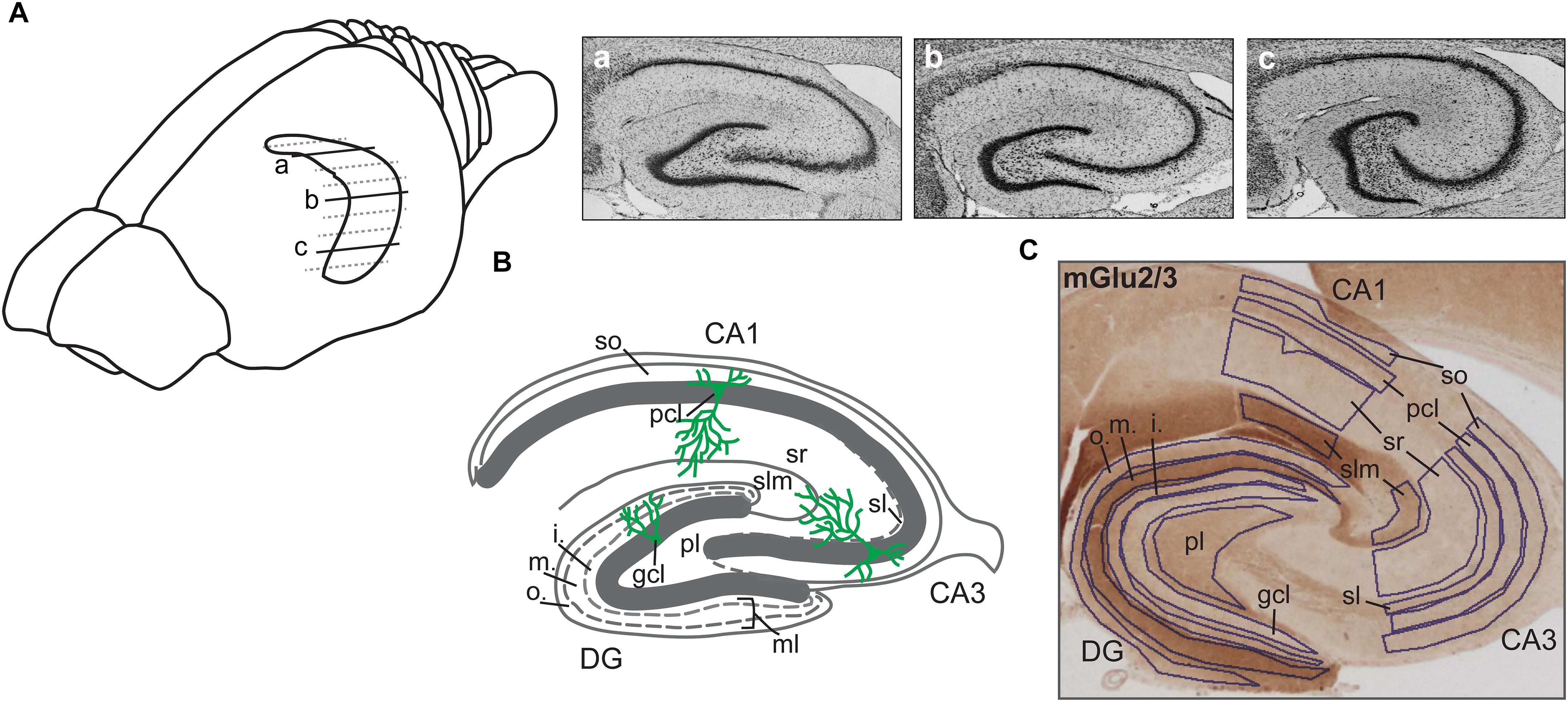
Figure 1. Illustration of hippocampal sections. (A) Drawing of the rat brain showing the horizontally sectioned hippocampus along its longitudinal axis. Nissl-stained sections correspond to dorsal (a), intermediate (b), and ventral (c) subdivisions. (B) Schematic illustration of the hippocampal transverse axis depicting its trisynaptic circuit with the laminar view. o., outer band of the ml of the DG; m., middle band of the ml of the DG; i., inner band of the ml of the DG; gcl, granule cell layer of the DG; ml, molecular layer of the DG; pl, polymorphic layer of the DG; so, Stratum oriens of CA3/CA1; pcl, pyramidal cell layer of CA3/CA1; sl, Stratum lucidum of the CA3; sr, Stratum radiatum of CA3/CA1; and slm, Stratum lacunosum-moleculare of CA3/CA1. (C) A representative example of the dorsal hippocampus showing the expression of mGlu2/3 receptors across its transverse axis. Laminal delineation across the DG, CA3, and CA1 regions is shown.
Immunohistochemistry
Antibody specificity was verified in our previous studies (Grüter et al., 2015; Dubovyk and Manahan-Vaughan, 2018a,b). Endogenous peroxidase was blocked by pretreating the free-floating brain sections in 0.3% H2O2 for 20 min. They were then rinsed in PBS and incubated with blocking solution containing 10% normal serum and 20% avidin in PBS with 0.2% Triton X-100 (PBS-Tx) for 90 min at room temperature (RT). Sections were incubated overnight at room temperature with primary antibodies: mGlu2/3 (rabbit polyclonal, 1:200; ab1553, Chemicon), mGlu5 (rabbit polyclonal, 1:200; ab5675, Millipore), GABAA receptor (mouse monoclonal, 1:400; mab341, Millipore), GABAB receptor 1 (mouse monoclonal, 1:250; ab55051, Abcam) or GAD67 (mouse monoclonal, 1:100; mab-5406, Millipore) in medium containing 1% normal serum in 0.2% PBS-Tx + 20% biotin. Sections were then rinsed in PBS and incubated with biotinylated goat anti-rabbit (1:500; BA-1000, Vector), horse anti-mouse (1:500; BA-2001, Vector) or horse anti-goat (1:500; BA-9500, Vector) antibodies in 1% normal serum in 0.1% PBS-Tx for 90 min at RT. Afterwards, sections were washed in PBS and incubated for 90 min at RT with ABC kit (PK-6100, Vector) in 1% normal serum in 0.1% PBS-Tx.
Staining with mGlu1 receptor antibodies required an additional amplification step with biotinylated tyramide for 20 min. Here, the sections were incubated for 5 days at 4°C with primary mGlu1 receptor antibodies (rabbit polyclonal, 1:400; ab82211, Abcam) in 1% BSA in 0.2% TBS-Tx. PBS was replaced with TBS, normal serum + PBS-Tx with bovine serum albumin + TBS-Tx, and one ABC reaction with two for 30 min each with amplification step in between. Here, sections were incubated with 10 μl b-tyramide + 10 μl 0.01% H2O2 in 1,000 μl of TBS for 20 min. Finally, the sections were washed in PBS and treated with diaminobenzidine and 0.01% H2O2 for approximately 10 min.
Quantitative Analysis
Regions of interest were defined using the rat brain atlas of Paxinos and Watson (1982) and Nissl staining where every 12th section throughout the whole hippocampus was stained with 0.1% Cresyl violet (c5042, Sigma) as a reference. Eleven areas of interest included: molecular layer (ml) of the dentate gyrus (DG); granule cell layer (gcl) of the DG; polymorphic layer (pl) of the DG; Stratum oriens (so) of CA3/CA1; pyramidal cell layer (pcl) of CA3/CA1; Stratum radiatum (sr) of CA3/CA1; and Stratum lacunosum-moleculare (slm) of CA3/CA1 on sections taken from the dorsal, intermediate and ventral hippocampal subdivisions. Due to the expression profile of mGlu2/3 receptors, a specific delineation of the ml of the DG into outer, middle and inner bands was possible. The Stratum lucidum (sl) of the CA3 could also be clearly identified and was therefore quantified, resulting in 15 areas of interest for the mGlu2/3 receptor (Figures 1B,C). Importantly, for background subtraction we used receptor-devoid tissue, which in the dorsal sections was the fimbria; in the intermediate sections was either the fimbria or the superior thalamic radiation; and in the ventral sections was the internal capsule. Pictures of stained sections were acquired with a light microscope (Leica DMR, Germany), equipped with a digital camera (MBF Bioscience) and stored in TIFF format. The regions of interest were analyzed at 2.5× lens magnification. The digitized high-resolution pictures were obtained using Neurolucida software (MBF Bioscience) and quantified using open-source ImageJ software (National Institutes of Health). Given that images were acquired with a RGB camera the ‘Color Deconvolution’ plugin in ImageJ was used to deconvolve the color information as well as to convert images to 8-bit format, thus increasing the dynamic range of the signal. R software was used to scale data from several independent stainings/plates using generalized residual sum of squares algorithm to account for batch variability in staining intensities (Kreutz et al., 2007; von der Heyde et al., 2014).
The amount of GAD67 positive cells was manually calculated in sections stained with anti-GAD67 antibodies. Quantification was limited to the DG, CA3, and CA1 regions across their respective laminal structures of the DH, IH, and VH sections.
Statistical Analysis
Data obtained in the immunohistochemical experiments were statistically analyzed by means of factorial analysis of variance (ANOVA) followed by Duncan’s post hoc test, which allowed detection of significant factors in a two-factor model. Here, the Vehicle and MK801 treatment data were considered as the TREATMENT factor (factor one), while the laminar organization of the trisynaptic circuit as the REGION factor (factor two). The cell count results were analyzed using the Students t-test. All significant differences were defined as p < 0.05 or p < 0.01. Values are expressed as mean values ± the standard error of the mean (SEM).
Results
To allow for detailed scrutiny of the hippocampus we studied the following hippocampal subcompartments: the molecular layer (ml), the granule cell layer (gcl), and the polymorphic layer (pl) of the dentate gyrus (DG), as well as the Stratum oriens (so), pyramidal cell layer (pcl), Stratum radiatum (sr), and Stratum lacunosum-moleculare (slm) of CA3/CA1. Sections from the dorsal hippocampus (DH), intermediate hippocampus (IH), and ventral hippocampus (VH) were examined (Figure 1). Expression of mGlu1, mGlu5, mGlu2/3, GABAA, and GABAB receptors was examined 1 week and 3 months after treatment with vehicle, or the NMDAR antagonist, MK801.
MGlu1 Expression Is Altered 1 Week, but Not 3 Months After MK801 Administration
One week after MK801 treatment, mGlu1 receptor protein levels were significantly changed in DH, and IH [multifactorial ANOVA, Treatment factor, DH: F(1,198) = 32.364, p < 0.001; IH: F(1,161) = 58.727, p < 0.001]. In the DH, protein expression was reduced in the ml, gcl, and pl of the DG and in the pcl of the CA1 (Figure 2A and Table 1). In contrast, the IH showed an up-regulation of mGlu1 receptor levels in the gcl of the DG, so, pcl and sr of the CA3; and pcl of the CA1. The VH remained unaltered [multifactorial ANOVA, Treatment factor, VH: F(1,165) = 3.209, p = 0.075] (Figure 2A and Table 1).
