Anticipatory Behavior for a Mealworm Reward in Laying Hens Is Reduced by Opioid Receptor Antagonism but Not Standard Feed Intake
- 1Animal Science, School of Environmental and Rural Science, Faculty of Science, Agriculture, Business and Law, University of New England, Armidale, NSW, Australia
- 2School of Science and Technology, Faculty of Science, Agriculture, Business and Law, University of New England, Armidale, NSW, Australia
- 3iMPACT Institute School of Medicine, Deakin University, Waurn Ponds, VIC, Australia
It is widely accepted that the absence of suffering no longer defines animal welfare and that positive affective experiences are imperative. For example, laying hens may be housed in environments that do not cause chronic stress but may lack particular resources that promote positive affective experiences, such as conspecifics or effective enrichment. Despite a consensus of how important positive affect is for animal welfare, they are difficult to identify objectively. There is a need for valid and reliable indicators of positive affect. Pharmacological interventions can be an effective method to provide insight into affective states and can assist with the investigation of novel indicators such as associated biomarkers. We aimed to validate a pharmacological intervention that blocks the subjective hedonistic phase associated with reward in laying hens via the administration of the non-selective (μ, δ, and κ) opioid receptor antagonist, nalmafene. We hypothesized that nonfood deprived, hens that did not experience a positive affective state when presented with a mealworm food reward due to the administration of nalmefene, would show minimal anticipatory and consummatory behavior when the same food reward was later presented. Hens (n = 80) were allocated to treatment groups, receiving either nalmefene or vehicle (0.9% saline) once or twice daily, for four consecutive days. An anticipatory test (AT) was performed on all days 30 min post-drug administration. Behavioral responses during the appetitive and consummatory phase were assessed on days 1, 3 and 4. Anticipatory behavior did not differ between treatment groups the first time hens were provided with mealworm food rewards. However, antagonism of opioid receptors reduced anticipatory and consummatory behavior on days 3 and 4. Feed intake of standard layer mash was not impacted by treatment, thus nalmefene reduced non-homeostatic food consumption but not homeostatic consumption. Behavioral observations during the AT provided no evidence that nalmefene treated hens were fearful, sedated or nauseous. The results suggest that we successfully blocked the hedonistic subjective component of reward in laying hens and provide evidence that this method could be used to investigate how hens perceive their environment and identify associated novel indicators to assess hen welfare.
Introduction
Most ethologists accept that animals possess a number of basic affective states (emotions) that reflect the animals’ needs or wants (Fraser and Duncan, 1998; Duncan, 2002). Affective states are critical evolutionary adaptions; negative affective states protect animals and positive affective states reinforce low-cost behaviors that have positive long-term implications (Fraser and Duncan, 1998; Spruijt et al., 2001). Affective states include behavioral, autonomic and subjective components (Boissy et al., 2007). It has been argued that how an animal feels (i.e., the subjective component of affective states) largely contributes to, or defines, animal welfare (Désiré et al., 2002). However, the inability to directly assess the subjective component of emotions has historically slowed the progress of animal welfare science. Yet, if it is accepted that emotional states have evolved to motivate behavior, insight into an animal’s affective state may be inferred by assessing animal preferences, aversions and priorities. Indeed, various authors have reported indirect measures that infer affective states, particularly negative affective states such as pain and fear (Colpaert et al., 2001; Forkman et al., 2007). Positive affective states have received less attention, although it is widely accepted that good welfare is fundamentally the presence of positive experiences not simply the absence of the negative (Désiré et al., 2002; Boissy et al., 2007).
Advances in our understanding of the reward system have identified three key components; affect, motivation, and learning that constantly interact in reward processing (Berridge and Robinson, 2003). These three psychological components of reward processing; hedonic value (affect), wanting (motivation), and learning have discriminable neural mechanisms. These processes occur throughout the reward cycle, and while learning occurs throughout the cycle, wanting tends to dominate the initial appetitive phase, whereas hedonic pleasure dominates the consummatory phase (Berridge and Kringelbach, 2015). As such a rewarding experience effectively reduces a motivational state by moving an animal closer towards the desired state, by either pursuing a stimulus with high hedonic value or reducing a negative affective state (van der Harst and Spruijt, 2007). Generally, consummatory behaviors such as eating, drinking, sex and social interactions are considered rewarding for most animals (van der Harst and Spruijt, 2007). Additionally, an animal’s evolutionary history may result in species-specific goal-directed (rewarding) behaviors and stimuli, such as exploration or grooming (Spruijt et al., 2001). The continuously developing field of affective neuroscience is providing evidence of the relationships between neurophysiology and goal-directed behaviors.
The neurobiology of consummatory behaviors is dissociated from the neurobiology of appetitive behaviors (Berridge, 1996; Spruijt et al., 2001; Boissy et al., 2007; Berridge and Kringelbach, 2015), such that the “liking” consummatory phase is regulated by opioids and the “wanting” appetitive phase is regulated by dopamine (Berridge and Robinson, 1998; Boissy et al., 2007; Zimmerman et al., 2011; Berridge and Kringelbach, 2015). Mesolimbic dopamine levels are not synonymous with hedonia, but rather sensorimotor processes involved in motivation and responses to conditioned rewarding stimuli (Salamone et al., 1997; Kelley et al., 2002). Thus, dopamine is not directly involved in liking (Berridge and Robinson, 1998; Berridge and Kringelbach, 2015). However, opioids do affect wanting; as opioids act indirectly or directly to stimulate dopaminergic ventral tegmental area (VTA) neurons or by increasing dopamine release at the level of the nucleus accumbens (NAc; Burgdorf and Panksepp, 2006). Interactions between the aforementioned neuronal systems demonstrate the complexity of the reward system and highlight that the subjective experience of reward is not mediated by one specific brain region or neurotransmitter.
Behavioral indicators of motivation include anticipatory behavior which is a behavioral change, typically an increase in activity, that precedes a predictable event (Krebs et al., 2017). Positive anticipatory behavior is goal-directed and dependent on expectations or predictions, thus anticipatory behavior is reflective of the combination of genetics and ontogeny that shape the animals’ subjective experience (Spruijt et al., 2001). van der Harst and Spruijt (2007) provide evidence that anticipatory behavior is displayed when rats are given a conditioned cue announcing a reward (an enriched cage or sexual contact), but is absent when conditioned cues announce negative or neutral stimuli (barren cage, or cylinder filled with water). As such, anticipatory behavior is likely reflective of an appetitive positive affective state associated with dopaminergic activity in the brain (van der Harst and Spruijt, 2007; Moe et al., 2011).
