- 1Department of Neurology, The First Affiliated Hospital, University of South China, Hengyang, China
- 2Department of Neurology, The Affiliated Nanhua Hospital, University of South China, Hengyang, China
- 3Institute of Neuroscience, Hengyang Medical College, University of South China, Hengyang, China
Background and Aim: Accumulation of β2-microglobulin (B2M), a systemic pro-aging factor, regulates negatively cognitive function. Hydrogen sulfide (H2S), a novel gas signaling molecule, exerts protection against cognitive dysfunction. Therefore, the present work was designed to explore whether H2S attenuates cognitive dysfunction induced by B2M and the underlying mechanism.
Materials and Methods: The cognitive function of rats was assessed by Y-maze, Novel object recognition (NOR), and Morris water maze (MWM) tests. The levels of autophagosome and autolysosome in hippocampus were observed by transmission electron microscopy. The expression of p62 protein in hippocampus was detected by western blot analysis.
Results: NaHS (a donor of H2S) significantly alleviated cognitive impairments in the B2M-exposed rats tested by Y-maze test, NOR test and MWM test. Furthermore, NaHS recovered autophagic flux in the hippocampus of B2M-exposed rats, as evidenced by decreases in the ratio of autophagosome to autolysosome and the expression of p62 protein in the hippocampus.
Conclusion: In summary, these data indicated that H2S attenuates B2M-induced cognitive dysfunction, involving in recovery of the blocked autophagic flux in the hippocampus, and suggested that H2S may be a novel approach to prevent B2M-induced cognitive dysfunction.
Introduction
β2-microglobulin (B2M), a component of major histocompatibility complex class 1 (MHC I) molecules, acts independently on its canonical immune function to regulate normal brain development, synaptic plasticity and related behaviors (Zijlstra et al., 1990; Huh et al., 2000; Jennifer et al., 2003; Boulanger and Shatz, 2004; Goddard et al., 2007; Shatz, 2009; Glynn et al., 2011; Hanmi et al., 2014). It is confirmed that systemic or local administration of B2M into hippocampus impairs the function of learning and memory, and there is a possibility to reverse B2M-induced cognitive defects when the excessive B2M is removed (Smith et al., 2015). The level of B2M is elevated in aging process (Yang et al., 2017), Alzheimer’s disease (AD) and HIV-associated dementia (Mcarthur et al., 1992; Brew et al., 1996; Carrette et al., 2003). In addition, increasing works have demonstrated that B2M is an independent risk factor for AD (Villeda et al., 2011; Lida et al., 2014). Thus, it is necessary for us to find approaches to antagonize B2M-induced cognitive dysfunction, which lead to the development of new strategies for treatment of AD.
Hydrogen sulfide (H2S), as the third gaseous signaling molecule (Łowicka and Beltowski, 2007; Szabo, 2007), plays an important role in learning and memory function (Whiteman et al., 2011). It has been demonstrated that H2S promotes the formation of hippocampal long-term potentiation (LTP) (Bliss and Collingridge, 1993; Abe and Kimura, 1996; Kimura, 2000), and attenuates cognitive deficits induced by lipopolysaccharide or beta-amyloid (Gong et al., 2011; Liu et al., 2015, 2016). Similarly, we have previously reported that H2S reverses homocysteine-induced impairments in learning and memory (Li et al., 2014, 2016). In the present work, we explored whether H2S ameliorates cognitive dysfunction caused by B2M in the rodent model.
Autophagy occurs as a cellular response to both extracellular stress conditions and intracellular signals and is essential for cellular growth and survival (Feng et al., 2018). The dynamic process of autophagy, termed “autophagic flux,” involves the rearrangement of subcellular membranes to sequester cytoplasm and organelles, which are delivered to the lysosome or vacuole to degrade and recycle (Levine and Kroemer, 2008). It has been proved that autophagic flux is closely related to presynaptic morphology, postsynaptic morphology and neuronal remodeling (Liang and Sigrist, 2018), associated with the enhancement of memory. The inhibition of autophagic flux was observed in mice with cognitive deficits, while activation of autophagic flux rescues these impairments of synaptic plasticity and cognition (Hara et al., 2006; Nikoletopoulou et al., 2017; Yan et al., 2018). Moreover, activating autophagy also alleviated cognitive dysfunction caused by chronic unpredictable mild stress and morphine (Gu et al., 2014; Pan et al., 2016). These findings suggest a potential protective role of autophagic flux in cognitive function. Interestingly, H2S regulates endoplasmic reticulum stress-mediated autophagy and autophagic neuronal cell death (Xie et al., 2017), which confirms the positive effect of H2S on regulating autophagic flux. Therefore, we evaluated whether H2S modulates the autophagic flux in the hippocampus of B2M-exposed rats.
In the present work, we have demonstrated that H2S ameliorates cognitive dysfunction induced by B2M and reverses the inhibition of autophagic flux in the hippocampus of B2M-exposed rats. These data suggested that H2S has the potential to ameliorate cognitive dysfunction induced by B2M, and may provide a new target for prevention of AD.
Materials and Methods
Experimental Schedule
The rats were pretreated with NaHS for 7 days and B2M was co-treated in the 8th day. After continuously treated with NaHS for 2 weeks, all rats were subjected to behavior tests. Finally, the hippocampal autophagy levels of rats were detected by Transmission electron microscopy (TEM) and Western blot (WB) (Figure 1).
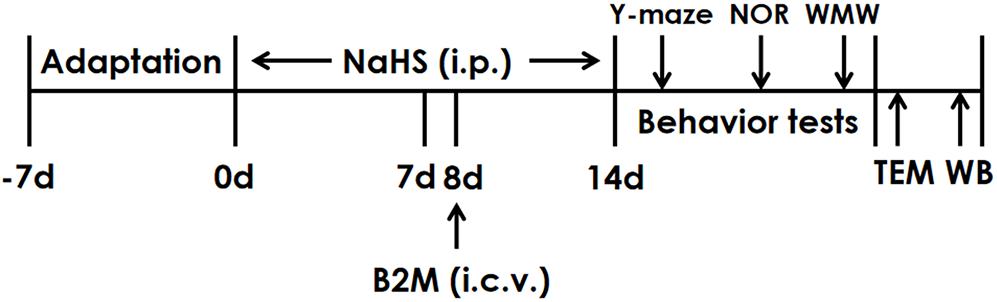
Figure 1. The schematic diagram of the experimental schedule. Y-maze, Y-maze test; NOR, Novel object recognition test; MWM, Morris water maze test; TEM, Transmission electron microscopy; WB, Western blot.
Animals
Adult male Sprague-Dawley (SD) rats (250–300 g) were obtained from the Hunan SJA Laboratory Animal Center (Changsha, Hunan, China). They were housed individually with free access to food and water under a normal 12 h light/dark schedule (lights on at 7:00 a.m.). Housing temperature was maintained at (22 ± 2)°C and relative humidity of 55 ± 5%. Before the beginning of the experiments, rats were allowed 7 days to adapt themselves to the housing conditions. According to the National Institutes of Health Guide for the Care and Use of Laboratory Animals, all the procedures were strictly implemented and approved by the Animal Use and Protection Committee of University of South China.
Reagents
Sodium hydrosulfide (NaHS, a donor of H2S) and β2-microglobulin were purchased from Sigma (Sigma, St. Louis, MO, United States). Anti-p62 antibody was purchased from Cell signaling Technology (Boston, MA, United States). Beta-actin antibody was purchased from Proteintech (Danvers, MA, United States).