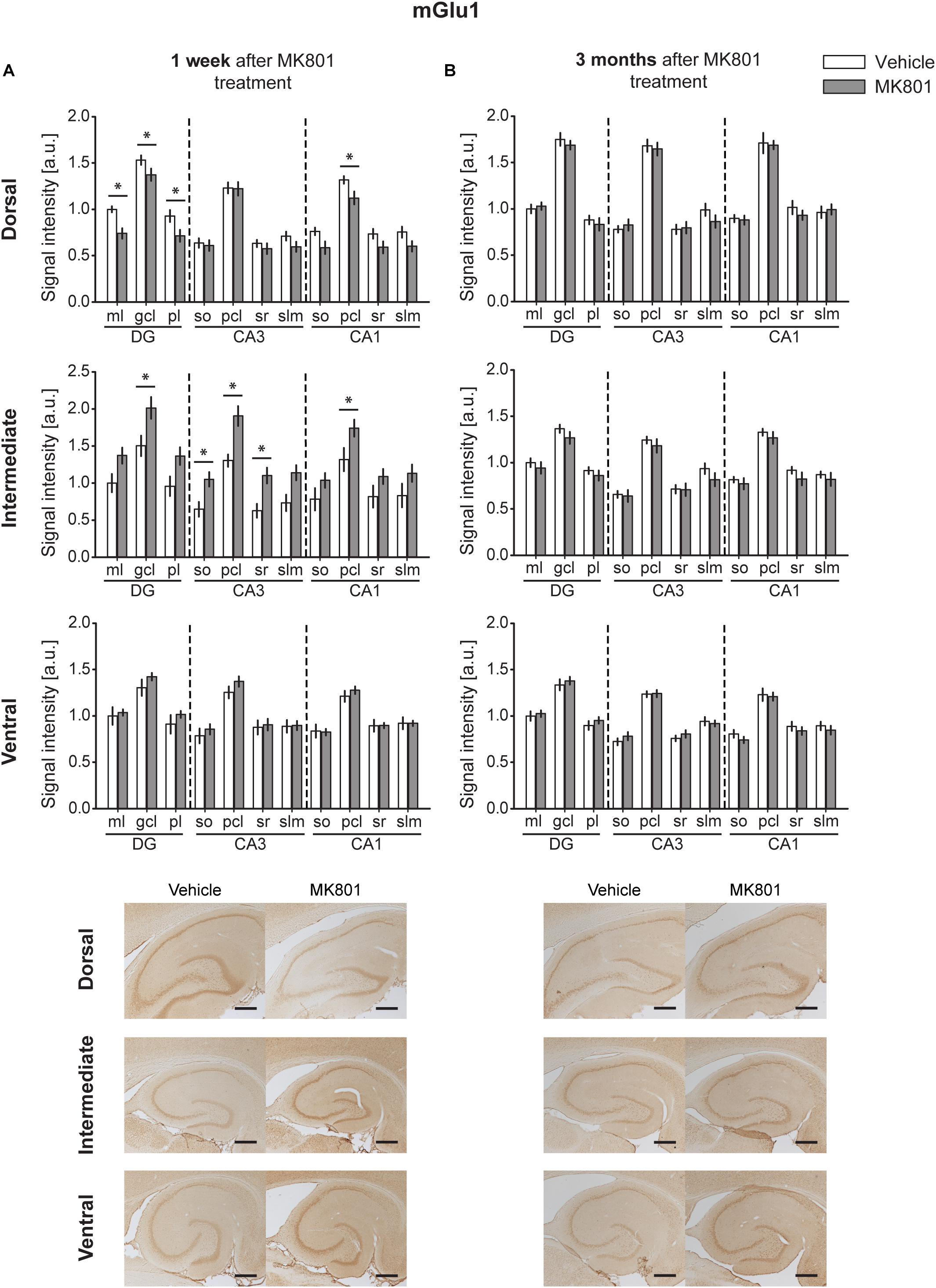
Figure 2. MK801 treatment leads to a transient change of mGlu1 receptor protein expression. Bar charts represent relative changes in protein levels 1 week and 3 months after MK801 treatment across the somato-dendritic layers of the transverse and longitudinal hippocampal axes. (A) mGlu1 receptor levels were down-regulated in the dorsal hippocampus, but up-regulated in the intermediate hippocampus 1 week after MK801 treatment. (B) No changes in mGlu1 receptor expression were detected 3 months after MK801 administration. Scale bar: 500 μm. Values expressed in arbitrary units (a.u.). Error bars indicate SEM. ∗p < 0.05 or ∗∗p < 0.01. ml, molecular layer of the DG; gcl, granule cell layer of the DG; pl, polymorphic layer of the DG; so, Stratum oriens of CA3/CA1; pcl, pyramidal cell layer of CA3/CA1; sr, Stratum radiatum of CA3/CA1; and slm, Stratum lacunosum-moleculare of CA3/CA1. Photomicrographs provide examples of mGlu1 receptor-stained sections from the dorsal, intermediate and ventral hippocampal subdivisions that originated from the same vehicle- or MK801-treated animal and correspond to 1 week (left) or 3 months (right) after treatment.
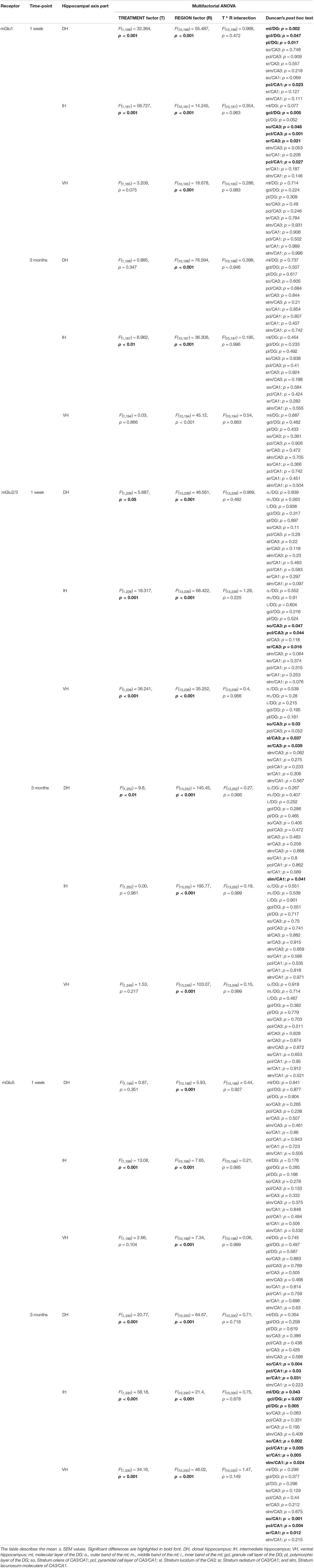
Table 1. An overview of statistically significant differences in the expression of metabotropic glutamatergic receptors.
Three months after MK801-treatment receptor expression was equivalent in hippocampi from MK801- and vehicle-treated animals [multifactorial ANOVA, Treatment factor, DH: F(1,198) = 0.885, p = 0.347; IH: F(1,187) = 6.962, p < 0.01; VH: F(1,194) = 0.03, p = 0.866] (Figure 2B and Table 1).
MGlu2/3 Levels Are Upregulated 1 Week After MK801 Treatment, With No Overall Changes Evident 3 Months After Treatment
A significant up-regulation in the levels of mGlu2/3 receptors was found 1 week after MK801 administration in the CA3 region of both the IH and VH [multifactorial ANOVA, Treatment factor, IH: F(1,238) = 18.317, p < 0.001; VH: F(1,238) = 36.241, p < 0.001]. The layers affected included so, pcl, and sr of the IH CA3; and so, sl and sr of the VH CA3 (Figure 3A and Table 1). The DH was unaffected [multifactorial ANOVA, Treatment factor, DH: F(1,238) = 5.687, p < 0.05].
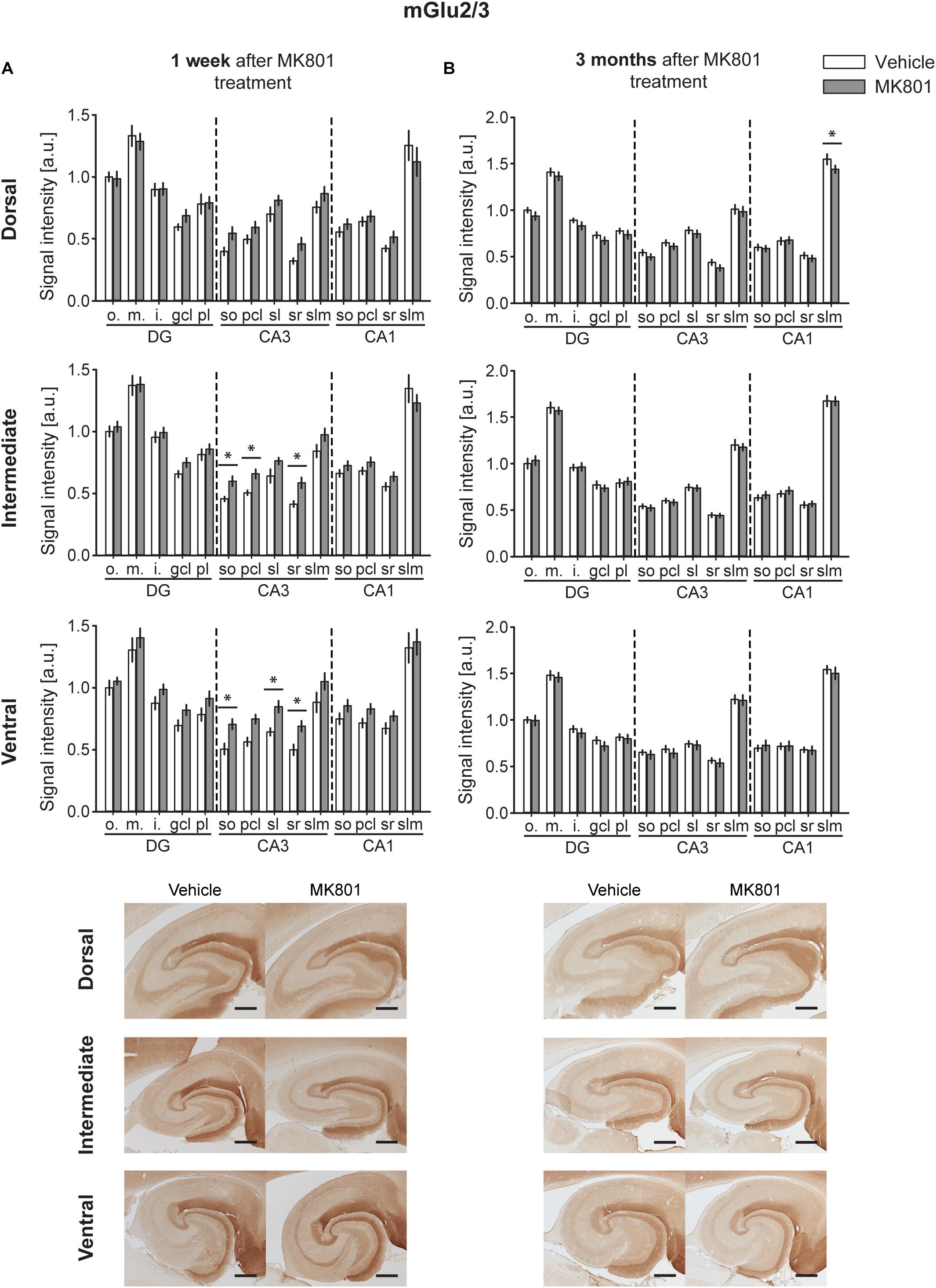
Figure 3. mGlu2/3 receptor levels are transiently up-regulated early after MK801 treatment. Bar charts represent relative changes in protein levels 1 week and 3 months after MK801 treatment across the somato-dendritic layers of the transverse and longitudinal hippocampal axes. (A) mGlu2/3 protein expression is significantly increased across the ventro-intermediate hippocampal CA3 region 1 week after MK801 treatment. (B) No overall changes were detected 3 months after MK801 application. Scale bar: 500 μm. Values expressed in arbitrary units (a.u.). Error bars indicate SEM. ∗p < 0.05. ml, molecular layer of the DG; gcl, granule cell layer of the DG; pl, polymorphic layer of the DG; so, Stratum oriens of CA3/CA1; pcl, pyramidal cell layer of CA3/CA1; sr, Stratum radiatum of CA3/CA1; and slm, Stratum lacunosum-moleculare of CA3/CA1. Photomicrographs provide examples of mGlu2/3 receptor-stained sections from the dorsal, intermediate and ventral hippocampal subdivisions that originated from the same vehicle- or MK801-treated animal and correspond to 1 week (left) or 3 months (right) after treatment.
Overall, no changes occurred in mGlu2/3 expression 3 months after treatment [multifactorial ANOVA, Treatment factor, DH: F(1,252) = 9.8, p < 0.01; IH: F(1,252) = 0.00, p = 0.961; VH: F(1,248) = 1.53, p = 0.217]. There was one exception to this, however: we detected a significant decrease in mGlu2/3 expression in the slm of the DH CA1 (Figure 3B and Table 1).