Pharmacological interventions have been an important part of providing evidence of subjective experiences of reward in animals (Peciña, 1998). Administering opioid receptor agonists or antagonists can alter affective states and subsequently behavioral responses, including feeding and social behavior, play, and self-grooming (Boissy et al., 2007). For example, opioid agonists have shown to increase behavioral expressions associated with pleasure after consumption of highly palatable sucrose solutions (Doyle et al., 1993; Cagniard and Murphy, 2009) or hyperphagia specifically related to palatability and macronutrient content (Rockwood and Reid, 1982; Marks-Kaufman et al., 1984; Parker et al., 1992; Levine et al., 1995; Woolley et al., 2006; Le Merrer et al., 2009). Such methods of blocking or enhancing certain receptors can provide insight into how rewards, or other stimuli, are perceived by animals.
Nalmefene (17-[cyclopropylmethyl]-4,5α-epoxy-6-methylenemorphinan-3, 14-diol) is a pure opioid antagonist, which blocks μ, δ, and κ opioid receptors (Glass et al., 1994). Opioid receptors are expressed primarily in the cortex, limbic system, and brain stem, however, some structures have higher expression of one receptor over the others (Le Merrer et al., 2009). Additionally, specific hotspots in various areas of the brain have been identified, that when specifically targeted, result in different impacts on subjective experiences (Kelley et al., 2002; Castro and Berridge, 2014). For example, stimulation of μ-opioid receptors in the rostrodorsal hotspot in the medial shell of the NAc has shown to increase the hedonistic component of reward in rats, evidenced by increased orofacial reactions to sucrose with no impact on standard food intake (Castro and Berridge, 2014). Nalmefene is long-acting, potent and has a high affinity to central nervous system opiate receptors relative to other available opioid antagonists such as naloxone (Glass et al., 1994). Nalmefene targets all three opioid receptors across various brain structures such that both the motivational and hedonistic components of reward are likely impacted. Human studies show that feed intake is reduced after the administration of nalmefene despite no difference in self-reported hunger (Yeomans et al., 1990). Further investigation showed the nalmefene specifically reduced the intake of fat and proteins, not carbohydrates, suggesting that the differences in consumption were likely related to the palatability of the food rather than influences of hunger, i.e., how much the subject liked the food provided (Yeomans et al., 1990).
Positive emotions can be categorized into three temporal states; past (post-consummatory satisfaction), present (pleasant sensory activity) and future (expectations of positive affective states; Boissy et al., 2007). There has been much work on the future temporal state (motivation) in the field of animal welfare science, however minimal investigation into satisfaction. We attempted to alter post-consummatory contentment by blocking the “liking” response to a novel food reward with the non-specific opioid receptor antagonist, nalmefene and assessed the success of the intervention by measuring the expectation when presented with the same reward in the future. We hypothesized that if hens had not experienced hedonistic value (due to the administration of nalmefene) they would show no, or minimal, anticipatory behavior, hyperphagia of palatable mealworms and no difference in non-homeostatic food consumption.
Whilst there has been considerable investigation into the assessment of negative affective states in laying hens (Forkman et al., 2007) assessments of positive affective states are lacking. As such, researchers, industry and producers do not have the tools required to comprehensively assess hen welfare. Such assessments would enable measurable improvements in housing, enrichment programs, and industry benchmarking programs. The first step to identifying novel indicators of hen welfare specifically related to positive affective states is to validate a method that disrupts the pathway associated with a specific affective state, for example, the positive effect of liking associated with reward. Thus, we aimed to validate a pharmacological model to investigate the subjective experience of pleasure, as a step towards identifying novel indicators of positive affective states in laying hens.
Animals, Materials and Methods
All animals and procedures used in this study were approved by the University of New England Animal Ethics Committee (Approval number 18-114).
Animals and Housing
Eighty adult Hy-line hens of 80 weeks of age were sourced from a commercial conventional cage enterprise and transported to a research facility at the University of New England (Armidale, NSW, Australia). Hens were individually housed in cages (50 × 54 cm) but were not visually isolated from each other. Hens were fed a commercially available layer mash (Norco, South Lismore, NSW, Australia) ad libitum and water was available at all times via nipple drinkers. Feed intake over the treatment period was calculated for each individual hen. Hens were provided with natural ventilation and lighting via two curtains that were raised at approximately 6:00 h and lowered at approximately 19:00 h. but were also provided with artificial lighting on a 16:8 L:D schedule (4:00–20:00 h) as per the recommendations for Hy-line hens of 80 weeks from the breed management guide (Hy-Line International, 2018). Hens were habituated to the housing conditions, feed and research staff for 7 days.
Treatments
Hens were randomly allocated to one of four treatment groups; nalmefene one dose daily (N1), nalmefene two doses daily (N2), saline one dose daily (C1) or saline two doses daily (C2). N1 and N2 hens received 0.5 ml of 0.4 mg/kg of nalmefene (17-[cyclopropylmethyl]-4, 5α-epoxy-6-methylenemorphinan-3,14-diol, nalmefene hydrochloride, 1B/220482, Tocris, Noble Park, Victoria, Australia) dissolved in 0.9% saline in the morning, administered via intramuscular injections into the pectoral muscle. The dose administered was based on the nalmefene dose-response study from Savory et al. (1989) on laying hen eating and drinking behavior. The half-life of nalmefene in humans is 12 h (Wang et al., 1998), but is unknown for hens. Therefore to determine if there were any negative welfare implications for hens dose with nalmefene every 12 h, e.g., theoretically keeping hens in a constant state of not experiencing reward the N2 treatments hens were dosed twice daily at 12-h intervals. N2 hens received an additional dose of 0.5 ml of nalmefene (0.2 mg/kg) 12 h after the morning injection. C2 hens were also dosed twice daily to control for any handling impacts. C1 and C2 hens received 0.5 ml 0.9% saline either once (C1) or twice (C2) daily at the same time and via same administration route as nalmafene treatment hens. Hens were dosed accordingly for four consecutive days.
Anticipatory Behavior
An anticipatory test (AT) was performed on all days from 1 to 4 (Figure 1), however, were only video recorded, and thus analyzed, on days 1, 3 and 4. Exactly 30 min after the morning injection and immediately after the feed was removed, five live mealworms in a transparent closed food container were presented at the front of their cage. The onset of action of nalmefene in hens was unknown so hens were tested based on the onset of action of nalmefene in humans after intramuscular administration, approximately 15 min (Dixon et al., 1986), with an additional 15 min lag to increase confidence in the likelihood that the nalmefene was altering hen state. Therefore, hens were tested 30 min after dosing. Hens could see the mealworms and could reach the container but could not access the mealworms due to the closed lid. Containers had two strips of green tape to provide a visual cue. After 1 min the lid was opened, and the hens were provided access to the mealworms, for a further 5 min. Visual contact with humans was restricted during the test excluding times when the mealworms were provided or the lid was removed and hens were visually isolated from other hens by placing a black curtain on either side of the cage. Hen behavior was recorded with a GoPro Hero 7 (GoPro, Carlsbad, CA, USA) for post-analysis. Latency to peck the container and number of pecks when the lid was closed was calculated, continuous observation for the whole 2 min, as an indicator of anticipation of the mealworm (reward). The number of pecks in the open container was quantified via continuous observations for the entire 5 min period as an indirect assessment of relative consumption.