Drug Treatment Protocols
A total of 1.68 mg or 5.6 mg of NaHS was dissolved in 1 mL of phosphate-buffered saline (PBS) to equal concentrations of NaHS 30 or 100 μmol/mL, respectively. A total of 0.3 μg of B2M was diluted in 3 μL PBS and intracerebroventricularly injected (i.c.v.) at a dose of 3 μL.
Lateral Ventricle Cannulation
Rats were firstly anesthetized with sodium pentobarbital (60 mg/kg, i.p., Sigma, St. Louis, MO, United States) and then placed in stereotaxic apparatus for operation. Using the coordinates (AP, −1.0 mm; ML, + 2 mm; DV, + 3 mm), a permanent guide cannula was aseptically and stereotaxically implanted into the right lateral ventricle of rats. After stereotaxic surgery, home-cage behaviors and wound healing of rats were monitored. It was an inclusion criterion that body weight recovered to no less than 90% of presurgery amount within 7 days.
Intracerebroventricular Injection
Under the control of micropump, 3 μl PBS and 3 μl B2M (0.1 μg/μl) were respectively injected into the lateral ventricle with an injection rate of 0.5 μl per min. In order to make sure that the entire injection had been delivered, the injection cannula was allowed to remain for additional 5 min before removed.
Y-Maze Test
The Y-maze is a Y-shaped platform that consists of three arms with 50 cm length and 10 cm width. And each arm was surrounded on three sides by 40 cm high walls. The three arms are connected in open ends centrally and positioned radially at 120° angles (Stoelting Co., Wood Dale, IL, United States). Each rat was placed at the center of the maze firstly and then allowed to explore freely for 5 min. Movement was recorded by an overhead camera and analyzed by the Any-maze software (SD Instruments, San Diego, CA, United States). Sequences of entering arm and numbers of entering each arm were recorded, respectively. Memory was defined by the frequency of alternately exploring different arms and was determined using the formula: total number of alternations/(total number of arm entries−2) × 100%.
Novel Objects Recognition (NOR) Test
The NOR test is consisted of three parts: adaptation, training and testing. Two days before training, rats were adapted to the arena (50 cm × 50 cm × 60 cm) for 5 min once a day without objects. During the training part, two different objects (A and B) were placed in the testing arena. Each rat was allowed to explore the objects for 5 min. The rat was considered to be exploring the object when its head was facing the object, or the rat was touching or sniffing the object. The total time spent exploring each object was recorded by same trained observer blind to treatments, and expressed as percentage of total exploration time. In the testing part, one identical and one novel objects (A and C) were used. A rat was allowed to explore the objects for 5 min, and the time spent exploring each object was recorded. The discrimination index = [(novel object−familiar object)/(novel object + familiar object)] × 100%, was used to measure the cognitive function of rats.
Morris Water Maze (MWM) Test
The water maze was a circular pool with diameter 180 cm and height 60 cm. The water temperature (23 ± 2)°C, light intensity, external cues in the room, and water opacity were rigorously reproduced. A transparent Plexiglas non-slippery platform (diameter 12.5 cm) was immersed under the water surface (2 cm) during acquisition phase. By a camera capture and video track software, swimming track of each rat was recorded and analyzed. The pool was divided by the software into four quadrants. As the distal spatial extra-maze cues for the rats to find the platform, several landmarks were fixed to the walls of the water maze room.
Acquisition Phase
The place navigation training consisted of four times of swimming per day for 5 days, and four start positions were randomly selected. And each rat was allowed a 120 s-swim to find the platform. Once the rat reached the platform, it was left 20 s on the platform, but if a rat did not reach the platform within 120 s, it was guided to the platform to remain for 20 s. The path and the escape latencies were recorded by an MT-200 Morris image motion system (Chengdu Taimeng Technology & Market Co., Chengdu, China).
Probe Phase
The probe test was performed after 24 h of the last swim on the 5th day. The platform was removed and each rat was allowed a free 120 s-swim. Rats were placed into the pool from another quadrant, which was opposite to the target quadrant, the former platform quadrant. The percentage of time spent in the target quadrant and the number of times that the same rat crossed the quadrant were determined.
Visible Platform Test
After the probe phase, visual, motor, and motivation skills were also tested with a visible platform test to eliminate the possible deficits of rats in sensorimotor processes. The platform was raised 2 cm above the water surface. The platform was moved to a novel quadrant in the pool at a fixed location for the four consisted trials, and the latency to reach the platform and the average speed were recorded.
Tissue Sampling
The hippocampal tissue is grinded and shredded in the tissue grinder after adding ice physiological water (nine times the weight of tissue). Then, the cell lysate and PMSF are added according to the proportion of 100:1, and fully lapped to 30 min. Finally, the liquid in the grinder is transferred into the EP tube and put into 4°C centrifuged for 10 min. The supernatant fluid was separated and packed. The BCA protein quantitative kit was quantified and stored at −20°C.
Western Blot Analysis for p62 Protein Expression
According to the molecular weight of p62, equivalent amount of proteins were run on SDS–PAGE gel (12% for p62). The electrophoresis was carried out with the constant pressure of 80 V. When the protein in the lanes ran to the junction point of the concentrating and the separating glue, the voltage was transformed into 120 V at constant pressure to continue the electrophoresis, and stopped until it ran to the bottom. According to the molecular weight of target protein, suitable size gel was cut. Then, “sandwich” (filter paper + glue + PVDF membrane + filter paper), wet transfer method constant flow membrane (300 mA, 30 min), the PVDF membrane is cut into the appropriate size and soaked in absolute methanol. After transferred to a PVDF membrane, the PVDF membrane was blocked using TBST buffer (50 mM Tris–HCl, pH 7.5, 150 mM NaCl, 0.05% Tween-20) containing 5% non-fat milk at room temperature for 2 h. Then the PVDF membrane was incubated by primary antibody of the target protein and internal reference protein (anti-p62, 1:1000; anti-beta-actin, 1:2000) overnight at 4°C. After the PVDF membrane was washed three times (10 min each time) with TBST, secondary antibody (1:5000) with 5% non-fat milk were added to incubate the PVDF membrane 2 h, and then washed for three times (10 min each time). Finally, the expression of protein p62 was analyzed by Image J on the gel imager.
Transmission Electron Microscopy Analysis for Hippocampal Autophagy Level of Rats
After all behavior experiments were completed, two rats of each group were anesthetized with the configured pentobarbital sodium. The brain tissues of rats were separated quickly. The blood clot and impurities on the surface of the brain tissue of the isolated rat were gently rinsed with ice physiological saline, and then the brain tissue was separated in the ice water. The hippocampal tissue was exposed to 5 pieces of hippocampal tissue of about 1–2 mm3 and fixed overnight at 4°C refrigerator. The following day, the sample was sent to Shanghai Zhicheng Biological Technology Co., Ltd., for sample embedding (after cleaning with 0.1 M phosphoric acid buffer for three times, then using 1% osmium acid at 4°C to fix 3 h). Then the buffer solution was cleaned three times, the ethanol was dehydrated, the epoxy propane was replaced, and the Spurr resin was soaked and buried. Finally it was polymerized at 70°C. The embedded blocks of different materials were sliced on the ultrathin section machine. The thickness of ultrathin sections was 70 nm, stained with Uranyl acetate and lead citrate, and observed and photographed under transmission electron microscope.