MGlu5 Expression Is Increased 3 Months After MK801 Application
One week after MK801-treatment, mGlu5 receptor expression was unchanged in the hippocampus [multifactorial ANOVA, Treatment factor, DH: F(1,198) = 0.87, p = 0.351; IH: F(1,198) = 13.08, p < 0.001; VH: F(1,198) = 2.66, p = 0.104] (Figure 4A and Table 1). By contrast, 3 months after treatment, a widespread up-regulation of mGlu5 receptor expression was evident across the entire CA1 region (dorsal, intermediate and ventral CA1) and in the intermediate DG compared to controls [multifactorial ANOVA, Treatment factor, DH: F(1,330) = 20.77, p < 0.001; IH: F(1,330) = 58.18, p < 0.001; VH: F(1,330) = 34.16, p < 0.001]. The layers affected included the so, pcl, and sr of the DH CA1, the so, pcl, and sr of the VH CA1, and the so, pcl, sr, and slm of the intermediate CA1. In addition, increased mGlu5 receptor expression occurred in the ml, gcl, and pl of the intermediate DG (Figure 4B and Table 1).
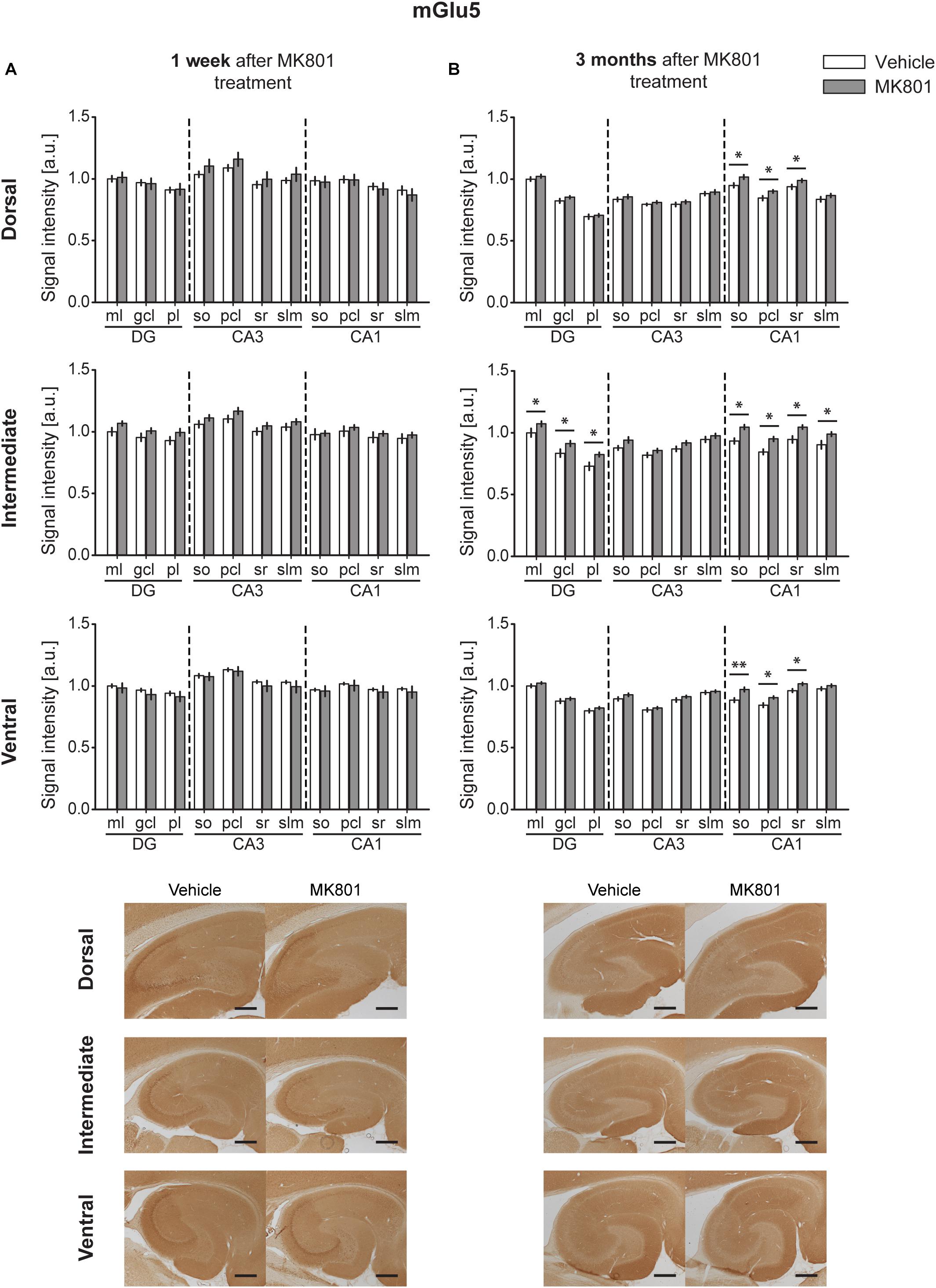
Figure 4. Late up-regulation of mGlu5 receptor levels occurs after MK801 treatment. Bar charts represent relative changes in protein levels 1 week and 3 months after MK801 treatment across the somato-dendritic layers of the transverse and longitudinal hippocampal axes. (A) mGlu5 receptor expression remained unaltered 1 week after MK801 treatment. (B) Three months after MK801 injection led to an extensive up-regulation of mGlu5 receptor levels across an entire hippocampus. Scale bar: 500 μm. Values expressed in arbitrary units (a.u.). Error bars indicate SEM. ∗p < 0.05. ml, molecular layer of the DG; gcl, granule cell layer of the DG; pl, polymorphic layer of the DG; so, Stratum oriens of CA3/CA1; pcl, pyramidal cell layer of CA3/CA1; sr, Stratum radiatum of CA3/CA1; and slm, Stratum lacunosum-moleculare of CA3/CA1. Photomicrographs provide examples of mGlu5 receptor-stained sections from the dorsal, intermediate and ventral hippocampal subdivisions that originated from the same vehicle- or MK801-treated animal and correspond to 1 week (left) or 3 months (right) after treatment.
GABAA Levels Remained Largely Unaltered Following MK801 Treatment
One week after MK801 treatment, a potent decrease in GABAA expression occurred in the pcl of the DH CA1 region (Figure 5A). No changes were found in any other subcompartment of the hippocampus [multifactorial ANOVA, Treatment factor, IH: F(1,187) = 8.805, p < 0.01; VH: F(1,183) = 8, p < 0.01] (Figure 5A and Table 2). Three months after treatment a significant increase in GABAA expression was evident in the slm of the DH CA3 region (Figure 5B). Expression levels in all other subcompartments were not different from controls at this time-point [multifactorial ANOVA, Treatment factor, IH: F(1,190) = 1.52, p = 0.219; VH: F(1,198) = 1.308, p = 0.254] (Figure 5B and Table 2).
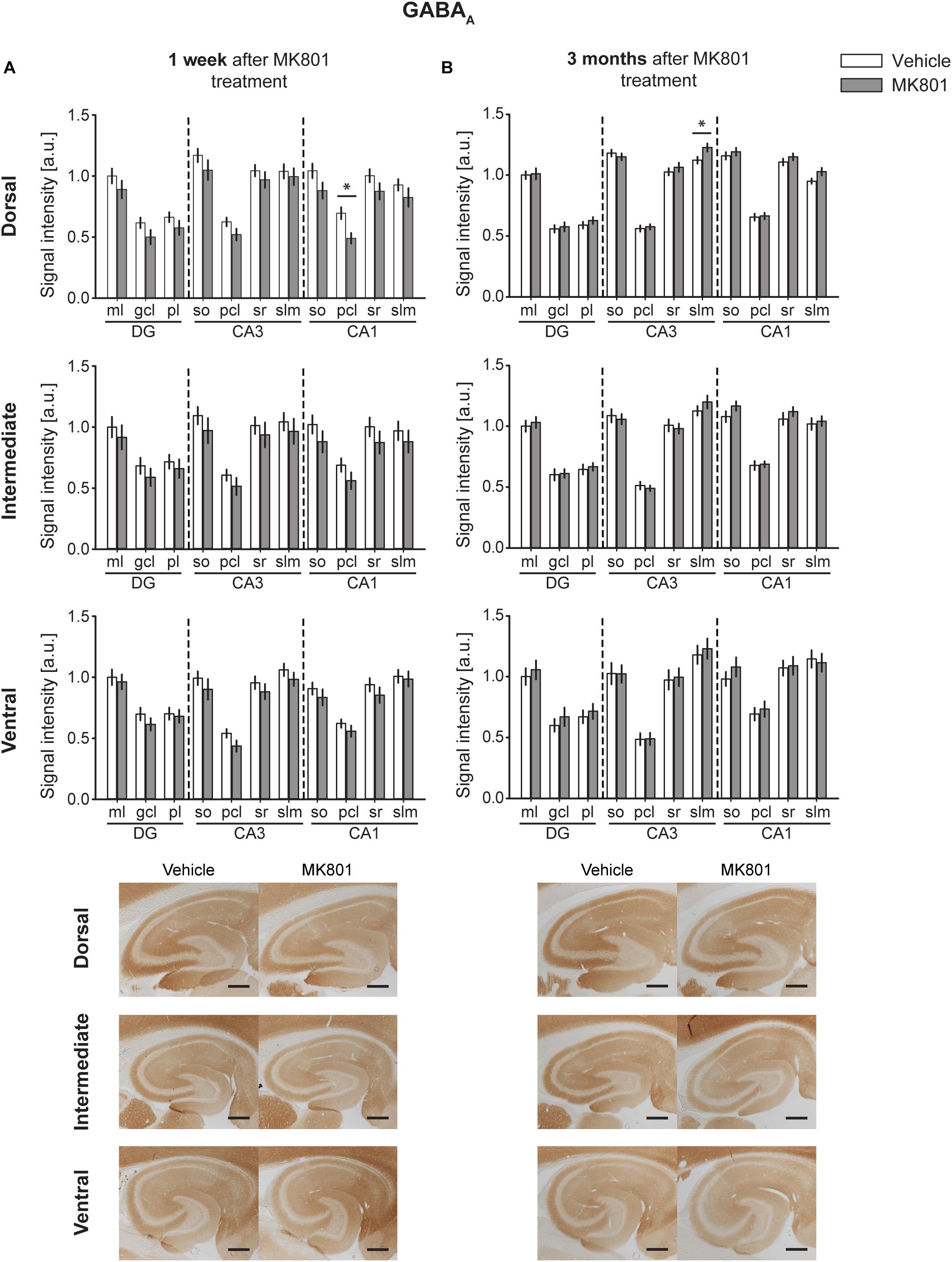
Figure 5. GABAA protein levels display no substantial changes 1 week or 3 months after MK801 treatment. Bar charts illustrate relative changes in receptor protein levels across the somato-dendritic layers of the transverse and longitudinal hippocampal axes. (A) No overall changes were detected 1 week after MK801 treatment. (B) Similarly, no overall changes were detected 3 months after MK801 treatment. Scale bar: 500 μm. Values expressed in arbitrary units (a.u.). Error bars indicate SEM. ∗p < 0.05. ml, molecular layer of the DG; gcl, granule cell layer of the DG; pl, polymorphic layer of the DG; so, Stratum oriens of CA3/CA1; pcl, pyramidal cell layer of CA3/CA1; sr, Stratum radiatum of CA3/CA1; and slm, Stratum lacunosum-moleculare of CA3/CA1. Photomicrographs provide examples of GABAA receptor-stained sections from the dorsal, intermediate and ventral hippocampal subdivisions that originated from the same vehicle- or MK801-treated animal, and correspond to 1 week (left) or 3 months (right) after treatment.