Figure 1. Experimental procedures including the time of dosing (−30 min) prior to the anticipatory test (AT) and behavioral time budget (BTB), subsequent dosing for C2 and N2 treatment hens and the procedures across consecutive days.
Hen behavior (Table 1) was analyzed by one trained observer using instantaneous scan sampling (Altmann, 1974). Behavior was recorded every 15 s for 2 min when hens were provided the closed mealworm container. The proportion of observations for each behavior was calculated over the 2-min for each day of testing.
Response to Novelty
Response to novelty was tested pre-treatment (day 1) and post-treatment (day 6) by placing a novel object (NO; pre-treatment: pink dog chew toy (20 cm × 4 cm); post-treatment: spiked yellow ball (10 cm diameter). Hens were tested in their home cages to avoid any handling effects. Hens were visually isolated from other hens by placing a black curtain on either side of the cage. NOs were placed on top of the hens’ feed tray and left for 10 min. Latency to peck the NO was assessed as an indicator of neophobia and exploration.
Statistics
All statistical analysis was performed with SPSS statistical software (v22, IBM Corporation, Armonk, NY, USA). Comparisons between treatments for the percentage of hens that pecked the open or closed box were analyzed with a Chi-Square model, multiple comparisons were corrected with the Bonferroni method. Censored data, including latency to peck the container or NO, were analyzed with Kaplan–Meier survival analysis and compared treatments on each test day. Handling treatment groups (i.e., the treatment groups that were handled and dosed twice daily; C2 and N2) within drug treatment (control or nalmefene) did not differ in latency to peck the closed container (p > 0.05) therefore these data were pooled to compare results within treatment group (control or nalmefene) across days. The data for pecks on the closed and open container did not meet the criteria for normality and therefore were analyzed with a non-parametric generalized linear model with a Poisson distribution with a log link function. Treatment, day and the interaction between treatment and day were included in the model as fixed factors. Post hoc comparisons were corrected with the least-squares difference method to account for multiple comparisons.
Behavioral Time Budget (BTB) data did not meet the criteria for normality, despite transformation attempts. Therefore, non-parametric Mann–Whitney comparisons were used to compare the effect of handling within treatment (e.g., control once daily compared to control twice daily) for each testing day. There were no differences between handling groups within treatment therefore data were pooled within treatment and comparisons between control and nalmefene treated hens for each testing day were compared using a two-tailed Mann–Whitney analysis. Feed intake data met the criteria for normality and was analyzed with an ANOVA comparing treatment groups.
Results
There was no difference in the proportion of hens that pecked the closed mealworm container between treatment groups on day 1 ( = 5.22, p = 0.156; Table 2). More C2 hens pecked the closed mealworm container than hens from both of the nalmefene treatment groups (N1 and N2) on day 3 ( = 17.1, p = 0.001; Table 2). More hens from both control groups (C1 and C2) pecked the closed mealworm container on day 4 than both nalmefene treatment groups ( = 24.4, p < 0.001; Table 2).

Table 2. Hen responses to the presentation of a closed transparent container containing live mealworms, including the percentage of hens within each treatment that pecked the closed container over time and latency (seconds) to peck the open container.
Control hens were quicker to peck the closed container over time ( = 39.3, p < 0.001; Figure 2A). The latency to peck the closed container over time did not differ for hens dosed with namelfene (p = 0.35; Figure 2B).
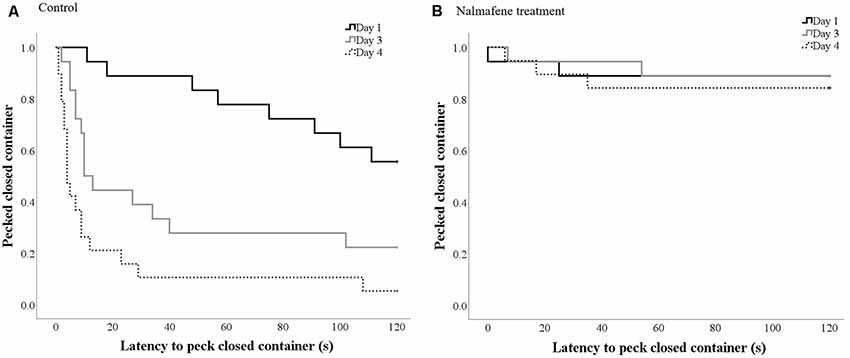
Figure 2. Kaplan-Meier curves indicating the proportion of control (A) and treatment (B) hens that pecked the closed container (y-axis) over time (seconds; x-axis) on each day of dosing/testing (day 1 solid black line; day 3 solid gray line; day 4 dotted gray line). Every time a hen-pecked the container, the probability on the y-axis drops.
Hens from the control groups were quicker to peck the closed mealworm container on all days than hens that received nalmefene (day 1: = 4.3, p = 0.038; day 3: = 17.15, p < 0.001; day 4: = 29.8, p < 0.001; Table 2).
The average number of pecks on the closed mealworm container increased over time (testing/dosing days), particularly for the control hens that were injected with saline twice daily ( = 7.7, p = 0.005; Figure 3). Although treatment hens pecked the closed container slightly more over time, they pecked the closed container fewer times than the control hens at all time points ( = 153.0, p < 0.001; Figure 3). Hens that were dosed with nalmefene twice daily pecked the closed container less than hens that were dosed with nalmefene only once a day on day 1 and 4 (p ≤ 0.02; Figure 3). Conversely, hens that were dosed twice daily with saline pecked at the closed container more than hens dosed once daily with saline on day 3 and day 4 (both p < 0.001; Figure 3).
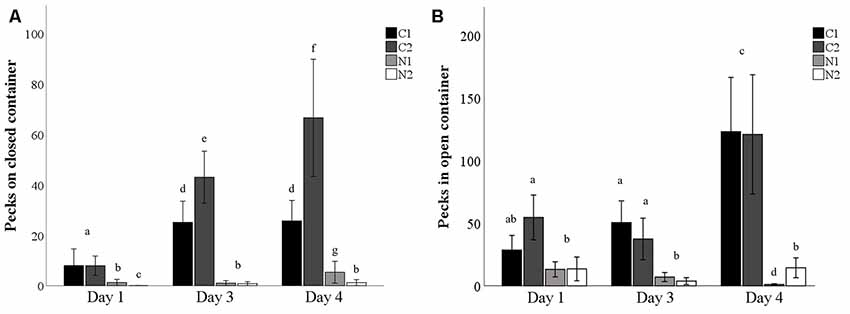
Figure 3. The number of pecks on a closed (A) and open (B) transparent container containing mealworms when presented to hens on day 1, 3 and 4 dosing either once (C1, N1) or twice (C2, N2) daily with saline (C1–black bar, C2–dark gray bar) or nalmefene (N1–light gray bar, N2–white bar) hens were presented with the container 30 min after dosing. Bars with differing subscript indicate significant differences between treatments and day of testing (p < 0.05).