Statistical Analysis
Statistical analysis was performed by SPSS soft version 20.0 (Chicago, IL, United States). Between-group effects on escape latency in the MWM task was analyzed by repeated-measures analysis of two-way ANOVA with group and time as the factors followed by the least significant difference (LSD) post hoc test. Statistical analyses of other parameters were carried out using one-way ANOVA followed by the LSD post hoc test. The data are expressed as the mean ± standard error of the mean (SEM), and P < 0.05 was considered statistically significant.
Results
H2S Improves the Cognitive Function of B2M-Treated Rats in Y-Maze Test
To investigate whether H2S mitigates the cognitive dysfunction of B2M-exposed rats, we examined the cognitive function of rats using the Y-maze test. As shown in Figure 2A, the correct rate of alternation in B2M-exposed rats was significantly lower than that in the control group, indicated that intracerebroventricular injection of B2M leads to impairment in the learning and memory of rats. However, treatment with NaHS (30 or 100 μmol/kg/d, i.p.) significantly increased the correct rate of alternation in the B2M-exposed rats (Figure 2A). In addition, the total times of rats entering each arm in the five groups was not statistically significant (Figure 2B). These results showed that H2S improves the cognitive ability of B2M-exposed rats.
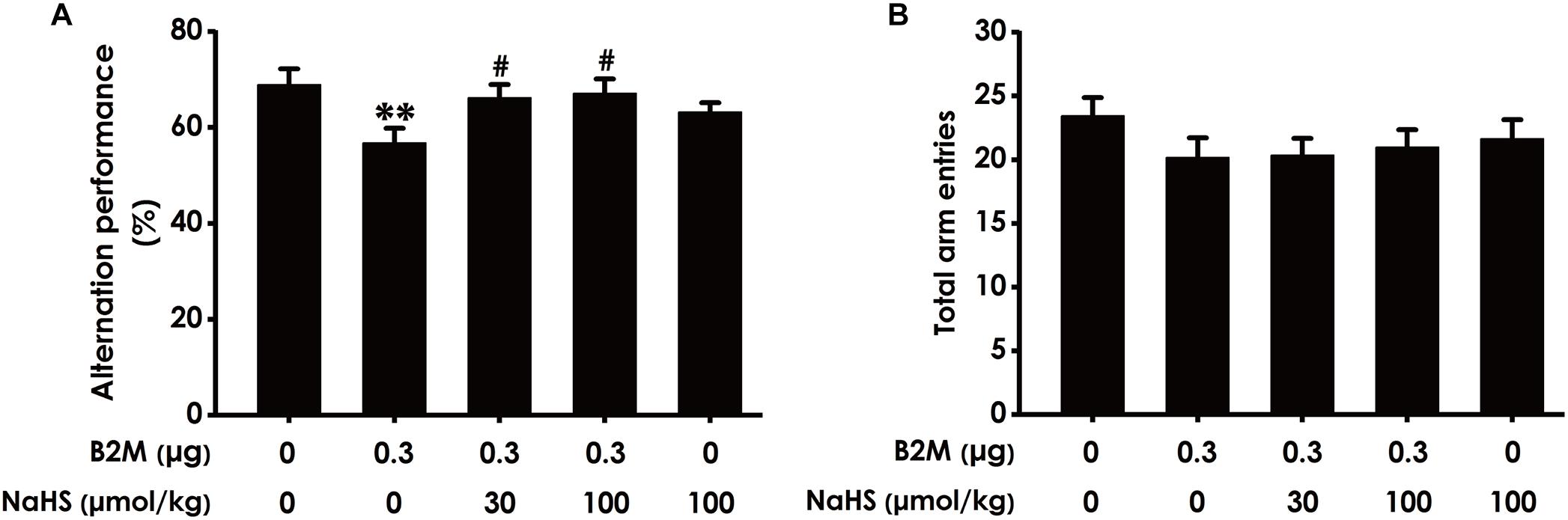
Figure 2. Effect of H2S on B2M-induced cognitive impairments of rats in Y-maze test. Seven days after intracerebroventricular administration of B2M (0.3 μg), the rats were submitted to the Y-maze test. The alternation performance (A) and the total arm entries (B) of rats in each group were recorded. Values were represented as mean ± SEM (n = 8–12); ∗∗P < 0.01, vs. control group; #P < 0.05, vs. B2M-treated alone group.
H2S Ameliorates the Cognitive Dysfunction of B2M-Exposed Rats in NOR Test
To further investigate whether H2S ameliorates the cognitive impairment in B2M-exposed rats, we also examined the cognitive function of rats using the NOR test. As shown in Figure 3A, the discrimination index in B2M-exposed rats was significantly decreased compared with the control group. However, NaHS (30 or 100 μmol/kg/d, i.p.) significantly increased the discrimination index of B2M-exposed rats. In addition, the total exploration time among these five groups had no significant difference (Figure 3B). Taken together, these data also suggested that H2S reverses the cognitive impairment induced by B2M.
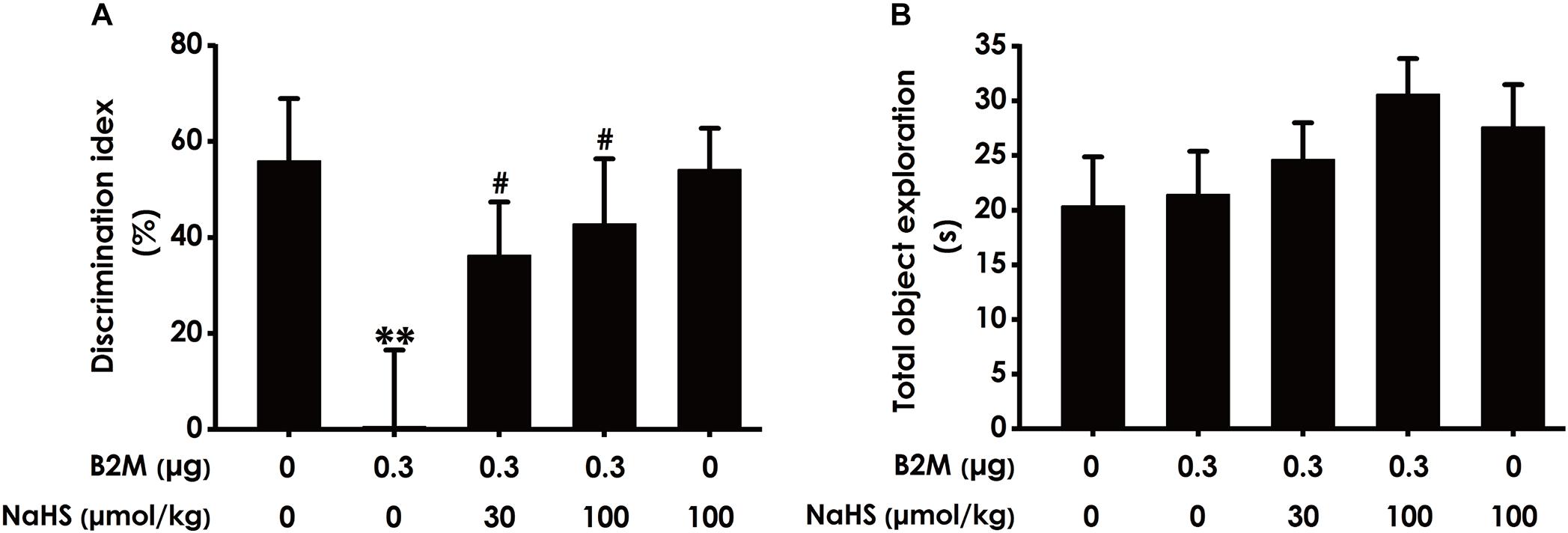
Figure 3. Effect of H2S on B2M-induced cognitive impairments of rats in Novel object recognition test. After the Y-maze test, the rats were submitted to the novel object recognition test. The discrimination index (A) and the total exploration time (B) of rats in each group were recorded. Values were represented as mean ± SEM (n = 8–12); ∗∗P < 0.01, vs. control group; #P < 0.05, vs. B2M-treated alone group.