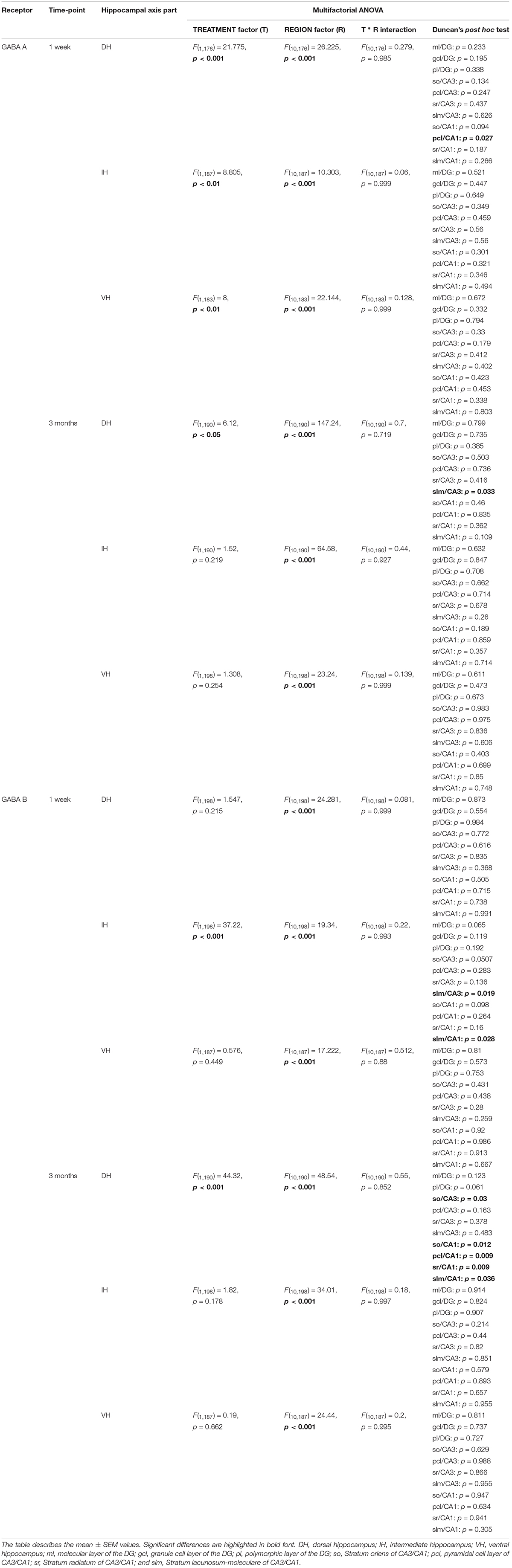
Table 2. An overview of statistically significant differences in the expression of GABAergic receptors.
GABAB Expression Is Downregulated After MK801 Application
One week following MK801 treatment, GABAB receptor changes occurred in the IH compared to controls (Figure 6A) [multifactorial ANOVA, Treatment factor, IH: F(1,198) = 37.22, p < 0.001]. Here, a significant down-regulation was seen in the so and slm of the IH CA3 and in the slm of the IH CA1 (Figure 6A and Table 2). Receptor expression in the DH and VH was equivalent to controls [multifactorial ANOVA, Treatment factor, DH: F(1,198) = 1.547, p = 0.215; VH: F(1,187) = 0.576, p = 0.449] (Table 2).
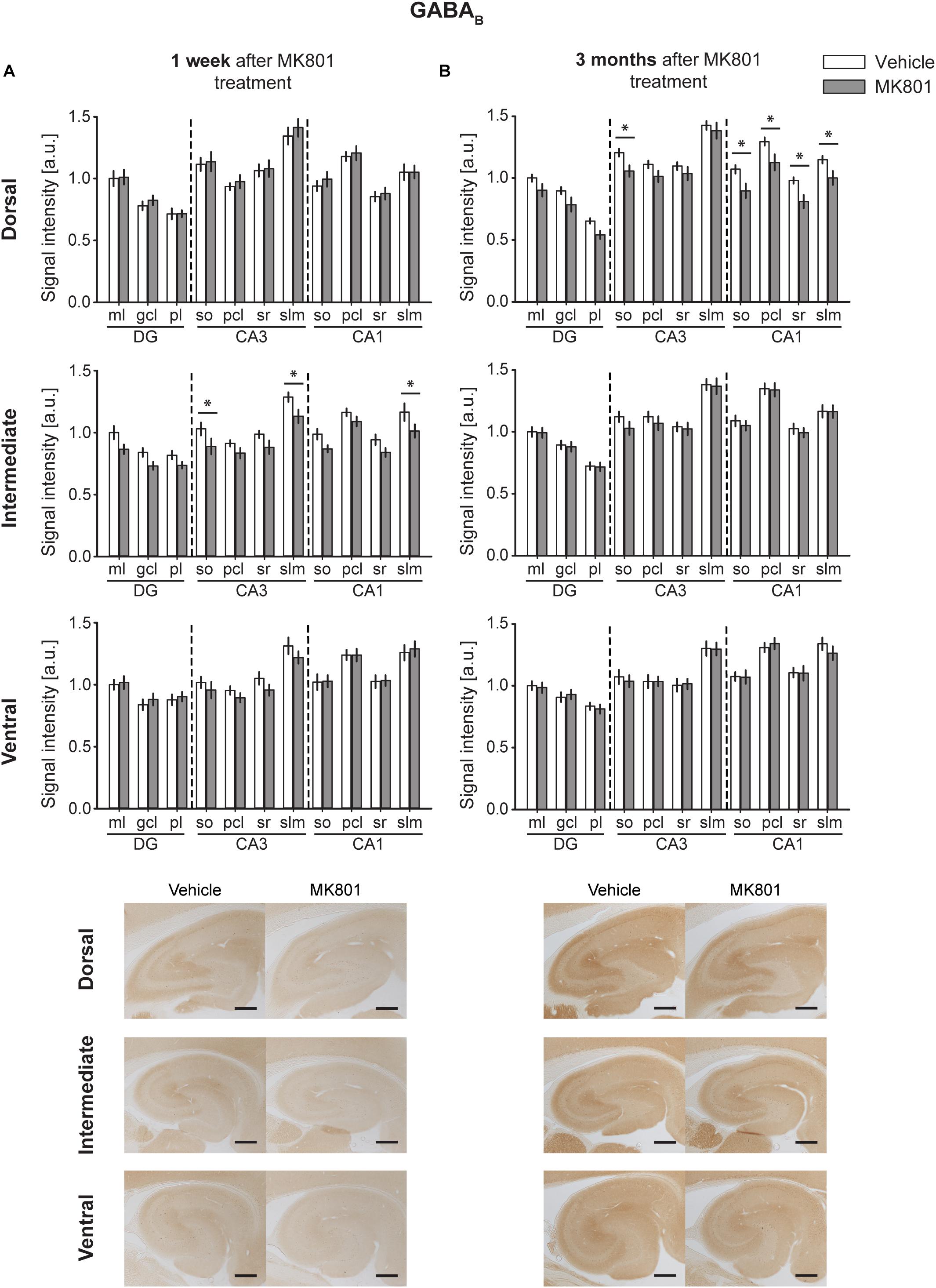
Figure 6. MK801 treatment leads to a down-regulation of GABAB receptor protein expression. Bar charts illustrate relative changes in receptor protein levels across the somato-dendritic layers of the transverse and longitudinal hippocampal axes. (A) A reduction of GABAB receptor expression was evident across the CA regions of the intermediate hippocampus 1 week after MK801 treatment. (B) Similarly, a reduction of GABAB receptor levels was detected across the dorsal CA regions 3 months after MK801 treatment. Scale bar: 500 μm. Values expressed in arbitrary units (a.u.). Error bars indicate SEM. ∗p < 0.05. ml, molecular layer of the DG; gcl, granule cell layer of the DG; pl, polymorphic layer of the DG; so, Stratum oriens of CA3/CA1; pcl, pyramidal cell layer of CA3/CA1; sr, Stratum radiatum of CA3/CA1; and slm, Stratum lacunosum-moleculare of CA3/CA1. Photomicrographs provide examples of GABAB receptor-stained sections from the dorsal, intermediate and ventral hippocampal subdivisions that originated from the same vehicle- or MK801-treated animal, and correspond to 1 week (left) or 3 months (right) after treatment.
Three months after MK801 treatment, no receptor changes were evident in the IH or VH [multifactorial ANOVA, Treatment factor, IH: F(1,198) = 1.82, p = 0.178; VH: F(1,187) = 0.19, p = 0.662] (Table 2), but a significant down-regulation in GABAB receptor levels was found across the entire DH cornus ammonis (Figure 6B): All layers (so, pcl, sr, and slm) of the CA1 and CA3 regions were significantly affected (Table 2).
MK801 Treatment Reduces the Amount of GAD67+ Cells in the Hippocampus
Our finding that GABAB receptor expression was potently downregulated 3 months after MK801-treatment might be explained by a loss of interneurons. To check this possibility, we assessed the number of GAD-expressing interneurons in the hippocampus 3 months after MK801 application (Figure 7). Here, we found a significant reduction in the amount of GAD67-positive cells in the DG (t-test, Vehicle: 69.21 ± 1.86 vs. MK801: 63.45 ± 1.86, p = 0.03, t = 2.185 with 90 degrees of freedom), CA3 (t-test, Vehicle: 54.06 ± 1.43 vs. MK801: 45.08 ± 1.4, p < 0.001, t = 4.471 with 88 degrees of freedom), and CA1 (t-test, Vehicle median: 52 vs. MK801 median: 45, Mann–Whitney U = 668, nV ehicle = 44, nMK801 = 47, p = 0.004) regions of the DH and in the CA3 (t-test, Vehicle: 51.27 ± 1.14 vs. MK801: 44.86 ± 1.27, p < 0.001, t = 3.736 with 88 degrees of freedom) and CA1 (t-test, Vehicle median: 41.5 vs. MK801 median: 36, Mann–Whitney U = 719, nV ehicle = 44, nMK801 = 46, p = 0.01) regions of the IH. No changes could be observed in the VH (t-test, DG, Vehicle: 53.78 ± 1.96 vs. MK801: 54.53 ± 2.08, p = 0.79, t = -0.263 with 83 degrees of freedom; CA3, Vehicle: 55.53 ± 1.55 vs. MK801: 54.84 ± 1.97, p = 0.78, t = 0.276 with 85 degrees of freedom), although a trend toward a decrease was detected in the CA1 region (t-test Vehicle: 43.19 ± 1.8 vs. MK801: 39.09 ± 1.75, p = 0.1, t = 1.628 with 84 degrees of freedom).
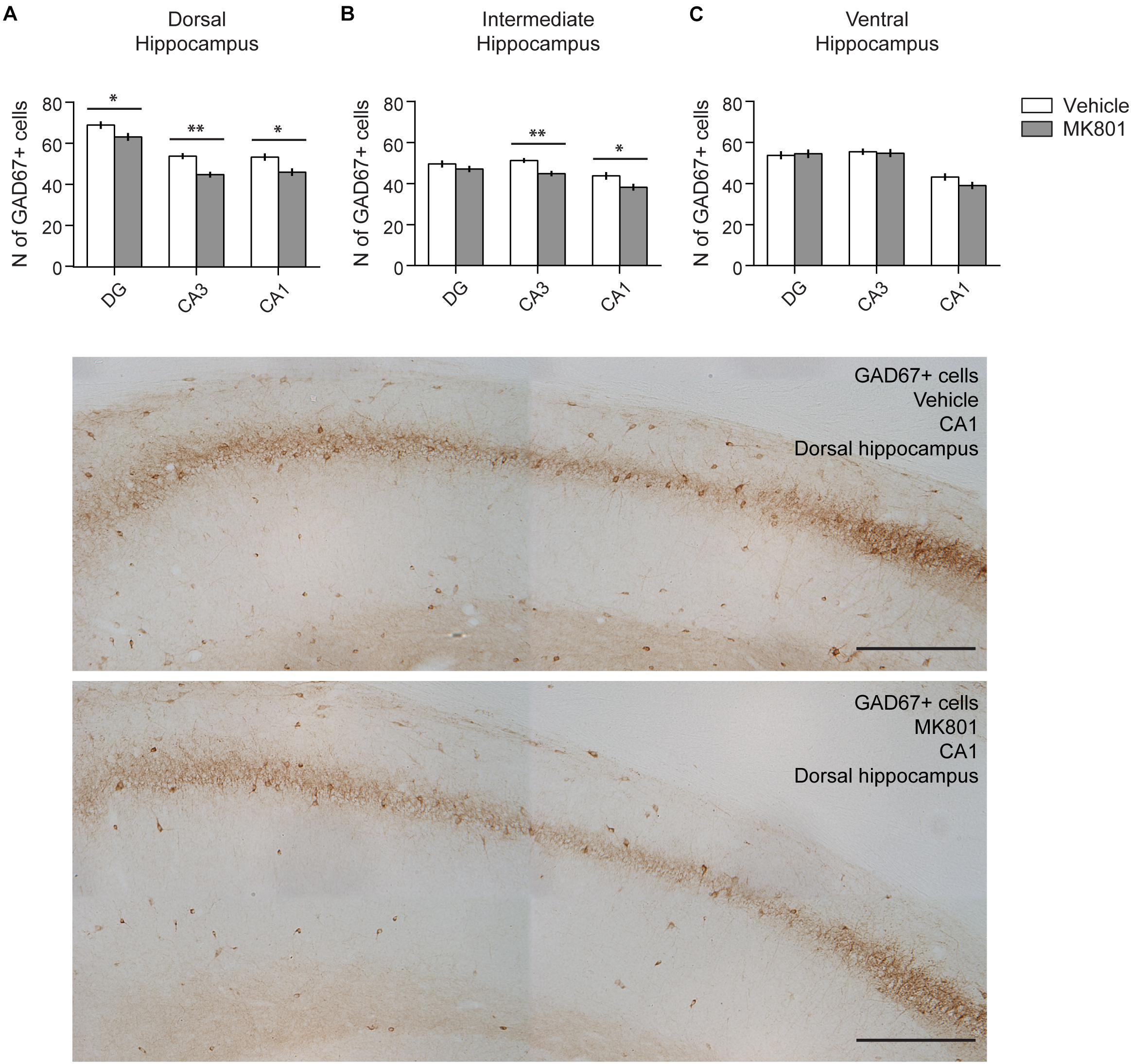
Figure 7. The amount of GAD67+ cells is reduced in the hippocampus after MK801 treatment. Bar charts summarize the number of interneurons 3 months after MK801 treatment across the transverse and longitudinal hippocampal axes: (A) dorsal, (B) intermediate, (C) ventral hippocampus. Whereas significant decreases were detected in subregions of the dorsal and intermediate hippocampus, no changes were observed in the ventral subdivision. Error bars indicate SEM. ∗p < 0.05, ∗∗p < 0.01. Examples of GAD67-stained sections from the dorsal, intermediate and ventral hippocampal subdivisions are shown below. Specifically, the CA1 region from vehicle and MK801-treated animals is shown. Scale bar: 300 μm.