The proportion of hens that pecked the open mealworm container did not change over time (p = 0.397; Table 3). More control hens pecked the open mealworm container than nalmefene treatment hens on day three ( = 21.7, p < 0.001; Table 3). Control hens were quicker to peck the open container than hens from the nalmefene treatment group on all days (day 1: = 5.9, p = 0.015; day 3: = 12.9, p < 0.001; day 4: = 18.2, p < 0.001; Table 3). Control hens were quicker to peck the open container over time ( = 39.3, p < 0.001; Table 3) there was no difference over time for nalmafene treatment hens (p = 0.35; Table 3). There was no interaction between treatment and day on the number of pecks in the open container (p = 0.645). However on all days, control hens pecked in the open container more than nalmefene treatment hens (F(3,95) = 6.6, p < 0.001; Figure 3).

Table 3. Hen responses to the presentation of an open transparent container containing live mealworms, including the percentage of hens within each treatment that pecked the open container and latency (seconds) to peck the open container.
Behavioral Time Budgets
When presented with a closed mealworm container for 2 min on day 1, hens spent most of the time alert (control 60.5 ± 5.6%; nalmefene hens 75.3 ± 6.0%; Table 4). However, control hens spent more time interacting with the container (day 1: z(2,36) = −2.7, p = 0.037; day 3: z(2,36) = −4.2, p < 0.001; day 4: z(2,36) = −4.5, p < 0.001) and more time standing than treatment hens on day 3 (z(2,36) = −2.9, p = 0.005) and day 4 (z(2,36) = −3.9, p < 0.001). There was no difference in time spent interacting with the container between treatment groups on day 1 (p = 0.790). Hens from both treatment groups were rarely immobile or resting during the test on any day (Table 4).
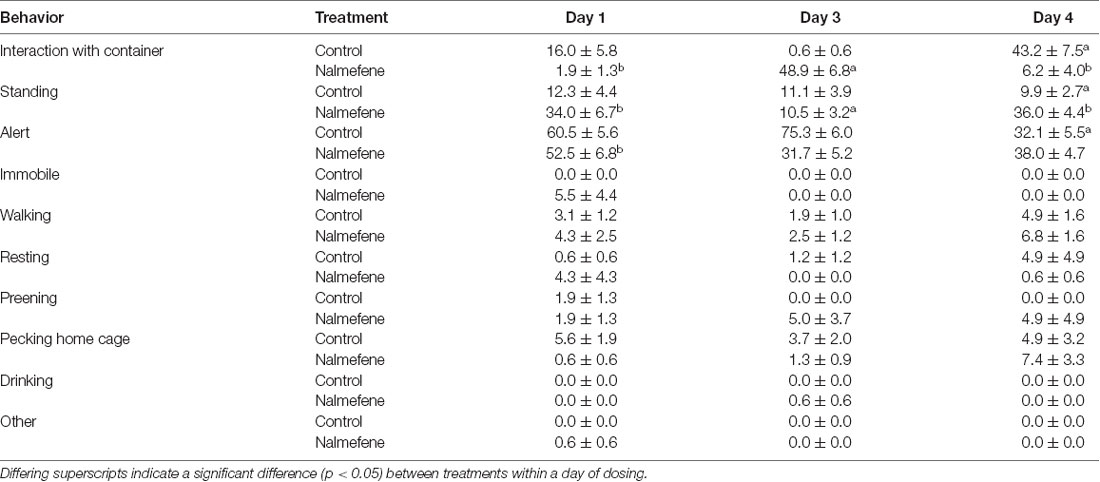
Table 4. Behavioral time budgets for hens administered with saline (control) or an opioid antagonist (nalmefene) after a closed container with five mealworms was presented in the home cage for 2 min.
Feed Intake
There was no statistical difference in feed intake over the trial period between any treatment groups, although numerically hens dosed twice daily with nalmefene consumed less than the other three treatment groups (Average feed intake: C1 281.2 ± 63.2 g; C2 217.6 ± 67.4 g; N1 229.7 ± 53.6 g; N2 83.2 ± 38.9 g; p = 0.114).
Response to Novelty
Only two hens touched the NO during the pre-treatment NO test, and there was no impact of treatment (p = 0.60). However post-treatment, control hens and hens that received nalmefene once daily were more likely to touch the NO (hens that touched the NO: C1 38.9% n = 7; C2 50.0%, n = 10; N1 23.5%, n = 4; N2 0.0%, n = 0; Figure 4) and to do so more quickly than hens that received nalmefene twice daily ( = 16.9, p = 0.001; Figure 4).
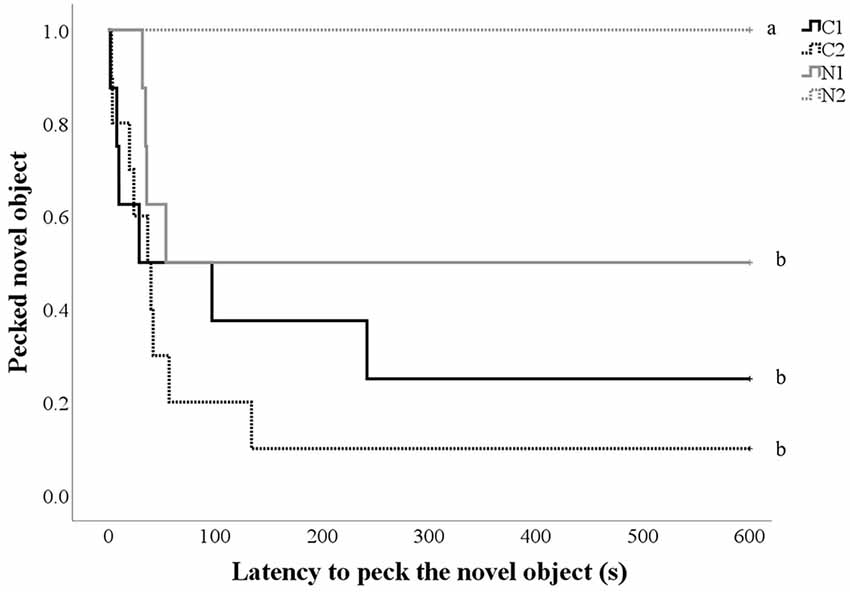
Figure 4. Kaplan-Meier curves indicating the proportion of control and treatment hens that pecked the novel object (NO; yellow spiked ball; y-axis) over time (seconds; x-axis) post-treatment. Every time a hen-pecked the NO, the probability on the y-axis drops. Hens received either a dose of saline (C1 and C2) or nalmefene (N1 and N2) for four consecutive days before the NO test was conducted, without the administration of any drug. Hens were dosed either once daily (C1 and N1) or twice daily (C2 and N2). The differing subscript is indicative of differences between treatment groups p < 0.05.