H2S Enhances Spatial Learning and Memory of B2M-Treated Rats in MWM Test
We also used the MWM test to investigate the protective role of H2S in the cognitive dysfunction of B2M-treated rats. The latency to find the platform in the acquisition phase is shown in Figures 4A–C. All five groups during the five training days exhibited a decrease in the escape latency (Figures 4A–C). B2M-treated alone rats exhibited significantly longer in escape latency in the 1st, 2nd, 4th, and 5th training day compared with the control group (Figure 4A), which implies a significant impairment of spatial learning in B2M-exposed rats. However, treatment with NaHS (30 or 100 μmol/kg/d, i.p.) significantly decreased the escape latency of B2M-treated alone rats in the 4th and 5th training day (Figure 4B). The escape latency in NaHS-treated alone rats was not significantly different from the control group (Figures 4C). Figure 4D shows the representative swimming tracks of rats searching for the underwater platform on the 1st and 5th training days. On the 1st training day, there was no difference of the distance in searching for the hidden platform among these five groups (Figure 4D). On the 5th training day, compared with the control group, B2M-treated alone rats exhibited a significant increase in the swimming distance, while the rats cotreated with NaHS (30 or 100 μmol/kg/d, i.p.) and B2M showed a significant decrease in the swimming distance compared with the B2M-treated alone group (Figure 4D).
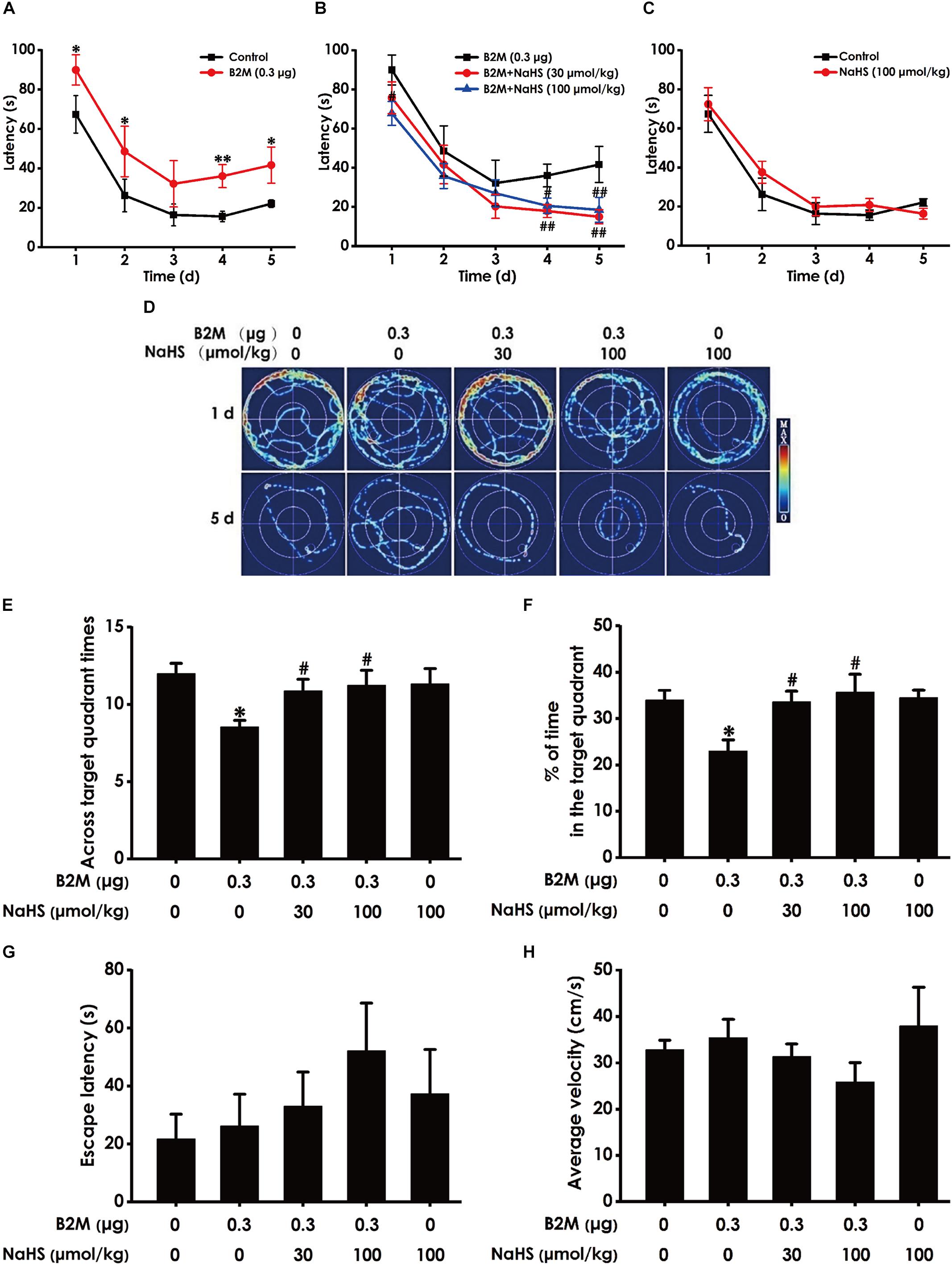
Figure 4. Effect of H2S on B2M-induced cognitive impairments of rats in Morris water maze test. After finishing NOR Test, the rats were tested by the Morris water maze test. Rats were submitted to acquisition phase of an invisible platform placed in a fixed location (target quadrant) with four times swim per day during 5 days. After finishing the acquisition phase, rats were submitted by the probe trail without platform, and then the visible platform test with platform. The latency time (A–C) and the swimming tracks (D) of rats searching for the underwater platform at the 1st to 5th training days were recorded. The times that rats crossed target quadrant (E) and the percentage of time spent in the target quadrant (F) in the probe trail were analyzed. The latency to reach the platform (G) and the average velocity of swimming (H) in the visible platform test were also recorded, respectively. Values were represented as mean ± SEM (n = 8–12); ∗P < 0.05, ∗∗P < 0.01, vs. control group; #P < 0.05, ##P < 0.01, vs. B2M-treated alone group.
In the probe phase, B2M-treated alone rats showed impaired memory, as evidenced by their significant decreases in the times of crossing the target quadrant (Figure 4E) and the time spent in the target quadrant (Figure 4F). However, NaHS (30 or 100 μmol/kg/d, i.p.) significantly increased the times that B2M-treated alone rats crossed the target quadrant (Figure 4E) and the time that B2M-treated alone rats spent in the target quadrant (Figure 4F). Together, these data suggested that H2S reverses the impairment in spatial learning and memory induced by B2M.