Discussion
In this study, we demonstrate that acute induction of hypoglutamatergic signaling in rats persistently alters the expression of several metabotropic glutamatergic and GABAergic receptors across the hippocampus. Importantly, the profile of rapid (1 week) changes differs from subsequent (3 month) changes. These alterations in receptor expression are also specific to the region (longitudinal axis), subregion (transverse axis) and layer of the hippocampus. In addition, 3 months after treatment, a significant reduction in GAD67-positive interneurons is evident in both the dorsal and the intermediate hippocampus.
In line with evidence for disturbed glutamatergic neurotransmission in schizophrenia and psychosis (Lesage and Steckler, 2010; Lin et al., 2012; Matosin and Newell, 2013), we observed an early impairment in the expression of mGlu1 receptors across the dorso-intermediate hippocampal axis, as well as an upregulation of expression in the intermediate hippocampus. An early up-regulation in mGlu2/3 levels across the ventro-intermediate CA3 region also occurred, whereas GABAB receptor expression was reduced in the intermediate hippocampus. Three months after MK801-treatment, an increase in mGlu5 expression was evident across the entire CA1 region and intermediate DG. At the same time-point a down-regulation in the levels of GABAB receptors occurred across the dorsal hippocampus. These changes can be expected to affect information transfer along the longitudinal axis of the hippocampus (Tóth and Freund, 1992; Andersen et al., 2000), and contribute to disturbed hippocampal-prefrontal cortex communication in psychosis (Heckers et al., 1998; Grüter et al., 2015; Hartung et al., 2016).
The changes in receptor expression that occurred 1 week after MK801-treatment may reflect a rapid adaptive response of the brain to the first episode of psychosis. This in turn, may contribute to the pathological steps that increase brain vulnerability toward disease progression and establishment. These changes are transient, however. Three months after treatment, expression of GABAA, mGlu1, and mGlu2/3 receptors had largely normalized, with the exception of a focal decrease of mGlu2/3 receptor expression in the Stratum lacunosum-moleculare of the dorsal CA1 region and a focal elevation of GABAA expression in the Stratum lacunosum-moleculare of the dorsal CA3 region. At this time-point, an extensive elevation of mGlu5 receptor expression had developed across the CA1 region of the entire dorsoventral axis. Strikingly, this result matches a recent post-mortem finding of increased expression of mGlu5 receptors in the hippocampal CA1 region of subjects with schizophrenia (Matosin et al., 2015), possibly suggesting an established phenotype of receptor changes in our animal model 3 months after MK801 administration.
The rodent model of a psychosis-like event that we used here involves an acute treatment of adults rats with the NMDAR antagonist, MK801 (Wozniak et al., 1996; Manahan-Vaughan et al., 2008a,b; Wiescholleck and Manahan-Vaughan, 2013a,b). It triggers widespread changes in NMDAR expression across the hippocampus (Dubovyk and Manahan-Vaughan, 2018b). NMDAR hypofunction in GABAergic interneurons appears to be an important component of models defining impairments in both, humans with psychosis and in relevant animal models (Belforte et al., 2010; Nakazawa et al., 2012; Snyder and Gao, 2013; Cohen et al., 2015; Jézéquel et al., 2018). It offers an explanation for a wide-range of schizophrenia’s positive, negative and cognitive symptoms. A primary alteration in glutamate-GABA levels and consequent destabilization of the levels of other neurotransmitters throughout the brain are believed to underlie these effects (Snyder and Gao, 2013; Perez and Lodge, 2014). Both glutamatergic and GABAergic neurotransmission are linked to a multitude of intrinsic sensory and cognitive processes such as perception, learning and memory (Kaila, 1994; Paulsen and Moser, 1998; Bettler et al., 2004; Malenka and Bear, 2004; Kullmann et al., 2005; Mukherjee and Manahan-Vaughan, 2013; Kesner and Rolls, 2015). Interestingly, both metabotropic glutamate and GABAergic receptors modulate NMDAR function, as well as glutamate and GABA release (Tu et al., 1999; Benquet et al., 2002; Heidinger et al., 2002; Marino and Conn, 2002; Bettler et al., 2004; Kullmann et al., 2005).
The changes in GABAB receptor expression that we detected, is in line with a reported decrease in GABAB receptor expression in the hippocampus of patients suffering from schizophrenia (Mizukami et al., 2000) and is especially interesting given the reported role of GABAB receptors in maintaining neuronal homeostasis in hippocampal networks (Vertkin et al., 2015). GABAB receptors regulate neuronal population firing rate through sensing of ambient GABA levels. This is then transduced into homeostatic changes in synaptic strength (Gassmann and Bettler, 2012; Degro et al., 2015). In addition to the loss of GABAB receptors, 3 months after MK801 treatment, a significant loss of hippocampal interneurons was also detected in our study. A loss of interneurons combined with a decrease of GABAB receptors can be expected to result in a loss of experience-dependent GABA release, of GABA sensitivity and inhibitory tonus in the hippocampus. In line with this, a decrease in GABAB receptors is associated with aberrantly increased neuronal activity in rodents (Schuler et al., 2001; Gassmann et al., 2004). Furthermore, elevations in hippocampal excitability, coupled to aberrant somatic immediate early gene expression and deficits in hippocampus-dependent learning occur several weeks after MK801 treatment of rats (Grüter et al., 2015).
Both mGlu1 (Aiba et al., 1994; Naie and Manahan-Vaughan, 2005; Gil-Sanz et al., 2008; Neyman and Manahan-Vaughan, 2008) and mGlu5 receptors are required for the persistence of hippocampal synaptic plasticity and hippocampus-dependent spatial memory (Naie and Manahan-Vaughan, 2004; Manahan-Vaughan and Braunewell, 2005; Popkirov and Manahan-Vaughan, 2011). In the hippocampus, mGlu1 receptors are predominantly expressed on interneurons (Baude et al., 1993; Ferraguti et al., 1998, 2004). Their activation evokes slow oscillatory inward currents and elevates the frequency of spontaneous inhibitory postsynaptic currents and their amplitude in principal cells (van Hooft et al., 2000; Mannaioni et al., 2001; Lapointe et al., 2004). Hippocampal interneurons dampen neuronal excitability via mGlu1 receptor signaling that increases activation of interneurons and GABA release, (van Hooft et al., 2000; Lapointe et al., 2004). Furthermore, mGlu5 receptors support information transfer through neuronal oscillations in the hippocampus (Bikbaev et al., 2008; Bikbaev and Manahan-Vaughan, 2017). Thus, the changes in expression of these receptors may underlie changes in synaptic plasticity and neuronal oscillations that have been described in the rodent model studied here (Kalweit et al., 2017), as well neuronal oscillations in patients who have experienced a first-episode (Uhlhaas and Singer, 2010).
‘Synaptic gain control’ determines how neurons respond to their inputs as well as the intrinsic excitation-inhibition balance in neural networks (Díez et al., 2017). We propose that changes in the relative balance and distribution of GABA and glutamate receptors underlie the loss of synaptic gain control that has been previously described in this animal model of psychosis (Grüter et al., 2015; Kalweit et al., 2017) and may possibly comprise a fundamental mechanism underlying loss of synaptic gain control as proposed to occur in patients suffering from psychosis (Adams et al., 2013; Ranlund et al., 2016; Díez et al., 2017).
Of the hippocampal subdivisions affected by MK801 treatment, the dorsal hippocampus was the most vulnerable and the ventral hippocampus was the most resistant. Functionally, the convergence of long-term receptor changes in the dorsal hippocampal CA1 region suggests that impairments in memory retrieval and prediction error function will develop (Schultz and Dickinson, 2000). This means that mismatches will occur between external sensory information and internal representations (Hasselmo, 2005; Ji and Maren, 2008). In our animal model, this is reflected by impairments of object recognition memory, object-place memory, sensory gating and spatial reference memory (Grüter et al., 2015; Manahan-Vaughan et al., 2008a). In psychotic patients, this might be reflected by attention and motivation being applied to unimportant events, the formation of erroneous thoughts, disrupted cognition, and possibly delusions (Corlett et al., 2009; Schobel et al., 2013).
In summary, our findings suggest that in an animal model of psychosis that is accompanied by both short-term changes in NMDAR expression and long-term NMDAR hypofunction (Dubovyk and Manahan-Vaughan, 2018a), rapid changes in the expression of metabotropic glutamate receptors and GABA receptors are superseded by an extensive long-term upregulation of mGlu5 receptors in the CA1 regions of the entire dorsoventral hippocampal axis, along with a decrease of GABAB receptor expression across all layers of the dorsal CA1 region and a loss of interneurons. The CA1 region is arguably the output structure of the hippocampus that engages in mismatch detection and has been proposed to be especially vulnerable to NMDAR hypofunction (Lisman and Otmakhova, 2001). Our findings indicate that this structure is particularly susceptible to changes elicited by the instigation of a first episode psychosis-like state in rodents that in turn triggers receptor expression changes that are similar to those reported in schizophrenic patients. We propose that these chronic changes in glutamatergic and GABAergic receptor expression comprise a primary mechanism underlying loss of synaptic gain control that is believed to underlie many features of psychosis including functional disconnectivity, abnormal neuronal oscillations and loss of sensory attenuation (Adams et al., 2013; Díez et al., 2017).
Ethics Statement
This study was carried out in accordance with the European Communities Council Directive of September 22nd, 2010 (2010/63/EU) for care of laboratory animals. Prior permission was obtained from the local authorities (Landesamt für Arbeitsschutz, Umwelt- und Naturschutz, Nordrhein-Westfalen).
Author Contributions
DM-V created the concept and strategy of the study. VD carried out the experiments. DM-V and VD conducted data analysis and interpretation, as well as the writing of the manuscript.
Funding
This project was supported by grants (SFB 874/B1, Project No: 122679504 and SFB1280/A4, Project No: 316803389) from the German Research Foundation (Deutsche Forschungsgemeinschaft, DFG) to DM-V.
Conflict of Interest Statement
The authors declare that the research was conducted in the absence of any commercial or financial relationships that could be construed as a potential conflict of interest.
Acknowledgments
We gratefully thank Ute Neubacher and Beate Krenzek for technical assistance and both Nadine Kollosch and Petra Küsener for animal care.
References
Adams, R. A., Stephan, K. E., Brown, H. R., Frith, C. D., and Friston, K. J. (2013). The computational anatomy of psychosis. Front. Psychiatry 4:47.