Discussion
We aimed to induce reward expectancy in hens by providing visual access to a mealworm container consecutively over 4 days. We expected to see indicators of anticipation in the hens that were expecting a reward. However, if hens had not experienced a positive affect associated with reward during previous experiences with mealworms (e.g., they did not like the mealworm due to blocked opioid receptors) such hens would show no indication that they expected (wanted) a reward (Berridge, 1996; Spruijt et al., 2001). Our results indicate that hens that received the non-specific opioid antagonist did not experience reward, as indicated by a reduction in anticipatory behavior when the visual cue was presented for 2 min before access to mealworms was provided and a reduction in the number of pecks to the open mealworm container, an indirect assessment of consumption, and no difference in homeostatic food consumption of standard layer mash. However, we take caution in the interpretation of these results as reward pathways are complex and off-target effects of nalmefene in laying hens are unknown.
Previous work has shown that sensitization of the reward system can be achieved via experiencing highly rewarding stimuli (Boissy et al., 2007). However, stressful experiences such as barren captive housing can also sensitize the reward system. For example, van der Harst et al. (2003a,b) provide evidence that rats housed in barren environments expressed more anticipatory behavior than rats kept in enriched environments. Conversely, chronically stressed animals often show symptoms of anhedonia and lose the ability to anticipate (van der Harst and Spruijt, 2007). As all of the hens in the current study were sourced from the same farm, housed in the same conditions throughout their early life and during the experiment, and no indicators of chronic stress were observed (e.g., abnormal behaviors or immobility) is it unlikely that negative previous experiences had any impact on the results.
Live mealworms were provided in the current study as these are preferred by hens over other foods, including wheat, cheese and sugary sweets (Bruce et al., 2003). Furthermore, hens will work for mealworms even if fully satiated (Moe et al., 2013), suggesting that hens find mealworms rewarding. We provide evidence that hens in the current study found mealworms rewarding as control hens approached the closed and open container faster from the 1st to the 4th day of testing. Consumption of food may be separated into homeostatic and non-homeostatic, either providing necessary nutritional uptake to sustain life or consumption driven by processes such as hedonics, respectively. Non-homeostatic consummatory behavior often leads to overconsumption (Olszewski and Levine, 2007).
Opioids play a central role in the hedonic evaluation of foods (Le Merrer et al., 2009) but also contribute to the incentive motivation to consume food, drugs and other rewards (Zhang et al., 2003; Grueter et al., 2012). We did not target specific opioid receptors, nor specific areas of the brain, therefore we likely impacted both the hedonistic and motivational component of the reward system. We do provide some evidence that we were able to disrupt the hedonistic component of reward, evident by a difference in mealworm consumption, albeit indirectly assessed, and no difference in the homeostatic consumption behavior of their standard layer mash feed over the course of the experiment. This approach of assessing homeostatic and non-homeostatic consummatory behavior, has been used with rats to identify specific hotspots in the brain that regulate the hedonistic component of reward (Rockwood and Reid, 1982; Marks-Kaufman et al., 1984; Levine et al., 1995; Castro and Berridge, 2014) For example, Castro and Berridge (2014) disassociated motivation to eat compared to sensory pleasure by stimulating specific hotspots in the NAc in rats which subsequently increased consumption of M&M candies but not standard rat chow. Additional methods that have aimed to separate the liking and wanting component of reward have used facial expressions, specifically orofacial responses to sweet or fatty food rewards in rats, humans and nonhuman primates (Berridge, 2000; Peciña and Berridge, 2005). Although, providing great insight into the subjective hedonic experience this method has not been validated, nor would be feasible, in particular species such as hens. Thus, there is a need to further develop methodology suitable for hens to differentiate the hedonistic and motivational component of reward, the anticipatory behavior reported in this study cannot provide such insight conclusively. Future investigations should consider assessing hen anticipatory behavior on subsequent days without the administration of nalmefene.
Whilst we found no significant difference in standard feed consumption between our treatment groups, N2 feed intake was numerically lower than the other treatment groups which are in agreement with Savory et al. (1989). The dose rates used in the current study were based on the Savory et al. (1989) study that provided evidence that feed intake was reduced at the dose rate of 0.4 mg/kg. The differences in methodological designs make it difficult to identify the cause of discrepancy between the two studies but may include differences in diet composition or the short term assessments of feed intake over 7 h post drug administration in the Savory et al. (1989) study compared to the total feed intake assessed over 4 days in the current study. Additionally, there were inconsistencies in the Savory et al. (1989) study, such that feed intake only statistically differed in the 1st, 3rd and 5th-h post-drug administration, but not the second or seventh. A better understanding of the impact of nalmefene on homeostatic and non-homoeostatic food consumption is required which may include both short and long term assessments of feeds that differ in palatability.
Wanting was assessed in the current study by behavioral indicators of anticipatory behavior. However, there was no evidence of anticipatory behavior as defined by Moe et al. (2009) “hens standing alert with head and neck stretched.” However, we did observe an increase in activity, similar to the increased frequency of head movements previously observed in laying hens (Zimmerman et al., 2011; Moe et al., 2013) and an increased focus towards the site where the reward was offered which is in agreement with anticipatory behavior in pigs (Petherick and Rutter, 1990), mink (Hansen and Jeppesen, 2004) and silver foxes (Moe et al., 2004). Further evidence of wanting was observed as control hens pecked the closed container more frequently which is likely indicative of motivation to access the reward. Such examples of increased “work” to access a reward has previously been observed in various laying hen operant conditioning trials (Lagadic and Faure, 1987; Olsson and Keeling, 2002). As anticipatory behavior is thought to reflect the animal’s perception of a stimulus we interpret the hen’s behavior to be an anticipation of the mealworm reward and conclude that control hens found the mealworms more rewarding than hens that were administered nalmefene. However, it must be noted that motivation (Le Merrer et al., 2009) or learning (Clissold and Pratt, 2014) may have also been directly impacted by nalmefene administration due to the off-target action of the drug on all opioid receptors.
We provide evidence that the administration of nalmefene (once or twice daily) successfully disrupted the opioid-mediated reward pathway in laying hens, in contrast to previous studies that used naloxone (Moe et al., 2013). Although previous work has shown that blocking opioid receptors with naloxone decrease the frequency of anticipatory behaviors in rodents (Spruijt et al., 2001; Woolley et al., 2006), it may not be as effective on hens. Indeed, previous research has shown that nalmefene was more effective in reducing feed intake in broiler chickens than naloxone (Savory et al., 1989). Additionally, naloxone has shown to have minimal effects on the behavior of other species including sheep (Verbeek et al., 2012) cattle (Rushen et al., 1999) and quail (Kostal and Kohutova, 2013).