After the probe phase, visible platform test is performed to examine the escape latency and average swimming speed of rats to exclude the possibility that is caused by the changes of vision and motor ability in the rats. There was no statistical difference in the escape latencies (Figure 4G) among all five groups in the visible platform test, and there was no significant difference in swimming speed (Figure 4H), which reflects that different vision or motor ability of rats does not contribute to the alterations of all indicators in probe trial.
H2S Reverses B2M–Inhibited Autophagic Flux in the Hippocampus of Rats
To investigate whether H2S-improved cognitive function in B2M-exposed rats is involved in the regulation of hippocampal autophagic flux, the number of autophagosome and autolysosome and the expression of p62 protein in the hippocampus were observed, respectively. Compared with the control group, the ratio of autophagosome to autolysosome (Figure 5A) and the expression level of p62 protein (Figure 5B) in B2M-exposed rats were significantly increased (P < 0.001), indicating that the hippocampal autophagic flux in B2M-exposed rats is blocked. After treatment with NaHS (30, 100 μmol/kg, i.p.), the ratio of autophagosome to autolysosome (Figure 5A) and the expression of p62 protein (Figure 5B) in the hippocampus of B2M-exposed rats were significantly decreased (P < 0.001), indicating that H2S restores the autophagic flux in the hippocampus of B2M-exposed rats.
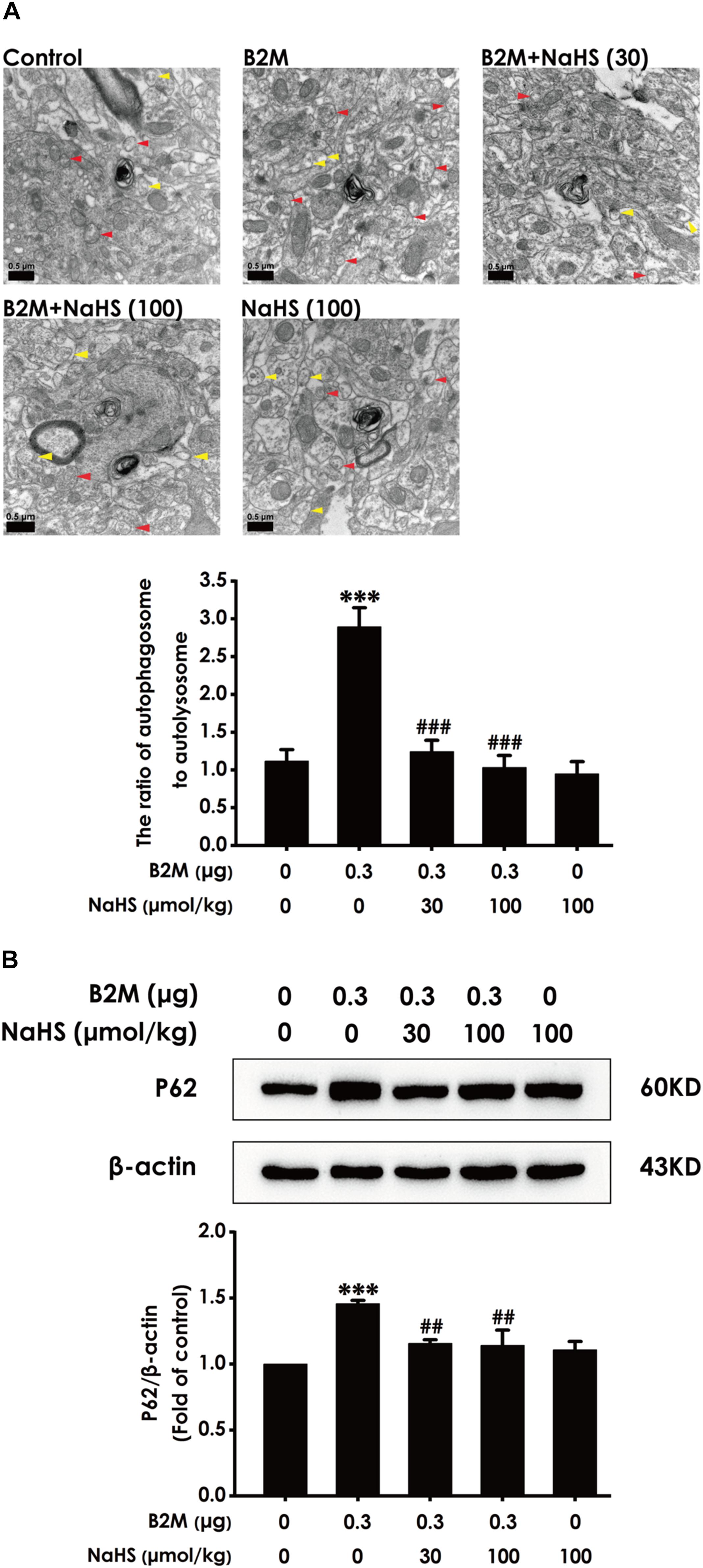
Figure 5. Effect of H2S on the hippocampal autophagic flux in B2M-exposed rats. After all behavioral experiments completed, the autophagy-associated markers in hippocampus were detected. (A) The number of autophagosome (Red arrowhead) and autolysosome (Yellow arrowhead) in the hippocampus was observed under transmission electron microscopy. (B) The expression of p62 was detected by Western blot analysis. Values were represented as mean ± SEM (n = 3); ∗∗∗P < 0.001, vs. control group; ##P < 0.01, ###P < 0.001 vs. B2M-treated alone group.
Discussion
The intentions of our study were to investigate the role of H2S in B2M-induced cognitive dysfunction and the underlying mechanisms. Our findings were as follows: (1) H2S attenuated cognitive dysfunction induced by B2M in rats; (2) H2S reversed the inhibition of autophagic flux in the hippocampus of rats treated with B2M. These results demonstrated that H2S ameliorates cognitive impairments induced by B2M, which is related to the recovery of hippocampal autophagic flux.
Exposure of B2M has certain toxicity in nervous system of human beings, recognized as a useful marker in early diagnosis and monitoring of brain metastasis in malignant disease (Alsobhi et al., 2007; Hansen et al., 2009). Research has shown that B2M, a systemic pro-aging factor, negatively regulates neurogenesis in the hippocampus (Smith et al., 2015). The interference with hippocampal neurogenesis is an important pathophysiological mechanism of cognitive dysfunction. Moreover, B2M plays an important role in synaptic plasticity and development of the mammalian nervous system (Lyupina et al., 2013). Clinical studies have reported that the level of B2M is elevated in cerebrospinal fluid of AD patients and HIV related dementia patients (Mcarthur et al., 1992; Carrette et al., 2003). Thus, these findings lead us to guess how the relationship is between B2M and cognitive impairments. In our present study, B2M-exposed rats showed cognitive defects in Y-maze test, NOR test, and MWM test. Our results are in agreement with the research that the elevation of B2M in the hippocampus is associated tightly with the dysfunction of learning and memory (Starkey et al., 2012). Just as B2M is an imperative negative factor in the nervous system of human beings, it is of certain scientific value to explore how to prevent and treat the damage to the cognitive function caused by B2M.