Adriano, F., Caltagirone, C., and Spalletta, G. (2012). Hippocampal volume reduction in first-episode and chronic schizophrenia: a review and meta-analysis. Neuroscientist 18, 180–200. doi: 10.1177/1073858410395147
Aiba, A., Chen, C., Herrup, K., Rosenmund, C., Stevens, C. F., and Tonegawa, S. (1994). Reduced hippocampal long-term potentiation and context-specific deficit in associative learning in mGluR1 mutant mice. Cell 79, 365–375. doi: 10.1016/0092-8674(94)90204-6
Alagarsamy, S., Saugstad, J., Warren, L., Mansuy, I. M., Gereau, I. V. R. W., and Conn, P. J. (2005). NMDA-induced potentiation of mGlu5 is mediated by activation of protein phosphatase 2B/calcineurin. Neuropharmacology 49, 135–145. doi: 10.1016/j.neuropharm.2005.05.005
Altinbilek, B., and Manahan-Vaughan, D. (2009). A specific role for group II metabotropic glutamate receptors in hippocampal long-term depression and spatial memory. Neuroscience 158, 149–158. doi: 10.1016/j.neuroscience.2008.07.045
Alvarez-Jimenez, M., Gleeson, J. F., Henry, L. P., Harrigan, S. M., Harris, M. G., Amminger, G. P., et al. (2011). Prediction of a single psychotic episode: a 7.5-year, prospective study in first-episode psychosis. Schizophr. Res. 125, 236–246. doi: 10.1016/j.schres.2010.10.020
Andersen, P., Soleng, A. F., and Raastad, M. (2000). The hippocampal lamella hypothesis revisited. Brain Res. 886, 165–171. doi: 10.1016/s0006-8993(00)02991-7
Arnold, O. H. (1999). Schizophrenia – a disturbance of signal interaction between the entorhinal cortex and the dentate dyrus? The contribution of experimental dibenamine psychosis to the pathogenesis of schizophrenia: a hypothesis. Neuropsychobiology 40, 21–32. doi: 10.1159/000026593
Avery, S. N., Rogers, B. P., and Heckers, S. (2018). Hippocampal network modularity is associated with relational memory dysfunction in schizophrenia. Biol. Psychiatry Cogn. Neurosci. Neuroimaging 3, 423–432. doi: 10.1016/j.bpsc.2018.02.001
Bak, N., Rostrup, E., Larsson, H. B., Glenthoj, B. Y., and Oranje, B. (2014). Concurrent functional magnetic resonance imaging and electroencephalography assessment of sensory gating in schizophrenia. Hum. Brain Mapp. 35, 3578–3587. doi: 10.1002/hbm.22422
Bast, T., and Feldon, J. (2003). Hippocampal modulation of sensorimotor processes. Prog. Neurobiol. 70, 319–345. doi: 10.1016/s0301-0082(03)00112-6
Bast, T., Wilson, I. A., Witter, M. P., and Morris, R. G. M. (2009). From rapid place learning to behavioral performance: a key role for the intermediate hippocampus. PLoS Biol. 7:e1000089. doi: 10.1371/journal.pbio.1000089
Baude, A., Nusser, Z., Roberts, J. D. B., Mulvihill, E., Mcllhinney, R. A. J., and Somogyi, P. (1993). The metabotropic glutamate receptor (mGluR1a) is concentrated at perisynaptic membrane of neuronal subpopulations as detected by immunogold reaction. Neuron 11, 771–787. doi: 10.1016/0896-6273(93)90086-7
Behrendt, R. P. (2010). Contribution of hippocampal region CA3 to consciousness and schizophrenic hallucinations. Neurosci. Biobehav. Rev. 34, 1121–1136. doi: 10.1016/j.neubiorev.2009.12.009
Belforte, J. E., Zsiros, V., Sklar, E. R., Jiang, Z., Yu, G., Li, Y., et al. (2010). Postnatal NMDA receptor ablation in corticolimbic interneurons confers schizophrenia-like phenotypes. Nat. Neurosci. 13, 76–83. doi: 10.1038/nn.2447
Benquet, P., Gee, C. E., and Gerber, U. (2002). Two distinct signaling pathways upregulate NMDA receptor responses via two distinct metabotropic glutamate receptor subtypes. J. Neurosci. 22, 9679–9686. doi: 10.1523/jneurosci.22-22-09679.2002
Bettler, B., Kaupmann, K., Mosbacher, J., and Gassmann, M. (2004). Molecular structure and physiological functions of GABAB receptors. Physiol. Rev. 84, 835–867.
Bikbaev, A., and Manahan-Vaughan, D. (2017). Metabotropic glutamate receptor, mGlu5, regulates hippocampal synaptic plasticity and is required for tetanisation-triggered changes in theta and gamma oscillations. Neuropharmacology 115, 20–29. doi: 10.1016/j.neuropharm.2016.06.004
Bikbaev, A., Neyman, S., Ngomba, R., Nicoletti, F., Conn, P. J., and Manahan-Vaughan, D. (2008). The metabotropic glutamate receptor, mGluR5, mediates the functional interaction between late-LTP, hippocampal network activity, and learning by a mechanism involving regulation of mGluR1 expression. PLoS One 3:e2155. doi: 10.1371/journal.pone.0002155
Borgwardt, S. J., McGuire, P. K., Aston, J., Gschwandtner, U., Pflüger, M. O., Stieglitz, R. D., et al. (2008). Reductions in frontal, temporal and parietal volume associated with the onset of psychosis. Schizophr. Res. 106, 108–114. doi: 10.1016/j.schres.2008.08.007
Bowie, C. R., and Harvey, P. D. (2005). Cognition in schizophrenia: impairments, determinants, and functional importance. Psychiatr. Clin. N. Am. 28, 613–633. doi: 10.1016/j.psc.2005.05.004
Bürgy, M. (2008). The concept of psychosis: historical and phenomenological aspects. Schizophr. Bull. 34, 1200–1210. doi: 10.1093/schbul/sbm136
Cheung, V., Chiu, C. P. Y., Law, C. W., Cheung, C., Hui, C. L. M., Chan, K. K. S., et al. (2010). Positive symptoms and white matter microstructure in never-medicated first episode schizophrenia. Psychol. Med. 41, 1709–1719. doi: 10.1017/S003329171000156X
Cohen, S. M., Tsien, R. W., Goff, D. C., and Halassa, M. M. (2015). The impact of NMDA receptor hypofunction on GABAergic neurons in the pathophysiology of schizophrenia. Schizophr. Res. 167, 98–107. doi: 10.1016/j.schres.2014.12.026
Compton, M. T., Fantes, F., Wan, C. R., Johnson, S., and Walker, E. F. (2015). Abnormal movements in first-episode, nonaffective psychosis: dyskinesias, stereotypies, and catatonic-like signs. Psychiatry Res. 226, 192–197. doi: 10.1016/j.psychres.2014.12.048
Connelly, W. M., Errington, A. C., Di Giovanni, G., and Crunelli, V. (2013). Metabotropic regulation of extrasynaptic GABAA receptors. Front. Neural Circ. 7:171. doi: 10.3389/fncir.2013.00171
Corlett, P. R., Honey, G. D., Krystal, J. H., and Fletcher, P. C. (2011). Glutamatergic model psychoses: prediction error, learning, and inference. Neuropsychopharmacology 36, 294–315. doi: 10.1038/npp.2010.163
Corlett, P. R., Krystal, J. H., Taylor, J. R., and Fletcher, P. C. (2009). Why do delusions persist? Front. Hum. Neurosci. 3:12. doi: 10.3389/neuro.09.012.2009
Davis, M. C., Horan, W. P., and Marder, S. R. (2014). Psychopharmacology of the negative symptoms: current status and prospects for progress. Eur. Neuropsychopharmacol. 24, 788–799. doi: 10.1016/j.euroneuro.2013.10.010
Degro, C. E., Kulik, A., Booker, S. A., and Vida, I. (2015). Compartmental distribution of GABAB receptor-mediated currents along the somatodendritic axis of hippocampal principal cells. Front. Synaptic Neurosci. 7:6. doi: 10.3389/fnsyn.2015.00006
Díez,Á, Ranlund, S., Pinotsis, D., Calafato, S., Shaikh, M., Hall, M. H., et al. (2017). Abnormal frontoparietal synaptic gain mediating the P300 in patients with psychotic disorder and their unaffected relatives. Hum. Brain Mapp. 38, 3262–3276. doi: 10.1002/hbm.23588
Dubovyk, V., and Manahan-Vaughan, D. (2018a). Less means more: the magnitude of synaptic plasticity along the hippocampal dorso-ventral axis is inversely related to the expression levels of plasticity-related neurotransmitter receptors. Hippocampus 28, 136–150. doi: 10.1002/hipo.22816
Dubovyk, V., and Manahan-Vaughan, D. (2018b). Time-dependent alterations in the expression of NMDA receptor subunits along the dorsoventral hippocampal axis in an animal model of nascent psychosis. ACS Chem. Neurosci. 9, 2241–2251. doi: 10.1021/acschemneuro.8b00017
Fanselow, M. S., and Dong, H. W. (2010). Are the dorsal and ventral hippocampus functionally distinct structures? Neuron 65, 1–20.
Ferraguti, F., Cobden, P., Pollard, M., Cope, D., Shigemoto, R., Watanabe, M., et al. (2004). Immunolocalization of metabotropic glutamate receptor 1a (mGluR1a) in distinct classes of interneuron in the CA1 region of the rat hippocampus. Hippocampus 14, 193–215. doi: 10.1002/hipo.10163
Ferraguti, F., Conquet, F., Corti, C., Grandes, P., Kuhn, R., and Knopfel, T. (1998). Immunohistochemical localization of the mGluR1β metabotropic glutamate receptor in the adult rodent forebrain: evidence for a differential distribution of mGluR1 splice variants. J. Comp. Neurol. 400, 391–407. doi: 10.1002/(sici)1096-9861(19981026)400:3<391::aid-cne8>3.0.co;2-3
Friston, K. J. (1998). The disconnection hypothesis. Schizophr. Res. 30, 115–125. doi: 10.1016/s0920-9964(97)00140-0
Gassmann, M., and Bettler, B. (2012). Regulation of neuronal GABAB receptor functions by subunit composition. Nat. Rev. 13, 380–394. doi: 10.1038/nrn3249
Gassmann, M., Shaban, H., Vigot, R., Sansig, G., Haller, C., Barbieri, S., et al. (2004). Redistribution of GABAB(1) protein and atypical GABAB responses in GABAB(2)-deficient mice. J. Neurosci. 24, 6086–6097. doi: 10.1523/jneurosci.5635-03.2004
Gil-Sanz, C., Delgado-Garcia, J. M., Fairen, A., and Gruart, A. (2008). Involvement of the mGluR1 receptor in hippocampal synaptic plasticity and associative learning in behaving mice. Cereb Cortex 18, 1653–1663. doi: 10.1093/cercor/bhm193
Gonzalez-Burgos, G., and Lewis, D. A. (2012). NMDA receptor hypofunction, parvalbumin-positive neurons, and cortical gamma oscillations in schizophrenia. Schizophr. Bull. 38, 950–957. doi: 10.1093/schbul/sbs010
Grüter, T., Wiescholleck, V., Dubovyk, V., Aliane, V., and Manahan-Vaughan, D. (2015). Altered neuronal excitability underlies impaired hippocampal function in an animal model of psychosis. Front. Behav. Neurosci. 9:117. doi: 10.3389/fnbeh.2015.00117
Guo, X., Li, J., Wei, Q., Fan, X., Kennedy, D. N., Shen, Y., et al. (2013). Duration of untreated psychosis is associated with temporal and occipitotemporal gray matted volume decrease in treatment naïve schizophrenia. PLoS One 8:e83679. doi: 10.1371/journal.pone.0083679
Häfner, H., Maurer, K., Löffler, W., Fätkenheuer, B., an der Heiden, W., Riecher-Rössler, A., et al. (1994). The epidemiology of early schizophrenia. Influence of age and gender on onset and early course. Br. J. Psychiatry 164, 29–38. doi: 10.1192/s0007125000292714
Haijima, S. V., Van Haren, N., Cahn, W., Koolschijn, P. C., Hulshoff, P. H., and Kahn, R. S. (2012). Brain volumes in schizophrenia: a meta-analysis in over 18 000 subjects. Schizophr. Bull. 39, 1129–1138. doi: 10.1093/schbul/sbs118
Hartung, H., Cichon, N., De Feo, V., Riemann, S., Schildt, S., Lindemann, C., et al. (2016). From shortage to surge: a developmental switch in hippocampal-prefrontal coupling in a gene-environment model of neuropsychiatric disorders. Cereb Cortex 26, 4265–4281. doi: 10.1093/cercor/bhw274
Hasselmo, M. E. (2005). The role of hippocampal regions CA3 and CA1 in matching entorhinal input with retrieval of associations between objects and context: theoretical comment on Lee et al. (2005). Behav. Neurosci. 119, 342–345. doi: 10.1037/0735-7044.119.1.342
Heckers, S., and Konradi, C. (2002). Hippocampal neurons in schizophrenia. J. Neural Transm. 109, 891–905. doi: 10.1007/s007020200073
Heckers, S., Rauch, S. L., Goff, D., Savage, C. R., Schacter, D. L., Fischman, A. J., et al. (1998). Impaired recruitment of the hippocampus during conscious recollection in schizophrenia. Nat. Neurosci. 1, 318–323. doi: 10.1038/1137
Heidinger, V., Manzerra, P., Wang, Z. Q., Strasser, U., Yu, S.-P., Choi, D. W., et al. (2002). Metabotropic glutamate receptor 1-induced upregulation of NMDA receptor current: mediation through the Pyk2/Src-family kinase pathway in cortical neurons. J. Neurosci. 22, 5452–5461. doi: 10.1523/jneurosci.22-13-05452.2002
Jézéquel, J., Lepleux, M., Kahn, R. S., Honnorat, J., Leboyer, M., and Groc, L. (2018). Molecular pathogenicity of anti-NMDA receptor autoantibody from patients with first-episode psychosis. Am. J. Psychiatry 175, 382–383. doi: 10.1176/appi.ajp.2017.17091053
Ji, J., and Maren, S. (2008). Differential roles for hippocampal areas CA1 and CA3 in the contextual encoding and retrieval of extinguished fear. Learn. Mem. 15, 244–251. doi: 10.1101/lm.794808
Kaila, K. (1994). Ionic basis of GABA A receptor channel function in the nervous system. Prog. Neurobiol. 42, 489–537. doi: 10.1016/0301-0082(94)90049-3
Kalweit, A. N., Amanpour-Gharaei, B., Colitti-Klausnitzer, J., and Manahan-Vaughan, D. (2017). Changer in neuronal oscillations accompany the loss of hippocampal LTP that occurs in an animal model of psychosis. Front. Behav. Neurosci. 11:36. doi: 10.3389/fnbeh.2017.00036
Kapur, S. (2003). Psychosis as a state of aberrant salience: a framework linking biology, phenomenology, and pharmacology in schizophrenia. Am. J. Psychiatry 160, 13–23. doi: 10.1176/appi.ajp.160.1.13
Keefe, R. S. E., and Harvey, P. D. (2012). Cognitive impairment in schizophrenia. Handb. Exp. Pharmacol. 213, 11–37.