Latency to peck the open mealworm container decreased over consecutive days of testing for control hens, but not treatment hens. Hens that received nalmefene waited more than 2 min, on average, before pecking at the open container and whilst this result may reflect a difference in motivation to consume the mealworms, it may simply be explained by an attraction to the live mealworm movement or impacts of satiety or the disruption of all opioid receptors rather than specific μ-opioid receptors that appear to regulate the hedonistic component of reward (Kelley et al., 2002; Castro and Berridge, 2014). However, differences in consumption of the mealworms could also be explained by unknown side effects of nalmefene administration which may include increased fearfulness, nausea, sedation or reduced curiosity. Indeed, various systems have been disrupted when specific opioid receptors are knocked out in mice; specifically motivation and anticipatory behavior in μ-receptor knockout mice (Kas et al., 2004), anxiety and depression in δ-receptor knockout mice (Filliol et al., 2000) or hallucinogenic activity when κ-opioid agonist are administered in mice (Yan and Roth, 2004). It is unlikely that nalmefene hens in the current study were sedated, as no differences in alertness or resting was observed between treatment and control hens. The NO test did suggest that nalmefene treated hens were more neophobic post-treatment than control hens, however this result may not be indicative of fearfulness. Forkman et al. (2007) suggest that the NO test is reflective of both inspective curiosity and neophobia (fearfulness). As there was no difference in immobility between treatment and control hens during the AT which is a typical behavioral indicator of fear in laying hens (Forkman et al., 2007), we suggest that the differences observed in the post-treatment NO test are more likely indicative of inspective curiosity. Although we cannot rule out that a reduction in motivation to explore in nalmefene treated hens was related to nausea induced by the drug (Cramer and Stanton, 2015), the NO test was performed when hens were not under the influence of the drug suggesting that previous experience and disruptions to the reward system may have had non-specific impacts on expectancy, exploration and other appetitive activities (Panksepp, 2005) or mood (Mendl et al., 2010). Further investigations are required and should consider assessing the impact of accessing non-food rewards to reduce the potential impact of homeostatic food motivations, or side effects from nalmefene that may have reduced the impact of motivation to eat.
Administration of either saline or nalmefene twice daily had an impact on anticipatory behavior compared to hens that were only dosed once daily. Rather than a nalmefene dose-response, this was likely an impact of handling as this effect was present in both nalmefene treated and control hens. Hens that were handled twice daily were likely less fearful of humans. These results likely reflect differences in human avoidance behavior when containers were delivered, or opened, by human experimenters, subsequently impacting latency to peck data and time available to interact with the container. This result serves as an important reminder of the impact of human researchers on subjects and the need to control for such impacts in experimental designs.
Hens treated with an opioid antagonist, nalmefene, showed less anticipatory behavior when a visual cue announcing the arrival of live mealworms was presented. Furthermore, nalmefene administration reduced non-homeostatic food consumption but not homeostatic consumption. Our results suggest that we successfully blocked the hedonistic subjective component of reward in laying hens and provides evidence that nalmefene could be used to further investigate how hens perceive their environment, specifically related to pleasurable experiences. However, nalmefene may have additionally, directly impacted the motivation to access food reward and further investigation into the impact of nalmefene on the liking component of reward compared to the wanting component warrants further investigation. As positive affective states are a critical aspect of animal welfare, understanding which environments and resources yield such experiences to hens will assist to develop science-based evidence to inform decisions on housing and effective enrichment programs for laying hens in commercial egg industries. Furthermore, the administration of nalmafene in laying hens may assist to identify associated novel indicators which can be implemented in on-farm welfare assessment programs to benchmark and safeguard hen welfare in a variety of contexts.
Data Availability Statement
The datasets generated for this study are available on request to the corresponding author.
Ethics Statement
The animal study was reviewed and approved by University of New England Animal Ethics Committee; Approval number 18-114.
Author Contributions
PT wrote the manuscript and conducted all analysis. PT and TC conducted the experiment. All authors helped with developing the concepts, methodologies, conceiving the experiments and contributed to manuscript writing.
Funding
This work was partially funded by Australian Eggs (grant number 1HS903DU).
Conflict of Interest
The authors declare that the research was conducted in the absence of any commercial or financial relationships that could be construed as a potential conflict of interest.
Acknowledgments
We would like to thank Ben Wade, Meagan Craven and Paul Hemsworth for their intellectual input into the design and interpretation of the study and Lara Fanning and Lachlan Thurtell for their help with the experimental work.
References
Altmann, J. (1974). Observational study of behavior: sampling methods. Behaviour 49, 227–266. doi: 10.1163/156853974x00534
Berridge, K. C. (1996). Food reward: brain substrates of wanting and liking. Neurosci. Biobehav. Rev. 20, 1–25. doi: 10.1016/0149-7634(95)00033-b
Berridge, K. C. (2000). Measuring hedonic impact in animals and infants: microstructure of affective taste reactivity patterns. Neurosci. Biobehav. Rev. 24, 173–198. doi: 10.1016/s0149-7634(99)00072-x
Berridge, K. C., and Kringelbach, M. L. (2015). Pleasure systems in the brain. Neuron 86, 646–664. doi: 10.1016/j.neuron.2015.02.018
Berridge, K. C., and Robinson, T. E. (1998). What is the role of dopamine in reward: hedonic impact, reward learning, or incentive salience? Addict. Behav. 28, 309–369. doi: 10.1016/s0165-0173(98)00019-8