Although H2S is an acknowledged toxic gas (Attene-Ramos et al., 2006), it has been recognized as a major biological messenger with in-depth research nearly two decades (Kimura et al., 2005). H2S have been discovered certain physiological concentration in the brain tissues of mice (Koike et al., 2017). Additionally, there is evidence to indicate that the toxic effect of H2S may be due to its high concentration (Su and Wong, 2017). Accumulating evidences confirmed that H2S produces antioxidant, anti-inflammatory, and antiapoptotic effects, which have relevance to the protective role of H2S in neurodegenerative diseases (Hideo, 2002; Li-Fang et al., 2011; Hideo et al., 2012). Moreover, it has been revealed that H2S facilitates long-term potentiation (LTP) and regulates intracellular calcium levels in the hippocampus, which are important processes in learning and memory (Nagpure and Bian, 2015). Previous works have demonstrated that H2S reverses learning and memory deficits of rats induced by homocysteine (Wei et al., 2014) and has a protective effect on beta-amyloid associated spatial memory impairments (Xuan et al., 2012). Hence, it has significant scientific value for us to elucidate the beneficial role of H2S in B2M-induced deficit of learning and memory function.
In this work, we found that administration of NaHS resulted in a conspicuous increase in the alternation performance in Y-maze test, a profound increase in the discrimination index in NOR test, and a significant increase in the crossing numbers and spending time in the target quadrant in WMW test in the B2M-exposed rats, suggesting that H2S alleviates the cognitive defects of B2M-exposed rats. Intriguingly, these results of three kinds of animal behaviors contribute to come up with this assumption that H2S may exhibit its protective effect in a concentration-dependent manner. In addition, it has been reported that H2S ameliorates cognitive dysfunction in streptozotocin-induced diabetic rats (Zou et al., 2017). Taken together, it is reasonable to believe that H2S prevents B2M-exposed rats from learning and memory impairments. More importantly, these findings also strengthen the importance of H2S in the aspect of improving cognitive function, and may provide a target for therapy of cognitive disorders.
Autophagy is the major intracellular degradation system by which cytoplasmic materials are delivered and degraded in the lysosome to maintain cellular renovation and homeostasis (Mizushima and Komatsu, 2011). Similarly to cell differentiation and death, autophagy is disturbed in multiple diseases, in which excessive or deficient autophagy may contribute to pathogenesis (Kroemer, 2015). Autophagic flux is a dynamic process in which cell substances are transferred to lysosomes for degradation and recycling (Levy et al., 2017). The low level of autophagy causes organelle and synaptic plasticity damage, leading to AD, PD and other neurodegenerative disease (Nixon, 2006). It has been reported that the inhibition of autophagic flux induces postsynaptic dysfunction and impairs memory function (Wang et al., 2019). Likewise, promoting autophagic flux could enhance cognitive performance (Puigoriolillamola et al., 2018). So we speculated that inhibited autophagic flux in hippocampus is inseparable from cognitive impairments of B2M-exposed rats. In this study, we found that the ratio of autophagosome to autolysosome and the expression of p62 protein showed an increasing trend in B2M-treated rats. These data demonstrated that B2M blocked the autophagic flux in the hippocampus of rats. Notably, the treatment of NaHS significantly reduced the ratio of autophagosome to autolysosome and the expression of p62 protein in B2M-treated rats, indicating that H2S reverses the inhibitory effect of B2M on autophagic flux in hippocampus. Interestingly, previous studies showed that H2S activates the autophagic flux in liver to reduce serum triglyceride level (Sun et al., 2015). In the colon cancer model, H2S regulates AMPK/mTOR pathway to induce protective autophagy (Wu et al., 2012). These previous studies provided a reasonable and strong evidence for our present results. Collectively, these results suggest that the recovery of hippocampal autophagic flux is an important mechanism involved in H2S antagonizing B2M-induced cognitive defects.
In summary, the present work has demonstrated that H2S improves the cognitive function and rescues the blockade of hippocampal autophagic flux in the B2M-exposed rats. Our data suggest that H2S reverses B2M-caused cognitive dysfunction by recovery of hippocampal autophagic flux. These findings establish the basis of our further study on the protective role of H2S through autophagy pathway and open a novel perspective that H2S might be a potential agent for protection against the cognitive impairment induced by B2M.
Data Availability Statement
All datasets generated for this study are included in the manuscript/supplementary files.
Ethics Statement
The animal study was reviewed and approved by the Animal Use and Protection Committee of University of South China.
Author Contributions
X-QT and WZ contributed conception and design of the study. X-QT and PZ guided and supervised the study. S-MC, Y-LY, DZ, Y-YT, and XK performed the experiments. S-MC, Y-LY, and DZ analyzed the data of the experiments. S-MC and Y-LY wrote the manuscript. All authors contributed to manuscript revision, read and approved the submitted version.
Funding
This study was supported by the National Natural Science Foundation of China (81671057 and 81771178), Science and Health Joint Project of Natural Science Foundation of Hunan Province (2019JJ80101), and Youth Project of Natural Science Foundation of Hunan Province (2019JJ50546).
Conflict of Interest
The authors declare that the research was conducted in the absence of any commercial or financial relationships that could be construed as a potential conflict of interest.
References
Abe, K., and Kimura, H. (1996). The possible role of hydrogen sulfide as an endogenous neuromodulator. J. Neurosci. 16, 1066–1071. doi: 10.0000/PMID8558235
Alsobhi, E. M., Hashim, I. A., Abdelaal, M. A., Aljifri, A. M., and Alshamy, A. M. (2007). Elevated cerebrospinal fluid beta-2 microglobulin as a tumor marker in a patient with myeloma of the central nervous system. Saudi Med. J. 28, 128–130. doi: 10.1016/j.revmed.2006.10.328
Attene-Ramos, M. S., Wagner, E. D., Plewa, M. J., and Gaskins, H. R. (2006). Evidence that hydrogen sulfide is a genotoxic agent. Mol. Cancer Res. 4, 9–14. doi: 10.1158/1541-7786.MCR-05-0126
Bliss, T. V. P., and Collingridge, G. L. (1993). A synaptic model of memory: long-term potentiation in the hippocampus. Nature 361, 31–39. doi: 10.1038/361031a0
Boulanger, L., and Shatz, C. (2004). Immune signalling in neural development, synaptic plasticity and disease. Nat. Rev. Neurosci. 5, 521–531. doi: 10.1038/nrn1428
Brew, B., Dunbar, N., Pemberton, L., and Kaldor, J. (1996). Predictive markers of AIDS dementia complex: CD4 cell count and cerebrospinal fluid concentrations of 2-microglobulin and neopterin. J. Infect. Dis. 174, 294–298. doi: 10.1093/infdis/174.2.294
Carrette, O., Demalte, I., Scherl, A., Yalkinoglu, O., Corthals, G. L., Burkhard, P., et al. (2003). A panel of cerebrospinal fluid potential biomarkers for the diagnosis of Alzheimer’s disease. Proteomics 3, 1486–1494.