Kehrer, C., Dugladze, T., Maziashvili, N., Wójtowicz, A., Schmitz, D., Heinemann, U., et al. (2007). Increased inhibitory input to CA1 pyramidal cells alters hippocampal gamma frequency oscillations in the MK-801 model of acute psychosis. Neurobiol. Dis. 25, 545–552. doi: 10.1016/j.nbd.2006.10.015
Kemp, A., and Manahan-Vaughan, D. (2007). Hippocampal long-term depression: master or minion in declarative memory processes? Trends Neurosci. 30, 111–118. doi: 10.1016/j.tins.2007.01.002
Keshavan, M. S., DeLisi, L. E., and Seidman, L. J. (2011). Early and broadly defined psychotic risk mental states. Schizophr. Res. 126, 1–10. doi: 10.1016/j.schres.2010.10.006
Kesner, R. P. (2013a). An analysis of the dentate gyrus function. Behav. Brain Res. 254, 1–7. doi: 10.1016/j.bbr.2013.01.012
Kesner, R. P. (2013b). A process analysis of the CA3 subregion of the hippocampus. Front. Cell Neurosci. 7:78. doi: 10.3389/fncel.2013.00078
Kesner, R. P., and Rolls, E. T. (2015). A computational theory of hippocampal function, and tests of the theory: new developments. Neurosci. Behav. Rev. 48, 92–147. doi: 10.1016/j.neubiorev.2014.11.009
Kim, J. S., Baek, J. H., Choi, J. S., Lee, D., Kwon, J. S., and Hong, K. S. (2011). Diagnostic stability of first-episode psychosis and predictors of diagnostic shift from non-affective psychosis to bipolar disorder: a retrospective evaluation after recurrence. Psychiatry Res. 188, 29–33. doi: 10.1016/j.psychres.2010.09.017
Kobayashi, K., Manabe, T., and Takahashi, T. (1996). Presynaptic long-term depression at the hippocampal mossy fiber-CA3 synapse. Science 273, 648–650. doi: 10.1126/science.273.5275.648
Kolomeets, N. S., Orlovskaya, D. D., and Uranova, N. A. (2007). Decreased numerical density of CA3 hippocampal mossy fiber synapses in schizophrenia. Synapse 61, 615–621. doi: 10.1002/syn.20405
Kreutz, C., Bartolome Rodriguez, M. M., Maiwald, T., Seidl, M., Blum, H. E., Mohr, L., et al. (2007). An error model for protein quantification. Bioinformatics 20, 2747–2753. doi: 10.1093/bioinformatics/btm397
Krystal, J. H., Karper, L. P., Seibyl, J. P., Freeman, G. K., Delaney, R., Bremner, J. D., et al. (1994). Subanesthetic effects of the noncompetitive NMDA antagonist, ketamine, in humans. Psychotomimetic, perceptual, cognitive, and neuroendocrine responses. Arch. Gen. Psychiatry 51, 199–214.
Kullmann, D. M., Ruiz, A., Rusakov, D. M., Scott, R., Semyanov, A., and Walker, M. C. (2005). Presynaptic, extrasynaptic and axonal GABA A receptors in the CNS: where and why? Prog. Biophys. Mol. Biol. 87, 33–46. doi: 10.1016/j.pbiomolbio.2004.06.003
Lahti, A. C., Koffel, B., LaPorte, D., and Tamminga, C. A. (1995). Subanesthetic doses of ketamine stimulate psychosis in schizophrenia. Neuropsychopharmacology 13, 9–19. doi: 10.1016/0893-133x(94)00131-i
Lapointe, V., Morin, F., Ratte, S., Croce, A., Conquet, F., and Lacaille, J. C. (2004). Synapse-specific mGluR1-dependent long-term potentiation in interneurons regulates mouse hippocampal inhibition. J. Physiol. 555, 125–135. doi: 10.1113/jphysiol.2003.053603
Larson, M. K., Walker, E. F., and Compton, M. T. (2010). Early signs, diagnosis and therapeutics of the prodromal phase of schizophrenia and related psychotic disorders. Expert Rev. Neurother. 10, 1347–1359. doi: 10.1586/ern.10.93
Lesage, A., and Steckler, T. (2010). Metabotropic glutamate mGlu1 receptor stimulation and blockade: therapeutic opportunities in psychiatric illness. Eur. J. Pharmacol. 639, 2–16. doi: 10.1016/j.ejphar.2009.12.043
Lin, C.-H., Lane, H.-Y., and Tsai, G. E. (2012). Glutamate signaling in the pathophysiology and therapy of schizophrenia. Pharmacol. Biochem. Behav. 100, 665–677. doi: 10.1016/j.pbb.2011.03.023
Linden, A. M., Baez, M., Bergeron, M., and Schoepp, D. D. (2006). Effects of mGlu2 or mGlu3 receptor deletions on mGlu2/3 receptor agonist (LY354740)-induced brain c-Fos expression: specific roles for mGlu2 in the amygdala and subcortical nuclei, and mGlu3 in the hippocampus. Neuropharmacology 51, 213–228. doi: 10.1016/j.neuropharm.2006.03.014
Lisman, J. E., and Otmakhova, N. A. (2001). Storage, recall, and novelty detection of sequences by the hippocampus: elaborating on the SOCRATIC model to account for normal and aberrant effects of dopamine. Hippocampus 11, 551–568. doi: 10.1002/hipo.1071.abs
Ludewig, K., Geyer, M. A., and Vollenweider, F. X. (2003). Deficits in prepulse inhibition and habituation in never-medicated, first-episode schizophrenia. Biol. Psychiatry 54, 121–128. doi: 10.1016/s0006-3223(02)01925-x
Manahan-Vaughan, D. (1998). Priming of group 2 metabotropic glutamate receptors facilitates induction of long-term depression in the dentate gyrus of freely moving rats. Neuropharmacology 37, 1459–1464. doi: 10.1016/s0028-3908(98)00150-6
Manahan-Vaughan, D., and Braunewell, K. H. (2005). The metabotropic glutamate receptor, mGluR5, is a key determinant of good and bad spatial learning performance and hippocampal synaptic plasticity. Cereb Cortex 15, 1703–1713. doi: 10.1093/cercor/bhi047
Manahan-Vaughan, D., von Haebler, D., Winter, C., Juckel, G., and Heinemann, U. (2008a). A single application of MK801 causes symptoms of acute psychosis, deficits in spatial memory, and impairment of synaptic plasticity in rats. Hippocampus 18, 125–134. doi: 10.1002/hipo.20367
Manahan-Vaughan, D., Wildförster, V., and Thomsen, C. (2008b). Rescue of hippocampal LTP and learning deficits in a rat model of psychosis by inhibition of glycine transporter-1 (GlyT1). Eur. J. Neurosci. 28, 1342–1350. doi: 10.1111/j.1460-9568.2008.06433.x
Mannaioni, G., Marino, M. J., Valenti, O., Traynelis, S. F., and Conn, P. J. (2001). Metabotropic glutamate receptors 1 and 5 differentially regulate CA1 pyramidal cell function. J. Neurosci. 21, 5925–5934. doi: 10.1523/jneurosci.21-16-05925.2001
Marek, G. J., Behl, B., Bespalov, A. Y., Gross, G., Lee, Y., and Schoemaker, H. (2010). Glutamatergic (N-methyl-D-aspartate receptor) hypofrontality in schizophrenia: too little juice or miswired brain? Mol. Pharmacol. 77, 317–326. doi: 10.1124/mol.109.059865
Marino, M. J., and Conn, P. J. (2002). Direct and indirect modulation of the N-methyl D-aspartate receptor. Curr. Drug Targets CNS Neurol. Disord. 1, 1–16. doi: 10.2174/1568007023339544
Matosin, N., Fernandez-Enright, F., Lum, J. S., Andrews, J. L., Engel, M., Huang, X.-F., et al. (2015). Metabotropic glutamate receptor 5, and its trafficking molecules Norbin and Tamalin, are increased in the CA1 hippocampal region of subjects with schizophrenia. Schizophr. Res. 166, 212–218. doi: 10.1016/j.schres.2015.05.001
Matosin, N., and Newell, K. A. (2013). Metabotropic glutamate receptor 5 in the pathology and treatment of schizophrenia. Neurosci. Behav. Rev. 37, 256–268. doi: 10.1016/j.neubiorev.2012.12.005
McGhie, A., and Chapman, J. (1961). Disorders of attention and perception in early schizophrenia. Br. J. Med. Psychol. 34, 103–116. doi: 10.1111/j.2044-8341.1961.tb00936.x
Mizukami, K., Sasaki, M., Ishikawa, M., Iwakiri, M., Hidaka, S., Shiraishi, H., et al. (2000). Immunohistochemical localization of γ-aminobutyric acidB receptor in the hippocampus of subjects with schizophrenia. Neurosci. Lett. 283, 101–104. doi: 10.1016/s0304-3940(00)00939-3
Mukherjee, S., and Manahan-Vaughan, D. (2013). Role of metabotropic glutamate receptors in persistent forms of hippocampal plasticity and learning. Neuropharmacology 66, 65–81. doi: 10.1016/j.neuropharm.2012.06.005
Naie, K., and Manahan-Vaughan, D. (2004). Regulation by metabotropic glutamate receptor 5 of LTP in the dentate gyrus of freely moving rats: relevance for learning and memory formation. Cereb Cortex 14, 189–198. doi: 10.1093/cercor/bhg118
Naie, K., and Manahan-Vaughan, D. (2005). Pharmacological antagonism of metabotropic glutamate receptor 1 regulates long-term potentiation and spatial reference memory in the dentate gyrus of freely moving rats via N-methyl-D-aspartate and metabotropic glutamate receptor-dependent mechanisms. Eur. J. Neurosci. 21, 411–421. doi: 10.1111/j.1460-9568.2005.03864.x
Nakazawa, K., Zsiros, V., Jiang, Z., Nakao, K., Kolata, S., Zhang, S., et al. (2012). GABAergic interneuron origin of schizophrenia pathophysiology. Neuropharmacology 62, 1574–1583. doi: 10.1016/j.neuropharm.2011.01.022
Neyman, S., and Manahan-Vaughan, D. (2008). Metabotropic glutamate receptor 1 (mGluR1) and 5 (mGluR5) regulate late phases of LTP and LTD in the hippocampal CA1 region in vitro. Eur. J. Neurosci. 27, 1345–1352. doi: 10.1111/j.1460-9568.2008.06109.x
Paulsen, O., and Moser, E. I. (1998). A model of hippocampal memory encoding and retrieval: GABAergic control of synaptic plasticity. Trends Neurosci. 21, 273–278. doi: 10.1016/s0166-2236(97)01205-8
Paxinos, G., and Watson, C. (1982). The Rat Brain in Stereotaxic Coordinates. San Diego, CA: Academic Press.