Berridge, K. C., and Robinson, T. E. (2003). Parsing reward. Trends Neurosci. 26, 507–513. doi: 10.1016/S0166-2236(03)00233-9
Boissy, A., Manteuffel, G., Jensen, M. B., Moe, R. O., Spruijt, B., Keeling, L. J., et al. (2007). Assessment of positive emotions in animals to improve their welfare. Physiol. Behav. 92, 375–397. doi: 10.1016/j.physbeh.2007.02.003
Bruce, E., Prescott, N., and Wathes, C. (2003). Preferred food rewards for laying hens in behavioural experiments. Br. Poult. Sci. 44, 345–349. doi: 10.1080/0007166031000085490
Burgdorf, J., and Panksepp, J. (2006). The neurobiology of positive emotions. Neurosci. Biobehav. Rev. 30, 173–187. doi: 10.1016/j.neubiorev.2005.06.001
Cagniard, B., and Murphy, N. P. (2009). Taste reactivity and its modulation by morphine and methamphetamine in C57BL/6 and DBA/2 mice. Physiol. Behav. 96, 412–420. doi: 10.1016/j.physbeh.2008.11.010
Castro, D. C., and Berridge, K. C. (2014). Opioid hedonic hotspot in nucleus accumbens shell: mu, delta and kappa maps for enhancement of sweetness “liking” and “wanting”. J. Neurosci. 34, 4239–4250. doi: 10.1523/JNEUROSCI.4458-13.2014
Clissold, K. A., and Pratt, W. E. (2014). The effects of nucleus accumbens μ-opioid and adenosine 2A receptor stimulation and blockade on instrumental learning. Behav. Brain Res. 274, 84–94. doi: 10.1016/j.bbr.2014.07.047
Colpaert, F. C., Tarayre, J. P., Alliaga, M., Slot, L. A. B., Attal, N., and Koek, W. (2001). Opiate self-administration as a measure of chronic nociceptive pain in arthritic rats. Pain 91, 33–45. doi: 10.1016/s0304-3959(00)00413-9
Cramer, M., and Stanton, A. (2015). Associations between health status and the probability of approaching a novel object or stationary human in preweaned group-housed dairy calves. J. Dairy Sci. 98, 7298–7308. doi: 10.3168/jds.2015-9534
Désiré, L., Boissy, A., and Veissier, I. (2002). Emotions in farm animals: a new approach to animal welfare in applied ethology. Behav. Processes 60, 165–180. doi: 10.1016/s0376-6357(02)00081-5
Dixon, R., Howes, J., Gentile, J., Hsu, H. B., Hsiao, J., Garg, D., et al. (1986). Nalmefene: intravenous safety and kinetics of a new opioid antagonist. Clin. Pharmacol. Ther. 39, 49–53. doi: 10.1038/clpt.1986.9
Doyle, T. G., Berridge, K. C., and Gosnell, B. A. (1993). Morphine enhances hedonic taste palatability in rats. Pharmacol. Biochem. Behav. 46, 745–749. doi: 10.1016/0091-3057(93)90572-b
Duncan, I. J. (2002). Poultry welfare: science or subjectivity? Br. Poult. Sci. 43, 643–652. doi: 10.1080/0007166021000025109
Filliol, D., Ghozland, S., Chluba, J., Martin, M., Matthes, H. W., Simonin, F., et al. (2000). Mice deficient for δ-and μ-opioid receptors exhibit opposing alterations of emotional responses. Nat. Genet. 25, 195–200. doi: 10.1038/76061
Forkman, B., Boissy, A., Meunier-Salaün, M.-C., Canali, E., and Jones, R. (2007). A critical review of fear tests used on cattle, pigs, sheep, poultry and horses. Physiol. Behav. 92, 340–374. doi: 10.1016/j.physbeh.2007.03.016
Fraser, D., and Duncan, I. J. (1998). ‘Pleasures’, ‘pains’ and animal welfare: toward a natural history of affect. Anim. Welf. 7, 383–396.
Glass, P., Jhaveri, R. M., and Smith, L. R. (1994). Comparison of potency and duration of action of nalmefene and naloxone. Anesth. Analg. 78, 536–541. doi: 10.1213/00000539-199403000-00021
Grueter, B. A., Rothwell, P. E., and Malenka, R. C. (2012). Integrating synaptic plasticity and striatal circuit function in addiction. Curr. Opin. Neurobiol. 22, 545–551. doi: 10.1016/j.conb.2011.09.009
Hansen, S. W., and Jeppesen, L. L. (2004). The anticipatory behaviours of mink expecting a positive or negative reward. Biol. Psychiatry Cogn. Neurosci. Neuroimaging 28, 19–23. Available online at: http://ifasanet.org/scientifur_integral_issues/vol28_3/Scientifur,%20Vol%2028,%20No%203.pdf#page=23
Hy-Line International. (2018). Ly-line management guide. Available online at: https://www.hyline.com/UserDocs/Pages/BRN_COM_ENG.pdf. Accessed November 2018.
Kas, M. J., van den Bos, R., Baars, A. M., Lubbers, M., Lesscher, H. M., Hillebrand, J. J., et al. (2004). Mu-opioid receptor knockout mice show diminished food-anticipatory activity. Eur. J. Neurosci. 20, 1624–1632. doi: 10.1111/j.1460-9568.2004.03581.x
Kelley, A. E., Bakshi, V. P., Haber, S. N., Steininger, T. L., Will, M. J., and Zhang, M. (2002). Opioid modulation of taste hedonics within the ventral striatum. Physiol. Behav. 76, 365–377. doi: 10.1016/s0031-9384(02)00751-5
Kostal, L., and Kohutova, A. (2013). “Reward-related behaviour in Japanese quail: the effects of environmental enrichment and dopamine and opioid receptors blockers (abst.),” in Behaviour 2013, Joint Meeting of the 33rd International Ethological Conference (IEC) and the Association for the Study of Animal Behaviour (ASAB), Abstract Book, 4th-8th August 2013; Newcastle-Gateshead, UK, 220.
Krebs, B. L., Torres, E., Chesney, C., Kantoniemi Moon, V., and Watters, J. V. (2017). Applying behavioral conditioning to identify anticipatory behaviors. J. Appl. Anim. Welf. Sci. 20, 155–175. doi: 10.1080/10888705.2017.1283225
Lagadic, H., and Faure, J.-M. (1987). Preferences of domestic hens for cage size and floor types as measured by operant conditioning. Appl. Anim. Behav. Sci. 19, 147–155. doi: 10.1016/0168-1591(87)90211-5
Le Merrer, J., Becker, J. A., Befort, K., and Kieffer, B. L. (2009). Reward processing by the opioid system in the brain. Physiol. Rev. 89, 1379–1412. doi: 10.1152/physrev.00005.2009
Levine, A. S., Weldon, D., Grace, M., Cleary, J., and Billington, C. J. (1995). Naloxone blocks that portion of feeding driven by sweet taste in food-restricted rats. Am. J. Physiol. 268, R248–R252. doi: 10.1152/ajpregu.1995.268.1.r248
Marks-Kaufman, R., Balmagiya, T., and Gross, E. (1984). Modifications in food intake and energy metabolism in rats as a function of chronic naltrexone infusions. Pharmacol. Biochem. Behav. 20, 911–916. doi: 10.1016/0091-3057(84)90016-9
Mendl, M., Burman, O. H., and Paul, E. S. (2010). An integrative and functional framework for the study of animal emotion and mood. Proc. Biol. Sci. 277, 2895–2904. doi: 10.1098/rspb.2010.0303
Moe, R., Kingsley-Smith, H., Kittilsen, S., and Bakken, M. (2004). “Anticipatory behaviour and emotional expressions in farmed silver foxes (Vulpes vulpes)-a new approach to animal welfare,” in Proceedings of the 38th International Congress of the International Society for Applied Ethology, Helsinki, Finland, 63.