Feng, A., Ling, C., Xinduo, L., Bing, W., Sanwu, W., Yu, Z., et al. (2018). Hydrogen sulfide protects human cardiac fibroblasts against H2O2-induced injury through regulating autophagy-related proteins. Cell Transplant. 27, 1222–1234. doi: 10.1177/0963689718779361
Glynn, M. W., Elmer, B. M., Garay, P. A., Xiao-Bo, L., Needleman, L. A., Faten, E. S., et al. (2011). MHCI negatively regulates synapse density during the establishment of cortical connections. Nat. Neurosci. 14, 442–451. doi: 10.1038/nn.2764
Goddard, C. A., Butts, D. A., and Shatz, C. J. (2007). Regulation of CNS synapses by neuronal MHC class I. Proc. Natl. Acad. Sci. U.S.A. 104, 6828–6833. doi: 10.1073/pnas.0702023104
Gong, Q., Wang, Q., Pan, L., Liu, X., Xin, H., and Zhu, Y. (2011). S-Propargyl-cysteine, a novel hydrogen sulfide-modulated agent, attenuates lipopolysaccharide-induced spatial learning and memory impairment: involvement of TNF signaling and NF-κB pathway in rats. Brain Behav. Immun. 25, 110–119. doi: 10.1016/j.bbi.2010.09.001
Gu, H. F., Nie, Y. X., Tong, Q. Z., Tang, Y. L., Zeng, Y., Jing, K. Q., et al. (2014). Epigallocatechin-3-gallate attenuates impairment of learning and memory in chronic unpredictable mild stress-treated rats by restoring hippocampal autophagic flux. PLoS One 10:e112683. doi: 10.1371/journal.pone.0117649
Hanmi, L., Brott, B. K., Kirkby, L. A., Adelson, J. D., Sarah, C., Feller, M. B., et al. (2014). Synapse elimination and learning rules co-regulated by MHC class I H2-Db. Nature 509, 195–200. doi: 10.1038/nature13154
Hansen, P. B., Kjeldsen, L., Dalhoff, K., and Olesen, B. (2009). Cerebrospinal fluid beta-2-microglobulin in adult patients with acute leukemia or lymphoma: a useful marker in early diagnosis and monitoring of CNS-involvement. Acta Neurol. Scand. 85, 224–227. doi: 10.1111/j.1600-0404.1992.tb04033.x
Hara, T., Nakamura, K., Matsui, M., Yamamoto, A., Nakahara, Y., Suzukimigishima, R., et al. (2006). Suppression of basal autophagy in neural cells causes neurodegenerative disease in mice. Nature 441, 885–889. doi: 10.1038/nature04724
Hideo, K., Norihiro, S., and Yuka, K. (2012). Hydrogen sulfide is a signaling molecule and a cytoprotectant. Antioxid. Redox Signal. 17, 45–57. doi: 10.1089/ars.2011.4345
Huh, G. S., Boulanger, L. M., Du, H., Riquelme, P. A., Brotz, T. M., and Shatz, C. J. (2000). Functional requirement for class I MHC in CNS development and plasticity. Science 290, 2155–2159. doi: 10.1126/science.290.5499.2155
Jennifer, L., Fabio, P., Ernie, C., Lisa, S., Jones, E. P., Toyoyuki, T., et al. (2003). Functional expression of murine V2R pheromone receptors involves selective association with the M10 and M1 families of MHC class Ib molecules. Cell 112, 607–618. doi: 10.1016/s0092-8674(03)00153-3
Kimura, H. (2000). Hydrogen sulfide induces cyclic AMP and modulates the NMDA receptor. Biochem. Biophys. Res. Commun. 267, 129–133. doi: 10.1006/bbrc.1999.1915
Kimura, H., Nagai, Y., Umemura, K., and Kimura, Y. (2005). Physiological roles of hydrogen sulfide: synaptic modulation, neuroprotection, and smooth muscle relaxation. Antioxid. Redox Signal. 7, 795–803. doi: 10.1089/ars.2005.7.795
Koike, S., Kawamura, K., Kimura, Y., Shibuya, N., Kimura, H., and Ogasawara, Y. (2017). Analysis of endogenous H2S and H2Sn in mouse brain by high-performance liquid chromatography with fluorescence and tandem mass spectrometric detection. Free Radic. Biol. Med. 113, 355–362. doi: 10.1016/j.freeradbiomed.2017.10.346
Kroemer, G. (2015). Autophagy: a druggable process that is deregulated in aging and human disease. J. Clin. Investig. 125, 1–4. doi: 10.1172/JCI78652
Levine, B., and Kroemer, G. (2008). Autophagy in the pathogenesis of disease. Cell 132, 27–42. doi: 10.1016/j.cell.2007.12.018
Levy, J. M. M., Towers, C. G., and Thorburn, A. (2017). Targeting autophagy in cancer. Nat. Rev. Cancer 17, 528–542. doi: 10.1038/nrc.2017.53
Li, M., Tang, J., Zhang, P., Li, X., Wang, C., Wei, H., et al. (2014). Disturbance of endogenous hydrogen sulfide generation and endoplasmic reticulum stress in hippocampus are involved in homocysteine-induced defect in learning and memory of rats. Behav. Brain Res. 262, 35–41. doi: 10.1016/j.bbr.2014.01.001
Li, M., Zhang, P., Wei, H., Li, M., Zou, W., Li, X., et al. (2016). Hydrogen Sulfide Ameliorates Homocysteine-Induced Cognitive Dysfunction by Inhibition of Reactive Aldehydes Involving Upregulation of ALDH2. Int. J. Neuropsychopharmacol. 20, 305–315. doi: 10.1093/ijnp/pyw103
Liang, Y., and Sigrist, S. J. (2018). Autophagy and proteostasis in the control of synapse aging and disease. Curr. Opin. Neurobiol. 48, 113–121. doi: 10.1016/j.conb.2017.12.006
Lida, K., Litterman, N. K., Schein, P. A., Miller, C. M., Loffredo, F. S., Wojtkiewicz, G. R., et al. (2014). Vascular and neurogenic rejuvenation of the aging mouse brain by young systemic factors. Science 344, 630–634. doi: 10.1126/science.1251141
Li-Fang, H., Ming, L., Peter Tsun, H. W., and Jin-Song, B. (2011). Hydrogen sulfide: neurophysiology and neuropathology. Antioxid. Redox Signal. 15, 405–419. doi: 10.1089/ars.2010.3517
Liu, H., Deng, Y., Gao, J., Liu, Y., Li, W., Shi, J., et al. (2015). Sodium hydrosulfide attenuates beta-amyloid-induced cognitive deficits and neuroinflammation via modulation of MAPK/NF-κB pathway in rats. Curr. Alzheimer Res. 12, 673–683. doi: 10.2174/1567205012666150713102326
Liu, Y., Deng, Y., Liu, H., Yin, C., Li, X., and Gong, Q. (2016). Hydrogen sulfide ameliorates learning memory impairment in APP/PS1 transgenic mice: a novel mechanism mediated by the activation of Nrf2. Pharmacol. Biochem. Behav. 150, 207–216. doi: 10.1016/j.pbb.2016.11.002
Łowicka, E., and Beltowski, J. (2007). Hydrogen sulfide (H2S) - the third gas of interest for pharmacologists. Pharmacol. Rep. 59, 4–24.
Lyupina, Y. V., Bogatyrev, M. E., Orlova, A. S., Marjukhnich, E. V., Kazansky, D. B., and Sharova, N. P. (2013). Proteasomes in the brain of β2-microglobulin knockout mice. Biochem. Biokhim. 78, 1124–1133. doi: 10.1134/S0006297913100064
Mcarthur, J. C., Nancesproson, T., Griffin, D. E., Hoover, D. R., Selnes, O. A., Miller, E. N., et al. (1992). The diagnostic utility of elevation in cerebrospinal fluid β2–microglobulin in HIV–1 dementia. Neurology 42, 1707–1712.