Perez, S. M., and Lodge, D. J. (2014). New approaches to the management of schizophrenia: focus on aberrant hippocampal drive of dopamine pathways. Drug Design Dev. Ther. 8, 887–896. doi: 10.2147/DDDT.S42708
Popkirov, S. G., and Manahan-Vaughan, D. (2011). Involvement of the metabotropic glutamate receptor mGluR5 in NMDA receptor-dependent, learning-facilitated long-term depression in CA1 synapses. Cereb Cortex 21, 501–509. doi: 10.1093/cercor/bhq093
Pöschel, B., and Manahan-Vaughan, D. (2007). Persistent ( > 24h) long-term depression in the dentate gyrus of freely moving rats is not dependent on activation of NMDA receptors, L-type voltage-gated calcium channels or protein synthesis. Neuropharmacology 52, 46–54. doi: 10.1016/j.neuropharm.2006.07.019
Ranlund, S., Adams, R. A., Díez,Á, Constante, M., Dutt, A., Hall, M. H., et al. (2016). Impaired prefrontal synaptic gain in people with psychosis and their relatives during the mismatch negativity. Hum. Brain Mapp. 37, 351–365. doi: 10.1002/hbm.23035
Riebe, I., Seth, H., Culley, G., Dosa, Z., Radi, S., Strand, K., et al. (2016). Tonically active NMDA receptors – a signalling mechanism critical for interneuronal excitability in the CA1 stratum radiatum. Eur. J. Neurosci. 43, 169–178. doi: 10.1111/ejn.13128
Ruby, E., Polito, S., McMahon, K., Gorovitz, M., Corcoran, C., and Malaspina, D. (2014). Pathways associating childhood trauma to the neurobiology of schizophrenia. Front. Psychol. Behav. Sci. 3:1–17.
Samudra, N., Ivleva, E. I., Hubbard, N. A., Rypma, B., Sweeney, J. A., Clementz, B. A., et al. (2015). Alterations in hippocampal connectivity across the psychosis dimension. Psychiatry Res. 233, 148–157. doi: 10.1016/j.pscychresns.2015.06.004
Schobel, S. A., Chaudhury, N. H., Khan, U. A., Paniagua, B., Styner, M. A., Asllani, I., et al. (2013). Imaging patients with psychosis and a mouse model establishes a spreading pattern of hippocampal dysfunction and implicates glutamate as a driver. Neuron 78, 81–93. doi: 10.1016/j.neuron.2013.02.011
Schoepp, D. D. (2001). Unveiling the functions of presynaptic metabotropic glutamate receptors in the central nervous system. J. Pharmacol. Exp. Ther. 299, 12–20.
Schuler, V., Lüscher, C., Blanchet, C., Klix, N., Sansig, G., Klebs, K., et al. (2001). Epilepsy, hyperalgesia, impaired memory, and loss of pre- and postsynaptic GABA(B) responses in mice lacking GABA(B(1)). Neuron 31, 47–58. doi: 10.1016/s0896-6273(01)00345-2
Schultz, W., and Dickinson, A. (2000). Neural coding of prediction errors. Annu. Rev. Neurosci. 23, 473–500.
Shen, W., Nan, C., Nelson, P. T., Ripps, H., and Slaughter, M. M. (2017). GABAB receptor attenuation of GABAA currents in neurons of the mammalian central nervous system. Physiol. Rep. 5, 1–11.
Shigemoto, R., Kinoshita, A., Wada, E., Nomura, S., Ohishi, H., Takada, M., et al. (1997). Differential presynaptic localization of metabotropic glutamate receptor subtypes in the rat hippocampus. J. Neurosci. 17, 7503–7522. doi: 10.1523/jneurosci.17-19-07503.1997
Snyder, M. A., and Gao, W. J. (2013). NMDA hypofunction as a convergence point for progression and symptoms of schizophrenia. Front. Cell. Neurosci. 7:31. doi: 10.3389/fncel.2013.00031
Stephan, K. E., Baldeweg, T., and Friston, K. J. (2006). Synaptic plasticity and dysconnection in schizophrenia. Biol. Psychiatry 59, 929–939. doi: 10.1016/j.biopsych.2005.10.005
Stephan, K. E., Friston, K. J., and Frith, C. D. (2009). Dysconnection in schizophrenia: from abnormal synaptic plasticity to failures of self-monitoring. Schizophr. Bull. 35, 509–527. doi: 10.1093/schbul/sbn176
Strange, B. A., Witter, M. P., Lein, E. S., and Moser, E. I. (2014). Functional organization of the hippocampal longitudinal axis. Nat. Rev. Neurosci. 15, 655–669. doi: 10.1038/nrn3785
Tóth, K., and Freund, T. F. (1992). Calbindin D28k-containing nonpyramidal cells in the rat hippocampus: their immunoreactivity for GABA and projection to the medial septum. Neuroscience 49, 793–805. doi: 10.1016/0306-4522(92)90357-8
Tu, J. C., Xiao, B., Naisbitt, S., Yuan, J. P., Petralia, R. S., Brakeman, P., et al. (1999). Coupling of mGluR/Homer and PSD-95 complexes by the Shank family of postsynaptic density proteins. Neuron 23, 583–592. doi: 10.1016/s0896-6273(00)80810-7
Uhlhaas, P. J., and Singer, W. (2010). Abnormal neural oscillations and synchrony in schizophrenia. Nat Rev Neurosci. 11, 100–113. doi: 10.1038/nrn2774
van Hooft, J. A., Giuffrida, R., Blatow, M., and Monyer, H. (2000). Differential expression of group I metabotropic glutamate receptors in functionally distinct hippocampal interneurons. J. Neurosci. 20, 3544–3551. doi: 10.1523/jneurosci.20-10-03544.2000
Velligan, D. I., and Alphs, L. D. (2008). Negative symptoms in schizophrenia: the importance of identification and treatment. Psychiatr. Times 25, 1–6.
Vertkin, I., Styr, B., Slomowitz, E., Ofir, N., Shapira, I., Berner, D., et al. (2015). GABAB receptor deficiency causes failure of neuronal homeostasis in hippocampal networks. Proc. Natl. Acad. Sci. U.S.A. 112, E3291–E3299. doi: 10.1073/pnas.1424810112
von der Heyde, S., Sonntag, J., Kaschek, D., Bender, C., Bues, J., and Wachter, A. (2014). RPPanalyzer toolbox: an improved R package for analysis of reverse phase protein array data. Biotechniques 57, 125–135. doi: 10.2144/000114205
Waldmeier, P. C., Kaupmann, K., and Urwyler, S. (2008). Roles of GABAB receptor subtypes in presynaptic auto- and heteroreceptor function regulating GABA and glutamate release. J. Neural Transm. 115, 1401–1411. doi: 10.1007/s00702-008-0095-7
Wiescholleck, V., and Manahan-Vaughan, D. (2012). PDE4 inhibition enchances hippocampal synaptic plasticity in vivo and rescues MK801-induced impairment of long-term potentiation and object recognition memory in an animal model of psychosis. Transl. Psychiatry 2:e89. doi: 10.1038/tp.2012.17
Wiescholleck, V., and Manahan-Vaughan, D. (2013a). Long-lasting changes in hippocampal synaptic plasticity and cognition in an animal model of NMDA receptor dysfunction in psychosis. Neuropharmacology 74, 48–58. doi: 10.1016/j.neuropharm.2013.01.001
Wiescholleck, V., and Manahan-Vaughan, D. (2013b). Persistent deficits in hippocampal synaptic plasticity accompany losses of hippocampus-dependent memory in a rat model of psychosis. Front. Integr. Neurosci. 7:12. doi: 10.3389/fnint.2013.00012
Wlodarczyk, A. I., Sylantyev, S., Herd, M. B., Kersante, F., Lambert, J. J., Rusakov, D. A., et al. (2013). GABA-independent GABAA receptor openings maintain tonic currents. J. Neurosci. 33, 3905–3914. doi: 10.1523/JNEUROSCI.4193-12.2013
Wöhrl, R., Eisenach, S., Manahan-Vaughan, D., Heinemann, U., and von Haebler, D. (2007). Acute and long-term effects of MK801 on direct cortical input evoked homosynaptic and heterosynaptic plasticity in the CA1 region of the female rat. Eur. J. Neurosci. 26, 2873–2883. doi: 10.1111/j.1460-9568.2007.05899.x
Wozniak, D. F., Brosnan-Watters, G., Nardi, A., McEwen, M., Corso, T. D., Olney, J. W., et al. (1996). MK-801 neurotoxicity in male mice: histologic effects and chronic impairment in spatial learning. Brain Res. 707, 165–179. doi: 10.1016/0006-8993(95)01230-3
Yin, D. M., Chen, Y. J., Sathyamurthy, A., Xiong, W. C., and Mei, L. (2012). Synaptic dysfunction in schizophrenia. Adv. Exp. Med. Biol. 970, 493–516. doi: 10.1007/978-3-7091-0932-8_22
Zemoura, K., Balakrishnan, K., Grampp, T., and Benke, D. (2018). Ca2+/Calmodulin-dependent protein kinase II (CaMKII) β-dependent phosphorylation of GABAB1 triggers lysosomal degradation of GABAB receptors via Mind Bomb-2 (MIB2)-mediated Lys-63-linked ubiquitination. Mol. Neurobiol. 56, 1293–1309. doi: 10.1007/s12035-018-1142-5
Keywords: psychosis, MK801, hippocampus, dorsoventral axis, mGlu receptors, GABA receptors, rodent
Citation: Dubovyk V and Manahan-Vaughan D (2019) Distinct Time-Course of Alterations of Groups I and II Metabotropic Glutamate Receptor and GABAergic Receptor Expression Along the Dorsoventral Hippocampal Axis in an Animal Model of Psychosis. Front. Behav. Neurosci. 13:98. doi: 10.3389/fnbeh.2019.00098
Received: 21 December 2018; Accepted: 23 April 2019;
Published: 08 May 2019.
Edited by:
Gregg Stanwood, Florida State University, United StatesReviewed by:
Juan Olaya, Neuroscience Research Australia (NeuRA), AustraliaDevon L. Graham, Florida State University College of Medicine, United States
Copyright © 2019 Dubovyk and Manahan-Vaughan. This is an open-access article distributed under the terms of the Creative Commons Attribution License (CC BY). The use, distribution or reproduction in other forums is permitted, provided the original author(s) and the copyright owner(s) are credited and that the original publication in this journal is cited, in accordance with accepted academic practice. No use, distribution or reproduction is permitted which does not comply with these terms.
*Correspondence: Denise Manahan-Vaughan, ZGVuaXNlLm1hbmFoYW4tdmF1Z2hhbkBydWIuZGU=; ZG12LWlnc25AcnViLmRl