Moe, R. O., Nordgreen, J., Janczak, A. M., Spruijt, B. M., and Bakken, M. (2013). Effects of signalled reward type, food status and a μ-opioid receptor antagonist on cue-induced anticipatory behaviour in laying hens (Gallus domesticus). Appl. Anim. Behav. Sci. 148, 46–53. doi: 10.1016/j.applanim.2013.08.001
Moe, R. O., Nordgreen, J., Janczak, A. M., Spruijt, B. M., Kostal, L., Skjerve, E., et al. (2011). Effects of haloperidol, a dopamine D2-like receptor antagonist, on reward-related behaviors in laying hens. Physiol. Behav. 102, 400–405. doi: 10.1016/j.physbeh.2010.12.008
Moe, R. O., Nordgreen, J., Janczak, A. M., Spruijt, B. M., Zanella, A. J., and Bakken, M. (2009). Trace classical conditioning as an approach to the study of reward-related behaviour in laying hens: a methodological study. Appl. Anim. Behav. Sci. 121, 171–178. doi: 10.1016/j.applanim.2009.10.002
Olsson, I., and Keeling, L. (2002). The push-door for measuring motivation in hens: laying hens are motivated to perch at night. Anim. Welf. 11, 11–19. Available online at: https://www.ufaw.org.uk/the-ufaw-journal/animal-welfare
Olszewski, P. K., and Levine, A. S. (2007). Central opioids and consumption of sweet tastants: when reward outweighs homeostasis. Physiol. Behav. 91, 506–512. doi: 10.1016/j.physbeh.2007.01.011
Panksepp, J. (2005). Affective consciousness: core emotional feelings in animals and humans. Conscious. Cogn. 14, 30–80. doi: 10.1016/j.concog.2004.10.004
Parker, L. A., Maier, S., Rennie, M., and Crebolder, J. (1992). Morphine- and naltrexone-induced modification of palatability: analysis by the taste reactivity test. Behav. Neurosci. 106, 999–1010. doi: 10.1037/0735-7044.106.6.999
Peciña, S., and Berridge, K. C. (2005). Hedonic hot spot in nucleus accumbens shell: where do μ-opioids cause increased hedonic impact of sweetness? J. Neurosci. 25, 11777–11786. doi: 10.1523/jneurosci.2329-05.2005
Peciña, S. M. (1998). Contrasting Roles of Mesostriatal Dopamine and Opioid Systems in Food “Wanting” and ”Liking”. Ann Arbor, MN: University of Michigan.
Petherick, J. C., and Rutter, S. M. (1990). Quantifying motivation using a computer-controlled push-door. Appl. Anim. Behav. Sci. 27, 159–167. doi: 10.1016/0168-1591(90)90015-6
Rockwood, G. A., and Reid, L. D. (1982). Naloxone modifies sugar-water intake in rats drinking with open gastric fistulas. Physiol. Behav. 29, 1175–1178. doi: 10.1016/0031-9384(82)90316-x
Rushen, J., Boissy, A., Terlouw, E., and de Passillé, A. (1999). Opioid peptides and behavioral and physiological responses of dairy cows to social isolation in unfamiliar surroundings. J. Anim. Sci. 77, 2918–2924. doi: 10.2527/1999.77112918x
Salamone, J., Cousins, M., and Snyder, B. (1997). Behavioral functions of nucleus accumbens dopamine: empirical and conceptual problems with the anhedonia hypothesis. Neurosci. Biobehav. Rev. 21, 341–359. doi: 10.1016/s0149-7634(96)00017-6
Savory, C., Gentle, M., and Yeomans, M. (1989). Opioid modulation of feeding and drinking in fowls. Br. Poult. Sci. 30, 379–392. doi: 10.1080/00071668908417159
Spruijt, B. M., van den Bos, R., and Pijlman, F. T. (2001). A concept of welfare based on reward evaluating mechanisms in the brain: anticipatory behaviour as an indicator for the state of reward systems. Appl. Anim. Behav. Sci. 72, 145–171. doi: 10.1016/s0168-1591(00)00204-5
van der Harst, J., Baars, A., and Spruijt, B. (2003a). Standard housing for rats is stressful as shown by enhanced sensitivity to rewards. Behav. Brain Res. 142, 151–156. doi: 10.1016/S0166-4328(02)00403-5
van der Harst, J., Fermont, P., Bilstra, A., and Spruijt, B. (2003b). Access to enriched housing is rewarding to rats as reflected by their anticipatory behaviour. Anim. Behav. 66, 493–504. doi: 10.1006/anbe.2003.2201
van der Harst, J., and Spruijt, B. (2007). Tools to measure and improve animal welfare: reward-related behaviour. Anim. Welf. 16, 67–73. Available online at: https://www.ufaw.org.uk/the-ufaw-journal/animal-welfare
Verbeek, E., Ferguson, D., de Monjour, P. Q., and Lee, C. (2012). Opioid control of behaviour in sheep: effects of morphine and naloxone on food intake, activity and the affective state. Appl. Anim. Behav. Sci. 142, 18–29. doi: 10.1016/j.applanim.2012.09.001
Wang, D. S., Sternbach, G., and Varon, J. (1998). Nalmefene: a long-acting opioid antagonist. Clinical applications in emergency medicine. J. Emerg. Med. 16, 471–475. doi: 10.1016/s0736-4679(98)00019-5
Woolley, J., Lee, B., and Fields, H. (2006). Nucleus accumbens opioids regulate flavor-based preferences in food consumption. Neuroscience 143, 309–317. doi: 10.1016/j.neuroscience.2006.06.067
Yan, F., and Roth, B. L. (2004). Salvinorin A: a novel and highly selective κ-opioid receptor agonist. Life Sci. 75, 2615–2619. doi: 10.1016/j.lfs.2004.07.008
Yeomans, M., Wright, P., Macleod, H., and Critchley, J. (1990). Effects of nalmefene on feeding in humans. Psychopharmacology 100, 426–432. doi: 10.1007/bf02244618
Zhang, M., Balmadrid, C., and Kelley, A. E. (2003). Nucleus accumbens opioid, GABaergic, and dopaminergic modulation of palatable food motivation: contrasting effects revealed by a progressive ratio study in the rat. Behav. Neurosci. 117, 202–211. doi: 10.1037/0735-7044.117.2.202
Keywords: welfare, nalmefene, poultry, affective states, hedonic, pleasure, goal-directed, pharmacological
Citation: Taylor PS, Hamlin AS and Crowley TM (2020) Anticipatory Behavior for a Mealworm Reward in Laying Hens Is Reduced by Opioid Receptor Antagonism but Not Standard Feed Intake. Front. Behav. Neurosci. 13:290. doi: 10.3389/fnbeh.2019.00290
Received: 14 October 2019; Accepted: 20 December 2019;
Published: 14 January 2020.
Edited by:
Raúl G. Paredes, National Autonomous University of Mexico, MexicoReviewed by:
Wendy Portillo, National Autonomous University of Mexico, MexicoLindsay Halladay, Santa Clara University, United States
Copyright © 2020 Taylor, Hamlin and Crowley. This is an open-access article distributed under the terms of the Creative Commons Attribution License (CC BY). The use, distribution or reproduction in other forums is permitted, provided the original author(s) and the copyright owner(s) are credited and that the original publication in this journal is cited, in accordance with accepted academic practice. No use, distribution or reproduction is permitted which does not comply with these terms.
*Correspondence: Peta S. Taylor, peta.taylor@une.edu.au