Mizushima, N., and Komatsu, M. (2011). Autophagy: renovation of cells and tissues. Cell 147, 728–741. doi: 10.1016/j.cell.2011.10.026
Nagpure, B. V., and Bian, J. (2015). Brain, learning, and memory: role of H2S in neurodegenerative diseases. Handb. Exp. Pharmacol. 230, 193–215. doi: 10.1007/978-3-319-18144-8_10
Nikoletopoulou, V., Sidiropoulou, K., Kallergi, E., Dalezios, Y., and Tavernarakis, N. (2017). Modulation of autophagy by BDNF underlies synaptic plasticity. Cell Metab. 26, 230.e5–e242.e5. doi: 10.1016/j.cmet.2017.06.005
Nixon, R. A. (2006). Autophagy in neurodegenerative disease: friend, foe or turncoat? Trends Neurosci. 29, 528–535. doi: 10.1016/j.tins.2006.07.003
Pan, J., He, L., Li, X., Li, M., Zhang, X., Venesky, J., et al. (2016). Activating autophagy in hippocampal cells alleviates the morphine-induced memory impairment. Mol. Neurobiol. 54, 1–15. doi: 10.1007/s12035-016-9735-3
Puigoriolillamola, D., Grinanferre, C., Vasilopoulou, F., Leiva, R., Vazquez, S., and Pallas, M. (2018). 11β-HSD1 inhibition by rl-118 promotes autophagy and correlates with reduced oxidative stress and inflammation, enhancing cognitive performance in SAMP8 mouse model. Mol. Neurobiol. 55, 8904–8915. doi: 10.1007/s12035-018-1026-8
Shatz, C. J. (2009). MHC class I: an unexpected role in neuronal plasticity. Neuron 64, 40–45. doi: 10.1016/j.neuron.2009.09.044
Smith, L. K., He, Y., Park, J., Bieri, G., Snethlage, C. E., Lin, K., et al. (2015). β2-microglobulin is a systemic pro-aging factor that impairs cognitive function and neurogenesis. Nat. Med. 21, 932–937. doi: 10.1038/nm.3898
Starkey, V. G., Kirk, C. A. V., Bixler, G. V., Imperio, C. G., Kale, V. P., Serfass, J. M., et al. (2012). Neuroglial expression of the MHCI pathway and PirB receptor Is Upregulated in the hippocampus with advanced aging. J. Mol. Neurosci. 48, 111–126. doi: 10.1007/s12031-012-9783-8
Su, J. C., and Wong, T. H. (2017). Hydrogen sulfide in stroke: protective or deleterious? Neurochem. Int. 105, 1–10. doi: 10.1016/j.neuint.2016.11.015
Sun, L., Zhang, S., Yu, C., Pan, Z., Liu, Y., Zhao, J., et al. (2015). Hydrogen sulfide reduces serum triglyceride by activating liver autophagy via the AMPK-mTOR pathway. Am. J. Physiol. Endocrinol. Metab. 309, e925–e935. doi: 10.1152/ajpendo.00294.2015
Szabo, C. (2007). Hydrogen sulphide and its therapeutic potential. Nat. Rev. Drug Discov. 6, 917–935. doi: 10.1038/nrd2425
Villeda, S. A., Luo, J., Mosher, K. I., Zou, B., Britschgi, M., Bieri, G., et al. (2011). The ageing systemic milieu negatively regulates neurogenesis and cognitive function. Nature 477, 90–94. doi: 10.1038/nature10357
Wang, B., Wu, Q., Lei, L., Sun, H., Michael, N., Zhang, X., et al. (2019). Long-term social isolation inhibits autophagy activation, induces postsynaptic dysfunctions and impairs spatial memory. Exp. Neurol. 311, 213–224. doi: 10.1016/j.expneurol.2018.09.009
Wei, H., Xu, J., Li, M., Tang, J., Zou, W., Zhang, P., et al. (2014). Hydrogen sulfide inhibits homocysteine-induced endoplasmic reticulum stress and neuronal apoptosis in rat hippocampus via upregulation of the BDNF-TrkB pathway. Acta Pharmacol. Sin. 35, 707–715. doi: 10.1038/aps.2013.197
Whiteman, M., Trionnaire, S. L., Chopra, M., Fox, B., and Whatmore, J. L. (2011). Emerging role of hydrogen sulfide in health and disease: critical appraisal of biomarkers and pharmacological tools. Clin. Sci. 121, 459–488. doi: 10.1042/CS20110267
Wu, Y. C., Wang, X. J., Yu, L., Chan, F. K., Cheng, A. S., Yu, J., et al. (2012). Hydrogen sulfide lowers proliferation and induces protective autophagy in colon epithelial cells. PLoS One 7:e37572. doi: 10.1371/journal.pone.0037572
Xie, L., Yu, S., Yang, K., Li, C., and Liang, Y. (2017). Hydrogen sulfide inhibits autophagic neuronal cell death by reducing oxidative stress in spinal cord ischemia reperfusion injury. Oxid. Med. Cell. Longev. 2017, 1–15. doi: 10.1155/2017/8640284
Xuan, A., Long, D., Li, J., Ji, W., Zhang, M., Hong, L., et al. (2012). Hydrogen sulfide attenuates spatial memory impairment and hippocampal neuroinflammation in beta-amyloid rat model of Alzheimer’s disease. J. Neuroinflamm. 9:202. doi: 10.1186/1742-2094-9-202
Yan, J., Porch, M. W., Courtvazquez, B., Bennett, M. V. L., and Zukin, R. S. (2018). Activation of autophagy rescues synaptic and cognitive deficits in fragile X mice. Proc. Natl. Acad. Sci. U.S.A. 115, e9707–e9716. doi: 10.1073/pnas.1808247115
Yang, R., Fu, S., Zhao, L., Zhen, B., Ye, L., Niu, X., et al. (2017). Quantitation of circulating GDF-11 and beta2-MG in aged patients with age-related impairment in cognitive function. Clin. Sci. 131, 1895–1904. doi: 10.1042/CS20171028
Zijlstra, M., Bix, M., Simister, N. E., Loring, J. M., Raulet, D. H., and Jaenisch, R. (1990). Beta 2-microglobulin deficient mice lack CD4-8+ cytolytic T cells. Nature 344, 742–746. doi: 10.1038/344742a0
Keywords: hydrogen sulfide, β2-microglobulin, cognitive dysfunction, autophagic flux, hippocampus
Citation: Chen S-M, Yi Y-L, Zeng D, Tang Y-Y, Kang X, Zhang P, Zou W and Tang X-Q (2019) Hydrogen Sulfide Attenuates β2-Microglobulin-Induced Cognitive Dysfunction: Involving Recovery of Hippocampal Autophagic Flux. Front. Behav. Neurosci. 13:244. doi: 10.3389/fnbeh.2019.00244
Received: 25 July 2019; Accepted: 04 October 2019;
Published: 25 October 2019.
Edited by:
Tamas Kozicz, Mayo Clinic, United StatesReviewed by:
Zhifang Dong, Chongqing Medical University, ChinaYasuyuki Ishikawa, Maebashi Institute of Technology, Japan
Copyright © 2019 Chen, Yi, Zeng, Tang, Kang, Zhang, Zou and Tang. This is an open-access article distributed under the terms of the Creative Commons Attribution License (CC BY). The use, distribution or reproduction in other forums is permitted, provided the original author(s) and the copyright owner(s) are credited and that the original publication in this journal is cited, in accordance with accepted academic practice. No use, distribution or reproduction is permitted which does not comply with these terms.
*Correspondence: Wei Zou, em91dzQxNUAxNjMuY29t; em91dzQxNUB1c2MuZWR1LmNu; Xiao-Qing Tang, dGFuZ3hxLXVzY0BxcS5jb20=; dGFuZ3hxLXVzY0B1c2MuZWR1LmNu
†These authors have contributed equally to this work